- 1School of Environmental Sciences, University of Guelph, Guelph, ON, Canada
- 2Sociedad Latinoamericana de Investigación en Abejas (Solatina), Montevideo, Uruguay
- 3USDA-ARS Bee Research Laboratory, Beltsville, MD, United States
- 4Consejo Nacional de Investigaciones Científicas y Técnicas (CCT CONICET La Plata) - Centro de Microbiología Básica y Aplicada (CEMIBA), Facultad de Ciencias Veterinarias, Universidad Nacional de La Plata, La Plata, Buenos Aires, Argentina
- 5Sección Etología, Facultad de Ciencias, Universidad de la República, Montevideo, Uruguay
- 6Instituto de Investigaciones en Producción Sanidad y Ambiente (IIPROSAM CONICET-UNMdP);Facultad de Ciencias Exactas y Naturales – UNMdP; Centro Científico Tecnológico Mar del Plata – CONICET; Centro de Asociación Simple CIC PBA, Mar del Plata, Argentina
- 7Centro de Investigaciones en Abejas Sociales, Facultad de Ciencias Exactas y Naturales, Universidad Nacional de Mar del Plata, Mar del Plata, Argentina
The honey bee (Apis mellifera) parasitic mite, Varroa destructor, is considered one of the main causes of colony losses in European honey bee (EHB) populations around the world. However, some EHB and Africanized honey bee (AHB) populations (derived from the African subspecies A. m. scutellata) that inhabit tropical and subtropical regions of the Americas, have survived varroa mite infestations in the absence of acaricide treatments. It is conceivable to expect that these honey bee populations, which have been subjected to natural selection over decades, would have developed resistance against V. destructor or possess pre-existing adaptations that allow them to survive mite parasitism. Here, we present a comprehensive literature review describing the spread of V. destructor and the honey bee populations occurring in Latin America (LA), and summarize the evidence of resistance of those populations to V. destructor. We also analyze reports describing the potential mechanisms of mite resistance and how they operate in those honey bee populations. Studies of a few EHB, as well as of numerous AHB populations exhibiting resistance to V. destructor in LA, unveil the existence of evolutionary adaptations that restrain V. destructor population growth and provide insight into the current host-parasite relationship. This review supports the notion that selective breeding of local honey bee populations from LA could be a viable strategy to manage varroa mite infestations in colonies.
1 Introduction
Western honey bees (Apis mellifera L.) are among the most important pollinators of flowering plants, playing a key role in food production and natural ecosystem maintenance (Klein et al., 2007; Lorenzo-Felipe et al., 2020). However, honey bees are exposed to a wide range of biotic and abiotic stressors such as pathogens and parasites, habitat fragmentation, pesticides, and environmental stressors, which have led to the loss of a significant number of colonies (Vanengelsdorp et al., 2009; Neumann and Carreck, 2010; Guzman-Novoa, 2016; Maggi et al., 2016; Requier et al., 2018). Currently, it is generally accepted that losses of honey bee colonies are a consequence of the synergy and interaction of multiple contributing factors, with the ectoparasitic mite Varroa destructor playing a major role (Guzman-Novoa et al., 2010; Nazzi et al., 2012; Hristov et al., 2020).
Varroa destructor (Anderson and Trueman, 2000) is an obligate parasite of two bee species, the Asian honey bee, Apis cerana, and the Western honey bee, A. mellifera. The Asian honey bee is the original host of V. destructor that has coexisted with the mite for a long time, which resulted in the development of mechanisms of defense in A. cerana that confer resistance to the bees against the parasite (Peng et al., 1987; Oldroyd, 1999; Rath, 1999). However, with the movement of European honey bees (EHB) to Asia, V. destructor switched hosts by the late 1950s (Delfinado, 1963), and rapidly spread throughout the world, which has led to high mite infestations and colony losses of its new host, particularly in countries of the Northern hemisphere where EHB populations are managed (Oldroyd, 1999; Rosenkranz et al., 2010; Morfin et al., 2023b).
Varroa destructor reproduces on honey bee brood and feeds mainly on bee fat body (Ramsey et al., 2019). The mite inhibits immune responses of parasitized bees (Koleoglu et al., 2017, Koleoglu et al., 2018) and is also known to be a very efficient vector of several honey bee viruses that are harmful to the bees, such as deformed wing virus (DWV) and others (Kevan et al., 2006; Martin et al., 2012; Wilfert et al., 2016; Reyes-Quintana et al., 2019; Beaurepaire et al., 2020). This association has led to parasite-pathogen synergistic effects on the health of parasitized bees (Gisder et al., 2009; Desai and Currie, 2016), a key cause of global colony losses (Dainat et al., 2012).
Most EHB populations are considered vulnerable to V. destructor and need chemical treatments to survive (Rosenkranz et al., 2010). Consequently, beekeepers rely heavily on the application of acaricides in their hives (Gregorc et al., 2018). However, the long-term use of these chemicals has resulted in widespread cases of mite-resistance to most of the compounds used (Milani, 1999; Elzen et al., 2000; Maggi et al., 2009; Medici et al., 2015; Mitton et al., 2022). Moreover, residues of acaricides are also frequently found in hive products like beeswax and honey (Mullin et al., 2010; Medici et al., 2020). Because of these acaricide-associated issues, an increasing number of researchers have focused their interest on the search for new mite management alternatives that promote sustainable beekeeping (Dietemann et al., 2012; Gregorc and Sampson, 2019). Thus, researchers shifted their interest to studying honey bee populations exhibiting natural resistance to V. destructor infestations in Europe and the Americas (Seeley, 2007; Locke, 2016; Le Conte et al., 2020; Mendoza et al., 2020; Mondet et al., 2020; Grindrod and Martin, 2021).
It has been shown that some honey bee populations that are not chemically-treated cope with V. destructor infestations through tolerance or resistance (Mondet et al., 2020). Resistance implies that a honey bee colony is able to restrain the population growth of V. destructor to a level that does not cause mortality or severe effects on the host health (Guzman-Novoa and Morfin, 2019). Tolerance for the case of this review is defined as the ability of host bees to withstand and survive high levels of mite parasitism. Accordingly, although some reports may use the term “tolerant bees”, most of the cases of Varroa-honey bee interaction studied demonstrate bee resistance. One potential problem associated with tolerant bees, is that they could endure a higher parasite load and could serve as a parasite reservoir for non-tolerant bees of susceptible colonies in the vicinity (Mitton et al., 2022).
Even though some honey bee populations have survived V. destructor infestations without treatment in many countries, one of the world’s largest populations of honey bees surviving varroa mite parasitism are the so-called Africanized honey bees (AHBs, derived from the African subspecies, A. m. scutellata) that are widespread in Latin America (LA). Furthermore, examples of EHB populations surviving the mite without treatment also occur in LA. These populations are therefore good models to investigate potential natural resistance mechanisms that allow the survival of honey bee colonies infested with V. destructor. Studies of this nature are valuable for the scientific community because they may shed light on matters regarding host-parasite interactions. They are also valuable for the beekeeping community because the knowledge generated from them may be applied to better design selective breeding programs to develop honey bee stocks resistant to the varroa mite.
This comprehensive review aims to describe the natural history of parasitism by V. destructor on A. mellifera in LA. Specifically, we have strived to summarize and analyze reports of honey bee populations that have survived mite parasitism in the absence of acaricide treatments, describing their potential mechanisms of resistance against the mite and how they operate.
2 Varroa destructor history and presence in Latin America
The Varroa genus comprises four different species of ecto-parasitic mites: Varroa jacobsoni, Varroa underwoodi, Varroa rindereri, and Varroa destructor (formerly known as Varroa jacobsoni), that originated in Southern Asia and have been parasitizing Apis cerana, the Asiatic honey bee, for centuries (Anderson and Trueman, 2000).
Varroa destructor spread from Asia and is now parasitizing honey bees in all continents. The Japanese haplotype of the mite was first introduced to the Americas when queen bees from Japan were imported into Paraguay in 1971 (De Jong and Gonçalves, 1981; De Jong et al., 1982). From Paraguay, varroa mites spread to Brazil by 1972 (Alves et al., 1978; De Jong et al., 1982; Castilhos et al., 2023) and to other South American countries shortly after that (Maggi et al., 2016). It is suspected that following the introduction from Japan, V. destructor was introduced multiple times to South America from Europe, but there is little documentation supporting this assumption (Maggi et al., 2016). In Central America, varroa mites were first detected in 1996 in Nicaragua (Düttmann et al., 2021), although they were probably introduced much earlier to that region since V. destructor had been found in Mexico in 1992 (Rodriguez et al., 1992), and in the Dominican Republic in 1995 (Serra et al., 2003). The mite was also introduced into Puerto Rico via swarms accidentally traveling on ships or through shipments of queens and bee packages (de Guzman et al., 1997). Figure 1 shows the dates of the first detection of V. destructor in different LA countries. Besides the Japanese haplotype of V. destructor, the more common Korean haplotype of the mite is also present in the Americas. The current distribution of V. destructor haplotypes in the Americas is fragmented, but recently published articles reported the Korean haplotype as the most prevalent in LA countries, suggesting that it may have been replacing the Japanese haplotype (Guerra et al., 2010; Maggi et al., 2012; Mendoza et al., 2020; Muntaabski et al., 2020; Düttmann et al., 2021; Reyes-Quintana et al., 2023). Nowadays, it appears that the distribution of the mite’s Japanese haplotype in LA is restricted to the Brazilian island of Fernando do Noronha and to Chile (Strapazzon et al., 2009; Guerra et al., 2010; Traynor et al., 2020). It is unknown how mites of the Korean haplotype reached the Americas, but they were first identified in Wisconsin, USA in 1987 (de Guzman et al., 1997), probably due to importations of queens from Europe.
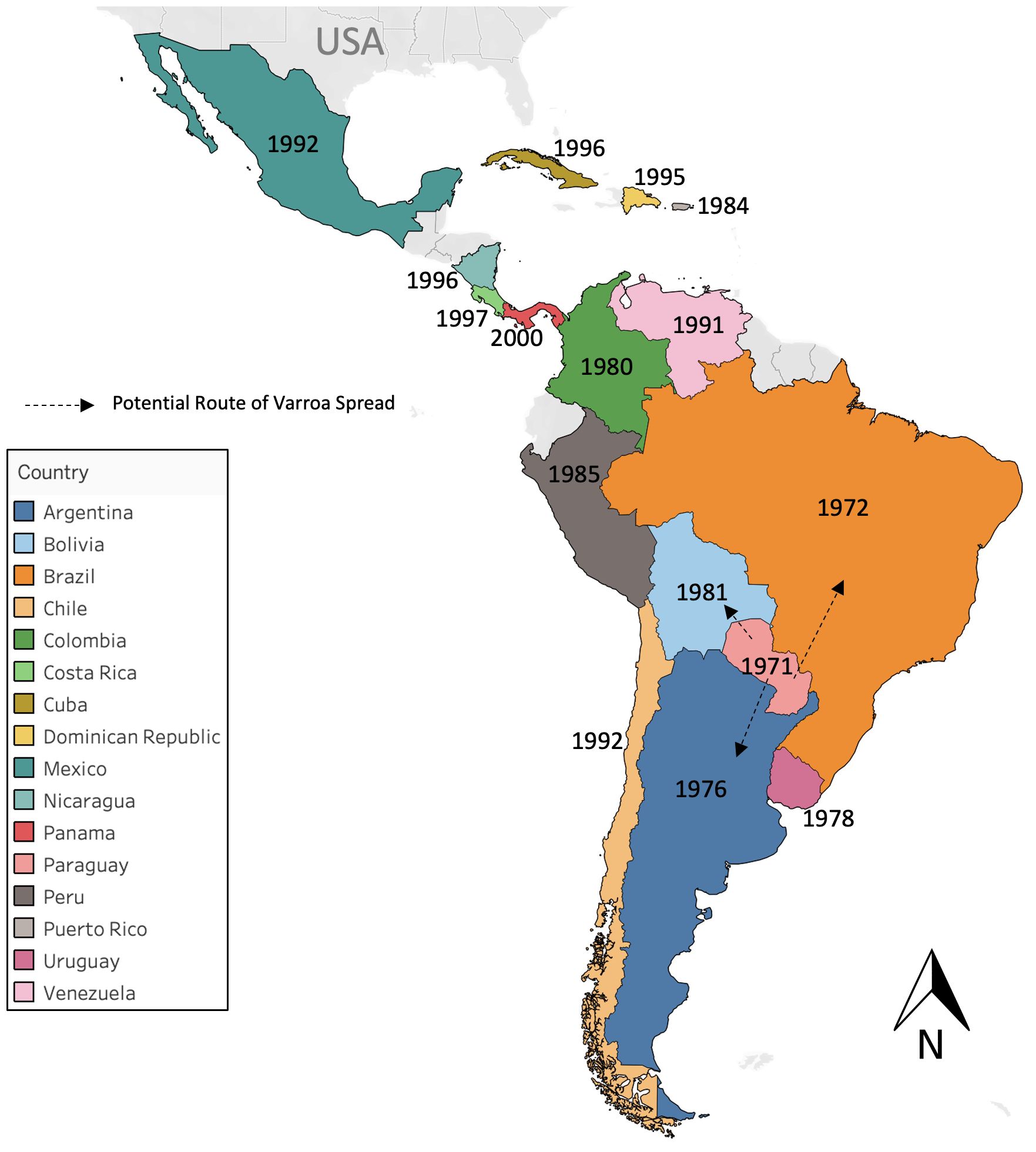
Figure 1 Year of introduction or first detection of Varroa destructor in different Latin American (LA) countries. Argentina (Montiel and Piola, 1976), Bolivia (Stearman, 1981), Brazil (Alves et al., 1978), Chile (Hinojosa and González, 2004), Colombia (Tibatá et al., 2017), Costa Rica (van Veen et al., 1998), Cuba (Demedio et al., 2002), Dominican Republic (Serra et al., 2003), Mexico (Rodriguez et al., 1992), Nicaragua (Düttmann et al., 2021), Panamá (Calderón et al., 2000) Paraguay (De Jong et al., 1982), Perú (Dávila et al., 1987), Puerto Rico (de Guzman et al., 1997), Uruguay (Invernizzi et al., 2011), Venezuela (Casanova and Perruolo, 1992). Countries of LA with no year in the map mean that no references indicating year of mite discovery or introduction could be found.
The association of V. destructor and honey bees in LA occurs under a complex scenario in which different haplotypes of the mite parasitize honey bee populations of different subspecies and ecotypes, that inhabit different climates and geographical landscapes. Biological traits of V. destructor from surviving colonies across LA have been documented in addition to the haplotype of the mite (see below). Moreover, many studies of varroa mite parasitism have been conducted in populations of different honey bee genotypes in which numerous health and productivity parameters have been measured (see below). Most of these studies have been carried out in Brazil, Argentina, Uruguay, and Mexico, as well as in a few other LA countries.
The varying impact of V. destructor on honey bee populations across the Americas might be in part explained by possible differences in virulence between different mite haplotypes. Researchers have postulated that the Korean haplotype of V. destructor is more virulent than the Japanese haplotype of the mite (de Guzman et al., 1997, de Guzman et al., 1998). However, to the best of our knowledge, no studies have clearly proven that the Korean haplotype of V. destructor is more virulent than the Japanese haplotype of the mite. Nevertheless, there is no doubt that geographic and historical data prove that mites characterized as Korean haplotype have more successfully spread and established in honey bee populations around the world than the Japanese one (Reyes-Quintana et al., 2023; Morfin et al., 2023b).
