- Biotactic Fisheries Research and Monitoring, Biotactic Inc., Kitchener, ON, Canada
Despite the importance of Great Lakes fisheries and the increasing popularity of dam removal as a method to restore river connectivity and increase fish passage, the adfluvial migration of Steelhead (Oncorhynchus mykiss) has been drastically understudied and only relatively few published studies have examined the impacts of dam removal on fish movement and timing. To help fill these knowledge gaps, spawning migrations of Great Lakes adfluvial Steelhead tagged in spring and fall were monitored for two years before and three years after removal of a dam that partially blocked upstream movement for 100 years. Removal of the dam not only reduced downstream delay and increased passage at the site of the dam removal itself, but increased travel speeds and increased passage at remaining upstream dams for both spring and fall run fish, underscoring the cumulative impact of successive dam passage on fish migration. Fall fish were most impacted by the dam removal and were able to pass not only the dam footprint, but, for the first time, were also able to pass both remaining upstream dams, allowing them to overwinter at locations closer to known spawning areas. For these fish, delay at the footprint was reduced from the order of 100+ days to < 1 hour and the number of days passage occurred compared to the number of days fish were present and blocked increased from 2% to 82%. The benefits of dam removal should ultimately equate to increases in fish production, as more critical habitat becomes reconnected and more fish are able to pass dams and arrive at spawning beds. The observation that fish are likely able to remain in better condition and retain more energy for continued migration and reproduction warrants further study.
1 Introduction
Motivated by changes in season, water temperature and flow, the upstream migrations of Rainbow trout are legendary and awe-inspiring. These fish can accelerate up to 59.7 m/s2 (Webb, 1975; Harper and Blake, 1990), can leap over 3 m high (Powers and Orsborn, 1985), can swim as far as 1500 km to reach natal spawning habitat (Good et al., 2005) and may spawn three to four times throughout their 7+ year lifespan (Scott and Crossman, 1998). Initially introduced into the Laurentian Great Lakes in 1874 (Biette et al., 1981), Rainbow trout have spread to nearly all available suitable habitats. While there is still widespread supplementation through various large and small scale hatchery stocking programs, naturalized self-recruiting populations have long been established throughout the Great Lakes system (Borgeson et al., 2020). Unlike anadromous Rainbow trout (Steelhead) that migrate from freshwater to the ocean and then return to freshwater to spawn, Rainbow trout within the Great Lakes watershed have adopted at least three life history strategies including river resident populations, lake resident populations and adfluvial populations that migrate from the Great Lakes into tributaries to spawn and back, without saltwater transitions. These adfluvial fish (hereafter also referred to as Steelhead) are generally larger than their resident counterparts (Kendall et al., 2015) and form a major component of the multi-billion dollar recreational fisheries of the Great Lakes (Kwain, 1981; Hansen et al., 1990; Melstrom and Lupi, 2013; Budnik, 2017). Despite the economic importance of this species within the Great Lakes and surrounding watersheds, surprisingly very little published research has focused on understanding adfluvial Steelhead migration timing or dam passage performance. The limited studies that have investigated dam passage through fish passage structures have calculated Steelhead attraction and passage efficiencies as 58% and 25%, respectively, at a vertical slot fishway on a tributary of Lake Ontario (115 PIT-tagged fish; Pratt et al., 2009) and 53% and 100%, respectively, at a nature-like fishway on a tributary of Georgian Bay in Lake Huron (30 radio-tagged fish; Bunt and Jacobson, 2019).
In stark contrast to these two short-term Great Lakes Steelhead studies, a vast number of comprehensive studies have been conducted on anadromous Steelhead populations within their native range on the west coast of North America. Perhaps the largest and most well-known studies are those on the Columbia and Snake Rivers where 2812 Steelhead were radio-tagged and movement across 6 dams was tracked from 1991 to 1995, and more recently where over 7832 Steelhead were radio-tagged and movement across 8 dams was tracked from 1996 to 2014. The multitude of publications stemming from this work, as well as other tagging studies in this system, have revealed details related to fish delay downstream from dams (English et al., 2006; Keefer et al., 2021), fishway effectiveness (Keefer et al., 2021), inter-dam movement rates (Keefer et al., 2004a; English et al., 2006; Salinger and Anderson, 2006; Keefer et al., 2021) and dam passage timing (Bjornn et al., 1995, Keefer et al., 2004a, Keefer et al., 2021) for early and late-arriving summer-run fish. Studied Steelhead populations have been found to have a mean dam passage efficiency of 97.9% and dam passage time of 15.1 hours (Keefer et al., 2021), and to move from 24 km/day to 4 to 8 km/day across a four dam and three reservoir hydrosystem stretch, depending on season and water temperature (Keefer et al., 2004a).
Despite impressive dam passage abilities and overall migratory speeds, images and reports of Steelhead repeatedly leaping into dam faces (Supplementary Videos S1-S4) and being visibly damaged by violent hydraulic jumps in spillways that force fish into solid objects (Ruggles and Murray, 1983; Cox et al., 2023), are unfortunately common. Even with fish passage structures, dams continue to block or at the very least delay fish movement with combined average attraction and passage efficiencies of only 62.3% and 51.5%, respectively, for 26 salmonid and non-salmonid fish species at four main types of fishways (Bunt et al., 2016). As such, dam removals have become an increasingly popular restoration method to achieve true river connectivity (Kemp, 2016; Bellmore et al., 2019). Prior to 2023, over 4900 barrier removals had been conducted in Europe and Asia, and over 1700 dam removals had been conducted in the United States (Duda and Bellmore, 2022). Unfortunately, the degree of change (i.e., biological benefit) resulting from the majority of these removals has not been fully documented (Foley et al., 2017), with only approximately 10% of the removals conducted in the United States studied, with approximately 50% of those published in scientific journals, and the remainder reported in various other formats (Bellmore et al., 2017).
To-date, the majority of published dam removal research has focussed on detailing the impact of the removal on river hydrology and geomorphology, with relatively few studies that investigated the impact of the removal on biological communities, such as fish (Bellmore et al., 2017). Those that have, primarily described changes in the river community using perhaps more basic metrics such as community composition, species richness, abundance or density (e.g., Catalano et al., 2007; Stanley et al., 2007; Maloney et al., 2008; Burroughs et al., 2010; Kornis et al., 2015; Magilligan et al., 2016; Poulos and Chernoff, 2017; Bubb et al., 2021). Furthermore, such studies often used methods that have provided information regarding only a snap-shot in time and/or space such as electrofishing, hydroacoustics, snorkeling or eDNA (e.g., Dorobek et al., 2015; Hogg et al., 2015; Duda et al., 2020; Scherelis et al., 2020; Huang et al., 2023; Jones et al., 2023; Kiffney et al., 2023; Whittum et al., 2023) and were often narrowly-scoped temporally, spatially or both (Bellmore et al., 2017; Foley et al., 2017; Whittum et al., 2023). These methods may not fully reflect fish responses, and do not reveal any direct information about fish movement and timing. In the Penobscot River in Maine, for example, where two dams were removed and fish passage structures at remaining dams were improved, annual counts of migratory species at the new lowermost dam (Milford Dam) post-removal far exceed fishway passage counts at the former lowermost dam (Veazie Dam) pre-removal, while electrofishing indicated only limited movement upstream of Milford Dam post lower dam removals (Whittum et al., 2023). While some tagging studies (PIT, radio, hydroacoustic) have been performed in this system, post-dam removal work has focussed on evaluating the new fish passage structure at Milford Dam (Izzo et al., 2016), with no information regarding movement rates with respect to just the dam removals. Two dams were also removed within the Elwha River in Washington, and radiotelemetry has revealed the expanding upstream spatial distribution of Bull Trout (Salvelinus confluentus) over several years post-removal (Brenkman et al., 2019). Formally complete barriers to movement, there was no pre-removal migration and thus no information on changes in movement timing and rates as a function of dam removal was available.
The Saugeen River is the largest Canadian tributary to Lake Huron, and supports perhaps the largest inland recreational salmonid fishery in Canada (Bunt and Jacobson, 2022a). The river is 160 km long, the first 101 km potentially accessible to migrating salmonids, assuming they can traverse several major dams present within the river stretch. One of these dams, the 100 year old Truax Dam, located in Walkerton, Ontario, is the second dam upstream from the river mouth, and was identified by angling club members and various local stakeholders as a partial barrier to fish migration with no effectively functional fish passage structure. In August 2019 this dam was removed as part of a long-term initiative to restore fish migration and enhance fish production in the watershed (Barnthouse et al., 2019; Bunt and Jacobson, 2022b). In order to fully document and monitor the impact of this removal on the connectivity of the Saugeen River, and the ability of adfluvial Steelhead to migrate to spawning grounds throughout the watershed, we conducted a long-term before-after radiotelemetry study that involved the collection of three seasons of telemetry data (two spring, one fall/winter) pre-dam removal and six seasons of telemetry data (three each spring and fall/winter) post-dam removal. We calculated several migration and fish passage metrics including dam passage delays, passage success and upstream inter-dam movement rates, at three Saugeen River dams, which included the dam that was removed as well as two additional upstream dams. Our goals were to 1) investigate changes in these metrics before versus after dam removal at the site of the removal itself and 2) determine if the dam removal affected the movement and passage of fish at remaining dams further upstream. This is the first detailed investigation of the impacts of a significant dam removal project on the temporal and spatial migration characteristics of Great Lakes adfluvial Steelhead, an important non-native species with high recreational fisheries value that is also the focus of considerable international management effort.
2 Materials and methods
2.1 Study area
The meandering and largely rural Saugeen River flows north downstream to Southampton, Ontario (Figure 1), and has a long history of dams being built, destroyed and rebuilt for milling, timberworks and hydropower. Denny’s Dam (Lat: -81.329293; Long: 44.504490), located five kilometers upstream from the river mouth in Southampton, Ontario, was originally constructed in 1870. Now owned and operated by the Ontario Ministry of Natural Resources and Forestry (OMNRF), the dam was rebuilt in 1970 to block the passage of invasive Sea lamprey (Petromyzon marinus) with the most recent rehabilitation, including modifications to an existing modified pool/baffle and orifice fishway, concluded in late 2018. Seventy-seven kilometers upstream, the Truax Dam (Lat: -81.141714; Long: 44.130115) in Walkerton, Ontario, was originally constructed from wood in 1852 and was converted to a 2.4 m high concrete structure in 1919. Despite having a sluiceway and being retrofitted with a culvert and baffle fishway in 2011, the fishway was largely ineffective and the dam was still identified by local stakeholders as a partial barrier to upstream fish passage. As such, it was removed in August 2019 (Supplementary Video S5). Two and a half kilometers further upstream, Carrick Dam (Lat: -81.135689; Long: 44.109513) was originally constructed in 1911 as a hydroelectric generating station and was decommissioned and partially removed in 1963. Originally just 3 m high, fish are currently able to pass through a large notch and sluice or jump and swim over the remaining deteriorating dam face. Ten kilometers upstream, Maple Hill Dam (Lat: -81.067499; Long: 44.142883), originally constructed in 1862, is a privately owned and operating hydroelectric dam outfitted with both a Denil fishway on one side of the river and a modified pool-and-weir fishway on the other. Five and a half kilometers upstream the Hanover Dam (Lat: -81.031838; Long: 44.160796), in Hanover, Ontario, is a complete barrier to all further upstream fish movement. Several tributaries join with the Saugeen River upstream from the site of the removal. Otter Creek, located 1 km further upstream from Carrick Dam, is the first coldwater high quality spawning tributary upstream from Walkerton and measures just ~2 km long until a dam blocks all further upstream fish movement. Three upstream tributaries join with the Saugeen River midway between Maple Hill and Hanover Dams - the Beatty Saugeen River measures 30.2 km long until a dam blocks all fish passage in Orchardville, Ontario, the South Saugeen River measures 23.5 km long until a dam blocks all fish passage in Ayton, Ontario and Meux Creek measures 7.2 km long until a dam blocks all fish passage in Neustadt, Ontario.
