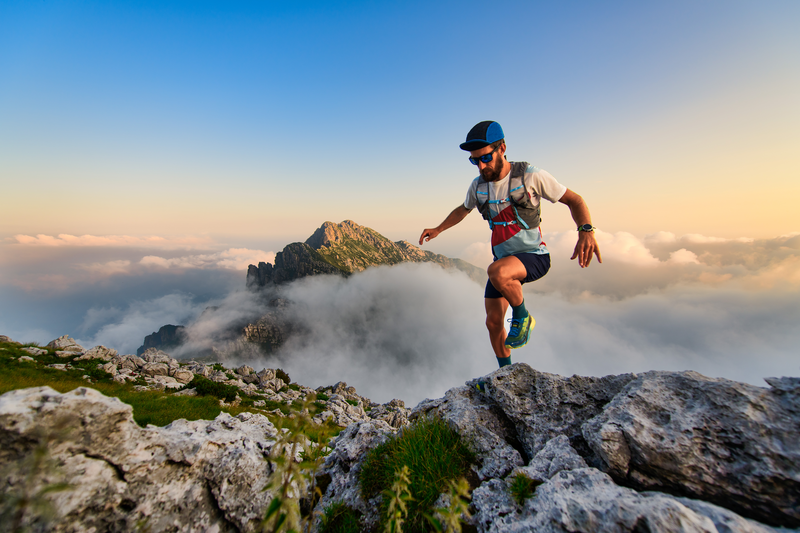
95% of researchers rate our articles as excellent or good
Learn more about the work of our research integrity team to safeguard the quality of each article we publish.
Find out more
PERSPECTIVE article
Front. For. Glob. Change , 23 June 2020
Sec. Tropical Forests
Volume 3 - 2020 | https://doi.org/10.3389/ffgc.2020.00070
This article is part of the Research Topic Improving Environmental Sustainability in Oil Palm: Linking Science, Policy, & Practice across the Tropics View all 18 articles
Tropical peatlands in Southeast Asia are important ecosystems that play a crucial role in global biogeochemical cycles, with a potential for strong climate feedback loops. The degradation of tropical peatlands due to the expansion of oil palm plantations and their impact on biodiversity and the carbon balance is a global concern. The majority of conversion of Southeast Asian peatlands to agriculture has been by smallholder oil palm farmers, who follow more varied cropping systems compared to industrial plantations, and have better scope for expansion of other alternative varied cropping systems if supported and encouraged. Using previously-published data on peat physicochemical properties, biodiversity and greenhouse gas emissions from small-holder oil palm plantations, we determined that prolonged oil palm monocropping for two generations would result in loss of carbon and peat functional properties that may lead to potential declassification of peatlands. We propose intercropping during the early stages of oil palm as a wise alternative for already-existing plantations in tropical peatlands to ameliorate some of the negative environmental impacts of oil palm on the physio-chemical properties of peat. However, we emphasize the need to more fully explore the sustainability of intercropping systems throughout the life cycle of palm plantations on peatlands, and integrate with current management practices. We also emphasize the further need for research to fully assess the impacts of oil palm intercropping compared to widely-practiced oil palm monocropping. Finally, we suggest changes in government certification policies to encourage intercropping practices by smallholders.
Tropical peatlands are important for their ecosystem services and distinct endemic biodiversity due to unique acidic, nutrient-poor, and waterlogged conditions (Sjögersten et al., 2011; Dohong et al., 2017). Anoxic conditions inhibit aerobic microbial activity and drive the accumulation of partially-decomposed plant organic materials, resulting in substantial carbon storage (Parish et al., 2008; Miettinen et al., 2012; Hodgkins et al., 2018). Under undisturbed conditions, tropical peatland acts as a long-term carbon sink and plays a crucial role in global carbon cycling, with the potential for strong climate feedback loops (Dommain et al., 2014; Sjögersten et al., 2014). Peatlands are frequently converted to agricultural production areas, which necessitates lowering of the water table by artificial drainage. Anthropogenic drainage switches peatlands from carbon sinks to carbon sources due to cessation of peat accumulation and enhanced aerobic peat decomposition (Hooijer et al., 2012; Dommain et al., 2018; Leifeld et al., 2019). This land use change is particularly widespread in Southeast Asian peatlands, where already 7.8 million ha (50%) were converted to agricultural areas by 2015 (Miettinen et al., 2016). Southeast Asia has the greatest proportion of tropical peatlands, with net carbon storage of 69 Gt (Page et al., 2011). This region also faces some of the highest rates of deforestation in the twenty first century (Hansen et al., 2013; Page and Hooijer, 2016). Approximately 25% of all forest degradation in the region occurs in peatlands (Lo and Parish, 2013). Oil palm, an important crop for food, cosmetic and energy needs, is behind much of this rapid change in the region (Miettinen and Liew, 2010; Koh et al., 2011). Oil palm is also expected to expand to other carbon rich tropical peatlands in the Americas and Africa with increasing global population and demand (Sayer et al., 2012).
