- 1Instituto de Ciências do Mar, Universidade Federal do Ceará, Fortaleza, Brazil
- 2Centre for Aquatic Environments, University of Brighton, Brighton, United Kingdom
- 3Institute of Agriculture and Environmental Sciences, Estonian University of Life Sciences, Tartu, Estonia
- 4Departamento de Geografia, Universidade Federal do Ceará, Fortaleza, Brazil
- 5Leibniz Centre for Tropical Marine Research, ZMT, Bremen, Germany
Brazilian mangroves cover about 11,100 km2 and provide a wide range of ecosystem services. Despite their importance, they are one of the most impacted ecosystems because of combined influences of climate change, pollution, and direct conversion and loss. A major driver of environmental impacts is shrimp farming and this is particularly acute in the semi-arid northeast of Brazil, where mangroves are constrained in a narrow band along ephemeral estuaries that are often impacted by multi-year droughts. Recent changes to Brazilian law, in particular the Forest Code, have weakened protection for mangroves and associated “apicum” (salt pan) ecosystems. In NE Brazil, most shrimp ponds are converted from mangrove-adjacent “apicuns” rather than the mangroves themselves with periodic hydrological connectivity through dammed channels, allowing the flushing of effluents. As a result, the main impacts on mangroves are typically indirect, because of pollution inputs from shrimp pond effluents and associated loss of ecosystem services including reductions in primary productivity, carbon storage capacity, resilience to other environmental stressors, their efficiency as estuarine filters, and biodiversity and abundance of subsistence use of marine species. Soil damage and infrastructure remaining after shrimp pond deactivation impairs mangrove recovery. This extends the duration of the damage and allows the occupation of degraded areas by other activities that can permanently impair ecosystem function. In this review, we address several aspects of the shrimp culture boom in NE Brazilian, their features and consequences, and the future of mangroves in the region considering climate change and rising poverty. Our conclusions on the practices and outcomes of shrimp farming in mangroves are likely to apply to areas with similar environmental settings, e.g., semiarid regions worldwide, and particularly in the Latin America and Caribbean region, and our findings can be taken into account to improve conservation and management of these forests at the least to a regional scale.
Introduction
Despite a slight reduction in forest loss rates mainly in the Americas, Africa and Australia (Friess et al., 2016, 2019; Hamilton and Casey, 2016) mangrove clearing and fragmentation continues, predominantly within Southeast Asia (80% of direct anthropogenic loss concentrated in Myanmar, Vietnam, Malaysia, Philippines, Thailand, and Indonesia), mainly due to the conversion of mangroves for aquaculture and agriculture [UNEP (United Nations Environment Programme), 2014; Hamilton and Casey, 2016; Bryan-Brown et al., 2020; Goldberg et al., 2020]. Between 2000 and 2016, anthropogenic impacts were responsible for 62% of the global mangrove area loss, with shrimp, rice and oil palm cultivation responsible for close to half of these global losses (Goldberg et al., 2020). Coastal erosion (mainly because of sea level rise and alterations to river dynamics) contributed to 27% of global losses (2000-2016) and is the second largest cause of global mangrove loss (Thomas et al., 2017; Goldberg et al., 2020). Unfortunately, little attention has been given to the degradation of mangrove functions, such as nutrient cycling, species composition and biomass allocation, which directly affects ecosystems services, since large scale monitoring using remote sensing techniques is seldom applicable and field observation requires relatively long monitoring periods to cover the natural variability of environmental processes (Sanyal et al., 2020).
There is still a large gap in the knowledge concerning the geochemical and biogeochemical responses of mangroves to degradation drivers (Lourenço et al., 2020). Approaches considering the land-ocean continuum, as well as the transfer processes involved, are still scarce and mostly consist of short-term analyses. In addition, long-term studies covering large geographical scales are scarce and threatened by economic instability of science funding in several countries harboring mangroves in the world’s tropical coasts (Lacerda et al., 2020). Of particular interest are studies that assess anthropogenic influences on the interaction between drainage basins and the continent-ocean interface, as well as impacts on ecosystem services, aimed at understanding the implications of global change on ecosystem functioning, conservation and sustainable development, the vulnerability of the continent-ocean interface, and threats to society through food security. The response of mangrove ecosystems to degradation drivers is frequently more intense and conspicuous in extreme environments, therefore, semiarid coastline are ideal sites for such studies.
Brazil has the third largest extent of mangroves extension in the world. Estimates of total area vary by 30%: 9,627 km2 (Giri et al., 2011), 9,940 km2 (Diniz et al., 2019), 10,123 km2 (Food and Agriculture Organization [FAO], 2007), 13,626 km2 (Lacerda, 2002), and 13,989 km2 (Instituto Chico Mendes de Conservação da Biodiversidade [ICMBio], 2018). Bunting et al. (2018) suggested 11,072 km2, as a more reliable figure based on detailed methodology and recent databases. The northeast coast bordering the semiarid hinterland spreads from about 2.7°S to 9.1°S and 41.5°W to 36.5°W, within the region “Northeast Brazil Large Marine Ecosystem,” under a Bs (semiarid) climate. Details of the biology, geology, geomorphology and oceanography of this sector of the Brazilian coast can be found in Ekau and Knoppers (1999); Knoppers et al. (1999). The region harbors about 690 km2 of mangroves, about 7% of the total Brazilian mangrove area (Figure 1), located in low-lying coastal plains that provide important environmental services for coastal populations (Instituto Chico Mendes de Conservação da Biodiversidade [ICMBio], 2018; Diniz et al., 2019). Due to the geographical location, semiarid climate, and resultant low terrestrial runoff to the continent-ocean interface, mangroves in this region are already under periodical environmental stress from natural drivers, including low annual rainfall, extended droughts and altered salinity (Marengo et al., 2018). As such, they are particularly sensitive to anthropogenic impacts from global (e.g., sea level rise; decreasing annual rainfall) (Godoy and Lacerda, 2015; Ward et al., 2016b; Ward and Lacerda, 2021) as well as regional drivers (e.g., damming; waste disposal) (Godoy et al., 2018; Loureiro and de Oliveira, 2019). For example, the combination of low continental runoff, river damming, increasing sea level and saline intrusion, results in mangroves in most world semiarid regions migrating landward (Ward and Lacerda, 2021).
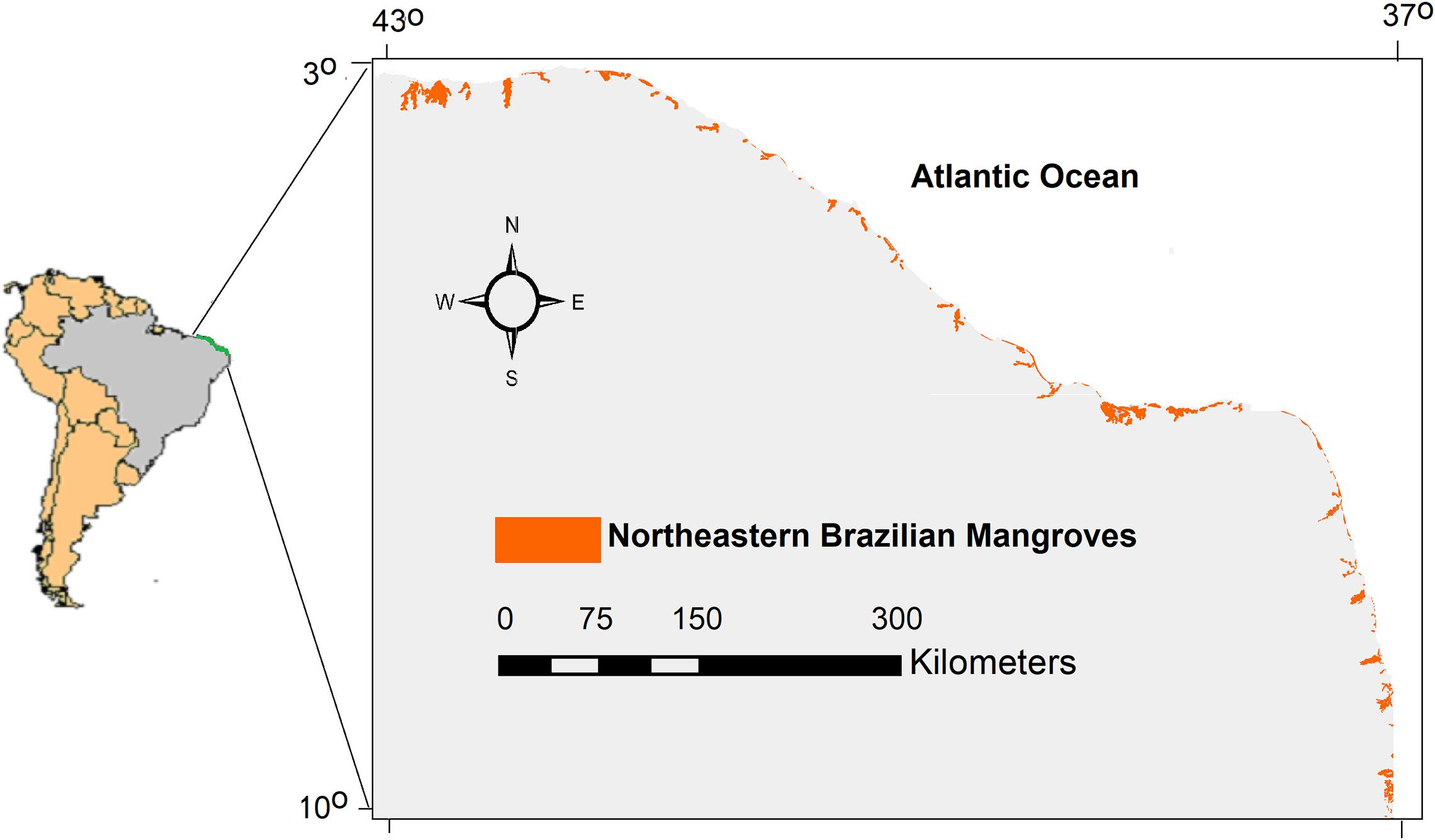
Figure 1. Distribution of mangroves along the Northeast of Brazil. Mangroves of the semiarid coast, where most aquaculture is established, are shown in red. Map modified from Maia et al. (2006), Instituto Chico Mendes de Conservação da Biodiversidade [ICMBio] (2018).
Mangrove expansion inland has been observed in most estuaries along semiarid coastlines and is characteristic of the Northeast coast of Brazil and this represents the most important adaptation to climate change and regional environmental change, whilst requiring the existence of a large buffer zone along the mangrove-land border (Godoy and Lacerda, 2015; Ferreira and Lacerda, 2016). Therefore, anthropogenic activities in these areas, such as urban and tourism expansion, agriculture and aquaculture may pose strong constraints to mangrove adaptation and even their survival in this region.
Brazil established a Forest Code in 1965, which not only considered mangroves as fully protected areas, but also included broad expanses of salt flats, locally known as “apicuns,” as integrated parts of the mangrove ecosystem. These flats stretch to the maximum influence of the tides or the extreme reaches of saline intrusion. In addition, coastal wetlands of the Northeast are exposed to long dry seasons and extended pluriannual drought (Marengo et al., 2018), facilitating the interpretation by developers and local authorities to acknowledge them as permanently dry habitats not considering the natural, broad temporal variation in water level as an inherent attribute of the system. This view, unfortunately, has led to a revision of the 1965 Forest Code following pressure from production sectors over the federal legislative chambers. This revision unlocked most of these flat plains to aquaculture and infrastructure development. Within the northeast region this “newly opened area” to development may reach over 6,000 km2 (Ferreira and Lacerda, 2016). In addition, the review of the old Forest Code has also allowed forest conversion in alleged “public utility” or “social interest” projects, even in permanent protection areas, such as mangroves (Oliveira-Filho et al., 2016). Further setbacks to mangrove conservation were triggered by Brazilian Government recently (Bernardino et al., 2021) abolishing a wider range of the protective legal framework, though suspended by judicial order.
Because of the strengthening aridity, caused by a decrease in annual rainfall and extended drought periods linked to climate change (Marengo et al., 2018), there is growing pressure on freshwater resources exacerbated by increasing human activity. This demand requires more dams and larger reservoirs, which have exacerbated the accelerated increase in sea level (Intergovernmental Panel on Climate Change [IPCC], 2019), and will result in a rapidly changing land-sea interface, where effects from human interventions are maximized. Therefore, conflicts between mangrove conservation and human occupation of salt flats are likely to escalate soon in this semiarid stretch of the northeast Brazilian coast, particularly related to shrimp farming, due to the accelerated rate of its development in the region. Also, there is a growing concern that these new exploitation areas can advance into the North Brazil mangroves, where around 70% of the country’s mangroves are located, including the largest continuous mangrove strip on the planet. This raises a global concern to their conservation. Similar temporal trends, practices and outcomes of shrimp farming have been seen in countless mangroves around the world, so our findings can be considered globally to monitor estuarine waters and improve the conservation and management of mangrove forests.
This review article provides a synthesis of the impacts of aquaculture on ecosystem service provision and the environmental condition of associated mangrove habitats in Northeast Brazil, providing a review of existing literature, with emphasis on semiarid regions, through an expert based approach. Care was taken to avoid non-refereed publications, as well as general publications on environmental impacts on mangroves that are not supported by field data and observations. For the specific case in the Jaguaribe estuary, the largest single area of shrimp farming in Brazil, where over 3.34 km2 of shrimp ponds has been built. We updated the remote sensing information to create a new map of the evolution of mangroves and shrimp farms in the area from 1992 to 2010, when aquaculture expansion was at its maximum, to show the little relationship between the increase of shrimp farms area and reduction in mangrove extension. The map is based on Landsat 5 images with 30-m spatial resolution. Images were obtained from the Brazilian National Institute for Space Research (INPE). Projection used was Universal Transverse Mercator (UTM), referenced to the horizontal geodetic datum SIRGAS 2000. Images were georeferenced using permanent reference points and the root mean square of geoprocessing error was less than 10m. Image vectorization was performed in ArcGIS 10 using a pixel-by-pixel supervised classification methodology. We hypothesize that rather than direct impacts on mangroves by deforestation and conversion, indirect impacts on ecosystem functioning are presently more significant, at least in semiarid climates.
Shrimp Farming Expansion in Northeast Brazil
The Pacific white shrimp Litopenaeus vannamei (Boone) is the most common species used in shrimp farming in the Latin America and Caribbean (LA&C) region. Although in the past century this activity was of minor environmental significance to mangroves throughout most of the continent, save for Ecuador, relative to other anthropogenic drivers, shrimp aquaculture has proliferated over the last three decades. From a few producer countries in 1990, to 22 out of 36 countries in the region were significant producers by 2017. The region’s total annual production and pond area increased from approximately 86,000 t and 25,000 ha in 1990 to over 766,000 t and about 200,000 ha, respectively, by 2017 (Food and Agriculture Organization [FAO], 2019). This growth ∼ 20% per year has resulted in shrimp aquaculture being the major driver of environmental impacts on LA&C mangroves (Lacerda et al., 2019). Additionally, this globalized system of aquaculture production is energy-intensive, induces pressure upon local ecosystems and is, in general, highly dependent on marine capture fisheries for aquafeed production (Ahmed and Thompson, 2019). Therefore, instead of supporting sustainable development, shrimp aquaculture, in many situations, intensifies ecological degradation by focusing on the production of a high-value commodity d, based on production-intensive systems (Longo et al., 2013).
