- 1British Antarctic Survey, Natural Environment Research Council, Cambridge, United Kingdom
- 2National Oceanography Centre, University of Southampton, Southampton, United Kingdom
Shallow water Antarctic marine macroepifaunal assemblages live in one of the most naturally disturbed marine environments due to the impact of icebergs scouring the seafloor. They are, however, amongst the least anthropogenically impacted assemblages and are afforded protection under the Antarctic Treaty system. When the British Antarctic Survey’s Rothera Research Station wharf needed extending to accommodate the newly constructed UK polar research vessel, the RRS Sir David Attenborough, a Comprehensive Environmental Evaluation (CEE) was conducted to assess the impact. The macroepifaunal likely to be impacted by the construction was surveyed through ROV videos of five transects, centered on the middle of the construction zone, from 10–100 m deep. A pre-construction survey was completed in March 2017, as part of the CEE impact assessment, and a post-build survey in 2022 (delayed from 2021, and reduced in scope, due to the COVID-19 pandemic). Sedimentation rates were also measured before and during construction and were high during the second summer when the wharf pilings were being back filled with crushed rock. The measured differences between pre- and post-construction assemblages were minor and were not reflected in the overall number of taxa (operational taxonomic units – OTU), or diversity, but there were subtle shifts in species composition. The largest differences in the macroepifauna were a reduction in the number of the common urchin, Sterechinus neumayeri, and seastar, Odontaster validus, and were within expected variability. The small changes detected in the macroepifauna indicate it was minimally impacted and/or recovered in the subsequent two years, therefore during wharf construction the accompanying mitigation measures were robust.
1 Introduction
The Antarctic and sub-Antarctic regions are often viewed as isolated from direct anthropogenic disturbance due to their remoteness (Rogers et al., 2020); however, Southern Ocean ecosystems have experienced major human impacts over many hundreds of years (see Bonner and Laws, 1964, Forcada and Staniland, 2009 and Rogers et al., 2020). There is a strong case, however, that Antarctic shallows (continental shelf >500 m) are among the least historically anthropogenically impacted ecosystems (Zwerschke et al., 2021), and therefore require protection and measures to prevent additional impact. Anthropogenic disturbance is regulated through the Antarctic Treaty system, and specifically the Protocol on Environmental Protection to the Antarctic Treaty (entered into force 1998). Signatories to the Protocol agreed to comply with the Annex I to the Protocol that requires a Comprehensive Environmental Evaluation (CEE) to be undertaken and notified to all Parties before the commencement of any project that is likely to have an impact considered to be greater than minor or transitory (see https://www.ats.aq/e/protocol.html).
The shallow Antarctic benthos is one of the most naturally disturbed environments on Earth, due to frequent iceberg scour disturbance (Smale, 2008). Iceberg scour is caused when the keel of an iceberg (or any ice of sufficient size) impacts the seafloor (Convey et al., 2014) causing mass mortality in the zoobenthos (Barnes, 2017). The frequency of iceberg scour is typically higher in the shallows and lower at depth, but with far greater impact per scour, due to the scarcity of larger icebergs (Barnes and Conlan, 2007). Iceberg scour, therefore, presents a particular challenge to disentangling any anthropogenic impact from the natural stochastic background of high intensity natural disturbance.
This study reports the findings of a CEE for the extension of the Biscoe Wharf at the British Antarctic Survey Rothera Research Station (67° 34’ S, 68° 08’ W) to accommodate the UK’s new Polar research vessel, the RSS Sir David Attenborough (Fothergill, 2018). This new vessel is longer and has a deeper draft than the vessels it replaced (Rogan-Finnemore et al., 2021) and so the wharf had to be extended to accommodate the ship (Figure 1).
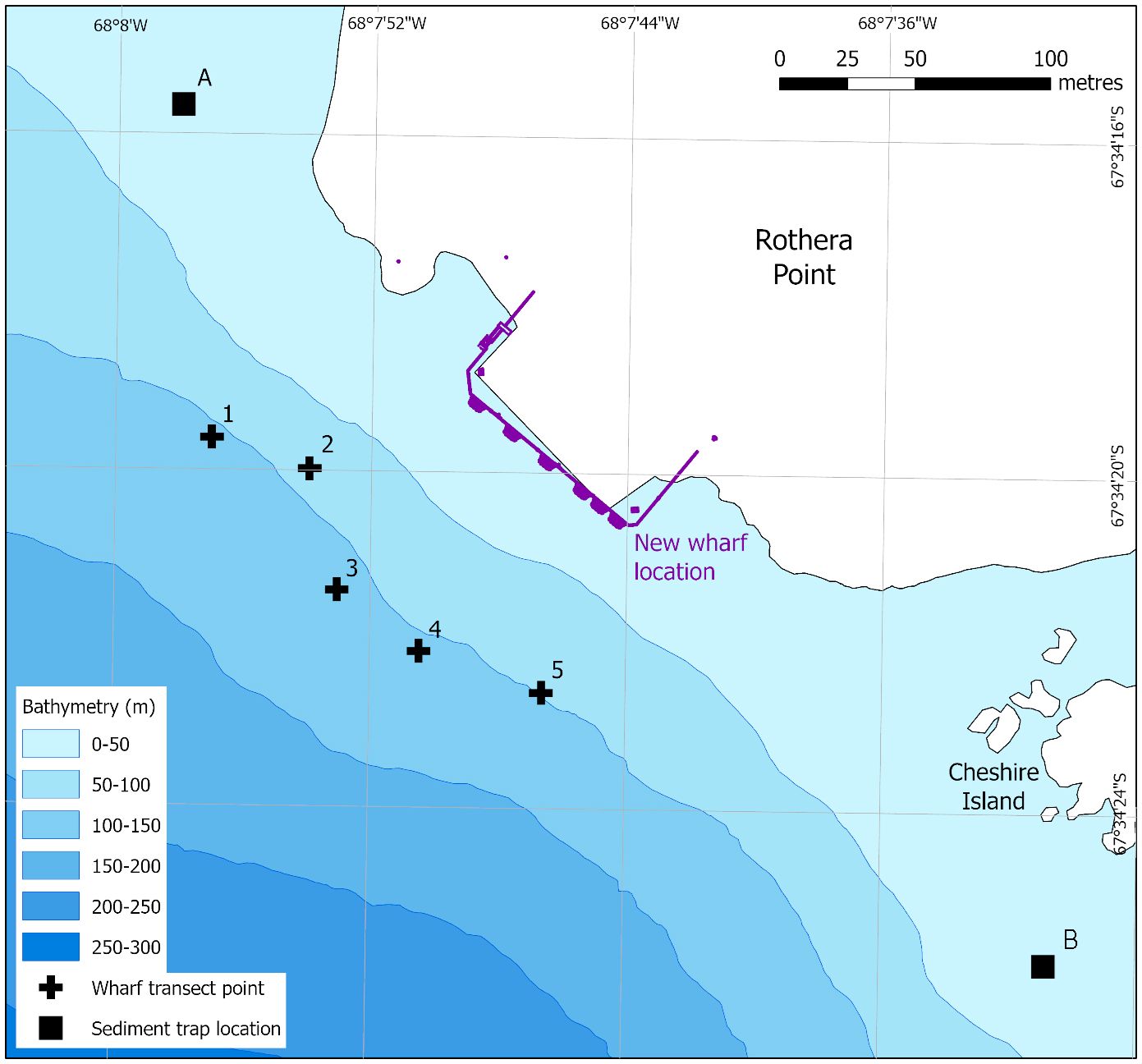
Figure 1 The location of the Biscoe Wharf extension at Rothera Research Station, showing the original and new (in purple) wharf footprint. Crosses 1–5 indicate the deepest point from which the five wharf transects started. The transects ran perpendicular to the wharf (NE), finishing at 10 m depth. A and B indicate locations of the two sediment traps. Coastline data from the SCAR Antarctic Digital Database, 2022. Bathymetry from Retallick et al. (2021).
