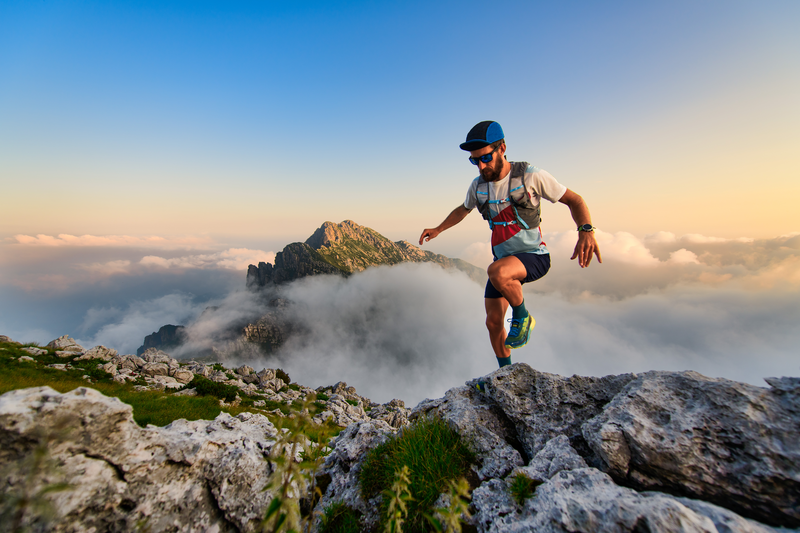
95% of researchers rate our articles as excellent or good
Learn more about the work of our research integrity team to safeguard the quality of each article we publish.
Find out more
SYSTEMATIC REVIEW article
Front. For. Glob. Change , 10 February 2020
Sec. Forest Disturbance
Volume 3 - 2020 | https://doi.org/10.3389/ffgc.2020.00007
This article is part of the Research Topic Invasions and Impacts of Exotic Species in Forests View all 6 articles
Invasive pathogens threaten the ability of forests globally to produce a range of valuable ecosystem services over time. However, the ability to detect such pathogen invasions—and thus to produce appropriate and timely management responses—is relatively low. We argue that a promising approach is to plan and manage forests in a way that increases their resilience to invasive pathogens not yet present or ubiquitous in the forest. This paper is based on a systematic search and critical review of empirical evidence of the effect of a wide range of forest management options on the primary and secondary infection rates of forest pathogens, and on subsequent forest recovery. Our goals are to inform forest management decision making to increase forest resilience, and to identify the most important evidence gaps for future research. The management options for which there is the strongest evidence that they increase forest resilience to pathogens are: reduced forest connectivity, removal or treatment of inoculum sources such as cut stumps, reduced tree density, removal of diseased trees and increased tree species diversity. In all cases the effect of these options on infection dynamics differs greatly amongst tree and pathogen species and between forest environments. However, the lack of consistent effects of silvicultural systems or of thinning, pruning or coppicing treatments is notable. There is also a lack of evidence of how the effects of treatments are influenced by the scale at which they are applied, e.g., the mixture of tree species. An overall conclusion is that forest managers often need to trade-off increased resilience to tree pathogens against other benefits obtained from forests.
Invasive species present significant threats to natural and planted forests (Wingfield et al., 2015; Liebhold et al., 2017; Muzika, 2017), and can, in combination with climate change, create “mega disturbances” which disrupt forests worldwide (Millar and Stephenson, 2015), leading to large ecological, economic, and social losses (Hill et al., 2019). While invasive species research often focuses on animal and plant invasions, forest ecosystems are also threatened by invasive microbial pathogens. Pathogens have the potential to disrupt timber and non-timber benefits provided by forests, and the need for a coordinated effort to tackle such invasive species is being increasingly recognized (Wingfield et al., 2015). In this context, to be considered invasive a pathogen does not need to be non-native to the region, but rather through an increase in its abundance produce a widespread negative impact on a given ecosystem (Warren, 2007; Carey et al., 2012).
Managing invasive pathogens presents unique challenges not associated with controlling invasive plants and animals, including insects. The cryptic nature of infection by pathogens, particularly at the beginning of their life cycles, means that many invasions remain undetected until trees become symptomatic, by which time the pathogen is often already widespread (Liebhold et al., 2017; Muzika, 2017). Even after infection has been detected, identification of the causal pathogen is normally reliant on examination of spores and/or DNA sequencing. As a result, many pathogens have remained unidentified or misidentified, restricting our capacity to manage invasions effectively (Wingfield et al., 2015, 2017). Genetic variation amongst microbial pathogens is greater than even that found between the plant and animal kingdoms, and as such these pathogens have highly varied life histories. This not only creates challenges in designing control measures for pathogens as a general invasive threat, but also in tackling individual pathogens, which can present unfamiliar life history traits (Wingfield et al., 2017). These complex and often still unknown life histories, as well as their potential for relatively rapid evolution, restrict our ability to predict which pathogens will become invasive, and how any invasion will progress (Ghelardini et al., 2017).
Tree pathogens threaten the ability of forests to deliver ecosystem services over the long-term. The importance of phytosanitary measures, such as quarantine, to reduce the risk of invasive tree pathogens reaching a country or a given forest have long been recognized (Wingfield et al., 2015). However, as rotation lengths from establishment to harvest of a forest tree crop generally last for several decades, the high rate of arrival of new pathogen species to locations around the world means that any newly established crop may potentially be subject to many new pathogens before it reaches maturity. This poses a particular challenge for forest managers. So far, management responses have largely been restricted to reactive measures taken after the presence of a given pathogen has been detected, by which time economic and ecological damage costs can rarely be avoided. This restriction on the ability of forest managers to respond until a specific pathogen has been detected raises the important question of whether and how forests should be planned and managed to maximize their resilience to the threat of future unknown pathogens.
To help address this challenge, a recent extension of epidemiological modeling (“epi-economic modeling”) has linked the economics of forest management practices to the impacts of tree pathogens across a range of primary and secondary infection rates and damage costs (Macpherson et al., 2017a,b, 2018). This approach is based on an epidemiological paradigm (Kleczkowski et al., 2019), whereby the population of trees is divided between healthy and susceptible individuals, and infected individuals. Mathematical models capturing the infection process can take different forms but the simplest equation is
with S (t) denoting the number of still healthy but susceptible trees, rp and rs the rate of primary and secondary infection, respectively, I (t) currently infected trees within the unit, and dI/dt the rate of appearance of new infections (Brassett and Gilligan, 1988).
The fundamental difference between primary and secondary infection is epidemiological. Primary infection is the invasion of the population of trees within the forest management or landscape-patch unit from an external source, e.g., an infected population of trees in another unit, and requires management at the boundary or beyond. Primary infections can also occur from a reservoir of inoculum in alternative hosts, or in soil or dead plant material when sites are replanted. Thus, the source is “external” to the population under threat albeit occupying the same parcel of land. Secondary infection is transmission from currently infected trees within the unit's population to its susceptible trees, driven by multiplication, dispersal, and infection of inoculum. Hence management activity in that forest unit can influence secondary infection and reduce epidemic spread. The rates of primary and secondary infections in Equation (1) capture the whole range of factors, including the susceptibility of individual trees to infection as well as the dispersal characteristics of the pathogen.
Fundamental to this paper is the recognition that resilience of a forest is linked to its response to invasions by forest pathogens. This response, in turn, is influenced by the management practices aimed at the prevention of such invasions, their control and, if control is unsuccessful, the mitigation of their effects. The modeling framework described above and its extensions, have successfully been used in describing spread and control of tree pathogens in forests (Macpherson et al., 2017a, 2018). However, application of such an approach to inform forest managers about how to increase forest resilience against future pathogen threats requires empirical evidence about the effects of forest management options on tree pathogen primary and secondary infection rates, and rates of forest recovery.
The key question in ecological and economic applications of the concept of resilience is “resilience of what, to what?” (Walker et al., 2010; Matsushita et al., 2018). The focus of this study is forests that are managed predominantly for timber production and in this case the most relevant concept of resilience is the one termed “engineering resilience,” which comprises two main components: “resistance” to the initial impact of a disturbance agent (in this case the invasion of a tree pathogen) and “recovery” toward the previous state or functioning of the forest ecosystem (Pimm, 1984; Holling, 1996; Grimm and Wissel, 1997; Newton and Cantarello, 2015). In the literature reviewed in our study, the measured response variables that give the best indication of “resistance” (at the scale of the forest ecosystem or stand) were level of individual tree infection or mortality, and the best indicators of “recovery” were rates of natural regeneration or stand-level growth rates of all surviving or subsequently established trees. We assume throughout the paper that managers are concerned with a single spatially contiguous unit of forest, because this is the scale at which most studies are carried out. However, the size of this unit may be highly variable, and we focus only on net increases or decreases in resilience. The majority of studies available for our review assumed that provisioning of timber was the ecosystem service of greatest importance resulting from the state or functioning of the forest.
The impacts of forest management on tree pathogens have been the subject of many recent reviews. Each has tended to focus on a single pathogen, such as white pine blister rust (Cronartium ribicola) (Schoettle and Sniezko, 2007; Hunt et al., 2010; Ostry et al., 2010; Zeglen et al., 2010), Phytophthora ramorum (Valachovic et al., 2010), ash dieback (Hymenoscyphus fraxineus) (Pautasso et al., 2013) or dothistroma needle blight (Dothistroma septosporum and D. pini) (Bulman et al., 2016). As a result, the findings have been highly variable, with recommendations largely dependent on the pathogen considered. Alternatively, pathogens have been considered alongside insect pests (Waring and O'Hara, 2005; Liebhold et al., 2017; Muzika, 2017) or other general threats to forest ecosystems (Jactel et al., 2009, 2017). Reviews focused on broader forest resistance to the threat of tree pathogens have been limited to considering only the effect of tree species mixtures on the spread of and damage caused by pathogens (Pautasso et al., 2005; Prospero and Cleary, 2017) or of more general principles of interactions and ecosystems services (Boyd et al., 2014). Overall, the local context of the forest and pathogen have been recognized as important in directing management responses.
The objective of the current study is to synthesize the evidence for the effect of forest management options on forest resilience to tree pathogens. The scope is broad, including all of the main categories of forest management variables and all tree pathogen species. However, animal pests, invasive plants and abiotic threats such as fire were excluded. A second objective is to forge an explicit link between forest resilience, forest design, or silvicultural management practices and epi-economic modeling grounded in plant and tree epidemiology (Macpherson et al., 2017a,b, 2018). Thus, we seek to assess the empirical evidence for the effect of each forest management option on the three key elements of primary infection, secondary infection and subsequent forest recovery, in order to inform forest management decision making to increase forest resilience, and to identify the most important evidence gaps to motivate future research.
We carried out a literature review using a systematic search method to identify published sources of empirical data on the relationship between forest management and resilience to tree pathogens. We conducted an initial search of the peer-reviewed literature through Web of Science, using search strings to identify papers on all of forest management, pests, pathogens or disease, and resilience, excluding medical papers and those concerned with food supply, using the Boolean search string of:
TS = ((((*forest* OR wood* OR tree*) AND (manage*)) OR silvicult*) AND (pest* OR disease* OR pathogen*) AND (exposure OR resist* OR recover* OR spread OR risk OR suscept* OR transmit* OR dispers* OR infect*) NOT (medicin* OR clinic* OR pharma* OR foodborne OR food-borne OR mycorrhizal OR biomedic* OR mosquito OR tick OR lyme* OR malaria*)) NOT SO = (medicin* OR clinic* OR pharma* OR biomedic*) NOT WC = (medicin* OR clinic* OR pharma* OR biomedic*)
This search (run on 27/06/2017) returned 3,534 papers. The papers were screened first by title, then abstract, and finally full text to identify papers reporting original empirical data on the effects of forest management on tree diseases caused by pests or pathogens, which retained 599 papers. We then excluded papers that only covered tree pests (362 papers), were only concerned with tropical forests (85 papers) or orchards (235 papers), were concerned with tree breeding (74 papers), or were entirely review (23 papers) or theoretical modeling (21 papers) studies (note papers may be present in more than one category). Removal of tree pests also included removing papers concerning insect vectors, because in the majority of cases the distinction between direct damage and vectoring of a pathogen could not be determined. This procedure retained 81 papers. As many forest management actions are not reported in the published literature, searches were also run in TREESEARCH, the research portal for the US Forest Service, using the following search string:
(disease OR fung* OR pathogen OR bacteri* OR oomycete OR virus) AND (((tree OR forest* OR wood) AND manage*) OR silvicult*)
and the UK Forestry Commission website, using the search string:
disease fung* pathogen bacteria* oomycete virus
Restrictions on the search terms for each search engine prevented identical searches from being carried out. The search in TREESEARCH returned 158 documents, of which 12 were identified as relevant and containing data. The Forestry Commission website returned 58 documents, of which three were identified as relevant and containing data. Together with literature identified from the reference lists of retained papers and identified reviews (nine papers), and further search terms added to account for fertilizer application (four papers), the final reference list contained 114 papers and reports. This list was further refined to include only papers whose reliability, robustness and applicability to forest management could be assured. Studies which were purely descriptive, lab-based or considered only pathogen presence, rather than impact, were excluded. The remaining 109 papers included within the review were scored for strength of evidence based on whether they were correlative or experimental, and whether single or multiple sites had been considered, within single or multiple forests.
We organized papers by management technique, treating each technique reported within a single paper independently, and then by pathogen type. We have included a broad range of management options, including forest design planning, site preparation for forest establishment, tree species diversity, silvicultural system and individual silvicultural actions. These categories were not pre-determined but were decided through reading the papers.
To assess the outcome of each management technique we classified the results of each study as strong positive or strong negative (relationship observed in all sites within the study), weak positive or weak negative (relationship observed in at least one site within the study, with no sites showing the opposite relationship), no relationship, or mixed (both positive and negative relationships observed across sites). A technique was therefore considered to have an overall positive or negative impact where multiple studies, or a robust single study across multiple forests, found the same result, and there were no robust studies reporting a contradictory result. If studies reported contradicting results, we considered the outcome of this technique to be mixed unless the results were weighted heavily in one direction, and the contradicting study was considered to be of low robustness. Where only a limited number of studies was available this was identified as a weakness in our conclusions. A fuller description of this critical appraisal of the studies is provided in the Supplementary Material, together with the full outputs of the search and critical appraisal in the Table 1.
