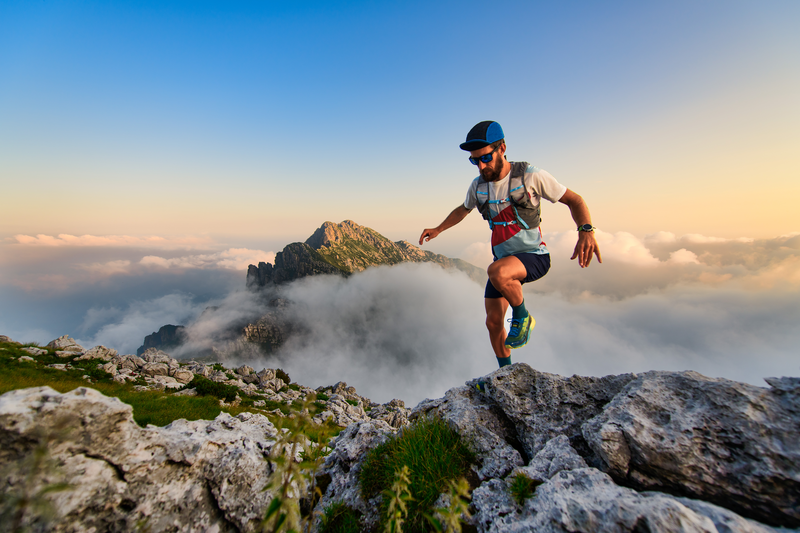
95% of researchers rate our articles as excellent or good
Learn more about the work of our research integrity team to safeguard the quality of each article we publish.
Find out more
ORIGINAL RESEARCH article
Front. For. Glob. Change , 24 July 2019
Sec. Tropical Forests
Volume 2 - 2019 | https://doi.org/10.3389/ffgc.2019.00033
This article is part of the Research Topic Improving Environmental Sustainability in Oil Palm: Linking Science, Policy, & Practice across the Tropics View all 18 articles
Oil palm plantations have expanded rapidly in recent decades, and are causing substantial impacts on tropical habitats and biodiversity. However, owing to its long lifespan (25–30 years), oil palm forms a much more varied and structurally-complex habitat than many other crops. This can include abundant understory vegetation and also epiphytes on palm trunks. However, the diversity of this plantation vegetation has been poorly studied, and there has been little consideration of the impacts of common plantation vegetation management practices on plant communities. We conducted a long-term vegetation management experiment that forms part of the Biodiversity and Ecosystem Function in Tropical Agriculture (BEFTA) Programme in Riau, Indonesia. We manipulated herbicide and manual cutting regimes within mature oil palm plantations to create three different understory complexity treatments (Reduced, Normal, and Enhanced vegetation) across replicated sets of plots. Plant communities were surveyed before and after experimental understory vegetation treatments began in three different microhabitats: within the middle of the plantation block (core), on the road edge (edge) and on oil palm trunks (trunk). Part of the sampling was also conducted during a drought event. We recorded 120 plant species, which comprised a mixture of native, non-native, “beneficial,” and “problem” species. We found substantial variation in plant communities between edge, core, and trunk microhabitats, indicating high levels of heterogeneity within the plantation. There were significant effects of varying understory treatment within both core and edge microhabitats, but no spillover of impacts into the trunk microhabitat. We also observed substantial impacts of drought on plant communities, with declines in either biomass, percentage cover, or richness seen across core, edge, and trunk microhabitats during low-rainfall periods. Our findings highlight the diversity of plant communities that can be supported within oil palm plantations, and the substantial impacts that management decisions, and also drought, can have on them. Given the role that diverse plant communities can have in supporting species in other groups, this is likely to have a significant impact on the wider plantation biodiversity. We suggest that plantation management strategies give greater consideration to within-plantation understory plant communities and choose more wildlife-friendly options where possible.
Global agriculture is rapidly expanding and intensifying to meet the needs of increased consumption and a growing human population (Tilman et al., 2001a, 2002; Godfray et al., 2010; Bonhommeau et al., 2013). Such change is happening fastest in the tropics, where there are still areas of natural habitat available for conversion, and socioeconomic conditions are encouraging rapid development (Laurance et al., 2014). In Southeast Asia, increasingly large areas are planted with oil palm (Elaeis guineensis)—a perennial crop used to produce palm oil. Area of oil palm plantations in Southeast Asia increased by 87% in the 10 years up to 2010 (Wilcove et al., 2013), and Indonesia and Malaysia are the world's leading producers of palm oil, together producing over 46 million tonnes annually—over 80% of the world's supply (Food and Agriculture Organisation (FAO), 2017). Palm oil is a highly versatile vegetable oil which can be used for cooking, processed foods, cosmetics, and biofuel production, and yields are very high compared to other oil crops (Wahid et al., 2005). However, despite its efficiency, the industry has had substantial environmental impacts, largely because most oil palm expansion to date has come at the expense of forest loss (Fitzherbert et al., 2008; Danielsen et al., 2009; Edwards D. P. et al., 2014; Gaveau et al., 2016). Southeast Asia has one of the highest rates of deforestation anywhere in the tropics, with 1.4% annual forest loss during the 1990s and 2000s (Sodhi et al., 2004). Nearly 70% of forest across Malaysia, Sumatra and Java had been lost by 2010 (Wilcove et al., 2013).
In comparison to tropical forest, oil palm plantations are structurally simplified and highly disturbed. Plantation establishment exposes soil and can make it vulnerable to compaction, erosion, and leaching (as described for land immediately after logging, in Clarke and Walsh, 2006). In peat soils there are also high levels of subsidence and peat oxidation when soils are exposed (Hooijer et al., 2012; Manning et al., 2019). In addition, chemical fertilisers and pesticides are frequently applied during the cultivation process (Corley and Tinker, 2003a). Oil palm trees are generally planted in monocultures, and in single age stands. When palms are under 10 years old, plantations have an open canopy, resulting in temperatures that are up to 6.5°C hotter, and significantly drier conditions than in old growth forest (Hardwick et al., 2015). By 22 years old, oil palm trees are about 13 m high with a more closed canopy and are better at buffering microclimate conditions (Luskin and Potts, 2011). Paths between planting rows, cleared circles around the bases of palms, and piles of dead fronds add some structural complexity at ground level. Nitrogen fixing cover crops are planted in young plantations to improve soil retention and fertility. In older plantations, cover crops have been outcompeted by other plants, and there is a deeper leaf litter layer and epiphyte abundances are twice as high as those in young palms (Luskin and Potts, 2011). However, after 25–30 years, palms are no longer as productive and so are cut down and replaced by young palms, once again simplifying the habitat conditions.
Studies that have investigated biodiversity in oil palm plantations have, almost universally, found lower biodiversity than in forest and communities that are heavily altered, even in relation to heavily logged forest (Fitzherbert et al., 2008; Koh and Wilcove, 2008; Danielsen et al., 2009; Foster et al., 2011; Edwards D. P. et al., 2014). Declines in birds, scavenger mammals and a wide range of insect taxa have been recorded (Edwards D. P. et al., 2014), along with shifts toward smaller-bodied, lower trophic level, and non-forest and invasive species (Brühl and Eltz, 2010; Senior et al., 2013; Edwards F. A. et al., 2014). Studies of plant communities within oil palm plantations have been very limited, with only three studies considering the ground flora (Danielsen et al., 2009; Rembold et al., 2017; Ashton-Butt et al., 2018), and only a handful that consider epiphyte communities (Piggott, 1980; Prescott et al., 2015; Böhnert et al., 2016), despite the region being a hotspot of plant diversity (Myers et al., 2000), and the likely importance of vegetation complexity for supporting animal communities. The terrestrial plant communities of plantations in Sumatra, Indonesia, have been found to be very species-poor compared to forest, with several major components of forest vegetation, including forest trees, lianas, epiphytic orchids and indigenous palms, completely absent (Danielsen et al., 2009). Assemblages have also been found to consist of low numbers of species, with low beta diversity, and to be dominated by herbaceous weeds (Rembold et al., 2017; Ashton-Butt et al., 2018). Only pteridophytes (ferns) have been found to be species-rich in oil palm plots, and in general, forest plant species were missing, with plant assemblages instead dominated by disturbance-tolerant and non-native species (Danielsen et al., 2009; Rembold et al., 2017). Studies of ephiphyte communities in oil palm have given mixed results, with diverse communities found in some studies (Piggott, 1980; Prescott et al., 2015), but species-poor communities at other sites (Böhnert et al., 2016), although this may be related to definitions of “epiphyte” differing between studies.
