- 1School of Biological Sciences, The University of Western Australia, Crawley, WA, Australia
- 2Department of Biodiversity, Conservation and Attractions, Kings Park Science, Kings Park, WA, Australia
- 3Centre for Engineering Innovation: Agriculture and Ecological Restoration, School of Agriculture and Environment, The University of Western Australia, Crawley, WA, Australia
Seed-based restoration in dryland environments is commonly limited by low and sporadic rainfall, extreme temperatures, and degraded soils. These challenges are exacerbated in dryland mine restoration sites where species are seeded onto mine waste substrates due to limited availability of topsoil. Native grasses are focal dryland and mine restoration species, though the ability to deliver seeds to targeted sites presents further constraints to seed-based restoration due to complex seed morphology and site attributes. Seed enhancement technologies (SETs) may help to mitigate environmental stressors and improving seed handling. In this study, SETs which promote seedling recruitment and/or address edaphic challenges while also improving seed morphology and handling were tested in four Australian native grasses (Cymbopogon ambiguus, C. obtectus, Eulalia aurea, and Eriachne obtusa). Flash flaming or acid digestion (depending on species), extruded seed pellets with or without the addition of topsoil, and flaming or acid digestion used in combination with extruded pellets (with or without topsoil) were evaluated on topsoil and mine waste substrates. Material treated with either flash flaming or acid digestion tended to produce similar maximum emergence (week 3), final emergence (week 14), and above-ground biomass (week 14) on both topsoil and mine waste when compared to the un-enhanced (‘control’) treatment. Extruded pellets enhanced emergence (maximum and final) on both soil types, though had no effect on biomass. Extruded pellets containing topsoil and/or when used in combination with flash flamed or acid digested florets, provided the most significant benefits to seedling emergence, particularly on mine waste soil. However, SETs were unable to mitigate the detrimental effects of mine waste substrates on seedling biomass. This study shows promise for improving seed-based restoration in post-mine and dryland environments using SETs, though also highlights potential limitations and the importance of understanding long-term (i.e., beyond emergence) plant recruitment outcomes in SET applications.
Introduction
Land degradation is prolific throughout dryland and rangeland systems globally due to overgrazing, weed invasion, cropping, and mining activities (Shackelford et al., 2021). In north-west Western Australia, for instance, over 230,000 ha of dryland restoration is required in post-mine sites, with this figure likely being larger due to undocumented clearing or abandoned mine sites (EPA, 2014; Lamb et al., 2015). Given the large scales across which plant reintroduction is required in drylands, seed-based restoration approaches have been identified as one of the most suitable techniques (Erickson et al., 2017; Christie et al., 2019; Shackelford et al., 2021; Jarrar et al., 2023). However, a wide range of challenges are encountered in dryland seed-based restoration resulting in plant establishment as low as <1% (James et al., 2011; Shackelford et al., 2021).
Low and sporadic rainfall, extreme temperatures, and degraded soils commonly limit seedling recruitment in dryland environments targeted for restoration (James et al., 2019; Masarei et al., 2020; Shackelford et al., 2021). Edaphic challenges are especially prolific in the context of post-mine site restoration where native species may be seeded or planted into mine waste, reconstructed, or degraded substrates (Muñoz-Rojas et al., 2016; Erickson et al., 2023). These substrates are often comprised of rock and mineral products which differ substantially in their chemical, biotic (i.e., absence of soil microbes), and structural attributes compared to topsoil (Lamb et al., 2015; Muñoz-Rojas et al., 2016; Masarei et al., 2020). Where topsoil has been stockpiled during mining operations, it is often available in limited quantities, and the quality may be compromised due to long storage periods (e.g., loss of soil biota and viability of soil seed bank) (Lamb et al., 2015; Muñoz-Rojas et al., 2016; Erickson et al., 2023).
Direct seeding in post-mine dryland environments is also commonly limited by seed availability and quality, and the ability to deliver seed to targeted sites (Merritt and Dixon, 2011; Masarei et al., 2021; Gibson-Roy et al., 2021a). Poor or unpredictable seed germination can result from seed dormancy mechanisms common among dryland species, low seed viability, and/or the lack of a suitable microsite (Erickson et al., 2017; Commander et al., 2020; Pedrini and Dixon, 2020). In addition, the seed morphology of many species may prevent seeds from passing through direct-seeding equipment (Gibson-Roy et al., 2021b; Berto et al., 2023), and conventional seeding equipment may be incompatible with site attributes such as terrain, soil structure, and slope (particularly in post-mine sites) (Masarei et al., 2019, 2021). The reduced ability to deliver seeds to targeted sites effectively (i.e., efficiently at an appropriate depth/location) increases the risk of seedling recruitment failure from seed which is already in limited supply (Masarei et al., 2021; Gibson-Roy et al., 2021b; Berto and Brown, 2022; Jarrar et al., 2023).
Seed enhancement technologies (SETs) are one possible solution for mitigating challenges associated with seed-based restoration in drylands and improving plant establishment outcomes (Madsen et al., 2016; Erickson et al., 2017, 2021). SETs are post-harvest seed treatments which can aid in improving seed handling, seedling recruitment, and/or tolerance of seeds and seedlings to environmental stress (Erickson et al., 2021). In particular, SETs which mitigate edaphic challenges and/or improve seed morphology or the ability to deliver seeds to targeted sites are of interest in dryland and post-mine restoration (Madsen et al., 2016; Erickson et al., 2019; Stock et al., 2020). Flash flaming, acid digestion, and extruded seed pellets (hereafter referred to as pellets or pelleting) are examples of SETs which may be able to deliver benefits to seed handling, germination and emergence, and in the instance of pelleting, overall recruitment success by provision of an enhanced microsite (Madsen et al., 2016; Erickson et al., 2021; Dadzie et al., 2022; Berto et al., 2023; Jarrar et al., 2023).
Flash flaming involves repeated, rapid passes of seeds via an open flame, while acid digestion involves the immersion of seeds into concentrated sulphuric acid. Both approaches reduce or remove hairs, appendages, and other structures surrounding the seed which are associated with poor seed handling (Erickson et al., 2021; Berto et al., 2023; Jarrar et al., 2023). These techniques have been linked to dormancy alleviation, and/or enhanced germination via fire-related germination cues (flash flaming) and/or scarification (acid digestion) (Stevens et al., 2015; Guzzomi et al., 2016; Berto et al., 2023; Jarrar et al., 2023). Pellets allow seeds to be incorporated into a soil matrix containing beneficial additives (e.g., microbes, growth promoting chemicals, nutrients) which can be extruded or moulded into various shapes and sizes to provide a valuable dispersal unit and microsite for seedling recruitment (Madsen et al., 2016; Gornish et al., 2019; Beveridge et al., 2020; Dadzie et al., 2022).
SETs hold particular relevance for native grasses which have been identified as key restoration species for post-mine sites in dryland ecosystems (Erickson et al., 2017; Shackelford et al., 2018; Christie et al., 2019; Jarrar et al., 2023). Seed-based approaches are essential for native grasses which are typically found across large scales and relatively high densities (Christie et al., 2019; Gibson-Roy et al., 2021b). However, direct seeding is commonly limited in native grasses due to extensive hairs, awns, or other appendages associated with the floret (i.e., palea and lemma which encase the seed) which prevent these species from readily passing through conventional seeding equipment (Loch, 1993; Pedrini et al., 2019; Berto et al., 2020). Low or asynchronous germination and emergence due to physiological dormancy is also common in native grasses and can result in poor recruitment in often narrow windows of opportunity in dryland environments (Erickson et al., 2017; Lewandrowski et al., 2017; Madsen et al., 2018). SETs like pelleting, flash flaming, or acid digestion are therefore ideal technologies for targeting seed handling and seedling recruitment challenges, and (in the instance of pelleting) may also aid in overcoming edaphic barriers common in mine restoration (Erickson et al., 2019; Stock et al., 2020; Berto et al., 2023).
This study tested flash flaming, acid digestion and pelleting with or without the addition of topsoil under field conditions in four native perennial grasses (Cymbopogon ambiguus A. Camus, C. obtectus S. T. Blake, Eulalia aurea (Bory) Kunth, and Eriachne obtusa R. Br; Appendix I; Supplementary Figure S1). As representative perennial grass components of the Pilbara region of Western Australia these species have high value in mine restoration and other re-seeding practices, though direct seeding efforts may be hampered by seed handling and/or germination and emergence challenges (Berto et al., 2023). Each species and SET were tested on both a reconstructed topsoil and mine waste substrate to determine the ability of the selected SETs to enhance seedling recruitment under optimal (topsoil) and challenging (mine waste) edaphic conditions. In particular, flash flaming and acid digestion were considered for their ability to improve emergence performance under a narrow recruitment window (achieved through mimicking an ecologically-informed rainfall regime), thus providing an advantage to seedling survival and growth. Pellets with or without topsoil were tested for possible microsite benefits, and to determine whether inoculation of topsoil within pellets could overcome limitations to seedling recruitment on the mine waste. Flash flaming and acid digestion were also tested in combination with seed pellets to determine whether additive benefits could be achieved.
Methods
Study design
This study tested the effects flash flaming or acid digestion (depending on the species) and extruded seed pellets composed of a “blank” mixture or a “topsoil” mixture (hereafter referred to as “blank pellets” and “topsoil pellets”, or “pellets” when referring to both). Both blank and topsoil pellets were tested with untreated control florets and florets treated with flash flaming or acid digestion. All SETs for each of the four study species were tested on the topsoil and mine waste substrates (Table 1).
The experiment was conducted at the Controlled Environment Facility (CEF) located in Newman, Western Australia (23°21’41.8”S, 119°42’26.1”E; Figures 1A, B). The CEF allows the trialling of different seed treatments and simulated rainfall regimes when sown in a range of reconstructed soil profiles whilst the natural conditions such as temperature, light, humidity, and wind of the location are maintained (Erickson et al., 2017, 2023). The facility contains 2 x 4 m planter beds containing different reconstructed soil types including topsoil, mine waste, and blends of these two (Erickson et al., 2017, 2023). For the present study the soil types used included a locally sourced topsoil representative of stockpiled material, and mine waste commonly extracted from the adjacent Mt Whaleback mine and nearby mine sites (Table 2).
