- 1Department of Biology, Wake Forest University, Winston-Salem, NC, United States
- 2Smithsonian Tropical Research Institute, Panama City, Panama
- 3Department of Environmental Science and Policy, University of California, Davis, Davis, CA, United States
- 4Department of Ecosystem Science and Management, University of Wyoming, Laramie, WY, United States
- 5Department of Plant Biology, Michigan State University, East Lansing, MI, United States
While woody stems are known to influence carbon and water dynamics, direct exchange with the atmosphere is seldom quantified, limiting our understanding of how these processes influence the exchange of mass and energy. The presence of woody stem chlorophyll in a diversity of climates and across a range of species suggests an evolutionary advantage to sustaining carbon assimilation and water relations through permeable stem tissue. However, no formal evaluation of this hypothesis has been performed. In this mini-review, we explore the interactions between woody stems and the atmosphere by examining woody stem photosynthesis and bark-atmosphere water exchange. Specifically, we address the following questions: (1) How do water and carbon move between the atmosphere and woody stems? (2) In what climate space is woody stem photosynthesis and bark water uptake advantageous? (3) How ubiquitous across plant families is woody stem photosynthesis and bark-atmosphere water exchange? In the literature, only seven species have been identified as exhibiting bark water uptake while over 300 species are thought to conduct woody stem photosynthesis. The carbon dioxide and water gained from these processes can offset respiration costs and improve plant water balance. These species span diverse biomes suggesting a broad prevalence of bark-atmosphere permeability. Finally, our results demonstrate that there may be an evolutionary component as demonstrated by a high Pagel’s lambda for the presence of stem photosynthesis. We end with recommendations for future research that explores how bark water and carbon interactions may impact plant function and mass flow in a changing climate.
Introduction
The global cycling of water and carbon is driven by small-scale forces that move these molecules through different pools in the environment. Plants play a major role in this cycling by absorbing and releasing both water and carbon through multiple plant organs. Most research has focused on leaves and roots as the integral locations where water and carbon fluxes occur while suberized tissue, such as stem bark, is stereotyped as an impermeable, enigmatic shield. Bark is known to protect plants from pathogens and fire (Morris and Jansen, 2016; Loram-Lourenco et al., 2020), provide mechanical support (Niklas, 1999), and transport and store various compounds (Lintunen et al., 2016; Ilek et al., 2021). The role of permeable bark in governing plant carbon and water budgets through direct exchange of these molecules with the atmosphere is largely considered trivial. Stems release carbon dioxide (respiration) and water as other plant surfaces do. The movement of these molecules can occur in the opposite direction too, but these phenomena are less studied. Justifiably, measured rates of stem photosynthesis and water uptake are generally small compared to total plant and ecosystem fluxes. Nevertheless, recent work has shown that bark carbon dioxide and water uptake can mitigate environmental stress by providing an additional subsidy of carbon and water (e.g., Teskey et al., 2008; Ávila et al., 2014; Earles et al., 2016). For example, stem net photosynthesis can range from 0.5 in Syringa vulgaris (Pilarski, 2002) to 43.9 μmol m–2s–1 in Bebbia juncea (Ávila-Lovera et al., 2019) and, across several species, compensate for all of the carbon that would otherwise be lost to the atmosphere through respiration. To date, at least 341 species have been empirically shown to exhibit bark water uptake or woody stem photosynthesis (7 for water, 334 for carbon; Supplementary Table 1). While this count is surely a subset of the total prevalence and significance of these processes, we leverage these studies to provide predictions and hypotheses about the broad role of bark in carbon and water exchange with the atmosphere.
Woody stem carbon dioxide uptake is largely considered to be an evolutionary relic because the earliest land plants primarily photosynthesized through their stems (Stewart and Rothwell, 1993; Nilsen, 1995). Woody stem assimilation of carbon dioxide can be classified into two types: stem net photosynthesis (Ávila et al., 2014), in which stems can directly absorb carbon dioxide from the atmosphere, and stem recycling photosynthesis, in which stems utilize carbon dioxide from internal respiration of root and stem tissue and often lack stomata on the stem (Nilsen, 1995; Ávila et al., 2014). Stem recycling photosynthesis can account for 7–126% of carbon dioxide refixation (Teskey et al., 2008; Ávila et al., 2014). The actual site of chlorophyll and woody stem photosynthesis can vary widely from the inner bark (living cortex) all the way to the pith (Cannon, 1908). Considerable work has explored carbon reassimilation dynamics within stems but only recently has research identified permeable bark as a novel source for water and carbon in woody species. Investigations of stem net photosynthesis has been largely limited by methods, because leaf chambers for gas exchange systems are not able to accommodate large stems or detect low fluxes.
The few studies that have explored this pathway find that carbon uptake is commonly facilitated by lenticels, stomata, cracks, or wounds (Grosse, 1997; Groh et al., 2002; Teskey and McGuire, 2005). The process of water uptake is less well understood, but likely occurs via symplastic pathways through hydrophilic regions of the phellem cell wall (Schönherr and Ziegler, 1980; Earles et al., 2016). The direct absorption of carbon and water from the atmosphere relies on similar bark structure and porosity meaning that species capable of carbon dioxide uptake likely can take up water as well. Liu et al. (2019), for instance, found that stem photosynthesis promoted bark water uptake and embolism repair in Salix matsudana. The interconnected exchange of carbon and water through permeable bark also suggests that, while bark water uptake is considerably understudied, hypotheses regarding this process may be developed from broad scale assessments of the more commonly studied stem photosynthesis.
