- 1School of Earth Science, Yunnan University, Kunming, China
- 2Shilin Scenic Spot Management Bureau, Kunming, China
- 3Yunnan International Joint Laboratory of Critical Mineral Resource, Kunming, China
This study considered Shilin World Geopark as the research object and constructed a landscape ecological risk assessment model based on the landscape pattern index by using remote sensing image data during five periods between 2000 and 2020. In addition, it analyzed the spatial and temporal changes of landscape ecological risk in the region. Spatial autocorrelation analysis was utilized to study spatial differences in the landscape ecological risk in the park. The results showed that during the study period, (1) cultivated land, forest land, and rocky desertification land were the main landscape types, different landscape types differed, and the area of rocky desertification land and building land increased by 37.47 km2 and 14.29 km2, respectively, while the area of cultivated land and grassland decreased significantly, with changes of 34.11 km2 and 18.67 km2; (2) landscape ecological risk of the park showed significant spatial differences, the ‘high–high’ risk areas have been concentrated mainly in the central and northern parts of the park, the ‘low–low’ risk areas have been concentrated in the central part and the southwest-southeast area of the park; and (3) landscape ecological risk of the geopark has been increasing, with the degree of landscape ecological risk being spatially positively correlated. The results of the study are of great significance for maintaining ecosystem health of the Shilin World Geopark and optimizing the ecological risk management of the park.
1 Introduction
As an ecosystem is the basis for human survival and development, maintaining ecosystem health is crucial for ensuring human health (Zeng et al., 1999; Ma et al., 2001). Scientific ecological risk management to maintain ecosystem health is a prerequisite for the harmonious development of human beings and the nature (Peng et al., 2007; Peng et al., 2015; Sahraoui et al., 2021). To effectively avoid, proactively adapt to, and comprehensively manage the ecological risk of a region and achieve the purpose of scientific management of regional ecological risk, a scientific and accurate evaluation of the ecological risk of a region from the perspective of the coupling of human beings and nature is essential (Cao and Shen, 1991; Huang et al., 2022). Ecological risk assessment serves as a basis for different environmental aspects such as ecological construction and environmental restoration, and its accuracy can have a direct impact on the management of regional ecological risks (Yang et al., 2007; Ayre and Landis, 2012). With the development of landscape ecology spatial heterogeneity and landscape pattern research in regional studies, and the continuous combination with ecological risk assessment, landscape ecological risk assessment has emerged (Zhang et al., 2012; Gong et al., 2020). Landscape ecological risk evaluation involves the analysis of correlation between landscape ecological processes and spatial patterns, providing a comprehensive description of multiple ecological risks in a region and facilitating spatial visualization, which constitutes a major branch of ecological risk evaluation on the regional scale (Qiu et al., 2007; Qiao et al., 2021; Rosero et al., 2023). Landscape ecological risk analysis not only highlights the influence of landscape pattern on ecological processes and ecological functions but also emphasizes the spatial and temporal heterogeneities of ecological risks and scale effects, thereby providing a theoretical basis for decision-making in regional integrated risk management (Peng et al., 2007; Ai et al., 2022).
Scholars in different research areas have conducted the landscape ecological risk assessments based on their evaluation purposes, related evaluation indicators, and method models (Peng et al., 2015; Yu et al., 2022). The primary evaluation targets are administrative regions and urban areas, followed by areas with intense anthropogenic activities and ecologically sensitive areas, including river basins (Liu et al., 2016; Cao et al., 2018), coastal areas (Chen et al., 2021), mining areas (Zhou et al., 2018), cities (Fang et al., 2014; Li et al., 2019), and wetlands (Zhang et al., 2019; Lou et al., 2020). Entropy method, comprehensive index method, model method(Chen and Li, 2017), and exposure-response method (Zhang et al., 2016) are the commonly employed evaluation methods.
