- 1Laboratório de Ecologia Vegetal, Programa de Pós-Graduação em Ecologia, Departamento de Ecologia, Instituto de Biociências, Universidade Federal do Rio Grande do Sul, Porto Alegre, Brazil
- 2Laboratório de Estudos em Vegetação Campestre, Programa de Pós-Graduação em Botânica, Instituto de Biociências, Universidade Federal do Rio Grande do Sul, Porto Alegre, Brazil
- 3Instituto Nacional de Pesquisas da Amazônia, Manaus, Brazil
Secondary forests originate from natural regeneration after fallow (succession) or restoration. Species assembly in these communities, which can affect ecosystem functions and successional trajectories, is very unpredictable. Trait-based trajectories can shed light on the recovery of ecosystem functions and enable predictions of how the regenerating communities will change with forest age. Regeneration communities are affected by initial conditions and also by canopy structure and functional traits that alter dispersers' attractiveness and coexistence mechanisms. Here we evaluated how community functional traits change over time and tested if functional diversity and composition of the established canopy, as well as the structure of the canopy and forest age, influence the functional structure of regenerating tree communities when compared to their reference forests. For this, we calculated dissimilarity in trait composition (community-weighted means) and in functional diversity of regenerating communities of each succession/restoration stand, using the tree stratum of nearby mature forests as baseline values. Functional trait information comprises leaf, wood density, and reproductive traits from tree species. Our community data contain information from natural successional forests and restoration sites, in the South-Brazilian Atlantic Forest. Predictor variables of functional dissimilarities were forest age, canopy structural variables, canopy functional composition, and functional diversity. Results showed leaf traits (leaf dry matter content, leaf nitrogen content, leaf nitrogen-phosphorus ratio) and seed mass varying with forest age. Canopy functional composition based on leaf traits and total basal area significantly predicted multiple trait functional dissimilarity between the regeneration component of secondary forests and their reference community values. Dissimilarity increased when the canopy was composed of species with more acquisitive traits. Difference in functional diversity was only influenced by forest age. Mid-stage secondary forests showed lower functional diversity than early-stage forests. Our results indicated the importance of canopy traits on the natural regeneration of secondary subtropical forests. If functional similarity with reference forests is a desired objective in order to recover ecosystem functions through natural regeneration, leaf functional traits of canopy trees that establish or are planted in degraded areas must be considered in the successional processes.
Introduction
Secondary forests are important for biodiversity conservation and ecosystem services, such as biomass stocks and water cycling, given the widespread deforestation and forest degradation processes in the tropics and subtropics (Chazdon et al., 2009). These forests are originated from natural regeneration processes after the abandonment of anthropogenic land-use types (succession) or after intentional actions to assist the recovery of ecosystems (restoration). Regeneration trajectories from both succession or restoration processes and the recovery of functioning and composition of these forests depend on ecosystem resilience, land-use history and landscape context, including many local and regional features (Holl and Aide, 2011; Crouzeilles et al., 2017; Holl et al., 2018). Even though many abiotic and biotic variables, as well as socio-economic context, are key drivers associated with the success of restoration strategies and regeneration trajectories (Pichancourt et al., 2014; Meli et al., 2017), biodiversity-based ecosystem services should be considered in restoration planning and monitoring to optimize forest restoration targets (Kollmann et al., 2016; Reij and Garrity, 2016; Rosenfield and Müller, 2020a). Ecosystem functioning and services are key goals in restoration projects, therefore biodiversity and trait-based researches have a high potential in informing the success of restoration efforts, embracing species parameters, interactions, and ecosystem functioning (Montoya et al., 2012; Rosenfield and Müller, 2019).
Successful regeneration of secondary forests should consider multifaceted parameters, including biodiversity, species composition, ecosystem functioning and services, to achieve a more holistic restoration. However, predicting secondary forest regeneration under natural conditions or even after active restoration actions is a big challenge. Even when considering the effect of distinct factors, studies from successional and restoration forests have shown that time is mandatory for tropical forest recovery (Holl et al., 2018), indicating that forest structure, species richness, and biomass are highly resilient in forests under natural regeneration processes (Martin et al., 2013; Zanini et al., 2014; Poorter et al., 2016; Meli et al., 2017; Rozendaal et al., 2019). Species composition, however, when compared to structural parameters or species richness, is the most unpredictable factor along succession, taking centuries to recover or even not recovering to similar pre-disturbances patterns at all (Chazdon, 2008; Liebsch et al., 2008; Rozendaal et al., 2019). Considering this and the importance of species characteristics and assemblies to ecosystem functions (Diaz et al., 2007), understanding and predicting the recovery of plant functional traits along the successional process of secondary forests is of most importance. A central question in recovering these forests is if secondary forests can achieve similar ecosystem functionality regardless of reaching similar values of former species composition. This is especially important in tropical and subtropical forests, where the number of rare species (with potential distinct and unique traits) is high when compared to the amount of common, abundant species.
Species establishment is a well-known barrier in early regeneration stages and, in forest restoration projects, planting seedlings is widely applied as a restoration tool (Holl and Brancalion, 2020). The choice of species to be used in these plantings can be a challenge, as they have distinct chances to survive and might further influence regeneration trajectories by altering the conditions for secondary species establishment or by determining coexistence mechanisms (Muscarella et al., 2017; Fernandes Neto et al., 2019; Charles, 2020). In areas of natural succession, earlier stages of forest under moist and wet conditions often have species that present a higher investment in leaf traits with high potential for acquiring resources, such as light and soil nutrients (e.g., high specific leaf area and leaf nitrogen content), and consequently grow faster (e.g., low wood density), quickly occupying the available space (Lohbeck et al., 2013; Boukili and Chazdon, 2017). However, under dry conditions, forests in earlier stages of succession, which are under more severe environmental conditions, are often dominated by species with conservative characteristics, showing denser wood stems and thicker leaves (Lohbeck et al., 2013; Poorter et al., 2019). Even though these general patterns are predictable to one extent, successional trajectories in the long-term might follow stochastic patterns or converge toward the functional composition of reference forests (Norden et al., 2015), which depends on the trait or function being analyzed (Boukili and Chazdon, 2017). Convergent trajectories regarding functional trait composition during forest development seem to prevail, but there are only few studies and less information about functional diversity trajectories along the successional time (Teixeira et al., 2020).
In addition to time and site conditions that influence community recovery trajectories, plant traits associated with the initial colonizers might influence the ongoing process (Mesquita et al., 2001), potentially causing priority effects (Fukami, 2015). Under natural regeneration, initial colonizers are influenced by land-use legacy and drive successional trajectories: colonizers of intensively used sites (e.g., pasture) arrest succession and trajectories might be unpredictable when compared to regeneration trajectories on sites that were clear-cut and abandoned without any type of soil-use (Mesquita et al., 2015; Fernandes Neto et al., 2019). Besides purely structural features of the canopy (e.g., height and cover), which affect microclimatic conditions associated with light and soil (Chazdon, 2008), morphological, physiological, and phenological traits of initial colonizers might influence the ongoing regeneration community, altering dispersal attractiveness (Viani et al., 2015) and coexistence mechanisms associated to growth and survival. Under the lens of priority effects, these mechanisms are associated with niche preemption and niche modification (Fukami, 2015). Some initial colonizers have traits that determine their own success in establishing and becoming dominant across time, also slowing down the colonization by other species with different traits (Jakovac et al., 2014; Fernandes Neto et al., 2019). This may result in stable states that retard the successional process toward reference conditions, whereas other traits can lead to community attributes that improve colonization by later species or ecosystem functions, thus speeding the successional process (Weidlich et al., 2018; Fernandes Neto et al., 2019). Forest regeneration success (trajectory and speed) might thus be associated with characteristics of first colonizers along the successional process (e.g., Werden et al., 2018), besides the already known influence of structural features, landscape, and abiotic conditions. Beyond the potential priority effects from first colonizers of community reassembly, the established forest canopy and their characteristics might continuously affect the regeneration mechanisms within forest dynamics (Muscarella et al., 2017).
By understanding the influence of some key traits of initial colonizers on forest regeneration patterns, relevant restoration actions can be employed. For example, by choosing tree species for planting through traits that may best influence subsequent regeneration trajectories, one could enhance the chance of restoring ecosystem functioning in a similar way as found in pre-disturbance communities, i.e., the target forest communities (Laughlin, 2014; Laughlin et al., 2018). Here we aim to assess if functional trait composition and diversity of regenerating tree communities in successional forests increase their similarity in relation to reference forests over time, also considering the characteristics of the established canopy as potential drivers of observed trajectories. More specifically, we are interested in evaluating (i) how community functional traits change with the age of secondary forests; and whether (ii) the functional diversity and composition of the established canopy, the structure of the canopy, or the time since forest abandonment influence the similarity of regenerating tree communities in relation to the functional structure of reference forests. This similarity was evaluated considering trait composition and functional diversity differences between each successional forest and their nearby reference forest. We are interested in possibly making predictions of trait-based trajectories of regenerating tree communities based on the leaf, reproductive, and wood characteristics of initial colonizers or planted trees (which are the main components of the established canopy), in order to recover reference baseline values of ecosystem functioning.
