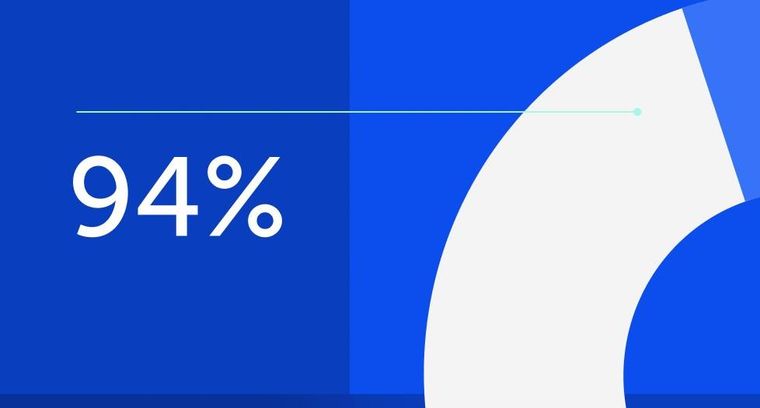
94% of researchers rate our articles as excellent or good
Learn more about the work of our research integrity team to safeguard the quality of each article we publish.
Find out more
ORIGINAL RESEARCH article
Front. Ecol. Evol., 04 April 2025
Sec. Conservation and Restoration Ecology
Volume 13 - 2025 | https://doi.org/10.3389/fevo.2025.1524202
Drylands cover approximately 40% of the global terrestrial area. These regions face multiple challenges, including water scarcity, soil erosion, and declining biodiversity, which significantly undermine soil fertility and ecosystem stability. Biological soil crusts (biocrusts) are widely recognized as key components in arid and semi-arid regions. Due to their unique structure and functions, biocrusts can significantly improve soil structure, enhance soil nutrient accumulation and cycling, and mitigate soil erosion, thereby providing critical support for ecological restoration and sustainable development. This study investigated variations in soil properties, bacterial and fungal community structures, and the relationships between soil properties and microbial communities in biocrusts under pine forests. These pine forests have been utilized for soil and water conservation over a ten-year period of ecological restoration in the Pisha sandstone area. Compared to bare ground, soil available phosphorus was 0.96 and 1.79 times higher in cyanobacterial and moss biocrusts, respectively, while soil available potassium was 1.44 and 2.34 times higher, respectively. These abundant soil nutrients provide a carbon source for microorganisms, promoting the growth and metabolic activity of soil microorganisms, thereby significantly enhancing soil microbial diversity and biomass. Biocrusts also enhanced soil urease and invertase activities. Soil microbial biomass carbon was 2.65 and 8.19 times higher in cyanobacterial biocrusts and moss biocrusts, respectively, compared to bare ground, and soil microbial biomass nitrogen was 5.05 and 11.07 times higher, respectively. Additionally, the Observed Species Richness Index and Abundance-based Coverage Estimator Index of bacterial and fungal microorganisms in moss biocrusts were significantly higher than those in bare ground and cyanobacterial biocrusts. Soil pH, copper, microbial biomass carbon, and microbial biomass nitrogen are key environmental factors influencing soil microbial communities. Biocrusts significantly improve soil quality in the Pisha sandstone area by enhancing the soil properties. This not only strengthens the stability and sustainability of the ecosystem but also provides critical support for improving ecosystem productivity and environmental quality.
Restorationists struggle with the global issue of arid land degradation because these regions are often characterized by severe soil erosion, sparse vegetation, and nutrient loss, which collectively undermine ecosystem resilience and sustainability. Pisha sandstone is called “cancer of Earth”, because the soil in the Pisha sandstone area is fragile and prone to soil erosion (Liu et al., 2023). Pisha sandstone is distributed in the middle of the Yellow River Basin, the northern part of the Loess Plateau (area; approximately 1.67 × 104 km2), which includes part of Inner Mongolia. This area was easily eroded to form the tertiary (Deng et al., 2021), a loose formation of sandstone, sandy shale, and muddy sandstone (Zhao et al., 2023). The Pisha sandstone area is among the key sources of Yellow River mud and coarse sand, with this area depositing 200 million tons of the yellow sediment in the Yellow River each year (Ma and Zhang, 2016). Up to 100 million tons coarse sediment was also deposited each year (Wang et al., 2021). The Pisha sandstone area in Zhungrun Banner has an arid climate with sparse and concentrated precipitation (Tian et al., 2023). Alkaline oxide is active in Pisha sandstone soil and is prone to erosion, which thus weakens the corrosion resistance property of this sandstone (Liang et al., 2019). In order to effectively reduce soil erosion, extensive afforestation has been carried out in the Pisha sandstone area. Pinus tabulaeformis, one of the native tree species in this region, is characterized by its drought resistance and tolerance to barren conditions. It provides ecological services such as soil and water conservation, carbon sequestration, oxygen release, and biodiversity conservation, as well as economic value through timber production (Li Z. et al., 2020). Due to these benefits, Pinus tabuliformis is widely planted in the region to conserve soil and water.