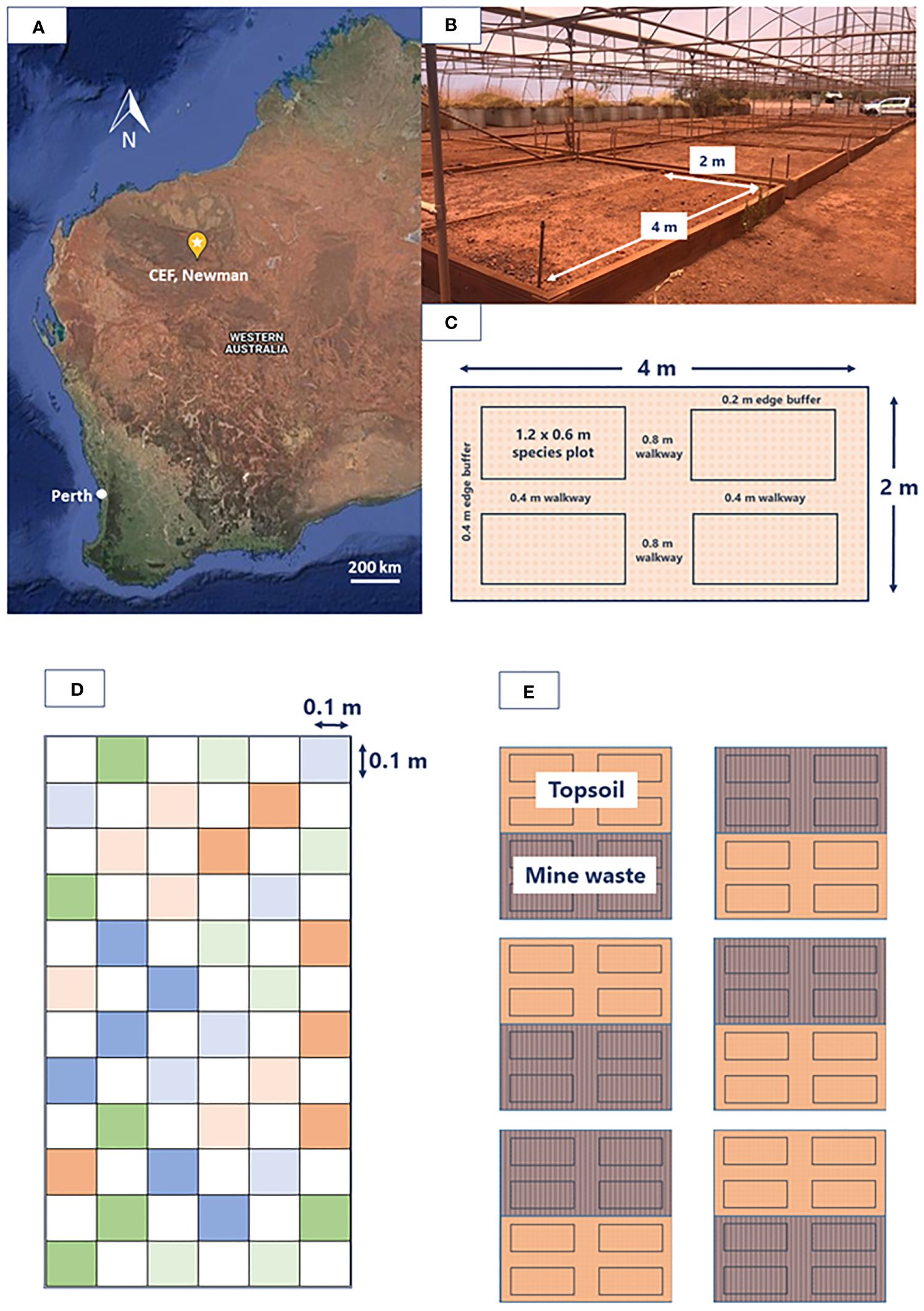
Figure 1 Study design details including (A) map showing the location of the Controlled Environment Facility (CEF) within Western Australia, (B) on ground image of the CEF highlighting the 2 x 4 m planter beds, (C) dimensions of each block and the respective species plots, (D) species plot broken down into 0.1 x 0.1 m seeding areas (colour coded to represent 6 replicates of each treatment) separated by buffer zones, (E) overall design showing species plots outlined within the six topsoil (TS) and six mine waste (MW) blocks.
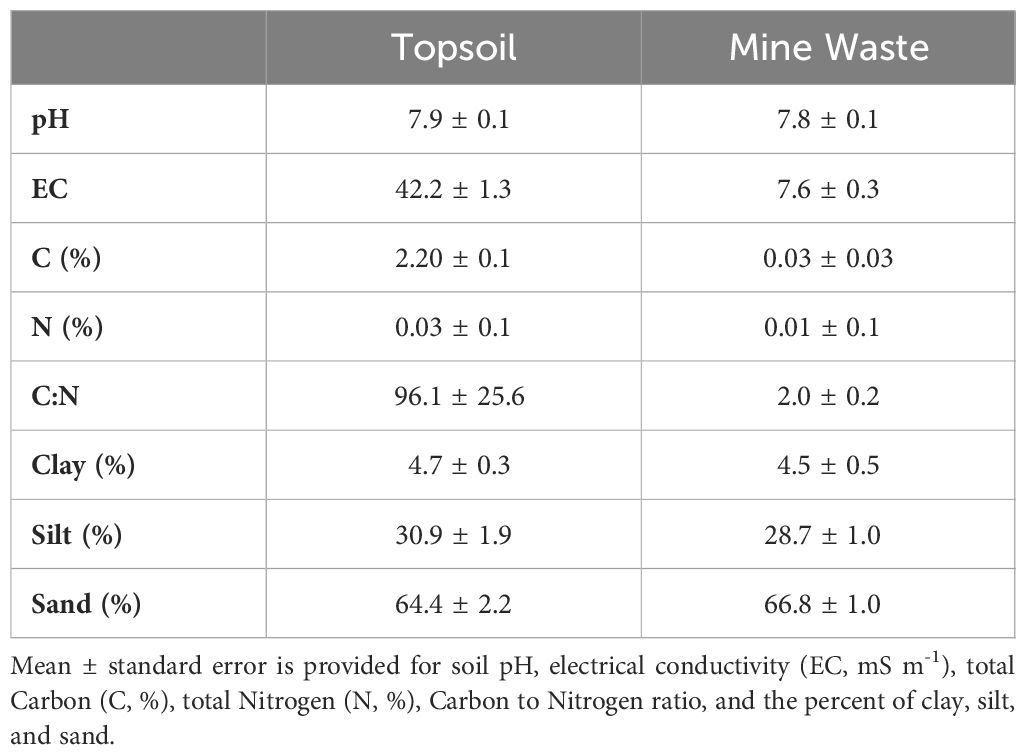
Table 2 Soil physicochemical properties of the topsoil and mine waste soils used in this study [values from Erickson et al. (2023)].
A total of six blocks (2 x 4 m planter beds) per soil type were established (12 blocks in total for the study; Figures 1C–E). Each block contained four 0.6 x 1.2 m plots (Figure 1C), randomly allocated one of each of the four study species. Within each species plot, each seed treatment was replicated six times and planted within a 0.1 x 0.1 m plot planting zone (totalling 36 planting zones per plot) (Figure 1D). Each planting zone was separated by a buffer zone of equal size. A total of 15 filled florets (i.e., florets containing a seed) were sown per replicate for each species to account for potential losses between the germination and emergence phase. Selection of filled florets was achieved by manually separating florets from non-target material (i.e., stalks, chaff), vacuum aspiration (‘Zig Zag’ Selecta, Machinefabriek BV, Enkhuizenm the Netherlands), and X-ray analysis (Faxitron MX-20 digital X-ray cabinet, Tucson, AZ, USA). Florets incorporated within a pellet were sown on the soil surface, while florets not contained within a pellet were buried at a depth of 5–10 mm. The experiment commenced on the 16th of February 2022 and ran for a duration of 14 weeks.
Study species
Each of the study species (Cymbopogon ambiguus, C. obtectus, Eulalia aurea, and Eriachne obtusa) are widely distributed rangeland grasses across the arid, semi-arid, sub-tropical and tropical zones of Australia (Appendix I; Supplementary Figure S1) where mean annual temperature and mean annual precipitation range between 14–29°C and 125–2000 mm, respectively (Appendix I; Supplementary Table S1). The external structures surrounding the seed (i.e., palea and lemma comprising the floret) have extensive hairs and/or awns (Figure 2) (Cavanagh et al., 2019), causing the florets to become easily entangled in one another in seed processing and direct seeding equipment. These inherent handling challenges were the main reasoning for selection in this study yet each of these species also have high value in ecosystem restoration [e.g., post-mine sites, degraded rangelands (Waters et al., 1997; Erickson et al., 2017; Ling et al., 2022)], as well as cultural and forage value (Lazarides, 2002). Seed collection information is available in Appendix I (Supplementary Table S1). Once received, florets were stored at 15°C and 15% relative humidity until experimental use.
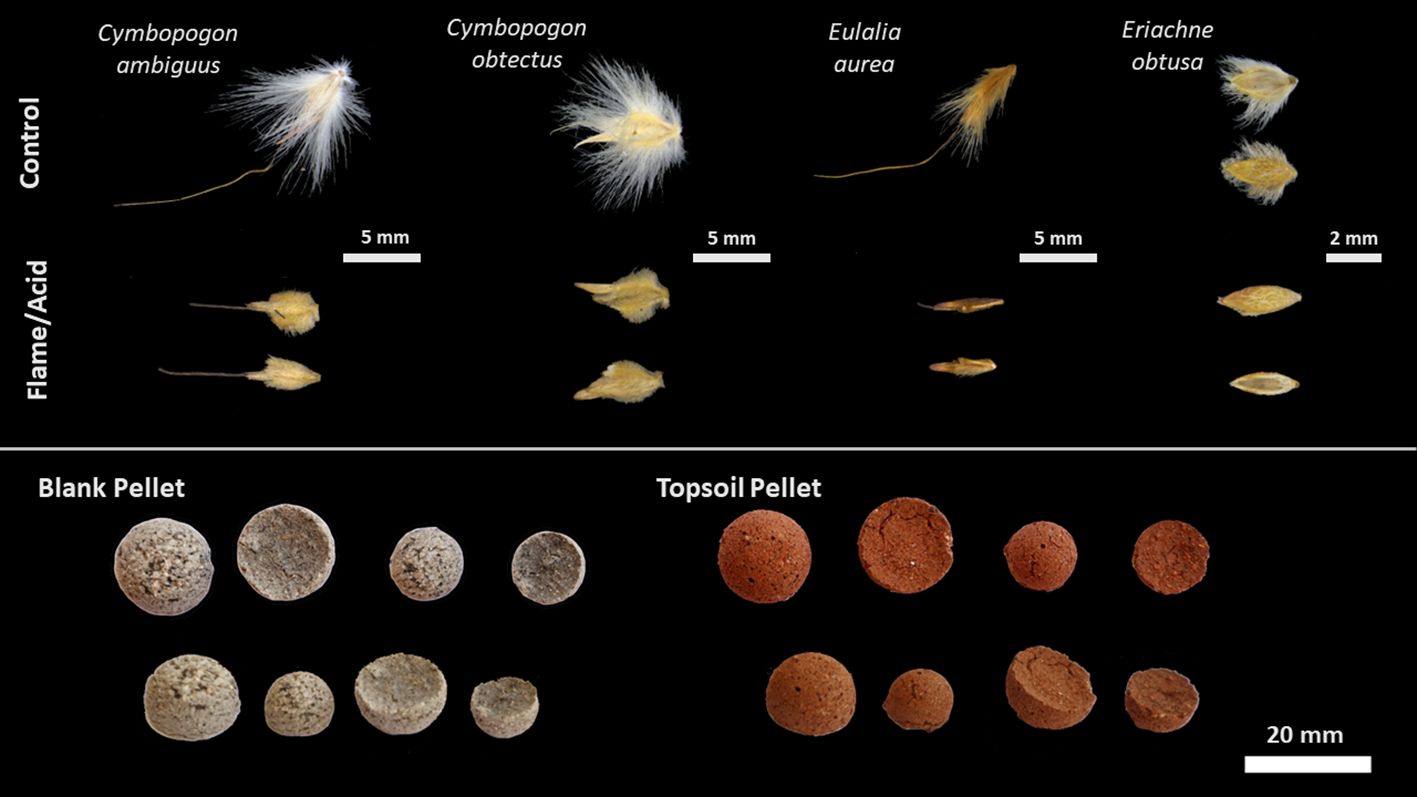
Figure 2 Images of untreated florets, flash flamed florets (of Cymbopogon ambiguus, C. obtectus, and Eulalia aurea) and acid treated florets (Eriachne obtusa only), and blank and topsoil pellets. Large (15 mm diameter) pellets were used for C. ambiguus and C. obtectus, while small (11 mm diameter) were used for E. aurea and E. obtusa. Individual scale bars are provided for each species and pellets.
