- 1Amsterdam Institute for Life and Environment (A-LIFE), Section Systems Ecology, Vrije Universiteit Amsterdam, Amsterdam, Netherlands
- 2Millennium Institute Biodiversity of Antarctic and Sub-Antarctic Ecosystems (BASE), Santiago, Chile
- 3Cape Horn International Center, Puerto Williams, Chile
- 4Universidad de Magallanes, Centro Universitario Cabo de Hornos, Puerto Williams, Chile
- 5British Antarctic Survey, Natural Environment Research Council, Cambridge, United Kingdom
- 6Department of Zoology, University of Johannesburg, Auckland Park, South Africa
Introduction: Elevation gradients are often used as a proxy for climate change as they allow comparisons of ecological responses over much larger temporal and spatial scales than is possible through experimental manipulations.
Methods: Here, we tested how microarthropod communities (Collembola and Acari) are affected by climatic differences between sea level and 600 m a.s.l. on Navarino Island, in the Magellanic sub-Antarctic ecoregion of southern Chile (mean annual temperatures of 5.6 vs 3.1°C, respectively). We quantified microarthropod abundance, richness and community trait characteristics in dominant moss (Racomitrium lanuginosum and Polytrichum strictum) and lichen (Usnea trachycarpa, Pseudocyphellaria freycinetii and Stereocaulon alpinum) vegetation growing at both elevations. These moss and lichen genera are characterized by large morphological differences and allow testing of how habitat characteristics affect microarthropod community response across elevation gradients.
Results: Collembola and Acari community composition differed between the low and high elevation sites. Total abundance levels of Acari were maintained in each habitat across elevation, whereas Collembola richness strongly declined (50%) at high elevation in the moss habitats. Acari community differences across elevation were driven by relative abundance changes whereas the Collembola community lost species at higher elevation. An anticipated decline of smaller eudaphic Collembola at high elevation was only observed in the moss Racomitrium, reflecting potentially lower temperature buffering capacity and shelter options compared to Polytrichum. Lichens mostly supported larger epigeic species irrespective of elevation. There were no consistent patterns linking microarthropod communities with habitat water holding capacity or water loss rates across the studied habitats and elevation.
Discussion: Habitat type and the genus of moss or lichen were associated with microarthropod community changes across elevation, including examples of declines, increases and no change. These findings highlight that community responses across gradients may not always relate to the generally hypothesized environmental variables (e.g. temperature variability) and that habitat characteristics should be taken into account when using elevation as a proxy for climate change.
Introduction
Polar regions are experiencing some of the most rapid warming rates on Earth, resulting in potentially large changes in vegetation composition, soil communities and soil process rates (Elmendorf et al., 2012; Myers-Smith et al., 2015; IPCC, 2023). Understanding ecosystem response to warming is crucial for understanding future feedbacks to global climate change. Microarthropods, such as springtails (Collembola) and mites (Acari), are soil biotic drivers of soil nutrient and carbon cycling (Seastedt, 1984; Filser, 2002; Kampichler and Bruckner, 2009) and changes in their abundance and community composition affect decomposition rates (Wall et al., 2008; Handa et al., 2014). Predicting microarthropod response to changes in climate is complicated as various factors change simultaneously in the soil biotic and abiotic environment, all of which can potentially promote or restrict individual growth and reproduction. In addition, some microarthropod species may be more responsive to direct changes in microclimate conditions (Makkonen et al., 2011; Bokhorst et al., 2012), while others respond to indirect climate-driven changes in vegetation and associated soil characteristics (Krab et al., 2010; Bokhorst et al., 2017). Untangling this context specificity across species response requires complementary approaches, and will help to anticipate challenges in polar ecosystems resulting from climate change.
Experimental warming studies show large and variable responses by microarthropods (Hodkinson et al., 1998; Convey et al., 2002; Bokhorst et al., 2008; Kardol et al., 2011). This variability in part reflects the context dependency on where these studies were performed. In addition, methodological drawbacks exist with experimental approaches in terms of realism (such as altering the frequency of temperature extremes) or affect multiple microclimate conditions (e.g., moisture deficits, wind speed and shade) (Bokhorst et al., 2013a). This can complicate our ability to determine the nature of causality behind response variables. Using comparisons across elevation as a proxy for climate differences provides an alternative approach to quantify climate change effects and allow assessment of ecological responses over much larger temporal and spatial scales than is possible through experimental manipulations (Hodkinson, 2005; Sundqvist et al., 2013). Such elevation approaches have been used to characterize microarthropod community changes, but often generate contrasting response patterns in terms of abundance and diversity (Lamoncha and Crossley, 1998; Loranger et al., 2001; Sadaka and Ponge, 2003; Jing et al., 2005; Hasegawa et al., 2006; Illig et al., 2010; Nash et al., 2013) or community changes that were habitat dependent (Bokhorst et al., 2018). Recent studies have suggested that springtail communities show a higher proportion of larger species and individuals with increasing elevation (Bokhorst et al., 2018; Wu et al., 2023; Hishi et al., 2024), which probably reflects selection against smaller species with vulnerability to freezing intensity and variability around zero degrees (freeze-thaw) found at higher elevation (Leinaas, 1983; Sømme, 1989; Bokhorst et al., 2012; Bokhorst et al., 2013b; van Dooremalen et al., 2013). Mite communities typically show a decline in abundance with altitude across major taxonomic groups (Bokhorst et al., 2018). To further untangle patterns of microarthropod distribution patterns across environmental gradients, studies can take advantage of habitat types that change as little as possible with elevation, such as provided by mosses and lichens.
Mosses and lichens are key players in shaping boreal and polar terrestrial microarthropod communities by providing habitat, water, food and buffering against weather extremes (Cornelissen et al., 2007; Blok et al., 2011; Bokhorst et al., 2014; Roos et al., 2021). However, moss and lichen species typically differ in the microarthropod communities they support (Bokhorst et al., 2015; Trekels et al., 2017; Roos et al., 2022), resulting from the way each moss and lichen species creates its own microhabitat (Stoy et al., 2012; van Zuijlen et al., 2020). Moss and lichen water content affects the type of microarthropods supported and higher water holding capacity often results in greater arthropod abundance (Booth and Usher, 1984; Bokhorst et al., 2015). Cryptogam water holding capacity is unlikely to change markedly across environmental gradients (Roos et al., 2019), but water loss rates may be slower in exposed areas, such as a montane plateaus, to prolong photosynthetic capacity (Pintado et al., 1997) which, in turn, provides a longer duration of moist environmental conditions for associated microarthropods. By comparing how microarthropod communities change across elevation within the same moss and lichen habitat, we can identify if microarthropod responses are driven by the overall changes in temperature conditions at higher elevation (environmental selection) or by habitat conditions created by the moss and lichen habitats.
Here we tested how Collembola and Acari community composition responded to changes in climate conditions across elevation, while retaining the same habitat structure provided by moss and lichen species that grew at both high and low elevation sites. The study was carried out on Navarino Island in the Magellanic sub-Antarctic ecoregion of Southern Chile, whose high elevation fell-field plateaus face similar environmental conditions to the core sub-Antarctic islands and parts of the Antarctic Peninsula region (Rosenfeld et al., 2020; Francisco et al., 2021). We hypothesized that: a) microarthropod abundance will decline at higher elevation as a result of the colder environmental conditions, b) microarthropod communities will be more strongly affected by elevation in lichen than moss habitat as mosses provide stronger buffering against environmental extremes (Stoy et al., 2012), and c) communities will shift toward larger sized Collembola at higher elevation.
Methods
The study site (Figure 1) was located on Navarino Island in southern Patagonia (55° 07’ S, 67° 66’ W). The low elevation sampling site (54° 94’ S, 67° 62’ W) was situated close to sea level at the edge of Nothofagus forest (mixed stands of N. pumilio and N. antarctica) intermixed with peatland (Sphagnum magellanicum). The high elevation sampling site was situated above the tree line (600 m. asl. 54° 97’ S, 67° 63’ W) on Cerro Bandera and comprised a typical fellfield community consisting of a mosaic of lichens and mosses intermixed with dwarf shrubs (Empetrum rubrum), cushion plants (Azorella spp.) and grasses, similar to sub-Arctic mountainous regions and sub-Antarctic islands (Greene, 1964; Upson et al., 2017). Mean annual soil temperatures are 5.6°C and 3.1°C for the low and high elevation sites, respectively, and 0.5°C and -2.2°C during winter (Table 1). Snow frequently occurs during summer months at the high elevation site, but melts out within a few days, while at sea level precipitation mostly falls as rain (Rozzi and Jimenez, 2013).
