- 1Departamento de Salud Ambiental, Instituto de Salud Pública de Chile, Santiago, Chile
- 2Laboratorio de Biología Molecular, Departamento de Bioquímica y Biología Molecular, Facultad de Ciencias Químicas y Farmacéuticas, Universidad de Chile, Santiago, Chile
- 3Laboratorio de Química de la Atmósfera y Radioquímica, Facultad de Ciencias Químicas y Farmacéuticas, Universidad de Chile, Santiago, Chile
Anthropogenic volatile organic compounds (AVOCs) represent the third largest contribution in gaseous emissions in the urban and peri-urban region of Santiago, the capital of Chile. Some of these compounds are toxic or mutagenic, cause serious damage to human health, and decrease plant growth and development. There is little international information related to atmospheric AVOCs in leaf content from trees exposed to specific sources of pollution, and to our knowledge, there is no research on this topic in Chile. The purpose of this work was to study the leaf content of AVOCs from the Organic Range of Gasoline (ORG: range 6–10 C) emitted by local traffic during the austral summer and spring seasons in leaves of two exotic tree species (Liriodendron tulipifera and Platanus × acerifolia). Leaf samples collected around 2 meters height above the ground were pulverized with a cryogenic mill and eleven chemical components were quantified (toluene, 1,2,4-trimethylbenzene (1,2,4-TMB), styrene, ethylbenzene, ortho, meta and para-xylenes, naphthalene, 1,3,5-trimethylbenzene (1,3,5-TMB), isopropylbenzene, and trichloroethene) using GC-MSD MSD. Benzene was detected but not quantified, because it was always under the quantification limit of the technique. Differences in concentrations were found for type of site exposure, season and tree species. The differences found in leaf content of AVOCs in P. × acerifolia exposed to vehicle traffic suggest that the concentration of these contaminants in leaves may be due to AVOC capturing. Considering the content of AVOCs in leaves and not in the whole individual tree, L. tulipifera presented a higher concentration of total AVOCs than P. × acerifolia for both seasons. The Prop-Equiv and OFP of L. tulipifera were very high in summer, being 13.6 and 14.8 times greater, respectively, than the corresponding values for P. × acerifolia.
Introduction
Anthropogenic volatile organic compounds (AVOCs) constitute the main precursors of tropospheric ozone, in conjunction with nitrogen oxide gaseous emissions from vehicles. Urban trees and forests provide multiple ecosystem services, including mitigation of air pollution by removing AVOCs. Fast-growing cities in developing countries are characterized by a disorganized spatial distribution, sprawling urban areas and increased vehicle traffic, which leads to poor air quality (Henríquez and Romero, 2019). Santiago, the capital of Chile, has a population of close to seven million inhabitants, where the large majority lives in areas with little vegetation, and only affluent neighborhoods in the northeast areas of the city have a close to adequate green infrastructure. Several factors contribute to high levels of air pollution in Santiago, including its dry and temperate region, location surrounded by mountains, a growing economy and densification of the city. Despite all technological improvements and policies developed since the 90's to tackle air pollution (MMA, 2016), the vehicle fleet and travel distances have progressively increased. On average, Santiago more than doubles the mean concentrations above the annual WHO recommendations (WHO, 2016), for both PM10 and PM2.5, especially during the autumn–winter period. Moreover, the Chilean normative for ozone (O3) is exceeded several days in the eastern part of the city during the spring-summer season (Préndez et al., 2019).
Motor vehicles are important emitters of ozone precursors, including particulate matter and both organic and inorganic gases, such as NOx. During the last years, Santiago has suffered a strong increase of the vehicle fleet. The period between 2014 and 2015 showed a growth rate of 2.71%, while in 2016 the city reached 4,960,946 vehicles, increasing 4% compared to 2015 (SINCA, 2017). Most vehicles (79.1%) corresponded to gasoline vehicles, 20.6% to diesel vehicles and only 0.3% corresponded to gas or electric vehicles (INE, 2018). Tropospheric O3 is an important secondary urban air pollutant formed as a result of atmospheric reactions of volatile organic compounds (VOCs), anthropogenic and/or biogenic and nitrogen oxides (NOx = NO + NO2) (Atkinson, 2000; Kansal, 2009; Préndez et al., 2013a; Guo et al., 2016). The Metropolitan Region vehicles emit around 23,000 tons of NOx per year (INE, 2018).
In urban areas, vehicle emissions (Shao et al., 2016; Li et al., 2017) are the main contributors to AVOCs. Fumes from liquid fuels containing aromatic compounds are emitted to the air; most of these aromatic compounds are added to gasoline for antiknock purposes, such as lead replacement (Karakitsios et al., 2007; Kountouriotis et al., 2014; Cui et al., 2018). In industrialized areas, high concentrations of AVOCs are mainly associated with petroleum refineries, petrochemical companies, petrol stations, commercial and industrial use of solvents, coal burning and even biomass burning (Karakitsios et al., 2007; Wang et al., 2015; Li et al., 2017).
Plants also emit VOCs; these biogenic VOCs (BVOCs) are an important source of some highly reactive pollutants derived from their eco-physiological functions (Kansal, 2009; Préndez et al., 2013a; Shao et al., 2016) that contribute to ozone formation. BVOCs include alkanes, alkenes, carbonyls, alcohols, esters, ethers, and acids (Kesselmeier and Staudt, 1999). The most important BVOCs are terpenes, the basic molecule of which is isoprene (2-methyl-1,3-butadiene, C5H8), and some monoterpenes.
The latest inventory of air pollutants in Santiago (Gramsch, 2014) revealed that emissions from VOCs corresponded to 97,028 t/year. Only 15% of the total VOCs correspond to BVOCs, which are mainly isoprene, monoterpenes and sesquiterpenes. Other gases are 2,3,2-MBO, methanol, acetone and CO. There is no specific information about benzene, toluene and xylenes (BTXs) or other benzene derivatives (Gramsch, 2014). To estimate the emissions from biogenic sources, the MEGAN2.1 program was used, including the latest improvements implemented in 2012 by the National Center for Atmospheric Research (Guenther et al., 2012). Préndez et al. (2019) experimentally quantified emissions of BVOCs from different urban tree species in Santiago, which corresponded mainly to terpenes (isoprene and monoterpenes).
Trees are an important part of the biomass of plants in urban settings and play an essential role in the population's quality of life. Several researchers in different continents, climates and socioeconomic conditions have reported the use of urban forests in the provision of ecosystem services. These forests cover the basic functions of the ecosystem, such as primary productivity (Costanza et al., 2007) and services, including the improvement of air quality (Escobedo et al., 2011), specially the removal of particulate matter (Dzierzanowski et al., 2011; Manes et al., 2016; Marando et al., 2016). Among these same functions, the advantage/disadvantage of emissions of BVOCs has been analyzed by Paoletti (2009), while Tallis et al. (2011) have shown that tree leaves can remove gases and aerosols from the atmosphere more efficiently than other surfaces. Therefore, a selection of species and an adequate location of urban vegetation can significantly improve air quality, not only by the direct effect on gases and atmospheric particulate matter, but also by generating local and regional ventilation (Hebbert and Webb, 2012). Therefore, the use of plants to improve air can be a cheaper, aesthetically pleasing, environmentally friendly, and sustainable process that can be used for a wide range of both organic and inorganic contaminants.
Globally, there is limited research on the likelihood of adsorption and/or absorption of atmospheric pollutants by tree leaves exposed to sources of pollution, specifically for AVOCs (Anyanwu and Kanu, 2006; Saphores and Li, 2012). To our knowledge, this information is non-existent in Chile. In the Metropolitan Region of Chile, where about one third of the population lives (around 7.1 million, INE, 2018), AVOCs represent the third source of gaseous emissions to the atmosphere after CO2 and CO (Gramsch, 2014). In Santiago, green areas tend to be concentrated in the more affluent areas of the city (Romero, 2019).
Trees remove VOCs and AVOCs through processes defined by the anatomical structure of their leaves and complex physiological processes. As reported by Brilli et al. (2018), the mechanisms of removal and retention of VOCs by leaves include stomata absorption, where VOCs enter through the stomata and metabolic degradation occurs, and a non-stomata adsorption, where adsorption of VOCs may depend on the composition of the leaf cuticle (Wararat et al., 2014). Hydrophobic or lipophilic substances can be absorbed directly by the cuticle or diffuse into the leaf through the cuticle (Libbert, 1974; Niinemets et al., 2014), while hydrophilic compounds, like gaseous and liquid contaminants may be absorbed by the cuticle to some extent (Agarwal et al., 2018). VOCs in the atmosphere enter the intercellular space through the stomata, depending on the physicochemical parameters of each VOC. Some VOCs are metabolized (enzymatically or non-enzymatically) in the cytoplasm of mesophyll cells, facilitating further partitioning of VOCs into the cells, which appears as a process of “uptake” or “absorption” of VOCs by plants. The uptake of VOCs leads to changes in cell metabolism; for example, the accumulation of glycosides or redox potential increase (Matsui, 2016).
