- Institute of Evolutionary Ecology and Conservation Genomics, Ulm University, Ulm, Germany
Introduction: Interactions between flowering plants and visiting wild bees are crucial for ensuring pollination and subsequent plant reproductive success. However, bee diversity in an area has rarely been recorded in relation to seed set in native plants. In this project, we investigated the effect of local wild bee communities on seed set in four common wild plant species.
Methods: Potted plants of Centaurea jacea, Cichorium intybus, Sinapis arvensis, and Salvia pratensis were placed for pollination experiments, in two distinct habitat types that we expected to show distinct bee communities, namely near-natural grassland and perennial wild flower strips, in Germany.
Results: Our results showed that near-natural grassland had a higher bee species richness and an increased seed set compared with flower strips that displayed a higher bee abundance in most study locations. Although we found effects of bee diversity on seed set, we did not detect a significant effect of bee abundance. Furthermore, the seed set in response to wild bee diversity differed across the plant species.
Discussion: We conclude that bee-friendly habitats ensure a high seed set in wild plants, but that the impact varies between different plant species.
1 Introduction
The majority of plant species in Germany have continually decreased in occurrence over the past six decades, with native plant species experiencing severe declines and with the highest relative losses in species that used to be moderately common (Eichenberg et al., 2020; Jansen et al., 2020). In the modern human-influenced landscape, agricultural land use intensity is steadily increasing (Sirami et al., 2019; Schils et al., 2022) concomitant with the increasing usage of fertilizers and pesticides and the overall homogenization of the landscape (Socher et al., 2013; Gossner et al., 2016). Agricultural intensification results in the population decrease of native plant species because of habitat loss (Robinson and Sutherland, 2002; Meyer et al., 2015). In addition to these direct drivers, pollinator reductions indirectly affect plant decline. About 78% of angiosperms are dependent on insect pollination (Ollerton et al., 2011), and these species show the strongest population declines (Biesmeijer et al., 2006).
Amongst pollinating insects, wild bees are particularly effective in providing pollination services (Garibaldi et al., 2013). The relationship between melittophilic plants and their pollinators is complex, and the interacting partners depend upon each other. When a visiting bee transfers pollen from another conspecific to the flower stigma, fertilization occurs and subsequently seeds develop. In plant species that depend on cross-pollination, bees therefore directly affect the next generation of their host plants (Turnbull et al., 2000). Bees themselves use flowers mainly as food sources and consequently depend upon them for their own reproduction (Zurbuchen and Müller, 2012; van der Meersch et al., 2022). This results in a loop with bees influencing the food resources for upcoming bee generations by shaping the next generations of plants.
Wild bee species differ in their phenology and in their preferences, including the degree of specialization for specific host plants, resulting in seasonal differences in wild bee communities and plant-bee interaction patterns within any one year (Westrich, 2019). Moreover, bee species richness is directly linked to the diversity of available plants (Zurbuchen and Müller, 2012; Kennedy et al., 2013). Semi-natural habitats that are flower-rich and -diverse act as hosts to the highest numbers of wild bee species, particularly rare and threatened species (Neumüller et al., 2020). In addition to local floral resources, the availability of nesting sites (Requier and Leonhardt, 2020) and the surrounding landscape structure are key factors for bee species diversity and abundance (Söderman et al., 2018; Beckmann et al., 2019; Herbertsson et al., 2021; Baden-Böhm et al., 2023). However, wild bees are negatively affected by modern agricultural management (Winfree et al., 2009), as this results in a loss of adequate habitats and restricts floral resource availability. The lack of food resources is one of the major reasons for bee decline (Biesmeijer et al., 2006; Potts et al., 2010; Scheper et al., 2014). Bees require large amounts of pollen to rear their offspring and are thus directly affected by the decrease of available flowering plants and the loss of flower-rich habitats (Müller et al., 2006). This particularly affects oligolectic (flower-specialized) bees that restrict their visits to a small range of plant species (Müller and Kuhlmann, 2008). The absence of specific host plants in a habitat leads to a lack of the corresponding oligolectic bee species, independently of other living conditions (Westrich, 2019). A decreasing number of wild bees results in fewer potential pollinators and lower pollination services to local flora, which in turn results in reduced food resources for bees. With these effects accumulating over several generations, substantial risks build up for both bees and plants.
The establishment of (wild) flower strips has become a popular measure for counteracting the loss of food resources of wild bees and for ensuring their pollination services in agricultural landscapes (Buhk et al., 2018; Albrecht et al., 2020; Hevia et al., 2021). By placing flower strips close to agricultural fields, crop yield increases because of higher pollinator availability (Tschumi et al., 2016; Dainese et al., 2019; Albrecht et al., 2020). However, this effect decreases with increasing distance to an ecological compensation area (Albrecht et al., 2007).
Crop pollination is essential for the provision of food for the increasing human population (Aizen and Harder, 2009). Thus, many studies have been performed in the context of the effect of bees on crop production for the resulting higher demands for human foodstuffs and on conservation measures to promote crop-pollinating bee species (Kremen et al., 2023; Osterman et al., 2023; Scheper et al., 2023). For example, small patches of native vegetation established within crop fields (prairie strips) increase flower resources for wild bees and positively influence the wild bee community (Kordbacheh et al., 2020) giving greater chances for bee-mediated plant pollination (Borchardt et al., 2023). Another example is, that the proximity to pollinator-supporting wild flower strips increases the quality and quantity of highbush blueberry yield (Blaauw and Isaacs, 2014a).
Nevertheless, the pollination service carried out by wild bees is not only important for the production of crops (Klein et al., 2007), but also ensures pollination in natural ecosystems (Steffan-Dewenter and Tscharntke, 1999; Blaauw and Isaacs, 2014b). Melittophilic plants highly depend on bees, and even plant species that do not solely depend on pollinators for reproduction can increase their seed set by up to 80% when pollinators providing cross-pollination are present (Rodger et al., 2021). The number of produced seeds depends on the number of bee visits and frequently on the bee species (Tobajas et al., 2024). Whereas many crops are pollinated by common and widespread bee species, diverse bees including rare and specialized ones are pollinators of many wild plant species (Garibaldi et al., 2013; Woodcock et al., 2019). However, plants species often differ in their pollinator assemblies. Flowers show specializations to restrict visits or, from the plant’s perspective, to filter out ineffective pollinators (Stang et al., 2009; Schwarz et al., 2021). Pollinator specialization can increase pollination efficiency through effective pollen transfer and reduced pollen loss (Fenster et al., 2004). This is important as pollen is a limiting factor in plant reproduction (Knight et al., 2005). The reproductive success of a plant is often measured as seed set, which can be used to quantify the efficiency of pollinators. In a meta-analysis on various wild flower species and their interrelationships with bees, seed set has been shown to be positively correlated to bee visits, although their effects differ vastly among plant species (Herbertsson et al., 2021). For example, bee species richness and abundance have a positive effect on the seed set of native grassland species such as Campanula glomerata, but not on Hypochaeris radicata (Albrecht et al., 2007). Salvia frutticosa and other wild species of Mediterranean scrubland had a reduced seed set after fires that negatively influence the solitary bee community and reduce visitation rates (Ne’eman et al., 2000). In addition to the quantity of seeds, their properties, such as weight, size, or seed coat robustness, can determine the dispersal, persistence, and establishment of the next plant generation (Saatkamp et al., 2019). A higher seed weight is linked to a more robust seed coat that provides protection for the embryo and enables it to survive in less favorable places and during unfavorable times (Niklas, 2008). It is also linked to the endospermic tissue, providing nutrients and the storage of energy required especially for the initial growth of the plantlet (Lamont and Groom, 2013). Therefore, dry seed weight is thought to be a good indicator of available resources to the seedling (Saatkamp et al., 2019).
The surrounding landscape also influences bee-mediated pollination events or seed set (Albrecht et al., 2020; Herbertsson et al., 2021; Ammann et al., 2024). Diverse structures (e.g., open structures for nesting, woody vegetation) and artificial pollinator-promoting structures (e.g., wild flower plantings) in the surrounding landscape have a positive effect on bee diversity (Neumüller et al., 2020; Neumüller et al., 2022), although, as mentioned above, these effects decrease with distance (Albrecht et al., 2007; Ekroos et al., 2015). Notwithstanding, few studies have focused on the impact of the occurring wild bee diversity within a habitat on seed set. Near-natural habitats or areas established as conservation measures for wild bees are thought to promote pollination within a habitat, but pollination success and its dependency on the wild bees of a habitat are only rarely determined for wild plants.
In this study, we have investigated the effect of various habitats and the presence of wild bees on the pollination efficiency of four wild plant species in Germany. Our main goal has been to reveal whether the bee community of a specific habitat is linked to a higher pollination efficiency as measured by seed weight. We have conducted pollination experiments on near-natural grasslands hosting a diverse flora and fauna, and on perennial wild flower strips established as conservation measures for wild bees within the BienABest project (www.bienabest.de) at nine study sites in southern Germany. Potted plants were placed in the field for three days to allow pollination by the bee community of the habitat. The bee abundance and species diversity within each habitat were monitored throughout the season as part of the wild bee monitoring scheme of the BienABest project. The selected plant species, namely Sinapis arvensis (Brassicaceae), Salvia pratensis (Lamiaceae), Centaurea jacea (Asteraceae), and Chichorium intybus (Asteraceae) differ in various traits such as phylogeny, blooming phenology, flower color, and flower morphology and are all visited by large numbers of various bee species (Zurbuchen and Müller, 2012; Kuppler et al., 2023). Specifically, we have asked the following questions. (1) Does the wild bee diversity differ between two habitats, namely near-natural grassland and perennial wild flower strips? (2) Does the wild bee diversity of the different habitats affect seed set across different plant species? (3) Do any effects on seed set differ between the tested plant species?
