- 1Department of Forestry Science and Biodiversity, Faculty of Forestry and Environment, Universiti Putra Malaysia, Serdang, Malaysia
- 2Institute of Tropical Forestry and Forest Products, Universiti Putra Malaysia, Serdang, Malaysia
- 3Department of Fish Ecology and Evolution, EAWAG Swiss Federal Institute of Aquatic Science and Technology, Center for Ecology, Evolution and Biogeochemistry, Dübendorf, Switzerland
- 4Biodiversity Unit, Institute of Bioscience, Universiti Putra Malaysia, Serdang, Malaysia
- 5School of Environmental and Geographical Sciences, University of Nottingham Malaysia, Semenyih, Malaysia
Large-scale oil palm agriculture has caused deforestation in the tropics, but also degrades stream water quality and reduces aquatic biodiversity. Though the outcomes of industrial-scale oil palm plantations for biodiversity have been explored extensively, the consequences of small-scale oil palm agriculture for freshwater macroinvertebrate fauna are poorly understood. Here, we explored the impacts of small-scale oil palm agriculture on aerial adult Odonata (the dragonflies and damselflies), which, due to their inherent sensitivity to habitat degradation, represent useful indicators of wider ecosystem health. We surveyed riparian corridors of man-made waterways in natural habitats converted into agricultural lands in both peat swamp and mangrove forest, comprising a total of 60 sampling units across a region of Peninsular Malaysia where such small-scale agricultural practices are widespread. We hypothesized that physicochemical water quality of oil palm waterways together with riparian vegetation influence Odonata species richness and composition. Our results revealed that Odonata species richness increased with dissolved oxygen, water temperature and vegetation cover, but decreased with water level, pH, and total dissolved solids. Species composition was influenced by both dissolved oxygen and pH. The present study provides valuable insights into the effects of small-scale oil palm agriculture for water quality of associated aquatic habitats, and subsequent responses of adult Odonata. Therefore, smallholders should reduce the use of chemical pesticides and fertilizers to improve the conservation value of oil palm waterways for both Odonata and aquatic fauna more generally, in order to be certified as biodiversity-friendly agriculture.
Introduction
Forest conversion to agricultural lands poses a major threat to biodiversity across terrestrial and aquatic ecosystems at multiple spatial scales (Mercer et al., 2014; Oliveira-Junior et al., 2015; Ghazali et al., 2016; Asmah et al., 2017). The expansion of oil palm plantations into tropical mixed-dipterocarp terra firma and fresh-water swamp forests affects terrestrial and river ecosystems through altered hydrology and habitat availability (Luke et al., 2016, 2017). In Malaysia, agricultural land is estimated to cover 10.31 Mha of the total land area with oil palm plantation representing at least 5.74 M ha (Kushairi, 2017). These major changes of land use characteristics have contributed to different species composition from the original composition of the community (Che Salmah et al., 1998, 2014; Kadoya et al., 2009; Wong et al., 2016; Yahya et al., 2017).
Despite the loss of natural ecosystems, human-modified landscapes are still often capable of sustaining considerable biodiversity (Koch et al., 2014; Luke et al., 2016). However, the occurrence of Odonata species in tropical agricultural lands, particularly oil palm production landscapes, is currently understudied. The expansion of oil palm plantations into tropical forests alters physical and biogeochemical inputs to streams, thereby modifying hydrological function (Carlson et al., 2014). Agriculture degrades streams by increasing non-point source (NPS) pollution from agrochemical applications runoff, impacting riparian and stream channel habitat, and altering water flow and quality, with wide-ranging implications for biological communities and ecosystems (Allan, 2004; Ouyang et al., 2014; Wu and Lu, 2019). For instance, low dissolved oxygen concentrations associated with organic pollution and eutrophication can reduce the survival of many aquatic fauna (Breitburg et al., 2003; Jones et al., 2006).
The key role of dragonflies and damselfies (the Odonata) as predators and their interactions with different organisms and habitat types makes them good ecological indicators (Knight et al., 2005; Miguel et al., 2017; Brito et al., 2018). In addition, the complex life-histories of the Odonata—wherein the larval stages are aquatic and the adult stages terrestrial—makes them vulnerable to anthropogenic stressors in both ecosystem types (Samways and Steytler, 1996; Cleary et al., 2004; Ferreras-Romero et al., 2009; Kutcher and Bried, 2014). Stressors such as variation in water quality can reduce species richness of Odonata, as most taxa are confined to small forest remnants that are under threat from a myriad of human activities (Orr, 2004; Kalkman et al., 2008). Odonata density is highly dependent on the type and structure of aquatic and terrestrial vegetation for their foraging sites (Remsburg, 2011; Dolnı et al., 2012; Dolný et al., 2014). Odonata fitness, however, is also associated with warming and contaminants during the aquatic larval stage with carry-over effects to adults stage (Stoks and Córdoba-Aguilar, 2012; Janssens et al., 2014). Agricultural practices on land adjacent to streams lead to soil erosion and subsequent runoff of fine sediments, nutrients, and pesticides, thus having major impacts on Odonata survival (Moore and Palmer, 2005).
Oliveira-Junior et al. (2015) suggested that agricultural activities appear to be the main factor determining changes in Odonata community composition and assemblages in the eastern Amazon, Brazil. Although Odonata are amphibiotic insects (Monteiro-Júnior et al., 2014), data from which to quantify the relationship between water quality and Odonata diversity are at present relatively scarce in the tropics. To date, only a few studies have indicated the importance of water quality for maintaining Odonata species, particularly aerial adult individuals in agricultural lands (Castillo et al., 2006; Rizo-Patrón et al., 2013; Mercer et al., 2014; Mendes et al., 2019, 2020). Studies in aquatic ecology and/or biodiversity in oil palm production landscapes are limited to waterbirds (Sulai et al., 2015; Hawa et al., 2016), fish (Giam et al., 2015; Wilkinson et al., 2018), Anurans (Gillespie et al., 2012; Faruk et al., 2013; Gallmetzer and Schulze, 2015), and macroinvertebrates (Oppel, 2006; Mercer et al., 2014; Cunha et al., 2015). Ndaruga et al. (2004) reported that significant changes in aquatic macroinvertebrate assemblages were primarily due to water quality rather than prevailing climatic conditions. Thus, agricultural drainage can impact water quality variables that affect Odonata, either directly, or indirectly via reductions in the availability of their preferred food resources (Elo et al., 2015).
The aim of the present study was to investigate the relationship between adult Odonata species richness and physicochemical water quality and aquatic vegetation structure in oil palm smallholdings. We hypothesized that species richness and community composition of Odonata varies with respect to physicochemical water quality and vegetation structure in oil palm waterways. Specifically, we predicted that (i): reduced water quality (here characterized by low dissolved oxygen (DO), high total dissolved solid (TDS), high pH and altered thermal regimes) and disturbed in-stream habitat leads to diminished species-level diversity in this taxon; and (ii): Both peat–swamp-forest–converted and mangrove-forest-converted smallholdings supporting similarly reduced Odonata biodiversity (i.e., species richness and abundance), due to comparable habitat degradation in both ecosystem types.
Materials and Methods
Study Area
The study was conducted in the municipality of Tanjung Karang (03°21.511′N, 101°13.163′E; area = 7,500 ha), west coast of Peninsular Malaysia (Figure 1). The daily temperature varies from 29 to 32°C and mean relative humidity of 65–70%. The highest temperature happened from April to June with the lowest relative humidity measured in June, July and September. Rainfall of about 58.6–240.0 mm per month was measured in the study area (Malaysian Meteorological Services Department). Permission to conduct fieldwork in smallholdings was granted by village heads and landowners. All study sites were located at <10 m above sea level, within coastal plain areas characterized by peat soil and flat terrain. The sizes of smallholdings in the study sites were less than 5 ha each and managed by independent smallholders. Man-made waterways have been established to prevent floodwater from entering cultivation areas during rainy seasons (primary monsoon, October to November; secondary monsoon, March to May) (Figure 2). The waterways were a common feature in the study area (Sulai et al., 2015). Oil palm has been commercially planted in this area since the 1970s (Sulai et al., 2015). Historically, the smallholding areas were converted from peat swamp (4439.67 ha) or mangrove forest (3097.71 ha).
