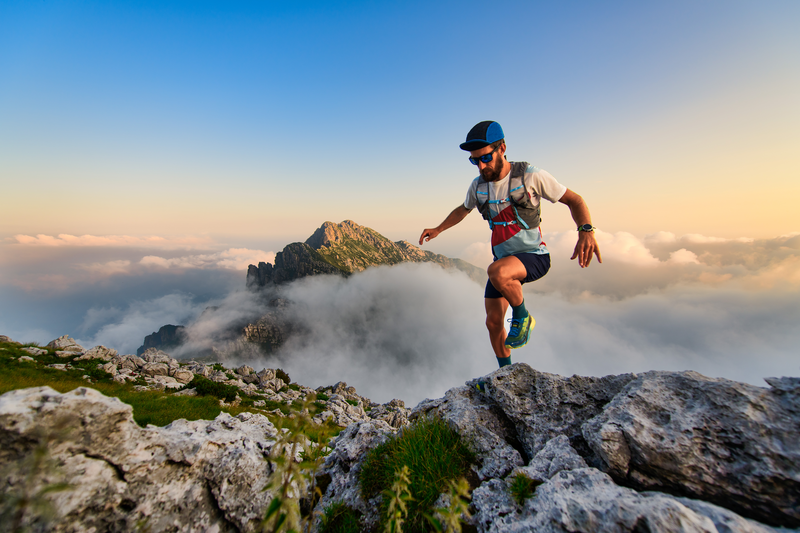
94% of researchers rate our articles as excellent or good
Learn more about the work of our research integrity team to safeguard the quality of each article we publish.
Find out more
REVIEW article
Front. For. Glob. Change , 24 March 2020
Sec. Forests and the Atmosphere
Volume 3 - 2020 | https://doi.org/10.3389/ffgc.2020.00030
This article is part of the Research Topic Urban Green Space and Air Quality View all 3 articles
It is well-acknowledged that plants in urban areas provide multiple ecosystem services. They contribute improving ambient quality and mitigating negative impacts of human presence, beautifying the anthropic environment, and promoting place identity and cultural heritage. However, the existence of plants in general, and trees in particular, cannot be considered independent on urban activities and infrastructures. Release of plant volatile compounds is profoundly affected in urban environments, in turn modifying plant relationships with other living organisms, both plants and animals, and affecting air chemistry and quality. Plants also interfere with stone artifacts, cultural and historical heritage. Plant-human coexistence requires precise and adequate managing measures, which have often been ignored in cities' government and planning. Plants and humans (and human infrastructures) are frequently considered as independent from each other and plant requirements are often disregarded, thus causing difficult or erroneous management and/or environmental damage. We review some of the most important ecosystem services provided by plants in urban environment, and also focus on possible negative effects of plants that may become relevant if urban vegetation is improperly managed and unintegrated in proper city planning, both of historical centers and of new towns or suburbs.
In human-driven ecosystems, such as urban areas where natural and artificial elements coexist, new relationships (both positive and negative) are established between plants, between plants and the man, animals or other organisms, and between plants and human artifacts. The fragile equilibrium regulating plant-urban environment interactions, and characterizing centuries of urbanization in large part of the inhabited world, is now further challenged by climate change.
Predictions on human population growth and sociological behavior call for a continuous increase of the urbanization process at the global scale (Goddard et al., 2010, 2013; Aronson et al., 2014; Shanahan et al., 2015; McDonnell and MacGregor-Fors, 2016; Alberti et al., 2017; Dobbs et al., 2017; among others). The more urban areas expand, the more natural environments become fragmented, as cities interrupt habitats and connectivity. Instead, urban “texture” results from a complex mosaic of built and vegetated patches. Only a fine-scaled land-cover classification, also based on remote sensing technology, can describe this kind of landscape heterogeneity. Green areas in the cities can be either remnants of native vegetation, often as yards or gardens, or transformed patches that may or may not represent local flora, and may include all-natural, semi-natural, and artificial ecological systems within and around the city (Goddard et al., 2010; Cilliers et al., 2013; Aronson et al., 2017; Lepczyk et al., 2017).
Here we review how plants in a fragile and artificial ecosystem, such as the urban environment, may perform multiple ecological functions and provide beneficial services for human well-being; and how these same features may also have negative consequences, especially if plants are not properly organized and managed (see Table 1 for a summarizing SWOT analysis). We further discuss how plant communities adapt to the human-transformed natural environment, and when they can represent problems to human artifacts and monuments. Finally, we consider possible influences and threats posed by climate change in plant-urban environment relationships.
Table 1. A SWOT (strengths, weaknesses, opportunities, and threats) analysis of plants in urban areas.
It is well known that plants in urban environments, particularly trees, provide several ecosystem services in different aspects of life. In general, from the environmental point of view, plant benefits include climatic regulation; uptake/reduction of CO2 and other greenhouse gases and pollutants; shading, which reduces urban heat island effects and is a general cooling factor, also intercepting incoming precipitations and avoiding dangerous water run-off while enriching water-table capacity (Alexandri and Jones, 2008; Edmondson et al., 2012; Maher et al., 2013; Livesley et al., 2016; Mori et al., 2016; Berland et al., 2017; Dobbs et al., 2017; Rahman et al., 2017; Weissert et al., 2017; Andoni and Wonorahardjo, 2018). Plants in densely urbanized areas also contribute to environmental protection from several hazards like strong winds, soil and slope erosion, torrential floods, landslides (Tanaka and Onai, 2017; Xiao et al., 2017; Pasha et al., 2018; Rey et al., 2019), and reduce traffic noise (Carrus et al., 2015; Shackleton et al., 2015). According to several results reviewed by Donovan (2017), benefits provided by plants (note: the author specifically refers to trees) change relative to their location within the urbanized area. For example, trees in parks and public areas with high air pollution, likely provide the greatest public-health benefits, whereas trees covering impervious surfaces have the greatest impact on storm-water management, and trees close to houses contribute mitigating summertime extreme temperatures. Modern city planning often uses plant species supposed to better fulfill environmental services, e.g., easy care, fast growth, small size, pollution resistance, while avoiding unfavorable effects, e.g., release of allergens. However, this may imply introducing novel plants whose long-term environmental impact should be carefully considered (see below).
While the interactions above are generally well-known and recognized, this general picture may need a more detailed discussion, as many more environmental benefits can be attributed to plants growing in urban environments, especially botanical gardens and historical parks (both public and private) (Figure 1 and Box 1 for a focus). Remnants of ancient historical gardens and botanical gardens, are characterized by the presence of native trees, and of exotic species introduced in the past centuries which are nowadays worth preserving for historical and educational purposes.
Figure 1. Villa “La Petraia” at Castello, near Florence, in a lunetta painted by Flemish Justus Utens between 1599 and 1602. Built in the sixteenth century as private estate of the Grand Dukes of Tuscany, the villa became property of the Italian state in the twentieth century and the park is now open to citizens and visitors (this picture is a public domain free of copyright).
Box 1. Focus on botanical gardens and public parks.
Initially founded as “physic gardens” for studying medicinal plants (middle of the sixteenth century), during European exploration and expansion in other continents (seventeenth to nineteenth century) botanical gardens became centers of trade for the introduction, acclimatization, and propagation of exotic flora. Since the second half of the twentieth century, with increased awareness of biodiversity, botanic gardens play an important role as repositories of plant diversity conservation (Krishnan and Novy, 2016).
It is worth to underline that the social/educational role is among the leading issues for establishing botanic gardens in developing countries, as the case of the nascent Palestine Institute of Sustainability and Palestine Museum of Natural History with botanical garden (Qumsiyeh et al., 2017).
Public urban parks have a different origin. The concept of urban park as an open space for community use arose in the nineteenth century, when population of both European and North American large cities tremendously increased. Under these circumstances, quality of life for many citizens was poor and unhealthy, with no access to natural spaces. In this period, landscape architects established public parks, which were considered as liminal space, marking the transition between the urbanized and the natural environment, areas where foster passive recreation and provide safe spaces for people. In 1843, architect Joseph Paxton designed what is considered the first urban park worldwide: Birkenhead Park in Liverpool. By studying his project few years later, in 1857, the American landscape architect Frederick Law Olmsted, together with Calvert Vaux, won a public design competition to build a new Central Park for fast-growing New York City (Figure 4). Unlike the European landscape architects that preceded him, Olmsted designed Central Park as a service to people, not as a contribution to art (Eisenman, 2013; Chow, 2016; Loughran, 2018).
From the point of view of human well-being, green areas integrated in the metropolitan context fulfill strong social relevance. Parks and gardens represent opportunities for relaxing and staying away from everyday routines; give positive impact on human social relationships; provide a place for recreation and meditation; contribute to make better life quality (Goddard et al., 2010; Ferrini and Fini, 2011; Luck et al., 2011; Dobbs et al., 2014; Carrus et al., 2015; Shackleton et al., 2015; Knez et al., 2018). Ultimately, they satisfy the definition of “restorative environments” for different ages, ethnics and genders (Razak et al., 2016; Carrus et al., 2017).
Indeed, most people in urbanized areas tend to perceive natural environments as more peaceful and restful than urban ones (van den Berg et al., 2007), and often seek for restoration of urban green spaces. In general, for park and garden visitors the most meaningful attributes are learning experience, stress relief and relaxation, improved quality of life (Wassenberg et al., 2015), and thermal comfort during extreme heat events (Lam et al., 2018). The complex of greenery in urban areas, commonly defined as “urban forestry” (see Glossary), contributes to provide human-related benefits and well-being. Citizens particularly appreciate mature trees (patriarchs), which can awaken emotions and peaceful sentiments, and tend to assign them symbolic, religious and ritual value, making these trees iconic landscape and heritage elements, and important landmarks in urban areas. Whenever old trees characterize with their presence particular places, they become part of the place identity, making it impossible to describe history and present condition of such places without their presence (Jones and Cloke, 2008). A few examples are given in Figure 2 where trees characterize the area because of their shape (Figure 2A) or majestic bearing (Figure 2C) which may even require complicated external support structures (Figure 2B). In several cultures, old trees are symbol of local history and cultural heritage, recalling historic events, and personalities (Blicharska and Mikusinski, 2014; Cavender and Donnelly, 2019; Box 2).
Figure 2. Examples of old trees characterizing with their presence particular places and becoming part of the place identity. (A) An extreme case of place-tree identification. In the town of Bolsena, central Italy, pine trees (Pinus pinea L.) are maintained in spite of their precarious equilibrium as “witness” of the anthropic landscape designed along the lakeshore. (B) In the historic Florentine garden “Boboli” an old Cedrus being a representative of the ancient flora of the garden itself is maintained through external protections. Whether or not such extreme example of protection is appropriate, this strategy is intended to meet citizens' and visitors' sentiment toward “patriarch” trees. (C) A “patriarch” tree (Castanea sativa Mill.) at Kew Gardens, London. All pictures by S. Fineschi.
Box 2. Focus on Parks of Remembrance.