Materials and Methods
Study Region
The study was conducted in the southern Brazilian Atlantic Forest domain. The most dominant climate in the region is classified as Cfa temperate humid climate, lacking a marked dry season, with hot summers above 22°C (Peel et al., 2007). In the eastern highlands, the climate type is Cfb, with the average temperature of the warmest month not exceeding 22°C, and the annual isotherm below 18°C. The southern Atlantic Forest encompasses different forest types such as the Atlantic moist forest, Araucaria forest (a mixed subtropical forest type located in the highlands), and seasonal forests, with high levels of beta diversity (Bergamin et al., 2017).
We included seven study sites from which we collected data on the regeneration tree communities in secondary forests and on reference tree communities (one for each site) in nearby mature forests. Secondary forests of four of our study sites consist of stands under natural succession after land abandonment (Zanini et al., 2014; Vicente-Silva et al., 2016), whereas the remaining three are sites undergoing ecological restoration after planting interventions of tree seedlings (Rosenfield and Müller, 2019) (Table 1). Planting of seedlings was conducted under legal requirement, using native species with distinct number of species and density (see Rosenfield and Müller, 2019). Forest stand age of restoration sites was determined by considering the regeneration time after the end of interventions, whereas for the stands under natural succession, the information was gathered through interviews and crosschecked with satellite images (Zanini et al., 2014; Vicente-Silva et al., 2016). Land-use history of the studied secondary forests was not intensive in terms of mechanization: Maquiné areas were used for slash-and-burn small subsistence agriculture; Pró-Mata areas were used for subsistence agriculture and pasture, and the restoration areas were formerly used for cattle grazing (Cachoeirinha), Eucalyptus plantation (Canela), and grape production (Santa Tereza). The age range of secondary forests, with the respective forest type and other relevant information of the study areas, are shown in Table 1. For each study site, we identified and surveyed a nearby reference site (old-growth or mature forest) to be used as baseline values of a desired tree functional structure (trait composition and functional diversity). For the reference forests, we set an approximated age since the interruption of human interventions.
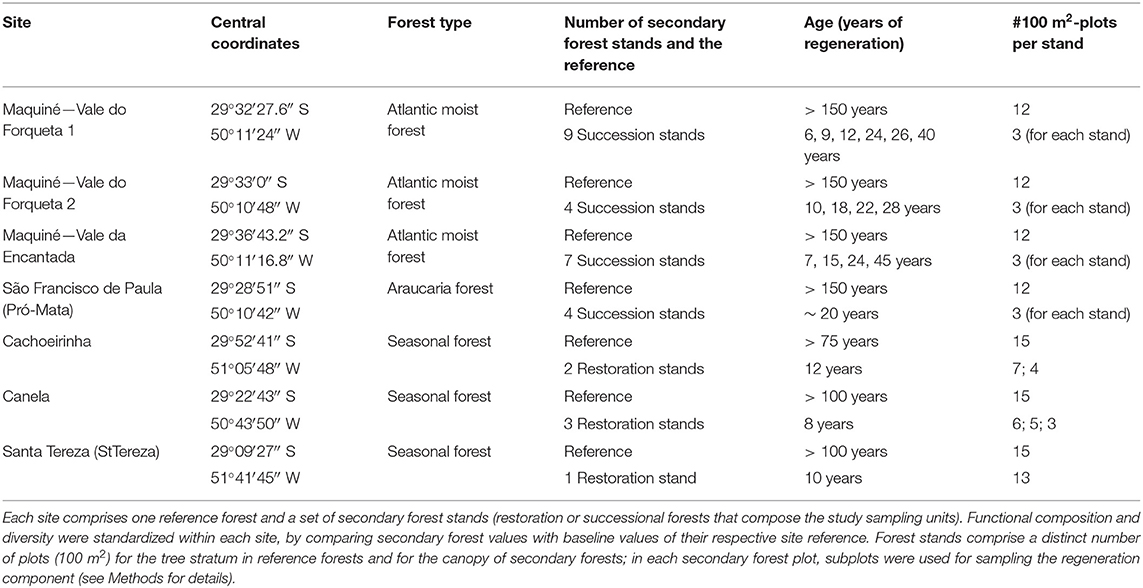
Table 1. General information for each study site in terms of site name, forest type, number of secondary forest stands evaluated, and stand age.
Data Sampling
In each study site, we surveyed tree and treelet species in both successional/restoration and reference forests. Reference forests were described by the tree stratum, including information of trees with diameter at breast height (DBH) ≥ 5 cm, in order to represent adult tree communities of mature forests. Secondary forests (successional/restoration stands) were described by the canopy (trees of the upper stratum, including all individuals with DBH ≥ 10 cm) and the regeneration component. The upper stratum of trees in secondary forests was defined as canopy, although we recognize that in very initial successional forests the crown of such individuals might not yet form a closed canopy. Structural characteristics of this stratum were also evaluated, including tree height, abundance and total basal area. By describing these features we intend to explicitly analyze the potential effect of these canopy trees (overstory) on the understory regeneration. The regeneration component was considered to be saplings from tree and treelet species with height ≥ 1.50 m and DBH < 5 cm.
Sampling design differed between study sites, so there is a variation in the number of stands and plots depending on the study site (Table 1). For the tree stratum in reference areas and the canopy of secondary forests, plots were 100 m2 in size, but the number of plots per stand varied depending on site characteristics. For the regeneration component, the sampling within each 100 m2 plot varied from 12 to 40 m2: in areas where restoration interventions were conducted (Cachoeirinha, Canela, and Santa Tereza), sampling consisted of three subplots of 4 m2 (i.e., 12 m2 per plot), while in the successional areas (Maquiné and São Francisco de Paula), sampling consisted of four subplots of 10 m2 in size (i.e., 40 m2 per plot). For our analysis, sampling units consisted of each secondary forest stand with a distinct age in each study site (i.e., six sampling units in restoration sites and 25 in successional sites; Table 1). Data from reference forests were only considered as baseline values, being used to calculate the dissimilarity in functional composition and diversity with the secondary forest stands within each study site (see Statistical analysis for more details). Data on species composition was averaged for number of individuals per 100 m2 per forest stand.
Functional Traits
We selected traits related to resource acquisition, structure, establishment, and reproduction. Resource acquisition traits are represented by leaf traits—leaf area (LA, cm2), specific leaf area (SLA, cm2/g), leaf dry-matter content (LDMC, mg/g), leaf nitrogen and phosphorus content (LNC and LPC, respectively, %), and the ratio of leaf nitrogen and leaf phosphorus content (LNC: LPC). These traits are related to photosynthetic assimilation, leaf stem structures, and nutrient concentration (Wright et al., 2004; Poorter et al., 2008). Wood density (WD, g/cm3) is related to stem structures, hydraulic conductance, and competitive ability (Poorter et al., 2008; Chave et al., 2014). Plant height is another key trait used to explore species competitive ability, however, in the present study, we did not include it as a trait because we used field-measured canopy height directly as a predictor variable in the models. Finally, reproductive traits are represented by seed mass (SM, g) and fruit size (FS, small: up to 5 mm of a mean size, medium: between 5.1 and 14 mm, large: larger than 14.1 mm (Githiru et al., 2002), which are related to seed dispersal and establishment (Pérez-Harguindeguy et al., 2013).
Leaf trait information was obtained from our Plant Ecology Lab at the Federal University of Rio Grande do Sul (LEVEG-UFRGS), which has a functional trait database based on field samples collected from individuals of the regional tree species pool that had been collected since 2009. Thus, for the present study, we consider trees measured within the region to calculate trait means per species. Collection and measurement protocols follow Pérez-Harguindeguy et al. (2013). Currently, all data is available under request from the TRY database (Kattge et al., 2020). Wood density was mostly obtained from the literature (Chave et al., 2014; Zimermann Oliveira et al., 2019), as well as tree reproductive traits (e.g., Sobral et al., 2006; Galetti et al., 2011; Seger et al., 2013). The list of species per study site and their mean trait values can be seen in Supplementary Table 6.
Statistical Analysis
The community-weighted mean of traits (CWM) was used as a functional composition measure (Garnier et al., 2004), while community functional diversity was represented by Rao's entropy index (Botta-Dukát, 2005). Before CWM and RaoQ calculation, we tested the correlation between functional traits. We excluded LPC due to its high correlation with LNC (r = 0.60) and retained the remaining traits in the analyses: LA, SLA, LDMC, LNC, LNC:LPC, WD, SM, and FS.
For objective (i), we calculated CWM values for each trait, based on a matrix of species abundances, and RaoQ for each sampling unit of secondary forests (regeneration component) and reference sites (tree stratum). We then tested the association between forest age and CWM values for each trait using simple linear regressions, standardizing trait values within each study site before fitting the models. The age of forest was log-scaled prior to the analysis.