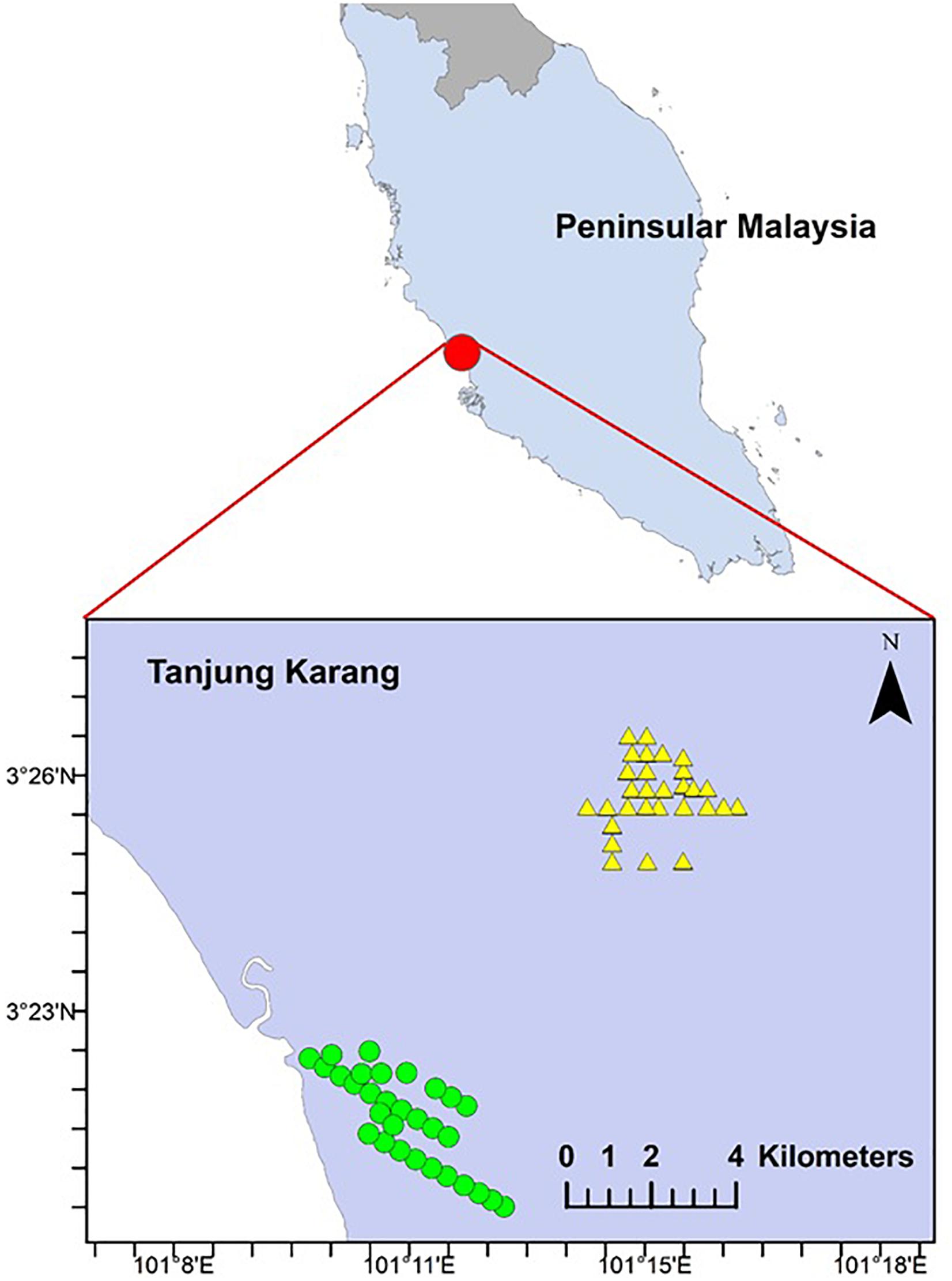
Figure 1. Map of the study area in Peninsular Malaysia and 60 sampling sites in Tanjung Karang. Mangrove forest-converted smallholdings and peat swamp forest-converted smallholdings are denoted by circle and triangle shape, respectively.
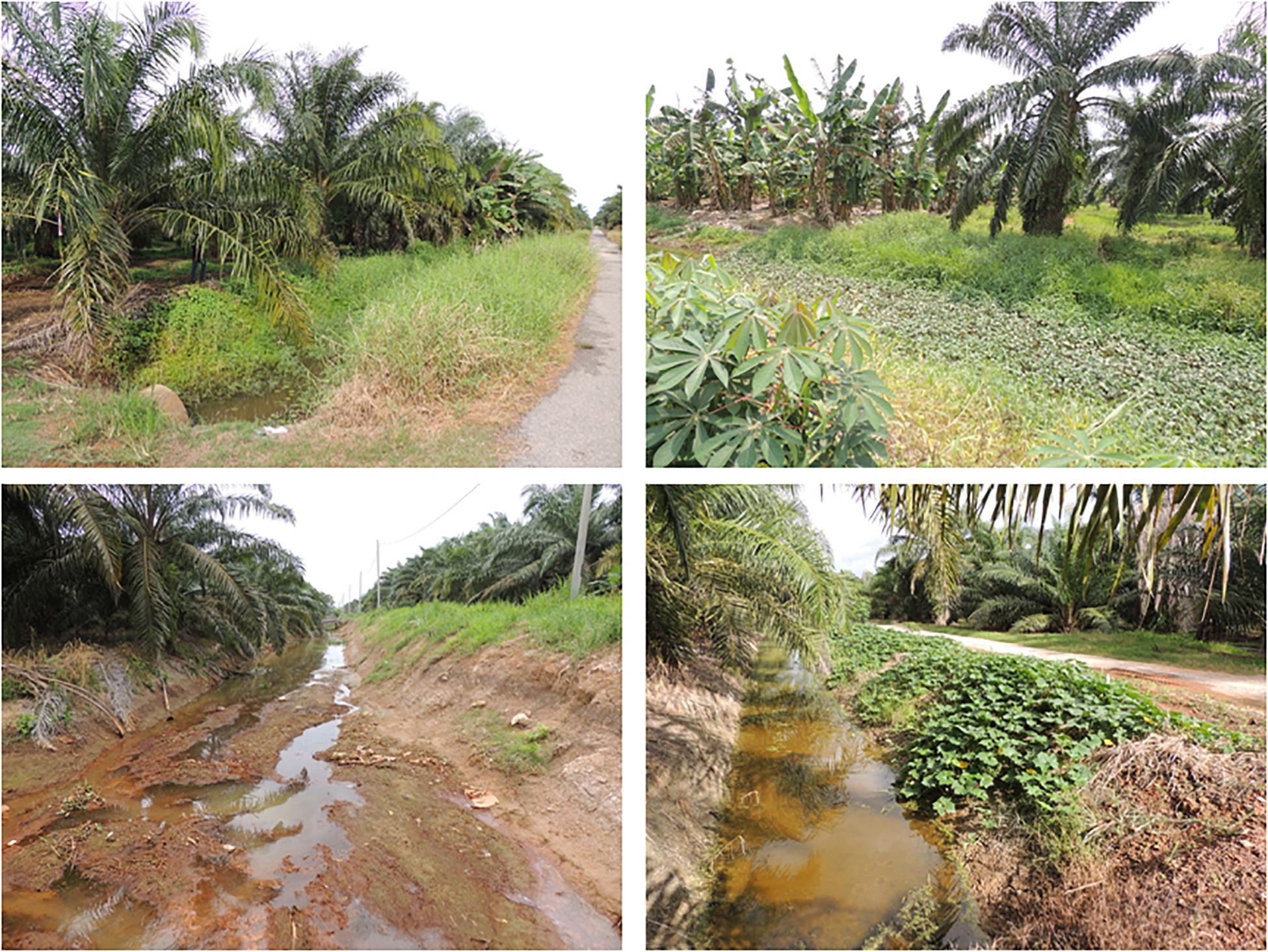
Figure 2. Typical man-made waterways at the study area. These waterways are built in oil palm smallholdings for flood-control and irrigation. Vegetation cover including non-aquatic and semi-aquatic weeds characterizes the waterways and provides places to perch from which Odonata can take off to capture prey and as a vantage point to patrol a territory.
We defined oil palm smallholdings as self-managed cultivation areas covering less than 50 ha that were owned by individual farmers (Azhar et al., 2011, 2015b; Oon et al., 2019a, b). Smallholdings typically support multiple-age stands of oil palm, in which oil palms are planted alongside other commercial plants (e.g., banana, cassava, coconut, pineapple, mangoes, and tapioca) (Amoah et al., 1995; Azhar et al., 2014, 2017; Razak et al., 2020). Patches of oil palms of different heights are common in smallholdings (Azhar et al., 2011). Oil palm stands (>25 years) were rarely removed in smallholdings and instead left to naturally die and decompose. Hence, smallholdings tend to be more heterogenous in terms of vegetation structure compared with plantation estates. Similarly, riparian vegetation can consist of remnants forest trees, replanted commercial plants and also weeds on streambank.
Sampling Design
We used a systematic survey design with a random start to ensure better spatial coverage and lower variance (Morrison et al., 2008; Thomas et al., 2010). We established 60 sampling points alongside oil palm waterways. Thirty sampling points were established in each historical land cover type (i.e., peat swamp forest-converted or mangrove forest-converted smallholdings) (Figure 1). To ensure independence of observations, each point was spaced at least 400 m apart. Geographic coordinates were also recorded for each site. Sampling was undertaken from March to September 2015.
Aerial Adult Odonata Sampling
The presence of adult insects at each sampling point was assessed based on observations recorded during a discrete 20 min period following Bried et al. (2012). We recorded the number of individuals observed within a 10 m radius of the chosen sampling point. To identify adult Odonata, we used specialized taxonomic keys for the region (Orr, 2003, 2005). We also captured individuals with an insect net when visual identification was not possible in the field. We recorded Odonata fauna encountered visually between 9 to 12.30 a.m. on a daily basis (during clear days). Sampling was repeated three times at each point.
Water Quality, Channel Morphology, and Vegetation Measurements
Physicochemical water quality variables were measured on the same day of Odanata sampling was done at each point. We used a Hanna Multimeter to measure temperature, salinity, pH, conductivity, dissolved oxygen (DO), turbidity, and total dissolved solids (TDS). Quadrats (25 m2) were established at each Odonata sampling point, to ensure every part of the sampling area had an equal chance of being sampled on each occasion (Sulai et al., 2015). We estimated the percentage of vegetation cover (inside the channel and on the bank) inside each quadrat. Similar to Odonata sampling, habitat sampling was repeated three times at each point. Water variables measured were summarized to provide descriptive statistical information (Table 1).