Particular cases are represented by war memorials, which symbolize our culture of remembrance. Military cemeteries and the surrounding landscape are commonly defined as “Parks of Remembrance.” Immediately after the First World War, these parks became areas where nature was considered the most appropriate way to celebrate the cult of fallen soldiers (Gough, 2000; Figure 5). Few decades later, the same criterion was applied for the military victims of Second World War.
The sacrifice of Hiroshima citizens on August 6th, 1945 led the Japanese government to the construction of the “Hiroshima Peace Commemorating City” that includes a Peace Park and a Peace Boulevard symbolizing the road to peace and forming a green belt around the city (Gough, 2000). A more recent example is represented by the social protest that took place in Istanbul at Taksin Square, a place dense of historic significance and symbolism for modern Turkey. After several urban planning interventions during the twentieth century, Taksim Square became the city most important aggregation center, a place where people could mix and participate equally to social and recreational activities. In 2013, a project for the re-organization of Gezi Park at Taksin Square that included uprooting several old trees, gave rise to the protest of environmental activists, which involved in few days the whole country. The Gezi Park protest represents a particular example of the symbolism of an urban place characterized by the presence of trees, which recalls the multitude of values, symbols, ideologies, and meanings hold by such places for the city and the urban community (Gül et al., 2014).
The beneficial role played by urban gardens on dwellers involves physical as well as aesthetic, recreational, and educational issues. Humans tend to transpose positive feeling of living in contact with natural environments from nature into urban greenery (Knez et al., 2018). This perspective somehow recalls a romanticized view of rural life, and idealizes nature in a romantic way. This vision characterized the Utopian movement during nineteenth and beginning of twentieth century, such as Howard's “Garden City,” Le Corbusier's “Radiant City,” and Lloyd Wright's “Broadacre City” (van den Berg et al., 2007). However, most studies on the “restorative” function of this kind of green areas are merely descriptive and do not focus on which mechanism can lead to such a positive outcome for people. Distinct socio-demographic categories might differently experience value, benefits and restoration deriving from green urban areas. Similarly, differences between city centers and peripheral suburbs might consistently influence the way citizens perceive benefits deriving from a green urban landscapes. According to Shackleton et al. (2015), trees in public and peripheral suburbs usually help people cope with lack of services and infrastructures. On the other hand, residents in historic city centers tend to ascribe high aesthetic value to trees, particularly those located within private gardens.
The effects of urban vegetation on human health can be direct or indirect. Direct benefits are those contributing to ameliorate life conditions of residents (e.g., reducing exposure to dangerous temperatures or pollutants); indirect benefits encompass other aspects of the physical human health, bringing people together in open spaces and spend time outdoor, where to meet, exercise, and practice sport activities, thus contributing to personal wellness and improving social connections. Indirect benefits also refer to improvements in the restoration of cognitive functions in response to viewing nature (Shanahan et al., 2015), to enhancement of cultural services, such as natural heritage, aesthetic value, “sense of place” (genius loci) (Dobbs et al., 2011, 2018). Ultimately, plants in urban environment contribute to generate an idealized feeling for nature increasing psychological, health and social well-being (Donovan, 2017; Vujcic et al., 2017).
Green environments included into hospital facilities (green plants and flowers, as well as water and other natural elements), are thought since long time to have beneficial effects on patients, despite the fact that the first report about a measurable effect of nature on health appeared relatively recently (Ulrich, 1984). This belief likely started because of the use of medicinal plants. This practice is more than 100,000 years old both in Eastern and Western cultures. For example, in European Middle Age monasteries until the nineteenth century the healing process was completely dependent on plants and herbs cultivated in enclosed gardens (cloisters). The therapeutic role of gardens in hospitals became less and less prevalent during the twentieth century, until the link between nature and healing was totally lost. Main changes occurred from 1950 to 1990, when the therapeutic value of access to nature disappeared from hospitals in most western countries. Hospitals were built like office buildings where air conditioning replaced natural ventilation and outdoor terraces and balconies disappeared; green areas succumbed to car streets and parking lots (Cooper Marcus, 2007). By the end of the century, this scenario changed again and the prevalent role of gardens in recovering from stress and illness is now acknowledged in several study cases. Since 1984 Ulrich published a series of seminal articles (Ulrich, 2002), reporting that patients in hospital rooms with a window view of trees could better recover from surgery than others laying without a view of green areas (Hartig and Cooper Marcus, 2006). A healing garden provides general benefits, thus serving as a restorative resource and an ecosystem service, helping patients, visitors, and staff alike to cope better with stress experienced in connection with their own or others' illness (Ulrich, 1984, 1992, 1999, 2002; Box 3). It is therefore somehow surprising that caretaking constructions built after industrial revolution in Europe often do not include appropriate green spaces and infrastructures.
Box 3. Focus on “Healing gardens.”
The therapeutic value of plants in general, and of trees in particular, is highlighted by the so called “healing gardens,” i.e., landscapes that allow for the improvement and restoration of an individual's mental and physical health. These gardens are appositely established in proximity or within hospitals and other healthcare facilities. They are addressed not only to patients, but also to visitors, staff, caregivers, themselves not physically hill, who might value a garden as a place of restoration. The concept of “Healing Garden” comprises both the place—the garden—and the process—the possibility for recovering health—, which intertwine each other (Hartig and Cooper Marcus, 2006).
After abolishment of psychiatric hospitals, former psychiatric asylums encounter different destinations and might be converted in parks of residential neighborhoods. In some cases, the new destination does not take track of the former one, thus making visitors unable to link their presence with healthcare facilities (e.g., Linden Grove established on former Sunnyside Hospital in UK, Joseph et al., 2013). In other cases, places formerly unexploited by most city residents were reconverted into urban parks and spaces open to the aggregation of citizens and groups, maintaining traces of the previous park destination. One of the most interesting case is Parco San Giovanni in Trieste, Italy, which also includes university departments, museums, and a rose garden.
Despite the extensive use of the term “biodiversity” in policy-making and science, this concept is often not well-recognized among people, which usually tend to confer biodiversity an aesthetic and/or emotional rather than a functional and economic value.
Promoting and accomplishing interdisciplinary studies (i.e., natural and social scientists working together) contribute to understand which is the role of urban biodiversity for human well-being (Muratet et al., 2015; Carrus et al., 2017), how people perceive and appreciate plant species richness and how it may be linked to ecological issues such as diversity protection and provision of ecosystem services.
Urbanized green areas often become islands and refuges for native animal and plant biodiversity. This important role of remnant vegetation and natural areas in urban planning was recently highlighted (Aronson et al., 2014). Trees in urban landscapes protect wildlife as they do in agricultural landscapes, supplying appropriate sites for food, shelter and nest. Plants are therefore of crucial importance for the survival of metropolitan fauna, particularly for the most mobile organisms, such as birds and bats (Fischer and Lindenmayer, 2002; Lumsden and Bennett, 2005; Barth et al., 2015). Botanic gardens, where flora is well-managed and highly diversified, represent a sheltered urban environment also for insects (Hofmann et al., 2018). The occurrence of wildlife in densely inhabited areas, particularly of songbirds, is highly appreciated by humans. Therefore, bird diversity and density are recognized as cultural value, increase well-being, and provide ecosystem services for city residents (Luck et al., 2011; Hedblom et al., 2017). The impact of urban gardens on biodiversity is further examined below with regards to some possible negative aspects.
The contribution of urban green areas to a more general concept of “biodiversity,” including cultural diversity and social integration (Campbell et al., 2016; Dobbs et al., 2018) is also important. Under this respect, the concept of “Third Landscape” proposed by Clément (2004) to define those spaces between urban and peri-urban areas that are not yet occupied by human activities is particularly of interest. As long as these environments are unutilized and left to natural landscape evolution, they can be considered as “genetic reservoirs of species diversity” (Clément, 2004).
An important tool to enhance biodiversity in urban environment is species reintroduction, which can be applied whenever connectivity between green patches is poor and rural habitats (present prior to urbanization) experienced strong modification (van Heezik and Seddon, 2018). However, successful species reintroduction depends on limiting factors such as presence and pressure of predators, habitat quality, and social factors.
From an ecological perspective, it should not be underestimated that urban green areas result from human initiatives led by city planning, heritage conservation, scientific purposes, or others. All of these foster and preserve biodiversity but have artificial origin, which may have unusual consequences on biodiversity protection and valorization. For example, green areas in cities usually host both autochthonous and non-autochthonous species, and native and non-native mutualistic, companion, or antagonistic organisms, such as pollinators, predators, and prays. Altogether, this implies a fragile equilibrium in which positive outcomes for biodiversity conservation and human well-being might be threatened by the potential spread of alien species (see below).
Further indirect benefits by urban green areas are the provision of services like food. Urban farming (see Glossary) is emerging as a major field to preserve green spaces in urban areas while providing a community benefit, an income to poor or unemployed residents, and a pleasant workspace for disabled people, therefore also fulfilling an important social goal (Meharg, 2016). Moreover, urban vegetable gardens and other farming systems may help shortening the food supply chain, thus avoiding costs and ecological problems associated to food transportation (e.g., road traffic and pollution) and conservation (e.g., refrigeration). See however below about possible constraints to urban farming in polluted urban areas.
The added value to residential areas is another important side benefit of well-managed green areas. For example, houses close and with access to well-maintained parks and gardens may have additional economic value (Park et al., 2017).
Appreciating the positive role and function of plants in urban areas seems somehow a straightforward issue that goes beyond any kind of controversial opinions. Why then urban forests, parks and gardens are not more widely planned, executed, and exploited worldwide? Indeed, a few negative interactions between plants (especially trees) and urban settings do occur, and are of concern (often exaggerated or misconceived) by residents, city-planners and policy-makers (Table 1). Easy examples are damages or accidents caused by tree falling in urban environment, or by means of transportation (automobiles, motorcycles, bicycles) accidentally impacting on large trees that pave streets and roads.
We will try to summarize some possibly negative plant features when growing in urban environments as it follows.
Several plant species commonly widespread in the urban areas produce and release in the environment substances that can be either toxic to humans, or can interfere with atmospheric chemistry and physics.
Some frequently planted species in urban and peri-urban green areas and streets worldwide are among the main agents of allergies that can adversely affect human health. They include cultivated trees (e.g., cypress, poplar) and wild weeds and herbs (e.g., Parietaria spp.). Allergic reactions to pollen or other substances released by plants (from irritation of the eyes and skin to severe asthmatic reactions) are becoming common among urban residents. Such allergies might have a great impact on health and generate environmental and socio-economic costs (Dobbs et al., 2014; Grote et al., 2016; Cariñanos et al., 2018, 2019; Kasprzyk et al., 2019; von Döhren and Haase, 2019).
