- 1MARE – Marine and Environmental Sciences Centre & ARNET – Aquatic Research Network, Laboratório Marítimo da Guia, Faculdade de Ciências Universidade de Lisboa, Cascais, Portugal
- 2Swire Institute of Marine Science, School of Biological Sciences, The University of Hong Kong, Hong Kong, Hong Kong SAR, China
- 3Departamento de Biologia Animal, Faculdade de Ciências Universidade de Lisboa, Lisbon, Portugal
- 4Hawai‘i Institute of Marine Biology, University of Hawai’i at Mānoa, Kāne‘ohe, HI, United States
Animal cognition is deeply influenced by interactions with the environment. A notable example of sophisticated cognition in the animal kingdom is described by the mutualistic relationship between cleaner fish and clients, where decision-making processes play a pivotal role in partner choice and fish survival. In this context, while extensive research has explored the cognition of the cleaner wrasse Labroides dimidiatus, it is important to note that studies on the cognition of other wrasse species and on its Caribbean counterparts, Elacatinus spp., are limited. Therefore, to extend our comprehension of cognition in cleaner fish with different evolutionary backgrounds, it is important to focus our attention on the genus Elacatinus spp. In this study, we used plexiglass plates as surrogates for clients and assessed the ability of cleaner gobies, Elacatinus oceanops, to solve a biological market task where prioritizing an ephemeral food plate over a permanent one would double the food reward. We varied cue-based decision-making using both ecologically relevant cues (plate, size, and color) and non-relevant ones (presentation side). Additionally, we tested their capacity for reversal learning, an indicator of complex cognitive abilities. Notably, cleaner gobies were able to solve the biological markets task when the distinguishing cue was a larger plate size. Given that these gobies tend to prioritize larger predatory clients in nature, our results align with their natural inclination. Consequently, further research, including studies involving wild individuals, is essential to elucidate the cognitive abilities of the studied species and their implications in the ecological context and evolutionary history.
1 Introduction
The survival and reproductive success of animals within their ecological niche are shaped by intricate interactions among behavior, ecological pressures, and natural selection (Duckworth, 2009). Evolutionary changes in individual fitness are primarily driven by behavioral mechanisms (Bateson and Gluckman, 2012). Simultaneously, an individual’s behavior is molded by both biotic and abiotic environmental factors (Healy and Braithwaite, 2000). In this context, cognitive abilities, defined as the capacity to process, internalize and act on information acquired from the environment (Shettleworth, 2001), stand as a crucial factor for animal survival and reproduction success (Sol et al., 2007; Cole et al., 2012; Ashton et al., 2018). Cognitive performance, which relies on brain functions with energetic demands, is proposed to vary due to phenotypic plasticity. This refers to the ability of a single genotype to exhibit different phenotypes (e.g., morphological behavioral, etc.) (Meyers and Bull, 2002; Fox et al., 2019) in response to changes in energy availability and environmental conditions (Maille and Schradin, 2017). In animals, behavior plasticity is a rapid response mechanism that mediates interactions between an individual and the environment (Cardoso et al., 2015). Consequently, the capacity of organisms to functionally adapt to new conditions is critical in determining their immediate success or failure in new contexts (Snell-Rood, 2013; Snell-Rood et al., 2018). Behavioral plasticity enables individuals of the same population to occupy diverse niches, facilitating better resource allocation, increased adaptation, and improved chances of survival (Svanbäck and Schluter, 2012). Such phenotypic plasticity enables animals to adapt their cognitive abilities in response to changes in energy availability and environmental demands (Buchanan et al., 2013). This variation in cognitive performance may impact decision-making processes, including partner choice and resource allocation. For instance, spatial discrimination abilities are more developed in environments where food availability is not immediate (White and Brown, 2015; Carbia and Brown, 2019). Conversely, in environments where predator threat is constant, cognitive skills related to perception and rapid response became more pronounced (Brown and Braithwaite, 2005). However, it is essential to note that dynamic environments may also impose costs on cognitive development, as maintaining flexibility can be energetically demanding.
The complex dynamics of social groups within animal communities also require sophisticated cognitive skills to recognize individuals, understand social hierarchies and interpret communicative signals (Fernald, 2017; Kappeler, 2019). Understanding animal cognition requires a study that investigates innate cognitive abilities and adaptations influenced by the ecological context and evolutionary processes (Cauchoix and Chaine, 2016; Szabo et al., 2022). Animals’ innate abilities can vary from basic perceptual processes to complex problem-solving abilities (Rowell et al., 2021). Investigating this aspect offers the basis for comprehending how animals interact with their environment and prompts questions about the adaptability and plasticity of cognitive abilities.
In this regard, coral reefs present unique challenges, due to their complex structure, high biodiversity, and fluctuating environmental conditions, requiring animals to develop adaptative strategies and specialized skills for survival (Dixon et al., 2015; Picq et al., 2016; van Oppen et al., 2018). While often underestimated, fish display remarkable cognitive capabilities essential for their survival and success, particularly in the context of avoiding predators and increasing survival (Brown and Braithwaite, 2005). These cognitive skills became particularly evident in mutualistic relationships, such as the one established between cleaner fish and their clients, where the ability to prioritize certain interactions over others suggests cognitive sophistication due to the complex social decision-making process involved (Triki et al., 2019).
Cleaning symbiosis is one of the most notable mutualisms in nature, and it play a crucial role in maintaining ecosystem dynamics on coral reefs (Côté, 2000). Reef fishes are usually infested by parasites that can cause irritation or pose a risk of diseases. To eliminate these parasites, reef fishes referred to as “clients” approach the “cleaning station”, allowing cleaner fish to remove parasites and dead skin from their bodies (Grutter, 1999; Vaughan et al., 2017). Through this interaction, there is an exchange of commodities between species where the cleaner fish gains a food source, and the client gets rid of parasites (Bshary and Noë, 2003).
