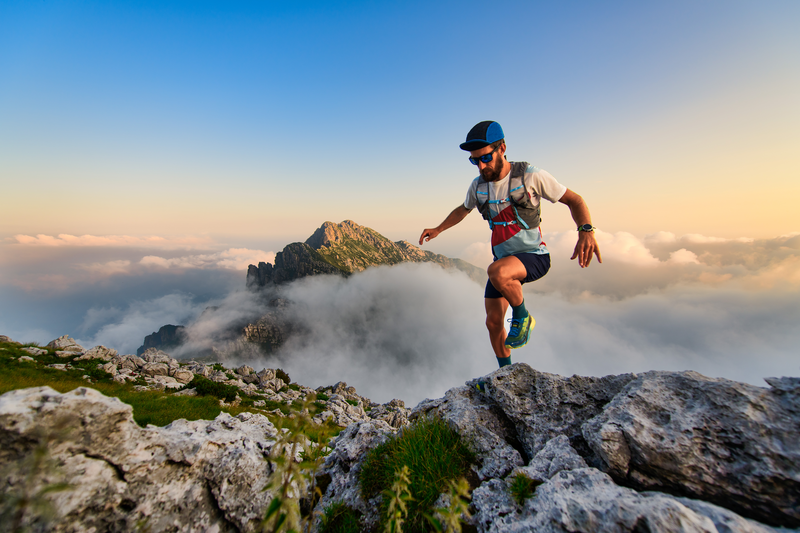
95% of researchers rate our articles as excellent or good
Learn more about the work of our research integrity team to safeguard the quality of each article we publish.
Find out more
ORIGINAL RESEARCH article
Front. Ecol. Evol. , 16 August 2024
Sec. Ecophysiology
Volume 12 - 2024 | https://doi.org/10.3389/fevo.2024.1440494
This article is part of the Research Topic The Adaptation, Plasticity and Extinction of Forest Plants to Climate Change: Mechanisms behind the Morphological, Physiological, Phenological and Ecological Traits View all 8 articles
Introduction: Bamboo is one of the fastest-growing plants on earth, and its young culms are formed by the elongation of internodes. However, the mathematical intricacies of its internode elongation are not well understood.
Methods: This study investigated the internode length growth of Phyllostachys edulis, Phyllostachys iridescens, and Pseudosasa amabilis at ten different culm height developmental stages (G1–G10).
Results and discussion: The tempo of internode elongation from the culm base to the tip generally followed a “slow-fast-slow” growth rhythm. The internode length and the serial number relationship showed a right-skewed curve. As the bamboo grows taller, the longest internode moves from the base to the middle of the culm. The relationship between relative internode number (RIN) and relative cumulative internode length (RCIL) displayed a typical S-shaped growth curve. The modified Brière (MBE) sigmoid equation achieved better goodness-of-fit than the logistic, power, and third-order functions in fitting the RIN-RCIL curves with the smallest average root mean square error (RMSE). The elongation rates of internodes varied not only with the growth of culm height, but also with the position of the bamboo culm from base to tip. In addition, as the bamboo grows in height, more internodes gradually contribute to the culm height growth. At G1 development stage, 24.01–38.23% of the internodes contributed 65.27–73.59% of the culm height, whereas at G10 stage, 49.28–61.07% of the internodes contributed 66.70–78.18% of the culm height. Our findings provide new insights into the mathematical characterization of bamboo internode elongation patterns involved in the rapid culm growth.
Bamboo belongs to the subfamily Bambusoideae of the family Gramineae and is an important non-timber forest resource as well as an important renewable biomass resource (van der Lugt and Vogtlander, 2015). The world has more than 70 genera and 1,300 species of bamboo, which are widely distributed in tropical and subtropical regions, covering 25 million hectares worldwide (Liese and Khöl, 2015). Bamboo is one of the most fast-growing plants on earth. Moso bamboo (Phyllostachys edulis (Carrière) J. Houz.) can grow almost a meter in a single day (Chen et al., 2022). Bamboo forests contribute to mitigating climate change by sequestering large amounts of atmospheric carbon, in a similar way that trees do (Sohel et al., 2015). Further, the culms of some bamboo species belonging to the perennial tall grass family are highly lignified and tough, and can be used for the production of paper, furniture, crafts, and building sectors (Silva et al., 2020). In addition, bamboo presents itself as an excellent sustainable substitute for wood and plastic. The traditional saying of the local farmers: “Without bamboo, the land dies” (Christanty et al., 1996). Bamboo’s utilization and climate change mitigation capabilities may be due to its fast-growing characteristics.
