- 1Centro de Investigación y Extensión Forestal Andino Patagónico (CIEFAP), Consejo Nacional de Investigaciones Científicas y Técnicas (CONICET), Esquel, Argentina
- 2Ministerio de la Producción, Rawson, Argentina
- 3Departamento de Ingeniería Forestal, Facultad de Ingeniería, Universidad Nacional de la Patagonia San Juan Bosco (UNPSJB), Esquel, Argentina
Reaching an equilibrium between timber production and biodiversity conservation is one of the aims increasingly pursued in forest plantations, since biodiversity favors plantation stability while contributing to the maintenance of native community structure and functions. This equilibrium is relevant for the Patagonian steppe, which has great diversity but low representation in protected areas. Residual slash management following pruning and thinning is necessary since the traditional practice of leaving slash on the floor may limit vegetation development and increase wildfire hazard. We assessed initial vegetation response to fuel reduction treatments in five exotic conifer plantations in the Patagonian forest-steppe ecotone. We implemented mastication, prescribed fire, and mastication plus prescribed fire fuel reduction treatments and compared initial vegetation richness, abundance, and composition among treatments and with that of an untreated control, having residues on the forest floor. After 3 years, we compared vegetation among fuel treatments and with the adjacent steppe at each site. We also compared residue cover among fuel treatments. Vegetation richness reached similar values in most fuel treatments a year after their implementation, whereas abundance gradually increased in all fuel reduction treatments; in general, the greatest abundance increase was in mastication. After 3 years, richness reached steppe values in most treatments (about 9 species/4 m2), whereas abundance did not (about 40% cover in the steppe and 5–25% in fuel treatments), and species composition similarity was low among treatments and steppe in all sites (Bray–Curtis index 0.1–0.6). Plant community composition was positively correlated with annual precipitation and initial residual biomass. In sites with less precipitation and initial residual biomass, native species predominated, and mastication had the lowest negative impact on initial vegetation recovery, whereas in sites with high proportion of exotic, vegetation recovery was led by exotics in all fuel reduction treatments; in a site having scarce vegetation within plantation, we did not detect any substantial change. Woody residues only decreased when their initial cover was >20%, and litter debris cover reloaded by the second year after fuel reduction. Our results suggest that mastication had the lowest negative short-term impact on native vegetation.
Introduction
Reaching an equilibrium between timber production and biodiversity conservation is one of the aims increasingly pursued in forest plantations, since biodiversity favors plantation stability while contributing to the maintenance of native community structure and functions (Carnus et al., 2006; Mori et al., 2013). Pruning and thinning are silvicultural practices that increase resource availability such as light and rainfall reaching the ground, promoting herb–shrub stratum vegetation development (Sonohat et al., 2004; Cummings and Reid, 2008). These practices improve timber quality, since they limit knot development and favor stem diameter increment (Daniel et al., 1979). However, woody debris generated by these practices are usually left on the site floor and may prevent normal vegetative growth by shading and covering it (Metlen et al., 2004; Kane et al., 2010), also creating a dry fuel layer that may increase fire intensity and risk (Agee and Skinner, 2005).
Conifer afforestations alter vegetation structure and fuel loads, modifying the fire regime of the ecosystems in which they are planted (Paritsis et al., 2018). In the Patagonian steppe, ponderosa pine (Pinus ponderosa) afforestations produce an increase in fine fuel amount and continuity that may increase the intensity of wildfires relative to those occurring naturally in this steppe (Paritsis et al., 2018). Although pruning and thinning reduce fuel vertical continuity (Agee and Skinner, 2005; Godoy et al., 2013), if woody and litter debris are left on the forest floor, flames may be longer and surface and crown spread rate faster (Paritsis et al., 2018). Thus, pruning and thinning without appropriate management of the generated residues may aggravate, rather than ameliorate, fire hazard (Agee and Skinner, 2005; Paritsis et al., 2018).
The most widely used fuel treatments aimed at reducing fuel buildup in fire-prone ecosystems besides thinning include prescribed fire and mastication. Prescribed fire consists of planned fires under controlled conditions; they are effective in reducing fire intensity and severity (de Ronde et al., 1990; Prichard et al., 2010; Fulé et al., 2012). However, mechanical treatments such as mastication should be considered where fire treatments are hazardous, for example near towns or other settlements, or in combination with prescribed fires (Busse et al., 2005; Kane et al., 2010; Quinn-Davidson and Varner, 2011; Defossé et al., 2015a). Mastication consists of shredding live or dead woody material and concentrating it in a dense layer on the forest floor. This treatment may reduce fire risk by reducing vertical fuel continuity and compacting surface fuels (Agee and Skinner, 2005).
Fuel management may have different effects on plant community. Thinning followed by prescribed fire usually results in an immediate increase in plant richness, with a high proportion of exotics, and a decrease in plant cover (Collins et al., 2007; Kane et al., 2010; Abella and Springer, 2015). Although mastication generates a dense fuel layer on the forest floor that could act as a barrier to vegetation germination and development (Kane et al., 2010), grasses and herbs usually recover their cover after mastication (Fornwalt et al., 2017). Subsequent fuel treatments such as prescribed fire may allow species to establish by releasing resources and clearing space. However, fire residence time and combustion depth may increase due to the presence of a dense fuel layer (Reiner et al., 2009), increasing soil temperatures, with the consequent damage to soil organisms (Busse et al., 2005). Thus, the choice of which fuel management technique is more appropriate should be based on both fire hazard reduction and ecosystem integrity (Agee and Skinner, 2005).
In the Patagonian steppe, fire is an important disturbance for plant community structure and function (Veblen et al., 2003; Ghermandi et al., 2004; Defossé et al., 2015b). The steppe plant community is fire resilient, since many species are resprouters and others form persistent seed banks (Ghermandi et al., 2004). Many of the predominant species in the above-ground vegetation are post-fire regrowing species such as the tussock grasses Pappostipa speciosa and Festuca pallescens, which have a post-fire survival higher than 60% (Gittins et al., 2011). The seed bank usually contains annual species, most of them exotic such as Myosotis stricta, Draba verna, Rumex acetosella (Rago et al., 2020), and some natives such as Boopis gracilis, Heliotropium paronychioides, and Nicotiana linearis, which usually appear briefly after fires (Ghermandi et al., 2004). However, if the plant community is already modified by fire-resistant conifer plantations, the addition of fire may result in different communities. For example, in a ponderosa pine afforestation in a shrub–steppe in Patagonia, exotic species richness and cover increased 5 years after a wildfire whereas native species recovery was low, probably due to the depleted vegetation within plantation before the fire (Nuñez and Raffaele, 2007). Thus, vegetation condition before fuel management may affect its response to fuel treatments (Dodson et al., 2008).
The Patagonian steppe has a rich diversity, but it has low representation in protected areas (Chehébar et al., 2013). Thus, conservation in forest plantations makes an important contribution to the maintenance of the native community's structure and functions. Through the study of vegetation richness, understood as the number of species per area, abundance, understood as species percentage cover, and composition, understood as species variety, it is possible to have a good measure of the community's diversity (Magurran, 1988). Moreover, the use of incidence-based indices such as Jaccard and abundance-based indices such as Bray–Curtis is good to assess species composition similarity among assemblages (Jost et al., 2011). Since pine plantations in Patagonia are in their first rotation, and fuel residues are commonly left on the site floor without further treatment, there is not much knowledge about their effect on fire regimes and plant community. To our knowledge, this is the first study to assess the initial vegetation response to fuel reduction treatments of pruning and thinning residues compared with that under traditional management, and with the natural ecosystem vegetation, in ponderosa pine plantations in Patagonia. Thus, this study is useful to get information about how to proceed with the implementation of fuel reduction treatments in Patagonian plantations. The fuel treatments considered were the traditional practice of leaving slash residues on the floor and three fuel reduction treatments: mastication, prescribed fire, and mastication followed by prescribed fire. We asked the following questions: (1) How do vegetation richness and abundance evolve for the first 3 years following fuel reduction treatments? (2) How are vegetation richness, abundance, and composition in fuel treatments 3 years after fuel reduction compared with natural vegetation? (3) Is the vegetation response similar among fuel treatments in sites presenting different site conditions? (4) How do residues and bare ground cover vary among fuel treatments following fuel reduction?
Materials and Methods
Study Area
The study area is located in north-west Chubut Province, Argentina (Figure 1). Phytogeographically, it belongs to the sub-Andean and Occidental districts of Patagonian Province (Cabrera, 1971). The sub-Andean district is characterized by a Festuca pallescens grass–steppe, which is gradually replaced, along a gradient of decreasing precipitation, up to the Occidental district characterized by a grass-shrub steppe dominated by bunchgrasses of the genera Festuca, Bromus, Hordeum, and Pappostipa and the shrubs Azorella prolifera, Adesmia volckmannii, and Berberis microphylla (Bertiller et al., 1995). Vegetation cover is between 25 and 50%, with different degradation levels caused by overgrazing during the last 100 years (Soriano, 1952; Defossé and Robberecht, 1987; Golluscio et al., 2009). The climate is Temperate-Mediterranean; summers are warm and dry, and winters are cool and wet (Köppen and Geiger, 1936). Ponderosa pine is planted in the area where annual precipitation varies from 700 mm in the West to 300 mm in the East (Bava et al., 2015). Originally, plantations were established in native Nothofagus dombeyi and Austrocedrus chilensis forests, where precipitation reaches up to 1,500 mm, but the replacement of native forests for exotic conifers is no longer allowed. Since Mediterranean climate dominates in this region, natural fires usually occur during the dry season, from October to April, especially from January to March (Oddi and Ghermandi, 2016). Fires are estimated to occur once every 9 years at landscape scale and ranging from 11 to 24 years at community scale (Oddi and Ghermandi, 2015).
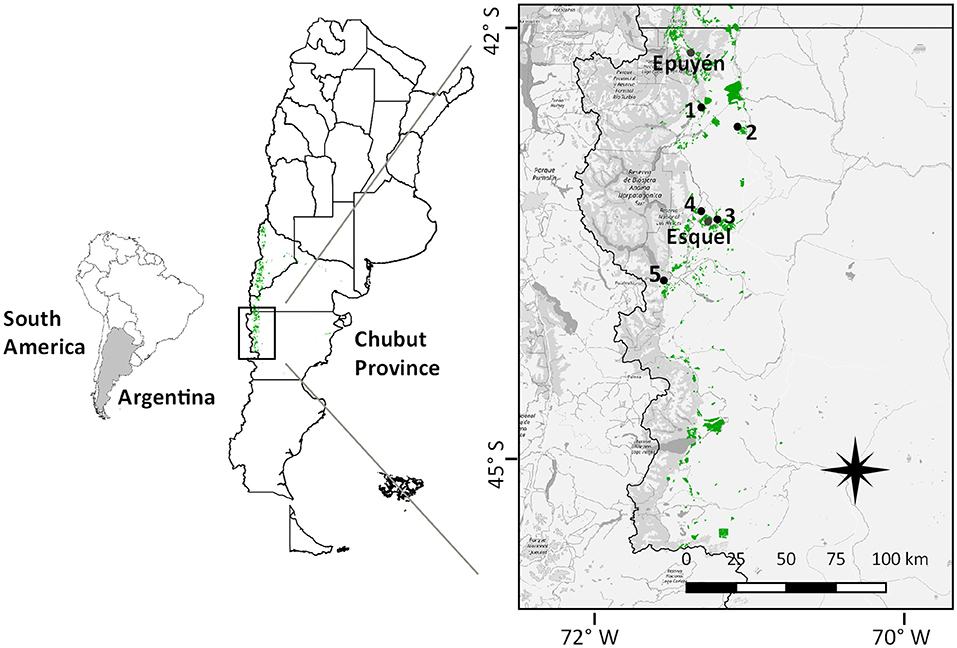
Figure 1. Conifer plantations location (green) along NW Chubut Province, Argentina, showing the five study sites. Andean–Patagonian forests distribution is represented in dark gray.