Very little is known about the genetic variability of V. destructor populations in LA. Most studies conducted in the past have used markers such as mitochondrial DNA fragment polymorphisms and sequences, as well as microsatellites (Morfin et al., 2023b). These markers are not very informative because of the quasi-clone nature of the mites, since they mate between siblings, resulting in reduced genetic variability. Ultimately, the best way to learn more about mite variants infesting honey bees in LA, will be through the use of full genome sequence comparisons. By analyzing full genomes, it will be possible to identify variants of V. destructor and compare them for their impact on different honey bee populations established in different environments. Therefore, the outcome of parasitism (level of damage to the colony or capability to survive to mite parasitism) should be analyzed considering the characteristics of the mite, the bee, and if possible, the environment in which the mite-bee interaction occurs.
3 Honey bee populations in Latin America
Apis mellifera is not native to the Americas; it was introduced to North and South America by European settlers about 250 years ago (Sheppard, 1989; Moritz et al., 2005). Therefore, the populations of EHBs found in the Americas descend from several subspecies of A. mellifera, including A. m. mellifera, A. m. ligustica, A. m. carnica, and A. m. caucasica, that were introduced from distinct European regions, and thus belong to different honey bee evolutionary lineages (Magnus and Szalanski, 2010; Bouga et al., 2011).
Honey bees descended from African subspecies exist in the Americas too. In LA, in particular, AHB populations descend from the African subspecies A. m. scutellata, which was introduced to Brazil in 1956 (Kerr, 1957) and later interbred with local populations of EHBs. This admixture led to the generation of a new hybrid bee (tropically adapted) that spread rapidly to all continental LA countries except Chile, and that has maintained its predominantly African genotype rather than its European genotype. Nevertheless, variation in Africanization levels exist in honey bee populations from different LA regions (Smith et al., 1989; Rinderer and Hellmich, 1991; Sheppard et al., 1991; Schneider et al., 2004; Domínguez-Ayala et al., 2016; Guzman-Novoa et al., 2020; Harpur et al., 2020). Populations of AHBs are not limited to the continental territories since they have been also found in Caribbean islands like Puerto Rico (Acevedo-Gonzalez et al., 2019) and the Dominican Republic (Nikaido et al., 2018).
Even though the tropically adapted AHB populations have spread throughout most of the Americas, their distribution and predominance, as well as their degree of Africanization, are not homogeneous across the different territories. For instance, the frequency of colonies having African mitochondrial DNA found in several comprehensive studies conducted relatively recently in different LA countries indicates that the Africanization of honey bee populations varies widely. It has been found to range from 5 to13% in one region of Argentina, south of 35° N latitude, to 80-100% in regions of Uruguay, Brazil, and Colombia. Intermediate Africanization frequency (25-70%) was found in colonies from several regions of Mexico (Table 1). Variation in Africanization frequency is also affected by climate and altitude, even within the same country. For example, Medina-Flores et al. (2015) and Ramos-Cuellar et al. (2023) reported that the Africanization frequency of honey bee colonies as determined by mitochondrial DNA varied between 12% and 87%. Colonies located at higher altitudes and in temperate climates were less frequently Africanized (temperate adapted) than colonies located in subtropical (tropically adapted) and low altitude regions (Medina-Flores et al., 2015; Ramos-Cuellar et al., 2023).
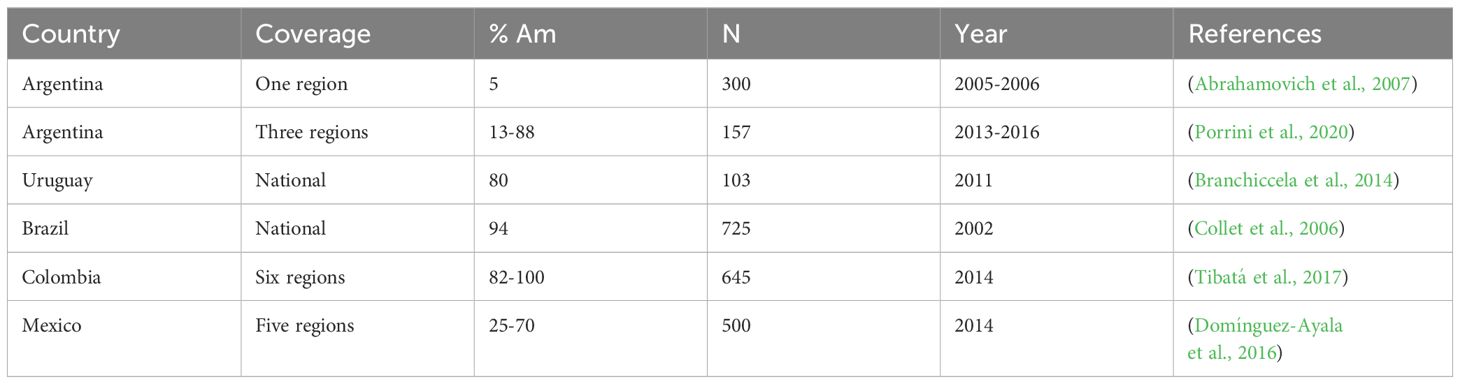
Table 1 Mean or range percentage of honey bee colonies classified as having African maternal descent by mitochondrial DNA haplotype (% Am) from comprehensive studies conducted in Latin American countries.
Despite the aforementioned studies, detailed, wide, and interregional molecular studies for AHB populations are lacking in LA. The majority of the studies on the ancestry of honey bees so far conducted in LA have been carried out in a few countries, and many of them are regional, which does not provide the range of Africanization that can be found in different areas of the same country. Additionally, a comprehensive genetic map of honey bee populations in LA remains to be completed, since most of the studies have been focused on the origin of the maternal lineages through the determination of mitochondrial haplotypes, ignoring the contribution of paternal heredity. This is because the use of nuclear markers of paternal inheritance is currently technically challenging (Chapman et al., 2015; Harpur et al., 2020). However, the combined use of mitotype determination and morphometric measurements provides a rough estimate of the degree of Africanization of honey bee populations.
As with other introduced and invasive species, A. mellifera adapted and established populations in different habitats in the Americas independently of human intervention. In fact, feral honey bee colonies, in particular those of AHBs, have had a strong impact on biodiversity and ecosystem services in the Americas because of their natural ability to adapt to tropical environments and to resist V. destructor parasitism (Guzman-Novoa et al., 2020). Nevertheless, despite their contribution to boost agricultural production in South and Central America by providing pollination services, AHBs also represent a threat to native bee populations (either by competing for resources or by the potential transmission of pathogens), and thus, indirectly affect pollination and plant diversity in the landscape (Roubik and Villanueva-Gutierrez, 2009; Portman et al., 2018; Guzman-Novoa et al., 2020).
4 Resistance of honey bee populations to Varroa destructor in Latin America
Several studies have concluded that chemical treatments to control V. destructor are not necessarily required to keep honey bee colonies alive in regions of LA because they show natural resistance to the mite. These regions generally coincide with areas where the majority of honey bee colonies are Africanized, although there are also examples of EHB populations that have survived without chemical treatments. Nevertheless, there is debate among researchers and beekeepers as to whether honey bee colonies surviving mite infestations in LA should be treated to maintain them as productive units.
4.1 Resistance of AHBs to Varroa destructor in Latin America
Studies so far conducted on V. destructor parasitism of honey bee colonies in LA have generally found that AHB colonies have lower varroa infestation levels (VIL) compared to other honey bee populations (e.g. EHBs), and that AHBs can survive V. destructor infestations without treatment (Rosenkranz et al., 2010; Castilhos et al., 2023; Morfin et al., 2023b).
High VIL of honey bee colonies were reported during the first years of the arrival of V. destructor in Brazil (Morse and Gonçalves, 1979). However, numerous studies conducted decades later in South America, Central America, and Mexico, reported low VIL, supporting the view that honey bee populations of primarily African descent are resistant to varroa mite infestations (Moretto et al., 1991, Moretto et al., 1995; Guzman-Novoa et al., 1999; Moretto and Mello, 1999; Rosenkranz, 1999; Calderón et al., 2000; Martin and Medina, 2004; Mondragón et al., 2005; Medina-Flores et al., 2014a; Giménez-Martínez et al., 2017; Tapia-González et al., 2019; Mendoza et al., 2020; Peixoto et al., 2021; Tibatá et al., 2021; Mendoza et al., 2022; Ramos-Cuellar et al., 2022; Castilhos et al., 2023).
The many AHB populations studied have consistently demonstrated low VIL (<5% on adult bees) in colonies of different countries, different environments, and in different seasons, which agrees with the definition of resistance to parasitism by V. destructor. Nevertheless, a few other studies conducted at the beginning of this century reported that in some regions of Brazil, the rates of varroa mite fertility, as well as the infestation rates of colonies, increased after 20 years of mite parasitism (Garrido et al., 2003; Carneiro et al., 2007). The results of these studies should be taken cautiously and not as a general representation of AHB populations in Brazil, because they were conducted with a limited number of colonies and in a few locations. Additionally, the VIL of those studies do not resemble the consistently low VIL of AHBs in other LA countries and in Brazil itself, in more recent studies, showing mean VIL lower than 5% (Santos et al., 2014; Peixoto et al., 2021). Furthermore, a comprehensive study analyzing VIL in AHB populations in Brazil from 1977 to 2020 concluded that despite regional variation in VIL, the mean mite infestation rate of AHB colonies has remained around 4.5% with a median of 3.8% in adult bees, during the last 45 years (Castilhos et al., 2023).
Varroa destructor is highly prevalent in colonies of countries with mostly EHB populations like the USA (97%) (Traynor et al., 2016; Abban et al., 2024), but also in those with predominantly AHB populations like Brazil (95.7%) (Santos et al., 2014), Colombia (92%) (Tibatá et al., 2021), and Mexico (84%) (Correa-Benítez et al., 2023). By comparison, lower V. destructor prevalence rates have been reported from countries with predominant EHB populations, such as Argentina (74%) and other LA countries (Maggi et al., 2016). Therefore, it seems that there is no association between the degree of Africanization of honey bee colonies and varroa mite prevalence, suggesting that other factors, including management practices (e.g., acaricide treatments, distance between hives and apiaries, migratory beekeeping, etc.) and climate, may be potentially affecting the mite’s prevalence (Goodwin et al., 2006; Nolan and Delaplane, 2017). Conversely, available evidence suggests a negative association between degree of Africanization and VIL. Regions where most colonies have African mitotype also have low VIL, as exemplified by the case of Colombia with more than 82% of colonies having African mitotype and 4.5% VIL (Tibatá et al., 2017, Tibatá et al., 2021). In Uruguay, it was demonstrated that the African morphometry of honey bees is negatively and significantly correlated with VIL of colonies (Giménez-Martínez et al., 2017). Furthermore, parallel studies conducted in Mexico demonstrated that colonies classified as Africanized by mitotype and morphotype, had significantly lower VIL than colonies classified as European. In one study, AHB colonies had VIL of 3.5%, compared to 5% of EHB colonies (Medina-Flores et al., 2014a). In a more recent study, colonies of African descent had 3% VIL vs. 8% for colonies of European descent in the same region (Ramos-Cuellar et al., 2023). Similarly, in a recent and large-scale (n = 4,039) study, a national VIL of 8.21% was reported in the USA, which overwhelmingly comprises EHBs (Abban et al., 2024).
4.2 Resistance of EHBs to Varroa destructor in Latin America
Although V. destructor is the main driver of colony losses in South American countries having a high proportion of temperate adapted EHB colonies such as Argentina, Uruguay, and Chile (Maggi et al., 2016), there are a few examples of EHB populations surviving varroa mite infestations in LA. Marcangeli et al. (1992) reported a population of Italian bees (A. m. ligustica) that had survived without acaricide treatment in a temperate climate South of Buenos Aires, Argentina. Reduced mite reproduction in this population was associated with low VIL. Later, Eguaras et al. (1995) studied another population of EHBs in the same region, and showed that colonies of that population maintained a constant low mite parasitism. More than twenty years later, Russo et al. (2020) reported a population of EHBs from Northern Argentina that has maintained low VIL and has survived mite infestations without treatment.
In the Dominican Republic, EHB populations that co-existed for several decades with V. destructor before the arrival of AHBs, showed varroa mite infestation levels not requiring acaricide treatment. Since these bees had been established in the Dominican Republic for a long time, with very few subsequent introductions, and with no artificial selection by the beekeepers, their presumed resistance to V. destructor seems to be the result of natural selection (Vandame and Palacio, 2010). A similar scenario was found in a population of EHBs from western Cuba. Rodríguez-Luis et al. (2022) characterized the population and claimed that the bees are resistant to V. destructor because their colonies have survived without treatments for over two decades.
Another example of EHB colonies that for decades have survived V. destructor parasitism without treatment is that of colonies from the Fernando de Noronha island in Brazil. A population of Italian honey bees parasitized with varroa mites was established in 1984 on the island (De Jong and Soares, 1997). These colonies were not treated with acaricides but had higher VIL and higher rates of mite fertility compared to colonies of AHBs kept in mainland Brazil (De Jong and Soares, 1997). It has been proposed that the ability of this Italian-derived honey bee population to survive despite having high VIL, is that they have developed tolerance to mite infestations, or that the mites on the island are less virulent than those parasitizing honey bees in mainland Brazil. The mites of Japanese haplotype that infest the bees on the island are considered less virulent than those found in mainland Brazil, which are of the Korean haplotype (Corrêa-Marques et al., 2002; Strapazzon et al., 2009). However, more studies on this population of EHBs are necessary to better understand why they have survived high loads of varroa mites.
Despite the above examples of EHB populations showing a degree of resistance to V. destructor, most colonies of European descent in parts of Uruguay, Argentina, and Chile, require chemical treatments to keep the colonies alive (Maggi et al., 2016).
4.3 Resistance to Varroa destructor vs. colony productivity in Latin America
Despite the abundant evidence of relative resistance of honey bee populations to V. destructor in LA, and that many populations survive without chemical treatments, the level of resistance to the mite does not seem to be high enough to prevent the reduction of colony honey yields as a consequence of varroa mite infestations, as has been demonstrated in several studies (Arechavaleta-Velasco and Guzman-Novoa, 2000; Uribe-Rubio et al., 2003; Medina-Flores et al., 2014b). Furthermore, it has been shown that acaricide treatment may additionally improve survival of mite-infested AHB colonies in Uruguay and Brazil (Invernizzi et al., 2011; Medici de Mattos and Chaud-Netto, 2012). Clearly, although natural resistance of honey bee populations to V. destructor in LA allows many colonies to survive, the level of resistance may not be sufficient to keep them as optimal productive units. However, despite being challenging, the approach of further selecting for Varroa-resistance in honey bee populations that have undergone natural selection, like those in LA, remains a promising non-chemical control of this harmful parasite that can be used together with other control measures.
5 Mechanisms of Varroa-resistance in honey bee populations in Latin America
Regardless of their tolerance or resistance adaptations, honey bees must cope with the two developmental phases of the mite. In the phoretic (dispersion) phase, female mites parasitize adult bees by feeding primarily on their fat body tissue and hemolymph (Ramsey et al., 2019), and use worker bees as transport vehicles to spread within the hive and between hives (Goodwin et al., 2006). In the reproductive phase, foundress female mites enter brood cells prior to being sealed and reproduce inside them, feeding on the brood within a protected environment (Rosenkranz et al., 2010; Traynor et al., 2020). So, under the phoretic phase, bees can resist mites by performing grooming behavior or by just tolerating them on their bodies (Mondet et al., 2020). Under the reproductive phase, adult bees may remove mites from cells through several mechanisms (e.g., hygienic behavior, recapping, etc.), or brood may resist them through mechanisms that inhibit or restrain mite reproduction (e.g., suppressed mite reproduction, short post-capping duration, low brood attractiveness, etc.), or simply tolerate the mites (Mondet et al., 2020). Given the severe damage that V. destructor inflicts to honey bee individuals and colonies, it would be expected that natural selection would quickly result in increased expression of different resistance traits, particularly in populations producing many generations of bees a year, and where beekeepers rely less on chemical treatments like in tropical regions of LA. The following mechanisms of resistance and their effects have been studied in honey bee populations in LA.