Oil palm is native to West Africa and was first introduced to Southeast Asia as an ornamental plant, but expanded to an industrial scale that covers most of the cultivated area in Malaysia and Indonesia (Sheil et al., 2009). Oil palm plantations contributed to 75% of peat forest loss in Peninsular Malaysia, Sumatra and Borneo, between 2007 and 2015 (Miettinen et al., 2016). Although most of these large-scale land-use changes are associated with industrial oil palm plantations, there is considerable cover from smallholder oil palm plantations in Southeast Asia (Miettinen et al., 2016). However, before 2010 small-holder plantations had a slightly greater cover than industrial plantations in the region (Wijedasa et al., 2018). These smallholder plantations generally follow less intensive management practices by comparison to industrial oil palm plantations, but practices can vary greatly between land owners (Azhar et al., 2011). The smallholder plantations generally lack infrastructure for water table monitoring and thus may have less intensive drainage than industrial plantations (Dommain et al., 2016). Whilst almost all of the industrial plantations are under monocropping, the smallholder plantations tend to plant other crops, with 39% of independent smallholders and 9% of managed smallholders in a recent survey planting another crop (intercropping) in their oil palm fields, out of 300 respondents in 6 different regions in Peninsular Malaysia (Saadun et al., 2018). In spite of industrial plantations' omission of intercropping due to the complexity in mechanizing farm management and potential lower yield of oil palm, smallholders tend to practice intercropping for subsistence and extra income between oil palm fruiting cycles (Nchanji et al., 2016). An extensive study covering 18,000 ha of smallholders plantations in Peninsular Malaysia by Yahya et al. (2017) found no significant differences between monocropping and intercropping systems in terms of understorey vegetation cover or height. However, canopy cover, epiphyte cover and oil palm age and height were significantly reduced in intercropping systems (Yahya et al., 2017).
Industrial oil palm plantations started in the late 1970s but did not expand to peatlands until more recently around 1990s and accelerated throughout the 2000s (Miettinen et al., 2012; Shevade and Loboda, 2019). From the land cover data provided by Miettinen et al. (2012, 2016), with average oil palm cropping cycle of 25–30 years (Luskin and Potts, 2011), it is very likely that most of the oil palm plantations currently in peatlands are in their first generation or in the early stages of a second generation. There is therefore a lack of environmental data describing changes in peat properties following the introduction of a second oil palm generation, as well as a more general sparsity of data on variations between cropping systems in peatlands. In this article, we review the impacts of prolonged oil palm monocropping and discuss oil palm intercropping systems as an environmentally wiser use alternative for oil palm monocropping in peatlands.
While there have been several recent efforts to restore disturbed peatlands back to peat swamp forest, a vast majority remains as agriculture, primarily on repetitive cycles of oil palm and acacia (Miettinen et al., 2016; Shevade and Loboda, 2019). Considering that there are increasing numbers of oil palm plantation in peatlands getting into their second generation, there is a need to understand the impacts of subsequent generations on peat properties and carbon storage. Furthermore, any approaches to mitigate the substantial carbon losses and minimize the impacts of continued cultivation should be viewed as a wiser (though not sustainable) management of peatlands in the longer term. This has significant consequences for smallholder farmers who regularly practice mixed and polyculture agriculture. As such, here we briefly discuss the ameliorating effects of intercropping (alley cropping) over monocropping on peat properties, resultant emissions of greenhouse gases, and biodiversity, putting the work of multiple studies in the context of management strategies for oil palm. Taken together, these studies also cast concern over the sustainability of peat in such plantations after two generations of monocropping, potentially leading to the gradual disappearance of peat in all such oil palm plantations on peatlands, and our findings suggest that intercropping may slow this process.
The FAO (1988) describes bulk density as arguably the most important intrinsic peat characteristic, as it is also a characteristic that is closely related to many other defining peat properties, and widely used for classification and categorization of peat. For the establishment of oil palm plantations, peatlands are drained, completely cleared of vegetation and usually compacted (Luskin and Potts, 2011). In addition, drainage exposes peat to oxygen, increasing decomposition and bulk density. Thus, it is unsurprising that first generation plantations have double the bulk density observed in other peat land uses, including drained, burnt peatlands and some agricultural open areas (Firdaus et al., 2010; Kononen et al., 2015; Tonks et al., 2017; Dhandapani et al., 2019a). The FAO (1988) describes the range for bulk density in tropical peatlands as between 0.05 and 0.5 g cm−3. The observed bulk density by Dhandapani et al. (2019a) in second generation monocropping at 0.43 g cm−3 is quite close to the FAO's described higher limit for tropical peat. However, in spite of all the studied agricultural sites being in one single peat dome and close together, only being few hundred meters away from each other, second generation intercropping system maintained the same bulk density as the first generation intercropping or lower (Table 1).
This significant change in bulk density may both influence, and be influenced by, other defining peat characteristics such as organic matter content and moisture retention. The loss of organic matter content due to increased decomposition over time, along with mechanical compaction in agriculture peatlands, results in reduced porosity and denser peat, showing a strong correlation between loss of organic matter content and increased bulk density (Tonks et al., 2017). Not surprisingly, second generation monocropping had decreased organic content and greatest bulk density among studied cropping systems. First generation plantations have similar level of organic matter content as secondary peat forest (Tonks et al., 2017; Dhandapani et al., 2019c). However, that dramatically changes when the monocropping is continued to 2nd generation (see example data in Table 1). This may be due to the increased drainage in monocropping possibly leading to peat subsidence, without any addition of peat forming organic material which would normally occur within a peat swamp forest (Yule and Gomez, 2009). Monthly monitoring of 2 oil palm and pineapple intercropping systems and 3 oil palm monocropping systems in the same peat dome from August 2018 to September 2019, show average water table for intercropping systems to be 50.6 ± 5.6 and for monocropping systems to be 69.1 ± 5.4 (Dhandapani and Evers, Unpublished). During the same monitoring period one of the other intercropping systems was converted to monocropping, with additional drainage ditches dug within the plantation during the conversion (Dhandapani et al. Unpublished). As a general practice in the Selangor region, intercropping do not contain additional ditches running within the plantations, unlike monocropping systems (see Dhandapani et al., 2019a,b) (Dhandapani, Pers. Obs.). Thus the practice of this less severe drainage in intercropping systems has the potential to mitigate some of the longer-term impacts (Dhandapani et al., 2019a). Whereas, owing to possible increased peat subsidence and the reduced, homogenous and easily degradable C input to soil from monocropping (Guillaume et al., 2016; Kerdraon et al., 2020), organic matter content in the second generation oil palm intercropping was ~54%, which does not meet the required 65% defined by the regional government organizations and followed by the Roundtable on Sustainable Palm Oil (RSPO) (Firdaus et al., 2010; RSPO, 2019). It also barely passes some other published definitions that describe 45% organic content requirement for tropical peatlands (Osaki et al., 2016). This specific property effectively declassifies the studied 2nd generation monocropping as non-peatland system for sustainability certification and government regulations.
