- 1Plant Ecology and Ecosystems Research, Albrecht von Haller Institute for Plant Sciences, University of Goettingen, Göttingen, Germany
- 2Chair of Ecophysiology and Vegetation Ecology, Julius von Sachs Institute of Biological Sciences, University of Würzburg, Würzburg, Germany
- 3Tropical Silviculture and Forest Ecology, University of Goettingen, Göttingen, Germany
- 4Plant Ecology, Justus Liebig University of Giessen, Giessen, Germany
- 5Department of Agrotechnology, Faculty of Agricultural Sciences, Tadulako University, Palu, Indonesia
The efficiency of the water transport system in trees sets physical limits to their productivity and water use. Although the coordination of carbon assimilation and hydraulic functions has long been documented, the mutual inter-relationships between wood anatomy, water use and productivity have not yet been jointly addressed in comprehensive field studies. Based on observational data from 99 Indonesian rainforest tree species from 37 families across 22 plots, we analyzed how wood anatomy and sap flux density relate to tree size and wood density, and tested their combined influence on aboveground biomass increment (ABI) and daily water use (DWU). Results from pairwise correlations were compared to the outcome of a structural equation model (SEM). Across species, we found a strong positive correlation between ABI and DWU. Wood hydraulic anatomy was more closely related to these indicators of plant performance than wood density. According to the SEM, the common effect of average tree size and sap flux density on the average stem increment and water use of a species was sufficient to fully explain the observed correlation between these variables. Notably, after controlling for average size, only a relatively small indirect effect of wood properties on stem increment and water use remained that was mediated by sap flux density, which was significantly higher for species with lighter and hydraulically more efficient wood. We conclude that wood hydraulic traits are mechanistically linked to water use and productivity via their influence on sap flow, but large parts of these commonly observed positive relationships can be attributed to confounding size effects.
Introduction
Water availability is probably the single most important determinant of tree size and productivity on a global scale (Moles et al., 2009; Šímová et al., 2019). Evolving an efficient vascular system designed for long-distance water transport allows trees to place their carbon assimilating foliage high above the ground (Sperry, 2003), thereby gaining a competitive advantage over shorter neighbors in the competition for light. The absence of frost and extended dry periods in tropical rainforest climates with their usually ample water supply has spurred the race to reach advantageous canopy positions. The reduced selection for a “safe” xylem in perennially wet climates allowed for the development of highly conductive vascular systems (Maherali et al., 2004; Mencuccini et al., 2015; Sanchez-Martinez et al., 2020). To enable a sufficiently high water supply to the canopy, the stem wood of tall and productive tropical trees is commonly composed of wide xylem vessels that facilitate the necessary high flow rates (Poorter et al., 2010; Schuldt et al., 2013; Kotowska et al., 2015; Hietz et al., 2016). Nonetheless, trees cannot escape the inevitable link between carbon assimilation and transpirative water loss (Brodribb et al., 2002; Santiago et al., 2004; Brodribb, 2009). Water supply needs to be secured over the entire length of the flow path, which ultimately limits the height growth potential (Koch et al., 2004; Liu et al., 2019). Although the coordination of productivity and hydraulic functioning has long been recognized, the underlying causal links often remain unresolved and the precise role of xylem vascular anatomy for the efficiency of the water transport system, tree water use and stem growth rate is hard to quantify (Hoeber et al., 2014). Nevertheless, the approach to use easy-to-measure plant functional traits for characterizing the hydraulic architecture of tropical trees and for understanding the coexistence and performance of tree species in communities is increasingly popular (Poorter et al., 2018; McDowell et al., 2019).
In ecosystems with ample soil water supply and low atmospheric evaporative demand, the capacity to transport water efficiently to the canopy is most likely a key factor limiting gas exchange and carbon assimilation. Consequently, physiological traits related to soil water access and water transport correlate positively with biomass increment (Tyree, 2003; Hajek et al., 2014; Smith and Sperry, 2014; Kotowska et al., 2015). Next to wood properties that determine hydraulic efficiency, wood density (WD) is another widely used property that might be indicative of the growth performance of trees (King et al., 2006; Chave et al., 2009; Poorter et al., 2010; Hoeber et al., 2014) and of water use dynamics (Bucci et al., 2004). Fast-growing pioneer tree species tend to have lower wood densities than slower-growing species from later successional stages, with consequences for their wood anatomical structure, construction cost and water transport properties (Apgaua et al., 2015; Eller et al., 2018). However, in evergreen tropical rainforests, several studies found wood density to vary largely independently of hydraulic traits (Baraloto et al., 2010; McCulloh et al., 2010; Fan et al., 2012; Schuldt et al., 2013; Kotowska et al., 2015; Grossiord et al., 2019), putting into question the predictive value of wood density.
Wood density, vessel diameter and hydraulic conductivity are traits increasingly used in dynamic vegetation models aiming to predict plant productivity and population dynamics across a broad range of environments (Powell et al., 2013, 2017; Anderegg, 2015; Maréchaux et al., 2015; Rowland et al., 2015; Xu et al., 2016; Griffin-Nolan et al., 2018). Yet, it is still a matter of debate as to whether and to what extent hydraulic traits and wood properties represent suitable proxies for characterizing carbon and water fluxes in trees in the absence of water limitation (Zanne et al., 2010; Fortunel et al., 2014). Studies that simultaneously measured wood density, hydraulic anatomy, tree water use, and aboveground biomass increment in species-rich tropical rainforests are virtually absent, but are essential to achieve a more mechanistic understanding of carbon assimilation and growth in trees.
Based on a dataset of 99 tropical tree species from lowland and pre-montane rainforests in Sumatra and Sulawesi (Indonesia), we studied how species averages of central tree structural and functional properties (tree height, stem diameter, wood density) are associated with wood anatomical and derived hydraulic traits, and how they interact to affect average sap flux density, daily water use and aboveground biomass increment. To illustrate that pairwise bivariate correlations are not sufficient to capture the complex relationships between these variables appropriately, we first analyse a set of well-established relationships with standardized major axis (SMA) regressions, and then contrast the outcome to the results of a structural equation model (SEM) based on a-priori hypotheses about causal relationships (cf. Figure 1). Our main hypotheses were that (i) an efficient xylem is a prerequisite for high area-based maximal sap flow rates, (ii) tree species with a higher water use have a higher biomass increment, and (iii) wood anatomical traits are more closely related to productivity and water use than wood density.
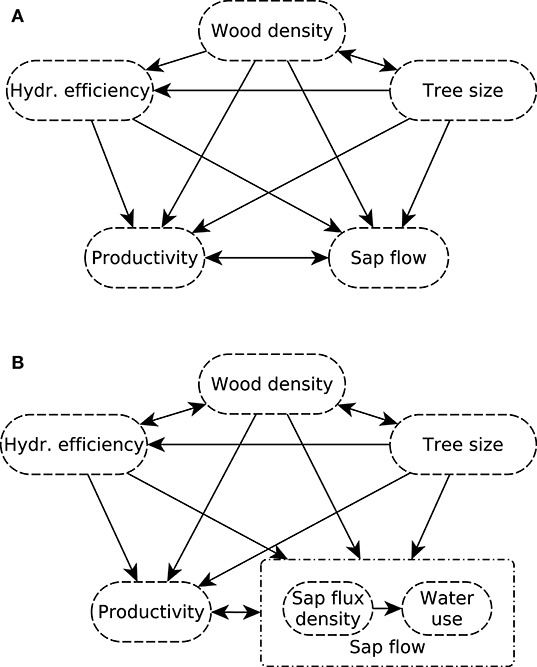
Figure 1. (A) Initial and (B) revised meta-model of the causal relations amongst structural characteristics and hydraulic traits, water use, and productivity. Correlative relationships are indicated by double-headed arrows, while functional relationships are indicated by single-headed arrows.
Materials and Methods
Study Sites
This study investigates stem sap flux, wood anatomy and aboveground biomass increment of 99 tropical tree species from 37 families using measurements from 265 tree individuals (Supplementary Table S1), combining data from two long-term studies in plot networks on the Indonesian islands Sumatra and Sulawesi comprising a total of 22 plots (Supplementary Figure S1). Structural variables (wood density, tree diameter, tree height) were recorded from every tree individual, while wood anatomy was measured on 238, sap flux density on 178, and biomass increment on 152 tree individuals, respectively (see Table 1 for details). All individuals were mature trees with DBH ≥ 10 cm. On Sumatra, sampled trees were randomly chosen in equal proportions from dominant and co-dominant trees grouped in three tree size classes: larger (DBH > 50 cm), medium (50–25 cm) and smaller diameter (10–25 cm). On Sulawesi, measurements were performed on 15 species from dominant families chosen to represent slow-growing mid-story species as well as fast-growing upper canopy species, both from late stages of forest succession.
On the island of Sumatra, the field measurements were conducted in 16 lowland rainforest sites in Jambi province (01°47′ - 02°09′ S, 102°34' - 103°20' E; 80 m a.s.l.), which were mixed dipterocarp forests in a close-to-natural state but subject to logging activities in the past, or secondary forests comprising a mixture of regrown native species (see Drescher et al., 2016). In the region, average annual temperature is 27.4 ± 1.0°C (data from 1991 to 2011; mean ± SD), and annual precipitation is 2,235 ± 385 mm. From June to September, the occurrence of a somewhat drier season (<120 mm monthly precipitation) is possible. Soil types in the region are sandy or clayey Acrisols (Allen et al., 2015). These study sites were part of EFForTS, a large collaborative research project on the ecological and socioeconomic consequences of rainforest transformation. On the island of Sulawesi, we used data from six study plots established in the frame of the STORMA project (see Hertel et al., 2009; Moser et al., 2014), situated in largely undisturbed old-growth rainforest at pre-montane elevation in the Pono Valley on the western boundary of Lore Lindu National Park close to Palu, Central Sulawesi province (01°29.6′ S, 120°03.4′ E; 1,050 m a.s.l.). The soils of this forest are Acrisols over metamorphic rocks. The climate is wet all around the year, with mean annual precipitation of 2,700 mm and mean annual air temperature of 20.8°C (Moser et al., 2014).
