- 1School of Biological, Earth and Environmental Sciences, Environmental Research Institute, University College Cork, Distillery Fields, Cork, Ireland
- 2Department of Palaeobiology, Swedish Museum of Natural History, Svante Arrhenius, Stockholm, Sweden
Plants and arthropods are primary drivers of terrestrial ecosystem function. Trace fossils of plant–arthropod interactions (PAIs) provide a unique window into assessing terrestrial ecosystem states through geological time and evaluating changes in herbivorous arthropod feeding guilds in the wake of global biotic crises. The end-Permian event (EPE; c. 252 Ma) resulted in the loss of keystone plant species from humid tropical and high-latitude ecosystems and the extinction of several major insect groups. The subsequent Early to Middle Triassic evinced diminished terrestrial productivity, punctuated by a series of second-order biotic crises that hindered recovery. Here, we survey records of Gondwanan Early to Middle Triassic floral assemblages for evidence of PAIs as an indication of ecosystem recovery following the EPE. We compiled a comprehensive dataset of fossil plant taxa and PAIs for lower Mesozoic strata of Gondwana, revealing an increase in specific and generic floral diversity from the Early to Middle Triassic. We noted a lack of PAIs reported from many localities with abundant fossil leaves, which might be interpreted to be a consequence of a post-EPE delay in the recovery of arthropod feeding guilds compared to the flora. However, by comparing floral assemblages between regions of Gondwana, our results also partly attribute the absence of PAIs to the relative paucity of palaeoichnological and palaeobotanical studies of this interval. To test for potential under-reporting of PAIs in the Triassic, we present a case study of the well-described Australian Middle Triassic Benolong Flora. In contrast to existing Australian Early to Middle Triassic PAI reports on only three plant specimens, this systematic investigation revealed 44 PAI traces comparable to published examples, hosted by 40 fossil plant fragments (7.77% of fragments assessed; N = 591). Margin-feeding traces constituted the dominant Functional Feeding Group (FFG) identified (23 examples: 3.72% of fragments assessed). Our review highlights several Early and Middle Triassic Gondwanan plant fossil-rich successions and existing collections that require further examination. We predict that investigations of these assemblages will greatly elucidate the relationships between rapidly changing environments during the Early and Middle Triassic and their effects on the plant and arthropod communities in the Southern Hemisphere.
1 Introduction
1.1 Plant–arthropod interactions in the fossil record
Plants (c. 380,000 extant species; Christenhusz and Byng, 2016) and terrestrial arthropods (c. 7 million extant species; Stork, 2018) constitute the most significant proportion of the lower trophic levels on which all higher levels of life on land depend, with their interactions playing a crucial role in terrestrial ecosystem nutrient cycling. Understanding and quantifying the relationships between arthropods and the plants with which they are associated can, therefore, be used to indicate the condition and evolution of terrestrial ecosystems (Labandeira, 2013). Today, arthropods can be observed directly interacting with plant organs, and specialized associations between particular plant and arthropod taxa can be identified. In fossil plant assemblages, traces of herbivory (consumption of live plant tissue), detritivory (feeding on dead tissues) and oviposition (egg-laying) left on or within plant organs provide evidence of fundamental behavioral interactions that formed the basis of ancient terrestrial food webs and ecosystem function (Labandeira and Currano, 2013).
Plant–arthropod interactions (PAIs), captured as trace fossils on plant tissues, record behaviors of arthropods that may not be deducible from their body fossils. Moreover, plants are relatively common in the fossil record and an arthropod may interact with many plants during its lifetime. So, the likelihood of a plant fragment exhibiting a PAI being preserved is greater than the likelihood of preserving an arthropod body fossil. Consequently, PAIs are important indicators of the richness and abundance of arthropods and herbivore guilds present in an ancient ecosystem (Labandeira and Wappler, 2023). Furthermore, fossil leaves are utilized in many palaeoecological studies as they are consistently more abundant and widespread than most other plant organs, such as wood and seeds and, therefore, provide more accessible records (Wilf, 2008). Morphological deterrents against arthropod herbivory, such as spines and hairs, have a much higher potential for preservation in the fossil record than chemical defenses. Their presence on plant fossils is evidence that arthropod interactions provided sufficient evolutionary pressure on plant taxa to favor selection of those plants investing resources in physical defenses (War et al., 2018).
Although detritivory is interpreted to have occurred since the initial establishment of complex terrestrial ecosystems in the late Silurian to Early Devonian (Kenrick et al., 2012; Garwood et al., 2020), the progression to herbivory is not extensively documented in the fossil record (Labandeira and Currano, 2013; Labandeira and Wappler, 2023). Probable arthropod coprolites (fossilized fecal pellets) containing spores and other fragments associated with late Silurian (412 Ma) and Early Devonian (390 Ma) land plants have been identified as the earliest documented evidence for the consumption of live tissues alongside dead plant matter (Edwards et al., 1995; Hagström and Mehlqvist, 2012; Labandeira and Wappler, 2023). The extraction of nutrients from plants via piercing and sucking of cortical stem tissues has been reported in Early Devonian vascular plants displaying lesions resulting from repeated insertion of stylet mouthparts (Banks and Colthart, 1993). By the Middle Devonian, several types of herbivory were represented on simple land plants: external tissue feeding, galling, and piercing and sucking (Labandeira et al., 2014).
Labandeira et al. (2007) published a guide focused primarily on trace fossil evidence of damage to foliage, consisting initially of 150 distinct Damage Types (DTs) almost all of which are probably linked to arthropods. The latest available updated compendium (as yet unpublished; C. Labandeira, pers. comm.) defines over 420 distinct damage types. These DTs are classified into a series of Functional Feeding Groups (FFGs): 1, hole feeding; 2, margin feeding; 3, skeletonization; 4, surface feeding; 5, oviposition; 6, piercing and sucking; 7, mining; 8, galling; 9, seed predation; 10, pathogenic damage; 11, boring; and 12, domatia construction. To these feeding and other interactive behaviors can be added palynophagy and nectarivory, which can be difficult to detect in the fossil record.
With a standardized classification scheme, the quantification of fossil leaves with invertebrate traces through time can be utilized to infer the state of health of the lower trophic levels, e.g., terrestrial ecosystem development and recovery before and after major environmental changes (Labandeira et al., 2018). The majority of mass extinction events were associated with worldwide changes in the climate, the most common and lethal of which were rapid warming episodes, or ‘hyperthermal events’ (Bond and Grasby, 2017). An understanding of how plant–arthropod interactions have responded to cases of past environmental destabilization will elucidate how terrestrial ecosystems evolve (Wilf et al., 2001; Labandeira, 2006; Wilf, 2008; Labandeira and Currano, 2013; Currano et al., 2016; Labandeira and Wappler, 2023; Zambon et al., 2023). Moreover, assessing the traits common to mass extinction survivors, and observing the progression of ecosystem inhabitants over time, enables the identification of potential patterns of recovery between plants and their dependent invertebrates throughout evolutionary history (Labandeira, 2006; Cariglino et al., 2021). This may then inform our predictions of how extant terrestrial ecosystems may be affected by modern rapid climate changes (Currano et al., 2016; Zambon et al., 2023).
1.2 Latest Permian to Middle Triassic climatic events
The transition from the Paleozoic to Mesozoic eras was punctuated by a series of climate-driven ecosystem shifts (Figure 1). The first of these was the end-Permian event (EPE, c. 252.2 Ma) that has been clearly linked to a major magmatic episode of the Siberian Traps Large Igneous Province (Burgess et al., 2017; Joachimski et al., 2019; Shen et al., 2023). The EPE hyperthermal event involved increases in atmospheric CO2 (Cui et al., 2021; Wu et al., 2023), temperature (Sun et al., 2012; Cui and Kump, 2015), wildfires (Vajda et al., 2020; Lu et al., 2022; Mays and McLoughlin, 2022), freshwater microbial blooms (Mays et al., 2021) and enhanced seasonality in precipitation (Fielding et al., 2019; Frank et al., 2021). A combination of these (and related) kill mechanisms during the EPE led to the highest biodiversity loss of any mass extinction within both the marine and continental realms (Stanley, 2016; Viglietti et al., 2021) including a globally significant floral and insect turnover (Anderson et al., 1999; Labandeira, 2005; Cascales-Miñana et al., 2016; Gastaldo et al., 2020; Schachat and Labandeira, 2021). Therefore, the Triassic fossil flora, entomofauna and their associated interactions can be used to inform patterns of recovery and continental ecosystem evolution in the aftermath of Earth’s greatest extinction event.
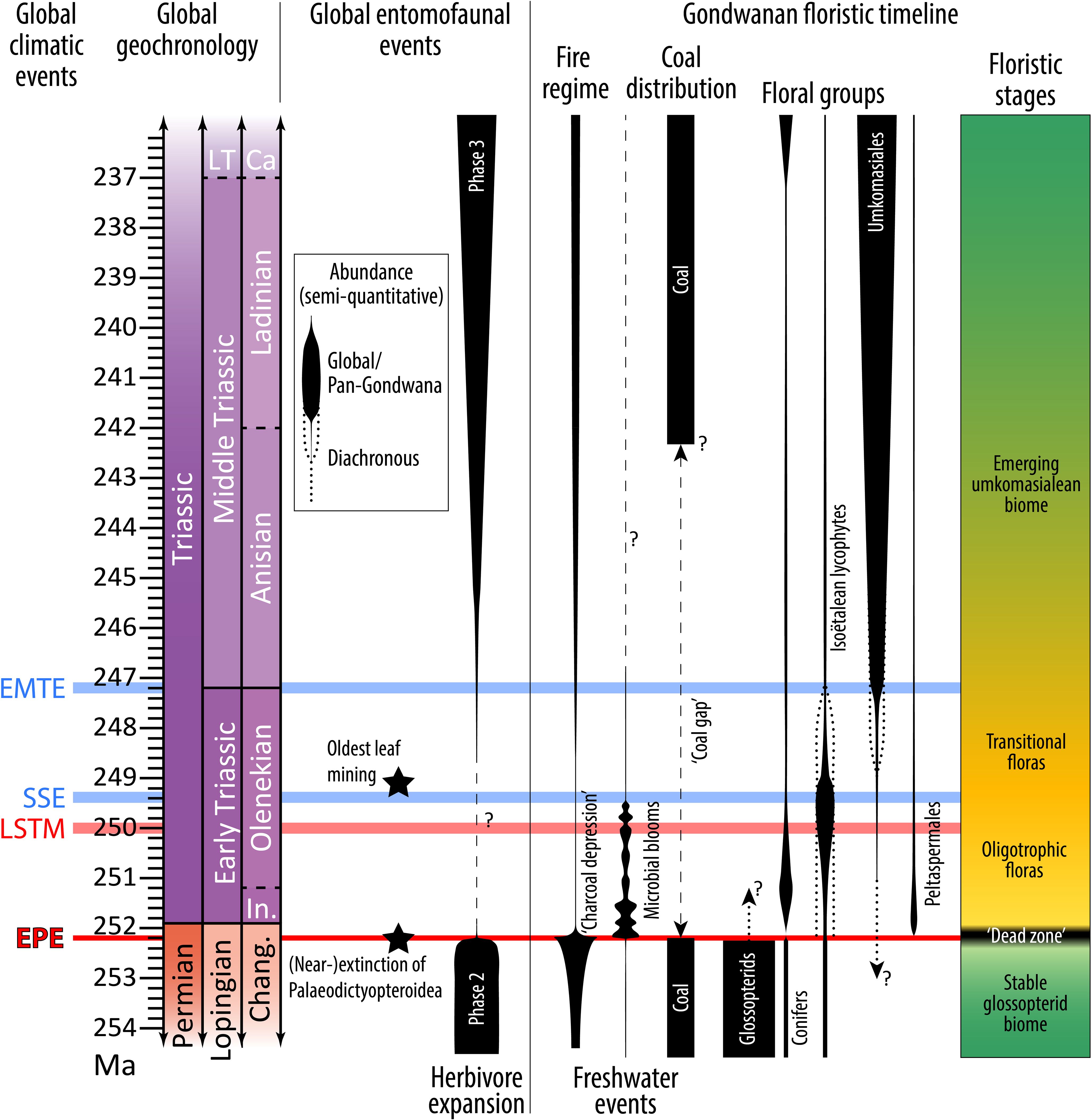
Figure 1. A summary of latest Permian to early Late Triassic global climatic, entomofaunal and Gondwanan floristic events. The widths of each bubble are indicative of semi-quantitative, relative abundances within each group, but not between groups. Plant–arthropod associations from Labandeira (2006). Evidence of Early Triassic mining from Krassilov and Karasev (2008), but precise age is unknown. Previously, Palaeodictyopteroidea were considered to have been totally extinguished at the EPE (Labandeira and Sepkoski, 1993), but Late Triassic occurrences of probable palaeodictyopterans have since been recovered (see Labandeira, 2005). Evidence of pre-EPE Dicroidium from Kerp et al. (2006) and Abu Hamad et al. (2008); for evidence of relictual glossopterids, see main text. Other floristic trends of Gondwana from Retallack (1980a), Anderson et al. (1999, 2007), Hermann et al. (2011), Schneebeli-Hermann et al. (2015) and Mays et al. (2020). Floristic stages after Spalletti et al. (2003); Mays et al. (2020) and Vajda et al. (2020). Fire regime interpretations from Mays and McLoughlin (2022). Freshwater events from Vajda et al. (2020) and Mays et al. (2021) but have only been reported from eastern Australia. Global climatic events: EPE, end-Permian event; LSTM, late Smithian thermal maximum; SSE, Smithian-Spathian event; EMTE, Early-Middle Triassic event. Additional abbreviations: Ca, Carnian Age; Chang., Changhsingian Age; In., Induan Age; LT, Late Triassic Epoch.
The post-EPE recovery of ecosystems is complicated by a series of Early Triassic climatic events (Figure 1). Three of these events have demonstrated major biotic impacts (in chronological order): 1, the late Smithian thermal maximum (c. 250 Ma; Sun et al., 2012; Du et al., 2022); 2, the Smithian–Spathian event (c. 249.2 Ma; Hermann et al., 2012a; Lindström et al., 2020); and 3, the Early–Middle Triassic event (c. 247.2 Ma; Hermann et al., 2012b). At least one of these (the late Smithian thermal maximum) has been linked to enhanced global warming (Du et al., 2022), possibly as a result of renewed Siberian Traps Large Igneous Province magmatism (Shen et al., 2019) although this is contested (Widmann et al., 2020). The latter two events (the Smithian–Spathian event and the Early–Middle Triassic event), however, seem to be associated with cooling trends (Romano et al., 2013; Widmann et al., 2020). Although magmatism has been indicated as the ultimate cause of both (Du et al., 2022; Saito et al., 2023), the precise mechanisms remain obscure. Regardless of the causes, it is highly likely that the climatic fluctuations hindered ecological recovery for millions of years following the EPE (Grauvogel-Stamm and Ash, 2005; Friesenbichler et al., 2021). A demonstration of this hindrance is the global absence of thick, economic coal deposits until the Middle Triassic (Veevers et al., 1994; Retallack et al., 1996); the likely oldest post-EPE coals in Gondwana are in the c. upper Anisian (Dolby and Balme, 1976) lower Mungaroo Formation on the Australian northwest shelf (Retallack et al., 1996; Zeng et al., 2019). The absence of dense vegetation represented by the coal gap was a probable contributor to the Early Triassic ‘charcoal depression’, an interval of relatively low wildfire prevalence (Figure 1; Mays and McLoughlin, 2022). These signs indicate that, although the main plant constituents of Gondwanan Middle and Upper Triassic coals (Umkomasiales, e.g., Dicroidium) had spread across the continent soon after the EPE, their abundances remained low until long after the major climatic fluctuations of the Early Triassic.
1.3 Ecosystem evolution after the end-Permian event (EPE)
Permian floral assemblages of Gondwana are overwhelmingly dominated by the remains of one group of broadleafed, arborescent seed plants, the glossopterids, alongside subsidiary lycopsids, sphenopsids, ferns, cycadaleans, ginkgoaleans, cordaitaleans, conifers and several seed plants of uncertain affinity (Anderson and Anderson, 1985; McLoughlin, 1992, 1994a, b; Prevec et al., 2009, 2010). With the extinction of glossopterids near the end of the Permian, new terrestrial ecosystems were slowly established and dominated by peltaspermalean, voltzialean conifer, pleuromeian/isoetalean lycophyte, and, eventually, umkomasialean (primarily Dicroidium) floras through the Early and Middle Triassic (Figure 1; Lele, 1974; Retallack, 1977; McLoughlin et al., 1997; Anderson et al., 1998). By the Middle Triassic, Dicroidium had filled the canopy plant niche left by the glossopterids until their own eventual extinction across Gondwana at the close of the Triassic, apart from a few potential relictual communities that persisted into the Early Jurassic in East Antarctica (Bomfleur et al., 2018).
Evidence for PAIs through the Permian of Gondwana has been broadly surveyed based on published literature (McLoughlin et al., 2021b) but there have been few quantitative studies of individual assemblages (Prevec et al., 2009, 2010). Labandeira (2006) identified four distinct phases of land plant–arthropod associations through deep time, with the EPE as the boundary between Herbivore Expansion Phases 2 and 3 (Figure 1). Most of the major functional feeding groups (FFGs) had evolved by the EPE, specifically during Herbivore Expansion 2, with the exception of mining and nectarivory (Labandeira, 2006; Schachat et al., 2014). Although putative evidence for leaf mining, has been reported on Glossopteris leaves from the Permian of South America (Adami-Rodrigues et al., 2004; Cariglino, 2018), these records are equivocal and, if present, mining was evidently extremely rare prior to the Triassic (McLoughlin and Santos, 2024). The oldest unequivocal leaf mining has been identified in leaves of the peltaspermalean seed fern Vjaznikopteris rigida from Lower Triassic strata of the Volga Basin in central Russia (Figure 1; Krassilov and Karasev, 2008).
Parallel to the floral timeline, the late Permian to Early Triassic was a pivotal evolutionary interval for insects when the Paleozoic Evolutionary Fauna was largely replaced by the Modern Evolutionary Fauna (terms sensu Labandeira, 2005). The floral and faunal turnovers of the EPE resulted in novel plant–arthropod associations being established by the Middle Triassic that characterize Herbivore Expansion Phase 3, but recorded instances of PAIs for the Early Triassic are sparse (Labandeira, 2006; Labandeira et al., 2018). Comparatively little is known about the transitional recovery flora and associated herbivores of the first five million years of the Triassic because there are relatively few well-preserved or well-documented fossil floras in continental deposits from the Early Triassic globally (Grauvogel-Stamm and Ash, 2005; Wappler et al., 2015). The Early Triassic spans a c. five-million-year interval for which published records of PAIs are, consequently, scarce (Labandeira et al., 2016; Cariglino et al., 2021; Labandeira and Wappler, 2023). Furthermore, the majority of existing PAI data from the Early Triassic come from deposits of the Northern Hemisphere (Krassilov and Karasev, 2008; Kustatscher et al., 2014; Labandeira and Wappler, 2023).
With the numerous and extreme environmental changes through the late Permian to the Middle Triassic, the plant and invertebrate components of terrestrial ecosystems were altered accordingly, and this is predicted to be reflected in the interactions recorded in fossil plant assemblages. Extinction and turnover of plant and invertebrate lineages, and their corresponding trophic interactions, are likely to have resulted in a reduced frequency of associated trace fossils prior to the recovery of these groups (Labandeira and Currano, 2013).
Building upon recent detailed surveys of Gondwanan Permian (Prevec et al., 2009, 2010; McLoughlin et al., 2021b), Late Triassic (Scott et al., 2004; Labandeira et al., 2018; Cariglino et al., 2021) and Jurassic (McLoughlin et al., 2015) PAIs, this review aims to document and quantify our current knowledge of PAIs on fossil leaves during the post-EPE recovery interval of the Early and Middle Triassic in the Southern Hemisphere. To this end, we conducted a comprehensive survey of published literature on austral fossil plant assemblages, with special emphasis on leaf floras, given their relative abundance and suitability as substrates of PAIs. A comparison of this data compilation to the results of a case study on a well-described Middle Triassic floral assemblage provides an independent gauge of the rate of PAI reporting. The review of the literature, and additional new case study, will: 1, assess the reliability of the present state of the fossil record as a gauge of terrestrial ecosystem health; 2, summarize the broad ecological stages represented by the plant–arthropod trace fossil records of the Early and Middle Triassic of Gondwana (with regional comparisons); 3, assess PAI presence in the eastern Australian Middle Triassic Benolong Flora, for which no herbivore damage has yet been reported; 4, establish a series of hypotheses regarding terrestrial ecosystem collapse and replacement that are testable with the late Paleozoic to early Mesozoic fossil record of plant–arthropod interactions; and 5, identify fossil assemblages for further research to address the gaps in our collective knowledge of Early to Middle Triassic Gondwanan floras and arthropod interactions.
2 Materials and methods
2.1 Data selection and database taxonomy
The literature evaluation and database creation (Supplementary Table S1) involved the following inclusion criteria: 1, fossil assemblages yielding plant material; 2, Early and Middle Triassic interpreted ages (including those with ages that may extend into the late Permian or Late Triassic); and 3, localities from the Gondwanan supercontinent. Some temporally relevant stratigraphic units bearing fossil flora from across the Southern Hemisphere were initially identified from the reports of Retallack (1980a), Anderson and Anderson (1993a, b), Escapa et al. (2011), Cariglino et al. (2021) and Moisan (2024). For every fossil plant assemblage identified, additional searches were conducted to identify any existing PAI records. The primary means of finding relevant papers was by using varied combinations of search terms in Google and Google Scholar. The search terms consisted of: “XX Formation” + “XX Basin” + “Triassic” + “fossil” + “plant” + “leaf”/“leaves” + “flora” + “palaeobotany” + “Dicroidium” + “insect”/“arthropod” + “damage”/“herbivory”; searches consisted of a combination of keywords with and without quotation marks.