In this mini-review, we survey the literature to demonstrate the prevalence and significance of bark in affecting woody stem carbon and water dynamics. We define woody stems as stems with secondary growth, i.e., having wood with a combination of living and non-living cells exterior to the cambium. Collectively, all the tissues beyond the cambium are known as bark. Our composite of all species where water or carbon dioxide uptake has occurred allows us to assess broad-scale environmental factors affecting bark permeability to these molecules. We then use two publicly available, large data sets from China (Prentice et al., 2011; Wang et al., 2018; accessed in TRY, Kattge et al., 2020) with information about the prevalence of stem photosynthesis to consider the biophysical environments and clades where we might expect significant bark carbon dioxide and water uptake to occur. These data allow us to hypothesize climates and contexts where researchers should explore the role of woody stem water and carbon uptake. With these new ideas, we hope to inspire colleagues to investigate stem gas and water exchange in their study systems and enhance our knowledge base of these understudied processes.
How Do Water and Carbon Move Between Woody Stems and the Atmosphere?
As with water movement throughout the soil-plant-atmosphere continuum, water is able to move radially through woody tissue based on water potential gradients. This movement can be bidirectional – water can be lost to evaporation or absorbed through bark water uptake. The few studies focused on bark water uptake investigated how water absorbed by bark affects xylem hydraulic function and whole plant water status (e.g., Earles et al., 2016). The amount of water mobilized and the directionality will depend on broad anatomical features such as the differences in space partitioning among vessel, fiber, and xylem parenchyma cell types, emergent hydraulic functional traits (e.g., wood density and capacitance; Pratt and Jacobsen, 2017), and environmental drivers such as soil water availability and vapor pressure deficit (VPD) (Laur and Hacke, 2014; Fontes et al., 2020). Stems with stomata have conductance values that range from 0.03 mmol m–2s–1 in Ruscus microglossum (cuticular conductance) (Pivovaroff et al., 2014) to 472.64 mmol m–2s–1 in B. juncea (maximum stomatal conductance) (Ávila-Lovera et al., 2019), which can result in substantial water loss. Stems without stomata have conductance values ranging from 0.86 to 12.98 mmol m–2s–1 (Wolfe, 2020). More recent research focused on the absorption of water by woody stems demonstrated that bark water uptake likely requires high relative humidity or wet surfaces but can improve plant water status and xylem hydraulic conductivity (Earles et al., 2016; Carmichael et al., 2020). While still sparse in number, the current literature suggests that bark water uptake likely benefits plant hydraulic functioning.
Woody stems that have the ability to photosynthesize have a protective tissue that is permeable to light and movement of carbon dioxide and water. Some species with secondary growth (i.e., secondary xylem or wood) delay periderm formation (Gibson, 1983) and maintain their epidermis with stomata during the lifetime of the stem (Lindorf et al., 2006). These stems appear green and exchange carbon dioxide and water regularly with the surrounding atmosphere. Similar to leaves, stem net photosynthesis responds to light availability, atmospheric carbon dioxide concentration, VPD, and air temperature (Nilsen, 1995). CO2 concentrations inside stems are an order of magnitude higher than atmospheric CO2 concentration: 0.1–26.3% in stems (Teskey et al., 2008) vs. ∼0.04% in air, hence most CO2 movement occurs from the stem to the atmosphere. However, when stem CO2 concentrations decreased to values lower than that of the atmosphere, carbon dioxide from the atmosphere can diffuse through the stem protective layers and be assimilated within the bark (Berveiller et al., 2007). Even in stems with an epidermis but closed stomata, water, and carbon dioxide can continue moving through the cuticle given sufficient concentration gradients (Ávila-Lovera et al., 2017).
The coordination of woody stem photosynthesis and bark water uptake is likely linked but rarely studied in tandem. One recent study on the coordination of woody stem photosynthesis and hydraulic traits showed that there is a positive relationship between stem photosynthetic rate and cuticular conductance to water (Ávila-Lovera et al., 2017). This suggests that structures enabling permeability in periderms and cuticles have the ability to influence carbon dioxide uptake, water loss, and potentially water uptake. Another study found that photosynthetic cells in the stems can increase the amount of water stored in the tissue through modification of the starch supply, influencing the osmotic potential of water through the bark (Liu et al., 2019). Bark conductance to water and carbon dioxide, and the environmental drivers that lead to bidirectional exchange will be key parameters for integrating carbon and water budgets. What remains unknown is the magnitude of these carbon and water fluxes, how prevalent they are, how they vary in time and space, and how they influence the whole-plant carbon and water economy.
In What Climate Space Are Bark Water Uptake and Woody Stem Photosynthesis Advantageous?
Stem net photosynthesis may benefit plant functioning by maintaining some carbon gain during periods of low or no leaf gas exchange (Nilsen and Sharifi, 1997) and by increasing water-use efficiency (Osmond et al., 1987). Stem water uptake may also benefit plants by promoting embolism repair (Earles et al., 2016; Liu et al., 2019). Consequently, permeable bark should be more common in climate conditions where these physiological effects are particularly advantageous. Photosynthetic woody stems should be beneficial in warm, dry environments where an additional source of carbon and, consequently, enhanced whole-plant water-use efficiency may be adaptive (e.g., high-latitude deserts; Ávila et al., 2014). Bark water uptake may also be adaptive in these ecosystems if bark wetting from small precipitation or fog/dew events facilitates localized hydraulic recovery despite a generally dry environment (Earles et al., 2016). For example, Earles et al. (2016) demonstrates that branch wetting from fog events improves plant water status in coastal redwood trees that experience summer drought. However, to our knowledge, this hypothesis has not been formally tested. Here, we explored if this is supported by mapping the occurrence of woody stem photosynthesis and bark water uptake in climatic niche space using Whittaker biome classifications (Whittaker, 1962). We created two figures that plotted: (1) occurrences of woody stem photosynthesis, bark conductance, and bark water uptake from our literature review, and (2) occurrences of reported stem photosynthesis from two datasets that surveyed the flora of China (Prentice et al., 2011; Wang et al., 2018).
