- 1College of Life Science, Xinjiang Normal University, Urumqi, China
- 2Xinjiang Key Laboratory of Special Species Conservation and Regulatory Biology, Xinjiang Normal University, Urumqi, China
Introduction: The ecological stoichiometric ratio of carbon, nitrogen, and phosphorus is an important index to understand the utilization and distribution of plant nutrients.
Method: To explore how leaf carbon, nitrogen, and phosphorus contents, along with the stoichiometric ratio of different life forms of plants, respond to variations in altitude and soil physical and chemical properties, leaves and soil samples were collected from different life forms of plants at different altitudes (1,100~1,700 m) within the Guozigou region of the forest. Subsequently, the contents and ratios of carbon, nitrogen, and phosphorus in the leaves, as well as the physicochemical properties of the soil, were determined.
Results: The results showed the following: (1) The three life forms of plants in the study area showed that the coefficient of variation of leaf carbon content was the smallest and the distribution was the most stable, while the coefficient of variation of carbon–nitrogen ratio was the largest. (2) Altitude had a significant effect on the carbon, nitrogen, and phosphorus contents of different life form of plants, among which the leaf nitrogen content of trees, shrubs, and herbs increased significantly with altitude (p < 0.01), the leaf phosphorus content of trees increased significantly with altitude (p < 0.01), and the leaf C:N of the three life form of plants decreased significantly with altitude (p < 0.01). The C:P of the arbor decreased significantly with altitude (p < 0.05), and the N:P of shrub and herb leaves increased significantly with altitude (p < 0.01). (3) Soil organic carbon and soil moisture content were the main environmental factors affecting the changes of carbon, nitrogen, and phosphorus in leaves of arbors, and nitrate nitrogen was the main environmental factor affecting the changes of carbon, nitrogen, and phosphorus in leaves of shrubs. Available phosphorus affected the changes of carbon, nitrogen, and phosphorus in the leaves of herbaceous plants.
Discussion: The results provide new insights into community-level biogeographical patterns and potential factors of leaf stoichiometry among plant life forms.
1 Introduction
Carbon, nitrogen, and phosphorus are crucial elements in plant growth and development and material cycling, with carbon as a structural substance influencing the carbon cycle of ecosystems and nitrogen and phosphorus as limiting nutrients for plant growth (Güsewell and Freeman, 2005). At the same time, there is a strong coupling between carbon, nitrogen, and phosphorus elements, which play essential roles in plant growth metabolism, regulation of physiological mechanisms, and ecosystem material and energy cycles (Ågren, 2004). Due to the significant differences in individual traits, different life forms and species adopt different life strategies to maintain their growth and reproduction (Cheng et al., 2021). Thus, their leaf carbon, nitrogen, and phosphorus contents differ in their response to altitude and soil nutrients. Changes in altitudinal gradient usually lead to changes in the reproduction, survival, material metabolism, and morphological and structural functions of plants. For example, Zhang X. L. et al. (2023) found that the leaf nitrogen and phosphorus contents of meadow plants in Wutai Mountain decreased with altitude. Chai et al. (2015) pointed out that the leaf phosphorus element of Quercus qinlingensis was higher in the high-altitude region. A series of changes in plant element content along the altitudinal gradient are the result of the long-term adaptation of plants to environmental heterogeneity. The “trade-off strategy” of plants determines the position of individuals in the community, which in turn affects the stable coexistence of multiple species. This not only helps to analyze the trade-offs of species at different elevational gradients but also helps to understand the differentiation of plant functions (Tayir et al., 2023).
As one of the origins of cultivated deciduous fruit trees in the world, the Tianshan wild fruit forest in Xinjiang is the largest and most primitive and dense wild fruit forest in Eurasia, and it is also one of the unique areas of biodiversity in China (Fan et al., 2020). It has the genetic diversity of various wild fruit trees and agricultural plants, which is of great significance to the sustainable development of agriculture (Liu et al., 2013). Wild fruit forests in the Tianshan Mountains are mainly distributed on slopes with an altitude of 1,100–1,700 m in western Xinjiang. They have steep slopes, thin soil layers, significant habitat differences, and are relatively fragile. Therefore, they are sensitive to external disturbances. Since the end of the 1950s, because of the large-scale development of wild fruit forests in Tianshan Mountains, the backward agricultural production mode has not been suitable for ecological protection, which has caused severe damage to the ecosystem of wild fruit forests in Tianshan Mountains, serious fruit tree diseases, and reduced species diversity (Hou and Xu, 2006). The main objectives of this study were: (1) What are the distribution characteristics of carbon, nitrogen, and phosphorus in the leaves of different life forms of plants in the wild fruit forests of Tianshan Mountain? (2) How do different life forms’ leaf carbon, nitrogen, and phosphorus change with altitude? (3) How do the physical and chemical properties of the soil affect the variation of leaf carbon, nitrogen, and phosphorus contents of different life forms of plants at different altitude gradients? Are the key factors influencing the leaf carbon, nitrogen, and phosphorus contents of different plant life forms the same? Therefore, based on the study of the coordination mechanism between the elemental content of plant leaves and the soil environment at different altitudes, this study attempts to explore the nutrient absorption strategies of different life forms of plants in this plant community and the critical environmental factors that drive the dynamic balance of nutrients.
2 Materials and methods
2.1 Overview of the study site
The study site is located in Guozigou, Yili, Xinjiang. The climate is characterized by a warm and humid “oceanic” climate, with an average annual air temperature of 10.4°C, yearly precipitation ranging from 260 mm to 800 mm, and an average of 2,898.4 h of sunshine per year (Cheng et al., 2020).
2.2 Sample plot setup
In July 2022, three transects were set from the bottom to the top of the mountain in the range of altitude where the distribution of plants in Guozigou, Yili, Xinjiang was relatively concentrated. Each transect was separated by 100 m. The range of altitude 1,100–1,700 m was selected as the research area, and the elevation of 100 m was increased in turn. A total of six altitude gradients (1,100–1,200 m, 1,200–1,300 m, 1,300–1,400 m, 1,400–1,500 m, 1,500–1,600 m, 1,600–1,700 m) were set. Three 50 m × 50 m arbor quadrats were set up in each elevation gradient, three shrub quadrats were set up in each arbor quadrat along the diagonal line, and three 1 m × 1 m herb quadrats were set up in the diagonal line of each shrub quadrat. A total of 18 arbor quadrats, 54 shrub quadrats, and 162 herb quadrats were set up (Table 1). In our study, we recognized that species differences may have an impact on leaf carbon, nitrogen, and phosphorus contents. Therefore, when we selected experimental plants, we tried to select some representative species in terms of ecological and physiological characteristics to better understand and compare their differences. At the same time, we also used appropriate statistical methods in data analysis to reduce the impact of species differences on the results. In order to minimize the impact of other environmental factors such as slope on the experimental results, we conducted a detailed geographical and ecological survey when selecting sampling sites to ensure that our sampling sites are distributed as much as possible in areas with similar slope, soil type, climatic conditions, and vegetation types.