Tree Morphological Characteristics
Tree height was measured with a Vertex III height meter (Haglöf, Långsele, Sweden) and tree diameter was recorded with diametric measuring tape at breast height (130 cm) or 10 cm above obstacles such as buttress roots. Stem sapwood samples with a diameter of 5.15 mm and a length between 4 and 7 cm were taken of each tree with an increment corer (Haglöf, Långsele, Sweden). Wood density (WD, g cm−3), defined as oven-dry weight over wet volume, was calculated for each core. The fresh volume of each sample was determined by Archimedes' principle, or by using the length and diameter of the core assuming a cylindrical shape (Schüller et al., 2013). Subsequently, samples were oven-dried for 48 h at 105°C before determining their dry weight.
Aboveground Biomass and Biomass Increment
Aboveground biomass was calculated with the R package BIOMASS (Réjou-Méchain et al., 2017) using the allometric equation of Chave et al. (2014) for tropical wet forests: AGB = 0.0673 × (WD × DBH2 × H)0.976, where AGB is the estimated aboveground biomass (kg), DBH the diameter at breast height (cm), H total tree height (m), and WD stem wood density (g cm−3). We measured diameter increment using dendrometer tapes (UMS GmbH, München, Germany) or conducted repeated measurements with measuring tapes at marked positions on the stem. To estimate plant growth performance, we calculated aboveground woody biomass increment (ABI; kg tree−1 yr−1) as the difference in tree biomass estimates (based on allometric regression models) over the measurement interval divided by the length of the measurement interval in years. Stem radial increment was monitored on trees equipped with dendrometers every 3 months from 2012 to 2017 in Sumatra and from 2007 to 2009 in Sulawesi, while a complete census of the remaining trees was conducted at the end of the study period. Tree height was measured only at the beginning of the study, as we expected the measurement error to be larger than height growth in the investigation period. The most extreme 2% of values recorded for AGB increment were excluded from further analysis, as they were most likely affected by measurement errors due to shifts in measurement positions on the stem.
Wood Anatomical and Derived Hydraulic Traits
For the xylem anatomical analysis, we used the outermost 4 cm of the sapwood from the increment cores. In total, 134 samples from Sumatra belonging to 80 species and 104 samples from Sulawesi belonging to 15 species were analyzed. The samples were stored in 70% ethanol and stained with safranin (1% in 50% ethanol, Merck, Darmstadt, Germany) before sectioning. Subsequently, 10–20 μm semi-thin cross-sections of the entire 4 cm sample were cut with a sliding microtome (G.S.L.1, WSL, Birmensdorf, Switzerland). Photographs of the sections were taken with a stereo-microscope with an automatic stage equipped with a digital camera (SteREOV20, Carl Zeiss MicroImaging GmbH, Göttingen, Gemany) at 100x magnification. Image processing was done with Adobe Photoshop CS6 (version 13.0.1, Adobe Systems Incorporated, USA) and the particle analysis-function of ImageJ v. 1.50e (Schneider et al., 2012). All vessels of a section (mean ± SD: 64.22 ± 25.03 mm2) were analyzed, yielding 100–500 measured vessels per sample. The hydraulically weighted average vessel diameter (Dh, μm) was calculated from the measured vessel diameters Di as Dh = ∑/∑ (Sperry et al., 1994). The potential hydraulic conductivity (Kp, kg m−1 MPa−1 s−1) of a wood segment was calculated based on Hagen-Poiseuille's law as Kp = (π × ρ × ∑)/(128 η × Axylem), where Di is the diameter of each single vessel i, η the water viscosity (1.002 × 10−9 MPa s) and ρ the density of water (998.2 kg m−3), both at 20°C, and Axylem (m2) the analyzed sapwood area.
Sap Flux Measurements and Water Use
To derive daily tree water use rates, xylem sap flux density (Js, g cm−2 d−1) was recorded with the thermal dissipation probe (TPD; Granier, 1985) technique [see Horna et al. (2011), Röll et al. (2019)]. Pairs of 20 mm long and 2 mm wide heating probes were inserted in northern and southern orientation into the sapwood at breast height (1.30 m) or above buttress roots; the upper probe was heated with a constant power of 0.2 W, resulting in a current of 120 mA. Subsequently, sensors were covered with reflective aluminum foil attached to isolative material and covered by plastic foil to minimize temperature gradients caused by radiation. The sensors were read by a CR1000 data logger (Campbell Scientific Inc., Logan, USA) and connected using an AM16/32 multiplexers, with data collected every 30 sec, averaged and logged at 10 min (Sumatra) or 30 min intervals (Sulawesi). Sap flux density was calculated according to the original empirical calibration coefficients given by Granier (1985) as Js = 119 × K1.231, where K is a temperature constant determined as K = (ΔT0 – ΔT)/ΔT, where ΔT0 is the maximum temperature difference at zero flux night-time conditions, and ΔT is the actual temperature difference between heated and reference probe. Several recent studies pointed out that using the original equation parameters given by Granier (1985) may result in a systematic underestimation of real flow, and recommend species-specific sensor calibration, which is hardly feasible in species-rich tropical forest settings (Steppe et al., 2010; Fuchs et al., 2017). However, a recent review of sap flow calibration studies concluded that notwithstanding this systematic bias, TDP sensors can be used when sap flow responses to environmental variables in relative terms are the primary focus of the study (Flo et al., 2019).
On Sumatra, sap flux density was measured for a minimum of 3 weeks on eight dominant and co-dominant tree individuals, which account for the major part of stand water use on each of the 16 plots, yielding 88 stems from 58 species in total. On Sulawesi, measurements were performed on up to 14 stems of each species on the six plots, which were continuously measured from July 2007 until April 2009, yielding 90 trees in total. We harmonized sap flux density by calculating the average of 3 representative days under similar evaporative demand were maximum values were reached. Sunny conditions with a daily average vapor pressure deficit (VPD) between 0.64 and 0.82 kPa (mean ± SD: 0.71 ± 0.09 kPa) were chosen to obtain consistent estimates of daily mean sap flux density (Js, g cm−2 d−1) for day-time hours (6 am to 7 pm). We chose this range for reasons of comparability because most trees had already reached their maximum flux at a VPD of 0.7 kPa (Supplementary Figures S2, S3). For details on microclimatic measurements in the respective study regions (i.e., air temperature and relative air humidity), see Drescher et al. (2016) for Sumatra and Horna et al. (2011) for Sulawesi.
To calculate daily water use per tree (DWU, kg d−1), Js was multiplied with the corresponding sapwood area (As, cm2). We used a subsample of 66 tree individuals belonging to 13 species covering a large range of tree height (9.5–81.0 m) and wood density values (0.340–0.682 g cm−3) for estimating As with dye injection to determine the maximum depth of hydraulically active xylem (e.g., Meinzer et al., 2001). During the morning hours and before the onset of high transpiration rates, a hole was drilled with an increment corer (Haglöf, Långsele, Sweden) and a plastic tube filled with a 0.1% indigocarmin dye solution attached to the stem and sealed with modeling clay (Terostat IX, Henkel, Düsseldorf, Germany). The tube was fixed to the stem in horizontal orientation but with a slight inclination in order to avoid a pressure gradient that might actively force the dye into the sapwood, and refilled if necessary. In the afternoon, a second core was extracted 5 cm above the first hole, and sapwood depth measured with a caliper. The resulting measurements of sapwood depth were used to establish a relationship between the conductive sapwood area and diameter at breast height (P < 0.0001, r2 = 0.75), which was used to predict the As of all trees equipped with sap flux sensors according to As = 3.4794 × exp[0.7801 × exp(0.4689 × ln(DBH))] (Supplementary Figure S4).
Statistical Analyses
All statistical analyses were performed in R v. 3.6.1 (R Core Team, 2019) in the framework of the tidyverse package (Wickham et al., 2019).
The relationship between Dh, WD and a series of growth-, water use- and size-related traits as well as the relationship between DWU and ABI were analyzed using standardized major axis (SMA) regressions of species averages of those variables using R package smatr v. 3.4-8 (Warton et al., 2012). As most of the variables in the dataset were strongly right-skewed, all traits except wood density were natural log-transformed prior to analysis.
The presumed causal relationships between tree size and various plant functional traits were analyzed with a structural equation modeling (SEM) approach based on the R package lavaan v. 0.6-3 (Rosseel, 2012). Following Grace et al. (2012), we first developed a meta-model that reflected our a priori theoretical expectations about the relationships between wood density, wood anatomical traits and tree size, and the way these variables affect biomass increment and sap flow (Figure 1A). Initial data inspection suggested that the dataset was more accurately characterized by separating between the (area-specific) sap flux density and the (tree-specific) overall daily water use, and replacing the causal link between wood density and wood anatomical properties by a correlative relationship (Figure 1B). We described “hydraulic efficiency” by a latent variable measured through vessel diameter Dh, vessel density VD and potential hydraulic conductivity Kp, and “tree size” by a latent variable linked to tree height and diameter. Biomass increment, water use and sap flux density were represented by the associated variables ABI, DWU, and Js, respectively, resulting in the causal diagram displayed in Supplementary Figure S5.