The tempo of height growth of bamboo culm generally follows the growth rhythm of “slow-fast-slow” (Song et al., 2016; Wang et al., 2016; Chen et al., 2022). Before the emergence of shoots from the soil, the number of nodes is almost definite. After that, internodes elongate by cell division and elongation of the intermediate meristematic issue (Liese and Weiner, 1996; Wang et al., 2021). The parenchyma cells within and between vascular bundles of bamboo internodes feature rapid elongation and are the basis for culm internode elongation (Zheng et al., 2022). He et al. (2002) reported that the parenchyma tissues of bamboo have two types of vertically positioned cells: the short and long parenchyma cells. The long parenchyma cells had thickened, polylamellate, and lignified walls, which were considered as an energy reservoir in the form of starch granules (Liese and Weiner, 1996). The short cells had dense cytoplasm, thin walls, and peroxidase activities, which were scattered among the long parenchyma cells (Parameswaran and Liese, 1980). A bamboo culm grows to its full height of 2 m to above 30 m within a period of few months (Liese and Weiner, 1996; Wang et al., 2016; Yen, 2016). In the beginning, the height of the bamboo culm grows slowly. Thereafter, the growth of the internodes under the soil stops and bamboo roots begin to grow into a root system. At the same time, the growth rate of the culm internodes accelerates until it reaches its peak. At the end, the top of the young culm bends, branches spread fast, and the growth of the young culm slows down till ending. As young culms are formed by the elongation of internodes. What is the elongation pattern of bamboo culm internodes?
The culm height depends on the length of the culms, and it varies from 2 to 37 m depending on the species’ character (McClure, 1966). Banik (1991) graphically represented the march of increase and decrease in the culm internode length of ten different bamboo species by plotting the internode length against its serial number from the culm base to the tip. In the studied bamboo species, the relationship between internode length and serial number indicates a right-skewed curve, which is usually steeper on the “left” side while flatter on the “right” side. The relationship between internode length and its serial number varies due to the variation in culm size (Higuchi, 1981). Inoue et al. (2012) further found that the relationship between the relative internode number (RIN, the internode number relativized by the total number of internodes) and the relative cumulated internode length (RCIL) of Moso bamboo should be described not by a power function but by a sigmoid function such as a third-order function. Inoue et al. (2017) demonstrated that the node distribution (RIN-RCIL relationship) of three bamboo species (P. edulis, Phyllostachys nigra var. henonis, and Phyllostachys nigra Munro) with different culm sizes can be represented by a single sigmoid curve. As a third-order sigmoid function, the Brière equation (BE) has been widely used to depict the effect of temperature on the development rate of insects (Brière et al., 1999; Shi et al., 2017). Jin et al. (2022) modified the BE by adding an additional parameter to further improve the elasticity of its data fitting and referred to the new equation as the modified Brière (MBE) equation. Their results showed that MBE achieved a good fit to plant height and biomass development data. Previous studies used three-parameter logistic equations and third-order polynomial functions to describe the RIN-RCIL relationship, but have not applied the MBE. Bamboo culms can grow to a full height of 2 m to above 30 m within a period of few months, and the culm height depends on the elongation of the internodes. How the relationship between internode length and serial number changes with culm height growth is not well understood.
In this study, the internode length growth of P. edulis, Phyllostachys iridescens C.Y.Yao & S.Y.Chen and Pseudosasa amabilis (McClure) Keng f. at different culm height stages were investigated. The objectives of the current study were (1) to understand the internode length growth pattern of these three bamboo species, (2) to test the validity of the MBE-sigmoid equation to fit the RIN-RCIL relationship, and (3) to explore the changes in the internode elongation characteristics with the development of culm height.
In this study, we examined three bamboo species with different culm heights: P. edulis, P. iridescens, and P. amabilis. Moso bamboo (P. edulis), a native bamboo species to China, is one of the largest bamboo species and on average approximately 18 m in tall and 11 cm in diameter at breast height (Kuehl, 2015). Moso bamboo is widely distributed in southern China and is one of the most widely distributed bamboo species in China (Shi et al., 2020). P. iridescens is native to southeastern China and grows primarily in warm temperate biomes, with culms 6–12 m tall and 6–10 cm in diameter, and is used as an ornamental plant and as a vegetable. P. Amabilis is also native to China with culms up to 6 m tall and 1.5–2.0 cm in diameter.