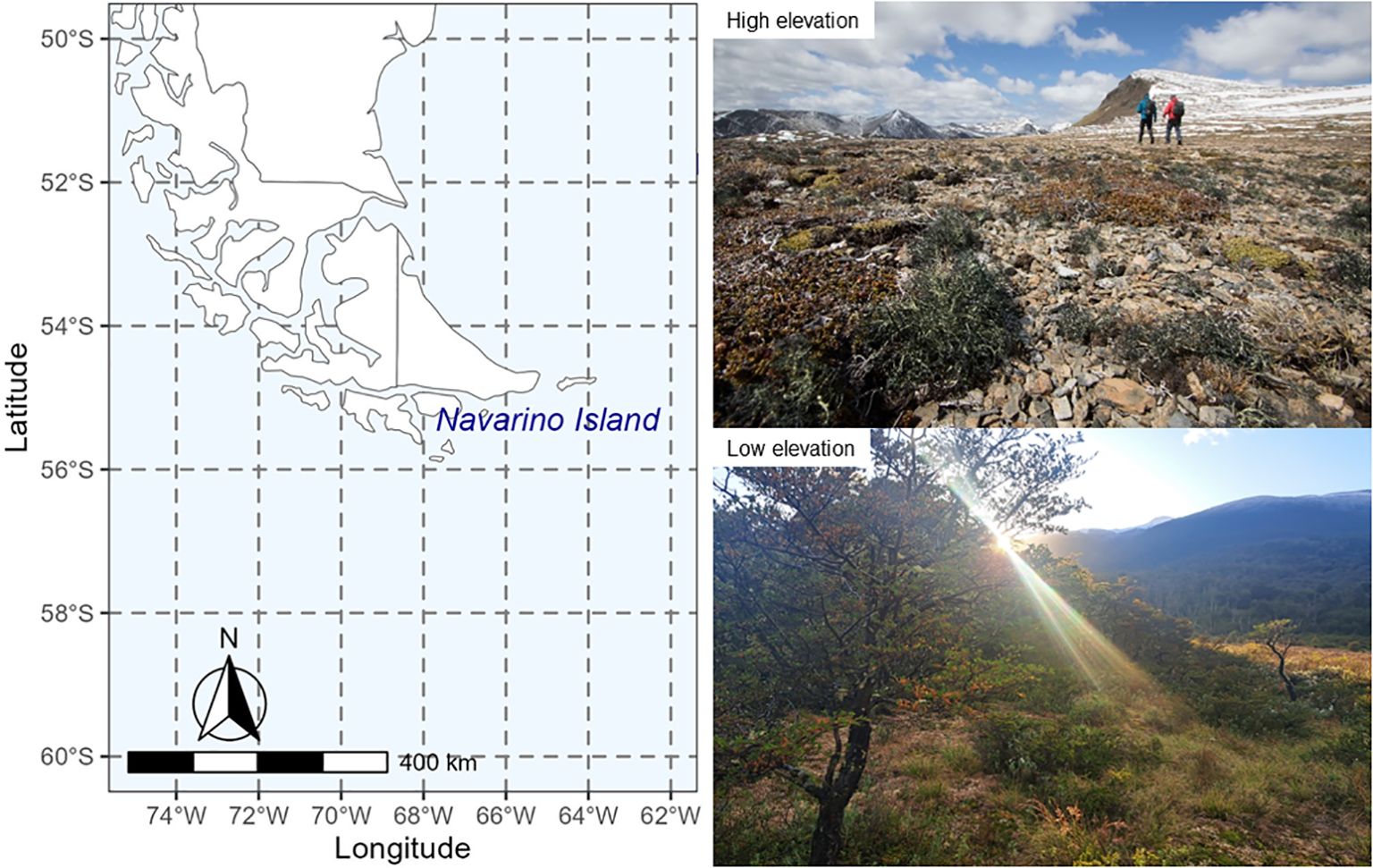
Figure 1. Map showing the location of Navarino Island in southern Patagonia (54° 97’ S, 67° 63’ W). The low elevation sampling site (lower right picture) was situated close to sea level at the edge of Nothofagus forest intermixed with peatland (Sphagnum magellanicum). The high elevation sampling site (upper right picture) was situated above the tree line (600 m. asl.) on Cerro Bandera and comprised a typical lichen fellfield community intermixed with dwarf shrubs and cushion plants.
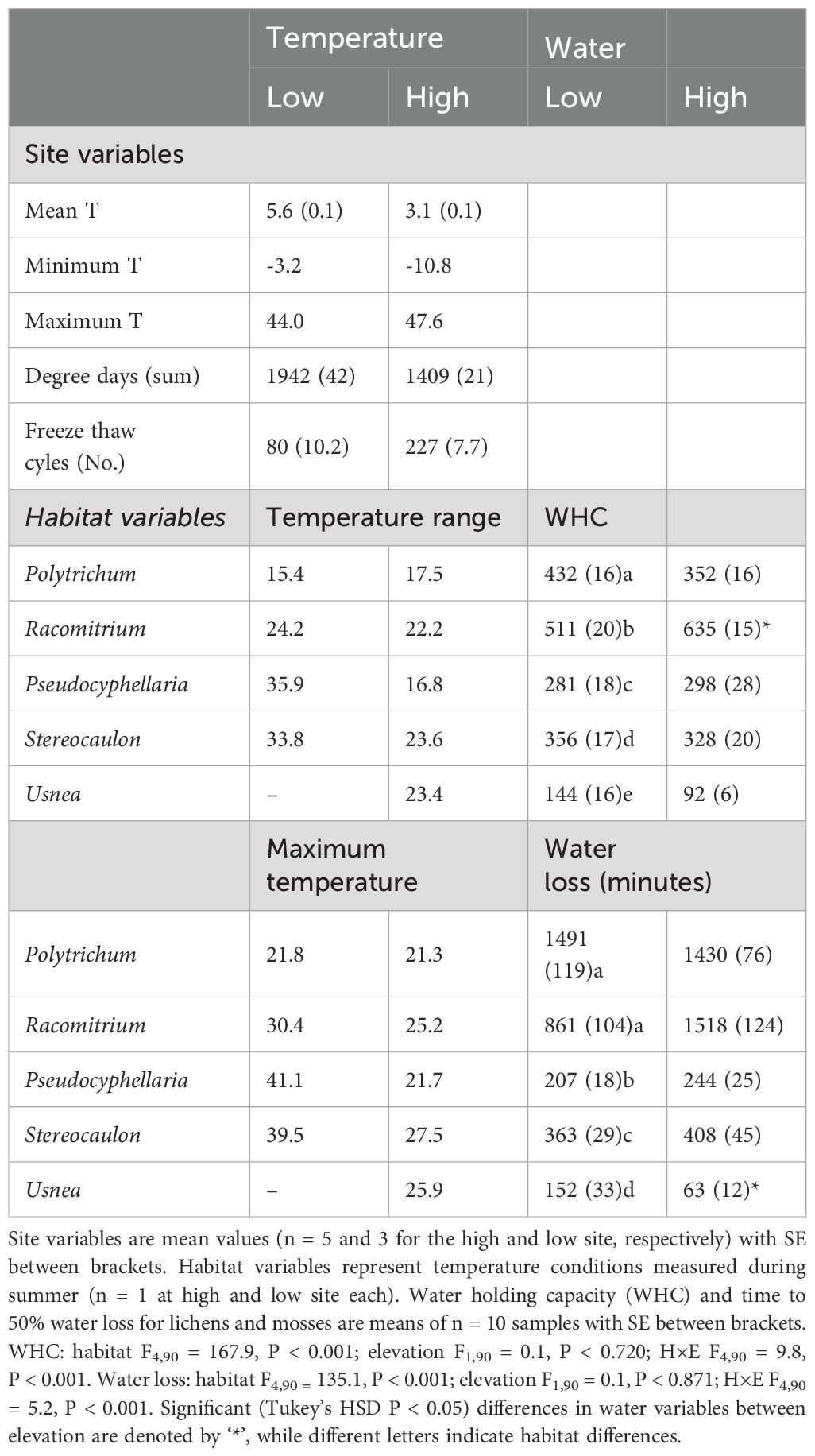
Table 1. Microclimate temperature conditions and water traits among different moss and lichen habitats at low and high field sites.
During the austral summer (22-29th January 2020) we collected dominant moss and lichen species that occur at both low (sea level) and high (600 m a.s.l.) elevation. Twenty clumps each of Racomitrium lanuginosum, Polytrichum strictum, Usnea trachycarpa, Pseudocyphellaria freycinetii and Stereocaulon alpinum from the low (n = 10) and high (n = 10) elevation study site. Henceforth these species will be referred to by their genus name alone. Polytrichum (Polytrichaceae, bog haircap moss) is an erect growing, acrocarpic, moss species preferring moist habitats (peatlands), which is also found in the maritime Antarctic and the Arctic. Its erect growth form creates a compact moss mat which can develop to tens of cm depth. Racomitrium (Grimmiaceae, woolly fringe moss) is a common moss species of montane and polar tundra (also in the maritime Antarctic) growing as large mats of loose horizontal shoots, across dry exposed rock and in boulder scree. Pseudocyphellaria (Peltigeraceae, speckle belly lichens) is a foliose lichen growing on tree trunks and boulders where it forms extensive mats. Although Peltigeraceae are present in Antarctica, the genus Pseudocyphellaria is not reported from the Arctic or Antarctic. Stereocaulon (Stereocaulaceae, snow lichens) is a fruticose grey-white lichen, with densely branched thalli covered with grainy outer edges, which also occurs in the maritime Antarctic. Usnea (Parmelicaceae, beard lichen) is pale grey-green fruticose lichen that resembles a miniature shrub. Lichens of this genus have a worldwide distribution with several species also occurring in Antarctica.