For objective (ii), we first log-transformed values of LA, SLA, LNC, LNC:LPC, and SM, and standardized all traits to zero mean and unit variance [using the “decostand” function in the vegan package (Oksanen et al., 2019)]. Following this procedure, with the matrices of species abundance per stand and species by traits, we calculated CWM and RaoQ values for (1) the tree stratum of the reference forests, (2) the regeneration component of the successional/restoration stands, and (3) the canopy of the successional/restoration stands, using the Syncsa package in R (Debastiani, 2020).
Functional composition and diversity of the tree stratum from reference forests were used to set baseline values (target) for each site (Table 1), whereas the regeneration component of the successional/restoration stands was used to set the status of secondary forest regeneration. Based on this, we calculated the dissimilarity between the regeneration component and the respective reference baseline (i.e., within each site) to evaluate how distant (or similar) from the target tree regeneration of secondary forest stands were. For CWM values, we used the pairwise Euclidean distance between each regeneration community and their reference (“CWM.dist”) to reflect the functional composition dissimilarity of tree regeneration communities in secondary forest stands with its target forest. For RaoQ values, we directly calculated the difference (RaoQ.diff) between the values found in each reference and regeneration stand: [RaoQ.diff = RaoQreference-RaoQregeneration]. This parameter thus shows if regenerating tree communities are reaching similar functional diversity values (near zero) or if they have lower (positive values) or higher (negative values) diversity than their reference forests. As the values of CWM.dist and RaoQ.diff consider comparisons within each site, we were able to control for differences associated to forest type, species pool, and regeneration process (succession or restoration), and only the differences in terms of functionality (composition and diversity) were considered as response variables in the statistical analyses. We repeated this procedure three times: with all traits analyzed together, with leaf traits only and with reproductive traits plus wood density (named “reproductive traits,” hereafter). Due to high correlations (r ≥ 0.70) between some of the variables generated by this procedure (see Supplementary Table 1), we selected the following response variables to be used in the models: CWM distance for leaf and reproductive traits (CWM.distleaf and CWM.distrep), and RaoQ difference for all traits (RaoQ.diffall).
Community data of the established canopy in successional/restoration stands was used to determine the predictor variables in our analysis, which were related to functional composition (CWM), diversity (RaoQ), and structure (total basal area, abundance, canopy mean height and variance). We conducted Principal Component Analyses (PCA) with canopy CWM traits in order to synthesize the main functional composition axes associated with this component. We performed three different PCAs: one including all traits, a second using only leaf traits, and a third with only reproductive traits. We then evaluated correlations between the first two axes of each PCA to select variables (functional composition axes) to be used in the models (see below). In order to meet model analysis requirements, we conducted log-transformation on stand age and square-root transformation on basal area and then standardized all predictors to mean zero and unit variance.
For all three response variables described above (CWM.distleaf, CWM.distrep, RaoQ.diffall), we fitted linear mixed models in order to evaluate the effect of predictor variables from the secondary forest canopy on regeneration trait compositional and diversity differences. Site (Table 1) was used as a random effect to control for local conditions. We first determined four main groups of predictor variables, each with a set of operational variables (Supplementary Table 2): Stand age (years after fallow or from the end of restoration activities), Canopy structure (structural variables), Functional composition (PCA axes originated from CWM values), and Functional diversity (RaoQ's values). Within each group of variables, we tested for correlations between variables (Supplementary Tables 3, 4) and only kept variables with r < 0.70. Predictor variables used in our full models were: stand age; basal area (canopy structure variables); PC1leaf, PC2leaf, and PC2rep (canopy functional composition variables); and RaoQall (canopy functional diversity variable). We additionally used the variance inflation factor (VIF) to test for multicollinearity among these variables before running the regression models of each response variable. We found no inflation among the chosen variables. We also accounted for the presence of spatial autocorrelation between our plots by fitting each model with and without an autocorrelation structure (i.e., linear, spherical, exponential, Gaussian, and rational quadratics relations based on local X and Y coordinates), and then selecting the model with the lowest AICc. This diagnosis showed no significant spatial structure regarding our response variables. Finally, regressions were conducted using the full model for each response variable and then running a backward selection (using the “stepAIC” function). The optimal model was considered the one with the lowest AICc (Burnham and Anderson, 2002). Variables considered significant within each optimal model were analyzed based on their confidence intervals. We performed all statistical analyses in R v.3.6.1 (R Core Team, 2019).
Results
The associations between forest age and functional composition or diversity showed a high range of variation in tree regeneration in secondary forests, even between forests with similar ages (Figure 1). Significant relationships between forest age and functional composition values were observed for leaf dry-matter content and seed mass (positive association) and leaf nitrogen content (negative association) (Figure 1). The ratio between nitrogen and phosphorus (LNP:LPC) also increased with stand age (with a marginally significant p-value of p = 0.057).
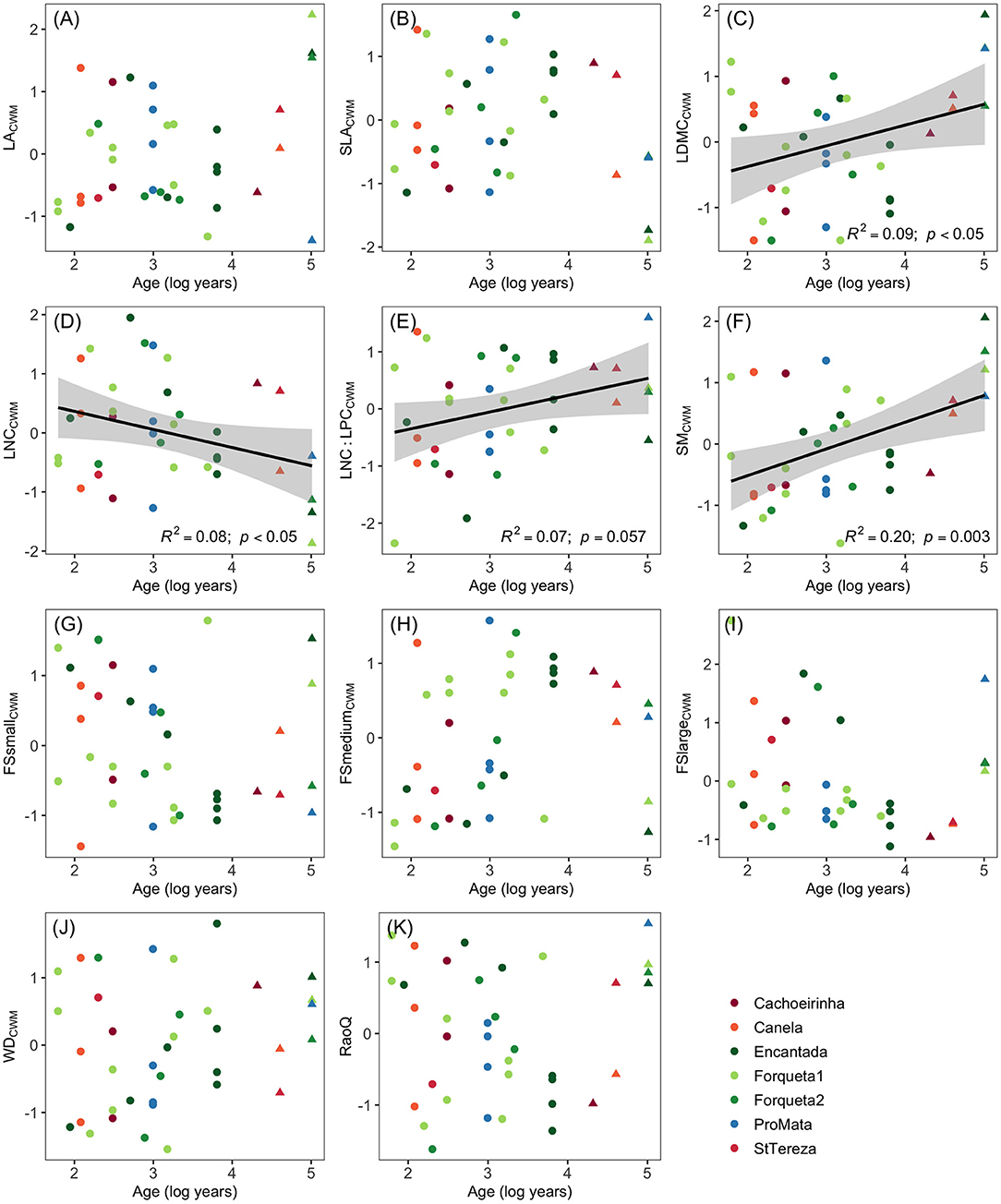
Figure 1. Scatter diagrams of functional trait composition (CWM: community-weighted means; A-J) and diversity (RaoQ: based on all traits; K) from the tree regeneration component of secondary forests of southern Brazilian Atlantic Forest against the age since regeneration started. Successional secondary forest sites are Encantada, Forqueta1, Forqueta2, and ProMata (green-blue colors), while restoration sites are Cachoeirinha, Canela, and StTereza (warm colors). Values for the tree stratum of reference forests are also given and correspond to the oldest forests in the right-hand side of axis x (indicated in triangles). Regression lines indicate significant associations (p <0.05 or p = 0.057 for (E); see text for further information). LA, leaf area; SLA, specific leaf area; LDMC, leaf dry matter content; LNC, leaf nitrogen content; LNC:LPC, leaf nitrogen-phosphorus ratio; SM, seed mass; FS, fruit size (sm: small; md: medium; lg: large); WD, wood density.