Volumetric moisture content was low in monocropping of both generations, with only second generation monocropping showing significant and big difference between seasons (Dhandapani et al., 2019a). The intercropping systems also showed significant seasonal reduction in moisture in the dry season (Dhandapani et al., 2019a). This may be due to increased evaporation in these systems with an open canopy than the first generation oil palm with a closed canopy. Even bigger differences in moisture between seasons for second generation monocropping may be due to the effect of high bulk density on water retention, as after an intermediate threshold, increased bulk density linearly relates to decreased moisture (Archer and Smith, 1973).
Oil palm monocropping have been reported by some studies to have lower CH4 emissions than natural peatlands, while others showing contradictory effect (Melling et al., 2005b; Hassler et al., 2015). Published reviews have not reported strong land-use effects, however lower emissions generally related to lower water table and lower litter addition in such peatlands (Hirano et al., 2009; Couwenberg et al., 2010). Similar studies for intercropping are scarce, which is evident from the published reviews of Greenhouse gas emissions or oil palm in tropical peatlands that do not include any oil palm intercropping (Couwenberg et al., 2010; Melling and Hansen, 2011; Evers et al., 2017), however some reviews have mentioned mixed croplands and shrub lands without a specific focus on oil palm intercropping (Hergoualc'h and Verchot, 2011, 2014). CH4 emissions in pineapple intercropping sites were higher than the monocropping sites, although they are relatively low in comparison to CO2 emissions, and their relative contribution to net greenhouse gas emissions is low (Figure 1). However, CH4 emissions in intercropping sites are still lower than 2 mg m−2hr−1 fluxes observed in primary peat swamp forest in Peninsular Malaysia (Dhandapani et al., 2019c). Considering these agricultural peatlands are drained, with oil palm monocropping having more severe drainage, there is a potential for greater methane emissions from drainage canals (Manning et al., 2019).
Figure 1. CO2 and CH4 fluxes from 1st and 2nd generation oil palm monoculture, 2nd generation oil palm and yam intercropping, and 2nd generation oil palm and pineapple intercropping. Data derived from Dhandapani et al. (2019a,b) containing 150 random measurement for each land use, excluding the known data (27 measurements) with autotrophic contributions in 1st generation oil palm monocropping (Dhandapani et al., 2019b). Means ± one SEM. Data in blue represents wet season and red represent dry season.
Other than our mentioned studies, there is general lack of research on the biogeochemical impacts of oil palm intercropping. Further studies on peat biogeochemistry of these landscapes are needed to fully assess the environmental impacts of oil palm intercropping relative to other land-uses, oil palm monocropping in particular.
Oil palm monocropping severely affect vertebrate and invertebrate biodiversity in South East Asia (Fitzherbert et al., 2008; Danielsen et al., 2009; Dhandapani, 2015). This is particularly important in tropical peatlands that are home to unique endemic biodiversity such as Borneo roundleaf bat, tri-colored langur etc. (Yule, 2010). Oil palm, being a non-native crop, has brought in several invasive pest species that adversely affect the endemic biodiversity (Dhandapani, 2015). It cannot be overstated that maintenance of healthy biodiversity is crucial for the long-term sustainability of an ecosystem and its services, including agricultural ecosystems (Dislich et al., 2017; Ashraf et al., 2018). Intercropping of oil palm plants with other crops automatically increased the plant diversity and provided an increased range of microhabitats that were beneficial for bats (Syafiq et al., 2016), invertebrates (Ghazali et al., 2016; Ashraf et al., 2018), and birds (Yahya et al., 2017). These studies included wide varieties of intercrops such as pineapple, banana, tapioca, sugarcane, corn and jackfruit, and included non-peat soils (Syafiq et al., 2016). Intercropping with flowering plants such as banana was particularly beneficial for frugivorous bats (Ghazali et al., 2016), even though they did not have a similar significant impacts on fruit feeding butterflies (Asmah et al., 2017). Moreover there was no big difference in community composition and abundance for some of the surveyed biodiversity groups (Ghazali et al., 2016; Asmah et al., 2017), nor a mention of intercropping supporting crucial forest species. However, all of those studies suggest that increased habitat complexity with intercropping or polyculture, as a better management practice than oil palm monocropping for improving biodiversity.
It is clear that second generation oil palm monocropping systems differ significantly from other peat land use, and continuation of such oil palm monocropping can quicken the potential declassification of such peatlands. We suggest that natural peatlands irrespective of their degree of degradation, should not be converted to oil palm monocropping, due to high peat carbon loss and changes in habitat characteristics. There is a need for a new classification unit for these transformed and newly mineralized ex-peatlands, which are going to increase in cover in coming decades with progressive generations of oil palm mono-culture.
Almost all of the oil palm intercropping is practiced by smallholders (Adila et al., 2017) (Dhandapani, pers. obs.), where the farmers see opportunity for additional income between the palm oil production cycles. However, small holding plantations and industrial plantations differ greatly in terms of manpower, availability of industrial equipment, financial spending and technical expertise, that may be the cause of lower yields in smallholder oil palm plantations (Azhar et al., 2011). Intercropping may not be a convenient option for industrial plantation which use industrial equipment for planting and harvesting. However, the total productivity of intercropping vs. monocropping oil palm plantations is not well-documented. Moreover, the price of crude oil palm has been decreasing since 2016, and intercropping may provide some extra income from the land for the smallholders, supporting their socio-economic sustainability.