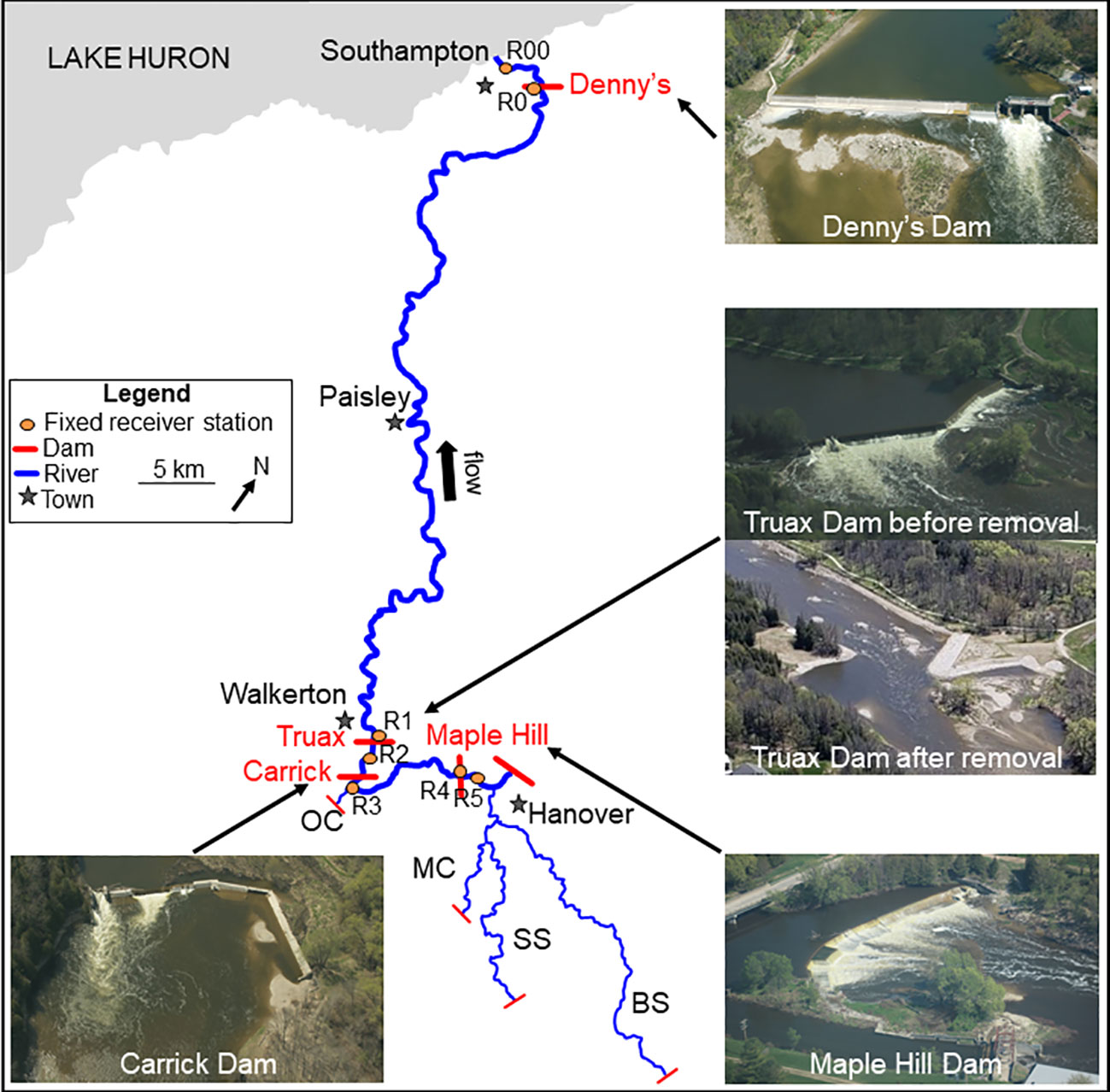
Figure 1 Saugeen River watershed, main-stem dams and the distribution of fixed receivers used for tracking Steelhead movement and passage. Thick blue lines indicate the main-stem of the Saugeen River. Tributaries are thin blue lines with OC Otter Creek, MC Meux Creek, SS South Saugeen River and BS Beatty Saugeen River.
2.2 Radiotelemetry
Each spring and fall from spring 2018 to 2022, fifty adult Steelhead were captured within the fishway trap at Denny’s Dam in Southampton and tagged. Due to COVID-19 restrictions, no fish were tagged in spring 2020. Only 18 fish were tagged in fall 2021, using transmitters returned from previous angler harvests. The Denny’s Dam fishway trap is operated by the Lake Huron Fishing Club and Ontario Steelheader volunteers. During tagging, volunteers transferred the fish from the trap to a holding pen equipped with recirculating river water located beside the fishway. After an approximate 20 minute recovery period, we individually removed fish from the holding pen and electro-sedated each fish with a low-voltage (9V) transdermal electrical nerve stimulation unit and conductive compression gloves (Durhack et al., 2020). Fish were quickly measured for total length (mm), weight (g) and girth (mm) before being partially submerged in a surgical tank. While still electro-sedated, our surgeon tagged each fish with an external anchor-tag and made a 1 cm incision posterior to the pelvic girdle. A radio-tag (Sigma Eight Inc. TX-PSC-I-450, 46x12 mm, 8.5 g, 3 year battery life expectancy) was then surgically implanted within the body cavity with the radio antenna threaded posterior to the incision before it was closed with a single simple interrupted suture. During the surgical process we recorded fish sex, origin (hatchery/wild) and condition (injuries/spawning state). After surgery was completed a digital photograph of each fish was taken and the fish was transferred to a temporary recovery tank with recirculating river water beside the surgical area. Processing and tagging of each individual, from removal from the holding pen to release into the recovery tank, was completed within ~3 minutes (based on timestamps of successive photographs). Fish were individually monitored within the recovery tank and were released ~15 m upstream of the dam by fishing club volunteers. Tagging occurred on April 23 and October 15 and 16 in 2018, April 26 and October 28 in 2019, October 17 and November 7 in 2020, April 10 and October 16 in 2021 and April 9 and 13 in 2022. No injuries or mortalities related to the tagging process were noted. All fish were tagged by the same surgeon, and all work was conducted under annual Licenses to Collect Fish for Scientific Purposes obtained from the Midhurst District Office of the OMNRF.
After release, we monitored fish movement with a combination of seven fixed receiver stations located at key points of passage within the main-stem of the Saugeen River (Figure 1), as well as aerial (small-aircraft) and land (truck and foot) mobile surveys. Range tests were conducted for each antenna during fixed receiver installation, and mobile receivers were calibrated with test tags throughout the study. We tracked tagged fish from October to March each fall/winter season (hereafter fall) and from March to June each spring season. While receivers (R) 1 - 4 were present and active since the initiation of the study in spring 2018, R00 was installed before the beginning of the fall 2021 season, R0 was installed before spring 2021 and R5 was installed before spring 2020. Receiver station R00 was located in Southampton harbor, 0.31 km upstream of the mouth with Lake Huron and 4.16 km downstream from Denny’s Dam, with one antenna directed upstream and one antenna directed downstream across the river. Receiver station R0 was located at the Denny’s Dam fishway, with one antenna installed at the top of the dam directed downstream to monitor fish arrival, one antenna located inside the fishway entrance and one antenna located upstream of the dam. Receiver station R1 was located ~180 m downstream of the Truax Dam, with one high gain antenna directed upstream across the river. Receiver station R2 was located ~1.95 km upstream of the Truax Dam and ~800 m downstream of Carrick Dam, with one antenna directed upstream and one antenna directed downstream. Receiver station R3 was located at the confluence with Otter Creek, ~850 m upstream of Carrick Dam, with one antenna directed across the Saugeen River and another antenna directed to monitor movement of fish into and out of the tributary. Receiver station R4 was installed at the Denil fishway at Maple Hill Dam ~ 9.99 km upstream of Otter Creek, with one antenna directed downstream and one antenna directed upstream of the dam. Lastly receiver station R5 was installed ~660 m upstream of the Maple Hill Dam, with one antenna that was directed downstream across the river. Data from R00 and R0 were not used within metric calculations, with data from R5 only serving to reinforce passage at Maple Hill Dam, as determined by the upstream antenna at R4. R1 remained online for 92.1% of the study period, R2 93.9%, R3 79.7%, R4 92.5% and R5 for 62.8% of the study period, with the main reasons for offline periods being power outages, particularly during the winter, and receiver malfunctions. Based on the known locations of fish when upstream receivers were offline, outages were not considered to have influenced the ability to detect dam arrival or passage; one exception of one period of R2 outage in spring 2019 is discussed below.
Our tracking team conducted a total of 21 aerial tracking surveys, four in spring 2018, three in fall 2018, three each in spring and fall 2019, one each in spring and fall 2020, four in spring 2021 and one each in fall 2021 and spring 2022. The area surveyed during each flight included from the mouth of the Saugeen River with Lake Huron to upstream of R5 as well as Otter Creek upstream to the passage barrier. Depending on likely fish distribution based on prior receiver downloads, flights often also surveyed further upstream within the main-stem of the Saugeen River to the Hanover Dam as well as the South Saugeen and Beatty Saugeen Rivers and Meux Creek tributaries upstream to passage barriers. Land based mobile tracking was conducted weekly to monthly throughout each season at Carrick Dam, river paths through public parks located 2 km downstream of the Truax Dam, in the town of Paisley, as well as along several trails downstream of Denny’s Dam between R0 and R00 and at the 12 river access points located between Walkerton and Southampton.
2.3 Migration metrics
We used the tracking data collected over the 1000 monitored days throughout the study period to calculate a series of migration metrics that detailed the movement of each tracked fish throughout the Saugeen River (Table 1). Metrics were calculated in minutes for the movement of spring-run fish within the season they were tagged (S), fall-run fish within the season they were tagged (F) and fall-run fish within the subsequent spring season (FS). Metrics 1 and 2 measured the delay of fish downstream of the Truax Dam/footprint and metrics 3 and 4 measured the delay of fish downstream of the Maple Hill Dam. Delay is defined as active downstream delay, which was the period when fish were present downstream of the dam/footprint attempting to continue their upstream movement. All fish that arrived and were detected on R1 were assumed to have attempted to pass the Truax Dam/footprint, and all fish that arrived and were detected on R4 were assumed to have attempted to pass Maple Hill Dam. Metrics 1 and 3, the overall period of delay at these dams, respectively, were calculated as the time between first and last detection at R1 or R4. Fall fish that were present downstream of the dam in both fall and spring were assigned F, with metric values coded FS only for those fish that first arrived and spent the entire period of delay downstream of the dam in the spring. Values for metrics 2 and 4, the final period of delay at the Truax Dam/footprint and Maple Hill Dam, respectively, were the same as metrics 1 and 3 if fish were continuously detected on R1 or R4 throughout the entire period they were delayed downstream. Fish that moved away from the dam (receivers) for a minimum of 2 days before returning were considered to have temporarily moved away from the dam, and were no longer considered actively delayed while attempting to pass upstream. In these cases, final delay was calculated as the last continuous period of time on R1 or R4 after a fish returned (akin to Keefer et al., 2004b). Final delays that occurred in the spring were assigned FS even if the fish was also present in the fall.