A summary of the structural variables used in the model and wood density (average values of each trait and number of replicates per species) is given in Supplementary Table S1. Before model fitting, all variables except wood density were natural log-transformed. Subsequently, all variables were centered and scaled to aid convergence. As the number of observations varied between species and the number of species was unevenly distributed between Sumatra and Sulawesi (Table 1), we decided to work with species-level averages of the observed variables to circumvent problems related to the nested data structure and to reduce the impact of missing data. The dataset used for model fitting included species-level averages of all available data of the 265 individuals from 99 species.
The model was fit with a robust estimator with Huber-White standard errors to accommodate deviations from multivariate normality, and using full information maximum likelihood to account for pairwise missing data. We started by fitting the model described in Supplementary Figure S5. Model adequacy was then evaluated by the inspection of modification indices (cf. Rosseel, 2012). Model fit was assessed by the scaled χ2-value and its corresponding P-value, Root Mean Square Error of Approximation (RMSEA) and Comparative Fit Index (CFI). Subsequent inspection of modification indices suggested that an important path was missing in the initial model based on Supplementary Figure S5, as this model did not account for the significant residual covariance between VD and Kp. After allowing for a correlation between these variables, there was no indication for missing paths and the model no longer showed significant deviations from the observed data (model χ2 = 15.39, df = 15, P = 0.442) and achieved a high goodness of fit (CFI = 0.999, RMSEA = 0.016).
Results
Unadjusted Relationships With Wood Anatomy and Wood Density
Species averages of wood anatomical and size-related variables were correlated with sap flux density, biomass increment, and water use (Figure 2). Wood density (WD), on the other hand, was only weakly associated with most other variables in the dataset (Figure 2). This is reflected in the results of the SMA regressions (Figure 3), which revealed a significant positive scaling relationship between hydraulically-weighted vessel diameter and biomass increment (ABI; r = 0.38, P < 0.001), daily water use (DWU; r = 0.56, P < 0.001), sap flux density (Js; r = 0.38, P < 0.001), and tree height (H; r = 0.39, P < 0.001), while their relationships to WD were insignificant with the exception of sap flux density (r = −0.30, P = 0.011; cf. Figure 3, Supplementary Table S2).
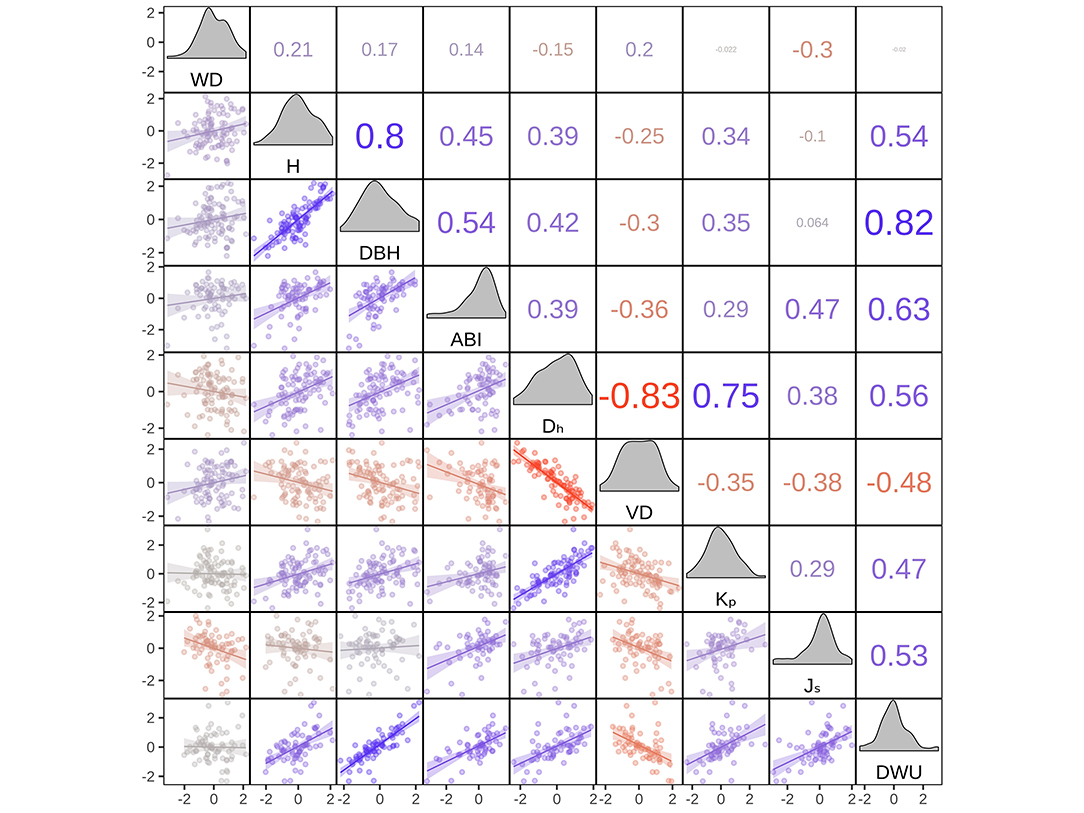
Figure 2. Pairwise Pearson correlation table (upper triangle), scatterplots (lower triangle), and density plots (diagonal panels) for the transformed variables used for model fitting. Shown are the species means of the ln–transformed (with the exception of WD), scaled and centered structural characteristics and hydraulic traits for n = 99 species, including trend lines from linear regression with 95% confidence intervals. Color and size indicate direction and strength of the correlation.
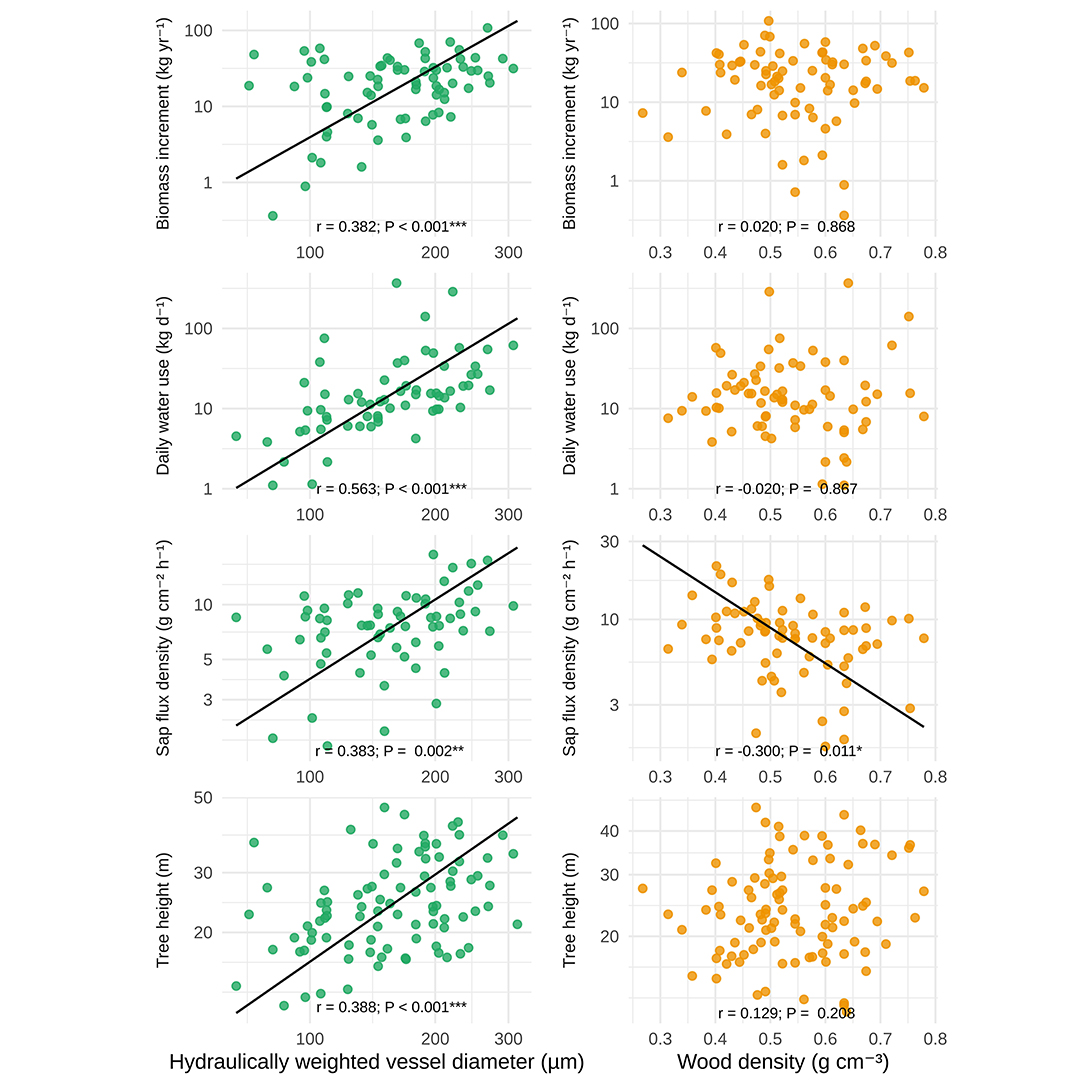
Figure 3. Results of standardized major axis regressions of species-level averages of biomass increment, daily water use, sap flux density, and tree height against hydraulically weighted vessel diameter and wood density.
Relationship Between Biomass Increment and Daily Water Use
ABI had a highly significant positive relationship with DWU (r = 0.63, P < 0.001; cf. Figures 2, 4), with a scaling coefficient of 1.271 (95% CI 1.028 – 1.572; cf. Supplementary Table S2). However, our structural equation model indicated that the strong correlation between the two variables was largely driven by their shared positive relationship with Js and average tree size (cf. Figures 2, 3). After accounting for the influence of these variables, no significant residual correlation remained between ABI and DWU (r = 0.145, z = 1.09, P = 0.28; Table 2, Figure 5).