Over the past three decades, Brazil, as in the broader LA&C region, L. vannamei aquaculture has expanded from a few hectares in 1990 to nearly 20,000 ha in 2018. This is spread over several coastal states, but the Northeast region corresponds to 98% of the country’s total shrimp production with 19,845 hectares of active ponds in 2018. Thus, the semiarid littoral region, reviewed here, corresponds to almost the whole of the country’s shrimp pond area. Recent annual production statistics for the Northeast region reached 70,500 t in 2015, but decreased to 45,500 t in 2018 (Instituto Brasileiro de Geografia e Estat stica [IBGE], 2018). This decrease was mostly caused by mortality because of viral diseases (Associação Brasileira dos Criadores de Camarão [ABCC], 2017; Carvalho and Martins, 2017). More recent estimates suggest a total area and production in 2020 of about 30,000 ha and 110,000 t (Freitas et al., 2017; Rocha, 2019), but there is high unreliability in those numbers.
In Northeast Brazil, during peak production years, export revenues reached US$ 240-270 million, making shrimp culture an extremely profitable activity, second only to sugar cane, surpassing traditional crops such as cashew nut and irrigated fruit agriculture (Costa and Sampaio, 2004; Sá et al., 2013). It has been claimed that the productive chain of intensive shrimp aquaculture can generate from 1.8 to 3.7 job ha–1, like the job demands of the irrigated fruit agriculture sector (Costa and Sampaio, 2004), but it can frequently be lower (0.6 job ha–1) (Monteiro et al., 2016). Farms in Rio Grande do Norte State have levels of 0.5 full-time job ha–1 to 1 job ha–1 during seasonal harvestings (pers. comm.). Indeed, in more than 75% of Brazilian farms (<10 ha in size) more than 41% of the employment is seasonal, and in extensive medium and large farms installed infrastructure requires fewer workers (Costa and Sampaio, 2004; Ministério da Pesca e da Agricultura [MPA], 2013). Whilst in some municipalities, aquaculture activity could contribute to municipal revenues, in general this contribution is mostly indirect and tax free (Sampaio et al., 2005, 2008). In several states, charged tariffs over the high water use of the activity are extremely low, including farms located in areas with chronic water shortages such as the semi-arid northeast coast (Monteiro et al., 2016). The contribution of shrimp farming to municipal GDP growth (Sampaio et al., 2005, 2008), does not necessarily mean direct employment nor development, since GDP growth is not a good indicator of development (Daly, 2005; Jackson, 2009). Some high productivity levels 30 t ha–1 y–1 (well above the national average of 2.56 t ha–1 y–1, however) (Tahim and Araújo Junior, 2014; Oliveira and Neto, 2019; Rocha, 2019) could generate (private) income estimated at more than 120,000 US$ ha–1 y–1 (shrimp local values at 2019), whilst the cumulative environmental damage to air, soil, water, biota and landscape can reach around 4.2 and 4.6 US$ million ha–1 (Ferreira and Lacerda, 2016), mainly through the high carbon (C) footprint due to deforestation of mangroves (Belettini et al., 2018; Ferreira et al., 2019; Nóbrega et al., 2019). Potential C emissions from the conversion of mangroves to shrimp ponds in Northeast Brazil are extremely significant, approximately 413 ± 94 MgC ha–1 (Kauffman et al., 2018). However, for now the well-established technology and a constantly growing market forecast for the demand for shrimp, suggests there will be a continuity of aquaculture activity in Northeast Brazil.
Impacts on Mangroves Forest Coverages
Globally, aquaculture is a significant driver of deforestation of mangroves (Friess et al., 2019). However, along the Northeast coast of Brazil, shrimp aquaculture is rather a driver of degradation, responsible for adversely affecting mangrove functioning through excess nutrient inputs (Sá et al., 2013; Marins et al., 2020), erosion of fringe mangrove forests (Godoy et al., 2018) and, to a lesser extent, illegal deforestation and conversion of mangroves to shrimp ponds (Ferreira and Lacerda, 2016).
Between 8% (Maia et al., 2006) and 10.5% (Instituto Chico Mendes de Conservação da Biodiversidade [ICMBio], 2018) of the total shrimp pond area is from mangrove deforestation. An unknown fraction of mangrove cover has been lost due to the opening of channels, changes in hydrological dynamics of coastal plains and sedimentation/erosion of tidal creeks and riverbanks. Since the Forest Code amendment requires no planning for decommissioning shrimp farms, many of the ponds deactivated in the northeast, due to disease and economic crises, are witnessing a new tide of transformation. Abandoned shrimp farms are being converted into cattle grazing areas, salt ponds and human occupation advances, rather than rehabilitating the original mangrove cover. This accelerates soil degradation and may eventually promote further expansion over the remnant mangroves (Nunes et al., 2011).
The semiarid northeast coast has less than 10% of the total mangrove area in Brazil (Instituto Chico Mendes de Conservação da Biodiversidade [ICMBio], 2018. Therefore, although only a small area of Northeast mangroves has been directly converted to aquaculture ponds (Maia et al., 2006; Instituto Chico Mendes de Conservação da Biodiversidade [ICMBio], 2018). This forest loss is proportionally more significant than in other mangrove regions, moreover, the coastal waters along the semiarid region are highly oligotrophic and most productivity depends on nutrient fluxes from the continent. Local artisanal fisheries, representing about 90% of the total catch of the region, are mostly based on mangrove associate species, such as crabs and oysters and other species dependent on mangroves for breeding, nursery and protection, mostly fish. Indeed, one hectare of preserved mangroves house around 5.1 t of mangrove crab Ucides cordatus and yield around 20 t of animal biomass per year including fishes, molluscs, and crustaceans (Instituto Brasileiro de Meio Ambiente [IBAMA] and Centro de Pesquisa e Conservação da Biodiversidade Marinha do Nordeste [CEPENE], 1994; Rocha Junior, 2011). This available food source makes a great difference for low-income families in the region, some of the poorest in the country. Conflicts between aquaculture and artisanal fishers have become frequent in the entire region.
A case study in the São Francisco estuary, based on local traditional fishers’ perception on the causes of the observed local mangrove-based fisheries decline, showed that about 90% of the respondents suggested that the primary reason for the observed decline in catch was the increase in the number of fishers working in the area. The second biggest impact, highlighted by 60% of the respondents, was considered to be shrimp farming. Only 13% of the respondents suggested the increase in shrimp farms as a possible solution for the decline in artisanal fisheries (Santos et al., 2017).
The lower Jaguaribe River estuary has the largest concentration of aquaculture activities in Northeast Brazil. Between 1992 and 2010, the total shrimp pond area increased from 230 ha in 1992 to nearly 21,600 ha in 2010 (Figure 2). The original mangrove cover of 700 ha has decreased by 210 ha over the same period, but the total mangrove extent in this estuary increased by 240 ha over the same period due to expansion into former mud/sand flat areas as a result of changes in the watershed influenced by global and regional drivers (Godoy and Lacerda, 2014; Godoy et al., 2018). This difference in mangrove extent is not a result of direct conversion, as seen in the Potengi estuary (Figure 3), but because of the indirect death of trees due to hydrological changes cause by pond construction and the opening of channels for incoming and outgoing water. Mangrove expansion linked to climate change and river damming has in part counterbalanced this reduction in extent (Godoy and Lacerda, 2015; Lacerda et al., 2020).
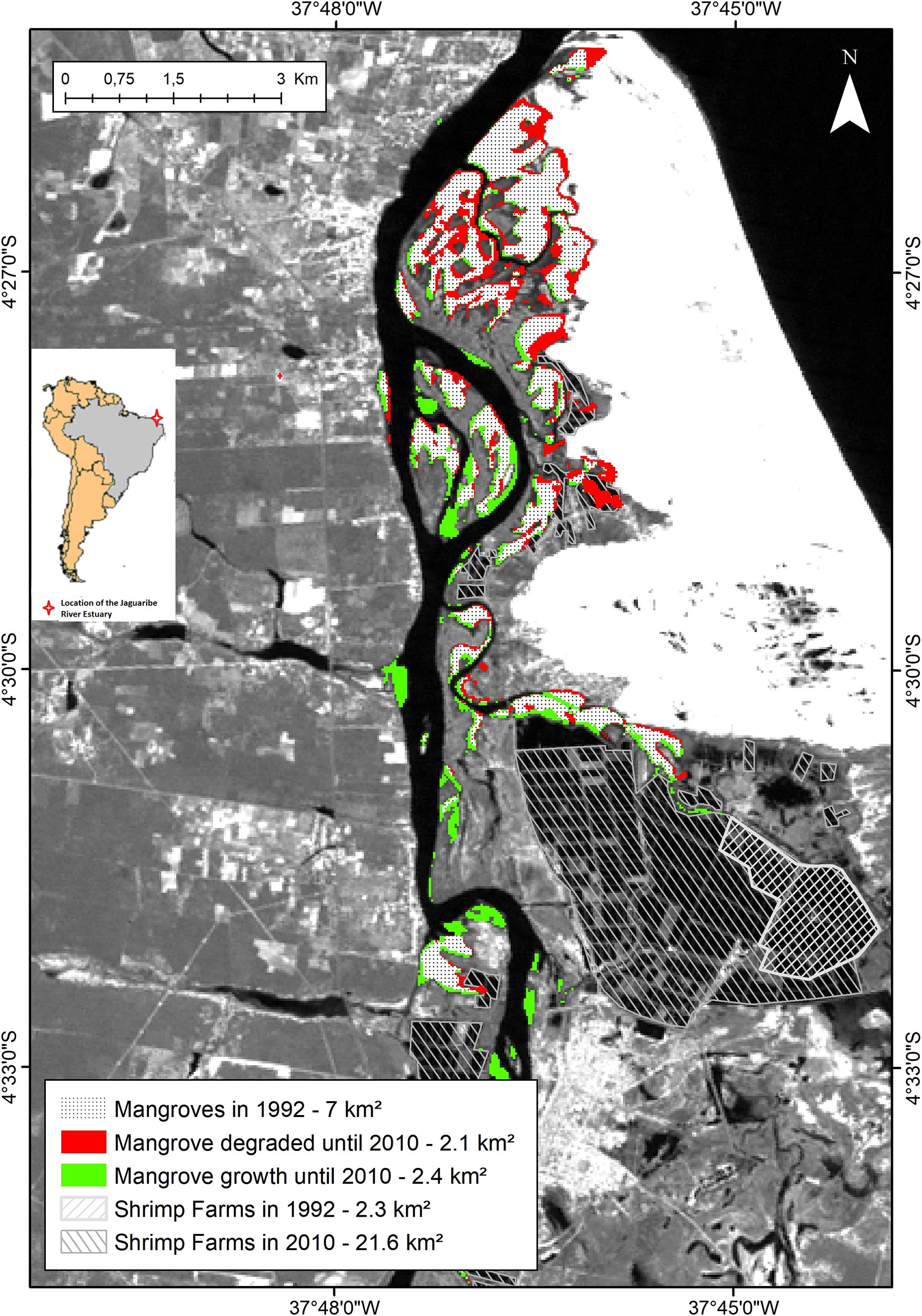
Figure 2. Evolution of mangrove and shrimp farm areas in the Jaguaribe River estuary, the largest concentration of aquaculture activities in Brazil, between 1992 and 2010, when most expansion of the activity occurred.
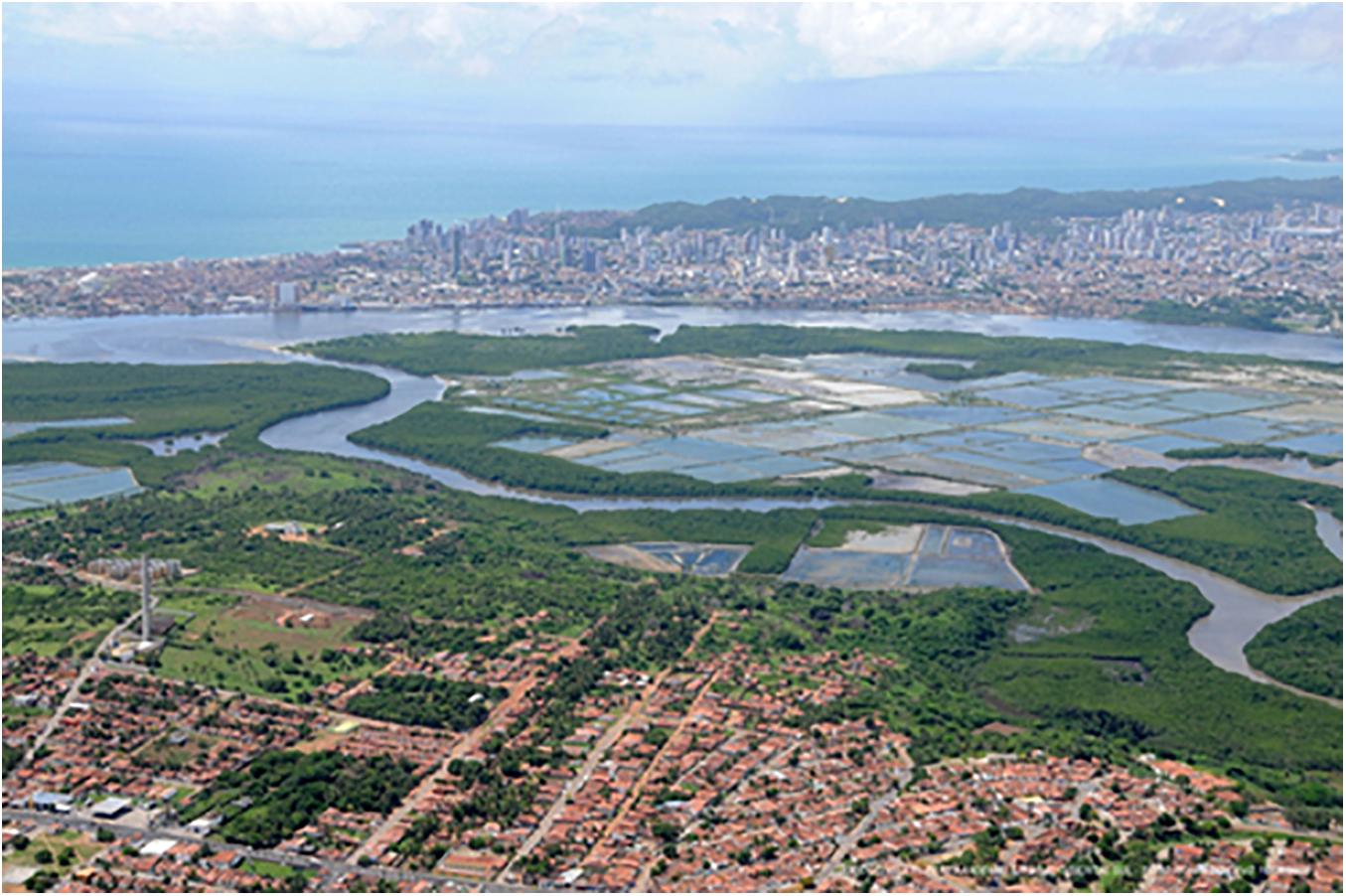
Figure 3. Large mangrove cleared areas by shrimp farms at Potengi Estuary, Rio Grande do Norte State (Northeast Brazil). The mangrove island at the center-right of the image was almost totally converted to ponds.
