- 1Department of Biology, Baylor University, Waco, TX, United States
- 2Center of Applied Ecology and Sustainability (CAPES), Pontificia Universidad Católica de Chile, Santiago, Chile
- 3Centro de Investigación Gaia Antártica (CIGA), Universidad de Magallanes, Punta Arenas, Chile
- 4Centro Internacional Cabo de Hornos (CHIC), Universidad de Magallanes, Puerto Williams, Chile
- 5Departamento de Áreas Silvestres Protegidas, Corporación Nacional Forestal, Puerto Aysén, Chile
- 6Patagonia on the Trail, Puerto Natales, Chile
- 7Instituto de Biología, Facultad de Ciencias, Universidad de Valparaíso, Valparaíso, Chile
- 8Centro Asistencial Docente y de Investigación, Universidad de Magallanes, Punta Arenas, Chile
- 9Private Practitioner, Región de Magallanes y Antártica Chilena, Porvenir, Chile
- 10Wildlife Conservation Society-Chile, Punta Arenas, Chile
- 11Marine and Environmental Sciences Department, Hampton University, Hampton, VA, United States
- 12Institute of Marine Sciences, University of California-Santa Cruz, Santa Cruz, CA, United States
Leopard seals have traditionally been considered Antarctic predators with a Southern Ocean distribution. Historically, sightings north of the Antarctic Polar Front were considered extralimital. However, recent studies suggest a significant presence of leopard seals in subantarctic regions. Here, we assess the spatial occurrence, residency status, and temporal trends of leopard seals in Chile using historical records, stranding reports, standardized monitoring data, photo-identification (photo ID) catalogs, and sightings from four research expeditions. We also characterize glaciers where sightings are concentrated, identifying glaciological and geomorphic attributes that prolong iceberg residency time, which is linked to high leopard seal concentrations. Based on these attributes, we evaluated other potential suitable glacial habitats in Patagonia. We obtained 438 sighting records of leopard seals from 1927 to 2023. Over the last 15 years, we documented a 4-18% annual increase in stranding events reported to national authorities. Most sightings (75%) were concentrated in two hotspots: National Park San Rafael Lagoon, located in Northern Patagonia, and Parry Fjord in Tierra del Fuego. Using photo ID catalogs, we identified 19 resident leopard seals, including 16 multi-year residents observed between 2010-2023 (10 in San Rafael, 6 in Tierra del Fuego) and 3 potential residents (observed multiple months in the same year in Tierra del Fuego). San Rafael monitoring data showed no inter-annual trend, but seasonal trends were observed. We also provide evidence of breeding in Chile, with records of at least 14 pups born and at least two females giving birth in multiple years. Our habitat characterization suggests that calving flux, fjord sinuosity, and fjord width variation are crucial for prolonging iceberg residency in hotspot areas. Based on these attributes, we identified 13 additional fjords in Patagonia as “very likely” suitable for leopard seals. Our study confirms that Patagonia is part of the species’ breeding distribution, shifting the paradigm that leopard seals are merely visitors north of the Antarctic Polar Front. Given the limited number of suitable glaciers in Chile and the potential impacts of climate change, our assessment highlights glacial retreat as a major threat for the ecosystem of this pagophilic marine apex predator in South America.
1 Introduction
Apex predators significantly affect ecosystem structure and function (Paine, 1980; Estes et al., 2011; Ripple et al., 2014). Changes in their abundance and distribution can trigger trophic cascades, affecting ecosystem-level processes such as prey population dynamics and nutrient cycling (Schmitz et al., 2010; Leo et al., 2019). Moreover, due to their high energetic demands and foraging efficiency, a small number of apex predators can rapidly modify the trophic composition of their habitat (Estes et al., 1998; Williams et al., 2004). Therefore, documenting the occurrence of apex predators, as well as monitoring changes in their populations, are crucial for understanding the trophic dynamics and functionality of ecosystems (Paine, 1980; Estes et al., 2011; Ripple et al., 2014).
Leopard seals (Hydrurga leptonyx, de Blainville, 1820) are one of the most conspicuous apex predators in the Southern Ocean (reviewed in Bester et al., 2017a). The species’ effective population size is estimated at ~24,000 individuals (Bender et al., 2023). They are known to have a broad, generalist diet, including both ectothermic (e.g., krill, cephalopods, fish) and endothermic prey (e.g., seabirds, seals; reviewed in Krause et al., 2020). As Southern Ocean apex predators, leopard seals exert strong top-down control on other Antarctic mesopredators, including Antarctic fur seals (Arctocephalus gazella) and penguin species (Boveng et al., 1998; Forcada et al., 2009; Schwarz et al., 2013; Krause et al., 2022) and are responsible for more predation on endothermic prey than any other pinniped worldwide (Walker et al., 1998; Casaux et al., 2009). For instance, Krause et al. (2022) showed that a small number of leopard seals at Cape Shirreff in the South Shetland Islands has caused the local colony of Antarctic fur seal to collapse. Moreover, leopard seal diet can vary according to life history traits (e.g., sex, age, and mass; Krause et al., 2020; Sperou et al., 2023) and seasonality (Hall-Aspland et al., 2005; Krause et al., 2020) and they also exhibit a high degree of individual behavioral plasticity, employing strategies such as ambush tactics, kleptoparasitism, scavenging, and group prey processing (Krause et al., 2015; Krause and Rogers, 2019; Robbins et al., 2019). Together, their varied diet and behavioral plasticity likely enhances leopard seals’ resilience to changes in prey availability and abundance.
Historically, leopard seals have been described as an Antarctic species predominately found in pack ice habitats around the Southern Ocean (Southwell et al., 2008; Meade et al., 2015; Bester et al., 2017a). In Antarctica, leopard seals are considered a pagophilic species that rely on sea ice for breeding, molting, and resting (Ray, 1970; Jessopp et al., 2004). However, leopard seals have long been observed in subpolar (and even ice-free) habitats across the South Atlantic, South Pacific, and Southern Indian Oceans, (reviewed in Bester et al., 2017b) and extensively observed in Patagonia (both Argentina and Chile; Rodrı́guez et al., 2003; Aguayo-Lobo et al., 2011; Cursach et al., 2011; Acevedo and Martinez, 2013; Acevedo et al., 2017). In Chile, some of the sightings have reached extreme northern latitudes such as the Juan Fernandez Archipelago and Easter Islands (Aguayo-Lobo et al., 2011; Hucke-Gaete et al., 2014; Stewart et al., 2020). Generally, sightings of leopard seals outside of the Southern Ocean have been considered as extralimital and were thought to be rare and/or isolated events.
Recently, research on leopard seals across the southern hemisphere has challenged the notion that leopard seals do not reside north of the Antarctic Polar Front (Hupman et al., 2020). Analyses combining historical records with contemporary sightings from subantarctic locations document the year-round presence of leopard seals in New Zealand, with more than 2,700 leopard seal sightings over the last eight centuries (1200-2018; Hupman et al., 2020). Furthermore, more recent data at both Marion Island—a subantarctic South African island—and South Australia suggest an increase in juvenile leopard seal sightings in the last two decades (Shaughnessy et al., 2023; de Bruyn and Ross, pers. comm.) compared to previous decades (Bester et al., 2006).
Similarly, in Chile, both historical and contemporary data demonstrate a centuries-long presence of leopard seals (Aguayo-Lobo et al., 2011; Torrejón et al., 2013; Stewart et al., 2020). For instance, anthropological evidence indicates that leopard seals have been occasional visitors to Easter Island (~3,500 km off the coast of Chile) since the arrival of Polynesians around 800 CE (Hucke-Gaete et al., 2014; Stewart et al., 2020). On the Chilean coast, a review by Aguayo-Lobo et al. (2011) provided compelling evidence of a nearly century-long periodic presence of leopard seals in Chile, documenting 118 sightings of leopard seals from 1927 to 2010. A later study documented a leopard seal in a Patagonian fjord of the Magallanes region in Chile making use of this location multiple times during five months, indicating potential residency (Acevedo and Martinez, 2013). Furthermore, observations of leopard seals since 2012 include sightings of adult-pup pairs and sexual behavior, indicating that mating and parturition also occur in Chilean waters (van der Linde et al., 2022; Kienle et al., 2024). Although these studies challenge the traditional view of leopard seals as episodic visitors in South America, there have been no systematic multi-year studies to examine occurrence and residency patterns of leopard seals in Chile, nor attempts to characterize their habitat outside Antarctica.
The main objective of this study was to determine the historical and current occurrence of leopard seals in Chile and assess residency patterns of individuals in areas with consistent sightings. We hypothesized that some leopard seals exhibit long-term site fidelity to ice-associated areas in Chile. To test this, we analyzed data from published historical sightings records, government reports, monitoring surveys, citizen science initiatives, and conducted boat-based expeditions to evaluate the spatial and temporal distribution of leopard seals from 1927 to 2023. In addition, we conducted a habitat characterization of leopard seals in Chile along with an assessment of potential habitats for the species across Patagonia. We hypothesized that at least half of the marine-terminating glaciers in Chile possess similar characteristics than those where most leopard seal sightings have been reported and may consist of additional suitable habitat. To test this, we characterized key glaciological and geomorphic attributes of marine-terminating glaciers where leopard seal sightings are most frequent and compared these with all other similar glaciers found throughout Chile. Our study thoroughly documents leopard seal occurrence, residency, and identify their potential habitats in Chile; we provide baseline information on the species distribution that would help to understand the impacts of climate change on this ice-dependent predator.
2 Methods
2.1 Historical sighting records
We conducted a literature review of peer-reviewed publications documenting the presence of leopard seals in Chile through Google Scholar and Web of Science using the following search terms: “leopard seals Chile”, “Hydrurga leptonyx Chile”, “leopard seals South America”, “Hydrurga leptonyx South America”, “leopard seals Patagonia”, “leopard seals Tierra del Fuego”, “leopard seals San Rafael”, and “leopard seals Easter Island” from 2009 to 2023. We completed literature searches in both English and Spanish. We searched for additional peer-reviewed publications and grey literature by reviewing the cited references of each publication. Sightings reported before 2009 were previously reviewed and reported by Aguayo-Lobo et al. (2011). We extracted these sightings from Aguayo-Lobo et al. (2011) with the following consideration: Aguayo-Lobo et al. (2011) added up the recorded number of animals from all sightings to provide a “total number of leopard seals” in Chile. However, their methodology could not determine if the same animals were counted multiple times because seals were not tagged, nor were photo-ID methods used to avoid duplicate counts. Therefore, here we considered every seal from each sighting event as an independent sighting rather than reporting the total number of animals per sighting as did Aguayo-Lobo et al. (2011).
From each publication, we extracted all relevant biological data reported on the individual’s life history (sex, age class, molting status), morphometrics (length), substrate (whether a seal was on land, ice, or in the water), location (name of the location, region, and coordinates transformed to decimal degrees), and date. We cross-referenced all data and identified, when possible, repeated individuals that were reported multiple times within the same publication or between publications. We also included any comment reported with each sighting. All sightings from our literature review and Aguayo-Lobo et al. (2011) are hereafter referred to as “Historical Records”.
2.2 New sighting records
We compiled new sighting records—records that have not been previously reported in peer-reviewed literature—and referred to as “New Records”. These new sighting records include strandings from government reports, monitoring data from San Rafael Lagoon National Park, photo-identification catalogs, and our expeditions.
2.2.1 Stranding data
Marine mammal stranding events in Chile are reported to the National Fisheries and Aquaculture Service of Chile (SERNAPESCA). These stranding events of dead or live animals are reported by citizens. We compiled all leopard seal sighting data publicly available online from SERNAPESCA (Sernapesca, 2023) from 2009 to 2023. SERNAPESCA stranding records up to 2009 were previously reviewed by Aguayo-Lobo et al. (2011).
2.2.2 San Rafael monitoring data
The National Park San Rafael Lagoon (henceforth “San Rafael”) located in the Aysén Region (46°40’0” S, 73°58’0” W) is administered by the National Forestry Corporation (CONAF). It is one of the two areas of Chile where leopard seals are frequently observed. In 2013, CONAF began the first and only systematic leopard seal monitoring program for leopard seals in Chile. Each month, a boat with at least two trained observers surveyed the San Rafael lagoon exclusively searching for leopard seals. They documented the presence/absence of leopard seals throughout the entire lagoon. During boat surveys, observers recorded the following information: total number of leopard seals, geographic coordinates, and substrate (i.e., whether each seal was on ice, land, or in the water). When possible, age class (pup, juvenile, adult) and sex were visually assessed. Photographs were collected opportunistically for each seal whenever possible. Due to weather conditions, monitoring was not consistent over time and in some years, it was not conducted every month. The San Rafael monitoring program has near-monthly occurrence records for leopard seals from January 2013 to March 2020. Monitoring was paused after March 2020 due to the COVID-19 pandemic and restarted irregularly in 2021. For this study, we only included data from the period where standardized monitoring was performed (January 2013 to March 2020).
2.2.3 Leopard seal photo-identification catalogs
Leopard seals have unique spot patterns and distinguishable scars that facilitate individual identification from photographs, a method called photo-identification (Photo ID; Forcada and Robinson, 2006; Hupman et al., 2020; Visser et al., 2022; Grabham et al., 2023). We used the photographic data of two Photo ID regional catalogs for identifying individual leopard seals in Chile. The first catalog was developed as part of a citizen science initiative conducted by CONAF in collaboration with tour operators that visit San Rafael. The second catalog was an initiative of this study, conducted in the Magallanes y Antártica Chilena Region of Chile to identify leopard seals in this region. To create these regional catalogs, photographs of leopard seals were solicited from tour operators, local scientists/conservationists, and the general public.
The San Rafael catalog published by CONAF in 2021 (henceforth “SR catalog”) was created for leopard seals exclusively observed in San Rafael. San Rafael is visited by hundreds of tourists year-round traveling with local tour operators. Thus, this catalog is the result of continuous sightings reported throughout the year and is composed of pictures collected from tour operators and tourists almost every month of the year.
The Tierra del Fuego catalog (henceforth “TF catalog”) was created from leopard seals observed across the southwest portion of Patagonia (between latitudes 48°36´S and 56°30´S and longitudes 66°25´ and 75°40´W). Although most of the sightings are concentrated in the Coastal Marine Protected Area of Multiple Uses (Spanish acronym: AMCP-MU) “Seno Almirantazgo” (Almirantazgo Sound, 54°31’0” S, 69°15’0” W), some of the sightings are outside this geographical area (but still within western Tierra del Fuego) therefore, the entire area is henceforth referred to as “Tierra del Fuego”.
We manually compared each leopard seal photograph to all others in our photographic database and used unique identifying spot patterns and scars to visually determine matches. A match was found when the same individual seal was documented in two or more images (or sets of images). Each image or set of images was visually inspected and manually manipulated if needed to maximize matching success; specifically, we cropped, and rotated each photo, and adjusted the exposure when necessary (Acevedo and Martinez, 2013; Cheeseman et al., 2017). Manual matching was validated by a second expert reviewer and if a disagreement occurred, a third expert reviewer decided. When available, we then extracted the location and dates from the metadata associated with each image and double checked the accuracy of the dates and locations with the photographer or project leaders of the initiatives. In pictures without location data, we estimated the coordinates using the location name provided by the photographer/project leaders. We used photographs to assign sex and age class when it was visually possible. Age class was simplified in three categories (pup, juvenile, adult) and estimated only when it was visually evident (pups: presence of lanugo or next to their mothers; juveniles: with no lanugo, unlikely to be accompanied by a mother, less than 2.0 meters, thin, and with minimum scars; and adults: over 2.5 meters with presence of several scars. Adapted from Laws, 1957; Forcada and Robinson, 2006; Hupman et al., 2020; van der Linde et al., 2022). We assigned each matched seal a unique identification number (Seal ID) and added them to each catalog. We added unmatched seals or leopard seals that did not have any matches to the database if there were high-quality, usable images that would allow for manually matching of the seal in the future as additional images are incorporated into each catalog.
We used the photographs of matched seals to identify resident individuals at each location. We classified a seal as ‘Resident’ if it was observed at least once per year across multiple years. We classified a seal as ‘Potential Resident’ if it was observed multiple months within a single year but not sighted in more than one year, and as ‘Undetermined’ if observers confirmed that the seal was not a match to any of the previously cataloged seals (thus, new to the catalog) and it had only been reported once. We assessed body condition (severe, poor, good, or excellent) of all cataloged individuals (i.e., Resident, Potential Resident and Undetermined) based on the last year with photographs available that included high resolution full-body profiles (following Hupman et al., 2020; see Supplementary Table 1).
