- 1Bio-Protection Research Centre, Lincoln University, Christchurch, New Zealand
- 2Plant Research International B.V., Wageningen, Netherlands
- 3AgResearch, Lincoln Science Centre, Christchurch, New Zealand
This contribution aimed to uncover the unique host plant-finding and spatial ecology of reproductive and diapausing Argentine stem weevil, Listronotus bonariensis, and its adaptive implications in Aotearoa-New Zealand pasture. Still-air two-armed olfactometers revealed the reproductive and diapausing L. bonariensis preferences for plant and weevil-derived volatiles. Winter-collected diapausing weevils were strongly attracted to host plant feeding damage by conspecifics and their frass. Such attraction disappeared when 20 stem weevils were added to the damaged ryegrass. This suggests that L. bonariensis on a damaged host plant above a certain density causes repellency probably avoiding over-exploitation of the host plant. Neither the weevils’ sex nor related physiological condition were found to impart any significant effects. Volatile organic compound (VOC) analysis showed that phenylacetaldehyde was the only de novo synthesised herbivore-induced volatile compound found resulting from diapausing L. bonariensis feeding damage. This study therefore found that the seasonal behaviour of L. bonariensis was very closely related to that of the rice water weevil Lissorhoptrus oryzophilus and its very similar responses to phenylacetaldehyde. In both cases the compound attracts spring populations (emerging from diapause) to their host plants and conspecifics. Thereafter, as with L. oryzophilus, L. bonariensis similarly on becoming reproductive, its sensitivity to phenylacetaldehyde ceases. Such close connection between L. bonariensis and L. oryzophilus seasonal responses to phenylacetaldehyde therefore greatly reenforces the concept of evolved L. bonariensis behaviour in its native range as being relict behaviour in New Zealand’s grassland ecosystem.
1 Introduction
The aim of this contribution was to learn more about the host plant-finding and spatial ecology of reproductive and diapausing Argentine stem weevil, Listronotus bonariensis (Kushel) (Coleoptera: Curculionidae) and their adaptive implications. Listronotus bonariensis is Aotearoa-New Zealand’s severest pest of high-performance pasture grasses, particularly the Lolium spp. L. (Poales: Poaceae). The weevil was first reported in New Zealand in late 1927 (Marshall, 1938), although its introduction has recently been shown to have been considerably earlier (Stewart et al., 2023). Moreover, its arrival was probably based on more than one introduction (Harrop et al., 2020). In New Zealand, adult population densities of up to 723 m-2 have been recorded (Barker and Addison, 1993). Damage is mainly caused by larval mining of the grass tillers and the destruction of establishing seedlings in newly-sown pastures.
The New Zealand ryegrass/clover pasture ecosystem is a partial transplant of the European ecosystem and is, therefore, species-sparse, with far simpler food-web assemblages than those in the European/Palearctic ‘parent’ ecosystems. This species’ paucity thus imparts little, if any, biotic resistance to invasive species such as L. bonariensis (e.g., Goldson et al., 2014, 2020; Tomasetto et al., 2017). In New Zealand, the weevil has been found to fly sporadically and weakly (Goldson, 1981a). Goldson et al. (1999) showed that typically, flight occurred at temperatures >19°C, RH <81% and windspeeds of <10.8 km h-1. Additionally, under exceptionally high temperatures and desiccating conditions (30.5°C), mass migration was found to occur, whereby all those weevils with developed flight muscles took to the wing (Goldson et al., 2023). The extent to which this behaviour occurs in the native range is uncertain.
Given the pest status of L. bonariensis, the parthenogenetic endoparasitoid Microctonus hyperodae Loan (Hymenoptera: Braconidae) was introduced into New Zealand in 1990 (Goldson et al., 1990) and led to useful suppression of the weevil (e.g., Goldson et al., 1998, 2011; Barker and Addison, 2006; Popay et al., 2011; Barker, 2013). This was in conjunction with Lolium perenne L. resistance imparted by infection with strains of Epichloë endophyte (Epichloë festucae var. lolii) (see Ferguson et al., 2019 for discussion).
Despite decades of research into the ecology and management of L. bonariensis, relatively little is known about semiochemical influences on the species’ spatial and reproductive behaviour. Hennessy et al. (2022), working with summer-collected, reproductive, L. bonariensis populations and using very similar olfactometer bioassays to those in this study, tested the weevil’s responses to the volatiles emitted by L. perenne infected with endophyte stains AR1 and WT (wild-type) (Caradus et al., 2013; Johnson et al., 2013) as well as those from endophyte-free plants. While these researchers found a positive L. bonariensis response to grass volatiles compared with that to moistened cotton wool, there was little, if any, weevil response to conspecific feeding damage in either the endophyte-free or endophyte-infected grasses. More broadly, Justus (2019) conducted a comprehensive still-air bioassay approach in Ohio (USA) to assess summer-collected carrot weevil Listronotus oregonensis (LeConte) responses to host plant-emitted volatiles. This researcher found that the weevil responded to both chopped carrot and parsley volatile organic compounds (VOCs) and concluded that these probably play an important role in the weevil’s host-plant finding.
By using still-air olfactometry, this contribution advances the understanding of the behavioural ecology of L. bonariensis and its possible evolutionary origins by extending the behavioural investigation conducted by Hennessy et al. (2022). This current contribution measured the responses of both diapausing and reproductive L. bonariensis to the presence of conspecifics and their feeding damage on endophyte-free Lolium multiflorum Lam.
2 Materials and methods
The plant-related volatiles discussed in this paper are referred to as volatile organic compounds (VOCs), except for the one that results from L. bonariensis feeding damage and frass, which is referred to as an HIPV (herbivore-induced plant volatile).
2.1 Experimental design
2.1.1 Weevil collection and maintenance
The diapausing weevil populations used in Treatment 1 were collected from Taiko (44°21’18.81” S, 171°3’17.95” E) on 1 March 2011, Upper Takaka (41°1’44.77” S, 172°49’19.80” E) on 16 March 2011, Lincoln (43°38’16.47” S, 172°26’30.61” E) on 28 April 2011, and North Otago on 30 May 2011. The reproductive weevils used in Treatment 2 were collected from Irwell (43° 42’ 34.71”S, 172° 22’ 21.10”E) on 21 September 2011, 17 and 24 October 2011, and 7, 14 and 22 November 2011. In all cases, these weevil collections were kept at Lincoln University, New Zealand and maintained for 1 to 3 weeks in clear containers (355 x 237 x 120 mm) in a controlled temperature room (18 ± 2°C under a 16:8-hour L:D photoperiod). The weevils were under this photoperiod for up to three weeks, and diapause was not broken, as confirmed by dissections after the bioassays. The food provided comprised greenhouse-grown non-endophytic ‘Tama’ Italian ryegrass (tetraploid L. multiflorum) grown in paperpots (Paperpots BS205, Lannen Tehtaat Oy Potma Ltd, Pello, Finland). This grass was presented as small bouquets prepared from two paperpots with their roots sealed in damp polyethylene bags. These bouquets were replaced weekly.
In all bioassays, each replicate consisted of 10 test weevils randomly selected from the field-collected populations, and they had been starved for 24 h prior to the assay. The weevils were not sexed, as field populations are known to approximate a 1:1 sex ratio (Goldson, 1979) and the process of handling them under a dissecting microscope was likely to disrupt their behaviour.
The treatments comprised a diapausing weevil population (Treatment 1) and a reproductive population (Treatment 2). Both Treatments were segmented into six replicated Subtreatments (with 10 weevils in each subtreatment replicate), these were then subjected to up to 15-29 runs as per Table 1. The results of this were then used to generate the HIPV/VOC contrasts to which the test weevil populations were subjected using the left and right arms of the olfactometer. The preparation of the Subtreatment HIPV/VOC sources is described below.
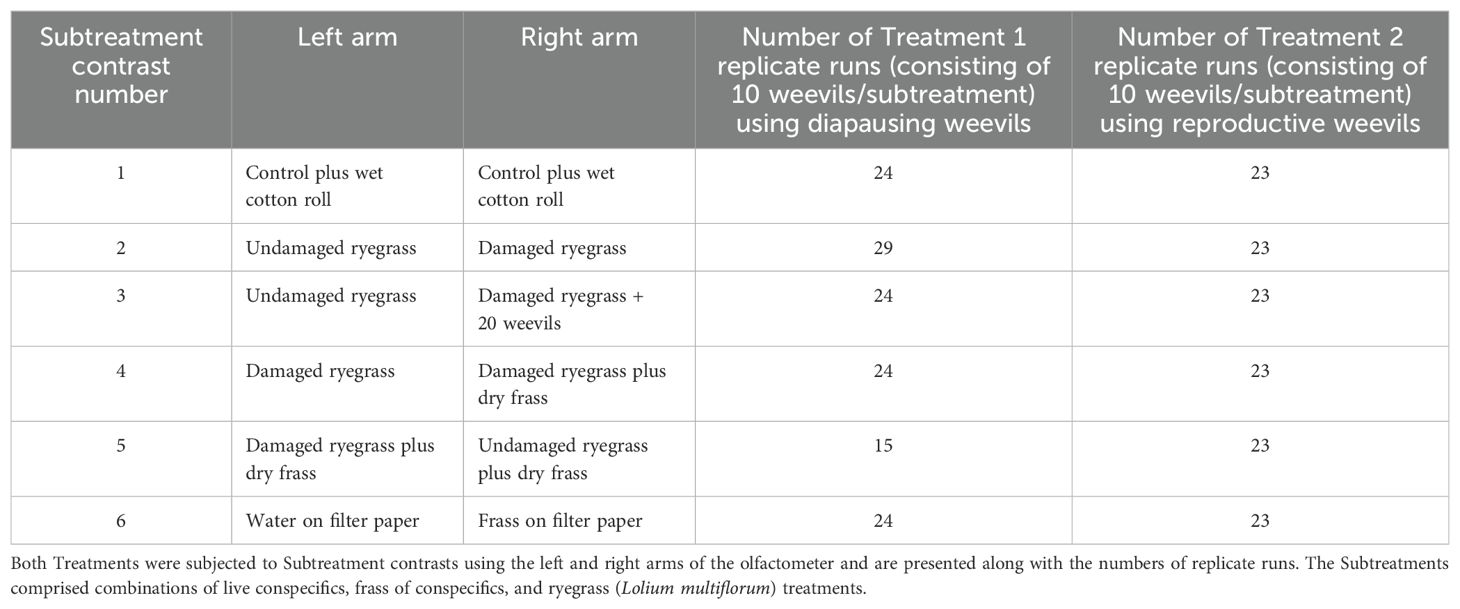
Table 1. Treatments 1 and 2, comprising the diapausing and reproductive Listronotus bonariensis populations, respectively.