The large variation in tree species, pathogens, and management techniques considered, as well as limited reporting of the particulars of management, prevented us from conducting a formal meta-analysis.
Our review of the literature revealed a clear bias toward a small number of highly damaging pathogens. Studies into Armillaria and Phytophthora species comprised 38% of all studies identified (17 and 21%, respectively), and we found no studies on the effects of forest management on bacteria or viruses. Studies mainly covered commercially valuable host tree species, with equal coverage of conifers and broadleaves (Table 1).
A geographical bias was also evident, with 59% of studies based in North America, and California and Oregon coastal forests alone accounting for 24%. The majority of the remaining papers originate from Europe (29%), with eight papers from Oceania and two from Asia. Studies reporting only from natural tropical forests had previously been excluded. Only a single paper (Cleary et al., 2013) reported results from more than one region (Table 1).
The response variables most commonly reported were mortality, disease incidence, and disease severity. The only indicator of forest recovery (defined in the section Introduction) that was widely reported was of subsequent tree growth rate, though this was often only measured over the short-term. There was minimal reporting of rates of tree natural regeneration. Studies generally reported outcomes in terms of symptoms of forest disease, and few papers considered the mechanisms connecting forest management to these outcomes. The distribution of studies amongst each forest management variable and each response variable is summarized in Table 2.
Table 2. Overview of published evidence of effects of forest management on resilience to tree diseases.
As explained above, the concept of primary infection [cf. Equation (1)] captures the pathways by which the pathogen enters the forest unit of interest. These primary infections can occur from other forest units, for example by wind or water movement of inoculum, from alternative hosts, by movement on machinery and other human-mediated activities, or by transmission from soil inoculum.
The importance of connectivity for the conservation of forest ecosystems at a landscape scale is well-recognized (Lindenmayer et al., 2006). However, connectivity can increase the risk of transmission of infection from one forest patch or unit to another, including from outside the region of interest. For tree pathogens connectivity does not just refer to spatial proximity, but also any connection through which inoculum may spread to a forest unit, such as via streams, wind, fog, animal (e.g., insects, fur, feathers) or human vectors (e.g., vehicles, recreation). The scale at which connectivity is important also varies with dispersal mechanism, and can be large in the case of flying animal and vehicle vectors. These “least cost” (resistance), but not necessarily shortest-distance, pathways have been shown to be important in models accounting for the spread of pathogens (Ellis et al., 2010).
The impacts of connectivity on tree diseases have predominantly been studied in coastal forests of California and Oregon (USA). Total forest area within a landscape, correlated with connectivity, predicted increases in incidence (Meentemeyer et al., 2008a) and severity of P. ramorum in Notholithocarpus densiflorus (tanoak) (Haas et al., 2011) and Umbellularia californica (California bay laurel) (Condeso and Meentemeyer, 2007; Meentemeyer et al., 2008a,b; Haas et al., 2011). Disease was considered only in these species because they represent the most prevalent hosts for P. ramorum within this landscape. Phytophthora ramorum incidence increased closer to streams in one site, suggesting that streams are also an important connectivity pathway for dispersal of this pathogen, though this relationship appears to depend on site topography and fog movement (Peterson et al., 2014). Connectivity through human vectors was related to higher concentrations of P. ramorum being isolated in soil from sites surrounded by larger human populations (Cushman and Meentemeyer, 2008), and increased mortality of Chamaecyparis lawsoniana (Port Orford cedar) caused by Phytophthora lateralis was found in sites intersected by a road (Jules et al., 2002). Phytophthora lateralis has been isolated from water used to wash vehicles and boots, providing further evidence of the importance of human vectors to the spread of this pathogen (Goheen et al., 2012). Within California and Oregon coastal forests, black stain root disease (Leptographium wageneri) is also concentrated at the roadside (Hessburg, 2001).
Forest connectivity via spatial proximity (Condeso and Meentemeyer, 2007; Meentemeyer et al., 2008a; Ellis et al., 2010; Haas et al., 2011), streams (Peterson et al., 2014; Havdova et al., 2017), or roads (Hessburg, 2001; Jules et al., 2002; Goheen et al., 2012) shows a consistent positive relationship with disease incidence and severity compared with less well-connected forests. However, the limited geographical range of these studies and their predominant focus on pathogen spread through soil restricts their applicability to other systems. There is also little mention of the effect of land cover in the matrix between forest patches that could affect pathogen dispersal. When addressing the increased risk of pathogen infection due to high connectivity, forest managers must also balance the extensive benefits that connected forests can have for biodiversity and some ecosystem services against increased vulnerability to disease (Lindenmayer et al., 2006). Future studies should identify and quantify pathogen transmission along different types of pathway connecting forest units and via different vectors in order to assess risks. This should help selection of management strategies to reduce the risk of primary infection of forest units.
Many tree pathogens, in particular root rots, can persist in soils following tree felling. Siting new plantations on previously forested sites may therefore increase the risk of infection due to high inoculum load in the soil. Here the soil acts as an “external” reservoir of inoculum for primary infection to initiate an epidemic in a newly planted tree population. However, research into the effects of previous land use is limited, due to the relative rarity of studies into forests established on previously non-forest land.
Naturally occurring U. californica trees on former grassland sites within Quercus forests in northern California had lower incidence of P. ramorum than U. californica growing in long-term forest areas (Meentemeyer et al., 2008a). However, this relationship was not reflected in Italian Abies alba (silver fir) plantations, where Heterobasidion annosum infection rate was significantly higher in forests established on former pastureland. Although A. alba planted on former pastureland was expected to be exposed to lower inoculum load, these trees were less healthy due to exposure to adverse environmental conditions resulting from previous intensive land use, potentially increasing susceptibility to disease (Puddu et al., 2003).
While previous land use could be expected to affect forest resilience to tree diseases, research on this is rare, and the findings amongst published studies are not consistent. This is likely to be due to the large variation in previous land use types, and particulars of previous land management, amongst the studies. However, such research is likely to increase in relevance for rotational forest systems, where the previous species planted in the unit may be considered. In some countries, including the UK, new forests are also being planted on land not forested in recent history in order to increase carbon capture in response to climate change, and natural tree regeneration is occurring due to abandonment of agricultural land (Poyatos et al., 2002).
Site preparation methods can either introduce pathogens into an area where they were not previously found or reduce forest resistance to primary infection and hence increase the initiation of local disease spread. Previously felled sites contain remnant stumps, root fragments and brash, which may be a source of primary infection through spread of pathogens over time (from a previous tree population to a new one). Nonetheless, stumps resulting from thinning or partial cutting can also act as a source of secondary infection within the current tree population. Although this coarse woody debris is important for forest biodiversity more generally (Hartley, 2002), it acts as a reservoir for many pests and pathogens, increasing exposure of newly established trees to inoculum. Stumps can also provide a nutrient source for inoculum of pathogens with saprotrophic activity. In response to this risk, site preparation may include stump removal or chemical treatment, in some cases accompanied by removal of part of the root system through raking. The origin of stumps can also be important for identifying the risks posed, with clearcut stumps having lower infection rates than stumps resulting from thinning (Bendz-Hellgren and Stenlid, 1998). Burning presents an alternative option to remove woody debris or reduce pathogen survival, but has other risks and environmental costs, such as to habitat quality or native fauna, or it can in fact increase disease incidence in the case of Rhizina undulata (Wingfield and Swart, 1994). In some cases site preparation also includes application of fertilizer, which may reduce impacts of pathogens on tree health through increasing tree nutrient concentrations, especially of calcium and magnesium (Anglberger and Halmschlager, 2003; Halmschlager and Katzensteiner, 2017). However, fertilizer application (especially of nitrogen and phosphorus) that increases tree growth rate can lead to nutrient inbalances that increase susceptibility to pathogens (Jactel et al., 2009).
Root rots have the highest potential for management through stump treatment and have unsurprisingly been the subject of the greatest number of studies. Methods of stump treatment may be physical or chemical. Infection of forest stands by H. annosum has long been known to be increased by any tree felling resulting in cut stumps that are susceptible to colonization from air-borne basidiospores (Woodward et al., 1998). The most extensive study of stump removal incorporated five sites from Canada and Scandinavia subject to infection by the conifer root rot pathogens H. annosum sensu lato (s.l.), Armillaria ostoyae and laminated root rot (Phellinus sulphurascens). The severity of infection and its contribution to mortality were monitored in stands that had been subject to removal of stumps, either as part of the whole tree or in a separate operation following felling, with raking to remove larger roots occurring in one site, compared with controls where no stump removal took place (Cleary et al., 2013). Stump removal was clearly associated with reduced disease incidence and tree mortality up to 21–50 years after treatment. One of these sites was then studied in more detail by Morrison et al. (2014), who confirmed that over 40 years after treatment, stump removal had reduced the rate of mortality in the next rotation of trees by 14% across all species. Notably, for Pseudotsuga menziesii (Douglas fir), Pinus contorta var. latifolia (lodgepole pine), Larix occidentalis (western larch), Thuja plicata (western red cedar), and Picea engelmannii (Engelmann spruce), stump removal reduced the mortality rate due to A. ostoyae and completely eliminated the occurrence of mortality in P. menziesii due to P. sulphurascens. These findings support those of a previous study of P. menziesii further south in Washington State and Oregon, where mortality due to P. sulphurascens was significantly reduced by pre-planting stump removal (Thies and Westlind, 2005).
There has been a long history of incidence of H. annosum in the plantations of Pinus sylvestris (Scots pine) and Pinus nigra ssp. laricio (Corsican pine) in Thetford Forest, UK. A series of experiments showed that colonization of stumps by air-borne basidiospores of H. annosum can be greatly reduced by stump treatment with spores of Phlebiopsis gigantea, a non-pathogenic basidiomycete. However, a series of long-term experiments have shown that only stump removal achieves adequate reduction in mortality into the second plantation rotation (Gibbs et al., 2002). The importance of removing stumps was further supported in studies of P. sylvestris infected by H. annosum in Sweden, where infection rate was higher in trees closer to infected stumps (Swedjemark and Stenlid, 1993). In contrast, in New Zealand Pinus radiata plantations infected by Armillaria novae-zelandiae, while inoculum load in stumps was high, this did not lead to greater infection within the forest after 19 years compared with forests where stumps were removed (Hood et al., 2002; Hood and Kimberley, 2009).
In addition to retained stumps, root fragments from felled trees can act as reservoirs of pathogen inoculum. Few studies have reported beneficial effects of root removal, although Shaw et al. (2012) found that greater intensity or thoroughness of removal of roots that acted as a source of A. ostoyae inoculum did significantly reduce the incidence of infection and resultant mortality in Pinus ponderosa after 35 years, though the study concluded that its benefits are unlikely to exceed its costs. In contrast, several studies have reported increases in infection following root removal. Negative impacts of mechanical root removal treatments can occur through increasing the dispersal of pathogen inoculum. Root raking has been found to move infected root fragments closer to the soil surface, although this was not associated with increased infection rates (Morrison et al., 1988, 2014). The incidence of western gall rust was found to be positively associated with sites that had undergone a variety of methods of mechanical site preparation to disrupt slash, forest floor and mineral soil layers, compared with control sites (Roach et al., 2015). Similar results were observed in Castanea dentata (American chestnut) infected by chestnut blight (Cryphonectria parasitica). In a study of plantations on reclaimed mine land in Ohio, USA, the site preparation treatments of deep ripping to 1 m depth resulted in a higher incidence of chestnut blight cankers on seedlings than in those plots plowed and disked to 30 cm depth, though this still exceeded the incidence of cankers for trees in control plots (Bauman et al., 2014).
Prescribed or natural fire reduces pre-planting inoculum load through either burning of stumps, root fragments and woody debris, or through killing of the pathogen due to high temperatures. However, results from burning are not consistent. Naturally occurring fires in Californian coastal redwood and mixed-evergreen forests led to reduced isolation rates of P. ramorum in symptomatic trees for 1 and 2 years following fire, however incidence of the pathogen increased by the second year, associated with the number of surviving symptomatic U. californica trees which acted as an inoculum reservoir (Beh et al., 2012). A prescribed burn treatment in a Pinus palustris (longleaf pine) forest in South Carolina, USA, was associated with increased mortality through H. annosum after 8–10 years, linked to reductions in tree health caused by the fire (Cram et al., 2010). Varied burning regimes in conifer forests in Ontario, Canada, had no impact on Armillaria sp. root rot (Whitney and Irwin, 2005).
Fertilizer application has mixed impacts on disease severity. Increased damage by twisting rust fungus (Melampsora pinitorqua) was found on Pinus pinaster (maritime pine) that had been fertilized with phosphorus compared with no fertilizer controls (Desprez-Loustau et al., 2016). However, fertilization of Picea abies (Norway spruce) did show a reduction in severity of infection by Sirococcus shoot blight (Sirococcus conigenus), linked to improved tree health (Anglberger and Halmschlager, 2003; Halmschlager and Katzensteiner, 2017). In P. menziesii seedlings fertilization with potassium and nitrogen had no impact on mortality due tolaminated root rot (Phellinus weirii) (Thies et al., 2006), and potassium, nitrogen and sulfur fertilizers had no impact on Armillaria spp. root disease in mixed conifer forests in Oregon (Filip et al., 2002).
Overall, removal or treatment of tree stumps as a source or receptor of pathogen inoculum has a positive effect on forest resilience to tree disease, through reduced infection of trees retained on the site or newly planted trees. However, studies are concentrated on root rots. Stumps, and other dead wood material, are also known to be important in survival of populations of a number of invertebrate forest pests, and there is an evidence gap about their significance as a source of inoculum of a wider range of pathogens with airborne spores that infect the shoots of trees. We found that studies of root fragment removal and burning give more mixed results and are under-researched. A future research priority is to assess the trade-offs between reducing inoculum levels using such treatments and the damage they cause to retained trees (e.g., through wounding), which can increase their susceptibility to infection.
Secondary infection [cf. Equation (1)] refers to transmission of a pathogen between trees within a region of interest (forest unit). Secondary infection therefore captures the direct transmission component of epidemics that depends upon the number of currently infected individuals. Although this typically relates to an outbreak situation between trees of a similar age, of particular concern for forest management is secondary infection from mature trees to seedlings, often planted to form the crop in the next forest rotation. Actions that increase environmental stress on a tree, thus reducing its vigor, are also likely to increase the rate of secondary infection.
