- 1North America Region Science, The Nature Conservancy, Minneapolis, MN, United States
- 2Reforestation, Nurseries, and Genetics Resources, USDA Forest Service, Portland, OR, United States
- 3John T. Harrington Forestry Research Center, New Mexico State University, Mora, NM, United States
- 4Sagebrush Sea Program, The Nature Conservancy, Bend, OR, United States
- 5Division of Forestry, Wisconsin Department of Natural Resources, La Crosse, WI, United States
- 6Natural Climate Solutions Science, The Nature Conservancy, Arlington, VA, United States
- 7Colorado Chapter, The Nature Conservancy, Boulder, CO, United States
- 8American Forests, Washington, DC, United States
- 9Department of Biology, University of New Mexico, Albuquerque, NM, United States
- 10Department of Ecosystem and Conservation Sciences, University of Montana, Missoula, MT, United States
- 11Department of Forest Management, University of Montana, Missoula, MT, United States
- 12Southern Forest Nursery Management Cooperative, School of Forestry and Wildlife Sciences, Auburn University, Auburn, AL, United States
- 13Forest Planning and Analysis, ArborGen Inc., Ridgeville, SC, United States
- 14USDA Forest Service, Washington, DC, United States
- 15Department of Forestry and Environmental Resources, North Carolina State University, Raleigh, NC, United States
- 16School of Forestry and Wildlife Sciences, Auburn University, Auburn, AL, United States
- 17Guldin Forestry LLC, Silver Spring, MD, United States
Large-scale global reforestation goals have been proposed to help mitigate climate change and provide other ecosystem services. To explore reforestation potential in the United States, we used GIS analyses, surveys of nursery managers and foresters, and literature synthesis to assess the opportunities and challenges associated with meeting proposed reforestation goals. We considered a scenario where 26 million hectares (64 million acres) of natural and agricultural lands are reforested by 2040 with 30 billion trees at an estimated cost of $33 ($24–$53) billion USD. Cost per hectare will vary by region, site conditions, and other factors. This scenario would require increasing the number of tree seedlings produced each year by 1.7 billion, a 2.3-fold increase over current nursery production levels. Additional investment (not included in the reforestation cost estimate) will be needed to expand capacity for seed collection, seedling production, workforce development, and improvements in pre- and post-planting practices. Achieving this scenario will require public support for investing in these activities and incentives for landowners.
Introduction
To constrain global warming, reductions in fossil fuels emissions are critical. In addition, we must also invest in strategies that remove carbon dioxide from the atmosphere (Masson-Delmotte et al., 2018). Reforestation is a promising opportunity to capture carbon dioxide while providing key ecosystem services including clean air and water (The White House, 2016; Griscom et al., 2017; Fargione et al., 2018; Domke et al., 2020). Enthusiasm for tree planting is gaining momentum, with multiple ambitious goals set forth to restore forest cover for climate mitigation (Griscom et al., 2017; Fuss et al., 2018; Bastin et al., 2019; Holl and Brancalion, 2020) and other environmental co-benefits such as soil stabilization, watershed protection, and wildlife habitat, among others (e.g., Bengston et al., 1999; Neary et al., 2009; Caldwell et al., 2014). These initiatives include the World Economic Forum's One Trillion Trees Initiative, the Bonn Challenge (Verdone and Seidl, 2017), the United Nations Decade on Ecosystem Restoration (Aronson et al., 2020), and the recently-formed United States One Trillion Trees Interagency Council (Federal Register, 2020). Reforestation could sequester an average of 6 metric tons of CO2 per hectare (5,355 pounds per acre) per year (Cook-Patton et al., 2020), but a full accounting of the climate mitigation benefits should also include other impacts (e.g., life cycle emissions, albedo, evapotranspiration; Bala et al., 2007; Kendall and McPherson, 2012; Zhang et al., 2020).
In the United States (US), tens of millions of hectares are potentially reforestable (Fargione et al., 2018; Cook-Patton et al., 2020; Domke et al., 2020; Strassburg et al., 2020). The area in need of reforestation through planting is growing. Currently, only about 31% of the lands that the US Department of Agriculture Forest Service (USFS) reforests are planted, while the rest are reforested through natural regeneration (Dumroese et al., 2019). On both public and private lands, however, increasing severity and size of wildfires (Parks and Abatzoglou, 2020) creates challenges for post-wildfire natural regeneration, thereby increasing the need for tree planting (North et al., 2019). Currently, the USFS is only able to reforest an estimated 20% of national forest lands requiring reforestation (Dumroese et al., 2019) and this gap is widening as the area burned by wildfire increases. The potential for restoring forest cover to these areas using natural regeneration will vary based on seed source proximity and abundance, climate, and land use. Most areas will likely require active planting with seedlings to recover forests within the 10–30-year timeframe of greenhouse gas (GHG) stabilization goals (Nave et al., 2019). The nation's capacity to handle a large increase in tree seedling production, planting, and care within this timeframe is largely unknown.
The most recent estimate (for 2019) of tree seedling production in the US is 1.3 billion (109) seedlings per year (Haase et al., 2020; Figure 1A). Long-term data is only available for the southern region, where most seedling production occurs (Figures 1B, 2). Seedling production peaked in the late 1980s with more than 2.6 billion seedlings per year in the southern US alone (Hernandez et al., 2016; Haase et al., 2020; Figure 1B). From its peak, national seedling production steadily declined to fewer than 1 billion following the 2008 recession. Causes for reductions in seedling production vary regionally and include market demand (i.e., the influence of timber prices on harvest volumes and subsequent planting), consolidation and liquidation of companies within the timber industry, and federal and state budget cuts to cost-share programs, such as the Conservation Reserve Program (Dumroese et al., 2005; Pike et al., 2018). Consequently, 28 private, industrial, state, and federal nurseries have shuttered since 1995 in the southern US alone, effectively reducing production by 650 million seedlings annually (equivalent to 46% of current production). Similar nursery closures have occurred throughout the rest of the US, including dozens of state and federal nurseries (Dumroese et al., 2005; National Association of State Foresters, 2016). In addition, the number of seed storage and processing facilities has steadily declined during the last several decades (Dumroese et al., 2005). While production levels have slowly climbed to the current (2019) level of about 1.3 billion (Haase et al., 2020; Figure 1B), they are still well-below levels needed to meet the growing reforestation demand.
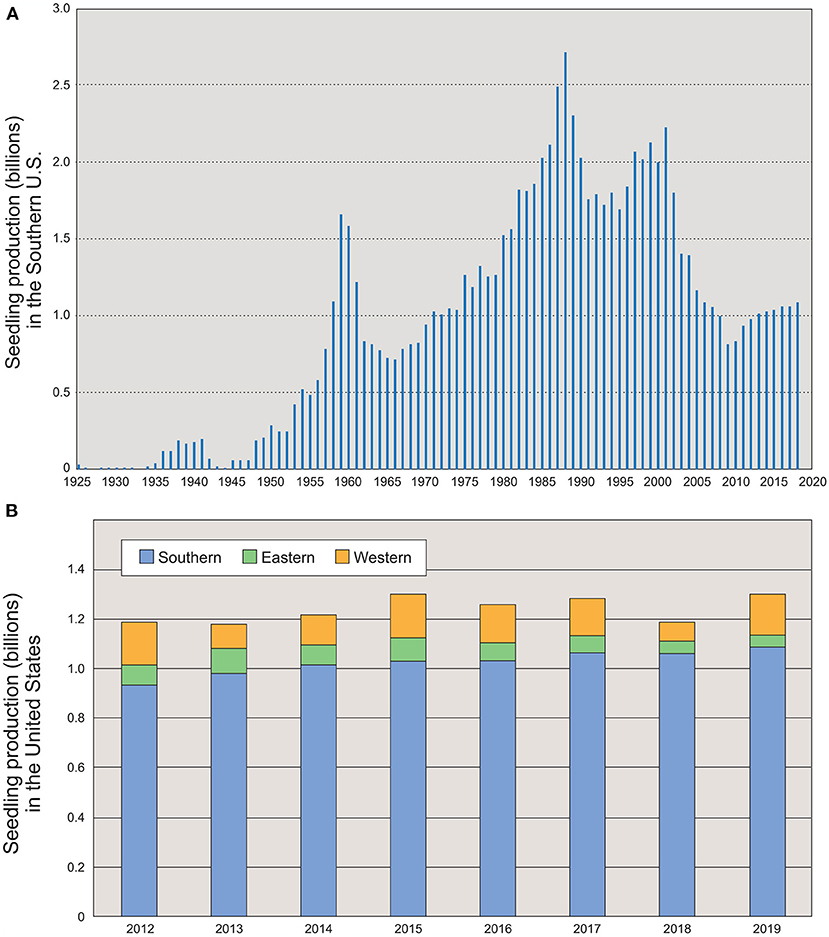
Figure 1. Variation in US seedling production over time. (A) Annual seedling production in the southern US since 1925 (adapted from Hernandez et al., 2016). (B) Seedling production by US region since 2012 (Haase et al., 2020).
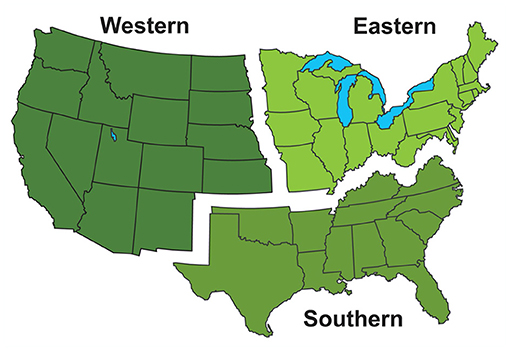
Figure 2. Nursery and reforestation surveys were summarized based on three regions of the US. We lacked information on the area of reforestation opportunity in Alaska and Hawaii, so those states were excluded from this analysis.
To accelerate reforestation, the entire “pipeline” for tree planting (i.e., seeds, nurseries, outplanting, and post-planting activities) would need to be scaled up, including seed collection and storage, nursery production, outplanting, and post-planting treatment and monitoring. Thus, identifying regional limitations and potential solutions is necessary for reforestation to be deployed at scale. Based on an estimate of reforestable land, a survey of nursery managers, a survey of foresters, and a synthesis of the available literature, we estimated how many seedlings would be required, compared that to current production, examined where potential limitations exist in the reforestation pipeline, and offered some potential solutions to projected limitations. Reforestation is generally defined as tree planting in previously forested areas. For the purposes of this paper, we use the term “reforestation” to refer to any tree planting that causes tree cover to increase to more than 25% on lands where forests historically occurred based on modeled potential vegetation (Rollins, 2009; Goward et al., 2016; Fargione et al., 2018; Cook-Patton et al., 2020). This includes lands that recently had forest cover, as well as lands that have been in a non-forest land use for an extended period. The results section presents the current situation and challenges to the reforestation pipeline in the US, based on survey results, GIS analyses, and literature synthesis; the discussion section presents solutions to these challenges.
Methods
Reforestable Area
Prior to 1630, the US had an estimated 414 million hectares (1 billion acres) of forest cover (Oswalt et al., 2014). Since then, forest cover has fluctuated because of extensive clearing for agriculture and human development, partially offset by reforestation of abandoned crop land and cleared forests; currently 310 million hectares (766 million acres) are covered by forests (Oswalt et al., 2019). While many cleared areas (e.g., productive croplands and urban cores) are unlikely to return to forest for economic and social reasons, other cleared areas may contain reforestation opportunities. These potential areas include: (1) unstocked or understocked forest land that is not independently recovering, for example after a fire (Sample, 2017; Dumroese et al., 2019; North et al., 2019; Cook-Patton et al., 2020; Domke et al., 2020); (2) marginal agricultural lands (Johnson et al., 2016; Nave et al., 2019), such as the 1.4 million hectares (3.5 million acres) abandoned in the 8 years between 2008 and 2016 (Lark et al., 2020), and (3) locations with high co-benefits, such as wildlife corridors, riparian areas, and floodplains that could provide habitat and improved water quality (Barnett et al., 2016; Dybala et al., 2019a,b; Keller and Fox, 2019).
A recent analysis identified reforestation potential across the contiguous US (Cook-Patton et al., 2020), finding up to 51.6 million hectares (128 million acres) of potential area for increased forest cover. We used a select portion of their identified area of opportunity on natural lands and marginal agricultural lands to estimate seedling needs across the US (Figure 3). We used the National Land Cover Dataset (NLCD) classification to define natural lands as shrub/scrub, grassland/herbaceous, deciduous forest, evergreen forest, and mixed forest. While areas identified as forest cover in NLCD may not seem to offer reforestation opportunities, we note that Cook-Patton et al. (2020) identified as reforestation opportunities only the portion having no tree cover (i.e., <25% cover) as identified in the North American Forest Dynamics dataset (Goward et al., 2016) and these areas were further statistically discounted by a visual inspection of imagery for 5,000 pixels to remove areas erroneously identified as reforestation opportunities. We modeled a large-scale scenario to determine needs in the reforestation pipeline, including: (1) 80% of the potential on natural lands, (2) 50% of the potential on marginal crop lands (defined by soils that severely limit agricultural production, which we refer to as “challenging soils” here; Soil Survey Staff, 2016), and (3) 50% of the potential on pasture land. Thus, the agricultural lands that we considered included both pasture (regardless of soil condition) and croplands (restricted to challenging soils; Cook-Patton et al., 2020). While the ability to reforest any parcel is uncertain, in aggregate, this probabilistic approach provides a relatively conservative estimate of the magnitude of the reforestation opportunity in the US. Uncertainties include landowner willingness, costs, labor, seed and seedling availability, herbivory, drought, fire, and climate change.