In contrast to the systematic monitoring efforts in San Rafael (see Section 2.2.2 above), the remote and isolated characteristics of Tierra del Fuego make monitoring leopard seals extremely difficult. As a result, no standardized monitoring program exists. Furthermore, tour operators visit this location sporadically, mostly during the austral summer. Since 2010 limited expeditions have been conducted every few years by the Wildlife Conservation Society (WCS) to the Almirantazgo Sound, where observers record leopard seal sightings when possible. However, these expeditions, are not comparable to the regular visits of the monitoring program in San Rafael.
The contrasting differences in the accessibility and monitoring efforts between San Rafael and Tierra del Fuego led us to incorporate the catalog sightings data on our sighting database following different criteria. Due to limited records from Tierra del Fuego, we include all sightings reported by both major tour operators and past WCS expeditions. In contrast, because San Rafael has a monitoring program, first we included all the monitoring data in our database and then compared the monitoring photographs with the SR catalog to identify known individuals when possible. Next, we used additional San Rafael sightings from the SR catalog to provide data for months where: (1) CONAF did not perform any monitoring due to weather/logistic constraints; or (2) CONAF monitoring efforts found no leopard seals in a given month but photos from tour operators confirmed the presence of one or more leopard seals from other days of that month. We applied these criteria to San Rafael to avoid including repeated records of the same individual within a month. This criterion was applied only to include records in our overall database as we aimed to ascertain the degree of residency of some of the individuals within each year. However, the additional data extracted from the catalogs was not included in the analysis of seasonal trends of the San Rafael standardized monitoring data (section 3.3).
2.2.4 Expeditions
Here we report new leopard seal sightings data from four recent expeditions in Chile that were targeting leopard seals: two to Tierra del Fuego and two to San Rafael. Chronologically, the first expedition was led by WCS to the Almirantazgo Sound, Tierra del Fuego in November 2021 (henceforth “TF-21”). The next two expeditions were to San Rafael in December 2022 (henceforth “SR-22”) and November 2023 (henceforth “SR-23”). The fourth expedition was to Tierra del Fuego in December 2023 (henceforth “TF-23”). During each expedition, we navigated the area daily and recorded total counts of leopard seals, geographic coordinates, habitat characteristics (i.e., whether the seal was on ice, land, or in the water) and visually assess age class/sex as was done for the catalogs. We identified known individuals from the SR and TF catalogs and our national photographic database when possible. All daily records of leopard seals were added to a compiled table of sightings except when an individual was observed multiple times on the same day.
We compiled the historical and new sightings data and associated metadata in a table of sightings (Supplementary Table 2). To visually assess the distribution of leopard seal sightings across Chile, we used the GPS coordinates for each record or estimated coordinates from Google Earth based on the name of the location provided. We then created a heatmap of all leopard seal sightings in Chile using ArcGIS Pro.
2.3 National and local population trends in leopard seal counts
We evaluated population trends in leopard seals using two datasets. First, we analyzed the 15 years of stranding data to examine temporal trends in the number of leopard seals reported across Chile by evaluating the rate of change of stranding events. We excluded records where the same individual was reported multiple times both temporally and spatially (e.g. same animal observed twice in a week in adjacent beaches). Second, we used the San Rafael standardized monitoring data to identify seasonal and inter-annual leopard seal trends at this location. To accomplish this, we used a model-based approach to generate estimates associated with temporal parameter effects, with confidence intervals based on Monte Carlo (MC) simulations to enhance the robustness of model parameters.
2.3.1 Rate of change of stranding events
We analyzed the rate of change of stranding events reported over time from the stranding data using a generalized linear model with a Poisson distribution. To ensure robust parameter estimation, we employed MC simulation with 10,000 iterations. This allowed us to estimate the uncertainty associated with the expected rate of change of stranding events. Additionally, we applied bias correction and acceleration to the resulting 95% confidence intervals (CI 95%) from the simulated distribution of parameter values to enhance the accuracy of our inferences.
2.3.2 Seasonal and inter-annual population trends in San Rafael
We analyzed seasonal variations in leopard seal counts at San Rafael using generalized additive models (GAMs) to delineate linear and non-linear relationships of seal counts with time. These models incorporated year and month as explanatory variables and the count of observed animals as our response variable. The effect of year was estimated as a linear variable. However, to capture non-linear seasonal monthly trends on the leopard seal counts the effect of month was estimated using a smooth function (penalized regression splines; Wood, 2011). The estimation of smoothing parameters was achieved through restricted maximum likelihood method, using cyclic penalized cubic regression splines as the basis to model cyclic temporal dimensions (Wood, 2017).
To characterize seasonal trends in terms of linear seasonal variations, we computed first derivatives using finite differences method from the predicted count of animals by using grid approximation, accompanied by CI 95% (Makowski et al., 2020). Robustness confidence intervals were estimated via bootstrap resampling using 10,000 MC simulations.
We evaluated inter-annual trends in leopard seal counts in San Rafael using GAMs. We computed the linear and quadratic effect of year on leopard seal counts using non-orthogonal polynomial contrasts, while holding constant the intra-annual seasonal effect estimated using cyclic splines based on the number of months that the monitoring was performed, which vary per year. This approach facilitated the exploration of potential linear and quadratic trends associated with variations in observed animal counts across years. Due to irregular and interrupted monitoring in 2020, we excluded this year from the analysis.
For both stranding and monitoring datasets, all analyses were performed using R (version 4.3.2). GAMs and corresponding model estimates were conducted using the mgcv and modelbased packages (Wood, 2017; Makowski et al., 2020). Complementary R packages were used for data visualization purposes (Wickham, 2016; Lüdecke et al., 2021).
2.4 Glacial habitat characterization
Most leopard seal sightings in Chile involve animals hauling out on icebergs produced by glaciers whose ice fronts are in contact with water (sea or lake), suggesting the importance of marine-terminating glaciers with high iceberg production (Aguayo-Lobo et al., 2011). Patagonia is the region that spans the southernmost tip of South America between ~40° and ~56° S. This territory exhibits the largest number of glaciers on the continent, including the largest icefields of the southern hemisphere outside Antarctica; the so-called Northern and Southern Patagonian Icefields (Soteres et al., 2023). Consequently, we focused our glacial habitat characterization on the glacial basins that produce icebergs in the two areas where leopard seals are most frequently sighted in Patagonia: the San Rafael glacier at San Rafael, and the Parry Fjord glaciers in Tierra del Fuego. In both places, the occurrence and local residence time of icebergs appear to be a critical leopard seals haul out substrate. Our methodological approach involves assessing iceberg production and identifying potential geomorphic traps (i.e., specific landforms and morphometric parameters within the glacial basins and fjords that can prolong the local presence of icebergs; Figure 1).
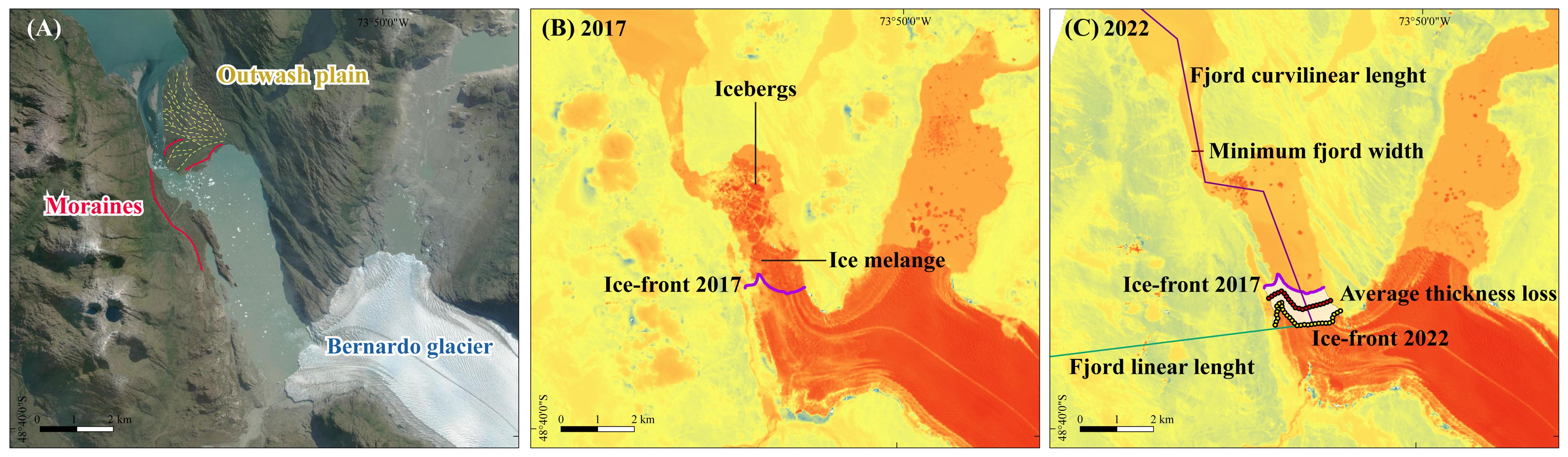
Figure 1. Schematic representation of variables used to calculate calving flux, fjord sinuosity, and fjord width in the study glaciers. (A) Satellite image illustrating geomorphic features (outwash plain and moraines) that affect the sinuosity and width of a fjord. Landsat 8 imagery in 2017 (B) and 2022 (C) with vectors used to calculate glacial and geomorphic attributes (list of Landsat 8 images used in ice flux calculation can be found in Supplementary Table 3).
Calving flux is the volume of ice detached from the front of water-terminating glaciers (i.e., marine-terminating and lake-terminating glaciers henceforth “marine-terminating glaciers”) per unit of time and it is considered a key metric to estimate ice loss due iceberg formation (Meier and Post, 1987; Evans et al., 2022). We calculated calving flux for the San Rafael glacier and Parry Fjord glaciers following Meier and Post (1987); see the detailed methodology in the Supplementary Methods in Supplementary Material).
Calving flux account for iceberg production but iceberg residence timing is also partly affected by local geomorphology and morphometry of the fjords, particularly sinuosity (i.e. the degree of linearity of a fjord) and fjord width variations often linked to the presence of glacial landforms within the fjords that could promote iceberg stranding. Therefore, we assessed fjord sinuosity following Vérité et al. (2023); see Supplementary Methods in Supplementary Material for details) and fjord width variations by calculating a ratio between the maximum and minimum width of each glacier (Figure 1; minimum and maximum values used can be found in Supplementary Table 4 in Supplementary Material).
We then produced a simplified geomorphic map that broadly depicts the spatial arrangement of the most prominent landforms of glacial origin, such as moraine ridges and outwash plains, for the San Rafael glacier and the Parry Fjord glacial basins. We analyzed satellite imagery (Figure 1) provided by Google Earth (2016 CNES/SPOT, ~15 m spatial resolution), ESRI Imagery (2017, TerraColor, ~15 m spatial resolution and SPOT, ~2.5 m spatial resolution), and ALOS PALSAR digital elevation models (~12.5 m spatial resolution) to examined basic morphometric attributes and to map local geomorphic features of the San Rafael glacier at San Rafael and Parry Fjord glaciers at Tierra del Fuego using ArcGIS 10.4. We followed the identification criteria used in prior geomorphic maps from Patagonia by Leger et al. (2020); Soteres et al. (2020) and Soteres et al. (2022). Preliminary maps based on remote data were subsequently ground-truthed during expeditions.
2.5 Glacial suitability analysis for leopard seals in Patagonia
We used the glaciological (calving flux) and geomorphic attributes (ice-marginal landforms, fjord sinuosity, and fjord width variations) calculated for the San Rafael and Parry Fjord glaciers as reference values to evaluate the suitability of other glacial basins and fjords as potential habitats for leopard seals across Patagonia. First, we identified marine-terminating glaciers in the region according to the Chilean glacier inventory (DGA, 2022) and checked them visually in recent satellite imagery to assure their ice fronts are still resting on water. We focused our efforts on the major icefields and ice caps in Patagonia, specifically (1) the Northern Patagonian Icefield (NPI; ~46.5-47.5°S), (2) the Southern Patagonian Icefield (SPI; ~48.3-51.5°S), (3) Gran Campo Nevado (GCN; ~52.8°S), (4) Isla Santa Inés (ISI; ~53.8°S), and (5) the Cordillera Darwin Icefield (CDI; ~54.6°S). Second, we calculated the calving flux, fjord sinuosity, and fjord width variations of all marine-terminating glaciers identified following the described methods of the previous section (See also Supplementary Methods and Supplementary Table 4 in Supplementary Material). Third, we calculated the added weighted mean of the normalized values for calving flux, width, and sinuosity for all marine-terminating glaciers, and applied a proportional calculated weighted estimates (CWE) for each parameter (i.e. calving flux, fjord width, and fjord sinuosity) that was determined based on the estimated influence of each parameter over the iceberg retention time in San Rafael and the Parry Fjord Glaciers. Finally, fjords were classified as “very likely,” “likely,” and “less likely” to host leopard seals, based on individual weighted mean values compared to the average weighted mean threshold determined from San Rafael Glacier and Parry Fjord.
3 Results
We documented 438 leopard seal sighting records in Chile from 1927 to 2023 (Supplementary Table 2). Two-thirds of the sightings were new records (66%, n=291), and one-third were historical records (34%, n=147). The new records included: (1) 15 years of SERNAPESCA stranding records (n=44), (2) San Rafael monitoring data (n=88), (3) Photo ID catalogs (n=108; SR catalog: 62 records, TF catalog: 46 records), and (4) expeditions data (n=51; TF-21: 4 records, TF-23: 15 records, SR-22: 12 records, SR-23: 20 records). From these sighting records, we were able to identify at least 41 leopard seal individuals that were observed two or more times.
Most leopard seal sightings (86%) were in Patagonia (between ~40° and ~56° S) where marine-terminating glaciers are found (Figure 2A). Two Patagonian locations accounted for 74% of sightings: San Rafael (15 historical and 183 new records) and Parry Fjord in Tierra del Fuego (63 historical and 63 new records). When substrate was reported for sightings in Patagonia (i.e. if the seal was observed on land, ice, or water), 84% of sightings were animals hauled out on floating ice.
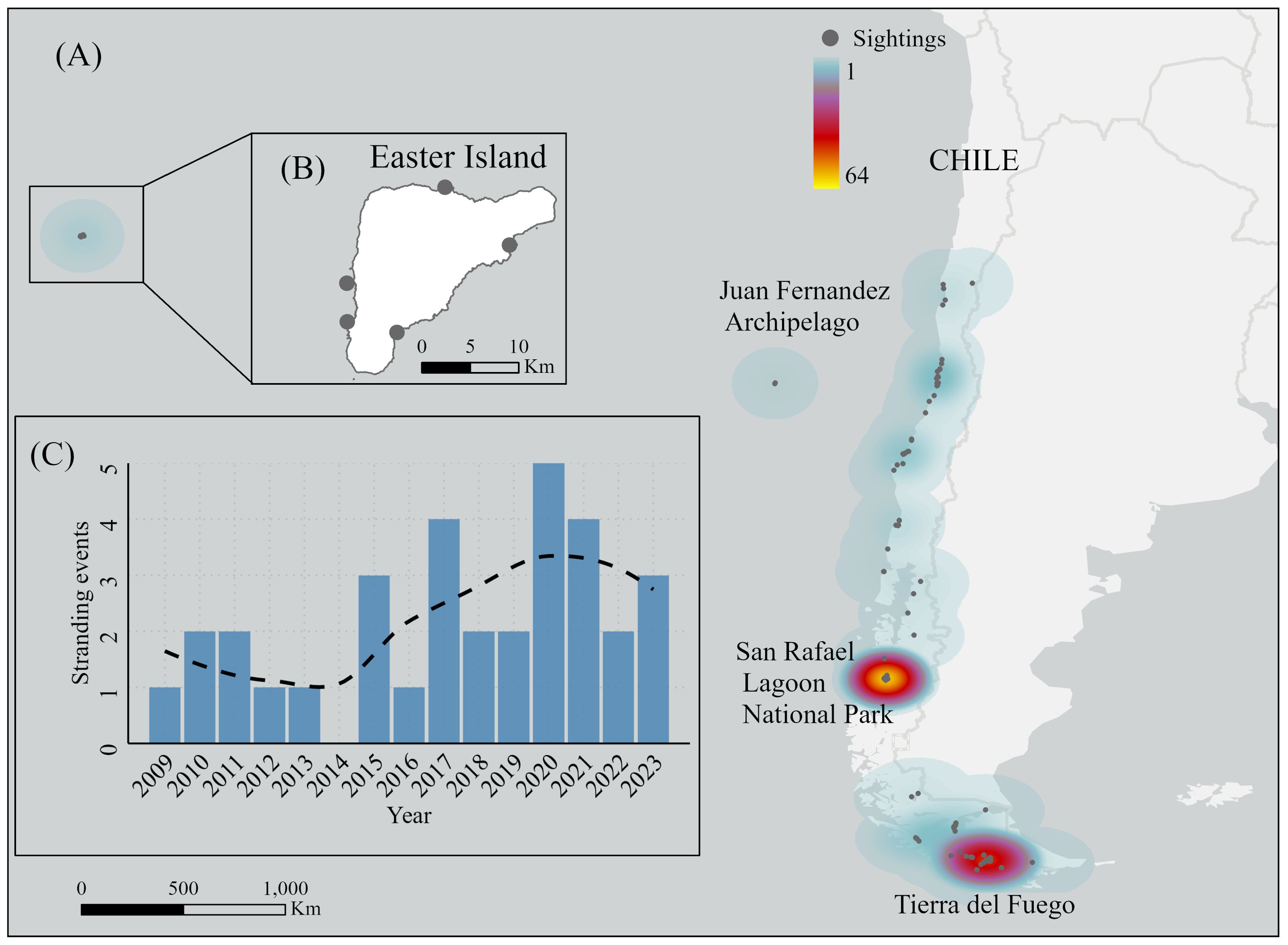
Figure 2. Historical and contemporary leopard seal sightings (gray circles) in Chile. (A) Heatmap of all 438 sightings, including those in Chilean oceanic insular territories (i.e., Juan Fernandez and Easter Island). (B) Map of Easter Island highlighting the northernmost sightings reported for Chile reviewed in Stewart et al. (2020) with some of the geolocations estimated from descriptions. (C) Annual leopard seal stranding events reported to the National Service of Fisheries and Aquaculture of Chile (SERNAPESCA) from 2009 to 2023. Stranding data are publicly available (Sernapesca, 2023). The dashed black line indicates the trend of the stranding data. Map Sources: Esri, DeLorme, HERE, MapmyIndia.
