- 1Forest Pathology Research Group, Department of Forest Botany and Tree Physiology, Faculty of Forest Sciences and Forest Ecology, University of Göttingen, Göttingen, Germany
- 2Section Mycology and Complex Diseases, Department of Forest Protection, Northwest German Forest Research Institute (NW-FVA), Göttingen, Germany
The ascomycete Sphaeropsis sapinea is the causal agent of the Diplodia Tip Blight disease on pines and other conifer species. This fungus has a symptomless endophytic life stage. Disease symptoms become visible when trees have been weakened by abiotic stress, usually related to warmer temperatures and drought. Currently, this disease is observed regularly in Scots pine (Pinus sylvestris) sites in parts of Europe, such as Germany, increasing dramatically in the last decade. Changes in climatic conditions will gradually increase the damage caused by this fungus, because it is favored by elevated temperature. Thus, host trees with reduced vitality due to climate change-related environmental stress are expected to be more susceptible to an outbreak of Diplodia Tip Blight disease. There is currently no established and effective method to control S. sapinea. This project aims to reveal the nature of the endophyte community of Scots pine. Utilizing the antagonistic core community of endophytes could serve as a novel tool for disease control. Results from this study provide a starting point for new solutions to improve forest health and counter S. sapinea disease outbreaks. We screened potential antagonistic endophytes against S. sapinea and infected Scots pine seedlings with the most common endophytes and S. sapinea alone and combination. The host was stressed by limiting access to water. The antagonism study revealed 13 possible fungi with the ability to inhibit the growth of S. sapinea in vitro, for example Sydowia polyspora. None of the tested co-infected fungi (Desmazierella acicola, Didymellaceae sp., Microsphaeropsis olivacea, Sydowia polyspora, and Truncatella conorum-piceae) showed strong necrosis development in vivo, even when host stress increased due to drought. However, the infection experiment demonstrated that drought conditions enhance the effect of the disease outbreak, triggering S. sapinea to cause more necrosis in the infected twigs.
Introduction
Climate change is a potential driver of adverse effects on forest health. With climate change, there is an increase in drought-associated stress, rendering trees more susceptible to threats such as pests and pathogens which can compromise their overall health (Bußkamp, 2018; Brodde et al., 2019; Terhonen et al., 2019b). Scots pine (Pinus sylvestris L.) is one of the most economically important forestry tree species in Europe. Changes in the environment, especially those related to drought, will affect Scots pine pathosystems. The ascomycete fungus Sphaeropsis sapinea (Fr.) Dyko & B. Sutton (≡ Diplodia sapinea (Fr.) Fuckel, Diplodia pinea (Desm.) J. Kickx f.) is recognized as the most widespread necrotrophic pathogen responsible for dramatic losses of Pinus species across the continents, causing a disease called Diplodia tip blight (Smith et al., 1996; Fabre et al., 2011; Bußkamp, 2018; Paez and Smith, 2018; Brodde et al., 2019). The correct name of this anamorphic Botryosphaeriaceae is in discussion and it is epityped (de Wet et al., 2003; Phillips et al., 2013), but the current name after Index Fungorum is still S. sapinea. The preferred name after the EPPO Global Database, however, is Diplodia sapinea1.
Recently, the disease Diplodia tip blight has been increasing in Germany, especially after the hot and dry years of 2018 and 2019 (Blumenstein et al., 2020). Sphaeropsis sapinea has a latent endophytic stage (Burgess et al., 2004; Flowers et al., 2006; CABI, 2019; Bußkamp et al., 2020; Terhonen et al., 2021) and disease symptoms become visible when trees have been weakened by stress, usually related to temperature and drought, allowing S. sapinea to switch its lifestyle from endophytic to pathogenic (Blodgett et al., 1997a; Stanosz et al., 2001; Blumenstein et al., 2020). The production of reactive oxygen species (e.g., hydrogen peroxide, H2O2) and the accumulation of free amino acids are common plant responses to drought (Sherwood et al., 2015). Because these drought-induced perturbations in metabolism also often occur during pathogenic attack, they may be partly responsible for an enhanced susceptibility to fungal pathogens (Desprez-Loustau et al., 2006; Sturrock et al., 2011; Sherwood et al., 2015) or lifestyle-switch. Nevertheless, it is not known in detail which fungal or host genetic factors are responsible in the lifestyle-switch of S. sapinea.
The term “endophyte” is used to describe microbes that live asymptotically inside plant tissues for the entire or at least a significant part of their life cycle without causing any clear negative harm to the host (Petrini, 1991; Saikkonen et al., 1998). Although endophytes in conifers have been studied intensively (e.g. Bußkamp, 2018; Bußkamp et al., 2020; e.g., Saikkonen et al., 1998; Sieber, 2007; Terhonen et al., 2019b), generally the interaction between different endophytes and their host remains poorly understood (Sieber and Grünig, 2013; Witzell et al., 2014; Compant et al., 2016; Terhonen et al., 2018; Witzell and Martín, 2018). Several endophytes are regarded as parasites tolerated by their host or as opportunistic or latent pathogens (Sanz-Ros et al., 2015). Endophytes can also act as primary colonizers and may remain in a physiological resting phase in their host. For example S. sapinea is a typical member of the fungal community of natural pruning of branches defined by Butin and Kowalski (1990). Growing inside their hosts’ tissues, they can form fruiting bodies and spores (Chapela and Boddy, 1988; Griffith and Boddy, 1988; Kehr, 1998; Osono, 2006; Oses et al., 2008; Sanz-Ros et al., 2015). The question is, how do endophytes overcome a host’s defenses and colonize them? This could either be due to the secretion of metabolites by the endophyte (Peters et al., 1998; Schulz et al., 2015, 2002) or by changing the phytohormone balance in the tree (Navarro-Meléndez and Heil, 2014). It is also conceivable that the endophyte defeats the metabolism of the tree by secreting lyzing enzymes (Schulz et al., 1998; Suryanarayanan et al., 2012). Sherwood et al. (2015) proposed a new model of fungal infection of plants based on interactions of the metabolisms of the free amino acid proline and H2O2 with drought. The authors showed that droughted Austrian pine (Pinus nigra Arnold) accumulated hydrogen peroxide in shoots. Hydrogen peroxide is toxic to S. sapinea, but the infection of the droughted Austrian pine with this pathogen led to a reduction in the H2O2-concentration in the host plants. S. sapinea is able to produce catalase and peroxidase in response to the oxidative stress by H2O2. Proline is a preferred nitrogen source for S. sapinea in vitro and it increased in the plant as well in response to drought as and in response to infection with S. sapinea. Sherwood et al. (2015) conclude from their results that the proline precursor, glutamate, protects S. sapinea from hydrogen peroxide damage.
In vitro, endophytes produce a variety of secondary metabolites such as herbicides, fungicides and antibiotics (Schulz et al., 2002; Kusari et al., 2012). The metabolites may serve the plant to maintain numerous balances: between endophytes and host, but also between other endophytic fungi and bacteria. The endophytic stage represents a balanced interaction between the fungus and its host. However, endophytic fungal species can become pathogens when this balance is disturbed or saprotrophs if the host dies (Müller and Krauss, 2005; Rodriguez et al., 2009; Bußkamp, 2018; Terhonen et al., 2016, 2019a).
Fungal endophytes are known to contribute to the health of plants, acting as growth promotors that synthesize phytohormones; in addition, they can potentially protect plants from pathogenic fungi by their anti-fungal activity (Witzell et al., 2014; Terhonen et al., 2018, 2019b). In conifer trees, it has been shown that inoculations with fungal endophytes protect the host from natural infection by Dothistroma septosporum (Dorogin) M. Morelet (Ridout and Newcombe, 2015). Consequently, several metabolites with antifungal properties have been isolated from foliar endophytes of Picea rubens Sarg. and Picea mariana (Mill.) Britt., E.E. Sterns & Poggenburg (McMullin et al., 2017). These results support the hypothesis that fungal endophytes may enhance the tolerance of the host tree to fungal pathogens (Sumarah et al., 2011, 2015; Richardson et al., 2014; Tanney et al., 2016; Oliva et al., 2021). Some endophytes can also be used as antagonists against potential pathogens (Arnold et al., 2003; Ganley et al., 2008; Rungjindamai et al., 2008; Martín et al., 2015). Whether an endophyte acts as an antagonist or competes with a particular pathogen, can be studied in vitro using so-called antagonism assays (Santamaría et al., 2012; Blumenstein, 2015; Romeralo et al., 2015; Bußkamp, 2018; Rigerte et al., 2019). These findings are extremely important as, in the future, the use of beneficial endophytes that can act as biocontrol agents against pathogens, in this case against S. sapinea, may be a valuable approach to disease control. Potential antagonistic fungi, such as Sydowia polyspora (Bref. & Tavel) E. Müll., Alternaria sp. and Epicoccum nigrum Link, were recently discussed in a study by Oliva et al. (2021).
Climate change could transform S. sapinea into a global threat to forest health, as the growth rate will be favored by climate warming (Fabre et al., 2011). Indirect effects (temperature, drought) are important because susceptibility of pines to S. sapinea is strongly enhanced by water stress (Blodgett et al.,1997a,b; Stanosz et al., 2001; Desprez-Loustau et al., 2006; Bußkamp, 2018). Hence, understanding how the lifestyle of fungi switches due to environmental stress is critical for deciphering the evolution of host–microbe interactions (Kuo et al., 2014). Fungal lifestyles are not in that sense stable but dynamic, and are likely to be influenced by the genetics of the fungal species, host factors and changing environments (Kuo et al., 2014). Endophytes may have evolved to switch their lifestyles to adapt different environmental conditions (Kuo et al., 2014). S. sapinea can be considered a very good model to test the direct effects of environmental changes on a fungal pathogen (lifestyle-switch), as well as indirect effects on the host susceptibility (stress). The high levels of Diplodia tip blight symptoms in forest stands are not due to a high abundance but rather depend on environmental conditions (Feci et al., 2002; Bußkamp, 2018; Blumenstein et al., 2020). The impact of climate change could shift the endophyte communities and will probably also affect the nature of fungal pathogens. Increased temperature may mean that host resistance to disease may be overcome more quickly as a result of rapid disease cycles. For S. sapinea, warmer temperatures will be suitable for accelerated growth and reproduction. To mitigate the impacts of climate change, understanding the factors that trigger development of forest tree disease epidemics will be essential.
The aims of this study on the pathosystem of S. sapinea and P. sylvestris were (1) to identify fungal Scots pine endophytes which may have the ability to antagonize the pathogen S. sapinea in vitro, (2) to test how the strength of drought-induced stress due to different levels of water availability effects potential disease symptoms, measured by necrosis length, in inoculation tests performed with S. sapinea and selected Scots pine endophytes in planta, and (3) to investigate whether there are potential remote influences between Scots pine endophytes and S. sapinea in an in vivo experiment. Aims 2 and 3 were tested in a greenhouse experiment. We infected Scots pine saplings with S. sapinea and selected endophytes from pine that are either reported to be latent pine pathogens or putative antagonistic endophytes. The host plants were inoculated with a single test strain or combined with different fungal strains on a separate twigs.
Materials and Methods
Test for Antagonistic Endophytes Against S. sapinea
Fungal Material: Isolates From Scots Pine Tips
In this study, 30 fungal isolates (two Sphaeropsis sapinea strains and 28 endophytes) were used for either the antagonism assay or inoculations (Table 1). The fungal strains chosen are regularly isolated from Scots pine tips in German forests according to Bußkamp et al. (2020). Origin and locations of the strains are described in Blumenstein et al. (2020). One strain (Didymellaceae sp., NW-FVA ID 5756) originated from a sample of the nursery pine plants (see section “Pre-experiment Detection of Endophytes Including Sphaeropsis sapinea in Plant Material”) that were used in this study for the antagonism assay.
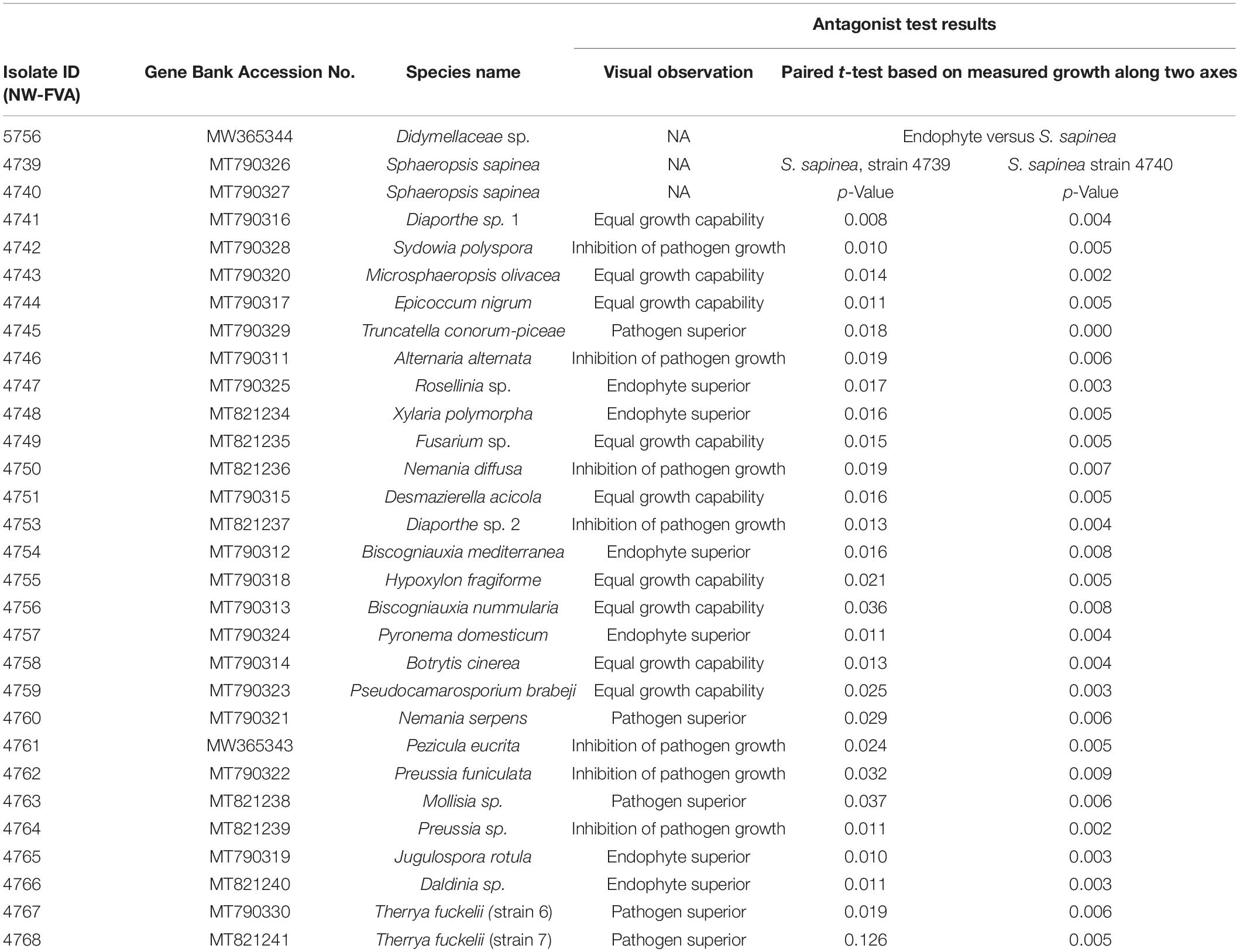
Table 1. All isolates used in antagonist study or/and inoculation study, their accession numbers and observations against S. sapinea.