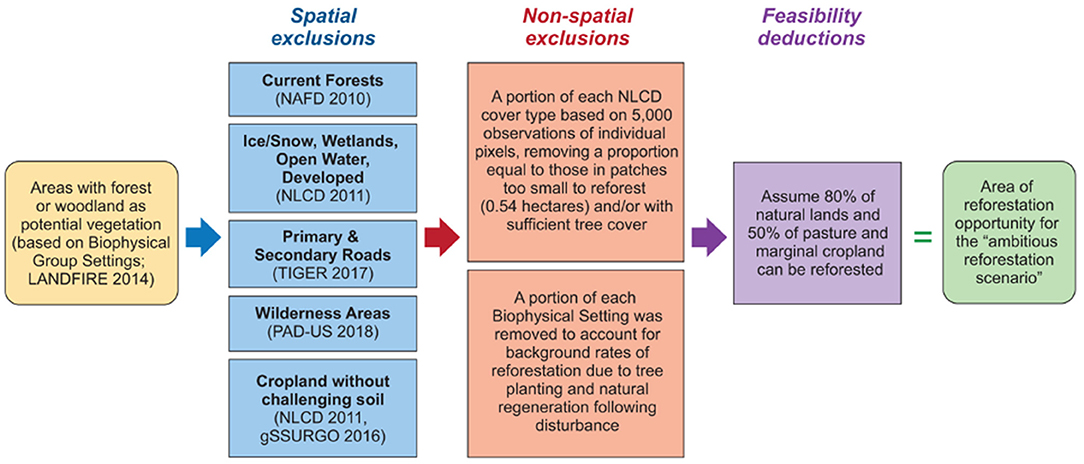
Figure 3. Using data from Cook-Patton et al. (2020), we used these criteria to quantify reforestation opportunities in the US. Datasets are referenced in parentheses where the year indicates the vintage of the data. Citations for these datasets are: LANDFIRE: (U. S. Department of the Interior Geological Survey, 2014); NAFD: (Goward et al., 2016); National Land Cover Dataset 2011: (Homer et al., 2015); TIGER: (US Census Bureau, 2017); Protected Area Database of the US (PAD-US): (U. S. Geological Survey Gap Analysis Project et al., 2018); gSSURGO: (Soil Survey Staff, 2016).
We did not include areas with the opportunity to plant urban trees because urban forestry faces different challenges. Urban plantings typically use older planting stock of different species and incur greater costs for planting and maintenance while generating unique benefits for home energy costs, air quality, property values, and mental health (e.g., Kroeger et al., 2018). Tree nurseries that supply seedlings for urban forestry may have distinct or additional challenges beyond those considered here.
Nursery and Reforestation Surveys
To better understand current nursery production and the possibilities for scaling up seedling production, we conducted a survey in May 2020 (hereafter “nursery survey”). We surveyed managers of all known nurseries in the western, eastern, and southern regions of the US (Figure 2) about their current production, maximum production capacity, seed sourcing, potential for expansion, and limitations to expansion (see Supplementary Material). Respondents (total = 111) were from federal (6), state (31), private (73), and tribal (1) nurseries across 37 states and produce bareroot seedlings (35), container seedlings (43), or both (33). This participation represents ~100% of federal, 75% of state, and 40% of private nurseries in the US, or about half the total estimated annual production in the country (based on Haase et al., 2020). We lacked information on the area of reforestation opportunity in Alaska and Hawaii, so those states were excluded from this analysis.
In June 2020, we conducted a second survey (hereafter “forester survey”) to better understand reforestation practices and costs, including stocktype choice, site preparation, and post-planting activities (See Supplementary Material). Respondent foresters (total = 70) were from federal (17), state (13), industrial private (11), non-industrial private (16), or non-profit (13) organizations and cumulatively conduct work in 44 states. The total number of reforestation practitioners in the US is unknown, so it is unclear how representative this sample is. We averaged responses across the same three regions used for the nursery survey.
To estimate the total seedlings needed for our reforestation scenario, we used the median stems per hectare for each of the three regions as reported in the forester survey to estimate the number of trees that would be planted on the reforestable area in each region. The number of these trees planted annually depends on the speed with which the reforestable area is planted. We estimated the number of trees required to be planted annually for two potential time horizons: from 2022 to 2040 and 2022 to 2030. We primarily focused on the 2040 timeframe to examine challenges along the reforestation pipeline, from seed collection to post-planting treatments. The 2030 scenario would require up-front investments in physical infrastructure and workforce development that would be difficult to justify if demand is not sustained beyond 2030. We asked respondents to estimate costs per hectare for seedlings, site preparation, planting, and post-planting treatments and summed across these four components to estimate total costs. When costs were missing for seedlings and planting, we used regional median costs. All dollar values in this paper are reported in 2020 USD.
Results
Reforestable Area
Our reforestation scenario across the contiguous 48 states included ~15.4 and 10.6 million hectares (38 and 26 million acres) for agricultural (marginal cropland and pasture) and natural lands, respectively, for a total of 26 million hectares (64 million acres; after Cook-Patton et al., 2020; Supplementary Table 1). Five states (Missouri, Kentucky, Tennessee, Virginia, and Arkansas) each had more than 700,000 hectares (1.7 million acres) of reforestation opportunities on agricultural lands, collectively comprising 29% of our nationwide estimate of opportunity on agricultural lands. Five states (Colorado, Montana, Idaho, New Mexico, and Utah) each had more than 700,000 hectares (1.7 million acres) of reforestation opportunities on natural lands, comprising 43% of our nationwide estimate of opportunity on natural lands.
Trees Required Per Year
Based on the forester survey results, we estimated planting density in each region. The median estimates were 741 stems per hectare (300 stems per acre) for western states, 1,344 stems per hectare (550 stems per acre) for eastern states, and 1,489 stems per hectare (603 stems per acre) for southern states. Based on a weighted average of reforestation opportunities in each region, this resulted in a national average of 1,162 stems per hectare (470 stems per acre; Supplementary Table 1). As a check, we compared these numbers with planting densities estimated by the USFS Forest Inventory and Analysis program from Haase et al. (2020), which yielded a weighted average of 1,308 stems per hectare (529 stems per acre)—that is 13% higher than our forester survey-based estimate. We used the more conservative number from the forester survey to estimate 30 billion trees required to reforest our 26 million hectares (64 million acres) reforestation scenario.
To estimate the number of trees required to be planted annually, we assumed that planting could begin in 2022 and would continue through 2040. This effort would require the planting of an additional 1.7 billion seedlings annually. These trees would need to be planted in addition to the 1.3 billion seedlings currently planted, which are almost exclusively planted on recently harvested or disturbed forests rather than the lands we have identified as opportunities to expand forest cover. Planting faster (to achieve the goal by 2030) would require 3.8 billion additional seedlings per year. Thus, we would need 3.0 or 5.1 billion seedlings per year to meet reforestation goals by 2040 or 2030, respectively.
Seed Collection and Storage
Increased seedling production for reforestation will require availability of viable, genetically appropriate seeds (Broadhurst et al., 2015, 2016; Gann et al., 2019). Tree nurseries acquire seeds from a variety of sources depending on species, production method, availability, cost, customer demand, end-use goals, and other considerations (Bonner and Karrfalt, 2008). Generally, seeds are obtained from specialized seed orchards and seed production areas (SPAs) or from the wild (Nevill et al., 2016; Erickson and Halford, 2020). Orchards and SPAs aim to produce seeds of higher quality and health potential (e.g., disease resistance) compared with wild-collected seeds and generally focus on commercial timber species. Due to decreasing investments in tree improvement during the past few decades, these seed resources have been declining, exposing gaps in the nation's tree seed supply (Wheeler et al., 2015). In the absence of orchards and SPAs, a high reliance is placed on harvesting seeds from wild stands or even seeds from urban trees, often with unknown genetic origin or quality.
Despite the growing demand for seeds across many geographies, guidance for collection, quality testing, and provenance reporting are often lacking (Ryan et al., 2008; Frischie et al., 2020). Successful seed collection programs rely on skilled collectors to identify, monitor, and access appropriate collection locations (Kelly, 1994; Hay and Probert, 2013; Whittet et al., 2016; Pike et al., 2020). Most seeds are only available for collection during limited windows of time, such that collectors require the knowledge to appropriately schedule collection efforts and mobilize resources. This is particularly important for species that exhibit seed masting behavior, for which seed production occurs sporadically across years and is difficult to predict (Gallego Zamorano et al., 2018). Chronic under-investment in skilled labor, infrastructure, and workforce training has, in many places, led to a limited capacity for seed collection, testing, and storage, which contributes to higher seed costs and limited inventory (Oldfield and Olwell, 2015; Broadhurst et al., 2016). In addition, it is becoming critical to understand how different seed sources may respond to shifting climate conditions and thus inform provenance selection and seed transfer consideration aimed at long-term reforestation success (Erickson et al., 2012; Jones, 2013). Many commercial seed collectors, however, operate across wide geographic areas and have limited information on the population-level genetics of a seed source (Kramer et al., 2019). Taken together, these factors contribute to a lack of a robust seed supply that impedes the ability to achieve reforestation goals (Jalonen et al., 2018). Species typically grown via vegetative propagation, such as cottonwood (Populus spp.) and willow (Salix spp.), face similar challenges around sourcing appropriate plant material.
In addition to the limited supply of seeds, the ability to properly clean, process, and store seeds requires specialized expertise, equipment, and storage facilities to ensure seed viability is maintained for years or decades (Bonner and Karrfalt, 2008; Frischie et al., 2020). The duration of seed storage that is biologically possible differs by tree species. Seeds of many species can be stored long-term, if appropriate storage facilities are available, while others (i.e., “recalcitrant” seeds) cannot be stored over multiple years (Bonner and Karrfalt, 2008; De Vitis et al., 2020). For species with recalcitrant seeds, in situ conservation in living collections, seed orchards, or SPAs are critical (Dickie and Pritchard, 2002; De Vitis et al., 2020).
Nationally, our nursery survey found that roughly 20% of seedlings are produced from wild-collected seeds. In southern and western regions of the US, most nurseries obtain <25% of their seeds from wild stocks, and a small percentage of nurseries rely heavily (>75%) on wild seed collection (Figure 4; Supplementary Table 2). In contrast, eastern nurseries generally rely more heavily on wild seed collection, with 87% of nurseries collecting at least 25% of their seeds from wild sources. The lower percentages of wild-collected seeds in the southern and western regions reflect the focus on orchard development for regionally important timber species, such as loblolly pine (Pinus palustris Mill.) and slash pine (P. elliottii Engelm.) in the south and Douglas-fir (Psuedotsuga menziesii Mirb. Franco) in the west (Wheeler et al., 2015). Species planted in low volumes, particularly hardwood species (e.g., oaks; Quercus spp.; Pike et al., 2018), generally do not have established orchards or SPAs and are typically grown from wild-collected seed. The higher use of oaks and other hardwoods explains the greater reliance on wild collection in the eastern region. However, heavy reliance on wild collection can create seed sourcing challenges. For example, many oak species, along with other hardwoods, produce recalcitrant seeds (Bonner and Karrfalt, 2008) making long-term seed storage difficult. Furthermore, many oak species also exhibit masting (Sork, 1993), which makes seed sourcing from wild collections unpredictable.
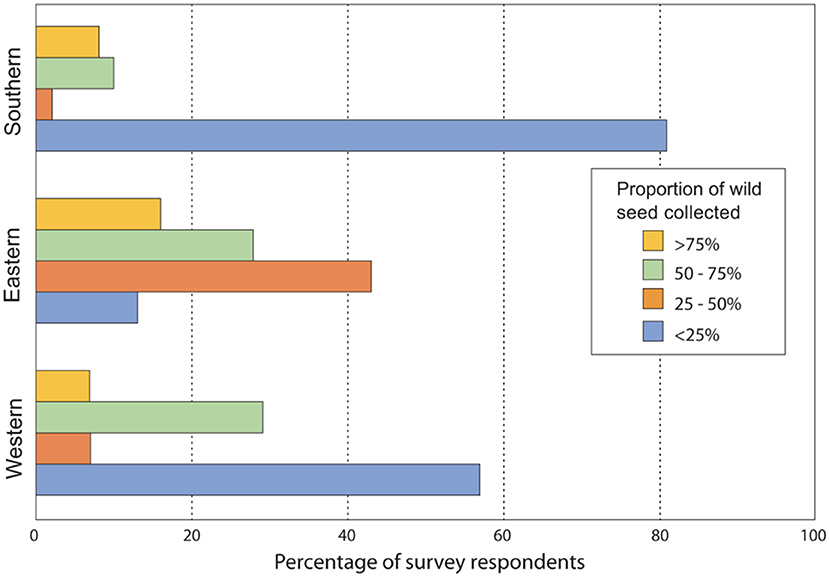
Figure 4. Percentage of seed that is collected from the wild (as opposed to from orchards or SPAs) as used by tree nurseries in three regions of the US. We excluded nurseries that reported at least 50% of their seeds coming from unknown sources. Sample size was 17, 33, and 50 for southern, eastern, and western regions, respectively.
