- 1Field Science Center, Faculty of Agriculture, Tokyo University of Agriculture and Technology, Fuchu, Japan
- 2School of Agricultural Sciences, Nagoya University, Nagoya, Japan
- 3Graduate School of Agricultural and Life Sciences, University of Tokyo, Nishitokyo, Japan
- 4Graduate School of Bioagricultural Sciences, Nagoya University, Nagoya, Japan
Vertical stratification of forests results in the occurrence of different arthropod assemblages between the vertical layers. Fallen arthropods from the canopy layers (i.e., “arthropod rain”) are additional food sources for predators thriving on the forest floor (FF). However, the abundances of arthropods are strongly affected by weather conditions and vertical stratification. Therefore, in this study, we investigated the vertical distribution of arthropod assemblages and effects of temperature and precipitation on the arthropod rain in a temperate conifer (Cryptomeria japonica) forest. Arthropods were collected by water-pan traps and trunk-sticky traps in the upper canopy (UC; 16 m), lower canopy (10 m), and FF (0.5 m). Among the fallen arthropods collected by water-pan traps, wandering detritivores, and herbivores were more abundant ranging from the FF to the UC, whereas the abundance of wandering predators (mainly spiders) was similar in the upper and lower canopies. However, detritivores, herbivores, and predators showed the highest abundance in the UC among the flying arthropods. Wandering arthropods moved upward from the FF toward the tree trunks more frequently than downward, indicating the importance of arthropod immigration from the ground to arboreal habitats. Temperature and precipitation had different effects on fallen and moving arthropods among different taxonomic groups. Flying arthropods were affected only by temperature, while wandering detritivores and herbivores were affected by precipitation and temperature. Thus, the abundance of wandering and flying arthropods differed among the vertical layers of a temperate conifer forest; additionally, arthropod rain was closely associated with weather conditions.
Introduction
Forests exhibit vertical stratification, and the dendritic pattern (i.e., tree trunks and branches) of trees serves as suitable habitat patches for arthropods. Arboreal arthropods occupy habitat patches, based on their different niches, such as flying or wandering within such habitat structures (Davis et al., 1997; Simon and Linsenmair, 2001; Su and Woods, 2001; Tanabe, 2002; Yoshida and Hijii, 2005b; Oguri et al., 2014); additionally, their population dynamics and community compositions are affected by the vertical stratification of forests (Basset et al., 2003; Nakamura et al., 2017). Wandering arthropods use arboreal habitat patches in the forest vertical layers by moving within the tree trunks (Hanula and Franzreb, 1998) or by aerial dispersal (Karasawa et al., 2005; Lindo, 2010; Bailey et al., 2018). Additionally, flying arthropods exhibit vertical distribution among different strata (Sutton and Hudson, 1980; Simon and Linsenmair, 2001; Tanabe, 2002; Leksono et al., 2005; Maguire et al., 2014).
Fallen arthropods from the canopy layers (i.e., “arthropod rain”) are additional food sources for predators thriving on the ground (Pringle and Fox-Dobbs, 2008; Rozanova et al., 2019) and in the streams (Kawaguchi and Nakano, 2001; Kawaguchi et al., 2003; Chan et al., 2008). Arthropod rain varies among different forest vertical layers, forest types (conifer vs. broad-leaved, evergreen vs. deciduous), and seasons. For example, many herbivore arthropods (hereafter referred to as herbivores) fall from the upper canopy (UC), which is characterized by living foliage, while detritivore arthropods (hereafter referred to as detritivores) fall from the lower canopy (LC), which is characterized by dead foliage. Thus, understanding the composition of arthropod rain and its source from the different canopy layers can reveal potential roles of energy inputs from the canopy into soil food webs (Goncharov et al., 2016; Potapov et al., 2016; Rozanova et al., 2019).
Moreover, weather conditions are known to influence arthropod dispersal (Greenstone, 1990; Delattre et al., 2013). For example, precipitation may increase or decrease arthropod activity on the forest floor (FF) (Pedigo, 1970; Lensing et al., 2005). Previous studies have reported that collembolans move upward from the FF to arboreal habitats during precipitation (Bowden et al., 1976; Bauer, 1979; Farrow and Greenslade, 1992). Rainwater also washes out arboreal arthropods, inhabiting the substrates of trees. Additionally, temperature predominately determines the activity and growth of arthropods, which are ectotherms, and affects the aerial dispersal of some arthropods (Johnson, 1969; Richter, 1970; Bishop, 1990). Therefore, weather conditions play an important role in determining the vertical distribution of arthropods and the arthropod rain in the canopy layers of forests.
Understanding the vertical distribution of arboreal arthropods and the factors affecting their movement among the vertical layers helps clarify the mechanisms and dynamics of arthropod assemblages in forests. Although many studies have reported on the vertical distribution of arthropods, the knowledge about the upward and downward movements of arboreal arthropods in the vertical structure of forests is limited. In the present study, we investigated the abundances of wandering and flying arthropods in the vertical layers of a temperate conifer forest using water-pan traps and trunk-sticky traps; additionally, we examined the effects of weather conditions on the arthropod rain in the forest. Surveys were conducted in a Japanese cedar (Cryptomeria japonica) plantation plot with much dead foliage in the LC layers (Yoshida and Hijii, 2006b). Detritivores, such as oribatid mites and collembolans, colonize both living and dead foliage of Japanese cedar trees (Yoshida and Hijii,2005a,b), and most of them move from the FF into the arboreal habitats (Yoshida and Hijii, 2005b, Yoshida and Hijii, 2006a). It is likely that such colonists will be easily washed out by rainwater from the arboreal substrates due to less morphological adaptation to arboreal life unlike truly arboreal species (Orivel et al., 2001; Karasawa and Hijii, 2008). Thus, we tested the following hypotheses in this study: (1) the wandering arthropods are more abundant at the lower traps that collect cumulatively fallen arthropods; (2) the flying arthropods (especially flying herbivores) are more abundant in the UC having much living foliage compared with the LC having much dead foliage; (3) the catch of wandering and flying arthropods, which are ectotherms, is influenced by temperature (i.e., the arthropod dispersal increases with increasing temperature in the temperate forest), while wandering detritivores, which are wingless and move from the FF, constitute a major component of arthropod rain and are strongly affected by precipitation since they are washed from the tree substrates by rainwater.
Materials and Methods
Study Sites
The study was conducted in a 36-year-old (as of 2006) Japanese cedar (C. japonica D. Don) plantation in the experimental forest plot of Nagoya University in central Japan (35°11′N, 137°33′E; 980 m.a.s.l., Supplementary Figure 1). The meteorological equipment near the stand (within 200 m) showed that mean annual precipitation and mean annual air temperature were 2,327 mm and 9.2°C, respectively (1995–2007). The stand density was approximately 2,000 trees ha–1, and the mean tree height of the trees in the stand was approximately 20 m (Yoshida and Hijii, 2006b). Since the tree canopy was closed, less understory vegetation grew on the FF, and thus the proportion of dead leaves and branches was high in the lower canopies (Yoshida and Hijii, 2006b). A 17-m observation tower was built to access the canopy in the stand. The weekly precipitation and mean air temperature observed during the study period are presented in Figure 1.