Chile is among the few countries with recorded sightings of leopard seal pups outside Antarctica (van der Linde et al., 2022). The earliest pup record is from 2012 (Acevedo et al., 2017). Here, we documented 14 pups (Tierra del Fuego: 4, San Rafael: 10). Eight of these pup records were reviewed by van der Linde et al. (2022), and six are from our new records (four from the San Rafael monitoring program, two from our expeditions). In Chile, all pup records occurred in Patagonia. Four pups were initially observed alive (one was later found dead), seven were reported dead, and three had no recorded status.
Of the 438 records of leopard seals in Chile, there were 404 confirmed age class records; 75% of these leopard seals were adults and 25% were immature individuals (i.e., pups and juveniles). In Patagonia specifically, there were 352 confirmed age class records; 82% (n = 289) of these leopard seals were adults and 18% (n =63) were immature individuals. In contrast, among the 52 age class records north of Patagonia, only 23% (n =12) were adults, and 77% (n =40) were immature, including the northernmost sightings from Easter Island (Figure 2B; Stewart et al., 2020).
3.1 Temporal trends of stranding data
Leopard seal stranding numbers in Chile are low. There was a mean of two leopard seal strandings per year, with a range from 0-5 leopard seals reported annually between 2013-2019 (Figure 2C). Bias corrected and accelerated intervals from MC samples estimated from simulation-based Poisson regression suggested a 4-18% increase in leopard seal stranding events rate annually; with 99.9% of the density mass of MC outcomes indicating a probability of > 0 and supporting an increase in the number of stranding events of leopard seals over time (Figure 3).
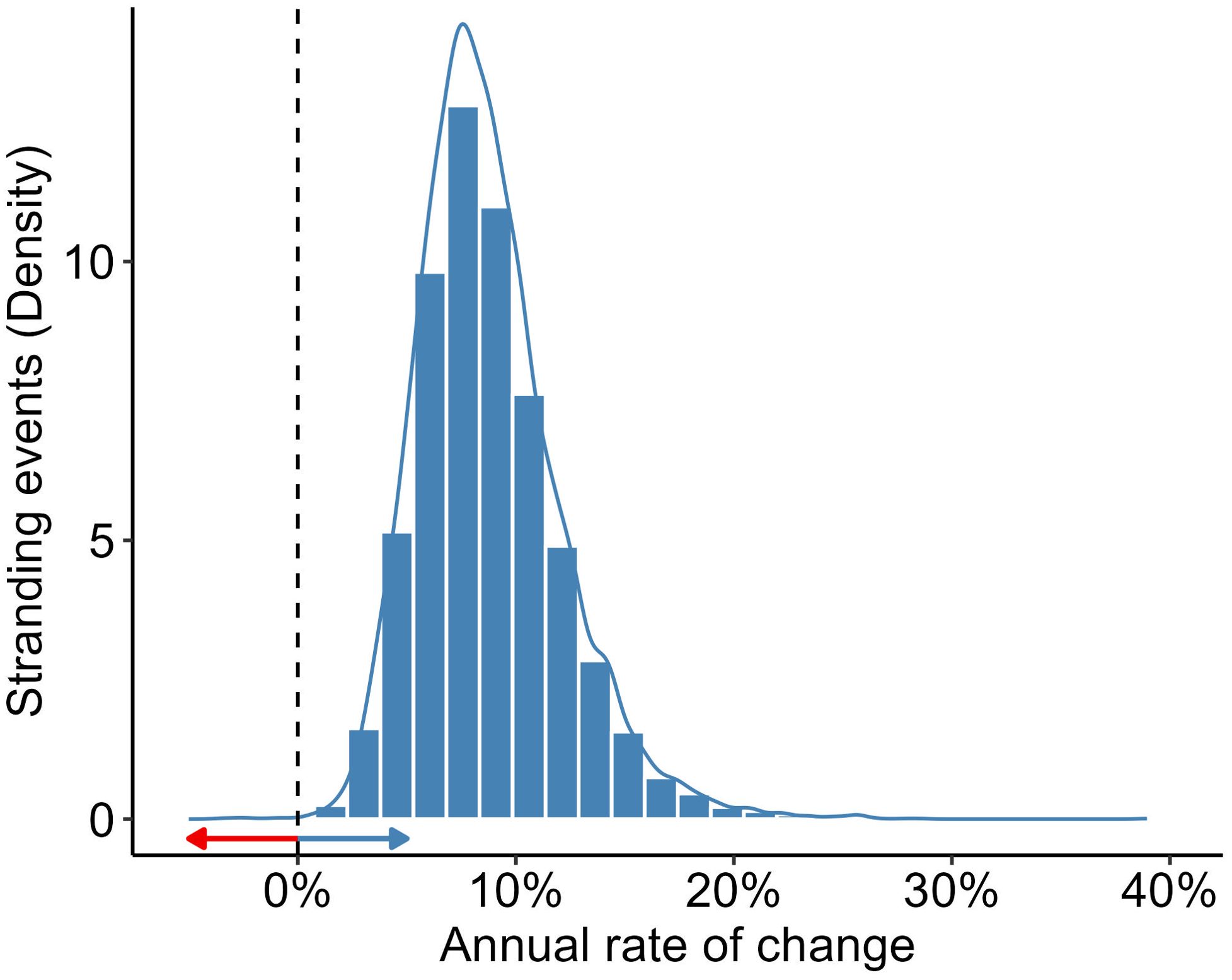
Figure 3. Probability distribution showing the annual rate of change in stranding events across Chile from Monte Carlo simulations of the Poisson model. The vertical dashed line indicates the null rate of change. The red arrow illustrates the probability of a decrease in stranding events (<0.1% of total Monte Carlo samples), while the blue arrow indicates the probability of an increase (>99.9% of total Monte Carlo samples).
3.2 Resident leopard seals
We reviewed ~2,500 photographs of leopard seals (catalogs and expedition photographs) from the two locations where most sightings were concentrated (i.e., San Rafael and Tierra del Fuego). 19 leopard seals had recurrent sightings over time (Table 1; Figure 4). After cross-referencing all images between San Rafael and Tierra del Fuego, we found no evidence of the same individuals visiting both locations. Both catalogs can be downloaded from the Supplementary Data in Supplementary Material.
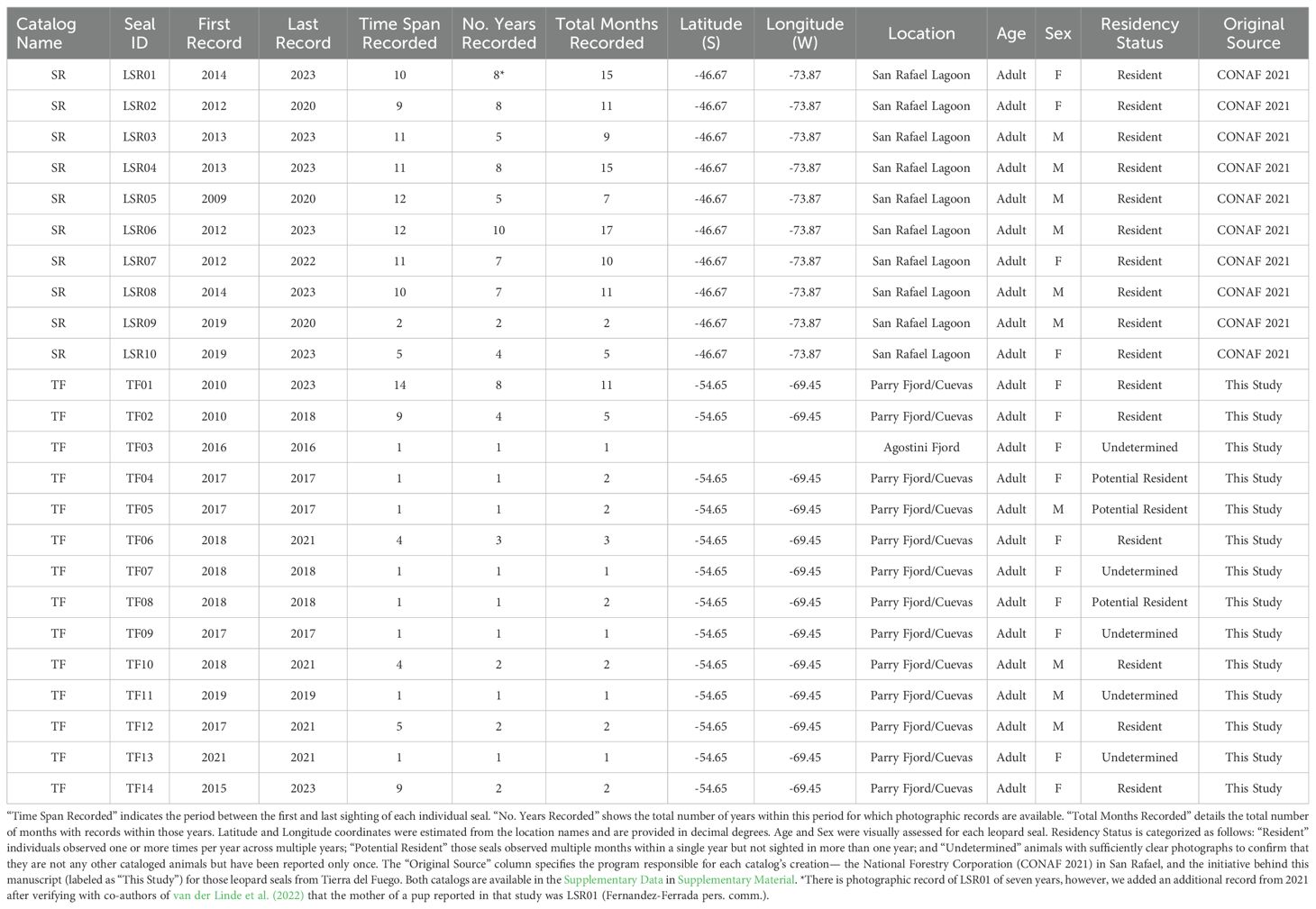
Table 1. Summary of sighting records and biological information compiled from the two catalogs of leopard seals from Chile and our four expeditions.
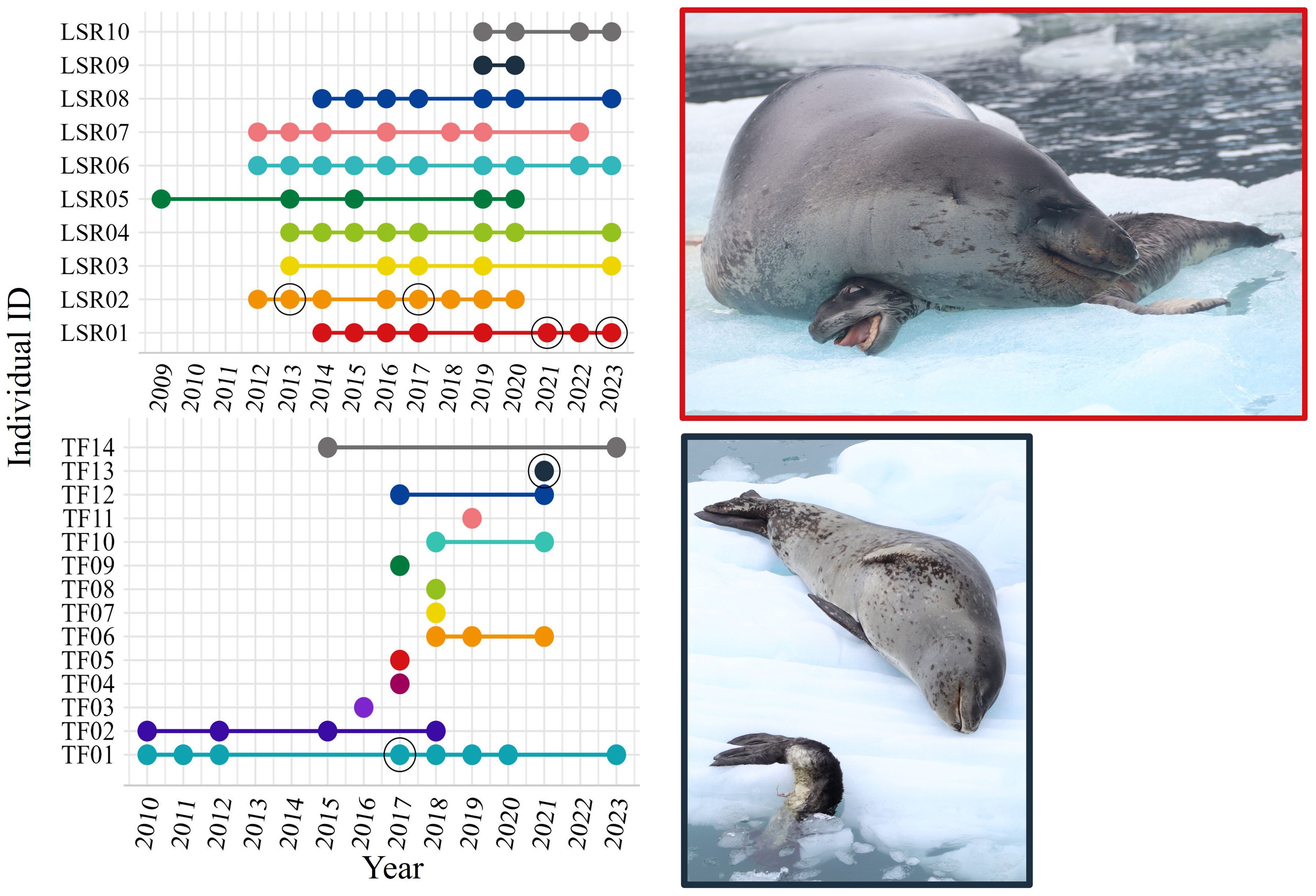
Figure 4. Annual presence of leopard seals in San Rafael (upper panel) from the photo-identification catalogs (until 2020) and expeditions (2021-2023). In the plots, each color represents a unique individual. Dots show years with photographic record of each individual. Colored lines connecting the dots represent the total time span that each seal has been observed. The 2021 sighting of LSR01 is the only data point that is not from the SR catalog or expeditions; the female was validated as the mother of a reported pup by van der Linde et al. (2022). Black unfilled circles show the year adult females were observed with pups. The right panels display photographs of the reported pups during our expeditions (both dead): the upper right picture is female LSR01 from San Rafael with her pup (SR-23), and the lower right picture is female TF13 from Tierra del Fuego with her pup (TF-21). Photo credits: Renato Borras-Chavez.
3.2.1 San Rafael
We reviewed ~1,500 photographs of leopard seals from San Rafael taken from 2009 to 2023. We identified 10 leopard seal individuals with recurrent sightings over time (Table 1; Figure 4; Supplementary Data in Supplementary Material) and classified all 10 individuals as residents. The sex ratio was nearly 1:1, with four females and six males. In years when body condition was assessed, all seals were in excellent body condition (Supplementary Table 1). The longest resident leopard seals were two males (LSR05 and LSR06). LSR05 was observed five different years from 2009-2020, and LSR06 was observed 10 different years from 2012-2023. Females LSR02 and LSR01 each gave birth at least twice (LSR02 in October 2013 and October 2017; LSR01 in October 2021 and October 2023; Figure 4). The only year with multiple pup sightings was in 2019 when two pups were sighted simultaneously but mothers were not identified. Both sightings were reported on the same day (January 14th, 2019) by the same observers. Pup records (n=10) were all reported from mid-October to the end of November (Supplementary Table 2).