In Northeast Brazil, the principal impacts from shrimp aquaculture on mangrove forest cover are indirect, as opposed to direct conversion as is more typical in other mangrove regions. A recent review of the environmental impacts associated with aquaculture in Mexico, predominantly also in a semiarid climate, highlighted the significance of indirect impacts, mostly from effluents altering environmental characteristics (Sosa-Villalobos et al., 2016). Hong et al. (2019) used multi-temporal Landsat data from a period of 30 years between 1988 and 2018 to reveal a decrease of 4,980 ha of dense mangrove forests (about 90% of the original vegetated area) and a decrease of 7,816 ha of sparse mangrove forests (about 55% of the original vegetated area), linked to an increase of 150,720 ha (5,024 ha year) of shrimp ponds in the SE Mekong River Delta, Vietnam. In Malaysia, about 11% of the dense mangrove forests and 21% increase of mudflats were lost between 1989 and 2017 along the Tumpat Kelantan littoral following the expansion of shrimp farms in the region (Rasid et al., 2019).
A global survey on the impacts of shrimp aquaculture on mangroves (Hamilton, 2013) showed direct mangrove deforestation in Brazil resulted in losses of about 13% of the total area, consistent to the figures discussed above, based on Maia et al. (2006), Instituto Chico Mendes de Conservação da Biodiversidade [ICMBio] (2018). In contrast, an average of 29.9% of Southeast Asian mangroves were converted to aquaculture ponds since 2001, with a high variation among the different countries (Murdiyarso et al., 2015; Richards and Friess, 2016). Area loss in Brazil is broadly comparable with Bangladesh (7%) and India (4%), but much lower than direct conversion rates observed in Vietnam (53%), Indonesia (48%), China and Ecuador (40%), and Thailand (19%). The time interval used in the research varied from country to country and was typically based on the first arrival of large-scale commercial aquaculture. The study showed a 51.9% loss of global mangrove area during the analyzed period, and estimated that commercial aquaculture accounted for 28% of the total mangrove loss (544,000 ha).
Altered Mangrove Functioning
Whilst not as evident as forest conversion, changes in mangrove functioning related to shrimp farming is more scarcely reported, particularly in semi-arid mangroves, where the shrimp farming is a strong driver of environmental changes, as well as regionally important economic activities (Queiroz et al., 2020). Major changes associated with shrimp farming relate to biomass allocation, forest architecture and health (Alatorre et al., 2016; Datta and Deb, 2017), species composition, cycling and mineralization of nutrients (Molnar et al., 2013; Nóbrega et al., 2013; Queiroz et al., 2020), accumulation of nutrients (Marins et al., 2020; Pérez et al., 2020; Queiroz et al., 2020), and C storages (Suárez-Abelenda et al., 2013; Ahmed et al., 2017; Nóbrega et al., 2019; Tian et al., 2019). The effect of shrimp farming on plant activity in mangroves has been investigated by direct measurements of ecological variables or indirectly through time series of the Normalized Difference Vegetation Index (NDVI). The basis of NDVI is the quantification of the amount of sun light reflectance at the canopy levels controlled by properties of pigments, water and C. Healthy vegetation absorbs sun light and reflects it back (Davaasuren and Meesters, 2012; Villoslada et al., 2020). Therefore, NDVI broadly compares photosynthetic activity and can be applied at spatial or temporal scales to monitor vegetation structure, phenology and biophysics (Wang et al., 2004), allowing monitoring of large stretches of mangroves.
Alatorre et al. (2016) showed the spatial relationship between the zones of greatest loss of mangroves and areas with a greater proliferation of shrimp farms in the Gulf of California, between 1990 and 2010. Over 30% percent of the total mangrove forest cover exhibited decreasing NDVI for the period associated with canopy degradation. Similarly, in the Northeast coast of Brazil, Marins et al. (2020) compared NDVI and P accumulation rates in two estuaries with different intensities of shrimp farming. A reduction of NDVI was obvious in the mangrove receiving effluents from the largest pond area. In addition, NDVI also decreases at the same pace as increasing pond area. A comparison of mangrove canopy structure in 2003 with 2017, in the Jaguaribe River, the location of Marins et al. (2020) study, is detailed in Supplementary Figures 1, 2. There was a 15% reduction in NDVI from 0.78 in 2003, to 0.65 in 2008, following an increase of 340 ha to 1,600 ha of shrimp pond area. NDVI decreased further to 0.2 and lower in 2017, when the area of shrimp farms located in the forest drainage basin increase 10-fold to about 3,400 ha. It is interesting to note that there was no clear conversion of mangroves to shrimp ponds, but the occupation of tidal flats behind the mangroves has apparently affected hydrological processes, resulting in decreasing health of the mangrove fringe (Supplementary Figure 1). Unfortunately, this reduction in health is not computed as mangrove area loss reported in the literature, suggesting that more than 8% to 10% of mangrove forest loss relative to shrimp pond area, normally accepted as converted, is affected by hydrological changes promoted by pond installation in this region. Considering these results and the areas with NDVI < 0.1 (Supplementary Figure 2), literally equivalent to bare soil, between 9.6 and 14.4% of the local mangrove total were significantly affected by shrimp aquaculture.
Nutrient Dynamics and Eutrophication
Eutrophication, i.e., the exposure of coastal waters to excess nutrients, is a major anthropogenic phenomenon, and has been associated with the release of aquaculture effluents in various areas worldwide (Herbeck et al., 2013; Nóbrega et al., 2013). In Northeast Brazil, major anthropogenic drivers of coastal eutrophication are sewage in metropolitan areas, and agri/aquaculture, cattle husbandry and shrimp production, in rural areas. Since aquaculture presents much higher emission factors for major nutrients than other rural-area activities (Table 1) and effluents are directly emitted into estuaries or coastal areas (Lacerda et al., 2019), wherever significant pond areas exist, intense shrimp aquaculture effluents are the most important driver of eutrophication. Notwithstanding the high emission factors verified in Northeast Brazil shrimp farms, they are still at the lower range compared to other important producers using intensive cultivation processes. For example, N and P emissions from intensive shrimp aquaculture in Hainan, China is about 10-times higher than values reported for Northeast Brazil (31.9 and 1.3 t km2 yr–1, respectively), like other large Asian producers (Herbeck et al., 2013).
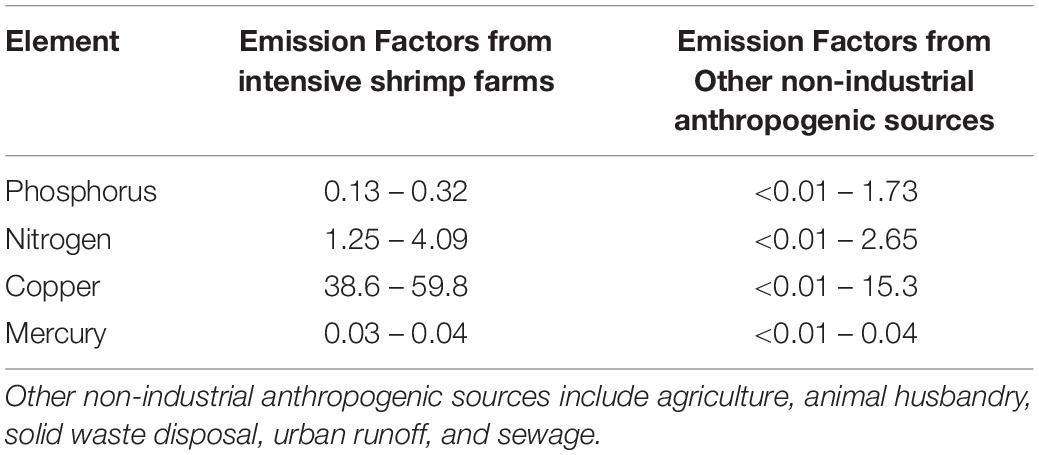
Table 1. Emission factors and total annual emission of nutrients (t km–2 year–1) and metals (kg km–2 year–1) from shrimp aquaculture in Northeast Brazil, after Lacerda et al. (2006a, b), Lacerda et al. (2011), Sá et al. (2013), León-Cañedo et al. (2017).
There is relatively little information on emission factors from aquaculture practices, thus the comparative figures between NE Brazil and China must be taken with care. However, in Latin America and the Caribbean, cultivated species and the technological packages are virtually the same. Also, as mentioned previously, most shrimp aquaculture in the region occurs under a semiarid climate. Therefore, the emission factors estimated for NE Brazil (Table 1) may be used to calculate emission loads at a regional level and may be significant to a worldwide evaluation of the relative contribution of shrimp farm effluents to coastal nutrients fluxes (Lacerda et al., 2006a, b).
The high reactivity of shrimp pond effluents, relative to agriculture and cattle husbandry facilitates rapid eutrophication. For example, in the Jaguaribe Estuary, a major shrimp production area in Ceará State, anthropogenic P emissions increased by 30% to 43.9 t yr–1, between 2001 and 2006, following the expansion of shrimp farms (Marins et al., 2011). Further expansion of local farms increased emissions to 69 t yr–1 in 2013 (Marins et al., 2020), representing over 60% of the total P emissions to the lower Jaguaribe Basin. Algal blooms are now frequently observed in this estuary (Figure 4).
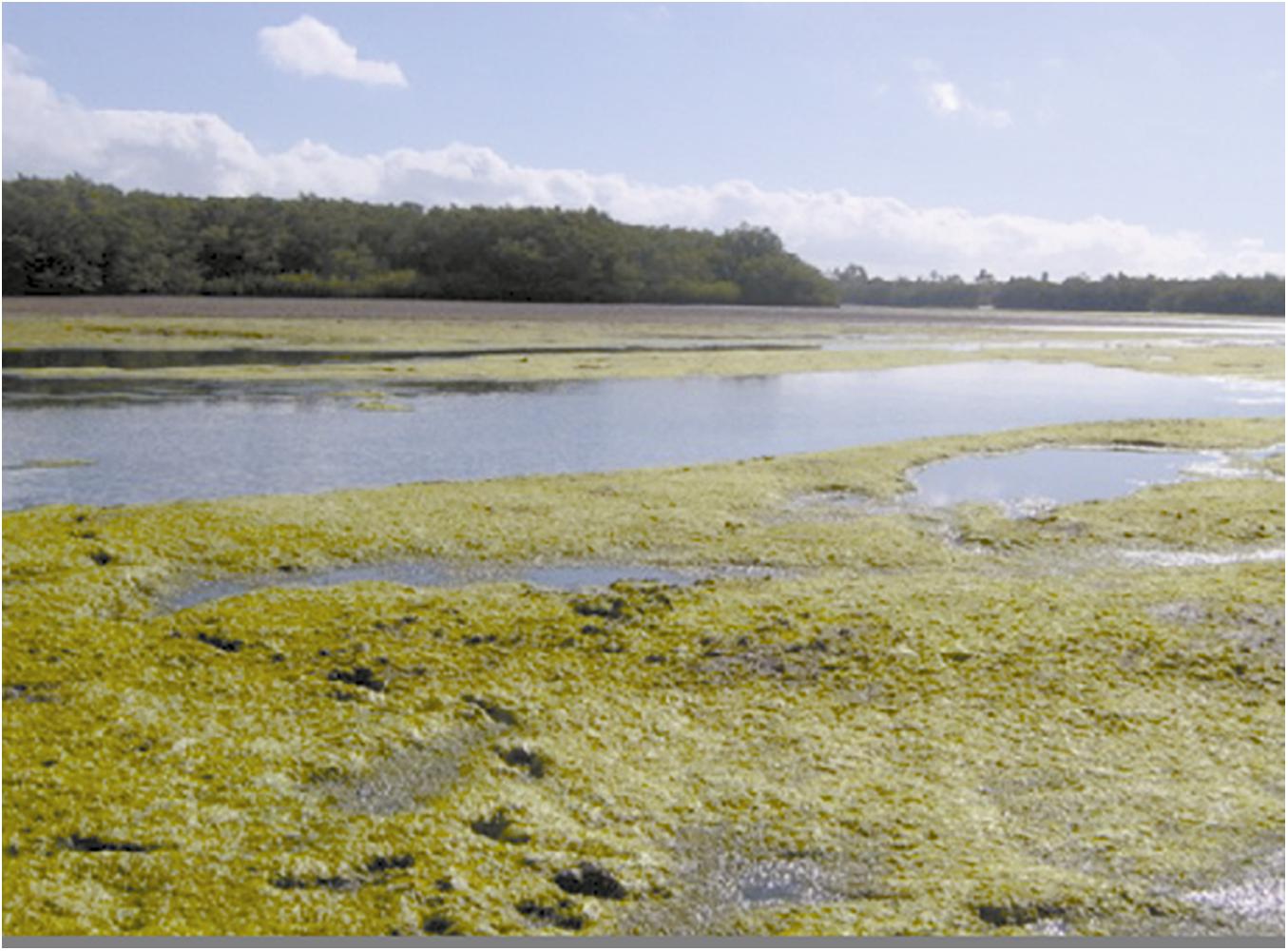
Figure 4. Extensive algal bloom resulting from excess nutrients from shrimp farm effluents in the Jaguaribe River Estuary, Northeast Brazil.
Apart from triggering eutrophication, shrimp farm effluents also affect mangroves by decreasing the ecosystem’s capacity to retain nutrients (Barcellos et al., 2019; Marins et al., 2020) and may increase mangrove C stock loss. Decreasing C accumulation capacity in mangrove soils is, in part, due to increasing organic matter decomposition by a bacterial community fueled by nutrient-rich aquaculture effluents and reducing pyritization due to increasing the concentration of strong electro acceptors, such as nitrates, which are also enriched in shrimp farm effluents (Suárez-Abelenda et al., 2013). Unfortunately, how this amount compares with losses from direct conversion of mangroves to shrimp ponds (58 - 82%) of the ecosystem C stocks (Kauffman et al., 2018), is still unaccountable.
A comparison of two estuaries in Brazil receiving effluents from urban sources and shrimp farms, with a pristine site, showed that shrimp farm effluents have stronger effects on mangroves than urban wastewater. The main cause for the difference in the degree of impact is the higher reactivity of P species from shrimp farm effluents, which are more enriched in exchangeable and soluble-P. Species of P in mangroves receiving wastewater are predominantly organic-P, oxide-P, and clay/Al-P (Barcellos et al., 2019).
