- 1Department of Environmental Systems Science, Institute of Terrestrial Ecosystems, Ecosystem Management, ETH Zürich, Zurich, Switzerland
- 2Center for International Forestry Research (CIFOR), Lima, Peru
- 3Department of Environmental Systems Science, Institute of Integrative Biology, Plant Ecological Genetics, ETH Zürich, Zurich, Switzerland
- 4International Institute of Social Studies (ISS), Erasmus University Rotterdam, The Hague, Netherlands
- 5Facultad de Ingeniería Forestal y Medio Ambiente, Universidad Nacional Amazónica de Madre de Dios (UNAMAD), Puerto Maldonado, Madre de Dios, Peru
- 6Instituto de Investigaciones de la Amazonia Peruana, Puerto Maldonado, Peru
- 7Bioversity International, Rome, Italy
Brazil nuts are an economically important non-timber forest product throughout the Amazon Basin, but the forests in which they grow are under threat of severe degradation by logging, road building, agricultural expansion, and forest fires. As a result, many Brazil nut trees grow within a mosaic of young secondary forest, primary forest remnants and agricultural fields. Little is known about the reproductive ecology and fruit production of Brazil nut in such degraded landscapes. Previous studies on Brazil nut productivity did not explicitly address forest degradation as a factor. In this study, we analyzed the extent to which Brazil nut fruit production is affected by the level of forest degradation. We collected 3 years of fruit production data of 126 Brazil nut trees occurring in degraded forest (the above-mentioned mosaics) and closed canopy (i.e., undegraded) forest in and around the Tambopata National Reserve in Madre de Dios, Peru. We analyzed the effect of forest degradation at two different levels: at the site type (i.e., degraded vs. undegraded forest) and the individual tree level (quantified as stand basal area and stem density around the individual Brazil nut trees). Stand basal area around the individual Brazil nut trees significantly positively influenced tree fruit production in all 3 years and stem density in year 2 and 3, with strongest effects in the 3rd year, and weakest effect in the 1st year, coinciding with an El Niño year. Trees in undegraded forest produced more fruits in the 2nd and 3rd year than trees in degraded forest (29.4% and 35.8% more, respectively), but not in the 1st year in which trees in undegraded forest produced 31.7% less fruits than trees in degraded forest. These within year effects were not significant, although the effects significantly differed between years. Our results show that forest degradation can affect Brazil nut fruit production, and suggest that the strength (and possibly the sign) of this effect might be different in (extreme) El Niño years. This illustrates the potential importance of restoring degraded forest to enhance resilience and protect the livelihoods of people depending on the Brazil nut trade.
Introduction
Non-timber forest products (NTFPs) provide livelihoods, food, medicine, income, and building materials to millions of people worldwide (Shackleton et al., 2011). At the global level, one of the economically most important NTFPs, almost exclusively harvested from wild populations, is the Brazil nut (the seeds of the canopy emergent Amazonian tree Bertholletia excelsa). Brazil nuts are harvested throughout the Amazon basin, largely in Bolivia, Brazil, and Peru (Guariguata et al., 2017). Across these three countries, forested landscapes, in which the Brazil nut trees grow, are rapidly degrading due to unplanned logging, illegal mining, trans-boundary road building, agricultural expansion, and forest fires (Foley et al., 2007; Davidson et al., 2012; Brando et al., 2014). Due to these processes, the landscape takes on a mosaic-type pattern, with patches of recently cleared areas, overgrown agricultural fields, secondary forest, and primary forest remnants in which large stems have been removed (Broadbent et al., 2008; Sun and Southworth, 2013). Within these mosaics, Brazil nut trees often remain in relative isolation, and are usually one of the few remaining large stems because they are legally protected from felling (Duchelle, 2009).
Although previous studies have shown that tropical forest degradation, fragmentation, and tree isolation from conspecifics can negatively affect tree fruit production (e.g., Ghazoul et al., 1998; Guariguata and Pinard, 1998; Ghazoul and McLeish, 2001; Lowe et al., 2005), the extent to which it affects reproductive success in Brazil nut trees remains largely unstudied. Previous studies on individual fruit production in Brazil nut trees across the Amazon Basin (Wadt et al., 2005; Kainer et al., 2007, 2014; Staudhammer et al., 2013; Thomas et al., 2017) have been conducted in closed-canopy forest, not explicitly addressing forest degradation as a contributing factor. Brazil nut trees are monoecious, self-incompatible, and insect pollinated (primarily by large bees; Maués, 2002, Cavalcante et al., 2012) thus making them vulnerable to reduced fruit set if forest degradation reduces pollen transfer and quality (Rocha and Aguilar, 2001; Wadt et al., 2015).
Here, we compare tree level estimates of fruit production of Brazil nut trees across undegraded (i.e., closed canopy) and severely degraded forest (partly cleared for agriculture) over 3 consecutive years in Madre de Dios, Peruvian Amazon. Knowledge on the effect of forest degradation on Brazil nut production may be important in order to gauge future Brazil nut availability across the landscape as this may have direct consequences for local livelihoods.
Materials and Methods
Study Species
B. excelsa naturally occurs throughout the Amazon Basin with highest adult densities in Brazil, Bolivia and Peru (Mori and Prance, 1990; Thomas et al., 2015; Levis et al., 2017). It can grow up to a height of 60 m and more than 3 m in diameter at breast height (DBH) and crown diameters of up to 40–60 m (Zuidema and Boot, 2002; Scoles and Gribel, 2011; Rockwell et al., 2015; Guariguata et al., 2017). It is an obligate outcrosser (Cavalcante et al., 2012). Although not confirmed yet for natural forest, several studies performed in Brazil nut plantations have shown that B. excelsa is primarily pollinated by several large bee species, including pollinators of the genera Xylocopa, Bombus, Epicharis and Eulaema (Maués, 2002; Cavalcante et al., 2012). Fruit maturation usually takes between 14 and 15 months (Maués, 2002). Fruit production of individual trees is influenced by DBH, crown diameter, crown form, liana load and crown illumination (Zuidema, 2003; Wadt et al., 2005; Kainer et al., 2007; Tonini et al., 2008; Rockwell et al., 2015). Individual fruits have a hard shell which contains 10 to 25 seeds (Peres et al., 2003). In closed canopy forest, probability of reproduction increases strongly once 40 cm DBH has been reached (Zuidema and Boot, 2002; Rockwell et al., 2015).
Tens of thousands of indigenous and local communities are involved in harvesting and commercialization of Brazil nuts thus playing an important role in forest conservation (Ortiz, 2002; Guariguata et al., 2017). Brazil nuts are one of the few Amazonian non-timber forest products with an important export market (Guariguata et al., 2017), which has been mentioned as one of the requisites for being a viable strategy for conservation and poverty reduction (Ros-Tonen and Wiersum, 2005). Once on the ground, fruits are opened in situ with a machete to extract the seeds, and empty fruit shells are generally piled up under the mother tree (Zuidema, 2003).