The research was carried out in P. edulis forests in Jiangning, Nanjing, China (118°41’18”E, 31°44’30”N) and P. iridescens and P. amabilis forests on the campus of Nanjing Forestry University, China (118°48’51”E, 32°04’59”N). In Jiangning, the annual mean temperature and precipitation in 2021 are 17.15°C and 1362.2 mm, and 16.98°C and 889.7 mm in 2022, respectively. On the campus of Nanjing Forestry University, the annual mean temperature and precipitation in 2021 are 17.36°C and 1343.7 mm, and 17.19°C and 867.1 mm in 2022, respectively. The climate data were based on records from the gridded CRU TS dataset with a spatial resolution of 0.5° (the CRU website: https://crudata.uea.ac.uk/cru/data/hrg/). The average culm diameters at breast height (1.3 m) of P. edulis, P. iridescens, and P. amabilis forests are approximately 9.0 cm, 6.1 cm, and 2.1 cm, respectively. Culms of P. edulis were collected between April and June 2021/2022 at ten development stages. Bamboo culm samples of P. iridescens and P. amabilis were collected at ten development stages between April and June 2022. We set the ten development stages of P. edulis and P. iridescens as G1: 1 m, G2: 2 m, G3: 3 m, G4: 4 m, G5: 5 m, G6: 6 m, G7: 7 m, G8: 8 m, G9: 9 m, and G10: 10 m. The ten development stages of P. amabilis are G1: 0.5 m, G2: 1.0 m, G3: 1.5 m, G4: 2.0 m, G5: 2.5 m, G6: 3.0 m, G7: 3.5 m, G8: 4.0 m, G9: 4.5 m, and G10: 5.0 m. Between 3 and 11 samples were collected for each bamboo species at each stage, totaling 121 bamboo culms (Table 1).
In spring, when the average culm height of new bamboo in the stand reached each stage, we collected the healthy and living culm samples and measured their culm height and internode length immediately after collection. The length of a culm segment between adjacent nodes is referred to as the internode length (IL, Figure 1). The internode of each culm sample was numbered from the culm base to the tip (IN, internode number).
Figure 1. Schematic explanation of changes in internode length along a culm at different culm height stages.
Since the culm heights on the same sampling day were often not exactly the same as the set values, the average culm heights of the collected P. edulis samples at the ten stages were G1 = 0.91 m, G2 = 2.08 m, G3 = 3.05 m, G4 = 4.01 m, G5 = 5.10 m, G6 = 5.86 m, G7 = 7.12 m, G8 = 8.10 m, G9 = 8.75 m and G10 = 11.04 m (Table 1). The mean culm heights of the collected P. iridescens at ten stages were G1 = 1.13 m, G2 = 2.13 m, G3 = 3.06 m, G4 = 4.20 m, G5 = 4.98 m, G6 = 5.76 m, G7 = 7.40 m, G8 = 8.20 m, G9 = 8.79 m and G10 = 10.14 m, respectively. The mean culm heights of the collected P. amabilis at ten stages were G1 = 0.67 m, G2 = 0.97 m, G3 = 1.55 m, G4 = 1.93 m, G5 = 2.54 m, G6 = 2.97 m, G7 = 3.46 m, G8 = 4.11 m, G9 = 4.50 m and G10 = 4.89 m, respectively.
To eliminate the effect of culm size, the method proposed by Higuchi (1981) was applied in this study. The average length of each internode was calculated for each growth stage (G1–G10) of each studied bamboo species. The internode number (IN) is relativized to the total number of internodes and is referred to as the relative internode number (RIN). The internode length cumulated from the base to a given internode is relativized by the total culm length referred to as the relative cumulative internode length (RCIL). The relationship between RIN and RCIL at ten different culm height development stages was fitted by the following equations:
MBE-sigmoid equation: the integral form of the modified Brière (MBE) equation:
Where y represents , and x represents the RIN. The a, m, and are the parameters. The represents the upper intersection between the curve and the x-axis.
For Equations 2–4, where y is the relative cumulated internode length (RCIL). The x represents the relative internode number (RIN). The a, b, c, and d are the parameters.
To evaluate the accuracy of the model fit, the root mean square error was calculated:
Where N represents the number of data points, and RSS is the residual sum of squares between the observed and predicted values. The Nelder-Mead algorithm (Nelder and Mead, 1965) was used to optimally minimize the RSS between observed and predicted values.
The “fitsigmoid” function in the “biogeom” package (Shi et al., 2022) was performed to fit the RIN-RCIL curve using the MBE-sigmoid (Equation 1) equation within an R environment (version 4.2.2). The “MbriereE” function (simplified version = 1) in the “biogeom” package was performed to fit the RIN-RCIL rate using the MBE equation. In addition, the RIN and RCIL of the right inflection point of the RIN-RCIL rate fitted by the MBE equation was plotted against the development stage. Other models (Equations 2–4) were conducted by the “nls” function in the “stats” package. The above calculations were based on the average length of each internode and each growth stage of each bamboo species studied.
The relationship between the longest internode length and its serial number (i.e., internode number) of each studied species was performed by a general linear regression model at the individual level. All the above analyses were implemented in the R software (version 4.2.2).