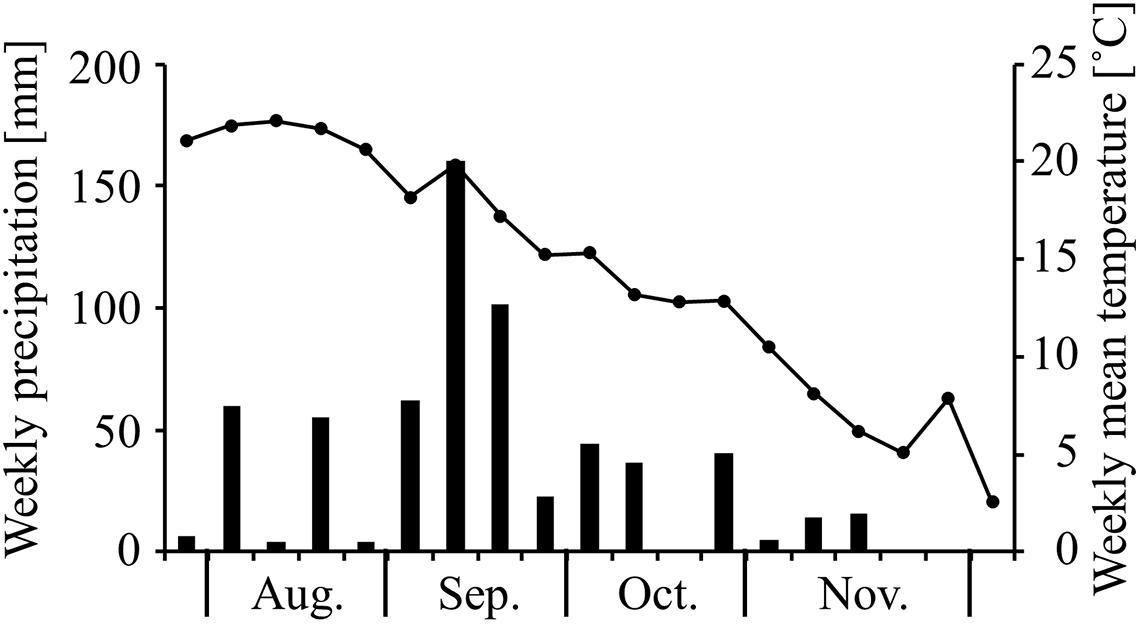
Figure 1. Temporal changes in weekly precipitation (solid bars) and weekly mean air temperature (solid circles) during the study period.
Arthropod Sampling
Water-pan traps and trunk-sticky traps were used for arthropod sampling. Plastic rectangular containers (0.073-m2 opening and 110-mm depth) were used as water pans to capture canopy arthropods along the vertical stratification of the stand. We placed five such water-pan traps, filled with 1 L of water and a small amount of detergent for preservation, at ca. 16 (UC), 10 (LC), and 0.5 m above the ground (near FF). The traps in the UCs and LCs were fixed with rubber bands on the edges of steel pipes protruding from the observation tower, and the traps near the FF were fixed to plastic boxes on the ground. We collected weekly arthropod samples through a 0.1-mm mesh sieved from July 25 to December 7, 2006. A total of 285 pan-trap samples [three vertical layers (UC, LC, and FF) × 5 replications × 19 sampling times] were collected.
A fly-catch sticky trap ribbon (Kamoi Kakoshi Co., Okayama, Japan) was used to collect arthropod samples from the tree trunks. The trap consisted of a strip of waterproof paper (270 mm × 50 mm) with adhesive on both sides. Similar to the water-pan traps, the sticky traps were fixed at ca. 10-m height (LC) using the footholds of steel pipes protruding from the observation tower and at 0.5-m height above the ground (near FF). The tree barks were stripped at each of the mentioned heights to provide a smooth surface where two sticky bands were wound, approximately 20 mm apart, around the trunk. This was done to differentiate between the arthropods moving downward from the upper trunk and those moving upward from the lower trunk. The traps were tightly fastened using tacks. We mounted a pair of sticky traps on the trunk at each height on each of the 10 different trees for a week of every month, i.e., August 10–17, September 21–28, October 19–26, and November 16–22, 2006. After the weekly period, we dissolved the adhesive on the sticky traps in kerosene to collect arthropods and segregated them by using a 0.1-mm mesh sieve. A total of 80 sticky-trap samples [2 vertical layers (UC and LC) × 2 band positions (upper and lower) × 5 replications × 4 sampling times] were collected.
Subsequently, we identified the arthropods sampled using the two traps to the order or suborder levels and counted them under a stereomicroscope (35× magnification). Furthermore, the arthropods were divided into four feeding groups (detritivores, predators, herbivores, and others) (following Moran and Southwood, 1982; Hijii, 1989; Stork and Blackburn, 1993). In our study, the detritivores included microbi-detritivores, fungivores, and epiphyte grazers since classification of the arthropods based on the feeding habits to the order and suborder level was difficult. We determined the feeding group of some orders and suborders that have multiple feeding groups based on the dominant family or species groups (e.g., leaf beetles for Coleoptera). Additionally, the arthropods were divided into “flying” and “wandering” groups with/without wings. Dipteran larvae were excluded from the water-pan trap samples since dipteran adults laid eggs in the traps. The density of arthropods collected by water-pan traps was expressed as the number of individuals per unit area per day (individuals m–2 day–1), while the density from the trunk-sticky traps was expressed as the number of individuals per unit trap per day (individuals trap–1 day–1).
Data Analysis
Generalized linear mixed models (GLMMs) with Poisson’s distributions and a log link were used to examine the effects of trap height (layer), temperature, and precipitation on the density of arboreal arthropods. We used the densities of the major taxonomic groups, feeding groups, and dispersal types (flying or wandering) as response variables; additionally, the type of layer, weekly mean temperature, and weekly precipitation were used as explanatory variables; and the replications of traps were treated as random effects. The post hoc comparisons between the layers (UC vs. LC, LC vs. FF) in terms of the densities of the major taxonomic groups of water-pan traps were made through estimated marginal means contrasts, using the emmeans package. For the analysis of the sticky trap, we used GLMMs with the density of arboreal arthropods as a response variable and the month of sampling, the layers, and the band position as the explanatory variables. We used likelihood ratio tests to evaluate the significance of the explanatory variables. Statistical analyses were conducted using the lme4 package for R v.3.6.2 (R Development Core Team, 2017).
