- 1Forestry and Game Management Research Institute, Jíloviště, Czechia
- 2Department of Game Management and Wildlife Biology, Faculty of Forestry and Wood Sciences, Czech University of Life Sciences Prague, Prague, Czechia
- 3Department of Ecology, Faculty of Environmental Sciences, Czech University of Life Sciences Prague, Prague, Czechia
- 4Norwegian Institute for Nature Research, Lillehammer, Trondheim, Norway
The long-term decline of the black grouse population throughout Europe is influenced by many factors that affect populations differently depending on the distribution range, from Central Europe to the Scandinavian countries. Therefore, we analyzed available literature sources to describe the main reasons for the population decline of this species and to suggest conservation measures. In total, 228 pieces of literature from 1955 to 2024 were analyzed for this study. Based on the literature analysis, it is clear that the drivers of population decline differ across the distributional range. In Central Europe, where the population is declining rapidly, habitat loss and forest fragmentation are crucial factors, as is the negative impact of tourism. In Scandinavia, where the population is gradually declining, decreasing breeding success and increasing chick mortality rates are generally considered the main negative factors. However, these factors also affect black grouse populations in Central Europe. It is crucial to acknowledge that a significant proportion of the contributing factors, such as predation and habitat loss, can be attributed to human activities. Therefore, it is necessary to emphasize that environmental protection should work hand in hand with wildlife managers to improve the situation.
1 Introduction
The black grouse (Lyrurus tetrix) is one of the forest grouses classified as a “least-concern species.” The European population is estimated at 2.45–4.08 million adult individuals (BirdLife International, 2022; IUCN, 2023). The native range extends from Great Britain through Russia to North Korea and from Fennoscandia to the Alps, with scattered populations in Central Europe (Cramp and Simmons, 1977; BirdLife International, 2022). Black grouse were also introduced to Canada and the United States of America, with the global population of adult individuals between 8–14 million (BirdLife International, 2022). However, the abundance varies considerably across the extension range worldwide. The black grouse is facing a population decline, especially in the countries of Western, Central and Eastern Europe, which have experienced a significant decrease in numbers over recent decades (Tomiałojć, 1990; Murin et al., 1994; Ludwig et al., 2009b; Merta et al., 2009; Chobot and Němec, 2017). In some countries, individuals are barely surviving (Höglund et al., 2007; Larsson et al., 2008; Watson and Moss, 2008; Segelbacher et al., 2014; Rutkowski et al., 2018). In Fennoscandia, the center of the European distribution range, the black grouse remains a hunted species despite a moderate long-term population decline (Spidsø et al., 1997; Storch, 2000, 2007; Jahren et al., 2016; Zhang et al., 2024).
The reasons for population declines vary throughout the distribution range, often differing on the local scale, due to the influence of many factors. The black grouse is a species with specific environmental requirements and is highly sensitive to its changes (Rolstad et al., 2009). Habitat loss, among other things, is considered one of the most important reasons for population decline, primarily in Central Europe (Doenecke and Niethammer, 1970; Loneux and Ruwet, 1997; Ludwig et al., 2009b; Gimmi et al., 2011; Strauß et al., 2018). Changes in population density lead to an alternative way of reproduction, where solitary lekking occurs, and mating success decreases (Höglund and Stohr, 1997; Geary et al., 2012; Nelli et al., 2016). Furthermore, there is a confirmed decrease in nesting success and an increase in the mortality of both chicks and adults (Spidsø et al., 1997; Storch, 2000, 2007; Merta et al., 2009; Pekkola et al., 2014; Viterbi et al., 2015; Jahren et al., 2016; Cukor et al., 2021). In general, habitat changes, predation, and changes in climatic conditions appear to be the main negative factors affecting black grouse. A considerable threat is also caused by tourism, recreation pressure, and other human activities, including forest management practices, which negatively affect both the reproduction period and the survival of individuals during the winter due to increased energy expenditures and much higher visibility to predators during the disturbances (Storch, 2000; Baltić et al., 2004; Arlettaz et al., 2007, 2013; Ludwig et al., 2008; Zeiler and Grünschachner-Berger, 2009; Immitzer et al., 2014; Formenti et al., 2015; Coppes et al., 2020; Tost et al., 2020). This review summarizes existing studies on the status of black grouse populations from Western and Central Europe to Fennoscandia. It identifies the current primary causes of the population decline. The partial aims of this study are (i) to summarize the available published studies dealing with the decline of the black grouse population, (ii) to point out the main reasons for the decline based on the literature, and (iii) to suggest options for the fundamental principles of population support.
2 Methods
This article focuses on the literature review of previously published sources on black grouse population dynamics and reasons for population decline. Online web search engines, including Web of Science, Scopus, Google Scholar, and ResearchGate, were used to gather information on the subject under study. Non-peer-reviewed sources (popular articles, media reports, unverified sources, etc.) were excluded from the study. The literature related to black grouse topic was searched based on a combination of the following keywords: black grouse, population dynamics, climate change, decline, land use change, mortality, survival rate, chick mortality, nest success, and predation. Document types were restricted to articles, books, conference proceedings, internet sources, statistics and other sources (including dissertations, technical reports, research reports, etc.), and the time period included years 1955-2024.
Based on the main issues addressed in the literature, we further divided the evaluated literature into three categories according to whether the negative impact on the black grouse population referenced in the articles can be reduced or eliminated by human management intervention. The literature in the first category indicates that an improvement can be observed in a relatively short period of time, potentially within a few years after the implementation of the measures (“short-term influence possible”). We have included topics related to human disturbance, tourism and habitat change in the first category because human disturbance and tourism can be reduced to a minimum in a relatively short period of time and habitat change is also feasible (of course, depending on the scale). The second category includes publications which consider reduction or elimination of the negative effects by management intervention within a short time-scale of a few years impossible (“short-term influence not possible”). In the second category, we have included topics related to population dynamics, reproduction, predation, parasites and climate change, because we believe they are impossible to solve and eliminate in the short time frame of a few years. The third category consists of publications, which do not directly address the negative impacts to black grouse populations. The third category includes behavioral topics, methodology, general information websites, genetics and acoustics studies and habitat catalogues (Figure 1). Our search returned 228 references included in this review, of which 176 were scientific articles from the Web of Science or Scopus databases, 22 were chapters from scientific books, eight were conference proceedings, four were internet sources, 11 were other sources (dissertations, technical reports, research reports, etc.) and seven sources were statistics on population dynamics. All sources found are listed in the references. The number of publications per year (Figure 2A) and the overview of the different types of sources are shown in Figure 2B.
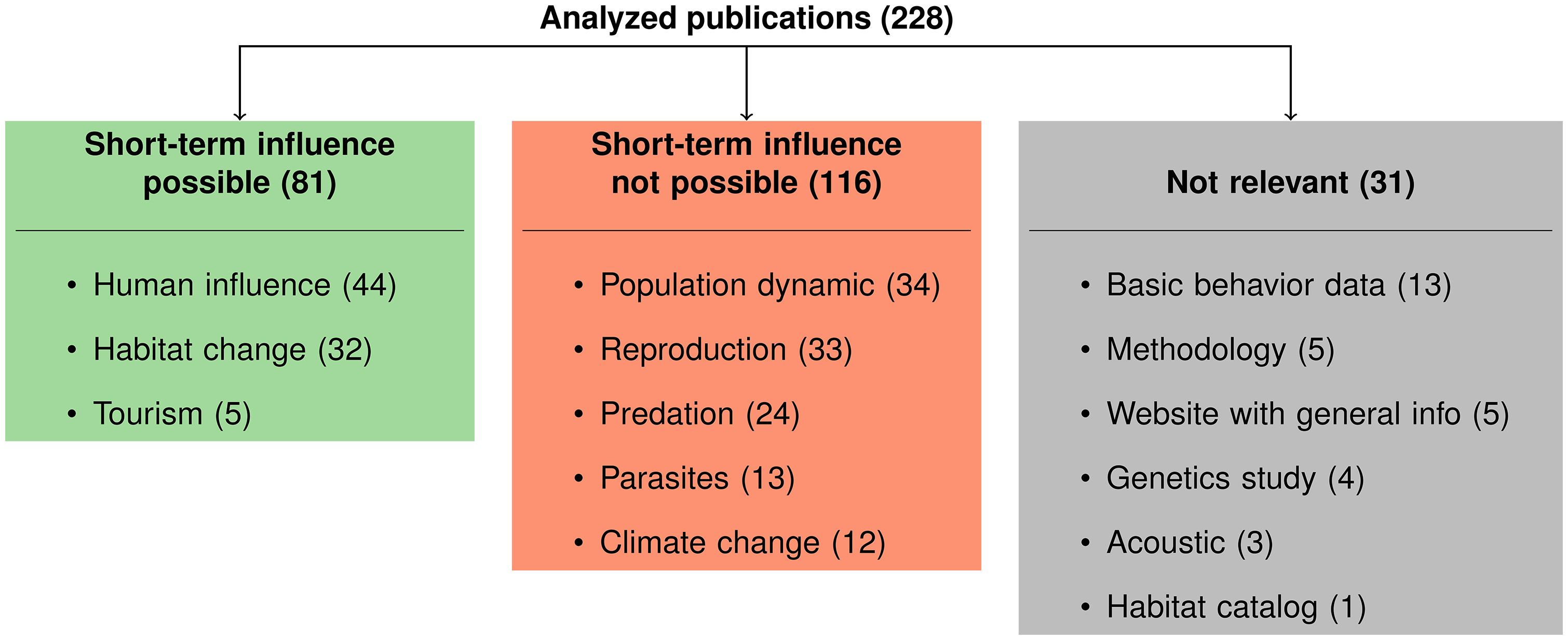
Figure 1. Division of article topics into three categories according to the possibility of human influence.
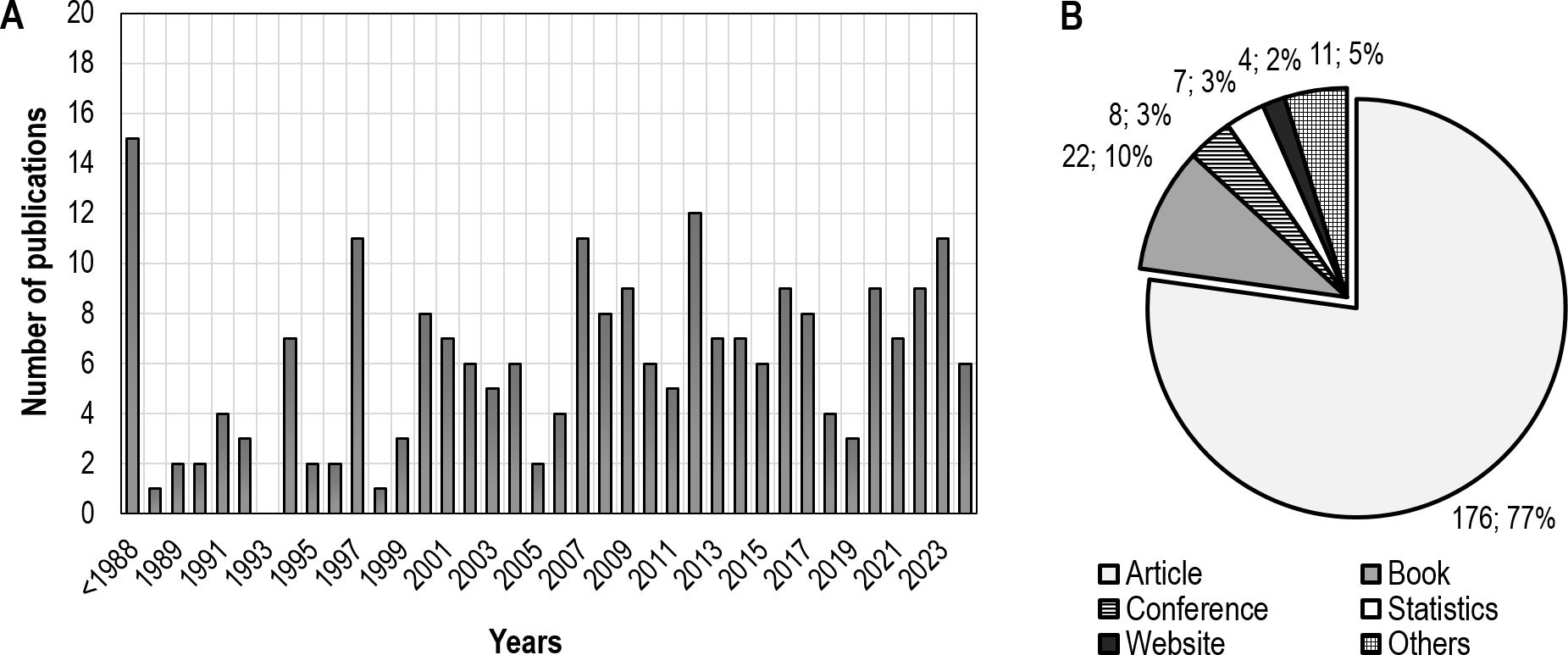
Figure 2. Temporal distribution of 228 literature sources from 1955 to 2024 on the black grouse population dynamics and reasons for population decline (A), and the proportion of references by source [scientific articles, professional books, conference proceedings, and other sources; (B)].
For European countries where black grouse is still a hunted species, hunting bag counts have been collected. The hunting bags data are considered as the most reliable data source from hunting statistics compared to the number of counted individuals (Kahlert et al., 2015). Hunting trends for black grouse in Sweden, Finland, Norway, Austria, and the Czechia alongside with particular linear regression results are shown in Figure 3. Each subplot contains p-value for hypothesis of slope equal to zero and R2 value. Computations were performed in R software (R Core Team, 2023), plot was created using its package ggplot2 (Wickham, 2016).