We envisage paludiculture that maintains high water table inhibits aerobic decomposition as a way forward in truly sustaining already converted peatlands. However, considering the current extensive cover of oil palm plantations in peatlands, intercropping may be a wise short term alternative. Existing smallholder plantations could be better managed by practicing intercropping and polyculture that reduces the need for drainage, and improves and diversifies carbon inputs because of inputs from the intercrops. However, it is highly unlikely that carbon inputs from any crops would support peat formation, considering even some of the forest species lack the chemical complexity needed for peat accumulation (Yule and Gomez, 2009). Moreover, Oil palm intercropping should be incentivised and encouraged for existing plantations in peatlands for prolonging peat organic matter properties under oil palm agriculture. Intercropping is, in the majority, practiced by smallholder plantations, yet certifications are mostly unaffordable for such smallholders (Azhar et al., 2017). Certification of smallholder oil palm produced from intercropping systems should therefore be incentivized or made more affordable for small holders, which may well be more “sustainable” than certified palm oil from industrial monocropping in peatlands. A change in policy to support easier certification for small holding plantations may have a significant impact, especially considering 40% of the world oil palm production comes from smallholding oil palm plantations (Azhar et al., 2017). However, smallholders commonly use fire as a management practice, which may offset any ameliorating properties of intercropping by reducing the C content to half and doubling the GHG emissions in fire affected areas compared to nearby unburnt areas (Dhandapani and Evers, unpublished). Additionally there is a need for increased awareness about the advantages of intercropping systems, as it is evident from the survey of Saadun et al. (2018) which showed that independent smallholders who were better educated had a higher percentage of intercropping in their plantations than lesser educated managed smallholders. Managed smallholders are also dependent on government subsidies and are subjected to several government restrictions on farm management and oil palm sales (Saadun et al., 2018). Intercropping should be promoted as a short-term alternative by the responsible government agencies to the managed smallholders and further subsidized.
This work indicates several future areas for research, First, paludiculture is widely discussed as a sustainable alternative to other drainage based agriculture in peatlands (Tata, 2019). Even though there are several native crops suitable for paludiculture, the environmental, economical and social impacts, and viability of transforming oil palm agriculture to paludiculture is yet to be fully researched. Second, oil palm in intercropping sites in the reported studies were below 3 years of age (Dhandapani et al., 2019a,b). Intercropping may not be possible when oil palm plants mature, the canopy closes, and light becomes restricted for the understorey. These intercropping systems should be assessed further if they are converted from intercropping to oil palm monocropping to understand whether intercropping at initial stages of oil palm is beneficial to peat properties and the environment in the longer term. Third, while the impacts of intercropping on CO2 and CH4 fluxes have been observed, there is no data available on the impacts of conversion on N2O fluxes. This represents a key knowledge gap because N2O has a global warming potential up to 298 times CO2 over a 100-year time scale, and both monocropping and intercropping sites are known to receive regular inputs of organic and inorganic fertilizers, frequently in excess of recommended application rates (Hashidoko et al., 2008; Oktarita et al., 2017). Fourth, root exudates and oxygen are key regulators of emissions in intact tropical peatlands (Hoyos-Santillan et al., 2016; Girkin et al., 2018a,b). However, it is unclear if the same processes apply in converted oil palm sites or the role of inputs from intercropping species. Fifth, broad-scale changes in organic matter chemistry have previously been reported on conversion of primary forest to oil palm plantations and between conversion stages (Tonks et al., 2017; Cooper et al., 2019). Organic matter properties have also been shown to vary significantly at small spatial scales within intact tropical peat swamp forests (Girkin et al., 2019). Dhandapani et al. (2019b) reported changes in surface peat properties, microbial phenotypic community structure and GHG emissions depending on sampling proximity to oil palm and other intercrops. However, the detailed impacts of intercropping and their rhizospheres on organic matter chemistry throughout the peat profile, and changes between palm oil generations remain to be explored. Sixth, several studies report higher total CO2 emissions in forest than in oil palm plantation (Melling et al., 2005a,c; Dhandapani et al., 2019a), however that might very well be due to the near 100% extra autotrophic contribution in the forest habitats (Hergoualc'h et al., 2017; Murdiyarso et al., 2017). There is an increasing need to differentiate heterotrophic and autotrophic CO2 emissions in total CO2 emissions, as only heterotrophic contribution accounts for long-term carbon loss, while autotrophic contribution is a product of root respiration in immediate photosynthetic cycle. This becomes increasingly difficult due to the limited number of studies reporting the autotrophic and heterotrophic contribution to total CO2 emissions in these complex ecosystems (Melling et al., 2013; Dariah et al., 2014; Matysek et al., 2017; Girkin et al., 2018c).
Thus, intercropping represents a management practice with high potential for ameliorating some adverse effects from oil palm plantations, although several key research questions remain to be addressed. There is also a need for more research to understand a variations in smallholder intercropping practices on local and regional level. However, it is clear that overall maintaining carbon stocks and preserving intact peatlands should be a priority.
The datasets generated for this study are available on request to the corresponding author.
SD contributed to all aspects of this article including idea, writing, all research, lab and field work. NG contributed with the idea, parts of writing, and in making figures and tables. SE contributed in improving the direction of the writing and general supervision of the work. KR and SS contributed in supervision of the work and editing the manuscript. All authors contributed to the article and approved the submitted version.
The authors declare that the research was conducted in the absence of any commercial or financial relationships that could be construed as a potential conflict of interest.
The data used in this article is from the research funded by Crops for the Future, Malaysia, and The School of Biosciences, University of Nottingham, UK. We also thank organizations such as Global Environment Centre, Selangor State Forestry Department, and local villagers, workers and land-owners who granted access and helped in various research activities in Malaysian peatlands that enabled us to write this article. We thank the reviewers for their useful comments.
