- 1College of Wildlife and Protected Area, Northeast Forestry University, Harbin, China
- 2Northeast Asia Biodiversity Research Center, Harbin, China
- 3Guangxi Forest Inventory and Planning Institute, Nanning, China
- 4Guangxi Darongshan Nature Reserve Management Department, Yulin, China
- 5Guangdong Key Laboratory of Animal Conservation and Resource Utilization, Guangdong Public Laboratory of Wild Animal Conservation and Utilization, Institute of Zoology, Guangdong Academy of Sciences, Guangzhou, China
Birds are sensitive to environmental changes and can drive range shifts rapidly due to their high mobility. Though previous studies have examined the associations between species traits and range shifts, whether species traits could still explain heterogeneity in shift directions remains poorly explored. Here, we compiled new bird records of China from 2000 to 2019 and analyzed species traits associated with apparent shift directions. We collected 350 provincial-level new records of birds belonging to 67 families of 22 orders. Of these, 32 are threatened, with 3 critically endangered, 11 endangered, and 18 vulnerable. Provinces in western China (i.e., Yunnan and Xizang) had relatively higher species richness of new recorded birds; this pattern was also reflected in the phylogenetic diversity we observed. In addition, provinces in northern China (i.e., Tianjin, Shandong, and Beijing) had relatively higher richness-controlled phylogenetic diversity. Phylogenetic overdispersion of new recorded bird communities was observed in 61.29% of provinces (19 of 31). The main shift directions indicated by new bird records were northward (with nearly 50% of birds moving NW, N and NE). Migration, hand-wing index (HWI), body mass, and range size are the four key factors that most significantly influence the shift directions in bird species, suggesting that bird movement toward newly suitable areas varies with species-specific traits. Together, these results demonstrate the importance of considering species ecological traits when predicting shift directions of birds.
Introduction
Analysis of new bird records is important for evaluating extinction risks, predicting the future changes in ecological communities, and undertaking actions to preserve species of conservation concern (Chen et al., 2011; Li et al., 2013; Liu et al., 2013; Renjifo et al., 2017). A recent meta-analysis has shown that the number of new bird records in China has increased yearly during the past two decades (Chen et al., 2021). This brings us opportunities to track the shifts in species distribution under environment change and manage species of conservation concern in a timely manner. For example, the first discovery of Pterorhinus courtoisi in Wuyuan region in the Jiangxi Province facilitated the construction of a protected area (Collar et al., 2021).
Previous analyses on range shifts have shown that birds tend to move to higher latitudes or higher elevations on a global scale (e.g., Du et al., 2009; Chen et al., 2011; Li et al., 2013; Neate-Clegg and Tingley, 2023), yet there is also considerable heterogeneity in the magnitude and direction of these shifts across different regions. For example, in eastern North America resident birds expand their ranges northward while neotropical migrants have shown a contraction at their southern ranges (Rushing et al., 2020), and North American migratory birds show considerable heterogeneity in shifts of breeding distributions, with 44% moving southward and 55% moving northward (McCaslin and Heath, 2020). In Europe, birds overwintering in Finland in arable land are shifting more northward compared to those inhabiting forest and rural areas (Bosco et al., 2022). This heterogeneity is typically associated with factors such as migratory behavior and winter geography (Rushing et al., 2020), protandry and supplemental cues (McCaslin and Heath, 2020), habitat types (Bosco et al., 2022), initial climate conditions and species’ range traits (traits of a species that are related to the range within which it naturally occurs, e.g., distance to the nearest continually occupied grid cell, range size, and distance to species’ center of gravity, Howard et al., 2023), ecological barriers (e.g., coastlines and mountains, Marjakangas et al., 2023), species’ traits (Martins et al., 2024). Among the many factors, climate change predominantly drives the present range shifts worldwide (e.g., Bateman et al., 2016; Hu et al., 2020; Antão et al., 2022). This trend underscores the profound impact of climate change on avian species, and the observed northward and upward movements are likely driven by a combination of factors including increasing temperatures (Tayleur et al., 2015; Hu et al., 2020), and changes in precipitation patterns (reviewed in Neate-Clegg and Tingley, 2023).
Species’ traits are also increasingly being used for predictions of range shifts (e.g., Estrada et al., 2016; Howard et al., 2023; Martins et al., 2024). For example, traits associated with dispersal ability (i.e., body size, migratory strategy and movement ability) are important predictors of the magnitude of range shift (reviewed in MacLean and Beissinger, 2017). Nonetheless, most of current analyses have focused on spatial distribution of new bird records and the potential causes of observed range shifts (e.g., Chen et al., 2021; Zhang et al., 2022), while little attention has been paid to the associations between bird species traits and range shifts (Yang et al., 2020). For example, ecological traits such as range size, habitat specificity (i.e., the number of habitats where a species has been documented), and trophic level have positive effects on species distribution; birds with higher trophic levels are more likely to exhibit range shifts in China, whereas body size was negatively associated with range shifts of Chinese birds (Yang et al., 2020). Indeed, species traits were considered important in improving the predictions of range shifts (Angert et al., 2011; Estrada et al., 2016; Howard et al., 2023).
However, compared with the evidence on the effects of species traits on range shifts of birds, whether species traits still explain heterogeneity in shift directions remains poorly explored (Auer and King, 2014; MacLean and Beissinger, 2017; Rushing et al., 2020). For example, migratory status (i.e., resident, or migratory species) was proved to drive differential shift directions (Rushing et al., 2020), with resident species shifting toward their northward range limits while migratory species retracting from their southern range limits. Moreover, ecological traits such as trophic niche, primary lifestyle, hand-wing index, range size and body mass are expected to influence shift directions (e.g., Niven et al., 2010; Reif and Flousek, 2012; Hallman et al., 2022; Laughlin and Pomara, 2023; Thompson et al., 2023). For instance, resident insectivorous birds have shifted northwards at higher rates than residents with generalist diets (Laughlin and Pomara, 2023), and land birds have a higher proportion of northward shifts (Niven et al., 2010). Additionally, the hand-wing index has strong explanatory power regarding the elevational shifts in birds (Hallman et al., 2022). Also, open habitat species are shifting to higher altitudes compared to forest species (Reif and Flousek, 2012). Unsurprisingly, species traits could strengthen predictions for future directions of range shifts and assist in the implementation of effective bird conservation strategies, so that additional investigation is required on whether species-level traits can be used to predict their responses to climate-induced range shifts.
In addition, shifts in bird distribution may also cause closely related species to colonize new habitats (Krosby et al., 2015), because species that are more ecologically similar tend to occur within similar habitats. For example, estimated net increase in species richness in certain regions of North America and northern Asia suggested that newly added species share close evolutionary relationships to each other (Voskamp et al., 2022). Thus, phylogenetic information can offer a novel perspective for predicting shifts in bird distribution. It helps us to consider whether closely related bird species tend to follow similar patterns in response to environmental changes and thus is valuable for guiding future efforts in monitoring, modeling, and conservation.