In addition to their biodiversity value, plant communities have the potential to provide valuable ecosystem services or even disservices in oil palm plantations that could affect oil palm yield. Presence of understory vegetation, including cover crops such as nitrogen-fixing Mucuna bracteata which are commonly planted in young plantations, can help reduce soil erosion, and increase soil fertility and water-holding capacity (Corley and Tinker, 2003a; Baligar and Fageria, 2007; Anderson, 2008). Cover crops can help to reduce soil temperature which, particularly in peat soils, can help to reduce the rate of microbial oxidation, thereby reducing CO2 emissions (Arifin et al., 2015). Cover crops can also help to reduce access of rhinoceros beetles (Oryctes rhinoceros, a pest species of young palms) to rotting logs that they use for breeding (Bedford, 1980). Understory vegetation, and particularly flowering plants, have also been shown to provide habitat for beneficial predators which can help to control populations of herbivores, such as bagworm (Pteroma pendula), that damage the oil palm (Kamarudin and Wahid, 2010; Hinsch, 2013). Conversely, presence of vegetation could reduce oil palm yield by providing shelter for pests, competing with palms for water or nutrients, or by hindering access to palms and reducing rates of harvesting. It is therefore necessary to investigate what plant species are present in plantations, and how to manage vegetation to strike the best balance between the needs of production whilst also maintaining as much biodiversity as possible. Studies have investigated the effects of epiphytes in oil palm plantations and found no loss of palm oil yield, but benefits for biodiversity where epiphytes are present (Koh, 2008; Prescott et al., 2015). However, no studies have yet investigated the effects of understory vegetation management on plant communities.
In this study we survey the plant communities present in different microhabitats within a mature oil palm plantation, experimentally assess the effects of differing understory management on plant communities, and investigate how plant communities change over time in relation to rainfall patterns. Specifically we consider three different microhabitats: the understory along the sides of access roads in the plantation, the understory in the core of a plantation block, and the trunk of oil palm trees. We also investigate the effects of three levels of understory vegetation management (“Reduced,” “Normal,” and “Enhanced” vegetation) which span a range of options currently used across the industry. We consider the effects on plant biomass, cover, species richness, and community composition.
The study was conducted in oil palm plantations in Riau Province, Sumatra, Indonesia (N0 55.559, E101 11.619) (Supplementary Figure 1), and measurements were taken during 2013 and 2014. The region has high average rainfall (>2400 mm/year), although during the course of this study, Riau was affected by an El Niño Southern Oscillation (ENSO)-related drought event (2014-2015). Measurements from nine rain gauges (each with a 100 cm2 collecting area) located across the concession area, indicated that mean rainfall in 2014 was lower than in 2013 (140.7 mm cf. 206.8 mm per month) although not significantly so (W = 96.5, p = 0.1658) (Supplementary Figure 2), but rainfall levels in the 6 months preceding 2014 data collections were significantly lower than those in the 6 months before 2013 data collections (99.4 mm cf. 288.1 mm per month; W = 35.5, p = 0.0064) [statistics generated using Mann-Whitney U tests (using R “stats”; R Core Team, 2016)].
The study area has mineral soils [oxic dystropepts and typic dystropepts (USDA classification)] and lowland tropical rainforest is the natural vegetation type, but following selective logging in the 1970s and clearance for oil palm plantations 1985-1995, very little natural vegetation remains. The oil palm plantation sites used in our study were planted between 1988 and 1993 and so were mature at the time of the study (2013-2014). The sites form part of an established research project: The Biodiversity and Ecosystem Function in Tropical Agriculture (BEFTA) Programme. This is a largescale, long term research programme run in collaboration with Sinar Mas Agro Resources and Technology Research Institute (SMARTRI) (the Research and Development entity for upstream activities of Golden Agri Resources) that tests the effects of plantation management strategies on biodiversity, ecosystem functioning, and oil palm yield. As part of this, the BEFTA Understory Vegetation Project is an experiment that investigates the impacts of understory vegetation complexity on oil palm ecosystems (Foster et al., 2014; www.oilpalmbiodiversity.com; Luke et al., submitted).
The BEFTA Understory Vegetation Project sites comprise eighteen 150 × 150 m (2.25 ha) plots, located in six triplets, each separated by at least one kilometre from any other replicate (Supplementary Figures 1, 3) (also Luke et al., submitted). Each triplet is located beside a road lined by a drainage ditch, and roads run through the spaces between plots. Each plot has a core 50 × 50 m area where the majority of data are collected, and a buffer region extending to 150 × 150 m which receives the same understory management treatment. The 150 × 150 m area contains a mixture of harvesting paths, weeded circles around palms, and windrows (including fronds piles and vegetation) (Ashton-Butt et al., 2018). All plots were managed in the same way, following the “Normal” business-as-usual practice outlined below, before treatments were started in February 2014. When treatments began one plot within each triplet was randomly assigned each of the following understory treatments:
1) “Normal” complexity understory vegetation—plots managed according to business-as-usual standard industry practice which includes herbicide treatment in circles around palm bases, along harvesting paths, and at yield bunch platforms, but leaving vegetation to grow naturally elsewhere within the plot. All plots were managed in this way pre-treatment, and so plots receiving this treatment acted as controls throughout the experiment.
2) “Reduced” complexity understory vegetation—involving use of herbicide to clear all understory vegetation within all areas of the plots.
3) “Enhanced” complexity understory vegetation—all areas of the plots left to grow naturally with no use of herbicides and only limited manual cutting to keep harvesting paths, and the circles at the bases of palms, open.
Herbicide application was conducted between three and five times per year, as necessary, to maintain a clear understory in Reduced plots, and cleared paths and palm circles in Normal plots. Manual cutting of paths and palm circles in the Enhanced plots began 1 year after treatments started, but then was done at the same frequency as herbicide application. Additional details about the herbicide mix used and the overall set-up of the BEFTA Understory Vegetation Project can be found in Luke et al. (submitted), Advento et al. (submitted).
Plant communities were surveyed once before (May-July 2013) and once after (August 2014) understory treatments began at each of the study plots (February 2014). Enhanced plots had therefore not yet had any manual cutting when post-treatment surveys were completed. Quadrats were placed within three different microhabitats inside each plot, and were all within the area that received the vegetation management treatment. The three different microhabitats were: (1) on the ground within the 1–2 m wide area between the drainage ditch near the plot edge and the access road at the border of the plot (“Edge”); (2) on the ground in the central 50 × 50 m core of the plot (including on harvesting path, palm circle, and windrow areas) (“Core”); and (3) wrapped around the trunk of oil palm trees (within the central 50 × 50 m area) at a randomly chosen height (1, 2, 3, or 4 m), accessed using a step ladder (“Trunk”) (Supplementary Figure 3). Quadrats were placed randomly in order to cover as full a range of the variation within microhabitat types as possible. Owing to the greater variability within the Core area, we placed ten quadrats in this microhabitat, whilst placing five at Edge locations, and five at Trunk locations in each plot. When the circumference of a trunk was <1 m, the remainder of the quadrat was wrapped around the next randomly chosen height section of the trunk. We chose to survey these three different microhabitats in order to consider the full range of plant habitat types within the plantation, and so gain an understanding of what might be found across the plantation as a whole. We were also interested in the effects of different levels of herbicide use and cutting on plant communities in the different microhabitats. This included whether impacts were localized to ground communities, or whether there were also indirect effects of herbicide use and cutting on the plants that grow on palm trunks, through impacts of pesticide spraying or changes in propagule levels following any change in the understory community.