Effects of tree species mixture, i.e., planting two or more species rather than a monoculture, or increasing tree species diversity, i.e., through the number of species planted together or as an indirect result of other silvicultural actions, on forest resilience to tree diseases have been extensively studied, with good coverage of both tree and pathogen species in sites across Europe and North America. Tree species diversity effects have been the subject of recent review papers. These recognize that greater diversity is associated with decreased tree mortality caused by pests and pathogens, identifying reduced access to hosts and increased distance between hosts as potential mechanisms for reducing secondary transmission as an epidemic progresses (Pautasso et al., 2005; Bauhus et al., 2017; Jactel et al., 2017; Prospero and Cleary, 2017), as well as potentially providing habitat for more species that deliver natural biocontrol (Bauhus et al., 2017). However, such results may be context-specific (Heybroek, 1982), and depend on whether the invading pathogen is a host specialist or generalist. We have included within this section observational studies of variation in pathogen incidence with tree species diversity as well as experimental studies with species mixtures. Their coverage is summarized in more detail in Tables 2, 3. Provenance and breeding of trees for resistance is also an important aspect of forest resilience to tree pathogens, but was outside the scope of this review.
There is general agreement across studies that increases in tree species diversity are associated with an increase in forest resilience with respect to invasive pathogens. In sites across Europe, more diverse forests were associated with lower levels of disease incidence (Nguyen et al., 2017). These findings are supported by experimental units of broadleaf trees in Germany, where fungal infections of the most susceptible tree species were reduced as species diversity increased (Hantsch et al., 2014a). This pattern was also recorded in studies in North America, where higher tree diversity was associated with lower occurrence of Fusarium sp. canker in Acer saccharum (sugar maple; Bergdahl et al., 2002), and higher tree species diversity was linked to a lower incidence of P. ramorum in Californian oak forests (Haas et al., 2011, 2016). Stands with higher tree diversity were also found to have lower mortality rates of P. menziesii caused by P. ramourm (Ramage et al., 2012). In an experiment in Germany, planted with individual tree-scale species combinations in 5 × 5 m plots, high tree species diversity in the same plot reduced the amounts of fungal infection in trees of Quercus petraea (sessile oak; Hantsch et al., 2014a). Only a single study contradicted these results, showing in Spain and Italy that Armillaria spp. presence and abundance in pure A. alba stands was lower than in mixed-species stands (Oliva et al., 2009).
Diversity amongst clones in monocultures can also affect resilience to tree disease. Willow rust (Melampsora epitea) causes decline in many short rotation Salix spp. (willow) crops. In an experiment in Northern Ireland, UK, McCracken and Dawson (1998) found that increasing the diversity of clones reduced mortality due to rust in the most susceptible clones only, but no further improvements in survival were seen when moving from 5- to 20-clone mixtures. Mixed-clone sites were also found to have later onset of disease during the first 4 years of growth, but showed no difference after this time (McCracken and Dawson, 1997). However, following observations of a subsequent experiment over two 3-year harvesting cycles, Begley et al. (2009) found no consistent effects of mixtures on reducing M. epitea on the most susceptible Salix genotype. They concluded that any benefit of mixture planting will be dependent on there being sufficient genetic diversity between the genotypes.
An important mechanism cited for the benefit of species mixture is the dilution of trees of species susceptible to a given pathogen by individuals of non-host species. However, experimental results of this effect are variable, and the specific composition of species in a mixture is found to be important. In an experimental study in Minnesota with seedlings of six conifer and four hardwood tree species planted in three mixtures differing in the proportion of individual tree species, the relative proportion of susceptible conifers or resistant broadleaves had a significant effect on mortality associated with Armillaria sp. root infection (Gerlach et al., 1997). These findings were supported by longer-term experiments in British Columbia, in which P. contorta var. latifolia had lower mortality, due predominantly to A. ostoyae, when grown in mixture with Betula papyrifera (paper birch) and T. plicata both of which have low susceptibility to this pathogen (Morrison et al., 2014). However, no benefit was found when growing it in mixture with the highly susceptible host species P. menziesii. In contrast, for 30-year-old P. menziesii no reduction in root pathogen-caused mortality rate was found from growing it in mixture with non-host species. Also in British Columbia, experimental removal of naturally regenerated broadleaves B. papyrifera and Populus tremuloides (quaking aspen) generally caused a 1.5- to 4-fold increase in mortality of planted P. menziesii for 3–5 years due to A. ostoyae infection, though the effect depended on removal method (Gerlach et al., 1997; Baleshta et al., 2005; Simard et al., 2005). In Southwest Lapland and in North Karelia, Finland, modeling of observational data showed that the incidence of M. pinitorqua on young P. sylvestris was greater in the presence of both Populus tremula (aspen, also a host species), and Salix spp. (willows, not known to be host species but said to be indicators of higher soil moisture and fertility) (Mattila et al., 2001; Mattila, 2002).
There is variation amongst conifer species in their susceptibility to H. annosum butt rot, and in southern Sweden the presence of less susceptible P. sylvestris was found to decrease the incidence of this pathogen in trees of the more susceptible P. abies (Linden and Vollbrecht, 2002). This effect increased notably up to a relative abundance of 50% of P. sylvestris but not above that proportion. Experimental plantings in Germany showed that, overall, tree species diversity in mixtures of 30 mono-specific 8 × 8 m sub-plots reduced the level of foliar pathogen infestation of susceptible Quercus spp. by Erysiphe alphitoides and E. hypophylla (powdery mildew) at the plot level (Hantsch et al., 2013). The presence of the highly susceptible Quercus spp. increased the plot-level pathogen load but resistant species such as P. abies decreased it.
Variation in the impacts of tree diversity on resistance to tree pathogens is likely related to tree species identity in the same forest unit (on a scale from individual adjacent trees up to ca. 50 m), with tree characteristics beyond simply host or non-host being important. Indeed, this may also be the main driver of any detected effects of species diversity within forest stands. Severity of infection of Fraxinus spp. to the pathogen H. fraxineus was highest in the presence of Quercus robur (pedunculate oak) and lowest in the presence of Acer spp. and Abies spp., both non-hosts. However, this result from an observational study of forests across the Czech Republic does not prove causation by the tree species themselves, but may be linked to variation in site environments (Havdova et al., 2017). In the rigorously-designed planted experiment studied by Hantsch et al. (2013), for trees of Tilia cordata (small-leaved lime) both fungal species richness and infestation level (predominantly of Passalora microsora and Asteromella tiliae) were reduced by tree diversity in the plot. For non-host tree species the effect of their proportion in the plot on infestation level in T. cordata varied from significantly positive (for Fagus sylvatica, European beech) to negative (for P. sylvestris and in some years for P. abies). Similarly, infestation of the leaves of Q. petraea increased with the proportion of Fraxinus excelsior (European ash) in the plot and decreased with P. abies. In a rehabilitated bauxite mine site in Western Australia, experimental mixed planting with non-host species reduced mortality of the susceptible host tree species Banksia grandis caused by soil inoculation with the pathogen Phytophthora cinnamomi only when grown in mixture with Acacia pulchella, but not with four other non-host Acacia species (D'Souza et al., 2004). The mechanism was suggested to be that mixture with A. pulchella reduced the soil inoculum level. These results provide strong evidence of the importance of tree species-identity effects.
In general, higher tree diversity improves forest resilience to tree diseases. However, a major mechanism in this effect has been found to be linked to the identity of the tree species present (i.e., species composition). Highly susceptible species show the largest reductions in pathogen presence and impact with increases in tree diversity. There is also evidence that greater benefit for such susceptible tree species can be obtained if they are mixed with trees not susceptible to the pathogen. It is important that future experiments are designed in a way that allows separation of effects due to species identity from those due to species diversity per se through careful consideration of species and mixtures tested. There would also be benefit in future studies determining the role of alternative mechanisms causing species mixture or diversity effects on pathogen infection, such as relative levels of inter- and intra-specific competition and their impacts on tree condition. While the resilience of mixed-species forests to individual host-specific tree pathogens may increase at the whole-forest ecosystem scale, the sum of components of these ecosystems are vulnerable to a larger number of tree pathogens than is the case for single-species forests. More work needs to be done to understand how the dynamics of different classes of pathogen affect the trade-off associated with the epidemiological risks and benefits of mixed cropping at different scales. Mixed-species forests generally increase the costs and complexity of management. Therefore, in reaching evidence-based forest management decisions, biological, and ecological considerations need to be combined with economic analysis that explicitly considers the multiple costs and benefits over time of alternative management responses to invasive pathogens.
For many tree species, planting or natural regeneration under shelterwood leads to better establishment than in open conditions (e.g., after clearcutting; Raymond and Bédard, 2017). This form of silviculture can also be beneficial for plant (Hannerz and Hanell, 1997) and bird (King and DeGraaf, 2000) biodiversity. Increased vigor of trees grown in shelterwood sites may increase resistance to tree disease, however the retained canopy trees may also act as a source of inoculum.
Across studies, the relative incidence of pathogen infection between shelterwood and clearcut sites shows high variation even within sites, and between tree and pathogen species. In study locations across the USA, Pinus strobus (eastern white pine) experienced either increase (Ostry, 2000), decrease (Katovich et al., 2004) or no change (Katovich et al., 2004) in incidence of C. ribicola when grown in shelterwood compared with clearcut sites. Across the same locations, Armillaria sp. root rot of P. strobus was less common in shelterwood than in clearcut sites (Ostry, 2000). A history of clearcutting increased the incidence of Neonectria ditissima in trees of Betula spp. (birch; Ward et al., 2010).
Amongst selection or retention systems, the size of felling gap has been suggested to influence forest resilience to tree diseases, but it is acknowledged that this varies with tree and pathogen species. The most rigorous experimental study was carried out in a pine forest in Minnesota (USA), in which Pinus resinosa, P. strobus, and P. banksiana (red, eastern white and jack pine) were planted within felling gaps of 0.3 and 0.1 ha, within plots with evenly-spaced retained overstorey trees or in an unfelled control. Incidence of shoot blight (predominantly Diplodia pinea) in dead seedlings was significantly less in the 0.3 ha gap treatment than in the control for both P. strobus and P. banksiana, but not for P. resinosa. In contrast, Armillaria solidipes incidence was significantly greater in one or both of the two gap sizes than the control for all three tree species. Gall rust (likely caused by Cronartium quercuum f. sp. banksianae) was observed only in P. banksiana, and its incidence was significantly greater in the small gaps than the control (Ostry et al., 2012). In no cases were any significant differences in pathogen incidence found amongst the two gap sizes and the evenly-spaced retention systems. Similar variability in response to silvicultural treatments was seen in an experimental study of Pinus radiata (Monterey pine) forest infected by Fusarium circinatum (a causal organism of pitch canker) in California. Here, incidence of the disease was greater in seedlings growing in intermediate-size (0.10 ha) gaps than in smaller (0.05 ha) or larger (0.20 ha) gaps (Ferchaw et al., 2013). However, gap size was not found to affect the odds of seedling survival. Tree position with respect to a long-term forest edge has been found to influence crown dieback caused by the pathogen H. fraxineus in retained trees of F. excelsior in an observational study following a tree harvest in Estonia (Rosenvald et al., 2015). The level of dieback and mortality resulting from H. fraxineus was less for trees adjacent to the pre-existing forest edge than those in the center of the harvest gap. Such effects of tree position need to be considered in studies of other pathogens and locations.
Understanding of the impacts of the range of alternative silvicultural systems on forest resilience to tree diseases is poor, with relatively few studies. It is not surprising that the available evidence shows little consistency across pathogen and tree species, given the wide variation in their transmission pathways and modes of infection. Transmission to seedlings of pathogens that spread via root contact is expected to be greater in shelterwood or other even-retention or small-gap selection systems. This effect may be less so for pathogens that disperse via airborne or water dispersal. Similarly, amongst trees, light-demanding species that show greatest vigor in open clearcut or large gap sites are likely to be less susceptible to infection in such site conditions. In contrast, more shade-tolerant species may be less susceptible in shelterwood or small gap systems where they are less vulnerable to environmental stress. However, such deductions, and in particular the net effects of any trade-offs between the effect of site conditions on the rate of pathogen infection and on the level of seedling vigor, need further empirical research. It can be expected that the net outcome will vary amongst tree and pathogen species.
Individual silvicultural systems differ from each other in several different component silvicultural operations and resulting stand conditions, which are addressed in turn in the sections below.
Differences in forest canopy cover at different stages of the forest growth cycle is one of the obvious distinctions amongst different silvicultural systems. It is also influenced by decisions over specific silvicultural operations, e.g., tree species selection, planting density, and thinning. Canopy cover affects microclimate, solar irradiation and air flow, all of which can alter the survival and dispersal of pathogens within a forest. Although it could not be distinguished as a separate effect in the reviewed literature, canopy cover would also be expected to affect movement of animal vectors of disease. We found only three studies explicitly investigating the impacts of canopy cover and their results conformed to the expectation for the different types of pathogen species, given that greater canopy cover is associated with higher air humidity, but lower sub-canopy wind speeds. Two studies in California mixed evergreen forest found a positive relationship between canopy cover and severity of infection by P. ramorum, a species whose dispersal and colonization is dependent on high humidity (Condeso and Meentemeyer, 2007; Ellis et al., 2010). In contrast, in British Columbia, C. ribicola, a species whose spores can disperse successfully through dry air, was reduced in sites with higher canopy cover (Campbell and Antos, 2000). As the effects of canopy cover clearly differ so much between different species it is not possible to draw conclusions across tree pathogens in general. In order to provide a stronger evidence base for the relative effect of different silvicultural systems in limiting the rate of secondary infection of tree pathogens, new research into the mechanisms by which canopy cover alters pathogen dispersal and infection is a high priority.
High tree density reduces the distance between potential host individuals and would therefore be expected to increase rates of pathogen spread by secondary infection within a forest. This effect is likely to vary among pathogen species, with a greater effect seen for pathogens that spread via root contact than for those with only airborne dispersal. Dispersal via animal vectors is also likely to be affected by tree density, though this could not be distinguished as a separate effect in the reviewed literature. Variation in total tree density can result from many causes, e.g., initial density of planting or natural regeneration or reduction in density due to intensity of thinning or other forms of selective felling. Reduction in density of individual host species can occur as a result of mixture with other species (reviewed in section Tree Species Mixture and Diversity). Studies that reported on the effects of thinning as an operation are reviewed in the following section Thinning.
