Response: Commentary: Large Trees Dominate Carbon Storage in Forests East of the Cascade Crest in the United States Pacific Northwest
- 1College of Forestry, Department of Forest Ecosystems and Society, Oregon State University College of Forestry, Corvallis, OR, United States
- 2College of Environment, School of Environmental and Forest Sciences, University of Washington College of the Environment, Seattle, WA, United States
- 3Applegate Forestry LLC, Corvallis, OR, United States
- 4Sustainable Northwest, Portland, OR, United States
A Commentary on
Large Trees Dominate Carbon Storage in Forests East of the Cascade Crest in the United States Pacific Northwest
by Mildrexler, D. J., Berner, L. T., Law, B. E., Birdsey, R. A., and Moomaw, W. R. (2020). Front. For. Glob. Change 3:594274. doi: 10.3389/ffgc.2020.594274
Introduction
The U.S. Forest Service (USFS) recently made revisions to an interim prohibition on cutting trees ≥53 cm diameter at breast height (DBH) in seasonally dry, fire-prone forests of eastern Oregon. This policy change is designed to allow cutting of young (<150 years) shade-tolerant fir ≥53 cm DBH to facilitate the conservation and recruitment of old (>150 years) shade-intolerant pine and larch (United States Department of Agriculture (USDA) Forest Service, 2020). Mildrexler et al. (2020) criticize this proposal based solely on evidence that large trees (i.e., trees ≥53 cm DBH) store more carbon than small trees (i.e., trees <53 cm DBH). Without any analysis of tree-, stand-, or landscape-scale carbon fluxes, Mildrexler et al. argue that forest-based climate change mitigation goals can best be served by maintaining prohibitions on cutting young trees ≥53 cm or even extending prohibitions to include trees as small as 30 cm DBH.
Mildrexler et al. err in assuming that prohibiting logging of relatively large but young shade-tolerant trees will enhance forest carbon storage over time in seasonally dry, fire-prone landscapes. Carbon stores in these forest communities are increasingly vulnerable to the combined effects of more than a century of fire exclusion and a warming climate (Hessburg et al., 2019). Mildrexler et al. disregard the ecological benefits of thinning projects that remove young shade-tolerant