In our nursery survey, larger nurseries tended to rely the least on wild collection, especially in the western and southern regions. On average across regions, nurseries that collected <25% wild seeds were 16 times larger (mean of 19.6 million seedlings per year, n = 19) than nurseries that collected more than 25% wild seeds (mean of 1.2 million seedlings per year, n = 37).
It is unclear how many additional seeds could be made available from orchards and SPAs. For example, collection could resume in older orchards where seed collection has ceased (North Carolina State University Cooperative Tree Improvement Program, 2020), although the availability of orchards and SPAs is limited to a relatively small number of commercial species. Therefore, near-term increases in seedling production to meet large-scale reforestation goals may disproportionately rely on increased wild seed collection, because orchards and SPAs require several years to establish and develop. If seed sourcing from orchards and SPAs remains unchanged, then wild seed collection would need to expand from currently sourcing about 0.3 billion seeds to about 2 billion seeds, a more than 6-fold increase. Increased wild seed collection will require additional trained seed collectors and may be complicated by increasing temporal variation due to a changing climate, especially for species that exhibit mast seeding (Koenig and Knops, 1998; Pearse et al., 2017). To supplement seed propagation, an increase in vegetative approaches such as somatic embryogenesis could be used to select and produce seedlings (Denchev and Grossnickle, 2019).
Based on our nursery survey, the average seed inventory per nursery would supply 3.8 and 1.4 years of conifer and hardwood species production, respectively, at current levels (Figure 5; Supplementary Table 2). This is insufficient to meet a more than 2-fold increase in annual seedling production, meaning that seed collection will have to be rapidly ramped up to meet the increased demand. Seed inventories vary regionally and depend on species-specific seed storage requirements, access to facilities to safely store seeds, adequate funding to support storage and infrastructure, and assurance that demand for a particular species will persist.
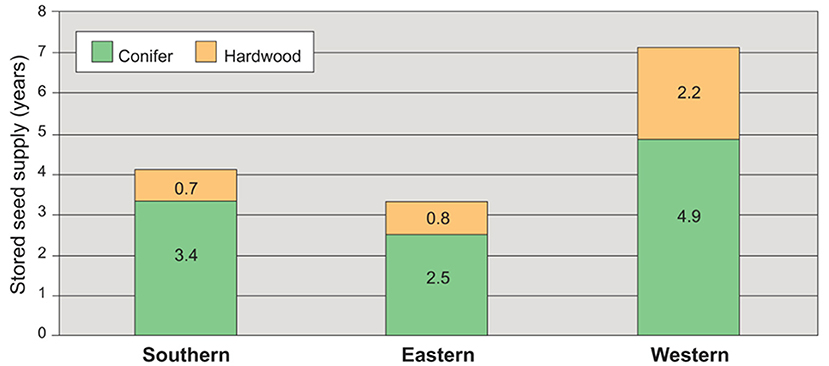
Figure 5. Estimated years of conifer and hardwood seed inventory available to support current (2020) seedling production levels in the US by region.
Tree Nurseries
Only 32% of nursery survey respondents currently produce at full capacity, with greater potential to boost capacity in federal and state nurseries compared with private nurseries. Federal nurseries could increase production by 151% and state nurseries by 74%, on average, to produce at full capacity, whereas private nurseries could increase by 21% (Supplementary Table 2). In our nursery survey results, private nurseries produce 80% of the seedlings; thus the average percentage increase to full capacity across all ownerships is 34%. Based on these results and extrapolation to all forest nurseries in the country, increasing all nurseries to maximum production would produce about 0.4 billion additional seedlings per year, about 25% of the additional 1.7 billion seedlings per year required in our large-scale reforestation scenario.
Further increasing production (i.e., beyond current maximum capacity) would require expansion of nursery infrastructure. Nurseries generating container seedlings tended to report a greater potential to expand nursery infrastructure than those producing bareroot seedlings. Of the survey respondents producing container seedlings, 61% reported the ability to expand beyond current maximum capacity by 10–100%, and 28% of those could double (or more) their current nursery size. In contrast, 57% of those producing bareroot seedlings have the ability to expand by 10–100%, and only 12% could double (or more) their current nursery size. Based on an average potential for production expansion of 94 and 47% for container and bareroot, respectively, we estimate an overall expansion potential of about 1.1 billion seedlings per year. Thus, current production (1.3 billion) combined with 0.4 billion from boosting production to maximum current capacity and 1.1 billion from nursery expansion beyond maximum capacity yields a total of 2.8 billion seedlings. This is 93% of the goal of 3.0 billion seedlings per year to meet reforestation goals by 2040.
While 76% of nursery survey respondents expressed a willingness to expand, 24% cited no desire to do so. In general, nursery respondents reported multiple limitations to expansion (Figure 6; Supplementary Table 2), with the biggest concern being insufficient workforce. Most nurseries have a year-round, core staff of 3–12 people and rely on seasonal crews of 30–100 workers during sowing, harvesting, sorting, and packing. Even without scaling up, labor shortages have been an increasing concern to accomplish seasonal nursery work due to remote nursery locations and competition with other agricultural operations (Shropshire, 2018; Trobaugh, 2018). Immigration policies also affect worker availability (Westerman, 2020). After labor, financial needs and market risks were the most frequently identified limitations to expanded production (Figure 6; Supplementary Table 2). Large, private nurseries generally grow seedlings on a contract basis. Public nurseries, especially state nurseries, and smaller private nurseries often grow a portion of their stock on a speculative basis, putting them at greater financial risk (e.g., Pike et al., 2018). Because it takes 1–3 years to produce seedlings, this uncertainty about demand and associated sales is a significant concern for scaling up.
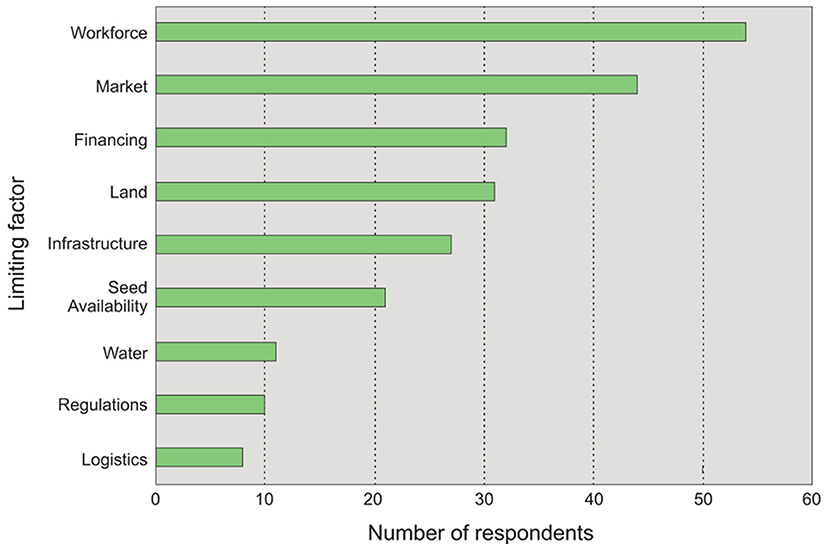
Figure 6. Relative importance of factors cited as limiting nursery production expansion, based on a 2020 survey of 111 US nurseries.
Public nurseries (federal and state) are also under various restrictions to prevent them from competing with private nurseries. For example, federal nurseries can only sell to other public entities. Regulations on state nurseries vary greatly by state and generally consist of one or more of the following restrictions on sales: a minimum sales amount (e.g., 25–100 seedlings per order); type of landowner (e.g., must have at least 5 acres [2 hectares]); intended use (e.g., conservation or reforestation only); and geographic area (e.g., must be within the state).
Outplanting
Outplanting is the permanent placement of nursery-grown seedlings on the reforestation site. The number of seedlings outplanted per year in the US is roughly equal to the number of seedlings produced by US nurseries plus the small amount (1.4%) imported from Canada (Haase et al., 2020). In general, outplanting efforts start with clearly defined objectives by the landowner or manager (e.g., wildlife habitat, timber production, carbon sequestration) and include site evaluation, species selection, stocktype specifications (i.e., type, size, and seedling morphological characteristics), genetic source, site preparation (e.g., vegetation control and soil conditioning), seedling transportation and storage, planting arrangements (i.e., density and distribution), planting techniques (i.e., hand or machine), and planting windows (Rose and Haase, 2006; Landis et al., 2010). An important consideration is to use seedlings grown from seeds collected from known provenance and genetics appropriate to the site's seed zone. Assisted migration strategies to mitigate climate change must also be considered (e.g., Joyce and Rehfeldt, 2017).
Based on our forester survey, current outplanting activities for site preparation and planting environment are summarized in Table 1. Survey respondents reported mechanical and chemical vegetation management as the most common site preparation practices across all three regions. Best management practices that promote successful reforestation vary by region and species. Although relatively few states have detailed guidelines on reforestation practices (e.g., Rose and Haase, 2006), many programs follow the Target Plant Concept, in which the nursery manager works directly with the client to plan and monitor outplanting activities to optimize seedling performance (Landis, 2011; Dumroese et al., 2016; Grossnickle and MacDonald, 2018). Failure to follow regionally specific reforestation guidelines can lead to increased mortality, as can poor stock quality, improper handling or transportation, poor planting techniques, and inappropriate timing (i.e., missing the planting window).
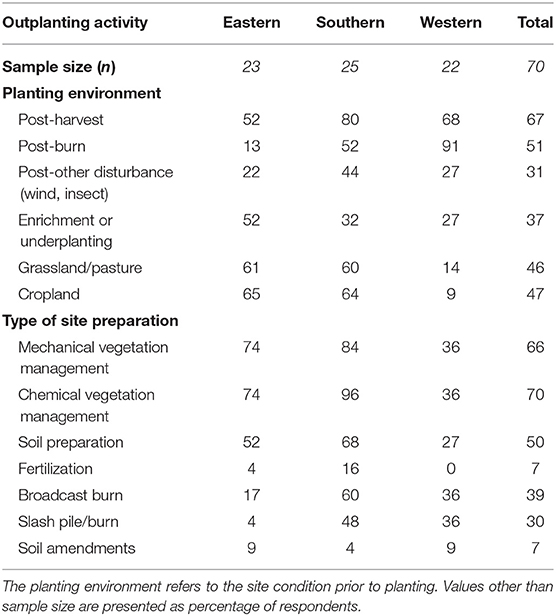
Table 1. Percentage of foresters implementing various outplanting activities in a typical reforestation effort by US region.
One of the greatest challenges to outplanting efforts is seedling mortality caused by water and heat stress, herbivory, disease, competing vegetation, or fire (Côté et al., 2004; Allen et al., 2010; Burney and Jacobs, 2013, 2018; Thyroff et al., 2019). Fire is a particular concern when seedlings are planted on warm, droughty sites in close proximity to downed woody material (North et al., 2019; Coop et al., 2020). Additionally, projected future climate conditions in some areas will create a mismatch between planted genotypes and the new climate. For example, warmer winters may cause some trees to prematurely lose cold hardiness, leading to freezing damage and decreased survival and growth (Kosiba et al., 2013).
Post-planting
While respondents to the forester survey reported substantial investments in site preparation practices prior to planting, most reported little investment into post-planting activities (Table 2). Lack of post-planting activities, also known as “plant and walk away,” can result in poor growth or survival of outplanted seedlings. A misplaced emphasis on how many trees are planted rather than how many survive and thrive can compromise reforestation success.
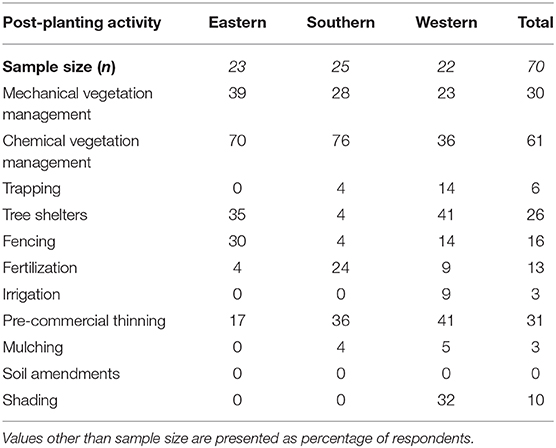
Table 2. Percentage of foresters implementing various post-planting activities in a typical reforestation effort by US region.