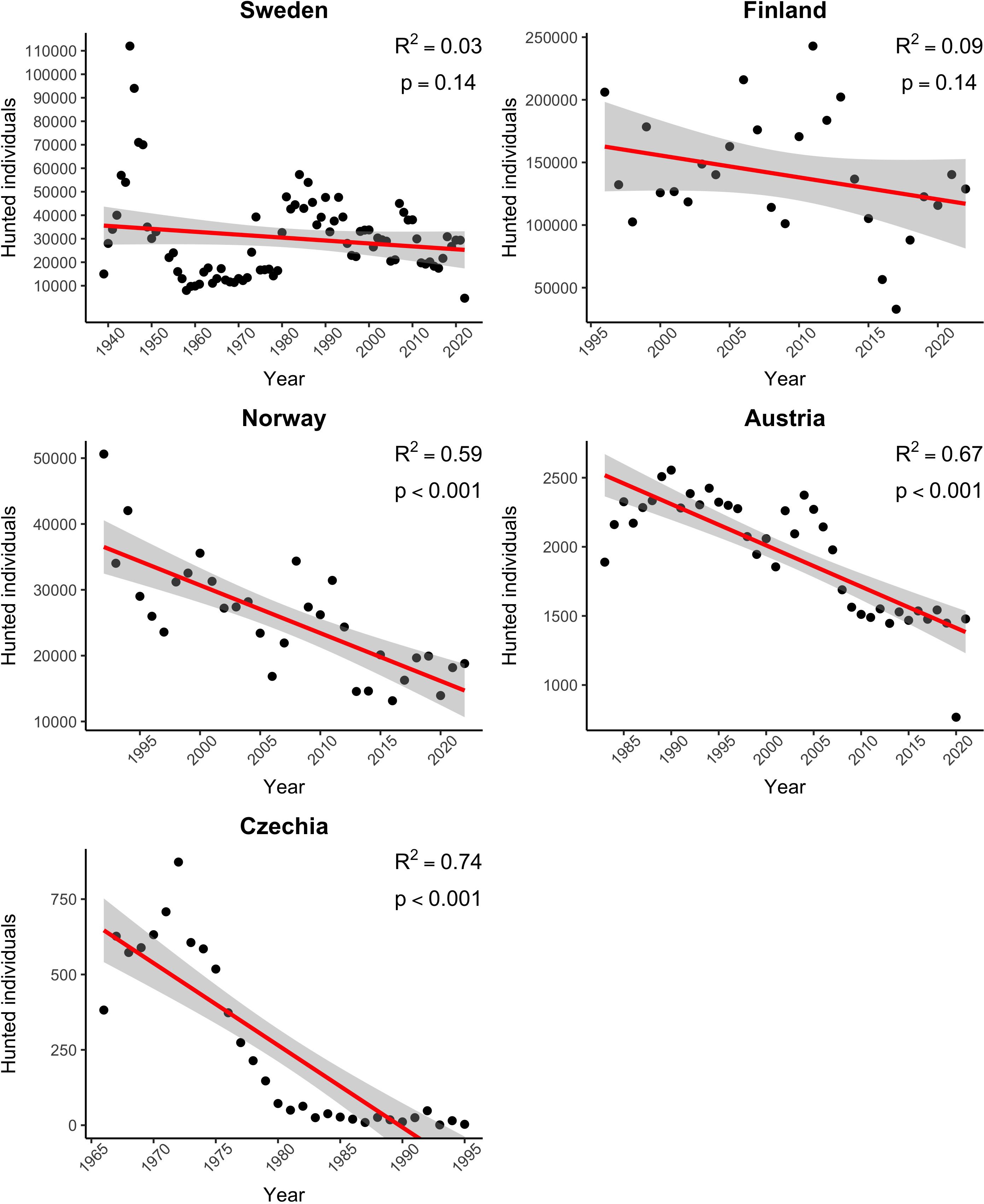
Figure 3. Declining trends in the number of black grouse hunted in Sweden, Finland, Norway, Austria, and Czechia (Statistik Austria-Federal Institution under Public Law, 2023; Statistics Finland, 2023; Svenska Jägareförbundet, 2023; Statistisk Sentralbyra, 2023; The Forest Management Institute Brandýs nad Labem, 2022).
3 Occurrence and population trends
In Europe, the breeding population of the black grouse is estimated to range between 792,000 and 1,030,000 calling/lekking males. However, there is a long-term population decline (European Environment Agency, 2016). European countries, where the black grouse is still present, can be roughly divided into three separate areas according to the population density. In the countries of Western, Central and Eastern Europe, the black grouse population is considered to be threatened. These countries include Austria, Italy, Czechia, Germany, Estonia, France, Belgium, Lithuania, Latvia, Poland, Belarus, Slovenia, Slovakia, and Great Britain (Ruwet et al., 1997; Danko et al., 2002; Sim et al., 2008; European Environment Agency, 2016; Dos Santos et al., 2021; Ķerus et al., 2021; Statistik Austria-Federal Institution under Public Law, 2023; The Forest Management Institute Brandýs nad Labem, 2022; Delcourt et al., 2023; Tomášek et al., 2023). Countries with few individuals or even zero population are Denmark, the Netherlands, and Romania (European Environment Agency, 2016; Elts et al., 2013).
On the other hand, stable populations with only moderate declines are reported from Finland, Sweden, and Norway (Statistics Finland, 2023; Svenska Jägareförbundet, 2023; Statistisk Sentralbyra, 2023). Although black grouse are still hunted there and population densities are sufficiently high, declines have been reported also in these Fennoscandian countries, mainly due to nest predation and an increase in mortality of chicks and subadults (Spidsø et al., 1997; Storch, 2000, 2007; Jahren et al., 2016).
There are also countries with only scattered information about their black grouse population, e.g., the history of records from Lithuania contains considerable data from 1948–2017, when the initial population size was more than 10 thousand black grouse, while the last record indicates only 1750 individuals (BirdLife International, 2023); available records in Ukraine cover the period between 1999 and 2007 when around 13,000 individuals were hunted (Ukrainian Nature Conservation Group, 2009). In Hungary, black grouse were hunted until the 19th century but also became extinct. The last record comes from 1966 (Pluhar et al., 2010). The same fate also befell the black grouse in Bulgaria, where, like in many other countries, it is registered on the Red List (Boev, 1997; Michev et al., 2011). Positive long-term growth of the black grouse population is taking place only in Latvia and Slovenia (except Fennoscandia). In other countries, the grouse is either stable or declining over the long term (European Environment Agency, 2016). Whether lesser or greater attention is paid to the black grouse in the countries mentioned above, the return, stabilization, or even an increase in its population is not easy, as it is a species that requires specific conditions to flourish.
4 Reproduction process
The stability of the black grouse population is directly dependent upon the reproduction process, which is influenced by the course of the mating performance itself (Jahren et al., 2016). At the beginning of the breeding season (March), but sometimes also in the autumn, promiscuous or polygamous males maintain typical leks (Baines, 1996; Rintamäki et al., 1999). Leks are usually found in open swamps, clearings, fields, or frozen lakes but never far from the forest (Koivisto, 1965; Alatalo et al., 1991; Rolstad et al., 2009). However, habitat selection is related to population density (Nelli et al., 2016). On the leks, females select males based on morphological, behavioral, or spatial characteristics (Alatalo et al., 1992; Höglund et al., 1997; Kokko et al., 1998; Rintamäki et al., 2001).
Female grouse choose the largest leks, and on them, the most dominant and vital males who fight the most (Alatalo et al., 1991, 1992, 1996; Hovi et al., 1994). As a result, only a few dominant males from the entire lek have the opportunity to mate, while most do not mate at all (Höglund and Alatalo, 1955; Lebigre et al., 2007). An interesting finding is that older females tend to mate with the same male as in previous years, even though the male is no longer the most vital (Rintamäki et al., 1995). Apparently, attractiveness also seems to play a role, related to the plucking of feathers from undertows during fights. Fewer plucked feathers indicate greater dexterity in the male, increasing its chances of success (Alatalo et al., 1991). On the other hand, ornaments of males, e.g., the length of their lyre-shaped tail and color of plumage, probably have only a complementary effect on the female’s choice (Höglund et al., 1992, 1994; Rintamäki et al., 1997, 2002; Siitari et al., 2007; Hämäläinen et al., 2012; Kervinen et al., 2016).
An essential part of the mating performance is the male acoustic manifestation. For male grouse, four types of vocalizations are typical (Cramp, 1983). These are resonant “rookooing,” similar to the cooing of a dove, a “hissing call,” further subdivided into four types (a wailing hiss, a hiss accompanying flight, a relatively long hissing call, and an arrhythmic hiss during fights), as well as tones of aggression and warning calls. Females emit cooing calls, tones during flight, and other sounds, which are divided into five types according to the situation (they relate to social contacts between individuals, with chicks, and, last but not least, warning calls). Rookooing is probably the most important during the mating performance, as females prefer leks with more than one male, and weaker male individuals may sometimes benefit from the presence of other rookooing males according to hotshot hypothesis (Hovi et al., 1997).
The lekking behavior is typical for well-established populations with high densities of birds. Males that move closer to the center of the lek become more successful (Kervinen et al., 2012). The selection of a place during the mating performance then depends on two factors: the position of the male on the imaginary social hierarchy and their patient waiting for a place to become available. High-ranking males maintain their territories for several years, but random shifts of weaker individuals are not excluded, as the stronger ones do not need to change their position to be closer to the center of the lek (Kokko et al., 1998). On the contrary, less preferred males take advantage of the eventual release of territorial positions, caused by mortality between seasons, to move closer to the center of the lek (Rintamäki et al., 1995). In Sweden, they noted a 15% probability of movement of males between leks, more so in younger individuals. It can be said, therefore, that grouse males show habitat fidelity during the mating season, but also across seasons. Thus, annual home ranges represent enlarged territories used during the mating season (Borecha et al., 2017). Leks are distributed irregularly at shorter distances of 1–2 km, according to habitat characteristics and the black grouse population density (Rolstad et al., 2009). The size of the lek can then affect its lifespan and resistance (Geary et al., 2012).
In the past, the leks were visited by large groups of males, but nowadays, the number is lower, and solitary males also appear (Höglund and Stohr, 1997). In recent years, there have been studies describing non-lekking populations in which males attract females with solitary displays (Höglund and Stohr, 1997). Non-lekking populations can be found, for example, in Sweden, Italy, Germany, and the Czechia (Höglund and Stohr, 1997; Zeitler, 2000; Angelstam, 2004; Svobodová et al., 2011b; Chamberlain et al., 2012; Rutkowski et al., 2018). Populations with solitary leks have lower genetic diversity than sites where birds aggregate at leks and may experience a reduction in effective population size and an overall decline in abundance (Svobodová et al., 2011b). Thus, the fragmented black grouse population in Central Europe seems to have lost genetic variation compared to those incomparably larger populations in Fennoscandia (Caizergues et al., 2003; Höglund et al., 2007; Svobodová et al., 2011b). Therefore, it seems that the low population density with non-lekking behavior represents an alarming risk for the black grouse population, not only from the point of lower and ineffective reproduction rate but also the risk for population sustainability from the perspective of genetic variability and black grouse conservation.
5 Factors affecting black grouse populations
5.1 Habitat loss
One of the crucial factors affecting black grouse populations is undisputably the loss of suitable habitat (Pearce-Higgins et al., 2007; Geary et al., 2015; Strauß et al., 2018; Tomášek et al., 2023). The authors agree that black grouse, like many other protected wildlife species, must deal with anthropogenic changes in habitats (Storch, 2000; Tost et al., 2020). For example, the abandonment of traditional extensive management (e.g. mowing or cattle grazing) of mountain meadows and pastures leads to the loss of suitable habitats through succession (MacDonald et al., 2000; Lešo et al., 2024). Habitat selection is a fundamental aspect of animal ecology, the understanding of which is essential for wildlife management and conservation (Northrup et al., 2013). Loss of suitable habitats can be a leading factor in time-delayed extinctions, as the current occurrence of species may instead reflect historical wetland cover and habitat configuration (Gimmi et al., 2011; Pearce-Higgins et al., 2016). In the past, grouse populations were dependent on extensive farming. As a result, most native subpopulations became extinct due to habitat loss caused by the large-scale conversion of heathland and peatland to forest, farmland, and pasture (Ludwig et al., 2009b). These phenomena began to occur most intensively around the 1950s and are considered the foremost causes of the loss of numerous grouse habitats from the Central European cultural landscape (Doenecke and Niethammer, 1970; Loneux and Ruwet, 1997). Until then, extensive land use with heathland and sheep farming provided optimal habitat conditions (Storch, 2007). Studies in Finland have shown that black grouse breeding success deteriorates as the proportion of agricultural land increases (Kurki et al., 2000) and the conditions for predators improve (Angelstam et al., 2000). Consequently, the renaturation of wet habitats not only within existing grouse habitats but also within dispersal distance is probably one of the most important measures to strengthen and increase black grouse metapopulations (Roos et al., 2016; Tost et al., 2022). Regarding forest habitat, when a new stand is established, it is possible to create new grouse sites, but, as the stand eventually closes, grouse leave it (Kurhinen et al., 2009). Therefore, it is recommended to produce smaller and more permanent forests, to focus on the protection of peatlands, and to carry out small-plot harvesting (White et al., 2013).
5.2 Changes in vegetation composition and structure
The demands on the structure and diversity of black grouse habitats are the subject of a long-term and multifaceted study (e.g., Müller, 1974, 1979; Scherzinger, 1976; Eiberle, 1976; Koch, 1978; Glänzer, 1980; Porkert, 1982; Klaus, 1991; Andrén, 1994; Baines, 1994; Glutz von Blotzheim et al., 1994; Calladine et al., 2002; Angelstam, 2004; Baines et al., 2007; Ludwig et al., 2008, 2009a, 2009b; Wegge and Kastdalen, 2008; Signorell et al., 2010; Patthey et al., 2012; Schweiger et al., 2012; Immitzer et al., 2014; White et al., 2015; Nelli et al., 2016; Strauß et al., 2018; Tost et al., 2020, 2022). These works show that a structural mosaic of both tree, shrub, and herb layers, as well as the relief of the soil, is crucial for the black grouse stability or even population increase. This structure allows grouse to use different properties of stands and soil in terms of microclimate, food opportunities, and shelter supply (Porkert, 1973; Šálek et al., 2004; Svobodová et al., 2004; Patthey et al., 2012). In the herb layer, the ratio of different age structures of Vacciniaceae (especially Vaccinium myrtillus, Vaccinium vitis-idaea, Vaccinium uliginosum) or Ericales (Calluna vulgaris, Erica tetralix, E. carnea) is of great importance (Porkert, 1982; Ludwig et al., 2010). Furthermore, interspersed areas covered with bryophytes and ferns, including some Cyperales and Juncales, or only with sedge, are crucial in the biotope structure (Porkert, 1973, 1976, 1980). From the point of forest stand management, small-scale management methods with natural renewal are preferred, which create a mosaic structure of stands (Poleno et al., 2009) and offer an optimal food and shelter in a suitable microclimate (Porkert, 1982, 1991).