Principal Component Analyses indicated functional composition patterns from the canopy of secondary forests and synthesize the differences according to leaf and reproductive traits (Figure 2). For leaf traits, the first PCA axis (PC1leaf) showed a variation from communities where trees have mostly acquisitive traits—higher LA, LNC and SLA (positive scores)—to communities with higher dominance of trees with conservative traits and small leaves, especially in restoration sites (warm colors), but also in some successional stands (Figure 2A). The second leaf trait axis (PC2leaf) was associated with tough leaves (higher LDMC) and higher limitation of nitrogen (LNC:LPC). For reproductive traits and wood density, large fruit size, and denser wood trees separated communities along the first PCA axis (PC1rep), whereas the second axis (PC2rep) represented a gradient from larger seed mass values (positive scores) to smaller fruits (Figure 2B). Axes PC1leaf, PC2leaf, and PC2rep constitute predictor variables used in the models that evaluated drivers of community regeneration dissimilarities with reference targets (see below).
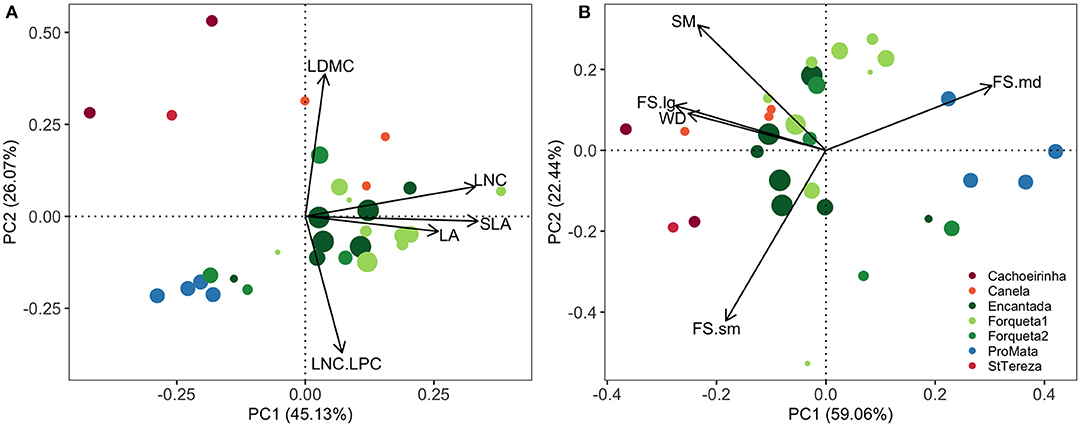
Figure 2. Ordination diagrams of Principal Component Analysis (PCA) for community-weighted means of leaf (A) and reproductive (B) traits of canopy trees in secondary forests of southern Brazilian Atlantic Forest. Successional secondary forest sites are Encantada, Forqueta1, Forqueta2, and ProMata (green-blue colors), while restoration sites are Cachoeirinha, Canela, and StTereza (warm colors). Circle sizes indicate an increase in the regeneration age of each forest stand. LA, leaf area; SLA, specific leaf area; LDMC, leaf dry matter content; LNC, leaf nitrogen content; LNC:LPC, leaf nitrogen-phosphorus ratio; SM, seed mass; FS, fruit size (sm: small; md: medium; lg: large); WD, wood density. The axes PC1 and PC2 of leaf traits, and PC2 of reproductive traits were considered as predictor variables of functional composition distance and diversity difference between the regeneration component of these secondary forests and their reference baseline (response variables: CWM.dist and RaoQ.diff in Figures 3, 4).
Results from linear mixed models indicated three predictors for CWM.distleaf, two for CWM.distrep, and four for RaoQ.diffall in their respective optimal models (Figure 3, Supplementary Table 5). The optimal model for CWM.distleaf showed a positive influence of PC1leaf (which represents an acquisitive strategy gradient), and a negative effect of basal area (Figure 4B) on the functional distance between secondary forest stands and their reference forests (Figure 4A). This indicates that the regeneration component of secondary forests is functionally more similar to their reference baseline when the canopy trees are composed by species with more conservative traits (lower LA, LNC, and SLA) and under forest stands with higher total basal area of canopy trees. The selected optimal model for CWM.distrep showed a negative influence of basal area on the functional composition distance (Figure 4C), evidencing a regeneration component functionally more similar to reference forests in communities characterized by larger trees on the upper stratum. The optimal model for functional diversity (RaoQ.diffall) indicated a significant positive association with stand age (Figures 3, 4D). Values of RaoQ.diffall close to zero indicate similar functional diversity between the regeneration component and their reference forest, whereas positive values indicate lower diversity in the regeneration component. Thus, regenerating tree communities of older secondary forests showed lower functional diversity than the observed in their reference forests and early secondary forests.
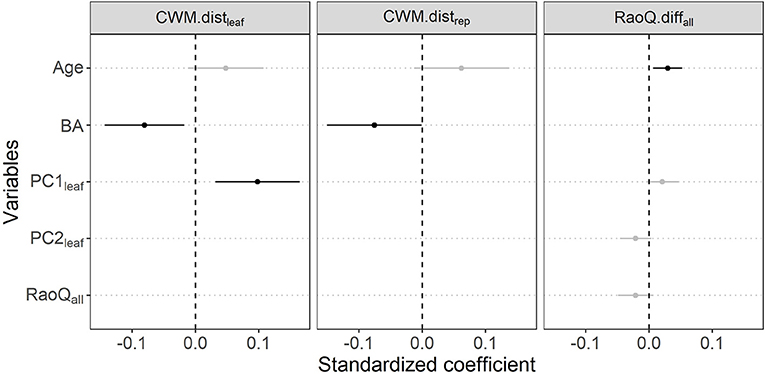
Figure 3. Standardized coefficients of optimal linear mixed models selected for each response variable considered for secondary forests in southern Brazilian Atlantic Forest. Response variables are functional traits composition distance (CWM.dist in terms of leaf or reproductive traits) and diversity difference (RaoQ.diff) between the regeneration component of secondary forest stands and their reference baseline. Selected predictor variables are the age of forest stand since regeneration started (Age) and parameters derived from the canopy of studied secondary forests: total basal area of canopy trees (BA), distinct spectra of strategies (PC1leaf and PC2leaf shown in Figure 2A, with a clear acquisitive strategy gradient in PC1), and the functional diversity based on all traits (RaoQ.all). Points are the average estimates of the models and bars correspond to 95% confident intervals (CI). Variables that do not overlap zero (indicated in black) have significant effects on the response variable.
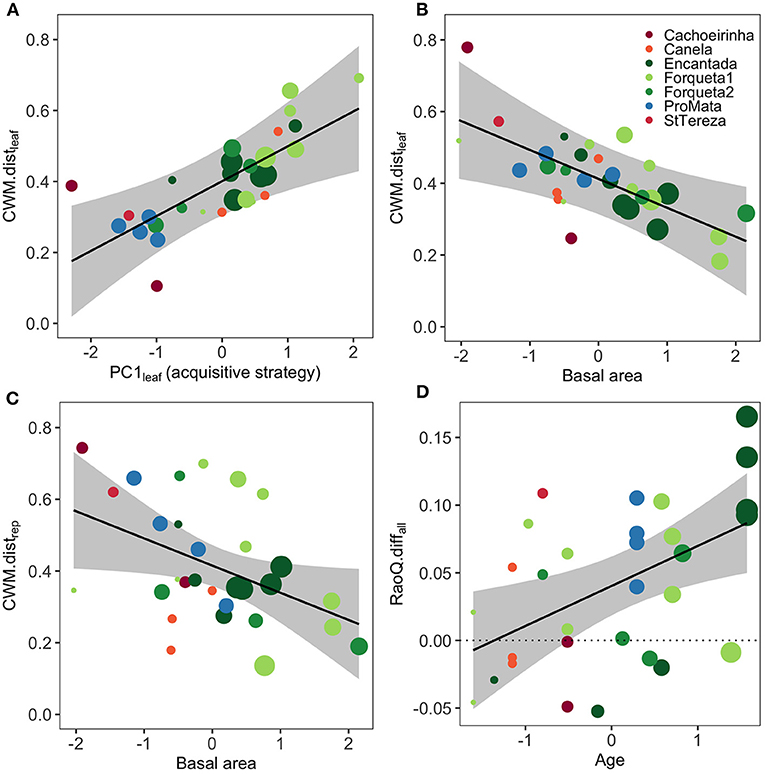
Figure 4. Scatterplots of significant predictor variables of functional trait composition distances (CWM.dist) and functional diversity differences (RaoQ.diff) between the regeneration component of secondary forest stands and their reference baseline in southern Brazilian Atlantic Forest. Successional secondary forest sites are Encantada, Forqueta1, Forqueta2, and ProMata (green-blue colors), while restoration sites are Cachoeirinha, Canela, and StTereza (warm colors). Optimal models are presented in Figure 3 and Supplementary Table 5. In plots (A–C), values in axis y indicate the Euclidean distance between community-weighted means (CWM) of leaf traits (CWM.distleaf) and reproductive plus wood density traits (CWM.distrep) of the regeneration component of secondary forests and their respective reference baseline (i.e., comparisons were restricted to each site, see Table 1). In plot (D), values in axis y indicate functional diversity differences: RaoQ.diffall = RaoQreference-RaoQregeneration; values close to zero suggest similar functional diversity between the regeneration component of secondary forest and their reference baseline. Regression lines indicate significant associations (p <0.05; see Supplementary Table 5). Circle sizes indicate an increase in the regeneration age of each forest stand.