Industrial reforestation programs typically invest in post-planting activities to ensure seedlings are “free-to-grow” above the competing vegetation and animal browse within the first few years, thereby protecting against losses and maximizing profits. For example, industrial reforestation programs often use browse protection measures (e.g., tree shelters, and repellents) and herbicide treatments to control competing vegetation (Ward and Stephens, 1995; Davis et al., 1999; Devine and Harrington, 2008; Maguire et al., 2009). Non-commercial reforestation efforts, however, often lack funding for post-planting activities, even though site conditions in these areas are typically challenging for seedling establishment, survival, and growth (North et al., 2019). Without some form of intervention, abiotic and biotic stress factors can result in planting failure on many reforestation sites (e.g., King and Keeland, 1999; Ouzts et al., 2015).
Investments in post-planting activities are shrinking. North et al. (2019) found that post-planting release treatments on National Forests in the western US have declined by more than 40% over the past 20 years. This decline is likely a function of workforce reductions, a shift in budget resources toward fire suppression, and other factors (North et al., 2019). When a lack of post-planting efforts results in wholesale failure of plantings, this represents a loss of resources invested into the entire reforestation pipeline, including seed sourcing, seedling production, and planting. Scaling-up reforestation would increase these challenges and require expansion of the workforce, expertise, and resources necessary for successful post-planting treatments.
An associated concern with post-planting activities is inadequate monitoring to gauge reforestation success, identify potential causes for failures, and remedy any identified shortcomings (Hobbs et al., 1992). Inadequate or absent monitoring of reforestation projects are due to (1) knowledge gaps on best practices for cost-effectively monitoring reforestation success, (2) limitations in funding and capacity, and (3) short-term planning that does not go beyond tree planting (Mansourian et al., 2017).
Planting Costs
Reforestation costs vary widely and are driven by regional differences in silvicultural prescriptions such as site-preparation, post-planting treatments, species (hardwood/conifer), stocktype (container/bareroot), and planting density. Our survey estimated median reforestation costs to be $788, $1,058, and $2,098 per hectare ($319, $428, and $849 per acre) for the southern, western, and eastern regions, respectively. Our survey estimated the 25th and 75th percentile (quartile) ranges to be $662–$1,006, $862–$1,290, $1,282–$4,319 per hectare ($268–$407, $349–$522, and $519–$1,748 per acre) for the southern, western, and eastern regions, respectively. Higher costs in the East are partly due to the greater expense of hardwood seedlings compared with conifers. Higher costs in the West are partly due to the higher price of seedlings grown in containers compared with seedlings grown bareroot, as the western region plants the highest proportion of container-grown seedlings. Lower costs in the South are partly due to the relatively inexpensive 1-year-old bareroot seedlings produced in those states. The USFS reports higher reforestation costs of $2,347 per hectare ($950 per acre) in California. While our survey did not allow us to estimate average costs specific to the state of California, this amount is roughly double the cost we estimated for the western region. We interpreted this as a real difference in costs between California and other states in the western region revealed by the higher-resolution (i.e., state-level vs. region-level) of the USFS data and therefore used the USFS estimate for California (and our surveyed estimates for the other 47 states).
We estimate that replanting all 26 million hectares (64 million acres) of our identified areas for reforestation opportunity would cost $33 billion, or an area-weighted national average of $1,262 per hectare ($511 per acre). Using the quartile range from the survey, the area-weighted national range is $24–53 billion. This cost includes seedlings, site-preparation, planting, and post-planting treatments until trees are considered free to grow. While it varies by region, site, and other variables, in general, the USFS estimates that 50% of their costs are for the planting crew, 29% for site preparation, 15% for seedlings, and 6% for contract administration and post-planting surveys and analysis (N. Balloffet, USFS, Forest & Rangeland Management & Vegetation Ecology, personal communication). The estimated cost does not include the required expansion of infrastructure and operations associated with seed collection, seed banks, and nursery production.
As the range of reported reforestation costs indicates, costs at individual sites can be much more expensive than median costs depending on site-conditions, planting methods, biotic and abiotic stressors, and the expense of seedlings and planting crews. The following factors were identified by survey respondents as determining the cost: slope; amount of slash; soil compaction; competition from invasive species or pasture grasses; remoteness; the extent to which site preparation is required; whether soil amendments are added; whether fencing is required for browse protection; whether shade shelters or irrigation are required to reduce water stress; whether planting or site preparation are done manually or mechanically; the size of the project (larger projects gain economies of scale); seedling species and stocktype; seasonal labor constraints; and time since harvest or disturbance (multiple years that allow growth of competing vegetation are more costly).
Discussion
A surge in reforestation efforts to meet ambitious goals will require increased support throughout the reforestation pipeline across all regions of the US. Our survey and analyses illustrate how a large-scale reforestation program requires more than doubling current seedling production and outplanting. Expansion efforts must address expected limitations, especially adequate seed supply, consistent market demand, and a sufficient and appropriately trained workforce for nursery and field work.
Overcoming Challenges to the Reforestation Pipeline
To successfully meet reforestation goals, capacity must be expanded across the entire pipeline, from seed collection and storage, to nursery production, to outplanting and post-planting treatments (Haase and Davis, 2017; Guy, 2020; Höhl et al., 2020). Several bottlenecks within the reforestation pipeline must be addressed to achieve this expansion (Figure 7). In the past, federal and state cost-share assistance programs have been effective funding mechanisms to support tree planting and increase productivity on non-industrial private forest lands (Haines, 1995) and similar programs could again be deployed to meet current goals.
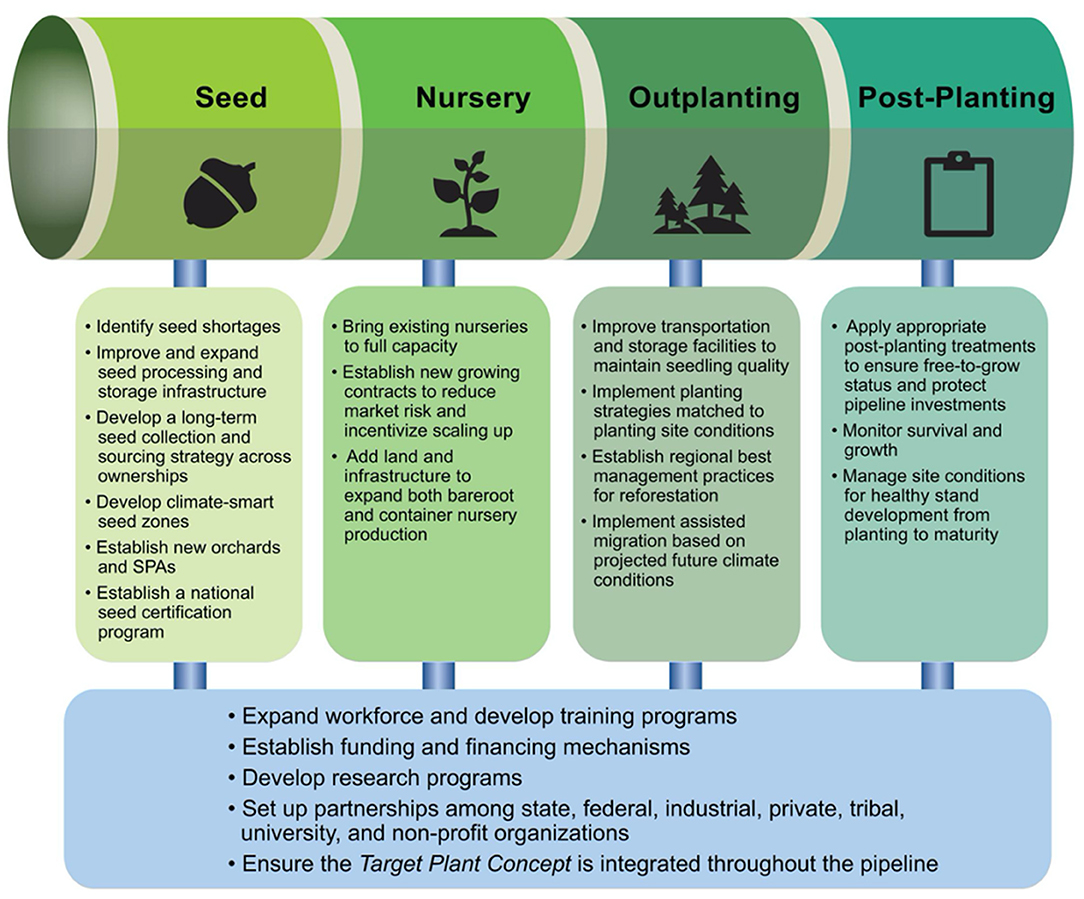
Figure 7. Actions needed to address challenges within the reforestation pipeline in order to implement an ambitious reforestation scenario.
Our surveys identified labor shortage as the single largest issue. Seasonal migrant workers have been the standard in nursery and forestry field work for many years, but the availability of this labor pool is influenced by immigration policies and competition within the agricultural sector. Historical precedent illustrates that federal programs to reduce unemployment and train workers can expand nurseries and tree planting. For example, nursery work and tree planting were once part of the Civilian Conservation Corps' (CCC) main activities (Dumroese et al., 2005). Similarly successful efforts have been undertaken in other countries (Vadell et al., 2016). The current goals to increase reforestation and the extreme unemployment levels created by the 2020 coronavirus pandemic could both be addressed by programs to develop a tree-planting workforce similar to the CCC (Kurtz, 2020; O'Mara, 2020). Below we discuss additional options for addressing pinch points along each stage of the reforestation pipeline.
Seed
To ensure an adequate tree seed supply, investments are needed to identify gaps and to build seed collection capacity and expertise (Jalonen et al., 2018; Barga et al., 2020; Erickson and Halford, 2020). For example, a useful model that could be expanded to address seed shortages is the “Assessment of Native Seed Needs and Capacities” conducted by the National Academies of Sciences Engineering Medicine (2020) though this effort currently targets non-tree species. Similarly, the Seeds of Success (SOS) program, led by the US Department of the Interior Bureau of Land Management (BLM) provides hands-on training and expertise in seed collection and conservation, although it also focuses on non-tree species (Barga et al., 2020). Expanding these efforts or conducting a similar systematic nation-wide effort focused on tree species would help to address seed shortages. Furthermore, the implementation and integration of seed certification programs, such as those in Europe and many US states (De Vitis et al., 2017; Association of Official Seed Certifying Agencies, 2018; Mainz and Wieden, 2019) to identify and track plant material along the supply chain would improve seed quality and planning capacity needed to meet the local and national reforestation goals outlined by the United Nations and similar initiatives (FAO UNEP, 2020). Any program to increase seed supplies must also include a seed testing component to ensure seed quality (Ryan et al., 2008; Erickson et al., 2012; Jones, 2013; Kramer et al., 2019; Frischie et al., 2020).
The development of seed zones and seed transfer guidelines for a number of species across the US, particularly for taxa that do not constitute high-value commercial species, represent a major advance in helping producers identify the most suitable genetic sources for a particular planting site (Cunningham, 1975; Alden, 1991; Bower et al., 2014; Erickson and Halford, 2020; Pike et al., 2020). For example, “Generalized Provisional Seed Zones” use climate data and ecoregional boundaries to delineate safe areas for seed transfer for seeds that lack species-specific transfer guidelines (Bower et al., 2014). Continued research is needed to understand how well these seed zones function and how this guidance may need to be refined in the face of a changing climate (Pike et al., 2020). Additionally, empirical research findings will need to be translated into practical decision support tools, like the “Climate-Smart Restoration Tool,” which helps match the climate adaptability of seeds with climate zones and conditions of candidate sites. To be effectively integrated into planning, such tools will need to be expanded to cover a wider range of species across the US (Cooke et al., 2019; Kilkenny et al., 2020).
Continued improvements to the genetic quality of the seed supply would also improve the outcome of reforestation efforts (Broadhurst et al., 2008; Alfaro et al., 2014; Jalonen et al., 2018; Erickson and Halford, 2020). In particular, tree improvement and forest genetics programs could further focus on physiological and restorative values such as drought, heat, insect, and disease tolerance, as well as adaptability to changing climatic conditions (Morgenstern and Wang, 2011; Wheeler et al., 2015; Nevill et al., 2016). While still in its infancy, genomic technologies for targeting disease resistance may play a bigger role in future seed sourcing decisions (Breed et al., 2019).
Nurseries
To increase seedling production beyond current production levels in the US, nurseries that currently produce below maximum capacity could bring their production up to full capacity, and nurseries that have the ability to expand beyond their current capacity could do so, either within their current footprint or onto additional lands. Additionally, new nurseries could open if the incentive to do so is sufficiently attractive and long-term markets are expected. Creative partnerships with other plant producers could result in mutually beneficial endeavors. For instance, legal cannabis production in some states has outweighed demand by as much as 50% (Crombie, 2019). Other plant production industries, such as landscaping, agriculture, or seasonal products, may also be underproducing and therefore underutilizing or abandoning existing facilities that could be used for tree seedling production.