The mosaic forest stands with a high proportion of open areas without tree vegetation are critically important, especially in the intensively managed forests of Central Europe (Klaus, 1991; Ludwig et al., 2009b; Wegge and Rolstad, 2011). Open places in forest ecosystems were related to peat bogs, moorlands, or other stands with high groundwater levels. Unfortunately, most of them were drained off in the 20th century (Paavilainen and Päivänen, 1995; Sjöberg and Ericson, 1997; Skaggs et al., 2016). Therefore, many habitats were destroyed by spontaneous succession after drying up and became unsuitable for black grouse, especially in the lekking season (Ludwig et al., 2010). Contrarily, another large-scale habitat change, which was positive from the black grouse point of view, was caused by the immission calamity in Central Europe between 1970 and 1995. There was a temporary creation of favorable habitats for the growth of grouse populations because of Norway spruce diebacks in mountain areas and the opening of forest stands (Porkert, 1982; Flousek, 1989; Vacek et al., 2007). As a result of the involvement of these stands in their artificial restoration, this effect waned at the beginning of the 21st century (Svobodová et al., 2011a). Moreover, open habitats with solitary trees or stands with a loose canopy (pine, spruce, larch, birch, and rowan) are suitable for grouse to use as lookouts during mating season, as a source of food in winter and spring (Porkert, 1982; Patthey et al., 2012; Strauß et al., 2018; Tost et al., 2022). Loose conifer forests also support the occurrence of anthills as a staple food for grouse chicks (Signorell et al., 2010; Schweiger et al., 2012).
Maintaining or restoring high-quality habitats in semi-natural and anthropogenic landscapes is a fundamental issue in conservation biology, as habitat quality is one of the main determinants of reproductive performance and survival (Pärt, 2001; Vickery et al., 2001; Hirzel and Le Lay, 2008; Patthey et al., 2012; Schweiger et al., 2012; Northrup et al., 2013; Immitzer et al., 2014; White et al., 2015; Tost et al., 2022; IUCN, 2023). Suitable habitats within Europe are relatively diverse for the given dwelling and vegetation conditions (Patthey et al., 2012). This is mainly a mosaic of shrubby stands, peat bogs, wetlands, meadows, extensive pastures, and loose forest stands, which closely follow each other in such a way as to ensure the availability of all essential environmental parameters for the grouse (Ludwig et al., 2009a; Rolstad et al., 2009; White et al., 2015; Tost et al., 2022). Considering the landscape characteristics, type of forest management, and acreage of protected areas, it is evident that Scandinavia offers an incomparably larger array of suitable habitats than Central Europe (Cukor et al., 2022). From a phytocoenological point of view, in Europe, these are mainly fens and transition bogs, highlands, alpine and subalpine scrub vegetation, peat cranberry forests, dry heaths of lowlands and uplands, dry heather forests of continental bogs and peat, and waterlogged spruces (Chytrý et al., 2010). Therefore, the basic prerequisite for the conservation of the black grouse as an endangered species is the effectual care of its listed habitats per the European Union Directive No. 92/43/EEC on the conservation of natural habitats, wild fauna and flora, and Directive No. 2009/147/EC on the conservation of wild birds.
5.3 Climate change
In recent decades, we have observed phenomena associated with climate change that have had adverse effects on black grouse populations. The evidence of climate change is clear and unmistakable worldwide. In the Czechia, for example, the mean annual air temperature has increased by 2°C between 1961 and 2021 as a result of climate change Český hydrometeorologický ústav (2022) and the temperatures in Southern and Central Europe are predicted to increase by a further 2°C by 2047 (Geßler et al., 2007). The changing quality, duration, and height of snow cover, temperature fluctuations, and uneven distribution of precipitation are particularly important factors for the black grouse (Prellwitz, 2002; Ludwig et al., 2006; Canonne et al., 2021). All the factors mentioned show fluctuations in environmental conditions, which have detrimental effects on the laying date, breeding success, chick survival, and the size of black grouse populations (Ludwig et al., 2006; Bocca et al., 2014; Barredo et al., 2020; Canonne et al., 2021).
Black grouses are well adapted to long and harsh winters by their feeding habits and their ability to dig snow burrows (Marjakangas, 1992). The primary causes of black grouse decline in the Alps are thought to be a long-term reduction in snow cover, changes in snow quality and increasing average winter temperatures (Canonne et al., 2021). Frequent temperature changes and recurring rainfall in winter combined with the snow melting and freezing again, create layers of frozen snow, or sometimes, ice, which the grouse cannot burrow through (Bocca et al., 2014). In this case, the grouse is forced to overwinter outside the snow burrows, where it is more likely to be seen by predators (Flousek, 2019). The length of the winter also affects the number of natural predators of grouse in the mountains, particularly martens and foxes (Bartoń and Zalewski, 2007). It is highly advisable to monitor the long-term trends in snow conditions at sites where black grouse are present and to carefully monitor the effects of these changes on their populations.
An earlier onset of spring can lead to earlier mating, earlier laying of eggs, and a temporary mismatch with the optimum time for the offspring to hatch (Ludwig et al., 2006; Wegge and Rolstad, 2017). The first clutches are often destroyed by adverse weather, such as the return of winter and frequent weather changes (Ludwig et al., 2006, 2010). For example, in a Scottish study (Moss et al., 2001), the decline in capercaillie breeding success between 1975 and 1999 was associated with the mid-April cooling period. Conversely, some studies do not support the general idea that a warmer climate will negatively affect the reproduction of birds and animals in northern latitudes (Virkkala et al., 2008; Wegge and Rolstad, 2017). Instead, Wegge and Rolstad (2017) found that the reproductive output of capercaillie and black grouse increased with warmer and earlier springs. However, the authors admit that a warmer climate may have negative effects, for example, on the survival of adult birds, which can moderate the positive effects on the reproductive process.
In recent years, climate change could have caused a mismatch between the timing of breeding and the amount of food available to chicks after they hatch (Visser et al., 2004). A warmer spring can lead to earlier nesting, but this timing may no longer coincide with the optimal amount of insects available, which could lead to poorer chick survival (Ludwig et al., 2006; Wegge et al., 2010). Another scenario can also take place. High spring temperatures can accelerate the development of invertebrate larvae, which presents a threat to chicks by transforming their primary food source into an imago (adult) before the chicks have had time to hatch. This leaves them with insufficient basic food.
Another challenge for black grouse can be recurring high summer temperatures. Prellwitz (2002) found that greater sage-grouse (Centrocercus urophasianus) are less vigilant at high temperatures (above 30°C) because they spend more time and energy cooling down, which reduces their vigilance against predators. There is also a risk of dehydration at high temperatures, especially in chicks (Prellwitz, 2002). Similar problems may be expected with black grouse. Black grouse populations can respond to changes in climate both locally and regionally, with these changes being more pronounced in coastal areas than inland areas (Kvasnes et al., 2010; Viterbi et al., 2015).
5.4 Adult mortality
In general, adult mortality has been adequately studied in the past decades (e.g., Warren and Baines, 2002; Svobodová et al., 2011a; Pekkola et al., 2014), and it is evident that adult mortality plays a critical role in the black grouse population dynamics and the current population decline. The black grouse survival probability was predominately studied by radiotelemetry of tagged individuals (Spidsø et al., 1997; Warren and Baines, 2002; Bowker et al., 2007; Svobodová et al., 2011a; Pekkola et al., 2014), which also offers acceptable solutions for evaluation of mortality causes.
Previously published studies have shown that the annual survival rate varies from year to year, ranging from 0.53 to 0.72 (Warren and Baines, 2002; Pekkola et al., 2014), but some studies have reported higher mortality rates, e.g., in Norway, 72% of radio-tagged birds died during a 12-month monitoring period (Spidsø et al., 1997). Differences in mortality rates are caused by several factors, including the age of birds, climatic conditions, the density of the black grouse population, and the abundance of predators with fluctuations between years. For example, Warren and Baines (2002) estimated an annual survival rate for adult individuals of 0.72 (for both sexes), but the survival rate of yearlings was significantly lower (0.46) in the same conditions. The lower survival of young birds was also confirmed by Pekkola et al. (2014) for the black grouse females in Finland. Moreover, the survival probability between adults and yearlings differs throughout the year. In winter (September–February), the survival rate can be 0.54 for yearlings and 0.73 for older individuals. In contrast, during the summer period (March–August), it can be 0.95 for yearlings and 0.84 for older birds (Warren and Baines, 2002). From the wider perspective, the mortality of adult females is most challenging for the black grouse population because this represents a high risk for the reproduction process. Black grouse females mature at one year of age and can have a potential lifespan of five to six years in the wild. Unfortunately, female predation most often occurs during the spring mating and subsequent egg laying and incubation (Willebrand, 1988; Pekkola et al., 2014), and again, there is a clear difference between the survival of young and older females (Pekkola et al., 2014).
Regarding the causes of black grouse mortality, predation is a principal proximate mortality reason for adult black grouse individuals (Tornberg et al., 2006; Pekkola et al., 2014; Sein and Väli, 2024). According to previously published research, it is evident that the proportion of avian and mammalian predators varies along the black grouse distribution range according to predator species composition and natural conditions. In a large study from Finland, where 253 females were radio-tagged, predation was confirmed as the primary cause of all-natural mortality. From the total proportion, the avian predators were responsible for 51.8% of caused mortality, and 26.7% was classified to mammals (Pekkola et al., 2014). This confirms the general assumption that raptors are the main predators of adult grouse (Spidsø et al., 1997; Warren and Baines, 2002; Tornberg et al., 2012; Pekkola et al., 2014). However, the exact determination of predator species involved can be problematic in relation to the decomposition of the found carcass of the monitored individual (Caro and Girling, 2006).
Nevertheless, more specific species composition of black grouse predators has been published in several studies. In the study from North Wales, raptors again played a crucial role in black grouse predation when the goshawk (Accipiter gentilis) and peregrine falcon (Falco peregrinus) were responsible for 64% of the predation caused, followed by the red fox (Vulpes vulpes), which was found to be a predator in the remaining 36% (Bowker et al., 2007). The goshawk was also identified as a primary predator in Norway, where almost half (46%) of the radio-tagged birds were killed by this predator, followed by pine marten (Martes martes; 23%), and the rest (31%) were killed by unknown predators (Spidsø et al., 1997). Those findings are supported by the fact that forest grouse generally represents the favorite prey species of the northern goshawk (Valkama et al., 2005; Tornberg et al., 2006, 2012). For example, in certain winters, female grouse are the optimal prey for male goshawks (Tornberg, 2000; Tornberg and Colpaert, 2001). The description of the main predators of black grouse is well-known in the Scandinavian countries where it is possible to tag a sufficient number of black grouse individuals, as was done in the studies mentioned above (Spidsø et al., 1997; Tornberg, 2000; Bowker et al., 2007; Pekkola et al., 2014).
On the other hand, the data from radiotelemetry monitoring of black grouse in Central Europe indicate that mammalian predators are the primary cause of adult mortality (Svobodová et al., 2011a). Data on causes of mortality are available, e.g., from the Czechia (Svobodová et al., 2011a). The results confirmed an extremely low survival rate of tagged male grouse. Only 21% of the male grouse survived one year. Eight of the 12 tagged males were killed by predators within one year (67%). Seven of these were killed by a mammalian predator (88%) that could not be accurately identified. These results suggest that the goshawk is not a significant threat to adult grouse in the Ore Mountains (Krušné hory) study area in the Czechia. On the contrary, the main predators were martens and foxes, which can be controlled by hunting (Svobodová et al., 2011a). Survival rates of 93 radio-tagged black grouse in two populations in the southern French Alps were higher for females than males and tended to be higher for adults than juveniles. Most adult deaths occurred in spring (females and males) or early summer (males). The causes of mortality were as follows: raptors 51%, mammals 27%, unidentified predators 11%, and hunting 11% (Caizergues and Ellison, 1997).
Black grouse populations have declined throughout most of Europe (Caizergues and Ellison, 1997; TenDen and Niewold, 2001; Jahren et al., 2016), making it difficult to capture and tag birds on their breeding grounds. For example, in the Czechia, between 2000–2003 when grouse telemetry was executed, the population in the study area was relatively stable, with an abundance of 1.2–3 grouse/100 ha (Svobodová et al., 2011a). However, due to a decline in the local populations, it will no longer be possible to conduct further adult telemetry studies in Central Europe. The populations are already so weak that any threats, including adult trapping, can significantly impact the local subpopulation, and therefore, cannot be risked.
5.5 Breeding success and chick mortality
Large-scale population declines have been the result of low levels of reproductive success (Ludwig et al., 2006; Jahren et al., 2016; Zbinden et al., 2022). A comprehensive study by Jahren et al. (2016) showed a dramatic decline in grouse breeding success between 1934 and 2014 in Fennoscandia and Central Europe. Jahren et al. (2016) found that breeding success in Fennoscandia declined from 0.9 to 0.55 for black grouse and from 0.89 to 0.35 for capercaillie between 1934 and the present. In Central Europe, the number of broods per female decreased for both species. Conversely, in the British Isles, there were no significant changes in black grouse brood frequencies between 1934 and 2002. These results suggest that black grouse populations in Britain have been more productive than elsewhere for some time (Jahren et al., 2016). The breeding success of black grouse populations studied in Switzerland was monitored from 1981 to 2020 (Zbinden et al., 2022). The results showed that the long-term average reproductive rate was 2.0 chicks/hen in northern Ticino and did not show any decline. However, reproductive rates in central/southern Ticino declined in the early years of the study period and then stabilized at the level of northern Ticino. Reproductive rates were highly variable from year to year and were influenced by a combination of weather and habitat parameters (Zbinden et al., 2022).
Successful reproduction is significantly affected by predation of nests, which occurs in the first days after their establishment (Merta et al., 2009). Martens, foxes and corvids, but also wild boars (Sus scrofa), are the most common predators of nests (Svobodová et al., 2004; Cukor et al., 2021). Studies focused on grouse predation show its high variability related to factors such as the number of predators, the nature of habitats, the population density or hunting management (Svobodová et al., 2004, 2011a; Merta et al., 2009; Cukor et al., 2021). However, grouse eggs are not a principal food source for predators but are only an alternative seasonal prey item. The main prey of mammalian grouse predators in the boreal zone are rodents, whose abundance fluctuates strongly between years (Hansson and Henttonen, 1989; Wegge and Storaas, 1990). Thus, breeding success may vary depending on the abundance of small mammals in a given area. If there are insufficient small mammals, grouse nests and chicks become alternative prey for mammalian predators (Šálek et al., 2004). The reproductive success of grouse is positively correlated with the abundance of the main prey (rodents) and is well documented by data from Scandinavia (Hansson and Henttonen, 1989; Kurki et al., 1997; Tornberg et al., 2012), whereas clear evidence from Central Europe is lacking (Ježková et al., 2014; Matysek et al., 2020).