Mangroves, like other tidal wetland vegetation, can decrease the impact of land-derived nutrient loads, by accumulation in soil and biomass (Ward et al., 2016a; Lima et al., 2020; Valiela et al., 2020). However, there is a critical level of nutrient load, meaning that there is a clear threshold to this filtering in mangroves (Valiela and Cole, 2002). In Northeast Brazil, an evaluation of the tidal exchange of suspended matter (TSS), total P (TP), soluble reactive P (SRP) and particulate P (Part-P), showed that the retention of P varies with the magnitude of shrimp farm effluents. In a site receiving effluents from nearly 3,000 ha of shrimp ponds, reaching from 1.2 to 5.2 kg hr–1 of total P, local mangroves could trap 40%, 45%, 47% and 70% of the TSS, TP, SRP, and Part-P, respectively, from the incoming high tidal flux. However, in another site, receiving P from only 10 ha of ponds (0.22 kg hr–1), tidal balances showed a much higher relative retention of the total influx, over 92% of the total tidal input of TSS and all P fractions. These results suggest that mangrove P accumulation capacity is significantly decreased with increasing nutrient inputs and this limits the potential of mangroves to act as a natural barrier to nutrient transport (Marins et al., 2020).
Similar results were observed by Queiroz et al. (2020) relative to Nitrogen (N) dynamics in sediments from mangroves affected by shrimp pond effluents in the Jaguaribe Estuary, Ceará State. The tidal balance of N through creeks showed that only 30% of the mineralized N remains stored in the sediment, whereas 70% was solubilized in tidal waters. Therefore, N mineralization may induce eutrophication by augmenting inorganic N bioavailability in mangroves receiving N-rich effluents from aquaculture, triggering increases in primary productivity.
Elemental ratios of nutrients in mangrove sediments were also highly affected by shrimp pond effluents. Total N content (13%) and C:N ratio (9.6) were much higher in sediments downstream of shrimp ponds than those from upstream sediments (TN = 3%, C:N = 4.2). Simultaneous analysis of aquafeeds used in the local shrimp farms also showed a high C:N ratio (8.0) and total N content (5%) (Zocatelli et al., 2007). Phosphorus (P) distribution is also affected by effluents. Concentrations of dissolved-P (3.1 μM and 5.6 μM) and particulate-P (2.1 – 6.5 μM and 1.3 – 11.9 μM) in mangrove tidal creeks receiving shrimp farm effluents are much higher than concentrations observed in mangrove creek waters not receiving aquaculture effluents, where average concentrations of dissolved-P (0.5 μM) and particulate-P (4.1 μM) were 8 to 1.5 times lower, respectively (Marins et al., 2020).
Similar results showing increasing nutrient content and changing elemental ratios related to shrimp farming have been reported in other areas worldwide. In Kandelia obovata forest sediments, along Jiulong River Estuary, in Fujian, China, shrimp pond effluents from 8-year-old farms were shown to have increased soil TOC and TP contributing to 30.0 to 33.6% of the total TOC within mangrove surface sediments (0 – 10 cm depth) (Tian et al., 2019).
Impacts of C losses from mangroves associated with conversion to shrimp aquaculture include the removal of above- and below-ground biomass, loss of soil organic C, and decreases in retention and sequestration through alterations to the hydrological regime and retention of allochthonous C as a result of both this, and removal of the vegetation canopy (e.g., periodically inundated roots and associated epiphytes). In fact, Arifanti et al. (2019) have suggested that conversion to aquaculture could result in C losses equivalent to 226 years’ worth of accumulation. It is not fully clear how mangroves will respond to restoration measures following aquaculture abandonment. Some studies have shown that there is a rapid increase in soil organic matter accumulation following mangrove restoration (Lunstrum and Chen, 2014; Osland et al., 2020) as well as above- and below-ground biomass (Charles et al., 2020). However, this will depend on the hydrological regime, soil surface micro-climate, inorganic and organic pollution levels, the relative spatiotemporal scales of mangrove vegetation change, sodicity, and sources of organic matter (whether recalcitrant or labile) (Suárez-Abelenda et al., 2013; Tran et al., 2015; Celis-Hernandez et al., 2020; Charles et al., 2020).
Reforested mangroves in arid climate zones typically sequester C in biomass 10 times slower than equivalent sites in high rainfall tropical zones (Sasmito et al., 2019). However, considering the generally low biomass of coastal zone vegetation in semiarid climates, frequently restricted to mangroves, the relatively lower C accumulation may still be significant at a regional level. As highlighted for many different regions such as the Gulf Coast (Sheppard et al., 2010; Saderne et al., 2018); East Africa (Benson et al., 2017); the Pacific (Alatorre et al., 2016); and the Caribbean coasts of Mexico (Adame et al., 2013).
The greatest impact from aquaculture on C stocks in mangroves is through organic soil losses from upper horizons to form ponds, where the bulk of C is stored (e.g., the top 1 m organic rich soil layer) (Kauffman et al., 2018). Kauffman et al. (2018) estimated that 67% of the soil organic C is stored in the upper 1m in mangroves in the semi-arid Northeast of Brazil and that C losses from the soil are likely to account for 81% of the C lost following conversion to aquaculture. Whilst shrimp ponds do start to store C in their soils following conversion, this is substantially less than adjacent mangrove areas due to decreases in C density in the soils, as well as reductions in accumulation rates (Eid et al., 2019). Aquaculture conversion contributes to higher C loss (72 ± 44 Mg C ha–1 or 83% ± 37%) in above and below biomass of mangroves, whereas abandoned ponds still release soil organic C and GHG continuously via both soil-water and soil-air interfaces. Regeneration of biomass levels in general last around 40 years, but soil levels of C take longer to return (Sasmito et al., 2019). It has been suggested that mangroves can lose up to 70% of their C through conversion to aquaculture, although where appropriate hydrological connectivity is maintained natural mangrove rehabilitation can occur, together with associated recovery of carbon stocks over time (Matsui et al., 2012; Elwin et al., 2019). Nam et al. (2016) suggest that, where hydrological connectivity is maintained, there is unlikely to be a substantial difference in carbon accumulation or stocks between planted and natural rehabilitation sites, which is important considering the increasing acknowledgment of this vital ecosystem service for climate change mitigation. However, where hydrological connectivity is not restored appropriately, it is likely that shrimp ponds will convert to unvegetated mud/sandflats, which typically contain less soil organic carbon and limited associated biomass than mangroves (Lunstrum and Chen, 2014).
Trace Metals Derived From Shrimp Farming
The high productivity of ponds depends on large amounts of aquafeeds and fertilizers to induce production, and strong aeration of ponds to avoid oxygen depletion. As a result, effluents are enriched in nutrients and organic matter from excess fertilizers, aquafeeds, ecdysis, and suspended matter due to erosion of pond walls by the aerators (Lacerda et al., 2006a). Due to the large amounts of aquafeeds used, impurities present in them as well as in other materials (e.g., fertilizers, lime, and chloride), such as trace metals, may also accumulate within the pond environment and thus be present in the effluents (Boyd and Massaut, 1999; Chou et al., 2002; Lacerda et al., 2009, 2011). Some trace elements, such as Cu and Hg, whose emission factors are particularly high relative to other anthropogenic sources (Table 1), are of environmental significance and represent an additional environmental threat. Another trace element of environmental significance is Zn, which has also shown to be affected by shrimp pond effluents (Silva et al., 2001) and seems to present high emission factors (León-Cañedo et al., 2017). Unfortunately, the one study, to our knowledge, that has analyzed Zn in Northeast Brazil mangroves observed an increase in Zn concentrations in oysters and sediments downstream of shrimp farm effluent outfalls (Silva et al., 2001).
Table 2 summarizes Hg and Cu concentrations in aquafeed and other products used in intensive shrimp aquaculture in NE Brazil, compared to concentrations found in sediments and suspended particles of the environment surrounding the farms. Shrimp farm emission factors (Table 1) result in annual emissions of 490 kgCu yr–1 and 0.35 kgHg yr–1 to Northeast Brazilian estuaries harboring shrimp farms. Aquafeeds and fertilizer are the principal sources of the metals to the aquaculture process. Notwithstanding, concentrations of Cu and Hg in farmed shrimp are low (40 μgCu g–1 and 17 ngHg g–1), posing no toxicological threat to human consumption. However, accumulation of both metals in exoskeleton compared to muscle tissue, suggest that detoxifying mechanisms are taking place and can thus impact shrimp growth and the economic efficiency of farms (Lacerda et al., 2006a, 2009; Soares et al., 2011).
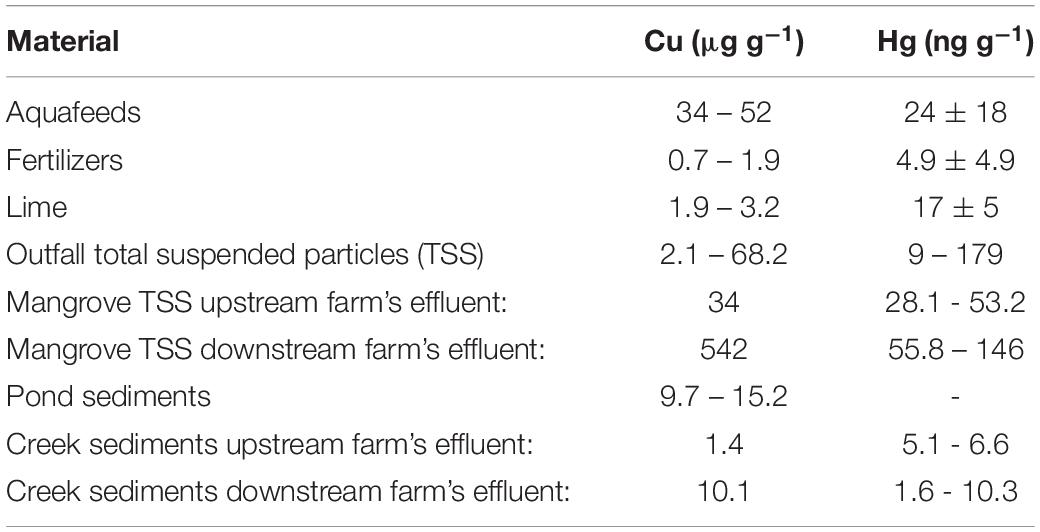
Table 2. Concentrations of Hg and Cu in aquafeeds and chemicals used in intensive shrimp farms in Northeast Brazil and environmental levels in local mangroves, from Lacerda et al. (2006a), Lacerda et al. (2011), Costa et al. (2013).
Cu and Hg distributions in surface sediments from a tidal creek receiving shrimp farm effluents confirm the importance of this source at the regional level and vertical distribution of metal concentrations in sediment cores suggest a recent increase contemporaneous with aquaculture development in the region. In a comparison between concentrations of Cu and Hg from similar areas at the Northeast coast lacking intensive shrimp culture, concentrations of Cu and Hg were up to five times higher in sediments and biota from estuaries with shrimp farms. The results summarized in Table 2, clearly show the effects of shrimp farm effluents on increasing environmental concentrations of Cu and Hg in deposited and suspended sediments.
Costa et al. (2013) observed an increase in Hg concentrations in sediment cores within a mangrove creek receiving shrimp farm effluents at the Jaguaribe River estuary in Northeast Brazil. Levels increased from background concentrations (<0.5 – 3.6 ng g–1) prior to the onset of shrimp farming to 7.2 – 11.7 ng g–1, in present day sediment layers. Concentrations in muscle tissues of mangrove catfish were also higher in the creek sector closer to shrimp farm effluents relative to those found further away in the main river course. In Todos os Santos Bay, Bahia State, Northeast Brazil, Hatje et al. (2019) suggested that upstream shrimp farms were a point source of Hg and possibly other trace metals to their downstream mangrove study site. This resulted in a clear increase in total Hg concentrations in both upstream and downstream sites closest to the effluent outfalls. They noted, however, that the spatial distribution of Hg concentrations implies an impact over a relatively small area.
Contrary to most sources of contaminants (agriculture, animal husbandry and solid waste disposal from urban areas) present in this sector of the Brazilian coast, effluents from shrimp farming are not released onto soils prior to reaching the estuary. Due to the high emission factors and direct input to the estuarine system, shrimp aquaculture is the major source of nutrients and metals in most rural areas of the Northeast coast.
Unfortunately, the few studies on the effects of trace metals on mangroves are based on observations in severe industrial and urban polluted mangroves, that receive very large loads of such contaminants. Most are performed under controlled conditions, to study the effects of trace metals on mangrove plants in detail. Most suggest a decrease in photosynthesis, growth, and biomass (Maiti and Chowdhury, 2013). Although field observation reports on the low capacity of mangrove plants to absorb most metals, the same may not be true for mangrove animals (Thành-Nho et al., 2019). Notwithstanding, a key issue regarding metal accumulation and eventual harmful effects on mangrove biota depend on metal abundance and availability (Marchand et al., 2011; Lacerda et al., 2020). Unfortunately, no study to our knowledge has observed the effects of aquaculture derived trace metals on mangroves and this is an urgent necessity in view of the rapid expansion of the activity and the high metal-complexing capacity of dissolved C present in shrimp farming effluents (Lacerda et al., 2006a, b, 2011; Hidayati et al., 2020).
Mangrove Services and Socioeconomic Impacts of Shrimp Farming
Mangroves goods and services provided to human populations are manifold: coastal protection (Marois and Mitsch, 2015; Veetil et al., 2019), estuarine filtration (Celis-Hernandez et al., 2020), local climate regulation (Crona, 2006; Neogi et al., 2016), C sequestration and storage (Lee et al., 2014; Mafi-Gholami et al., 2018), fisheries (Aburto-Oropeza et al., 2008; Hutchison et al., 2014; Carrasquilla-Henao and Juanes, 2017), habitat for biodiversity (Nagelkerken et al., 2008; Barbier et al., 2011), and cultural values to local communities (Queiroz et al., 2017). In Northeast Brazil, the importance of these services is heightened by pressing socioeconomic hardship faced by a large part of the population, including poverty and hunger (Ottonelli and Mariano, 2014; Caldas and Sampaio, 2015) and precarious employment (da Silva Filho and de Queiroz, 2011). Added to these issues is a history of environmental degradation that dates back to the early decades after the Portuguese invasion (Machado, 2008).
Initial settlement by the Portuguese in Northeast Brazil was focused on the coastal areas of the region, where mangroves are located. Countrywide, this is still reflected today: a quarter of Brazil’s population live on the coast. Alongside numerous large-scale enterprises on the Northeast coast, such as high-environmental-impact ports (Koening et al., 2002; Ferreira et al., 2012), and tourist resorts (Cardoso, 2005; Sousa et al., 2016), the Northeast coast is populated by fishing communities that are highly dependent on the goods and services locally provided by mangroves, especially food from fisheries (Vasconcellos et al., 2007). Per year, one hectare of preserved mangroves can yield 20 tons of animal biomass (Rocha Junior, 2011) and generate around US$ 40,000 of economic value from fisheries (Aburto-Oropeza et al., 2008).