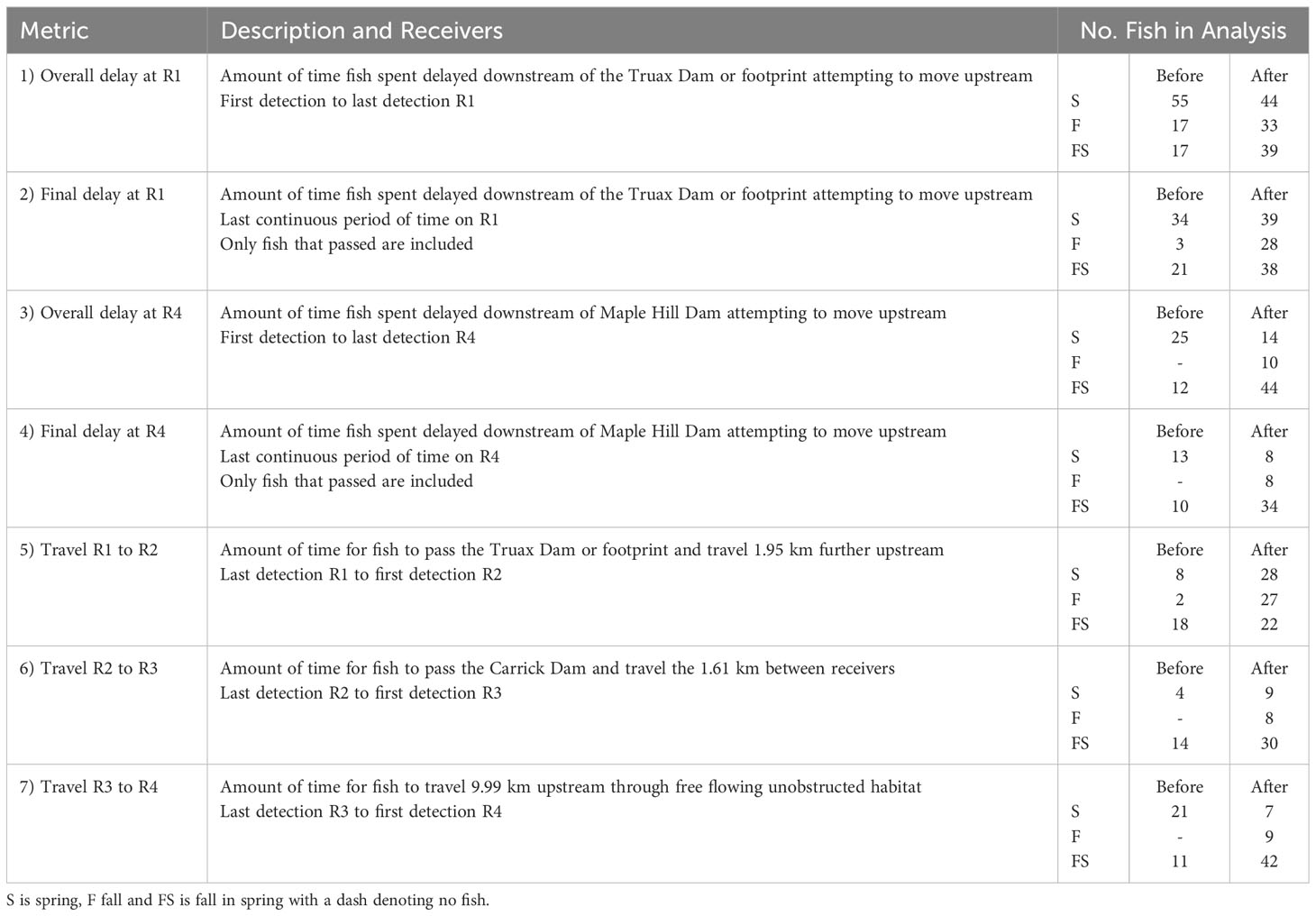
Table 1 Description of calculated migration metrics and the number of fish and their coding used in statistical analyses.
Metrics 5, 6 and 7 quantified the time required for fish to move upstream through the Saugeen River. Metric 5 measured the amount of time fish took to travel from R1 downstream of the Truax Dam/footprint to R2 upstream of the dam/footprint. Metric 6 calculated the amount of time fish took to travel from R2 downstream to R3 upstream of Carrick Dam. Metric 7 calculated the amount of time fish took to travel from R3 to R4, an open water stretch between dams after fish passed both Truax and Carrick Dam locations. Metrics were calculated as the time from the last detection on the downstream receiver to the first detection on the upstream receiver. In several instances, fish moved between pairs of receivers more than once. Only the time of the initial movement was considered. For travel metrics, fall fish that were present at the downstream receiver in the fall but the upstream receiver in the spring were assigned FS, with values coded F only those fish that were on both receivers in the fall.
2.4 Passage success and environmental conditions
We calculated passage success (%) at the Truax Dam/footprint, Carrick Dam and Maple Hill Dam as the number of individuals detected passing upstream of each of these locations divided by the number of individuals detected arriving downstream, multiplied by 100. Again, all fish that arrived at R1 and R4 were assumed to have arrived downstream of the respective dams, and to have been actively attempting to pass upstream. All fish that were detected on R2 were assumed to have likewise arrived at, and have been attempting to pass, Carrick Dam. Passage at each dam was calculated considering all fish that were present within a season, where the same fall fish could be included in calculations of passage success of both F and FS if it did not pass in the fall. For fish that passed the dams multiple times, only the first successful passage, and the season in which it occurred, was included. A metric of daily passage likelihood (%) was calculated that combined overall active downstream delay and passage success. For each day that fish were detected on either R1 downstream of the Truax Dam/footprint or R4 downstream of the Maple Hill Dam, passage likelihood was calculated by dividing the number of fish that passed the dam by the number of fish that were present and attempted to pass upstream.
In order to account for the influence of environmental conditions on fish delay and passage, we obtained river discharge data (m3/s) within the Saugeen River from the Environment Canada Hydrometric monitoring station 02FC002 located 4.14 km upstream from Carrick Dam and 6.66 km downstream from Maple Hill Dam. Water temperature (°C) was monitored using a HOBO Tidbit temperature logger initially installed 0.20 km downstream from the Truax Dam on June 7, 2018. No water temperature data were available for the spring 2018 monitoring period. River discharge was recorded every 5 minutes and water temperature was recorded every hour. Both were converted into daily average values.
2.5 Statistical analyses
To determine if the removal of the Truax Dam had an effect on the downstream delay or upstream movement rates of S, F or FS fish groups, we conducted analyses testing for significant differences in metric values before (spring 2018 – spring 2019) versus after the dam removal (fall 2019 – spring 2022) separately for each season. Metric data for each fish group were first explored to see if they met the underlying assumptions of parametric tests. As normality and homogeneity of variance were often violated even after the removal of identified outliers and data transformation, exact Wilcoxon-Mann-Whitney tests were performed, where significant outcomes should be interpreted as before or after data having dominance over the other. Effect size was determined by calculating Vargha and Delany’s A (Vargha and Delaney, 2000). The direction of the dominance relationship can be interpreted from the provided figures as well as group medians and, when significant, quartiles. While analyses regarding overall delay metrics 1 and 2 included all individuals that arrived at the Truax Dam/footprint and Maple Hill Dam, respectively, analyses regarding final delay metrics 3 and 4 only included individuals that ultimately passed the respective dams. Significant differences in passage success at each dam before versus after the removal of the Truax Dam were tested for using 2 x 2 contingency tables and, due to small sample sizes, log-likelihood ratio G-tests.
Multiple regression models were used to determine if differences in dam passage success and delay for S, F or FS fish before versus after dam removal were a function of the dam removal itself, or instead related to differences in the environmental conditions encountered between time periods. The metric daily passage likelihood was used as the dependent variable and dam presence (classification: before or after removal), daily average river discharge (m3/s) and daily average water temperature (°C; continuous) were used as predictors. Complete models as well as those testing the effects of all subsets of predictors were analyzed and Akaike information criterion (AIC) scores were used to select the best-fit model. As no temperature data were available for the spring 2018 monitoring period, S fish from this period were not included in the multiple regression models.
All analyses were performed with R version 4.0.3 (R Core Team, 2020) with the exception of AIC model selection which was performed using the AICcmodavg package (Mazerolle, 2023) in R version 4.3.2 (R Core Team, 2023). Assumptions testing was performed using the rstatix package (Kassambara, 2023), exact Wilcoxon-Mann-Whitney tests were performed using the coin package (Hothorn et al., 2008), Vargha and Delany’s A was calculated using the effsize package (Torchiano, 2020), G-tests were performed using the DescTools package (Signorell et al., 2020) and combined violin boxplots were produced using the ggplot2 package (Wickham, 2016). All results were considered significant at p-value ≤ 0.05.
3 Results
We radio-tagged 368 fish from spring 2018 to spring 2022 (Table 2). In the seasons in which 50 fish were tagged, an average of 89.7% of newly tagged fish were tracked (detected) within the season in which they were tagged (range fall 2020: 84% - spring 2022: 98%). Only 72% of the 18 newly tagged fish were tracked in fall 2021. Of the 368 fish, only 21 (5.7%) were never tracked across any monitoring period. As 14 of these fish were tagged prior to the installation of downstream receivers R0 and R00, these fish likely emigrated from the tagging site back to Lake Huron without detection. Unreported harvests or tag failure may also account for a subset of these individuals.
3.1 Active dam delay
Fish were significantly less delayed downstream from the footprint while actively attempting to continue their upstream migration after the Truax Dam was removed (Figures 2A, B). The median time of the overall period of delay (metric 1) for S fish before the dam was removed was 10.9 hours (25% and 75% quartiles: 2.0 – 31.8 hours), while after the dam removal the median delay was 0.3 hours (0.2 – 1.9 hours; Z=-4.832, A=0.217, p<0.000). For FS fish the median time of overall delay was 159.9 hours (31.6 hours – 13.4 days) before, compared to 0.5 hours (0.2 – 0.7 hours; Z=-5.650, A=0.022, p<0.000) after removal, and for F fish the median time was 141.6 days (17.2 – 149.6 days) compared to only 0.8 hours (0.3 – 1.1 hours; Z=-5.377, A=0.032, p<0.000), respectively. The same pattern occurred with the period of final delay before fish ultimately passed the dam or footprint (metric 2), with S fish delayed a median of 8.2 hours (1.3 – 17.2 hours) before passing while the dam was present, however only 0.3 hours (0.2 – 0.4 hours) after it was removed (Z=-5.200, A=0.146, p<0.000). For FS fish this delay decreased from a median of 153.0 hours (3.5 hours – 13.4 days) to 0.5 hours (0.2 – 0.7 hours; Z=-5.613, A=0.056, p<0.000), and for F this delay decreased from a median of 44.4 hours (1.0 – 71.2 hours) to 0.7 hours (0.3 – 1.0 hours; Z=-2.273, A=0.095, p=0.017).
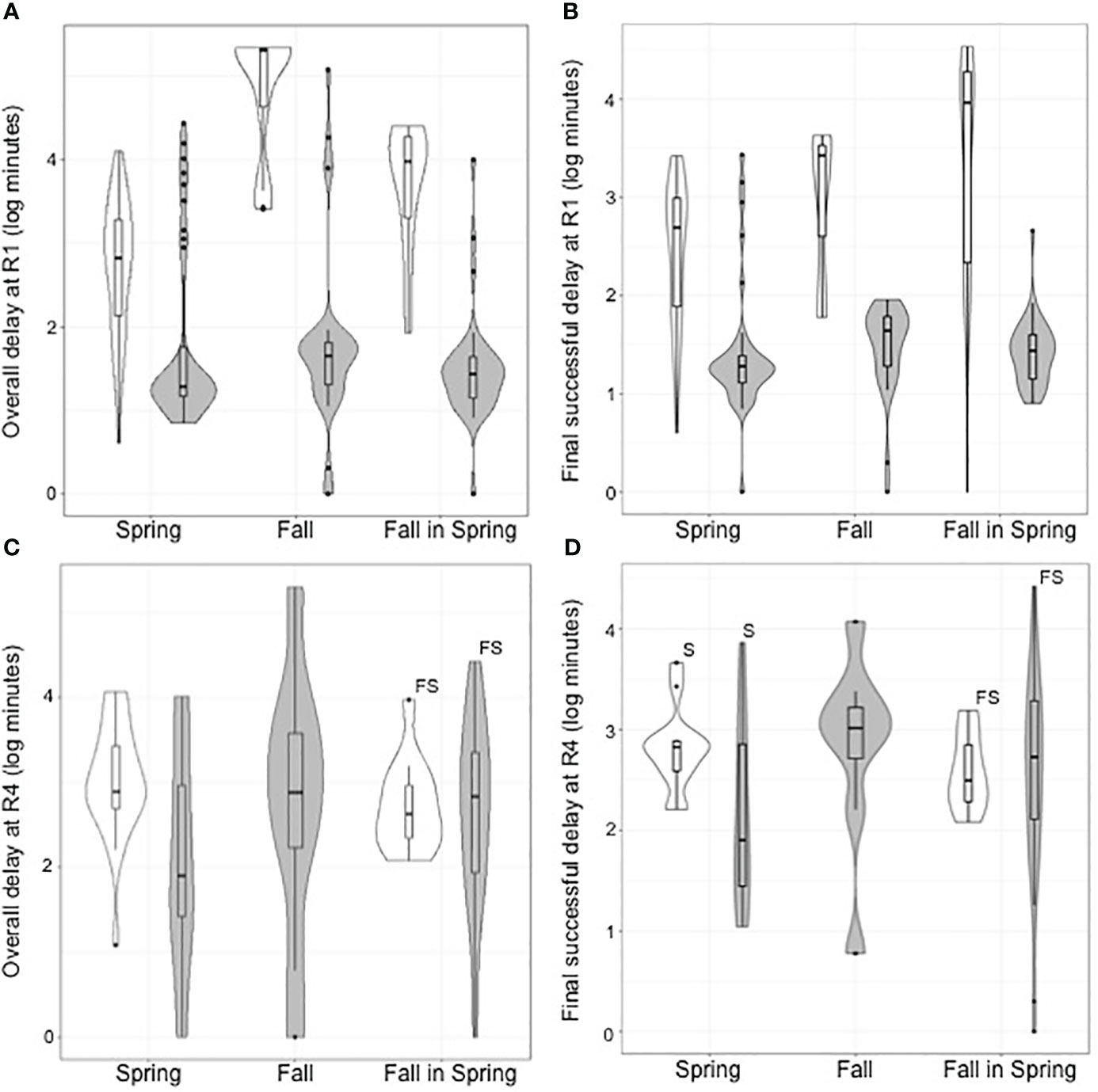
Figure 2 Combined violin and boxplots with exact Wilcoxon-Mann-Whitney test results for delays in Steelhead passage downstream of Truax Dam and Maple Hill Dam before (white) and after (gray) dam removal. (A) is metric 1 and (B) metric 3 at the Truax Dam and (C) is metric 2 and (D) metric 4 at Maple Hill Dam. Matching letters denote non-statistically significant differences at p=0.05.