Woody stem photosynthesis was widespread across Whittaker biomes for both our literature review (Figure 1), and the Prentice et al. (2011) and Wang et al. (2018) datasets (Figure 2). As expected, woody stem photosynthesis reported within our literature review was prevalent across warm, dry biomes such as temperate grasslands/deserts and subtropical deserts. However, this phenomenon was also observed across tropical seasonal forest/savanna, woodland/shrubland, boreal forest, temperate seasonal forest, and temperate rainforest biomes, and spanned a climate range of 6.7–248.1 cm of mean annual precipitation (MAP) and −2.2–28.8°C of mean annual temperature (MAT). Stem photosynthesis reported by Prentice et al. (2011) and Wang et al. (2018) showed a similar distribution across climate space but did not occur in temperate rain forest or subtropical desert (MAP 1.5–186.8 cm and MAT −5.3 to 22.9°C). Bark water uptake, which was investigated in far fewer studies from our literature review (seven species; Supplementary Table 1), occurred in temperate grassland/desert, woodland/shrubland, temperate seasonal forest, and tropical seasonal forest/savanna (MAP 48.7–131.8 mm and MAT 4.3–20.2°C). While there is bias in the spatial sampling of these biomes, these results demonstrate that stem photosynthesis and bark water uptake occur across a wide range of temperature and rainfall environments and are thus likely more prevalent than previously considered.
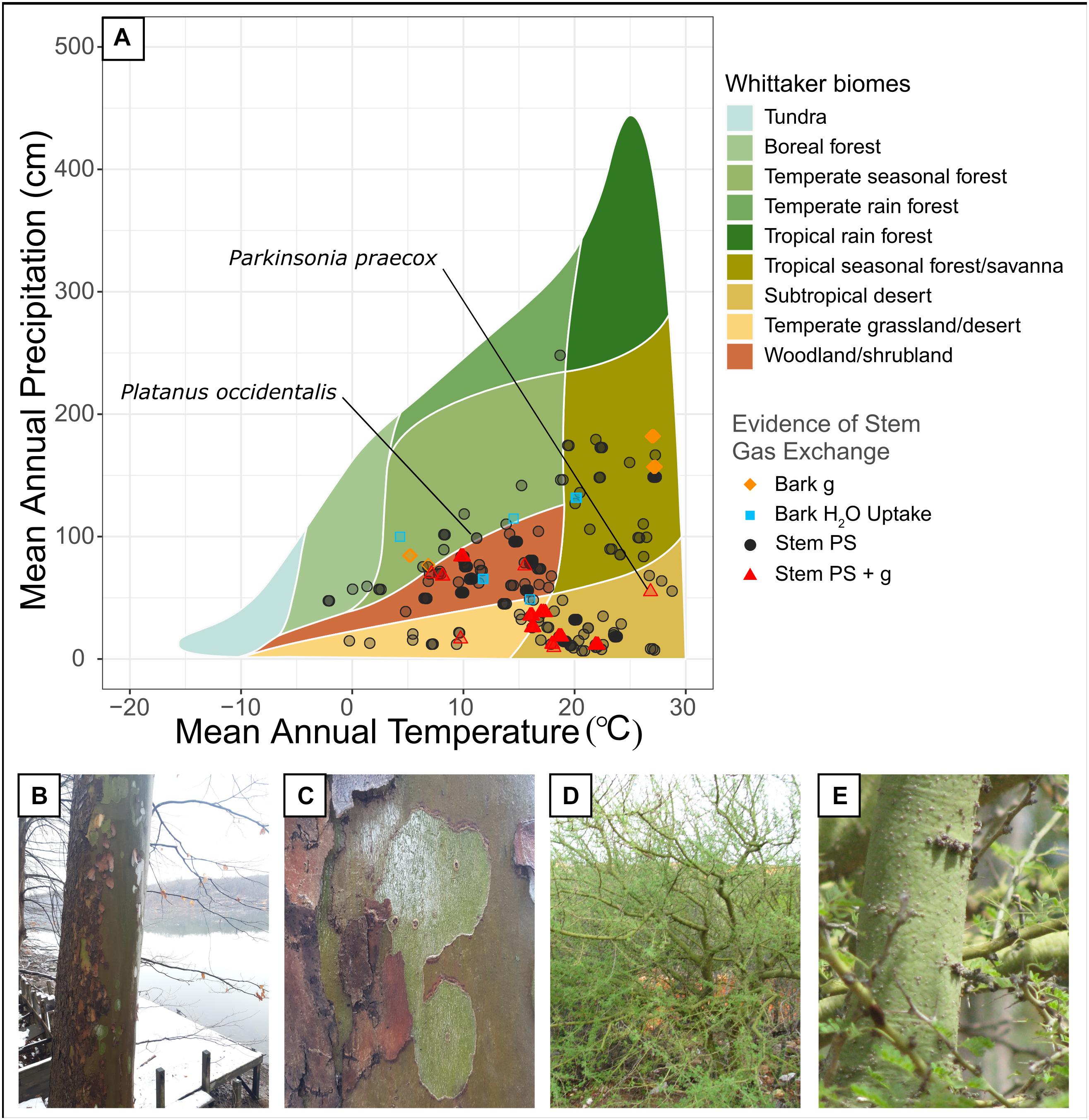
Figure 1. (A) Whittaker plot showing the biomes where stem carbon dioxide and water exchange have been quantified (data come from Supplementary Table 1). Shown are observations where only bark photosynthesis was observed (including both net CO2 uptake and CO2 reassimilation, “Stem PS”), bark photosynthesis and bark conductance were both observed (“Stem PS + g”), only bark conductance was observed (“Bark g”), and bark water uptake was observed (“Bark H2O Uptake”). Observations were plotted as semi-transparent points in order to improve clarity of clustered points. (B,C) Sycamore tree (Platanus occidentalis) with green, deciduous bark, photographed at Cheat Lake, West Virginia (photos by N. Emery). (D,E) Foothill Palo Verde (Parkinsonia praecox) with bright green bark, photographed in a xerophytic woodland in Venezuela (photos by W. Tezara).
