- 1British Antarctic Survey, Biodiversity Evolution and Adaptation, Natural Environment Research Council, Cambridge, United Kingdom
- 2Scottish Association for Marine Science, Oban, United Kingdom
- 3Royal Botanic Garden Edinburgh, Edinburgh, United Kingdom
- 4Swedish Museum of Natural History, Stockholm, Sweden
- 5University of Johannesburg, Johannesburg, South Africa
- 6Millennium Institute Biodiversity of Antarctic and Sub-Antarctic Ecosystems (BASE), Santiago, Chile
- 7Cape Horn International Center (CHIC), Puerto Williams, Chile
The Southern Ocean benthos is remarkably rich and diverse, and managed under a complexity of treaties and conventions, further complicated by geopolitical boundaries. Traditionally, conservation management is largely informed by species lists augmented, when data are available, by known vulnerability of the taxa. Species presence absence database resources are valuable tools with proven and positive management outcomes, however, in a vast, difficult to access and thus understudied region such as the Southern Ocean, there are large gaps in knowledge regarding the ecology, ecophysiology, life history and even species identity. Conservation biogeography identifies regions of conservation concern, rather than a species-by-species approach, but also relies on the availability of high-quality presence data from species lists and thus both approaches are undermined when species lists are inaccurate or species in general are poorly described. In addition, the data provide a snapshot of the current species diversity and have inadequate power to identify the processes underlying the patterns uncovered. Identifying historical processes common to shaping diversity (species or genetic) can be generalized across assemblages and regions, providing a more robust basis for conservation policy and decisions. In this study, largely based on consideration of Southern Ocean ophiuroids, we discuss the challenges inherent in using species lists, the power and limitations of genetic analyses, and revisit previous suggestions of building a spatial model of diversity that includes underlying evolutionary relationships transcending the simple species diversity approach, and that is applicable to assemblages, rather than just to individual taxa.
1 Introduction
The Southern Ocean region and its rich natural resources are governed and managed by various international treaties and conventions further complicated by geopolitical boundaries. These agreements generally adopt a precautionary approach to management, conservation and exploitation, with areas south of 60 degrees of latitude falling under the Antarctic Treaty governance. However, areas under individual national governments are managed according to their specific interests and specific policy pressures. Where population sizes of species in assemblages are abundant, widespread and well connected, poor management or strong exploitation from small regional territories is unlikely to negatively impact the wider assemblage elements. However, small, regionally localized and isolated species or populations are vulnerable to pressures at small regional scales, whether they be anthropogenic or stochastic. Given that many of the nation states with territories or interests in the Southern Ocean are signatories of the Convention on Biological Diversity that explicitly defines biodiversity as including within and between species variation (Article 2), it is essential that some understanding of the biological systems is generated in order to best approach conservation management if required.
The benthic faunal assemblage of the Southern Ocean is traditionally viewed as “Antarctic”, with some influence from the South American shelf fauna around the sub-Antarctic islands (Hedgpeth, 1969; Griffiths et al., 2009). Under this paradigm, there is little need for concern regarding the small, regionally managed locations in the Southern Ocean as any regional assemblages in decline at this scale can be continually seeded from the vast and well-connected populations surrounding them. However, the Southern Ocean benthos is very difficult to study, and as a result poorly understood in regard to any aspect of its ecology, ecophysiology, even species identity. The past 20 years have seen a change in the way biodiversity is assessed and a wealth of genetic data is challenging the widespread assumption of a general “Antarctic” fauna (Linse et al., 2007; Leese and Held, 2008; Havermans et al., 2011; Dietz et al., 2015; Dömel et al., 2017; Guzzi et al., 2022). Rather than well-connected assemblages with large population sizes, genetic data – largely mitochondrial sequences – imply that biogeographically structured populations may be the norm, potentially with many cryptic or currently unrecognized species, or at least isolated populations following independent evolutionary trajectories.
The resources available for assessing diversity patterns used in first pass conservation assessment across the vast and relatively unknown Southern Ocean largely consist of species lists such as the Global Biodiversity Information Facility (GBIF, 2024) and the International Union for Conservation of Nature’s (IUCN) Red List (IUCN Red List, 2024), the latter augmented by information regarding each species’ vulnerability status (Hilton-Taylor and Brackett, 2000; Rodrigues et al., 2006; Vié et al., 2009). These are demonstrably valuable resources (Heberling et al., 2021) and freely available online. Their full value relies heavily on the extent of data being collected (Huettmann, 2020) and its quality (Abe, 2005; Zizka et al., 2020; Rocha-Ortega et al., 2021). Unreliable taxonomic identification is certainly a significant issue, but a more pressing and perhaps damaging challenge is given by formal taxonomic descriptions that are inadequate for subsequent confident identification or that inadvertently underestimate species diversity. These taxonomic limitations will overestimate species distributions, population sizes and connectivity, the three key elements that inform assessment of a species’ vulnerability status. Genetic techniques can ameliorate these limitations, but associating genetic diversity with species diversity is difficult and controversial (Blaxter, 2004; DeSalle et al., 2005; Ebach and Holdrege, 2005; Cook et al., 2010). Complicating this further is the ongoing debate regarding what a species is and its relevance in biology (Aldhebiani, 2018; Stanton et al., 2019). With species lists requiring accurate species descriptions, many available species descriptions are currently inadequate in relation to the distribution of genetic diversity, and genetic diversity in turn not reflected in species descriptions required for species lists, a problem exists that is set to confound efforts to assess diversity patterns essential for raising conservation awareness.