Some of the identified fossil assemblages have poor age controls, and the chronostratigraphic boundaries of some lithostratigraphic units may extend beyond the target interval (Middle to Upper Triassic) but contain some beds of relevant age. These instances are indicated in the database (Supplementary Tables S1, S2). Some sources report Lower Triassic stratigraphic units containing specimens typical of pre-EPE late Permian plant assemblages, such as Glossopteris (in Australia, Jordan, Madagascar and South Asia). Although some of these recorded fossil assemblages are likely to be of Early Triassic age, the formations that contain these beds may: 1, have poor age constraints; 2, span the Permian-Triassic boundary; and/or 3, the identified fossils may be stratigraphically misplaced. Some specimens documented by previous authors may also have been misidentified. Resolving these possibilities is beyond the scope of the present study. Some records of plant assemblages have not been assigned to a particular lithostratigraphic unit; instead, they were listed according to their positions relative to identifiable units, or the localities at which they were found. For some units indicated by previous authors to contain plant macrofossils, it was not possible to locate all the primary literature. In such cases, non-peer reviewed conference abstracts, and unpublished doctoral and bachelor’s theses were utilized, and these were indicated in the database. Despite these efforts, some units included in the database host very few confirmed species- or genus-level records, and the authors that have indicated additional macrofossils have been cited.
Lithostratigraphic units were grouped into time bins: latest Permian (Lopingian)–Early Triassic (LP–ET), Early Triassic only (ET), Early to Middle Triassic (ET–MT), Middle Triassic only (MT) and Middle to Late Triassic (MT–LT). Assemblages of more uncertain age spanning the Early to Late Triassic (ET–LT) were not included in analyses (Supplementary Table S2).
In addition to leaves, all macrofossil plant materials reported in the compiled literature were entered into the database, including wood, reproductive organs, seeds, and unidentifiable fragments (Supplementary Table S1); plant microfossil records (micro-/megaspores and pollen) were not included. Owing to their prevalence in the fossil record, and their diverse and diagnostic forms, this study focused primarily on fossil leaf damage types. Records of Early to Middle Triassic woods and seeds are sparse, and these data were not compiled systematically herein. Similarly, this review deals with damage caused primarily by terrestrial invertebrate species and excludes any diagnostic herbivory, detritivory or incidental damage caused to plant remains by aquatic invertebrate taxa (Philippe et al., 2022).
For every stratigraphic unit, each distinct report of a plant taxon was entered as one record in the database. Duplicate reports attributed to the same formation (e.g., reports of the same species collected in separate sampling expeditions) were combined into one record (Supplementary Table S1). In an attempt to standardize the database with other eastern Australian Early to Middle Triassic floras and minimize the potential for taxonomic inflation, classifications of taxa higher than genus rank follow the scheme used for description of the Nymboida flora (eastern Australia), which has been documented extensively (Holmes, 2000, 2001a, 2003; Holmes and Anderson, 2005a, b, 2007; 2008, 2013a, 2022; Holmes et al., 2010). Other plant species, genera and higher rank taxa were included in the database with the original designations utilized by the cited authors; however, designations were updated in cases where typographical errors were identified. Consequently, there is some potential for taxonomic inflation as some genera and species may have been reassigned in subsequent studies. For example, Holmes and Anderson (2005a) designated the following genera as junior synonyms of Dicroidium: Dicroidiopsis, Diplasiophyllum, Johnstonia, Tetraptilon, Xylopteris and Zuberia. Misidentifications of specimens and leaf features (incorrect taxa or pseudotraces) by previous authors may also be included.
For temporal and spatial comparison of floral composition, records were organized into fourteen groups in accordance with the aforementioned taxonomic designations: bryophytes, lycophytes, ferns and sphenophytes (spore producers); bennettitaleans, conifers, cordaitaleans (sensu the original authors), cycads, ginkgophytes, gnetopsids, ‘seed ferns’ (i.e., Umkomasiales, Peltaspermales and affiliated groups), gymnosperm incertae sedis (seed producers); and other taxa of uncertain affinity incertae sedis. In contrast to the “incertae sedis” group that was defined by uncertainty in higher taxonomic affinity of the genera by the original authors, higher taxonomic affinities for 61 plant records, reported only as a genus or genus + species by the cited sources, were not able to be identified using the search protocol outlined above and were placed into an additional group denoted by a question mark “?”.
In many cases, the plant fossils were not identifiable to species level and, instead, are only reported to genus or even higher taxonomic levels (referred to as suprageneric records herein). Records containing indications of uncertainty in the genus or species identifications, or taxa in open nomenclature (“sp.”, “spp.”, “cf.”, “aff.”, “?”, etc.) were included in the database and subsequent analyses where they were informative of floral diversity. There are 191 plant fossil records where the number of species discussed was unclear and thus were not included in analyses of counts of species reports from each locality (Supplementary Table S3): 129 suprageneric records (including three records where two genera were indicated as equally probable), and 62 records only specified to genus level, 11 of which were reported as “spp.” indicating the presence of at least one distinct fossil-species from a given genus.
2.2 PAI case study
To determine whether the currently reported levels of arthropod herbivore damage are representative of Triassic assemblages across Gondwana, we also conducted a case study of the Middle Triassic Benolong Flora of the Napperby Formation in the Gunnedah Basin, Australia (Supplementary Table S4). The Benolong Flora is from a site at Ugothery, near Benolong, New South Wales. All specimens investigated in this study (N = 591) are housed in the Australian Museum Palaeontological Collection, Sydney, Australia. For data collection, all hand specimens c. ≤40 cm long were photographed using a Canon 80D camera with a 50 mm Canon macro lens. Dust and loose particles were removed from the specimens prior to imaging using an air blower brush tool. Photography of each sample was conducted under three light conditions: one ‘ambient’ high-angle light condition, and two conditions of low-angle light with orthogonal light directions. For each light condition, a series of photos were taken at slightly different focal lengths. Each photo series was then merged into a composite focused image using Helicon Focus v.8.2.2. Individual hand specimens were typically imaged with a consistent field of view and, hence, focal length (notwithstanding the minor length changes for the composite image-stitching outlined above). However, average focal length varied between samples (c. 30–100 cm), thus resulting in an inconsistent spatial resolution.
All fossils identifiable as individual plant fragments were included in the analyses. For each rock surface, the number of distinct plant fragments was counted, and assessed for PAIs using the DT/FFG categories described by Labandeira et al. (2007) and later unpublished additions (C. Labandeira, pers. comm.). Physical herbivore defenses, such as hairs or spines, were also documented.
Artefacts that could be misidentified as PAIs include abiotic or non-herbivore biotic damage to the plant prior to fossilization, the effects of taphonomic processes, extraction marks, overlying sediment, and adhering dust. The presence of reaction tissue—produced by the living plant around the damaged area to limit tissue infection—was utilized to more confidently identify biotic damage inflicted on living plant tissue. In this way, traces of herbivory were distinguishable from detritivore damage on dead plant material and subsequent taphonomic artefacts. Furthermore, stereotypy, the repeated pattern of size, shape and position of damage, was used to distinguish PAI evidence from abiotic environmental damage to the plant (Labandeira et al., 2016; Currano et al., 2021). Imaging the fossil using low-angle light from orthogonal directions highlighted low-relief, positive and negative topographic features on the rock surfaces, allowing for areas where fossil layers were absent or covered by overlying sediment to be distinguished from plant fossil areas removed by herbivory.
Additional potential confounding variables include the difficulty in accurately counting specimens on rock surfaces with multiple overlapping plant fragments. Moreover, over the course of examining 591 plant fragments, it is possible that the accuracy of the assessment improved due to increased familiarity with the DT guide and assessors’ skills in recognizing more subtle PAIs and in excluding pseudotraces. As noted by Thomas and Hill (2023), none of the images used to assign a PAI to the DT categories of Labandeira et al. (2007) is of an Australian specimen. Moreover, most of the specimens illustrated by Labandeira et al. (2007) are: 1, of much younger material than the Middle Triassic Benolong Flora; and 2, consist almost exclusively of angiosperms, a group of plants for which there is no unequivocal Middle Triassic evidence. Hence, some variation in the expression of these FFGs is expected and a significant proportion of PAIs identified were not confidently assignable to a DT, but were referred only to an FFG.
3 Results
3.1 Database: localities and stratigraphic units
Eighty-nine stratigraphic units yielding 1559 plant records were included in the Gondwanan Early–Middle Triassic fossil database (Figure 2; Supplementary Tables S1, S2): six from Africa, three from Antarctica, two from Jordan, fifty from Oceania, twenty-three from South America, and five from South Asia.
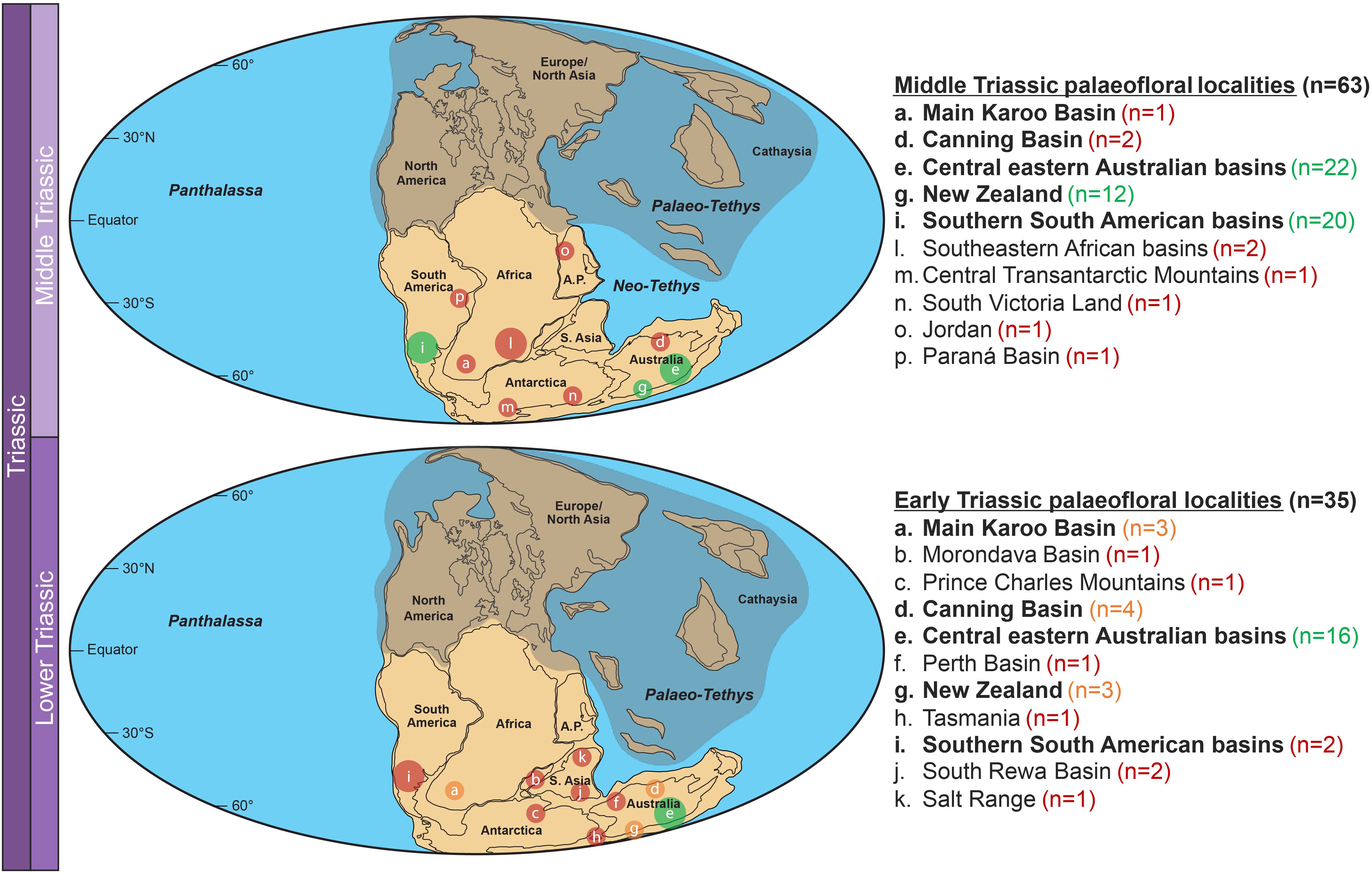
Figure 2. Distribution maps of Early and Middle Triassic Gondwanan basins indicating the numbers of localities (n) included in the plant fossil database. Green circles indicate >10 localities, orange circles indicate 3–10 localities, red circles indicate <3 localities. Regions with high concentrations of basins have been combined for simplicity and are represented by larger circles. Central eastern Australian basins: the Bowen, Gunnedah, Lorne, Nymboida and Sydney basins and the Esk and Warialda troughs. Southern South American basins: the Cuyo/Cuyana, El Tranquilo, Los Menucos and San Rafael basins of Argentina and the Antofagasta, Atacama, Coquimbo and Valparaíso regions of Chile. Southeastern African basins: the Luangwa and Ruhuhu basins. Basins in bold have relevant localities across both the Early and Middle Triassic. Fossiliferous units spanning the Early to Middle Triassic have been duplicated into both age categories as they likely represent transitional floras and may contain assemblages from either, or both the Early and Middle Triassic.
3.1.1 Late Permian to Early Triassic assemblages
Fossil leaves of latest Permian to earliest Triassic age (i.e., close in age to the EPE) are represented in South Asia from the Mianwali Formation (Schneebeli-Hermann et al., 2015) and the Nidpur beds and adjacent strata (Bose and Srivastava, 1971; Banerji et al., 1976; Srivastava, 1988; Bhowmik and Parveen, 2014). Stratigraphic units of other Gondwanan regions that span this interval are: the Puesto Tscherig Formation (Argentina) and the Sakamena Formation (Madagascar) (Carpentier, 1935, 1936; Artabe, 1985a, b; Spalletti et al., 1999; Falco et al., 2020). This time bin contains 96 records (including seven genus-only/“spp.” records and eight suprageneric records).
The Jordanian Umm Irna Formation, of putative latest Permian age (LP; Supplementary Table S2), hosts abundant Dicroidium and the floral records and PAI reports were included in the plant fossil database for comparison (57 plant records including one suprageneric record) (Abu Hamad et al., 2008, 2017; Blomenkemper et al., 2018, 2019, 2020, 2021; Kerp et al., 2021, 2024). However, there are no strata that extend into the specified Early to Middle Triassic age range and so the floral records were not included in any further analyses.
3.1.2 Early Triassic assemblages
The majority of records of Early Triassic leaves are from Australian strata: the Bald Hill Claystone, Bulgo Sandstone, Munmorah Conglomerate, Tuggerah Formation, a single species from the Banks Wall Sandstone, and two plant assemblages with imprecise stratigraphic provenance, are all from the Sydney Basin (Raggatt, 1969; Helby, 1970; Balme and Helby, 1973; Retallack, 1980a). In addition to the Sydney Basin localities, there is also the Camden Head Claystone and Grants Head Formation (one reported species) from the Lorne Basin, the Arcadia Formation from the Bowen Basin, the Blina Shale and Millyit Sandstone from the Canning Basin, the Kockatea Shale from the Perth Basin, and the Knocklofty Formation from Tasmania (White and Yeates, 1976; Ash, 1979; Holmes and Ash, 1979; Chaloner and Turner, 1987; Retallack, 1995, 1997; Cantrill and Webb, 1998; Haig et al., 2015). Non-Australian Early Triassic assemblages include those from the Flagstone Bench Formation (Ritchie Member) in Antarctica and the Katberg and Verkykerskop formations from South Africa (McLoughlin et al., 1997; Gastaldo et al., 2005; Gastaldo and Bamford, 2023). This investigation did not identify any records of plant macrofossil assemblages of definitive Early Triassic age from the Arabian Peninsula, South America or South Asia.
This time bin contains 139 records (including 16 genus-only records and 27 suprageneric records).
3.1.3 Late Early to early Middle Triassic assemblages
The fossil-bearing stratigraphic units that may span the Lower to Middle Triassic boundary in Gondwana are largely from Australia: the Burralow, Garie, Gosford and Terrigal formations of the Sydney Basin, the Clematis Sandstone and Glenidal Formation of the Bowen Basin, and the Culvida and Erskine sandstones from the Canning Basin (White, 1965; Goldbery, 1969; Raggatt, 1969; White, 1971; White and Yeates, 1976; Retallack, 1977; Ash, 1979; Retallack, 1980a; Chaloner and Turner, 1987; Holmes, 2001b; McLoughlin, 2011a). The Murihiku Supergroup from New Zealand includes the Potiki Siltstone and the Wairuna Peak Beds of this age, along with some leaf fossil occurrences not allocated to a stratigraphic unit (Retallack, 1985). The palaeoflora-bearing Burgersdorp Formation (South Africa) and the Puesto Vera Formation (Argentina) have also been attributed to the Early to Middle Triassic (Anderson and Anderson, 1985; Artabe, 1985b, a; Bamford, 1999; Spalletti et al., 1999; Hancox et al., 2002; Bamford, 2004; Gastaldo et al., 2005; Falco et al., 2020; Hancox et al., 2020).
This time bin contains 159 records (including seven genus-only/”spp.” records and 15 suprageneric records).
3.1.4 Middle Triassic assemblages
Fossil leaves confidently ascribed to the Middle Triassic are also described predominantly from Australian sedimentary basins. Fossiliferous units of this interval include the Gragin Conglomerate and Gunnee Beds from the Warialda Trough, the Napperby Formation from the Gunnedah Basin, the Ashfield Shale, Bringelly Shale, Brookvale Shale, Hawkesbury Sandstone and the Newport Formation from the Sydney Basin, the Moolayember and Teviot formations from the Bowen Basin, the Basin Creek Formation from the Nymboida Basin, and the Bryden and Esk formations, together with a single record from the Neara Volcanics in the Esk Trough (Walkom, 1924, 1928; White, 1965; Branagan, 1969; Jell, 1969; Lovering and McElroy, 1969; White, 1969, 1971; Bourke et al., 1977; Rigby, 1977; Retallack, 1980a; Holmes, 1982; Playford et al., 1982; Webb, 1982; Webb and Holmes, 1982; Webb, 2001). Also from the Sydney Basin, another small assemblage reported as “Mittagong Formation or basal Ashfield Shale” by Retallack (1980a, p. 426), and one unit yielding only one species, the Minchinbury Sandstone, were included (Lovering and McElroy, 1969). From New Zealand, the Black Jacks Formation and the Tank Gully Coal Measures are assigned to the Middle Triassic (Retallack, 1980b, 1983), along with the remaining Murihiku Supergroup units and unspecified assemblages (Retallack, 1985): The North Etal Group, the North Peak (Jacobs River Member) and Pears formations, an assemblage “southeast of Mautaura island” (p. 11), an assemblage “above the Franklin Conglomerate” (p. 9) and the ‘Tauringatura Group’ (p. 13), the latter two with only one species reported each. The remaining Middle Triassic strata are: 1, the Mukheiris Formation from Jordan (Abu Hamad et al., 2019), and; 2, the Barreal, Cerro de Las Cabras, Corral de Piedra, Monina, Montaña, Paramillos, and Quebrada de los Fósiles formations from Argentina (Brea et al., 2008; Cariglino et al., 2016, 2018; Bodnar et al., 2019; Pedernera et al., 2019; Drovandi et al., 2020; Pedernera et al., 2022).
This time bin contains 582 records (including 19 genus-only/“spp.” records and 39 suprageneric records).