2 Materials and methods
2.1 Study sites
The pollination experiments were performed within agricultural areas at nine different study sites in south-west Germany (see Figure 1A and Supplementary Table S1). The study sites were part of the BienABest project (www.bienabest.de; see Neumüller et al., 2020 and Neumüller et al., 2021 for the BienABest study design and establishment of study plots). The BienABest project aimed to safeguard the ecosystem service of pollination and to enhance wild bee diversity in agricultural landscapes. Flower strips were established as conservation measures at various sites in Germany, accompanied by extensive wild bee monitoring during the years 2018 to 2022.
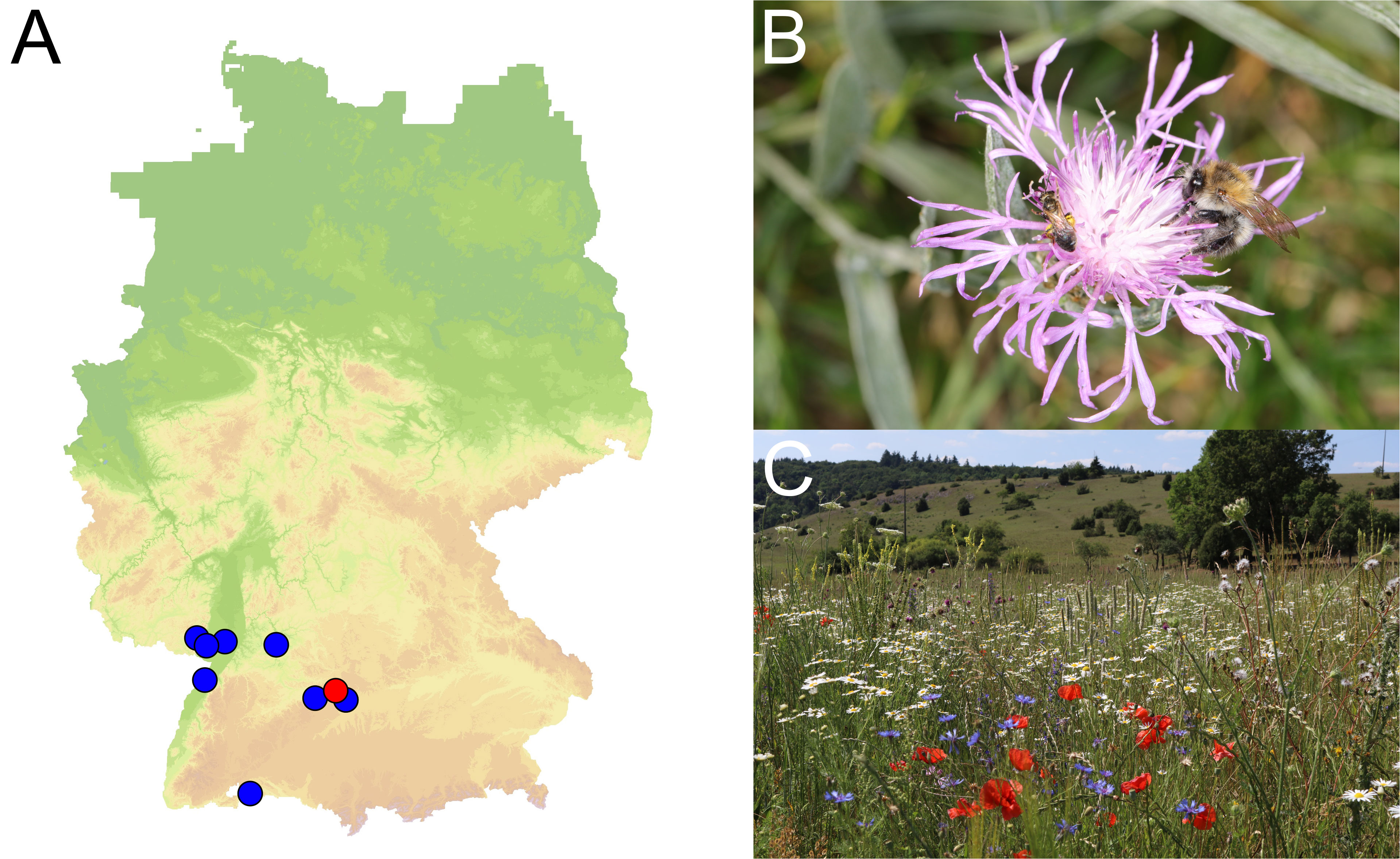
Figure 1 (A) Map showing the nine study sites located south-west Germany. Each dot represents a site. Within each site, a total of four plots of 0.3 ha each were investigated. Two plots were located within near-natural grassland areas, and two were identical to the wild flower strips implemented for the BienABest wild bee monitoring. (B) Halictus tumulorum and Bombus pascuorum bees on the study species Centaurea jacea. (C) Example of a study site on the Swabian Alb (red dot on map 1A): established wild flower planting with Cichorium intybus in the foreground, near-natural grassland plots in the background. [Map © GeoBasis-DE / BKG 2018; photos H. Burger].
The study sites extended over 180 km latitudinally and 175 km longitudinally. The landscape of each site was an agricultural matrix of arable land and/or intensively-used grassland and incorporated bodies of semi-natural grassland. At each study site, 0.3-ha plots were established on various habitats within a 500-m radius. Two plots each of the two different habitat types were used at each study site, resulting in 36 plots (2 x 2 plots at each of the nine study sites) for the pollination experiments and observed bee data. The pollination experiments were performed on study plots with two different habitat types within each study site: near-natural habitats and perennial wild flower strips (Figure 1C). Near-natural habitat plots were placed in extensively managed grassland such as calcareous grassland, mostly in nature conservation areas. The semi-natural grasslands were characterized by a high diversity of native flowering plants and were rich in diverse structures (e.g., open soil for nesting or woody vegetation). The habitats were managed by occasional grazing by sheep and met the needs of a diverse spectrum of naturally occurring flora and fauna. The wild flower strips were established on tilled arable land in 2017 by using a seed mixture consisting of ca. 50 plant species (autochthonous seeds, provided by Rieger-Hofmann, Blaufelden, Germany). The seed mixture consisted of annual and perennial plant species that are regionally native or cultivated plant species and included the study plant species used in the pollination experiments. Half of each wild flower strip was mown once a year at peak bloom in June as a measure to increase plant diversity and to induce a second bloom, prolonging the blooming season to promote pollinators. When the experiments of this project were performed in the year 2020, the wild flower strips were well established, with mainly perennial plants flowering. On average, 38 plant species (minimum 26, maximum 42 species) from the seed mixtures were established in each flower strip. The flower cover varied between seasons but was well pronounced when pollination experiments were performed.
2.2 Plant species
The four different bee-pollinated plant species (Figure 2) were common species occurring at all study sites and were part of the established seed mixture. The species differed in floral cues and phenology (Figure 2) and required cross pollination for seed set (see control plants in pollination experiments). The species were chosen to be frequently visited by a broad spectrum of both generalist and specialist wild bees (Figure 1B; Kuppler et al., 2023).
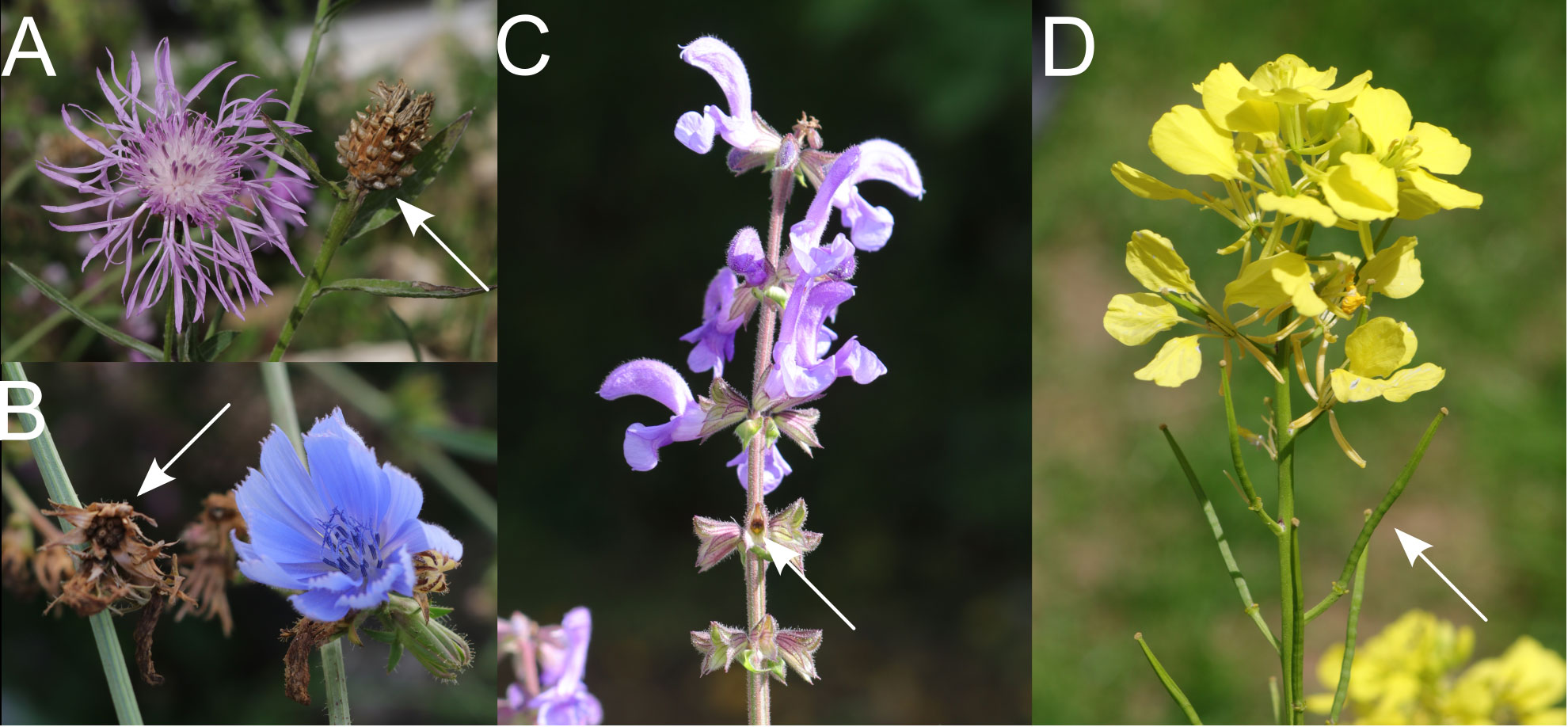
Figure 2 Flowers of the four different plant species used in the pollination experiments: Centaurea jacea (A), Chichorium intybus (B), Salvia pratensis (C), and Sinapis arvensis (D). White arrows indicate ripening seed structures. [Photos H. Burger].
