- 1Key Laboratory of the Ministry of Education for Coastal and Wetland Ecosystems, College of the Environment & Ecology, Xiamen University, Xiamen, China
- 2Administrative Office of Fujian Xiongjiang Huangchulin National Nature Reserve, Minqing, China
Quercus chungii is a tree species endemic to subtropical areas of China, rare and endangered in Fujian Province. Minqing Huangchulin National Nature Reserve was specifically established to preserve Q. chungii forests with severe logging history. How the composition of Q. chungii communities changed after the initiation of this conservation practice remains unknown. Understanding the successional trajectories of Q. chungii communities is crucial for conserving and restoring Q. chungii forests and similar secondary forests with logging history in subtropical areas. In this study, we assessed how species and phylogenetic composition of Q. chungii communities changed, and whether these temporal trajectories were primarily contributed by stochastic processes. We established two sites composed by Q. chungii adult trees in the nature reserve in 2013, and measured their species composition in 2013 and 2023, respectively. We found that the communities undergone significant species replacement and reordering from 2013 to 2023, thus leading to high temporal β diversity. These temporal changes in community composition were primarily the results of deterministic ecological processes based on the results of null model analyses. A larger contribution from nestedness than turnover to the temporal β diversity suggested that the change in species richness caused by the loss or gain of species was more important than species turnover in driving the compositional change. A larger contribution from balanced abundance variations than abundance gradient to the temporal β diversity suggested that there was no significant consistent increase or decrease in species abundance during this decade. The target species Q. chungii significantly increased in abundance and basal area, making this species rank among one of the most dominant species in the communities after a decade, indicating the effectiveness of the nature reserve in conserving Q. chungii communities. However, the over-reliance on resprouts may reduce the genetic diversity and increase the risks from natural enemies, thus limiting the future development of Q. chungii. We conclude that although Q. chungii communities undergone rapid recovery from logging disturbance, continuing efforts in studying its population structures and dynamics and their driving factors are crucial for restoring and conserving Q. chungii communities.
1 Introduction
Deforestation threatens biodiversity in forest ecosystems and the substantial ecosystem services they sustain (Hansen et al., 2013; Hoang and Kanemoto, 2021; Wang et al., 2023). These threats are extremely severe in tropical and subtropical areas containing both high forest biodiversity and human disturbances (Hansen et al., 2013). Southern China experienced a severe wave of forest logging in the 20th century (Zhang, 2019). After the 1980s, increasing efforts, like establishment of nature reserves, have been implemented to restore and conserve these disturbed secondary forests (Li et al., 2010; Tong et al., 2023). Studying how species diversity and community composition change after logging can improve our understanding of forest successional trajectories after anthropogenic disturbance and provide critical guidance for forest restoration (McDowell et al., 2020; Liang et al., 2023; Sanjeewani et al., 2024). Quercus chungii is a tree species of Fagaceae, endemic to China, rare and endangered in Fujian Province (Jiang et al., 2019; Sun et al., 2021). This oak species has the capacity to establish dominant communities within the forests and contribute significantly to high biomass production (Wang et al., 2011; Liu et al., 2004). The Q. chungii forest community was a typical representative of the evergreen broad-leaved forest at the southern edge of the mid-subtropical zone of China (Li et al., 2010). They serve as an important habitat and a crucial food source for various terrestrial wildlife (Huang et al., 2010; Li et al., 2010). However, due to excessive logging for their high-quality timber in the history and the rapid expansion of plantation forests, the area of natural Q. chungii forest drastically reduced, resulting in fragmented and scattered distributions in southern China. Previous work has conducted systematic survey of Q. chungii populations, forest communities, and seed production (Chen, 2004; Huang et al., 2010; Li et al., 2010; Wang et al., 2011; Zhong et al., 2024). However, how the composition of these forest communities has changed since the initiation of this conservation practice, and the driving processes behind these changes, remain unknown, yet they are crucial for conserving and restoring Q. chungii and its associated ecosystems.
Anthropogenic disturbances, like post-logging, may cause complicated impacts on natural successional processes, depending on both the properties of disturbances and communities (Kariuki et al., 2006; Dalmaso et al., 2020; Mahayani et al., 2020; Rubio-Cuadrado et al., 2024). Q. chungii possesses strong capacity to sprout from stumps (Supplementary Figure S1), which may facilitate secondary succession after logging disturbances. However, difficulties in seed regeneration and high age-specific death probabilities at the early developmental stages may seriously hamper its natural succession (Huang et al., 2011; Wang et al., 2011; Sun et al., 2021; Zhong et al., 2024).
Disentangling the primary processes driving the successional processes can enhance the prediction of temporal trajectories of disturbed forests and may provide important intervention clues to facilitate forest restoration. Multiple community assembly processes can drive the temporal successional trajectories of forest communities following logging disturbances (Connell, 1978; Kariuki et al., 2006; Avalos, 2019; Dalmaso et al., 2020; Rubio-Cuadrado et al., 2024), such as composition of regional species pools, immigration and emigration of species, habitat filtering, and local interactions between species with different life-history strategies (e.g., shade tolerance) (Vellend, 2010; HilleRisLambers et al., 2012; van der Sande et al., 2016; Avalos, 2019; Chapman et al., 2021; Rubio-Cuadrado et al., 2024). Some of these processes (e.g., abiotic environmental filtering and biotic interactions) can be deterministic and make the successional trajectories more predictable (Tilman, 1982; Liu et al., 2012; Kraft et al., 2015; Liu et al., 2015). Stochastic processes (e.g., dispersal and chance events) may increase the uncertainty of the successional trajectories (Hubbell, 2001). Identifying the specific determinators in driving the assembly processes can guide the restoration efforts. For example, if substantial gain or loss of species is the primary driver, more efforts should be focused on the assessment of the potential impacts of gained or lost species on focal species.
Null model analysis and additive partitioning of temporal diversity are two powerful tools to disentangle the relative contributions of community assembly processes to the successional dynamics. Null model compares species compositions observed v.s. simulated from randomization processes, which disentangles the relative contribution of stochastic from deterministic processes (Chase, 2010; Swenson et al., 2011; Kraft et al., 2011; Chase et al., 2020). This approach has been widely used to better understand community assembly processes in post-disturbance forests (Myers et al., 2015; Osazuwa-Peters et al., 2015). Another way to assess the temporal changes in species composition along successional trajectories is to measure temporal diversity and its additive components (Baselga, 2010; Dalmaso et al., 2020; Villa et al., 2021). The diversity between two temporal points of a community can be partitioned to temporal species turnover and nestedness. Temporal species turnover refers to the replacement of species in one community at a time by different species in the same community at another time, while nestedness captures the gain or loss of species in a community from one to another times. This partitioning can improve the understanding of mechanisms underlying changes in community diversity and composition (Baselga, 2010, 2013).
This study focuses on the decadal dynamics of Q. chungii communities at two sites of the Fujian Minqing Huangchulin National Nature Reserve. The objectives of this study are to assess how and why the species and phylogenetic composition of Q. chungii forest communities have changed. We analyzed the temporal changes in woody plant species diversity, phylogenetic diversity and composition between 2013 and 2023. We disentangled the contributions from stochastic v.s. deterministic processes by conducting null model analyses. We assessed the relative importance of different assembly processes to the temporal compositional variation by conducting additive partitioning of diversity. We hypothesize that (1) the secondary Q. chungii forests increase in plant species and phylogenetic diversity from 2013 to 2023; (2) the recovery of Q. chungii leads to a large change in species and phylogenetic composition and high temporal diversity; (3) changes in biotic and abiotic environment induced by the recovery of Q. chungii may further induce rapid replacement of existing species and make relevant deterministic processes dominate the successional processes. This research will enhance the understanding of the mechanisms driving secondary forest succession after logging, and provide guidance for the conservation and restoration of Q. chungii and its associated communities and ecosystems.
2 Materials and methods
2.1 Study site
Currently, relatively concentrated natural Q. chungii communities are only found in Minqing County, Fujian Province (Li et al., 2010; Wang et al., 2011). A specific nature reserve (Fujian Minqing Huangchulin Nature Reserve) in this area was established in 1985 to better conserve Q. chungii and its associated communities and ecosystems (Li et al., 2010). Therefore, we conducted this research in this nature reserve.