2.1.2 Still-air olfactometer
This study sought to measure the movement of diapausing and reproductive L. bonariensis in response to both weevil- and plant-derived volatiles. For this purpose, a classical Y-shaped olfactometer (e.g., Giordanengo et al., 1993) was initially used, but produced no results (the weevils did not move much, despite horizontal and vertical set-ups, and light and dark conditions). Instead, a two-choice still-air olfactometer was employed based on that used by van Tol et al. (2002) (Figure 1), who investigated the behavioural responses of Otiorhynchus sulcatus F. (Coleoptera: Curculionidae) to volatiles. As shown in Figure 1, the equipment comprised a glass 90-mm diameter Petri dish with two 10-mm holes in its lid. Short glass tubes were positioned over each hole (10-mm diameter; 15-mm length). A larger glass cylinder (20-mm diameter; 40-mm length) was centred over each of these tubes and on top of each of these was positioned a glass ‘HIPV cup’ (length 15 mm; diameter 67 mm) into which the test material was placed. A piece of nylon gauze (mesh 1 mm) was clamped between the glass cylinder and the cup to act as a separator to prevent the weevils from moving up into the VOC/HIPV source material.
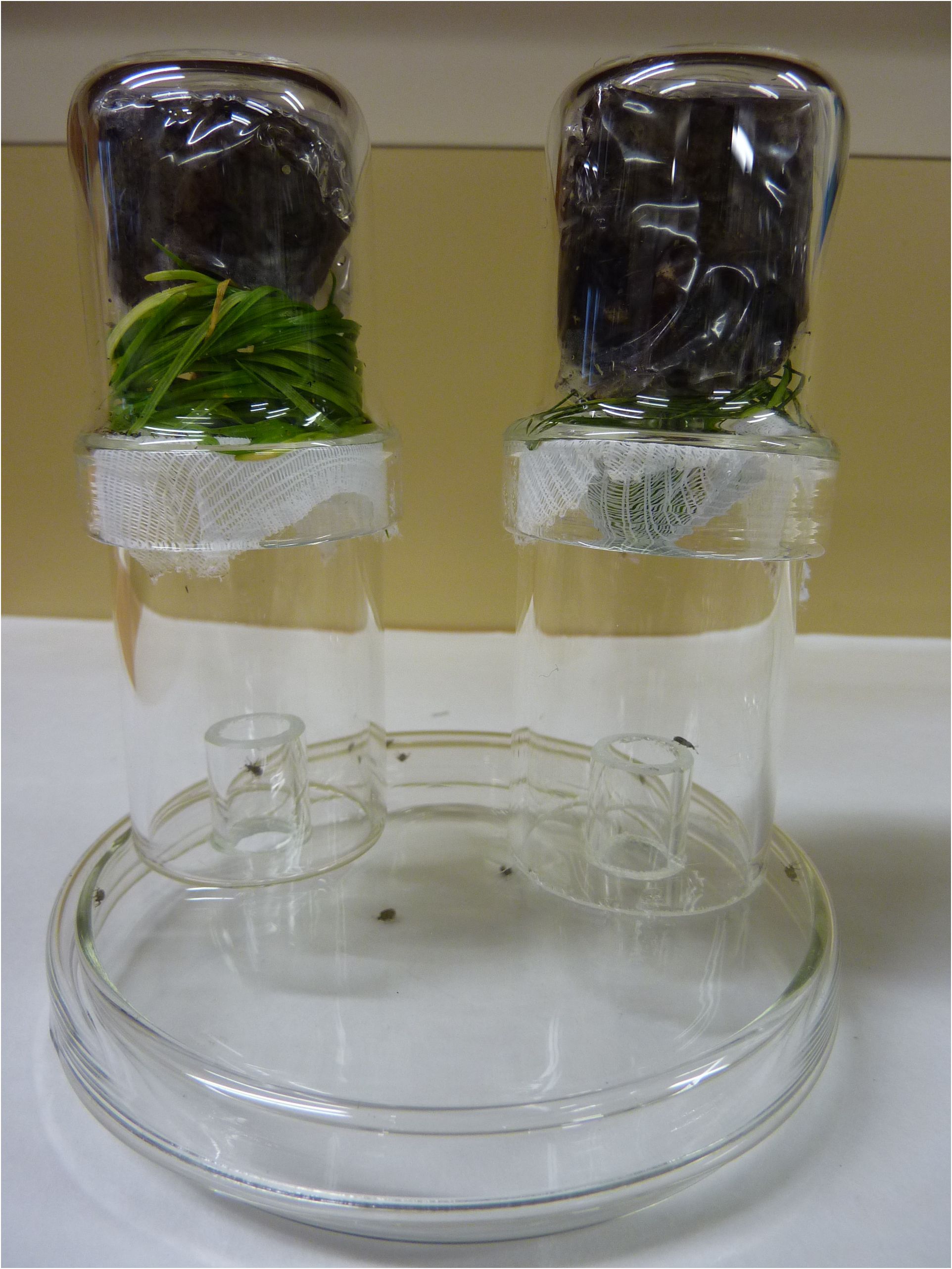
Figure 1. The two-choice still-air olfactometer used in this study. Weevils released in the Petri dish can choose to enter one of either of the cylinders through the short glass tubes on top of the Petri dish lid. Nylon gauze allowed diffusion of volatiles but prevented the weevils from entering the ‘herbivore-induced plant volatiles/volatile organic compound’ cups on top of the cylinders.
In all cases, before the trial started and to allow the volatiles to diffuse, the olfactometers, with the volatile sources in place, were left for 1 hour under ambient light at 20°C. At the onset of the Subtreatment contrasts, ten 24-h starved weevils were released in the Petri dish at the base and were left to move towards either of the VOC/HIPV sources (as depicted in Figure 1). Those weevils that landed on their backs were set upright to eliminate any potential variability. The numbers of replicate runs for all of the Subtreatment contrasts in Treatment 1 and Treatment 2 are given in Table 1. Each replicate run commenced 4–6 h after scotophase, when the weevils are actively searching for food and when most eggs are laid (Barratt et al., 1995), and continued for 4 hours thereafter. The Petri dishes were turned 90° between runs to prevent set-up bias. During the bioassay, the entire assembly was placed under a non-airtight black wooden cover, which itself was covered with black plastic. At the end of a bioassay, the weevils in each ‘arm’ of the olfactometer were collected and immediately frozen. The olfactometers were then washed in warm water with phosphate-free RBS pF (Borghgraef s.a.- nv, Belgium), rinsed with 70% ethanol and oven-dried at 120°C overnight, except for the Petri dish lids because this treatment would melt the glue. Instead these were air-dried overnight, as were the mesh separators.
2.1.3 Plant material and frass preparation as subtreatment HIPV/VOC sources
Damaged ryegrass: 48 hours before the bioassays, plant material from a single paperpot was removed, and 75% of the root material was excised. The remaining truncated root material was then sealed in a wet polyethylene bag with the foliage still intact. This was then placed into a polycarbonate container (235 x 170 x 80 mm), into which 20 weevils were added and maintained at 18–22°C and 16:8 L:D photoperiod for 48 hours. Thereafter, the weevils were removed, leaving only the leaf damage and frass.
Damaged ryegrass + 20 weevils: 48 hours before the bioassays, plant material from a single paperpot was removed, and 75% of the root material was excised. The remaining truncated root material was then sealed in a wet polyethylene bag with the foliage still intact. This was then placed in a polycarbonate container (235 x 170 x 80 mm), to which 20 weevils were added and maintained at 18–22°C and 16:8 L:D photoperiod. The 20 weevils remained in place on the damaged ryegrass foliage for the entire bioassay.
Washed undamaged ryegrass: To reduce the possibility of extraneous volatile influences, 48 hours before the bioassays plant material from a single paperpot was removed and 75% of the root material excised. The remaining truncated root material was then sealed in a wet polyethylene bag with the foliage still intact. The foliage was soaked in water for one minute and then gently washed (agitated/rubbed lightly with fingers, so as not to damage the leaves) four times with ultrapure water (Barnstead™ Nanopure™, California, USA). These plants were then placed between paper towels to dry.
Washed damaged ryegrass: 48 hours before the bioassays, plant material from a single paperpot was removed and 75% of the root material excised. The remaining truncated root material was then sealed in a wet polyethylene bag with the foliage still intact. This was then placed in a (235 x 170 x 80 mm) polycarbonate container to which 20 weevils had been added and maintained at 18–22°C and 16:8 L:D photoperiod for 48 hours. The weevils were then removed, and the foliage was soaked in water for one minute and then gently washed (agitated/rubbed lightly with fingers, so as not to damage the leaves) four times with ultrapure water to remove any frass and other weevil-related substances but not organic compounds. These plants were then placed between paper towels to dry.
Dry frass collection: Dry frass was collected from the population maintenance containers on the same day as the assay and kept in a glass Petri dish until use. When required, this was added to the ryegrass in the olfactometer VOC/HIPV cup at a rate of 0.01g.
Frass in suspension: Frass was collected from the population maintenance containers. Twenty-four hours before the assay, 500 µl of ultrapure water was added to 0.01g of the dry frass and thoroughly mixed in a Petri dish. The mixture was then maintained at 4°C until two hours before assay commencement when 100 µl of the suspension was added to a strip of grade 1 filter paper (Whatman®, GE Healthcare Life Sciences, UK) which was placed in the VOC/HIPV cup. A strip of filter paper with 100 µl ultrapure water added was used as the opposite odour for this subtreatment.
The ryegrass was placed into the olfactometer along with the polyethylene bag in all treatments.
2.1.4 Dissection of responsive weevils
The frozen test weevils were dissected to ascertain whether their physiological condition influenced weevil choice preferences. The following variables were assessed: parasitism status, sex, sexual maturity (stage 1–3 for males and 0–5 for females), mated or unmated, wing muscle size index (0–3), the presence of teratocytes (indicative of parasitism) and the presence of eggs, or β carotene crystals, indicative of late oöcyte absorption (Goldson, 1983).