Seed enhancement application
Selection of flash flaming in C. ambiguus, C. obtectus, and E. aurea, and acid digestion in E. obtusa was based on previous testing which found these treatments were best suited to improving seed morphology while maintaining or improving germination in the respective species [see Berto et al. (2023)]. Pellets were tested as an alternative method of improving dispersal ability (which does not require manipulation of floret morphology), and for potential benefits to seedling recruitment via an improved ‘pelleted’ microsite and/or the delivery of beneficial compounds (in the instance of topsoil pellets). Flame + pellet and acid + pellet treatments were tested to determine whether additive benefits could be achieved through these combination treatments (e.g., germination and emergence benefits associated with flaming or acid digestion and pellet microsite, and/or enhanced survival and growth from pellet microsite and/or beneficial components of topsoil).
Flash flaming
Following the methods of Berto et al. (2023), a 1 L sample of floret material was flamed for 10 minutes at 110 ± 10°C for C. ambiguus and C. obtectus and at 150 ± 10°C for E. aurea with a small torch [sensu Ling et al. (2019)] using the custom built flaming machine “MK1” [described in Erickson et al. (2019)]. The temperature where the stream of floret material intercepts the flame was monitored at regular intervals using a laser thermometer (Ozito, Bangholme Australia).
Acid digestion
Acid digestion was performed for E. obtusa only using a 50% concentration solution of sulphuric acid (H2SO4) for an exposure time of 2.5 min [see Berto et al. (2023)]. Concentrated sulphuric acid (reagent grade 98%; Sigma Chemicals, Willetton, Western Australia) was dissolved in reverse osmosis (RO) water. Approximately 200 ml of dry floret material was placed into a conical flask before adding 100 ml of the sulphuric acid solution. The flask was swirled continuously throughout each treatment to ensure rapid and thorough coverage of all floret material. Treated material was immediately neutralised in sodium hydrogen carbonate solution (8.4 g L-1 NaHCO3, Sigma-Aldrich, St Louis, USA) and rinsed thoroughly in RO water before being dried for a minimum of 48 h at 15°C and 15% relative humidity.
Extruded pellets
Untreated florets and flamed or acid treated florets were incorporated into seed pellets (Figure 2). Blank pellets were made using a mixture of sand, biochar, diatomaceous earth, bentonite clay, fungicide (Captan), and a liquid binder solution (Opadry®) (details in Appendix II; Supplementary Table S2). Topsoil pellets were made using a 1:1 (v/v) blend of the blank pellet mixture and locally sourced topsoil (as used in the planter beds) processed through a 2 mm sieve. These materials provide a balanced blend of different particle sizes which could aid in creating a beneficial microsite via improved soil structure, water holding capacity (from clay components), nutrient and microbe availability (from clay, carbon, and topsoil components). For both blank and topsoil pellets, the dry materials were combined and mixed thoroughly before gradually mixing through the binder until a smooth, wet mixture was achieved (i.e., dry ingredients reached maximum moisture-holding capacity but weren’t so wet that moisture pooling occurred). Pellets were created by spreading a wet soil mixture into hemispherical moulds with a diameter of 15 mm and 11 mm (corresponding to volumes of approx. 1 ml and 0.5 ml) for large (C. ambiguus and C. obtectus) and small (E. aurea and E. obtectus) seeded species, respectively (Figure 2). A single filled floret was pressed gently into the centre of each pellet. Pellets were dried at ambient temperatures of 25–30°C for 24 h.
Irrigation
Simulated rainfall in the CEF is achieved via a fully automated, pressurised watering system which applies water at a rate of 10 mm per h−1 (MP 2000 sprinkler heads; Hunter MP Rotator®, Hunter Industries, San Marcos, CA, USA). In the first week of the experiment, a typical wet-season rainfall scenario was created by delivering discrete events of 30 mm, every second day, over four separate events (e.g., 4 events x 30 mm = 120 mm total). Follow up irrigation events of 30 mm were supplied once per week for the next three weeks of the experiment (90 mm total), then a single irrigation event of 30 mm once per month for the remaining two months of the experiment (overall total water supply of 270 mm throughout the experiment). The initial pulse irrigation event and subsequent infrequent events were selected based on previous studies which have tested a range of rainfall scenarios typical of cyclonic summer rainfall events experienced in this region (Lewandrowski et al., 2017; Stock et al., 2020; Erickson et al., 2023).
Data collection and analysis
The number of emerged seedlings were counted at weeks 3 (maximum emergence) and 14 (final emergence). Upon completion of the experiment (14 weeks), above-ground biomass was harvested and dried at 70°C for 3d before being weighed. Total above-ground biomass was divided by the number of seedlings within a given replicate to provide average seedling above-ground biomass. HOBO® data loggers (HOBO® RX3000 Data Logger; Onset Computer Corporation, Bourne, MA, USA) were used to monitor ambient temperature (°C), relative humidity (RH; %), dew point (°C), and photosynthetically active radiation (PAR; uE) (Appendix III, Supplementary Figure S2).
All data were analysed in R Studio (version 4.3.0; R Core Team, 2023). Linear mixed-effects regression models (lmer and glmer functions in lme4 package in R) was used to examine the effects of SET, soil, and the interaction SET * soil on emergence and biomass where applicable. In addition, week and the interaction SET * week were considered to assess survival of emerged seedlings between week 3 and 14. Block was included as a random effect but was removed if it did not significantly improve model fit (in which case a generalised linear model (glm function) was used in the absence of random effects). Where zero scores were common for emergence, zero-inflated beta-binomial mixed-effects hurdle models (glmmTMB package) were used instead (Appendix IV, Supplementary Table S3). Biomass models were weighted using the inverse variance to account for unequal sample sizes. Q-Q Normal plots and plot of residuals were used to check model assumptions. Predictor variable significance was determined using type III ANOVA (Anova function from car package in R). Estimated marginal means and pairwise comparisons (p-values adjusted using the Benjamini-Hochberg method) were obtained using the emmeans package.
Results
Emergence and survival
Emergence (i.e., seedling counts converted to percentage) was significantly affected by SET and the interaction between SET and soil in all species (p < 0.01; Appendix V, Supplementary Table S4). Soil significantly affected emergence in C. obtectus and E. aurea (p < 0.01). Week was a significant predictor of emergence counts in all species (p < 0.05) except for C. ambiguus. The interaction between SET and week was also a significant predictor of emergence in E. aurea and E. obtusa (p < 0.01).
In C. ambiguus, pairwise comparisons showed that the blank pellet treatment tended to result in lower maximum (week 3) and average (i.e., averaged across week) emergence compared to the control and flame treatments when tested on topsoil (by 24–27%, p < 0.05; Figure 3; Table 3; Appendix VI, Supplementary Table S5). On mine waste, the topsoil pellet treatment improved final (i.e., emerged seedlings at week 14) and average emergence (by 34–48%, p < 0.05) and the flame + topsoil pellet treatment improved average emergence (by 36%, p < 0.01) in C. ambiguus compared to the control (Figure 3; Table 3; Supplementary Table S5). SET comparisons averaged across soil type were not significant for maximum and final emergence in C. ambiguus, but average emergence was lower for the blank pellet treatment compared to all treatments which included flaming (by 17–23%, p < 0.05; Supplementary Table S6). Emergence was higher on topsoil compared to mine waste in C. ambiguus for the control (by 21–52% for maximum, final, and average, p < 0.01) and flaming (by 22% for average only, p < 0.05) treatments only (Supplementary Table S7). Significant declines between maximum and final emergence counts were only present for the control when averaged across soil type in C. ambiguus (by 24%, p < 0.05; Supplementary Table S8).