The nature reserve is located in the western part of Minqing County, Fujian Province, China (26°15′29″ N ~26°22′41″N, 118°39′38″ E ~ 118°51′19″ E, with an elevation range of 100 ~ 595 m) and covering an area of 12,513.3 hectares. The reserve has a mid-subtropical maritime monsoon climate characterized by warmth and humidity. The annual average temperature is 17.5°C, with an average annual sunshine duration of 1,871.4 hours, a frost-free period of 294 days, and an average annual precipitation of 1,400 to 1,900 mm. The forest soil is predominantly mountainous red soil, with small areas of mountainous yellow soil. The soil layer is interspersed with a large number of semi-weathered granite rocks. The soil is acidic with a relatively shallow layer (Li et al., 2010). One of the main function of this reserve is to specifically protect Q. chungii and its associated communities and ecosystems (Li et al., 2010).
2.2 Community survey
We established two permanent sites in the nature reserve in 2013. Both sites are located within regions that are characterized by either high population density or the presence of the largest Q. chungii stems, and both possess a recorded history of logging activities predating 1985. Site 1, located near Tangxia village, was among the areas with most dense distribution of Q. chungii in the nature reserve. Site 2, located at Fengshiling, contained lower density of Q. chungii. We established sixteen 10 m × 10 m plots at Site 1, and six 10 m × 10 m plots at Site 2. We measured every tree with a DBH ≥ 2.5 cm within the plots and recorded its species name and growth status both in 2013 and 2023. We acknowledged that using larger-area forest plots would allow more robust investigation of the forest community composition and dynamics. However, the initial survey a decade ago based on 10 m × 10 m plots constrained our comparative analyses to this small scale.
We used bootstrap resampling approach to derive the average and its uncertainty of the temporal difference in basal areas and abundances for the 15 species with highest importance values (see below) at each site. That is, we resampled the 10 m × 10 m plots with replacement, calculated the average temporal difference in basal areas and abundances for each species at each site, and repeated this process for 10,000 times. We constructed the 95% CI (confidence interval) of the mean differences from the bootstrap.
2.3 Species relative importance
We used four indices to assess the relative importance of species within communities: species abundance, species dominance, species frequency, and species importance value. Species abundance refers to the number of individuals of the same species within a plot. Species dominance represents the sum of the basal areas of all the stems of a given species. Species frequency is the proportion of plots where a species is present. Species importance value (IV) is calculated as the average of relative abundance (RA), relative dominance (RD), and relative frequency (RF) of the species (Zhang, 2005).
2.4 Species diversity and community composition dynamics
We used Hill numbers, or effective species numbers, (q = 0, 1, and 2) to measure species diversity (Equations 1-4). Hill numbers provide a comprehensive measure of species diversity, accounting for both species richness and abundance while allowing for the evaluation of the influence of rare and abundant species based on the chosen value of q (Chao et al., 2014). The formula for calculating Hill numbers is as follows:
In the formula above, i represents the i-th species, S is the total number of species, and denotes the relative abundance of the i-th species. When q = 0, the Hill number equals species richness and does not consider the relative abundances of species. When q = 1, the formula yields the Shannon entropy index (i.e., equals the exponential of the Shannon-Wienner index), which represents the diversity of more common species within the community. When q = 2, it represents the diversity of more dominant species within the community (i.e., equals one minus the inverse of Simpson index) (Hsieh et al., 2016). We conducted Student’s t-test to compared the significant difference in Hill numbers between 2013 and 2023 for each site.
Hill numbers can also be used to measure differences in species composition over time, known as β diversity (Chao et al., 2014) (Equations 5-7):
We calculated β diversity per plot pair within each site. As we lost the exact spatial information of plots in 2013, we randomly pair the plots between 2013 and 2023 separately for each site. Finally, we calculated the average and 95% CI of β diversity values across all possible pairs.
We conducted null model analyses to disentangle the contributions of deterministic from stochastic processes to temporal changes in community composition. For each plot, we randomly sample species from species pool of corresponding site. Species’ selection probability was determined by its relative abundance within each site and the overall abundance within each plot (Gotelli, 2000; Chase et al., 2011). These analyses were conducted separately for Hill numbers with q values 0, 1, and 2. In each null model, we performed 999 random samplings, and calculated the average β diversity across pairs (βrandom). This average value was then standardized against the observed average β diversity calculated from all observed pairs, resulting in the SES (standardized effect size) of β diversity (Equation 8). The specific calculation formula is as follows:
Where represents the average β diversity of all observed pairs. Mean () and sd () respectively denote the mean and standard deviation of the β diversity obtained from 999-times random simulations. The SES β diversity index indicates the degree to which species composition deviates from stochastic change, with values closer to 0 indicating a closer resemblance to stochastic process. An absolute value larger than 1.96 indicates significant deviation from stochastic process, thus possible important contribution from deterministic processes (Swenson et al., 2011).
To explore the community assembly processes during the last decade, we performed additive partitioning analyses of the temporal β diversity, using the R package “betapart” (Baselga and Orme, 2012). First, we decomposed species incidence-based β diversity index (Sørensen index, βsor) into spatial turnover (βsim) and nestedness (βnes) (Baselga, 2010) (Equations 9-11):
Where a represents the number of species that are shared between two plots being compared; b represents the number of species present in the first plot but absent in the second; c represents the number of species found in the second plot but not in the first. βsim represents the spatial turnover of species and βnes represents the loss or gain of species due to nestedness.
Second, we decomposed the abundance-based β diversity index (Bray-Curtis index, βBC) into a balanced abundance variation (βBC-bal) and an abundance gradient (βBC-gra) (Baselga, 2013) (Equations 12-18):
Where xij represents the abundance of species i in plot j, xik represents the abundance of species i in plot k. Therefore, A is the minimum number of individuals for each species that exist in both plots j and k, while B and C are the number of individuals specific to plots j and k, respectively. βBC-bal describes a situation where, in one location, individuals of certain species are replaced by an equal number of individuals of different species in another location, the total abundance remains largely balanced, but the species composition changes. βBC-gra describes a gradient in which species composition changes due to the loss of some individuals, resulting in one location’s species becoming a subset of another location. This change is typically accompanied by a decrease in total abundance, meaning that the number of individuals of certain species decreases in a given area.
2.5 Phylogenetic diversity and composition
We used two indices to measure phylogenetic diversity of each plot, Faith’s phylogenetic diversity (PD) and mean pairwise distance (MPD). Faith’s PD reflects the sum of branch lengths occupied by all species within a community on the phylogenetic tree (Faith, 1992), while MPD represents the average phylogenetic distance between pairs of species within the community. We performed Student’s t-test to compare the significant difference in phylogenetic diversity between 2013 and 2023. The phylogenetic tree was constructed by “V.PhyloMaker2” package (Jin and Qian, 2022) using all 67 woody species in this study.
We calculated phylogenetic β diversity between plots in 2013 and 2023 to assess how phylogenetic composition changed during this period. This metric was calculated by Phylosor function from the R package “Picante” (Kembel et al., 2010). It represents the proportion of shared phylogenetic tree branch lengths between two communities (Bryant et al., 2008). We calculated the average value () across all possible pairs within each site. We also conducted null model analyses to disentangle the relative contribution of deterministic from stochastic processes in driving the phylogenetic composition change. We performed the null models with the Phylosor.rnd function, by randomly shuffling species at the tips of the phylogenetic tree 999 times, while keeping species richness constant within each plot. For each randomization, we calculated the average β diversity value across all pairs, denoted as . We calculated the standardized effect size of phylogenetic diversity (SES.Phylosor index) using the following equation (Equation 19):
Where represents the observed average phylogenetic β diversity calculated from the real data; mean () and sd () denote the mean and standard deviation of β diversities obtained through random simulations, respectively. A SES.Phylosor value close to 0 indicates that stochastic processes are important in driving the changes in community phylogenetic composition. An absolute value larger than 1.96 indicates significant deviation from stochastic process, thus possible important contribution from deterministic processes (Swenson et al., 2011).
All the above analyses were performed in R 4.3.1 (R Development Core Team, 2023). The bootstrap method was implemented by using the “boot” package (Canty and Ripley, 2022), and the graphs were created using the “ggplot2” package (Wickham, 2016).