Upstream of both Truax Dam and Carrick Dam, spring fish were also less delayed downstream of Maple Hill Dam after the Truax Dam was removed (Figures 2C, D). The overall period of delay for S fish downstream of Maple Hill Dam (metric 3) was a median of 12.8 hours (7.9 – 47.8 hours) when the Truax Dam was present, however it was only 1.5 hours (0.4 – 43.5 hours) after the dam was removed (Z=-2.357, A=0.270, p=0.017), with the final delay of fish before ultimately passing (metric 4) reduced from a median time of 11.2 hours before, compared to 1.5 hours after dam removal (Z=-1.594, A=0.288, p=0.117). Although not significantly different before versus after, delay times increased for FS fish post-removal with a median time of 7.1 hours for overall delay downstream of Maple Hill Dam before, and 11.3 hours after (Z=0.020, A=0.502, p=0.988), and a median time of 5.3 hours for final delay before successfully passing before, and 9.1 hours after (Z=0.280, A=0.529, p=0.788), dam removal. No F fish arrived at the Maple Hill Dam prior to the Truax Dam removal.
3.2 Dam passage success
As expected, the passage success of Steelhead at the Truax footprint increased significantly after the dam was removed (Figures 3A–C). Passage increased from 61.8% to 86.7% (G=8.167, p=0.004) for S fish, 72.4% to 90.5% (G=3.934, p=0.047) for FS fish, and increased from only 17.6% to 84.8% (G=22.491, p<0.000) for F fish. While passage was also significantly different at Carrick Dam for S (91.2% compared to 66.7%; G=6.835, p=0.009), interpreted passage success pre-dam removal during spring 2019 was impacted at both Truax and Carrick Dams by a key 6 day period wherein 10 S individuals arrived at R1 while R2 was offline. Passage success of these fish at the Truax Dam was therefore only able to be based on detections at R3 upstream of Carrick Dam and the arrival of these fish at Carrick Dam was pre-determined by their successful passage and detection on R3. Passage success at Truax Dam was likely, therefore, under-represented and at Carrick Dam was likely over-represented. Considering instead only spring 2018 passage information for each of these dams, passage at the Truax footprint still increased from 63.0% to 86.7% (G=5.343, p=0.021) and passage at Carrick Dam was no longer significantly different (82.4% compared to 66.7%; G=1.514, p=0.219) before versus after removal. While not statistically significant, passage at Carrick Dam increased post-Truax Dam removal for both F (G=1.693, p=0.193) and FS fish (G=3.570, p=0.059; Figures 3B, C). Passage at Maple Hill Dam increased from the arrival of zero F fish pre-Truax Dam removal to the passage of 80% of F fish post-removal (Figure 3B); a G-test could not be performed due to zeros in the pre-removal data. There was no difference in the passage success of either S (G=0.243, p=0.622) or FS (G=0.507, p=0.476) at this dam. Removal of the Truax Dam also appeared to have increased the number of times fish could successfully pass the dams that they subsequently encountered. Pre-removal, three fish passed the dam twice, while post-dam removal, 12 fish passed the footprint twice, four fish passed three times and one passed four times. One fish also passed Carrick Dam twice and four fish passed Maple Hill Dam twice post-Truax Dam removal.
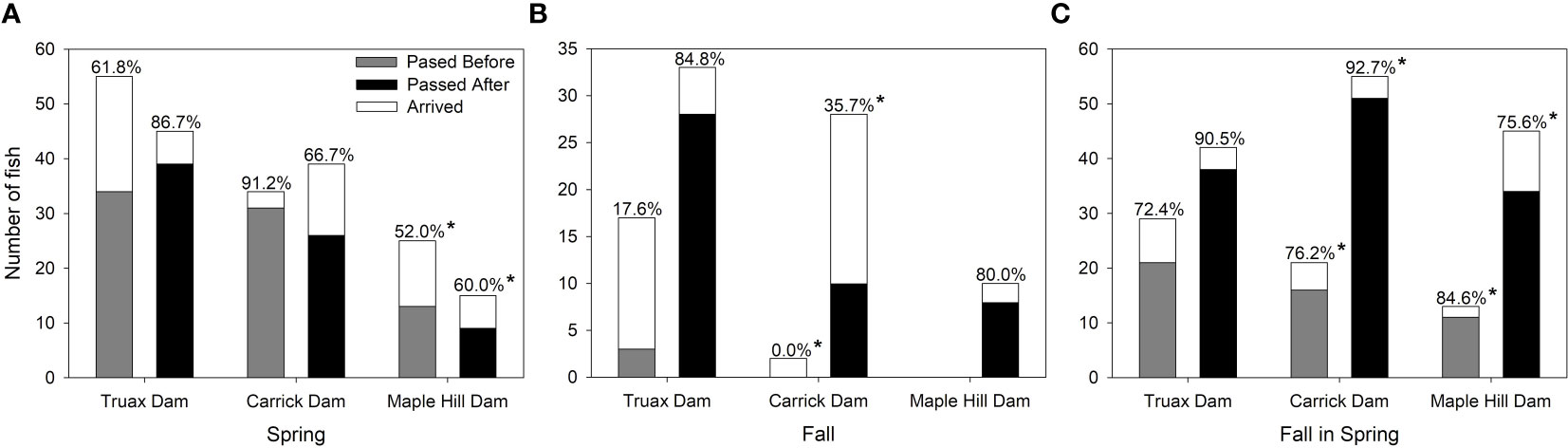
Figure 3 Passage success at the Truax Dam/footprint, Carrick Dam and Maple Hill Dam for (A) Spring fish, (B) Fall fish and (C) Fall fish in the spring. Numbers above each bar show the passage success (%) of fish. Matching * denote non-statistically significant differences at p=0.05 based on G-tests.
The decreased delay and increased passage success of Steelhead at the Truax footprint appeared to be a function of the dam removal itself, with the 4 highest ranked multiple regression models including dam as a predictor and the 3 lowest ranked models based only on water temperature and/or river discharge (Table 3). With respect to S, fish were present downstream of the dam for 15 days and passed on 8 of these days while the dam was present (spring 2019 only), and were present for an average of 17 days, passing on an average of 11 days after the dam was removed. Out of the 7 tested models, the best-fit based on AIC scores included both dam presence and water temperature as predictors (F(2,46)=7.425, p=0.002, R2adj=0.211), with daily passage likelihood reduced at warmer temperatures (Table 4). More effectively blocked by the presence of the dam, F were present downstream for 124 days and passed on only 3 days pre-removal, and were present for an average of only 11 days and passed on 9 days post-removal. An outlier that was detected on R1 continuously for 85 days post-removal in fall 2019 was removed from the analysis. The model including dam presence and river discharge as predictors was selected as the best-fit (F(2,148)=252.60, p<0.000, R2adj=0.770), with daily passage likelihood higher with increased flow (Table 4). Lastly FS fish were present downstream of the dam for 47 days and passed on 14 days pre-removal, and were present on an average of 21 days and passed on an average of 17 days post-removal. The model including all possible predictors was selected as the best-fit (F(3,87)=25.970, p<0.000, R2adj=0.454) based on AIC model selection. Note that as only 18 fish were tagged in fall 2021 the number of days when these fish were present and passed were not included in calculations of post-removal averages; these fish were included in all other analyses and calculations.
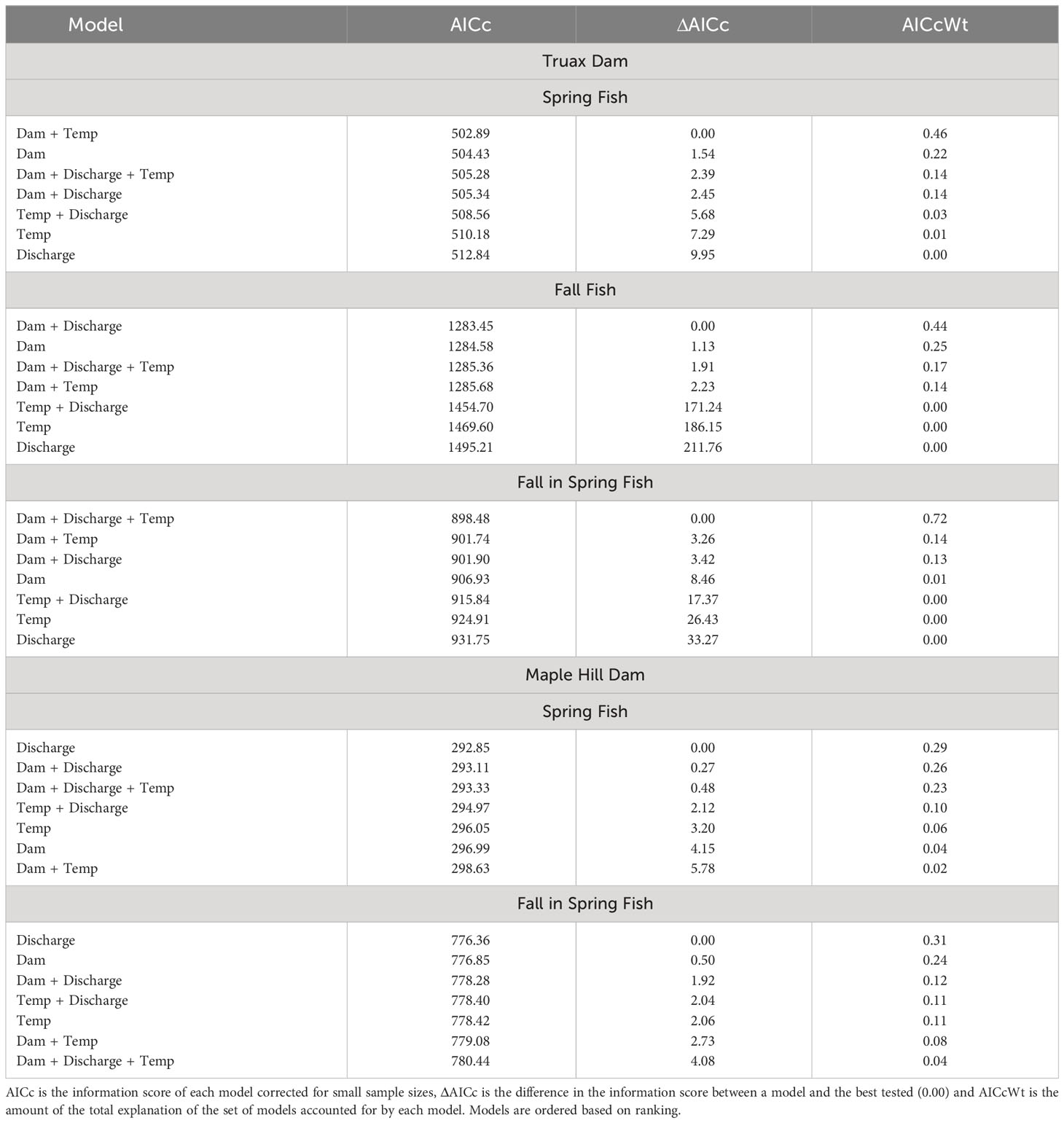
Table 3 Results of AIC model selection for tested multiple regression analyses of the daily passage likelihood (%) of fish at the Truax Dam/footprint and Maple Hill Dam as a function of combinations of dam presence (classification: before or after removal) and daily average river discharge (Discharge, m3/s) and water temperature (Temp, °C; continuous) predictors.