In this study, we analyzed more than 400 provincial-level new bird records in China published within the past 20 years to obtain range shift patterns. The results provide a comprehensive review of bird ranges in China. Specifically, we asked the following questions: 1) Where are newly recorded birds concentrated in China and what are the corresponding patterns of phylogenetic structure? 2) which species-level traits are closely linked with the expansion directions of newly recorded birds?
Methods
Data collection
China extends across parts of the Palearctic and Indomalayan realms, ranging in latitude from 3°51′N to 53°33′N, including tropical monsoon, sub-tropical monsoon, temperate monsoon, temperate continental, and mountain climates (Domrös and Peng, 2012). China’s complex and diverse habitats harbor an extremely rich bird diversity (1505 bird species; Zheng, 2023), which constitute up to 13.79% of bird species worldwide (Gill et al., 2021). In this study, we collected new bird records in each province of China through the China National Knowledge Infrastructure (https://www.cnki.net/), selecting all databases except those for patents and almanacs. We searched all peer-reviewed scientific literature published from 2000 to 2019 using the following topic search terms: (China* OR province name) AND (bird* OR avian OR wild bird) AND (new occurrence records* OR new range OR new sighting OR new habitat) (accessed January 2020) (Supplementary Table 1 lists the Chinese translations for these search terms). A total of 350 newly recorded bird species were found.
We extracted 9 ecological traits commonly linked to shifts in distribution from the AVONET database (Tobias et al., 2022): hand–wing index (ratio of the distance between the tip of the first secondary feather and the tip of the longest primary feather to the total wing chord), body mass (g), habitat (forest, wetland, woodland, grassland, marine, human-modified, coastal, riverine, rock, shrubland, or desert), habitat density (dense, semi-open, or open), migration (sedentary, partially migratory, or migratory), trophic level (herbivore, omnivore, carnivore, or scavenger), trophic niche (herbivore terrestrial, aquatic predator, omnivore, herbivore aquatic, granivore, frugivore, invertivore, vertivore, scavenger, or nectarivore), primary lifestyle (terrestrial, aquatic, generalist, insessorial, or aerial), and range size (km2) (Tobias et al., 2022).
We constructed a phylogenetic tree for the study species by pruning the global phylogenetic tree of birds produced by BirdTree (http://birdtree.org) using the “Hackett All Species” option, which employs a set of 10,000 trees, each containing 9,993 operational taxonomic units (Jetz et al., 2012). We sampled 5,000 pseudo-posterior distributions and estimated the maximum clade credibility tree using mean node heights provided by the TreeAnnonator v1.10.4 software and the BEAST package (Drummond and Rambaut, 2007). The resulting tree was used for all subsequent phylogenetic analyses (Figure 1).
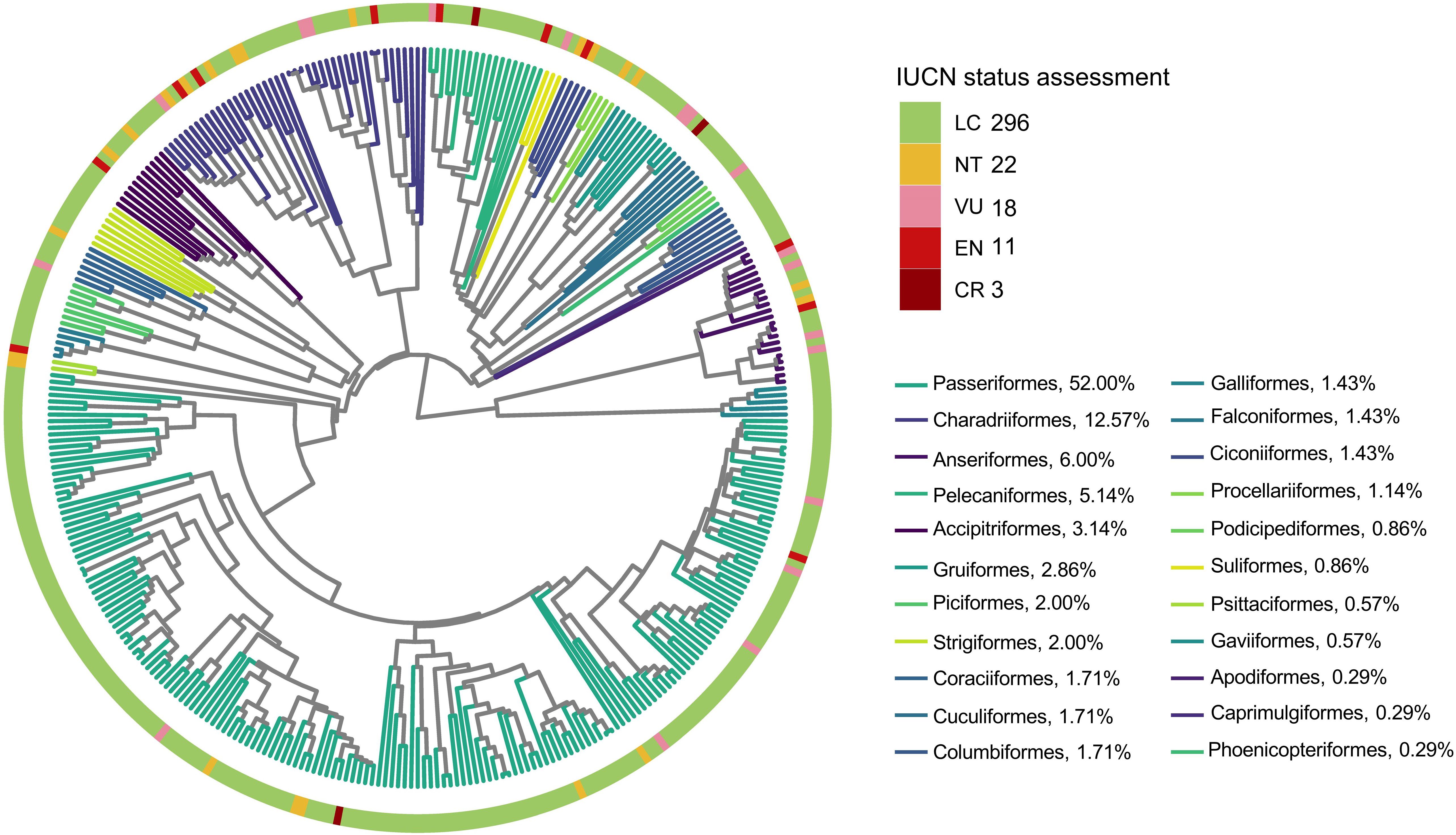
Figure 1 Phylogenetic tree of all new bird records, and the proportion of species within each order relative to the total number of species.