In addition to the cuticle and stomata that constitute barriers to gaseous diffusion, cell walls, membranes and mesophyll cells cause another type of resistance to gaseous diffusion. Mesophyllic resistance mostly depends on the solubility of gaseous pollutants, gas-liquid diffusion, and leaf size geometry, and are influenced by some environmental factors, such as wind speed (Khan and Abbasi, 2000).
Both BVOCs and AVOCs are precursors of tropospheric ozone. The theoretical contribution of ozone precursors can be calculated based on the Ozone Formation Potential (OFP). This OFP depends on the photochemical reactivity of the different VOCs with atmospheric OH radicals and other reactive species. A greater content of AVOCs in trees leaves is expected to remove more precursors that generate ozone in the urban environments, expressed in terms of propylene equivalents (Prop-equiv) and OFP, thereby improving air quality.
The aim of this work was to quantify the content of AVOCs emitted from motor vehicles in leaves from Platanus and Liriodendrum trees and evaluate their performance in terms of improving urban air quality, in relation to their proximity to vehicle emissions. The studied species were selected in a field context of the city of Santiago because they correspond to the most common trees lining main avenues with heavy vehicle traffic. Two locations with similar levels of vehicle emissions were selected and the leaf content of VOCs in two common exotic tree species was measured. In Santiago, Platanus orientalis, P. occidentalis, and P. × acerifolia are found, and these plane trees are common in the Vitacura commune. Liriodendrum tulipifera is common along streets with vehicle traffic in the La Florida commune.
Materials and Methods
Sampling Sites
The Metropolitan Region (33.5°S, 70.8°W) is a closed basin between the Andes to the east and the Coastal Range to the west, the Chacabuco mountain range to the north, and the Cantillana mountain range to the south. The mountains surrounding the central valley reach altitudes between 1,000 and 6,000 m asl and the basin of the city has a gentle slope from west to east, from ~450 m asl to over 1,000 m asl. During most of the year, the climate of the region is affected by synoptic systems, where the Southeast Pacific Subtropical Anticyclone is the dominant one. This anticyclone generates a persistent subsidence thermal inversion during the cold months, weak airflows, and semi-arid climatic conditions. Hence, ventilation at the basin is poor and mainly generated by local breeze systems. Wind intensity and direction vary seasonally, with a southwest regime being dominant throughout the year (Ulriksen, 1993). Four seasons are clearly distinguished: summer (December 21–March 21), autumn (March 21–June 21), winter (June 21–September 21), and spring (September 21–December 21).
Three sites of sampling sites were selected: two with similar high vehicle traffic and a third in an urban park, as explained below and shown in Figure 1. At the first site, samples of well-developed sunny leaves and directly exposed to high vehicle traffic (operational speed of 26.5 km/h, UOCT, 2019) were taken from twenty individuals of L. tulipifera (n = 20) along Vicuña Mackenna avenue (VM Avenue) in the La Florida commune. At the second site, and in similar conditions as the first site (operational speed of 29.5 km/h, UOCT, 2019) samples were taken from fourteen individuals of Platanus (n = 14) along Vitacura avenue (V Avenue), in the Vitacura commune. At both sites, the trees are in close proximity to a permanent flow of vehicles and exposed to fumes at a distance of <2 m. At the third site, well-developed sunny leaves were collected from twenty individuals of L. tulipifera (n = 20), located in an urban park called Parque Bicentenario (B Park), without internal motor vehicle traffic, located in the Vitacura commune. The same individuals were sampled in two campaigns carried out in the austral summer (February) and spring (November) of 2017. Each sampling campaign at each site took ~6 h. In all cases, the selected leaves of each individual were collected in a height range of 1.7 and 2.5 meters all along the perimeter for each individual, until completing ~30 g of foliar biomass, corresponding to around 40 leaves. All leaf samples were immediately frozen in situ in dry ice and stored at −20°C until analysis. This range of height corresponds to the criterion used for aerosol sampling for health purposes by the international monitoring (USEPA, 1989). From each sample of 30 g, a subset of 10 g leaf material was selected at random for chemical analysis.
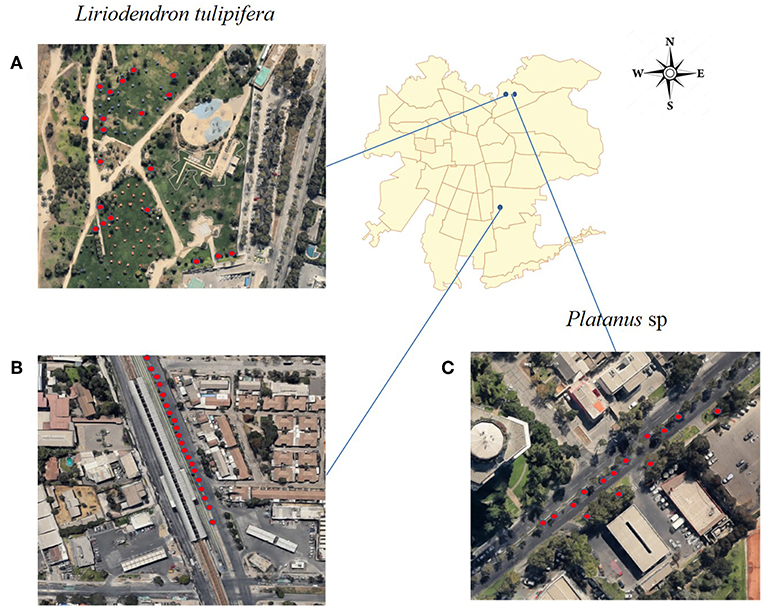
Figure 1. Sampling sites in Santiago, Chile. (A) B Park (L. tulipifera); (B) VM Avenue (L. tulipifera); and (C) V Avenue (Platanus sp). Red dots identify individuals sampled at each site.
Chemical Analysis
Leaf samples were milled using a cryogenic mill (CryoMill Retsch). Each sample of 2 g was placed in a 20 ml glass vial with PTFE/silicone septum. HS-SPME extraction was performed using a GC CombiPAL 80 Agilent, containing a fiber assembly with 65 μm Polydimethylsiloxane/Divinylbenzene (PDMS/DVB) coating (Supelco). Conditioning was performed according to the manufacturer's instructions, in the GC injection port at 250°C for 30 min prior to use. Extraction time of the analytes was 50 min at 70°C and temperature and desorption time was 5 min at 250°C at the injection port. A blank fiber experiment was carried out to ensure the absence of contaminants in the fiber.
Sample analysis was performed with an Agilent Technologies 7890A GC coupled with an Agilent technologies 5975C mass spectrometer (MS) in the selective ion monitoring (SIM) mode splitless injection onto a HP-5MS (30 m × 0.25 mm i.d, 0.25 μm film thickness); a capillary column was used with a He flow 1 mL/min as carrier gas. The temperature of the injection port was set at 250°C and the temperature of the column was programmed at 35°C. The mass selective detector (MS) was used for mass spectral identification and quantification of the GC–HS-SPME components at a MS ionizations voltage of 70 eV. Eleven AVOCs were determined and quantified (styrene, 1,2,4-TMB, naphthalene, trichloroethene, 1,3,5-TMB, isopropylbenzene, ethylbenzene, toluene, and ortho, meta, para-xylenes) (standard provided by Chem Service) in the organic range of gasoline (ORG). An ααα-trifluorotoluene (standard provided by Supelco) internal standard was used. Quantification was performed by means of a calibration curve of nine concentration levels (in ng/g) of the leaf matrix.
Ozone Formation Potential (OFP)
In this work, we used the propylene equivalent concentration (Prop-Equiv) and the maximum incremental reactivity (MIR), as reported by Cai et al. (2010), Wu et al. (2017) and Kumar et al. (2018) to calculate OFP. The following equation was used to calculate the equivalent propylene concentration for each individual AVOC (Chameides et al., 1992):
where:
Prop-Equiv(i): Concentration of AVOC(i) on an OH-reactivity based scale normalized to the reactivity of C3H6, expressed in ppbC.
conc(i): Concentration of AVOC expressed in ppbC.
