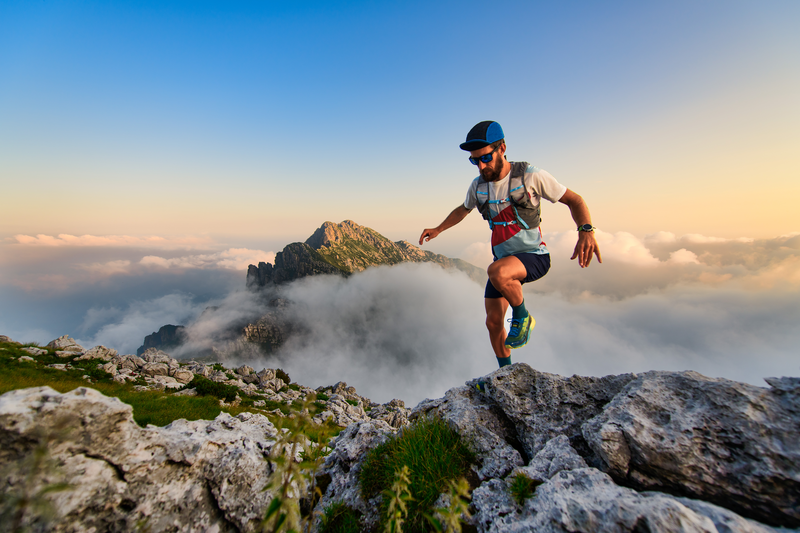
94% of researchers rate our articles as excellent or good
Learn more about the work of our research integrity team to safeguard the quality of each article we publish.
Find out more
ORIGINAL RESEARCH article
Front. For. Glob. Change , 28 February 2020
Sec. Fire and Forests
Volume 3 - 2020 | https://doi.org/10.3389/ffgc.2020.00010
This article is part of the Research Topic Impact of Fire on Biodiversity View all 4 articles
Fire in tropical forests increases tree mortality, degrades forest structure, and reduces carbon stocks. Currently, there are large gaps in understanding how fire affects understory forest structure and composition, interactions with fire recurrence, and long-term impacts. Understanding these changes is critical to evaluate the present and future response of tropical forests to fire. We studied post-fire changes in understory regeneration in forests in Mato Grosso State, southern Amazonia, Brazil, aiming to answer the following questions: (i) does forest structure (basal area) and tree community composition vary with fire frequency and time since the last fire? (ii) does the response differ among strata (e.g., sapling, larger trees)? (iii) are changes in diversity associated with changes in forest structure? We surveyed trees and lianas in previously structurally intact forests that underwent selective logging, followed by different fire histories, including 5 and 16 years after once-burned, 5 years after three times burned, and unburned (control). Overall, species composition (abundance, richness, and number of families) and diversity were highest for the unburned treatment and lowest for the recurrent burned areas. Fire frequency negatively affected plant structure and basal area; basal area of small, medium, and large plants declined significantly by more than 50% in the most frequently burned areas. Richness was positively related to basal area in the three times burned sites and in the 16 years regenerating site for all strata. Our results demonstrate the negative influence of frequent fires on both the composition and structure of small trees in Amazonian forest. These changes to the cohort of small-sized trees may persist and have long-term impacts on forest structure, affecting the capacity, and direction of forest recovery. With wildfire widespread across the region and increasing in frequency, fire may negatively affect tree diversity in remaining selectively logged forests, and affect regional carbon cycling with consequences for the global vegetation carbon sink.
Logging in the Brazilian Amazon damaged 10,000−20,000 km2 y−1 of tropical forests in the early 2000's and understory fires burned ca. 85,000 km2 of standing forest from 1999 to 2010 (Asner et al., 2005; Morton et al., 2013). From 2004 to 2018, 150,937 km2 of Legal Amazonia was deforested through clear cut and fire (Prodes, http://www.obt.inpe.br/). In the Amazon Basin, fires have been associated with roads, pastures, agriculture, and logging (Feldpausch et al., 2004; Alencar et al., 2006; Cochrane and Barber, 2009).
Forest fires in the Amazon Basin are one of the most important drivers of tropical forest degradation, especially recently. In the Brazilian Amazon, fire effects on tropical forests were first studied in the 1980's due to concerns of land clearing from wildfires (Uhl et al., 1981, 1982). While evidence of past fire has been reported for some old-growth Amazonian forests from charcoal radiocarbon dating and estimates of soil pyrogenic carbon storage (Sanford et al., 1985; Goulart et al., 2017; Koele et al., 2017), it is unclear whether past fire led to the selection of fire-adapted traits among Amazon forest tree species (Massi et al., 2017). If plant communities were adapted to past fires in Amazon forests, current burning would impact these differently (Brando et al., 2012). Modern undisturbed moist forests rarely burn (Uhl and Serrao, 1998); however, forests that have burned once are more likely to burn again (Cochrane, 1999; Zarin et al., 2005). Fire impacts vary according to vegetation composition and structure, and with fire regime (intensity, frequency, rate of spread: Silva et al., 2005).
Fires occurring over recent decades in Amazonia have affected forest dynamics and structure by increasing tree mortality (Gerwing, 2002; Barlow and Peres, 2008; Silvério et al., 2013; Balch et al., 2015; Massad et al., 2015). Fires in tropical forests generally cause extensive top-kill in small trees (Hoffmann et al., 2009) and leaf-fall in larger diameter trees, allowing increased light to the forest floor. This higher light availability together with lower air humidity causes dryness in the forest environment, decreasing tree cover development with time, and influencing species richness (Fu et al., 2013). Frequent fires are especially dangerous because they affect forest regeneration through alteration of the seed bank (Kennard et al., 2002) and through the isolation of populations, making fire-tolerant species more abundant and fire-sensitive species may disappear (Cochrane and Barber, 2009; Brando et al., 2012). In southeastern Amazonian, forest stem density recovered within a year after a first burn, but after repeated fires, increased mortality, and decreased regeneration by seedlings led to a reduction in tree and liana densities (Balch et al., 2015). There is little information on changes in Amazon forest composition and structure after burning, especially on the mortality of saplings and seedlings. These groups most affected by fire (Barlow et al., 2003) play a critical role in post-fire recovery. Thus, studying lower forest stratum allows understanding the consequences of disturbance on short- and long-term forest dynamics and recovery.
One of the main interests in studying fire in Amazonia has been the effect of fire on forest carbon storage and cycling. Amazonian forests store ca. 100 Pg C in trees (Feldpausch et al., 2012), contribute to global net productivity (Brienen et al., 2015), and affect global climate (Fearnside, 2018). In drought years, forest fires have the potential to turn the Amazon Basin into a net C source (Aragão et al., 2018; Metcalfe et al., 2018; Silva Junior et al., 2019). Fire and drought effects are not restricted to upland forests. Riparian forests have large biomass loss over periods spanning drought and fire, losing ca. −3.3 Mg ha−1 year−1 (Nogueira et al., 2019). Following fire, recovery of biomass and forest structure is likely to be impeded by abundant lianas and the high incidence of crown and stem damage in the residual stands (Gerwing, 2002) and the infestation of exotic grasses in highly disturbed sites (Silvério et al., 2013).
Fires may also affect the species composition of forests because trees species suffer varying rates of mortality from fire (Brando et al., 2014; Massad et al., 2015). In southern Amazonia, diversity in triennially burned forest was more similar to unburned than in annually burned forest, and the community composition of triennially burned forest was intermediate between unburned and annually burned areas (Massad et al., 2015). Changes in forest composition may also affect the capacity of forests to recover and store carbon, resulting in multiple alternative stable states with varying carbon storage and diversity when a site is disturbed. However, little is known about these potential changes in Amazon tree biodiversity and carbon cycling despite the increasing frequency of fires over recent decades (Brando et al., 2014; Barlow et al., 2016).
Southern Amazonia is undergoing changes making these forests more fire prone. Selective logging is pervasive throughout the region, which is then followed by the northward advancing “arc of deforestation” and agricultural frontier, with fire from associated anthropogenic activities (Souza et al., 2013) having enormous impacts on the remaining forest ecosystems (Morton et al., 2013; Alencar et al., 2015). In El Niño dry years, the area that burned increased (Marengo et al., 2011; Doughty et al., 2015; Chen et al., 2017; Liu et al., 2017; Prodes, http://www.obt.inpe.br/). The region lacks intact forests, having the highest rates of fire detection and selective logging of Amazonia (Hummel et al., 2010). Protection from fire is particularly urgent in this region due to threats from land-use change and proximity to the savanna, a fire-dependent biome (Massad et al., 2015). In the last few years, studies have focused on understanding fire impacts on Amazonian vegetation (Barlow and Peres, 2008; Silvério et al., 2013; Balch et al., 2015; Massad et al., 2015), but one of the main challenges remains to understanding how Amazonian species will respond to a more frequent fire disturbance scenario (Schwenk et al., 2009). It is expected that disturbed and altered ecosystems will be less diverse and have less ecological functionality and ecosystem services, with some species becoming locally extinct (Potter et al., 2013).