In vitro Study: Antagonism Assay
In order to identify endophytic fungal isolates that may have the ability to antagonize the pathogen S. sapinea inside the pine trees’ tissue (in planta), the interactions of 27 isolated pine endophytes (Blumenstein et al., 2020) against two different S. sapinea strains were investigated by means of a paired-growth assay (antagonism assay in vitro) as described in Rigerte et al. (2019). The ability of an endophyte to antagonize the pathogen was determined based on the inhibition level (defined as pathogen growth with and without the endophyte) over a given period of time. This was achieved by assessing and measuring the concurrent growth of the pathogens on 1.5% Malt-Yeast-Peptone (MYP-agar, after Langer (1994)) nutrient medium (pH = 6) and the assessment by eye of the growth behavior of the paired fungal strains. The latter observations were categorized as follows: (1) Inhibition of S. sapinea growth (Figure 1A), (2) endophyte superior = the endophyte has overgrown and inhibited the mycelium of S. sapinea (Figure 1B), (3) Equal growth capability = the tested endophyte and S. sapinea did not overgrow or obviously inhibit each other (Figure 1C), and (4) S. sapinea superior = S. sapinea inhibited and may also have overgrown the tested endophyte strain (Figure 1D). The endophyte and pathogen were placed opposite each other on the surface of MYP agar plates within 2.1 cm of the perimeter of the petri dish (Figure 2; Rigerte et al., 2019). Such pairings were prepared in triplicate. Control plates containing only the pathogen (located identically on the plate as in the paired setup) were also prepared. All these plates were then incubated under the same conditions: room temperature (ca. 22°C) and diffuse daylight. Growth was assessed 3, 7, and 10 days after inoculation. Measurements were performed with a ruler for both the endophytes and the pathogens in the antagonism test plate. For analysis of the data and to determine whether the presence of an endophyte had an effect to the pathogen, a t-test to compare the pathogens’ growth (alpha direction, Figure 2) after 10 days with the growth of the control was performed with SPSS version 26.0 (IBM Corporation, New York, United States).
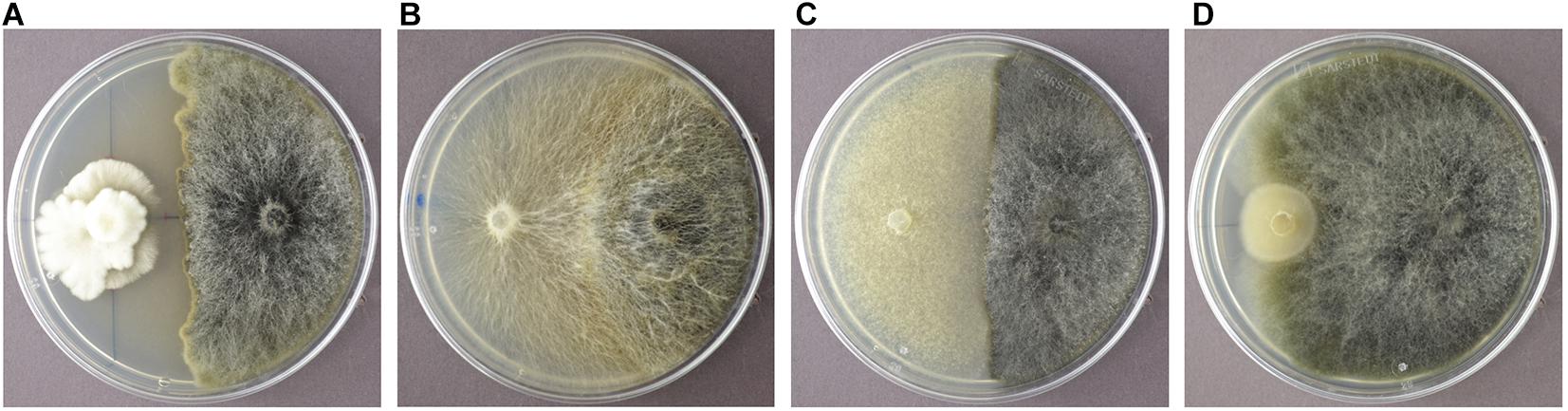
Figure 1. Examples of the different reactions of the endophyte (white mycelium in examples A-D) during the antagonism assays growing in dual culture with S. sapinea (brownish-gray morphology). (A) Nemania diffusa (4750) vs. S. sapinea: inhibition of S. sapinea growth; (B) Biscogniauxia mediterranea (4754) vs. S. sapinea: endophyte superior; (C) Botrytis cinerea (4758) vs. S. sapinea: equal growth; (D) Mollisia sp. (4763) vs. S. sapinea: S. sapinea superior.
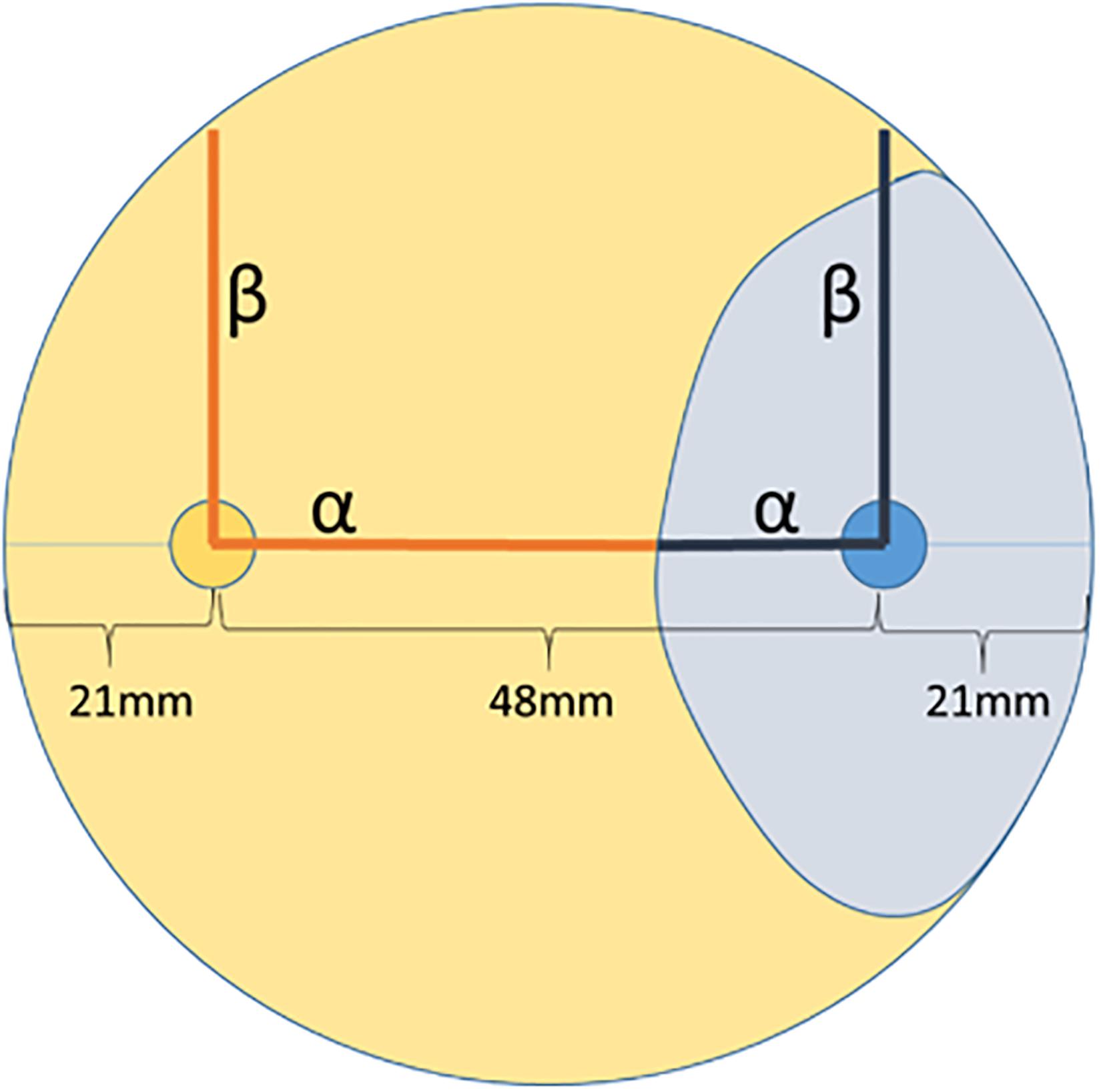
Figure 2. Arrangement of the two-paired culture assay of one fungus (e.g., endophyte) in orange and the other fungus (e.g., pathogen) in blue. Measurement was along the alpha and beta axes. The light orange and blue areas suggest possible growth areas of the fungi and how their growth could be measured.
Greenhouse-Study
Four endophytes, Desmazierella acicola Lib., Microsphaeropsis olivacea (Bonord.) Höhn., Sydowia polyspora, Truncatella conorum-piceae (Tubeuf) Steyaert, and S. sapinea were chosen for the greenhouse inoculation experiment. We compared the infection capacity of these fungi under variable water content on infected pine trees both alone and in combination. Combined infections were set up so that different twigs of the plants were infected with different fungi, to test for possible remote synergistic effects of the endophytic fungi on S. sapinea. Additionally, one endophyte (Didymellaceae sp.) was included in the study that was isolated with the highest frequency in pre-experiment detection of endophytes (section “Pre-experiment Detection of Endophytes Including Sphaeropsis sapinea in Plant Material”).
Plant Material
The plant material consisted of 1008 2-year-old, apparently healthy and vital, Scots pine (P. sylvestris) seedlings purchased from Niedersächsische Landesforsten Services GmbH (seeds originated from the collin Black Forest area, Germany) and LIECO GmbH & Co KG. Seedlings were potted into plastic containers arranged in trays with six containers / tray. These were filled with fertilized peat (Flora gard, TKS®2 Instant Plus and PERLIGRAN® Extra 2-6mm, Hermann Meyer KG, Rellingen, Germany). Containers were placed on tables covered with plastic sheets where excess water could accumulate and be absorbed later. The seedlings were acclimatized to the greenhouse conditions for 30-days prior to receiving water according to their treatment group (section “Experimental Design of the Green-House Study”), during the initial period they received tap water as required to maintain moist soil. No additional fertilization was given during the experiment.
Pre-experiment Detection of Endophytes Including Sphaeropsis sapinea in Plant Material
Six seedlings were examined for pre-colonization of the internal woody tissues by S. sapinea and to reveal the endophyte community. The methodology followed the procedures described in Bußkamp et al. (2020). The complete stem and all shoots of each plant were investigated by culture-based isolation of endophytes. Needles were removed and the tips und stem were surface sterilized (1 min in 70% ethanol/5 min 4% sodium hypochlorite/1 min 70% ethanol) and cut into segments (0.5 cm). Three segments were plated on a petri dish filled with 1.5% MYP-agar. In total, 457 woody plant segments were plated (Ø 76 segments, min. 50, max. 122). Additionally, discolored or dead needles that were found occasionally were checked for infection with S. sapinea. Discolored needles (n = 6 needles, = 62 plated tissue segments) were treated according to the same procedure as the woody plant part, except with a shorter 1 min sterilization in 4% sodium hypochlorite. Dead needles were incubated in a moist chamber for potential formation of pycnidia by S. sapinea. After 7 and 14 days all petri dishes were checked for the presence of S. sapinea and other outgrowing endophytes.
DNA Extraction, PCR, and Sequencing
Before inoculations, the DNA was extracted from 150 mg of the homogenized mycelium sample as described in Keriö et al. (2020). 1000 μl of PVP extraction buffer (1 M NaCl, 100 mM Tris-Hcl, 10 mM EDTA, 2% PVP (w/v)) was added to a 1.5 ml Eppendorf tube with 0.3 g of ground mycelium sample. After incubation at 65°C for 15 min, the sample was centrifuged 5000 rpm for 10 min. The supernatant was transferred into a 1.5 ml tube (ca. 500 μl). One volume of SDS (1% SDS (w/v), 0.5 M KCl) was added. The sample was vortexed for 20 s and centrifuged at 15 000 rpm for 10 min. The supernatant (ca.700 μl) was transferred into a 1.5 ml tube. 0.85 volume of isopropanol was added and mixed by inversion for 20 s, followed by centrifuging for 10 min at 15 000 rpm. The supernatant was removed by pouring it away and the pellet was washed with 200 μl of cold 70% ethanol. After centrifuging at 15 000 rpm for 5 min, ethanol was removed and the pellet dried for 15 min at 65°C. The pellet was re-suspended in nuclease free water (50 μl).
Taq DNA polymerase (Qiagen) was used for PCR amplification of ITS regions with the primer pair ITS1-F and ITS4 (White et al., 1990; Gardes and Bruns, 1993). Briefly, the PCR protocol was as follows: 1X PCR Buffer, 200 μM dNTP, 0.5 μM primer 1, 0.5 μM primer 2, 100 ng template DNA, 0.2 U/μL DNA polymerase; the reaction was adjusted to 25 μL with autoclaved MQ H2O. The PCR conditions used were 94°C for 3 min; 30 cycles of 94°C for 30 s, 55°C for 1 min, 72°C for 1 min, and 72°C for 10 min. Possible contaminants were determined with a negative control using sterile water as a template in both PCR protocols. StainIN REDTM Nucleic Acid Stain was used to confirm DNA amplicons on a 1.5% agarose gel and the visual detection was undertaken with ultraviolet transillumination. ITS region PCR products were purified and sequenced using the ITS4 primer at Microsynth SEQLAB (Göttingen, Germany).