In the Caribbean, cleaner gobies Elacatinus spp. provide an essential function to the ecosystem through cleaning mutualism (Côté and Soares, 2011; Vaughan et al., 2017). Here, cleaner gobies engage in cleaning with a wide variety of clients, including potentially threatening predators (Soares et al., 2007). Despite evidence of partner choice in natural observation (Soares et al., 2008, 2013; Dunkley et al., 2019), the cognitive abilities of cleaning gobies have been poorly investigated.
Partner-choice-derived cognition has been widely tested in cleaner fishes using a learning paradigm based on a fish’s capacity to solve discriminatory two-choice tasks, referred to in this context as partner-choice tasks (Noë and Hammerstein, 1994; Mazzei et al., 2019; Triki et al., 2019; Truskanov et al., 2021; Bshary and Noë, 2023), inspired by the principles of biological market theory (Noë and Hammerstein, 1995; Hammerstein and Noë, 2016; Noë, 2016; Bshary and Noë, 2023). This theory provides insight into how organisms make decisions based on trading and cooperation observed in human economic markets. Within the context of cleaning mutualism, where the clients act as consumers and the cleaners as service providers, these ecological interactions resemble transactions in a marketplace (Hammerstein and Noë, 2016). These services are analogous to goods or resources of a traditional market, with clients benefiting from removing ectoparasites. Cleaner gobies prioritize their clients based on specific cues determining the order in which cleaning services are provided (Noë and Hammerstein, 1994; Triki et al., 2019).
In our experiment, we applied this principle to assess the cognitive abilities of cleaning gobies, specifically their decision-making skills and learning capacity, by testing their ability to solve a partner-choice task. Elacatinus oceanops, commonly known as neon gobies, stand as a good model for this study due to their dedicated cleaning behavior and reliance on sophisticated cognitive mechanisms to prioritize clients based on visual cues (Côté and Soares, 2011). Previous studies on cleaner wrasse (Labroides dimidiatus) and capuchin monkeys in biological market task variations highlighted the importance of cue type for solving these tasks (Salwiczek et al., 2012; Gingins and Bshary, 2016; Prétôt et al., 2016). Similarly, cleaner gobies prioritize clients with higher parasite loads, often indicated by larger body size (Sikkel et al., 2004; Soares et al., 2007), making them an ideal species to study cue-based decision-making.
The task required them to make decisions mirroring their ecological context. As extensively tested, the learning process in cleaner fish can be investigated using plexiglass plates offering food as proxies of real clients (Salwiczek et al., 2012; Gingins and Bshary, 2016; Triki et al., 2019). Specifically, the fish were presented with two plates and were asked to choose one of them. Giving priority to an ephemeral food plate (representing a client) over a permanent plate doubled the food reward (Bshary and Grutter, 2002; Triki et al., 2019). To understand if cleaner gobies are able to solve partner choice cognitive tests, we tested captive-bred dedicated cleaner gobies Elacatinus oceanops, commonly known as neon goby, for their capacity to solve a biological market test. We varied cue-based decision-making using both ecologically relevant cues (plate size and color) and non-relevant ones (presentation side). Notably, size and color are considered ecologically relevant cues because they mirror essential client characteristics, such as species identity. In their natural habitat, cleaner gobies rely on visual cues like size to identify clients with higher ectoparasite loads, which is crucial for their feeding, providing efficient cleaning services and shaping decision-making strategies. Additionally, we tested their capacity for reversal learning, an indicator of complex cognitive abilities.
2 Material and methods
2.1 Study species and experimental design
Cleaner gobies, Elacatinus oceanops, were bred in an ornamental aquaculture facility and transported to the aquatic facilities of Laboratório Marítimo da Guia (Cascais, Portugal) by TMC-Iberia. A total of 13 cleaner gobies were individually housed and acclimated to the experimental setup by feeding them mysid shrimps spread on Plexiglas plates.
The study took place from November 2022 to January 2023. We adapted our experimental design from the biological market theory experiment introduced by Bshary and Grutter, 2002. This design has been widely replicated (e.g., Wismer et al., 2014; Mazzei et al., 2019; Paula et al., 2019; Triki et al., 2019), and further modified by Wismer et al. (2019). In our setup, individual aquariums were divided into two sections by a transparent partition (see Figure 1). The fish were kept in the smaller section while experimental Plexiglass plates were introduced into the larger section. When trials began, the partition was removed, giving the gobies access to two Plexiglass plates, each with a small amount of mysid shrimp (approximately 0.001 g). One plate was ephemeral and removed if not chosen first, while the other was permanent, remaining in the aquarium for the entire duration of the trial (around 2 min.), regardless of the gobies’ foraging decisions.
