- 1Experimental Center of Forestry in North China, Chinese Academy of Forestry, Beijing, China
- 2National Permanent Scientific Research Base for Warm Temperate Zone Forestry of Jiulong Mountain in Beijing, Beijing, China
- 3Chinese Academy of Forestry, Beijing, China
- 4Qinghai Provincial Investigation, Design & Research Institute of Water Conservancy & Hydropower Co., Ltd., Xining, China
Introduction: Planted forests are of great significance in reducing wind erosion and controlling degraded land, and are the main measure to improve the ecological environment in arid and semi-arid areas. Afforestation is mainly based on tall trees, but forest belts consume a large amount of water, bringing significant pressure to the environmental carrying capacity. While shrubs generally consume less water than do trees, it remains understudied what role shrubs play in configuration of shelterbelts in arid areas.
Methods: In this study, we conducted wind tunnel simulation experiments with the common used afforestation tree species Populus popularis and shrub species Salix psammophila. We set up single- or double-belt pure forest and mixed tree/shrub configurations, to analyze their effects on the wind speed, flow field, and wind prevention efficiency of different forest belt types.
Results: The results showed that: 1) the wind erosion resistance of the double-belt shelter forest was stronger than that of a single-belt forest. 2) Shelterbelts consisting of trees had a strong resistance to airflow disturbances, but their protective effect was reflected mainly in the canopy layer, with poor near-surface protection. 3) Shelterbelts consisting of shrubs had a good protective effect within effective height, and the wind speed in the double-shrub forest belt was 77% of that in the single-tree forest belt. 4) The wind prevention efficiency within the shrub forest belt was better than that of the tree forest belt, with wind prevention efficiency exceeding 40% in most double-shrub forests. Shrubs as a supplement to trees could increase the minimum wind prevention efficiency by up to 30%.
Discussion: Therefore, the role of shrubs should be emphasized in afforestation in arid areas, especially in areas that need near-surface protection.
1 Introduction
Arid regions account for 41% of the global land area and support approximately 40% of the global population (United Nations Convention to Combat Desertification, 2008; Wang et al., 2015). The ecosystems in arid and semi-arid areas are fragile and highly sensitive to climate change and human activities (Tomasella et al., 2018; Oktay et al., 2021; Zhou et al., 2023). Wind erosion is a common form of soil erosion that occurs in arid and semi-arid regions (Bruno et al., 2018; Zhang et al., 2019). It causes serious natural disasters and environmental problems, leading to extensive desertification and land degradation that severely constrain the sustainable development of the local society, economy, and ecology (Mganga et al., 2018;. Lüttschwager and Jochheim, 2020; Travis et al., 2023).
In arid and semi-arid areas, large-scale afforestation activities are considered as the main measure to improve the ecological environment (Deng et al., 2013; Zhu and Song, 2021). Planted forests can alleviate wind and sand disasters, change the physical properties of underlying surfaces, affect the water cycle of microclimates and the redistribution of water resources, and effectively improve land productivity in farmland, grasslands, oases, and other areas (Atangana et al., 2014; Jaskulska and Jaskulska, 2017; Zara et al., 2022; Howlader, 2023). However, the roots of planted forests can absorb soil moisture at different depths (Jia and Shao, 2014; Wafa et al., 2019), and seriously affect soil moisture conditions through transpiration, infiltration, and interception, leading to a decrease of the entire soil profile and even totally dry soil layers (Wang et al., 2011; Su and Shangguan, 2019). Meanwhile, soil moisture content in turn can affect the growth and development of vegetation, leading to the formation of “little-old-man tree” in arid areas, and making it more difficult for herbaceous plants and other species in afforestation areas to survive (Cao et al., 2011; Butz et al., 2018). This has brought significant pressure to the environmental carrying capacity of arid and semi-arid areas (Maximiliane et al., 2023; Qiu et al., 2023), and also leads to controversy over afforestation measures (Ma et al., 2020; Teng et al., 2022).
Water condition is an important factor restricting the sustainable development of the ecological environment in arid and semi-arid areas (McVicar et al., 2010; Wang et al., 2013; Thivakaran et al., 2020; Yi et al., 2023). Therefore, it is necessary to balance the ecological compensation benefits of forest belts and the ecological pressure on water resource consumption in arid areas. One widely suggested management approach is to thin the existing planted forests to conserve water resources and to maintain plantation forestland sustainability (Meng et al., 2022). However, it is difficult to reverse the current situation of water deficit and poor forest growth conditions just by thinning. Instead, the water consumption should be considered before afforestation in arid and semi-arid areas. At present, the selection of plant species for planted forests is mainly based on tall trees, while shrubs are an important component of native vegetation in arid and semi-arid areas. Compared to trees, shrubs have weaker transpiration and consume less water than do trees, and should thus play an important role in configuration of shelterbelt in arid areas. However, it remains understudied how shrubs affect the wind erosion protection of shelterbelt in arid areas. In this study, we carried out wind tunnel simulation experiments with shelterbelts consisting of trees and shrubs under various wind conditions to address two research questions: 1) What are the wind erosion protection characteristics of trees and shrubs? 2) What role do shrubs play in planted forests?
2 Materials and methods
2.1 Wind tunnel experiments
Wind tunnel experiments were conducted in Jiufeng Sandstorm Physics Laboratory of Beijing Forestry University. The experimental system is composed of three parts: the wind tunnel body, a three-dimensional measurement system, and wind speed measurement equipment. The wind tunnel is a standard, direct-current wind tunnel, with a total length of 24 m and seven sections (Figure 1). An electric motor provides power to the fan blades, allowing airflow to enter the transition section. The stabilization section uses a honeycomb-shaped wire mesh to disperse large masses of air, making the airflow more uniform. When the airflow passes through the contracted section, it accelerates due to a reduction in the cross-section as it enters the experimental section. The length of the experimental section is 12 m, with sharp wedges (having a right-angled triangular pyramid shape, with a height of 20 cm and a length of 5 cm on the bottom inclined side) and rough elements (4 cm height × 5 cm width × 6 cm length) arranged at the front end to adjust the wind speed profile. The cross-sectional area is 0.6 m × 0.6 m (Figure 1), and the boundary layer is 10 cm. After passing through the experimental section, the airflow traverses the diffusion section.