Biological soil crust (biocrust) is a special biological community composed of cyanobacteria, lichens and mosses that are distributed on the soil surfaces of these plantations (Elbert et al., 2012). Before the establishment of plantations, biocrusts already existed, but after the construction of artificial forests, these biocrusts have developed significantly. They can resist drying and extreme temperature (Li et al., 2022), produce chemical substances, and undergo asexual reproduction (Warren et al., 2019). Biocrusts cover approximately 12% of the global land surfaces (Rodriguez-Caballero et al., 2022). Being a crucial biological component of semiarid ecosystems, biocrusts are involved in biogeochemical carbon, nitrogen, phosphorus and sulfur (C, N, P, and S respectively) cycling in ecosystems and are affected by light, temperature, and humidity (Baumann et al., 2019; Wang Q. et al., 2022; Zhou et al., 2020; Qi et al., 2021), as well as soil fauna and microorganisms. Under the sufficient moisture conditions, biocrusts exhibit higher growth rates and biomass (Ding et al., 2023), and when precipitation is higher, the carbon fixation capabilities of cyanobacteria biocrustes and moss biocrusts are enhanced (Zhang C. P. et al., 2018). Climate warming has reduced the coverage of lichen-dominated biocrusts while promoting the growth of moss biocrusts (Ladrón de Guevara et al., 2018). Moreover, higher summer temperatures lead to a decline in the species diversity and coverage of nitrogen-fixing lichen biocrusts (Finger-Higgens et al., 2022). Biocrusts play a significant role in controlling soil erosion by regulating soil erodibility. In cyanobacterial crusts, Cyanobacteria with carbonic anhydrase activity can enhance sand consolidation through microbial carbonate precipitation, thereby alleviating soil erosion (Mai et al., 2024). Cyanobacteria, Chloroflexi, Deinococcota, and Patescibacteria in the biocrusts can secrete extracellular polymeric substances, thereby enhancing the soil resistance to erosion (Wang et al., 2024). The development of biocrusts significantly reduces soil erodibility. Their thickness exerts the most critical influence by directly bonding or binding soil particles and aggregates, thereby enhancing the soil resistance to erosion (Zhu et al., 2024). Biocrusts improve soil nutrients and moisture, thereby increasing soil enzyme activity, promoting microbial growth and helping to maintain spatial heterogeneity in the landscape (Riveras-Muñoz et al., 2022). Biocrust successions are typically categorized into four stages: bare ground, cyanobacterial crusts, lichen biocrusts, and moss crusts. The effects of biocrusts on soil nutrients and microbial communities vary among different successional stages (Hui et al., 2022). The metabolic pathways of the biocrust microbiome contribute to biogeochemical processes, thus augmenting the physicochemical properties of topsoil. The different morphological, anatomical, and physiological characteristics of biocrusts determine their effects on soil nutrients and water cycling (Housman et al., 2006; Li J. et al., 2020). Biocrusts increase soil nutrient such as alkali hydrolyzed nitrogen (AN) and available phosphorus (AP); increase the activities of soil enzymes such as urease (URE) and catalase (CAT); and decrease soil pH compared with bare ground. In biocrusts, the levels of soil nutrients are higher in the later stages of succession (moss crusts) than in the early stages of succession (cyanobacterial crusts, mixed biocrusts, and bare ground) (Kakeh et al., 2018). The content of soil nutrients and microbial biomass increases with the succession of biocrusts (Niu et al., 2017). Soil organic carbon (SOC) content of moss crusts was higher than that of cyanobacterial crusts, and the SOC content mixed crusts was higher than that of single-organism crusts (Xu et al., 2022). The total soil phosphorus (TP) content, total soil nitrogen (TN) content, and URE activity significantly decreased one year after moss crusts were removed compared with intact crusts (Cheng et al., 2021). In lichen biocrusts, species such as S. cartilaginea, D. diacapsis, and F. bracteata increase soil moisture and nutrient content but reduce soil pH. The decomposition of lichen biocrusts benefits the cycling of SOC, and enhances the activity of soil acid phosphatase (Ghiloufi et al., 2023). Additionally, Various ecological functions of biocrusts are related to the abundance and diversity of microorganisms. Xiao and Veste (2017) reported that the number of operational taxonomic units (OTUs) of bacteria and fungi was higher in moss crusts in the Loess Plateau than in bare ground. The diverse soil microorganisms contribute to soil fertility and play a vital role in ecosystem functioning. In biocrusts, the relationship between soil nutrients and microorganisms is crucial for ecosystem conservation and functioning. The presence of biocrusts promotes the recruitment and growth of soil microorganisms. The biocrusts enhance the metabolic activities and organic matter decomposition, facilitate nutrient cycling and enhance soil structure and stability, thereby reducing soil erosion and improving soil fertility. Soil nutrients directly affect the activity and diversity of microorganisms, which in turn influence the overall functioning of ecosystems (She et al., 2024). Therefore, the rational management of biocrust nutrients and the protection of microbial communities are key strategies for maintaining ecosystem health and sustainability. The effects of biocrusts on soil nutrient indicators and microbial communities were still unclear in the Pisha sandstone area. Therefore, the objectives of this study were (1) to investigate the effects of biocrusts (cyanobacteria biocrusts and moss biocrusts) on soil properties in the Pisha sandstone area in Pinus tabuliformis plantations used for soil and water conservation over ten years; (2) to conduct the effects of biocrusts on the composition of soil microbial communities (bacteria and fungi) in the Pisha sandstone area; and (3) to explore the relationship between soil properties and bacterial and soil microbial communities in the Pisha sandstone area.
The study area is located in Soil and Water Conservation Park of Zhungrun Banner, Erdos, Inner Mongolia, China (110.58 °E, 39.79 °N) (Supplementary Figure S1). The mean annual temperature is 6°C–9°C, and the precipitation ranges from 380 to 420 mm, concentrated in the July–September period. The terrain is undulating and has ravines, and the soil in this area is severely eroded by wind and water. The soil in the Pisha sandstone area is mostly composed of weathered Pisha sandstone, loess and wind-formed sand, with coarse particles, large voids and loose soil structure. The area primarily has artificial vegetation, and the key tree species are pines (Pinus tabuliformis), P. sylvestris var, Armeniaca sibica, etc. The predominant shrub species are Caragana korshinski, Hippophae rhamnoides, etc., and the main herbaceous species are Leymus chinensis, Thymus mongolicus, and Heteropappus altaicus. The soil collected in this study was under the pines in the Soil and Water Conservation Park. The pines were planted in 2013 with a row spacing of 3 m × 4 m. The cyanobacteria- and moss-dominated biocrusts are distributed under the pines in soil.
Biocrust soil samples were collected in the Pinus tabuliformis plantations in July 2022. All samples were collected using a soil drill (0–5 cm) in a serpentine manner. Soil samples were collected from the bare ground (CK), cyanobacterial crusts (PC), and moss crusts (PM) in the study area with four replicates (Supplementary Figures S1c–e). The collected soil samples were sieved (2mm) and divided into 3 portions. The first part of the soil sample was placed in liquid nitrogen and used for DNA extraction and high-throughput sequencing (16s and ITS), the second sample was placed in a refrigerator at −4°C and used for determining soil enzyme activity. The third sample was used for determining soil nutrient indicators. We extracted DNA from soil samples and sent them to Gene Denovo Biotechnology (Guangzhou, China) for high-throughput sequencing (Illumina Hiseq). The ssequencing data for microbial communities were deposited in the NCBI database. (Accession numbers PRJNA1148314 for bacterial microorganisms and PRJNA1148338 for fungal microorganisms). The method of soil nutrient indicators was shown in Table 1.