5.1 Hygienic behavior, Varroa sensitive hygiene, and recapping
The term hygienic behavior has been used to describe the ability of adult honey bees to detect and remove dead or diseased brood from capped cells (Guzman-Novoa and Morfin, 2019). The behavioral sequence of uncapping and removing the brood is the same whether the brood is diseased, mite-infested, or dead (Boecking and Dreschner, 1991; Spivak and Danka, 2021), and this motor pattern may be triggered by the detection of different odorants associated with the health status of the brood (Mondet et al., 2015; Wagoner et al., 2019). Hygienic behavior is a trait highly influenced by genetic effects, and therefore, heritable (Rothenbuhler, 1964; Lapidge et al., 2002; Scannapieco et al., 2017; Harpur et al., 2019).
Hygienic behavior may be associated to resistance of honey bees against V. destructor. However, the scientific reports about the relationship between hygienic behavior, VIL, and V. destructor population growth in honey bee populations in LA, are somewhat inconsistent. For example, Aumeier et al. (2000) reported a higher between-colony variation for removal behavior of Varroa-infested brood in AHB colonies than in Carniolan honey bee (A. m. carnica) colonies, and concluded that hygienic behavior might not be a factor associated with resistance to varroa mites. Similarly, Medina-Flores et al. (2014b) did not find significant differences in VIL between colonies of AHBs that expressed high and low rates of hygienic behavior. Nevertheless, numerous other studies conducted in LA have shown that in general, AHBs are more hygienic than EHBs. For example, Guerra et al. (2000) reported that in their study, AHB colonies removed 51% of artificially mite-infested brood, while Italian-Africanized hybrid colonies removed only 25% of the parasitized brood. In another study with AHB colonies, Moretto et al. (2006) found that the uncapping rate of brood cells naturally infested with varroa mites was 3.5-fold higher than that of non-infested cells. These results suggest that AHBs are able to recognize, open, and remove brood from cells infested with V. destructor. It is possible that the AHB populations of the studies by Guerra et al. (2000) and Moretto et al. (2006) had displayed Varroa-sensitive hygiene (VSH), which is a specialized type of hygienic behavior (see below). Similarly, when AHB colonies were compared with colonies of Italian bees from Fernando de Noronha island in Brazil, the former colonies were found to be significantly more efficient at removing infested brood (61% vs. 35%, respectively), even though the population of Italian bees on the island had been exposed to, and had survived V. destructor infestations without treatment for more than a decade (Guerra et al., 2000; Corrêa-Marques et al., 2002). Vandame et al. (2002) also reported that in their study conducted in Mexico, EHBs removed 8% of mite-infested brood, while AHBs removed 32.5% of infested brood. In a more recent study, Tapia-González et al. (2021) found that the highest frequency of colonies expressing elevated rates of hygienic behavior occurred in areas where honey bee populations were highly Africanized.
Based on the notion that AHBs appear to be more hygienic than EHBs, several studies conducted in LA have analyzed the hypothesis that AHBs express a degree of hygienic behavior high enough to maintain V. destructor populations below damage thresholds, which could in part explain their relative resistance to the mite. For example, Schafaschek et al. (2019) found high correlations between hygienic behavior and low mite reproduction rates in AHB colonies and proposed that hygienic behavior interferes with V. destructor population dynamics (Schafaschek et al., 2019). Nevertheless, other studies have concluded that hygienic behavior is insufficient to significantly restrain V. destructor infestations. For instance, Vandame et al. (2002) proposed that hygienic behavior represents a heavy cost for AHB colonies, as removing pupae results in a lower number of adult bees. The authors of the study speculated that the removal of infested brood is beneficial to the colonies up to a threshold, above which, the cost of removal becomes greater than the benefit. Similar conclusions were reached by Mondragón et al. (2005), who claimed that hygienic behavior could not explain well the mite population dynamics in AHB colonies in Mexico. Although it is evident that in general AHB colonies express higher rates of hygienic behavior than EHB colonies, the relative contribution of hygienic behavior to the resistance of AHBs against V. destructor is still unclear.
Less than two decades ago, a new term was coined to describe a specialized type of hygienic behavior, “Varroa-sensitive hygiene” (VSH) (Harris, 2007). This behavior is performed by worker bees that are highly sensitive to the presence of varroa mites under capped cells, particularly those that are undergoing reproduction (Ibrahim and Spivak, 2004; Harbo and Harris, 2005). Bees expressing VSH readily uncap infested cells and remove mites and the parasitized brood (Harris, 2007), thus, restraining V. destructor reproduction and population growth. Several candidate genes have been identified for VSH (Tsuruda et al., 2012), which further indicates that this trait is heritable. Hygienic bees are more sensitive to odorant compounds from mite-parasitized brood than non-hygienic bees, and that triggers their uncapping and removal behaviors (Chakroborty et al., 2015). Some of these compounds include oleic acid, tritriacontene, and brood ester pheromone (Mondet et al., 2016; McAfee et al., 2018).
To our knowledge, there are no studies specifically aimed at analyzing VSH in LA honey bee populations, although research conducted before the behavior was characterized, probably recorded the effect of VSH bees in AHB populations in Brazil (Corrêa-Marques and De Jong, 1998; Guerra et al., 2000; Moretto et al., 2006). This is not a minor point, considering the inconsistent results reported on general hygienic behavior as previously described. Also, different results from different studies may arise as a consequence of using different methods of evaluating the behavior. The freeze-killed brood or pin-test methods used to score general hygienic behavior fail to identify colonies that may display VSH, which is measured differently (Danka et al., 2013; Mondet et al., 2020). Some studies conducted with EHBs confirmed that honey bee populations selected and bred for high hygienic behavior using the freeze-killed method, displayed lower mite levels than control colonies (Spivak and Reuter, 1998; Ibrahim and Spivak, 2006). However, new studies have concluded that the sole use of the freeze-killed brood or pin-test assays does not appear to be sufficiently selective for Varroa-resistance (Mondet et al., 2020). In this context, studies on VSH in honey bee populations in LA and other regions are warranted.
Recapping is a worker bee behavior that involves the re-sealing of Varroa-infested cells that are previously uncapped without injure to the host brood (Villa et al., 2009). This behavior could alter mite fertility and fecundity, particularly because the mortality of mite offspring is higher in recapped cells than in normally mite-infested, but otherwise not opened cells (Harris et al., 2012). Recapping of cells has been observed at high frequency in honey bee colonies selected for VSH (Harris, 2008), which suggests that it may be a component of VSH. However, other studies have shown that bees of unselected populations recapped a higher proportion of Varroa-infested cells than bees displaying high rates of VSH (de Guzman et al., 2015). Recently, Martin et al. (2020) reported high levels of cell opening and re-capping in AHB and A. m. capensis colonies than in EHB colonies, and proposed that this behavior could be a mechanism of resistance against V. destructor in different honey bee populations. In addition to AHBs, a few EHB colonies from western Cuba that had survived V. destructor parasitism for more than 20 years, demonstrated high rates of recapping behavior of mite infested cells (77%). Moreover, the bees of these colonies removed 80% of mites from parasitized cells and mite fertility was low. These traits were found in a naturally evolved population of honey bees that had not been treated with acaricides, indicating the potential effect of natural selection (Rodríguez-Luis et al., 2022). Nevertheless, despite the studies that suggest that recapping behavior may be a relevant mechanism of mite resistance, the relative contribution of recapping to varroa mite resistance in honey bee colonies warrants further studies. Very few studies on this matter have been conducted and thus, knowledge about the relationship of the behavior and VIL in honey bee colonies is still needed to determine its relative contribution to Varroa-resistance, particularly for honey bee populations in LA.
5.2 Grooming behavior
Grooming behavior, the ability of bees to remove dust or pollen, as well as mites from their own bodies (auto-grooming) or from the body of other bees (allo-grooming), using legs and mandibles (Boecking and Spivak, 1999), is a trait that contributes to varroa mite resistance in the Asiatic honey bee, A. cerana (Peng et al., 1987; Büchler et al., 1992). Grooming behavior is also expressed in A. mellifera, but at a lower frequency than in A. cerana (Fries et al., 1996). Bees from colonies that are resistant to V. destructor tend to perform intense grooming instances when exposed to the mite and are more successful at removing and injuring mites than bees from mite-susceptible colonies (Guzman-Novoa et al., 2012; Invernizzi et al., 2016; Morfin et al., 2020a).
Grooming behavior is influenced by genetic effects and therefore, presumed to be heritable. Moretto et al. (1993) using direct observation of grooming instances of mite-parasitized bees calculated a heritability index of 0.71 for this trait (Moretto et al., 1993). Moreover, candidate genes influencing grooming behavior have been pinpointed in AHB and EHB populations in different countries of the Americas (Arechavaleta-Velasco et al., 2012; Hamiduzzaman et al., 2017; Morfin et al., 2023a), which indicates genetic and heritable effects on the behavior.
Populations of AHBs from Brazil (Moretto et al., 1993, Moretto et al., 1995; Moretto and Mello, 1999; Corrêa-Marques et al., 2000), Mexico (Arechavaleta-Velasco and Guzman-Novoa, 2001; Mondragón et al., 2005; Guzman-Novoa et al., 2012), Puerto Rico (Rivera-Marchand et al., 2012), and Uruguay (Invernizzi et al., 2016; Mendoza et al., 2020), have shown low VIL associated to high proportions of damaged mites recovered from the bottom board of hives, suggesting an effect of grooming behavior on the resistance of honey bee colonies to V. destructor in LA. For example, one study conducted in Mexico found indirect evidence of grooming superiority of AHBs over EHBs. The proportion of injured mites collected from sticky papers installed on the bottom board of hives, explained most of the variation in V. destructor population growth, and there was a high negative correlation between V. destructor population growth and number of injured mites (r = - 0.76) (Arechavaleta-Velasco and Guzman-Novoa, 2001). Mondragón et al. (2005) also reported a significant negative correlation between VIL and proportion of injured mites (r= - 0.51) in the AHB colonies of their one-year study. In another study, Guzman-Novoa et al. (2012) compared AHB and EHB colonies for injured mites. In EHB colonies, 16% of the mites collected from sticky boards were injured, whereas more than 26% of them were damaged in AHB colonies (Guzman-Novoa et al., 2012). Likewise, Invernizzi et al. (2016) reported rates of mutilated mites recovered from hive bottom boards of 18% and 29% for Italian and AHB colonies, respectively. Moreover, Junkes et al. (2007) found a continuously increasing number of live and dead mites dropped on the bottom board of hives as the amount of brood of AHB colonies decreased over time, suggesting the effect of bees’ grooming behavior. Furthermore, Moretto (2002) analyzed rates of live and dead mite drop in broodless AHB and Carniolan honey bee colonies. The daily proportion of fallen mites was three times higher in AHB colonies than in Carniolan honey bee colonies, further supporting the conclusion that grooming behavior was responsible for the differences in mite drop since the total mite population of the tested colonies was parasitizing adult bees (Moretto, 2002). Grooming behavior assessed by the proportion of injured mites has also been associated to low VIL in a few EHB populations in LA. For example, Eguaras et al. (1995) and Russo et al. (2020) found evidence of high expression of grooming behavior as the main mechanism involved in the low VIL and survival of EHB colonies in Argentina.
Clearly, grooming behavior as determined by the proportion of injured mites, seems to be a robust trait to select for honey bee resistance against V. destructor. Therefore, researchers in LA have recommended evaluating this trait for breeding programs aimed at increasing resistance of A. mellifera to V. destructor infestations (Moretto et al., 1995; Guzman-Novoa et al., 1996, Guzman-Novoa et al., 2012; Russo et al., 2020). In fact, selecting honey bees for increased varroa mite mutilation has been very successful in a breeding program conducted in the USA (Andino and Hunt, 2011; Morfin et al., 2020b). This breeding program produced a honey bee genotype that has demonstrated resistance to V. destructor and has resulted in significantly higher colony survival rates than those of control colonies in the studies. Vandame et al. (2002) also found that grooming behavior was more intense in AHBs than in EHBs, but the authors considered this difference insufficient to maintain V. destructor infestations below colony treatment thresholds.
Most of the above-mentioned studies were conducted in colonies where grooming behavior was evaluated by estimating body damage rates of mites recovered from the bottom board of hives. This is an indirect method for evaluating grooming behavior (Guzman-Novoa et al., 2024) that not only indicates active grooming by adult bees but may also indicate VSH performed by bees that damage and drop mites when uncapping parasitized cells (Boecking and Spivak, 1999; Kirrane et al., 2018). For example, Corrêa-Marques et al. (2000) found a significant and negative correlation between mite leg mutilation and VIL in bee brood, but not in VIL of adult bees, suggesting a VSH effect. Mite damage may also be caused by wax moths and other pests (Boecking and Spivak, 1999). Consequently, researchers must be cautious when using indirect methods to evaluate honey bee grooming behavior at the colony level, because a number of the mites collected from hive bottom boards actually come from brood cells (Lobb and Martin, 1997; Rosenkranz et al., 1997) rather than from active bee grooming. It is therefore likely that a portion of the damaged mites found in mite-resistant honey bee colonies result from the cleaning of mite-parasitized cells by hygienic bees, or caused by wax moth infestations, or by other pests.
Despite the drawbacks of indirect methods for assessing the grooming behavior of honey bees, significant correlations have been found between mite removal rates in laboratory tests and mutilated mites collected from hive bottom boards. These correlations have been found in both EHB and AHB populations (Andino and Hunt, 2011; Guzman-Novoa et al., 2012; Invernizzi et al., 2016; Morfin et al., 2020b), indicating that mite mutilation may be an acceptable proxy for partially evaluating grooming behavior of honey bees, as well as other potential mechanisms of resistance such as VSH working in synergy with grooming behavior.
Direct methods of evaluating grooming behavior have been used in studies conducted with workers of EHB and AHB colonies. Most methods imply direct observation of the bees confined in Petri dishes or observation hives (Moretto et al., 1993; Aumeier, 2001; Invernizzi et al., 2016; Morfin et al., 2020a), which is time consuming but more reliable than using indirect methods. When AHBs have been evaluated for grooming behavior using direct methods, they have shown to be more efficient groomers than EHBs in terms of mite removal success in some studies (Moretto et al., 1993; Aumeier, 2001; Guzman-Novoa et al., 2012). For example, higher proportions of AHBs groomed, and initiated grooming instances significantly faster than EHBs in studies conducted in Mexico (Guzman-Novoa et al., 2012; Morfin et al., 2020a). These results agree with findings from a study conducted in Brazil, in which AHBs removed seven times more mites from their bodies than Italian bees (Moretto et al., 1993). Conversely, a study conducted in Uruguay reported no differences in the proportion of dislodged mites between AHBs and EHBs, but AHBs performed more grooming instances than EHBs (Invernizzi et al., 2016). The rate of mite removal success in AHBs has been attributed to a more frequent and intense grooming compared to Varroa-susceptible or EHB genotypes (Aumeier, 2001; Guzman-Novoa et al., 2012; Hamiduzzaman et al., 2017; Morfin et al., 2020a).
5.3 Mite non-reproduction related mechanisms
Mite non-reproduction (MNR) was initially called suppressed mite reproduction (SMR) because it was believed that traits expressed by honey bee brood had an inhibitory effect on the ability of V. destructor to reproduce (Harbo and Harris, 1999). This trait was measured by determining the proportion of mites that were infertile (Harbo and Harris, 2003). Ritter and De Jong (1984) compared reproductive parameters of V. destructor in EHB colonies kept in Europe and in AHB colonies kept in Brazil. They found that a proportion of foundress female mites did not reproduce when infesting brood of the two bee genotypes, but the rate of MNR was twice as high in AHB colonies compared to EHB colonies. Also in Brazil, Camazine (1986) analyzed varroa mite reproduction on AHB and EHB brood by introducing combs containing larvae of both bee types in mite-infested colonies. He found that in a common environment, 35% less mites reproduced on AHB brood, compared to EHB brood. Additional studies also reported that reduced V. destructor fertility appears to be an important factor involved in the resistance of AHBs to this mite in Brazil (Rosenkranz and Engels, 1994; Rosenkranz, 1999). Conversely, studies conducted in Mexico reported mite fertility rates above 80% and up to 96% in AHB colonies (Guzman-Novoa et al., 1999; Medina and Martin, 1999; Vandame et al., 1999; Medina et al., 2002; Mondragón et al., 2005), which are similar to those of EHB colonies. However, VIL were lower in AHB colonies than in EHB colonies in those studies.