The wind speed in the wind tunnel is adjustable over the range of 3–42 m·s-1, and the effective experimental cross-sectional wind speed fluctuation is less than 1.5%. The three-dimensional measurement system can move freely throughout the experimental section to obtain wind speed measurements at different positions and heights within the experimental section, effectively creating a three-dimensional measurement grid. The measurement system has a movement accuracy of 1 mm. The wind speed measurement equipment includes a hot film anemometer (IFA300, TSI, Shoreview, MN, USA) and a hot wire anemometer (KIMO, Montpon-Ménestérol France).
2.2 Model preparation
Populus popularis is a commonly used afforestation tree species in northern China, which has the characteristics of rapid growth and strong drought resistance. Salix psammophila is a common shrub species distributed in arid and semi-arid areas in China, and has the characteristics of drought resistance, barren resistance and saline-alkali resistance. This study takes P. popularis and S. psammophila as the research species and adopted a simulation tree model with a scaling ratio of 1:100. The model parameters are set according to the commonly used configuration models for planted forests (Table 1). The tree height was set at 10 cm, the height-to-crown base was 3 cm, and the crown diameter was 3 cm. The shrub height was 2.5 cm and its crown diameter was 2 cm. The thickness of the boundary layer at the bottom of the experimental section was 25 cm, which exceeded the height of the model. The constrictivity of the forest belt model was less than 5%, which met the requirements of similar conditions for wind tunnel simulations. The configurations of the forest belts included single- and double-belt forests (Figure 2). In the double-belt configuration, the two rows were arranged in a triangular pattern and numbered separately.

Figure 2 Schematic diagram of forest belt setting (A) Single-belt forest. (B) Double-belt forest. (C) Mixed tree and shrub forest.
2.3 Wind speed measurements
Wind speed measurements were conducted along the longitudinal sections and horizontall plane of the experimental section. The distribution of the measurement points is shown in Figure 3. The layout range of longitudinal measurement points spanned from 30 cm in front of the tree line to 100 cm behind the tree line, with a spacing interval of 2 cm. The lowest measurement point was 1 cm, and the highest measurement point was 21 cm. The layout range of horizontal measurement points started from 50 cm behind the forest belt, with a spacing of 2 cm and a height of 2 cm. In combination with meteorological data in arid areas, we set the experimental wind speed as 8 m/s (The measured height is 2m). The minimum Reynolds number under the experimental conditions was 4.8 × 105. At each measurement point, wind speed was measured with a KIMO hot wire anemometer for 10 s, and the average value was calculated as the wind speed at that measurement point.
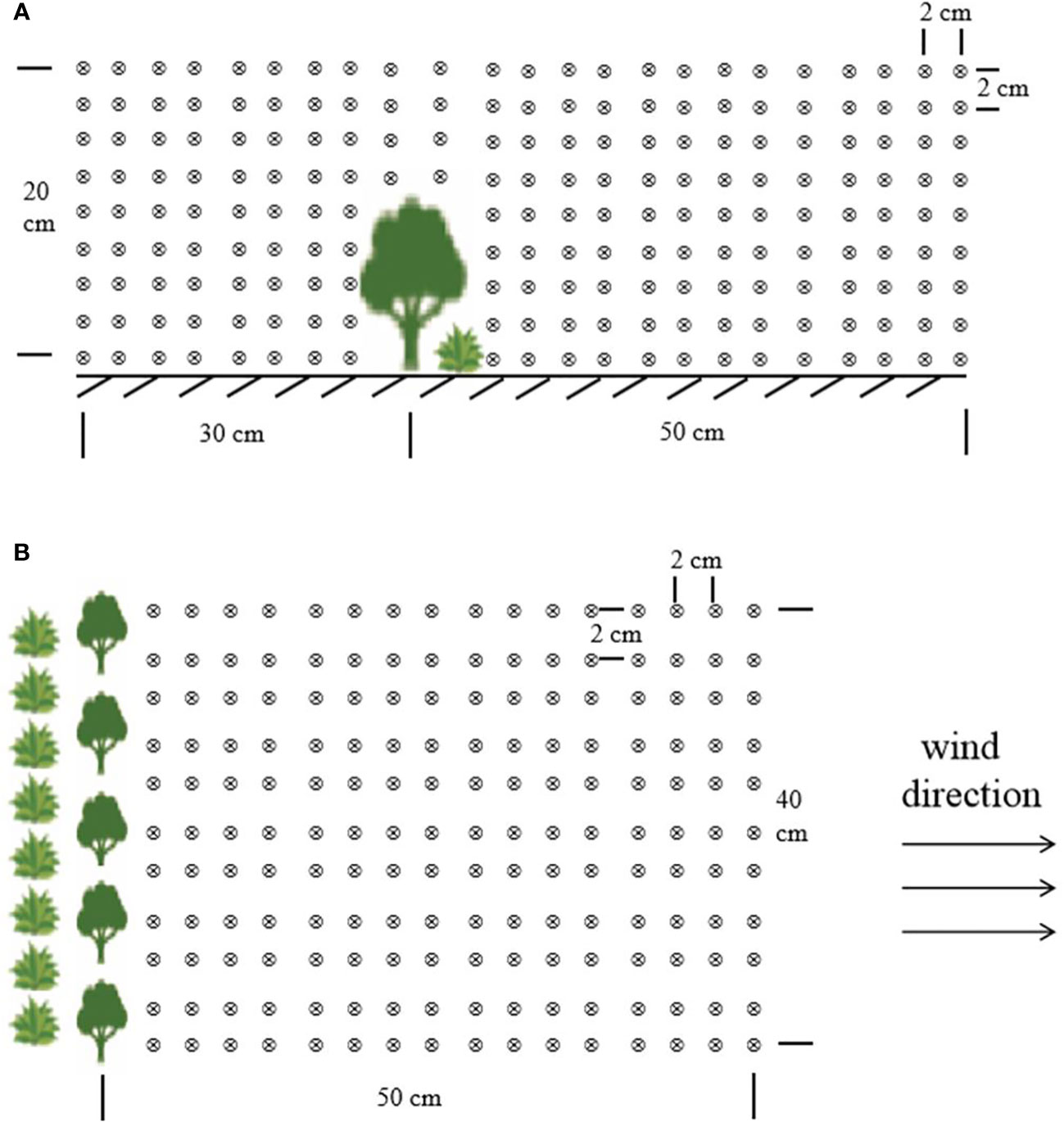
Figure 3 Layout of wind speed observation points in the wind tunnel. (A) Schematic diagram of longitudinal section measurement points. (B) Schematic diagram of horizontal measurement points.
