- 1Institute for Anatomy I, Medical Faculty and University Hospital Düsseldorf, Heinrich-Heine-University, Düsseldorf, Germany
- 2Institute of Animal Welfare and Animal Husbandry, Friedrich-Loeffler-Institut Celle, Federal Institute of Animal Health, Celle, Germany
- 3Institute of Veterinary Pathology, Faculty of Veterinary Medicine, Leipzig University, Leipzig, Germany
- 4Bruno Duerigen Institute, Scientific Poultry Yard, Rommerskirchen, Germany
Introduction: Crested chickens show abnormalities in their anatomy of the skull, endocranium, and brain (including cerebral elongation) and can be appropriate model systems for neuroanatomical evolution, brain–skull integration, and skull and brain deformities. Here, we give a detailed comprehensive description of the skull of crested chickens using the example of the Padovana chicken, including ontogenetic aspects and an allometric analysis of their brain size.
Methods: In total, 109 chickens of two different strains of the Padovana chicken were hatched together. All animals were X-rayed weekly during growth. Nine juvenile (ready for hatch) and 22 adult skulls were processed for histology and morphological descriptions, and a further 20 individuals were processed for brain analysis.
Results: At hatching, all chicks were already crested, and a distinctive bony protuberance was first observed at the age of 4 weeks. Juvenile chickens exhibit either an open neurocranium or a protuberance. In the adult skull, foramina of different sizes can be found in the frontal bone, but no completely open neurocrania are observed in juveniles. Particularly in Padovana with cranial protuberances, several peculiarities can be observed in the os mesethmoidale, os nasale, os praemaxillare, orbit, and cranial fossae. Additionally, the brain of Padovana with cranial protuberances looks drawn in length with the shape of an hourglass and showed significantly larger encephalization indices than plain-headed breeds, topped only by another crested chicken breed.
Discussion: Investigations on chickens with cerebral elongation may facilitate the understanding of skull and brain dysplasia and may provide meaningful insights into cerebral hernia development. Additionally, crested breeds, combined with standard chickens, form a promising comparative system for investigating the emergence of novel brain and skull morphologies.
1 Introduction
Domestic chickens are spread all over the world and are the most widely used poultry species of all. In the course of domestication, many different chicken breeds have emerged, and in addition to the commercially used strains with high egg or meat production, there are also many non-commercial breeds that are bred only for the pleasure of their breeder. This so-called fancy poultry shows a high genetic, morphological, and/or behavioral variability. There are breeds with altered feathering (curly feathers, feather crests, foot feathering, and elongated tail feathers), with morphological characteristics (dwarfism, gigantism, shortened legs, rumplessness, and missing or enlarged combs) or with behavioral peculiarities (breeds without breeding instinct, fighting/game breeds, and breeds with special crowing behavior) (Mehlhorn and Rehkämper, 2013). In addition, all of these breeds are available in many different colors, a typical phenomenon of domestication (Sheppy, 2011). Through selective measures, breeders can increase the frequency of carriers of desirable traits within an animal population within a few generations, especially in domesticated birds with their typically short generation cycles. Domestication can serve as a generalized model of evolution, and domestic chickens can be considered as well-adapted to their ecological niche “man” (Rehkämper et al., 2008).
The integument of the head is especially disposed for the formation of feather crests (Herre and Röhrs, 1990), and crested chickens have been popular with poultry fanciers for several hundred years (Hagenbach, 1839). A fully developed crest is typical of several breeds, including Houdans, White Crested Polish (WCP), and Padovana, and is a result of mutation and intense selective breeding. Often, the phenotype shows a degree of sexual dimorphism, with males exhibiting more voluminous crests than females. The bizarre appearance of crested breeds is associated with abnormalities in the anatomy of the skull, endocranium, and brain and has therefore long attracted the attention of scientists and opened a wide field for scientific research under various points of view, e.g., morphogenesis, pathogenesis, and etiology (Mayr, 2018). Before molecular biological methods were developed, it was assumed that the full crest in domestic chickens was encoded by an incompletely dominant autosomal gene (Requate, 1959). In 2012, two studies described the crest phenotype in chickens in detail: first, Wang et al. (2012) focused on Silkie chickens, whose feathers show a hairy structure in combination with a well-developed feather crest but not so extremely elongated feathers as other full-crested breeds. They confirmed that the crest shows an autosomal incompletely dominant mode of inheritance with location on the E22C19W28 linkage group and ectopic expression of HOXC8 in the cranial skin during embryonic development. In addition, they noticed that the crest is usually associated with cerebral hernia. Second, Yoshimura et al. (2012) focused on WCP, described the phenomenon of cerebral hernia, and analyzed the inheritance and ontogenetic process of cerebral hernia in this chicken breed. Their genetic analysis revealed that cerebral hernia is controlled by a single autosomal recessive gene and is closely associated with crest formation. Furthermore, their morphological analysis of brain structures in the progenies showed a significant enlargement of the brain cavity, particularly after 15 days of incubation. The authors suggested that this enlargement at later stages of embryos may be the main cause of cerebral hernia. The manifestation of cerebral hernia in crested chicken should be already distinctive at hatching, and it was observed that the protuberance on the top of the head was ambiguous before 11 days of incubation (E11) and became distinct from E12 onward (Yoshimura et al., 2012). However, among crested chickens, two phenotypes of feather crests can be distinguished: the helmet-shaped, forward-inclining feather crests as, for example, in the Appenzeller Chicken and the full crests as, for example, in the Houdan, WCP, or Padovana (Bartels, 2003). Anomalies of the skull or cerebral hernia can only be observed in full-crested chickens. Here, anomalies of the skull in the form of protuberant and vaulted frontal bones, deformations of the nasal and intermaxillary bones, and other cranial skeletal elements are common and long-known (Pallas, 1767; Sandifort, 1793; Blumenbach, 1812, Hagenbach, 1839, Tegetmeier, 1856; Klatt, 1910; Requate, 1959; Somes, 1990). In 2021, Li et al. used whole-genome sequencing and extended the knowledge of the genetic background again. They revealed that the crest phenotype, and also susceptibility to cerebral hernia, is caused by a 195-bp duplication of an evolutionarily conserved sequence located in the intron of HOXC10 on chromosome 33.
WCP (Figure 1) is still the most astonishing and best-studied example of crested chicken. It has one of the longest histories of pure breeding among chickens and is special in many more aspects than its appearance (Brothwell, 1979; Tiemann and Rehkämper, 2008; Tiemann and Rehkämper, 2009). As in other full-crested breeds, the feather crest of WCP is placed on a bony protuberance of frontal bones. Mostly, this protuberance is not completely calcified and can be, if it is very distinct, comparable to that in a human exencephalocele (Figure 1). It has already been shown that the external morphology of its brain under the distinctively shaped skull is different from that of other chicken breeds (Frahm and Rehkämper, 1998; Rehkämper et al., 2003; Yoshimura et al., 2012; Mehlhorn and Caspers, 2020; Watanabe et al., 2023). The forebrain and the hindbrain seemed to be more separated from each other, and the cerebral hemispheres were extruded into the anterodorsal region of the skull, giving the brain the shape of an hourglass. Endocranial reconstructions based on micro-CT images showed that WCP brains differ from White Leghorn chicken brains in they possess a larger cerebrum and smaller cerebellum and medulla (Watanabe et al., 2023). Allometric comparison of WCP brains and brain structures even revealed that the size of the brain and major brain regions are significantly altered compared to that of several uncrested domestic chicken breeds (Frahm and Rehkämper, 1998). Additionally, WCP shows peculiarities in brain composition and behavior (Rehkämper et al., 2003; Tiemann and Rehkämper, 2009). This was confirmed by Tao et al. (2020). However, it has to be considered that the latter investigated a chicken model of cerebral hernia consisting of chickens with cerebral hernia phenotype without specifying the origin or the breed of these chickens. Additionally, it has to be considered that the term “cerebral hernia” is not used uniformly and is not clearly defined. Most authors designate the upthrusting of cerebral hemispheres into the anterodorsal region of the skull as cerebral hernia (e.g., Yoshimura et al., 2012), whereas Tao et al. (2020) emphasized that it is the protrusion of cerebral hemispheres into the unsealed skull since the skull is often incompletely calcified (often caused by the unsealed frontal skull combined with misplaced sphenoid bone).
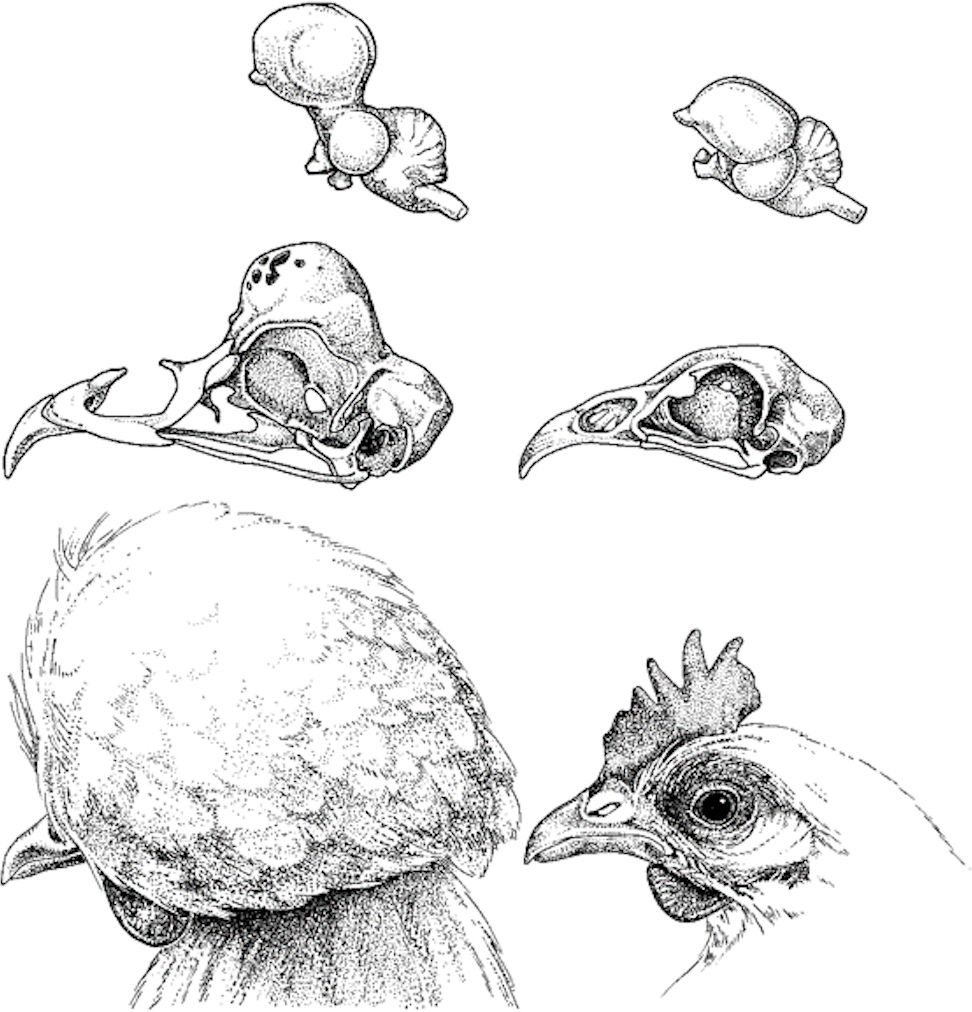
Figure 1 Portraits of skull and brain of a White Crested Polish (left) and a crestless laying hen (right).
Verdiglione and Rizzi (2018) conducted a detailed morphological study on the skull of the Padovana chicken, another crested chicken breed that often shows cranial protuberances and originates from Italy. Next to an upthrusted brain and enlarged frontal bones, the authors also observed two more cranial variations in the nasal and pre-maxilla bones. The frontal processes of the nasal bone are quite large, and the nasal processes of the pre-maxilla are not joined together to constitute a single bone tissue along the dorsal beak’s middle line but leave an open space. Consequently, the nostrils are partially without bone support and are placed rather high on the beak profile, looking wide, flat, and elastic. Generally, due to the presence of the bony protuberance, the height of the skull, frontal bone height, and frontal bone height/skull width ratio were higher than those in an uncrested chicken or crossbreeds between crested and uncrested chickens (Verdiglione and Rizzi, 2018). Tao et al. (2020) also described that the location of the sphenoid bone changed from a moderate angle to the horizon in wild-type chickens to a nearly vertical angle in chickens with cerebral hernia. The alteration of the sphenoid bone lifted the upper telencephalon and was possibly the major cause of the distinctive external brain morphology of crested chickens. Additionally, Tao et al. (2020) showed that the number and density of neurons, their appearance, and the size and shape of the specified regions in the telencephalon were significantly changed in chickens with cerebral hernia. Although these studies give a good insight into the skull and brain morphology of crested chicken, there is still a need for more research. Particularly, the ontogeny of cerebral hernia provides open questions, and it is not yet clear whether the situation is the same in crested chickens without cranial protuberances. Additionally, investigations on chickens with cerebral hernia may facilitate the understanding of skull and brain dysplasia and may provide meaningful insights into cerebral hernia development. Crested chickens can be appropriate model systems for brain and skull evolution, integration, and particularly deformities.
Thus, we would like to give a detailed anatomical description of crested chicken with and without cerebral hernia and a short insight into the recent discussion of how this morphological curiosity may develop. For the detailed description, we analyzed two different colorings of the crested chicken breed Padovana (Figure 2), which are known to exhibit different skull characteristics. Black-colored Padovana chickens are known for their distinct bony protuberance, and chamois-colored Padovana chickens show a similar-sized crest but with inconspicuous cranial protuberances. To complete the picture, we also examined their brains and investigated whether the brain sizes of chickens with and without cerebral hernia differ from those of other chicken breeds. We analyzed both crested and uncrested breeds and also specimens of the red junglefowl, the common ancestor of all domesticated chickens.