The aim of our study was to evaluate fire effects on small individuals (seedlings and saplings < 10 cm diameter) of trees and lianas species in forests affected by fire in southern Amazonia. We addressed the following questions: (i) do changes in vegetation structure (i.e., basal area) and community composition (e.g., species richness and diversity) vary with fire frequency and time since the last fire? (ii) does the response differ among strata (e.g., sapling, larger trees)? (iii) are these changes in forest composition associated with changes in forest structure (using basal area loss of large trees as a proxy for fire severity)?
The study took place in nine seasonal evergreen forest sites (Ivanauskas et al., 2008) in southern Amazonia in the municipality of Feliz Natal, Mato Grosso, Brazil (Lat: −12.38 and Long: −54.92, Figure 1). Soils are dystrophic red-yellow Latosols following the Brazilian classification (BRASIL and IBGE, 2009). The Köppen climate class is Am with an average temperature of 26°C, a mean annual precipitation (P) of 2,512 mm, and with a strong dry season (4 months with <100 mm of rainfall); the wet season takes place from November to March (Alvares et al., 2013). Despite 43% of the Brazilian Amazon biome experiencing significant negative maximum cumulative water deficit (MCWD) anomalies during the study year of 2015, the Feliz Natal region had positive MCWD anomalies (Aragão et al., 2018). The MCWD corresponds to the maximum value of the accumulated water deficit (Aragão et al., 2007) based on the estimate that moist tropical forest canopies transpire ~100 mm/month, a mean evapotranspiration value obtained from ground measurements for Amazonia. When monthly precipitation is <100 mm, the forest enters into water deficit conditions. The annual MCWD for a given area is the most negative value of the climatological water deficit among all months in a given year.
Figure 1. Study sites in southern Amazonia in Mato Grosso State, in the municipality of Feliz Natal, showing the distribution of nine study sites, with four plots per site. Sites were classified as “not-burned (T04, T05, and T06)”, “burned-1999 (T07, T08, and T09)”, “burned only once, in 2010 (T03),” and “Burned-3x (burned three times in 2006, 2010, and 2013) (T01 and T02)”.
Fieldwork was carried out in August 2015 in nine selectively logged forest sites where data of previous fires were available. Forest plots were classified according to fire frequency and year burned, namely Not-Burned (NB), Burned-1999 (burned one time in 1999), Burned-2010 (burned one time in 2010) and Burned-3x (burned three times in 2006, 2010, and 2013) (Table 1). Data of fire frequency and period were obtained from local inhabitants and from Landsat satellite data, which confirmed the fire occurrence in the areas. We established four permanent plots of 50 × 50 m per forest site, each plot separated by 100 m, where small trees, woody lianas, and large trees were sampled in a nested design for 1 ha total of surveyed forest per area (Figure 2). Diameter (D) measurement and species identification within the nested plots were as follows: (i) 50 × 50 m, all large trees (named LT) ≥ 35 cm D were surveyed, (ii) 5 × 50 m, all medium trees (MT) 10–34.9 cm D; (iii) 2 × 50 m, small trees and woody lianas (SML) 5–9.9 cm D; (iv) 2 × 10 m, saplings (SP) 2.5–4.9 cm diameter at ground height (DG); (v) and 1 × 5 m plot, seedlings (SE) < 2.5 cm DG (Figure 2). Smaller plants were measured with a ruler and caliper and for larger diameter plants, we used a measuring tape to the nearest millimeter.
Table 1. Area characteristics of burned and unburned forests and number of plots sampled (T01–T09) in Mato Grosso, southern Amazonia, Brazil.
Figure 2. Sampling plot and nested subplot distribution. Each transect consisted of four 50 × 50 m plots composed of five subplots corresponding to the separate strata: 1 × 5 m, Seedling (SE); 2 × 10 m, Saplings (SP); 2 × 50 m, Small Trees and Lianas (SML); 5 × 50 m, Medium Trees (MT); 50 × 50 m, Large Trees (LT).
Large trees (≥ 10 cm D) were identified in the field by local parataxonomists. For trees < 10 D, vouchers were collected for unknown species and later compared to herbarium specimens. Plant material was identified and fertile specimens were archived at the Universidade do Estado de Mato Grosso (UNEMAT) Herbarium. For plant family classification, we used the Angiosperm Phylogeny Group IV (APG IV, 2016) and Brazil Flora List (http://www.floradobrasil.jbrj.gov.br).
As this research was not conducted in prescribed fire experiments, fire intervals, severity and timing were not controlled. Thus, we used the change in total basal area as an indicator of fire severity. The random locations of wildfire and limited access to install plots in farms across the region created challenges to establish a large number of experimental replicates (Oksanen, 2001). For purposes of analyzing the effect of time since last fire, we applied a conservative estimate of at least 50 years to the non-burned site, because these sites had no evidence of fire scars on trunks or fire scars in satellite data and AMS radiocarbon dating indicates that structurally intact forests in the region have not burned for at least 100 years (T. Feldpausch unpublished data).
To evaluate differences between richness and diversity (Shannon Wiener index) in treatments (NB, B1-1999, B1-2010, and B-3x) and strata (SE, SP, SML, MT, and LT), we used the interpolation and extrapolation method in iNEXT (an R package modified from the original version supplied in the Supplement of Chao et al., 2014; Hsieh et al., 2016). This analysis allows calculating the diversity of samples by extrapolating up to twice the size of the plot abundance or a specified size, and also allows evaluating diversity in terms of sample adequacy associated with 95% confidence intervals (Chao and Jost, 2012).
We estimated plant stem density (ha−1) and basal area (sum of the cross-section area of the trunk in m2 ha−1) on a per hectare basis for each nested plot. Skewness tests were used to evaluate the distribution of basal area among strata and treatments. To examine differences in plant basal area according to treatment, we performed Kruskal-Wallis tests for each stratum. Analyses of covariance (ANCOVA) were used to evaluate whether richness, diversity (Shannon index), number of families and abundance of plant species were related to basal area and treatments. In these analyses, the average basal area per plot/stratum/treatment was used. We tested the assumptions of homogeneity, normality, linearity, and regression homogeneity. Analyses are thought to be robust when data have different group sizes and when samples are unbalanced. In these analyses, we used the mean basal area per plot/stratum/treatment. The relationship between plant richness, basal area, and time since the last fire in each stratum was examined with linear and non-linear regression. Due to non-linearity between richness and basal area, we used non-linear regression (nls procedures in R) through asymptotic models.
All analyses were performed in R statistical software version 3.5.0 (R Core Team, 2017) using the following packages: ggplot2 (Wickham, 2016), dplyr (Wickham et al., 2019), gridExtra (Auguie, 2017), vegan (Oksanen et al., 2019), FSA (Ogle et al., 2019), MASS (Venables and Ripley, 2002), moments (Komsta and Novomestky, 2015), and dunn.test (Dinno, 2017).
Across all study sites, we sampled a total of 1,561 individual plants, distributed in 149 species from 49 families. The most abundant species sampled (n > 50 individuals) were Tachigali cf. glauca (8%, n = 129), Protium sagotianum (6%, n = 98), Trattinnickia glaziovii (4%, n = 70), Passiflora sp. (4%, n = 67), Hirtella gracilipes (3%, n = 56), Doliocarpus dentatus (3%, n = 55), Cheiloclinium cognatum (3%, n = 54), and Qualea paraensis (3%, n = 50). The most frequent species, i.e., found in all four treatments (B-3x, B1-1999, B1-2010, NB) were Abuta grandifolia, Anemopaegma sp., Aniba parviflora, Ocotea glomerata, Passiflora sp., Qualea paraensis, and Trattinnickia glaziovii.