Here we share a perspective for an alternative strategy to reliance on presence/absence species lists, based on a proven technique that uses information relating to the processes that underly geographic patterns observed in genetic sequence data (Taberlet and Bouvet, 1994; Moritz and Faith, 1998; Emerson et al., 2001). Shared historical processes can be inferred from genetic data of selected target species across a landscape. Such processes are likely to have been experienced by all species in any given assemblage. Recognition of this provides this provides a model that can help inform managers of the geographic extent of diversity, the size of local populations and the connectivity between populations, allowing inference of the potential for resilience in any given species or population. The benefit of such an approach is that it circumvents the need for accurate “traditional” species identifications. At the same time, it helps define potential species boundaries, highlights patterns of variation along the species continuum, and provides both geographic, ecological and evolutionary information regarding population (and by extension, assemblage) resilience.
As conservation management and policy are currently tightly bound to formally described species, traditional taxonomy is time intensive and relies on a rapidly dwindling skill pool (Wägele et al., 2011; Saucède et al., 2020), the number of putative new species “discovered” in molecular studies vastly exceeds the rate at which new species descriptions are possible. This creates a profound challenge that undermines the key importance of the IUCN Red List and the multitude of conventions it informs. In essence the bulk of the diversity on Earth is likely to be a large underestimate, and while the focus of conservation efforts is directed on a species-by-species basis, this is at a cost to regional assemblages holding unappreciated, unique diversity.
2 The species challenge: do species matter?
The term “species” is used frequently in biology and by non-biologists referring to biological units. Very few would argue with this and yet an acceptable definition, or concept, of a “species” remains elusive leading to a long running debate that has been described as “…sometimes boring and seemingly fruitless, but is not wholly futile” (Simpson, 1951). The debate continues with the concept of a species recognized as fundamental to biology, but controversial amongst biologists (Wheeler and Meier, 2000). There are currently at least 28 competing species concepts (Wilkins, 2018), each providing arguments justifying the specific groupings as recognized by the responsible authors. The challenge, with so many views on what a species may be, and the variety of concepts created, is that they are often incompatible in their outcomes, with individuals or populations being included in or excluded from a given species depending on which definition is adopted. Underlying this debate is the fact that the process of speciation is a continuum over time, that the time taken to “speciate” differs among species, the species or population under examination may be anywhere along the speciation continuum and the species concept criterion applied may also vary along that continuum. For example, where ecological or sexual selection is strong, such as with cichlid fish (Barluenga and Meyer, 2004), speciation as determined by discrete morphology or ecology may be readily apparent, fitting the ecological and morphological species concept, and yet the same groupings may be impossible to detect using genetic sequence data, thereby not satisfying the phylogenetic, Hennigian or cladistic species concept.
To enable meaningful comparison of species across space and time, species concepts matter (Cracraft, 2000), especially within a management context. The term “species” is critical for conservation biology, as it has been almost universally adopted as the base level of diversity. Of particular note in this context is the IUCN Red List, widely adopted by international conventions and treaties such as the Convention on International Trade in Endangered Species (CITES), Convention on Migratory Species (CMS), Convention on Biological Diversity (CBD), Ramsar Convention and Intergovernmental Science-policy Platform on Biodiversity and Ecosystem Services (IPBES). The Red List influences resource allocation (e.g. Global Environment Facility) and informs conservation planning (e.g. Key Biodiversity Areas; IUCN Red List, 2022). The central criterion required before initiating a Red List assessment is that the unit being assessed is “at or below the species level” (IUCN Red List Categories and Criteria V3.1 second edition, Preamble, paragraph 1), confirming that the base level of diversity is the species, with the recognition that further subdivision is possible.
On this basis, “species” do matter, specifically formally described species. This leads to the question: to what extent does the accurate parameterization of a species affect conservation biology, particularly in the light of confusing concepts and definitions? Answering this question is long and complex and depends on perspective and context. In the context of biodiversity management across a large realm managed by multiple states, treaties and conventions, such as the Southern Ocean, we suggest that any consequences of overestimating diversity based on “species” assemblages would be negligible, whereas underestimating diversity could be catastrophic.
3 Does DNA hold the solution?
The advent of DNA sequencing and phylogenetic reconstruction has resulted in an understanding of the systematic structure of the tree of life (Li et al., 2021) far exceeding the expectations of the evolutionary biologists of previous generations (Dobzhansky, 1935). In some groups, the use of multiple genetic markers and sophisticated analyses has resulted in very high resolution of relationships. Here, we focus as an exemplar on the work being carried out on class Ophiuroidea, known as brittle stars (O’Hara et al., 2017), where phylogenetic relationships have been reconciled with previously unappreciated informative morphological characters (Thuy and Stöhr, 2016) to formally restructure the taxonomy of the class (O’Hara et al., 2018). However, taking genetic inference at face value, without independent supporting evidence, can lead to the proposal of false relationships and incorrect interpretations.
The start of the twenty-first century saw a movement towards DNA based taxonomy, species delimitation and identification (Tautz et al., 2002; Wiens and Penkrot, 2002; Tautz et al., 2003; Blaxter, 2004; Hebert and Gregory, 2005). With the investment of substantial funding, initiatives such as the International Barcode of Life (International Barcode of Life – Illuminate Biodiversity, 2024) have resulted in many thousands of studies contributing data to resources such as the Barcode of Life Database (Ratnasingham and Hebert, 2007), which currently displays a total of 255,000 animal species with sequence data, relating to 1,046,000 “BINS” or putative DNA barcode species from 15,910,000 DNA barcodes (http://www.boldsystems.org accessed 28th February 2024). Whether this is an appropriate strategy for determining species or not is debatable, but the publicly available resource of a DNA sequence alongside a georeferenced specimen that has been unambiguously identified is precious, widely comparable and growing. There has been considerable resistance to the concept of DNA barcoding and its use in taxonomy (Mallet and Willmott, 2003; DeSalle et al., 2005; Ebach and Holdrege, 2005; Will et al., 2005). A synopsis of this debate is that DNA barcoding as a step in taxonomic discovery is useful but with exceptions. Incomplete lineage sorting and introgression can confuse genetic signals. Furthermore, population theory suggests that large, stable populations will hold large amounts of diversity over long periods of time (Pfaffelhuber et al., 2011; Nordborg, 2019), which could be confused with deeply divergent clades on a phylogeny. For a large number of well-characterized species, mitochondrial DNA sequences do indeed accurately define individual species groups (Hogg and Hebert, 2004; Rock et al., 2008; Ward et al., 2008; Jossart et al., 2021), but results and interpretations should be viewed with caution (Havermans et al., 2010, 2011).