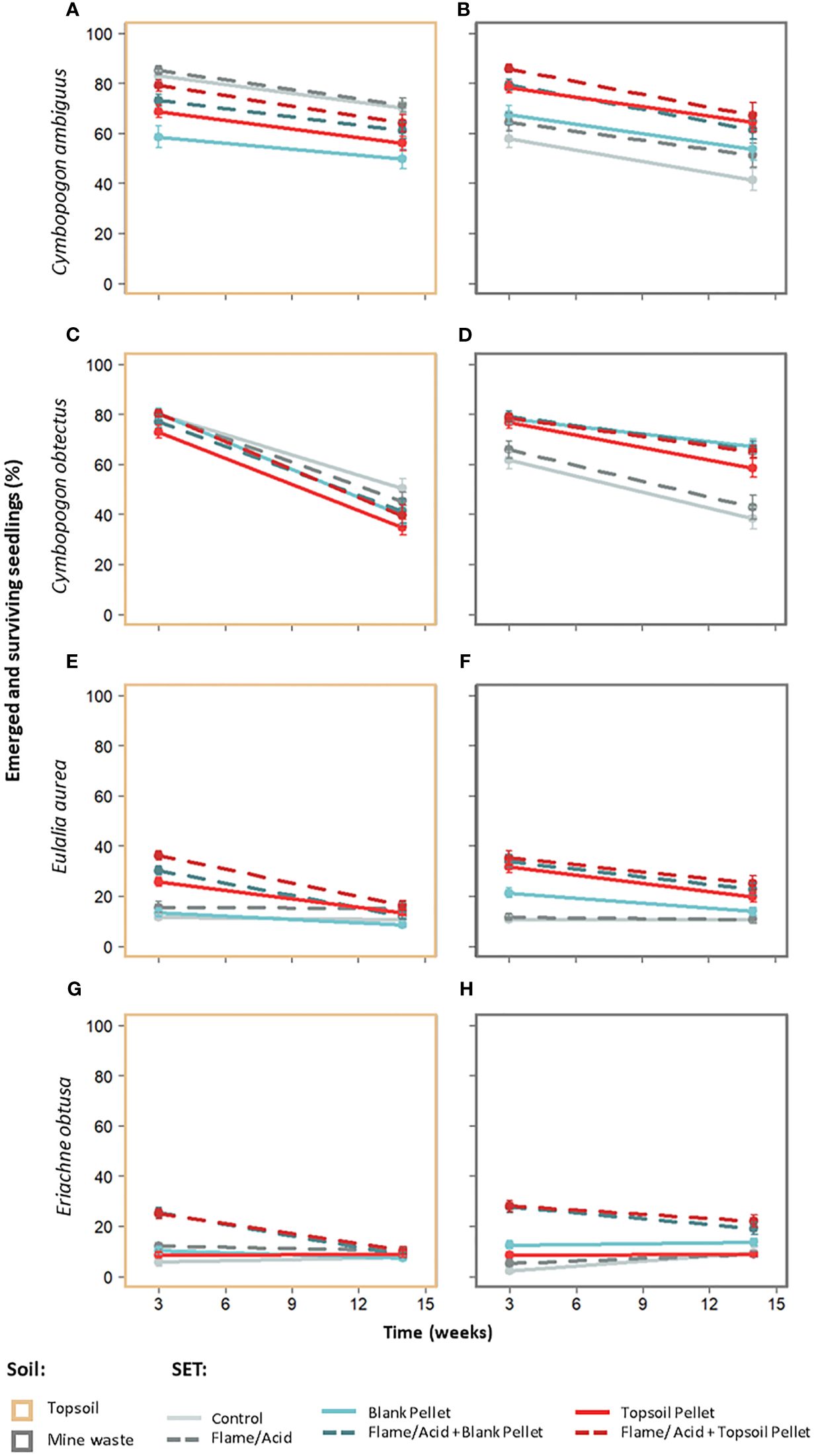
Figure 3 Emerged seedlings (%) for each SET at week 3 (maximum emergence) and week 14 (survival) on topsoil (A, C, E, G) and mine waste (B, D, F, H) for Cymbopogon ambiguus (A, B), C. obtectus (C, D), Eulalia aurea (E, F), and Eriachne obtusa (G, H). True mean and standard error are shown (see Appendix VI, Supplementary Table S9 for model estimates).
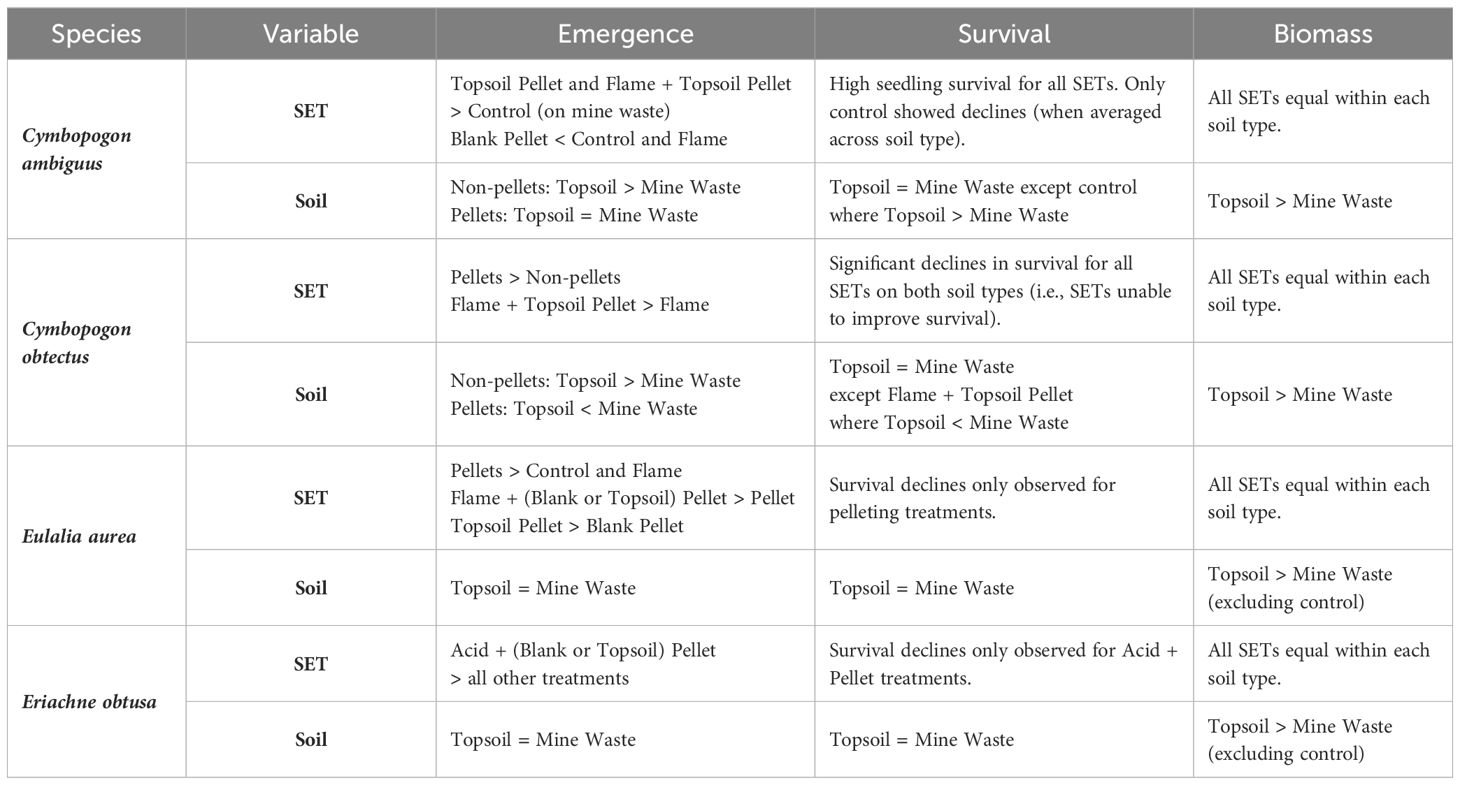
Table 3 Summary of the general trends and key overall effects on emergence and biomass for the SETs tested in each study species.
In C. obtectus, pairwise comparisons of SETs were not significant on both soil types for maximum emergence. Final and average emergence, however, were generally higher for pellet treatments compared to the control (by 31–67%, p < 0.05), and for the flame + topsoil pellet compared to flaming treatment (by 14–25%, p < 0.05) (Figure 3; Table 3; Supplementary Table S5). SET comparisons for maximum, final and average (i.e., averaged across week) emergence were not significant for C. obtectus when averaged across soil type (Supplementary Table S6). Emergence in C. obtectus was higher on mine waste compared to topsoil for the flame + topsoil pellet (by 2–54%, p < 0.05; maximum, final, and average emergence), blank pellet (by 28%, p < 0.05; average emergence), and flame + blank pellet treatments (by 21%, p < 0.05; average emergence), and higher on topsoil compared to mine waste for the control (by 29%, p < 0.05; average emergence) (Supplementary Table S7). Final emergence was lower than maximum emergence (i.e., survival declined) for all SETs on topsoil (by 36–69%, p < 0.05), mine waste (by 10–66%, p < 0.05) and when averaged across soil type (by 31–56%, p < 0.05) in C. obtectus (Supplementary Table S8).
Pairwise comparisons for E. aurea showed that the control and flaming tended to have lower maximum and average emergence compared to pelleting treatments (except the blank pellet treatment in some instances) on both soil types and when averaged across soil type (by 6–24%, p < 0.05), though maximum and average emergence were lower for the blank pellet compared to all other pellet treatments (by 7–19%, p < 0.01), and for the topsoil pellet compared to flame + topsoil pellet treatment (by 5–9%, p < 0.05, on topsoil only) (Figure 3; Table 3; Supplementary Tables S5, S6). Final emergence in E. aurea tended to be higher for the flame + topsoil pellet treatment compared to the control, flame, and blank pellet treatments depending on soil type (by 6–9%, p < 0.05), and for all pellet (except blank pellet) treatments compared to flaming on mine waste (by 5–10%, p < 0.05) (Figure 3; Table 3; Supplementary Tables S5–6). Emergence (maximum, final, and average) was similar for each SET compared with itself across topsoil and mine waste for E. aurea (Supplementary Table S7). Final emergence was lower than maximum emergence (i.e., survival declined) in E. aurea for pelleting treatments only on both soil types and when averaged across soil type (by 6–17%, p < 0.05; Supplementary Table S8).
In E. obtusa, pairwise comparisons showed that maximum and average emergence tended to be higher for the acid + blank pellet and acid + topsoil pellet treatments compared to all other treatments across both soil types and when averaged across soil type (by 6–20%, p < 0.05; Figure 3; Table 3; Supplementary Tables S5, S6). Final emergence was higher for the acid + topsoil pellet compared to the acid treatment and topsoil pellet treatment on mine waste and when averaged across soil type (by 3–9%, p < 0.05), and for the acid + blank pellet compared to topsoil pellet treatment on mine waste (by 6%, p < 0.05) (Figure 3; Table 3; Supplementary Tables S5, S6). Emergence (maximum, final, and average) was similar for each SET compared with itself across topsoil and mine waste for E. obtusa (Supplementary Table S7). Declines in survival between maximum and final emergence for E. obtusa were only observed for the acid + blank pellet and acid + topsoil pellet treatments on both soil types and when averaged across soil type (by 9–14%, p < 0.001; Supplementary Table S8).
Biomass
SET was a significant predictor of seedling biomass only in C. obtectus (p < 0.001), while soil was a significant predictor of seedling biomass for all four study species (p < 0.05) (Appendix V; Supplementary Table S4). Pairwise comparisons of SETs on each soil type and when averaged across soil type yielded no significant results, even in C. obtectus (despite SET being a significant model predictor) (Figure 4; Table 3; Appendix VI, Supplementary Table S10). When each SET was compared against itself across soil type, seedling biomass was higher on topsoil compared to mine waste for all SETs in C. ambiguus (by 0.1473–0.1875 g = 1.7–3.8 times heavier, p < 0.001), and for all SETs except the control in E. aurea (by 0.0438–0.0692 g = 1.4–5.9 times heavier, p < 0.01), and E. obtusa (by 0.0246–0.0446 g = 2.6–4.6 times heavier, p < 0.01) (Figure 4; Table 3; Supplementary Table S11). Pairwise comparisons of seedling biomass for each SET across soil type were not significant in C. obtectus (despite soil being a significant model predictor) (Figure 4; Table 3; Supplementary Table S11).