Study Region
We conducted the study between January 2017 and March 2019 within and around the Jorge Chavez area of the Tambopata National Reserve (about 2,747 km2) in the Department of Madre de Dios, Peru. Madre de Dios is characterized by lowland evergreen rainforest, and contains about 1.2–2.6 million ha of Brazil nut rich forest (Chávez et al., 2012). Annual rainfall in this area ranges between 2,500 and 3,500 mm with a distinctive rainy season from December to March (Rockwell et al., 2015). In Madre de Dios, Brazil nut tree density varies between 0.5 and 1.5 adult (40 cm DBH or greater) tree per hectare (Rockwell et al., 2015). Brazil nut trees flower between November and February and ripe fruits fall between December and March (Ortiz, 2002; Rockwell et al., 2015). The harvesting of Brazil nuts in Peru represented an estimated export value of 66 million USD in 2018 (ADEX, 2019). Since 2000, nut harvesting within the National Reserve Tambopata is regulated through government-sanctioned forest concessions in which timber extraction is prohibited (Willem et al., 2019).
Study Design
General Setup
Our study was designed to analyze the effects of forest degradation at both the site and individual tree level. We compare individual tree fruit production between two categories of degradation: undegraded forest (i.e., closed canopy) and degraded forest (i.e., partly cleared for agriculture) using four replicates for each (see section Site selection for details). Because heterogeneity in degradation occurs across and within sites, we also compared individual tree fruit production to level of forest degradation (in terms of SBA and stem density) in a 50 m radius around individual Brazil nut trees within all sites. This also allowed us to test for non-linearity in the relation between fruit production and forest degradation.
Site Selection
In January 2017, we selected four degraded forest sites in the study region (with site size varying between 3.4 and 29.1 ha, Figures 1, 2). These had partly been deforested between 8 and 20 years before the start of the study for conversion to pastureland and small-scale agriculture. Four paired, adjacent closed canopy forest sites (hereafter defined as undegraded) were also selected (Figure 1). The undegraded sites varied between 22.2 and 40.8 ha in size. Each pair was considered an “area” (area A–D). We chose to keep the distance between degraded and undegraded forest sites relatively short (i.e., in between 0.2 and 5.8 km) in all cases (Figure 1), to minimize biophysical variation. The degraded vs. undegraded forest sites differed significantly in stand basal area and stem density (see the Results section).
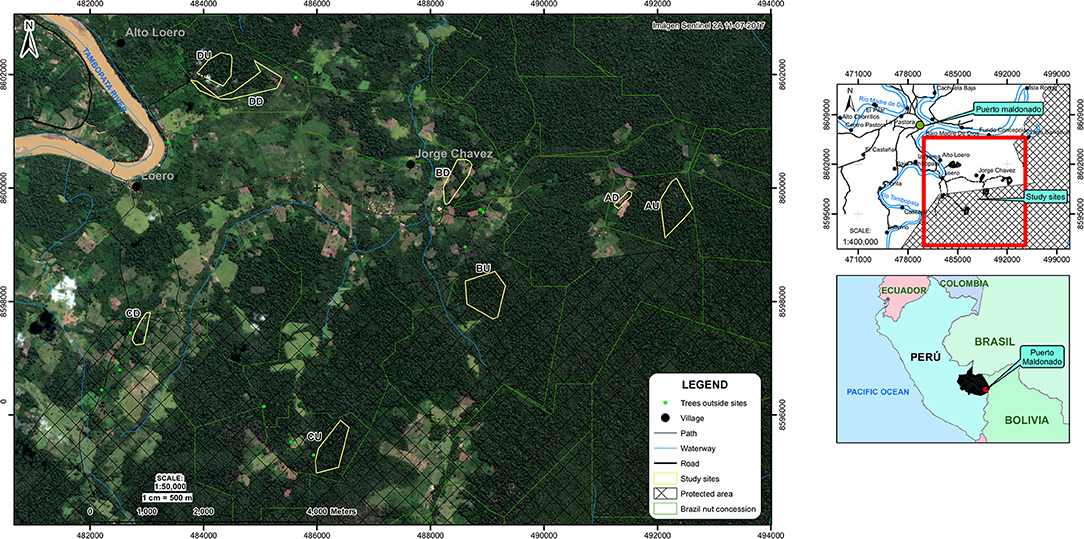
Figure 1. Location research sites. The sites ending in “U” or “D” are the undegraded and degraded forest sites respectively, located in areas A–D. Sources: Esri, INEI, MTC, IGN, SERNANP, DSFLPR-MDD, GERFOR-MDD, SENTINEL 2A.
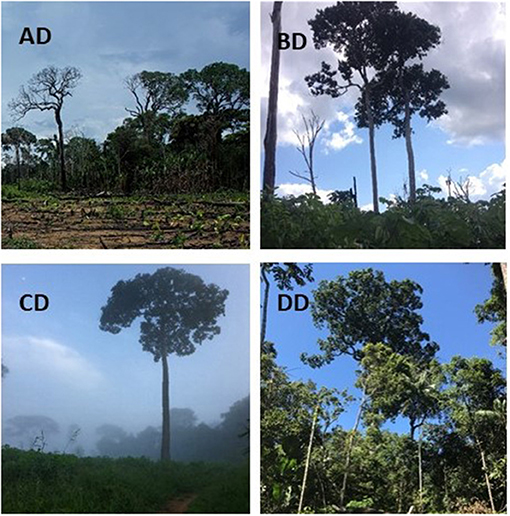
Figure 2. Photos of the four degraded forest sites (AD, BD, CD, and DD). Note that heterogeneity within sites was large (see Figure 3), and photos are therefore not representative of the entire site. Photos by Gabriela Wiederkehr Guerra.
In all degraded sites, Brazil nut trees were relatively isolated, immersed in a mosaic of young secondary forest, primary forest remnants, and agricultural fields (Figures 1, 2). Three of the four sites were in active agricultural use at the time of the study (manioc, upland rice, and maize), with annual burnings. Only one site had been entirely abandoned half a year before the start of our study, and was previously in use for manioc, rice, and cattle. Sites were selected based on willingness of concessionaires and owners to allow access and were considered representative for the majority of sites in the wider area with an agricultural history. Time since deforestation in the degraded sites was assessed by interviewing landowners and concessionaires. We did not detect any logging stumps in our undegraded forest sites.