Diversity indices
We estimated the taxonomic and phylogenetic diversity of newly recorded birds in each province of China. Taxonomic diversity was calculated as the number of new records in each province (SR), and phylogenetic diversity was estimated as Faith’s phylogenetic diversity index (PD) (Faith, 1992). Given that PD is correlated with SR because these metrics sum across species, we calculated the standardized effect size (SES) of PD using null models to account for the effects of species richness. Specifically, we applied a null model that randomly sampled species 999 times from all the species recorded across all the provinces, while maintaining a constant level of species richness in each province (Jarzyna et al., 2021).
To determine the phylogenetic structure of the communities, we calculated the standardized effect size (SES) of mean pairwise phylogenetic distance (MPD) for each province. The SES.MPD was calculated as follows: (Obs – Exp)/SDexp where Obs is the observed MPD, Exp is the average value of 999 random null model simulations, and SDexp is the associated standard deviation (SD). When SES.MPD < 0, the phylogenetic structure is clustered and environment filtering may drive the community assembly, whereas SES.MPD > 0 indicates overdispersion, such that competitive exclusion may drive community structure (Webb et al., 2002); SES.MPD > 1.96 or SES.MPD < −1.96 indicates significant overdispersion or clustering, respectively (P < 0.05).
Data analyses
When a new bird record was affected by uncertainty in taxonomic identification or in the geographic origin of the recorded bird, and when the original range of a certain species and its new record (i.e., the arrival point) are spaced by one or more provinces, we regarded the point within the species’ original range which is the nearest to the new record as the bird’s initial range and used this location to measure its shift directions. Shift directions were determined from the initial range, including only areas within China (Mackinnon et al., 2000), to the centroid of the colonized province (the geometric centroid of the new occurrence sites), and classified as east, west, south, north, northeast, northwest, southeast, or southwest (See Supplementary Figure 1 for a detailed illustration showing how we determine the shift directions). The shift directions were measured in degrees using the Ovital Map v8.9.4 (Beijing Yuansheng Huawang Software Co., Ltd, https://www.ovital.com/download/) and Google Maps software (Google LLC, http://www.google.cn/maps). Subsequently, these degree measurements were converted to radians: with the zero-reference point aligned to the east, the direction of northeast, for instance, was designated by an angle of 1.75π radians. In the subsequent analysis, the radians value served as the response variable, while the nine ecological traits considered were utilized as predictor variables.
Because a single species can shift its range in different directions, for each species, we entered each ecological trait the same number of times as the number of directions for that species. Then, we obtained a table with 485 rows (each row represents one shift direction; some birds may only have one shift direction, but there are also birds with multiple shift directions) and 10 columns (1 radian column and 9 ecological trait columns). Based on preliminary comparisons between generalized linear models (GLMs) and phylogenetic generalized least-squares models (Orme et al., 2013), we found that phylogenetic information has little influence on the results. Therefore, we performed subsequent analyses without considering phylogenetic effects. Further, we chose the random forest (RF) algorithm to explore the relative importance of each considered ecological trait in determining the shift directions for newly recorded bird species because it does not require strict assumptions about the data and, compared to GLMs, it can better handle issues such as multicollinearity and non-linear relationships (Breiman, 2001). In the RF model, the response variable was the shift directions (in radians), while the predictor variables were the nine ecological traits. To assess the relative importance of each ecological trait, we used the average of the percentage increase in mean squared error (%IncMSE) within RF models built after permuting the values of the considered trait, with a higher importance value suggesting that trait to be more influential than others in explaining the directions of range shifts for newly recorded birds. Random forest regression models were built using the “randomForest” package (Liaw and Wiener, 2002) in the R v4.2.1 software (R Core Team, 2021).
Results
Distribution of new bird records
A total of 350 species were recorded in China during 2000–2019, belonging to 67 families of 22 orders. Among these species, 32 are classified as Threatened, including 3 that are critically endangered, 11 endangered, and 18 vulnerable. Additionally, there are 22 near-threatened species and 296 of least concern (Figure 1). Most of the new records were Passeriformes (182 species; 52%), followed by Charadriiformes (44 species; 12.57%) and Anseriformes (21 species; 6%).
Specifically, western China (i.e., Yunnan and Xizang) had relatively higher species richness of new recorded birds (Figure 2A); we observed similar patterns in phylogenetic diversity (Figure 2B). In addition, northern China (i.e., Tianjin, Shandong, and Beijing) had relatively higher richness-controlled phylogenetic diversity (Figure 2C). Most provinces (19 of 31) had positive but not statistically significant SES.MPD values, indicating an overdispersed phylogenetic structure of bird communities for species with new records (Figure 2D).
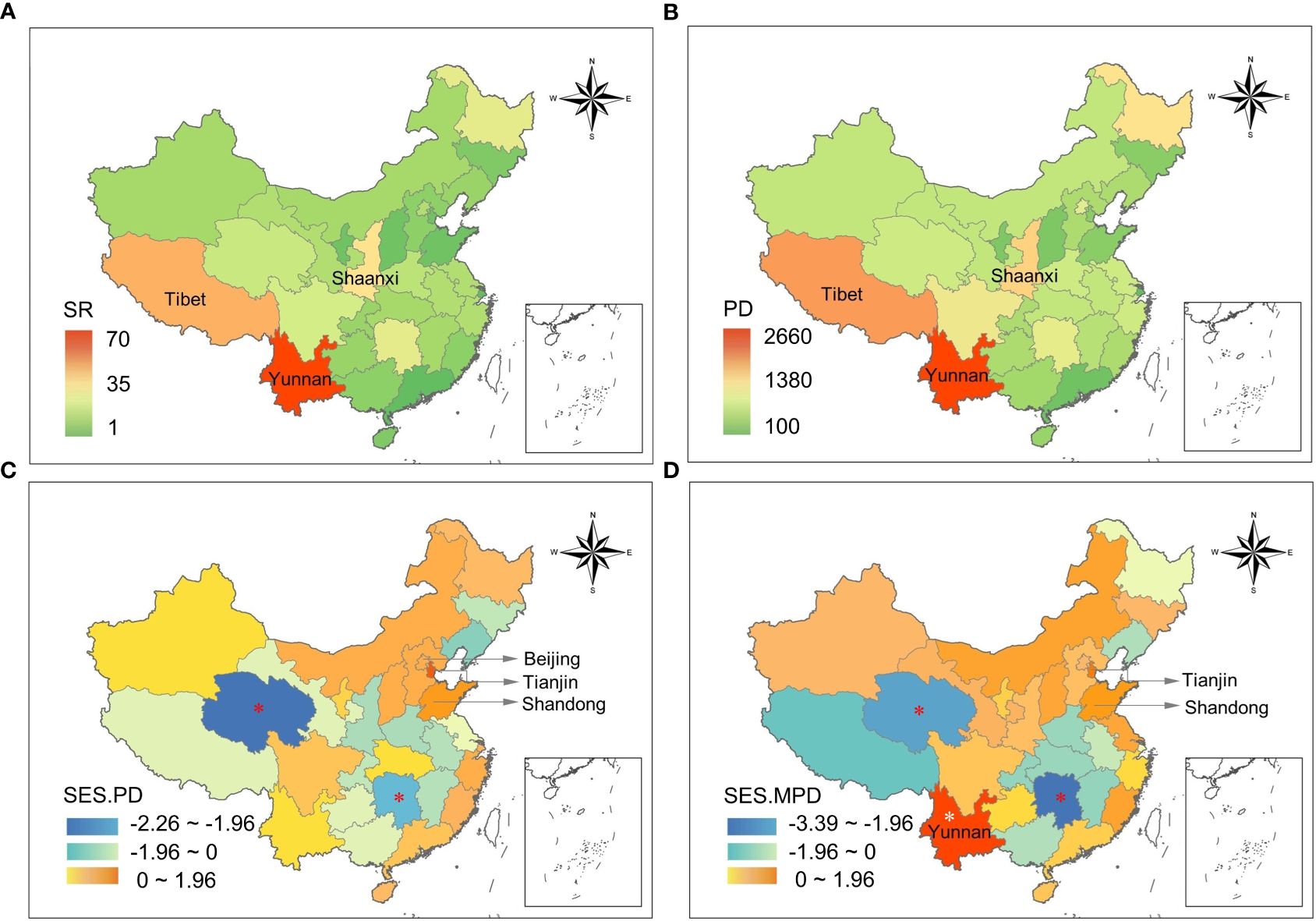
Figure 2 (A) Species richness (SR), (B) phylogenetic diversity (PD), (C) standardized effect size of phylogenetic diversity (SES.PD) and (D) standardized effect size of mean pairwise phylogenetic distance (SES.MPD) for new bird records in each province of China. Province names were added to the three ones with highest values of species richness, PD, SES.PD and SES.MPD, respectively. The asterisk (*) indicates the provinces where SES.PD (C) or SES.MPD (D) are less than -1.96 or greater than +1.96, indicating significant phylogenetic clustering or overdispersion.