Many studies agree that post-hatch mortality of grouse is high, especially during the first year of life, and that predation is the primary cause of this mortality (Hannon and Martin, 2006; Park et al., 2008). Predation explained 68% of total chick mortality during the first month after hatching, with up to 90% of predation by avian predators in Wales (Johnstone and Lindley, 2003). Also, in Finland, avian predators pose a threat to grouse chicks, especially in years when rodents are scarce (Reif et al., 2001, 2004). During the breeding season, hawks predated about 6% of grouse chicks there, while buzzards predated about 4% (Tornberg et al., 2012). Approximately 36% of females lost their chicks within the first ten days after hatching, based on telemetric monitoring of chicks from 81 successfully hatched nests in Finland (Ludwig et al., 2010). However, the probability of chick survival, in this case, did not depend on the local abundance of martens and foxes during the previous winter, so the authors conclude that the chief cause of chick mortality here was not predation risk. In this study, the authors identify that the most salient predictor of chick survival is the bilberry cover, which provides food for the chicks as well as safe shelter from predators (Ludwig et al., 2010), and that food availability for chicks is particularly important during the post-hatching period (Wegge et al., 2022). Other limiting factors for black grouse chicks are unfavorable weather conditions, especially sudden temperature changes, low temperatures or snowfall during the critical post-hatching period, which have a major impact on their survival rate and therefore impact to whole reproduction process (Rotelli et al., 2021).
5.6 Parasites and diseases
Parasites are another reason for the decline in the number of black grouse. Although they do not cause pronounced and sudden population declines, they have shown to affect population dynamics and the survivability of grouse (Isomursu et al., 2017). A higher parasitic incidence is observed in artificially reared individuals (Sokól and Pluta, 2022) compared to individuals in the wild (Strauß et al., 2022), while the decreasing population density and distribution of the grouse is characterized by a decrease in parasitic diseases (Coates et al., 2017). For an individual, parasites can increase the stress hormone level, as it affects the organism’s resistance (Berto and Lopes, 2020). The most common group of grouse parasites are coccidia, specifically protozoa from the genus Eimera (Jankovská et al., 2012; Sokól and Galecki, 2018; Sokól and Koziatek-Sadlowska, 2020; Tizzani et al., 2021; Sokól and Pluta, 2022; Strauß et al., 2022) and the coccidia Cryptosporidium baileyi, first recorded in England in 2010 (Coldwell et al., 2012). There are also nematodes in grouse, e.g., the species Capillaria caudinflata (Formenti et al., 2013; Fanelli et al., 2020; Tizzani et al., 2021; Sokól and Pluta, 2022), Trichostrongylus tenuis, Heterakis spp., and Syngamus trachea (Jankovská et al., 2012; Strauß et al., 2022) and, last but not least, flatworms and borers (Jankovská et al., 2012; Tizzani et al., 2021).
5.7 Recreation pressure and human disturbances
Disturbance caused by human outdoor recreation and tourism activities is increasingly viewed as threatening to wildlife (Storch, 2013). An inescapable factor negatively affecting the distribution and abundance of black grouse individuals is human activity, associated, for example, with the construction of wind farms in areas that may largely overlap with locations suitable for black grouse (Zeiler and Grünschachner-Berger, 2009; Coppes et al., 2020). These construction projects mainly cause a change in the distribution and use of grouse leks in the given location. For example, the construction of a wind power plant itself did not affect the behavior of male black grouse in the lek sites in the Austrian Alps, however, the number of lekking males in the monitored lek site decreased from 12 recorded individuals before the construction to zero two years after construction (Zeiler and Grünschachner-Berger, 2009). A similar reduction of lekking males was recorded in Scotland, when before and during the construction of the wind farm, they recorded nine and eight males, respectively, yet throughout the two years after the construction, during the monitoring of the same lek site, only four females were found, but no lekking males (Percival et al., 2018). On the other hand, Zwart et al. (2015) did not record a significant decline in males after the construction of a wind farm at seven lek sites in Scotland. The lek sites were originally located within 500 m (n = 4, median distance = 250 m) of the wind farm but moved up to 803 m (median distance) further from the wind turbines. In addition, this structure located near the lek and breeding area can also directly cause the mortality of black grouse individuals (Wöss and Zeiler, 2003; Zeiler and Grünschachner-Berger, 2009; Coppes et al., 2020). For example, in Austria, within two years, mortality was recorded in five individuals, which was most likely caused by a collision with the tower of the wind power plant itself, not with the wind turbines, as this species usually flies only up to approx. 27 m above the ground (Zeiler and Grünschachner-Berger, 2009). However, it is still not clear whether there is a higher number of collisions and thus increased mortality, which could negatively affect the abundance of the local population of this species (Coppes et al., 2020).
Human activities are also causing other significant land-use changes. On the one hand, traditional grazing on forest pastures is being abandoned, leading to the overgrowth of these habitats with scrub and forest. On the other hand, outdoor winter recreation (as well as summer recreation) is critically expanding. Ski infrastructure is damaging fragile mountain habitats and snow sports causing disturbance and stress to wildlife (Patthey et al., 2012; Storch, 2013) which is, in case of black grouse, essential to minimize energy expenditures of overwintering birds. Negative impacts of human disturbance have been documented on the reproduction success (Ingold, 2005; Storch, 2013; Rutkowski et al., 2018) but also on the distribution and survival of adult individuals of the black grouse. These effects are resulting to the stress and energy expenditure associated with tourism in the form of outdoor activities, skiing, mountain biking, walking in nature, photography or gathering forest fruits (Storch, 2000; Baltić et al., 2004; Arlettaz et al., 2007, 2013; Immitzer et al., 2014; Formenti et al., 2015; Jäger et al., 2020; Tost et al., 2020). In addition, every disturbance means the necessity of unexpected movement of threaten individuals and therefore also increased risk of crash to the iron fence used in forestry or other obstacles in the terrain (Baines and Andrew, 2003). Long-term tourism in a given location can also cause a change in the behavior of males, who may, for this reason, switch to a solitary lekking pattern (Zeitler, 2000). For example, Arlettaz et al. (2007) found sustained increases in the stress hormone corticosterone over three days of up to 60% in three captive individuals exposed to a single daily disturbance during the experiment. Similar results were obtained by Formenti et al. (2015), who found a higher level of stress hormones in winter in locations that were more frequently used by people for winter sports. Due to this, the black grouse can exert more energy for thermoregulation after leaving their snow burrows (igloos), but also for escape in wintering individual or when searching for and creating new snow burrows. This activity, in turn, increases the probability of detection by a predator (Arlettaz et al., 2007; Formenti et al., 2015). Additionally, Baltić et al. (2004) found that disturbed birds increased their foraging duration by up to 23% during the morning, while predation risk for these disturbed individuals increased by up to 12%. Immitzer et al. (2014), in turn, found that the presence of used hiking trails during the summer reduced the probability of black grouse occurrence in potentially suitable habitats within 50 m by up to 93%. Similar results were also found in the study by Tost et al. (2020), who also noted the effect of tourist routes on the distribution of black grouse. Marked individuals avoided heavily used trails, and the use of these habitats varied throughout the day, with the presence of marked individuals near the trail mostly recorded at night and dawn, but grouse avoided these habitats during the peak human activity phase around midday and afternoon (Tost et al., 2020). As can be seen from these studies, human activity can impose a significant effect on the physiology, behavior, and demography of the black grouse, causing, together with other factors, the reduction of the population of this species throughout Europe.
6 Key actions to support black grouse populations
6.1 Forest management
In times of ongoing climate change, carbon sequestration by forests is a crucial factor for mitigation (Bellassen and Luyssaert, 2014; Cukor et al., 2017; Vacek et al., 2023a). On the other hand, extensive afforestation (especially moorlands) harms black grouse populations (Baines, 1996; Pearce-Higgins et al., 2007; White et al., 2015). As grouse have adapted to dynamic environments with changing mosaics of different successional stages, they are sensitive to anthropogenically homogenized and human-disturbed habitats (Angelstam, 2004; Ludwig et al., 2008; Immitzer et al., 2014; Tost et al., 2020).
In terms of forest management and silvicultural practices, small-scale management practices using shelterwood methods and selection principles, preferably with natural regeneration, create a mosaic structure of stands which is favored by grouse (Poleno et al., 2009; Vacek et al., 2023b). The recommended measures include a reduction in planting density (Baines et al., 2000; Scridel et al., 2017), tree planting in smaller patches over a longer time period, creation of small-scale heterogenous forest mosaics with the loose stand canopy and locally open landscapes (Grant et al., 2009; Tost et al., 2022; Mazziotta et al., 2024). It is also important to restore and promote wet habitats, peat bogs, and waterlogged forest stands with the specific tree species composition, including alders (Alnus glutinosa, A. viridis) and willows (Salix caprea, S. cinerea, S. aurita) (Ramanzin et al., 2000; White et al., 2015). Furthermore, the reduction of tree natural regeneration and of young trees, (pioneer vegetation), preservation of solitary trees, such as birches (Betula pendula, B. pubescens), rowan (Sorbus aucuparia), pines (Pinus sylvestris, P. cembra, P. uncinata), larch (Larix decidua), and juniper (Juniperus communis) as food sources and shelter (Sim et al., 2008; Patthey et al., 2012; Bocca et al., 2014), thinning of forest stand edges and creation of transition gradients over several hundred meters (Sim et al., 2008), mixing pastures with habitat elements, such as loose shrub formations for cover (Zeitler, 2003; Signorell et al., 2010; Tost et al., 2022). Finally, reducing fencing in the forest to protect regeneration from game damage to prevent collisions leading to grouse mortality (Catt et al., 1994; Baines and Andrew, 2003; Stevens et al., 2012) and improving habitat connectivity (Andrén, 1994).
The ensuing steps in local grouse research are focusing on vegetation microhabitats by examining in detail the species composition and evaluating management practices, e.g., heathland burning and sheep grazing, which significantly affect forest regeneration and its tree and shrub species composition (Wegge and Kastdalen, 2008; Patthey et al., 2012). In addition, the overlap between the grouse habitats and the habitats of the most common predators is currently being investigated, thereby identifying conflict zones (Signorell et al., 2010). Some isolated and very small-scale forest planting on open ground may have a significant effect for predator attractiveness. Paradoxically, the decline of spruce stands over the last 20 years, particularly as a result of storm damage and bark beetle outbreaks, may have helped black grouse by accelerating the creation of clearings and the transformation of conifer monocultures into mosaic stands that suit them (Thorn et al., 2016). The current development of unmanned aerial vehicles (UAVs), or simply ‘drones’, offers an innovative way of efficiently mapping changes in black grouse habitat, as drones can be used to monitor larger areas, including those that are difficult to access by humans, which can help us better plan management measures (Han et al., 2017).
6.2 Game management
Game management includes several activities that can significantly help the black grouse population conservation. Primarily, the hunting of their main mammalian predators such as red fox, beech marten (Martes foina) and pine marten, European badger (Meles meles), but also occasional nest predators, such as wild boar should be intensified in designated black grouse areas. Invasive predator species, such as the raccoon dog (Nyctereutes procyonoides) and raccoon (Procyon lotor), are also a significant threat, and their numbers in grouse habitats are increasing every year (Cove and O’Connell, 2022; Holopainen et al., 2024). Reducing the abundance of these predators has a positive direct effect on black grouse breeding success and adult survival (Grant et al., 2009). However, it is imperative to focus on all mammalian predators on the site, not only foxes, for example because compensatory recruitment of unhunted predators would occur almost immediately, as shown in the study by Smedshaug et al. (1999). Hunting large ungulates also means leaving unnecessary entrails in forest ecosystems, inadvertently encouraging scavengers and predators to consume the remains and return to the area. In addition, high concentrations of wild boar and deer (family Cervidae) disturb black grouse and by grazing on bilberry vegetation, they also negatively affect the availability of food resources and safe cover for chicks. Thus, reducing high numbers of wildlife not only contributes to improved black grouse survival but also leads to a fundamental change in habitat suitability (Cole et al., 2012).
Another very effective measure, often not applied in practice, is a strict ban on feeding game animals in black grouse areas. Overfeeding has a direct impact on the abundance of target species, which are attracted to certain areas due to that. In upland areas, wild boars are often overfed, which leads to a higher predation rate of grouse nests in the feeding sites. This fact has been demonstrated by experiments with artificial nests (Selva et al., 2014; Oja et al., 2015; Cukor et al., 2021).
However, the primary risk of overfeeding is the potential indirect impact on the populations of the grouse’s main predator species. The food offered (even of plant origin) is also consumed by rodents, which increases the likelihood of the main predators being present in the area. This relationship was demonstrated in a study carried out in Poland (Selva et al., 2014). The probability of predation on artificial nests placed near feeding sites was 30% higher than on randomly placed artificial nests in the forest. In addition, artificial nests near feeding sites were found quickly by predators. When comparing photo-trap records, it was also found that feeding sites attracted more potential predators of grouse nests (82% of records) than the target species (8%, the rest of the records being other species). In this study, the authors confirmed evidence of increased predation pressure on artificially laid nests within 1 km of feeding sites (Selva et al., 2014). The study by Oja et al. (2015) also confirmed that the predation risk decreases with increasing distance from the feeding site. In addition, the predation risk level is affected after the end of the feeding period. In the case of the cessation of feeding at the feeding site, the risk of predation was higher for another four years. Therefore, it can be concluded that recently abandoned feeding sites still pose an increased risk of predation on black grouse nests and possibly chicks (Oja et al., 2015). The feeding of game animals to reduce their numbers must, therefore, be carried out in pre-selected places and to a limited extent. Otherwise, wild boar, as well as deer and other animals, may be concentrated in locations where they would not naturally appear (Ježek et al., 2013). Therefore, the feeding of game animals should not be allowed in core black grouse areas, especially during the breeding and winter seasons.
6.3 Monitoring populations
Monitoring the distribution, abundance, density, and presence or absence of the watched species are critical indicators describing how populations respond to environmental changes (Williams et al., 2002; Egwumah et al., 2017). Systematic and long-term monitoring of changes and trends in populations can effectively help protect declining or endangered species, document the effectiveness of conservation measures, and also reveal negative impacts on populations of monitored species associated with anthropogenic activity (Goldsmith, 2012). That is why monitoring the abundance and distribution of the black grouse has been—and still is—a vital tool that contributes to the conservation of this species throughout Europe.