Tree Selection
Within all degraded sites, all Brazil nut trees with DBH > 40 cm were mapped and tagged (number of trees varied between 10 and 17). An equal number of trees were randomly selected in each paired, undegraded forest site, in which a minimum distance to forest edge of 100 m was maintained to minimize edge effects. The R base function “sample” (R Development Core Team, 2014) was used to randomly select these trees from the total number of trees present in the undegraded forest sites from lists of coordinates of all Brazil nut trees within the concessions. A few trees in site AU (Figure 1) had high liana loads (i.e., lianas covering part of the crown), which was attributed to temporary abandonment of the concession due to change of ownership, according to the current concession owner. We chose to exclude these trees if they were randomly selected and instead selected the closest adjacent tree without liana loads, because liana loads are known to negatively influence fruit production (Rockwell et al., 2015). Brazil nut trees with conspecific neighbors within a 30 m range were also excluded/not selected to avoid overlapping crowns which would make fruit counting difficult at the individual level (which was the case for two trees in the degraded sites). In November 2017, 16 more trees were selected from land with similar characteristics because additional trees were required for another study (3 trees were selected within the undegraded sites and 10 and 3 trees just outside the degraded and undegraded sites, respectively, Figure 1). In total, we selected 126 trees > 40 cm DBH of which 117 were reproductive (i.e., fruits were found under the tree in at least 1 of the 3 years of our study).
Data Collection
Forest Structure
To characterize vegetation structure, we established, at the center of each of the 126 Brazil nut trees within our sites, north–south and east–west transects of 5 m × 50 m. All live and standing dead trees within these transects of DBH > 10 cm were tallied. From this data, stand basal area (SBA), stem density, deadwood SBA (DSBA) and deadwood stem density (DSD) around each Brazil nut tree were estimated by dividing total SBA, stem density, DSBA and DSD by the total area of the plot. Only alive stems were included in the SBA and stem density calculations. Standing deadwood was recorded because deadwood provides nesting sites for one of the main pollinators of the Brazil nut tree, Xylocopa frontalis (Freitas and Oliveira-Filho, 2003).
Brazil Nut Tree Characteristics
For all 126 Brazil nut trees DBH, crown diameter, crown illumination index and tree damage were determined. DBH was measured at 1.15 m above the ground with measuring tape. Crown diameter was measured from north to south and from east to west and then averaged. An inclinometer was used to determine the exact positions of the edge of the crowns. Crown illumination index was quantified following methods as in Keeling and Phillips (2007). Any trees with visible fire damage, missing branches and/or incomplete crowns were considered as “damaged”.
Brazil Nut Tree Fruit Production
Fruit production per tree was quantified in March 2017, 2018, and 2019 by counting the number of empty fruits under each sampled tree after being piled up by Brazil nut collectors (only counting the lower halves of the fruits). In addition, any mature fruits remaining in the crown (bound to eventually fall the same year) were counted by using binoculars, and unopened fruits were searched for under the crown of the tree. For the trees that were added to the study in November 2017, fruit production of the two first census years was determined at the same moment (i.e., March 2018), by counting both the fruits that were opened or unharvested in the current and the previous year (fruits from different years can easily be distinguished due to decay of the outer shell of the fruits over time). In cases in which piles of empty fruits consisted of fruits from multiple trees, if there was any doubt about the origin of the pile of empty fruits (i.e., which mother tree), or if some fruits were known to be stolen (after consulting with collectors), the corresponding tree was excluded from further analysis (12, 6, and 12 trees in total in census years 1, 2, and 3, respectively).
Statistical Analysis
Site Type Differences in Forest Structure and Brazil Nut Tree Characteristics
We first tested for differences between undegraded and degraded forest in terms of forest structure (i.e., SBA, stem density, DSBA, and DSD) and Brazil nut tree characteristics (i.e., tree damage, crown illumination index, DBH, and crown diameter). For this, we used mixed effect regression analysis with site included as random effect. We fitted a model for each of the tree characteristics and forest structure variables. The model for tree damage was fitted using a binomial distribution, that for crown illumination index using ordinal logistic regression. Other models were fitted using a normal distribution and REML estimation. Significance of degradation level was determined using ANOVA comparison between the model with and without the site type term (for which models in analyses with normal distribution were re-fitted using Maximum Likelihood estimation).
Effect of Forest Degradation on Brazil Nut Fruit Production
We then analyzed the effect of forest degradation on individual tree fruit production. As explained above, we used measures at two different levels of forest degradation, i.e., forest degradation at the site level (i.e., degraded vs. undegraded forest) and forest degradation around the individual Brazil nut trees (i.e., SBA and stem density around the individual trees). Because SBA and stem density significantly differed between degraded and undegraded sites (see Results section), we performed separate regression analyses for these variables.
Analyses were performed using generalized mixed effect all subset Poisson regression analysis. Model construction and optimal model selection were performed following the steps described in Zuur et al. (2009). Measured Brazil nut tree characteristics and DSBA and DSD were included in all beyond optimal models. We included quadratic and square root terms of SBA and stem density in the beyond optimal models of SBA and stem density, respectively, to test for non-linearity in the corresponding relation. Census year, interactions between site type and census year, and linear and quadratic terms of DBH and mean crown diameter were included in all beyond optimal models. Year 1 was used as reference year in all beyond optimal models, and undegraded forest as reference site type in the site type beyond optimal model.
Optimal random effects structures of the beyond optimal models were for each model selected based on lowest AIC from three random effect structures: (1) individual tree within site, (2) individual tree within area, and (3) individual tree and a random interaction between area and site type/SBA/SD. After selection of the optimal random effect structure of the beyond optimal model, fixed effects were selected based on lowest AIC and degrees of freedom within the Δ AIC <2 range. The selected models were refitted with the other years (i.e., year 2 and 3) and site type (i.e., degraded forest) as reference year/site type.
Used Software
All analyses were performed in R (R Development Core Team, 2014). The ordinal logistic model was fitted using the package ordinal (Christensen, 2019). All other models were fitted using the lme4 package (Bates et al., 2014), and all subset fixed effect selection was performed using the dredge function of the MuMIn package (Barton, 2015).
Results
Forest Structure of Sites and Brazil Nut Tree Characteristics
As a whole, undegraded and degraded forest sites differed in forest structure. Average SBA varied between 20.2 and 26.3 m2ha−1 among undegraded forest sites and between 8.7 and 11.9 m2ha−1 among degraded forest sites. Stem density varied between 391.9 and 500.0 stems ha−1 among undegraded forest sites, and between 187.0 and 222.8 stems ha−1 among degraded forest sites. We found SBA and stem density both to be significantly higher in undegraded forest sites compared to degraded forest (Figure 3, p = 6.64e-06 and ΔAIC = −18.30 for SBA, p = 7.62e-06, and ΔAIC = −18.03 for stem density). Overall, SBA was estimated to be a factor 2.3 higher in undegraded than in degraded forest sites (model estimations of 23.1 m2ha−1 compared to 10.1 m2ha−1, respectively). Stem density was estimated to be a factor 2.1 higher in undegraded forest sites than in degraded sites (model estimations of 435.7 stems ha−1 compared to 203.2 stems ha−1, respectively). DSBA area DSD around individual trees did not significantly differ between undegraded and degraded forest (p = 0.249 and 0.105, respectively). Brazil nut tree crown illumination was higher is degraded forest (i.e., a lower crown illumination index, p = 1.38e-10) while other Brazil nut tree characteristics (i.e., tree damage, DBH, and crown diameter) did not differ significantly (p = 0.678, 0.660, and 0.367, respectively).