Adila, N., Sasidhran, S., Kamarudin, N., Puan, C. L., Azhar, B., and Lindenmayer, D. B. (2017). Effects of peat swamp logging and agricultural expansion on species richness of native mammals in Peninsular Malaysia. Basic Appl. Ecol. 22, 1–10. doi: 10.1016/j.baae.2017.04.002
Archer, J. R., and Smith, P. D. (1973). “The relation between bulk density, available water capacity and air capacity of soils,” J.R. Archer and P.D. SmithJ. Soil Sci.23 (4), 475–480 (1972). J. Terramechanics 10:61. doi: 10.1111/j.1365-2389.1972.tb01678.x
Ashraf, M., Zulkifli, R., Sanusi, R., Tohiran, K. A., Terhem, R., Moslim, R., et al. (2018). Alley-cropping system can boost arthropod biodiversity and ecosystem functions in oil palm plantations. Agric. Ecosyst. Environ. 260, 19–26. doi: 10.1016/j.agee.2018.03.017
Asmah, S., Ghazali, A., Syafiq, M., Yahya, M. S., Peng, T. L., Norhisham, A. R., et al. (2017). Effects of polyculture and monoculture farming in oil palm smallholdings on tropical fruit-feeding butterfly diversity. Agric. For. Entomol. 19, 70–80. doi: 10.1111/afe.12182
Azhar, B., Lindenmayer, D. B., Wood, J., Fischer, J., Manning, A., Mcelhinny, C., et al. (2011). The conservation value of oil palm plantation estates, smallholdings and logged peat swamp forest for birds. For. Ecol. Manage 262, 2306–2315. doi: 10.1016/j.foreco.2011.08.026
Azhar, B., Saadun, N., Prideaux, M., and Lindenmayer, D. B. (2017). The global palm oil sector must change to save biodiversity and improve food security in the tropics. J. Environ. Manage 203, 457–466. doi: 10.1016/j.jenvman.2017.08.021
Cooper, H. V., Vane, C. H., Evers, S., Aplin, P., Girkin, N. T., and Sjögersten, S. (2019). From peat swamp forest to oil palm plantations: the stability of tropical peatland carbon. Geoderma 342, 109–117. doi: 10.1016/j.geoderma.2019.02.021
Couwenberg, J., Dommain, R., and Joosten, H. (2010). Greenhouse gas fluxes from tropical peatlands in south-east Asia. Glob. Chang. Biol. 16, 1715–1732. doi: 10.1111/j.1365-2486.2009.02016.x
Danielsen, F., Beukema, H., Burgess, N. D., Parish, F., Brühl, C. A., Donald, P. F., et al. (2009). Biofuel plantations on forested lands: double jeopardy for biodiversity and climat plantaciones de biocombustible en terrenos boscosos: doble peligro para la biodiversidad y el clima. Conserv. Biol. 23, 348–358. doi: 10.1111/j.1523-1739.2008.01096.x
Dariah, A., Marwanto, S., and Agus, F. (2014). Root- and peat-based CO2 emissions from oil palm plantations. Mitig. Adapt. Strateg. Glob. Chang. 19, 831–843. doi: 10.1007/s11027-013-9515-6
Dhandapani, S. (2015). “Biodiversity loss associated with oil palm plantations in Malaysia: serving the need versus saving the nature,” in 4th International Conference on Biodiversity (Las Vegas, NV: Journal of Ecosystem and Ecography).
Dhandapani, S., Ritz, K., Evers, S., and Sjögersten, S. (2019a). Environmental impacts as affected by different oil palm cropping systems in tropical peatlands. Agric. Ecosyst. Environ 276, 8–20. doi: 10.1016/j.agee.2019.02.012
Dhandapani, S., Ritz, K., Evers, S., and Sjögersten, S. (2019b). GHG emission under different cropping systems in some Histosols of Malaysia. Geoderma Reg. 18:e00229. doi: 10.1016/j.geodrs.2019.e00229
Dhandapani, S., Ritz, K., Evers, S., Yule, C. M., and Sjögersten, S. (2019c). Are secondary forests second-rate? comparing peatland greenhouse gas emissions, chemical and microbial community properties between primary and secondary forests in Peninsular Malaysia. Sci. Total Environ. 655, 220–231. doi: 10.1016/j.scitotenv.2018.11.046
Dislich, C., Keyel, A. C., Salecker, J., Kisel, Y., Meyer, K. M., Auliya, M., et al. (2017). A review of the ecosystem functions in oil palm plantations, using forests as a reference system. Biol. Rev. 92, 1539–1569. doi: 10.1111/brv.12295
Dohong, A., Aziz, A. A., and Dargusch, P. (2017). A review of the drivers of tropical peatland degradation in South-East Asia. Land Use Policy 69, 349–360. doi: 10.1016/j.landusepol.2017.09.035
Dommain, R., Couwenberg, J., Glaser, P. H., Joosten, H., and Suryadiputra, I. N. N. (2014). Carbon storage and release in Indonesian peatlands since the last deglaciation. Quat. Sci. Rev. 97, 1–32. doi: 10.1016/j.quascirev.2014.05.002
Dommain, R., Dittrich, I., Giesen, W., Joosten, H., Rais, D. S., Silvius, M., et al. (2016). “Ecosystem services, degradation and restoration of peat swamps in the South East Asian tropics,” in Peatland Restoration and Ecosystem Services: Science, Policy and Practice, eds A. Bonn, H. Joosten, M. Evans, R. Stoneman, and T. Allott (Cambridge: Cambridge University Press), 253–288. doi: 10.1017/CBO9781139177788.015
Dommain, R., Frolking, S., Jeltsch-Thommes, A., Joos, F., Couwenberg, J., and Glaser, P. H. (2018). A radiative forcing analysis of tropical peatlands before and after their conversion to agricultural plantations. Glob. Chang. Biol. 24, 5518–5533. doi: 10.1111/gcb.14400
Evers, S., Yule, C. M., Padfield, R., O'reilly, P., and Varkkey, H. (2017). Keep wetlands wet: the myth of sustainable development of tropical peatlands - implications for policies and management. Glob. Chang. Biol. 23, 534–549. doi: 10.1111/gcb.13422
FAO (1988). Nature and Management of tropical peat soils [Online]. Food and Agriculture Organization of the United Nations. Available online at: http://www.fao.org/3/x5872e/x5872e00.htm#Contents (accessed October 25, 2019).