3.1.5 Middle to Late Triassic assemblages
Formations with strata indicated to extend from the Middle Triassic into the Upper Triassic are located predominantly in South America, the vast majority of which have uncertain age controls: 1, the Cañadón Largo Formation from the El Tranquilo Basin (Herbst, 1988; Jalfin and Herbst, 1995; Gnaedinger and Herbst, 1998a, b, 1999; Troncoso et al., 2000; Herbst et al., 2001; Herbst and Gnaedinger, 2002; Adami-Rodrigues et al., 2008; Villalva, 2017; Gnaedinger et al., 2023; Villalva et al., 2023); 2, the Agua de la Zorra, Cortaderita (Don Raúl Member), Agua de Los Pajaritos and Potrerillos formations from the Cuyo/Cuyana Basin in Argentina (Ottone et al., 2011; Cariglino et al., 2016; Lara et al., 2017; Bodnar et al., 2019; Pedernera et al., 2019); 3, the El Bordo and El Mono beds, and the Pular, Tuina, Guanaco Sonso, Las Breas and El Puquén formations of Chile (Azcárate and Fasola, 1970; Breitkreuz et al., 1992; Lutz et al., 1999; Troncoso and Herbst, 1999; Herbst, 2000; Herbst and Troncoso, 2000; Melchor and Herbst, 2000; Troncoso et al., 2000; Gnaedinger and Herbst, 2001; Herbst and Gnaedinger, 2002; Gnaedinger and Herbst, 2004a, b; Niemeyer et al., 2008; Moisan, 2024); and 4, the Santa Maria Formation of Brazil (Guerra-Sommer and Cazzulo-Klepzig, 2000; Cenci, 2013; Barboni and Dutra, 2015; Barboni et al., 2016; Cenci and Adami-Rodrigues, 2017; Cenci et al., 2019). Very few plant species have been identified from the Tuina Formation and El Mono beds in this review. Similarly, only four species were reported from the Ntawere Formation in Zambia, and only one plant species was identifiable from the Manda Formation of Tanzania, which is poorly dated but suggested to be within this age range (Dixey, 1937; Lacey and Smith, 1970). The rich plant assemblages from the permineralized peat (and associated beds) of the upper Fremouw Formation of the Transantarctic Mountains (Schopf, 1978; Stubblefield and Taylor, 1986; Perovich and Taylor, 1989; Taylor et al., 1989; Millay and Taylor, 1990; Pigg, 1990; Delevoryas et al., 1992; Meyer-Berthaud et al., 1993; Taylor et al., 1994; Del Fueyo et al., 1995; Yao et al., 1995, 1997; Axsmith et al., 1998; Phipps et al., 2000; Klavins et al., 2002; Rothwell et al., 2002; Cúneo et al., 2003; Klavins et al., 2003; Kellogg and Taylor, 2004; Klavins et al., 2004; Retallack et al., 2005; Hermsen et al., 2006, 2007a, b; Decombeix et al., 2010; Schwendemann et al., 2010; Bergene et al., 2013; Decombeix et al., 2014) have commonly been assigned to the Middle (or even Lower) Triassic (Taylor et al., 1986, 1993). However, palynoassemblages from these beds are preferable to the Alisporites Assemblage Zone C or Australian APT4 zone, indicating a possible Carnian (Late Triassic) age (Farabee et al., 1990). The Antarctic Lashly Formation and the Long Gully Formation of New Zealand have also been assigned to this geochronological interval (Retallack, 1981; Escapa et al., 2011; Bomfleur et al., 2013b).
This time bin contains 444 records (including ten genus-only/“spp.” records and 33 suprageneric records).
3.1.6 Assemblages of Early to Late Triassic or uncertain age
There are three remaining formations in the database, indicated to be in the range of Lower to Upper Triassic without more precise stratigraphic resolution: the Chilean San Felix Formation (Mohr and Schöner, 1985; Bell and Suarez, 1994; Troncoso et al., 2000; Salazar et al., 2013) and the Indian Panchet and upper Kamthi formations (Bose and Banerji, 1974; Banerji et al., 1976; Banerji and Bose, 1977; Pal and Ghosh, 1997; Pal et al., 2010, 2014; Saxena et al., 2019; Ghosh et al., 2021). As plant fossil records from these formations (82 records, including three genus-only/“spp.” records and six suprageneric records) cannot be grouped into any particular time bin or used for comparison across the Early to Middle Triassic, they were not included in the analyses.
Additional units in India are possibly referable to the Middle Triassic (e.g., the Pali, Parsora, and Panchmarhi formations) but age constraints are currently insufficient for confident assignment of fossil assemblages from these units to any particular time bin (Joshi et al., 2014).
3.2 Floral composition
Gondwanan terrestrial ecosystems were dominated by glossopterid gymnosperms throughout most of the Permian (McLoughlin, 2011b). The end-Permian event in Gondwana was marked by the demise of glossopterids (Figure 1) and many associated free-sporing and gymnospermous plants (Anderson et al., 1998; Retallack et al., 2005; Lindström and McLoughlin, 2007; Fielding et al., 2019; Gastaldo and Bamford, 2023). Reports of glossopterids surviving the end-Permian extinction event and persisting into the earliest Triassic are equivocal. Records of glossopterids in units close to or spanning the Permian-Triassic boundary collated in the present database derive from: 1, the Umm Irna Formation (LP; Jordan; Kerp et al., 2021); 2, the Sakamena Formation (LP–ET; Madagascar; Carpentier, 1935, 1936); 3, the Munmorah Conglomerate, Blina Shale, Millyit Sandstone (ET; Australia) the Erskine Sandstone (ET–MT; Australia) (Raggatt, 1969; White and Yeates, 1976); and 4, several units in India, including the Nidpur beds and associated strata, the Mianwali Formation (all LP–ET), and the Panchet and Kamthi formations (ET–LT) (Supplementary Table S1; Banerji et al., 1976; Banerji and Bose, 1977; Srivastava, 1988; Pal and Ghosh, 1997; Pal et al., 2010; Schneebeli-Hermann et al., 2015; Saxena et al., 2019). Additional Indian strata hosting putative Glossopteris of possible Triassic age are the Pali, Hinjir, Maitur, Parsora, and Hirapur/“Upper Kamthi” formations (Maheshwari, 1992; Shah, 2000; Ghosh et al., 2016; Shah, 2021); however, these records may represent misidentifications of typical Triassic taxa, such as Gontriglossa. With the exception of the Munmorah Conglomerate and Blina Shale, Dicroidium has also been reported from these same strata (Carpentier, 1935, 1936; Raggatt, 1969; Bose and Srivastava, 1971; Bose and Banerji, 1974; Banerji et al., 1976; White and Yeates, 1976; Srivastava, 1988; Maheshwari, 1992; Retallack, 1995; Pal and Ghosh, 1997; Shah, 2000; Pal et al., 2014; Schneebeli-Hermann et al., 2015; Saxena et al., 2019; Kerp et al., 2021). Srivastava et al. (2010) also reported Dicroidium associated with Glossopteris from Lower Permian strata of the Barakar Formation, however, the putative Dicroidium specimens were later reinterpreted as peltaspermalean foliage (Srivastava et al., 2011). Other putative co-occurrences of Dicroidium and Glossopteris recorded in this database may include conflations of pre-EPE Permian and post-EPE Permian or Triassic plant assemblages that have been grouped into the same formation or have become stratigraphically misplaced by faulted juxtapositioning of beds of different ages (Tiwari and Ram-Awatar, 1990). Although we cannot exclude the possibility that some glossopterids persisted as post-EPE survivors, this conclusion awaits verification. No Glossopteris has yet been identified in any of the Antarctic or South American Triassic units included in this database, although Rigby and Schopf (1969) report the co-occurrence of these taxa in an Antarctic assemblage they determined to be equivalent to the Panchet Stage (no formation indicated).
Later in the Early Triassic, phases of alternating dominance by pleuromeian lycopsids, voltzialean conifers and Dicroidium (Umkomasiales), together with spikes in charcoal abundance, indicate repeated fluctuations in environmental conditions (Retallack, 1977; Helby et al., 1987; Mays et al., 2020; Mays and McLoughlin, 2022). By the end of the Early Triassic, Umkomasiales had become established as the dominant gymnosperm group across southern Gondwana (Figures 1, 3; Retallack, 1977; Anderson and Anderson, 2003).
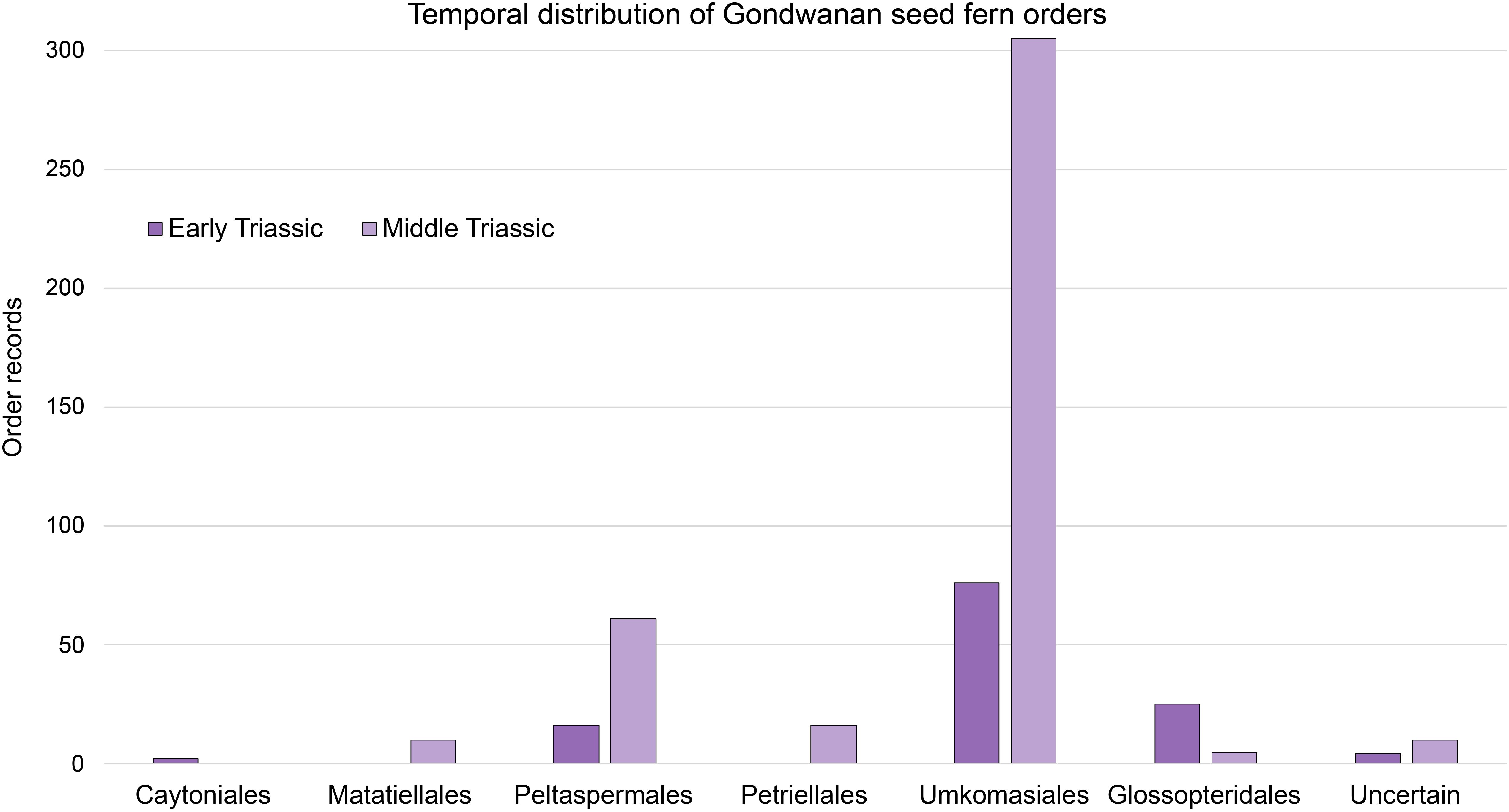
Figure 3. Gondwanan Early and Middle Triassic seed fern diversity. The number of records of each order in the plant fossil database is reported. The “uncertain” group consists of fossil records where authors have indicated affinity to seed ferns, but for which designations of order were not specified. Seed fern records from assemblages spanning Early to Middle Triassic age have been included in both age categories: Early Triassic (n=123); Middle Triassic (n=407).
Through the Middle Triassic, Umkomasiales diversified and retained dominance of the Gondwanan temperate vegetation (Retallack, 1977; Anderson and Anderson, 1985; Taylor et al., 1993). They were associated with a broad range of sphenophytes, ferns, broad-leafed conifers, cycads, bennettitopsids, peltasperms, voltzialean conifers and various gymnosperms of uncertain affinity (Figures 3, 4; Walkom, 1928; Anderson and Anderson, 1985; Taylor et al., 1993; McLoughlin et al., 2018b). Overall land-plant diversity continued to increase in the austral temperate zone (>35°S) through the Middle and Late Triassic (Anderson and Anderson, 1989, 2003), before suffering depletion and replacement of key taxa during the end-Triassic extinction event (Anderson et al., 1998; Kustatscher et al., 2018).
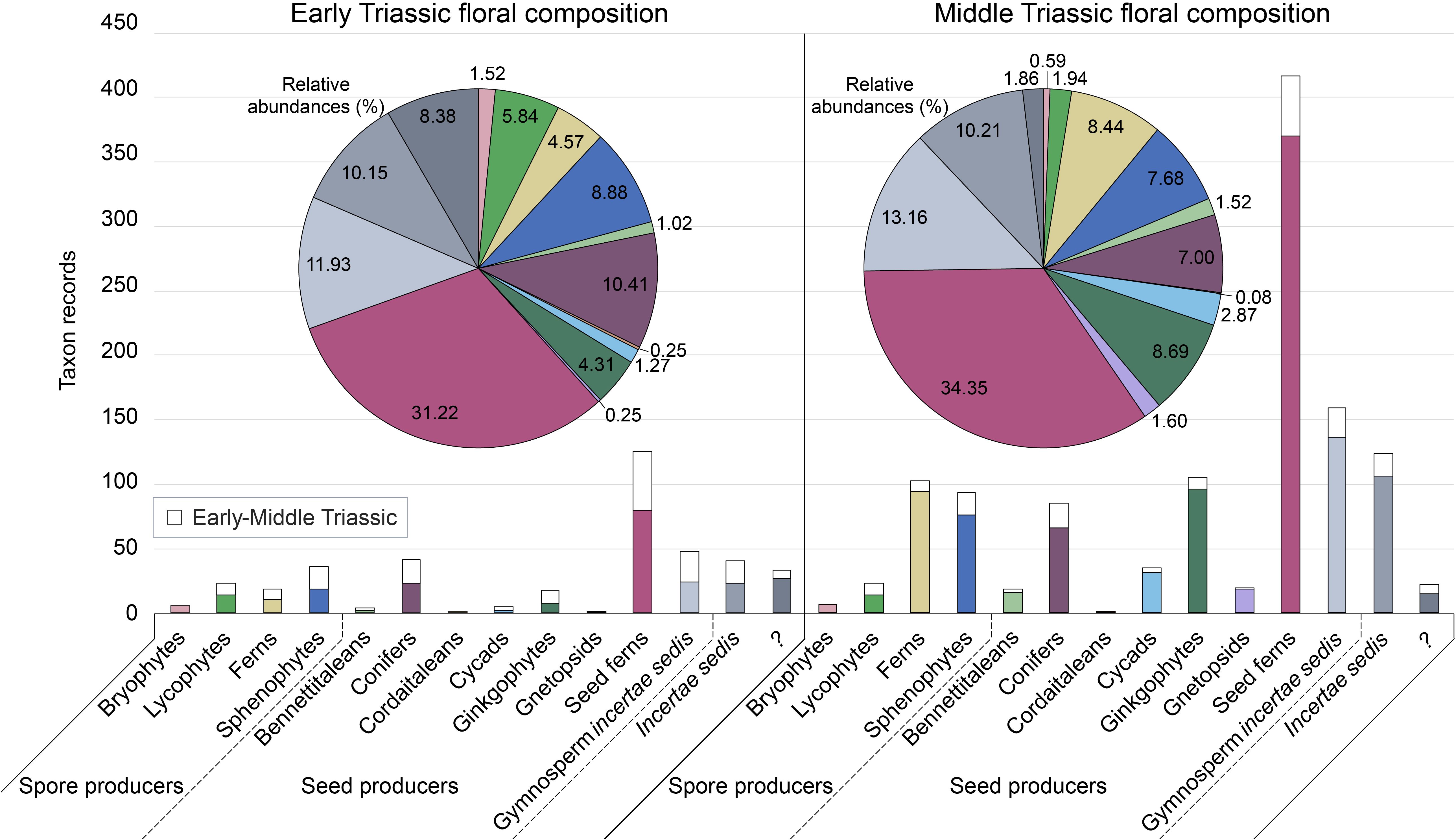
Figure 4. Comparison of Gondwanan Early and Middle Triassic floral composition. The number of records of each higher taxonomic group in the plant fossil database is reported. Uncoloured bars represent Early to Middle Triassic plant fossil records, with equal values above the data for both the Early Triassic and Middle Triassic age categories. Higher taxonomic groups follow the same sequence for all charts: bryophytes; lycophytes; ferns; sphenophytes; bennettitaleans; conifers; cordaitaleans; cycads; ginkgophytes; gnetopsids; seed ferns; gymnosperm incertae sedis; incertae sedis; “?”. The “?” group consists of plant fossil genera for which higher taxonomic designations were unable to be identified in this review, in contrast to the incertae sedis group defined by uncertainty in higher taxonomic affinity of the genera. Pie chart values represent proportional contributions of each higher taxonomic group to the total floral records in that age category (in %). Plant fossil records from assemblages spanning Early to Middle Triassic age have been included in the pie charts of both age categories; total Early Triassic records (n=394); total Middle Triassic records (n=1185).
A floral transition is evidenced in the present database by the higher proportion of bryophytes, lycophytes, sphenophytes and conifers in the Early Triassic compared to the Middle Triassic, whereas ferns, bennettitaleans, cycads, ginkgophytes, gnetopsids and gymnosperms of uncertain affinity constitute a greater proportion of Middle Triassic diversity than in the Early Triassic assemblages (Figure 4). Seed ferns, including umkomasialeans and peltasperms, although dominant in both Early and Middle Triassic assemblages, constitute a greater proportion of Middle Triassic total species richness, demonstrating their continued diversification (Figures 3–5). The total number of plant species reported increases in the Middle Triassic, particularly in Oceania and South America (Figures 3–5, Supplementary Figure S1; Supplementary Table S3). This likely represents a combination of recovery in species diversity after the EPE, and increased collection and taxonomic identification efforts for Middle Triassic assemblages compared to those of the Early Triassic.
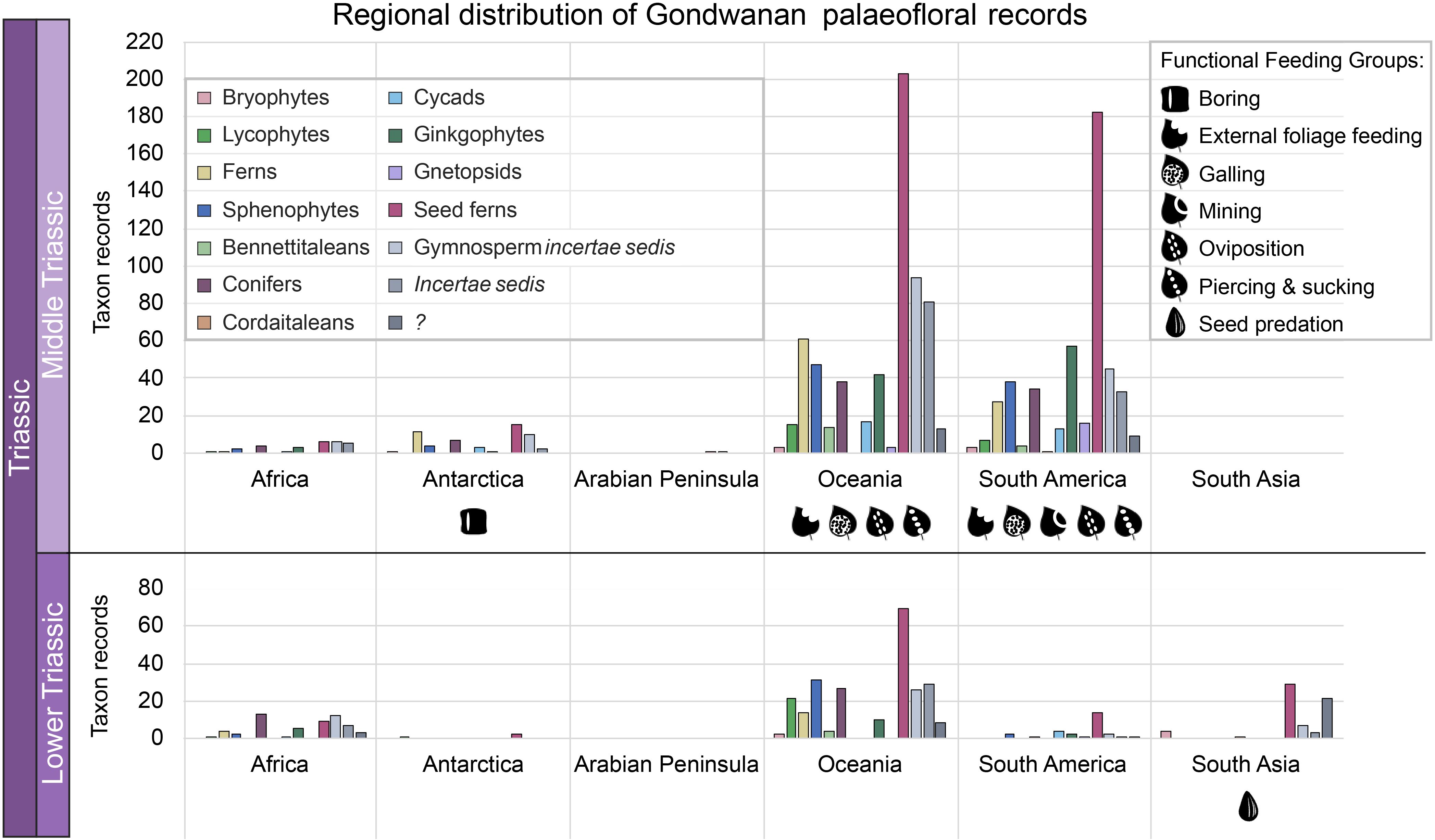
Figure 5. Gondwanan Early and Middle Triassic floral composition by region. The number of records of each higher taxonomic group in the plant fossil database is reported. Higher taxonomic groups follow the same sequence for both charts: bryophytes, lycophytes, ferns, and sphenophytes (spore producers); bennettitaleans, conifers, ‘cordaitaleans’, cycads, ginkgophytes, gnetopsids, seed ferns, and gymnosperm incertae sedis (seed producers); incertae sedis; “?”. The “?” group consists of plant fossil genera for which higher taxonomic designations were unable to be identified in this review, in contrast to the incertae sedis group defined by uncertainty in higher taxonomic affinity of the genera. Plant fossil records from assemblages spanning Early to Middle Triassic age have been included in both age categories. Early Triassic taxon records: Africa (n=57); Antarctica (n=54); Arabian Peninsula (n=0); Oceania (n=241); South America (n=28); South Asia (n=65). Middle Triassic taxon records: Africa (n=29); Antarctica (n=54); Arabian Peninsula (n=2); Oceania (n=631); South America (n=469); South Asia (n=0). Functional feeding groups of reported plant–arthropod interactions are indicated beneath the chart for each region.