Statistical Analyses
All statistical analyses were performed in GenStat version 15 (VSN International). To assess the overall sampling effort, we compared the observed species richness with the Chao 1 bias correction estimator for the species richness in Estimates version 9.1 (Colwell et al., 2004). To take into account imperfect detection of rare species, we also used ACE (Abundance Coverage-based Estimator) (Colwell and Coddington, 1994). Ten samples were used as the recommended upper limit for rare or infrequent species. Expected species accumulation curves were generated using Microsoft Excel. To contrast Odonata species richness and abundance between peat swamp forest-converted (n = 30) and mangrove forest-converted smallholdings (n = 30), we used two-sample t-test. Prior to the analysis, we conducted Shapiro-Wilk test and Bartlett’s test to check for normality and homogeneity of variance, respectively. As species richness and abundance did not meet the assumptions of parametric statistical tests (i.e., data are not normally distributed and variances are not equal across groups), we square root-transformed both of them.
The relationship between Odonata species richness and habitat characteristics (e.g., physicochemical water quality and aquatic vegetation structure) was determined using Generalized Linear Models (GLMs) (Schall, 1991). To verify model assumptions, we plotted residuals versus fitted values, versus each covariate in the model and versus each covariate not in the model. Water quality variables, vegetation cover, historical land cover and sampling month were registered as explanatory variables in GLMs. Regression models predicting richness or diversity in aquatic habitats based on abiotic variables explained greater variance than did those predicting abundance (Therriault and Kolasa, 1999). We used a log-link function assuming a Poisson distribution to fit the models. Because the response variables (i.e., count data) exhibited over-dispersion, Poisson models were used as the preferred model. The dispersion parameter of Poisson models had been assumed to be fixed. In this case, the dispersion parameter was fixed at 1. We conducted Spearman correlation tests to determine multicollinearity among the predictor variables. Only one of each pair of highly correlated explanatory variables were included in the analysis, as collinearity can distort model estimation (| r| > 0.7) (Dormann et al., 2013). Conductivity (coefficient of correlation, r = −0.725) and salinity (coefficient of correlation, r = −0.911) were therefore excluded, while total dissolved solids remained in the model. We did not include historical land cover type (peat swamp forest-converted or mangrove forest-converted) as a predictor variable because it is obviously characterized by pH and salinity. Akaike Information Criterion (AIC) tests were conducted to select the most parsimonious models (Burnham and Anderson, 2002) and models with the lowest AIC scores were chosen. Because the number of samples/number of parameters = 24.86 < 40 for our models, we used corrected AIC (Hurvich and Tsai, 1989; Burnham and Anderson, 2002). Under this criterion, the chosen model is the one that minimizes the Kullback-Leibler distance between the model and the truth (Burnham and Anderson, 2002). We also reported the percentage of explained deviance of each competing model (Burnham and Anderson, 2002). We computed Akaike weights to provide a measure of model selection uncertainty.
SIMPER analysis was used to determine the contribution of each species and differentiate Odonata assemblages (Clarke and Warwick, 2001). The cut–off for species contribution to each assemblage was fixed at 90%. BIO-ENV analysis was used to determine the environmental factors most associated with Odonata assemblages (Clarke and Warwick, 2001). Computation in this analysis was based on the Spearman–rank correlation method (Number of permutations = 999). SIMPER and BIO-ENV analyses were conducted in Primer version 6 (PRIMER-E Ltd.).
Results
Throughout the sampling period, we recorded a total of 1,217 adult Odonata, representing 24 species, 22 genera, and four families, comprising mostly dragonfly (Anisoptera) taxa (Table 2). Conversely, only five damselflies (Zygoptera) species were recorded. We recorded four forest species. These include Rhyothemis phyllis, Lathrecista asiatica, Nannophya pygmaea, and Anax guttatus. Based on the Chao 1 and ACE estimators of “true” species richness, our sampling effort yielded 94.90 and 91.03% of the “true” Odonata species richness, respectively (Figure 3). Our results revealed no significant difference in Odonata species richness (t = −1.07; p = 0.285) between peat swamp forest-converted (mean ± SE = 2.90 ± 0.16) and mangrove forest-converted smallholdings (mean ± SE = 2.60 ± 0.23). Similarly, we did not detect significant differences in Odonata abundance (t = 1.53; p = 0.127) between peat swamp forest-converted (mean ± SE = 20.51 ± 1.37 individuals) and mangrove forest-converted smallholdings (mean ± SE = 25.61 ± 3.03 individuals).
The most parsimonious model for Odonata was one that included dissolved oxygen, water temperature, vegetation cover, water level, pH, and total dissolved solids (Table 3). This model revealed that Odonata species richness increased with dissolved oxygen, water temperature, and vegetation cover (Figure 4). However, Odonata species richness decreased with increasing water level, pH, and total dissolved solids (Figures 5, 6). In total, the model accounted for 72.1% of the Akaike weights in the model set and explained 58.55% of the variation in Odonata species richness. Model validation indicated no problems. The following equation describes this model:
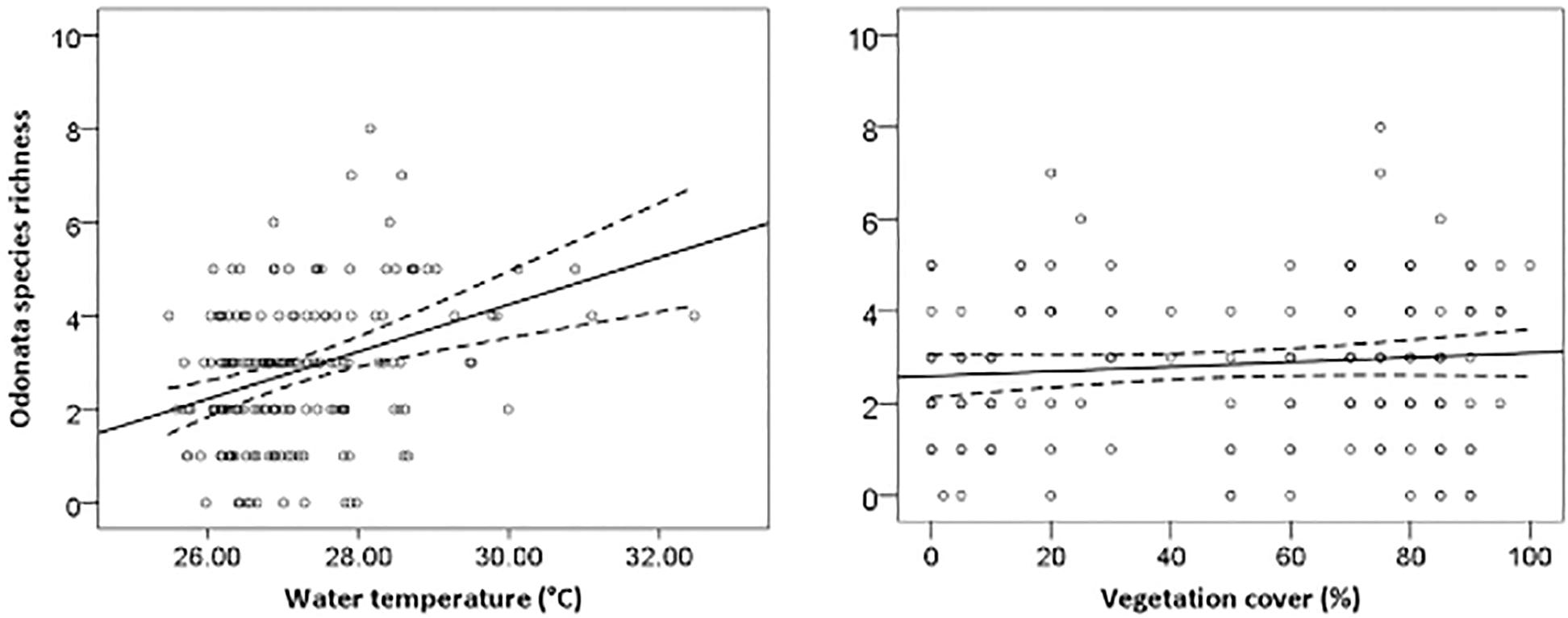
Figure 4. Responses of Odonata species richness to water temperature (°C) and vegetation cover (%). Based on GLMs, scatter plots have 95% confidence intervals (dashed) on the regression (solid) line.