Moreover, emission of Biogenic Volatile Organic Compounds (BVOCs) by plants plays a crucial role in biosphere-atmosphere interactions. BVOCs (especially isoprenoids, which are produced and emitted during the day) react with other compounds released in the atmosphere as consequence of human activities (particularly NOx and ozone). The result of this reaction may have a cleansing effect on the atmosphere, or more often (e.g., when anthropogenic NOx are high) can increment aerosols and particles, therefore contributing to negatively affect air quality (Goldstein and Galbally, 2007; Zhao et al., 2018). Many tree species that are favorites in urban settings for their rapid growth emit a range of BVOCs (e.g., poplars and deciduous oaks are strong isoprene emitters, Loreto and Fineschi, 2015, while live oaks are major emitters of monoterpenes, Loreto et al., 2009). BVOC impact on the urban atmosphere depends on anthropogenic emissions as noted before (= plants do not pollute). However, BVOC emissions also scale across species, taxonomic status and other “life history traits” (see Glossary, and including e.g., leaf and plant age) and should be accurately monitored when associated to anthropogenic pollution. Scientific literature on BVOCs and their importance both in rural lands and urban areas is rich and comprehensive (for detailed reviews: Calfapietra et al., 2013; Grote et al., 2016).
In addition to interfering with the atmospheric chemistry, BVOCs play also an important role in interactions between plants and animals, which are usually determined by both visual and olfactory signals. BVOC rapid scavenging by reaction with anthropogenic emissions, and the formation of a range of secondary volatile compounds which do not serve the same purpose as primary BVOCs, can therefore disturb and interrupt plant-animal communication and mutualistic interaction, also affecting plant signaling consequent to stress endurance (Jürgens and Bischoff, 2017).
The presence of large old plants may, in some cases, bring to negative consequences in urban environments. City residents usually are disturbed by plant aging processes (e.g., trees dropping dead branches or deadwood falling on the ground), and tall trees near houses may cause shading (e.g., winter shade of evergreen trees) or problems for building maintenance, e.g., clogging drainage pipes with falling leaves. Moreover, large branches falling from trees are a hazard for public safety sometimes causing injuries and death of people who are accidentally hit. Tree fall is becoming more and more frequent and worrisome because of increasing tree age, and insufficient maintenance of city green areas but also because exposure to stress induced by climate change (both abiotic and biotic) make trees more vulnerable (Mullaney et al., 2015). Finally, plants growing near traffic areas can decrease road and traffic light visibility and increase the risk for traffic accidents (Lyytimäki and Sipilä, 2009; Blicharska and Mikusinski, 2014; Cariñanos et al., 2018), and falling leaves may contribute to make worse road conditions, especially when combined with bad weather events (e.g., making slippery roads and pavements during and after rainfalls).
Trees play an important role in controlling water regime and reducing wind damage. However, their potential in urban storm water and wind management also depends on the species and the life stage. If winds are too strong, plants oscillate until branches, or the whole trees, fall or are even uprooted. It is important to understand how plants have adapted to their wind environment and evaluate the impact wind damage can have on individual plants and plant communities in urban ecosystems, including an assessment of economic importance of wind damage. Trees shedding leaves, particularly older and bigger ones, can better survive severe winter wind storms. However, stem or branch injuries, which facilitate insects and fungi attacks, reduce the mechanic resistance of trees to strong wind and threaten the life of plants and the benefit they are supposed to provide (Tello et al., 2005). Despite the social value and the potential catastrophic impact of urban tree falling (e.g., building damage, human deaths or injuries, and impact on service such as electricity and transport) urban trees have been less studied than trees growing in forests far from cities (Gardiner et al., 2016; Berland et al., 2017; Cavender and Donnelly, 2019).
Archaeological and historical sites are often integrated in urban environments where stone monuments and vegetation (from trees to mosses), and microorganisms, interact (Cicinelli et al., 2018). Indeed, depending on composition, exposure, and porosity of the substrate, plant and microflora communities can grow on different habitats, both vertical and horizontal, such as walls, ruins, rocky surfaces, sculptures, and stone and marble artifacts (Ceschin et al., 2016).
In rock decay processes, both physical and chemical mechanisms are involved. The first step of decay is stone alteration caused by synergic physical actions (rain, wind, sunlight, and freezing/thawing cycles). Consequently, the stone surface becomes progressively rough and characterized by the formation of micro fractures. The result is a loss of cohesion of stone material and the general weakening of the superficial structural strength, which makes stones a suitable living environment for plants and microorganisms (Tiano, 2002). Growth of living organisms may degrade heritage monuments, leading to aesthetic and structural deterioration. Esthetic damages, such as color and/or shape modification, are caused by biofilm formation; mechanical and chemical damages occur at the surface as well as in the inner zone of the artifacts and can bring in the long term to the monument decay (Scheerer et al., 2009; Toreno et al., 2018; Cicinelli et al., 2019).
Damage to stones caused by living organisms is often referred to as bioweathering or “biodeterioration” (see Glossary). Under particular climatic conditions, such as high humidity, fungi are extremely erosive and may penetrate inside stone, marble, and antique glass, and cyanobacteria, algae and lichens may also contribute to the weathering of stone (Sterflinger et al., 2018). Some organisms, particularly those interacting with higher plants (e.g., at the root system level), have a high biodeterioration potential, which leads to the formation of pits and causes problems for the conservation of cultural heritage. Among higher plants, trees may bring to most negative consequences to historic monuments. Tree-stone interactions occur at belowground and aboveground level. Belowground, trees influence soil characteristics (hydrology, chemistry, structure) through the root system, which can develop both in depth and laterally for several meters. If tree roots grow in mortars between stones (the zones with lower resistance) they may cause cracking and damages to monuments, and further stone weathering. In archaeological sites where buildings have been buried and rebuilt in piles over the centuries, as is the case in Rome, tree roots can damage the walls because of the short distance between the vaults of ancient buildings and the present ground level. Tree stability may also be threatened by poor root anchorage to unstable stones; fall of trees as consequence of strong winds may cause serious injuries to monuments. Moreover, tree crowns may obscure the view of the whole site and alter its context and landscape (Crow and Moffat, 2005). Plants growing too close to buried monuments, e.g., catacombs or temples may cause detachment of frescos or damage to walls (Caneva et al., 1991, 2006; Caneva, 1999).
In some cases, urban green spaces evoke fear of crime. Urban green areas such as public parks are often perceived as unsafe environments because they become meeting point for drug dealers and consumers, as well as refuge for socially marginalized and homeless people, which are often recognized as dangerous.
However, usually there is not a single attribute influencing fear, rather a complex interaction of the environment with other attributes (users' age and gender, social factors, and physical factors) occurs. Elder people and women, particularly during night-time, tend to be more fearful of being victimized than younger and male individuals; ethnic minorities, which experienced previous direct or indirect victimization, feel insecure in urban green spaces. Social factors like poor social integration contribute to increase fear of crime. Physical factors including disorders, signs of negligence, graffiti, trash, damaged property, and poor lighting also play a role in adding insecurity in green spaces in the cities. Alcohol consumption, noise nuisance, intimidation, abusive language, offensive behavior, vandalism, further contribute to produce higher levels of perceived insecurity in parks and urban gardens (Lyytimäki and Sipilä, 2009; Maruthaveeran and Konijnendijk van den Bosch, 2014; Stefanizzi and Verdolini, 2019). Unmaintained vegetation is also negatively considered as unpleasant and ugly, and might induce sense of fear; whereas, unmanaged parks and gardens that are not intensively cared (e.g., prairie-like) may host high biodiversity (Lyytimäki and Sipilä, 2009).
The control on urban green areas is often very difficult and expensive for municipalities, which may leave public parks and garden unattended, in turn promoting any kind of illegal activities, as well as the colonization of parks by homeless people and, more recently, by refugees illegally entering the country. These negative features also contribute to increase perception of insecurity in residents and human fears related to personal safety.
Other types of ecosystem functions might be negatively perceived by humans, such as the presence in urban parks of undesired wild or semi-wild animals (i.e., rats, bats, mice, wild dogs, and larger mammals such as wild-hogs). Particularly when searching for food (e.g., from trash bins) or defending cubs, these animals may cause fear, anxiety or may even, albeit rarely, attack people. Often, these animals are vectors of pathologies that can affect both humans and domestic animals, e.g., migratory birds carrying avian influenza, or cats and dogs carrying rabies or tick-borne diseases such as Lyme disease. Other animals can induce health epidemics indirectly (e.g., squirrels falling into water towers) (Lyytimäki and Sipilä, 2009; Dobbs et al., 2011).
A final, though very important, possible negative consequence of bad management of plants in urban areas, is the human-mediated introduction of invasive alien species. Botanical gardens, in particular, might have been responsible for the deliberate or accidental introduction of invasive alien plants, facilitating plant invasions, thus conflicting with their own plant conservation and protection functions. Obviously, humans play the most relevant role in the process of inadvertent escape of species from botanical gardens. Appropriate and specialist management is required in order to identify the steps that potentially change living collections from ornamental to detrimental (Hulme, 2011).
The urban green landscape is often the first contact point for exotic pests accidentally introduced when planting exotic trees in urban areas. Once established in urban environments, dispersal of introduced pests can proceed to natural forest landscapes or planted forests (Pautasso et al., 2015; Bradshaw et al., 2016; Paap et al., 2017; Potgieter, 2019). Moreover, plants in cities become friendly environment for exotic animals, particularly birds, like parrots, which easily breed in urban tree cavities, or monk parakeets, whose populations are present and are growing exponentially in the Mediterranean cities (Postigo et al., 2017; Diamond and Ross, 2019). Ring-necked parakeet is one of the most invasive birds worldwide and can easily exploit anthropogenic habitats that are inefficiently used by native birds, as in the case of several urban environments. This parakeet is particularly aggressive in competing with native species for nest-site preferences (Hernández-Brito et al., 2014).
The choice of exotic flora is often determined by requirements that make these trees more suited in modern city planning (e.g., for their aesthetic value like blossom cherry, or for producing less debris and compounds that may annoy people like allergenics, or resins). In this way, both a biodiversity reduction of native flora, and a biodiversity increase due to alien species introduction can occur. Wrong choice of plant species might have serious consequences and finally result in social, environmental and economic damage. The main tree negative features and a list of possible solutions to problems caused by tree interactions in urban areas are summarized in Table 2.
In modified ecosystems, such as urban landscapes, habitat loss and/or fragmentation, which represent a major threat for biodiversity, are more frequent than in non-urban environments. Discontinuity, patch size, shape, and heterogeneity are factors that strongly determine the potential for biodiversity conservation. Most green spaces in cities are small and highly fragmented, thus they may not fulfill requirements for wildlife natural processes such as spontaneous plant reproduction, pollinators' survival, bird nesting, resources provision for long-distance moving animals. When urbanization process grows, patch density generally increases, whereas patch size, and consequently landscape connectivity, decrease (Goddard et al., 2010; Figure 3). While heterogeneity may positively affect the urban landscape, for example by increasing plant species diversity, fragmentation interrupts habitat connectivity, i.e., zones of continuous ecological conditions required by many living organisms, particularly non-mobile or less mobile ones.
Figure 3. A schematic representation of possible fragmentation patterns of green areas (parks, garden) in expanding urban areas. Examples range from the pattern of (A), where fragmentation is limited and large green areas almost come into contact, to the pattern of (C) where, as indicated in the text, increasing fragmentation and decreasing connectivity may result in alteration or reduction of ecosystem services offered by green areas (see also Table 1). (B) Represents an intermediate pattern.