Sinapis arvensis (L.) (Figure 2D) is an annual Brassicaceae flowering from May onward. For experiments, S. arvensis seeds (Rieger-Hofmann GmbH, Blaufelden, Germany) were sown into 16 x 16 cm flowering pots with 2 to 3 individuals per pot.
Salvia pratensis (L.) (Figure 2C) is a perennial Lamiaceae blooming from May to August. It is self-compatible but requires pollinators for pollen transfer (Moughan et al., 2021). Its flowers are specialized for pollination by large bees, e.g., bumblebees, and are rarely visited by insects other than bees (Moughan et al., 2021). This species can produce a maximum of 4 seeds per floret.
Both Asteraceae species, i.e., Centaurea jacea (L.) (Figure 2A), and Cichorium intybus (L.) (Figure 2B), are perennial and bloom from June/July until the end of season. Bees are the most abundant visitors of Centaurea jacea (Hirsch et al., 2003). All perennial species (S. pratensis, C. jacea, C. intybus) were cultivated in 16 x 16 cm flower pots with single plants per pot in the plant-rearing area of the Botanical Garden Ulm for at least one year prior to the experiments.
2.3 Pollination experiment
Experiments were performed in the spring and midsummer of 2020. Immediately before starting to bloom, plants were placed in pollinator-excluding cages of fine mesh to ensure that flowers could only be pollinated during the field experiments. When in full bloom, plants were brought into the study sites to be pollinated by the local bee community. Some plants remained in the flight cages as controls for self-pollination; no seed set occurred in the controls. The two different blooming seasons resulted in two different flower pairings. For the spring experiments, 4 pots of S. pratensis and 6 pots of S. arvensis, and for the summer experiments, 4 individuals of C. jacea and C. intybus were placed in the field per habitat type per study site, respectively. The plants were left on the study plots for 72h when sunny weather was forecast. All plant pots for one plot were placed on two trays (ca. 35 cm x 50 cm x 4 cm) and were located at least 1.5 m from the edges of the plots. The plants were provided with at least 2.5 l water per plant tray to avoid drought stress during the experiments. Subsequently, plants were moved back into the mesh cage where they were checked regularly for seed maturation. Seeds were harvested when flowers or siliques turned dry and were then spread out to dry to avoid the growth of mold. The seed number was then counted and weighed using accurate weighing scales (accuracy of 0.1 mg).
For S. arvensis, 10 siliques per plant were chosen, and their intact seeds (round, with a diameter of at least 1 mm) were extracted manually and weighed to obtained an average weight per seed. For plants with fewer siliques, all siliques were measured. All seeds of the respective plant were then counted and weighed, and the average weight of intact seeds was calculated. As S. pratensis has a constant number of 4 seeds per flower, only the number of intact seeds of ten flowers were counted, and the relevant seeds were weighed. Again, the total number of flowers per plant was counted, and the seeds were retrieved, sorted, and weighed. For the Asteraceae species, intact seeds were broken off the dried flowers and processed as described above.
2.4 Bee data
Wild bee monitoring data for the season of 2020 were received from the BienABest project (for detailed information on bee monitoring see Neumüller et al., 2020; Neumüller et al., 2022 and Verein Deutscher Ingenieure e.V, 2023). Between April and September 2020, five sampling events took place per plot covering the entire season at intervals of 3 to 4 weeks, starting with the flowering of the Taraxacum sect ruderalis dandelion. Each sampling event consisted of two 25 min transect walks per plot, one in the morning and one in the afternoon, during which collectors were able to move freely within the plot. Sampling was performed by wild bee experts to guarantee reliable species determination. All observed bee individuals (males and females) were caught with an entomological net, except for those that could be determined at first sight, and were identified to species level in the field. Individuals that could not identified in the field were taken to the laboratory and identified using a binocular microscope and standard literature. Sampling was conducted only during sunny weather (cloud cover maximum 30%), at temperatures higher than 10°C and under low wind conditions.
This method for the bee survey was undertaken with the aim of recording high numbers of bee species and floral resources for wild bees with standardized monitoring methods according to Verein Deutscher Ingenieure e.V, 2023 and represents a trade-off between the highest standardization and the recording of the complete species inventory in an appropriate period of time. The methodology was additionally verified by Herrera-Mesías et al., 2022.
2.5 Statistical analysis
All analyses were performed using R (version 4.2.2, The R Foundation for Statistical Computing). The nearest weather station for each study site was determined, and the corresponding weather data for each day and site was retrieved from the Deutscher Wetter Dienst (DWD, www.dwd.de; Supplementary Table S1).
2.5.1 Bee data analyses and predictions
Individual-based species accumulation curves were calculated from the BienABest wild bee monitoring data to compare the species richness and the abundance of bees recorded on the two habitat types by using the R package iNEXT (Hsieh et al., 2016). This package was also used to calculate Shannon indices H’ for the whole year, taking into consideration the habitat type on each study site. The Shannon index H’ gives overall diversity while taking the species richness and the abundance for each species into account. As it combines these two factors of the bee community, it also provides an indication of the relevance of rare species in our analyses.
In order to predict bee abundance and diversity for each date (first entire day of the 72 h pollination experiment), generalized additive models (GAM) were constructed using the ‘mgcv’ R package (Wood, 2012). Models were based on BienABest wild bee monitoring data and weather data. We included the day and the maximum temperature as smoothing factors and the plot ID, the study site, and the habitat type as variables in the models (see Supplementary Table S2). We then used the predict() function in order to predict the species richness and abundance of bees for each of the days and plots of the pollination experiment. These predictions are used as independent variables in the further analyses.
2.5.2 Seed set analyses
Linear mixed models (LMMs) for the seed weight were constructed using the lm function in the lme4 package (Bates et al., 2015). We made a total of 399 measurements of seed weight for all plant species. For better compatibility between plant species, a quotient was calculated by dividing the seed weight of individual measurements by the maximum observed value within each species. These values for relative seed weight allowed better comparisons between species.
First, three models for seed set analyses were designed as models with relative seed weight as the dependent variable. One of the three bee variables (species richness, abundance, or Shannon H’) were used as an independent variable in the three global models. Furthermore, we included the plant species and habitat type (wild flower strip or near-natural grassland) as variables and the study site as a random effect in all models.
As a result of the significances of the global models, six plant-species-specific generalized linear models (GLMs) were designed for species richness and the Shannon index H’ (for each of the 3 tested plant taxa) in which the daily mean temperature of the experimental day was added as a continuous factor, and the study site was added as a random factor to the models. Because of the statistical results of the global models (see pairwise tests for each model in Supplementary Tables S3 and S4) and considerations of the biology of the plants, C. intybus and C. jacea were treated as one group (“Asteraceae”) in these analyses. Therefore, for Asteraceae models, plant species remained as a random factor.
3 Results
3.1 Bee diversity of habitats
A total of 10,922 bee individuals of 210 bee species were recorded, which could be grouped into 4,572 individuals of 187 bee species on near-natural grassland and 6,350 individuals of 163 bee species on wild flower strips (Figure 3), resulting in a significant difference of species richness between habitat types (non-overlapping confidence intervals). Of these 210 species, ca. 68% (143 species) overlapped, 47 species only occurred on near-natural grassland, and 23 species occurred only on wild flower strips. The average number of individuals per species was higher on wild flower strips (mean ± SD [lowest value, highest value]: 38.9 ± 117.4 [1, 1119] individuals/species, 40 singletons) than on near-natural grassland (24.4 ± 70.6 [1, 561] individuals/species, 39 singletons). Extremely abundant species (more than 100 individuals in total) made up ca. 61% of bee individuals observed on near-natural grassland (10 species, most abundant Bombus terrestris bumblebees) and ca. 75% of bee individuals observed on wild flower strips (15 species, most abundant B. lapidarius bumblebees). Shannon diversities H’ of each habitat type within the individual study sites indicated an overall high diversity with average indices of H’ = 2.73 (SD: 0.37; [1.69, 3.33]) for near-natural grassland and H’ = 2.79 (SD: 0.41; [1.77, 3.33]) for wild flower strips.