3 Results
3.1 Wind speed profile characteristics
The leeward wind profile of different forest belts is shown in Figure 4. The presence of the forest belts significantly changed the structural characteristics of the wind speed profile. The configuration mode of mixed trees and shrubs in front of the forest belt provided the best wind prevention effect, followed by the double-belt trees, both of which were significantly better than the other configurations. Three times the height of the tree in front of the forest belt and the configuration modes of a single-belt tree forest and double-belt shrub forest had a reducing effect on wind speed within the height range of 5–10 cm. The wind speed difference between the other ranges and the control was small, while the single-belt shrub forest was consistent with the control wind speed at different height ranges, indicating that the shrub forest had no effect on wind speed here. Corresponding to the height of the tree in front of the forest belt, the wind speed of different configuration modes of the forest belt was lower than that at three times the tree height in the same forest belt, and the wind speed was lower than the control wind speed within the range of 0–10 cm. Except for the mixed forest of tree and shrub and the double-belt tree forest, the wind speed of the other configurations increased gradually above the control wind speed after exceeding a height of 15 cm. The wind speed profile at different positions behind the forest belt was similar. The wind speed of the forest belt with shrubs increased with the height; the wind speed decreased significantly within the range of shrub height. However, the tree forest belt showed a pattern in which the wind speed decreased at first and then increased due to the height-to-crown base. The protective effect of the double-belt tree forest was better than that of the single-belt forest. The windbreak effect of the mixed forest belt with tree and shrub was not as good as that of the two-row tree forest; however, this was compensated for by the protection of areas below the height of the branches. The wind speed of forest belts with different configurations exceeded the control wind speed within the tree height range.
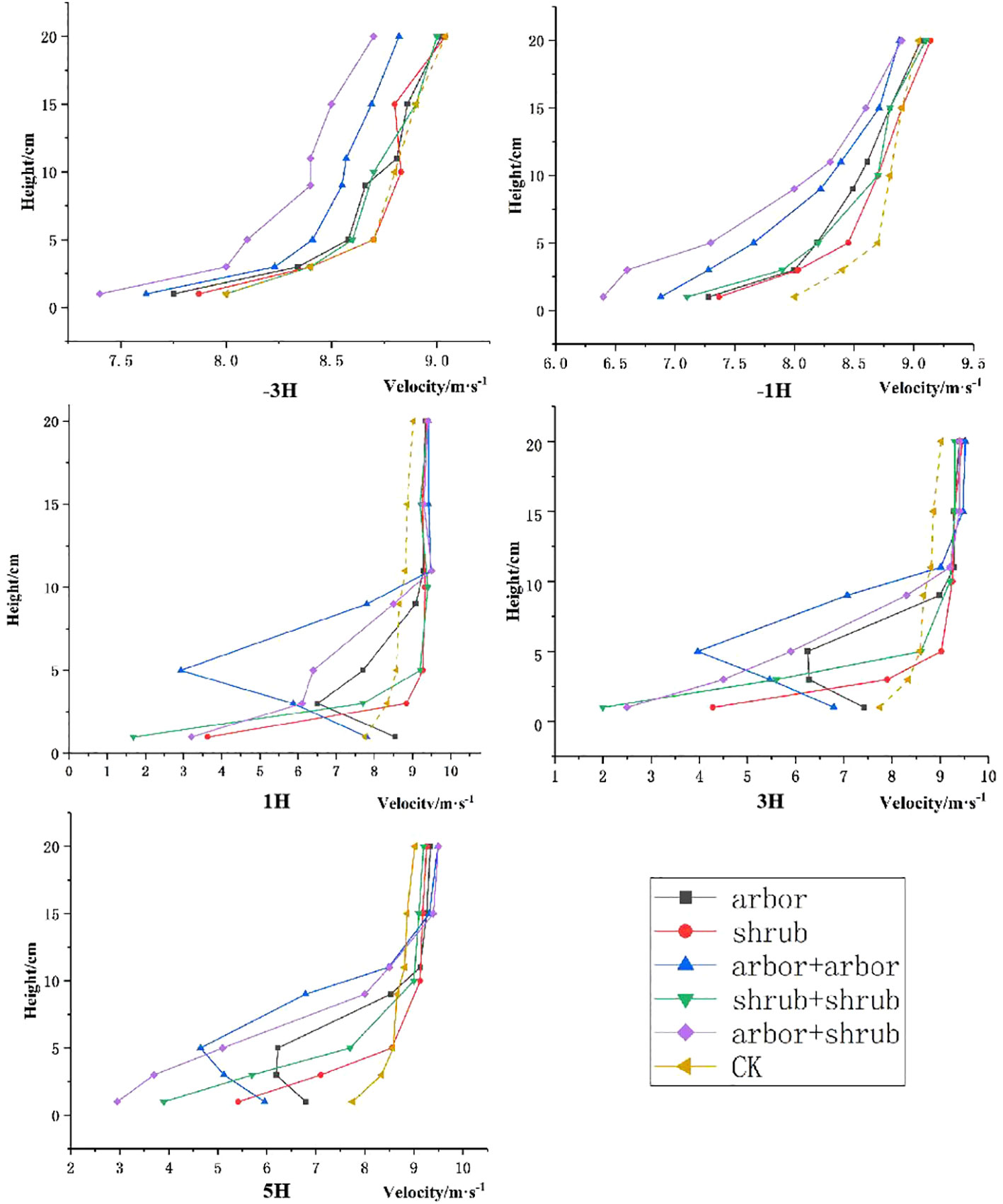
Figure 4 Wind speed profiles at different locations (H represents the height of the tree, negative values represent the distance in front of the forest belt, CK means no forest belt).