Figure 4. View of Central Park, New York, designed as city public park by landscape architects Frederick Law Olmsted and Calvert Vaux and established in 1857 (http://www.zzdyjyw.com/2018/11/15/central-park/).
Figure 5. Cypress trees surrounding the monument to the fallen soldiers of World War I in the cloister of the Franciscan Basilica di Santa Croce (the Basilica of the Holy Cross) in Florence. Each tree is dedicated to one fallen soldier (in this case to Gold Medallists), according to the concept of “Park of Remembrance.” Picture by S. Fineschi.
Coexistence of plants and animals is of particular interest in urban areas and plays an important role for the vulnerable equilibrium between living organisms in human managed ecosystems. Even if cities still host high diversity of species, the density of both plants and animals has declined during the last decades in urban areas. Several studies deal with impacts of urbanization and habitat loss on animal population diversity. Anthropogenic features, mostly land cover, rather than non-anthropogenic factors (such as geography, climate, and topography) are responsible for decreasing species density, indicating that vegetation structure plays a crucial role for animal conservation in urbanized areas; Aronson et al. (2014) pointed it out in the case of birds. Other evidences report that recently developed peripheries, poorly vegetated or characterized by exotic plant species, which provide less adequate resources, host a lower bird diversity (White et al., 2005; Evans et al., 2009; Fontana et al., 2011; Shwartz et al., 2013; Taylor et al., 2013; Barth et al., 2015).
Habitat loss and fragmentation, invasive alien species, as well as changes in land-use and management intensity, climate change, stress from parasite and pesticides, represent the major threats also for other animals, such as insects, which are among the most threatened species, both in urban and agricultural environments (Potts et al., 2016; Hofmann et al., 2018).
Climate change is increasingly challenging entire ecosystems at planetary level, and plants (unable to escape unfavorable meteorological events) are among the most sensitive organisms to climate variations. In temperate regions of the world, the trends toward higher temperatures, lower precipitations, and increasing occurrence of extreme events (see for example the very recent—winter 02019-2020—Ciara storm in northern and central Europe) are important factors that may limit vegetation growth, productivity and ecosystem services. In most fragile areas, climate change factors may even bring to catastrophic events such as biodiversity loss, migrations, desertification, and alien organism invasions.
Plants of urban environments are useful to mitigate climate, as previously mentioned. However, in urban environments climate change superimposes anthropogenic negative factors (e.g., pollution, heat island effect, soil scarcity and consequent scarce rooting and poor water interception and availability) threatening plant life. Therefore, plants of urban environments should be carefully selected to adapt to the combination of stresses to which they will be exposed, now, and under future conditions. The choice of urban tree flora characterized by best adaptive potential to changing environmental conditions is crucial for fulfilling other ecosystem services such as habitat restoration and delivering aesthetical values. For example, drought-tolerant (xeric) trees will have higher performance under water-limited situations and might contribute to urban cooling through shading; choosing plants selected from natural dryland habitats results in optimized water-use strategies. In the same way, drought tolerant plants, e.g., succulents, should be preferred for green roof planning (Emilsson and Sang, 2017).
Urban planning in response to present and forthcoming climate change starts now to be perceived as an important issue, and planning green infrastructures is particularly relevant. For example, cities are increasingly replacing plant species that are sensitive to drought and are therefore suffering recurrent and more severe drought stress periods (e.g., plane trees) with more resistant or adaptable plant species that will guarantee plant ecosystem service (such as shade and consequent temperature reduction, or pollution uptake and control) also under more extreme whether constraints (e.g., Celtis).
Particularly important is the selection of plant species that offer best ecosystem services under future climate conditions. This selection is of course different depending on estimated impacts of climate change at regional scale. Plants respond to environmental changes at the species level (species' life traits) and at the genotype level (single genotype performance and fitness). Under predicted future climatic changes, ultimately plant communities (species abundance and composition), will be rearranged and eventually experience local extinction. For this reason, genotype and plant species choice in urban planning is an opportunity to steer toward mitigation of the impacts of climate change using nature-based solutions (Espeland and Kettenring, 2018). For example, in the Mediterranean areas there may be a need to select species resistant to drought or salinization, thus responding to problems of water scarcity that will exceedingly plague these regions. Plants thriving when using reclaimed wastewaters and in poor soils will also be usefully employed, as the imperative scope is to fight desertification and soil loss in these areas. On the other hand, in boreal areas where climate change will be responsible for more temperate conditions we may be able to use soon plants of temperate regions, exploiting suitable traits such as deciduousness, fast growth and excellent photosynthetic properties, in turn serving nature-based uptake of CO2 and anthropogenic pollutants.
The impact of global change on urban farming deserves an even more careful attention. Urban food must be safe and healthy, which is apparently a contradiction when considering the constraints (particularly soil and air pollution) characterizing urban environments. City and country policies are trying to minimize pollution episodes in urban areas, with successful examples worldwide, especially in megacities (e.g., Beijing). However, increasing urbanization, birth rate, and human pressure, particularly in developing countries, together with inadequate environmental criteria for urban planning, represents a persistent limitation for urban farming. Ultimately, plants alone will unlikely be able to mitigate negative outcomes of human activities and consumption of natural resources, although wise planning of adapted plant species for changing urban conditions is mandatory to maintain sufficient ecosystem services by urban vegetation.
Literature on managing and planning cities under the observation lens of ecology and sustainability is rich, and deals on how to face the major challenges of the twenty-first century: further increase of urbanization, rising pollution and waste, escalation of soil and other natural resources consumption because of building and trade activities. Moreover, several studies report about environmental hazards caused by climate change such as summer heat and drought, as well as winter extreme events, which seriously affect urban resilience and make life quality worse. In this regard, ecosystem service-based approaches, which utilize green infrastructures, provide benefits not only ecologically and socially required, but also economically advantageous (Lynch, 2016; Lafortezza et al., 2017). Sustainable urban development should be based on green infrastructure panning strategies, which utilize urban woodlands, street trees, private and public gardens, roof gardens, and urban parks, including blue areas. Results show that local governments that incorporate many green infrastructures planning strategies have more success in providing ecosystem services over time (Lynch, 2016). Further, sustainable urban planning should consider interdisciplinary approaches to improve green spaces and educate citizens about the importance of green areas in urban life; approaches that provide ecosystem benefit to the society also include inter-linked networks of urban green and natural, or semi-natural, areas (Anguluri and Narayan, 2017).
However, urban green infrastructures are frequently heavily impacted by surrounding residential and commercial areas, a drawback that is often overlooked by city planners (Hostetler et al., 2011).
According to Artmann et al. (2019), sustainable urban development should develop “smart-compact-green cities,” thus a framework that links the concepts of smart growth and green infrastructure. Moreover, urban planning and management should avoid the “one-size-fits-all approach” and adapt in a refined manner to local conditions (Jim et al., 2018). All processes connected with the continuous growth of human population worldwide result in loss of urban green space, habitat connectivity, and biodiversity within cities, indicating the need of innovative concepts in urban design aimed to develop more sustainable, more resilient and healthier cities (Sanesi et al., 2017; Russo and Cirella, 2018). Green corridors that connect isolated remnant habitat patches can increase connectivity and provide ecosystem services in cities (Zhang et al., 2019). The United Nations Sustainable Development Goals (SDG) underline the role of green areas in making cities healthier and more livable for people, with particular attention to women, children, older persons and persons with disabilities (Goal 11. Make cities and human settlements inclusive, safe, resilient, and sustainable) (United Nations, 2015). In particular, trees provide benefits that help cities and countries meet 15 of the 17 internationally supported United Nations Sustainable Development Goals (Turner-Skoff and Cavender, 2019).
Maintaining and appropriately managing existing urban parks and green areas is the first strategy that policymakers and local administrations should pursue. Protecting trees in cities is an investment for the future. Preserving existing trees is not sufficient, and planting new trees without precise programs for their survivorship and healthy growth, which strongly depends on cultural care, improvement of site conditions, and continuous maintenance, is not a wise strategy. Indeed, tree plantation without rigorous planning methodology, technical criteria, and appropriate choice of species (i.e., functional type of plant) can limit plant potential to supply environmental services, and can enhance maintenance costs (Cavender and Donnelly, 2019; Núñez-Florez et al., 2019).
Prior to the 1990s, scientific studies on nature within cities were considered unworthy, unless solving problems that threatened human well-being (McDonnell, 2011; McDonnell and MacGregor-Fors, 2016). Urban ecology (see Glossary) application to city planning and management developed as an inter- and trans-disciplinary discipline in the 1990s and early 2000s. It links different expertise, making natural scientists, and ecologists cooperate with economists, sociologists and landscape architects. The common goal of these interdisciplinary studies is to make urban environments livable and resilient; studies have three perspectives: bioecology, planning and design, and education. The emergence of urban ecology answered to the need of a “science of cities” for understanding cities as a complex system where the human and the natural components are coupled. Urban scientists introduced mathematical rigor to the exploration of common urban properties, thus making new insights for city planners and policy makers available (Alberti et al., 2018).
Building solutions such as green roofs, green façades, and vertical green are urban ecology strategies that link architectural design and ecological solutions. These structures improve building efficiency and provide environmental benefits, such as reducing heat island effect, improving air quality, saving energy, regulating building temperatures, adsorbing noise, dust and smog, and reducing storm water runoff (Perini et al., 2011; Taleghani, 2017; Baraldi et al., 2018; Velázquez et al., 2019). Green roofs may be intensive, which include shrubs and trees and require deep soil media, or extensive, consisting of only herbaceous perennial or annual plants, and requiring minimal maintenance. Because of easy care and low maintenance costs, extensive green roofs are more common (Baraldi et al., 2018).
Vertical greening can be achieved in different ways. Green façades utilize climbers, evergreen, or deciduous, attached themselves directly to the building surface (as in traditional architecture), or supported by steel cables. Living walls, also called green walls and vertical gardens, consist of modular panels, contain their own soil, and utilize balanced nutrient solutions to provide all or part the plant's food and water requirements (Perini et al., 2011).
In some cases, for example in the city of Copenhagen, municipalities made implementation of green roofs mandatory in urban planning; in the case of Buenos Aires, a special law was established for regulating the incorporation of green roofs in buildings. Even roofs of old building can be covered with vegetation, if appropriate measures are used (Viecco et al., 2018).
Some local governments are working together with citizens for participatory environmental-friendly city planning. There is increasing need among urban dwellers for the creation of healthier and more sustainable cities; ecologists, designers, building, and city managers should operate in synergy with stakeholders to update and improve the way we conceive the needs of modern human agglomerations (McDonnell and MacGregor-Fors, 2016). Under this perspective, urban residents are encouraged to interact with and to utilize public green spaces and to experience different benefits derived by green environments (Mahmoudi Farahani and Maller, 2018).
Current urban ecosystems are experiencing a multitude of environmental threats, that make them extremely fragile, and seriously weaken their resilience and buffer (mitigation) potential. The mechanisms linking urban development patterns to rapid evolutionary changes in wildlife (animal, plants, and microorganisms), which are part of the ecosystem and play important functional roles, remain particularly elusive (Alberti et al., 2017).