3 Results
3.1 Species relative importance and its temporal dynamics
We found significant changes in community composition between 2013 and 2023 at both Site 1 and Site 2 (Figures 1, 2; Supplementary Tables S1-S3). In 2013, we found 41 species and 414 woody plants with DBH 2.5cm at the two sites. Daphniphyllum oldhamii and Castanopsis fargesii had the largest importance values at Sites 1 and 2, respectively, according to the species rank abundance curves (Figure 1). The focal species, Quercus chungii, ranked third and ninth in the importance values at Sites 1 and 2, respectively (Figure 1; Supplementary Table S1). In 2023, we found 47 species and 329 woody plants (DBH 2.5cm) at the two sites. The Q. chungii had the largest importance value, abundance and basal area at Site 1 (Figure 1; Supplementary Table S2), due to its increasement in abundance and basal area (Supplementary Table S3). The importance value of Q. chungii also increased slightly at Site 2 (Figure 1; Supplementary Tables S2, S3). Compared to 2013, 22 and 6 species disappeared from Sites 1 and 2, respectively, while 17 and 15 species recruited into Sites 1 and 2 (Figures 3A, B). D. oldhamii, one of the most dominant species in 2013 at both sites, declined significantly in abundance, basal area and frequency in 2023 (Figures 1, 2; Supplementary Tables S1-S3).
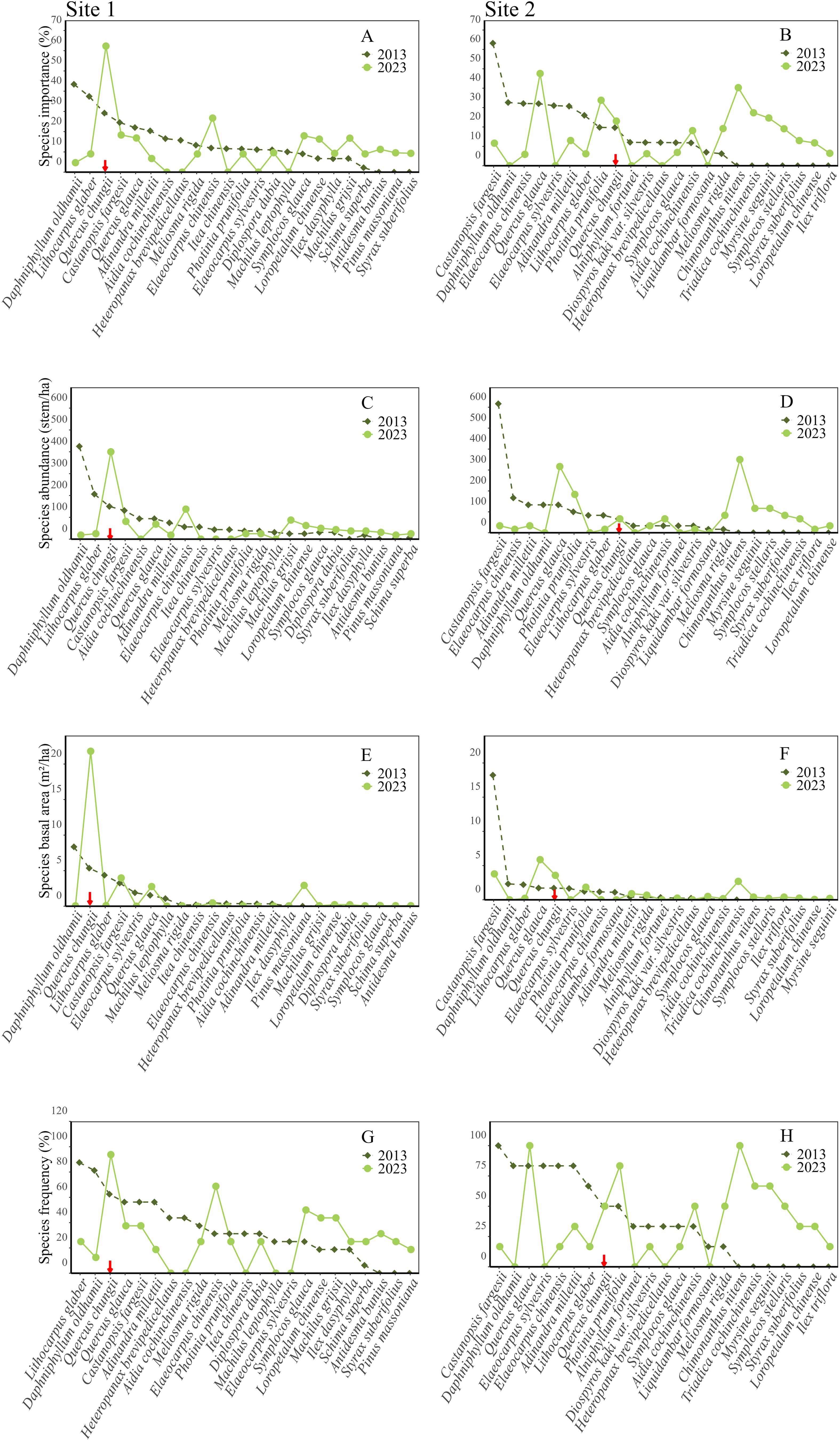
Figure 1. Substantial changes in importance values (A, B), abundances (C, D), basal areas (E, F) and frequencies (G, H) of common woody species between 2013 (black points and curves) and 2023 (green points and curves) at Site 1 (A, C, E, G) and Site 2 (B, D, F, H), respectively. Species are arranged in descending order based on their corresponding values in 2013 and then compared to the species in 2023. Q. chungii is highlighted with a red arrow. The figure lists and compares the top 15 species with the highest importance values at Site 1 or Site 2.
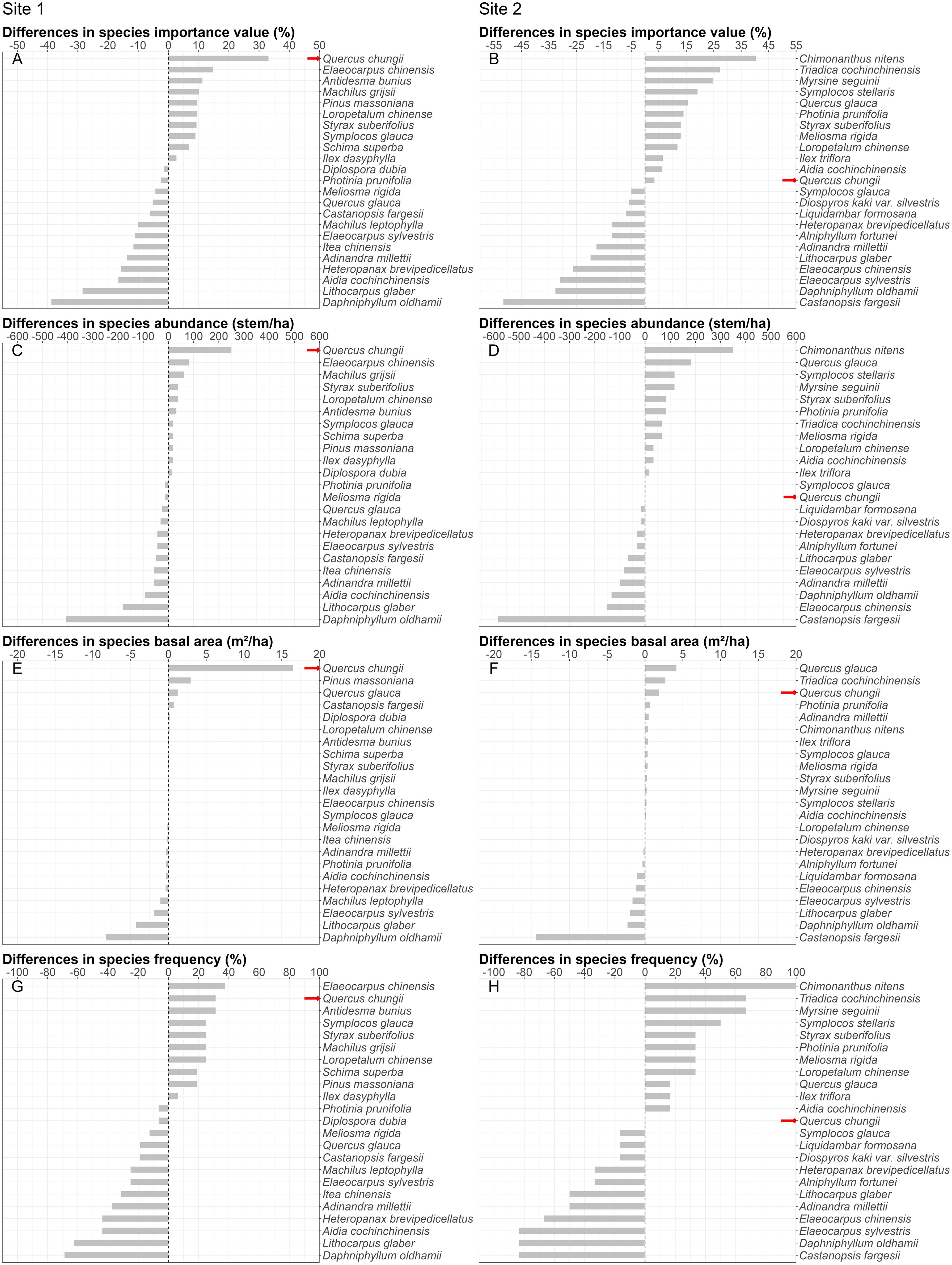
Figure 2. Q. chungii (indicated as red arrows) shows obvious increasement in its importance values (A, B), abundances (C, D), basal areas (E, F) and frequencies (G, H) between 2013 and 2023 among the common woody species at Site 1 (first column), but less obvious at Site 2 (second Column). Note that positive values indicate that the corresponding values of the species increases over time, and negative values indicate the opposite. The species in Figure 1 are arranged in descending order, based on the magnitude of their respective values, from the greatest increase to the greatest decrease.