Discussion
In this study, we show that functional leaf trait composition and total basal area of the established canopy, as well as forest age, determine the functional patterns of natural tree regeneration in the understory of secondary subtropical forests (ranging from 6 to 45 years old). Leaf attributes of the upper stratum trees in early to intermediate secondary forests, mainly composed by early colonizers in successional forests and/or planted trees in restoration sites, determine how similar the regenerating trees communities are when compared to the observed functional composition patterns in reference mature forests. Community traits similarity was higher in sites where there is a higher amount of canopy tree basal area. We additionally show that the functional diversity of regenerating communities was lower in mid-successional forests (20-45 years) when compared with younger stands and with reference forests. Considering that functional similarity between regenerating communities and their reference forests is the desired target in order to recover ecosystem functions of the formerly existing forests, leaf functional traits of canopy trees that early colonize degraded areas and/or are planted for restoration must be considered as important features in the successional process, especially for restoration purposes.
Functional Composition and Diversity of Regenerating Trees Across Forest Age
Our results indicate an overall dispersion of trait community-weighted means in the successional forests, without clear trajectories along the age of successional forests for many individual traits, and also for functional diversity. However, seed mass, leaf dry-matter content, and the proportion of nitrogen and phosphorus on leaves (both LNC and LNC:LPC) showed significant relationships with forest age. Seed mass had the strongest relationship, showing a continuous increase in community mean values with forest age. This is in accordance with other papers that found a decrease in the proportion of small-seeded species with increased forest age (Warring et al., 2016). Large or heavier seeds are usually found in intermediate to advanced stages of succession, as they are usually associated with animal dispersal (Tabarelli et al., 2008), which takes longer to recover after disturbance. Leaf traits LDMC, LNC, and LNC:LPC also evidenced an expected change toward mature forest communities, showing a prevalence of more conservative attributes across successional time in moist tropical forests (Lohbeck et al., 2013; Fernandes Neto et al., 2019). Leaf nitrogen-phosphorus ratio increases with forest age, evidencing a potential nitrogen limitation in initial successional forests and a phosphorus shortage mainly observed in old-growth forests (Zeng et al., 2017). This ratio can be a useful indicator of nutrient limitation for productivity (Townsend et al., 2007). However, the transition state between nitrogen and phosphorus limitation is a ratio of 14–16, and our community values ranged from 11.7 to 15.8 (mean = 14.1), indicating almost no limitation for phosphorus, but maybe for nitrogen in some early-stage communities. For the other traits studied here (leaf area, SLA, fruit size, and WD), we found a large variation across the studied forests, which prevented us to evidence any trend with forest age. One could improve community assembly predictions and understanding of natural regeneration process along with forest age development, by considering intraspecific trait variability. Plant functional traits vary among individuals of the same species, especially when environmental conditions change (Albert et al., 2010; Siefert et al., 2015), but here we were not able to account for intraspecific variability within the studied species.
Time since abandonment in secondary succession and/or after restoration interventions is crucial for natural regeneration processes in tropical and subtropical forests. Tree stem density, biomass, height, and species richness can achieve similar values to old-growth forests in 30 to 50 years of forest regeneration (Guariguata and Osterag, 2001; Letcher and Chazdon, 2009; Poorter et al., 2016; Rozendaal et al., 2019), however successional trajectories can be either deterministic or stochastic. Temporal dynamic of successional trajectories varies widely within and between sites under similar land-use history, challenging the predictability even for structural features (e.g., stem density and basal area) in highly diverse tropical forests (Norden et al., 2015). This unpredictability might be associated with plot identity (i.e., involving processes that vary under local environmental conditions and interactions that occur inside each plot), which can overlap the contribution of age on successional dynamics (Norden et al., 2015). Random assembly patterns might be expected due to the high diversity of tropical and subtropical forests per se and to the often-patchy recruitment patterns of species (Fridley, 2013), especially when we think of low-abundant or rare species that predominate in these forest communities.
Determinants of Similarities in Functional Composition and Diversity Between Regenerating Trees and Reference Forests
Tree regenerating communities were more similar to reference forest values in terms of leaf traits as the canopy of established communities was composed mostly by species with conservative plant strategies: lower values of SLA, LA, and LNC. A study that experimentally tested distinct sets of planting trees (animal-dispersed vs. wind-dispersed tree species), aiming to restore tropical forests in Mexico, did not find differences in phylogenetic and functional diversity of early communities of recruits (eight years old), assuming that the initial composition did not influence community assembly (Li et al., 2018). Animal-dispersed species are often pointed out as an important component of forest recovery, due to their potential in attracting dispersers from nearby forest remnants and thus increasing the proportion of propagules' arrival (Boukili and Chazdon, 2017; Meli et al., 2017); leaf trait composition, however, is less frequently considered. Here, we found consistent influence of leaf attributes of the canopy on the functional composition of tree regenerating communities. Leaf functional traits of initial colonizers and/or of planted trees affect abiotic conditions—by altering shading patterns and nutrient content in litter and on the soil surface (Poorter, 2009; Rosenfield and Müller, 2020b; Teixeira et al., 2020)—and biotic interactions associated to competition for light and the use of soil resources (Kunstler et al., 2016; Muscarella et al., 2017). These conditions are relevant for mechanisms of tree establishment and growth and might be more relevant than the dispersal/arrival of individuals for the regeneration of secondary forests toward reference baseline values. Dispersal seems not to be a barrier to forest regeneration where the surrounding landscape still have forest remnants to serve as font of propagules and habitat for dispersers (Meiners et al., 2015), and our studied areas do not seem to be limited in terms of propagules arriving from surrounding forests (Zanini et al., 2014; Vicente-Silva et al., 2016; Rosenfield and Müller, 2019).
Functional composition distances between the regeneration component and the reference forests were also associated with the total basal area of the canopy stratum, a parameter of forest structure. Secondary forest stands with a greater sum of basal area of upper trees (canopy) had a regeneration component more similar to the expected target than less structured forests (i.e., with lower values of total basal area). The similarity was greater both in terms of leaf traits and reproductive plus wood density traits, indicating a secondary forest trajectory toward the expected target in terms of the functional composition and, consequently, ecosystem functionality. The functional composition of forest communities is often associated with ecosystem processes and temporal dynamics, representing the effect of the attributes of the most abundant species (mass-ratio theory), instead of the variability of traits (niche complementarity hypothesis), on ecosystem functions (Garnier et al., 2004; Van der Sande et al., 2018; Bordin and Müller, 2019; Rosenfield and Müller, 2020b). Greater similarity with respect to functional composition in these secondary forests may indicate that important ecosystem functions associated with these traits, and encompassing different compartments of the system (e.g., nutrient cycling, trophic interactions), may be recovering as well (Rosenfield and Müller, 2020a). Less structured secondary forests often fail to offer adequate conditions, such as more shadowed environments, for the establishment of secondary tree species (Holl et al., 2018). Regenerating trees in such communities might be composed by species with distinct strategies, e.g., pioneer species, thus increasing the distance from reference forests in terms of leaf and reproductive traits.
Differences in functional diversity values of tree regeneration communities in secondary forests when compared to reference values were predicted by forest age. The best model included functional composition and diversity variables, but only age was significant and with a low predictability power. Forest age was positively associated with the differences in RaoQ, indicating that early-stages of succession (6–12 years) have more similar values to reference forests (points close to zero located in the left side of Figure 4D) than mid-stage secondary forests (15–45 years), that still showed lower values of functional diversity in the regeneration component (positive values of RaoQ.diff; right side of Figure 4D). This is partially in accordance with a study that found decreasing values of functional diversity along succession (from 10 to 40 years) in tropical forests, but higher functional diversity in old-growth forests (Lasky et al., 2014). In this study, the pattern of decreasing functional diversity along succession was found when analyzing all traits together in a single index (similar to what was performed in our study), however diversity values based on single traits showed slight increases with stand-age (Lasky et al., 2014). In our study, RaoQ for all traits was highly correlated with RaoQ calculated for leaf traits and reproductive traits separately, so the observed pattern was consistent among the sets of chosen traits and not skewed by one or another selected set (Zhu et al., 2017).