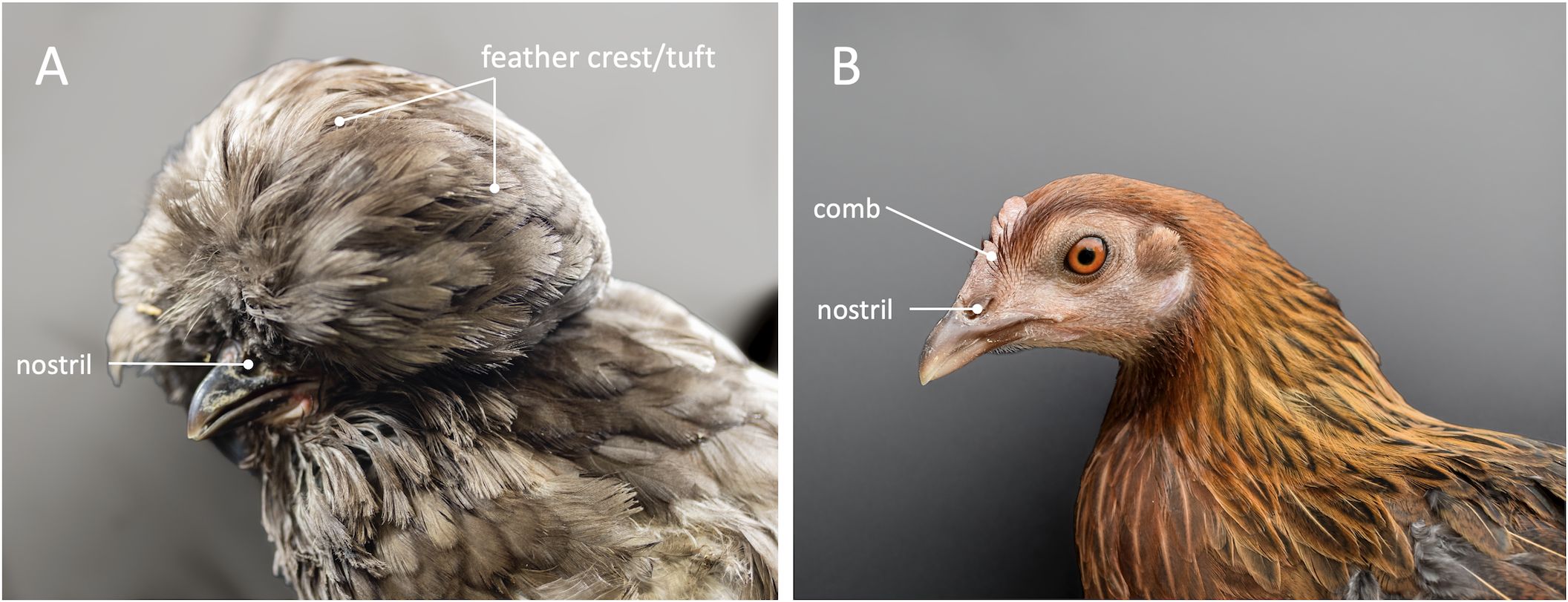
Figure 2 Portraits of a hen of the crested chicken breed Padovana (A) and a hen of the uncrested red junglefowl breed (B).
2 Materials and methods
2.1 Animals
In total, 177 eggs of two different strains of Padovana chickens (98 black-colored Pblack and 79 chamois-colored Pcham, Gallus gallus f.d.) of four different breeders were hatched using a common incubator (Petersime Vision Type 96, Zulte, Belgium). Padovana chickens originated approximately 500 years ago in Italy, and the first Padovana arrived in Germany at the end of the 19th century via Italy and the Netherlands (Schmidt and Proll, 2020). Both colors are widespread and popular with German fancy poultry breeders and show distinct crests.
The number of fertilized eggs, hatched chicks, and unhatched chickens was recorded. Eggs that did not hatch were opened 3 days after the end of hatching time, and the habitus and skull of extant embryos were investigated. Additionally, nine of these unhatched chicks were fixed in formaldehyde solution and histologically processed with hematoxylin and eosin (H&E) staining (see Section 2.2). Hatched chickens were reared all together in an indoor pen littered with wood shaving at the Friedrich-Loeffler-Institut, Institute of Animal Welfare and Animal Husbandry. After reaching the age of 6 weeks, they were allowed outside during the day. Commercial chick feed was used until their sixth week of life, and then commercial pullet feed and water were supplied ad libitum. All hatched individuals were X-rayed once a week from the 4th to 14th weeks of life (see Section 2.3). After they were fully grown, six of the animals were randomly selected for further examinations (see below), and the majority of the other animals were given to breeders. A total of 16 animals (seven Pblack and nine Pcham, all males) remained, were X-rayed again, and then also processed for histology. Morphological skull descriptions were executed on six adult Padovana chickens (Pblack, one male and one female; Pcham, one male and three females) and two (female) individuals of red junglefowl. They were based on mediosagittal views, macerated skulls (see Section 2.4), and the abovementioned X-ray images. Red junglefowl hens originated in the Poultry Research Center, Rommerskirchen, and correspond as close as possible to the wild type of red junglefowl (e.g., just one to two clutches of eggs per year, hen feathering of the cock in the non-breeding season). Additionally, a macerated skull of a WCP cock of the Poultry Research Center, Rommerskirchen, was included in the analysis for comparative purposes.
For the allometric approach (see below), a further 20 individuals of Padovana (Pblack, six males and six females; Pcham, four males and four females) and 11 individuals of red junglefowl (five males and six females) were used (see Section 2.5).
All applicable international, national, and/or institutional guidelines for the care and use of animals were followed. The study was approved by the Committee on the Ethics of Animal Experiments of the state of Lower Saxony, Germany (AZ 33.19-42502-05-20A499) and of North Rhine-Westphalia, Germany (O/51/1999).
2.2 Histology
The formaldehyde-fixed skulls of either complete hatchlings (n = 9) or skinned adult chickens (seven Pblack and nine Pcham, all males) were sliced into 10 coronal sections using a water-cooled bandsaw equipped with a 0.2-mm diamond saw band (E300, EXAKT Advanced Technologies GmbH, Norderstedt, Germany). The slices were decalcified using an EDTA-based decalcifying solution (Osteosoft, Merck KGaA, Darmstadt, Germany), automatically dehydrated in a graded series of alcohol (Donatello, Diapath, Martinengo, Italy), and embedded in paraffin (Paraplast, Engelbrecht, Edermünde, Germany). The paraffin-embedded tissues were cut into 3–4-µm thin slices using a sliding microtome (SM2010 R, Leica, Wetzlar, Germany). The sections were mounted on glass slides and stained with H&E, using the staining protocol of Romeis et al. (2010). All slides were scanned using a slide scanner (Axioscan 7, Carl Zeiss Microscopy, Jena, Germany) equipped with a ×20 magnifying objective (Plan-Apochromat 20x/0.45, Carl Zeiss Microscopy, Jena, Germany) and examined histologically using ZEISS ZEN 3.4 blue edition software (Carl Zeiss Microscopy GmbH, Jena, Germany). It was recorded whether a cranial protuberance was present and additionally the number and size of cranial foramina. The proportion of animals with protuberance was determined using Fisher’s exact test.
2.3 X-ray pictures
All hatched individuals were X-rayed once a week from the 4th to 14th weeks of life.
The X-ray images were taken using the X-ray generator WDT Blueline 1040 HF (Wirtschaftsgenossenschaft Deutscher Tierärzte eG, Garbsen, Germany), the digital flat panel detector Thales Piximum 2430 EZ wireless (Thales Electron Devices S.A., Velizy-Villacoublay, France), and a laptop, which were transported in an X-ray case Leonardo DR mini (Oehm und Rehbein GmbH, Rostock, Germany).
As described by Eusemann et al. (2018), the non-anesthetized chickens were gently placed on their left side on a digital flat-panel detector (Thales Pixium 2430 EZ wireless, Thales Electron Devices S.A., Vélizy-Villacoublay, France). From their 4th to 8th weeks of life, the chicks were wrapped in rolls of paper during the X-rays, as they were too small to be held in place. When they were 9 weeks old until the end of the experiment, they were fixated on the hands of the experimenter. The center of the radiation field was located directly above the center of the crosshairs. The images were recorded at 50.0 kV and 2 mAs for each chicken.
2.4 Osteological analysis of skull morphology
For the maceration process, the heads of the animals were first skeletonized carefully by removing feathers and skin by sparing the beak. Afterward, specimens were lysed in warm water for a minimum of 96 hours before being placed into the maceration solution (5% sodium carbonate decahydrate, Acros Organics, Geel, Belgium) at 35°C–45°C in a heating oven (UE400, Memmert, Schwabach, Germany). After a minimum of 10 days of maceration followed by irrigation in distilled water, the specimens were degreased in ethanol (TechniSolv, VWR Chemicals, Darmstadt, Germany) and dried for further examination. For accurate osteometric measurements of the macerated skulls, a digital caliper with an accuracy of 0.01 mm (Hoffmann Group, Munich, Germany) was used. Up to two specimens per breed were sagittally split using a table band saw (MBS 240/E, Proxxon, Wecker, Luxembourg) for a detailed analysis and a better overview of the inner structures of the chicken skulls.
2.5 Allometric analysis of brain size
For the allometric approach, body weight measures and the brains of 20 Padovana and 11 red junglefowl chickens (see above) were collected and compared with the body and brain data of eight other domestic chicken breeds (n = 80) from our existing avian brain collection. These “fancy” breeds are well-defined according to the German Standard of Perfection (Deutscher Rassegeflügelstandard) and show a long and distinct breeding history. They cover a large range of major groups of domestic chickens: Bantams (Japanese Bantams, Peking Bantams), Mediterranean chickens (Red Leghorns), Crested chickens (WCPs, Bredas), chickens originally used as game birds (Malay), and those with a peculiar phenotype, such as tailless Araucanas and Silkie chickens with silky feathers. All individuals originated from private poultry breeders and were extensively kept in aviaries or gardens and small groups with free-ranging possibilities. We paid attention to a balanced distribution of sexes. Regarding the red junglefowl, we again paid attention to the use of animals that correspond as close as possible to the wild type of red junglefowl (e.g., just one to two clutches of eggs per year, hen feathering of the cock in the non-breeding season). All used Padovana chickens were randomly selected from the abovementioned hatch and equally distributed by sex. After their body weights were determined, individuals were sacrificed by an overdose of pentobarbital (Fagron, Glinde, Germany) and subsequently perfused with saline solution and paraformaldehyde (Merck, Darmstadt, Germany) via the left ventricle. By inspecting the intestines, we ascertained that the animals had not been fattened or showed signs of nutrient deficiency. Brains were removed and weighed immediately (<60 min) to capture the fresh brain weight after perfusion (Stephan et al., 1981).
The difference in body size between Padovana chickens and the other fancy chicken breeds makes an allometric approach necessary when brain sizes are to be compared (Snell, 1892, Dubois, 1897; Harvey, 1988; Stephan et al., 1988; Striedter, 2005). This method includes the calculation of a regression line slope that expresses the brain size/body size relationship. To obtain reliable slopes, the data should originate from a sample that covers a reasonable body weight range and whose individual members are part of a biologically significant group, for example, a taxonomic unit. Both criteria are given with the inclusion of all 111 investigated individuals. There is a reasonable body weight range, and all individuals belong to the taxon G. gallus. The relationship between the brain and body weight is represented best by the following allometric formula:
where y represents the brain weight, b is the intercept of the allometric regression with the abscissa, x is the body weight, and a is the slope of the regression (Snell, 1892). This approach allowed us to compare brain sizes independently of the different body sizes. We compared the fresh brain weight of Padovana chickens to that of all other chickens by carrying out a regression analysis and calculating allometric encephalization indices (EIs). To calculate these indices, we divided the actual brain weight of an individual by its predicted brain mass obtained from the regression (Stephan et al., 1986). All points on the regression line represent an EI of 1.0, so an EI of 2.0 would mean that a brain was twice as heavy as the predicted weight based on the data.
To determine differences in the whole data set, we first applied the Kruskal–Wallis one-way analysis of variance (ANOVA). In the case of significance, we used Student’s t-test to compare EIs or the Mann–Whitney U rank sum test in cases of non-normal distribution. The level of significance was 5%. The software package SigmaPlot/SigmaStat version 12.0 (Systat Software Inc., Chicago, IL, USA) was used for all statistical calculations.
3 Results
Of the Padovana eggs, 75.7% were fertilized (134 of 177), and the hatching rate was 81.3%. Seven chicks died in the first 18 days of incubation (5.2%), and 18 chicks did not hatch at all (13.4%).
3.1 Comparative morphology of the skull and the brain during ontogenesis
At hatching, all chicks were already crested. The X-ray images showed, generally, that Pcham chickens have at most a minor cranial protuberance, both as chicks and as adults, whereas Pblack chickens already have a distinctive protuberance from the age of a few weeks (Figure 3).
