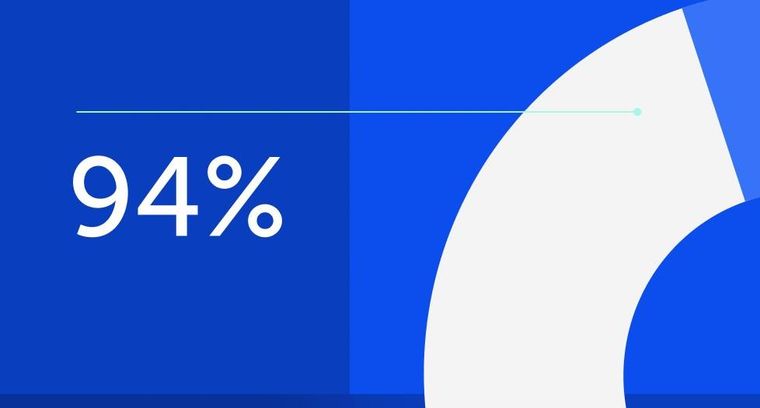
94% of researchers rate our articles as excellent or good
Learn more about the work of our research integrity team to safeguard the quality of each article we publish.
Find out more
ORIGINAL RESEARCH article
Front. For. Glob. Change, 21 July 2020
Sec. Fire and Forests
Volume 3 - 2020 | https://doi.org/10.3389/ffgc.2020.00082
This article is part of the Research TopicImpact of Fire on BiodiversityView all 4 articles
The trajectory and recovery time of fire-disturbed forests depend on the capacity of seedlings and resprouts to get established over time. Here, we investigated the mechanisms associated with fire effects on post-fire regeneration in the context of a large-scale fire experiment located in southeastern Amazonia. Specifically, we tested the hypothesis that recurrent understory fires reduce forest regeneration capacity by reducing the recruitment of seedlings, the viability of the soil seed bank, the seed rain, and the resprouts. The experiment was comprised of three 50-ha plots: an unburned control, and two plots that were experimentally burned three (triennially) or six (annually) times between 2004 and 2010. Seedlings represented 2.4 and 0.6% of the seed rain and soil seed bank in the Control and burned treatment plots, respectively. Compared to the control, the triennial burns caused more reduction than the annual ones in seed rain (−42 vs. −10%) and seed bank (−78 vs. −50%). These fire-related reduction in seed rain in both treatments explain most of the low post-fire density of seedlings (0.4 and 0.8 ind/m2, respectively), compared with the Control (2.9 ind/m2). The 3-year interval between fires contributed to highest mortality of seeds stored in the soil than in the annual burn treatment, where fires were less severe. However, there were 5.5 times more resprouting in the plot burned triennially compared with the one burned annually. In both treatments, though, the number of resprouts declined over time. In conclusion, seeds from both seed rain and seed bank and resprouting were negatively affected by both fire frequencies, reducing the likelihood of native species recovery by altering the regeneration pathways. This situation is expected to be increasingly common in southeastern Amazon forests, particularly under warmer and dryer climate conditions.
Human activity is the main ignition source for Amazon wildfires. People use fire as a management tool, to clean deforested fields, to manage pasturelands, or in slash-and-burn agricultural systems (Nepstad et al., 2001; Lindenmayer et al., 2009; Brando et al., 2019). Although dense forests can maintain high levels of moisture underneath their canopies, therefore reducing litter flammability, extreme droughts and forest degradation can disrupt this natural firebreak. When this disruption occurs, management fires often escape into primary forests and cause fire-related tree mortality, especially along forest edges. Wildfires have been widespread over the past decades along the “Arc of deforestation” in Amazonia (Nepstad et al., 2001, 2008; Aragão et al., 2018), where droughts, sources of ignition, and forest degradation are all common. Brazil is the sixth-biggest emitter of greenhouse gases on the planet, with 1.9 billion tons of carbon dioxide emissions in 2018 and 44% was connected to land-use change (Sistema de Estimativas de Emissões e Remoções de Gases de Efeito Estufa (SEEG), 2020). Following climate and land-use changes in the coming decades, wildfires are expected to become even more common (Brando et al., 2020), with important implications for forest carbon dynamics and biodiversity.
Post fire recovery of Amazon forests depends on the capacity of reproductive trees to resist fire and of seedlings and resprouts to get established. On one hand, Amazon tree communities are highly susceptible to fires; their thin bark often exposes cambium cells to damaging levels of fire-related heat (Brando et al., 2012; Staver et al., 2019). While some larger, thicker-barked trees survive low-to-medium intensity fires over the short-term, fire-related wounds to trees' roots, stems, and vascular systems are expected to substantially reduce trees' lifespans and cause prolonged forest degradation (Barlow et al., 2003; Staver et al., 2019). On the other hand, tropical forest communities are resilient to a range of disturbances. This suggests that post-fire regeneration, originated from seed rain, seed bank, and resprouting, can lessen some of the negative ecological effects caused by fires. Therefore, an important knowledge gap is whether and in what extent these fire-disturbed forests can recover. Indeed, fire-disturbed forests are expected to partially or fully recover their structure, diversity, and functions over time (Alencar et al., 2006; Balch et al., 2008; Morton et al., 2013; Brando et al., 2019), but assessments of the effects of understory fires on forest regeneration processes is still scarce. Thus, quantify the regeneration diversity and abundance can shed some light on the capacity of forests to recover some of their fundamental properties after wildfires (Coe et al., 2013; Brando et al., 2019).