The internode elongation patterns of the three bamboo species at ten different growth stages are shown in Figure 2. In general, the three species showed a similar pattern, i.e., as the number of internodes increased from culm base to tip, the internode length showed a tendency to increase first and then decrease. The relationship between the internode length and the serial number showed a right-skewed curve, with the peaks of the curves gradually shifting from the “left” side to the center as the development stage of bamboo culm height progressed from G1 to G10. In addition, there was a significant linear positive relationship between the serial number of the longest internodes and the culm length (Figure 3). The number of internodes with the longest length increased linearly as the culm length increased.
Figure 2. The relationship between the internode number and the internode length at ten culm height developmental stages (G1–G10) of P. edulis (A), P. iridescens (B), P. amabilis (C). Each point represents the average value of internode length for each internode and each stage.
Figure 3. A linear relationship between the serial number of the longest internode and the culm length of P. edulis (A), P. iridescens (B), P. amabilis (C). Each point represents the serial number of the internode with the longest length of each bamboo culm studied.
Figure 4; Supplementary Figure S1 depict the RIN-RCIL relationships at ten growth stages for the three bamboo species studied. The RIN-RCIL relationships of P. edulis, P. iridescens, and P. amabilis were consistent with an S-shaped curve, indicating that the tempo of internode length of bamboo culms generally follows the growth rhythm of “slow-fast-slow” from culm base to tip. Furthermore, as the height of the bamboo culm increased, the number of internodes that are in the fast-growing stage increased. The RMSE of the three studied species at ten development stages ranged from 0.0044 to 0.1476 (Figure 5; Supplementary Table S1; Equation 5). The power function had the highest mean RMSE among the four equations (P. edulis = 0.1026, P. iridescens = 0.1085, P. amabilis = 0.1104). The mean RMSE of the third-order function was the second highest (P. edulis = 0.0343, P. iridescens = 0.0328, P. amabilis = 0.0429). This was followed by the logistic function with mean RMSE values of 0.0172, 0.0162, and 0.0220 for P. edulis, P. iridescens, and P. amabilis, respectively. The MBE-sigmoid equation showed the lowest RMSE values with P. edulis = 0.0150, P. iridescens = 0.0154, and P. amabilis = 0.0131. The RIN-RCIL rates fitted with the MBE equation are shown in Figure 6. The MBE equation reflects the growth rate of RCIL to RIN as a derivative function, and its integral over a certain interval exhibits the length of an internode. The RIN-RCIL rate curves again verified that the number of internodes in the rapid growth stage increased as the height of the bamboo cum increased. The maximum rate of the relative cumulative internode length was higher for culms in the early height growth stage compared to the later growth stage. The relationship between RCIL/RIN at the right inflection point of the RIN-RCIL rate curve fitted by the MBE equation and the development stage is shown in Figure 7. As the bamboo culm height developed from G1 to G10, the RIN at the right inflection point increased significantly (R2 = 0.5894, p < 0.001), while the positive correlation between the RCIL at the right inflection point and the growth stage (R2 = 0.2406, p < 0.01) was weaker compared to that of RIN.
Figure 4. The relationship between the relative internode number (RIN) and the relative cumulative internode length (RCIL) of P. edulis (A), P. iridescens (B), P. amabilis (C).
Figure 5. The root mean square error (RMSE) values obtained for P. edulis, P. iridescens, and P. amabilis using MBE-sigmoid, logistic, power, and third-order equations. The horizontal solid line indicates the median, the red asterisk indicates the mean, and the whiskers extend to 1.5 times the interquartile range from the top and bottom of the box.
Figure 6. The growth rate of relative cumulative internode length (RCIL) against relative internode number (RIN) was fitted by the MBE equation for P. edulis (A), P. iridescens (B), and P. amabilis (C).
Figure 7. Changes in (A) relative cumulative internode length (RCIL) and (B) relative internode number (RIN) at the right inflection points of the RIN-RCIL rate curves with development stage (G1–G10). Each point indicates RCIL or RIN at the right inflection point of the RIN-RCIL rate curve in Figure 6.
This study shows that as the serial number of internodes increased from culm base to tip, the internode length of P. edulis, P. iridescens, and P. amabilis showed a tendency to increase first and then decrease (Figure 2). Consistent with the results of previous studies, the relationship between internode length and its serial number of the studied three bamboo species showed a right-skewed curve (Banik, 1991; Cheng et al., 2017; Tsuyama et al., 2017; Shimada et al., 2021). Further, as the culm height increased (from G1 to G10), the peaks of the curves gradually shifted from the “left” side to the center. Although the three bamboo species differ in size, they exhibited similar relationships between internode length and internode number. A significant linear positive relationship was found between the serial number of the longest internodes and the culm length, which indicates that the internode with the longest length moves from the base to the middle of the culm as the bamboo grows taller (Table 1; Figure 3). At the entire bamboo stem, the lower internodes grow and develop before the upper ones. In the early stage of culm development (i.e., spring of the first growing season), the degree of lignification and cell development is higher in basal internodes than in upper ones (Cui et al., 2012; Tsuyama et al., 2017).