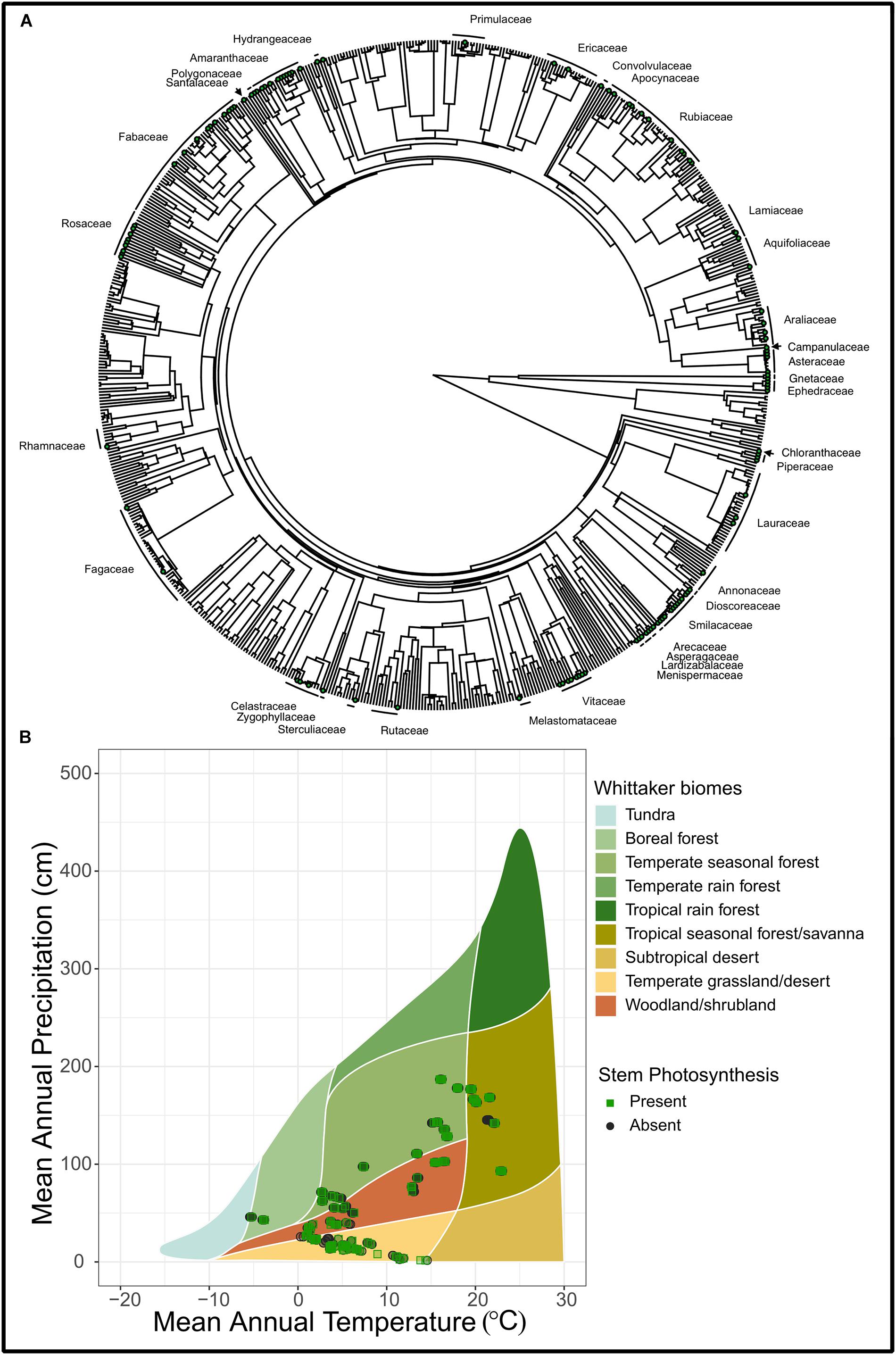
Figure 2. (A) A phylogenetic tree of 685 species from Prentice et al. (2011) and Wang et al. (2018) that demonstrate stem photosynthesis. Green points represent species with stem photosynthesis and families that have at least a single species are labeled on the outside of the tree. (B) A Whittaker plot illustrating the climatic space of stem photosynthesis. Shown are observations where stem photosynthesis was recorded as present (“Present”) and those where stem photosynthesis was recorded as absent (“Absent”). Out of a total of 719 species in this plot, stem photosynthesis was present in 125 species and absent in 594 species. Observations were plotted as semi-transparent points in order to improve clarity of clustered points.
While woody stem photosynthesis likely occurs in most biomes, broad-scale climate factors such as MAP and MAT might not be good predictors of where this phenomenon occurs. Rather, variability in small-scale environmental factors (e.g., topography, soil texture, water availability in the rhizosphere, and light availability) may be more important environmental drivers for bark carbon dioxide uptake. Although a large number of observations occurred in subtropical desert and woodland/shrublands, these data do not necessarily indicate that woody stem photosynthesis is more likely to occur in this climate space simply because much of the existing woody stem photosynthesis research has occurred in these ecosystems (e.g., Ehleringer et al., 1987; Comstock et al., 1988; Tinoco-Ojanguren, 2008; Pivovaroff et al., 2016; Ávila-Lovera et al., 2019). Likewise, few studies have investigated bark water uptake (e.g., Mayr et al., 2014; Earles et al., 2016; Yu et al., 2018; Liu et al., 2019), inherently limiting its known distribution across climate space. Given that stem carbon and water uptake should be linked through permeable periderms and cuticles (Ávila-Lovera et al., 2017), stem water uptake likely occurs in similar climate space as woody stem photosynthesis. This notion is further supported by our observation that stem conductance was reported within a similar climate space as stem photosynthesis (Figure 1). This analysis opens up new opportunities for researchers to explore the interwoven processes of woody stem photosynthesis and bark water uptake across different biomes and ecological gradients to better understand their global frequency and distribution, and to determine the role these processes may play in mediating plant responses to a changing climate.