3.3 PAI record of Gondwana
Of the 85 stratigraphic units included in the Gondwanan Early–Middle Triassic analyses (Supplementary Table S1), only twelve (14.1%) yielded PAIs reported in the surveyed literature (Table 1).
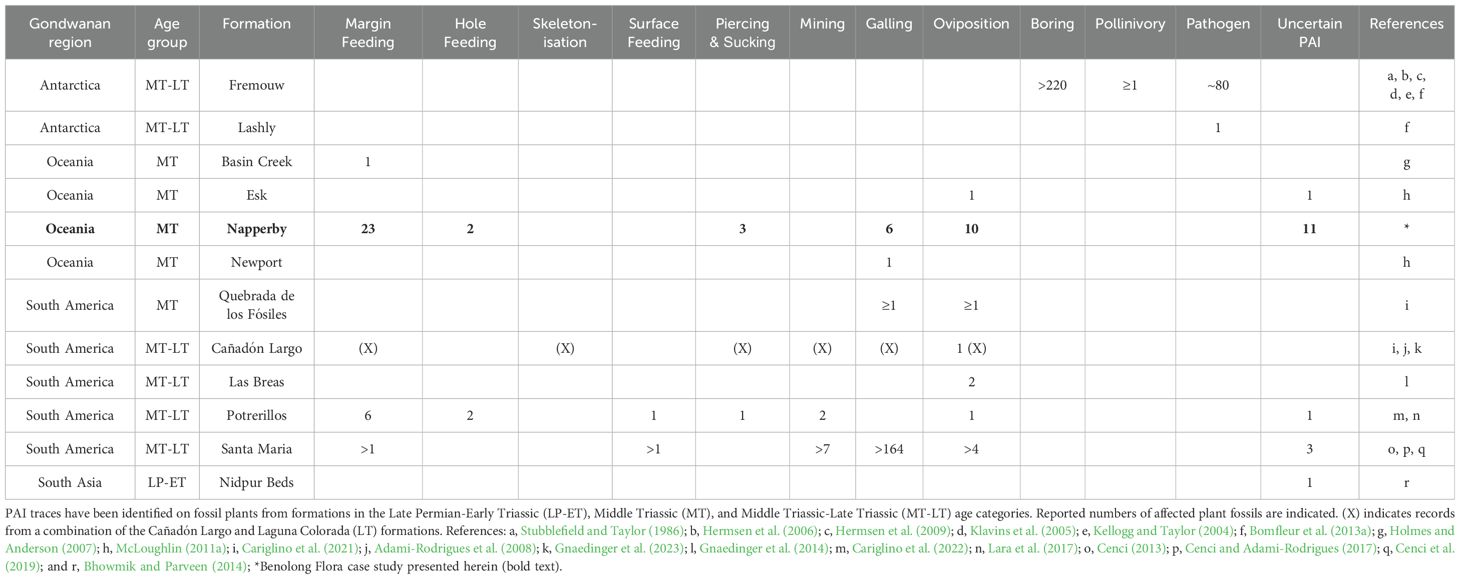
Table 1. Records of plant–arthropod interactions (PAIs) from formations in the Gondwanan plant fossil database.
3.3.1 Africa
We could find no reports of insect damage from Early to Middle Triassic African floral assemblages. Labandeira et al. (2018) noted the conspicuous absence of galls from a collection of 1386 plant specimens of the Burgersdorp Formation (ET–MT).
3.3.2 Antarctica
The majority of PAI records from the late Middle to early Late Triassic of Antarctica derive from the Fremouw Formation, with several organ types affected, although no PAIs have yet been found on leaves. These trace fossils include evidence of boring—likely caused by oribatid mites—in 220 specimens of stems, petioles and roots including a petiole of the fern Antarctipteris (Kellogg and Taylor, 2004). This assemblage also yielded insect coprolites containing pollen located in the cycad pollen cone Delemaya spinulosa (Klavins et al., 2005). Additionally, Hermsen et al. (2006, 2009) identified feeding galleries in stems of the cycad Antarcticycas schopfii of the Fremouw assemblage, which was likely caused by wood-boring arthropod larvae. Fungal damage has also been observed in almost eighty specimens of Araucarioxylon-type wood and one specimen of the coniferous ovulate organ Parasciadopitys from this formation (Stubblefield and Taylor, 1986; Bomfleur et al., 2013a). Bomfleur et al. (2013a) also reported fungal hyphae on one coniferous leaf of Heidiphyllum from the Lashly Formation of the same age. However, as noted above, the ages of the upper Fremouw and Lashly formations may be Late Triassic.
3.3.3 Arabian peninsula
Hole feeding on three Dicroidium irnensis cuticles from the late Permian Umm Irna Formation (Jordan) was identified by McLoughlin et al. (2021a). However, no definitive Triassic PAIs have yet been reported.
3.3.4 Oceania
This review identified three Middle Triassic Australian formations hosting examples of PAI traces on fossil plants. Holmes and Anderson (2007) identified margin feeding on a leaf apex of the ginkgoalean Sphenobaiera densinerva from the Basin Creek Formation (MT). McLoughlin (2011a) recognized oviposition scars, possible margin feeding and either hole feeding or surface feeding on a leaf of Taeniopteris parvilocus from the Esk Formation (MT) and galling on a Dicroidium odontopteroides leaf from the Newport Formation (MT). The present study describes several newly identified PAIs from the Benolong Flora (Napperby Fm, MT); see section 3.4 below for more details.
3.3.5 South America
Permian and Triassic PAI records from South America were extensively compiled and reviewed by Gnaedinger et al. (2014), Cariglino et al. (2021) and Romero-Lebrón et al. (2022). An updated summary of these occurrences is provided below (in stratigraphic order).
The Argentinian Quebrada de los Fósiles Formation (MT) yielded gall-bearing leaves of the seed fern Ptilozamites longifolia (possibly = Kurtziana sp.) and oviposition traces on the frond of another seed fern of uncertain affinity (Cariglino et al., 2020, 2021).
Adami-Rodrigues et al. (2008) identified PAIs from the Argentinian El Tranquilo Group, including the Cañadón Largo Formation (MT–LT), and the Late Triassic Laguna Colorada Formation. That study listed several types of external foliage feeding, together with piercing and sucking, mining, galling and oviposition. PAIs were identified on sphenophytes, ginkgoaleans, conifers, gymnosperms of uncertain affinity and umkomasialean seed ferns, with fossils of the latter group hosting the majority of documented PAIs (Adami-Rodrigues et al., 2008; Cariglino et al., 2021). However, it was not clear which floral remains and their associated PAIs were recovered from strata of definitively Middle versus Late Triassic age. Gnaedinger et al. (2023) identified oviposition scars on a stem of the sphenopsid Equisetites lateralis, also from the Cañadón Largo Formation.
Two occurrences of oviposition traces were reported from two Taeniopteris sp. leaves of the Las Breas Formation, Chile (MT–LT; Gnaedinger et al., 2014).
The flora of the Potrerillos Formation from Argentina (MT–LT) hosts several PAIs. Lara et al. (2017) assessed 56 plant specimens, reporting 11 specimens hosting PAI traces: margin feeding on leaves of the umkomasialeans Dicroidium argenteum, D. odontopteroides, Johnstonia stelzneriana and the matatiellalean Kurtziana brandmayri, hole-feeding traces on leaves of Johnstonia sp. and Yabeiella wielandii, surface feeding and piercing-and-sucking traces on leaves of uncertain affinity, and oviposition traces on an indeterminate sphenophyte stem. Cariglino et al. (2022) identified mines on leaves of the conifer Heidiphyllum cacheutense and the gnetalean Yabeiella wielandii, with possible oviposition or a fungal spot identified alongside the mine in one Y. wielandii leaf.
The leaf floras of the Santa Maria Formation of Brazil (MT–LT) have been the most extensively investigated assemblages of any Gondwanan locality, with the most PAI records in the database herein (Cenci, 2013; Cenci and Adami-Rodrigues, 2017; Cenci et al., 2019). Cenci (2013) noted that, of a total of 414 plant samples from the Santa Maria Formation, 149 (36%) hosted evidence of PAIs: 135 umkomasialean leaves (52 external foliage feeding (EFF) traces, seven mines, 578 galls), 11 ginkgoalean leaves (three mines, 49 galls), one voltzialean leaf (one mine), eight equisetalean stems (one mine, 22 galls) and nine incertae sedis (one EFF, two mines, 10 galls). Cenci and Adami-Rodrigues (2017) also found galls to be especially common in this unit, with 164 gall-bearing specimens from their sample of 414 (39.6%), apparently representing the same dataset as that employed by Cenci (2013). The vast majority of the galls were identified on umkomasialean leaves. Six-hundred and fifty-nine individual galls were identified across umkomasialean, ginkgoalean, equisetalean and incertae sedis taxa, including leaves of Dicroidium odontopteroides, Dicroidium sp., Sphenobaiera sp. and Taeniopteris sp., an umkomasialean seed, and a stem of the sphenophyte Neocalamites. Cenci et al. (2019) assessed 550 plant specimens, and reported oviposition (six DTs), surface feeding (three DTs), piercing and sucking (four DTs), mining (two DTs), margin feeding (one DT) and hole feeding (one DT). They found that the majority of PAI traces identified were hosted by the dominant umkomasialeans of the Santa Maria Formation, though the ginkgoaleans displayed the greatest proportion of affected specimens.
3.3.6 South Asia
To our knowledge, records of PAIs from this region are almost entirely absent for the Early to Middle Triassic. Bhowmik and Parveen (2014) reported evidence of potential plant-insect interactions from the Indian Nidpur beds (LP–ET). They suggested that clumped pollen grains inside the pollen chamber of the pteridospermous seed Gopadispermum papillatus, indicate the possibility of fluid feeding, palynophagy and insect pollination in the seeds, although such pollen characters are also common in anemophilous plants (Benson, 1908; McLoughlin et al., 2018a). Ghosh et al. (2015) reported galls on Dicroidium leaves from the Parsora Formation (of unspecified Triassic age and, therefore, not included in the database).
3.4 Case study: Benolong Flora
Five hundred and ninety-one fossil leaf fragments were assessed from the Benolong Flora (Supplementary Table S4). Fifty-five potential traces of plant–arthropod interaction were identified on forty-nine plant fragments (8.29% of fragments assessed; Figures 6A, 7). Forty-four of these PAI traces (78.6%), hosted by 40 plant fragments (1.1 PAIs per affected fragment), matched the DT categories described by Labandeira et al. (2007). Margin feeding was the most common FFG, with 23 examples referable to established DTs (identified on 3.72% of plant fragments; Figures 6B, 7A, B; Table 1); this was more than twice as common as oviposition (identified on 1.69% of plant fragments) and all other recognized (non-ambiguous) FFGs combined (Figures 6B, 7C–H; Table 1). However, margin-feeding traces may be more identifiable on compression fossils than other FFGs as they do not require relief to be observed and are especially visible where there is a high degree of contrast between the fossil and the host sedimentary matrix. Eleven traces were not consistent with any established DTs or had characters in common with DTs of multiple FFGs (e.g., resembling features of various hole feeding, oviposition or piercing and sucking DTs). In such cases, these were recorded as ‘ambiguous’.
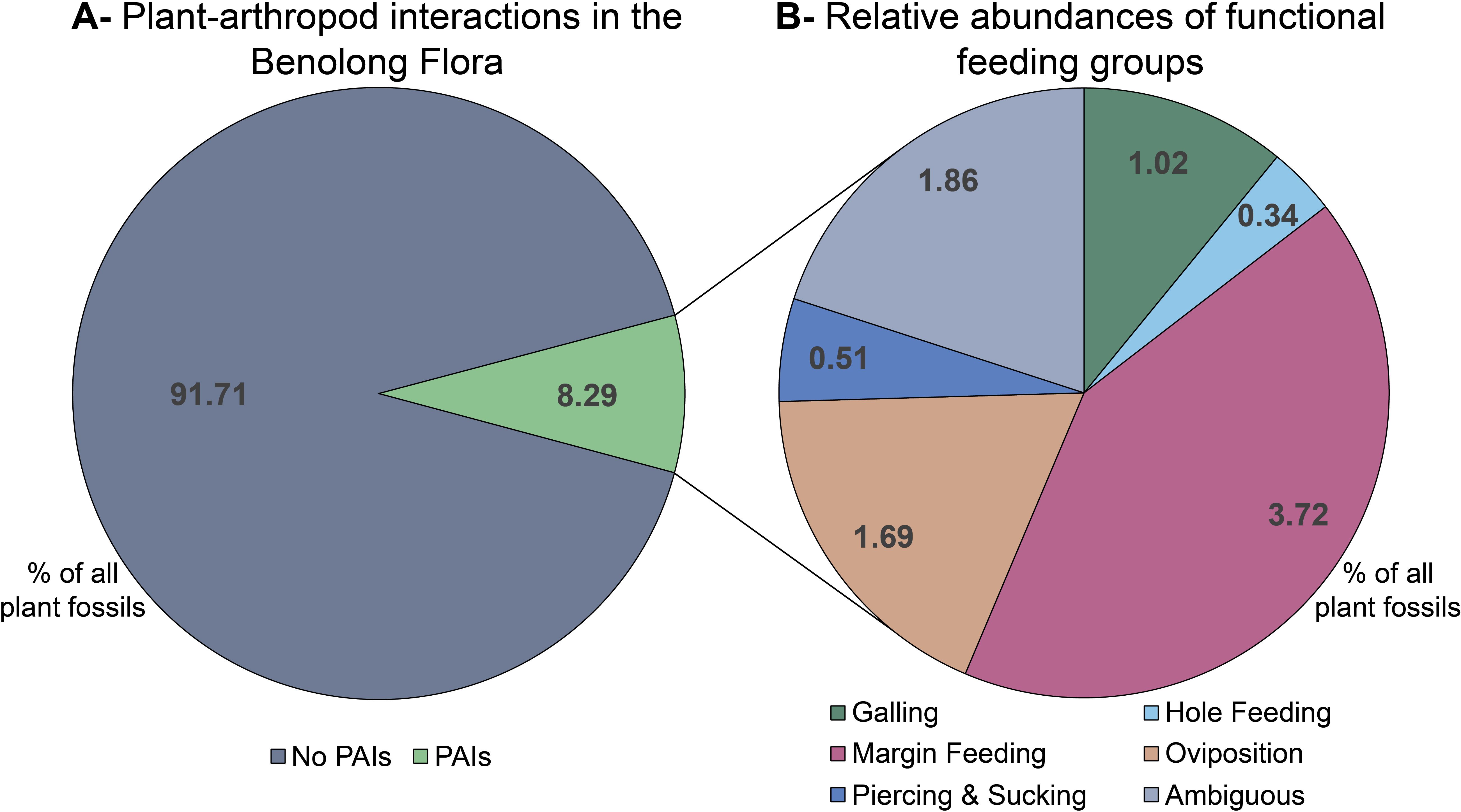
Figure 6. (A) Percentage of Benolong Flora fossil fragments hosting plant–arthropod interaction (PAI) traces relative to the total sample (n=591); (B) Proportions of functional feeding groups from identified PAI trace fossils (n=55). Values indicate percentages of plant fragments hosting each functional feeding group relative to the total sample.
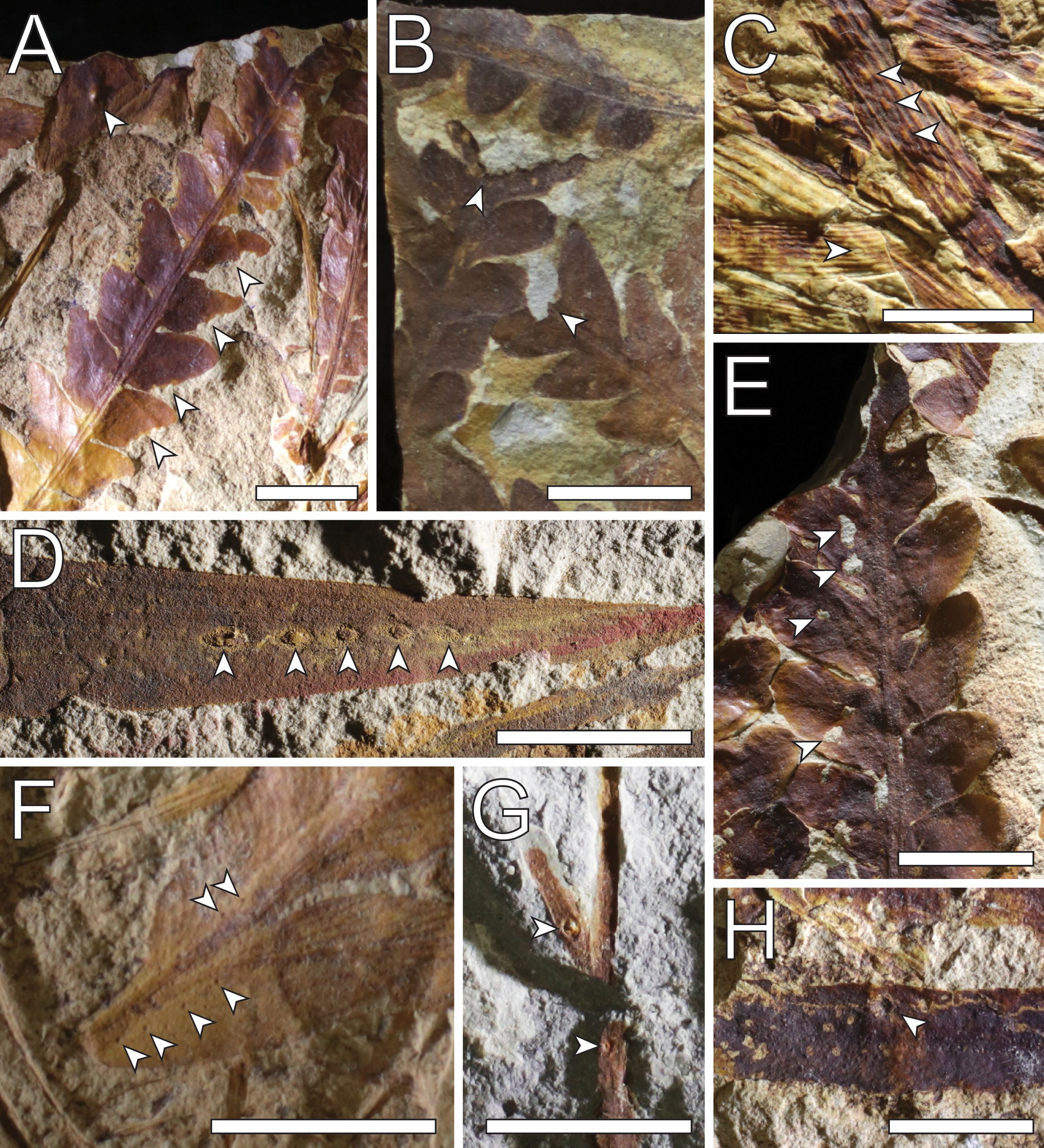
Figure 7. Plant–arthropod interaction traces on Benolong Flora fossil fragments. (A) gall (top arrow) and margin feeding on two fragments of Dicroidium sp. (F61131); (B) apical margin feeding on two fragments of Dicroidium odontopteroides (F61138); (C) oviposition scars on two fragments of Heidiphyllum elongatum (F61501); (D) oviposition scars on Heidiphyllum elongatum (F50237); (E) possible hole feeding on Dicroidium odontopteroides (F61140); (F) piercing and sucking scars on Dicroidium sp. leaf (F61143); (G) gall (top arrow) and an uncertain feature (F61129); and (H) gall on seed fern rachis (F61130). Scale bars: 1 cm.
Preservational artefacts resembling leaf mine traces (Supplementary Figure S2A) and leaf features with atypical textures and surficial patterns (Supplementary Figures S2B–D), potentially of biotic origin but not able to be categorized into any FFG using the guide images, were not included in the analysis. One fossilized structure resembled a gall with possible radiating partitions (Supplementary Figure S2E); however, this feature was not located on any observable plant remains and had the same color as the host sediment and so was also excluded from analyses. Other leaf features that highlighted the potential for the inflation of identified PAIs included a semicircular line of discoloration on the margin of another leaf fossil, suggestive of pathogenic, possibly fungal, damage (Supplementary Figure S2F resembles DT058) and the presence of pseudotraces, such as fern sori resembling the texture of a gall (Supplementary Figure S2G resembles DT106). Twenty-seven fossil fragments of leaves and stems displayed textural evidence of hairs or spines.