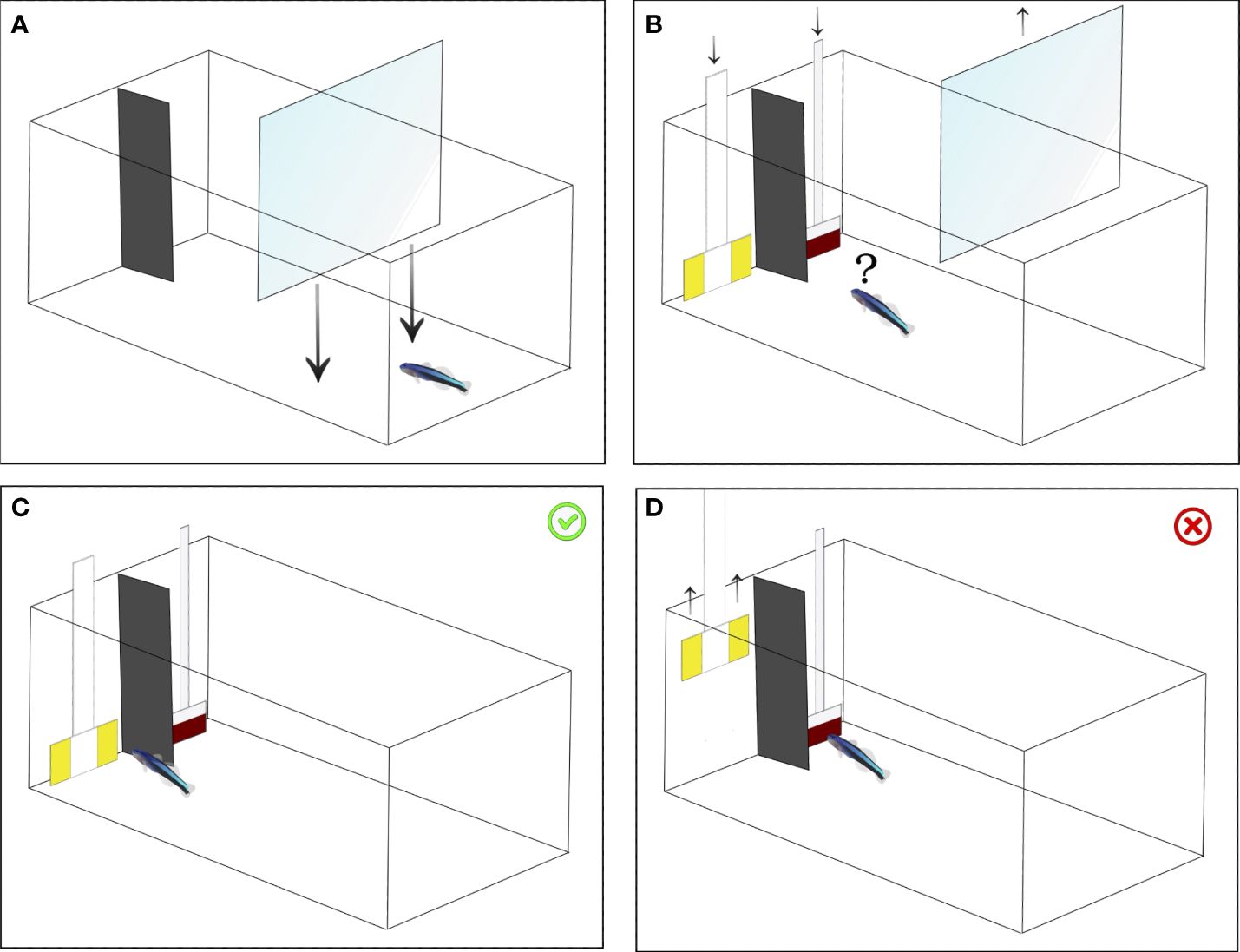
Figure 1 Test procedure. (A) The fish was initially guided into one side of the aquarium using a transparent partition. (B) Subsequently, the transparent partition was removed, allowing the fish to forage freely. (C) In the event of the fish choosing the correct plate, both plates remained in the aquarium, granting access to the food reward. (D) Conversely, if the fish opted for the incorrect plate, the opposing plate was promptly removed, denying access to the reward.
In this study, the established protocol was followed with one key difference: the method of indicating which plate was ephemeral varied by test, using either size, color, or spatial cues. Colors and sizes were selected according to previous research conducted by the authors and cleaner fish literature (Cheney et al., 2008; Soares et al., 2016; Paula et al., 2019). The choice of color was based on contrast and brightness since clients can change from light to dark colors when posing at cleaning stations (Caves, 2021). The different size was used based on the evidence that cleaners may assess parasite load (Arnal et al., 2000) or predation (Côté and Soares, 2011) using client body size. For all tests except those involving spatial cues, the placement of the ephemeral plate in the aquarium (either left or right) was balanced and semi-randomized across 10 trials. A lottery system ensured the ephemeral plate appeared on each side 50% of the time, without being on the same side more than three times consecutively. The number of trials it took for the cleaner fish to learn to feed from the ephemeral plate first was monitored. The learning criterion was deemed met if the cleaners chose the ephemeral plate first in at least 9 out of 10 consecutive trials, 8 out of 10 trials in two consecutive sessions, or 7 out of 10 trials in three consecutive sessions. Each cleaner fish was exposed to all cue types in the same order: plate size, then a reversal, followed by color, another reversal, spatial cues, and a final reversal. Each fish participated in one session per day, with each session comprising 10 trials, totaling 100 trials per cue type.
2.1.1 Test 1: variation in size
Two Plexiglas plates, one large (7 cm x 4 cm) and one small (4 cm x 3 cm), characterized by two different colors and patterns (i.e., two yellow vertical stripes and one brown horizontal stripe; see Supplementary Figure 1), were used. The larger plate represented the ephemeral plate (correct choice), and the small one represented the permanent (wrong choice). The presentation side of each plate size was balanced and randomized across the 100 trials.
2.1.2 Test 2: variation in color
The second test started 60 days after the first. During the 60 days, fish were fed with white plexiglass plates without any color or size information to desensitize any learning patterns from the first test. Plates of equal dimensions were presented in colors and patterns matching those of Test 1. The plate positions (left or right) were balanced and randomized across the 100 trials.
2.1.3 Test 3: spatial cue
The test involved associating a specific location (left or right side of the tank) with the food reward. Two white Plexiglas plates of equal size were used. The correct plate location (left or right) was randomized, as in Tests 1 and 2.
2.1.4 Reversal learning
Following a 12-day break after each cue test, the fish that successfully completed the initial test were subjected to a reversal test. In these tests, the cue associated with the ephemeral plate was switched for each type of cue: size, color, and spatial. The procedures remained the same, except for a key change: the behavior of the plates was reversed. The plate that was previously ephemeral (removed if not chosen first) now remained in the tank permanently, regardless of the fish’s choice, while the plate that was previously permanent became the ephemeral one. This reversal was implemented abruptly to assess the fish’s adaptability to changes in cue associations.
The time frame of the tests is indicated in Supplementary Table 1.