Experimental Design and Fuel Treatments
We selected five stands of ponderosa pine plantations, four of them located in the steppe and one in an area corresponding to native forest. Plantations' age ranges from 18 to 22 years, and they covered at least 30 ha, which is considered a representative size for Patagonian plantations (Bava et al., 2015). All of them had undergone at least one pruning and one thinning carried out 2–4 years before fuel reduction treatment implementation. Some heterogeneity among sites was observed, mainly given by the natural regional heterogeneity (Soriano, 1956), their grazing history before plantation, and their forest structure. The precipitation data for each site was obtained from the WorldClim platform (Fick and Hijmans, 2017). Initial biomass of the residues generated by previous pruning and thinning was obtained through the dry weight estimation of the pre-treatment forest floor biomass present in ten 1-m2 plots at each site (Lederer unpublished data). History of use was based on personal communication and on-site observations (Table 1). Soil properties varied among sites, such as pH (6–6.6) and organic matter (3.5–10%) (Lederer, unpublished data). To characterize forest structure, we established a 400-m2 circular plot in each stand. We sampled the diameter of all ponderosa pines at breast height (1.3 m) and four trees for total and initial crown height. With the overstory tree data, we calculated several forest structural variables, such as the Reineke density index (Reineke, 1933; Table 1). In each plantation, a 1-ha area was delimited to establish a complete randomized design, which consisted of 16 plots (144 m2 each). One fuel treatment replicate was assigned to each plot, giving a total of four replicates for each of the four fuel treatments. In the four plantations located in the steppe, an adjacent 1-ha area with natural vegetation was delimited, where four 144-m2 plots were selected for natural vegetation sampling (Figure 2).
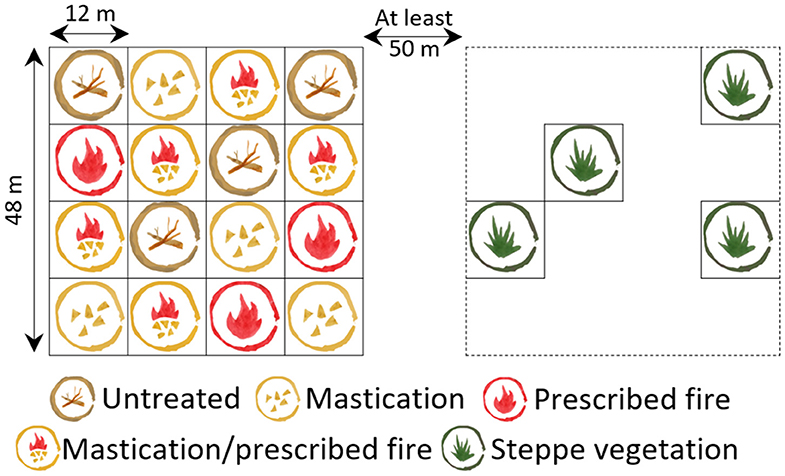
Figure 2. Original design scheme representing four replicates per fuel treatment within the plantation on the left, and four replicates per steppe vegetation on the right.
There were four fuel treatments: the traditional practice of leaving slash residues on the forest floor (UNTREATED) and three fuel reduction treatments, mastication (MAST), prescribed fire (RX), and mastication followed by prescribed fire (MAST/RX) (Figure 3). The adjacent area having natural vegetation was selected as a reference condition of the natural ecosystem (STEPPE). Mastication was implemented in winter, between July and October, using a rotary drum style masticating head with teeth, mounted on a crawler. Prescribed fire was implemented in spring, between October and November, after the wet season, in both RX and MAST/RX plots, through the backing fire technique.
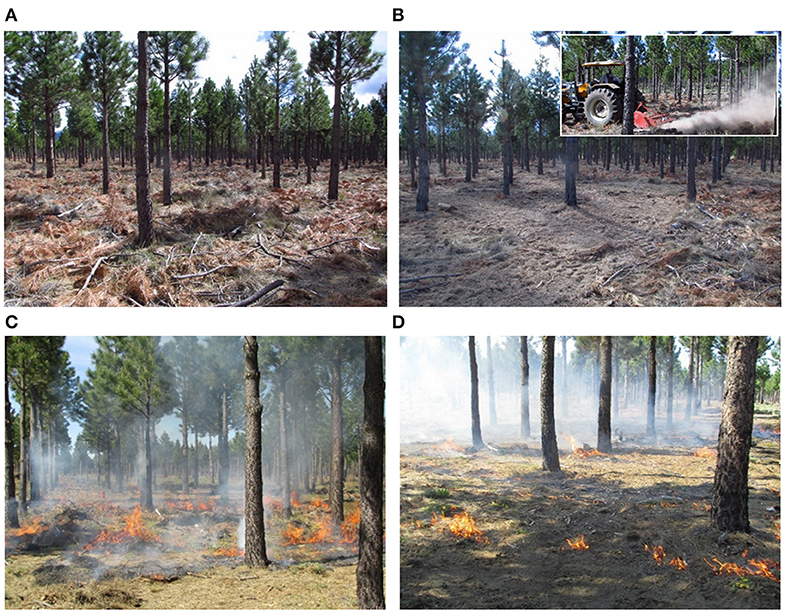
Figure 3. Images of fuel treatments: residues on the floor (UNTREATED) (A), mastication (MAST) (B), prescribed fire (RX) (C), and mastication plus prescribed fire (MAST/RX) (D).
The dates on which we implemented the treatments varied due to weather conditions required for treatments and because of the addition of new study sites in subsequent years (Table 2). Vegetation sampling started the same year after fuel reduction treatments were implemented in some sites and a year after fuel reduction in others. On some sites, it was not possible to complete burnings since appropriate weather conditions limited the burning time; thus, some sites had fewer than four replicates in RX and MAST/RX treatments. Furthermore, some plots were disturbed after treatment implementation, so, we did not consider them in the subsequent measurements. In the third year, all UNTREATED plots were disturbed in site 3. We therefore used second-year measurements for the third year in that site, due to the minimal variation we detected between the first and second years on UNTREATED plots.
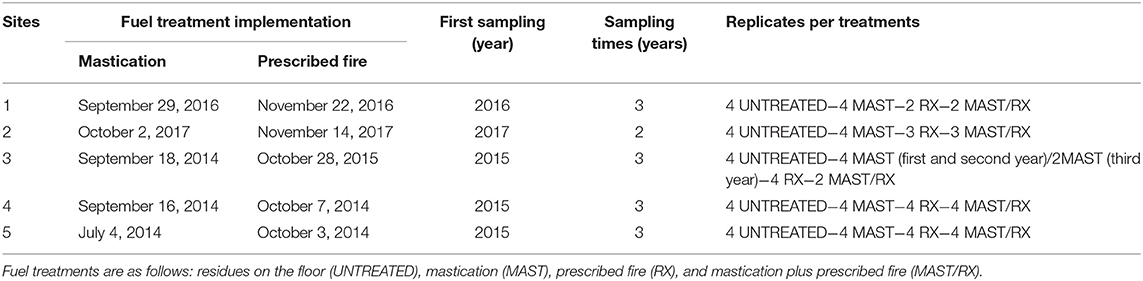
Table 2. Details of fuel treatment implementation, vegetation samplings, and replicates per treatments at each study site.
Data Collection and Classification
For sampling vegetation, we established a 4-m2 (2 × 2 m) permanent plot in the center of each plot, in each site, where we sampled total vascular plant species composition and estimated their aerial percentage cover. We sampled vegetation for 3 consecutive years in the fuel treatment plots and once in the adjacent steppe plots. To better characterize plant assemblage, we also surveyed species composition of the complete plot, except within a 1-m buffer of each treatment edge to avoid contact areas among different treatments (100 m2 per plot) (Supplementary Material 1). Unidentified species in the field were identified in the laboratory according to Correa (1969–1999) and Zuloaga et al. (2019). Likewise, we used the same 4-m2 vegetation plots for sampling residues and assessing bare ground cover. We estimated percentage cover of pine cones and branches, litter debris (including small masticated branches in MAST and MAST/RX treatments), and bare ground.
We classified the identified species by origin into “natives” and “exotics” and by growth form into “trees,” shrubs and sub-shrubs as “shrubs,” non-grass perennial herbs as “perennial herbs,” “perennial grasses,” annual and biannual herbs as “annual herbs,” and “annual grasses,” according to Zuloaga et al. (2019). We estimated each origin and growth form group richness and abundance, and total richness and abundance for each permanent 4-m2 plot, and then we used them as response variables. We considered the unidentified species only for total abundance.
Statistical Analyses
Vegetation Richness and Abundance Response for 3 Years After Fuel Reduction Treatments
Due to site-specific conditions and differences in treatment implementation among sites, we first analyzed each site independently. To compare total, by origin, and by growth form richness and abundance responses among fuel treatments and time since their implementation, we used linear mixed models. We used a factorial design (4 × 3); we treated the “fuel treatment” factor (with four levels: UNTREATED, MAST, RX, and MAST/RX) and the “year” factor (with three levels: first, second, and third) as fixed effects and plots as random effect. Plots were correlated through the order one autoregressive model. Normality assumption was evaluated through Shapiro–Wilk test, and homoscedasticity assumption through residuals. When heteroscedasticity was detected, we carried out a second test modeling it. We compared both models, and if p < 0.05, we selected the one with the lowest Akaike (AIC) value or the simplest one. When differences were detected (p < 0.05), we carried out Fisher's least-significant difference (LSD) contrasts. We performed the analysis only for those richness variables with at least two species and abundance variables with at least 1% cover in some of the treatments. Lower values were interpreted as variable presence or absence in the treatment. To carry out these analyses, we used the software InfoStat (Di Rienzo et al., 2018). InfoStat implements an interface of R platform (R Core Team, 2018) for estimation of linear mixed models through the functions gls and lme from Non-linear Mixed-Effects Models library (Pinheiro and Bates, 2004; Di Rienzo et al., 2017).
Vegetation Richness, Abundance, and Composition Response to Fuel Treatments Compared With Natural Vegetation
To compare the same variables among fuel treatments and natural vegetation, we used last year sampling data, and we performed variance analyses (ANOVA). We modeled the “fuel treatment and steppe” factor (with five levels: UNTREATED, MAST, RX, MAST/RX, and STEPPE) as fixed effect. Normality assumption was evaluated through the Shapiro–Wilk test, and homoscedasticity assumption through residuals. When differences (p < 0.05) were detected, we carried out LSD Fisher contrasts. As in the previous analyses, we performed them only for those richness variables with at least 2 species, and abundance variables with at least 1% cover in some of the treatments. To carry out these analyses, we used the software InfoStat (Di Rienzo et al., 2018). To evaluate species composition similarity among fuel treatments and natural vegetation from the last sampling year, we used Jaccard and Bray–Curtis indices at site level. We estimated both indices through vegdist function from Vegan library (Oksanen et al., 2018) in the software R version 3.5.2 (R Core Team, 2018).
Vegetation Response Patterns to Fuel Treatments 3 Years After Their Implementation
To evaluate vegetation response patterns among fuel treatments and natural vegetation across sites, we described the last sampling year richness and abundance response patterns observed among sites. To compare plant community similarity, we performed a permutational multivariate analysis of variance using distance matrices (PERMANOVA). We used Bray's distance, based on species abundance at plot level from the last sampling year. Factors were “fuel treatment and steppe” (with five levels: UNTREATED, MAST, RX, MAST/RX, and STEPPE) and “site” (with five levels: site 1, site 2, site 3, site 4, and site 5). We added a dummy variable to include plots with zero abundance values in the analysis. Dummy variables not only are useful to include zero abundance plots but also allow to show similarity among plots with low abundance (Clarke et al., 2006). To visualize the results, we displayed plant community data, through combination of factor levels, graphically in ordination space using a non-metric multidimensional scaling (NMDS) based on species abundance with Bray's distance, obtaining an interaction plot. To correlate vegetation response with fuel treatments at each site with some explanatory variables, we ran a redundancy analysis (RDA). We used the species abundance data from the last sampling year for each fuel treatment at each site. The possible explanatory variables were forest structure variables, annual precipitation, and years of grazing exclusion (Table 1). Before the analysis, the collinearity of explanatory variables was tested, and highly correlated variables (correlation value that exceeded 0.6) were excluded from the analysis. To perform PERMANOVA, NMDS, and RDA analyses, we used the functions adonis, metaMDS, and rda, respectively, from Vegan library (Oksanen et al., 2018) in the software R, version 3.5.2 (R Core Team, 2018).
Residues and Bare Ground Cover in Fuel Treatments
To compare residue and bare ground cover percentage among fuel treatments, we used the first and last sampling years for these variables in each site. We performed ANOVAs to compare the fuel treatment factor, for each year. We carried out the same analyses described for the comparison among fuel treatments and natural vegetation.