However, monitoring methods are diverse (Haider et al., 2024). The most commonly used method is based on the visual and acoustic recording of male grouse by a given observer in the field (Hancock et al., 1999; Dumont et al., 2019; Policht et al., 2019). Monitoring of lekking males is carried out two hours before and no later than two hours after sunrise (Cayford and Walker, 1991; Canonne et al., 2021) and in suitable weather conditions, to avoid distortion or underestimation of the abundance of the monitored population (Hancock et al., 1999; Canonne et al., 2021). Another method, used mainly in northern regions, is counting grouse on pre-marked linear transects with the help of trained dogs that systematically search the surroundings on both sides of the transect and with the help of a mobile application that automatically recalculates the detected abundance for the entire area of interest (e.g., Wegge and Rolstad, 2011; Cukor et al., 2020). Among the non-invasive methods of monitoring black grouse is the observing of local lek sites using camera traps. Compared to traditional monitoring methods, large amounts of data can easily be collected over a relatively long period. For example, Gregersen and Gregersen (2014) found that black grouse males visit lek sites most of the year, primarily searching for food, lekking, or simply socializing. With the help of camera traps, it is relatively easy to monitor populations of this species without increased disturbance by nearby observers of lek habitats (Gregersen and Gregersen, 2014). Another non-invasive monitoring tool can be passive acoustic monitoring using a recording device. This method can significantly reduce the risk of overestimating the population and lead to a more accurate determination of the abundance of the monitored populations of black grouse (Hambálková et al., 2021). A more suitable signal for this method is a hissing call, which, unlike rookooing, does not overlap each other and is easier to filter out in acoustic software, as it is located at higher sound frequencies (Hambálková et al., 2021). Acoustic performance also has the potential for distinguishing individual subpopulations of the black grouse across its range (Hambálková et al., 2023). One of the reasons why the differentiation of acoustic performance can occur is the isolation of subpopulations. Monitoring and evaluating acoustic variability have the potential to non-invasively assess changes in population across its distributional range.
7 Conclusions
According to our review analysis, it is clear that the status of black grouse in Europe can be characterized by population declines across almost the entire range. However, the main causes vary according to the specific conditions in each country. Central Europe is typically affected most by habitat loss, where black grouse still occur mainly in mountainous habitats and moorlands. In addition, published studies have highlighted increasing predation rates and the impact of human activities, including tourism. Hunting management is another aspect that needs revision in Central European countries where supplementary feeding of wild boar and other ungulates is a common and typical practice, e.g., in the Czechia, attracting not only the target species but also predators such as red fox or marten. In the case of the Scandinavian countries, where black grouse is a commonly hunted species, the gradual population decline can be attributed to decreasing breeding success and increasing chick mortality, with less impact from habitat changes and tourism.
Generally speaking, it is clear that the causes of black grouse decline vary across Europe, with different environmental conditions and human impacts on nature. The positive finding is that many of the identified factors responsible for population decline could be influenced by conservation measures. However, it is important to stress that environmental protection should work hand in hand with wildlife managers to improve these conditions. It is essential that conservation efforts and research continue, particularly in areas where black grouse are rapidly declining and at risk of extinction.
Author contributions
LH: Data curation, Writing – original draft, Writing – review & editing. JC: Conceptualization, Methodology, Supervision, Writing – original draft, Writing – review & editing. KB: Data curation, Writing – original draft, Writing – review & editing. RŠ: Data curation, Writing – original draft, Writing – review & editing. ZV: Visualization, Writing – original draft, Writing – review & editing. SV: Writing – original draft, Writing – review & editing. VS: Writing – original draft, Writing – review & editing. RL: Data curation, Visualization, Writing – review & editing. OA: Writing – review & editing, Supervision.
Funding
The author(s) declare financial support was received for the research, authorship, and/or publication of this article. The research was financially supported by the Ministry of Agriculture of the Czech Republic, institutional support MZE-RO0123 and also by project RAGO (n. 3201200001) supported by Norwegian funds.
Acknowledgments
The authors thank Jitka Šišáková, an expert in the field, and Richard Lee Manore, a native speaker, for checking English and to the two reviewers for their constructive comments.
Conflict of interest
The authors declare that the research was conducted in the absence of any commercial or financial relationships that could be construed as a potential conflict of interest.
Publisher’s note
All claims expressed in this article are solely those of the authors and do not necessarily represent those of their affiliated organizations, or those of the publisher, the editors and the reviewers. Any product that may be evaluated in this article, or claim that may be made by its manufacturer, is not guaranteed or endorsed by the publisher.
References
Alatalo R. V., Burke T., Dann J., Hanotte O., Höglund J., Lundberg A., et al. (1996). Paternity, copulation disturbance and female choice in lekking black grouse. Anim. Behav. 52, 861–873. doi: 10.1006/anbe.1996.0234
Alatalo R. V., Höglund J., Lundberg A. (1991). Lekking in the black grouse— a test of male viability. Nature 352, 155–156. doi: 10.1038/352155a0
Alatalo R. V., Höglund J., Lundberg A., Sutherland W. J. (1992). Evolution of black grouse leks: female preferences benefit males in larger leks. Behav. Ecol. 3, 53–59. doi: 10.1093/beheco/3.1.53
Andrén H. (1994). Effects of habitat fragmentation on birds and mammals in landscapes with different proportions of suitable habitat: a review. Oikos 71, 355–366. doi: 10.2307/3545823
Angelstam P. (2004). Habitat thresholds and effects of forest landscape change on the distribution and abundance of black grouse and Capercaillie. Ecol. Bull. 51, 173–187. doi: 10.2307/20113307
Angelstam P., Runfors O., Mikusinski G., Seiler C. (2000). Long-term dynamics of three types of black grouse habitat in the centre and at the edge of the distribution range in Sweden 1850 – 2000. Cah. d’Ethologie 20, 165–190.
Arlettaz R., Patthey P., Baltic M., Leu T., Schaub M., Palme R., et al. (2007). Spreading free-riding snow sports represent a novel serious threat for wildlife. Proc. R. Soc B Biol. Sci. 274, 1219–1224. doi: 10.1098/rspb.2006.0434
Arlettaz R., Patthey P., Braunisch V. (2013). “Impacts of outdoor winter recreation on Alpine wildlife and mitigation approaches: a case study of the black grouse,” in The Impacts of Skiing and Related Winter Recreational Activities on Mountain Environments Eds. Rixen C., Rolando A. (Bentham eBooks, Bussum, NL), 137–154.
Baines D. (1994). Seasonal differences in habitat selection by black grouse Tetrao tetrix in the northern Pennines, England. Ibis. 136, 39–43. doi: 10.1111/j.1474-919X.1994.tb08129.x
Baines D. (1996). The implications of grazing and predator management on the habitats and breeding success of black grouse Tetrao tetrix. J. Appl. Ecol. 33, 54–62. doi: 10.2307/2405015
Baines D., Andrew M. (2003). Marking of deer fences to reduce frequency of collisions by woodland grouse. Biol. Conserv. 110, 169–176. doi: 10.1016/S0006-3207(02)00185-4
Baines D., Blake K., Calladine J. (2000). Reversing the decline: a review of some black grouse conservation projects in the United Kingdom. Cah. d’Ethologie 20, 217–234.
Baines D., Warren P., Richardson M. (2007). Variations in the vital rates of black grouse Tetrao tetrix in the United Kingdom. Wildl. Biol. 13, 109–116. doi: 10.2981/09096396(2007)13[109:VITVRO]2.0.CO;2
Baltić M., Mettaz S., Baltić M., Jenni-Eiermann S., Arlettaz R., Palme R. (2004). Impact of human disturbance on Alpine wildlife in winter: stress, activity and energetics in the endangered black grouse Tetrao tetrix. Universität Bern, Bern, (CH.
Barredo J. I., Mauri A., Caudullo G. (2020). Impacts of Climate Change in European Mountains – Alpine Tundra Habitat Loss and Treeline Shifts under Future Global Warming (Luxembourg: Publications Office of the European Union). doi: 10.2760/653658
Bartoń K., Zalewski A. (2007). Winter severity limits red fox populations in Eurasia. Glob. Ecol. Biogeogr. 16, 281–289. doi: 10.1111/j.1466-8238.2007.00299.x
Bellassen V., Luyssaert S. (2014). Carbon sequestration: Managing forests in uncertain times. Nature 506, 153–155. doi: 10.1038/506153a
Berto B. P., Lopes C. W. G. (2020). Coccidia of wild birds as ecological biomarkers: some approaches on parasite-host-environment interaction. J. Parasitol. 106, 707–713. doi: 10.1645/19-148
BirdLife International (2022). Species factsheet: Lyrurus tetrix. Available online at: http://www.birdlife.org (Accessed June 21, 2022).
BirdLife International (2023). Lithuanian Ornithological Society Available online at: https://birdlife.lt/ (Accessed September 18, 2023).
Bocca M., Caprio E., Chamberlain D., Rolando A. (2014). The winter roosting and diet of black grouse Tetrao tetrix in the north-western Italian Alps. J. Ornithol. 155, 183–194. doi: 10.1007/s10336-013-1000-1
Boev Z. (1997). The black grouse, Tetrao tetrix (L. 1758) (Tetraonidae, Aves), a disappeared species in Bulgaria (Paleolithic and Neolithic records). Anthropozoologica 25–26, 643–646.
Borecha D. E., Willebrand T., Nielsen O. K. (2017). Lek site defines annual spatial use of male black grouse (Tetrao tetrix). Ornis Fenn. 94, 150–160. doi: 10.51812/of.133920
Bowker G., Bowker C., Baines D. (2007). Survival rates and causes of mortality in black grouse Tetrao tetrix at Lake Vyrnwy, North Wales, UK. Wildl. Biol. 13, 231–237. doi: 10.2981/0909-6396(2007)13[231:SRACOM]2.0.CO;2
Caizergues A., Ellison L. (1997). Survival of black grouse Tetrao tetrix in the French Alps. Wildl. Biol. 3, 177–188. doi: 10.2981/wlb.1997.022
Caizergues A., Rätti O., Helle P., Rotelli L., Ellison L., Rasplus J.-Y. (2003). Population genetic structure of male black grouse (Tetrao tetrix L.) in fragmented vs. continuous landscapes. Mol. Ecol. 12, 2297–2305. doi: 10.1046/j.1365-294X.2003.01903.x
Calladine J., Baines D., Warren P. (2002). Effects of reduced grazing on population density and breeding success of black grouse in northern England. J. Appl. Ecol. 39, 772–780. doi: 10.1046/j.1365-2664.2002.00753.x
Canonne C., Montadert M., Besnard A. (2021). Drivers of black grouse trends in the French Alps: The prevailing contribution of climate. Divers. Distrib. 27, 1338–1352. doi: 10.1111/ddi.13242
Caro T., Girling S. (2006). Antipredator Defenses in Birds and Mammals. (Chicago: Chicago University Press).
Catt D. C., Dugan D., Green R. E., Moncrieff R., Moss R., Picozzi N., et al. (1994). Collisions against fences by woodland grouse in Scotland. For. Int. J. For. Res. 67, 105–118. doi: 10.1093/forestry/67.2.105
Cayford J. T., Walker F. (1991). Counts of male black grouse Tetrao tetrix in North Wales. Bird Study 38, 80–86. doi: 10.1080/00063659109477072
Český hydrometeorologický ústav. (2022). (Czech Hydrometeorological Institute). Available online at: https://www.chmi.cz/. (Accessed April 21, 2022).
Chamberlain D. E., Bocca M., Migliore L., Caprio E., Rolando A. (2012). The dynamics of alternative male mating tactics in a population of black grouse Tetrao tetrix in the Italian Alps. J. Ornithol. 153, 999–1009. doi: 10.1007/s10336-012-0821-7
Chobot K., Němec M. (2017). Red List of Threatened Species of Czech Republic: Vertebrates Available online at: https://portal.nature.cz/publik_syst/ctihtmlpage.php?what=1264 (Accessed November 26, 2021).
Chytrý M., Kučera T., Kočí M., Grulich V., Lustyk P. (2010). Katalog biotopů České republiky [Catalog of biotopes of the Czech Republic] 2nd Edn (Prague: Agentura ochrany přírody a krajiny ČR).
Coates A., Barnett L. K., Hoskin C., Phillips B. L. (2017). Living on the edge: parasite prevalence changes dramatically across a range edge in an invasive gecko. Am. Nat. 189, 178–183. doi: 10.1086/689820
Coldwell L., Caldow G., Holliman A., Mearns R., Errington H., Giles M., et al. (2012). Cryptospordium baileyi in wild red grouse with “bulgy eye.“. Vet. Rec. 170, 603–604. doi: 10.1136/vr.e3940
Cole J., Gordon J., Fraser A., Boles Y., O’Brien M., Grant M. (2012). A review of management prescriptions for black grouse Tetrao tetrix in Britain: An update and revision (Edinburgh: RSPB, SNH, Forestry Commission Scotland, GWCT).
Coppes J., Braunisch V., Bollmann K., Storch I., Mollet P., Grunschachner-Berger V., et al. (2020). The impact of wind energy facilities on grouse: a systematic review. J. Ornithol. 161, 1–15. doi: 10.1007/s10336-019-01696-1
Cove M., O’Connell A. (2022). “Global review of the effects of small carnivores on threatened species,” in Small Carnivores: Evolution, Ecology, Behaviour, and Conservation Eds. Do Linh San E., Sato J. J., Belant J. L., Somers M. J. (John Wiley & Sons Ltd, Hoboken, NJ), 471–488.
Cramp S. (1983). Handbook of the birds of Europe, the Middle East and North Africa : The Birds of the Western Palearctic. Vol. 3, Waders to Gulls (Oxford: Oxford University Press).
Cramp S., Simmons K. E. L. (1977). Handbook of the Birds of Europe, the Middle East and North Africa: The Birds of the Western Palearctic. Vol. 1: Ostrich to Ducks (Oxford: Oxford University Press).
Cukor J., Eriksen L. F., Linda R., Andersen O. (2020). Role myslivců v ochraně a zodpovědném managementu tetřívka obecného v Norsku. Svět Myslivosti 3, 26–28.
Cukor J., Havránek F., Hambálková L., Vacek Z., Vacek S. (2022). Metodika úprav lesních ekosystémů ve vztahu k podpoře populací tetřívka obecného. Certifikované metodiky pro praxi, Lesnický průvodce 4 (Strnady: Výzkumný ústav lesního hospodářství a myslivosti). Methodology for Forest Ecosystems Improvement in Relation to Supporting of Black Grouse Populationv.v.i.