3.2 Wind speed flow field along the longitudinal section of the wind direction
The wind speed statistics along the longitudinal direction after flowing through different forest belts are shown in Table 2. The effective protection height of the double-belt tree forest was the highest, exceeding the height of the tree itself, followed by that of the single-belt tree forest and the mixed tree and shrub forest belt. The effective protective height of the shrub belt was lower than that of the tree forest, but exceeded the height of the shrubs themselves. Within the effective protective height, the average wind speed behind the forest belt showed a pattern of two rows of forest belts being lower than that of the single forest belt, and the shrub forest being lower than that of the tree forest. The single-belt tree forest had the highest average wind speed, which was 1.29 times the lowest average wind speed of the double-belt shrub forest. Compared with the double-belt pure forest, the mixed forest of tree and shrub had a larger protective area than the shrub forest, and better protection capabilities than the tree forest, with the lowest average wind speed in the area behind the forest belt.
The interpolation simulation of the longitudinal wind speed flow field in different configuration modes is shown in Figure 5. The airflow formed a distinct low wind-speed zone after passing through the forest belt, and the wind speed decreased significantly within the height range of the forest belt. The airflow above the forest belt experienced an acceleration effect, in which the wind speed exceeded that in front of the forest belt. There were multiple eddies in the wind-speed flow field of the pure tree forest belt, and the air disturbance was strong. Due to the presence of height-to-crown base, the near-surface wind speed was higher, and the protective effect at the height of the canopy layer was better. Within the effective protective height, the wind speed behind the single-belt tree forest was mainly within the range of 6.5–8.0 m/s, whereas the wind speed behind the double-belt tree forest ranged from 4.0 to 7.0 m/s. The air disturbance in forest belts with shrubs was not as severe as in tree forests, and the airflow was more stable after passing through the forest belts. The wind speed after traversing the single-belt shrub forest ranged from 4.5 to 7.0 m/s, whereas the wind speed after the double-belt shrub forest was 1.0 to 6.0 m/s. The mixed forest of tree and shrub had a high protective height; the wind speed near the surface was reduced significantly, thus combining the advantages of tree and shrub forest belts in which the wind speed behind the forest belt ranged from 2.5 to 7.0 m/s.
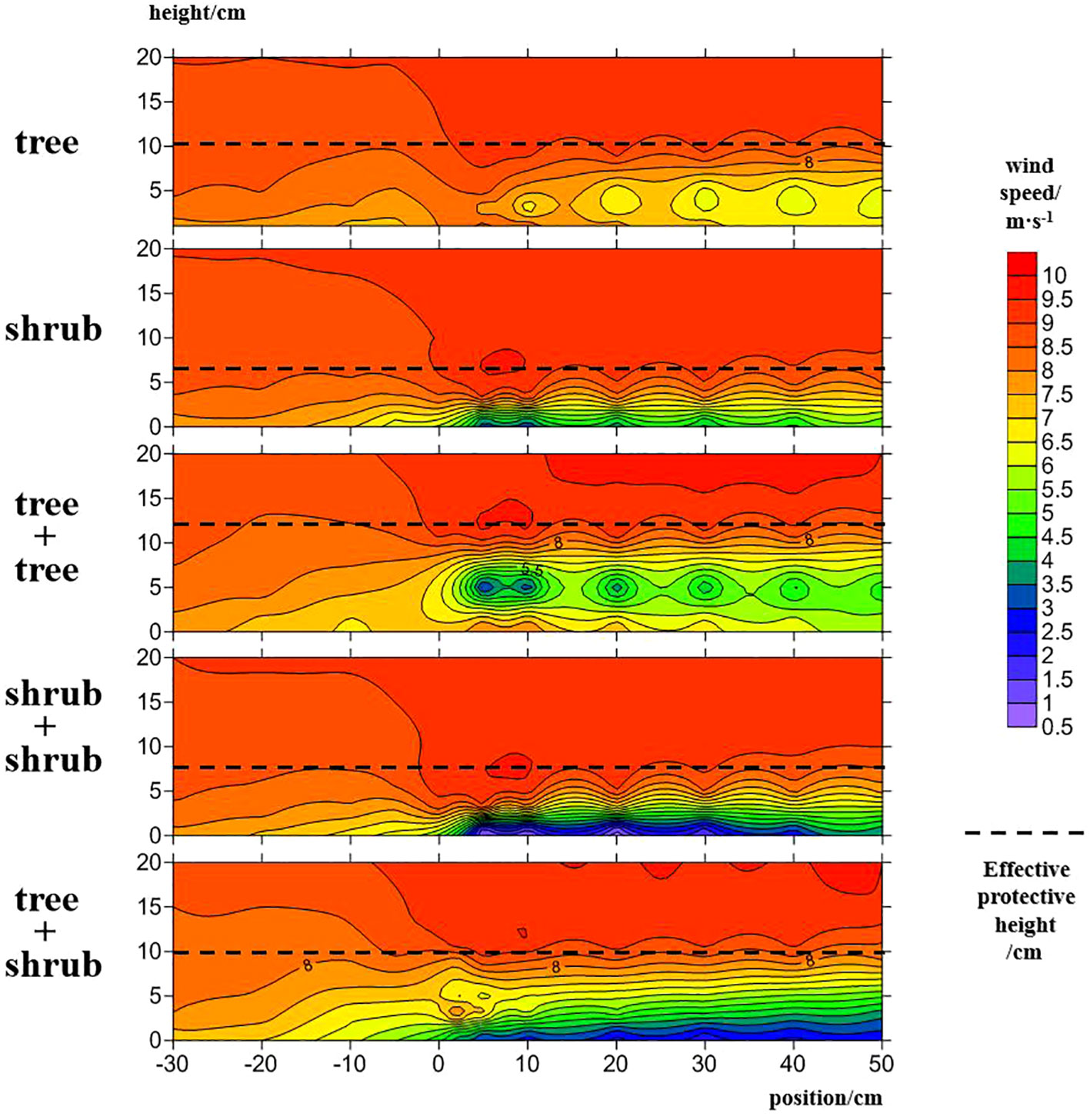
Figure 5 Wind speed flow field at longitudinal sections of different forest belts (the horizontal coordinate 0 represents the position of the forest belt, and a negative value represents the front of the forest belt).