How Ubiquitous Across Plant Families Is Woody Stem Photosynthesis and Bark-Atmosphere Water Exchange?
Permeable stems are likely the ancestral state of all terrestrial vascular plants, as some of the earliest land plants conducted photosynthesis exclusively from stems (Stewart and Rothwell, 1993; Nilsen, 1995). However, as plants evolved woody tissue and secondary phloem (inner bark), this permeability might have been replaced with thicker, more robust bark, which inherently decreases light transmission (Rosell et al., 2015). Within species, younger, more light-exposed stems tend to have higher chlorophyll content (Pfanz et al., 2002), suggesting that bark permeability is age-related and light interception may be a primary factor limiting the retention of this trait (Pfanz and Aschan, 2001, Aschan and Pfanz, 2003). For example, Rosell et al. (2015) found all 85 species studied had photosynthesis in small stems but only 43 of those species retained this trait in main trunks. In our data set, the presence of stem photosynthesis exists across a diversity of plant families (Figure 2). Whether this is a reversal to an ancestral trait or convergent evolution requires further investigation.
We conducted a phylogenetic analysis using Pagel’s λ to determine if stem photosynthesis had a phylogenetic signal across a broad range of woody species. We only analyzed woody plant species based on life form designation within the data sets (see Supplementary Methods 1). Our phylogenetic analysis of 685 woody plant species (Prentice et al., 2011; Wang et al., 2018) indicates that stem photosynthesis is clustered across the tree with some families containing many species exhibiting stem photosynthesis and others with few (Figure 2). This is demonstrated by the high Pagel’s λ (λ = 0.87, p < 0.0001) which indicates a significant phylogenetic signal in stem photosynthesis (Pagel, 1999). A significant phylogenetic signal indicates that the similarity between species is related to their phylogenetic relatedness (Losos, 2008). Both mapping the stem photosynthesis trait and estimating Pagel’s λ suggest that relatives resemble each other more than they resemble species sampled at random from the tree (Blomberg and Garland, 2002). The uneven distribution of stem photosynthesis across the vascular plant phylogeny suggests that multiple evolutionary events may have led to permeable bark tissue that facilitates carbon dioxide and water exchange (Nilsen, 1995; Gibson, 1996; Ávila-Lovera and Ezcurra, 2016). Thus, retaining bark permeability in mature stems may indeed provide a functional advantage for woody plants. However, a few limitations should be noted. First, it is possible that the analysis cannot separate stem photosynthesis from stem and plant size. In other words, plants that are smaller would have smaller stems and thus be more likely to be registered as having photosynthetic stems. Secondly, we cannot know from the data set whether the species exhibit stem recycling photosynthesis or if they take up carbon dioxide from the atmosphere although both types improve carbon balance of plants. Ultimately, this large data set provides great power for analysis but also comes with limitations of extensive inference. This leaves many fascinating questions around stem photosynthesis and the potential prevalence of bark water uptake. Future work that investigates bark water uptake across a broad range of species and climate space would also be able to utilize these data to reveal novel insights regarding the prevalence of bark water uptake across the woody plant phylogeny and its functional significance.
Similar to the biome analysis, we find stem photosynthesis represented across a broad swath of species, ranging from gymnosperms (Ephedraceae and Gnetaceae, 269–104 Mya) to Asteraceae (91–36 Mya). Out of a total of 719 species reported in the data set, stem photosynthesis was present in 125 species and absent in 594 species. Trees, shrubs, and vines were all represented including temperate 30 m tall trees (Machilus yunnanensis, Lauraceae), desert shrubs (Atraphaxis bracteata, Polygonaceae), and tropical climbing vines (Psychotria serpens, Rubiaceae). The widespread prevalence of stem photosynthesis across our woody plant phylogeny suggests that this trait is likely advantageous and possibly evolves under different abiotic and biotic pressures. Given the similar constraints of carbon dioxide and water exchange with the atmosphere, we infer that stem permeability is adaptive as well.
Unanswered Questions and Conclusions
Across biomes, climates, and clades, stems absorb water and photosynthesize. Bark water and carbon dioxide exchange can repair embolisms (Earles et al., 2016), reduce localized water stress, recover up to 100% of respired carbon, and can even result in a net carbon gain (Teskey et al., 2008). But as with most scientific endeavors, new discovery opens up many new unanswered questions. First and foremost, methods that increase the precision and throughput capacity of quantifying carbon and water uptake would dramatically increase research capacity. This should include exploring new tracers, engineering new sensors to detect uptake, and permanent modification to current gas exchange chambers. Additional mechanistic and functional questions fall into two broad categories: (1) the structural and microclimatic conditions that drive woody stem water–carbon exchange and consequences for physiological functioning, and (2) the implications for these processes on ecosystem water–carbon budgets, natural selection, and climate change.