2.3 Sample collection
In each sample plot, five well-grown, healthy adult individuals (diameter at breast height > 5 cm) were selected as sampling plants, and 10 moth-free, unbroken leaves were randomly collected from different directions. The altitude, latitude, longitude, and other information of each sampling point were recorded through GPS localization (Table 2).
Five sampling points were evenly selected in each plot by the diagonal sampling method. Soil samples of 0~20 cm depth were collected with soil drills at each sampling point. After removing impurities such as litter and leaves in the soil, we put them into fresh-keeping bags and sent them back to the laboratory for subsequent treatment.
2.4 Sample determination
2.4.1 Leaf nutrient determination
The plant leaf samples were dried at 65°C for 24 h, ground in a mortar, and passed through a 60-mesh sieve. The C content of leaves was determined by potassium dichromate volumetric-external heating method, the N content of leaves was measured by perchloric acid-sulfuric acid digestion method, and the P content of leaves was determined by acid solubilization-molybdenum antimony colorimetric method.
2.4.2 Determination of soil physical and chemical properties
Referring to the soil nutrient testing standards (Bao, 2000), soil bulk weight was determined by the ring knife method; soil salinity was determined by the residue drying-mass method; soil water content = (fresh weight − dry weight)/fresh weight × 100%; soil organic carbon was determined by the volumetric method using potassium dichromate with external heating; soil total nitrogen was determined by the semi-micro Kjeldahl method; soil total phosphorus was determined by the sodium hydroxide melting-molybdenum antimony colorimetric method; available phosphorus was determined by the 0.5-mol·L−1 NaHCO3 method; soil total phosphorus was measured by sodium hydroxide fusion-molybdenum antimony colorimetric method; available phosphorus was measured by the 0.5 mol·L−1 NaHCO3 method; nitrate nitrogen was measured by phenol disulphonic acid colorimetric method; and ammonium nitrogen was measured by 2 mol·L−1 KCl leaching-distillation method.
2.5 Data analysis
Data were processed through Excel version 2021, and experimental data were tested for normality (S-W test) using SPSS 26.0. Data that were not normal were log-transformed to satisfy Chi-square and normality; a one-way ANOVA and significance of difference test (Duncan’s method) were performed; and the data in the graphs and charts are the mean ± standard error of the mean. Origin 2021 software was used for graphing, and correlation analysis was performed using the R4.0.1 ggcorr package (Huang et al., 2020).
3 Analysis of results
3.1 Descriptive statistics of carbon, nitrogen, and phosphorus in leaves of plants of different life forms
As shown in Table 3, the leaf carbon content (LCC) of the three life forms of plants ranged from 262.58 to 591.59, leaf nitrogen content (LNC) from 12.04 to 48.77, leaf phosphorus content (LPC) from 0.81 to 3.71, carbon-to-nitrogen ratio (C:N) from 8.47 to 39.99, carbon–phosphorus ratio (C:P) from 90.63 to 528.00, and nitrogen-to-phosphorus ratio (N:P) from 6.69 to 29.15. The carbon, nitrogen, and phosphorus contents of the leaves of the three types of plants were somewhat different. The mean values of LCC and C:N were higher in shrubs than in arbors and herbs; LNC and LPC were more elevated in herbs than in shrubs and herbs; and C:P and N:P were higher in arbors than in shrubs and herbs. Comparing the distribution of leaf carbon, nitrogen, and phosphorus contents of different life forms, it was found that the distribution of leaf carbon contents of the three life forms was the most stable with the smallest coefficient of variation, while the coefficient of variation of the carbon-to-nitrogen ratio was the largest.
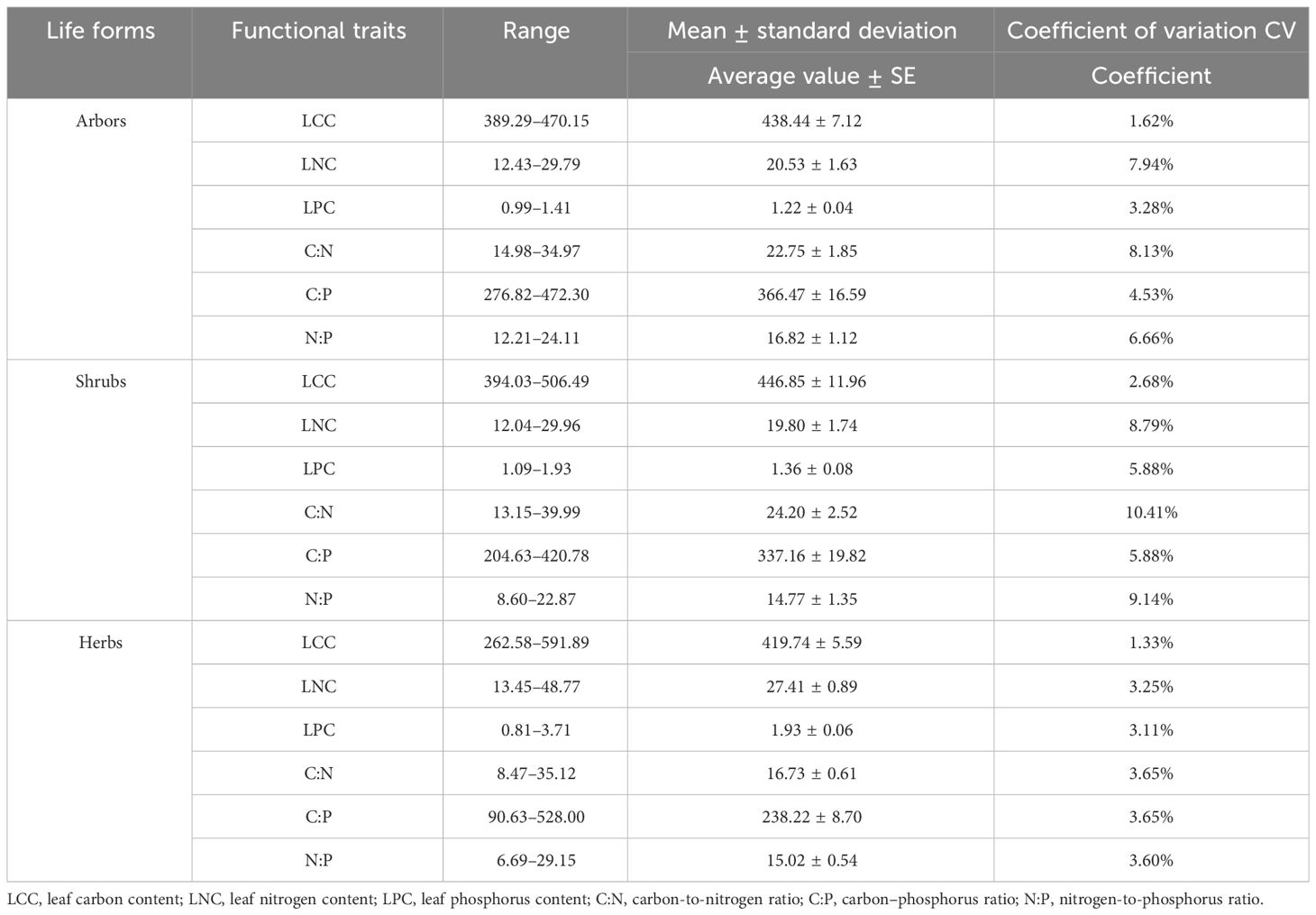
Table 3 Characteristics of carbon, nitrogen, and phosphorus distribution in leaves of different life forms.