The sea floor adjacent to the Biscoe Wharf (~10 m depth) is subject to high natural disturbance from frequent and consistent iceberg scour (e.g., see Smale et al., 2006). Further from the wharf, the sea floor drops steeply away into deep water, at an approximate 45° angle. The Biscoe wharf experiences periods of seasonal sea ice, long period light and darkness during austral summer and winter respectively and stable temperatures year round, as has been reported from approximately 30 years of year round measurements (review available here: Venables et al., 2023) Due to the bathymetry of the site, it was also important to measure any impact on macroepifauna composition at depths below the site of wharf construction as rockfall and increased sedimentation into the water column could have impacted the deeper macroepifaunal assemblage. At these greater depths, where the sea floor is exposed to lower rates of iceberg scour disturbance, the macroepifaunal assemblage consists of a slower growing, less disturbance-resilient, species (Robinson et al., 2021). Deeper water assemblages may, therefore, not be able to recover as rapidly as the more disturbance-tolerant species found in the shallows (Robinson et al., 2020, 2022).
Across the study area, previous studies (see Robinson et al., 2021) have found that between 10–30 m depth, the macroepifauna are dominated by mobile grazers, coincident with highest abundances of encrusting and macroalgae species. Between 40 and 60 m depth there is a combination of sessile suspension feeders including ascidians and anthozoans, as well as mobile grazers and scavengers such as ophiuroids and asteroids. At depths between 70–100 m sessile suspension feeders dominate in particular bryozoans and porifera. The macroepifaunal assemblages however show no distinct depth zonation, with multiple species having wide depth ranges that can encompass 10–100 m depth and high spatial heterogeneity (Smale et al., 2008), with the general trends described above often overlapping and combining. In this study, we aimed to measure the impact of the extension of the Biscoe Wharf construction on the surrounding Antarctic benthos (Figure 2). To describe any changes to macroepifauna composition pre- and post-construction video transects were recorded adjacent to, and alongside, the wharf construction sites. Due to the rocky substratum and depth of the site meiofauna and infauna assemblages were not sampled. Sediment traps were also deployed to monitor any changes in sedimentation rates associated with construction. Any changes detected were considered within the context of the naturally high levels of iceberg disturbance and natural macroepifauna variability seen within this environment (Robinson et al., 2021).
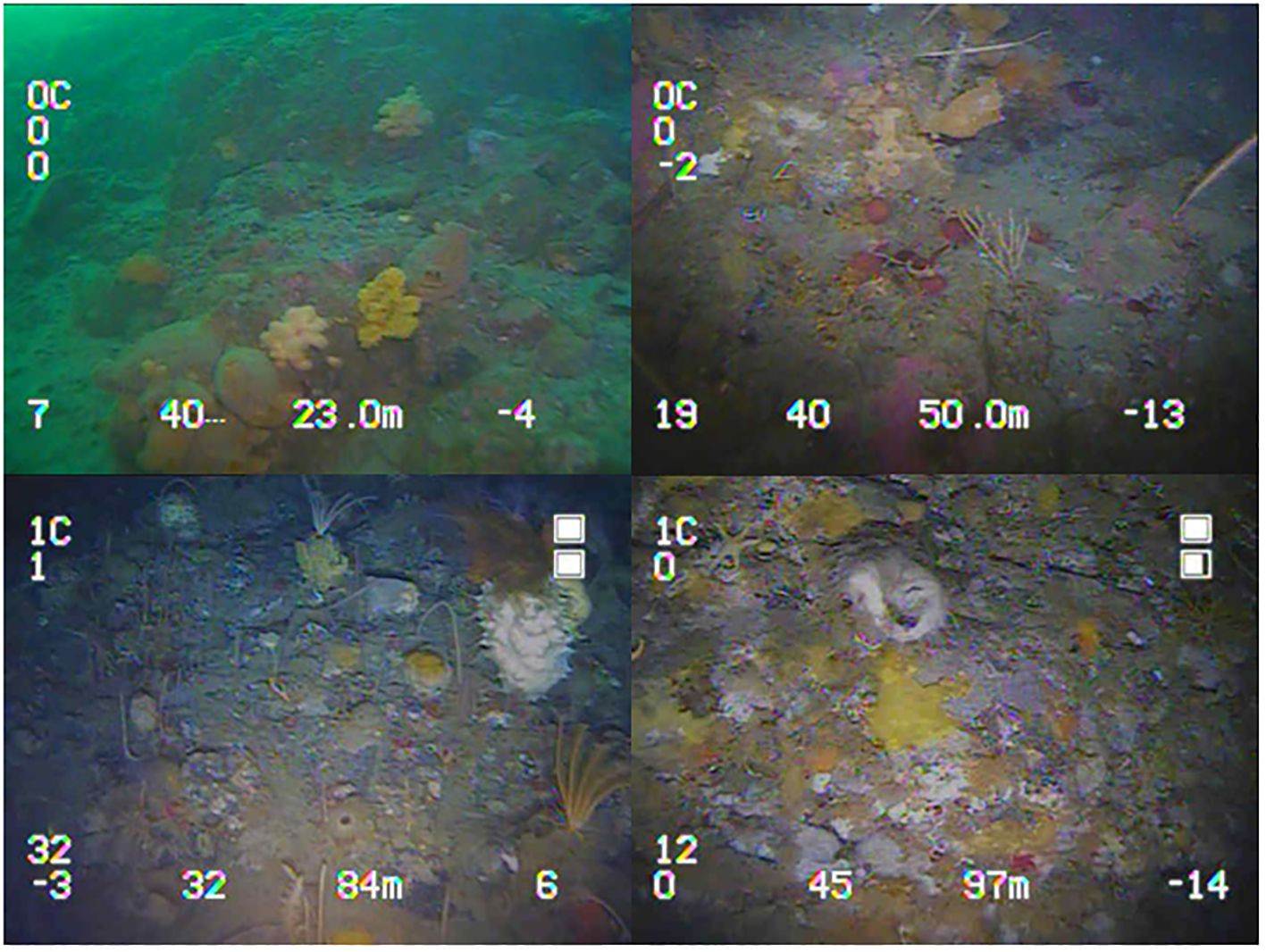
Figure 2 Frames from the video of the benthic macroepifaunal assemblages captured during ROV wharf surveys, from top right moving clockwise 23 m, 50 m, 97 m and 84 m depth.