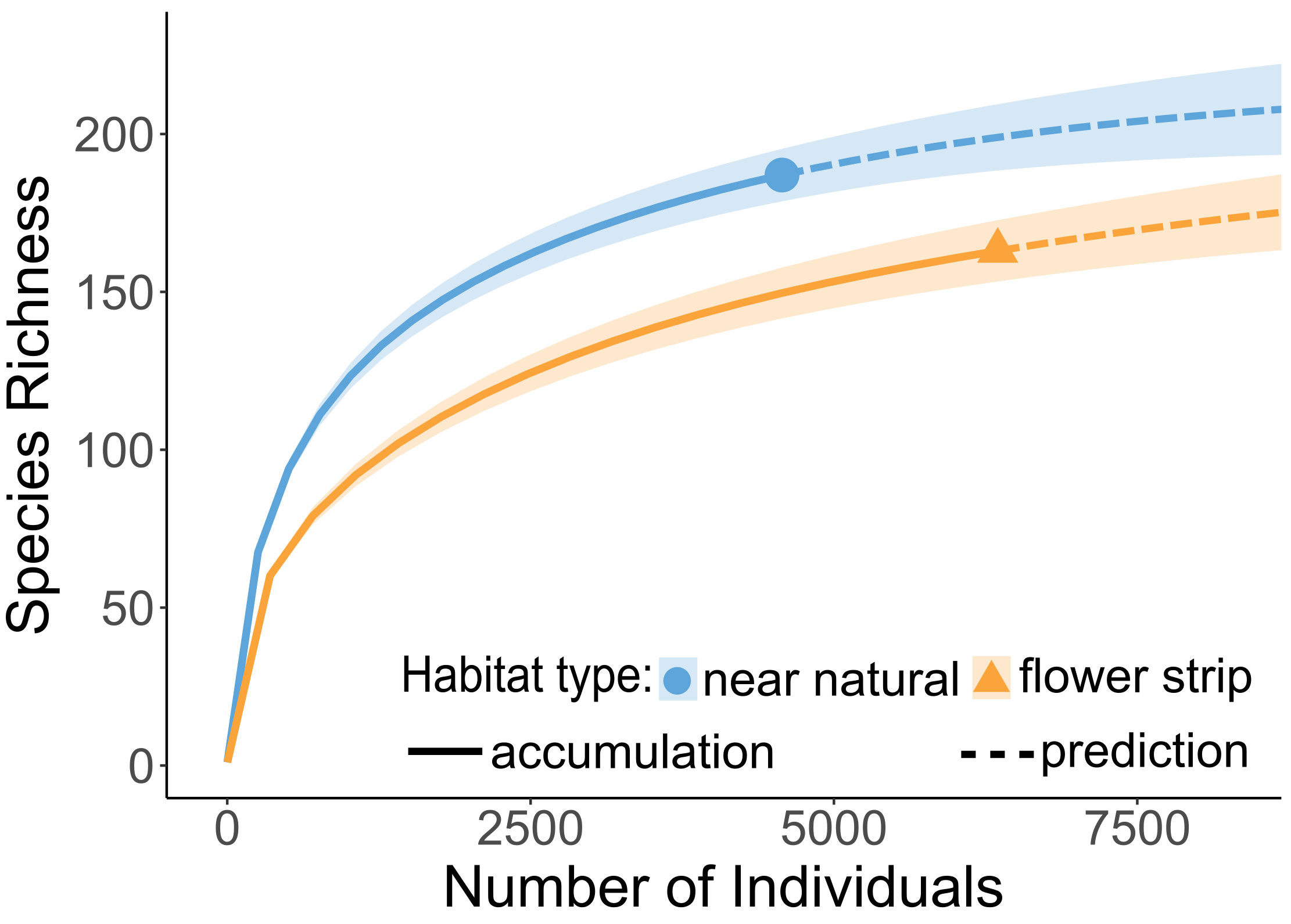
Figure 3 Individual-based randomized species accumulation curves comparing wild bee richness and abundance between the two habitat types of near-natural grassland and wild flower strips on the study sites.
3.2 Overall seed set
The global models across all plant species showed that the standardized seed weight was significantly positively correlated with the bee species richness of the experimental day (Figure 4B and Table 1) and with the Shannon index H’ of the entire season (Figure 4A and Table 1). Furthermore, both models revealed significant differences between the habitat types, with near-natural habitats outperforming wild flower strips in both cases and differences among the plant species (Table 1).
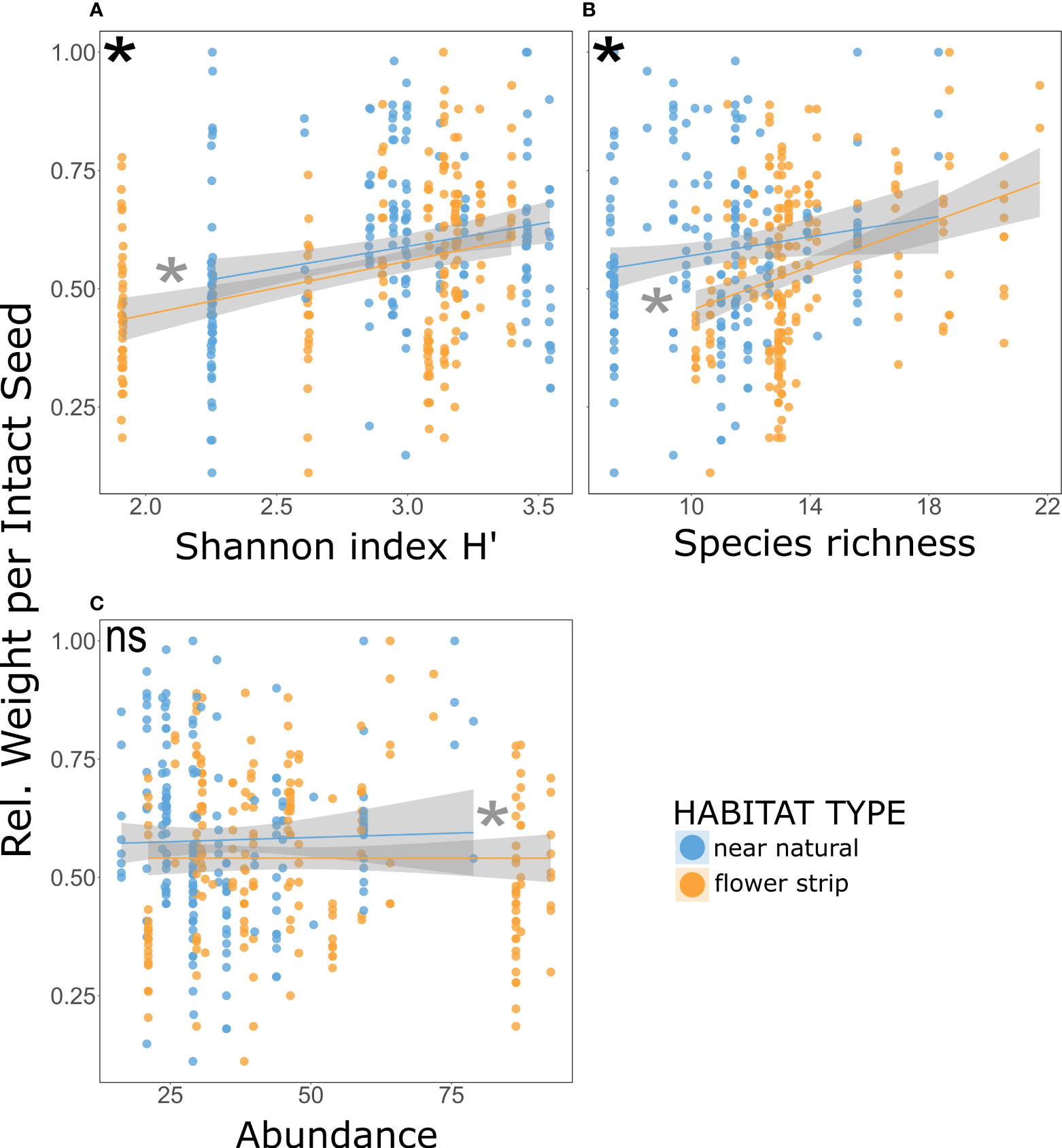
Figure 4 Correlation between the relative weight per intact seed on the two habitat types and the Shannon index H’ (A), the species richness (B), and abundance (C). Within each global model, black asterisks indicate a significant effect for the bee variable, and gray asterisks indicate a significant effect of the habitat type (GLM, p<0.05). Non-significant differences are indicated by 'ns' (p>0.05).
When testing for the dependency of standardized seed weight on the bee abundance, we found no significant effect, although here again, we determined significant differences between the habitat types, with near-natural habitats outperforming wild flower strips, and among the various plant species (Figure 4C and Table 1).
3.3 Species-specific seed sets
Because of significant differences between plant species in the global models, we performed pairwise comparisons: no significant differences between the two Asteraceae species, C. intybus and C. jacea, were revealed, but these species showed significant differences from S. arvensis and S. pratensis, which again did not exhibit significant differences from each other (see Supplementary Tables S3 and S4).
For the Asteraceae specific models, we found a significant positive relationship for greater seed weight with greater Shannon index H’ and bee species richness (Figure 5 and Table 2). Neither of the models showed a significant difference between the habitat types (Figure 5 and Table 2). For the S. pratensis Shannon index H’ model, we observed a significant positive relationship for the Shannon index H’ and a difference between the habitat types with near-natural habitats again outperforming wild flower strips (Figure 5 and Table 2). Similar results were obtained for the S. pratensis species richness model (Figure 5 and Table 2).