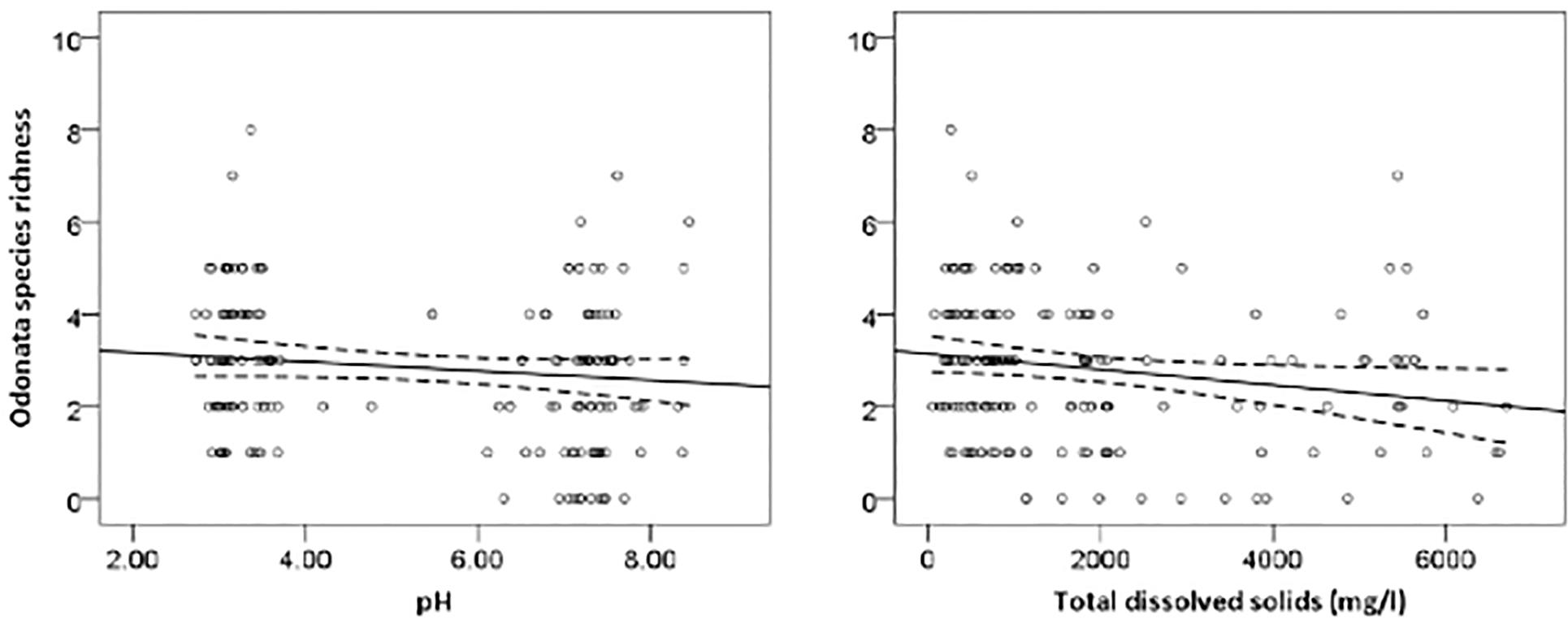
Figure 5. Responses of Odonata species richness to water pH and total dissolved solids (mg/l). Based on GLMs, scatter plotshave 95% confidence intervals (dashed) on the regression (solid) line.
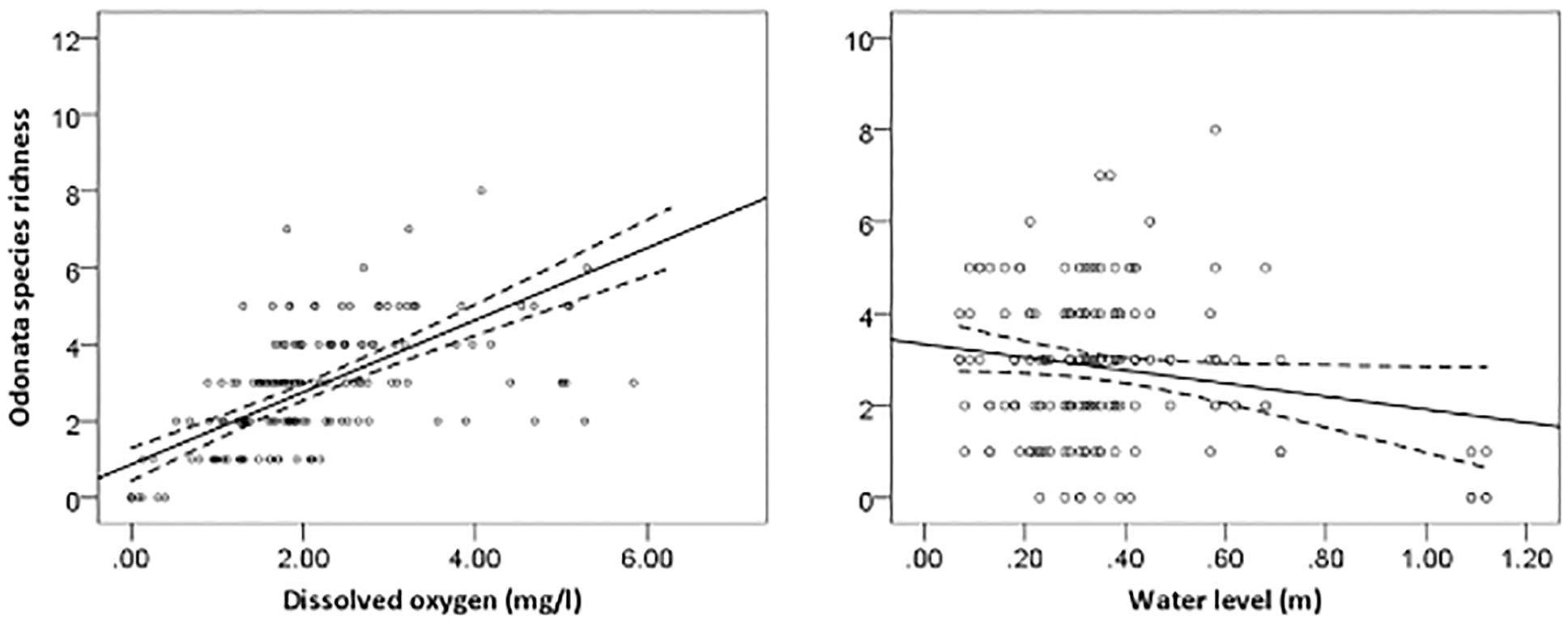
Figure 6. Responses of Odonata species richness to dissolved oxygen (mg/l) and water level (m). Based on GLMs, scatter plots have 95% confidence intervals (dashed) on the regression (solid) line.
SP0.2565xDO−2.375xWL−0.1439xpH−0.001xTDS0.116xWT0.004xVC−5.120, where SP = Species Richness, DO = Dissolved Oxygen, WL = Water Level, pH = Water pH, TDS = Total Dissolved Solids, WT = Water Temperature, VC = Vegetation Cover.
A relatively small number of species dominated the Odonata community in oil palm smallholdings, with ten out of a total of 24 of the species responsible for > 90% of the individuals recorded (Table 4). Species composition was significantly associated with dissolved oxygen and pH (r = 0.387; p = 0.001).
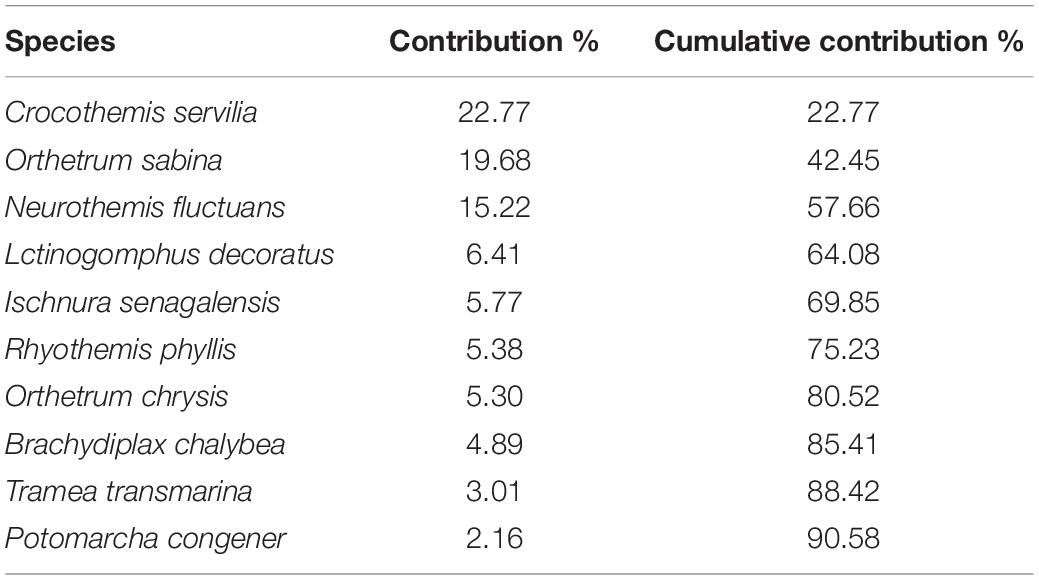
Table 4. Odonata species that together composed over 90% of the assemblages (based on abundance) observed in sampled oil palm waterways.
Discussion
Our results reveal that, despite disturbance and habitat degradation, oil palm waterways still appear to contribute considerably to freshwater biodiversity within small-scale agricultural habitats. This finding reinforces the importance of maintaining man-made waterbodies for conservation outcomes in oil palm production landscapes (Sulai et al., 2015). Our data are comparable with Abdul et al. (2017) who recorded 22 Odonate species at larval stage, collected from a river which passes through an oil palm plantation in the northern part of Peninsular Malaysia. However, our study suggested that Odonata communities supported by oil palm waterways are impoverished in comparison to natural forests. Prior to forest conversion, as many as 78 Odonate species from 12 families were likely to exist in the study sites (Norma-Rashid et al., 2001), with Dow et al. (2012) reporting 50 Odonate species from nine families in comparable natural peat swamp forest on the east coast of Peninsular Malaysia. Despite considerably reduced diversity in the oil palm smallholding waterways studied here, Anisopteran diversity was still appreciable, though characterized by more tolerant, generalist species compared to those recorded from undisturbed swamp habitats. Whilst we reveal that smallholdings may retain relatively high Anisoptera species richness despite extensive habitat disturbance, they host few species of typically more sensitive Zygoptera taxa (Júnior et al., 2015; Oliveira-Junior et al., 2015; Oliveira-Junior and Juen, 2019). Oliveira-Junior et al. (2015) suggested that Anisoptera are likely to be associated with degraded environments such as oil palm plantations. By contrast, Zygoptera are likely to indicate undisturbed environments such natural forests. With respect to land use modification, our findings are consistent with Sulai et al. (2015) who report that species richness and abundance did not differ significantly between peat swamp forest-converted and mangrove forest-converted smallholdings, with this likely associated with the comparable loss of original forest vegetation and associated habitat degradation in both ecosystem types.