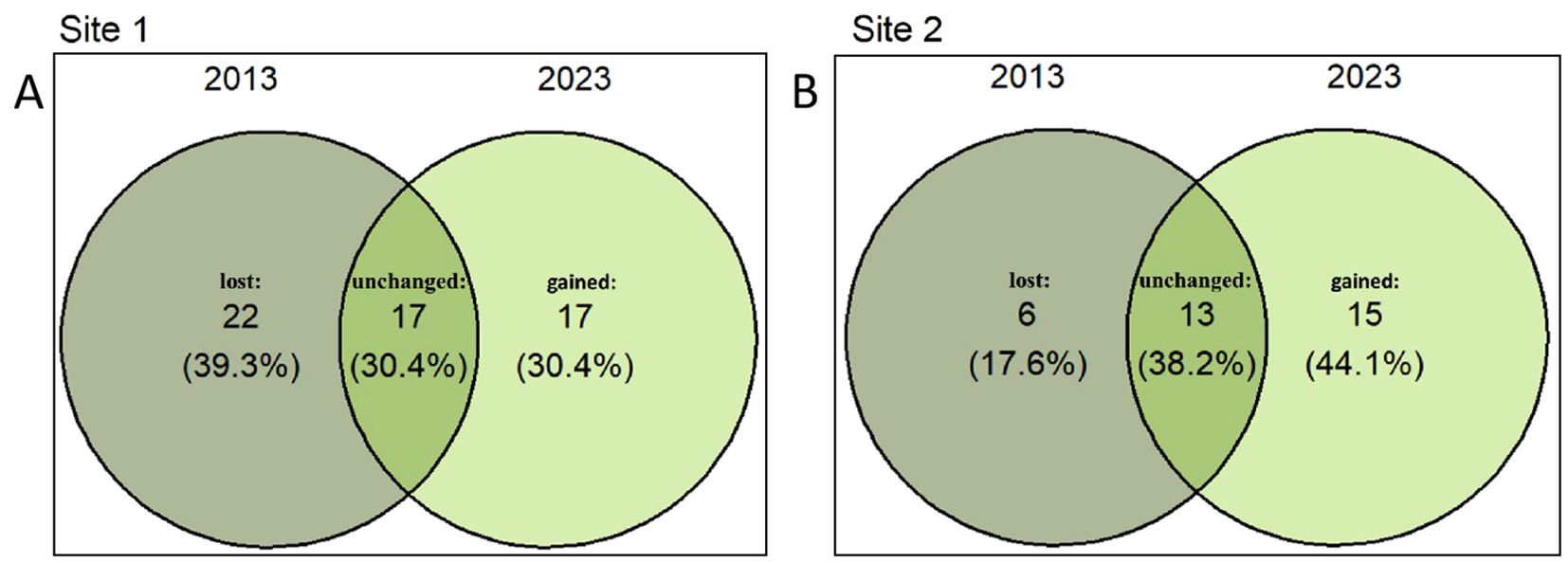
Figure 3. Communities experienced substantial gain and loss of species at Sites 1 (A) and 2 (B) over a decade. The numbers within parentheses indicate the percentage of species counts in 2013 and 2023, relative to the total species count across both years.
3.2 Temporal change in species diversity and composition
The significant turnover in species composition between 2013 and 2023 led to the large values of temporal β diversity over time (Supplementary Table S4). This temporal change in species composition was primarily contributed from deterministic rather than stochastic process at both sites (Figure 4). The contribution of nestedness to Sørensen index was more significant than species turnover at both sites (Figure 5A), indicating that the loss of existing species or the gain of new species in 2023 relative to 2013 was the primarily driver of changes in species composition. The contribution of balanced abundance variation to Bray-Curtis index was more important than abundance gradient at both sites (Figure 5B), implying that there was no significant consistent increase or decrease in species abundances over time. However, species diversity did not vary over the decade in neither site (Supplementary Figure S2).
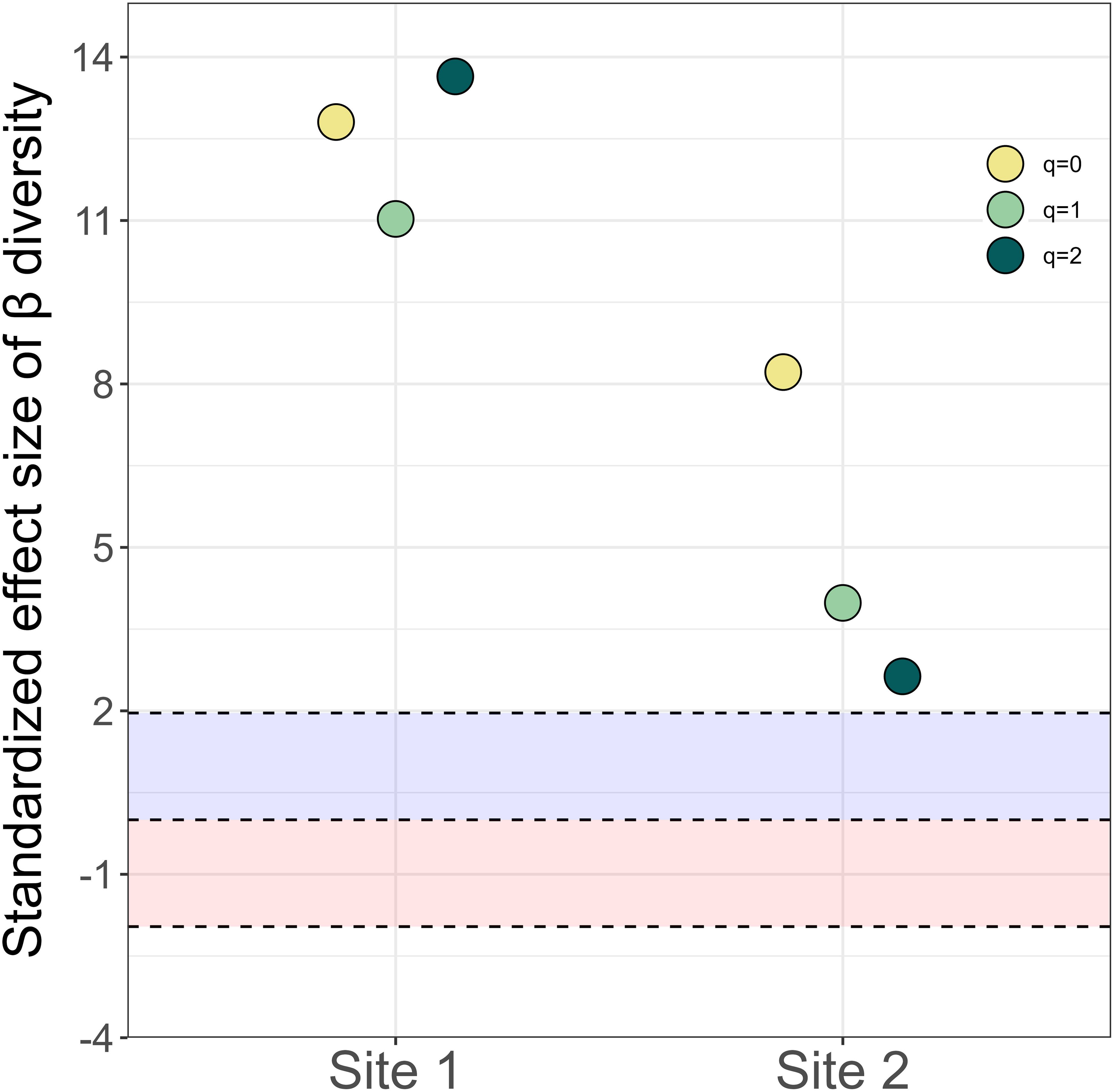
Figure 4. Standardized effect sizes of temporal diversity of species composition between 2013 and 2023 deviate significantly from random expectation from null model analyses. The shades of blue and red represent the ranges of 1.96 and -1.96, respectively.