The ITS sequences were extracted with the open source software ITSx to separate the ITS1 and ITS2 subregions from the fungal ITS sequences (Bengtsson-Palme et al., 2013). The ITS1 and ITS2 sequences were used for BLASTN (Zhang et al., 2000) searches against GenBank/NCBI (Sayers et al., 2011) to provide taxonomic identification. The sequences with ≥98% similarity and a query coverage ≥97% were set to constrict species level (Arnold and Lutzoni, 2007; Koukol et al., 2012) and confirmed the morphological identification (Table 1).
Experimental Design of the Green-House Study
The experiment was conducted at the University of Göttingen, Germany (51°33′28.4″ N 9°57′30.5″ E) from mid-March until August 2019. The 1008 seedlings were randomly block-assigned to waterproof tables in four water treatment groups: the optimal water content (100%) and addition of water equivalent to 75%, 50%, and 25% of the optimal amount (Linnakoski et al., 2019; Terhonen et al., 2019b). The optimal amount of water was considered to be sufficient to maintain moist soil (not soaked), which was measured regularly with a soil moisture meter. Fungal isolates were plated on 2% Malt Extract Agar (MEA) and grown at 21°C for 2 weeks prior to the experimental inoculations. Three months after the planting and two months after the start of water availability treatments, the trees were inoculated (Table 2): 768 trees were infected with one species (= one tip per tree, I): S. sapinea (25 per water treatment group), T. conorum-piceae (25 per treatment group), M. olivacea (25 per treatment group), Didymellaceae sp. (25 per treatment group), Sy. polyspora (25 per treatment group), D. acicola (25 per treatment group), mock-inoculated controls with an agar plug (20 per treatment group), and 22 per water treatment group were left entirely untreated. A total of 240 trees were inoculated with four fungi, one on each tip (Table 2):
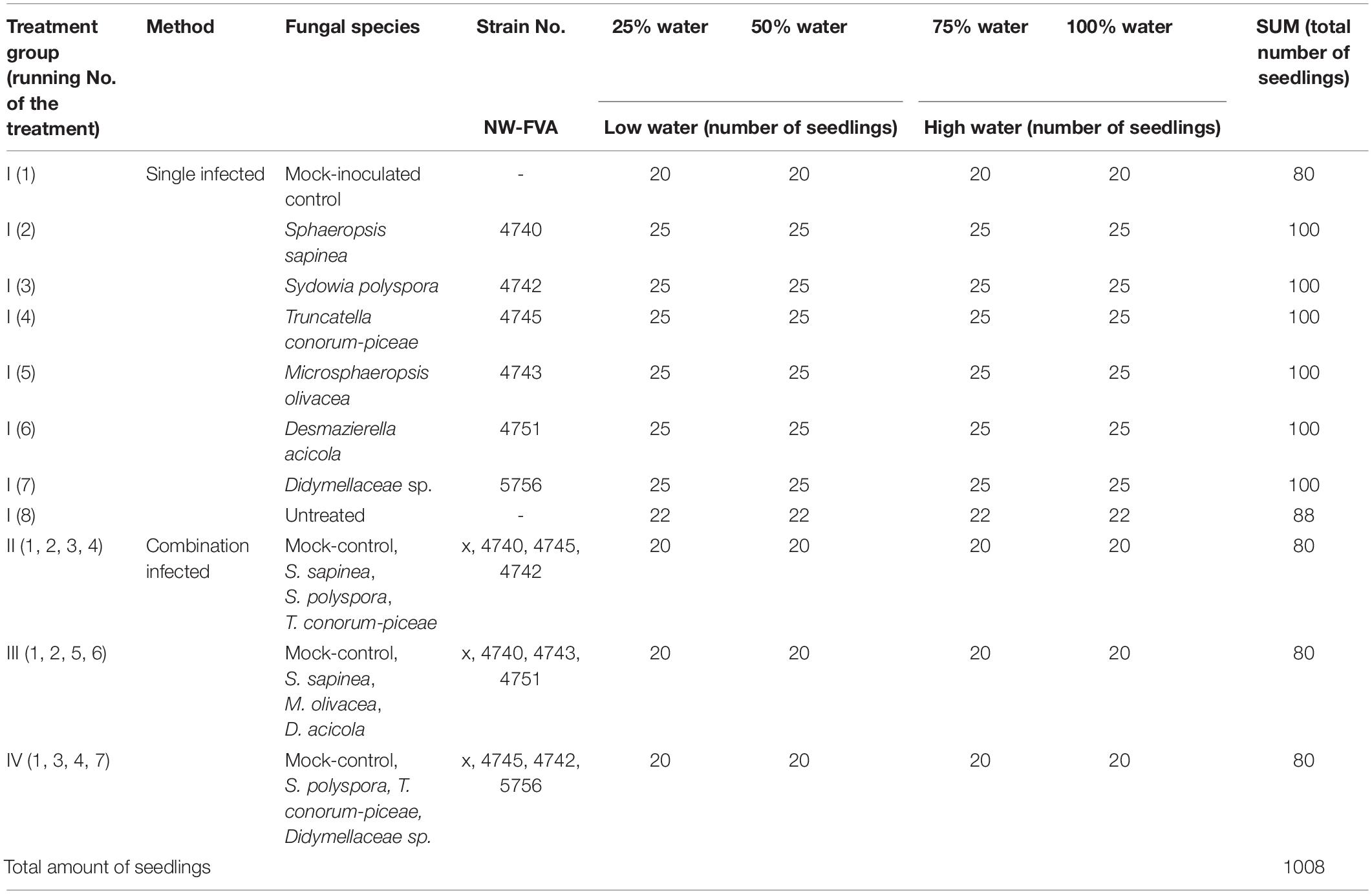
Table 2. Total amount of seedlings and the number of the infections of Scots pine seedlings in different water treatments.
• treatment group II; mock-control, S. sapinea, T. conorum-piceae, S. polyspora (20 per treatment group);
• treatment group III; mock-control, S. sapinea, M. olivacea, D. acicola (20 per treatment group) and
• treatment group IV; mock-control, Didymellaceae sp., T. conorum-piceae, Sy. polyspora (20 per treatment group).
Inoculations were on a first-year shoot. A sterile Ø 3 mm cork-borer was used to punch through hyphae (fungi or control, 2% MEA) in order to get a round-shaped plug. One 1 cm tip of a side shoot per seedling or four tips of four side-shoots (combination-infections), were cut off with a scissor. The agar plug was placed onto the exposed surface, with the mycelium facing the cut, and sealed with Parafilm® (Figure 3).
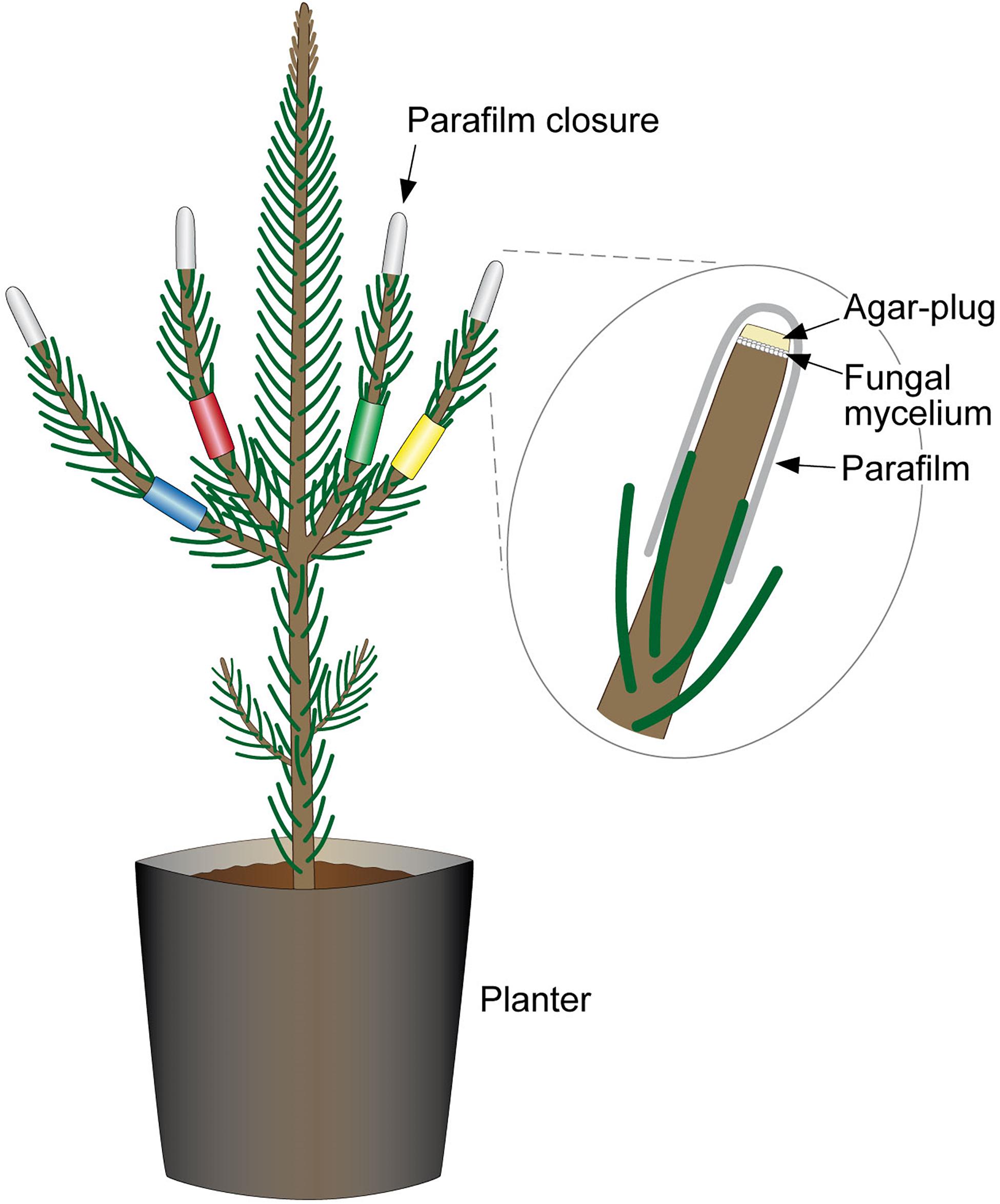
Figure 3. Combined-infection of pine seedlings. Each of the side-shoots is color-labeled to indicate the different fungal strains with which pine was infected. The labeling allowed differentiation during necrosis measurements and re-isolations. Excised tips were replaced with a mycelium-covered agar plug and were sealed with Parafilm®.
The inoculation experiment ran for 66 days. Since seedling water intake varied with the ambient temperature, we continuously monitored the amounts of water applied, in order to maintain the essential level in the 100% watering treatment group, considered to be the optimal amount. The moisture for each treatment was measured with a soil moisture meter for the same seedlings throughout the experiment. The water amounts needed to be adjusted to the increasing temperatures during the growing season in 2019. At the beginning of the experiment, each seedling in the optimum (100%) water treatment group was given 60 ml of water three times per week, and each seedling in the drought stress water treatment group (25%) was given 15 ml of water three times per week. After 2 weeks, the watering regimes were modified to 120 ml for the 100% water treatment group, and 30 ml × 3 for the 25% water treatment groups. After 5 weeks, the water level was modified again to 180 ml × 3 and 45 ml × 3, respectively. The 75% and 50% water treatment groups received the corresponding amounts per seedling relative to the optimal. Water quantities were increased in July and maintained at that level until August.
Data Collection and Post-experiment Detection of Fungal Species
The lesion lengths (nearest 0.001 mm) were measured with a stereomicroscope (Stemi 508, Carl Zeiss Microscopy GmbH, Jena, Germany) with an attached camera (Axiocam ERc5s, Carl Zeiss Microscopy GmbH, Jena, Germany) using the freely available software Labscope (Carl Zeiss Microscopy GmbH, Jena, Germany). First, the bark was gently peeled to expose the necrosis in the phloem and then measured. The lesion length was measured only in the vertical direction. Dead twigs were removed from necrosis length analysis and analyzed separately. After measurements, five randomly chosen seedlings (in total 35 seedlings) were selected from each fungal or control treatment, to confirm the infection and to guarantee the fulfillment of Koch’s postulates. Pieces from the interface of necrosis and healthy tissue were surface sterilized (as described above) and plated onto a 1.5% MYP plate. The fungi were first identified by morphology, followed by DNA extraction and ITS region sequencing to confirm identity (as described above). The sequences obtained were aligned with BLASTN (Zhang et al., 2000) to confirm that the isolate was the same as used in infections (Table 3).
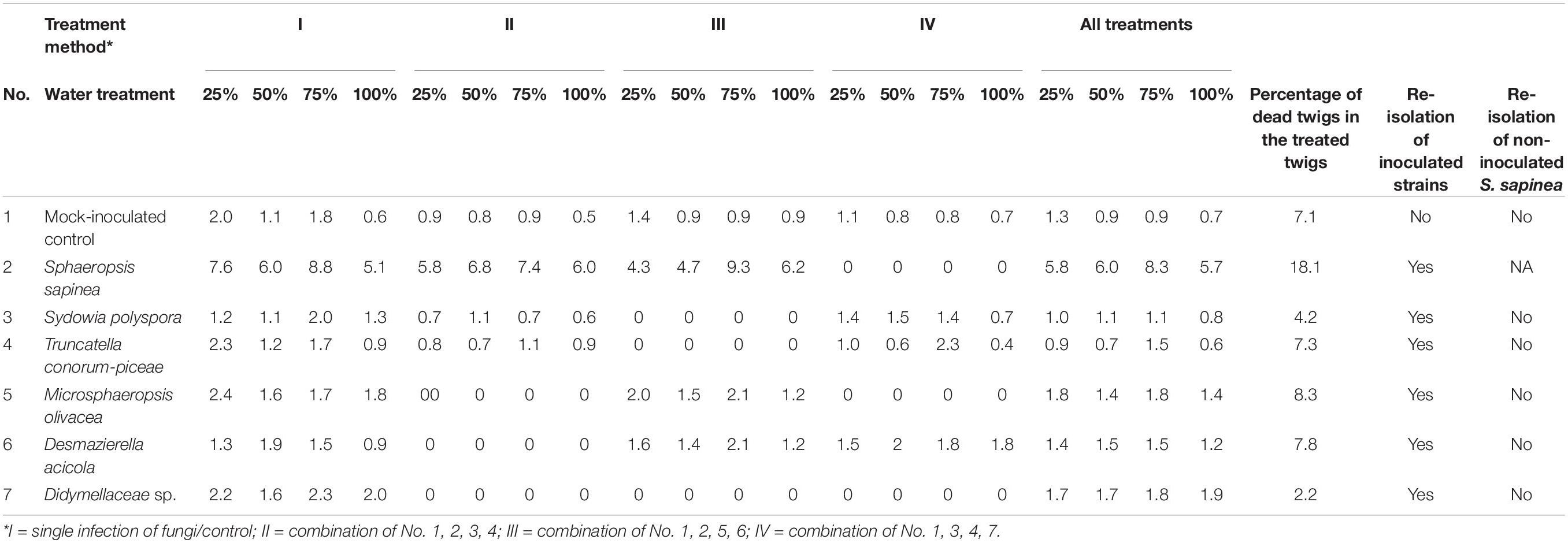
Table 3. Greenhouse-experiment: Mean necrosis length in mm, percentage of dead twigs, and results of re-isolation.