To determine the drivers of woody stem water and carbon exchange, studies need to simultaneously explore woody stem structural variation combined with microclimatic drivers that will affect these fluxes. Similar to leaves, specific bark area is positively related to stem photosynthetic rate (Cernusak and Marshall, 2000; Berveiller et al., 2007; Ávila-Lovera et al., 2017), meaning that long and thin stems have higher rates than thick, short stems. Age is another factor that affects the ability of stems to conduct photosynthesis, with increasingly older stems having lower photosynthetic capacity (Nilsen et al., 1989; Aschan et al., 2001). But to what extent do specific bark area and age affect bark water uptake and do these relationships hold across broad bark anatomies of the plant kingdom? From there, understanding the implications of bark permeability and other traits to whole plant function will also be important to unravel. For example, does a dew event in the midst of a summer drought improve plant hydraulic function and photosynthetic rates through direct bark uptake? Are the water and carbon balances of woody stems tightly linked to leaf carbon and water dynamics? Additionally, is bark permeability to water and carbon comparable and consistent across all contexts? What microclimatic conditions promote bark water and carbon uptake? Do these processes tend to occur in similar environmental conditions or at separate moments, allowing for plants to maximize carbon and water balance at different times? Many of these questions are beginning to be explored, with fascinating results, but a more holistic understanding is still needed.
Finally, even fewer studies have explored bark water and carbon uptake with a focus on larger scale implications. While bark water and carbon fluxes are small compared to ecosystem budgets, there are likely periods where they play a disproportionate role. Can we identify when woody stem exchange is important and use that information to refine ecosystem models? How much water can be absorbed by bark in different species? Here we find a phylogenetic pattern to woody stem photosynthesis, but it is still unclear if this leads to a competitive advantage for some plant species. Do the clear benefits to carbon or water balance lead to some greater likelihood for survival and reproduction? If so, has this trait been lost and regained in certain clades and what are the conditions driving the reemergence? All of these questions should also be considered within the context of climate change. As ecosystems experience more variable rainfall patterns, temperatures, and frequencies of extreme events (e.g., drought, fire, and flood), how will that affect the role of bark exchange in species persistence? Will this alternative strategy for maintaining physiological function drive ecosystem resilience following extreme events? These questions all push at the boundaries of our understanding and will continue to reveal new linkages between plant and ecosystem processes.
Author Contributions
All authors listed have contributed equally and made a substantial, direct and intellectual contribution to the work, and approved if for publication.
Conflict of Interest
The authors declare that the research was conducted in the absence of any commercial or financial relationships that could be construed as a potential conflict of interest.
Publisher’s Note
All claims expressed in this article are solely those of the authors and do not necessarily represent those of their affiliated organizations, or those of the publisher, the editors and the reviewers. Any product that may be evaluated in this article, or claim that may be made by its manufacturer, is not guaranteed or endorsed by the publisher.
Acknowledgments
We would like to genuinely thank all contributions to the two data sets that were used to conduct our analyses. This paper would not be possible without the data sets in Prentice et al. (2011) and Wang et al. (2018). We also would like to acknowledge that some of these data were accessed through the TRY database requests 12687 and 12688. We are thankful for the time, support, and conversations had with each other as fellow early-career scientists. We would also like to acknowledge our grant support: USDA-NIFA Award 2020-67014-30916 (ZC Berry), USDA-NIFAAward 2020-67014-30915 (E Avila-Lovera), Smithsonian Tropical Research Institute Earl S. Tupper Postdoctoral Fellowship (E Avila-Lovera), and DOE TES Award DESC0019037 (K O’K).
Supplementary Material
The Supplementary Material for this article can be found online at: https://www.frontiersin.org/articles/10.3389/ffgc.2021.675299/full#supplementary-material
Supplementary Table 1 | List of species that exhibit evidence of bark CO2 uptake (Bark PS), bark conductance (Bark g), bark CO2 uptake, and bark conductance (Bark PS + g), and bark water uptake. Also shown are corresponding citations and GPS coordinates of observation, when available.
Supplementary Methods 1 | Description of the methods used to conduct the literature review, estimate phylogenetic signal and trait mapping, and to classify stems as “photosynthetic” in the China Plant Trait Database.
References
Aschan, G., and Pfanz, H. (2003). Non-foliar photosynthesis–a strategy of additional carbon acquisition. Flora-Morphol. Distrib. Funct. Ecol. Plants 198, 81–97. doi: 10.1078/0367-2530-00080
Aschan, G., Wittmann, C., and Pfanz, H. (2001). Age-dependent bark photosynthesis of aspen twigs. Trees 15, 431–437. doi: 10.1007/s004680100120
Ávila, E., Herrera, A., and Tezara, W. (2014). Contribution of stem CO2 fixation to whole-plant carbon balance in nonsucculent species. Photosynthetica 52, 3–15. doi: 10.1007/s11099-014-0004-2
Ávila-Lovera, E., and Ezcurra, E. (2016). “Stem-succulent trees from the Old and New World tropics,” in Tropical tree physiology, eds G. Goldstein and L. S. Santiago (Switzerland: Springer), 45–65. doi: 10.1007/978-3-319-27422-5_3
Ávila-Lovera, E., Haro, R., Ezcurra, E., and Santiago, L. S. (2019). Costs and benefits of photosynthetic stems in desert species from southern California. Funct. Plant Biol. 46, 175–186. doi: 10.1071/fp18203
Ávila-Lovera, E., Zerpa, A. J., and Santiago, L. S. (2017). Stem photosynthesis and hydraulics are coordinated in desert plant species. New Phytol. 216, 1119–1129. doi: 10.1111/nph.14737
Berveiller, D., Kierzkowski, D., and Damesin, C. (2007). Interspecific variability of stem photosynthesis among tree species. Tree Physiol. 27, 53–61. doi: 10.1093/treephys/27.1.53
Blomberg, S. P., and Garland, T. (2002). Tempo and mode in evolution: phylogenetic inertia, adaptation and comparative methods. J. Evol. Biol. 15, 899–910. doi: 10.1046/j.1420-9101.2002.00472.x
Cannon, W. A. (1908). The topography of the chlorophyll apparatus in desert plants. Washington, DC: Carniege Institution of Washington.