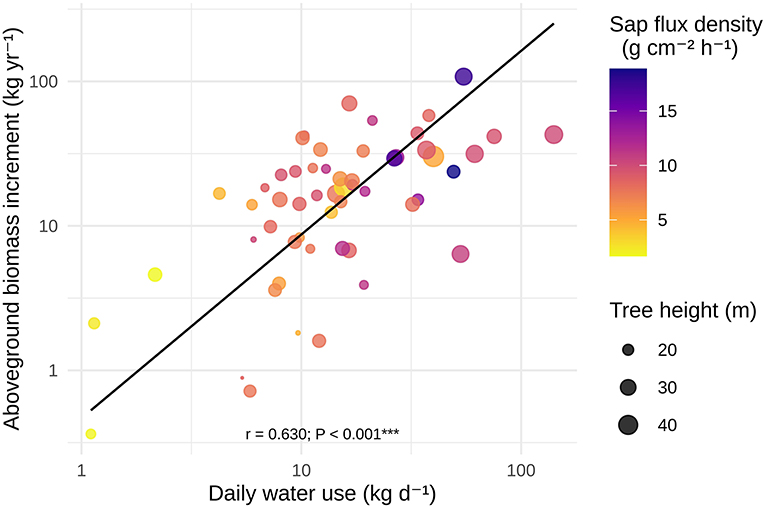
Figure 4. Results of standardized major axis regressions of species-level averages of biomass increment vs. daily water use. Color: average sap flux density; size: average tree height.
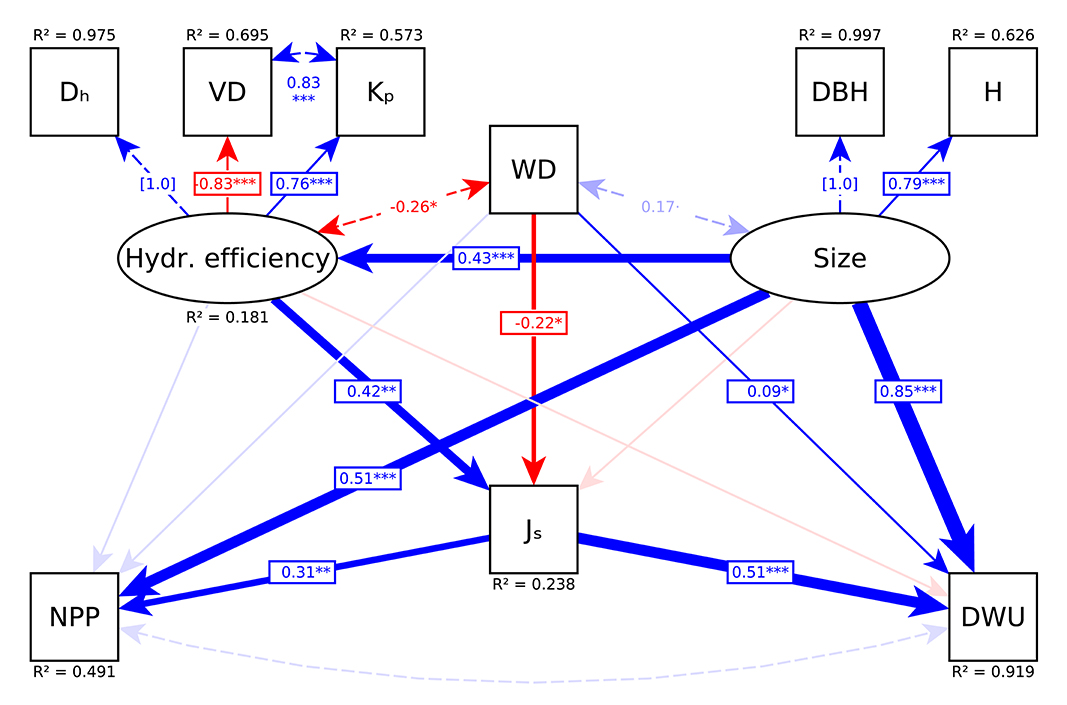
Figure 5. Path diagram of the final model (model χ2 = 15.39, df = 15, P = 0.442; CFI = 0.999, RMSEA = 0.016). Shown are causal relationships with scaled path coefficients (one-sided arrows), residual correlations (two-sided dashed arrows), and explained variances *P < 0.05, **P < 0.01, and ***P < 0.001. Positive links are displayed in blue, negative links in red and insignificant links in transparent color. Paths with coefficients fixed to one for computational reasons (Dh and DBH) are displayed as dashed arrows. See Table 1 for abbreviations.
Determinants of Sap Flux Density and Water Use
We found Js to be significantly higher for species with higher hydraulic efficiency (standardized path coefficient 0.42, z = 3.21, P = 0.001; Table 2, Figure 5), and lower for species with a lower wood density (−0.22, z = −2.08, P = 0.038; Table 2, Figure 5). While average tree size did not have a direct effect on Js, we found an indirect size effect mediated by hydraulic efficiency (0.43, z = 3.817, P < 0.001; Table 2, Figure 5), indicating that larger tree species had higher sap flux rates because of their significantly higher hydraulic efficiency. In either case, the low fraction of 23.8% of explained variance in Js (Table 2, Figure 5) shows that differences in tree size, WD and wood anatomical variables only explained a small fraction of the interspecific differences in Js.
In total, the model explained 91.9% of the variance in DWU (Table 2, Figure 5). As expected, DWU increased highly significantly with tree size (0.85, z = 12.64, P < 0.001; Table 2, Figure 5) and Js (0.51, z = 9.943, P < 0.001; Table 2, Figure 5). After accounting for the effects of these two factors, no significant direct effect of hydraulic efficiency on DWU remained, indicating that the positive association between DWU and wood anatomical traits (cf. Figures 2, 3) was fully explained by their effect on Js. However, there was a small but significant positive effect of WD on DWU (0.09, z = 2.37, P = 0.018; Table 2, Figure 5) (i.e., given the same values of Js and tree size, trees with harder wood had a slightly higher water use).
Determinants of Plant Productivity
As anticipated, average tree size had a highly significant positive effect on the rate of aboveground biomass accumulation (0.51, z = 4.30, P < 0.001; Table 2, Figure 5). After accounting for this size effect, there was no evidence for direct effects of hydraulic efficiency and WD on ABI. However, species of a given average size had a significantly higher ABI when they had higher sap flux densities (0.31, z = 3.01, P = 0.003; Table 2, Figure 5), indicating that the effect of both WD and wood hydraulic properties on ABI was entirely mediated through their effect on Js. Together, the predictor variables explained 49.1% of the variance in ABI (Table 2, Figure 5).
Discussion
Drivers of Productivity-Water Use-Relationships
In line with our expectations, we found aboveground biomass increment to be tightly positively associated with water use across a wide range of tropical tree species in this sample of Southeast Asian trees. We further show that differences in average tree size and sap flux density are sufficient to fully explain the relationship between aboveground biomass increment (ABI) and daily water use (DWU): at a given average size, tree species that can achieve higher sap flux rates use more water and grow faster. In turn, sap flux density was confirmed to be significantly higher in trees that possess lighter sapwood with a higher hydraulic efficiency. This reaffirms the importance of an efficient vascular system as a prerequisite for high productivity and water use (cf. Tyree, 2003; Brodribb, 2009). Although these findings are merely supportive of previously discussed concepts, to our knowledge we are the first to demonstrate this interdependence in a comprehensive observational dataset of both wood anatomical and empirically determined hydraulic traits of tropical rainforest species, and to combine it with growth measurements conducted for the same species.
The single most important predictor for the biomass production and water use of a tree species was its average size, highlighting the repeatedly documented central role of large trees for the productivity and water cycles of tropical forests (cf. Slik et al., 2013; Stephenson et al., 2014; Bastin et al., 2015, 2018; Lutz et al., 2018). This is not surprising as both carbon assimilation and transpiration directly depend on foliage area, which scales with tree mass (cf. Enquist et al., 1999; West, 1999; Brown et al., 2004). Furthermore, larger trees typically have access to higher light intensities (Coble and Cavaleri, 2014), which is a key variable for tropical tree growth (King et al., 2005; Rüger et al., 2011, 2012), and are exposed to higher evaporative demand, which enhances water use (Horna et al., 2011). In tropical forest with high vapor pressure deficits at midday hours, trees thereby seem to rely on internal water storage to meet this high demand during the course of a day (James et al., 2003; Meinzer et al., 2003; Scholz et al., 2011), which is again size-dependent and permits larger trees to have their maximum of photosynthetic activity at later times (Meinzer et al., 2001). In consequence, growth and water turnover are highly coordinated (Smith and Sperry, 2014), as larger trees assimilate more carbon (Stephenson et al., 2014) and use more water (Wullschleger et al., 1998; Meinzer et al., 2005; Horna et al., 2011; Kunert et al., 2017; Link et al., 2020). The strong size dependence of important aspects of tree carbon and water relations has the potential to mask or confound relationships between other variables in studies focusing on functional traits. In correspondence, many recent studies have highlighted the great importance of including plant size as a covariate when studying relationships between tree functional traits (e.g., Hietz et al., 2016; Gleason et al., 2018; Lechthaler et al., 2019; Šímová et al., 2019). Indeed, we observed a meaningful influence of average tree size on wood anatomical properties related to hydraulic efficiency. These observations are in agreement with other studies showing that larger tropical trees possess wider vessels and higher potential conductivity, both at the stem base and in distal twigs (Poorter et al., 2010; Zach et al., 2010; Schuldt et al., 2013; Hietz et al., 2016). Tree height in particular has been singled out as the putative main driver of conduit dimensions (Coomes et al., 2007; Rosell et al., 2017; Olson et al., 2018; Fajardo et al., 2020). Interestingly, average tree size was virtually unrelated to sap flux density, indicating that the exposition of taller tree species to higher light levels and vapor pressure deficits (Horna et al., 2011; Schuldt et al., 2011) was fully offset by these changes in hydraulic anatomy along the flow path.