4 Repeated patterns in genes and geography
Given the difficulties surrounding defining a species, the challenges of describing species and general uncertainty in assigning species using molecular techniques, a change in perspective is required. Patterns shared across taxa indicate shared historical events, in which case they probably represent the structure of assemblages (Moritz and Faith, 1998). The subdiscipline that specifically addresses the spatial distribution of genetic diversity is known as phylogeography (Avise et al., 1987; Avise, 2000). When analyses of co-distributed species are involved it is termed comparative phylogeography (Bermingham and Moritz, 1998). Linking gene trees, or evolutionary relationships, to geography provides historical information relevant to the formation of discrete spatial distributions (Taberlet et al., 1998; Hewitt, 2004). The information generated from such analyses transcends that of species diversity or richness (Taberlet et al., 2012) as it spans the divergence continuum from individuals to species and beyond.
Benthic species diversity is high in the Southern Ocean (Arntz et al., 1994; Brey et al., 1994; Clarke and Johnston, 2003) with representatives of the class Ophiuroidea (Figure 1) often the most abundant in terms of numbers and biomass (Martín-Ledo and López-González, 2013; Sands et al., 2013). Several studies have identified cryptic diversity in Southern Ocean ophiuroids (Hunter and Halanych, 2008; Martín-Ledo et al., 2013; Sands et al., 2015; Galaska et al., 2017a, b; Jossart et al., 2019; Sands et al., 2021). For example, the sub-Antarctic species Ophiuroglypha lymani Ljungman, 1871 forms several island-specific clades, including one in which its sister species, the Antarctic shelf endemic Ophiuroglypha carinifera Koehler, 1901 is subsumed (Sands et al., 2015). Similarly, Astrotoma agassizii Lyman, 1875 has several discrete clades across the Southern Ocean, one which includes its sister species Astrotoma drachii Guille, 1979 (Jossart et al., 2019).
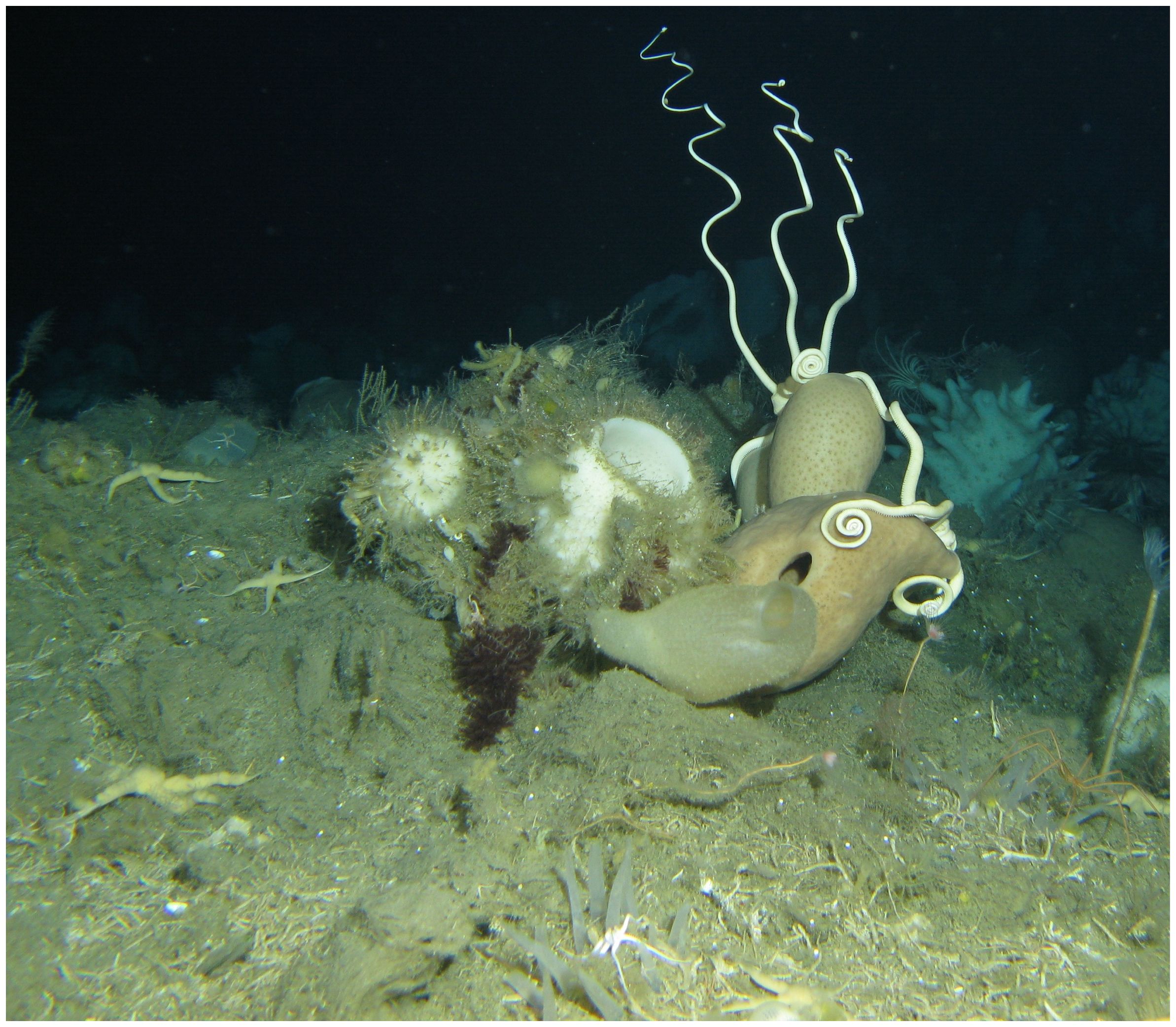
Figure 1 Image of the sea floor from the Eastern Weddell Sea at a depth of ~300m. The large brittle stars attached to hexactinellid sponges are Astrotoma agassizii, the brittle stars covered in Iophon sponge symbiont are Ophioplinthus gelida, and the white brittle star in the foreground is Ophiostiera antarctica. The photograph was taken using an ROV by Tomas Lundäv and used with permission.