2 Materials and methods
2.1 Macroepifauna video transects
A pre-construction video survey was completed in March 2017 to provide baseline assemblage data for the CEE. A post build survey was completed in March 2022 to assess the impact of the construction on the benthic assemblage. This survey was delayed by one year, from 2021, and reduced to 50% of the original scope, due to constraints resulting from the COVID-19 pandemic (Hughes and Convey, 2020). The survey was conducted using a Deeptrekkor DTG2 ROV (Remotely Operated Vehicle) which recorded video transects from 0–100 m depth along five transects centered on the wharf construction zone and covering the area that was expected to be impacted by the construction (Figure 1). Video was recorded on the DTG2’s internal 1/3 Sony CCD colour 700HD camera, lit by the inbuilt 1350 lumen lights with an additional pair of SOLA600 lights providing a total of 2550 lumen.
From the videos, abundance was scored in the range 0–10 by summing the presence or absence of each OTU (Operational Taxonomic Unit)in every one meter increment, within each 10 m depth interval. For example, OTUs present at every meter within the 10–20 m depth interval would be scored 10, whereas OTUs present at only three depths would be scored 3. This provided a rapid and repeatable way of measuring abundance, while preventing hyper-abundant OTUs, or species that occur in clusters, from dominating the analysis.
For each sample all mega and macrofauna were identified to the lowest possible taxonomic resolution; however, due to no specimens being retrieved for identification and the constraints of video resolution, a reduced and modified OTU guide was adopted (see Supplementary Table S1 – OTU guide). The benthic biota of the study area has been well-characterised, many collected and an extensive catalogue of high-quality benthic images has been created, and so OTUs in video images could be identified and grouped together, based on their morphology. However, it was not always possible to identify OTUs to species level, as some species have a similar morphology, and would require collection or closer examination to differentiate.
2.2 Sedimentation rates
Sediment traps were deployed and collected by SCUBA divers to measure if the construction activity added sediment to the water column (Supplementary Figure S1). Traps were deployed two locations either side of the construction zone in July 2017 (locations A: 67°S 34.261, 068°W 07.967 and location B: 67°S 34.430, 068°W 07.514) (Figure 1). Sediment traps were placed at 30 m depth, to reduce the chances of them being hit by icebergs and were designed to have a small profile within the water column without a surface buoy that would be at risk of entanglement by seasonal sea ice and icebergs. By reducing the sediment trap profile in the water and allowing a degree of flexibility in the mooring itself, only a direct hit by icebergs on the anchor point (a concrete weight) would destroy the sediment trap, thereby reducing the risk of sample loss. Despite these precautions, the western trap (location A) was destroyed by an iceberg after redeployment in October 2018 so only pre-construction sedimentation data were available from this trap. The south-eastern sediment trap (location B) remained in place until it was removed in May 2021. Once collected the sediment dry mass (after 24 h at 60°C) and ash free dry mass (after 24 h at 480°C) were measured.
2.3 Macroepifauna biodiversity
Pre- and post- construction diversity was determined by comparing OTU richness, the number of morphotypes identified within each sample and the Shannon-Wiener diversity index (Pielou, 1966). A Friedman’s non-parametric two-way ANOVA was implemented due to the non-parametric and non-balanced constraints of the post-construction samples for both OTU richness and Shannon-Wiener diversity index across depth. Both OTU richness data and Shannon-Wiener diversity index data, were averaged across depth to accommodate the requirements of the Friedman’s test, which was performed using R v4.3.2.
2.4 Multivariate analysis
Multivariate analysis was used to describe the macroepifauna composition change between pre- and post-construction surveys between 10–100 m depth using Primer 7 with the PERMANOVA+ software package (Clarke and Gorley, 2015). An nMDS plot was constructed to describe the variance between all samples after 999 permutations using a Bray-Curtis similarity matrix. nMDS stress, a measure of how representative nMDS is of the underlying high dimension data, was reported at 0.12, which is considered a fair presentation of the underlying data. Hierarchical cluster analysis was overlayed on top of the nMDS to demonstrate groups sharing 45% similarity. Two-way ANOSIM tests were implemented to test macroepifauna composition change for pre- and post-construction and across depth, with SIMPER (SIMilarity PERcentage) tests investigating which taxa were responsible for any dissimilarity (Supplementary Table S2).
3 Results
3.1 Macroepifauna biodiversity and composition
Both pre- and post-construction diversity metrics, i.e., OTU richness and Shannon-Wiener diversity index, describe a general unimodal trend across depth peaking ~70 m depth (Figure 3). Species OTU richness (χ2 = 12.0, df = 8, P-values = 0.15; Friedman test) and Shannon-Wiener diversity index values (χ2 = 13.07, df = 8, P-values = 0.11; Friedman test) were not significantly different during the pre- and post-construction. Pre- and post-construction values had a similar peak diversity of 42 and 40 OTU richness, respectively. Nine unique OTUs and 12 unique OTUs were found in the pre- and post-construction surveys, respectively. All occurred with low abundance, i.e., < 10 counts, except for ALG005 and ALG006 which are both species of macroalgae.