Results
The abundances of wandering and flying arthropods were 29,407 and 8,153, respectively. Collembola (detritivores) was the most dominant taxonomic group among the wandering arthropods (Supplementary Table 1), while winged arthropods belonging to the orders Diptera (detritivores) and Hymenoptera (predators) dominated the flying arthropods (Supplementary Table 2). Among the fallen (wandering) arthropods collected by water-pan traps, wandering detritivores and herbivores were more abundant in the LC layers than in the UC layers. The abundance of wandering predators was lower in LC than in UC, although the abundances were not significantly different between the two layers (p > 0.05; Figure 2 and Table 1). Wandering spiders, which are the most dominant group among the wandering predators, were significantly more abundant in UC than in LC (Supplementary Table 3). Among the flying arthropods, detritivores, and predators had the highest abundance in UC, while the abundances of herbivores did not significantly differ between UC and LC (p > 0.05; Figure 2 and Table 1).
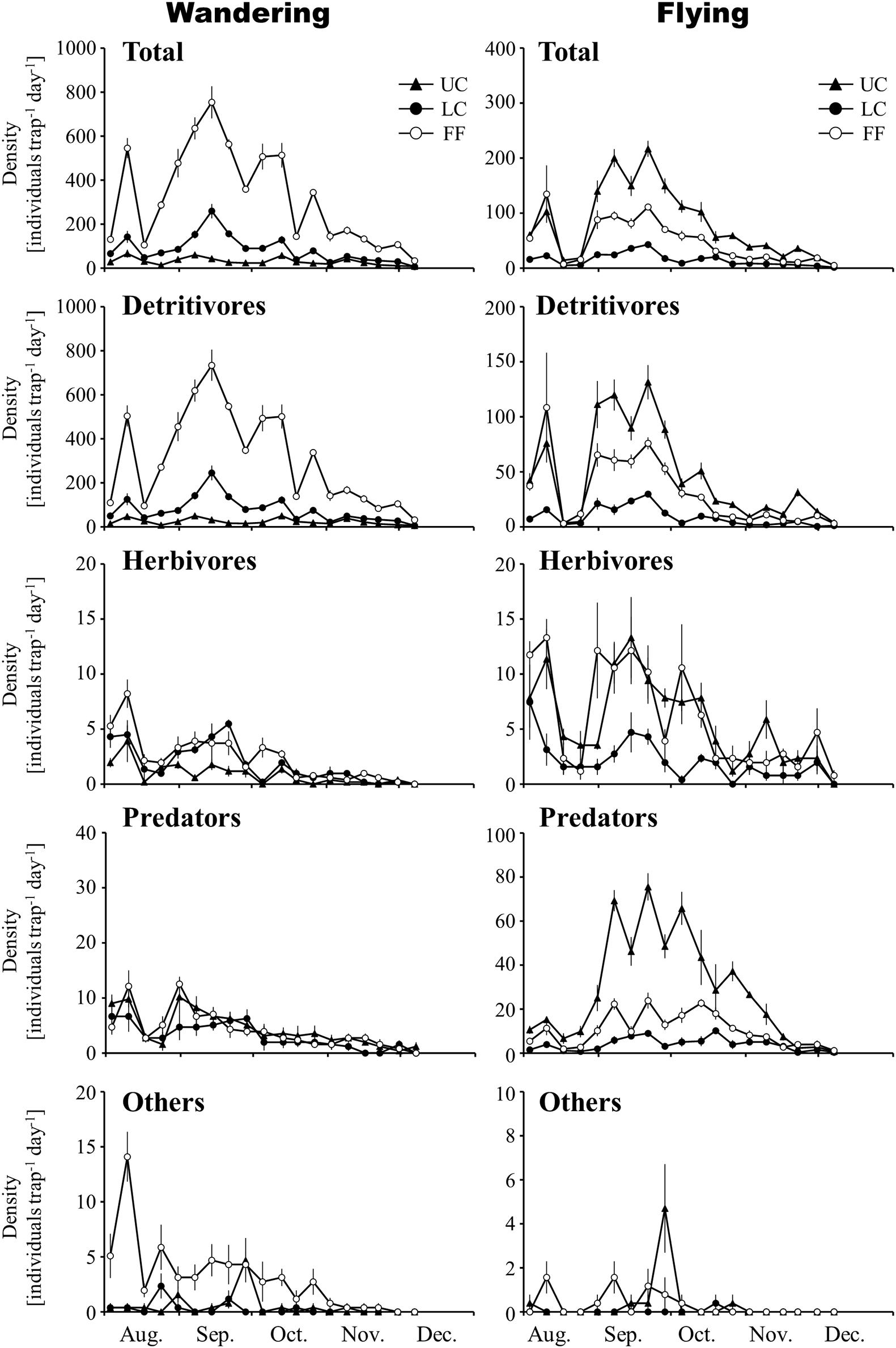
Figure 2. Temporal changes in the density of arthropod groups collected by water-pan traps in the vertical strata of a Japanese cedar (Cryptomeria japonica) forest. Values represent mean ± standard error. UC, upper canopy; LC, lower canopy; FF, forest floor.
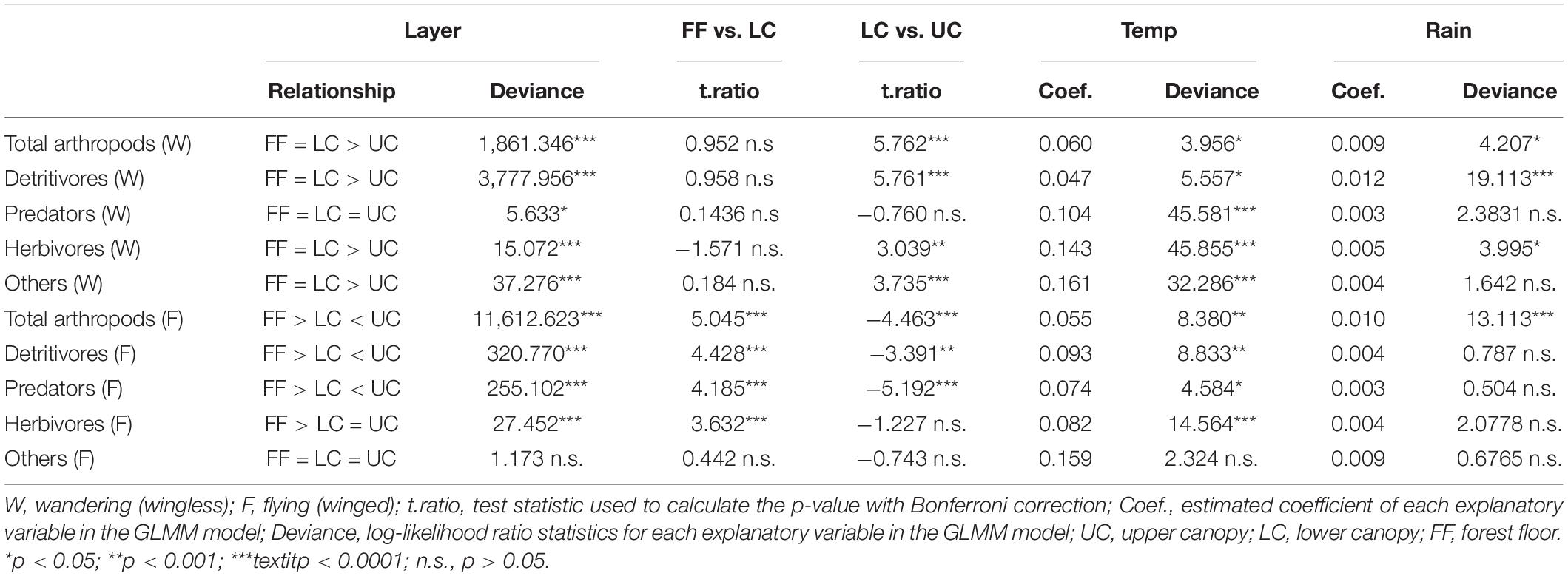
Table 1. Summary results of generalized linear mixed models (GLMMs) that incorporated the effects of trap height (Layer), different layers, temperature (Temp), and precipitation (Rain) on the abundance of feeding groups collected by water-pan traps (a total of 285 samples) in a Japanese cedar (Cryptomeria japonica) forest.