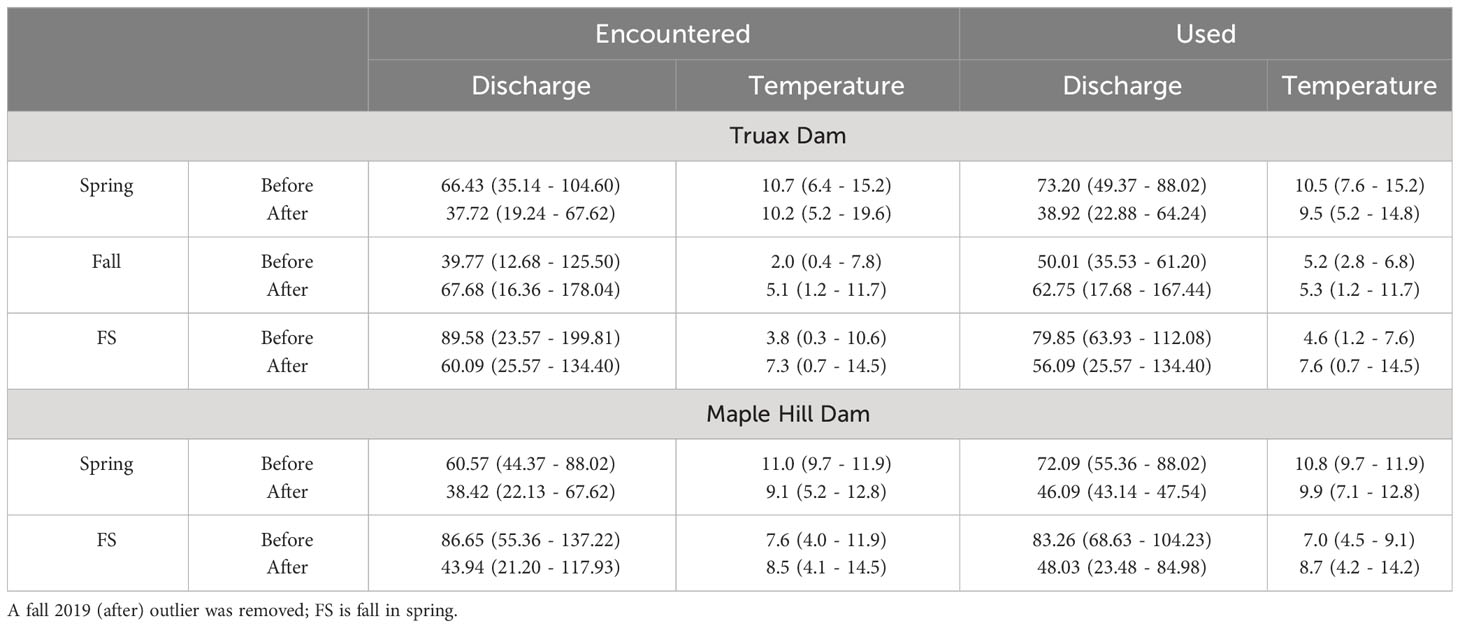
Table 4 Daily average river discharge (m3/s) and water temperature (°C) when fish were present and delayed downstream of the Truax Dam/footprint or Maple Hill Dam (encountered) compared to the river conditions on days when passage at the footprint or dam occurred (used) for each fish group before and after dam removal with means and ranges in brackets.
In contrast to the Truax footprint, delay and passage success of fish at Maple Hill Dam appeared to be primarily a function of river discharge (Tables 3, 4). S fish were present downstream of the dam for 9 days and passed on 4 of these days while the Truax Dam was present (spring 2019 only), and were present for an average of 11 days and passed on an average of 4 days after the Truax Dam was removed. Out of the 7 tested models, the best-fit based on AIC model selection included only discharge as a predictor (F(1,28)=4.167, p=0.051, R2adj=0.098). Note, however, that only one of the seven models tested was significant and this model included discharge, temperature and dam presence as predictors (F(3,26)=3.134, p=0.043, R2adj=0.181). With respect to FS fish, individuals were present downstream of Maple Hill Dam for 12 days and passed on 6 days pre-Truax Dam removal, and were present for an average of 31 days and passed on an average of 11 days post-removal (not including fall 2021). Again the best-fit model only included discharge as a predictor (F(1,74)=2.065, p=0.155, R2adj=0.014); none of the seven tested models were significant.
3.3 Upstream movement rates
Not only were fish less delayed downstream while passage success increased after compared to before the Truax Dam was removed, but the amount of time fish took to travel upstream also decreased (Figure 4). The travel times of all S, F and FS fish from R1 to R2 (metric 5), which includes passage time across the Truax Dam or footprint, decreased significantly post-removal (Figure 4A). Travel time decreased from a median of 39.3 hours (20.0 – 135.4 hours) before to a median of 3.2 hours after removal for S (1.9 – 22.7 hours; Z=-3.045, A=0.143, p=0.001), and a median of 9.3 hours (7.5 – 26.9 hours) to 2.3 hours for FS (1.8 – 3.4 hours; Z=-4.269, A=0.104, p<0.000), respectively. Only two F fish arrived at R2 pre-removal, and required 27.9 and 35.5 hours to travel the distance between receivers. After removal, the 28 fish that arrived traveled between receivers in a median time of 4.4 hours (3.2 – 17.4 hours; Z=-1.980, A=0.074, p=0.044).
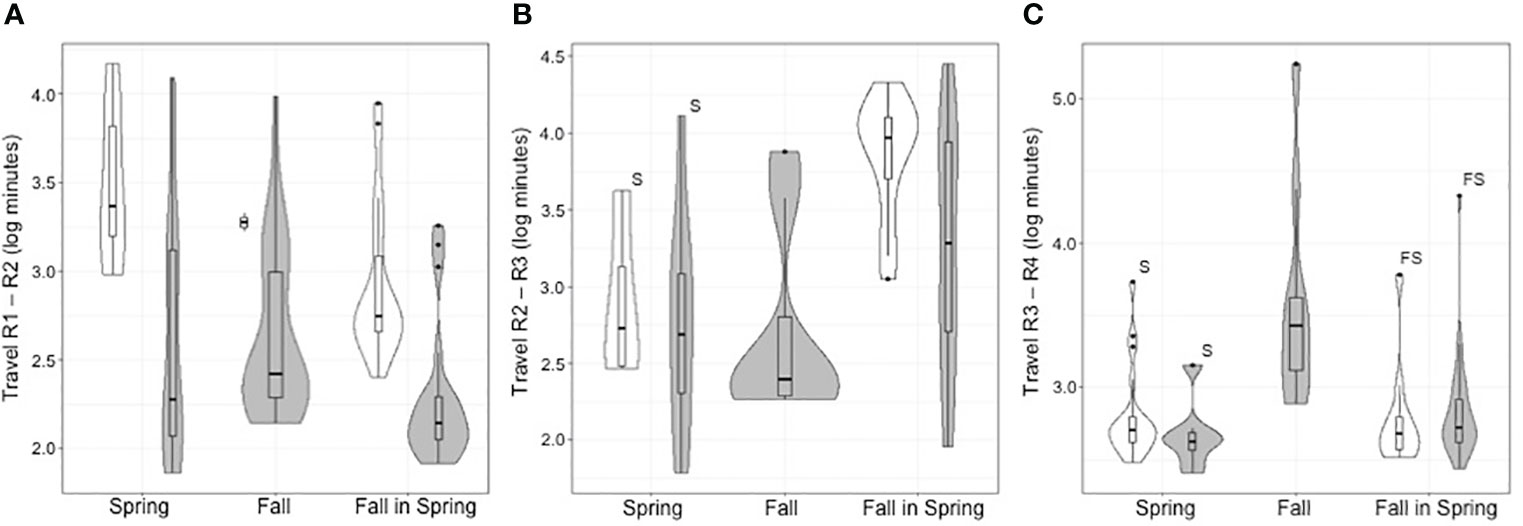
Figure 4 Combined violin and boxplots with exact Wilcoxon-Mann-Whitney test results for upstream movement of Steelhead between fixed receiver stations before (white) and after (gray) dam removal. (A) is metric 5, movement from downstream to upstream of the Truax Dam/footprint, (B) metric 6, movement from downstream to upstream of Carrick Dam and (C) metric 7, movement within the 9.99km open water stretch between Carrick and Maple Hill Dams. Matching letters denote non-statistically significant differences at p=0.05.
As no F fish passed Carrick Dam pre-removal, no before-after comparisons could be made for travel times between further upstream receivers for this group of fish (Figures 4B, C). Only the movement of FS fish from R2 to R3 (metric 6), which includes passage at Carrick Dam, was significantly reduced post-Truax Dam removal, with fish traveling this distance in a median time of 157.2 hours (67.0 hours – 9.1 days) compared to 32.1 hours, respectively (7.2 – 154.0 hours; Z=-2.520, A=0.262, p=0.011). Travel time from R3 to R4 (metric 7) was not significantly different (8.0 compared to 8.8 hours; Z=0.965, A=0.595, p=0.345). Neither travel time from R2 to R3 (Z=-0.154, A=0.472, p=0.940), or R3 to R4 (Z=-1.035, A=0.367, p=0.316) were significantly different as a function of dam removal for S fish. Median times were 10.2 and 8.2 hours, and 8.4 and 7.0 hours before versus after removal, respectively.
4 Discussion
This study provides a unique description of the migration speed and dam passage performance of a Great Lakes adfluvial Steelhead population and, more importantly, documents the long-term (5 years) spatial and temporal changes in fish movement patterns before and after removal of a dam that had long been identified as a partial barrier to upstream migration. As expected, delay was reduced, and passage success increased at the site of the dam removal itself. Calculated inter-receiver movement metrics were also reduced and dam passage success also increased at locations and dams further upstream, underscoring the cumulative impact of successive dam passage on fish migration (Dean et al., 2023).
4.1 Changes at the site of dam removal
The presence of dams can lead to extended periods of downstream delay, causing fish to remain relatively stationary while unable to continue their upstream migration. Such periods of delay can have significant biological and physiological consequences (McLaughlin et al., 2013), including increased risk of predation (Schilt, 2007), increased opportunities for over-exploitation by anglers (Bunt and Jacobson, 2022a), exposure to elevated water temperatures causing thermal stress (Rubenstein, 2021), increased energy store utilization (Izzo et al., 2016; Rubenstein et al., 2022), as well as possible overall migration failure (Caudill et al., 2007). Removal of the Truax Dam significantly reduced the amount of downstream delay experienced by fish, and increased passage success at the dam footprint. Fall fish were the most impacted, with active downstream delay decreased from 100+ days to under an hour, and passage success at the footprint increased 4.8-fold. Such changes were on the order of 100+ hours to under an hour of delay for fall fish in the spring, with passage increased 1.3-fold at the footprint, while for spring fish, downstream delay decreased from 10+ hours to under an hour, and passage increased 1.4-fold. Differences in the orders of magnitude of delay and success were likely related to differences in underlying migration motivation between these groups. Fall fish were tagged in October and overwinter in the watershed and do not spawn until the spring, (i.e., precocious or premature migrants, Quinn et al., 2015), while spring fish were tagged in April and spawn within the same season, often within the same month (Bunt and Jacobson, 2022b).