Carmichael, M. J., White, J. C., Cory, S. T., Berry, Z. C., and Smith, W. K. (2020). Foliar water uptake of fog confers ecophysiological benefits to four common tree species of southeastern freshwater forested wetlands. Ecohydrology 13:e2240.
Cernusak, L., and Marshall, J. (2000). Photosynthetic refixation in branches of western white pine. Funct. Ecol. 14, 300–311. doi: 10.1046/j.1365-2435.2000.00436.x
Comstock, J. P., Cooper, T. A., and Ehleringer, J. R. (1988). Seasonal patterns of canopy development and carbon gain in nineteen warm desert shrub species. Oecologia 75, 327–335. doi: 10.1007/bf00376933
Earles, J. M., Sperling, O., Silva, L. C., McElrone, A. J., Brodersen, C. R., North, M. P., et al. (2016). Bark water uptake promotes localized hydraulic recovery in coastal redwood crown. Plant Cell Environ. 39, 320–328. doi: 10.1111/pce.12612
Ehleringer, J. R., Comstock, J. P., and Cooper, T. A. (1987). Leaf-twig carbon isotope ratio differences in photosynthetic-twig desert shrubs. Oecologia 71, 318–320. doi: 10.1007/bf00377301
Fontes, C. G., Fine, P. V. A., Wittmann, F., Bittencourt, P. R. L., Piedade, M. T. F., Higuchi, N., et al. (2020). Convergent evolution of tree hydraulic traits in Amazonian habitats: implications for community assemblage and vulnerability to drought. New Phytol. 228, 106–120. doi: 10.1111/nph.16675
Gibson, A. C. (1983). Anatomy of photosynthetic old stems of nonsucculent dicotyledons from North American deserts. Bot. Gaz. 144, 347–362. doi: 10.1086/337383
Groh, B., Hübner, C., and Lendzian, K. J. (2002). Water and oxygen permeance of phellems isolated from trees: the role of waxes and lenticels. Planta 215, 794–801. doi: 10.1007/s00425-002-0811-8
Grosse, W. (1997). “Gas transport of trees,” in Trees: contributions to modern tree physiology, eds H. Rennenberg, W. Escrich, and H. Ziegler (Leiden: Backhuys), 57–74.
Ilek, A., Siegert, C. M., and Wade, A. (2021). Hygroscopic contriutions to bark water storage and controls exerted by internal bark stucture over water vapor absorption. Trees 35, 831–843. doi: 10.1007/s00468-021-02084-0
Kattge, J., Boenisch, G., and Diaz, S. (2020). TRY plant trait database - enhanced coverage and open access. Glob. Change Biol. 26, 119–188.
Laur, J., and Hacke, U. G. (2014). Exploring P icea glauca aquaporins in the context of needle water uptake and xylem refilling. New Phytol. 203, 388–400. doi: 10.1111/nph.12806
Lindorf, H., Parisca, L. D., and Rodríguez, P. (2006). Botánica. Clasificación, estructura, reproducción., 2nd. edition. Caracas: Ediciones de la Biblioteca de la Universidad Central de Venezuela.
Lintunen, A., Paljakka, T., Jyske, T., Peltoniemi, M., Sterck, F., Von Arx, G., et al. (2016). Osmolality and non-structural carbohydrate composition in the secondary phloem of trees across a latitudinal gradient in Europe. Front. Plant Sci. 7:726. doi: 10.3389/fpls.2016.00726
Liu, J., Gu, L., Yu, Y., Huang, P., Wu, Z., Zhang, Q., et al. (2019). Corticular photosynthesis drives bark water uptake to refill embolized vessels in dehydrated branches of Salix matsudana. Plant Cell Environ. 42, 2584–2596. doi: 10.1111/pce.13578
Loram-Lourenco, L., Farnese, F. D. S., Sousa, L. F. D., Alves, R. D. F. B., Andrade, M. C. P. D., Almeida, S. E. D. S., et al. (2020). A structure shaped by fire, but also water: ecological consequences of the variability in bark properties across 31 species from the brazilian cerrado. Front. Plant Sci. 10:1718. doi: 10.3389/fpls.2019.01718
Losos, J. B. (2008). Phylogenetic niche conservatism, phylogenetic signal and the relationship between phylogenetic relatedness and ecological similarity among species. Ecol. Lett. 11, 995–1003. doi: 10.1111/j.1461-0248.2008.01229.x
Mayr, S., Schmid, P., Laur, J., Rosner, S., Charra-Vaskou, K., Dämon, B., et al. (2014). Uptake of water via branches helps timberline conifers refill embolized xylem in late winter. Plant Physiol. 164, 1731–1740. doi: 10.1104/pp.114.236646
Morris, H., and Jansen, S. (2016). Secondary xylem parenchyma–from classical terminology to functional traits. Iawa J. 37, 1–15. doi: 10.1163/22941932-20160117
Nilsen, E. T. (1995). “Stem photosynthesis: extent, patterns, and role in plant carbon economy,” in Plant stems: physiology and functional morphology, ed. B. L. Gartner (San Diego: Academic Press), 223–240. doi: 10.1016/b978-012276460-8/50012-6
Nilsen, E. T., and Sharifi, M. R. (1997). Carbon isotopic composition of legumes with photosynthetic stems from Mediterranean and desert habitats. Am. J. Bot. 84, 1707–1713. doi: 10.2307/2446469
Nilsen, E. T., Meinzer, F. C., and Rundel, P. W. (1989). Stem photosynthesis in Psorothamnus spinosus (smoke tree) in the Sonoran Desert of California. Oecologia 79, 193–197. doi: 10.1007/bf00388478
Osmond, C. B., Smith, S. D., Gui-Ying, B., and Sharkey, T. D. (1987). Stem photosynthesis in a desert ephemeral. Eriogonum inflatum. Oecologia 72, 542–549. doi: 10.1007/bf00378980
Pagel, M. (1999). Inferring the historical patterns of biological evolution. Nature 401, 877–884. doi: 10.1038/44766
Pfanz, H., and Aschan, G. (2001). The existence of bark and stem photosynthesis in woody plants and its significance for the overall carbon gain. An eco-physiological and ecological approach in Progress in botany. Berlin: Springer, 477–510.