2.2 Statistical analysis
Survival analyses were conducted for each test using the R package “survival” (Therneau and Grambsch, 2000) to analyze time event data (number of trials to solve the task) and compare tests with different ecological relevance for the study species. The survival analysis was fitted using the Cox proportional hazard model (“coxph”). Initially, a survival standard object was constructed (“surv”), and subsequently, to assess statistically significant differences in the learning performances among the tests, a non-parametric long-rank test was performed (“survdiff”). This test allowed us to compare the survival curves representing the task completion over time. To validate the assumption of proportional hazards, a Schoenfeld test (“cox.zph”) was conducted. Following this, the residuals were graphically examined (“ggcoxzph”) to identify any time-dependent effects of the “correct” variable on the hazard.
3 Ethical note
Animal experimentation met the ASAB guidelines for the ethical treatment of animals. The experiments were conducted under the approval of Faculdade de Ciências da Universidade de Lisboa animal welfare body (ORBEA-FCUL, ref: 04/2023)
4 Results
Out of the initial 13 E. oceanops individuals, 13 were tested for size cues and 12 for color and spatial cues, as one fish died during the experiment (see Table 1; see Supplementary Table 2 for individual performance). Among them, 10 (77%) successfully solved the task using the plate size as a cue (Figure 2A, χ2 = 11.55, p = 0.003). Subsequently, with both color and spatial cues, only 2 (17%) passed the test. However, none of the gobies successfully solved the reversal version of any cue (Figure 2B).
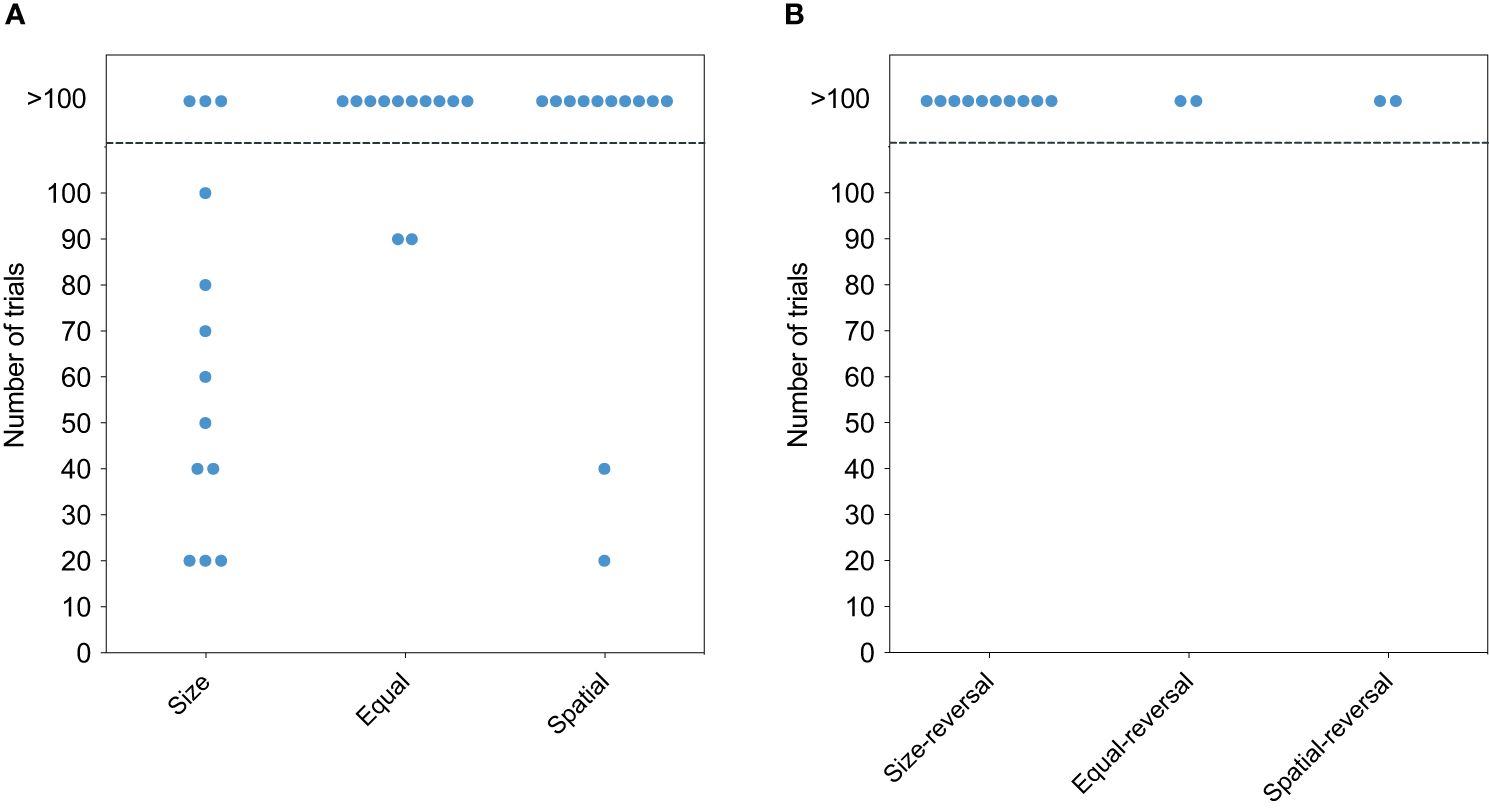
Figure 2 Performance of cleaners in the cognitive tests. (A) The number of trials required for cleaners to establish a significant preference for the visitor plate. Each circle represents the performance of one individual. Individuals above the dashed line did not complete the task within the maximum of 100 trials. Cue type, namely size, colour and spatial, is represented on the y-axis. (B) The number of trials required for successful cleaners to reject their established preference and learn to prefer the reversal cue.
The survival analyses, conducted using the Cox proportional hazard model, revealed significant differences in learning performances among the tests (χ2 = 15.8, p < 0.0001). Pairwise comparisons indicated that when individuals were tested with the same size plate and during the spatial discrimination test, they exhibited significantly lower hazard rates compared to those tested with plates of different sizes (p = 0.00734; p = 0.01114, respectively).