Our regression models (i.e., GLMs) indicated that Odonata species richness was influenced by both physicochemical water quality variables and within-channel and bankside vegetation structure. This in contrast to a previous a study (Jacobsen, 2008), where multiple regression analyses exploring the influence of several predictor variables revealed that oxygen saturation had the greatest (and the only statistically significant effect) on density-corrected richness. However, we did find that Odonata species richness and composition was positively related to dissolved oxygen, partially corroborating this finding. Similarly, previous studies (de Paiva Silva et al., 2010; Pryke et al., 2015) reported dissolved oxygen was an important determinant for Odonata species richness, and macroinvertebrate diversity indices more generally correlate inversely with TDS, pH and turbidity but positively with dissolved oxygen (Ndaruga et al., 2004). Some macroinvertebrates showed sensitivity to low oxygen conditions, and lethal effects were observed at low DO (i.e., <20%) saturation levels for a number of species (Connolly et al., 2004). The sensitivity to oxygen conditions is likely to be associated with the locations for oviposition and predation (Kietzka et al., 2017).
Our result indicated that Odonata species richness declined with increasing water level and pH. Williams et al. (2004) reported that water level was the main environmental factor linked to the gradual change in species assemblage between rivers, streams and ditches. Drainage may result in a decrease in pH (Laine et al., 1995). Indeed, pH is an important attribute affecting Odonata community composition more generally (Johansson and Brodin, 2003). Acidic waters with low pH values can cause adverse effects on dragonflies, particularly on the developmental time and hatching success of eggs (Punzo, 1988; Pollard and Berrill, 1992). Although Odonata are typically relatively tolerant to low pH, it may affect survival of some species (Corbet, 2004). In addition, acid-stressed regions are suitable habitats for specialists, which do not propagate in neutral waters (Hudson and Berrill, 1986). Rising water level may affect the zone where submerged plant roots form an important habitat type in oil palm waterways that become unavailable for Odonata and their prey specifically invertebrates.
Odonata species richness increased with rising water temperature in oil palm waterways. Many Odonates prefer warmer and shallow water for their ovipositional sites (Michiels and Dhondt, 1990), and higher water temperatures can promote increased rates of egg hatching and reduce predation on adult Odonata (Wolf and Waltz, 1988). Streams draining oil palm plantations often have markedly higher water temperatures compared to forest streams (Carlson et al., 2014). However, our findings are in contrast to patterns typically encountered in temperate ecosystems with, for example, Durance and Ormerod (2009) reporting that the abundance of macroinvertebrate families can decline with increasing temperature. In addition to water quality, inevitable changes in vegetation patterns affect aquatic invertebrates (Elo et al., 2015). Vegetation structure served as a good indicator of habitat requirements for dragonflies and damselflies, thereby partially predicting species diversity (Remsburg and Turner, 2009). Vegetation structure benefits Odonata through resource availability and shelter (Buchwald, 1992), with positive relationships between Odonata and plant species richness observed at multiple spatial scales (Honkanen et al., 2011). Moreover, the height of key plant species also determines Odonate diversity, emphasizing the importance of vegetation structure for fliers and perchers (Lee Foote and Rice Hornung, 2005).
The use of agrochemicals (e.g., high rate of fertilizer application) to sustain crop productivity is one of the leading causes of water quality impairment in oil palm waterways (Khatun et al., 2017). Oil palm smallholders and plantation managers should be wary of eutrophication in oil palm smallholdings, due to consequent reductions in total dissolved oxygen in waterways. Moreover, eutrophication may limit sunlight availability for aquatic autotrophs due to the proliferation of heterotophic fungi and the decay of algae on waterbody surfaces (de Paiva Silva et al., 2010; Burchett and Burchett, 2011). Excessive nutrient loads, primarily elevated nitrogen and phosphorus levels, can accumulate in slower moving waterways due to surface water run-off in poorly managed farmland (Burchett and Burchett, 2011). The present study recommended establishment of buffer strips to reduce the effects of eutrophication. Buffer strips greatly improve water quality of agricultural streams by reducing nutrient leaching in groundwater and surface water run-off, even though they comprise little of the total catchment area (Vought et al., 1995). Moreover, they may act to buffer streams from a host of catchment-wide anthropogenic stressors; even relatively small areas of near-stream forest cover may increase ecosystem resilience and stability, acting to boost aquatic macroinvertebrate diversity and production (Thomas et al., 2016). This may be especially important in structurally degraded monocultural landscapes, whereby the increased habitat complexity and resource availability facilitated by buffer strips can help promote diversity in macroinvertebrate taxa, including Odonates.
Our results provide valuable information on which to assess the importance of man-made waterways as aquatic habitat for Odonata in oil palm production landscapes. Waterway management is particularly important to guarantee profitable agricultural production. Well-maintained waterways can increase yield while maintaining aquatic insect biodiversity, particularly in the case of sensitive groups such as Odonata. Most Odonata species encountered belong to the family Libellulidae as the larger-bodied adults can thermoregulate efficiently under warmer waterways condition in oil palm sites (Luke et al., 2017). The narrower channels in oil palm sites also lead to higher organic matter input through sediment deposition that promotes higher vegetation density (Singh et al., 2015). The applications of chemical pesticides and fertilizers in oil palm smallholding can, however, negatively impact Odonata, as chemical runoff can limit juvenile growth, development and survival (Dinh Van et al., 2014). Herbicide overuse removes undergrowth cover completely, which protects the soil against erosion caused by surface runoff in oil palm plantations (Tohiran et al., 2017; Darras et al., 2019). Another runoff carries away topsoil into the waterways, causing sedimentation and adversely affects the water quality. Odonata sensitivity to water quality thus provides a useful indicator for ecosystem quality more broadly. In return, biodiversity may help to buffer aquatic ecosystems against the ecological impacts of nutrient pollution (Cardinale, 2011). Despite the strength and consistency of our findings, our data are inherently limited to effects on adult Odonata taxa.
Conclusion
Our findings support evidence that human-induced disturbances such as oil palm expansion in the tropics resulted in pronounced changes in the taxonomical composition of the Odonata. Our data suggests that adult odonates have highly specific habitat requirements with respect to physicochemical water quality of oil palm waterways and riparian vegetation. Water quality appears to be key in driving resultant variation in Odonata biodiversity in oil palm production landscapes. By maintaining water quality in oil palm waterways, these man-made aquatic habitats may conserve Odonata communities in oil palm production landscapes. Future studies need to gather evidence related to improved waterway management. Unpolluted waterways in oil palm production landscapes may reduce ecological disturbances that affect odonate survival. It is essential to highlight that riparian vegetated buffer strips in oil palm smallholdings can differ depending on the type of farm management. Establishment of buffer strips along waterways can provide beneficial impact for soil and water quality while maintaining biodiversity in oil palm smallholdings. As there is no law enforcement in the study area, some smallholders consistently spray the buffer strips with chemical herbicides to clear them for oil palm planting.
In general, if agricultural practices intend to limit disturbances from oil palm cultivation, proper waterway management could help to improve the value of oil palm smallholdings as part of wider conservation-agricultural matrices (Azhar et al., 2015a; Sulai et al., 2015). As such, we suggest self-managed oil palm farming should aim to maintain water quality and physical habitat structure in plantation waterways, and minimize the use of chemical herbicides and fertilizers which flow into the river when it rained (Abdul et al., 2017), in order to be certified as biodiversity-friendly agriculture (e.g., Roundtable on Sustainable Palm Oil and Malaysian Sustainable Palm Oil). We recommend that smallholders should be given more information regarding biodiversity-friendly practices to help mitigate the negative impacts of intensive oil palm monoculture farming with respect to aquatic habitat management.
Data Availability Statement
The raw data supporting the conclusions of this article will be made available by the authors upon request.
Author Contributions
MI and AG carried out the experiment. AN wrote the manuscript with support from ST. SN helped supervise the project. BA conceived the original idea and supervised the project. All authors contributed to the article and approved the submitted version.
Funding
This project was funded by the Universiti Putra Malaysia (grant no. GP-IPM/2017/9584900).
Conflict of Interest
The authors declare that the research was conducted in the absence of any commercial or financial relationships that could be construed as a potential conflict of interest.
Acknowledgments
We thank the oil palm smallholders in Tanjung Karang, Selangor and staff of Institute of Bioscience, Universiti Putra Malaysia for assisting us in the field during data collection.