Mangroves, as nurseries for fisheries resources, directly or indirectly support more than 1 million people in Brazil (Prates et al., 2012). When analyzed in connection with the ecosystems where they live and obtain their livelihoods, these fishing communities form social-ecological systems. This intrinsic dependence of local populations on mangroves makes them particularly vulnerable whenever mangrove goods and services are threatened or reduced by loss or damage to the mangroves.
Since shrimp farming is largely responsible for the degradation of mangroves in Northeast Brazil, consequently it is also responsible for the loss of related ecosystem goods and services that constitute the livelihoods of vulnerable populations that live on the Northeast coast. Some benefits can be locally provided by this activity, e.g., an increase and stability of employment and of income, leading to increased municipal revenue and improved living conditions in Northeast Brazil (Sampaio et al., 2008). Conversely, another study showed that only a very low percentage of the local population work in shrimp farms in the region (Safadi, 2018). It is likely that the few benefits are fully outweighed by the environmental impacts detailed in this review. And although some shrimp farms implement measures to lessen environmental impacts, efforts are mostly concentrated on increasing technical production efficiency when compared to those that aim to improve environmental quality (Araújo et al., 2018).
Queiroz et al. (2013) associated the rapid growth of shrimp farming in Ceará, Northeast Brazil, with an environmental and socioeconomic degradation of the mangrove system, which reduces the availability of services and compromises the socioenvironmental sustainability for the medium-and long-term. Impacts include increases in poverty, lack of land, food insecurity, displacement of local communities, water contamination, and poor working conditions. In Bahia state, for example, the lack of public protection policies has allowed the establishment of shrimp farms without sustainable productive alternatives that consider employment and food production (Dias et al., 2012). The perception of fishers regarding shrimp farming show that local communities do not necessarily enjoy the possible benefits from shrimp farming and are impacted by this activity due to the devastation of mangroves, impediment of passage, damage to fishing, among others. In traditional fishing communities in Northeast Brazil, typically only 20% of the local population worked on shrimp farms, yet the whole community is impacted by the activities (Safadi, 2018).
Alternatives to traditional shrimp farming practices have been shown to provide positive social outcomes. In Asian countries, mixed mangrove shrimp systems are sometimes used, where ponds are located in the ditches between the rows of mangrove trees that have been planted on platforms where water is exchanged only when needed for the management of the shrimp and other aquatic organisms (Bosma et al., 2014). These have a lower capital requirement compared to other shrimp farming systems, livelihood diversification through polyculture, provision of regular income and recognition as an organic farming practice (Bosma et al., 2014).
While it has been suggested that proper planning and management and alternative practices could help lessen the negative impacts of shrimp farming, it is essential to highlight that, like many other socioeconomic activities performed under the current capitalist mode of production, there is no evidence that it is possible to produce shrimp that can be sold in domestic or international markets in a competitive and upscalable way and, at the same time, generate socioeconomic benefits that clearly compensate for the social and environmental externalities generated by the activity. To quote Queiroz et al. (2013): “Shrimp aquaculture obeys the logic of appropriation of space generating socio-environmental consequences and compromising the flux of ecosystem services produced by mangroves.”
The social-environmental impacts of shrimp farming have also been detected in other mangroves worldwide. These include the privatization of water and common-use public lands, the expulsion of ancestral fishing and indigenous populations, deforestation of mangroves, water contamination, depletion of fish stocks, salinization of aquifers, and loss of biodiversity, impacting food security and subsistence for mangrove populations (Polidoro et al., 2010).
At the local level, and in close collaboration with fishing and other extractive communities, the implementation of protected areas seems to be a key conservation strategy, especially by preventing or strongly regulating the installation of shrimp ponds. Restoration projects, as explained above, could potentially help lessen the negative impacts generated by shrimp farming. Local legislation could also help hinder degradational shrimp farming practices, but, in Brazil, these are subjected to federal regulation, which, as we already mentioned, was recently changed and, since those changes, exposes “apicum” areas to even greater threats (Schaeffer-Novelli et al., 2012; Ferreira and Lacerda, 2016; Borges et al., 2017).
Restoration of Deactivated Ponds
An estimated 1.4 million hectares of mangroves were lost in the world due to shrimp culture at the beginning of the 21st century (Valiela et al., 2020). By 2010, Aide et al. (2013) estimated a loss of 91.400 hectares of mangroves in LA&C alone, from which around 54,600 hectares were lost to shrimp aquaculture (Ahmed et al., 2018). Conversion of mangroves to aquaculture ponds and subsequent restoration has been undertaken in many countries including Sri Lanka, Thailand, Philippines, Indonesia, Brazil (Stevenson et al., 1999; López-Portillo et al., 2017) and other countries with unpublished data, like Ecuador and some Caribbean nations. Mangroves are resilient ecosystems that can self-repair in natural conditions. Depending on the level of degradation, abandoned shrimp ponds, if opened to input of tides and estuarine waters, can self-recuperate in 15 to 30 years and this seems to be the first and most effective solution to commence restoration (Stevenson et al., 1999; Matsui et al., 2010; Primavera et al., 2011; López-Portillo et al., 2017). Eliminating impairing or stressing factors e.g., dams or altered hydrology allows the influx of estuarine water to the ponds, and can supply areas with waterborne propagules, enabling recovery.
However, the effectiveness of this strategy and the time necessary to observe successful restoration will depend on the magnitude of the alterations in soil physicochemical features, such as acidification by sulfate oxidation (due to soil intervention by pond construction), desiccation, erosion or hardening/compaction, as well as hydrology (Stevenson et al., 1999; Di Nitto et al., 2013). Soil elevation and the lack of hydrological connectivity are factors that impair mangrove recovery (Wodehouse and Rayment, 2019). Leaving dams and enclosures of ponds after shrimp and salt work enterprise abandonment is a common problem that impairs the deposition of waterborne propagules and the repair of soil features, delaying mangrove recovery, sometimes by decades (Reis-Neto et al., 2019).
Artificial restoration is often necessary when the degradation of edaphic or hydrological conditions impairs the settling and development of propagules, when the homeostasis of the system has been disrupted, or where there is a need for rapid restoration (Lewis and Streever, 2000; Lewis, 2005; Ferreira et al., 2015). The best management practice is to restore a varied set of native species present before the degradation. Matsui et al. (2010) opened the banks of an abandoned shrimp pond and planted four native mangroves, among them Rhizophora apiculata and R. mucronata, and in six years, another fifteen mangrove species colonized the area. However, in sites where monospecific forests were cleared, or when only one species can cope with the soil/hydrological conditions, the planting of such specific species can be a shortcut to stand development, allowing the conditions necessary for the development of other mangrove species. Avicennia germinans has already been used for restoration in hypersaline soils in degraded mangrove areas in semi-arid estuaries (Toledo et al., 2001; Flores-Verdugo et al., 2015). Rhizophora spp. have been used extensively for their ecological tolerance, survival rate, rapid growth and easy planting, and these species can rapidly develop and restore some mangrove functionality, while other trees usually colonize later (Ferreira et al., 2015; López-Portillo et al., 2017; Eddiwan, 2018). Where many mangrove species are required, it is preferable to plant several species during initial restoration activities (Primavera et al., 2011).
Sometimes restoration of shrimp culture damage is costly, and in Brazil, offenders are not obliged to pay it. Brazil, like several other developing countries, suffers from a lack of political will to enforce environmental laws and increase monitoring of mangroves. Mangrove restoration of former shrimp ponds faces several socio-political difficulties. Community engagement/co-management arrangements can help improve organization and raise awareness and create a sense of responsibility over natural resources that are crucial to restoration success (Primavera et al., 2011; Ferreira and Lacerda, 2016; Borges et al., 2017; Hai et al., 2020). After the destruction of more than 75% of their mangroves, the Philippines initiated a special committee to integrate and rationalize programs of mangrove conservation, protection and restoration (Primavera and Esteban, 2008), and this could be a measure to formulate specific policies and protect mangroves in other countries, including Brazil.
In the Northeast of Brazil, several pond areas remain abandoned. Sea level rise is starting to enable the recovery of mangroves, as we observe in an experimental restoration in an abandoned saltpan constructed over an “apicum” (or salt flats) in the Pacoti estuary (Ceará State). More than three decades after deactivation, the saltpans have been partially colonized by A. germinans, because of increased sea level promoting overtopping the dikes that enclose the area. In a test to assess the influence of hydrological restoration on the planted Rhizophora mangle, increased influx of saline water resulted in high survival rates as well as an influx of natural seedlings of Laguncularia racemosa and A. germinans, which established in two years (pers. comm.). This showed that these abandoned ponds, and several similar level “apicum” areas, are subjected to natural (and sometimes rapid) regeneration if hydrological connectivity is restored. This suggests that these “apicum” wetlands are really part of the mangrove ecosystem and offer suitable mangrove refuges in the face of sea level rise. This also adds to the increased concern regarding recent Brazilian legislation, which puts at risk thousands of “apicum” areas by allowing their conversion to “productive” spaces such as shrimp ponds (Ferreira and Lacerda, 2016; Borges et al., 2017).
Mangrove restoration can also be used to return a range of ecosystem services including C sequestration and storage and sustainable fish, crabs or shrimp breeding (Fitzgerald, 2000; Lewis and Gilmore, 2007; Bosma et al., 2014; Ahmed et al., 2018; Ferreira et al., 2019). Shrimp production as practiced presently in Brazil shows the consequences of privatizing public environmental patrimony (and profit) while socializing environmental damage, because the abandonment of ponds leaves areas that formerly provided a range of goods and services as brownfield waste sites.
Upscaling the Results
In Northeast Brazil, direct conversion of mangroves into shrimp farming ponds is less significant than in other LA&C and Asian countries. However, indirect effects on mangrove functioning, as reported by decreasing canopy health through NDVI estimates based on satellite images, reduction of sediment and nutrient accumulation capacity, as well as increasing GHG emissions from affected areas occur wherever adjacent mangrove areas are occupied by shrimp aquaculture. This makes it difficult to use experience from other major shrimp producer countries to forecast scenarios of the impacts from the activity on mangroves in Northeast Brazil.
Emission factors of nutrients and some trace metals of environmental significance are, in some cases, orders of magnitude higher from intensive shrimp aquaculture than other anthropogenic activities. This and the direct emission of effluents in mangrove creeks and estuaries, has been associated with eutrophication and contamination of sediments and biota in several world regions. Unfortunately, notwithstanding their significance for environmental health, trace metal contamination from shrimp farm effluents has received only minor attention from researchers, and no regulation from environmental authorities.
Since northeastern mangroves represents only 10% of Brazil’s total area of these forests, conservation of existing forests and restoration of degraded mangroves and associated goods and services, is an urgent task both for the environment and local human populations. Restoration of mangroves requires systematic planning, societal awareness and commitment, public policies to shelter, coordinate and undertake mangrove restoration/rehabilitation, together with robust scientific knowledge to support successful schemes.
The recent changes in Brazilian forestry legislation, which resulted in opening “apicum” areas adjacent to mangroves for aquaculture development, allow for an intensification of environmental impacts to mangroves, and a real and present threat to the survival of traditional local populations that are still highly dependent on mangrove goods and services in Northeast Brazil. To fully understand this threat, however, future research should be based on valuation surveys and participatory methods, designed and consistent with international studies, such as focus groups and participant observation, in order to gather information and actively involve traditional local communities.
Finally, the major observations in this review apply not only to the NE Brazil region, although this sector of the Brazilian coast is responsible for the near totality of shrimp aquaculture in the country, but to other similar environmental settings. Major producers (Ecuador, Brazil, and Mexico) of aquaculture shrimp in Latin America and the Caribbean, use the same species (L. vannamei) and farms are preferentially located in semiarid coasts; therefore observations are likely to apply at a regional scale. In addition, other degradation drivers on mangroves, such as global warming, agriculture runoff and changing hydrology, will trigger similar environmental impacts as those from shrimp aquaculture reviewed here. Supporting integrated multinational initiatives to study indirect effects of aquaculture, rather than solely the effects on mangrove extension loss and gains should be a priority.
Author Contributions
LL, RW, and AF conceived and wrote the manuscript. MG, RB, and AA contributed respectively to the remote sensing mapping and the analysis of human drivers, impacts and response, and had participated in the writing and reviewing the original manuscript. All authors contributed to the article and approved the submitted version.
Funding
This study was supported by grants from the CNPq, Brazil, Proc. no. 405.244/2018-5 and 309.718/2016-3 to LL.
Conflict of Interest
The authors declare that the research was conducted in the absence of any commercial or financial relationships that could be construed as a potential conflict of interest.
Supplementary Material
The Supplementary Material for this article can be found online at: https://www.frontiersin.org/articles/10.3389/ffgc.2021.653096/full#supplementary-material
Supplementary Figure 1 | Impact on mangrove forest canopy health by increasing adjacent shrimp farm area. Note that direct conversion of mangroves into ponds is relatively small, but degradation of forest cover is widespread. Jaguaribe River estuary, Ceará State, NE Brazil.
Supplementary Figure 2 | Impact on mangrove forest canopy health by increasing adjacent shrimp farm area. Note that degradation areas present NDVI similar to bare soil. Jaguaribe River estuary, Ceará State, NE Brazil. Data from 2003 and 2008 from Marins et al. (2020); and for 2017, estimated in this study.
References
Aburto-Oropeza, O., Ezcurra, E., Danemann, G., Valdez, V., Murray, J., and Sala, E. (2008). Mangroves in the Gulf of California increase fishery yields. Proc. Nat. Acad. Sci. U.S.A. 105, 10456–10459. doi: 10.1073/pnas.0804601105
Adame, M. F., Kauffman, J. B., Medina, I., Gamboa, J. N., Torres, O., Caamal, J., et al. (2013). Carbon stocks of tropical coastal wetlands within the karstic landscape of the Mexican Caribbean. PLoS One 8:e56569. doi: 10.1371/journal.pone.0056569
Ahmed, N., Cheung, W., Thompson, S., and Glaser, M. (2017). Solutions to blue carbon emissions: shrimp cultivation, mangrove deforestation and climate change in coastal Bangladesh. Ma. Policy 82, 68–75. doi: 10.1016/j.marpol.2017.05.007
Ahmed, N., and Thompson, S. (2019). The blue dimensions of aquaculture: a global synthesis. Sci. Total Environ. 652, 851–861. doi: 10.1016/j.scitotenv.2018.10.163
Ahmed, N., Thompson, S., and Glaser, M. (2018). Integrated mangrove-shrimp cultivation: potential for blue carbon sequestration. Ambio 47, 441–452. doi: 10.1007/s13280-017-0946-2
Aide, T. M., Clark, M. L., Grau, H. R., López-Carr, D., Levy, M. A., Redo, D., et al. (2013). Deforestation and reforestation of Latin America and the Caribbean (2001–2010). Biotropica 45, 262–271. doi: 10.1111/j.1744-7429.2012.00908.x
Alatorre, L. C., Sanchez-Carrillo, S., Miramontes-Beltrán, S., Medina, R. J., Torres-Olave, M. E., Bravo, L. C., et al. (2016). Temporal changes of NDVI for qualitative environmental assessment of mangroves: shrimp farming impact on the health decline of the arid mangroves in the Gulf of California (1990-2010). J. Arid Environ. 125, 98–109. doi: 10.1016/j.jaridenv.2015.10.010
Araújo, A. M. M., Araújo, R. C. P. D., Carvalho, R. M., and Reis, L. D. R. (2018). Análise das boas práticas de manejo na carcinicultura e seus efeitos sobre a produtividade no Estado do Ceará. Arq. Ciênc. Mar. 51, 7–25. doi: 10.32360/acmar.v51i1.20387
Arifanti, V. B., Kauffman, J. B., Hadriyanto, D., Murdiyarso, D., and Diana, R. (2019). Carbon dynamics and land use carbon footprints in mangrove-converted aquaculture: the case of the Mahakam Delta. Indonesia. For. Ecol. Manag. 432, 17–29. doi: 10.1016/j.foreco.2018.08.047A
Associação Brasileira dos Criadores de Camarão [ABCC], (2017). Os Riscos que Ameaçam a Carcinicultura Brasileira e Ações em Curso para Superá-lo. Natal: ABCC.