The densities of wandering arthropods (7.4–65.4 individuals m–2 day–1 in the UC, 6.3–258.7 individuals m–2 day–1 in the LC, and 33.3–753.4 individuals m–2 day–1 near the FF) and flying arthropods (4.7–216.8 individuals in the UC, 1.6–43.1 individuals in the LC, and 5.1–134.6 individuals near the FF) collected by water-pan traps exhibited a similar temporal trend. The densities of both were maximum in September in the three layers (Figure 2). In contrast, among the arthropods collected by trunk-sticky traps, the densities of the wandering predators (0.1–1.9 individuals trap–1 day–1), wandering herbivores (0.0–3.8 individuals m–2 day–1), and flying arthropods (0.0–9.2 individuals m–2 day–1) decreased in September, and the density of wandering detritivores (1.6–37.0 individuals m–2 day–1) decreased in November (Figures 3, 4). Additionally, the abundances of both wandering and flying arthropods significantly differed between the LC and FF (p < 0.05; Table 2 and Figures 3, 4). Both wandering and flying arthropods (especially detritivores and herbivores) showed more upward migration (caught on the lower sticky bands) than downward migration (caught on the upper bands) (p < 0.05; Table 2 and Figures 3, 4).
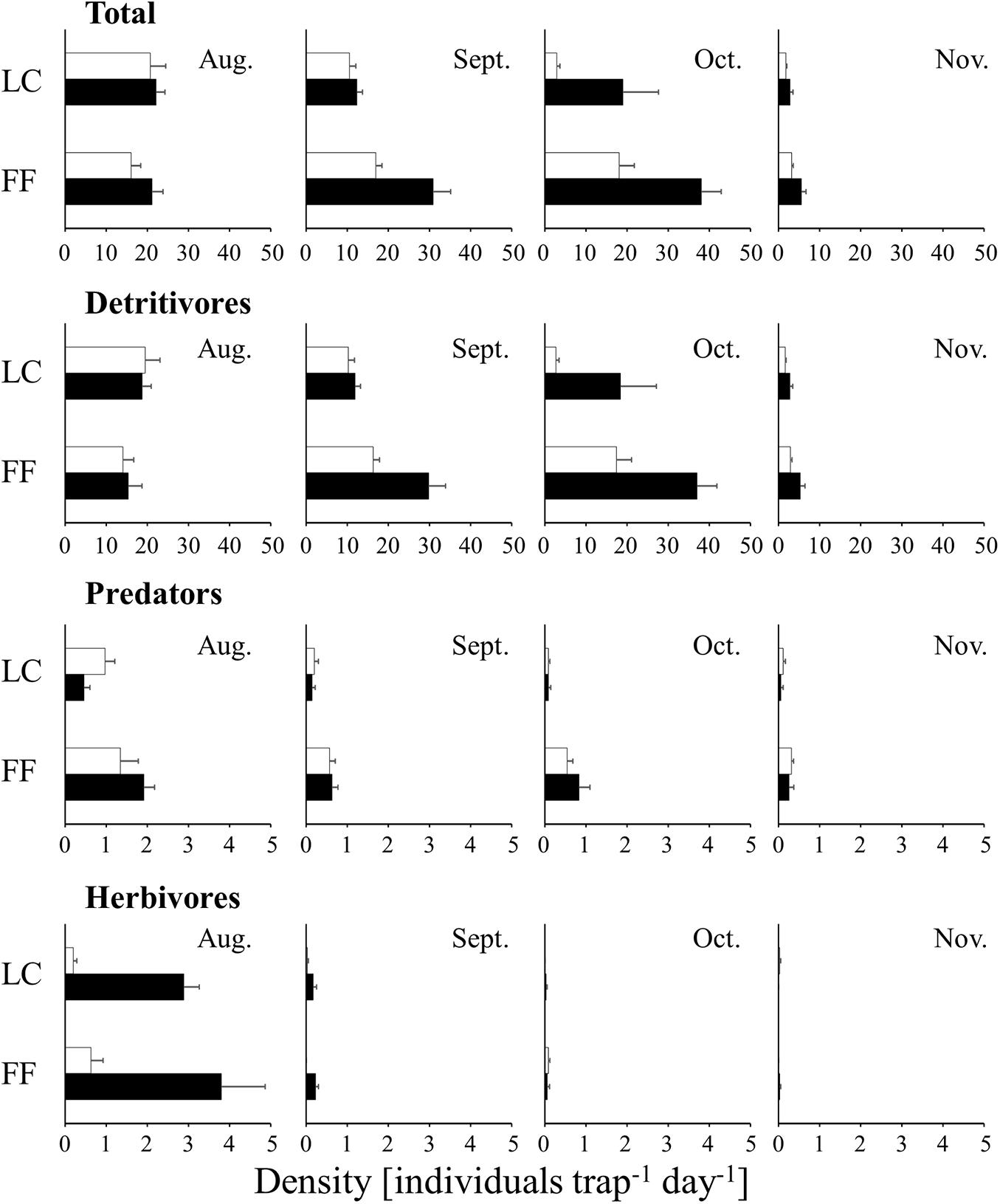
Figure 3. Temporal changes in the density of wandering arthropods collected by trunk-sticky traps in the vertical strata of a Japanese cedar (Cryptomeria japonica) forest. The black and white bars indicate upper and lower sticky bands, respectively. Values represent mean ± standard error. LC, lower canopy; FF, forest floor.