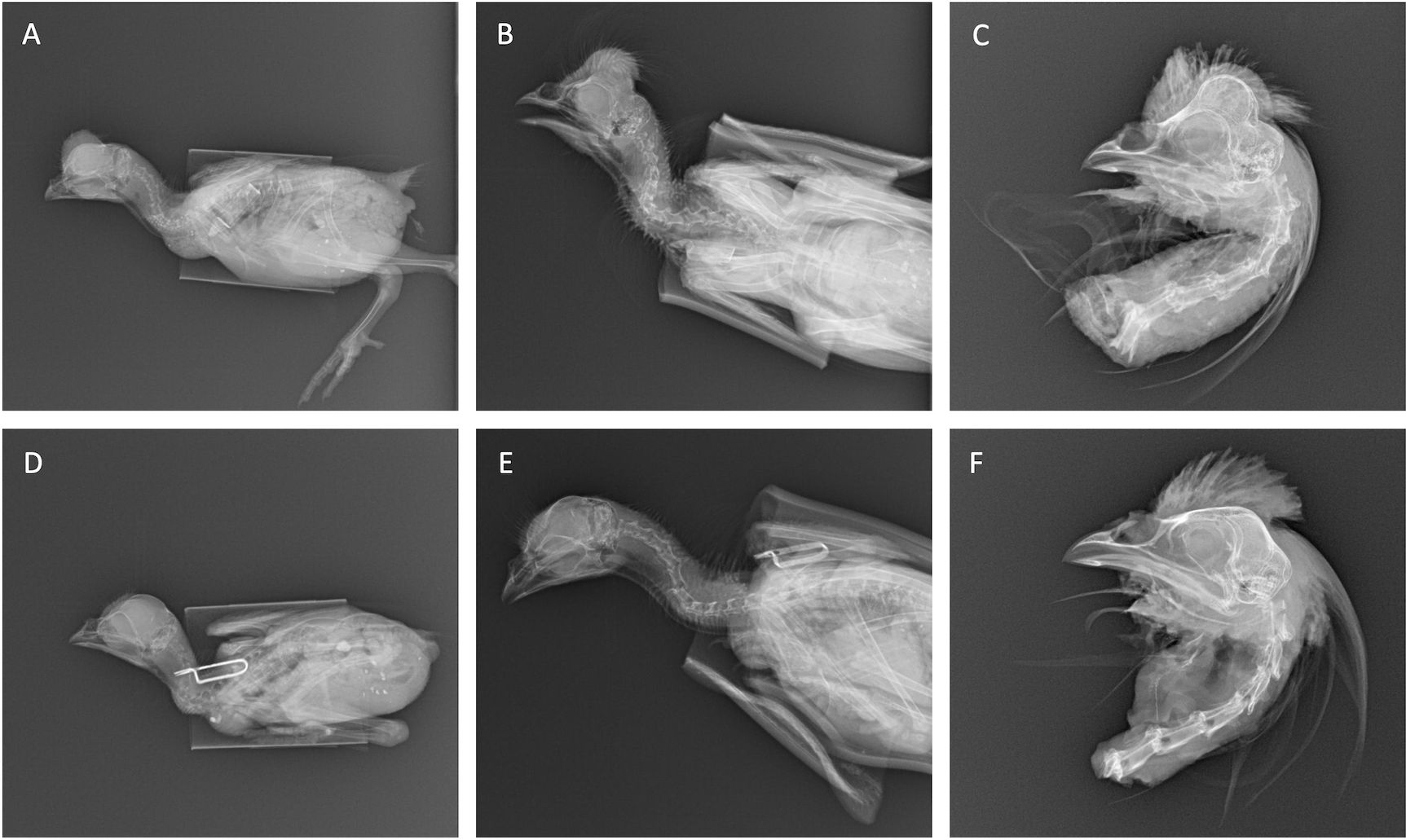
Figure 3 X-ray images of a black-colored (Pblack, A–C) and chamois-colored (Pcham, D–F) Padovana cocks at the age of 5 weeks (A, D), 9 weeks (B, E), and 17 months (C, F). Animals in panels (A, B, D, E) were stuck in a paper roll for fixation during the taking of the radiographs.
Figure 4 shows a mediosagittal view of the skull of (A) a red junglefowl hen and (B) a black-colored Padovana hen with a typical cranial protuberance. The brain of the Padovana hen shows an hourglass shape (Figure 4B). A significantly increased proportion of 81.25% of the analyzed adult Padovana chickens (13 out of n = 16) displayed a cranial protuberance as compared to 0% of the juvenile chickens (Fisher’s exact test, p = 0.0159, Supplementary Table S1). The center of the protuberance was located within a coronal section at the height of the caudal end of the occipital cortical lobes and the midbrain. Macroscopic and histopathologic examinations revealed a thick and complete osseous neurocranium in this location. In general, the cranial protuberances were covered by feathered bonnets, inducing the characteristic exterior of the Padovana lineage (Figure 4B).
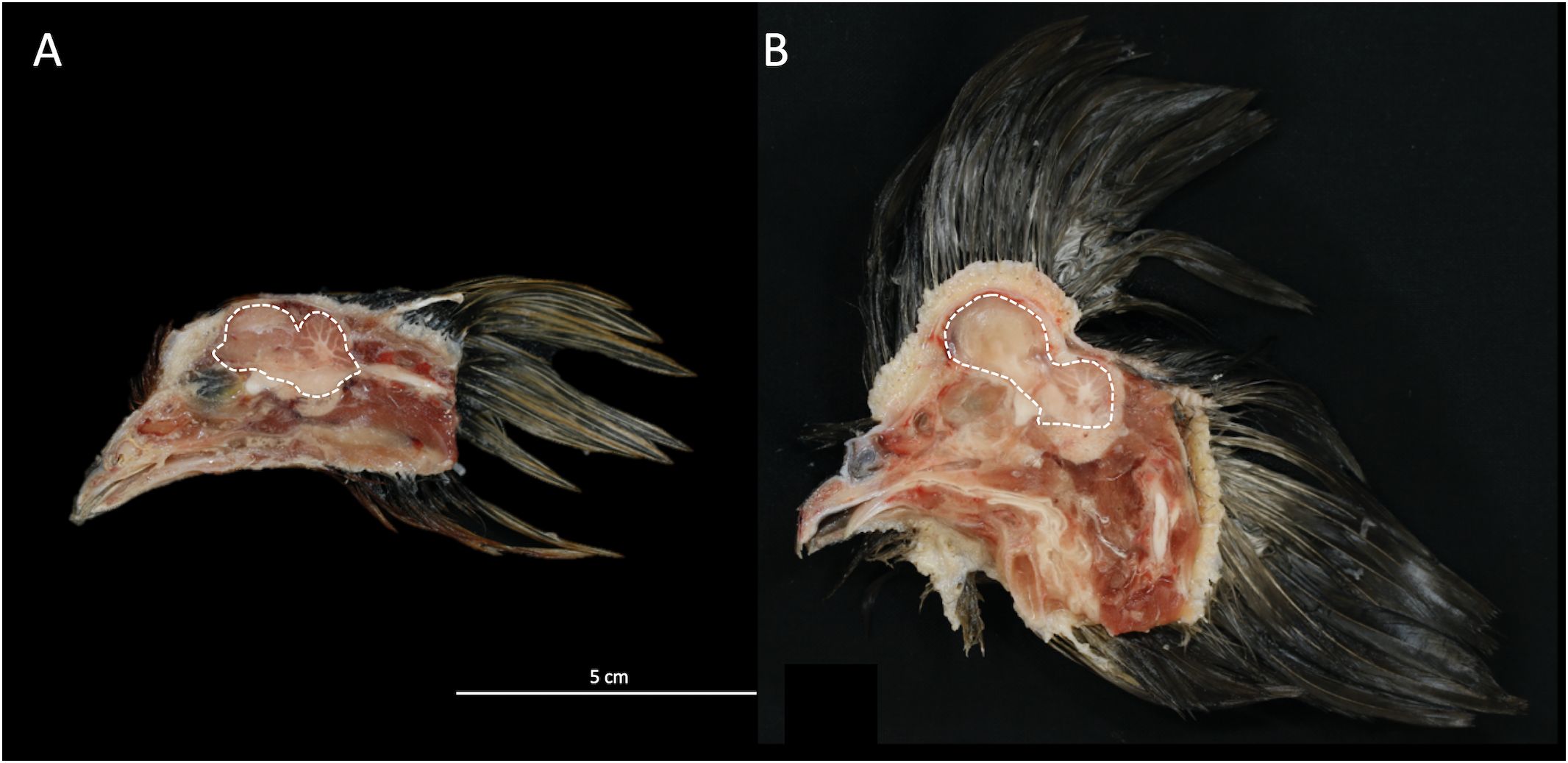
Figure 4 Mediosagittal view of the skull of (A) a red junglefowl hen and (B) a black-colored Padovana hen with cranial protuberance. The white dotted line contours the shape of the brain (telencephalon, mesencephalon, and cerebellum); note the hourglass shape of the Padovana chicken’s brain.
In contrast, a significantly increased proportion of 88.89% of the juvenile chickens showed foramina/fontanella in the cranial vault as compared to 0% of the adults (Fisher’s exact test, p ≤ 0.0001; Figure 5, Supplementary Table S1). The center of the unclosed part of the vault was located in the midline within a coronal section on the height of the cranial frontal cortical lobes and the eyes. In those chickens with open cranial vaults, a maximum diameter of the foramina of 3.413 ± 0.6312 mm was measured.
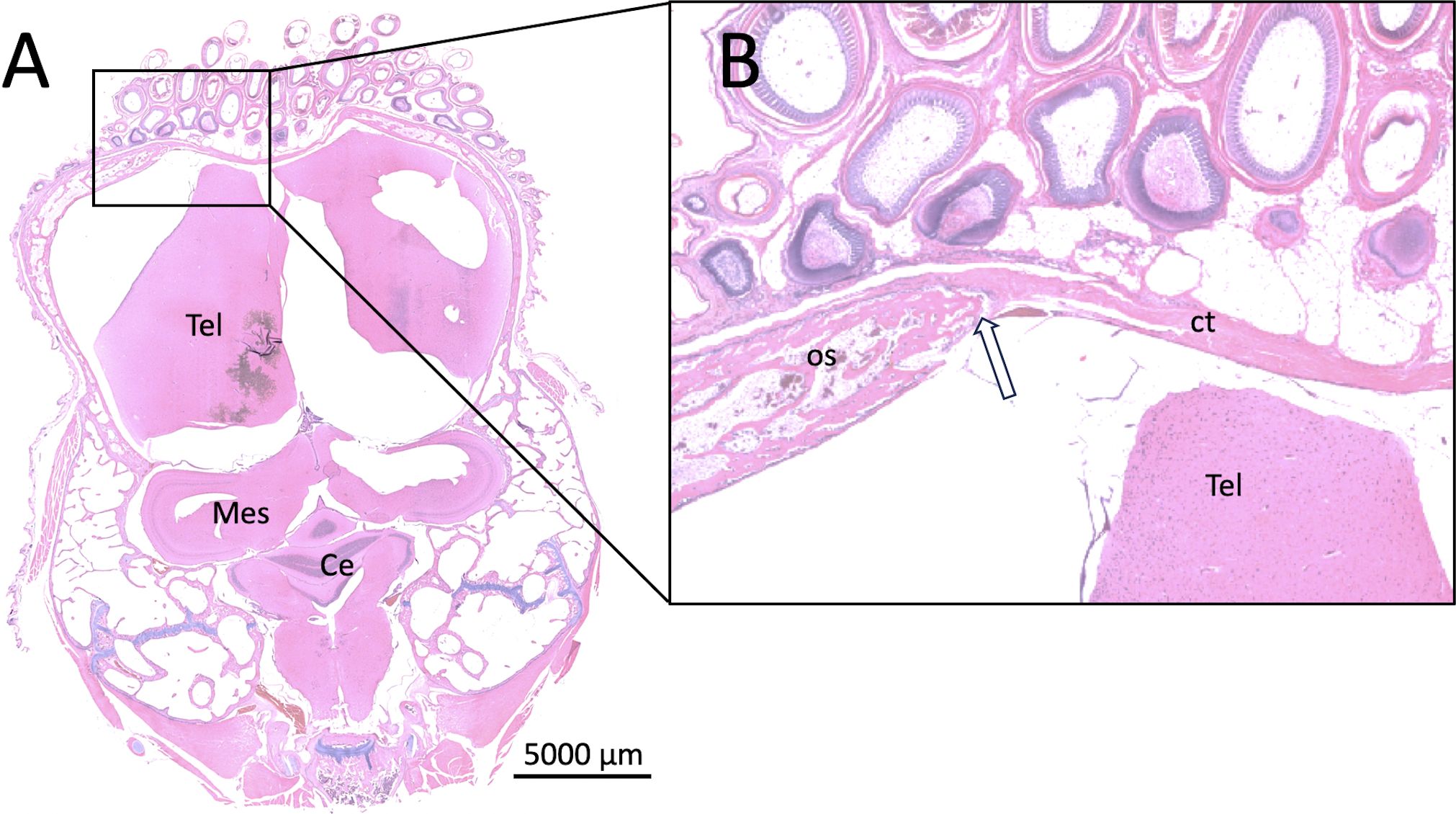
Figure 5 Frontal view of the skull of a Padovana chick at the age of hatching on the level of the optic tectum and the beginning of the cerebellum (A, H&E staining). The cutout (B) shows the open fontanella. Ce, cerebellum; Mes, mesencephalon; Tel, telencephalon; os, ossified part of the frontal bone; ct, connective tissue that closes the developing cranial vault. White arrow indicates transition zone between ossified and non-ossified frontal bone.
Histopathological examination of the skin, subcutis, dura mater, bones, and brain showed no further pathological findings in any of the animals.
3.2 Detailed osteological analysis of skull morphology
The macerated skulls of Padovana chickens were analyzed with a focus on the cranial vault, the area around the orbit, and the rostral part of the upper beak. In the following, osteological findings are listed, which stood out in comparison with red junglefowl and partially with WCP. Our qualitative investigations did not reveal any sex differences.
3.2.1 Os frontale
The most noticeable feature of the skull of both the Padovana chicken and the WCP is the bony dome, also called protuberance. This protuberance is formed by the os frontale, centrally located, and can be very variable in size and expression. It seems to be that the osseous basis of the protuberance depends on the size of the protuberance. Small-sized protuberances show a rather broad-based transition of the rest of the skull into the bony protuberance. In cases of a strong protuberance, a clear boundary of the base is found (Figures 6–9). Furthermore, the overall shape in the cases of strongly developed protuberances is comparable to the shape of a shifted parabola when viewed from the lateral. The protuberance seems to be tilted rather rostrally so that the vertex of the prominence is not centered on the basis (Figures 7–9). As a result, the rostral angle between the protuberance and the anteriorly situated viscerocranium is smaller than the dorsal angle between the protuberance and the rest of the posteriorly situated neurocranium, especially the os parietale.