Soil indicators and diversity indices of microbial communities were analyzed through one-way analysis of variance (ANOVA) by using SPSS 24.0 software. The analysis of significance was performed using the Least Significant Difference (LSD) method (α = 0.05). Before these analyses were performed, data were tested for normality using the chi-square test and transformed, where necessary. The data were visualized, and the correlation between soil nutrient indicators was analyzed using Origin 2024. By integrating the genetic information of species from three major databases, namely IMG, KEGG, and PATRIC, phenotypic changes in the soil microbial communities were analyzed using PICRUSt2 and FunGuild. A diversity index (Observed Species Richness Index, Shannon-Wiener Index, Simpson’s Diversity Index, Chao1 Richness Estimator Index, Abundance-based Coverage Estimator Index and Pielou’s Evenness Index) of microbial communities was calculated from the Omicsmart cloud platform(http://www.omicsmart.com; Zhang et al., 2021).
The presence of biological soil crusts (biolcrusts) significantly increases the content of mineral elements and nutrients such as nitrogen, phosphorus, potassium and carbon in soil (Table 2). Soil sodium oxide (Na2O), copper (Cu), zinc (ZN) and available potassium (AK) were higher in the cyanobacterial crusts (PC) and moss crusts (PM) than in bare ground (CK) (P < 0.05). Soil AK was 143.51% and 233.62% higher, respectively, in PC and PM than in CK. PM was overall better than PC in enhancing the nutrient content, which significantly increased the soil content of alkali hydrolyzed nitrogen (AN), total soil nitrogen (TN), total soil phosphorus (TP), SOC, and soil organic matter (SOM) nutrients compared to CK and PC (P < 0.05), with the greatest increase in AN, were 231.27% and 121.14% higher compared to CK and PC, respectively. PC also greatly increased the microbial biomass in the soil, with microbial biomass carbon (MBC) and microbial biomass nitrogen (MBN) increasing 8.19 and 11.07 times, respectively, in PM compared to CK (P < 0.05).
Soil urease activity (URE) was significantly increased in PC and PM as compared to CK. Soil invertase activity (INV) was significantly higher in PM than in CK and PC. Soil INV was 4.35 and 1.82 times higher in PM than in CK and PC, respectively. Soil calcium oxide (CaO), pH, and catalase activity (CAT) were significantly decreased in PC and PM, with CAT enzyme activity decreased the most, by 48.60% and 74.0% compared to CK, respectively. The presence of biocrusts significantly changed the distribution pattern of soil nutrients and played an important role in soil fertility.
Biocrusts increased the richness and diversity of microbial communities in the soil (Supplementary Tables S1, S2). The Observed Species Richness (Sobs), Chao1 Richness Estimator Index (Chao 1) and Abundance-based Coverage Estimator Index (ACE) of bacterial microorganisms and the Sobs of fungal microorganisms in PM were significantly higher than those of CK and PC, but the Pielou’s Evenness Index (Pielou) of fungal microorganisms in PC was significantly lower than that of CK and 13% lower than that of CK.
The dominant phyla in the bacterial community were Proteobacteria (15.93%–20.17%), Acidobacteria (11.12%–24.23%), Cyanobacteria (1.28%–25.97%), Bacteroidetes (6.68%–14.46%), and Actinobacteria (7.40%–15.73%). The PC and PM significantly increased the relative abundance of Cyanobacteria and Bacteroidetes in the soil compared to CK, while significantly decreasing the relative abundance of Acidobacteria, Actinobacteria and Gemmatimonadetes in the soil (Figure 1a; Supplementary Table S3).
Figure 1. Top 10 bacterial (a) and fungal (b) community structures at the phylum level and their relative abundenced in soil biological crusts. CK, control without soil biological crust; PC, Cyanobacterial biological crust; PM, moss biological crust.
Among the fungal communities, Ascomycota had the highest relative abundance (71.67%–80.90%), followed by Basidiomycota (9.39%–13.97%) and Glomeromycota (0.05%–9.16%). The PC and PM significantly reduced the relative abundance of Glomeromycota in the soil compared to CK, with PC and PM reducing it by 195.43 times and 76.69 times, respectively, while other fungal phyla did not show significant differences among different treatments (Figure 1b; Supplementary Table S4).
The top 10 soil bacterial functional genes were all related to metabolism (Figure 2a). Among them carbohydrate metabolism, amino acid metabolism, metabolism of cofactors and vitamins, metabolism of terpenoids and polyketides, and metabolism of other amino acidsde were higher in abundance, totaling more than 50% in each treatment. Based on the fungal nutritional mode, the monotrophic fungal types were pathotrophs, saprotrophs, and symbiotrophs. The fungi with composite nutrient types were pathotroph–saprotroph, pathotroph–saprotroph–symbiotroph, pathotroph–symbiotroph, and saprotroph–symbiotroph (Figure 2b).
Figure 2. Functional abundance of bacterial (a) and fungal (b) community compositions. CK, control without soil biological crust; PC, Cyanobacterial biological crust; PM, moss biological crust.
The Cu, pH, CaO and MBN were the key environmental factors affecting bacterial and fungal microbial communities, explaining 95.08% of the bacterial microbial community and 95.45% of the fungal microbial community, respectively (Figures 3a, b). As key environmental factors, soil CaO showed significant positive correlations with Gemmatimonadetes and significant negative correlations with Cyanobacteria. The pH, CAT showed significant positive correlation with Acidobacteria, Actinobacteria, Gemmatimonadetes and Verrucomicrobia, while it showed significant negative correlation with Cyanobacteria, Bacteroidetes. The soil pH also showed a significant positive correlation with Glomeromycota, but soil Na2O, Cu and MBN showed a significant negative correlation with Glomeromycota (Figure 4).
Figure 3. Redundancy analysis of the relationship between soil properties and microbial communities of bacteria (a) and fungi (b). CK, control without soil biological crust; PC, Cyanobacterial biological crust; PM, moss biological crust.
Figure 4. Correlation analysis between soil nutrient indicators and microbial communities of bacteria (a) and fungi (b). *P < 0.05; **P < 0.01; ***P < 0.001. CK, control without soil biological crust; PC, Cyanobacterial biological crust; PM, moss biological crust.
In the correlation analysis of soil nutrient indicators (Table 3), the soil indicators MBC and MBN, which measure the size of soil microbial communities and their activities, generally showed significant positive correlations with soil nitrogen nutrients (AN and TN), enzyme activities (URE and CAT), carbon fractions (SOC, DOC, and SOM), AP and AK, but showed significant negative correlations with pH (Table 3).