Cukor J., Linda R., Andersen O., Eriksen L. F., Vacek Z., Riegert J., et al. (2021). Evaluation of spatio-temporal patterns of predation risk to forest grouse nests in the Central European mountain regions. Animals 11, 316. doi: 10.3390/ani11020316
Cukor J., Vacek Z., Linda R., Bílek L. (2017). Carbon sequestration in soil following afforestation of former agricultural land in the Czech Republic. Cent. Eur. For. J. 63, 97–104. doi: 10.1515/forj-2017-0011
Delcourt J., Hambuckers A., Vangeluwe D., Poncin P. (2023). Fifty years of spring censuses in black grouse (Lyrurus tetrix) in the High Fens (Belgium): did the rabies vaccination has a negative impact on a fox prey population? Eur. J. Wildl. Res. 69, 24. doi: 10.1007/s10344-023-01642-w
Doenecke M., Niethammer G. (1970). Bestandsänderungen des Birkwildes und die Wandlung der Bodennutzung im westlichen Münsterland im Verlauf der letzten 100 Jahre. Z. Jagdwiss. 16, 97–115. doi: 10.1007/BF01891889
Dos Santos V., Bernard-Laurent A., Ménoni E., Montadert M., Novoa C., Amblard B., et al. (2021). “Suivi des galliformes de montagne alpins et pyrénéens,” in Bilan de la décennie 2010-2019 (Observatoire Des Galliformes de Montagne, Sévrier).
Dumont L., Lauer E., Zimmermann S., Roche P., Auliac P., Sarasa M. (2019). Monitoring black grouse Tetrao tetrix in Isere, northern French Alps: cofactors, population trends and potential biases. Anim. Biodivers. Conserv. 42, 227–244. doi: 10.32800/abc.2019.42.0227
Egwumah F. A., Egwumah P. O., Edet D. I. (2017). Paramount roles of wild birds as bioindicators of contamination. Int. J. Avian Wildl. Biol. 2, 194–200. doi: 10.15406/ijawb.2017.02.00041
Eiberle K. (1976). Zur Analyse eines Auerwildbiotops im Schweizerischen Mittelland. Forstwissenschaftliches Cent. 95, 108–124. doi: 10.1007/BF02741022
Elts J., Leito A., Leivits A., Luigujõe L., Mägi E., Nellis R., et al (2013). Status and numbers of Estonian birds, 2008–2012. Hirundo. 26, 80–112.
European Environment Agency (2016). Report under the Article 12 of the Birds Directive Period 2008-2012, Tetrao tetrix tetrix. Available online at: https://projects.eionet.europa.eu/article-12-birds-directive/library/2008-2012-reporting/species-factsheets/tetrao-tetrix-tetrix (Accessed September 13, 2016).
Fanelli A., Menardi G., Chiodo M., Giordano O., Ficetto G., Bessone M., et al. (2020). Gastroenteric parasite of wild Galliformes in the Italian Alps: implication for conservation management. Parasitology 147, 471–477. doi: 10.1017/S003118201900177X
Flousek J. (1989). Impact of industrial emissions on bird populations breeding in mountain spruce forests in Central Europe. Ann. Zool. Fennici 26, 255–263.
Flousek J. (2019). Přežijí krkonošští tetřívci rok 2040? [Will the Black Grouse from Krkonoše Mountains survive the year 2040]? Ochr. přírody 74, 6–9.
Formenti N., Viganó R., Bionda R., Ferrari N., Trogu T., Lanfranchi P., et al. (2015). Increased hormonal stress reactions induced in an Alpine black grouse (Tetrao tetrix) population by winter sports. J. Ornithol. 156, 317–321. doi: 10.1007/s10336-014-1103-3
Formenti N., Viganò R., Rotelli L., Ferrari N., Cerutti M. C., Lanfranchi P. (2013). Effect of suboptimal environment and host age on helminth community of black grouse (Tetrao tetrix). Eur. J. Wildl. Res. 59, 351–358. doi: 10.1007/s10344-012-0681-8
Geßler A., Keitel C., Kreuzwieser J., Matyssek R., Seiler W., Rennenberg H. (2007). Potential risks for European beech (Fagus sylvatica L.) in a changing climate. Trees 21, 1–11. doi: 10.1007/s00468-006-0107-x
Geary M., Fielding A. H., Marsden S. J. (2012). The anatomy of population change in a black grouse population 1992-2008. Oecologia 168, 73–81. doi: 10.1007/s00442-011-2062-5
Geary M., Fielding A. H., Marsden S. J. (2015). Both habitat change and local lek structure influence patterns of spatial loss and recovery in a black grouse population. Popul. Ecol. 57, 421–431. doi: 10.1007/s10144-015-0484-3
Gimmi U., Lachat T., Bürgi M. (2011). Reconstructing the collapse of wetland networks in the Swiss lowlands 1850–2000. Landsc. Ecol. 26, 1071–1083. doi: 10.1007/s10980-011-9633-z
Glänzer U. W. (1980). [amp]]Uuml;ber die Auswirkung von Landnutzungsänderungen auf Tierbiotope, dargestellt am Beispiel des Birkhuhns (Tetrao tetrix) in Bayern. Verh. Ges. Ökol. 5, 151–162.
Glutz von Blotzheim U. N., Bauer K., Bezzel E. (1994). Handbuch der vögel mitteleuropas. Band 5: Galliformes und Guiformes 2nd ed (Wiesbaden: Aula-Verlag).
Goldsmith F. B. (2012). Monitoring for Conservation and Ecology (Dordrecht: Springer Science & Business Media).
Grant M. C., Cowie N., Donald C., Dugan D., Johnstone I., Lindley P., et al. (2009). Black grouse response to dedicated conservation management. Folia Zool. 58, 195.
Gregersen H., Gregersen F. (2014). Wildlife cameras effectively survey Black Grouse Lyrurus tetrix leks. Ornis Nor. 37, 1–6. doi: 10.15845/on.v37i0.595
Haider M., Steixner R., Zeni T., Vallant S., Lentner R., Schlick-Steiner B. C., et al. (2024). The influence of sample size on two approaches to estimate black grouse Lyrurus tetrix population size using non-invasive sampling methods. Ibis 166, 55–68. doi: 10.1111/ibi.v166.1
Hämäläinen A., Alatalo R. V., Lebigre C., Siitari H., Soulsbury C. D. (2012). Fighting behaviour as a correlate of male mating success in black grouse Tetrao tetrix. Behav. Ecol. Sociobiol. 66, 1577–1586. doi: 10.1007/s00265-012-1411-7
Hambálková L., Policht R., Cukor J., Hart V., Ševčík R. (2023). Black grouse hissing calls show geographic variability across the distribution area. Animals 13, 1844. doi: 10.3390/ani13111844
Hambálková L., Policht R., Horák J., Hart V. (2021). Acoustic individuality in the hissing calls of the male black grouse (Lyrurus tetrix). PeerJ 9, e11837. doi: 10.7717/peerj.11837
Han Y. G., Yoo S. H., Kwon O. (2017). Possibility of applying unmanned aerial vehicle (UAV) and mapping software for the monitoring of waterbirds and their habitats. J. Ecol. Environ. 41, 1–7. doi: 10.1186/s41610-017-0040-5
Hancock M., Baines D., Gibbons D., Etheridge B., Shepherd M. (1999). Status of male black grouse Tetrao tetrix in Britain in 1995-96. Bird Study 46, 1–15. doi: 10.1080/00063659909461110
Hannon S., Martin K. (2006). Ecology of juvenile grouse during the transition to adulthood. J. Zool. 269, 422–433. doi: 10.1111/j.1469-7998.2006.00159.x
Hansson L., Henttonen H. (1989). Rodents, predation and wildlife cycles. Finnish Game Res. 46, 26–33.
Hirzel A. H., Le Lay G. (2008). Habitat suitability modelling and niche theory. J. Appl. Ecol. 45, 1372–1381. doi: 10.1111/j.1365-2664.2008.01524.x
Höglund J., Alatalo R. V., Lundberg A. (1992). The effects of parasites on male ornaments and female choice in the lek-breeding black grouse (Tetrao tetrix). Behav. Ecol. Sociobiol. 30, 71–76. doi: 10.1007/BF00173942
Höglund J., Alatalo R. V., Lundberg A., Rätti O. (1994). Context-dependent effects of tail-ornament damage on mating success in black grouse. Behav. Ecol. 5, 182–187. doi: 10.1093/beheco/5.2.182
Höglund J., Johansson T., Pelabon C. (1997). Behaviourally mediated sexual selection: Characteristics of successful male black grouse. Anim. Behav. 54, 255–264. doi: 10.1006/anbe.1996.0459
Höglund J., Larsson J. K., Jansman H. A. H., Segelbacher G. (2007). Genetic variability in European black grouse (Tetrao tetrix). Conserv. Genet. 8, 239–243. doi: 10.1007/s10592-006-9158-1
Höglund J., Stohr S. (1997). A non-lekking population of black grouse Tetrao tetrix. J. Avian Biol. 28, 184–187. doi: 10.2307/3677312
Holopainen S., Selonen V., Krüger H., Kotanen J., Laaksonen T., Miettinen E., et al. (2024). Forest habitat loss and human land use alter predation of artificial ground nests. For. Ecol. Manage. 561, 121858. doi: 10.1016/j.foreco.2024.121858
Hovi M., Alatalo R. V., Halonen M., Lundberg A. (1997). Responses of male and female black grouse to male vocal display. Ethology 103, 1032–1041. doi: 10.1111/j.1439-0310.1997.tb00145.x
Hovi M., Alatalo R. V., Höglund J., Lundberg A., Rintamäki P. T. (1994). Lek centre attracts black grouse females. Proc. R. Soc London. Ser. B Biol. Sci. 258, 303–305. doi: 10.1098/rspb.1994.0177
Immitzer M., Nopp-Mayr U., Zohmann M. (2014). Effects of habitat quality and hiking trails on the occurrence of black grouse (Tetrao tetrix L.) at the northern fringe of alpine distribution in Austria. J. Ornithol. 155, 173–181. doi: 10.1007/s10336-013-0999-3
Ingold P. (2005). Freizeitaktivitaöten im Lebensraum der Alpentiere (Bern: Haupt Verlag). Konfliktbereiche zwischen Mensch und Tier.
Isomursu M., Helle P., Rätti O. (2017). Intestinal parasites as potential factors in the dynamics of a fluctuating forest grouse community. Ann. Zool. Fennici 54, 301–313. doi: 10.5735/086.054.0503
IUCN (2023). The IUCN Red List of Threatened Species. Version 2023-1 Available online at: https://www.iucnredlist.org (Accessed December 29, 2023).
Jäger H., Schirpke U., Tappeiner U. (2020). Assessing conflicts between winter recreational activities and grouse species. J. Environ. Manage. 276, 111194. doi: 10.1016/j.jenvman.2020.111194
Jahren T., Storaas T., Willebrand T., Moa P. F., Hagen B. R. (2016). Declining reproductive output in capercaillie and black grouse-16 countries and 80 years. Anim. Biol. 66, 363–400. doi: 10.1163/15707563-00002514
Jankovská I., Bejček V., Langrová I., Valek P., Vadlejch J., Čadková Z. (2012). Black grouse in Czech Republic and its parasites. Helminthologia 49, 78–81. doi: 10.2478/s11687-012-0016-z
Ježek M., Kušta T., Holá M., Červený J. (2013). GPS telemetrie černé zvěře. Závěrečná zpráva (Prague: Česká zemědělská univerzita v Praze, Fakulta lesnická a dřevařská).
Ježková M., Svobodová J., Kreisinger J. (2014). Dynamics of rodent abundance and ground-nest predation risks in forest habitats of Central Europe: No evidence for the alternative prey hypothesis. Folia Zool. 63, 269–280. doi: 10.25225/fozo.v63.i4.a6.2014
Johnstone I., Lindley P. (2003). The proximate causes of black grouse breeding failure in Wales (Bangor: RSPB report to CCW (CCW contract science report No. 600).
Kahlert J., Fox A. D., Heldbjerg H., Asferg T., Sunde P. (2015). Functional responses of human hunters to their prey - why harvest statistics may not always reflect changes in prey population abundance. Wildl. Biol. 21, 294–302. doi: 10.2981/wlb.00106
Ķerus V., Dekants A., Aunins A., Mārdega I. (2021). Latvijas Ligzdojošo Putnu Atlanti 1980 - 2017 (Riga: Latvijas Ornitoloģijas Biedrība).
Kervinen M., Alatalo R. V., Lebigre C., Siitari H., Soulsbury C. D. (2012). Determinants of yearling male lekking effort and mating success in black grouse (Tetrao tetrix). Behav. Ecol. 23, 1209–1217. doi: 10.1093/beheco/ars104
Kervinen M., Lebigre C., Soulsbury C. D. (2016). Simultaneous age-dependent and age-independent sexual selection in the lekking black grouse (Lyrurus tetrix). J. Anim. Ecol. 85, 715–725. doi: 10.1111/1365-2656.12496
Klaus S. (1991). Effects of forestry on grouse populations: case studies from the Thuringian and Bohemian Forests, Central Europe. Scand. J. Ornithol. 22, 218–223. doi: 10.2307/3676593
Koch N. (1978). Hazel grouse and heath hens from Hohen Rone (Canton of Zug, Switzerland). J. For. suisse = Schweizerische Z. fur Forstwes. 129, 897–933.
Koivisto I. (1965). Behaviour of the black grouse, Lyrurus tetrix (L.), during the spring display. Finn. Game Res. 26, 1–60.
Kokko H., Lindström J., Alatalo R. V., Rintamäki P. T. (1998). Queuing for territory positions in the lekking black grouse (Tetrao tetrix). Behav. Ecol. 9, 376–383. doi: 10.1093/beheco/9.4.376
Kurhinen J., Danilov P., Gromtsev A., Helle P., Linden H. (2009). Patterns of black grouse, Tetrao tetrix distribution in northwestern Russia at the turn of the millennium. Folia Zool. 58, 168–172.
Kurki S., Helle P., Lindén H., Nikula A. (1997). Breeding success of black grouse and Capercaillie in relation to mammalian predator densities on two spatial scales. Oikos 79, 301–310. doi: 10.2307/3546014
Kurki S., Nikula A., Helle P., Lindén H. (2000). Landscape fragmentation and forest composition effects on grouse breeding success in boreal forests. Ecology 81, 1985–1997. doi: 10.2307/177287
Kvasnes M. A. J., Storaas T., Pedersen H. C., Bjork S., Nilsen E. B. (2010). Spatial dynamics of Norwegian tetraonid populations. Ecol. Res. 25, 367–374. doi: 10.1007/s11284-009-0665-7
Larsson J. K., Jansman H. A. H., Segelbacher G., Höglund J., Koelewijn H. P. (2008). Genetic impoverishment of the last black grouse (Tetrao tetrix) population in the Netherlands: detectable only with a reference from the past. Mol. Ecol. 17, 1897–1904. doi: 10.1111/j.1365-294X.2008.03717.x
Lebigre C., Alatalo R. V., Siitari H., Parri S. (2007). Restrictive mating by females on black grouse leks. Mol. Ecol. 16, 4380–4389. doi: 10.1111/j.1365-294X.2007.03502.x
Lešo P., Demko M., Krištín A., Ridzoň J., Karaska D., Chavko J., et al. (2024). Červená kniha ohrozených druhov vtákov Slovenska (Banská Bystrica: Štátna ochrana prírody SR).