Moreover, the analyses revealed significant time-dependent effects of the “correct” variable on the hazard, suggesting deviations from the proportional hazard assumption (Schoenfeld test, p < 0.05). Specifically, this time-dependent effect was significant only in the size discrimination task (Schoenfeld test, p < 0.05).
5 Discussion
Understanding the mechanisms behind the diversity in decision-making rules is crucial for grasping how natural selection shapes social behaviors in response to environmental needs. Our study focused on whether cleaner gobies, Elacatinus oceanops, could successfully complete three discriminatory two-choice tasks in a biological market scenario by employing various cue-based decision strategies. We observed that cleaner gobies could successfully accomplish this test when specific cues, particularly size, were utilized but failed when cues such as color were included. These findings emphasize the critical significance of accurately identifying salient cues, such as size, to test cognitive performances and determine the ability of cleaner gobies to complete a biological market task.
Despite the potential involvement of other cues, such as odor, our experiment shows the paramount importance of size in cleaner gobies’ decision-making processes. When size was the cue, a significant majority of cleaner gobies (77%) successfully solved the test by choosing the larger plate, indicating the universal recognition of size as an informative cue. In their natural habitat, size is a practical cue, as larger fish typically host more ectoparasites (Grutter, 1995; Grutter and Poulin, 1998), and gobies show a preference for clients with a higher parasite load (Sikkel et al., 2004; Soares et al., 2007). Additionally, observations revealed that gobies prioritize predator clients, often characterized by larger body sizes. Prioritizing predators aids gobies in reducing the negative impact of a potential threat to the other fish, encouraging the clients to return promptly, and facilitating the mutualistic exchange of benefits (Soares et al., 2007). Another important aspect is the cleaning dependence of the species study. Elacatinus oceanops is a dedicated cleaner, exhibiting behavior that requires sophisticated mechanisms. Their ability to solve the test where the decision-making criteria are associated with the size might reflect the precision with which their cognitive skills have adapted to meet their needs. This not only highlights the adaptability of their cognitive abilities but also underscores the significant role played by the feeding behavior for which they were naturally selected.
However, in our study cleaner gobies struggled to use color cues effectively. This unexpected result contradicts previous assumptions about the importance of color in species recognition during cleaning interactions. While parasite loads and mucus quality vary not only with the size of the fish but also across different species (Grutter, 1994; Arnal et al., 2001), our findings suggest a reliance on size rather than color or patterns in the decision-making process of cleaner gobies. This result underlines the importance of size as a salient cue in the learning process of this fish, also considering the observation in an experiment conducted with a closely related cleaner goby species, Elacatinus prochilos (Mazzei et al., 2019), where gobies exhibited limited learning abilities to discriminatory tasks involving different patterns but same colors and size. The reliance on size cues over color or pattern cues may reflect the importance of the physical characteristics of clients.
Furthermore, in contrast to their proficiency with ecologically relevant cues like size and their related species E. prochilos, cleaner gobies displayed a notable lack of cognitive ability in a test unrelated to their natural ecological context, the spatial cue (Test 3). In this test, the fish were required to distinguish between spatial arrangements to successfully complete the test. Unlike the tests involving size cues, where the gobies could rely on their innate or learned ecological behaviors, the spatial test demanded a different kind of cognitive processing. We propose two possible explanations for the observed lack of flexible cognition. Firstly, the spatial discrimination test likely posed a challenge because it required the gobies to utilize spatial memory skills, which are not as directly linked to their natural behaviors. In their natural habitat, cleaner gobies are more likely to rely on visual cues like size and color to identify clients with higher ectoparasite loads, which is crucial for their feeding and survival. However, navigating and remembering spatial configurations does not have a direct bearing on these essential tasks. Another plausible explanation could be a consequence of the experimental procedure. The fish were exposed to different tasks over a period of three months. This prolonged training period might have resulted in decreased motivation and a potential failure in successfully navigating the spatial discrimination task.
While these findings may indicate low cognitive flexibility in cleaner gobies, it is essential to note potential confounding factors, including using fish raised in captivity. Studies have shown that individuals raised in not complex environments may exhibit different and lower cognitive performance than their wild counterparts raised in complex and enriched environments due of environmental experiences (Wismer et al., 2019). Furthermore, the importance of testing species in their natural habitat is underscored by previous research (Bräuer et al., 2020; Salena et al., 2021), highlighting the impact of artificial selection and life experiences on cognition.
Given the potential variance in the a lack impact of natural selection between laboratory and wild environments, the observed low capacity in cognitive abilities and the absence of flexibility may be attributed to biases inherent in laboratory conditions. Moreover, the experimental setup, notably the two-week gap between tasks, and potential sequence effects might have impacted the strength of the association between stimuli and reinforcement. This choice might have influenced the performances of the fish. Therefore, the apparent challenges in task-solving proficiency could arise from factors such as prolonged training regimens, diminished motivation, and stress induced by the test procedures. Furthermore, the inability to learn subsequent discriminations does not necessarily signify inferior cognitive capacity, as environmental and motivational variables can impact task execution.
However, the fact that these gobies demonstrated cognitive skills in tests closely resembling their natural ecological scenarios indicates an inherent, specialized learning mechanism. This tendency of cleaner gobies to effectively process and respond to cues relevant to their natural behaviors, despite their lack of direct environmental experience, emphasizes the potential for a latent cognitive framework shaped by evolutionary history. While it is understood that animal cognition is shaped by a complex interplay of various factors, including both biotic and abiotic elements, genetics, and evolutionary processes, our findings particularly highlight the significant role of evolutionary influences. This underscores the profound impact of evolutionary history on shaping innate cognitive abilities in animals, particularly in relation to ecological relevancy.
While our findings hold significance on the innate cognitive skills of cleaner gobies, further research incorporating wild populations is essential to comprehensively understand their holistic cognitive abilities. Studying these fish in a laboratory setting, especially those reared individually, primarily sheds light on their innate cognitive skills. However, extending this research to include wild populations of cleaner gobies could offer additional insights and a deeper understanding of the species’ cognitive capabilities. Conducting similar tests in the wild would not only help determine if their ability to utilize other cues, such as color or patterns, could be enhanced through natural cleaning interactions, but it would also provide a more holistic view of their cognitive skills. In their natural habitats, cleaner gobies must continuously adapt their behavior to the ever-changing environmental conditions.