To eliminate the effect of culm size, the internode number was relativized to the total number of internodes as the relative internode number (RIN), and the internode length cumulated from base to a given internode was relativized by the total culm length as the relative cumulative internode length (RCIL; Higuchi, 1981; Inoue et al., 2012, 2017). The RIN-RCIL relationship for each species at ten development stages displayed a typical S-shaped growth curve (sigmoid growth curve, Figure 4; Supplementary Figure S1). Jin et al. (2022) illustrated that the additional parameter δ of the modified Brière (MBE) equation significantly enhanced the adaptability in S-shaped curve fitting. Compared to the Brière (BE) equation, this additional parameter of the MBE equation expands the ability to fit S-shaped curves with varying curvatures for its integral form, allowing the MBE equation to demonstrate excellent fitting performance in a wide range of materials. In our study, the MBE-sigmoid equation achieved better goodness-of-fit than logistic, power, and third-order functions in fitting RIN-RCIL curves, with the lowest average RMSE for all studied bamboo species (Figure 5; Supplementary Table S1). In addition, the MBE-sigmoid equation showed better stability and reliability, with the smallest standard deviation of RMSE between different growth stages within each studied species compared with other functions, e.g., for P. iridescens, the standard deviation of the MBE-sigmoid, logistic, power, and third-order functions are 0.0022, 0.0069, 0.0197 and 0.0129.
The tempo of internode elongation from the culm base to the tip of P. edulis, P. iridescens, and P. amabilis generally follows a growth rhythm of “slow-fast-slow” (Figures 4, 6). This is consistent with previous studies on P. edulis, Phyllostachys nigra var. henonis and Phyllostachys nigra Munro growing in Japan (Inoue et al., 2012, 2017; Shimada et al., 2021), P. edulis in Anhui province, China (Wu et al., 2023), and Pseudosasa amabilis McClure in Jiangsu province, China (Cheng et al., 2017). In the early stage of culm development (the first growing season in spring), the RIN-RCIL rate fitted by the MBE equation showed a left-leaning curve (Figure 6), which suggests that the new internodes formed from the apex are shorter and undergo a slow elongation phase, and the lower internodes begin and complete elongation earlier than the upper internodes. As the development stage of bamboo culm height progressed, the internode with the longest length moved from the lower part of the bamboo culm to the middle, indicating that there are differences in the elongation rates of different internodes in the bamboo culm. That is, the elongation rates of the same internode at different culm height development stages are different, and the elongation rates of different internodes at the same culm height development stage are also diverse. By observing the RIN at the right inflection points of the RIN-RCIL rate curves, we found that after entering the G1 development stage, 24.01–38.23% of the internodes began to elongate rapidly to promote the height growth of bamboo culms (Supplementary Table S2). Furthermore, by extracting the RCIL at the right inflection points of the RIN-RCIL rate curves, we can find that during the G1 development stage, the cumulative length of the rapidly growing internodes (accounting for 24.01–38.23% of all internodes) contributes 65.27–73.59% culm height (Supplementary Table S2). As the bamboo height growth proceeded, more and more internodes gradually contributed to the growth of culm height. By the G10 development stage, the proportion of internodes participating in culm height growth reached 49.28–61.07%, and the cumulative length of rapid-growing internodes contributed 66.70–78.18% of the total culm height. Bamboo, as one of the most fast-growing plants on earth, can reach a height of up to 30 m in a few months and grow almost a meter in a single day (Liese and Weiner, 1996; Wang et al., 2016; Yen, 2016; Chen et al., 2022). The straight, hollow, and tapering structural characteristics of bamboo culms composed of nodes and internodes contribute to mechanical support during rapid height growth (Liese and Tang, 2015; Lorenzo and Mimendi, 2020; Kanahama and Sato, 2023). We speculate that during the early stage of culm development, the cumulative length of the lower part internodes contributes the majority of the total culm height, which may help support rapid cell division of the shorter upper internodes. In addition, in the diaphragm, the net-like structure of the vascular bundle systems consisting of horizontal and axial vascular bundles, not only contributes to mechanical properties, such as bending and crack resistance during rapid height growth, but also facilitates the tangential and axial transport of water and nutrients (Peng et al., 2014; Palombini et al., 2020; Li et al., 2021; Huang et al., 2023). We speculate that the structure of longer internodes in the lower part and shorter internodes in the upper part, as well as the structure with sparse node distribution at the mid-culm and dense near the ends of the culm may facilitate the uptake of water and nutrients from the culm base to tip. Bamboo development consists of multiple stages from cell division to elongation, maturation, and lignification (Wang et al., 2021). The results of this study validate previous findings that cells of the lower part internodes are more mature, longer, and more lignified than those in the upper part of bamboo culm (Cui et al., 2012; Tsuyama et al., 2017; Tao et al., 2020). In addition, the growth hormones auxins, cytokinins, and gibberellins accumulated at the shoot apex of P. edulis, whereas the stress hormones ABA, salicylic acid, and jasmonic acid are predominantly present in the lower part of the shoot (Gamuyao et al., 2017). However, within the internode, the cells transition from an active division phase at the base to a cell elongation phase in the upper part, with a gradual thickening of the cell wall. Within the internode, cell growth-related hormones are higher at the base compared to the upper part, leading to faster cell division (Wang et al., 2021), and the dense and small cells in the lower part may support the elongation of the upper cells. The growth pattern of bamboo, i.e., the lower internodes of the entire culm grow and develop before the upper internodes, and the opposite pattern occurs within the internodes, is the basis for the rapid height growth of bamboo. In future research, an in-depth understanding of the mathematical relationship between cell division and elongation will help to understand the mechanism of rapid height growth in bamboo.