KOH(i): Rate constant for the reaction of compound i.*
KOH(C3H6): Rate constant for the reaction of C3H6 (propylene) with OH, respectively.
* As reported by Atkinson and Arey (2003).
The Ozone Formation Potential (OFP) for individual AVOC(i) using the MIR method (Carter, 1994), was defined by the following equation:
where:
OFP(i): Ozone formation potential of individual AVOCi, expressed in μg/m3.
conc(i): Concentration of AVOC expressed in ppbC.
MIRcoeff(i): Maximum incremental reactivity coefficient of compoundi, expressed in g O3/g VOC.
Statistical Analysis
All analyses were performed using the Minitab 16 statistical software. Variables were tested for normality and homogeneity of variance, and transformations were made as necessary to meet the underlying statistical assumptions of the models used. A two-way analysis of variance (ANOVA) was used to test the main and interactive effects of species and seasons on AVOC emissions. The assertion of statistically significant differences between two groups of data (same species exposed to different sources of contamination and different species exposed to the same source) was supported by Fisher tests and p-value.
Results
Variability of Total Concentration of AVOCs Leaf Content Due to the Pollution Source
The following AVOCs were quantified: toluene, 1,2,4-trimethylbenzene (1,2,4-TMB), styrene, ethylbenzene, ortho, meta, and para-xylenes, naphthalene, 1,3,5-trimethylbenzene (1,3,5-TMB), isopropylbenzene, and trichloroethene. Benzene was detected but not quantified, because it was always under the quantification limit of the technique. There were statistically significant differences between the total concentrations of AVOCs leaf content in leaves from L. tulipifera individuals exposed to high (VM Avenue) and low (B Park) emissions of vehicles. The mean values of total concentrations of AVOCs in summer were 267.9 ± 92.4 ng of C (153.6 ± 50.7 ng/g) and 184.7 ± 58.8 ng of C (102.5 ± 32.2 ng/g), respectively (Fobs = 10.74; p-value = 0.002). In spring, concentrations were 255.0 ± 127.9 ng of C (144.7 ± 70.1 ng/g) and 172.4 ± 52.6 ng of C (99.3 ± 28.7 ng/g), respectively (Fobs = 7.17; p-value = 0.011). Total concentrations of AVOCs content in leaves of Platanus individuals (V Avenue) exposed to high emissions of vehicles presented mean concentrations of 103.0 ± 24.1 ng of C (56.6 ± 13.1 ng/g) in summer and 186.7 ± 91.8 ng of C (103.0 ± 50.5 ng/g) in spring (Figure 2).
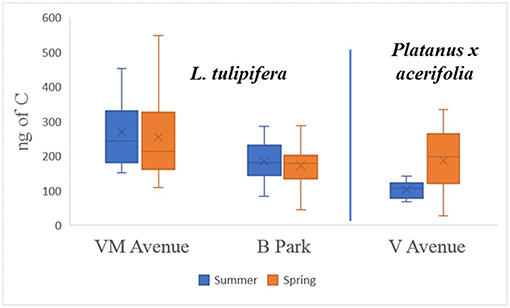
Figure 2. Total concentrations of AVOCs leaf content in L. tulipifera and Platanus x acerifolia at the sampling sites VM Avenue, B Park, and V Avenue, both in summer and in spring. Concentrations are expressed as ng of C. In the boxes: X = arithmetic mean; horizontal line = median.
Figure 3 shows the leaf content of chemical compounds in individuals of L. tulipifera located along the high traffic VM Avenue and the low exposure B Park. The highest concentration was found for toluene in both seasons (summer and spring). Trichloroethene and isopropylbenzene were below detection limits at both sites and during both seasons, while 1,2,4-TMB levels were below detection limits in summer at the B Park. In most cases, a greater concentration of chemical species was observed in leaves sampled at the VM Avenue than at the B Park. There were statistically significant differences in toluene levels between VM avenue and B Park in summer, but not in spring. 1,2,4-TMB, styrene, xylenes and naphthalene show statistically significant differences between VM Avenue and B Park in both seasons (Fobs > Fcri, p < 0.05). 1,3,5-TMB shows statistically significant differences between VM Avenue and B Park in spring, but not in summer.
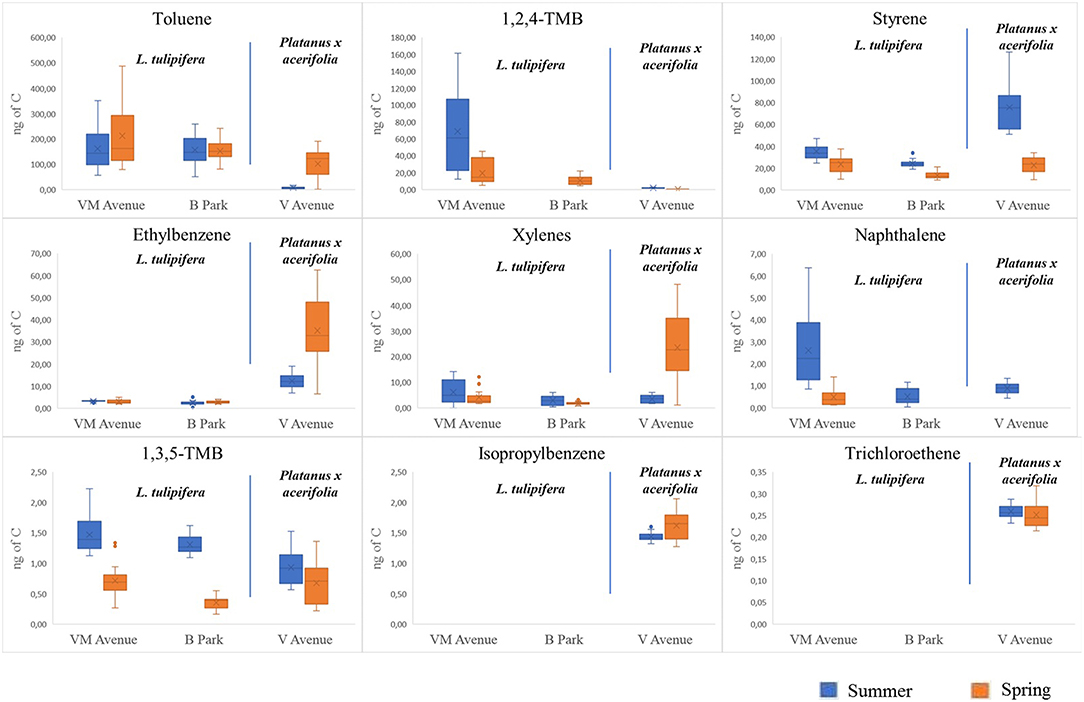
Figure 3. Concentrations of individual AVOCs leaf content by the leaves of L. tulipifera and Platanus x acerifolia at the sampling sites VM Avenue, B Park, and V Avenue in both summer and spring. Concentrations are expressed as ng of C. In the boxes: X = arithmetic mean; horizontal line = median.
Seasonal Variability of Concentrations of AVOCs
Table 1 shows the statistical results of AVOCs in foliar material at the three sampling sites (VM Avenue, V Avenue and B Park) during the two seasons. Ethylbenzene (VM Avenue and B Park), toluene (VM Avenue), and trichloroethene (V Avenue) did not show statistically significant differences in samples for both seasons (Fobs < Fcri and p-value > 0.05).
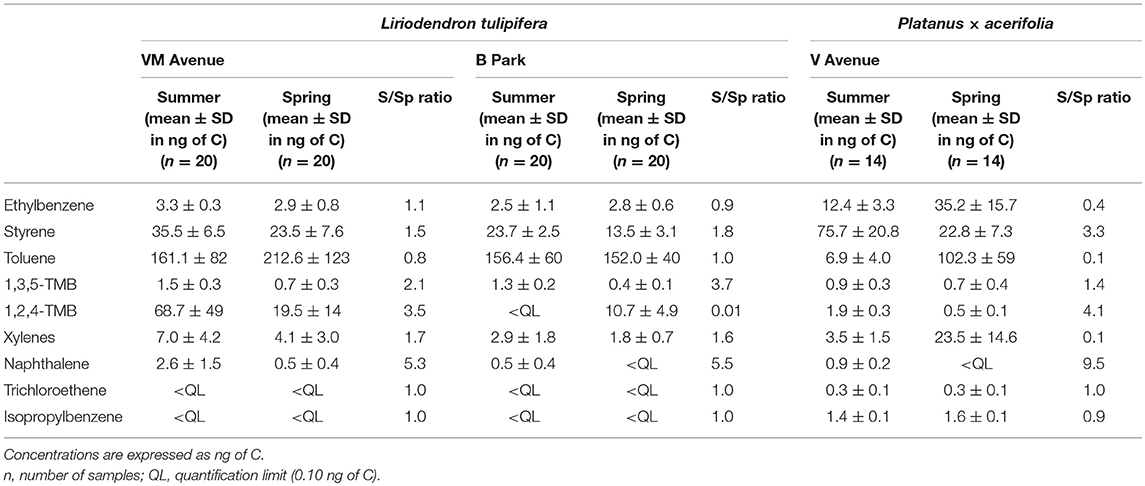
Table 1. Seasonal variability of selected AVOCs at the three sampling sites (VM Avenue, V Avenue, and B Park).