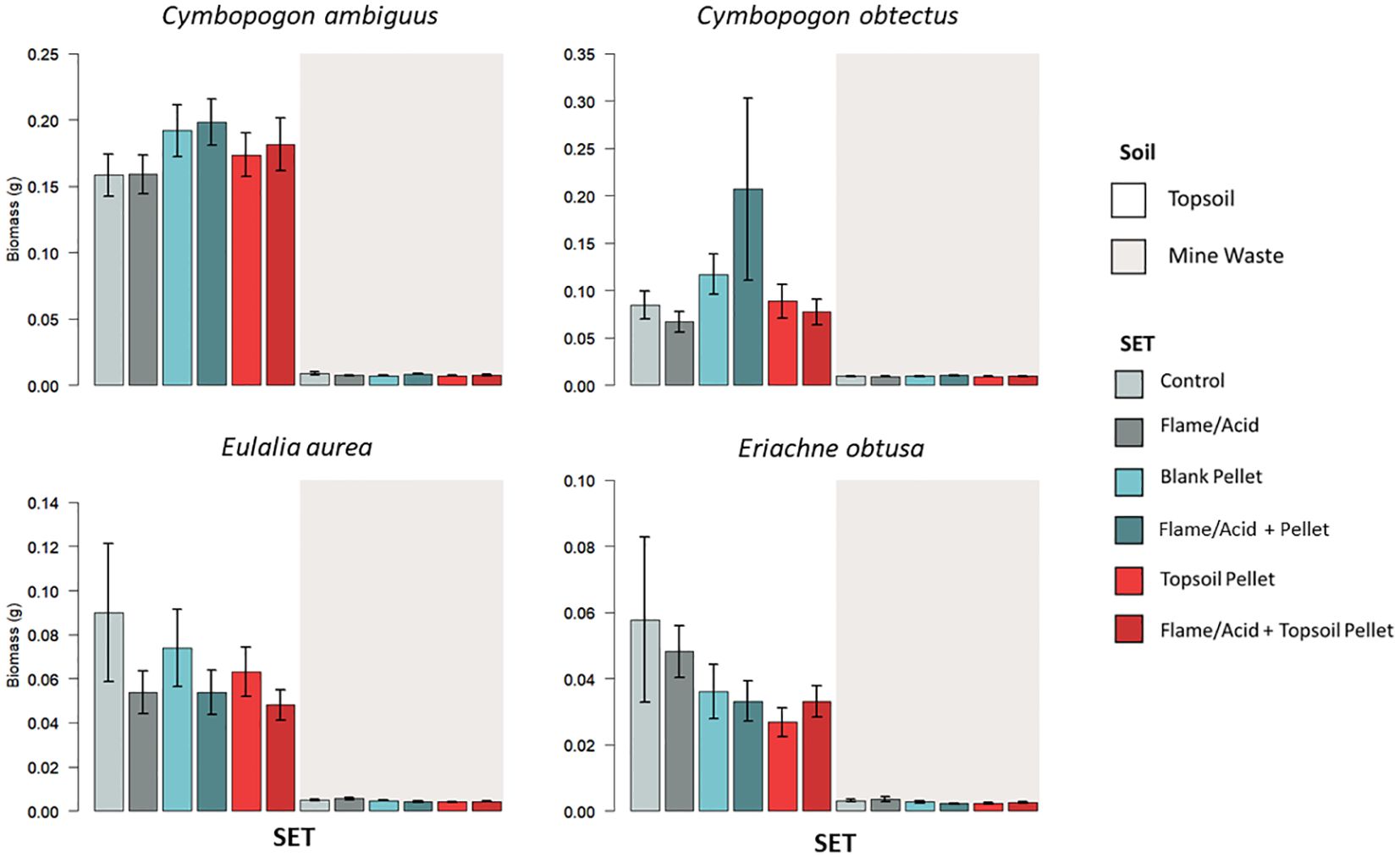
Figure 4 Average seedling biomass (g) for each SET on topsoil and mine waste. Options for “Flame” (flash flaming) in the key correspond to C. ambiguus, C. obtectus, and E. aurea, while “Acid” (acid digestion) corresponds to E. obtusa only. True mean and standard error are shown (see Appendix VI, Supplementary Table S12 for model estimates).
Discussion
Seed-based restoration in dryland environments, particularly in mined landscapes, is commonly limited by harsh environmental conditions (i.e., degraded soil, low rainfall, extreme heat, poor microsite availability) resulting in poor seedling recruitment, as well as seed handling challenges (Erickson et al., 2019; Shackelford et al., 2021; Erickson et al., 2023). SETs which enhance emergence and/or provide a favourable microsite while improving seed handling may help to mitigate some of these significant challenges (Madsen et al., 2016; Erickson et al., 2017). Understanding the extent to which SETs might benefit seedling recruitment at different life-stages and on different soil types commonly encountered in post-mine restoration can help to guide and optimise future SET development and implementation. This study highlights clear benefits to seedling emergence and early survival provided by SETs, with these benefits becoming especially pronounced on reconstructed mine waste substrates. Pellets, particularly with the inclusion of topsoil and/or when used in combination with flash flaming or acid digestion, provided the most significant benefits to seedling emergence on mine waste substrates. However, the benefits of SETs were largely limited to the emergence phase, with the effects being absent for early (week 14) above-ground biomass. The selected SETs show promise for improving seed-based restoration at the emergence phase while also increasing the efficacy of resource use via precision delivery of topsoil and ability to deliver seeds to site (i.e., greater seeding efficiency due to improved seed morphology).
SET effects - can SETs provide a recruitment advantage?
SETs may increase the ability of seeds to utilise often narrow and infrequent windows of opportunity for recruitment typical of dryland environments (Pedrini et al., 2020; Jarrar et al., 2023). For example, faster germination or emergence resulting from SET application may mean seedlings emerge sooner during a pulse rainfall event (e.g., cyclone) and are better able to establish roots to a depth sufficient for survival compared to untreated seeds (Pedrini et al., 2020; Eshleman and Riginos, 2023). Higher maximum germination and emergence resulting from SET application may also allow for a larger number of surviving seedlings compared to untreated seeds, even where survival rates are similar (Kildisheva et al., 2020). An early recruitment advantage (via enhanced germination and emergence) increases the ability of seedlings to utilise temporally limited resources, which may in turn confer to longer-term benefits to plant establishment (Vaughn and Young, 2015).
Flash flaming and acid digestion have previously been found to enhance maximum germination and germination rate in a range of native grasses, including the selected study species (Stevens et al., 2015; Ling et al., 2022; Berto et al., 2023). In this study, there was little evidence to suggest that flaming or acid digestion (used in isolation) benefitted emergence compared to untreated florets (i.e., maximum emergence was similar to the control, subsequently flaming and acid treatments did not result in higher survival or biomass).
Seed pellets are often associated with delayed or reduced emergence (Clenet et al., 2019; Gornish et al., 2019), which could become particularly problematic in dryland environments if rapid emergence is desirable during a narrow recruitment window. For example, Donovan et al. (2023) found that maximum emergence was three-fold higher for non-pelleted compared to pelleted Artemesia tridentata seed, and Munro et al. (2023) found that pellets reduced emergence in Acacia pulchella. By contrast, Baughman et al. (2023) found that pellets resulted in similar or increased seedling counts for native (US) rangeland grasses Elymus elymoides, Poa secunda, and Pseudoroegneria spicata. Similarly in this study, maximum and final emergence counts for pellets were often greater than the control, suggesting pellets did not restrict emergence (except in the instance of blank pellets for C. ambiguus on topsoil). The addition of topsoil (at 1:1 v/v) tended to further enhance emergence outcomes, particularly for E. aurea (whether tested on mine waste or topsoil), and C. ambiguus on mine waste. Final seedling counts were higher for pellet compared to non-pellet treatments in a number of instances due to improved survival (e.g., pellet treatments on mine waste in Cymbopogon sp.) or higher maximum emergence (e.g., acid + topsoil pellet on mine waste in E. obtusa). This highlights the value of maximising seedling emergence and/or survival via SETs to increase the odds of plant establishment.
Pellet hardness and compaction owing to the materials (e.g., clay content) and/or extrusion method are often considered as possible reasons for delayed or reduced seedling emergence from pelleting treatments (Clenet et al., 2019; Gornish et al., 2019; Erickson et al., 2021). The structure of the soil mixture used in pellets in this study may have allowed for better water penetration and/or retention compared to the substrates on which they were tested, a key consideration given that moisture availability is critical in dryland, post-mine restoration (Erickson et al., 2023). In addition, pellet production for restoration has often utilised an industrial pasta/dough machine to extrude the soil matrix containing seeds through a die, risking excessive compression and/or damage to seeds (Clenet et al., 2019; Brown et al., 2021). The pellet production method used in this study (using silicone moulds) eliminates the risk of compression and seed damage and therefore provides a valuable and efficient alternative for pellet production.
An additional key finding for emergence outcomes in this study were the additive benefits to emergence often found when flamed or acid treated florets were used in pellets (i.e., flame/acid + pellet > flame/acid or pellet). In this instance, the pellet microsite may have facilitated the expression of flaming and acid digestion emergence benefits. This was especially pronounced in E. obtusa where acid digestion and pellets when used in isolation had neutral effects on emergence, but significant benefits to emergence were seen when used in combination. There are a limited number of studies which consider the use of SETs in combination due to the increased resource investment associated with applying more than one SET, and the challenge of disentangling the effects of SET when applied in combination (Beveridge et al., 2020; Berto et al., 2021, 2024). However, it has also been recognised that applying a single SET may be redundant if it only addresses one of multiple challenges to seed-based restoration (Berto et al., 2021; Erickson et al., 2021; Berto and Brown, 2022). This concept is supported by the dependence of flaming and acid digestion on pellets to provide emergence benefits in this study.
The enhanced emergence outcomes for pellets, however, did not translate to improved seedling growth (biomass). Overall, this suggests that SET benefits were largely restricted to emergence and early survival in this study, and that these benefits weren’t so significant as to provide a longer-term or ongoing advantage. Multiple studies acknowledge that seed germination and seedling emergence present two of the most critical bottlenecks to plant establishment in seed-based restoration efforts (Merritt et al., 2007; James et al., 2011). However, once these bottlenecks are addressed (e.g., via dormancy break treatments or SETs), other challenges (e.g., soil effects) may arise which prevent successful plant establishment, thus highlighting the need to focus on long-term outcomes.