There is strong evidence that both Astrotoma agassizii and Ophiuroglypha lymani represent species complexes. In these two examples, this results in increased overall diversity while individual population sizes have decreased. Both species groups appear to have congruent phylogeographic structure – in that it appears that clades are specific to island shelf regions and the Antarctic shelf region (Sands et al., 2015; Jossart et al., 2019). Most interesting perhaps is the sister-species/outgroup problem as, in both cases, the sister-species appears to be an element of the species complex rather than an outgroup, and this pattern has been found again with respect to Amphiura belgicae Koehler, 1900 and A. eugeniae Ljungman, 1867, its likely sister-species. (Sands et al. This Volume). This again suggests shared demographic histories and provides a starting point for comparative analyses.
5 A generalized model of genetic variation across the Southern Ocean
The concept that regions of conservation value can be identified based on co-distribution of discrete genetic variation between species is not new (Faith, 1992, 1993). Over the past 30 years the field has matured (Garrick et al., 2015; Edwards et al., 2022; McGaughran et al., 2022), with the cost of laboratory work much reduced and the sophistication and sensitivity of analyses greatly increased. Based on some of the above citations we can generalize that the island shelves around the Southern Ocean, particularly South Georgia, appear to contain discrete genetic types distinct from those around the Antarctic (Linse et al., 2007; Sands et al., 2015; Jossart et al., 2019). This is the basis of a model and more taxa should be added to strengthen it. The effects of the shared historical process may not be recorded in the genetic regions of all members of an assemblage, as life history difference in particular having a strong influence on retained polymorphisms (Avise, 2009; da Silva Ribeiro et al., 2020). For example, decapods with high dispersal capacity show a Southern Ocean wide panmictic distribution (Raupach et al., 2010; Dambach et al., 2016). Most individuals across the Southern Ocean are from species with some developmental stage with high dispersal capacity, but most “species” tend to have low dispersal in general (Poulin et al., 2002). For this reason choosing an appropriate taxon that is likely to have retained ancestral signal in its DNA is important for adequately building and testing a model such as we are suggesting.
Choosing taxa likely to hold historical information is one consideration. Others are the sample size and geographic spread of the study collection (Ruiz-García et al., 2021). Sufficient sample size within each region is required to compare the diversity of genetic types within a region as well as between regions. For example, in Sands et al. (2015) the included samples of Ophiuroglypha lymani from the South Sandwich Islands and Bouvetøya were distinctly divergent from other regions sampled. However, the sample sizes of these two regions were three and two respectively. If sample sizes for all regions were equally small there would be little confidence in their distinctness, and it is the larger sample sizes of the target regions in this study that indicate they are likely to be discrete populations. Broad geographic collections are more likely to hold more power for accurate inference. The studies comparing Astrotoma agassizii from Patagonian shelf and Antarctic shelf (Hunter and Halanych, 2008; Galaska et al., 2017a) identified divergences between populations, but the true extent of the diversity within A. agassizii was not appreciated until samples were collected and analyzed from across the Southern Ocean (Jossart et al., 2019).
Using gene trees for demographic inference using a single marker is prone uncertainty and risks over interpretation (Knowles and Maddison, 2002). The ability to use multiple genetic markers as a method of within-group replication is a powerful option for refining demographic inference for a single species (Brito and Edwards, 2009; Garrick et al., 2015; Edwards et al., 2022). However, the costs and complexity associated with the huge amount of data collected for these types of studies are substantial. For the purpose of this particular exercise – descriptive model of spatial diversity of the Southern Ocean – we suggest that it is by replicating species groups rather than loci that power and rigor can be obtained. Southern Ocean collection expeditions are infrequent, usually geographically limited, and costly in time, man power and environmental cost. Collections are not selective, so it is likely that they include a substantial number of different taxa from which to build relatively cost effective comparative studies. Inference from single marker gene trees should be treated with caution, but they are still a powerful tool in their own right (Avise, 2009) and once a comparative model can be generated, more definitive, if expensive, methods can and should be used to test the models rigor.
6 Conclusions
The diversity of the Southern Ocean benthic fauna, described as remarkably rich (Clarke and Johnston, 2003), is underestimated. The reliance of species lists and databases obscures the true extent of diversity, fails to capture the uniqueness of shelf assemblages and does nothing to promote awareness of small, isolated populations likely in need of conservation management. Furthermore, it ignores the genetic variation within species that is within the definition of Biological Diversity according to the Convention on Biological Diversity Article 2. A model developed on a series of phylogeographic studies of benthic taxa sampled across the Southern Ocean would quickly identify patterns and hint at shared processes underlying genetic diversity, providing a robust tool that highlights regions conservation priorities.