3.2 Response of carbon, nitrogen, and phosphorus to altitude in different life forms
As shown in Figure 1, the leaf carbon, nitrogen, and phosphorus contents of different life forms of plants varied with altitude. Among them, shrubs’ and herbs’ LCC showed a decreasing trend with altitude, while the opposite was true for arbors, and the LCC of the three life forms did not vary significantly (p > 0.05) with change. Arbors’, shrubs’, and herbs’ LNC showed a very significant (p < 0.01) increasing trend with altitude, and herbs’ LNC content was higher than that of arbors’ and shrubs’. As the altitude rises, arbors’ LPC showed a very significant change (p < 0.01), and shrubs’ and herbs’ LPC did not change significantly (p > 0.05). Leave’s C:N of the three life forms showed a very significant (p < 0.01) decreasing trend with altitude. Arbors’ C:P showed a significant decreasing trend with altitude (p < 0.05), while shrubs’ and herbs’ C:P did not change significantly (p > 0.05). With increasing altitude, shrubs’ and herbs’ leaf N:P showed a very significant increasing trend (p < 0.01), while arbors’ leaf N:P did not change significantly (p > 0.05).
3.3 Effect of soil physical and chemical properties on leaf carbon, nitrogen, and phosphorus
As shown in Figure 2A, arbors’ LCC was significantly negatively correlated with TOC (p < 0.05), and LPC was very significantly positively correlated with LNC (p < 0.01). C:N was significantly negatively correlated with LNC and LPC (p < 0.01). C:P was very considerably negatively correlated with LNC and LPC (p < 0.01) and very significantly positively correlated with PH and C:N (p < 0.01). N:P was very considerably negatively correlated with LNC (p < 0.01) and very significantly positively correlated with C:N (p < 0.01).
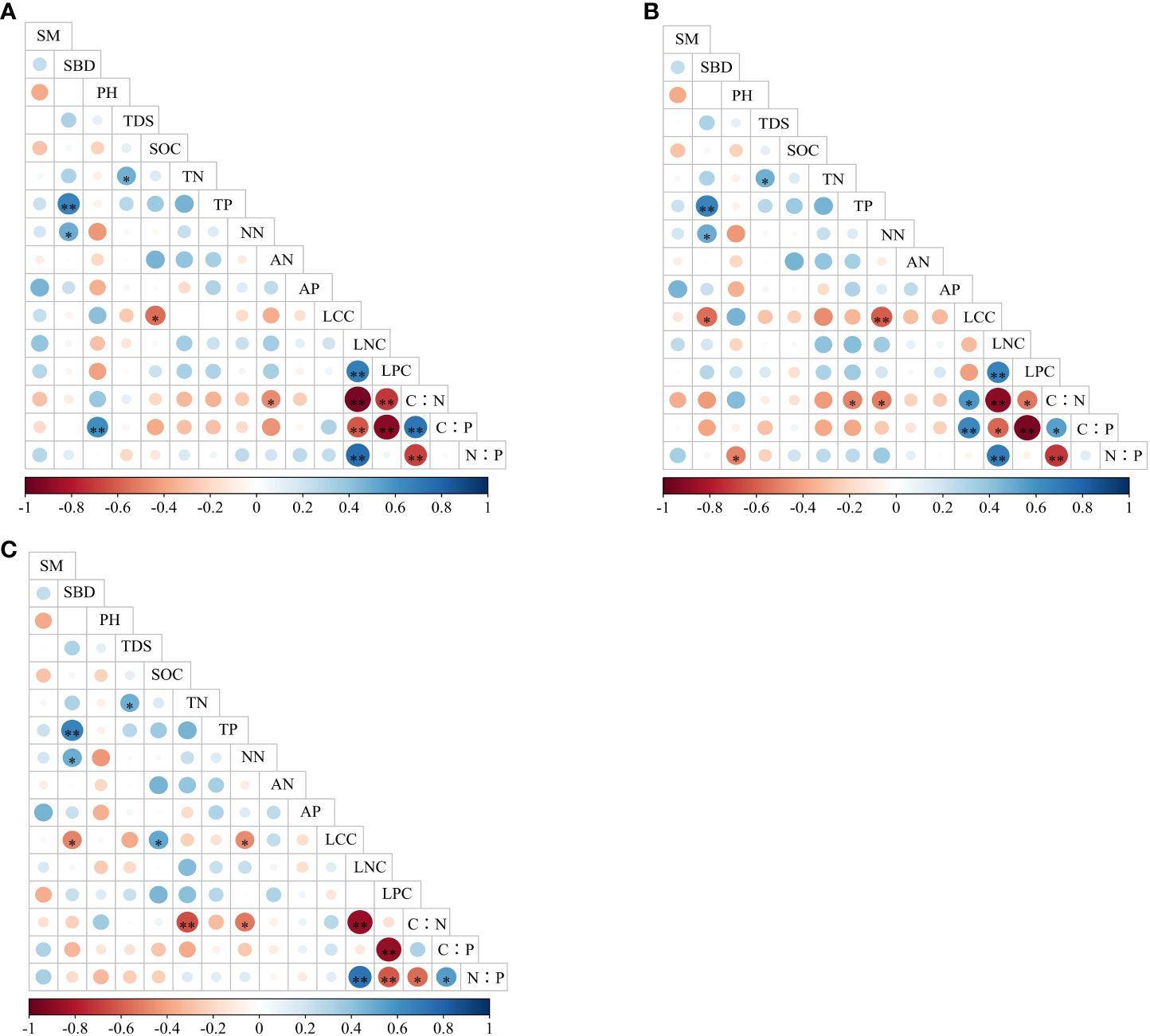
Figure 2 Correlation analysis between different living plants and soil physicochemical properties. (A) Arbors. (B) Shrubs. (C) Herbs.