Surveys were conducted by a trained botanist (DP) and included assessments of percentage cover, biomass and species richness within 1 m2 quadrats. Percentage cover of each species, and total species richness within each quadrat were assessed visually in the field. Biomass of each species and total vegetation biomass within the quadrat (g) were assessed by collecting the vegetation from within the quadrat (cut at ground/trunk level), drying it at 70°C for 1–2 days until it reached constant mass, and then weighing it.
Based on information in the literature (Holtum, 1966; Merlier and Montegut, 1982; Soerjani and Kostermans, 1987; Barnes and Chan, 1990) and expertise of an oil palm plantation botanist (DP), plants were categorised according to whether they were native or non-native to Indonesia, and whether they were considered a problem (allelopathic or a competitor) or beneficial (parasitoid host, nitrogen fixer, or maintain soil moisture) to oil palm trees by plantation managers (Supplementary Table 1). If categories were unclear, we included them in total richness, biomass, and percentage cover measures, but did not include them in analyses that considered traits. All subsequent analyses were conducted on these categories of data (Native, Non-native, Problem, and Beneficial) as well as on total numbers.
All statistical analyses were conducted in R statistical package version 3.3.0 (R Core Team, 2016). To assess differences in plant communities between microhabitats (Edge, Core, and Trunk) we ran mixed effects models in the package “lme4” (Bates et al., 2015) using just the pre-treatment data. We used microhabitat (Edge, Core, or Trunk) as the explanatory variable in each model, along with the response variables, error distributions, model types, and transformations listed in Supplementary Table 2. In each case (1|Plot) was included as a random effect to account for non-independence of samples within each plot. To illustrate the number of shared and non-shared species between the three different microhabitats (Edge, Core and Trunk) during normal understory management we used the package “eulerr” (Larsson, 2019) to produce a Venn diagram using species lists from the pre-treatment survey. To give an indication of which species were most dominant within each microhabitat, we produced plots of mean biomass per quadrat, made up of the stacked biomasses of the five most dominant species (by biomass) within each habitat, and “Other” species.
Using both pre- and post-treatment data, but subset according to microhabitat, we ran linear mixed effects models to test for effects of understory treatment on plant communities. All models were run using the package “lme4” (Bates et al., 2015) with Gaussian error distributions. Response variables are listed in Supplementary Table 3, whilst the explanatory variable in each case was the effect of conducting the experimental treatments (coded as Treatment*Before After). Biomass and richness data were Log10+1 transformed, whilst percentage cover data were Arcsine square root transformed. Issues with non-independence both within triplets, and by taking repeat measures at the same sites were accounted for in each model using a random effect (1|Triplet/Before After). We used the package “eulerr” (Larsson, 2019), using post-treatment data, to produce Venn diagrams to show the number of shared and non-shared species between different understory treatments after treatment. To give an indication of which species were most dominant within each microhabitat across the different treatments we produced plots of mean biomass per quadrat, made up of the stacked biomasses of the five most dominant species (by biomass) within each habitat, and “Other” species.
To assess whether there were changes in the plant community over time that were independent of the experimental treatments, we ran linear mixed effects models (lmer, Gaussian error distributions, in “lme4”; Bates et al., 2015) using pre- and post-treatment data from just the Normal plots. These maintained the same treatment throughout the experiment, and so any significant differences in these plots are likely to be caused by other factors. Data were subsetted according to microhabitat. Response variables are listed in Supplementary Table 4, whilst the explanatory variable in each case was collection time point (Before After). Plot was included as a random effect (1|Plot). Plant groupings (Native, Non-native, Problem, and Beneficial) were not tested separately because sample size was too small.
In all models, residuals were checked for normality and homoscedasticity. We used log-likelihood ratio comparisons with null models to generate P-values to assess the significance of differences in responses in relation to explanatory variables. In the case of comparisons between microhabitats we also ran Tukey post-hoc tests (using the glht function in the package “multcomp”; Hothorn et al., 2008) to test which of the different microhabitats were significantly different from each other. For the tests of effects of the understory vegetation treatments, we used lsmeans tests from the package “lsmeans” (Lenth, 2016) to assess which treatments were different from one another post-treatment, and also which treatments showed a change between pre- and post-treatment. Unless otherwise stated, we used the library “ggplot2” (Wickham, 2009; with reference to Chang, 2013) to plot results.
We recorded a total of 120 different species from 41 different families (Supplementary Table 1). Ninety three species were flowering plants (54 Dicotyledons, 39 Monocotyledons), and 27 species were ferns (Pteridophyta). Of these, 44 species could be classified as definitely native, and 66 as definitely non-native. Seventeen of the 120 species are classified as beneficial by plantation managers because of their role as parasitoid host plants, nitrogen fixers, or in helping to maintain soil moisture. In contrast, 87 species are considered to be problem species that are either allelopathic or compete with the oil palms (Supplementary Table 1).
Plant growth and communities varied substantially between Edge, Core and Trunk microhabitats. We found that plant species richness, biomass, and percentage cover were significantly higher in understory quadrats (both Core and Edge) than in quadrats on oil palm trunks (Figure 1; Supplementary Table 2). Within understory quadrats, Edge quadrats had significantly higher total plant species richness, biomass, and percentage cover than Core plantation quadrats (Figure 1; Supplementary Table 2).
Figure 1. Vegetation within 1 m2 quadrats across different microhabitats (understory at plot edge, “Edge”; understory at plot core, “Core”; and oil palm trunk, “Trunk”) within BEFTA Understory Vegetation Project experimental plots during the pre-treatment phase of the experiment. During this time, all plots were being managed using Normal understory vegetation management practices. Panel (A) shows total plant species richness per quadrat; (B) total plant biomass per quadrat; (C) total plant percentage cover per quadrat. Boxplots show median and interquartile range, with outliers being shown as circles. Mean values are shown by black diamonds. Significant differences between microhabitats are indicated by asterisks: ***p < 0.001, **p < 0.01. Refer to Supplementary Table 1 for full values.
Edge and Core quadrats had higher species richness, biomass and percentage cover of Native, Non-native, Problem and Beneficial plants than Trunk quadrats. Edge quadrats had higher Native plant species richness, biomass and percentage cover; higher Non-native plant species richness and biomass; higher Problem plant species richness; and also higher Beneficial plant species richness, biomass and percentage cover than in Core plots (Supplementary Table 2). Notably, percentage cover of Non-native plants, percentage cover of Problem plants, and biomass of Problem plants, showed no significant differences between Core and Edge quadrats, despite Edge quadrats generally supporting more plants than Core quadrats (Supplementary Table 2).
In terms of species composition, Edge microhabitat contained the most unique species (36), and the highest predicted number of species of the three microhabitats. The average Edge quadrat was dominated by the ferns Nephrolepis biserrata, Asplenium longissimum, and Dicranopteris linearis, and the flowering plants Asystasia gangetica subsp. micrantha (referred to as Asystasia micrantha hereafter), and Borreria latifolia. However, nearly 25% of the biomass in average Edge quadrats was made up of species other than these top five (Figure 2). Core microhabitat quadrats contained 18 unique species, and their overall predicted species richness approached that of the Edge quadrats. As in Edge quadrats, Nephrolepis biserrata, Asystasia micrantha, and Asplenium longissimum also made up the majority of the biomass, but other species made up a much lower proportion of the plant biomass present (only ~14% of biomass was made up of species other than these three dominant species) (Figure 2). Trunk microhabitats had the lowest number of unique species (8), low predicted overall species numbers, and were dominated by ferns. Vittaria ellongata made up over half of the biomass within average Trunk quadrats, with Microsorrum punctatum, Goniophlebium percussum, Asplenium longissimum, and Asplenium glaucophyllum making the next biggest contributions to biomass (Figure 2).