Within the urban texture, plant occurrence characterizes different typologies of open spaces, such as historical and newly planned green areas (both private and public), archaeological sites, botanical gardens, and new generation green infrastructures. Each of these spaces requires specific conservation measures that imply interdisciplinary expertise and above all, cooperation between responsible authorities, specialists, stakeholders, and the citizenship. Until now, this winning strategy has been poorly applied. More often landscape- and environmental-friendly solutions are modestly regarded in city planning. Moreover, social and economic inequalities, which heavily differentiate developed and developing countries, give urban green areas planning different priority and importance.
The authors equally contributed to the preparation of this article, in all its parts.
The authors declare that the research was conducted in the absence of any commercial or financial relationships that could be construed as a potential conflict of interest.
Aguinagalde, I., Hampe, A., Mohanty, A., Martín, J. P., Duminil, J., and Petit, R. J. (2005). Effects of life-history traits and species distribution on genetic structure at maternally inherited markers in European trees and shrubs. J. Biogeogr. 32, 329–339 doi: 10.1111/j.1365-2699.2004.01178.x
Alberti, M., Correa, C., Marzluff, J. M., Hendry, A. P., Palkovacs, E. P., Gotanda, K. M., et al. (2017). Global Urban signatures of phenotypic change in animal and plant populations. Proc. Natl. Acad. Sci. U.S.A. 114, 8951–8956. doi: 10.1073/pnas.1606034114
Alberti, M., McPhearson, T., and Gonzalez, A. (2018). “Embracing Urban complexity,” in Urban Planet: Knowledge Towards Sustainable Cities, eds T. Elmqvist, X. Bai, N. Frantzeskaki, C. Griffith, D. Maddox, T. McPhearson, S. Parnell, P. Romero-Lancao, D. Simon, and M. Watkins (Cambridge: Cambridge University Press, 45–67.
Alexandri, E., and Jones, P. (2008). Temperature decrease in a Urban canyon due to green walls and green roofs in diverse climates. Build. Environ. 43, 480–493. doi: 10.1016/j.buildenv.2006.10.055
Allsopp, D., Seal, K., and Gaylarde, C. (2004). Introduction to Biodeterioration. New York, NY: Cambridge Univ Press. 1–10.
Andoni, H., and Wonorahardjo, S. (2018). A review on mitigation technologies for controlling Urban heat Island effect in housing and settlement areas. IOP Conf. Ser. Earth Environmental Sci. 152:012027. doi: 10.1088/1755-1315/152/1/012027
Anguluri, R., and Narayan, P. (2017). Role of green space in Urban planning: outlook towards smart cities. Urban For. Urban Gree. 25, 58–65. doi: 10.1016/j.ufug.2017.04.007
Aronson, M. F. J., La Sorte, F. A., Nilon, C. H., Katti, M., Goddard, M. A., Lepczyk, C. A., et al. (2014). A global analysis of the impacts of Urbanization on bird and plant diversity reveals key anthropogenic drivers. Proc. Biol. Sci. 281:20133330. doi: 10.1098/rspb.2013.3330
Aronson, M. F. J., Lepczyk, C. A., Evans, K. L., Goddard, M. A., Lerman, S. B., MacIvor, J. S., et al. (2017). Biodiversity in the city: key challenges for Urban green space management. Front. Ecol. Environ. 15, 189–196. doi: 10.1002/fee.1480
Artmann, M., Kohlera, M., Meinela, G., Ganb, J., and Iojac, I.-C. (2019). How smart growth and green infrastructure can mutually support each other - a conceptual framework for compact and green cities. Ecol. Indic. 96, 10–22. doi: 10.1016/j.ecolind.2017.07.001
Baraldi, R., Neri, L., Costa, F., Facini, O., Rapparini, F., and Carriero, G. (2018). Ecophysiological and micromorphological characterization of green roof vegetation for Urban mitigation. Urban For. Urban Gree. 37, 24–32. doi: 10.1016/j.ufug.2018.03.002
Barth, B. J., FitzGibbon, S. I., and Wilson, R. S. (2015). New Urban developments that retain more remnant trees have greater bird diversity. Landscape Urban Plan. 136, 122–129 doi: 10.1016/j.landurbplan.2014.11.003
Berland, A., Shiflett, S. A., Shuster, W. D., Garmestani, A. S., Goddard, H. C., Herrmann, D. L., et al. (2017). The role of trees in Urban stormwater management. Landscape Urban Plan. 162, 167–177. doi: 10.1016/j.landurbplan.2017.02.017
Blicharska, M., and Mikusinski, G. (2014). Incorporating social and cultural significance of large old trees in conservation policy. Conserv. Biol. 28, 1558–1567. doi: 10.1111/cobi.12341
Bradshaw, C. J., Leroy, B., Bellard, C., Roiz, D., Albert, C., Fournier, A., et al. (2016). Massive yet grossly underestimated global costs of invasive insects. Nat. Commun. 7:12986. doi: 10.1038/ncomms12986
Calfapietra, C., Fares, S., Manes, F., Morani, A., Sgrigna, G., Loreto, F., et al. (2013). Role of biogenic volatile organic compounds (BVOC) emitted by Urban trees on ozone concentration in cities: a review. Environ. Pollut. 183, 71–80. doi: 10.1016/j.envpol.2013.03.012
Campbell, L. K., Svendsen, E. S., Sonti, N. F., and Johnson, M. L. (2016). A social assessment of Urban Parkland: analyzing park use and meaning to inform management and resilience planning. Environ. Sci. Policy 62, 34–44. doi: 10.1016/j.envsci.2016.01.014
Caneva, G. (1999). A botanical approach to the planning of archaeological, parks in Italy. Conserv. Manage. Archaeol. 3, 127–134. doi: 10.1179/135050399793138590
Caneva, G., Ceschin, S., and De Marco, G. (2006). Mapping the risk of damage from tree roots for the conservation of archaeological sites: the case of the Domus Aurea, Rome. Conserv. Manage. Archaeol. 7, 163–170. doi: 10.1179/135050306793137403
Caneva, G., Galotta, G., Cancellieri, L., and Savo, V. (2009). Tree roots and damages in the Jewish catacombs of Villa Torlonia (Roma). J. Cult. Herit. 10, 53–62. doi: 10.1016/j.culher.2008.04.005
Caneva, G., Nugari, M. P., and Salvadori, O. (1991). Biology in the Conservation of Works of Arts. Rome: International Centre for the Study of the Preservation and Restoration of Cultural Property (ICCROM).
Cariñanos, P., Calaza, P., Hiemstra, J., Pearlmutter, D., and Vilhar, U. (2018). The role of Urban and peri-Urban forests in reducing risks and managing disasters. Unasylva 69, 53–58.
Cariñanos, P., Grilo, F., Pinho, P., Casares-Porcel, M., Branquinho, C., Acil, N., et al. (2019). Estimation of the allergenic potential of Urban trees and Urban parks: towards the healthy design of Urban green spaces of the future. Int. J. Environ. Res. Public Health 16, 1–12. doi: 10.3390/ijerph16081357
Carrus, G., Scopelliti, M., Lafortezza, R., Colangelo, G., Ferrini, F., Salbitano, F., et al. (2015). Go greener, feel better? The positive effects of biodiversity on the well-being of individuals visiting Urban and peri-Urban green areas. Landscape Urban Plan. 134, 221–228. doi: 10.1016/j.landurbplan.2014.10.022
Carrus, G., Scopelliti, M., Panno, A., Lafortezza, R., Colangelo, G., Pirchio, S., et al. (2017). A different way to stay in touch with ‘Urban nature': the perceived restorative qualities of botanical gardens. Front. Psychol. 8:914. doi: 10.3389/fpsyg.2017.00914
Cavender, N., and Donnelly, G. (2019). Intersecting Urban forestry and botanical gardens to address big challenges for healthier trees, people, and cities. Plants People Planet 1, 315–322. doi: 10.1002/ppp3.38
Ceschin, S., Bartoli, F., Salerno, G., Zuccarello, V., and Caneva, G. (2016). Natural habitats of typical plants growing on ruins of Roman archaeological sites (Rome, Italy). Plant Biosyst. 150, 866–875. doi: 10.1080/11263504.2014.990536
Chow, K. (2016). Park politics: political influences on frederick law olmsted & the creation of central park (Honors theses). Paper 819. Available online at: https://digitalcommons.colby.edu/honorstheses/819 (accessed November 20, 2019).
Cicinelli, E., Benelli, F., Bartoli, F., Traversetti, L., and Caneva, G. (2019). Trends of plant communities growing on the etruscan tombs (Cerveteri, Italy) related to different management practices. Plant Biosyst. 154, 158–164. doi: 10.1080/11263504.2019.1578286
Cicinelli, E., Salerno, G., and Caneva, G. (2018). An assessment methodology to combine the preservation of biodiversity and cultural heritage: the San Vincenzo al volturno historical site (Molise, Italy). Biodivers. Conserv. 27, 1073–1093. doi: 10.1007/s10531-017-1480-z
Cilliers, S., Cilliers, J., Lubbe, R., and Siebert, S. (2013). Ecosystem services of Urban green spaces in African countries – perspectives and challenges. Urban Ecosyst. 16, 681–702. doi: 10.1007/s11252-012-0254-3
Crow, P., and Moffat, A. J. (2005). The management of the archaeological resource in UK wooded landscapes: an environmental perspective. Conserv. Manage. Archaeol. 7, 103–116. doi: 10.1179/135050305793137512
Diamond, J. M., and Ross, M. S. (2019). Exotic parrots breeding in Urban tree cavities: nesting requirements, geographic distribution, and potential impacts on cavity nesting birds in southeast Florida. Avian Res. 10:39. doi: 10.1186/s40657-019-0176-3
Dobbs, C., Eleuterio, A. A., Amaya, J. D., Montoya, J., and Kendal, D. (2018). The benefits of Urban and peri-Urban forestry Unasylva 69, 22–29.
Dobbs, C., Escobedo, F. J., and Zipperer, W. C. (2011). A framework for developing Urban forest ecosystem services and goods indicators. Landscape Urban Plan 99, 196–206. doi: 10.1016/j.landurbplan.2010.11.004
Dobbs, C., Kendal, D., and Nitschke, C. R. (2014). Multiple ecosystem services and disservices of the Urban forest establishing their connections with landscape structure and sociodemographics. Ecol. Indic. 43, 44–55. doi: 10.1016/j.ecolind.2014.02.007
Dobbs, C., Nitschke, C., and Kendal, D. (2017). Assessing the drivers shaping global patterns of Urban vegetation landscape structure. Sci. Total Environ. 592, 171–177. doi: 10.1016/j.scitotenv.2017.03.058
Donovan, G. H. (2017). Including public-health benefits of trees in Urban forestry decision making. Urban For. Urban Green. 22, 120–123. doi: 10.1016/j.ufug.2017.02.010
Duminil, J., Fineschi, S., Hampe, A., Jordano, P., Salvini, D., Vendramin, G. G., et al. (2007). Can population genetic structure be predicted from life history traits? Am. Nat. 169, 662–672. doi: 10.1086/513490
Edmondson, J. L., Davies, Z. G., McHugh, N., Gaston, K. J., and Leake, J. R. (2012). Organic carbon hidden in Urban ecosystems. Sci. Rep. 2:963. doi: 10.1038/srep00963
Eisenman, T. S. (2013). Frederick law olmsted, green infrastructure, and the evolving city. J. Plan. Hist. 12, 287–311. doi: 10.1177/1538513212474227
Emilsson, T., and Sang, Å. O. (2017). “Impacts of climate change on Urban Areas and nature-based solutions for adaptation,” in Nature-Based Solutions to Climate Change Adaptation in Urban Areas - Linkages Between Science, Policy and Practice, eds N. Kabisch, H. Korn, J. Stadler, and A. Bonn (Cham: Springer Open), 15–27.