Many hypotheses have been proposed to explain why a percentage of varroa mites do not reproduce in parasitized brood. One explanation is that VSH bees quickly remove mites actively reproducing in cells, leaving a few of them that do not reproduce. However, VSH cannot explain all NMR. Brood effects may inhibit mite reproduction too, as shown in some studies (Behrens et al., 2011; Broeckx et al., 2019). This trait is shared by several honey bee populations that demonstrate resistance to V. destructor (Rosenkranz et al., 2010; Nganso et al., 2018). One of the mechanisms involved in inhibiting mite reproduction on the brood of some honey bee genotypes appears to be linked to larval ecdysone titers. The mite requires ecdysone obtained from the larva to initiate vitellogenesis. If the larva does not produce enough ecdysone, this could affect mite reproduction (Conlon et al., 2019; Mondet et al., 2020). Additionally, other larval compounds could also be involved in inhibiting mite reproduction (Frey et al., 2013; Broeckx et al., 2019).
Low mite fecundity is consistently found in presumed Varroa-resistant populations of honey bees across LA countries. The low mite fecundity rates found in these bee populations are mainly associated to a reduced production of viable female mite progeny in colonies of the resistant bees. Viable female mites are those that can reach the adult stage and are able to mate (Medina and Martin, 1999; Corrêa-Marques et al., 2003). The reduced number of viable offspring per reproductive female mite is similarly low in honey bee colonies from several LA countries. For example, the proportion of viable female offspring of V. destructor in AHB colonies in Mexico, Costa Rica, and Brazil, ranged between 0.30 and 0.40 in different studies (Medina and Martin, 1999; Calderón et al., 2003; Corrêa-Marques et al., 2003). Fewer viable female mites are produced in AHB colonies compared to EHB colonies, mainly because higher mite progeny mortality, particularly of males, occurs in AHB colonies (Medina and Martin, 1999; Medina et al., 2002). Similarly, Mondragón et al., 2006) concluded that mite offspring mortality is an important component in the resistance of AHBs to V. destructor because it reduces the number of mated female offspring produced per mother mite. In Costa Rica, Calderón et al. (2012) found that less than 38% of female mites invading cells containing AHB larvae produced viable offspring, compared to 65% in drone cells. They also found that almost 24% of the male mite offspring died in worker cells. The authors concluded that male mite mortality and absence of male mites in infested cells, are among the major factors contributing to the production of many unviable female mites in AHB colonies, and therefore to the resistance of these bees to V. destructor. A study conducted in Kenya further confirmed that low levels of V. destructor infestations are associated with low rates of viable mite offspring in A. m. scutellata colonies (Nganso et al., 2018).
The reasons why many mites’ progeny die in presumed Varroa-resistant honey bee colonies are not well understood. It has been proposed that larvae movements during pupation injure or reduce the feeding activity of mites on the host, or that mite eggs are damaged due to movements when the prepupa molts into a pupa (Calderón et al., 2009; Calderón et al., 2012). Mite population growth may be limited by these potential mechanisms, but there is still growth because foundress mites are able to complete up to three reproductive cycles (Martin and Kemp, 1997), and because mites produce more progeny in drone brood than in worker brood (Calderón et al., 2007).
5.4 Brood attractiveness
Another factor that may affect the reproductive potential and abundance of varroa mites in AHB and EHB colonies is the relative attractiveness of their larvae to the parasites. Varroa mites are attracted to cells about to be capped by pheromones and cuticular hydrocarbons produced by the larvae (Le Conte et al., 1989; Trouiller et al., 1991). In a study in which larvae and bees of the two genotypes were co-fostered in common colonies that were heavily infested with V. destructor, mite infestation rates were twice as high in EHB brood than in AHB brood, although mite reproduction did not vary between EHB and AHB infested brood (Guzman-Novoa et al., 1996). Similar results were reported by Aumeier et al. (2002) after comparing the attractiveness of AHB and EHB worker larvae to varroa mites. These results suggest that the attractiveness of AHB larvae may not have a direct impact on V. destructor reproductive rate. However, it may affect the reproductive potential of V. destructor by modifying the choice of the mite to infest a given cell, which could slow down the mite’s population growth in AHB colonies (Junqueira et al., 2004).
5.5 Other mechanisms of resistance
Other potential mechanisms of resistance to varroa mites in AHB populations have been suggested, but the results of studies comparing AHBs and EHBs are insufficient, inconclusive, or contradictory. One such presumed mechanism is brood cell size. Some studies have concluded that the narrower brood cells of AHBs reduce the infestation and reproductive rates of V. destructor (Message and Gonçalves, 1995; Piccirillo and De Jong, 2003, Piccirillo and De Jong, 2004; Maggi et al., 2010). However, studies with EHBs have not found an effect of cell size on V. destructor population growth (Berry et al., 2010; Coffey et al., 2010). Another such mechanism is short brood capping duration, but studies conducted in LA have not demonstrated a significant relationship between length of the brood capping stage and varroa mite resistance in honey bee populations (Guzman-Novoa et al., 1996). Similarly, in Africa, the post-capping period of A. m. scutellata in worker brood was in average, less than 15 hours shorter than that of A. m. carnica (Moritz, 1985), which may not be long enough to have a significant impact on restraining V. destructor population growth. Swarming frequency affects V. destructor population levels and growth in honey bee colonies because the bees leaving the original nest carry more than one third of the colony’s mites (Fries et al., 2003; Loftus et al., 2016). Additionally, swarmed colonies with no brood, expose mites to the grooming behavior of bees, and the same occurs with the original colony when it experiences brood interruption due to the swarming event. Colonies of AHBs tend to swarm significantly more frequently than EHB colonies (Schneider et al., 2004) and thus, this is a factor contributing to lower rates of varroa mite infestations in AHB colonies compared with EHB colonies in LA. Absconding behavior, the abandoning of a nest by most of the adults of a honey bee colony, is frequently observed in bees of A. m. scutellata descent (Schneider et al., 2004). This behavior might also reduce to some degree mite infestation levels of the absconding swarm. However, the absconding bees likely carry most of the mites with them when they leave, because nests are abandoned with few brood cells present in them (Winston et al., 1979; Cheruiyot et al., 2020). There are not LA studies analyzing the effect of this bee behavior on varroa mite populations, something that warrants further investigation. Similarly, no studies in LA have analyzed the population growth of V. destructor in honey bee colonies of different genotypes during times of the year of reduced brood production. In addition to the above, colony size could affect varroa mite population dynamics, however, studies conducted in several LA regions have found that colonies of African and European descent that are established in hives in the same locations do not significantly vary in size (Medina-Flores et al., 2014a, Medina-Flores et al., 2019). Table 2 summarizes the mechanisms that are thought to confer resistance against V. destructor to honey bee populations in LA.
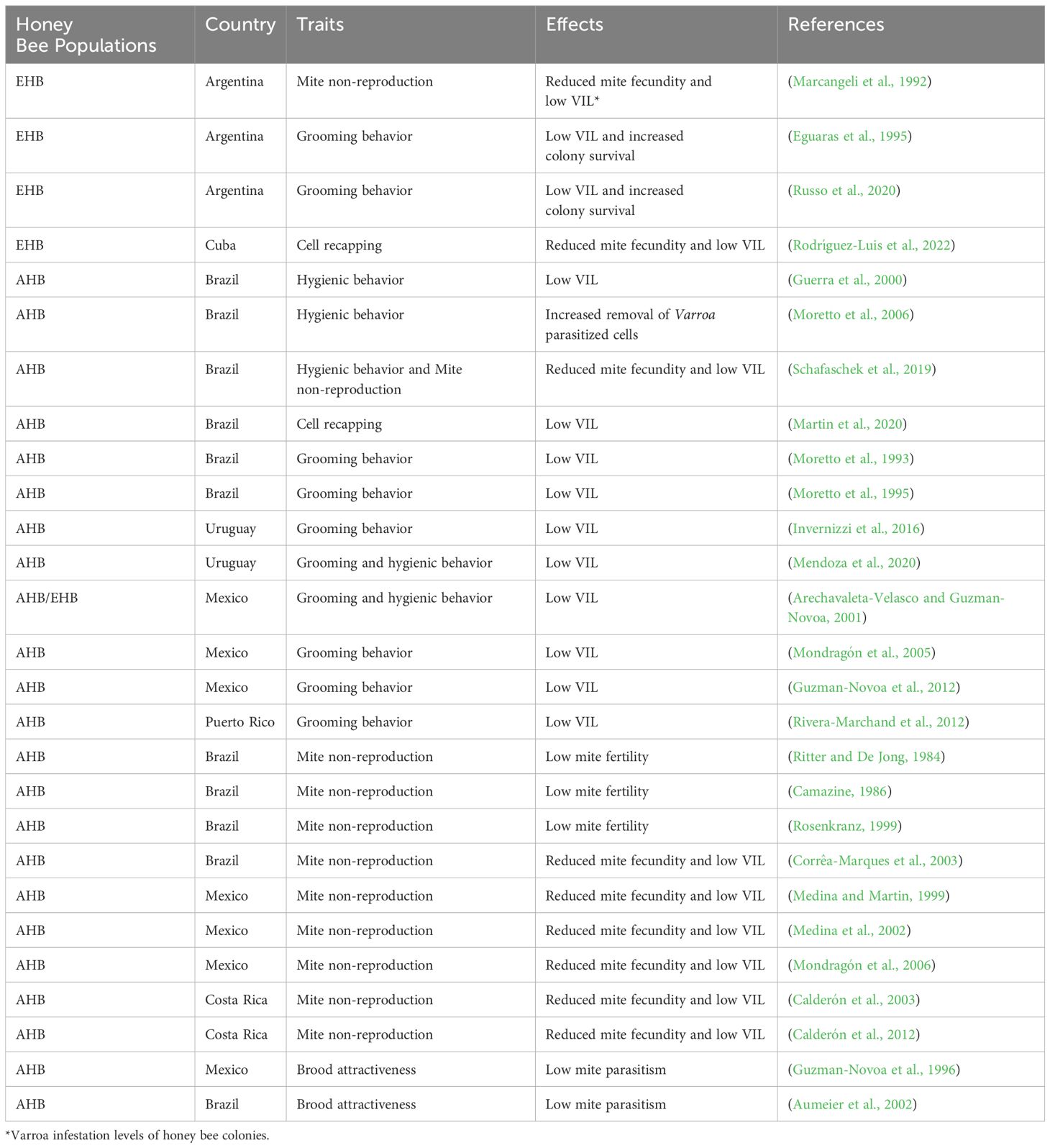
Table 2 Traits and effects associated to Varroa destructor resistance in European (EHB) and Africanized (AHB) honey bee populations in Latin America.
6 Final remarks and future research
In this review, aspects of V. destructor haplotypes and A. mellifera populations interacting in LA, as well as evidence of resistance to the mite, and mechanisms of resistance of some honey bee genotypes, are discussed. The Korean haplotype of V. destructor predominates infesting populations of honey bees in LA, but not much more is known about other variants of the mite, and how they differ in virulence in honey bee populations in the Americas. Accordingly, more genetic studies of the mite are needed, particularly using full genome sequence comparisons. By analyzing full genomes, it will be possible to compare different populations of V. destructor and correlate them with their impact on honey bee colonies in different regions of LA.
The existing populations of honey bees in LA are mainly derived from several subspecies of EHBs, as well as from the African subspecies A. m. scutellata that admixed with the existing population of EHB subspecies, which resulted in the generation of a hybrid population of neotropical bees (AHBs). These tropically adapted bees have demonstrated resistance to V. destructor by maintaining low VIL in their colonies. Evidence of resistance to the mite in some temperate adapted bees (EHB populations) has also been documented in several LA countries.
The main mechanisms known to be involved in providing resistance to honey bee populations against V. destructor in LA, include hygienic and grooming behavior, as well as MNR related mechanisms, mainly low mite fecundity, and more specifically, reduced production of fertile, mite female progeny. Other mechanisms that could be associated to V. destructor-resistance in populations of honey bees in LA may include VSH and recapping behavior of the bees. However, these mechanisms are still poorly understood and studied in LA scenarios. Therefore, research on these potential mechanisms of resistance of honey bees to varroa mites is warranted. Although it is known that some mechanisms are important contributors to the overall resistance of honey bees to V. destructor (Figure 2), it is critical that future research unveils the relative contribution of each of the several mechanism that confer Varroa-resistance to honey bees. Researchers have wondered about whether the traits that seem to confer resistance to honey bee populations in LA are pre-existing adaptations that allowed the bees to cope with the mites, or whether they evolved and increased in expression with time after honey bees interacted with V. destructor in regions where colonies have not been treated with acaricides for many years. The comparison of different honey bee populations from LA (mainly EHB and AHB populations), suggests the existence of conserved evolutionary pre-adaptations that restrain V. destructor population growth, especially in AHBs. Since AHB populations have successfully thrived over decades with no treatments against V. destructor, it is conceivable to presume that these populations have successfully used pre-adaptations to confront V. destructor parasitism. Populations of AHBs have consistently demonstrated lower VIL across years and seasons compared with EHBs in different LA countries. Therefore, it is probable that some characteristics inherited from A. m. scutellata, such as increased grooming and Varroa-specific hygienic behavior could have made AHBs initially more resistant to the mite (Moretto et al., 1993; Guerra et al., 2000; Cheruiyot et al., 2018). Moreover, it also would be expected that AHB and EHB populations developed further adaptations or increased the expression of pre-existing adaptations to counteract V. destructor parasitism if not treated with acaricides for a long time.