2.1.5 HIPV collection and GC-MS analysis
Dynamic headspace collection of volatiles was carried out using a modified push-pull system as described by Turlings et al. (2004) and Rostás and Eggert (2008). Undamaged ryegrass was prepared as above, with one paperpot per plastic bag. Damaged ryegrass (1 paperpot) with its roots in a polyethylene bag was damaged for 65 h by 20 reproductive L. bonariensis in a plastic container at 20°C and 16:8 L:D. Four replicates of each treatment were produced and placed in glass VOC/HIPV -source vessels. Filtered air (activated charcoal filter, 400 cc, Alltech, Deerfield, IL, USA) originating from a compressed air cylinder was pushed into the vessel at a rate of 300 mL min-1. A vacuum pump (ILMVAC GmbH, Germany) pulled air out at 200 mL min-1 through a trapping filter containing 30-mg SuperQ (ARS Inc., Gainesville, FL, USA). Empty VOC/HIPV source vessels were also sampled to check for background contamination. Before each experiment, the trapping filters were cleaned by rinsing with 1 mL of methylene chloride.
Trapped volatile compounds were eluted from the filter with 150 µL of methylene chloride, and 200-ng methyl jasmonate (Sigma-Aldrich, Australia) was added as an internal standard. The sample mixtures were separated using a Shimadzu GCMS-QP2010 (Shimadzu Corporation, Japan) gas chromatograph/mass spectrometer fitted with a Restek Rxi-1ms fused silica capillary column (30.0-m x 0.25-mm i.d. x 0.25 μm, Bellefonte, PA, USA). From each sample, 3 µL were injected in a pulsed splitless mode at a temperature of 220°C and with a pulse of 168 kPa for 40 s. The oven temperature was held at 50°C for 3 min, then raised to 320°C, and held at this temperature for 8 min. Helium was used as carrier gas with a flow rate of 1.5 mL min-1. Compounds were identified using GCMS solution v. 2.72 (Shimadzu Corporation, Japan) software with the NIST 11 mass spectrum library, the software MassFinder4/Terpenoids library (Hochmuth Scientific Software, Hamburg, Germany) as well as by comparison with standard compounds. The quantification of plant-derived volatiles was obtained by comparing the compounds’ area to the area of the internal standard.
2.1.6 Statistical analysis
Based on the olfactometer results and considering each of the paired subtreatment comparisons, the weevils’ preference for one subtreatment treatment over the other was analysed using a one-sample binomial exact test. This test compared the observed proportion of weevils found in each VOC/HIPV subtreatment arm against the null hypothesis that the proportion in each should be 0.5. Each test was applied to only those weevils which made a choice between the paired Subtreatments; those that remained undecided (i.e., no choice) were excluded from the analysis.
Based on its physiological condition, the individual weevil’s preference for each subtreatment paired contrast was coded as a binary variable, with 1 if one physiological characteristic was chosen and 0 if the other treatment was chosen. Then, these binary preference data were analysed using a generalised linear model (GLM) with binomial distributions using a logit link function. However, for each setting in Treatment 1 and Treatment 3, only limited GLM analyses could be usefully performed. This was because the population needed to be partitioned according to the physiological characteristics being considered, resulting in small sample sizes. For example, sexual maturity could only be scored for non-parasitised weevils, and teratocyte presence could only be scored for parasitised weevils. Likewise, the β-carotene crystals, eggs and mated status applied only to the females. Blocking was applied where there were sufficient degrees of freedom for proper analysis.
The volatiles from damaged and undamaged plants and their quantities were compared using the Mann-Whitney U test because data were not normally distributed. All statistical analyses were performed using Genstat 14 (VSNI, 2019).
3 Results
3.1 Overall population responses
Figures 2 and 3 summarise the subtreatment responses shown by Treatments 1 and 2.
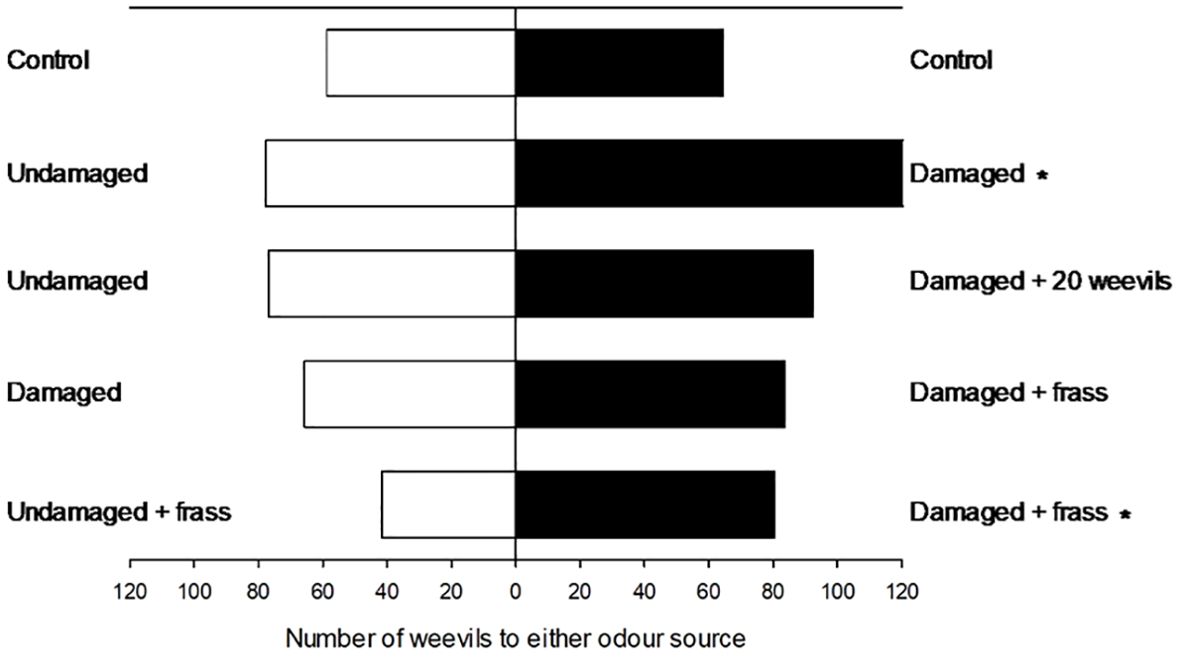
Figure 2. The significant behavioural responses (indicated by * at p<0.05) shown by diapausing Listronotus bonariensis to the Subtreatment ‘herbivore-induced plant volatiles/volatile organic compound’ contrasts as presented in Table 1 in a still-air olfactometer.
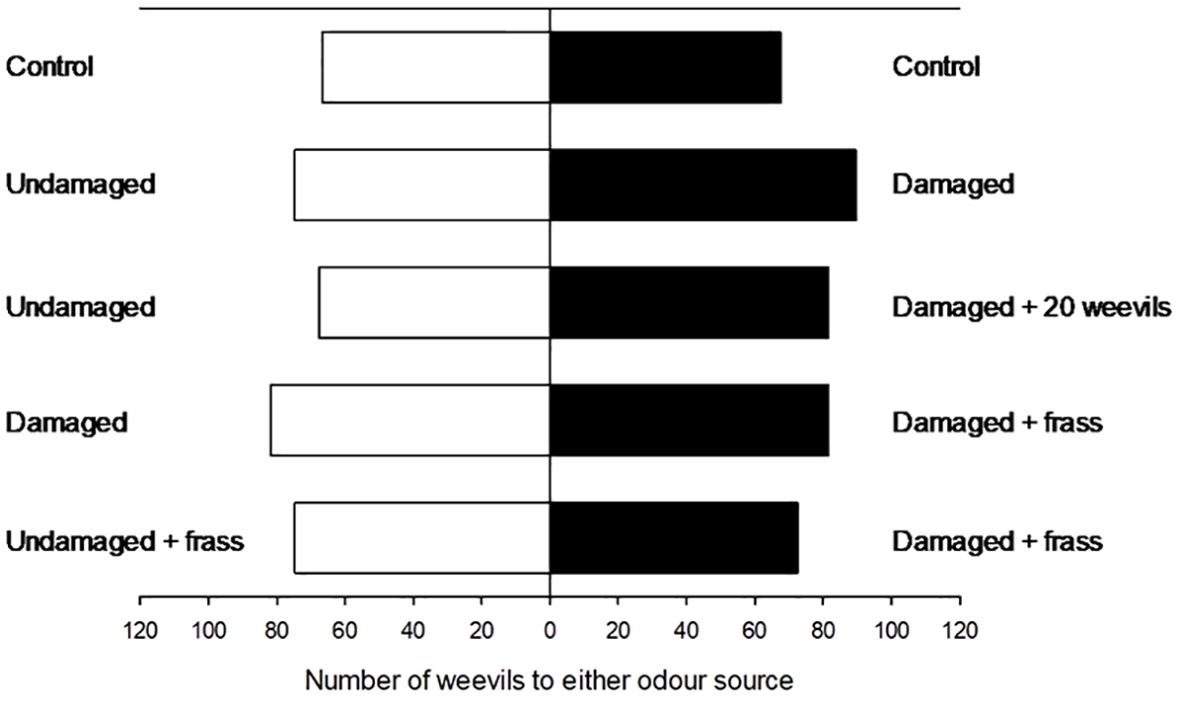
Figure 3. The negligible behavioural responses shown by reproductive Listronotus bonariensis to the Subtreatment ‘herbivore-induced plant volatiles/volatile organic compound’ contrasts as presented in Table 1 in a still-air olfactometer.
3.1.1 Treatment 1: diapausing weevils
Damaged ryegrass (Subtreatment 2) was significantly attractive to the test weevils (Figure 2; Table 2). Subtreatment 5, comprising frass and weevil feeding damage combined, was also significantly attractive to the test weevils, although this showed no greater weevil response than those shown in Subtreatment 2. Frass in suspension (Subtreatment 6) was also significantly attractive to the diapausing weevils.