Espeland, E. K., and Kettenring, K. M. (2018). Strategic plant choices can alleviate climate change impacts: a review. J. Environ. Manage. 222, 316–324. doi: 10.1016/j.jenvman.2018.05.042
Evans, K. L., Newson, S. E., and Gaston, K. J. (2009). Habitat influences on Urban avian assemblages. Ibis 151, 19–39. doi: 10.1111/j.1474-919X.2008.00898.x
Ferrini, F., and Fini, A. (2011). Sustainable management techniques for trees in the Urban areas. JBES 1, 1–20.
Fischer, J., and Lindenmayer, D. B. (2002). The conservation value of paddock trees for birds in a variegated landscape in southern New South Wales. 1. species composition and site occupancy patterns. Biodivers. Conserv. 11, 807–832. doi: 10.1023/A:1015371511169
Fontana, S., Sattler, T., Bontadina, F., and Moretti, M. (2011). How to man-age the Urban green to improve bird diversity and community structure. Landscape Urban Plan. 101, 278–285. doi: 10.1016/j.landurbplan.2011.02.033
Gardiner, B., Berry, P., and Moulia, B. (2016). Review: wind impacts on plant growth, mechanics and damage. Plant Sci. 245, 94–118. doi: 10.1016/j.plantsci.2016.01.006
Goddard, M. A., Dougill, A. J., and Benton, T. G. (2010). Scaling up from gardens: biodiversity conservation in Urban environments. Trends Ecol. Evol. 25, 90–98. doi: 10.1016/j.tree.2009.07.016
Goddard, M. A., Dougill, A. J., and Benton, T. G. (2013). Why garden for wildlife? Social and ecological drivers, motivations and barriers for biodiversity management in residential landscapes. Ecol. Econom. 86, 258–273. doi: 10.1016/j.ecolecon.2012.07.016
Goldstein, A. H., and Galbally, I. E. (2007). Known and unexplored organic constituents in the earth's atmosphere much remains to be learned about the sources, structure, chemistry, and fate of gas-phase and aerosol organic compounds. Environ. Sci. Technol. 41, 1515–1521. doi: 10.1021/es072476p
Gough, P. (2000). From heroes' groves to parks of peace: landscapes of remembrance, protest and peace. Landscape Res. 25, 213–228. doi: 10.1080/713684669
Grote, R., Samson, R., Alonso, R., Amorim, J. H., Cariñanos, P., Churkina, G., et al. (2016). Functional traits of Urban trees: air pollution mitigation potential. Front. Ecol. Environ. 14, 543–550. doi: 10.1002/fee.1426
Gül, M., Dee, J., and Cünük, C. N. (2014) Istanbul's Taksim Square Gezi Park: the place of protest the ideology of place. J. Archit. Urban 38, 63–72. doi: 10.3846/20297955.2014.902185
Hamrick, J. L., and Godt, M. J. W. (1996). Effects of life history traits on genetic diversity in plant species. Philos. Trans. R. Soc. Biol. Sci. 351, 1291–1298. doi: 10.1098/rstb.1996.0112
Hartig, T., and Cooper Marcus, C. (2006). Essay healing gardens—places for nature in health care. Lancet 368, S36–S37. doi: 10.1016/S0140-6736(06)69920-0
Hedblom, M., Knez, I., and Gunnarsson, B. (2017). “Bird diversity improves the well-being of city residents,” in Ecology and Conservation of Birds in Urban Environments, eds E. Murgui and M. Hedblom (Heidelberg: Springer), 287–306.
Helms, J. A. (1998). The Dictionary of Forestry. The Society of American Foresters. Bethesda: Society of American Foresters.
Hernández-Brito, D., Carrete, M., Popa-Lisseanu, A. G., Ibáñez, C., and Tella, J. L. (2014). Crowding in the city: losing and winning competitors of an invasive bird. PLoS ONE 9:e100593. doi: 10.1371/journal.pone.0100593
Hofmann, M. M., Fleischmann, A., and Renner, S. S. (2018). Changes in the bee fauna of a German botanical garden between 1997 and 2017, attributable to climate warming, not other parameters. Oecologia 187, 701–706. doi: 10.1007/s00442-018-4110-x
Hostetler, M., Allen, W., and Meurk, C. (2011). Conserving Urban biodiversity? Creating green infrastructure is only the first step. Landscape Urban Plan. 100, 369–371. doi: 10.1016/j.landurbplan.2011.01.011
Hueck, H. J. (1968). “The biodetrioration of materials – an appraisal,” in Biodeterioration of Materials, eds A. H. Walters and J. S. Elphic (London: Elsevier), 6–12.
Hulme, P. E. (2011). Addressing the threat to biodiversity from botanic gardens. Trends Ecol. Evol. 26, 168–174. doi: 10.1016/j.tree.2011.01.005
Jim, C. Y., Konijnendijk van den Bosch, C., and Chen, W. Y. (2018). Acute challenges and solutions for Urban forestry in compact and densifying cities. J. Urban Plann. Dev. 2018:4018025. doi: 10.1061/(ASCE)UP.1943-5444.0000466
Jones, O., and Cloke, P. (2008). “Chapter 5: Non-human agencies: trees in place and time,” in Material Agency, eds C. Knappett and L. Malafouris (New York, NY: Springer Science + Business Media, LLC), 79–95. doi: 10.1007/978-0-387-74711-8_5
Joseph, A., Kearns, R., and Moon, G. (2013). Re-imagining psychiatric asylum spaces through residential redevelopment: strategic forgetting and selective remembrance. Hous. Stud. 28, 135–153. doi: 10.1080/02673037.2013.729270
Junier, P., and Joseph, E. (2017). Microbial biotechnology approaches to mitigating the deterioration of construction and heritage materials. Microb. Biotechnol. 10, 1145–1148. doi: 10.1111/1751-7915.12795
Jürgens, A., and Bischoff, M. (2017). Changing odour landscapes: the effect of anthropogenic volatile pollutants on plant–pollinator olfactory communication. Funct. Ecol. 31, 56–64. doi: 10.1111/1365-2435.12774
Kasprzyk, I., Cwik, A., Borycka, K., Wójcik, T., and Cariñanos, P. (2019). Allergenic pollen concentrations in the air of Urban parks in relation to their vegetation. Urban For. Urban Green. 46:126486. doi: 10.1016/j.ufug.2019.126486
Knez, I., Ode Sang, Å., Gunnarsson, B., and Hedblom, M. (2018). Wellbeing in Urban greenery: the role of naturalness and place identity. Front. Psychol. 9:491. doi: 10.3389/fpsyg.2018.00491
Krishnan, S., and Novy, A. (2016). The role of botanic gardens in the twenty-first century. CAB Rev. 11, 1–10. doi: 10.1079/PAVSNNR201611023
Lafortezza, R., Chen, J., Konijnendijk van den Bosch, C., and Randrup, T. B. (2017). Nature-based solutions for resilient landscapes and cities. Environ. Res. 165, 431–441. doi: 10.1016/j.envres.2017.11.038
Lam, C. K. C., Loughnan, M., and Tapper, N. (2018). Visitors' perception of thermal comfort during extreme heat events at the Royal Botanic Garden Melbourne. Int. J. Biometeorol. 62, 97–112. doi: 10.1007/s00484-015-1125-4
Lepczyk, C. A., Aronson, M. F. J., Evans, K. L., Goddard, M. A., Lerman, S. B., and MacIvor, J. S. (2017). Biodiversity in the city: fundamental questions for understanding the ecology of Urban green spaces for biodiversity conservation. Bio Sci. 67, 799–807. doi: 10.1093/biosci/bix079
Livesley, S. L., McPherson, E. G., and Calfapietra, C. (2016) The Urban forest ecosystem services: impacts on Urban water, heat, pollution cycles at the tree, street, city scale. J. Environ. Qual. 45, 119–124. doi: 10.2134/jeq2015.11.0567
Loreto, F., Bagnoli, F., and Fineschi, S. (2009). One species, many terpenes: matching chemical and biological diversity. Trends Plant Sci. 14, 416–420. doi: 10.1016/j.tplants.2009.06.003
Loreto, F., and Fineschi, S. (2015). Reconciling functions and evolution of isoprene emission in higher plants. New Phytol. 206, 578–582. doi: 10.1111/nph.13242
Loughran, K. (2018). Urban parks and Urban problems: an historical perspective on green space development as a cultural fix. Urban Stud. 1–18. doi: 10.1177/0042098018763555
Loveless, M. D., and Hamrick, J. L. (1984). Ecological determinants of genetic structure in plant populations. Ann. Rev. Ecol. Syst. 15, 65–95. doi: 10.1146/annurev.es.15.110184.000433
Luck, G. W., Davidson, P., Boxall, D., and Smallbone, L. (2011). Relations between Urban bird and plant communities and human well-being and connection to nature. Conserv. Biol. 25, 816–826. doi: 10.1111/j.1523-1739.2011.01685.x
Lumsden, L. F., and Bennett, A. F. (2005). Scattered trees in rural landscapes: foraging habitat for insectivorous bats in south-eastern Australia. Biol. Conserv. 122, 205–222. doi: 10.1016/j.biocon.2004.07.006
Lynch, A. J. (2016). Is it good to be green? Assessing the ecological results of county green infrastructure planning. J. Plan. Educ. Res. 36, 90–104. doi: 10.1177/0739456X15598615
Lyytimäki, J., and Sipilä, M. (2009). Hopping on one leg – the challenge of ecosystem disservices for Urban green management. Urban For. Urban Green. 8, 309–315. doi: 10.1016/j.ufug.2009.09.003
Maher, B. A., Ahmed, I. A. M., Davison, B., Karloukovski, V., and Clarke, R. (2013). Impact of roadside tree lines on indoor concentrations of traffic-derived particulate matter. Environ. Sci. Technol. 47, 13737–13744. doi: 10.1021/es404363m
Mahmoudi Farahani, L., and Maller, C. (2018). Perceptions and preferences of Urban greenspaces: a literature review and framework for policy and practice. Landscape Online 61, 1–22. doi: 10.3097/LO.201861
Maruthaveeran, S., and Konijnendijk van den Bosch, C. C. (2014). A socio-ecological exploration of fear of crime in Urban green spaces – a systematic review. Urban For. Urban Green. 13, 1–18. doi: 10.1016/j.ufug.2013.11.006
McDonnell, M. J. (2011). “The history of Urban ecology,” in Urban Ecology: Patterns, Processes, and Applications, ed J. Niemelä (Oxford: Oxford University Press), 5–13.