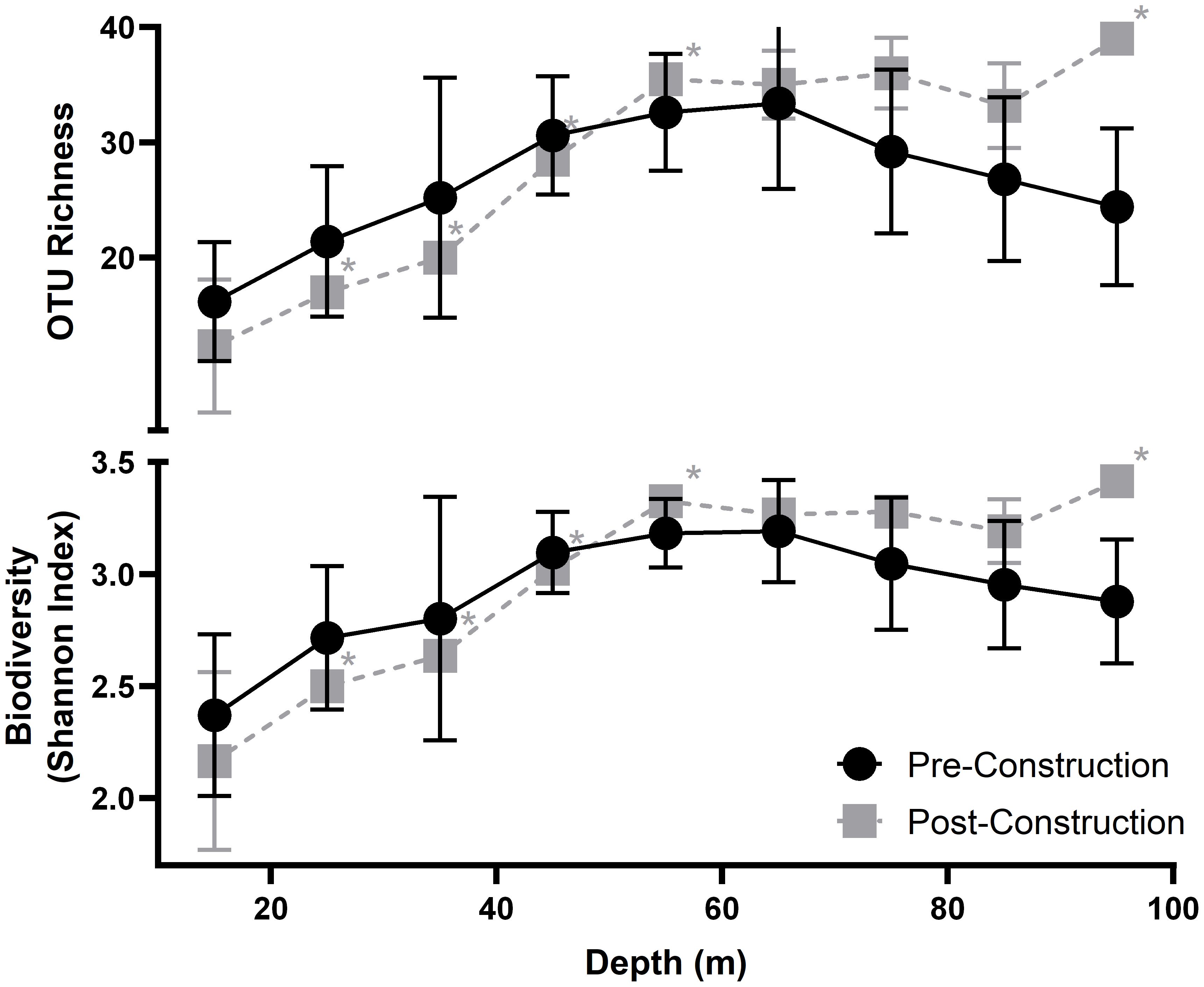
Figure 3 Average OTU richness (top) and Average Shannon-Wiener diversity index (bottom) measured across each 10 m depth interval; points are placed at the mid-point of each transect. Pre-construction data are shown in black and post-construction in grey, with the errors bars showing standard deviation. Asterisks indicated averages with <3 transects and no error bars.
Across the study area the macroepifauna was characterised by mobile grazers and scavengers between 10–30 m depth, in particular Nacella concinna (GAS001), Sterechinus neumayeri (ECH001) and Odontaster validus (AST001) (Figure 4). Between 40 and 60 m depth a mixture of groups including suspension feeders including Primnoella sp. (ANT001) and Cnemidocarpa verrucosa (ASC009), as well as grazers and scavengers such as S. neumayeri (ECH001). Calcareous pink encrusting algae (ALG002), was dominate at all depth between 10–50m depth. At 60 m to 100 m depth, sessile suspension feeders were found in higher abundances including Perinsiana littoralis (POL002), yellow encrusting porifera (POR001), and encrusting and foliose bryozoans (BRY001–BYR003), these bryozoan OTUs do contain multiple different species … As observed in previous studies (see Smale et al., 2008) there was no clear depth zonation, within the Antarctic benthos with assemblages overlapping at various depths, within the above description of the overall trend. Individual species also could demonstrate wide depth ranges, for example Nacella concinna (GAS001) a species of grazing limpet which dominated at 10 m depth could be found, in low abundances, in the deepest samples (100 m depth).