Data availability statement
The original contributions presented in the study are included in the article/supplementary material. Further inquiries can be directed to the corresponding author.
Author contributions
CS: Conceptualization, Writing – original draft, Writing – review & editing. WG-C: Conceptualization, Supervision, Writing – review & editing. SS: Supervision, Writing – review & editing. BN: Supervision, Writing – review & editing. PC: Supervision, Writing – review & editing.
Funding
The author(s) declare that no financial support was received for the research, authorship, and/or publication of this article.
Acknowledgments
We thank Drs Jasmine Lee, David Barnes and a reviewer for thoughts and comments on the text.
Conflict of interest
The authors declare that the research was conducted in the absence of any commercial or financial relationships that could be construed as a potential conflict of interest.
The author(s) declared that they were an editorial board member of Frontiers, at the time of submission. This had no impact on the peer review process and the final decision.
Publisher’s note
All claims expressed in this article are solely those of the authors and do not necessarily represent those of their affiliated organizations, or those of the publisher, the editors and the reviewers. Any product that may be evaluated in this article, or claim that may be made by its manufacturer, is not guaranteed or endorsed by the publisher.
References
Abe W. (2005). Insuetifurca austronipponica, a new tardigrade (Eutardigrada : Macrobiotidae) from Kagoshima, Southern Japan. Zool. Sci. 22, 1295–1299. doi: 10.2108/zsj.22.1295
Aldhebiani A. Y. (2018). Species concept and speciation. Saudi J. Biol. Sci. 25, 437–440. doi: 10.1016/j.sjbs.2017.04.013
Avise J. C. (2009). Phylogeography: retrospect and prospect. J. Biogeogr. 36, 3–15. doi: 10.1111/j.1365-2699.2008.02032.x
Avise J. C., Arnold J., Ball R. M., Bermingham E., Lamb T., Neigel J. E., et al. (1987). Intraspecific phylogeography: The mitochondrial DNA bridge between population genetics and systematics. Ann. Rev. Ecol. Syst. 18, 489–522. doi: 10.1146/annurev.es.18.110187.002421
Barluenga M., Meyer A. (2004). The Midas cichlid species complex: incipient sympatric speciation in Nicaraguan cichlid fishes? Mol. Ecol. 13, 2061–2076. doi: 10.1111/j.1365-294X.2004.02211.x
Bermingham E., Moritz C. (1998). Comparative phylogeography - concepts and applications. Mol. Ecol. 7, 367–369. doi: 10.1046/j.1365-294x.1998.00424.x
Blaxter M. L. (2004). The promise of a DNA taxonomy. Philos. Trans. R. Soc Lond. B. Biol. Sci. 359, 669–679. doi: 10.1098/rstb.2003.1447
Brey T., Klages M., Dahm C., Gorny M., Gutt J., Hain S., et al. (1994). Antarctic benthic diversity. Nature 368, 297–297. doi: 10.1038/368297a0
Brito P. H., Edwards S. V. (2009). Multilocus phylogeography and phylogenetics using sequence-based markers. Genetica 135, 439–455. doi: 10.1007/s10709-008-9293-3
Clarke A., Johnston N. M. (2003). Antarctic marine benthic diversity. Oceanogr. Mar. Biol. Annu. Rev. 41, 47–114.
Cook L. G., Edwards R. D., Crisp M. D., Hardy N. B. (2010). Need morphology always be required for new species descriptions? Invertebr. Syst. 24, 322–326.
Cracraft J. (2000). Species concepts in theoretical and applied biology: a systematic debate with consequences.
Dambach J., Raupach M. J., Leese F., Schwarzer J., Engler J. O. (2016). Ocean currents determine functional connectivity in an Antarctic deep-sea shrimp. Mar. Ecol. 37, 1336–1344. doi: 10.1111/maec.12343
da Silva Ribeiro T., Batalha-Filho H., Silveira L. F., Miyaki C. Y., Maldonado-Coelho M. (2020). Life history and ecology might explain incongruent population structure in two co-distributed montane bird species of the Atlantic Forest. Mol. Phylogenet. Evol. 153, 106925. doi: 10.1016/j.ympev.2020.106925
DeSalle R., Egan M. G., Siddall M. (2005). The unholy trinity: taxonomy, species delimitation and DNA barcoding. Philos. Trans. R. Soc B Biol. Sci. 360, 1905–1916. doi: 10.1098/rstb.2005.1722
Dietz L., Arango C. P., Dömel J. S., Halanych K. M., Harder A. M., Held C., et al. (2015). Regional differentiation and extensive hybridization between mitochondrial clades of the Southern Ocean giant sea spider Colossendeis megalonyx. Open Sci. 2, 140424. doi: 10.1098/rsos.140424
Dobzhansky T. (1935). A critique of the species concept in biology. Philos. Sci. 2, 344–355. doi: 10.1086/286379
Dömel J. S., Melzer R. R., Harder A. M., Mahon A. R., Leese F. (2017). Nuclear and Mitochondrial Gene Data Support Recent Radiation within the Sea Spider Species Complex Pallenopsis patagonica. Front. Ecol. Evol. 4. doi: 10.3389/fevo.2016.00139
Ebach M. C., Holdrege C. (2005). DNA barcoding is no substitute for taxonomy. Nature 434, 697–697. doi: 10.1038/434697b
Edwards S. V., Robin V. V., Ferrand N., Moritz C. (2022). The evolution of comparative phylogeography: putting the geography (and more) into comparative population genomics. Genome Biol. Evol. 14, evab176. doi: 10.1093/gbe/evab176
Emerson B. C., Paradis E., Thébaud C. (2001). Revealing the demographic histories of species using DNA sequences. Trends Ecol. Evol. 16, 707–716. doi: 10.1016/S0169-5347(01)02305-9
Faith D. (1993). Biodiversity and systematics: the use and misuse of divergence information in assessing taxonomic diversity. Pac Cons Biol. 1, 53–57.