As shown in Figure 2B, shrubs’ LCC showed a very significant negative correlation (p < 0.01) with nitrate nitrogen and a significant negative correlation (p < 0.05) with SBD. LPC showed a very significant positive correlation (p < 0.01) with LNC. C:N showed a significant negative correlation (p < 0.05) with LPC, nitrate nitrogen, and phosphorus as a whole, a very significant negative correlation (p < 0.01), and a significantly positive correlation with LCC (p < 0.05). C:P was significantly positively correlated with C:N (p < 0.05), significantly positively correlated with LCC (p < 0.01), significantly negatively correlated with LNC (p < 0.05), and significantly negatively correlated with LPC (p < 0.01). N:P was significantly negatively correlated with PH (p < 0.05), very significantly negatively correlated with C:N (p < 0.01), and very significantly positively correlated with LNC (p < 0.01).
As shown in Figure 2C, the herbs’ LCC was significantly positively correlated with SOC (p < 0.05) and significantly negatively correlated with nitrate nitrogen and SBD (p < 0.05). C:N was significantly negatively correlated with LNC and TN (p < 0.01). C:P was significantly negatively correlated with LPC (p < 0.01). N:P was significantly positively correlated with C:P (p < 0.05), very significantly positively correlated with LNC (p < 0.01), very significantly negatively correlated with C:N (p < 0.05), and very significantly negatively correlated with LPC (p < 0.01).
The study conducted a DCA analysis to analyze the carbon, nitrogen, and phosphorus contents of leaves of arbors, shrubs, and herbs at different altitudes in the study area concerning soil physicochemical characteristics. As shown in Table 4, the maximum sorting axis length was less than 3.0, so RDA was used to analyze the relationship between soil physicochemical characteristics and leaf carbon, nitrogen, and phosphorus.

Table 4 DCA analysis of carbon, nitrogen, and phosphorus contents of leaves and soil physicochemical properties of different living plants.
Combining Figure 3A and Table 5, sorting axes 1 and 2 explained 57.18% and 28.98% of the variation in carbon, nitrogen, and phosphorus contents of arbor leaves, respectively, with a cumulative explanation of 86.16% for the first two axes. As seen from Table 3 and Figure 3C, soil moisture content (SM), soil bulk density (SBD), soil organic carbon (SOC), total Nitrogen (TN), total Phosphorus (TP), Nitrate nitrogen (NN), ammonium nitrogen (AN), and Available Phosphorus (AP) were positively correlated with axis 1, while potential of hydrogen (PH) and total dissolved solids (TDS) were negatively correlated with axis 1. Among them, the carbon, nitrogen, and phosphorus contents of arborvitae leaves were mainly significantly (p < 0.05) affected by SOC and SM. The effects of soil physical and chemical properties on the carbon, nitrogen, and phosphorus contents of arbor leaves were, in descending order: SOC > SM = PH > TN > AN > TP > NN > TDS > AP > SBD.
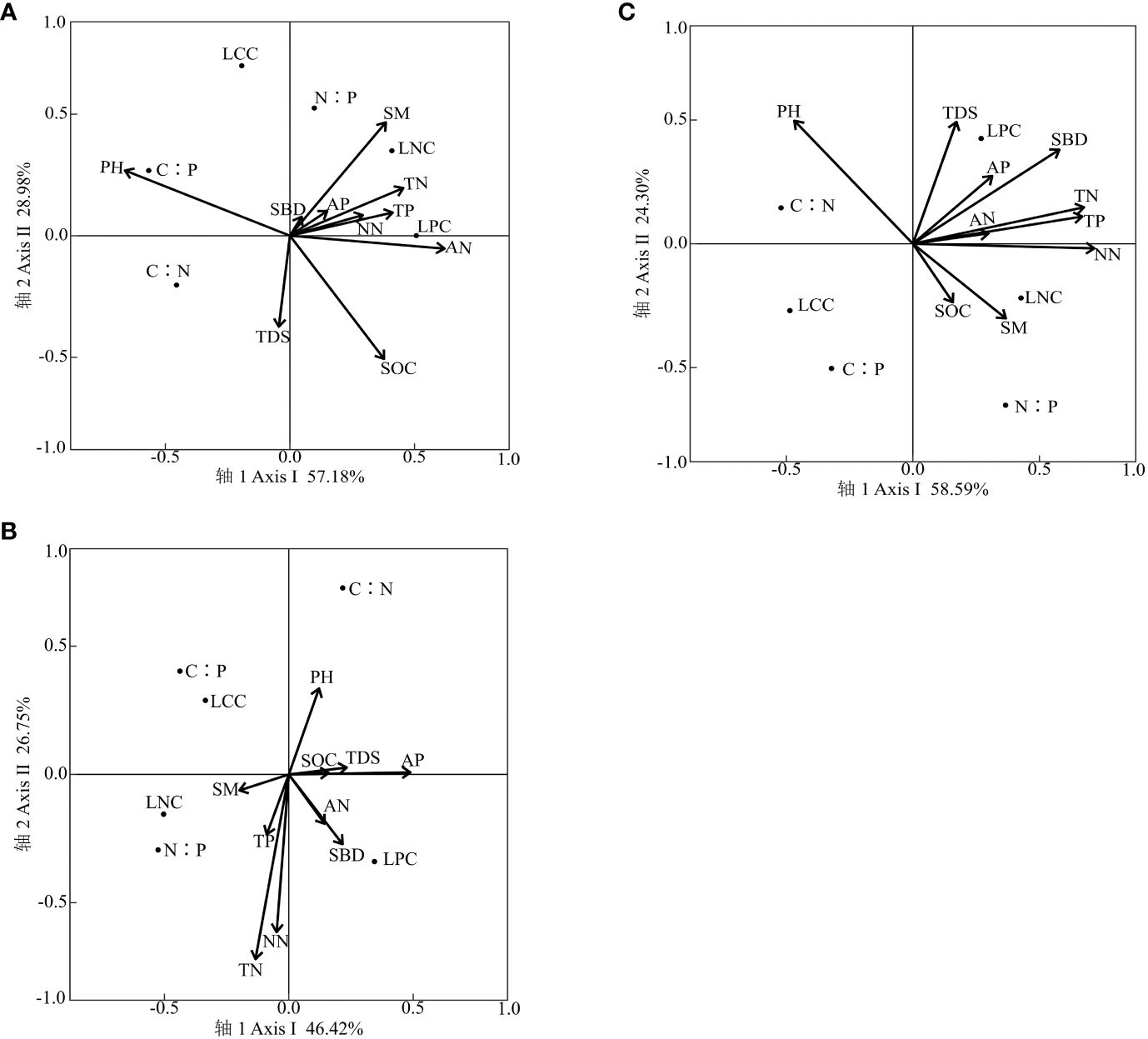
Figure 3 Carbon, nitrogen, and phosphorus content of leaves of different living plants and soil RDA analysis. (A) Arbors. (B) Shrubs. (C) Herbs.