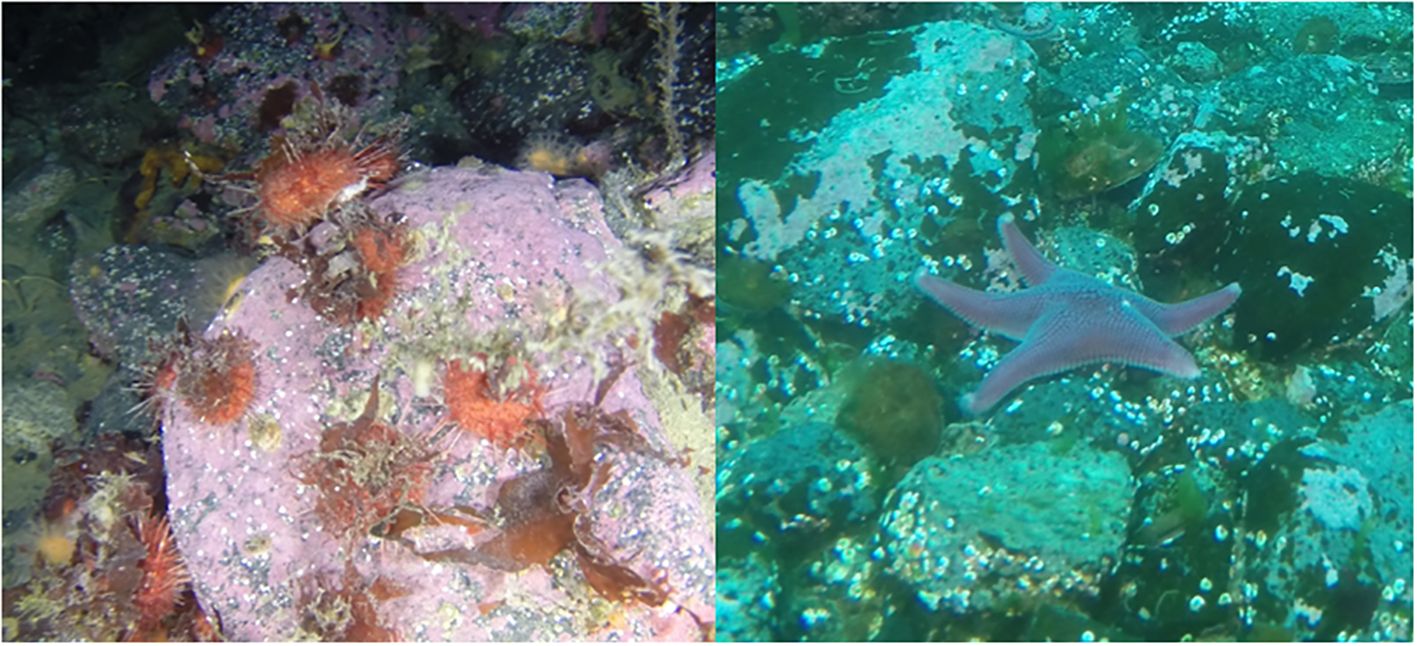
Figure 4 Specimen images of Sterechinus neumayeri (ECH001) (left) and Odontaster validus (AST001) (right), from survey are in front of Biscoe Wharf at Rothera Research station.
3.2 Multivariate analysis
Across all depths there was a high degree of overlap between samples, indicating similar macroepifauna composition amongst those samples, with the greatest degree of overlap between pre- and post-construction observed at depths greater than 50 m (Figure 5). While the differences in assemblages between depths (F8,78 = 9.1, P < 0.01; Table 1) and transects (F5,78 = 4.4, P < 0.01) remained the same after construction (i.e., there were no interaction terms), there were small but significant differences between the assemblages measured pre- and post-construction (F1,78 = 5.3, P = 0.03). The biggest difference between pre- and post-construction assemblages was attributed to a reduction in both Sterchinus neumayeri and Odontaster validus (Figure 4) after the construction, with SIMPER attributing 5.4 and 4.2%, respectively, of the difference to these OTUs (Supplementary Table S2).
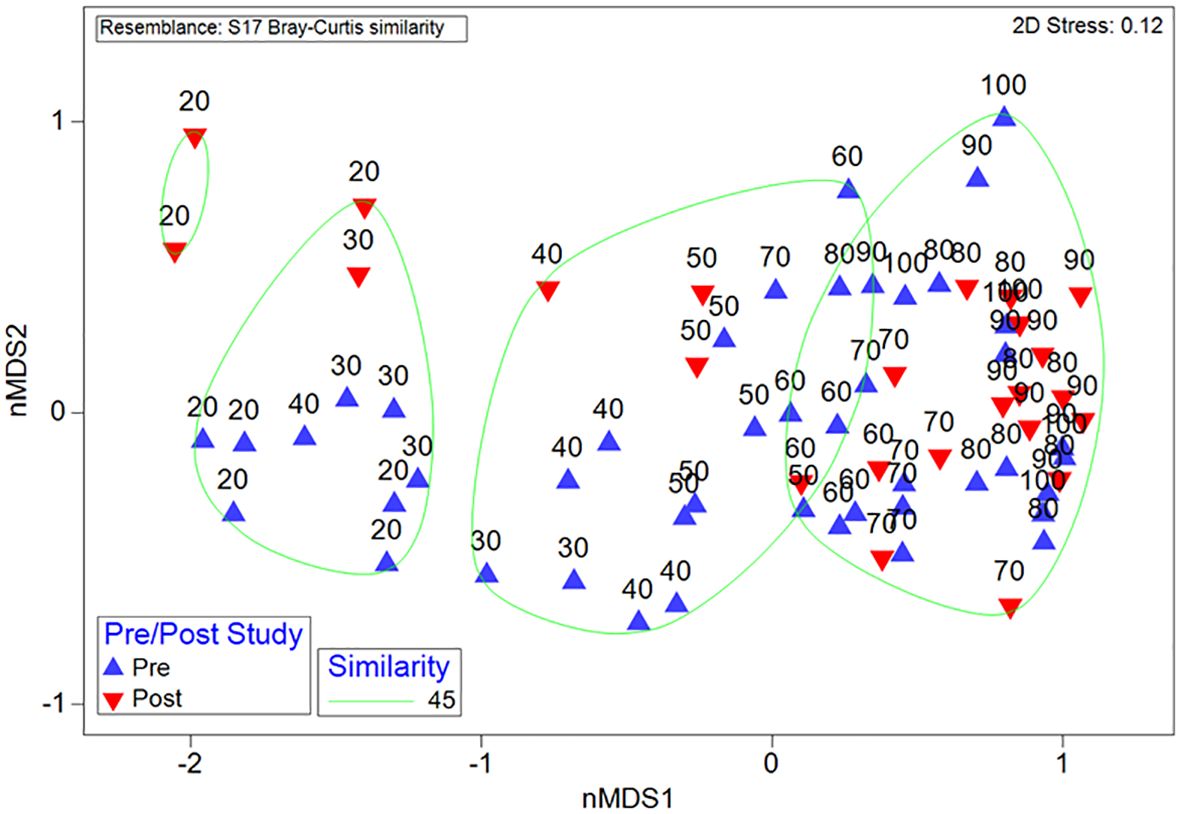
Figure 5 Macroepifauna structure from pre- and post-construction surveys, displayed with non-metric MultiDimensional Scaling (nMDS) with similarity bands based on hierarchical cluster analysis. Samples consist of 10 m depth intervals; samples are labelled with the greatest depth in the range (i.e., ‘20’ represents the transect with the 10–20 m depth range).