3.2.2 Tierra del Fuego
We reviewed ~1,000 photographs from Tierra del Fuego between 2010 and 2023. We identified nine leopard seals with recurrent sightings over time (Table 1; Figure 4; Supplementary Data in Supplementary Material). We identified six residents, three potential residents, and five undetermined individuals. The longest resident recorded was a female (TF01) observed in eight different years over a 14-year period (2010-2023). We identified more females than males at this location (females: 10, males: 4). All seals were in excellent body condition in all years when body condition was assessed (Supplementary Table 2). Female TF01 gave birth in November 2017, and female TF13 gave birth in November 2021 (Figure 4). Pup records (n=4) were all reported between the beginning of October to beginning of December (Supplementary Table 1).
3.3 Standardized monitoring data of San Rafael
3.3.1 Seasonal trends
We analyzed monthly trends on the observed number of seals in San Rafael from 2013 to 2019. The highest number of leopard seals reported during one monitoring day was seven (January 2019). After accounting for between-year differences, we found a non-linear effect (p = 0.003), suggesting seasonal intra-annual variations in the number of leopard seals (Figure 5). Specifically, the non-linear seasonal pattern reveals two distinct intra-annual trends. First, there was a significant tendency of seal counts to decrease from March (Month = 3.2, linear slope β = -0.31, CI95% [-0.67, -0.00]) to May (Month = 5.3, β = -0.29, CI95%[-0.61, -0.01]), with counts likely remaining low throughout the austral winter (Figure 5). Second, we found a significant tendency of seal counts to increase in late August (Month = 8.5, linear slope β = 0.27, CI95%[0.00, 0.55]) to late October (Month = 9.8, β = 0.40, CI95%[0.00, 0.83]). These higher counts persisted through late austral spring (November-December; Figure 5).
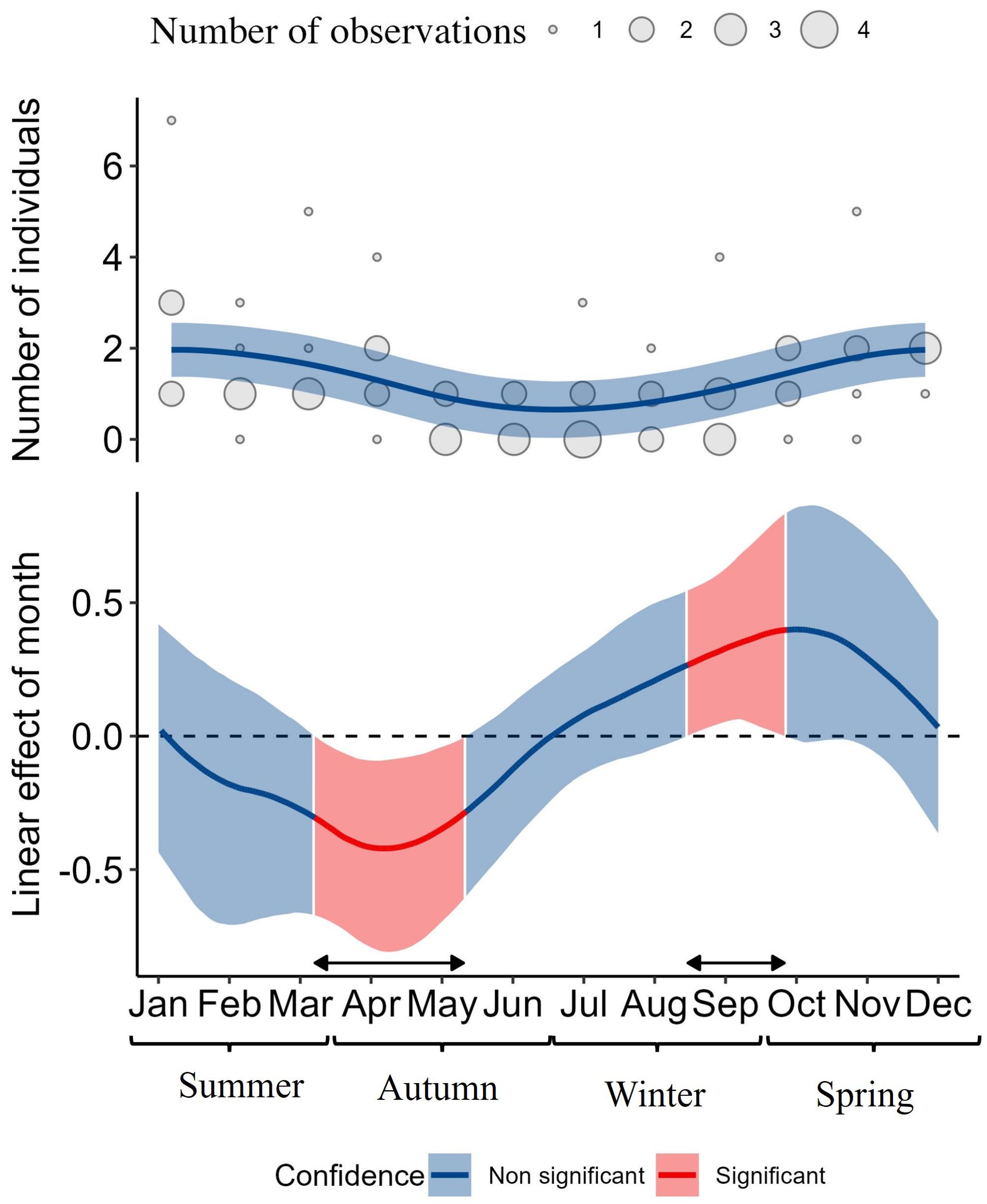
Figure 5. Seasonal variation and rate of change in leopard seal counts based on San Rafael standardized monitoring data. The upper panel displays the change in leopard seal counts across seasons. The lower panel illustrates the slope of the seasonal rate of change in the predicted number of leopard seals based on the first derivative of the fitted Generalized Additive Model (GAM) shown in the upper panel. In the lower panel, red areas indicate time segments where 95% of the Monte Carlo simulations for the first derivative of the GAM did not include the null effect, indicating a significant linear effect during these periods.
3.3.2 Inter-annual trends
When analyzing the inter-annual trends from the San Rafael monitoring data, we found no significant negative (β = -0.17, CI95% [-0.98, 0.63], p = 0.666) or positive quadratic or linear trends (β = 0.28, CI95% [-0.56, 1.11], p = 0.513) even after accounting for seasonal variations (Figure 6).
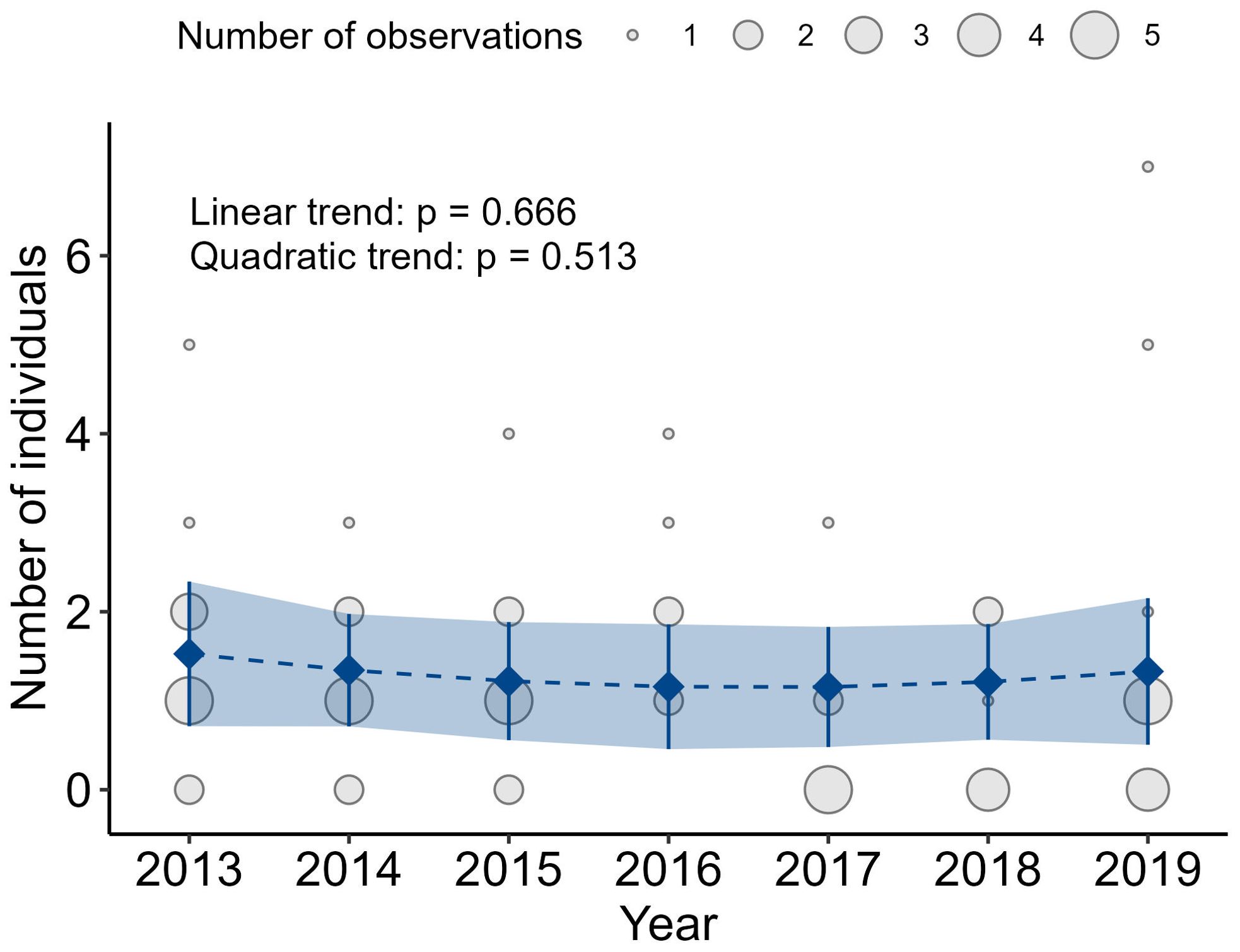
Figure 6. Inter-annual variations in the San Rafael monitoring data from 2013 to 2019. After accounting for intra-annual (seasonal) variation and based on non-orthogonal polynomial contrasts up to the second order, the fitted model shows no trends in the increase or decrease of individual numbers of leopard seals in San Rafael.
3.4 Glacial habitat characterization
The contrasting characteristics between the San Rafael glacier in the NPI and the glaciers in Parry Fjord in the CDI — particularly the Darwin and Cuevas glaciers — make these locations ideal for identifying characteristics that may define optimal glacial habitats for leopard seals (Figure 7A). Located at the northern (San Rafael) and southern (Parry Fjord glaciers) limits of Patagonia these glaciers experience different climate conditions, glaciological regimes, and topographical features (Sagredo and Lowell, 2012; Sagredo et al., 2014).
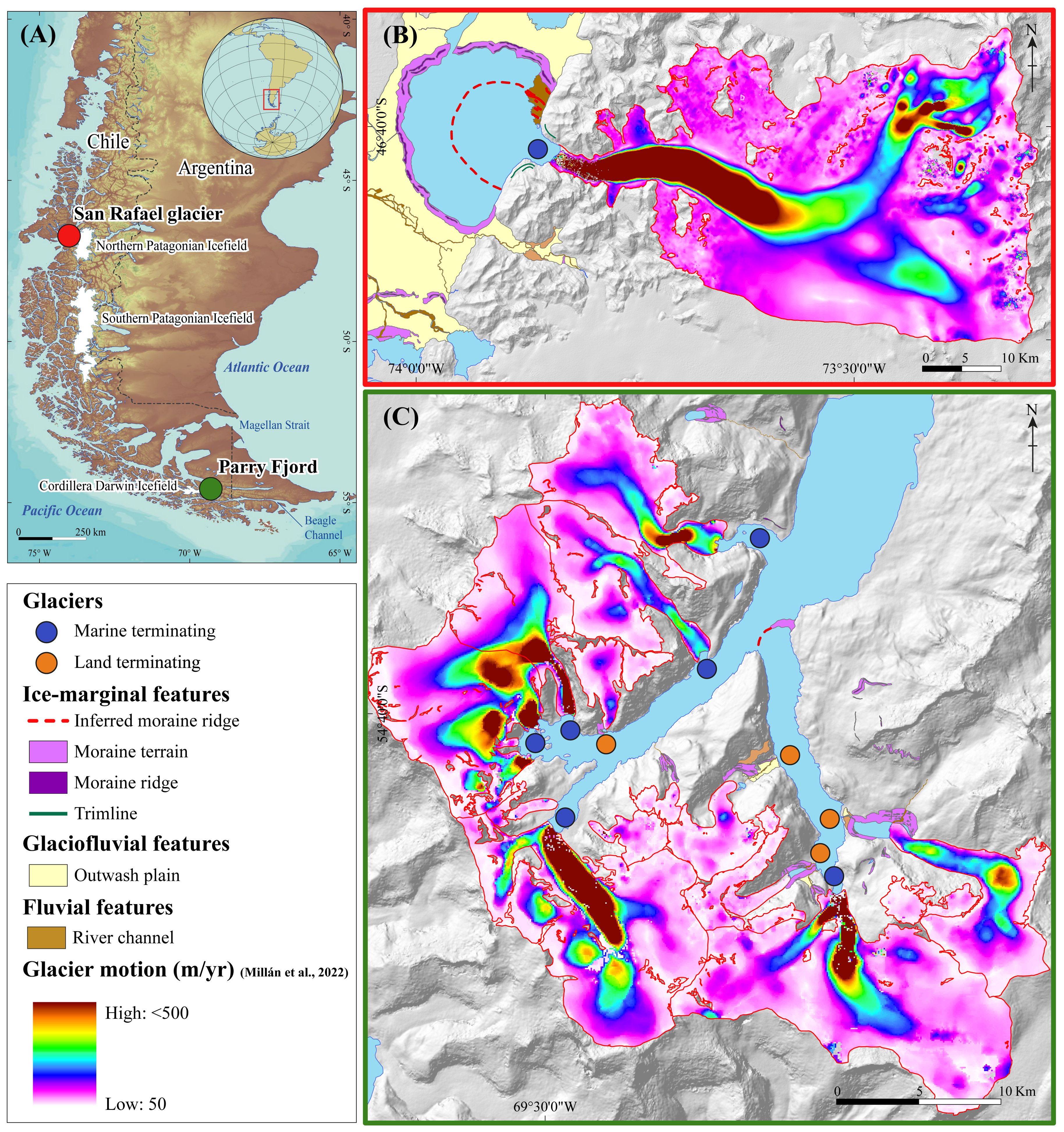
Figure 7. Characterization of the San Rafael and the Parry Fjord glaciers. (A) Locations of the San Rafael and the Parry Fjord glaciers in Patagonia. (B, C) are simplified geomorphic maps of the San Rafael and Parry Fjord glaciers, respectively. Glaciers flowing towards the main water bodies are outlined based on the Chilean Glacier Inventory (DGA, 2022). Surface glacier velocity is depicted in graded colors, with dark red indicating faster areas and white indicating slower areas of ice motion (Millán et al., 2022).
3.4.1 San Rafael glacier
The San Rafael glacier, flowing westwards from the NPI, is the northernmost marine-terminating glacier in Patagonia (Figure 7B). The local geomorphology features well-preserved arcuate moraine ridges known as “Témpanos” moraines, deposited following successive ice advances during the Holocene (Harrison et al., 2012). Extensive outwash plains extend westwards from these moraines, most likely connecting the Taitao peninsula with the mainland. This landform assembly creates a proglacial basin occupied by the fan-like-shaped San Rafael Lagoon, which receives inflow from the San Rafael glacier and drains into the main fjord through a narrow and shallow channel known as “Canal Témpanos”. Recent geodetic measurements have estimated ice velocity in the glacier front ranging from ~7200 to 6900 m/yr, making it one of the fastest glaciers in the region and, in the middle latitudes of the Southern Hemisphere as well (Mouginot and Rignot, 2015; Millán et al., 2022). First-order estimations revealed that the San Rafael glacier calving flux reached ~2.7 Km3/yr between 2017 and 2022 (Supplementary Table 4). Given the geographic setting of the San Rafael Lagoon, the distance between the glacier front and the Pacific Ocean is relatively short and linear, so the sinuosity is low (0.31; Supplementary Table 4). Furthermore, the drainage width is very narrow, so the change between the width of the proglacial basin and the main fjord is very high (0.86; Supplementary Table 4). In the San Rafael glacier, normalized values of glaciological and geomorphic attributes indicates that changes in fjord width (~52%) and calving flux (~34%) are the major contributing factors to iceberg residence time, while sinuosity (~14%) plays a secondary role.