Figure 2. Plant community composition within different microhabitats (understory at plot edge, “Edge”; understory at plot core, “Core”; and oil palm trunk, “Trunk”) within BEFTA Understory Vegetation Project experimental plots during the pre-treatment phase of the experiment. During this time, all plots were being managed using business-as-usual vegetation management practices (Normal vegetation). Panel (A) shows the total number of plant species found within, and shared between, different microhabitats. Note that the collection effort was double in the Core plots compared to Edge and Trunk. Note also that the species total reported here (110) does not match the grand total from the whole study (120) because only pre-treatment data are used in this figure. Panel (B) shows interpolated and extrapolated species accumulation curves for the different microhabitats. Panel (C) shows the relative dominance of different plant species within each microhabitat by showing the total mean plant biomass (±SE) per 1m2 quadrat split across the five most dominant (by biomass) plant species, and “Other” plant species. The species name “Asystasia gangetica subsp. micrantha” has been shortened to Asystasia micrantha within the figure. We have included a colour blind friendly version of this figure in Supplementary Materials.
Within Edge quadrats, there were no significant differences in species richness per quadrat (Total, and all sub-groupings) (Figure 3; Supplementary Table 3), or in predicted overall species richness (Figure 4) as a result of changing the understory treatment during the experiment. There was, however, an overall decline over time that was seen in all the plots (Figure 3; Supplementary Tables 3, 4). On the other hand, Edge plot biomass and percentage cover (Total, and all sub-groupings) showed significant differences across treatments, with declines seen predominantly within the Reduced treatment understory plots. There were also significant decreases in total percentage cover, Problem percentage cover, and Problem biomass in Normal plots compared to Enhanced plots as a result of treatment differences (Figure 3; Supplementary Table 3). The most common species (by contribution to biomass) in Edge quadrats across all three treatments were Nephrolepis biserrata, Asystasia micrantha, and Borreria latifolia. However, a much higher percentage of the total biomass was made up by other species within Enhanced plot quadrats than in Normal plots, and in turn, both had higher levels of other species than Reduced plots. Edge quadrats in Enhanced plots had a total of 14 unique species, whilst Normal plots had 11, and Reduced plots had 4, although predicted overall species richness values were similar for all treatments (Figure 4; Supplementary Table 1).
Figure 3. Measures of vegetation within three different microhabitats (understory at plot edge, “Edge”; understory at plot core, “Core”; oil palm trunk, “Trunk”) across different BEFTA Understory Vegetation Project plots (“Enhanced”-shown by green circles; “Normal”-shown by blue triangles; “Reduced”-shown by red squares) both before and after experimental treatments were initiated. During the “Before” time-period all plots were managed using Normal levels of understory vegetation management, but during the “After” time-period, management was adjusted according to treatment type. Results for Edge microhabitats are shown in panels (A–C); Core microhabitats are shown in panels (D–F); and Trunk are shown in panels (G–I). Panels (A,D,G) show mean species richness (±SE) per 1m2 quadrat. Panels (B,E,H) show mean biomass (g) (±SE) per 1m2 quadrat. Panels (C,F,I) show mean percentage cover (±SE) per 1m2 quadrat. Note that the scales are different across panels. Effects of changing understory vegetation management as part of the BEFTA Understory Vegetation Project are indicated under “Treatment effect,” with asterisks showing that there was a significant change in the response variable as a result of the experimental treatments beginning (***p < 0.001; **p < 0.01; *p < 0.05; ns, not significant) (refer to Supplementary Table 3 for full results). Changes in the response variable over time are indicated under “Change over time,” with asterisks showing that there was a significant change in the response variable in Normal plots between Before and After time-periods (***p < 0.001; **p < 0.01; *p < 0.05; ns, not significant) (refer to Supplementary Table 4 for full results).
Figure 4. Plant community composition within different microhabitats (understory at plot edge, “Edge”; understory at plot core, “Core”; and oil palm trunk, “Trunk”) within BEFTA Understory Vegetation Project experimental plots during the post-treatment phase of the experiment. During this time, a third of the plots were managed according to business-as-usual vegetation management (Normal vegetation); a third were managed more intensively (Reduced vegetation); and a third were managed less intensively (Enhanced vegetation). Results for Edge microhabitats are shown in (A–C); Core microhabitats are shown in (D–F); and Trunk are shown in (G–I). Panels (A,D,G) show the total number of plant species found within, and shared between, different treatments. Note that the collection effort was double in the Core plots compared to Edge and Trunk. Panels (B,E,H) show interpolated and extrapolated species accumulation curves for the different treatments. Note that the scales are different across panels. The legend in (H) also applies to (B) and (E). Panels (C,F,I) show the relative dominance of different plant species within each treatment in terms of the total mean plant biomass (±SE) per 1m2 quadrat split across the five most dominant (by biomass) plant species, and “Other” plant species. Note that the scales are different across panels. The species name “Asystasia gangetica subsp. micrantha” has been shortened to Asystasia micrantha within the figure. We have included a colour blind friendly version of this figure in Supplementary Materials.
Within Core quadrats, understory treatment had a significant effect on all measures of plant species richness, biomass and percentage cover (Total, Native, Non-native, Problem, and Beneficial), with substantial reductions in plant communities in the Reduced plots compared to Normal and Enhanced plots. In addition, there was also a significantly lower percentage cover of problem species in Normal plots relative to Enhanced plots (Figure 3; Supplementary Table 3). Predicted species richness was higher in Enhanced plots than in Normal, which in turn had higher predicted richness than Reduced. However, numbers of unique species were the same in Enhanced and Normal plots (13 each), but were much lower in Reduced (3). Core quadrats in Enhanced and Normal plots were heavily dominated (in terms of biomass) by Asystasia micrantha and Nephrolepis biserrata. Core quadrats in Reduced plots had very low levels of biomass, but the most dominant species within these was Asystasia micrantha. Biomass of other species was low across all treatments (Figure 4; Supplementary Table 1).
There were substantial declines in species richness, biomass and percentage cover in Trunk quadrats over the course of the study, but in almost all cases this trend was the same across treatments (Figure 3; Supplementary Table 3). The only exception to this was a significant change in Non-native plant biomass as a result of treatment, with declines in Non-native plant biomass seen in Trunk quadrats within Enhanced vegetation plots (Supplementary Table 3). Trunk quadrats across all treatments were largely dominated by the species Vitarria ellongata, Microsorrum punctatum, and Goniophlebium percussum, and biomass of other species was low across all treatments. Trunk quadrats in Normal plots contained nine unique species, whilst Enhanced and Reduced plots supported three unique species each, but overall predicted species richness was very similar across all three treatments (Figure 4; Supplementary Table 1).
Several measures of plant communities within Normal plots showed significant changes during the course of the 2013 and 2014 data collection at the BEFTA Understory Vegetation Project, indicating changes in vegetation over time that were independent of understory treatment.
Within Edge quadrats, we found a significant decline in total species richness (8.2–5.8) (Figure 3; Supplementary Table 4). The dominant species (by biomass) remained largely similar pre- and post-treatment with Nephrolepis biserrata and Asystasia micrantha dominating throughout. However, Borreira latifolia and Axonopus compressus became more abundant post-treatment, whilst Asplenium longissimum declined in dominance (Figures 2, 4). In Core quadrats we observed significant decreases in total plant biomass (from an average of 115.3−67.7g per plot) and percentage cover (67.7–59.0%) (Figure 3; Supplementary Table 4). Trends in community composition over time in the Core quadrats were similar to those in the Edge quadrats, with continued dominance of Nephrolepis biserrata and Asystasia micrantha throughout, but declines in dominance of Asplenium longissimum (Figures 2, 4). Trunk quadrats showed significant decreases in total species richness and percentage cover over time (3.1–1.6; 37.6–23.5%) (Figure 3; Supplementary Table 4). Vittaria ellongata and Microsorrum punctatum were equally dominant (by biomass) pre-treatment, but M.punctatum showed substantial declines, and V.ellongata substantial increases in dominance post-treatment (Figures 2, 4).