The well-developed biocrusts beneath the artificial Pinus tabuliformis enhance the stability of soil aggregates by secreting organic matter and forming mycelial networks, while also creating a solid protective layer on the soil surface. This protective layer reduces the direct erosion caused by wind and water, thereby lowering the risk of soil and water loss. These processes provide favorable conditions for the restoration and conservation of the ecosystem in the Pisha sandstone area. The CaO content was 49.25% and 44.76% lower in PC and PM, respectively, than in bare ground. Pisha sandstone was mainly composed of kaolinite, quartz, feldspar, and other minerals. It was characterized by low diagenesis and poor mechanical strength (Zhang et al., 2023). The CaO was the main oxide components in the Pisha sandstone. When the CaO came into contact with water, it underwent disintegration, leading to soil erosion and loss in the surface layer (Zhang et al., 2024). The coverage of biocrusts could form a protective layer on the soil surface (Kheirfam and Roohi, 2020), reducing the intense reaction between CaO and soil moisture, thereby decreasing soil erosion. Biocrusts could form mycelium within the soil, and the mycelium can secrete polysaccharides and other substances that promote the formation of soil aggregates, improving soil physical and chemical properties and preventing soil erosion (Vieira et al., 2025). As biocrusts develop, soil erosion decreases and soil nutrients increase, which in turn supports the further development of more biocrusts. This virtuous cycle not only enhances the soil resistance to erosion but also lays the foundation for the long-term stability and functional recovery of ecosystems.
Biocrusts significantly enhance soil nutrient content by efficiently capturing dust and performing photosynthesis, which increases soil organic matter and promotes the fixation and cycling of carbon, nitrogen, and phosphorus nutrients. In this study, the soil mineral elements and nutrients in moss biocrusts (PM) were significantly increased as compared with bare ground (CK). On the one hand, moss biocrusts were more efficient in capturing dust, enhancing photosynthesis, and fixing nutrients, thereby promoting the accumulation of soil nutrients (Zhang Y. et al., 2022). On the other hand, biocrusts secreted extracellular polymers, such as polysaccharides. These polymers increase the cation exchange capacity of the soil, thereby retaining available nutrients (Harper and Belnap, 2001). Biocrusts influenced the biogeochemistry of soils through C and N fixation (Ferrenberg et al., 2022). In this study, biocrusts significantly increased soil AN, SOC, and AP. Biocrusts were the primary nitrogen (N) source in sandy lands (Tucker et al., 2020). Soil N inputs, transformations, and gaseous losses vary in different biocrust types, which increases nitrogen effectiveness (Zhou et al., 2020). The proportions of unstable and moderately unstable phosphorus are higher in biocrust soils than in bare ground. Moreover, biocrusts are a key player in P cycling as they convert stabilized P to unstable, readily bioavailable P (Baumann et al., 2017). They are also a pivotal source of carbon inputs to ecosystems. According to Li et al. (2012), biocrusts have higher carbon gains in summer, because they are more likely to meet the photosynthesis requirements of optimal temperature and light intensity in the moist summer than in spring and fall. They also have high water-holding and light-trapping capacities, high chlorophyll content, and thus high carbon input (Zhang C. P. et al., 2018). The existence of biocrusts reduced soil pH, possibly because biocrusts, such as lichens, can secrete organic acids, thereby lowering soil pH (Concostrina-Zubiri et al., 2013). Additionally, soil pH may also decrease because biocrusts increase the infiltration potential of soil, leading to percolation of soil ions into the soil and a drop in soil surface pH (Kakeh et al., 2018). Biocrusts protect the surface soil land, by enhancing soil nutrients and improving soil structure, provide favorable conditions for vegetation growth. The growth of vegetation further strengthens soil stability and reduces water and soil erosion. This process contributes to the restoration and conservation of ecosystems.
Biocrusts enhance organic matter content, improve soil conditions, and promote nutrient cycling, thereby increasing enzyme activities. Biocrusts play a vital role in the restoration of degraded ecosystems, the enhancement of soil quality, and the maintenance of ecological balance. In this study, the activities of URE enzyme were higher in PM and PC than in CK. This is due to the increase in the extent of biocrusts, which are associated with higher nutrient and moisture contents in the soil. The photosynthetic efficiency of biocrusts in the late succession stage is higher than that in the early succession stage, significantly increasing the content of organic matter and polysaccharides in the soil (Gypser et al., 2016). These organic materials provide abundant carbon sources for soil microbial activity, promoting microbial growth and metabolic processes. As microbial activity increases, the synthesis and secretion of soil enzymes also rise (Niu et al., 2017). The soil URE and INV enzymes in soil were associated with carbon and nitrogen cycling in soils (Zhang W. et al., 2018; Dostos et al., 2022). These processes are essential for maintaining soil fertility and ecosystem functioning. In this study, the SOM, TN, and TP contents were significantly higher in biocrusts than in bare ground, which corresponds to the aforementioned results. Biocrusts enhance the water retention capacity of the surface by capturing airborne silt and particles, among them, moss biocrusts have a higher particle capture ability than cyanobacterial biocrusts. Additionally, moss biocrusts absorb water through the hair-like tips on their leaves, further improving the soil water retention capacity and thereby enhancing soil moisture content (Zhang Y. et al., 2022). However, the CAT activity was significantly lower in biocrusts than in bare ground. This may be a result of the freeze–thaw cycle, which changes the temperature and water content of the soil surface and affects soil enzyme activity (Zhang et al., 2023). These mechanisms not only improve soil quality but also provide critical conditions for vegetation restoration and ecosystem stability, playing a vital protective role in preventing soil nutrient loss and soil degradation.
Biocrusts enhanced soil nutrients content and water retention, thereby promoting the growth and metabolic activities of soil microbial communities. The synergistic action of these dominant phyla facilitates the accumulation and cycling of soil nutrients, which was of great significance for the restoration and stabilization of the Pisha standstone area. Organic matter and nutrients were rich carbon sources for microorganisms (Zhang J. et al., 2022), which promoted the growth of microorganisms. Biocrusts could recruit microorganisms in the soil (Maier et al., 2018), Successional stages of biocrusts affected soil microbial communities, leading to the formation of specific microbial communities in different biocrusts (Glaser et al., 2022). Proteobacteria, Acidobacteria, and Cyanobacteria were the dominant bacterial phyla in biocrusts. By secreting polysaccharides and other organic materials, Cyanobacteria could improve soil water retention and stability (Maier et al., 2018). In addition, Cyanobacteria provided carbon and nitrogen sources for other microorganisms during the formation of biocrusts, promoting microbial community replenishment and diversity (Wang L. et al., 2022). Acidobacteria could decompose complex organic matter in the soil to release nutrients. Proteobacteria was also involved in the cycling of carbon, nitrogen, and phosphorus in the soil (Araujo et al., 2020). Ascomycota and Basidiomycota dominated the soil fungal community. In this study, the relative abundance of Ascomycota was 23.37% and 11.88% higher in PC and PM, respectively, than in CK. Ascomycota could promote soil nitrogen cycling (Aanderud et al., 2018). Basidiomycota exhibited strong adaptive capacity and participated in material decomposition and cycling in ecosystems (Wang et al., 2023). This corresponded to the higher soil nutrients content observed beneath biocrusts in this study.