Wood Anatomical Traits Outperform Wood Density as Predictors of Plant Growth and Water Use
In accordance with previous findings, our observations show that growth and water use are more closely related to the water transport capacity in the vascular system—mainly driven by larger average vessel diameters—than to wood density (Russo et al., 2010; Hoeber et al., 2014; Eller et al., 2018). As hypothesized, we found a significant link between wood anatomy and sap flux density on the species level. This effect was sufficiently large to explain the association of growth and water use with anatomical traits remaining after controlling for average tree size, demonstrating that the frequently reported relationship between productivity and wood properties is in fact caused by the limiting effect of these anatomical features on plant hydraulic conductance and water transport (cf. Brodribb, 2009). To our knowledge, our study is the first to confirm this relationship across a high number of broad-leaved tree species, although wood anatomical properties may critically influence sap flow calibration performance (Xie and Wan, 2018; Flo et al., 2019).
In principle, natural selection should favor the development of higher hydraulic efficiency per sapwood area to save xylem construction and maintenance costs (McCulloh and Sperry, 2005; Gleason et al., 2016). However, the advantage of large vessels and an efficient vascular network likely trades off with the vulnerability of conduits to embolize, which puts a practical limit on vessel dimensions (Tyree et al., 1994; Wheeler et al., 2005; Hajek et al., 2014). As trees in humid tropical forests are neither constrained by freeze-thaw embolism nor prolonged periods of soil desiccation, their maximum xylem hydraulic efficiency is expected to be high (Schuldt et al., 2013; Gleason et al., 2016; Liu et al., 2019).
A positive influence of vessel size (and thus larger potential hydraulic conductivity) on growth rates has repeatedly been demonstrated (Poorter et al., 2010; Hietz et al., 2016; Gleason et al., 2018). Wood density, on the other hand, is known to be associated with most structural and functional wood properties (Chave et al., 2009). However, it ultimately constitutes an emergent property of wood structure rather than an unambiguous functional trait, with a large range of combinations of wood anatomical features resulting in the same wood density (Ziemińska et al., 2013, 2015). Thus, wood density is at least partially decoupled from wood anatomical traits (Zanne et al., 2010; Fan et al., 2012; Schuldt et al., 2013).
In tropical trees, harder wood tends to be associated with lower growth rates (e.g., Muller-Landau, 2004; King et al., 2006; Poorter et al., 2010; Rüger et al., 2012; Hoeber et al., 2014). Our results indicate that this relationship is a consequence of the negative relationship of wood density with both hydraulic efficiency and sap flux density, while wood density had no direct causal effect on ABI and only a minor effect on DWU. Due to the much closer mechanistic link of wood anatomical traits to Js, their larger effect size on Js compared to wood density (WD) is not surprising. As WD does not have a direct mechanistic link to water transport, the observed effect on Js most likely results from a correlation with wood anatomical attributes that were not covered by our latent variable for hydraulic efficiency [e.g., pit membrane characteristics (Choat et al., 2008; Jansen et al., 2009; Li et al., 2016)]. It is further notable that in agreement with the null model of Francis et al. (2017), no direct WD effects on biomass increment could be identified. The lack of WD effects on productivity may be a consequence of assessing it on a biomass increment basis, which implicitly accounts for construction costs. It is worth noting that WD and hydraulic efficiency together only explain a comparatively low fraction of variance in sap flux density in our dataset (r2 = 0.24, cf. Figure 5), which puts a limit on the magnitude of their indirect effect on biomass increment and water use. If, as our data suggest, a large part of the correlation between biomass increment, water use and wood properties results from their common dependence on tree size, the question arises whether their importance for parameterizing dynamic vegetation and earth system models and for understanding responses to environmental change (e.g., Fisher et al., 2018; Griffin-Nolan et al., 2018; Olson et al., 2018; Mencuccini et al., 2019) may be overstated.
Accounting for Confounding Size Effects in Ecological Datasets
The present study illustrates that focusing on pairwise relationships between plant traits without accounting for the complex, multivariate nature of ecological datasets can result in fundamentally different interpretations. Our approach highlights that many commonly observed plant performance-hydraulic trait relationships may be attributed to a confounding effect of tree size (cf. Poorter et al., 2010; Fan et al., 2012; Hietz et al., 2016; Gleason et al., 2018). The latter influences the hydraulic efficiency of the vascular system through its effects on a species' wood anatomical properties, while simultaneously being strongly associated with biomass increment and water use. To be able to interpret functional plant trait relationships in the context of plant performance, it is therefore necessary to account for the effects of tree size, which has been reportedly neglected in the past. A common strategy to avoid confounding size effects is to compare species under “optimal” growth conditions and at maturity, when species are more likely to express their full trait potential (Poorter and Bongers, 2006; Pérez-Harguindeguy et al., 2013). However, the definition of both “mature” trees and “optimal” growth conditions across various ecosystems remains disputable, especially since observed hydraulic adaptations are inevitably interlinked with climate and the maximum potential height a tree can reach (Liu et al., 2019; Medeiros et al., 2019; Šímová et al., 2019). Given the intrinsic complexity of ecological datasets, where tree size is only one of many potential confounders, it may often be preferable to explicitly account for the network of relationships between variables. Adopting a structural equation modeling approach did not only enable us to account for the aforementioned size effects, but also permitted to differentiate between direct and indirect effects, and to test the hypothesis that our model as a whole is consistent with the observed data.
Conclusions
Our study provides empirical evidence that the biomass increment and water use of tropical trees are highly coordinated. We show that for tree species of a given average size, differences in stem increment and water use are explained by hydraulic adjustments resulting from differences in wood structure. The highly significant relationship between wood anatomy and sap flux density and the significant effect of the latter on growth provide evidence that the vascular water transport system indeed can be considered as a “functional backbone of terrestrial productivity” (Brodribb, 2009). However, their explanatory power is dwarfed by the magnitude of size effects, illustrating the importance of average tree size as a driver of forest productivity. This highlights the need to account for confounding size effects when interpreting species-level relationships between size-related variables such as water use and biomass increment.
Data Availability Statement
The raw data supporting the conclusions of this article will be made available by the authors, without undue reservation.
Author Contributions
BS, DHe, DHö, and CL developed the original research questions. MK, P-AW, and GM carried out the biomass increment and structural measurements, and MK, BS, and RL the wood anatomical analyses. AR and BS collected the sap flow data. RL performed the data analysis and statistical interpretation in close collaboration with MK and BS. MK and RL wrote the first version of the manuscript, which was discussed and revised by all authors. All authors contributed to the article and approved the submitted version.
Funding
This study was financed by the German Research Foundation (DFG) in the framework of the research projects EFForTS (CRC990) and STORMA (CRC552). Roman Link was funded by DFG grant number SCHU 2935/1-1.
Conflict of Interest
The authors declare that the research was conducted in the absence of any commercial or financial relationships that could be construed as a potential conflict of interest.
Acknowledgments
We thank village leaders, local plot owners, PT REKI, authorities of the Bukit Duabelas National Park, the Lore Lindu National Park for granting access, and the Indonesian Research Foundation (LIPI) for research permissions. We further thank our counterparts and local assistants for support with fieldwork and administration, and Katja Rembold, Fabian Brambach, and Heike Culmsee for species identification. This study was conducted with research permits No. 2704/IPH.1/KS.02/X1/2012, No. 15/EXT/SIP/FRP/SM/II/2013, and No. 251/SIP/FRP/E5/Dit.KI/VII/2016 issued by the FRP-Kemenristek. An earlier version of this study has been published as part of the Ph.D. thesis of RL (Link, 2020).