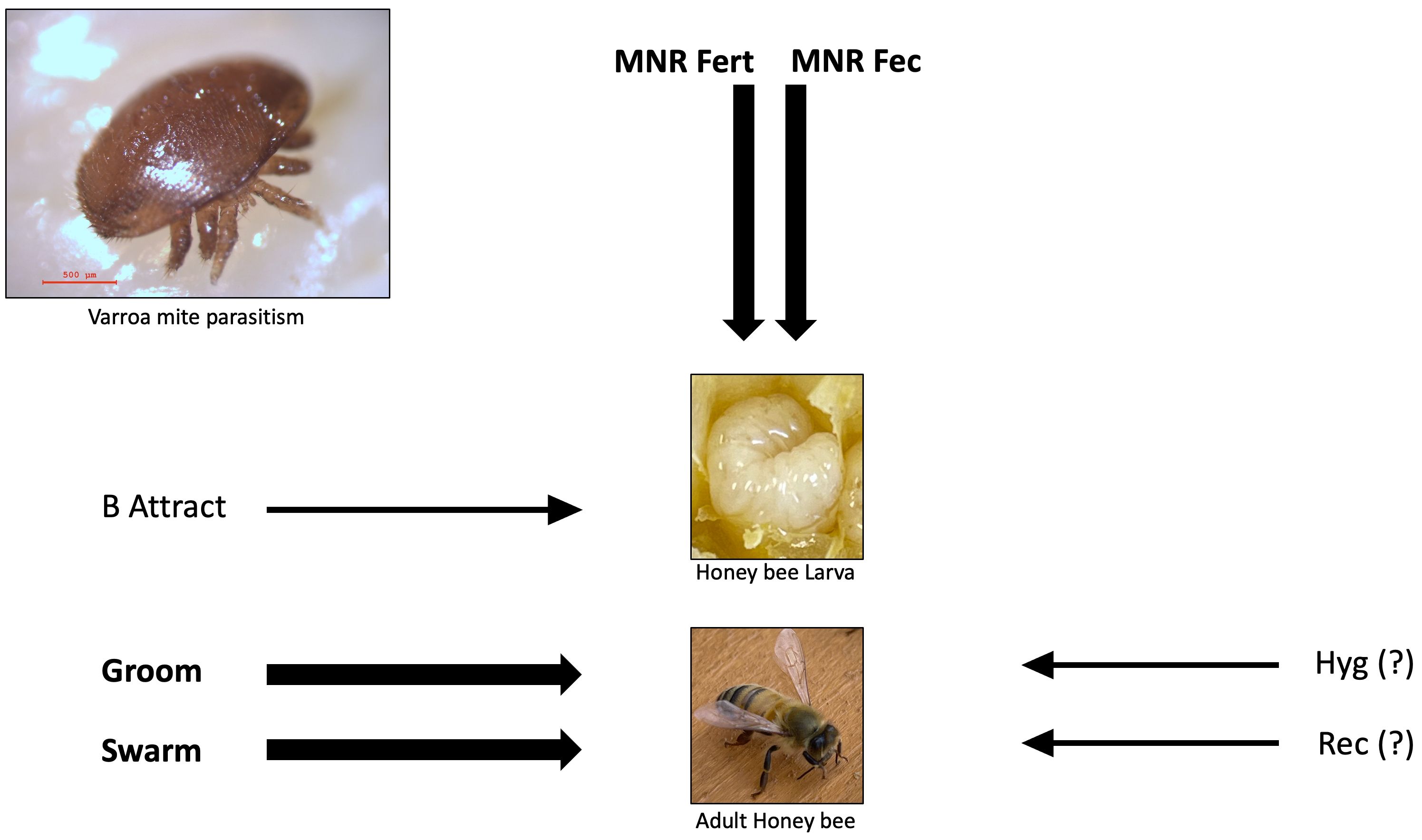
Figure 2 Predominant mechanisms of resistance to the parasitic mite Varroa destructor in honey bee populations in Latin America, and whether they operate on the brood or adult bees. The thickness of the arrows indicates whether a mechanism has a high, or a moderate or unknown contribution to mite resistance as arbitrarily inferred from an extensive review of the currently available literature. A thick line indicates a high contribution, while a thin line indicates moderate or unknown (?) contribution. The mechanisms depicted are mite non reproduction affecting fertility (MNR Fert), mite non reproduction affecting fecundity (MNR Fec), hygienic behavior (Hyg), cell recapping (Rec), brood attractiveness (B Attract), frequent swarming (Swarm), and grooming behavior (Groom).
It is likely that the alleles for resistance traits such as grooming and hygienic behaviors, as well as brood effects on MNR, were pre-adaptations that existed at higher frequencies in bees of African ancestry than in bees of European ancestry, which has resulted in honey bee populations in LA that are relatively more resistant to V. destructor compared to populations in Europe or North America. However, very few studies using genomic techniques have been carried out to identify candidate genes associated with resistance traits to V. destructor in honey bee populations. Examples of such studies conducted with honey bee populations in LA, include the discovery of Neurexin, a gene highly associated with intense grooming behavior (Arechavaleta-Velasco et al., 2012), and genes associated with hygienic behavior (Scannapieco et al., 2017). Nevertheless, more studies on the genetic basis of Varroa-resistance mechanisms, particularly genomic studies in honey bee populations of LA are warranted. These studies could lead to the identification of molecular markers that could be used for marker-assisted selection (MAS) in selective breeding programs. Additionally, it is critical to more accurately measure the heritability of traits involved in providing mite resistance to honey bees.
Except for hygienic and grooming behavior, other mechanisms of resistance to V. destructor that have been studied in honey bee populations surviving mite infestations in LA are still poorly understood. A better understanding about how different mechanisms provide resistance to honey bee populations is important for the scientific and beekeeping communities. For the scientific community, unraveling how natural selection has operated to select certain resistance traits will shed light in the complex parasite-host interaction between honey bees and a harmful parasitic mite. For the beekeeping community, understanding how and how much different mechanisms provide resistance to the bees against V. destructor, and whether or not these traits are heritable, is relevant to establish the basis of selective breeding programs aimed at developing resistant stocks of honey bees for the control of V. destructor infestations.
Many questions remain to be answered regarding the reproductive biology of V. destructor parasitizing honey bee populations in LA and other regions of the world. For example, is there a difference in the number of mite reproductive cycles occurring in resistant and susceptible bee genotypes?, is there a difference in the time that varroa mites spend during their phoretic phase when parasitizing resistant or susceptible bee genotypes?, what causes mite offspring to die in brood cells?, when measuring NMR related traits like mite fertility and fecundity, are we also indirectly measuring other traits conferring resistance to honey bees against the mite like VSH or cell recapping?, what proportion of the fallen and mutilated mites recovered from the bottom board of hives are a consequence of natural mortality, grooming behavior, VSH, or other causes?, how much colony swarming or absconding frequency affects the population dynamics of V. destructor in mite resistant and susceptible honey bee genotypes?
The honey bee populations showing resistance to V. destructor in LA have traits that seem to work additively or synergistically to allow colony survival without acaricide treatments. However, the relative contribution of each of the mechanisms of resistance should be investigated to strategically incorporate them into selective breeding programs. This review supports the notion that selective breeding of local honey bee populations from LA could be a viable strategy to manage varroa mite infestations in their colonies.
Author contributions
EG-N: Writing – original draft. MC: Writing – original draft, Conceptualization. MA: Writing – review & editing. FJR: Writing – review & editing. CI: Writing – review & editing. GFL: Writing – review & editing. MM: Writing – original draft.
Funding
The author(s) declare that no financial support was received for the research, authorship, and/or publication of this article.
Conflict of interest
The authors declare that the research was conducted in the absence of any commercial or financial relationships that could be construed as a potential conflict of interest.
Publisher’s note
All claims expressed in this article are solely those of the authors and do not necessarily represent those of their affiliated organizations, or those of the publisher, the editors and the reviewers. Any product that may be evaluated in this article, or claim that may be made by its manufacturer, is not guaranteed or endorsed by the publisher.
References
Abban S., Smith B., Corona M., Cook S. C., Evans J. D., Chen Y., et al. (2024). Prevalence and distribution of Varroa destructor and Nosema spp. in symptomatic honey bee colonies across the USA from 2015 to 2022. Sci. Rep. 14, 1726. doi: 10.1038/s41598-024-51514-9
Abrahamovich A. H., Atela O., de la Rúa P., Galián J. (2007). Assessment of the mitochondrial origin of honey bees from Argentina. J. Apic. Res. 46, 191–194. doi: 10.1080/00218839.2007.11101391
Acevedo-Gonzalez J. P., Galindo-Cardona A., Avalos A., Whitfield C. W., Rodriguez D. M., Uribe-Rubio J. L. (2019). Colonization history and population differentiation of the honey bees (Apis mellifera L.) in Puerto Rico. Ecol. Evol. 9, 10895–10902. doi: 10.1002/ece3.5330
Alves S. B., Flechtmann C. H., Rosa A. E. (1978). Varroa jacobsoni oudeman(Acari, mesostigmata, varroidae) also in Brazil. Ecossistema 3, 78–779.
Anderson D. L., Trueman J. W. (2000). Varroa jacobsoni (Acari: Varroidae) is more than one species. Exp. Appl. Acarol. 24, 165–189. doi: 10.1023/A:1006456720416
Andino G. K., Hunt G. J. (2011). A scientific note on a new assay to measure honey bee mite-grooming behavior. Apidologie 42, 481–484. doi: 10.1007/s13592-011-0004-1
Arechavaleta-Velasco M. E., Alcala-Escamilla K., Robles-Rios C., Tsuruda J. M., Hunt G. J. (2012). Fine-scale linkage mapping reveals a small set of candidate genes influencing honey bee grooming behavior in response to Varroa mites. PloS One 7, e47269. doi: 10.1371/journal.pone.0047269
Arechavaleta-Velasco M. E., Guzman-Novoa E. (2000). Producción de miel de colonias de abejas (Apis mellifera L.) tratadas y no tratadas con fluvalinato contra Varroa jacobsoni Oudemans en Valle de Bravo, Estado de Mexico. Vet. Mex 31, 381–384.
Arechavaleta-Velasco M. E., Guzman-Novoa E. (2001). Relative effect of four characteristics that restrain the population growth of the mite Varroa destructor in honey bee (Apis mellifera) colonies. Apidologie 32, 157–174. doi: 10.1051/apido:2001121
Aumeier P. (2001). Bioassay for grooming effectiveness towards Varroa destructor. Apidologie 32, 81–90. doi: 10.1051/apido:2001113
Aumeier P., Rosenkranz P., Francke W. (2002). Cuticular volatiles, attractivity of worker larvae and invasion of brood cells by Varroa mites. A comparison of Africanized and European honey bees. Chemoecology 12, 65–75. doi: 10.1007/s00049-002-8328-y
Aumeier P., Rosenkranz P., Gonçalves L. S. (2000). A comparison of the hygienic response of Africanized and European (Apis mellifera carnica) honey bees to Varroa-infested brood in tropical Brazil. Genet. Mol. Biol. 23, 787–791. doi: 10.1590/S1415-47572000000400013
Büchler R., Drescher W., Tornier I. (1992). Grooming behavior of Apis cerana, Apis mellifera and Apis dorsata and its effect on the parasitic mites Varroa jacobsoni and Tropilaelaps clareae. Exp. Appl. Acarol. 16, 313–319. doi: 10.1007/BF01218573
Beaurepaire A., Piot N., Doublet V., Antunez K., Campbell E., Chantawannakul P. (2020). Diversity and global distribution of viruses of the western honey bee, Apis mellifera. Insects 11, 239. doi: 10.3390/insects11040239
Behrens D., Huang Q., Geßner C., Rosenkranz P., Frey E., Locke B. (2011). Three QTL in the honey bee Apis mellifera L. suppress reproduction of the parasitic mite Varroa destructor. Ecol. Evol. 1, 451–458. doi: 10.1002/ece3.17
Berry J. A., Owens W. B., Delaplane K. S. (2010). Small-cell comb foundation does not impede Varroa mite population growth in honey bee colonies. Apidologie 41, 40–44. doi: 10.1051/apido/2009049
Boecking O., Dreschner W. (1991). Response of Apis mellifera L colonies infested with Varroa jacobsoni Oud. Apidologie 22, 237–241. doi: 10.1051/apido:19910308
Boecking O., Spivak M. (1999). Behavioral defenses of honey bees against Varroa jacobsoni Oud. Apidologie 30, 141–158. doi: 10.1051/apido:19990205
Bouga M., Alaux C., Bienkowska M., Büchler R., Carreck N. L., Cauia E. (2011). A review of methods for discrimination of honey bee populations as applied to European beekeeping. J. Apic. Res. 50, 51–84. doi: 10.3896/IBRA.1.50.1.06
Branchiccela B., Aguirre C., Parra G., Estay P., Zunino P., Antúnez K. (2014). Genetic changes in Apis mellifera after 40 years of Africanization. Apidologie 45, 752–756. doi: 10.1007/s13592-014-0293-2
Broeckx B. J. G., De Smet L., Blacquière T., Maebe K., Khalenkow M., Van Poucke M. (2019). Honey bee predisposition of resistance to ubiquitous mite infestations. Sci. Rep. 9, 7794. doi: 10.1038/s41598-019-44254-8
Calderón R. A., Fallas N., Zamora L. G., Van Veen J. W., Sanchez L. A. (2009). Behavior of varroa mites in worker brood cells of Africanized honey bees. Exp. Appl. Acarol. 49, 329–338. doi: 10.1007/s10493-009-9266-y
Calderón R. A., Ortiz A., Aparisio B., Ruiz M. T. (2000). Varroa in Panama: detection, spread and prospects. Bee World 81, 126–128. doi: 10.1080/0005772X.2000.11099482
Calderón R. A., Sommeijer M. J., De Ruijter A., Van Veen J. W. (2003). The reproductive ability of Varroa destructor in worker brood of Africanized and hybrid honey bees in Costa Rica. J. Apic. Res. 42, 65–67. doi: 10.1080/00218839.2003.11101095
Calderón R. A., Ureña S., van Veen J. W. (2012). Reproduction of Varroa destructor and offspring mortality in worker and drone brood cells of Africanized honey bees. Exp. Appl. Acarol. 56, 297–307. doi: 10.1007/s10493-012-9518-0
Calderón R. A., Zamora L. G., Van Veen J. W., Quesada M. V. (2007). A comparison of the reproductive ability of Varroa destructor (Mesostigmata: Varroidae) in worker and drone brood of Africanized honey bees (Apis mellifera). Exp. Appl. Acarol. 43, 25–32. doi: 10.1007/s10493-007-9102-1
Camazine S. (1986). Differential reproduction of the mite, Varroa jacobsoni (Mesostigmata: Varroidae), on Africanized and European honey bees (Hymenoptera: Apidae). Ann. Entomol. Soc Am. 79, 801–803. doi: 10.1093/aesa/79.5.801
Carneiro F. E., Torres R. R., Strapazzon R., Ramírez S. A., Guerra J. C. Jr., Koling D. F., et al. (2007). Changes in the reproductive ability of the mite Varroa destructor (Anderson e Trueman) in Africanized honey bees (Apis mellifera L.) (Hymenoptera: Apidae) colonies in southern Brazil. Neotrop. Entomol. 36, 949–952. doi: 10.1590/S1519-566X2007000600018
Casanova O., Perruolo G. (1992). Parasitic diseases of the honey bee Apis mellifera in Tachir, Venezuela. Vida Apícola 54, 20–24.
Castilhos D., Polesso A. M., da Silva A. C. F., dos Santos A. B., de Carvalho Lopes A. T., de Oliveira Filho A. A., et al. (2023). Varroa destructor infestation levels in Africanized honey bee colonies in Brazil from 1977 when first detected to 2020. Apidologie 54, 5. doi: 10.1007/s13592-022-00984-9
Chakroborty N. K., Bienefeld K., Menzel R. (2015). Odor learning and odor discrimination of bees selected for enhanced hygienic behavior. Apidologie 46, 499–514. doi: 10.1007/s13592-014-0342-x
Chapman N. C., Harpur B. A., Lim J., Rinderer T. E., Allsopp M. H., Zayed A. (2015). A SNP test to identify Africanized honeybees via proportion of African ancestry. Mol. Ecol. Resour. 15, 1346–1355. doi: 10.1111/1755-0998.12411
Cheruiyot S. K., Kahuthia-Gathu R., Mbugi J. P., Muli E., Lattorff H. M. G. (2020). Population abundance of Varroa destructor and its effects on Apis mellifera scutellata colonies in Kenya. Exp. Appl. Acarol. 82, 171–184. doi: 10.1007/s10493-020-00548-5
Cheruiyot S. K., Lattorff H. M. G., Kahuthia-Gathu R., Mbugi J. P., Muli E. (2018). Varroa-specific hygienic behavior of Apis mellifera scutellata in Kenya. Apidologie 49, 439–449. doi: 10.1007/s13592-018-0570-6
Coffey M. F., Breen J., Brown M. J. F., McMullan J. B. (2010). Brood-cell size has no influence on the population dynamics of Varroa destructor mites in the native western honey bee, Apis mellifera mellifera. Apidologie 41, 522–530. doi: 10.1051/apido/2010003
Collet T., Ferreira K. M., Arias M. C., Soares A. E. E., Del Lama M. A. (2006). Genetic structure of Africanized honeybee populations (Apis mellifera L.) from Brazil and Uruguay viewed through mitochondrial DNA COI–COII patterns. Heredity 97, 329–335. doi: 10.1038/sj.hdy.6800875
Conlon B. H., Aurori A., Giurgiu A. I., Kefuss J., Dezmirean D. S., Moritz R. F. A. (2019). A gene for resistance to the Varroa mite (Acari) in honey bee (Apis mellifera) pupae. Mol. Ecol. 28, 2958–2966. doi: 10.1111/mec.15080
Correa-Benítez A., Anguiano-Baez R., Heneidi-Zeckua A., Dávalos-Flores J. L., Peña-Haaz N. T., Pérez-Martínez E. E., et al. (2023). Prevalence of adult honey bee (Apis mellifera L.) pests and pathogens in the five beekeeping regions of Mexico. Animals 13, 1734. doi: 10.3390/ani13111734
Corrêa-Marques M. H., De Jong D. (1998). Uncapping of worker bee brood, a component of the hygienic behavior of Africanized honey bees against the mite Varroa jacobsoni Oudemans. Apidologie 29, 283–289. doi: 10.1051/apido:19980307
Corrêa-Marques M. H., De Jong D., Rosenkranz P., Gonçalves L. S. (2002). Varroa-tolerant Italian honey bees introduced from Brazil were not more efficient in defending themselves against the mite Varroa destructor than Carniolan bees in Germany. Genet. Mol. Res. 1, 153–158.