Post-fire seedling recruitment—the prevalent regeneration mode of tropical forests—likely compensates some losses in forest diversity associated with fire disturbance (Kennard et al., 2002; Hoffmann et al., 2003; Balch et al., 2013). However, this recovery capacity may decline with death of reproductive trees caused by fire, leading to a decline in seed sources. Although seeds stored in the soil (the seedbank) can enable seedling recruitment, those seeds tend to be short-lived (i.e., <3 years), thus highly dependent on the seed rain, and relatively vulnerable to high-intensity, recurrent fires (Clark et al., 1999; Bond and Midgley, 2003; Wright et al., 2005; Norden et al., 2007; Poorter et al., 2010). However, when seed-to-seedling recruitment failures, resprouts (root, basal, or epicormic resprouting) shows to be another important regeneration source, contributing to post-fire recovery and maintenance of diversity (Poorter et al., 2010). It remains unclear, however, how fire intensity and frequency affect tree resprouting as a recovery mechanism.
Amazon surface fires vary in extent, frequency, and intensity. How these metrics interact has important effects on post-fire trajectories of Amazon forests. When fires occur less frequently but are more intense (Balch et al., 2008), post-fire mortality of large trees tend to increase disproportionally (Brando et al., 2014). Because larger individuals tend to produce more seeds than small ones, high-intensity fires can substantially reduce seed production and associated regeneration. In addition, when fires occur less frequently, we expect more fuel accumulation, higher fuel continuity, and higher burned area—an important predictor of fire-related seedling and sapling mortality (Balch et al., 2011, 2015). Although high fire frequency may also kill reproductive trees and drive changes in seed sources, these fires are likely to be less intense. However, frequent fires can still substantially affect forest regeneration by killing seedlings, resprouts and seeds in the seed bank (Montibeller-Santos, 2014; Cury, 2016). Few studies though have quantified how different fire frequencies and intensity affect tropical forest regeneration.
Overall, understory fires are expected to be stronger at forest edges, where post-fire reduction in seedling and soil seed bank abundance are likely to be higher, both by direct killing of seeds and seedlings and by reduced seed rain, which in turn is caused by increased tree mortality. The delayed mortality of large trees following fire (Silvério et al., 2018) may also contribute to changes in the forest microclimate and thus strongly influence seed germination and seedling establishment (Clark et al., 1999; Cochrane and Schulze, 1999; Cochrane, 2003; Peres et al., 2003; Wright et al., 2005; Barlow and Peres, 2006; Balch et al., 2015).
Here, we assessed how changes in fire frequency and edge distance affect forest regeneration and two associated processes: seed rain and storage of seeds in the soil. We studied these processes in a large burning experiment conducted in a transitional forest, between Amazonia and Brazilian Cerrado. Our main predictions are that: (1) fire drives reduction in forest regeneration capacity by reducing the number of regenerating plants and the seed rain and seed bank, with stronger effects where fires are more frequent; (2) forest regeneration processes (seedlings, resprouts, seed rain, and seed bank) are more severely impacted near the forest edges; (3) post fire forest regeneration mode shifts from seedling to resprouting.
Beginning in 2004, a large-scale burning experiment (150-ha) was conducted in a transitional forest, located between Amazon rainforest and Brazilian Cerrado (Savanna), in a privately owned agricultural holding (80,000-ha), in Mato Grosso state, Brazil (13°04′35″ S; 52°23′08″ W; Figure 1). The farm was partially cleared (~50%) for pasture in the 1980s and was converted for soybean cultivation between 2003 and 2008.
Figure 1. Sampling design of a long-term fire experiment in Mato Grosso state, southeastern Amazon Basin. Lines indicate the regeneration plots and seed rain mesh traps distributed from agriculture edge to forest interior (0–750 m). The “x” indicates soil seed bank sampling points. B0 (control) was not burned, B3 was burned three times with 3 years interval, and B6 was burned annually, six times.
The soil is classified as dystrophic red-yellow Oxisols by Instituto Brasileiro de Geografia e Estatística (IBGE) (2009), being deep, well-drained and with low natural fertility (Instituto Brasileiro de Geografia e Estatística (IBGE), 2007). According to Köppen's classification, the climate is tropical Aw, with an average annual precipitation of 1,770 mm (data from 2005 to 2011; Rocha et al., 2014), a rainy season extending from September to April, and a dry season between May and August, when monthly rainfall is typically below 10 mm (Rocha et al., 2014).
The vegetation is classified as Evergreen Seasonal Forest, with low deciduousness on dry season (Instituto Brasileiro de Geografia e Estatística (IBGE), 2012). Due to the proximity of the Brazilian Cerrado this forest is also known as “transitional forest” (Kunz et al., 2009). The forest has a relatively low canopy height (averaging 20 m) and lower plant diversity in comparison with the wetter forests of the central Amazon (Balch et al., 2008). Furthermore, about 97 species of trees and lianas ≥10 cm diameter at 1.3 m stem diameter breast height (DBH) were identified over the entire 150-ha experimental area (Balch et al., 2011). The nine most common species accounted for 50 percent of the Vegetation Importance Index (Balch et al., 2008, 2011), and more than 74% of tree and shrub species also occur in the adjacent Cerrado vegetation (Cury, 2016).
The experimental site was located in a forest with no history of logging or previous fires. The experimental area of 1.5 × 1.0 km (150-ha; Figure 1) was subdivided into three 50-ha plots: an unburned Control (B0), a plot burned three times at 3-year interval (B3; burned in 2004, 2007, and 2010), and a plot burned six times (B6), with annual experimental fires from 2004 to 2010 (except for 2008). The controlled fires were conducted toward the end of the dry season (August) and were carried out by a large team of researchers, students, volunteers, technicians, firefighters, farm workers, among others. To know more about the site description, the study design, aboveground biomass, canopy height, canopy density, and the burning experiment with the ignition technique that were used to burn the plots (see Balch et al., 2008).