Data Analysis
Data (water content and necrosis length) were analyzed using SPSS version 26.0 (IBM Corporation, New York, United States). A generalized linear model (GLM) was constructed to evaluate the fixed effects of inoculation method (control/fungal species) and combination (single or combined infection) under different water treatments (25%, 50%, 75%, and 100%) on necrosis length in tips. Initial fixed explanatory variables in the necrosis length model included inoculation method (categorical value), water treatment (categorical value), and combination (categorical value). Tray (categorical) was set as random factor in the model. The necrosis lengths in statistically different treatments were further assessed by Tukey HSD test for two-samples assuming equal variances. Differences were considered statistically significant if the p-value was below the threshold of 0.01. Similarly, the water content during different time points (each week) between water treatments was assessed by ONE-WAY-ANOVA and Tukey HSD test for two-samples.
The generalized linear model (glm) in R version 3.5.1 (R Core Team, 2019) was run for twig mortality as with the results of the water treatment / fungal inoculation. Further one-way-ANOVA (aov) was conducted and Tukey’s HSD test was used to examine the differences between groups (in water/fungal treatment).
Results
In vitro Study: Antagonism Assay
Four different kinds of interaction between the Scots pine endophytes and S. sapinea strains were observed (Figure 1 and Table 1). About a quarter of the tested endophytes (26%, Alternaria alternata (Fr.) Keissl., Diaporthe sp. 2, Nemania diffusa (Sowerby) Gray, Pezicula eucrita (P. Karst.) P. Karst., Preussia sp., Preussia funiculate (Preuss) Fuckel, and S. polyspora, Figure 1A) inhibited the growth of S. sapinea. Twenty-two percent of all tested endophytes were superior in growth versus S. sapinea (Figure 1B, Biscogniauxia mediterranea (De Not.) Kuntze, Jugulospora rotula (Cooke) N. Lundq., Daldinia sp. Pyronema domesticum (Sowerby) Sacc., Rosellinia sp., and Xylaria polymorpha (Pers.) Grev.). A third of the tested strains (33%, Biscogniauxia nummularia (Bull.) Kuntz, Botrytis cinerea Pers., D. acicola, Diaporthe sp. 1, E. nigrum, Fusarium sp., Hypoxylon fragiforme (Pers.) J. Kickx f., and M. olivacea) showed equal growth capability (Figure 1C and Table 1). Nineteen percent of endophytes tested against S. sapinea were inferior (= S. sapinea was superior; Figure 1D, Mollisia sp., Nemania serpens (Pers.) Gray, T. conorum-piceae, and Therrya fuckelii (Rehm) Kujala). All of the endophytes had statistical effect with respect at least to one of the S. sapinea strains after ten days (Table 1).
Sphaeropsis sapinea, strain 4740, had higher p-Values (presence of endophytes had stronger effect). If stricter rules for the p-value had been applied and only lower p-values accepted (p < 0.01), only two endophytes would have exhibited statistically significant inhibition (Sydowia polyspora and Diaporthe sp. 2). Sy. polyspora (Figure 4A), M. olivacea (Figure 4B), T. conorum-piceae (Figure 4C), and D. acicola (Figure 4D) were chosen for inoculation studies because of their frequent endophytic occurrence in P. sylvestris.
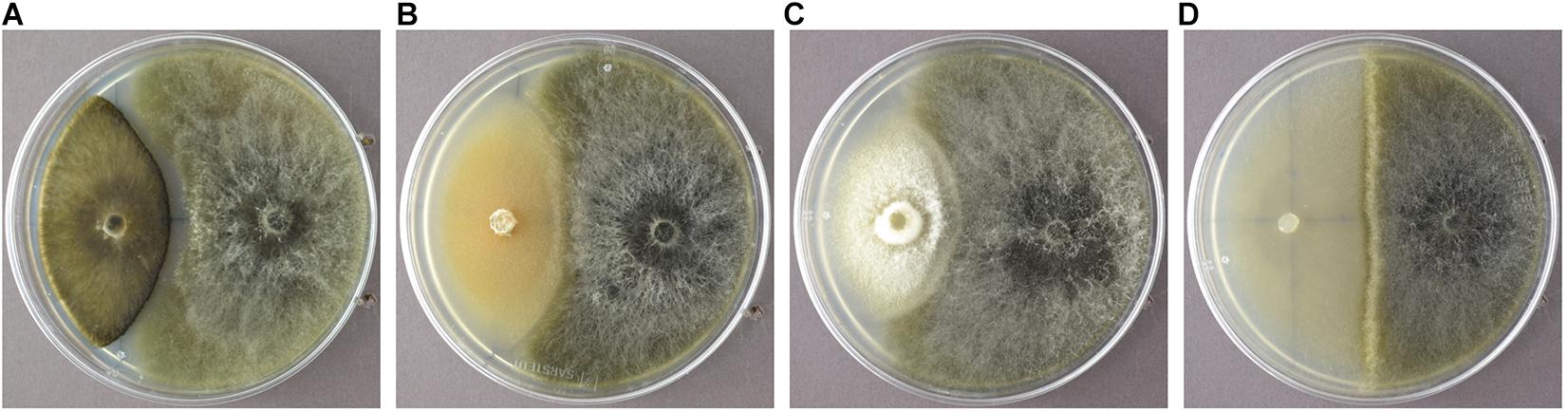
Figure 4. Sydowia polyspora (A), Microsphaeropsis olivacea (B), Truncatella conorum-piceae (C), and Desmazierella acicola (D) were the endophytes chosen for the infection experiment.
In planta Study: Greenhouse Inoculations
Pre-experiment Detection of Sphaeropsis sapinea and the Fungal Isolates
Eighteen different endophytic fungal species were isolated from stems and shoots of the six studied nursery pine trees (Table 4); these included Alternaria spp., Diaporthe spp., E. nigrum, M. olivacea, Sy. polyspora, and T. conorum-piceae. The most frequently isolated species was identified as Didymellaceae sp. (NW-FVA ID 5756) and it occurred in all tested trees and 30.9% of all analyzed shoot segments. S. sapinea was not isolated from asymptomatic woody tissues of those pines nor detected in any discolored or dead needles that were attached to the plants.
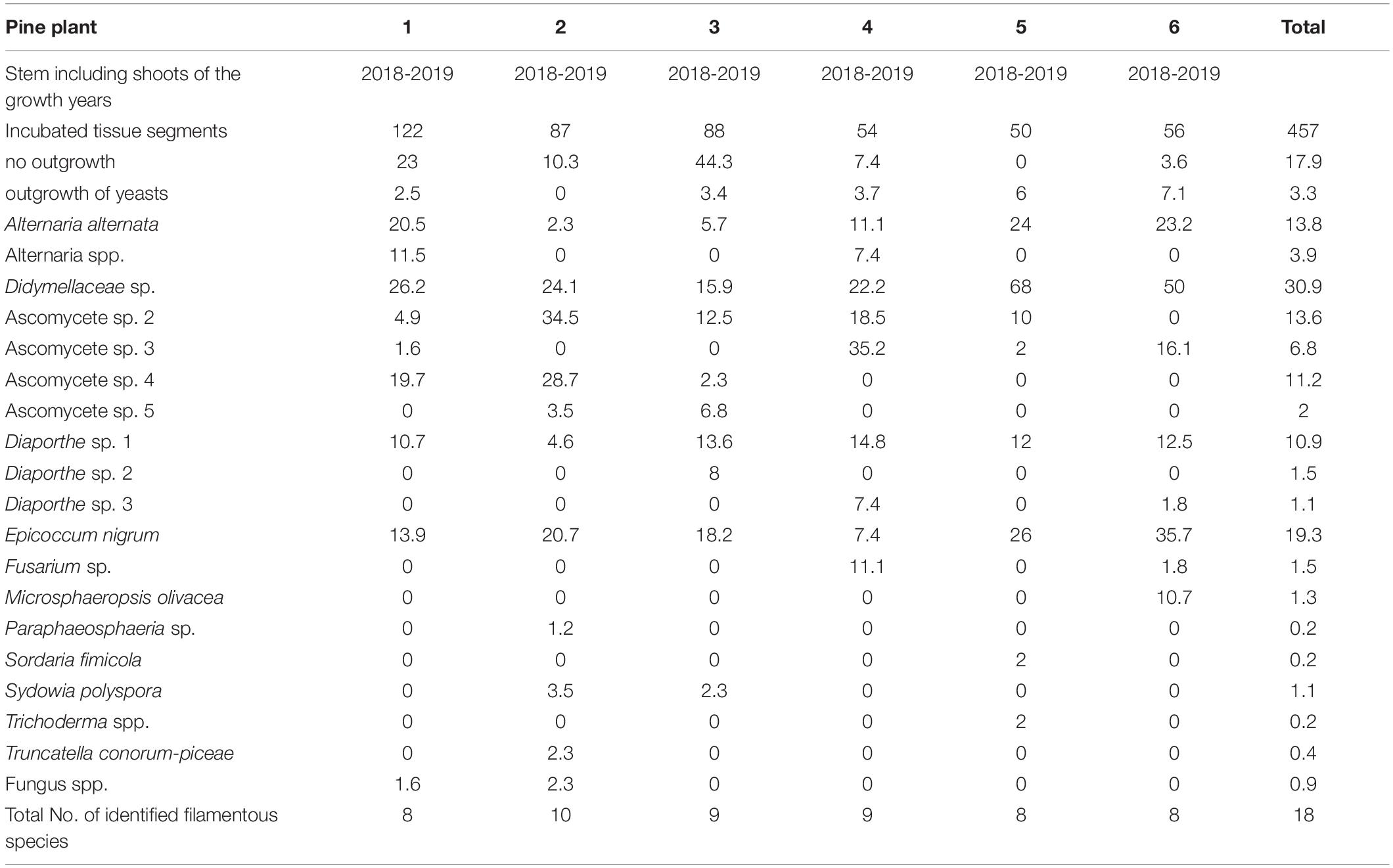
Table 4. Frequency as percent of twig-inhabiting fungal endophytes isolated from two-year-old Pinus sylvestris.
Soil Water Content
At week 3, the soil moisture in the 25% water content treatment was statistically lower than in the 50% (p = 0.008) and 100% (p = 0.010) treatments (Figure 5). After 4 weeks the soil moisture in the 100% treatment was statistically higher than in the 25%, 50%, and 75% treatments. Similarly, the soil moisture in the 25% treatment was lower than the soil moisture of the other treatment groups (all p-Values 0.00, except for 100% versus 50% p = 0.003). The soil moisture in the 50% and 75% treatments was, overall, the same throughout the study. In the middle of the experiment (week 5), the soil moisture in the 25% treatment was statistically lower than in the 100% (p = 0.00), 75% (p = 0.003) and 50% (p = 0.035) treatments. The same results were obtained for week 6. At week 7, the soil moisture in the 25% treatment was statistically lower than in the 100% p = 0.00 and 75% (p = 0.001) treatments and the soil moisture in the 75% treatment was statistically lower than in the 100% treatment (p = 0.013). Similarly, the soil moisture in the 50% treatment was lower than in the 100% treatment (p = 0.035). At week 8, the soil moisture in the 75% treatment was still statistically lower than in the 100% treatment (p = 0.019), and the soil moisture in the 25% treatment was lower than in all other groups: 100% p = 0.000, 75% p = 0.04, 50% p = 0.028. At week 9 the soil moisture in the 25% group was again lower than in the other groups (100% p = 0.000, 75% p = 0.009, 50% p = 0.024), the soil moisture in the 50% (p = 0.017) and 75% (p = 0.011) treatments was lower than in the 100% treatment. At the end of the study (week 10), the soil moisture in the 25% treatment was statistically lower (p = 0.042, 0.001, and 0.00) than the 50%, 75%, and 100% treatments.
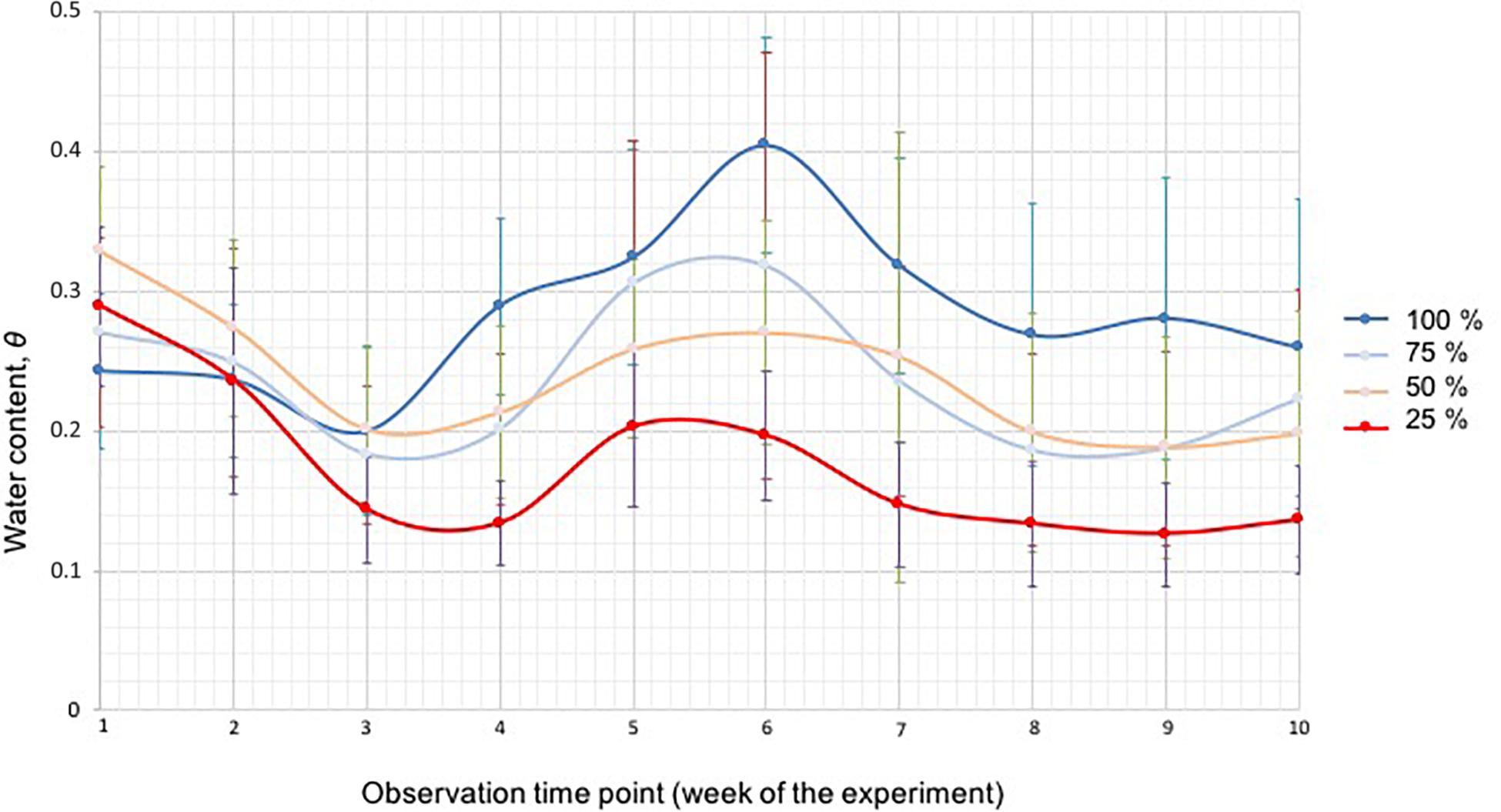
Figure 5. The soil moisture of the four different water treatment groups (100% blue, 75% light blue, 50% green, and 25% red) measured with a soil moisture meter, shown as water content ⊖ for each week during the duration of the experiment. The bars represent standard deviation.