Combining Figure 3B and Table 6, sorting axes 1 and 2 explained 58.59% and 24.30% of the variation in the carbon, nitrogen, and phosphorus contents of shrub leaves, respectively, with a cumulative explanation of 82.89% for the first two axes. As shown in Table 3 and Figure 3B, AN, AP, TDS, SM, SBD, SOC, TN, TP, and NN were positively correlated with axis 1, and PH was negatively correlated with axis 1. Among them, the shrub leaves’ carbon, nitrogen, and phosphorus contents were mainly significantly affected by SBD and NN (p < 0.05). The effects of soil physical and chemical properties on carbon, nitrogen, and phosphorus contents of shrub leaves were, in descending order, SBD > NN > TP > TN > PH > SM > AP > TDS > AN > SOC.
Combining Figure 3C and Table 7, we can see that sorting axes 1 and 2 explained 46.42% and 26.75% of the variation in carbon, nitrogen, and phosphorus contents of herb leaves, respectively, and the cumulative explanation rate of the first two axes amounted to 73.17%. As can be seen from the table and figure, PH, TDS, SBD, SOC, AN, and AP were positively correlated with axis 1, while SM, TN, TP, and NN were negatively correlated with axis 1. Among them, the carbon, nitrogen, and phosphorus contents of herb leaves were mainly significantly affected by AP (p < 0.05). The effects of soil physical and chemical properties on the carbon, nitrogen, and phosphorus contents of herb leaves were, in descending order: AP > AN > SOC > TP > SBD > NN > TN > TDS > SM > PH.
4 Discussion
4.1 Characteristics of carbon, nitrogen, and phosphorus distribution in the leaves of different life forms of plants
The altitude gradient is a comprehensive environmental factor composed of temperature, light, moisture, radiation, and other factors. Habitat conditions at different altitude gradients have particular differences in how species adapt to the environment, thus producing a series of adaptive changes (Zhao et al., 2023). Plant leaves’ carbon, nitrogen, and phosphorus contents can reflect nutrient utilization efficiency and allocation strategies (Zhang et al., 2022). The leaf carbon content of the three life-forming plants in this study was lower than the global average (Reich and Oleksyn, 2004), which indicates that the plant carbon sequestration capacity in the study area is weak. The nitrogen and phosphorus contents of herb leaves in this study were higher than those of arbors and shrubs, which is consistent with the findings of Güsewell (2004). This is because herb plants are characterized by a faster growth rates and shorter life cycles compared with other plants (Thompson et al, 1997), which makes the absorption efficiency of nitrogen and phosphorus elements by leaves higher than that of other plants. The carbon-to-nitrogen and carbon-to-phosphorus ratios of plant leaves can, to some extent, reflect the efficiency of carbon sequestration in the absorption of nutrients by plants (Wang H. et al., 2023). The carbon-to-nitrogen and carbon-to-phosphorus ratios of shrub leaves in this study were higher than the global average; the opposite was true for herbs, which indicated that during the growing season, shrubs in the study area had a higher efficiency of element utilization. Herb plant leaves improved their photosynthetic capacity by storing nitrogen and phosphorus to proliferate (Li et al., 2023).
In this study, the carbon-to-nitrogen ratio of arbor leaves was higher than that of herbs. Since the growth rate of arbors was lower than that of herbs, arbors reduced the photosynthetic rate by increasing the carbon content in the leaves to improve their tolerance to high temperatures and drought (Wang J. J. et al., 2023). According to Koerselman and Meuleman (1996), when the nitrogen-to-phosphorus ratio of plant leaves is greater than 16, plant growth is mainly restricted by phosphorus, and when the nitrogen-to-phosphorus ratio is between 14 and 16, plant growth may be limited by both nitrogen and phosphorus; when the nitrogen–phosphorus ratio is less than 14, plant growth is mainly determined by nitrogen. The range of N:P ratios of plant leaves in the study area showed that plant growth was unilaterally and jointly limited by nitrogen and phosphorus, which may be related to the heterogeneous environments at different altitudes.
Different plants have different adaptations to the environment, so there are some differences in the internal stability of their carbon, nitrogen, and phosphorus contents (Liu et al., 2018). In this study, we found that the coefficient of variation for leaf carbon content was the smallest among different plant life forms, showing a specific internal stability. This finding is consistent with observations from Wang et al. (2021) on the carbon content of oil pine leaves. They believed that carbon acts as the main component of the cytoskeleton, and thus has strong stability. Additionally, our results support the study of Cao et al. (2020), who studied the carbon, nitrogen, and phosphorus content of Echinocactus gluteus leaves. It is also the same as the study of Cao et al. (2020) on plant leaves’ carbon, nitrogen, and phosphorus, which attributed to plants’ carbon uptake, mainly through photosynthesis, to fix carbon dioxide in the air. Therefore, the carbon content of plant leaves is more stable. The leaf C:N ratio primarily reflects the physiological regulation mechanism of plants (Zuo et al., 2021). The coefficients of variation of leaf C:N ratios were the largest in this study, which may be attributed to the enormous changes in the nitrogen content of plants due to the differences in habitats at different altitudes, leading to the large variability of C:N ratios.
4.2 Leaf carbon, nitrogen, and phosphorus response to altitude in different life forms of plants
Changes in the elevation gradient affect many environmental factors, and as the elevation gradient increases, light intensity increases. At the same time, temperature, air pressure, and carbon dioxide concentration decrease, and water and heat conditions in the environment are redistributed (Liu et al., 2020). As environmental conditions change, leaf functional traits of different life forms vary along the altitudinal gradient (Wang Z. H. et al., 2023). The leaf carbon content of the three plant life forms in this study did not change significantly with elevation, which is consistent with observations by He et al. (2023) and other studies. This is because carbon, as the main component of the cytoskeleton of plant leaves, is sensitive to changes in environmental stress; when plants are under adverse stressors like drought, high temperature, or other pressures, they improve their adaptive capacity by storing carbon in their leaves (Elser et al., 2000). However, the study area is located in the Yili River valley, which is endowed with a warm and humid “oceanic” climate (Li et al., 2016), which does not cause drought stress to plants in this area. Therefore, the carbon content is relatively stable. In this study, the nitrogen and phosphorus content of tree and shrub leaves increased with altitude, which is consistent with the observations of Han et al. (2005). According to the “temperature–plant physiological hypothesis” (Reich and Oleksyn, 2004), the nitrogen–phosphorus regulation mechanism of plants is mainly related to temperature. As the ambient temperature decreases with altitude, the efficiency of nitrogen-rich enzymes and phosphorus-rich RNA in cells is inhibited, and plants need to increase leaf nitrogen and phosphorus content to compensate for the increase in carbon content. Leaf nitrogen and phosphorus levels compensate for the decrease in physiological activity of the organism caused by low temperatures. Therefore, to some extent, the leaf nitrogen and phosphorus content of plants showed a significant upward trend with altitude. The present study showed that the phosphorus content of herbs was opposite that of trees and shrubs, which to some extent reflected the differences in survival strategies of plants with different lifestyles. Herbs adopted a “conservative strategy”. They adapted to the low temperature and high radiation environment at high altitudes by lowering the P content of their leaves.