Although press articles often refer to oil palm plantations as “green deserts”—monocultures of palms with little ability to support other species—we found a diverse and thriving plant community made up of 120 species from 41 different families, including many native species, and high numbers of species that can be found in forest habitats. While these numbers are undoubtedly low compared to the rainforest that the plantations have replaced (a study in neighbouring Jambi found that oil palm plantations had 77% fewer plant species than forest sites; Rembold et al., 2017), and the assemblage contained a high number of non-native and “problem” species, there is nonetheless substantial biodiversity represented within plantations, with many more species present than are supported by other high-yielding tropical crop systems (e.g., rice paddies, Luo et al., 2014).
High levels of plant diversity may bring benefits for functioning and productivity within plantations. Experiments in grasslands have shown that systems with higher levels of plant diversity are more productive, and have more efficient use of nutrients and lower levels of leaching (Tilman et al., 1996, 2001b). In addition, high plant diversity in tropical ecosystems is closely linked to high diversity of both herbivore and non-herbivore arthropod groups (Chung et al., 2000; Basset et al., 2012). Through food web linkages, this in turn allows other animals to be supported. This gives increased potential for additional ecosystem services and functions that are provided by animals to develop within a system, including those important for agricultural production, such as pest control and soil processing (Fragoso et al., 1997; Brussaard et al., 2007; Turner and Hinsch, 2018). If managed effectively, a more complex plant system has substantial potential to bring benefits for both biodiversity and production.
A first step toward more effective crop management is to develop a greater understanding of what vegetation is present within plantations. We found that understory habitat on the edge of plantation blocks had more abundant and diverse plant communities than understory habitat within the core of plantation blocks. The community was also less dominated by just a few species or by non-native and “problem” species than the core plots. The plot edge supported twice the number of unique species than the core habitat, and over four times the number of unique species found on trunks. The “Edge” habitat was alongside a road at the edge of the plantation block, meaning that it was exposed to higher levels of light, and lower levels of harvesting disturbance, including application of herbicides, manual cutting for paths, or trampling by harvesters. It is likely that this allowed a more diverse community to develop, including species that are more light-demanding, or less disturbance-tolerant, as well as allowing greater levels of natural re-seeding over time. Indeed, the forest specialist fig tree species Ficus benjamina was found in edge habitat, but not in core areas.
In comparison to Edge habitat, understory quadrats in the core of the plantation had lower species richness, biomass, and cover of plant species, and were more dominated by just a few species. The majority of the biomass within Core quadrats was made up of the fast-growing, shade-tolerant ferns Nephrolepis biserrata and Asplenium longissimum, and fast-growing flowering plant Asystasia micrantha, which is considered to be a weed and can quickly swamp other vegetation. However, Core habitat did support a number of unique species that were not found in other microhabitats and so added plant diversity to the system as a whole. Although, it must be noted that the majority were deemed to be “problem” species, and many were non-native. The limited other studies that have considered plant communities within core areas of oil palm plantations have also found that they were dominated by herbaceous weeds, ferns, and non-native species, with forest-specialised species being absent (Danielsen et al., 2009; Rembold et al., 2017; Ashton-Butt et al., 2018).
Oil palm trunks provided another valuable additional microhabitat for plants. Trunk habitat is created through the build-up of organic matter around the frond bases that remain attached to the trunks after fronds have been cut as part of the harvesting process. The volume of “soil” available is therefore very low, giving limited potential for rooting, uptake of nutrients, or storage of water, and most likely higher variations in temperature than in the understory. Therefore, a smaller area was available for plants to establish, and only specialised epiphytic plants, and a subset of ground species that are able to adapt to an epiphytic lifestyle, were able to grow on palm trunks. This meant that species richness, biomass, and cover were substantially lower on trunks than in understory quadrats. However, eight species were only found in the Trunk habitat and, in comparison to the majority of other crop systems, this is a unique additional habitat which increases the complexity of the ecosystem. Indeed, oil palm epiphyte communities have also been found to be species rich at other sites (Piggott, 1980; Prescott et al., 2015). In addition, epiphytes such as the large Asplenium nidus ferns have been found to support very high levels of arthropod diversity in tropical ecosystems, including within oil palm systems (Ellwood et al., 2002; Ellwood and Foster, 2004; Turner and Foster, 2009; Fayle et al., 2010).
Plots that were exposed to the highest levels of vegetation management had much lower levels of all types of plant biomass and percentage cover in Core and Edge habitat. However, there was no change in biomass or cover in Trunk microhabitats as a result of the experiment. Species richness estimates were similar across treatments for Edge and Trunk microhabitats, but Reduced plots had lower species richness in Core habitat compared to the other treatments. Across all three microhabitats, Normal and Enhanced plots had largely similar plant assemblages, except in Edge habitat where Enhanced plots supported more unique species and a greater mix of species than Normal plots. However, Normal plots had a significantly lower percentage cover of problem species than Enhanced plots. Trunk plant communities largely showed no change as a result of understory vegetation management, except that there was a reduction in the biomass of non-native plants in Enhanced plots.
The few studies that have been conducted on understory oil palm systems also indicate that differences in management can have substantial effects on plant communities. Higher numbers of species (219) were found in the less intensively-managed smallholder oil palm systems studied by Rembold et al. (2017) including 84 that were also found in forest sites, whilst in weeded circles and windrow areas of intensively managed plantations, only 45 species were found (Ashton-Butt et al., 2018). Similarly, in our study, high use of herbicides in the Reduced vegetation treatment largely eradicated the plant community within Edge and Core understory areas. This not only caused loss of the problem plants, at which herbicide application is aimed, but also losses of beneficial or native plants which managers may wish to protect. Such substantial losses of biomass and cover are likely to have large impacts on the structure of the habitat, shading, and amount of fodder that the plants provide for other wildlife in the ecosystem, thereby having an impact on the wider plantation biodiversity (e.g., evidence from multiple taxa in a review of UK-based studies, Marshall et al., 2003; and on specific taxa in oil palm plantations, Nájera and Simonetti, 2010; Pardo et al., 2018; Hood et al., submitted; Spear et al., submitted).
The more moderate business-as-usual practice of spraying only around the bases of palms and along paths (Normal vegetation treatment), led to more moderate declines in plant biomass and cover than in Reduced plots, but also lower levels of problem species than in Enhanced plots. Where management was relaxed in Enhanced plots, problem species increased in number, with little sign of beneficial species showing similar increases. This suggests that more targeted management may be required to specifically control problem species while allowing the rest of the plant community to develop. Alternatively, it may be that beneficial species take longer to respond and that these too could increase in number in the Enhanced plots over longer time periods.
Species richness in Core plot areas was heavily affected by experimental treatment, with approximately a quarter of the plant species not being found in Normal plots, and approximately half not being found in Reduced plots compared to Enhanced. This represents a substantially lower level of plant diversity in the system, with potential knock-on impacts for other species that use the plants and for ecosystem functioning in the system, e.g., for birds (Nájera and Simonetti, 2010), and activity of leopard cats (Hood et al., submitted). In comparison to the core plot area, Edge microhabitat in Enhanced plots supported more unique species, and a greater mix of species, but were not significantly different from Normal or Reduced plots in terms of overall species richness. This suggests that many Edge species are able to survive the herbicide levels that reach them in the Reduced and Normal treatments, and that reduced intensity management can encourage a more even mix of species. However, the fact that richness was not higher overall in Enhanced plots may indicate a lack of ability for the Edge community to rebound and diversify following a relaxation of management, perhaps due to a lack of source seeds, or because of the continued dominance of a few species, making establishment of other species difficult.
The lack of differences in Trunk plant communities between the different experimental treatment plots indicates that their growth and survival are not negatively affected by the use of herbicides in the understory. Although some plantations follow a management policy of cutting epiphytes from palm trunks, this is not current practice in the plantations within the local area of our study site. Therefore, although relatively less species-rich than understory communities, Trunk plant communities represent a system that is protected, to some extent, from understory spraying and may support a host of other species that are less tolerant to disturbance. Indeed, epiphytes in oil palm plantations have been reported to house a considerable number and diversity of arthropod species (Turner and Foster, 2009; Fayle et al., 2010), and removing them from palm trunks has been found to bring no benefits for yield (Prescott et al., 2015).