We found only one study testing the relationship between tree density and the incidence of a pathogen species that spreads through root contact. In Minnesota, USA, broadleaf and conifer seedlings were planted in several species mixtures in recently logged sites at four different densities, ranging from 0.25 to 2 m spacing. In this study the effect of closer spacing on mortality was not significant (Gerlach et al., 1997). Airborne pathogens have been subject to much more extensive study. The intensity of P. ramorum infection increased in an observational study of mixed evergreen stands in California with higher densities of the three primary host species (Dillon et al., 2014). This positive relationship between tree density and pathogen incidence or impact has been observed for a range of other tree and airborne pathogen species and locations, including crown dieback of F. excelsior due to H. fraxineus in forests across the Czech Republic (Havdova et al., 2017), mortality of P. sylvestris due to snow blight (Phacidium infestans) in Sweden (Burdon et al., 1992), and infection level by M. pinitorqua of both P. sylvestris in Southwest Lapland and in Northern Karelia, Finland (Mattila et al., 2001; Mattila, 2002), and P. pinaster in France (Desprez-Loustau and Wagner, 1997). However a number of other studies find no relationship between pathogen incidence and tree density (McCracken and Dawson, 1998; Bishaw et al., 2003; Piirto and Valkonen, 2005).
High tree densities increase susceptibility to a broad range of tree pathogens, both those spread by root contact and airborne spores, although this effect is not universal, with many studies showing no relationship. It is likely that the relationship between tree density and pathogen prevalence is not linear but characterized by thresholds at both low and high densities. For most pathogen species forests with a high load are unlikely to see changes in pathogen spread through reduction in tree density, as the probability of secondary infection is likely to remain high even with relatively large distances between trees. Similarly, once distance between trees exceeds the normal dispersal distance of a pathogen, further increases in distance would be expected to have a smaller effect. We found no clear evidence of effects of forest structure per se, though many studies did report on the progression of disease during the development of planted stands. Priorities for future applied research would be to improve understanding of the mechanisms of tree density effects and identify thresholds in tree density related to pathogen load. In considering the role of tree density as a factor in species diversity effects on susceptibility to pathogens, an important source of evidence from future research would be to distinguish the influence of absolute tree density from that of the relative density of individual species and from the effect of forest structure (e.g., tree size heterogeneity). Thus, research should specifically compare the effects of reducing host species density by increased spacing in monoculture vs. dilution by planting in mixture with non-host species.
Thinning may be carried out as a planned action to increase production of the highest value timber from a forest, to improve other components of stand condition, or in response to damaging disturbance events, including tree pathogen outbreaks. In the latter case, thinning can take the form of salvage cutting, where dead or dying trees are removed, or sanitation cutting, which targets trees highly susceptible to disease, with the intention of reducing forest inoculum load. The latter type of thinning to remove susceptible trees will be considered in the next section Diseased Tree Removal. Thinning to improve growth or other components of tree vigor, through reduction in tree density (section Tree Density), could also be expected to improve resilience to tree diseases. However, studies show a large variation in forest response to thinning actions. Negative impacts could be attributed to the resulting stumps, whose cut surfaces are susceptible to infection (compare section Site Preparation), wounding of remaining trees, or due to increased traffic within managed areas, increasing pathogen spread by vectors (Jules et al., 2002; Cushman and Meentemeyer, 2008; Goheen et al., 2012).
Armillaria sp. root rots have been the best studied pathogen with regards to thinning impacts, with studies consistently finding evidence that thinning increases pathogen infection. Weights of Armillaria sp. isolated from the soil increased with past thinning intensity in A. alba stands in the Spanish Pyrenees (Oliva et al., 2009) and incidence of A. ostoyae infection was higher in experimental units that had been thinned for a range of conifer species in British Columbia, compared with paired unthinned stands (Morrison et al., 2001). This result was also observed in experimental P. menziesii plantations in Oregon (Rosso and Hansen, 1998) and Idaho (Entry et al., 1991), as well as for A. luteobubalina infection of Eucalyptus diversicolor (karri) in Western Australia, with no increased growth rate of trees retained after thinning (Robinson, 2003). In P. radiata plantations in New Zealand thinning also increased stand-level infection of retained trees by A. novae-zelandiae partly through infection from stumps, however the incidence of infection diminished as the stumps decomposed, leaving no effect of thinning after 6 years (Hood et al., 2002; Hood and Kimberley, 2009).
Studies of other species of root rot also predominantly show an increase in infection with thinning. In 15 year old P. menziesii stands in northern California, incidence of L. wageneri was much higher in thinned than in unthinned stands (Harrington et al., 1983). This finding was confirmed in a much more extensive survey of P. menziesii plantations in southwest Oregon, which found that incidence of L. wageneri was significantly higher in thinned than unthinned stands, though this effect was not apparent in all the studied forests (Hessburg, 2001). In an experimental study of P. abies stands in Sweden, the probability of stump infection was much higher following thinning in the summer than the winter (Thor and Stenlid, 2005). This pattern persisted following a second thinning of these plots (Oliva et al., 2010). However, infection rates following summer thinning were greatly reduced by a range of chemical and biological (spores of P. gigantea) treatments of the cut stumps (Thor and Stenlid, 2005), and plots with stumps treated with urea had much lower overall mortality (Oliva et al., 2008). Only a single study recorded a decrease in infection following thinning, with reduced mortality of P. ponderosa due to L. wageneri 10 years after experimental thinning in north-eastern California (Otrosina et al., 2007). Thinning may also be accompanied by measures to remove root fragments, particularly where thinning was carried out with the intention of tackling root rots. However, intensive root removal can be associated with wounding the roots of retained trees, which increased the risk of infection of P. tremuloides by Armillaria spp. (Pankuch et al., 2003).
Thinning has highly variable effects on tree diseases besides root rots. The most frequently studied pathogens infecting tree shoots have been dothistroma needle blight of Pinus spp. caused by D. septosporum and D. pini. A comprehensive review of management and control of these pathogens was provided by Bulman et al. (2016). They found that in Australia, Chile, New Zealand and USA, reducing stand density by thinning reduced disease levels. However, ongoing experiments in the generally wetter climates of Great Britain and British Columbia have not shown a notable effect on disease incidence. No benefits were found of thinning for control of these pathogens, or Lecanosticta acicula, in P. radiata plantations in northern Spain (de Urbina et al., 2017). Thinning was reported to reduce damage of P. contorta var. latifolia due to E. harknessii across 27 plantations in southeastern British Columbia (Roach et al., 2015). However, no significant effect of thinning of P. contorta var. latifolia on incidence of E. harknessii infection had been found in a previous multi-site study in British Columbia, though thinning was associated with a large increase in the incidence of infection by stalactiform blister rust (Cronartium coleosporioides; van der Kamp, 1994). Similarly, in Idaho, thinning was related to an increase in the number of new lethal infections per tree of Pinus monticola (western white pine) by C. ribicola 5 years after treatment (Hungerford et al., 1982). In an experiment in a forest in Missouri, USA, where a range of oak species are subject to “oak decline” that may be caused by a range of root pathogens or insects, thinning in the form of “improvement harvests” (selective cutting to remove trees that were declining and to reduce tree density) did not significantly alter the incidence of oak decline after 10 (Meadows et al., 2013) or 14 years (Dwyer et al., 2007; Meadows et al., 2013).
As well as being a legacy of the harvesting of mature trees, stumps are also present throughout growing stands as a result of thinning operations. Chemical or biological treatment of stumps resulting from thinning can be effective at reducing pathogen incidence, as is the case for final harvest tree stumps (section Site Preparation). In Sweden, following thinning, the proportion of P. abies stump area colonized by H. annosum after 6–7 weeks was reduced by 88–99% in stumps treated with either 35% urea solution, 5% disodium octaborate tetrahydrate solution or spores of Phlebiopsis gigantea, compared with untreated stumps (Thor and Stenlid, 2005).
In the majority of cases, forests that have undergone thinning have a higher incidence of tree disease than unthinned sites. However, results are variable, even within the same site, pathogen or tree species. Such variation likely arises not from thinning itself, but from other changes within the forest associated with thinning regimes. Pathogen loads can increase due to increased movement of machinery and human vectors into a forest to carry out thinning (Jules et al., 2002; Cushman and Meentemeyer, 2008; Goheen et al., 2012) and wounding of stems and roots of retained trees that can provide entry points for pathogens. More complex effects can be mediated by changes in forest species composition and structural composition resulting from thinning. Therefore, to provide a more robust basis for management recommendations, future studies should focus on identifying and accounting for the sources of this variation, and determining how impacts vary during the course of a pathogen invasion.
Removal of diseased trees is often one of the criteria applied for tree selection in thinning of diseased stands. In some cases it is the sole focus of a control programme, either restricted to trees already showing disease symptoms or extended to trees considered to be at high risk of infection, e.g., because of their species and proximity to diseased trees. The effectiveness of this measure has been assessed in a number of studies, though not through rigorous experimentation. Examples include the spatial spread of Dutch elm disease (Ophiostoma novo-ulmi) in New Zealand (Ganley and Bulman, 2016) and Gotland Island, Sweden (Menkis et al., 2016), and P. ramorum in the coastal forests of Oregon (Kanaskie et al., 2006). In 35-year-old coppiced Castanea sativa (sweet chestnut) in Italy, thinning that targeted the cutting of infected stems did result in a reduction in the severity of damage due to C. parasitica 2 years later (Amorini et al., 2001). In all of these studies, while some evidence was found that removal of infected and adjacent trees slowed the spread of the pathogen, it had only delayed, rather than prevented, eventual infection. The cryptic nature of the pathogens that prevents sufficiently early identification of infected trees to enable their removal before they become a source of inoculum, and the occasional occurrence of long-distance inoculum dispersal events through vectors, e.g., human or animal movement, are among the major constraints. The potential for removal of diseased trees to disrupt natural biocontrol, e.g., hypovirulence of C. parasitica caused by virus infection of the fungus, merits future research.
Pruning of lateral branches is usually carried out to improve timber quality by reducing knots in the subsequent radial wood growth. Analogous to thinning, pruning may also be carried out to reduce pathogen incidence by targeting infected or susceptible damaged branches or to reduce sub-canopy humidity in the forest. However, pruning wounds also create potential sites for pathogen entry and, as with thinning, pruning operations may increase traffic and potential of cross-infection on tools, acting as vectors of pathogens.
Positive impacts of pruning Pinus spp. on resistance to C. ribicola rust have been largely consistent across North America. In Idaho pruning of P. monticola in addition to thinning greatly reduced the total number of new lethal and non-lethal infections per tree after 5 years compared with thinning-only treatment and controls (Hungerford et al., 1982). Incidence and severity of C. ribicola infection of young P. strobus was reduced by preventative pruning of susceptible lower branches in sites across the eastern USA (Ostry, 2000). Pruning of infected branches of P. strobus also reduced the incidence of disease and tree mortality in Quebec, Canada (Lavallee, 1991).
For other pathogens, results of pruning have not been so positive. Pruning increased F. circinatum canker symptoms in P. radiata plantations in Cantabria, Spain, which was attributed to the role of pruning wounds in permitting the pathogen to infect the tree (Bezos et al., 2012). Pruning increased D. pinea infection in P. radiata trees in New Zealand that were experimentally inoculated, with a large increase in infection rates with intensity of pruning (percentage of crown removed; Chou and MacKenzie, 1988). Infection by D. pinea and by Seiridium cardinale was also positively associated with pruning of Cupressus sempervirens (cypress) trees in Israel (Madar et al., 1991). No effect of pruning was observed on control of D. septosporum, D. pini, or L. acicula in P. radiata plantations in northern Spain (de Urbina et al., 2017), nor Armillaria spp. infection in New Zealand P. radiata plantations (Hood et al., 2002). For stands of P. abies in Baden-Württemburg, Germany, careful pruning of branches up to 10 m height was found to produce only a low risk of wood deterioration, however it did lead to an increase in heartwood infection by a range of pathogens, especially Nectria fuckeliana (Metzler, 1997). The review of studies in Australia and New Zealand on Dothistroma spp. infection of Pinus spp. by Bulman et al. (2016) reported mixed results of pruning, particularly beyond short-term impacts.
Shoot removal to reduce multiple stems to a single stem was carried out on Acacia mangium and A. crassicarpa plantation trees in South Africa, and was followed by experimental inoculation by pathogenic Ceratocystis acaiivora and Lasiodiplodia theobromae fungi (Tarigan et al., 2011). Careful pruning resulted in reduced lesion size compared with trees pruned less carefully, causing tearing of the bark, which suffered infection from naturally spreading spores even if not inoculated. Pathogen impacts are of particular concern for short-rotation coppice systems with fast-growing trees that are particularly susceptible to infection. In an experiment in Northern Ireland, McCracken and Dawson (2003) found that coppicing produced mixed results amongst genotypes of Salix spp. In one genotype, levels of the M. epitea rust pathogen were much higher in the first 3-year harvest cycle than during the second cycle. However, for a number of other genotypes, M. epitea infection was more severe on the regrowth from freshly coppiced stools.
Phytophthora ramorum is able to persist in, and produce spores from, resprouted stumps (effectively increasing the inoculum load available for secondary infection within a plantation thus generating secondary infection). A benefit of sprout cutting was shown, as isolation of P. ramorum from the sprouts growing from cut stumps of U. californica was reduced in sprouts that had been cut 1-year previously compared with those left to grow for 7 years, however there was no treatment effect for sprouts growing on stumps of N. densiflorus (Valachovic et al., 2013a).
Pruning, coppicing and shoot removal have a highly variable impact on resilience of forests to tree pathogens. Some of the literature on the subject points to an increase in susceptibility caused by pruning wounds and increased vector or air movement of the pathogen within the forest. However, other studies show a decrease in susceptibility to some pathogens, linked to removal of susceptible branch material and reduced sub-canopy humidity. There is a lack of experimental studies that enable testing of these mechanisms and their trade-offs. While pruning is less common as a forest management practice than is thinning, it should be a priority for future studies. There is good potential to link knowledge of the effects of pruning practice on tree pathogens in arboriculture with the evidence required to inform forest management. A priority is to understand more about what controls the risk of entry into pruning wounds of the main airborne pathogens of commercial tree species.