To expand beyond current maximum capacity requires more land and facilities. Bareroot nurseries are ideally located on relatively flat land with favorable soil conditions, as well as essential infrastructure, such as tractors and implements for sowing, culturing, lifting, packing, and storing seedlings prior to shipment to planting sites. Container nurseries also require relatively flat land, construction of outdoor growing compounds, shadehouses, or greenhouses, storage facilities, and specialized equipment. Growing space needs will vary depending on local target species, growing densities, and stocktypes. For example, hardwood seedlings tend to be grown at much lower densities compared with conifer seedlings. All nurseries need access to a reliable, abundant, and clean water source and installation of an effective irrigation system as well as structures for administration, processing, and storage. Planning for a significant surge in tree seedling production over the next 10–15 years should also include a plan for longer term adjustments to changes in future demand.
Increased seedling production must not be done at the expense of seedling quality. For example, simply sowing more seeds in bareroot beds is not an option as seedling growing density significantly impacts seedling quality. Using the Target Plant Concept (Dumroese et al., 2016), nursery-grown seedlings must be matched to the conditions of the outplanting site and thus be morphologically balanced and physiologically ready to grow and thrive after outplanting (Grossnickle and South, 2017). Adopting a national nursery certification program aimed at producing high-quality seedlings may be helpful in conjunction with scaling up efforts.
Outplanting
Improving regional and state reforestation guidelines, as well as education and training programs for land managers and tree planters, would help reduce the risk of outplanting failures, particularly on harsh sites. Following such guidelines can reduce seedling mortality due to improper handling/transportation, poor planting techniques, and inappropriate timing (i.e., missing the planting window). Guidance also helps ensure that species and genotypes are matched with the climate and environmental conditions of the outplanting site.
Increasing access to temporary storage facilities and transportation units (i.e., covered and/or refrigerated trailers or vans) can protect seedlings from threats such as temperature extremes, desiccation, and rough handling (Landis et al., 2010). Access to this type of equipment and facilities could be developed as a cooperative model among regional organizations. Additionally, it is important to refine planting windows based on shifting climate conditions to reduce risks to seedlings and optimize survival and growth after outplanting (Landis et al., 2010).
Future research efforts should focus on outplanting strategies that could increase reforestation success and decrease associated costs. For example, ground-based robotics and unmanned aerial vehicles for both planting trees and direct seeding are potential new technologies (Aghai and Manteuffel-Ross, 2020). Another promising strategy is nucleation planting, where trees are planted in clusters that provide future seed sources for colonizing unplanted areas. This approach can mimic natural regeneration processes and provide landscape-scale heterogeneity which can improve habitat quality and reduce future disturbance size or severity (Corbin and Holl, 2012). For example, landscape heterogeneity can reduce the spread of pests and diseases (e.g., mountain pine beetle; Raffa et al., 2008) and reduce the size of high-severity burn patches (Hessburg et al., 2019).
Post-planting
Evaluation of post-planting survival, growth, tree density, and tree cover through field monitoring and remote sensing enables land managers to mitigate any issues, ensure that planted trees reach a “free to grow” status, and learn from projects that succeed vs. fail (McDonald et al., 2016). Additionally, monitoring efforts provide an opportunity to assess the overall performance of the pipeline (connected to the Target Plant Concept) and refine the process to improve future efforts. Post-planting activities may eventually progress into young stand management activities, such as pre-commercial thinning, depending on ownership objectives.
While post-planting activities are often more expensive and likely to receive the least amount of attention of the four pipeline components (Table 2), investments in the final stage of the pipeline protects prior investments into seed collection and storage, nursery production, and outplanting. Furthermore, additional accounting for the full suite of benefits trees provide, including carbon capture, protection of clean water, and other ecosystem services (Lal et al., 2009; Stanturf et al., 2014; Keller and Fox, 2019; Ovando and Brouwer, 2019) can help justify post-planting monitoring and maintenance costs (Hamrick and Gallant, 2018). Water quality is an especially promising avenue for potential payments for ecosystem services. For example, in a nationwide survey of over 1,000 US households, respondents were more willing to pay for water quality than habitat, flood control, or landscape esthetics (Aguilar et al., 2018).
Costs
We estimated the planting costs for 26 million hectares at $33 ($24–$53) billion, but this estimate does not include additional investments in infrastructure necessary to expand pipeline capacity in seed collection and storage, nursery production, outplanting, and post-planting activities. These costs may be substantial, and while many promising paths for scaling up the reforestation pipeline exist, large federal and state incentives and technical assistance will be required to achieve the goals identified here. Studies have shown that forest and other rural landowners respond to incentive payments and plant more trees for timber production than simple market forces would generate (De Steiguer, 1984; Royer and Moulton, 1987; English et al., 1997; Daniels et al., 2010). Currently, when reforestation occurs on private lands, private landowners typically bear most reforestation costs even with government cost-share programs, which could be increased to further offset these costs. Additionally, incentive programs can be effective for prompting tree planting for production of ecosystem services such as wildlife habitat, carbon storage, sustainable conservation of forestlands, and other forest-based amenities (Jacobson et al., 2009; Tian et al., 2015; Snyder et al., 2019; Chizmar et al., 2021). Increased efficiencies and economies of scale that help reduce costs would also make it more feasible to meet ambitious reforestation goals (Corbin and Holl, 2012; Corbin et al., 2016; Almeida et al., 2019; Aghai and Manteuffel-Ross, 2020). Incentive programs and other funding mechanisms must account for cost variations due to location, stocktype, terrain, and other factors; therefore, a simple “one-dollar-per-tree” approach is often inadequate and cannot be applied universally.
Uncertainties in Reforestable Areas
The area of land we are able to reforest will depend greatly on the costs and returns of tree planting, public incentives, and landowner willingness (Claytor et al., 2018). Several biophysical factors may also limit the need or ability to reforest the entire 26 million hectares (64 million acres) classified in our analysis as reforestable. In addition, while our analysis restricts reforestation opportunities to places that were historically forested, which excludes natural grasslands, shrublands, and woodlands, care should be taken not to adversely impact species of conservation concern that are dependent on non-forest habitat.
Planting in pastures faces challenges associated with appropriate species selection, site-preparation to remove competing vegetation and reduce soil compaction, and may have mortality associated with water stress in more arid environments if not irrigated (Löf et al., 2012; Cunningham et al., 2015). The 14 million hectares (35 million acres) of pastures in our reforestation scenario comprise only about 8% of the 178 million hectares (440 million acres) of grazed lands in the US (2017 Ag Census https://quickstats.nass.usda.gov/). In some areas, trees could be planted on pasture either by ceasing grazing and converting completely to forest or by introducing silvopastoral systems, which allows continued use of the land for livestock production (Barlow et al., 2016; Jose and Dollinger, 2019).
Another area of concern is semi-arid forests at low elevations, which provide marginal conditions for reforestation due to drought and heat stress (Kolb and Robberecht, 1996). In these areas, alternating periods of cool/wet and hot/dry conditions result in expansion and contraction of low-elevation forests (League and Veblen, 2006; Rother and Veblen, 2017; Davis et al., 2019). Tree seedlings cannot tolerate stress as well as mature trees, especially in hotter and drier environments (Dobrowski et al., 2015). Climate change can exacerbate this issue as the climate continues to deviate from the more favorable historic conditions that enabled the mature trees to establish (Rodman et al., 2020). The combination of disturbance and ongoing climate change may necessitate a shift in the composition of genotypes, ecotypes, and/or species (Coop et al., 2020). Reforestation goals in these areas may benefit from assisted population, range, and/or species migration (Williams and Dumroese, 2013). In some cases, shifting restoration goals to non-forest vegetation may be more practical.
Climate change also contributes to the increasing size and frequency of wildfires (Seidl et al., 2017), with a direct relationship between the area and intensity of fires and increasing temperature (Abatzoglou and Williams, 2016; Westerling, 2016; Parks and Abatzoglou, 2020). Current rates of reforestation are already inadequate to keep up with the “backlog” of burned areas that are not naturally regenerating, and climate change is expected to exacerbate this by increasing the size and intensity of wildfire (Dumroese et al., 2019). This is likely to lead to an increase in the area in need of reforestation, in addition to the 26 million hectares that we identified in our large-scale scenario. This climate-driven increase in the likelihood of fire also has the potential to increase mortality of young trees, thereby reinforcing a non-tree dominated ecosystem (Enright et al., 2015; Turner et al., 2019; Keyser et al., 2020). This risk is increased if post-burn plantings are high-density, because any subsequent fires are likely to burn at higher severity in the plantings than in surrounding unmanaged areas (Thompson et al., 2007; Zald and Dunn, 2018). Thus, it is critical to consider the likelihood of future fire when designing the density and spatial arrangement of post-fire plantings (North et al., 2019).
Conclusion
We considered a scenario where 26 million hectares (64 million acres) of natural and agricultural lands are reforested by 2040 with 30 billion trees at an estimated cost of $33 ($24–$53) billion dollars. This scenario would require increasing the number of tree seedlings produced each year by 1.7 billion, a 2.3-fold increase over current nursery production levels. Meeting this or similarly ambitious reforestation goals will require expanded capacity for seed collection, nursery production, workforce development, and improvements in pre- and post-planting treatment practices. Historically, in the US and elsewhere, such investments have successfully provided the labor and plant materials necessary to increase forest cover on the landscape (Vadell et al., 2016; Dumroese et al., 2019) and provide benefits to society for biodiversity, clean water, soil stabilization, recreation, and fiber.
Data Availability Statement
The original contributions generated for the study are included in the article/Supplementary Material, further inquiries can be directed to the corresponding author.
Author Contributions
All authors contributed to the writing and editing of the manuscript.
Funding
Partial funding for this project was provided by the Paul and June Rossetti Foundation.
Conflict of Interest
RG is employed by the company Guldin Forestry LLC and RD is employed by Arborgen, Inc.
The remaining authors declare that the research was conducted in the absence of any commercial or financial relationships that could be construed as a potential conflict of interest.
Acknowledgments
We acknowledge Elizabeth Bowersock, Lori Mackey, Andrew Nelson, Chelsea Silva, Zhao Ma, and Dan Majka for help in conducting surveys, Jim Marin for producing the figures, and two reviewers for significantly improving the manuscript.
Supplementary Material
The Supplementary Material for this article can be found online at: https://www.frontiersin.org/articles/10.3389/ffgc.2021.629198/full#supplementary-material
References
Abatzoglou, J. T., and Williams, A. P. (2016). Impact of anthropogenic climate change on wildfire across western US forests. Proc. Natl. Acad. Sci. U.S.A. 113, 11770–11775. doi: 10.1073/pnas.1607171113
Aghai, M., and Manteuffel-Ross, T. (2020). Enhanced direct seedling efforts with unmanned aerial vehicle (UAV) “swarms” and seed technology. Tree Plant. Notes 63, 32–48. Available online at: https://rngr.net/publications/tpn/63-2/enhancing-direct-seeding-efforts-with-unmanned-aerial-vehicle-uav-201cswarms201d-and-seed-technology
Aguilar, F. X., Obeng, E. A., and Cai, Z. (2018). Water quality improvements elicit consistent willingness-to-pay for the enhancement of forested watershed ecosystem services. Ecosyst. Serv. 30, 158–171. doi: 10.1016/j.ecoser.2018.02.012
Alden, J. N. (1991). Provisional Tree Seed Zones and Transfer Guidelines for Alaska. Portland, OR: U.S. Department of Agriculture, Forest Service, Pacific Northwest Research Station, General Technical Report PNW-GTR-270, 35. doi: 10.2737/PNW-GTR-270
Alfaro, R. I., Fady, B., Vendramin, G. G., Dawson, I. K., Fleming, R. A., Sáenz-Romero, C., et al. (2014). The role of forest genetic resources in responding to biotic and abiotic factors in the context of anthropogenic climate change. For. Ecol. Manag. 333, 76–87. doi: 10.1016/j.foreco.2014.04.006
Allen, C. D., Macalady, A. K., Chenchouni, H., Bachelet, D., McDowell, N., Vennetier, M., et al. (2010). A global overview of drought and heat-induced tree mortality reveals emerging climate change risks for forests. For. Ecol. Manag. 259, 660–684. doi: 10.1016/j.foreco.2009.09.001
Almeida, D. R. A., Broadbent, E. N., Zambrano, A. M. A., Wilkinson, B. E., Ferreira, M. E., Chazdon, R., et al. (2019). Monitoring the structure of forest restoration plantations with a drone-lidar system. Int. J. Appl. Earth Obs. Geoinformation 79, 192–198. doi: 10.1016/j.jag.2019.03.014
Aronson, J., Goodwin, N., Orlando, L., Eisenberg, C., and Cross, A. T. (2020). A world of possibilities: six restoration strategies to support the United Nation's Decade on Ecosystem Restoration. Restor. Ecol. 28, 730–736. doi: 10.1111/rec.13170
Association of Official Seed Certifying Agencies (2018). Seed Certifying Agencies. Available online at: https://www.aosca.org/seed-certifying-agencies/ (accessed October 19, 2020).