The structure of soil microbial communities is influenced by multiple factors, including soil properties, mineral elements, pH, enzyme activity, and nutrient supply. These factors collectively maintain the stability of soil ecosystems by regulating microbial growth, metabolic activities, and nutrient cycling. The presence of mineral elements was often accompanied by changes in soil pH. In alkaline soils, the bioavailability of mineral elements was reduced, which in turn impacted microbial growth and activity (Kenarova et al., 2014; Lammel et al., 2018). Soil microbial communities were correlated with soil AK. On the one hand, soil AK provides nutrients for microbial growth. On the other hand, it mitigated the toxicity of heavy metals in the soil, thereby protecting soil microorganisms (You et al., 2023). Naz et al. (2022) reported that metabolic processes in microbial communities were highly susceptible to pH and thus mediate nutrient availability in soil. The relative abundance of microbial communities was significantly correlated with soil CAT enzyme activity. This is because microbial growth and metabolic activities were enhanced under nutrient-rich soil conditions, which increased the activity of soil enzymes (Huang et al., 2022). The CAT enzyme was related to the cycling of soil N nutrients (Wang et al., 2018). The increase of soil mineral elements, pH, enzyme activity and increasing nutrient supply can promote the growth of soil microorganisms, thereby enhancing the stability and productivity of soil ecosystems. Moreover, biocrusts stabilized soil moisture, regulated pH, and provided organic matter, offered favorable growth conditions for microbial communities, thereby further improving the soil microenvironment and promoting soil health and ecological balance.
Biological soil crusts (biocrusts) can significantly improve soil properties and enhance the diversity of soil microorganisms, particularly under Pinus tabuliformis that have been restored for over a decade in the Pisha sandstone area. The presence of biocrusts protects the surface soil by preventing water and soil erosion. Biocrusts can improve soil properties, including AN AP andAK, These abundant soil nutrients provide sufficient carbon sources and nutrients for microbial activities, promoting the growth and metabolic processes of microbial communities. As a result, biocrusts enhance soil microbial biomass and increase microbial diversity. The enhanced metabolic activities of soil microorganisms lead to significant increases in the activities of urease and invertase. Soil nutrients are key factors in maintaining soil fertility and ecosystem functions. The increase in soil nutrients in the Pisha sandstone area is conducive to plant growth, thereby reducing soil erosion. This provides support for the development of rapid biocrust restoration techniques in the Pisha sandstone areas, which can contribute to the sustainable development of artificial forest ecosystems.
The datasets presented in this study can be found in online repositories. The names of the repository/repositories and accession number(s) can be found in the article/Supplementary Material.
QL: Formal Analysis, Methodology, Validation, Writing – original draft, Writing – review & editing. MR: Conceptualization, Data curation, Formal Analysis, Investigation, Methodology, Project administration, Software, Validation, Visualization, Writing – original draft, Writing – review & editing. RW: Conceptualization, Data curation, Formal Analysis, Funding acquisition, Investigation, Methodology, Project administration, Resources, Software, Supervision, Validation, Visualization, Writing – original draft, Writing – review & editing. ZM: Data curation, Formal Analysis, Investigation, Methodology, Software, Writing – review & editing. MT: Data curation, Formal Analysis, Investigation, Methodology, Software, Writing – review & editing. YT: Data curation, Formal Analysis, Methodology, Software, Writing – review & editing. LY: Conceptualization, Funding acquisition, Supervision, Writing – review & editing. YF: Conceptualization, Funding acquisition, Investigation, Project administration, Supervision, Writing – review & editing.
The author(s) declare that financial support was received for the research and/or publication of this article. The authors gratefully acknowledge funding from the Major Special Foundation of Science and Technology Plan of Inner Mongolia (No. 2021ZD00804, 2023YFSH0025, and 2024JBGS0007), National Key Research and Development Program (No. 2024YFD13003023) and the Project for Young Talent Scientists of Inner Mongolia (No. NMGIRT2316).
The authors gratefully acknowledge funding from the Major Special Foundation of Science and Technology Plan of Inner Mongolia (No. 2021ZD00804, 2023YFSH0025, and 2024JBGS0007), National Key Research and Development Program (No. 2024YFD13003023) and the Project for Young Talent Scientists of Inner Mongolia (No. NMGIRT2316).
AuthorS LY and YF were employed by the company Inner Mongolia M-Grass Ecology and Environment Group Co., Ltd.
The remaining authors declare that the research was conducted in the absence of any commercial or financial relationships that could be construed as a potential conflict of interest.
The author(s) declare that no Generative AI was used in the creation of this manuscript.
All claims expressed in this article are solely those of the authors and do not necessarily represent those of their affiliated organizations, or those of the publisher, the editors and the reviewers. Any product that may be evaluated in this article, or claim that may be made by its manufacturer, is not guaranteed or endorsed by the publisher.