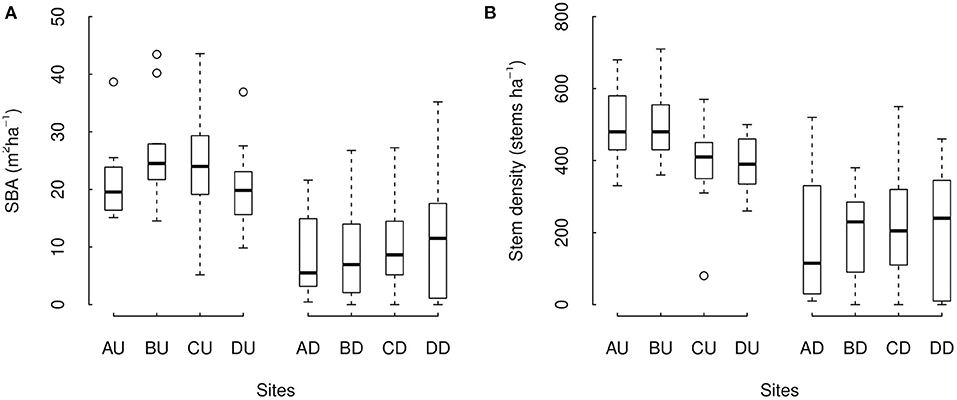
Figure 3. Stand basal area (A) and stem density (B) across sites with different levels of degradation. Sites AU-DU correspond to the undegraded forest sites, and AD–DD to the degraded sites in the areas A–D. Boxes are the interquartile range (IQR), black lines in the middle of boxes are medians, whiskers are the extreme data point with 1.5 × IQR.
Forest Degradation and Inter-annual Fruit Production
Site-Type Level Effects
We did not find a significant overall effect of forest degradation on fruit production, but we did find a significant interaction between the effect of forest degradation and year (ΔAIC = −1.97, p = 0.8633, and ΔAIC = −823.0 and p = <2.2e-16, respectively), indicating that the effect of degradation strongly differed among years. Our statistical model estimated fruit production of individual trees with average crown diameter to be 29.4% and 35.8% higher in undegraded forest compared to degraded forest in census years 2 and 3, respectively, and 31.7% lower in year 1 (Figure 4). However, these within year differences between degraded and undegraded forest were not significant (p = 0.609, 0.634, and 0.571 for years 1, 2, and 3, respectively, Supplementary Table 1).
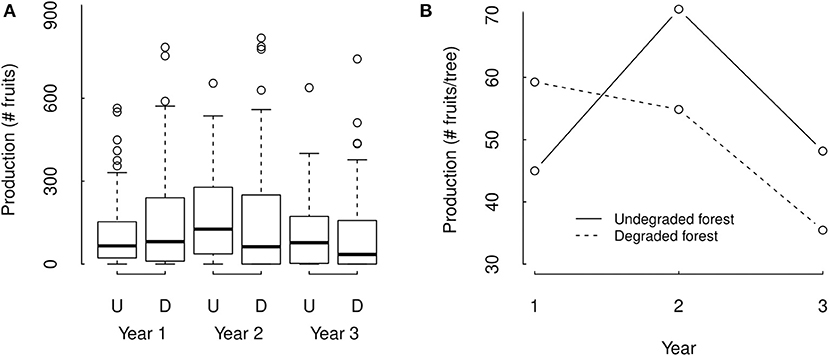
Figure 4. Three years data (A) and statistical model estimations (B) of individual Brazil nut tree fruit production in undegraded (U) and degraded forest (D) in the Tambopata National Reserve and its buffer zone in Madre de Dios, Amazonian Peru. In panel (A), boxes are the interquartile range (IQR), black lines in the middle of boxes are medians, whiskers are the extreme data point with 1.5 × IQR. The statistical model estimations in panel (B) represent estimations for an individual tree with average DBH and tree damage of trees present in our dataset, and were obtained with mixed effect all subset Poisson regression analysis.
Tree fruit production significantly differed among years (ΔAIC = 1314.7 and p = < 2.2e-16). Within undegraded forest, tree fruit production was estimated to be 57.7% and 47.3% higher in the second year compared to 1st and 3rd year (p = < 2e-16 for both) and 6.6% lower in the 1st year compared to the 3rd year (p = 0.0001, Figure 4 and Supplementary Table 1). Within degraded forest the trend was different, with tree fruit production in degraded forest estimated to be 8.0% and 67.1% higher in the 1st year compared to the 2nd and 3rd year (p = 3.38e-08 and < 2e-16, respectively), and the second and third year also significantly differing in fruit production (p = < 2e-16, Figure 3 and Supplementary Table 1).
Individual tree within site was selected as optimal random effect structure (i.e., inclusion of area nor an interaction between area and site type significantly improved the model, ΔAIC = 2.348 and 1.058, respectively), indicating that the effect of forest degradation on tree fruit production did not significantly differ between the four study areas. Furthermore, we found a positive quadratic effect of DBH on tree fruit production, and a negative effect of tree damage (p = 0.0060, and 0.0118, respectively, Supplementary Table 1). Other explanatory variables that were included in the beyond-optimal model (i.e., crown diameter, DSBA, and DSD), were not selected by the all subset regression (i.e., did not improve the model with ΔAIC > 2).
The results of the analysis of the effect of site type on tree fruit production clearly show that fruit production varies among years within both degraded and undegraded forest. Furthermore, it suggests that forest degradation affects individual tree Brazil nut production yet with interannual variation in the size and sign of the effect. However, as the within year effects of forest degradation were not significant, this cannot be concluded with certainty based on our analysis.
Individual Tree Level Effects
In separate analyses, we found a significant effect of SBA around individual trees on tree fruit production and significant interannual variations in this effect (i.e., a statistical model with SBA and interactions between SBA and year significantly improved our statistical model, ΔAIC = −7.2, p = 0.0025, and ΔAIC = −476.0, p = < 2.2e-16, respectively). The effect of SBA was positive and significant within all 3 years (p = 0.0180, 0.0018, and 0.0002), strongest in year 3 and weakest in year 1 (p = < 2.2e16 and < 2.2e-16, respectively, Figure 5A and Supplementary Table 2). The effects of stem density around trees on tree fruit production were similar (Figure 5B), with significant overall effects of stem density and significant interannual variations in this effect (AIC – ΔAIC = 6.91, p = 0.0028 and ΔAIC = 1,289.52, p = < 2.2e-16, respectively), effects being positive and significant in two of the 3 years (p = 0.0533, 0.00267, and 2.18e-05 for year 1, 2, and 3, respectively), and strongest effect in year 3 and weakest in year 1 (p = < 2.2e16, Figure 5B and Supplementary Table 2). Quadratic and square root terms of SBA did not significantly improve the SBA models (ΔAIC = 1.961, p = 0.844 and ΔAIC = 0.771, p = 0.267, respectively), nor did a quadratic or square root term of stem density significantly improve the stem density model (ΔAIC 1.558, p = 0.5062 and ΔAIC = 0.36360, p = 0.2011, respectively). Our results provide clear evidence of positive effects of SBA and stem density around individual Brazil nut trees on fruit production, but not for non-linearity in these relations. Furthermore, our results show that the strength of these relations can vary between years.