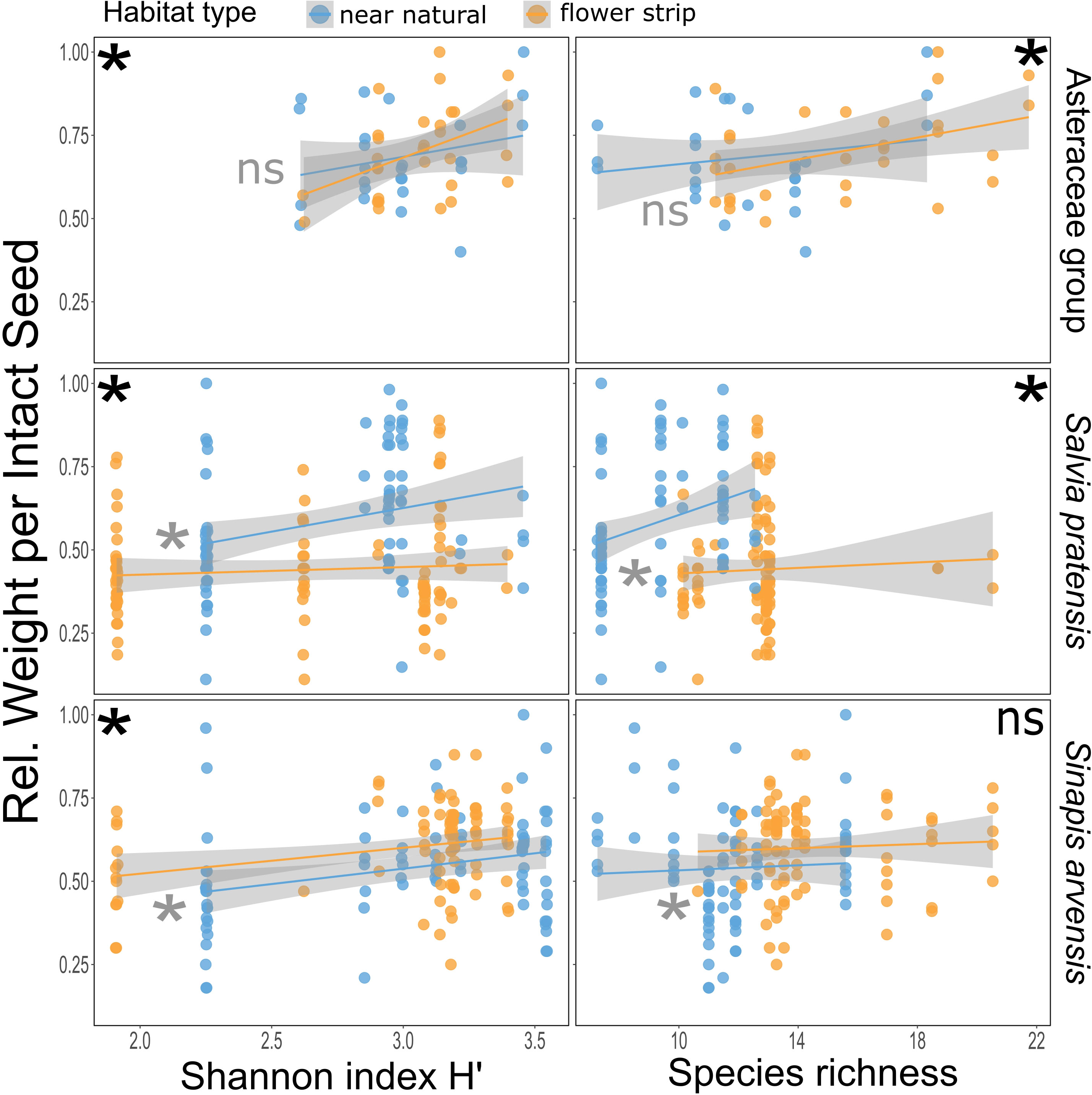
Figure 5 Comparison of relationships between bee diversity (Shannon index H’ for the entire season left, species richness for experimental day right) and seed weight between the plant taxa (Asteraceae group (Centaurea jacea and Cichorium intybus), Salvia pratensis, and Sinapis arvensis). Within each model, black asterisks indicate a significant effect of the bee variable, and gray asterisks indicate a significant effect of the habitat type (GLM, p<0.05). Non-significant differences are indicated by 'ns' (p>0.05).
Significant differences were also found between habitat types for both S. arvensis models, with plants on wild flower strips performing better than on near-natural habitats (Figure 5 and Table 2). Nevertheless, only the Shannon index H’ was significantly positively correlated to seed weight, whereas species richness was not (Figure 5 and Table 2).
4 Discussion
The examined study locations showed an overall high wild bee diversity, which resulted in high seed sets in four wild plant species that are important host plants of wild bees. We found significant differences across the habitats of near-natural grasslands and flower strips and the effects of bee species richness and Shannon index H’ on the seed weight but did not find such an effect of bee abundance. The results suggest that the wild bee diversity in a habitat influence the seed set of native plant species and consequently influence the reproductive success of these plants.
4.1 Increased seed sets in near-natural habitats
The study locations of our pollination experiments hosted a high bee diversity and abundance. Higher species richness was found on near-natural grassland. Permanent habitats such as near-natural grasslands are normally rich in flowers and also provide diverse nesting sites for wild bees, thus explaining the high bee diversities (Requier and Leonhardt, 2020). In comparison, wild flower strips generally had a higher bee abundance while being inhabited by fewer species. Despite these differences, the Shannon index H’ was not significantly different over the two habitat types and across all study sites. The relatively high Shannon index H’ indicated the high proportions of intermediate and rare species (Peet, 1974). The wild bee monitoring data and previous analyses of the bee diversity at the study locations (Neumüller et al., 2020) confirmed the occurrence of rare species that are often threatened according to the German Red List of wild bees (Westrich et al., 2011). We also found a substantial overlap of bee species between both habitats. These findings indicate that the artificially created flower strips were highly attractive for wild bees and can be considered as a successful conservation measure for improving the availability of floral resources within a region. Many commercially available seed mixtures with high proportions of annual non-native plants often only increase the abundance of a few abundant bee species (Albrecht et al., 2020). In contrast, the floral resources of the examined flower strips were sustainably relatively flower-rich over several years because of an effective mowing regime and the use of a species-rich seed mixture (Neumüller et al., 2021). The perennial flower strips still provided valuable food resources including important pollen hosts of wild bees (Kuppler et al., 2023), and the high flower cover resulted in a high bee abundance. Nevertheless, permanent habitats such as near-natural grassland hosted the highest numbers of bee species. These habitats, which were extensively managed, were highly diverse in flowers and also provided diverse nesting sites for wild bees, factors that explain high bee diversities (Requier and Leonhardt, 2020).
The high numbers of bee species found in near-natural grasslands might explain the significant influence of the habitat type on the seed setting rates. We found a significant influence of the habitat type, with higher seed setting rates in near-natural grassland compared with those on flower strips. Previous studies have shown that structure-rich landscapes have a positive effect on bee diversity (Kennedy et al., 2013; Neumüller et al., 2020) and, consequently, on seed set (Albrecht et al., 2020). Nevertheless, increasing distances to pollinator-friendly habitats have a negative effect on plant reproductive success (Steffan-Dewenter and Tscharntke, 1997; Steffan-Dewenter et al., 2001), and high proportions of arable land in a landscape, often combined with decreased structure richness, can negatively affect the number of produced seeds (Söderman et al., 2018; Herbertsson et al., 2021; Ammann et al., 2024). In contrast to these previous studies, we have not analyzed effects at the landscape scale, as the two habitat types were in close proximity at a study site and therefore were surrounded by the same landscape within the flight distance of most wild bee species (Steffan-Dewenter et al., 2002). In our study, the bee community was determined on 0.3 ha plots established within different habitats. Although the previous studies mentioned above did not directly monitor bee diversity in the surrounding landscape elements, we can draw similar conclusions from our results. We conclude that both the preservation of near-natural habitats as a reservoir of wild plant species diversity and the conservation of reproduction-assuring pollinators are of great importance for seed setting.
4.2 Bee diversity affects seed set
In our pollination experiments, we found increases in seed set with greater wild bee diversity (species richness and Shannon index H’) across habitats and study sites. Although the bee species richness was calculated for the experimental day and specific plot, the Shannon index H’ represents the bee diversity of the entire season. We thus compared two bee parameters and included two different time-scale approaches. We were able to show that both parameters significantly influenced the seed weight, as greater species richness and a greater Shannon index H’ resulted in greater seed set, and both seemed to be a suitable measure for seed set prediction. Both parameters allowed conclusions to be made from monitoring data without the performance of time-consuming pollination experiments and direct observations of bee visits. The studies reviewed by Garibaldi et al., 2013 and the meta-analysis by Woodcock et al., 2019 have especially particularly highlighted the important role of wild bees in pollination and have shown that the visits of diverse wild bee species, in particular, explain the pollination success. For example, distinct bee assemblages increased the seed set of Helianthus anuus by up to 45% (Mallinger et al., 2019).
Wild bee diversity highly depends on the structure of the habitat, but the effects of the habitat type on pollination success and its dependency on wild bees have previously often been measured only indirectly as effects of surrounding landscape elements. For example, the seed set of Raphanus sativus is increased by flower strips in the surroundings (Albrecht et al., 2007) and, for the seed set of Sinapis arvensis, by near-natural habitats. Although these previous studies have not directly monitored the wild bee community of the habitats, as carried out in our study, the results support our findings: habitats that naturally host or are established to promote high bee diversities increase the seed set in wild plant species. Concurrently, pollinator diversity, in addition to bees and thus resulting niche complementarity, has been shown to impact fruit set positively (Albrecht et al., 2012; Magrach et al., 2021), but this was not a focus of our study.
As the chosen plant species benefit from cross-pollination by bees and are highly attractive to generalists and specialist bees, we assume that the higher seed weights are attributable to cross-pollination by diverse bee species. Seed weight is particularly linked to the initial growth of the plantlet (Lamont and Groom, 2013). In this phase, the fitness of the plantlet in its surroundings is crucial for its survival. Survival is assured, for example, by its genotypic characteristics such as higher heterozygosity presumably arising from cross-pollination, adaptability resulting from cross-pollination, and its fast growth as a competitive advantage (e.g., competition for light by out-growing other plants). We conclude that the high bee diversities of our habitat types provides the necessary pollination services to maintain the next generations of study plant species, which are important host plants of diverse bee species.
4.3 High bee abundance does not increase seed set
In addition to bee diversity, a greater bee visitation rate has been reported to result in a higher seed set (Herbertsson et al., 2021). As a high bee abundance leads to more potential flower visits, we expected to observe this effect in our experiments. However, this was not confirmed by our study. The bee abundance at the study sites was mostly driven by a few widespread bee species that occurred in high numbers, such as the bumblebees Bombus lapidarius and B. terrestris. An effective seed set thus seems not to be strongly affected by abundant bee species. More specialized visitors that are often part of species-rich wild bee communities can, in contrast, greatly improve pollination (Mallinger et al., 2019). Similarly, we found an increase in pollination via higher bee diversity, which can largely explain the seed sets in the studied plant species. The foraging behavior and functional traits of intermediate or rare bee species are more important than high abundancies of common bee species. An alternative explanation for the non-significant effect of bee abundance might be that a saturation in pollination occurs (Morris et al., 2010). This means that at least the minimum bee abundance needed for pollination was assured in both our habitats. On the other hand, over-pollination attributable to an excess of bee visits did not seem to be a factor in our experiments, as this would have resulted in a decreased seed set (Sáez et al., 2014; Garratt et al., 2021).