The main shift directions indicated by new bird records were northward, with nearly 50% of birds moving NE (19.59%), NW (18.76%) and N (10.52%). (Figure 3). For threatened birds, their main shift directions were still northward (totaling 43.48%, including North 8.70%, Northeast 13.04%, Northwest 21.74%, Supplementary Figure 2). Passeriformes species shifted mainly to the northeast (21.19%), southeast (16.95%), and east (15.25%), whereas non-Passeriformes species shifted mainly to the northwest (23.69%), northeast (18.07%), and west (18.07%). Charadriiformes species shifted mainly to the northwest (27.54%), west (26.09%), and northeast (14.49%).
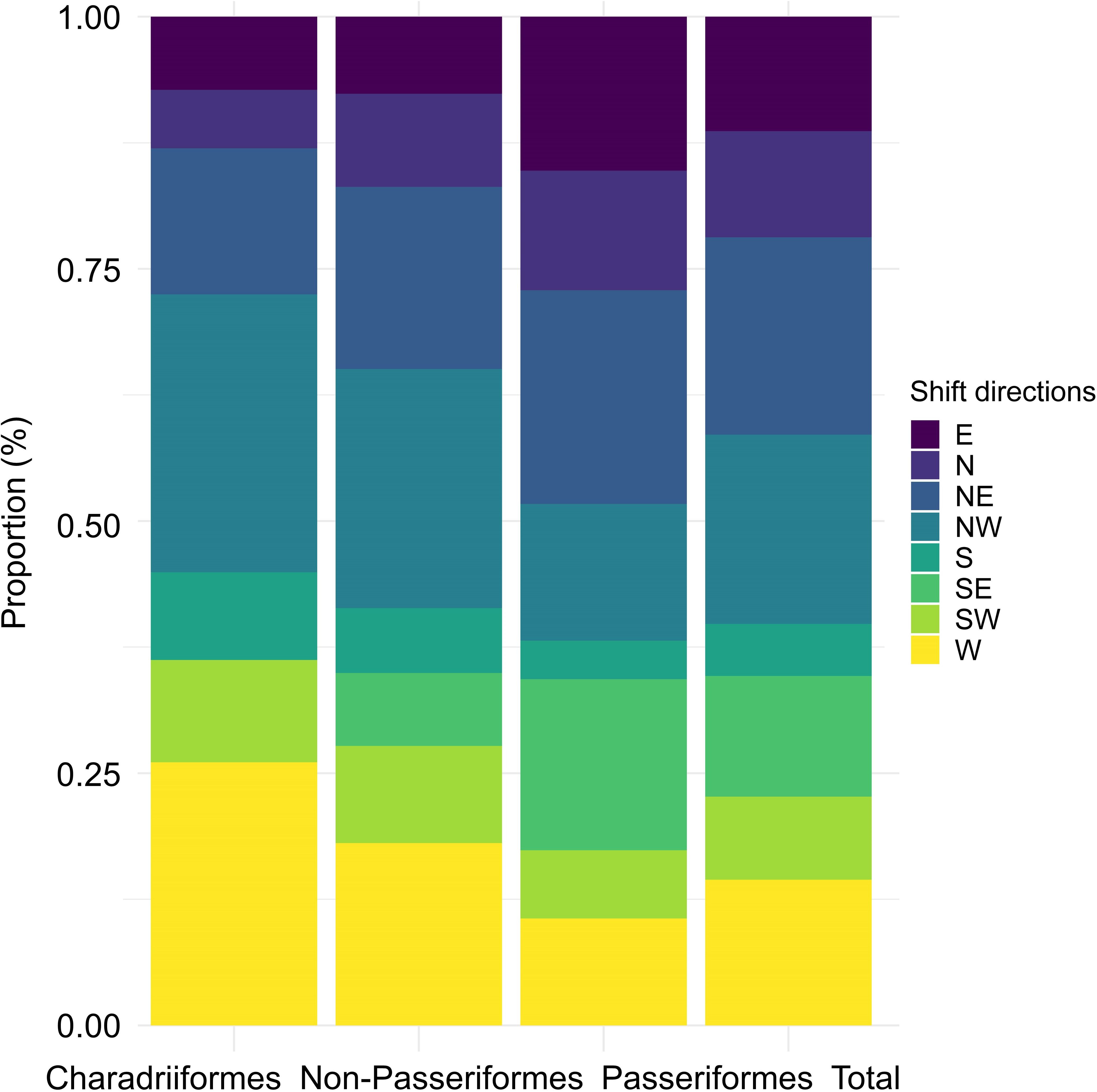
Figure 3 Proportions of Charadriiformes, Non-Passeriformes, Passeriformes and total species in eight shift directions among provincial-level new records in China.
Newly recorded birds were mainly observed in forests (39.59%) and wetlands (24.74%) (Supplementary Figure 3). At the trophic level, they were found to be mostly carnivorous (69.07%). Upon examining their trophic niche, invertivorous (37.32%) and aquatic predators (30.10%) were the two most common groups. In terms of the primary lifestyle, the insessorial (40.00%) and terrestrial (36.49%) groups were the two most prevalent (Supplementary Figure 3).