We observed substantial changes in plant communities between the first and second survey periods which were independent of the experimental treatments. Across the majority of treatments, there was a general trend for declines in species richness, biomass, and percentage cover of plants across all microhabitats. Considering the Normal plots in particular, whose vegetation management remained constant throughout the study, we observed significant declines in Core understory total biomass and total percentage cover, Edge understory species richness, and Trunk species richness and percentage cover.
Plants are dependent on water for growth, and tropical rainforest plants in particular have few adaptations for drought and so can die back substantially during periods of low rainfall. The second survey period was after a period of much lower rainfall than the first survey period as a result of an El Niño-related drought event that affected the region in 2014 and 2015. During the drier second survey period, plants within the Core plantation area may have been in particularly severe competition with the palms for water, driving declines in plant biomass and cover. Oil palms are highly water demanding and grow best in areas with ~2,000–2,500 mm of rainfall per year, with no marked dry season (Corley and Tinker, 2003b). They ideally need at least 100 mm of rain per month (Corley and Tinker, 2003b) and their yield has been found to be reduced by 10–21% during drought periods (Suresh, 2013). Indeed, in particular we observed a decline in the dominance of the fast-growing fern Asplenium longissimum which typically grows in moist areas (Holtum, 1966). Although the Edge habitat areas were likely to be in relatively lower competition from the palms, they were also in more exposed environments, potentially making the effects of the drought more marked. Similar to Core plantation areas, the fern Asplenium longissimum declined in dominance post-treatment, making way for increased growth of the open habitat specialist Borreira latifolia and the grass Axonopus compressus, both of which are considered to be beneficial species for the plantation (as a provider of nectar for beneficial insects, and as a cover crop, respectively). Loss of plant growth as a result of drought could well have impacts on wider biodiversity that are similar to those seen under intense understory vegetation management practices, with declines in animal communities that can be supported, and potentially therefore, functions and services that can be provided (e.g., see Spear et al., submitted).
Trunk plant assemblages showed sharp declines between pre- and post-treatment time periods, suggesting that they were particularly negatively affected by the drought period. Epiphytes rely on getting water from rainfall rather than groundwater supplies, and their limited “soil” systems give little potential for water storage. Although some obligate epiphyte species may have adaptations to drought conditions, many of the species found on the trunks were “opportunistic” epiphytes which were also found growing on the ground, and even amongst obligate epiphytes, some species were less able to withstand low rainfall levels (discussed for Australian rainforest systems in Kitching and Arthur, 1993). The epiphytic ferns Vittaria ellongata and Microsorrum punctatum were equally dominant (by biomass) pre- and post-treatment, but highly water demanding M.punctatum showed substantial declines, whilst drought-tolerant V. ellongata showed substantial increases in dominance post-treatment.
The onset of the El Niño-related drought event may partly explain the Enhanced plots' apparent inability to substantially regrow after vegetation management practices were relaxed. Shortage of water would have impeded new growth. In addition, because post-treatment surveys were completed only 6 months after treatments began, there may have been little time for the community to have responded to the relaxation of management techniques—a problem potentially compounded further by the lack of rainfall.
We found 120 plant species in total, with varied communities found within different plantation microhabitats. Therefore, in comparison to many other crop systems, oil palm plantations can be very diverse and heterogeneous habitats for plants. The community was, however, vulnerable to the effects of drought, with substantial declines in cover, biomass, and species richness seen during the course of our study which correlated with a period of El Niño-related drought in our region. Our experimental results indicate that the strategies that are used to manage understory vegetation also have a substantial effect on plant species richness, biomass, and percentage cover of understory species. Use of herbicides at the highest levels led to almost complete loss of plant cover and biomass within Core and Edge habitats, which is likely to have substantial impacts on their ability to support diverse fauna and ecosystem functions. Avoiding use of herbicides led to much more abundant plant communities, with higher species richness in core plot areas, and a greater mix of species being dominant in plantation edges. However, there were higher levels of problem species in the Enhanced minimal-management plots, indicating that no-spraying management could result in a rise of problem species. Therefore, a more balanced application of herbicides may represent the best approach for keeping some species in check, while also allowing the development of a more diverse understory. In addition to benefits for plant biodiversity, reducing use of herbicides could help to reduce impacts on non-target species, reduce the risks of water pollution, and improve the health of plantation workers, therefore helping to meet a range of sustainability guidelines laid out by certification bodies such as the RSPO (Roundtable on Sustainable Palm Oil, 2018). In addition, following more sustainable vegetation management practices could help to decrease plantation management costs, potentially providing a win-win for both production and environmental protection.
The raw data supporting the conclusions of this manuscript will be made available by the authors, without undue reservation, to any qualified researcher.
ET, WF, and JS designed the manipulation experiment, and ADA, AAA, MN, TR, SP, and J-PC maintained it. ET, WF, JS, and DP designed the study. DP led the data collection, including lab-based evaluation of species, with assistance from ADA, AAA, RP, SP, S, J-PC, and MN. SL performed the data analysis and wrote the manuscript. All authors commented on the manuscript.
This work was funded by The Isaac Newton Trust Cambridge, Golden Agri Resources, and the Natural Environment Research Council [grant number NE/P00458X/1].
Co-authors listed with a SMARTRI affiliation were employed by SMARTRI, a research division of Golden Agri Resources, at the time of the study. The wider BEFTA Programme is co-funded by Golden Agri Resources, with matched funds from the Isaac Newton Trust, Cambridge. However, there is an MOU in place that protects the intellectual freedom and data-use for all researchers working on the project. The Programme therefore represents a collaboration between the University of Cambridge and an oil palm company, ensuring that results are readily disseminated to inform best management practices, but maintaining academic independence.
The remaining authors declare that the research was conducted in the absence of any commercial or financial relationships that could be construed as a potential conflict of interest.
We thank RISTEK for permission to establish the BEFTA Understory Vegetation Project and to conduct research in Indonesia (permit numbers 426/SIP/FRP/SM/XI/2012, 72/EXT/SIP/FRP/SM/IX/2013, 44/EXT/SIP/FRP/SM/IX/2014). We thank Pt. Ivo Mas Tunggal and Golden Agri Resources, and Sinar Mas Agro Resources Technology Research Institute (SMARTRI) for allowing us to conduct research in their plantations, and we are grateful to the staff of SMARTRI for their help with fieldwork. We thank two reviewers for their suggested improvements to the manuscript.
The Supplementary Material for this article can be found online at: https://www.frontiersin.org/articles/10.3389/ffgc.2019.00033/full#supplementary-material
Anderson, J. M. (2008). Eco-friendly approaches to sustainable palm oil production. J. Oil Palm Res. 127–142.
Arifin, Atmojo, S. W., Setyono, P., and Dewi, W. S. (2015). Temperature Effect Investigation toward Peat Surface CO2 Emissions by Planting Leguminous Cover Crops in Oil Palm Plantations in West Kalimantan. J. Agric. Sci. Technol. B 5, 170–183. doi: 10.17265/2161-6264/2015.03B.002
Ashton-Butt, A., Aryawan, A. A. K., Hood, A. S. C., Naim, M., Purnomo, D., Suhardi, et al. (2018). Understory vegetation in oil palm plantations benefits soil biodiversity and decomposition rates. Front. For. Glob. Change 1:10. doi: 10.3389/ffgc.2018.00010
Baligar, V. C., and Fageria, N. K. (2007). Agronomy and physiology of tropical cover crops. J. Plant Nutr. 30, 1287–1339. doi: 10.1080/01904160701554997
Barnes, D. E., and Chan, L. G. (1990). Common Weeds of Malaysia and Their Control. Kuala Lumpur: Ancom Berhad.