Corrêa-Marques M. H., Issa M. R. C., De Jong D. D. (2000). Classification and quantification of damaged Varroa jacobsoni found in the debris of honey bee colonies as criteria for selection? Am. Bee J. 140, 820–824.
Corrêa-Marques M. H., Medina L. M., Martin S. J., De Jong D. (2003). Comparing data on the reproduction of Varroa destructor. Genet. Mol. Res. 2, 1–6.
Dainat B., Evans J. D., Chen Y. P., Gauthier L., Neumann P. (2012). Dead or alive: deformed wing virus and Varroa destructor reduce the life span of winter honeybees. Appl. Environ. Microbiol. 78, 981–987. doi: 10.1128/AEM.06537-11
Danka R. G., Rinderer T. E., Spivak M., Kefuss J. (2013). Comments on:”Varroa destructor, research avenues towards sustainable control. J. Apic. Res. 52, 69–71. doi: 10.3896/IBRA.1.52.2.14
Dávila N. M., Ortiz M. S., Martínez P. P., Risco M. (1987). Ensayo sobre control de Varroa jacobsoni en colmenas de Lima. Rev. Peru. Entomol. 30, 74–76.
de Guzman L. I., Rinderer T. E., Frake A. M., Kirrane M. J. (2015). Brood removal influences fall of Varroa destructor in honey bee colonies. J. Apic. Res. 54, 216–225. doi: 10.1080/00218839.2015.1117294
de Guzman L. I., Rinderer T. E., Stelzer J. A. (1997). DNA evidence of the origin of Varroa jacobsoni Oudemans in the Americas. Biochem. Genet. 35, 327–335. doi: 10.1023/A:1021821821728
de Guzman L. I., Rinderer T. E., Stelzer J. A., Anderson D. (1998). Congruence of RAPD and mitochondrial DNA markers in assessing Varroa jacobsoni genotypes. J. Apicult. Res. 37, 49–51. doi: 10.1080/00218839.1998.11100955
De Jong D., Morse R. A., Eickwort G. C. (1982). Mite pests of honey bees. Annu. Rev. Entomol. 27, 229–252. doi: 10.1146/annurev.en.27.010182.001305
De Jong D., Soares A. E. E. (1997). An isolated population of italian bees that has survived Varroa jacobsoni infestation without treatment for over 12 years. Am. Bee J. 137, 742–745.
Delfinado M. (1963). Mites of the honeybee in south east asia. J. Apic. Res. 2, 113–114. doi: 10.1080/00218839.1963.11100070
Demedio J., Sanabria J. L., Roque E., Peñate I., Cartas J. (2002). Reporte del acaro parasito Varroa destructor (Anderson & Trueman) en colmenas (Apis mellifera L.) de una zona de la provincia de la Habana. Rev. Prod. Anim. 14, 37–42.
Desai S. D., Currie R. W. (2016). Effects of wintering environment and parasite–pathogen interactions on honey bee colony loss in north temperate regions. PloS One 11, e0159615. doi: 10.1371/journal.pone.0159615
Dietemann V., Pflugfelder J., Anderson D., Charrière J. D., Chejanovsky N., Dainat B. (2012). Varroa destructor: research avenues towards sustainable control. J. Apic. Res. 51, 125–132. doi: 10.3896/IBRA.1.51.1.15
Domínguez-Ayala R., Moo-Valle H., May-Itzá W. J., Medina-Peralta S., Quezada-Euán J. J. G. (2016). Stock composition of northern Neotropical honey bees: mitotype and morphotype diversity in Mexico (Hymenoptera: Apidae). Apidologie 47, 642–652. doi: 10.1007/s13592-015-0414-6
Düttmann C., Flores B., Sheleby-Elías J., Castillo G., Osejo H., Bermudez S., et al. (2021). Morphotype and haplotype identification of Varroa destructor (Acari: Varroidae), and its importance for apiculture in Nicaragua. Exp. Appl. Acarol. 83, 527–544. doi: 10.1007/s10493-021-00603-9
Eguaras E., Marcangeli K., Oppedisano N., Fernandez N. (1995). Mortality and reproduction of Varroa jacobsoni in resistant colonies of honey bees (Apis mellifera) in Argentina. Bee Sci. 3, 174–178.
Elzen P. J., Baxter J. R., Spivak M., Wilson W. T. (2000). Control of Varroa jacobsoni Oud. resistant to fluvalinate and amitraz using cumaphos. Apidologie 31, 437–441. doi: 10.1051/apido:2000134
Frey E., Odemer R., Blum T., Rosenkranz P. (2013). Activation and interruption of the reproduction of Varroa destructor is triggered by host signals (Apis mellifera). J. Invertebr. Pathol. 113, 56–62. doi: 10.1016/j.jip.2013.01.007
Fries I., Hansen H., Imdorf A., Rosenkranz P. (2003). Swarming in honey bees (Apis mellifera) and Varroa destructor population development in Sweden. Apidologie 34, 389–397. doi: 10.1051/apido:2003032
Fries I., Huazhen W., Wei S., Jin C. S. (1996). Grooming behavior and damaged mites (Varroa jacobsoni) in Apis cerana and Apis mellifera ligustica. Apidologie 27, 3–11. doi: 10.1051/apido:19960101
Garrido C., Rosenkranz P., Paxton R. J., Gonçalves L. S. (2003). Temporal changes In Varroa destructor fertility and haplotype in Brazil. Apidologie 53, 535–541. doi: 10.1051/apido:2003041
Giménez-Martínez P., Mendoza Y., Invernizzi C., Fuselli S., Alonso-Salces R., Fernández-Iriarte P., et al. (2017). Morphometric correlation between Apis mellifera morphotypes (Hymenoptera) and Varroa destructor (Acari) from Uruguay. J. Apic. Res. 56, 122–129. doi: 10.1080/00218839.2017.1287998
Gisder S., Aumeier P., Genersch E. (2009). Deformed wing virus: replication and viral load in mites (Varroa destructor). J. Gen. Virol. 90, 463–467. doi: 10.1099/vir.0.005579-0
Goodwin R. M., Taylor M. A., Mcbrydie H. M., Cox H. M. (2006). Drift of Varroa destructor-infested worker honey bees to neighboring colonies. J. Apic. Res. 45, 155–156. doi: 10.1080/00218839.2006.11101335
Gregorc A., Alburaki M., Sampson B., Knight P. R., Adamczyk J. (2018). Toxicity of selected acaricides to honey bees (Apis mellifera) and varroa (Varroa destructor Anderson and Trueman) and their use in controlling varroa within honey bee colonies. Insects 9, 55. doi: 10.3390/insects9020055
Gregorc A., Sampson B. (2019). Diagnosis of varroa mite (Varroa destructor) and sustainable control in honey bee (Apis mellifera) colonies—a review. Diversity 11, 243. doi: 10.3390/d11120243
Grindrod I., Martin S. J. (2021). Parallel evolution of Varroa resistance in honey bees: a common mechanism across continents? Proc. Biol. Sci. 288, 20211375. doi: 10.1098/rspb.2021.1375
Guerra J. C., Gonçalves L. S., De Jong D. (2000). Africanized honey bees (Apis mellifera L.) are more efficient at removing worker brood artificially infested with the parasitic mite Varroa jacobsoni Oudemans than are Italian bees or Italian/Africanized hybrids. Genet. Mol. Biol. 23, 89–92. doi: 10.1590/S1415-47572000000100016
Guerra J. C., Issa M. R., Carneiro F. E., Strapazzon R., Moretto G. (2010). RAPD identification of Varroa destructor genotypes in Brazil and other regions of the Americas. Genet. Mol. Res. 9, 303–308. doi: 10.4238/vol9-1gmr696
Guzman-Novoa E. (2016). “Colony collapse disorder and other threats to honey bees,” in One health case studies: addressing complex problems in a changing world. Eds. Cork S., Hall D. C., Liljebjelke K. (Sheffield, UK: 5M Publishing Ltd), 204–216.
Guzman-Novoa E., Eccles L., Calvete Y., Mcgowan J., Kelly P. G., Correa-Benítez A. (2010). Varroa destructor is the main culprit for the death and reduced populations of overwintered honey bee (Apis mellifera) colonies in Ontario, Canada. Apidologie 41, 443–450. doi: 10.1051/apido/2009076
Guzman-Novoa E., Emsen B., Unger P., Espinosa-Montaño L. G., Petukhova T. (2012). Genotypic variability and relationships between mite infestation levels, mite damage, grooming intensity, and removal of Varroa destructor mites in selected strains of worker honey bees (Apis mellifera L.). J. Invertebr. Pathol. 110, 314–320. doi: 10.1016/j.jip.2012.03.020
Guzman-Novoa E., Morfin N. (2019). “Disease resistance in honey bees (Apis mellifera L.) at the colony and individual levels,” in Comprehensive biotechnology. Ed. Moo-Young M. (Oxford, UK: Elsevier), 811–817. doi: 10.1016/B978-0-444-64046-8.00254-8
Guzman-Novoa E., Morfin N., Dainat B., Williams G. R., van der Steen J., Correa-Benítez A., et al. (2024). Standard methods to estimate strength parameters, flight activity, comb construction, and fitness of Apis mellifera colonies 2.0. J. Apic. Res., 1–22. doi: 10.1080/00218839.2024.2329853
Guzman-Novoa E., Morfin N., de la Mora A., Macías-Macías J. O., Tapia-González J. M., Contreras-Escareño F. (2020). The process and outcome of the Africanization of honey bees in Mexico: lessons and future directions. Front. Ecol. Evol. 8. doi: 10.3389/fevo.2020.608091
Guzman-Novoa E., Sánchez A., Page R. E., García T. (1996). Susceptibility of European and Africanized honeybees (Apis mellifera L.) and their hybrids to Varroa jacobsoni Oud. Apidologie 27, 93–103. doi: 10.1051/apido:19960204
Guzman-Novoa E., Vandame R., Arechavaleta-Velasco M. E. (1999). Susceptibility of European and Africanized honey bees (Apis mellifera L.) to Varroa jacobsoni Oud. in Mexico. Apidologie 30, 173–182. doi: 10.1051/apido:19990207
Hamiduzzaman M. M., Emsen B., Hunt G. J., Subramanyam S., Williams C. E., Tsuruda J. M., et al. (2017). Differential gene expression associated with honey bee grooming behavior in response to Varroa mites. Behav. Genet. 47, 335–344. doi: 10.1007/s10519-017-9834-6
Harbo J. R., Harris J. W. (1999). Selecting honey bees for resistance to Varroa jacobsoni. Apidologie 30, 183–196. doi: 10.1051/apido:19990208
Harbo J. R., Harris J. W. (2003). An evaluation of commercially produced queens that have the SMR trait. Am. Bee J. 143, 213–216.
Harbo J. R., Harris J. W. (2005). Suppressed mite reproduction explained by the behavior of adult bees. J. Apic. Res. 44, 21–23. doi: 10.1080/00218839.2005.11101141
Harpur B. A., Guarna M. M., Huxter E., Higo H., Moon K. M., Hoover S. E. (2019). Integrative genomics reveals the genetics and evolution of the honey bee’s social immune system. Genome Biol. Evol. 11, 937–948. doi: 10.1093/gbe/evz018
Harpur B. A., Kadri S. M., Orsi R. O., Whitfield C. W., Zayed A. (2020). Defense response in Brazilian honey bees (Apis mellifera scutellata × spp.) Is underpinned by complex patterns of admixture. Genome Biol. Evol. 12, 1367–1377. doi: 10.1093/gbe/evaa128
Harris J. W. (2007). Bees with Varroa sensitive hygiene preferentially remove mite infested pupae aged ≤ five days post capping. J. Apic. Res. 46, 134–139. doi: 10.1080/00218839.2007.111013
Harris J. W. (2008). Effect of brood type on Varroa-sensitive hygiene by worker honey bees (Hymenoptera: Apidae). Ann. Entomol. Soc Am. 101, 1137–1144. doi: 10.1603/0013-8746-101.6.1137
Harris J. W., Danka R. G., Villa J. D. (2012). Changes in infestation, cell cap condition, and reproductive status of Varroa destructor (Mesostigmata: Varroidae) in brood exposed to honey bees with Varroa sensitive hygiene. Ann. Entomol. Soc Am. 105, 512–518. doi: 10.1603/AN11188
Hinojosa A., González D. (2004). Prevalencia de parásitos en Apis mellifera L. en colmenares del secano costero e interior de la VI Región, Chile. Parasitol. Latinoam. 59, 137–141. doi: 10.4067/S0717-77122004000300008
Hristov P., Shumkova R., Palova N., Neov B. (2020). Factors associated with honey bee colony losses: A mini-review. Vet. Sci. 7, 166. doi: 10.3390/vetsci7040166
Ibrahim A., Spivak M. (2004). The relationship between the suppression of mite reproduction (SMR) and hygienic behavior. Am. Bee J. 144, 406.
Ibrahim A., Spivak M. (2006). The relationship between hygienic behavior and suppression of mite reproduction as honey bee (Apis mellifera) mechanisms of resistance to Varroa destructor. Apidologie 37, 31–40. doi: 10.1051/apido:2005052
Invernizzi C., Antúnez K., Campa J., Harriet J., Mendoza Y., Santos E., et al. (2011). Situación sanitaria de las abejas melíferas en Uruguay. Veterinaria 47, 15–27.
Invernizzi C., Zefferino I., Santos E., Sánchez L., Mendoza Y. (2016). Multilevel assessment of grooming behavior against Varroa destructor in Italian and Africanized honey bees. J. Apic. Res. 54, 321–327. doi: 10.1080/00218839.2016.1159055
Junkes L., Guerra J., J. C. V., Moretto G. (2007). Varroa destructor mite mortality rate according to the amount of worker broods in Africanized honey bee (Apis mellifera L.) colonies. Acta Sci. Biol. Sci. 29, 305–308.
Junqueira E., Piccirillo G. A., De Jong D. (2004). A semi-natural bioassay for host-finding behavior of Varroa destructor. Am. Bee J. 144, 625–627.
Kevan P. G., Hannan M. A., Ostiguy A., Guzman-Novoa E. (2006). A summary of the varroa-virus disease complex in honey bees. Am. Bee J. 146, 694–697.