Loneux M., Ruwet J.-C. (1997). Evolution des populations du tétras lyre Tetrao tetrix L. en Europe: un essai de synthèse TT - Evolution of black grouse (Tetrao tetrix) populations in Europe: an overview. Cah. d’Ethologie 17, 287.
Ludwig G. X., Alatalo R. V., Helle P., Linden H., Lindstrom J., Siitari H. (2006). Short- and long-term population dynamical consequences of asymmetric climate change in black grouse. Proc. R. Soc B Biol. Sci. 273, 2009–2016. doi: 10.1098/rspb.2006.3538
Ludwig G., Alatalo R. V., Helle P., Siitari H. (2010). Individual and environmental determinants of early brood survival in black grouse Tetrao tetrix. Wildl. Biol. 16, 367–378. doi: 10.2981/10-013
Ludwig T., Storch I., Gärtner S. (2009a). Large-scale land use change may explain bird species declines in semi-natural areas: the case of black grouse population collapse in Lower Saxony, Germany. J. Ornithol. 150, 871–882. doi: 10.1007/s10336-009-0410-6
Ludwig T., Storch I., Graf R. F. (2009b). Historic landscape change and habitat loss: the case of black grouse in Lower Saxony, Germany. Landsc. Ecol. 24, 533–546. doi: 10.1007/s10980-009-9330-3
Ludwig T., Storch I., Wuebbenhorst J. (2008). How the black grouse was lost: historic reconstruction of its status and distribution in Lower Saxony (Germany). J. Ornithol. 149, 587–596. doi: 10.1007/s10336-008-0306-x
MacDonald D., Crabtree J. R., Wiesinger G., Dax T., Stamou N., Fleury P., et al. (2000). Agricultural abandonment in mountain areas of Europe: environmental consequences and policy response. J. Environ. Manage. 59, 47–69. doi: 10.1006/jema.1999.0335
Marjakangas A. (1992). Winter activity patterns of the black grouse Tetrao tetrix. Ornis Fenn. 69, 184–192. doi: 10.1007/BF01651657
Matysek M., Gwiazda R., Zięba F., Klimecki M., Mateja R., Krzan P. (2020). High tourism activity alters the spatial distribution of Hazel grouse (Tetrastes bonasia) and predation on artificial nests in a high-mountain habitat. Ornis Fenn. 97, 53–63. doi: 10.51812/of.133966
Mazziotta A., Lindén A., Eyvindson K., Bianchi S., Kangas A., Melin M., et al. (2024). Unraveling the characteristic spatial scale of habitat selection for forest grouse species in the boreal landscape. For. Ecol. Manag 563, 122008. doi: 10.1016/j.foreco.2024.122008
Merta D., Bobek B., Furtek J., Kolecki M. (2009). Distribution and number of black grouse, Tetrao tetrix in southwestern Poland and the potential impact of predators upon nesting success of the species. Folia Zool. 58, 159–167.
Michev T., Boev Z., Kambourova N. (2011).Red list of the birds of Bulgaria. In: Proceedings of the Jubilee National Scientific Conference with International Participation “The Man and the Universe” (Smolyan, Bulgaria) (Accessed 6–8 October).
Moss R., Oswald J., Baines D. (2001). Climate change and breeding success: decline of the Capercaillie in Scotland. J. Anim. Ecol. 70, 47–61. doi: 10.1111/j.1365-2656.2001.00473.x
Müller F. (1974). Die wichtigsten Ergebnisse 10jähriger Auer-wild-Forschung im hessischen Bergland. Allg. Forst- und Jagdzeitung 29, 834–836.
Müller F. (1979). “A 15-year study of a Capercaillie lek in thewestern Rhön-mountains (W. Germany),” in Proceedings of the International Grouse Symposium I Ed. Lovel T. W. I.(Norfolk, Suffolk, UK: Journal of the World Pheasant Association), 120–130.
Murin B., Krištín A., Darolová A., Danko Š., Kropil R. (1994). Početnosť hniezdnych populácií vtákov na Slovensku. Sylvia 30, 97–105.
Nelli L., Murru M., Meriggi A. (2016). Effects of density on lek-site selection by black grouse Tetrao tetrix in the Alps. Bird Study 63, 187–195. doi: 10.1080/00063657.2016.1180503
Northrup J. M., Hooten M. B., Anderson C. R., Wittemyer G. (2013). Practical guidance on characterizing availability in resource selection functions under a use-availability design. Ecology 94, 1456–1463. doi: 10.1890/12-1688.1
Oja R., Zilmer K., Valdmann H. (2015). Spatiotemporal effects of supplementary feeding of wild boar (Sus scrofa) on artificial ground nest depredation. PLoS One 10, e0135254. doi: 10.1371/journal.pone.0135254
Park K., Graham K., Calladine J., Wernham C. (2008). mpacts of birds of prey on gamebirds in the UK: a review. Ibis 150, 9–26. doi: 10.1111/j.1474-919X.2008.00847.x
Pärt T. (2001). The effects of territory quality on age-dependent reproductive performance in the northern wheatear, Oenanthe oenanthe. Anim. Behav. 62, 379–388. doi: 10.1006/anbe.2001.1754
Patthey P., Signorell N., Rotelli L., Arlettaz R. (2012). Vegetation structural and compositional heterogeneity as a key feature in Alpine black grouse microhabitat selection: conservation management implications. Eur. J. Wildl. Res. 58, 59–70. doi: 10.1007/s10344-011-0540-z
Pearce-Higgins J. W., Grant M. C., Robinson M. C., Haysom S. L. (2007). The role of forest maturation in causing the decline of black grouse Tetrao tetrix. Ibis 149, 143–155. doi: 10.1111/j.1474-919X.2006.00623.x
Pearce-Higgins J. W., Wright L. J., Grant M. C., Douglas D. J. (2016). The role of habitat change in driving black grouse Tetrao tetrix population declines across Scotland. Bird Study 63, 66–72. doi: 10.1080/00063657.2015.1134439
Pekkola M., Alatalo R. V., Pöysä H., Siitari H. (2014). Seasonal survival of young and adult black grouse females in boreal forests. Eur. J. Wildl. Res. 60, 477–488. doi: 10.1007/s10344-014-0809-0
Percival S., Percival T., Lowe T. (2018). Minnygap wind farm: post-construction phase breeding bird surveys 2018 (year 2) (Durham: Ecology Consulting Report to Renewable Energy Systems Ltd).
Pluhar D., Kaknics L., Juhasz L., Szendrei L. (2010). Breeding black grouse in artificial conditions in Hungary. Stud. Univ. Vasile Goldis Arad Ser. Stiint. Vietii 20, 35–39.
Poleno Z., Vacek S., Podrázský V., Remeš J., Štefančík I., Mikeska M., et al. (2009). Pěstování lesů III. Praktické postupy pěstování lesů (Kostelec nad Černými lesy: Lesnická práce, s.r.o). Silviculture III. Practical Methods in Silviculture.
Policht R., Hart V., Goncharov D., Surový P., Hanzal V., Červený J., et al. (2019). Vocal recognition of a nest-predator in black grouse. PeerJ 7, e6533. doi: 10.7717/peerj.6533
Porkert J. (1973). K významu kapradí pro tetřevy a tetřívky. Acta Musei Reginaehrad. Ser. A. Sci. Nat. 8, 23–34.
Porkert J. (1976). Methoden zur Untersuchung der Fortpflanzungsbiologie bedrohter Tetraoniden-Populationen (Aves: Galliformes). Věstnik Československá společnosti Zool 40, 41–52.
Porkert J. (1982). Ke strukturálním změnám biotopů tetřevovitých (Tetraonidae) v hřebenových partiích východních Sudet a jejich vztahu k imisím škodlivin transportovaných srážkovými vodami. Opera Corcontica 19, 165–182.
Porkert J. (1991). Hoarfrost deposits as a factor contributing to the extinction of tetraonids in the eastern Sudetes. Scand. J. Ornithol 22, 292–293. doi: 10.2307/3676607
Prellwitz D. M. (2002). Greater sage-grouse at Bowdoin National Wildlife Refuge, Montana. Intermt. J. Sci. 8, 115–116.
Ramanzin M., Fuser S., Bottazzo M. (2000). Summer habitat selection by black grouse in the Belluno province (Eastern Italian Alps). Cah. d’Ethologie 20, 461–472.
R Core Team (2023). R: A Language and Environment for Statistical Computing (Vienna, Austria: R Foundation for Statistical Computing). Available at: https://www.R-project.org/.
Reif V., Tornberg R., Huhtala K. (2004). Juvenile grouse in the diet of some raptors. J. Raptor Res. 38, 243–249.
Reif V., Tornberg R., Jungell S., Korpimäki E. (2001). Diet variation of Common Buzzards in Finland supports the alternative prey hypothesis. Ecography (Cop.) 24, 267–274. doi: 10.1111/j.1600-0587.2001.tb00199.x
Rintamäki P. T., Alatalo R. V., Höglund J., Lundberg A. (1995). Male territoriality and female choice on black grouse leks. Anim. Behav. 49, 759–767. doi: 10.1016/0003-3472(95)80208-8
Rintamäki P. T., Alatalo R. V., Höglund J., Lundberg A. (1997). Fluctuating asymmetry and copulation success in lekking black grouse. Anim. Behav. 54, 265–269. doi: 10.1006/anbe.1996.0434
Rintamäki P., Håstad O., Odeen A., Höglund J., Lundberg A. (2002). Sexual selection, colour perception and coloured leg rings in grouse (Tetraonidae). Avian Sci. 2, 145–152.
Rintamäki P. T., Höglund J., Alatalo R. V., Lundberg A. (2001). Correlates of male mating success on black grouse (Tetrao tetrix L.) leks. Ann. Zool. Fennici 38, 99–109.
Rintamäki P. T., Karvonen E., Alatalo R. V., Lundberg A. (1999). Why do black grouse males perform on lek sites outside the breeding season? J. Avian Biol. 30, 359–366. doi: 10.2307/3677008
Rolstad J., Wegge P., Sivkov A. V., Hjeljord O., Storaunet K. O. (2009). Size and spacing of grouse leks: comparing capercaillie (Tetrao urogallus) and black grouse (Tetrao tetrix) in two contrasting Eurasian boreal forest landscapes. Can. J. Zool 87, 1032–1043. doi: 10.1139/Z09-093
Roos S., Donald C., Dugan D., Hancock M. H., O’Hara D., Stephen L., et al. (2016). Habitat associations of young black grouse Tetrao tetrix broods. Bird Study 63, 203–213. doi: 10.1080/00063657.2016.1141167
Rotelli L., Bionda R., Zbinden N., Schaub M. (2021). Chick survival and hunting are important drivers for the dynamics of two Alpine black grouse Lyrurus tetrix populations. Wildl. Biol. 2021, wlb.00874. doi: 10.2981/wlb.00874
Rutkowski R., Pałucki A., Dulisz B., Ciach M., Nowak-Zyczyńska Z., Kowalewska K. (2018). Conservation genetics of the black grouse Tetrao tetrix in Poland - distribution of genetic diversity among the last populations. Acta Ornithol 53, 181–204. doi: 10.3161/00016454ao2018.53.2.008
Ruwet J.-C., Fontaine S., Houbart S. (1997). Inventory, description and evolution of black grouse (Tetrao tetrix) arenas, and evolution of cocks numbers from 1966 to 1997 in the Belgian Hautes-Fagnes plateau. Cahiers d’Ethologie 17, 137–287.
Šálek M., Svobodová J., Bejček V., Albrecht T. (2004). Predation on artificial nests in relation to the numbers of small mammals in the Krušné hory Mts, the Czech Republic. Folia Zool 53, 312–318.
Scherzinger W. (1976). Rauhfuss-Hühner. Schriftenreihe des Bayerischen Staatsministeriums für Ernährung, Landwirtschaft und Forsten. Natl. Bayer. Wald Heft 2, 1–85.
Schweiger A. K., Nopp-Mayr U., Zohmann M. (2012). Small-scale habitat use of black grouse (Tetrao tetrix L.) and rock ptarmigan (Lagopus muta helvetica Thienemann) in the Austrian Alps. Eur. J. Wildl. Res. 58, 35–45. doi: 10.1007/s10344-011-0537-7
Scridel D., Groom J. D., Douglas D. J. T. (2017). Native woodland creation is associated with increase in a black grouse Lyrurus tetrix population. Bird Study 64, 70–83. doi: 10.1080/00063657.2016.1273879
Segelbacher G., Strand T. M., Quintela M., Axelsson T., Jansman H. A. H., Koelewijn H. P., et al. (2014). Analyses of historical and current populations of black grouse in Central Europe reveal strong effects of genetic drift and loss of genetic diversity. Conserv. Genet. 15, 1183–1195. doi: 10.1007/s10592-014-0610-3
Sein G., Väli Ü. (2024). Diet composition explains interannual fluctuations in reproductive performance in a lowland golden eagle population. Diversity 16, 69. doi: 10.3390/d16010069
Selva N., Berezowska-Cnota T., Elguero-Claramunt I. (2014). Unforeseen effects of supplementary feeding: ungulate baiting sites as hotspots for ground-nest predation. PLoS One 9, e90740. doi: 10.1371/journal.pone.0090740
Signorell N., Wirthner S., Patthey P., Schranz R., Rotelli L., Arlettaz R. (2010). Concealment from predators drives foraging habitat selection in brood-rearing Alpine black grouse Tetrao tetrix hens: habitat management implications. Wildl. Biol. 16, 249–257. doi: 10.2981/09-028
Siitari H., Alatalo R. V., Halme P., Buchanan K. L., Kilpimaa J. (2007). Color signals in the black grouse (Tetrao tetrix): Signal properties and their condition dependency. Am. Nat. 169, S81–S92. doi: 10.1086/510140
Sim I. M. W., Eaton M. A., Setchfield R. P., Warren P. K., Lindley P. (2008). Abundance of male black grouse Tetrao tetrix in Britain in 2005, and change since 1995-96. Bird Study 55, 304–313. doi: 10.1080/00063650809461536
Sjöberg K., Ericson L. (1997). Mosaic boreal landscapes with open and forested wetlands. Ecol. Bull. 46, 48–60.