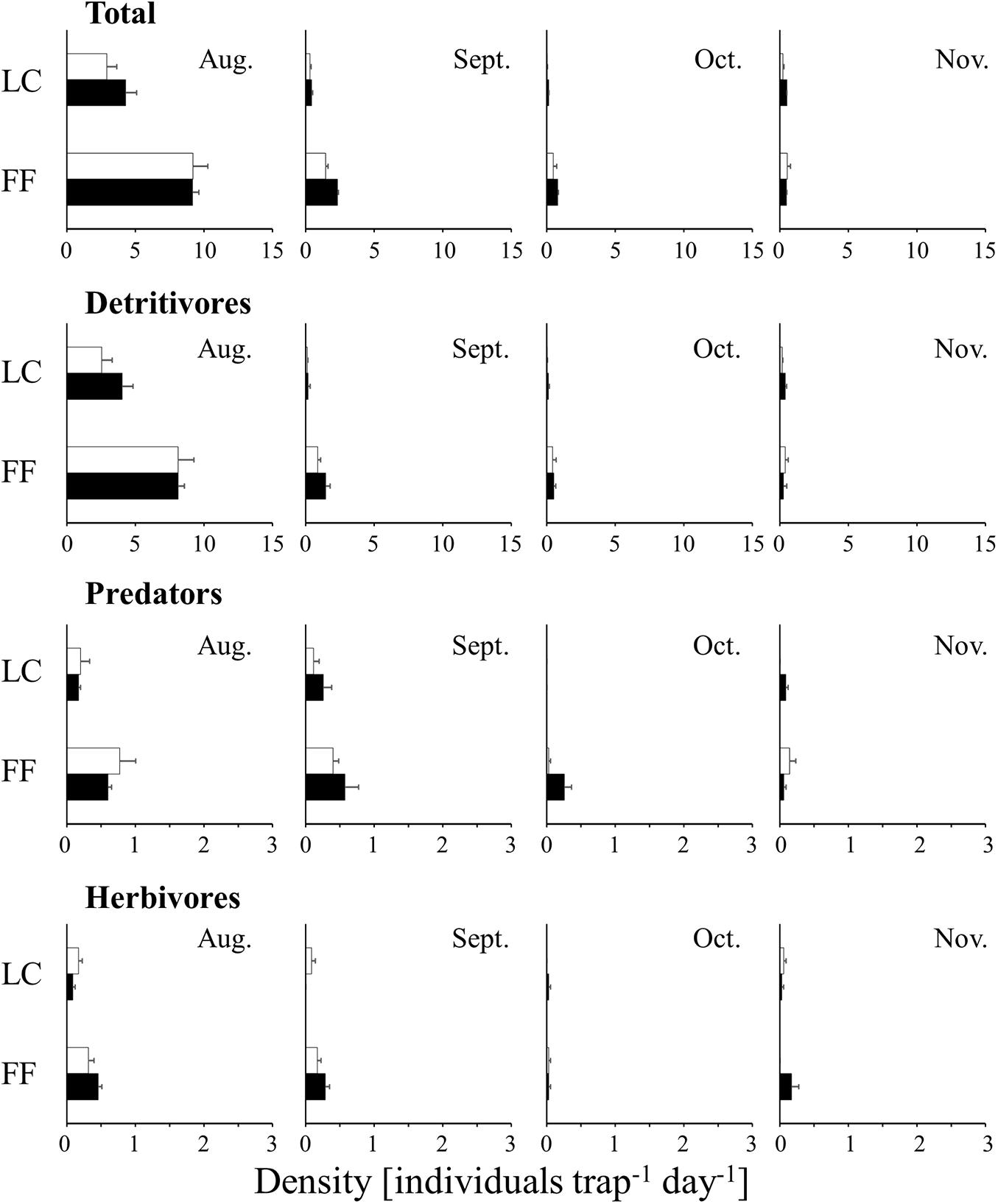
Figure 4. Temporal changes in the density of flying arthropods collected by trunk-sticky traps in the vertical strata of a Japanese cedar (Cryptomeria japonica) forest. The black and white bars indicate upper and lower sticky bands, respectively. Values represent mean ± standard error. LC, lower canopy; FF, forest floor.
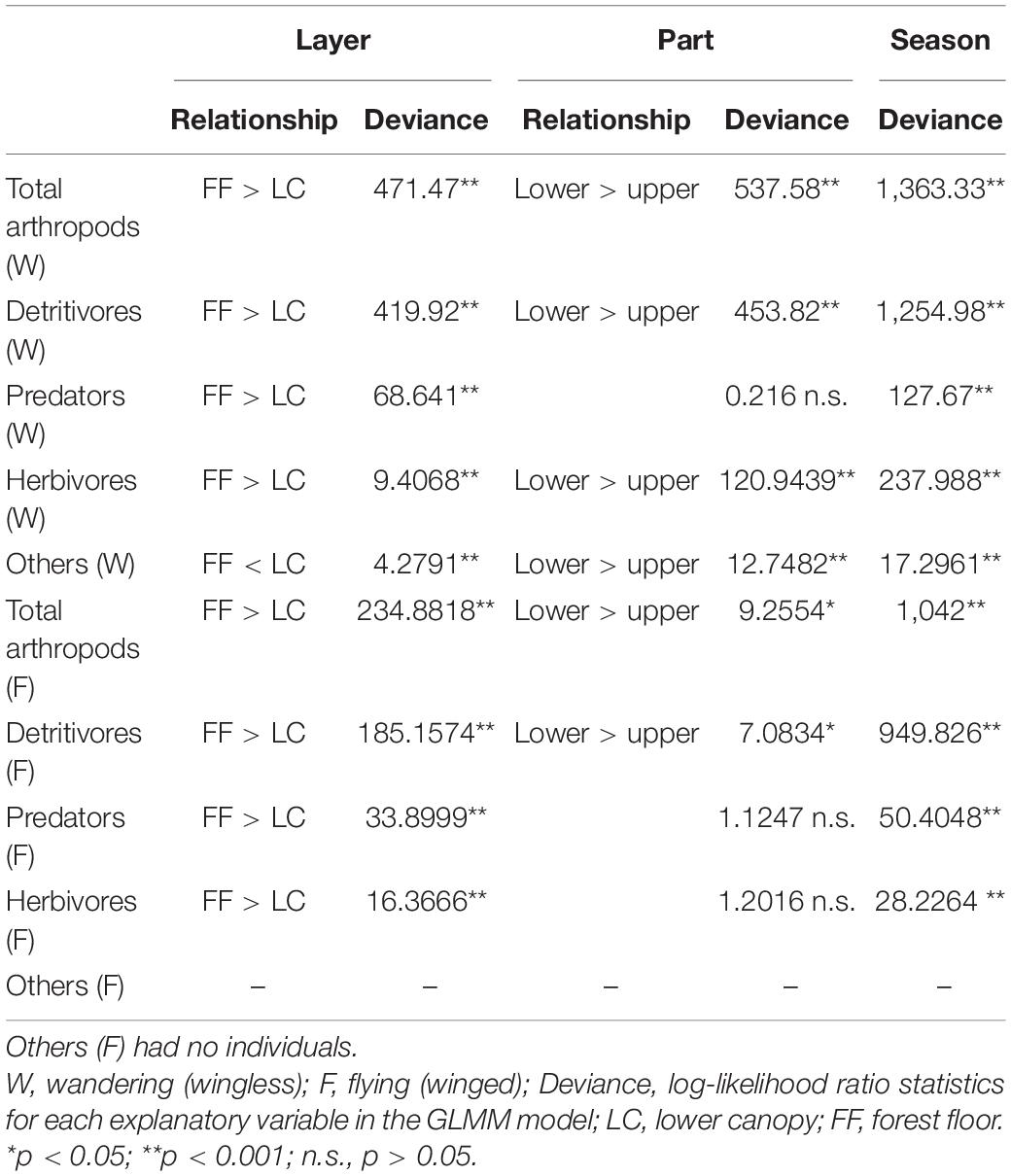
Table 2. Summary of the results of the generalized linear mixed models (GLMMs) that incorporated the effects of trap height (Layer), trap parts (Part), and seasons (Season) on the abundance of feeding groups collected by trunk-sticky traps (a total of 80 samples) in a Japanese cedar (Cryptomeria japonica) forest.