3.4.2 Parry Fjord glaciers
The Parry Fjord glaciers are part of the CDI located in Tierra del Fuego, southernmost Patagonia. It is composed by two coalescing glacial valleys flooded by the ocean, the Parry fjord to the West and the Seno Cuevas to the East (Figure 7C). The Parry Fjord valley has at least six marine-terminating and one land-terminating glaciers. Satellite-derived ice motion measurements indicate that the marine-terminating glaciers move at velocities ranging from ~200 to 1750 m/yr, whereas the land-terminating glacier reaches velocities of ~30 m/yr (Millán et al., 2022). The Seno Cuevas is drained by only one marine-terminating glacier, the Cuevas glaciers, which reaches maximum velocities of ~1630 m/yr. However, there are several land-terminating glaciers that most likely transitioned from a marine to a terrestrial proglacial environment in the past millennia or centuries (Strelin et al., 2008; Menounos et al., 2020; Reynhout et al., 2021). At the mouth of the Seno Cuevas, we identified the remnants of a moraine ridge closing the fjord (Figure 7C). The Darwin glacier in Parry Fjord and the Cuevas glacier in Seno Cuevas—both locations with the highest record of leopard seals of the region—present the highest calving rate of their respective areas reaching ~0.15 and ~0.13 Km3/yr between 2017 and 2022, respectively. Altogether glaciers of Parry Fjord (glaciers 45 through 51 of Supplementary Table 4) flowing towards the ocean exhibit a combined calving flux of ~0.48 Km3/yr. Sinuosity of the Parry Fjord range from moderate to high (0.55 average; Supplementary Table 4). The average width ratio of local glaciers is very high (0.83) denoting that, geomorphic features such as moraine crest and subaequous bedrock knobs, are frequent in the area (Supplementary Table 4). In Parry Fjord, normalized values of fjord width (~57%) and fjord sinuosity (~35%) indicate that local geomorphology is the key factor for prolonged iceberg residence, while calving flux (~7%) exerts a secondary control on iceberg residence.
Given that leopard seal sighting records have historically been concentrated in San Rafael and Parry Fjord, we utilized the combined normalized values of the analyzed glaciological and geomorphic attributes of these two areas as references to assess the suitability of other fjords in Patagonia as leopard seal potential habitats. We calculated the weighted mean of these attributes for each location; San Rafael and Parry Fjord glaciers (Table 2). With these results, we hypothesized that calving flux, fjord sinuosity and fjord width ratio all contribute to increase iceberg residence time and, consequently, higher chances of being visited/inhabited by leopard seals.
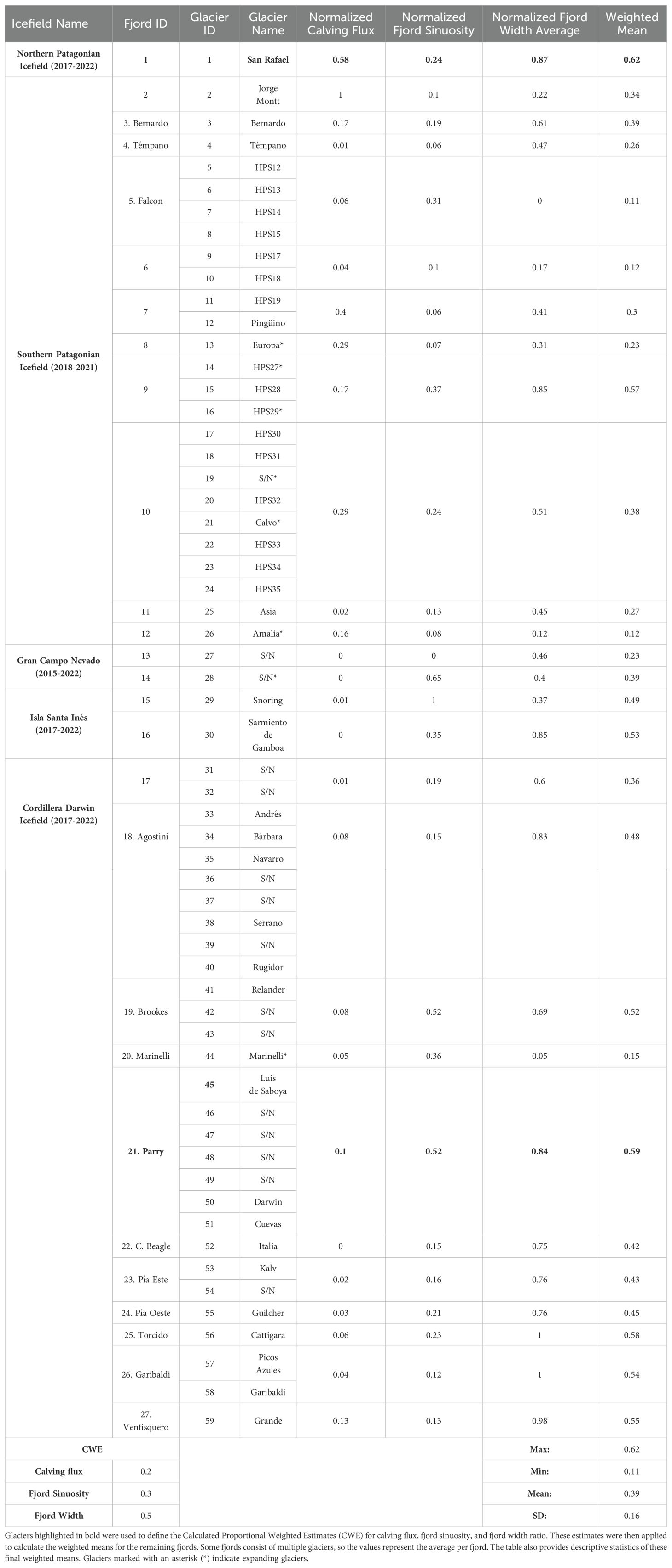
Table 2. Normalized glaciological and geomorphic attributes of marine-terminating glaciers in Patagonia (calving flux, fjord sinuosity, and width ratio).
When considering the normalized values of glaciological and geomorphic attributes at both San Rafael and Parry Fjord together, the width ratio emerged as the primary factor contributing to prolonged iceberg residence at both locations, accounting for 50% of the observed variance. In contrast, calving flux played a more significant role in San Rafael (34%) compared to Parry Fjord (7%), while sinuosity was less influential in San Rafael (14%) but contributed considerably more in Parry Fjord (35%). Therefore, when evaluating other suitable fjords in Chile (discussed in section 3.5) we assigned a Calculated Proportional Weighted Estimate (CWE) to each calculated weighted mean of all fjords of 0.5 to width and 0.2 and 0.3 to calving flux and sinuosity respectively (Table 2).
3.5 Glacial suitability analysis for leopard seal in Patagonia
According to the Chilean Glacier Inventory (DGA, 2022), we identified 59 marine-terminating glaciers grouped within 27 fjords along the Pacific coast of Patagonia (Figure 8). In recent years, the surface area of these glaciers has receded at varying rates, ranging from 0.63 km²/yr at Jorge Montt Glacier to nearly 0 km²/yr at an unnamed minor glacier in the Parry Fjord (Supplementary Table 4). Exceptionally, the Europa Glacier has expanded by 0.20 km² between 2018 and 2021. Individually, Jorge Montt Glacier exhibits the highest calving flux in Patagonia, reaching 4.61 km³/yr, followed by San Rafael Glacier at 2.69 km³/yr, Pinguino Glacier at 1.47 km³/yr, and Europa Glacier at 1.35 km³/yr (Supplementary Table 4). All these glaciers exceed the regional average calving flux of 0.29 km³/yr.
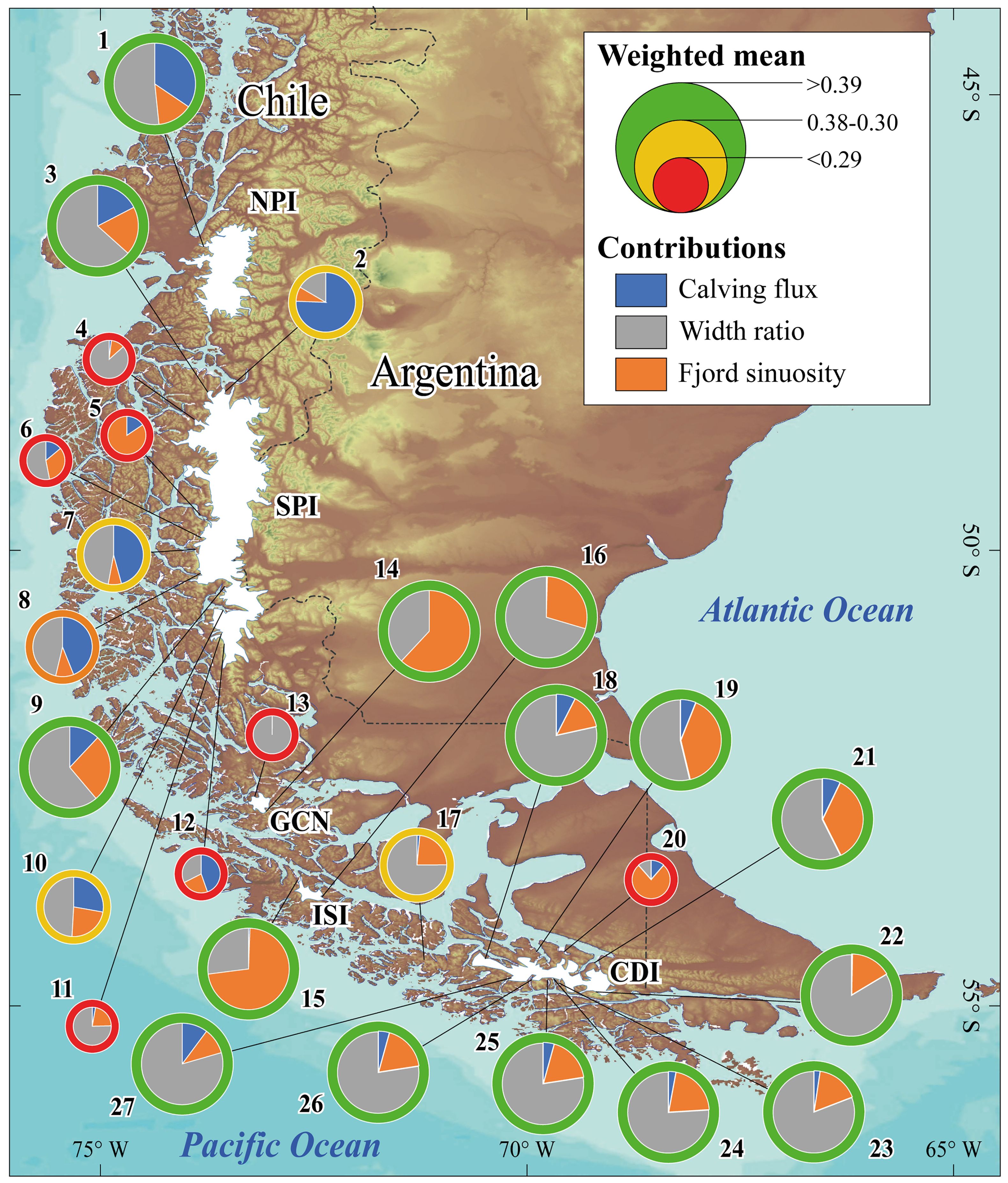
Figure 8. Fjords hosting marine-terminating glaciers of Patagonia. The figure displays pie charts corresponding to the fjord IDs listed in Table 2. The color and size of each pie chart indicate the likelihood of the site to host leopard seals; fjords were categorized as “very likely” when their weighted mean was equal or above 0.39, and “likely” or “less likely” between 0.38 and 0.30 and less than 0.29, respectively. Within the pie charts, different colors represent the contributions of calving flux (blue), fjord width ratio (grey), and fjord sinuosity (orange) to the potential of each glacier as habitat for leopard seals.
Despite the geographical complexity of the Patagonian archipelago, regional fjords generally show low sinuosity, ranging from 0.16 to 0.99, with a mean of 0.34 (Supplementary Table 4). Many fjords exhibit geomorphic features, such as moraines and outwash plains, that create “geomorphic traps” enhancing iceberg residence time. The width ratios of these fjords vary from 0.23 to 0.96, with a mean of 0.68 (Supplementary Table 4). The normalized weighted mean of glaciological and geomorphic attributes in Patagonian fjords, aligned with values calculated for the San Rafael Glacier and those hosted in Parry Fjord, ranges from 0.62 to 0.11, averaging 0.39 (Table 2). Fjords were classified as “very likely,” “likely,” and “less likely” to host leopard seals if their weighted means were equal to or above 0.39, between 0.38 and 0.30, and below 0.29, respectively (Figure 8 and Table 2).
From 27 fjords encompassing all 59 marine-terminating glaciers, we found 13 fjords—in addition to San Rafael and Parry Fjord— with potential long-lasting iceberg residence and therefore classified as “very likely” suitable for leopard seals (Figure 8; see values in Table 2). In the SPI, Bernardo (fjord ID 3; 0.39) and the fjord hosting the HPS27, HPS28 and HPS29 (fjord ID 9; 0.57). To the south, in the GCN, the S/N glacier (fjord ID 14; 0.39) and the Snoring and Sarmiento de Gamboa glaciers in the ISI (fjord ID 15; 0.49 and fjord ID 16; 0.53, respectively). In the CDI at Tierra del Fuego, the Agostini Fjord (fjord ID 18; 0.48), Relander glacier and two glaciers without name (fjord ID 19; 0.52), Italia glacier (fjord ID 22 C. Beagle; 0.42), Pía Fjord (fjord ID 23; 0.43 and 24; 0.45), Torcido Fjord (fjord ID 25; 0.58), Garibaldi Fjord (fjord ID 26; 0.54), Grande glacier (27; 0.55). 4 fjords were classified as “likely” habitats for leopard seals: the Jorge Montt glacier (fjord ID 2; 0.34), Pingüino Fjord (fjord ID 7; 0.30), HPS30 Fjord (fjord ID 10; 0.38), and S/N Glacier in the South Patagonian Icefield (fjord ID 17; 0.36).
4 Discussion
Leopard seals have been observed across most regions of Chile: from Easter Island in the north/west to Tierra del Fuego in the south. Aguayo-Lobo et al. (2011) first compiled historical records of leopard seals to show that this species is a regular member of the marine fauna of southern Chile. Then, Acevedo and Martinez (2013) provided the first evidence of a potential multi-month resident leopard seal individual in Tierra del Fuego. Both studies hypothesized the existence of resident aggregations of leopard seals in Patagonia. Here, we provide evidence to support this hypothesis through multiyear observations of the same leopard seal individuals. Specifically, we confirmed two distinct resident aggregations of leopard seals in Chile: one in San Rafael and one in Parry Fjord. There was no evidence that the same individuals were at both sites. Our results also show that these resident aggregations in Patagonia have existed for decades at least.
We documented an increase in pups and stranding events reported over the past 10-15 years in Chile (van der Linde et al., 2022; this study). Together, these pup records, as well as recent observations of leopard seal sexual behavior in San Rafael (Kienle et al., 2024), show that breeding is not limited to Antarctic pack ice as previously suggested (Bester et al., 2017a). Therefore, Patagonia should be considered as an integral part of the species breeding distribution range rather than continuing to report leopard seal sightings as occasional or extralimital.
4.1 Leopard seal distribution north from the Antarctic Polar Front
Leopard seal sightings have increased in Chile and other areas north of the Antarctic Polar Front. Our study reports 438 sightings in Chile, with 86% of sightings concentrated in Patagonia. When we correct and transform Aguayo-Lobo et al. (2011) “total animals reported” to individual sightings (118 records), we found a 271% increase in sightings from 2009-2023 compared to pre-2009 (Aguayo-Lobo et al., 2011). Similarly, Hupman et al. (2020) documented an increase in leopard seal sightings in New Zealand, from 19 records in 2000 to 781 records in 2018. Systematic monitoring at Marion Island also suggests an increase in leopard seals, from 19 immature animals reported over 25 years (1980-2005; Bester et al. (2006) to almost double over the last 15 years (2005-2020; De Bruyn and Ross, pers. comm.). Increasing numbers have also been reported in South Australia with a rate of 1.2 seals per year between 1979-2009 to 2.8 seals per year between 2017-2022 (Shaughnessy et al., 2023). The increase in leopard seal sightings north of the Polar Front may be driven by a shift in distribution patterns, with more seals potentially crossing the Antarctic Convergence and/or an increase in their abundance in subantarctic areas.