Figure 2 shows no variation in terms of total concentrations of AVOCs (ΣAVOCs), in each season for L. tulipifera from B Park (Fobs = 2.80; Fcri = 4.10; p-value = 0.103). A different behavior was observed for L. tulipifera from VM Avenue (Fobs = 12.53; p-value = 0.001) and for Platanus sp from V Avenue samples (Fobs = 11.08; p-value = 0.003), with greater total concentration of AVOCs in spring than in summer, mainly due to the low amount of ethylbenzene, toluene and xylene leaf content in summer (Figure 2).
Figure 4 shows the relative concentrations, in percentages of leaf content, of different AVOCs by leaves, when comparing the profiles of L. tulipifera at both sites in spring and summer. In summer, the main leaf content of chemical compounds along VM Avenue were toluene, styrene and 1,2,4-TMB, while at B park only toluene and styrene leaf content was quantified; 1,2,4-TMB and naphthalene were not detected in the foliar material.
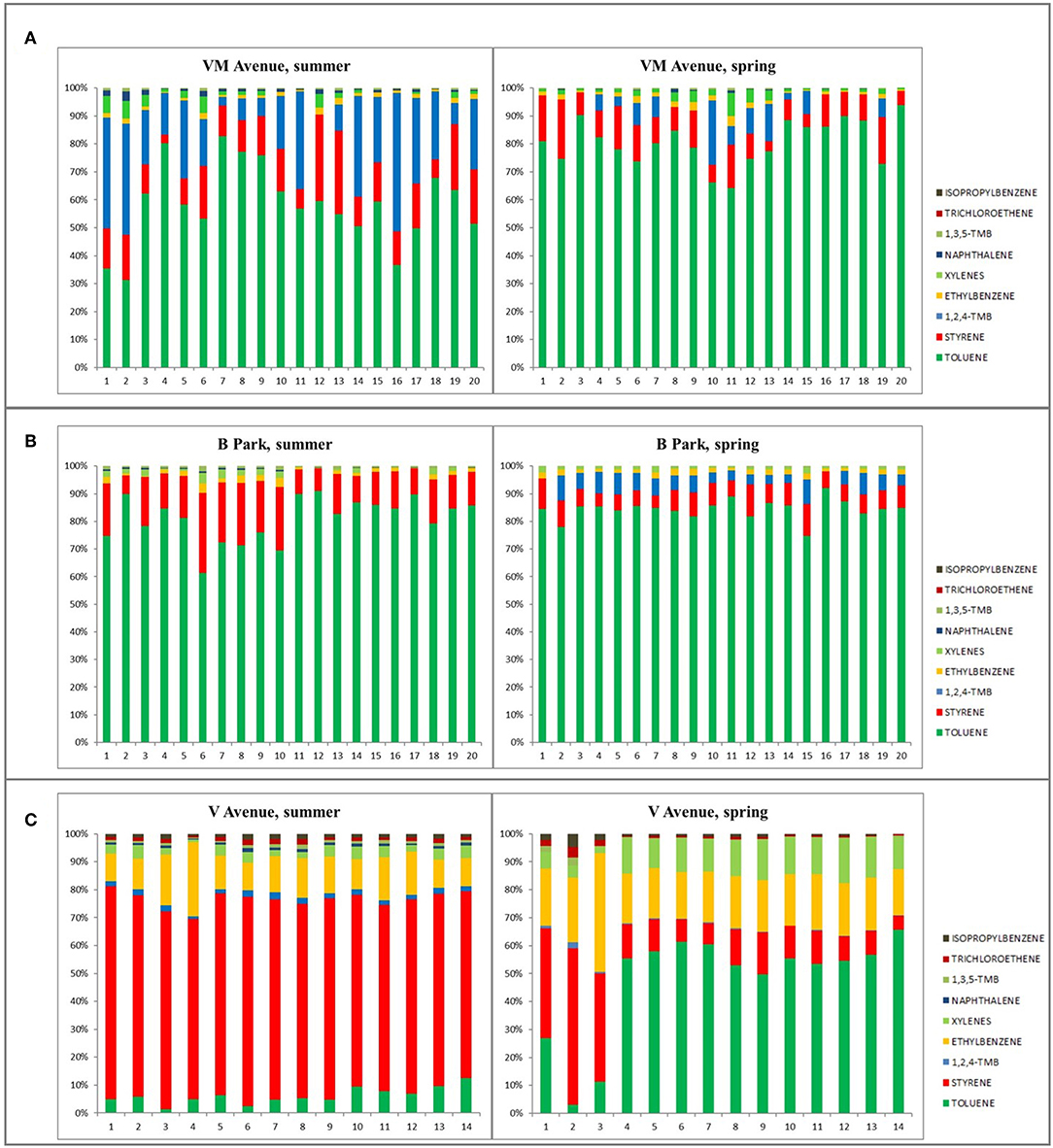
Figure 4. Concentration profiles of AVOCs, in relative percentages, for each sampling site in summer and spring: (A) VM Avenue (L. tulipifera), (B) B Park (L. tulipifera), and (C) V Avenue (Platanus sp).
There was a large heterogeneity of AVOCs in leaf content among Platanus sp samples collected in spring (see Figure 4C), which was not explained by the location of each individual along V Avenue. In order to determine if all Platanus trees sampled corresponded to the same species, a genetic analysis was performed. Therefore, the species or presence of Platanus hybrids was determined by analyzing the internal transcribed spacer (ITS) subregion of the nuclear rDNA cistron.
DNA extraction yields ranged between 90 and 220 ng/L and an amplicon of ~650 bp was obtained for all samples. A single nucleotide polymorphism at the relative position 120 allowed discriminating between P. orientalis and the hybrid P. × acerifolia. This position is always “G” for P. orientalis, while a double signal of T or G is present in the hybrid P. × acerifolia. Analysis of 13 of the Platanus samples presented the “T” variant, defining their assignment to P. × acerifolia. Sample V7 did not amplify. Sample V14 presented five additional polymorphisms; however, the overall genetic profile was compatible with both P. orientalis and P. × acerifolia, and not indicative of P. occidentalis (Figure 5).
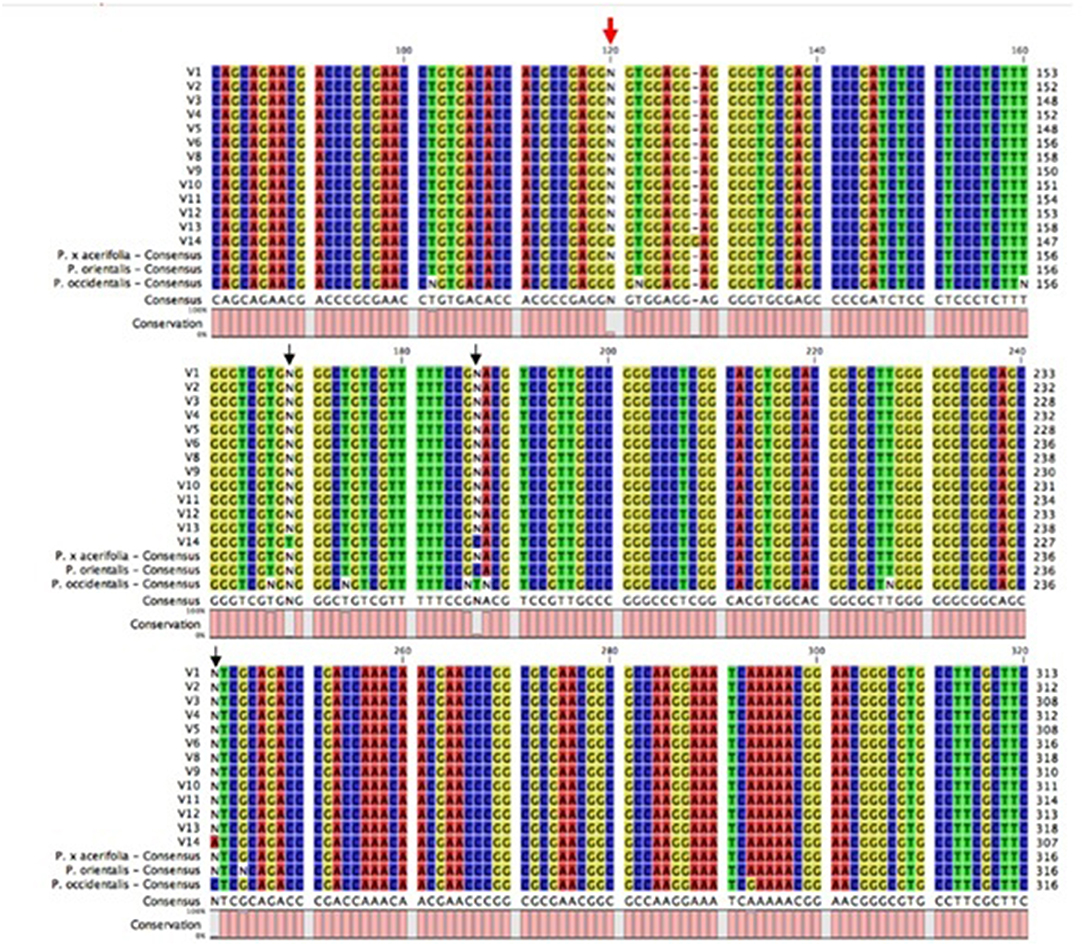
Figure 5. Comparison of the ITS nuclear rDNA region from the Platanus samples and published P. × acerifolia, P. orientalis, and P. occidentalis consensus sequences. A red arrow at the relative position 120 shows the discriminating polymorphism. Small black arrows indicate other polymorphisms.