The processes described above in terms of primary and secondary infection capture the first element of forest resilience, its resistance to an invading pathogen. The second element, the capacity of the forest to recover, is discussed in this section. As explained above, we considered rates of tree growth and natural regeneration following the onset of pathogen infection, which were the only measures of the recovery of the forest ecosystem reported in the reviewed studies. Within our working definition of resilience, we did not include changes in pathogen inoculum or infection level in the ecosystem as measures of recovery, in order to avoid mixing up “cause” and “effect.” The capacity for forest ecosystem recovery can be assessed over a wide range of temporal and spatial scales. For entire managed forests it is extremely likely that, over the long term, the decisions of forest managers will be crucial in determining the rate and trajectory of forest recovery. Gibbs et al. (2002) provide an insightful account of how successive generations of managers have experimented and adapted the management of a forest (Thetford, UK) to promote recovery and longer-term forest resilience to the threat posed by H. annosum. A number of studies have investigated at a smaller and shorter-term scale the effect of individual management actions on the capacity for forest recovery from pathogen impacts through natural regeneration or tree growth. They have researched some of the forest management options already considered in terms of their impact on primary and secondary infection (above), and we review their evidence on forest recovery below, with a summary in Table 2.
In a large experiment in British Columbia forests subject to infection by A. ostoyae and P. sulphurascens, mixed species plots had lower basal area after 40 years growth compared with monocultures, but the effect on stem diameter and on dominant tree height was variable (Morrison et al., 2014). Interpretation of other experimental studies in British Columbia crosses over between the effects of species mixture and of thinning as a treatment. In British Columbia forests where P. menziesii is subject to infection by A. ostoyae, mean diameter increment and height:diameter ratio increased significantly in stands where B. papyrifera was removed or partly thinned (Baleshta et al., 2005). This was linked to increases in light and soil moisture levels caused by the thinning. In higher altitude British Columbia forests infested with A. ostoyae, the diameter growth of planted P. menziesii was 27% greater after experimental removal of the naturally regenerated broadleaves B. papyrifera and P. tremuloides compared with untreated controls, and the increase was greater with higher intensity removal treatments, however height growth was not significantly affected (Gerlach et al., 1997; Baleshta et al., 2005; Simard et al., 2005). In a second experiment, diameter growth of P. contorta var. latifolia (but not P. menziesii) was increased by removal of broadleaves. These results indicate the importance of the identity of tree species in a mixture for tree growth recovery from pathogen infection.
In a complex mixture experiment of many Salix spp. varieties in a short rotation coppice system in Northern Ireland subject to the M. epitea rust pathogen, tree growth rate was invariably greater in mixtures compared with monoculture, even when a majority of varieties in the mixture were killed by the pathogen (McCracken et al., 2001). However, in a subsequent experimental study of Salix viminalis genotypes subject to infection by M. epitea, whilst at the harvest at the end of the first 3-year growth cycle mixtures showed a higher yield compared with monocultures, this difference did not persist to the harvest at the end of the second cycle (Begley et al., 2009).
Inoculum removal prior to planting has had mixed effects on subsequent tree growth. A large-scale experiment in a British Columbia forest infested with the root pathogens P. sulphurascens and A. ostoyae showed that stump removal increased plot basal area by an average of 1.3 times after 40 years of growth of the rotation following treatment, and increased dominant tree height, but did not alter stem diameter (Morrison et al., 2014). In forests infested by P. sulphurascens in Washington State and Oregon, pre-planting stump removal produced mixed results on the growth of P. menziesii (Thies and Westlind, 2005). It increased seedling height in two study sites, and reduced the final measured volume at one site, but there were no significant effects at the other sites studied.
There are insufficient studies of alternative silvicultural systems to draw any clear conclusions about the implications for recovery. In P. radiata forest infected by F. circinatum in California, gap size was positively associated with seedling height and diameter growth rates, showing a pattern that did not correspond to that of the variation in disease incidence with gap size (Ferchaw et al., 2013). In mixed conifer forest in Oregon subject to infection by A. ostoyae, experimental harvesting treatments of group selection and shelterwood were compared with unharvested forest (Filip et al., 2010). Diameter growth of retained trees 10 years after infection by A. ostoyae was not significantly altered by the silvicultural harvesting treatments, and there were no consistent effects on the density of natural regeneration amongst species.
In a P. monticola forest infected by C. ribicola in Washington State, tree height at 16 years of age was not significantly affected by tree spacing over a range from 3 to 5 m, however it was lower with very close spacing (2 m) and very wide spacing (6 m) (Bishaw et al., 2003). Tree diameter increased with spacing from 2 to 5 m. Plot basal area and volume decreased with spacing over the whole range from 2 to 6 m. Thus, response to variation in tree density differed amongst measures of forest growth. Results from this single study of one tree-pathogen species combination do not provide a sufficient basis for any generalization.
Studies of thinning impacts have consistently shown that it results in increased tree growth rates in infected stands sufficient to promote forest recovery. In P. radiata plantations in New Zealand subject to severe infection by A. novae-zelandiae, thinning treatments were followed by long-term increase in growth (and assumed associated resistance) in the retained trees at a level sufficient to counter the effects of increased inoculum potential following treatment (Hood and Kimberley, 2009). As a result, it is expected that thinning of these diseased stands will not lead to any reduction in final crop volume. Similarly, in coppiced C. sativa forest infected by C. parasitica in Italy, a thinning treatment that targeted the cutting of infected stems increased the growth rate of the retained stems, resulting in the same stand volume growth rate as pre-thinning (Amorini et al., 2001). In Mississippi, USA, where red oaks (Quercus spp.) are infected with the canker decay fungus Inonotus hispidus, experimental improvement thinning that removed smaller and diseased trees significantly increased the diameter growth of the retained trees (Meadows et al., 2013).
One study was notable for providing evidence of natural regeneration as a process of forest recovery, but its results were mixed. In British Columbia selective cutting, a silvicultural treatment somewhat akin to thinning, in forests infested with A. ostoyae, resulted in a large increase in rates of subsequent natural regeneration of a range of conifer species, compared with uncut control plots, but in only two of the four studied forest sites. Less than 30% of the naturally regenerated trees were killed by the pathogen in these two sites (Morrison et al., 2001).
Published studies on forest resilience to tree diseases have uneven coverage with regard to geographical locations, management options, and pathogen species. The majority of studies have been restricted to a single forest area, while larger-scale studies often find inconsistent results across locations. This patchy coverage, and a lack of detail in reporting of the management options tested or the scale of their effects, hampers our ability to produce a systematic assessment of the similarities and differences in the impacts of management on tree resilience to different tree pathogens. Insufficient evidence is provided to enable comparison of effectiveness between options. Individual studies are limited to considering a single, or small number of related, pathogens, and therefore do not provide an adequate evidence base for forest managers who need to decide how best to increase forest resilience against multiple known and unknown future threats. Determining general conclusions to best inform forest management in the face of such a diversity of (and likely increasing pressure from) future pathogen risks is therefore challenging. Most management actions have been responsive, seeking to combat specific pathogens that are either established in a forest or new outbreaks after they have reached a region. Interventions thus tend to focus on reducing sources of inoculum or the rate of secondary infection, including the transmission of inoculum and the susceptibility of trees. Because most studies have researched forests managed for timber production, their evidence should not simply be extrapolated to forests managed for other benefits (for which different measures of resilience, linked to other ecosystem services, would be more relevant).
Although the published studies included in this review were very uneven in their coverage and did not produce consistent results, they provided stronger evidence of the benefit of certain management options for forest resilience to pathogens. The reduction of primary infection by limiting the connectivity of forest units and by the removal or treatment of stumps during site preparation, and the reduction of secondary infection by planting mixed species forests, are the management options with the strongest evidence for improving forest resilience to pathogens. Despite this, in each case the effects are strongly modified by the particular methods used and tree species involved, so this evidence can only be taken as a first indication to inform management decision-making. Forest managers must also consider the scale of the effect of each management option and trade-offs with other impacts on the forest system, such as the effects on environmental conditions and thus tree health and vigor.
Commercial timber production is the dominant management objective in the studied forests, with the need to reduce the risk to this posed by tree pathogens and pests increasingly recognized. However, even in commercial forests biodiversity conservation is also an increasingly important objective. Therefore, the potential negative impacts of connectivity on risk of spread of pathogens and pests needs to be weighed against the demonstrated benefits of higher connectivity for biodiversity (Lindenmayer et al., 2006). For many tree pathogens, connectivity through vehicular and human movement may be more significant than a continuity of habitat cover, depending on how specific pathogens spread. Therefore, controlling primary infection rate via human vectors may provide a reduction in functional connectivity for some pathogens, with little impact on other organisms. Stump removal as a method to reduce sources of pathogen innocuum also presents an interesting trade-off with habitat management for the benefit of biodiversity. Dead wood such as stumps are recognized as important habitat components for a wide range of forest biodiversity, including many forest specialist species (Hartley, 2002). Providing adequate evidence to inform the management response to this trade-off is also a research priority.
Perhaps the most consistent finding of the reviewed research, supported by multiple studies across a range of pathogen species, although still not ubiquitous, is that the rate of secondary infection of trees of a given species is reduced if these trees are growing in a mixed, species-diverse forest rather than a monoculture. In this sense, higher species diversity has an insurance value against future income risks due to disease, which will be positively valued by risk-averse forest managers (Finger and Buchmann, 2015). However, this outcome depends on the species within the mixture, with only non-host species increasing forest resilience to pathogens. In fact, the presence of secondary host species can make infection worse and may increase the rate of primary infection (Power and Mitchell, 2004). This finding points to a need to extend the importance of tree species selection in strategies to combat tree diseases. Beyond considering the susceptibility of candidate primary crop species to pathogen species known to be present in the region (or at risk of arriving during the forest rotation), forest managers also need to consider the potential role of each tree species in a mixed forest for how it may influence the risk of infection (by a broad range of pathogens) of the other crop species present.
In seeking to achieve the most economically efficient solution to exploiting the benefits of higher tree species diversity for increasing forest system resilience to tree pathogens, a key consideration is the spatial scale at which such mixing occurs. If the economic benefit of including within the forest a portfolio of different tree crop species can be achieved by establishing large monoculture blocks of each species, then this may only cause a small increase in management costs compared with a whole-forest monoculture. However, knowledge of ecological mechanisms would suggest that the larger the monoculture blocks the smaller will be the ecological benefit through diluting the individual trees of susceptible species. It is also possible that resilience of susceptible trees is increased due to interaction with other tree species, which is unlikely to occur if mixing takes place only at the landscape scale (Bauhus et al., 2017). It is therefore striking that we found no studies reporting results on the effect of the spatial scale of tree species mixing on rates of pathogen infection. Similarly, for the studies that researched at a landscape scale, we did not find any that reported the effects of the heterogeneity (e.g., in tree species composition) between adjacent forest patches. These evidence gaps limit our ability to draw detailed conclusions regarding how mixed-species forests should be designed to maximize this benefit, and we therefore identify these as high priorities for future research.
With reference to recovery of forest ecosystems, there are a number of studies of the effects of silvicultural treatments on the growth of mostly conifer crop trees in pathogen-infested forests. The results generally indicate that tree growth increased following silvicultural treatments, irrespective of the fact that the studies were carried out in forests where the trees were subject to pathogen infection. However, there are very few studies reporting on the effect of silvicultural treatments on forest recovery through natural regeneration.
The findings of this review have several implications for epidemiological modeling of emergence, spread, and persistence of tree pathogens and for capturing the resilience of forests in response to such threats. However, we identified many important evidence gaps in the empirical literature that should be a priority for new primary research to fill. Our review aimed at providing a foundation for linking the processes and parameters used in models, specifically the epidemiological components of primary and secondary infection, and the ecological components of forest recovery, to the published observational and experimental data. This has several important implications for this area of modeling. Firstly, while our review showed the importance of tree species mixture effects, most models consider a forest comprising only a single host species (Kleczkowski et al., 2019). Secondly, our review showed the importance of connectivity between forest units. While there has been significant progress in recent years on spatial and meta-population modeling of plant pathogens, to our knowledge these have not yet been combined with ecological models to improve understanding of the trade-offs between the benefits of connectivity for biodiversity (Lindenmayer et al., 2006) and the reduction of pathways for pathogens to spread between and within forests. Thirdly, in models the effects of management options on epidemiological processes are often described as a simple reduction of primary or secondary infection rate. In reality, many of these processes may involve non-linearities and threshold behavior, though these have not been thoroughly studied. Fourthly, our findings on the importance of persistent inoculum for certain pathogens, from reservoirs such as tree stumps and root fragments of felled trees, suggest that infection rates in a forest unit may be “path-dependent,” for example on the forest unit's history of infection and control options adopted, with implications for the structure of models. Fifthly, the implications of the reviewed empirical studies for epidemiological modeling are limited by their generally small sample size and, in many cases, weakness in the capacity of the experimental design to test the influence of environmental variables and interaction effects.
We hope that this paper will contribute to a dialogue between forest managers and ecologists on one hand and epidemiological and bioeconomic modelers on the other, to establish criteria for experimentation that can be used to better parameterize models and rigorously test their results.
All datasets generated for this study are included in the article/Supplementary Material.
JH conceived the study. AK, NH, CG, and JH acquired funding for the project. JH and MR designed the study. MR carried out data collection, performed the analysis and led the drafting of the manuscript, with input from JH. All authors discussed and interpreted the results and contributed to the writing of the final manuscript.
This work is from the project titled Modeling economic impact and strategies to increase resilience against tree disease outbreaks. This is one of seven projects in the Tree Health and Plant Biosecurity Initiative (phase 2) funded by BBSRC, Defra, ESRC, Forestry Commission, NERC, and Scottish Government. The Rural & Environment Science & Analytical Services Division of the Scottish Government provided supporting capacity to MR for final editing of the paper.
The authors declare that the research was conducted in the absence of any commercial or financial relationships that could be construed as a potential conflict of interest.
The authors thank the other members of the FOREMOD project team (Chris Quine, Morag Macpherson, Ciara Dangerfield, and Oleg Sheremet) for valuable discussions and insights which have contributed to the development of this paper in many ways.