Faith D. P. (1992). Conservation evaluation and phylogenetic diversity. Biol. Conserv. 61, 1–10. doi: 10.1016/0006-3207(92)91201-3
Galaska M. P., Sands C. J., Santos S. R., Mahon A. R., Halanych K. M. (2017a). Crossing the divide: admixture across the antarctic polar front revealed by the brittle star astrotoma agassizii. Biol. Bull. doi: 10.1086/693460
Galaska M. P., Sands C. J., Santos S. R., Mahon A. R., Halanych K. M. (2017b). Geographic structure in the Southern Ocean circumpolar brittle star Ophionotus victoriae (Ophiuridae) revealed from mtDNA and single-nucleotide polymorphism data. Ecol. Evol. 7, 475–485. doi: 10.1002/ece3.2617
Garrick R. C., Bonatelli I. A. S., Hyseni C., Morales A., Pelletier T. A., Perez M. F., et al. (2015). The evolution of phylogeographic data sets. Mol. Ecol. 24, 1164–1171. doi: 10.1111/mec.13108
GBIF (2024). Available online at: https://www.gbif.org/ (Accessed February 29, 2024).
Griffiths H. J., Barnes D. K. A., Linse K. (2009). Towards a generalized biogeography of the Southern Ocean benthos. J. Biogeogr. 36, 162–177. doi: 10.1111/j.1365-2699.2008.01979.x
Guille A. (1979). Astrotoma drachi, nouvelle espéce bathyale d’Ophiuride, Gorgonocephalidae des ïles Philippines. Vie Milieu, 437–442.
Guzzi A., Alvaro M. C., Danis B., Moreau C., Schiaparelli S. (2022). Not all that glitters is gold: barcoding effort reveals taxonomic incongruences in iconic ross sea stars. Diversity 14, 457. doi: 10.3390/d14060457
Havermans C., Nagy Z., Sonet G., De Broyer C., Martin P. (2010). Incongruence between molecular phylogeny and morphological classification in amphipod crustaceans: A case study of Antarctic lysianassoids. Mol. Phylogenet. Evol. 55, 202–209. doi: 10.1016/j.ympev.2009.10.025
Havermans C., Nagy Z. T., Sonet G., De Broyer C., Martin P. (2011). DNA barcoding reveals new insights into the diversity of Antarctic species of Orchomene sensu lato (Crustacea: Amphipoda: Lysianassoidea). Deep Sea Res. Part II Top. Stud. Oceanogr. 58, 230–241. doi: 10.1016/j.dsr2.2010.09.028
Heberling J. M., Miller J. T., Noesgaard D., Weingart S. B., Schigel D. (2021). Data integration enables global biodiversity synthesis. Proc. Natl. Acad. Sci. 118, e2018093118. doi: 10.1073/pnas.2018093118
Hebert P. D. N., Gregory T. R. (2005). The promise of DNA barcoding for taxonomy. Syst. Biol. 54, 852–859. doi: 10.1080/10635150500354886
Hedgpeth J. W. (1969). Introduction to Antarctic zoogeography. Distribution of selected groups of marine invertebrates in waters south of 35° S. (New York: American Geographical Society), 1–29.
Hewitt G. M. (2004). The structure of biodiversity – insights from molecular phylogeography. Front. Zool. 1, 4. doi: 10.1186/1742-9994-1-4
Hogg I. D., Hebert P. D. N. (2004). Biological identification of springtails (Hexapoda : Collembola) from the Canadian Arctic, using mitochondrial DNA barcodes. Can. J. Zool.-Rev. Can. Zool. 82, 749–754. doi: 10.1139/z04-041
Huettmann F. (2020). “The Forgotten Data: A Rather Short but Deep Story of Museums and Libraries in HKH and Similar Information Sources in Support of the Global Biodiversity Information System (GBIF.org) and Model-Predictions for Improved Conservation Management,” in Hindu Kush-Himalaya Watersheds Downhill: Landscape Ecology and Conservation Perspectives. Eds. Regmi G. R., Huettmann F. (Springer International Publishing, Cham), 497–520. doi: 10.1007/978-3-030-36275-1_25
Hunter R. L., Halanych K. M. (2008). Evaluating Connectivity in the Brooding Brittle Star Astrotoma agassizii across the Drake Passage in the Southern Ocean. J. Hered. 99, 137–148. doi: 10.1093/jhered/esm119
International Barcode of Life – Illuminate Biodiversity (2024). Available online at: https://ibol.org/ (Accessed March 7, 2024).
IUCN Red List (2022). The IUCN Red List of threatened species. Available online at: https://www.iucnredlist.org/en (Accessed August 29, 2022).
IUCN Red List (2024). The IUCN Red List of threatened species. Available online at: https://www.iucnredlist.org/en (Accessed March 4, 2024).
Jossart Q., Kochzius M., Danis B., Saucède T., Moreau C. V. E. (2021). Diversity of the Pterasteridae (Asteroidea) in the Southern Ocean: a molecular and morphological approach. Zool. J. Linn. Soc 192, 105–116. doi: 10.1093/zoolinnean/zlaa097
Jossart Q., Sands C. J., Sewell M. A. (2019). Dwarf brooder versus giant broadcaster: combining genetic and reproductive data to unravel cryptic diversity in an Antarctic brittle star. Heredity 1. doi: 10.1038/s41437-019-0228-9
Knowles L. L., Maddison W. P. (2002). Statistical phylogeography. Mol. Ecol. 11, 2623–2635. doi: 10.1046/j.1365-294X.2002.01637.x
Koehler R. (1900). Note préliminaire sur les Echinides et les Ophiures de l’Expédition antarctique belge … impr, Hayez.