3.3 Sedimentation rates
Sediment settlement remained below 5 mg day between July 2017 and May 2020, for both settlement traps and remained at this level throughout the first construction period between December 2018 and March 2019 (Supplementary Figure S2). A large pulse in sedimentation was recorded in the south-eastern sediment trap (location B), during the final measurement period February 2020 to May 2021; however, as the western sediment trap (at location A) was lost to iceberg scour, this observation is the only result available (Figure 6). The pulse in sedimentation coincided with the backfilling of the wharf pilings with crushed rocks during the second construction period October 2019 to March 2020.
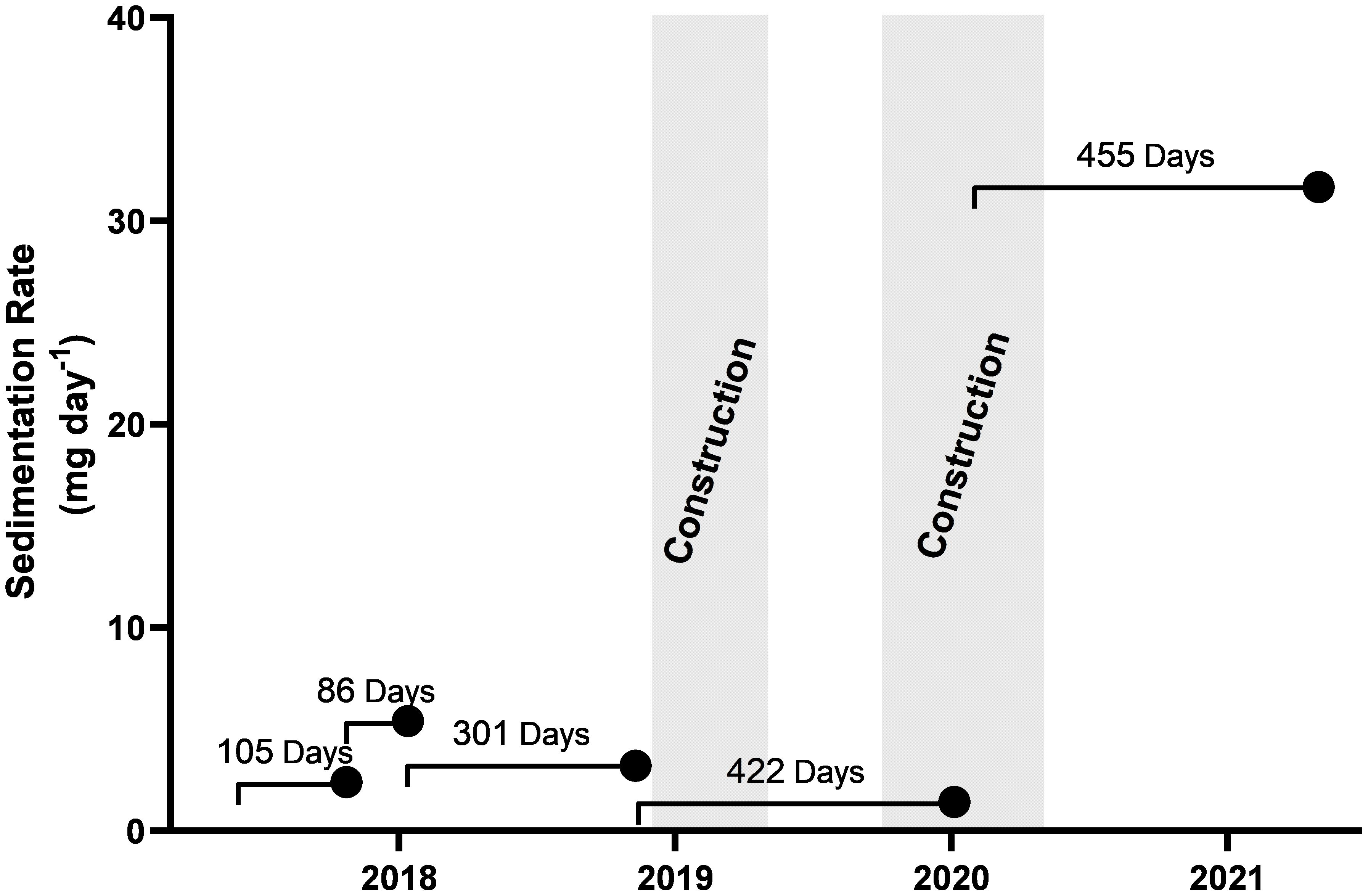
Figure 6 Sedimentation rate recorded by sediment trap B. Each point indicates the retrieval date of the sediment trap and the line represents the deployment time and date. Sedimentation rates were averaged across the entire deployment. The two periods of construction activity between Dec-2018 to May-2019 and Oct-2019 to May-2020 are represented in grey.
4 Discussion
There were small differences in the assemblage on the steep rocky slope in front of the Biscoe Wharf between the pre- and post-construction survey. The largest difference was a small (9%) shift in community composition driven by a reduction in the presence of the sea urchin, Sterechinus neumayeri, and the seastar, Odontaster validus, in the shallows (10–20 m depth). These species are mobile and are known to migrate into recent iceberg scours to scavenge on the carcases and organic material generated by the impact (Robinson et al., 2020). The reduction in these two species is contrary to what we would expect from a site recently disturbed from construction, however in the two years since the impact, the high recovery capability (resilience) of the macroepifauna in the shallows (Robinson et al., 2020; Zwerschke et al., 2021) would have likely led to return to pre-construction state from a local disturbance event, such as the wharf construction. The natural and frequent impacts of iceberg scours within this area (Smale et al., 2006, 2008) could account for the variation in S. neumayeri and O. validus. With similar variations in abundance detected within the same area from previous studies (Robinson et al., 2021).
Macroalgae species, such as OTUs ALG005 and ALG006, were only found in the post-construction survey, but are particularly variable in presence and abundance in the polar environment, as they rely on downwelling light levels that can be restricted or even blocked, by sea ice (Vernet et al., 2008). Many Antarctic species also have episodic recruitment events (e.g., sponges; Dayton et al. (2016)) and the shift in macroalgal presence is likely to represent one such recruitment event (Barnes and Souster, 2011) and with additional removal due frequent iceberg scours increasing natural variability.