The Supplementary Material for this article can be found online at: https://www.frontiersin.org/articles/10.3389/fevo.2025.1524202/full#supplementary-material
Aanderud Z. T., Smart T. B., Wu N., Taylor A. S., Zhang Y., Belnap J. (2018). Fungal loop transfer of nitrogen depends on biocrust constituents and nitrogen form. Biogeosciences 15, 3831–3840. doi: 10.5194/bg-15-3831-2018
Araujo R., Gupta V. V. S. R., Reith F., Bissett A., Mele P., Franco C. M. M. (2020). Biogeography and emerging significance of Actinobacteria in Australia and northern Antarctica soils. Soil Biol. Biochem. 146, 107805. doi: 10.1016/j.soilbio.2020.107805
Baumann K., Glaser K., Mutz J. E., Karsten U., MacLennan A., Hu Y., et al. (2017). Biological soil crusts of temperate forests: Their role in P cycling. Soil Biol. Biochem. 109, 156–166. doi: 10.1016/j.soilbio.2017.02.011
Baumann K., Siebers M., Kruse J., Eckhardt K. U., Hu Y., Michalik D., et al. (2019). Biological soil crusts as key player in biogeochemical P cycling during pedogenesis of sandy substrate. Geoderma 338, 145–158. doi: 10.1016/j.geoderma.2018.11.034
Bhatti S., Baig J. A., Kazi T. G., Afridi H. I., Pathan A. A. (2019). Macro and micro mineral composition of Pakistani common spices: a case study. J. Food Measure. Character. 13, 2529–2541. doi: 10.1007/s11694-019-00173-w
Cheng C., Gao M., Zhang Y. D., Long M., Wu Y., Li X. (2021). Effects of disturbance to moss biocrusts on soil nutrients, enzyme activities, and microbial communities in degraded karst landscapes in southwest China. Soil Biol. Biochem. 152, 108065. doi: 10.1016/j.soilbio.2020.108065
Concostrina-Zubiri L., Huber-Sannwald E., Martínez I., Flores Flores J. L., Escudero A. (2013). Biological soil crusts greatly contribute to small-scale soil heterogeneity along a grazing gradient. Soil Biol. Biochem. 64, 28–36. doi: 10.1016/j.soilbio.2013.03.029
Deng L., Wang T., Luo W., He L., Liang Z. (2021). Effects of a compound microbial agent and plants on soil properties, enzyme activities, and bacterial composition of Pisha sandstone. Environ. Sci. pollut. Res. Int. 28, 53353–53364. doi: 10.1007/s11356-021-14533-x
Ding Y., Geng Y., Zhou W., Li D. (2023). Habitat-specific environmental factors regulate the spatial variability of biological soil crust microbial communities on the Qinghai-Tibet Plateau. Sci. Total Environ. 901, 165937. doi: 10.1016/j.scitotenv.2023.165937
Dostos T., Kapagianni P. D., Monokrousos N., Stamou G. P., Papatheodorou E. M. (2022). Spatial heterogeneity of Cladonia rangiformis and Erica spp. induces variable effects on soil microbial communities which are most robust in bare-soil microhabitats. Web Ecol. 22, 21–31. doi: 10.5194/we-22-21-2022
Elbert W., Weber B., Burrows S., Steinkamp J., Büdel B., Andreae M. O., et al. (2012). Contribution of cryptogamic covers to the global cycles of carbon and nitrogen. Nat. Geosci. 5, 459–462. doi: 10.1038/ngeo1486
Ferrenberg S., Tucker C. L., Reibold R., Howell A., Reed S. C. (2022). Quantifying the influence of different biocrust community states and their responses to warming temperatures on soil biogeochemistry in field and mesocosm studies. Geoderma 409, 115633. doi: 10.1016/j.geoderma.2021.115633
Finger-Higgens R., Duniway M. C., Fick S., Geiger E.L., Hoover D. L., Pfennigwerth A. A., et al. (2022). Decline in biological soil crust N-fixing lichens linked to increasing summertime temperatures. Proc. Natl. Acad. Sci. United States America 119, e2120975119. doi: 10.1073/pnas.2120975119
Garcia G. V., Campos M. E., Wyngaard N., Reussi-Calvo N. I., San Martino S., Covacevich F., et al. (2021). Anaerobically mineralized nitrogen within macroaggregates as a soil health indicator. CATENA 198, 105034. doi: 10.1016/j.catena.2020.105034
Ghiloufi W., Yun J., Kim J., Lee J., Kang H. (2023). The influences of lichens on soil physico-chemical properties, enzymes and microbes are species specific: Insights from South Mediterranean arid ecosystem. Appl. Soil Ecol. 182, 104656. doi: 10.1016/j.apsoil.2022.104656
Glaser K., Albrecht M., Baumann K., Overmann J., Sikorski J. (2022). Biological soil crust from mesic forests promote a specific bacteria community. Front. Microbiol. 13, 76 9767–769767. doi: 10.3389/fmicb.2022.769767
Gou X. L., Hu J., Chen Y. J., Wei X., Du Z., Zhou Q. (2019). The effect of artificial vegetation recovery on the soil nutrients and enzyme activities in subhumid desert land on the southeast Qinghai-Tibetan Plateau, China. Ecol. Eng. 139, 105528–105528. doi: 10.1016/j.ecoleng.2019.06.023
Gypser S., Herppich B. W., Fischer T., Lange P., Veste M. (2016). Photosynthetic characteristics and their spatial variance on biological soil crusts covering initial soils of post-mining sites in Lower Lusatia, NE Germany. Flora 220, 103–116. doi: 10.1111/nph.15000
Harper K. T., Belnap J. (2001). The influence of biological soil crusts on mineral uptake by associated vascular plants. J. Arid Environ. 47, 347–357. doi: 10.1006/jare.2000.0713
Housman D. C., Powers H. H., Collins A. D., Belnap J. (2006). Carbon and nitrogen fixation differ between successional stages of biological soil crusts in the Colorado Plateau and Chihuahuan Desert. J. Arid Environ. 66, 620–634. doi: 10.1016/j.jaridenv.2005.11.014
Huang H. Y., Tian D., Zhou L. H., Su H., Ma S., Feng Y., et al. (2022). Effects of afforestation on soil microbial diversity and enzyme activity: A meta-analysis. Geoderma 423, 115961. doi: 10.1016/j.geoderma.2022.115961
Hui R., Zhao R. M., Liu L. C., Li X. (2022). Effect of snow cover on water content, carbon and nutrient availability, and microbial biomass in complexes of biological soil crusts and subcrust soil in the desert. Geoderma 406, 115505. doi: 10.1016/j.geoderma.2021.115505
Kakeh J., Gorji M., Sohrabi M., Tavili A., Pourbabaee A. A. (2018). Effects of biological soil crusts on some physicochemical characteristics of rangeland soils of Alagol, Turkmen Sahra, NE Iran. Soil Tillage Res. 181, 152–159. doi: 10.1016/j.still.2018.04.007
Kenarova A., Radeva G., Traykov I., Boteva S. (2014). Community level physiological profiles of bacterial communities inhabiting uranium mining impacted sites. Ecotoxicol. Environ. Saf. 100, 226–232. doi: 10.1016/j.ecoenv.2013.11.012
Kheirfam H., Asadzadeh F. (2020). Accelerating the formation of biological soil crusts in the newly dried-up lakebeds using the inoculation-based technique. Sci. Total Environ. 98, 136036. doi: 10.1016/j.scitotenv.2019.136036
Lammel D. R., Barth G., Ovaskainen O., Cruz L. M., Zanatta J .A., Ryo M., et al. (2018). Direct and indirect effects of a pH gradient bring insights into the mechanisms driving prokaryotic community structures. Microbiome 6, 106. doi: 10.1186/s40168-018-0482-8
Ladrón de Guevara M., Gozalo B., Raggio J., Lafuente A., Prieto M., Maestre F. T. (2018). Warming reduces the cover, richness and evenness of lichen-dominated biocrusts but promotes moss growth. New Phytol. 220, 811–823.