Barbier, E. B., Hacker, S. D., Kennedy, C., Koch, E. W., Stier, A. C., and Silliman, B. R. (2011). The value of estuarine and coastal ecosystem services. Ecol. Monogr. 81, 169–193. doi: 10.1890/10-1510.1
Barcellos, D., Queiroz, H. M., Nóbrega, G. N., Oliveira Filho, R. L., Santaella, S. T., Otero, X. L., et al. (2019). Phosphorus enriched effluents increase eutrophication risks for mangrove systems in northeastern Brazil. Mar. Pollut. Bull. 142, 58–63. doi: 10.1016/j.marpolbul.2019.03.031
Belettini, F., Seiffert, W. Q., Lapa, K. R., Vieira, F. N., Espírito Santo, C. M., and Arana, L. A. V. (2018). Carbon footprint in commercial cultivation of marine shrimp: a case study in southern Brazil. R. Bras. Zootec. 47,e 20160353, 1–5. doi: 10.1590/rbz4720160353
Benson, L., Glass, L., Jones, T. G., Ravaoarinorotsihoarana, L., and Rakotomahazo, C. (2017). Mangrove carbon stocks and ecosystem cover dynamic in Southwest Madagascar and the implications for local management. Forests 8:190. doi: 10.3390/f8060190
Bernardino, A. F., Nóbrega, G. N., and Ferreira, T. O. (2021). Consequences of terminating mangrove’s protection in Brazil. Mari. Policy 125:104389. doi: 10.4236/nr.2013.48065
Borges, R., Ferreira, A. C., and Lacerda, L. D. (2017). Systematic planning and ecosystem-based management as strategies to reconcile mangrove conservation with resource use. Front. Mar. Sci. 4:353. doi: 10.3389/fmars.2017.00353
Bosma, R. H., Nguyen, T. H., Siahainenia, A. J., Tran, H. T. P., and Tran, H. N. (2014). Shrimp-based livelihoods in mangrove silvo-aquaculture farming systems. Rev. Aquacult. 6, 1–18. doi: 10.1111/raq.12072
Boyd, C. E., and Massaut, L. (1999). Risks associated with the use of chemicals in pond aquaculture. Aquacult. Eng. 20, 113–132. doi: 10.1016/S0144-8609(99)00010-2
Bryan-Brown, D. N., Connolly, R. M., Richards, D. R., Adame, F., Friess, D. A., and Brown, C. J. (2020). Global trends in mangrove forest fragmentation. Scient. Rep. 10, 1–8.
Bunting, P., Rosenqvist, A., Lucas, R. M., Rebelo, L.-M., Hilarides, L., Thomas, N., et al. (2018). The Global Mangrove Watch - A new 2010 global baseline of mangrove extent. Remote Sens. 10:1669. doi: 10.3390/rs10101669
Caldas, R. M., and Sampaio, Y. S. B. (2015). Pobreza no nordeste brasileiro: uma análise multidimensional. Rev. Econ. Contempor. 19, 74–96. doi: 10.1590/198055271914
Cardoso, R. C. (2005). Dimensões Sociais do Turismo Sustentável: Estudo Sobre a Contribuição dos Resorts de Praia Para o Desenvolvimento das Comunidades Locais. Tese (Doutorado em Administração de Empresas). Bela Vista: Programa de Pós-Graduação da Escola de Administração de Empresas de São Paulo da Fundação Getúlio Vargas, 264.
Carrasquilla-Henao, M., and Juanes, F. (2017). Mangroves enhance local fisheries catches: a global meta-analysis. Fish Fisher 18, 79–93.
Carvalho, R. A. A., and Martins, P. C. C. (2017). Caracterização da atividade de carcinicultura no Vale do Rio Açu. Rio Grande do Norte Brasil. Holos 2, 96–107.
Celis-Hernandez, O., Giron-Garcia, P. M., Ontiveros-Cuadras, J., Canales-Delgadillo, J., Pérez-Ceballos, R., Ward, R. D., et al. (2020). Environmental risk of heavy metals in mangrove ecosystems: an assessment of natural vs oil and urban inputs. Sci. Tot. Environ. 730:138643. doi: 10.1016/j.scitotenv.2020.138643
Charles, S. P., Kominoski, J. S., Armitage, A. R., Guo, H., Weaver, C. A., and Pennings, S. C. (2020). Quantifying how changing mangrove cover affects ecosystem carbon storage in coastal wetlands. Ecology 101:e02916. doi: 10.1002/ecy.2916
Chou, C. L., Haya, K., Paon, L. A., Burridge, L., and Moffatt, J. D. (2002). Aquaculture-related trace metals in sediments and lobsters and relevance to environmental monitoring program ratings for nearfield effects. Mar. Pollut. Bull. 44, 1259–1268. doi: 10.1016/S0025-326X(02)00219-9
Costa, E. F., and Sampaio, Y. (2004). Direct and indirect job generation in the farmed shrimp production chain. Aquacult. Econ. Manag. 8, 143–155. doi: 10.1080/13657300409380359
Costa, B. G. B., Soares, T. M., Torres, R. F., and Lacerda, L. (2013). Mercury distribution in a mangrove tidal creek affected by intensive shrimp farming. Bull. Environ. Contamin. Toxicol. 90, 537–541. doi: 10.1007/s00128-012-0957-4
Crona, B. I. (2006). Of Mangroves and Middlemen: A Study of Social and Ecological Linkages in a Coastal Community. PhD Thesis. Stockholm: Stockholm University.
da Silva Filho, L. A., and de Queiroz, S. N. (2011). Recuperação econômica e emprego formal: avaliação para o Nordeste brasileiro entre 2000 e 2008. Perspec. Econ. 7, 42–54. doi: 10.4013/1297
Daly, H. E. (2005). Economics in a Full World. Sci. Am. 293, 100–107. doi: 10.1038/scientificamerican0905-100
Datta, D., and Deb, S. (2017). Forest structure and soil properties of mangrove ecosystems under different management scenarios: experiences from the intensely humanized landscape of Indian Sunderbans. Ocean Coast. Manag. 140, 22–33. doi: 10.1016/j.ocecoaman.2017.02.022
Davaasuren, N., and Meesters, H. W. G. (2012). Extent and Health of Mangroves in Lac Bay Bonaire Using Satellite Data. Wageningen: IMARES - Institute for Marine Resources & Ecosystem Studies, 64. Report number C190/11.
Di Nitto, D., Erftemeijer, P. L. A., van Beek, J. K. L., Dahdouh-Guebas, F., Higazi, L., Quisthoudt, K., et al. (2013). Modelling drivers of mangrove propagule dispersal and restoration of abandoned shrimp farms. Biogeosciences 10, 5095–5113. doi: 10.5194/bg-10-5095-2013
Dias, H. M., Soares, M. L. G., and Neffa, E. (2012). Conflitos socioambientais: o caso da carcinicultura no complexo estuarino Caravelas - Nova Viçosa/Bahia-Brasil. Amb. Soc. 15, 111–130. doi: 10.1590/S1414-753X2012000100008
Diniz, C., Cortinhas, L., Nerino, G., Rodrigues, J., Sadeck, L., Adami, M., et al. (2019). Brazilian mangrove status: three decades of satellite data analysis. Remote Sens. 11:808. doi: 10.3390/rs11070808
Eddiwan, K. (2018). Success level of mangrove tree planting real in Kepulauan Meranti district, Riau, Indonesia. J. Aquac. Mar. Biol. 7, 13–218. doi: 10.15406/jamb.2018.07.00211
Eid, E., Arshad, M., Shaltout, K., El-Sheikh, M., Alfarhan, A., Picó, Y., et al. (2019). Effect of the conversion of mangroves into shrimp farms on carbon stock in the sediment along the southern Red Sea coast, Saudi Arabia. Environ. Res. 176:108536. doi: 10.1016/j.envres.2019.108536
Ekau, W., and Knoppers, B. A. (1999). An introduction to the pelagic system of the East and Northeast Brazilian shelf. Arch. Fish. Mar. Res. 47, 113–132.
Elwin, A., Bukoski, J. J., Jintana, V., Robinson, E. J. Z., and Clark, J. M. (2019). Preservation and recovery of mangrove ecosystem carbon stocks in abandoned shrimp ponds. Sci. Rep. 9:18275. doi: 10.1038/s41598-019-54893-6
Ferreira, A. C., Bezerra, L. E. A., and Mathews-Cascon, H. (2019). Aboveground stock in a restored Neotropical mangrove: influence of management and brachyuran crab assemblage. Wetlands Ecol. Manag. 27, 223–242.
Ferreira, A. C., Ganade, G., and Attayde, J. L. (2015). Restoration versus natural regeneration in a neotropical mangrove: effects on plant biomass and crab communities. Ocean. Coast. Manag. 110, 38–45. doi: 10.1016/j.ocecoaman.2015.03.006
Ferreira, A. C., and Lacerda, L. D. (2016). Degradation and conservation of Brazilian mangroves, status and perspectives. Ocean Coast. Manag. 125, 38–46. doi: 10.1016/j.ocecoaman.2016.03.011
Ferreira, A. N., Beretta, M., Júnior, M., and de Oliveira, P. (2012). Avaliação do impacto da dragagem sobre associação fitoplanctônica do porto de Aratu, Baía de Todos os Santos, Bahia. Arq. Cienc. Mar. 45, 30–46. doi: 10.32360/acmar.v45i1.141
Fitzgerald, W. J. (2000). “Integrated mangrove forest and aquaculture systems in Indonesia,” in Mangrove-Friendly Aquaculture: Proceedings of the Workshop on Mangrove-Friendly Aquaculture organized by the SEAFDEC Aquaculture Department, January 11-15, 1999, eds J. H. Primavera, L. M. B. Garcia, M. T. Castaños, and M. B. Surtida Iloilo City, 21–34.
Flores-Verdugo, F., Zebadua-Penagos, F., and Flores-de-Santiago, F. (2015). Assessing the influence of artificially constructed channels in the growth of afforested black mangrove (Avicennia germinans) within an arid coastal region. J. Environ. Manag. 160, 113–120.
Food and Agriculture Organization [FAO] (2007). The World’s Mangroves 1980–2005. FAO Forest Paper 153. Rome: Food and Agriculture Organization of the United Nations.
Food and Agriculture Organization [FAO] (2019). Fishery Statistical Collections. Global Aquaculture Production. Rome: Food and Agriculture Organization of the United Nations.
Freitas, D. M., Ramos, A. L. A., Sano, E. E., Resende, K. B., Fumi, M., Oliveira, F. F. G., et al. (2017). “Mapeamento de manguezais e carcinicultura do Brasil com base em imagens dos satélites Landsat-8 OLI e RapidEye (ano-base: 2013),” in Anais do 18° Congr. Bras. Sens. Remoto, May 28-31, 2017, São Paulo, 1–4.
Friess, D. A., Rogers, K., Lovelock, C. E., Krauss, K. W., Hamilton, S. E., Lee, S. Y., et al. (2019). The state of the world’s mangrove forests: past, present, and future. Annu. Rev. Environ. Resour. 44, 89–115. doi: 10.1146/annurev-environ-101718-033302
Friess, D. A., Thompson, B. S., Brown, B., Amir, A. A., Cameron, C., Koldewey, H. J., et al. (2016). Policy challenges and approaches for the conservation of mangrove forests in Southeast Asia. Conser. Biol. 30:933949. doi: 10.1111/cobi.12784
Giri, C., Ochieng, E., Tieszen, I., Zhu, Z., Singh, A., Loveland, T., et al. (2011). Status and distribution of mangrove forests of the world using Earth observation satellite data. Glob. Ecol. Biogeogr. 20, 154–159.
Godoy, M. D. P., and Lacerda, L. D. (2014). River-island morphological response to basin Land-use changes within the Jaguaribe river estuary. NE Brazil. J. Coast. Res. 30, 399–410. doi: 10.2112/JCOASTRES-D-13-00059.1
Godoy, M. D. P., and Lacerda, L. D. (2015). Mangroves response to climate change: a review of recent findings on mangrove extension and distribution. An. Acad. Bras. Ciênc. 87, 651–667.
Godoy, M. D. P., Meireles, A. J. A., and Lacerda, L. D. (2018). Mangrove response to land use change in estuaries along the semiarid coast of ceará. Brazil. J. Coast. Res. 34, 524–533.
Goldberg, L., Lagomasino, D., Thomas, N., and Fatoyinbo, T. (2020). Global decline in human-driven mangrove loss. Glob Change Biol. 26:58445855. doi: 10.1111/gcb.15275
Hai, N. T., Dell, B., Phuong, V. T., and Harper, R. J. (2020). Towards a more robust approach for the restoration of mangroves in Vietnam. Ann. Forest Sci. 77:18. doi: 10.1007/s13595-020-0921-0
Hamilton, S. (2013). Assessing the role of commercial aquaculture in displacing mangrove forest. Bull. Mar. Sci. 89, 585–601. doi: 10.5343/bms.2012.1069
Hamilton, S. E., and Casey, D. (2016). Creation of a high spatio-temporal resolution global database of continuous mangrove forest cover for the 21st century (CGMFC-21). Glob. Ecol. Biogeog. 25, 729–738. doi: 10.1111/geb.12449
Hatje, V., Andrade, R. L. B., Jesus, R. M., Masqu, P., Andrade, J. B., and Santos, A. C. S. S. (2019). Historical records of mercury deposition in dated sediment cores reveal the impacts of the legacy and present-day human activities in Todos os Santos Bay. Northeast Brazil. Mar. Pollut. Bull. 145, 396–406. doi: 10.1016/j.marpolbul.2019.06.041
Herbeck, L. S., Unger, D., Wu, Y., and Jennerjahn, T. C. (2013). Effluent, nutrient and organic matter export from shrimp and fish ponds causing eutrophication in coastal and back-reef waters of NE Hainan, tropical China. Cont. Shelf Res. 57, 92–104. doi: 10.1016/j.csr.2012.05.006
Hidayati, N. V., Prudent, P., Asia, L., Vassalo, L., Torre, F., and Widowati, I., et al. (2020). Assessment of the ecological and human health risks from metals in shrimp aquaculture environments in Central Java, Indonesia. Environ. Sci. Pollut. Res. 27, 41668–41687. doi: 10.1007/s11356-020-09967-8
Hong, H. T. C., Avtar, R., and Fuji, M. (2019). Monitoring changes in land use and distribution of mangroves in the southeastern part of the Mekong River Delta, Vietnam. Trop. Ecol. 60, 552–565. doi: 10.1007/s42965-020-00053-1
Hutchison, J., Spalding, M., and Ermgassen, P. (2014). The Role of Mangroves in Fisheries Enhancement. London: The Nature Conservancy and Wetlands Internacional, 54.