Individual lichen thalli (c. 5 cm × 5 cm) were collected by hand, ensuring at least 50 cm distance between thalli. Mosses were collected using a 5 cm diameter, maximum depth of 5 cm, corer with a minimum of 1 m distance between cores. Mosses and lichens were obtained from patches dominated by the target species, to minimize potential edge effects. Each sample was immediately sealed in a sampling pot with lid and microarthropod extraction was started as soon as possible upon return to the field station (<5 h). Species replicate samples (n = 10) were always collected on the same day, but logistical practicalities prevented sampling all species across both study sites at the same day. However, given the short duration of the sampling campaign (7 d), we assume that any time differences between low and high site sampling dates (1-5 d) and species (3-6 d) did not affect microarthropod abundances.
A Tullgren heat extractor was used to collect Collembola, Acari and spiders (Araneae) from the vegetation samples. Extractions took place over a minimum of 48 h until the cryptogam habitat was dry, and the extracted animals were immediately preserved in ethanol (70%). Collembola species were identified to genus or species level following Heckman (2001) and grouped into life-form groups according to their known vertical distributions across soil-litter profiles: in soil, eudaphic; in litter, hemi-edaphic; on plants, epigeic; (Gisin, 1943). To determine whether Collembola species with specific traits would be differentially affected by elevation, community weighted trait means (CWM) were calculated (de Bello et al., 2011). For each trait the relative abundance of a Collembola species was multiplied by its trait value, and then summed over all species in the community. For Collembola we used the traits of life form eudaphic (trait value 1), hemidaphic (trait value 2) and epigeic (trait value 3), and CWM body length (mm). Trait information was obtained from the literature (Gisin, 1943; Fjellberg, 1998; Heckman, 2001). Acari were identified to major groups (Oribatida, Mesotigmata and Prostigmata-Astigmata) and, where possible, to family level. All spiders were counted, but not compared across functional trait groups as this type of information is lacking. All abundance data were expressed as individuals per gram oven dried substrate (70°C 48 h). Collembola species and Acari group frequency across sampled habitats are presented in Supplementary Table 1.
To determine if habitats differently buffer against environmental conditions, we measured hourly temperature and light conditions of each moss and lichen habitat at the high and low elevation site for 48 h in late January, using HOBO pendants (UA 002-64). Logger placement among mosses resulted in the logger being buried and not exposed to direct illumination, while among lichen thalli the loggers were partly exposed to direct illumination. Due to logger limitation there was no replication of microclimate logging at the respective elevation sites; the logger placed in Usnea at the low elevation site malfunctioned. For each habitat type (species) we calculated daily mean temperature, temperature range and the minimum and maximum temperature. Although the microclimate recording took place over only a short mid-summer period, it reflects the temperature and light conditions soil invertebrates are exposed to when living among these moss and lichen habitats. Year-round temperature and light data were obtained using HOBO pendants (UA 002-64) placed on the soil at the high (n = 5) and low (n = 3) elevation site.
Moss and lichen water holding capacity (WHC) was quantified by comparing the weight difference between fully hydrated and oven dried (70°C 48 h) tissue samples. Full hydration was achieved by wetting lichen thalli and moss plugs and placing them in a closed jar with moist tissue paper for 24 h. A thin layer (1-2 cm) of water was added to the moss plugs as these can take up substantially more water than lichens. To quantify water loss rates, fully hydrated samples were weighed at regular intervals (1.5-3 h) under standardized laboratory settings at room temperature for 7 h. Water loss rates followed a linear or exponential decline and the time to 50% water loss was calculated as a response variable (water retention time) for each individual moss and lichen sample.
Statistical analyses
A factorial ANOVA was used to test how microarthropod abundance (total, Collembola, Acari, spider, species and groups), taxonomic richness, community weighted trait values, water holding capacity (WHC) and water retention time were affected by habitat (n = 5 species) and elevation (low vs. high). We quantified how Collembola and Acari community composition (based on abundance) differed between elevation in each habitat using Bray-Curtis distance and compared habitat differences using the adonis-method from the R ‘vegan package’ (Oksanen, 2015). To identify effects of changing water conditions across elevation within each habitat we assessed the correlation (Pearson) of habitat WHC and water retention time with microarthropod abundance and richness. All data were log- or square root-transformed to improve homogeneity of variances. All analyses were performed using R (R-Core-Team, 2023). We used the online iNterpolation and EXTrapolation tool to check if sampling size was sufficient capture microarthropod diversity (Supplementary Figure S1) (Chao et al., 2014). This type of sampling curve plots the diversity estimates with respect to sample size. Although we tested for moss and lichen habitat differences in microarthropod composition measures, the results and discussion primarily focus on elevation comparisons between moss and lichen habitats.
Results
Microclimate differences
Mean annual soil temperature was 2.5°C lower at high elevation, and this was also reflected in lower moss (3.0 - 3.6°C) and lichen (5.4 - 5.7°C) summer temperatures (Table 1). The high elevation site was exposed to nearly three times higher frequency of freeze-thaw events annually, and experienced a larger temperature range due to colder winter and warmer summer temperatures (Table 1). The summer temperature range at the low elevation site was on average 7°C higher among the moss and lichen habitats compared to the high elevation site. Moss temperature range was about 10-20°C lower than that of lichens at low elevation, whereas this differences was reduced at high elevation (Table 1, Supplementary Figures 2, 3). Water holding capacity and water retention time were highest in the two moss habitats compared to the lichens, with the latter decreasing in the following order: Stereocaulon > Pseudocyphellaria > Usnea. Racomitrium had a 24% higher WHC at high elevation, and Usnea from high elevation lost water more than two times more rapidly than did specimens from low elevation. Other moss and lichen habitats did not differ in WHC or water retention time between high and low elevation (Table 1).
Microarthropod abundance and richness responses
Total microarthropod abundance was affected by elevation but not consistently across moss and lichen habitats, and a similar pattern was observed for Collembola and Acari abundance and taxonomic richness (Table 2). Collembola abundance was lower at high elevation in Racomitrium (64%) and Usnea (84%) compared to the low elevation site, as predicted, but no significant differences were observed among the other moss and lichen habitats. Acari abundance was 92% lower among Usnea at high elevation (Figures 2A, B). Collembola species richness was reduced by 50% at high elevation in Polytrichum, Racomitrium and Usnea, as predicted, while a doubling of Acari taxonomic richness was observed in Polytrichum at high elevation (Figures 2C, D). Spider abundance was low overall and spiders were absent from moss but not lichen at high elevation.
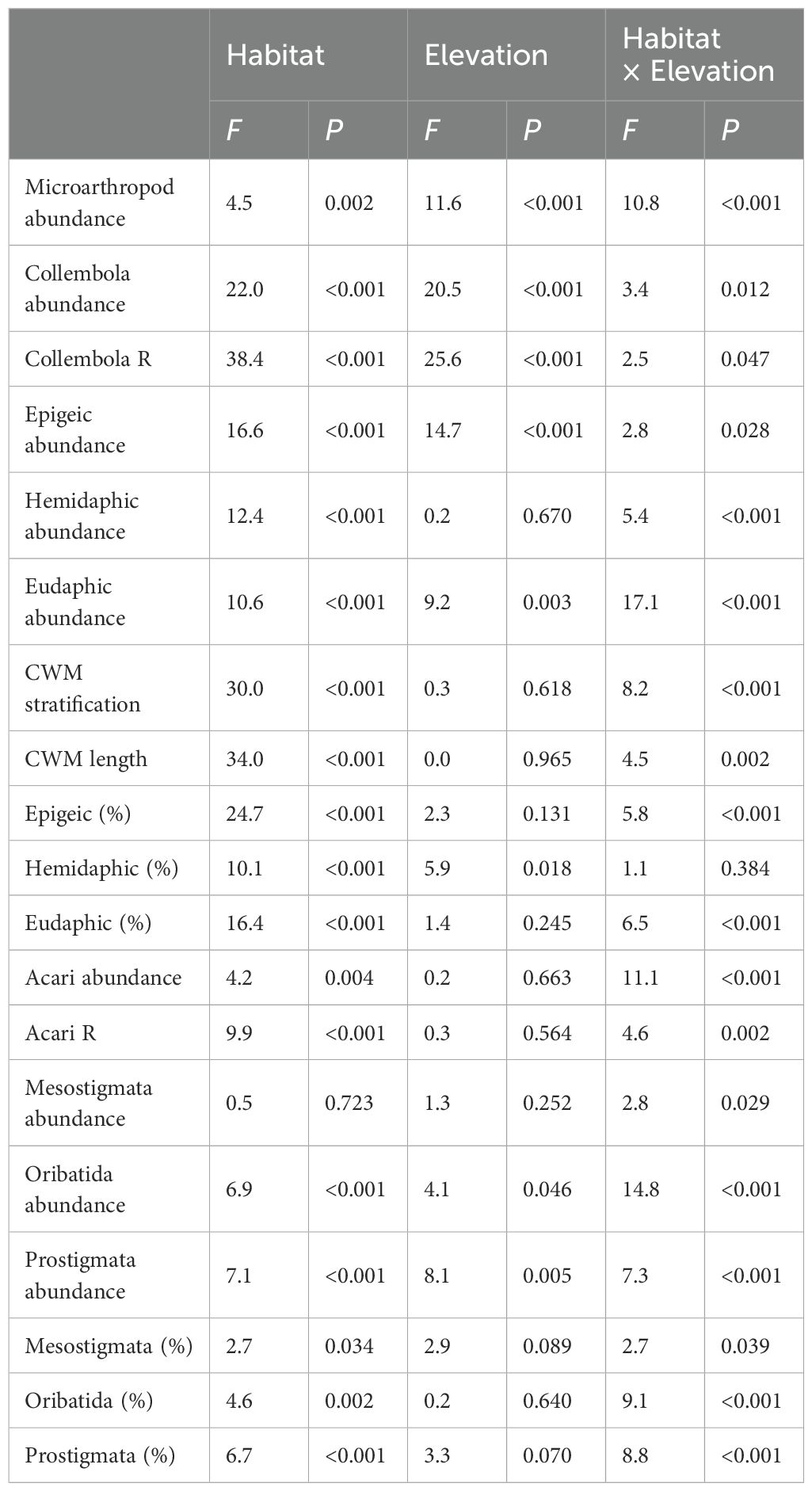
Table 2. ANOVA results (F and P-values) of micro-arthropod abundance, taxonomic richness and community weighted mean traits between different lichen and moss habitats across low and high elevation.