The tempo of internode elongation from the culm base to the tip of P. edulis, P. iridescens and P. amabilis generally follows a “slow-fast-slow” growth rhythm. The relationship between internode length and serial number of the three bamboo species studied showed a right-skewed curve. Furthermore, as the culm height increased from G1 to G10, the peaks of the curves gradually shifted from the “left” side to the center, i.e., the longest internode moved from the base to the middle of the culm. The RIN-RCIL relationship at ten development stages of each species displayed a typical S-shaped growth curve, and the MBE-sigmoid equation achieved better goodness of fit than logistic, power, and third-order functions in fitting the RIN-RCIL curves with the smallest average RMSE. By observing the RIN and RCIL at the right inflection points of the RIN-RCIL rate curves, we found that as the bamboo height growth proceeded, more internodes gradually contributed to the culm height growth. This study improves the understanding of the mathematics involved in the pattern of bamboo internode elongation during rapid height growth.
The raw data supporting the conclusions of this article will be made available by the authors, without undue reservation.
JT: Investigation, Formal analysis, Methodology, Visualization, Writing – review & editing. XL: Investigation, Writing – review & editing. QL: Investigation, Writing – review & editing. KM: Investigation, Writing – review & editing. WH: Conceptualization, Methodology, Supervision, Funding acquisition, Visualization, Writing – original draft, Writing – review & editing.
The author(s) declare financial support was received for the research, authorship, and/or publication of this article. The work was supported by the National Natural Science Foundation of China (No. 32271973) and the Natural Science Foundation of Jiangsu Province, China (No. BK20220413).
We are grateful to Yuying Yang, Yongyan Ding, and Jinfeng Wang who participated in the sample collection and measurement.
The authors declare that the research was conducted in the absence of any commercial or financial relationships that could be construed as a potential conflict of interest.
All claims expressed in this article are solely those of the authors and do not necessarily represent those of their affiliated organizations, or those of the publisher, the editors and the reviewers. Any product that may be evaluated in this article, or claim that may be made by its manufacturer, is not guaranteed or endorsed by the publisher.
The Supplementary Material for this article can be found online at: https://www.frontiersin.org/articles/10.3389/fevo.2024.1440494/full#supplementary-material
Banik R. L. (1991). Biology and propagation of bamboos of Bangladesh (Dhaka: University of Dhaka). Ph.D. thesis.
Brière J.-F., Pracros P., Le Roux A.-Y., Pierre J.-S. (1999). A novel rate model of temperature-dependent development for arthropods. Environ. Entomol. 28, 22–29. doi: 10.1093/ee/28.1.22
Chen M., Guo L., Ramakrishnan M., Fei Z., Vinod K. K., Ding Y., et al. (2022). Rapid growth of Moso bamboo (Phyllostachys edulis): Cellular roadmaps, transcriptome dynamics, and environmental factors. Plant Cell 34, 3577–3610. doi: 10.1093/plcell/koac193
Cheng L., Hui C., Reddy G. V. P., Ding Y., Shi P. (2017). Internode morphometrics and allometry of Tonkin Cane Pseudosasa amabilis. Ecol. Evol. 7, 9651–9660. doi: 10.1002/ece3.3483
Christanty L., Mailly D., Kimmins J. P. (1996). Without bamboo, the land dies”: Biomass, litterfall, and soil organic matter dynamics of a Javanese bamboo talun-kebun system. For. Ecol. Manage. 87, 75–88. doi: 10.1016/S0378-1127(96)03834-0