Instituto Brasileiro de Geografia e Estat stica [IBGE] (2018). Pesquisa da Pecuária Municipal. Instituto Brasileiro de Geografia e Estatística. Rio de Janeiro: IBGE.
Instituto Brasileiro de Meio Ambiente [IBAMA] and Centro de Pesquisa e Conservação da Biodiversidade Marinha do Nordeste [CEPENE] (1994). Relatório da Reunião do Grupo Permanente de Estudos do Caranguejo-uçá. São Luís: IBAMA, 53.
Instituto Chico Mendes de Conservação da Biodiversidade [ICMBio] (2018). Atlas dos Manguezais do Brasil. Brasília: Instituto Chico Mendes de Conservação da Biodiversidade do Ministério do Meio Ambiente. Brasília, DF.
Intergovernmental Panel on Climate Change [IPCC] (2019). The Ocean and the Cryosphere in a Changing Climate. Summary for Policymakers. Monaco: Intergovernmental Panel on Climate Change.
Kauffman, J. B., Bernardino, A. F., Ferreira, T. O., Bolton, N. W., Gomes, L. E. O., and Nobrega, G. N. (2018). Shrimp ponds lead to massive loss of soil carbon and greenhouse gas emissions in northeastern Brazilian mangroves. Ecol. Evol. 8, 5530–5540. doi: 10.1002/ece3.4079
Knoppers, B., Ekau, W., and Figueiredo, A. G. (1999). The coast and shelf of east and northeast Brazil and material transport. Geo-Mar. Lett. 19, 171–178. doi: 10.1007/s003670050106
Koening, M. L., Eskinazi-Leça, E., Neumann-Leitão, S., and Macêdo, S. J. (2002). Impactos da construção do Porto de Suape sobre a comunidade fitoplanctônica no estuário do rio Ipojuca (Pernambuco-Brasil). Acta Bot. Bras. 16, 407–420. doi: 10.1590/S0102-33062002000400004
Lacerda, L. D. (2002). Mangrove Ecosystems: Function and Management. Berlin: Springer, doi: 10.1007/978-3-662-04713-2
Lacerda, L. D., Borges, R., and Ferreira, A. C. (2019). Neotropical mangroves: conservation and sustainable use in a scenario of global climate change. Aquat. Conser. Mar. Freshwat. Ecosys. 29, 1347–1364. doi: 10.1002/aqc.3119
Lacerda, L. D., Marins, R. V., and Dias, F. J. S. (2020). An arctic paradox: response of fluvial hg inputs and bioavailability to global climate change in an extreme coastal environment. Front. Earth Sci. 8:93. doi: 10.3389/feart.2020.00093
Lacerda, L. D., Santos, J. A., and Lopes, D. V. (2009). Fate of copper in intensive shrimp farms: bioaccumulation and deposition in pond sediments. Braz. J. Biol. 69, 851–858. doi: 10.1590/S1519-69842009000400012
Lacerda, L. D., Santos, J. A., and Madrid, R. M. (2006a). Copper emission factors from intensive shrimp aquaculture. Mar. Pollut. Bull. 52, 1823–1826. doi: 10.1016/j.marpolbul.2006.09.019
Lacerda, L. D., Vaisman, A. G., Maia, L. P., Cunha, E., and Silva, C. A. R. (2006b). Relative importance of nitrogen and phosphorus emissions from shrimp farming and other anthropogenic sources for six estuaries along the NE Brazilian coast. Aquaculture 253, 433–446. doi: 10.1016/j.aquaculture.2005.09.005
Lacerda, L. D., Soares, T. M., Costa, B. G. B., and Godoy, M. D. P. (2011). Mercury emission factors from intensive shrimp aquaculture and its relative importance to the Jaguaribe River Estuary. NE Brazil. Bull. Environ. Cont. Toxicol. 87, 657–661. doi: 10.1007/s00128-011-0399-4
Lee, S. Y., Primavera, J. H., Dahdouh-Guebas, F., McKee, K., Bosire, J. O., Cannicci, S., et al. (2014). Ecological role and services of tropical mangrove ecosystems: a reassessment. Glob. Ecol. Biogeogr. 23, 726–743.
León-Cañedo, J. A., Alarcón-Silvas, S. G., Fierro-Sañudo, J. F., Mariscal-Lagarda, M. M., Díaz-Valdés, T., and Páez-Osuna, F. (2017). Assessment of environmental loads of Cu and Zn from intensive inland shrimp aquaculture. Environ. Monit. Assess. 189, 69–77.
Lewis, R. R. III (2005). Ecological engineering for successful management and restoration of mangrove forests. Ecol. Eng. 24, 403–418. doi: 10.1016/j.ecoleng.2004.10.003
Lewis, R. R., and Streever, W. (2000). Restoration of Mangrove Habitat. Vicksburg, MS: US Army, Corps of Engineers, Waterways Experiment Station. Tech Note ERDC TN-WRP-VN-RS-3.
Lewis, R., and Gilmore, R. G. (2007). Important considerations to achieve successful mangrove forest restoration with optimum fish habitat. Bull. Mar. Sci. 18, 823–837.
Lima, M., Ward, R., and Joyce, C. (2020). Environmental drivers of carbon stocks in temperate seagrass meadows. Hydrobiologia 847, 1773–1792.
Longo, S. B., Clark, B., and York, R. (2013). The globalization of ecologically intensive aquaculture (1984-2008). J. Environ. Stud. Sci. 3, 297–305. doi: 10.1007/s13412-013-0124-1
López-Portillo, J., Lewis, R. R. III, Saenger, P., Rovai, A., Koedam, N., Dahdouh-Guebas, F., et al. (2017). “Chapter 10. mangrove forest restoration and rehabilitation,” in Mangrove Ecosystems: A Global Biogeographic Perspective Structure, Function, and Services, eds V. H. Rivera-Monroy, S. Y. Lee, E. Kristensen, and R. R. Twilley (Berlin: Springer), 301–345. doi: 10.1007/978-3-319-62206-4
Loureiro, C. V., and de Oliveira, C. F. (2019). Os aspectos socioeconômicos do manguezal do rio Coreaú e sua relação com a sustentabilidade ambiental. Com. Ciênc. Tecnol. 13, 78–83.
Lourenço, R. A., Combi, T., da Rosa Alexandre, M., Sasaki, S. T., Zanardi-Lamardo, E., and Yogui, G. T. (2020). Mysterious oil spill along Brazil’s northeast and southeast seaboard (2019–2020): trying to find answers and filling data gaps. Mar. Pollut. Bull. 156:111219. doi: 10.1016/j.marpolbul.2020.111219
Lunstrum, A., and Chen, L. (2014). Soil carbon stocks and accumulation in young mangrove forests. Soil Biol. Biochem. 75, 223–232. doi: 10.1016/j.soilbio.2014.04.008
Machado, M. R. (2008). O processo histórico do desmatamento do Nordeste Brasileiro: impactos ambientais e atividades econômicas. Revist. Geogr. 23, 123–134.
Mafi-Gholami, D., Zenner, E., Jaafari, A., and Ward, R. (2018). Modeling multi-decadal mangrove leaf area index in response to drought along the semi-arid southern coasts of Iran. Sci. Tot. Environ. 656, 1326–1336. doi: 10.1016/j.scitotenv.2018.11.462
Maia, L. P., Lacerda, L. D., Monteiro, L. H. U., and Souza, G. M. (2006). Atlas dos Manguezais do Nordeste do Brasil: Avaliação das Áreas de Manguezais dos Estados do Piauí, Ceará, Rio Grande do Norte, Paraíba e Pernambuco. Fortaleza: Secretaria do meio Ambiente do Estado do Ceará.
Maiti, S. K., and Chowdhury, A. (2013). Effects of anthropogenic pollution on mangrove biodiversity: a review. J. Environ. Protec. 4, 1428–1434. doi: 10.4236/jep.2013.412163
Marchand, C., Allenbach, M., and Lallier-Vergès, E. (2011). Relationships between heavy metals distribution and organic matter cycling in mangrove sediments (Conception Bay, New Caledonia). Geoerma 1160, 444–456. doi: 10.1016/j.geoderma.2010.10.015
Marengo, J. A., Alves, L. M., Alvala, R. C. S., Cunha, A. P., Brito, S., and Moraes, O. L. L. (2018). Climatic characteristics of the 2010-2016 droughts in the semiarid Northeast Brazil region. An. Acad. Bras. Ciên. 90, 1973–1985. doi: 10.1590/0001-3765201720170206
Marins, R. V., Lacerda, L. D., Araújo, I. C. S., Fonseca, L. V., and Silva, A. T. F. (2020). Phosphorus and suspended matter retention in mangroves affected by shrimp farm effluents in NE Brazil. An. Acad. Bras. Ciênc. 92:e20200758. doi: 10.1590/0001-3765202020200758
Marins, R. V., Paula Filho, F. J., Eschrique, S. A., and Lacerda, L. D. (2011). Anthropogenic sources and distribution of phosphorus in sediments from the Jaguaribe River estuary, NE, Brazil. Braz. J. Biol. 71, 673–678. doi: 10.1590/S1519-69842011000400011
Marois, D. E., and Mitsch, W. J. (2015). Coastal protection from tsunamis and cyclones provided by mangrove wetlands – a review. Inter. J. Biodiver. Sci. Ecosys. Serv. Manag. 11, 71–83. doi: 10.1080/21513732.2014.997292
Matsui, N., Morimune, K., Meepol, W., and Chukwamdee, J. (2012). Ten year evaluation of carbon stock in mangrove plantation reforested from an abandoned shrimp pond. Forests 3, 431–444. doi: 10.3390/f3020431
Matsui, N., Suekumi, J., Nogami, M., Havanond, S., and Salikul, P. (2010). Mangrove rehabilitation dynamics and soil organic carbon changes as a result of full hydraulic restoration and re-grading of a previously intensively managed shrimp pond. Wetl. Ecol. Manag. 18, 233–242. doi: 10.1007/s11273-009-9162-6
Ministério da Pesca e da Agricultura [MPA] (2013). Levantamento da Infraestrutura Produtiva e Dos Aspectos Tecnológicos, Econômicos, Sociais e Ambientais da Carcinicutura Marinha no Brasil em 2011. MPA, 77.
Molnar, N., Welsh, D. T., Marchand, C., Deborde, J., and Meziane, T. (2013). Impacts of shrimp farm effluent on water quality, benthic metabolism and N dynamics in a mangrove forest (New Caledonia). Estuar. Coast. Shelf. Sci. 117, 12–21. doi: 10.1016/j.ecss.2012.07.012
Monteiro, L. S., Borges, D. A., Studart, T. M. C., Campos, J. N. B., and Mota, F. S. B. (2016). Calculation method for charging water on shrimp farming. Rev. Bras. Rec. Híd. 21, 789–796. doi: 10.1590/2318-0331.011615006
Murdiyarso, D., Purbopuspito, J., Kauffman, J., Taberima, B. S., and Kurnianto, S. (2015). The potential of Indonesian mangrove forests for global climate change mitigation. Nat. Clim. Change 5, 1089–1092. doi: 10.1038/nclimate2734
Nagelkerken, I., Blaber, S. J. M., Bouillon, S., Green, P., Haywood, M., Kirton, L. G., et al. (2008). The habitat function of mangroves for terrestrial and marine fauna: a review. Aquat. Bot. 89, 155–185.
Nam, V. N., Sasmito, S. D., Murdiyarso, D., Purbopuspito, J., and MacKenzie, R. A. (2016). Carbon stocks in artificially and naturally regenerated mangrove ecosystems in the Mekong Delta. Wetl. Ecol. Manag. 24, 231–244. doi: 10.1007/s11273-015-9479-2
Neogi, S. B., Dey, M., Lutful Kabir, S. M., Masum, S. J. H., Kopprio, G. A., Yamasaki, S., et al. (2016). Sundarban mangroves: diversity, ecosystem services and climate change impacts. Asian J. Med. Biol. Res. 2, 488–507. doi: 10.3329/ajmbr.v2i4.30988
Nóbrega, G. N., Ferreira, T. O., Neto, M. S., Mendonça, E. S., Romero, R. E., and Otero, X. L. (2019). The importance of blue carbon soil stocks in tropical semiarid mangroves: a case study in Northeastern Brazil. Environ. Earth Sci. 78, 1–10. doi: 10.1007/s12665-019-8368-z
Nóbrega, G. N., Ferreira, T. O., Romero, R. E., Neto, M. S., Mendonça, E. S., and Otero, X. L. (2013). Iron and sulfur geochemistryin semi-arid mangrove soils (Ceará, Brazil) in relation to seasonal changes and shrimp farming effluents. Environ. Monit. Assess. 185, 7393–7407. doi: 10.1007/s10661-013-3108-4
Nunes, A. J. P., Madrid, R. M., and Andrade, T. P. (2011). Carcinicultura Marinha no Brasil: Passado, Presente e Futuro. Panor. Aq ic. 21, 26–33.
Oliveira, A. G. J., and Neto, R. (2019). Cultivo de camarão marinho em águas de baixa salinidade: uma realidade na carcinicultura com o camarão marinho L. vannamei na Paraíba. Rev. ABCC 1, 54–56.