Results
Vegetation Richness and Abundance Response for 3 Years After Fuel Reduction Treatments
Throughout all sites, fuel treatments, and years, total richness varied between 1.5 and 11.5 species/4 m2, and total abundance varied between 0.2 and 27.9% cover. In the plantation of site 4, richness was <2 species/4 m2 and abundance was <1% cover before fuel reduction implementation, and we did not detect any substantial change after it. For this reason, we did not perform any statistical analysis of this site to compare richness and abundance response among fuel treatments and time since their implementation. Thus, no results are shown in any table and figure for this site. Vegetation richness and abundance showed some different responses at each site for the first 3 years following fuel reduction treatments (Table 3, for more statistical details see Supplementary Material 2).
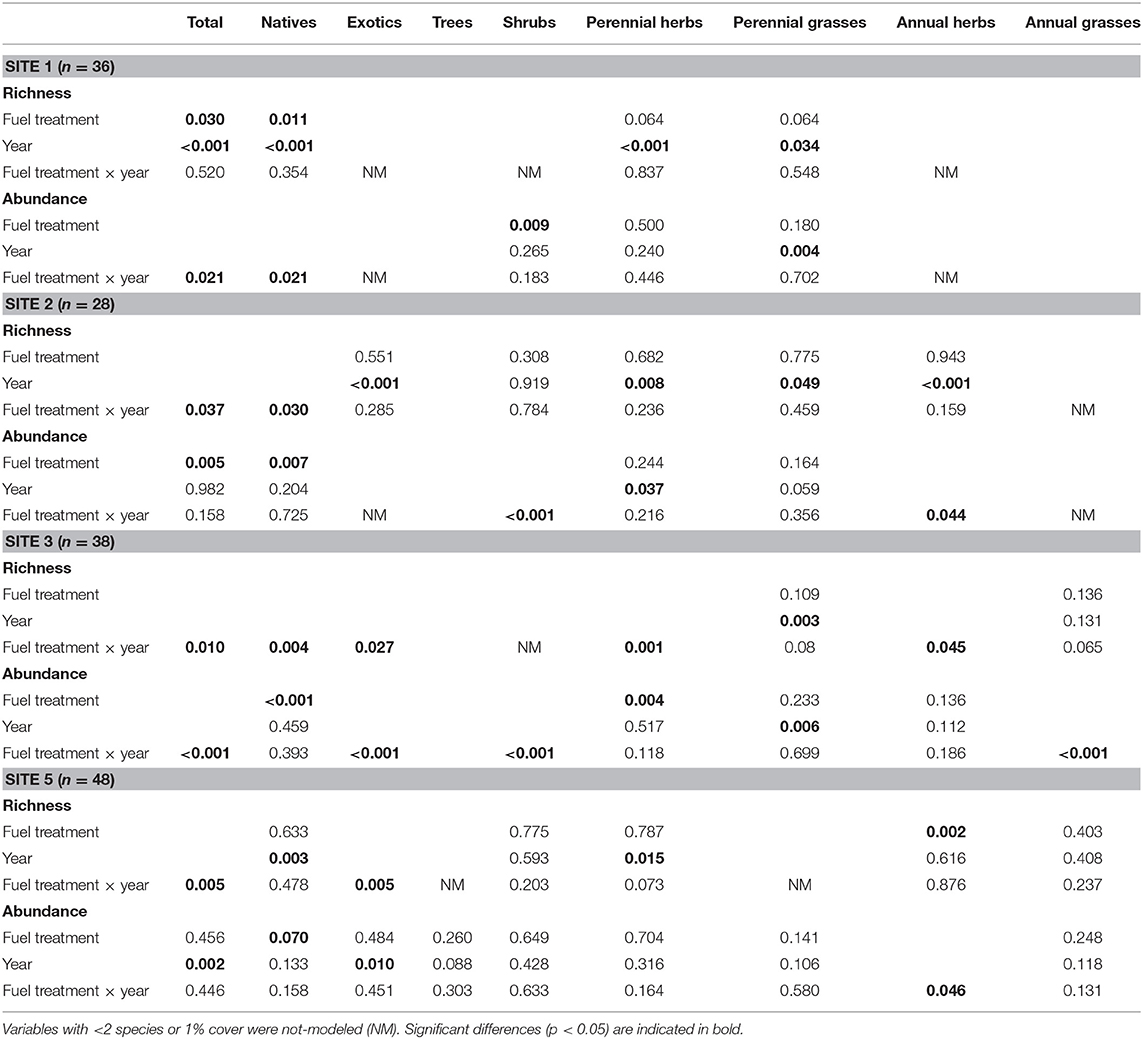
Table 3. P-values for fuel treatment and year factors main effect, and their interaction for richness and abundance variables at each site.
Total richness only showed differences among fuel treatments in site 1, where it was greater in MAST than in MAST/RX and RX (Figures 4A,C,E). On the other sites, total richness increased from the first to the second year in all fuel reduction treatments in site 2 (Figures 5A,C) and in RX in site 3 (Figures 6A,C,E), and it decreased in RX in site 5 (Figures 7A,C,E). Furthermore, total richness increased from the second to the third year in UNTREATED in site 5. Regarding origin, native richness was lower in RX than in UNTREATED in site 1, and it was lower in RX than in UNTREATED and MAST the first year in site 3. In contrast, exotic richness increased from the first to the second year in all fuel treatments in site 2, and in MAST in site 3, although it decreased again the third year in site 3. Also, exotic richness was greater the first and second years in all fuel reduction treatments than in UNTREATED in site 5, whereas the third-year differences were only detected between RX and UNTREATED. Regarding growth form, perennial grass richness increased from the first to the second or third year in all fuel treatments in sites 1, 2, and 3. Perennial herb richness also increased from the first to the second or third year in all fuel treatments in sites 1 and 2, but it increased only in RX in site 3. Annual herb richness increased from the first to the second year in RX in site 3, and it was greater in all fuel reduction treatments than in UNTREATED in site 5.
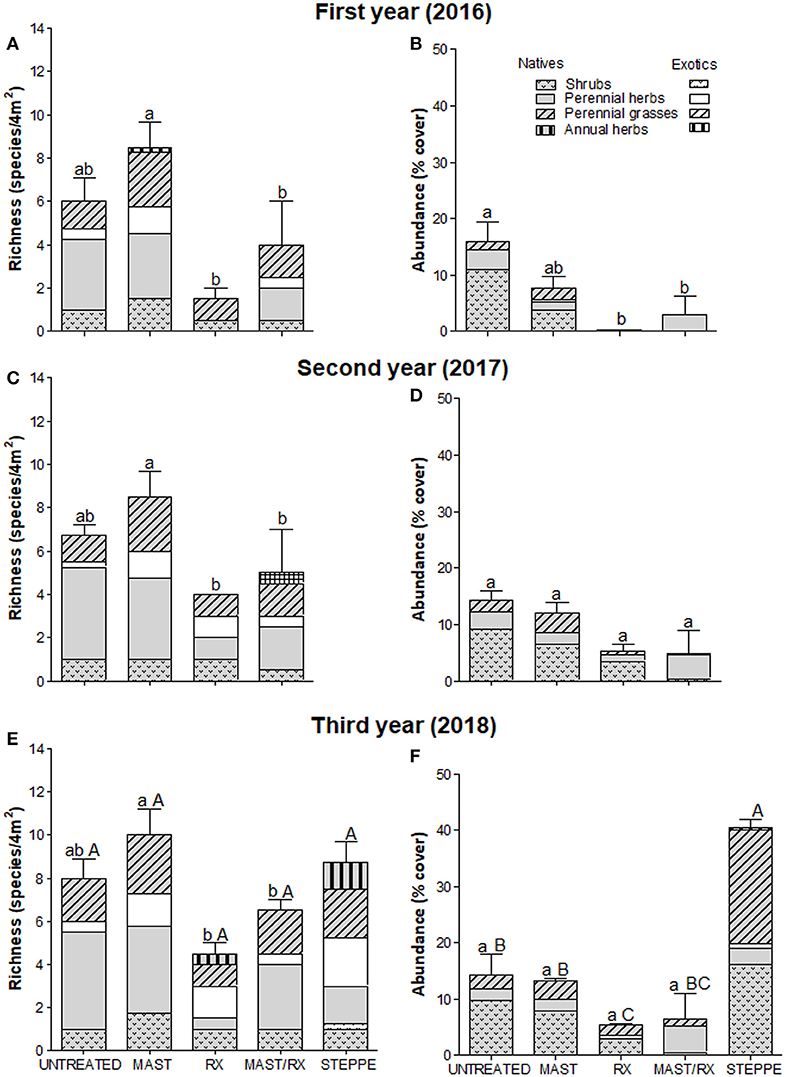
Figure 4. Vegetation richness (A,C,E) and abundance (B,D,F) by origin and growth form in each fuel treatment for the first (A,B), the second (C,D), and the third (E,F) years; steppe vegetation variables are included for the third year in site 1. Bars indicate total richness and abundance SE, respectively. Different small letters indicate differences among fuel treatments for each year (differences among years for each fuel treatment are not represented), and capital letters indicate differences among fuel treatments and steppe. Treatments are as follows: residues on the floor (UNTREATED), mastication (MAST), prescribed fire (RX), mastication plus prescribed fire (MAST/RX), and steppe vegetation (STEPPE).
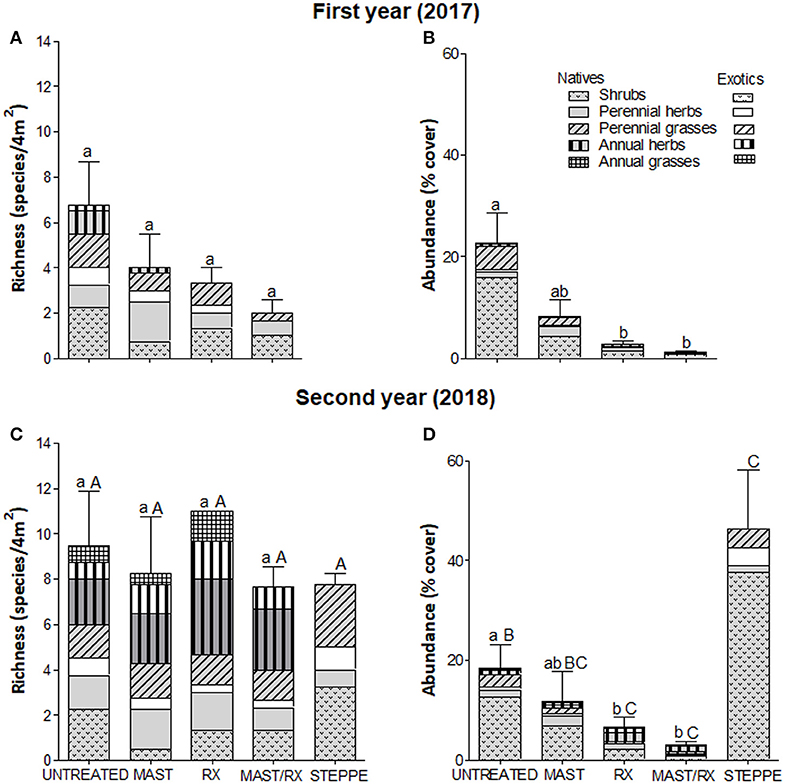
Figure 5. Vegetation richness (A,C) and abundance (B,D) by origin and growth form in each fuel treatment for the first (A,B) and the second (C,D) years; steppe vegetation variables are included for the second year in site 2. Bars indicate total richness and abundance SE, respectively. Different small letters indicate differences among fuel treatments for each year (differences among years for each fuel treatment are not represented), and capital letters indicate differences among fuel treatments and steppe. Treatments are as follows: residues on the floor (UNTREATED), mastication (MAST), prescribed fire (RX), mastication plus prescribed fire (MAST/RX), and steppe vegetation (STEPPE).