Kirrane M. J., de Guzman L. I., Whelan P. M., Frake A. M., Rinderer T. E. (2018). Evaluations of the removal of Varroa destructor in Russian honey bee colonies that display different levels of Varroa sensitive hygienic activities. J. Insect. Behav. 31, 283–297. doi: 10.1007/s10905-018-9672-2
Klein A. M., Vaissière B. E., Cane J. H., Steffan-Dewenter I., Cunningham S. A., Kremen C., et al. (2007). Importance of crop pollinators in changing landscapes for world crops. Proc. Biol. Sci. 274, 303–313. doi: 10.1098/rspb.2006.3721
Koleoglu G., Goodwin P. H., Reyes-Quintana M., Hamiduzzaman M. M., Guzman-Novoa E. (2017). Effect of Varroa destructor, wounding and varroa homogenate on gene expression in brood and adult honey bees. PloS One 12, e0169669. doi: 10.1371/journal.pone.0169669
Koleoglu G., Goodwin P. H., Reyes-Quintana M., Hamiduzzaman M. M., Guzman-Novoa E. (2018). Varroa destructor parasitism reduces hemocyte concentrations and prophenol oxidase gene expression in bees from two populations. Parasitol. Res. 117, 1175–1183. doi: 10.1007/s00436-018-5796-8
Lapidge K. L., Oldroyd B. P., Spivak M. (2002). Seven suggestive quantitative trait loci influence hygienic behavior of honey bees. Naturwissenschaften 89, 565–568. doi: 10.1007/s00114-002-0371-6
Le Conte Y., Arnold G., Trouiller J., Masson C., Chappe B., Ourisson G. (1989). Attraction of the parasitic mite varroa to the drone larvae of honey bees by simple aliphatic esters. Science 245, 638–639. doi: 10.1126/science.245.4918.638
Le Conte Y., Meixner M. D., Brandt A., Carreck N. L., Costa C., Mondet F. (2020). Geographical distribution and selection of European honey bees resistant to Varroa destructor. Insects 11, 873. doi: 10.3390/insects11120873
Lobb N., Martin S. (1997). Mortality of Varroa jacobsoni Oudemans during or soon after the emergence of worker and drone honeybees Apis mellifera L. Apidologie 28, 367–374. doi: 10.1051/apido:19970604
Locke B. (2016). Natural Varroa mite-surviving Apis mellifera honeybee populations. Apidologie 47, 467–482. doi: 10.1007/s13592-015-0412-8
Loftus J. C., Smith M. L., Seeley T. D. (2016). How honey bee colonies survive in the wild: Testing the importance of small nests and frequent swarming. PloS One 11, e0150362. doi: 10.1371/journal.pone.0150362
Lorenzo-Felipe I., Blanco C., Corona M. (2020). Impact of apoidea (Hymenoptera) on the world’s food production and diets. Ann. Entomol. Soc Am. 113, 407–424. doi: 10.1093/aesa/saaa016
Maggi M., Antúnez K., Invernizzi C., Aldea P., Vargas M., Negri P. (2016). Honeybee health in south america. Apidologie 47, 835. doi: 10.1007/s13592-016-0445-7
Maggi M., Medici S., Quintana S., Ruffinengo S., Marcángeli J., Gimenez Martinez P., et al. (2012). Genetic structure of Varroa destructor populations infesting Apis mellifera colonies in Argentina. Exp. Appl. Acarol. 56, 309–318. doi: 10.1007/s10493-012-9526-0
Maggi M. D., Ruffinengo S. R., Damiani N., Sardella N. H., Eguaras M. J. (2009). First detection of Varroa destructor resistance to coumaphos in Argentina. Exp. Appl. Acarol. 47, 317–320. doi: 10.1007/s10493-008-9216-0
Maggi M., Ruffinengo S., Negri P., Eguaras M. (2010). Resistance phenomena to amitraz from populations of the ectoparasitic mite Varroa destructor of Argentina. Parasitol. Res. 107, 1189–1192. doi: 10.1007/s00436-010-1986-8
Magnus R., Szalanski A. L. (2010). Genetic evidence for honey bees (Apis mellifera L.) of middle eastern lineage in the United States. Sociobiology 55, 285–296.
Marcangeli J. A., Eguaras M. J., Fernandez N. A. (1992). Reproduction of Varroa jacobsoni (Acari: Mesostigmata: Varroidae) in temperate climates of Argentina. Apidologie 23, 57–60. doi: 10.1051/apido:19920106
Martin S. J., Hawkins G. P., Brettell L. E., Reece N., Correia-Oliveira M. E., Allsopp M. H. (2020). Varroa destructor reproduction and cell re-capping in mite-resistant Apis mellifera populations. Apidologie 51, 369–381. doi: 10.1007/s13592-019-00721-9
Martin S. J., Highfield A. C., Brettell L., Villalobos E. M., Budge G. E., Powell M., et al. (2012). Global honey bee viral landscape altered by a parasitic mite. Science 336, 1304–1306. doi: 10.1126/science.1220941
Martin S. J., Kemp D. (1997). Average number of reproductive cycles performed by Varroa jacobsoni in honey bee (Apis mellifera) colonies. J. Apic. Res. 36, 113–123. doi: 10.1080/00218839.1997.11100937
Martin S. J., Medina L. M. (2004). Africanized honeybees have unique tolerance to Varroa mites. Trends Parasitol. 20, 112–114. doi: 10.1016/j.pt.2004.01.001
McAfee A., Chapman A., Iovinella I., Gallagher-Kurtzke Y., Collins T. F., Higo H., et al. (2018). A death pheromone, oleic acid, triggers hygienic behavior in honey bees (Apis mellifera L.). Sci. Rep. 8, 5719. doi: 10.1038/s41598-018-24054-2
Medici S. K., Blando M., Sarlo E., Maggi M., Espinosa J. P., Ruffinengo S. (2020). Pesticide residues used for pest control in honeybee colonies located in agroindustrial areas of Argentina. Int. J. Pest. Manage. 66, 163–172. doi: 10.1080/09670874.2019.1597
Medici S. K., D. M. M., Sarlo E. G., Ruffinengo S., Marioli J. M., Eguaras M. J. (2015). The presence of synthetic acaricides in beeswax and its influence on the development of resistance in Varroa destructor. J. Apic. Res. 54, 267–274. doi: 10.1080/00218839.2016.1145407
Medici de Mattos I., Chaud-Netto J. (2012). Analysis of mortality in Africanized honey bee colonies with high levels of infestation by Varroa destructor. Sociobiology 59, 369–380. doi: 10.13140/2.1.2254.2723
Medina L. M., Martin S. J. (1999). A comparative study of Varroa jacobsoni reproduction in worker cells of honey bees (Apis mellifera) in England and Africanized bees in Yucatan, Mexico. Exp. Appl. Acarol. 23, 659–667. doi: 10.1023/A:1006275525463
Medina L. M., Martin S. J., Espinosa-Montaño L., Ratnieks F. L. (2002). Reproduction of Varroa destructor in worker brood of Africanized honey bees (Apis mellifera). Exp. Appl. Acarol. 27, 79–88. doi: 10.1023/A:1021579113907
Medina-Flores C. A., Guzman-Novoa E., Aguilera Soto J. I., López Carlos M. A., Medina Cuéllar S. E. (2019). Condiciones poblacionales y alimenticias de colonias de abejas melíferas (Apis mellifera) en tres regiones del altiplano semiárido de México. Rev. Mex. Cienc. Pecu. 10, 199–211. doi: 10.22319/rmcp.v10i1.4387
Medina-Flores C. A., Guzman-Novoa E., Aréchiga-Flores C. F., Gutierrez H., Aguilera J. I. (2014b). Honey production and Varroa destructor infestation of Africanized honey bees (Apis mellifera) with high and low hygienic behavior. Rev. Mex. Cienc. Pecu. 5, 157–170.
Medina-Flores C. A., Guzman-Novoa E., Hamiduzzaman H. H., Aguilera-Soto J., Lopez-Carlos M. A. (2015). Africanization of honey bees (Apis mellifera) in three climatic regions of northern Mexico. Vet. Mex. 2, 1–9. doi: 10.21753/vmoa.2.4.353
Medina-Flores C. A., Guzman-Novoa E., Hamiduzzaman M. M., Aréchiga-Flores C. F. (2014a). Africanized honey bees (Apis mellifera) have low infestation levels of the mite Varroa destructor in different ecological regions in Mexico. Genet. Mol. Res. 13, 7282–7293. doi: 10.4238/2014.February.21.10
Mendoza Y., Santos E., Clavijo-Baquett S., Invernizzi C. (2022). A reciprocal transplant experiment confirmed mite-resistance in a honey bee population from Uruguay. Vet. Sci. 9, 596. doi: 10.3390/vetsci9110596
Mendoza Y., Tomasco I. H., Antúnez K., Castelli L., Branchiccela B., Santos E. (2020). Unraveling honey bee-Varroa destructor interaction: multiple factors Involved in differential resistance between two Uruguayan populations. Vet. Sci. 7, 116. doi: 10.3390/vetsci7030116
Message D., Gonçalves L. S. (1995). Effect of the size of worker brood cells of Africanized honey bees on infestation and reproduction of the ectoparasitic mite Varroa jacobsoni Oud. Apidologie 26, 381–386. doi: 10.1051/apido:19950503
Milani N. (1999). The resistance of Varroa jacobsoni Oud. to acaricides. Apidologie 30, 229–234. doi: 10.1051/apido:19990211
Mitton G. A., Meroi Arcerito F., Cooley H., Fernández de Landa G., Eguaras M. J., Ruffinengo S. R., et al. (2022). More than sixty years living with Varroa destructor: a review of acaricide resistance. Int. J. Pest. Manage., 1–18. doi: 10.1080/09670874.2022.2094489
Mondet F., Alaux C., Severac D., Rohmer M., Mercer A. R., Le Conte Y. (2015). Antennae hold a key to Varroa-sensitive hygiene behavior in honey bees. Sci. Rep. 5, 10454. doi: 10.1038/srep10454
Mondet F., Beaurepaire A., McAfee A., Locke L., Alaux C., Blanchard S. (2020). Honey bee survival mechanisms against the parasite Varroa destructor: a systematic review of phenotypic and genomic research efforts. Int. J. Parasitol. 50, 433–447. doi: 10.1016/j.ijpara.2020.03.005
Mondet F., Kim S. H., de Miranda J. R., Beslay D., Y. L. C., Mercer A. R. (2016). Specific cues associated with honey bee docial defense against Varroa destructor infested brood. Sci. Rep. 6, 25444. doi: 10.1038/srep25444
Mondragón L., Martin S., Vandame R. (2006). Mortality of mite offspring: a major component of Varroa destructor resistance in a population of Africanized bees. Apidologie 37, 67–74. doi: 10.1051/apido:2005053
Mondragón L., Spivak M., Vandame R. (2005). A multifactorial study of the resistance of honeybees Apis mellifera to the mite Varroa destructor over one year in Mexico. Apidologie 36, 345–358. doi: 10.1051/apido:2005022
Montiel J. C., Piola G. A. (1976). “A new enemy of bees,” in Varroasis a honey bee disease. Ed. Harnaj V. (Bucharest, Romania: Apimondia Publishing House), 37–38.
Moretto G. (2002). Mortality of Varroa destructor in broodless Africanized and carnica honey bee (Apis mellifera L.) colonies. Interciencia 27, 702–704.
Moretto G., Gonçalves L. S., De Jong D. (1993). Heritability of Africanized and European honey bee defensive behavior against the mite Varroa jacobsoni. Brazil J. Genet. 16, 71–77.
Moretto G., Gonçalves L. S., De Jong D., Bichuette M. Z. (1991). The effects of climate and bee race on Varroa jacobsoni Oud infestations in Brazil. Apidologie 22, 197–203. doi: 10.1051/apido:19910303
Moretto G., Guerra J. C. V., Bittencourt C. V. (2006). Uncapping activity of Apis mellifera L. (Hymenoptera: Apidae) towards worker brood cells infested with the mite Varroa destructor Anderson & Trueman (Mesostigmata: Varroidae). Neotrop. Entomol. 35, 299–301. doi: 10.1590/S1519-566X2006000300002
Moretto G., Mello L. J. (1999). Varroa jacobsoni infestation of adult Africanized and Italian honey bees (Apis mellifera) in mixed colonies in Brazil. Genet. Mol. Biol. 22, 121–123. doi: 10.1590/S1415-47571999000300006
Moretto G., Pillati A., Goncalves L. S., Cassini F. L. (1995). Reduction of Varroa infestations in the state of Santa Catarina, in Southern Brazil. Am. Bee J. 135, 498–500.
Morfin N., Espinosa-Montaño L. G., Guzman-Novoa E. (2020a). A direct assay to assess self-grooming behavior in honey bees (Apis mellifera L.). Apidologie 51, 892–897. doi: 10.1007/s13592-020-00769-y
Morfin N., Given K., Evans M., Guzman-Novoa E. (2020b). Grooming behavior and gene expression of the Indiana “mite-biter” honey bee stock. Apidologie 51, 267–275. doi: 10.1007/s13592-019-00710-y
Morfin N., Goodwin P. H., Guzman-Novoa E. (2023b). Varroa destructor and its impacts on honey bee biology. Front. Insect Sci. 1. doi: 10.3389/frbee.2023.1272937
Morfin N., Harpur B. A., de la Mora A., Guzman-Novoa E. (2023a). Breeding honey bees (Apis mellifera L.) for low and high Varroa destructor population growth: Gene expression of bees performing grooming behavior. Front. Insect. Sci. 3. doi: 10.3389/finsc.2023.951447
Moritz R. F. A. (1985). Heritability of the postcapping stage in Apis mellifera and its relation to varroatosis resistance. J. Hered. 76, 267–270. doi: 10.1093/oxfordjournals.jhered.a110090
Moritz R. F., Härtel S., Neumann P. (2005). Global invasions of the western honeybee (Apis mellifera) and the consequences for biodiversity. Ecoscience 12, 289–301. doi: 10.2980/i1195-6860-12-3-289.1
Morse R. A., Gonçalves L. S. (1979). Varroa disease, a threat to world beekeeping. Glean Bee Cult. 107, 79–81.
Mullin C. A., Frazier M., Frazier J. L., Ashcraft S., Simonds R., Vanengelsdorp D. (2010). High levels of miticides and agrochemicals in North American apiaries: implications for honey bee health. PloS One 5, e9754. doi: 10.1371/journal.pone.0009754
Muntaabski I., Russo R. M., Liendo M. C., Palacio M. A., Cladera J. L., Lanzavecchia S. B., et al. (2020). Genetic variation and heteroplasmy of Varroa destructor inferred from ND4 mtDNA sequences. Parasitol. Res. 119, 411–421. doi: 10.1007/s00436-019-06591-5
Nazzi F., Brown S. P., Annoscia D., Del Piccolo F., Di Prisco G., Varricchio P., et al. (2012). Synergistic parasite-pathogen interactions mediated by host immunity can drive the collapse of honeybee colonies. PloS Pathog. 8, e100273. doi: 10.1371/journal.ppat.1002735
Neumann P., Carreck N. L. (2010). Honey bee colony losses. J. Apic. Res. 49, 1–6. doi: 10.3896/IBRA.1.49.1.01
Nganso B. T., Fombong A. T., Yusuf A. A., Pirk C. W. W., Stuhl C., Torto B. (2018). Low fertility, fecundity and numbers of mated female offspring explain the lower reproductive success of the parasitic mite Varroa destructor in African honeybees. Parasitology 145, 1633–1639. doi: 10.1017/S0031182018000616
Nikaido S. S., Villalobos E. M., Castillo N. R., Cubero Murillo A., Rodríguez J. M., Aracena R. M. (2018). Detection of africanized bees in the Dominican republic. J. Apic. Res. 57, 351–353. doi: 10.1080/00218839.2018.1447838
Nolan M. P., Delaplane K. S. (2017). Distance between honey bee Apis mellifera colonies regulates populations of Varroa destructor at a landscape scale. Apidologie 48, 8–16. doi: 10.1007/s13592-016-0443-9
Oldroyd B. P. (1999). Coevolution while you wait: Varroa jacobsoni, a new parasite of western honeybees. Trends Ecol. Evol. 14, 312–315. doi: 10.1016/S0169-5347(99)01613-4
Peixoto C. M., Correia-Oliveira M. E., de Lima Silva F., de Oliveira Ramos C. E. C., Lopes de Carballo C. A. (2021). Varroa destructor in Apis mellifera colonies in Brazil. J. Apic. Res. 63, 480–486. doi: 10.1080/00218839.2021.1960746
Peng Y. S., Fang Y. Z., Xu S. Y., Ge L. S. (1987). The resistance mechanism of the Asian honey bee, Apis cerana Fabr., to an ectoparasitic mite, Varroa jacobsoni Oudemans. J. Invertebr. Pathol. 49, 54–60. doi: 10.1016/0022-2011(87)90125-X
Piccirillo G. A., De Jong D. (2003). The influence of brood comb cell size on the reproductive behavior of the ectoparasitic mite Varroa destructor in Africanized honey bee colonies. Genet. Mol. Res. 2, 36–42.