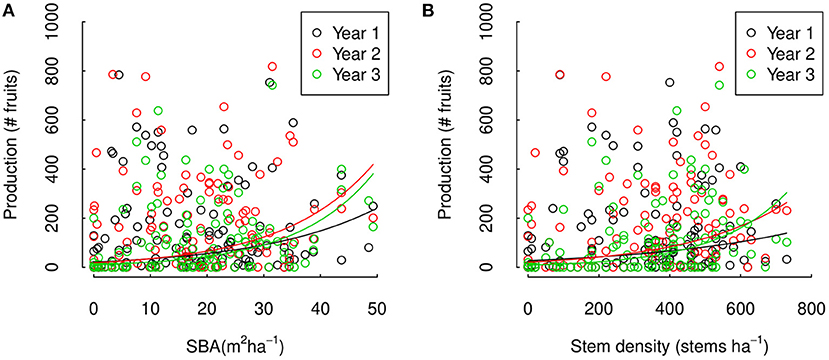
Figure 5. Three years Brazil nut tree fruit production across a (A) SBA and (B) stem density gradient in the Tambopata National Reserve and its buffer zone in Madre de Dios, Amazonian Peru. The data points represent fruit counts of individual Brazil nut trees in our study sites, compared to the SBA and stem density in a 50 m radius around the corresponding Brazil nut tree. The lines are model estimations obtained with mixed effect Poisson regression analysis for trees with average DBH and tree damage within our sites.
Individual tree within site was selected as optimal random effect structure of both the SBA and stem density model (i.e., inclusion of area nor a random interaction between area and SBA/stem density significantly improved the models, ΔAIC = 1.294 and 3.226, respectively, for the SBA model and 2.822 and 1.484, respectively, for the stem density model), indicating that the effect of SBA and stem density on fruit production did not significantly differ between areas. Tree damage was found to negatively affect individual fruit production in both the SBA and the stem density model (p = 0.0210 and 0.0121, respectively), and fruit production was positively related to DBH in both models (p = 0.00936 and 0.0107, Supplementary Table 2). Other explanatory variables that were included in the beyond-optimal model (i.e., DSBA/DSD, crown diameter and crown illumination index), were not selected by the all subset regression.
Discussion
The Effect of Forest Degradation on Individual Tree Fruit Production
Our analysis revealed that individual Brazil nut tree fruit production in our four study areas was influenced by forest degradation both in terms of site type (i.e., degraded vs. undegraded forest) and forest structure around individual Brazil nut trees, with significant interannual variations (i.e., strongest effect in year 3 and weakest in year 1, and an estimated positive effect in year 1 at the site type level). However, within year effects of degradation at the site type level were not significant.
A possible explanation for the non-significant within year effects could be the large heterogeneity within the degraded forest sites, with part of the Brazil nut trees in the degraded sites growing within forest patches with SBA and stem density comparable to that of trees growing in undegraded forest (Figure 3). Our results of the effect of SBA and stem density around individual trees on fruit production are concurrent with the idea that the negative effects on production are highest at highest level of degradation (i.e., no stems around the tree). Therefore, the patchy structure of our sites could have contributed to within site variation in fruit production. This could also explain differences with estimations in Rocha and Aguilar (2001), who found inflorescences on the dry forest tree Enterolobium cyclocarpum in Costa Rica to be seven times more likely to set fruits when growing in continuous forest compared to trees growing in pastures, while the biggest difference between undegraded and degraded forest in our study was of a factor 1.4 in the 3rd year. Furthermore, the higher fruit production in degraded forest than in undegraded forest in the 1st year is seemingly contradictory to the negative effect on fruit production of forest structure around individual Brazil nut trees in this year. This supports the notion that other attributes of degradation than small scale forest structure could influence fruit production.
Our choice to exclude liana infested Brazil nut trees from one of the closed canopy forest sites could theoretically have led to a small overestimation in fruit production in the corresponding site. However, the effect of none of the degradation measures on fruit production significantly differed between areas, indicating that results were likely not strongly affected by the choice to exclude liana loaded trees from one of the sites. We cannot exclude the potential effects of fruit removal by agoutis (Dasyprocta spp., Mori and Prance, 1990) on recorded tree fruit production (which was not included in our study). However, a study in Bolivia did not find any effect of forest degradation on probability of seed dispersal by agoutis (van Leur, 2002), which suggests that Agouti activity is unlikely to be a significant factor influencing our estimates of the effect of forest degradation on Brazil nut fruit production.
Which Mechanisms Could Explain Our Results?
An association between pollinator abundance and forest degradation could explain the decline in fruit production of individual trees, but our methods do not allow an investigation of this. Forest degradation and logging have been shown to affect pollination services; through reduced total habitat, edge effects and associated changes in micro climate. Reduced connectivity has been shown to affect pollination, generally lowering pollen quality (i.e., due to reduced outcrossing rates) and quantity (Hadley and Betts, 2012; Stangler et al., 2015). For example, Chiriboga-Arroyo et al. (2020) found less genetic diversity and more inbreeding in seedlings compared to adults, depending on the level of forest degradation, and Wadt et al. (2015) found correlated mating to be significantly higher in Brazil nut trees growing in pasture compared to trees growing natural forest, suggesting bees forage over a narrower neighborhood of conspecifics in pastures. However, opposite effects have also been found in other systems, Ismail et al. (2012) found isolated trees to receive more diverse pollen pools in a canopy emergent tree in coffee landscapes in India. Presence of suitable pollinator nesting sites can increase pollination services (Ghazoul, 2005). However, we did not find any significant effect of deadwood stem density or deadwood SBA on fruit production, nor did it differ between undegraded and degraded forest. Further, a relationship between pollination services and level of forest degradation does not explain the strong differences in effect size of forest degradation on fruit production in the 1st year of our study compared to the other 2 years, nor does it explain the interannual variations in fruit production that we observed both in degraded and in undegraded forest. Alternative or additional unexplored mechanisms presumably caused the observed differences between undegraded and degraded forest and among years.