4.4 Differences in seed set between plant species
The pollination experiments revealed that the bee diversity differently impacted the seed set of the four studied wild plant species. In all represented taxa, the seed set was positively correlated with greater Shannon indices H’ and, in two models, also to bee species richness, although the effects depended, in some of the plant species, on the habitat type.
4.4.1 Sinapis arvensis
Sinapis arvensis plays an important role as a floral resource for wild bees in spring (Kuppler et al., 2023) because of the limited availability of flowering herbaceous plants early in the season. Although the nectar reward lies relatively deeply within the flowers, the petals are flexible, and the nectar and pollen is easy to exploit regardless of bee morphology. This result in visits by a broad variety of bee species including widespread ones that are often abundant in agricultural landscapes. We found that the seed set in S. arvensis was only slightly influenced by greater bee-species richness and the Shannon index H’ indicating sufficient pollination events, even by a few bee species occurring in sufficient numbers. This also supports our finding that S. arvensis performs better in wild flower strips, which have been shown to exhibit higher bee abundances compared with the flower strips in our rarefaction analyses.
The effective pollination of S. arvensis flowers by bees has also been demonstrated in previous studies. Sinapis plants are annuals and easy to breed probably explaining the use of this native plant species in contrast to others frequently used in pollination experiments. Previous studies have shown landscape effects with decreasing seed set as a result of increasing distances to near-natural grassland (Steffan-Dewenter and Tscharntke, 1997; Steffan-Dewenter and Tscharntke, 1999). Moreover, these studies have shown that wild bee abundance is a better predictor for S. arvensis seed weight per plant than that of honey bees alone (Steffan-Dewenter and Tscharntke, 1999). Other members of the Brassiceae, i.e., the widely cultivated Brassica napus is highly dependent on bees for pollination. Osmini mason bees, O. cornifrons, and O. lignaria have been shown to increase seed set in flight cage experiments (Abel et al., 2003). Not only analyses of bee visitor identity, but also the determination of the pollen deposition of visiting species are needed to identify the most effective pollinating species of S. arvensis and other Brassicaceae species.
4.4.2 Centaurea jacea and Cichorium intybus
The Asteraceae species Centaurea jacea and Cichorium intybus bloom during peak bee activity in high and late summer and might be crucial resources for nectar and pollen for a broad spectrum of bee species. Based on the ranking of bee visits analyzed by Kuppler et al., 2023, C. jacea has the highest visitor richness of the four wild flower species used in this study. High numbers of visiting bee species suggest that the flowers have a more generalist pollination system (with several bee species as effective pollinators) and do not restrict access to floral rewards to a small visitor subset. Asteraceae species are also among the most important host plants for oligolectic bees (Zurbuchen and Müller, 2012), which are often specialized on host plants that are frequently visited by other bee species as reliable food sources. Our results indicate that Asteraceae are the only group without a habitat-specific effect, and thus, we assume that they are the least affected by specific bee species compositions because of their overall high attractiveness for bees. Although the Shannon index H’ did not appear to play a crucial role, seed set for both plants was higher with greater bee diversity. Again, the results emphasize that bee-diverse habitats are needed to ensure effective pollination and to conserve plant diversity.
In agreement with our findings, an effect of landscape complexity, which normally coincides with high bee diversities, has been shown to affect seed set in C. jacea (Steffan-Dewenter et al., 2001). However, other studies on the pollination efficiency in C. jacea and C. intybus are rare. The florets of Crepis sancta, another Asteraceae species with a similar morphology, lie closer to the center of the inflorescence and have been demonstrated to be more prone to fertilization by homogenic pollen, with the resulting seeds therefore being not as well adapted for dispersal as seeds from the outer florets (Cheptou et al., 2001). This results in certain intra-floral and intra-plant differences of seed traits, which should be considered in future experiments, e.g., by only evaluating outer seeds to measure seed set.
4.4.3 Salvia pratensis
The seed set of Salvia pratensis was most strongly affected by habitat types, with near-natural habitats performing significantly better than the experimental plots located on wild flower strips. Salvia pratensis attracts fewer bee species compared with the other study plants (Kuppler et al., 2023). Instead, the pollination system of Salvia is described as being specialized for large bees, mostly bumblebees (Moughan et al., 2021). The exploitation of Salvia flowers requires a distinct body size for entering the flowers, with small bees being unable to trigger the floral mechanism, and a sufficient tongue length for reaching the nectar in the deep corolla (Claβen-Bockhoff et al., 2003; Reith et al., 2007). We have observed high numbers of bumble bee individuals in both habitats, a result that seems to contradict the significant effects of habitat type. However, the seed set in S. pratensis not only depends on the number of visiting bumblebees, but also on bee traits (Zhang and Claßen-Bockhoff, 2019), again indicating that some bee species or individuals are more effective than others. In addition to bumblebees, Anthophora spp. or Lasioglossum xanthopus are, for example, regular S. pratensis visitors, both having a large body size. Interactions between S. pratensis and these bee species and the higher diversity of Bombus species have only been found in the near-natural grassland in the examined study locations. Thus, the pollinator requirements of S. pratensis seem to be more specific than expected.
5 Conclusion
In this pollination study, we have determined the effects of local bee communities, recorded on the habitat scale, on the reproduction of four wild plant species that are known to be attractive to wild bees. We have shown that high-quality habitats, particularly near-natural grasslands, with a (resulting) high bee diversity have a positive effect on the reproductive success of all tested plant species. Perennial flower strips can promote diverse bee species in agricultural landscapes, although near-natural grasslands host the highest bee diversities resulting in high seed sets. We conclude that the preservation of diverse wild bee communities and suitable wild bee habitats is indispensable for the indirect promotion of native plants that have suffered severe declines over the last few decades.
Data availability statement
The raw data supporting the conclusions of this article will be made available by the authors, without undue reservation.
Ethics statement
The manuscript presents research on animals that do not require ethical approval for their study.
Author contributions
KH: Writing – original draft, Formal analysis, Investigation, Methodology, Visualization. MA: Conceptualization, Supervision, Writing – review & editing. HB: Conceptualization, Supervision, Writing – original draft, Writing – review & editing.
Funding
The author(s) declare financial support was received for the research, authorship, and/or publication of this article. KH thanks the Deutsche Bundesstiftung Umwelt (reference number 20019/614) for her PhD student scholarship.
Acknowledgments
We gratefully acknowledge A. Szabo for help during fieldwork and seed counting, A. Mayr for input concerning model building, and T. Jones for linguistic advice. We thank the gardeners of the Botanical Garden of Ulm University for their support in the cultivation and care of our plants. We are grateful to the BienABest project for the opportunity to perform the experiments on the study sites and for providing the bee data. We thank two reviewers for their thoughtful comments and dedicated input on an earlier version of this manuscript.
Conflict of interest
The authors declare that the research was conducted in the absence of any commercial or financial relationships that could be construed as a potential conflict of interest.
Publisher’s note
All claims expressed in this article are solely those of the authors and do not necessarily represent those of their affiliated organizations, or those of the publisher, the editors and the reviewers. Any product that may be evaluated in this article, or claim that may be made by its manufacturer, is not guaranteed or endorsed by the publisher.