Li C. M., Dong J. L., Zhao S. B., Liu H., Yao W., Wang L. (2018). Development of low cost supplementary cementitious materials utilizing thermally activated Pisha sandstone. Construct. Build. Mater. 174, 484–495. doi: 10.1016/j.conbuildmat.2018.04.111
Li Q., Hu C. X., Yang H. J. (2022). Responses of cyanobacterial crusts and microbial communities to extreme environments of the stratosphere. Microorganisms 10, 1252–1252. doi: 10.3390/microorganisms10061252
Li X. R., Zhang P., Su Y.G., Jia R. L. (2012). Carbon fixation by biological soil crusts following revegetation of sand dunes in arid desert regions of China: A four-year field study. CATENA 97, 119–126. doi: 10.1016/j.catena.2012.05.009
Li J. Y., Jin X. Y., Zhang X. C., Chen L., Liu J. L., Zhang H. M., et al. (2020). Comparative metagenomics of two distinct biological soil crusts in the Tengger Desert, China. Soil Biol. Biochem. 140, 107637. doi: 10.1016/j.soilbio.2019.107637
Li Z., Xiao J., Lu G., Sun W., Ma C., Jin Y, et al. (2020). Productivity and profitability of Larix principis-rupprechtii and Pinus tabuliformis plantation forests in Northeast China. For. Policy Econ. 121, 102314. doi: 10.1016/j.forpol.2020.102314
Liang Z. S., Wu Z. R., Yao W. Y., Noori M., Yang C., Xiao P., et al. (2019). Pisha sandstone: Causes, processes and erosion options for its control and prospects. Int. Soil Water Conserv. Res. 7, 1–8. doi: 10.1016/j.iswcr.2018.11.001
Liu W. F., Huang Z., Guo Z. X., López-Vicente M., Wang Z., Wu G.L., et al. (2023). A nature-based solution to reduce soil water vertical leakage in arid sandy land. Geoderma 438, 116630. doi: 10.1016/j.geoderma.2023.116630
Ma W. M., Zhang X. C. (2016). Effect of Pisha sandstone on water infiltration of different soils on the Chinese Loess Plateau. J. Arid Land 8, 331–340. doi: 10.1007/s40333-016-0122-8
Mai Z. M., Chen Q. Q., Wang L., Zhang J., Cheng H., Su H., et al. (2024). Bacterial carbonic anhydrase-induced carbonates mitigate soil erosion in biological soil crusts. J. Environ. Manage. 352, 120085. doi: 10.1016/j.jenvman.2024.120085
Maier S., Tamm A., Wu D. M., Caesar J., Grube M., Weber B. (2018). Photoautotrophic organisms control microbial abundance, diversity, and physiology in different types of biological soil crusts. ISME J. 12, 1032–1046. doi: 10.1038/s41396-018-0062-8
Naz M., Dai Z., Sajid H., Tariq M., Danish S., Khan I. U., et al. (2022). The soil pH and heavy metals revealed their impact on soil microbial community. J. Environ. Manage. 321, 115770. doi: 10.1016/j.jenvman.2022.115770
Niu J. P., Yang K., Tang Z. J., Wang Y. (2017). Relationships between soil crust development and soil properties in the desert region of North China. Sustainability 9, 725–739. doi: 10.3390/su9050725
Qi J. H., Liu Y. B., Wang Z. R., Zhao L., Zhang W., Wang Y., et al. (2021). ariations in microbial functional potential associated with phosphorus and sulfur cycling in biological soil crusts of different ages at the Tengger Desert, China. Appl. Soil Ecol. 165, 104022. doi: 10.1016/j.apsoil.2021.104022
Qi L., Pokharel P., Chang S. X., Zhou P., Niu H., He X., et al. (2020). Biochar application increased methane emission, soil carbon storage and net ecosystem carbon budget in a 2-year vegetable–rice rotation, Agriculture. Ecosyst. Environ. 292, 106831. doi: 10.1016/j.agee.2020.106831
Riveras-Muñoz N., Seitz S., Witzgall K., Rodríguez V., Kühn P., Mueller C.W., et al. (2022). Biocrust-linked changes in soil aggregate stability along a climatic gradient in the Chilean Coastal Range. Soil 8, 717–731. doi: 10.5194/soil-8-717-2022
Rodriguez-Caballero E., Stanelle T., Egerer S., Cheng Y., Su H., Canton Y., et al. (2022). Global cycling and climate effects of aeolian dust controlled by biological soil crusts. Nat. Geosci. 15, 458–463. doi: 10.1038/s41561-022-00942-1
She S., Tao Y., Zhu J., Ye W., Hou L., Fu Y., et al. (2024). Spatial distribution and succession of microbial communities in biological soil crusts as affected by microtopography factors in the Granite Tailing Areas of Macheng, China. Catena 247, 108532. doi: 10.1016/j.catena.2024.108532
Tian X. J., Tian P., Zhao G. J., Gómez J.A., Guo J., Mu X., et al. (2023). Sediment source tracing during flood events in the Huangfu River basin in the northern Loess Plateau, China. J. Hydrol. 620, 129540. doi: 10.1016/j.jhydrol.2023.129540
Tucker C., Ferrenberg S., Reed S. C. (2020). Modest residual effects of short-term warming, altered hydration, and biocrust successional state on dryland soil heterotrophic carbon and nitrogen cycling. Front. Ecol. Evol. 8, 467157. doi: 10.3389/fevo.2020.467157
Vieira K. C., Marascalchi N. M., Rozmoš M., Benada O., Belova V., Jansa J. (2025). Arbuscular mycorrhizal fungal highways–What, how and why? Soil Biol. Biochem. 202, 109702.