The origin of leopard seals north of the Polar Front has been widely discussed (reviewed in Bester, 2021; Stewart et al., 2020). For example, early reports for the Indian Ocean and Australasian sub-Antarctic waters suggested that leopard seals migrate north during the winter expansion of Antarctic pack ice (Rounsevell and Eberhard, 1980; Bester and Roux, 1986; Bester et al., 1995). Similarly, in Chile, researchers have suggested that leopard seals potentially migrate from the Western Antarctic Peninsula (WAP) as sea ice expands northward (Stewart et al., 2020). While leopard seals can travel long distances equivalent to those separating southern Chile and the WAP (~1,000 km; tagged leopard seals in the WAP are capable of travelling more than 1,500 km, Kienle et al., 2022; Staniland et al., 2018), most instrumented seals from Antarctica showed movement patterns restricted to a few hundred kilometers (Rogers et al., 2005; Kienle et al., 2022). In addition, consistent multi-year/multi-month observations of resident leopard seals in Patagonia (this study), along with increasing reports of newborns in subantarctic areas (van der Linde et al., 2022; this study), support the hypothesis that there are local resident aggregations of leopard seals in some subpolar locations (Aguayo-Lobo et al., 2011; Acevedo and Martinez, 2013).
Historically, leopard seals found north of the Polar Front were described as being in poor condition and usually as immature individuals (Siniff and Stone, 1985; Rounsevell and Pemberton, 1994; Bester et al., 2006). For instance, juvenile leopard seals have been reported in large numbers at Macquarie Island every 4-5 years, likely due to a northward winter dispersal in response to conditions in the pack ice to the south (Testa et al., 1991). Here, however, resident leopard seals in both San Rafael and Tierra del Fuego were in excellent body condition, and most seals from Patagonia (82%) were adults with only a few (18%) immature individuals. Similarly, in New Zealand, leopard seals were predominantly adults in good to excellent body condition (Hupman et al., 2020). The presence of adults in good body condition regularly observed north of the Polar Front indicates that Patagonia, and potentially New Zealand, provide secure habitats and abundant resources necessary for leopard seals to thrive.
Furthermore, Rounsevell and Pemberton (1994) hypothesized that the number of immature leopard seals increases with decreasing latitude. Like previous studies, we also identified changes in leopard seal age class across Chile. North of San Rafael in Chile, leopard seal sightings are mostly of immature individuals (82%). Sightings of these immature individuals that included mentions of their health status showed that 74% of these leopard seals were in suboptimal condition (out of 19 records, 14 were reported either poor, emaciated, injured, or dead). Similarly, in South Australia most leopard seal sightings are of juvenile animals reported in fair/thin body conditions (Shaughnessy et al., 2023). Further, the cyclical increase in leopard seals at Macquarie Island are mostly the result of immature individuals dispersing north, likely driven by poor environmental conditions (Testa et al., 1991). Our data therefore support the hypothesis that age class is correlated with latitude, but we found that, in Chile, this pattern starts much further north of the Polar Front than proposed in other locations (Rounsevell and Pemberton, 1994; Testa et al., 1991; van den Hoff et al., 2005; Bester et al., 2006).
4.2 Long term temporal trends in Chile
It is arguable whether leopard seal aggregations have increased or remained stable in San Rafael and Tierra del Fuego. In San Rafael, the first official historical record of leopard seals was of three individuals in 1979 (Torres et al., 1979; reviewed in Aguayo-Lobo et al., 2011). Afterwards, there was a 30-year absence of records (1979-2009) where no sightings were reported from San Rafael. Now, at least 10 unique leopard seals have been identified from >195 sighting records. Although this could suggest a potential local increase of leopard seals in San Rafael, the San Rafael monitoring data have shown a stable leopard seal aggregation, with no major increase in seal counts between 2013-2019 (Figure 6). This leads us to call for caution when interpreting sightings data. The lack of pre-2009 records in San Rafael may have been due to a reduced number of private tour companies operating before 2013, leading to no sightings reported during this time, rather than a true absence of leopard seals.
Similarly in Parry Fjord, only nine records of leopard seals existed before 2010 (Aguayo-Lobo et al., 2011) compared to >55 new sighting records after 2010. Moreover, at Parry Fjord, tour operators report that not observing leopard seals during their visits is rare (Moreno, pers. comm.). Like San Rafael, the recent increase in sightings in Parry Fjord may also reflect the rise of the tourism industry, leading to more people visiting the area. However, leopard seal aggregations have been reported there since 1971 (Markham, 1971; n=4) with numbers similar to our most recent expedition in the area (TF-23; n=6), suggesting that numbers may be at least stable at this location.
The overall stranding data did show a positive annual rate of change within the last two decades in Chile (Figure 3). This data indicates an overall increase in the number of leopard seals inhabiting Chile in the last 15 years. Stranding reports are more reliable than sighting records as they do not require photographic validation and are often verified by trained personnel. An increase in leopard seal numbers along the Chilean coast aligns with the expanding distribution and reappearance of other pinnipeds on the Pacific coast after centuries of exploitation. For example, southern elephant seals are recolonizing the eastern South Pacific where they were heavily depleted during the sealing era (Acevedo et al., 2016, 2019). As in our study, Cárcamo et al. (2019) showed an increase in elephant seal sighting records along the coast of Chile. Additionally, South American fur seals (Arctocephalus australis) and South American sea lions (Otaria flavescens) populations are increasing in key breeding areas of South America, including Chile (Rodrigues et al., 2018; Milano et al., 2020; Sepúlveda et al., 2021).
4.3 San Rafael: a unique case study
The leopard seals at San Rafael may constitute an isolated population from other aggregations found in Chile. There are ten resident leopard seals at this location. Eight seals have been continuously observed over the last ten years, and only two new individuals (LSR09 and LSR10) were reported in recent years (2019-2020). In addition, our two most recent expeditions to San Rafael did not find new seals beyond those already reported. In contrast, we continuously obtain sighting reports of new individuals at Parry Fjord. For instance, our latest ten day expedition (TF-23) found four new individuals never reported before. We have also found consistency in the seasonality of individuals from San Rafael, stressing their resident nature. There is a seasonal trend, with numbers tending to decrease during the austral autumn (march–may) and increasing during the austral spring (August–October). The slight tendency of higher number of seals during the spring aligns with the hypothesized breeding season for the species in locations where residents are found (between September-November; van der Linde et al., 2022). Specifically, 8 of 10 pups of San Rafael were recorded between mid-October and early December with two resident females giving birth in San Rafael at least twice (LSR01 and LSR02). Moreover, evidence of mating behavior has been recently reported in San Rafael (Kienle et al., 2024). The temporal overlap among individuals in San Rafael, the lack of evidence of transient individuals, and the consistency in annual life history cyclic patterns such as reproduction, suggest that the seals living in San Rafael and nearby areas may be isolated from the rest of Patagonia.
During the Last Glacial Maximum (globally around 26-19 kya), the abundance of leopard seals in Antarctic increased likely driven by the abundance of ice (Bender et al., 2023). Similarly, the Patagonian ice sheet also expanded, covering much of southern Andes of Chile and Argentina; this was followed by a progressive fragmentation of the Patagonian ice sheet into today’s multiple ice caps (Davies et al., 2020). The NPI separated from the SPI around 12.6-11.3 kya (Thorndycraft et al., 2019; Vásquez et al., 2022), isolating the NPI where the San Rafael Lagoon formed sometime after ~9.5 kya (Harrison et al., 2012). Furthermore, the San Rafael glacier constitutes the only marine-terminating glacier in the NPI. The nearest glacier, Jorge Montt Glacier, is not easily accessible from San Rafael. Leopard seals would need to swim ~630 km to reach the Jorge Montt Glacier. While leopard seals can travel long distances (Staniland et al., 2018), most tend to remain close to their origin (Rogers et al., 2005; Kienle et al., 2022). Altogether, this support the idea that leopard seals of the NPI may have been isolated during the separation of the NPI from the SPI with the San Rafael glacier being today’s only glacier producing icebergs in the region.
The potentially isolated leopard seal population of the NPI was then severely decimated by the sealing industry during the 18th and 19th centuries, likely causing a local demographic reduction. The peak sealing period in the mid-19th century focused on southern sea lions also included southern elephant seals and leopard seals (Torrejón et al., 2013). Historical accounts indicate a significant reduction of all pinniped species by the end of the 19th century, including leopard seals. The navy captain E. Simpson mentioned “(…) there was another seal larger than southern sea lions but smaller than elephant seals called leopards due to their spot patterns, and they have also disappeared from the region” (Simpson, 1875). The progressive fragmentation of the Patagonian Ice Sheet after the Last Glacial Maximum, as well as the significant reduction of leopard seals during the sealing era, supports our hypothesis of a potential isolated leopard seal aggregation in San Rafael with little flux of new animals—at least— in the last decades. Genetic analyses are needed to assess inbreeding levels in San Rafael and compare the genetic make-up of these animals to other aggregations in Chile and elsewhere. These assessments will help clarify the history of this population and levels of past and contemporary gene flow among leopard seal aggregations.
4.4 Identifying potential habitats for leopard seals in Chile
Based on our characterization of the San Rafael and Parry Fjord glaciers, we find that fjord width ratio, which accounts for geomorphic traps (e.g., moraine ridges, outwash plains), is key to controlling residence of local icebergs (the most important substrate for leopard seals in Chile). Our simplified calving flux estimation for the San Rafael glacier (~2.7 km³/yr) in the NPI and the Darwin and Cuevas glaciers (0.15 and 0.13 km³/yr) in the CDI aligns with previous estimates of the same glaciers in the last decades (Koppes et al., 2011; Melkonian et al., 2013). More broadly, our regional calving flux estimation is consistent with previous estimates for Patagonian marine-terminating glaciers (Koppes et al., 2011; Melkonian et al., 2013; Schaefer et al., 2015). The minor differences observed between our values and those from the studies mentioned, may be explained by the challenges in outlining glacier ice fronts due to the lack of cloud-free satellite imagery and ice mélange (i.e., mixture of icebergs, sea-ice and snow) in the ice front. More important, glaciological parameters database assume uncertainties of ~30% in ice velocity estimations, and 25-35% for ice thicker than 100 m and >50% for thinner ice (Millán et al., 2022). Thus, we suggest that our calving flux assessments can be considered as a qualitative indicator.
Geomorphic attributes are crucial for iceberg retention at regional scale. Patagonian marine-terminating glaciers and fjords in the NPI and SPI show higher contributions from calving flux, while southern ice masses (GCN, ISI, and CDI) are more influenced by morphometric attributes and the existence of landforms closing fjords. However, winter sea ice formation in several fjords of CDI, including Marinelli and Parry Fjords (Salame et al., 2020), may compensate for limited calving flux.
Our habitat model aligns well with the leopard seal sighting records, thereby suggesting additional fjords and glaciers across Patagonia that should be explored in search of leopard seals. While most sighting records are concentrated in San Rafael and Parry Fjord, other locations are consistent with fjords predicted by our model as “very likely” suitable habitats. We identified 13 additional fjords in Patagonia (out of 27 fjords that included 59 glaciers in total) as “very likely” suitable habitats for leopard seal with many of them matching areas where leopard seals have also been reported. For instance, Agostini Fjord and Ballena Sound (fjords 18 and 15 in Figure 8) are both identified as very likely suitable habitats for leopard seals, and the historic records show that each location has had 11 leopard seal sighting records, including multiyear records (Aguayo-Lobo et al., 2011; Acevedo and Martinez, 2013, and this study). Similarly, Pia Sound (4 reported sightings near fjords 23 and 24), Helado Sound (2 sightings near Sarmiento Glacier, fjord 16), and the Beagle Channel (1 sighting near Italia Glacier, fjord 22) were all classified as “very likely” habitats for leopard seals.
Because of the challenges in identifying Patagonian glaciers, the 59 marine-terminating glaciers evaluated should be considered a minimum number of iceberg-prone glaciers existing in Patagonia. Overall, intense calving flux, accounting for iceberg production, and the existence of geomorphic landforms in the fjords—particularly moraines and outwash plains that create iceberg traps—are critical factors when suitable habitats for leopard seals are evaluated in Patagonia. Furthermore, other factors such as prey availability also play an important role defining suitable location for leopard seals, however, resources availability seems not to be a problem in Patagonia (Aguayo-Lobo et al., 2011; Friedlander et al., 2021; Castilla et al., 2023). The fact that 76% of sighting records were on icebergs, and the consistent match between our predicted suitable fjords and historical sighting locations, support the idea that iceberg availability and residency are crucial variables for evaluating suitable habitats for leopard seals in the Chilean fjords.
4.5 The role of leopard seals as apex predators
Apex predator populations are rapidly changing in response to widespread environmental changes (Roff et al., 2018). Their presence or absence can lead to significant restructuring of ecosystems (e.g., Wilmers and Post, 2006; Knopff et al., 2014; Jonsen et al., 2019). Introducing new predators often results in a redistribution and potential shift in local food web structures (Johnson et al., 2007; Letnic et al., 2009; Sherman et al., 2020), while the suppression of apex predators can trigger ecosystem restructuring through various ecophysiological pathways (e.g. Leo et al., 2019; Hammerschlag et al., 2022). This highlights the importance of monitoring the distribution of apex predators such as leopard seals.
We document the long-term presence of 10 resident leopard seals in San Rafael and 6-9 in Tierra del Fuego. While these numbers may seem low, small numbers of apex predators can significantly impact prey species abundance (Williams et al., 2004; Krause et al., 2022). For instance, fewer than 40 killer whales may be responsible of the decline of the Steller sea lion (Eumetopias jubatus) population in the Aleutian Islands and a pod of only five individuals could account for the regional decline of sea otters (Williams et al., 2004). Leopard seals are effective and efficient apex predators (Hiruki et al., 1999; Krause et al., 2015) and cause drastic reductions in their prey populations (Forcada et al., 2009). At Cape Shirreff in the WAP, leopard seals consumed on average 2.5 Antarctic fur seal pups per day during the 2004-2005 breeding season (Vera et al., 2004). The annual predation rate over Antarctic fur seal pups increased from 7.1% to 73.8% between 2002-2020, with a mean predation rate of nearly 70% annually since 2010. The average maximum number of leopard seals responsible for this high predation rate was only 20 individuals per year between 2011 and 2020 with this small average number contributing to the local population collapse of Antarctic fur seals at Cape Shirreff (Krause et al., 2022). This highlights the importance of monitoring occurrence of apex predators and distinguishing resident from transient individuals (movement patterns) to help predict changes in prey abundance and local food web dynamics (Andrews and Harvey, 2013).
We provide baseline data on the historical resilience of leopard seals in Patagonia. However, assessing the short- and long-term ecological role of leopard seals in these ecosystems is critical and requires information on their movement patterns, diet, and local behavioral flexibility. Our results confirmed that leopard seals are a permanent component of the food web in Patagonian ecosystems and enhanced our understanding of leopard seal ecology by providing evidence of their distribution and residency beyond Antarctica.
4.6 Conclusions
We present evidence that multiple resident leopard seal aggregations exist in Chile and confirm that their breeding distribution is not limited to Antarctic pack ice (Bester et al., 2017a). Specifically, we show long-term residence of leopard seals in two locations of Patagonia (San Rafael and Parry Fjord), evidenced by multiyear observations of adults. Additionally, we report an increase in stranding events and newborn sightings over the last 15 years, suggesting an overall growing population in Chile. Although the origin of these animals remains unknown, continued monitoring of leopard seals, particularly of newborn and juvenile animals, is essential, as including reproductive success in species distribution models can lead to more accurate predictions of population growth (Chuine and Beaubien, 2001).
Global warming is rapidly impacting the cryosphere (Schneider et al., 2020) especially in Patagonia, where marine-terminating glaciers are increasingly transitioning to land-terminating glaciers (Dussaillant et al., 2019). Consequently, this will cause a decrease in the number of potential habitats for leopard seals and likely impact their distribution. For instance, cyclical increases of juvenile leopard seal numbers in locations north of the Polar Front— likely due to changes in sea ice extent in the south—is an example of how the absence of sea ice may influence the dispersal of these animals (Testa et al., 1991). Similarly, climate change driven responses are already shaping the distribution of other pagophilic apex predators such as polar bears in the Arctic (Wiig et al., 2008; Sahanatien and Derocher, 2012). Future studies should conduct comprehensive habitat suitability analyses combining oceanographic variables, iceberg characteristics, local prey availability, and species movement patterns to assess the impact of climate change on ice-dependent apex predators’ occurrence and distribution.