Supplementary material
The Supplementary Material for this article can be found online at: https://www.frontiersin.org/articles/10.3389/fevo.2024.1343885/full#supplementary-material
References
Abel C. A., Wilson R. L., Luhman R. L. (2003). Pollinating efficacy of Osmia cornifrons and Osmia lignaria subsp. lignaria (Hymenoptera: Megachilidae) on three Brassicaceae species grown under field cages. J. Entomol. Sci. 38, 545–552. doi: 10.18474/0749-8004-38.4.545
Aizen M. A., Harder L. D. (2009). The global stock of domesticated honey bees is growing slower than agricultural demand for pollination. Curr. Biol. 19, 915–918. doi: 10.1016/j.cub.2009.03.071
Albrecht M., Duelli P., Müller C. B., Kleijn D., Schmid B. (2007). The Swiss agri-environment scheme enhances pollinator diversity and plant reproductive success in nearby intensively managed farmland. J. Appl. Ecol. 44, 813–822. doi: 10.1111/j.1365-2664.2007.01306.x
Albrecht M., Kleijn D., Williams N. M., Tschumi M., Blaauw B. R., Bommarco R., et al. (2020). The effectiveness of flower strips and hedgerows on pest control, pollination services and crop yield: a quantitative synthesis. Ecol. Lett. 23, 1488–1498. doi: 10.1111/ele.13576
Albrecht M., Schmid B., Hautier Y., Müller C. B. (2012). Diverse pollinator communities enhance plant reproductive success. Proc. Biol. Sci. 279, 4845–4852. doi: 10.1098/rspb.2012.1621
Ammann L., Bosem-Baillod A., Herzog F., Frey D., Entling M. H., Albrecht M. (2024). Spatio-temporal complementarity of floral resources sustains wild bee pollinators in agricultural landscapes. Agric. Ecosyst. Environ. 359, 108754. doi: 10.1016/j.agee.2023.108754
Baden-Böhm F., Dauber J., Thiele J. (2023). Biodiversity measures providing food and nesting habitat increase the number of bumblebee (Bombus terrestris) colonies in modelled agricultural landscapes. Agric. Ecosyst. Environ. 356, 108649. doi: 10.1016/j.agee.2023.108649
Bates D., Mächler M., Bolker B., Walker S. (2015). Fitting linear mixed-effects models using lme4. J. Stat. Soft. 67, 1–48. doi: 10.18637/jss.v067.i01
Beckmann M., Gerstner K., Akin-Fajiye M., Ceauşu S., Kambach S., Kinlock N. L., et al. (2019). Conventional land-use intensification reduces species richness and increases production: A global meta-analysis. Glob. Chang. Biol. 25, 1941–1956. doi: 10.1111/gcb.14606
Biesmeijer J. C., Roberts S. P. M., Reemer M., Ohlemüller R., Edwards M. E., Peeters T., et al. (2006). Parallel declines in pollinators and insect-pollinated plants in Britain and the Netherlands. Science 313, 351–354. doi: 10.1126/science.1127863
Blaauw B. R., Isaacs R. (2014a). Flower plantings increase wild bee abundance and the pollination services provided to a pollination-dependent crop. J. Appl. Ecol. 51, 890–898. doi: 10.1111/1365-2664.12257
Blaauw B. R., Isaacs R. (2014b). Larger patches of diverse floral resources increase insect pollinator density, diversity, and their pollination of native wildflowers. Basic. Appl. Ecol. 15, 701–711. doi: 10.1016/j.baae.2014.10.001
Borchardt K. E., Kadelka C., Schulte L. A., Toth A. L. (2023). An ecological networks approach reveals restored native vegetation benefits wild bees in agroecosystems. Biol. Conserv. 287, 110300. doi: 10.1016/j.biocon.2023.110300
Buhk C., Oppermann R., Schanowski A., Bleil R., Lüdemann J., Maus C. (2018). Flower strip networks offer promising long term effects on pollinator species richness in intensively cultivated agricultural areas. BMC Ecol. 18, 55. doi: 10.1186/s12898-018-0210-z
Cheptou P. O., Lepart J., Escarre J. (2001). Differential outcrossing rates in dispersing and non-dispersing achenes in the heterocarpic plant Crepis sancta (Asteraceae). Evol. Ecol. 15, 1–13. doi: 10.1023/A:1011961905525
Claβen-Bockhoff R., Wester P., Tweraser E. (2003). The staminal lever mechanism in Salvia L. (Lamiaceae) - a review. Plant Biol. Stutt) 5, 33–41. doi: 10.1055/s-2003-37973
Dainese M., Martin E. A., Aizen M. A., Albrecht M., Bartomeus I., Bommarco R., et al. (2019). A global synthesis reveals biodiversity-mediated benefits for crop production. Sci. Adv. 5, eaax0121. doi: 10.1126/sciadv.aax0121
Eichenberg D., Bowler D. E., Bonn A., Bruelheide H., Grescho V., Harter D., et al. (2020). Widespread decline in central european plant diversity across six decades. Glob. Chang. Biol. 27, 1097–1110. doi: 10.1111/gcb.15447
Ekroos J., Jakobsson A., Wideen J., Herbertsson L., Rundlöf M., Smith H. G. (2015). Effects of landscape composition and configuration on pollination in a native herb: a field experiment. Oecologia 179, 509–518. doi: 10.1007/s00442-015-3370-y
Fenster C. B., Armbruster W. S., Wilson P., Dudash M. R., Thomson J. D. (2004). Pollination syndromes and floral specialization. Annu. Rev. Ecol. Evol. Syst. 35, 375–403. doi: 10.1146/annurev.ecolsys.34.011802.132347
Garibaldi L. A., Steffan-Dewenter I., Winfree R., Aizen M. A., Bommarco R., Cunningham S. A., et al. (2013). Wild pollinators enhance fruit set of crops regardless of honey bee abundance. Science 339, 1608–1611. doi: 10.1126/science.1230200
Garratt M. P. D., de Groot G. A., Albrecht M., Bosch J., Breeze T. D., Fountain M. T., et al. (2021). Opportunities to reduce pollination deficits and address production shortfalls in an important insect-pollinated crop. Ecol. Appl. 31, e02445. doi: 10.1002/eap.2445
Gossner M. M., Lewinsohn T. M., Kahl T., Grassein F., Boch S., Prati D., et al. (2016). Land-use intensification causes multitrophic homogenization of grassland communities. Nature 540, 266–269. doi: 10.1038/nature20575
Herbertsson L., Ekroos J., Albrecht M., Bartomeus I., Batáry P., Bommarco R., et al. (2021). Bees increase seed set of wild plants while the proportion of arable land has a variable effect on pollination in European agricultural landscapes. Pl. Ecol. Evol. 154, 341–350. doi: 10.5091/plecevo.2021.1884
Herrera-Mesías F., Bause C., Ogan S., Burger H., Ayasse M., Weigand A. M., et al. (2022). Double-blind validation of alternative wild bee identification techniques: DNA metabarcoding and in vivo determination in the field. JHR 93, 189–214. doi: 10.3897/jhr.93.86723
Hevia V., Carmona C. P., Azcárate F. M., Heredia R., González J. A. (2021). Role of floral strips and semi-natural habitats as enhancers of wild bee functional diversity in intensive agricultural landscapes. Agric. Ecosyst. Environ. 319, 107544. doi: 10.1016/j.agee.2021.107544
Hirsch M., Pfaff S., Wolters V. (2003). The influence of matrix type on flower visitors of Centaurea jacea L. Agric. Ecosyst. Environ. 98, 331–337. doi: 10.1016/S0167-8809(03)00093-8
Hsieh T. C., Ma K. H., Chao A. (2016). iNEXT: an R package for rarefaction and extrapolation of species diversity (Hill numbers). Methods Ecol. Evol. 7, 1451–1456. doi: 10.1111/2041-210X.12613
Jansen F., Bonn A., Bowler D. E., Bruelheide H., Eichenberg D. (2020). Moderately common plants show highest relative losses. Conserv. Lett. 13 (1), e12674. doi: 10.1111/conl.12674
Kennedy C. M., Lonsdorf E., Neel M. C., Williams N. M., Ricketts T. H., Winfree R., et al. (2013). A global quantitative synthesis of local and landscape effects on wild bee pollinators in agroecosystems. Ecol. Lett. 16, 584–599. doi: 10.1111/ele.12082
Klein A.-M., Vaissière B. E., Cane J. H., Steffan-Dewenter I., Cunningham S. A., Kremen C., et al. (2007). Importance of pollinators in changing landscapes for world crops. Proc. Biol. Sci. 274, 303–313. doi: 10.1098/rspb.2006.3721
Knight T. M., Steets J. A., Vamosi J. C., Mazer S. J., Burd M., Campbell D. R., et al. (2005). Pollen limitation of plant reproduction: pattern and process. Annu. Rev. Ecol. Evol. Syst. 36, 467–497. doi: 10.1146/annurev.ecolsys.36.102403.115320
Kordbacheh F., Liebman M., Harris M. (2020). Strips of prairie vegetation placed within row crops can sustain native bee communities. PloS One 15, e0240354. doi: 10.1371/journal.pone.0240354
Kremen C., Wu J., Beckmann M., Bulling M., Garibaldi L., Krisztin T., et al. (2023). Biodiversity-production feedback effects lead to intensification traps in agricultural landscapes. preprint 00, 0. doi: 10.21203/rs.3.rs-2923694/v1
Kuppler J., Neumüller U., Mayr A. V., Hopfenmüller S., Weiß K., Prosi R., et al. (2023). Favourite plants of wild bees. Agric. Ecosyst. Environ. 342, 108266. doi: 10.1016/j.agee.2022.108266
Lamont B. B., Groom P. K. (2013). Seeds as a source of carbon, nitrogen, and phosphorus for seedling establishment in temperate regions: a synthesis. AJPS 04, 30–40. doi: 10.4236/ajps.2013.45A005
Magrach A., Molina F. P., Bartomeus I. (2021). Niche complementarity among pollinators increases community-level plant reproductive success. PeerJ 1. doi: 10.24072/pcjournal.1
Mallinger R. E., Bradshaw J., Varenhorst A. J., Prasifka J. R. (2019). Native solitary bees provide economically significant pollination services to confection sunflowers (Helianthus annuus L.) (Asterales: Asteraceae) grown across the northern great plains. J. Econ. Entomol. 112, 40–48. doi: 10.1093/jee/toy322
Meyer S., Bergmeier E., Becker T., Wesche K., Krause B., Leuschner C. (2015). Detecting long-term losses at the plant community level - arable fields in Germany revisited. Appl. Veg. Sci. 18, 432–442. doi: 10.1111/avsc.12168
Morris W. F., Vázquez D. P., Chacoff N. P. (2010). Benefit and cost curves for typical pollination mutualisms. Ecology 91, 1276–1285. doi: 10.1890/08-2278.1
Moughan J., McGinn K. J., Jones L., Rich T. C. G., Waters E., Vere N. (2021). Biological flora of the british isles: Salvia pratensis. J. Ecol. 109, 4171–4190. doi: 10.1111/1365-2745.13805
Müller A., Diener S., Schnyder S., Stutz K., Sedivy C., Dorn S. (2006). Quantitative pollen requirements of solitary bees: Implications for bee conservation and the evolution of bee–flower relationships. Biol. Conserv. 130, 604–615. doi: 10.1016/j.biocon.2006.01.023
Müller A., Kuhlmann M. (2008). Pollen hosts of western palaearctic bees of the genus Colletes (Hymenoptera: Colletidae): the Asteraceae paradox. Biol. J. Linn. Soc. 95, 719–733. doi: 10.1111/j.1095-8312.2008.01113.x
Ne’eman G., Dafni A., Potss S. G. (2000). The effect of fire on flower visitation rate and fruit set in four core-species in east Mediterranean scrubland. Plant Ecol. 146, 97–104. doi: 10.1023/A:1009815318590
Neumüller U., Burger H., Krausch S., Blüthgen N., Ayasse M. (2020). Interactions of local habitat type, landscape composition and flower availability moderate wild bee communities. Landsc. Ecol. 35, 2209–2224. doi: 10.1007/s10980-020-01096-4
Neumüller U., Burger H., Mayr A. V., Hopfenmüller S., Krausch S., Herwig N., et al. (2022). Artificial nesting hills promote wild bees in agricultural landscapes. Insects 13 (8), 726. doi: 10.3390/insects13080726
Neumüller U., Burger H., Schwenninger H.-R., Hopfenmüller S., Krausch S., Weiß K., et al. (2021). Prolonged blooming season of flower plantings increases wild bee abundance and richness in agricultural landscapes. Biodivers. Conserv. 30, 3003–3021. doi: 10.1007/s10531-021-02233-4
Niklas K. J. (2008). “Embryo morphology and seedling evolution,” in Seedling Ecology and Evolution. Eds. Leck M. A., Simpson R. L., Parker V. T. (Cambridge: Cambridge University Press), 103–129.