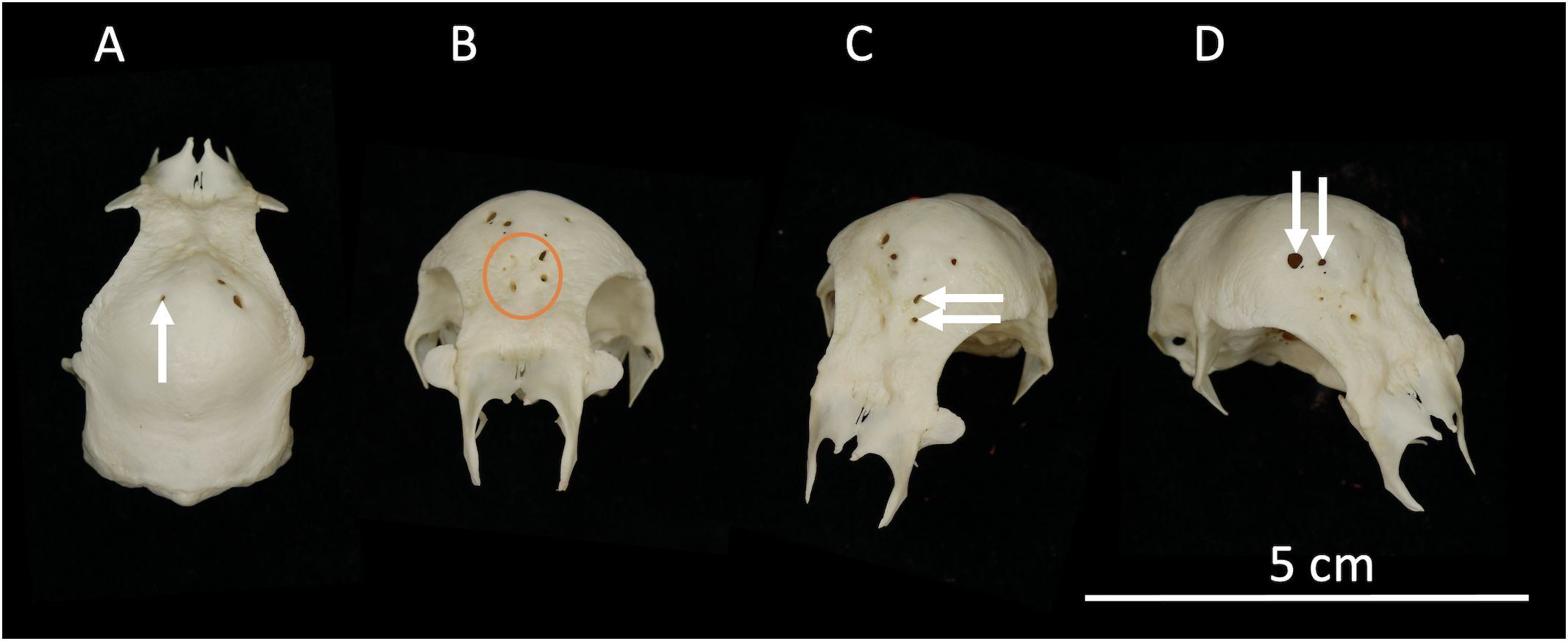
Figure 6 Cranial (A), rostral (B), and rostrolaterocranial (C, D) views on the macerated skull of an adult female chamois-colored Padovana chicken with a moderate cranial protuberance. Note the foramina of different sizes mainly in the rostral region of the cranial protuberance with a maximum diameter of 1.5 mm (arrows). Note also the clustering of smaller foramina at the fusion area between os frontale and os prefrontale (orange circle).
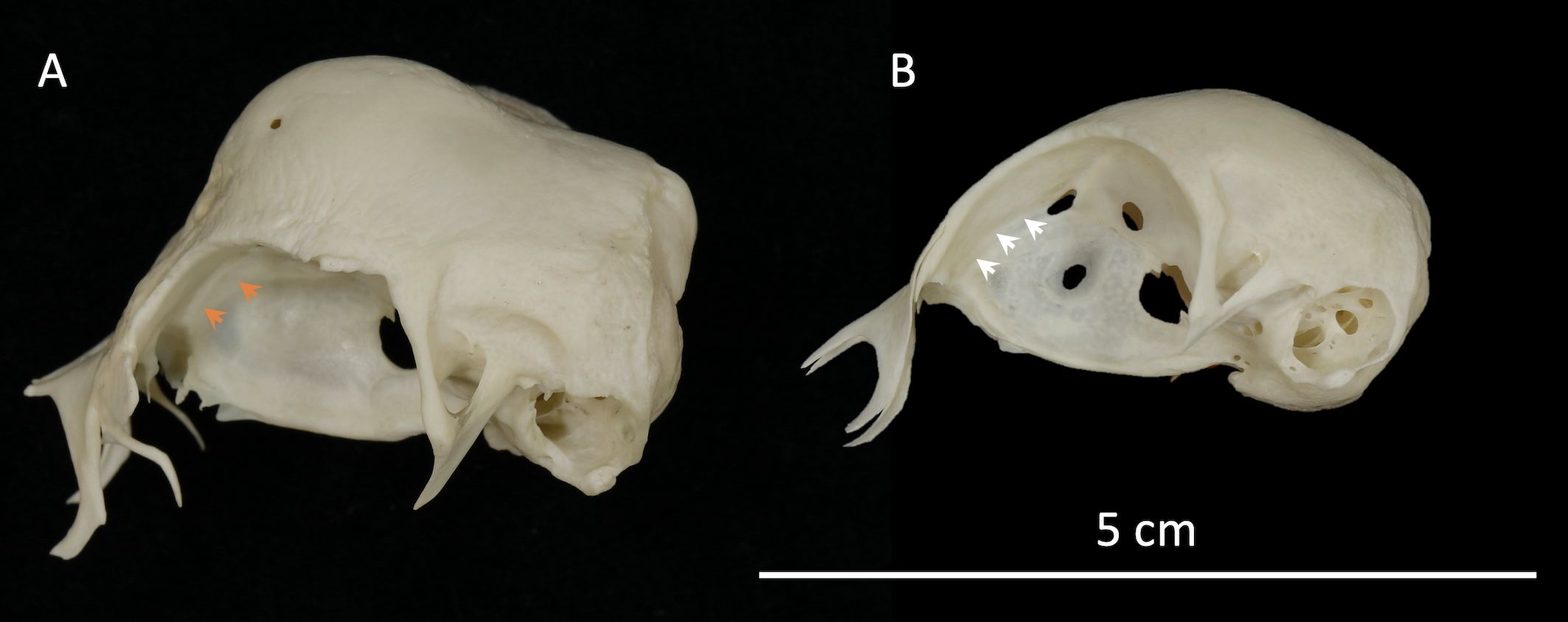
Figure 7 Skull of (A) a chamois-colored Padovana hen with moderate cranial protuberance and (B) a red junglefowl hen in lateral view. Note the strong osseous rostrocranial part of the interorbital septum (lamina dorsalis ossis mesethmoidalis) of the Padovana chicken in (orange arrowheads), forming an osseous strengthening of the mesethmoidal bone. Note also the lesser strong build os mesethmoidale of the red junglefowl (white arrowheads).
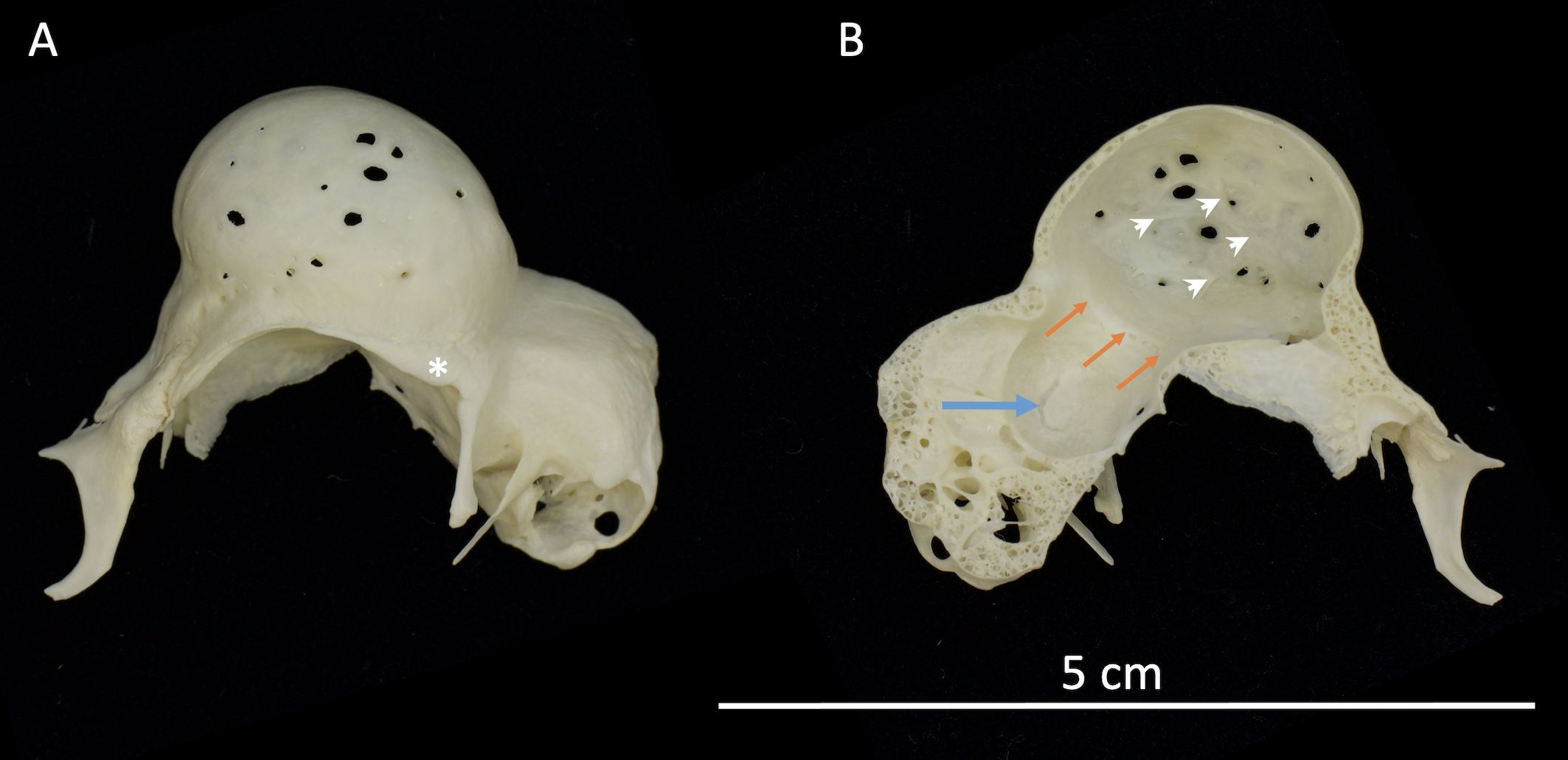
Figure 8 (A) Lateral and (B) medial views of a macerated and sagitally split skull of an adult black-colored Padovana hen. Note the foramina of different sizes, sparing the dorsal region of the cranial protuberance, and the irregularities of the tabula interna between the different foramina (arrowheads). Also note the strong sulcus arteriosus of the middle cranial fossa (blue arrow), the strong osseous ridge dividing the fossa cerebri into a rostral and dorsal part (orange arrows), and the osseous strengthening of the posterocranial supraorbital margin (asterisk).
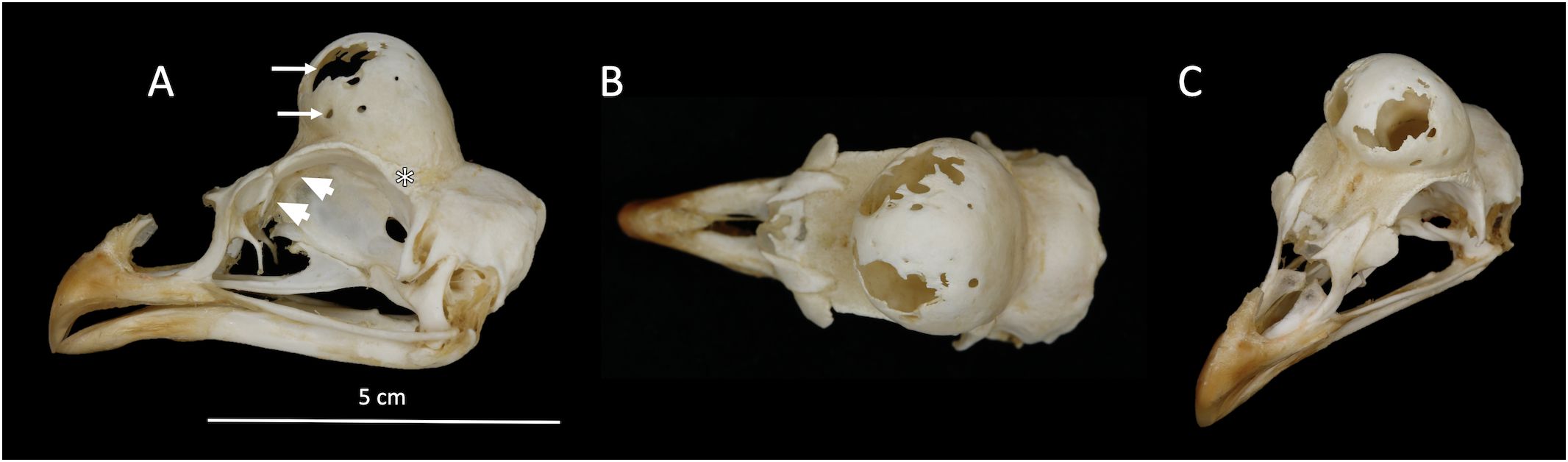
Figure 9 (A) Lateral, (B) cranial, and (C) rostrolaterocranial views of a macerated skull of a White Crested Polish cock. Note the bilateral symmetric position of the extended foramina (arrows) of different sizes, sparing the dorsal region of the cranial protuberance. Take note also of the osseous ridge strengthening the posterocranial supraorbital margin (asterisk) and the strengthened osseous rim of the mesethmoidal bone (arrowheads).