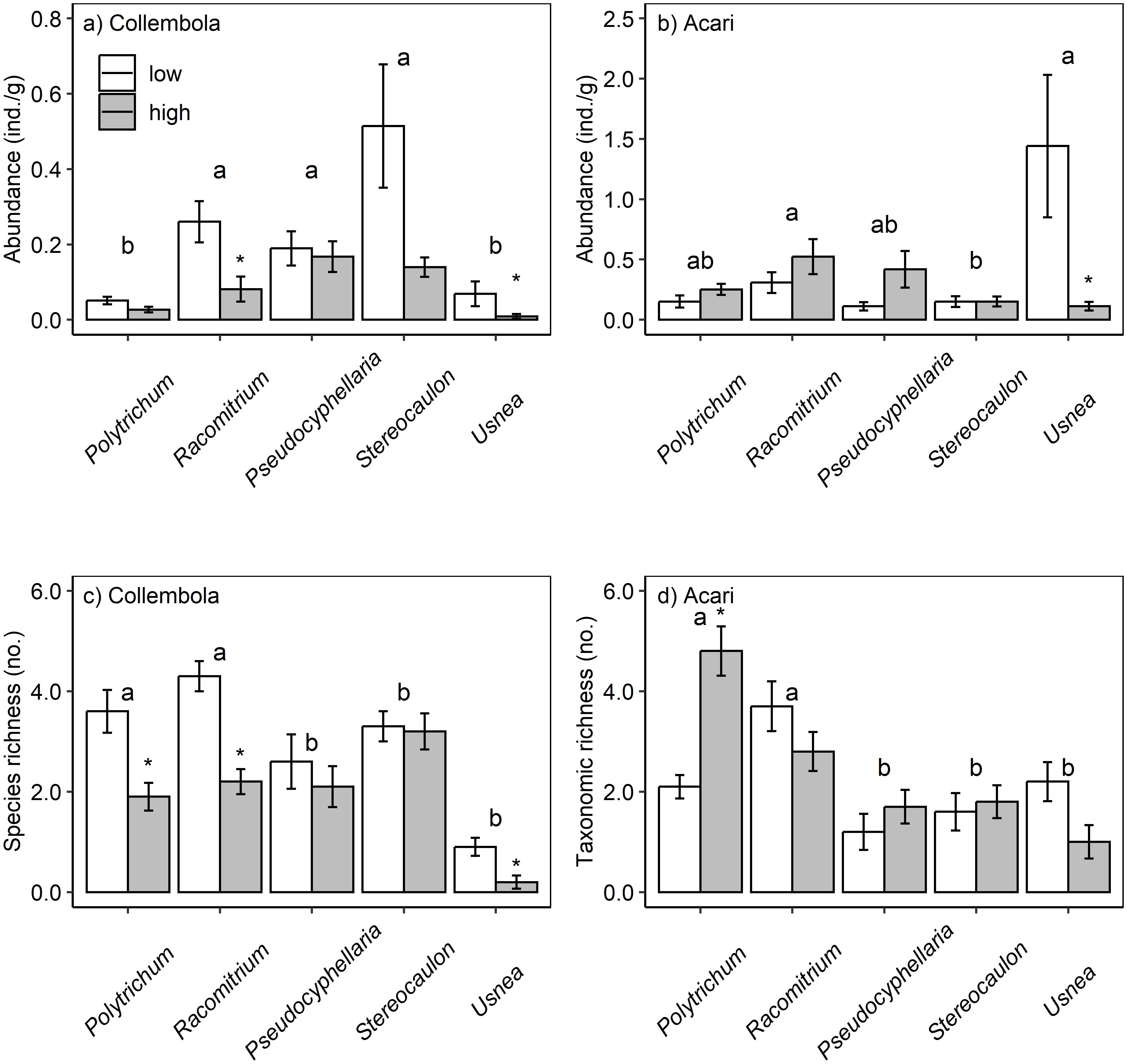
Figure 2. Micro-arthropod abundance (A, B) and taxonomic richness (C, D) across elevation and moss and lichen habitats. Mosses: Polytrichum and Racomitrium, lichens: Pseudocyphellaria, Stereocaulon and Usnea. Each bar is the mean of n = 10 replicates with SE as error bars. * denotes significant differences between elevation, while different letters indicate habitat differences.
There was no consistent Collembola size response with elevation across habitats (Figures 3A, B). Epigeic Collembola abundance was decreased (84%) at high elevation in Stereocaulon (Supplementary Figure 4A). Eudaphic Collembola were absent at high elevation in Racomitrium, and hemidaphic Collembola abundance was reduced (62%) in this moss, resulting in a community with larger species (Figures 3A, B). No elevation effects were observed for any of the other moss or lichen habitats on these Collembola groups (Supplementary Figures 4B, C). Abundance of mesostigmatid mites was not consistently affected across elevation. Oribatid mite abundance was reduced (99%) at higher elevation in Usnea, while prostigmatid mite abundance increased ten-fold in Racomitrium at high elevation.
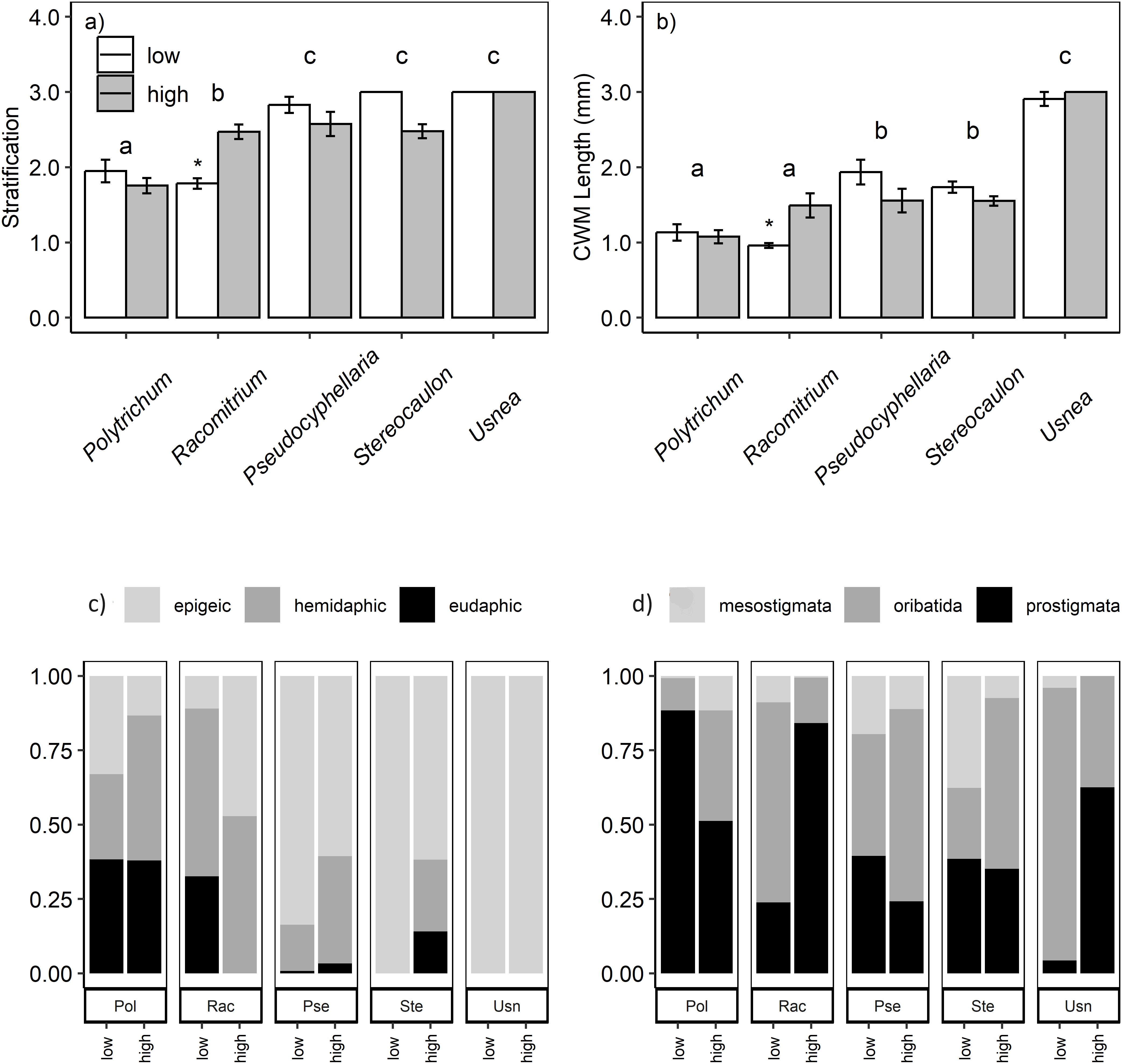
Figure 3. Micro-arthropod community composition in different habitats across elevation. Collembola community weighted mean vertical stratification (A), and community weighted mean length (B). The lower panels show the proportion of Collembola groups (C) and major Acari taxonomic groups (D). mosses: polytrichum and racomitrium, lichens: pseudocyphellaria, stereocaulon and usnea. Bars show mean values of n = 10 replicates per habitat type with SE as error bars. Lowercase letters indicate significant community differences between mosses and lichens.