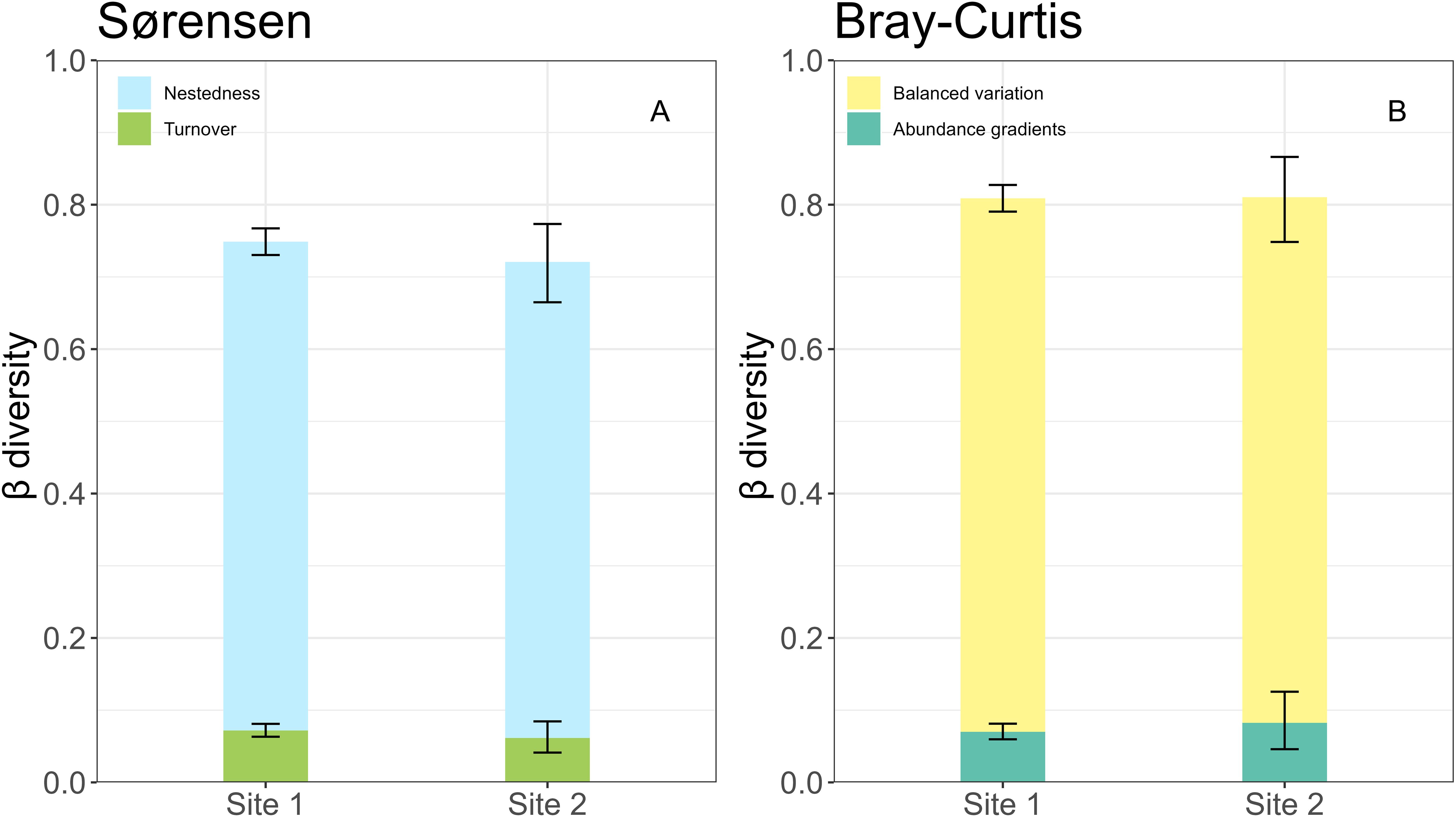
Figure 5. Nestedness and balanced variation are more important than turnover and abundance gradients in contributing the temporal β diversity of species composition based on Sørensen (A) and Bray-Curtis indices (B), respectively. The error bars represent the 95% CIs, derived from a bootstrap method with 10,000 repetitions.
3.3 Temporal change in phylogenetic diversity and composition
Faith’s PD did not differ significantly over the period at either site (Figure 6). The mean pairwise distance in 2023 increased significantly (t = -5.01, P< 0.01) than those in 2013 at Site 2 but not at Site 1 (Figure 6). The absolute values of standardized phylogenetic β diversity over time were smaller than 1.96 (Supplementary Figure S3), indicating that there was no strong evidence to reject the hypothesis that the changes in phylogenetic composition were mainly driven by stochastic processes.
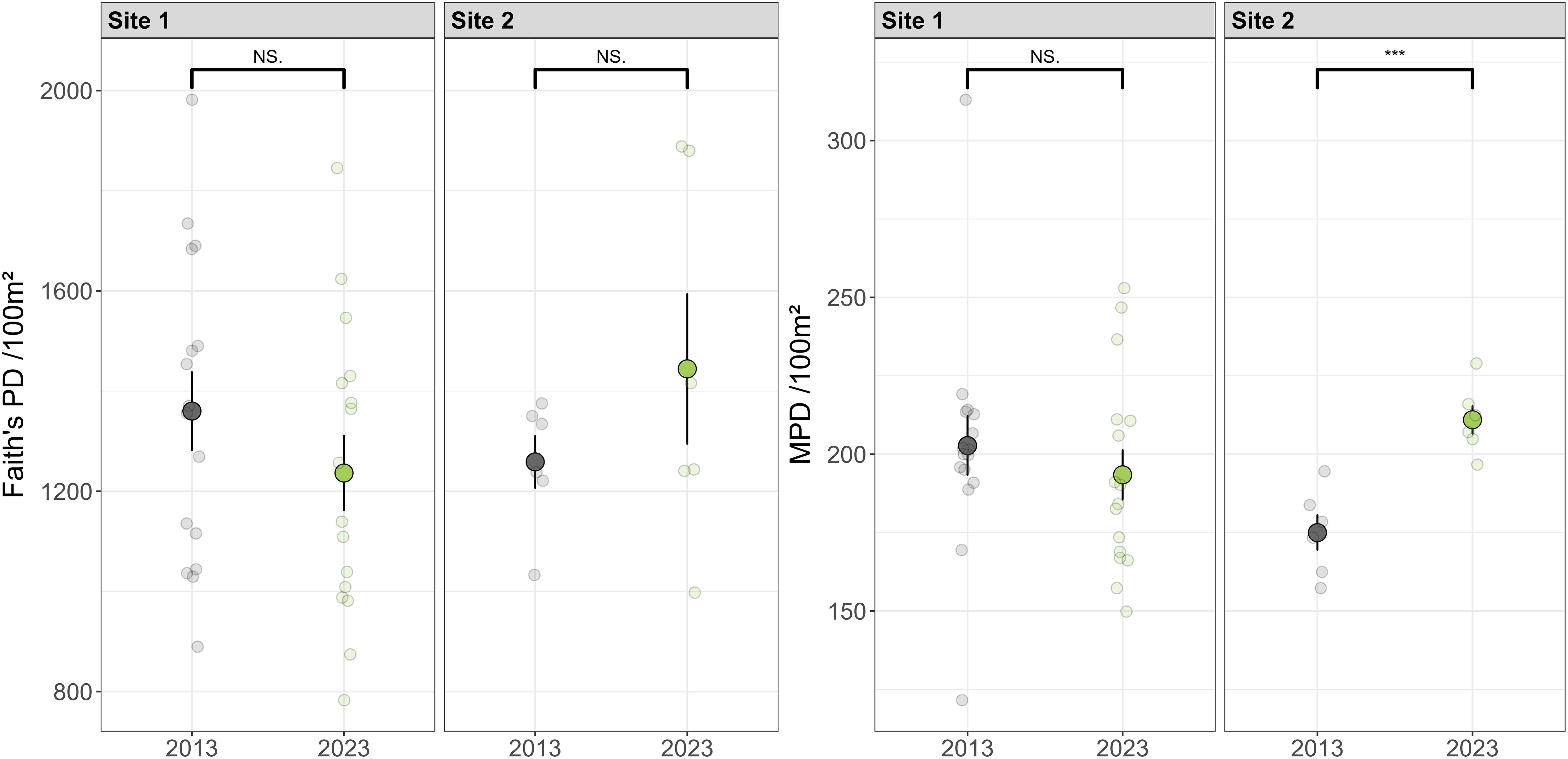
Figure 6. Faith’s phylogenetic diversity (PD) indices do not show significant changes over the decade, while the mean pairwise phylogenetic distances (MPD) significantly increase at Site 2 but not at Site 1. Transparent scatter points represent diversity values of each plot. Solid points represent mean values across plots per year. Error bars represent standard errors. “***” indicates a significant difference in diversity values between 2013 and 2023 (p < 0.001, from Student’s t-test), while “NS.” indicates nonsignificant difference.