Pfanz, H., Aschan, G., Langenfeld-Heyser, R., Wittmann, C., and Loose, M. (2002). Ecology and ecophysiology of tree stems: corticular and wood photosynthesis. Naturwissenschaften 89, 147–162. doi: 10.1007/s00114-002-0309-z
Pilarski, J. (2002). Diurnal and seasonal changes in the intensity of photosynthesis in stems of lilac (Syringa vulgaris L.). Acta Physiol. Plant. 24, 29–36. doi: 10.1007/s11738-002-0018-4
Pivovaroff, A. L., Pasquini, S. C., De Guzman, M. E., Alstad, K. P., Stemke, J. S., and Santiago, L. S. (2016). Multiple strategies for drought survival among woody plant species. Funct.Ecol. 30, 517–526. doi: 10.1111/1365-2435.12518
Pivovaroff, A. L., Sharifi, R., Scoffoni, C., Sack, L., and Rundel, P. (2014). Making the best of the worst of times: traits underlying combined shade and drought tolerance of Ruscus aculeatus and Ruscus microglossum (Asparagaceae). Funct. Plant Biol. 41, 11–24. doi: 10.1071/fp13047
Pratt, R. B., and Jacobsen, A. L. (2017). Conflicting demands on angiosperm xylem: Tradeoffs among storage, transport and biomechanics. Plant Cell Environ. 40, 897–913. doi: 10.1111/pce.12862
Prentice, I. C., Meng, T., Wang, H., Harrison, S. P., Ni, J., and Wang, G. (2011). Evidence of a universal scaling relationship for leaf CO2 drawdown along an aridity gradient. New Phytol. 190, 169–180. doi: 10.1111/j.1469-8137.2010.03579.x
Rosell, J. A., Castorena, M., Laws, C. A., and Westoby, M. (2015). Bark ecology of twigs vs. main stems: functional traits across eighty-five species of angiosperms. Oecologia 178, 1033–1043. doi: 10.1007/s00442-015-3307-5
Schönherr, J., and Ziegler, H. (1980). Water permeability of Betula periderm. Planta 147, 345–354. doi: 10.1007/bf00379844
Stewart, W. N., and Rothwell, G. W. (1993). Paleobotany and the evolution of plants. Cambridge, MA: Cambridge University Press.
Teskey, R. O., and McGuire, M. A. (2005). CO 2 transported in xylem sap affects CO 2 efflux from Liquidambar styraciflua and Platanus occidentalis stems, and contributes to observed wound respiration phenomena. Trees 19, 357–362. doi: 10.1007/s00468-004-0386-z
Teskey, R. O., Saveyn, A., Steppe, K., and Mcguire, M. A. (2008). Origin, fate and significance of CO2 in tree stems. New Phytol. 177, 17–32. doi: 10.1111/j.1469-8137.2007.02286.x
Tinoco-Ojanguren, C. (2008). Diurnal and seasonal patterns of gas exchange and carbon gain contribution of leaves and stems of Justicia californica in the Sonoran Desert. J. Arid. Environ. 72, 127–140. doi: 10.1016/j.jaridenv.2007.06.004
Wang, H., Harrison, S. P., Prentice, I. C., Yang, Y., Bai, F., Togashi, H. F., et al. (2018). The China plant trait database: Toward a comprehensive regional compilation of functional traits for land plants. Ecology 99:500. doi: 10.1002/ecy.2091
Whittaker, R. H. (1962). Classification of natural communities. Bot. Rev. 28, 1–239. doi: 10.1007/bf02860872
Wolfe, B. T. (2020). Bark water vapour conductance is associated with drought performance in tropical trees. Biol. Lett. 16:20200263. doi: 10.1098/rsbl.2020.0263
Keywords: woody stem interaction with the atmosphere, woody stem photosynthesis, phylogenetic signal, water flux, carbon flux, bark water uptake
Citation: Berry ZC, Ávila-Lovera E, De Guzman ME, O’Keefe K and Emery NC (2021) Beneath the Bark: Assessing Woody Stem Water and Carbon Fluxes and Its Prevalence Across Climates and the Woody Plant Phylogeny. Front. For. Glob. Change 4:675299. doi: 10.3389/ffgc.2021.675299
Received: 02 March 2021; Accepted: 25 June 2021;
Published: 27 July 2021.
Edited by:
John T. Van Stan, Georgia Southern University, United StatesReviewed by:
Brett Wolfe, Louisiana State University Agricultural Center, United StatesSalli F. Dymond, University of Minnesota Duluth, United States
Copyright © 2021 Berry, Ávila-Lovera, De Guzman, O’Keefe and Emery. This is an open-access article distributed under the terms of the Creative Commons Attribution License (CC BY). The use, distribution or reproduction in other forums is permitted, provided the original author(s) and the copyright owner(s) are credited and that the original publication in this journal is cited, in accordance with accepted academic practice. No use, distribution or reproduction is permitted which does not comply with these terms.
*Correspondence: Z. Carter Berry, zcberry@gmail.com
†These authors have contributed equally to this work