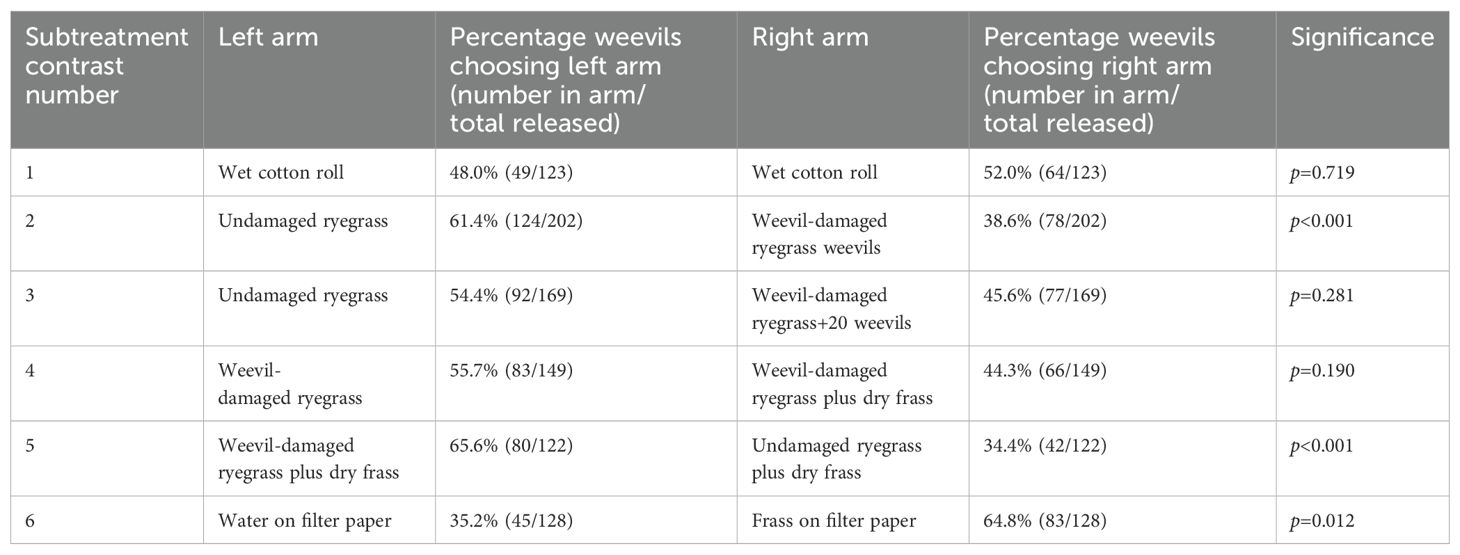
Table 2. Summary of the diapausing Listronotus bonariensis response to the ‘herbivore-induced plant volatiles/volatile organic compound’ treatments as shown in Table 1 in a still-air olfactometer.
An important aspect of this study was apparent in Subtreatment 3, which had the same contrast as Subtreatment 2 (undamaged versus damaged grass) except for the addition of 20 weevils (Figure 2; Table 2). This addition resulted in the damaged ryegrass becoming no more attractive than the undamaged ryegrass treatment in Subtreatment 2; the addition of 20 weevils offset the attractiveness of the damaged grass.
3.1.1.1 The effect of L. bonariensis physiological condition on responses to HIPV contrasts
Despite the clear L. bonariensis movement responses found in Treatment 1 (diapausing weevils), subtreatment contrasts 2, 5, and 6 in Treatment 1, showed by dissection little evidence that the presence of parasitism, oöcyte resorption (β carotene crystals) or mated condition had had any effects on weevil response. However, there was some evidence that the degree of developmental maturity may have had some effect. Subtreatment 5 showed that a significantly higher percentage (82.4% (14/17)) of unparasitised female weevils at level 3 were more strongly attracted to the frass than their unparasitised counterparts at level 2 (20%) (p=0.023).
Generally, the significance of these results probably needs to be treated with some caution in that once the overall population was partitioned according to the physiological state of the test populations, the resulting sample sizes were small.
3.1.2 Treatment 2: reproductive weevils
This study found no significant response of reproductive L. bonariensis populations at all to the VOC/HIPVs produced by the conspecific weevil populations, frass, and host plant feeding (p>0.05 in all cases) (Figure 3). This is highly consistent with the findings of Hennessy et al. (2022) who in their very similar olfactometer-based experiment found weak if any responses, by the reproductive L. bonariensis populations.
3.2 VOCs and HIPV collection and GC-MS analysis
Head-space analysis resulting from L. bonariensis feeding damage indicated de novo synthesis of the HIPV phenylacetaldehyde; of the VOCs, the weevil-damaged ryegrass also showed significant increases in the monoterpenes (Z)-β-ocimene, (E)- β-ocimene, along with the diterpene neophytadiene (Figure 4). Weevil feeding damage also increased release rates of the green leaf volatiles 2-hexenal and cis-3-hexen-1-ol, but these increases were comparatively small (Figure 4; Supplementary Material).
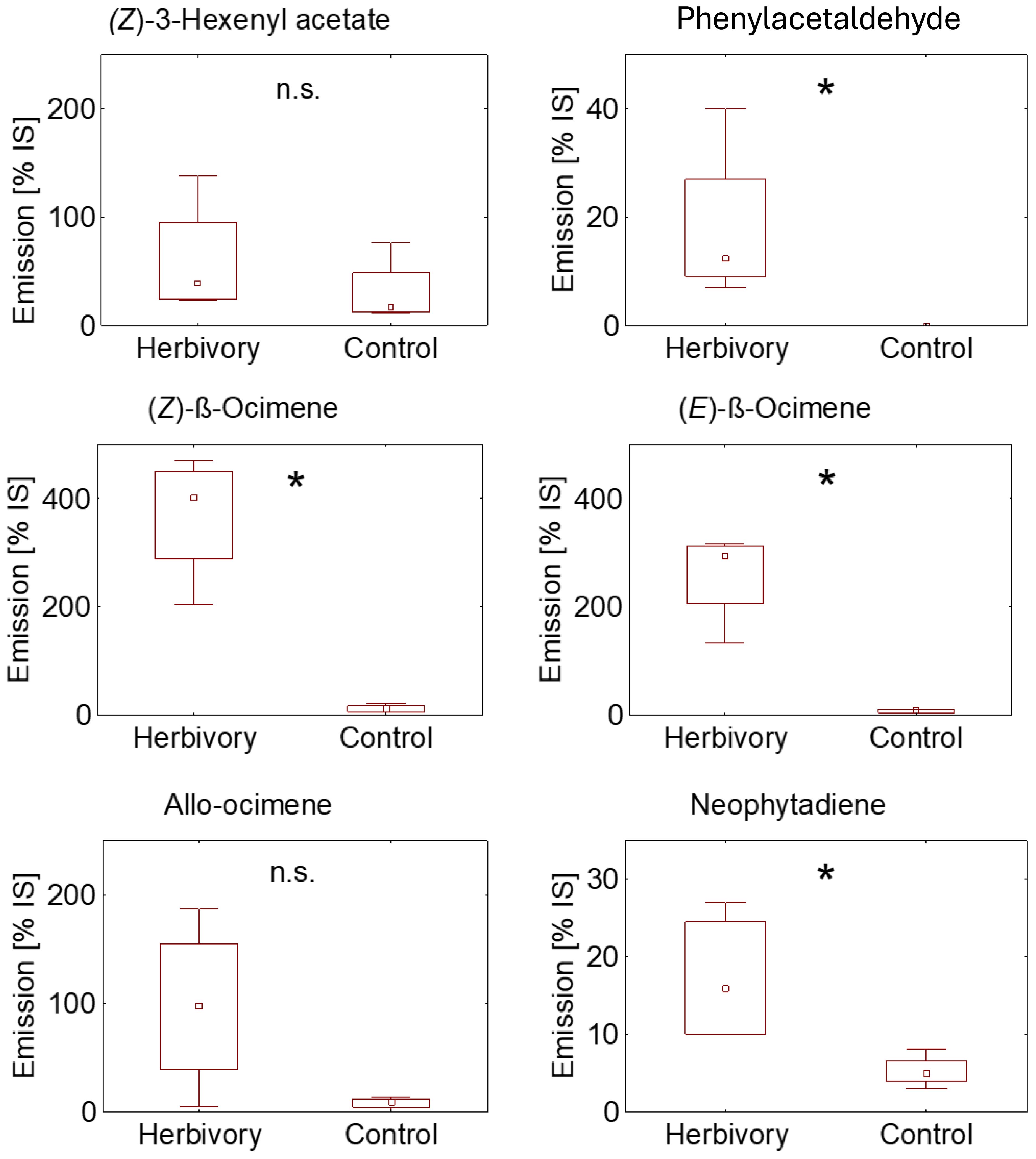
Figure 4. Relative emission rates of Lolium multiflorum ‘Tama’ volatiles presented as percentages of the internal standard (IS). Herbivory = plants were subjected to damage by 20 diapausing Listronotus bonariensis individuals for 65 hours and subsequently removed prior to volatile collection. Control = undamaged L. multiflorum plants. The box plot illustrates the median with interquartile range (25th and 75th quartiles), while whiskers indicate extreme values. Mann-Whitney U Test, n = 4, *p < 0.05, n.s., not significant.
4 Discussion
4.1 Olfactometer-based behavioural responses of L. bonariensis
There is an extensive body of literature that considers the interactions between insects and plants via various combinations of VOCs and HIPVs. Significantly, the HIPV mix can vary in quality and quantity compared with the VOC blends released by undamaged hosts (Schoonhoven et al., 2005). Plant HIPVs are typically more readily detected by responding species than VOCs, with the insect-induced blend conveying additional information about, for example, the presence of conspecific aggregations of host plants or intense competition for plant resources. Significant to this study, such characteristics may influence insects’ foraging decisions, leading to attraction to or repellency from the HIPV source (e.g., Kalberer et al., 2001; Meiners et al., 2005; Ballhorn et al., 2013; Aartsma et al., 2017; Menacer et al., 2023). This study has shown that diapausing populations of L. bonariensis, (Treatment 1) were, in the main, strongly attracted to feeding damage by conspecifics. Conversely, in the case of the reproductive populations, there was little if any response.