Wang Y., Hao J. J., Gou L. J., Zhao L., Chai B., Jia T. (2023). Fungal community characteristics and driving factors in Bothriochloa ischaemum litter in a copper mining area. Fungal Biol. 127, 1426–1438. doi: 10.1016/j.funbio.2023.10.008
Wang L., Li J., Zhang S. (2022). A comprehensive network integrating signature microbes and crucial soil properties during early biological soil crust formation on tropical reef islands. Front. Microbiol. 13, 831710. doi: 10.3389/fmicb.2022.831710
Wang L., Li J., Zhang S., Huang Y., Ouyang Z., Mai Z. (2024). Biological soil crust elicits microbial community and extracellular polymeric substances restructuring to reduce soil erosion on a tropical island in the South China Sea. Mar. Environ. Res. 197, 106449. doi: 10.1016/j.marenvres.2024.106449
Wang S. Q., Li. T. X., Zheng Z. C. (2018). Effects of tea plantation age on soil aggregate-associated C- and N-cycling enzyme activities in the hilly areas of Western Sichuan, China. Catena 171, 145–153. doi: 10.1016/j.catena.2018.07.010
Wang Q., Zhang Q. Y., Hna Y. C., Zhang D., Zhang C.C., Hu C. (2022). Carbon cycle in the microbial ecosystems of biological soil crusts. Soil Biol. Biochem. 171, 108729. doi: 10.1016/j.soilbio.2022.108729
Wang J. P., Zhen Q., Xin J. X., Wang Y., Zhang X. (2021). Phosphorus removal from aqueous solution using Al-modified Pisha sandstone. J. Clean. Product. 308, 127255. doi: 10.1016/j.jclepro.2021.127255
Warren S. D., St Clair L. L., Stark L. R., Lewis L.A., Pombubpa N., Kurbessoian T. (2019). Reproduction and dispersal of biological soil crust organisms. Front. Ecol. Evol. 7, 344. doi: 10.3389/fevo.2019.00344
Wu Y., Ren H. P., Wang X., Shen Z.Yang Z. (2020). The relationship between physical and chemical characteristics and the loss of pisha sandstones. J. Phys.: Conf. Ser. 1637, 12068. doi: 10.1088/1742-6596/1637/1/012068
Xiao B., Veste M. (2017). Moss-dominated biocrusts increase soil microbial abundance and community diversity and improve soil fertility in semi-arid climates on the Loess Plateau of China. Appl. Soil Ecol. 117-118, 165–177. doi: 10.1016/j.apsoil.2017.05.005
Xu H. K., Zhang Y. J., Shao X. Q., Liu N. (2022). Soil nitrogen and climate drive the positive effect of biological soil crusts on soil organic carbon sequestration in drylands: A Meta-analysis. Sci. Total Environ. 803, 150030. doi: 10.1016/j.scitotenv.2021.150030
You A., Tang J., Shu J. X., Xiao Y., Ai Y., Liu X., et al. (2023). The impacts of soil properties and heavy metals on soil microbial communities in the artificial soils on railway rock-cut slopes. J. Soils Sediments 23, 1820–1831. doi: 10.1007/s11368-022-03420-8
Zhang Y. D., Gao M., Yu C. Y., Zhang H., Yan N., Wu Q., et al. (2022). Soil nutrients, enzyme activities, and microbial communities differ among biocrust types and soil layers in a degraded karst ecosystem. CATENA 212, 106057. doi: 10.1016/j.catena.2022.106057
Zhang Y., Li L., Liu L., Zhang S., Zhao W., Ren Y., et al. (2024). Spatiotemporal variation and driving force of gully erosion in the Pisha sandstone area. Front. Environ. Sci. 12, 1472175. doi: 10.3389/fenvs.2024.1472175
Zhang Q., Li J. W., Zhang S. J., Li Y., Wu N., Zhou X., et al. (2023). Differentiate responses of soil nutrient levels and enzymatic activities to freeze-thawing cycles in different layers of moss-dominated biocrusts in a temperate desert. Front. Plant Sci. 14, 1137754. doi: 10.3389/fpls.2023.1137754
Zhang C. P., Niu D. C., Song M. L., et al. (2018). Effects of rainfall manipulations on carbon exchange of cyanobacteria and moss-dominated biological soil crusts. Soil Biol. Biochem. 124, 24–31. doi: 10.1016/j.soilbio.2018.05.021
Zhang W., Qiao W. J., Gao D. X., Dai Y., Deng J., Yang G., et al. (2018). Relationship between soil nutrient properties and biological activities along a restoration chronosequence of Pinus tabulaeformis plantation forests in the Ziwuling Mountains. CATENA 161, 85–95. doi: 10.1016/j.catena.2017.10.021
Zhang J., Xu M., Xu M. X. (2022). Characterising the diversity and functionality of the microbial community within biocrusts associated with different vegetation communities and soil habitats. Appl. Soil Ecol. 175, 104458. doi: 10.1016/j.apsoil.2022.104458
Zhang Q., Zhong D., Sun R., Li Y., Wu N., Zhou X., et al. (2021). Prevention of loperamide induced constipation in mice by KGM and the mechanisms of different gastrointestinal tract microbiota regulation. Carbohydr. Polym. 256, 117418. doi: 10.1016/j.carbpol.2020.117418
Zhao Y. B., Yang C. Y., Qu F., Wu Z., Ding K., Liang Z. (2023). Effect of wet-dry cycles on the mechanical performances and microstructure of Pisha sandstone. Molecules 28, 2533–2533. doi: 10.3390/molecules28062533
Zhou X. B., Tao Y., Yin B. F., Tucker C., Zhang Y (2020). Nitrogen pools in soil covered by biological soil crusts of different successional stages in a temperate desert in Central Asia. Geoderma 366, 114166. doi: 10.1016/j.geoderma.2019.114166
Keywords: enzyme activity, microbial community, soil property, crust, Pisha sandstone
Citation: Le Q, Ru M, Weibo R, Min Z, Wentao M, Ting Y, Yaling L and Feng Y (2025) Biological crusts increase soil microbial biomass and alter bacterial and fungal community compositions under pine restored over ten years in a Pisha sandstone soil. Front. Ecol. Evol. 13:1524202. doi: 10.3389/fevo.2025.1524202
Received: 07 November 2024; Accepted: 17 March 2025;
Published: 04 April 2025.
Edited by:
Hanxi Wang, Harbin Normal University, ChinaReviewed by:
Justin Bastow, Eastern Washington University, United StatesCopyright © 2025 Le, Ru, Weibo, Min, Wentao, Ting, Yaling and Feng. This is an open-access article distributed under the terms of the Creative Commons Attribution License (CC BY). The use, distribution or reproduction in other forums is permitted, provided the original author(s) and the copyright owner(s) are credited and that the original publication in this journal is cited, in accordance with accepted academic practice. No use, distribution or reproduction is permitted which does not comply with these terms.
*Correspondence: Ren Weibo, cndlaWJvMjAyMkAxNjMuY29t
†These authors have contributed equally to this work
Disclaimer: All claims expressed in this article are solely those of the authors and do not necessarily represent those of their affiliated organizations, or those of the publisher, the editors and the reviewers. Any product that may be evaluated in this article or claim that may be made by its manufacturer is not guaranteed or endorsed by the publisher.
Research integrity at Frontiers
Learn more about the work of our research integrity team to safeguard the quality of each article we publish.