Bala, G., Caldeira, K., Wickett, M., Phillips, T. J., Lobell, D. B., Delire, C., et al. (2007). Combined climate and carbon-cycle effects of large-scale deforestation. Proc. Natl. Acad. Sci. U.S.A. 104, 6550–6555. doi: 10.1073/pnas.0608998104
Barga, S. C., Olwell, P., Edwards, F., Prescott, L., and Leger, E. A. (2020). Seeds of success: a conservation and restoration investment in the future of U.S. lands. Conserv. Sci. Pract. 2:e209. doi: 10.1111/csp2.209
Barlow, R. J., Hunt, S., and Kush, J. S. (2016). “The silviculture of silvopasture,” in Proceedings of the 18th Biennial Southern Silvicultural Research Conference. e-Gen. Tech. Rep. SRS-212, eds. C. J. Schweitzer, W. K. Clatterbuck, and C. M. Oswalt (Asheville, NC: U.S. Department of Agriculture, Forest Service, Southern Research Station), 285–287.
Barnett, A., Fargione, J., and Smith, M. P. (2016). Mapping trade-offs in ecosystem services from reforestation in the Mississippi Alluvial Valley. BioScience 66, 223–237. doi: 10.1093/biosci/biv181
Bastin, J.-F., Finegold, Y., Garcia, C., Mollicone, D., Rezende, M., Routh, D., et al. (2019). The global tree restoration potential. Science 365, 76–79. doi: 10.1126/science.aax0848
Bengston, D. N., Fan, D. P., and Celarier, D. N. (1999). A new approach to monitoring the social environment for natural resource management and policy: the case of US national forest benefits and values. J. Environ. Manage. 56, 181–193. doi: 10.1006/jema.1999.0278
Bonner, F. T., and Karrfalt, R. P., (eds.). (2008). The Woody Plant Seed Manual, Agriculture Handbook 727. Washington, DC: U.S. Department of Agriculture, Forest Service, 1223.
Bower, A. D., St. Clair, J. B., and Erickson, V. (2014). Generalized provisional seed zones for native plants. Ecol. Appl. 24, 913–919. doi: 10.1890/13-0285.1
Breed, M. F., Harrison, P. A., Blyth, C., Byrne, M., Gaget, V., Gellie, N. J. C., et al. (2019). The potential of genomics for restoring ecosystems and biodiversity. Nat. Rev. Genet. 20, 615–628. doi: 10.1038/s41576-019-0152-0
Broadhurst, L., Driver, M., Guja, L., North, T., Vanzella, B., Fifield, G., et al. (2015). Seeding the future – the issues of supply and demand in restoration in Australia. Ecol. Manag. Restor. 16, 29–32. doi: 10.1111/emr.12148
Broadhurst, L. M., Jones, T. A., Smith, F. S., North, T., and Guja, L. (2016). Maximizing seed resources for restoration in an uncertain future. BioScience 66, 73–79. doi: 10.1093/biosci/biv155
Broadhurst, L. M., Lowe, A., Coates, D. J., Cunningham, S. A., McDonald, M., Vesk, P. A., et al. (2008). Seed supply for broadscale restoration: maximizing evolutionary potential. Evol. Appl. 1, 587–597. doi: 10.1111/j.1752-4571.2008.00045.x
Burney, O. T., and Jacobs, D. F. (2013). Ungulate herbivory of boreal and temperate forest regeneration in relation to seedling mineral nutrition and secondary metabolites. New For. 44, 753–768. doi: 10.1007/s11056-013-9381-9
Burney, O. T., and Jacobs, D. F. (2018). Species selection – a fundamental silvicultural tool to promote forest regeneration under high animal browsing pressure. For. Ecol. Manag. 408, 67–74. doi: 10.1016/j.foreco.2017.10.037
Côté, S. D., Rooney, T. P., Tremblay, J.-P., Dussault, C., and Waller, D. M. (2004). Ecological impacts of deer overabundance. Annu. Rev. Ecol. Evol. Syst. 35, 113–147. doi: 10.1146/annurev.ecolsys.35.021103.105725
Caldwell, P., Muldoon, C., Ford Miniat, C., Cohen, E., Krieger, S., Sun, G., et al. (2014). Quantifying the Role of National Forest System Lands in Providing Surface Drinking Water Supply for the Southern United States. Asheville, NC: U.S. Department of Agriculture, Forest Service, Southern Research Station, 135. doi: 10.2737/SRS-GTR-197
Chizmar, S., Parajulli, R., Bardon, R., and Cubbage, F. (2021). State cost-share programs for forest landowners in the southern United States: a review. J. For. 119:fvaa054. doi: 10.1093/jofore/fvaa054
Claytor, H. S., Clark, C. D., Lambert, D. M., and Jensen, K. L. (2018). Cattle producer willingness to afforest pastureland and sequester carbon. For. Policy Econ. 92, 43–54. doi: 10.1016/j.forpol.2018.03.004
Cooke, B., Richardson, B., Kilkenny, F., Clair, B. S., Finch, D., and Prendeville, H. (2019). Getting Climate-Smart With Seeds: How a New Software Tool Helps Prepare Landscapes for Expected Future Conditions. Fort Collins, CO: U.S. Department of Agriculture, Forest Service, Rocky Mountain Research Station, Science You Can Use Bulletin, Issue 35, 11.
Cook-Patton, S. C., Gopalakrishna, T., Daigneault, A., Leavitt, S. M., Platt, J., Scull, S. M., et al. (2020). Lower cost and more feasible options to restore forest cover in the contiguous United States for climate mitigation. One Earth 3, 739–752. doi: 10.1016/j.oneear.2020.11.013
Coop, J. D., Parks, S. A., Stevens-Rumann, C. S., Crausbay, S. D., Higuera, P. E., Hurteau, M. D., et al. (2020). Wildfire-driven forest conversion in Western North American landscapes. BioScience 70, 659–673. doi: 10.1093/biosci/biaa061
Corbin, J. D., and Holl, K. D. (2012). Applied nucleation as a forest restoration strategy. For. Ecol. Manag. 265, 37–46. doi: 10.1016/j.foreco.2011.10.013
Corbin, J. D., Robinson, G. R., Hafkemeyer, L. M., and Handel, S. N. (2016). A long-term evaluation of applied nucleation as a strategy to facilitate forest restoration. Ecol. Appl. 26, 104–114. doi: 10.1890/15-0075
Crombie, N. (2019). Marijuana Supply far Outweighs Demand in Oregon's Legal Market, New Study Says. The Oregonian. Available online at: https://www.oregonlive.com/news/g66l-2019/02/d05137b73d8055/marijuana-supply-far-outweighs-demand-in-oregons-legal-market-new-study-says.html (accessed October 20, 2020).
Cunningham, R. A. (1975). Provisional Tree and Shrub Seed Zones for the Great Plains. Fort Collins, CO: U.S. Department of Agriculture, Forest Service, Rocky Mountain Forest and Range Experiment Station RM-150, 15. doi: 10.5962/bhl.title.99997
Cunningham, S. C., Mac Nally, R., Baker, P. J., Cavagnaro, T. R., Beringer, J., Thomson, J. R., et al. (2015). Balancing the environmental benefits of reforestation in agricultural regions. Perspect. Plant Ecol. Evol. Syst. 17, 301–317. doi: 10.1016/j.ppees.2015.06.001
Daniels, S. E., Kilgore, M. A., Jacobson, M. G., Greene, J. L., and Straka, T. J. (2010). Examining the compatibility between forestry incentive programs in the US and the practice of sustainable forest management. Forests 1, 49–64. doi: 10.3390/f1010049
Davis, K. T., Dobrowski, S. Z., Higuera, P. E., Holden, Z. A., Veblen, T. T., Rother, M. T., et al. (2019). Wildfires and climate change push low-elevation forests across a critical climate threshold for tree regeneration. Proc. Natl. Acad. Sci. U.S.A. 116, 6193–6198. doi: 10.1073/pnas.1815107116
Davis, M. A., Wrage, K. J., Reich, P. B., Tjoelker, M. G., Schaeffer, T., and Muermann, C. (1999). Survival, growth, and photosynthesis of tree seedlings competing with herbaceous vegetation along a water-light-nitrogen gradient. Plant Ecol. 145, 341–350. doi: 10.1023/A:1009802211896
De Steiguer, J. E. (1984). Impact of cost-share programs on private reforestation investment. For. Sci. 30, 697–704. doi: 10.1016/0022-1910(84)90033-7
De Vitis, M., Abbandonato, H., Dixon, K. W., Laverack, G., Bonomi, C., and Pedrini, S. (2017). The european native seed industry: characterization and perspectives in grassland restoration. Sustainability 9:1682. doi: 10.3390/su9101682
De Vitis, M., Hay, F. R., Dickie, J. B., Trivedi, C., Choi, J., and Fiegener, R. (2020). Seed storage: maintaining seed viability and vigor for restoration use. Restor. Ecol. 28, S249–S255. doi: 10.1111/rec.13174
Denchev, P., and Grossnickle, S. C. (2019). Somatic embryogenesis for conifer seedling production: the biology of scaling. Reforesta 7, 109–137. doi: 10.21750/REFOR.7.08.70
Devine, W. D., and Harrington, C. A. (2008). Influence of Four Tree Shelter Types on Microclimate and Seedling Performance of Oregon White Oak and Western Redcedar. Portland, OR: U.S. Department of Agriculture, Forest Service. General Technical Report PNW-GTR 576, Pacific Northwest Research Station, 35. doi: 10.2737/PNW-RP-576
Dickie, J. B., and Pritchard, H. W. (2002). “Systematic and evolutionary aspects of desiccation tolerance in seeds,” in Desiccation and Survival in Plants: Drying Without Dying, eds. M. Black and H. W. Pritchard (Wallingford: CABI Publication), 239–259. doi: 10.1079/9780851995342.0239
Dobrowski, S. Z., Swanson, A. K., Abatzoglou, J. T., Holden, Z. A., Safford, H. D., Schwartz, M. K., et al. (2015). Forest structure and species traits mediate projected recruitment declines in western US tree species. Glob. Ecol. Biogeogr. 24, 917–927. doi: 10.1111/geb.12302
Domke, G. M., Oswalt, S. N., Walters, B. F., and Morin, R. S. (2020). Tree planting has the potential to increase carbon sequestration capacity of forests in the United States. Proc. Natl. Acad. Sci. U.S.A. 117, 24649–24651. doi: 10.1073/pnas.2010840117
Dumroese, R. K., Balloffet, N., Crockett, J. W., Stanturf, J. A., and Nave, L. E. (2019). A national approach to leverage the benefits of tree planting on public lands. New For. 50, 1–9. doi: 10.1007/s11056-019-09703-2
Dumroese, R. K., Landis, T. D., Barnett, J. P., and Burch, F. (2005). Forest service nurseries: 100 years of ecosystem restoration. J. For. 103, 241–247.
Dumroese, R. K., Landis, T. D., Pinto, J. R., Haase, D. L., Wilkinson, K. W., and Davis, A. S. (2016). Meeting forest restoration challenges: using the target plant concept. Reforesta 1, 37–52. doi: 10.21750/REFOR.1.03.3
Dybala, K. E., Matzek, V., Gardali, T., and Seavy, N. E. (2019a). Carbon sequestration in riparian forests: a global synthesis and meta-analysis. Glob. Change Biol. 25, 57–67. doi: 10.1111/gcb.14475
Dybala, K. E., Steger, K., Walsh, R. G., Smart, D. R., Gardali, T., and Seavy, N. E. (2019b). Optimizing carbon storage and biodiversity co-benefits in reforested riparian zones. J. Appl. Ecol. 56, 343–353. doi: 10.1111/1365-2664.13272
English, B. C., Bell, C. D., Wells, G. R., and Roberts, R. K. (1997). Stewardship incentives in forestry: participation factors in Tennessee. South. J. Appl. For. 21, 5–10. doi: 10.1093/sjaf/21.1.5
Enright, N. J., Fontaine, J. B., Bowman, D. M., Bradstock, R. A., and Williams, R. J. (2015). Interval squeeze: altered fire regimes and demographic responses interact to threaten woody species persistence as climate changes. Front. Ecol. Environ. 13, 265–272. doi: 10.1890/140231
Erickson, V., Aubry, C., Berrang, P., Blush, T., Bower, A., Crane, B., et al. (2012). Genetic Resource Management and Climate Change: Genetic Options for Adapting National Forests to Climate Change. Washington, DC: U.S. Department of Agriculture, Forest Service, Forest Management, 19.
Erickson, V. J., and Halford, A. (2020). Seed planning, sourcing, and procurement. Restor. Ecol. 28, S219–S227. doi: 10.1111/rec.13199
FAO and UNEP (2020). The State of the World's Forests 2020. Forests, Biodiversity and People. Rome: FAO and UNEP.
Fargione, J. E., Bassett, S., Boucher, T., Bridgham, S. D., Conant, R. T., Cook-Patton, S. C., et al. (2018). Natural climate solutions for the United States. Sci. Adv. 4:eaat1869. doi: 10.1126/sciadv.aat1869
Federal Register (2020). Establishing the one trillion trees interagency council (Executive Order 13955). Off. Fed. Regist. 85, 65643–65645.