Cui K., He C., Zhang J., Duan A., Zeng Y. (2012). Temporal and spatial profiling of internode elongation-associated protein expression in rapidly growing culms of bamboo. J. Proteome Res. 11, 2492–2507. doi: 10.1021/pr2011878
Gamuyao R., Nagai K., Ayano M., Mori Y., Minami A., Kojima M., et al. (2017). Hormone distribution and transcriptome profiles in bamboo shoots provide insights on bamboo stem emergence and growth. Plant Cell Physiol. 58, 702–716. doi: 10.1093/pcp/pcx023
He X., Suzuki K., Kitamura S., Lin J., Cui K., Itoh T. (2002). Toward understanding the different function of two types of parenchyma cells in bamboo culms. Plant Cell Physiol. 43, 186–195. doi: 10.1093/pcp/pcf027
Higuchi K. (1981). Changes in internode length and its mid-diameter on bamboo species. J. Japanese Forestry Soc. 63, 379–382. doi: 10.11519/jjfs1953.63.10_379
Huang W., Yang Y., Fu H., Ding Y. (2023). Application of magnetic resonance imaging (MRI) technology in the characterization of microstructure and moisture content of young Moso bamboo. Chin. J. Appl. Ecol. 34, 83–91. doi: 10.13287/j.1001-9332.202301.040
Inoue A., Kuraoka K., Kitahara F. (2012). Mathematical expression for the relationship between internode number and internode length for bamboo, Phyllostachys pubescens. J. Forestry Res. 23, 435–439. doi: 10.1007/s11676-012-0281-1
Inoue A., Tochihara S., Sato M., Shima H. (2017). Universal node distribution in three bamboo species (Phyllostachys spp.). Trees 31, 1271–1278. doi: 10.1007/s00468-017-1546-2
Jin J., Quinn B. K., Shi P. (2022). The modified Brière equation and its applications. Plants 11, 1769. doi: 10.3390/plants11131769
Kanahama T., Sato M. (2023). Plant strategies for greatest height: Tapering or hollowing. Sci. Rep. 13, 18158. doi: 10.1038/s41598-023-45468-7
Kuehl Y. (2015). “Resources, yield, and volume of bamboos,” in Bamboo: The Plant and its Uses. Eds. Liese W., Khöl M. (Springer International Publishing, Cham), 91–111. doi: 10.1007/978-3-319-14133-6_4
Li S., Yang S., Shang L., Liu X., Ma J., Ma Q., et al. (2021). 3D visualization of bamboo node’s vascular bundle. Forests 12, 1799. doi: 10.3390/f12121799
Liese W., Köhl M. (Eds.) (2015). Bamboo: the plant and its uses (Cham: Springer International Publishing). doi: 10.1007/978-3-319-14133-6
Liese W., Tang T. K. H. (2015). “Properties of the bamboo culm,” in Bamboo: The Plant and its Uses. Eds. Liese W., Köhl M. (Springer International Publishing, Cham), 227–256. doi: 10.1007/978-3-319-14133-6_8
Liese W., Weiner G. (1996). Ageing of bamboo culms. A review. Wood Sci. Technol. 30, 77–89. doi: 10.1007/BF00224958
Lorenzo R., Mimendi L. (2020). Digitisation of bamboo culms for structural applications. J. Build. Eng. 29, 101193. doi: 10.1016/j.jobe.2020.101193
McClure F. A. (1966). The Bamboos: A Fresh Perspective (Cambridge, MA: Harvard University Press). doi: 10.4159/harvard.9780674428713
Nelder J. A., Mead R. (1965). A simplex method for function minimization. Comput. J. 7, 308–313. doi: 10.1093/comjnl/7.4.308
Palombini F. L., Nogueira F. M., Junior K. W., Paciornik S., Mariath J. E. D. A., De Oliveira B. F. D. (2020). Biomimetic systems and design in the 3D characterization of the complex vascular system of bamboo node based on X-ray microtomography and finite element analysis. J. Mater. Res. 35, 842–854. doi: 10.1557/jmr.2019.117
Parameswaran N., Liese W. (1980). Ultrastructural aspects of bamboo cells. Cellul. Chem. Technol. 14, 587–609.