Firdaus, M. S., Gandaseca, S., Ahmed, O. H., and Majid, N. M. A. (2010). Effect of converting secondary tropical peat swamp forest into oil palm plantation on selected peat soil physical properties. Am. J. Environ. Sci. 6, 402–405. doi: 10.3844/ajessp.2010.402.405
Fitzherbert, E. B., Struebig, M. J., Morel, A., Danielsen, F., Brühl, C. A., Donald, P. F., et al. (2008). How will oil palm expansion affect biodiversity? Trends Ecol. Evol. 23, 538–545. doi: 10.1016/j.tree.2008.06.012
Ghazali, A., Asmah, S., Syafiq, M., Yahya, M. S., Aziz, N., Peng, T. L., et al. (2016). Effects of monoculture and polyculture farming in oil palm smallholdings on terrestrial arthropod diversity. J. Asia Pac. Entomol. 19, 415–421. doi: 10.1016/j.aspen.2016.04.016
Girkin, N. T., Turner, B. L., Ostle, N., Craigon, J., and Sjögersten, S. (2018a). Root exudate analogues accelerate CO2 and CH4 production in tropical peat. Soil Biol. Biochem. 117, 48–55. doi: 10.1016/j.soilbio.2017.11.008
Girkin, N. T., Turner, B. L., Ostle, N., and Sjögersten, S. (2018b). Composition and concentration of root exudate analogues regulate greenhouse gas fluxes from tropical peat. Soil Biol. Biochem. 127, 280–285. doi: 10.1016/j.soilbio.2018.09.033
Girkin, N. T., Turner, B. L., Ostle, N., and Sjögersten, S. (2018c). Root-derived CO2 flux from a tropical peatland. Wetl. Ecol. Manag. 26, 985–991. doi: 10.1007/s11273-018-9617-8
Girkin, N. T., Vane, C. H., Cooper, H. V., Moss-Hayes, V., Craigon, J., Turner, B. L., et al. (2019). Spatial variability of organic matter properties determines methane fluxes in a tropical forested peatland. Biogeochemistry 142, 231–245. doi: 10.1007/s10533-018-0531-1
Guillaume, T., Holtkamp, A. M., Damris, M., Brümmer, B., and Kuzyakov, Y. (2016). Soil degradation in oil palm and rubber plantations under land resource scarcity. Agric. Ecosyst. Environ. 232, 110–118. doi: 10.1016/j.agee.2016.07.002
Hansen, M. C., Potapov, P. V., Moore, R., Hancher, M., Turubanova, S. A., Tyukavina, A., et al. (2013). High-resolution global maps of 21st-century forest cover change. Science 342, 850–853. doi: 10.1126/science.1244693
Hashidoko, Y., Takakai, F., Toma, Y., Darung, U., Melling, L., Tahara, S., et al. (2008). Emergence and behaviors of acid-tolerant Janthinobacterium sp that evolves N2O from deforested tropical peatland. Soil Biol. Biochem. 40, 116–125. doi: 10.1016/j.soilbio.2007.07.014
Hassler, E., Corre, M. D., Tjoa, A., Damris, M., Utami, S. R., and Veldkamp, E. (2015). Soil fertility controls soil-atmosphere carbon dioxide and methane fluxes in a tropical landscape converted from lowland forest to rubber and oil palm plantations. Biogeoscience 12, 5831–5852. doi: 10.5194/bgd-12-9163-2015
Hergoualc'h, K., Hendry, D. T., Murdiyarso, D., and Verchot, L. V. (2017). Total and heterotrophic soil respiration in a swamp forest and oil palm plantations on peat in Central Kalimantan, Indonesia. Biogeochemistry 135, 203–220. doi: 10.1007/s10533-017-0363-4
Hergoualc'h, K., and Verchot, L. V. (2011). Stocks and fluxes of carbon associated with land use change in Southeast Asian tropical peatlands: a review. Global Biogeochem. Cycles 25:13. doi: 10.1029/2009GB003718
Hergoualc'h, K., and Verchot, L. V. (2014). Greenhouse gas emission factors for land use and land-use change in Southeast Asian peatlands. Mitig. Adapt. Strateg. Glob. Chang. 19, 789–807. doi: 10.1007/s11027-013-9511-x
Hirano, T., Jauhiainen, J., Inoue, T., and Takahashi, H. (2009). Controls on the carbon balance of tropical peatlands. Ecosystems 12, 873–887. doi: 10.1007/s10021-008-9209-1
Hodgkins, S. B., Richardson, C. J., Dommain, R., Wang, H., Glaser, P. H., Verbeke, B., et al. (2018). Tropical peatland carbon storage linked to global latitudinal trends in peat recalcitrance. Nat. Commun. 9:3640. doi: 10.1038/s41467-018-06050-2
Hooijer, A., Page, S., Jauhiainen, J., Lee, W. A., Lu, X. X., Idris, A., et al. (2012). Subsidence and carbon loss in drained tropical peatlands. Biogeosciences 9, 1053–1071. doi: 10.5194/bg-9-1053-2012
Hoyos-Santillan, J., Craigon, J., Lomax, B. H., Lopez, O. R., Turner, B. L., and Sjögersten, S. (2016). Root oxygen loss from Raphia taedigera palms mediates greenhouse gas emissions in lowland neotropical peatlands. Plant Soil 404, 47–60. doi: 10.1007/s11104-016-2824-2
Kerdraon, D., Drewer, J., Chung, A. Y. C., Majalap, N., Slade, E. M., Bréchet, L., Wallwork, A., Castro-Trujillo, B., and Sayer, E. J. (2020). Litter Inputs, but Not Litter Diversity, Maintain Soil Processes in Degraded Tropical Forests – A Cross-Continental Comparison. Front. For. Glob. Change 2. doi: 10.3389/ffgc.2019.00090
Koh, L. P., Miettinen, J., Liew, S. C., and Ghazoul, J. (2011). Remotely sensed evidence of tropical peatland conversion to oil palm. Proc. Natl. Acad. Sci. U.S.A. 108:5127. doi: 10.1073/pnas.1018776108
Kononen, M., Jauhiainen, J., Laiho, R., Kusin, K., and Vasander, H. (2015). Physical and chemical properties of tropical peat under stabilised land uses. Mires and Peat 16, 1–13.