We found Brazil nut production at the individual tree level to be significantly highest in year 1 in degraded forest and in year 2 in undegraded forest. Interannual variation is climate is one possible explanation for this. Fruit ripening in B. excelsa requires 14–15 months (Maués, 2002). Therefore, fruit fall during early 2017 (the 1st year of our study) dates back to fruit ripening throughout 2016, which was a strong El Niño year (Jiménez-Muñoz et al., 2016) across Western Amazonia. Climatological data from Puerto Maldonado meteorological station (about 10 km from our study areas) reveals that annual rainfall was only 2,032 mm in 2016, compared to 2,285 mm on average over the last 5 years, and 2,327 and 2,428 in 2017 and 2018, respectively (SENAMHI, 2019). The low rainfall in 2016 might have caused high water vapor deficits, which could have caused higher rates of tree fruit abortion (Augspurger, 1983; Gunarathne and Perera, 2014). Rainfall during the flowering period corresponding to our first census year (i.e., the flowering period of November 2015–February 2016), was also relatively low (263.5 mm compared to 289.1 and 328.8 mm average monthly rainfall in November 2016/2017–February 2017/2018, respectively). Drought stress preceding and during flowering might have caused trees to produce less and/or abort flowers, leading to lower fruit production (Borchert, 1994). A strong reduction in population level fruit production of B. excelsa following a dry El Niño year was reported in Bolivia (Zuidema, 2003). However, interannual variations in rainfall do not directly explain the relatively high fruit production in year 1 in degraded forest, and the associated weaker effect of forest degradation. This could be explained by differences in competition for water. Lower stem density and stand basal area, as found in our severely degraded sites, are known to reduce water competition in forests (Giuggiola et al., 2013). Likely, water availability was not a limiting factor for fruit production in the relatively wet 2nd and 3rd year of our study, but was limiting in the relatively dry first (i.e., the el Niño) year. Brazil nut trees growing in areas with a relatively low stem density could have had a relative advantage compared to individuals growing in closed canopy forest in terms of water competition, providing a plausible explanation for the relatively high fruit production of trees in severely degraded forest in the first census year.
Conclusions and Implications
Our study suggests that forest degradation can have negative effects on Brazil nut production at the tree level, but that the strength of this effect varies between years and could potentially be positive in some years. Our analysis also highlights the difficulties in applying categorical variables to forest degradation, when heterogeneity of this degradation can be high over relatively small spatial scales. Both the effect of forest degradation and high inter-annual variability in fruit production (the second of which has also been observed in other studies Kainer et al., 2007; Rockwell et al., 2015) may have implications for sustainability of the Brazil nut industry, including the livelihoods of the Brazil nut collectors. Fragmented landscapes impacted by anthropogenic (e.g., agriculture and logging) and natural (e.g., drought) disturbances are expected to be the trend throughout the Amazon Basin (Broadbent et al., 2008; Oliveira et al., 2019). The negative effect of forest degradation on Brazil nut fruit production implies that anthropogenic disturbances could affect Brazil nut collectors' livelihoods. Furthermore, extreme climate events are likely to become more frequent throughout the Amazon basin (Marengo et al., 2016), which could exacerbate these issues.
Based on our research, we cannot determine the frequency by which the years with differences in fruit production and degradation effects occur, but the intercurrence of a very dry El Niño year with the year in which the effect of forest degradation on fruit production was weakest and fruit production in closed canopy forest was lowest, suggests that forest degradation, and climate fluctuations could have interacting effects. A more in-depth analysis of the relation between Brazil nut production, climate fluctuations and landscape degradation with multiple sites across the Amazon Basin and multiple years of data could help reveal if this is indeed the case and shed light on the main drivers and mechanisms behind these relations. More generally, with the increased recognition of the role of non-timber forest products and tree-based foods to support resilient tropical forest landscapes (Jansen et al., 2020), it will be important to understand better the critical factors shaping interannual variation in fruit production across landscapes with different degradation levels.
Data Availability Statement
The datasets generated for this study are available on request to the corresponding author.
Author Contributions
The idea for the study was conceived by CJK and MRG, the methodology was designed by MJ, CJK, MRG, FC-A, FMVQ, and EAQ. Data was collected by MJ, FV, and EA and data analysis and interpretation was performed by MJ and MRG. MJ led the writing of the manuscript. All authors contributed critically to the drafts and gave final approval for publication.
Funding
The research was financially supported by the COOP program of the ETH Zurich World Food System Center. FC-A was financially supported by ETH Zurich (grant number ETH-1516-1).
Conflict of Interest
The authors declare that the research was conducted in the absence of any commercial or financial relationships that could be construed as a potential conflict of interest.
Acknowledgments
We thank SERNANP and AIDER for providing access to the Tambopata National Reserve and for continuous logistic support (including the support of the Jorge Chavez check point park rangers), all concessionaires for allowing access to their concessions and their willingness to collaborate with our project, Alessia Capurso and Daniel Navarro for designing and executing the collection of part of the forest structure data, Edwin Corrimanya, Analí Escalante, Saraí Vargas, Piher Maceda, Manuel Huinga, and Sufer Baezfor for help with collection of data, Daniel Navarro for help with creating Figure 1 and Cara Rockwell for providing comments on an earlier version of the manuscript.
Supplementary Material
The Supplementary Material for this article can be found online at: https://www.frontiersin.org/articles/10.3389/ffgc.2020.525533/full#supplementary-material
References
Augspurger, C. K. (1983). Phenology, flowering synchrony, and fruit set of six neotropical shrubs. Biotropica 15, 257–267. doi: 10.2307/2387650
Bates, D., Mächler, M., Bolker, B., and Walker, S. (2014). Fitting linear mixed-effects models using lme4. arXiv:1406.5823. doi: 10.18637/jss.v067.i01
Borchert, R. (1994). Water status and development of tropical trees during seasonal drought. Trees 8, 115–125. doi: 10.1007/BF00196635
Brando, P. M., Balch, J. K., Nepstad, D. C., Morton, D. C., Putz, F. E., Coe, M. T., et al. (2014). Abrupt increases in Amazonian tree mortality due to drought-fire interactions. Proc. Natl. Acad. Sci. U. S. A. 111, 6347–6352. doi: 10.1073/pnas.1305499111
Broadbent, E. N., Asner, G. P., Keller, M., Knapp, D. E., Oliveira, P. J., and Silva, J. N. (2008). Forest fragmentation and edge effects from deforestation and selective logging in the Brazilian Amazon. Biol. Conserv. 141, 1745–1757. doi: 10.1016/j.biocon.2008.04.024
Cavalcante, M., Oliveira, F., Maués, M., and Freitas, B. (2012). Pollination requirements and the foraging behavior of potential pollinators of cultivated Brazil nut (Bertholletia excelsa Bonpl.). trees in central Amazon rainforest. Psyche J. Entomol. 2012:978019. doi: 10.1155/2012/978019
Chávez, A., Guariguata, M. R., Cronkleton, P., Menton, M., Capella, J. L., Araujo, J. P., et al. (2012). Superposición Espacial en la Zonificación de Bosques en Madre de Dios: Implicaciones Para la Sostenibilidad del Recurso Castañero. Center for International Forestry Research (CIFOR), Bogor, Indonesia.