Ozone Formation Potential (OFP)
Table 2 shows the concentration of Prop-Equiv and the OFP calculated for AVOCs content in leaves from the studied sites in summer and spring. L. tulipifera individuals located at VM Avenue presented higher total and individual values, compared to L. tulipifera individuals located at B Park and the individuals of P. × acerifolia located at V Avenue (except for ethylbenzene and styrene). Additionally, the concentrations of Prop-Equiv and OFP for individuals sampled were higher in summer than in spring.
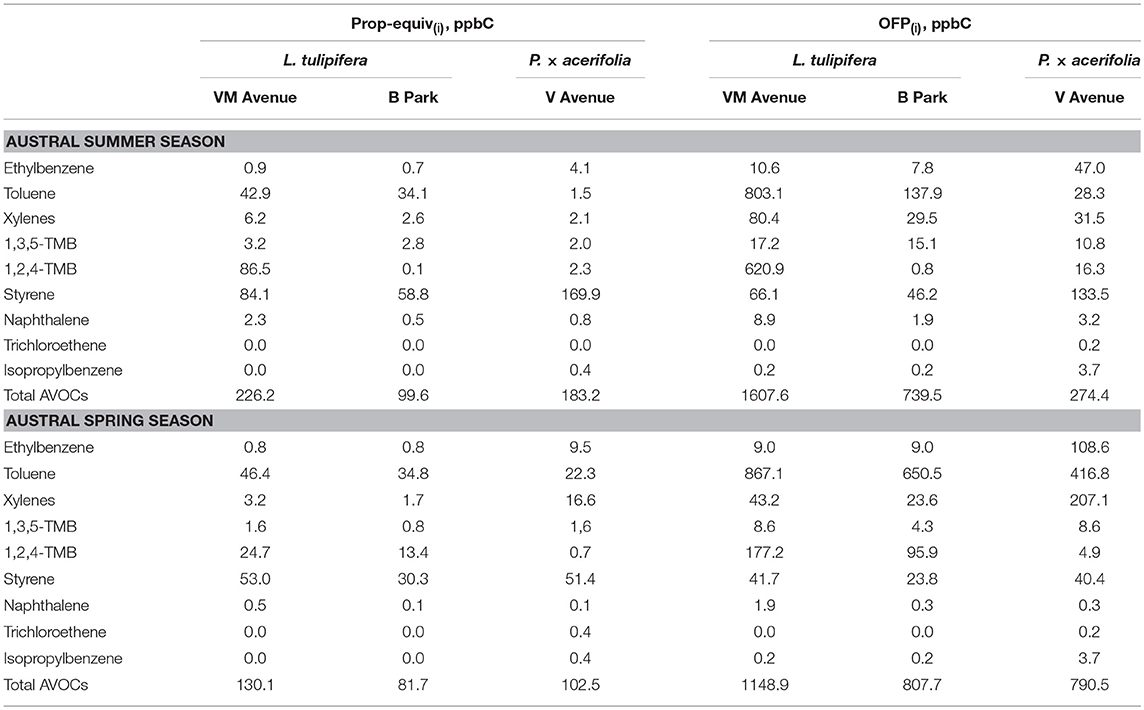
Table 2. Prop-equiv(i) concentration (ppbC) and OFP(i) (ppbC) of AVOCs at VM Avenue, B Park and V Avenue sites, for austral summer season, and austral spring.
Discussion
Many VOCs, including benzene, toluene and xylene are emitted by anthropogenic and biogenic sources. Annual global emissions of biogenic VOCs reported by Wiedinmyer et al. (2004) are mainly isoprene (250–750 TgC yr−1), followed by methanol, various terpenes and very low concentrations of toluene (0.4–2 TgC yr−1), in contrast to high toluene concentrations indicative of anthropogenic VOCs. Misztal et al. (2015) have reported that benzenoids from plants contribute in a similar proportion than AVOCs emitted by fossil fuels to atmospheric emissions in native forests located at the north and northeast regions of the United States. The proportion of BVOCs and AVOCs depends on climate conditions, urban development, plant cover and many other factors. Measurements of trace gas emissions of the small Mediterranean shrub Halimium halimifolium have revealed a broad range of BVOCs under controlled laboratory conditions, emitting terpenes, trimethylbenzene, ethylphenol, and toluene, indicating de novo BVOC biosynthesis of these compounds (Fasbender et al., 2018). Under field conditions, either in natural or urban settings, benzenoid emissions may vary significantly. In Santiago, Chile, a large city set in a temperate Mediterranean climate, AVOCs correspond to 85% of all VOCs emitted and only 15% are BVOCs (Gramsch, 2014). BVOCs are secondary metabolites that play an important role in the defense response to heat and drought, and complement other defense mechanisms, such as antioxidant enzymes (Tattini et al., 2015). Platanus × acerifolia, a high isoprene-emitting species, is frequently used as an ornamental plant in urban areas of the Mediterranean basin (Tattini et al., 2015) and other temperate regions exposed to the combined effect of high light, high temperature, and drought. Liriodendrum tulipifera is native to the United States and has been introduced in Chile because of its ornamental value and fast growth. The absorption mechanism and further degradation and metabolism of either AVOCs or BVOCs has not been studied in these two exotic arboreal species under the climate and pollution conditions prevalent in Santiago, and was beyond the scope of this analysis.
Platanus sp
The Platanus individuals located on V Avenue presented greater content of ethylbenzene, toluene and xylenes in spring than in summer (S/Sp <1). The heterogeneity observed in concentrations of AVOC leaf content by the individual Platanus specimens was unexpected.
In Santiago, plane trees P. orientalis, P. occidentalis, and the hybrid P. × acerifolia are found. Due to the distinctly variable AVOC concentrations in samples from trees 1, 2, and 3 compared to samples from specimens 4 to 14, we hypothesized that the different trees might correspond to phenotypically similar, but genetically distinct Platanus accessions. Therefore, a genetic test that amplifies the nuclear ribosomal internal transcribed spacer (ITS) region allowed discriminating between the different Platanus species and the P. × acerifolia hybrid. This region of high copy number sequences is frequently utilized for phylogenetic analyses at the genus and species levels (Coleman, 2003), is inherited biparentally and useful to determine hybrid speciation (Álvarez and Wendel, 2003), and therefore, valuable in this study. The results of this analysis indicated that all sampled trees correspond to the P. × acerifolia hybrid and thus, the observed differences are not due to a differential genetic background. More details in Supplementary Material. At present, we lack an explanation for the observed differences in AVOC leaf content, except that individuals 1, 2, and 3 corresponded to older and larger trees. It is known that the emitted BVOCs vary according to development, as reported for other arboreal species (Préndez et al., 2013b); however, we did not expect to detect differences in AVOC leaf content depending on the age of individual trees. In hindsight, it is not unreasonable to speculate that age-related metabolic changes do not only modulate emissions, but may also affect AVOC content. Therefore, we cannot exclude active metabolic processes involving AVOC capturing.