The data collection process in this case study, which involved systematic photography of an entire floral assemblage, is inherently limited by the resolution of the images. Even in cases where small specimens were imaged, evidence of smaller PAIs, such as piercing and sucking, was difficult to distinguish from preservational artefacts and adhering dust on the rock surface. Another potential source of error of this approach is the inconsistent focal length, hence spatial resolution, of images taken of separate specimens. At the maximum focal length, as used for the largest hand specimens, much less detail is captured on the image, resulting in lower levels of confidence in distinguishing PAIs from artefacts where these are not verified by microscopic inspection. For increased validity of inter-sample comparisons, we recommend a consistent, high-resolution field-of-view and stereomicroscopic evaluation of each specimen. Despite the limitations, we report abundant PAIs from this case study of a relatively small Middle Triassic fossil assemblage. These findings clearly demonstrate marked under-reporting of arthropod trace fossils from the post-EPE recovery phase, as discussed below.
4 Discussion
4.1 Patterns of Early to Middle Triassic plant–arthropod interactions in Gondwana
4.1.1 Temporal trends and gaps
With the inclusion of the 13 Early to Middle Triassic localities into the data of both age groups, only one of 35 localities from the Early Triassic group reported any PAIs (2.9% of localities), and eleven of 63 localities from the Middle Triassic group (17.5% of localities) yielded PAIs (Figure 2; Table 1; Supplementary Table S2). Our survey highlights the distinct paucity of arthropod trace fossil records from Early Triassic floras across Gondwana, with only one pteridospermous seed hosting putative arthropod traces reported from the Nidpur beds of India (Table 1; Supplementary Table S1). In contrast, at least 544 Middle and Middle-to-Late Triassic plant fragments from eleven localities have been confirmed to host PAI traces, from ten of eleven categories of FFG: margin feeding (5.70% of quantifiable reports); hole feeding (0.74%); surface feeding (0.37%); piercing and sucking (0.74%); mining (1.65%); galling (31.62%); oviposition (3.68%); boring (40.44%); palynophagy (0.18%); pathogenic/fungal damage (14.89%). The remaining FFG, skeletonization, has been recognized but is possibly of Late Triassic age (Table 1; Supplementary Table S1).
Austral temperate floras were fundamentally restructured after the end-Permian extinction event. Earliest Triassic vegetation consisted of low-diversity open forests dominated by lepidopterid peltasperms and scale-leafed voltzialean conifers in the upper story, and pleuromeian lycopsids, sphenopsids, and ferns in the understory (Retallack, 1980a; Mays et al., 2020; Vajda et al., 2020; McLoughlin et al., 2021a). There are 247 plant species across 118 genera reported from the Gondwanan Early Triassic, and 608 species from 200 genera identified from the Middle Triassic (Supplementary Table S1). Whereas the latest Permian to Early Triassic PAI was identified on a seed fern taxon, the Middle to Late Triassic PAIs are distributed across ferns, sphenophytes, conifers, cycads, ginkgophytes, seed ferns, and plants of uncertain taxonomic affinity (Supplementary Table S1). This disparity may be due to the relatively low productivity and slow recovery of the immediate post-EPE flora compared to the Middle Triassic. Low productivity and low diversity of vegetation would have contributed to fewer Early Triassic PAIs by: 1, producing fewer leaves for potential burial and 2, sustaining a smaller and less diverse population of arthropods. The difficulty in confidently identifying feeding traces on small needle- and scale-shaped leaves (e.g., voltzialean leaves and pleuromeian microphylls), owing to their diminutive size and commonly dense occurrence, may have implications for assessing damage frequency in the Early Triassic, when leaves of this type were proportionately more common (Figure 4). The absence of coals immediately post-EPE (Retallack et al., 1996) may provide another, economic, bias for the observed trends, as historic coal-mining efforts have produced a significant proportion of known plant fossil collections.
4.1.2 Geographic trends and gaps
Strata from South America and Oceania contain the vast majority of Lower and Middle Triassic palaeobotanical assemblages (Figure 2; Supplementary Table S2), floral diversity (Figure 5; Supplementary Figure S1; Supplementary Table S3) and PAIs (Figure 5; Table 1), constituting 75% of Gondwanan PAI-reporting localities and suggesting a major sampling bias towards these regions. Of the 23 South American localities included in the plant fossil database, five (21.7%) contain PAI traces attributable to at least seven FFG categories, whereas in Oceania only four of 50 localities (8%) host reported PAIs, in at least five FFGs. As both regions report sufficiently high numbers of plant species (Supplementary Table S3), this observed difference in the number of recorded FFGs from localities in Oceania versus South America likely represents a bias in effort towards South American arthropod trace fossil investigations. The PAI investigations of South America constitute the majority of quantitative PAI assessments included in the database (Potrerillos and Santa Maria formations in particular; all MT–LT; Table 1), however, there is a notable lack of Lower Triassic fossiliferous strata reported from South America (Supplementary Tables S2, S3). As discussed in section 4.1.1, the disparity in floral productivity between the Early and Middle Triassic is likely to impact the probability of finding PAIs over these two time intervals, and the greater proportion of Lower Triassic strata in Oceania suggests another potential reason for the observed South American PAI report abundance.
The Lashly Formation (Antarctica; MT–LT) yielded one leaf hosting fungal hyphae, the Fremouw Formation (Antarctica; MT–LT) and the Nidpur beds (India; LP-ET) do not report any PAIs on leaves at all, and the Umm Irna Formation (Jordan; late Permian) reports only hole-feeding on one species of Dicroidium to make a combined total of four FFGs across these three Gondwanan regions (possibly five, as it was not clear what type of interaction was occurring on the Nidpur seed). There is clearly great potential in advancing research on the Early Triassic floras and PAIs of these broad regions.
To the authors’ knowledge, the Benolong Flora investigation presented herein represents the first quantitative PAI assessment of an Early or Middle Triassic macrofloral assemblage from Oceania. More PAI traces were identified in the Benolong Flora than the majority of other assemblages in the database, except for the well-studied Santa Maria Formation fossil suites, and the quantitative investigations undertaken on wood specimens of the Fremouw Formation (Table 1); both of these assemblages are possibly Late Triassic in age. Furthermore, higher FFG diversity was identified in the Benolong Flora than any Antarctic, Arabian, Oceanian, and Indian localities, and three out of five of the South American stratigraphic units (Table 1). These findings indicate a high likelihood of under-reporting and under-investigation of PAIs for Triassic strata across the majority of Gondwana and clearly demonstrates how a concerted investigation can greatly increase the reported number of PAIs.
4.2 Future work and recommendations
Although there has been increased interest in PAI investigations in recent years, this compilation of data indicates large temporal and spatial gaps in our knowledge of plant–arthropod interactions during this interval of Gondwanan history. The pattern of gaps in the database can be informative, since they are not equally distributed in time or space, and our understanding of ancient plant–arthropod interactions is almost entirely dependent on the accuracy of identification and completeness of the plant fossil record.
The variation in floral composition between the Early to Middle Triassic resulted in major changes in the representation of shapes, sizes, and densities of leaves and other organs. The arthropod populations surviving in these evolving plant communities may have resulted in taxonomically specialized plant–arthropod interactions and spatiotemporal variations in FFG abundances. Taxonomic trends in FFG distribution on plants are difficult to discern from the current post-EPE PAI record, since specific plant–arthropod associations have not yet been reported in sufficient detail in most cases. Although some affected taxa and species were described in the reports included in this review, many PAIs/FFGs identified were not linked to specific plant taxa outside of individual instances in the figured specimens, and the numbers of affected leaves/organs per FFG were not clearly stated in many cases.
Thomas and Hill (2023) identified significant under-reporting of insect damage from plant fossils illustrated in the figures of Cenozoic publications, owing, in part, to the tendency for palaeobotanical researchers to preferentially illustrate undamaged leaves in their papers. This highlights the necessity for greater awareness of, and emphasis on, the reporting of PAIs by researchers conducting these plant taxonomic studies. Therefore, increased efforts in describing new localities and floral assemblages, revisiting existing palaeobotanical collections and publications, and conducting PAI assessments would greatly increase available data and the reliability of any trends observed.
More extensive Early Triassic Gondwanan floral assemblage identification, collection, taxonomic description and PAI assessment would enable more accurate comparisons to Middle Triassic PAI records, and the determination of ecological recovery trends from this interval. Description of more floral assemblages from Middle Triassic strata of Africa, Antarctica, South Asia and the Arabian Peninsula would also provide a more representative pan-Gondwanan assessment of PAI spatiotemporal trends. Oceania hosts the majority of described palaeobotanical records in this database; however, PAIs from the Australasian flora are relatively understudied compared to South American assemblages. Eastern Australia in particular, hosts a thick succession of continental upper Permian to Middle Triassic stratigraphic units (Herbert, 1997; Fielding et al., 2021; McLoughlin et al., 2021a; Fielding et al., 2022) and an abundance of fossil plant-bearing strata (e.g., in the Bowen, Nymboida and Sydney basins and the Esk Trough; Walkom, 1924; White, 1965; Raggatt, 1969; White, 1969, 1971; Rigby, 1977; Retallack, 1980a; Playford et al., 1982; Cantrill and Webb, 1998; Holmes and Anderson, 2013b) that represent useful targets for tracking the evolution of Gondwanan Permian–Triassic plant and arthropod interactions.
Detailed quantification of arthropod damage (e.g., DT/FFG intensity and diversity; Schachat et al., 2022) is not currently practical for this time interval of Gondwana. With larger datasets resulting from detailed taxonomic assessment of more floral assemblages, and increased reporting of insect damage types, more quantitative analyses can then be conducted. A more accurate understanding of the changes in relative abundances of plant groups over time will contextualize implications for the PAI record (e.g., preferential or specialized arthropod interactions with specific plant groups, DT/FFG preservation biases between leaf types).
To this end, it is recommended that a standardized approach for the assessment and reporting of PAIs is implemented, necessitating approaches that accurately identify, record and report PAI data. Variation in preservational conditions between floral assemblages (e.g., impressions vs coalified compressions) may influence the detectability of various DTs and FFGs, potentially leading to reporting biases in DT frequency. Similarly, different PAI assessment techniques may be better for highlighting particular FFGs (e.g., analyzing images vs hand specimens, using microscopy, low-angle lighting, UV light, and X-ray tomography). Incorporating the DT code of Labandeira et al. (2007; unpublished additions) into PAI assessments will standardize records and increase confidence in DT identification. The ‘sampling unit’ per individual PAI recorded should be clearly defined as either one rock specimen, one plant fossil, or one plant organ (e.g., one leaf). Furthermore, a standard of quantitative reporting of findings should be established: for each DT, the average number of traces per plant sampling unit, the total number of sampling units involved, and the affected plant taxa should be reported; for each FFG, the DT diversity and distribution across plant taxa could then be compared (see Labandeira et al., 2018).
Addressing these concerns will also enable researchers to contrast trends in plant–arthropod interactions from Gondwana to those of the Laurasian and Tethyan regions for a global perspective on the terrestrial ecological response to late Permian to Middle Triassic climatic instability.
5 Conclusions
This review summarizes our present understanding of an essential component of terrestrial ecosystem function following the most severe extinction event in Earth’s history. The compilation of Early and Middle Triassic fossil leaf floras of Gondwana and their associated arthropod traces presented herein demonstrates:
1. The present state of the published fossil record is temporally and spatially biased, providing an unreliable gauge of terrestrial ecosystem changes during the end-Permian event recovery in Gondwana. These spatial and temporal biases, particularly the patchiness and under-representation of PAI reporting, need to be addressed before the available data can be meaningfully assessed for quantitative ecological trends.
2. The reliability of the Gondwanan PAI record is compromised by two main biases. Firstly, observational biases, which include a geographical skew against Gondwanan collections in favor of those from Laurasia and the Tethys regions, and within Gondwana, a greater representation of assemblages from South America and Oceania. There is also a stratigraphic observational bias, particularly against Lower Triassic strata, which have historically received less attention owing to their scarcity of fossils and coal. Secondly, preservational biases will tend to facilitate the identification of PAIs in certain styles of fossilization and in specific types of leaves or plant organs over others (e.g., broad vs scale-like leaves). In addition to these biases, an absence of standardized data collection currently limits meaningful comparisons across the region.
3. By mitigating the limiting factors above, it may in the future be possible to utilize the PAI record of Gondwana to construct distinctive ecological and evolutionary trends during the recovery period following the end-Permian event.
4. In contrast to existing PAI reports from the Australian Early to Middle Triassic (that documented three fossil plant specimens hosting one PAI trace each), our systematic investigation of the Benolong Flora revealed 44 PAI traces consistent with published DTs, hosted by 40 fossil plant fragments. This demonstrates that a concerted quantitative investigation can greatly increase the number of PAIs identified on an assemblage and reaffirms the likelihood of under-reporting of PAIs for similarly overlooked Gondwanan sites.
5. We propose several key next steps to address the demonstrable gaps in our collective knowledge of Early to Middle Triassic Gondwanan floras and arthropod interactions. Specifically, we advocate for establishment of a standardized approach for assessing and reporting PAIs that will facilitate accurate identification, recording and reporting of PAI data. Finally, we highlight that the excellent Early and Middle Triassic macrofloral assemblages from age-controlled, and readily accessible localities in eastern Australia offer a remarkable opportunity to track the long-term patterns of recovery in plant and arthropod herbivores from before the end-Permian extinction through the environmental crises of the Early to Middle Triassic.
Data availability statement
The original contributions presented in the study are included in the article/Supplementary Material. Further inquiries can be directed to the corresponding author/s.
Author contributions
H-AT: Conceptualization, Funding acquisition, Investigation, Methodology, Writing – original draft, Writing – review & editing. SM: Conceptualization, Funding acquisition, Methodology, Supervision, Writing – original draft, Writing – review & editing. CM: Conceptualization, Funding acquisition, Methodology, Software, Supervision, Writing – original draft, Writing – review & editing.
Funding
The author(s) declare financial support was received for the research, authorship, and/or publication of this article. This project was supported by an Irish Research Council Government of Ireland Postgraduate Scholarship awarded to H-AT (GOIPG/2022/2071), a Swedish Research Council (VR) grant (2022-03920) awarded to SM, and research grant 13/RC/2092_P2 from Science Foundation Ireland’s Research Centre in Applied Geosciences (13/RC/2092_P2) awarded to CM.
Acknowledgments
We would like to thank Matthew McCurry, Patrick Smith, Graham McLean and Ailie MacKenzie for access to and assistance with the Benolong specimens in the Australian Museum Palaeontological Collection. Special thanks are given to Keith Holmes and Heidi Anderson for their input and insights regarding the Middle Triassic plant records of eastern Australia, including the Benolong Flora. We thank Editor Sandra Schachat, Zhou Feng, Bárbara Cariglino and one anonymous reviewer for their helpful insights that have improved the manuscript.
Conflict of interest
The authors declare that the research was conducted in the absence of any commercial or financial relationships that could be construed as a potential conflict of interest.
Publisher’s note
All claims expressed in this article are solely those of the authors and do not necessarily represent those of their affiliated organizations, or those of the publisher, the editors and the reviewers. Any product that may be evaluated in this article, or claim that may be made by its manufacturer, is not guaranteed or endorsed by the publisher.
Supplementary material
The Supplementary Material for this article can be found online at: https://www.frontiersin.org/articles/10.3389/fevo.2024.1419254/full#supplementary-material
References
Abu Hamad A. M. B., Al-Saqarat B., Gonçalves C. V., Spiekermann R., Jasper A., Uhl D. (2019). The first record of Dicroidium from the Triassic palaeotropics based on dispersed cuticles from the Anisian Mukheiris Formation of Jordan. Paläontologische Z. 93, 487–498. doi: 10.1007/s12542-019-00470-1
Abu Hamad A. M. B., Blomenkemper P., Kerp H., Bomfleur B. (2017). Dicroidium bandelii sp. nov. (corystospermalean foliage) from the Permian of Jordan. Paläontologische Z. 91, 641–648. doi: 10.1007/s12542-017-0384-2
Abu Hamad A. M. B., Kerp H., Vörding B., Bandel K. (2008). A Late Permian flora with Dicroidium from the Dead Sea region, Jordan. Rev. Palaeobotany Palynol. 149, 85–130. doi: 10.1016/j.revpalbo.2007.10.006
Adami-Rodrigues K., Gnaedinger S., Gallego O. (2008). “Registro de interações inseto-planta do grupo El Tranquilo (Triássico Superior) Provincia de Santa Cruz, Patagonia Argentina,” in Simposio Brasileiro de Paleobotânica e Palinologia Boletim de Resumos, Florianópolis, Brazil, 12, 1.
Adami-Rodrigues K., Iannuzzi R., Pinto I. D. (2004). Permian plant-insect interactions from a Gondwana flora of southern Brazil. J. Fossils Strata. 51, 106–125. doi: 10.18261/9781405169851-2004-07
Anderson J. M., Anderson H. M. (1985). Palaeoflora of Southern Africa: Prodromus of South African Megafloras, Devonian to Lower Cretaceous (Palaeoflora of Southern Africa) (Rotterdam: A.A. Balkema).
Anderson J. M., Anderson H. M. (1989). Palaeoflora of Southern Africa. Molteno Formation (Triassic). Volume 2. Gymnosperms (excluding Dicroidium) (Rotterdam: A. A. Balkema).
Anderson J. M., Anderson H. M. (1993a). Terrestrial flora and fauna of the Gondwana Triassic: Part 1- Occurrences. Bull. New Mexico Museum Natural History Sci. 3, 3–12.
Anderson J. M., Anderson H. M. (1993b). Terrestrial flora and fauna of the Gondwana Triassic: Part 2- Co-evolution. Bull. New Mexico Museum Natural History Sci. 3, 13–25.
Anderson J. M., Anderson H. M. (2003). Heyday of the Gymnosperms: Systematics and Biodiversity of the Late Triassic Molteno Fructifications, Strelitzia (Pretoria: National Botanical Institute).
Anderson J. M., Anderson H. M., Archangelsky S., Bamford M., Chandra S., Dettmann M., et al. (1999). Patterns of Gondwana plant colonisation and diversification. J. Afr. Earth Sci. 28, 145–167. doi: 10.1016/S0899-5362(98)00083-9
Anderson J. M., Anderson H. M., Cleal C. J. (2007). Brief History of the Gymnosperms: Classification, Biodiversity, Phytogeography and Ecology (Pretoria: South African National Biodiversity Institute).
Anderson J. M., Anderson H. M., Cruickshank A. (1998). Late Triassic ecosystems of the Molteno/Lower Elliot biome of southern Africa. Palaeontology 41, 387–421.
Artabe A. E. (1985a). Estudio sistemático de la Tafoflora triásica de Los Menucos, Provincia de Río Negro, Argentina. Parte I, Sphenophyta, Filicophyta y Pteridospermophyta. Ameghiniana 22, 3–22.
Artabe A. E. (1985b). Estudio sistemático de la Tafoflora triásica de Los Menucos, Provincia de Río Negro, Argentina. Parte II. Cycadophyta, Ginkgophyta y Coniferophyta. Ameghiniana 22, 159–180.
Ash S. R. (1979). Skilliostrobus gen. nov., a new lycopsid cone from the Early Triassic of Australia. Alcheringa 3, 73–89. doi: 10.1080/03115517908619087
Axsmith B. J., Taylor T. N., Taylor E. L. (1998). Anatomically preserved leaves of the conifer Notophytum krauselii (Podocarpaceae) from the Triassic of Antarctica. Am. J. Bot. 85, 704–713. doi: 10.2307/2446541
Azcárate V., Fasola A. (1970). Sobre formas nuevas para la flora triásica de Los Molles. Boletín del Museo Nacional Hist. Natural 29, 249–270.
Balme B. E., Helby R. J. (1973). Floral modifications at the Permian-Triassic boundary in Australia, in: The Permian and Triassic Systems and their Mutual Boundary, Memoir. Can. Soc. Petroleum Geologists pp, 433–444.
Bamford M. (1999). Permo-Triassic fossil woods from the South African Karoo Basin. Palaeontologia Africana 35, 25–40.