The seed rain was sampled biweekly for 36 months, starting after the last fire event in September 2010 until August 2013. We collected seed rain using 0.5 m2 mesh traps (N = 90 per 50-ha plot) placed systematically throughout the experimental plots, from the cleared edge to the forest interior (0, 10, 15, 30, 50, 100, 250, 500, and 750 m; Figure 1). Traps were suspended ~1 m above the forest floor adjacent to regeneration sub-plots (described below). Number of seeds was quantified, and all seeds were identified to species or at least genera by means of comparison with a reference seed collection.
Two years after the 2010 fires, 120 soil samples were taken from the burned treatments and the control (N = 40 per plot) using a cylinder with 20 cm diameter and 5 cm deep. The samples were collected at 0, 30, 100, 500, and 750 m from forest edge. One soil sample was composed by a mix of two cores taken from <2 m away from the regeneration sub-plot (Figure 1). Samples were then stored in polythene bags placed in Styrofoam boxes, in a shaded cover for 1 week. Afterward, the soil was spread in perforated plastic trays, keeping soil layer height up to 3 cm, and placed in a greenhouse watered twice a day. Four control trays containing only sterilized sand were randomly distributed among the samples to control potential contamination from seeds dispersed through the greenhouse. Seedlings were weekly censored for the period of 1 year. All individuals that emerged were counted, identified through comparison with seedlings, juveniles and adult materials previously collected during fieldwork. Non-identified seedlings were transplanted into pots and grown until a larger size to further identification. Six months after the beginning of the experiment, the soil was overturned to promote the germination of seeds that were deeply buried. This method was adapted from Roberts (1981).
The focus of this study was the woody vegetation: therefore, all seedlings, saplings and resprouts of tree, shrub and liana species were recorded in 90 0.5 m2 sub-plots per 50-ha plot. Sub-plots were distributed systematically adjacent to seed traps, from the edge to the forest interior (Figure 1). All plants taller than 5 cm and ≤ 1 cm DBH (diameter at breast height or 1.30 m above ground) were tagged and identified. The regeneration mode (i.e., individuals originating from seed germination or root, basal, or epicormic resprouts) was also recorded. Whenever needed, small excavations near the plant base were done to confirm the resprouting origin. Censuses were conducted annually in 2011, 2012, and 2013. If a given individual was present in one inventory and not in the next, it was considered dead. Plants that were present in those inventories but lacked living structures and had no resprouting were recorded as dead. Plant species were identified using the botanical collection of IPAM (Amazon Environmental Research Institute) and the Herbarium NX at Mato Grosso State University at Nova Xavantina. Species names followed the Missouri Botanical Garden Tropicos® database (www.tropicos.org; Table S1).
To evaluate the degree in which fire frequency (triennially and annually burned) affect species diversity, we analyzed patterns of species richness and abundance using individual-based species accumulation curves for seeds from seed rain, seeds stored in the soil, seedlings and resprouts. Also, structure and composition of communities (i.e., seed rain, seed bank, seedlings and resprouts) were analyzed using non-metric multidimensional scaling (NMDS). The NMDS was generated from a Bray-Curtis dissimilarity matrix and a posteriori analysis of similarities (ANOSIM) was used to test whether there are significant differences among burned plots and the control, and to analyze whether structure and composition of seedling assemblages were similar to that in the seed rain and seed bank samples. We also analyzed species abundance by means of Whittaker plots for seeds and regenerating woody plants.
A generalized linear model (GLM) was used to account for differences in the species richness and abundance among edge distances (nine distances for seed rain, seedlings and resprouts; five distances for seed bank), and also among years (2011, 2012, 2013, except for seed bank that was collected only in 2012). GLM was performed using Poisson distribution, indicated for counts with Quasi-Poisson correction when data presented variance higher than average.
Diversity index of seedlings, resprouts, seed rain and seed bank in the Table S3 was performed using the Shannon Index, the most commonly used, although it considers equal weight between rare and abundant species; and Simpson's diversity index (D) that provides a good estimate of diversity in small population sizes (Magurran, 1988). We also calculated Pielou's evenness (equitability).
All analyses were performed using R (R Core Team, 2014) and the package Vegan (Oksanen et al., 2013).
We collected a total of 39,356 seeds spread across 67 species (Tables S1, S2): 15,911 (59 species) in B0; 9,135 (52 species) in B3; and, 14,310 (52 species) in B6 (Figure 2). The seed rain density in the Control (118 seeds/m2) was higher than in B3 (68 seeds m2) but similar compared to B6 (106 seeds m2; Figure 2). Species richness in the seed rain was also significantly higher in the Control than in the burned plots (see the Species Accumulation Curves; Figure 3A). In addition, according to our NMDS analysis, the structure and composition of the community in the seed rain were similar between the two burned treatments, and both differed from the Control (ANOSIM: R = 0.07; P = 0.001). Sloanea eichleri was the most abundant species in B3, accounting for 50% of the total number of seeds in seed rain in this treatment; Xylopia frutescens was the most abundant species in B6 (Figure 4A).
Figure 2. Contribution of seed rain, seed bank, seedlings, and resprouts for forest regeneration, 3 years after fire exclusion. Species richness (S), density (ind/m2), and recruiting rates (% of seedlings by total of seeds arriving) and annual mortality rates (%). B0 (control) was not burned, B3 was burned three times with 3 years interval, and B6 was burned annually, six times.
Figure 3. Individual-based species accumulation curves for seed rain (A), seed bank (B), seedlings (C), and resprouts (D). B0 (control) was not burned, B3 was burned three times with 3 years interval, and B6 was burned annually, six times. B0 shows 95% confidence interval.