3.3 Horizontal wind speed flow field behind the different forest belts
The horizontal wind speed flow field after interpolation simulation of different forest belts is shown in Figure 6. The wind flow field of single-belt shrub and double-belt shrub forest had similar structural characteristics, both formed a clear low wind-speed zone and then the wind speed increased gradually, but the effect of wind speed reduction in double-belt shrub was more obvious. In addition, compared with the wind flow field at the higher position, the wind speed reduction effect near the surface was better, and the low wind speed area was dominated by the blue area. The structural characteristics of the wind flow field were similar at different heights when air flow passed through pure trees. After passing through the single-belt tree forest, the airflow formed a high wind speed zone due to the “narrow tube effect”. The contour line in the downwind zone was parallel to the wind direction, indicating that the airflow was relatively stable and the wind speed had not changed. The airflow that passed through the double-belt tree forest formed a high wind-speed zone within the range of the tree height. However, unlike the single-belt tree forest, the wind speed decreased gradually in the wind direction. Unlike shrub forests, the wind speed near the surface of trees was higher than that at high places due to the acceleration of “narrow tube effect” between trunks. After the airflow passed through the mixed forest of tree and shrub, a large area of low wind speed was formed behind the forest belt, and the near surface wind speed was significantly lower than the higher position. The reduction degree of wind speed and the area of low wind speed in the mixed forest of tree and shrub at different heights were better than those of other forest belts.
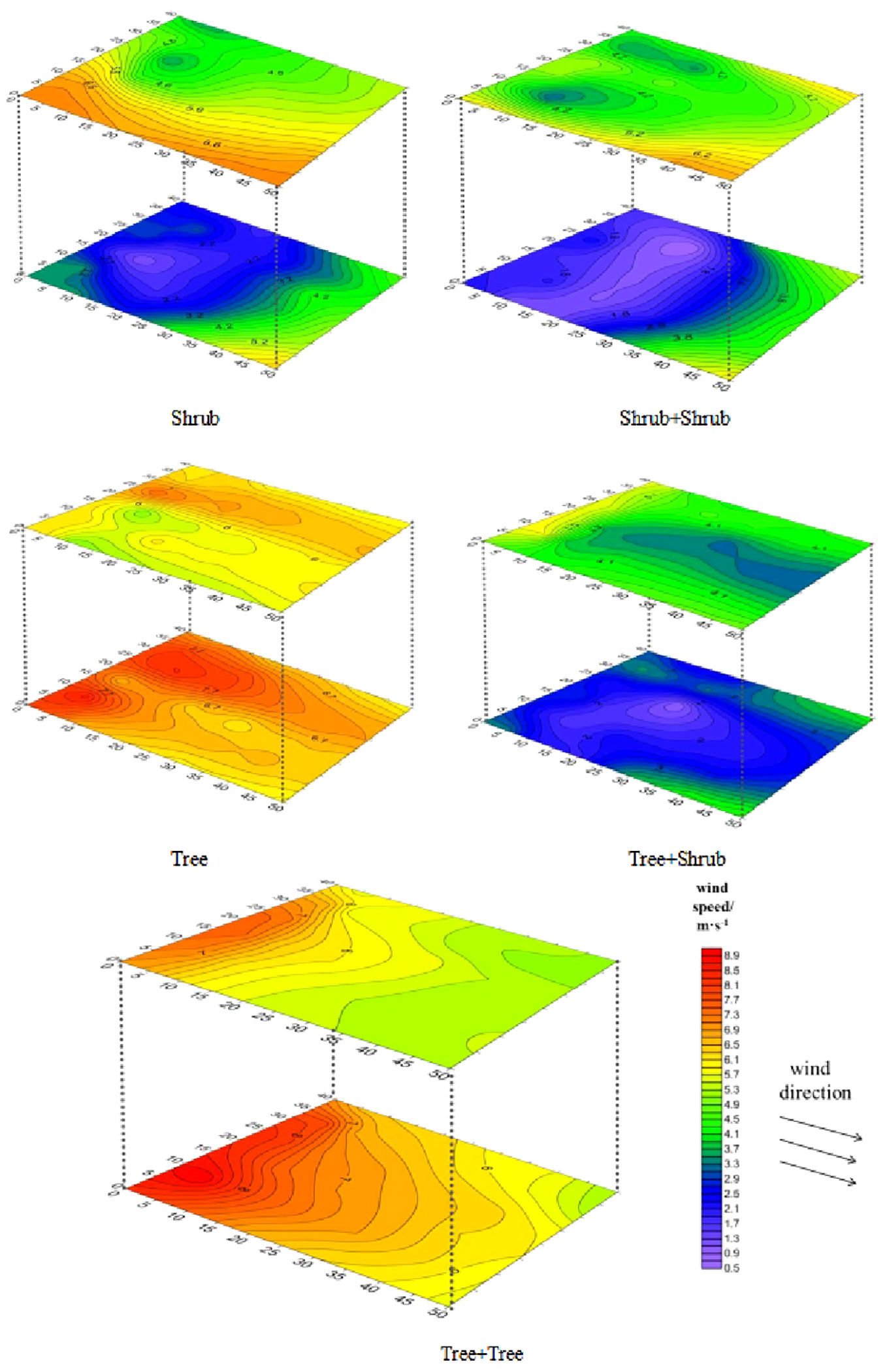
Figure 6 Horizontal wind speed flow field behind different forest belts configurations: In each panel, the measurement height of the upper and lower figures was 3 cm and 1 cm, respectively. The meaning of arrows is “wind direction”, which has been indicated in the figure 6.