Bamford M. K. (2004). Diversity of the woody vegetation of Gondwanan southern Africa. Gondwana Res. 7, 153–164. doi: 10.1016/S1342-937X(05)70314-2
Banerji J., Bose M. N. (1977). Some Lower Triassic plant remains from Asansol region, India. Palaeobotanist 24, 202–210. doi: 10.54991/jop.1975.986
Banerji J., Maheshwari H. K., Bose M. N. (1976). Some plant fossils from the Gopad River Section near Nidpur, Sidhi District, Madhya Pradesh | Journal of Palaeosciences. Palaeobotanist 23, 59–71. doi: 10.54991/jop.1974.952
Banks H. P., Colthart B. J. (1993). Plant-animal-fungal interactions in Early Devonian trimerophytes from Gaspé, Canada. Am. J. Bot. 80, 992–1001. doi: 10.1002/j.1537-2197.1993.tb15325.x
Barboni R., Dutra T. L. (2015). First record of Ginkgo-related fertile organs (Hamshawvia, Stachyopitys) and leaves (Baiera, Sphenobaiera) in the Triassic of Brazil, Santa Maria formation. J. South Am. Earth Sci. 63, 417–435. doi: 10.1016/j.jsames.2015.08.001
Barboni R., Dutra T. L., Faccini U. F. (2016). Xylopteris (Frenguelli) stipanicic & Bonetti in the Middle-Upper Triassic (Santa maria formation) of Brazil. Ameghiniana 53, 599–622. doi: 10.5710/AMGH.11.07.2016.2897
Bell C. M., Suarez M. (1994). The sedimentation and tectonics of a marine fan-delta developed on an active continental margin: the Triassic San Félix Formation in the Andes of northern Chile. J. South Am. Earth Sci. 7, 403–413. doi: 10.1016/0895-9811(94)90024-8
Benson M. (1908). On the contents of the pollen chamber of a specimen of Lagenostoma ovoides. Botanical Gazette 45, 409–412. doi: 10.1086/329595
Bergene J. A., Taylor E. L., Taylor T. N. (2013). Dordrechtites arcanus sp. nov., an anatomically preserved gymnospermous reproductive structure from the Middle Triassic of Antarctica. Int. J. Plant Sci. 174, 250–265. doi: 10.1086/668792
Bhowmik N., Parveen S. (2014). Fossilized pollination droplet in a new seed genus from the Middle Triassic of Nidpur, India. Acta Palaeontologica Polonica 59, 491–503. doi: 10.4202/app.2012.0026
Blomenkemper P., Abu Hamad A., Bomfleur B. (2019). Cryptokerpia sarlaccophora gen. et sp. nov., an enigmatic plant fossil from the Late Permian Umm Irna Formation of Jordan. Paläontologische Z. 93, 479–485. doi: 10.1007/s12542-019-00466-x
Blomenkemper P., Bäumer R., Backer M., Abu Hamad A., Wang J., Kerp H., et al. (2021). Bennettitalean leaves from the Permian of equatorial Pangea—The early radiation of an iconic Mesozoic gymnosperm group. Front. Earth Sci. 9. doi: 10.3389/feart.2021.652699
Blomenkemper P., Kerp H., Abu Hamad A., Bomfleur B. (2020). Contributions towards whole-plant reconstructions of Dicroidium plants (Umkomasiaceae) from the Permian of Jordan. Rev. Palaeobotany Palynol. 278, 104210. doi: 10.1016/j.revpalbo.2020.104210
Blomenkemper P., Kerp H., Abu Hamad A., DiMichele W. A., Bomfleur B. (2018). A hidden cradle of plant evolution in Permian tropical lowlands. Science 362, 1414–1416. doi: 10.1126/science.aau4061
Bodnar J., Iglesias A., Colombi C. E., Drovandi J. M. (2019). Stratigraphical, sedimentological and palaeofloristic characterization of the Sorocayense Group (Triassic) in Barreal depocenter, San Juan Province, Argentina. Andean Geology 46, 567–603. doi: 10.5027/andgeoV46n3-3127
Bomfleur B., Blomenkemper P., Kerp H., McLoughlin S. (2018). “Polar regions of the Mesozoic–Paleogene greenhouse world as refugia for relict plant groups.” In Transformative Paleobotany: Papers to Commemorate the Life and Legacy of Thomas N. Taylor. Eds, Krings M., Harper C. J., Cúneo N. R., Rothwell G. W. Elsevier, Amsterdam, 593–611. doi: 10.1016/B978-0-12-813012-4.00024-3
Bomfleur B., Decombeix A.-L., Escapa I., Schwendemann A., Axsmith B. (2013a). Whole-plant concept and environment reconstruction of a Telemachus conifer (Voltziales) from the Triassic of Antarctica. Int. J. Plant Sci. 174, 425–444. doi: 10.1086/668686
Bomfleur B., Escapa I., Serbet R., Taylor E., Taylor T. (2013b). A reappraisal of Neocalamites and Schizoneura (fossil Equisetales) based on material from the Triassic of East Antarctica. Alcheringa 37, 1–17. doi: 10.1080/03115518.2013.764693
Bond D. P. G., Grasby S. E. (2017). On the causes of mass extinctions. Palaeogeogr. Palaeoclimatol. Palaeoecol. 478, 3–29. doi: 10.1016/j.palaeo.2016.11.005
Bose M. N., Banerji J. (1974). Some fragmentary plant remains from the Lower Triassic of Auranga Valley, District Palamau. Palaeobotanist 23, 139–144. doi: 10.54991/jop.1974.959
Bose M. N., Srivastava S. C. (1971). The genus Dicroidium from the Triassic of Nidpur, Madhya Pradesh, India. J. Palaeosciences 19, 41–51. doi: 10.54991/jop.1970.852
Bourke D. J., Gould R. E., Helby R., Morgan R., Retallack G. J. (1977). Floral evidence for a Middle Triassic age of the Gunnee Beds and Gragin Conglomerate, near Delungra, New South Wales. J. Proc. R. Soc. New South Wales 110, 33–40. doi: 10.5962/p.361068
Branagan D. F. (1969). “V. The Sydney Basin—Hawkesbury Sandstone—fauna and flora,” in The Geology of New South Wales. Ed. Packham G. H. (Sydney: Geological Society of Australia), 415–417.
Brea M., Artabe A. E., Spalletti L. A. (2008). Ecological reconstruction of a mixed Middle Triassic forest from Argentina. Alcheringa 32, 365–393. doi: 10.1080/03115510802417760
Breitkreuz C., Helmdach F.-F., Kohring R., Mosbrugger V. (1992). Late Carboniferous intra-arc sediments in the north Chilean Andes: Stratigraphy, paleogeography and paleoclimate. Facies 26, 67–80. doi: 10.1007/BF02539794
Burgess S. D., Muirhead J. D., Bowring S. A. (2017). Initial pulse of Siberian Traps sills as the trigger of the end-Permian mass extinction. Nat. Commun. 8, 164. doi: 10.1038/s41467-017-00083-9
Cantrill D. J., Webb J. A. (1998). Permineralized pleuromeid lycopsid remains from the Early Triassic Arcadia Formation, Queensland, Australia. Rev. Palaeobotany Palynol. 102, 189–211. doi: 10.1016/S0034-6667(98)80005-5
Cariglino B. (2018). Patterns of insect-mediated damage in a Permian Glossopteris flora from Patagonia (Argentina). Palaeogeogr. Palaeoclimatol. Palaeoecol. 507, 39–51. doi: 10.1016/j.palaeo.2018.06.022
Cariglino B., Moisan P., Lara M. B. (2021). The fossil record of plant-insect interactions and associated entomofaunas in Permian and Triassic floras from southwestern Gondwana: A review and future prospects. J. South Am. Earth Sci. 111, 103512. doi: 10.1016/j.jsames.2021.103512
Cariglino B., Monti M., Zavattieri A. M. (2018). A Middle Triassic macroflora from southwestern Gondwana (Mendoza, Argentina) with typical Northern Hemisphere elements: Biostratigraphic, palaeogeographic and palaeoenvironmental implications. Rev. Palaeobotany Palynol. 257, 1–18. doi: 10.1016/j.revpalbo.2018.06.004
Cariglino B., Prevec R., Lara M. B., Zavattieri A. M. (2022). Evidence of insect leaf mining on Late Triassic (Carnian) gymnosperms from Argentina. Rev. Palaeobotany Palynol. 305, 104749. doi: 10.1016/j.revpalbo.2022.104749
Cariglino B., Zavattieri A., Lara M. B. (2020). Insect galls on Triassic pteridosperms from the Quebrada de los Fósiles Formation (Puesto Viejo Group, Mendoza, Argentina). Presented at the 1° Reunión Virtual de Comunicaciones de la Asociación Paleontológica Argentina, Buenos Aires.
Cariglino B., Zavattieri A. M., Gutiérrez P. R., Balarino M. L. (2016). The paleobotanical record of the Triassic Cerro de las Cabras Formation at its type locality, Potrerillos, Mendoza (Uspallata Group): An historical account and first record of fossil flora. Ameghiniana 53, 184–204. doi: 10.5710/AMGH.17.12.2015.2952
Carpentier A. (1935). Études paléobotaniques sur le Groupe de la Sakoa et le Groupe de la Sakamena (Madagascar). Annales Géologiques du Service Des. Mines Madagascar 5, 7–32.
Carpentier A. (1936). Additions a l’etude de la flore du groupe de la Sakamena (Madagascar). Annales Géologiques du Service Des. Mines Republic Malagasy 6, 7–11.
Cascales-Miñana B., Diez J. B., Gerrienne P., Cleal C. J. (2016). A palaeobotanical perspective on the great end-Permian biotic crisis. Historical Biol. 28, 1066–1074. doi: 10.1080/08912963.2015.1103237
Cenci R. (2013). Registro de interações inseto-planta em níveis do Triássico, membro Passo das Tropas, formação Santa Maria, Bacia do Paraná, Rio Grande do Sul. (Brazil: Trabalho Acadêmico, Universidade Federal de Pelotas), 73.
Cenci R., Adami-Rodrigues K. (2017). Record of gall abundance as a possible episode of radiation and speciation of galling insects, Triassic, Southern Brazil. Rev. Bras. Paleontologia 20, 279–286. doi: 10.4072/rbp.2017.3.01
Cenci R., Adami-Rodrigues K., Horodyski R. S., Villegas-Martín J. (2019). “Plant-insect interactions of the Passo das Tropas outcrop, Santa Maria Formation, southern Brazil (Late Ladinian),” in Hallesches Jahrbuch Für Geowissenschaften. Presented at the 3rd International Conference of Continental Ichnology. Hallesches Jahrbuch Für Geowissenschaften, Beiheft, Germany, 46, 8–10.
Chaloner W. G., Turner S. (1987). An enigmatic Triassic lycopod axis from Australia. Rev. Palaeobotany Palynol. 51, 51–58. doi: 10.1016/0034-6667(87)90019-4
Christenhusz M. J. M., Byng J. W. (2016). The number of known plants species in the world and its annual increase. Phytotaxa 261, 201. doi: 10.11646/phytotaxa.261.3.1
Cui Y., Kump L. R. (2015). Global warming and the end-Permian extinction event: Proxy and modeling perspectives. Earth-Science Rev. 149, 5–22. doi: 10.1016/j.earscirev.2014.04.007
Cui Y., Li M., van Soelen E. E., Peterse F., Kürschner W. M. (2021). Massive and rapid predominantly volcanic CO2 emission during the end-Permian mass extinction. Proc. Natl. Acad. Sci. United States America 118, e2014701118. doi: 10.1073/pnas.2014701118
Cúneo N. R., Taylor E. L., Taylor T. N., Krings M. (2003). In situ fossil forest from the upper Fremouw Formation (Triassic) of Antarctica: paleoenvironmental setting and paleoclimate analysis. Palaeogeogr. Palaeoclimatol. Palaeoecol. 197, 239–261. doi: 10.1016/S0031-0182(03)00468-1
Currano E., Azevedo Schmidt L., Maccracken G., Swain A. (2021). Scars on fossil leaves: An exploration of ecological patterns in plant–insect herbivore associations during the Age of Angiosperms. Palaeogeogr. Palaeoclimatol. Palaeoecol. 582, 110636. doi: 10.1016/j.palaeo.2021.110636
Currano E. D., Laker R., Flynn A. G., Fogt K. K., Stradtman H., Wing S. L. (2016). Consequences of elevated temperature and pCO2 on insect folivory at the ecosystem level: perspectives from the fossil record. Ecol. Evol. 6, 4318–4331. doi: 10.1002/ece3.2203
Decombeix A.-L., Bomfleur B., Taylor E., Taylor T. (2014). New insights into the anatomy, development, and affinities of corystosperm trees from the Triassic of Antarctica. Rev. Palaeobotany Palynol. 203, 22–34. doi: 10.1016/j.revpalbo.2014.01.002
Decombeix A.-L., Klavins S. D., Taylor E. L., Taylor T. N. (2010). Seed plant diversity in the Triassic of Antarctica: A new anatomically preserved ovule from the Fremouw Formation. Rev. Palaeobotany Palynol. 158, 272–280. doi: 10.1016/j.revpalbo.2009.09.008
Delevoryas T., Taylor T. N., Taylor E. L. (1992). A marattialean fern from the Triassic of Antarctica. Rev. Palaeobotany Palynol. 74, 101–107. doi: 10.1016/0034-6667(92)90140-C
Del Fueyo G. M., Taylor E. L., Taylor T. N., Cúneo N. R. (1995). Triassic wood from the Gordon Valley, central Transantarctic Mountains, Antarctica. IAWA J. 16, 111–126. doi: 10.1163/22941932-90001398
Dixey F. (1937). The geology of part of the Upper Luangwa Valley, north-eastern Rhodesia. Q. J. Geological Soc. London 93, 52–76. doi: 10.1144/GSL.JGS.1937.093.01-04.05
Dolby J. H., Balme B. E. (1976). Triassic palynology of the Carnarvon Basin, Western Australia. Rev. Palaeobotany Palynol. 22, 105–168. doi: 10.1016/0034-6667(76)90053-1
Drovandi J. M., Correa G. A., Bodnar J., Colombi C. E., Coturel E. P., Morel E. M. (2020). A new paleofloristic assemblage from the Cuyana Basin (Agua de los Pajaritos depocenter), Argentina and its paleobiogeographic and paleoenvironmental implications. J. South Am. Earth Sci. 104, 102819. doi: 10.1016/j.jsames.2020.102819
Du Y., Algeo T. J., Tian L., Chu D., Shi W., Li C., et al. (2022). A massive magmatic degassing event drove the Late Smithian Thermal Maximum and Smithian–Spathian boundary mass extinction. Global Planetary Change 215, 103878. doi: 10.1016/j.gloplacha.2022.103878
Edwards D., Selden P. A., Richardson J. B., Axe L. (1995). Coprolites as evidence for plant–animal interaction in Siluro–Devonian terrestrial ecosystems. Nature 377, 329–331. doi: 10.1038/377329a0
Escapa I. H., Taylor E. L., Cúneo R., Bomfleur B., Bergene J., Serbet R., et al. (2011). Triassic floras of Antarctica: Plant diversity and distribution in high paleolatitude communities. PALAIOS 26, 522–544. doi: 10.2110/palo.2010.p10-122r
Falco J. I., Bodnar J., Del Río D. (2020). Revisión estratigráfica del Grupo Los Menucos, Pérmico Tardío - Triásico Temprano del Macizo Nordpatagónico, provincia de Río Negro, Argentina. Rev. la Asociación Geológica Argent. 77, 530–550.
Farabee M. J., Taylor E. L., Taylor T. N. (1990). Correlation of Permian and Triassic palynomorph assemblages from the central Transantarctic Mountains, Antarctica. Rev. Palaeobotany Palynol. 65, 257–265. doi: 10.1016/0034-6667(90)90075-T
Fielding C. R., Frank T. D., McLoughlin S., Vajda V., Mays C., Tevyaw A. P., et al. (2019). Age and pattern of the southern high-latitude continental end-Permian extinction constrained by multiproxy analysis. Nat. Commun. 10, 385. doi: 10.1038/s41467-018-07934-z
Fielding C. R., Frank T. D., Savatic K., Mays C., McLoughlin S., Vajda V., et al. (2022). Environmental change in the late Permian of Queensland, NE Australia: The warmup to the end-Permian Extinction. Palaeogeogr. Palaeoclimatol. Palaeoecol. 594, 110936. doi: 10.1016/j.palaeo.2022.110936
Fielding C. R., Frank T. D., Tevyaw A. P., Savatic K., Vajda V., McLoughlin S., et al. (2021). Sedimentology of the continental end-Permian extinction event in the Sydney Basin, eastern Australia. Sedimentology 68, 30–62. doi: 10.1111/sed.12782
Frank T. D., Fielding C. R., Winguth A. M. E., Savatic K., Tevyaw A., Winguth C., et al. (2021). Pace, magnitude, and nature of terrestrial climate change through the end-Permian extinction in southeastern Gondwana. Geology 49, 1089–1095. doi: 10.1130/G48795.1
Friesenbichler E., Hautmann M., Bucher H. (2021). The main stage of recovery after the end-Permian mass extinction: taxonomic rediversification and ecologic reorganization of marine level-bottom communities during the Middle Triassic. PeerJ 9, e11654. doi: 10.7717/peerj.11654
Garwood R. J., Oliver H., Spencer A. R. T. (2020). An introduction to the Rhynie Chert. Geological Magazine 157, 47–64. doi: 10.1017/S0016756819000670
Gastaldo R. A., Adendorff R., Bamford M., Labandeira C. C., Neveling J., Sims H. (2005). Taphonomic trends of macrofloral assemblages across the Permian-Triassic boundary, Karoo Basin, South Africa. PALAIOS 20, 479–497. doi: 10.2110/palo.2004.P04-62
Gastaldo R. A., Bamford M. K. (2023). The influence of taphonomy and time on the paleobotanical record of the Permian–Triassic transition of the Karoo Basin (and elsewhere). J. Afr. Earth Sci. 204, 104960. doi: 10.1016/j.jafrearsci.2023.104960
Gastaldo R. A., Bamford M., Calder J., DiMichele W. A., Iannuzzi R., Jasper A., et al. (2020). “The coal farms of the late Paleozoic,” in Nature through Time: Virtual Field Trips through the Nature of the Past, Springer Textbooks in Earth Sciences, Geography and Environment. Eds. Martinetto E., Tschopp E., Gastaldo R. A. (Springer International Publishing, Cham), 317–343. doi: 10.1007/978-3-030-35058-1_13
Ghosh A. K., Chatterjee R., Pramanik S., Kar R. (2021). “Radiation of flora in the Early Triassic succeeding the End Permian Crisis: Evidences from the Gondwana Supergroup of Peninsular India,” in Mesozoic Stratigraphy of India: A Multi-Proxy Approach. Eds. Banerjee S., Sarkar S. (Cham: Springer International Publishing), 87–113. doi: 10.1007/978-3-030-71370-6_3
Ghosh A., Kar R., Chatterjee R. (2015). Leaf galls on Dicroidium hughesii (Feistmantel) Lele from the Triassic of India—a new record. Alcheringa 39, 92–98. doi: 10.1080/03115518.2015.958285
Ghosh A., Kar R., Chatterjee R. (2016). Reassessment of the macroflora of the Parsora Formation with remarks on the age connotation. J. Palaeontological Soc. India 61, 225–238.
Gnaedinger S. C., Adami-Rodrigues K., Gallego O. F. (2014). Endophytic oviposition on leaves from the Late Triassic of northern Chile: Ichnotaxonomic, palaeobiogeographic and palaeoenvironment considerations. Geobios 47, 221–236. doi: 10.1016/j.geobios.2014.06.003
Gnaedinger S., Herbst R. (1998a). La flora triásica del Grupo El Tranquilo, provincia de Santa Cruz, (Patagonia). Parte V: Pteridospermae. Ameghiniana. 35, 33–52.
Gnaedinger S., Herbst R. (1998b). La flora triásica del Grupo El Tranquilo, provincia de Santa Cruz, Patagonia. Parte V: Pteridophylla. Ameghiniana. 35, 53–65.
Gnaedinger S., Herbst R. (1999). La flora triásica del Grupo El Tranquilo, provincia de Santa Cruz, Patagonia. Parte VI: Ginkgoales. Ameghiniana. 36, 281–296.
Gnaedinger S., Herbst R. (2001). Pteridospermas triásicas del Norte Chico de Chile. Ameghiniana. 38, 281–298.
Gnaedinger S., Herbst R. (2004a). Pteridophylla Triásicas del Norte de Chile. II. Géneros DeJerseya Herbst, Linguifolium (Arber) Retallack and Yabeiella Oishi. Rev. Del Museo Argentino Cienc. Naturales 6, 49–59. doi: 10.22179/REVMACN
Gnaedinger S., Herbst R. (2004b). Pteridophylla Triásicas del Norte de Chile. I. El género Taeniopteris Brongniart. Rev. Del Museo Argentino Cienc. Naturales 41, 91–110.
Gnaedinger S., Villalva A. S., Zavattieri A. M. (2023). Triassic Equisetites lateralis Phillips with strobilus in organic connection from Patagonia of Argentina and endophytic oviposition insect scars. Rev. Palaeobotany Palynol. 317, 104964. doi: 10.1016/j.revpalbo.2023.104964
Goldbery R. (1969). Geology of the Western Blue Mountains Vol. 20 (Sydney, Australia: Department of Mines. Geological Survey on New South Wales).
Grauvogel-Stamm L., Ash S. R. (2005). Recovery of the Triassic land flora from the end-Permian life crisis. Comptes Rendus Palevol 4, 593–608. doi: 10.1016/j.crpv.2005.07.002
Guerra-Sommer M., Cazzulo-Klepzig M. (2000). The Triassic taphoflora from Paraná Basin, southern Brazil: An overview. Rev. Bras. Geociências 30, 480–485. doi: 10.25249/0375-7536.2000303481485
Hagström J., Mehlqvist K. (2012). The dawn of terrestrial ecosystems on Baltica: First report on land plant remains and arthropod coprolites from the Upper Silurian of Gotland, Sweden. Palaeogeogr. Palaeoclimatol. Palaeoecol. 317–318, 162–170. doi: 10.1016/j.palaeo.2012.01.001
Haig D. W., Martin S. K., Mory A. J., McLoughlin S., Backhouse J., Berrell R. W., et al. (2015). Early Triassic (early Olenekian) life in the interior of East Gondwana: mixed marine–terrestrial biota from the Kockatea Shale, Western Australia. Palaeogeogr. Palaeoclimatol. Palaeoecol. 417, 511–533. doi: 10.1016/j.palaeo.2014.10.015
Hancox J., Brandt D., Reimold W., Koeberl C., Neveling J. (2002). “Permian-Triassic boundary in the northwest Karoo Basin: Current stratigraphic placement, implications for basin development models, and search for evidence of impact,” in Catastrophic Events and Mass Extinctions: Impacts and Beyond: Boulder, Colorado, Geological Society of America Special Paper, Boulder, Colorado. Eds. Koeberl C., MacLeod K. G., 429–444. doi: 10.1130/0-8137-2356-6.429
Hancox P. J., Neveling J., Rubidge B. S. (2020). Biostratigraphy of the Cynognathus Assemblage Zone (Beaufort Group, Karoo Supergroup), South Africa. South Afr. J. Geology 123, 217–238. doi: 10.25131/sajg.123.0016
Helby R. (1970). A Biostratigraphy of the Late Permian and Triassic of the Sydney Basin (Australia: University of Sydney), 513.