As expected, reduced delay and increased passage were related to the physical removal of the dam structure and could not be solely accounted for by differences in river discharge or water temperature within the Saugeen River during the before (spring 2018 - spring 2019) and after (fall 2019 - spring 2022) dam-removal study periods. As in Izzo et al. (2016), the movement of fish across the river stretch that included the Truax Dam (metric 5) more closely resembled the movement of fish within an open water stretch within the river (metric 7) post-removal. Travel speeds for S fish increased from a median of 0.05 to 0.61 km/hour, compared to open water travel speeds from 1.19 to 1.43 km/hour, and for FS fish travel speed increased from a median of 0.21 to 0.85 km/hour, compared to open water speeds from 1.14 to 1.25 km/hour. No F fish were able to move upstream as far as the open water portion pre-removal, however travel speeds across the Truax footprint increased from a median of 0.06 to 0.44 km/hour post-removal. Travel speeds still remained slower across the footprint than within the open water river segment, likely due to gradient and water velocity differences between these stretches. The open water portion of the river generally consisted of slow-moving deep pool habitat, while the location of the footprint, as well as the area within the former 1.5 km dam headpond, had re-established natural riffle-pool sequences with complex shallow high flow habitat (Bunt and Jacobson, 2022b). Although the scale of their migration differs, for comparison, in the naturally flowing Nass and Skeena Rivers in British Columbia, west coast anadromous Steelhead were found to move upstream at a rate of 0.16 and 0.50 to 0.67 km/hour, respectively (English et al., 2006), and passed through reservoirs associated with lower Columbia and Snake River dams at rates ranging from 0.92 to 1.71 km/hour (Keefer et al., 2004a).
Taken together, the significantly reduced downstream delay and increased travel speeds after the Truax Dam was removed suggest that not only were a greater number of fish able to pass the footprint and reach locations further upstream, but that these fish were likely also in better condition. Passing fish no longer experienced the potential physical trauma of multiple failed attempts to jump through the sluice of a concrete structure, or underwent the additional stress and energy expenditure from being held at one location for 100+ days. This likely translated into the increased travel speeds and dam passage success that was found at locations further upstream of the dam post-removal. Among iteroparous species such as Steelhead, repercussions of excess energy depletion may even extend beyond a single spawning season, with the incurred debt not easily compensated for without needing to sacrifice production to balance future somatic growth and gonadal development (Fenkes et al., 2016). Rubenstein et al. (2022) suggested that dam removal may increase iteroparity exactly as a function of such increased energy availability and improved fish condition.
4.2 The impact of downstream removal on upstream movement and passage
As with at the site of the dam removal, fall fish showed the greatest degree of change in their passage success and movement at upstream locations, with tagged fish able to pass remaining dams for the first time after the downstream dam was removed. Neither of the only two fish that arrived at Carrick Dam (2.61 km upstream) were able to pass pre-removal, however 35% of the 28 fish that arrived after removal of the Truax Dam were able to pass. Perhaps even more biologically significant, with potential implications for future spawning and production, 80% of the fish that were able to arrive at Maple Hill Dam (13.55 km upstream) in the fall were also able to pass this location post-downstream dam removal. Steelhead within the Saugeen River, as on the west coast (Keefer et al., 2008; Fuchs et al., 2021), tended to remain somewhat stationary from December to February, with the majority resuming active upstream migration during March at the onset of warmer water temperatures. When the Truax Dam was present, the majority of fall fish overwintered in the river downstream from this dam with the first passage of a fall-tagged fish at Maple Hill Dam not occurring until April 13, after fish had been reclassified from F to FS in the spring in March. After the Truax Dam was removed, passage at Maple Hill Dam occurred as early as October 25, with these fish able to overwinter at upstream locations closer to spawning areas used in the spring. These fish were likely present and able to spawn in such areas before spring-run fish arrived. The observation that reduced downstream delay can change the migration timing of when fish arrive at and pass upstream of remaining dams has also been noted in other dam removal studies (Fjeldstad et al., 2011; Izzo et al., 2016).
The case for increased movement rates and passage at upstream dams was perhaps less clear for the other two groups of fish, S and FS. Indeed, the impact of the removal of the Truax Dam may have been somewhat obscured by differences in spring river conditions between the before and after dam removal study periods. Unlike at the footprint, river discharge more greatly accounted for daily passage likelihood at Maple Hill Dam than the presence of the Truax Dam, with the average encountered discharge while fish were delayed downstream during the before study period generally double those encountered after the dam was removed. Such differences in flow likely influenced the ability of fish to use the Denil fishway at this dam, as reduced water flows negatively impact fishway attraction, as well as flow dynamics across Denil fishway baffles (Conley, 2021). River discharge also likely influenced the passage of S fish at Carrick Dam, with the average encountered discharge again doubled during the before removal study period (average before 80.90 m3/s, after 39.82 m3/s). As fish pass Carrick Dam through either a large notch or by jumping and swimming over the remaining deteriorating dam face (Supplementary Video S3), passage at this location was likely facilitated by the higher discharges in the period before removal. Passage success of FS at Carrick Dam still increased post-removal. Average encountered river discharges differed less between study periods (average before 84.66 m3/s, after 67.96 m3/s), with FS fish able to pass this dam earlier post-Truax removal, often in March closer to spring freshets and before spring fish were tagged.
Note that the dates that fish were present directly downstream of Carrick Dam, and thus the encountered river conditions presented above, are general estimates only. Unlike at Truax and Maple Hill Dams, no receiver was able to be installed directly at this location due to concerns with equipment security, based on the high degree of public use and observed vandalism in the area downstream of the dam. As no spawning habitat was present within the river between R2 800 m downstream and the dam, all fish that were detected on R2 were assumed to have continued their upstream movement and to have ultimately arrived at Carrick Dam; the exact date of this arrival is unknown. Note also that all water temperature and river discharge data used within this study were obtained from single sources, a temperature logger installed 0.20 km downstream from the Truax Dam/footprint and an Environment Canada Hydrometric monitoring station located within the open water stretch 4.14 km upstream from Carrick Dam and 6.66 km downstream from Maple Hill Dam. As such, the reported river conditions are not specific to the conditions at each dam and are not the actual flows encountered by fish within the notches, sluices or fishway. River condition data were used for comparative purposes only, to investigate relative differences between study periods which may have influenced dam passage, and cannot be interpreted as the specific flow and temperature windows that fish used to pass each of these locations.
The differences in the environmental conditions between study periods likely favored increased dam passage pre-dam removal, and as in other studies (e.g., Martin et al., 2013), the change in post-removal conditions impacted the apparent success of the removal. Indeed, dams present even more of a barrier during reduced river water flows, a situation that is likely to become even more of a common occurrence moving forward due to climate change (Kukula and Bylak, 2022; Yoder and Raymond, 2022; Zarri et al., 2022). As passage still increased for S at Maple Hill Dam, for F at Carrick and Maple Hill Dams and for FS at Carrick Dam, these results strongly suggest that removal of the Truax Dam positively impacted the movement of fish at further upstream locations, with the degree of this benefit potentially larger and more evident if the environmental conditions had remained more comparable between study periods. Importantly, while passage success percentage may not have increased for FS at Maple Hill Dam, the greater number of fish passing the downstream footprint and Carrick Dam translated into an increase in the actual number of fish that were able to pass upstream. An average of 3 additional F fish and 5 additional FS fish passed Maple Hill Dam for every 50 fall-run fish tagged and released ~90 km downstream at Denny’s Dam. As run sizes within the Saugeen River likely number in the thousands (Borgeson et al., 2020), this translates into an ecologically significant increase in the number of fish potentially accessing and spawning within upstream tributaries considering just the fall-run fish alone.
4.3 Far-reaching implications
Removal of the Truax Dam in the Saugeen River significantly increased the passage of migrating Great Lakes Steelhead at remaining upstream dams, and impacted the timing of this passage such that fish may reach and be able to utilize upstream spawning areas earlier in the season, when conditions may remain more favorable (i.e., before the onset of low flows and increased water temperatures). Unlike other studies where passage was completely blocked prior to dam removal (e.g., Burroughs et al., 2010; Kornis et al., 2015; Brenkman et al., 2019), and/or other systems where remaining dams were also altered to help ensure increased upstream passage (e.g., Hogg et al., 2015; Watson et al., 2018; Whippelhauser, 2021), the Truax Dam was only a partial barrier prior to removal and Carrick and Maple Hill Dams remained unchanged. While studies where dams completely block passage are interested in upstream recolonization, and in a way can more easily achieve and demonstrate success, the aim of partial barrier removals is to increase upstream movement from a non-zero baseline (Brewitt, 2016). While improvements may not appear as dramatic, they can still have large ecological impacts. In systems with multiple partial barriers, the fact that these impacts can be achieved with the modification of only one location, and may not also require costly improvements or additional removals at other sites, should further reinforce the potential value of even comparatively smaller-scale dam removal projects.
The presence of multiple dams, with and without functional fishways that block or at the very least delay fish passage to varying degrees within Great Lakes tributaries, is unfortunately common and widespread. Approximately 2,400 tributaries enter the Great Lakes, with 270,000 potential barriers, including 3,954 dams, that fragment habitat and prevent migratory fish from reaching more than 70% of the tributary channel length, with more than 100,000 barriers on channels that are large enough to prevent access to spawning habitat for migratory fishes (Moody et al., 2017; Zielinski and Freiburger, 2021). As of 2023, approximately 70% of Lake Huron tributaries, mostly on the American side, are disconnected from the lake (Environment and Climate Change Canada, 2022). Even with the provision of the most well-intended fishways, recent reviews and meta-analyses on fish passage (Noonan et al., 2012; Bunt et al., 2016) suggest that the best long-term solution to restore river connectivity is dam removal (Kemp, 2016). As described by Dean et al. (2023), in rivers where consecutive dams affect fish migration, it is the lowermost dam that contributes most to the overall cumulative negative impact. While we recognize that lowermost dams/barriers are considered to be necessary, and are contentiously used throughout the Great Lakes basin to block invasive species such as Sea lamprey (Zielinski and Freiburger, 2021), gradual de-fragmentation and opening of river corridors may occur naturally to some degree as existing dams decay in the coming decades. While managers will then be tasked with either repair or demolition, proactive strategic dam removal may greatly help to expedite and ensure the full reopening and reconnecting of hundreds of tributaries throughout the Great Lakes in a biologically significant way. A reduction in the number of migratory barriers, and by extension reduction in delay during upstream migration, means that overall fish production should increase as more critical habitat becomes reconnected, available and more accessible. With more energy retained for reproduction, removals could easily extrapolate to an increase in annual fish production that would be measured in the millions of kilograms per year, potentially offsetting the need for the many small and large scale hatchery stocking programs currently implemented throughout the Great Lakes.
From a fisheries perspective, dam removal restores riverine habitat, returns flow and thermal regimes to undammed conditions, and eliminates barriers that either delay or block upstream fish passage. As dams continue to be removed, it should be noted that well planned long-term before-and-after monitoring studies are needed and produce valuable data that continue to demonstrate the diverse and widespread benefits of dam removal (Kibler et al., 2011; Brewitt, 2016; Foley et al., 2017). While not always available (e.g., Raabe and Hightower, 2014), before dam-removal (baseline) data is strictly necessary to quantify and draw conclusions regarding the benefit of removals of partial barriers in particular. Researchers monitoring partial barrier removals may also consider the benefits of tagging studies such as the one detailed here, where information on the change in the amount of downstream delay experienced by fish attempting to pass upstream can be collected. This provides additional support and context for discussions regarding improved passage and potentially reduced physiological strain. In the Great Lakes, the focus should be on measuring changes in fish production after dam removal, considering impacts on the temporal differentiation of seasonal fish runs, as well as the likely consequences of future climate change scenarios.
Data availability statement
The raw data supporting the conclusions of this article will be made available by the authors, without undue reservation.