Species composition values (abundance, richness and number of families) and diversity were highest for the Not-Burned treatment and lowest for the B-3x (except for species richness) (Table 2). The SP stratum had, in general, the lowest species composition values (except for the number of families) and diversity (Figure 3). The SE had a high abundance of individuals and MT had high diversity. Values of richness and abundance were highest in the seedling stratum and lowest for saplings. No plant species was found in all five strata, and only Endlicheria ruforamula was sampled in four of them (SE, SP, MT, and LT). Species richness was similar in Not-Burned and B1-1999, whereas B1-2010 and B-3x showed low richness and were different from the other treatments (Figure 3). With the exception of the sapling size-class, species richness of all strata increased with time since last fire (Figure 4 and Table 1S).
Table 2. Descriptive data of species occurring in all four treatments and all five strata for the burned and unburned plots in southern Amazonia, Brazil.
Table 3. Analysis of covariance relating plant richness, diversity (Shannon index), number of families and number of individual plants with basal area and treatments (fire regime).
Figure 3. Diversity estimates for all vegetation strata for different fire frequency and time since burned [NB, B1-1999, B1-2010, and B-3x (2006, 2010, and 2013)]. Analyses were conducted separately for each vegetation strata (A) SE, (B) SP, (C) SML, (D) MT, and (E) LT, and (F) for all strata together. LT stands for adult trees, MT to medium trees, SML for small trees and woody lianas, SP for saplings and SE for seedlings. Continuous and dashed lines refer to observed and extrapolated sample coverage, and the area around the curve represents a 95% confidence interval.
Figure 4. Relationship in each stratum between richness and time since the last fire (years). In non-linear models fitted using (nls procedure in R) with asymptotic models, the equation was conducted separately for each stratum of vegetation (A) SE, (B) SP, (C) SML, (D) MT, and (E) LT, and (F) for all strata together. LT stands for large trees, MT for medium trees, SML for small trees and woody lianas, SP for seedlings, and SE for seedlings. Only curves that showed significant differences were plotted.
Total basal area (m2 ha−1) varied significantly among the treatments (B-3x, B1-2010, B1-1999, and NB) (Figure 5). For the SE and SP classes, the basal area did not show significant differences among treatments (Figure 5A). For the SML, LT and all strata combined classes, the basal area was lower for the B-3x compared to the B1-1999 and NB classes (Figures 5C,E,F). For the MT size-class, basal area was lower in the B1-2010 compared to the other classes and there were no individuals in the B-3x treatment (Figures 5D). The basal area of the plants in the control area was not consistently larger compared to other treatments for many size classes. The basal area increased with time since the last fire for the SML and larger size classes and for all combined size classes, but there was no significant relationship between the predictors and the response variable for SE and SP (Figure 6 and Table 2S).
Figure 5. Basal area (m2 ha−1) in each stratum and according to fire regimes. Analyses were conducted separately for each vegetation strata (A) SE, (B) SP, (C) SML, (D) MT, (E) LT, and (F) for all strata together. LT stands for large trees, MT for medium trees, SML for small trees and woody lianas, SP for saplings, and SE for seedlings. The p-values indicate whether comparisons were statistically significant according to Kruskal–Wallis tests, and the lowercase letters above bars show differences among treatments. The line crossing bars indicate the median, the solid square denotes the mean, the boxes account for the 25th and 75th percentiles and vertical lines are the minimum and maximum values.
Figure 6. Relationship in each stratum between basal area (m2 ha−1) and time since last fire. Non-linear models adjusted using the procedure (nls in R) with asymptotic models, the equation was performed separately for each stratum of vegetation (A) SE, (B) SP, (C) SML, (D) MT, (E) LT, and (F) for all strata together. LT stands for large trees, MT for medium trees, SML for small trees and woody lianas, SP for seedlings and SE for seedlings. Only curves that showed significant differences were plotted.
Richness, diversity (Shannon index), number of families and number of plants were all affected by basal area. Richness and diversity were also affected by treatment (Table 3). Considering all treatments and all trees combined, species richness increased with total plot basal area in non-burned and 1999-burned sites (Figure 7F). For the LT size-class, variation in species richness was positively and significantly related to the basal area for all treatments (Figure 7E). Richness of all size classes increased with basal area in Not-Burned and B1-1999 sites (Figures 7A–E). However, for sites burned recently one-time and burned three times only some of the size classes showed significant relationships between richness and basal area (Figures 7B,C,E). Qualea paraensis and Tetragastris altissima were species that contributed the most for total basal area of sites (Table 4).
Figure 7. Relationship between richness and basal area. Analyses were conducted separately for each vegetation strata (A) SE, (B) SP, (C) SML, (D) MT, (E) LT, and (F) for all strata together.
Table 4. Species that contributed most to basal area (m2 ha−1) in each treatment and associated abundance.
This study represents one of the first results to show determinants of changes in forest understory species composition and structure following regeneration from single and recurring wildfires in southern Amazonia. Our main finding is that three-times burned forests underwent major reductions in species richness, changes in species compositions, and degradation of forest structure by basal area loss, which have major implications for the future of rainforest regeneration. Changes in tree basal area due to fire was a strong predictor of changes in species richness, i.e., the loss by fire of large trees which contribute most to basal area also affects composition of understory species. Our results suggest that basal area change due to wildfire degradation could be a useful proxy to gauge changes in species composition after frequent burnings. An experimental burn study in southeastern Amazon showed fire susceptibility of large trees (Brando et al., 2012, 2014; Balch et al., 2015). Therefore, protecting forests from wildfires is essential to preserve both carbon storage and diversity, especially given the sensitivity of these forests to ongoing drought-fire interactions (Nogueira et al., 2019). Our results highlight the ecological implications of repeated fire in the forest-savanna transition which is exposed to the greatest seasonal water deficits of Amazonian and to recurrent drought (Feldpausch et al., 2016), fragmentation (Vedovato et al., 2016), and wildfire (Aragão et al., 2018).
Overall, species composition (abundance, richness, and number of families) and diversity were highest for the unburned forests and lowest for the frequently burned areas, showing that fire frequency is associated with changes in floristic composition and biodiversity in Amazon forests. Amazonian forest species are not well-adapted to tolerate water stress and to resist fires, especially small individuals, and forest tree species are less tolerant of fire than savanna species (Hoffmann et al., 2012). Fire in tropical forests (gallery forest) causes extensive top-kill to small trees (Hoffmann et al., 2009), reducing the abundance, and richness of regenerating plants by killing young individuals (Oliveira et al., 2014). When forests are subjected to fire, community composition may change, allowing species with traits for fire resistance such as thick bark to dominate (Balch et al., 2015). Repeated fire return, such as in the three times burned areas, might even affect the composition of more fire-adapted systems (for example tropical savannas: Brando and Durigan, 2005).
We found that Non-Burned areas (NB) and the 1999 burned sites (B1-1999) were similar in richness and diversity and that the 2010 burned sites (B1-2010) had intermediate plant richness and diversity (between NB and B-3x). The largest differences in richness and diversity occurred between the tree (high richness and diversity) and sapling strata. Our results from southern Amazonia corroborate those from eastern Amazonia showing that the more a forest burns, the more it differentiates from the original composition and structure (Barlow and Peres, 2008). Degradation of tropical forests results in more open formations dominated by a few large remnant forest trees, short-lived pioneers, exotic grasses, and low diversity of native herbaceous species (Veldman and Putz, 2011). Degraded forests are prone to exotic invasion (D'Antonio and Vitousek, 1992; Cochrane, 1999) because they provide space for rapid colonization of invasive species (Hobbs et al., 2006). We did find invasive grasses (Brachiaria sp.) in the three times burned forests.
Few studies have shown what happens in tropical forests after understory fire, especially understory regeneration (Barlow et al., 2007). There is little tendency for convergence in community composition of Amazon rainforest, as succession does not follow a single, largely deterministic trajectory over time (Longworth et al., 2014). Our results confirm a lack of convergence, by showing that no plant species was found in all five strata. Furthermore, only seven species were common among the three fire frequencies; and, species that contributed more to basal area per area were different in each fire treatment.
For the few floristic studies following understory fire in the Amazon Basin, the main focus has been monitoring fire frequency and the immediate consequences of fire on plant species (Nepstad et al., 1999, 2002; Cochrane and Laurance, 2002; Balch et al., 2008, 2013, 2015; Staver et al., 2011). Longer-term studies have focused on regrowth following deforestation and pasture abandonment, e.g., in a 25-year study, Amazon rainforest regenerated stem density and biomass following clear cut and pasture land-use (using fire); however, species accumulation and ecosystem services were limited (Mesquita et al., 2015). Chazdon et al. (2016) showed that young secondary forests (<20 years) store more carbon than older forests, but only after 100 years did the forests develop high species richness. Thus, our study is one of few to evaluate fire effects as interactions between changes in composition and vegetation structure, especially for smaller plants.