The most common Platanus trees in Santiago are P. × acerifolia, and the samples analyzed correspond to this widely planted hybrid tree. Therefore, data obtained in this work is probably representative of AVOC leaf content by the bulk of Platanus trees in Santiago. Plane trees are one of the most common exotic trees, with a relative abundance of 2.3% at the city level (Hernández and Villaseñor, 2018), although all plane trees are planted along main avenues and hence, are one of the main urban species exposed to vehicle emissions.
Liriodendron tulipifera
We have not found reports on benzenoid emissions from or captured by Liriodendron in the literature. Our data indicate three concentration ranges in the leaf content of AVOCs of L. tulipifera (Figure 3): (1) toluene, 1,2,4 TMB and styrene (10–100 of ng of C); (2) ethylbenzene, xylenes, naphthalene and 1,3,5 trimethylbenzene (in the order of ng of C); (3) trichloroethane and isopropylbenzene were not quantified because they were below the detection limit of the technique used. All chemical compounds reported are present in emissions from vehicle traffic; however, vehicles are not the only source of these pollutants. For example, naphthalene is present in the combustion of tobacco and wood, manufacture of paints, varnishes and agents for leather tanning. Styrene is present in cigarette smoke, released by the use of photocopiers and the manufacturing industry at concentrations of 0.06–4.6 ppb in air. Xylenes are used in paints, varnishes, products that prevent corrosion, cleaning products and as solvents; xylene concentrations of 1–30 ppb are reported in air. Toluene is widely used in paint thinners, adhesives, paint varnishes and gasoline, at concentrations of 1–35 ppbv in air (ATSDR, 2019). For these reasons, the sampling was performed with leaves directly exposed to vehicle traffic at the V. Mackenna (VM Avenue) site.
The total concentration of AVOCs leaf content (Figure 2) by L. tulipifera on VM Avenue was statistically higher in summer and in spring than the corresponding concentrations found in the Bicentennial Park (B Park, low exposure to vehicle traffic), except that at B Park ethylbenzene and 1,2,4-TMB presented greater AVOC leaf content in spring than in summer. Toluene remained unchanged. AVOCs leaf content of L. tulipifera in B Park evidences a background level of pollution characteristic of urban activities, according to Kumar et al. (2018).
The standard deviations detected in spring were higher than in summer. According to Brilli et al. (2018), who studied the use of plants for improvement of indoor air quality, this could be due to the leaf content of VOCs through two mechanisms: stomatal absorption and cuticular adsorption, dependent on the process of greater vegetative growth that occurs in spring.
Toluene, 1,2,4-TMB and styrene presented the highest values for PFO and Prop-equiv (Table 2), which measure the contribution of different precursors to the atmosphere. The difference in benzenoid concentrations between the VM Avenue and B Park sites is related to different concentrations of ozone precursors present in leaves from each site, and therefore could indicate an eventual decrease of tropospheric ozone.
Interspecies Variability
A comparison of leaf content of the individual concentrations of AVOCs in both tree species near the source of pollution (Figure 3) showed a greater concentrations of ethylbenzene and xylenes (spring), styrene (summer), trichloroethene and isopropylbenzene (summer and spring) by P. × acerifolia, while L. tulipifera presented higher concentrations of toluene (spring), xylenes, naphthalene, 1,2,4-TMB, and 1,3,5-TMB (summer). No statistically significant differences were observed for styrene and 1,3,5-TMB by leaves in spring by both species.
Clear differences were observed in the AVOC leaf content profiles presented in Figures 4A,C: leaves collected in summer yielded mainly toluene, 1,2,4-TMB and styrene by L. tulipifera, and styrene and ethylbenzene by P. × acerifolia. In spring L. tulipifera leaves contained mainly toluene and styrene, while P. × acerifolia AVOC leaf content consisted mainly of toluene, ethylbenzene, xylenes and styrene. The differences in the response associated with AVOCs in leaves of L. tulipifera and P. × acerifolia may be related to the cuticular composition of the leaves of each species, affecting the adsorption of VOCs, as described by Wararat et al. (2014).
In terms of total concentrations of AVOCs (Figure 2), in summer L. tulipifera leaves contained 2.7 times more than P. × acerifolia leaves (Fobs = 42.1, p-value = 7.03 × 10−7) in terms of ng of C under field conditions with similar vehicle traffic. The same trend was observed in spring, when L. tulipifera leaves presented 1.4 times more AVOCs than P. × acerifolia leaves (Fobs = 7.17; p-value = 0.011), presenting statistically significant differences in all cases. It is important to point out that these results imply AVOC content at the level of leaf tissue, and that these values cannot be extrapolated directly to the performance of individual trees or species.
Prop-Equiv and OFP
We used the concentration values of Prop-Equiv and OFP to associate that greater AVOC content by leaves means lower generation of ozone in the urban environment.
Liriodendron tulipifera leaves presented a higher leaf content of total AVOCs than Platanus × acerifolia leaves in summer and spring. Even considering an eventual biogenic contribution of benzenoids by the studied species, it is possible to introduce the use of OFP and Prop-Equiv to estimate the decrease of ozone formation precursors in the atmosphere. In general, L. tulipifera showed a greater capacity to improve air quality than P. × acerifolia, presenting higher values in terms of total Prop-Equiv concentration (1.23 and 1.26 times for summer and spring, respectively) and total OFP (5.9 times in summer and 1.5 times in spring).
An important group of compounds for atmospheric chemistry is BTXs (benzene, toluene, and xylenes). In this work, benzene was not quantified because it was under the detection limits of the technique. However, concentrations of the TX compounds were high in the studied trees that were close to the source of pollution. Of note, the Prop-Equiv and OFP of L. tulipifera were very high in summer, being 13.6 and 14.8 times greater, respectively, than the corresponding values for P. × acerifolia. Therefore, when measuring the contribution of leaves, L. tulipifera contributes more to improving air quality than P. × acerifolia, because it decreases the amount of precursors that participate in the formation of tropospheric ozone.
Conclusion
Understanding how AVOCs contribute to environmental pollution is important in order to mitigate the effects of vehicle traffic and create more sustainable cities for the growing urban populations. The present study was performed under field conditions in an urban setting with a Mediterranean climate. The eastern part of Santiago showed high ozone concentrations during the spring-summer months. As a secondary pollutant, ozone reduction is closely linked to the decrease of its precursors: VOCs and NOx. A natural way to reduce VOCs is to find urban tree species that efficiently remove these precursors from the atmosphere. To avoid the contribution of other chemical compound sources (range of aromatic components of gasoline), we studied the most common tree species directly exposed to vehicle traffic.
In this case, the study considered two species that are frequently planted along streets and avenues of the city: L. tulipifera and P. × acerifolia. The leaf content of AVOCs in these urban arboreal species was measured for the first time in Chile. To our knowledge, the emission of benzenoids by L. tulipifera has not been reported in the international literature. The large difference found between concentrations in leaves exposed to the high vehicle traffic of Av. Vicuña Mackenna (VM Avenue) and the low exposure to vehicle traffic in the Bicentennial Park (B Park), suggests that the values obtained for the B Park could correspond either to AVOCs or to emission of these compounds from the leaves.
The high values determined for Prop-equiv and OFP of 1,2,4 TMB, toluene, and styrene and their high content in leaves of L. tulipifera, showed that this tree has a higher potential than P. × acerifolia as a tool to cooperate with atmospheric decontamination processes in urban environments, as a first step to evaluate their decontamination potential. In order to extend these observations to the level of individual trees, the foliar surface of each tree has to be considered. Calculations of the Leaf Area Index (LAI) of individual trees and urban tree cover are in progress, in order to estimate the effective removal of AVOCs from vehicle emissions by different tree species in Santiago. Further steps will imply measurements of LAI in both species in order to compare their relative efficiency in the removal of AVOCs.
The two exotic species analyzed in this study are deciduous trees. During the austral winter, the main pollutant in Santiago corresponds to particulate matter, coincident with the season when these species lack leaves and therefore, do not contribute to the removal of this pollutant from urban air. However, during the austral spring, ozone is the main pollutant in Santiago. Under the studied field conditions, P. × acerifolia removes less AVOCs than L. tulipifera, measured in terms of leaf mass. Finding the most suitable arboreal species that are able to contribute to the improvement of the air quality of Santiago with criteria of sustainability will imply a long-term task.