Chiriboga-Arroyo, F., Jansen, M., Bardales-Lozano, R., Ismail, S. A., Thomas, E., García, M., et al. (2020). Genetic threats to the Forest Giants of the Amazon: Habitat degradation effects on the socio-economically important Brazil nut tree (Bertholletia excelsa). Plants People Planet. 1–17. doi: 10.1002/ppp3.10166
Christensen, R. H. B. (2019). Ordinal-Regression Models for Ordinal Data. R Package Version 2019.12-10. Available online at: https://CRAN.R-project.org/package=ordinal
Davidson, E. A., de Araújo, A. C., Artaxo, P., Balch, J. K., Brown, I. F., Bustamante, M. M., et al. (2012). The Amazon basin in transition. Nature 481, 321. doi: 10.1038/nature10717
Duchelle, A. E. (2009). Conservation and Livelihood Development in Brazil Nut-Producing Communities in a Tri-National Amazonian Frontier, Dissertation, University of Florida.
Foley, J. A., Asner, G. P., Costa, M. H., Coe, M. T., DeFries, R., Gibbs, H. K., et al. (2007). Amazonia revealed: forest degradation and loss of ecosystem goods and services in the Amazon Basin. Front. Ecol. Environ. 5, 25–32. doi: 10.1890/1540-9295(2007)5[25:ARFDAL]2.0.CO;2
Freitas, B. M., and Oliveira-Filho, J. d. (2003). Ninhos racionais para mamangava (Xylocopa frontalis) na polinização do maracujá-amarelo (Passiflora edulis). Ciência Rural 33, 1135–1139. doi: 10.1590/S0103-84782003000600021
Ghazoul, J. (2005). Buzziness as usual? Questioning the global pollination crisis. Trends Ecol. Evol. 20, 367–373. doi: 10.1016/j.tree.2005.04.026
Ghazoul, J., Liston, K. A., and Boyle, T. (1998). Disturbance-induced density-dependent seed set in Shorea siamensis (Dipterocarpaceae), a tropical forest tree. J. Ecol. 86, 462–473. doi: 10.1046/j.1365-2745.1998.00270.x
Ghazoul, J., and McLeish, M. (2001). “Reproductive ecology of tropical forest trees in logged and fragmented habitats in Thailand and Costa Rica,” Tropical Forest Canopies: Ecology and Management, K. E. Linsenmair, A. J. Davis, B. Fiala, M. R. Speight (Dordrecht: Springer), 335–345.
Giuggiola, A., Bugmann, H., Zingg, A., Dobbertin, M., and Rigling, A. (2013). Reduction of stand density increases drought resistance in xeric Scots pine forests. For. Ecol. Manage. 310, 827–835. doi: 10.1016/j.foreco.2013.09.030
Guariguata, M. R., Cronkleton, P., Duchelle, A. E., and Zuidema, P. A. (2017). Revisiting the 'cornerstone of Amazonian conservation': a socioecological assessment of Brazil nut exploitation. Biodivers. Conserv. 26, 2007–2027. doi: 10.1007/s10531-017-1355-3
Guariguata, M. R., and Pinard, M. A. (1998). Ecological knowledge of regeneration from seed in neotropical forest trees: implications for natural forest management. For. Ecol. Manag. 112, 87–99. doi: 10.1016/S0378-1127(98)00318-1
Gunarathne, R., and Perera, G. (2014). Climatic factors responsible for triggering phenological events in Manilkara hexandra (Roxb.). Dubard., a canopy tree in tropical semi-deciduous forest of Sri Lanka. Trop. Ecol. 55, 63–73.
Hadley, A. S., and Betts, M. G. (2012). The effects of landscape fragmentation on pollination dynamics: absence of evidence not evidence of absence. Biol. Rev. 87, 526–544. doi: 10.1111/j.1469-185X.2011.00205.x
Ismail, S. A., Ghazoul, J., Ravikanth, G., Uma Shaanker, R., Kushalappa, C., and Kettle, C. J. (2012). Does long-distance pollen dispersal preclude inbreeding in tropical trees? Fragmentation genetics of D ysoxylum malabaricum in an agro-forest landscape. Mol. Ecol. 21, 5484–5496. doi: 10.1111/mec.12054
Jansen, M., Guariuata, M., Raneri, J., Ickowitz, A., and Kettle, C. (2020). Food for thought: the underutilized potential of tropical tree-based foods for 21 century sustainable food systems. Br. Ecol. Soc. 2, 1006–1020. doi: 10.1002/pan3.10159
Jiménez-Muñoz, J. C., Mattar, C., Barichivich, J., Santamaría-Artigas, A., Takahashi, K., Malhi, Y., et al. (2016). Record-breaking warming and extreme drought in the Amazon rainforest during the course of El Niño 2015-2016. Sci. Rep. 6:33130. doi: 10.1038/srep33130
Kainer, K. A., Wadt, L. H., and Staudhammer, C. L. (2014). Testing a silvicultural recommendation: Brazil nut responses 10 years after liana cutting. J. Appl. Ecol. 51, 655–663. doi: 10.1111/1365-2664.12231
Kainer, K. A., Wadt, L. H. O., and Staudhammer, C. L. (2007). Explaining variation in Brazil nut fruit production. For. Ecol. Manag. 250, 244–255. doi: 10.1016/j.foreco.2007.05.024
Keeling, H. C., and Phillips, O. L. (2007). A calibration method for the crown illumination index for assessing forest light environments. For. Ecol. Manag. 242, 431–437. doi: 10.1016/j.foreco.2007.01.060
Levis, C., Costa, F. R., Bongers, F., Peña-Claros, M., Clement, C. R., Junqueira, A. B., et al. (2017). Persistent effects of pre-Columbian plant domestication on Amazonian forest composition. Science 355, 925–931. doi: 10.1126/science.aal0157
Lowe, A., Boshier, D., Ward, M., Bacles, C., and Navarro, C. (2005). Genetic resource impacts of habitat loss and degradation; reconciling empirical evidence and predicted theory for neotropical trees. Heredity 95, 255. doi: 10.1038/sj.hdy.6800725
Marengo, J. A., Williams, E. R., Alves, L. M., Soares, W. R., and Rodriguez, D. A. (2016). “Extreme seasonal climate variations in the Amazon basin: droughts and floods,” in Interactions between Biosphere, Atmosphere and Human Land Use in the Amazon Basin, eds L. Nagy, B. R. Forsberg, and P. Artaxo (Berlin; Heidelberg: Springer), 55–76.
Maués, M. M. (2002). “Reproductive phenology and pollination of the Brazil nut tree (Bertholletia excelsa Humb. and Bonpl. Lecythidaceae) in Eastern Amazonia,” in Pollinating Bees: The Conservation Link Between Agriculture and Nature, eds P. Kevan and V. L. Imperatriz Fonseca (Brasilia: Ministry of Environment), 245–254.
Mori, S., and Prance, G. T. (1990). Taxonomy, ecology, and economic botany of the Brazil nut (Bertholletia excelsa Humb. and Bonpl.: Lecythidaceae). Adv. Econ. Bot. 8, 130–150.