Figure 4. Whittaker's plot (rank-relative abundance diagram) of woody species (trees and lianas) sampled from the seed rain (A), seed bank (B), seedlings (C), and resprouts (D). The two more abundant species by treatment were identified in the plot. B0 (control) was not burned, B3 was burned three times with 3 years interval, and B6 was burned annually, six times.
Species richness in the seed rain was lower near the edge than in the forest interior in both burned plots, with a stronger effect in B3 than in B6 (Table S4; Figure 5A). In contrast, in the Control plot species richness was similar between the forest edge and interior (Table S4; Figure 5A). Seed rain density was also lower at the forest edge and up to 500 and 750 m into the forest in B3 and B6, respectively. In the Control, edge effects on seed density were observed only in the first 15 m from the forest border. The number of species and density of seeds in the seed rain were constant along the years in both burned treatments and in the Control.
Figure 5. Number of woody species sampled from seed rain (A), seed bank (B), seedlings (C), and resprouts (D) by distance from forest edge. B0 (control) was not burned, B3 was burned three times with 3 years interval, and B6 was burned annually, six times. Standard error bars. Asterisks indicate significant differences between distances (10–750 m) with the edge (0 m; GLM; P < 0.05).
In soil seed bank samples, we recorded 2,330 viable seeds from 34 species (Tables S1, S2): 1,352 in the Control (30 species; 538 seeds/m2); 310 in B3 (17 species; 123 seeds/m2); and 668 in B6 (18 species; 266 seeds/m2; Figure 2). Species accumulation curve showed that species richness was higher in the Control than in B6, but similar between B6 and B3 (Figure 3B). The structure and composition of the soil seed bank community was also affected by the experimental fires, with significant differences between the Control and burned plots (ANOSIM: R = 0.33; P = 0.001). Miconia was the most abundant genus in the seed bank, with its five species contributing 84, 92, and 95% of the total seeds in the seed bank in the Control, B3 and B6, respectively (Figure 4B). Surprisingly, despite being the most abundant species in the seed bank, no species from the genus Miconia was found among the regeneration.
Edge distance influenced the species richness and abundance of seeds in the seed bank in both burned treatments. Compared with the forest interior, we observed lower species richness in the seed bank samples in B3 and B6 up to 500 and 100 m (Table S4; Figure 5B), respectively. Also, the abundance of seeds was reduced up to 500 m from edge in B3 (GLM, P < 0.004), and along all distances in B6, presenting no significant differences between 0 and 750 m.
Summarizing, we observed a large reduction in seed availability in both fire treatments. The species richness and the number of seeds of both seed sources (seed rain and seed bank) were reduced, for example, decreased 45 and 13% of seeds, respectively, in B3 and B6, compared with B0 (Figure 2).
Overall, we registered 114 species in the four sampling components (seed rain, seed bank, seedlings, and resprouts; Table S1). From this total, only four species were represented in all components (Amaioua guianensis, Doliocarpus spatulifolius, Solanum mauritianum, and Tachigali vulgaris); and 55 in only one component (Table S1). These results suggest that regeneration occurred via multiple pathways.
The sampled regeneration (seedlings and resprouts) comprised 72 species and 739 individuals. From all species, 65 were found in the Control, 44 in B3, and 18 in B6 (Figure 2). Summing the seedlings and resprouts together, the density of these small individuals was higher in the Control (3.3 ind/m2) than in B3 (1.2 ind/m2) and B6 (0.9 ind/m2; Figure 2). Evaluating only seedlings, the species accumulation curves indicate that species richness also decreased with increasing fire frequency (55 species in the Control; 23 in B3; and 18 in B6 (Figure 3C). However, the density of seedlings was 87% lower in B3 (49 individuals; 0.4 ind/m2) and 73% lower in B6 (106 individuals; 0.8 ind/m2), compared with Control (391 individuals; 2.9 ind/m2; Figure 2).
Interestingly, seeds in the seed rain (from 2011 to 2013) and soil seed bank samples (2012), only 2.4% resulted in new seedlings in the Control, vs. 0.6–0.7% in the burned plots (Figure 2). Also, our analysis showed that the seedlings samples were more similar to the seed rain samples [ANOSIM; R = 0.35 (B3) and 0.36 (B6)] than to the seed bank [ANOSIM; R = 0.41 (B3) and 0.64 (B6)] in both burned plots (Figure 6).
Figure 6. Analysis of similarities (ANOSIM), based on Non-metric multidimensional scaling (NMDS–Bray-Curtis similarity index) comparing the similarities of the structure and species composition of seed source samples (seed rain and seed bank) and seedlings. B0 (control) was not burned, B3 was burned three times with 3 years interval, and B6 was burned annually, six times.
B3 had two times more species and 5.5 times more live stems of resprouts than B6 (Figures 2, 3D). This is important to explain why the sum of regeneration stems (i.e., counting seedlings and resprouts) presented more than twice the number of species and individuals in B3 than in B6. Pyrostegia venusta, the most abundant liana in B3, was present both as seedlings and resprouts (Figures 4C,D).
When looking at distance from forest edge, both burned treatments exhibited reduced species richness of seedlings along all distances. A different pattern was registered in the Control, which increased gradually the number of species beginning at 30 m from the edge (Figure 5C). Resprouting species richness had no difference in the Control regardless of edge distance. Contrasting, B3 increased the number of species with regrowth of stems in all distances (Figure 5D).