A large pulse of sediment was recorded during the final measurement period, which started during the second half of the construction in 2020. This coincided with the backfilling of the wharf pilings with crushed rocks. The sediment traps gave an average measure of sedimentation over their whole period of underwater deployment but it is likely that the increased sedimentation came in a pulse from the rock as it was dumped into the water behind the pilings. No shifts in the overall pattern of macroepifaunal assemblages with depth was observed either between pre and post construction surveys or from previous data collected from this site, including amongst the sessile filter feeding assemblages. The sessile filter feeding assemblage includes a diverse range of taxa such as Perinsiana littoralis (POL002), yellow encrusting porifera (POR001), and encrusting and foliose bryozoans (BRY001–BYR003), which were found at high abundance at depth (70–100 m depth). These macroepifaunal communities would be expected to have the greatest vulnerability to increased sedimentation, detected during construction (Riegl and Branch, 1995; Tompkins-MacDonald and Leys, 2008; Bannister et al., 2012). However, with no knowledge of the local current regime, the impact for remote down-current filter feeding assemblages is unknown. While a single point measurement does not support speculation on the wider impacts of this sedimentation event, it does indicate that those undertaking extended construction activities should consider putting measures in place to mitigate the impact of such marine sedimentation events.
The mitigating measures undertaken during construction of the wharf included the reducing the footprint of the new wharf, while still accommodating the larger vessel and choosing a design to lower the amount of seabed milling and preparation that was required (drilling or changing seabed topography) (Fothergill, 2018). These mitigation measures were designed to reduce the direct loss of benthic habitat, reduce rock/boulder displacement (rock falls), and decrease sediment input into the marine environment.
Due to the delay of the post-construction survey, this report cannot comment on the initial impact of the wharf construction, only that any impact was transient and had become indistinguishable from natural state two years after construction had finished. Below 30 m depth, there was also no significant shift in macroepifauna composition, this is despite macroepifaunal groups with lesser recovery capability, such as Stylocordyla chupachups (POR016), yellow encrusting porifera (POR001) and Phorbas areolatus (POR026), dominating at greater depths (i.e., 70–100 m; Robinson, 2021). Any impact on deeper zoobenthos, e.g., due to rock falls or sediment smothering, would have been particularly evident, even two years after construction. Elsewhere, similar impacts from naturally occurring iceberg scours have been detectable a decade after the initial impact (Gutt et al., 1996). Supporting the conclusion that the mitigating efforts were successful and the environment outside the footprint remains unchanged.
The Antarctic and sub-Antarctic benthos remain some of the least human impacted macroepifauna assemblages on Earth (Rogers et al., 2020). It is therefore important to consider how to mitigate and measure any anthropogenic impact within the Southern Ocean. The monitoring project, although constrained as a consequence of the COVID-19 pandemic, demonstrates the low to negligible impact that the Biscoe Wharf extension has had on the local marine environment, which indicates that the various mitigation measures enacted were successful (Fothergill, 2018). When considering best practices and procedures for future monitoring of construction on seafloor biodiversity within Antarctica, we suggest the implementation of comprehensive monitoring regime, including monitoring of impacts during the construction phase and monitoring of the wider area. However, this study demonstrates the practical need for long term science to understand variability within the marine environment and how the understanding of the underlying mechanisms controlling biodiversity can be applied to quantifying the impact of anthropogenic activities.
Data availability statement
The original contributions presented in the study are included in the article/Supplementary Material, further inquiries can be directed to the corresponding author.
Ethics statement
The manuscript presents research on animals that do not require ethical approval for their study.
Author contributions
BR: Conceptualization, Data curation, Formal analysis, Investigation, Methodology, Software, Supervision, Visualization, Writing – original draft, Writing – review & editing. KH: Conceptualization, Funding acquisition, Project administration, Resources, Writing – review & editing. DS: Funding acquisition, Project administration, Supervision, Writing – review & editing. SM: Formal analysis, Funding acquisition, Investigation, Project administration, Resources, Supervision, Validation, Visualization, Writing – original draft, Writing – review & editing.
Funding
The author(s) declare financial support was received for the research, authorship, and/or publication of this article. Funding for BR came from the Antarctic Infrastructure Modernisation program at the British Antarctic Survey and the NERC SPITFIRE doctoral training partnership at the University of Southampton. KH and SM were funded through NERC funding to the British Antarctic Survey.
Acknowledgments
The authors acknowledge the expert support of the marine team at the British Antarctic Survey’s Rothera Research Station, but particularly Ryan Mathews. This paper is a contribution to the ‘Human Impacts and Sustainability’ research theme of the SCAR Scientific Research Programme ‘Integrated Science to Inform Antarctic and Southern Ocean Conservation’ (Ant-ICON).
Conflict of interest
The authors declare that the research was conducted in the absence of any commercial or financial relationships that could be construed as a potential conflict of interest.
The author(s) declared that they were an editorial board member of Frontiers, at the time of submission. This had no impact on the peer review process and the final decision
Publisher’s note
All claims expressed in this article are solely those of the authors and do not necessarily represent those of their affiliated organizations, or those of the publisher, the editors and the reviewers. Any product that may be evaluated in this article, or claim that may be made by its manufacturer, is not guaranteed or endorsed by the publisher.
Supplementary material
The Supplementary Material for this article can be found online at: https://www.frontiersin.org/articles/10.3389/fevo.2024.1383362/full#supplementary-material
References
Bannister R. J., Battershill C. N., De Nys R. (2012). Suspended sediment grain size and mineralogy across the continental shelf of the great barrier reef: Impacts on the physiology of a coral reef sponge. Continental Shelf Res. 32, 86–95.
Barnes D. K. A. (2017). Iceberg killing fields limit huge potential for benthic blue carbon in Antarctic shallows. Glob Chang Biol. 23, 2649–2659. doi: 10.1111/gcb.13523
Barnes D. K., Conlan K. E. (2007). Disturbance, colonization and development of Antarctic benthic communities. Philos. Trans. R Soc. Lond B Biol. Sci. 362, 11–38. doi: 10.1098/rstb.2006.1951
Barnes D. K. A., Souster T. (2011). Reduced survival of Antarctic benthos linked to climate-induced iceberg scouring. Nat. Climate Change 1, 365–368. doi: 10.1038/nclimate1232
Bonner W. N., Laws R. M. (1964). Seals and sealing. In: Priestly R., Adu R. J., du G., Robin Q. (editors). Antarctic research. London: Butterworth, 163–190.
Convey P., Chown S. L., Clarke A., Barnes D. K., Bokhorst S., CummingS V., et al. (2014). The spatial structure of Antarctic biodiversity. Ecol. Monogr. 84, 203–244. doi: 10.1890/12-2216.1
Clarke K. R., Gorley R. N. (2015). “Getting started with PRIMER v7,” in PRIMER-e: plymouth, (Plymouth Marine Laboratory) 20, 1.