In general, the patterns of plant attractiveness found in this study are similar to those found in other Listronotus spp. McGraw et al. (2011), using olfactometry, found that the annual bluegrass weevil L. maculicollis (Kirby) was attracted to damaged Poa annua L. Justus (2019) reported that L. oregonensis was attracted to carrot and parsley in both olfactometer studies and field trappings. Other curculionid species where attraction occurs include the tree-of-heaven trunk weevil, Eucryptorrhynchus brantii (Harold) (Sun et al., 2023), the tea weevil Myllocerinus aurolineatus (Voss) (Sun et al., 2010), the plum curculio Conotrachelus nenuphar (Herbst) (Leskey and Prokopy, 2001), the boll weevil Anthonomus grandis Boheman (e.g., Magalhães et al., 2012), the black vine weevil O. sulcatus (Van Tol et al., 2004), and the cranberry weevil Anthonomus musculus Say (Szendrei et al., 2011).
The emission of the HIPVs triggered by L. bonariensis damage of the L. multiflorum plants (Figure 4) in this study was typical of plant HIPV emissions measured elsewhere (e.g., Dudareva et al., 2006; Farré-Armengol et al., 2017). Furthermore, Figure 4 shows how the detected insect-elicited responses were much greater than those of the controls indicating little (if any) effect resulting from the initial handling of the test plant material. Of particular interest amongst the HIPV treatment responses was the de novo detection of phenylacetaldehyde (Figure 4) which is known to occur in response to insect feeding across a range of plant species (e.g., El-Sayed et al., 2008, 2016, 2018; Gutensohn et al., 2011). Zhang et al. (2023) have shown that phenylacetaldehyde induced by the rice water weevil, Lissorhoptrus oryzophilus Kuschel feeding on rice seedlings is attractive to the incipiently reproductive spring populations of the weevil when migrating from their overwintering sites into the emerging rice crop (Saito et al., 2005). This has the effect of attracting more conspecifics onto the plants (Zhang et al., 2023). Significantly, these workers then determined that the L. oryzophilus responsiveness to phenylacetaldehyde ceased when the weevils entered their summer reproductive phase. This life history is strikingly similar to that found in L. bonariensis except that in New Zealand the adults diapause in the pasture itself (e.g., Goldson, 1981b). As with L. oryzophilus the present study has shown that late diapausing/spring L. bonariensis populations showed strong responses to the HIPVs including phenylacetaldehyde (Figure 4) whereas, as with L. oryzophilus, this almost completely disappeared in the summer reproductive populations (Figure 3). This result explains the lack of L. bonariensis responsiveness found by Hennessy et al. (2022) who worked on only reproductive populations of the weevil. The adaptive implications of this seasonal pattern are discussed below. Notably many of the c.100 species of Listronotus occupy the similar semiaquatic habitats as L. oryzophilus (e.g., Blatchley and Leng, 1916; Lloyd, 1966; O’Brien, 1977).
The cessation of attraction to the VOC/HIPV treatments shown by diapausing L. bonariensis (Treatment 1) to damaged ryegrass and frass after the addition of 20 conspecifics (Figure 2) is consistent with the generally observed population dispersion as L. bonariensis populations increase. Barlow et al. (1994), conducted an analysis of field populations of L. bonariensis in the South Island of New Zealand and showed that the adult weevils were aggregated at low densities but trended towards randomness when approaching c. 400 m-2. Barker and Addison (1989) found a similar pattern in the North Island of New Zealand and McNeill et al. (1998) tested weevils confined to field plots and again, found evidence of negative density dependent oviposition. Probably also related to this, Prestidge et al. (1987) found that there was never more than 3% of ryegrass tillers in any sample showing multiple L. bonariensis ovipositional sites. Pilkington and Springett (1988) noted that the proportion of weevil ovipositional holes plugged with frass increased with increasing weevil density and suggested that there was an epideictic pheromone associated with this. Goldson et al. (2011) using a life-table approach, found that the realised fecundity of the emergent first summer generation of L. bonariensis was inversely related to the density of adults per ryegrass tiller. Such population density dependent responses in L. bonariensis populations could go some way towards explaining the very strong negative influence that caging has on L. bonariensis reproductivity, including the resorption of oöcytes (Goldson, 1981b; Vereijssen and Goldson, 2016).
More generally, the pattern of population density-related behaviour in L. bonariensis is similar to the observation of Tansey et al. (2005) who showed that on leafy spurge (Euphorbia spp. near esula), the HIPVs arising from flea beetle, Aphthona nigriscutis Foudas, damage ranged from attractive to repellant depending on their concentration. Similarly, Ballhorn et al. (2013) noted that lima bean plants (Phaseolus lunatus L.), when emitting low HIPVs, attracted males of the chrysomelids Gynandrobrotica guerreroensis Jacoby and Ceratoma ruficornis Olivier to facilitate mating. At higher concentrations of the HIPVs, they became repellent (Ballhorn et al. (2013).
Studies elsewhere have shown that the sex and physiological condition of insects influence their responses to HIPVs (e.g., Bernays and Chapman, 1994; Davidson et al., 2006; McGraw et al., 2011; Ballhorn et al., 2013). However, sparse evidence for this in this contribution is probably not definitive in that when the L. bonariensis population was partitioned into the various physiological categories for analysis, small sample sizes became problematic.
4.2 Possible relict behaviour in L. bonariensis populations in New Zealand
The L. bonariensis behaviour observed in this contribution may well reflect relict aspects of the natural history of L. bonariensis in its native range, in a way similar to that found by Goldson and Emberson (1980) and Goldson (1981b) when considering the species’ diapause seasonality. These workers showed that the New Zealand weevil populations entered diapause throughout the country triggered by a critical photoperiod of 12.3:11.7 hours L:D. While of no apparent adaptive advantage in New Zealand, Goldson and Emberson (1980) concluded that this behaviour had occurred as a result of evolutionary pressures in the species’ native range. Published peer-reviewed literature relating to the biology and ecology of L. bonariensis (and M. hyperodae) in South America is sparse (e.g., Loan and Lloyd, 1974; Ahmad, 1978). However, there exist nine, sometimes untitled, mimeographed reports to the Commonwealth Institute of Biological Control (CIBC and its antecedents) written by DC Lloyd and later, Lloyd and Ahmad, between 1945 and 1973. This detailed compendium is based on field and laboratory studies describing the ecology and biology of L. bonariensis at various altitudes and latitudes in temperate South America. In the Bariloche area in Argentina (altitude 890m; latitude 41.13°S; longitude 71.3°W) the reproductive season of L. bonariensis was initially observed by Lloyd and Ahmad (1972) and Loan and Lloyd (1974) to extend from October to December, with adult weevils emerging in February overwintering and becoming reproductive in the following spring. Subsequently, Ahmad (1978) extended this reproductive season of L. bonariensis from September to February, noting that the weevil overwintered in diapause, as found in New Zealand by Goldson (1981b). Lloyd and Ahmad (1972) also observed a second summer generation of the weevil at latitudes c. 35°S in the Buenos Aires and Montevideo areas and asserted that this life history probably occurred in latitudes 31°S to 28°S. This pattern is also very close to that which has been observed in New Zealand (e.g. Goldson, 1981b; Barker et al., 1989; Goldson et al., 2011). Moreover, Lloyd (1966) also noted that a third L. bonariensis generation was known to occur in favourable conditions such as those in Santiago, Chile (altitude 520m, latitude 33.45°S, longitude 70.68°W) again this is similar to that observed in the warmer northern parts of New Zealand (e.g., Barker et al., 1989). Although Lloyd (1966) reported a very wide distribution of L. bonariensis in South America, latitudes 17°S and 51°S and from sea level to 3660 m, he and Lloyd and Ahmad (1972) contended that the weevil’s native range is likely to be in the Andean intermontane valleys known as ‘mallines’, which comprise cool wet meadows (Figure 5). These ecosystems extend from c. 36° to 56°S (e.g., Mazzoni and Rabassa, 2013) and have been comprehensively described (e.g., Squeo et al., 2006; Mazzoni and Rabassa, 2013; Curcio et al., 2023). The native Gramineae found in these ecosystems include Festuca spp., Poa lanuginosa Poir. and Hordeum comosom J.Presl (Gaitán et al., 2011) but notably, there were no Lolium spp. in the indigenous ecosystem (Frakes, 1973). Further, Mazzoni and Rabassa (2013) have also observed that variability within the mallines ecosystem occurs in very small areas, depicting high intrinsic habitat heterogeneity. Based on these descriptions it can be argued that suitable L. bonariensis host plants in the weevil’s native range were likely to have been sparsely and widely distributed, supporting low weevil population densities (Goldson, personal observation). It is probable that the weevil has subsequently become more widely distributed, as described by Lloyd (1966), with the arrival of ‘European’ agricultural systems based on exotic host plant species, especially Gramineae, which are more palatable to the weevil than the native host plants such as Paspalum gayanum E. Desv. and Sertaria geniculatea (Lam.) P. Beauv (Lloyd, 1966).
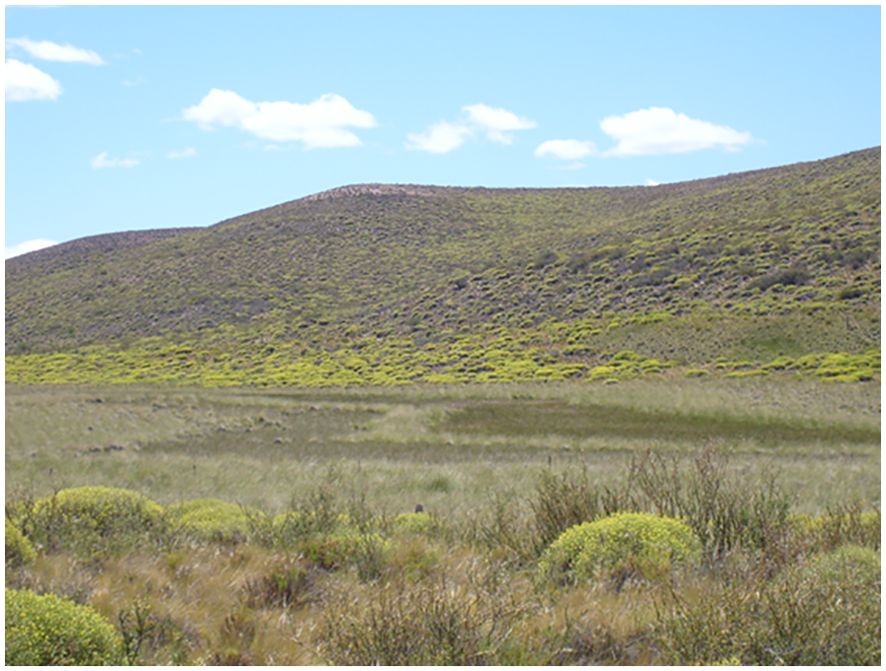
Figure 5. Photograph of a ‘mallines’ ecosystem (wet meadows) in Argentina, from where it is thought Listronotus bonariensis originates. These areas are wet and cool Andean high-fertility intermontane valleys extending between 36° and 56°S. Photograph by Andrea Soledad Enriquez, INTA, Argentina.