We noticed that in the second year after fires (2012) the seedling species richness increased in B3, as well as the density in B3 and B6 (Figure 7A). In 2012, only two species accounted for 90% of all seedlings recruited in B6 treatment, with Pouteria ramiflora alone accounting for 66.5% and Amaioua guianensis for 23.5%. In contrast, resprouts showed a gradual reduction through the years in both burned plots, losing species and density in the third year after fire exclusion in 2013 (Figure 7B). The Control had no resprouting species and density variation through the years (Figures 7A,B). Finally, seedling mortality was similar among plots, around 29% per plot per year. On the other hand, resprout mortality was similar between Control (19.7%) and B3 plot (17.6%), but higher in B6 (36.9%; Figure 2).
Figure 7. Temporal assessment (2011, 2012, 2013) of seedlings (A) and resprouts (B) density and species richness. B0 (control) was not burned, B3 was burned three times with 3 years interval, and B6 was burned annually, six times. Asterisks indicate differences with the first year (P < 0.05).
Experimental fires caused substantial reduction in the abundance and diversity of seedlings in both fire treatments by reducing the seed rain, killing seedlings and impoverishing the seed bank. We also observed decreasing post-fire resprouting due complex fire effects. These results suggest that recurrent fires potentially threaten all regeneration modes, reducing forest postfire recovery ability. The differences in regeneration patterns along forest edge-to-interior gradients also suggest that forest fragmentation, by creating more edges, can further reduce post-fire forest regeneration (Silvério et al., 2018). Along these highly flammable edges, high-intensity fires can cause substantial reduction in seedlings, resprouts, seed rain, and seed bank. Although forest regeneration may recover over longer time spans (Brando et al., 2014; Balch et al., 2015), it is unclear how the observed changes in regeneration associated with fire will influence future plant communities.
The first hypothesis about reduction in forest regeneration capacity by reducing the number of regenerating plants and the seed rain and seed bank in the treatment burned six times annually was partially corroborated. The most likely explanation for this result is differences in fire intensity between the two treatments. The longer fire interval in the treatment burned three times triennially, allowed increased fire intensity and severity during the droughts of 2007 and 2010 (Brando et al., 2014; Balch et al., 2015). As a result, both seed sources (seed rain and seed bank) and the pool of resprouting stems experienced sharper declines, especially at the forest edges, where the fires were even more intense (Kennard et al., 2002; Barlow and Peres, 2006; Brando et al., 2014; Montibeller-Santos, 2014; Ribeiro and Borghetti, 2014). Furthermore, post-fire tree mortality was lower in the high fire frequency plot (Brando et al., 2019). This helps to explain why the seedling assemblage was more similar with the seed rain than with the soil seed bank community. Thus, corroborating Barlow and Peres (2006), local fruit production represents an important source of viable seeds for post-fire forest recovery, at least during the earliest stages of regeneration.
Our prediction that post-fire forest regeneration was lower at the forest edge was soundly confirmed. Our results showed that seedlings and seed sources (seed rain and seed bank) were lower within the first 100 m from the agricultural field neighboring the treatment plots. These patterns probably result from the increased dry plant material and lower humidity along forest edges. These characteristics can trigger intense and severe fires (Brando et al., 2014) causing death of large fruiting trees (Barlow and Peres, 2006) and reduction of seeds stored in the soil (Melo et al., 2007). Moreover, three interdependent processes could also contribute to these results: reduced seed-dispersing animal abundance near the edge (Peres et al., 2003); increased seed predation and increased seedling herbivory (Carvalho et al., 2012; Massad et al., 2013); and seed dehydration (Daws et al., 2005).
Our prediction that regeneration mode is more dependent on resprouting than seedling germination received strong support. We noted that resprouts increased in density just after fire (2011) in the plot burned three times, particularly near the forest edge. As a result, there was increased species diversity in that treatment compared with the plot burned six times. As reported in previous studies, vegetative growth is an adaptive trait allowing response to disturbance and therefore, is a key process for rapid forest recovery (Hoffmann et al., 2003; Poorter et al., 2010). However, we observed that resprouting stem density and species richness declined over time in both burned treatments, especially where fires were more frequent. The possible explanation for this pattern is that the repeated fire events killed the resprouting bank or reduced the capacity of plants to allocate non-structural carbohydrate storage for vegetative growth (Hoffmann et al., 2003). This is compatible with the two-fold greater mortality in the plot burned six times annually (37%) than in the plot burned three times triennially (17.6%) and suggest a major limitation for the recovery of transitional forests after the occurrence of fires.
The future trajectory of burned forests strongly depends on how species regenerate in response to one or more fires. According to the prediction that pioneer species would prevail in the soil seed bank and in the regeneration after fires (Garwood, 1989; Montibeller-Santos, 2014), we expected to find some similarity between soil seed bank and seedlings. The high abundance of Miconia sp. in the soil seed bank could indicate a high potential for this species to dominate the early succession after the fires (Dalling et al., 1998; Filho et al., 2005). However, we observed no seedlings of this genus in both burned treatments. Moreover, the seed rain was a driver for the first pulse of regeneration, with several species being present in the seed rain and as seedlings (e.g., Amaioua guianensis, Pouteria ramiflora). We speculate that there are at least three explanations for this result. First, species-specific traits may promote rapid post-fire germination and could be an advantage for some desiccation-sensitive species coming from seed rain, with higher seed nutrient reserve (e.g., Ocotea sp., Pouteria ramiflora, Mabea fistulifera, Virola sebifera, Sacoglottis guianensis), allowing reduction in seed exposure to predation and desiccation (Daws et al., 2005). Second, seedlings with small seeds (e.g., Miconia spp.) may be outcompeted, particularly by fast-spreading invasive grasses near to the forest edge (Snyder and Chesson, 2003; Reid and Holl, 2013; Silvério et al., 2013; Balch et al., 2015).