Peng G., Jiang Z., Liu X., Fei B., Yang S., Qin D., et al. (2014). Detection of complex vascular system in bamboo node by X-ray μCT imaging technique. Holzforschung 68, 223–227. doi: 10.1515/hf-2013-0080
Shi P., Fan M., Ratkowsky D. A., Huang J., Wu H., Chen L., et al. (2017). Comparison of two ontogenetic growth equations for animals and plants. Ecol. Modell. 349, 1–10. doi: 10.1016/j.ecolmodel.2017.01.012
Shi P., Gielis J., Quinn B. K., Niklas K. J., Ratkowsky D. A., Schrader J., et al. (2022). ‘biogeom’: An R package for simulating and fitting natural shapes. Ann. N. Y. Acad. Sci. 1516, 123–134. doi: 10.1111/nyas.14862
Shi P., Preisler H. K., Quinn B. K., Zhao J., Huang W., Röll A., et al. (2020). Precipitation is the most crucial factor determining the distribution of Moso bamboo in Mainland China. Glob. Ecol. Conserv. 22, e00924. doi: 10.1016/j.gecco.2020.e00924
Shimada N., Munekata N., Tsuyama T., Matsushita Y., Fukushima K., Kijidani Y., et al. (2021). Active transport of lignin precursors into membrane vesicles from lignifying tissues of bamboo. Plants 10, 2237. doi: 10.3390/plants10112237
Silva M. F., Menis-Henrique M. E. C., Felisberto M. H. F., Goldbeck R., Clerici M. T. P. S. (2020). Bamboo as an eco-friendly material for food and biotechnology industries. Curr. Opin. Food Sci. 33, 124–130. doi: 10.1016/j.cofs.2020.02.008
Sohel Md. S.I., Alamgir M., Akhter S., Rahman M. (2015). Carbon storage in a bamboo (Bambusa vulgaris) plantation in the degraded tropical forests: Implications for policy development. Land Use Policy 49, 142–151. doi: 10.1016/j.landusepol.2015.07.011
Song X., Peng C., Zhou G., Gu H., Li Q., Zhang C. (2016). Dynamic allocation and transfer of non-structural carbohydrates, a possible mechanism for the explosive growth of Moso bamboo (Phyllostachys heterocycla). Sci. Rep. 6, 25908. doi: 10.1038/srep25908
Tao G., Ramakrishnan M., Vinod K. K., Yrjälä K., Satheesh V., Cho J., et al. (2020). Multi-omics analysis of cellular pathways involved in different rapid growth stages of Moso bamboo. Tree Physiol. 40, 1487–1508. doi: 10.1093/treephys/tpaa090
Tsuyama T., Shimada N., Motoda T., Matsushita Y., Kijidani Y., Fukushima K., et al. (2017). Lignification in developing culms of bamboo Sinobambusa tootsik. J. Wood Sci. 63, 551–559. doi: 10.1007/s10086-017-1651-2
van der Lugt P., Vogtlander J. G. (2015). The environmental impact of industrial bamboo products: Life-cycle assessment and carbon sequestration. 2nd edition Vol. 35 (The International Network for Bamboo and Rattan). INBAR Technical Report. Available online at: https://pure.tudelft.nl/ws/portalfiles/portal/15386531/1489458449_1.pdf.
Wang K., Zhang Y., Zhang H., Lin X., Xia R., Song L., et al. (2021). MicroRNAs play important roles in regulating the rapid growth of the Phyllostachys edulis culm internode. New Phytol. 231, 2215–2230. doi: 10.1111/nph.17542
Wang Y., Bai S., Binkley D., Zhou G., Fang F. (2016). The independence of clonal shoot’s growth from light availability supports moso bamboo invasion of closed-canopy forest. For. Ecol. Manage. 368, 105–110. doi: 10.1016/j.foreco.2016.02.037
Wu C., Bai Y., Cao Z., Xu J., Xie Y., Zheng H., et al. (2023). Plasticity in the morphology of growing bamboo: A bayesian analysis of exogenous treatment effects on plant height, internode length, and internode numbers. Plants 12, 1713. doi: 10.3390/plants12081713
Yen T. (2016). Culm height development, biomass accumulation and carbon storage in an initial growth stage for a fast-growing moso bamboo (Phyllostachy pubescens). Bot. Stud. 57, 10. doi: 10.1186/s40529-016-0126-x
Keywords: internode length, culm height growth, modified Brière equation, Phyllostachys edulis, Phyllostachys iridescens, Pseudosasa amabilis
Citation: Tan J, Liu X, Li Q, Ma K and Huang W (2024) Variation in internode length patterns: a data analysis of internode length and serial number in three bamboo species. Front. Ecol. Evol. 12:1440494. doi: 10.3389/fevo.2024.1440494
Received: 29 May 2024; Accepted: 29 July 2024;
Published: 16 August 2024.
Edited by:
Tingting Mei, Zhejiang A&F University, ChinaCopyright © 2024 Tan, Liu, Li, Ma and Huang. This is an open-access article distributed under the terms of the Creative Commons Attribution License (CC BY). The use, distribution or reproduction in other forums is permitted, provided the original author(s) and the copyright owner(s) are credited and that the original publication in this journal is cited, in accordance with accepted academic practice. No use, distribution or reproduction is permitted which does not comply with these terms.
*Correspondence: Weiwei Huang, d2hAbmpmdS5lZHUuY24=
†These authors have contributed equally to this work
Disclaimer: All claims expressed in this article are solely those of the authors and do not necessarily represent those of their affiliated organizations, or those of the publisher, the editors and the reviewers. Any product that may be evaluated in this article or claim that may be made by its manufacturer is not guaranteed or endorsed by the publisher.
Research integrity at Frontiers
Learn more about the work of our research integrity team to safeguard the quality of each article we publish.