The stoichiometric properties of carbon, nitrogen, and phosphorus in leaves can reflect plant adaptation strategies to habitats and patterns of nitrogen and phosphorus nutrient limitation (Wardle et al., 2004). Carbon-to-nitrogen and carbon-to-phosphorus ratios reflect the efficiency of nutrient uptake and utilization by plants (Wang et al., 2017). In this study, we found that the leaf carbon-to-nitrogen and carbon-to-phosphorus ratios of the three plant life forms decreased significantly with altitude. It showed that the nitrogen and phosphorus utilization efficiency of trees, shrubs, and herbs in the study area gradually decreased with increasing altitude. The nitrogen and phosphorus content of leaves at high altitudes was significantly higher than that at low altitudes, which may be attributed to the fact that the temperature at high altitudes is lower, the plant’s heat demand is limited, and the plant, to maintain normal life activities during the growing season, increases the photosynthetic and respiratory rates by increasing the content of nutrient elements in the leaves (Mooney and Billings, 1961).
The leaf nitrogen–phosphorus ratio can reflect the growth regulation mechanism of plants (Treutter, 2006), and the leaf nitrogen–phosphorus ratio of the three living plants in this study showed a significant increasing trend with altitude, which is similar to the findings of Müller et al. (2017). The growth rate hypothesis states that a low nitrogen–phosphorus ratio predicts a high growth rate and that plants allocate more phosphorus to ribosomal RNA to meet the rapid synthesis of proteins by ribosomes and to maintain the rapid growth of plants (Elser et al., 2003). Nitrogen–phosphorus ratios in the leaves of plants of different life forms increase with elevation, suggesting that the emphasis at low elevations is on self-growth. As altitude increases, the rate of plant growth slows. Koerselman and Meuleman (1996) suggested that when the nitrogen–phosphorus ratio of plant leaves is greater than 16, plant growth is mainly limited by phosphorus; when the nitrogen–phosphorus ratio is between 14 and 16, plant growth may be determined by a combination of nitrogen and phosphorus; and when the nitrogen–phosphorus ratio is less than 14, plant growth is mainly limited by nitrogen.
In this study, the nitrogen–phosphorus ratio of the leaves of the three plant life forms was less than 14 at 1,100~1,300 m above sea level, indicating that plant growth was more susceptible to nitrogen limitation at this altitude range. This is because the photosynthetic capacity of plants is higher at low altitudes than at high altitudes. The nitrogen content of plant leaves affects the photosynthetic capacity of plants. More nitrogen is used to build photosynthetic organs such as chloroplasts, which are therefore more susceptible to nitrogen limitation (Zhang X. et al., 2023, Zhang X. L. et al., 2023), which is basically consistent with the findings of Gong et al. (2020). At 1,300~1,400 m, leaf N:P ratios ranged from 14 to 16, indicating that plants at this altitude were co-limited by nitrogen and phosphorus. At 1,400~1,700 m, the leaf N:P ratio was greater than 16, indicating that the growth of community plants in the study area was more sensitive to phosphorus, which was consistent with the findings of Aerts and Chapin (1999), who reported that the development of forest communities in subtropical regions was mainly limited by soil P. This is because the only directly available phosphorus in the free state provided by the environment to plants is available phosphorus, which is less compared to the immediately available nitrogen (ammonium nitrogen and nitrate nitrogen).
4.3 Effect of soil physical and chemical properties on leaf carbon, nitrogen, and phosphorus
As an essential source of nutrient uptake by plants, the level of elemental content of the soil plays a crucial role in plant growth (Wang Y. et al., 2023). Due to the different survival strategies of plants with different life forms, the dependence on other elements in the soil is also foreign. In this study, it was found that the leaf carbon, nitrogen, and phosphorus of trees were significantly and positively correlated with soil organic carbon and soil moisture content, which is because the trees distributed in the study area are mainly deciduous trees, which pay more attention to leaf growth and have a higher photosynthetic capacity and nutrient uptake efficiency, which makes the leaves accumulate more carbon (Osada, 2017). Water, as one of the raw materials for leaf photosynthesis, is ultimately absorbed from the root conduit into the leaf (Yu et al., 2019), so soil water content is positively correlated with leaf water content. In shrub leaves, there was a significant positive correlation between carbon, nitrogen, phosphorus, and nitrate nitrogen. Herbs were positively affected by available phosphorus because the elements nitrogen and phosphorus are critical factors limiting plant growth in the ecosystem (Tong et al., 2019). The higher the content of available phosphorus and ammonium nitrogen in the soil, the more vigorously the plant is involved in physiological metabolism such as photosynthesis, respiration, and energy storage and transfer, which can promote the growth and development of the root system of the plant rooted in the deep soil to obtain water and improve the ability of plants to adapt to the environment (Tang et al., 2018).
5 Conclusions
In this paper, it was found that the nitrogen content and nitrogen–phosphorus ratio of shrubs and herbs in the study area increased significantly with the increase in altitude, and the carbon–nitrogen ratio decreased significantly with the increase in altitude. The contents of nitrogen and phosphorus in the leaves of tree plants and the ratio of carbon to nitrogen increased significantly with the increase of altitude, and the ratio of carbon to phosphorus decreased significantly with the increase in altitude. The soil physical and chemical factors affecting the content of carbon, nitrogen, and phosphorus in the leaves of different life forms are different. The carbon, nitrogen, and phosphorus in the leaves of trees are mainly affected by soil water content, pH, and organic carbon. The carbon, nitrogen, and phosphorus in the leaves of shrubs are mainly affected by soil bulk density and nitrate nitrogen. The carbon, nitrogen, and phosphorus in the leaves of herbaceous plants are mainly affected by soil-available phosphorus. The results also provide valuable information and support for vegetation restoration and protection measures.