3.4 Wind prevention efficiency of the different forest belts
The wind protection efficiency of different forest belts was calculated, and the results are shown in Figure 7. The double-belt shrub forest and the mixed tree and shrub forest had wind protection efficiency exceeding 30%, and the efficiency distribution was relatively uniform. The wind prevention efficiency of double-belt shrub ranged from 40% to 80%, while that of mixed forests of tree and shrub was mainly within the range of 30% and 70%. The wind prevention efficiencies of single-belt and double-belt forest were poor, with a minimum wind prevention efficiency of only 10%. Among them, the wind prevention efficiency of single-belt shrub ranged from 20% to 50%, single-belt tree forest from 10% to 50%. The wind prevention efficiency of double-belt tree forest from 20% to 40%, and the range of 30–40% accounted for 66% of the distribution.
4 Discussion
4.1 Protective effects of different forest belts
The windbreak effect of a shelterbelt depends on the wind energy consumption of the forest canopy, and the effect is affected by tree height, crown width, and other factors (Zastrow, 2019; Cheng et al., 2020; Torita and Masaka, 2020). This study shows that the tree forest has played a good role in wind protection, and has a high protection height and strong airflow disturbance ability (Figure 5). The wind speed after the tree forest belt gradually decreases within the experimental range and there is no trend of recovery (Figure 6), indicating that the tree forest has a longer effective protection distance. Sai (Sai et al., 2021) reached the same conclusion that trees and shrubs have different effects on airflow, tree belts have a stratification effect on airflow, while shrub belts have a lifting effect on airflow. The shrub forest belts have better protective effects within effective protective heights (Figures 5, 6). The taller the vegetation, the longer the protection distance of the forest belt will be, consistent with Wu (Wu et al., 2013). High porosity reduces the protection effect, and low porosity can form strong eddy currents in the leeward side of the forest belt, shortening the protection distance (Dong et al., 2007), and we came to a similar conclusion. The presence of under-branch height in a tree forest leads to higher forest belt sparsity (Table 1), while the short-fat” nature of shrubs leads to lower forest belt sparsity, which is also the main reason for the difference in wind protection effects between the two types of forest belts. It should be noted that in our study the model was made based on the characteristics of trees with leaves. In arid and semi-arid regions, the defoliation period of forest belts is usually a period of strong winds. A further increase in the sparsity of broad-leaved trees after defoliation leads to a continuous decrease in their protective effect. Therefore, attention should be paid to the supplement of shrub species in afforestation.
4.2 Protective effects near the surface
China has a large population, and the relationship between people and land is tense. Therefore, a large number of farmlands, grassland and other production land are distributed in arid and semi-arid areas. For these scenes, near-surface wind erosion protection is critical, such as preventing soil particles from being eroded by wind in degraded grassland, reducing wind speed near the surface in farmland to avoid lodging of crops. Shrub belts can not only reduce the wind speed near the surface, but also cover the ground with branches to form a protective layer, making them more suitable in arid areas. Shrubs can effectively increase the organic matter content of soil. Soil C content and C stocks were on average 148% and 117% greater in shrub-encroached grassland soil (Phesheya et al., 2019). In addition, shrubs can also intercept soil particles, form nebkhas, produce fertilizer island effect, and contribute to the improvement of soil physical and chemical properties and the change of microbial community structure (Wang et al., 2010; Cao et al., 2016). In summary, shrub belts should play an important role in afforestation of arid areas.
5 Conclusions
After passing through the forest belt, the structural characteristics of the airflow change significantly. The tree forest belt has a large protective area and strong airflow disturbance ability, and the protective effect of canopy is stronger than that of height-to-crown base. Although the protection area of shrub forest is smaller than that of tree forest, its protection effect is better within the effective protection height, and its protection effect on the near-surface is more obvious than that of trees. Further, when mixed with tree forest, shrubs can make up for the shortcomings of poor protection effect in the height-to-crown base of tree forest. In conclusion, the role of shrub forest should be emphasized in afforestation in arid areas, especially in areas that need near-surface protection.
Data availability statement
The raw data supporting the conclusions of this article will be made available by the authors, without undue reservation.
Author contributions
SZ: Conceptualization, Formal analysis, Funding acquisition, Methodology, Writing – original draft, Writing – review & editing. WY: Funding acquisition, Methodology, Writing – original draft. YYu: Methodology, Project administration, Writing – original draft. YZ: Project administration, Supervision, Writing – original draft. WW: Data curation, Writing – original draft. LW: Data curation, Writing – original draft. YYa: Data curation, Software, Writing – original draft. HW: Data curation, Visualization, Writing – original draft.
Funding
The author(s) declare financial support was received for the research, authorship, and/or publication of this article. This study was supported by the National Natural Science Foundation of China (No. 32001375), the Science and Technology Program for Innovative Talent granted by the National Forestry and Grassland Administration of China (No.2019132604) and the Science and Technology Popularization Project of National Forestry and Grassland Administration (No.[2019]34).
Conflict of interest
LW was employed by the company Qinghai Provincial Investigation, Design & Research Institute of Water Conservancy & Hydropower Co., Ltd.