Author Contributions
MA: assembly and validation of the analytical technique, quantification of the chemical species absorbed in the leaves of the trees, discussion of results. DS: supervised and procured funding for genetic analyses and participated in the writing of the manuscript. MB: performed genetic analysis. BP-A: supervised genetic analysis and performed analysis of genetic data. JV: statistical support. CE: graphic and bibliographic support, discussion of results. MP: general design of the research and discussion of results and wrote the manuscript.
Conflict of Interest Statement
The authors declare that the research was conducted in the absence of any commercial or financial relationships that could be construed as a potential conflict of interest.
Acknowledgments
Authors acknowledge project UREDES Consolidación-URC-026/17, VID, Universidad de Chile for financial support and thank reviewers for their useful comments and suggestions to improve this manuscript significantly.
Supplementary Material
The Supplementary Material for this article can be found online at: https://www.frontiersin.org/articles/10.3389/ffgc.2019.00042/full#supplementary-material
References
Agarwal, P., Sarkar, M., Chakraborty, B., and Banerjee, T. (2018). “Phytoremediation of air pollutants: prospects and challenges,” in Phytomanagement of Polluted Sites: Market Opportunities in Sustainable Phytoremediation, eds V. C. Pandey and K. Bauddh (Oxford, UK: Elsevier Inc.), 221–241. doi: 10.1016/B978-0-12-813912-7.00007-7
Álvarez, I., and Wendel, J. F. (2003). Ribosomal ITS sequences and plant phylogenetic inference. Mol. Phylogenet. Evol. 29, 417–434. doi: 10.1016/S1055-7903(03)00208-2
Anyanwu, E. C., and Kanu, I. (2006). The role of urban forest in the protection of human environmental in geographically prone unpredictable hostile weather conditions. Int. J. Environ. Sci. Technol. 3, 197–201. doi: 10.1007/BF03325926
Atkinson, R. (2000). Atmospheric chemistry of VOCs and NOX. Atmos. Environ. 34, 2063–2101. doi: 10.1016/S1352-2310(99)00460-4
Atkinson, R., and Arey, J. (2003). Atmospheric degradation of volatile organic compounds. Chem. Rev. 103, 4605–4638. doi: 10.1021/cr0206420
ATSDR (2019). Agency for Toxic Substances and Disease Registry. Available online at: http://www.atsdr.cdc.gov (accessed April 28, 2019).
Brilli, F., Fares, S., Ghirardo, A., de Visser, P., Calatayud, V., Muñoz, A., et al. (2018). Plants for sustainable improvement of indoor air quality. Trends Plant Sci. 23, 507–512. doi: 10.1016/j.tplants.2018.03.004
Cai, C.-J., Geng, F.-H., Tie, X.-X., Yu, Q., Li, P., and Zhou, G.-Q. (2010). Characteristics of ambient volatile organic compounds (VOCs) measured in Shanghai, China. Sensors 10, 7843–7862. doi: 10.3390/s100807843
Carter, W. P. L. (1994). Development of ozone reactivity scales for volatile organic compounds. Air Waste 44, 881–899. doi: 10.1080/1073161X.1994.10467290
Chameides, W. L., Fehsfeld, F., Rodgers, M. O., Cardelino, C., Martinez, J., Parrish, D., et al. (1992). Ozone precursor relationships in the ambient atmosphere. J. Geophys. Res. 97, 6037–6055. doi: 10.1029/91JD03014
Coleman, A. W. (2003). ITS2 is a double-edged tool for eukaryote evolutionary comparisons. Trends Genetics. 19, 370–375. doi: 10.1016/S0168-9525(03)00118-5
Costanza, R., Fisher, B., Mulder, K., Liu, S., and Christopher, T. (2007). Biodiversity and ecosystem services: a multi-scale empirical study of the relationship between species richness and net primary production. Ecol. Econ. 61, 478–491. doi: 10.1016/j.ecolecon.2006.03.021
Cui, L., Wang, X. L., Ho, K. F., Gao, Y., Liu, C., Hang Ho, S. S., et al. (2018). Decrease of VOC emissions from vehicular emissions in Hong Kong from 2003 to 2015: results from a tunnel study. Atmos. Environ. 177, 64–74. doi: 10.1016/j.atmosenv.2018.01.020
Dzierzanowski, K., Popek, R., Gawronska, H., Sæbø, A., and Gawronski, S. W. (2011). Deposition of particulate matter of different size fractions on leaf surfaces and in waxes of urban forest species. Int. J. Phytoremediation 13, 1037–1046. doi: 10.1080/15226514.2011.552929
Escobedo, F. J., Kroeger, T., and Wagner, J. E. (2011). Urban forests and pollution mitigation: analyzing ecosystem services and disservices. Environ. Pollut. 159:2078–2087. doi: 10.1016/j.envpol.2011.01.010
Fasbender, L., Yáñez-Serrano, A. M., Kreuzwieser, J., Dubbert, D., and Werner, C. (2018). Real-time carbon allocation into biogenic volatile organic compounds (BVOCs) and respiratory carbon dioxide (CO2) traced by PTR-TOF-MS, 13CO2 laser spectroscopy and 13C-pyruvate labelling. PLoS ONE 13:e0204398. doi: 10.1371/journal.pone.0204398
Gramsch, E. (2014). Estudio “Actualización y sistematización del inventario de emisiones de contaminantes atmosféricos en la Región Metropolitana”. Departamento de Física, Universidad de Santiago de Chile. Available online at: https://sustempo.com/website/wp-content/uploads/2015/07/Inventario-de-emisiones-RM_USACH_2014.pdf (accessed January 8, 2019).
Guenther, A.B., Jiang, X., Heald, C.L., Sakulyanontvittaya, T., Duhl, T., Emmons, L. K., et al. (2012). The model of emissions of gases and aerosols from nature version 2.1 (MEGAN2.1): an extended and updated framework for modeling biogenic emissions. Geosci. Model Dev. 5, 1471–1492. doi: 10.5194/gmd-5-1471-2012
Guo, H., Ling, Z. H., Cheng, H., Simpson, I., Lyu, X., Wang, X., et al. (2016). Tropospheric volatile organic compounds in China. Sci. Total Environ. 574, 1021–1043. doi: 10.1016/j.scitotenv.2016.09.116
Hebbert, M., and Webb, B. (2012). “Towards a liveable urban climate – lessons from Stuttgart,” in Liveable Cities: Urbanising World (ISOCARP 07), eds C. Gossop, and S. Nan (London; New York, NY: Routledge, 132–149 (Chapter 7).
Henríquez, C., and Romero, H. (2019). “Introduction,” in Urban Climates in Latin America, eds C. Henríquez and H. Romero (Switzerland AG: Springer Nature, 1–14 (Chapter 1). doi: 10.1007/978-3-319-97013-4
Hernández, H. J., and Villaseñor, N. R. (2018). Twelve-year change in tree diversity and spatial segregation in the Mediterranean city of Santiago, Chile. Urban Forestry and Urban Greening. 29, 10–18. doi: 10.1016/j.ufug.2017.10.017
INE (2018). Instituto Nacional de Estadísticas. Available onlINEat: http://www.ine.cl (accessed January 8, 2019).