Data availability statement
The raw data supporting the conclusions of this article will be made available by the authors, without undue reservation.
Ethics statement
The animal study was approved by Subsecretary of Fisheries and Aquaculture of Chile (SUBPESCA). Permit PINV E-2022-394 and the CONAF Authorization N° XI-21-2022. The study was conducted in accordance with the local legislation and institutional requirements.
Author contributions
RB-C: Conceptualization, Data curation, Formal analysis, Funding acquisition, Investigation, Methodology, Project administration, Resources, Software, Supervision, Validation, Visualization, Writing – original draft, Writing – review & editing. RS: Conceptualization, Data curation, Formal analysis, Investigation, Methodology, Validation, Visualization, Writing – original draft, Writing – review & editing. GG: Conceptualization, Investigation, Methodology, Resources, Writing – review & editing. FM: Conceptualization, Data curation, Methodology, Writing – review & editing. NF-F: Conceptualization, Data curation, Investigation, Methodology, Validation, Writing – review & editing. MC-A: Formal analysis, Visualization, Writing – original draft, Writing – review & editing. FMA: Resources, Validation, Writing – review & editing. CD: Data curation, Resources, Validation, Writing – review & editing. CA: Data curation, Resources, Validation, Writing – review & editing. NB: Data curation, Investigation, Writing – review & editing. ES: Funding acquisition, Investigation, Resources, Writing – review & editing. CB: Funding acquisition, Investigation, Writing – review & editing. MG: Investigation, Resources, Writing – review & editing. AG: Investigation, Writing – review & editing. MD: Investigation, Writing – review & editing. SK: Funding acquisition, Investigation, Resources, Supervision, Writing – review & editing.
Funding
The author(s) declare financial support was received for the research, authorship, and/or publication of this article. The work was partially funded by NSF OPP #2146068 and the David and Lucille Packard Foundation. Work was also partially funded by the AMNH Lerner Gray Memorial Fund and the American Philosophical Society Lewis and Clark Fund for Exploration and Field Research grant awarded to ES.
Acknowledgments
The authors thank all tour operators, photographers, conservationists, and citizens in general who contribute to this work with their photographic material from both San Rafael and Tierra del Fuego (a list of photographers and tour operators that were major contributors can be found in Supplementary Table 5). Authors also thank all CONAF personnel for their logistic support in San Rafael, particularly park rangers Hector Marchant and Sandro Campos. We acknowledge the continuous hard work of SERNAPESCA behind the stranding data evaluated in this manuscript. RS thanks to ANID FONDECYT postdoctoral grant #3220537 and ANID BASAL CHIC #FB210018. RB-C thanks ANID PIA/BASAL FB0002. Finally, RB-C appreciates the help of Yanira Belmar for initial contact with monitoring organizations.
Conflict of interest
The authors declare that the research was conducted in the absence of any commercial or financial relationships that could be construed as a potential conflict of interest.
Publisher’s note
All claims expressed in this article are solely those of the authors and do not necessarily represent those of their affiliated organizations, or those of the publisher, the editors and the reviewers. Any product that may be evaluated in this article, or claim that may be made by its manufacturer, is not guaranteed or endorsed by the publisher.
Supplementary material
The Supplementary Material for this article can be found online at: https://www.frontiersin.org/articles/10.3389/fevo.2024.1448098/full#supplementary-material
References
Acevedo J., Aguayo-Lobo A., Brito J., Torres D., Cáceres B., Vila A., et al. (2016). Review of the current distribution of southern elephant seals in the eastern south pacific. New Z. J. Mar. Freshw. Res. 50, 240–258. doi: 10.1080/00288330.2015.1132746
Acevedo J., González A., Garthe S., González I., Gómez R., Aguayo-Lobo A. (2017). Births of leopard seals Hydrurga leptonyx in southern Chile. Polar Biol. 40, 713–717. doi: 10.1007/s00300-016-1968-0
Acevedo J., Martinez F. (2013). Residence of the leopard seal in the Magellan strait: A potential sub-Antarctic population inhabiting the waters of southern Chile? Polar Biol. 36, 453–456. doi: 10.1007/s00300-012-1275-3
Acevedo J., Vargas R., Torres D., Aguayo-Lobo A. (2019). Northerly births of the southern elephant seal (Mirounga leonina) in their former southeast pacific distribution. Aquat. Mammals 45, 293–298. doi: 10.1578/AM.45.3.2019.293
Aguayo-Lobo A., Acevedo J., Brito J. L., Acuña G. P., Bassoi M., Secchi E. R., et al. (2011). Presence of the leopard seal, Hydrurga leptonyx (de Blainville 1820), on the coast of Chile: An example of the Antarctica-South America connection in the marine environment. Oecologia Australis 15, 69–85. doi: 10.4257/oeco.2011.1501.07
Andrews K. S., Harvey C. J. (2013). Ecosystem-level consequences of movement: Seasonal variation in the trophic impact of a top predator. Mar. Ecol. Prog. Ser. 473, 247–260. doi: 10.3354/meps10095
Bender A. N., Krause D. J., Goebel M. E., Hoffman J. I., Lewallen E. A., Bonin C. A. (2023). Genetic diversity and demographic history of the leopard seal: A Southern Ocean top predator. PloS One 18, e0284640. doi: 10.1371/journal.pone.0284640
Bester M. N. (2021). Status of pinnipeds on mid-Atlantic ridge islands, South Atlantic Ocean. Polar Biol. 44, 865–871. doi: 10.1007/s00300-021-02838-z
Bester M. N., Bester W. A., Wege M., Schofield R. A., Glass T. A. (2017b). Vagrant leopard seal at Tristan da Cunha Island, South Atlantic. Polar Biol. 40, 1903–1905. doi: 10.1007/s00300-017-2073-8
Bester M. N., Bornemann H., McIntyre T. (2017a). “Antarctic marine mammals and sea ice,” in Sea ice. Ed. Thomas D. N. (The Atrium, Southern Gate, Chichester, West Sussex, UK: John Wiley & Sons, Ltd), 534–555.
Bester M. N., Erickson A. W., Ferguson J. W. H. (1995). Seasonal change in the distribution and density of seals in the pack ice off princess Martha coast, Antarctica. Antarctic Sci. 7, 357–364. doi: 10.1017/S0954102095000502
Bester M., Hofmeyr G., Kirkman S., Chauke L., De Bruyn P., Ferreira S., et al. (2006). The leopard seal at Marion Island, vagrant or seasonal transient?. South Afr. J. Wildlife Research-24-month delayed Open Access 36, 195–198. doi: abs/10.10520/EJC117238
Bester M. N., Roux J. P. (1986). Summer presence of leopard seals Hydrurga leptonyx at the Courbet Peninsula, Iles Kerguelen. South Afr. J. Antarctic Res. 16, 29–32.
Boveng P. L., Hiruki L. M., Schwartz M. K., Bengtson J. L. (1998). Population growth of Antarctic fur seals: Limitation by a top predator, the leopard seal? Ecology 79, 2863–2877. doi: 10.1890/0012-9658(1998)079[2863:PGOAFS]2.0.CO;2
Cárcamo D., Pizarro M., Orellana M., Muñoz L., Pavez G., Sepúlveda M., et al. (2019). Are southern elephant seals re-invading mid-latitude grounds? New sightings and first birth records off the Chilean coast. Polar Biol. 42, 433–440. doi: 10.1007/s00300-018-2433-z
Casaux R., Baroni A., Ramón A., Carlini A., Bertolin M., DiPrinzio C. Y. (2009). Diet of the leopard seal Hydrurga leptonyx at the Danco coast, Antarctic peninsula. Polar Biol. 32, 307–310. doi: 10.1007/s00300-008-0567-0
Castilla J. C., Armesto Zamudio J. J., Martı́nez-Harms M. J., Tecklin D. (2023). Conservation in Chilean Patagonia: Assessing the state of knowledge, opportunities, and challenges. (Cham, Switzerland: Springer Nature; Santiago, Chile: Ediciones UC) 504.
Cheeseman T., Johnson T., Southerland K., Muldavin N. (2017). Happywhale: Globalizing marine mammal photo identification via a citizen science web platform (Santa Cruz, CA, USA: Happywhale). Rep. SC/67b/PH 2.
Chuine I., Beaubien E. G. (2001). Phenology is a major determinant of tree species range. Ecol. Lett. 4, 500–510. doi: 10.1046/j.1461-0248.2001.00261.x
Cursach J. A., Rau J. R., Ojeda J., Vilugrón J., Tobar C., Oyarzún C., et al. (2011). Diversidad de aves y mamı́feros marinos en bahı́a san Pedro, costa de Purranque, centro-sur de Chile. Gayana (Concepción) 75, 146–154. doi: 10.4067/S0717-65382011000200003
Davies B. J., Darvill C. M., Lovell H., Bendle J. M., Dowdeswell J. A., Fabel D., et al. (2020). The evolution of the Patagonian ice sheet from 35 ka to the present day (PATICE). Earth-Science Rev. 204, 103152. doi: 10.1016/j.earscirev.2020.103152
DGA (2022). “Inventario Público de Glaciares,” in Dirección General de Aguas. Available at: https://dga.mop.gob.cl/Paginas/InventarioGlaciares.aspx.
Dussaillant I., Berthier E., Brun F., Masiokas M., Hugonnet R., Favier V., et al. (2019). Two decades of glacier mass loss along the Andes. Nat. Geosci. 12, 802–808. doi: 10.1038/s41561-019-0432-5
Estes J. A., Terborgh J., Brashares J. S., Power M. E., Berger J., Bond W. J., et al. (2011). Trophic downgrading of planet earth. Science 333, 301–306. doi: 10.1126/science.1205106
Estes J. A., Tinker M. T., Williams T. M., Doak D. F. (1998). Killer whale predation on sea otters linking oceanic and nearshore ecosystems. science 282, 473–476. doi: 10.1126/science.282.5388.473
Evans E., Fraser A. D., Cook S., Coleman R., Joughin I. (2022). An observation-based approach to calculating ice-shelf calving mass flux. Remote Sens. Environ. 272, 112918. doi: 10.1016/j.rse.2022.112918
Forcada J., Malone D., Royle J. A., Staniland I. J. (2009). Modelling predation by transient leopard seals for an ecosystem-based management of Southern Ocean fisheries. Ecol. Model. 220, 1513–1521. doi: 10.1016/j.ecolmodel.2009.03.020
Forcada J., Robinson S. L. (2006). Population abundance, structure and turnover estimates for leopard seals during winter dispersal combining tagging and photo-identification data. Polar Biol. 29, 1052–1062. doi: 10.1007/s00300-006-0149-y
Friedlander A. M., Ballesteros E., Goodell W., Hüne M., Muñoz A., Salinas-de-León P., et al. (2021). Marine communities of the newly created Kawésqar National Reserve, Chile: From glaciers to the Pacific Ocean. PloS One 16, e0249413. doi: 10.1371/journal.pone.0249413
Grabham A. A., van der Linde K., Visser I. N., Nelson X. J. (2023). Initial insights into leopard seal moult in Aotearoa New Zealand. New Z. J. Ecol. 47, 3553. doi: 10.20417/nzjecol.47.3553
Hall-Aspland S. A., Rogers T. L., Canfield R. B. (2005). Stable carbon and nitrogen isotope analysis reveals seasonal variation in the diet of leopard seals. Mar. Ecol. Prog. Ser. 305, 249–259. doi: 10.3354/meps305249
Hammerschlag N., Fallows C., Meÿer M., Seakamela S. M., Orndorff S., Kirkman S., et al. (2022). Loss of an apex predator in the wild induces physiological and behavioural changes in prey. Biol. Lett. 18, 20210476. doi: 10.1098/rsbl.2021.0476
Harrison S., Glasser N. F., Duller G. A., Jansson K. (2012). Early and mid-Holocene age for the Témpanos moraines, Laguna San Rafael, Patagonian Chile. Quaternary Sci. Rev. 31, 82–92. doi: 10.1016/j.quascirev.2011.10.015
Hiruki L. M., Schwartz M. K., Boveng P. L. (1999). Hunting and social behaviour of leopard seals (Hydrurga leptonyx) at seal island, south Shetland islands, Antarctica. J. Zoology 249, 97–109. doi: 10.1111/j.1469-7998.1999.tb01063.x
Hucke-Gaete R., Aguayo-Lobo A., Yancovic-Pakarati S., Flores M. (2014). Marine mammals of Easter Island (Rapa Nui) and Salas y Gómez Island (Motu Motiro Hiva), Chile: A review and new records. Latin Am. J. Aquat. Res. 42, 743–751. doi: 10.3856/vol42-issue4-fulltext-5
Hupman K., Visser I. N., Fyfe J., Cawthorn M., Forbes G., Grabham A. A., et al. (2020). From vagrant to resident: Occurrence, residency and births of leopard seals (Hydrurga leptonyx) in New Zealand waters. New Z. J. Mar. Freshw. Res. 54, 1–23. doi: 10.1080/00288330.2019.1619598
Jessopp M., Forcada J., Reid K., Trathan P., Murphy E. (2004). Winter dispersal of leopard seals (Hydrurga leptonyx): Environmental factors influencing demographics and seasonal abundance. J. Zoology 263, 251–258. doi: 10.1017/S0952836904005102
Johnson C. N., Isaac J. L., Fisher D. O. (2007). Rarity of a top predator triggers continent-wide collapse of mammal prey: Dingoes and marsupials in Australia. Proc. R. Soc. B: Biol. Sci. 274, 341–346. doi: 10.1098/rspb.2006.3711
Jonsen I., McMahon C., Patterson T., Auger-Méthé M., Harcourt R., Hindell M., et al. (2019). Movement responses to environment: Fast inference of variation among southern elephant seals with a mixed effects model. Ecology 100, e02566. doi: 10.1002/ecy.2019.100.issue-1
Kienle S. S., Bonin C. A., Gómez G., Goebel M. E., Donke M., Sperou E. S., et al. (2024). First paired observations of sexual behavior and calls in wild leopard seals. Polar Biol. 47 (10), 1025–1037. doi: 10.1007/s00300-024-03275-4
Kienle S. S., Goebel M. E., LaBrecque E., Borras-Chavez R., Trumble S. J., Kanatous S. B., et al. (2022). Plasticity in the morphometrics and movements of an Antarctic apex predator, the leopard seal. Front. Mar. Sci. 9, 976019. doi: 10.3389/fmars.2022.976019
Knopff A. A., Knopff K. H., Boyce M. S., Clair C. C. S. (2014). Flexible habitat selection by cougars in response to anthropogenic development. Biol. Conserv. 178, 136–145. doi: 10.1016/j.biocon.2014.07.017
Koppes M., Conway H., Rasmussen L., Chernos M. (2011). Deriving mass balance and calving variations from reanalysis data and sparse observations, Glaciar San Rafael, northern Patagonia 1950–2005. Cryosphere 5, 791–808. doi: 10.5194/tc-5-791-2011
Krause D. J., Bonin C. A., Goebel M. E., Reiss C. S., Watters G. M. (2022). The rapid population collapse of a key marine predator in the northern Antarctic peninsula endangers genetic diversity and resilience to climate change. Front. Mar. Sci. 8. doi: 10.3389/fmars.2021.796488
Krause D. J., Goebel M. E., Kurle C. M. (2020). Leopard seal diets in a rapidly warming polar region vary by year, season, sex, and body size. BMC Ecol. 20, 1–15. doi: 10.1186/s12898-020-00300-y
Krause D. J., Goebel M. E., Marshall G. J., Abernathy K. (2015). Novel foraging strategies observed in a growing leopard seal (Hydrurga leptonyx) population at Livingston Island, Antarctic peninsula. Anim. Biotelemetry 3, 1–14. doi: 10.1186/s40317-015-0059-2
Krause D. J., Rogers T. L. (2019). Food caching by a marine apex predator, the leopard seal (Hydrurga leptonyx). Can. J. zoology 97, 573–578. doi: 10.1139/cjz-2018-0203
Laws R. (1957). On the growth rate of the leopard seal Hydrurga leptonyx (de blainville 1820). Saugetierkundliche Mitt. 5, 49–55.