Soil effects – can SETs improve seedling recruitment on mine waste?
Emergence was largely unrestricted by soil type for the species selected in this study. SETs tended to improve emergence and survival on both soil types, forming reasonable justification for their adoption in restoration efforts. However, the lack of SET effects on seedling biomass within each soil type, and the significant difference in seedling biomass across each soil type, suggest that the SETs applied in this study are unable to aid in overcoming the significant challenges the mine waste substrate presented to plant establishment.
Amending mine waste substrates with topsoil (or other amendments) has been shown to improve seedling recruitment and plant establishment in mine site restoration (Kneller et al., 2018; Commander et al., 2020; Erickson et al., 2023), though the limited supply of topsoil may mean that these techniques are not always feasible over larger scales (Lamb et al., 2015). Precision delivery of topsoil to seedlings via pellets was considered as an alternative to overcoming challenges associated with seeding into mine waste substrates in this study. Pellets, particularly those containing topsoil (1:1 v/v) tended to enhance emergence (and occasionally survival) on mine waste compared to non-pelleted florets and compared to pellet treatments tested on topsoil. In a number of instances, emergence on topsoil was higher for topsoil pellets compared to the control (e.g., E. aurea, E. obstusa), suggesting that pellet microsite benefits exceeded the benefits of seeding untreated florets into pure topsoil. However, pellets (with or without topsoil) were unable to overcome bottlenecks to seedling growth (biomass) on mine waste soil, with above-ground biomass being strongly negatively impacted by mine waste compared to topsoil substrates.
Conceptually, the precision delivery of topsoil or other microbial amendments (via pellets) can provide seedlings with nutrients and microbes critical to seedling establishment in addition to providing pellet microsite benefits (Alfonzetti et al., 2023). The introduction of valuable soil biota (e.g., via topsoil contained within pellets) can provide significant benefits to seedling recruitment on mine waste soils which lack essential microbial communities (Muñoz-Rojas et al., 2018; Dadzie et al., 2022; Schultz et al., 2022). The poor growth (biomass) on the mine waste compared to topsoil substrate for seedlings from topsoil pellets in this study, however, suggests that the benefits of topsoil could not be achieved via pellets on mine waste. Given the small volume of the pellets used in this study (0.5–1 ml volume), it is possible that topsoil quantities were insufficient to provide prolonged benefits to seedling growth. While studies have shown that it is possible to establish microbial communities in post-mine environments using dilute application of inoculants [e.g., 1:100 biocrust to soil used by Schultz et al. (2022)] and over time frames [e.g., within 6 weeks of inoculation observed by Rezasoltani et al. (2023) and 12 weeks observed by Roman et al. (2018)] comparable with this study, it is possible that the unique site and mine waste substrate characteristics used in this study exacerbate the challenge of re-establishing soil microbial communities. Furthermore, the age and quality of topsoil used in the topsoil pellets may have affected the microbial activity and potential for colonisation (Lamb et al., 2015; Williams et al., 2019).
SET effects tended to be consistent on both soil types (i.e., flaming and acid digestion had similar emergence and pellets enhanced emergence compared to the control on both soil types). In the instance of C. ambiguus, however, interactions between SET and soil were observed. In this case, emergence counts tended to be lower for the control and flamed florets on mine waste compared to topsoil, while pellets had similar emergence counts across the two soil types. This indicates that the control and flamed florets may have been more vulnerable to the detrimental environment of the mine waste substrate, and/or that the mine waste substrate was more inhospitable than the topsoil substrate, creating a recruitment barrier such as a physical crust that prevented seedling emergence. Again, this illustrates the promising microsite benefits provided by pellets to mitigate an edaphic challenge which would otherwise contribute to an emergence bottleneck.
Seed morphology and handling
Although flaming and acid digestion used in isolation did not always enhance emergence, survival or biomass when compared to the control, these treatments were able to successfully improve seed morphology while maintaining seedling recruitment potential. Flaming and acid digestion pose a potential risk to seeds, if applied excessively, as these SETs can result in the removal or partial disintegration of floret structures surrounding the seed (Pedrini et al., 2019; Barberis et al., 2023; Berto et al., 2023). With this partial or complete removal, excessive treatment application can chemically alter and/or physically damage seeds which may impede seedling emergence and growth (Kelly and Van Staden, 1985; Loch et al., 1996; Pedrini et al., 2019; Barberis et al., 2023). Furthermore, the structures surrounding the seed may be important in providing protection from predation, physical damage during mechanical seeding and processing, and environmental stressors (e.g., suboptimal temperatures) (Loch, 1993; Cavanagh et al., 2019; Berto et al., 2023). The findings of this study highlight that, when applied effectively, flash flaming and acid digestion can improve seed morphology without making seeds more vulnerable to potential stressors in the environment (e.g., extreme heat, degraded soil) and/or resulting in compromised growth. A subsequent benefit of this finding may be that flamed or acid treated florets can be mechanically seeded with greater efficacy and precision, increasing the likelihood of seeds being positioned at a soil depth or location most conducive to seedling growth and survival (Masarei et al., 2021; Gibson-Roy et al., 2021b; Ling et al., 2022).
Pellets also provided a valuable dispersal unit which effectively concealed the challenging seed morphology of the study species. The increased size and shape of these pellets may also prove to be beneficial for native grass seed which, like other species with small seed mass and high wind resistance (created by structures surrounding the seed), are often challenging to disperse effectively and are easily lost from targeted sites (Gornish et al., 2019; Hoose et al., 2019; Jarrar et al., 2023). In addition, the compatibility of pellets with surface-sowing may allow for successful aerial (e.g., drone) seeding, a particularly important consideration in mine-restoration where site attributes (e.g., terrain, soil rock content, slope) are often unsuited to conventional seeding equipment (Masarei et al., 2019, 2021). The large-scale uptake of and ability to handle pellets may still be limited in some instances due the significant weight and volume increases of pellets compared to non-pelleted seed (Erickson et al., 2021). However, it may be possible to somewhat mitigate these logistical constraints if seeding rates can be reduced as a result of pelleting techniques sufficiently enhancing plant establishment outcomes and reducing seed losses for small seeded or highly wind-resistant species.
Conclusions and future directions
The SETs selected in this study, in particular pellets, show great promise for overcoming seed dispersal and seedling recruitment challenges on both topsoil and mine waste substrates. The enhanced benefits of pellets containing topsoil and/or using flaming and acid digestion in combination with pellets provides a proof of concept for precision delivery of topsoil, and additive benefits of combination SETs. However, there are still a number of considerations for the feasibility, improvement, and scalability of these treatments.
Higher emergence following SET application was highlighted as a way to capitalise on limited resources (i.e., pulse rainfall events), thus improving growth and survival. However, the counter scenario is that increased emergence associated with SET treatments could mean seedlings emerge at times not conducive to survival. In these instances, delayed germination and emergence can be favourable (Lewandrowski et al., 2018; Richardson et al., 2019). Because environmental variables (e.g., rainfall events) are often challenging to predict in restoration contexts, several studies have suggested the value of using a mixture of SET treated and untreated seed when implementing seed-based restoration as a means of bet-hedging (Davies et al., 2018; Brown et al., 2021).
A further challenge which persists is the inability of the selected SETs to provide long-term benefits. Pellet benefits observed for maximum and final emergence did not confer an advantage for seedling growth (biomass). Most significantly, precision delivery of topsoil via pellets was unable to overcome the limitations to seedling growth associated with the mine waste substrate. Increasing the volume of topsoil and/or the inclusion of other beneficial additives may allow prolonged benefits of precision topsoil delivery. A potential challenge is that pellets may become too thick for seedlings to readily emerge, and optimal seed positioning within pellets may become essential for seedling emergence (Baughman et al., 2021; Brown et al., 2023; Lieurance et al., 2024). Field-deployed extruded pellets provide an alternative which allows larger volumes of pellet material (e.g., topsoil or other amendments) to be delivered with minimal increases in pellet thickness (Stock et al., 2020). These large ‘pat’ shaped pellets may also further enhance the pellet microsite benefits observed in this study by increasing the size of the microsite and therefore warrant further investigation in overcoming longer-term limitations to plant establishment (e.g., seedling growth) on mine waste substrates.
An additional consideration for future assessment of long-term SET effects (particularly on mine waste substrates) is monitoring of root development. The present study only considered above-ground biomass to assess seedling growth. Multiple studies have highlighted the importance of root development for successful plant establishment (Larson et al., 2020), particularly for native grasses in dryland environments (Fort et al., 2013; Ferguson et al., 2015; Leger et al., 2019). Below-ground biomass may therefore be a more informative outcome variable. In particular, isolating the stage of seedling development at which soil effects overwhelm SET effects would be valuable in developing suitable treatments to address this barrier to plant establishment.
It should also be acknowledged that the rainfall regime utilised in this study represents a high/above average rainfall scenario for the targeted environment (Lewandrowski et al., 2017; Erickson et al., 2023). Given that water availability is the most critical driver of seedling recruitment in dryland, post-mine environments (Erickson et al., 2023), the absence of soil effects on emergence in this study may be explained by the high watering regime used. Future efforts would benefit from testing under a lower rainfall regime to understand the broader range of effects of these SETs and soils on seedling recruitment.
Finally, the scalability of the SETs tested in this study is a key consideration for their uptake and implementation. Flash flaming is already being adopted at scale in mine-site rehabilitation in the Pilbara (Erickson et al., 2017, 2019), and extruded pelleting techniques have been widely implemented in agriculture making them readily scalable (Brown et al., 2021; Svejcar et al., 2021; Jarrar et al., 2023). However, acid digestion techniques are currently laborious, costly (e.g., compared to flaming), and present a risk to the technician (Berto et al., 2023). Engineering solutions will be necessary to enable to scaled application of this technique. Combination SET treatments have not yet been adopted at scale as they are poorly studied and the increased cost of application further reduces their appeal (Berto et al., 2021; Erickson et al., 2021; Berto et al., 2024). However, combination SET treatments may become unavoidable in situations where multiple barriers to seed-based restoration exist. In these contexts, applying a particular SET in isolation may achieve little to no effect whereas applying multiple SETs could have additive benefits, as supported by the data in this study. Therefore, the increased investment in applying combination SETs may result in greater returns and restoration success compared to applying a single SET and will continue to be an important consideration in future SET development and uptake.
Data availability statement
The original contributions presented in the study are included in the article/Supplementary Material. Further inquiries can be directed to the corresponding author.