Kansal, A. (2009). Sources and reactivity of NMHCs and VOCs in the atmosphere: a review. J. Hazard. Mater. 166, 17–26. doi: 10.1016/j.jhazmat.2008.11.048
Karakitsios, S. P., Delis, V. K., Kassomenos, P. A., and Pilidis, G. A. (2007). Contribution to ambient benzene concentrations in the vicinity of petrol stations: estimation of the associated health risk. Atmos. Environ. 41, 1889–1902. doi: 10.1016/j.atmosenv.2006.10.052
Kesselmeier, J., and Staudt, M. (1999). Biogenic volatile organic compouds (VOC): an overview on emission, physiology and ecology. J. Atmos. Chem. 33, 23–88. doi: 10.1023/A:1006127516791
Khan, F. I., and Abbasi, S. A. (2000). Attenuation of gaseous pollutants by greenbelts. Environ. Monit. Assess. 64, 457–475. doi: 10.1023/A:1006278000352
Kountouriotis, A., Aleiferis, P. G., and Charalambides, A. G. (2014). Numerical investigation of VOC levels in the area of petrol stations. Sci. Total Environ. 470, 1205–1224. doi: 10.1016/j.scitotenv.2013.10.064
Kumar, A., Singh, D., Kumar, K., Singh, B. B., and Kumar, J. V. (2018). Distribution of VOCs in urban and rural atmospheres of subtropical India: temporal variation, source attribution, ratios, OFP and risk assessment. Sci. Total Environ. 613–614, 492–501. doi: 10.1016/j.scitotenv.2017.09.096
Li, B., Ho, S. S. H., Xue, Y., Huang, Y., Wang, L., Cheng, Y., et al. (2017). Characterizations of volatile organic compounds (VOCs) from vehicular emissions at roadside environment: the first comprehensive study in Northwestern China. Atmos. Environ. 161, 1–12. doi: 10.1016/j.atmosenv.2017.04.029
Manes, F., Marando, F., Capotorti, G., Blasi, C., Salvatori, E., Fusaro, L., et al. (2016). Regulating ecosystem services of forests in ten Italian metropolitan cities: air quality improvement by PM10 and O3 removal. Ecol. Indic. 67, 425–440. doi: 10.1016/j.ecolind.2016.03.009
Marando, F., Salvatori, E., Fusaro, L., and Manes, F. (2016). Removal of PM10 by forests as a nature-based solution for air quality improvement in the metropolitan city of Rome. Forests. 7:150. doi: 10.3390/f7070150
Matsui, K. (2016). Portion of plant airborne communication is endorsed by uptake and metabolism of volatile organic compounds. Curr. Opin. Plant Biol. 32, 24–30. doi: 10.1016/j.pbi.2016.05.005
Misztal, C.N., Hewitt, J., Wildt, J.D., Blande, A.S., Eller, D., Fares, S., et al. (2015). Atmospheric benzenoid emissions from plants rival those from fossil fuels. Sci. Rep. 5:12064. doi: 10.1038/srep12064
MMA (2016). Informe Final para la Gestión de Episodios Críticos de Contaminación Atmosférica por Material Particulado Respirable (MP10), Periodo 2016. Secretaria Regional Ministerial (SEREMI) del Medio Ambiente, Región Metropolitana. Ministerio del Medio Ambiente. Gobierno de Chile. Retrieved from: http://portal.mma.gob.cl/wp-content/uploads/2017/01/INFORME-_GEC_Fin-2016_EFA.pdf
Niinemets, Ü., Fares, S., Harley, P., and Jardine, K.J. (2014). Bidirectional exchange of biogenic volatils with vegetation: emission sources, reactions, breakdown and deposition. Plant Cell Environ. 37, 1790–1809. doi: 10.1111/pce.12322
Paoletti, E. (2009). Ozone and urban forests in Italy. Environ. Pollu. 157, 1506–1512. doi: 10.1016/j.envpol.2008.09.019
Préndez, M., Araya, M., Criollo, C., Egas, C., Farías, I., Fuentealba, R., et al. (2019). “Urban trees and its relationships with air pollution by particulate matter and ozone in Santiago de Chile,” in Chapter 8: Urban Climates in Latin America, eds C. Henríquez, and H. Romero (Cham: Springer Nature Switzerland AG), 167–206. doi: 10.1007/978-3-319-97013-4_8
Préndez, M., Carvajal, V., Corada, K., Morales, J., Alarcón, F., and Peralta, H. (2013a). Biogenic volatile organic compounds from the urban forest of the Metropolitan Region, Chile. Environ. Pollut. 183, 143–150. doi: 10.1016/j.envpol.2013.04.003
Préndez, M., Corada, K., and Morales, J. (2013b). Emission factors of biogenic volatile organic compounds in various stages of growth present in the urban forest of the Metropolitan Region, Chile. Res. J. Chem. Environ. 17, 108–116. Available online at: https://www.scopus.com/inward/record.uri?eid=2-s2.0-84886913189&partnerID=40&md5=f20c25a3da64e278af06d56c7a513bc7
Romero, H. (2019). “Urban climates and the challenge of sustainable development of Chilean cities,” in Urban Climates in Latin America, eds C. Henríquez, and H. Romero (Cham: Springer), 167–206. doi: 10.1007/978-3-319-97013-4_9
Saphores, J., and Li, W. (2012). Landscape and urban planning estimating the value of urban green areas: a hedonic pricing analysis of the single-family housing market in Los Angeles, CA. Landsc. Urban Plan. 104, 373–387. doi: 10.1016/j.landurbplan.2011.11.012
Shao, P., An, J., Xin, J., Wu, F., Wang, J., Ji, D., et al. (2016). Source apportionment of VOCs and the contribution to photochemical ozone formation during summer in the typical industrial area in the Yangtze River Delta, China. Atmos. Res. 176–177, 64–74. doi: 10.1016/j.atmosres.2016.02.015
SINCA (2017). Sistema de Información Nacional de Calidad del Aire. Gobierno de Chile. Available online at: http://sinca.mma.gob.cl/index.php/region/index/id/M (accessed December 10, 2019).
Tallis, M., Taylor, G., Sinnett, D., and Freer-Smith, P. (2011). Estimating the removal of atmospheric particulate pollution by the urban tree canopy of London, under current and future environments. Landsc. Urban Plan. 103,129–138. doi: 10.1016/j.landurbplan.2011.07.003
Tattini, M., Loreto, F., Fini, A., Guidi, L., Brunetti, C., Velikova, V., et al. (2015). Isoprenoids and phenylpropanoids are part of the antioxidant defense orchestrated daily by drought-stressed Platanus × acerifolia plants during Mediterranean summers. New Phytol. 207, 613–626. doi: 10.1111/nph.13380
Ulriksen, P. (1993). “Factores meteorológicos de la contaminación atmosférica de Santiago,” in Chapter 2: Contaminación Atmosférica de Santiago: Estado Actual y Soluciones, eds H. Sandoval, M. Préndez, and P. Ulriksen (Santiago de Chile: Universidad de Chile y Comisión Especial de Descontaminación de la Región Metropolitana), 37–60.
UOCT (2019). Operational Unit of Traffic Control, Ministry of Transport of Chile. Available online at: http://www.mtt.gob.cl/pyd/uoct (accessed April 28, 2019).
USEPA (1989). Network Design and Site Exposure Criteria for Nonmethane Organic Hydrocarbons. Office Air Quality Planning and Standards, SYSAPP-89/138. Research Triangle Park, NC: United States Environmental Protection Agency.
Wang, H., Wang, Q., Chen, J., Chen, C., Huang, C., Qiao, L., et al. (2015). Do vehicular emissions dominate the source of C6-C8 aromatics in the megacity Shanghai of eastern China? J. Environ. Sci. 27, 290–297. doi: 10.1016/j.jes.2014.05.033
Wararat, S., Parinda, S., Sirintip, A., Polawat, K., Atcharaphan, W., Anuchit, S., et al. (2014). Uptake of toluene and ethylbenzene by plants: removal of volatile indoor air contaminants. Ecotoxicol. Environ. Saf. 102, 147–151. doi: 10.1016/j.ecoenv.2014.01.032
WHO (2016). Ambient Air Pollution: A Global Assessment of Exposure and Burden Of Disease. World Health Organization. Retrieved from: http://www.who.int/phe/health_topics/outdoorair/databases/cities/en/Z
Wiedinmyer, C., Guenther, A., Harley, P., Hewitt, N., Geron, C., Artaxo, P., et al. (2004). “Globals organic emissions from vegetation,” in Emissions of Atmospheric Trace Compounds, eds C. Granier, P. Artaxo, and C. Reeves (Dordrecht: Kluwer Academic Publishers), 115–170. doi: 10.1007/978-1-4020-2167-1_4
Keywords: ecosystem services, ozone formation potential, vehicle emissions, Santiago de Chile, urban trees
Citation: Araya M, Seelenfreund D, Buscaglia M, Peña-Ahumada B, Vera J, Egas C and Préndez M (2019) Assessment of Anthropogenic Volatile Organic Compounds in Leaves of Two Urban Tree Species in Santiago de Chile. Front. For. Glob. Change 2:42. doi: 10.3389/ffgc.2019.00042
Received: 01 February 2019; Accepted: 16 July 2019;
Published: 06 August 2019.
Edited by:
Silvano Fares, Council for Agricultural and Economics Research, ItalyReviewed by:
Ana Maria Yáñez-Serrano, University of Freiburg, GermanyLeonardo Montagnani, Free University of Bozen-Bolzano, Italy
Copyright © 2019 Araya, Seelenfreund, Buscaglia, Peña-Ahumada, Vera, Egas and Préndez. This is an open-access article distributed under the terms of the Creative Commons Attribution License (CC BY). The use, distribution or reproduction in other forums is permitted, provided the original author(s) and the copyright owner(s) are credited and that the original publication in this journal is cited, in accordance with accepted academic practice. No use, distribution or reproduction is permitted which does not comply with these terms.
*Correspondence: Margarita Préndez, mprendez@ciq.uchile.cl