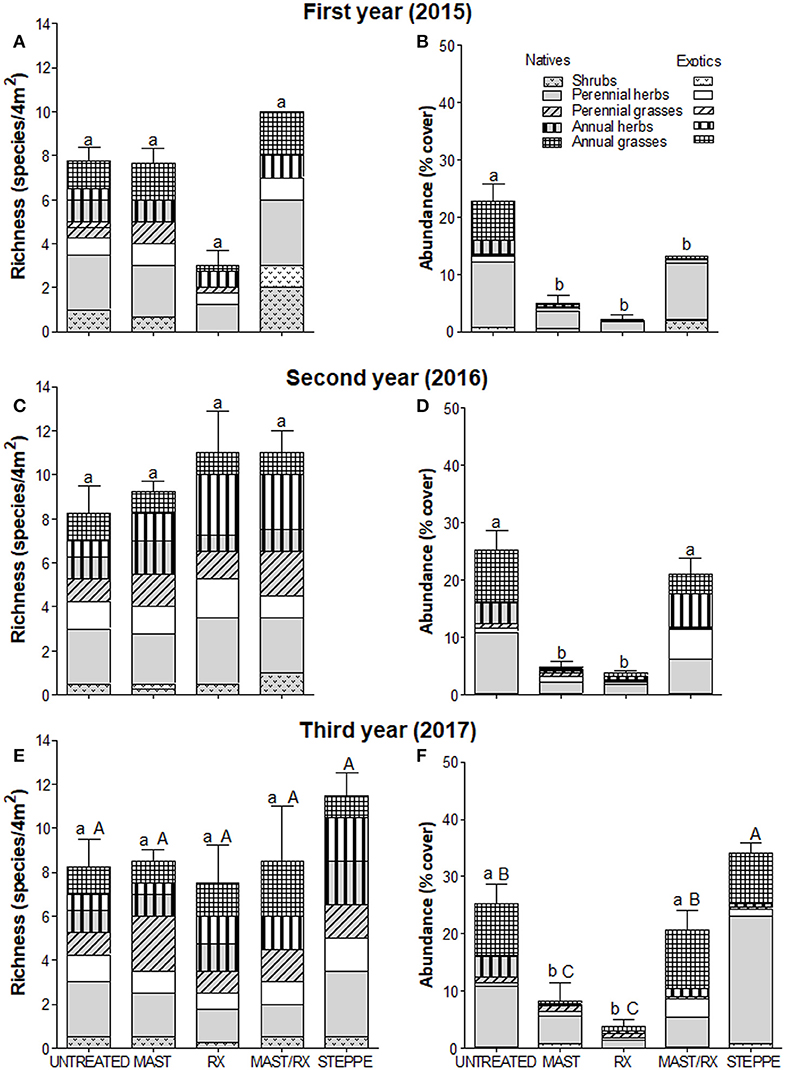
Figure 6. Vegetation richness (A,C,E) and abundance (B,D,F) by origin and growth form in each fuel treatment for the first (A,B), the second (C,D), and the third (E,F) years; steppe vegetation variables are included for the third year in site 3. Bars indicate total richness and abundance SE, respectively. Different small letters indicate differences among fuel treatments for each year (differences among years for each fuel treatment are not represented), and capital letters indicate differences among fuel treatments and steppe. Treatments are as follows: residues on the floor (UNTREATED), mastication (MAST), prescribed fire (RX), mastication plus prescribed fire (MAST/RX), and steppe vegetation (STEPPE).
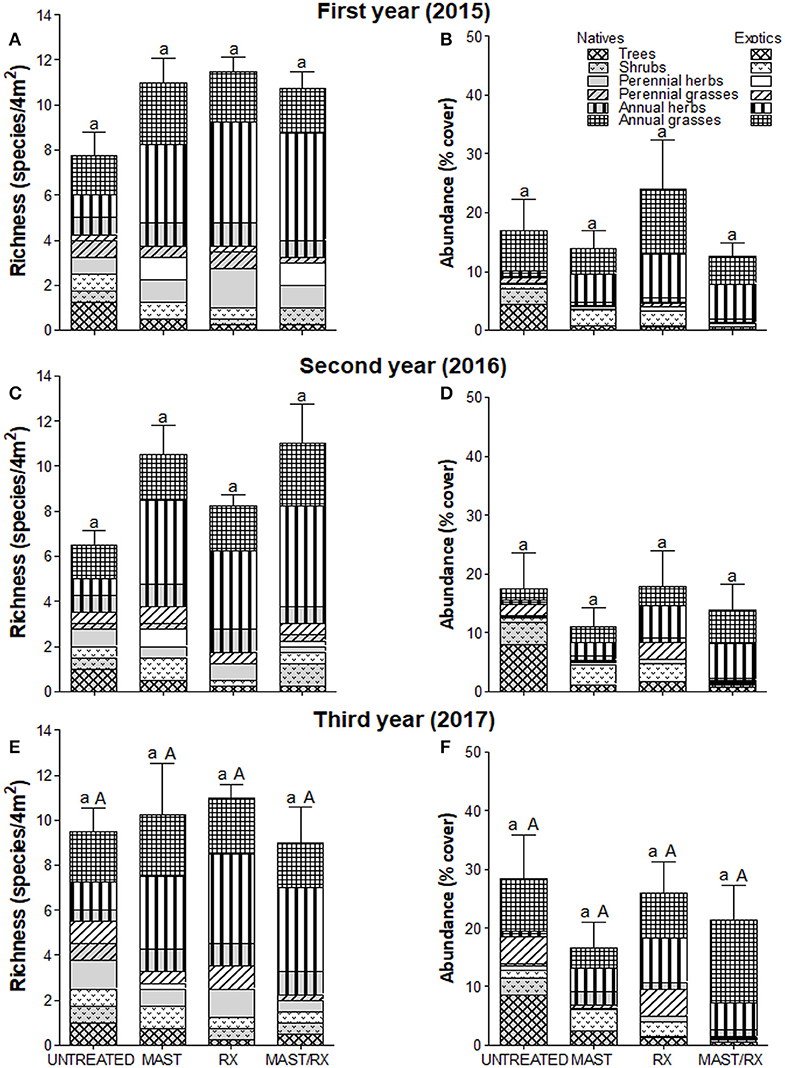
Figure 7. Vegetation richness (A,C,E) and abundance (B,D,F) by origin and growth form in each fuel treatment for the first (A,B), the second (C,D), and the third (E,F) years in site 5. Bars indicate total richness and abundance SE, respectively. Different small letters indicate differences among fuel treatments for each year (differences among years for each fuel treatment are not represented), and capital letters indicate differences among fuel treatments for the third year. Treatments are as follows: residues on the floor (UNTREATED), mastication (MAST), prescribed fire (RX), mastication plus prescribed fire (MAST/RX), and steppe vegetation (STEPPE).
Total abundance showed differences among fuel treatments in all sites except site 5 (Figures 4–6, 7B,D,F). MAST/RX and RX had the lowest total abundance the first year in sites 1 and 2, and it remained low the second year in site 2. The three fuel reduction treatments had lower total abundance than UNTREATED the first year in site 3, and it remained low the following years in MAST and RX, but it increased the second year in MAST/RX, due to an increase in exotic species. Native abundance showed the same pattern as total abundance in sites 1 and 2, since native cover was close to 100% total cover, whereas it was greater in UNTREATED than in all fuel reduction treatments in site 5. Regarding growth form, shrub abundance was greater in UNTREATED and MAST than in MAST/RX in site 1. Perennial grass abundance showed an increase the third year in all fuel treatments in sites 1 and 3. Perennial herb abundance increased the second year in all fuel treatments in site 2, and it was greater in UNTREATED and MAST/RX than in the other fuel treatments in site 3. Annual grass abundance increased the second year in UNTREATED and the third year in MAST in site 3. Finally, annual herb abundance increased the second year in MAST in site 2, and it was greater in RX than in UNTREATED only the first year in site 5.
Vegetation Richness, Abundance, and Composition Response to Fuel Treatments Compared With Natural Vegetation
Throughout all sites, and fuel treatments and steppe, 3 years after fuel reduction implementation total richness varied between 4.5 and 11 species/4 m2, and total abundance varied between 1.6 and 50.8% cover. Since vegetation was scarce before fuel reduction implementation in the plantation of site 4, we only briefly mentioned its steppe's composition. In site 5, we could not find a representative natural vegetation area; thus, we compared richness, abundance, and composition only among fuel treatments.
Total richness did not show differences among the levels of the fuel treatment and steppe factor in any site, but some differences were detected regarding origin and growth form (Table 4, Figures 4E, 5C, 6E, 7E). Native richness was lower in RX than in the other fuel treatments and steppe in site 1, and it was lower in RX and MAST/RX than in STEPPE in site 3. Regarding growth form, shrub richness was the greatest in STEPPE in site 2. Perennial herb richness showed the same response as native richness in site 3, whereas perennial grass richness was greater in UNTREATED than in MAST and MAST/RX in site 5. Annual herb richness was greater in the three fuel reduction treatments than in UNTREATED, it was not recorded in STEPPE in site 2, and it was greater in RX and MAST/RX than in UNTREATED in site 5.
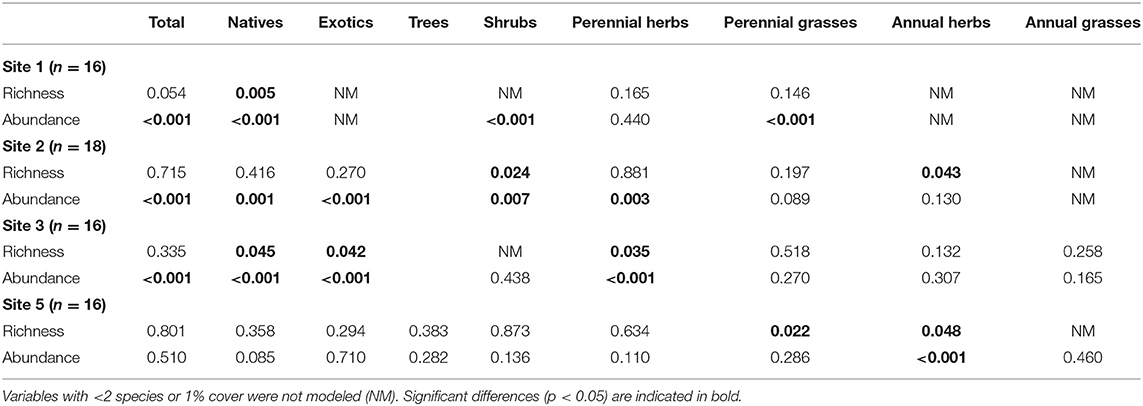
Table 4. P-values for fuel treatment and steppe factor for richness and abundance variables at each site.
Total abundance was greater in STEPPE than in the fuel treatment in all sites with steppe (Table 4, Figures 4F, 5D, 6F). Additionally, it was greater in UNTREATED and MAST than in RX in site 1, in UNTREATED than in MAST/RX and RX in site 2, and in UNTREATED and MAST/RX than in MAST and RX in site 3. Native abundance showed the same responses as total abundance in sites 1 and 2, whereas in site 3 it was greater in STEPPE than in UNTREATED, and that of UNTREATED was greater than that of the fuel reduction treatments. Exotic abundance was greater in STEPPE than in all fuel treatments in site 2, whereas it was greater in STEPPE, MAST/RX, and UNTREATED than in MAST and RX in site 3. Regarding growth form, shrub abundance was greater in STEPPE and UNTREATED in site 1, and in STEPPE in site 2. Perennial herb abundance was greater in STEPPE in sites 1 and 3, and in STEPPE and MAST in site 2. Annual herb abundance only showed differences in site 5, where it was greater in the three fuel reduction treatments than in UNTREATED (Figure 7F).
Regarding composition, in site 1, STEPPE was more similar to MAST (Jaccard 0.6, Bray–Curtis 0.4). In the STEPPE, Pappostipa speciosa (native perennial grass) and Junellia tridactylites (native shrub) were predominant. These species were also predominant in UNTREATED, MAST, and RX, but their abundances were lower in these treatments than in STEPPE. In MAST/RX they were also present, but Oreopolus glacialis (native perennial herb) was predominant. In site 2, even though STEPPE had low similarity with all fuel treatments, it was more similar to UNTREATED and MAST (Jaccard 0.3, Bray–Curtis 0.3, for both). Berberis microphylla and Discaria articulata (native shrubs) were predominant in STEPPE. These species were present in most of the fuel treatments, although their abundances were low. STEPPE had many shrubs, perennial herbs, and perennial grasses with abundance >1% cover, whereas fuel treatments had also many species, but their abundances were lower than 1% cover. In the fuel treatments, we recorded many annual herbs and grasses not recorded in the STEPPE. In site 3, STEPPE was more similar to UNTREATED (Jaccard 0.5, Bray–Curtis 0.6). In STEPPE, Acaena splendens (native perennial herb) and Bromus tectorum (exotic annual grass) were predominant, but their abundances in the fuel treatments were lower than a half. RX had many species, but all of them had low abundance. In MAST/RX Vulpia myuros, Bromus tectorum (exotic annual grasses), Acaena splendens (native perennial herb), and Rumex acetosella (exotic perennial herb) were predominant. In site 4, Festuca pallescens (native perennial grass) and Acaena pinnatifida (native perennial herb) were predominant in the STEPPE, and Senecio filaginoides (native shrub), Rumex acetosella, and Acaena splendens had also great abundance. Although some species were present in the fuel treatments, they had scarce abundance. Among fuel treatments, UNTREATED had more species, followed by MAST/RX. In site 5, the greatest similarity was between MAST and RX (Jaccard 0.7, Bray–Curtis 0.5). In UNTREATED Schinus patagonicus (native tree), Bromus sterilis (exotic annual grass), and Arrhenatherum elatius (exotic perennial grass) were predominant. In the fuel reduction treatments, the abundance of these species decreased, and Verbascum thapsus, Cardus thoermeri, Galium aparine (exotic annual herbs), and Collomia biflora (native annual herb) were predominant. In RX and MAST/RX, Holcus lanatus (exotic annual grass) was also predominant.