McDonnell, M. J., and MacGregor-Fors, I. (2016). The ecological future of cities. Science 352, 936–938. doi: 10.1126/science.aaf3630
Miller, R. W., Hauer, R. J., and Werner, L. P. (2015). Urban Forestry: Planning and Managing Urban Greenspaces, 3rd Edn. Long Grove, IL: Waveland Press, Inc.
Mori, J., Fini, A., Burchi, G., and Ferrini, F. (2016). Carbon uptake and air pollution mitigation of different evergreen shrub species. Arboric. Urban. For. 42, 329–345.
Mougeot, L. J. A. (2000). Urban Agriculture: Definition, Presence, Potentials and Risks, and Policy Challenges. Cities Feeding, People Series Report 31. Ottawa, ON: International Development Research Centre.
Mullaney, J., Lucke, T., and Trueman, S. J. (2015). A review of benefits and challenges in growing street trees in paved Urban environments. Landscape Urban Plan. 134, 157–166. doi: 10.1016/j.landurbplan.2014.10.013
Muratet, A., Pellegrini, P., Dufour, A. B., Arrif, T., and Chiron, F. (2015). Perception and knowledge of plant diversity among Urban park users Landscape Urban Plan. 137, 95–106. doi: 10.1016/j.landurbplan.2015.01.003
Núñez-Florez, R., Pérez-Gómez, U., and Fernández-Méndez, F. (2019). Functional diversity criteria for selecting Urban trees. Urban For. Urban Green. 38, 251–266. doi: 10.1016/j.ufug.2019.01.005
Nybom, H., and Bartish, I. V. (2000). Effects of life history traits and sampling strategies on genetic diversity estimates obtained with RAPD markers in plants. Perspect. Plant Ecol. Evol. Syst. 3, 93–114. doi: 10.1078/1433-8319-00006
Paap, T., Burgess, T. I., and Wingfield, M. J. (2017). Urban trees: bridge-heads for forest pest invasions and sentinels for early detection. Biol. Invasions 19, 3515–3526. doi: 10.1007/s10530-017-1595-x
Park, J. H., Lee, D. K., Park, C., Kim, H. G., Jung, T. Y., and Kim, S. (2017). Park accessibility impacts housing prices in Seoul. Sustainability 9:185. doi: 10.3390/su9020185
Pasha, G. A., Tanaka, N., Yagisawa, J., and Achmad, F. N. (2018). Tsunami mitigation by combination of coastal vegetation and a backward-facing step. Coast. Eng. J. 60, 104–125. doi: 10.1080/21664250.2018.1437014
Pautasso, M., Schlegel, M., and Holdenrieder, O. (2015). Forest health in a changing world. Microb. Ecol. 69, 826–842. doi: 10.1007/s00248-014-0545-8
Perini, K., Ottelé, M., Fraaij, A. L. A., Haas, E. M., and Rai, R. (2011). Vertical greening systems and the effect on air flow and temperature on the building envelope. Build. Environ. 46, 2287–2294. doi: 10.1016/j.buildenv.2011.05.009
Petit, R. J., Duminil, J., Fineschi, S., Hampe, A., Salvini, D., and Vendramin, G. G. (2005). Comparative organisation of chloroplast, mitochondrial and nuclear diversity in plant populations. Mol. Ecol. 14, 689–701. doi: 10.1111/j.1365-294X.2004.02410.x
Postigo, J. L., Shwartz, A., Strubbe, D., and Muñoz, A. M. (2017). Unrelenting spread of the alien monk parakeet Myiopsitta monachus in Israel. Is it time to sound the alarm? Pest. Manag. Sci. 73, 349–353. doi: 10.1002/ps.4349
Potgieter, L. (2019). Assessing the impacts of invasive alien plants on Urban ecosystem services (dissertation). Stellenbosch University, Stellenbosch, South Africa.
Potts, S. G., Imperatriz-Fonseca, V., Ngo, H. T., Aizen, M. A., Biesmeijer, J. C., Breeze, T. D., et al. (2016). Safeguarding pollinators and their values to human well-being. Nature 540, 220–229. doi: 10.1038/nature20588
Poulsen, M. N., Neff, R. A., and Winch, P. J. (2017). The multifunctionality of Urban farming: perceived benefits for neighbourhood improvement. Local Environ. 22, 1411–1427. doi: 10.1080/13549839.2017.1357686
Qumsiyeh, M., Handal, E., Chang, J., Abualia, K., Najajreh, M., and Abusarhan, M. (2017). Role of museums and botanical gardens in ecosystem services in developing countries: case study and outlook. Int. J. Environ. Stud. 74, 340–350. doi: 10.1080/00207233.2017.1284383
Rahman, F. A., Aziz, M. M. A., Saidur, R., Bakar, W. W. A., Hainin, M. R., Putrajaya, R., et al. (2017). Pollution to solution: capture and sequestration of carbon dioxide (CO2) and its utilization as a renewable energy source for a sustainable future. Renew. Sust. Ener. Rev. 71, 112–126. doi: 10.1016/j.rser.2017.01.011
Razak, M. A. W. A., Othman, N., and Nazir, N. N. M. (2016). Connecting people with nature: Urban park and human well-being. Procedia Soc. Behav. Sci. 222, 476–484. doi: 10.1016/j.sbspro.2016.05.138
Rey, F., Bifulco, C., Bischetti, G. B., Bourrier, F., De Cesare, G., Florineth, F., et al. (2019). Soil and water bioengineering: practice and research needs for reconciling natural hazard control and ecological restoration. Sci. Total Environ. 648, 1210–1218. doi: 10.1016/j.scitotenv.2018.08.217
Russel, J. C., and Blackburn, T. M. (2017a). Invasive alien species: denialism, disagreement, definitions, and dialogue. Trends Ecol. Evol. 32, 312–314. doi: 10.1016/j.tree.2017.02.005
Russel, J. C., and Blackburn, T. M. (2017b). The rise of invasive species denialism. Trends Ecol. Evol. 32, 3–6. doi: 10.1016/j.tree.2016.10.012
Russo, A., and Cirella, G. T. (2018). Modern compact cities: how much greenery do we need? Int. J. Environ. Res. Public Health 15:2180. doi: 10.3390/ijerph15102180
Sanesi, G., Colangelo, G., Lafortezza, R., Clavo, E., and Davies, C. (2017). Urban green infrastructure and Urban forests: a case study of the Metropolitan Area of Milan. Landscape Res. 42, 164–175. doi: 10.1080/01426397.2016.1173658
Scheerer, S., Ortega-Morales, O., and Gaylarde, C. (2009). Microbial deterioration of stone monuments-an updated overview. Adv. Appl. Microbiol. 66, 97–139. doi: 10.1016/S0065-2164(08)00805-8
Shackleton, S., Chinyimba, A., Hebinck, P., Shackleton, C., and Kaoma, H. (2015). Multiple benefits and values of trees in Urban landscapes in two towns in northern South Africa. Landscape Urban Plan. 136, 76–86. doi: 10.1016/j.landurbplan.2014.12.004
Shanahan, D. F., Lin, B. B., Bush, R., Gaston, K. J., Dean, G. H., Barber, E., et al. (2015). Toward improved public health outcomes from Urban nature. Am. J Public Health. 105, 470–477. doi: 10.2105/AJPH.2014.302324
Shwartz, A., Muratet, A., Simon, L., and Julliard, R. (2013). Local and management variables outweigh landscape effects in enhancing the diversity of different taxa in a big metropolis. Biol. Conserv. 157, 285–292. doi: 10.1016/j.biocon.2012.09.009
Specht, K., Siebert, R., Hartmann, I., Freisinger, U. B., Sawicka, M., Werner, A., et al. (2014). Urban agriculture of the future: an overview of sustainability aspects of food production in and on buildings. Agric. Hum. Values 31, 33–51. doi: 10.1007/s10460-013-9448-4
Stefanizzi, S., and Verdolini, V. (2019). Bordered communities: the perception of insecurity in five European cities. Qual. Quant. Int. J. Methol. 53, 1165–1186. doi: 10.1007/s11135-018-0810-x
Sterflinger, K., Little, B., Pinar, G., Pinzari, F., de los Rios, A., and Gu, J. D. (2018). Future directions and challenges in biodeterioration research on historic materials and cultural properties. Int. Biodeter. Biodegr. 129, 10–12. doi: 10.1016/j.ibiod.2017.12.007
Taleghani, M. (2017). Outdoor thermal comfort by different heat mitigation strategies- A review. Renew. Sust. Energ. Rev. 81, 2011–2018. doi: 10.1016/j.rser.2017.06.010
Tanaka, N., and Onai, A. (2017). Mitigation of destructive fluid force on buildings due to trapping of floating debris by coastal forest during the great east Japan tsunami. Landscape Ecol. Eng. 13, 131–144. doi: 10.1007/s11355-016-0308-4
Taylor, L., Taylor, C., and Davis, A. (2013). The impact of Urbanisation on avian species: the inextricable link between people and birds. Urban Ecosyst. 16, 481–498. doi: 10.1007/s11252-012-0283-y
Tello, M.-L., Tomalak, M., Siwecki, R., Gaper, J., Motta, E., and Mateo-Sagasta, E. (2005). “Biotic Urban growing condition – threats, pests and diseases,” in Urban Forests and Trees, eds C. C. Konijnendijk, K. Nilsson, T. B. Randrup, and J. Schipperijn (Berlin: Springer), 325–365.
Tiano, P. (2002). Biodegradation of Cultural Heritage: Decay, Mechanisms and Control Methods. Seminar Article Held at The Universidade Nova De Lisboa, Departamento De Concervacão E Restauro, 7–12. Available online at: http://www.arcchip.cz/w09/w09_tiano. pdf (accessed May 11, 2019).
Toreno, G., Isola, D., Meloni, P., Carcangiu, G., Selbmann, L., Onofri, S., et al. (2018). Biological colonization on stone monuments: a new low impact cleaning method. J. Cult. Herit. 30, 100–109. doi: 10.1016/j.culher.2017.09.004
Turner-Skoff, J. B., and Cavender, N. (2019). The benefits of trees for livable and sustainable communities. Plants People Planet 1, 323–335. doi: 10.1002/ppp3.39
Ulrich, R. S. (1984). View through a window may influence recovery from surgery. Science 224, 420–421. doi: 10.1126/science.6143402.
Ulrich, R. S. (1992). Effects of interior design on wellness: theory and recent scientific research. J. Health. Care Inter. Des. 3, 97–109.
Ulrich, R. S. (1999). “Effects of gardens on health outcomes: theory and research,” in Healing Gardens: Therapeutic Benefits and Design Recommendations, eds C. Cooper Marcus and M. Barnes (New York, NY: John Wiley & Sons), 27–86.
Ulrich, R. S. (2002). “Health benefits of gardens in hospitals,” in Paper for conference, Plants for People International Exhibition Floriade.