Community responses
Collembola community composition differed between the low and high elevation sites for four of the five sampled cryptogams, the exception being Usnea (Table 3, Figure 3). A similar pattern was observed for Acari community composition but no difference was found in Pseudocyphellaria. The proportion of epigeic Collembola increased with elevation in Racomitrium. Eudaphic Collembola species disappeared from Racomitrium at high elevation, but not from Polytrichum. The proportion of hemiedaphic Collembola was greater at high elevation (39% ± 5) compared to low sites (21% ± 5). The proportion of Oribatida declined at higher elevation in Racomitrium and a similar trend was found in Usnea (Figure 3D). The proportion of Prostigmata increased with elevation in both Racomitrium and Usnea but not in the other moss and lichen habitats. Mesostigmata were unaffected by elevation in any of the moss and lichen habitats. A small number of species were only observed at one elevation: the Collembola Entomobrya lanuginosa, Setanodosa sp. and Sminthurinus patagonicus were only observed at low elevation while a Friesea sp. was only observed at high elevation (Table 4). No such patterns were observed among the Acari families/groups (Table 5).
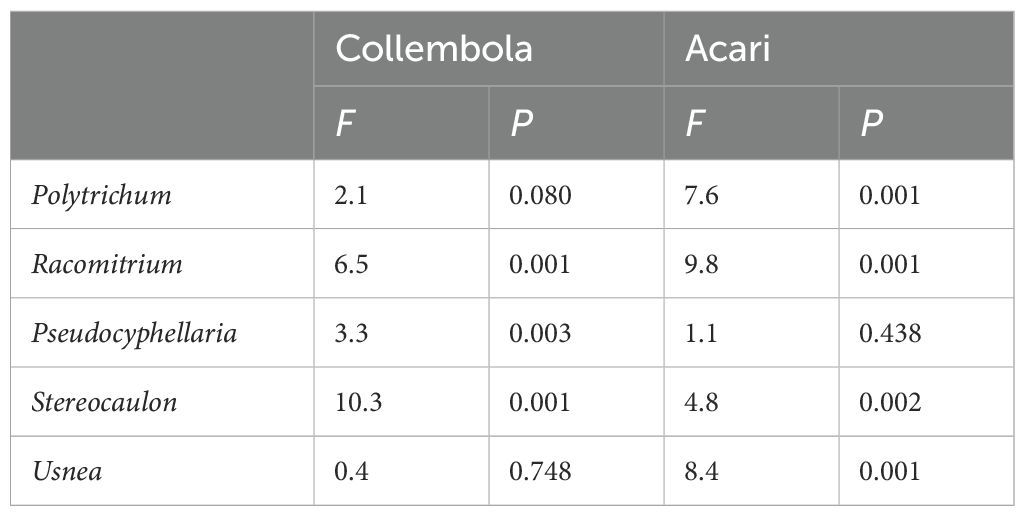
Table 3. ANOVA output (F and P-values) community composition following Bray Curtis comparison of Collembola and Acari composition between low and high elevation for each moss and lichen habitat.
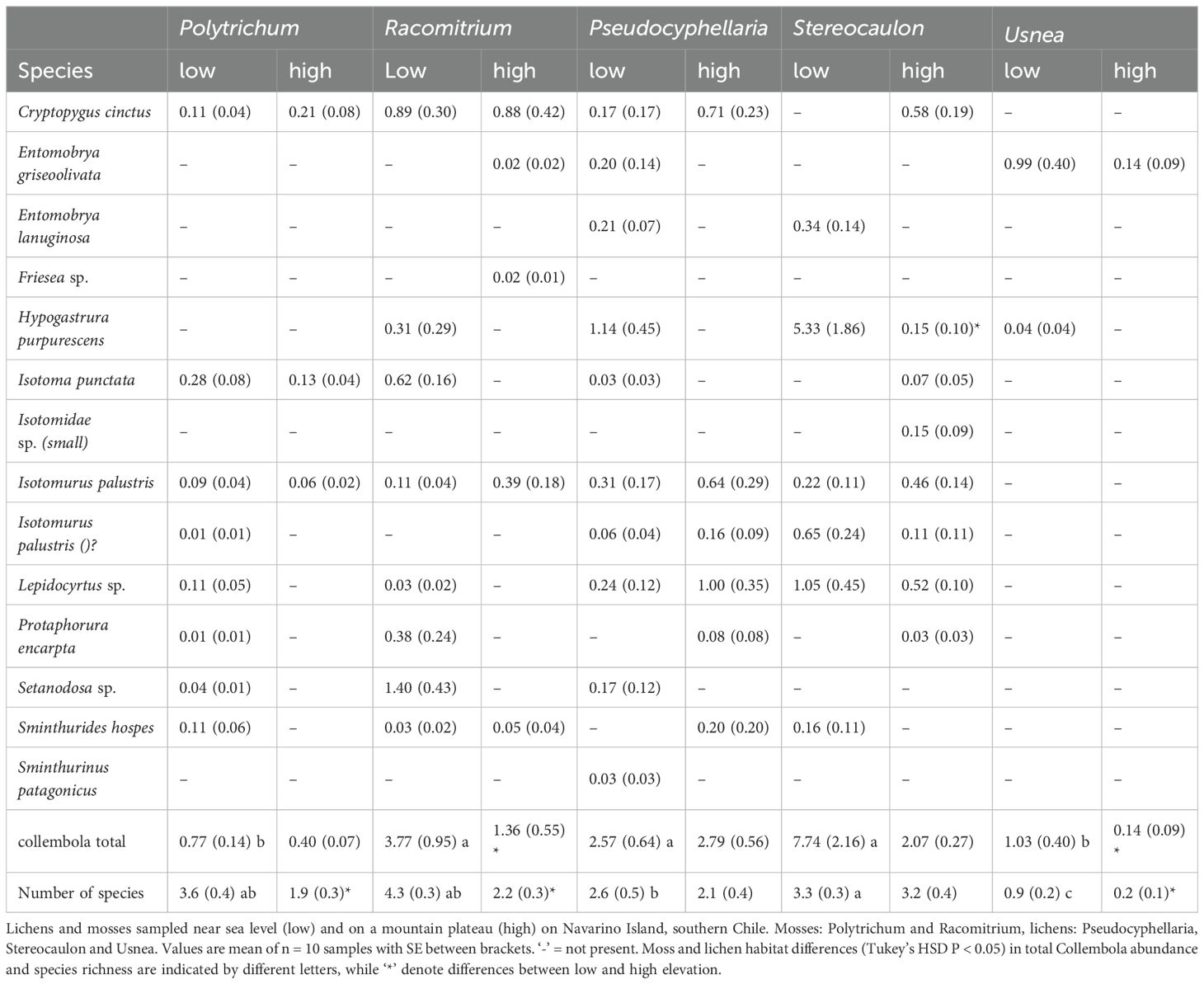
Table 4. Collembola species abundance (ind./g) across different moss and lichen habitat and elevation.
There were significant correlations between microarthropod (group) abundance and habitat WHC and water retention times. However, microarthropod abundance was never positively correlated, as anticipated. In addition, for the moss habitats these correlations resulted from a clear grouping of WHC and retention time between low and high elevation (Supplementary Figures 5–8). Total Acari abundance was negatively related to Stereocaulon WHC (R = -0.546, P = 0.027). Oribatida abundance was negatively related to Stereocaulon water retention time (R = -0.632, P = 0.007). Epigeic Collembola showed a negative correlation with Polytrichum water retention time (R = -0.459, P = 0.042).