References
Abdul, N. H., Rawi, C. S. M., Ahmad, A. H., and Al-Shami, S. A. (2017). Effect of environmental disturbances on odonata assemblages along a tropical polluted river. Ekológia (Bratislava) 36, 388–402. doi: 10.1515/eko-2017-0030
Allan, J. D. (2004). Landscapes and riverscapes: the influence of land use on stream ecosystems. Annu. Rev. Ecol. Evol. Syst. 35, 257–284. doi: 10.1146/annurev.ecolsys.35.120202.110122
Amoah, F. M., Nuertey, B. N., Baidoo-Addo, K., Oppong, F. K., Osei-Bonsu, K., and Asamoah, T. E. O. (1995). Underplanting oil palm with cocoa in Ghana. Agrofor. Syst. 30, 289–299. doi: 10.1007/bf00705215
Asmah, S., Ghazali, A., Syafiq, M., Yahya, M. S., Peng, T. L., Norhisham, A. R., et al. (2017). Effects of polyculture and monoculture farming in oil palm smallholdings on tropical fruit-feeding butterfly diversity. Agric. For. Entomol. 19, 70–80. doi: 10.1111/afe.12182
Azhar, B., Lindenmayer, D. B., Wood, J., Fischer, J., Manning, A., McElhinny, C., et al. (2011). The conservation value of oil palm plantation estates, smallholdings and logged peat swamp forest for birds. For. Ecol. Manage. 262, 2306–2315. doi: 10.1016/j.foreco.2011.08.026
Azhar, B., Puan, C. L., Aziz, N., Sainuddin, M., Adila, N., Samsuddin, S., et al. (2015a). Effects of in situ habitat quality and landscape characteristics in the oil palm agricultural matrix on tropical understory birds, fruit bats and butterflies. Biodivers. Conserv. 24, 3125–3144. doi: 10.1007/s10531-015-1005-6
Azhar, B., Puan, C. L., Zakaria, M., Hassan, N., and Arif, M. (2014). Effects of monoculture and polyculture practices in oil palm smallholdings on tropical farmland birds. Basic Appl. Ecol. 15, 336–346. doi: 10.1016/j.baae.2014.06.001
Azhar, B., Saadun, N., Prideaux, M., and Lindenmayer, D. B. (2017). The global palm oil sector must change to save biodiversity and improve food security in the tropics. J. Environ. Manage. 203, 457–466. doi: 10.1016/j.jenvman.2017.08.021
Azhar, B., Saadun, N., Puan, C. L., Kamarudin, N., Aziz, N., Nurhidayu, S., et al. (2015b). Promoting landscape heterogeneity to improve the biodiversity benefits of certified palm oil production: evidence from Peninsular Malaysia. Glob. Ecol. Conserv. 3, 553–561. doi: 10.1016/j.gecco.2015.02.009
Breitburg, D. L., Adamack, A., Rose, K. A., Kolesar, S. E., Decker, B., Purcell, J. E., et al. (2003). The pattern and influence of low dissolved oxygen in the Patuxent River, a seasonally hypoxic estuary. Estuaries 26, 280–297. doi: 10.1007/bf02695967
Bried, J. T., Hager, B. J., Hunt, P. D., Fox, J. N., Jensen, H. J., and Vowels, K. M. (2012). Bias of reduced-effort community surveys for adult Odonata of lentic waters. Insect Conserv. Divers. 5, 213–222. doi: 10.1111/j.1752-4598.2011.00156.x
Brito, J. G., Martins, R. T., Oliveira, V. C., and de Paula, F. R. (2018). Biological indicators of diversity in tropical streams: congruence in the similarity of invertebrate assemblages. Ecol. Indic. 85, 85–92. doi: 10.1016/j.ecolind.2017.09.001
Buchwald, R. (1992). Vegetation and dragonfly fauna–characteristics and examples of biocenological field studies. Vegetatio 101, 99–107.
Burchett, S., and Burchett, S. (2011). Introduction to Wildlife Conservation in Farming. West Sussex: John Wiley & Sons.
Burnham, K. P., and Anderson, D. R. (2002). Model Selection and Multimodel Inference: a Practical Information-Theoretic Approach. Berlin: Springer Science & Business Media.
Cardinale, B. J. (2011). Biodiversity improves water quality through niche partitioning. Nature 472, 86–89. doi: 10.1038/nature09904
Carlson, K. M., Curran, L. M., Ponette-González, A. G., Ratnasari, D., Lisnawati, N., Purwanto, Y., et al. (2014). Influence of watershed-climate interactions on stream temperature, sediment yield, and metabolism along a land use intensity gradient in Indonesian Borneo. J. Geophys. Res. Biogeosci. 119, 1110–1128. doi: 10.1002/2013jg002516
Castillo, L. E., Martínez, E., Ruepert, C., Savage, C., Gilek, M., Pinnock, M., et al. (2006). Water quality and macroinvertebrate community response following pesticide applications in a banana plantation, Limon, Costa Rica. Sci. Total Environ. 367, 418–432. doi: 10.1016/j.scitotenv.2006.02.052
Che Salmah, M. R., Al-Shami, S. A., Madrus, M. R., and Ahmad, A. H. (2014). Biological and ecological diversity of aquatic macroinvertebrates in response to hydrological and physicochemical parameters in tropical forest streams of Gunung Tebu, Malaysia: implications for ecohydrological assessment. Ecohydrology 7, 496–507. doi: 10.1002/eco.1368
Che Salmah, M. R., Hassan, S. T. S., Abu Hassan, A., and Ali, A. B. (1998). Influence of physical and chemical factors on the larval abundance of Neurothemis tullia (Drury)(Odonata: Libellulidae) in a rain fed rice field. Hydrobiologia 389, 193–202.
Clarke, K. R., and Warwick, R. M. (2001). Change in Marine Communities: an Approach to Statistical Analysis and Interpretation. Plymouth: PRIMER-E, 2.
Cleary, D. F., Mooers, A. Ø, Eichhorn, K. A., Van Tol, J., De Jong, R., and Menken, S. B. (2004). Diversity and community composition of butterflies and odonates in an ENSO-induced fire affected habitat mosaic: a case study from East Kalimantan, Indonesia. Oikos 105, 426–448. doi: 10.1111/j.0030-1299.2004.12219.x
Colwell, R. K., and Coddington, J. A. (1994). Estimating terrestrial biodiversity through extrapolation. Philos. Trans. R. Soc. B 345, 101–118. doi: 10.1098/rstb.1994.0091
Colwell, R. K., Mao, C. X., and Chang, J. (2004). Interpolating, extrapolating and comparing incidence-based species accumulation curves. Ecology 85, 2717–2727. doi: 10.1890/03-0557
Connolly, N. M., Crossland, M. R., and Pearson, R. G. (2004). Effect of low dissolved oxygen on survival, emergence, and drift of tropical stream macroinvertebrates. J. N. Am. Benthol. Soc. 23, 251–270. doi: 10.1899/0887-3593(2004)023<0251:eoldoo>2.0.co;2
Corbet, P. S. (2004). Dragonflies: Behaviour and Ecology of Odonata (Revised Edition). Colchester: Harley Books.
Cunha, E. J., de Assis Montag, L. F., and Juen, L. (2015). Oil palm crops effects on environmental integrity of Amazonian streams and Heteropteran (Hemiptera) species diversity. Ecol. Indic. 52, 422–429. doi: 10.1016/j.ecolind.2014.12.024
Darras, K. F., Corre, M. D., Formaglio, G., Tjoa, A., Potapov, A., Brambach, F., et al. (2019). Reducing fertilizer and avoiding herbicides in oil palm plantations—ecological and economic valuations. Front. For. Glob. Change 2:65. doi: 10.3389/ffgc.2019.00065
de Paiva Silva, D., De Marco, P., and Resende, D. C. (2010). Adult odonate abundance and community assemblage measures as indicators of stream ecological integrity: a case study. Ecol. Indic. 10, 744–752. doi: 10.1016/j.ecolind.2009.12.004
Dinh Van, K., Janssens, L., Debecker, S., and Stoks, R. (2014). Temperature and latitude specific individual growth rates shape the vulnerability of damselfly larvae to a widespread pesticide. J. Appl. Ecol. 51, 919–928. doi: 10.1111/1365-2664.12269
Dolnı, A., Harabiš, F., Bárta, D., Lhota, S., and Drozd, P. (2012). Aquatic insects indicate terrestrial habitat degradation: changes in taxonomical structure and functional diversity of dragonflies in tropical rainforest of East Kalimantan. Trop. Zool. 25, 141–157. doi: 10.1080/03946975.2012.717480
Dolný, A., Harabiš, F., and Mižičová, H. (2014). Home range, movement, and distribution patterns of the threatened dragonfly Sympetrum depressiusculum (Odonata: Libellulidae): a thousand times greater territory to protect? PLoS One 9:e100408. doi: 10.1371/journal.pone.0100408
Dormann, C. F., Elith, J., Bacher, S., Buchmann, C., Carl, G., Carre, G., et al. (2013). Colinearity: a review of method to deal with it and a simulation study evaluating their performance. Ecography 36, 27–46.