Williams et al. (1994) used Random Amplified Polymorphic DNA (RAPD) analyses of the various weevil populations collected in South America, Australia and New Zealand to determine that the New Zealand populations were likely to have originated in the River Plate area. Later, Harrop et al. (2020) used genotyping-by-sequencing (GBS) analysis to determine the genetics of L. bonariensis populations distributed throughout New Zealand. These workers concluded that a likely scenario is that there were two separate introductions from the same South American source population into regions north and south of New Zealand’s Main Divide and that thereafter there had been some migration between the two populations. While the exact locations of where the New Zealand populations came from have yet to be definitively resolved, the observations made by the CIBC researchers are probably correct, given that Bariloche is relatively close to the mallines ecosystems. That the weevil evolved in these ecosystems is also arguably reflected by the life history of its parasitoid M. hyperodae, which has been the subject of extensive research into what are taken to be its own relict characteristics, given its relatively recent introduction into New Zealand (e.g., Goldson et al., 1990, 1995; McNeill et al., 1998; Phillips and Baird, 2001; Phillips and Kean, 2017). Under laboratory conditions, the parasitoid has been shown to have low fecundity of 48 ± 8 eggs and a long life span of 21 ± 4 days (Goldson et al., 1995) coupled with very high searching efficiency (Barlow et al., 1993). Related to this and significantly when weevil hosts are abundant, 71% of the parasitoid’s ovipositional effort occurs within the first three days after eclosion and with this, 41% of the parasitoid’s lifespan occurs after the cessation of oviposition. Taken together, these characteristics point to M. hyperodae being adapted to spending a large proportion of its life searching for patchy and sparse distributions of L. bonariensis; i.e., the parasitoid’s ovipositional effort is time-limited (Phillips and Kean, 2017) and as discussed by Goldson et al. (1995) this again reflects features of the mallines ecosystem.
The absence of olfactometer responsiveness of the reproductive populations of L. bonariensis (Treatment 2) may, at first sight be counterintuitive, but this may reflect the nature of the weevil’s primitive ecosystem. In view of the mallines habitat’s latitude and altitude, there are short growing seasons. For example in Pilcaniyeu, which is at northern end of the mallines distribution (altitude; 900 m latitude 41.12°S; longitude 70.72°W), the growing season is only 2.1 months (67 days), from around 18 December to around 22 February (https://weatherspark.com/y/26504/Average-Weather-in-Pilcaniyeu-Argentina-Year-Round) Given this limitation, the short growing season could well lead to extensive L. bonariensis mortality owing to the population’s incomplete development prior to the onset of cold conditions. Under such conditions there would therefore be adaptive value in L. bonariensis commencing reproductive development as soon as temperatures permit in the early spring. This would optimise the chances of the still pre-reproductive L. bonariensis populations locating sparsely distributed host plants with conspecific populations required for feeding and reproduction. Arguably, with L. bonariensis, this requirement could possibly be effected by the damaged plant’s emission of phenylacetaldehyde (Figure 4). As discussed above, such ecology is very reminiscent of the phenylacetaldehyde-stimulated L. oryzophilus spring migration into rice crops at a time of ovarian development after which responsiveness declines in the summer generation (Saito et al., 2005; Zhang et al., 2023).
Listronotus bonariensis is known to be able to disperse rapidly into resown pastures in New Zealand (e.g., Prestidge et al., 1989; Popay et al., 2011) in a manner similar to the observation of Justas and Long (2019), working with L. oregonensis using baited traps and (2011) observed movement of L. maculicollis towards mechanically damaged turfgrasses (McGraw et al., 2011). While the situation is different in New Zealand, it is also interesting to note that after diapause in the spring (even with an obvious abundance of host plants available), those L. bonariensis with developed flight musculature were 20 times more likely to fly than at any other time of the year (Goldson et al., 1999).
The cessation of the damaged plants’ attractiveness when 20 weevils were added (Table 2) is again consistent with the relict behaviour hypothesis, whereby the species needs to avoid the overexploitation of the widely distributed plant hosts. Typically, L. bonariensis larval mining of one larva destroys 3 - 8 ryegrass tillers during instar development (Ferguson et al., 2019). This limitation of conspecific recruitment is reminiscent of the findings of Tansey et al. (2005) and Ballhorn et al. (2013), where insect responses to HIPVs varied according to their intensity.
4.3 Conclusion
This study has demonstrated unequivocally that diapausing L. bonariensis is significantly attracted to conspecific frass and feeding damage on L. multiflorum foliage. This may, in part, be the result of the only identified de novo synthesised HIPV, phenylacetaldehyde, which initially attracted the weevils to the damaged plants. Once established on their host plants, the weevils had become reproductive and ceased to respond to the phenylacetaldehyde. These characteristics were very similar to those observed in the closely-related L. oryzophilus (Saito et al., 2005; Zhang et al., 2023) in rice fields.
That the loss of attractiveness to weevil feeding damage and frass when 20 L. bonariensis were added to the test sample can be interpreted to be a relict response to prevent the over exploitation of a scarcely distributed host plants.
Taken together, the results of this olfactometric study strongly suggests L. bonariensis relict behaviour reflecting the species adaptation to the short growing season and sparsely distributed host plants in the species’ mallines native range. Such responses by diapausing L. bonariensis are in marked contrast to the minimal response shown by reproductive weevils.
Data availability statement
The original contributions presented in the study are publicly available. This data can be found here: Zenodo, accession 14064202.
Ethics statement
The manuscript presents research on animals that do not require ethical approval for their study.
Author contributions
JV: Conceptualization, Collected data, Methodology, Writing - review & editing, Writing – original draft. SG: Conceptualization, Funding acquisition, Supervision, Writing – original draft, Writing – review & editing. MR: Conceptualization, Formal analysis, Investigation, Methodology, Writing – original draft. RT: Conceptualization, Investigation, Methodology, Writing – original draft. CK: Data curation, Formal analysis, Investigation, Writing – review & editing, Writing – original draft.
Funding
The author(s) declare financial support was received for the research, authorship, and/or publication of this article. This study was funded by an AGMARDT Postdoctoral Fellowship (JV), AgResearch New Zealand and the Bio-Protection Research Centre at Lincoln University.
Acknowledgments
The authors are indebted to Mark McNeill (AgResearch Lincoln) for his practical advice in conducting the experiments. We also thank John Proffitt (AgResearch Lincoln) for collecting Argentine stem weevil samples and Brent Richards and Leona Meachen (Lincoln University) for maintaining the ryegrass cultures. David Saville (Saville Stats) provided valuable statistical advice. Dr Andrea Soledad Enriquez (INTA) kindly provided the photograph of the mallines environment.
Conflict of interest
The authors declare that the research was conducted in the absence of any commercial or financial relationships that could be construed as a potential conflict of interest.
Publisher’s note
All claims expressed in this article are solely those of the authors and do not necessarily represent those of their affiliated organizations, or those of the publisher, the editors and the reviewers. Any product that may be evaluated in this article, or claim that may be made by its manufacturer, is not guaranteed or endorsed by the publisher.
Supplementary material
The Supplementary Material for this article can be found online at: https://www.frontiersin.org/articles/10.3389/fevo.2024.1470023/full#supplementary-material
References
Aartsma Y., Bianchi F. J. J. A., van der Werf W., Poelman E. H., Dicke M. (2017). Herbivore-induced plant volatiles and tritrophic interactions across spatial scales. New Phytol. 216, 1054–1063. doi: 10.1111/nph.14475
Ahmad R. (1978). Note on breeding the Argentine stem weevil Hyperodes bonariensis [Col.: Curculionidae] and its egg parasite Patasson atomarius [Hym.: Mymaridae. BioControl 23, 161–162. doi: 10.1007/bf02371722
Ballhorn D. J., Kautz S., Heil M. (2013). Distance and sex determine host plant choice by herbivorous beetles. PloS One 8, e55602. doi: 10.1371/journal.pone.0055602
Barker G. M. (2013). Biology of the introduced biocontrol agent Microctonus hyperodae (Hymenoptera: Braconidae) and its host Listronotus bonariensis (Coleoptera: Curculionidae) in northern New Zealand. Environ. Entomology 42, 902–914. doi: 10.1603/EN11248
Barker G. M., Addison P. J. (1989). Sampling Argentine stem weevil, Listronotus bonariensis (Kushel), populations in pasture: the soil-dwelling stages. New Z. J. Agric. Res. 32, 95–103. doi: 10.1080/00288233.1989.10423482
Barker G. M., Addison P. J. (1993). “Argentine stem weevil populations and damage in ryegrass swards of contrasting Acremonium infection,” in Proceedings of the 6th Australasian Conference on Grassland Invertebrate Ecology. 161–168 (New Zealand: AgResearch Hamilton).
Barker G. M., Addison P. J. (2006). Early impact of endoparasitoid Microctonus hyperodae (Hymenoptera: Braconidae) after its establishment in Listronotus bonariensis (Coleoptera: Curculionidae) populations of northern New Zealand pastures. J. Economic Entomology 99, 273–287. doi: 10.1603/0022-0493-99.2.273
Barker G. M., Pottinger R. P., Addison P. J. (1989). Population dynamics of the Argentine stem weevil (Listronotus bonariensis) in pastures of Waikato, New Zealand. Agric. Ecosyst. Environ. 26, 79–115. doi: 10.1016/0167-8809(89)90021-2
Barlow N. D., Goldson S. L., McNeill M. R., Proffitt J. R. (1993). “Measurement of the attack behaviour of the parasitoid Microctonus hyperodae as a classical biological control agent of Argentine stem weevil Listrontous bonariensis,” in Proceedings of the Sixth Australasian Conference on Grassland Invertebrate Ecology, (Ruakura Agric Centre Hamilton. 326–330 (New Zealand: AgResearch Hamilton, New Zealand).