The hypotheses of disturbance-mediated coexistence, such as herbivory or a small physical disturbance, that generally leads to high diversity (Sousa, 1984) may not apply to fire in tropical forests, especially recurrent burnings. In southern Amazonian forests, species richness of all strata (except saplings) significantly declined with fire frequency increase. Similar results were found for seasonally dry tropical forest landscape evaluating plant species diversity in Central America (Gillespie et al., 2000) and woody plant diversity in India (Dattaraja et al., 2018) subjected to frequent fires. The decline in species richness may be related to the elimination of fire-intolerant species.
Fire frequency also affected forest structure (Figure 5). Plants from the most frequently burned areas showed the lowest basal areas. The structure of plant communities in many natural ecosystems is largely determined by disturbances, which occur frequently, but on small scales (White, 1979). Our finding of a right-skewed basal area data distribution corroborates the reverse J-shaped curves reported for other disturbed forests in the Amazon Basin (Oliveira-Filho et al., 1997; Oliveira et al., 2016). Additionally, the low basal area values for the forest burned in 2010 may have been caused by a severe fire that happened in that year, worsened by a severe drought (Gatti et al., 2014; Feldpausch et al., 2016), with forest recuperation subsequently hindered.
Within the B-3x treatment, basal area was lower for the small to larger size strata but not for the SE and SP strata. Recurring fires (as B-3x) change forest microclimate, facilitating colonization and establishment of invasive grasses, favoring new, more intense, fires to re-enter the area (Miranda et al., 2013) and killing small and medium size native woody individuals (Hoffmann et al., 2004; Brando et al., 2014). As a consequence of regeneration, seedlings density of a few generalist species may increase, and competition for light, water, and minerals may suppress their increment.
Ecological studies have found that increased plant species diversity results in increased productivity (Tilman et al., 1996; Waide et al., 1999) and increased carbon storage (Strassburg et al., 2010), especially in small scale observations (Sullivan et al., 2017). Our results corroborate these findings for carbon storage for all strata and treatments, except for three times burned forests. Elaborate mechanisms such as niche complementarity or reduced interspecific competition compared with intraspecific competition (Tilman et al., 2014) may explain such positive relations between diversity (expressed here by plant richness, Shannon Index and number of families) and basal area (a proxy for C storage). Field observations showed that in highly disturbed sites, one or two opportunistic plant species were able to grow and cover the area, but richer sites (regarding species richness) were found in better conservation status areas.
Basal area of small, medium, and large trees significantly declined (by more than 50%) with fire frequency increase; but the frequent fires had little effect on seedlings and saplings. Reductions in basal area were also observed for stems < 30 cm D of stands burned once in central Amazonia (increase of 63.2% in loss of basal area compared to non-burned: Andrade et al., 2019) and on trees > 50 cm D of three times burned sites in northern Amazonia (reduction of about 54% basal area: Martins et al., 2012). In seasonally dry tropical forest in India, woody plants were apparently unaffected by fire frequency, which may be related to dominance by larger individuals (Dattaraja et al., 2018).
Changes in composition were associated with changes in forest structure (Figure 7). In general, we found LT (Large Trees) with higher diversity and species richness in non-burned and 16 years old burned forests, while the other four strata (SE, SP, SML, and LT) had lower and similar values for these parameters. Burning kills seedlings and saplings (Oliveira et al., 2014) and small trees (Hoffmann et al., 2009), reducing their density (Balch et al., 2011) and consequently, diversity. Small plants are fire sensitive, because of reduced diameter, canopy base height, thin bark, and less dense wood (Brando et al., 2012; Balch et al., 2013). Many studies in savanna ecosystems have shown that it is advantageous growing a thicker stem (escape diameter: Lawes et al., 2011), protecting buds, and the vascular cambium and being buffered against fire heat (Uhl and Kauffman, 1990; Lawes et al., 2011). We are not aware of studies investigating the minimum diameter needed for Amazon forest species to survive burnings, but large trees ≥ 35 cm D in this study were the only to escape the effects of fire on reducing diversity.
No plant species was found in all five strata, and only Endlicheria ruforamula was sampled in four strata (SE, SP, MT, and LT) indicating that burning may be causing floristic changes by premature succession (Xaud et al., 2013), where humid-environment specialist species are lost and generalists with high dispersal efficiency dominate the smallest size classes (Solar et al., 2015). That may be observed in the highly abundant seedlings stratum (SE) where we found Cheiloclinium cognatum, Doliocarpus dentatus, Passiflora sp., Protium sagotianum, Tachigali cf. glauca as the most abundant species. Additionally, the great importance of Passiflora sp., Mabea fistulifera (a pioneer species) and Trattinnickia glaziovii (found in forest-savanna transition zones) in the B-3x forests indicates that these forests are following a new regenerating pathway, possibly with some specialist species in terms of fire adaptations. In fact, none of the hyperdominant species of the Amazon tree flora (Ter Steege et al., 2013) were species that contributed most to basal area in each burned-frequency site, indicating that the hyperdominance found across Amazonia is not related to fire.
During the first decades following natural disturbances, succession proceeds under high rates of increasing species richness, density of stems, and basal area of trees (Finegan, 1996). Our results of positive relations between richness and basal area for most strata in B1-1999 sites and non-burned forests corroborate that and indicate that these forests are going through their natural succession pathways rather than succession stalling (e.g., Tymen et al., 2016). Although, as fire frequency increases, succession may deviate from this general pattern (Barlow and Peres, 2008). With no fundamental changes in climate and/or soil nutrient pools, most burned forests recover biomass within decades; but, with fire frequency becoming higher as our results suggest, the potential trajectory of burned tropical forests might be a transition to a new state (Brando et al., 2019).
One of the important questions for forest management and conservation in Amazonia is the degree to which forests can regenerate and conserve biodiversity following disturbance by selective logging and fire. We found that 16 years of regeneration allowed burned forests to develop forest structure, richness, and diversity similar to unburned forests. However, richness similarity was not expressed in all the five strata (especially saplings), showing that these forests might be vulnerable to future environmental changes. Our results support findings from other regions of Amazonia that recovery after forest degradation may take decades (Barlow and Peres, 2008), but only if forests remain protected against recurrent fires. Additionally, El Niño and other drought events observed in the Amazon Basin (and in the studied sites) in 1997/1998, 2005, and 2007 (while not a major drought year for all of the Amazon, it seemed to be important for the study region based on the number of fires there: Morton et al., 2013), 2010 and 2015/2016 (Chen et al., 2017; Liu et al., 2017; Silva Junior et al., 2019) can reduce tree growth (Vlam et al., 2014), carbon assimilation and storage, biomass and productivity (Duffy et al., 2015; Feldpausch et al., 2016), promoting tree mortality (Phillips et al., 2009; Balch et al., 2015) and ultimately, making fire probability higher.
This research was not conducted in prescribed fire experiments; therefore, we do not know the conservation status of these forests before the burnings. We also could not control for the fire timing, a factor known to influence savanna regeneration. While the four plot replicates per site could be considered a form of pseudoreplication (van Mantgem et al., 2001), plots were separated by 100 m, which is sufficient to be an independent sampling unit (Gotelli and Ellison, 2011). This necessary limitation to increase experimental replication (Oksanen, 2001) was due to the random nature of the wildfire sites available and being unable to obtain permission from additional landholders in the region to access forests.
We stress that even the non-burned forest sites were not free from disturbance, as they may have undergone selective logging and drought effects. Climate change-related drought events intensified burning in the Amazon Basin, resulting in vegetation degradation (Brando et al., 2014; Aragão et al., 2018). We found that fire frequency was associated with changes in plant structural complexity (namely basal area), that there were differences in community composition (species richness, number of species, and diversity) between areas (burned and not-burned) and that those differences were associated with different strata. Thus, recurring fires may change forest species composition and even drive forests toward a biotic homogenization dominated by a few habitat generalists, resulting in fewer compositional differences among sites (Mckinney and Lockwood, 1999). More attention to environmental policies related to fire control and fire prevention for the Amazon region is needed to protect forest biodiversity.