Correlates of range expansion direction in newly recorded birds
The random forest analysis identified migration, hand–wing index (HWI), body mass, and range size as the top four significant ecological traits influencing range shift direction (i.e., measured range shift radians) (Figure 4). Further examination of their relationships indicated that body mass exerts a negative effect on radians, whereas HWI and range size have positive effects on radians. In examining the correlation between migratory behavior and radians, sedentary species show a relatively higher frequency of northward shift (105 occurrences), followed by migratory species with 78 occurrences (Supplementary Figure 4).
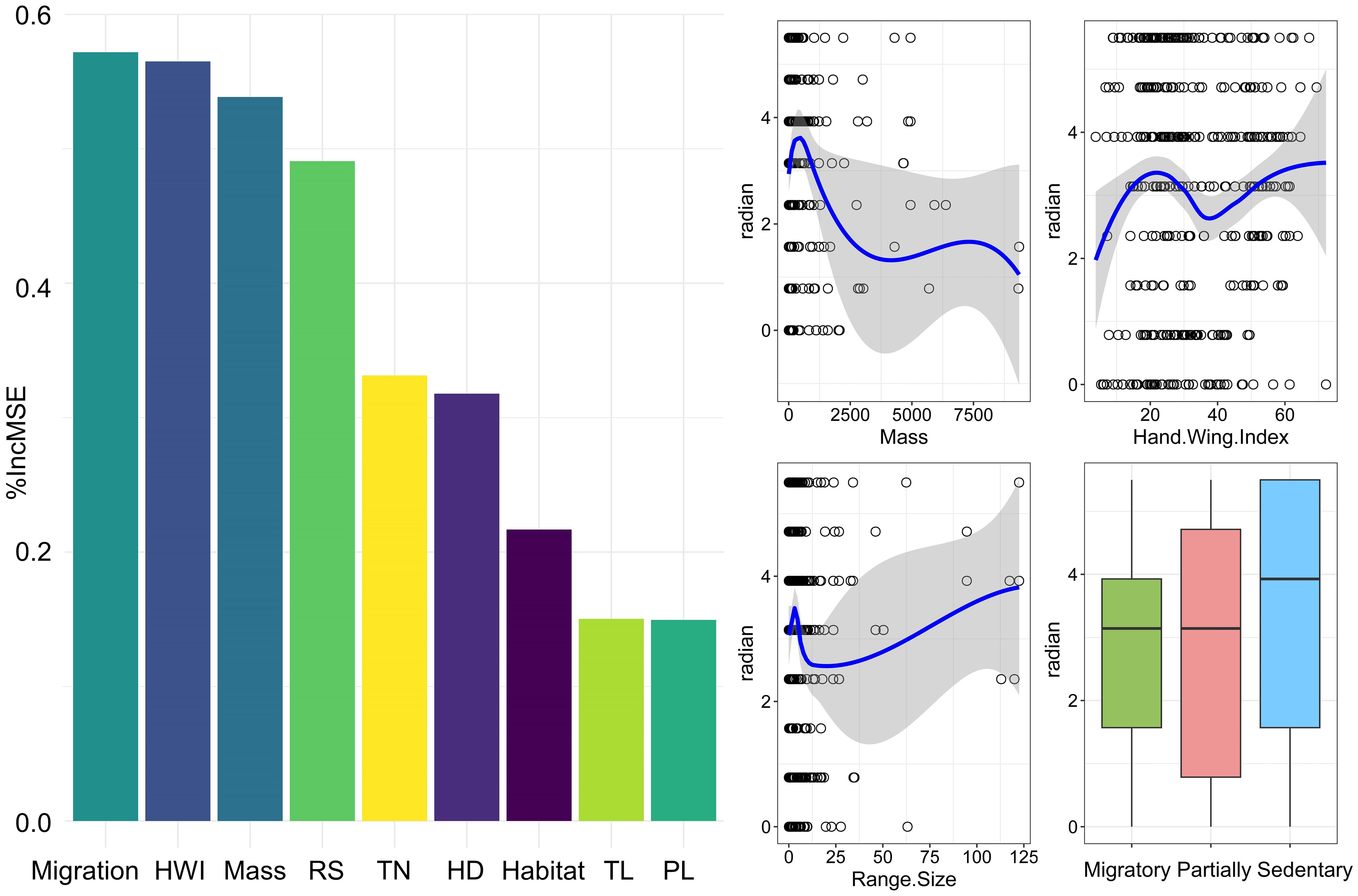
Figure 4 Ecological trait importance assessed by %IncMSE in random forest model (left panel), and the relationships between the top 4 most important traits and the radian (right panel). HWI, hand-wing index; Mass, body mass; RS, range size; TN, trophic niche; HD, habitat density; TL, trophic level; PL, primary lifestyle.
Discussion
In this study, we examined new bird species records in China during 2000–2019 and analyzed their shift directions. The new bird records showed heterogeneous distribution; however, shift directions were mainly northward (48.87% of all records). Migration, HWI, body mass, and range size are the critical factors influencing the shift directions of newly recorded birds, reflecting trait-dependent colonization of new suitable habitats.
Spatial distribution of new bird records
Our findings showed that the new bird records were predominantly northward, which is consistent with the established pattern of range shifts, a widely observed phenomenon (e.g., Howard et al., 2023; Marjakangas et al., 2023; Martins et al., 2024). However, we also identified shifts toward the west, southeast (SE), and east, which contributed to 37.73% of the total shifts. It is acknowledged that the northward shift is commonly related to climate change and the species’ poleward ranges response to rising temperatures. Nonetheless, it should be noted that the distribution of shifts is influenced by diverse factors, including ecological traits (McCaslin and Heath, 2020), habitat types (Bosco et al., 2022), ecological barriers (Marjakangas et al., 2023), and others (Thompson et al., 2023). In addition, large proportion of the newly recorded birds are forest species, which may be a consequence of the expansion of forested areas from 1980 to 2019 in China (Yu et al., 2022). Furthermore, the majority of these newly recorded birds are carnivorous (69.07%), which is consistent with the large proportion of this trophic level among China’s bird species (more than 60% out of 1,505 species; Zheng, 2023).
Previous analyses showed that there are two potential hotspots of new bird records in China, namely four western provinces (southwest provinces including Xizang, Yunnan, and Guangxi, as well as the northwest province of Xinjiang), and four coastal provinces (i.e., Hebei, Tianjin, Fujian and Taiwan) (Liu et al., 2013). Accordingly, we found that western provinces have relatively higher newly recorded bird species compared to the rest of the country. This is probably because these provinces have high bird species diversity, and border other countries such that birds may easily extend their ranges into China. In addition, the rapid development of birdwatching in recent years has offered greater opportunities for new bird records (Ma et al., 2013; Walther and White, 2018), particularly in these regions.