Ethics statement
Ethical approval was not required for the study involving animals in accordance with the local legislation and institutional requirements because this research was conducted by a private research company. The methodology was reviewed prior to acquisition of a scientific collection permit that was approved by the Ontario Ministry of Natural Resources and Forestry.
Author contributions
CB: Conceptualization, Data curation, Funding acquisition, Investigation, Methodology, Project administration, Resources, Supervision, Visualization, Writing – original draft, Writing – review & editing. BJ: Data curation, Formal analysis, Investigation, Methodology, Writing – original draft, Writing – review & editing.
Funding
The author(s) declare that financial support was received for the research, authorship, and/or publication of this article. This study was funded by Bruce Power LP.
Acknowledgments
This five year study was funded by Bruce Power LP (BP) and is part one of a multi-component 10 year evaluation of the Truax Dam removal project. The authors wish to graciously thank the following individuals and organizations that directly or indirectly contributed to this project: Jody Scheifley (OMNRF), Francis Chua (BP), Cherie-Lee Fietsch (BP), Dave Snider (BP), April Patterson (BP), Kristen Coleman (BP), Rick Baldwin (BP), Jeremy Webster, Tyler Scruton, Roger Klein, Dana Eddy, Tim Fernandes, Rick Auiler, Dan Nydam, Jess Nelson, Andy Plater (Owen Sound Flight Services), Jess Leger, Al Crawford, Ryan and Marsha Cartright, Doug and Jane Fidler, Dave and Dana Van De Vyvere, Tom and Suzanne Wingfield, Jim McLay, Grant McAlpine, Kelly Mullen, Kodey Buchanan and the Lake Huron Fishing Club, Ontario Steelheaders, OMNRF Lake Huron Management Unit, all of the anglers who recaptured and reported tagged fish and landowners who granted us access for tracking.
Conflict of interest
Authors CB and BJ were employed by the company Biotactic Inc.
Publisher’s note
All claims expressed in this article are solely those of the authors and do not necessarily represent those of their affiliated organizations, or those of the publisher, the editors and the reviewers. Any product that may be evaluated in this article, or claim that may be made by its manufacturer, is not guaranteed or endorsed by the publisher.
Supplementary material
The Supplementary Material for this article can be found online at: https://www.frontiersin.org/articles/10.3389/fevo.2024.1346712/full#supplementary-material
References
Barnthouse L. W., Fietsch C.-L., Snider D. (2019). Quantifying restoration offsets at a nuclear power plant in Canada. Environ. Manage. 64, 593–607. doi: 10.1007/s00267-019-01214-2
Bellmore J. R., Duda J. J., Craig L. S., Greene S. L., Torgersen C. E., Collins M. J., et al. (2017). Status and trends of dam removal research in the United States. WIREs Water 4, e1164. doi: 10.1002/wat2.1164
Bellmore J. R., Pess G. R., Duda J. J., O’Connor J. E., East A. E., Foley M. M., et al. (2019). Conceptualizing ecological responses to dam removal: If you remove it, what’s to come? Biosci 69, 26–39. doi: 10.1093/biosci/biy152
Biette R. M., Dodge D. P., Hassinger R. L., Stauffer T. M. (1981). Life history and timing of migrations and spawning behavior of rainbow trout (Salmo gairdneri) populations of the Great Lakes. Can. J. Fish. Aquat. Sci. 38, 1759–1771. doi: 10.1139/f81-224
Bjornn T. C., Hunt J. P., Tolotti K. R., Keniry P. J., Ringe R. R. (1995). Migration of adult chinook salmon and steelhead past dams and through reservoirs in the lower Snake River and into tributaries-1993. Tech. Rep. 95–1 (Portland, Oregon: U.S. Army Corps of Engineers).
Borgeson D. J., Gonder D., Green R. G. (2020). ““Status of introduced salmonines in Lake Huron in 2018”,” in The state of Lake Huron in 2018. Eds. Riley S. C., Ebener M. P. (Ann Arbor, Michigan: Great Lakes Fisheries Commission), 107–125. Available at: http://www.glfc.org/pubs/SpecialPubs/Sp20_01.pdf.
Brenkman S. J., Peters R. J., Tabor R. A., Geffre J. J., Sutton K. T. (2019). Rapid recolonization and life history responses of bull trout following dam removal in Washington’s Elwha River. N. Am. J. Fish. Manage. 39, 560–573. doi: 10.1002/nafm.10291
Brewitt P. K. (2016). Do the fish return? A qualitative assessment of anadromous Pacific salmonids’ upstream movement after dam removal. Northwest Sci. 90, 433–449. doi: 10.3955/046.090.0405
Bubb D. H., Birnie-Gauvin K., Tummers J. S., Aarestrup K., Jepsen N., Lucas M. C. (2021). Short-term effects of low-head barrier removals on fish communities and habitats. Front. Ecol. Evol. 9. doi: 10.3389/fevo.2021.697106
Budnik R. R. (2017). Assessment of site-fidelity and straying in Lake Erie Steelhead trout (Bowling Green, Ohio: Bowling Green State University).
Bunt C. M., Castro-Santos T., Haro A. (2016). Reinforcement and validation of the analyses and conclusions related to fishway evaluation data from Bunt et al.: ‘Performance of fish passage structures at upstream barriers to migration’. River Res. Applic. 32, 2125–2137. doi: 10.1002/rra.3095.
Bunt C., Jacobson B. (2019). Rainbow Trout migration and use of a nature-like fishway at a Great Lakes tributary. N. Am. J. Fish. Manage. 39, 460–467. doi: 10.1002/nafm.10285
Bunt C., Jacobson B. (2022a). The impact of the COVID-19 pandemic on a recreational rainbow trout (Oncorhynchus mykiss) fishery. Environ. Biol. Fish. 105, 499–507. doi: 10.1007/s10641-022-01250-8
Bunt C., Jacobson B. (2022b). Saugeen River Truax dam 2022: Post dam-removal year 3 fish biomass monitoring and near-field habitat mapping. Unpublished report submitted to Bruce Power LP. (Kitchener, Ontario: Biotactic Inc.).
Burroughs B. A., Hayes D. B., Klomp K. D., Hansen J. F., Mistak J. (2010). The effects of the stronach dam removal on fish in the Pine River, Manistee County, Michigan. T. Am. Fish. Soc 139, 1595–1613. doi: 10.1577/T09-056.1
Catalano M. J., Bozek M. A., Thomas D., Pellett D. (2007). Effects of dam removal on fish assemblage structure and spatial distributions in the baraboo river, wisconsin. N. Am. J. Fish. Manage. 27, 519–530. doi: 10.1577/M06-001.1
Caudill C. C., Daigle W. R., Keefer M. L., Boggs C. T., Jepson M. A., Burke B. J., et al. (2007). Slow dam passage in adult Columbia River salmonids associated with unsuccessful migration: delayed negative effects of passage obstacles or condition-dependent mortality? Can. J. Fish Aquat. Sci. 64, 979–995. doi: 10.1139/f07-065
Conley M. E. (2021). Small scale Denil development for use in headwater streams in southwest Montana (Bozeman, Montana: Montana State University).
Cox R. X., Kingsford R. T., Suthers I., Felder S. (2023). Fish injury from movements across hydraulic structures: A review. Water 15, 1888. doi: 10.3390/w15101888
Dean E. M., Infante D. M., Yu H., Cooper A., Wang L., Ross J. (2023). Cumulative effects of dams on migratory fishes across the conterminous United States: Regional patterns in fish responses to river network fragmentation. River Res. Applic. 39, 1736–1748. doi: 10.1002/rra.4173
Dorobek A., Sullivan S. M. P., Kautza A. (2015). Short-term consequences of lowhead dam removal for fish assemblages in an urban river system. River Syst. 21, 125–139. doi: 10.1127/rs/2015/0098
Duda J. J., Bellmore J. R. (2022). ““Dam removal and river restoration”,” in Encyclopedia of Inland Waters. Eds. Tockner K., Mehner T. (Oxford, United Kingdom: Elsevier Ltd.), 10.
Duda J. J., Hoy M. S., Chase D. M., Pess G. R., Brenkman S. J., McHenry M. M., et al. (2020). Environmental DNA is an effective tool to track recolonizing migratory fish following large-scale dam removal. Environ. DNA 3, 121–141. doi: 10.1002/edn3.134
Durhack T. C., Jeffrey J. D., Enders E. C. (2020). In search of an anesthesia alternative for field-based research. Aquaculture 525, 735285. doi: 10.1016/j.aquaculture.2020.735285
English K. K., Robichaud D., Sliwinski C., Alexander R. F., Koski W. R., Nelson T. C., et al. (2006). Comparison of adult Steelhead migrations in the Mid-Columbia hydrosystem and in large naturally flowing British Columbia rivers. T. Am. Fish. Soc 135, 739–754. doi: 10.1577/T05-043.1
Environment and Climate Change Canada and U.S. Environmental Protection Agency. (2022). State of the Great Lakes 2022 Technical Report. Available online at: https://binational.net/2022/07/29/sogl-edgl-2022/.
Fenkes M., Shiels H. A., Fitzpatrick J. L., Nudds R. L. (2016). The potential impacts of migratory difficulty, including warmer waters and altered flow conditions, on the reproductive success of salmonid fishes. Comp. Biochem. Phys. A 193, 11–21. doi: 10.1016/j.cbpa.2015.11.012
Fjeldstad H., Barlaup B. T., Stickler M., Gabrielsen S.-E., Alfredsen K. (2011). Removal of weirs and the influence on physical habitat for salmonids in a Norwegian river. River Res. Applic. 28, 753–763. doi: 10.1002/rra.1529
Foley M. M., Bellmore J. R., O’Connor J. E., Duda J. J., East A. E., Grant G. E., et al. (2017). Dam removal: Listening in. Water Resour. Res. 53, 5229–5246. doi: 10.1002/2017WR020457
Fuchs N. T., Caudill C. C., Murdoch A. R., Truscott B. L. (2021). Overwintering distribution and post spawn survival of Steelhead in the upper columbia river basin. N. Am. J. Fish. Manage. 41, 757–774. doi: 10.1002/nafm.10585
Good T. P., Waples R. S., Adams P. (2005). Updated status of federally listed ESUs of West Coast salmon and Steelhead. NOAA Technical Memo NMFS-NWFSC-66 (Washington, D. C.: U.S. Department of Commerce, National Oceanic and Atmospheric Administration).
Hansen M. J., Schultz P. T., Lasee B. A. (1990). Changes in Wisconsin’s Lake Michigan salmonid sport fishery 1969-1985. N. Am. J. Fish. Manage. 10, 442–457. doi: 10.1577/1548-8675(1990)010<0442:CIWSLM>2.3.CO;2
Harper D. G., Blake R. W. (1990). Fast-start performance of rainbow trout Salmo gairdneri and northern pike Esox lucius. J. Exp. Biol. 150, 321–342. doi: 10.1242/jeb.150.1.321
Hogg R. S., Coghlan S. M. Jr., Zydlewski J., Gardner C. (2015). Fish community response to a small-stream dam removal in a Maine coastal river tributary. T. Am. Fish. Soc 144, 467–479. doi: 10.1080/00028487.2015.1007164
Hothorn T., Hornik K., Van De Wiel M. A., Zeileis A. (2008). Implementing a class of permutation tests: The coin package. J. Stat. Software 28, 1–23. doi: 10.18637/jss.v028.i08
Huang C. S., Legett H. D., Plough L. V., Aguilar R., Fitzgerald C., Gregory B., et al. (2023). Early detection and recovery of river herring spawning habitat use in response to a mainstem dam removal. PloS One 18, e0284561. doi: 10.1371/journal.pone.0284561
Izzo L. K., Maynard G. A., Zydlewski J. (2016). Upstream movements of Atlantic salmon in the lower Penobscot river, Maine following two dam removals and fish passage modifications. Mar. Coast. Fish. 8, 448–461. doi: 10.1080/19425120.2016.1185063
Jones A. C., Meiners S. J., Effort-Fanta E., Thomas T., Smith S. C. F., Colombo R. E. (2023). Low-head dam removal increases functional diversity of stream fish assemblages. River Res. Applic. 39, 3–20. doi: 10.1002/rra.4063
Kassambara A. (2023). Rstatix: Pipe-friendly framework for basic statistical tests (R package version 0.7.2). Published on the Comprehensive R Archive Network (CRAN). Available at: https://CRAN.R-project.org/package=rstatix.