Leifeld, J., Wüst-Galley, C., and Page, S. (2019). Intact and managed peatland soils as a source and sink of GHGs from 1850 to 2100. Nat. Clim. Chang. 9, 945–947. doi: 10.1038/s41558-019-0615-5
Lo, J., and Parish, F. (2013). Peatlands and Climate Change in Southeast Asia. Selangor: Global Environment Centre.
Luskin, M. S., and Potts, M. D. (2011). Microclimate and habitat heterogeneity through the oil palm lifecycle. Basic Appl. Ecol. 12, 540–551. doi: 10.1016/j.baae.2011.06.004
Manning, F. C., Kho, L. K., Hill, T. C., Cornulier, T., and Teh, Y. A. (2019). Carbon emissions from oil palm plantations on peat soil. Front. For. Glob. Change 2:37. doi: 10.3389/ffgc.2019.00037
Matysek, M., Evers, S., Samuel, M. K., and Sjogersten, S. (2017). High heterotrophic CO2 emissions from a Malaysian oil palm plantations during dry-season. Wetl. Ecol. Manage. 26, 415–424. doi: 10.1007/s11273-017-9583-6
Melling, L., Hatano, R., and Goh, K. J. (2005a). Global warming potential from soils in tropical peatlands of Sarawak, Malaysia. Phyton 45, 275–284. Available online at: https://www.semanticscholar.org/paper/Global-warming-potential-from-soils-in-tropical-of-Melling-Hatano/81a47c7e163b5d87dc2081b412eb0e7ac29b44d2
Melling, L., Tan, C. S. Y., Goh, K. J., and Hatano, R. (2013). Soil microbial and root respirations from three ecosystems in tropical Peatland Of Sarawak, Malaysia. J. Oil Palm Res 25, 44–57. Available online at: http://palmoilis.mpob.gov.my/publications/joprv25april2013-LuiLie.pdf
Melling, L., and Hansen, I. E. (2011). Greenhouse gas exchange of tropical peatlands- a review. J. Oil Palm Res. 23, 1087–1095. Available online at: http://palmoilis.mpob.gov.my/publications/joprv23aug2011-Lulie.pdf
Melling, L., Hatano, R., and Goh, K. J. (2005b). Methane fluxes from three ecosystems in tropical peatland of Sarawak, Malaysia. Soil Biol. Biochem. 37, 1445–1453. doi: 10.1016/j.soilbio.2005.01.001
Melling, L., Hatano, R., and Goh, K. J. (2005c). Soil CO2 flux from three ecosystems in tropical peatland of Sarawak, Malaysia. Tellus B 57, 1–11. doi: 10.3402/tellusb.v57i1.16772
Miettinen, J., Hooijer, A., Tollenaar, D., Malins, C., Vernimmen, R., Shi, C., et al. (2012). Historical Analysis and Projection of Oil Palm Plantation Expansion on Peatland in Southeast Asia. Washington, DC: International Council on Clean Transportation.
Miettinen, J., and Liew, S. C. (2010). Degradation and development of peatlands in Peninsular Malaysia and in the islands of Sumatra and Borneo since 1990. Land Degrad. Dev. 21, 285–296. doi: 10.1002/ldr.976
Miettinen, J., Shi, C. H., and Liew, S. C. (2016). Land cover distribution in the peatlands of Peninsular Malaysia, Sumatra and Borneo in 2015 with changes since 1990. Glob. Ecol. Conserv. 6, 67–78. doi: 10.1016/j.gecco.2016.02.004
Murdiyarso, D., Saragi-Sasmito, M. F., and Rustini, A. (2017). Greenhouse gas emissions in restored secondary tropical peat swamp forests. Mitig. Adapt. Strateg. Glob. Chang. 24, 507–520. doi: 10.1007/s11027-017-9776-6
Nchanji, Y. K., Nkongho, R. N., Mala, W. A., and Levang, P. (2016). Efficacy of oil palm intercropping by smallholders: case study in South-West Cameroon. Agrofor. Syst. 90, 509–519. doi: 10.1007/s10457-015-9873-z
Oktarita, S., Hergoualc'h, K., Anwar, S., and Verchot, L. V. (2017). Substantial N2O emissions from peat decomposition and N fertilization in an oil palm plantation exacerbated by hotspots. Environ. Res. Lett. 12:104007. doi: 10.1088/1748-9326/aa80f1
Osaki, M., Hirose, K., Segah, H., and Helmy, F. (2016). “Tropical peat and peatland definition in Indonesia,” in Tropical Peatland Ecosystems, eds M. Osaki, and N. Tsuji (Tokyo: Springer Japan), 137–147. doi: 10.1007/978-4-431-55681-7_9
Page, S. E., and Hooijer, A. (2016). In the line of fire: the peatlands of Southeast Asia. Philos. Trans. R. Soc. Lond., B, Biol. Sci. 371:20150176. doi: 10.1098/rstb.2015.0176
Page, S. E., Rieley, J. O., and Banks, C. J. (2011). Global and regional importance of the tropical peatland carbon pool. Glob. Chang. Biol. 17, 798–818. doi: 10.1111/j.1365-2486.2010.02279.x
Parish, F., Sirin, A., Charman, D., Joosten, H., Minayeva, T., Silvius, M., et al. (2008). Assessment on Peatlands, Biodiversity and Climate Change. Selangor; Wageningen: Global Environment Centre and Wetlands International.