Overall, species composition (abundance, richness, and number of families) and diversity were highest for the unburned treatment and lowest for the recurrent burned areas. Fire frequency negatively affected plant structure and basal area. Richness was positively related to basal area in the three times burned sites and in the 16 years regenerating site for all strata. Our results demonstrate the negative influence of frequent fires on both the composition and structure of small trees in Amazonian forest. These changes to the cohort of small-sized trees, especially in forests burned more than once, may persist, and have long-term impacts on forest structure, affecting the capacity and direction of forest recovery. To conserve diversity and carbon storage, protecting burned forests from subsequent burning is as important as preventing unburned forest from burning, e.g., given at least two decades, once-burned forests can recover, at least partially, the diversity, complexity of floristic composition, and forest structure found in unburned forests. Without such protection, the large-scale and increasing frequency of fire in Amazonia is reducing tree diversity and may affect regional carbon cycling with consequences for the global vegetation carbon sink.
The datasets generated for this study are available on request to the corresponding author.
NP, TF, KM, BSM, BM-J, and MK conceived the study and planned the fieldwork. NP, KM, and TF carried out the fieldwork. NP, KM, RF, and EO identified the species at the herbarium. DN and ES contributed to data analysis. NP led writing the manuscript with assistance from KM and TF. All authors provided critical feedback and helped shape the analysis and manuscript.
Financial support was provided by the Coordination of Improvement of Personnel in Higher Education, Brazil (CAPES) through a Science without Borders grant to TF (PVE 177/2012) and NERC (NE/N011570/1). Fieldwork was supported by the Sustainable Landscapes Brazil Project operated by the USFS and EMBRAPA with financial support from USAID and US Department of State and a SilvaCarbon grant to MK and TF. The National Council of Science and Technology, Brazil (CNPq) is acknowledged for a productivity grant (bolsa produtividade PQ) for BM-J and BSM, for a Postdoctoral fellowship to DN, and the financial support to the projects PELD (403725/2012-7) and PPBio (457602/2012-0).
The authors declare that the research was conducted in the absence of any commercial or financial relationships that could be construed as a potential conflict of interest.
We gratefully acknowledge financial support from CAPES, CNPq, NERC, USAID, the US Department of State, and SilvaCarbon. We thank the Sustainable Landscapes Brazil Project operated by the USFS and EMBRAPA for assistance with field logistics and for providing the large-tree dataset and funding. We thank William Magnusson for comments on a draft of the manuscript, the UNEMAT lab staff for their help, Aliança da Terra for assisting with contacting farmers, and the farmers of Feliz Natal, MT for providing access to their land.
The Supplementary Material for this article can be found online at: https://www.frontiersin.org/articles/10.3389/ffgc.2020.00010/full#supplementary-material
Alencar, A., Brando, P. B., and Asner, G. P. (2015). Landscape fragmentation, severe drought, and the new amazon forest fire regime. Ecol. Soc. Am. 25, 1493–1505. doi: 10.1890/14-1528.1
Alencar, A., Nepstad, D., and Del Carmen Vera-Diaz, M. (2006). Forest understory fire in the Brazilian amazon in ENSO and non-ENSO years: area burned and committed carbon emissions. Earth Interact. 10:EI150. doi: 10.1175/EI150.1
Alvares, C. A., Stape, J. L., Sentelhas, P. C., Gonçalves, J. L., and Sparovek, G. (2013). Köppen's climate classification map for Brazil. Meteorolo. Zeitschrift 22, 711–728. doi: 10.1127/0941-2948/2013/0507
Andrade, D. F. C., Gama, J. R. V., Ruschel, A. R., Melo, L. O., Avila, A. L., and Carvalho, J. O. P. (2019). Post-fire recovery of a dense ombrophylous forest in amazon. An. Acad. Bras. Ciênc. 91:e20170840. doi: 10.1590/0001-3765201920170840
Aragão, L. E. O. C., Anderson, L. O., Fonseca, M. G., Rosan, T. M., Vedovato, L. B., Wagner, F. H., et al. (2018). Decline of amazon deforestation carbon emissions. Nat. Commun. 9:536. doi: 10.1038/s41467-017-02771-y
Aragão, L. E. O. C., Malhi, Y., Roman-Cuesta, R. M., Saatchi, S., Anderson, L. O., and Shimabukuro, Y. E. (2007). Spatial patterns and fire response of recent amazonian droughts. Geophys. Res. Lett. 34:L07701. doi: 10.1029/2006GL028946
Asner, G. P., Knapp, D. E., Broadbent, E. N., Oliveira, P. J. C., Keller, M., and Silva, J. N. (2005). Selective logging in the Brazilian amazon. Science 310, 480–482. doi: 10.1126/science.1118051
Auguie, B. (2017). gridExtra: Miscellaneous Functions for “Grid” Graphics. R package version 2.3. Available online at: https://CRAN.R-project.org/package=gridExtra
Balch, J. K., Brando, P. M., Nepstad, D. C., Coe, M. T., Silvério, D., Massad, T. J., et al. (2015). The susceptibility of southeastern amazon forests to fire: insights from a large-scale burn experiment. Bioscience 65, 893–905. doi: 10.1093/biosci/biv106
Balch, J. K., Massad, T. J., Brando, P. M., Nepstad, D. C., and Curran, L. M. (2013). Effects of high-frequency understorey fires on woody plant regeneration in southeastern amazonian forests. Philos. Trans. R. Soc. B Biol. Sci. 368:20120157. doi: 10.1098/rstb.2012.0157
Balch, J. K., Nepstad, D. C., Brando, P. M., Curran, M., Portela, O., Carvalho, O., et al. (2008). Negative fire feedback in a transitional forest of southeastern amazonia. Glob. Chang. Biol. 14, 2276–2287. doi: 10.1111/j.1365-2486.2008.01655.x
Balch, J. K., Nepstad, D. C., Curran, L. M., Brando, P. M., Portela, O., Guilherme, P., et al. (2011). Size, species, and fire behavior predict tree and liana mortality from experimental burns in the Brazilian amazon. For. Ecol. Manage. 261, 68–77. doi: 10.1016/j.foreco.2010.09.029
Barlow, J., Gardner, T. A., Araujo, I. S., Ávila-Pires, T. C., Bonaldo, A. B., Costa, J. E., et al. (2007). Quantifying the biodiversity value of tropical primary, secondary, and plantation forests. Proc. Natl. Acad. Sci. U.S.A. 104, 18555–18560. doi: 10.1073/pnas.0703333104
Barlow, J., Lagan, B. O., and Peres, C. A. (2003). Morphological correlates of fire-induced tree mortality in a central amazonian forest. J. Trop. Ecol. 19, 291–299. doi: 10.1017/S0266467403003328
Barlow, J., Lennox, G. D., Ferreira, J., Berenguer, E., Lees, A. C., Mac Nally, R., et al. (2016). Anthropogenic disturbance in tropical forests can double biodiversity loss from deforestation. Nature 535:144–147. doi: 10.1038/nature18326
Barlow, J., and Peres, C. A. (2008). Fire-mediated dieback and compositional cascade in an amazonian forest. Philos. Trans. R. Soc. Lond. B. Biol. Sci. 363, 1787–1794. doi: 10.1098/rstb.2007.0013
Brando, P. M., Balch, J. K., Nepstad, D. C., Morton, D. C., Putz, F. E., Coe, M. T., et al. (2014). Abrupt increases in amazonian tree mortality due to drought-fire interactions. Proc. Natl. Acad. Sci. U.S.A. 111, 6347–6352. doi: 10.1073/pnas.1305499111
Brando, P. M., and Durigan, G. (2005). Changes in cerrado vegetation after disturbance by frost (São Paulo State, Brazil). Plant Ecol. 175, 205–215. doi: 10.1007/s11258-005-0014-z
Brando, P. M., Nepstad, D. C., Balch, J. K., Bolker, B., Christman, M. C., Coe, M., et al. (2012). Fire-induced tree mortality in a neotropical forest: the roles of bark traits, tree size, wood density and fire behavior. Glob. Chang. Biol. 18, 630–641. doi: 10.1111/j.1365-2486.2011.02533.x
Brando, P. M., Paolucci, L., Ummenhofer, C. C., Ordway, E. M., Hartmann, H., Cattau, M. E., et al. (2019) Droughts, wildfires, and forest carbon cycling: a pantropical synthesis. Annu. Rev. Earth Planet. Sci. 47, 555–581. doi: 10.1146/annurev-earth-082517-010235
BRASIL, and IBGE. (2009). Environmental Information, Pedology and Maps. Available online at: https://geoftp.ibge.gov.br/informacoes_ambientais/ (accessed December 12, 2017).