trees to enhance the resistance of old shade-intolerant trees that can store carbon over longer periods in the face of a warming climate (Henson et al., 2013; Bradford and Bell, 2017; Stephens et al., 2020). The errors, oversights, and misrepresentations in Mildrexler et al. summarized below and in Table 1 make this study an unsuitable basis for evaluating policy change.
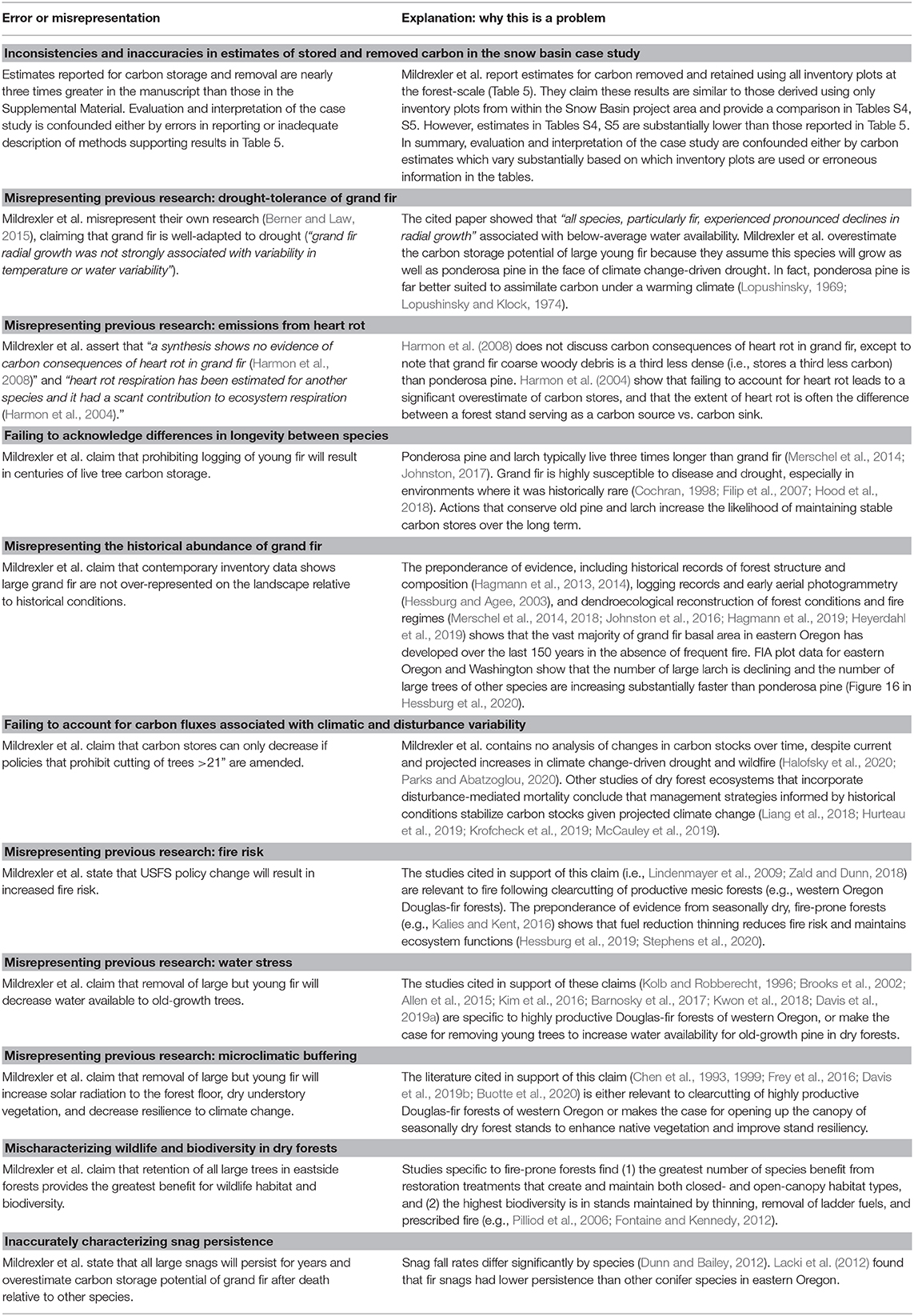
Table 1. Summary of key errors and misrepresentations in Mildrexler et al. (2020).
Mildrexler et al. Misrepresent Forest Ecology and Carbon Dynamics in Seasonally Dry Forests
At the heart of Mildrexler et al.'s argument is the conviction that current carbon stocks can be maintained, and even more carbon can be stored in seasonally dry forests of eastern Orgon if thinning is limited to trees <53 cm DBH (or, alternately, 30 cm DBH). This argument ignores the fact that current carbon stores in eastern Oregon forests accumulated because fire was effectively excluded from the landscape for more than a century (Parks et al., 2015; Reilly et al., 2017; Haugo et al., 2019). In particular, the number of shade-tolerant fir ≥53 cm DBH increased substantially over the last century as a consequence of fire exclusion (Hagmann et al., 2013, 2014; Merschel et al., 2014; Johnston, 2017; Johnston et al., 2018). Mildrexler et al. ignore research showing that dry forests have overshot their carbon-carrying capacity and that thinning treatments, although they reduce carbon stocks in the short term, will tend to stabilize carbon stocks over multi-decadal time scales in the face of a warming climate (e.g., Hurteau et al., 2019; Krofcheck et al., 2019). Mildrexler et al. assert without evidence that large shade-tolerant fir are not over-represented on the landscape and that forests of eastern Oregon have “low future climatic vulnerability.” But deepening drought and increasing fire extent and severity throughout eastern Oregon (Reilly et al., 2017; Parks and Abatzoglou, 2020) have made it clear that much of the carbon currently stored on this landscape is increasingly vulnerable to loss over the next several decades if stand densities remain at their current levels (Halofsky et al., 2018; Kerns et al., 2018; Stephens et al., 2020).