Regarding phylogenetic dimension of diversity, we did not find that the closely related species are more likely to inhabit new areas; i.e., we observed less phylogenetic clustering than expected. Indeed, there is mixed evidence that range shifts tend to occur more frequently in closely related species (e.g., Chunco, 2014; Krosby et al., 2015). For example, Krosby et al. (2015) argued that there is a lower probability of range overlap among closely related species, and that such overlap would be more common in the tropics. In addition, we found pervasive overdispersed phylogenetic structure across provinces but only one southwestern province (i.e., Yunnan province) exhibited significant overdispersion. This may be related to various factors, with elevation being one possible factor that could play a role in explaining the patterns of phylogenetic overdispersion/clustering. For example, previous studies have shown that elevation indeed drives changes in phylogenetic structure, shifting from being overdispersed at lower elevations toward being clustered at higher elevations (Xu et al., 2017; Hanz et al., 2019), and high elevation heterogeneity could be another important factor inducing phylogenetic overdispersion. By contrast, Qinghai and Hunan Provinces exhibited significant clustering, likely due to the homogeneity of their habitats. Specifically, Qinghai Province is dominated by alpine grasslands and desert areas (Zhou, 2006). In Hunan Province, the newly recorded species were concentrated in the Hupingshan National Nature Reserve, which covers a relatively small geographic area and therefore contains a relatively small number of habitat types.
Correlates of range expansion direction
Range shifts in birds can differ according to migratory status (Rushing et al., 2020). Indeed, northward range shifts have primarily occurred for species wintering in temperate areas and they may be due to increasing winter temperatures that allow species to survive at higher latitudes. Our results indicate that sedentary birds tend to shift more northward (Supplementary Figure 4), which is generally consistent with the findings that resident birds expand along their northern margin while the ranges of migratory species have contracted at their southern margin (Rushing et al., 2020). This is probably because the factors that determine range limits vary with migratory status (Hovick et al., 2016), as temperature affect more directly resident birds during winter season, thus making them more prompt to shift their range, while migratory species show high site-fidelity during the breeding season (Hu et al., 2020; Rushing et al., 2020; Lehikoinen et al., 2021; Liang et al., 2021). However, due to the limited research conducted on the relationship between migratory status and shift direction, more comprehensive studies are needed to yield more definitive findings.
Body mass had a negative effect on the shift directions, indicating that larger birds tend to expand to the east, southeast, south, and southwest (see Supplementary Figure 5 for radian values and their corresponding directions). Given that body mass is associated with a range of factors including resource availability and species richness (Olson et al., 2009), whether the observed associations between body mass and shift directions are due to the direct influence of body mass or the indirect impact of factors associated with body mass on shift directions requires further investigation. In addition, both hand-wing index and range size had positive effects on the directions of distribution shifts. That is, birds with stronger flight capabilities and those with larger range sizes are more inclined to move northward. Given that the power of generalization of species’ traits is weak (Thompson et al., 2023), it is difficult to find general patterns in the relationship between ecological traits and shift directions, as range shifts are influenced by multiple factors or their interactions, such as species traits, environmental preferences and ecological barriers (e.g., Bosco et al., 2022; Marjakangas et al., 2023; Thompson et al., 2023), rather than by a single factor.
Caveats and limitations
Although our research has identified some general trends or factors that influence bird movements, we should also recognize that the presence of vagrant birds in new records introduces unpredictability, which can interfere with drawing general conclusions. However, understanding the traits of these birds can still provide valuable insights into broader ecological and environmental patterns, which can contribute to our understanding of species’ responses to changing environments, particularly relevant in the context of climate change and habitat fragmentation. Future research should aim to eliminate the impact of vagrants through continuous monitoring, which can increase the accuracy of the results. In addition, the rapid development of birdwatching in recent years has increased the opportunities for recording new bird species (Ma et al., 2013; Walther and White, 2018), particularly in the four western and four coastal provinces that are hotspots for biodiversity. This increased activity might obscure the actual factors contributing to these new discoveries. Finally, publication bias could also affect our results, as some species potentially receive more attention than others, and this disparity is likely to have impacts on the observed results. Future research should strive for comprehensive data resources to achieve more accurate results.
Conclusion
In conclusion, our study offers a comprehensive perspective on the distribution patterns of newly recorded bird species across China, revealing that western regions harbor greater species richness and phylogenetic diversity (as indicated by PD), while northern regions display higher richness-controlled phylogenetic diversity (as indicated by SES.PD). We observed a widespread pattern of phylogenetic overdispersion and a notable trend of northward range shifts among newly recorded bird species. These shifts are significantly influenced by key ecological traits such as migration status, hand-wing index (HWI), body mass, and range size, with sedentary species showing a particular propensity for northward movement. Our results underscore the complex interplay between species traits and shift directions, emphasizing the necessity for an integrative approach that considers ecological traits when modeling and predicting species range shifts in response to environmental changes.
Further, 32 of the newly recorded species are classified as Threatened. The range shifts of these endangered birds have, to some extent, formed a form of ex-situ conservation, which is an important strategy for preserving biodiversity. By monitoring and managing the habitats of these species, we can better understand the factors driving their range shifts and implement effective conservation measures to ensure their survival in the face of environmental changes.
Data availability statement
The original contributions presented in the study are included in the article/Supplementary Material. Further inquiries can be directed to the corresponding author.
Author contributions
ZFD: Conceptualization, Data curation, Formal analysis, Investigation, Methodology, Resources, Software, Validation, Visualization, Writing – original draft, Writing – review & editing, Funding acquisition, Supervision. XX: Conceptualization, Data curation, Formal analysis, Funding acquisition, Investigation, Methodology, Project administration, Resources, Supervision, Validation, Visualization, Writing – original draft, Writing – review & editing. XW: Writing – review & editing. XL: Writing – review & editing. FL: Data curation, Writing – review & editing. ZWD: Writing – review & editing. YL: Writing – review & editing. QL: Writing – review & editing.
Funding
The author(s) declare financial support was received for the research, authorship, and/or publication of this article. This work was supported by the Fundamental Research Funds for the Central Universities (grant numbers 2572022BE02), National Natural Science Foundation of China (grant numbers 32170485, 31501867), Guangdong Basic and Applied Basic Research Foundation (grant numbers 2024A1515011411) and Forestry Administration of Guangdong Province (grant numbers DFGP Project of Fauna of Guangdong-202115).
Acknowledgments
We would like to thank Xiaobin Qin and Wenshuang Bao for support and help with advising and revising the manuscript. We also thank two reviewers for constructive feedback that allowed us to improve the paper.
Conflict of interest
The authors declare that the research was conducted in the absence of any commercial or financial relationships that could be construed as a potential conflict of interest.
Publisher’s note
All claims expressed in this article are solely those of the authors and do not necessarily represent those of their affiliated organizations, or those of the publisher, the editors and the reviewers. Any product that may be evaluated in this article, or claim that may be made by its manufacturer, is not guaranteed or endorsed by the publisher.