Helby R., Morgan R., Partridge A. D. (1987). A palynological zonation of the Australian Mesozoic. Memoirs Assoc. Australas. Palaeontologists 4, 1–94.
Herbert C. (1997). Sequence stratigraphic analysis of Early and Middle Triassic alluvial and estuarine facies in the Sydney Basin, Australia. Aust. J. Earth Sci. 44, 125–143. doi: 10.1080/08120099708728299
Herbst R. (1988). La flora Triasica del Grupo El Tranquilo, provincia de Santa Cruz (Patagonia). Parte II. Filicopsida Ameghiniana 25, 365–379.
Herbst R. (2000). The Ituzaingó Formation (Pliocene). Stratigraphy and distribution. The Neogene of Argentina. INSUGEO Geological Correlation Ser. 14, 181–190.
Herbst R., Gnaedinger S. (2002). Kurtziana Frenguelli (Pteridospermae? Incertae sedis) and Alicurana nov. gen. (Cycadopsida) from the Triassic and Early Jurassic of Argentina and Chile. Ameghiniana 39, 331–341.
Herbst R., Troncoso A. (2000). Las Cycadophyta del Triásico de las Formaciones La Ternera y El Puquén (Chile). Ameghiniana 37, 283–292.
Herbst R., Troncoso A., Gnaedinger S. (2001). Rochipteris nov. gen. leaf incertae sedis (= Chiropteris pro parte) Upper Triassic of Argentina and Chile. Ameghiniana 38, 257–269.
Hermann E., Hochuli P. A., Bucher H., Brühwiler T., Hautmann M., Ware D., et al. (2011). Terrestrial ecosystems on North Gondwana following the end-Permian mass extinction. Gondwana Res. 20, 630–637. doi: 10.1016/j.gr.2011.01.008
Hermann E., Hochuli P. A., Bucher H., Brühwiler T., Hautmann M., Ware D., et al. (2012a). Climatic oscillations at the onset of the Mesozoic inferred from palynological records from the North Indian Margin. J. Geological Society London 169, 227–237. doi: 10.1144/0016-76492010-130
Hermann E., Hochuli P. A., Bucher H., Roohi G. (2012b). Uppermost Permian to Middle Triassic palynology of the Salt Range and Surghar Range, Pakistan. Rev. Palaeobotany Palynol. 169, 61–95. doi: 10.1016/j.revpalbo.2011.10.004
Hermsen E. J., Taylor T. N., Taylor E. L. (2007a). A voltzialean pollen cone from the Triassic of Antarctica. Rev. Palaeobotany Palynol. 144, 113–122. doi: 10.1016/j.revpalbo.2006.07.006
Hermsen E. J., Taylor E. L., Taylor T. N. (2009). Morphology and ecology of the Antarcticycas plant. Rev. Palaeobotany Palynol. 153, 108–123. doi: 10.1016/j.revpalbo.2008.07.005
Hermsen E. J., Taylor T. N., Taylor E. L., Stevenson D. (2006). Cataphylls of the Middle Triassic cycad Antarcticycas schopfii and new insights into cycad evolution. Am. J. Bot. 93, 724–738. doi: 10.3732/ajb.93.5.724
Hermsen E. J., Taylor T. N., Taylor E. L., Stevenson D. W. (2007b). Cycads from the Triassic of Antarctica: Permineralized cycad leaves. Int. J. Plant Sci. 168, 1099–1112. doi: 10.1086/518840
Holmes W. B. K. (1982). The Middle Triassic flora from Benolong, near Dubbo, central-western New South Wales. Alcheringa 6, 1–33. doi: 10.1080/03115518208565416
Holmes W. B. K. (2000). The Middle Triassic megafossil flora of the Basin Creek Formation, Nymboida Coal Measures, NSW, Australia. Part 1: Bryophyta, Sphenophyta. Proc. Linn. Soc. New South Wales 122, 43–68.
Holmes W. B. K. (2001a). The Middle Triassic megafossil flora of the Basin Creek Formation, Nymboida Coal Measures, New South Wales, Australia. Part 2. Filicophyta. Proc. Linn. Soc. New South Wales 123, 39–87.
Holmes W. B. K. (2001b). Equisetalean plant remains from the Early to Middle Triassic of New South Wales, Australia. Records Aust. Museum 53, 9–20. doi: 10.3853/j.0067-1975.53.2001.1320
Holmes W. B. K. (2003). The Middle Triassic megafossil flora of the Basin Creek Formation, Nymboida Coal Measures, New South Wales, Australia. Part 3. Fern-like foliage. Proc. Linn. Soc. New South Wales 124, 53–108.
Holmes W. B. K., Anderson H. M. (2005a). The Middle Triassic megafossil flora of the Basin Creek Formation, Nymboida Coal Measures, New South Wales, Australia. Part 4. Umkomasiaceae. Dicroidium and affiliated fructifications. Proc. Linn. Soc. New South Wales 126, 1–37.
Holmes W. B. K., Anderson H. M. (2005b). The Middle Triassic megafossil flora of the Basin Creek Formation, Nymboida Coal Measures, New South Wales, Australia. Part 5. The genera Lepidopteris, Kurtziana, Rochipteris and Walkomiopteris. Proc. Linn. Soc. New South Wales 126, 39–79.
Holmes W. B. K., Anderson H. M. (2007). The Middle Triassic megafossil flora of the Basin Creek Formation, Nymboida Coal Measures, New South Wales, Australia. Part 6. Ginkgophyta. Proc. Linn. Soc. New South Wales 128, 155–200.
Holmes W. B. K., Anderson H. M. (2008). The Middle Triassic megafossil flora of the Basin Creek Formation, Nymboida Coal Measures, New South Wales, Australia. Part 7. Cycadophyta. Proc. Linn. Soc. New South Wales 129, 113–149.
Holmes W. B. K., Anderson H. M. (2013a). The Middle Triassic megafossil flora of the Basin Creek Formation, Nymboida Coal Measures, New South Wales, Australia. Part 9. The Genera Heidiphyllum, Voltziopsis, Rissikia and affiliated cones, and ? Yabeiella. Proc. Linn. Soc. New South Wales 135, 55–76.
Holmes W. B. K., Anderson H. M. (2013b). “A synthesis of the rich Gondwana Triassic megafossil flora from Nymboida, Australia,” in The Triassic System: New Developments in Stratigraphy and Paleontology, Bulletin. Eds. Tanner L. H., Spielmann J. A., Lucas S. G. (Albuquerque: New Mexico Museum of Natural History and Science), 296–305.
Holmes W. B. K., Anderson H. M. (2022). The Middle Triassic megafossil flora of the Basin Creek Formation, Nymboida Coal Measures, New South Wales, Australia. Part 10. Miscellaneous foliar (Rochipteris) and fertile genera (Fraxinopsis, Tomiostrobus, Umkomasia, Weltrichia). Proc. Linn. Soc. New South Wales 144, 79–101. doi: 10.3316/informit.641266671258529
Holmes W. B. K., Anderson H. M., Webb J. A. (2010). The Middle Triassic megafossil flora of the Basin Creek Formation, Nymboida Coal Measures, New South Wales, Australia. Part 8. The genera Nilssonia, Taeniopteris, Linguifolium, Gontriglossa and Scoresbya. Proc. Linn. Soc. New South Wales 131, 1–26.
Holmes W. B. K., Ash S. R. (1979). An Early Triassic megafossil flora from the Lorne Basin, New South Wales. Proc. Linn. Soc. New South Wales 103, 47–70.
Jalfin G. A., Herbst R. (1995). The Triassic flora of the El Tranquilo Group, province of Santa Cruz (Patagonia). Ameghiniana 32, 211–229.
Joachimski M. M., Alekseev A. S., Grigoryan A., Gatovsky Y. (2019). Siberian Trap volcanism, global warming and the Permian-Triassic mass extinction: New insights from Armenian Permian-Triassic sections. GSA Bull. 132, 427–443. doi: 10.1130/B35108.1
Joshi A., Tewari R., Agnihotri D. (2014). Plant diversity in the Kamthi Formation of India: A review. Palaeobotanist 63, 127–136. doi: 10.54991/jop.2014.328
Kellogg D. W., Taylor E. L. (2004). Evidence of oribatid mite detritivory in Antarctica during the late Paleozoic and Mesozoic. J. Paleontol. 78, 1146–1153. doi: 10.1666/0022-3360(2004)078<1146:EOOMDI>2.0.CO;2
Kenrick P., Wellman C. H., Schneider H., Edgecombe G. D. (2012). A timeline for terrestrialization: consequences for the carbon cycle in the Palaeozoic. Philos. Trans. R. Soc. B: Biol. Sci. 367, 519–536. doi: 10.1098/rstb.2011.0271
Kerp H., Abu Hamad A., Vörding B., Bandel K. (2006). Typical Triassic Gondwanan floral elements in the Upper Permian of the paleotropics. Geology 34, 265–268. doi: 10.1130/G22187.1
Kerp H., Blomenkemper P., Abu Hamad A., Bomfleur B. (2021). The fossil flora of the Dead Sea region, Jordan - A late Permian garden of delights. J. Palaeosciences 70, 135–158. doi: 10.54991/jop.2021.12
Kerp H., Krause K. K., Hamad A. A., Bomfleur B. (2024). Early Marattiaceae from the late Permian Umm Irna Formation, Jordan. Rev. Palaeobotany Palynol. 322, 105015. doi: 10.1016/j.revpalbo.2023.105015
Klavins S. D., Kellogg D. W., Krings M., Taylor E. L., Taylor T. N. (2005). Coprolites in a Middle Triassic cycad pollen cone: evidence for insect pollination in early cycads? Evolutionary Ecol. Res. 7, 479–488.
Klavins S. D., Taylor E. L., Krings M., Taylor T. N. (2003). Gymnosperms from the Middle Triassic of Antarctica: The first structurally preserved cycad pollen cone. Int. J. Plant Sci. 164, 1007–1020. doi: 10.1086/378662
Klavins S. D., Taylor T. N., Taylor E. L. (2002). Anatomy of Umkomasia (Corystospermales) from the Triassic of Antarctica. Am. J. Bot. 89, 664–676. doi: 10.3732/ajb.89.4.664
Klavins S. D., Taylor T. N., Taylor E. L. (2004). Matoniaceous ferns (Gleicheniales) from the Middle Triassic of Antarctica. J. Paleontol. 78, 211–217. doi: 10.1666/0022-3360(2004)078<0211:MFGFTM>2.0.CO;2
Krassilov V., Karasev E. (2008). First evidence of plant - arthropod interaction at the Permian - Triassic boundary in the Volga Basin, European Russia. Alavesia 2, 47–52.
Kustatscher E., Ash S., Karasev E., Pott C., Vajda V., Yu J., et al. (2018). “Flora of the Late Triassic.” In The Late Triassic World: Earth in a Time of Transition. Ed. Tanner L. Topics in Geobiology, Vol. 46. Ed. Tanner L. H. (Cham: Springer International Publishing), 545–622. doi: 10.1007/978-3-319-68009-5_13
Kustatscher E., Franz M., Heunisch C., Reich M., Wappler T. (2014). Floodplain habitats of braided river systems: depositional environment, flora and fauna of the Solling Formation (Buntsandstein, Lower Triassic) from Bremke and Fürstenberg (Germany). Palaeobiodiversity Palaeoenvironments 94, 237–270. doi: 10.1007/s12549-014-0161-0
Labandeira C. C. (2005). The fossil record of insect extinction: New approaches and future directions. Am. Entomologist 51, 14–29. doi: 10.1093/ae/51.1.14
Labandeira C. C. (2006). The four phases of plant-arthropod associations in deep time. Geologica Acta 4, 409–438.
Labandeira C. C. (2013). A paleobiologic perspective on plant–insect interactions. Curr. Opin. Plant Biol. 16, 414–421. doi: 10.1016/j.pbi.2013.06.003
Labandeira C. C., Anderson J. M., Anderson H. M. (2018). “Expansion of arthropod herbivory in Late Triassic South Africa: The Molteno Biota, Aasvoëlberg 411 Site and Developmental Biology of a Gall,” in The Late Triassic World: Earth in a Time of Transition, Topics in Geobiology, Vol. 46. Ed. Tanner L. H. (Springer International Publishing, Cham), 623–719. doi: 10.1007/978-3-319-68009-5_14
Labandeira C. C., Currano E. D. (2013). The fossil record of plant-insect dynamics. Annu. Rev. Earth Planetary Sci. 41, 287–311. doi: 10.1146/annurev-earth-050212-124139
Labandeira C. C., Kustatscher E., Wappler T. (2016). Floral assemblages and patterns of insect herbivory during the Permian to Triassic of northeastern Italy. PloS One 11, e0165205. doi: 10.1371/journal.pone.0165205
Labandeira C. C., Sepkoski J. J. (1993). Insect diversity in the fossil record. Science 261, 310–315. doi: 10.1126/science.11536548
Labandeira C. C., Tremblay S. L., Bartowski K. E., VanAller Hernick L. (2014). Middle Devonian liverwort herbivory and antiherbivore defence. New Phytol. 202, 247–258. doi: 10.1111/nph.12643
Labandeira C. C., Wappler T. (2023). Arthropod and pathogen damage on fossil and modern plants: Exploring the origins and evolution of herbivory on land. Annu. Rev. Entomol. 68, 341–361. doi: 10.1146/annurev-ento-120120-102849
Labandeira C. C., Wilf P., Johnson K., Marsh F. (2007). Guide to Insect (and Other) Damage Types on Compressed Plant Fossils. Version 3.0 (Washington, D.C: Smithsonian Institution).
Lacey W. S., Smith C. S. (1970). “Karroo floras from the upper Luangwa Valley, Zambia,” in Proceedings of the Second International Gondwana Symposium, Johannesburg, South Africa. 571–574.
Lara M. B., Cariglino B., Zavattieri A. M. (2017). Palaeoenvironmental interpretation of an Upper Triassic deposit in southwestern Gondwana (Argentina) based on an insect fauna, plant assemblage, and their interactions. Palaeogeogr. Palaeoclimatol. Palaeoecol. 476, 163–180. doi: 10.1016/j.palaeo.2017.03.029
Lele K. M. (1974). Late Palaeozoic and Triassic floras of India and their relation to the floras of Northern and Southern hemispheres. Palaeobotanist 23, 89–115. doi: 10.54991/jop.1974.956
Lindström S., Bjerager M., Alsen P., Sanei H., Bojesen-Koefoed J. (2020). The Smithian–Spathian boundary in North Greenland: implications for extreme global climate changes. Geological Magazine 157, 1547–1567. doi: 10.1017/S0016756819000669
Lindström S., McLoughlin S. (2007). Synchronous palynofloristic extinction and recovery after the end-Permian event in the Prince Charles Mountains, Antarctica: Implications for palynofloristic turnover across Gondwana. Rev. Palaeobotany Palynol. 145, 89–122. doi: 10.1016/j.revpalbo.2006.09.002
Lovering J. F., McElroy C. T. (1969). “V. The Sydney Basin- Wianamatta Group,” in The Geology of New South Wales. Ed. Packham G. H. (Sydney: Geological Society of Australia), 417–423.
Lu J., Wang Y., Yang M., Zhang P., Bond D. P. G., Shao L., et al. (2022). Diachronous end-Permian terrestrial ecosystem collapse with its origin in wildfires. Palaeogeogr. Palaeoclimatol. Palaeoecol. 594, 110960. doi: 10.1016/j.palaeo.2022.110960
Lutz A., Crisafulli A., Herbst R. (1999). Gymnospermous woods from the Upper Triassic of northern Chile. J. Palaeosciences 48, 31–38. doi: 10.54991/jop.1999.1289
Maheshwari H. K. (1992). Provincialism in Gondwana floras. Palaeobotanist 40, 101–127. doi: 10.54991/jop.1991.1769
Mays C., McLoughlin S. (2022). End-Permian burnout: The role of Permian-Triassic wildfires in extinction, carbon cycling, and environmental change in eastern Gondwana. PALAIOS 37, 292–317. doi: 10.2110/palo.2021.051
Mays C., McLoughlin S., Frank T. D., Fielding C. R., Slater S. M., Vajda V. (2021). Lethal microbial blooms delayed freshwater ecosystem recovery following the end-Permian extinction. Nat. Commun. 12, 5511. doi: 10.1038/s41467-021-25711-3
Mays C., Vajda V., Frank T. D., Fielding C. R., Nicoll R. S., Tevyaw A. P., et al. (2020). Refined Permian–Triassic floristic timeline reveals early collapse and delayed recovery of south polar terrestrial ecosystems. GSA Bull. 132, 1489–1513. doi: 10.1130/B35355.1
McLoughlin S. (1992). Late Permian plant megafossils from the Bowen Basin, Queensland, Australia: Part 1. Palaeontographica Abteilung B -Stuttgart- 228, 105–149.
McLoughlin S. (1994a). Late Permian plant megafossils from the Bowen Basin, Queensland, Australia: Part 2. Palaeontographica Abteilung B -Stuttgart- 231, 1–29.
McLoughlin S. (1994b). Late Permian plant megafossils from the Bowen Basin, Queensland, Australia: Part 3. Palaeontographica Abteilung B -Stuttgart- 231, 31–62.
McLoughlin S. (2011a). New records of leaf galls and arthropod oviposition scars in Permian–Triassic Gondwanan gymnosperms. Aust. J. Bot. 59, 156–169. doi: 10.1071/BT10297
McLoughlin S. (2011b). Glossopteris - insights into the architecture and relationships of an iconic Permian Gondwanan plant. J. Botanical Soc. Bengal 65, 93–106.
McLoughlin S., Bomfleur B., Drinnan A. (2018a). “Pachytestopsis tayloriorum gen. et sp. nov., an anatomically preserved glossopterid seed from the Lopingian of Queensland, Australia,” in Transformative Paleobotany: Papers to Commemorate the Life and Legacy of Thomas N. Taylor. Eds. Krings M., Harper C. J., Cúneo N. R., Rothwell G. W. (Amsterdam: Elsevier), 155–178. doi: 10.1016/B978-0-12-813012-4.00009-7
McLoughlin S., Lindström S., Drinnan A. N. (1997). Gondwanan floristic and sedimentological trends during the Permian–Triassic transition: new evidence from the Amery Group, northern Prince Charles Mountains, East Antarctica. Antarctic Sci. 9, 281–298. doi: 10.1017/S0954102097000370
McLoughlin S., Martin S. K., Beattie R. (2015). The record of Australian Jurassic plant–arthropod interactions. Gondwana Res. 27, 940–959. doi: 10.1016/j.gr.2013.11.009
McLoughlin S., Nicoll R. S., Crowley J. L., Vajda V., Mays C., Fielding C. R., et al. (2021a). Age and paleoenvironmental significance of the Frazer Beach Member—A new lithostratigraphic unit overlying the End-Permian Extinction Horizon in the Sydney Basin, Australia. Front. Earth Sci. 8. doi: 10.3389/feart.2020.600976
McLoughlin S., Pott C., Sobbe I. H. (2018b). The diversity of Australian Mesozoic bennettitopsid reproductive organs. Palaeobiodiversity Palaeoenvironments 98, 71–95. doi: 10.1007/s12549-017-0286-z
McLoughlin S., Prevec R., Slater B. J. (2021b). Arthropod interactions with the Permian Glossopteris flora. J. Palaeosciences 70, 43–133. doi: 10.54991/jop.2021.11
McLoughlin S., Santos A. A. (2024). Excavating the fossil record for evidence of leaf mining. New Phytol. 242, 2391–2393. doi: 10.1111/nph.19652
Melchor R., Herbst R. (2000). Sedimentology of the El Puquén Formation (Upper Triassic, central Chile) and the new plant Mollesia melendeziae gen. et sp. nov. (Pteridophylla, incertae sedis). Ameghiniana 37, 477–485.
Meyer-Berthaud B., Taylor T. N., Taylor E. L. (1993). Petrified stems bearing Dicroidium leaves from the Triassic of Antarctica. Palaeontology 36, 337–356.