The USFS's proposal to allow cutting of some large but young shade-tolerant trees is designed to restore ecosystem resilience to fire and drought and increase the resistance (and long-term carbon storage potential) of shade-intolerant old-growth trees, especially ponderosa pine. Old-growth ponderosa pine has extensive heartwood and exceptional drought, insect, and fire tolerance when freed from competition with fast-growing shade-tolerant fir with high leaf area and transpiration demands (Hessburg et al., 2020). Mildrexler et al. assert that extant populations of young shade-tolerant fir can provide “centuries of long-term carbon storage” and that removal of relatively large young trees facilitated by Forest Service policy change represents a net emission to the atmosphere over all spatial and temporal scales. In fact, relative to the old pine and larch they endanger, large young fir that were off-limits to removal are far more prone to heart rot, which results in significant greenhouse gas emissions (Aho, 1977; Covey et al., 2012). They are also far more prone to mortality from drought, insects, and root diseases than pine. A number of studies investigating mortality of grand fir in eastern Oregon report 100% mortality of large fir over 10–20 years of observations (i.e., Cochran, 1998; Filip et al., 2007).
Throughout their paper, Mildrexler et al. assert that prohibitions on cutting large but young fir in eastern Oregon convey significant benefits to wildlife, water quality, and fire and drought resilience. But the literature cited in support of these claims either speaks to management of old-growth trees in highly productive mesic forests of western Oregon or actually makes the case for the USFS's proposal to remove large but young fir to reduce competition with fire- and drought-tolerant old-growth pine and larch. There is little doubt that conserving the most productive structurally complex older forests in western Oregon achieves carbon storage, water quality, and wildlife habitat benefits without risking uncharacteristically extensive mortality from fire and drought (Halofsky et al., 2018). But in seasonally dry forests of eastern Oregon, research demonstrates that providing a wide range of wildlife habitat, protecting old-growth trees, and enhancing stream and watershed health is best achieved by judicious removal of young trees, including large shade-tolerant trees, that established while fire was excluded from the landscape (Lehmkuhl et al., 2007; Fontaine and Kennedy, 2012; Hessburg et al., 2020).
Discussion
Avoiding catastrophic effects of rising global temperatures is the most important challenge facing human civilization (IPCC, 2018). Forests have an important role in sequestering carbon to offset anthropogenic emissions. For instance, deferring harvest or increasing rotation ages in mesic forests currently below their carbon storage capacity has tremendous potential for offsetting emissions (Hudiburg et al., 2009). But relying on seasonally dry, fire-prone stands that are currently well above historical levels of aboveground tree carbon is likely to destabilize carbon stocks and forfeit the multiple ecological benefits associated with restoration treatments, especially as the climate warms (Hurteau et al., 2016; Liang et al., 2018; Foster et al., 2020; Stephens et al., 2020). We urge policy makers to rely on comprehensive and accurate accounts of carbon dynamics when crafting policy for dry forests.
Author Contributions
JJ, RH, SS, AM, JF, and KJ contributed research and writing. All authors contributed to the article and approved the submitted version.
Conflict of Interest
NJ was employed by Applegate Forestry.
The remaining authors declare that the research was conducted in the absence of any commercial or financial relationships that could be construed as a potential conflict of interest.
Acknowledgments
The authors are indebted to Harold Zald, Matt Reilly, the associate editor, and two reviewers for helpful comments that improved a draft manuscript.
References
Aho, P. E. (1977). Decay of grand fir in the Blue Mountains of Oregon and Washington. USDA Forest Service Research Paper PNW-229, 18.