Dow, R. A., Ng, Y. F., and Choong, C. Y. (2012). Odonata of Sungai Bebar, Pahang, Malaysia, with four species recorded for the first time from mainland Asia. J. Threat. Taxa 4, 2417–2426. doi: 10.11609/jott.o3041.2417-26
Durance, I., and Ormerod, S. J. (2009). Trends in water quality and discharge confound long-term warming effects on river macroinvertebrates. Freshw. Biol. 54, 388–405. doi: 10.1111/j.1365-2427.2008.02112.x
Elo, M., Penttinen, J., and Kotiaho, J. S. (2015). The effect of peatland drainage and restoration on Odonata species richness and abundance. BMC Ecol. 15:11. doi: 10.1186/s12898-015-0042-z
Faruk, A., Belabut, D., Ahmad, N., Knell, R. J., and Garner, T. W. (2013). Effects of oil-palm plantations on diversity of tropical anurans. Conserv. Biol. 27, 615–624. doi: 10.1111/cobi.12062
Ferreras-Romero, M., Marquez-Rodriguez, J., and Ruiz-Garcia, A. (2009). Implications of anthropogenic disturbance factors on the Odonata assemblage in a Mediterranean fluvial system. Int. J. Odonatol. 12, 413–428. doi: 10.1080/13887890.2009.9748354
Gallmetzer, N., and Schulze, C. H. (2015). Impact of oil palm agriculture on understory amphibians and reptiles: a Mesoamerican perspective. Glob. Ecol. Conserv. 4, 95–109. doi: 10.1016/j.gecco.2015.05.008
Ghazali, A., Asmah, S., Syafiq, M., Yahya, M. S., Aziz, N., Tan, L. P., et al. (2016). Effects of monoculture and polyculture farming in oil palm smallholdings on terrestrial arthropod diversity. J. Asia Pac. Entomol. 19, 415–421. doi: 10.1016/j.aspen.2016.04.016
Giam, X., Hadiaty, R. K., Tan, H. H., and Wilcove, D. S. (2015). Mitigating the impact of oil-palm monoculture on freshwater fishes in Southeast Asia. Conserv. Biol. 29, 1357–1367. doi: 10.1111/cobi.12483
Gillespie, G. R., Ahmad, E., Elahan, B., Evans, A., Ancrenaz, M., Goossens, B., et al. (2012). Conservation of amphibians in Borneo: relative value of secondary tropical forest and non-forest habitats. Biol. Conserv. 152, 136–144. doi: 10.1016/j.biocon.2012.03.023
Hawa, A., Azhar, B., Top, M. M., and Zubaid, A. (2016). Depauperate avifauna in tropical peat swamp forests following logging and conversion to oil palm agriculture: evidence from mist-netting data. Wetlands 36, 899–908. doi: 10.1007/s13157-016-0802-3
Honkanen, M., Sorjanen, A. M., and Mönkkönen, M. (2011). Deconstructing responses of dragonfly species richness to area, nutrients, water plant diversity and forestry. Oecologia 166, 457–467. doi: 10.1007/s00442-010-1846-3
Hudson, J., and Berrill, M. (1986). Tolerance of low pH exposure by the eggs of Odonata (dragonflies and damselflies). Hydrobiologia 140, 21–25. doi: 10.1007/bf00006725
Hurvich, C. M., and Tsai, C. L. (1989). Regression and time series model selection in small samples. Biometrika 76, 297–307. doi: 10.1093/biomet/76.2.297
Jacobsen, D. (2008). Low oxygen pressure as a driving factor for the altitudinal decline in taxon richness of stream macroinvertebrates. Oecologia 154, 795–807. doi: 10.1007/s00442-007-0877-x
Janssens, L., Dinh Van, K., Debecker, S., Bervoets, L., and Stoks, R. (2014). Local adaptation and the potential effects of a contaminant on predator avoidance and antipredator responses under global warming: a space-for-time substitution approach. Evol. Appl. 7, 421–430. doi: 10.1111/eva.12141
Johansson, F., and Brodin, T. (2003). Effects of fish predators and abiotic factors on dragonfly community structure. J. Freshw. Ecol. 18, 415–423. doi: 10.1080/02705060.2003.9663977
Jones, J. C., Reynolds, J. D., and Raffaelli, D. (2006). “Environmental variables,” in Ecological Census Techniques: a Handbook, 2nd Edn, ed. J. W. Sutherland (Cambridge: Cambridge University Press).
Júnior, P. D. M., Batista, J. D., and Cabette, H. S. R. (2015). Community assembly of adult odonates in tropical streams: an ecophysiological hypothesis. PLoS One 10:e0123023. doi: 10.1371/journal.pone.0123023
Kadoya, T., Suda, S. I., and Washitani, I. (2009). Dragonfly crisis in Japan: a likely consequence of recent agricultural habitat degradation. Biol. Conserv. 142, 1899–1905. doi: 10.1016/j.biocon.2009.02.033
Kalkman, V. J., Clausnitzer, V., Dijkstra, K. D. B., Orr, A. G., Paulson, D. R., and van Tol, J. (2008). Global diversity of dragonflies (Odonata) in freshwater. Hydrobiologia 595, 351–363. doi: 10.1007/978-1-4020-8259-7_38
Khatun, R., Reza, M. I. H., Moniruzzaman, M., and Yaakob, Z. (2017). Sustainable oil palm industry: the possibilities. Renew. Sustain. Energy Rev. 76, 608–619. doi: 10.1016/j.rser.2017.03.077
Kietzka, G. J., Pryke, J. S., and Samways, M. J. (2017). Aerial adult dragonflies are highly sensitive to in-water conditions across an ancient landscape. Divers. Distrib. 23, 14–26. doi: 10.1111/ddi.12493
Knight, T. M., Mccoy, M. W., Chase, J. M., Mccoy, K. A., and Holt, R. D. (2005). Trophic cascades across ecosystems. Nature 437, 880–883.
Koch, K., Wagner, C., and Sahlén, G. (2014). Farmland versus forest: comparing changes in Odonata species composition in western and eastern Sweden. Insect Conserv. Divers. 7, 22–31. doi: 10.1111/icad.12034
Kushairi, D. (2017). Overview of the Malaysian Oil Palm Industry 2016. MPOB Palm Oil Statistics. Malaysia: Malaysian Palm Oil Board.
Kutcher, T. E., and Bried, J. T. (2014). Adult Odonata conservatism as an indicator of freshwater wetland condition. Ecol. Indic. 38, 31–39. doi: 10.1016/j.ecolind.2013.10.028
Laine, J., Vasander, H., and Laiho, R. (1995). Long-term effects of water level drawdown on the vegetation of drained pine mires in southern Finland. J. Appl. Ecol. 32, 785–802. doi: 10.2307/2404818
Lee Foote, A., and Rice Hornung, C. L. (2005). Odonates as biological indicators of grazing effects on Canadian prairie wetlands. Ecol. Entomol. 30, 273–283. doi: 10.1111/j.0307-6946.2005.00701.x
Luke, S. H., Barclay, H., Bidin, K., Chey, V. K., Ewers, R. M., Foster, W. A., et al. (2016). The effects of catchment and riparian forest quality on stream environmental conditions across a tropical rainforest and oil palm landscape in Malaysian Borneo. Ecohydrology 10, e1827. doi: 10.1002/eco.1827
Luke, S. H., Dow, R. A., Butler, S., Vun Khen, C., Aldridge, D. C., Foster, W. A., et al. (2017). The impacts of habitat disturbance on adult and larval dragonflies (Odonata) in rainforest streams in Sabah, Malaysian Borneo. Freshw. Biol. 62, 491–506. doi: 10.1111/fwb.12880
Mendes, T. P., Amado, L. L., Ribeiro, R. A. B., and Juen, L. (2020). Morphological diversity of Odonata larvae (Insecta) and abiotic variables in oil palm plantation areas in the Eastern Amazon. Hydrobiologia 847, 161–175. doi: 10.1007/s10750-019-04079-y
Mendes, T. P., Benone, N. L., and Juen, L. (2019). To what extent can oil palm plantations in the Amazon support assemblages of Odonata larvae? Insect Conserv. Divers. 12, 448–458. doi: 10.1111/icad.12357
Mercer, E. V., Mercer, T. G., and Sayok, A. K. (2014). Effects of forest conversions to oil palm plantations on freshwater macroinvertebrates: a case study from Sarawak, Malaysia. J. Land Use Sci. 9, 260–277. doi: 10.1080/1747423x.2013.786149
Michiels, N. K., and Dhondt, A. A. (1990). Costs and benefits associated with oviposition site selection in the dragonfly Sympetrum danae (Odonata: Libellulidae). Anim. Behav. 40, 668–678. doi: 10.1016/s0003-3472(05)80696-7
Miguel, T. B., Oliveira-Junior, J. M. B., Ligeiro, R., and Juen, L. (2017). Odonata (Insecta) as a tool for the biomonitoring of environmental quality. Ecol. Indic. 81, 555–566. doi: 10.1016/j.ecolind.2017.06.010
Monteiro-Júnior, C. S., Juen, L., and Hamada, N. (2014). Effects of urbanization on stream habitats and associated adult dragonfly and damselfly communities in central Brazilian Amazonia. Landsc. Urban Plan. 127, 28–40. doi: 10.1016/j.landurbplan.2014.03.006
Moore, A. A., and Palmer, M. A. (2005). Invertebrate biodiversity in agricultural and urban headwater streams: implications for conservation and management. Ecol. Appl. 15, 1169–1177. doi: 10.1890/04-1484
Morrison, M. L., Block, W. M., Strickland, M. D., Collier, B. A., and Peterson, M. J. (2008). Wildlife Study Design. New York, NY: Springer.
Ndaruga, A. M., Ndiritu, G. G., Gichuki, N. N., and Wamicha, W. N. (2004). Impact of water quality on macroinvertebrate assemblages along a tropical stream in Kenya. Afr. J. Ecol. 42, 208–216. doi: 10.1111/j.1365-2028.2004.00516.x
Norma-Rashid, Y., Mohd-Sofian, A., and Zakaria-Ismail, M. (2001). Diversity and distribution of Odonata (dragonflies and damselflies) in the fresh water swamp lake Tasek Bera, Malaysia. Hydrobiologia 459, 135–146.