Brienen, R. J. W., Phillips, O. L., Feldpausch, T. R., Gloor, E., Baker, T. R., Lloyd, J., et al. (2015). Long-term decline of the amazon carbon sink. Nature 519, 344–348. doi: 10.1038/nature14283
Chao, A., Gotelli, N. J., Hsieh, T. C., Sander, E. L., Ma, K. H., Colwell, R. K., et al. (2014). Rarefaction and extrapolation with Hill numbers: a unified framework for sampling and estimation in biodiversity studies. Ecol. Monogr. 84, 45–67. doi: 10.1890/13-0133.1
Chao, A., and Jost, L. (2012). Coverage-based rarefaction and extrapolation: standardizing samples by completeness rather than size. Ecology 93, 2533–2547. doi: 10.1890/11-1952.1
Chazdon, R. L., Broadbent, E. N., Rozendaal, D. M. A., Bongers, F., Zambrano, A. M. A., Aide, T. M., et al. (2016). Carbon sequestration potential of second-growth forest regeneration in the Latin American tropics. Sci. Adv. 2:e1501639. doi: 10.1126/sciadv.1501639
Chen, L., Li, T., Wang, B., and Wang, L. (2017). Formation mechanism for 2015/16 super el niño. Sci. Rep. 7, 1–10. doi: 10.1038/s41598-017-02926-3
Cochrane, M. A. (1999). Forest fire, deforestation and landcover change in the Brazilian amazon. Jt. Fire Sci. Conf. Work. 1985, 1–7.
Cochrane, M. A., and Barber, C. P. (2009). Climate change, human land use and future fires in the amazon. Glob. Chang. Biol. 15, 601–612. doi: 10.1111/j.1365-2486.2008.01786.x
Cochrane, M. A., and Laurance, W. F. (2002). Fire as a large-scale edge effect in amazonian forests. J. Trop. Ecol. 18, 311–325. doi: 10.1017/S0266467402002237
D'Antonio, C. M., and Vitousek, P. M. (1992). Biological Invasions by exotic grasses, the grass/fire cycle, and global change. Annu. Rev. Ecol. Evol. Syst. 23, 63–87. doi: 10.1146/annurev.es.23.110192.000431
Dattaraja, H. S., Pulla, S., Suresh, H. S., Nagaraja, M. S., Murthy, C. A. S., and Sukumar, R. (2018). Woody plant diversity in relation to environmental factors in a seasonally dry tropical forest landscape. J. Veg. Sci. 29, 704–714. doi: 10.1111/jvs.12652
Dinno, A. (2017). dunn.test: Dunn's Test of Multiple Comparisons Using Rank Sums. R package version 1.3.5. Available online at: https://CRAN.R-project.org/package=dunn.test
Doughty, C. E., Metcalfe, D. B., Girardin, C. A. J., Amézquita, F. F., Cabrera, D. G., Huasco, W. H., et al. (2015). Drought impact on forest carbon dynamics and fluxes in amazonia. Nature. 519, 78–82. doi: 10.1038/nature14213
Duffy, P. B., Brando, P., Asner, G. P., and Field, C. B. (2015). Projections of future meteorological drought and wet periods in the amazon. Proc. Natl. Acad. Sci. U.S.A. 112, 13172–13177. doi: 10.1073/pnas.1421010112
Fearnside, P. M. (2018). O texto que segue é um PREPRINT. please cite as: favor citar como: fearnside, P. M. 2018. challenges Brazilian amazonia. Sustain. Dev. 26, 141–149. doi: 10.1002/sd.1725
Feldpausch, T. R., Lloyd, J., Lewis, S. L., Brienen, R. J. W., Gloor, M., Monteagudo Mendoza, A., et al. (2012). Tree height integrated into pantropical forest biomass estimates. Biogeoscience 9, 3381–3403. doi: 10.5194/bg-9-3381-2012
Feldpausch, T. R., Phillips, O. L., Brienen, R. J. W., Gloor, E., Lloyd, J., Lopez-Gonzalez, G., et al. (2016). Amazon forest response to repeated droughts. Glob. Biogeochem. Cycles 30, 964–982. doi: 10.1002/2015GB005133
Feldpausch, T. R., Rondon, M. A., Fernandes, E. C. M., Riha, S. J., and Wandelli, E. (2004). Carbon and nutrient accumulation in secondary forests regenerating on pastures in central amazonia. Ecol. App. 14, S164–S176. doi: 10.1890/01-6015
Finegan, B. (1996) Pattern and process in neotropical secondary rain forests: the first 100 years of succession. Tree 11, 119–124. doi: 10.1016/0169-5347(96)81090-1
Fu, R., Yin, L., Li, W., Arias, P. A., Dickinson, R. E., Huang, L., et al. (2013). Increased dry-season length over southern amazonia in recent decades and its implication for future climate projection. Proc. Natl. Acad. Sci. U.S.A. 110, 18110–18115. doi: 10.1073/pnas.1302584110
Gatti, L. V., Gloor, M., Miller, J. B., Doughty, C. E., Malhi, Y., Domingues, L. G., et al. (2014). Drought sensitivity of amazonian carbon balance revealed by atmospheric measurements. Nature 506, 76–80. doi: 10.1038/nature12957
Gerwing, J. J. (2002). Degradation of forests through logging and fire in the eastern Brazilian. Amazon For. Ecol. Manage. 157, 131–141. doi: 10.1016/S0378-1127(00)00644-7
Gillespie, T. W., Grijalva, A., and Farris, C. N. (2000). Diversity, composition, and structure of tropical dry forests in Central America. Plant Ecol. 147, 37–47. doi: 10.1023/A:1009848525399
Gotelli, N. J., and Ellison, A. M. (2011). Princípios de Estatística em Ecologia. Porto Alegre: Artmed, 528.
Goulart, A. C., Macario, K. D., Scheel-Ybert, R., Alves, E. Q., Bachelet, C., Pereira, B. B., et al. (2017). Charcoal chronology of the amazon forest: a record of biodiversity preserved by ancient fires. Quat. Geochronol. 41, 180–186. doi: 10.1016/j.quageo.2017.04.005
Hobbs, R. J., Arico, S., Aronson, J., Baron, J. S., Bridgewater, P., Cramer, V., et al. (2006). Novel ecosystems: theoretical and management aspects of the new ecological world order. Glob. Ecol. Biogeogr. 15, 1–7. doi: 10.1111/j.1466-822X.2006.00212.x
Hoffmann, W. A., Adasme, R., Haridasan, M., De Carvalho, M. T., Geiger, E. L., Pereira, M. A. B., et al. (2009). Tree topkill, not mortality, governs the dynamics of savanna-forest boundaries under frequent fire in central Brazil. Ecology 90, 1326–1337. doi: 10.1890/08-0741.1
Hoffmann, W. A., Geiger, E. L., Gotsch, S. G., Rossatto, D. R., Silva, L. C. R., Lau, O. L., et al. (2012) Ecological thresholds at the savanna-forest boundary: how plant traits, resources and fire govern the distribution of tropical biomes. Ecol. Lett. 15, 759–768. doi: 10.1111/j.1461-0248.2012.01789.x
Hoffmann, W. A., Lucatelli, V. M. P. C., Silva, F. J., Azeuedo, I. N. C., Albuquerque, A. M. S., Moreira, S. P., et al. (2004). Impact of the invasive alien grass. Divers. Distrib. 10, 99–103. doi: 10.1111/j.1366-9516.2004.00063.x
Hsieh, T. C., Ma, K. H., and Chao, A. (2016). iNEXT: an R package for rarefaction and extrapolation of species diversity (hill numbers). Methods Ecol. Evol. 7, 1451–1456. doi: 10.1111/2041-210X.12613
Hummel, A. C., Alves, M. V. D. S., Pereira, D., Veríssimo, A., and Santos, D. (2010). A Atividade Madeireira na Amazônia Brasileira: Produção, Receita e Mercados. Serviço Florest. Bras. Inst. do Homem e Meio Ambient. da Amaz.