Author contributions
BB: Conceptualization, Data curation, Formal analysis, Methodology, Writing – original draft, Writing – review & editing. AR: Conceptualization, Methodology, Resources, Supervision, Writing – review & editing. TE: Conceptualization, Funding acquisition, Methodology, Project administration, Resources, Supervision, Writing – review & editing.
Funding
The author(s) declare financial support was received for the research, authorship, and/or publication of this article. The authors acknowledge financial support from the Research Training Program (RTP) scholarship, BHP, the Australian Research Council’s Linkage Program (project no. LP 170100075), and the Department of Industry, Innovation and Science’s Global Innovation Linkages grant program (GIL53873).
Acknowledgments
BB thanks the University of Western Australia, Kings Park Science (Department of Biodiversity, Conservation and Attractions), and the Centre for Engineering Innovation: Agriculture and Ecological Restoration (UWA) for providing support and facilities during this PhD research, and the Restoration Seedbank Initiative (BHP Western Australia Iron Ore Community Development Project (contract no. 8 600 048 550) for supplying seed. BB would additionally like to thank E. Stock, and M. Masarei for their contributions to the maintenance of this experiment.
Conflict of interest
The authors declare that the research was conducted in the absence of any commercial or financial relationships that could be construed as a potential conflict of interest.
Publisher’s note
All claims expressed in this article are solely those of the authors and do not necessarily represent those of their affiliated organizations, or those of the publisher, the editors and the reviewers. Any product that may be evaluated in this article, or claim that may be made by its manufacturer, is not guaranteed or endorsed by the publisher.
Supplementary material
The Supplementary Material for this article can be found online at: https://www.frontiersin.org/articles/10.3389/fevo.2024.1405649/full#supplementary-material
References
Alfonzetti M., Tetu S. G., Mills C. H., Gallagher R. V. (2023). Assessing the efficacy of extruded seed pellets and microbial amendments for native revegetation. Restor. Ecol. 31, e13857. doi: 10.1111/rec.13857
Barberis D., Turner S., Pedrini S. (2023). Overcoming germination constraints in seven grass species for seed-based restoration in the Australian monsoonal tropics. Restor. Ecol. 32, e14064. doi: 10.1111/rec.14064
Baughman O. W., Eshleman M. M., Griffen J., Rios R., Boyd C., Kildisheva O. A., et al. (2023). Assessment of multiple herbicide protection seed treatments for seed-based restoration of native perennial bunchgrasses and sagebrush across multiple sites and years. PloS One 18, e0283678. doi: 10.1371/journal.pone.0283678
Baughman O. W., Griffen J., Kerby J., Davies K. W., Clenet D., Boyd C. (2021). Herbicide protection pod technology for native plant restoration: one size may not fit all. Restor. Ecol. 29, e13323. doi: 10.1111/rec.13323
Berto B., Brown V. S. (2022). 10 years to restore the planet: a seedy situation. Restor. Ecol. 31, e13755. doi: 10.1111/rec.13755
Berto B., Erickson T. E., Ritchie A. L. (2020). Flash flaming improves flow properties of mediterranean grasses used for direct seeding. Plants 9, 1699. doi: 10.3390/plants9121699
Berto B., Erickson T. E., Ritchie A. L. (2023). Improving seed morphology and germination potential in Australian native grasses using seed enhancement technologies. Plants 12, 2432. doi: 10.3390/plants12132432
Berto B., Ritchie A. L., Erickson T. (2021). Seed-enhancement combinations improve germination and handling in two dominant native grass species. Restor. Ecol. 29, e13275. doi: 10.1111/rec.13275
Berto B., Ritchie A. L., Erickson T. (2024). The effects of seed enhancements on plant establishment in native grasses: a meta-analysis. Appl. Veg. Sci. 27, e12774. doi: 10.1111/avsc.12774
Beveridge F. C., Williams A., Adkins S. W. (2020). Seed enhancement technologies to improve germination and emergence of Australian native Poaceae. Seed Sci. Res. 30, 293–303. doi: 10.1017/S0960258520000276
Brown V. S., Erickson T. E., Merritt D. J., Madsen M. D., Hobbs R. J., Ritchie A. L. (2021). A global review of seed enhancement technology use to inform improved applications in restoration. Sci. Total Environ. 798, 149096. doi: 10.1016/j.scitotenv.2021.149096
Brown V. S., Ritchie A. L., Stevens J. C., Hanks T. D., Hobbs R. J., Erickson T. E. (2023). Seed positioning in extruded pellets: does it matter? Restor. Ecol. 31, e13784. doi: 10.1111/rec.13784
Cavanagh A. M., Godfree R. C., Morgan J. W. (2019). An awn typology for Australian native grasses (Poaceae). Aust. J. Bot. 67, 309–334. doi: 10.1071/BT18216
Christie G., Horner B., Scanlon A. T., Lemon J., Williams B. (2019). “A ground up approach to revegetation in the arid zone,” in Mine Closure 2019: Proceedings of the 13th International Conference on Mine Closure. Eds. Fourie A. B., Tibbett M. (Australian Centre for Geomechanics, Perth, Western Australia).
Clenet D. R., Davies K. W., Johnson D. D., Kerby J. D. (2019). Native seeds incorporated into activated carbon pods applied concurrently with indaziflam: a new strategy for restoring annual-invaded communities? Restor. Ecol. 27, 738–744. doi: 10.1111/rec.12927
Commander L. E., Merino-Martín L., Elliott C. P., Miller B. P., Dixon K., Stevens J. (2020). Demographic, seed and microsite limitations to seedling recruitment in semi-arid mine site restoration. Plant Soil 457, 113–129. doi: 10.1007/s11104-019-04081-2
Dadzie F., Moles A., Erickson T., Slavich E., Muñoz-Rojas M. (2022). Native bacteria and cyanobacteria can influence seedling emergence and growth of native plants used in dryland restoration. J. Appl. Ecol. 59, 2983–2992. doi: 10.1111/1365-2664.14293
Davies K. W., Boyd C. S., Madsen M. D., Kerby J., Hulet A. (2018). Evaluating a seed technology for sagebrush restoration across an elevation gradient: support for bet hedging. Rangeland Ecol. Manage. 71, 19–24. doi: 10.1016/j.rama.2017.07.006
Donovan C., Eshleman M. M., Riginos C. (2023). Delayed seeding and nutrient amendment seed enhancement technology: potential to improve sagebrush establishment? Restor. Ecol. 32, e14046. doi: 10.1111/rec.14046
EPA (2014). “Cumulative environmental impacts of development in the Pilbara region” (Perth, Western Australia: Environmental Protection Authority of Western Australia).
Erickson T., Dwyer J. M., Dalziell E. L., James J. J., Munoz-Rojas M., Merritt D. (2023). Unpacking the recruitment potential of seeds in reconstructed soils and varying rainfall patterns. Aust. J. Bot. 71, 353–370. doi: 10.1071/BT22141
Erickson T., Kildisheva O., Baughman O., Breed M., Ruiz-Talonia L., Brown V., et al. (2021). “Florabank Guidelines Module 12 - Seed Enhancement Technologies,” in Florabank Guidelines – best practice guidelines for native seed collection and use, 2nd edn. Ed. Commander L. E. (Florabank Consortium, Australia).
Erickson T. E., Munoz-Rojas M., Guzzomi A. L., Masarei M., Ling E., Bateman A. M., et al. (2019). “A case study of seed-use technology development for Pilbara mine site rehabilitation,” in Proceedings of the 13th International Conference on Mine Closure. Eds. Fourie A. B., Tibbett M. (Australian Centre for Geomechanics, Perth, Australia).
Erickson T. E., Muñoz-Rojas M., Kildisheva O., Stokes B., A. White S., L. Heyes J., et al. (2017). Benefits of adopting seed-based technologies for rehabilitation in the mining sector: A Pilbara perspective. Aust. J. Bot. 65, 646–660. doi: 10.1071/BT17154
Eshleman M. M., Riginos C. (2023). Potential to improve wyoming big sagebrush establishment with a root-enhancement seed technology. Rangeland Ecol. Manage. 88, 143–151. doi: 10.1016/j.rama.2023.03.002
Ferguson S. D., Leger E. A., Li J., Nowack R. S. (2015). Natural selection favors root investment in native grasses during restoration of invaded fields. J. Arid Environ. 116, 11–17. doi: 10.1016/j.jaridenv.2015.01.009
Fort F., Jouany C., Cruz P. (2013). Root and leaf functional trait relations in Poaceae species: implications of differing resource-acquisition strategies. J. Plant Ecol. 6, 211–219. doi: 10.1093/jpe/rts034
Gibson-Roy P., Hancock N., Broadhurst L., Driver M. (2021a). Australian native seed sector characteristics and perceptions indicate low capacity for upscaled ecological restoration: insights from the Australian Native Seed Report. Restor. Ecol. 29, e13428. doi: 10.1111/rec.13428
Gibson-Roy P., Zwiersen T., Millsom D. A., Driver M., Berryman T., Logie S., et al. (2021b). “FloraBank Guidelines Module 14 - Direct Seeding,” in Florabank Guidelines – best practice guidelines for native seed collection and use, 2nd edn. Ed. Commander L. E. (Florabank Consortium, Australia).
Gornish E., Arnold H., Fehmi J. (2019). Review of seed pelletizing strategies for arid land restoration. Restor. Ecol. 27, 1206–1211. doi: 10.1111/rec.13045
Guzzomi A. L., Erickson T. E., Ling K. Y., Dixon K. W., Merritt D. J. (2016). Flash flaming effectively removes appendages and improves the seed coating potential of grass florets. Restor. Ecol. 24, S98–S105. doi: 10.1111/rec.12386
Hoose B. W., Call R. S., Bates T. H., Anderson R. M., Roundy B. A., Madsen M. D. (2019). Seed conglomeration: a disruptive innovation to address restoration challenges associated with small-seeded species. Restor. Ecol. 27, 959–965. doi: 10.1111/rec.12947
James J. J., Sheley R. L., Leger E. A., Adler P. B., Hardegree S. P., Gornish E. S., et al. (2019). Increased soil temperature and decreased precipitation during early life stages constrain grass seedling recruitment in cold desert restoration. J. Appl. Ecol. 56, 2609–2619. doi: 10.1111/1365-2664.13508
James J. J., Svejcar T. J., Rinella M. J. (2011). Demographic processes limiting seedling recruitment in arid grassland restoration. J. Appl. Ecol. 48, 961–969. doi: 10.1111/jpe.2011.48.issue-4
Jarrar H., El-Keblawy A., Ghenai C., Abhilash P. C., Bundela A. K., Abideen A., et al. (2023). Seed enhancement technologies for sustainable dryland restoration: Coating and scarification. Sci. Total Environ. 904, 161150. doi: 10.1016/j.scitotenv.2023.166150
Kelly K. M., Van Staden J. (1985). Effect of acid scarification on seed coat structure, germination and seedling vigour of Aspalathus linearis. J. Plant Physiol. 121, 37–45. doi: 10.1016/S0176-1617(85)80089-4
Kildisheva O. A., Dixon K. W., Silveira F. A. O., Chapman T., Di Sacco A., Mondoni A., et al. (2020). Dormancy and germination: making every seed count in restoration. Restor. Ecol. 28, S256–S265. doi: 10.1111/rec.13140
Kneller T., Harris R. J., Bateman A., Muñoz-Rojas M. (2018). Native-plant amendments and topsoil addition enhance soil function in post-mining arid grasslands. Sci. Total Environ. 621, 744–752. doi: 10.1016/j.scitotenv.2017.11.219
Lamb D., Erskine P. D., Fletcher A. (2015). Widening gap between expectations and practice in Australian minesite rehabilitation. Ecol. Manage. Restor. 16, 186–195. doi: 10.1111/emr.12179
Larson J. E., Anacker B. L., Wanous S., Funk J. L. (2020). Ecological strategies begin at germination: Traits, plasticity and survival in the first 4 days of plant life. Funct. Ecol. 34, 968–979. doi: 10.1111/1365-2435.13543
Lazarides M. (2002). “Economic attributes of Australian grasses,” in Flora of Australia, Volume 43 Poaceae 1: Introduction and Atlas. (Melbourne, Australia: CSIRO).