Our results show that recurrent fires can have lasting effects on potential trajectories of tropical forests. Not only fires have major direct effects on the diversity and structure of tropical forests, but also on the capacity of these forests to recover its diversity. We showed that frequent fires can drive reduction in both abundance and species richness of seed rain, seed bank, seedlings, and resprouts, and that this reduction is stronger at forest edges. Monitoring burned areas is essential to understand how the biodiversity will change in the future. Specially now when the deregulation in the current Brazilian environmental legislation will lead to the acceleration in deforestation, forest fragmentation and occurrence of fires.
The datasets generated for this study are available on request to the corresponding author.
PB and JB conceived the burning experiment. RC, JT, and PB conceived the sampling design. RC, CM-S, and PB carried out the experiment in the field. RC wrote the manuscript with support from JB, PB, and JT. All authors contributed to the final version of the manuscript.
We thank IPAM and Londrina State University for financial support. RC benefited from a CNPq grant (#248491/2013-0) during internship term at University of Colorado-Boulder and JT from a CNPq research grant (#309244/2015-3). PB and JB acknowledged financial support from the National Science Foundation (NSF) Macrosystems and the National Council for Scientific and Technological Development (productive grant). IPAM staff, specially R. M. Quintino, S. Nascimento, A. R. Coelho, and D. N. Costa, provided invaluable help in fieldwork. D. Silvério, R. Andrade, C. Oliveira-Santos, C. C. C. Oliveira, A. L. Cavalheiro, R. P. Scervino, T. D. Lagasse, and N. M. S. Cury provided helpful suggestions on an earlier draft of this paper.
The authors declare that the research was conducted in the absence of any commercial or financial relationships that could be construed as a potential conflict of interest.
The Supplementary Material for this article can be found online at: https://www.frontiersin.org/articles/10.3389/ffgc.2020.00082/full#supplementary-material
Alencar, A., Nepstad, D. C., and Diaz, M. C. V. (2006). Forest understory fire in the Brazilian Amazon in ENSO and non-ENSO years: area burned and committed carbon emissions. Earth Interact. 10, 1–17. doi: 10.1175/EI150.1
Aragão, L. E. O. C., Anderson, L. O., Fonseca, M. G., Rosan, T. M., Vedovato, L. B., Wagner, F. H., et al. (2018). 21st Century drought-related fires counteract the decline of Amazon deforestation carbon emissions. Nat. Commun. 9:536. doi: 10.1038/s41467-017-02771-y
Balch, J. K., Brando, P. M., Nepstad, D. C., Coe, M. T., Silvério, D., Massad, T. J., et al. (2015). The susceptibility of southeastern Amazon Forests to fire: insights from a large-scale burn experiment. BioScience 65, 953–905. doi: 10.1093/biosci/biv106
Balch, J. K., Massad, T. J., Brando, P. M., Nepstad, D. C., and Curran, L. M. (2013). Effects of high-frequency understorey fires on woody plant regeneration in southeastern Amazonian forests. Philos. Trans. R. Soc. B. 368:20120157. doi: 10.1098/rstb.2012.0157
Balch, J. K., Nepstad, D. C., Brando, P. M., Curran, L., Portela, O., Carvalho, O., et al. (2008). Negative fire feedback in a transitional forest of southeastern Amazonia. Glob. Chang. Biol. 14, 2276–2287. doi: 10.1111/j.1365-2486.2008.01655.x
Balch, J. K., Nepstad, D. C., Curran, L. M., Brando, P. M., Portela, O., Guilherme, P., et al. (2011). Size, species, and fire behavior predict tree and liana mortality from experimental burns in the Brazilian Amazon. For. Ecol. Manage. 261, 68–77. doi: 10.1016/j.foreco.2010.09.029
Barlow, J., and Peres, C. A. (2006). Effects of single and recurrent wildfires on fruit production and large vertebrate abundance in a central Amazonian forest. Biodivers. Conserv. 15. 985–1012. doi: 10.1007/s10531-004-3952-1
Barlow, J., Peres, C. A., Lagan, B. O, and Haugaasen, T. (2003). Large tree mortality and the decline of forest biomass following Amazonian wildfires. Ecol. Lett. 6, 6–8. doi: 10.1046/j.1461-0248.2003.00394.x
Bond, W. J., and Midgley, J. J. (2003). The evolutionary ecology of sprouting in woody plants. Int. J. Plant Sci. 164, 103–114. doi: 10.1086/374191
Brando, P. M., Balch, J. K., Nepstad, D. C., Morton, D. C., Putz, F. E., Coe, M. T., et al. (2014). Abrupt increases in Amazonian tree mortality due to drought-fire interactions. Proc. Natl. Acad. U.S.A.111, 6347–6352. doi: 10.1073/pnas.1305499111
Brando, P. M., Nepstad, D. C., Balch, J. K., Bolker, B., Christman, M. C., Coe, M., et al. (2012). Fire-induced tree mortality in a neotropical forest: the roles of bark traits, tree size, wood density and fire behavior. Global Change Biol. 18, 630–641. doi: 10.1111/j.1365-2486.2011.02533.x
Brando, P. M., Paolucci, L., Ummenhofer, C. C., Ordway, E. M., Hartmann, H., Cattau, M. E., et al. (2019). Droughts, wildfires, and forest carbon cycling: a pantropical synthesis. Annu. Rev. Earth Planet. Sci. 47, 555–581. doi: 10.1146/annurev-earth-082517-010235
Brando, P. M., Soares-Filho, B., Rodrigues, L, Assunção, A., Morton, D., and Tuchschneider, D. (2020) The gathering firestorm in southern Amazonia. Sci. Adv. 6:eaay1632. doi: 10.1126/sciadv.aay1632.