Necrosis Length and Dead Twigs
The untreated control plants stayed healthy during the experiment and no dead twigs were observed. In the mock-inoculated control 7.1% of the twigs died. The inoculation with S. sapinea led to the highest shoot mortality of inoculated Scots pine twigs in the greenhouse study (Figure 6; Table 3). Over all treatment groups these necroses caused complete die-off of 18% of all inoculated twigs (n = 260) compared to 1.2-8.3% for individual inoculated endophyte and 7.1% for twigs inoculated with the mock community. S. sapinea killed 31.9% of inoculated twigs in the 25% water treatment, 27.7% in both the 50% and the 75% water treatments and only 13% in the 100% water treatment (Supplementary Table 1). After M. olivacea inoculation, 8.3% of the twigs were dead, followed by D. acicola (7.8%), T. conorum-piceae (7.3%), mock-inoculated control (7.1%), Sy. polyspora (4.2%), and Didymellaceae sp. (2.2%).
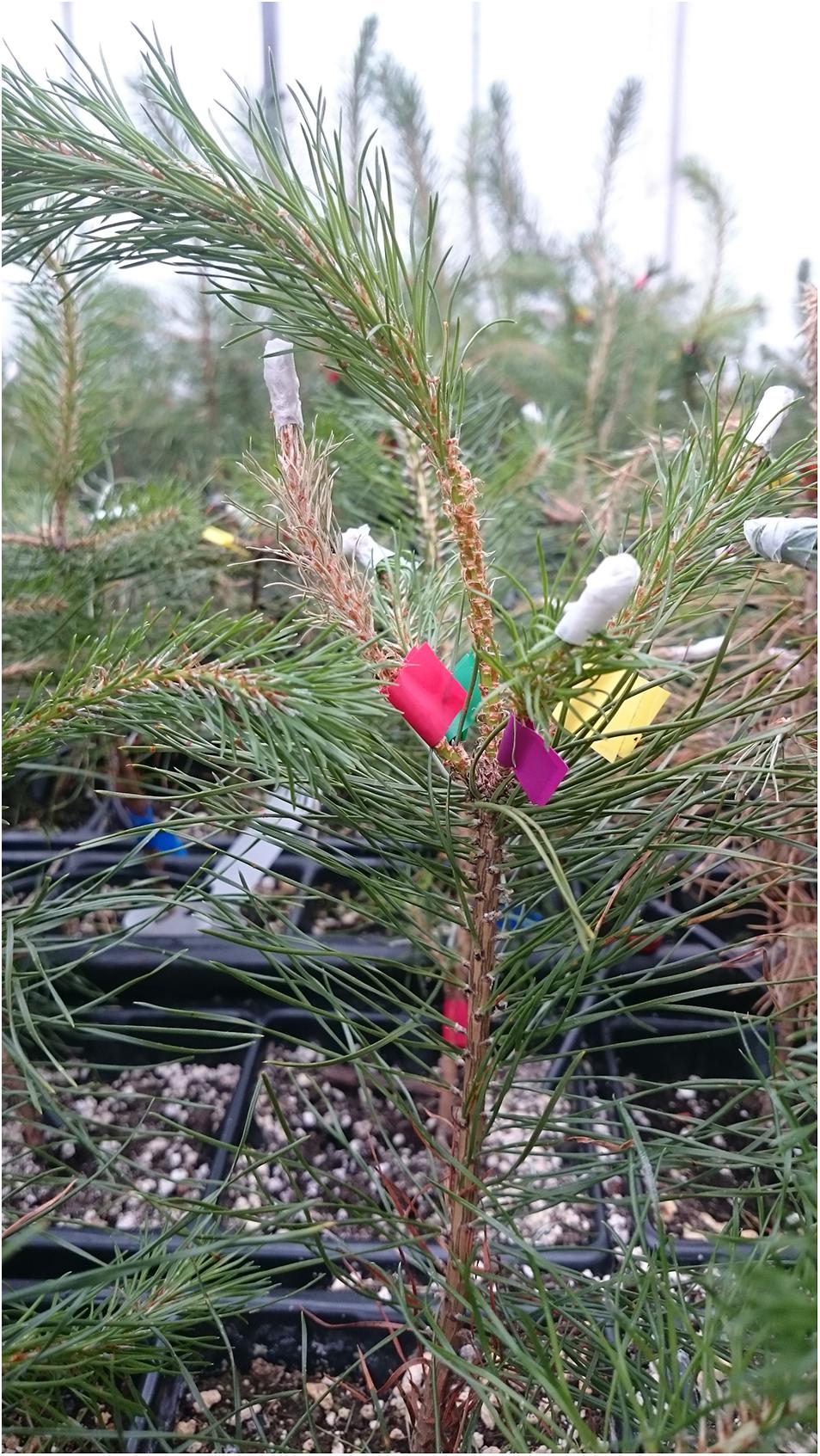
Figure 6. Pine shoot with combined infections at the end of the experiment. The red labeled side-shoot was infected with S. sapinea and shows full necrosis.
The necrosis length model for endophytes and pathogen (GLM) showed that necrosis size (length) was statistically affected by treatment (fungal species) and water availability but not by the combination used (single infection or several in one plant) (Table 5). Statistical differences are listed only in Table 6 and omitted from Figure 7. Statistical differences were found between S. sapinea (Figure 7 and Table 6) and all other inoculation treatments in all water treatments. Similarly, necrosis caused by Didymellaceae sp. was statistically higher than the mock-control and T. conorum-piceae in the 100% water treatment. Necrosis length was statistically higher (mock-control) in the 25% water treatment than in the 100% treatment (Figure 7 and Table 6). The necrosis was greatest statistically in the 75% water treatment compared to the 100% (for S. sapinea and T. conorum-piceae) (Figure 7 and Table 6). Similarly, for S. sapinea inoculation, the necrosis was statistically higher in the 75% compared to the 25% water treatment (Figure 7 and Table 6).
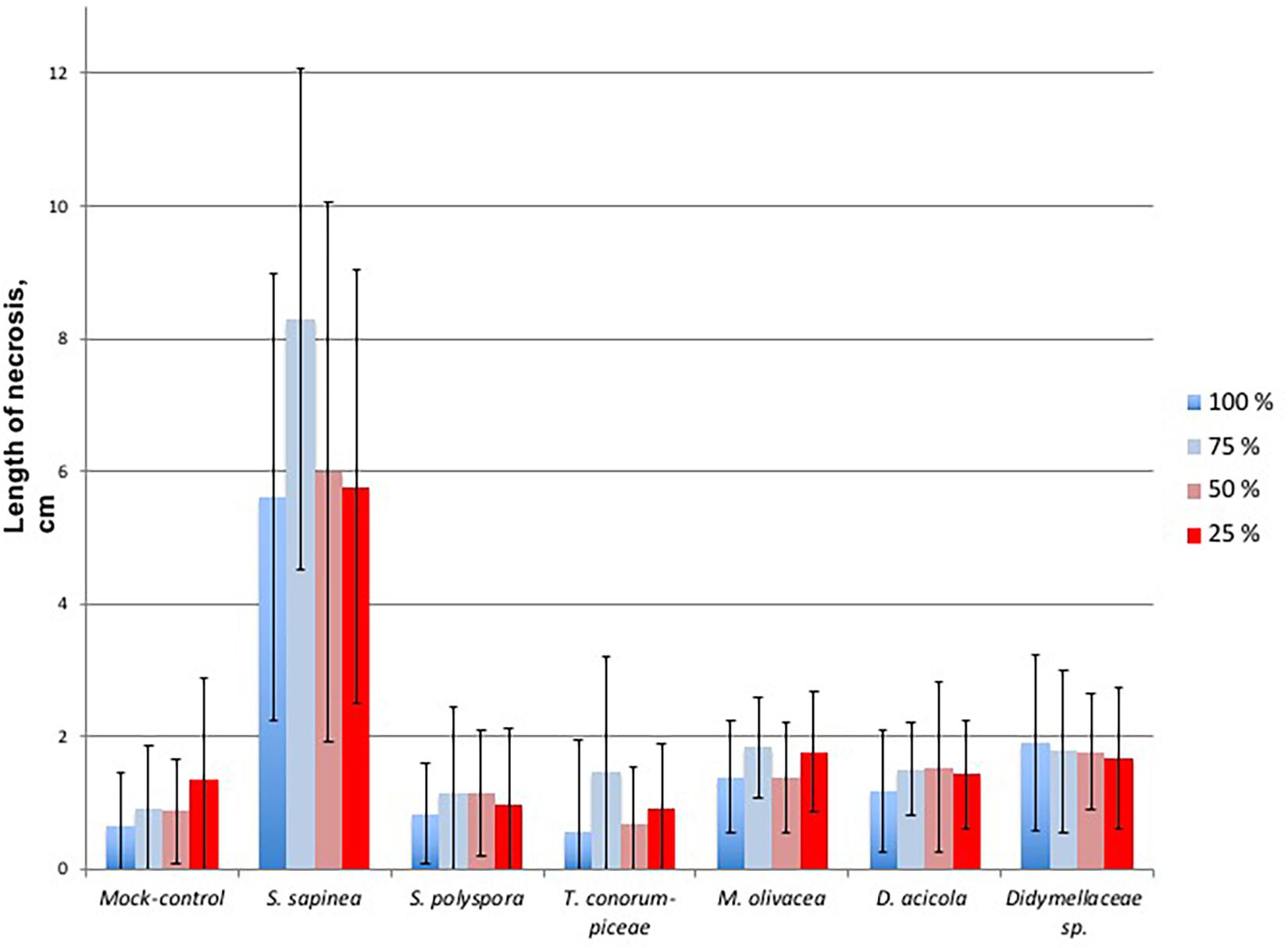
Figure 7. Necrosis length of the single infected shoots per fungal isolate, each in relation to the water treatment groups, where blue is 100% water treatment, light blue 75%, light red 50%, and red 25%. The bars represent standard deviation.
The generalized linear model showed that the number of dead twigs was affected by fungal inoculation due to S. sapinea (p = 0.0079, Supplementary Figure 1A). The water treatment also affected twig mortality (p = 0.05). The Tukey HSD test showed that the mortality of the twigs was statistically higher in the 25% (p = 0.03) and 50% (p = 0.03) water treatments compared to the 100% water treatment (Supplementary Figure 1B).
The water treatment (drought) affected the mortality of the twigs in the S. sapinea treatment (Supplementary Figure 2). The number of dead twigs was higher in 25% compared to the 100% water treatment (p = 0.04, Supplementary Figure 2). Drought, caused by the reduced water treatment, was not found to have any impact on the number of dead twigs in other fungal/control treatments.
Re-isolations of Inoculated Fungi
Sphaeropsis sapinea did not grow out from the surface sterilized samples of the mock-controls. From all inoculated samples, the respective fungus was isolated 66 days after inoculation and identified as the original inoculated species (Table 3).
Discussion
The forest pathosystems’ behavior can be unpredictable in the future due to changes in the environment that favor fungal pathogens rather than the hosts’ vitality. Similarly, this might have unknown effects on host trees core fungal endophytes. We found that abiotic stress, here defined as drought, increased the aggressiveness of S. sapinea (no. of dead twigs, necrosis length) but not the other tested endophytes. In case of S. sapinea, the negative impact can be expected to increase. Similarly, we observed different modes of competition between other endophytes against S. sapinea. Overall these results indicate that other endophytes in Scots pine tissues (especially in twigs) can play a role in the disease development of “Diplodia Tip Blight.” Hypothetically, favoring certain tree microbiome in tree health would produce effective, durable, and environmentally friendly control method against severe disease outbreaks.
Antagonism Studies
Scots pine endophytes and S. sapinea interacted with each other in various ways as demonstrated by the in vitro antagonism assays. Four interaction categories were found: about half of the tested strains were able to either inhibit S. sapinea in vitro or were superior in their growth towards S. sapinea. Similar results were presented by Bußkamp (2018). In our study, thirteen species could be considered potential antagonists against S. sapinea in vitro, because they had either a faster growth than S. sapinea or inhibited the pathogen’s development. This partly corresponds with the results of Bußkamp (2018), who found 22% of the tested 89 endophytic strains inhibited the growth of S. sapinea. A contactless inhibition of S. sapinea was observed for 26% of the endophytes in our study (e.g., A. alternata and Pe. eucrita). Chemical antagonism can be assumed when a fungus reacts to the presence of an opponent fungus with an inhibition zone between the two fungal colonies. One fungus might excrete secondary metabolites that inhibit the opponent fungus (Schulz et al., 2002). Secondary metabolites should be extracted and tested to see whether the same reaction towards the pathogen can be observed, in order to determine whether a certain metabolite can inhibit growth (Tellenbach et al., 2013). S. sapinea, could not penetrate the barrier surrounding one of those endophytes.