Keefer M. L., Boggs C. T., Peery C. A., Caudill C. C. (2008). Overwintering distribution, behavior, and survival of adult summer steelhead: Variability among Columbia River populations. N. Am. J. Fish. Manage. 28, 81–96. doi: 10.1577/M07-011.1
Keefer M. L., Jepson M. A., Clabough T. S., Caudill C. C. (2021). Technical fishway passage structures provide high passage efficiency and effective passage for adult Pacific salmonids at eight large dams. PloS One 16, e0256805. doi: 10.1371/journal.pone.0256805
Keefer M. L., Peery C., Bjornn T. C., Jepson M. A., Stuehrenberg L. C. (2004a). Hydrosystem, dam, and reservoir passage rates of adult Chinook Salmon and Steelhead in the Columbia and Snake Rivers. T. Am. Fish. Soc 133, 1413–1439. doi: 10.1577/T03-223.1
Keefer M. L., Peery C. A., Jepson A., Stuehrenberg L. C. (2004b). Upstream migration rates of radio-tagged adult chinook salmon in riverine habitats of the Columbia River basin. J. Fish. Biol. 65, 1126–1141. doi: 10.1111/j.0022-1112.2004.00522.x
Kemp P. S. (2016). Meta-analyses, metrics and motivation: Mixed messages in the fish passage debate. River Res. Applic. 32, 2116–2124. doi: 10.1002/rra.3082
Kendall N. W., McMillan J. R., Sloat M. R., Buehrens T. W., Quinn T. P., Pess G. R., et al. (2015). Anadromy and residency in Steelhead and rainbow trout (Oncorhynchus mykiss): a review of the processes and patterns. Can. J. Fish. Aquat. Sci. 72, 319–342. doi: 10.1139/cjfas-2014-0192
Kibler K. M., Tullos D. D., Kondolf G. M. (2011). Learning from dam removal monitoring: Challenges to selecting experimental design and establishing significance of outcomes. River Res. Applic. 27, 967–975. doi: 10.1002/rra.1415
Kiffney P. M., Lisi P. J., Liermann M., Naman S. M., Anderson J. H., Bond M. H., et al. (2023). Colonization of a temperate river by mobile fish following habitat reconnection. Ecosphere 14, e4336. doi: 10.1002/ecs2.4336
Kornis M. S., Weidel B. C., Powers S. M., Diebel M. W., Cline T. J., Fox J. M., et al. (2015). Fish community dynamics following dam removal in a fragmented agricultural stream. Aquat. Sci. 77, 465–480. doi: 10.1007/s00027-014-0391-2
Kukula K., Bylak A. (2022). Barrier removal and dynamics of intermittent stream habitat regulate persistence and structure of fish community. Sci. Rep. 12, 1512. doi: 10.1038/s41598-022-05636-7
Kwain W.-H. (1981). Population dynamics and exploitation of rainbow trout in Stokely Creek, eastern Lake Superior. T. Am. Fish. Soc 110, 210–215. doi: 10.1577/1548-8659(1981)110<210:PDAEOR>2.0.CO;2
Magilligan F. J., Nislow K. H., Kynard B. E., Hackman A. M. (2016). Immediate changes in stream channel geomorphology, aquatic habitat, and fish assemblages follow dam removal in a small upland catchment. Geomorphology 252, 158–170. doi: 10.1016/j.geomorph.2015.07.027
Maloney K. O., Dodd H. R., Butler S. E., Wahl D. H. (2008). Changes in macroinvertebrate and fish assemblages in a medium-sized river following a breach of a low-head dam. Freshw. Biol. 53, 1055–1068. doi: 10.1111/j.1365-2427.2008.01956.x
Martin B. A., Hewitt D. A., Ellsworth C. M. (2013). Effects of Chiloquin Dam on spawning distribution and larval emigration of lost river, shortnose, and Klamath largescale suckers in the Williamson and Sprague Rivers, U.S.Geological Survey Open-File Report 2013-1039. (Klamath Falls, Oregon: U.S. Geological Survey).
Mazerolle M. J. (2023). AICcmodavg: Model selection and multimodel inference based on (Q) AIC (c) (R package version 2.3.3). Published on the Comprehensive R Archive Network (CRAN). Available at: https://CRAN.R-project.org/package=AICcmodavg.
McLaughlin R. L., Smyth E. R. B., Castro-Santos T., Jones M. L., Koops M. A., Pratt T. C., et al. (2013). Unintended consequences and trade-offs of fish passage. Fish Fish. 14, 580–604. doi: 10.1111/faf.12003
Melstrom R. T., Lupi F. L. (2013). Valuing recreational fishing in the Great Lakes. N. Am. J. Fish. Manage. 33, 1184–1193. doi: 10.1080/02755947.2013.835293
Moody A. T., Neeson T. M., Wangen S. T., Dischler F., Diebel M. W., Milt A., et al. (2017). Pet project or best project? Online decision support tools for prioritizing barrier removals in the great lakes and beyond. Fisheries 42, 57–65. doi: 10.1080/03632415.2016.1263195
Noonan M. J., Grant J. W. A., Jackson C. D. (2012). A quantitative assessment of fish passage efficiency. Fish Fish. 13, 450–464. doi: 10.1111/j.1467-2979.2011.00445.x
Poulos H. M., Chernoff B. (2017). Effects of dam removal on fish community interactions and stability in the Eightmile River system, Connecticut, USA. Environ. Manage. 59, 249–263. doi: 10.1007/s00267-016-0794-z
Powers P. D., Orsborn J. F. (1985). Analysis of barriers to upstream fish migration. An investigation of the physical and biological conditions affecting fish passage success at culverts and waterfalls. BPA Fisheries Project. Project 82-14. Final Report, Part 4 (Portland, Oregon: Bonneville Power Administration).
Pratt T. C., O’Connor L. M., Hallett A., McLaughlin R. L., Katopodis C., Hayes D. B., et al. (2009). Balancing habitat fragmentation and control of invasive species: Enhancing selective fish passage at Sea Lamprey control barriers. T. Am. Fish. Soc 138, 652–665. doi: 10.1577/T08-118.1
Quinn T. P., McGinnity P., Reed T. E. (2015). The paradox of “premature migration” by adult anadromous salmonid fishes: patterns and hypotheses. Can. J. Fish. Aquat. Sci. 73, 1015–1030. doi: 10.1139/cjfas-2015-0345
Raabe J. K., Hightower J. E. (2014). Assessing distribution of migratory fishes and connectivity following complete and partial dam removals in a North Carolina river. N. Am. J. Fish. Manage. 34, 955–969. doi: 10.1080/02755947.2014.938140
R Core Team. (2020). R: a language and environment for statistical computing (Vienna, Austria: R Foundation for Statistical Computing). Available at: https://www.R-project.org/.
R Core Team. (2023). R: a language and environment for statistical computing (Vienna, Austria: R Foundation for Statistical Computing). Available at: https://www.R-project.org/.
Rubenstein S. R. (2021). Energetic Impacts of Passage Delays in Migrating Adult Atlantic Salmon. (Orono, Maine: The University of Maine).
Rubenstein S. R., Peterson E., Christman P., Zydlewski J. D. (2022). Adult Atlantic salmon (Salmo salar) delayed below dams rapidly deplete energy stores. Can. J. Fish. Aquat. Sci. 80, 170–182. doi: 10.1139/cjfas-2022-000
Ruggles C. P., Murray D. G. (1983). “A review of fish response to spillways,” in Can. Tech. Rep. Fish. Aquat. Sci. No. 1172 (Government of Canada, Fisheries and Oceans, Halifax, NS, Canada).
Salinger D. H., Anderson J. J. (2006). Effects of water temperature and flow on adult salmon migration swim speed and delay. T. Am. Fish. Soc 135, 188–199. doi: 10.1577/T04-181.1
Scherelis C., Zydlewski G. B., Brady D. C. (2020). Using hydroacoustics to relate fluctuations in fish abundance to river restoration efforts and environmental conditions in the Penobscot River, Maine. River Res. Applic. 36, 234–246. doi: 10.1002/rra.3560
Schilt C. R. (2007). Developing fish passage and protection at hydropower dams. Appl. Anim. Behav. Sci. 104, 295–325. doi: 10.1016/j.applanim.2006.09.004
Scott W. B., Crossman E. J. (1998). Freshwater fishes of Canada (Oakville, ON: Galt House Publications Ltd).
Signorell A., Aho K., Alfons A., Anderegg N., Aragon T., Arachchige C., et al. (2020). DescTools: Tools for descriptive statistics (R package version 0.99.39). Published on the Comprehensive R Archive Network (CRAN). Available at: https://CRAN.R-project.org/package=DescTools.
Stanley E. H., Catalano M. J., Mercado-Silva N., Orr C. H. (2007). Effects of dam removal on brook trout in a Wisconsin stream. River Res. Applic. 23, 792–798. doi: 10.1002/rra.1021
Torchiano M. (2020). _effsize: Efficient effect size computation (R package version 0.8.1). Published on the Comprehensive R Archive Network (CRAN). Available at: https://CRAN.R-project.org/package=effsize.
Vargha A., Delaney H. D. (2000). A critique and improvement of the CL common language effect size statistics of McGraw and Wong. J. Educ. Behav. Stat. 25, 101–132. doi: 10.3102/10769986025002101
Watson J. M., Coghlan S. M. Jr., Zydlewski J., Hayes D. B., Kiraly I. A. (2018). Dam removal and fish passage improvement influence fish assemblages in the Penobscot River, Maine. T. Am. Fish. Soc 147, 525–540. doi: 10.1002/tafs.10053
Webb P. W. (1975). Hydrodynamics and energetics of fish propulsion. Bull. Fish. Res. Bd. Can. 190, 1–159. Available at: https://waves-vagues.dfo-mpo.gc.ca/library-bibliotheque/1486.pdf
Whippelhauser G. (2021). Recovery of diadromous fishes: A Kennebec River case study. T. Am. Fish. Soc 150, 277–290. doi: 10.1002/tafs.10292
Whittum K. A., Zydlewski J. D., Coghlan S. M. Jr., Hayes D. B., Watson J., Kiraly I. (2023). Fish assemblages in the Penobscot River: A decade after dam removal. Mar. Coast. Fish. 15, e1022. doi: 10.1002/mcf2.10227
Yoder J., Raymond C. (2022). Climate change and stream flow: Barriers and Opportunities. Preliminary project report to the Washington State Department of Ecology (Olympia, Washington: Water Resources Program).
Zarri L. J., Palkovacs E. P., Post D. M., Therkildsen N. O., Flecker A. S. (2022). The evolutionary consequences of dams and other barriers for riverine fishes. BioScience 72, 431–448. doi: 10.1093/biosci/biac004
Keywords: adfluvial migration, dam removal, fish passage, movement rates, passage delay, rainbow trout, Steelhead
Citation: Bunt CM and Jacobson B (2024) Adfluvial migration and passage of Steelhead before and after dam removal at a major Great Lakes tributary. Front. Ecol. Evol. 12:1346712. doi: 10.3389/fevo.2024.1346712
Received: 29 November 2023; Accepted: 21 February 2024;
Published: 12 March 2024.
Edited by:
Arnaldo Marín, University of Murcia, SpainReviewed by:
Jeffrey J. Duda, US Geological Survey, Western Fisheries Research Center, United StatesMatthew Keefer, University of Idaho, United States
Copyright © 2024 Bunt and Jacobson. This is an open-access article distributed under the terms of the Creative Commons Attribution License (CC BY). The use, distribution or reproduction in other forums is permitted, provided the original author(s) and the copyright owner(s) are credited and that the original publication in this journal is cited, in accordance with accepted academic practice. No use, distribution or reproduction is permitted which does not comply with these terms.
*Correspondence: Christopher M. Bunt, Y2J1bnRAYmlvdGFjdGljLmNvbQ==
†These authors have contributed equally to this work and share first authorship