The Supplementary Material for this article can be found online at: https://www.frontiersin.org/articles/10.3389/ffgc.2020.00007/full#supplementary-material
Amorini, E., Manetti, M. C., Turchetti, T., Sansotta, A., and Villani, F. (2001). Impact of silvicultural system on Cryphonectria parasitica incidence and on genetic variability in a chestnut coppice in Central Italy. For. Ecol. Manag. 142, 19–31. doi: 10.1016/S0378-1127(00)00337-6
Anglberger, H., and Halmschlager, E. (2003). The severity of Sirococcus shoot blight in mature Norway spruce stands with regard to tree nutrition, topogrpahy and stand age. For. Ecol. Manag. 177, 221–230. doi: 10.1016/S0378-1127(02)00440-1
Baleshta, K. E., Simard, S. W., Guy, R. D., and Chanway, C. R. (2005). Reducing paper birch density increases Douglas-fir growth rate and Armillaria root disease incidence in southern interior British Columbia. For. Ecol. Manag. 208, 1–13. doi: 10.1016/j.foreco.2004.07.076
Bauhus, J., Forrester, D., Gardiner, B., Jactel, H., Vallejo, R., and Pretzsch, H. (2017). “Ecological stability of mixed-species forests,” in Mixed-Species Forests - Ecology and Management, eds H. Pretzsch, D. I. Forrester, and J. Bauhus (Verlag: Heidelberg), 337–382. doi: 10.1007/978-3-662-54553-9_7
Bauman, J. M., Keiffer, C. H., and McCarthy, B. C. (2014). Growth performance and chestnut blight incidence (Cryphonectria parasitica) of backcrossed chestnut seedlings in surface mine restoration. N. Forests 45, 813–828. doi: 10.1007/s11056-014-9439-3
Begley, D., McCracken, A. R., Dawson, W. M., and Watson, S. (2009). Interaction in Short Rotation Coppice willow, Salix viminalis genotype mixtures. Biomass Bioenergy 33, 163–173. doi: 10.1016/j.biombioe.2008.06.001
Beh, M. M., Metz, M. R., Frangioso, K. M., and Rizzo, D. M. (2012). The key host for an invasive forest pathogen also facilitates the pathogen's survival of wildfire in California forests. N Phytol. 196, 1145–1154. doi: 10.1111/j.1469-8137.2012.04352.x
Bendz-Hellgren, M., and Stenlid, J. (1998). Effects of clear-cutting, thinning, and wood moisture content on the susceptibility of Norway spruce stumps to Heterobasidion annosum. Canad. J. Forest Res. 28, 759–765. doi: 10.1139/x98-043
Benedict, W. V. (1981). History of White Pine Blister Rust Control - A Personal Account. Washington, DC: Department of Agriculture Forest Service.
Bergdahl, D. R., Sendak, P. E., Tobi, D. R., Bove, J. R., and Tritton, L. M. (2002). Incidence of annual canker of sugar maple studied with the aid of a geographic information system. North. J. Appl. Forest. 19, 73–79. doi: 10.1093/njaf/19.2.73
Bezos, D., Lomba, J. M., Martinez-Alvarez, P., Fernandez, M., and Diez, J. J. (2012). Effects of pruning in Monterrey pine plantations affected by Fusarium circinatum. Forest Syst. 21, 481–488. doi: 10.5424/fs/2012213-02262
Bishaw, B., DeBell, D. S., and Harrington, C. A. (2003). Patterns of survival, damage, and growth for western white pine in a 16-year-old spacing trial in western Washington. West. J. Appl. Forest. 18, 35–43. doi: 10.1093/wjaf/18.1.35
Blodgett, J. T., Kruger, E. L., and Stanosz, G. R. (1997). Sphaeropsis sapinea and water stress in a red pine plantation in central Wisconsin. Phytopathology 87, 429–434. doi: 10.1094/PHYTO.1997.87.4.429
Boyd, I. L., Freer-Smith, P. H., Gilligan, C. A., and Godfray, H. C. (2014). The consequence of tree pests and diseases for ecosystem services. Science 342:1235773. doi: 10.1126/science.1235773
Brassett, P. R., and Gilligan, C. A. (1988). A model for primary and secondary infection in botanical epidemics. Zeitschrift für Pflanzenkrankheiten und Pflanzenschutz 95, 352–360.
Bulman, L. S. (2008). Pest detection surveys on high-risk sites in New Zealand. Austr. Forest. 71, 242–244. doi: 10.1080/00049158.2008.10675042
Bulman, L. S., Bradshaw, R. E., Fraser, S., Martin-Garcia, J., Barnes, I., Musolin, D. L., et al. (2016). A worldwide perspective on the management and control of Dothistroma needle blight. Forest Pathol. 46, 472–488. doi: 10.1111/efp.12305
Burdon, J. J., Wennstrom, A., Ericson, L., Muller, W. J., and Morton, R. (1992). Density dependent mortality in Punis sylvestris cause by snow blight pathogen Phacidium infestans. Oecologia 90, 74–79. doi: 10.1007/BF00317811
Campbell, E. A., and Antos, J. (2000). Distribution and severity of white pine blister rust and mountain pine beetle on whitebark pine in British Colombia. Canad. J. Forest Res. 30, 1051–1059. doi: 10.1139/x00-020
Carey, M., Sanderson, B., Barnas, K., and Olden, J. (2012). Native invaders - challenges for science, management, policy, and society. Front. Ecol. Environ. 10, 373–381. doi: 10.1890/110060
Chou, C. M., and MacKenzie, M. (1988). Effect of pruning intensity and season on Diplodia pinea infection of Pinus radiata stem through pruning wounds. Euro. J. Forest Pathol. 18, 437–444. doi: 10.1111/j.1439-0329.1988.tb00233.x
Cleary, M. R., Arhipova, N., Morrison, D. J., Thomsen, I. M., Sturrock, R. N., Vasaitis, R., et al. (2013). Stump removal to control root disease in Canada and Scandinavia: a synthesis of results from long-term trials. For. Ecol. Manag. 290, 5–14. doi: 10.1016/j.foreco.2012.05.040
Condeso, T. M., and Meentemeyer, R. K. (2007). Effects of landscape heterogeneity on the emerging forest disease sudden oak death. J. Ecol. 95, 364–375. doi: 10.1111/j.1365-2745.2006.01206.x
Cram, M. S., Shea, D., and Forbus, K. (2010). “Case study to examine the effects of a growing-season burn and Annosum root disease on mortality in a longleaf pine stand,” in Proceedings of the 14th Biennial Souther Silivicultural Research Conference (Asheville, NC).
Crone, M., McComb, J. A., O'Brien, P. A., and Hardy, G. E. S. (2014). Host removal as a potential control method for Phytophthora cinnamomi on severely impacted black gravel sites in the jarrah forest. Forest Pathol. 44, 154–159. doi: 10.1111/efp.12080
Cushman, J. H., and Meentemeyer, R. K. (2008). Multi-scale patterns of human activity and the incidence of an exotic forest pathogen. J. Ecol. 96, 766–776. doi: 10.1111/j.1365-2745.2008.01376.x
Davidson, J. M., Patterson, H. A., Wickland, A. C., Fichtner, E. J., and Rizzo, D. M. (2011). Forest type influences transmission of Phytophthora ramorum in California Oak Woodlands. Phytopathology 101, 492–501. doi: 10.1094/PHYTO-03-10-0064
de Urbina, E. O., Mesanza, N., Aragones, A., Raposo, R., Elvira-Recuenco, M., Boque, R., et al. (2017). Emerging needle blight diseases in atlantic pinus ecosystems of Spain. Forests 8:18. doi: 10.3390/f8010018
Desprez-Loustau, M. L., Aguayo, J., Dutech, C., Hayden, K. J., Husson, C., Jakushkin, B., et al. (2016). An evolutionary ecology perspective to address forest pathology challenges of today and tomorrow. Ann Forest Sci. 73, 45–67. doi: 10.1007/s13595-015-0487-4
Desprez-Loustau, M. L., and Wagner, K. (1997). Influence of silvicultural practices on twisting rust infection and damage in maritime pine, as related to growth. For. Ecol. Manag. 98, 135–147. doi: 10.1016/S0378-1127(97)00079-0
Dillon, W. W., Haas, S. E., Rizzo, D. M., and Meentemeyer, R. K. (2014). Perspectives of spatial scale in a wildland forest epidemic. Euro. J. Plant Pathol. 138, 449–465. doi: 10.1007/s10658-013-0376-3
D'Souza, N. K., Colquhoun, I. J., Shearer, B. L., and Hardy, G. E. S. (2004). The potential of five Western Australian native Acacia species for biological control of Phytophthora cinnamomi. Austr. J. Bot. 52, 267–279. doi: 10.1071/BT03089
Dwyer, J. P., Kabrick, J. M., and Wetteroff, J. (2007). Do improvement harvests mitigate oak decline in Missouri Ozark forests? North. J. Appl. Forest. 24, 123–128. doi: 10.1093/njaf/24.2.123
Ellis, A. M., Vaclavik, T., and Meentemeyer, R. K. (2010). When is connectivity important? A case study of the spatial pattern of sudden oak death. Oikos 119, 485–493. doi: 10.1111/j.1600-0706.2009.17918.x
Entry, J. A., Cromack, K., Kelsey, R. G., and Martin, N. E. (1991). Response of douglas-fir to infection by Armillaria ostoyae after thinning or thinning plus fertilisation. Phytopathogy 81, 682–689. doi: 10.1094/Phyto-81-682
Ferchaw, V. A. L., Goldsworthy, E., Pinkerton, J., Yun, D. I., Lund, U. J., Mark, W., et al. (2013). Management strategies for pitch canker infected Ano Nuevo stands of Monterey pine. For. Ecol. Manag. 308, 101–115. doi: 10.1016/j.foreco.2013.07.032
Fichtner, E. J., Rizzo, D. M., Kirk, S. A., and Webber, J. F. (2011). Root infections may challenge management of invasive Phytophthora spp. in UK Woodlands. Plant Dis. 95, 13–18. doi: 10.1094/PDIS-03-10-0236
Filip, G. M., Ganio, L. M., Oester, P. T., Mason, R. R., and Wickman, B. E. (2002). Ten-year effect of fertilization on tree growth and mortality associated with armillaria root disease, fir engravers, dwarf mistletoe, and western spruce budworm in Northeastern Oregon. West. J. Appl. Forest. 17, 122–128. doi: 10.1093/wjaf/17.3.122
Filip, G. M., Maffei, H. M., Chadwick, K. L., and Max, T. A. (2010). Armillaria root disease-caused tree mortality following silvicultural treatments (shelterwood or group selection) in an oregon mixed-conifer forest: insights from a 10-year case study. West. J. Appl. Forest. 25, 136–143. doi: 10.1093/wjaf/25.3.136
Finger, R., and Buchmann, N. (2015). An ecological economic assessment of risk-reducing effects of species diversity in managed grasslands. Ecol. Econ. 110, 89–97. doi: 10.1016/j.ecolecon.2014.12.019
Ganley, R. J., and Bulman, L. S. (2016). Dutch elm disease in New Zealand: impacts from eradication and management programmes. Plant Pathol. 65, 1047–1055. doi: 10.1111/ppa.12527
Gerlach, J. P., Reich, P. B., Puettmann, K., and Baker, T. (1997). Species, diversity, and density affect tree seedling mortality from Armillaria root rot. Canad. J. Forest Res. 27, 1509–1512. doi: 10.1139/x97-098
Ghelardini, L., Luchi, N., Pecori, F., Pepori, A. L., Danti, R., Della Rocca, G., et al. (2017). Ecology of invasive forest pathogens. Biol. Invas. 19, 3183–3200. doi: 10.1007/s10530-017-1487-0
Gibbs, J. N., Greig, B. J. W., and Pratt, J. E. (2002). Fromes root rot in thetford forest, east anglia: past, present and future. Forestry 75, 191–202. doi: 10.1093/forestry/75.2.191
Goheen, D. J., Mallams, K., Betlejewski, F., and Hansen, E. (2012). Effectiveness of vehicle washing and roadside sanitation in decreasing spread potential of port-orford-cedar root disease. West. J. Appl. Forest. 27, 170–175. doi: 10.5849/wjaf.11-011
Greene, T. A., Reemts, C. M., and Appel, D. N. (2008). Efficacy of basal girdling to control oak wilt fungal mat production in Texas Red Oak (Quercus buckleyi) in Central Texas. South. J. Appl. Forest. 32, 168–172. doi: 10.1093/sjaf/32.4.168
Grimm, V., and Wissel, C. (1997). Babel, or the ecological stability discussions: an inventory and analysis of terminology and a guide for avoiding confusion. Oecologia 109, 323–334. doi: 10.1007/s004420050090
Haas, S. E., Cushman, J. H., Dillon, W. W., Rank, N. E., Rizzo, D. M., and Meentemeyer, R. K. (2016). Effects of individual, community, and landscape drivers on the dynamics of a wildland forest epidemic. Ecology 97, 649–660. doi: 10.1890/15-0767.1
Haas, S. E., Hooten, M. B., Rizzo, D. M., and Meetenmeyer, R. K. (2011). Forest species diversity reduces disease risk in a generalist plant pathogen invasion. Ecol. Lett. 14, 1108–1116. doi: 10.1111/j.1461-0248.2011.01679.x
Hagle, S. K., and Grasham, J. L. (1988). Biological and Economic Feasibility of Pruning and Excising White Pines for Blister Rust Control. United States Department of Agriculture Forest Service.
Halmschlager, E., and Katzensteiner, K. (2017). Vitality fertilisation balanced tree nutrition and mitigated severity of Sirococcus shoot blight on mature Norway spruce. For. Ecol. Manag. 389, 96–104. doi: 10.1016/j.foreco.2016.12.019
Hannerz, M., and Hanell, B. (1997). Effects on the flora in Norway spruce forests following clearcutting and shelterwood cutting. For. Ecol. Manag. 90, 29–49. doi: 10.1016/S0378-1127(96)03858-3
Hantsch, L., Bien, S., Radatz, S., Braun, U., Auge, H., and Bruelheide, H. (2014a). Tree diversity and the role of non-host neighbour tree species in reducing fungal pathogen infestation. Journal of Ecology 102, 1673–1687. doi: 10.1111/1365-2745.12317
Hantsch, L., Braun, U., Haase, J., Purschke, O., Scherer-Lorenzen, M., and Bruelheide, H. (2014b). No plant functional diversity effects on foliar fungal pathogens in experimental tree communities. Fung. Divers. 66, 139–151. doi: 10.1007/s13225-013-0273-2
Hantsch, L., Braun, U., Scherer-Lorenzen, M., and Bruelheide, H. (2013). Species richness and species identity effects on occurrence of foliar fungal pathogens in a tree diversity experiment. Ecosphere 4, 1–12. doi: 10.1890/ES13-00103.1
Harrington, T. C., Reinhart, C., Thornburgh, D. A., and Cobb, F. W. Jr. (1983). Association of black-stain root disease with precommercial thinning of Douglas-fir. Forest Sci. 29, 12–14.