Koehler R. (1901). Expédition Antarctique Belge. Résultats du vogage de S. Y. “Belgica” en 1897-98-99. Echinides Ophiures. Zoologie, 1–42.
Leese F., Held C. (2008). Identification and characterization of microsatellites from the Antarctic isopod Ceratoserolis trilobitoides: nuclear evidence for cryptic species. Conserv. Genet. 9, 1369–1372. doi: 10.1007/s10592-007-9491-z
Li Y., Shen X.-X., Evans B., Dunn C. W., Rokas A. (2021). Rooting the animal tree of life. Mol. Biol. Evol. 38, 4322–4333. doi: 10.1093/molbev/msab170
Linse K., Cope T., Lörz A.-N., Sands C. J. (2007). Is the Scotia Sea a centre of Antarctic marine diversification? Some evidence of cryptic speciation in the circum-Antarctic bivalve Lissarca notorcadensis (Arcoidea: Philobryidae). Polar Biol. 30, 1059–1068. doi: 10.1007/s00300-007-0265-3
Ljungman A. V. (1871). Om tvänne nya arter Ophiurider. Öfversigt Af Kongl Vetensk.-Akad. Förh. Stockh. 27, 471–475.
Lyman T. (1875). Ophiuridae and Astrophytidae: including those dredged by the late Dr. William Stimpson (University Press).
Mallet J., Willmott K. (2003). Taxonomy: renaissance or tower of babel? Trends Ecol. Evol. 18, 57–59. doi: 10.1016/S0169-5347(02)00061-7
Martín-Ledo R., López-González P. J. (2013). Brittle stars from Southern ocean (Echinodermata: ophiuroidea). Polar Biol., 1–16. doi: 10.1007/s00300-013-1411-8
Martín-Ledo R., Sands C. J., López-González P. J. (2013). A new brooding species of brittle star (Echinodermata: Ophiuroidea) from Antarctic waters. Polar Biol. 36, 115–126. doi: 10.1007/s00300-012-1242-z
McGaughran A., Liggins L., Marske K. A., Dawson M. N., Schiebelhut L. M., Lavery S. D., et al. (2022). Comparative phylogeography in the genomic age: Opportunities and challenges. J. Biogeogr. 49, 2130–2144. doi: 10.1111/jbi.14481
Moritz C., Faith D. P. (1998). Comparative phylogeography and the identification of genetically divergent areas for conservation. Mol. Ecol. 7, 419–429. doi: 10.1046/j.1365-294x.1998.00317.x
O’Hara T. D., Hugall A. F., Thuy B., Stöhr S., Martynov A. V. (2017). Restructuring higher taxonomy using broad-scale phylogenomics: The living Ophiuroidea. Mol. Phylogenet. Evol. 107, 415–430. doi: 10.1016/j.ympev.2016.12.006
O’Hara T. D., Stöhr S., Hugall A. F., Thuy B., Martynov A. (2018). Morphological diagnoses of higher taxa in Ophiuroidea (Echinodermata) in support of a new classification. Eur. J. Taxon. doi: 10.5852/ejt.2018.416
Pfaffelhuber P., Wakolbinger A., Weisshaupt H. (2011). The tree length of an evolving coalescent. Probab. Theory Relat. Fields 151, 529–557. doi: 10.1007/s00440-010-0307-6
Poulin E., Palma A. T., Féral J.-P. (2002). Evolutionary versus ecological success in Antarctic benthic invertebrates. Trends Ecol. Evol. 17, 218–222. doi: 10.1016/S0169-5347(02)02493-X
Ratnasingham S., Hebert P. D. N. (2007). BOLD: the barcode of life data system (http://www.barcodinglife.org). Mol. Ecol. Notes 7, 355–364. doi: 10.1111/j.1471-8286.2007.01678.x
Raupach M., Thatje S., Dambach J., Rehm P., Misof B., Leese F. (2010). Genetic homogeneity and circum-Antarctic distribution of two benthic shrimp species of the Southern Ocean, Chorismus antarcticus and Nematocarcinus lanceopes. Mar. Biol. 157, 1783–1797. doi: 10.1007/s00227-010-1451-3
Rocha-Ortega M., Rodriguez P., Córdoba-Aguilar A. (2021). Geographical, temporal and taxonomic biases in insect GBIF data on biodiversity and extinction. Ecol. Entomol. 46, 718–728. doi: 10.1111/een.13027
Rock J., Costa F. O., Walker D. I., North A. W., Hutchinson W. F., Carvalho G. R. (2008). DNA barcodes of fish of the scotia sea, Antarctica indicate priority groups for taxonomic and systematics focus. Antarct. Sci. 20, 253–262. doi: 10.1017/S0954102008001120
Rodrigues A. S., Pilgrim J. D., Lamoreux J. F., Hoffmann M., Brooks T. M. (2006). The value of the IUCN Red List for conservation. Trends Ecol. Evol. 21, 71–76. doi: 10.1016/j.tree.2005.10.010
Ruiz-García M., Jaramillo M. F., Sánchez-Castillo S., Castillo M. I., Pinto C. M., Shostell J. M. (2021). “Effects of Sample Size in the Determination of the True Number of Haplogroups or ESUs Within a Species with Phylogeographic and Conservation Purposes: The Case of Cebus albifrons in Ecuador, and the Kinkajous and Coatis Throughout Latin America,” in Molecular Ecology and Conservation Genetics of Neotropical Mammals. Eds. Nardelli M., Túnez J. I. (Springer International Publishing, Cham), 101–148. doi: 10.1007/978-3-030-65606-5_6
Sands C. J., Griffiths H. J., Downey R. V., Barnes D. K. A., Linse K., Martín-Ledo R. (2013). Observations of the ophiuroids from the West Antarctic sector of the Southern Ocean. Antarct. Sci. 25, 3–10. doi: 10.1017/S0954102012000612