4 Discussion
In this study, we found that the Q. chungii communities experienced significant changes in species composition for a decade, after previous logging disturbance. This temporal compositional changes was primarily the results of the loss of existing species or the colonization of new species, and the balanced variation in species abundances, driven by deterministic ecological processes. Specifically, the focal species, Q. chungii, increased in its dominance, due to significant increase in abundance and basal area. These findings have important implications for the conservation of Q. chungii and its associated forest communities and ecosystems.
We found that the dominant species transited from C. fargesii and D. oldhamii in 2013 to Q. chungii and Q. glauca in 2023. This transition may be related to the changes in light availability in the understory and species differentiation in shade tolerance. During the recovery process of a community, the growth of trees and canopies reduce the light availability in forest understories. In this case, shade-tolerant, late successional species are superior competitors to light-demanding, early succession species (Kariuki et al., 2006; Avalos, 2019). This hypothesis was supported by a previous studies in Fagaceae forests with cutting history as well (Rubio-Cuadrado et al., 2024), but only partly supported in this study. The overall basal area indeed increased for 0.495 m2 at Site 1. But there was no evidence to support a higher shade tolerance for Q. chungii or C. fargesii and D. oldhamii. Instead, we suspected that the increased dominance of Q. chungii may be related to its strong resprouting capacity (Chen, 2004; Wang et al., 2011). We found that many big Q. chungii individuals in the research areas were resprouted from previously cut big trunks (Supplementary Figure S1). Also, many existing seedlings and saplings resprouted from roots of large adult trees, but not from seeds. This strong sprouting ability can enable its fast recovery after disturbances (Fujii et al., 2010). This rapid recovery facilated by sprouting was also found in other Fagaceae trees (Fujii et al., 2010). This raises the important question about the relative importance of seed v.s. non-seed recruitmental strageties to restoring Fagaceae and other forests. However, over reliance on resprouting recruits can reduce the genetic diversity of the population and increase the risk of insect herbivory and pathogen infestation, especially given its already high dominance in the communities (Gilbert and Webb, 2007; Liu et al., 2012, 2015). This implies potential risks for the future population development. It also suggests that continuing efforts in monitoring the population dynamics of Q. chungii and investigating its relationships with pests and pathogens would be important in future research. Assisted seed dispersal or inhibited seed predation in the nature reserve can be effective strategies to facilitate the further recovery and maintenance of Q. chungii populations. Collecting seeds from multiple remote areas would be essential for either preventing a decline in or enhancing genetic diversity.
Changes of dominant species in a community can significantly affect the ecosystem structures and functions (Garcia Florez et al., 2017; Ediriweera et al., 2020; Tian et al., 2022; Rubio-Cuadrado et al., 2024; Wu et al., 2024). This species used to be among the most important canopy tree species in this nature reserve (Chen, 2004; Li et al., 2010; Wang et al., 2011), thus contributing significantly to the primary productivity and carbon sequestration to its forest ecosystem (Grime, 1998). Seeds of Q. chungii are also important foods for rodents and boars as about 46% of its seeds were consumed by animals (Huang et al., 2010). Changes in Q. chungii may have significant impacts on the food web structures and energy flows within ecosystems.
The temporal changes in species composition was primarily the results of the loss of many existing species or the gain of new species, and the balanced variation in species abundances. These results imply that the Q. chungii communities were still far away from stability after disturbance decades ago, but experienced dynamic regional immigration and emigration, and local species interactions. This was also reflected in our null model analyses, in which we found that the temporal compositional changes were primarily contributed by deterministic ecological processes. These results also suggest that perspectives and strategies based on multiple spatial scales would be important for the successful restoration and conservation of Q. chungii communities. For example, an important next step could be to identify potential strong competitors from surrounding regions. These could be species from later successional stages in this subtropical area and have a good share with the niche with Q. chungii, such as the Fagaceae species C. fargesii usually dominated in nearby closed canopy forests. However, we did not find significant changes in the species diversity and Faith’s PD. Additional potential explanations could be that the recovery of biodiversity in disturbed forests can be a very long process (Dalmaso et al., 2020; Poorter et al., 2021).
In addition to biotic interactions, abiotic environmental filtering can also be important processes driving the spatio-temporal distribution of species and communities. To obtain a more complete picture of driving assembly processes, future studies may benefit from measuring and assessing the join effects of environmental variables, species interactions, dispersal and sampling bias on the observed patterns from across multiple spatial scales.
5 Conclusions
In this study, we found significant changes in the species composition of Q. chungii forest communities with logging history over a decade. These temporal changes are primarily the result of from both regional and local deterministic processes. This suggests that successful restoration and conservation of Q. chungii communities require perspectives and strategies that incorporate multiple spatial scales. The target species (Q. chungii) ranked among the most dominant species within the communities after a decade which suggests the effectiveness of conservation practice by the nature reserve particularly established for this species. However, the strong resprouting ability of Q. chungii may have facilitated its fast recovery and dominance, but may also increase its future population development risks due to its reduced genetic diversity and increased pressures from natural enemies. This highlights the importance of continuing to monitor its genetic diversity, assisting in the dispersal of seeds from different genetic sources, and investigating multi-trophic interactions for the healthy development of Q. chungii populations. Our study helps to reveal the mechanisms driving the successional dynamics of Q. chungii communities, and provides important implications for the restoration and conservation of Q. chungii and its associated communities and ecosystems.
Data availability statement
The datasets and R code that supporting the findings of this study are available in the figshare at https://doi.org/10.6084/m9.figshare.27825258.v3.
Author contributions
WL: Conceptualization, Data curation, Investigation, Methodology, Supervision, Writing – original draft, Writing – review & editing. XZ: Conceptualization, Data curation, Formal analysis, Investigation, Methodology, Writing – original draft. ZL: Funding acquisition, Investigation, Methodology, Writing – review & editing. YH: Investigation, Methodology, Writing – review & editing. XC: Investigation, Writing – review & editing. LH: Investigation, Writing – review & editing. YW: Investigation, Writing – review & editing. YC: Conceptualization, Funding acquisition, Investigation, Writing – review & editing.
Funding
The author(s) declare financial support was received for the research, authorship, and/or publication of this article. This work was supported by the Funds of Study on Biodiversity in Huangchulin Nature Reserve ((350124)FJKT(GK)2022017), the National Natural Science Foundation of China (32071536) and the Fundamental Research Funds for the Central Universities of China (20720240091).