Supplementary Material
The Supplementary Material for this article can be found online at: https://www.frontiersin.org/articles/10.3389/ffgc.2020.598759/full#supplementary-material
References
Allen, K., Corre, M. D., Tjoa, A., and Veldkamp, E. (2015). Soil nitrogen-cycling responses to conversion of lowland forests to oil palm and rubber plantations in Sumatra, Indonesia. PLoS ONE 10:e0133325. doi: 10.1371/journal.pone.0133325
Anderegg, W. R. L. (2015). Spatial and temporal variation in plant hydraulic traits and their relevance for climate change impacts on vegetation. New Phytol. 205, 1008–1014. doi: 10.1111/nph.12907
Apgaua, D. M. G., Ishida, F. Y., Tng, D. Y. P., Laidlaw, M. J., Santos, R. M., Rumman, R., et al. (2015). Functional traits and water transport strategies in lowland tropical rainforest trees. PLoS ONE 10:e0130799. doi: 10.1371/journal.pone.0130799
Baraloto, C., Paine, C. E. T., Poorter, L., Beauchene, J., Bonal, D., Domenach, A.-M., et al. (2010). Decoupled leaf and stem economics in rain forest trees. Ecol. Lett. 13, 1338–1347. doi: 10.1111/j.1461-0248.2010.01517.x
Bastin, J.-F., Barbier, N., Réjou-Méchain, M., Fayolle, A., Gourlet-Fleury, S., Maniatis, D., et al. (2015). Seeing Central African forests through their largest trees. Sci. Rep. 5:13156. doi: 10.1038/srep13156
Bastin, J.-F., Rutishauser, E., Kellner, J. R., Saatchi, S., Pélissier, R., Hérault, B., et al. (2018). Pan-tropical prediction of forest structure from the largest trees. Glob. Ecol. Biogeogr. 27, 1366–1383. doi: 10.1111/geb.12803
Brodribb, T. J. (2009). Xylem hydraulic physiology: the functional backbone of terrestrial plant productivity. Plant Sci. 177, 245–251. doi: 10.1016/j.plantsci.2009.06.001
Brodribb, T. J., Holbrook, N. M., and Gutierrez, M. V. (2002). Hydraulic and photosynthetic co-ordination in seasonally dry tropical forest trees. Plant Cell Environ. 25, 1435–1444. doi: 10.1046/j.1365-3040.2002.00919.x
Brown, J. H., Gillooly, J. F., Allen, A. P., Savage, V. M., and West, G. B. (2004). Toward a metabolic theory of ecology. Ecology 85, 1771–1789. doi: 10.1890/03-9000
Bucci, S. J., Goldstein, G., Meinzer, F. C., Scholz, F. G., Franco, A. C., and Bustamante, M. (2004). Functional convergence in hydraulic architecture and water relations of tropical savanna trees: from leaf to whole plant. Tree Physiol. 24, 891–899. doi: 10.1093/treephys/24.8.891
Chave, J., Coomes, D., Jansen, S., Lewis, S. L., Swenson, N. G., and Zanne, A. E. (2009). Towards a worldwide wood economics spectrum. Ecol. Lett. 12, 351–366. doi: 10.1111/j.1461-0248.2009.01285.x
Chave, J., Réjou-Méchain, M., Búrquez, A., Chidumayo, E., Colgan, M. S., Delitti, W. B., et al. (2014). Improved allometric models to estimate the aboveground biomass of tropical trees. Glob. Chang. Biol. 20, 3177–3190. doi: 10.1111/gcb.12629
Choat, B., Cobb, A. R., and Jansen, S. (2008). Structure and function of bordered pits: new discoveries and impacts on whole-plant hydraulic function. New Phytol. 177, 608–626. doi: 10.1111/j.1469-8137.2007.02317.x
Coble, A. P., and Cavaleri, M. A. (2014). Light drives vertical gradients of leaf morphology in a sugar maple (Acer saccharum) forest. Tree Physiol. 34, 146–158, doi: 10.1093/treephys/tpt126
Coomes, D. A., Jenkins, K. L., and Cole, L. E. S. (2007). Scaling of tree vascular transport systems along gradients of nutrient supply and altitude. Biol. Lett. 3, 87–90. doi: 10.1098/rsbl.2006.0551
Drescher, J., Rembold, K., Allen, K., Beckschäfer, P., Buchori, D., Clough, Y., et al. (2016). Ecological and socio-economic functions across tropical land use systems after rainforest conversion. Philos. Trans. R. Soc. B Biol. Sci. 371:20150275. doi: 10.1098/rstb.2015.0275
Eller, C. B., Barros, F., de, V., Bittencourt, P. R. L., Rowland, L., Mencuccini, M., et al. (2018). Xylem hydraulic safety and construction costs determine tropical tree growth. Plant Cell Environ. 41, 548–562. doi: 10.1111/pce.13106
Enquist, B. J., West, G. B., Charnov, E. L., and Brown, J. H. (1999). Allometric scaling of production and life-history variation in vascular plants. Nature 401, 907–911. doi: 10.1038/44819
Fajardo, A., Martínez-Pérez, C., Cervantes-Alcayde, M. A., and Olson, M. E. (2020). Stem length, not climate, controls vessel diameter in two trees species across a sharp precipitation gradient. New Phytol. 225, 2347–2355. doi: 10.1111/nph.16287
Fan, Z.-X., Zhang, S.-B., Hao, G.-Y., Ferry Slik, J. W., and Cao, K.-F. (2012). Hydraulic conductivity traits predict growth rates and adult stature of 40 Asian tropical tree species better than wood density: Xylem traits and tree growth. J. Ecol. 100, 732–741. doi: 10.1111/j.1365-2745.2011.01939.x
Fisher, R. A., Koven, C. D., Anderegg, W. R., Christoffersen, B. O., Dietze, M. C., Farrior, C. E., et al. (2018). Vegetation demographics in earth system models: a review of progress and priorities. Glob. Chang. Biol. 24, 35–54. doi: 10.1111/gcb.13910
Flo, V., Martinez-Vilalta, J., Steppe, K., Schuldt, B., and Poyatos, R. (2019). A synthesis of bias and uncertainty in sap flow methods. Agric. Forest Meteorol. 271, 362–374. doi: 10.1016/j.agrformet.2019.03.012
Fortunel, C., Ruelle, J., Beauchêne, J., Fine, P. V. A., and Baraloto, C. (2014). Wood specific gravity and anatomy of branches and roots in 113 Amazonian rainforest tree species across environmental gradients. New Phytol. 202, 79–94. doi: 10.1111/nph.12632
Francis, E. J., Muller-Landau, H. C., Wright, S. J., Visser, M. D., Iida, Y., Fletcher, C., et al. (2017). Quantifying the role of wood density in explaining interspecific variation in growth of tropical trees. Glob. Ecol. Biogeogr. 26, 1078–1087. doi: 10.1111/geb.12604
Fuchs, S., Leuschner, C., Link, R., Coners, H., and Schuldt, B. (2017). Calibration and comparison of thermal dissipation, heat ratio and heat field deformation sap flow probes for diffuse-porous trees. Agric. Forest Meteorol. 244-245, 151–161. doi: 10.1016/j.agrformet.2017.04.003
Gleason, S. M., Stephens, A. E. A., Tozer, W. C., Blackman, C. J., Butler, D. W., Chang, Y., et al. (2018). Shoot growth of woody trees and shrubs is predicted by maximum plant height and associated traits. Funct. Ecol. 32, 247–259. doi: 10.1111/1365-2435.12972
Gleason, S. M., Westoby, M., Jansen, S., Choat, B., Hacke, U. G., Pratt, R. B., et al. (2016). Weak tradeoff between xylem safety and xylem-specific hydraulic efficiency across the world's woody plant species. New Phytol. 209, 123–136. doi: 10.1111/nph.13646
Grace, J. B., Schoolmaster, D. R., Guntenspergen, G. R., Little, A. M., Mitchell, B. R., Miller, K. M., et al. (2012). Guidelines for a graph-theoretic implementation of structural equation modeling. Ecosphere 3, 1–44. doi: 10.1890/ES12-00048.1
Granier, A. (1985). Une nouvelle méthode pour la mesure du flux de sève brute dans le tronc des arbres. Ann. Sci. Forest. 42, 193–200. doi: 10.1051/forest:19850204
Griffin-Nolan, R. J., Bushey, J. A., Carroll, C. J. W., Challis, A., Chieppa, J., Garbowski, M., et al. (2018). Trait selection and community weighting are key to understanding ecosystem responses to changing precipitation regimes. Funct. Ecol. 32, 1746–1756. doi: 10.1111/1365-2435.13135
Grossiord, C., Christoffersen, B., Alonso-Rodríguez, A. M., Anderson-Teixeira, K., Asbjornsen, H., Aparecido, L. M. T., et al. (2019). Precipitation mediates sap flux sensitivity to evaporative demand in the neotropics. Oecologia. 191, 519–530. doi: 10.1007/s00442-019-04513-x
Hajek, P., Leuschner, C., Hertel, D., Delzon, S., and Schuldt, B. (2014). Trade-offs between xylem hydraulic properties, wood anatomy and yield in populus. Tree Physiol. 34, 744–756. doi: 10.1093/treephys/tpu048
Hertel, D., Moser, G., Culmsee, H., Erasmi, S., Horna, V., Schuldt, B., and Leuschner, C.H. (2009). Below- and above-ground biomass and net primary production in a paleotropical natural forest (Sulawesi, Indonesia) as compared to neotropical forests. For. Ecol. Manage. 258, 1904–1912. doi: 10.1016/j.foreco.2009.07.019
Hietz, P., Rosner, S., Hietz-Seifert, U., and Wright, S. J. (2016). Wood traits related to size and life history of trees in a Panamanian rainforest. New Phytol. 213, 170–180. doi: 10.1111/nph.14123
Hoeber, S., Leuschner, C., Köhler, L., Arias-Aguilar, D., and Schuldt, B. (2014). The importance of hydraulic conductivity and wood density to growth performance in eight tree species from a tropical semi-dry climate. For. Ecol. Manage. 330, 126–136. doi: 10.1016/j.foreco.2014.06.039
Horna, V., Schuldt, B., Brix, S., and Leuschner, C. (2011). Environment and tree size controlling stem sap flux in a perhumid tropical forest of Central Sulawesi, Indonesia. Ann. For. Sci. 68, 1027–1038. doi: 10.1007/s13595-011-0110-2
James, S. A., Meinzer, F. C., Goldstein, G., Woodruff, D., Jones, T., Restom, T., et al. (2003). Axial and radial water transport and internal water storage in tropical forest canopy trees. Oecologia 134, 37–45. doi: 10.1007/s00442-002-1080-8
Jansen, S., Choat, B., and Pletsers, A. (2009). Morphological variation of intervessel pit membranes and implications to xylem function in angiosperms. Am. J. Bot. 96, 409–419. doi: 10.3732/ajb.0800248
King, D. A., Davies, S. J., Supardi, M. N. N., and Tan, S. (2005). Tree growth is related to light interception and wood density in two mixed dipterocarp forests of Malaysia. Funct. Ecol. 19, 445–453. doi: 10.1111/j.1365-2435.2005.00982.x
King, D. A., Davies, S. J., Tan, S., Noor, N. S., and Md. (2006). The role of wood density and stem support costs in the growth and mortality of tropical trees. J. Ecol. 94, 670–680. doi: 10.1111/j.1365-2745.2006.01112.x
Koch, G. W., Sillett, S. C., Jennings, G. M., and Davis, S. D. (2004). The limits to tree height. Nature 428, 851–854. doi: 10.1038/nature02417
Kotowska, M. M., Hertel, D., Rajab, Y. A., Barus, H., and Schuldt, B. (2015). Patterns in hydraulic architecture from roots to branches in six tropical tree species from cacao agroforestry and their relation to wood density and stem growth. Front. Plant Sci. 6:191. doi: 10.3389/fpls.2015.00191
Kunert, N., Aparecido, L. M. T., Wolff, S., Higuchi, N., Santos, J., dos Araujo, A. C., et al. (2017). A revised hydrological model for the Central Amazon: the importance of emergent canopy trees in the forest water budget. Agric. Forest Meteorol. 239, 47–57. doi: 10.1016/j.agrformet.2017.03.002
Lechthaler, S., Turnbull, T. L., Gelmini, Y., Pirotti, F., Anfodillo, T., Adams, M. A., et al. (2019). A standardization method to disentangle environmental information from axial trends of xylem anatomical traits. Tree Physiol. 39, 495–502. doi: 10.1093/treephys/tpy110
Li, S., Lens, F., Espino, S., Karimi, Z., Klepsch, M., Schenk, H. J., et al. (2016). Intervessel pit membrane thickness as a key determinant of embolism resistance in angiosperm xylem. IAWA J. 37, 152–171. doi: 10.1163/22941932-20160128
Link, R. M. (2020). The Role of Tree Height and Wood Density for the Water Use, Productivity and Hydraulic Architecture of Tropical Trees. (PhD Thesis). Georg-August-University Göttingen. Göttingen, Germany.