Oliveira-Filho, R. R., Rovai, A. S., Menghini, R. P., Coelho-Júnior, C., Novelli, Y. S., and Cintrón, G. (2016). On the impact of the Brazilian Forest Code on mangroves: a comment to Ferreira and Lacerda (2016). Ocean Coast. Manag. 132, 36–37. doi: 10.1016/j.ocecoaman.2016.08.003
Osland, M. J., Feher, L. C., Spivak, A. C., Nestlerode, J. A., Almario, A. E., Cormier, N., et al. (2020). Rapid peat development beneath created, maturing mangrove forests: ecosystem changes across a 25-yr chronosequence. Ecol. Appl. 30:e02085. doi: 10.1002/eap.2085
Ottonelli, J., and Mariano, J. L. (2014). Pobreza multidimensional nos municípios da Região Nordeste. Rev. Admin. Públ. 48, 1253–1279. doi: 10.1590/0034-76121724
Pérez, A., Machado, W., Gutiérrez, D., Saldarriaga, M., and Sanders, C. (2020). Shrimp farming influence on carbon and nutrient accumulation within Peruvian mangroves sediments. Estuar. Coast. Shelf Sci. 243:106879. doi: 10.1016/j.ecss.2020.106879
Polidoro, B. A., Carpenter, K. E., Collins, L., Duke, N. C., Ellison, A. M., and Ellison, J. C. (2010). The loss of species: mangrove extinction risk and geographic areas of global concern. PLoS One 5:e10095. doi: 10.1371/journal.pone.0010095
Prates, A. P. L., Gonçalves, M. A., and Rosa, M. R. (2012). Panorama da Conservação dos Ecossistemas Costeiros e Marinhos no Brasil. Brasília: MMA, 152.
Primavera, J. H., and Esteban, J. M. A. (2008). A review of mangrove rehabilitation in the Philippines: successes, failures and future prospects. Wetl. Ecol. Manag. 16, 345–358. doi: 10.1007/s11273-008-9101-y
Primavera, J. H., Rollon, R. N., and Samson, M. S. (2011). “Chapter 10.10 the pressing challenges of mangrove rehabilitation: pond reversion and coastal protection,” in Ecohydrology and Restoration. Treatise on Estuarine and Coastal Science, Vol. 10, eds L. Chicharo and M. Zalewski (Amsterdam: Elsevier), 217–244.
Queiroz, L., Rossi, S., Meireles, J., and Coelho, C. (2013). Shrimp aquaculture in the federal state of Ceará, 1970–2012: trends after mangrove forest privatization in Brazil. Ocean Coast. Manag. 73, 54–62.
Queiroz, L. D. S., Ferreira, T. O., Tanigushi, C. A. K., Barcelos, D., Nascimento, J. C., Nóbrega, G. N., et al. (2020). Nitrogen mineralization and eutrophication risks in mangroves receiving shrimp farming effluents. Environ. Sci. Pollut. Res. 27, 34941–34950. doi: 10.1007/s11356-020-09720-1
Queiroz, L. D. S., Rossi, S., Calvet, L., Ruiz-Mallén, I., García-Betorz, S., Salvà-Prat, J., et al. (2017). Neglected ecosystem services: highlighting the socio-cultural perception of mangroves in decision-making processes. Ecos. Serv. 26, 137–145. doi: 10.1016/j.ecoser.2017.06.013
Rasid, S. A., Jemali, N. J. N., and Majid, N. K. S. A. (2019). Detection of vegetation losses in shrimp farming area using remote sensing technique. J. Trop. Resour. Sustain. Sci. 7, 103–107.
Reis-Neto, A. S., Andrade, A. J., and Lignon, M. C. (2019). Natural regeneration of the mangrove vegetation on abandoned salt ponds in ceará, in the semi-arid region of Northeastern Brazil. Diversity 11, 1–15. doi: 10.3390/d11020027
Richards, D. R., and Friess, D. A. (2016). Rates and drivers of mangrove deforestation in Southeast Asia, 2000–2012. Proc. Nat. Acad. Sci. U.S.A. 12, 344–349. doi: 10.1073/pnas.1510272113
Rocha, I. (2019). Análise da Produção aquícola mundial e das oportunidades para o Brasil. Rev. da ABCC 2, 8–13.
Rocha Junior, J. M. (2011). Avaliação Ecológico-Econômica do Manguezal de Macau/RN e a Importância da Aplicação de Práticas Preservacionistas pela Indústria Petrolífera Local. Dissertação (Mestrado). Rio Grande do Norte: Universidade Federal do Rio Grande do Norte, 100.
Sá, T. D., Sousa, R. R., Rocha, I. R. C. B., Lima, G. C., and Costa, F. H. F. (2013). Brackish shrimp farming in Northeastern Brazil: the environmental and socio-economic impacts and sustainability. Nat. Resour. 4, 538–550. doi: 10.4236/nr.2013.48065
Saderne, V., Cusack, M., Almahasheer, H., Serrano, O., Masqué, P., Arias-Ortiz, A., et al. (2018). Accumulation of carbonates contributes to coastal vegetated ecosystems keeping pace with sea level rise in an arid region (Arabian Peninsula. ). J. Geophys. Res. Biogeosci. 123:1498e1510. doi: 10.1029/2017JG004288
Safadi, V. T. (2018). Impactos Socioambientais da Carcinicultura nos Povoados Ponta dos Mangues e Boca da Barra, Pacatuba/SE. Trabalho de conclusão de Curso. São Cristóvão: Universidade Federal de Sergipe.
Sampaio, Y., Costa, E. F., and Sampaio, E. A. B. R. (2008). Impactos socioeconômicos do cultivo de camarão marinho em municípios selecionados do Nordeste brasileiro. Rev. Econ. Sociol. Rural. 46, 1015–1042. doi: 10.1590/S0103-20032008000400005
Sampaio, Y. S. B., Costa, E. F., Albuquerque, E., and Sampaio, B. R. (2005). Impactos Sócio-econômicos do cultivo do camarão marinho em municípios selecionados do nordeste brasileiro. Rev. ABCC 7, 21–30.
Santos, L. C. M., Gasalla, M. A., Dahdouh-Guebas, F., and Bitencourt, M. D. (2017). Socio-ecological assessment for environmental planning in coastal fishery areas: a case study in Brazilian mangroves. Ocean Coast. Manag. 138, 60–69. doi: 10.1016/j.ocecoaman.2017.01.009
Sanyal, P., Ray, R., Paul, M., Gupta, V. K., Acharya, A., Bakshi, S., et al. (2020). Assessing the dynamics of dissolved organic matter (DOM) in the coastal environments dominated by mangroves, Indian Sundarbans. Front. Earth Sci. 8:218. doi: 10.3389/feart.2020.00218
Sasmito, S. D., Taillardat, P., Clendenning, J. N., Cameron, C., Friess, D. A., Murdiyarso, D., et al. (2019). Effect of land-use and land-cover change on mangrove blue carbon: a systematic review. Glob. Change Biol. 25, 4291–4302. doi: 10.1111/gcb.14774
Schaeffer-Novelli, Y., Rovai, A. S., Coelho-Jr, C., Menghini, R. P., and de Almeida, R. (2012). Alguns Impactos do PL 30/2011 Sobre os Manguezais Brasileiros. Código Florestal e a Ciência: o que Nossos Legisladores Ainda Precisam Saber. Comitê Brasil: Brasília, 2012.
Sheppard, C., Al-Husiani, M., Al-Jamali, F., Al-Yamani, F., Baldwin, R., Bishop, J., et al. (2010). The gulf: a young sea in decline. Mar. Pollut. Bull. 60, 13–38. doi: 10.1016/j.marpolbul.2009.10.017
Silva, C. A. R., Rainbow, P. S., and Smith, B. D. (2001). Biomonitoring of trace metal contamination in the Potengi estuary, Natal (Brazil), using the oyster Crassostrea rhizophorae, a local food source. Water Res. 35, 4072–4078. doi: 10.1016/S0043-1354(01)00144-0
Soares, T. M., Coutinho, D. A., Lacerda, L. D., Moraes, M. O., and Rebelo, M. F. (2011). Mercury accumulation and metallothionein expression from aquafeeds by Litopenaeus vannamei Boone, 1931 under intensive aquaculture conditions. Braz. J. Biol. 71, 131–137. doi: 10.1590/S1519-69842011000100019
Sosa-Villalobos, C., Castañeda-Chávez, M. R., Amaro-Espejo, I. A., Galaviz-Villa, I., and Lango-Reynoso, F. (2016). Diagnosis of the current state of aquaculture production systems with regard to the environment in México. Lat. Am. J. Aquat. Res. 44, 193–201. doi: 10.3856/vol44-issue2-fulltext-1
Sousa, P. G., Matias, E. M., and Selva, V. S. F. (2016). Do turismo residencial aos complexos turísticos imobiliários: a apropriação da zona costeira do nordeste brasileiro pela atividade turística imobiliária. Amb. Soc. 19, 177–198. doi: 10.1590/1809-4422ASOC141673V1932016
Stevenson, N. J., Lewis, R. R., and Burbridge, P. R. (1999). “Disused shrimp ponds and mangrove rehabilitation,” in An International Perspective on Wetland Rehabilitation, ed. W. Streever (Berlin: Springer Science & Business Media), 277–297.
Suárez-Abelenda, M., Ferreira, T. O., Camps-Arbestain, M., Rivera-Monroy, V. H., Macías, F., Nuto-Nóbrega, G., et al. (2013). The effects of nutrient-rich effluents from shrimp farming on mangrove soil carbon storage and geochemistry under semi-arid climate conditions in northern Brazil. Geoderma 213, 551–559. doi: 10.1016/j.geoderma.2013.08.007
Tahim, E. F., and Araújo Junior, I. F. (2014). A carcinicultura do nordeste brasileiro e sua inserção em cadeias globais de produção: foco nos APLs do Ceará. Rev. Econ. Sociol. Rural. 52, 567–586. doi: 10.1590/S0103-20032014000300009
Thành-Nho, N., Marchand, C., Strady, E., Huu-Phat, N., and Nhu-Trang, T.-T. (2019). Bioaccumulation of some trace elements in tropical mangrove plants and snails (Can Gio. Vietnam). Environ. Pollut. 248, 635–645. doi: 10.1016/j.envpol.2019.02.041
Thomas, N., Lucas, R., Bunting, P., Hardy, A., Rosenqvist, A., and Simard, M. (2017). Distribution and drivers of global mangrove forest change, 19962010. PLoS One 12:19962010. doi: 10.1371/journal.pone.0179302
Tian, Y., Chen, G., Lu, H., Zhu, H., and Ye, Y. (2019). Effects of shrimp pond effluents on stocks of organic carbon, nitrogen and phosphorus in soils of Kandelia obovata forests along Jiulong River Estuary. Mar. Pollut. Bull. 149:110657. doi: 10.1016/j.marpolbul.2019.110657
Toledo, G., Rojas, A., and Bashan, Y. (2001). Monitoring of black mangrove restoration with nursery-reared seedlings on an arid coastal lagoon. Hydrobiologia 444, 101–109.
Tran, D. B., Hoang, T. V., and Dargusch, P. (2015). An assessment of the carbon stocks and sodicity tolerance of disturbed Melaleuca forests in Southern Vietnam. Carbon Balance Manag. 10, 15. doi: 10.1186/s13021-015-0025-6
UNEP (United Nations Environment Programme) (2014). in The Importance of Mangroves to People: A Call to Action, eds J. van Bochove, E. Sullivan, and T. Nakamura (Cambridge: United Nations Environment Programme World Conservation Monitoring Centre,), 128.
Valiela, I., and Cole, M. L. (2002). Comparative evidence that salt marshes and mangroves may protect seagrass meadows from land-derived nitrogen loads. Ecosystems 5, 92–102. doi: 10.1007/s10021-001-0058-4
Valiela, I., Juman, R., Asmath, H., Hanacek, D., Lloret, J., Elmstrom, E., et al. (2020). Water quality, nutrients, and stable isotopic signatures of particulates and vegetation in a mangrove ecosystem exposed to past anthropogenic perturbations. Reg. Stud. Mar. Sci. 35:101208. doi: 10.1016/j.rsma.2020.101208
Vasconcellos, M., Diegues, A. C., and Sales, R. D. (2007). “. Limites e possibilidades na gestão da pesca artesanal costeira. Nas redes da pesca artesanal, 1, 15-84,” in 2015. Aspectos Econômicos das Cidades de Baixo Carbono: Recife, eds A. Gouldson, S. Colenbrander, A. Sudmant, F. McAnulla, and Y. O. de Sousa (Brazil: University of Leeds), 88.
Veetil, B., Ward, R., Quang, N., Trang, N., and Giang, T. (2019). Mangroves of Vietnam: historical development, current state of research and future threats. Estuar. Coast. Shelf Sci. 218, 212–236. doi: 10.1016/j.ecss.2018.12.021
Villoslada, M., Bergamo, T., Ward, R., Burnside, N., Joyce, C., and Sepp, K. (2020). Fine scale plant community assessment in coastal meadows using UAV based multispectral data. Ecol. Indicators 111:105979. doi: 10.1016/j.ecolind.2019.105979
Wang, J., Rich, P. M., Price, K. P., and Kettle, W. D. (2004). Relations between NDVI and tree productivity in the central Great Plains. Inter. J. Rem. Sens. 25, 3127–3138. doi: 10.1080/0143116032000160499
Ward, R., Burnside, N., Joyce, C., and Sepp, K. (2016a). Importance of micro-topography in determining plant community distribution in Baltic coastal wetlands. J. Coas. Res. 32, 1062–1070.
Ward, R., Friess, D., Day, R., and Mackenzie, R. (2016b). Impacts of climate change on global mangrove ecosystems: a regional comparison. Ecosys. Health Sustainab. 2, 1–25. doi: 10.1002/ehs2.1211
Ward, R., and Lacerda, L. D. (2021). “Responses of mangrove ecosystems to sea level change,” in Dynamic Sedimentary Environment of Mangrove Coasts, eds D. Friess and F. Sidik (Netherlands: Elsevier), 235–253.
Wodehouse, D., and Rayment, M. B. (2019). Mangrove area and propagule number planting targets produce sub-optimal rehabilitation and afforestation outcomes. Estuar. Coast. Shelf Sci. 222, 91–102. doi: 10.1016/j.ecss.2019.04.003
Keywords: aquaculture, eutrophication, deforestation, human impacts, nutrients, blue carbon
Citation: de Lacerda LD, Ward RD, Godoy MDP, de Andrade Meireles AJ, Borges R and Ferreira AC (2021) 20-Years Cumulative Impact From Shrimp Farming on Mangroves of Northeast Brazil. Front. For. Glob. Change 4:653096. doi: 10.3389/ffgc.2021.653096
Received: 13 January 2021; Accepted: 29 March 2021;
Published: 23 April 2021.
Edited by:
Sigit Sasmito, National University of Singapore, SingaporeReviewed by:
Jacob Bukoski, University of California, Berkeley, United StatesDaniel Friess, National University of Singapore, Singapore
Marília Cunha-Lignon, São Paulo State University, Brazil
Alexander Pérez, Universidad Peruana Cayetano Heredia, Peru
Copyright © 2021 de Lacerda, Ward, Godoy, de Andrade Meireles, Borges and Ferreira. This is an open-access article distributed under the terms of the Creative Commons Attribution License (CC BY). The use, distribution or reproduction in other forums is permitted, provided the original author(s) and the copyright owner(s) are credited and that the original publication in this journal is cited, in accordance with accepted academic practice. No use, distribution or reproduction is permitted which does not comply with these terms.
*Correspondence: Luiz Drude de Lacerda, ldrude@pq.cnpq.br; orcid.org/0000-0002-3496-0785