Acknowledgments
We greatly acknowledge Fengying Jiang, Xiuzhe Li, Xiaoxiao Liu, Yuchun Xiao and Lixi Lin who assisted with field work. We greatly acknowledge Yiyang An for helpful discussion.
Conflict of interest
The authors declare that the research was conducted in the absence of any commercial or financial relationships that could be construed as a potential conflict of interest.
Publisher’s note
All claims expressed in this article are solely those of the authors and do not necessarily represent those of their affiliated organizations, or those of the publisher, the editors and the reviewers. Any product that may be evaluated in this article, or claim that may be made by its manufacturer, is not guaranteed or endorsed by the publisher.
Supplementary material
The Supplementary Material for this article can be found online at: https://www.frontiersin.org/articles/10.3389/fevo.2024.1486853/full#supplementary-material
References
Avalos G. (2019). Shade tolerance within the context of the successional process in tropical rain forests. Rev. Biol. Trop. 67, 53–77. doi: 10.15517/rbt.v67i2SUPL.37206
Baselga A. (2010). Partitioning the turnover and nestedness components of beta diversity. Glob. Ecol. Biogeogr. 19, 134–143. doi: 10.1111/j.1466-8238.2009.00490.x
Baselga A. (2013). Separating the two components of abundance-based dissimilarity: balanced changes in abundance vs. abundance gradients. Methods Ecol. Evol. 4, 552–557. doi: 10.1111/2041-210X.12029
Baselga A., Orme C. D. L. (2012). Betapart: an R package for the study of beta diversity. Methods Ecol. Evol. 3, 808–812. doi: 10.1111/j.2041-210X.2012.00224.x
Bryant J. A., Lamanna C., Morlon H., Kerkhoff A. J., Enquist B. J., Green J. L. (2008). Microbes on mountainsides: Contrasting elevational patterns of bacterial and plant diversity. PNAS. 105, 11505–11511. doi: 10.1073/pnas.0801920105
Chao A., Gotelli N. J., Hsieh T. C., Sander E. L., Ma K. H., Colwell R. K., et al. (2014). Rarefaction and extrapolation with Hill numbers: a framework for sampling and estimation in species diversity studies. Ecol. Monogr. 84, 45–67. doi: 10.1890/13-0133.1
Chapman C. A., Galan-Acedo C., Gogarten J. F., Hou R., Lawes M. J., Omeja P. A., et al. (2021). A 40-year evaluation of drivers of African rainforest change. For. Ecosyst. 8, 66. doi: 10.1186/s40663-021-00343-7
Chase J. M. (2010). Stochastic community assembly causes higher biodiversity in more productive environments. Science 328, 1388–1391. doi: 10.1126/science.1187820
Chase J. M., Blowes S. A., Knight T. M., Gerstner K., May F. (2020). Ecosystem decay exacerbates biodiversity loss with habitat loss. Nature 584, 238–243. doi: 10.1038/s41586-020-2531-2
Chase J. M., Kraft N. J. B., Smith K. G., Vellend M., Inouye B. D. (2011). Using null models to disentangle variation in community dissimilarity from variation in α-diversity. Ecosphere 2, 1–11. doi: 10.1890/ES10-00117.1
Chen S. (2004). Changes in species diversity of plants in Cyclobalanopsis chungii forest during the course of restoration. J. Zhejiang forestry college. 21, 258–262. doi: 1000-5692(2004)03-0258-05
Connell J. H. (1978). Diversity in tropical rain forests and coral reefs. Curr. Contents/Agric. Biol. Environ. Sci. 199, 1302–1310. doi: 10.1126/science.199.4335.1302
Dalmaso C. A., Marques M. C. M., Higuchi P., Zwiener V. P., Marques R. (2020). Spatial and temporal structure of diversity and demographic dynamics along a successional gradient of tropical forests in southern Brazil. Ecol. Evol. 10, 3164–3177. doi: 10.1002/ece3.5816
Ediriweera S., Bandara C., Woodbury D. J., Mi X. C., Gunatilleke I. A. U. N., Gunatilleke C. V. S., et al. (2020). Changes in tree structure, composition, and diversity of a mixed-dipterocarp rainforest over a 40-year period. For. Ecol. Manage. 458, 117764. doi: 10.1016/j.foreco.2019.117764
Faith D. P. (1992). Conservation evaluation and phylogenetic diversity. Biol. Conserv. 61, 1–10. doi: 10.1016/0006-3207(92)91201-3
Fujii S., Kubota Y., Enoki T. (2010). Long-term ecological impacts of clear-fell logging on tree species diversity in a subtropical forest, southern Japan. J. For. Res. 15, 289–298. doi: 10.1007/s10310-010-0191-z
Garcia Florez L., Vanclay J. K., Glencross K., Nichols J. D. (2017). Understanding 48 years of changes in tree diversity, dynamics and species responses since logging disturbance in a subtropical rainforest. For. Ecol. Manage. 393, 29–39. doi: 10.1016/j.foreco.2017.03.012
Gilbert G. S., Webb C. O. (2007). Phylogenetic signal in plant pathogen-host range. PNAS 104, 4979–4983. doi: 10.1073/pnas.0607968104
Gotelli N. J. (2000). Null model analysis of species co-occurrence patterns. Ecology 81, 2606–2621. doi: 10.1890/0012-9658(2000)081[2606:NMAOSC]2.0.CO;2
Grime J. P. (1998). Benefits of plant diversity to ecosystems: immediate, filter and founder effects. J. Ecol. 86, 902–910. doi: 10.1046/j.1365-2745.1998.00306.x
Hansen M. C., Potapov P. V., Moore R., Hancher M., Turubanova S. A., Tyukavina A., et al. (2013). High-resolution global maps of 21st-century forest cover change. Science 342, 850–853. doi: 10.1126/science.1244693
HilleRisLambers J., Adler P. B., Harpole W. S., Levine J. M., Mayfield M. M. (2012). Rethinking community assembly through the lens of coexistence theory. Annu. Rev. Ecol. Evol. Syst. 43, 227–248. doi: 10.1146/annurev-ecolsys-110411-160411
Hoang N. T., Kanemoto K. (2021). Mapping the deforestation footprint of nations reveals growing threat to tropical forests. Nat. Ecol. Evol. 5, 845–853. doi: 10.1038/s41559-021-01417-z
Hsieh T. C., Ma K. H., Chao A. (2016). iNEXT: An R package for rarefaction and extrapolation of species diversity (Hill numbers). Methods Ecol. Evol. 7, 1451–1456. doi: 10.1111/2041-210X.12613
Huang Y., Ma X., Ye G., Zhuang K., Chen J., Huang H. (2011). Community characteristics of natural forest of Cyclobalanopsis chungii on Yunzhong Mountain. J. Fujian Coll. For. 31, 304–308. doi: 10.13324/j.cnki.jfcf.2011.04.014
Huang Y., Ma X., Zhuang K., Liu M., Huang D. (2010). Seed rain and soil seed bank of Cyclobalanopsis chungii forest in minqing,fujian province. J. Trop. Subtrop. Bot. 18, 68–74. doi: 1005-3395(2010)01-0068-07
Hubbell S. P. (2001). The unified neutral theory of biodiversity and biogeography (Princeton: Princeton University Press).
Jiang X., Xu G., Deng M. (2019). Spatial genetic patterns and distribution dynamics of the rare oak Quercus chungii: Implications for biodiversity conservation in Southeast China. Forests 10, 821. doi: 10.3390/f10090821
Jin Y., Qian H. (2022). V.PhyloMaker2: An updated and enlarged R package that can generate very large phylogenies for vascular plants. Plant Divers. 44, 335–339. doi: 10.1016/j.pld.2022.05.005
Kariuki M., Kooyman R. M., Smith R. G. B., Wardell-Johnson G., Vanclay J. K. (2006). Regeneration changes in tree species abundance, diversity and structure in logged and unlogged subtropical rainforest over a 36-year period. For. Ecol. Manage. 236, 162–176. doi: 10.1016/j.foreco.2006.09.021
Kembel S. W., Cowan P. D., Helmus M. R., Cornwell W. K., Morlon H., Ackerly D. D., et al. (2010). Picante: R tools for integrating phylogenies and ecology. Bioinformatics 26, 1463–1464. doi: 10.1093/bioinformatics/btq166
Kraft N. J. B., Adler P. B., Godoy O., James E. C., Fuller S., Levine J. M. (2015). Community assembly, coexistence and the environmental filtering metaphor. Funct. Ecol. 29, 592–599. doi: 10.1111/1365-2435.12345
Kraft N. J., Comita L. S., Chase J. M., Sanders N. J., Swenson N. G., Crist T. O., et al. (2011). Disentangling the drivers of β diversity along latitudinal and elevational gradients. Science 333, 1755–1758. doi: 10.1126/science.1208584
Li Z., Chen X., Liu C. (2010). Comprehensive scientific investigation report of Fujian Xiongjiang Huangchulin Nature Reserve (Xiamen: Xiamen University Press).