Leger T. P., Hein A. S., Bingham R. G., Martini M. A., Soteres R. L., Sagredo E. A., et al. (2020). The glacial geomorphology of the rı́o Corcovado, Río Huemul and Lago Palena/General Vintter valleys, northeastern Patagonia (43 s, 71 w). J. Maps 16, 651–668. doi: 10.1080/17445647.2020.1794990
Leo V., Reading R. P., Gordon C., Letnic M. (2019). Apex predator suppression is linked to restructuring of ecosystems via multiple ecological pathways. Oikos 128, 630–639. doi: 10.1111/oik.2019.v128.i5
Letnic M., Koch F., Gordon C., Crowther M. S., Dickman C. R. (2009). Keystone effects of an alien top-predator stem extinctions of native mammals. Proc. R. Soc. B: Biol. Sci. 276, 3249–3256. doi: 10.1098/rspb.2009.0574
Lüdecke D., Patil I., Ben-Shachar M. S., Wiernik B. M., Waggoner P., Makowski D. (2021). see: An R package for visualizing statistical models. J. Open Source Software 6, 3393. doi: 10.21105/joss.03393
Makowski D., Ben-Shachar M. S., Patil I., Lüdecke D. (2020). Estimation of model-based predictions, contrasts and means (CRAN). Available at: https://github.com/easystats/modelbased.
Markham B. J. (1971). Observation on the elephant seal and the leopard seal seals on Brecknock Peninsula (Alberto M. de Agostini National Park). Tierra del Fuego. Anales Instituto la Patagonia 2, 160–163.
Meade J., Ciaglia M. B., Slip D. J., Negrete J., Márquez M. E. I., Mennucci J., et al. (2015). Spatial patterns in activity of leopard seals Hydrurga leptonyx in relation to sea ice. Mar. Ecol. Prog. Ser. 521, 265–275. doi: 10.3354/meps11120
Meier M., Post A. (1987). Fast tidewater glaciers. J. Geophysical Research: Solid Earth 92, 9051–9058. doi: 10.1029/JB092iB09p09051
Melkonian A., Willis M., Pritchard M., Rivera A., Bown F., Bernstein S. (2013). Satellite-derived volume loss rates and glacier speeds for the cordillera Darwin icefield, Chile. Cryosphere 7, 823–839. doi: 10.5194/tc-7-823-2013
Menounos B., Maurer L., Clague J. J., Osborn G. (2020). Late Holocene fluctuations of Stoppani glacier, southernmost Patagonia. Quaternary Res. 95, 56–64. doi: 10.1017/qua.2019.87
Milano V. N., Grandi M. F., Schiavini A. C., Crespo E. A. (2020). Sea lions (Otaria flavescens) from the end of the world: Insights of a recovery. Polar Biol. 43, 695–706. doi: 10.1007/s00300-020-02672-9
Millán R., Mouginot J., Rabatel A., Morlighem M. (2022). Ice velocity and thickness of the world’s glaciers. Nat. Geosci. 15, 124–129. doi: 10.1038/s41561-021-00885-z
Mouginot J., Rignot E. (2015). Ice motion of the Patagonian icefields of South America: 1984–2014. Geophysical Res. Lett. 42, 1441–1449. doi: 10.1002/2014GL062661
Paine R. T. (1980). Food webs: Linkage, interaction strength and community infrastructure. J. Anim. Ecol. 49, 667–685. doi: 10.2307/4220
Reynhout S. A., Kaplan M. R., Sagredo E. A., Aravena J. C., Soteres R. L., Schwartz R., et al. (2021). Holocene glacier history of northeastern cordillera Darwin, southernmost South America (55° s). Quaternary Res. 105, 166–181. doi: 10.1017/qua.2021.45
Ripple W. J., Estes J. A., Beschta R. L., Wilmers C. C., Ritchie E. G., Hebblewhite M., et al. (2014). Status and ecological effects of the world’s largest carnivores. Science 343, 1241484. doi: 10.1126/science.1241484
Robbins J. R., Poncet D., Evans A. R., Hocking D. P. (2019). A rare observation of group prey processing in wild leopard seals (Hydrurga leptonyx). Polar Biol. 42, 1625–1630. doi: 10.1007/s00300-019-02542-z
Rodrigues P., Seguel M., Gutiérrez J., Pavés H., Verdugo C. (2018). Genetic connectivity of the South American fur seal (Arctocephalus australis) across Atlantic and pacific oceans revealed by mitochondrial genes. Aquat. Conservation: Mar. Freshw. Ecosyst. 28, 315–323. doi: 10.1002/aqc.2870
Rodrı́guez D., Bastida R., Morón S., Heredia S., Loureiro J. (2003). Occurrence of leopard seals in northern Argentina. Latin Am. J. Aquat. Mammals 2, 51–54. doi: 10.5597/lajam00031
Roff G., Brown C. J., Priest M. A., Mumby P. J. (2018). Decline of coastal apex shark populations over the past half century. Commun. Biol. 1, 223. doi: 10.1038/s42003-018-0233-1
Rogers T. L., Hogg C. J., Irvine A. (2005). Spatial movement of adult leopard seals (Hydrurga leptonyx) in Prydz Bay, Eastern Antarctica. Polar Biol. 28, 456–463. doi: 10.1007/s00300-004-0703-4
Rounsevell D., Eberhard I. (1980). Leopard seals, Hydrurga leptonyx (Pinnipedia), at Macquarie Island from 1949 to 1979. Wildlife Res. 7, 403–415. doi: 10.1071/WR9800403
Rounsevell D., Pemberton D. (1994). The status and seasonal occurrence of leopard seals, Hydrurga leptonyx, in Tasmanian waters. Aust. Mammalogy 17, 97–102. doi: 10.1071/AM94010
Sagredo E., Lowell T. (2012). Climatology of Andean glaciers: A framework to understand glacier response to climate change. Global Planetary Change 86, 101–109. doi: 10.1016/j.gloplacha.2012.02.010
Sagredo E. A., Rupper S., Lowell T. V. (2014). Sensitivities of the equilibrium line altitude to temperature and precipitation changes along the Andes. Quaternary Res. 81, 355–366. doi: 10.1016/j.yqres.2014.01.008
Sahanatien V., Derocher A. E. (2012). Monitoring sea ice habitat fragmentation for polar bear conservation. Anim. Conserv. 15, 397–406. doi: 10.1111/j.1469-1795.2012.00529.x
Salame C., Gonzalez I., Gomez-Fell R., Jaña R., Arigony-Neto J. (2020). First record of the occurrence of sea ice in the cordillera Darwin fjords (54 s), Chile. Ann. Glaciology 61, 472–482. doi: 10.1017/aog.2021.3
Schaefer M., Machguth H., Falvey M., Casassa G., Rignot E. (2015). Quantifying mass balance processes on the southern Patagonia icefield. Cryosphere 9, 25–35. doi: 10.5194/tc-9-25-2015
Schmitz O. J., Hawlena D., Trussell G. C. (2010). Predator control of ecosystem nutrient dynamics. Ecol. Lett. 13, 1199–1209. doi: 10.1111/j.1461-0248.2010.01511.x
Schneider C., Braun M., Schaefer M., Grießinger J., Casassa G. (2020). Editorial: climate impacts on glaciers and biosphere in fuego-patagonia. Front. Earth Sci. 8. doi: 10.3389/feart.2020.00091
Schwarz L. K., Goebel M. E., Costa D. P., Kilpatrick A. M. (2013). Top-down and bottom-up influences on demographic rates of Antarctic fur seals Arctocephalus gazella. J. Anim. Ecol. 82, 903–911. doi: 10.1111/jane.2013.82.issue-4
Sepúlveda M., Carrasco P., Quiñones R. (2021). Long-term abundance dynamics of the south American sea lion (Otaria flavescens) in the most important breeding colony in central Chile. Austral Ecol. 46, 1277–1286. doi: 10.1111/aec.13086
Sernapesca (2023). Stranding events reports. Available online at: https://www.sernapesca.cl/informacion-utilidad/registro-de-varamientos/. (Accessed [February 10th, 2023]).
Shaughnessy P. D., Tomo I., Gibbs S. E., Kemper C. M., Stemmer D. (2023). Records of leopard seals Hydrurga leptonyx ashore in south Australia 2017–2022. Aust. Mammalogy 46, AM22041. doi: 10.1071/AM22041
Sherman C. S., Heupel M. R., Moore S. K., Chin A., Simpfendorfer C. A. (2020). When sharks are away, rays will play: Effects of top predator removal in coral reef ecosystems. Mar. Ecol. Prog. Ser. 641, 145–157. doi: 10.3354/meps13307
Simpson E. (1875). Esploraciones hechas por la corbeta Chacabuco al mando del capitan de fragata D. Enrique M. Simpson en los archipiélagos de las Guaitecas, Chonos i Taitao (Santiago, Chile: Anuario Hidrográfico de la Marina de Chile I), 3–147.
Siniff D., Stone S. (1985). “The role of the leopard seal in the tropho-dynamics of the Antarctic marine ecosystem,” in Antarctic nutrient cycles and food webs (Berlin, Heidelberg: Springer), 555–560.
Soteres R. L., Peltier C., Kaplan M. R., Sagredo E. A. (2020). Glacial geomorphology of the strait of Magellan ice lobe, southernmost Patagonia, South America. J. Maps 16, 299–312. doi: 10.1080/17445647.2020.1736197
Soteres R. L., Riquelme F. M., Sagredo E. A., Kaplan M. R. (2023). (Paleo) glacier studies in Patagonia over the past decades, (1976–2020): A bibliometric perspective based on the Web of Science. J. South Am. Earth Sci. 122, 104173. doi: 10.1016/j.jsames.2022.104173
Soteres R. L., Sagredo E. A., Moreno P. I., Lowell T. V., Alloway B. V. (2022). Glacial geomorphology of the central and southern Chilotan archipelago (42.2° s–43.5° s), northwestern Patagonia. J. Maps 18, 151–167. doi: 10.1080/17445647.2021.2008538
Southwell C., Paxton C. G., Borchers D., Boveng P., Rogers T., de la Mare W. K. (2008). Uncommon or cryptic? Challenges in estimating leopard seal abundance by conventional but state-of-the-art methods. Deep Sea Research Part I. Oceanographic Res. Papers 55, 519–531. doi: 10.1016/j.dsr.2008.01.005
Sperou E. S., Crocker D. E., Borras-Chavez R., Costa D. P., Goebel M. E., Kanatous S. B., et al. (2023). Large and in charge: Cortisol levels vary with sex, diet, and body mass in an Antarctic predator, the leopard seal. Front. Mar. Sci. 10, 1179236. doi: 10.3389/fmars.2023.1179236
Staniland I. J., Ratcliffe N., Trathan P. N., Forcada J. (2018). Long term movements and activity patterns of an Antarctic marine apex predator: The leopard seal. PloS One 13, e0197767. doi: 10.1371/journal.pone.0197767
Stewart B. S., Grove J. S., Kühlem A., Flores M. (2020). Recent and historic occurrences of leopard seals (Hydrurga leptonyx) at easter island (Rapa Nui), Eastern Polynesia. Polar Biol. 43, 1899–1902. doi: 10.1007/s00300-020-02741-z
Strelin J., Casassa G., Rosqvist G., Holmlund P. (2008). Holocene glaciations in the ema glacier valley, monte Sarmiento massif, Tierra del Fuego. Palaeogeography Palaeoclimatology Palaeoecol. 260, 299–314. doi: 10.1016/j.palaeo.2007.12.002
Testa J. W., Oehlert G., Ainley D. G., Bengtson J. L., Siniff D. B., Laws R. M., et al. (1991). Temporal variability in Antarctic marine ecosystems: periodic fluctuations in the phocid seals. Can. J. Fisheries Aquat. Sci. 48, 631–639. doi: 10.1139/f91-081
Thorndycraft V. R., Bendle J. M., Benito G., Davies B. J., Sancho C., Palmer A. P., et al. (2019). Glacial lake evolution and Atlantic-Pacific drainage reversals during deglaciation of the Patagonian ice sheet. Quaternary Sci. Rev. 203, 102–127. doi: 10.1016/j.quascirev.2018.10.036
Torrejón F., Bizama G., Araneda A., Aguayo M., Bertrand S., Urrutia R. (2013). Descifrando la historia ambiental de los archipiélagos de Aysén, Chile: El influjo colonial y la explotación económica-mercantil republicana (siglos XVI-XIX). Magallania (Punta Arenas) 41, 29–52. doi: 10.4067/S0718-22442013000100002
Torres D., Yañez J., Catán P. (1979). Mamíferos Marinos de Chile. Antecedentes y Situación Actual. Biología Pesquera (Chile) 11, 49–81.
van den Hoff J., Fraccaro R., Mitchell P., Field I., Mcmahon C., Burton H., et al. (2005). Estimating body mass and condition of leopard seals by allometrics. J. Wildlife Manage. 69, 1015–1023. doi: 10.2193/0022-541X(2005)069[1015:EBMACO]2.0.CO;2
Visser I. N., van der Linde K., Richard S. E., Cooper T. E., Hardie T. M., Bout R., et al. (2022). Can a leopard seal, Hydrurga leptonyx de Blainville, 1820 (Mammalia Phocidae), change its spots? (Spoiler alert: at least one adult female can). Biodiversity J. 13, 685–693. doi: 10.31396/Biodiv.Jour.2022.13.3.685.693
van der Linde K., Visser I. N., Bout R., Krause D. J., Forcada J., Siniff D., et al. (2022). A review of leopard seal (Hydrurga leptonyx) births and pups using a standardized age-class classification system. Polar Biol. 45, 1193–1209. doi: 10.1007/s00300-022-03053-0
Vásquez A., Flores-Aqueveque V., Sagredo E., Hevia R., Villa-Martı́nez R., Moreno P. I., et al. (2022). Evolution of glacial lake C during the last glacial termination, central Chilean Patagonia (47°s). Front. Earth Sci. 10, 817775. doi: 10.3389/feart.2022.817775
Vera C., Vargas R., Torres D. (2004). El impacto de la foca leopardo en la población de cachorros de lobo fino antártico en cabo Shirreff, Antártica, temporada 2003-2004. Boletín del Instituto Antártico Chileno 23, 19–22.
Vérité J., Ravier É., Bourgeois O., Bessin P., Pochat S. (2023). New metrics reveal the evolutionary continuum behind the morphological diversity of subglacial bedforms. Geomorphology 427, 108627. doi: 10.1016/j.geomorph.2023.108627
Walker T., Boyd I. L., McCafferty D., Huin N., Taylor R., Reid K. (1998). Seasonal occurrence and diet of leopard seals (Hydrurga leptonyx) at bird island, South Georgia. Antarctic Sci. 10, 75–81. doi: 10.1017/S0954102098000108
Wickham H. (2016). ggplot2: Elegant graphics for data analysis (New York: Springer-Verlag). Available at: https://ggplot2.tidyverse.org.
Wiig Ø., Aars J., Born E. W. (2008). Effects of climate change on polar bears. Sci. Prog. 91, 151–173. doi: 10.3184/003685008x324506
Williams T. M., Estes J. A., Doak D. F., Springer A. M. (2004). Killer appetites: Assessing the role of predators in ecological communities. Ecology 85, 3373–3384. doi: 10.1890/03-0696
Wilmers C. C., Post E. (2006). Predicting the influence of wolf-provided carrion on scavenger community dynamics under climate change scenarios. Global Change Biol. 12, 403–409. doi: 10.1111/j.1365-2486.2005.01094.x
Wood S. N. (2011). Fast stable restricted maximum likelihood and marginal likelihood estimation of semiparametric generalized linear models. J. R. Stat. Soc. (B) 73, 3–36. doi: 10.1111/j.1467-9868.2010.00749.x
Keywords: Hydrurga leptonyx, pagophilic species, Antarctic seal, Southern Ocean pinnipeds, Patagonia biodiversity, species distribution, glaciers
Citation: Borras-Chavez R, Soteres RL, Gómez-González G, Martínez F, Fernández-Ferrada N, Castillo-Aguilar M, Moreno Azua F, Dougnac C, Arredondo C, Brown N, Sperou ES, Bonin CA, Goebel ME, Guerrero AI, Donke M and Kienle SS (2024) Occurrence, residency, and habitat characterization of leopard seals in Chile. Front. Ecol. Evol. 12:1448098. doi: 10.3389/fevo.2024.1448098
Received: 12 June 2024; Accepted: 16 September 2024;
Published: 31 October 2024.
Edited by:
Peter Convey, British Antarctic Survey (BAS), United KingdomReviewed by:
David Ainley, H.T. Harvey & Associates, United StatesDavide Tamagnini, Sapienza University of Rome, Italy
Copyright © 2024 Borras-Chavez, Soteres, Gómez-González, Martínez, Fernández-Ferrada, Castillo-Aguilar, Moreno Azua, Dougnac, Arredondo, Brown, Sperou, Bonin, Goebel, Guerrero, Donke and Kienle. This is an open-access article distributed under the terms of the Creative Commons Attribution License (CC BY). The use, distribution or reproduction in other forums is permitted, provided the original author(s) and the copyright owner(s) are credited and that the original publication in this journal is cited, in accordance with accepted academic practice. No use, distribution or reproduction is permitted which does not comply with these terms.
*Correspondence: Renato Borras-Chavez, renato_borras-chavez@baylor.edu