In the mediosagittal view of the Padovana skull, a distinct diploe is visible only in the dorsal region of the frontal prominence (Figure 8B). In the anterior region of the os frontale, the tabulae externa and interna lie directly on each other. This finding is also observed in the red junglefowl, but here, the posterior area of the protuberance without diploe is much shorter, and the anterior part of the frontal bone, which forms the rostral termination of the brain capsule, is again provided with a diploe unlike in the Padovana chicken. Additionally, the Padovana chicken shows a distinct change in the internal relief of the cranial roof. The inner side of the skull roof in the area of the osseous prominence does not show a homogeneous tabula interna but many small elongated bone bridges between the foramina in this area (Figure 8B).
The bony protuberance of the Padovana chicken is further characterized by many small foramina (Figures 6–8). These can be found mainly in the rostral and partly in the intermedial part of the protuberance. This is the same in the WCP specimen (Figure 9).
The area of the Crista frontalis interna, a vertically drawing bony ridge in the median plane of the skull, appears to be spared by the formation of the foramina in both the Padovana and the WCP so that this area is always left without such foramina. The maximum diameter of the existing foramina amounts to 1.5 mm, most of them show a rather circular shape, and they mainly lie in the transition toward the beak area of the prefrontal bone (Figure 6). These foramina are accompanied by an irregular inner surface of the cranial vault (tabula interna) in the same area (Figure 8B). The WCP skull shows much more pronounced foramina; they are perforations with a maximum width of 14.2 mm and are more irregular/amorphous than a circular shape (Figure 9).
3.2.2 Os mesethmoidale
The frontal region of the orbital septum, the os mesethmoidale, shows a strongly developed lamina dorsalis ossis mesethmoidalis (Figure 7A). This osseous overbuilding separates the septum from the os frontale and its rostral extensions and forms a clear cranial border of the septum. Such a strong development of the lamina dorsalis can be observed not only in the red junglefowl (Figure 7B) but also in the Pblack and WCP skulls (Figures 8, 9).
3.2.3 Upper beak: os praemaxillare and os nasale
The os praemaxillare and os nasale of the examined Padovana chickens showed several peculiarities when compared to the red junglefowl (Figure 10).
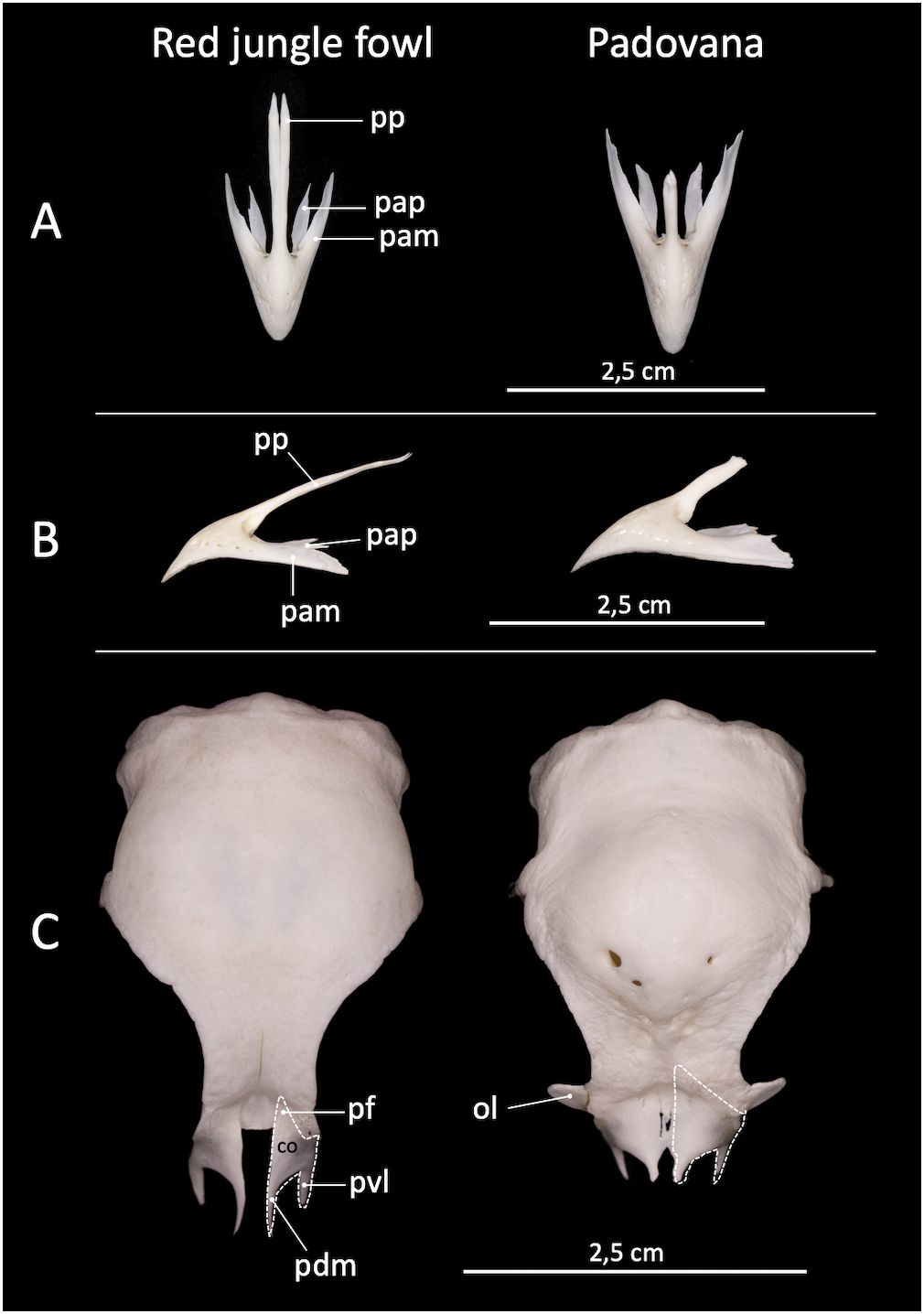
Figure 10 Ossa praemaxillaria of a red junglefowl hen (left) and a chamois-colored Padovana hen (right) in cranial (A) and lateral (B) views. (C) Cranial view on the frontal cranial vault and ossa nasalia (dotted line: os nasale sinistrum). co, corpus ossis nasalis; ol, os lacrimale; pam, pars maxillaris ossis praemaxillaris; pap, pars palatina ossis praemaxillaris; pdm, processus dorsomedialis/maxillaris ossis nasalis; pf, processus frontalis ossis nasalis; pp, processus praenasalis/frontalis ossis praemaxillaris; pvl, processus ventrolateralis/praemaxillaris ossis nasalis. Note: The ossa lacrimalia in the red junglefowl are not fused with the ossa frontalia and are missing in panel (C) This is a common phenomenon observed in macerated chicken skulls and was not interpreted as a major difference between the examined chicken breeds.
The bilateral formed os praemaxillare of the chicken is different from that of most other non-avian vertebrates, the main element of the upper jaw apparatus. It is, instead of the os maxillare, the basis for the upper beak and has three different elements, processus praenasalis, pars maxillaris, and pars palatina, which are analyzed:
(1) The processus praenasalis of the red junglefowl is a long, gracile, and straight osseous element of the upper beak (Figures 10A, B). It points toward the frontal bone (therefore also named processus frontalis MARINELLI) and gets in contact with the frontal bones’ most rostral area. It lies medial to the praemaxillar/dorsomedial process of the nasal bone and fills the gap between the nasal bones of both sites (see below). The praenasal processes of the praemaxillar bone of both sites are separated by a thin fissure. The same process but of the Padovana chicken is a shortened and stubby process with a tubercular tip (Figures 10A, B), pointing but not reaching the median portion of the frontal bone. Further on, the thin fissure that separates both processes of the red junglefowl’s praemaxilla cannot be observed in the Padovana chicken’s skull.
(2) The pars maxillaris of the praemaxillar bone is the basis of the beak tip and its lateral edge and points toward the proximal osseous elements of the upper beak, the os maxillare, os jugale, and os quadratojugale (Figure 10C). No main differences between the partes maxillares of red junglefowl and Padovana chickens have been found.
(3) The third element, the pars palatina, is the smallest part of the praemaxilla, lies medial to the latter with a similar course, and forms the osseous basis of the rostral palatum. When comparing the partes palatinae of both chicken breeds, the pars palatina of the Padovana chicken is sturdy, whereas that of the red junglefowl beak is of a more lanceolate morphology (Figures 10A, B).
The chicken’s nasal bone contains a body, corpus ossis nasalis, from which three osseous processes arise (Figure 10C). The corpus ossis nasalis of the Padovana chicken is of a broadened characteristic. Due to the expansion in width, especially medially, the corpora of both sites reach each other in the median line and show a small area of synostosis. In the red junglefowl, both ossa nasalia are completely separate from each other (Figure 10C). Beneath the body, also the upper dorsal process, processus frontalis, which points toward the os frontale, seems widened and stout when compared to conditions of the red junglefowl skull. On closer examination of both rostral processes, processus praemaxillaris (sive dorsomedialis MARINELLI) and processus maxillaris (sive ventrolateralis MARINELLI, Figure 10C), it becomes apparent that both processes at the Padovana chicken are less in length. The dorsomedial process, which points toward the os praemaxillare, ends with a nodular thickened tip.
When looking at the upper beak, particularly, the changes in the os nasale and os praemaxillare have the effect of generating a gap between the two bones, which becomes clear when viewed from the lateral (Figure 11). In contrast to WCP and Padovana chickens (Figures 11A–C), the upper beak of the red junglefowl is closed in this area by an osseous arch consisting of the dorsomedial MARINELLI processes and the processus praenasales of the ossa praemaxillaria (Figure 11D).
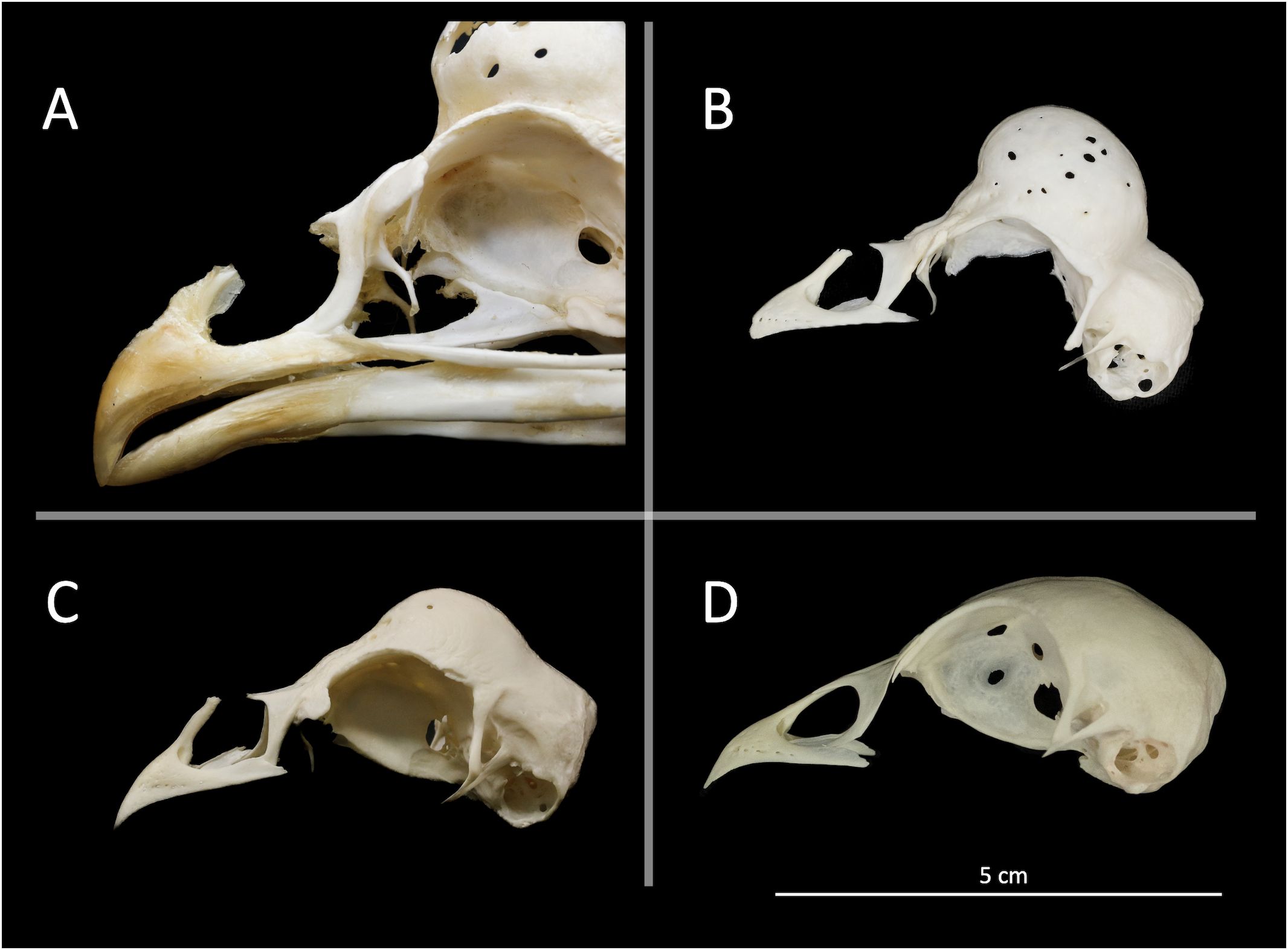
Figure 11 Osseous apparatus of the upper beak with focus on the os praemaxillare and os nasale; lateral view on (half-)macerated chicken skulls of a White Crested Polish cock (A), a black-colored Padovana hen with strong bony protuberance (B), a chamois-colored Padovana hen with moderate protuberance (C), and a red junglefowl hen with no protuberance of the os frontale (D).