Hartley, M. J. (2002). Rationale and methods for conserving biodiversity in plantation forests. For. Ecol. Manag. 155, 81–95. doi: 10.1016/S0378-1127(01)00549-7
Havdova, L. Z. D., Romportl, D., Peskova, V., and Cerny, K. (2017). Environmental and silvicultural characteristics influencing the extent of ash diebakc in forest stands. Baltic Forest. 23, 168–182.
Hessburg, P. (2001). Association of black stain root disease with roads, skid trails, and precommercial thinning in southwest Oregon. West. J. Appl. Forest. 16, 127–135. doi: 10.1093/wjaf/16.3.127
Heybroek, H. M. (1982). “Monoculture versus mixture: interactions between susceptible and resistant trees in a mixed stand,” Proceedings the Third International Workshop on the Genetics of Host-Parasite Interactions In Forestry (Wageningen).
Hill, L., Jones, G., Atkinson, N., Hector, A., Hemery, G., and Brown, N. (2019). The £15 billion cost of ask dieback in Britian. Curr. Biol. 29, 315–316. doi: 10.1016/j.cub.2019.03.033
Holling, C. S. (1996). “Engineering resilience versus ecological resilience,” in Engineering Within Ecological Constraints, ed P.C. Schulze (Washington, DC: National Academy Press), 31–43.
Holzmueller, E. J., Jose, S., and Jenkins, M. A. (2008). The relationship between fire history and an exotic fungal disease in a deciduous forest. Oecologia 155, 347–356. doi: 10.1007/s00442-007-0908-7
Hood, I. A., and Kimberley, M. O. (2009). Impact of armillaria root disease and the effect of thinning in a late-rotation Pinus radiata plantation. Forest Pathol. 39, 415–427. doi: 10.1111/j.1439-0329.2009.00602.x
Hood, I. A., Kimberley, M. O., Gardner, J. F., and Sandberg, C. J. (2002). Armillaria root disease of Pinus radiata in New Zealand. Influence of thinning and pruning. N Zeal. J. Forest. Sci. 32, 116–132.
Hungerford, R. D., Williams, R. E., and Marsden, M. A. (1982). Thinning and Pruning Western White Pine: A Potential for Reducing Mortality Due to Blister Rust. Ogden, UT: United States Department of Agriculture Forest Service.
Hunt, R. S., Geils, B. W., and Hummer, K. E. (2010). White pines, ribes, and blister rust: integration and action. Forest Pathol. 40, 402–417. doi: 10.1111/j.1439-0329.2010.00665.x
Jactel, H., Bauhus, J., Boberg, J., Bonal, D., Castagneyrol, B., Gardiner, B., et al. (2017). Tree diversity drives forest stand resistance to natural disturbances. Curr. Forest. Rep. 3, 223–243. doi: 10.1007/s40725-017-0064-1
Jactel, H., Nicoll, B. C., Branco, M., Gonzalez-Olabarria, J. R., Grodzki, W., Langstrom, B., et al. (2009). The influences of forest stand management on biotic and abiotic risks of damage. Ann. Forest Sci. 66:2009054. doi: 10.1051/forest/2009054
Jules, E. S., Kauffman, M. J., Ritts, W. D., and Carroll, A. L. (2002). Spread of an invasive pathogen over a variable landscape: A nonnative root rot on Port Orford Cedar. Ecology 83, 3167–3181. doi: 10.1890/0012-9658(2002)083[3167:SOAIPO]2.0.CO;2
Kaitera, J. (2002). Short-term effect of thinning on pinus sylvestris damage and sporulation caused by Cronartium flaccidum. Scand. J. Forest Res. 17, 158–165. doi: 10.1080/028275802753626809
Kanaskie, A., Osterbauer, N., McWilliams, M., Goheen, E., Hansen, E., and Sutton, W. (2006). “Eradication of Phytophthora ramorum in Oregon forests - status after 3 years,” in Proceedings of the Sudden Oak Death Second Science Symposium: the State of our Knowledge.
Katovich, S., O'Brien, J. G., Mielke, M. E., and Ostry, M. (2004). “Restoration and management of Eastern white pine within high blister rust hazard zones in the lake states,” in USDA Forest Service Proceeding (Fort Collins, CO), 135–145.
King, D. I., and DeGraaf, R. M. (2000). Bird species diversity and nesting success in mature, clearcut and shelterwood forest in northern New Hampshire, USA. For. Ecol. Manag. 129, 227–235. doi: 10.1016/S0378-1127(99)00167-X
Kleczkowski, A., Hoyle, A., and McMenemy, P. (2019). One model to rule them all? Modelling approaches across OneHealth for human, animal and plant epidemics. Philos. Trans. R. Soc. B 374:20180225. doi: 10.1098/rstb.2018.0255
Kwon, T. S., Shin, J. H., Lim, J. H., Kim, Y. K., and Lee, E. J. (2011). Management of pine wilt disease in Korea through preventative silvicultural control. For. Ecol. Manag. 261, 562–569. doi: 10.1016/j.foreco.2010.11.008
Lavallee, A. (1991). “White pine blister rust control in a 5 year old eastern white pine plantation at Vercheres, Quebec (1984-88 results),” Proceedings of the IUFRO Rusts of Pine Working Party Conference (Banff, AB).
Leak, W. B. (2006). Fifty-year impacts of the beech bark disease in the Bartlett Experimental Forest, New Hampshire. North. J. Appl. Forest. 23, 141–143. doi: 10.1093/njaf/23.2.141
Liebhold, A. M., Brockerhoff, E. G., Kalisz, S., Nuñez, M. A., Wardle, D. A., and Wingfield, M. J. (2017). Biological invasions in forest ecosystems. Biol. Invasions. 19, 3073–3077. doi: 10.1007/s10530-017-1458-5
Linden, M. L., and Vollbrecht, G. (2002). Sensitivirt of Picea abies to Butt Rot in pure stands and mixed stands with Pinus sylvestric in Southern Sweden. Silva Fennica 36, 767–778. doi: 10.14214/sf.519
Lindenmayer, D. B., Franklin, J. F., and Fischer, J. (2006). General management principles and a checklist of strategies to guide forest biodiversity conservation. Biol. Conserv. 131, 433–445. doi: 10.1016/j.biocon.2006.02.019
Macpherson, M. F., Kleczkowski, A., Healey, J. R., and Hanley, N. (2017a). Payment for multiple forest benefits alters the effect of tree disease on optimal forest rotation length. Ecol. Econ. 134, 82–94. doi: 10.1016/j.ecolecon.2017.01.008
Macpherson, M. F., Kleczkowski, A., Healey, J. R., and Hanley, N. (2018). The effects of disease on optimal forest rotation: a generalisable analytical framework. Environ. Res. Econ. 70, 565–588. doi: 10.1007/s10640-016-0077-4
Macpherson, M. F., Kleczkowski, A., Healey, J. R., Quine, C. P., and Hanley, N. (2017b). The effects of invasive pests and pathogens on strategies for forest diversification. Ecol. Model. 350, 87–99. doi: 10.1016/j.ecolmodel.2017.02.003
Madar, Z., Solel, Z., Sztejnberg, A., and Kimchi, M. (1991). Effect of pruning of trunk side-branches of cypress on infection by seiridium-cardinale and diplodia-pinea F-SP cupressi. For. Ecol. Manag. 44, 255–260. doi: 10.1016/0378-1127(91)90012-K
Martin, J., Cabezas, J., Buyolo, T., and Paton, D. (2005). The relationship between Cerambyx spp. damage and subsequent Biscogniauxia mediterranum infection on Quercus suber forests. For. Ecol. Manag. 216, 166–174. doi: 10.1016/j.foreco.2005.05.027
Matsushita, K., Taki, H., Yamane, F., and Asano, K. (2018). Shadow value of ecosystem resilience in complex natural land as a wild pollinator habitat. Am. J. Agr. Econ. 100, 829–843. doi: 10.1093/ajae/aax075
Mattila, U. (2002). The risk of pine twisting rust damage in young Scots pines: a multilevel logit model approach. For. Ecol. Manag. 165, 151–161. doi: 10.1016/S0378-1127(01)00657-0
Mattila, U., Jalkanen, R., and Nikula, A. (2001). The effects of forest structure and site characteristics on probability of pine twisting rust damage in young Scots pine stands. For. Ecol. Manag. 142, 89–97. doi: 10.1016/S0378-1127(00)00342-X
McCracken, A. R., and Dawson, W. M. (1997). Growing clonal mixtures of willow to reduce effect of Melampsora epitea var. epitea. Euro. J. Forest Pathol. 27, 319–329. doi: 10.1111/j.1439-0329.1997.tb01086.x
McCracken, A. R., and Dawson, W. M. (1998). Short rotation coppic willow in Northern Ireland since 1973: development of the use of mixtures in the control of foliar rust (Melampsora spp.). Euro. J. Forest Pathol. 28, 241–250. doi: 10.1111/j.1439-0329.1998.tb01179.x
McCracken, A. R., and Dawson, W. M. (2003). Rust disease (Melamspora epitea) of willow (Salix spp.) grown in short rotation coppice (SRC) in inter- and intra-species mixtures. Ann. Appl. Biol. 143, 381–393. doi: 10.1111/j.1744-7348.2003.tb00308.x
McCracken, A. R., Dawson, W. M., and Bowden, G. (2001). Yield response of willow (Salix) grown in mixtures in short rotation coppice (SRC). Biomass Bioenergy 21, 311–319. doi: 10.1016/S0961-9534(01)00046-0
Meadows, J. S., Leininger, T. D., Montwe, D., and Nebeker, T. E. (2013). “Thinning to improve growth, bole quality, and forest health in an Inonotus hispidus infected red oak-sweetgum stand in the Mississippi delta: 10 year results,” in Proceedings of the 15th Biennial Southern Silvicultural Research Conference (Asheville, NC).
Meentemeyer, R. K., Rank, N. E., Anacker, B. L., Rizzo, D. M., and Cushman, J. H. (2008a). Influence of land-cover change on the spread of an invasive forest pathogen. Ecol. Appl. 18, 159–171. doi: 10.1890/07-0232.1
Meentemeyer, R. K., Rank, N. E., Shoemaker, D. A., Oneal, C. B., Wickland, A. C., Frangioso, K. M., et al. (2008b). Impact of sudden oak death on tree mortality in the Big Sur ecoregion of California. Biol. Invas. 10, 1243–1255. doi: 10.1007/s10530-007-9199-5
Menkis, A., Ostbrant, I. L., Wagstrom, K., and Vasaitis, R. (2016). Dutch elm disease on the island of Gotland: monitoring disease vector and combat measures. Scand. J. For. Res. 31, 237–241. doi: 10.1080/02827581.2015.1076888
Metzler, B. (1997). Quantitative assessment of fungal colonisation in Norway spruce after green pruning. Euro. J. Forest Pathol. 27, 1–11. doi: 10.1111/j.1439-0329.1997.tb00848.x
Millar, C. I., and Stephenson, N. L. (2015). Temperate forest health in an era of emerging megadisturbance. Science 349, 823–826. doi: 10.1126/science.aaa9933
Morrison, D. J., Cruickshank, M. G., and Lalumiere, A. (2014). Control of laminated and Armillaria root diseases by stump removal and tree species mixtures: Amount and cause of mortality and impact on yield after 40 years. For. Ecol. Manag. 319, 75–98. doi: 10.1016/j.foreco.2014.02.007
Morrison, D. J., Pellow, K. W., Nemec, A. F. L., Norris, D. J., and Semenoff, P. (2001). Effects of selective cutting on the epidemiology of armillaria root disease in the southern interior of British Columbia. Canad. J. Forest Res. 31, 59–70. doi: 10.1139/x00-144
Morrison, D. J., Wallis, G. W., and Weir, L. C. (1988). Control of Armillaria and Phellinus Root Diseases: 20 Year Results From the Skimikin Stump Removal Experiment. Victoria, BC: Canadian Forestry Service.
Muzika, R. M. (2017). Opportunities for silviculture in management and restoration of forests affected by invasive species. Biol. Invas. 19, 3419–3435. doi: 10.1007/s10530-017-1549-3
Nebeker, T. E., Leininger, T. D., and Meadows, J. S. (1999). “Silvicultural practices in forests of the Southern United States: Insect and disease considerations,” in Tenth Biennial Southern Silvicultural Research Conference (Shreveport, LA).