Temperature and precipitation had varying effects among the different taxonomic groups of arthropods collected by water-pan traps. Flying arthropods were affected only by temperature, while wandering detritivores and herbivores were affected by precipitation and temperature (Table 1).
Discussion
The results of the water-pan trap method showed that the arthropod rain mainly consisted of wandering detritivores (collembolans and mites) in the studied temperate conifer forest. This was in agreement with the findings of the previous study in temperate forests (Rozanova et al., 2019). Furthermore, wandering detritivores and herbivores were more abundant in the lower two layers, thus proving that our first hypothesis, which stated that the abundance of wandering arthropods is more at the lower traps that collects fallen arthropods, is true. However, the density of wandering predators (spiders) was lower in the LC layer than the UC layer. A previous study, which used foliage beating method in the same stand, showed that spider densities at foliage level did not differ between the two layers (Oguri et al., 2014). The variability in the results could be attributed to the dispersal activity (ballooning) because spiders living in the higher strata of vegetation and depending on web-building to catch their prey are suggested to have more opportunities to ballooning dispersal due to more frequent silk production (Bonte et al., 2003).
Flying detritivores (winged arthropods belonging to the order Diptera) and predators (winged arthropods belonging to the order Hymenoptera) were more abundant in the UC than the other two layers, while the abundance of flying herbivores did not significantly differ between UC and LC. Therefore, the results of flying detritivores and predators except for the flying herbivores support the second hypothesis that flying arthropods would be more abundant in UC than in the LC. A similar tendency was observed in the previous studies, which proved that dipterans and hymenopterans exhibited higher activity in the upper canopies compared with the lower canopies in tropical and temperate forests (Sutton and Hudson, 1980; Simon and Linsenmair, 2001; Maguire et al., 2014). For example, some dipteran and hymenopteran species mate and swarm near tree crowns (Morgan, 1968; Downes, 1969). The reason dipteran adults were less abundant in the LC with much dead foliage is unknown. However, a foliage washing survey in the same stand found that dipteran larvae were more abundant in the dead foliage of the conifer canopies (Yoshida and Hijii, 2005a). This suggests that flying dipterans change the habitat use among their developmental stage vertically.
Conversely, fewer herbivores were collected by water-pan traps in UC than expected despite the presence of large quantities of living foliage. However, previous studies that used chemical knockdown and foliage clipping methods reported that many herbivores belonging to orders Hemiptera and Thysanoptera were observed in the canopy of Japanese cedar forests (Hijii, 1989; Hijii et al., 2001). High abundance of predators in UC was due to the presence of sufficient herbivores (preys). Thus, the low abundance of flying herbivores observed in UC in this study would be either because they are majorly sessile (e.g., Coccoidea) or because they were dispersed on a smaller scale within the living foliage of a single layer, rather than between the layers.
Furthermore, our findings indicated that the number of wandering arthropods moving upward was more than that moving downward toward the FF, indicating the importance of immigration by arthropods from the FF to arboreal habitats. Some species of wandering arthropods are known to lay eggs on the FF and then move into trees during the larval and adult stages (Yoshida and Hijii, 2005b, Yoshida and Hijii, 2006a, 2014 for Collembola). In addition to this migration behavior, dispersal for colonizing new habitats might be another factor driving upward movement toward trees. For example, Lindo and Winchester (2007) reported that many ground-dwelling arthropods expand their habitat ranges to several meters above ground level on tree trunks. This immigration tendency of wandering arthropods and flying arthropods from the FF to arboreal habitats might increase the similarity of arthropod assemblages in the arboreal and forest-floor strata. Additionally, this tendency may sustain the arboreal food-web structure through the presence of ground-dwelling predators (e.g., spiders and harvestmen) and prey (e.g., collembolans) (Miyashita et al., 2003; Haraguchi et al., 2013).
Arthropod rain (falling of wandering detritivores and herbivores) was closely associated with precipitation and temperature fluctuations. The findings supported our third hypothesis that the wandering herbivores and detritivores are influenced by precipitation, whereas flying arthropods are influenced only by temperature. Additionally, the ballooning of wandering spiders is associated with air temperature (Richter, 1970; Bishop, 1990). Bishop (1990) indicated that spider ballooning occurred after a decline in air temperature after the warming of the ground, which provides upward air currents. Moreover, wandering arthropods, such as Acari and Collembola, can be washed out of the tree substrates by rainwater. After precipitation, however, tree trunks are moist, which serve as suitable habitats for arthropods, resulting in their consequent upward movement to the arboreal habitats (Bowden et al., 1976; Bauer, 1979; Farrow and Greenslade, 1992). Subsequently, increased tree climbing by arthropods would increase the dispersal of arthropods by wind currents or would promote their falling from the arboreal habitats, thus leading to arthropod rain. In our study, the dispersal patterns of majority of the arthropod groups were observed to be influenced by temperature. Furthermore, temperature had a significant effect on detritivores, herbivores, and predators, indicating that predators responded directly to temperature and indirectly to an increased abundance of potential prey.
Conclusion
In conclusion, the abundances of fallen and moving arthropods differed among the vertical layers of a temperate conifer forest, and the arthropod rain was closely associated with weather conditions. The results that showed the upward and downward movements of arboreal arthropods among the vertical layers reveal the spillover effects of arboreal arthropods in the vertical structure of forests. Several studies have suggested that an arboreal-prey subsidy by abiotic physical conveyor (gravity) (i.e., arthropod rain) has important functional roles in trophic dynamics of the recipient system (ground surface) in forests (Pringle and Fox-Dobbs, 2008; Goncharov et al., 2016; Potapov et al., 2016; Rozanova et al., 2019). Thus, the findings of the study highlight the dependence of falling-prey subsidies into FF on the weather conditions and the importance of arthropod immigration from the ground to arboreal habitats. The arthropod movement among the vertical layers will be significantly affected by climate change. Climate warming leads to increased abundances of forest arthropods (Logan et al., 2003; Robinet and Roques, 2010). However, in the case that it causes drier conditions (i.e., low and infrequent precipitation), it should decrease the upward movement of wandering detritivores from the FF to the arboreal habitats, thus resulting in a decrease in arthropod rain within the forests.
Data Availability Statement
The original contributions presented in the study are included in the article/Supplementary Material, further inquiries can be directed to the corresponding author/s.
Author Contributions
TY and NH formed and designed the field survey and wrote the manuscript. TY and YK performed the field survey. YF analyzed the data. All authors contributed to the article and approved the submitted version.