The results found for functional diversity patterns can be related to transient community stages, where pioneer and secondary tree species co-occur in the regeneration component of early-stage successional forests without the dominance of only a few species. This leads to functional diversity values to be similar to the values found in the tree stratum of reference forests. High rates of species arrival and the absence of severe abiotic or biotic filters, arresting establishment, might be contributing to high functional diversity values in the early-stage secondary forests studied here. Along successional time, some shade-tolerant tree and treelets (e.g., Cupania vernalis, Casearia silvestris, Mollinedia shottiana, Piper aduncum, Psychotria suterela) eventually become more dominant in mid-stage forests, due to high colonization rate and low mortality in the understory. This dominance affects RaoQ values, which considers pair-wise traits' distance weighted by species abundance (Botta-Dukát, 2005). As self-thinning processes occur (Stephenson et al., 2011), decreasing the dominance of these species, there should be an increase in functional diversity, increasing the similarity with reference forests. However, functional diversity does not often vary across succession (e.g., Lohbeck et al., 2012; Böhnke et al., 2014), as the turnover of species along the regeneration time can maintain similar patterns of trait dispersion within communities.
Conclusion
A great portion of current subtropical and tropical forest coverage comprises secondary forests, which increases the role of these forests for biodiversity conservation and the provision of ecosystem services (Chazdon et al., 2009; Martin et al., 2013; Pichancourt et al., 2014). Even though many studies have shown that the recovery of forest structure parameters and even species diversity is possible in a relatively short time of succession (e.g., Guariguata and Osterag, 2001; Letcher and Chazdon, 2009; Poorter et al., 2016), species composition is hard to recover or restore (e.g., Dent et al., 2013; Suganuma et al., 2014; Rozendaal et al., 2019). Understanding whether functional composition and diversity of regenerating tree communities increase in similarity when compared to reference forests is essential in order to evaluate the potential return of ecosystem functions and services from these secondary forests. Our study offers an opportunity to understand the effect of first colonizers and/or planted species in the functionality patterns of regenerating tree communities in the understory of secondary forests originated from both passive and active restoration processes.
We provided some perspectives about the importance of forest structure and leaf trait composition of canopy species in the functional patterns of the future tree component of secondary forests (i.e., tree regeneration component). These perspectives go beyond any potential influence of climatic and soil conditions, as they concern natural regeneration of forests under sites that differ in terms of environment and species pool. Leaf trait composition of trees from the upper stratum influences the similarity of ongoing successional communities, indicating potential priority effects of initial assemblies on natural regeneration processes. Thus, despite the great potential of natural regeneration in tropical and subtropical moist forests, under the scope of ecological restoration, practitioners should consider leaf attributes that characterize plants with acquisitive strategies that grow fast to compose the set of species for early-stage communities, by planting or by thinning in the first years of recovery. This might turn initial canopy structure more similar to the observed in natural successional forests and might accelerate the increment of tree stem basal area. As we saw, forest stands with higher basal area of canopy trees can improve the functional similarity of regenerating trees with the expected functional target. In addition, the presence of species with conservative strategies in the canopy of early and intermediate successional forests seems to improve similarities of tree regeneration communities with target functional patterns; thus, species with such strategies (low SLA, LA, LNC) should also be considered in restoration plantings, especially after the planting of fast-growing species. Finally, the influence of recovery time on the regeneration of functional patterns along the successional and restoration processes is uncertain, making the monitoring of the tree regeneration component necessary to inform if, when, and where secondary forests are becoming functionally similar to their reference forest ecosystems.
Data Availability Statement
The data analyzed in this study is subject to the following licenses/restrictions: Most data are available as Supplementary Material; additional information might be available under request to the authors. Requests to access these datasets should be directed to Sandra Cristina Müller,c2FuZHJhLm11bGxlckB1ZnJncy5icg==.
Author Contributions
SM conceived the idea of the paper. RB, KB, JK, and MR participated in the collection of field data. SM, MR, KB, and JK performed statistical analyses. MR and KB prepared the figures. SM and MR led the writing of the manuscript. All authors contributed to the collection and compilation of functional trait data, discussed the results and thoroughly revised the manuscript, and contributed critically to the drafts and gave final approval for publication.
Funding
This study was financed in part by the Coordenação de Aperfeiçoamento de Pessoal de Nível Superior—Brazil (CAPES) through the Programa de Excelência Acadêmica (PROEX) and the Portal de Periódicos, by the Conselho Nacional de Desenvolvimento Científico e Tecnológico (CNPq grant number 309659/2019-1 to SCM) and by the Fundação de Amparo à Pesquisa do Estado do Rio Grande do Sul (FAPERGS process 2218–2551/12-2).
Conflict of Interest
The authors declare that the research was conducted in the absence of any commercial or financial relationships that could be construed as a potential conflict of interest.
Acknowledgments
We would like to thank all colleagues that helped collect data but especially K.J. Zanini, J. Vicente-Silva, G. Seger, M. Molz, A. F. Boeni, D. Fonseca, J. Schuler, R. Porciuncula, T. Toma, D.C. Morales, C. Basilio, and R.C. Picolotto. We also like to thank three reviewers and the editors of the manuscript for all comments and suggestions made on a previous version.
Supplementary Material
The Supplementary Material for this article can be found online at: https://www.frontiersin.org/articles/10.3389/ffgc.2021.572864/full#supplementary-material
References
Albert, C. H., Thuiller, W., Yoccoz, N. G., Douzet, R., Aubert, S., and Lavorel, S. (2010). A multi-trait approach reveals the structure and the relative importance of intra- vs. interspecific variability in plant traits. Funct. Ecol. 24, 1192–1201. doi: 10.1111/j.1365-2435.2010.01727.x
Bergamin, R. S., Bastazini, V. A. G., Vélez-Martin, E., Debastiani, V., Zanini, K. J., Loyola, R., and Müller, S. C. (2017). Linking beta diversity patterns to protected areas: lessons from the Brazilian Atlantic Rainforest. Biodiver. Conserv. 26, 1557–1568. doi: 10.1007/s10531-017-1315-y
Böhnke, M., Kröber, W., Welk, E., Wirth, C., and Bruelheide, H. (2014). Maintenance of constant functional diversity during secondary succession of a subtropical forest in China. J. Veg. Sci. 25, 897–911. doi: 10.1111/jvs.12114
Bordin, K. M., and Müller, S. C. (2019). Drivers of subtropical forest dynamics: the role of functional traits, forest structure and soil variables. J. Veg. Sci. 30, 1164–1174. doi: 10.1111/jvs.12811
Botta-Dukát, Z. (2005). Rao's quadratic entropy as a measure of functional diversity based on multiple traits. Journal of Vegetation Science 16, 533–540. doi: 10.1111/j.1654-1103.2005.tb02393.x
Boukili, V. K., and Chazdon, R. L. (2017). Environmental filtering, local site factors and landscape context drive changes in functional trait composition during tropical forest succession. Perspectives in Plant Ecology, Evolution and Systematics 24, 37–47. doi: 10.1016/j.ppees.2016.11.003
Burnham, K. P., and Anderson, D. R. (2002). Model selection and multimodel inference: A practical information-theoretic approach. Springer.
Charles, L. S. (2020). Plant functional traits and species selection in tropical forest restoration. Trop. Conserv. Sci. 11:157. doi: 10.1177/1940082918784157
Chave, J., Réjou-Méchain, M., Búrquez, A., Chidumayo, E., Colgan, M. S., Delitti, W. B. C., et al. (2014). Improved allometric models to estimate the aboveground biomass of tropical trees. Glob. Change Biol. 20, 3177–3190. doi: 10.1111/gcb.12629
Chazdon, R. L. (2008). “Chance and determinism in tropical forest succession,” in Tropical Forest Community Ecology, eds. W. P. Carson and S. A. Schnitzer. (Oxford: Wiley-Blackwell Publishing), 384–408.
Chazdon, R. L., Peres, C. A., Dent, D., Sheil, D., Lugo, A. E., Lamb, D., et al. (2009). The potential for species conservation in tropical secondary forests. Conserv. Biol. 23, 1406–1417. doi: 10.1111/j.1523-1739.2009.01338.x
Crouzeilles, R., Ferreira, M. S., Chazdon, R. L., Lindenmayer, D. B., Sansevero, J. B. B., Monteiro, L., et al. (2017). Ecological restoration success is higher for natural regeneration than for active restoration in tropical forests. Sci. Adv. 3:e1701345. doi: 10.1126/sciadv.1701345
Debastiani, V. (2020). Analysis of Functional and Phylogenetic Patterns in Metacommunities. SYNCSA package. 1.3.4 ed. Porto Alegre.