Barlow N. D., Proffitt J. R., Goldson S. L. (1994). “The distribution of adult Argentine stem weevils in Canterbury dryland pastures,” in Proceedings of the New Zealand Plant Protection Conference. 210–214. doi: 10.30843/nzpp.1994.47.11098
Barratt B. I. P., Evans A. A., Ferguson C. M. (1995). “Circadian patterns of oviposition and feeding in Listronotus bonariensis (Kuschel) (Coleoptera: Curculionidae) in the laboratory,” in Proceedings of the NZ Plant Protection Conference, Vol. 48. 219–223. doi: 10.30843/nzpp.1995.48.11485
Bernays E. A., Chapman R. E. (1994). “Behavior: the process of host-plant selection,” in Host-plant selection by phytophagous insects (Springer, Boston, MA), 95–165. doi: 10.1007/978-0-585-30455-7_5
Blatchley W. S., Leng C. W. (1916). Rhynchophora or weevils of north eastern America (Indianapolis, USA: Nature Publishing Company).
Caradus J., Lovatt S., Belgrave B. (2013). “Adoption of forage technologies,” in Proceedings of the New Zealand grassland association, 39–44. Available at: https://www.nzgajournal.org.nz/index.php/ProNZGA/article/download/2917/2545.
Curcio M., Irisarri G., García Martínez G., Oesterheld M. (2023). Trends of aboveground net primary productivity of Patagonian meadows, the omitted ecosystem in desertification studies. Remote Sens. 15, 2531. doi: 10.3390/rs15102531
Davidson M. M., Butler R. C., Teulon D. A. (2006). Starvation period and age affect the response of female Frankliniella occidentalis (Pergande)(Thysanoptera: Thripidae) to odor and visual cues. J. Insect Physiol. 52, 729–736. doi: 10.1016/j.jinsphys.2006.03.013
Dudareva N., Negre F., Nagegowda D. A., Orlova I. (2006). Plant volatiles: Recent advances and future perspectives. Crit. Rev. Plant Sci. 25, 417–440. doi: 10.1080/07352680600899973
El-Sayed A. M., Byers J. A., Manning L. M., Rgens A. J., Mitchell V. J., Suckling D. M. (2008). Floral scent of Canada thistle and its potential as a generic insect attractant. J. Economic Entomology 101, 720–727. doi: 10.1093/jee/101.3.720
El-Sayed A. M., Knight A. L., Basoalto E., Suckling D. M. (2018). Caterpillar-induced plant volatiles attract conspecific herbivores and a generalist predator. J. Appl. Entomology 142, 495–503. doi: 10.1111/jen.12495
El-Sayed A. M., Knight A. L., Byers J. A., Judd G. J. R., Suckling D. M. (2016). Caterpillar-induced plant volatiles attract conspecific adults in nature. Sci. Rep. 6, 37555. doi: 10.1038/srep37555
Farré-Armengol G., Filella I., Llusià J., Peñuelas J. (2017). [amp]]beta;-Ocimene, a key floral and foliar volatile involved in multiple interactions between plants and other organisms. Molecules 22, 1148. doi: 10.3390/molecules22071148
Ferguson C. M., Barratt B. I., Bell N., Goldson S. L., Hardwick S., Jackson M., et al. (2019). Quantifying the economic cost of invertebrate pests to New Zealand’s pastoral industry. New Z. J. Agric. Res. 62, 255–315. doi: 10.1080/00288233.2018.1478860
Frakes R. V. (1973). “The ryegrasses,” in in forages: the science of grassland agriculture. Eds. Heath M. E., Barnes R. F., Metcalfe D. S. (Iowa State University Press, Ames, IA), 307–313.
Gaitán J. J., López C. R., Bran D. E. (2011). Vegetation composition and its relationship with the environment in mallines of north Patagonia, Argentina. Wetlands Ecol. Manage 19, 121–130. doi: 10.1007/s11273-010-9205-z
Giordanengo P., Brun L. O., Frerot B. (1993). Evidence for allelochemical attraction of the coffee berry borer, Hypothenemus hampei, by coffee berries. J. Chem. Ecol. 19, 763–769. doi: 10.1007/BF00985007
Goldson S. L. (1979). The reproductive seasonality and morphology of Argentine stem weevil, Hyperodes bonariensis Kuschel (Coleoptera: Curculionidae) and the effect of host grasses on its pre-reproductive development (Christchurch, New Zealand: Lincoln College, University of Canterbury). Available at: https://researcharchive.lincoln.ac.nz/handle/10182/1721.
Goldson S. L. (1981a). Non-reproductive determination of “migratory“ flight in Argentine stem weevils, Listronotus bonariensis. Physiol. Entomology 6, 283–288. doi: 10.1111/J.1365-3032.1981.TB00272.X
Goldson S. L. (1981b). Reproductive diapause in the Argentine stem weevil, Listronotus bonariensis (Kuschel) (Coleoptera: Curculionidae), in New Zealand. Bull. Entomological Res. 71, 275–287. doi: 10.1017/S0007485300008300
Goldson S. L. (1983). Implications of oöcyte resorption bodies in the ovarioles of Argentine stem weevil, Listronotus bonariensis (Coleoptera: Curculionidae). New Z. J. Zoology 10, 111–116. doi: 10.1080/03014223.1983.10423896
Goldson S. L., Barker G. M., Chapman H. M., Popay A. J., Stewart A. V., Caradus J. R., et al. (2020). Severe insect pest impacts on New Zealand pasture: the plight of an ecological outlier. J. Insect Sci. 20, 17. doi: 10.1093/jisesa/ieaa018
Goldson S. L., Barron M. C., Kean J. M., van Koten C. (2011). Argentine stem weevil (Listronotus bonariensis, Coleoptera: Curculionidae) population dynamics in Canterbury, New Zealand dryland pasture. Bull. Entomological Res. 101, 295–303. doi: 10.1017/S0007485310000507
Goldson S. L., Emberson R. M. (1980). Relict diapause in an introduced weevil in New Zealand. Nature 286, 489–490. doi: 10.1038/286489a0
Goldson S. L., McNeill M. R., Bana M., Olaniyan O., Popay A. J., Barratt B. I., et al. (2023). Implications of sampling bias and population behaviour in the study of parasitoid-based biocontrol of Listronotus bonariensis in New Zealand’s exotic pasture ecosystems. New Z. J. Agric. Res. 66, 374–388. doi: 10.1080/00288233.2022.2084121
Goldson S. L., McNeill M. R., Proffitt J. R., Hower A. A. (1995). An investigation into the reproductive characteristics of Microctonus hyperodae (Hym.: Braconidae), a parasitoid of Listronotus bonariensis (Col.: Curculionidae). Entomophaga 40, 413–426. doi: 10.1007/BF02373729
Goldson S. L., McNeill M. R., Stufkens M. W., Proffitt J. R., Pottinger R. P., Farrell J. A. (1990). “Importation and quarantine of Microctonus hyperodae a South American parasitoid of Argentine stem weevil,”. Ed. Popay A. J. (New Zealand Weed and Pest Control Society, Pacific Park Hotel, Dunedin, New Zealand), 334–338. Available at: http://www.nzpps.org/journal/43/nzpp_433340.pdf.
Goldson S. L., Proffitt J. R., Baird D. B. (1998). The bionomics of Listronotus bonariensis (Coleoptera: Curculionidae) in Canterbury, New Zealand. Bull. Entomological Res. 88, 415–423. doi: 10.1017/S0007485300042152
Goldson S. L., Proffitt J. R., Baird D. B. (1999). Listronotus bonariensis (Coleoptera: Curculionidae) flight in Canterbury, New Zealand. Bull. Entomological Res. 89, 423–431. doi: 10.1017/S0007485399000553
Goldson S., Tomasetto F., Popay A. (2014). Biological control against invasive species in simplified ecosystems: its triumphs and emerging threats. Curr. Opin. Insect Sci. 5, 50–56. doi: 10.1016/j.cois.2014.09.003
Gutensohn M., Klempien A., Kaminaga Y., Nagegowda D. A., Negre-Zakharov F., Huh J.-H., et al. (2011). Role of aromatic aldehyde synthase in wounding/herbivory response and flower scent production in different Arabidopsis ecotypes. Plant J. 66, 591–602. doi: 10.1111/j.1365-313X.2011.04515.x
Harrop T. W., Le Lec M. F., Jauregui R., Taylor S. E., Inwood S. N., van Stijn T., et al. (2020). Genetic diversity in invasive populations of Argentine stem weevil associated with adaptation to biocontrol. Insects 11, 441. doi: 10.3390/insects11070441
Hennessy L. M., Popay A. J., Glare T. R., Finch S. C., Cave V. M., Rostás M. (2022). Olfactory responses of Argentine stem weevil to herbivory and endophyte-colonisation in perennial ryegrass. J. Pest Sci. 95, 263–277. doi: 10.1007/s10340-021-01375-2
Johnson L. J., de Bonth A. C., Briggs L. R., Caradus J. R., Finch S. C., Fleetwood D. J., et al. (2013). The exploitation of epichloae endophytes for agricultural benefit. Fungal Diversity 60, 171–188. doi: 10.1007/s13225-013-0239-4
Justus E. J. (2019). New tools to assess carrot weevil behavioral ecology: Still-air bioassay and degree-day activity model for Ohio (Ohio, USA: The Ohio State University). Available online at: https://etd.ohiolink.edu/acprod/odb_etd/etd/r/1501/10?clear=10&p10_accession_num=osu1562155921546993 (Accessed November 2, 2023).
Justus E. J., Long E. Y. (2019). Biology and management of the carrot weevil (Coleoptera: Curculionidae) in North America. J. Integrated Pest Manage. 10, 8. doi: 10.1093/jipm/pmz007
Kalberer N. M., Turlings T. C. J., Rahier M. (2001). Attraction of a leaf beetle (Oreina cacaliae) to damaged host plants. J. Chem. Ecol. 27, 647–661. doi: 10.1023/A:1010389500009
Leskey T. C., Prokopy R. J. (2001). Adult plum curculio (Coleoptera: Curculionidae) attraction to fruit and conspecific odors. Ann. Entomological Soc. America 94, 275–288. doi: 10.1603/0013-8746(2001)094[0275:APCCCA]2.0.CO;2
Lloyd D. C. (1966). Natural enemies of the stem weevil Hyperodes bonariensis Kuschel in South America (London, United Kingdom: Commonwealth Institute of Biological Control).