In conclusion, our study highlights the critical role of incorporating cue salience within an ecological context for understanding the variations in social decision-making processes. By examining how specific cues influence cognition, we can better appreciate the intricate balance between innate abilities and learned behaviors in shaping social decision rules. This approach not only enriches our comprehension of the evolutionary and environmental factors driving these variations but opens up new avenues for exploring the complex interplay between an organism’s innate predispositions and its adaptive responses to ecological demands.
Data availability statement
The datasets presented in this study can be found in online repositories. The names of the repository/repositories and accession number(s) can be found below: FigShare, 10.6084/m9.figshare.26121952.
Ethics statement
The animal study was approved by Órgão Responsável pelo Bem-Estar dos Animais (ORBEA) da Faculdade de Ciências da Universidade de Lisboa. The study was conducted in accordance with the local legislation and institutional requirements.
Author contributions
MR: Conceptualization, Formal analysis, Investigation, Methodology, Visualization, Writing – original draft, Writing – review & editing. MC: Formal analysis, Investigation, Writing – review & editing. BP: Investigation, Writing – review & editing. DR: Investigation, Writing – review & editing. JP: Conceptualization, Data curation, Formal analysis, Funding acquisition, Investigation, Methodology, Project administration, Resources, Supervision, Validation, Visualization, Writing – original draft, Writing – review & editing.
Funding
The author(s) declare financial support was received for the research, authorship, and/or publication of this article. This work was supported by FCT-Fundação para a Ciência e Tecnologia, I.P, within the project grant PTDC/BIA-BMA/0080/2021 -ChangingMoods to JP (DOI: https://doi.org/10.54499/PTDC/BIA-BMA/0080/2021), the scientific employment stimulus program 2021.01030.CEECIND (DOI: https://doi.org/10.54499/2021.01030.CEECIND/CP1654/CT0003) to JP, the strategic project UIDB/04292/2020 granted to MARE and project LA/P/0069/2020 granted to the Associate Laboratory ARNET.
Acknowledgments
We would like to acknowledge all members of the Behavioral Ecology and Evolution group who contributed with comments and help throughout this work.
Conflict of interest
The authors declare that the research was conducted in the absence of any commercial or financial relationships that could be construed as a potential conflict of interest.
The author(s) declared that they were an editorial board member of Frontiers, at the time of submission. This had no impact on the peer review process and the final decision
Publisher’s note
All claims expressed in this article are solely those of the authors and do not necessarily represent those of their affiliated organizations, or those of the publisher, the editors and the reviewers. Any product that may be evaluated in this article, or claim that may be made by its manufacturer, is not guaranteed or endorsed by the publisher.
Supplementary material
The Supplementary Material for this article can be found online at: https://www.frontiersin.org/articles/10.3389/fevo.2024.1375835/full#supplementary-material
References
Arnal C., Côté I. M., Morand S. (2001). Why clean and be cleaned? The importance of client ectoparasites and mucus in a marine cleaning symbiosis. Behav. Ecol. Sociobiology 51, 1–7. doi: 10.1007/s002650100407
Arnal C., Côté I. M., Sasal P., Morand S. (2000). Cleaner-client interactions on a Caribbean reef: influence of correlates of parasitism. Behav. Ecol. Sociobiology 47, 353–358. doi: 10.1007/s002650050676
Ashton B. J., Ridley A. R., Edwards E. K., Thornton A. (2018). Cognitive performance is linked to group size and affects fitness in Australian magpies. Nature 554, 364–367. doi: 10.1038/nature25503
Bateson P., Gluckman P. (2012). Plasticity and robustness in development and evolution. Int. J. Epidemiol. 41, 219–223. doi: 10.1093/ije/dyr240
Bräuer J., Hanus D., Pika S., Gray R., Uomini N. (2020). Old and new approaches to animal cognition: there is not “One cognition. J. Intell. 8, 28. doi: 10.3390/jintelligence8030028
Brown C., Braithwaite V. A. (2005). Effects of predation pressure on the cognitive ability of the poeciliid Brachyraphis episcopi. Behav. Ecol. 16, 482–487. doi: 10.1093/beheco/ari016
Bshary R., Grutter A. S. (2002). Experimental evidence that partner choice is a driving force in the payoff distribution among cooperators or mutualists: the cleaner fish case. Ecol. Lett. 5, 130–136. doi: 10.1046/j.1461-0248.2002.00295.x
Bshary R., Noë R. (2003). Biological markets: the ubiquitous influence of partner choice on the dynamics of cleaner fish-client reef fish interactions. Genet. Cultural Evol. Cooperation 9, 167–184. doi: 10.7551/mitpress/3232.003.0011
Bshary R., Noë R. (2023). A marine cleaning mutualism provides new insights in biological market dynamics. Philos. Trans. R. Soc. B: Biol. Sci. 378, 20210501. doi: 10.1098/rstb.2021.0501
Buchanan K. L., Grindstaff J. L., Pravosudov V. V. (2013). Condition dependence, developmental plasticity, and cognition: implications for ecology and evolution. Trends Ecol. Evol. 28, 290–296. doi: 10.1016/j.tree.2013.02.004
Carbia P. S., Brown C. (2019). Environmental enrichment influences spatial learning ability in captive-reared intertidal gobies (Bathygobius cocosensis). Anim. Cogn. 22, 89–98. doi: 10.1007/s10071-018-1225-8
Cardoso S. D., Teles M. C., Oliveira R. F. (2015). Neurogenomic mechanisms of social plasticity. J. Exp. Biol. 218, 140–149. doi: 10.1242/jeb.106997
Cauchoix M., Chaine A. S. (2016). How can we study the evolution of animal minds? Front. Psychol. 7. doi: 10.3389/fpsyg.2016.00358
Caves E. M. (2021). The behavioural ecology of marine cleaning mutualisms. Biol. Rev. 96, 2584–2601. doi: 10.1111/brv.12770
Cheney K. L., Grutter A. S., Marshall N. J. (2008). Facultative mimicry: cues for colour change and colour accuracy in a coral reef fish. Proc. R. Soc. B: Biol. Sci. 275, 117–122. doi: 10.1098/rspb.2007.0966
Cole E. F., Morand-Ferron J., Hinks A. E., Quinn J. L. (2012). Cognitive ability influences reproductive life history variation in the wild. Curr. Biol. 22, 1808–1812. doi: 10.1016/j.cub.2012.07.051
Côté I. M. (2000). Evolution and ecology of cleaning symbioses in the sea. Oceanography Mar. Biol. 38, 311–355. https://api.semanticscholar.org/CorpusID:83110787.