Dayton P., Jarrell S., Kim S., Thrush S., Hammerstrom K., Slattery M., et al. (2016). Surprising episodic recruitment and growth of Antarctic sponges: implications for ecological resilience. J. Exp. Mar. Biol. Ecol. 482, 38–55. doi: 10.1016/j.jembe.2016.05.001
Forcada J., Staniland I. J. (2009). “Antarctic fur seal Arctocephalus gazella,” in Encyclopedia of marine mammals 2nd ed., eds. Perrin W. F., Würsig B., Thewissen J. G.M. (London: Academic Press), 36–42.
Fothergill C. (2018). Final comprehensive environmental evaluation for rother wharf reconstruction & Coastal stabilisation. Available online at: https://www.bas.ac.uk/about/Antarctica/environmental-protection/environmental-policy-and-management/environmental-impact-assessments-eias-in-Antarctica/eias-for-proposed-activities-in-Antarctica-prepared-by-the-uk/ (Accessed 08/12/2023).
Gutt J., Starmans A., Dieckmann G. (1996). Impact of iceberg scouring on polar benthic habitats. Mar. Ecol. Prog. Ser. 137, 311–316. doi: 10.3354/meps137311
Hughes K. A., Convey P. (2020). Implications of the COVID-19 pandemic for Antarctica. Antarctic Sci. 32, 426–439. doi: 10.1017/S095410202000053X
Pielou E. (1966). Shannon’s formula as a measurement of species diversity: it’s use and disuse. Am. Nat. 100, 463–465. doi: 10.1086/282439
Retallick K., Van Landeghem K., Fremand A., Howard F., Sands C., Roman-Gonzalez A., et al. (2021). Seafloor bathymetry of Sheldon Cove, Börgen Bay and Marian Cove, merged and gridded from EM122 multibeam echosounder data collected for the project NE/P003087/1 (2017–2020) (Version 1.0) (NERC EDS UK Polar Data Centre). doi: 10.5285/1B4AB7BC-4272-4B16-A642-565E40544B0A
Riegl B., Branch G. M. (1995). Effects of sediment on the energy budgets of four scleractinian (Bourne 1900) and five alcyonacean (Lamouroux 1816) corals. J. Exp. Mar. Biol. Ecol. 186, 259–275.
Robinson B. J. (2021). Disturbance ecology of the shallow Antarctic Benthos. (Doctoral dissertation). University of Southampton.
Robinson B. J., Barnes D., Grange L., Morley S. (2021). Intermediate ice scour disturbance is key to maintaining a peak in biodiversity within the shallows of the Western Antarctic Penisula. Sci. Rep. 11, 16712.
Robinson B. J., Barnes D. K., Grange L. J., Morley S. A. (2022). The extremes of disturbance reduce functional redundancy: Functional trait assessment of the shallow Antarctic benthos. Front. Mar. Sci. 8, 2149. doi: 10.3389/fmars.2021.797112
Robinson B. J., Barnes D. K., Morley S. A. (2020). Disturbance, dispersal and marine assemblage structure: A case study from the nearshore Southern Ocean. Mar. Environ. Res., 105025. doi: 10.1016/j.marenvres.2020.105025
Rogan-Finnemore M., Ojeda M., Acosta J. M. P., Bretel P., Browne N., Flått S., et al. (2021). Icebreaking polar class research vessels: New Antarctic fleet capabilities. Polar Rec. 57, e46. doi: 10.1017/S003224742100067X
Rogers A., Frinault B., Barnes D., Bindoff N., Downie R., Ducklow H., et al. (2020). Antarctic futures: An assessment of climate-driven changes in ecosystem structure, function, and service provisioning in the Southern Ocean. Annu. Rev. Mar. Sci. 12, 87–120. doi: 10.1146/annurev-marine-010419-011028
Smale D. A. (2008). Continuous benthic community change along a depth gradient in Antarctic shallows: evidence of patchiness but not zonation. Polar Biol. 31, 189–198. doi: 10.1007/s00300-007-0346-3
Smale D. A., Barnes D. K. A., Fraser K. P. P. (2006). The influence of depth, site exposure and season on the intensity of iceberg scouring in nearshore Antarctic waters. Polar Biol. 30, 769–779. doi: 10.1007/s00300-006-0236-0
Smale D. A., Brown K. M., Barnes D. K., Fraser K. P., Clarke A. (2008). Ice scour disturbance in Antarctic waters. Science 321, 371. doi: 10.1126/science.1158647
Tompkins-MacDonald G. J., Leys S. P. (2008). Glass sponges arrest pumping in response to sediment: implications for the physiology of the hexactinellid conduction system. Mar. Biol. 154, 973–984.
Venables H., Meredith M. P., Hendry K. R., Hoopen P. T., Peat H., Chapman A., et al. (2023). Sustained year-round oceanographic measurement from Rother Research Station, Antarctica 1997–2017. Sci. Data 10, 265. doi: 10.1038/s41597-023-02172-5
Vernet M., Martinson D., Iannuzzi R., Stammerjohn S., Kozlowski W., Sines K., et al. (2008). Primary production within the sea-ice zone west of the Antarctic Peninsula: I—Sea ice, summer mixed layer, and irradiance. Deep Sea Res. Part II: Topical Stud. Oceanography 55, 2068–2085. doi: 10.1016/j.dsr2.2008.05.021
Keywords: disturbance, Antarctic, Antarctic Treaty, environmental impact, construction
Citation: Robinson BJO, Hughes KA, Seaton D and Morley SA (2024) Measuring the impact of wharf construction on the Antarctic benthos. Front. Ecol. Evol. 12:1383362. doi: 10.3389/fevo.2024.1383362
Received: 07 February 2024; Accepted: 05 June 2024;
Published: 24 June 2024.
Edited by:
Julieta Orlando, University of Chile, ChileReviewed by:
Conxita Avila, University of Barcelona, SpainGiorgio Castellan, National Research Council (CNR), Italy
Copyright © 2024 Robinson, Hughes, Seaton and Morley. This is an open-access article distributed under the terms of the Creative Commons Attribution License (CC BY). The use, distribution or reproduction in other forums is permitted, provided the original author(s) and the copyright owner(s) are credited and that the original publication in this journal is cited, in accordance with accepted academic practice. No use, distribution or reproduction is permitted which does not comply with these terms.
*Correspondence: Ben J. O. Robinson, YmpvckBlY29zLmF1LmRr
†Present address: Ben J. O. Robinson, Marine Biodiversity and Experimental Ecology, Department of Ecoscience, Aarhus University, Roskilde, Denmark