Frischie, S., Miller, A. L., Pedrini, S., and Kildisheva, O. A. (2020). Ensuring seed quality in ecological restoration: native seed cleaning and testing. Restor. Ecol. 28, S239–S248. doi: 10.1111/rec.13217
Fuss, S., Lamb, W. F., Callaghan, M. W., Hilaire, J., Creutzig, F., Amann, T., et al. (2018). Negative emissions—part 2: costs, potentials and side effects. Environ. Res. Lett. 13:063002. doi: 10.1088/1748-9326/aabf9f
Gallego Zamorano, J., Hokkanen, T., and Lehikoinen, A. (2018). Climate-driven synchrony in seed production of masting deciduous and conifer tree species. J. Plant Ecol. 11, 180–188. doi: 10.1093/jpe/rtw117
Gann, G. D., McDonald, T., Walder, B., Aronson, J., Nelson, C. R., Jonson, J., et al. (2019). International principles and standards for the practice of ecological restoration. second edition. Restor. Ecol. 27, S1–S46. doi: 10.1111/rec.13035
Goward, S. N., Huang, C., Zhao, F., Schleeweis, K., Rishmawi, K., Lindsey, M., et al. (2016). NACP NAFD Project: Forest Disturbance History From Landsat, 1986-2010. Oak Ridge, TN: ORNL DAAC.
Griscom, B. W., Adams, J., Ellis, P. W., Houghton, R. A., Lomax, G., Miteva, D. A., et al. (2017). Natural climate solutions. Proc. Natl. Acad. Sci. U.S.A. 114, 11645–11650. doi: 10.1073/pnas.1710465114
Grossnickle, S. C., and MacDonald, J. E. (2018). Why seedlings grow: influence of plant attributes. New For. 49, 1–34. doi: 10.1007/s11056-017-9606-4
Grossnickle, S. C., and South, D. B. (2017). Seedling quality of southern pines: influence of plant attributes. Tree Plant. Notes 60, 29–40. Available online at: https://rngr.net/publications/tpn/60-2/seedling-quality-of-southern-pines-influence-of-plant-attributes
Haase, D. L., and Davis, A. S. (2017). Developing and supporting quality nursery facilities and staff are necessary to meet global forest and landscape restoration needs. Reforesta 4, 69–93. doi: 10.21750/REFOR.4.06.45
Haase, D. L., Pike, C., Enebak, S., Mackey, L., Ma, Z., and Silva, C. (2020). Forest nursery seedling production in the United States—Fiscal year 2019. Tree Plant. Notes 63, 26–31. Available online at: https://rngr.net/publications/tpn/63-2/forest-nursery-seedling-production-in-the-united-states2014fiscal-year-2019
Haines, T. (1995). Federal and state forestry cost-share assistance programs: structure, accomplishments, and future outlook. New Orleans, LA: U.S. Department of Agriculture, Forest Service, Southern Forest Experiment Station, 24. doi: 10.2737/SO-RP-295
Hamrick, K., and Gallant, M. (2018). Voluntary Carbon Markets Insights: 2018 Outlook and First-Quarter Trends. Washington, DC: Ecosyst. Marketpl. For. Trends.
Hay, F. R., and Probert, R. J. (2013). Advances in seed conservation of wild plant species: a review of recent research. Conserv. Physiol. 1:cot030. doi: 10.1093/conphys/cot030
Hernandez, G., Pike, C., Haase, D. L., Eneback, S., Ma, Z., Clarke, L., et al. (2016). Forest nursery seedling production in the United States-fiscal year 2015. Tree Plant. Notes 59, 20–24. Available online at: https://rngr.net/publications/tpn/59-2/tree-planting-in-the-south-1925-to-2012
Hessburg, P. F., Miller, C. L., Parks, S. A., Povak, N. A., Taylor, A. H., Higuera, P. E., et al. (2019). Climate, environment, and disturbance history govern resilience of western North American forests. Front. Ecol. Evol. 7, 1–27. doi: 10.3389/fevo.2019.00239
Hobbs, S. D., Tesch, S. D., Owston, P. W., Stewart, R. E., and Tappeiner II, J. C. (Eds.). (1992). Reforestation Practices in Southwestern Oregon and Northern California. Corvallis, OR: Forest Research Laboratory, Oregon State University.
Höhl, M., Ahimbisibwe, V., Stanturf, J. A., Elsasser, P., Kleine, M., and Bolte, A. (2020). Forest landscape restoration—what generates failure and success? Forests 11:938. doi: 10.3390/f11090938
Holl, K. D., and Brancalion, P. H. S. (2020). Tree planting is not a simple solution. Science 368, 580–581. doi: 10.1126/science.aba8232
Homer, C. G., Dewitz, J., Yang, L., Jin, S., Danielson, P., Xian, G. Z., et al. (2015). Completion of the 2011 National Land Cover Database for the conterminous United States – Representing a decade of land cover change information. Photogramm. Eng. Remote Sens. 81, 345–354.
Jacobson, M. G., Greene, J. L., Straka, T. J., Daniels, S. E., and Kilgore, M. A. (2009). Influence and effectiveness of financial incentive programs in promoting sustainable forestry in the South. South. J. Appl. For. 33, 35–41. doi: 10.1093/sjaf/33.1.35
Jalonen, R., Valette, M., Boshier, D., Duminil, J., and Thomas, E. (2018). Forest and landscape restoration severely constrained by a lack of attention to the quantity and quality of tree seed: insights from a global survey. Conserv. Lett. 11:e12424. doi: 10.1111/conl.12424
Johnson, K. A., Dalzell, B. J., Donahue, M., Gourevitch, J., Johnson, D. L., Karlovits, G. S., et al. (2016). Conservation Reserve Program (CRP) lands provide ecosystem service benefits that exceed land rental payment costs. Ecosyst. Serv. 18, 175–185. doi: 10.1016/j.ecoser.2016.03.004
Jones, T. A. (2013). Ecologically appropriate plant materials for restoration applications. BioScience 63, 211–219. doi: 10.1525/bio.2013.63.3.9
Jose, S., and Dollinger, J. (2019). Silvopasture: a sustainable livestock production system. Agrofor. Syst. 93, 1–9. doi: 10.1007/s10457-019-00366-8
Joyce, D. G., and Rehfeldt, G. E. (2017). Management strategies for black spruce (Picea mariana (Mill.) B.S.P.) in the face of climate change: climatic niche, clines, climatypes, and seed transfer. For. Int. J. For. Res. 90, 594–610. doi: 10.1093/forestry/cpx018
Keller, A. A., and Fox, J. (2019). Giving credit to reforestation for water quality benefits. PLoS ONE 14:e0217756. doi: 10.1371/journal.pone.0217756
Kelly, D. (1994). The evolutionary ecology of mast seeding. Trends Ecol. Evol. 9, 465–470. doi: 10.1016/0169-5347(94)90310-7
Kendall, A., and McPherson, E. G. (2012). A life cycle greenhouse gas inventory of a tree production system. Int. J. Life Cycle Assess. 17, 444–452. doi: 10.1007/s11367-011-0339-x
Keyser, A. R., Krofcheck, D. J., Remy, C. C., Allen, C. D., and Hurteau, M. D. (2020). Simulated increases in fire activity reinforce shrub conversion in a southwestern US forest. Ecosystems 23, 1702–1713. doi: 10.1007/s10021-020-00498-4
Kilkenny, F. F., Richardson, B. A., and St. Clair, B. (2020). United States Department of Agriculture Forest Service Pacific Northwest Research Station. Clim.-Smart Restor. Tool. Available online at: https://climaterestorationtool.org/csrt/ (accessed December 17, 2020).
King, S. L., and Keeland, B. D. (1999). Evaluation of reforestation in the lower Mississippi River alluvial valley. Restor. Ecol. 7, 348–359. doi: 10.1046/j.1526-100X.1999.72029.x
Koenig, W. D., and Knops, J. M. H. (1998). Scale of mast-seeding and tree-ring growth. Nature 396, 225–226. doi: 10.1038/24293
Kolb, P. F., and Robberecht, R. (1996). High temperature and drought stress effects on survival of Pinus ponderosa seedlings. Tree Physiol. 16, 665–672. doi: 10.1093/treephys/16.8.665
Kosiba, A. M., Schaberg, P. G., Hawley, G. J., and Hansen, C. F. (2013). Quantifying the legacy of foliar winter injury on woody aboveground carbon sequestration of red spruce trees. For. Ecol. Manag. 302, 363–371. doi: 10.1016/j.foreco.2013.03.006
Kramer, A. T., Crane, B., Downing, J., Hamrick, J. L., Havens, K., Highland, A., et al. (2019). Sourcing native plants to support ecosystem function in different planting contexts. Restor. Ecol. 27, 470–476. doi: 10.1111/rec.12931
Kroeger, T., McDonald, R. I., Boucher, T., Zhang, P., and Wang, L. (2018). Where the people are: current trends and future potential targeted investments in urban trees for PM10 and temperature mitigation in 27 U.S. Cities. Landsc. Urban Plan. 177, 227–240. doi: 10.1016/j.landurbplan.2018.05.014
Lal, H., Delgado, J. A., Gross, C. M., Hesketh, E., McKinney, S. P., Cover, H., et al. (2009). Market-based approaches and tools for improving water and air quality. Environ. Sci. Policy 12, 1028–1039. doi: 10.1016/j.envsci.2009.05.003
Landis, T. D. (2011). “The target plant concept-a history and brief overview,” in National Proceedings: Forest and Conservation Nursery Associations - 2010. Proc RMRS-P-65, eds. L. E. Riley, D. L. Haase, and J. R. Pinto (Fort Collins, CO: U.S. Department of Agriculture, Forest Service, Rocky Mountain Research Station), 61–66.
Landis, T. D., Dumroese, R. K., and Haase, D. L. (2010). The Container Tree Nursery Manual, Volume 7, Seedling Processing, Storage, and Outplanting. Washington, DC: U. S. Department of Agriculture, Forest Service, Agricultural Handbook, 674.
Lark, T. J., Spawn, S. A., Bougie, M., and Gibbs, H. K. (2020). Cropland expansion in the United States produces marginal yields at high costs to wildlife. Nat. Commun. 11:4295. doi: 10.1038/s41467-020-18045-z
League, K., and Veblen, T. (2006). Climatic variability and episodic Pinus ponderosa establishment along the forest-grassland ecotones of Colorado. For. Ecol. Manag. 228, 98–107. doi: 10.1016/j.foreco.2006.02.030
Löf, M., Dey, D. C., Navarro, R. M., and Jacobs, D. F. (2012). Mechanical site preparation for forest restoration. New For. 43, 825–848. doi: 10.1007/s11056-012-9332-x
Maguire, D. A., Mainwaring, D. B., Rose, R., Garber, S. M., and Dinger, E. J. (2009). Response of coastal Douglas-fir and competing vegetation to repeated and delayed weed control treatments during early plantation development. Can. J. For. Res. 39, 1208–1219. doi: 10.1139/X09-032
Mainz, A. K., and Wieden, M. (2019). Ten years of native seed certification in Germany – a summary. Plant Biol. 21, 383–388. doi: 10.1111/plb.12866
Mansourian, S., Stanturf, J. A., Derkyi, M. A. A., and Engel, V. L. (2017). Forest landscape restoration: increasing the positive impacts of forest restoration or simply the area under tree cover? Restor. Ecol. 25, 178–183. doi: 10.1111/rec.12489
Masson-Delmotte, V., Zhai, P., Pörtner, H.-O., Roberts, D., Skea, J., Shukla, P. R., et al. (2018). Global Warming of 1.5 OC: an IPCC Special Report on the Impacts of Global Aarming of 1.5° C Above Pre-Industrial Levels and Related Global Greenhouse Gas Emission Pathways, in the Context of Strengthening the Global Response to The threat of Climate Change, Sustainable Development, and Efforts to Eradicate Poverty. Geneva: World Meteorological Organization.
McDonald, T., Jonson, J., and Dixon, K. W. (2016). National standards for the practice of ecological restoration in Australia. Restor. Ecol. 24, S1–S32. doi: 10.1111/rec.12359
Morgenstern, E. K., and Wang, B. S. P. (2011). Trends in forest depletion, seed supply, and reforestation in Canada during the past four decades. For. Chron. 77, 1014–1021. doi: 10.5558/tfc771014-6
National Academies of Sciences Engineering Medicine (2020). An Assessment of the Need for Native Seeds and the Capacity for Their Supply: Interim Report. Washington, DC: The National Academies Press, 73.
National Association of State Foresters (2016). National Survey of State Operated Tree Seedling Nurseries and Tree Improvement Programs. Washington, DC: The National Association of State Foresters, 28.