Côté M., Soares M. C. (2011). Gobies as cleaners. In: Patzner R., Van T. J., Kovacic M., Kapoor B. (eds) The biology of gobies. 525–551. (Boca Raton, FL: CRC Press).
Dixon G. B., Davies S. W., Aglyamova G. V., Meyer E., Bay L. K., Matz M. V. (2015). Genomic determinants of coral heat tolerance across latitudes. Science 348, 1460–1462. doi: 10.1126/science.1261224
Duckworth R. A. (2009). The role of behavior in evolution: a search for mechanism. Evolutionary Ecol. 23, 513–531. doi: 10.1007/s10682-008-9252-6
Dunkley K., Ioannou C. C., Whittey K. E., Cable J., Perkins S. E. (2019). Cleaner personality and client identity have joint consequences on cleaning interaction dynamics. Behav. Ecol. 30, 703–712. doi: 10.1093/beheco/arz007
Fernald R. D. (2017). Cognitive skills and the evolution of social systems. J. Exp. Biol. 220, 103–113. doi: 10.1242/jeb.142430
Fox R. J., Donelson J. M., Schunter C., Ravasi T., Gaitán-Espitia J. D. (2019). Beyond buying time: The role of plasticity in phenotypic adaptation to rapid environmental change. In. Philos. Trans. R. Soc. B: Biol. Sci. 374, 1768). doi: 10.1098/rstb.2018.0174
Gingins S., Bshary R. (2016). The cleaner wrasse outperforms other labrids in ecologically relevant contexts, but not in spatial discrimination. Anim. Behav. 115, 145–155. doi: 10.1016/j.anbehav.2016.02.022
Grutter A. S. (1994). Spatial and temporal variations of the ectoparasites of seven reef fish species from Lizard Island and Heron Island, Australia. Marine Ecology Progress Series. 115, 21–30. doi: 10.3354/meps115021
Grutter A. (1995). Relationship between cleaning rates and ectoparasite loads in coral reef fishes. Mar. Ecol. Prog. Ser. 118, 51–58. doi: 10.3354/meps118051
Grutter A., Poulin R. (1998). Intraspecific and interspecific relationships between host size and the abundance of parasitic larval gnathiid isopods on coral reef fishes. Mar. Ecol. Prog. Ser. 164, 263–271. doi: 10.3354/meps164263
Hammerstein P., Noë R. (2016). Biological trade and markets. Philos. Trans. R. Soc. B: Biol. Sci. 371, 20150101. doi: 10.1098/rstb.2015.0101
Healy S., Braithwaite V. (2000). Cognitive ecology: a field of substance? Trends Ecol. Evol. 15, 22–26. doi: 10.1016/S0169-5347(99)01737-1
Kappeler P. M. (2019). A framework for studying social complexity. Behav. Ecol. Sociobiology 73, 13. doi: 10.1007/s00265-018-2601-8
Maille A., Schradin C. (2017). Ecophysiology of cognition: How do environmentally induced changes in physiology affect cognitive performance? Biol. Rev. 92, 1101–1112. doi: 10.1111/brv.12270
Mazzei R., Lampe M., Ohnesorge A., Pajot A., Soares M. C., Bshary R. (2019). Ecological differences in the facultative Caribbean cleaning goby Elacatinus prochilos do not predict learning performance in discriminatory two-choice tasks. Anim. Cogn. 22, 1039–1050. doi: 10.1007/s10071-019-01295-w
Meyers L. A., Bull J. J. (2002). Fighting change with change: adaptive variation in an uncertain world. Trends Ecol. Evol. 17, 551–557. doi: 10.1016/S0169-5347(02)02633-2
Noë R. (2016). How do biological markets compare to the markets of economics? Available at: https://mpra.ub.uni-muenchen.de/72509/.