Supplementary material
The Supplementary Material for this article can be found online at: https://www.frontiersin.org/articles/10.3389/fevo.2024.1415268/full#supplementary-material
References
Angert A. L., Crozier L. G., Rissler L. J., Gilman S. E., Tewksbury J. J., Chunco A. J. (2011). Do species’ traits predict recent shifts at expanding range edges? Ecol. Lett. 14, 677–689. doi: 10.1111/j.1461-0248.2011.01620.x
Antão L. H., Weigel B., Strona G., Hällfors M., Kaarlejärvi E., Dallas T., et al. (2022). Climate change reshuffles northern species within their niches. Nat. Clim Change 12, 587–592. doi: 10.1038/s41558-022-01381-x
Auer S. K., King D. I. (2014). Ecological and life-history traits explain recent boundary shifts in elevation and latitude of western North American songbirds. Global Ecol. Biogeogr 23, 867–875. doi: 10.1111/geb.12174
Bateman B. L., Pidgeon A. M., Radeloff V. C., VanDerWal J., Thogmartin W. E., Vavrus S. J., et al. (2016). The pace of past climate change vs. potential bird distributions and land use in the United States. Glob Change Biol. 22, 1130–1144. doi: 10.1111/gcb.13154
Bosco L., Xu Y., Deshpande P., Lehikoinen A. (2022). Range shifts of overwintering birds depend on habitat type, snow conditions and habitat specialization. Oecologia 199, 725–736. doi: 10.1007/s00442-022-05209-5
Chen I. C., Hill J. K., Ohlemüller R., Roy D. B., Thomas C. D. (2011). Rapid range shifts of species associated with high levels of climate warming. Science 333, 1024–1026. doi: 10.1126/science.1206432
Chen W., Qian R. E., Hu C. C., Liu W., Zhang C. L., Xu H. G. (2021). New bird records in China from 2000 to 2018. J. Ecol. Rural. Environ. 37, 904–908. doi: 10.19741/j.issn.1673-4831.2020.0535
Chunco A. J. (2014). Hybridization in a warmer world. Ecol. Evol. 4, 2019–2031. doi: 10.1002/ece3.1052
Collar N., Robson C., Sharpe C. J. (2021). “Blue-crowned Laughingthrush (Pterorhinus courtoisi), version 1.1,” in Birds of the world. Eds. del Hoyo J., Elliott A., Sargatal J., Christie D. A., de Juana E. (Cornell Lab of Ornithology, Ithaca, NY, USA). doi: 10.2173/bow.buclau1.01.1
Drummond A. J., Rambaut A. (2007). BEAST: Bayesian evolutionary analysis by sampling trees. BMC Evol. Biol. 7, 1–8. doi: 10.1186/1471-2148-7-214
Du Y., Zhou F., Shu X. L., Li Y. L. (2009). The impact of global warming on China avifauna. Acta Zootaxonomica Sin. 34, 664–674. doi: 10.3969/j.issn.1000-0739.2009.03.049
Estrada A., Morales-Castilla I., Caplat P., Early R. (2016). Usefulness of species traits in predicting range shifts. Trends Ecol. Evol. 31, 190–203. doi: 10.1016/j.tree.2015.12.014
Faith D. P. (1992). Conservation evaluation and phylogenetic diversity. Biol. Conserv. 61, 1–10. doi: 10.1016/0006-3207(92)91201-3
Gill F., Donsker D., Rasmussen P. (2021). IOC world bird list (v 11.2). Available online at: http://www.worldbirdnames.org/.
Hallman T. A., Guélat J., Antoniazza S., Kéry M., Sattler T. (2022). Rapid elevational shifts of Switzerland's avifauna and associated species traits. Ecosphere 13, e4194. doi: 10.1002/ecs2.4194
Hanz D. M., Böhning-Gaese K., Ferger S. W., Fritz S. A., Neuschulz E. L., Quitián M., et al. (2019). Functional and phylogenetic diversity of bird assemblages are filtered by different biotic factors on tropical mountains. J. Biogeogr 46, 291–303. doi: 10.1111/jbi.13489
Hovick T. J., Allred B. W., McGranahan D. A., Palmer M. W., Elmore R. D., Fuhlendorf S. D. (2016). Informing conservation by identifying range shift patterns across breeding habitats and migration strategies. Biodivers Conserv. 25, 345–356. doi: 10.1007/s10531-016-1053-6
Howard C., Marjakangas E. L., Morán-Ordóñez A., Milanesi P., Abuladze A., Aghababyan K., et al. (2023). Local colonisations and extinctions of European birds are poorly explained by changes in climate suitability. Nat. Commun. 14, 4304. doi: 10.1038/s41467-023-39093-1
Hu R., Gu Y. Y., Luo M., Lu Z., Wei M., Zhong J. (2020). Shifts in bird ranges and conservation priorities in China under climate change. PloS One 15, e0240225. doi: 10.1371/journal.pone.0240225
Jarzyna M. A., Quintero I., Jetz W. (2021). Global functional and phylogenetic structure of avian assemblages across elevation and latitude. Ecol. Lett. 24, 196–207. doi: 10.1111/ele.13631
Jetz W., Thomas G. H., Joy J. B., Hartmann K., Mooers A. O. (2012). The global diversity of birds in space and time. Nature 491, 444–448. doi: 10.1038/nature11631
Krosby M., Wilsey C. B., McGuire J. L., Duggan J. M., Nogeire T. M., Heinrichs J. A., et al. (2015). Climate-induced range overlap among closely related species. Nat. Clim Change 5, 883–886. doi: 10.1038/nclimate2699
Laughlin A. J., Pomara L. Y. (2023). Winter range shifts and their associations with species traits are heterogeneous in eastern North American birds. Ornithology 140, ukad027. doi: 10.1093/ornithology/ukad027
Lehikoinen A., Lindström Å., Santangeli A., Sirkiä P. M., Brotons L., Devictor V., et al. (2021). Wintering bird communities are tracking climate change faster than breeding communities. J. Anim. Ecol. 90, 1085–1095. doi: 10.1111/1365-2656.13433
Li X. Y., Liang L., Gong P., Liu Y., Liang F. F. (2013). Bird watching in China reveals bird distribution changes. Chin. Sci. Bull. 58, 649–656. doi: 10.1007/s11434-012-5458-7
Liang J., Peng Y. H., Zhu Z. Q., Li X., Xing W., Li X. D., et al. (2021). Impacts of changing climate on the distribution of migratory birds in China: Habitat change and population centroid shift. Ecol. Indic 127, 107729. doi: 10.1016/j.ecolind.2021.107729
Liaw A., Wiener M. (2002). Classification and regression by randomForest. R news 2, 18–22. doi: 10.1016/j.ecolind.2021.107729
Liu Y., Wei Q., Dong L., Lei J. (2013). On an update of recent new bird records in China. Chin. J. Zool 48, 752–759. doi: 10.13859/j.cjz.2013.05.016
Ma Z., Cheng Y., Wang J., Fu X. (2013). The rapid development of birdwatching in mainland China: a new force for bird study and conservation. Bird Conserv. Int. 23, 259–269. doi: 10.1017/S0959270912000378
MacKinnon J. R., Phillipps K., He F. (2000). A field guide to the birds of China. (Changsha: Hunan Education Publishing House)
MacLean S. A., Beissinger S. R. (2017). Species’ traits as predictors of range shifts under contemporary climate change: A review and meta-analysis. Global Change Biol. 23, 4094–4105. doi: 10.1111/gcb.13736
Marjakangas E. L., Bosco L., Versluijs M., Xu Y., Santangeli A., Holopainen S., et al. (2023). Ecological barriers mediate spatiotemporal shifts of bird communities at a continental scale. P Natl. Acad. Sci. U.S.A. 120, e2213330120. doi: 10.1073/pnas.2213330120
Martins P. M., Anderson M. J., Sweatman W. L., Punnett A. J. (2024). Significant shifts in latitudinal optima of North American birds. P Natl. Acad. Sci. U.S.A. 121, e2307525121. doi: 10.1073/pnas.2307525121
McCaslin H. M., Heath J. A. (2020). Patterns and mechanisms of heterogeneous breeding distribution shifts of North American migratory birds. J. Avian Biol. 51. doi: 10.1111/jav.02237
Neate-Clegg M. H. C., Tingley M. W. (2023). Building a mechanistic understanding of climate-driven elevational shifts in birds. PloS Climate 2, e0000174. doi: 10.1371/journal.pclm.0000174
Niven D. K., Butcher G. S., Bancroft G. T. (2010). Northward shifts in early winter abundance. Am. Birds 63, 10–15.