3.2.4 Fossa cerebri and cranial fossae
The fossa cerebri of the Padovana chicken is clearly divided into two sections, which correspond to the clear distinction of the skull base into a fossa cranii rostralis and fossa cranii media (Figure 8B). These two sections are separated by a distinctly projecting bony ridge. This sturdily built osseous ridge divides the fossa cerebri in a collar-like manner (Figure 8B).
In the red junglefowl, this separation can also be observed, but the dividing osseous ridge is much thinner and seems much more characterized by lightweight construction.
Furthermore, in Padovana chickens, it is noticeable that in the region of the middle cranial fossa, a strongly pronounced sulcus arteriosus can be observed (Figure 8B).
The posterior cranial fossa does not seem to be further subdivided by the structural changes of the cranial vault.
3.2.5 The orbit
The Margo supraorbitalis of the posterocranial area of the orbit in Padovana chicken is extended laterocaudally and thus forms a thickening in this area (Figure 8A). Here, the bony mass of the protuberance broadens the skull laterally to form this bony thickening that is rough on the surface. Such formation of the Margo supraorbitalis can be also observed at the orbit of the WPC (Figure 9) but not at the orbit of the red junglefowl (Figure 7).
3.3 Allometric analysis of brain size
Brains of adult Padovana chickens with cranial protuberances showed a similar shape as observed in WCP (Figures 4B, 12A). The forebrain and the hindbrain seemed to be more separated from each other, and the whole brain looked drawn in length. Padovana chickens without a cranial protuberance did not show this morphological alteration (Figure 12B). Average body weights, brain masses, and allometric size indices of Padovana chicken, red junglefowl (Figures 2B, 12C), and eight other domestic chicken breeds are given in Table 1. EIs of Padovana with and without cranial protuberances did not differ significantly (t = −0.932, df = 18, p = 0.182). Kruskal–Wallis ANOVA of EIs showed a significant difference between analyzed chicken breeds (H = 49.40, df = 10, p < 0.001), but single comparisons did not reveal that Padovana chickens show significantly different EIs for brain mass compared to the pooled data of other chicken breeds (Figure 13; Padovana with protuberance, T = 632.00, p = 0.461; Padovana without protuberance, T = 545.00, p = 0.881). The breeds whose brain mass stands out are WCP (T = 1058.00, p = 0.001) and Red Leghorn (T = 177.00, p = 0.001). WCP showed the highest EIs of all investigated breeds (1.269 ± 0.16) and Red Leghorn the lowest (0.91 ± 0.04). However, after excluding WCP data, Padovana with protuberance showed a significantly larger EI than pooled plain-headed breeds (t = 1.864, df = 99, p = 0.033, Padovana without protuberance, t = −0.375, df = 99, p = 0.354).
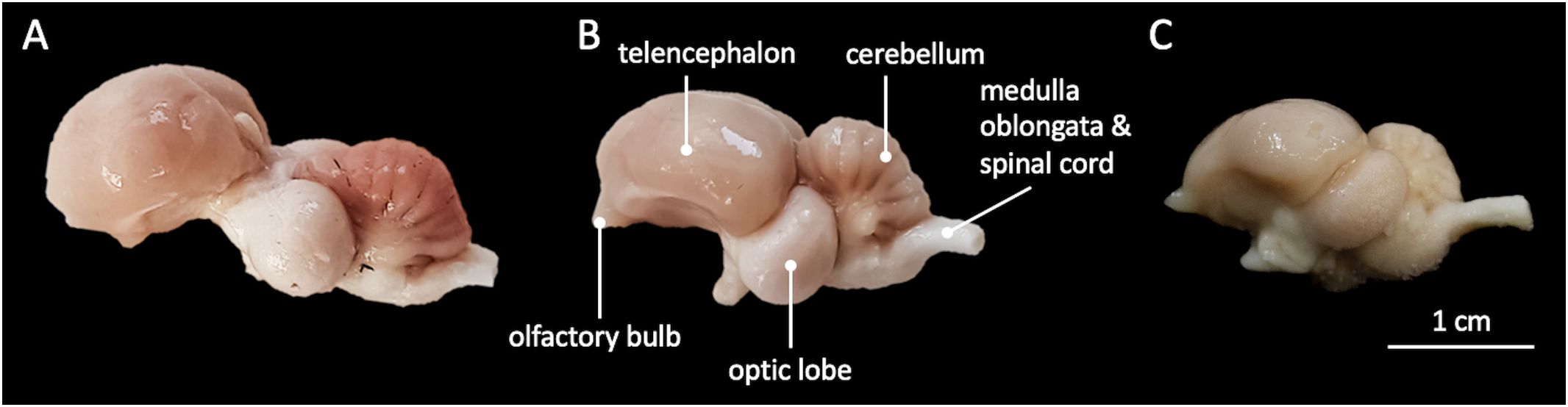
Figure 12 Brains of Padovana hens with (A, Pblack) and without (B, Pcham) cranial protuberance and a red junglefowl hen (C).
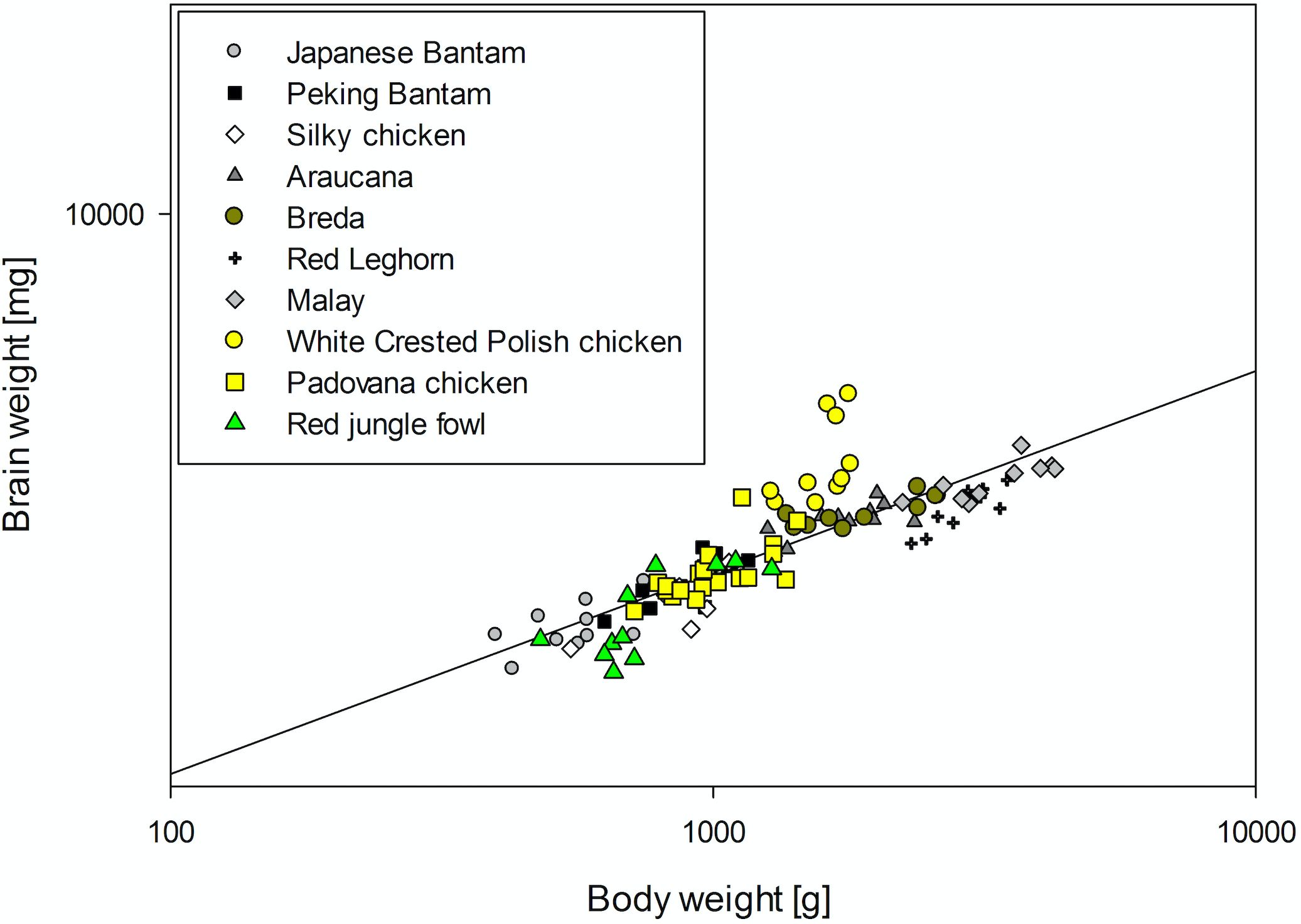
Figure 13 Fresh brain weight (mg) of nine domestic chicken breeds and Padovana chicken (divided into individuals with and without cranial protuberance) in relation to body weight (g). Allometric size indices are given in Table 1.
4 Discussion
4.1 Skull morphology
The characteristic feather crest of crested chicken is associated with a cranio-dorsal displacement of the telencephalon, inducing an hourglass-shaped morphology of the brain within a skull with a cranial protuberance (often called “cerebral hernia”). In this study, we investigated this phenomenon in detail and described the osseous basis of this peculiar skull formation in the crested chicken breed Padovana.
The osseous domes or protuberances of the examined crested chickens are based exclusively on symmetrical alterations of the ossa frontalia. This osseous part of the neurocranium is lifted at its anterior portion, forms in the sagittal plane a non-parabolic protuberance, and turns into the narrow-formed os parietale. These relations are in line with earlier detailed observations on osseous alterations on crested chicken skulls by Hagenbach (1839). What also has been documented by Hagenbach and can be confirmed by our study is the fact that the expression of osseous protuberances is a non-binary phenomenon with gradual characteristics, so different grades of protuberances can be observed when examining different individuals of the same breed. In extreme cases, the ossa frontalia only form a crown-like rim but are absent in the area of the expected vault, so the neurocranium is exposed in a crater-like manner. Even the median bony stripe that we can observe in all our examined animals vanishes under these conditions. Illustrations of these extreme cases can be found at Verdiglione and Rizzi (2018). Our histological processing of incompletely closed cranial vaults of juvenile Padovana chickens suggests that these areas are comparable to the open fontanella. Here, only the dura mater and connective tissue, which correspond to the periost of the chicken’s cranium as well as superficial layers of skin, protect the cerebrum. We have to assume that these juvenile conditions are conserved at the crater-like cranial vaults of adult chickens that show a complete lack of bones due to absent ossification in the area of the potential protuberance. However, in these cases, we observed that at least partial ossification of the frontal bone always occurred, so the skull is present in this area but in all cases perforated with a different amount and extent of foramina.
It is known that, at hatching, the WCP was already crested. However, Yoshimura et al. (2012) described that nearly all parts of the frontal bone were still membranous at this time and that the forebrain region protruded under the dura mater. In adult WCP, the membranous area became ossified, and the cranium was upthrusted. In Padovana chickens, it seems to be that the situation is similar. However, not all of our analyzed chicks showed membranous frontal bones compared with the open fontanella at the age of hatching. There is the assumption that particularly chickens with developing cranial protuberances show this feature. In our hatchlings, we could not differentiate between black- and chamois-colored individuals; thus, it could be possible that individuals with closed vaults were chamois-colored specimens that did not develop a distinct cranial protuberance. Based on these findings, we assume that the development of the ossa frontalia in crested chickens shows a heterogeneous growth with a later ossification of its dorsomedial portions. This is in line with the results of the Jollie (1957) examination of White Leghorn chicken skulls, that the median region of the frontal bones of G. gallus is the last of the cranial vault that ossifies. An explanation of this growth pattern is given by Erdmann (1940), who stated that the ossa frontalia of birds is the result of a proper rostral os frontale and a second dorsal osseous element, the os postfrontale mediale. This accessory part of the developing frontal bone can be also found in reptilia, where the lateral pendant, os postfrontale laterale, also plays a role in the development of the cranial vault. The Anlage of the chickens’ frontal bones therefore consists of a minimum of two ossification centers, whereas the dorsal portion contributes to the development of the crested chicken’s protuberance and may show ossification defects in cases of non-closed cranial vaults. These malformations, especially the extreme cases with crater-like defects, should not be mixed up with another feature of the cranial vault of reptilia, especially found in Lacertilia like Lacerta viridis: the parietal foramen (Wiedersheim, 1909). This median situated foramen can be found between the bilateral symmetric Anlage of the parietal bone and is not given when looking at the case of crested chickens with frontal protuberances.
The observation of Hagenbach (1839) that the cranial protuberances of hens are of a higher grade of expression than those of cocks can be confirmed by an overall qualitative impression of the X-ray documentation in our study. We cannot confirm that the protuberance grows with age, as claimed by Schwarze (1966), but we have not examined very old animals. However, since bone and brain growth is actually completed by 6 months of age, we do not think it is likely.