RSPO (2019). Revision of RSPOOrganic & Peat Soil Classification. Roundtable for Sutainable Palm Oil. Avaliable online at: https://rspo.org/principles-and-criteria-review/revision-of-rspo-organic-and-peat-soil-classification (accessed October 25, 2019).
Saadun, N., Lim, E. A. L., Esa, S. M., Ngu, F., and Awang, F. (2018). Socio-ecological perspectives of engaging smallholders in environmental friendly palm oil certification schemes. Land Use Policy 72, 333–340. doi: 10.1016/j.landusepol.2017.12.057
Sayer, J., Ghazoul, J., Nelson, P., and Boedhihartono, A. K. (2012). Oil palm expansion transforms tropical landscapes and livelihoods. Glob. Food Sec. Agric. Policy Econ. Environ. 1, 114–119. doi: 10.1016/j.gfs.2012.10.003
Sheil, D., Casson, A., Meijaard, E., Van Noordwijk, M., Gaskell, J., Sunderland-Groves, J., et al. (2009). The Impacts And Opportunities of Oil Palm In Southeast Asia; What Do We Know? What Do We Need To Know? (Indonesia: CIFOR).
Shevade, V. S., and Loboda, T. V. (2019). Oil palm plantations in Peninsular Malaysia: determinants and constraints on expansion. PLoS ONE 14:e0210628. doi: 10.1371/journal.pone.0210628
Sjögersten, S., Black, C. R., Evers, S., Hoyos-Santillan, J., Wright, E. L., and Turner, B. L. (2014). Tropical wetlands: a missing link in the global carbon cycle? Global Biogeochem. Cycles 28, 1371–1386. doi: 10.1002/2014GB004844
Sjögersten, S., Cheesman, A. W., Lopez, O., and Turner, B. L. (2011). Biogeochemical processes along a nutrient gradient in a tropical ombrotrophic peatland. Biogeochemistry 104, 147–163. doi: 10.1007/s10533-010-9493-7
Syafiq, M., Atiqah, A. R. N., Ghazali, A., Asmah, S., Yahya, M. S., Aziz, N., et al. (2016). Responses of tropical fruit bats to monoculture and polyculture farming in oil palm smallholdings. Acta Oecol. 74, 11–18. doi: 10.1016/j.actao.2016.06.005
Tata, H. L. (2019). Paludiculture: can it be a trade-off between ecology and economic benefit on peatland restoration? IOP Conf. Ser. Earth Environ. Sci. 394:012061. doi: 10.1088/1755-1315/394/1/012061
Tonks, A. J., Aplin, P., Beriro, D. J., Cooper, H., Evers, S., Vane, C. H., et al. (2017). Impacts of conversion of tropical peat swamp forest to oil palm plantation on peat organic chemistry, physical properties and carbon stocks. Geoderma 289, 36–45. doi: 10.1016/j.geoderma.2016.11.018
Wijedasa, L. S., Sloan, S., Page, S. E., Clements, G. R., Lupascu, M., and Evans, T. A. (2018). Carbon emissions from South-East Asian peatlands will increase despite emission-reduction schemes. Glob. Chang. Biol. 24, 4598–4613. doi: 10.1111/gcb.14340
Yahya, M. S., Syafiq, M., Ashton-Butt, A., Ghazali, A., Asmah, S., and Azhar, B. (2017). Switching from monoculture to polyculture farming benefits birds in oil palm production landscapes: evidence from mist netting data. Ecol. Evol. 7, 6314–6325. doi: 10.1002/ece3.3205
Yule, C. M. (2010). Loss of biodiversity and ecosystem functioning in Indo-Malayan peat swamp forests. Biodivers. Conserv. 19, 393–409. doi: 10.1007/s10531-008-9510-5
Keywords: tropical peatlands, oil palm plantations, intercropping, carbon dioxide, methane, biodiversity, palm oil certification
Citation: Dhandapani S, Girkin NT, Evers S, Ritz K and Sjögersten S (2020) Is Intercropping an Environmentally-Wise Alternative to Established Oil Palm Monoculture in Tropical Peatlands? Front. For. Glob. Change 3:70. doi: 10.3389/ffgc.2020.00070
Received: 30 October 2019; Accepted: 18 May 2020;
Published: 23 June 2020.
Edited by:
Eleanor Slade, Nanyang Technological University, SingaporeReviewed by:
René Dommain, Smithsonian Institution, United StatesCopyright © 2020 Dhandapani, Girkin, Evers, Ritz and Sjögersten. This is an open-access article distributed under the terms of the Creative Commons Attribution License (CC BY). The use, distribution or reproduction in other forums is permitted, provided the original author(s) and the copyright owner(s) are credited and that the original publication in this journal is cited, in accordance with accepted academic practice. No use, distribution or reproduction is permitted which does not comply with these terms.
*Correspondence: Selvakumar Dhandapani, c2NjYWx2YUBnbWFpbC5jb20=
Disclaimer: All claims expressed in this article are solely those of the authors and do not necessarily represent those of their affiliated organizations, or those of the publisher, the editors and the reviewers. Any product that may be evaluated in this article or claim that may be made by its manufacturer is not guaranteed or endorsed by the publisher.
Research integrity at Frontiers
Learn more about the work of our research integrity team to safeguard the quality of each article we publish.