Olson V. A., Davies R. G., Orme C. D. L., Thomas G. H., Meiri S., Blackburn T. M., et al. (2009). Global biogeography and ecology of body size in birds. Ecol. Lett. 12, 249–259. doi: 10.1111/j.1461-0248.2009.01281.x
Orme D., Freckleton R., Thomas G., Petzoldt T., Fritz S., Isaac N., et al. (2013). The caper package: comparative analysis of phylogenetics and evolution in R 5, 1–36. R package version.
R Core Team. (2021). R: A language and environment for statistical computing 5, 1–36. Foundation for Statistical Computing, Vienna, Austria. Available online at: https://www.R-project.org/.
Reif J., Flousek J. (2012). The role of species’ ecological traits in climatically driven altitudinal range shifts of central European birds. Oikos 121, 1053–1060. doi: 10.1111/j.1600-0706.2011.20008.x
Renjifo L. M., Repizo A., Ruiz-Ovalle J. M., Ocampo S., Avendaño J. E. (2017). New bird distributional data from Cerro Tacarcuna, with implications for conservation in the Darién highlands of Colombia. Bull. Br. Ornithologists’ Club 137, 46–66. doi: 10.25226/bboc.v137i1.2017.a7
Rushing C. S., Royle J. A., Ziolkowski D. J. Jr., Pardieck K. L. (2020). Migratory behavior and winter geography drive differential range shifts of eastern birds in response to recent climate change. P Natl. Acad. Sci. U.S.A. 117, 12897–12903. doi: 10.1073/pnas.2000299117
Tayleur C., Caplat P., Massimino D., Johnston A., Jonzén N., Smith H. G., et al. (2015). Swedish birds are tracking temperature but not rainfall: evidence from a decade of abundance changes. Global Ecol. Biogeogr 24, 859–872. doi: 10.1111/geb.12308
Thompson L., Wells K., Galiana N., Lurgi M. (2023). Joint effects of species traits and environmental preferences on range edge shifts of British birds. Global Ecol. Biogeogr 32, 2085–2099. doi: 10.1111/geb.13752
Tobias J. A., Sheard C., Pigot A. L., Devenish A. J. M., Yang J., Sayol F., et al. (2022). AVONET: morphological, ecological and geographical data for all birds. Ecol. Lett. 25, 581–597. doi: 10.1111/ele.13898
Voskamp A., Hof C., Biber M. F., Böhning-Gaese K., Hickler T., Niamir A., et al. (2022). Projected climate change impacts on the phylogenetic diversity of the world's terrestrial birds: more than species numbers. P Roy Soc. B-Biol Sci. 289, 20212184. doi: 10.1098/rspb.2021.2184
Walther B. A., White A. (2018). The emergence of birdwatching in China: history, demographics, activities, motivations, and environmental concerns of Chinese birdwatchers. Bird Conserv. Int. 28, 337–349. doi: 10.1017/S0959270917000557
Webb C. O., Ackerly D. D., McPeek M. A., Donoghue M. J. (2002). Phylogenies and community ecology. Annu. Rev. Ecol. Evol. S 33, 475–505. doi: 10.1146/annurev.ecolsys.33.010802.150448
Xu J., Chen Y., Zhang L., Chai Y., Wang M., Guo Y., et al. (2017). Using phylogeny and functional traits for assessing community assembly along environmental gradients: A deterministic process driven by elevation. Ecol. Evol. 7, 5056–5069. doi: 10.1002/ece3.3068
Yang X., Wang Y., Si X., Feng G. (2020). Species traits linked with range shifts of Chinese birds. Glob Ecol. Conserv. 21, e00874. doi: 10.1016/j.gecco.2019.e00874
Yu Z., Ciais P., Piao S., Houghton R. A., Lu C., Tian H., et al. (2022). Forest expansion dominates China’s land carbon sink since 1980. Nat. Commun. 13, 5374. doi: 10.1038/s41467-022-32961-2
Zhang M., Tian C. P., Che X. L., Zhao Y. Y., Chen S. W., Zhou X., et al. (2022). New bird records in Guangdong Province and their correlation with natural and social-economic factors. Biodivers Sci. 30, 21396. doi: 10.17520/biods.2021396
Zheng G. (2023). A checklist on the classification and distribution of the birds of China. 4th ed. (Beijing: Science Press).
Keywords: phylogenetic diversity, ecological traits, shift directions, new bird records, China
Citation: Xing X, Wang X, Li X, Lan F, Deng Z, Li Y, Li Q and Ding Z (2024) Where are the provincial-level new records in China from the past 20 years, and which traits determine their shift directions? Front. Ecol. Evol. 12:1415268. doi: 10.3389/fevo.2024.1415268
Received: 10 April 2024; Accepted: 19 July 2024;
Published: 06 August 2024.
Edited by:
Francesco Cerasoli, University of L’Aquila, ItalyReviewed by:
Jérémy Cours, University of Jyväskylä, FinlandChiara Bettega, Museo delle Scienze, Italy
Copyright © 2024 Xing, Wang, Li, Lan, Deng, Li, Li and Ding. This is an open-access article distributed under the terms of the Creative Commons Attribution License (CC BY). The use, distribution or reproduction in other forums is permitted, provided the original author(s) and the copyright owner(s) are credited and that the original publication in this journal is cited, in accordance with accepted academic practice. No use, distribution or reproduction is permitted which does not comply with these terms.
*Correspondence: Zhifeng Ding, ZGluZ3poZkAxNjMuY29t