Nave, L. E., Walters, B. F., Hofmeister, K. L., Perry, C. H., Mishra, U., Domke, G. M., et al. (2019). The role of reforestation in carbon sequestration. New For. 50, 115–137. doi: 10.1007/s11056-018-9655-3
Neary, D. G., Ice, G. G., and Jackson, C. R. (2009). Linkages between forest soils and water quality and quantity. For. Ecol. Manag. 258, 2269–2281. doi: 10.1016/j.foreco.2009.05.027
Nevill, P. G., Tomlinson, S., Elliott, C. P., Espeland, E. K., Dixon, K. W., and Merritt, D. J. (2016). Seed production areas for the global restoration challenge. Ecol. Evol. 6, 7490–7497. doi: 10.1002/ece3.2455
North Carolina State University Cooperative Tree Improvement Program (2020). 64th Annual Report. Raleigh, NC: Tree Improvement Program, 58.
North, M. P., Stevens, J. T., Greene, D. F., Coppoletta, M., Knapp, E. E., Latimer, A. M., et al. (2019). Tamm review: reforestation for resilience in dry western U.S. forests. For. Ecol. Manag. 432, 209–224. doi: 10.1016/j.foreco.2018.09.007
Oldfield, S., and Olwell, P. (2015). The right seed in the right place at the right time. BioScience 65, 955–956. doi: 10.1093/biosci/biv127
O'Mara, C. (2020). 7.7 Million Young People are Unemployed. We Need a New 'Tree Army.'. N. Y. Times. Available online at: https://www.nytimes.com/2020/05/18/opinion/coronavirus-unemployment-youth.html (accessed October 20, 2020).
Oswalt, S. N., Smith, W. B., Miles, P. D., and Pugh, S. A. (2014). Forest Resources of the United States, 2012: A Technical Document Supporting the Forest Service 2010 Update of the RPA Assessment. Washington, DC: U.S. Department of Agriculture, Forest Service, Washington Office, General Technical Report WO-91, 218. doi: 10.2737/WO-GTR-91
Oswalt, S. N., Smith, W. B., Miles, P. D., and Pugh, S. A. (2019). Forest Resources of the United States, 2017: A Technical Document Supporting the Forest Service 2020 RPA Assessment. Washington, DC: U.S. Department of Agriculture, Forest Service, General Technical Report WO-97, 223. doi: 10.2737/WO-GTR-97
Ouzts, J., Kolb, T., Huffman, D., and Sánchez Meador, A. (2015). Post-fire ponderosa pine regeneration with and without planting in Arizona and New Mexico. For. Ecol. Manag. 354, 281–290. doi: 10.1016/j.foreco.2015.06.001
Ovando, P., and Brouwer, R. (2019). A review of economic approaches modeling the complex interactions between forest management and watershed services. For. Policy Econ. 100, 164–176. doi: 10.1016/j.forpol.2018.12.007
Parks, S. A., and Abatzoglou, J. T. (2020). Warmer and drier fire seasons contribute to increases in area burned at high severity in western US forests from 1985 to 2017. Geophys. Res. Lett. 47:e2020GL089858. doi: 10.1029/2020GL089858
Pearse, I. S., LaMontagne, J. M., and Koenig, W. D. (2017). Inter-annual variation in seed production has increased over time (1900–2014). Proc. R. Soc. B Biol. Sci. 284:20171666. doi: 10.1098/rspb.2017.1666
Pike, C., Potter, K. M., Berrang, P., Crane, B., Baggs, J., Leites, L., et al. (2020). New seed-collection zones for the eastern United States: the eastern seed zone forum. J. For. 118, 444–451. doi: 10.1093/jofore/fvaa013
Pike, C., Warren, J., and Coggeshall, M. (2018). Trends in production of hardwood tree seedlings across the northeast United States from 2008 to 2016. Tree Plant. Notes 61, 18–25. Available online at: https://rngr.net/publications/tpn/61-1/trends-in-production-of-hardwood-tree-seedlings-across-the-northeast-united-states-from-2008-to-2016
Raffa, K. F., Aukema, B. H., Bentz, B. J., Carroll, A. L., Hicke, J. A., Turner, M. G., et al. (2008). Cross-scale drivers of natural disturbances prone to anthropogenic amplification: the dynamics of bark beetle eruptions. BioScience 58, 501–517. doi: 10.1641/B580607
Rodman, K. C., Veblen, T. T., Battaglia, M. A., Chambers, M. E., Fornwalt, P. J., Holden, Z. A., et al. (2020). A changing climate is snuffing out post-fire recovery in montane forests. Glob. Ecol. Biogeogr. 29, 2039–2051. doi: 10.1111/geb.13174
Rollins, M. G. (2009). LANDFIRE: a nationally consistent vegetation, wildland fire, and fuel assessment. Int. J. Wildland Fire 18, 235–249. doi: 10.1071/WF08088
Rose, R., and Haase, D. L. (2006). Guide to Reforestation in Oregon. Corvallis, OR: College of Forestry, Oregon State University, 48.
Rother, M. T., and Veblen, T. T. (2017). Climate drives episodic conifer establishment after fire in dry ponderosa pine forests of the Colorado Front Range, USA. Forests 8:159. doi: 10.3390/f8050159
Royer, J. P., and Moulton, R. J. (1987). Reforestation incentives: tax incentives and cost sharing in the South. J. For. 85, 45–47.
Ryan, N., Laverack, G., and Powell, A. (2008). Establishing quality control in UK wildflower seed production. Seed Test. Int. 135, 49–53.
Sample, V. A. (2017). Potential for additional carbon sequestration through regeneration of nonstocked forest land in the United States. J. For. 115, 309–318. doi: 10.5849/jof.2016-005
Seidl, R., Thom, D., Kautz, M., Martin-Benito, D., Peltoniemi, M., Vacchiano, G., et al. (2017). Forest disturbances under climate change. Nat. Clim. Change 7, 395–402. doi: 10.1038/nclimate3303
Shropshire, S. (2018). H-2A for Nurseries. Digger Mag. Available online at: http://www.diggermagazine.com/h-2a-for-nurseries/ (accessed October 20, 2020).
Snyder, S. A., Butler, B. J., and Markowski-Lindsay, M. (2019). Small-area family forest ownerships in the USA. Small-Scale For. 18, 127–147. doi: 10.1007/s11842-018-9410-9
Soil Survey Staff (2016). Gridded Soil Survey Geographic (gSSURGO) Database for the Conterminous United States. Washington, DC:United States Department of Agriculture, Natural Resources Conservation Service. Available online at: https://gdg.sc.egov.usda.gov/ (accessed October 20, 2020).
Sork, V. L. (1993). “Evolutionary ecology of mast-seeding in temperate and tropical oaks (Quercus spp.),” in Frugivory and Seed Dispersal: Ecological and Evolutionary Aspects Advances in Vegetation Science, eds. T. H. Fleming and A. Estrada (Dordrecht: Springer), 133–147. doi: 10.1007/978-94-011-1749-4_9
Stanturf, J. A., Palik, B. J., and Dumroese, R. K. (2014). Contemporary forest restoration: a review emphasizing function. For. Ecol. Manag. 331, 292–323. doi: 10.1016/j.foreco.2014.07.029
Strassburg, B. B. N., Iribarrem, A., Beyer, H. L., Cordeiro, C. L., Crouzeilles, R., Jakovac, C. C., et al. (2020). Global priority areas for ecosystem restoration. Nature 586, 724–729. doi: 10.1038/s41586-020-2784-9
The White House (2016). United States Mid-Century Strategy for Deep Decarbonization. Washington, DC, 111.
Thompson, J. R., Spies, T. A., and Ganio, L. M. (2007). Reburn severity in managed and unmanaged vegetation in a large wildfire. Proc. Natl. Acad. Sci. U.S.A. 104, 10743–10748. doi: 10.1073/pnas.0700229104
Thyroff, E. C., Burney, O. T., and Jacobs, D. F. (2019). Herbivory and competing vegetation interact as site limiting factors in maritime forest restoration. Forests 10:950. doi: 10.3390/f10110950
Tian, N., Poudyal, N. C., Hodges, D. G., Young, T. M., and Hoyt, K. P. (2015). Understanding the factors influencing nonindustrial private forest landowner interest in supplying ecosystem services in Cumberland Plateau, Tennessee. Forests 6, 3985–4000. doi: 10.3390/f6113985
Trobaugh, J. (2018). Contracting, communication, and pricing trends for forest seedlings. Tree Plant. Notes 61, 126–133. Available online at: https://rngr.net/publications/tpn/61-2/contracting-communication-and-pricing-trends-for-forest-seedlings
Turner, M. G., Braziunas, K. H., Hansen, W. D., and Harvey, B. J. (2019). Short-interval severe fire erodes the resilience of subalpine lodgepole pine forests. Proc. Natl. Acad. Sci. U.S.A. 116, 11319–11328. doi: 10.1073/pnas.1902841116
US Census Bureau (2017). TIGER/Line Shapefiles. Available online at: https://www.census.gov/geographies/mapping-files/time-series/geo/tiger-line-file.html (accessed October, 20, 2020).
U. S. Department of the Interior Geological Survey (2014). Landfire Biophysical Settings. Landfire. Available online at: https://landfire.gov/vegetation.php (accessed December, 23, 2020).
U. S. Geological Survey Gap Analysis Project Croft, M., Johnson, L., and Prior-Magee, J. S. (2018). Protected Areas Database of the United States (PAD-US) 2.0 Denver, CO.
Vadell, E., de-Miguel, S., and Pemán, J. (2016). Large-scale reforestation and afforestation policy in Spain: a historical review of its underlying ecological, socioeconomic and political dynamics. Land Use Policy 55, 37–48. doi: 10.1016/j.landusepol.2016.03.017
Verdone, M., and Seidl, A. (2017). Time, space, place, and the Bonn Challenge global forest restoration target. Restor. Ecol. 25, 903–911. doi: 10.1111/rec.12512
Ward, J. S., and Stephens, G. R. (1995). “Protection of tree seedlings from deer browsing,” in Proceedings, 10th Central Hardwood Forest Conference, Morganstown, WV, USA, eds. K. W. Gottschalk and S. L. C. Fosbroke (Radnor, PA: U.S. Department of Agriculture, Forest Service, Northeastern Forest Experiment Station, General Technical Report NE-197), 507–514.
Westerling, A. L. (2016). Increasing western US forest wildfire activity: sensitivity to changes in the timing of spring. Philos. Trans. R. Soc. B Biol. Sci. 371:20150178. doi: 10.1098/rstb.2015.0178
Westerman, B. (2020). The Forest Industry is Essential — so are the Employees Who Work in it. The Hill. Available online at: https://thehill.com/blogs/congress-blog/politics/512648-the-forest-industry-is-essential-so-are-the-employees-who-work (accessed October 20, 2020).
Wheeler, N. C., Steiner, K. C., Schlarbaum, S. E., and Neale, D. B. (2015). The evolution of forest genetics and tree improvement research in the United States. J. For. 113, 500–510. doi: 10.5849/jof.14-120
Whittet, R., Cottrell, J., Cavers, S., Pecurul, M., and Ennos, R. (2016). Supplying trees in an era of environmental uncertainty: identifying challenges faced by the forest nursery sector in Great Britain. Land Use Policy 58, 415–426. doi: 10.1016/j.landusepol.2016.07.027
Williams, M. I., and Dumroese, R. K. (2013). Preparing for climate change: forestry and assisted migration. J. For. 111, 287–297. doi: 10.5849/jof.13-016
Zald, H. S. J., and Dunn, C. J. (2018). Severe fire weather and intensive forest management increase fire severity in a multi-ownership landscape. Ecol. Appl. 28, 1068–1080. doi: 10.1002/eap.1710
Keywords: afforestation, tree planting, nurseries, seedlings, land use
Citation: Fargione J, Haase DL, Burney OT, Kildisheva OA, Edge G, Cook-Patton SC, Chapman T, Rempel A, Hurteau MD, Davis KT, Dobrowski S, Enebak S, De La Torre R, Bhuta AAR, Cubbage F, Kittler B, Zhang D and Guldin RW (2021) Challenges to the Reforestation Pipeline in the United States. Front. For. Glob. Change 4:629198. doi: 10.3389/ffgc.2021.629198
Received: 13 November 2020; Accepted: 11 January 2021;
Published: 04 February 2021.
Edited by:
Marco Mina, Université du Québec à Montréal, CanadaReviewed by:
Celine Boisvenue, Canadian Forest Service, CanadaKasten Dumroese, United States Forest Service (USDA), United States
Copyright © 2021 Fargione, Haase, Burney, Kildisheva, Edge, Cook-Patton, Chapman, Rempel, Hurteau, Davis, Dobrowski, Enebak, De La Torre, Bhuta, Cubbage, Kittler, Zhang and Guldin. This is an open-access article distributed under the terms of the Creative Commons Attribution License (CC BY). The use, distribution or reproduction in other forums is permitted, provided the original author(s) and the copyright owner(s) are credited and that the original publication in this journal is cited, in accordance with accepted academic practice. No use, distribution or reproduction is permitted which does not comply with these terms.
*Correspondence: Joseph Fargione, jfargione@tnc.org