Vegetation Response Patterns to Fuel Treatments 3 Years After Their Implementation
Three years after fuel reduction treatment implementation (2 years post-treatment in site 2), total richness was similar among sites in all fuel treatments and steppe (Figure 8A). In contrast, total abundance was variable among and within sites; it was greater in the STEPPE than in the fuel treatments, in all sites with steppe (Figure 9A). Regarding origin, the STEPPE in sites 1, 2, and 3 had greater native than exotic richness and abundance proportions (Figures 8B,C, 9B,C). However, in site 3 such proportion was close to 1, whereas in site 5 exotic proportion was greater in all fuel reduction treatments. Among fuel treatments, sites 1 and 2 had lower total abundance in those treatments involving fire, whereas in site 3 MAST/RX had greater total abundance than MAST and RX, but it was due to exotic species, and in site 5 total abundance was similar among all fuel treatments. Regarding growth form, both richness and abundance proportions varied among and within sites (Figures 8D–I, 9D–I). In general, the growth forms predominant in the STEPPE had lower proportion in fuel treatments, in all sites. Although this pattern was found for both richness and abundance proportions, it was more apparent for abundance proportion. For example, shrub abundance proportion was greater in the STEPPE, followed by UNTREATED, and then the fuel reduction treatments, in site 2. A similar pattern was found for perennial herbs in site 3, and perennial grasses in site 1. Concerning annual herbs and grasses, these groups had greater abundance proportion in sites 3 and 5, corresponding mainly to exotic species.
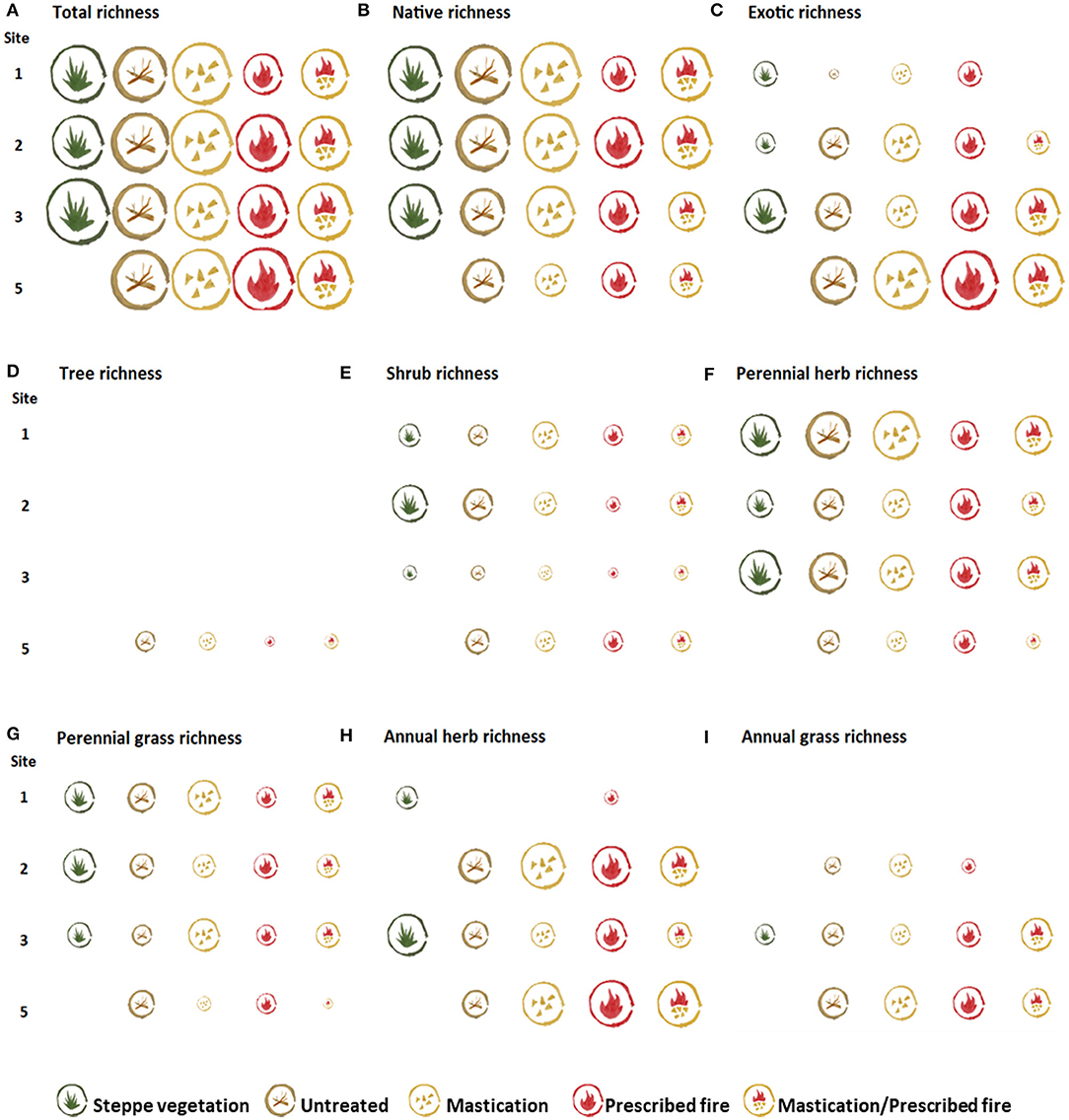
Figure 8. Representation of total richness (A), and by origin (B,C) and growth form (D–I), in the steppe vegetation and fuel treatments the last sampling year in each study site. Icon size corresponds to variables' mean value (ranging from 0.25 to 11.50 species/4m2) for each treatment.
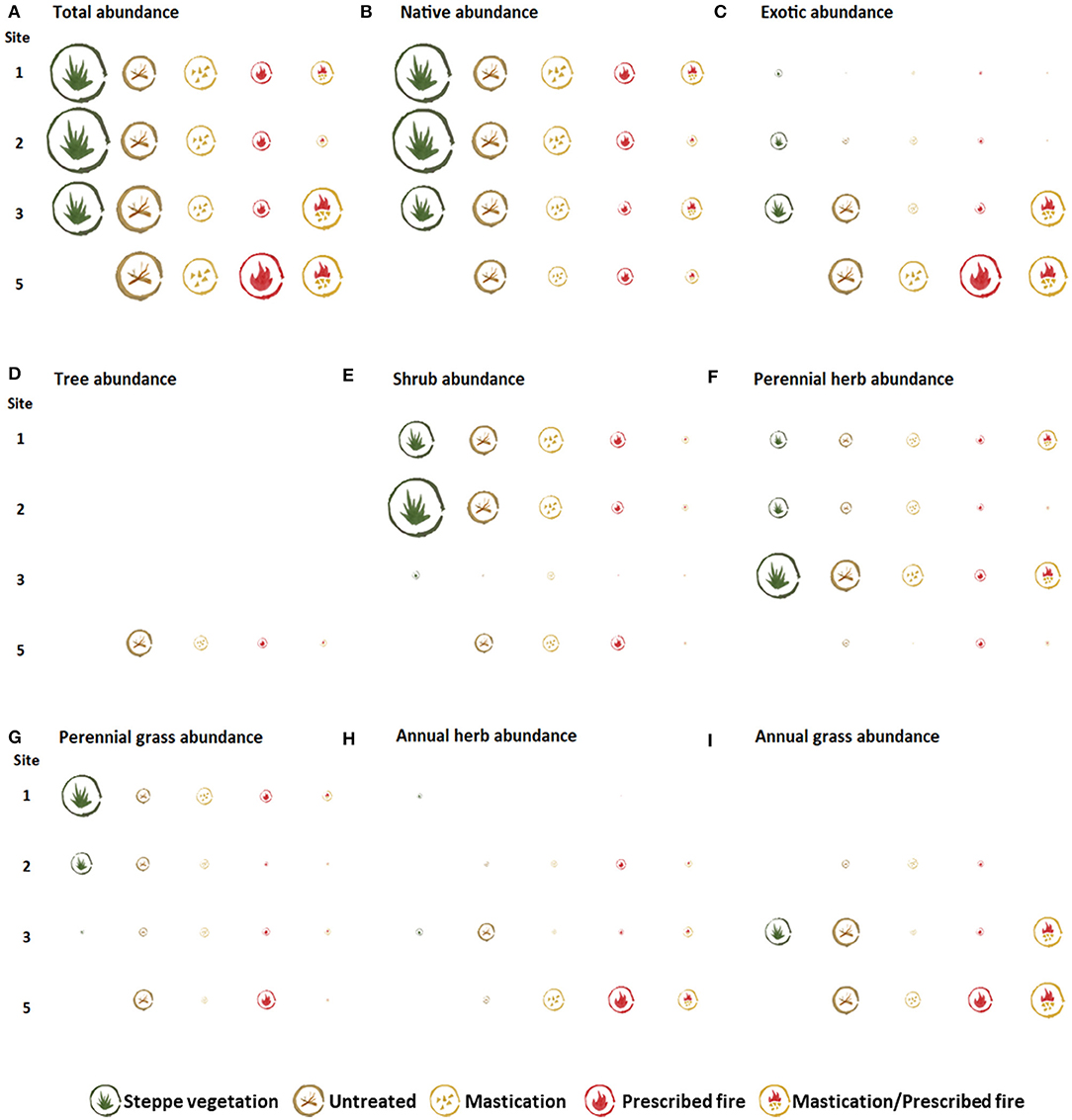
Figure 9. Representation of total abundance (A), and by origin (B,C) and growth form (D–I), in the steppe vegetation and fuel treatments the last sampling year in each study site. Icon size corresponds to variables' mean value (ranging from 0.50 to 50.75% cover) for each treatment.
Plant community was different among sites (R2 = 0.34, p = 0.001), and although differences were detected for the fuel treatment and steppe factor (R2 = 0.08, p = 0.001), a low variability was explained by this factor. Besides, an interaction between site and fuel treatment and steppe factors was detected (R2 = 0.21, p = 0.001), indicating that the plant community among fuel treatments and steppe varied among sites. NMDS ordination (Figure 10A) shows that site 5 is the most dissimilar one, and fuel treatments appear to be, in general, more similar among them than to the STEPPE in each site, whereas sites are more similar in their respective fuel treatments than in their respective STEPPE plant communities. Within each site, the greatest dissimilarity among STEPPE and fuel treatments is in site 4, and the greatest similarity among STEPPE and fuel treatments is in site 1, which shows the greatest similarity between STEPPE and MAST. Site 2 shows the greatest similarity between UNTREATED and MAST treatments, whereas site 3 shows the greatest similarity between MAST and RX, and STEPPE is more similar to MAST/RX. Site 5 appears to be more similar among their fuel reduction treatment communities than to the UNTREATED plant community. Considering plant community response to fuel treatments at each site related to site characteristics, about 15% of plant community variance is explained by the first two RDA axes (Figure 10B). The first axis is strongly and positively correlated with annual precipitation, covering 9.75% of the variance, whereas the second is positively correlated with initial residual biomass, covering 5.25% of the variance. Another good explanatory variable was years of grazing exclusion, but since it was strongly and negatively correlated with initial residual biomass (correlation value −0.81), we excluded it from the analysis to avoid collinearity. Fuel treatments' plant communities from site 5 are positively correlated with the first axis, with the greatest precipitation values, and those of site 3 are correlated with the second axis, with the greatest initial residual biomass values, whereas those of sites 1 and 2 are negatively correlated with both axes. In addition, exotic species are more positively associated with the axes, being more common in sites with greater precipitation and initial biomass, whereas native species are negatively associated with these axes.