Carvalho, K. S., Balch, J., and Moutinho, P. (2012). Influências de Atta spp (Hymenoptera: Formicidae) na recuperação da vegetação pós-fogo em floresta de transição amazônica. Acta Amaz. 42, 81–88. doi: 10.1590/S0044-59672012000100010
Clark, J. S., Beckage, B., Camill, P., Cleveland, B., HilleRislambers, J., Lichter, J., et al. (1999). Interpreting recruitment limitation in forests. Am. J. Bot. 86, 1–16. doi: 10.2307/2656950
Cochrane, M. A., and Schulze, M. D. (1999). Fire as a recurrent event in tropical forests of the eastern Amazon: effects on forest structure, biomass, and species composition. Biotropica 31, 2–16. doi: 10.1111/j.1744-7429.1999.tb00112.x
Coe, M. T., Marthews, T. R., Costa, M. H., Galbraith, D. R., Greenglass, N. L., Imbuzeiro, H. M. A., et al. (2013). Deforestation and climate feedbacks threaten the ecological integrity of south – southeastern Amazonia. Philos. Trans. R. Soc. B 368:20120155. doi: 10.1098/rstb.2012.0155
Cury, R. T. S. (2016). Respostas da vegetação a diferentes frequências e intensidades de incêndios no sudeste da Bacia Amazônica (Ph.D. dissertation). Universidade Estadual de Londrina, Londrina, Brazil.
Dalling, J. W., Swaine, M. D., and Garwood, N. C. (1998). Dispersal patterns and seed bank dynamics of pioneer trees in moist tropical forest. Ecology. 79, 564–578. doi: 10.1890/0012-9658(1998)079[0564:DPASBD]2.0.CO;2
Daws, M. I., Garwood, N. C., and Pritchard, H. W. (2005). Traits of recalcitrant seeds in a semi-deciduous tropical forest in Panama: some ecological implications. Funct. Ecol. 19, 874–885. doi: 10.1111/j.1365-2435.2005.01034.x
Filho, N. L., Sena, S., and Rodrigues, G. (2005). Variações espaço-temporais no estoque de sementes do solo na floresta amazônica. Acta Amaz. 43, 305–314. doi: 10.1590/S0044-59672013000300006
Garwood, N. C. (1989). “Tropical soil seed banks: a review,” in Ecology of Soil Seed Bank, eds M. A. Leck, V. T. Parker, and R. L. Simpson (San Diego, CA: Academic Press), 149–209. doi: 10.1016/B978-0-12-440405-2.50014-2
Hoffmann, W. A., Orthen, B., and Nascimento, P. K. V. (2003). Comparative fire ecology of tropical savanna and forest trees. Funct. Ecol. 17, 720–726. doi: 10.1111/j.1365-2435.2003.00796.x
Instituto Brasileiro de Geografia e Estatística (IBGE) (2007). Manual Técnico de Pedologia, 2nd Edn. Rio de Janeiro: IBGE.
Instituto Brasileiro de Geografia e Estatística (IBGE) (2009). Mapa de Solos do Brasil, 1st Edn. Available online at: http://mapas.ibge.gov.br/en/tematicos/solos (accessed February 14, 2014).
Instituto Brasileiro de Geografia e Estatística (IBGE) (2012). Manual Técnico da Vegetação Brasileira, 2nd Edn. Rio de Janeiro: IBGE.
Kennard, D. K., Gould, K., Putz, F. E., Fredericksen, T. S., and Morales, F. (2002). Effect of disturbance intensity on regeneration mechanisms in a tropical dry forest. For. Ecol. Manage. 162, 197–208. doi: 10.1016/S0378-1127(01)00506-0
Kunz, S. H., Ivanauskas, N. M., Martins, S. V., Silva, E., and Stefanello, D. (2009). Análise da similaridade florística entre florestas do Alto Rio Xingu, da Bacia Amazônica e do Planalto Central. Rev. Bras. Bot. 4, 725–736. doi: 10.1590/S0100-84042009000400011
Lindenmayer, D. B., Hunter, M. L., Burton, P. J., and Gibbons, P. (2009). Effects of logging on fire regimes in moist forests. Conserv. Lett. 2, 271–277. doi: 10.1111/j.1755-263X.2009.00080.x
Magurran, A. E. (1988). Ecological Diversity and its Measurement. Princeton, NJ: Princeton University Press. doi: 10.1007/978-94-015-7358-0
Massad, T. J., Balch, J. K., Davidson, E. A., Brando, P. M., Mews, C. L., Porto, P., et al. (2013). Interactions between repeated fire, nutrients, and insect herbivores affect the recovery of diversity in the southern Amazon. Oecologia 172, 219–229. doi: 10.1007/s00442-012-2482-x
Melo, A. C. G., Durigan, G., and Gorenstein, M. R. (2007). Efeito do fogo sobre o banco de sementes em faixa de borda de Floresta Estacional Semidecidual, SP, Brasil. Acta Bot. Bras. 21, 927–934. doi: 10.1590/S0102-33062007000400017
Montibeller-Santos, C. (2014). Os efeitos de incêndios recorrentes sobre o banco de sementes da floresta de transição Amazônia-Cerrado (Master's thesis), Universidade Estadual de Londrina, Londrina, Brazil.