Allen, C. D., Breshears, D. D., and McDowell, N. G. (2015). On underestimation of global vulnerability to tree mortality and forest die-off from hotter drought in the Anthropocene. Ecosphere 6:129. doi: 10.1890/ES15-00203.1
Barnosky, A. D., Hadly, E. A., Gonzalez, P., Head, J., Polly, P. D., Lawing, A. M., et al. (2017). Merging paleobiology with conservation biology to guide the future of terrestrial ecosystems. Science 355:eaah4787. doi: 10.1126/science.aah4787
Berner, L. T., and Law, B. E. (2015). Water limitations on forest carbon cycling and conifer traits along a steep climatic gradient in the Cascade Mountains, Oregon. Biogeosciences 12, 6617–6635. doi: 10.5194/bg-12-6617-2015
Bradford, J. B., and Bell, D. M. (2017). A window of opportunity for climate-change adaptation: easing tree morality by reducing forest basal area. Front. Ecol. Environ. 15, 11–17. doi: 10.1002/fee.1445
Brooks, J. R., Meinzer, F. C., Coulombe, R., and Gregg, J. (2002). Hydraulic redistribution of soil water during summer drought in two contrasting Pacific Northwest coniferous forests. Tree Physiol. 22, 1107–1117. doi: 10.1093/treephys/22.15-16.1107
Buotte, P. C., Law, B. E., Ripple, W. J., and Berner, L. T. (2020). Carbon sequestration and biodiversity co-benefits of preserving forests in the western United States. Ecol. Appl. 30:e02039. doi: 10.1002/eap.2039
Chen, J., Franklin, J. F., and Spies, T. A. (1993). Contrasting microclimates among clearcut, edge, and interior of old-growth Douglas-fir forest. Agric. For. Meteorol. 63, 219–237. doi: 10.1016/0168-1923(93)90061-l
Chen, J., Saunders, S. C., Crow, T. R., Naiman, R. J., Brosofske, K. D., Mroz, G. D., et al. (1999). Microclimate in forest ecosystem and landscape ecology. BioScience 49, 288–297. doi: 10.2307/1313612
Cochran, P. H. (1998). Examples of Mortality and Reduced Annual Increments of White fir Induced by Drought, Insects, and Disease at Different Stand Densities, Vol. 525. US Department of Agriculture, Forest Service, Pacific Northwest Research Station, Portland, OR.
Covey, K. R., Wood, S. A., Warren, R. J., Lee, X., and Bradford, M. A. (2012). Elevated methane concentrations in trees of an upland forest. Geophys. Res. Lett. 39. doi: 10.1029/2012GL052361
Davis, K. T., Dobrowski, S. Z., Higuera, P. E., Holden, Z. A., Veblen, T. T., Rother, M. T., et al. (2019a). Wildfires and climate change push low-elevation forests across a critical climate threshold for tree regeneration. Proc. Natl. Acad. Sci. U.S.A. 116, 6193–6198. doi: 10.1073/pnas.1815107116
Davis, K. T., Dobrowski, S. Z., Holden, Z. A., Higuera, P. E., and Abatzoglou, J. T. (2019b). Microclimatic buffering in forests of the future: the role of local water balance. Ecography 42, 1–11. doi: 10.1111/ecog.03836
Dunn, C. J., and Bailey, J. D. (2012). Temporal dynamics and decay of coarse wood in early seral habitats of dry-mixed conifer forests in Oregon's Eastern Cascades. For. Ecol. Manage. 276, 71–81. doi: 10.1016/j.foreco.2012.03.013
Filip, G. M., Maffei, H., and Chadwick, K. L. (2007). Forest health decline in a central Oregon mixed-conifer forest revisited after wildfire: a 25-year case study. West. J. Appl. For. 22, 278–284. doi: 10.1093/wjaf/22.4.278
Fontaine, J. B., and Kennedy, P. L. (2012). Meta-analysis of avian and small-mammal response to fire severity and fire surrogate treatments in US fire-prone forests. Ecol. Appl. 22, 1547–1561. doi: 10.1890/12-0009.1
Foster, D. E., Battles, J. J., Collins, B. M., York, R. A., and Stephens, S. L. (2020). Potential wildfire and carbon stability in frequent-fire forests in the Sierra Nevada: trade-offs from a long-term study. Ecosphere 11:e03198. doi: 10.1002/ecs2.3198
Frey, S. J. K., Hadley, A. S., Johnson, S. L., Schulze, M., Jones, J. A., and Betts, M. G. (2016). Spatial models reveal the microclimate buffering capacity of old-growth forests. Sci. Adv. 2:e1501392. doi: 10.1126/sciadv.1501392
Hagmann, R. K., Franklin, J. F., and Johnson, K. N. (2013). Historical structure and composition of ponderosa pine and mixed-conifer forests in south-central Oregon. For. Ecol. Manage. 304, 492–504. doi: 10.1016/j.foreco.2013.04.005