Skaggs R. W., Tian S., Chescheir G. M., Amatya D. M., Youssef M. (2016). “Forest drainage,” in Forest hydrology: Processes, Management, and Applications Eds. Amatya D. M., William T. M., Bren L., de Jong C. (CABI, Wallingford, UK), 124–140.
Smedshaug C., Selås V., Lund S., Sonerud G. (1999). The effect of a natural reduction of red fox Vulpes vulpes on small game hunting bags in Norway. Wildl. Biol. 5, 157–166. doi: 10.2981/wlb.1999.020
Sokól R., Galecki R. (2018). The resistance of Eimeria spp. to toltrazuril in black grouse (Lyrurus tetrix) kept in an aviary. Poult. Sci. 97, 4193–4199. doi: 10.3382/ps/pey296
Sokól R., Koziatek-Sadlowska S. (2020). Changes in the corticosterone level in tooting male black grouse (Tetrao tetrix) infected with Eimeria spp. Poult. Sci. 99, 1306–1310. doi: 10.1016/j.psj.2019.10.074
Sokól R., Pluta P. (2022). Endoparasites in western capercaillies (Tetrao urogallus) and black grouse (Tetrao tetrix) kept in various types of aviaries. J. Wildl. Dis. 58, 114–121. doi: 10.7589/JWD-D-21-00017
Spidsø T. K., Hjeljord O., Dokk J. G. (1997). Seasonal mortality of black grouse Tetrao tetrix during a year with little snow. Wildl. Biol. 3, 205–209. doi: 10.2981/wlb.1997.025
Statistics Finland (2023). Hunting Available online at: https://stat.fi/en/statistics/riisaa (Accessed September 18, 2023).
Statistik Austria-Federal Institution under Public Law (2023). Statistical Database STATcube by Bundesanstalt Statistik Österreich Available online at: https://www.statistik.at/datenbanken/statcube-statistische-datenbank (Accessed September 18, 2023).
Statistisk Sentralbyra (2023). Small game and roe deer hunting Available online at: https://www.ssb.no/ (Accessed September 18, 2023).
Stevens B. S., Reese K. P., Connelly J. W., Musil D. D. (2012). Greater sage-grouse and fences: Does marking reduce collisions? Wildl. Soc Bull. 36, 297–303. doi: 10.1002/wsb.142
Storch I. (2000). Conservation status and threats to grouse worldwide: an overview. Wildl. Biol. 6, 195–204. doi: 10.2981/wlb.2000.016
Storch I. (2007). Conservation status of grouse worldwide: an update. Wildl. Biol. 13, 5–12. doi: 10.2981/0909-6396(2007)13[5:CSOGWA]2.0.CO;2
Storch I. (2013). Human disturbance of grouse - Why and when? Wildl. Biol. 19, 390–403. doi: 10.2981/13-006
Strauß E., Maistrelli C., Strube C., Ludwig T., Siebert U. (2022). Prevalence of gastrointestinal parasites in a lowland black grouse population in Central Europe. J. Ornithol 163, 1025–1037. doi: 10.1007/s10336-022-02006-y
Strauß E., Tost D., Ratsch C., Kulow J., Stolter C., Wormanns S., et al. (2018). Bestandsentwicklung und Nahrungsökologie des Birkhuhns Tetrao tetrix in Niedersachsen. Der Ornithologische Beobachter 115, 261–280.
Svenska Jägareförbundet. (2023). Viltövervakning Available online at: https://jagareforbundet.se/vilt/viltovervakning/ (Accessed September 18, 2023).
Svobodová J., Albrecht T., Šálek M. (2004). The relationship between predation risk and occurrence of black grouse (Tetrao tetrix) in a highly fragmented landscape:: An experiment based on artificial nests. Ecoscience 11, 421–427. doi: 10.1080/11956860.2004.11682851
Svobodová J., Bejček V., Málková P., Šťastný K. (2011a). Nízké přežívání tetřívků obecných (Tetrao tetrix) v sukcesních stadiích lesních porostů Krušných hor. [Low survival rate of black grouse (Tetrao tetrix) in maturating forest growths in the Krušné hory Mts. Sylvia 47, 77–89.
Svobodová J., Segelbacher G., Höglund J. (2011b). Genetic variation in black grouse populations with different lekking systems in the Czech Republic. J. Ornithol 152, 37–44. doi: 10.1007/s10336-010-0543-7
TenDen P. G. A., Niewold F. J. J. (2001). The black grouse in the Netherlands: monitoring the last ()? surviving population. Cah. d'Ethol 20, 299–310.
The Forest Management Institute Brandýs nad Labem (2022). Myslivecká evidence za ČR (Game Management Records for Czech Republic). Available online at: https://www.uhul.cz/mapy-a-data/myslivecka-evidence-za-cr/ (Accessed December 28, 2022).
Thorn S., Werner S. A., Wohlfahrt J., Bässler C., Seibold S., Quillfeldt P., et al. (2016). Response of bird assemblages to windstorm and salvage logging—Insights from analyses of functional guild and indicator species. Ecol. Indic 65, 142–148. doi: 10.1016/j.ecolind.2015.06.033
Tizzani P., Fanelli A., Belleau E. (2021). Gastrointestinal parasites of black grouse Lyrurus tetrix: A long-term study, (1986-2019) in the French Alps. Res. Vet. Sci. 137, 163–169. doi: 10.1016/j.rvsc.2021.05.005
Tomášek V., Volf O., Weidinger K., Svobodová J., Rolečková B., Hájková P., et al. (2023). Tetřívek - Poslední šance? (Vrchlabí: Správa KRNAP).
Tornberg R. (2000). Effect of changing landscape structure on the predator-prey interaction between goshawk and grouse University of Oulu, Oulu (FI.
Tornberg R., Colpaert A. (2001). Survival, ranging, habitat choice and diet of the Northern goshawk Accipiter gentilis during winter in Northern Finland. Ibis 143, 41–50. doi: 10.1111/j.1474-919X.2001.tb04168.x
Tornberg R., Korpimäki E., Byholm P. (2006). Ecology of the Northern goshawk in Fennoscandia. Stud. Avian Biol. 31, 141–157.
Tornberg R., Reif V., Korpimäki E. (2012). What explains forest grouse mortality: predation impacts of raptors, vole abundance, or weather conditions? Int. J. Ecol. 375260, 375260. doi: 10.1155/2012/375260
Tost D., Ludwig T., Strauss E., Jung K., Siebert U. (2022). Habitat selection of black grouse in an isolated population in northern Germany-the importance of mixing dry and wet habitats. PeerJ 10, e14161. doi: 10.7717/peerj.14161
Tost D., Strauss E., Jung K., Siebert U. (2020). Impact of tourism on habitat use of black grouse (Tetrao tetrix) in an isolated population in northern Germany. PLoS One 15, 19. doi: 10.1371/journal.pone.0238660
Ukrainian Nature Conservation Group (2009). Numbers, distribution and bagging of black grouse (Lyrurus tetrix) Available online at: https://uncg.org.ua/en/cadastre/monitoring-of-numbers-distribution-and-bagging-of-game-species/numbers-distribution-and-bagging-of-black-grouse-lyrurus-tetrix/ (Accessed January 26, 2009).
Vacek S., Matějka K., Simon J., Malík V., Schwarz O., Podrázský V., et al. (2007). Zdravotní stav a dynamika lesních ekosystémů Krkonoš pod stresem vyvolaným znečištěním ovzduší. [Health Status and Dynamics of Forest Ecosystems under Air Pollution Stress in the Giant Mts.] (Kostelec nad Černými lesy: Lesnická práce, s.r.o).
Vacek S., Poleno Z., Vacek Z., Podrázský V., Remeš J., Štefančík I., et al. (2023a). Přírodě blízké pěstování lesů. [Close to Nature Silviculture] (Prague: Česká zemědělská univerzita v Praze, Fakulta lesnická a dřevařská).
Vacek Z., Vacek S., Cukor J. (2023b). European forests under global climate change: review of tree growth processes, crises and management strategies. J. Environ. Manage 332, 117353. doi: 10.1016/j.jenvman.2023.117353
Valkama J., Korpimäki E., Arroyo B., Beja P., Bretagnolle V., Bro E., et al. (2005). Birds of prey as limiting factors of gamebird populations in Europe: a review. Biol. Rev. Camb. Philos. Soc. 80, 171–203. doi: 10.1017/S146479310400658X
Vickery J. A., Tallowin J. R., Feber R. E., Asteraki E. J., Atkinson P. W., Fuller R. J., et al. (2001). The management of lowland neutral grasslands in Britain: effects of agricultural practices on birds and their food resources. J. Appl. Ecol. 38, 647–664. doi: 10.1046/j.1365-2664.2001.00626.x
Virkkala R., Heikkinen R., Leikola N., Luoto M. (2008). Projected large-scale range reductions of northern-boreal land bird species due to climate change. Biol. Conserv. 141, 1343–1353. doi: 10.1016/j.biocon.2008.03.007
Visser M. E., Both C., Lambrechts M. M. (2004). Global climate change leads to mistimed avian reproduction. Adv. Ecol. Res. 35, 89–110. doi: 10.1016/S0065-2504(04)35005-1
Viterbi R., Imperio S., Alpe D., Bosser-Peverelli V., Provenzale A. (2015). Climatic control and population dynamics of black grouse (Tetrao tetrix) in the Western Italian Alps. J. Wildl. Manage 79, 156–166. doi: 10.1002/jwmg.810
Warren P. K., Baines D. (2002). Dispersal, survival and causes of mortality in black grouse Tetrao tetrix in northern England. Wildl. Biol. 8, 91–97. doi: 10.2981/wlb.2002.013
Wegge P., Kastdalen L. (2008). Habitat and diet of young grouse broods: resource partitioning between capercaillie (Tetrao urogallus) and black grouse (Tetrao tetrix) in boreal forests. J. Ornithol 149, 237–244. doi: 10.1007/s10336-007-0265-7
Wegge P., Moss R., Rolstad J. (2022). Annual variation in breeding success in boreal forest grouse: Four decades of monitoring reveals bottom-up drivers to be more important than predation. Ecol. Evol. 2022, 9327. doi: 10.1002/ece3.9327
Wegge P., Rolstad J. (2011). Clearcutting forestry and Eurasian boreal forest grouse: Long-term monitoring of sympatric capercaillie Tetrao urogallus and black grouse T. tetrix reveals unexpected effects on their population performances. For. Ecol. Manage 261, 1520–1529. doi: 10.1016/j.foreco.2011.01.041
Wegge P., Rolstad J. (2017). Climate change and bird reproduction: warmer springs benefit breeding success in boreal forest grouse. Proc. R. Soc B Biol. Sci. 284, 20171528. doi: 10.1098/rspb.2017.1528
Wegge P., Storaas T. (1990). Nest loss in capercaillie and black grouse in relation to the small rodent cycle in southeast Norway. Oecologia 82, 527–530. doi: 10.1007/BF00319796
Wegge P., Vesterås T., Rolstad J. (2010). Does timing of breeding and subsequent hatching in boreal forest grouse match the phenology of insect food for the chicks? Ann. Zool. Fennici 47, 251–260. doi: 10.5735/086.047.0403
White P. J. C., Warren P., Baines D. (2013). Forest expansion in Scotland and its potential effects on black grouse Tetrao tetrix conservation. For. Ecol. Manage 308, 145–152. doi: 10.1016/j.foreco.2013.07.038
White P. J. C., Warren P., Baines D. (2015). Habitat use by black grouse Tetrao tetrix in a mixed moorland-forest landscape in Scotland and implications for a national afforestation strategy. Bird Study 62, 1–13. doi: 10.1080/00063657.2014.1000261
Wickham H. (2016). Data Analysis BT - ggplot2: Elegant Graphics for Data Analysis (New York: Springer-Verlag).
Willebrand T. (1988). Demography and ecology of a black grouse (Tetrao tetrix L.) population. Disciplinary Domain of Science and Technology Uppsala University, Acta Universitatis Upsaliensis, Uppsala (SE.
Williams B., Nichols J., Conroy M. (2002). Analysis and Management of Animal Populations 1st Edn (Cambridge: Academic Press).
Wöss M., Zeiler H. (2003). Building projects in black grouse habitats-assessment guidelines. Sylvia 39, 87–96.
Zbinden N., Korner-Nievergelt F., Tettamanti F., Keller V. (2022). Long-term trends of reproductive success of black grouse Lyrurus tetrix in the southern Swiss Alps in relation to changes in climate and habitat. Wildl. Biol. 2022, e01022. doi: 10.1002/wlb3.01022
Zeiler H. P., Grünschachner-Berger V. (2009). Impact of wind power plants on black grouse, in Alpine regions. Folia Zool. 58, 173–182.
Zeitler A. (2000). Human disturbance, behaviour and spatial distribution of black grouse in skiing areas in the Bavarian Alps. Cah. d’Ethologie 20, 381–402.
Zeitler A. (2003). Maintaining black grouse wintering habitats by Alpine pasture management plans. Sylvia 39, 97–102.
Zhang C., Lu Z., Zhuang H., Zhou J., Zhang Y., Lv X., et al. (2024). Identification of potential suitable areas and conservation priority areas for representative wild animals in the Greater and Lesser Khingan Mountains. Ecol. Evol. 14, e11600. doi: 10.1002/ece3.11600
Keywords: Tetraonidae, predation, nest success, climate change, habitat loss, biodiversity conservation
Citation: Hambálková L, Cukor J, Brynychová K, Ševčík R, Vacek Z, Vacek S, Skoták V, Linda R and Andersen O (2024) Black grouse (Lyrurus tetrix) population status, reasons for decline and potential conservation measures from Western and Central Europe to Fennoscandia: a literature review. Front. Ecol. Evol. 12:1452317. doi: 10.3389/fevo.2024.1452317
Received: 20 June 2024; Accepted: 13 September 2024;
Published: 30 September 2024.
Edited by:
Jakub Drimaj, Mendel University in Brno, CzechiaReviewed by:
Łukasz Kajtoch, Polish Academy of Sciences, PolandRudolf Kropil, Technical University of Zvolen, Slovakia
Copyright © 2024 Hambálková, Cukor, Brynychová, Ševčík, Vacek, Vacek, Skoták, Linda and Andersen. This is an open-access article distributed under the terms of the Creative Commons Attribution License (CC BY). The use, distribution or reproduction in other forums is permitted, provided the original author(s) and the copyright owner(s) are credited and that the original publication in this journal is cited, in accordance with accepted academic practice. No use, distribution or reproduction is permitted which does not comply with these terms.
*Correspondence: Richard Ševčík, sevcik@vulhm.cz