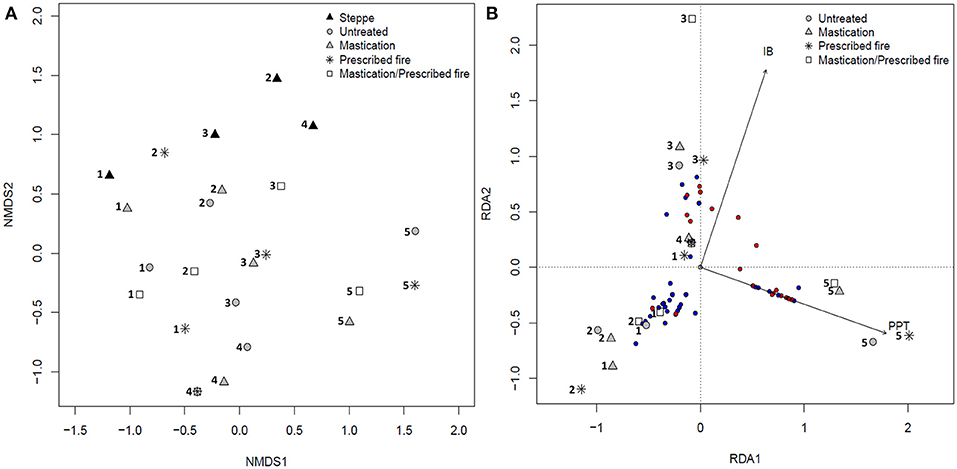
Figure 10. Two-dimensional non-metric multidimensional scaling (NMDS) ordination (A) of vegetation 3 years after fuel reduction implementation in fuel treatments and steppe in all sites (1, 2, 3, 4, and 5) in the two-way factorial design (fuel treatments and steppe × sites); stress = 0.19. Biplot of the first and second axes of the redundancy analysis (RDA) ordination (B) of vegetation 3 years after fuel reduction implementation in fuel treatments in all sites. Circles are species, indicating natives (blue) and exotics (red). Significant explanatory variables are annual precipitation (PPT) and initial residual biomass (IB). Variable covered by the first axis is 9.75%, and that by the second is 5.25%.
Residues and Bare Ground Cover in Fuel Treatments
Cone and branch cover was different among fuel treatments in sites 3, 4, and 5 the second and third years. In all these sites, cone and branch cover was greater in UNTREATED than in the other treatments, except MAST in site 3 the second year, and MAST and RX in site 5 the second and third years, respectively, which did not show differences to UNTREATED (Figures 11A,D,G,J,M). Litter debris cover was different among fuel treatments in only the first year in site 1, being lower in RX than in the other treatments. Although site 5 had a p < 0.05 for the first year, no LSD Fisher differences were detected, probably due to high variability (Figures 11B,E,H,K,N). Bare ground cover was different among fuel treatments in sites 1, 2, 3, and 4, whereas in site 5 none of the treatments had bare ground cover (Figures 11C,F,I,L). In site 1, bare ground was greater in RX than in the other treatments. In site 2, bare ground was greater in RX, followed by MAST/RX the first year, and greater in both treatments the second year, than in the other treatments. In site 3, although LSD Fisher did not detect differences, UNTREATED had about 0% cover the second and third years, and about 12 to 33% cover in the other treatments. In site 4, bare ground was different among treatments the second year, in which MAST/RX and RX covers were greater than UNTREATED, having about 0%, and intermediate values in MAST.
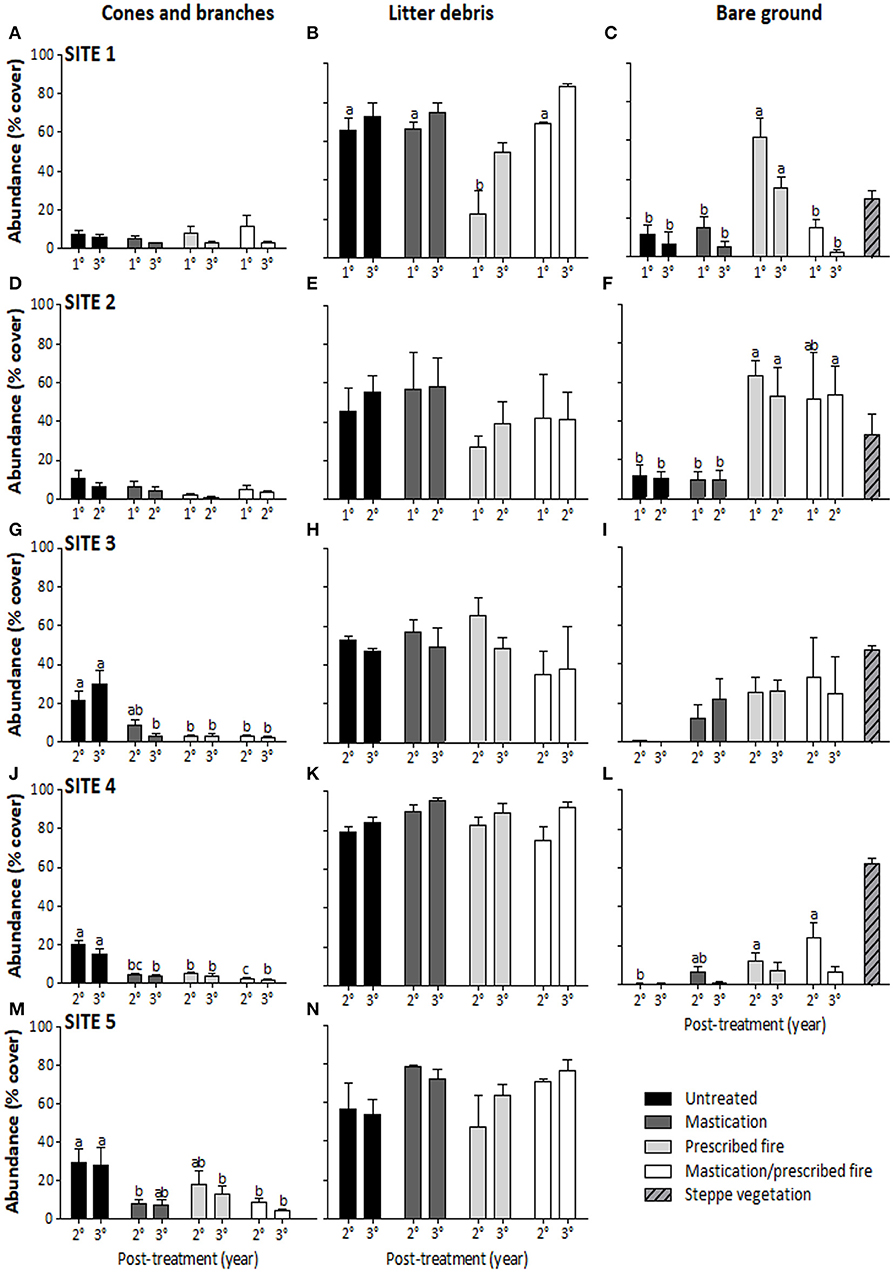
Figure 11. Cones and branches (A,D,G,J,M), litter debris (B,E,H,K,N), and bare ground (C,F,I,L) cover in 2 years of sampling for each fuel treatment in sites 1 (A–C), 2 (D–F), 3 (G–I), 4 (J–L), and 5 (M,N). Bars indicate SE, and letters indicate significant differences (p < 0.05) among fuel treatments.
Discussion
Vegetation was affected by fuel reduction treatments in ponderosa pine plantations located in NW Patagonia. We found plant response differences among fuel treatments and years, which varied among sites due to site-specific conditions. During the first 3 years after fuel reduction implementation, total richness and abundance did not exceed, although sometimes reached, the UNTREATED values in any site. Three years post-treatment, total richness reached STEPPE values in most fuel reduction treatments, whereas total abundance was always greater in the STEPPE. Plant community similarity was low among fuel treatments and compared to the STEPPE. Sites were compositionally different, due to natural heterogeneity, different history of use, and different plantation structure. Although any fuel treatment improved UNTREATED vegetation conditions, similar trends in vegetation response among sites allow us to consider MAST as the fuel reduction treatment with the lowest negative short-term impact on vegetation. This treatment, compared with UNTREATED, has the plus that may reduce fire hazard.
Native species usually remain constant after fuel reduction treatments, whereas exotics commonly increase in those treatments involving fire (Abella and Springer, 2015). However, this general pattern may vary depending on treatment specificities and pre-treatment vegetation conditions (Stephens et al., 2012). In the four analyzed sites, total, and native richness reached UNTREATED values in MAST immediately or after a year following fuel reduction. This could be due to germination from the soil seed bank, which could be stimulated by the mastication process, and resprouter species that persisted after treatment. In contrast, total richness recovery delayed in RX and MAST/RX in sites 1 and 3, whereas native richness did not reach UNTREATED values either in RX in sites 1 and 3 or in MAST/RX in site 3. It might be possible that fire had more severe effects on vegetation, causing resprouter organism death (Ghermandi et al., 2013), and litter burning caused seed elimination (Facelli and Pickett, 1991). Similar results were found in a mixed conifer forest in California, USA, where native richness recovered 1 year after mastication, whereas fire-involved treatments delayed or limited its recovery (Collins et al., 2007).
The low exotic richness found in all fuel treatments, even in the STEPPE, in site 1, could be explained by years of grazer exclusion. In site 2, exotics, mainly annual herbs and grasses, increased their number the second year (2017), in all fuel treatments. Given that the increase also happened in the UNTREATED, we cannot interpret it as a response to fuel reduction treatments, but it might have been caused by inter- or intra-annual variations (Korb and Fulé, 2008). No annual herbs and grasses were recorded in the STEPPE; however, this sector was sampled in 2016 that had an atypical winter with low precipitations. Year 2017, instead, had abundant precipitations, which might have favored annual species emergence (Yan et al., 2015). In site 5, we observed the same inter-annual variation for exotic abundance; most of them were annual herbs and grasses. In site 3, the high exotic proportion found in all fuel treatments and steppe could be due to overgrazing, which might have caused a high level of degradation, as evidenced by Acaena splendens predominance (Bertiller and Bisigato, 1998). In site 5, an early increase in exotic richness occurred in all fuel reduction treatments, exceeding UNTREATED values, and a delayed increase in native species. This could be since natural vegetation in site 5 was not steppe vegetation, but remnants of Austrocedrus chilensis native forest, which burned in 1987. Besides, this site was grazed, for at least 10 years before this study. Native forests that have been grazed in the region usually have fewer native species and more exotics, especially if there was a canopy cover reduction (Vázquez, 2002).
Different plant cover responses to fuel reduction treatments may be possible, since they depend on many factors such as pre-treatment species composition and cover (Dodson et al., 2008), treatment implementation season (Knapp et al., 2007), and treatment effects on soil properties (Owen et al., 2009), among others. Total plant cover reached UNTREATED values immediately in MAST in sites 1 and 2, in all fuel reduction treatments in site 5, and the second year in MAST/RX in site 3. In sites 1 and 2, the increase corresponded to native species, since about 100% of total cover was natives in all fuel treatments and steppe. In sites 3 and 5, where exotic abundance was about 28 and 54% in UNTREATED, respectively, and about 30% in STEPPE in site 3, the total abundance increase corresponded to exotic species. The different proportion in exotic cover increase was related to the different levels of degradation sites had.
Regarding growth form, herbs, and grasses usually recover their cover soon, while shrubs usually need more time, after fuel reduction treatments (Schwilk et al., 2009; Abella and Springer, 2015). Although we did not detect significant differences in perennial group abundance among fuel reduction treatments, all of them showed a general trend to delay reaching UNTREATED values in those treatments involving fire, especially for native perennial species. Perennial herb and grass abundance showed a trend to recover their cover slower or not to reach UNTREATED values in RX and MAST/RX. Only in site 3 perennial herb abundance was greater in UNTREATED and MAST/RX than in RX; however, exotic proportion was higher in MAST/RX. Shrub abundance reached UNTREATED values in MAST in site 1, while it tended to reach UNTREATED values in MAST in site 2. In site 5, although shrub abundance was not different among fuel treatments, most of them were natives in UNTREATED, while there were more exotics in the fuel reduction treatments. Besides, site 5 was the only site having tree cover, which tended to decrease in fuel reduction treatments. The apparent more severe effect of RX and MAST/RX treatments on native perennial abundance recovery, most of them resprouters (Ghermandi et al., 2004), might have been caused by burning season. Prescribed fire was implemented in the early season, between October and November, after winter precipitations and when most species are initiating or reaching the growing peak. At that time, plant tissues have more moisture content, so less heat is necessary to kill them (Wright, 1970). Moreover, when growing season initiates, root carbohydrate reserves are reduced due to their allocation to the aerial portion of plants, resulting in a reduction of resprouting capacity (Bowen and Pate, 1993; Robertson and Hmielowski, 2014). Furthermore, plantation canopy may provoke aboveground and belowground biomass reduction, affecting resprouting even more. Aboveground biomass reduction may result in less tiller density in some perennial grasses, exposing axillar buds to fire damage (Moore et al., 2019), whereas belowground biomass reduction may result in a less carbohydrate reserve, which may be further reduced since shade generated by the canopy may reduce photosynthate production, delaying plant recovery (Bowen and Pate, 1993). An alternative for evaluation is late-season burning, when plant species are at the end of the growing season and the carbohydrate reserve in roots increases. However, if fuel load is high, more intense fires may be generated due to the fact that the soil is dryer, leading to deeper heat penetration into the soil, which may affect perennial species buds and rhizomes (Knapp et al., 2007). It could be possible that mastication affected resprouting capacity less than fires, since it was previously implemented, between July and October, when carbohydrate reserves in roots were greater. Similar results of plant cover recovery in the short term after mastication were recorded in a ponderosa pine (Kane et al., 2010) and in Pinus edulis and Juniperus osteosperma forests (Owen et al., 2009). These authors found that recovery was led by resprouting (Kane et al., 2010) and that it correlated with soil stability and moisture, which remained after mastication (Owen et al., 2009).