Hagmann, R. K., Franklin, J. F., and Johnson, K. N. (2014). Historical conditions in mixed-conifer forests on the eastern slopes of the northern Oregon Cascade Range, USA. For. Ecol. Manage. 330, 158–170. doi: 10.1016/j.foreco.2014.06.044
Hagmann, R. K., Merschel, A. G., and Reilly, M. J. (2019). Historical patterns of fire severity and forest structure and composition in a landscape structured by frequent large fires: Pumice Plateau ecoregion, Oregon, USA. Landscape Ecol. 34, 551–568. doi: 10.1007/s10980-019-00791-1
Halofsky, J. E., Peterson, D. L., and Harvey, B. J. (2020). Changing wildfire, changing forests: the effects of climate change on fire regimes and vegetation in the Pacific Northwest, USA. Fire Ecol. 16:4. doi: 10.1186/s42408-019-0062-8
Halofsky, J. S., Donato, D. C., Franklin, J. F., Halofsky, J. E., Peterson, D. L., and Harvey, B. J. (2018). The nature of the beast: examining climate adaptation options in forests with stand-replacing fire regimes. Ecosphere 9:e02140. doi: 10.1002/ecs2.2140
Harmon, M. E., Bible, K., Ryan, M. G., Shaw, D. C., Chen, H., Klopatek, J., et al. (2004). Production, respiration, and overall carbon balance in an old-growth Pseudotsuga-Tsuga forest ecosystem. Ecosystems 7, 498–512. doi: 10.1007/s10021-004-0140-9
Harmon, M. E., Woodall, C. W., Fasth, B., and Sexton, J. (2008). Woody detritus density and density reduction factors for tree species in the united states: a synthesis. Gen. Tech. Rep. NRS-29. Newtown Square, PA: U.S. Department of Agriculture, 84.
Haugo, R. D., Kellogg, B. S., Cansler, C. A., Kolden, C. A., Kemp, K. B., Robertson, J. C., et al. (2019). The missing fire: quantifying human exclusion of wildfire in Pacific Northwest forests, USA. Ecosphere 10:e02702. doi: 10.1002/ecs2.2702
Henson, P., Thrailkill, J., Glenn, B., Woodbridge, B., and White, B. (2013). Using ecological forestry to reconcile spotted owl conservation and forest management. J. For. 111, 433–437. doi: 10.5849/jof.13-072
Hessburg, P. F., and Agee, J. K. (2003). An environmental narrative of Inland Northwest United States forests, 1800-2000. For. Ecol. Manage. 178, 23–59. doi: 10.1016/S0378-1127(03)00052-5
Hessburg, P. F., Charnley, S., Wendel, K. L., White, E. M., Singleton, P. H., Peterson, D. W., et al. (2020). The 1994 Eastside Screens Large-Tree Harvest Limit: Review of Science Relevant to Forest Planning 25 Years Later. Gen. Tech. Rep. PNW-GTR-990. Portland, OR: US Department of Agriculture, Forest Service, Pacific Northwest Research Station. 114, 990.
Hessburg, P. F., Miller, C. L., Povak, N. A., Taylor, A. H., Higuera, P. E., Prichard, S. J., et al. (2019). Climate, environment, and disturbance history govern resilience of western North American forests. Front. Ecol. Evolut. 7, 239–237. doi: 10.3389/fevo.2019.00239
Heyerdahl, E. K., Loehman, R. A., and Falk, D. A. (2019). A multi-century history of fire regimes along a transect of mixed-conifer forests in central Oregon, U.S.A. Can. J. For. Res. 49, 76–86. doi: 10.1139/cjfr-2018-0193
Hood, S., Abrahamson, I., and Cansler, A. C. (2018). “Fire resistance and regeneration characteristics of Northern Rockies tree species,” in Fire Effects Information System. U.S. Department of Agriculture, Forest Service, Rocky Mountain Research Station, Missoula Fire Sciences Laboratory (Producer). Available online at: https://www.fs.fed.us/database/feis/pdfs/other/FireResistRegen.html (accessed December 18, 2020).
Hudiburg, T., Law, B., Turner, D. P., Campbell, J., Donato, D., and Duane, M. (2009). Carbon dynamics of Oregon and Northern California forests and potential land-based carbon storage. Ecol. Appl. 19, 163–180. doi: 10.1890/07-2006.1
Hurteau, M. D., Liang, S., Martin, K. L., North, M. P., Koch, G. W., and Hungate, B. A. (2016). Restoring forest structure and process stabilizes forest carbon in wildfire-prone southwestern ponderosa pine forests. Ecol. Appl. 26, 382–391. doi: 10.1890/15-0337
Hurteau, M. D., North, M. P., Koch, G. W., and Hungate, B. A. (2019). Opinion: Managing for disturbance stabilizes forest carbon. Proc. Natl. Acad. Sci. U.S.A. 116, 10193–10195. doi: 10.1073/pnas.1905146116
IPCC (2018). Special Report on Global Warming of 1.5°C (SR1.5). (Intergovernmental Panel on Climate Change.