Several of the tested endophytes in this study over-grew the S. sapinea mycelium. This ability might indicate a stronger capacity to metabolize nutrients. The faster growth and better ability to utilize nutrients are two strategies with clear advantages during competition (Mgbeahuruike et al., 2011). The fungi Sy. polyspora, Xylaria sp. and Diaporthe sp. are typical endophytes in Scots pine (Bußkamp et al., 2020). If their growth or infection in the host tissues could be promoted, in theory they could provide strong competition against pathogens in nature (Terhonen et al., 2019a). A co-occurrence analysis of shoot-inhabiting endophytes in mature pines by Oliva et al. (2021) identified a cluster of species that was negatively correlated with S. sapinea and could be potential antagonists. The most negatively correlated was an Alternaria species, accompanied by E. nigrum and Sy. polyspora. Similarly, we show that Sy. polyspora had antagonistic capability against S. sapinea. Oliva et al. (2021) provided some evidence that the competition between S. sapinea and other endophytes for stress-related metabolites could prevent the growth of S. sapinea and development of Diplodia tip blight symptoms. Our results support this conclusion as the tested endophytes (e.g., Sy. polyspora) sharing the same niche (in this case MYP plates) with S. sapinea could inhibit the fungus’ growth in vitro. The co-infection of the studied Scots pines with different endophytes together with S. sapinea on different twigs did not cause a visible increase in the host plant’s immune system in planta. In our study, we did not aim at directly inhibiting S. sapinea growth but rather testing for an influence (lower necrosis caused by S. sapinea) on the host plant as a consequence of the combined infections (e.g., induced systemic resistance due to the presence of an endophyte).
Several studies indicate that fungal endophytes may increase their host plant’s immune system in vivo (e.g., Witzell and Martín, 2018). Ganley et al. (2008) demonstrated that Pinus monticola Douglas gained resistance against Cronartium ribicola J.C. Fisch. as a result of prior infection with certain endophytes. Mejía et al. (2008) were able to enhance Theobroma cacao L. plants’ defense against herbivore and pathogen attacks, by inoculating the leaves with the endophyte Colletotrichum tropicale E.I. Rojas, S.A. Rehner & Samuels. The host plant’s defense was enhanced due to a priming effect caused by the endophytes, increasing the expression of suites of host genes involved in in plant defense pathways or e.g., cell wall development (Mejía et al., 2008). Similar results were found by Raghavendra and Newcombe (2013); Martínez-Arias et al. (2019) with endophytic fungi on Populus species. Enhancement of resistance to Dutch elm disease by elm endophytes has been recently described by Martínez-Arias et al. (2021). The combination infections did not impact the necrosis length, indicating no activation of systematic resistance. The inoculation method in our study was very aggressive (mycelium) and perhaps, infecting S. sapinea later than the endophytes in the host would have produced different results. Similarly, mimicking more natural infection methods through conidia infections would give more legitimate results.
The remaining tested strains in the antagonism assays showed either neutral (33% of strains with equal growth capability) interaction or were inferior to S. sapinea. Therefore, they do not appear to be suitable potential antagonists against S. sapinea. Pairing with equal growth capability may indicate that the endophytes’ and S. sapineas’ growth was not disturbed by each other, meaning no antagonism took place. In the host, this might mean the fungi can grow together and neither specifically reacts to the presence of the other. S. sapinea has a comparatively high growth rate (Bußkamp, 2018) and therefore, it can be assumed that it has a fast metabolism to use the available nutrients. Is not surprising that S. sapinea was found to be superior and occupied a larger area of the plate more rapidly than several more slow growing endophytic test partners, such as T. conorum-piceae, N. serpens, Mollisia sp. and two Th. fuckelii strains. When the Scots pine host is weakened due to drought stress for example, the common endophytes inside the tree could be disadvantaged while S. sapinea can become more established as secondary pathogen and occupy more tissues faster in the host, thus outcompeting these inferior endophytes. This could be one reason why S. sapinea is present in higher numbers in diseased sites (Bußkamp et al., 2020).
Pre-colonization of the Trees With Fungal Community
The pre-examination of the nursery Scots pines indicated a relatively small fungal community. No S. sapinea could be detected from the tested seedlings prior to the experiments, whereas the other fungal strains used for the greenhouse-infections were found to be present. We concluded that these species belong to the naturally observed Scots pine endophyte community (Blumenstein et al., 2020; Bußkamp et al., 2020). This corresponds to results of Bihon et al. (2011) who found that S. sapinea was not an endophyte of healthy seedlings collected from greenhouses and nurseries. The composition of endophytes isolated from two-year-old Scots pine plants in this study was dominated by A. alternata and E. nigrum, and was typical of young pines from tree nurseries (own unpublished data). In contrast to intensive studies on the fungal endophytic community of Scots pine shoots of mature trees (Blumenstein et al., 2020; Bußkamp et al., 2020; Oliva et al., 2021), the endophyte α-diversity of the tested two-year old Scots pines was lower. In total, only 18 filamentous, ascomycetous endophytes (8 – 10 species per seedling) were isolated in this study, whereas 103 different species (5 – 22 isolated per tree) were found in mature pine shoots in a study by Bußkamp et al. (2020). Foliar fungal endophyte assemblages have been shown to vary between the developmental stage of the host (mature vs. seedling (Helander et al., 2011; Koukol et al., 2012; Skaltsas et al., 2019). The core foliar endophytes seem to be the same in seedlings and mature Scots pine (Blumenstein et al., 2020; Bußkamp et al., 2020) but community compositions and species differences are probably related to the age of the host (Taudière et al., 2018).
The genesis of the Scots pine endophytic community is poorly known. There are hints that composition of the endophytic community is mainly determined by the host species and organ type (Peršoh, 2013), health condition, composition of the forest trees, and vegetation which is growing in the surroundings of the host tree (Peršoh et al., 2010; Nguyen et al., 2016; Bußkamp, 2018). The latter factor may explain why plants could be species-poorer in a nursery field than in a mixed forest. Horizontal transmission from mature trees in forest stands is probably the main source for young trees to obtain endophyte inocula, as shown for Pinus patula Schltdl. & Cham. And S. sapinea (Bihon et al., 2011). Additionally, the composition of endophytes depends on the age of the tissue (Fröhlich et al., 2000; Arnold and Herre, 2003; Terhonen et al., 2011, 2019a; Bußkamp, 2018), which determines whether an endophyte community can accumulate over years or if it represents only annual accumulation, such as in leaves. Peršoh (2013) found that one- and three-year-old pine stem sections had different assemblies of endophytic fungi, supporting the theory that forest trees mainly accumulate their endophytic community as the infection rate tends to increase with age (Guo et al., 2008; Deckert and Peterson, 2011).
Interaction of Endophytes With S. sapinea Infection
Necrosis of the Scots pine was marginal when infected with D. acicola, Didymellaceae sp., M. olivacea, Sy. polyspora, and T. conorum-piceae. These fungi (excluding S. sapinea) were most likely already endophytically present in the Scots pine plants before inoculation (based on pre-examination). The fact that D. acicola (anamorph Verticicladium trifidum Preuss) did not cause higher necrosis compared to the control is not surprising, as it is a typical twig endophyte on Pinus spp. in Europe (Petrini and Fisher, 1988; Kowalski and Kehr, 1992; Kowalski and Zych, 2002; Bußkamp, 2018) and lives endophytically in pine needles (Kendrick, 1962). This saprotrophic species also colonizes needles after fall (Ponge, 1991) and ascocarps can be found on dead blackened leaves. No pathogenic behavior has ever been described and it is one of the most common fungi growing in pine leaf litter all over the world (Martinoviæ et al., 2016). The tested Didymellaceae sp. was not identified to the species level based on the ITS analysis. The Didymellaceae is a species rich, cosmopolitan family, assigned to the Pleosporales and includes genera such as Didymella, Leptosphaerulina, Macroventuria, Monascostroma, Platychora and moreover some species of Phoma and Ascochyta (Zhang et al., 2009). Most species of the Didymellaceae are associated with dicotyledon plants and mostly hemibiotrophic and saprobic (Zhang et al., 2009) or pathogens, causing leaf and stem lesions (Chen et al., 2017). M. olivacea (basionym: Coniothyrium olivaceum Bonord.) has been described from Pinus plants in very few publications (Petrini and Fisher, 1988; Kowalski and Kehr, 1992; Bußkamp et al., 2020). Petrini and Fisher (1988) described M. olivacea as a typical endophytic colonizer of conifers. Bußkamp et al. (2020) were able to confirm this observation as it was the third most common fungus isolated from pine twigs and they found it in nearly all studied stands in Germany. In contrast, Kowalski and Kehr (1992), isolated the fungus endophytically only from very few pine branches. M. olivacea is known to be plurivorous and was found on twigs and branches of other tree species (e.g., Hormazabal et al., 2005), causing brown spine rot of Camelthorn (Alhagi maurorum Medik.) (Razaghi and Zafari, 2016).
Sydowia polyspora and T. conorum-piceae, which can be conifer pathogens (Sutton and Waterston, 1970; Heydeck, 1991; Talgø et al., 2010; Pan et al., 2018), did not cause strong necrosis on the twigs. The reason may be that they are specialized true endophytes of Scots pine (Bußkamp et al., 2020) and the host-fungi continuum is balanced. Sy. polyspora is widely distributed all over the world (Muñoz-Adalia et al., 2017; Pan et al., 2018). As an endophyte it has a high consistency and frequency in Scots pine twigs (Sanz-Ros et al., 2015; Blumenstein et al., 2020; Bußkamp et al., 2020). Predominately, Sy. polyspora lives saprophytically on dead plant material but also occurs as a secondary pathogen on previously damaged needles and twigs (Heydeck, 1991), as wound pathogen and blue stain-fungus (Sutton and Waterston, 1970), or as causal agent of current season needle necrosis (CSNN) on true fir (Abies spp.) (Talgø et al., 2010). Ascomata and pycnidia appear on dead pine branches and needles (Gremmen, 1977). Cleary et al. (2019) suggest that this fungus could benefit from climate change as additional stresses are added to the host so that Sy. polyspora can become a more commonly opportunistic pathogen. Here we show that the necrosis caused by Sy. polyspora in Scots pine is not affected by drought. In a study by Bußkamp (2018), Sy. polyspora did not show antagonistic behavior in dual culture with S. sapinea so the observed antagonism in this study might be strain related. T. conorum-piceae (≡ Pestalotia conorum-piceae Tubeuf) is also a frequently occurring twig endophyte of Scots spine with high consistency in Germany (Bußkamp et al., 2020). Mainly, it is a decomposer of pre-damaged needles of pine and is saprotrophic. However, T. conorum-piceae is also known as a secondary pathogen of pine and spruce needles and cones (Landeskompetenzzentrum Forst Eberswalde (LFE) 2015). The fungus is hardly described in the literature, but due to the fact that it is very abundant (Blumenstein et al., 2020; Bußkamp et al., 2020), it is important to gain more knowledge about this species in future studies. Other allied Amphisphaeriaceae such as Pestalotiopsis species can be primary causal agents of spots, blights and diebacks of various host plants. For example, the very common Pestalotiopsis funerea (Desm.) Steyaert, with a worldwide distribution, causes damping off, root and collar rots of seedlings, needle blight, tip-blight, twig dieback, and stem canker of conifers, especially Cupressaceae (Sinclair and Lyon, 2005).
The twig mortality was highest in the 25% water group and different statistically from the 100% treatment. This aspect is important with regard to the disease development in mature trees, as the twig mortality and thus the tree health, increases due to drought. Other studies have shown that water availability can affect the growth of pathogens in conifer trees during water stress. Heterobasidion species were able to increase necrosis under water stress (Terhonen et al., 2019b). Similarly, the blue stain fungus Endoconidiophora polonica (Siemaszko) Z.W. de Beer, T.A. Duong & M.J. Wingf. caused greater necrosis and mortality in Pi. abies seedlings with low water availability compared to those with high water availability (Linnakoski et al., 2017). Even a 25% decrease in water was able to trigger the activity of S. sapinea. The necrosis caused by S. sapinea was statistically higher in all water treatments compared to other fungi, indicating that host stress can particularly benefit this opportunistic pathogen.
Overall, the findings of our greenhouse-study demonstrate how severe the infection of S. sapinea is in drought stressed Scots pines, evidenced by high twig mortality and necrosis. The other fungi with the potential to become pathogens (as described above) did not benefit from host stress. In conclusion, pine endophytes showing antagonism against S. sapinea and with no detrimental effect to the host could be applied as inoculants to facilitate host protection. We will plan new research to find innovative and effective ways of utilizing the beneficial tree mycobiome in the context of future challenges. Sphaeropsis sapinea is a high-risk Scots pine pathogen because the disease severity (mortality of twigs, necrosis length) is increasing under drought stress. Climate change, leading to more severe and prolonged drought, will therefore lead to more outbreaks of the disease “Diplodia Tip Blight” in the future.
Data Availability Statement
The raw data supporting the conclusions of this article will be made available by the authors, without undue reservation.
Author Contributions
KB planned and performed the greenhouse experiment. JB and GL performed the isolation of the endophytes and the dual-culture study. RS and NPR contributed to the greenhouse experiment, the measurements of the necrosis, and the re-isolation of the fungi. ET contributed to the study design and analyzed the data. KB wrote the first draft. KB, JB, GL, and ET contributed to the final version of the manuscript. All authors contributed to the article and approved the submitted version.
Funding
The study was funded by the Faculty of Forest Sciences and Forest Ecology of the University of Göttingen and the Northwest German Forest Research Institute, Göttingen, Germany. We acknowledge support by the Open Access Publication Funds of the Göttingen University.
Conflict of Interest
The authors declare that the research was conducted in the absence of any commercial or financial relationships that could be construed as a potential conflict of interest.
Acknowledgments
We thank Annette Ihlemann from the NW-FVA for her contribution to the study during the antagonism assay and Etta Paar for helping with the illustrations. We also thank David Răscuţoi from the Forest Pathology group of University of Göttingen for taking care of the trees and helping with the infections.
Supplementary Material
The Supplementary Material for this article can be found online at: https://www.frontiersin.org/articles/10.3389/ffgc.2021.655769/full#supplementary-material
Supplementary Figure 1 | Boxplot representing the mortality of the twigs due to fungal (A) and water (B) treatment.
Supplementary Figure 2 | Boxplot presenting number of dead twigs after Sphaeropsis sapinea treatment in different water treatments.
Supplementary Table 1 | Number of dead twigs in the end of the greenhouse-experiment.
Footnotes
References
Arnold, A. E., and Herre, E. A. (2003). Canopy cover and leaf age affect colonization by tropical fungal endophytes: ecological pattern and process in Theobroma cacao (Malvaceae). Mycologia 95, 388–398. doi: 10.1080/15572536.2004.11833083
Arnold, A. E., and Lutzoni, F. (2007). Diversity and host range of foliar fungal endophytes: are tropical leaves biodiversity hotspots? Ecology 88, 541–549.