When compared to other poultry, the ossa frontalia of crested chickens show significantly different behaviors in their formation. The frontal bones of, e.g., Anser spp., may show a concavity, which can be under morphological considerations contrasted to the convex protuberance of G. gallus f.d. as a depressio frontalis (Baumel and Club, 1993).
Regarding the morphology of the upper beak, the most striking difference between red junglefowl and the examined crested chicken skulls is the unclosed connection between the nasal bone and praemaxilla that is due to the regression of nasal and praemaxillar processes. The result of that malformation is an opened apertura piriformis (terminology by Wiedersheim, 1909) and a lack of an osseous substructure for the nostrils, which have been already described in crested breeds by Verdiglione and Rizzi (2018) and Stange et al. (2018) before. In contrast to the latter and in line with the former, we were able to describe these alterations of the upper beak and the cranial vault in the same individuals. According to that, the statement of Stange et al. (2018), that the skeletal malformations of crested chickens show abnormalities of either the cranial vault or the upper beak, cannot be confirmed.
The formation of the protuberance and the further alterations of the chickens’ viscerocranium (e.g., thickening of the lamina dorsalis ossis mesethmoidalis and open apertura piriformis) result in region-specific changes of the cranial skeleton. However, we do not think that these malformations have a decisive influence on the kinetics of the crested chicken’s skull, as these morphological modifications take place outside the prokinetic chain of the jaw-palatal apparatus. This functional unit consists according to Starck (1979) of os quadratum, arcus zygomaticus, and the palate and is therefore not affected by the observed alterations.
Our qualitative observations are in line with those of Verdiglione and Rizzi (2018), who showed that the height of the skull and the ratio of the frontal bone to the width of the skull are greater in chickens with bony protuberances. Additionally, our results are mostly in line with those of Stange et al. (2018), who also showed that the main differences between domestic chicken breeds can be found when comparing the osseous elements of the cranial vault and praemaxilla-nasal region. Crested breeds like WCP, Appenzeller Spitzenhaubenhuhn, crested individuals of Araucana chickens, and also the Padovana chickens of our study are extreme examples of these differences.
4.2 Brain size
In addition to the morphological observations, we investigated the brain size of crested Padovana chickens with and without cranial protuberances and red junglefowl and compared them allometrically with that of eight other chicken breeds including WCP. The relative brain size of Padovana chickens did not differ from that of other chickens in contrast to WCP, which showed a significantly higher relative brain size compared to all other chicken breeds. WCP are known for their distinct cranial protuberances (Frahm and Rehkämper, 1998), and it seems to be that WCP showed the most extreme form of skull (and brain) alterations among all members of the taxon G. gallus. Maybe this is due to their long breeding history. WCP has one of the longest histories of pure breeding among chickens (Hagenbach, 1839). Frahm and Rehkämper (1998) already showed that WCP has outstanding brain size and brain composition. It has not only larger brain weights than other breeds but also a larger net brain volume. This enlargement is partly due to enlarged ventricles; also, the optic tract, diencephalon, telencephalon in total, apicale hyperpallium, densocellular hyperpallium, mesopallium, and nidopallium are significantly enlarged in this breed (Frahm and Rehkämper, 1998). We cannot say anything about the brain composition of our investigated Padovana chickens, but after excluding WCP from the analysis, Padovana chickens with cranial protuberances also showed a significantly larger relative brain size compared to the other breeds. This may indicate that bony protuberances in general induce an enlargement of the brain. Whether Padovana also shows an altered brain composition is still to be investigated. Tao et al. (2020) also described brain alterations in cerebral hernia-type chickens, namely, a decreased density of neurons, a shifted pallidum, elongated hippocampus, expanded mesopallium and nidopallium, and reduced hyperpallium. However, their investigations were done on juvenile chicks (28 days old), and their investigations were partly just descriptive. It was not clear whether the authors really compared the same atlas layers. Nevertheless, these results also confirm that crested chicken brains are peculiar.
Interestingly, there are no brain size differences in the red junglefowl, the common ancestor of all chicken breeds. Following the “regression hypothesis” of Hemmer (1983), it would be expected that specimens of red junglefowl show larger relative brain sizes compared to domestic chickens. According to the “regression hypothesis”, domesticated animals showed not only a reduction in behavioral patterns but also a reduction in brain size due to a decline in environmental appreciation. However, here, we could show that red junglefowl chickens exhibit rather small brains with the second lowest EI, which is in contrast to Kawabe et al. (2017), who described a distinctly larger relative brain volume in red junglefowl than in domestic fowl. Our findings are more in line with the “adaptation hypothesis”, which considers domestication as a dynamic evolutionary process in which all changes due to the changing conditions of a man-made environment have an adaptive characteristic (Rehkämper et al., 1988; Rehkämper et al., 2008). Additionally, other studies also described rather small relative brains in red junglefowl compared with larger brains (structures) in domesticated chickens (Henriksen et al., 2016; Racicot et al., 2021). Thus, domestication does not necessarily lead to smaller brains, and our data from Padovana and WCP showed that even an increase in brain size can be observed. However, it should be remembered that our red junglefowl is the original state of the wild junglefowl but has been bred and kept in captivity. Purebred red junglefowl can still be found in Asia, but mostly as a hybrid of wild jungle fowl and village chickens (Tixier-Boichard et al., 2011; Bosse, 2019). Additionally, there are also likely more chicken species included in the domestic chicken than only red junglefowl (G. gallus), such as Gallus sonneratii, Gallus varius, and Gallus lafayettii. Gene flow between wild jungle fowl and village chickens still takes place in some regions of Southeast Asia, showing that domestication is still an ongoing process (Tixier-Boichard et al., 2011; Miao et al., 2013; Bosse, 2019).
Direct comparisons between the wild and domestic chickens are difficult and rare, and as far as we know, there are no studies on the detailed brain morphology of the red junglefowl available.
4.3 The feather crest and cerebral hernia
It is obvious that the feather crest is without intrinsic adaptive value for the affected birds. The persistence of such hereditary plumage alterations in a domestic population is exclusively due to the fact that they are attractive to breeders (Bartels, 2003). Of course, feather crests can obstruct the view (Figure 2A), and it cannot be excluded that the pain-sensitive dura mater, which is more exposed under cranial foramina, can lead to impaired welfare. However, so far, there are no studies on this, and no studies have shown that the crest or cerebral hernia has a negative impact on brain size or cognitive abilities. The crest and the accompanying enlargement of the skull lead to a larger brain compared to other chicken breeds. However, the consequences of this larger brain for the animals are not yet known. Behavioral studies have not been able to show an advantage in cognitive or learning behavior (Tiemann and Rehkämper, 2007).
By analyzing and interpreting the skull anatomy of crested poultry, it is important to consider that there are different kinds of skull alterations in, e.g., chickens and ducks. As in crested chickens, the crest of crested ducks consists of proportionally enlarged contour feathers. However, usually, it is situated more parietal and on a cushion of fat and connective tissue if the feather crests are voluminous. The underlying calvaria are often not closed in crested ducks and show one big opening, located in the intersection of the sutura supraoccipitoparietalis, sutura interparietalis, and sutura frontoparietalis and the caudal region of the ossa parietalia (Bartels, 2003; Mehlhorn and Rehkämper, 2010). Crested ducks often show enlarged ventricles as WCP, but in crested ducks, it is due to intracranial fat bodies. These fat bodies can have an impact on brain composition and the flow of cerebrospinal fluid because of the resulting compression of the brain (Cnotka et al., 2007, Cnotka et al., 2008; Mehlhorn and Rehkämper, 2010). Additionally, crested ducks often show behavioral abnormalities like motor incoordination, e.g., demonstrated by a tottering walk. These behavioral deficits cannot be explained by the existence of a fat body, but they could be explained by functional suboptimal cerebella and tegmental, which are significantly reduced in crested ducks (Cnotka et al., 2008; Mehlhorn and Rehkämper, 2010). In contrast to the duck, the osseous protuberance of the chickens is only formed by alterations of the frontal bone, and there are no intracranial fat bodies or motor deficits known from crested chickens as observed in crested ducks.
The suitability of the term “cerebral hernia/herniation”, as used by several authors in this context (e.g., Yoshimura et al., 2012; Verdiglione and Rizzi, 2018; Watanabe et al., 2023), is certainly questionable, as cerebral herniation in the medical sense is usually understood to be a dislocation of parts of the brain in pre-existing foramina of the skull (especially foramen magnum) or under dural duplications. This dislocation usually causes acutely severe neurological symptoms and damage. This pathogenesis initially has nothing to do with the phenomenon of the morphological differences observed here between chickens with “cerebral hernia” and those without. These morphological differences are rather seen as anatomical variants or breed characteristics. We therefore believe that the term cerebral hernia/herniation should not be used in this context, as there are no clearly pathological clinical conditions associated with it. Alternatively, we suggest the term “cerebral elongation” to take into account the change in brain morphology without implying a potential pathogenetic factor.
That the abnormal development of cerebral elongation leads to pre- and post-natal mortalities (Tao et al., 2020) cannot be confirmed by our study. A hatching rate of almost 82% is quite acceptable and not worse than in other fancy poultry breeds (Anderson Brown, 1988). Tao et al. (2020) also postulated that the membranous skull is prone to mechanical pressure and injury, resulting in unconsciousness and large economic losses in the poultry industry. They assumed that all their results indicate that the brain is injured with dysfunction in chickens with cerebral hernia. In our opinion, there is no evidence that the brains of cerebral hernia-type chickens are damaged in general. However, we cannot exclude limitations such as defective or restricted flight behavior based on the morphological findings only. This requires a systematic behavioral study. In addition, the economic losses in the poultry industry should be moderate because crested chickens do not exist there.
In contrast to our findings, Verdiglione and Rizzi (2018) described bony protuberances in chamois-colored Padovana. However, these differences can be caused by a different breeding history of chamois-colored Padovana in Italy and Germany. However, they also described differences between different strains (chamois-colored and silver-colored). Thus, it does not seem uncommon for different colored strains of a breed to differ in more than just their color. The authors also find sex dimorphism in the skull morphology such as frontal bone height/skull width ratio. According to this, a difference in brain size might also have been possible. We tested our brain indices accordingly but did not find significant differences between males and females. Therefore, we did not further separate our results by sex. Our skulls for morphological descriptions did not show obvious differences between males and females, but one explanation could be that we have only examined a small number of skulls in a qualitative way. Anyway, it seems to be that there are no differences in brain sizes, even when the skulls of male and female crested chickens may differ. Generally, it has to be considered that German Padovana chickens have been bred in Germany for over a hundred years, and it cannot be ruled out that the German strains now differ strongly from the Italian strains.
Genetic studies in recent years (Yoshimura et al., 2012; Li et al., 2021) have produced some findings on the genetic determination of feather crest formation and brain elongation with associated skull variations in fowls. Li et al. (2021) showed that although the crest phenotype is caused by a 195-bp duplication in the intron of HOXC10 in eight typical crested chicken breeds, this mutation is not sufficient to cause cerebral herniation. They referred to Silkie chicken, where this mutation has been found, but the brain and skull malformations are not present. The breed (German) chamois-colored Padovana, which was not included in the study of Li et al. (2021), is another example of a chicken breed with a feather crest (and probably this mutation) but without (distinct) cerebral hernia. There is the assumption that cerebral hernia is exclusively found in birds carrying the Cr2 or Cr3 alleles and with a large crest (like Polish chicken), but not in small-crested breeds like Silkie chicken carrying the Cr1 allele (Li et al., 2021). In our opinion, it would be interesting to investigate whether and to what extent chamois- and black-colored Padovana chickens differ in their alleles.
Regarding the morphological origin of cerebral hernia or cerebral elongation, there is much uncertainty. The increased accumulation and unbalanced amount of cerebrospinal fluid observed by other authors (Hutt, 1949; Tao et al., 2020) is discussed as a possible causative factor for the development of the elongation of the brain. The underlying mechanisms may be 1) the expansion of the brain by positive pressure and 2) the initiation and proliferation of neuroepithelial cells and neurons (Yoshimura et al., 2012). Another concept is the assumption that enlarged blood vessels observed in the lower dermis and a thickened upper dermis in the cranial skin of crested chickens not only cause the development of crest feathers but also affect deeper tissues and may cause cerebral hernia (Li et al., 2021).
The current state of research can be concluded that some genetic causes of the development of crest, brain elongation, and skull deformities have already been identified. However, the resulting (patho-)morphological changes in particular require further in-depth investigation. Further morphological studies could shed more light on this issue.
4.4 Final considerations
In summary, we confirm that crested chickens are special in many aspects. There are studies that even suggest that, e.g., WCP is on its way to becoming a new species (Tiemann and Rehkämper, 2012). Here, we described the peculiarities of the skull and the brain size in detail and revealed that there are some modifications in several cranial bones. Regarding the brain, crested chickens show an altered and unique brain configuration and a tendency to enlarged brains. Novel brain morphologies as observed here could be a compelling non-mammalian model system for understanding how unique brain morphologies evolve across vertebrates and how novel brain morphology originate (Watanabe et al., 2023). The accessibility and phylogenetic proximity of crested chicken breeds, combined with other domestic chicken breeds, are further arguments for their utility in investigating novel brain morphologies.
Data availability statement
The original contributions presented in the study are included in the article/Supplementary Material. Further inquiries can be directed to the corresponding author.
Ethics statement
The animal study was approved by Committees on the Ethics of Animal Experiments of the state of Lower Saxony and of North Rhine-Westphalia, Germany. The study was conducted in accordance with the local legislation and institutional requirements.
Author contributions
MW-V: Formal analysis, Investigation, Visualization, Writing – original draft, Writing – review & editing. SP: Conceptualization, Investigation, Resources, Writing – review & editing. MS: Investigation, Writing – review & editing. MF: Conceptualization, Investigation, Resources, Writing – review & editing. RU: Investigation, Resources, Writing – review & editing. JM: Conceptualization, Formal analysis, Investigation, Project administration, Resources, Supervision, Validation, Writing – original draft, Writing – review & editing.