Data availability statement
The raw data supporting the conclusions of this article will be made available by the authors, without undue reservation.
Author contributions
XJ: Data curation, Writing – original draft. LW: Visualization, Writing – review & editing. JR: Visualization, Writing – review & editing. XP: Formal analysis, Writing – review & editing. HL: Project administration, Writing – review & editing.
Funding
The author(s) declare financial support was received for the research, authorship, and/or publication of this article. This work was supported by the National Natural Science Foundation of China (No. 32160090) and the Third Xinjiang Scientific Expedition Program (2022xjkk150506).
Conflict of interest
The authors declare that the research was conducted in the absence of any commercial or financial relationships that could be construed as a potential conflict of interest.
Publisher’s note
All claims expressed in this article are solely those of the authors and do not necessarily represent those of their affiliated organizations, or those of the publisher, the editors and the reviewers. Any product that may be evaluated in this article, or claim that may be made by its manufacturer, is not guaranteed or endorsed by the publisher.
References
Aerts R., Chapin F. S. (1999). The Mineral Nutrition of Wild Plants Revisited:A Reevaluation of Processes and Patterns (Boston: Advances in Ecological Research:Academic Press). doi: 10.1016/S0065-2504(08)60016-1
Ågren G. I. (2004). The C:N:P stoichiometry of autotrophs:theory and observations. Ecol. Lett. 7, 185–191. doi: 10.1016/j.envexpbot.2017.05.013
Cao J. J., Wang X. Y., Adamowski J. F., Biswas A., Liu C. F., Chang Z. Q., et al. (2020). Response of leaf stoichiometry of Oxytropis ochrocephala to elevation and slope aspect. Catena. 194, 104772. doi: 10.1016/j.catena.2020.104772
Chai Y. F., Zhang X. F., Yue M., Liu X., Li Q., Shang H. L., et al. (2015). Leaf traits suggest different ecological strategies for two Quercus species along an altitudinal gradient in the Qinling Mountains. J. For. Res. 20, 501–513. doi: 10.1007/s10310-015-0496-z
Cheng X. Q., Tai P., Li Z. Z., Wang T., Han H. R., Epstein H. E. (2021). Effects of environmental factors on plant functional traits across different plant life forms in a temperate forest ecosystem. New Forests 53, 125–142. doi: 10.1007/s11056-021-09847-0
Cheng J. H., XJ S. H. I., Fan P. R., Zhou X. B., Sheng J. D., Zhang Y. M. (2020). Relationship of species diversity between overstory trees and understory herbs along the environmental gradients in the Tianshan Wild Fruit Forests, Northwest China. Arid Land 12, 618–629. doi: 10.1007/s40333-020-0055-0
Elser J. J., Acharya K., Kyle M., Cotner J., Makino J., Markow J., et al. (2003). Growth rate-stoichiometry couplings in diverse biota. Ecol. Lett. 6, 936–943. doi: 10.1046/j.1461-0248.2003.00518.x
Elser J. J., Fagan W. F., Denno R. F., Dobberfuhl D. R., Folarin A., Huberty A., et al. (2000). Nutritional constraints in terrestrial and freshwater food webs. Nature 408, 578–580. doi: 10.1038/35046058
Fan H. J., Lai F. B., Cao J. R., Chen S. J., Xu Y. (2020). Spatial and temporal evolution of landslide landscapes and ecological risk prediction in the wild fruit forest area of Tianshan Mountain. J. Mountain Geology 38, 231–240. doi: 10.1016/j.ecolind.2021.107454
Gong H., Li Y. Y., Yu T., Zhang S. H., Gao J., et al. (2020). Soil and climate effects on leaf nitrogen and phosphorus stoichiometry along elevational gradients. Global Ecol. Conserv. 23, e01138. doi: 10.1016/j.gecco.2020.e01138
Güsewell S. (2004). N:P ratios in terrestrial plants:variation and functional significance. New Phytol. 164, 243–266. doi: 10.3390/agronomy13020411
Güsewell S., Freeman C. (2005). Nutrient limitation and enzyme activities during litter decomposition of nine wetland species in relation to litter N:P ratios. Funct. Ecol. 19, 582–593. doi: 10.1111/j.1365–2435.2005.01002.x
Han W., Fang J., Guo D., Zhang Y. (2005). Leaf nitrogen and phosphorus stoichiometry across 753 terrestrial plant species in China. New Phytol. 168, 377–385. doi: 10.1111/j.1469-8137.2005.01530.x
He X. L., Ma J., Jin M., Li Z. (2023). Characteristics and controls of ecological stoichiometry of shrub leaf in the alpine region of northwest China. Catena 224, 107005. doi: 10.1016/j.catena.2023.107005
Hou B., Xu Z. (2006). Studies on wild fruit arbors and their relatives in Yili, China. Arid Zone Res. 03, 453–458. doi: 10.13866/j.azr.2006.03.013
Huang H. Y., Zhou L., Chen J., Wei T. (2020). ggcor: Extended tools for correlation analysis and visualization. Rpackage version 0.9.7. Github. Available at: https://rdrr.io/github/houyunhuang/ggcor/.