Funding
This study was supported by the Japanese Society for the Promotion of Science under Grants 16K07775, 18H02223, and 19K06121.
Conflict of Interest
The authors declare that the research was conducted in the absence of any commercial or financial relationships that could be construed as a potential conflict of interest.
Publisher’s Note
All claims expressed in this article are solely those of the authors and do not necessarily represent those of their affiliated organizations, or those of the publisher, the editors and the reviewers. Any product that may be evaluated in this article, or claim that may be made by its manufacturer, is not guaranteed or endorsed by the publisher.
Acknowledgments
We thank Y. Imaizumi, N. Yamaguchi, and N. Takabe for providing assistance with the fieldwork.
Supplementary Material
The Supplementary Material for this article can be found online at: https://www.frontiersin.org/articles/10.3389/ffgc.2021.672601/full#supplementary-material
References
Bailey, R. I., Molleman, F., Vasseur, C., Woas, S., and Prinzing, A. (2018). Large body size constrains dispersal assembly of communities even across short distances. Sci. Rep. 8, 1–12. doi: 10.1038/s41598-018-29042-0
Basset, Y., Hammond, P. M., Barrios, H., Holloway, J. D., and Miller, S. E. (2003). “Vertical stratification of arthropod assemblages,” in Arthropods of Tropical Forests: Spatio-temporal dynamics and resource use in the canopy, eds Y. Basset, V. Novotny, S. E. Miller, and R. L. Kitching (Cambridge: Cambridge University Press), 17–27.
Bauer, T. (1979). Die Feuchtigkeit als steuernder Faktor für das Kletterverhalten von Collembolen. Pedobiologia 19, 165–175.
Bishop, L. (1990). Meteorological aspects of spider ballooning. Environ. Entomol. 19, 1381–1387. doi: 10.1093/ee/19.5.1381
Bonte, D., Vandenbroecke, N., Lens, L., and Maelfait, J.-P. (2003). Low propensity for aerial dispersal in specialist spiders from fragmented landscapes. Proc. R. Soc. Lond. B. 270, 1601–1607. doi: 10.1098/rspb.2003.2432
Chan, E. K. W., Zhang, Y., and Dudgeon, D. (2008). Arthropod ‘rain’ into tropical streams: the importance of intact riparian forest and influences on fish diets. Mar. Freshw. Res. 59, 653–660. doi: 10.1071/MF07191
Davis, A. J., Huijbregts, J., Kirk-Spriggs, A. H., Krikken, J., and Sutton, S. L. (1997). “The ecology and behavior of arboreal dung beetles in Borneo,” in Canopy arthropods, eds N. E. Stork, J. Adis, and R. K. Didham (London: Chapman and Hall), 453–476.
Delattre, T., Baguette, M., Burel, F., Stevens, V. M., Quénol, H., and Vernon, P. (2013). Interactive effects of landscape and weather on dispersal. Oikos 122, 1576–1585. doi: 10.1111/j.1600-0706.2013.00123.x
Downes, J. A. (1969). The swarming and mating flight of Diptera. Annu. Rev. Entomol. 14, 271–298. doi: 10.1146/annurev.en.14.010169.001415
Farrow, R. A., and Greenslade, P. (1992). A vertical migration of Collembola. Entomologist 111, 38–45.
Goncharov, A. A., Tsurikov, S. M., Potapov, A. M., and Tiunov, A. V. (2016). Short-term incorporation of freshly fixed plant carbon into the soil animal food web: field study in a spruce forest. Eco. Res. 31, 923–933. doi: 10.1007/s11284-016-1402-7
Greenstone, M. H. (1990). Meteorological determinants of spider ballooning: the roles of thermals vs. the vertical windspeed gradient in becoming airborne. Oecologia 84, 164–168. doi: 10.1007/BF00318267
Hanula, J., and Franzreb, K. (1998). Source, distribution and abundance of macroarthropods on the bark of longleaf pine potential prey of the red-cockaded woodpecker. Forest Ecol. Manage. 102, 89–102. doi: 10.1016/S0378-1127(97)00119-9
Haraguchi, T. F., Uchida, M., Shibata, Y., and Tayasu, I. (2013). Contributions of detrital subsidies to aboveground spiders during secondary succession, revealed by radiocarbon and stable isotope signatures. Oecologia 171, 935–944. doi: 10.1007/s00442-012-2446-1
Hijii, N. (1989). Arthropod communities in a Japanese cedar (Cryptomeria japonica D. Don) plantation: abundance, biomass and some properties. Ecol. Res. 4, 243–260. doi: 10.1007/BF02348446
Hijii, N., Umeda, Y., and Mizutani, M. (2001). Estimating density and biomass of canopy arthropods in coniferous plantations: an approach based on a tree-dimensional parameter. Forest Ecol. Manage. 144, 147–157. doi: 10.1016/S0378-1127(00)00367-4
Karasawa, S., Gotoh, K., Sasaki, T., and Hijii, N. (2005). Wind-based dispersal of oribatid mites (Acari: Oribatida) in a subtropical forest in Japan. J. Acarol. Soc. Jpn. 14, 117–122. doi: 10.2300/acari.14.117
Karasawa, S., and Hijii, N. (2008). Vertical stratification of oribatid (Acari: Oribatida) communities in relation to their morphological and life-history traits and tree structure in a subtropical forest in Japan. Ecol. Res. 23, 57–69. doi: 10.1007/s11284-007-0337-4
Kawaguchi, Y., and Nakano, S. (2001). Contribution of terrestrial invertebrates to the annual resource budget for salmonids in forest and grassland reaches of a headwater stream. Freshw. Biol. 46, 303–316. doi: 10.1046/j.1365-2427.2001.00667.x
Kawaguchi, Y., Taniguchi, Y., and Nakano, S. (2003). Terrestrial invertebrate inputs determine the local abundance of stream fishes in a forested stream. Ecology 84, 701–708.
Leksono, A. S., Takada, K., Koji, S., Nakagoshi, N., Anggraeni, T., and Nakamura, K. (2005). Vertical and seasonal distribution of flying beetles in a suburban temperate deciduous forest collected by water pan trap. Insect Sci. 12, 199–206. doi: 10.1111/j.1005-295X.2005.00025.x
Lensing, J. R., Todd, S., and Wise, D. H. (2005). The impact of altered precipitation on spatial stratification and activity-densities of springtails (Collembola) and spiders (Araneae). Ecol. Entomol. 30, 194–200. doi: 10.1111/j.0307-6946.2005.00669.x
Lindo, Z. (2010). “Communities of Oribatida associated with litter input in western red cedar tree crowns: Are moss mats ‘magic carpets’ for oribatid mite dispersal?,” in Trends in Acarology: proceedings 12th international congress of acarology, eds M. W. Sabelis and J. Bruin (Dordrecht: Springer), 143–148. doi: 10.1007/978-90-481-9837-5_22
Lindo, Z., and Winchester, N. N. (2007). Resident corticolous oribatid mites (Acari: Oribatida): decay in community similarity with vertical distance from the ground. Écoscience 14, 223–229.