Liang M., Han T., Ma J., Li R., Yang Y., Qiu X., et al. (2023). Response of temperate forest ecosystem services to rainfall: A case study in the forest nature reserves of northern China. Front. Ecol. Evol. 11. doi: 10.3389/fevo.2023.1132396
Liu X., Etienne R. S., Liang M., Wang Y., Yu S. (2015). Experimental evidence for an intraspecific Janzen-Connell effect mediated by soil biota. Ecology 96, 662–671. doi: 10.1890/14-0014.1
Liu X., Liang M., Etienne R. S., Wang Y., Staehelin C., Yu S. (2012). Experimental evidence for a phylogenetic Janzen-Connell effect in a subtropical forest. Ecol. Lett. 15, 111–118. doi: 10.1111/j.1461-0248.2011.01715.x
Liu A., Liu C., Ma X. (2004). Study on biomass production of Cyclobalanopsis chungii plantation. J. Fujian Coll. For. 24, 294–297. doi: 10.13324/j.cnki.jfcf.2004.04.002
Mahayani N. P. D., Slik F. J. W., Savini T., Webb E. L., Gale G. A. (2020). Rapid recovery of phylogenetic diversity, community structure and composition of Bornean tropical forest a decade after logging and post-logging silvicultural interventions. For. Ecol. Manage. 476, 118467. doi: 10.1016/j.foreco.2020.118467
McDowell N. G., Allen C. D., Anderson-Teixeira K., Aukema B. H., Bond-Lamberty B., Chini L., et al. (2020). Pervasive shifts in forest dynamics in a changing world. Science 368, eaaz9463. doi: 10.1126/science.aaz9463
Myers J. A., Chase J. M., Crandall R. M., Jiménez I. (2015). Disturbance alters beta-diversity but not the relative importance of community assembly mechanisms. J. Ecol. 103, 1291–1299. doi: 10.1111/1365-2745.12436
Osazuwa-Peters O. L., Jiménez I., Oberle B., Chapman C. A., Zanne A. E. (2015). Selective logging: do rates of forest turnover in stems, species composition and functional traits decrease with time since disturbance? - A 45 year perspective. For. Ecol. Manage. 357, 10–21. doi: 10.1016/j.foreco.2015.08.002
Poorter L., Craven D., Jakovac C. C., van der Sande M. T., Amissah L., Bongers F, et al. (2021). Multidimensional tropical forest recovery. Science 374, 1370–1376. doi: 10.1126/science.abh3629
R Development Core Team (2023). R: a Language and environment for statistical computing (Vienna: R foundation for statistical computing). Available at: http://www.r-project.org (accessed March 15, 2023).
Rubio-Cuadrado A., Alberdi I., Cañellas I., Montes F., Rodríguez-Calcerrada J., López R., et al. (2024). Twenty years of population dynamics in European beech-oak forest at their rear range margin anticipate changes in its structure and composition. For. Ecosyst. 11, 100197. doi: 10.1016/j.fecs.2024.100197
Sanjeewani N., Samarasinghe D., Jayasinghe H., Ukuwela K., Wijetunga A., Wahala S., et al. (2024). Variation of floristic diversity, community composition, endemism, and conservation status of tree species in tropical rainforests of Sri Lanka across a wide altitudinal gradient. Sci. Rep. 14, 2090. doi: 10.1038/s41598-024-52594-3
Sun X. Q., Song Y. G., Ge B. J., Dai X. L., Kozlowski G. (2021). Intermediate epicotyl physiological dormancy in the recalcitrant seed of Quercus chungii F.P.Metcalf with the elongated cotyledonary petiole. Forests 12, 263. doi: 10.3390/f12030263
Swenson N. G., Anglada-Cordero P., Barone J. A. (2011). Deterministic tropical tree community turnover: evidence from patterns of functional beta diversity along an elevational gradient. P. R. Soc B-Biol. Sci. 278, 877–884. doi: 10.1098/rspb.2010.1369
Tian L., Letcher S. G., Ding Y., Zang R. (2022). A ten-year record reveals the importance of tree species' habitat specialization in driving successional trajectories on Hainan Island, China. For. Ecol. Manage. 507, 120027. doi: 10.1016/j.foreco.2022.120027
Tilman D. (1982). Resource competition and community structure (Princeton: Princeton University Press).
Tong X., Brandt M., Yue Y., Zhang X., Fensholt R., Ciais P., et al. (2023). Reforestation policies around 2000 in southern China led to forest densification and expansion in the 2010s. Commun. Earth Environ. 4, 260. doi: 10.1038/s43247-023-00923-1
van der Sande M. T., Arets E. J. M. M., Pena-Claros M., de Avila A. L., Roopsind A., Mazzei L., et al. (2016). Old-growth Neotropical forests are shifting in species and trait composition. Ecol. Monogr. 86, 228–243. doi: 10.1890/15-1815.1
Vellend M. (2010). Conceptual synthesis in community ecology. Q. Rev. Biol. 85, 183–206. doi: 10.1086/652373
Villa P. M., Martins S. V., Diniz É.S., Neto S. N., Neri A. V., Pinto-Junior H. V., et al. (2021). Taxonomic and functional beta diversity of woody communities along Amazon forest succession: The relative importance of stand age, soil properties and spatial factor. For. Ecol. Manage. 482, 118885. doi: 10.1016/j.foreco.2020.118885
Wang Y., Hollingsworth P. M., Zhai D., West C. D., Green J. M. H., Chen H., et al. (2023). High-resolution maps show that rubber causes substantial deforestation. Nature 623, 340–346. doi: 10.1038/s41586-023-06642-z
Wang Y., Wu P., Wang R., Ma Q., Zou X. (2011). Community characteristics of Cyclobalanopsis chungii forest in Mingqing Nature Reserve. J. Fujian Agric. For. Univ. (Nat. Sci. Ed.) 40, 37–42. doi: 10.13323/j.cnki.j.fafu(nat.sci.).2011.01.015
Wu A., Xiong X., Zhou G., Barmon M., Li A., Tang X., et al. (2024). Climate change-related biodiversity fluctuations and composition changes in an old-growth subtropical forest: A 26-yr study. Sci. Total Env. 914, 169899. doi: 10.1016/j.scitotenv.2024.169899
Zhang D. (2019). China's forest expansion in the last three plus decades: Why and how? For. Policy Econ. 98, 75–81. doi: 10.1016/j.forpol.2018.07.006
Keywords: beta diversity, community composition, Quercus chungii, secondary forest, temporal change
Citation: Li W, Zhong X, Li Z, Huang Y, Chen X, Huang L, Wang Y and Chen Y (2024) Decadal changes in species composition of rare oak Quercus chungii forests with logging history. Front. Ecol. Evol. 12:1486853. doi: 10.3389/fevo.2024.1486853
Received: 30 August 2024; Accepted: 26 November 2024;
Published: 13 December 2024.
Edited by:
Pavel Kindlmann, Charles University, CzechiaReviewed by:
Chao Huang, Jiangxi Agricultural University, ChinaYunquan Wang, Zhejiang Normal University, China
Copyright © 2024 Li, Zhong, Li, Huang, Chen, Huang, Wang and Chen. This is an open-access article distributed under the terms of the Creative Commons Attribution License (CC BY). The use, distribution or reproduction in other forums is permitted, provided the original author(s) and the copyright owner(s) are credited and that the original publication in this journal is cited, in accordance with accepted academic practice. No use, distribution or reproduction is permitted which does not comply with these terms.
*Correspondence: Wenbin Li, bHdiQHhtdS5lZHUuY24=
†These authors have contributed equally to this work and share first authorship