Millay M., Taylor T. N. (1990). New fern stems from the Triassic of Antarctica. Rev. Palaeobotany Palynol. 62, 41–64. doi: 10.1016/0034-6667(90)90016-C
Mohr B., Schöner F. (1985). Eine obertriassische Dicroidium-Flora südöstlich Alto del Carmen, Región de Atacama (Chile). Neues Jahrbuch für Geologie und Paläontologie 6, 368–379. doi: 10.1127/njgpm/1985/1985/368
Moisan P. (2024). Devonian to Triassic paleobotanical record from Chile: An overview of the micro- and macrofossil record and fossil localities. Rev. Palaeobotany Palynol. 320, 105014. doi: 10.1016/j.revpalbo.2023.105014
Niemeyer H., Zavattieri A. M., Ballent S., Zamuner A., Gallego O. (2008). Triassic age of the continental Pular Formation, Sierra de Almeida, Antofagasta, northern Chile. Rev. geológica Chile 35, 147–161. doi: 10.4067/S0716-02082008000100007
Ottone E. G., Avellaneda D., Koukharsky M. (2011). Plantas Triásicas y su Relación Con el Volcanismo en la Formación Agua de la Zorra, Provincia de Mendoza, Argentina. Ameghiniana 48, 177–188. doi: 10.5710/AMGH.v48i2(312
Pal P. K., Ghosh A. K. (1997). Megafloral zonation of Permian-Triassic sequence in the Formation, Talcher Coalfield, Orissa. Palaeobotanist 46, 81–87. doi: 10.54991/jop.1997.1319
Pal P. K., Ghosh A., Kar R., Singh R. S., Sarkar M., Chatterjee R. (2014). Reappraisal of the genus Dicroidium Gothan from the Triassic sediments of India. J. Palaeosciences 63, 137–155. doi: 10.54991/jop.2014.327
Pal P. K., Srivastava A. K., Ghosh A. K. (2010). Plant fossils of Maitur Formation: possibly the ultimate stage of Glossopteris flora in Raniganj Coalfield, India. Palaeobotanist 59, 33–45. doi: 10.54991/jop.2010.186
Pedernera T. E., Mancuso A. C., Ottone E. G. (2022). Triassic paleoclimate and paleofloristic trends of southwestern Gondwana (Argentina). J. South Am. Earth Sci. 116, 103852. doi: 10.1016/j.jsames.2022.103852
Pedernera T. E., Ottone E. G., Mancuso A. C., Benavente C. A., Abarzúa Cutroni F. D. (2019). Syn-eruptive taphoflora from the Agua de la Zorra Formation (Upper Triassic) Cuyana Basin, Mendoza, Argentina. Andean Geology 46, 604–628. doi: 10.5027/andgeoV46n3-3228
Perovich N. E., Taylor E. L. (1989). Structurally preserved fossil plants from Antarctica. IV. Triassic ovules. Am. J. Bot. 76, 992–999. doi: 10.1002/j.1537-2197.1989.tb15079.x
Philippe M., McLoughlin S., Strullu-Derrien C., Bamford M., Kiel S., Nel A., et al. (2022). Life in the woods: Taphonomic evolution of a diverse saproxylic community within fossil woods from Upper Cretaceous submarine mass flow deposits (Mzamba Formation, southeast Africa). Gondwana Res. 109, 113–133. doi: 10.1016/j.gr.2022.04.008
Phipps C. J., Axsmith B. J., Taylor T. N., Taylor E. L. (2000). Gleichenipteris antarcticus gen. et sp. nov. from the Triassic of Antarctica. Rev. Palaeobotany Palynol. 108, 75–83. doi: 10.1016/S0034-6667(99)00034-2
Pigg K. B. (1990). Anatomically preserved Dicroidium foliage from the central Transantarctic Mountains. Rev. Palaeobotany Palynol. 66, 129–145. doi: 10.1016/0034-6667(90)90031-D
Playford G., Rigby J., Archibald D. (1982). A Middle Triassic flora from the Moolayember Formation, Bowen Basin, Queensland. Geological Survey Queensland Publ. 380, 1–52.
Prevec R., Gastaldo R. A., Neveling J., Reid S. B., Looy C. V. (2010). An autochthonous glossopterid flora with latest Permian palynomorphs and its depositional setting in the Dicynodon Assemblage Zone of the southern Karoo Basin, South Africa. Palaeogeogr. Palaeoclimatol. Palaeoecol. 292, 391–408. doi: 10.1016/j.palaeo.2010.03.052
Prevec R., Labandeira C. C., Neveling J., Gastaldo R. A., Looy C. V., Bamford M. (2009). Portrait of a Gondwanan ecosystem: A new late Permian fossil locality from KwaZulu-Natal, South Africa. Rev. Palaeobotany Palynol. 156, 454–493. doi: 10.1016/j.revpalbo.2009.04.012
Raggatt H. G. (1969). “V. The Sydney Basin– northwest margin and Goulburn Valley– macroflora and fauna,” in The Geology of New South Wales. Ed. Packham G. H. (Sydney: Geological Society of Australia), 405–407.
Retallack G. J. (1977). Reconstructing Triassic vegetation of eastern Australasia: a new approach for the Biostratigraphy of Gondwanaland. Alcheringa 1, 247–277. doi: 10.1080/03115517708527763
Retallack G. J. (1980a). “Late Carboniferous to Middle Triassic megafossil floras from the Sydney Basin,” in A Guide to the Sydney Basin. Geological Survey of New South Wales, Australia. Bulletin, 26, 384–430.
Retallack G. J. (1980b). Middle Triassic megafossil plants and trace fossils from Tank Gully, Canterbury, New Zealand. J. R. Soc. New Z. 10, 31–63. doi: 10.1080/03036758.1980.10426550
Retallack G. J. (1981). Middle Triassic megafossil plants from Long Gully, near Otematata, north Otago, New Zealand. J. R. Soc. New Z. 11, 167–200. doi: 10.1080/03036758.1981.10421836
Retallack G. J. (1983). Middle Triassic megafossil marine algae and land plants from near Benmore Dam, southern Canterbury, New Zealand. J. R. Soc. New Z. 13, 129–154. doi: 10.1080/03036758.1983.10415325
Retallack G. J. (1985). Triassic fossil plant fragments from shallow marine rocks of the Murihiku Supergroup, New Zealand. J. R. Soc. New Z. 15, 1–26. doi: 10.1080/03036758.1985.10421741
Retallack G. J. (1995). An early Triassic fossil flora from Culvida Soak, Canning Basin, Western Australia. J. R. Soc. Western Aust. 78, 57–66.
Retallack G. J. (1997). Earliest Triassic origin of Isoetes and quillwort evolutionary radiation. J. Paleontol. 71, 500–521. doi: 10.1017/S0022336000039524
Retallack G. J., Jahren A. H., Sheldon N. D., Chakrabarti R., Metzger C. A., Smith R. M. H. (2005). The Permian–Triassic boundary in Antarctica. Antarctic Sci. 17, 241–258. doi: 10.1017/S0954102005002658
Retallack G. J., Veevers J. J., Morante R. (1996). Global coal gap between Permian–Triassic extinction and Middle Triassic recovery of peat-forming plants. GSA Bull. 108, 195–207. doi: 10.1130/0016-7606(1996)108<0195:GCGBPT>2.3.CO;2
Rigby J. F. (1977). New collections of Triassic plants from the Esk Formation, southeast Queensland. Queensland Government Min. J. 78, 320–325.
Rigby J. F., Schopf J. M. (1969). “Stratigraphic implications of Antarctic paleobotanical studies,” in International Union of Geological Sciences Symposium, United Nations Educational, Scientific and Cultural Organisation, Buenos Aires, 1967. UNESCO, Paris. 91–106.
Romano C., Goudemand N., Vennemann T. W., Ware D., Schneebeli-Hermann E., Hochuli P. A., et al. (2013). Climatic and biotic upheavals following the end-Permian mass extinction. Nat. Geosci. 6, 57–60. doi: 10.1038/ngeo1667
Romero-Lebrón E., Robledo J. M., Delclòs X., Petrulevičius J. F., Gleiser R. M. (2022). Endophytic insect oviposition traces in deep time. Palaeogeogr. Palaeoclimatol. Palaeoecol. 590, 110855. doi: 10.1016/j.palaeo.2022.110855
Rothwell G. W., Taylor E., Taylor T. (2002). Ashicaulis woolfei n. sp.: Additional evidence for the antiquity of osmundaceous ferns from the Triassic of Antarctica. Am. J. Bot. 89, 352–361. doi: 10.3732/ajb.89.2.352
Saito R., Kaiho K., Tian L., Takahashi S. (2023). Frequent high-temperature volcanic combustion events delayed biotic recovery after the end-Permian mass extinction. Earth Planetary Sci. Lett. 614, 118194. doi: 10.1016/j.epsl.2023.118194
Salazar E., Coloma F., Creixell C. (2013). Geología del área El Tránsito-Lagunillas: Región de Atacama. Servicio Nacional de Geología y Minería, Subdirección Nacional de Geología. Carta Geología de Chile, Serie Geología Básica, Chile, Vol. 149.
Saxena A., Singh K. J., Cleal C. J., Chandra S., Goswami S., Shabbar H. (2019). Development of the Glossopteris flora and its end Permian demise in the Tatapani–Ramkola Coalfield, Son–Mahanadi Basin, India. Geological J. 54, 2472–2494. doi: 10.1002/gj.3307
Schachat S. R., Labandeira C. C. (2021). Are insects heading toward their first mass extinction? Distinguishing turnover from crises in their fossil record. Ann. Entomological Soc. America 114, 99–118. doi: 10.1093/aesa/saaa042
Schachat S. R., Labandeira C. C., Gordon J., Chaney D., Levi S., Halthore M. N., et al. (2014). Plant-insect interactions from Early Permian (Kungurian) Colwell Creek Pond, north-central Texas: The early spread of herbivory in riparian environments. Int. J. Plant Sci. 175, 855–890. doi: 10.1086/677679
Schachat S. R., Payne J. L., Boyce C. K., Labandeira C. C. (2022). Generating and testing hypotheses about the fossil record of insect herbivory with a theoretical ecospace. Rev. Palaeobotany Palynol. 297, 104564. doi: 10.1016/j.revpalbo.2021.104564
Schneebeli-Hermann E., Kürschner W. M., Kerp H., Bomfleur B., Hochuli P. A., Bucher H., et al. (2015). Vegetation history across the Permian–Triassic boundary in Pakistan (Amb section, Salt Range). Gondwana Res. 27, 911–924. doi: 10.1016/j.gr.2013.11.007
Schopf J. M. (1978). An unusual osmundaceous specimen from Antarctica. Can. J. Bot. 56, 3083–3095. doi: 10.1139/b78-371
Schwendemann A., Taylor T., Taylor E., Krings M., Osborn J. (2010). “Modern traits in early Mesozoic sphenophytes: The Equisetum-like cones of Spaciinodum collinsonii with in situ spores and elaters,” in Plants in Mesozoic Time: Morphological Innovations, Phylogeny, Ecosystems (Indianapolis: Indiana University Press), 15–33.
Scott A. C., Anderson J. M., Anderson H. M. (2004). Evidence of plant–insect interactions in the Upper Triassic Molteno Formation of South Africa. J. Geological Soc. 161, 401–410. doi: 10.1144/0016-764903-118
Shah B. (2000). Revision of age of Parsora Formation from plant assemblages in Karkati area, Johilla-son Valley, Rewa Basin, M.P. Indian Minerals 54, 223–232.
Shah B. A. (2021). Diagenesis and genesis of clay minerals in the Triassic sandstones of the Panchet and Parsora formations, Damodar–Son Basin, India. J. Sedimentary Environments 6, 255–265. doi: 10.1007/s43217-021-00055-6
Shen J., Algeo T. J., Planavsky N. J., Yu J., Feng Q., Song H., et al. (2019). Mercury enrichments provide evidence of Early Triassic volcanism following the end-Permian mass extinction. Earth-Science Rev. 195, 191–212. doi: 10.1016/j.earscirev.2019.05.010
Shen J., Chen J., Yu J., Algeo T. J., Smith R. M. H., Botha J., et al. (2023). Mercury evidence from southern Pangea terrestrial sections for end-Permian global volcanic effects. Nat. Commun. 14, 6. doi: 10.1038/s41467-022-35272-8
Spalletti L. A., Artabe A. E., Morel E. M. (2003). Geological factors and evolution of southwestern Gondwana Triassic plants. Gondwana Res. 6, 119–134. doi: 10.1016/S1342-937X(05)70648-1
Spalletti L. A., Artabe A. E., Morel E. M., Brea M. (1999). Biozonación paleoflorística y cronoestratigrafía del Triásico Argentino. Ameghiniana 36, 419–451.
Srivastava S. C. (1988). Stratigraphic position and age of plant bearing Nidpur beds. Palaeobotanist 36, 154–160. doi: 10.54991/jop.1987.1573
Srivastava A. K., Krassilov V. A., Agnihotri D. (2011). Peltasperms in the Permian of India and their bearing on Gondwanaland reconstruction and climatic interpretation. Palaeogeogr. Palaeoclimatol. Palaeoecol. 310, 393–399. doi: 10.1016/j.palaeo.2011.07.030
Srivastava A., Srivastava R., Agnihotri D. (2010). Dicroidium: no more a Triassic Gondwana index fossil. Nat. Precedings, 1–6. doi: 10.1038/npre.2010.4777.1
Stanley S. M. (2016). Estimates of the magnitudes of major marine mass extinctions in Earth history. Proc. Natl. Acad. Sci. United States America 113, E6325–E6334. doi: 10.1073/pnas.1613094113
Stork N. E. (2018). How many species of insects and other terrestrial arthropods are there on Earth? Annu. Rev. Entomol. 63, 31–45. doi: 10.1146/annurev-ento-020117-043348
Stubblefield S. P., Taylor T. N. (1986). Wood decay in silicified gymnosperms from Antarctica. Botanical Gazette 147, 116–125. doi: 10.1086/337577
Sun Y., Joachimski M. M., Wignall P. B., Yan C., Chen Y., Jiang H., et al. (2012). Lethally hot temperatures during the Early Triassic greenhouse. Science 338, 366–370. doi: 10.1126/science.1224126
Taylor T. N., Fueyo G. M. D., Taylor E. L. (1994). Permineralized seed fern cupules from the Triassic of Antarctica: implications for cupule and carpel evolution. Am. J. Bot. 81, 666–677. doi: 10.1002/j.1537-2197.1994.tb15501.x
Taylor E. L., Taylor T. N., Collinson J. W. (1986). Paleoenvironment of Lower Triassic plants from the Fremouw Formation. Antarctic J. U.S. 21, 26–27.
Taylor E. L., Taylor T. N., Collinson J. W. (1989). Depositional setting and paleobotany of Permian and Triassic permineralized peat from the central Transantarctic Mountains, Antarctica. Int. J. Coal Geology 12, 657–679. doi: 10.1016/0166-5162(89)90068-2
Taylor T. N., Taylor E. L., Del Fueyo G. M. (1993). “Permineralized Triassic plants from Antarctica,” in The Nonmarine Triassic Bulletin. Eds. Lucas S. G., Morales M. (Albuquerque: New Mexico Museum of Natural History and Science), 457–460.
Thomas M., Hill R. (2023). Insect damage on fossil leaves in Cenozoic Australia: A largely unreported palaeo-record. Rev. Palaeobotany Palynol. 310, 104841. doi: 10.1016/j.revpalbo.2023.104841
Tiwari R. S., Ram-Awatar (1990). Palyno-dating of Nidpur beds, son graben, madhya pradesh. Palaeobotanist 38, 105–121. doi: 10.54991/jop.1989.1645
Troncoso A., Gnaedinger S., Herbst R. (2000). Heidiphyllum, Rissikia y Desmiophyllum (Pinophyta, Coniferales) en el Triásico del norte chico de Chile y sur de Argentina. Ameghiniana 37, 119–125.
Troncoso A., Herbst R. (1999). Ginkgoales del Triásico del norte de Chile. Rev. geológica Chile 26, 255–271. doi: 10.4067/S0716-02081999000200007
Vajda V., McLoughlin S., Mays C., Frank T. D., Fielding C. R., Tevyaw A., et al. (2020). End-Permian (252 Mya) deforestation, wildfires and flooding—An ancient biotic crisis with lessons for the present. Earth Planetary Sci. Lett. 529, 115875. doi: 10.1016/j.epsl.2019.115875
Veevers J. J., Powell C., Collinson J. W., López-Gamundí O. R. (1994). “Synthesis,” in Permian-Triassic Pangean Basins and Foldbelts Along the Panthalassan Margin of Gondwanaland, GSA Memoirs, Vol. 184. Eds. Veevers J. J., Powell C. (Boulder, Colorado: Geological Society of America), 331–353. doi: 10.1130/MEM184-p331
Viglietti P. A., Benson R. B. J., Smith R. M. H., Botha J., Kammerer C. F., Skosan Z., et al. (2021). Evidence from South Africa for a protracted end-Permian extinction on land. Proc. Natl. Acad. Sci. United States America 118, e2017045118. doi: 10.1073/pnas.2017045118
Villalva A. S. (2017). Análisis de una megaflora seleccionada equisetales y pteridospermales del grupo El Tranquilo, triásico, Santa Cruz, Argentina. Presented at the XXIII XXIII Comunicaciones Científicas y Tecnológicas, Universidad Nacional del Nordeste. Secretaria General de Ciencia y Técnica, Universidad Nacional del Nordeste, Corrientes, Argentina.
Villalva A. S., Gnaedinger S., Zavattieri A. M. (2023). Systematic and organ relationships of Neocalamites (Halle) Vladimirovicz, and Nododendron (Artabe and Zamuner) emend. from the Triassic of Patagonia. Palaeobiogeographic, palaeoenvironments and palaeoecology considerations. Review of Palaeobotany and Palynology. Rev. Palaeobotany Palynol. 316, 104939. doi: 10.1016/j.revpalbo.2023.104939
Walkom A. B. (1928). Fossil plants from the Esk district, Queensland. Proc. Linn. Soc. New South Wales 53, 458–468.
Wappler T., Kustatscher E., Dellantonio E. (2015). Plant-insect interactions from Middle Triassic (late Ladinian) of Monte Agnello (Dolomites, N-Italy)-initial pattern and response to abiotic environmental pertubations. PeerJ. 3, e921. doi: 10.7717/peerj.921
War A. R., Taggar G. K., Hussain B., Taggar M. S., Nair R. M., Sharma H. C. (2018). Plant defense against herbivory and insect adaptations. AoB Plants. 10, ply037. doi: 10.1093/aobpla/ply037
Webb J. A. (1982). Triassic species of Dictyophyllum from eastern Australia. Alcheringa 6, 79–91. doi: 10.1080/03115518208566988
Webb J. A. (2001). A new marattialean fern from the Middle Triassic of eastern Australia. Proc. Linn. Soc. New South Wales 123, 215–224.
Webb J. A., Holmes W. B. K. (1982). Three new thalloid fossils from the Middle Triassic of eastern Australia. Proc. R. Soc. Queensland 93, 83–88.
White M. E. (1965). Report on 1964 Plant Fossil Collections Vol. 101 (Australia: Bureau of Mineral Resources, Geology and Geophysics).
White M. E. (1969). Report on 1968 Collection of Plant Fossils from the Moolayember and Teviot Formations Vol. 51 (Australia: Bureau of Mineral Resources, Geology and Geophysics).
White M. E. (1971). Report on 1969 Collection of Plant Fossils from the Moolayember Formation and Clematis Sandstone Vol. 104 (Australia: Bureau of Mineral Resources, Geology and Geophysics).
White M. E., Yeates A. N. (1976). Plant Fossils from the Northeastern Part of the Canning Basin, Western Australia Vol. 18 (Canberra, Australia: Records of the Bureau of Mineral Resources, Geology and Geophysics).
Widmann P., Bucher H., Leu M., Vennemann T., Bagherpour B., Schneebeli-Hermann E., et al. (2020). Dynamics of the largest carbon isotope excursion during the Early Triassic biotic recovery. Front. Earth Sci. 8, 1–16. doi: 10.3389/feart.2020.00196
Wilf P. (2008). Insect-damaged fossil leaves record food web response to ancient climate change and extinction. New Phytol. 178, 486–502. doi: 10.1111/j.1469-8137.2008.02395.x
Wilf P., Labandeira C. C., Johnson K. R., Coley P. D., Cutter A. D. (2001). Insect herbivory, plant defense, and early Cenozoic climate change. Proc. Natl. Acad. Sci. United States America 98, 6221–6226. doi: 10.1073/pnas.111069498
Wu Y., Cui Y., Chu D., Song H., Tong J., Dal Corso J., et al. (2023). Volcanic CO2 degassing postdates thermogenic carbon emission during the end-Permian mass extinction. Sci. Adv. 9, eabq4082. doi: 10.1126/sciadv.abq4082
Yao X., Taylor T. N., Taylor E. L. (1995). The corystosperm pollen organ Pteruchus from the Triassic of Antarctica. Am. J. Bot. 82, 535–546. doi: 10.1002/j.1537-2197.1995.tb15675.x
Yao X., Taylor T. N., Taylor E. L. (1997). A taxodiaceous seed cone from the Triassic of Antarctica. Am. J. Bot. 84, 343–354. doi: 10.2307/2446008
Zambon R., Denayer J., Prestianni C. (2023). Plant-insect interactions in the Selandian (early Paleocene) Gelinden fossil flora (Belgium) and what they mean for the ecosystems after the Cretaceous-Paleogene mass extinction. Palaeogeogr. Palaeoclimatol. Palaeoecol. 619, 111524. doi: 10.1016/j.palaeo.2023.111524
Keywords: End-Permian extinction, fossil, Gondwana, palaeobotany, palaeoecology, plant-herbivore interactions, post-extinction recovery, Triassic
Citation: Turner H-A, McLoughlin S and Mays C (2024) Comprehensive survey of Early to Middle Triassic Gondwanan floras reveals under-representation of plant–arthropod interactions. Front. Ecol. Evol. 12:1419254. doi: 10.3389/fevo.2024.1419254
Received: 17 April 2024; Accepted: 05 August 2024;
Published: 11 September 2024.
Edited by:
Sandra Schachat, Stanford University, United StatesReviewed by:
Barbara Cariglino, National Scientific and Technical Research Council (CONICET), ArgentinaZhuo Feng, Yunnan University, China
Copyright © 2024 Turner, McLoughlin and Mays. This is an open-access article distributed under the terms of the Creative Commons Attribution License (CC BY). The use, distribution or reproduction in other forums is permitted, provided the original author(s) and the copyright owner(s) are credited and that the original publication in this journal is cited, in accordance with accepted academic practice. No use, distribution or reproduction is permitted which does not comply with these terms.
*Correspondence: Holly-Anne Turner, hollyaturner@ucc.ie