The remaining authors declare that the research was conducted in the absence of any commercial or financial relationships that could be construed as a potential conflict of interest.
Publisher’s note
All claims expressed in this article are solely those of the authors and do not necessarily represent those of their affiliated organizations, or those of the publisher, the editors and the reviewers. Any product that may be evaluated in this article, or claim that may be made by its manufacturer, is not guaranteed or endorsed by the publisher.
References
Atangana A., Khasa D., Chang S., Degrande A. (2014). Agroforestry for soil conservation. Tropical Agroforestry. (Netherlands: Springer), 203–216. doi: 10.1007/978-94-007-7723-1
Bruno L., Fransos D., Giudice A. L. (2018). Solid barriers for windblown sand mitigation: Aerodynamic behavior and conceptual design guidelines. J. Wind Eng. Ind. Aerodyn. 173, 79–90. doi: 10.1016/j.jweia.2017.12.005
Butz P., Hölscher D., Cueva E., Graefe S. (2018). Tree water use patterns as influenced by phenology in a dry forest of Southern Ecuador. Front. Plant Sci. 9. doi: 10.3389/fpls.2018.00945
Cao C., Abulajiang Y., Zhang Y., Feng S., Wang T., Ren Q., et al. (2016). Assessment of the effects of phytogenic nebkhas on soil nutrient accumulation and soil microbiological property improvement in semi-arid sandy land. Ecol. Engineering. 91, 582–589. doi: 10.1016/j.ecoleng.2016.03.042
Cao S., Chen L., Shankman D., Wang C., Wang X., Zhang H. (2011). Excessive reliance on afforestation in China's arid and semi-arid regions: lessons in ecological restoration. Earth Sci. Rev. 104, 240–245. doi: 10.1016/j.earscirev.2010.11.002
Cheng H., Liu C., Kang L. (2020). Experimental study on the effect of plant spacing, number of rows and arrangement on the airflow field of forest belt in a wind tunnel. J. Arid Environments. 178, 104169. doi: 10.1016/j.jaridenv.2020.104169
Deng R., Wang W., Li Y., Zhao D. (2013). A retrieval and validation method for shelterbelt vegetation fraction. J. Forestry Res. 24, 357–360. doi: 10.1007/s11676-013-0360-y
Dong Z. B., Luo W. Y., Qian G. Q., Wang H. T. (2007). A wind tunnel simulation of the mean velocity fields behind upright porous fences. Agric. For. Meteorology. 146, 82–93. doi: 10.1016/j.agrformet.2007.05.009
Howlader A. (2023). Determinants and consequences of large-scale tree plantation projects: Evidence from the Great Plains Shelterbelt Project. Land Use Policy 132, 106785. doi: 10.1016/j.landusepol.2023.106785
Jaskulska R., Jaskulska J. (2017). Efficiency of old and young shelterbelts in reducing the contents of nutrients in Luvisols. Agriculture Ecosyst. Environment. 240, 269–275. doi: 10.1016/j.agee.2017.02.033
Jia Y., Shao M. (2014). Dynamics of deep soil moisture in response to vegetational restoration on the Loess Plateau of China. J. Hydrology. 519, 523–531. doi: 10.1016/j.jhydrol.2014.07.043
Lüttschwager D., Jochheim H. (2020). Drought primarily reduces canopy transpiration of exposed beech trees and decreases the share of water uptake from deeper soil layers. Forests. 11, 537. doi: 10.3390/f11050537
Ma H., Zhu Q., Zhao W. (2020). Soil water response to precipitation in different micro-topographies on the semi-arid Loess Plateau, China. J. Forestry Res. 34, 245–256. doi: 10.1007/s11676-018-0853-9
Maximiliane M., Julia E., Katja T. (2023). Plant community productivity and soil water are not resistant to extreme experimental drought in temperate grasslands but in the understory of temperate forests. Sci. Total Environment. 891, 164625. doi: 10.1016/j.scitotenv.2023.164625
McVicar T., Van N., Li L., Wen Z., Yang Q., Li R., et al. (2010). Parsimoniously modelling perennial vegetation suitability and identifying priority areas to support China's re-vegetation program in the Loess Plateau: matching model complexity to data availability. For. Ecol. Management. 259, 1277–1290. doi: 10.1016/j.foreco.2009.05.002
Meng L., Cui Z., Huang Z., Esteban M., Dunkerley D., Wu G. (2022). Thinning effects on forest production and rainfall redistribution: Reduced soil water deficit and improved sustainability of semiarid plantation forestlands. Land Degradation Dev. 16, (33):3163–3173. doi: 10.1002/ldr.4379
Mganga K., Nyariki D., Musimba N., Amwata D. (2018). Determinants and rates of land degradation: application of stationary time-series model to data from a semi-arid environment in Kenya. J. Arid Land. 10, 1–11. doi: 10.1007/s40333-017-0036-0
Oktay Y., Derya E., Murat S., Bilal C., Bülent T., Hüseyin D. (2021). Restoration success in afforestation sites established at different times in arid lands of Central Anatolia. For. Ecol. Management. 503, 119808. doi: 10.1016/j.foreco.2021.119808
Phesheya D., Vusi M., Masibonge G., Louis T., Lerato S. (2019). Chemical stabilisation of carbon stocks by polyvalent cations in plinthic soil of a shrub-encroached savanna grassland, South Africa. Catena. 181, 104088. doi: 10.1016/j.catena.2019.104088
Qiu D., Zhu G., Lin X., Jiao Y., Lu S., Liu J., et al. (2023). Dissipation and movement of soil water in artificial forest in arid oasis areas: Cognition based on stable isotopes. Catena. 228, 107178. doi: 10.1016/j.catena.2023.107178
Sai K., Zhao Y., Bao Y., Liu. C., Ding G., Gao G. (2021). Wind-tunnel tests study of shelter effects of deciduous farmland shelterbelts in arid and semi-arid areas. Trans. Chin. Soc. Agric. Engineering. 37, 157–165. doi: 10.11975/j.issn.1002-6819.2021.05.018