Lloyd D. C., Ahmad R. (1972). Investigations on natural enemies of some pasture and alfalfa insects in Argentina. Commonwealth Institute Biol. Control CAB South Am. Station Rep.
Loan C. C., Lloyd D. C. (1974). Description and field biology of Microctonus hyperodae Loan, n. sp. (Hymenoptera: Braconidae, Euphorinae) a parasite of Hyperodes bonariensis in South America (Coleoptera: Curculionidae). Entomophaga 19, 7–12. doi: 10.1007/BF02371504
Magalhães D. M., Borges M., Laumann R. A., Sujii E. R., Mayon P., Caulfield J. C., et al. (2012). Semiochemicals from herbivory induced cotton plants enhance the foraging behavior of the Cotton boll weevil, Anthonomus grandis. J. Chem. Ecol. 38, 1528–1538. doi: 10.1007/s10886-012-0216-5
Marshall G. A. (1938). New curculionidae (Col.) from New Zealand. Trans. R. Soc. New Z. 67, 316–340.
Mazzoni E., Rabassa J. (2013). Types and internal hydro-geomorphologic variability of mallines (wet-meadows) of Patagonia: Emphasis on volcanic plateaus. J. South Am. Earth Sci. 46, 170–182. doi: 10.1016/j.jsames.2011.08.004
McGraw B. A., Rodriguez-Saona C., Holdcraft R., Szendrei Z., Koppenhöfer A. M. (2011). Behavioral and electrophysiological responses of Listronotus maculicollis (Coleoptera: Curculionidae) to volatiles from intact and mechanically damaged annual bluegrass. Environ. Entomology 40, 412–419. doi: 10.1603/en10266
McNeill M. R., Baird D. B., Goldson S. L. (1998). Evidence of density-dependent oviposition behaviour by Listronotus bonariensis (Coleoptera: Curculionidae) in Canterbury pasture. Bull. Entomological Res. 88, 527–536. doi: 10.1080/09583159650039548
Meiners T., Hacker N., Anderson P., Hilker M. (2005). Response of the elm leaf beetle to host plants induced by oviposition and feeding: the infestation rate matters. Entomologia Experimentalis Applicata 115, 171–177. doi: 10.1111/j.1570-7458.2005.00280.x
Menacer K., Hervé M. R., Marie Cortesero A., Aujames T., Anton S. (2023). Sex- and maturity-dependent antennal detection of host plant volatiles in the cabbage root fly, Delia radicum. J. Insect Physiol. 146, 104500. doi: 10.1016/j.jinsphys.2023.104500
O’Brien C. W. (1977). The semiaquatic weevil genus Listronotus in Mexico and Central America (Coleoptera: Curculionidae: Cylindrorhininae). Ann. Entomological Soc. America 70, 804–814. doi: 10.1093/aesa/70.5.804
Phillips C. B., Baird D. B. (2001). Geographic variation in egg load of Microctonus hyperodae Loan (Hymenoptera: Braconidae) and its implications for biological control success. Biocontrol Sci. Technol. 11, 371–380. doi: 10.1080/09583150120055781
Phillips C. B., Kean J. M. (2017). Response of parasitoid egg load to host dynamics and implications for egg load evolution. J. Evolutionary Biol. 30, 1313–1324. doi: 10.1111/jeb.13095
Pilkington S., Springett B. P. (1988). “Do epideictic pheromones play a role in Argentine stem weevil oviposition?,” in Proceedings of the 5th Australasian Conference on Grassland Invertebrate Ecology. 243–245.
Popay A. J., McNeill M. R., Goldson S. L., Ferguson C. M. (2011). The current status of Argentine stem weevil (Listronotus bonariensis) as a pest in the North Island of New Zealand. New Z. Plant Prot. 64, 55–62. doi: 10.30843/nzpp.2011.64.5962
Prestidge R. A., Barker G. M., Pottinger R. P. (1987). Observations on Argentine stem weevil mortalities in Volcanic Plateau pastures. New Z. Entomologist 10, 64–68. doi: 10.1080/00779962.1987.9722512
Prestidge R. A., van der Zijpp S., Gault P. (1989). Effect of Argentine stem weevil on vigour of ‘Grasslands Roa’ tall fescue under dairying in the Waikato. New Z. J. Agric. Res. 32, 291–297. doi: 10.1080/00288233.1989.10423464
Rostás M., Eggert K. (2008). Ontogenetic and spatio-temporal patterns of induced volatiles in Glycine max in the light of the optimal defence hypothesis. Chemoecology 18, 29–38. doi: 10.1007/s00049-007-0390-z
Saito T., Hirai K., Way M. O. (2005). The rice water weevil, Lissorhoptrus oryzophilus Kuschel (Coleoptera: Curculionidae). Appl. Entomol. Zool. 40, 31–39. doi: 10.1303/aez.2005.31
Schoonhoven L. M., Van Loon J. J., Dicke M. (2005). Insect-plant biology. 2nd (Oxford, UK: Oxford university press).
Squeo F. A., Warner B. G., Aravena R., Espinoza D. (2006). Bofedales: high altitude peatlands of the central Andes [Bofedales: turberas de alta montaña de los Andes centrales. Rev. chil. hist. Nat. 79, 245–255. doi: 10.4067/S0716-078X2006000200010
Stewart A. V., Goldson S. L., Marris J. W., Rubenstein J. M., Rolston M. P. (2023). Analytical purity of old New Zealand forage seed samples and detection of fungal and insect contaminants including ryegrass endophyte and Argentine stem weevil. New Z. J. Agric. Res. 66, 270–283. doi: 10.1080/00288233.2022.2066140
Sun X., Song W., Guo W., Wang S., Wen J. (2023). The influencing factors of aggregation behavior of Tree-of-Heaven trunk weevil, Eucryptorrhynchus brandti (Harold)(Coleoptera: Curculionidae). Insects 14, 253. doi: 10.3390/insects14030253
Sun X.-L., Wang G.-C., Cai X.-M., Jin S., Gao Y., Chen Z.-M. (2010). The tea weevil, Myllocerinus aurolineatus, is attracted to volatiles induced by conspecifics. J. Chem. Ecol. 36, 388–395. doi: 10.1007/s10886-010-9771-9
Szendrei Z., Averill A., Alborn H., Rodriguez-Saona C. (2011). Identification and field evaluation of attractants for the cranberry weevil, Anthonomus musculus Say. J. Chem. Ecol. 37, 387–397. doi: 10.1007/s10886-011-9938-z
Tansey J. A., McClay A. S., Cole D. E., Keddie B. A. (2005). Evidence for the influence of conspecific chemical cues on Aphthona nigriscutis (Coleoptera: Chrysomelidae) behaviour and distribution. Biocontrol 50, 343–358. doi: 10.1007/s10526-004-1232-3
Tomasetto F., Tylianakis J. M., Reale M., Wratten S., Goldson S. L. (2017). Intensified agriculture favors evolved resistance to biological control. Proc. Natl. Acad. Sci. 114, 3885–3890. doi: 10.1073/pnas.1618416114
Turlings T. C., Davison A. C., Tamò C. (2004). A six-arm olfactometer permitting simultaneous observation of insect attraction and odour trapping. Physiol. Entomology 29, 45–55. doi: 10.1111/j.1365-3032.2004.0362.x
Van Tol R. W. H. M., Van Dijk N., Sabelis M. W. (2004). Host plant preference and performance of the vine weevil Otiorhynchus sulcatus. Agric. For. Entomology 6, 267–278. doi: 10.1111/j.1461-9555.2004.00232.x
Van Tol R. W. H. M., Visser J. H., Sabelis M. W. (2002). Olfactory responses of the vine weevil, Otiorhynchus sulcatus, to tree odours. Physiol. Entomology 27, 213–222. doi: 10.1046/J.1365-3032.2002.00288.X
Vereijssen J., Goldson S. L. (2016). Caging overrides effects of density in laboratory-based physiological estimates of reproductivity in Listronotus bonariensis (Coleoptera: Curculionidae). Austral Entomology 55, 154–162. doi: 10.1111/aen.12163
Williams C. L., Goldson S. L., Baird D. B., Bullock D. W. (1994). Geographical origin of an introduced insect pest, Listronotus bonariensis (Kuschel), determined by RAPD analysis. Heredity 72, 412–419. doi: 10.1038/hdy.1994.57
Keywords: Listronotus bonariensis, still-air olfactometry, HIPVs, ryegrass, diapause, relict behaviour, Lissorhoptrus oryzophilus, phenylacetaldehyde
Citation: Vereijssen J, van Tol R, Rostás M, van Koten C and Goldson S (2024) Olfactometer-measured responses of Listronotus bonariensis (Kuschel) (Coleoptera: Curculionidae) to feeding-induced grass volatiles and conspecific frass as influenced by the weevil’s seasonality. Front. Ecol. Evol. 12:1470023. doi: 10.3389/fevo.2024.1470023
Received: 24 July 2024; Accepted: 30 October 2024;
Published: 19 November 2024.
Edited by:
Andrea Sciarretta, University of Molise, ItalyReviewed by:
Rob Morrison, Agricultural Research Service (USDA), United StatesMarco Colacci, University of Molise, Italy
Copyright © 2024 Vereijssen, van Tol, Rostás, van Koten and Goldson. This is an open-access article distributed under the terms of the Creative Commons Attribution License (CC BY). The use, distribution or reproduction in other forums is permitted, provided the original author(s) and the copyright owner(s) are credited and that the original publication in this journal is cited, in accordance with accepted academic practice. No use, distribution or reproduction is permitted which does not comply with these terms.
*Correspondence: Stephen Goldson, c3RlcGhlbi5nb2xkc29uQGFncmVzZWFyY2guY28ubno=
†Present address: Jessica Vereijssen, The New Zealand Institute for Plant and Food Research Limited, Christchurch, New Zealand Michael Rostás, Department for Crop Sciences, Georg-August-Universität Göttingen, Göttingen, Germany Rob van Tol, Bug Research Consultancy, Maastricht, Netherlands