Leger E. A., Atwater D. Z., James J. J. (2019). Seed and seedling traits have strong impacts on establishment of a perennial bunchgrass in invaded semi-arid systems. J. Appl. Ecol. 56, 1343–1354. doi: 10.1111/1365-2664.13367
Lewandrowski W., Erickson T. E., Dalziell E. L., Stevens J. C. (2018). Ecological niche and bet-hedging strategies for Triodia (R.Br.) seed germination. Ann. Bot. 121, 367–375. doi: 10.1093/aob/mcx158
Lewandrowski W., Erickson T. E., Dixon K. W., Stevens J. C. (2017). Increasing the germination envelope under water stress improves seedling emergence in two dominant grass species across different pulse rainfall events. J. Appl. Ecol. 54, 997–1007. doi: 10.1111/1365-2664.12816
Lieurance P., Mills C., Tetu S., Gallagher R. (2024). Putting seed traits into pellets: using seed mass data to improve seed encapsulation technology for native plant revegetation. J. Appl. Ecol. 61, 847–858. doi: 10.1111/1365-2664.14611
Ling E., Guzzomi A. L., Merritt D. J., Renton M., Erickson T. E. (2019). “Flash flaming technology shows promise to improve seed-based rehabilitation outcomes,” in Proceedings of the 13th International Conference on Mine Closure. Eds. Fourie A. B., Tibbett M. (Australian Centre for Geomechanics, Perth).
Ling E., Masarei M., Guzzomi A., Merritt D., Renton M., Erickson T. (2022). Flash flaming is a valid seed enhancement for a diverse range of species and seed morphologies. Seed Sci. Technol. 50, 387–405. doi: 10.15258/sst.2022.50.3.10
Loch D. S. (1993). Improved handling of chaffy grass seeds: options, opportunities and value. Trop. Grasslands 27, 314–326.
Loch D. S., Johnston P. W., Jensen T. A., Harvey G. L. (1996). Harvesting, processing, and marketing Australian native grass seeds. New Z. J. Agric. Res. 39, 591–599. doi: 10.1080/00288233.1996.9513218
Madsen M. D., Davies K. W., Boyd C. S., Kerby J. D., Svejcar T. J. (2016). Emerging seed enhancement technologies for overcoming barriers to restoration. Restor. Ecol. 24, S77–S84. doi: 10.1111/rec.12332
Madsen M. D., Svejcar L., Radke J., Hulet A. (2018). Inducing rapid seed germination of native cool season grasses with solid matrix priming and seed extrusion technology. PloS One 13, e0204380. doi: 10.1371/journal.pone.0204380
Masarei M., Astfalck L. C., Guzzomi A. L., Merritt D. J., Erickson T. E. (2020). Soil rock content influences the maximum seedling emergence depth of a dominant arid zone grass. Plant Soil 450, 497–509. doi: 10.1007/s11104-020-04493-5
Masarei M., Guzzomi A. L., Merritt D. J., Erickson T. E. (2019). Factoring restoration practitioner perceptions into future design of mechanical direct seeders for native seeds. Restor. Ecol. 27, 1251–1262. doi: 10.1111/rec.13001
Masarei M. I., Erickson T. E., Merritt D. J., Hobbs R. J., Guzzomi A. L. (2021). Engineering restoration for the future. Ecol. Eng. 159, 106103. doi: 10.1016/j.ecoleng.2020.106103
Merritt D. J., Dixon K. W. (2011). Restoration seed banks — a matter of scale. Science 332, 424–425. doi: 10.1126/science.1203083
Merritt D. J., Turner S. R., Clarke S., Dixon K. W. (2007). Seed dormancy and germination stimulation syndromes for Australian temperate species. Aust. J. Bot. 55, 336–344. doi: 10.1071/BT06106
Muñoz-Rojas M., Chilton A., Liyanage G., Erickson T., Merritt D., Neilan B., et al. (2018). Effects of indigenous soil cyanobacteria on seed germination and seedling growth of arid species used in restoration. Plant Soil 429, 91–100. doi: 10.1007/s11104-018-3607-8
Muñoz-Rojas M., Erickson T., Martini D., Dixon K., Merritt D. (2016). Climate and soil factors influencing seedling recruitment of plant species used for dryland restoration. SOIL 2, 287–298. doi: 10.5194/soil-2-287-2016
Munro T. P., Ritchie A. L., Erickson T. E., Nimmo D. G., Price J. N. (2023). Activated carbon seed technologies provide some protection to seedlings from the effects of post-emergent herbicides. Restor. Ecol. 31, e13875. doi: 10.1111/rec.13875
Pedrini S., Balestrazzi A., Madsen M. D., Bhalsing K., Hardegree S. P., Dixon K. W., et al. (2020). Seed enhancement: getting seeds restoration-ready. Restor. Ecol. 28, S266–S275. doi: 10.1111/rec.13184
Pedrini S., Dixon K. W. (2020). International principles and standards for native seeds in ecological restoration. Restor. Ecol. 28, S286–S303. doi: 10.1111/rec.13155
Pedrini S., Lewandrowski W., Stevens J. C., Dixon K. W. (2019). Optimising seed processing techniques to improve germination and sowability of native grasses for ecological restoration. Plant Biol. 21, 415–424. doi: 10.1111/plb.12885
R Core Team (2023). “R: A language and environment for statistical computing” (Vienna, Austria: R Foundation for Statistical Computing).
Rezasoltani S., Champagne P., Mann V. (2023). Improvement in mine tailings biophysicochemical properties by means of cyanobacterial inoculation. Waste Biomass Valorization 15, 1689–1699. doi: 10.1007/s12649-023-02195-4
Richardson W. C., Badrakh T., Roundy B. A., Aanderud Z. T., Petersen S. L., Allen P. S., et al. (2019). Influence of an abscisic acid (ABA) seed coating on seed germination rate and timing of Bluebunch Wheatgrass. Ecol. Evol. 9, 7438–7447. doi: 10.1002/ece3.5212
Roman J. R., Roncero-Ramos B., Chamizo S., Rodriguez-Caballero E., Canton Y. (2018). Restoring soil functions by means of cyanobacteria inoculation: Importance of soil conditions and species selection. Land Degradation Dev. 29, 3184–3193. doi: 10.1002/ldr.3064
Schultz N. L., Sluiter I. R. K., Allen G. G., MaChado-de-Lima N. M., Munoz-Rojas M. (2022). Biocrust amendments to topsoils facilitate biocrust restoration in a post-mining arid environment. Front. Microbiol. 13. doi: 10.3389/fmicb.2022.882673
Shackelford N., Miller B., Erickson T. (2018). Restoration of open-cut mining in semi-arid systems: A synthesis of long-term monitoring data and implications for management. Land Degradation Dev. 29, 944–1004. doi: 10.1002/ldr.2746
Shackelford N., Paterno G. B., Winkler D. E., Erickson T. E., Leger E. A., Svejcar L. N., et al. (2021). Drivers of seedling establishment success in dryland restoration efforts. Nat. Ecol. Evol. 5, 1283–1290. doi: 10.1038/s41559-021-01510-3
Stevens J., Chivers I., Symons D., Dixon K. (2015). Acid-digestion improves native grass seed handling and germination. Seed Sci. Technol. 43, 313–317. doi: 10.15258/sst.2015.43.2.19
Stock E., Standish R. J., Muñoz-Rojas M., Bell R. W., Erickson T. E. (2020). Field-deployed extruded seed pellets show promise for perennial grass establishment in arid zone mine rehabilitation. Front. Ecol. Evol. 8. doi: 10.3389/fevo.2020.576125
Svejcar L. N., Brown V. S., Ritchie A. L., Davies K. W., Svejcar T. J. (2021). A new perspective and approach to ecosystem restoration: a seed enhancement technology guide and case study. Restor. Ecol. 30, e13615. doi: 10.1111/rec.13615
Vaughn K. J., Young T. P. (2015). Short-term priority over exotic annuals increases the initial density and longer-term cover of native perennial grasses. Ecol. Appl. 25, 791–799. doi: 10.1890/14-0922.1
Waters C., Loch D., Johnston P. (1997). The role of native grasses and legumes for land revegetation in central and eastern Australia with particular reference to low rainfall areas. Trop. Grasslands 31, 304–310.
Keywords: acid digestion, dryland restoration, seed enhancement technologies, extruded pellets, flash flaming, seed-based restoration, topsoil
Citation: Berto B, Ritchie AL and Erickson TE (2024) Seed enhancements to improve direct-seeding outcomes for native grasses in mine restoration. Front. Ecol. Evol. 12:1405649. doi: 10.3389/fevo.2024.1405649
Received: 23 March 2024; Accepted: 10 June 2024;
Published: 04 July 2024.
Edited by:
Ajay Sharma, Auburn University, United StatesReviewed by:
Akasha May Faist, University of Montana, United StatesOlga A. Kildisheva, The Nature Conservancy, United States
Copyright © 2024 Berto, Ritchie and Erickson. This is an open-access article distributed under the terms of the Creative Commons Attribution License (CC BY). The use, distribution or reproduction in other forums is permitted, provided the original author(s) and the copyright owner(s) are credited and that the original publication in this journal is cited, in accordance with accepted academic practice. No use, distribution or reproduction is permitted which does not comply with these terms.
*Correspondence: Bianca Berto, bianca.berto@research.uwa.edu.au