Link, R. M., Fuchs, S., Arias Aguilar, D., Leuschner, C., Castillo Ugalde, M., Valverde Otarola, J. C., et al. (2020). Tree height predicts the shape of radial sap flow profiles of Costa-Rican tropical dry forest tree species. Agric. Forest Meteorol. 287:107913. doi: 10.1016/j.agrformet.2020.107913
Liu, H., Gleason, S. M., Hao, G., Hua, L., He, P., Goldstein, G., et al. (2019). Hydraulic traits are coordinated with maximum plant height at the global scale. Sci. Adv. 5:eaav1332. doi: 10.1126/sciadv.aav1332
Lutz, J. A., Furniss, T. J., Johnson, D. J., Davies, S. J., Allen, D., Alonso, A., et al. (2018). Global importance of large-diameter trees. Glob. Ecol. Biogeogr. 27, 849–864. doi: 10.1111/geb.12747
Maherali, H., Pockman, W. T., and Jackson, R. B. (2004). Adaptive variation in the vulnerability of woody plants to xylem cavitation. Ecology 85, 2184–2199. doi: 10.1890/02-0538
Maréchaux, I., Bartlett, M. K., Sack, L., Baraloto, C., Engel, J., Joetzjer, E., et al. (2015). Drought tolerance as predicted by leaf water potential at turgor loss point varies strongly across species within an Amazonian forest. Funct. Ecol. 29, 1268–1277. doi: 10.1111/1365-2435.12452
McCulloh, K., Sperry, J. S., Lachenbruch, B., Meinzer, F. C., Reich, P. B., and Voelker, S. (2010). Moving water well: comparing hydraulic efficiency in twigs and trunks of coniferous, ring-porous, and diffuse-porous saplings from temperate and tropical forests. New Phytol. 186, 439–450. doi: 10.1111/j.1469-8137.2010.03181.x
McCulloh, K. A., and Sperry, J. S. (2005). Patterns in hydraulic architecture and their implications for transport efficiency. Tree Physiol. 25, 257–267. doi: 10.1093/treephys/25.3.257
McDowell, N. G., Brodribb, T. J., and Nardini, A. (2019). Hydraulics in the 21 st century. New Phytol. 224, 537–542. doi: 10.1111/nph.16151
Medeiros, J. S., Lens, F., Maherali, H., and Jansen, S. (2019). Vestured pits and scalariform perforation plate morphology modify the relationships between angiosperm vessel diameter, climate and maximum plant height. New Phytol. 221, 1802–1813. doi: 10.1111/nph.15536
Meinzer, F. C., Bond, B. J., Warren, J. M., and Woodruff, D. R. (2005). Does water transport scale universally with tree size? Funct. Ecol. 19, 558–565. doi: 10.1111/j.1365-2435.2005.01017.x
Meinzer, F. C., Goldstein, G., and Andrade, J. L. (2001). Regulation of water flux through tropical forest canopy trees: do universal rules apply? Tree Physiol. 21, 19–26. doi: 10.1093/treephys/21.1.19
Meinzer, F. C., James, S. A., Goldstein, G., and Woodruff, D. (2003). Whole-tree water transport scales with sapwood capacitance in tropical forest canopy trees. Plant Cell Environ. 26, 1147–1155. doi: 10.1046/j.1365-3040.2003.01039.x
Mencuccini, M., Manzoni, S., and Christoffersen, B. (2019). Modelling water fluxes in plants: from tissues to biosphere. New Phytol. 222, 1207–1222. doi: 10.1111/nph.15681
Mencuccini, M., Minunno, F., Salmon, Y., Martínez-Vilalta, J., and Höltt,ä, T. (2015). Coordination of physiological traits involved in drought-induced mortality of woody plants. New Phytol. 208, 396–409. doi: 10.1111/nph.13461
Moles, A. T., Warton, D. I., Warman, L., Swenson, N. G., Laffan, S. W., Zanne, A. E., et al. (2009). Global patterns in plant height. J. Ecol. 97, 923–932. doi: 10.1111/j.1365-2745.2009.01526.x
Moser, G., Schuldt, B., Hertel, D., Horna, V., Coners, H., Barus, H., et al. (2014). Replicated throughfall exclusion experiment in an Indonesian perhumid rainforest: wood production, litter fall and fine root growth under simulated drought. Glob. Chang. Biol. 20, 1481–1497. doi: 10.1111/gcb.12424
Muller-Landau, H. C. (2004). Interspecific and inter-site variation in wood specific gravity of tropical trees. Biotropica. 36, 20–32. doi: 10.1646/02119
Olson, M. E., Soriano, D., Rosell, J. A., Anfodillo, T., Donoghue, M. J., Edwards, E. J., et al. (2018). Plant height and hydraulic vulnerability to drought and cold. Proc. Natl. Acad. Sci. U. S. A. 115, 7551–7556. doi: 10.1073/pnas.1721728115
Pérez-Harguindeguy, N., Díaz, S., Garnier, E., Lavorel, S., Poorter, H., Jaureguiberry, P., et al. (2013). New handbook for standardised measurement of plant functional traits worldwide. Aust. J. Bot. 61, 167–234. doi: 10.1071/BT12225
Poorter, L., and Bongers, F. (2006). Leaf traits are good predictors of plant performance across 53 rain forest species. Ecology 87, 1733–1743. doi: 10.1890/0012-9658(2006)87[1733:LTAGPO]2.0.CO;2
Poorter, L., Castilho, C. V., Schietti, J., Oliveira, R. S., and Costa, F. R. C. (2018). Can traits predict individual growth performance? a test in a hyperdiverse tropical forest. New Phytol. 219, 109–121. doi: 10.1111/nph.15206
Poorter, L., McDonald, I., Alarcón, A., Fichtler, E., Licona, J.-C., Peña-Claros, M., et al. (2010). The importance of wood traits and hydraulic conductance for the performance and life history strategies of 42 rainforest tree species. New Phytol. 185, 481–492. doi: 10.1111/j.1469-8137.2009.03092.x
Powell, T. L., Galbraith, D. R., Christoffersen, B. O., Harper, A., Imbuzeiro, H. M. A., Rowland, L., et al. (2013). Confronting model predictions of carbon fluxes with measurements of Amazon forests subjected to experimental drought. New Phytol. 200, 350–365. doi: 10.1111/nph.12390
Powell, T. L., Wheeler, J. K., Oliveira, A. A. R., de Costa, A. C. L., da Saleska, S. R., Meir, P., et al. (2017). Differences in xylem and leaf hydraulic traits explain differences in drought tolerance among mature Amazon rainforest trees. Glob. Chang. Biol. 23, 4280–4293. doi: 10.1111/gcb.13731
R Core Team (2019). R: A Language and Environment for Statistical Computing. Available online at: https://www.R-project.org/ (accessed October 29, 2019).