Plant community composition may be affected by fuel reduction treatments (Kane et al., 2010); however, pre-treatment and site-specific conditions may lead to compositional differences after fuel reduction treatments (Dodson et al., 2008). Plant community composition similarity was medium to low among all fuel treatments and steppe, in all four analyzed sites. Jaccard's index showed low composition similarity, which was accentuated when considering specific abundances, with Bray–Curtis's index. Since plot size was small, two opposite consequences may have happened: species composition could have been underestimated, while edge effect may have been great, accelerating colonization rate through seed dispersal from adjacent areas (Harper et al., 2005). Thus, larger treatment areas would be important to have more accurate plant community representation and recovery rates. However, many undetected species in permanent plots (4-m2) were detected in total plots (100-m2) suggesting that many native steppe species remain in the plantations' herb–shrub stratum, even after fuel reduction treatments. Besides, given the short term of this study, and the steppe species dynamics and persistence strategies, most plants probably recovered by stem basis resprouting, regrowth of tillers, or germination from the seed bank (Ghermandi et al., 2004; Rago et al., 2020), so that seed dispersal influence, although important, might have been relatively minor. When considering plant community in all sites together, a clear separation among sites was detected; the main differentiation was among site 5 and the other sites. Differences among sites 1, 2, 3, and 4 were led by the STEPPE, whereas an apparent homogenization among plantations was observed, with different levels of plant community similarity among fuel treatments in each site. Plant community similarity in fuel treatments may be led by their low plant cover. We found that some of the variables explaining the differences in vegetation response to fuel treatments among sites were annual precipitation and initial residual biomass. Years of grazing exclusion was another good explanatory variable, negatively correlated with initial residual biomass. However, the variability explained by the RDA ordination was low (15%), indicating that fuel treatments affected vegetation response and probably other explanatory variables that have not been measured. For example, soil properties, slope, and exposition, among others, should be included in future studies to get a better understanding of possible vegetation responses to fuel reduction treatments. It is important to highlight that fuel reduction treatments, commonly implemented in conifer forests in North America, generally result in canopy reduction due to upper vegetation stratum elimination, leading to more light reaching the ground and stimulating understory vegetation development (Abella and Springer, 2015; Fornwalt et al., 2017). In the studied plantations, although thinning had been previously applied, the fuel reduction treatments analyzed did not result in canopy opening; thus, it was not expectable to find a substantial increase in plant cover, compared to the UNTREATED. However, plant cover usually decreases in the initial stages after fuel reduction, and from 5 years post-treatments it starts to increase (Abella and Springer, 2015). The plant cover increase detected among years in the fuel reduction treatments allows us to expect that this trend might continue in the following years.
Residual slash following pruning and thinning may decrease vegetation by burying plants (Metlen et al., 2004; Kane et al., 2010). The effect of fuel reduction treatments on cone and branch reduction was significant only in sites 3, 4, and 5, where their cover was 20% greater in the UNTREATED. These sites also had the highest initial fuel loads. Litter debris cover was >50% in most sites and fuel treatments, but it only decreased to about 22% cover in RX in site 1 the first year post-treatment, whereas in site 2, litter cover was about 25% in RX. However, these values increased the second and third years, suggesting that fine fuel reload may be fast and should be considered to determine burning frequency, depending on the fuel load amount that is wanted to keep on site, generally to try to maintain the pool of nutrients that otherwise, and with more frequent fires, would be lost. Besides, mineral soil exposure after fire-involved treatments may favor plant recruitment and establishment, since available space increase may reduce plant competition (Collins et al., 2007; Kane et al., 2010). Bare ground cover was greater in RX in site 1, and in RX and MAST/RX in sites 2 and 4. However, in the third year, a trend to increase ground cover was found on account of litter or plant cover. Due to the trend in plant cover increase in RX and MAST/RX and being these treatments the ones having more bare ground, it could be expected that plant cover continues to increase the following years.
This study glimpses the possible effects of mastication, prescribed fire, and their combination on plant community, which should be considered for the implementation of fuel management in pine plantations. Although steppe plant species are adapted to frequent fires, and expected to recover after mastication or prescribed fires, our results suggest that their recovery capacity is affected, since native plant community is affected by plantations. Mastication seems to be the fuel reduction treatment with the lowest negative effect on vegetation. However, it is important to consider that although this technique reduces vertical fuel continuity, it does not reduce total fuel load. Furthermore, site-specific conditions may lead to vegetation responses. In plantations where herb–shrub stratum vegetation is scarce, in general due to high stand density and initial fuel load, prescribed fire may be the most effective technique to reduce fuel loads, whereas seeding and planting herb–shrub stratum species should be considered if vegetation restoration is an aim. In plantations where natural vegetation before plantation establishment corresponded to degraded native forests, it could be important to consider exotic species management and to evaluate long-term recuperation of native shrubs and trees. In plantations degraded by overgrazing, recovery of native cover may be associated with degradation level, therefore requiring more than 3 years to recover when it is high.
Data Availability Statement
The raw data supporting the conclusions of this article will be made available by the authors, without undue reservation, to any qualified researcher.
Author Contributions
GD and NL designed the fuel reduction study. GD, NL, and MR executed the fuel reduction treatments. MR, GD, and MU designed the vegetation sampling. MR executed the sampling and species identification. MR and MU designed the published data analyses and conducted the statistical analyses. MR, MU, and GD contributed in writing the manuscript.
Funding
This study was financed through a grant from Unidad para el Cambio Rural (UCAR, PIA No. 14066) and a grant from the National Scientific and Technical Research Council of Argentina (CONICET, PUE 4116/16).
Conflict of Interest
The authors declare that the research was conducted in the absence of any commercial or financial relationships that could be construed as a potential conflict of interest.
Acknowledgments
We thank plantations' owners, Esquel Municipality, and Army and INTA administrations, who allowed us to do the experiment and sampling. We thank Servicio Provincial de Manejo del Fuego staff for conducting the prescribed fires and Marcela Godoy and Jorge Bonansea for helping in the implementation of treatments. We appreciate Paula Ines Castro aid with the graphics design, and we thank María Laura Besio for proofreading. We acknowledge CONICET and SCTeIP-Chubut for their support through a Ph.D. scholarship to MR.
Supplementary Material
The Supplementary Material for this article can be found online at: https://www.frontiersin.org/articles/10.3389/ffgc.2020.00055/full#supplementary-material
References
Abella, S. R., and Springer, J. D. (2015). Effects of tree cutting and fire on understory vegetation in mixed conifer forests. For. Ecol. Manage. 335, 281–299. doi: 10.1016/j.foreco.2014.09.009
Agee, J. K., and Skinner, C. N. (2005). Basic principles of forest fuel reduction treatments. For. Ecol. Manage. 211, 83–96. doi: 10.1016/j.foreco.2005.01.034
Bava, J., Loguercio, G., Orellana, I. A., and Ríos, F. (2015). Evaluación Ambiental Estratégica. Una visión sobre dónde y cómo forestar. Esquel: CIEFAP-FUNDFAEP.
Bertiller, M. B., and Bisigato, A. (1998). Vegetation dynamics under grazing disturbance. the state-and-transition model for the Patagonian steppes. Ecol. Austral. 8, 191–199.
Bertiller, M. B., Elissalde, N. O., Rostagno, C. M., and Defossé, G. E. (1995). Environmental patterns and plant distribution along a precipitation gradient in western Patagonia. J. Arid Environ. 29, 85–97. doi: 10.1016/S0140-1963(95)80066-2
Bowen, B. J., and Pate, J. S. (1993). The significance of root starch in post-fire shoot recovery of the resprouter Stirlingia latifolia R. Br. (Proteaceae). Ann. Bot. 72, 7–16. doi: 10.1006/anbo.1993.1075
Busse, M. D., Hubbert, K. R., Fiddler, G. O., Shestak, C. J., and Powers, R. F. (2005). Lethal soil temperatures during burning of masticated forest residues. Int. J. Wildl. Fire 14, 267–276. doi: 10.1071/WF04062
Cabrera, A. L. (1971). Fitogeografía de la República Argentina. Boletín la Soc. Argentina Botánica 14:25.
Carnus, J. M., Parrotta, J., Brockerhoff, E. G., Arbez, M., Jactel, H., Kremer, A., et al. (2006). Planted forests and biodiversity. J. For. 104, 65–77. doi: 10.1093/jof/104.2.65
Chehébar, C., Novaro, A., Iglesias, G., Walker, S., Funes, M., Tammone, M., et al. (2013). Identificacion de áreas de importancia para la biodiversidad en la estepa y el monte de Patagonia. Buenos Aires: Wildlife Conservation Society, INIBIOMA, The Nature Conservancy.
Clarke, K. R., Somerfield, P. J., and Chapman, M. G. (2006). On resemblance measures for ecological studies, including taxonomic dissimilarities and a zero-adjusted Bray-Curtis coefficient for denuded assemblages. J. Exp. Mar. Bio. Ecol. 330, 55–80. doi: 10.1016/j.jembe.2005.12.017
Collins, B. M., Moghaddas, J. J., and Stephens, S. L. (2007). Initial changes in forest structure and understory plant communities following fuel reduction activities in a Sierra Nevada mixed conifer forest. For. Ecol. Manage. 239, 102–111. doi: 10.1016/j.foreco.2006.11.013
Correa, M. N. (1969–1999). Flora Patagónica, Colección científica. Buenos Aires: Instituto Nacional de Tecnología Agropecuaria.
Cummings, J., and Reid, N. (2008). Stand-level management of plantations to improve biodiversity values. Biodivers. Conserv. 17, 1187–1211. doi: 10.1007/s10531-008-9362-z
Daniel, T. W., Helms, J. A., and Baker, F. S. (1979). Principles of Silviculture. New York, NY: McGraw-Hill.
de Ronde, C., Goldammer, J. G., Wade, D. D., and Soares, R. V. (1990). “Prescribed fire in industrial pine plantations,” in Fire in the Tropical Biota – Ecosystem Process and Global Challenges, ed J. G. Goldammer (Berlin: Springer Verlag), 216–272. doi: 10.1007/978-3-642-75395-4_12
Defossé, G. E., Godoy, M. M., Bianchi, L. O., Lederer, N. S., and Kunst, C. (2015b). “Fire history, fire ecology and management in Argentine Patagonia: from ancient times to nowadays” in Current International Perspectives on Wildland Fires, Mankind and the Environment, eds B. Leblon, and M. E. Alexander (New York, NY: Nova Science Publishers), 177–209.
Defossé, G. E., Godoy, M. M., and Guglielmin, D. A. (2015a). “El manejo y la prevención de los incendios forestales,” in Manual de Buenas Prácticas Para El Manejo De Plantaciones Forestales En El Noroeste De La Patagonia, eds L. Chauchard, C. Frugoni, and C. Nowak (Buenos Aires: Proyecto Conservación de la Biodiversidad en Paisajes Productivos Forestales - GEF TF 090118, Ministerio de Agroindustria Presidencia de la Nación), 371–387.
Defossé, G. E., and Robberecht, R. (1987). Patagonia: range management at the end of the World. Rangelands 9, 106–109.