Su B., Shangguan Z. (2019). Decline in soil moisture due to vegetation restoration on the Loess Plateau of China. Land Degradation Dev. 3, 290–299. doi: 10.1002/ldr.3223
Teng Y., Zhan J., Su M., Wu Y., Zhang Y., Yu C., et al. (2022). Identifying prioritized afforestation types in ecologically vulnerable zones of Northern China considering reducing water consumption and increasing carbon sequestration. Ecol. Indicators. 145, 109734. doi: 10.1016/j.ecolind.2022.109734
Thivakaran G., Sharma S., Chowdhury A., Murugan A. (2020). Status, structure and environmental variations in semi-arid mangroves of India. J. Forestry Res. 31, 163–173. doi: 10.1007/s11676-018-0793-4
Tomasella J., Vieira R., Barbosa A., Rodriguez D., Santana M., Sestini M. (2018). Desertification trends in the northeast of Brazil over the period 2000-2016. Int. J. Appl. Earth Obs. Geoinform. 73, 197–206. doi: 10.1016/j.jag.2018.06.012
Torita H., Masaka K. (2020). Influence of planting density and thinning on timber productivity and resistance to wind damage in Japanese larch (Larix kaempferi) forests. J. Environ. Management. 268, 110298. doi: 10.1016/j.jenvman.2020.110298
Travis W., Seth M., Saroj D., Nicolas P., Michael C. (2023). Synergistic soil, land use, and climate influences on wind erosion on the Colorado Plateau: Implications for management. Sci. Total Environment. 893, 164605. doi: 10.1016/j.scitotenv.2023.164605
United Nations Convention to Combat Desertification. (2008). Desertification—coping with today’s global challenges in the context of the strategy of the UNCCD, p9.
Wafa A., Lesley K., Steve G., Peter K., Brent C. (2019). The historical basis and future options for native plant-species in the hyper-arid forests of Abu. Land Use Policy. 88, 104186. doi: 10.1016/j.landusepol.2019.104186
Wang T., Xue X., Zhou L., Guo J. (2015). Combating aeolian desertification in northern China. Land Degrad. Dev. 26, 118–132. doi: 10.1002/ldr.2190
Wang X., Zhang C., Zhang J., Hua T., Lang L., Zhang X., et al. (2010). Nebkha formation: Implications for reconstructing environmental changes over the past several centuries in the Ala Shan Plateau, China. Palaeogeography Palaeoclimatology Palaeoecology. 297, 697–706. doi: 10.1016/j.palaeo.2010.09.020
Wang Y., Shao M., Liu Z. (2013). Vertical distribution and influencing factors of soil water content within 21-m profile on the Chinese Loess Plateau. Geoderma. 193, 300–310. doi: 10.1016/j.geoderma.2012.10.011
Wang Y., Shao M., Zhu Y., Liu Z. P. (2011). Impacts of land use and plant characteristics on dried soil layers in different climatic regions on the Loess Plateau of China. Agric. For. Meteorology. 151, 437–448. doi: 10.1016/j.agrformet.2010.11.016
Wu T., Yu M., Wang G., Wang Z., Duan X., Dong Y., et al. (2013). Effects of stand structure on wind speed reduction in a Metasequoia glyptostroboides shelterbelt. Agroforestry Systems. 87, 251–257. doi: 10.1007/s10457-012-9540-6
Yi H., Wang Y., Lou Y., Han X. (2023). Effects of vegetation restoration on the hydrological regimes of the Chinese loess plateau: A comparative analysis of forested and less-forested catchments. Forests. 14, 1199. doi: 10.3390/f14061199
Zara E., Thomas P., Mark A., Daniel M. (2022). Shelterbelt species composition and age determine structure: Consequences for ecosystem services. Agriculture Ecosyst. Environment. 329, 107884. doi: 10.1016/j.agee.2022.107884
Zastrow M. (2019). China’s tree-planting drive could falter in a warming world. Nature. 573, 474–475. doi: 10.1038/d41586-019-02789-w
Zhang S., Ding G., Yu M., Gao G., Zhao Y., Wang L., et al. (2019). Application of boundary layer displacement thickness in wind erosion protection evaluation: Case study of a salix psammophila sand barrier. Int. J. Environ. Res. Public Health. 16, 592. doi: 10.3390/ijerph16040592
Zhou D., Si J., He X., Jia B., Zhao C., Wang C., et al. (2023). The relationship between vegetation and soil moisture reveals the vegetation carrying capacity threshold—A case study of a Haloxylon ammodendron plantation in the Alxa desert, China. Front. Environ. Sci. 11. doi: 10.3389/fenvs.2023.1054195
Keywords: arid and semi-arid areas, selection strategy, wind speed flow field, wind tunnel simulation, windproof efficiency
Citation: Zhang S, Yuan W, Yu Y, Zhang Y, Wang W, Wang L, Yang Y and Wang H (2024) Shrubs plays an important role in configuration of shelterbelt in windy and sandy areas. Front. Ecol. Evol. 12:1347714. doi: 10.3389/fevo.2024.1347714
Received: 04 December 2023; Accepted: 15 February 2024;
Published: 06 March 2024.
Edited by:
Zhongju Meng, Inner Mongolia Agricultural University, ChinaReviewed by:
Xinping Liu, Chinese Academy of Sciences (CAS), ChinaHuijie Xiao, Beijing Forestry University, China
Copyright © 2024 Zhang, Yuan, Yu, Zhang, Wang, Wang, Yang and Wang. This is an open-access article distributed under the terms of the Creative Commons Attribution License (CC BY). The use, distribution or reproduction in other forums is permitted, provided the original author(s) and the copyright owner(s) are credited and that the original publication in this journal is cited, in accordance with accepted academic practice. No use, distribution or reproduction is permitted which does not comply with these terms.
*Correspondence: Weijie Yuan, yuanwj@caf.ac.cn