Piccirillo G. A., De Jong D. (2004). Old honey bee brood combs are more infested by the mite Varroa destructor than are new brood combs. Apidologie 35, 359–364. doi: 10.1051/apido:2004022
Porrini L. P., Quintana S., Brasesco C., Porrini M. P., Garrido P. M., Eguaras M. J. (2020). Southern limit of Africanized honey bees in Argentina inferred by mtDNA and wing geometric morphometric analysis. J. Apic. Res. 59, 648–657. doi: 10.1080/00218839.2019.1681116
Portman Z. M., Tepedino V. J., Tripodi A. D., Szalanski A. L., Durham S. L. (2018). Local extinction of a rare plant pollinator in Southern Utah (USA) associated with invasion by Africanized honey bees. Biol. Invasions 20, 593–606. doi: 10.1007/s10530-017-1559-1
Ramos-Cuellar A. K., de la Mora A., Contreras-Escareño F., Morfin N., Tapia-González J. M., Macías-Macías J. O., et al. (2022). Genotype, but not climate, affects the resistance of honey bees (Apis mellifera) to viral infections and to the mite Varroa destructor. Vet. Sci. 9, 358. doi: 10.3390/vetsci9070358
Ramos-Cuellar A. K., de la Mora A., Contreras-Escareño F., Morfin N., Tapia-González J. M., Macías-Macías J. O., et al. (2023). Altitudinal effects on the africanization of honey bee colonies (Apis mellifera L.) in Jalisco, Mexico. Ecosist. Recur. Agropec. 10, e3457. doi: 10.19136/era.a10n3.3457
Ramsey S. D., Ochoa R., Bauchan G., Gulbronson C., Mowery J. D. (2019). Varroa destructor feeds primarily on honey bee fat body tissue and not hemolymph. Proc. Natl. Acad. Sci. U. S. A. 116, 1792–1801. doi: 10.1073/pnas.1818371116
Rath W. (1999). Co-adaptation of Apis cerana Fabr. and Varroa jacobsoni Oud. Apidologie 30, 97–110. doi: 10.1051/apido:19990202
Requier F., Antúnez K., Morales C. L., Aldea Sánchez P., Castilhos D., Garrido P. M., et al. (2018). Trends in beekeeping and honey bee colony losses in Latin America. J. Apic. Res. 57, 657–662. doi: 10.1080/00218839.2018.1494919
Reyes-Quintana M., Espinosa-Montaño L. G., Prieto-Merlos D., Koleoglu G., Petukhova T., Correa-Benítez A. (2019). Impact of Varroa destructor and deformed wing virus on emergence, cellular immunity, wing integrity and survivorship of Africanized honey bees in Mexico. J. Invertebr. Pathol. 164, 43–48. doi: 10.1016/j.jip.2019.04.009
Reyes-Quintana M., Goodwin P. H., Correa-Benítez A., Pelaez-Hernández R., Guzman-Novoa E. (2023). Genetic variability of the honey bee mite, Varroa destructor, from a humid continental climatic region of Canada, and temperate and tropical climatic regions of Mexico. Exp. Appl. Acarol. 91, 541–559. doi: 10.1007/s10493-023-00848-6
Rinderer T. E., Hellmich R. L. (1991). “The process of africanization,” in The African honey bee. Eds. Spivak M., Fletcher D. J. C., Breed M. D. (Boulder CO: Westview Press), 95–117. doi: 10.1525/ahu.2004.29.1.95
Ritter W., De Jong D. (1984). Reproduction of Varroa jacobsoni O. @ in Europe, the middle East and tropical South America. J. Appl. Entomol. 98, 55–57. doi: 10.1111/j.1439-0418.1984.tb02684.x
Rivera-Marchand B., Oskay D., Giray T. (2012). Gentle Africanized bees on an oceanic island. Evol. Appl. 5, 746–756. doi: 10.1111/j.1752-4571.2012.00252.x
Rodríguez-Luis A., Grindrod I., Webb G., Piñeiro A. P., Martin S. J. (2022). Recapping and mite removal behavior in Cuba: home to the world's largest population of Varroa-resistant European honeybees. Sci. Rep. 12, 15597. doi: 10.1038/s41598-022-19871-5
Rosenkranz P. (1999). Honey bee (Apis mellifera L.) tolerance to Varroa jacobsoni Oud. in South America. Apidologie 30, 159–172. doi: 10.1051/apido:19990206
Rosenkranz P., Aumeier P., Ziegelmann B. (2010). Biology and control of Varroa destructor. J. Invertebr. Pathol. 103, S96–119. doi: 10.1016/j.jip.2009.07.016
Rosenkranz P., Engels W. (1994). Infertility of Varroa jacobsoni females after invasion into Apis mellifera worker brood as a tolerance factor against Varroatosis. Apidologie 25, 402–411. doi: 10.1051/apido:19940407
Rosenkranz P., Fries I., Boecking O., Stürmer M. (1997). Damaged Varroa mites in the debris of honey bee (Apis mellifera L) colonies with and without hatching brood. Apidologie 28, 427–437. doi: 10.1051/apido:19970609
Rothenbuhler W. C. (1964). Behavior genetics of nest cleaning in honey bees. I. Responses of four inbred lines to disease-killed brood. Anim. Behav. 12, 578–584. doi: 10.1016/0003-3472(64)90082-X
Roubik D. W., Villanueva-Gutierrez R. (2009). Invasive Africanized honey bee impact on native solitary bees: a pollen resource and trap nest analysis. Biol. J. Linn. Soc Lond. 98, 152–160. doi: 10.1111/j.1095-8312.2009.01275.x
Russo R. M., Liendo M. C., Landi L., Pietronave H., Merke J., Fain H., et al. (2020). Grooming behavior in naturally Varroa-resistant Apis mellifera colonies from north-central Argentina. Front. Ecol. Evol. 8. doi: 10.3389/fevo.2020.590281
Santos L., Alves M., Message D., Pinto F., Silva M., Teixeira E. (2014). Honey bee health in apiaries in the Vale do Paraíba, Saão Paulo State, Southeastern Brazil. Sociobiology 61, 307–312. doi: 10.13102/sociobiology.v61i3.307-312
Scannapieco A. C., Mannino M. C., Soto G., Palacio M. A., Cladera J. L., Lanzavecchia S. B. (2017). Expression analysis of genes putatively associated with hygienic behavior in selected stocks of Apis mellifera L. from Argentina. Insect Soc 64, 485–494. doi: 10.1007/s00040-017-0567-6
Schafaschek T. P., Hickel E. R., de Oliveira C. A., de Toledo V. D. (2019). Infestation and Reproduction of Varroa destructor Anderson and Trueman and hygienic behavior in colonies of Apis mellifera L. (Africanized honeybee) with queens of different genetic origins. Sociobiology 66, 448–456. doi: 10.13102/sociobiology.v66i3.3444
Schneider S. S., DeGrandi-Hoffman G., Smith D. R. (2004). The African honey bee: factors contributing to a successful biological invasion. Annu. Rev. Entomol. 49, 351–376. doi: 10.1146/annurev.ento.49.061802.123359
Seeley T. D. (2007). Honey bees of the Arnot Forest: a population of feral colonies persisting with Varroa destructor in the northeastern United States. Apidologie 38, 19–29. doi: 10.1051/apido:2006055
Serra C. A., Jorge P. E., Abud-Antún A. J., Alvarez P., Perguero B. (2003). “Invasive alien species in the Dominican Republic: their impact and strategies to manage introduced pests,” in 39th Annual Meeting Caribbean, Grenada, West Indies, Caribbean Food Crops Society, July 13-18, 2003. doi: 10.22004/ag.econ.256720
Sheppard W. S. (1989). A history of the introduction of honey bee races into the United States: Part I. Am. Bee J. 129, 617–619.
Sheppard W. S., Rinderer T. E., Mazzoli J. A., Stelzer J. A., Shimanuki H. (1991). Gene flow between African and European derived honey bee populations in Argentina. Nature 349, 782–784. doi: 10.1038/349782a0
Smith D. R., Taylor O. R., Brown W. M. (1989). Neotropical Africanized honey bees have African mitochondrial DNA. Nature 339, 213–215. doi: 10.1038/339213a0
Spivak M., Danka R. G. (2021). Perspectives on hygienic behavior in Apis mellifera and other social insects. Apidologie 52, 1–16. doi: 10.1007/s13592-020-00784-z
Spivak M., Reuter G. S. (1998). Performance of hygienic honey bee colonies in a commercial apiary. Apidologie 29, 291–302. doi: 10.1051/apido:19980308
Strapazzon R., Carneiro F. E., Guerra J. C. V. Jr., Moretto G. (2009). Genetic characterization of the mite Varroa destructor (Acari: Varroidae) collected from honey bees Apis mellifera (Hymenoptera, Apidae) in the state of Santa Catarina, Brazil. Genet. Mol. Res. 8, 990–997. doi: 10.4238/vol8-3gmr567
Tapia-González J. M., Alcazar-Oceguera G., Macías-Macías J. O., Contreras-Escarenão F., Tapia-Rivera J. C., Petukhova T., et al. (2019). Varroosis in honey bees in different environmental and regional conditions of Jalisco, Mexico. Ecosist. Recur. Agropec. 6, 243–251. doi: 10.19136/era.a6n17.2018
Tapia-González J. M., León-Mantecón T., Contreras-Escareño F., Macías-Macías J., Tapia-Rivera J., Guzman-Novoa E. (2021). Climatic, regional and bee brood quantity influences on the hygienic behavior of Apis mellifera. Abanico Vet. 11, 1–16. doi: 10.21929/abavet2021.20
Tibatá V. M., Arias E., Corona M., Ariza-Botero F., Figueroa-Ramírez J., Junca H. (2017). Determination of the Africanized mitotypes in populations of honey bees (Apis mellifera L.) of Colombia. J. Apic. Res. 57, 219–227. doi: 10.1080/00218839.2017.1409065
Tibatá V. M., Sanchez A., Palmer-Young E., Junca H., Solarte V. M., Madella S., et al. (2021). Africanized honey bees in Colombia exhibit high prevalence but low level of infestation of Varroa mites and low prevalence of pathogenic viruses. PloS One 16, e0244906. doi: 10.1371/journal.pone.0244906
Traynor K. S., Mondet F., de Miranda J. R., Techer M., Kowallik V., Oddie M., et al. (2020). Varroa destructor: A complex parasite, crippling honey bees worldwide. Trends Parasitol. 36, 592–606. doi: 10.1016/j.pt.2020.04.004
Traynor K. S., Rennich K., Forsgren E., Rose R., Pettis J., Kunkel G., et al. (2016). Multiyear survey targeting disease incidence in US honey bees. Apidologie 47, 325–347. doi: 10.1007/s13592-016-0431-0
Trouiller J., Arnold G., Le Conte Y., Masson C., Chappe B. (1991). Temporal pheromonal and kairomonal secretion in the brood of honeybees. Naturwissenschaften 78, 368–370. doi: 10.1007/BF01131612
Tsuruda J. M., Harris J. W., Bourgeois L., Danka R. G., Hunt G. J. (2012). High-resolution linkage analyses to identify genes that influence Varroa sensitive hygiene behavior in honey bees. PloS One 7, e48276. doi: 10.1371/journal.pone.0048276
Uribe-Rubio J. L., Guzman-Novoa E., Hunt G. J., Correa-Benítez A., Zozaya J. A. (2003). The effect of Africanization on honey production, defensive behavior and size of honeybees (Apis mellifera L.) in the Mexican high plateau. Vet. Mex OA 34, 47–59. doi: 10.21753/vmoa.2.4.353
Vandame R., Colin M. E., Otero-Colina G. (1999). Africanized honeybees tolerance to Varroa in Mexico: mite infertility is not the main tolerance factor. Apiacta 34, 12–20.
Vandame R., Morand S., Colin M. E., Belzunces L. P. (2002). Parasitism in the social bee Apis mellifera: quantifying costs and benefits of behavioral resistance to varroa mites. Apidologie 33, 433–455. doi: 10.1051/apido:2002025
Vandame R., Palacio M. A. (2010). Preserved honey bee health in Latin America: a fragile equilibrium due to low-intensity agriculture and beekeeping? Apidologie 41, 243–255. doi: 10.1051/apido/2010025
Vanengelsdorp D., Evans J. D., Saegerman C., Mullin C., Haubruge E., Nguyen B. K., et al. (2009). Colony collapse disorder: a descriptive study. PloS One 4, e6481. doi: 10.1371/journal.pone.0006481
van Veen J., Calderon R. A., Cubero Murillo A., Arce Arce H. G. (1998). Varroa jacobsoni in Costa Rica: detection, spread and treatment with formic acid. Bee World 79, 5–10. doi: 10.1080/0005772X.1998.11099370
Villa J. D., Danka R. G., Harris J. W. (2009). Simplified methods of evaluating colonies for levels of Varroa sensitive hygiene (VSH). J. Apic. Res. 48, 162–167. doi: 10.3896/IBRA.1.48.3.03
Wagoner K., Spivak M., Hefetz A., Reams T., Rueppell O. (2019). Stock-specific chemical brood signals are induced by Varroa and Deformed Wing Virus, and elicit hygienic response in the honey bee. Sci. Rep. 9, 8753. doi: 10.1038/s41598-019-45008-2
Wilfert L., Long G., Leggett H. C., Schmid-Hempel P., Butlin R., Martin S. J., et al. (2016). Deformed wing virus is a recent global epidemic in honeybees driven by Varroa mites. Science 351, 594–597. doi: 10.1126/science.aac9976
Keywords: Apis mellifera, Varroa destructor, adaptation, Africanized honey bee, mechanisms of resistance, host-parasite, Latin America
Citation: Guzman-Novoa E, Corona M, Alburaki M, Reynaldi FJ, Invernizzi C, Fernández de Landa G and Maggi M (2024) Honey bee populations surviving Varroa destructor parasitism in Latin America and their mechanisms of resistance. Front. Ecol. Evol. 12:1434490. doi: 10.3389/fevo.2024.1434490
Received: 17 May 2024; Accepted: 15 July 2024;
Published: 06 August 2024.
Edited by:
Roberto Romani, University of Perugia, ItalyReviewed by:
Michael Lattorff, University of KwaZulu-Natal, South AfricaDavid R. Tarpy, North Carolina State University, United States
Copyright © 2024 Guzman-Novoa, Corona, Alburaki, Reynaldi, Invernizzi, Fernández de Landa and Maggi. This is an open-access article distributed under the terms of the Creative Commons Attribution License (CC BY). The use, distribution or reproduction in other forums is permitted, provided the original author(s) and the copyright owner(s) are credited and that the original publication in this journal is cited, in accordance with accepted academic practice. No use, distribution or reproduction is permitted which does not comply with these terms.
*Correspondence: Miguel Corona, miguel.corona@usda.gov