Basset, Y., Cizek, L., Cuénoud, P., Didham, R. K., Guilhaumon, F., Missa, O., et al. (2012). Arthropod diversity in a tropical forest. Science 338, 1481–1484. doi: 10.1126/science.1226727
Bates, D., Mächler, M., Bolker, B., and Walker, S. (2015). Fitting linear mixed-effects models using lme4. J. Stat. Softw. 67, 1–48. doi: 10.18637/jss.v067.i01
Bedford, G. O. (1980). Biolog, ecology, and control of palm rhinoceros beetles. Annu. Rev. Entomol. 25, 309–339. doi: 10.1146/annurev.en.25.010180.001521
Böhnert, T., Wenzel, A., Altenhövel, C., Beeretz, L., Tjitrosoedirdjo, S. S., Meijide, A., et al. (2016). Effects of land-use change on vascular epiphyte diversity in Sumatra (Indonesia). Biol. Conserv. 202, 20–29. doi: 10.1016/j.biocon.2016.08.008
Bonhommeau, S., Dubroca, L., Le Pape, O., Barde, J., Kaplan, D. M., Chassot, E., et al. (2013). Eating up the world's food web and the human trophic level. Proc. Natl. Acad. Sci. U.S.A. 110, 20617–20620. doi: 10.1073/pnas.1305827110
Brühl, C. A., and Eltz, T. (2010). Fuelling the biodiversity crisis: species loss of ground-dwelling forest ants in oil palm plantations in Sabah, Malaysia (Borneo). Biodivers. Conserv. 19, 519–529. doi: 10.1007/s10531-009-9596-4
Brussaard, L., de Ruiter, P. C., and Brown, G. G. (2007). Soil biodiversity for agricultural sustainability. Agric. Ecosyst. Environ. 121, 233–244. doi: 10.1016/j.agee.2006.12.013
Chung, A. Y., Eggleton, P., Speight, M. R., Hammond, P. M., and Chey, V. K. (2000). The diversity of beetle assemblages in different habitat types in Sabah, Malaysia. Bull. Entomol. Res. 90, 475–496. doi: 10.1017/S0007485300000602
Clarke, M., and Walsh, R. (2006). Long-term erosion and surface roughness change of rain-forest terrain following selective logging, Danum Valley, Sabah, Malaysia. Catena 68, 109–123. doi: 10.1016/j.catena.2006.04.002
Corley, R. H. V., and Tinker, P. B. (eds.). (2003a). “Care and maintenance of oil palms,” in The Oil Palm, 4th Ed. (Oxford: Blackwell Publishing), 287–326. doi: 10.1002/9780470750971
Corley, R. H. V., and Tinker, P. B. (eds.). (2003b). “The climate and soils of the oil palm-growing regions,” in The Oil Palm, 5th Ed. (Oxford: Blackwell Publishing), 53–88. doi: 10.1002/9781118953297.ch3
Danielsen, F., Beukema, H., Burgess, N. D., Parish, F., Brühl, C. A., Donald, P. F., et al. (2009). Biofuel plantations on forested lands: double jeopardy for biodiversity and climate. Conserv. Biol. 23, 348–358. doi: 10.1111/j.1523-1739.2008.01096.x
Edwards, D. P., Magrach, A., Woodcock, P., Ji, Y., Lim, N. T.-L, Edwards, F. A., et al. (2014). Selective-logging and oil palm: multitaxon impacts, biodiversity indicators, and trade-offs for conservation planning. Ecol. Appl. 24, 2029–2049. doi: 10.1890/14-0010.1
Edwards, F. A., Edwards, D. P., Larsen, T. H., Hsu, W. W., Benedick, S., Chung, A., et al. (2014). Does logging and forest conversion to oil palm agriculture alter functional diversity in a biodiversity hotspot? Anim. Conserv. 17, 163–173. doi: 10.1111/acv.12074
Ellwood, M. D. F., and Foster, W. A. (2004). Doubling the estimate of invertebrate biomass in a rainforest canopy. Nature 429, 549–551. doi: 10.1038/nature02560
Ellwood, M. D. F., Jones, D. T., and Foster, W. A. (2002). Canopy ferns in lowland dipterocarp forest support a prolific abundance of ants, termites, and other invertebrates. Biotropica 34, 575–583. doi: 10.1111/j.1744-7429.2002.tb00576.x
Fayle, T. M., Turner, E. C., Snaddon, J. L., Chey, V. K., Chung, A. Y. C., Eggleton, P., et al. (2010). Oil palm expansion into rain forest greatly reduces ant biodiversity in canopy, epiphytes and leaf-litter. Basic Appl. Ecol. 11, 337–345. doi: 10.1016/j.baae.2009.12.009
Fitzherbert, E. B., Struebig, M. J., Morel, A., Danielsen, F., Brühl, C. A., Donald, P. F., et al. (2008). How will oil palm expansion affect biodiversity? Trends Ecol. Evol. 23, 538–545. doi: 10.1016/j.tree.2008.06.012
Food Agriculture Organisation (FAO) (2017). United Nations, Statistics Division. Available online at: http://www.fao.org/faostat/en/#data/RL
Foster, W. A., Snaddon, J. L., Advento, A. D., Agung, A. A., Barclay, H., Caliman, J.-P., et al. (2014). The biodiversity and ecosystem function in tropical agriculture (BEFTA) Project. The Planter, Kuala Lumpur 90, 581–591.
Foster, W. A., Snaddon, J. L., Turner, E. C., Fayle, T. M., Cockerill, T. D., Ellwood, M. D., et al. (2011). Establishing the evidence base for maintaining biodiversity and ecosystem function in the oil palm landscapes of South East Asia. Philos. Trans. R. Soc. Lond. B Biol. Sci. 366, 3277–3291. doi: 10.1098/rstb.2011.0041
Fragoso, C., Brown, G. G., Patrón, J. C., Blanchart, E., Lavelle, P., Pashanasi, et al. (1997). Agricultural intensification, soil biodiversity and agroecosystem function in the tropics: the role of earthworms. Appl. Soil Ecol. 6, 17–35. doi: 10.1016/S0929-1393(96)00154-0
Gaveau, D. L., Sheil, D., Husnayaen, Salim, M. A., Arjasakusuma, S., Ancrenaz, M., et al. (2016). Rapid conversions and avoided deforestation: examining four decades of industrial plantation expansion in Borneo. Sci. Rep. 6:32017. doi: 10.1038/srep32017
Godfray, H. C. J., Crute, I. R., Haddad, L., Lawrence, D., Muir, J. F., Nisbett, N., et al. (2010). The future of the global food system. Philos. Trans. R. Soc. Lond. B Biol. Sci. 365, 2769–2777. doi: 10.1098/rstb.2010.0180
Hardwick, S. R., Toumi, R., Pfeifer, M., Turner, E. C., Nilus, R., and Ewers, R. M. (2015). The relationship between leaf area index and microclimate in tropical forest and oil palm plantation: Forest disturbance drives changes in microclimate. Agric. For. Meteorol. 201, 187–195. doi: 10.1016/j.agrformet.2014.11.010
Hinsch, J. (2013). Substituting Pesticides With Biodiversity - The Potential for Biocontrol in Oil Palm Plantations. Copenhagen: University of Copenhagen.
Hooijer, A., Page, S., Jauhiainen, J., Lee, W. A., Lu, X. X., Idris, A., et al. (2012). Subsidence and carbon loss in drained tropical peatlands. Biogeosciences 9, 1053–1071. doi: 10.5194/bg-9-1053-2012
Hothorn, T., Bretz, F., and Westfall, P. (2008). Simultaneous inference in general parametric models. Biometrical J. 50, 346–363. doi: 10.1002/bimj.200810425
Kamarudin, N., and Wahid, M. B. (2010). Interactions of the bag worm, Pteroma pendula (Lepidoptera: Psychidae), and its natural enemies in an oil palm plantation in Perak. J. Oil Palm Res. 22, 758–764.
Kitching, R. L., and Arthur, M. (1993). The biodiversity of arthropods from Australian rain forest canopies: summary of projects and impact of drought. Selbyana 14, 29–35.