Ivanauskas, N. M., Monteiro, R., and Rodrigues, R. R. (2008). Classificação fitogeográfica das florestas do alto rio xingu. Acta Amaz. 38, 387–402. doi: 10.1590/S0044-59672008000300003
Kennard, D. K., Gould, K., Putz, F. E., Fredericksen, T. S., and Morales, F. (2002). Effect of disturbance intensity on regeneration mechanisms in a tropical dry forest. For. Ecol. Manage. 162, 197–208. doi: 10.1016/S0378-1127(01)00506-0
Koele, N., Bird, M., Haig, J., Marimon-Junior, B. H., Marimon, B. S., Phillips, O. L., et al. (2017). Amazon basin forest pyrogenic carbon stocks: first estimate of deep storage. Geoderma 306, 237–243. doi: 10.1016/j.geoderma.2017.07.029
Komsta, L., and Novomestky, F. (2015). moments: Moments, Cumulants, Skewness, Kurtosis and Related Tests. R package version 0.14. Available online at: https://CRAN.R-project.org/package=moments
Lawes, M. J., Richards, A., Dathe, J., and Midgley, J. J. (2011). Bark thickness determines fire resistance of selected tree species from fire-prone tropical savanna in north Australia. Plant Ecol. 212, 2057–2069. doi: 10.1007/s11258-011-9954-7
Liu, J., Bowman, K. W., Schimel, D. S., Parazoo, N. C., Jiang, Z., Lee, M., et al. (2017). Contrasting carbon cycle responses of the tropical continents to the 2015–2016 el niño. Science 358, 191–198. doi: 10.1126/science.aam5690
Longworth, J. B., Mesquita, R. C. G., Bentos, T. V., Moreira, M. P., Massoca, P. E. S., and Williamson, G. B. (2014). Shifts in dominance and species assemblages over two decades in alternative successions in central amazonia. Biotropica 46, 529–537. doi: 10.1111/btp.12143
Marengo, J. A., Tomasella, J., Alves, L. M., Soares, W. R., and Rodriguez, D. A. (2011). The drought of 2010 in the context of historical droughts in the amazon region. Geophys. Res. Lett. 38, 1–5. doi: 10.1029/2011GL047436
Martins, F. S. R. V., Xaud, H. A. M., Santos, J. R., and Galvão, L. S. (2012). Effects of fire on above-ground forest biomass in northern Brazilian amazon. J. Trop. Ecol. 28, 591–601. doi: 10.1017/S0266467412000636
Massad, T. J., Balch, J. K., Mews, C. L., Porto, P., Marimon-Junior, B. H., Mota Quintino, R., et al. (2015). Early recruitment responses to interactions between frequent fires, nutrients, and herbivory in the southern amazon. Oecologia 178, 807–817. doi: 10.1007/s00442-015-3259-9
Massi, K. G., Bird, M., Marimon, B. S., Marimon, B. H. Jr., Nogueira, D. S., Oliveira, E. A., et al. (2017). Does soil pyrogenic carbon determine plant functional traits in amazon basin forests? Plant Ecol. 218, 1047–1062. doi: 10.1007/s11258-017-0751-9
Mckinney, M. L., and Lockwood, J. L. (1999). Biotic homogenization: a few winners replacing many losers in the next mass extinction. Trends Ecol. Evol. 14, 450–453. doi: 10.1016/S0169-5347(99)01679-1
Mesquita, R. C. G., Massoca, P. E. S., Jakovac, C. C., Bentos, T. V., and Williamson, G. B. (2015). Amazon rain forest succession: stochasticity or land-use legacy? Bioscience 65, 849–861. doi: 10.1093/biosci/biv108
Metcalfe, D. B., Rocha, W., Balch, J. K., Brando, P. M., Doughty, C. E., and Malhi, Y. (2018) Impacts of fire on sources of soil CO2 efflux in a dry amazon rain forest. Glob. Change Biol. 24, 3629–3641. doi: 10.1111/gcb.14305
Miranda, A. C., Miranda, H. S., Fátima, I., De Dias, O., Miranda, A. C., Miranda, H. S., et al. (2013). Soil and air temperatures during prescribed cerated fires in Central Brazil soil and air temperatures during prescribed cerrado fires in Central Brazil. J. Trop. Ecol. 9, 313–320. doi: 10.1017/S0266467400007367
Morton, D. C., Le Page, Y., DeFries, R., Collatz, G. J., and Hurtt, G. C. (2013). Understorey fire frequency and the fate of burned forests in southern amazonia. Philos. Trans. R. Soc. Lond. B. Biol. Sci. 368, 1–8. doi: 10.1098/rstb.2012.0163
Nepstad, D., McGrath, D., Alencar, A., Barros, A. C., Carvalho, G., Santilli, M., et al. (2002). Frontier governance in amazonia. Science 295, 629–631. doi: 10.1126/science.1067053
Nepstad, D. C., Verissimo, A., Alencar, A., Nobre, C., Lima, E., Lefebvre, P., et al. (1999). Large-scale impoverishment of amazonian forests by logging and fire. Nature 398, 505–508. doi: 10.1038/19066
Nogueira, D. S., Marimon, B. S., Marimon-Junior, B. H., Oliveira, E. A., Morandi, P., Reis, S. M., et al. (2019). Impacts of fire on forest biomass dynamics at the southern amazon edge. Environ. Conserv. 48, 285–292. doi: 10.1017/S0376892919000110
Ogle, D. H., Wheeler, P., and Dinno, A. (2019). FSA: Fisheries Stock Analysis. R package version 0.8.25. Available online at: https://github.com/droglenc/FSA
Oksanen, J., Blanchet, F. G., Friendly, M., Kindt, R., Legendre, P., McGlinn, D., et al. (2019). vegan: Community Ecology Package. R package version 2.5-5. Available online at: https://CRAN.R-project.org/package=vegan
Oksanen, L. (2001). Logic of experiments in ecology: is pseudoreplication a pseudoissue? Oikos 94, 27–38. doi: 10.1034/j.1600-0706.2001.11311.x
Oliveira, J. C., Vieira, I. C. G., Almeida, A. S., and Silva Junior, C. A. (2016). Floristic and structural status of forests in permanent preservation areas of moju river basin, amazon region. Braz. J. Biol. 76, 912–927. doi: 10.1590/1519-6984.04415
Oliveira, M. T., Damasceno-Junior, G. A., Pott, A., Filho, A. C. P., Suarez, Y. R., and Parolin, P. (2014). Regeneration of riparian forests of the Brazilian pantanal under flood and fire influence. For. Ecol. Manag. 331, 256–263. doi: 10.1016/j.foreco.2014.08.011
Oliveira-Filho, A. T., Márcio de Mello, J., and Scolforo, J. R. S. (1997). Effects of past disturbance and edges on tree community structure and dynamics within a fragment of tropical semideciduous forest in south-eastern Brazil over a five-year period (1987–1992). Plant Ecol. 131, 45–66. doi: 10.1023/A:1009744207641
Phillips, O. L., Aragão, L. E. O. C., Lewis, S. L., Fisher, J. B., Lloyd, J., López-González, G., et al. (2009). Drought sensitivity of the amazon rainforest. Science 323, 1344–1347. doi: 10.1126/science.1164033
Potter, K. A., Arthur Woods, H., and Pincebourde, S. (2013). Microclimatic challenges in global change biology. Glob. Chang. Biol. 19, 2932–2939. doi: 10.1111/gcb.0.12257
R Core Team (2017). R: A Language and Environment for Statistical Computing. Vienna: R Foundation for Statistical Computing.