Sands C. J., O’Hara T., Barnes D. K. A., Martín-Ledo R. (2015). Against the flow: evidence of multiple recent invasions of warmer continental shelf waters by a Southern Ocean brittle star. Evol. Popul. Genet. 3. doi: 10.3389/fevo.2015.00063
Sands C. J., O’Hara T. D., Martín-Ledo R. (2021). Pragmatic assignment of species groups based on primary species hypotheses: the case of a dominant component of the Southern ocean benthic fauna. Front. Mar. Sci. 8. doi: 10.3389/fmars.2021.723328
Saucède T., Eléaume M., Jossart Q., Moreau C., Downey R., Bax N., et al. (2020). Taxonomy 2.0: computer-aided identification tools to assist Antarctic biologists in the field and in the laboratory. Antarct. Sci., 1–13. doi: 10.1017/S0954102020000462
Stanton D. W. G., Frandsen P., Waples R. K., Heller R., Russo I.-R. M., Orozco-terWengel P. A., et al. (2019). More grist for the mill? Species delimitation in the genomic era and its implications for conservation. Conserv. Genet. 20, 101–113. doi: 10.1007/s10592-019-01149-5
Taberlet P., Bouvet J. (1994). Mitochondrial DNA polymorphism, phylogeography, and conservation genetics of the brown bear ursus arctos in Europe. Proc. R. Soc B Biol. Sci. 255, 195–200.
Taberlet P., Fumagalli L., Wustsaucy A. G., Cosson J. F. (1998). Comparative phylogeography and postglacial colonization routes in Europe. Mol. Ecol. 7, 453–464. doi: 10.1046/j.1365-294x.1998.00289.x
Taberlet P., Zimmermann N. E., Englisch T., Tribsch A., Holderegger R., Alvarez N., et al. (2012). Genetic diversity in widespread species is not congruent with species richness in alpine plant communities. Ecol. Lett. 15, 1439–1448. doi: 10.1111/ele.12004
Tautz D., Arctander P., Minelli A., Thomas R. H., Vogler A. P. (2002). DNA points the way ahead in taxonomy. Nature 418, 479. doi: 10.1038/418479a
Tautz D., Arctander P., Minelli A., Thomas R. H., Vogler A. P. (2003). A plea for DNA taxonomy. Trends Ecol. Evol. 18, 70–74. doi: 10.1016/S0169-5347(02)00041-1
Thuy B., Stöhr S. (2016). A new morphological phylogeny of the ophiuroidea (Echinodermata) accords with molecular evidence and renders microfossils accessible for cladistics. PloS One 11, e0156140. doi: 10.1371/journal.pone.0156140
Vié J.-C., Hilton-Taylor C., Pollock C., Ragle J., Smart J., Stuart S. N., et al. (2009). “The IUCN Red List: a key conservation tool,” in Wildl. Chang. World–An Anal. 2008 IUCN Red List Threat. Species, vol. 1.
Wägele H., Klussmann-Kolb A., Kuhlmann M., Haszprunar G., Lindberg D., Koch A., et al. (2011). The taxonomist - an endangered race. A practical proposal for its survival. Front. Zool. 8, 25. doi: 10.1186/1742-9994-8-25
Ward R. D., Holms B. H., O’Hara T. D. (2008). DNA barcoding discriminates echinoderm species. Mol. Ecol. Resour. 8, 1202–1211. doi: 10.1111/j.1755-0998.2008.02332.x
Wheeler Q., Meier R. (2000). Species Concepts and Phylogenetic Theory: A Debate (Columbia University Press).
Wiens J. J., Penkrot T. A. (2002). Delimiting species using DNA and morphological variation and discordant species limits in spiny lizards (Sceloporus). Syst. Biol. 51, 69–91. doi: 10.1080/106351502753475880
Will K. W., Mishler B. D., Wheeler Q. D. (2005). The perils of DNA barcoding and the need for integrative taxonomy. Syst. Biol. 54, 844–851. doi: 10.1080/10635150500354878
Keywords: conservation biology, historical demographics, IUCN Red List, comparative phylogeography, Ophiuroidea, Southern Ocean
Citation: Sands CJ, Goodall-Copestake WP, Stöhr S, Narayanaswamy BE and Convey P (2024) Comparative phylogeography, a tool to increase assessment efficiency of polar assemblage resilience and vulnerability. Front. Ecol. Evol. 12:1409618. doi: 10.3389/fevo.2024.1409618
Received: 30 March 2024; Accepted: 10 June 2024;
Published: 17 July 2024.
Edited by:
Mauro Fois, University of Cagliari, ItalyReviewed by:
Paolo Casula, Independent researcher, Cagliari, ItalyCopyright © 2024 Sands, Goodall-Copestake, Stöhr, Narayanaswamy and Convey. This is an open-access article distributed under the terms of the Creative Commons Attribution License (CC BY). The use, distribution or reproduction in other forums is permitted, provided the original author(s) and the copyright owner(s) are credited and that the original publication in this journal is cited, in accordance with accepted academic practice. No use, distribution or reproduction is permitted which does not comply with these terms.
*Correspondence: Chester J. Sands, cjsan@bas.ac.uk