Arnold, A. E., Mejía, L. C., Kyllo, D., Rojas, E. I., Maynard, Z., Robbins, N., et al. (2003). Fungal endophytes limit pathogen damage in a tropical tree. Proc Natl Acad Sci U.S.A. 100:15649. doi: 10.1073/pnas.2533483100
Bengtsson-Palme, J., Ryberg, M., Hartmann, M., Branco, S., Wang, Z., Godhe, A., et al. (2013). Improved software detection and extraction of ITS1 and ITS2 from ribosomal ITS sequences of fungi and other eukaryotes for analysis of environmental sequencing data. Methods Ecol. Evol. 4, 914–919. doi: 10.1111/2041-210X.12073
Bihon, W., Slippers, B., Burgess, T., Wingfield, M. J., and Wingfield, B. D. (2011). Diverse sources of infection and cryptic recombination revealed in South African Diplodia pinea populations. Fungal Biol. 116, 112–120. doi: 10.1016/j.funbio.2011.10.006
Blodgett, J. T., Kruger, E. L., and Stanosz, G. R. (1997a). Effects of moderate water stress on disease development by Sphaeropsis sapinea on Red Pine. Phytopathology 87, 422–428. doi: 10.1094/PHYTO.1997.87.4.422
Blodgett, J. T., Kruger, E. L., and Stanosz, G. R. (1997b). Sphaeropsis sapinea and water stress in a Red Pine plantation in Central Wisconsin. Phytopathology 87, 429–434. doi: 10.1094/PHYTO.1997.87.4.429
Blumenstein, K. (2015). Endophytic Fungi in Elms: Implications for the Integrated Management of Dutch elm Disease. Alnarp: Swedish University of Agricultural Sciences.
Blumenstein, K., Bußkamp, J., Langer, G. J., Langer, E. J., and Terhonen, E. (2020). The opportunistic pathogen Sphaeropsis Sapinea is found to be one of the most abundant fungi in symptomless and diseased Scots Pine in Central-Europe. BMC Plant Biol. doi: 10.21203/rs.3.rs-48366/v1
Brodde, L., Adamson, K., Julio Camarero, J., Castaño, C., Drenkhan, R., Lehtijärvi, A., et al. (2019). Diplodia tip blight on its way to the North: drivers of disease emergence in Northern Europe. Front. Plant Sci. 9:1818. doi: 10.3389/fpls.2018.01818
Burgess, T. I., Wingfield, M. J., and Wingfield, B. D. (2004). Global distribution of Diplodia pinea genotypes revealed using simple sequence repeat (SSR) markers. Aust. Plant Pathol. 33, 513–519. doi: 10.1071/AP04067
Butin, V. H., and Kowalski, T. (1990). Die natürliche astreinigung und ihre biologischen Voraussetzungen. Eur. J. Forest Pathol. 20, 44–54. doi: 10.1111/j.1439-0329.1990.tb01272.x
Bußkamp, J. (2018). Schadenserhebung, Kartierung und Charakterisierung des “Diplodia-Triebsterbens“der Kiefer, insbesondere des endophytischen Vorkommens in den klimasensiblen Räumen und Identifikation von den in Kiefer (Pinus sylvestris) vorkommenden Endophyten. Kassel: Universität Kassel.
Bußkamp, J., Langer, G. J., and Langer, E. J. (2020). Sphaeropsis sapinea and fungal endophyte diversity in twigs of Scots pine (Pinus sylvestris) in Germany. Mycol. Progress 19, 985–999. doi: 10.1007/s11557-020-01617-0
CABI (2019). Sphaeropsis Sapinea (Sphaeropsis Blight) [WWW Document]. Invasive Species Compendium. Available online at: https://www.cabi.org/isc/datasheet/19160 (accessed November 13, 2019).
Chapela, I. H., and Boddy, L. (1988). Fungal colonization of attached beech branches. New Phytol. 110, 47–57. doi: 10.1111/j.1469-8137.1988.tb00236.x
Chen, Q., Hou, L. W., Duan, W. J., Crous, P. W., and Cai, L. (2017). Didymellaceae revisited. Stud. Mycol. 87, 105–159. doi: 10.1016/j.simyco.2017.06.002
Cleary, M., Oskay, F., Doğmuş, H. T., Lehtijärvi, A., Woodward, S., and Vettraino, A. M. (2019). Cryptic risks to forest biosecurity associated with the global movement of commercial seed. Forests 10:459. doi: 10.3390/f10050459
Compant, S., Saikkonen, K., Mitter, B., Campisano, A., and Mercado-Blanco, J. (2016). Editorial special issue: soil, plants and endophytes. Plant Soil 405, 1–11. doi: 10.1007/s11104-016-2927-9
de Wet, J., Burgess, T., Slippers, B., Preisig, O., Wingfield, B. D., and Wingfield, M. J. (2003). Multiple gene genealogies and microsatellite markers reflect relationships between morphotypes of Sphaeropsis sapinea and distinguish a new species of Diplodia. Mycol. Res. 107, 557–566. doi: 10.1017/s0953756203007706
Deckert, R. J., and Peterson, R. L. (2011). Distribution of foliar fungal endophytes of Pinus strobus between and within host trees. Can. J. Forest Res. 30, 1436–1442. doi: 10.1139/x00-078
Desprez-Loustau, M.-L., Marçais, B., Nageleisen, L.-M., Piou, D., and Vannini, A. (2006). Interactive effects of drought and pathogens in forest trees. Ann. For. Sci. 63, 597–612. doi: 10.1051/forest:2006040
Fabre, B., Piou, D., Desprez-Loustau, M.-L., and Marçais, B. (2011). Can the emergence of pine Diplodia shoot blight in France be explained by changes in pathogen pressure linked to climate change? Glob. Change Biol. 17, 3218–3227.
Feci, E., Battisti, A., Capretti, P., and Tegli, S. (2002). An association between the fungus Sphaeropsis sapinea and the cone bug Gastrodes grossipes in cones of Pinus nigra in Italy. Forest Pathol. 32, 241–247. doi: 10.1046/j.1439-0329.2002.00286.x
Flowers, J., Hartman, J. R., and Vaillancourt, L. J. (2006). Histology of Diplodia pinea in diseased and latently infected Pinus nigra shoots. Forest Pathol. 36, 447–459.
Fröhlich, J., Hyde, K. D., and Petrini, O. (2000). Endophytic fungi associated with palms. Mycol. Res. 104, 1202–1212.
Ganley, R. J., Sniezko, R. A., and Newcombe, G. (2008). Endophyte-mediated resistance against white pine blister rust in Pinus monticola. Forest Ecol. Manag. 255, 2751–2760. doi: 10.1016/j.foreco.2008.01.052
Gardes, M., and Bruns, T. D. (1993). ITS primers with enhanced specificity for basidiomycetes – application to the identification of mycorrhizae and rusts. Mol. Ecol. 2, 113–118. doi: 10.1111/j.1365-294X.1993.tb00005.x
Gremmen, J. (1977). Fungi colonizing living and dead tissue of Pinus sylvestris and P. nigra. Kew Bull. 31, 455–460. doi: 10.2307/4119386
Griffith, G. S., and Boddy, L. (1988). Fungal communities in attached ash (Fraxinus excelsior) twigs. Trans. Br. Mycol. Soc. 91, 599–606. doi: 10.1016/S0007-1536(88)80033-0
Guo, L.-D., Huang, G.-R., and Wang, Y. (2008). Seasonal and tissue age influences on endophytic fungi of Pinus tabulaeformis (Pinaceae) in the Dongling Mountains. Beijing. J. Integr. Plant Biol. 50, 997–1003. doi: 10.1111/j.1744-7909.2008.00394.x
Helander, M., Wäli, P., Kuuluvainen, T., and Saikkonen, K. (2011). Birch leaf endophytes in managed and natural boreal forests. Can. J. Forest Res. 36, 3239–3245. doi: 10.1139/x06-176
Heydeck, P. (1991). Nadelschädigung und triebsterben in verbindung mit Sclerophoma pithyophila (Corda) Höhn. Der. Wald. 41:142.
Hormazabal, E., Schmeda-Hirschmann, G., Astudillo, L., Rodríguez, J., and Theoduloz, C. (2005). Metabolites from Microsphaeropsis olivacea, an endophytic fungus of Pilgerodendron uviferum. Z. Naturforsch. C. J. Biosci. 60, 11–21. doi: 10.1515/znc-2005-1-203
Kehr, R. (1998). Zur bedeutung pilzlicher endophyten bei waldbäumen. Mitt. Biol. Bundesanst. Land Forstwirtsch. Berlin Dahlem 349, 8–30.
Kendrick, W. (1962). Biological aspects of the decay of Pinus sylvestris leaf litter. Nova Hedwigia 4, 313–342.
Keriö, S., Terhonen, E., and LeBoldus, J. (2020). Safe DNA-extraction protocol suitable for studying tree-fungus interactions. Bio Protocol 10:e3634. doi: 10.21769/BioProtoc.3634
Koukol, O., Kolařík, M., Kolářová, Z., and Baldrian, P. (2012). Diversity of foliar endophytes in wind-fallen Picea abies trees. Fungal Divers. 54, 69–77. doi: 10.1007/s13225-011-0112-2
Kowalski, T., and Kehr, R. (1992). Endophytic fungal colonization of branch bases in several forest tree species. Sydowia 44, 137–168.
Kowalski, T., and Zych, P. (2002). Fungi isolated from living symptomless shoots of Pinus nigra growing in different site conditions. Österr. Z. Pilzk. 11, 107–116.
Kuo, H.-C., Hui, S., Choi, J., Asiegbu, F. O., Valkonen, J. P. T., and Lee, Y.-H. (2014). Secret lifestyles of Neurospora crassa. Sci. Rep. 4:5135. doi: 10.1038/srep05135
Kusari, S., Hertweck, C., and Spiteller, M. (2012). Chemical ecology of endophytic fungi: origins of secondary metabolites. Chem. Biol. 19, 792–798. doi: 10.1016/j.chembiol.2012.06.004
Langer, G. J. (1994). Die Gattung Botryobasidium DONK (Corticiaceae, Basidiomycetes), Bibliotheca Mycologica. Stuttgart: J. Cramer, 459.
Linnakoski, R., Kasanen, R., Dounavi, A., and Forbes, K. (2019). Forest health under climate change: effects on tree resilience, and pest and pathogen dynamics. Front. Plant Sci. 10:1157. doi: 10.3389/fpls.2019.01157
Linnakoski, R., Sugano, J., Junttila, S., Pulkkinen, P., Asiegbu, F. O., and Forbes, K. M. (2017). Effects of water availability on a forestry pathosystem: fungal strain-specific variation in disease severity. Sci. Rep. 7:13501. doi: 10.1038/s41598-017-13512-y
Martín, J. A., Macaya-Sanz, D., Witzell, J., Blumenstein, K., and Gil, L. (2015). Strong in vitro antagonism by elm xylem endophytes is not accompanied by temporally stable in planta protection against a vascular pathogen under field conditions. Eur. J. Plant Pathol. 142, 185–196. doi: 10.1007/s10658-015-0602-2
Martínez-Arias, C., Macaya-Sanz, D., Witzell, J., and Martín, J. A. (2019). Enhancement of Populus alba tolerance to Venturia tremulae upon inoculation with endophytes showing in vitro biocontrol potential. Eur. J. Plant Pathol. 153, 1031–1042. doi: 10.1007/s10658-018-01618-6
Martínez-Arias, C., Sobrino-Plata, J., Ormeño-Moncalvillo, S., Gil, L., Rodríguez-Calcerrada, J., and Martín, J. A. (2021). Endophyte inoculation enhances Ulmus minor resistance to Dutch elm disease. Fungal Ecol. 50:101024. doi: 10.1016/j.funeco.2020.101024
Martinoviæ, T., Koukol, O., and Hirose, D. (2016). Distinct phylogeographic structure recognized within Desmazierella acicola. Mycologia 108, 20–30. doi: 10.3852/14-291
McMullin, D. R., Green, B. D., Prince, N. C., Tanney, J. B., and Miller, J. D. (2017). Natural products of Picea endophytes from the Acadian Forest. J. Nat. Prod. 80, 1475–1483. doi: 10.1021/acs.jnatprod.6b01157
Mejía, L. C., Rojas, E. I., Maynard, Z., Bael, S. V., Arnold, A. E., Hebbar, P., et al. (2008). Endophytic fungi as biocontrol agents of Theobroma cacao pathogens. Biol. Control 46, 4–14. doi: 10.1016/j.biocontrol.2008.01.012
Mgbeahuruike, A. C., Sun, H., Fransson, P., Kasanen, R., Daniel, G., Karlsson, M., et al. (2011). Screening of Phlebiopsis gigantea isolates for traits associated with biocontrol of the conifer pathogen Heterobasidion annosum. Biol. Control 57, 118–129. doi: 10.1016/j.biocontrol.2011.01.007
Müller, C. B., and Krauss, J. (2005). Symbiosis between grasses and asexual fungal endophytes. Curr. Opin. Plant Biol. Biotic Interact. 8, 450–456. doi: 10.1016/j.pbi.2005.05.007
Muñoz-Adalia, E. J., Sanz-Ros, A. V., Flores-Pacheco, J. A., Hantula, J., Diez, J. J., Vainio, E. J., et al. (2017). Sydowia polyspora dominates fungal communities carried by two tomicus species in Pine plantations threatened by Fusarium circinatum. Forests 8:127. doi: 10.3390/f8040127
Navarro-Meléndez, A. L., and Heil, M. (2014). Symptomless endophytic fungi suppress endogenous levels of salicylic acid and interact with the jasmonate-dependent indirect defense traits of their host, lima bean (Phaseolus lunatus). J. Chem. Ecol. 40, 816–825. doi: 10.1007/s10886-014-0477-2
Nguyen, D., Boberg, J., Ihrmark, K., Stenström, E., and Stenlid, J. (2016). Do foliar fungal communities of Norway spruce shift along a tree species diversity gradient in mature European forests? Fungal Ecol. 23, 97–108. doi: 10.1016/j.funeco.2016.07.003
Oliva, J., Ridley, M., Redondo, M. A., and Caballol, M. (2021). Competitive exclusion amongst endophytes determines shoot blight severity on pine. Funct. Ecol. 35, 239–254. doi: 10.1111/1365-2435.13692
Oses, R., Valenzuela, S., Freer, J., Sanfuentes, E., and Rodriguez, J. (2008). Fungal endophytes in xylem of healthy Chilean trees and their possible role in early wood decay. Fungal Divers. 33, 77–86.