Sanford, R. L. Jr., Saldarriaga, J., Clark, K. E., Uhl, C., and Herrera, R. (1985). Amazon rain-forest fires. Science 227, 53–55. doi: 10.1126/science.227.4682.53
Schwenk, K., Padilla, D. K., Bakken, G. S., and Full, R. J. (2009). Grand challenges in organismal biology. Integr. Comp. Biol. 49, 7–14. doi: 10.1093/icb/icp034
Silva Junior, C. H. L., Anderson, L. O., Silva, A. L., Almeida, C. T., Dalagnol, R., Pletsch, M. A. J. S., et al. (2019). Fire responses to the 2010 and 2015/2016 amazonian droughts. Front. Earth Sci. 7:97. doi: 10.3389/feart.2019.00160
Silva, V. F., Oliveira Filho, A. T., Venturin, N., Carvalho, W. A. C., and Gomes, J. B. V. (2005). Impacto do fogo no componente arbóreo de uma floresta estacional semidecídua no município de ibituruna, mg, Brasil. Acta Bot. Brasilica 19, 701–716. doi: 10.1590/S0102-33062005000400005
Silvério, D. V., Brando, P. M., Balch, J. K., Putz, F. E., Nepstad, D. C., Oliveira-Santos, C., et al. (2013). Testing the amazon savannization hypothesis: fire effects on invasion of a neotropical forest by native cerrado and exotic pasture grasses. Philos. Trans. R. Soc. Lond. B. Biol. Sci. 368, 1–8. doi: 10.1098/rstb.2012.0427
Solar, C. R. R., Barlow, J., Ferreira, J., Berenguer, E., Lees, A. C., Thomson, J. R., et al. (2015). How pervasive is biotic homogenization in human-modified tropical forest landscapes? Ecol. Lett. 18, 1108–1118. doi: 10.1111/ele.12494
Sousa, W. P. (1984). The role of disturbance in natural communities. Ann. Rev. Ecol. Syst. 15, 353–391. doi: 10.1146/annurev.es.15.110184.002033
Souza, C. M. Jr., Siqueira, J. V., Sales, M. H., Fonseca, A. V., Ribeiro, J. G., Numata, I., et al. (2013). Ten-year landsat classification of deforestation and forest degradation in the Brazilian Amazon 5, 5493–5513. doi: 10.3390/rs5115493
Staver, A. C., Archibald, S., and Levin, S. A. (2011). The global extent and determinants of savanna and forest as alternative biome states. Science 334, 230–232. doi: 10.1126/science.1210465
Strassburg, B. B. N., Kelly, A., Balmford, A., Davies, R. G., Gibbs, H. K., Lovett, A., et al. (2010). Global congruence of carbon storage and biodiversity in terrestrial ecosystems. Conserv. Lett. 3, 98–105. doi: 10.1111/j.1755-263X.2009.00092.x
Sullivan, M. J. P., Talbot, J., Lewis, S. L., Phillips, O. L., Qie, L., Begne, S. K., et al. (2017). Diversity and carbon storage across the tropical forest biome. Scienti. Rep. 7:39102. doi: 10.1038/srep39102
Ter Steege, H., Pitman, N. C., Sabatier, D., Baraloto, C., Salomão, R. P., Guevara, J. E., et al (2013) Hyperdominance in the amazonian tree flora. Science 342, 325–334. doi: 10.1126/science.1243092
Tilman, D., Isbell, F., and Cowles, J. M. (2014). Biodiversity and ecosystem functioning. Annu. Rev. Ecol. Evol. Syst. 45, 471–493. doi: 10.1146/annurev-ecolsys-120213-091917
Tilman, D., Wedin, D., and Knops, J. (1996). Productivity and sustainability influenced by biodiversity in grassland ecosystems. Nature 379, 718–720. doi: 10.1038/379718a0
Tymen, B., Réjou-Méchain, M., Dalling, J. W., Fauset, S., Feldpausch, T. R., Norden, N., et al. (2016). Evidence for arrested succession in a liana-infested amazonian forest. J. Ecol. 104, 149–159. doi: 10.1111/1365-2745.12504
Uhl, C., Clark, H., Clark, K., and Maquirino, P. (1982). Successional patterns associated with slash-and-burn agriculture in the upper rio negro region of the amazon basin. Biotropica 14, 249–254. doi: 10.2307/2388082
Uhl, C., Clark, H., Clark, K., and Murphy, P. (1981). Early plant succession after cutting and burning in the upper Rio negro region of the amazonian basin. J. Ecol. 69, 631–649. doi: 10.2307/2259689
Uhl, C., and Kauffman, J. B. (1990) Deforestation, fire susceptibility, potential tree responses to fire in the eastern amazon. Ecology 71, 437–449. doi: 10.2307/1940299
Uhl, C., and Serrao, E. A. S. (1998). Abandoned pastures in eastern amazonia. J. Ecol. 76, 663–681. doi: 10.2307/2260566
van Mantgem, P. J., Schwartz, M. W., and Keifer, M. (2001). Monitoring fire effects for managed burns and wildfires: coming to terms with pseudoreplication. Nat. Areas J. 21, 266–273.
Vedovato, L. B., Fonseca, M. G., Arai, E., Anderson, L. O., and Aragão, L. E. O. C. (2016). The extent of 2014 forest fragmentation in the Brazilian Amazon. Reg. Environ. Change 16, 2485–2490. doi: 10.1007/s10113-016-1067-3
Veldman, J. W., and Putz, F. E. (2011) Grass-dominated vegetation, not species-diverse natural savanna, replaces degraded tropical forests on the southern edge of the amazon basin. Biol. Cons. 144, 1419–1429. doi: 10.1016/j.biocon.2011.01.011
Venables, W. N., and Ripley, B. D. (2002). Modern Applied Statistics with, 4th Edn. New York, NY: Springer. doi: 10.1007/978-0-387-21706-2
Vlam, M., Baker, P. J., Bunyavejchewin, S., and Zuidema, P. A. (2014). Temperature and rainfall strongly drive temporal growth variation in Asian tropical forest trees. Oecologia 174, 1449–1461. doi: 10.1007/s00442-013-2846-x
Waide, R. B., Willig, M. R., Steiner, C. F., Mittelbach, G., Gough, L., Dodson, S. I., et al. (1999). The relationship between productivity and species richness. Annu. Rev. Ecol. Evol. Syst. 30, 257–300. doi: 10.1146/annurev.ecolsys.30.1.257
White, P. S. (1979). Pattern, process and natural disturbance in vegetation. Bot. Rev. 45, 230–299. doi: 10.1007/BF02860857
Wickham, H. (2016). ggplot2: Elegant Graphics for Data Analysis. New York, NY: Springer-Verlag. doi: 10.1007/978-3-319-24277-4_9
Wickham, H., François, R., Henry, L., and Müller, K. (2019). dplyr: A Grammar of Data Manipulation. R package version 0.8.3. Available online at: https://CRAN.R-project.org/package=dplyr
Xaud, H. A. M., Martins, F. S. R. V., and Santos, J. R. (2013). Tropical forest degradation by mega-fires in the northern Brazilian amazon. For. Ecol. Manage. 294, 97–106. doi: 10.1016/j.foreco.2012.11.036
Keywords: carbon, basal area, diversity, regeneration, resilience, richness, seedling, selective logging
Citation: Prestes NCCdS, Massi KG, Silva EA, Nogueira DS, de Oliveira EA, Freita R, Marimon BS, Marimon-Junior BH, Keller M and Feldpausch TR (2020) Fire Effects on Understory Forest Regeneration in Southern Amazonia. Front. For. Glob. Change 3:10. doi: 10.3389/ffgc.2020.00010
Received: 01 September 2019; Accepted: 23 January 2020;
Published: 28 February 2020.
Edited by:
Imma Oliveras, University of Oxford, United KingdomReviewed by:
Olga Viedma Sillero, University of Castilla La Mancha, SpainCopyright © 2020 Prestes, Massi, Silva, Nogueira, de Oliveira, Freitag, Marimon, Marimon-Junior, Keller and Feldpausch. This is an open-access article distributed under the terms of the Creative Commons Attribution License (CC BY). The use, distribution or reproduction in other forums is permitted, provided the original author(s) and the copyright owner(s) are credited and that the original publication in this journal is cited, in accordance with accepted academic practice. No use, distribution or reproduction is permitted which does not comply with these terms.
*Correspondence: Ted R. Feldpausch, dC5yLmZlbGRwYXVzY2hAZXhldGVyLmFjLnVr
†ORCID: Nayane Cristina Candida Dos Santos Prestes orcid.org/0000-0001-8229-8231
Estevão Alves Silva orcid.org/0000-0002-1650-8605
Denis Silva Nogueira orcid.org/0000-0001-8893-7903
Edmar Almeida de Oliveira orcid.org/0000-0002-6446-3376
Renata Freitag orcid.org/0000-0003-1461-5834
Klécia Gili Massi orcid.org/0000-0003-1823-7965
Beatriz Schwantes Marimon orcid.org/0000-0003-3105-2914
Ben Hur Marimon-Junior orcid.org/0000-0002-6359-6281
Michael Keller orcid.org/0000-0002-0253-3359
Ted R. Feldpausch orcid.org/0000-0002-6631-7962
Disclaimer: All claims expressed in this article are solely those of the authors and do not necessarily represent those of their affiliated organizations, or those of the publisher, the editors and the reviewers. Any product that may be evaluated in this article or claim that may be made by its manufacturer is not guaranteed or endorsed by the publisher.
Research integrity at Frontiers
Learn more about the work of our research integrity team to safeguard the quality of each article we publish.