United Nations (2015). Transforming Our World: The 2030 Agenda For Sustainable Development. ARES 701.
van den Berg, A. E., Hartig, T., and Staats, H. (2007). Preference for nature in Urbanized societies: stress, restoration, and the pursuit of sustainability. J. Soc. Issues 63, 79–96. doi: 10.1111/j.1540-4560.2007.00497.x
van Heezik, Y., and Seddon, P. J. (2018). Animal reintroductions in peopled landscapes: moving towards urban-based species restorations in New Zealand. Pac. Conserv. Biol. 24, 349–359. doi: 10.1071/PC18026
Velázquez, J., Anza, P., Gutierrez, J., Sánchez, B., Hernando, A., and García-Abril, A. (2019). Planning and selection of green roofs in large Urban areas. Application to madrid metropolitan area. Urban For. Urban Green. 4, 323–334. doi: 10.1016/j.ufug.2018.06.020
Viecco, M., Vera, S., Jorquera, H., Bustamante, W., Gironás, J., Dobbs, C., et al. (2018). Potential of particle matter dry deposition on green roofs and living walls vegetation for mitigating Urban atmospheric pollution in semiarid climates. Sustainability 10:2431. doi: 10.3390/su10072431
von Döhren, P., and Haase, D. (2019). Risk assessment concerning Urban ecosystem disservices: the example of street trees in Berlin, Germany. Ecosyst. Serv. 40:101031. doi: 10.1016/j.ecoser.2019.101031
Vujcic, M., Tomicevic-Dubljevic, J., Grbic, M., Lecic-Tosevski, D., Vukovic, O., and Toskovic, O. (2017). Nature based solution for improving mental health and well-being in Urban areas. Environ. Res. 158, 385–392. doi: 10.1016/j.envres.2017.06.030
Wassenberg, C. L., Goldenberg, M. A., and Soule, K. E. (2015). Benefits of botanical garden visitation: a means-end study. Urban For. Urban Green. 14, 148–155. doi: 10.1016/j.ufug.2015.01.002
Weissert, L. F., Salmond, J. A., and Schwendenmann, L. (2017). Photosynthetic CO2 uptake and carbon sequestration potential of deciduous and evergreen tree species in an Urban environment. Urban Ecosyst. 20, 663–674. doi: 10.1007/s11252-016-0627-0
White, J. G., Antos, M. J., Fitzsimons, J. A., and Palmer, G. C. (2005). Non-uniform bird assemblages in Urban environments: the influence of streetscape vegetation. Landscape Urban Plan. 71, 123–135. doi: 10.1016/j.landurbplan.2004.02.006
Xiao, H., Huang, J., Ma, Q., Wan, J., Li, L., Peng, Q., and Rezaeimalek, S. (2017). Experimental study on the soil mixture to promote vegetation for slope protection and landslide prevention. Landslides 14, 287–297. doi: 10.1007/s10346-015-0634-x
Zhang, Z., Meerow, S., Newell, J. P., and Lindquist, M. (2019). Enhancing landscape connectivity through multifunctional green infrastructure corridor modeling and design. Urban For Urban Green. 38, 305–317. doi: 10.1016/j.ufug.2018.10.014
Zhao, S., Yu, Y., Yin, D., Qin, D., He, J., and Dong, L. (2018). Spatial patterns and temporal variations of six criteria air pollutants during (2015) to (2017) in the city clusters of Sichuan Basin, China. Sci. Total Environ. 624, 540–557. doi: 10.1016/j.scitotenv.2017.12.172
Alien (or “exotic” or “introduced”) species are those whose presence in a region is attributable to human actions that have enabled them to overcome barriers to their natural dispersal. According to the Secretariat of the Convention on Biological Diversity (2002) a species (or lower taxon) is considered alien when introduced outside its natural past or present distribution; this definition refers also to any part, gametes, seeds, eggs, or propagules of such species that might survive and subsequently reproduce. When an alien species becomes “invasive” it acquires automatically an unfavorable meaning. The IUCN defines Invasive Alien Species (IAS) as “introduced by man into places out of their natural range of distribution, where they become established and disperse, generating a negative impact.” However, disagreement can arise from the use of this definition of IAS, which includes an explicit statement about impact; in fact, a species might be invasive because it has a wide distribution without generating negative impacts. This evidence should induce doubt over the current negative perception toward alien species. The terms “alien” and “invasive” are not synonymous, and should not be used or interpreted interchangeably. In the same way, natural invasions and biological invasions by alien species are different processes. A species might expand its range to new locations through its own dispersal mechanisms and strategies, thus giving rise to a process of natural invasion or colonization. On the other hand, biological invasions by alien species occur after a species is transported by humans outside its native range (Russel and Blackburn, 2017a,b).
Outdoor stone artifacts are exposed to several environmental constrains, including living organisms, which can settle and spread on and into the rock materials. Different biological (and environmental) processes cause degradation or structural damage to heritage materials: bioweathering, which refers to rocks and minerals; biodeterioration (organic substrates) and biocorrosion (metals) (Junier and Joseph, 2017). The term “biodeterioration” was coined in the late 60's by Hueck (1965, 1968) to describe “any undesirable change in a material brought about by the vital activities of organisms” Allsopp et al., 2004; Sterflinger et al., 2018).
Plants produce and emit in the atmosphere thousands of biogenic volatile organic compounds (BVOCs). Opposite to primary or basic metabolites (i.e., necessary for the survival of the cells), these chemical compounds are recognized as secondary metabolites; they may occur in special, differentiated cells and are not necessary for the cells themselves, but may be useful for the plant as a whole. Emission of BVOCs has its maximum in summer; in contrast to anthropogenic VOC (AVOCs), BVOCs are usually very abundant and reactive, and once emitted in the atmosphere they play a crucial role in the interaction between biosphere and atmosphere, e.g., entering cycles of ozone and particle formation.
Life history traits or ecological attributes are species-specific characters, i.e., measurable aspects of the phenotype affecting the demography of the species. Each species is characterized by a set of life-history and ecological features, which have significant effects on the partitioning of genetic diversity within and among populations.
Compared to purely morphological, behavioral, or physiological traits, life history traits have an especially strong effect on overall fitness. They can also vary widely between closely related species or even among populations of the same species (Loveless and Hamrick, 1984; Hamrick and Godt, 1996; Nybom and Bartish, 2000; Aguinagalde et al., 2005; Petit et al., 2005; Duminil et al., 2007).
Main LHT measured in plant populations genetic studies comprise:
— Breeding system (autogamous, mixed mating, outcrossed)
— Floral morphology (hermaphroditic, monoecious, dioecious)
— Mode of reproduction (obligate apomixis, facultative apomixis, sexual reproduction)
— Pollination mechanism (mediated by insects, birds, bats, wind)
— Seed dispersal (gravity, animal-ingested, animal-attached, wind-mediated)
— Seed dormancy (present, absent)
— Floral phenology (populations asynchronous, populations seasonal, synchronous)
— Life cycle (annual, short-lived, long-lived)
— Successional stage (early, late)
— Geographic range (endemic, narrow, regional, widespread)
— Population size (large and stable, small and stable, fluctuating size)
— Population density (high, low)
— Population spatial distribution (patchy, uniform).
Urban texture is a commonly accepted expression in the architecture disciplines. It might be used to indicate urban geometry, in particular the width of streets, their orientation, spacing, intersection. Urban green areas are part of the heterogeneous urban texture, which is the most complex and heterogeneous landscapes on earth, composed by a mosaic of patches under dynamic change. City patch may include houses and outbuildings, the paved surfaces of streets, walks, and driveways, and vegetation cover consisting of trees and a ground layer (grasses and herbs).
In many cities, particularly in developing countries, urban agriculture contributes to the food security. It fulfills the necessity to provide part of the food needed by the household, and limits dependence on the commercially processed food. Urban agriculture comprises very diverse agricultural productions, though the major cultures are grain, root, vegetable, aromatic and medicinal herbs and fruit crops, and livestock of all shapes and sizes (Mougeot, 2000). In developed countries, urban farming represents a particular type of urban agriculture, which focuses on entrepreneurial food production and serves multiple functions in neighborhoods (Poulsen et al., 2017). Public projects based on urban farming bring several benefits, such as physical improvement of degraded space, production of local food, increasing of social connectedness, improving youth education and development, and creating employment opportunities. Urban farming offers opportunities resulting from the use and recycling of resources; moreover, it represents a tool to establish a small-scale resource saving system. In spite of positive aspects, some urban agriculture practices require high standards of technology, maintenance, operation, and investment, in addition to free space that is very limited in many cities (Specht et al., 2014).
In the 1920s the Chicago School of urban sociology pioneered the use of ecological theory and terms to describe the structure and function of cities. Over time, the term “urban ecology” brought a diversity of meanings to mind. In the early 1970s the UNESCO Man and the Biosphere Program (MAB) funded the first integrated urban ecology research, bringing together natural sciences, engineering/planning, and social sciences. Each of these sciences has its own terminology, methodologies and objectives, thus resulting in an assortment of definitions and meanings for the term urban ecology (McDonnell, 2011).
Urban forestry is a concept that links the urban location and the urban function of the forest or tree. It was developed in North America during the 1960s as innovative approach for managing natural resources in urban environments. Miller et al. (2015) define urban forestry “as the sum of street trees, residential trees, park trees, and greenbelt vegetation.” It also includes trees in unused land, trees in transportation corridors, trees in gardens and backyards, both public and private, and forests on watershed lands private (Goddard et al., 2013; Cavender and Donnelly, 2019). A further definition, which also implies the human perception of green spaces, is that by (Helms, 1998), which defined urban forestry as “the art, science, and technology of managing trees and forest resources in and around urban community ecosystems for the physiological, sociological, economic, and aesthetic benefits trees provide society.”
Keywords: ecosystem services, global change, green area typologies in cities, green urban planning and management, positive (negative) plant-human interactions, urban trees
Citation: Fineschi S and Loreto F (2020) A Survey of Multiple Interactions Between Plants and the Urban Environment. Front. For. Glob. Change 3:30. doi: 10.3389/ffgc.2020.00030
Received: 30 November 2019; Accepted: 02 March 2020;
Published: 24 March 2020.
Edited by:
Rüdiger Grote, Karlsruhe Institute of Technology (KIT), GermanyReviewed by:
Leonardo Montagnani, Free University of Bozen-Bolzano, ItalyCopyright © 2020 Fineschi and Loreto. This is an open-access article distributed under the terms of the Creative Commons Attribution License (CC BY). The use, distribution or reproduction in other forums is permitted, provided the original author(s) and the copyright owner(s) are credited and that the original publication in this journal is cited, in accordance with accepted academic practice. No use, distribution or reproduction is permitted which does not comply with these terms.
*Correspondence: Silvia Fineschi, c2lsdmlhLmZpbmVzY2hpQGNuci5pdA==
Disclaimer: All claims expressed in this article are solely those of the authors and do not necessarily represent those of their affiliated organizations, or those of the publisher, the editors and the reviewers. Any product that may be evaluated in this article or claim that may be made by its manufacturer is not guaranteed or endorsed by the publisher.
Research integrity at Frontiers
Learn more about the work of our research integrity team to safeguard the quality of each article we publish.