Oliveira, A., Soares-Filho, B., Costa, M., Lima, L., Garcia, R., Rajão, R., et al. (2019). Bringing economic development for whom? An exploratory study of the impact of the Interoceanic Highway on the livelihood of smallholders in the Amazon. Landsc. Urban Plann. 188, 171–179. doi: 10.1016/j.landurbplan.2019.04.025
Ortiz, E. (2002). “Brazil nut (Bertholletia excelsa),” in Tapping the Green Market: Certification and Management of Non-Timber Forest Products, eds A. Guillen, S. A. Laird, P. Shanley, A. R. Pierce (London: Earthscan), 61–74.
Peres, C. A., Baider, C., Zuidema, P. A., Wadt, L. H., Kainer, K. A., Gomes-Silva, D. A., et al. (2003). Demographic threats to the sustainability of Brazil nut exploitation. Science 302, 2112–2114. doi: 10.1126/science.1091698
R Development Core Team (2014). R: A Language and Environment for Statistical Computing. Vienna: R Foundation for Statistical Computing.
Rocha, O. J., and Aguilar, G. (2001). Reproductive biology of the dry forest tree Enterolobium cyclocarpum (Guanacaste) in Costa Rica: a comparison between trees left in pastures and trees in continuous forest. Am. J. Bot. 88, 1607–1614. doi: 10.2307/3558405
Rockwell, C. A., Guariguata, M. R., Menton, M., Quispe, E. A., Quaedvlieg, J., Warren-Thomas, E., et al. (2015). Nut production in Bertholletia excelsa across a logged forest mosaic: implications for multiple forest use. PLoS One 10:e0135464. doi: 10.1371/journal.pone.0135464
Ros-Tonen, M. A., and Wiersum, K. F. (2005). The scope for improving rural livelihoods through non-timber forest products: an evolving research agenda. For. Trees Livelihoods 15, 129–148. doi: 10.1080/14728028.2005.9752516
Scoles, R., and Gribel, R. (2011). Population structure of Brazil nut (Bertholletia excelsa, Lecythidaceae) stands in two areas with different occupation histories in the Brazilian Amazon. Hum. Ecol. 39, 455–464. doi: 10.1007/s10745-011-9412-0
Shackleton, S., Delang, C. O., and Angelsen, A. (2011). “From subsistence to safety nets and cash income: exploring the diverse values of non-timber forest products for livelihoods and poverty alleviation,” in Non-Timber Forest Products in the Global Context, eds S. Shackleton, C. Shackleton, and P. Shanley (Berlin: Springer), 55–81.
Stangler, E. S., Hanson, P. E., and Steffan-Dewenter, I. (2015). Interactive effects of habitat fragmentation and microclimate on trap-nesting Hymenoptera and their trophic interactions in small secondary rainforest remnants. Biodivers. Conserv. 24, 563–577. doi: 10.1007/s10531-014-0836-x
Staudhammer, C. L., Wadt, L. H., and Kainer, K. A. (2013). Tradeoffs in basal area growth and reproduction shift over the lifetime of a long-lived tropical species. Oecologia 173, 45–57. doi: 10.1007/s00442-013-2603-1
Sun, J., and Southworth, J. (2013). Remote sensing-based fractal analysis and scale dependence associated with forest fragmentation in an Amazon tri-national frontier. Remote Sens. 5, 454–472. doi: 10.3390/rs5020454
Thomas, E., Alcázar Caicedo, C., McMichael, C. H., Corvera, R., and Loo, J. (2015). Uncovering spatial patterns in the natural and human history of Brazil nut (Bertholletia excelsa) across the Amazon Basin. J. Biogeogr. 42, 1367–1382. doi: 10.1111/jbi.12540
Thomas, E., Valdivia, J., Alcázar Caicedo, C., Quaedvlieg, J., Wadt, L. H. O., and Corvera, R. (2017). NTFP harvesters as citizen scientists: Validating traditional and crowdsourced knowledge on seed production of Brazil nut trees in the Peruvian Amazon. PLoS ONE. 12:e0183743. doi: 10.1371/journal.pone.0183743
Tonini, H., Kaminski, P. E., and da Costa, P. J. P. A.B. (2008). Relação da produção de sementes de castanha-do-brasil com características morfométricas da copa e índices de competição. Pes. Agropec. Brasil. 43, 1509–1516. doi: 10.1590/S0100-204X2008001100009
van Leur, H. (2002). Effects of Habitat on Spatial Dispersal of Brazil Nuts (Bertholletia excelsa), MSc Thesis, Utrecht University.
Wadt, L. d,.O, Baldoni, A., Silva, V., Campos, T.d., Martins, K., et al. (2015). Mating system variation among populations, individuals and within and among fruits in Bertholletia excelsa. Silvae Genet. 64, 248–259. doi: 10.1515/sg-2015-0023
Wadt, L. H., Kainer, K. A., and Gomes-Silva, D. A. (2005). Population structure and nut yield of a Bertholletia excelsa stand in Southwestern Amazonia. For. Ecol. Manag. 211, 371–384. doi: 10.1016/j.foreco.2005.02.061
Willem, H. V., Ingram, V. J., and Guariguata, M. R. (2019). Brazil nut forest concessions in the Peruvian Amazon: success or failure. Int. For. Rev. 21, 254–265. doi: 10.1505/146554819826606540
Zuidema, P. A. (2003). Ecology and Management of the Brazil Nut Tree (Bertholletia excelsa). Promab.
Zuidema, P. A., and Boot, R. G. (2002). Demography of the Brazil nut tree (Bertholletia excelsa) in the Bolivian Amazon: impact of seed extraction on recruitment and population dynamics. J. Trop. Ecol. 18, 1–31. doi: 10.1017/S0266467402002018
Keywords: Bertholletia excelsa, Madre de Dios, non-timber forest products, Peru, Tambopata National Reserve
Citation: Jansen M, Guariguata MR, Chiriboga-Arroyo F, Quaedvlieg J, Vargas Quispe FM, Arroyo Quispe E, García Roca MR, Corvera-Gomringer R and Kettle CJ (2021) Forest Degradation and Inter-annual Tree Level Brazil Nut Production in the Peruvian Amazon. Front. For. Glob. Change 3:525533. doi: 10.3389/ffgc.2020.525533
Received: 09 January 2020; Accepted: 15 December 2020;
Published: 21 January 2021.
Edited by:
Mirjam Ros, University of Amsterdam, NetherlandsReviewed by:
Stephen Perz, University of Florida, United StatesTerence C. Sunderland, University of British Columbia, Canada
Copyright © 2021 Jansen, Guariguata, Chiriboga-Arroyo, Quaedvlieg, Vargas Quispe, Arroyo Quispe, García Roca, Corvera-Gomringer and Kettle. This is an open-access article distributed under the terms of the Creative Commons Attribution License (CC BY). The use, distribution or reproduction in other forums is permitted, provided the original author(s) and the copyright owner(s) are credited and that the original publication in this journal is cited, in accordance with accepted academic practice. No use, distribution or reproduction is permitted which does not comply with these terms.
*Correspondence: Merel Jansen, bWVyZWxqYW5zZW4yJiN4MDAwNDA7Z21haWwuY29t