Noë R., Hammerstein P. (1994). Biological markets: supply and demand determine the effect of partner choice in cooperation, mutualism and mating. Behav. Ecol. Sociobiology 35, 1–11. doi: 10.1007/BF00167053
Noë R., Hammerstein P. (1995). Biological markets. Trends Ecol. Evol. 10, 336–339. doi: 10.1016/S0169-5347(00)89123-5
Paula J. R., Baptista M., Carvalho F., Repolho T., Bshary R., Rosa R. (2019). The past, present and future of cleaner fish cognitive performance as a function of CO 2 levels. Biol. Lett. 15, 20190618. doi: 10.1098/rsbl.2019.0618
Picq S., McMillan W. O., Puebla O. (2016). Population genomics of local adaptation versus speciation in coral reef fishes (Hypoplectrus spp, Serranidae). Ecol. Evol. 6, 2109–2124. doi: 10.1002/ece3.2028
Prétôt L., Bshary R., Brosnan S. F. (2016). Comparing species decisions in a dichotomous choice task: adjusting task parameters improves performance in monkeys. Anim. Cogn. 19, 819–834. doi: 10.1007/s10071-016-0981-6
Rowell M. K., Pillay N., Rymer T. L. (2021). Problem solving in animals: proposal for an ontogenetic perspective. Animals 11, 866. doi: 10.3390/ani11030866
Salena M. G., Turko A. J., Singh A., Pathak A., Hughes E., Brown C., et al. (2021). Understanding fish cognition: a review and appraisal of current practices. In Anim. Cogn. 24, 395–406). doi: 10.1007/s10071-021-01488-2
Salwiczek L. H., Prétôt L., Demarta L., Proctor D., Essler J., Pinto A. I., et al. (2012). Adult cleaner wrasse outperform capuchin monkeys, chimpanzees and orang-utans in a complex foraging task derived from cleaner – client reef fish cooperation. PloS One 7, e49068. doi: 10.1371/journal.pone.0049068
Shettleworth S. J. (2001). Animal cognition and animal behaviour. Anim. Behav. 61, 277–286. doi: 10.1006/anbe.2000.1606
Sikkel P. C., Cheney K. L., Côté I. M. (2004). In situ evidence for ectoparasites as a proximate cause of cleaning interactions in reef fish. Anim. Behav. 68, 241–247. doi: 10.1016/j.anbehav.2003.10.023
Snell-Rood E. C. (2013). An overview of the evolutionary causes and consequences of behavioural plasticity. Anim. Behav. 85, 1004–1011. doi: 10.1016/j.anbehav.2012.12.031
Snell-Rood E. C., Kobiela M. E., Sikkink K. L., Shephard A. M. (2018). Mechanisms of plastic rescue in novel environments. Annual review of ecology, evolution, and systematics. 49 (1), 331–354. doi: 10.1146/annurev-ecolsys-110617-062622
Soares M. C., Bshary R., Cardoso S. C., Côté I. M. (2008). Does competition for clients increase service quality in cleaning gobies? Ethology 114, 625–632. doi: 10.1111/j.1439-0310.2008.01510.x
Soares M. C., Cardoso S. C., Côté I. M. (2007). Client preferences by Caribbean cleaning gobies: food, safety or something else? Behav. Ecol. Sociobiology 61, 1015–1022. doi: 10.1007/s00265-006-0334-6
Soares M. C., Cardoso S. C., Nicolet K. J., Côté I. M., Bshary R. (2013). Indo-Pacific parrotfish exert partner choice in interactions with cleanerfish but Caribbean parrotfish do not. Anim. Behav. 86, 611–615. doi: 10.1016/j.anbehav.2013.06.017
Soares M. C., Paula J. R., Bshary R. (2016). Serotonin blockade delays learning performance in a cooperative fish. Anim. Cogn. 19, 1027–1030. doi: 10.1007/s10071-016-0988-z
Sol D., Székely T., Liker A., Lefebvre L. (2007). Big-brained birds survive better in nature. Proc. R. Soc. B: Biol. Sci. 274, 763–769. doi: 10.1098/rspb.2006.3765
Svanbäck R., Schluter D. (2012). Niche specialization influences adaptive phenotypic plasticity in the threespine stickleback. Am. Nat. 180, 50–59. doi: 10.1086/666000
Szabo B., Valencia-Aguilar A., Damas-Moreira I., Ringler E. (2022). Wild cognition – linking form and function of cognitive abilities within a natural context. Curr. Opin. Behav. Sci. 44, 101115. doi: 10.1016/j.cobeha.2022.101115
Therneau T. M., Grambsch P. M. (2000). Modeling survival data: extending the cox model (New York: Springer).
Triki Z., Wismer S., Rey O., Ann Binning S., Levorato E., Bshary R. (2019). Biological market effects predict cleaner fish strategic sophistication. Behav. Ecol. 30, 1548–1557. doi: 10.1093/beheco/arz111
Truskanov N., Emery Y., Porta S., Bshary R. (2021). Configural learning by cleaner fish in a complex biological market task. Anim. Behav. 181, 51–60. doi: 10.1016/j.anbehav.2021.08.023
van Oppen M. J. H., Bongaerts P., Frade P., Peplow L. M., Boyd S. E., Nim H. T., et al. (2018). Adaptation to reef habitats through selection on the coral animal and its associated microbiome. Mol. Ecol. 27, 2956–2971. doi: 10.1111/mec.14763
Vaughan D. B., Grutter A. S., Costello M. J., Hutson K. S. (2017). Cleaner fishes and shrimp diversity and a re-evaluation of cleaning symbioses. Fish Fisheries 18, 698–716. doi: 10.1111/faf.12198
White G. E., Brown C. (2015). Cue choice and spatial learning ability are affected by habitat complexity in intertidal gobies. Behav. Ecol. 26, 178–184. doi: 10.1093/beheco/aru178
Wismer S., Pinto A. I., Triki Z., Grutter A. S., Roche D. G., Bshary R. (2019). Cue-based decision rules of cleaner fish in a biological market task. Anim. Behav. 158, 249–260. doi: 10.1016/j.anbehav.2019.09.013
Keywords: cleaning mutualism, cognition, cue, Elacatinus oceanops, biological market
Citation: Ranucci M, Court M, Pereira BP, Romeo D and Paula JR (2024) Cleaner gobies can solve a biological market task when the correct cue is larger. Front. Ecol. Evol. 12:1375835. doi: 10.3389/fevo.2024.1375835
Received: 24 January 2024; Accepted: 04 July 2024;
Published: 22 July 2024.
Edited by:
Anindita Bhadra, Indian Institute of Science Education and Research Kolkata, IndiaReviewed by:
Elia Gatto, University of Ferrara, ItalyMystera M Samuelson, University of Nebraska Medical Center, United States
Copyright © 2024 Ranucci, Court, Pereira, Romeo and Paula. This is an open-access article distributed under the terms of the Creative Commons Attribution License (CC BY). The use, distribution or reproduction in other forums is permitted, provided the original author(s) and the copyright owner(s) are credited and that the original publication in this journal is cited, in accordance with accepted academic practice. No use, distribution or reproduction is permitted which does not comply with these terms.
*Correspondence: Maddalena Ranucci, mranucci@ciencias.ulisboa.pt; José Ricardo Paula, jrpaula@ciencias.ulisboa.pt