Di Rienzo, J. A., Casanoves, F., Balzarini, M. G., Gonzalez, L., Tablada, M., and Robledo, C. W. (2018). InfoStat. Centro de Transferencia InfoStat, FCA, Universidad Nacional de Córdoba, Argentina. Available online at: http://www.infostat.com.ar (accessed November 5, 2018).
Di Rienzo, J. A., Macchiavelli, R., and Casanoves, F. (2017). Modelos Lineales Mixtos Aplicaciones en InfoStat. Córdoba: Grupo Infostat.
Dodson, E. K., Peterson, D. W., and Harrod, R. J. (2008). Understory vegetation response to thinning and burning restoration treatments in dry conifer forests of the eastern Cascades, USA. For. Ecol. Manage. 255, 3130–3140. doi: 10.1016/j.foreco.2008.01.026
Facelli, J., and Pickett, S. (1991). Plant litter: its dynamics and effects on plant community structure. Bot. Rev. 57, 1–32. doi: 10.1007/BF02858763
Fick, S. E., and Hijmans, R. J. (2017). Worldclim 2: new 1-km spatial resolution climate surfaces for global land areas. Int. J. Climatol. 37, 4302–4315. doi: 10.1002/joc.5086
Fornwalt, P. J., Rocca, M. E., Battaglia, M. A., Rhoades, C. C., and Ryan, M. G. (2017). Mulching fuels treatments promote understory plant communities in three Colorado, USA, coniferous forest types. For. Ecol. Manage. 385, 214–224. doi: 10.1016/j.foreco.2016.11.047
Fulé, P. Z., Crouse, J. E., Roccaforte, J. P., and Kalies, E. L. (2012). Do thinning and/or burning treatments in western USA ponderosa or Jeffrey pine-dominated forests help restore natural fire behavior? For. Ecol. Manage. 269, 68–81. doi: 10.1016/j.foreco.2011.12.025
Ghermandi, L., Gonzalez, S., Lescano, M. N., and Oddi, F. (2013). Effects of fire severity on early recovery of Patagonian steppes. Int. J. Wildl. Fire 22, 1055–1062. doi: 10.1071/WF12198
Ghermandi, L., Guthmann, N., and Bran, D. (2004). Early post-fire succession in northwestern Patagonia grasslands. J. Veg. Sci. 15, 67–76. doi: 10.1111/j.1654-1103.2004.tb02238.x
Gittins, C., Ghermandi, L., and Bran, D. (2011). Studying the post-fire performance of tussock grasses in Patagonia: survival, biomass production and early competition. J. Arid Environ. 75, 986–990. doi: 10.1016/j.jaridenv.2011.05.005
Godoy, M. M., Defossé, G. E., Bianchi, L. O., Davel, M. M., and Withington, T. E. (2013). Fire-caused tree mortality in thinned Douglas-fir stands in Patagonia, Argentina. Int. J. Wildl. Fire 22, 810–814. doi: 10.1071/WF12107
Golluscio, R. A., Austin, A. T., García Martínez, G. C., Gonzalez-Polo, M., Sala, O. E., and Jackson, R. B. (2009). Sheep grazing decreases organic carbon and nitrogen pools in the patagonian steppe: combination of direct and indirect effects. Ecosystems 12, 686–697. doi: 10.1007/s10021-009-9252-6
Harper, K. A., Macdonald, S. E., Burton, P. J., Chen, J., Brosofske, K. D., Saunders, S. C., et al. (2005). Edge influence on forest structure and composition in fragmented landscapes. Conserv. Biol. 19, 768–782. doi: 10.1111/j.1523-1739.2005.00045.x
Jost, L., Chao, A., and Chazdon, R. L. (2011). “Compositional similarity and β (beta) diversity”, in Biological Diversity Frontiers in Measurement and Assessment, eds A. E. Magurran, and B. J. McGill (New York, NY: Oxford University Press), 66–84.
Kane, J. M., Morgan Varner, J., Knapp, E. E., and Powers, R. F. (2010). Understory vegetation response to mechanical mastication and other fuels treatments in a ponderosa pine forest. Appl. Veg. Sci. 13, 207–220. doi: 10.1111/j.1654-109X.2009.01062.x
Knapp, E. E., Schwilk, D. W., Kane, J. M., and Keeley, J. E. (2007). Role of burning season on initial understory vegetation response to prescribed fire in a mixed conifer forest. Can. J. For. Res. 37, 11–22. doi: 10.1139/x06-200
Köppen, W., and Geiger, R. (1936). Das geographische System der Klimate. Berlin: Verlag von Gebrüder Borntraeger.
Korb, J. E., and Fulé, P. Z. (2008). Intra- and interannual vegetation change: Implications for long-term research. Restor. Ecol. 16, 5–11. doi: 10.1111/j.1526-100X.2007.00256.x
Magurran, A. E. (1988). Ecological Diversity and Its Measurement. New York, NY: Princeton University Press. doi: 10.1007/978-94-015-7358-0
Metlen, K. L., Fiedler, C. E., and Youngblood, A. (2004). Understory response to fuel reduction treatments in the blue mountains of northeastern Oregon. Northwest Sci. 78, 175–185. Available online at: https://www.fs.usda.gov/treesearch/pubs/20383.
Moore, N. A., Camac, J. S., and Morgan, J. W. (2019). Effects of drought and fire on resprouting capacity of 52 temperate Australian perennial native grasses. New Phytol. 221, 1424–1433. doi: 10.1111/nph.15480
Mori, A. S., Furukawa, T., and Sasaki, T. (2013). Response diversity determines the resilience of ecosystems to environmental change. Biol. Rev. 88, 349–364. doi: 10.1111/brv.12004
Nuñez, M. A., and Raffaele, E. (2007). Afforestation causes changes in post-fire regeneration in native shrubland communities of northwestern Patagonia, Argentina. J. Veg. Sci. 18, 827–834. doi: 10.1111/j.1654-1103.2007.tb02599.x
Oddi, F. J., and Ghermandi, L. (2015). Dendroecological potential of shrubs for reconstructing fire history at landscape scale in Mediterranean-type climate grasslands: the case of Fabiana imbricata. Dendrochronologia 33, 16–24. doi: 10.1016/j.dendro.2014.11.002
Oddi, F. J., and Ghermandi, L. (2016). Fire regime from 1973 to 2011 in north-western Patagonian grasslands. Int. J. Wildl. Fire 25, 922–932. doi: 10.1071/WF15211
Oksanen, J., Blanchet, F. G., Friendly, M., Kindt, R., Legendre, P., McGlinn, D., et al. (2018). Vegan: Community Ecology Package. Version 2.5-3. Retrieved from: https://cran.r-project.org/web/packages/vegan/index.html (accessed November 5, 2018).
Owen, S. M., Sieg, C. H., Gehring, C. A., and Bowker, M. A. (2009). Above- and belowground responses to tree thinning depend on the treatment of tree debris. For. Ecol. Manage. 259, 71–80. doi: 10.1016/j.foreco.2009.09.044
Paritsis, J., Landesmann, J. B., Kitzberger, T., Tiribelli, F., Sasal, Y., Quintero, C., et al. (2018). Pine plantations and invasion alter fuel structure and potential fire behavior in a Patagonian forest-steppe ecotone. Forests 9, 1–16. doi: 10.3390/f9030117
Pinheiro, J. C., and Bates, D. M. (2004). Mixed-Effects Models in S and S-PLUS. New York, NY: Springer. doi: 10.1007/b98882
Prichard, S. J., Peterson, D. L., and Jacobson, K. (2010). Fuel treatments reduce the severity of wildfire effects in dry mixed conifer forest, Washington, USA. Can. J. For. Res. 40, 1615–1626. doi: 10.1139/X10-109
Quinn-Davidson, L. N., and Varner, J. M. (2011). Impediments to prescribed fire across agency, landscape and manager: an example from northern California. Int. J. Wildl. Fire 21, 210–218. doi: 10.1071/WF11017
R Core Team (2018). R: A Language and Environment for Statistical Computing. Vienna: R Foundation for Statistical Computing. Available online at: https://www.r-project.org (accessed November 5, 2018).
Rago, M. M., Urretavizcaya, M. F., Orellana, I. A., and Defossé, G. E. (2020). Strategies to persist in the community: soil seed bank and aboveground vegetation in Patagonian pine plantations. Appl. Veg. Sci. 23, 254–265. doi: 10.1111/avsc.12482
Reineke, H. L. (1933). Perfecting a stand-density index for even- aged forests. J. Agric. Res. 46, 627–638.
Reiner, A. L., Vaillant, N. M., Fites-Kaufman, J. A., and Dailey, S. N. (2009). Mastication and prescribed fire impacts on fuels in a 25-year old ponderosa pine plantation, southern Sierra Nevada. For. Ecol. Manage. 258, 2365–2372. doi: 10.1016/j.foreco.2009.07.050
Robertson, K. M., and Hmielowski, T. L. (2014). Effects of fire frequency and season on resprouting of woody plants in southeastern US pine-grassland communities. Oecologia 174, 765–776. doi: 10.1007/s00442-013-2823-4
Schwilk, D. W., Keeley, J. E., Knapp, E. E., McIver, J. D., Bailey, J. D., Fettig, C. J., et al. (2009). The National Fire and Fire Surrogates Study: effects of alternative fuel reduction methods on forest vegetation structure and fuels. Ecol. Appl. 19, 285–304. doi: 10.1890/07-1747.1
Sonohat, G., Balandier, P., and Ruchaud, F. (2004). Predicting solar radiation transmittance in the understory of even-aged coniferous stands in temperate forests. For. Chron. 85, 583–592. doi: 10.1051/forest:2004061
Soriano, A. (1952). La regeneración de la vegetación en las Zonas Aridas. Cienc. Invest. 8, 544–549.
Soriano, A. (1956). Los distritos florísticos de la Provincia Patagónica. Rev. Invest. Agríc. 10, 323–348.
Stephens, S. L., McIver, J. D., Boerner, R. E. J., Fettig, C. J., Fontaine, J. B., Hartsough, B. R., et al. (2012). The effects of forest fuel-reduction treatments in the United States. Bioscience 62, 549–560. doi: 10.1525/bio.2012.62.6.6
Vázquez, D. (2002). Multiple effects of introduced mammalian herbivores in a temperate forest. Biol. Invasions 22, 255–267. doi: 10.1023/A:1020522923905
Veblen, T. T., Kitzberger, T., Raffaele, E., and Lorenz, D. C. (2003). “Fire history and vegetation changes in northern Patagonia, Argentina,” in Fire and Climatic Change in Temperate Ecosystems of the Western Americas, Ecological Studies, eds T. T. Veblen, W. Baker, G. Montenegro, and T. W. Swetnam (New York, NY: Springer Verlag), 265–295.
Wright, H. A. (1970). A method to determine heat-caused mortality in bunchgrasses. Ecology 51, 582–587. doi: 10.2307/1934038
Yan, H., Liang, C., Li, Z., Liu, Z., Miao, B., He, C., et al. (2015). Impact of precipitation patterns on biomass and species richness of annuals in a dry steppe. PLoS ONE 10:e0125300. doi: 10.1371/journal.pone.0125300
Zuloaga, F. O., Morrone, O., and Belgrano, M. (2019). Catálogo de las plantas vasculares del cono sur. Avaliable online at: http://www.darwin.edu.ar/Proyectos/FloraArgentina/fa.htm (accessed September 2, 2019).
Keywords: Patagonia, plant community, fuel management, afforestation, prescribed fire, mastication, steppe
Citation: Rago MM, Urretavizcaya MF, Lederer NS and Defossé GE (2020) Plant Community Response to Forest Fuel Management in Patagonian Pine Plantations. Front. For. Glob. Change 3:55. doi: 10.3389/ffgc.2020.00055
Received: 13 December 2019; Accepted: 14 April 2020;
Published: 27 May 2020.
Edited by:
Michele Salis, National Research Council (CNR), ItalyReviewed by:
Fermín J. Alcasena Urdíroz, United States Forest Service (USDA), United StatesCarolyn Hull Sieg, United States Forest Service (USDA), United States
Copyright © 2020 Rago, Urretavizcaya, Lederer and Defossé. This is an open-access article distributed under the terms of the Creative Commons Attribution License (CC BY). The use, distribution or reproduction in other forums is permitted, provided the original author(s) and the copyright owner(s) are credited and that the original publication in this journal is cited, in accordance with accepted academic practice. No use, distribution or reproduction is permitted which does not comply with these terms.
*Correspondence: María Melisa Rago, bWVsaXNhcmFnb0BnbWFpbC5jb20=