Discussion
Explaining and predicting microarthropod abundance and diversity patterns is one of the many challenges in ecology (Hodkinson, 2005). Our data showed that the colder and higher freezing frequency conditions of higher elevation did not consistently affect moss- and lichen-associated microarthropod communities, as hypothesized. Instead, abundance and diversity responses to elevation were dependent on habitat type, as also reported in a study of whole ecosystem change in sub-Arctic landscapes (Bokhorst et al., 2018). However, the community changes, or lack thereof, were also features of different habitats within the high elevation fell-field vegetation, indicating that small spatial scale heterogeneity affects community response within an ecosystem type. This was also apparent at the microarthropod species level, where species losses in one microhabitat at higher elevation were compensated by gains in a different microhabitat, thereby retaining the overall species pool, reflecting the relative roles of the spatial scale and heterogeneity of environmental factors on soil fauna community assembly (Widenfalk et al., 2016). Polar microarthropod community responses to climate change will, therefore, be habitat dependent.
Elevation effect on microarthropod abundance
The high elevation study site was exposed to lower temperatures and a higher frequency of freezing and thawing, also during the summer growing season, which is generally expected to negatively affect the abundance of ectothermic organisms (Sinclair et al., 2003a). However, the studied moss and lichen habitats maintained similar Collembola and Acari population sizes across elevation. This contrasts with patterns observed across elevation in other montane regions, where Acari abundance has been reported to decline with elevation while Collembola abundance increased (Cutz-Pool et al., 2010; Bokhorst et al., 2018; Wu et al., 2023). Such differences may reflect habitat turnover changes across elevation (Cutz-Pool et al., 2010; Wu et al., 2023), substrate quantity and quality (Bokhorst et al., 2018), or differences in climate condition changes with elevation between studies. The current study sites have an annual mean temperature 2-3°C higher than those reported by Bokhorst et al. (2018) and larger microarthropod community differences may occur across greater elevation differences. However, the high frequency of freeze-thaw cycles (227 events at the high elevation site compared to 80 at the low site) are also detrimental for growth and survival (Bale et al., 2001; Sinclair et al., 2003b; Sinclair and Chown, 2005), and can be sufficient to induce equivalent changes in microarthropod abundance. The studied moss and lichen habitats appear, in most cases, to buffer micro-arthropod abundance patterns against temperature contrasts across elevation gradients, indicating that the population changes reported in other studies may in part result from larger habitat characteristic changes rather than changes in temperature conditions per se.
Micro-arthropod taxonomic richness was often reduced at higher elevation in the sampled habitats, indicating that fewer species were able to survive there, consistent with our prediction as well as reported in other studies of microarthropod communities across elevational gradients (Cutz-Pool et al., 2010; Illig et al., 2010; Bokhorst et al., 2018; Wu et al., 2023). However, total microarthropod diversity was sustained in the high-elevation landscape by the presence of the different moss and lichen species. These patterns may reflect physiological tolerance limits for species survival at this colder site with greater temperature fluctuations resulting in larger microarthropod population variability (Kuznetsova, 2006).
Responses in mosses and lichens
There was no support for our second hypothesis that microarthropod communities would change more among lichens than mosses, as the latter would provide stronger buffering against environmental variability (Stoy et al., 2012). Instead, almost all Collembola and Acari communities differed across elevation, irrespective of moss or lichen habitat. In addition, abundance differences were minimal between habitat types, despite clear differences in potential water status (Table 1), an important factor behind cryptogam-associated micro-arthropod communities (Booth and Usher, 1984; Bokhorst et al., 2015). Microarthropods most likely move across the vegetation mosaic at the high elevation site, from high population densities in favorable habitats to others, and so affect population and diversity patterns. Although, this study presents community data at a single point in time, there were clear habitat-specific microarthropod communities that likely derive from mobility and selection for a suitable habitat. Acari community differences primarily reflected changes in relative abundance instead of taxonomic group turnover between low and high elevation. These Acari community changes with elevation were inconsistent across moss and lichen habitats and the specific taxonomic groups involved, reflecting the context-dependency of Acari response to changes in environmental conditions (Nielsen et al., 2010; Wissuwa et al., 2012; Bokhorst et al., 2017). Collembola community differences between low and high elevation resulted from species losses, especially among the mosses, and turnover at higher elevation. The fruticose lichen Usnea supported only few species, and the Collembola species observed (Entomobrya griseoolivata and Hypogastrura purpurescens) at both elevations are generalist species commonly observed across many environments (Fjellberg, 1998, 2007). These results indicate that habitat type has a potentially strong role in shaping microarthropod community changes across environmental gradients and influences the conclusions that can be derived from such studies.
Community trait response
There was limited support for our third hypothesis, that larger-sized animals would dominate communities at higher elevation except in Racomitrium, where there was a clear increase in community weighted mean length at higher elevation which resulted from the loss of eudaphic Collembola. This community response has been observed in other elevation studies (Cutz-Pool et al., 2010; Bokhorst et al., 2018; Hishi et al., 2024), and most likely reflects a vulnerability of eudaphic species to the colder and more variable montane weather (van Dooremalen et al., 2013; Bokhorst et al., 2018). Interestingly this decline in eudaphic and smaller species was not observed in Polytrichum, possibly suggesting stronger temperature buffering capacity than Racomitrium (Table 1). The lichen habitats did not support eudaphic species to any great extent and, therefore, the associated microarthropod community is already adapted to ‘harsher’ conditions, with greater CWM-length and dominance of surface-dwelling species, irrespective of elevation. Overall, species identity and associated traits can provide insights into community shifts across elevation, but this cannot be easily disentangled from the habitat type under study. Future research should consider species physiological limits and the functional role (biotic and abiotic control) of microhabitats for microarthropods.
Conclusions
Many biotic and abiotic conditions change across elevation gradients (Hodkinson, 2005; Sundqvist et al., 2013), and associated changes in plant community structure and soil properties are underlying factors that shape animal communities, while at the same time making it hard to identify causal links with micro-arthropod patterns. By targeting the same habitat across elevation we attempted here to shed light on potential causal factors for micro-arthropod abundance and community changes across contrasting temperature conditions. Moss and lichen nutrient content was not taken into consideration but, given the isolation from industrial or agricultural activities and absence of bird colonies near the sampling sites that could provide major nutrient inputs (Bokhorst et al., 2019; van der Vegt and Bokhorst, 2024; Zmudczyńska-Skarbek et al., 2024), it is unlikely that this played a role in the observed microarthropod patterns across elevation. Overall, the responses seen appeared highly context-dependent, in that some lichen habitats maintained population levels even under colder conditions, while moss-associated communities appeared more responsive to changes associated with elevation. These differences appear to be driven by the habitat characteristics and the type of organisms associated with each habitat. While elevation gradients can provide useful insights into how ecosystem properties and the contained communities may change, the underlying causes for specific target groups may not always be obvious and care should be taken when extrapolating to other habitats and regions.
Data availability statement
The original contributions presented in the study are publicly available. This data can be found here: https://npdc.nl/dataset/b20d02d3-63b4-5e8d-9b00-08bda0049337.
Ethics statement
The manuscript presents research on animals that do not require ethical approval for their study.
Author contributions
SB: Conceptualization, Formal analysis, Investigation, Methodology, Writing – original draft, Writing – review & editing. TC: Funding acquisition, Writing – review & editing. RM: Investigation, Resources, Writing – review & editing. PC: Conceptualization, Funding acquisition, Writing – review & editing. RA: Conceptualization, Funding acquisition, Investigation, Writing – review & editing.
Funding
The author(s) declare that financial support was received for the research, authorship, and/or publication of this article. This study was funded by the Netherlands Polar Programme (ALWPP2016.006). PC is supported by core funding from the Natural Environment Research Council to the British Antarctic Survey’s ‘Biodiversity, Evolution, and Adaptation’ team.
Acknowledgments
We are grateful for the hospitality offered by the Cape Horn International Center in Puerto Williams, Chile, during our fieldwork. We are grateful for the constructive feedback from three reviewers on an earlier version of this manuscript.
Conflict of interest
The authors declare that the research was conducted in the absence of any commercial or financial relationships that could be construed as a potential conflict of interest.
The handling editor MM and reviewer CT declared a past co-authorship with the author PC.
The author(s) declared that they were an editorial board member of Frontiers, at the time of submission. This had no impact on the peer review process and the final decision.
Publisher’s note
All claims expressed in this article are solely those of the authors and do not necessarily represent those of their affiliated organizations, or those of the publisher, the editors and the reviewers. Any product that may be evaluated in this article, or claim that may be made by its manufacturer, is not guaranteed or endorsed by the publisher.