Funding
The author(s) declare that no financial support was received for the research, authorship, and/or publication of this article.
Acknowledgments
We would like to thank Andreas Prescher (MOCA, RWTH Aachen) for his advice and support of the maceration process, Kay Körner (Institute for Anatomy I, HHU) for taking the photographs of the Padovana hens and the skulls, the animal caretaker of the Friedrich-Loeffler-Institut, and Silke Werner and Franziska Suerborg for their technical assistance.
Conflict of interest
The authors declare that the research was conducted in the absence of any commercial or financial relationships that could be construed as a potential conflict of interest.
Publisher’s note
All claims expressed in this article are solely those of the authors and do not necessarily represent those of their affiliated organizations, or those of the publisher, the editors and the reviewers. Any product that may be evaluated in this article, or claim that may be made by its manufacturer, is not guaranteed or endorsed by the publisher.
Supplementary material
The Supplementary Material for this article can be found online at: https://www.frontiersin.org/articles/10.3389/fevo.2024.1389382/full#supplementary-material
References
Bartels T. (2003). Variations in the morphology, distribution, and arrangement of feathers in domesticated birds. JEZ B 298, 91–108. doi: 10.1002/jez.b.28
Baumel J. J., Club N. O. (1993). Handbook of avian anatomy: Nomina Anatomica Avium. 2nd ed (Cambridge: Nuttal Ornithological Club).
Blumenbach J. F. (1812). “Jo. Frid. Blumenbachii de anomalis et vitiosis quibusdam nisus formativi aberrationibus commentatio recitata d. XI. Jul. 1812,” in Commentationes societatis regiae scient. Gött. Recentiores classis physicae (Göttingen), 1811–1813. Vol. II, ad a.
Bosse M. (2019). No “doom“ in chicken domestication? PloS Genet. 15, e1008089. doi: 10.1371/journal.pgen.1008089
Brothwell D. (1979). Roman evidence of a crested form of domestic fowl, as indicated by a skull showing associated cerebral hernia. J. Arch. Sci. 6, 291–293. doi: 10.1016/0305-4403(79)90007-4
Cnotka J., Frahm H. D., Mpotsaris A., Rehkämper G. (2007). Motor incoordination, intracranial fat bodies, and breeding strategy in Crested ducks (Anas platyrhynchos f.d.). Poult. Sci. 86, 1850–1855. doi: 10.1093/ps/86.9.1850
Cnotka J., Tiemann I., Frahm H. D., Rehkämper G. (2008). Unusual brain composition in Crested Ducks (Anas platyrhynchos f.d.)—including its effect on behavior and genetic transmission. Brain Res. Bull. 76, 324–328. doi: 10.1016/j.brainresbull.2008.03.009
Dubois E. (1897). Uber die Abhängigkeit des Hirngewichtes von der Körpergröße bei den Säugetieren. Arch. Anthropol. 25, 1–28.
Erdmann K. (1940). Zur Entwicklungsgeschichte der Knochen im Schädel des Huhnes bis zum Zeitpunkt des Ausschlüpfens aus dem Ei. Z. für Morphologie und Ökologie der Tiere 36, 315–400. doi: 10.1007/BF00406236
Eusemann B. K., Sharifi A. R., Patt A., Reinhard A. K., Schrader L., Petow S. (2018). Influence of a sustained release deslorelin acetate implant on reproductive physiology and associated traits in laying hens. Front. Physiol. 9, 1846. doi: 10.3389/fphys.2018.01846
Frahm H. D., Rehkämper G. (1998). Allometric comparison of the brain and brain structures in the white crested polish chicken with uncrested domestic chicken breeds. Brain Behav. Evol. 52, 292–307. doi: 10.1159/000006574
Hagenbach E. (1839). “Untersuchungen über den Hirn- und. Schädelbau der sogenannten Hollenhühner,” in Archiv für Anatomie, Physiologie und Wissenschaftliche Medizin. Ed. Müller J. (Verlag Von Veit Et Comp., Berlin), 311–331.
Harvey P. (1988). “Allometric analysis and brain size,” in Intelligence and evolutionary biology, vol. 17 . Eds. Jerison H. J., Jerison I. (Springer, Berlin), 199–210.
Hemmer H. (1983). Domestication: the decline of environmental appreciation (Cambridge: Cambridge University Press).
Henriksen R., Johnsson M., Andersson L., Jensen P. (2016). The domesticated brain: genetics of brain mass and brain structure in an avian species. Sci. Rep. 6, 34031. doi: 10.1038/srep34031
Herre W., Röhrs M. (1990). Haustiere – zoologisch gesehen (Stuttgart: Fischer). doi: 10.1007/978-3-642-39394-5
Hutt F. B. (1949). “Variations in the plumage: variations in the length of feathers: Crest, Cr,” in Genetics of the fowl: the classic guide to poultry breeding and chicken genetics (Norton Creek Press, Blodgett, OR), 127–128.
Jollie M. T. (1957). The head skeleton of the chicken and remarks on the anatomy of this region in other birds. J. Morphology 100, 389–436. doi: 10.1002/jmor.1051000302
Kawabe S., Tsunekawa N., Kudo K., Tirawattanawanich C., Akishinonomiya F., Endo H. (2017). Morphological variation in brain through domestication of fowl. J. Anat. 231, 287–297. doi: 10.1111/joa.12623
Li J., Lee M. O., Davis B. W., Wu P., Li S. M. H., Chuong C. M., et al. (2021). The crest phenotype in domestic chicken is caused by a 197 bp duplication in the intron of HOXC10. G3 11, jkaa048. doi: 10.1093/g3journal/jkaa048
Mayr G. (2018). A survey of casques, frontal humps, and other extravagant bony cranial protuberances in birds. Zoomorphology 137, 457–472. doi: 10.1007/s00435-018-0410-2
Mehlhorn J., Caspers S. (2020). The effects of domestication on the brain and behavior of the chicken in the light of evolution. Brain Behav. Evol. 95, 287–301. doi: 10.1159/000516787
Mehlhorn J., Rehkämper G. (2010). Brain alterations, their impact on behavior and breeding strategy in Crested Ducks (Anas platyrhynchos f. d.). Arch. Geflügelkunde 74, 203–209.
Mehlhorn J., Rehkämper G. (2013). Some remarks on bird’s brain and behavior under the constraints of domestication. ISRN Evolutionary Biol., 460580. doi: 10.5402/2013/460580
Miao Y. W., Peng M. S., Wu G. S., Ouyang Y. N., Yang Z. Y., Yu N., et al. (2013). Chicken domestication: an updated perspective based on mitochondrial genomes. Heredity 110, 277–282. doi: 10.1038/hdy.2012.83
Pallas P. S. (1767). “Spicilegia zoologica – quibus novae imprimis et obscurae animalium species iconibus, descriptionibus atque commentariis illustrantur,” in Tom. 1, fasc. IV.
Racicot K. J., Popic C., Cunha F., Wright D., Henriksen R., Iwaniuk A. N. (2021). The cerebellar anatomy of red junglefowl and white leghorn chickens: insights into the effects of domestication on the cerebellum. R. Soc Open Sci. 8, 211002. doi: 10.1098/rsos.211002
Rehkämper G., Frahm H. D., Cnotka J. (2008). Mosaic evolution and adaptive brain component alteration under domestication seen on the background of evolutionary theory. Brain Behav. Evol. 71, 115–126. doi: 10.1159/000111458
Rehkämper G., Haase E., Frahm H. D. (1988). Allometric comparison of brain weight and brain structure volumes in different breeds of the domestic pigeon, Columba livia f.d. (fantails, homing pigeons, strassers). Brain Behav. Evol. 31, 141–149. doi: 10.1159/000116581
Rehkämper G., Kart E., Frahm H. D., Werner C. W. (2003). Discontinuous variability of brain composition among domestic chicken breeds. Brain Behav. Evol. 61, 59–69. doi: 10.1159/000069352
Requate H. (1959). Federhauben bei Vögeln. Eine genetische und entwicklungsphysiologische Studie zum Problem der Parallelbildungen. Z. Wiss. Zool. 162, 191–313.
Romeis B., Aescht E., Mulisch M. (2010). Romeis mikroskopische technik. 18. ed (Heidelberg: Spektrum Akad. Verlag).
Sandifort E. (1793). Museum anatomicum academiae Lugduno-Batavae (Vol. 1. Leiden: Luchtmans). doi: 10.5962/bhl.title.120555
Schwarze E. (1966). Kompendium der Veterinär-Anatomie – Band V: Anatomie des Hausgeflügels (Jena: Gustav Fischer).
Sheppy A. (2011). The colour of domestication and the designer chicken. Opt. Laser Technol. 43, 295–301. doi: 10.1016/j.optlastec.2009.02.003
Snell E. (1892). Die Abhängigkeit des Hirngewichtes von dem Körpergewicht und den geistigen Fähigkeiten. Arch. Psych. 23, 436–446. doi: 10.1007/BF01843462
Somes R. G. (1990). “Mutations and major variants of muscles and skeleton in chickens,” in Poultry breeding and genetics. Ed. Crawford R. D. (Elsevier, Amsterdam), 209–237.
Stange M., Núñez-León D., Sánchez-Villagra M. R., Jensen P., Wilson L. A. B. (2018). Morphological variation under domestication: how variable are chickens? R. Soc. Open Sci. 5, 180993. doi: 10.1098/rsos.180993
Starck D. (1979). Vergleichende Anatomie der Wirbeltiere auf evolutionsbiologischer Grundlage (Berlin: Springer Verlag). doi: 10.1007/978-3-642-67159-3
Stephan H., Baron G., Frahm H. D. (1988). “Comparative size of brains and brain components,” in Comparative primate biology. Eds. Steklis H. D., Erwin J. (Alan R. Liss, New York), 1–38.
Stephan H., Baron G., Frahm H. D., Stephan M. (1986). Comparison of the size of brains and brain structures of mammals. Z. Mikrosk. Anat. Forsch. 100, 189–212.
Stephan H., Frahm H. D., Baron G. (1981). New and revised data on volumes of brain structures in insectivores and primates. Folia Primatol 35, 1–29. doi: 10.1159/000155963
Tao Y., Zhou X., Zheng X., Li S., Mou C. (2020). Deciphering the forebrain disorder in a chicken model of cerebral hernia. Genes 11, 1008. doi: 10.3390/genes11091008
Tegetmeier W. B. (1856). On the remarkable pecularities existing in the skulls of the feather-crested variety of the domestic fowl, now known as the Polish. Proc. Zool. Soc 24, 366–368.
Tiemann I., Rehkämper G. (2007). Rasseunterschiede bei Hausḧhnern (Gallus gallus f.d.) bei klassischer und operanter Konditionierung in der Skinnerbox. Acta Biol. Benrodis 13, 231–40.
Tiemann I., Rehkämper G. (2008). Breed-specific companions-inter-individual distances reflect isolating mechanisms within domesticated chickens (Gallus gallus f.d.). Brain Res. Bull. 76, 300–383. doi: 10.1016/j.brainresbull.2008.03.013
Tiemann I., Rehkämper G. (2009). Effect of artificial selection on female choice among domesticated chickens Gallus gallus f.d. Poult. Sci. 88, 1948–1954. doi: 10.3382/ps.2008-00250
Tiemann I., Rehkämper G. (2012). Evolutionary pets: off-spring numbers reveal speciation process in domesticated chickens. PloS One 7, e41453. doi: 10.1371/journal.pone.0041453
Tixier-Boichard M., Bed’hom B., Rognon X. (2011). Chicken domestication: from archeology to genomics. C. R. Biol. 334, 197–204. doi: 10.1016/j.crvi.2010.12.012
Verdiglione R., Rizzi C. (2018). A morphometrical study on the skull of Padovana chicken. Ital. J. Anim. Sci. 17, 785–796. doi: 10.1080/1828051X.2017.1412810
Wang Y., Gao Y., Imsland F., Gu X., Feng C., Liu R., et al. (2012). The crest phenotype in chicken is associated with ectopic expression of HOXC8 in cranial skin. PloS One 7, e34012. doi: 10.1371/journal.pone.0034012
Watanabe A., Marshall A. S., Gignac P. M. (2023). Dumbbell shaped brains of Polish crested chickens as a model system for the evolution of novel brain morphologies. J. Anat. 243, 421–430. doi: 10.1111/joa.13883
Keywords: crested chicken, Padovana, skull anatomy, brain, brain size, cerebral hernia, cranial protuberance
Citation: Wolf-Vollenbröker M, Petow S, Schmidbauer M, Fellmin M, Ulrich R and Mehlhorn J (2024) Detailed analysis of skull morphology and brain size in crested Padovana chicken (Gallus gallus f.d.). Front. Ecol. Evol. 12:1389382. doi: 10.3389/fevo.2024.1389382
Received: 21 February 2024; Accepted: 23 April 2024;
Published: 13 May 2024.
Edited by:
Megan G. Davey, University of Edinburgh, United KingdomReviewed by:
Violeta Trejo Reveles, University of Edinburgh, United KingdomElisabetta Versace, Queen Mary University of London, United Kingdom
Copyright © 2024 Wolf-Vollenbröker, Petow, Schmidbauer, Fellmin, Ulrich and Mehlhorn. This is an open-access article distributed under the terms of the Creative Commons Attribution License (CC BY). The use, distribution or reproduction in other forums is permitted, provided the original author(s) and the copyright owner(s) are credited and that the original publication in this journal is cited, in accordance with accepted academic practice. No use, distribution or reproduction is permitted which does not comply with these terms.
*Correspondence: Michael Wolf-Vollenbröker, d29sZnZvbGxAaGh1LmRl