Koh, L. P. (2008). Can oil palm plantations be made more hospitable for forest butterflies and birds? J. Appl. Ecol. 45, 1002–1009. doi: 10.1111/j.1365-2664.2008.01491.x
Koh, L. P., and Wilcove, D. S. (2008). Is oil palm agriculture really destroying tropical biodiversity? Conserv. Lett. 1, 60–64. doi: 10.1111/j.1755-263X.2008.00011.x
Larsson, J. (2019). eulerr: Area-Proportional Euler and Venn Diagrams with Ellipses. R package version 5.1.0. Available online at: https://cran.r-project.org/package=eulerr
Laurance, W. F., Sayer, J., and Cassman, K. G. (2014). Agricultural expansion and its impacts on tropical nature. Trends Ecol. Evol. 29, 107–116. doi: 10.1016/j.tree.2013.12.001
Lenth, R V. (2016). Least-squares means: the R package lsmeans. J. Stat. Softw. 69, 1–33. doi: 10.18637/jss.v069.i01
Luo, Y., Fu, H., and Traore, S. (2014). Biodiversity conservation in rice paddies in China: toward ecological sustainability. Sustainability 6, 6107–6124. doi: 10.3390/su6096107
Luskin, M. S., and Potts, M. D. (2011). Microclimate and habitat heterogeneity through the oil palm lifecycle. Basic Appl. Ecol. 12, 540–551. doi: 10.1016/j.baae.2011.06.004
Manning, F. C., Kho, L. K., Hill, T. C., Cornulier, T., and The, Y. A. (2019). Carbon emissions from oil palm plantations on peat soil. Front. For. Glob. Change. doi: 10.3389/ffgc.2019.00037
Marshall, E. J. P., Brown, V. K., Boatman, N. D., Lutman, P. J. W., Squire, G. R., and Ward, L. K. (2003). The role of weeds in supporting biological diversity within crop fields. Weed Res. 43, 77–89. doi: 10.1046/j.1365-3180.2003.00326.x
Merlier, H., and Montegut, J. (1982). Adventices Tropicales Flore aux Stades Plantule et Adulte de 123 Espèces Africaines ou Pantropicales. République Française Ministèreministère Des Relations Extérieures-Coopération Et Développement.
Myers, N., Mittermeier, R. A., Mittermeier, C. G., da Fonseca, G. A. B., and Kent, J. (2000). Biodiversity hotspots for conservation priorities. Nature 403, 853–858. doi: 10.1038/35002501
Nájera, A., and Simonetti, J. A. (2010). Can oil palm plantations become bird friendly? Agrofor. Syst. 80, 203–209. doi: 10.1007/s10457-010-9278-y
Pardo, L. E., Campbell, M. J., Edwards, W., Clements, G. R., and Laurance, W. F. (2018). Terrestrial mammal responses to oil palm dominated landscapes in Colombia. PLoS ONE 13:e197539. doi: 10.1371/journal.pone.0197539
Piggott, A. G. (1980). The fern flora of oilpalm plantations in West Malaysia. Fern. Gaz. 12, 93–102.
Prescott, G. W., Edwards, D. P., and Foster, W. A. (2015). Retaining biodiversity in intensive farmland: epiphyte removal in oil palm plantations does not affect yield. Ecol. Evol. 5, 1944–1954. doi: 10.1002/ece3.1462
Rembold, K., Mangopo, H., Tjitrosoedirdjo, S. S., and Kreft, H. (2017). Plant diversity, forest dependency, and alien plant invasions in tropical agricultural landscapes in Sumatra. Biol. Conserv. 213, 234–242. doi: 10.1016/j.biocon.2017.07.020
Senior, M. J. M., Hamer, K. C., Bottrell, S., Edwards, D. P., Fayle, T. M., Lucey, J. M., et al. (2013). Trait-dependent declines of species following conversion of rain forest to oil palm plantations. Biodivers. Conserv. 22, 253–268. doi: 10.1007/s10531-012-0419-7
Sodhi, N. S., Koh, L. P., Brook, B. W., and Ng, P. K. L. (2004). Southeast Asian biodiversity: an impending disaster. Trends Ecol. Evol. 19, 654–660. doi: 10.1016/j.tree.2004.09.006
Soerjani, M., and Kostermans, A. J. G. H. (1987). Weeds of Rice in Indonesia. Jakarta: Balai Pustaka.
Suresh, K. (2013). “Adaptation and mitigation strategies for climate-resilient oil palm,” in Climate-Resilient Horticulture: Adaptation and Mitigation Strategies, eds H. Singh, N. Rao, and K. Shivashankar (Springer), 199–211. doi: 10.1007/978-81-322-0974-4_18
Tilman, D., Cassman, K. G., Matson, P. A., Naylor, R., and Polasky, S. (2002). Agricultural sustainability and intensive production practices. Nature 418, 671–677. doi: 10.1038/nature01014
Tilman, D., Fargione, J., Wolff, B., D'Antonio, C., Dobson, A., Howarth, R., et al. (2001a). Forecasting agriculturally driven global environmental change. Science 292, 281–284. doi: 10.1126/science.1057544
Tilman, D., Reich, P. B., Knops, J., Wedin, D., Mielke, T., and Lehman, C. (2001b). Diversity and productivity in a long-term grassland experiment. Science 294, 843–846. doi: 10.1126/science.1060391
Tilman, D., Wedin, D., and Knops, J. (1996). Productivity and sustainability influenced by biodiversity in grassland ecosystems. Nature 379, 718–720. doi: 10.1038/379718a0
Turner, E. C., and Foster, W. A. (2009). The impact of forest conversion to oil palm on arthropod abundance and biomass in Sabah, Malaysia. J. Trop. Ecol. 25, 23–30. doi: 10.1017/S0266467408005658
Turner, E. C., and Hinsch, J. (2018). “Integrated pest management in sustainable palm oil production,” in Achieving Sustainable Cultivation of Oil Palm, ed A. Rival (Cambridge: Burleigh Dodds Science Publishing Limited), 93–113. doi: 10.19103/AS.2017.0018.18
Wahid, M. B., Abdullah, S. N. A., and Henson, I. E. (2005). Oil palm — achievements and potential. Plant Prod. Sci. 8, 288–297. doi: 10.1626/pps.8.288
Wickham, H. (2009). ggplot2: Elegant Graphics for Data Analysis. New York, NY: Springer. doi: 10.1007/978-0-387-98141-3
Keywords: biodiversity, epiphytes, oil palm, plantation management, plants, Southeast Asia, sustainability, tropical agriculture
Citation: Luke SH, Purnomo D, Advento AD, Aryawan AAK, Naim M, Pikstein RN, Ps S, Rambe TDS, Soeprapto, Caliman J-P, Snaddon JL, Foster WA and Turner EC (2019) Effects of Understory Vegetation Management on Plant Communities in Oil Palm Plantations in Sumatra, Indonesia. Front. For. Glob. Change 2:33. doi: 10.3389/ffgc.2019.00033
Received: 22 February 2019; Accepted: 10 June 2019;
Published: 24 July 2019.
Edited by:
Rory Padfield, University of Leeds, United KingdomReviewed by:
Bambang Irawan, Jambi University, IndonesiaCopyright © 2019 Luke, Purnomo, Advento, Aryawan, Naim, Pikstein, Ps, Rambe, Soeprapto, Caliman, Snaddon, Foster and Turner. This is an open-access article distributed under the terms of the Creative Commons Attribution License (CC BY). The use, distribution or reproduction in other forums is permitted, provided the original author(s) and the copyright owner(s) are credited and that the original publication in this journal is cited, in accordance with accepted academic practice. No use, distribution or reproduction is permitted which does not comply with these terms.
*Correspondence: Sarah H. Luke, c2FyYWguaC5sdWtlQGdtYWlsLmNvbQ==
†These authors have contributed equally to this work
Disclaimer: All claims expressed in this article are solely those of the authors and do not necessarily represent those of their affiliated organizations, or those of the publisher, the editors and the reviewers. Any product that may be evaluated in this article or claim that may be made by its manufacturer is not guaranteed or endorsed by the publisher.
Research integrity at Frontiers
Learn more about the work of our research integrity team to safeguard the quality of each article we publish.