Dent, D. H., DeWalt, S. J., and Denslow, J. S. (2013). Secondary forests of central Panama increase in similarity to old-growth forest over time in shade tolerance but not species composition. J. Veg. Sci. 24, 530–542. doi: 10.1111/j.1654-1103.2012.01482.x
Diaz, S., Lavorel, S., de Bello, F., Quetier, F., Grigulis, K., and Robson, M. (2007). Incorporating plant functional diversity effects in ecosystem service assessments. Proc. Natl. Acad. Sci. U.S.A. 104, 20684–20689. doi: 10.1073/pnas.0704716104
Fernandes Neto, J. G., Costa, F. R. C., Williamson, G. B., and Mesquita, R. C. G. (2019). Alternative functional trajectories along succession after different land uses in central Amazonia. J. Appl. Ecol. 56, 2472–2481. doi: 10.1111/1365-2664.13484
Fridley, J. D. (2013). Successional convergence, stochastic assembly and the future of tropical forests. J. Veg. Sci. 24, 415–416. doi: 10.1111/jvs.12056
Fukami, T. (2015). Historical contingency in community assembly: integrating niches, species pools, and priority effects. Ann. Rev. Ecol. Evol. Syst. 46, 1–23. doi: 10.1146/annurev-ecolsys-110411-160340
Galetti, M., Pizo, M. A., and Morellato, L. P. C. (2011). Diversity of functional traits of fleshy fruits in a species-rich Atlantic rain forest. Biota Neotropica 11, 181–193. doi: 10.1590/S1676-06032011000100019
Garnier, E., Cortez, J., Billès, G., Navas, M.-L., Roumet, C., Debussche, M., et al. (2004). Plant functional markers capture ecosystem properties during secondary succession. Ecology 85, 2630–2637. doi: 10.1890/03-0799
Githiru, M., Lens, L., Bennur, L. A., and Ogol, C. P. K. O. (2002). Effects of site and fruit size on the composition of avian frugivore assemblages in a fragmented Afrotropical forest. Oikos 96, 320–330. doi: 10.1034/j.1600-0706.2002.960214.x
Guariguata, M. R., and Osterag, R. (2001). Neotropical secondary forest succession: changes in structural and functional characteristic. Forest Ecol. Manag. 148, 185–196. doi: 10.1016/S0378-1127(00)00535-1
Holl, K. D., and Aide, T. M. (2011). When and where to actively restore ecosystems? Forest Ecol. Manag. 261, 1558–1563. doi: 10.1016/j.foreco.2010.07.004
Holl, K. D., and Brancalion, P. H. S. (2020). Tree planting is not a simple solution. Science 368, 580–581. doi: 10.1126/science.aba8232
Holl, K. D., Reid, J. L., Oviedo-Brenes, F., Kulikowski, A. J., and Zahawi, R. A. (2018). Rules of thumb for predicting tropical forest recovery. Appl. Veg. Sci. 21, 669–677. doi: 10.1111/avsc.12394
Jakovac, A. C. C., Bentos, T. V., Mesquita, R. C. G., and Williamson, G. B. (2014). Age and light effects on seedling growth in two alternative secondary successions in central Amazonia. Plant Ecol. Diver. 7, 349–358. doi: 10.1080/17550874.2012.716088
Kattge, J., Bönisch, G., Díaz, S., Lavorel, S., Prentice, I. C., Leadley, P., et al. (2020). TRY plant trait database – enhanced coverage and open access. Glob. Change Biol. 26, 119–188. doi: 10.1111/gcb.14904
Kollmann, J., Meyer, S. T., Bateman, R., Conradi, T., Gossner, M. M., de Souza Mendonça, M. Jr, et al. (2016). Integrating ecosystem functions into restoration ecology—recent advances and future directions. Restor. Ecol. 24, 722–730. doi: 10.1111/rec.12422
Kunstler, G., Falster, D., Coomes, D. A., Hui, F., Kooyman, R. M., Laughlin, D. C., et al. (2016). Plant functional traits have globally consistent effects on competition. Nature 529, 204–207. doi: 10.1038/nature16476
Lasky, J. R., Uriarte, M., Boukili, V. K., Erickson, D. L., John Kress, W., and Chazdon, R. L. (2014). The relationship between tree biodiversity and biomass dynamics changes with tropical forest succession. Ecol. Lett. 17, 1158–1167. doi: 10.1111/ele.12322
Laughlin, D. C. (2014). Applying trait-based models to achieve functional targets for theory-driven ecological restoration. Ecol. Lett. 17, 771–784. doi: 10.1111/ele.12288
Laughlin, D. C., Chalmandrier, L., Joshi, C., Renton, M., Dwyer, J. M., and Funk, J. L. (2018). Generating species assemblages for restoration and experimentation: a new method that can simultaneously converge on average trait values and maximize functional diversity. Methods Ecol. Evol. 9, 1764–1771. doi: 10.1111/2041-210x.13023
Letcher, S. G., and Chazdon, R. L. (2009). Rapid recovery of biomass, species richness, and species composition in a forest chronosequence in Northeastern Costa Rica. Biotropica 41, 608–617.
Li, L., Cadotte, M. W., Martínez-Garza, C., de la Peña-Domene, M., and Du, G. (2018). Planting accelerates restoration of tropical forest but assembly mechanisms appear insensitive to initial composition. J. Appl. Ecol. 55, 986–996. doi: 10.1111/1365-2664.12976
Liebsch, D., Marques, M. C. M., and Goldenberg, R. (2008). How long does the Atlantic Rain Forest take to recover after a disturbance? Changes in species composition and ecological features during secondary succession. Biol. Conserv. 141, 1717–1725.
Lohbeck, M., Poorter, L., Lebrija-Trejos, E., Martínez-Ramos, M., Meave, J. A., Paz, H., et al. (2013). Successional changes in functional composition contrast for dry and wet tropical forest. Ecology 94, 1211–1216. doi: 10.1890/12-1850.1
Lohbeck, M., Poorter, L., Paz, H., Pla, L., van Breugel, M., Martínez-Ramos, M., et al. (2012). Functional diversity changes during tropical forest succession. Persp. Plant Ecol. Evol. Syst. 14, 89–96. doi: 10.1016/j.ppees.2011.10.002
Martin, P. A., Newton, A. C., and Bullock, J. M. (2013). Carbon pools recover more quickly than plant biodiversity in tropical secondary forests. Proc. R. Soc. 280:20132236. doi: 10.1098/rspb.2013.2236
Meiners, S. J., Cadotte, M. W., Fridley, J. D., Pickett, S. T. A., and Walker, L. R. (2015). Is successional research nearing its climax? New approaches for understanding dynamic communities. Funct. Ecol. 29, 154–164. doi: 10.1111/1365-2435.12391
Meli, P., Holl, K. D., Rey Benayas, J. M., Jones, H. P., Jones, P. C., Montoya, D., et al. (2017). A global review of past land use, climate, and active vs. passive restoration effects on forest recovery. PLoS ONE 12:e0171368. doi: 10.1371/journal.pone.0171368
Mesquita, R. C. G., Ickes, K., Ganade, G., and Williamson, G. B. (2001). Alternative successional pathways in the Amazon Basin. J. Ecol. 89, 528–537. doi: 10.1046/j.1365-2745.2001.00583.x
Mesquita, R. C. G., Massoca, P. E. S., Jakovac, C. C., Bentos, T. V., and Williamson, G. B. (2015). Amazon rain forest succession: stochasticity or land-use legacy? BioScience 65, 849–861. doi: 10.1093/biosci/biv108
Montoya, D., Rogers, L., and Memmott, J. (2012). Emerging perspectives in the restoration of biodiversity-based ecosystem services. Trends Ecol. Evol. 27, 666–672. doi: 10.1016/j.tree.2012.07.004
Muscarella, R., Lohbeck, M., Martínez-Ramos, M., Poorter, L., Rodríguez-Velázquez, J. E., van Breugel, M., et al. (2017). Demographic drivers of functional composition dynamics. Ecology 98, 2743–2750. doi: 10.1002/ecy.1990
Norden, N., Angarita, H. A., Bongers, F., Martínez-Ramos, M., Granzow-de la Cerda, I., van Breugel, M., et al. (2015). Successional dynamics in Neotropical forests are as uncertain as they are predictable. Proc. Natl. Acad. Sci. U.S.A. 112, 8013–8018. doi: 10.1073/pnas.1500403112
Oksanen, J., Blanchet, F. G., Friendly, M., Kindt, R., Legendre, P., McGlinn, D., et al (2019). vegan: Community Ecology Package. R package version 2.5-6. Available online at: https://cran.r-project.org, https://github.com/vegandevs/vegan.