Osono, T. (2006). Role of phyllosphere fungi of forest trees in the development of decomposer fungal communities and decomposition processes of leaf litter. Can. J. Microbiol. 52, 701–716. doi: 10.1139/w06-023
Paez, C. A., and Smith, J. A. (2018). First report of Diplodia sapinea and Diplodia scrobiculata causing an outbreak of tip blight on slash pine in Florida. Plant Dis. 102:1657. doi: 10.1094/PDIS-07-17-1093-PDN
Pan, Y., Ye, H., Lu, J., Chen, P., Zhou, X.-D., Qiao, M., et al. (2018). Isolation and identification of Sydowia polyspora and its pathogenicity on Pinus yunnanensis in Southwestern China. J. Phytopathol. 166, 386–395. doi: 10.1111/jph.12696
Peršoh, D. (2013). Factors shaping community structure of endophytic fungi–evidence from the Pinus-Viscum-system. Fungal Divers. 60, 55–69.
Peršoh, D., Melcher, M., Flessa, F., and Rambold, G. (2010). First fungal community analyses of endophytic ascomycetes associated with Viscum album ssp. austriacum and its host Pinus sylvestris. Fungal Biol. 114, 585–596.
Peters, S., Draeger, S., Aust, H.-J., and Schulz, B. (1998). Interactions in dual cultures of endophytic fungi with host and nonhost plant calli. Mycologia 90, 360–367. doi: 10.1080/00275514.1998.12026919
Petrini, O. (1991). “Fungal endophytes of tree leaves,” in Microbial Ecology of Leaves, Brock/Springer Series in Contemporary Bioscience, eds J. H. Andrews and S. S. Hirano (New York, NY: Springer), 179–197. doi: 10.1007/978-1-4612-3168-4_9
Petrini, O., and Fisher, P. J. (1988). A comparative study of fungal endophytes in xylem and whole stem of Pinus sylvestris and Fagus sylvatica. Trans. Br. Mycol. Soc. 91, 233–238. doi: 10.1016/S0007-1536(88)80210-9
Phillips, A. J. L., Alves, A., Abdollahzadeh, J., Slippers, B., Wingfield, M. J., Groenewald, J. Z., et al. (2013). The Botryosphaeriaceae: genera and species known from culture. Stud. Mycol. 76, 51–167.
Ponge, J. F. (1991). Succession of fungi and fauna during decomposition of needles in a small area of Scots pine litter. Plant Soil 138, 99–113. doi: 10.1007/BF00011812
R Core Team (2019). R: A Language and Environment for Statistical Computing. Vienna: R Foundation for Statistical Computing.
Raghavendra, A. K. H., and Newcombe, G. (2013). The contribution of foliar endophytes to quantitative resistance to Melampsora rust. New Phytol. 197, 909–918. doi: 10.1111/nph.12066
Razaghi, P., and Zafari, D. (2016). First report of Microsphaeropsis olivacea causing brown spine rot on Alhagi Maurorum in Iran. J. Plant Pathol. 98:377. doi: 10.4454/JPP.V98I2.044
Richardson, S. N., Walker, A. K., Nsiama, T. K., McFarlane, J., Sumarah, M. W., Ibrahim, A., et al. (2014). Griseofulvin-producing Xylaria endophytes of Pinus strobus and Vaccinium angustifolium: evidence for a conifer-understory species endophyte ecology. Fungal Ecol. 11, 107–113. doi: 10.1016/j.funeco.2014.05.004
Ridout, M., and Newcombe, G. (2015). The frequency of modification of Dothistroma pine needle blight severity by fungi within the native range. Forest Ecol. Manag. 337, 153–160. doi: 10.1016/j.foreco.2014.11.010
Rigerte, L., Blumenstein, K., and Terhonen, E. (2019). New R-Based methodology to optimize the identification of root endophytes against Heterobasidion parviporum. Microorganisms 7:102. doi: 10.3390/microorganisms7040102
Rodriguez, R. J., White, J. F. Jr., Arnold, A. E., and Redman, R. S. (2009). Fungal endophytes: diversity and functional roles. New Phytol. 182, 314–330. doi: 10.1111/j.1469-8137.2009.02773.x
Romeralo, C., Santamaria, O., Pando, V., and Diez, J. J. (2015). Fungal endophytes reduce necrosis length produced by Gremmeniella abietina in Pinus halepensis seedlings. Biol. Control 80, 30–39.
Rungjindamai, N., Pinruan, U., Hattori, T., and Choeyklin, R. (2008). Molecular characterization of basidiomycetous endophytes isolated from leaves, rachis and petioles of the oil palm, Elaeis guineensis, in Thailand. Fungal Divers. 33, 139–161.
Saikkonen, K., Faeth, S. H., Helander, M., and Sullivan, T. J. (1998). Fungal endophytes: a continuum of interactions with host plants. Annu. Rev. Ecol. Syst. 29, 319–343. doi: 10.1146/annurev.ecolsys.29.1.319
Santamaría, O., Smith, D. R., and Stanosz, G. R. (2012). Interaction between Diplodia pinea or Diplodia scrobiculata and fungal endophytes isolated from pine shoots. Can. J. For. Res. 42, 1819–1826. doi: 10.1139/x2012-132
Sanz-Ros, A. V., Müller, M. M., San Martín, R., and Diez, J. J. (2015). Fungal endophytic communities on twigs of fast and slow growing Scots pine (Pinus sylvestris L.) in northern Spain. Fungal Biol. 119, 870–883. doi: 10.1016/j.funbio.2015.06.008
Sayers, E. W., Barrett, T., Benson, D. A., Bolton, E., Bryant, S. H., Canese, K., et al. (2011). Database resources of the National Center for Biotechnology Information. Nucleic Acids Res. 39, D38–D51. doi: 10.1093/nar/gkq1172
Schulz, B., Boyle, C., Draeger, S., Römmert, A.-K., and Krohn, K. (2002). Endophytic fungi: a source of novel biologically active secondary metabolites. Mycol. Res. 106, 996–1004. doi: 10.1017/S0953756202006342
Schulz, B., Guske, S., Dammann, U., and Boyle, C. (1998). Endophyte-Host Interactions. II. Defining Symbiosis of the Endophyte-Host Interaction. Philadelphia, PA: Symbiosis.
Schulz, B., Haas, S., Junker, C., Andrée, N., and Schobert, M. (2015). Fungal endophytes are involved in multiple balanced antagonisms. Curr. Sci. 109, 39–45.
Sherwood, P., Villari, C., Capretti, P., and Bonello, P. (2015). Mechanisms of induced susceptibility to Diplodia tip blight in drought-stressed Austrian pine. Tree Physiol. 35, 549–562. doi: 10.1093/treephys/tpv026
Sieber, T. N. (2007). Endophytic fungi in forest trees: are they mutualists? Fungal Biol. Rev. Fungal Endophytes 21, 75–89. doi: 10.1016/j.fbr.2007.05.004
Sinclair, W. A., and Lyon, H. H. (2005). Diseases of Trees and Shrubs, 2nd Edn. Ithaca, NY: Comstock Publishing.
Skaltsas, D. N., Badotti, F., Vaz, A. B. M., Silva, F. F., da Gazis, R., Wurdack, K., et al. (2019). Exploration of stem endophytic communities revealed developmental stage as one of the drivers of fungal endophytic community assemblages in two Amazonian hardwood genera. Sci. Rep. 9:12685. doi: 10.1038/s41598-019-48943-2
Smith, H., Wingfield, M., Crous, P., and Coutinho, T. A. (1996). Sphaeropsis sapinea and Botryosphaeria dothidea endophytic in Pinus spp. and Eucalyptus spp. in South Africa. S. Afr. J. Bot. 62, 86–88. doi: 10.1016/S0254-6299(15)30596-2
Stanosz, G. R., Blodgett, J. T., Smith, D. R., and Kruger, E. L. (2001). Water stress and Sphaeropsis sapinea as a latent pathogen of red pine seedlings. New Phytol. 149, 531–538. doi: 10.1046/j.1469-8137.2001.00052.x
Sturrock, R. N., Frankel, S. J., Brown, A. V., Hennon, P. E., Kliejunas, J. T., Lewis, K. J., et al. (2011). Climate change and forest diseases. Plant Pathol. 60, 133–149. doi: 10.1111/j.1365-3059.2010.02406.x
Sumarah, M. W., Kesting, J. R., Sørensen, D., and Miller, J. D. (2011). Antifungal metabolites from fungal endophytes of Pinus strobus. Phytochemistry 72, 1833–1837. doi: 10.1016/j.phytochem.2011.05.003
Sumarah, M. W., Walker, A. K., Seifert, K. A., Todorov, A., and Miller, J. D. (2015). “Screening of fungal endophytes isolated from Eastern White Pine deedles,” in The Formation, Structure and Activity of Phytochemicals, Recent Advances in Phytochemistry, ed. R. Jetter (Cham: Springer International Publishing), 195–206. doi: 10.1007/978-3-319-20397-3_8
Suryanarayanan, T. S., Thirunavukkarasu, N., Govindarajulu, M. B., and Gopalan, V. (2012). Fungal endophytes: an untapped source of biocatalysts. Fungal Divers. 54, 19–30. doi: 10.1007/s13225-012-0168-7
Sutton, B. C., and Waterston, J. M. (1970). Sydowia polyspora CMI Descriptions of Pathogenic Fungi and Bacteria. CAB International, Wallingford, 1–2.
Talgø, V., Chastagner, G., Thomsen, I. M., Cech, T., Riley, K., Lange, K., et al. (2010). Sydowia polyspora associated with current season needle necrosis (CSNN) on true fir (Abies spp.). Fungal Biol. 114, 545–554. doi: 10.1016/j.funbio.2010.04.005
Tanney, J. B., McMullin, D. R., Green, B. D., Miller, J. D., and Seifert, K. A. (2016). Production of antifungal and antiinsectan metabolites by the Picea endophyte Diaporthe maritima sp. nov. Fungal Biol. Integr. Taxon. Uncovering Fungal Divers. 120, 1448–1457. doi: 10.1016/j.funbio.2016.05.007
Taudière, A., Bellanger, J.-M., Carcaillet, C., Hugot, L., Kjellberg, F., Lecanda, A., et al. (2018). Diversity of foliar endophytic ascomycetes in the endemic Corsican pine forests. Fungal Ecol. 36, 128–140. doi: 10.1016/j.funeco.2018.07.008
Tellenbach, C., Sumarah, M. W., Grünig, C. R., and Miller, J. D. (2013). Inhibition of Phytophthora species by secondary metabolites produced by the dark septate endophyte Phialocephala europaea. Fungal Ecol. 6, 12–18. doi: 10.1016/j.funeco.2012.10.003
Terhonen, E., Babalola, J., Kasanen, R., Jalkanen, R., and Blumenstein, K. (2021). Sphaeropsis sapinea found as symptomless endophyte in Finland. Silva Fenn. 55:13. doi: 10.14214/sf.10420
Terhonen, E., Blumenstein, K., Kovalchuk, A., and Asiegbu, F. O. (2019a). Forest tree microbiomes and associated fungal endophytes: functional roles and impact on forest health. Forests 10:42. doi: 10.3390/f10010042
Terhonen, E., Kovalchuk, A., Zarsav, A., and Asiegbu, F. O. (2018). “Biocontrol potential of forest tree endophytes,” in Endophytes of Forest Trees: Biology and Applications, Forestry Sciences, eds A. M. Pirttilä and A. C. Frank (Cham: Springer International Publishing), 283–318. doi: 10.1007/978-3-319-89833-9_13
Terhonen, E., Langer, G. J., Bußkamp, J., Răscuţoi, D. R., and Blumenstein, K. (2019b). Low water availability increases necrosis in Picea abies after artificial inoculation with fungal root rot pathogens Heterobasidion parviporum and Heterobasidion annosum. Forests 10:55. doi: 10.3390/f10010055
Terhonen, E., Marco, T., Sun, H., Jalkanen, R., Kasanen, R., Vuorinen, M., et al. (2011). The effect of latitude, season and needle-age on the mycota of Scots pine (Pinus sylvestris) in Finland. Silva Fenn. 45:104. doi: 10.14214/sf.104
Terhonen, E., Sipari, N., and Asiegbu, F. O. (2016). Inhibition of phytopathogens by fungal root endophytes of Norway spruce. Biol. Control 99, 53–63. doi: 10.1016/j.biocontrol.2016.04.006
White, T. J., Bruns, T., Lee, S., and Taylor, J. (1990). “Amplification and direct sequencing of fungal ribosomal RNA genes for phylogenetics,” in PCR Protocols, eds M. A. Innis, D. H. Gelfand, J. J. Sninsky, White, and J. Thomas (San Diego, CA: Academic Press), 315–322. doi: 10.1016/B978-0-12-372180-8.50042-1
Witzell, J., and Martín, J. A. (2018). “Endophytes and forest health,” in Endophytes of Forest Trees: Biology and Applications, Forestry Sciences, eds A. M. Pirttilä and A. C. Frank (Cham: Springer International Publishing), 261–282. doi: 10.1007/978-3-319-89833-9_12
Witzell, J., Martín, J. A., and Blumenstein, K. (2014). “Ecological aspects of endophyte-based biocontrol of forest diseases,” in Advances in Endophytic Research, eds V. C. Verma and A. C. Gange (New Delhi: Springer India), 321–333. doi: 10.1007/978-81-322-1575-2_17
Zhang, Y., Schoch, C. L., Fournier, J., Crous, P. W., de Gruyter, J., Woudenberg, J. H. C., et al. (2009). Multi-locus phylogeny of Pleosporales: a taxonomic, ecological and evolutionary re-evaluation. Stud. Mycol. 64, 85–102. doi: 10.3114/sim.2009.64.04
Keywords: Diplodia tip blight, climate change, antagonism, infection, drought, Sphaeropsis sapinea, Sydowia polyspora
Citation: Blumenstein K, Bußkamp J, Langer GJ, Schlößer R, Parra Rojas NM and Terhonen E (2021) Sphaeropsis sapinea and Associated Endophytes in Scots Pine: Interactions and Effect on the Host Under Variable Water Content. Front. For. Glob. Change 4:655769. doi: 10.3389/ffgc.2021.655769
Received: 19 January 2021; Accepted: 26 April 2021;
Published: 24 May 2021.
Edited by:
Caterina Villari, University of Georgia, United StatesReviewed by:
Treena Burgess, Murdoch University, AustraliaBenoit Marçais, INRA Centre Nancy-Lorraine, France
Copyright © 2021 Blumenstein, Bußkamp, Langer, Schlößer, Parra Rojas and Terhonen. This is an open-access article distributed under the terms of the Creative Commons Attribution License (CC BY). The use, distribution or reproduction in other forums is permitted, provided the original author(s) and the copyright owner(s) are credited and that the original publication in this journal is cited, in accordance with accepted academic practice. No use, distribution or reproduction is permitted which does not comply with these terms.
*Correspondence: Eeva Terhonen, terhonen@uni-goettingen.de