Johnston, J. D. (2017). Forest succession along a productivity gradient following fire exclusion. For. Ecol. Manag. 392, 45–57. doi: 10.1016/j.foreco.2017.02.050
Johnston, J. D., Bailey, J. D., and Dunn, C. J. (2016). Influence of fire disturbance and biophysical heterogeneity on pre-settlement ponderosa pine and mixed conifer forests. Ecosphere 7, 1–19. doi: 10.1002/ecs2.1581
Johnston, J. D., Dunn, C. J., Vernon, M. J., Bailey, J. D., Morrisette, B. A., and Morici, K. (2018). Restoring historical forest conditions in a diverse inland Pacific Northwest landscape. Ecosphere 9:e02400. doi: 10.1002/ecs2.2400
Kalies, E. L., and Kent, L. L. Y. (2016). Tamm review: are fuel treatments effective at achieving ecological and social objectives? A systematic review. For. Ecol. Manage. 375, 84–95. doi: 10.1016/j.foreco.2016.05.021
Kerns, B. K., Powell, D. C., Mellmann-Brown, S., Carnwath, G., and Kim, J. B. (2018). Effects of projected climate change on vegetation in the Blue Mountains ecoregion, USA. Clim. Serv. 10, 33–43. doi: 10.1016/j.cliser.2017.07.002
Kim, Y., Still, C. J., Hanson, C. V., Kwon, H., Greer, B. T., and Law, B. E. (2016). Canopy skin temperature variations in relation to climate, soil temperature, and carbon flux at a ponderosa pine forest in central Oregon. Agr. For. Meteorol. 226, 161–173. doi: 10.1016/j.agrformet.2016.06.001
Kolb, P. F., and Robberecht, R. (1996). High temperature and drought stress effects on survival of Pinus ponderosa seedlings. Tree Physiol. 16, 665–672. doi: 10.1093/treephys/16.8.665
Krofcheck, D. J., Remy, C. C., Keyser, A. R., and Hurteau, M. D. (2019). Optimizing forest management stabilizes carbon under projected climate and wildfires. J. Geophys. Res. Biogeosci. 124, 3075–3087. doi: 10.1029/2019JG005206
Kwon, H., Law, B. E., Thomas, C. K., and Johnson, B. G. (2018). The influence of hydrological variability on inherent water use efficiency in forests of contrasting composition, age, and precipitation regimes in the Pacific Northwest. Agric. For. Meteorol. 249, 488–500. doi: 10.1016/j.agrformet.2017
Lacki, M. J., Baker, M. D., and Johnson, J. S. (2012). Temporal dynamics of roost snags of long-legged myotis in the Pacific Northwest, USA. J. Wildlife Manage. 76, 1310–1316. doi: 10.1002/jwmg.376
Lehmkuhl, J. F., Kennedy, M., Ford, E. D., Singleton, P. H., Gaines, W. L., and Lind, R. L. (2007). Seeing the forest for the fuel: integrating ecological values and fuels management. For. Ecol. Manage. 246, 73–80. doi: 10.1016/j.foreco.2007.03.071
Liang, S., Hurteau, M. D., and Westerling, A. L. (2018). Large-scale restoration increases carbon stability under projected climate and wildfire regimes. Front. Ecol. Environ. 16, 207–212. doi: 10.1002/fee.1791
Lindenmayer, D. B., Hunter, M. L., Burton, P. J., and Gibbons, P. (2009). Effects of logging on fire regimes in moist forests. Conserv. Lett. 2, 271–277. doi: 10.1111/j.1755-263x.2009.00080.x
Lopushinsky, W. (1969). Stomatal closure in conifer seedlings in response to leaf moisture stress. Botanical Gazette 130, 258–263.
Lopushinsky, W., and Klock, G. O. (1974). Transpiration of conifer seedlings in relation to soil water potential. For. Sci. 20, 181–186.