Peel, M. C., Finlayson, B. L., and McMahon, T. A. (2007). Updated world map of the Köppen-Geiger climate classification. Hydrol. Earth Syst. Sci. 11, 1633–1644. doi: 10.5194/hess-11-1633-2007
Pérez-Harguindeguy, N., Díaz, S., Garnier, E., Lavorel, S., Poorter, H., Jaureguiberry, P., et al. (2013). New handbook for standardised measurement of plant functional traits worldwide. Austr. J. Bot. 61, 167–234. doi: 10.1071/BT12225
Pichancourt, J.-B., Firn, J., Chadès, I., and Martin, T. G. (2014). Growing biodiverse carbon-rich forests. Global Change Biol. 20, 382–393. doi: 10.1111/gcb.12345
Poorter, L. (2009). Leaf traits show different relationships with shade tolerance in moist versus dry tropical forests. New Phytologist 181, 890–900. doi: 10.1111/j.1469-8137.2008.02715.x
Poorter, L., Bongers, F., Aide, T. M., Almeyda Zambrano, A. M., Balvanera, P., Becknell, J. M., et al. (2016). Biomass resilience of Neotropical secondary forests. Nature 530, 211–214. doi: 10.1038/nature16512
Poorter, L., Rozendaal, D. M. A., Bongers, F., de Almeida-Cortez, J. S., Almeyda Zambrano, A. M., Álvarez, F. S., et al. (2019). Wet and dry tropical forests show opposite successional pathways in wood density but converge over time. Nat. Ecol. Evol. 3, 928–934. doi: 10.1038/s41559-019-0882-6
Poorter, L., Wright, S. J., Paz, H., Ackerly, D. D., Condit, R., Ibarra-Manriquez, G., et al. (2008). Are functional traits good predictors of demographic rates? Evidence from five Neotropical forests. Ecology 89, 1908–1920. doi: 10.1890/07-0207.1
R Core Team (2019). R: A Language and Environment for Statistical Computing. Vienna: R Foundation for Statistical Computing.
Reij, C., and Garrity, D. (2016). Scaling up farmer-managed natural regeneration in Africa to restore degraded landscapes. Biotropica 48, 834–843. doi: 10.1111/btp.12390
Rosenfield, M. F., and Müller, S. C. (2019). Assessing ecosystem functioning in forests undergoing restoration. Restor. Ecol. 27, 158–167. doi: 10.1111/rec.12828
Rosenfield, M. F., and Müller, S. C. (2020a). Ecologia funcional como ferramenta para planejar e monitorar a restauração ecológica de ecossistemas. Oecol. Austr. 24, 550–565, doi: 10.4257/oeco.2020.2403.02
Rosenfield, M. F., and Müller, S. C. (2020b). Plant traits rather than species richness explain ecological processes in subtropical forests. Ecosystems 23, 52–66. doi: 10.1007/s10021-019-00386-6
Rozendaal, D. M. A., Bongers, F., Aide, T. M., Alvarez-Dávila, E., Ascarrunz, N., Balvanera, P., et al. (2019). Biodiversity recovery of Neotropical secondary forests. Sci. Adv. 5:eaau3114. doi: 10.1126/sciadv.aau3114
Seger, G. D. S., Duarte, L. D. S., Debastiani, V. J., Kindel, A., and Jarenkow, J. A. (2013). Discriminating the effects of phylogenetic hypothesis, tree resolution and clade age estimates on phylogenetic signal measurements. Plant Biology 15, 858–867. doi: 10.1111/j.1438-8677.2012.00699.x
Siefert, A., Violle, C., Chalmandrier, L., Albert, C. H., Taudiere, A., Farjado, A., et al. (2015). A global meta-analysis of the relative extent of intraspecific trait variation in plant communities. Ecol. Lett. 18, 1406–1419. doi: 10.1111/ele.12508
Sobral, M., Jarenkow, J. A., Brack, P., Irgang, B. E., Larocca, J., and Rodrigues, R. S. (2006). Flora Arbórea e Arborescente do Rio Grande do Sul, Brasil. Porto Alegre: Novo Ambiente.
Stephenson, N. L., van Mantgem, P. J., Bunn, A. G., Bruner, H., Harmon, M. E., O'Connell, K. B., et al. (2011). Causes and implications of the correlation between forest productivity and tree mortality rates. Ecol. Monogr. 81, 527–555. doi: 10.1890/10-1077.1
Suganuma, M. S., Assis, G. B., and Durigan, G. (2014). Changes in plant species composition and functional traits along the successional trajectory of a restored patch of Atlantic Forest. Comm. Ecol. 15, 27–36. doi: 10.1556/ComEc.15.2014.1.3
Tabarelli, M., Lopes, A. V., and Peres, C. A. (2008). Edge-effects drive Tropical Forest fragments towards an early-successional system. Biotropica 40, 657–661. doi: 10.1111/j.1744-7429.2008.00454.x
Teixeira, H. M., Cardoso, I. M., Bianchi, F. J. J. A., da Cruz Silva, A., Jamme, D., and Peña-Claros, M. (2020). Linking vegetation and soil functions during secondary forest succession in the Atlantic forest. Forest Ecol. Manag. 457:117696. doi: 10.1016/j.foreco.2019.117696
Townsend, A. R., Cleveland, C. C., Asner, G. P., and Bustamante, M. M. C. (2007). Controls over foliar N:P ratios in tropical rain forests. Ecology 88, 107–118. doi: 10.1890/0012-9658(2007)88[107:cofnri]2.0.co;2
Van der Sande, M. T., Arets, E. J. M. M., Peña-Claros, M., Hoosbeek, M. R., Cáceres-Siani, Y., van der Hout, P., et al. (2018). Soil fertility and species traits, but not diversity, drive productivity and biomass stocks in a Guyanese tropical rainforest. Funct. Ecol. 32, 461–474. doi: 10.1111/1365-2435.12968
Viani, R. A. G., Vidas, N. B., Pardi, M. M., Castro, D. C. V., Gusson, E., and Brancalion, P. H. S. (2015). Animal-dispersed pioneer trees enhance the early regeneration in Atlantic Forest restoration plantations. Nat. Conser. 13, 41–46. doi: 10.1016/j.ncon.2015.03.005
Vicente-Silva, J., Bergamin, R. S., Zanini, K. J., Pillar, V. D., and Müller, S. C. (2016). Assembly patterns and functional diversity of tree species in a successional gradient of Araucaria forest in Southern Brazil. Nat. Conser. 14, 67–73. doi: 10.1016/j.ncon.2016.09.006
Warring, B., Cardoso, F. C. G., Marques, M. C. M., and Varassin, I. G. (2016). Functional diversity of reproductive traits increases across succession in the Atlantic forest. Rodriguésia 67, 321–333. doi: 10.1590/2175-7860201667204
Weidlich, E. W. A., von Gillhaussen, P., Max, J. F. J., Delory, B. M., Jablonowski, N. D., Rascher, U., et al. (2018). Priority effects caused by plant order of arrival affect below-ground productivity. J. Ecol. 106, 774–780. doi: 10.1111/1365-2745.12829
Werden, L. K., Alvarado, J. P, Zarges, S., Calderón, M. E, Schilling, E. M., Gutiérrez L, M., et al. (2018). Using soil amendments and plant functional traits to select native tropical dry forest species for the restoration of degraded Vertisols. J. Appl. Ecol. 55, 1019–1028. doi: 10.1111/1365-2664.12998
Wright, I. J., Reich, P. B., Westoby, M., Ackerly, D. D., Baruch, Z., Bongers, F., et al. (2004). The worldwide leaf economics spectrum. Nature 428, 821–7. doi: 10.1038/nature02403
Zanini, K. J., Bergamin, R. S., Machado, R. E., Pillar, V. D., and Müller, S. C. (2014). Atlantic rain forest recovery: successional drivers of floristic and structural patterns of secondary forest in Southern Brazil. J. Veg. Sci. 25, 1056–1068. doi: 10.1111/jvs.12162
Zeng, Y., Fang, X., Xiang, W., Deng, X., and Peng, C. (2017). Stoichiometric and nutrient resorption characteristics of dominant tree species in subtropical Chinese forests. Ecol. Evol. 7, 11033–11043. doi: 10.1002/ece3.3527
Zhu, L., Fu, B., Zhu, H., Wang, C., Jiao, L., and Zhou, J. (2017). Trait choice profoundly affected the ecological conclusions drawn from functional diversity measures. Sci. Rep. 7:3643. doi: 10.1038/s41598-017-03812-8
Keywords: community-weighted means, functional diversity, succession, restoration, Atlantic Forest, South Brazil
Citation: Müller SC, Bergamin RS, Bordin KM, Klipel J and Rosenfield MF (2021) Canopy Leaf Traits, Basal Area, and Age Predict Functional Patterns of Regenerating Communities in Secondary Subtropical Forests. Front. For. Glob. Change 4:572864. doi: 10.3389/ffgc.2021.572864
Received: 15 June 2020; Accepted: 05 January 2021;
Published: 29 January 2021.
Edited by:
Jess K. Zimmerman, University of Puerto Rico, Puerto RicoReviewed by:
Alexandre Siminski, Federal University of Santa Catarina, BrazilJ. Aaron Hogan, Florida International University, United States
Copyright © 2021 Müller, Bergamin, Bordin, Klipel and Rosenfield. This is an open-access article distributed under the terms of the Creative Commons Attribution License (CC BY). The use, distribution or reproduction in other forums is permitted, provided the original author(s) and the copyright owner(s) are credited and that the original publication in this journal is cited, in accordance with accepted academic practice. No use, distribution or reproduction is permitted which does not comply with these terms.
*Correspondence: Sandra Cristina Müller, c2FuZHJhLm11bGxlckB1ZnJncy5icg==