Supplementary material
The Supplementary Material for this article can be found online at: https://www.frontiersin.org/articles/10.3389/fevo.2024.1440649/full#supplementary-material
References
Bale J. S., Worland M. R., Block W. (2001). Effects of summer frost exposures on the cold tolerance strategy of a sub-Antarctic beetle. J. Insect Physiol. 47, 1161–1167. doi: 10.1016/S0022-1910(01)00097-X
Blok D., Heijmans M., Schaepman-Strub G., van Ruijven J., Parmentier F. J. W., Maximov T. C., et al. (2011). The cooling capacity of mosses: Controls on water and energy fluxes in a Siberian tundra site. Ecosystems 14, 1055–1065. doi: 10.1007/s10021-011-9463-5
Bokhorst S., Huiskes A. H. L., Convey P., Bodegom P. M. v, Aerts R. (2008). Climate change effects on soil arthropod communities from the Falkland Islands and the Maritime Antarctic. Soil Biol. Biochem. 40, 1547–1556. doi: 10.1016/j.soilbio.2008.01.017
Bokhorst S., Phoenix G. K., Bjerke J. W., Callaghan T. V., Huyer-Brugman F., Berg M. P., et al. (2012). Extreme winter warming events more negatively impact small rather than large soil fauna: shift in community composition explained by traits not taxa. Global Change Biol. 18, 1152–1162. doi: 10.1111/j.1365-2486.2011.02565.x
Bokhorst S., Huiskes A. H. L., Aerts R., Convey P., Cooper E. J., Dalen L., et al. (2013a). Variable temperature effects of Open Top Chambers at polar and alpine sites explained by irradiance and snow depth. Global Change Biol. 19, 64–74. doi: 10.1111/gcb.2012.19.issue-1
Bokhorst S., Metcalfe D. B., Wardle D. A. (2013b). Reduction in snow depth negatively affects decomposers but impact on decomposition rates is substrate dependent. Soil Biol. Biochem. 62, 157–164. doi: 10.1016/j.soilbio.2013.03.016
Bokhorst S., Wardle D. A., Nilsson M.-C., Gunda M. J. (2014). Impact of understory mosses and dwarf shrubs on soil micro-arthropods in a boreal forest chronosequence. Plant Soil 379, 121–133. doi: 10.1007/s11104-014-2055-3
Bokhorst S., Asplund J., Kardol P., Wardle D. A. (2015). Lichen physiological traits and growth forms affect communities of associated invertebrates. Ecology 96, 2394–2407. doi: 10.1890/14-1030.1
Bokhorst S., Kardol P., Bellingham P. J., Kooyman R. M., Richardson S. J., Schmidt S., et al. (2017). Responses of communities of soil organisms and plants to soil aging at two contrasting long-term chronosequences. Soil Biol. Biochem. 106, 69–79. doi: 10.1016/j.soilbio.2016.12.014
Bokhorst S., Wardle D. A., Nilsson M.-C., Gundale M. J. (2018). Contrasting responses of springtails and mites to elevation and vegetation type in the sub-Arctic. Pedobiologia 67, 57–64. doi: 10.1016/j.pedobi.2018.02.004
Bokhorst S., Convey P., Aerts R. (2019). Nitrogen inputs by marine vertebrates drive abundance and richness in Antarctic terrestrial ecosystems. Curr. Biol. 29, 1721–1727. doi: 10.1016/j.cub.2019.04.038
Booth R. G., Usher M. B. (1984). Arthropod communities in a Maritime Antarctic Moss-Turf habitat: Effects of the Physical and Chemical Environment. J. Anim. Ecol. 53, 879–893. doi: 10.2307/4665
Chao A., Gotelli N. J., Hsieh T. C., Sander E. L., Ma K. H., Colwell R. K., et al. (2014). Rarefaction and extrapolation with Hill numbers: a framework for sampling and estimation in species diversity studies. Ecol. Monogr. 84, 45–67. doi: 10.1890/13-0133.1
Convey P., Pugh P. J. A., Jackson C., Murray A. W., Ruhland C. T., Xiong F. S., et al. (2002). Response of Antarctic terrestrial microarthropods to long-term climate manipulations. Ecology 83, 3130–3140. doi: 10.1890/0012-9658(2002)083[3130:ROATMT]2.0.CO;2
Cornelissen J. H. C., Lang S. I., Soudzilovskaia N. A., During H. J. (2007). Comparative cryptogam ecology: A review of bryophyte and lichen traits that drive biogeochemistry. Ann. Bot. 99, 987–1001. doi: 10.1093/aob/mcm030
Cutz-Pool L. Q., Palacios-Vargas J. G., Cano-Santana Z., Castano-Meneses G. (2010). Diversity patterns of Collembola in an elevational gradient in the NW slope of Iztaccihuatl volvano, State of Mexico, Mexico. Entomol. News 121, 249–261. doi: 10.3157/021.121.0306
de Bello F., Lavorel S., Albert C. H., Thuiller W., Grigulis K., Dolezal J., et al. (2011). Quantifying the relevance of intraspecific trait variability for functional diversity. Methods Ecol. Evol. 2, 163–174. doi: 10.1111/j.2041-210X.2010.00071.x
Elmendorf S. C., Henry G. H. R., Hollister R. D., Bjork R. G., Bjorkman A. D., Callaghan T. V., et al. (2012). Global assessment of experimental climate warming on tundra vegetation: heterogeneity over space and time. Ecol. Lett. 15, 164–175. doi: 10.1111/j.1461-0248.2011.01716.x
Filser J. (2002). The role of Collembola in carbon and nitrogen cycling in soil. Pedobiologia 46, 234–245. doi: 10.1078/0031-4056-00130
Fjellberg A. (1998). The Collembola of Fennoscandia and Denmark Part 1: Poduromorpha (Brill: Leiden).
Fjellberg A. (2007). The Collembola of Fennoscandia and Denmark Part 2: Entomobryomorpha and Symphypleona (Brill: Leiden).
Francisco, Francisco A., López D., Grego R. D., Buma B., Carvajal D., et al. (2021). Climatic gradients and their high influences on terrestrial ecosystems of the Cape Horn Biosphere Reserve, Chile (Anales del Instituto de la Patagonia), 49.
Gisin H. (1943). [amp]]Ouml;kologie und lebensgmeinschaften der Collembolen im Schweizer exkursiongebiet Basels. Rev. Suisse. Zool. 50, 131–224.
Greene S. W. (1964). “The vascular flora of South Georgia,” in British Antarctic Survey Scientific Reports, 45.
Handa I. T., Aerts R., Berendse F., Berg M. P., Bruder A., Butenschoen O., et al. (2014). Consequences of biodiversity loss for litter decomposition across biomes. Nature 509, 218–221. doi: 10.1038/nature13247
Hasegawa M., Ito M. T., Kitayama K. (2006). Community structure of oribatid mites in relation to elevation and geology on the slope of Mount Kinabalu, Sabah, Malaysia. Eur. J. Soil Biol. 42, 191–196. doi: 10.1016/j.ejsobi.2006.07.006
Heckman C. W. (2001). Encyclopedia of South American aquatic insects: Collembola (Dordrecht: Springer-Science+Business Media).
Hishi T., Uemori K., Tashiro N., Nakamura T. (2024). Asymmetric environmental selection on intraspecific body size in Collembola communities along an elevational gradient in northern Japan. Eur. J. Soil Biol. 120, 103596. doi: 10.1016/j.ejsobi.2024.103596
Hodkinson I. D. (2005). Terrestrial insects along elevation gradients: species and community responses to altitude. Biol. Rev. 80, 489–513. doi: 10.1017/S1464793105006767
Hodkinson I. D., Webb N. R., Bale J. S., Block W., Coulson S. J., Strathdee A. T. (1998). Global change and Arctic ecosystems: Conclusions and predictions from experiments with terrestrial invertebrates on Spitsbergen. Arctic. Alpine. Res. 30, 306–313. doi: 10.2307/1551978
Illig J., Norton R. A., Scheu S., Maraun M. (2010). Density and community structure of soil- and bark-dwelling microarthropods along an altitudinal gradient in a tropical montane rainforest. Exp. Appl. Acarol. 52, 49–62. doi: 10.1007/s10493-010-9348-x
IPCC (2023). Climate Change 2021 – The Physical Science Basis: Working Group I Contribution to the Sixth Assessment Report of the Intergovernmental Panel on Climate Change (Cambridge: Cambridge University Press).