Oliveira-Junior, J. M. B., and Juen, L. (2019). The Zygoptera/Anisoptera ratio (Insecta: Odonata): a new tool for habitat alterations assessment in Amazonian streams. Neotrop. Entomol. 48, 552–560. doi: 10.1007/s13744-019-00672-x
Oliveira-Junior, J. M. B., Shimano, Y., Gardner, T. A., Hughes, R. M., Marco Júnior, P., and Juen, L. (2015). Neotropical dragonflies (Insecta: Odonata) as indicators of ecological condition of small streams in the eastern Amazon. Austral Ecol. 40, 733–744. doi: 10.1111/aec.12242
Oon, A., Mohd Shafri, H. Z., Lechner, A. M., and Azhar, B. (2019a). Discriminating between large-scale oil palm plantations and smallholdings on tropical peatlands using vegetation indices and supervised classification of LANDSAT-8. Int. J. Remote Sens. 40, 7312–7328. doi: 10.1080/01431161.2019.1579944
Oon, A., Ngo, K. D., Azhar, R., Ashton-Butt, A., Lechner, A. M., and Azhar, B. (2019b). Assessment of ALOS-2 PALSAR-2L-band and Sentinel-1 C-band SAR backscatter for discriminating between large-scale oil palm plantations and smallholdings on tropical peatlands. Remote Sens. Appl. Soc. Environ. 13, 183–190. doi: 10.1016/j.rsase.2018.11.002
Oppel, S. (2006). Comparison of two Odonata communities from a natural and a modified rainforest in Papua New Guinea. Int. J. Odonatol. 9, 89–102. doi: 10.1080/13887890.2006.9748266
Orr, A. G. (2003). A Guide to the Dragonflies of Borneo: their Identification and Biology. Kota Kinabalu: Natural History Publications (Borneo).
Orr, A. G. (2004). Critical species of Odonata in Malaysia, Indonesia, Singapore and Brunei. Int. J. Odonatol. 7, 371–384. doi: 10.1080/13887890.2004.9748222
Orr, A. G. (2005). Dragonflies of Peninsular Malaysia and Singapore. Kota Kinabalu: Natural History Publications (Borneo).
Ouyang, W., Song, K., Wang, X., and Hao, F. (2014). Non-point source pollution dynamics under long-term agricultural development and relationship with landscape dynamics. Ecol. Indic. 45, 579–589. doi: 10.1016/j.ecolind.2014.05.025
Pollard, J. B., and Berrill, M. (1992). The distribution of dragonfly nymphs across a pH gradient in south-central Ontario lakes. Can. J. Zool. 70, 878–885. doi: 10.1139/z92-125
Pryke, J. S., Samways, M. J., and De Saedeleer, K. (2015). An ecological network is as good as a major protected area for conserving dragonflies. Biol. Conserv. 191, 537–545. doi: 10.1016/j.biocon.2015.07.036
Punzo, F. (1988). Effects of low environmental pH and temperature on hatching and metabolic rates in embryos of Anax junius drury (Odonata: Aeshnidae) and the role of hypoxia in the hatching process. Comp. Biochem. Physiol. C Comp. 91, 333–336. doi: 10.1016/0742-8413(88)90038-2
Razak, S. A., Saadun, N., Azhar, B., and Lindenmayer, D. B. (2020). Smallholdings with high oil palm yield also support high bird species richness and diverse feeding guilds. Environ. Res. Lett. 15:094031. doi: 10.1088/1748-9326/aba2a5
Remsburg, A. (2011). Relative influence of prior life stages and habitat variables on dragonfly (Odonata: Gomphidae) densities among lake sites. Diversity 3, 200–216. doi: 10.3390/d3020200
Remsburg, A. J., and Turner, M. G. (2009). Aquatic and terrestrial drivers of dragonfly (Odonata) assemblages within and among north-temperate lakes. J. N. Am. Benthol. Soc. 28, 44–56. doi: 10.1899/08-004.1
Rizo-Patrón, F. V., Kumar, A., Colton, M. B. M., Springer, M., and Trama, F. A. (2013). Macroinvertebrate communities as bioindicators of water quality in conventional and organic irrigated rice fields in Guanacaste, Costa Rica. Ecol. Indic. 29, 68–78. doi: 10.1016/j.ecolind.2012.12.013
Samways, M. J., and Steytler, N. S. (1996). Dragonfly (Odonata) distribution patterns in urban and forest landscapes, and recommendations for riparian management. Biol. Conserv. 78, 279–288. doi: 10.1016/s0006-3207(96)00032-8
Schall, R. (1991). Estimation in generalized linear-models with random effects. Biometrika 78, 719–727. doi: 10.1093/biomet/78.4.719
Singh, M., Malhi, Y., and Bhagwat, S. (2015). Aboveground biomass and tree diversity of ri- parian zones in an oil palm-dominated mixed landscape in Borneo. J. Trop. For. Sci. 27, 227–239.
Stoks, R., and Córdoba-Aguilar, A. (2012). Evolutionary ecology of Odonata: a complex life cycle perspective. Annu. Rev. Entomol. 57, 249–265. doi: 10.1146/annurev-ento-120710-100557
Sulai, P., Nurhidayu, S., Aziz, N., Zakaria, M., Barclay, H., and Azhar, B. (2015). Effects of water quality in oil palm production landscapes on tropical waterbirds in Peninsular Malaysia. Ecol. Res. 30, 941–949. doi: 10.1007/s11284-015-1297-8
Therriault, T. W., and Kolasa, J. (1999). Physical determinants of richness, diversity, evenness and abundance in natural aquatic microcosms. Hydrobiologia 412, 123–130.
Thomas, L., Buckland, S. T., Rexstad, E. A., Laake, J. L., Strindberg, S., Hedley, S. L., et al. (2010). Distance software: design and analysis of distance sampling surveys for estimating population size. J. Appl. Ecol. 47, 5–14. doi: 10.1111/j.1365-2664.2009.01737.x
Thomas, S. M., Griffiths, S. W., and Ormerod, S. J. (2016). Beyond cool: adapting upland streams for climate change using riparian woodlands. Glob. Change Biol. 22, 310–324. doi: 10.1111/gcb.13103
Tohiran, K. A., Nobilly, F., Zulkifli, R., Maxwell, T., Moslim, R., and Azhar, B. (2017). Targeted cattle grazing as an alternative to herbicides for controlling weeds in bird-friendly oil palm plantations. Agronomy for Sustainable Development 37, 62.
Vought, L. B. M., Pinay, G., Fuglsang, A., and Ruffinoni, C. (1995). Structure and function of buffer strips from a water quality perspective in agricultural landscapes. Landsc. Urban Plan. 31, 323–331. doi: 10.1016/0169-2046(94)01057-f
Wilkinson, C. L., Yeo, D. C. J., Tan, H. H., Fikri, A. H., and Ewers, R. M. (2018). The availability of freshwater fish resources is maintained across a land-use gradient in Sabah, Borneo. Aquat. Conserv. Mar. Freshw. Ecosyst. 28, 1044–1054. doi: 10.1002/aqc.2920
Williams, P., Whitfield, M., Biggs, J., Bray, S., Fox, G., Nicolet, P., et al. (2004). Comparative biodiversity of rivers, streams, ditches and ponds in an agricultural landscape in Southern England. Biological Conservation 115, 329–341. doi: 10.1016/s0006-3207(03)00153-8
Wolf, L. L., and Waltz, E. C. (1988). Oviposition site selection and spatial predictability of female white-faced dragonflies (Leucorrhinia intacta) (Odonata: Libellulidae). Ethology 78, 306–320. doi: 10.1111/j.1439-0310.1988.tb00240.x
Wong, M. K., Tsukamoto, J., Yusuyin, Y., Tanaka, S., Iwasaki, K., and Tan, N. P. (2016). Comparison of soil macro-invertebrate communities in Malaysian oil palm plantations with secondary forest from the viewpoint of litter decomposition. For. Ecol. Manage. 381, 63–73. doi: 10.1016/j.foreco.2016.09.011
Wu, J., and Lu, J. (2019). Landscape patterns regulate non-point source nutrient pollution in an agricultural watershed. Sci. Total Environ. 669, 377–388. doi: 10.1016/j.scitotenv.2019.03.014
Keywords: aquatic biodiversity, dragonflies, damselflies, habitat degradation, smallholdings, water quality
Citation: Ishak M, Norhisham AR, Thomas SM, Nurhidayu S, Ghazali A and Azhar B (2021) Physicochemical Properties as Driver of Odonata Diversity in Oil Palm Waterways. Front. For. Glob. Change 4:613064. doi: 10.3389/ffgc.2021.613064
Received: 01 October 2020; Accepted: 04 May 2021;
Published: 07 June 2021.
Edited by:
Geertje M. F. Van Der Heijden, University of Nottingham, United KingdomReviewed by:
Lenize Calvão, Universidade Federal do Amapá, BrazilKarina Silva, Federal University of Pará, Brazil
Copyright © 2021 Ishak, Norhisham, Thomas, Nurhidayu, Ghazali and Azhar. This is an open-access article distributed under the terms of the Creative Commons Attribution License (CC BY). The use, distribution or reproduction in other forums is permitted, provided the original author(s) and the copyright owner(s) are credited and that the original publication in this journal is cited, in accordance with accepted academic practice. No use, distribution or reproduction is permitted which does not comply with these terms.
*Correspondence: Ahmad R. Norhisham, Norhisham_razi@upm.edu.my