Newton, A. C., and Cantarello, E. (2015). Restoration of forest resilience: an achievable goal? N. Forests 46, 645–668. doi: 10.1007/s11056-015-9489-1
Nguyen, T. P., Wright, E. P., Nguyen, T. T., Schuiling-Veninga, C. C., Bijlsma, M. J., Nguyen, T. B., et al. (2016). Cost-effectiveness analysis of screening for and managing identified hypertension for cardiovascular disease prevention in Vietnam. PLoS ONE 11:e155699. doi: 10.1371/journal.pone.0155699
Nguyen, T. V., Park, Y. S., Jeoung, C. S., Choi, W. I., Kim, Y. K., Jung, I. H., et al. (2017). Spatially explicit model applied to pine wilt disease dispersal based on host plant infestation. Ecol. Model. 353, 54–62. doi: 10.1016/j.ecolmodel.2016.10.022
Oliva, J., Samils, N., Johansson, U., Bendz-Hellgren, M., and Stenlid, J. (2008). Urea treatment reduced Heterobasidion annosum sl root rot in Picea abies after 15 years. For. Ecol. Manag. 255, 2876–2882. doi: 10.1016/j.foreco.2008.01.063
Oliva, J., Suz, L. M., and Colinas, C. (2009). Ecology of Armillaria species on silver fir (Abies alba) in the Spanish Pyrenees. Ann. Forest Sci. 66:2009046. doi: 10.1051/forest/2009046
Oliva, J., Thor, M., and Stenlid, J. (2010). Long-term effects of mechanized stump treatment against Heterobasidion annosum root rot in Picea abies. Canad. J. Forest Res. 40, 1020–1033. doi: 10.1139/X10-051
Ostry, M. (2000). Restoration of white pine in Minnesota, Wisconsin, and Michigan. Hort Techol. 10, 542–543. doi: 10.21273/HORTTECH.10.3.542
Ostry, M. E., Laflamme, G., and Katovich, S. A. (2010). Silvicultural approaches for management of eastern white pine to minimize impacts of damaging agents. Forest Pathol. 40, 332–346. doi: 10.1111/j.1439-0329.2010.00661.x
Ostry, M. E., Moore, M. J., Kern, C. C., Venette, R. C., and Palik, B. J. (2012). Multiple diseases impact survival of pine species planted in red pine stands harvested in spatially variable retention patterns. For. Ecol. Manag. 286, 66–72. doi: 10.1016/j.foreco.2012.08.017
Otrosina, W. J., Kliejunas, J. T., Smith, S., Cluck, D. R., Sung, S. S., and Cook, C. D. (2007). Black stain root disease studies on Ponderosa pine- Parameters and disturbanc treatments affecting infection and mortality. Acta Silva. Lign. Hung. Spec. Edn, 247–251.
Pankuch, J. M., Blenis, P. V., Lieffers, V. J., and Mallett, K. I. (2003). Fungal colonization of aspen roots following mechanical site preparation. Canad. J. Forest Res. 33, 2372–2379. doi: 10.1139/x03-172
Pautasso, M., Aas, G., Queloz, V., and Holdenrieder, O. (2013). European ash (Fraxinus excelsior) dieback - a conservation biology challenge. Biol. Conserv. 158, 37–49. doi: 10.1016/j.biocon.2012.08.026
Pautasso, M., Holdenrieder, O., and Stenlid, J. (2005). “Susceptibility to fungal pathogens of forests differing in tree diversity,” in Forest Diversity and Function, eds M. Scherer-Lorenzen, C. H. Körner, and E. D. Schulze (Berlin; Heidelberg: Springer), 63–289. doi: 10.1007/3-540-26599-6_13
Peacock, L., Hunter, T., Turner, H., and Brain, P. (2001). Does host genotype diversity affect the distribution of insect and disease damage in willow cropping systems? J. Appl. Ecol. 38, 1070–1081. doi: 10.1046/j.1365-2664.2001.00655.x
Peterson, E., Hansen, E., and Kanaskie, A. (2014). Spatial relationship between Phytophthora ramorum and roads or streams in Oregon tanoak forests. For. Ecol. Manag. 312, 216–224. doi: 10.1016/j.foreco.2013.10.002
Piirto, D. D., and Valkonen, S. (2005). Structure and development of pitch canker infected Monterey pine stands at Ano Nuevo, California. For. Ecol. Manag. 213, 160–174. doi: 10.1016/j.foreco.2005.03.026
Pimm, S. L. (1984). The complexity and stability of ecosystems. Nature 307, 321–326. doi: 10.1038/307321a0
Pleysier, C. E., Bayliss, K. L., Dell, B., and Hardy, G. E. S. (2006). Temperature, humidity, wounding and leaf age influence the development of Alternaria alternata lesions on leaves of Paulownia fortunei. Austr. Plant Pathol. 35, 329–333. doi: 10.1071/AP06030
Power, A. G., and Mitchell, C. E. (2004). Pathogen spillover in disease epidemics. Am. Natur. 164, S79–S89. doi: 10.1086/424610
Poyatos, R., Latron, J., and Llorens, P. (2002). Land use and land cover change after agricultural abandonment. Mount. Res. Dev. 23, 362–368. doi: 10.1659/0276-4741(2003)023[0362:LUALCC]2.0.CO;2
Prospero, S., and Cleary, M. (2017). Effects of host variability on the spread of invasive forest diseases. Forests 8, 1–21. doi: 10.3390/f8030080
Puddu, A., Luisi, N., Capretti, P., and Santini, A. (2003). Environmental factors related to damage by Heterobasidion abietinum in Abies alba forests in Southern Italy. For. Ecol. Manag. 180, 37–44. doi: 10.1016/S0378-1127(02)00607-2
Ramage, B. S., Forrestel, A. B., Moritz, M. A., and O'Hara, K. L. (2012). Sudden oak death disease progression across two forest types and spatial scales. J. Vegetat. Sci. 3, 151–163. doi: 10.1111/j.1654-1103.2011.01340.x
Raymond, P., and Bédard, S. (2017). The irregular shelterwood system as an alternative to clearcutting to achieve compositional and structural objectives in temperate mixedwood stands. For. Ecol. Manag. 398, 91–100. doi: 10.1016/j.foreco.2017.04.042
Richter, B. S., Benson, D. M., and Ivors, K. L. (2011a). Microbial profiling of cultural systems for suppression of phytophthora root rot in fraser fir. Plant Dis. 95, 537–546. doi: 10.1094/PDIS-03-10-0238
Richter, B. S., Ivors, K., Shi, W., and Benson, D. M. (2011b). Cellulase activity as a mechanism for suppression of phytophthora root rot in mulches. Phytopathology 101, 223–230. doi: 10.1094/PHYTO-04-10-0125
Roach, W. J., Simard, S. W., and Sachs, D. L. (2015). Evidence against planting lodgepole pine monocultures in the cedar-hemlock forests of southeastern British Columbia. Forestry 88, 345–358. doi: 10.1093/forestry/cpv005
Robinson, R. M. (2003). Short-term impact of thinning and fertilizer application on Armillaria root disease in regrowth karri (Eucalyptus diversicolor F. Muell.) in Western Australia. For. Ecol. Manag. 176, 417–426. doi: 10.1016/S0378-1127(02)00231-1
Ronnberg, J. (2000). Logging operation damage to roots of clear-felled Picea abies and subsequent spore infection by Heterobasidion annosum. Silva Fennica 34, 29–36. doi: 10.14214/sf.642
Rosenvald, R., Drenkhan, R., Riit, T., and Lohmus, A. (2015). Towards silvicultural mitigation of the European ash (Fraxinus excelsior) dieback: the importance of acclimated trees in retention forestry. Canad. J. Forest Res. 45, 1206–1214. doi: 10.1139/cjfr-2014-0512
Rosso, P., and Hansen, E. (1998). Tree vigour and the susceptibility of Douglas fir to Armillaria root disease. Forest Pathol. 28, 43–52. doi: 10.1111/j.1439-0329.1998.tb01164.x
Sakamoto, K., Tamesa, T., Yukio, T., Tokuhisa, Y., Maeda, Y., and Oka, M. (2016). Risk factors and managements of bile leakage after hepatectomy. World J. Surg. 40, 182–189. doi: 10.1007/s00268-015-3156-8
Santini, A., Pepori, A., Ghelardini, L., and Capretti, P. (2008). Persistence of some pine pathogens in coarse woody debris and cones in a Pinus pinea forest. For. Ecol. Manag. 256, 502–506. doi: 10.1016/j.foreco.2008.05.010
Schoettle, A. W., and Sniezko, R. A. (2007). Proactive intervention to sustain high-elevation pine ecosystems threatened by white pine blister rust. J. For. Res. 12, 327–336. doi: 10.1007/s10310-007-0024-x
Shaw, C. G., Omdal, D. W., Ramsey-Kroll, A., and Roth, L. F. (2012). Inoculum reduction measures to manage armillaria root disease in a severely infected stand of ponderosa pine in south-Central Washington: 35-year results. West. J. Appl. Forest. 27, 25–29. doi: 10.1093/wjaf/27.1.25
Simard, S. W., Hagerman, S. M., Sachs, D. L., Heineman, J. L., and Mather, W. J. (2005). Conifer growth, Armillaria ostoyae root disease, and plant diversity responses to broadleaf competition reduction in mixed forests of southern interior British Columbia. Canad. J. Forest Res. 35, 843–859. doi: 10.1139/x05-010
Staudhammer, C. L., Jokela, E. J., and Martin, T. A. (2009). Competition dynamics in pure- versus mixed-family stands of loblolly and slash pine in the southeastern United States. Canad. J. Forest Res. 39, 396–409. doi: 10.1139/X08-184
Swedjemark, G., and Stenlid, J. (1993). Population dynamics of the root rot fungus Heterobasidion annosum following thinning of Picea abies. Oikos 247–254. doi: 10.2307/3544811
Tarigan, M., Wingfield, M. J., van Wyk, M., Tjahjono, B., and Roux, J. (2011). Pruning quality affects infection of Acacia mangium and A. crassicarpa by Ceratocystis acaciivora and Lasiodiplodia theobromae. Southern Forests 73, 187–191. doi: 10.2989/20702620.2011.639498
Thies, W. G., Kelsey, R. G., Westlind, D. J., and Madsen, J. (2006). Potassium fertilizer applied immediately after planting had no impact on Douglas-fir seedling mortality caused by laminated root rot on a forested site in Washington State. For. Ecol. Manag. 229, 195–201. doi: 10.1016/j.foreco.2006.03.028
Thies, W. G., and Westlind, D. J. (2005). Stump removal and fertilization of five Phellinus weirii-infested stands in Washington and Oregon affect mortality and growth of planted Douglas-fir 25 years after treatment. For. Ecol. Manag. 219, 242–258. doi: 10.1016/j.foreco.2005.08.050
Thor, M., and Stenlid, J. (2005). Heterobasidion annosuminfection ofPicea abiesfollowing manual or mechanized stump treatment. Scand. J. Forest Res. 20, 154–164. doi: 10.1080/02827580510008338
Valachovic, Y., Cobb, R. C., Rizzo, D., Twieg, B., Lee, C., and Glebocki, R. (2013a). “Is stump sprout treatment necessary to effectively control Phytophthora ramorum in California;s wildlands, in Proceedings of the Sudden Oak Death Fifth Science Symposium (Albany, NY).
Valachovic, Y., Lee, C., Marshall, J., and Scanlon, H. (2010). “Forest treatment strategies for Phytophthora ramorum,” in Proceedings of the Sudden Oak Death Fourth Science Symposium (Santa Cruz, CA), 239.
Valachovic, Y., Lee, C., Twieg, B., Rizzo, D., Cobb, R. C., and Glebocki, R. (2013b). “Suppression of Phytophthora ramorum infestation through silvicultural treatment in California's north coast,” in Proceedings of the Sudden Oak Death Fifth Science Symposium (Albany, NY).
van der Kamp, B. J. (1994). Lodgepole pine stem disease and management of stand density in the British Colombia interior. Forest. Chron. 70, 773–779. doi: 10.5558/tfc70773-6
Walker, B., Pearson, L., Harris, M., Maler, K. G., Li, C., Biggs, R., et al. (2010). Incorporating resilience in the assessment of inclusive wealth. Environ. Res. Econ. 45, 183–202. doi: 10.1007/s10640-009-9311-7
Ward, J. S., Anagnostakis, S., and Ferrandino, F. J. (2010). Nectria canker incidence on birch (Betula spp.) in Connecticut. North. J. Appl. Forest. 27, 85–91. doi: 10.1093/njaf/27.3.85
Waring, K. M., and O'Hara, K. L. (2005). Silvicultural strategies in forest ecosystems affected by introduced pests. For. Ecol. Manag. 209, 27–41. doi: 10.1016/j.foreco.2005.01.008
Warren, C. (2007). Perspectives on the 'alien' vs 'native' species debate: a critique of concepts, language and practice. Progr. Hum. Geogr. 31, 427–446. doi: 10.1177/0309132507079499
Whitney, R. D., and Irwin, R. N. (2005). Comparison of Armillaria root disease on burned and unburned, harvested sites in Ontario. Forest. Chron. 81, 56–60. doi: 10.5558/tfc81056-1
Wingfield, M. J., Brockerhoff, E. G., Wingfield, B. D., and Slippers, B. (2015). Planted forest health: The need for a global strategy. Science 349, 832–836. doi: 10.1126/science.aac6674
Wingfield, M. J., Slippers, B., Wingfield, B. D., and Barnes, I. (2017). The unified framework for biological invasions: a forest fungal pathogen perspective. Biol. Invas. 19, 3201–3214. doi: 10.1007/s10530-017-1450-0
Wingfield, M. J., and Swart, W. J. (1994). Integrated management of forest tree diseasesin South-Africa For. Ecol. Manag. 65, 11–16. doi: 10.1016/0378-1127(94)90253-4
Woodward, S., Stenlid, J., Karjalainen, R., and Huttermann, A. (1998). Heterobasidion annosum Biology, Ecology, Impact and Control. Wallingford, CT: CAB International.
Keywords: tree disease, epidemiology, forestry, pathogen, silviculture, forest management, invasive species, species diversity
Citation: Roberts M, Gilligan CA, Kleczkowski A, Hanley N, Whalley AE and Healey JR (2020) The Effect of Forest Management Options on Forest Resilience to Pathogens. Front. For. Glob. Change 3:7. doi: 10.3389/ffgc.2020.00007
Received: 25 June 2019; Accepted: 16 January 2020;
Published: 10 February 2020.
Edited by:
Alberto Santini, Italian National Research Council, ItalyReviewed by:
Rose Marie Muzika, Carnegie Museum of Natural History, United StatesCopyright © 2020 Roberts, Gilligan, Kleczkowski, Hanley, Whalley and Healey. This is an open-access article distributed under the terms of the Creative Commons Attribution License (CC BY). The use, distribution or reproduction in other forums is permitted, provided the original author(s) and the copyright owner(s) are credited and that the original publication in this journal is cited, in accordance with accepted academic practice. No use, distribution or reproduction is permitted which does not comply with these terms.
*Correspondence: Michaela Roberts, TWljaGFlbGEucm9iZXJ0c0BodXR0b24uYWMudWs=
Disclaimer: All claims expressed in this article are solely those of the authors and do not necessarily represent those of their affiliated organizations, or those of the publisher, the editors and the reviewers. Any product that may be evaluated in this article or claim that may be made by its manufacturer is not guaranteed or endorsed by the publisher.
Research integrity at Frontiers
Learn more about the work of our research integrity team to safeguard the quality of each article we publish.