Koerselman W., Meuleman A. F. (1996). The vegetation N:P ratio:a new tool to detect the nature of nutrient limitation. J. Appl. Ecol. 33, 1441–1450. doi: 10.2307/2404783
Li M., Hu X., Miao X. J., Zhang Q. P., Fan G. Q., Zhang S. K., et al. (2016). Genetic diversity of wild apricot populations in the Ili River Valley based on SRAP markers. J. Horticulture 43, 1980–1988. doi: 10.1007/s10722–013-9996-x
Li Y. T., Yu Y. H., Song Y. P. (2023). Leaf functional traits of zanthoxylum planispinum 'Dintanensis' Plantations with different planting combinations and their responses to soil. Forests 14, 468. doi: 10.3390/f14030468
Liu R. H., Liang S. C., Long W. X., Jiang Y. (2018). Variations in leaf functional traits across ecological scales in riparian plant communities of the Lijiang River, Guilin, Southwest China. Trop. Conserv. Sci. 11. doi: 10.1177/1940082918804680
Liu W., Li Z., Qi D. H. (2020). Variation in leaf traits at different altitudes reflects the adaptive strategy of plants to environmental changes. Ecol. Evol. 10, 8166–8175. doi: 10.1002/ece3.6519
Liu Y., Zhang X. F., Zhao Y., Gan Y. P. (2013). Population structure and its dynamics of wild Prunus divaricata in Xinjiang Northwest China. Chin. J. Ecol. 32 (7), 1762–1769. doi: 10.13292/j.1000-4890.2013.0391
Mooney H. A., Billings W. D. (1961). Comparative physiological ecology of arctic and alpine populations of oxyria digyna. Ecol. Monogr. 31, 1–29. doi: 10.2307/1950744
Müller M., Oelmann Y., Schickhoff U., Böhner J., Scholten T. (2017). Himalayan arborsline soil and foliar C:N:P stoichiometry indicate nutrient shortage with elevation. Geoderma 291, 21–32. doi: 10.1016/J.GEODERMA.2016.12.015
Osada N. (2017). Relationships between the timing of budburst, plant traits, and distribution of 24 coexisting woody species in a warm-temperate forest in Japan. Am. J. Bot. 104. doi: 10.3732/ajb.1600444
Reich P. B., Oleksyn J. (2004). Global patterns of plant leaf N and P in relation to temperature and latitude. Proc. Natl. Acad. Sci. U.S.A. 101, 11001–11006. doi: 10.1073/pnas.0403588101
Tang Z. Y., Xu W. T., Zhou G. Y., Bai Y. F., Li J. X., Tang X. L., et al. (2018). Patterns of plant carbon, nitrogen, and phosphorus concentration in relation to productivity in China's terrestrial ecosystems. Proc. Natl. Acad. Sci. 115, 4033–4038. doi: 10.1073/pnas.1700295114
Tayir M., Dai Y., Shi Q. D., Abdureyim A., Erkin F., Huang W. Y. (2023). Distinct leaf functional traits of Tamarix chinensis at different habitats in the hinterland of the Taklimakan desert. Front. Plant Sci. 13. doi: 10.3389/fpls.2022.1094049
Thompson K., Parkinson J. A., Band S. R., Soencer R. E. (1997). A comparative study of leaf nutrient concentrations in a regional herbaceous flora. New Phytol. 136, 679–689. doi: 10.1046/j.1469-8137.1997.00787.x
Tong R., Zhou B., Jiang L., Ge X. G., Cao Y. H., Yang Z. Y. (2019). Leaf Nitrogen and Phosphorus Stoichiometry of Chinese fir Plantations across China:A Meta-Analysis. Forests 10, 945. doi: 10.3390/f10110945
Treutter D. (2006). Significance of flavonoids in plant resistance:a review. Environ. Chem. Lett. 4, 147–157. doi: 10.1007/s10311-006-0068-8
Wang L., Jing X., Han J., et al. (2021). How C:N:P stoichiometry in soils and carbon distribution in plants respond to forest age in a Pinus tabuliformis plantation in the mountainous area of eastern Liaoning Province, China. PeerJ. 9, e11873. doi: 10.7717/peerj.11873
Wang J. J., Liang Y. J., Wang G. A., et al. (2023). Leaf nitrogen and phosphorus stoichiometry and its response to geographical and climatic factors in a tropical region:Evidence from Hainan Island 411. doi: 10.3390/agronomy13020411
Wang M., Liu G. H., Jin T. T., Li Z. S., Gong L., Wang H., et al. (2017). Age-related changes of leaf traits and stoichiometry in an alpine shrub (Rhododendron agglutinatum) along altitudinal gradient. J. Mountain Sci. 14. doi: 10.1007/s11629–016-4096-y
Wang H., Sun T., Liu Y., Liu W., Xiao H. L. (2023). Stoichiometric characteristics and homeostasis of leaf nitrogen and phosphorus responding to different water surface elevations in hydro-fluctuation zone of the Three Gorges Reservoir. Aquat Sci. 85, 80. doi: 10.1007/s00027-023-00977-5
Wang Y., Wu F., Li X., Li C. C., Zhao Y. K., Gao Y. X., et al. (2023). Effects of plants and soil microorganisms on organic carbon and the relationship between carbon and nitrogen in constructed wetlands. Environ. Sci. pollut. Res. Int. 30 (22), 62249–62261. doi: 10.1007/s11356-023-26489-1
Wardle D. A., Walker L. R., Bardgett R. D. (2004). Ecosystem properties and forest decline in contrasting long-term chronosequences. Science. 305, 509–513. doi: 10.1126/science.1098778
Yu Z. Y., Wang X., Meng X. T., Zhang X. L., Wu D. Q., Liu H. J., et al. (2019). SPAD prediction model of rice leaves considering the characteristics of water spectral absorption. Spectrosc. Spectral Anal. 39. doi: 10.3964/j.issn.1000–0593(2019)08–2528-05
Zhang C., Zeng F. P., Zeng Z. X., Du H., Zhang L. J., Su L., et al. (2022). Carbon, nitrogen and phosphorus stoichiometry and its influencing factors in karst primary forest. Forests 13 (12), 1990. doi: 10.3390/f13050755
Zhang X., Hu H., Li F. L., Huang L., Bao W. K. (2023). Within leaf nitrogen allocation regulates the photosynthetic behavior of xerophytes in response to increased soil rock fragment content. Plant Physiol. Biochem. 200, 107753. doi: 10.1016/j.plaphy.2023.107753
Zhang X. L., Qin H. P., Zhang Y. B., Niu J. J., Wang Y. J., Shi L. J. (2023). Driving factors of community-level leaf stoichiometry patterns in a typical temperate mountain meadow ecosystem of northern China. Front. Plant Sci. 14. doi: 10.3389/fpls.2023.1141765
Zhao L. J., Gao R. H., Liu J. Q., Liu L., Li R. J., Men L. N., et al. (2023). Effects of environmental factors on the spatial distribution pattern and diversity of insect communities along altitude gradients in Guandi Mountain, China. Insects 14. doi: 10.3390/insects14030224
Keywords: stoichiometric ratio, life forms, altitude, Tianshan, leaf functional traits
Citation: Jia X, Wu L, Ren J, Peng X and Lv H (2024) Response of carbon, nitrogen, and phosphorus in leaves of different life forms to altitude and soil factors in Tianshan wild fruit forest. Front. Ecol. Evol. 12:1368185. doi: 10.3389/fevo.2024.1368185
Received: 10 January 2024; Accepted: 03 May 2024;
Published: 24 May 2024.
Edited by:
Shuai Ouyang, Central South University Forestry and Technology, ChinaReviewed by:
Xiuqing Nie, Chinese Academy of Forestry, ChinaRen You, Washington State University, United States
Copyright © 2024 Jia, Wu, Ren, Peng and Lv. This is an open-access article distributed under the terms of the Creative Commons Attribution License (CC BY). The use, distribution or reproduction in other forums is permitted, provided the original author(s) and the copyright owner(s) are credited and that the original publication in this journal is cited, in accordance with accepted academic practice. No use, distribution or reproduction is permitted which does not comply with these terms.
*Correspondence: Haiying Lv, lvhyxj@163.com