Réjou-Méchain, M., Tanguy, A., Piponiot, C., Chave, J., and Hérault, B. (2017). BIOMASS : an R package for estimating above-ground biomass and its uncertainty in tropical forests. Methods Ecol. Evol. 8, 1163–1167. doi: 10.1111/2041-210X.12753
Röll, A., Niu, F., Meijide, A., Ahongshangbam, J., Ehbrecht, M., Guillaume, T., et al. (2019). Transpiration on the rebound in lowland Sumatra. Agric. Forest Meteorol. 274, 160–171. doi: 10.1016/j.agrformet.2019.04.017
Rosell, J. A., Olson, M. E., and Anfodillo, T. (2017). Scaling of xylem vessel diameter with plant size: causes, predictions, and outstanding questions. Curr. Forest. Rep. 3, 46–59. doi: 10.1007/s40725-017-0049-0
Rosseel, Y. (2012). lavaan : an r package for structural equation modeling. J. Stat. Softw. 48:110515. doi: 10.18637/jss.v048.i02
Rowland, L., Lobo-do-Vale, R. L., Christoffersen, B. O., Melém, E. A., Kruijt, B., Vasconcelos, S. S., et al. (2015). After more than a decade of soil moisture deficit, tropical rainforest trees maintain photosynthetic capacity, despite increased leaf respiration. Glob. Chang. Biol. 21, 4662–4672. doi: 10.1111/gcb.13035
Rüger, N., Berger, U., Hubbell, S. P., Vieilledent, G., and Condit, R. (2011). Growth strategies of tropical tree species: disentangling light and size effects. PLoS ONE 6:e25330. doi: 10.1371/journal.pone.0025330
Rüger, N., Wirth, C., Wright, S. J., and Condit, R. (2012). Functional traits explain light and size response of growth rates in tropical tree species. Ecology 93, 2626–2636. doi: 10.1890/12-0622.1
Russo, S. E., Jenkins, K. L., Wiser, S. K., Uriarte, M., Duncan, R. P., and Coomes, D. A. (2010). Interspecific relationships among growth, mortality and xylem traits of woody species from New Zealand: tree growth, mortality and woody traits. Funct. Ecol. 24, 253–262. doi: 10.1111/j.1365-2435.2009.01670.x
Sanchez-Martinez, P., Martínez-Vilalta, J., Dexter, K. G., Segovia, R. A., and Mencuccini, M. (2020). Adaptation and coordinated evolution of plant hydraulic traits. Ecol. Lett. 23, 1599–1610. doi: 10.1111/ele.13584
Santiago, L. S., Goldstein, G., Meinzer, F. C., Fisher, J. B., Machado, K., Woodruff, D., et al. (2004). Leaf photosynthetic traits scale with hydraulic conductivity and wood density in Panamanian forest canopy trees. Oecologia 140, 543–550. doi: 10.1007/s00442-004-1624-1
Schneider, C. A., Rasband, W. S., and Eliceiri, K. W. (2012). NIH Image to ImageJ: 25 years of image analysis. Nat. Methods 9, 671–675. doi: 10.1038/nmeth.2089
Scholz, F. G., Phillips, N. G., Bucci, S. J., Meinzer, F. C., and Goldstein, G. (2011). “Hydraulic capacitance: biophysics and functional significance of internal water sources in relation to tree size,” in Size- and Age-Related Changes in Tree Structure and Function, eds F. C. Meinzer, B. Lachenbruch, and T. D. Dawson (Dordrecht: Springer).
Schuldt, B., Leuschner, C., Brock, N., and Horna, V. (2013). Changes in wood density, wood anatomy and hydraulic properties of the xylem along the root-to-shoot flow path in tropical rainforest trees. Tree Physiol. 33, 161–174. doi: 10.1093/treephys/tps122
Schuldt, B., Leuschner, C., Horna, V., Moser, G., Köhler, M., van Straaten, O., et al. (2011). Change in hydraulic properties and leaf traits in a tall rainforest tree species subjected to long-term throughfall exclusion in the perhumid tropics. Biogeosciences 8, 2179–2194. doi: 10.5194/bg-8-2179-2011
Schüller, E., Martínez-Ramos, M., and Hietz, P. (2013). Radial gradients in wood specific gravity, water and gas content in trees of a mexican tropical rain forest. Biotropica 45, 280–287. doi: 10.1111/btp.12016
Šímová, I., Sandel, B., Enquist, B. J., Michaletz, S. T., Kattge, J., Violle, C., et al. (2019). The relationship of woody plant size and leaf nutrient content to large-scale productivity for forests across the Americas. J. Ecol. 107, 2278–2290. doi: 10.1111/1365-2745.13163
Slik, J. W. F., Paoli, G., McGuire, K., Amaral, I., Barroso, J., Bastian, M., et al. (2013). Large trees drive forest aboveground biomass variation in moist lowland forests across the tropics: large trees and tropical forest biomass. Glob. Ecol. Biogeogr. 22, 1261–1271. doi: 10.1111/geb.12092
Smith, D. D., and Sperry, J. S. (2014). Coordination between water transport capacity, biomass growth, metabolic scaling and species stature in co-occurring shrub and tree species. Plant Cell Environ. 37, 2679–2690. doi: 10.1111/pce.12408
Sperry, J. S. (2003). Evolution of water transport and xylem structure. Int. J. Plant Sci. 164, S115–S127. doi: 10.1086/368398
Sperry, J. S., Nichols, K. L., Sullivan, J. E. M., and Eastlack, S. E. (1994). Xylem embolism in ring-porous, diffuse-porous, and coniferous trees of northern utah and interior alaska. Ecology 75, 1736–1752. doi: 10.2307/1939633
Stephenson, N. L., Das, A. J., Condit, R., Russo, S. E., Baker, P. J., Beckman, N. G., et al. (2014). Rate of tree carbon accumulation increases continuously with tree size. Nature. 507, 90–93. doi: 10.1038/nature12914
Steppe, K., De Pauw, D. J. W., Doody, T. M., and Teskey, R. O. (2010). A comparison of sap flux density using thermal dissipation, heat pulse velocity and heat field deformation methods. Agric. Forest Meteorol. 150, 1046–1056. doi: 10.1016/j.agrformet.2010.04.004
Tyree, M. T. (2003). Hydraulic limits on tree performance: transpiration, carbon gain and growth of trees. Trees 17, 95–100. doi: 10.1007/s00468-002-0227-x
Tyree, M. T., Davis, S. D., and Cochard, H. (1994). Biophysical perspectives of xylem evolution: is there a tradeoff of hydraulic efficiency for vulnerability to dysfunction? IAWA J. 15, 335–360. doi: 10.1163/22941932-90001369
Warton, D. I., Duursma, R. A., Falster, D. S., and Taskinen, S. (2012). smatr 3– an R package for estimation and inference about allometric lines. Methods Ecol. Evol. 3, 257–259. doi: 10.1111/j.2041-210X.2011.00153.x
West, G. B. (1999). The fourth dimension of life: fractal geometry and allometric scaling of organisms. Science 284, 1677–1679. doi: 10.1126/science.284.5420.1677
Wheeler, J. K., Sperry, J. S., Hacke, U. G., and Hoang, N. (2005). Inter-vessel pitting and cavitation in woody Rosaceae and other vesselled plants: a basis for a safety versus efficiency trade-off in xylem transport. Plant Cell Environ. 28, 800–812. doi: 10.1111/j.1365-3040.2005.01330.x
Wickham, H., Averick, M., Bryan, J., Chang, W., McGowan, L. D., François, R., et al. (2019). Welcome to the tidyverse. J. Open Source Softw. 4:1686. doi: 10.21105/joss.01686
Wullschleger, S. D., Meinzer, F. C., and Vertessy, R. A. (1998). A review of whole-plant water use studies in trees. Tree Physiol. 18, 499–512. doi: 10.1093/treephys/18.8-9.499
Xie, J., and Wan, X. (2018). The accuracy of the thermal dissipation technique for estimating sap flow is affected by the radial distribution of conduit diameter and density. Acta Physiol. Plant. 40:88. doi: 10.1007/s11738-018-2659-y
Xu, X., Medvigy, D., Powers, J. S., Becknell, J. M., and Guan, K. (2016). Diversity in plant hydraulic traits explains seasonal and inter-annual variations of vegetation dynamics in seasonally dry tropical forests. New Phytol. 212, 80–95. doi: 10.1111/nph.14009
Zach, A., Schuldt, B., Brix, S., Horna, V., Culmsee, H., and Leuschner, C. (2010). Vessel diameter and xylem hydraulic conductivity increase with tree height in tropical rainforest trees in Sulawesi, Indonesia. Flora 205, 506–512. doi: 10.1016/j.flora.2009.12.008
Zanne, A. E., Westoby, M., Falster, D. S., Ackerly, D. D., Loarie, S. R., Arnold, S. E. J., et al. (2010). Angiosperm wood structure: global patterns in vessel anatomy and their relation to wood density and potential conductivity. Am. J. Bot. 97, 207–215. doi: 10.3732/ajb.0900178
Ziemińska, K., Butler, D. W., Gleason, S. M., Wright, I. J., and Westoby, M. (2013). Fibre wall and lumen fractions drive wood density variation across 24 Australian angiosperms. AoB Plants 5:plt046. doi: 10.1093/aobpla/plt046
Keywords: functional traits, growth rate, hydraulic efficiency, net primary production, sap flux density, structural equation modeling, wood anatomy
Citation: Kotowska MM, Link RM, Röll A, Hertel D, Hölscher D, Waite P-A, Moser G, Tjoa A, Leuschner C and Schuldt B (2021) Effects of Wood Hydraulic Properties on Water Use and Productivity of Tropical Rainforest Trees. Front. For. Glob. Change 3:598759. doi: 10.3389/ffgc.2020.598759
Received: 25 August 2020; Accepted: 14 December 2020;
Published: 26 January 2021.
Edited by:
Nikolaos M. Fyllas, University of the Aegean, GreeceReviewed by:
Simone Aparecida Vieira, Campinas State University, BrazilBradley Christoffersen, The University of Texas Rio Grande Valley, United States
Copyright © 2021 Kotowska, Link, Röll, Hertel, Hölscher, Waite, Moser, Tjoa, Leuschner and Schuldt. This is an open-access article distributed under the terms of the Creative Commons Attribution License (CC BY). The use, distribution or reproduction in other forums is permitted, provided the original author(s) and the copyright owner(s) are credited and that the original publication in this journal is cited, in accordance with accepted academic practice. No use, distribution or reproduction is permitted which does not comply with these terms.
*Correspondence: Martyna M. Kotowska, bWFydHluYS5rb3Rvd3NrYUBiaW9sb2dpZS51bmktZ29ldHRpbmdlbi5kZQ==; Bernhard Schuldt, YmVybmhhcmQuc2NodWxkdEBwbGFudC1lY29sb2d5LmRl
†These authors have contributed equally to this work