Logan, J. A., Régnière, J., and Powell, J. A. (2003). Assessing the impacts of global warming on forest pest dynamics. Front. Ecol. Environ. 1:130–137. doi: 10.1890/1540-92952003001[0130:ATIOGW]2.0.CO;2
Maguire, D. Y., Robert, K., Brochu, K., Larrivée, M., Buddle, C. M., and Wheeler, T. A. (2014). Vertical stratification of beetles (Coleoptera) and flies (Diptera) in temperate forest canopies. Environ. Entomol. 43, 9–17. doi: 10.1603/EN13056
Miyashita, T., Takada, M., and Shimazaki, A. (2003). Experimental evidence that aboveground predators are sustained by underground detritivores. Oikos 103, 31–36. doi: 10.1034/j.1600-0706.2003.12586.x
Moran, V. C., and Southwood, T. R. E. (1982). The guild composition of arthropod communities in trees. J. Anim. Eol. 51, 289–306. doi: 10.2307/4325
Morgan, F. D. (1968). Bionomics of Siricidae. Annu. Rev. Entomol. 13, 239–256. doi: 10.1146/annurev.en.13.010168.001323
Nakamura, A., Kitching, R. L., Cao, M., Creedy, T. J., Fayle, T. M., Freiberg, M., et al. (2017). Forest and their canopies: achievements and horizons in canopy science. Trends Ecol. Evol. 32, 438–451. doi: 10.1016/j.tree.2017.02.020
Oguri, H., Yoshida, T., Nakamura, A., Soga, M., and Hijii, N. (2014). Vertical stratification of spider assemblages in two conifer plantations in central Japan. J. Arachnol. 42, 34–43. doi: 10.1636/P13-73.1
Orivel, J., Malherbe, M. C., and Dejean, A. (2001). Relationships between pretarsus morphology and arboreal life in ponerine ants of the genus Pachycondyla (Formicidae: Ponerinae). Ann. Entomol. Soc. Am. 94, 449–456. doi: 10.1603/0013-8746(2001)094[0449:RBPMAA]2.0.CO;2
Pedigo, L. P. (1970). Activity and local distribution of surface-active Collembola (Insecta): I. woodland populations. Am. Mid. Nat. 83, 107–118. doi: 10.2307/2424010
Potapov, A. M., Goncharov, A. A., Tsurikov, S. M., Tully, T., and Tiunov, A. V. (2016). Assimilation of plant-derived freshly fixed carbon by soil collembolans: Not only via root? Pedobiologia 59, 189–193. doi: 10.1016/j.pedobi.2016.07.002
Pringle, R. M., and Fox-Dobbs, K. (2008). Coupling of canopy and understory food webs by ground-dwelling predators. Ecol. Lett. 11, 1328–1337. doi: 10.1111/j.1461-0248.2008.01252.x
R Development Core Team (2017). R: A language and environment for statistical computing. Vienna: R Foundation and statistical computing.
Richter, C. J. J. (1970). Aerial dispersal in relation to habitat in eight wolf spider species (Pardosa, Araneae, Lycosidae). Oecologia 5, 200–214. doi: 10.1007/BF00344884
Robinet, C., and Roques, A. (2010). Direct impacts of recent climate warming on insect populations. Integr. Zool. 5, 132–142. doi: 10.1111/j.1749-4877.2010.00196.x
Rozanova, O., Tsurikov, S. M., Tiunov, A. V., and Semenina, E. E. (2019). Arthropod rain in a temperate forest: Intensity and composition. Pedobiologia 75, 52–56. doi: 10.1016/j.pedobi.2019.05.005
Simon, U., and Linsenmair, K. E. (2001). “Arthropods in tropical oaks: differences in their spatial distributions within tree crowns,” in Tropical Forest Canopies: Ecology and Management, eds K. E. Linsenmair, A. J. Davis, B. Fiala, and M. R. Speight (Dordrecht: Springer), 179–191. doi: 10.1007/978-94-017-3606-0_14
Stork, N. E., and Blackburn, T. M. (1993). Abundance, body size and biomass of arthropods in tropical forest. Oikos 67, 483–489. doi: 10.2307/3545360
Su, J. C., and Woods, S. A. (2001). Importance of sampling along a vertical gradient to compare the insect fauna in managed forests. Environ. Entomol. 30, 400–408. doi: 10.1603/0046-225X-30.2.400
Sutton, S. L., and Hudson, P. J. (1980). The vertical distribution of small flying insects in the lowland rain forest of Zaïre. Zool. J. Linn. Soc. Lond. 68, 111–123. doi: 10.1111/j.1096-3642.1980.tb01921.x
Tanabe, S. (2002). Between-forest variation in vertical stratification of drosophilid populations. Ecol. Entomol. 27, 720–731. doi: 10.1046/j.1365-2311.2002.00469.x
Yoshida, T., and Hijii, N. (2005a). The composition and abundance of microarthropod communities on arboreal litter in the canopy of Cryptomeria japonica trees. J. Forest Res. 10, 35–42. doi: 10.1007/s10310-004-0098-7
Yoshida, T., and Hijii, N. (2005b). Vertical distribution and seasonal dynamics of arboreal collembolan communities in a Japanese cedar (Cryptomeria japonica D. Don) plantation. Pedobiologia 49, 425–434. doi: 10.1016/j.pedobi.2005.05.001
Yoshida, T., and Hijii, N. (2006a). Seasonal distribution of Xenylla brevispina (Collembola) in the canopy and soil habitat of a Cryptomeria japonica plantation. Pedobiologia 50, 235–242. doi: 10.1016/j.pedobi.2005.12.002
Yoshida, T., and Hijii, N. (2006b). Spatiotemporal distribution of aboveground litter in a Cryptomeria japonica plantation. J. Forest Res. 11, 419–426. doi: 10.1007/s10310-006-0235-6
Keywords: canopy, arthropod abundance, trunk-sticky trap, water-pan trap, arthropod rain, weather conditions
Citation: Yoshida T, Kusunoki Y, Fukano Y and Hijii N (2021) Vertical Distribution of Arthropod Assemblages and the Effects of Weather Conditions on Arthropod Rain in a Temperate Conifer Forest. Front. For. Glob. Change 4:672601. doi: 10.3389/ffgc.2021.672601
Received: 26 February 2021; Accepted: 28 June 2021;
Published: 23 July 2021.
Edited by:
Louise A. Ashton, The University of Hong Kong, Hong KongReviewed by:
Claire Gely, James Cook University, AustraliaFrederico Neves, Federal University of Minas Gerais, Brazil
Copyright © 2021 Yoshida, Kusunoki, Fukano and Hijii. This is an open-access article distributed under the terms of the Creative Commons Attribution License (CC BY). The use, distribution or reproduction in other forums is permitted, provided the original author(s) and the copyright owner(s) are credited and that the original publication in this journal is cited, in accordance with accepted academic practice. No use, distribution or reproduction is permitted which does not comply with these terms.
*Correspondence: Tomohiro Yoshida, yoshitom@cc.tuat.ac.jp