Ollerton J., Winfree R., Tarrant S. (2011). How many flowering plants are pollinated by animals? Oikos 120, 321–326. doi: 10.1111/j.1600-0706.2010.18644.x
Osterman J., Benton F., Hellström S., Luderer-Pflimpfl M., Pöpel-Eisenbrandt A.-K., Wild B. S., et al. (2023). Mason bees and honey bees synergistically enhance fruit set in sweet cherry orchards. Ecol. Evol. 13, e10289. doi: 10.1002/ece3.10289
Peet R. K. (1974). The measurement of species diversity. Annu. Rev. Ecol. Syst. 5, 285–307. doi: 10.1146/annurev.es.05.110174.001441
Potts S. G., Biesmeijer J. C., Kremen C., Neumann P., Schweiger O., Kunin W. E. (2010). Global pollinator declines: trends, impacts and drivers. Trends Ecol. Evol. 25, 345–353. doi: 10.1016/j.tree.2010.01.007
Reith M., Baumann G., Classen-Bockhoff R., Speck T. (2007). New insights into the functional morphology of the lever mechanism of Salvia pratensis (Lamiaceae). Ann. Bot. 100, 393–400. doi: 10.1093/aob/mcm031
Requier F., Leonhardt S. D. (2020). Beyond flowers: including non-floral resources in bee conservation schemes. J. Insect Conserv. 24, 5–16. doi: 10.1007/s10841-019-00206-1
Robinson R. A., Sutherland W. J. (2002). Post-war changes in arable farming and biodiversity in Great Britain. J. Appl. Ecol. 39, 157–176. doi: 10.1046/j.1365-2664.2002.00695.x
Rodger J. G., Bennett J. M., Razanajatovo M., Knight T. M., van Kleunen M., Ashman T.-L., et al. (2021). Widespread vulnerability of flowering plant seed production to pollinator declines. Sci. Adv. 7, eabd3524. doi: 10.1126/sciadv.abd3524
Saatkamp A., Cochrane A., Commander L., Guja L. K., Jimenez-Alfaro B., Larson J., et al. (2019). A research agenda for seed-trait functional ecology. New Phytol. 221, 1764–1775. doi: 10.1111/nph.15502
Sáez A., Morales C. L., Ramos L. Y., Aizen M. A. (2014). Extremely frequent bee visits increase pollen deposition but reduce drupelet set in raspberry. J. Appl. Ecol. 51, 1603–1612. doi: 10.1111/1365-2664.12325
Scheper J., Badenhausser I., Kantelhardt J., Kirchweger S., Bartomeus I., Bretagnolle V., et al. (2023). Biodiversity and pollination benefits trade off against profit in an intensive farming system. Proc. Natl. Acad. Sci. U.S.A. 120, e2212124120. doi: 10.1073/pnas.2212124120
Scheper J., Reemer M., van Kats R., Ozinga W. A., van der Linden G. T. J., Schaminée J. H. J., et al. (2014). Museum specimens reveal loss of pollen host plants as key factor driving wild bee decline in The Netherlands. Proc. Natl. Acad. Sci. U.S.A. 111, 17552–17557. doi: 10.1073/pnas.1412973111
Schils R. L., Bufe C., Rhymer C. M., Francksen R. M., Klaus V. H., Abdalla M., et al. (2022). Permanent grasslands in Europe: Land use change and intensification decrease their multifunctionality. Agric. Ecosyst. Environ. 330, 107891. doi: 10.1016/j.agee.2022.107891
Schwarz B., Dormann C. F., Vázquez D. P., Fründ J. (2021). Within-day dynamics of plant-pollinator networks are dominated by early flower closure: an experimental test of network plasticity. Oecologia 196, 781–794. doi: 10.1007/s00442-021-04952-5
Sirami C., Gross N., Baillod A. B., Bertrand C., Carrié R., Hass A., et al. (2019). Increasing crop heterogeneity enhances multitrophic diversity across agricultural regions. Proc. Natl. Acad. Sci. U.S.A. 116, 16442–16447. doi: 10.1073/pnas.1906419116
Socher S. A., Prati D., Boch S., Müller J., Baumbach H., Gockel S., et al. (2013). Interacting effects of fertilization, mowing and grazing on plant species diversity of 1500 grasslands in Germany differ between regions. Basic. Appl. Ecol. 14, 126–136. doi: 10.1016/j.baae.2012.12.003
Söderman A. M., Irminger Street T., Hall K., Olsson O., Prentice H. C., Smith H. G. (2018). The value of small arable habitats in the agricultural landscape: Importance for vascular plants and the provisioning of floral resources for bees. Ecol. Indic. 84, 553–563. doi: 10.1016/j.ecolind.2017.09.024
Stang M., Klinkhamer P. G. L., Waser N. M., Stang I., van der Meijden E. (2009). Size-specific interaction patterns and size matching in a plant-pollinator interaction web. Ann. Bot. 103, 1459–1469. doi: 10.1093/aob/mcp027
Steffan-Dewenter I., Münzenberg U., Bürger C., Thies C., Tscharntke T. (2002). Scale-dependent effects of landscape context on three pollinator groups. Ecology 83, 1421–1432. doi: 10.1890/0012-9658(2002)083[1421:SDEOLC]2.0.CO;2
Steffan-Dewenter I., Münzenberg U., Tscharntke T. (2001). Pollination, seed set and seed predation on a landscape scale. Proc. Biol. Sci. 268, 1685–1690. doi: 10.1098/rspb.2001.1737
Steffan-Dewenter I., Tscharntke T. (1997). Bee diversity and seed set in fragmented habitats. Acta Hortic. 437, 231–234. doi: 10.17660/ActaHortic.1997.437.24
Steffan-Dewenter I., Tscharntke T. (1999). Effects of habitat isolation on pollinator communities and seed set. Oecologia 121, 432–440. doi: 10.1007/s004420050949
Tobajas E., Domínguez-García V., Molina F. P., Bartomeus I. (2024). Pollinator asynchrony drives the temporal stability of flower visitation rates, but not of plant reproductive success. J. Ecol. 122, 4–13. doi: 10.1111/1365-2745.14216
Tschumi M., Albrecht M., Bärtschi C., Collatz J., Entling M. H., Jacot K. (2016). Perennial, species-rich wildflower strips enhance pest control and crop yield. Agric. Ecosyst. Environ. 220, 97–103. doi: 10.1016/j.agee.2016.01.001
Turnbull L. A., Crawley M. J., Rees M. (2000). Are plant populations seed-limited? A review of seed sowing experiments. Oikos 88, 225–238. doi: 10.1034/j.1600-0706.2000.880201.x
van der Meersch V., Billaud O., San Cristobal M., Vialatte A., Porcher E. (2022). Landscape floral resources provided by rapeseed correlate with next-year reproduction of cavity-nesting pollinators in a national participatory monitoring program. Landsc. Ecol. 37, 551–565. doi: 10.1007/s10980-021-01353-0
Verein Deutscher Ingenieure e.V. (2023). Biodiversität - Standardisierte bestandsschonende Erfassung von Wildbienen für ein Langzeitmonitoring Blatt 1 (Düsseldorf, Germany: Technologies of Life Sciences).
Westrich P., Frommer U., Mandery K., Riemann H., Ruhnke H., Saure C., et al. (2011). Rote Liste und Gesamtartenliste der Bienen (Hymenoptera, Apidae) Deutschlands (Bonn-Bad Godesberg: Bundesamt für Naturschutz).
Winfree R., Aguilar R., Vázquez D. P., LeBuhn G., Aizen M. A. (2009). A meta-analysis of bees’ responses to anthropogenic disturbance. Ecology 90, 2068–2076. doi: 10.1890/08-1245.1
Wood S. (2012). mgcv: Mixed GAM Computation Vehicle with GCV/AIC/REML smoothness estimation. Available at: http://cran.r-project.org/web/packages/mgcv/index.html.
Woodcock B. A., Garratt M. P. D., Powney G. D., Shaw R. F., Osborne J. L., Soroka J., et al. (2019). Meta-analysis reveals that pollinator functional diversity and abundance enhance crop pollination and yield. Nat. Commun. 10, 1481. doi: 10.1038/s41467-019-09393-6
Zhang B., Claßen-Bockhoff R. (2019). Sex-differential reproduction success and selection on floral traits in gynodioecious Salvia pratensis. BMC Plant Biol. 19, 375. doi: 10.1186/s12870-019-1972-y
Keywords: pollination, near-natural grassland, wild flower strip, plant reproduction, wild bee
Citation: Heuel KC, Ayasse M and Burger H (2024) Bee-diverse habitats positively affect seed set in wild plant species. Front. Ecol. Evol. 12:1343885. doi: 10.3389/fevo.2024.1343885
Received: 24 November 2023; Accepted: 22 January 2024;
Published: 06 February 2024.
Edited by:
Kris Braman, University of Georgia, United StatesReviewed by:
Michael Cunningham-Minnick, University of Massachusetts Amherst, United StatesBodie Pennisi, University of Georgia, United States
Copyright © 2024 Heuel, Ayasse and Burger. This is an open-access article distributed under the terms of the Creative Commons Attribution License (CC BY). The use, distribution or reproduction in other forums is permitted, provided the original author(s) and the copyright owner(s) are credited and that the original publication in this journal is cited, in accordance with accepted academic practice. No use, distribution or reproduction is permitted which does not comply with these terms.
*Correspondence: Kim C. Heuel, kim.heuel@gmail.com