Morton, D. C., Le Page, Y., DeFries, R., Collatz, G. J., and Hurtt, G. C. (2013). Understorey fire frequency and the fate of burned forests in southern Amazonia. Philos. Trans. R. Soc. B 368:20120163. doi: 10.1098/rstb.2012.0163
Nepstad, D., Carvalho, G., Barros, A. C., Alencar, A., Capobianco, J. P., Bishop, J., et al. (2001). Road paving, fire regime feedbacks, and the future of Amazon forests. For. Ecol. Manage. 154, 395–407. doi: 10.1016/S0378-1127(01)00511-4
Nepstad, D. C., Stickler, C. M., Soares-Filho, B., and Merry, F. (2008). Interactions among Amazon land use, forests and climate: prospects for a near-term forest tipping point. Philos. Trans. R. Soc. B. 363, 1737–1743. doi: 10.1098/rstb.2007.0036
Norden, N., Chave, J., Caubère, A., Châtelet, P., Ferroni, N., Forget, P. M., et al. (2007). Is temporal variation of seedling communities determined by environment or by seed arrival? A test in a neotropical forest. J. Ecol. 95, 507–516. doi: 10.1111/j.1365-2745.2007.01221.x
Oksanen, J., Blanchet, F. G., Kindt, R., Legendre, P., Minchin, P. R., O'Hara, R. B., et al. (2013). Vegan: Community Ecology Package. R package version 2.3–1. Available online at: http://CRAN.R-project.org/package=vegan (accessed July 07, 2020)
Peres, C. A., Barlow, J., and Haugaasen, T. (2003). Vertebrate responses to surface wildfires in a central Amazonian forest. Oryx 37, 1–13. doi: 10.1017/S0030605303000188
Poorter, L., Kitajima, K., Mercado, P., Chubiña, J., Melgar, I., and Prins, H. H. T. (2010). Resprouting as a persistence strategy of tropical forest trees: relations with carbohydrate storage and shade tolerance. Ecology 91, 2613–2627. doi: 10.1890/09-0862.1
R Core Team (2014). R: A Language and Environment for Statistical Computing. Vienna: R Foundation for Statistical Computing. Available online at: http://www.R-project.org
Reid, J., and Holl, K. (2013). Arrival ≠ Survival. Rest. Ecol. 21, 135–155. doi: 10.1111/j.1526-100X.2012.00922.x
Ribeiro, L. C., and Borghetti, F. (2014). Comparative effects of desiccation, heat shock and high temperatures on seed germination of savanna and forest tree species. Austral Ecol. 39, 267–278. doi: 10.1111/aec.12076
Roberts, H. A. (1981). “Seed banks in soils,” in Advances in Applied Biology (Cambridge: Academic Press), 55.
Rocha, W., Metcalfe, D. B., Doughty, C. E., Brando, P. M., Silvério, D., Halladay, K., et al. (2014). Ecosystem productivity and carbon cycling in intact and annually burnt forest at the dry southern limit of the Amazon rainforest (Mato Grosso, Brazil). Plant Ecol. Divers. 7, 25–40. doi: 10.1080/17550874.2013.798368
Silvério, D. V., Brando, P. M., Balch, J. K., Putz, F. E., Nepstad, D. C., Bustamante, M. M. C., et al. (2013). Testing the Amazon savannization hypothesis: fire effects on invasion of a neotropical forest by native Cerrado and exotic pasture grasses. Philos. Trans. R. Soc. B 368:20120427. doi: 10.1098/rstb.2012.0427
Silvério, D. V., Brando, P. M., Bustamante, M. M. C., Putz, F. E., Marra, D. M., Levick, S. R., et al. (2018). Fire, fragmentation, and windstorms: a recipe for tropical forest degradation. J. Ecol. 107, 656–667. doi: 10.1111/1365-2745.13076
Sistema de Estimativas de Emissões e Remoções de Gases de Efeito Estufa (SEEG) (2020). Nota Técnica - Impacto da Pandemia de Covid-19 Nas Emissões de Gases de Efeito Estufa no Brasil. Available online at: http://www.observatoriodoclima.eco.br/wp-content/uploads/2020/05/SEEG-OC_Nota_Tecnica_Covid19_Final.pdf (accessed May 24, 2020).
Snyder, R. E., and Chesson, P. (2003). Local dispersal can facilitate coexistence in the presence of permanent spatial heterogeneity. Ecol. Lett. 6, 301–309. doi: 10.1046/j.1461-0248.2003.00434.x
Staver, A. C., Brando, P. M., Barlow, J., Morton, D. C., Paine, C. E. T., Malhi, Y., et al. (2019). Thinner bark increases sensitivity of wetter Amazonian tropical forests to fire. Ecol. Lett. 23, 99–106. doi: 10.1111/ele.13409
Keywords: deforestation, global climate change, resprout, seed rain, soil seed bank, understory fire
Citation: Cury RTdS, Montibeller-Santos C, Balch JK, Brando PM and Torezan JMD (2020) Effects of Fire Frequency on Seed Sources and Regeneration in Southeastern Amazonia. Front. For. Glob. Change 3:82. doi: 10.3389/ffgc.2020.00082
Received: 06 December 2019; Accepted: 12 June 2020;
Published: 21 July 2020.
Edited by:
Francisco Seijo, National University of Patagonia San Juan Bosco, ArgentinaReviewed by:
Blanca Cespedes, New Mexico Highlands University, United StatesCopyright © 2020 Cury, Montibeller-Santos, Balch, Brando and Torezan. This is an open-access article distributed under the terms of the Creative Commons Attribution License (CC BY). The use, distribution or reproduction in other forums is permitted, provided the original author(s) and the copyright owner(s) are credited and that the original publication in this journal is cited, in accordance with accepted academic practice. No use, distribution or reproduction is permitted which does not comply with these terms.
*Correspondence: Roberta Thays dos Santos Cury, cnRzY3VyeUBnbWFpbC5jb20=
Disclaimer: All claims expressed in this article are solely those of the authors and do not necessarily represent those of their affiliated organizations, or those of the publisher, the editors and the reviewers. Any product that may be evaluated in this article or claim that may be made by its manufacturer is not guaranteed or endorsed by the publisher.
Research integrity at Frontiers
Learn more about the work of our research integrity team to safeguard the quality of each article we publish.