Jing S., Solhøy T., Huifuà W., Vollan I., Rumei X. (2005). Differences in Soil Arthropod Communities along a High Altitude Gradient at Shergyla Mountain, Tibet, China. Arctic. Antarctic. Alpine. Res. 37, 261–266. doi: 10.1657/1523-0430(2005)037[0261:DISACA]2.0.CO;2
Kampichler C., Bruckner A. (2009). The role of microarthropods in terrestrial decomposition: a meta-analysis of 40 years of litterbag studies. Biol. Rev. 84, 375–389. doi: 10.1111/j.1469-185X.2009.00078.x
Kardol P., Reynolds W. N., Norby R. J., Classen A. T. (2011). Climate change effects on soil microarthropod abundance and community structure. Appl. Soil Ecol. 47, 37–44. doi: 10.1016/j.apsoil.2010.11.001
Krab E. J., Oorsprong H., Berg M. P., Cornelissen J. H. C. (2010). Turning northern peatlands upside down: disentangling microclimate and substrate quality effects on vertical distribution of Collembola. Funct. Ecol. 24, 1362–1369. doi: 10.1111/j.1365-2435.2010.01754.x
Kuznetsova N. A. (2006). Long-term dynamics of Collembola in two contrasting ecosystems. Pedobiologia 50, 157–164. doi: 10.1016/j.pedobi.2005.12.004
Lamoncha K. L., Crossley D. A. (1998). Oribatid mite diversity along an elevation gradient in a southeastern Appalachian forest. Pedobiologia 42, 43–55. doi: 10.1016/S0031-4056(24)00390-1
Leinaas H. P. (1983). Winter strategy of surface dewlling Collembola. Pedobiologia 25, 235–240. doi: 10.1016/S0031-4056(23)05928-0
Loranger G., Bandyopadhyaya I., Razaka B., Ponge J. F. (2001). Does soil acidity explain altitudinal sequences in collembolan communities? Soil Biol. Biochem. 33, 381–393. doi: 10.1016/S0038-0717(00)00153-X
Makkonen M., Berg M. P., Hal van J. R., Callaghan T. V., Press M. C., Aerts R. (2011). Traits explain the responses of sub-arctic Collembola community to climate manipulation. Soil Biol. Biochem. 43, 377–384. doi: 10.1016/j.soilbio.2010.11.004
Myers-Smith I. H., Elmendorf S. C., Beck P. S. A., Wilmking M., Hallinger M., Blok D., et al. (2015). Climate sensitivity of shrub growth across the tundra biome. Nat. Clim. Change 5, 887–891. doi: 10.1038/nclimate2697
Nash M. A., Griffin P. C., Hoffmann A. A. (2013). Inconsistent responses of alpine arthropod communities to experimental warming and thermal gradients. Climate Res. 55, 227–237. doi: 10.3354/cr01136
Nielsen U. N., Osler G. H. R., Campbell C. D., Burslem D., van der Wal R. (2010). The influence of vegetation type, soil properties and precipitation on the composition of soil mite and microbial communities at the landscape scale. J. Biogeogr. 37, 1317–1328. doi: 10.1111/j.1365-2699.2010.02281.x
Pintado A., Valladares F., Sancho L. G. (1997). Exploring phenotypic plasticity in the lichen Ramalina capitata: Morphology, water relations and chlorophyll content in north- and south-facing populations. Ann. Bot. 80, 345–353. doi: 10.1006/anbo.1997.0453
R-Core-Team (2023). R: A language and environment for statistical computing (Vienna: R Foundation for Statistical Computing).
Roos R. E., van Zuijlen K., Birkemoe T., Klanderud K., Lang S. I., Bokhorst S., et al. (2019). Contrasting drivers of community-level trait variation for vascular plants, lichens and bryophytes across an elevational gradient. Funct. Ecol. 33, 2430–2446. doi: 10.1111/1365-2435.13454
Roos R. E., Asplund J., van Zuijlen K. (2021). Covered by a blanket of lichens: how mat-forming lichens affect microclimate and ecological processes. A commentary on: ‘Lichens buffer tundra microclimate more than the expanding shrub Betula nana’. Ann. Bot. 128, i–iii.
Roos R. E., Birkemoe T., Bokhorst S., Wardle D. A., Asplund J. (2022). The importance of foundation species identity: A field experiment with lichens and their associated micro-arthropod communities. Basic. Appl. Ecol. 62, 45–54. doi: 10.1016/j.baae.2022.04.004
Rosenfeld S., Convey P., Contador T., Rendoll J., Poulin E., Maturana C., et al. (2020). “Magallanes, laboratorio del cambio global,” in Estrecho de Magallanes: Tres Descubrimientos, Museo de Chileno de Arte Precolombino. Eds. Rozzi R., Morello F., M. F., Aldunate C. (Colección Santander, Santiago Chile), 176–193.
Rozzi R., Jimenez J. E. (2013). Sub-Antarctic Magellanic Ornithology: The first decade of bird studies at Omora Ethnobotanical Park, Cape Horn Biosphere Reserve. University of North Texas Press.
Sadaka N., Ponge J. F. (2003). Soil animal communities in holm oak forests: influence of horizon, altitude and year. Eur. J. Soil Biol. 39, 197–207. doi: 10.1016/j.ejsobi.2003.06.001
Seastedt T. R. (1984). The role of microarthropods in decomposition and mineralization processes. Annu. Rev. Entomol. 29, 25–46. doi: 10.1146/annurev.en.29.010184.000325
Sinclair B. J., Chown S. L. (2005). Deleterious effects of repeated cold exposure in a freeze-tolerant sub-Antarctic caterpillar. J. Exp. Biol. 208, 869–879. doi: 10.1242/jeb.01455
Sinclair B. J., Addo-Bediako A., Chown S. L. (2003a). Climatic variability and the evolution of insect freeze tolerance. Biol. Rev. 78, 181–195. doi: 10.1017/S1464793102006024
Sinclair B. J., Vernon P., Klok C. J., Chown S. L. (2003b). Insects at low temperatures: an ecological perspective. Trends Ecol. Evol. 18, 257–262. doi: 10.1016/S0169-5347(03)00014-4
Sømme L. (1989). Adaptations of terrestrial arthropods to the alpine environment. Biol. Rev. 64, 367–407. doi: 10.1111/j.1469-185X.1989.tb00681.x
Stoy P. C., Street L. E., Johnson A. V., Prieto-Blanco A., Ewing S. A. (2012). Temperature, heat flux, and reflectance of common Subarctic mosses and lichens under field conditions: Might changes to community composition impact climate-relevant surface fluxes? Arctic. Antarctic. Alpine. Res. 44, 500–508. doi: 10.1657/1938-4246-44.4.500
Sundqvist M. K., Sanders N. J., Wardle D. A. (2013). Community and ecosystem responses to elevational gradients: processes, mechanisms, and insights for global change. Annu. Rev. Ecol. Evol. Syst. 44, 261–280. doi: 10.1146/annurev-ecolsys-110512-135750
Trekels H., Driesen M., Vanschoenwinkel B. (2017). How do patch quality and spatial context affect invertebrate communities in a natural moss microlandscape? Acta Oecol. 85, 126–135.
Upson R., Myer B., Floyd K., Lee J., Clubbe C. P. (2017). Field guide to the introduced flora of South Georgia (Kew: Royal Botanical Gardens, Kew).
van der Vegt W., Bokhorst S. (2024). Bird traits and their nutrient impact on terrestrial invertebrate populations. Polar. Biol. 47, 821–832. doi: 10.1007/s00300-023-03161-5
van Dooremalen C., Berg M. P., Ellers J. (2013). Acclimation responses to temperature vary with vertical stratification: implications for vulnerability of soil-dwelling species to extreme temperature events. Global Change Biol. 19, 975–984. doi: 10.1111/gcb.2013.19.issue-3
van Zuijlen K., Roos R. E., Klanderud K., Lang S. I., Asplund J. (2020). Mat-forming lichens affect microclimate and litter decomposition by different mechanisms. Fungal Ecol. 44, 100905. doi: 10.1016/j.funeco.2019.100905
Wall D. H., Bradford M. A., John M. G. S., Trofymow J. A., Behan-Pelletier V., Bignell D. D. E., et al. (2008). Global decomposition experiment shows soil animal impacts on decomposition are climate-dependent. Global Change Biol. 14, 2661–2677. doi: 10.1111/j.1365-2486.2008.01672.x
Widenfalk L. A., Malmström A., Berg M. P., Bengtsson J. (2016). Small-scale Collembola community composition in a pine forest soil - Overdispersion in functional traits indicates the importance of species interactions. Soil Biol. Biochem. 103, 52–62. doi: 10.1016/j.soilbio.2016.08.006
Wissuwa J., Salamon J. A., Frank T. (2012). Effects of habitat age and plant species on predatory mites (Acari, Mesostigmata) in grassy arable fallows in Eastern Austria. Soil Biol. Biochem. 50, 96–107. doi: 10.1016/j.soilbio.2012.02.025
Wu Y., Xie Z., Wan Z., Ji Q.-Q., Yang J., Chen T.-W., et al. (2023). Elevational changes in canopy Collembola community composition are primarily driven by species turnover on Changbai Mountain, northeastern China. Biodivers Conserv. 32, 4853–4872.
Keywords: Collembola, moss, lichen, microclimate, Acari (mites)
Citation: Bokhorst S, Contador T, Mackenzie R, Convey P and Aerts R (2024) Habitat type controls microarthropod community changes across a Magellanic sub-Antarctic elevation gradient. Front. Ecol. Evol. 12:1440649. doi: 10.3389/fevo.2024.1440649
Received: 29 May 2024; Accepted: 30 August 2024;
Published: 23 September 2024.
Edited by:
Marco A. Molina-Montenegro, University of Talca, ChileReviewed by:
Cristian Torres, University of the Bío Bío, ChileMarie Louise Davey, Norwegian Institute for Nature Research (NINA), Norway
Hugo A. Benítez, Universidad Católica del Maule, Chile
Copyright © 2024 Bokhorst, Contador, Mackenzie, Convey and Aerts. This is an open-access article distributed under the terms of the Creative Commons Attribution License (CC BY). The use, distribution or reproduction in other forums is permitted, provided the original author(s) and the copyright owner(s) are credited and that the original publication in this journal is cited, in accordance with accepted academic practice. No use, distribution or reproduction is permitted which does not comply with these terms.
*Correspondence: Stef Bokhorst, cy5mLmJva2hvcnN0QHZ1Lm5s