McCauley, L. A., Robles, D. M., Wooley, T., Marshall, R. M., Kretchun, A., and Gori, D. F. (2019). Large-scale forest restoration stabilizes carbon under climate change in Southwest United States. Ecol. Appl. 29:8. doi: 10.1002/eap.1979
Merschel, A. G., Heyerdahl, E. K., Spies, T. A., and Loehman, R. A. (2018). Influence of landscape structure, topography, and forest type on spatial variation in historical fire regimes, Central Oregon, USA. Landscape Ecol. 33, 1195–1209. doi: 10.1007/s10980-018-0656-6
Merschel, A. G., Spies, T. A., and Heyerdahl, E. K. (2014). Mixed-conifer forests of central Oregon: effects of logging and fire exclusion vary with environment. Ecol. Appl. 24, 1670–1688. doi: 10.1890/13-1585.1
Mildrexler, D. J., Berner, L. T., Law, B. E., Birdsey, R. A., and Moomaw, W. R. (2020). Large trees dominate carbon storage in forests east of the cascade crest in the United States Pacific Northwest. Front. For. Glob. Change 3:127. doi: 10.3389/ffgc.2020.594274
Parks, S. A., and Abatzoglou, J. T. (2020). Warmer and drier fire seasons contribute to increases in area burned at high severity in western US forests from 1985-2017. Geophys. Res. Lett. 47:e2020GL089858. doi: 10.1029/2020GL089858
Parks, S. A., Miller, C., Parisien, M. A., Holsinger, L. M., Dobrowski, S. Z., and Abatzoglou, J. (2015). Wildland fire deficit and surplus in the western United States, 1984–2012. Ecosphere 6, 1–13. doi: 10.1890/ES15-00294.1
Pilliod, D. S., Bull, E. L., Hayes, J. L., and Wales, B. C. (2006). Wildlife and Invertebrate Response to Fuel Reduction Treatments in Dry Coniferous Forests of the Western United States: A Synthesis. Gen. Tech. Rep. RMRS-GTR-173. Fort Collins, CO: U.S. Department of Agriculture, Forest Service, Rocky Mountain Research Station, 34 .
Reilly, M. J., Dunn, C. J., Meigs, G. W., Spies, T. A., Kennedy, R. E., Bailey, J. D., et al. (2017). Contemporary patterns of fire extent and severity in forests of the Pacific Northwest, USA (1985–2010). Ecosphere 8:e01695. doi: 10.1002/ecs2.1695
Stephens, S. L., Westerling, A. L., Hurteau, M. D., Peery, M. Z., Schultz, C. A., and Thompson, S. (2020). Fire and climate change: conserving seasonally dry forests is still possible. Front. Ecol. Environ. 18, 354–360. doi: 10.1002/fee.2218
United States Department of Agriculture (USDA) Forest Service (2020). Forest Plans Amendment. Forest Management Direction for Large Diameter Trees in Eastern Oregon. Environmental Assessment - Preliminary. USDA, Forest Service, Pacific Northwest Region. Portland, OR, 174. Available online at: https://www.fs.usda.gov/nfs/11558/www/nepa/113601_FSPLT3_5332338.pdf (accessed December 2020).
Keywords: carbon storage, climate change mitigation, dry forests, eastern Oregon, eastside screens, forest restoration, 21-inch rule
Citation: Johnston JD, Hagmann RK, Seager ST, Merschel AG, Franklin JF and Johnson KN (2021) Commentary: Large Trees Dominate Carbon Storage in Forests East of the Cascade Crest in the United States Pacific Northwest. Front. For. Glob. Change 4:653774. doi: 10.3389/ffgc.2021.653774
Received: 15 January 2021; Accepted: 26 February 2021;
Published: 23 March 2021.
Edited by:
Marco Mina, Université du Québec à Montréal, CanadaReviewed by:
Dario Martin-Benito, Centro de Investigación Forestal (INIA), SpainKerry Kemp, The Nature Conservancy, United States
Copyright © 2021 Johnston, Hagmann, Seager, Merschel, Franklin and Johnson. This is an open-access article distributed under the terms of the Creative Commons Attribution License (CC BY). The use, distribution or reproduction in other forums is permitted, provided the original author(s) and the copyright owner(s) are credited and that the original publication in this journal is cited, in accordance with accepted academic practice. No use, distribution or reproduction is permitted which does not comply with these terms.
*Correspondence: James D. Johnston, james.johnston@oregonstate.edu