Karst area is one of the main ecologically sensitive zones in the world, and the study of its ecological environment is a popular topic in geoscience research (Yuan, 1997; Yuan, 2001). Southwest China exhibits the most concentrated karst distribution in the world. Because of the low soil-making and surface water-holding capacity of karst, in addition to the large population, less available land, contradictions between humans and environment, expansion of cities, and unreasonable utilization of resources, the ecological environment in southwest China has degraded considerably, resulting in the prominence of environmental problems such as rocky desertification (Cai, 1996; Chen, 2017). The ecosystem in karst area is complex and sensitive; thus, evaluating the landscape ecological risk in this area can provide valuable insights for implementing preventive measures. Shilin World Geopark, located at the center of southwest karst area, is the only karst landform scenic spot in the subtropical plateau mountainous area in the world. It was listed in the first batch of national geoparks in 2001, and in 2007, it was designated as the ‘Karst in South China’ heritage site and included in the World Heritage List by UNESCO.
Considering Shilin World Geopark as the research area, this study uses the related theories of landscape ecology. Based on GIS and RS technologies and using Landsat remote sensing images from 2000 to 2020 as the main data source, the present study analyses the spatiotemporal changes of landscape pattern. The findings of this study provide scientific insights for understanding the spatial distribution characteristics and changing rules of landscape ecological risks in Shilin World Geopark and constructing a landscape ecological risk evaluation model, thereby providing a scientific basis for park ecological risk management.
Shilin World Geopark exhibits almost all karst forms in the world, which is of high value for geological research due to the antiquity, complexity, multi-phase, and diversity of karst development and evolution (Chen, 2018; Chen et al., 2018), the study results of the research on landscape pattern change and landscape ecological risk are of great significance for maintaining the ecosystem health of the geopark and optimizing the ecological risk management of the park. And this study did not quantitatively analyze the factors affecting regional landscape ecological risk, such as human activities and the economy, due to data collection, etc., and the authors will conduct a more in-depth investigation of the mechanisms affecting landscape ecological risk in a subsequent study.
2 General situation of study area
Shilin World Geopark is located in Shilin Yi Autonomous County (referred to as ‘Shilin County’ in text), which is 76 km away from Kunming, the capital of Yunnan Province. It is located between 103°16′ 43″-103°28′ 28″ east longitude and 24°56′ 47″-24°37′ 30″ north latitude (Figure 1), including Shilin town and lake-town, both of which fall under the jurisdiction of Shilin County. The park covers the whole area of Shilin County, spanning approximately 35.7 km from north to south and 19.8 km from east to west, with a total area of approximately 369.4 km2. The terrain generally inclines downward from the east to the west, with an altitude between 1650 m and 2201 m. This area is characterized by diverse karst landforms including mainly karst hills and depressions and Shilin landforms (Liu, 2020). Owing to its low latitude, the park falls in the monsoon climate zone of the subtropical plateau, with nearly equal periods of rain and heat, and the annual average temperature and rainfall are 16°C and 939.5 mm, respectively. The water area system in the park comprises a surface water area and underground river, named the Nanpan River basin. The main soil types are red soil, yellow brown soil, purple soil, impact soil, and paddy soil. The region has unique karst features, with a thin soil cover and exposed bedrock in most areas.
3 Data sources and research methods
3.1 Data source and preprocessing
Using the geospatial data cloud website (www.gscloud.cn), this study uses the freely downloadable landsat8 OLI and Landsat5 TM data products with the row number 129/43. The data range of this row number of Landsat images covers Shilin County, Kunming City, and Yunnan Province, and the images of Shilin World Geopark can be obtained by cutting this row number of remote sensing images using ArcGIS10.7 software. This study employs the remote sensing image data of Shilin World Geopark during five periods from 2000 to 2020, with a resolution of 30 m. In the study area, vegetation growth is vigorous during summer (from May to August) due to equal periods of rain and heat. Considering the effect of rainfall and cloud cover on the interpretation of remote sensing images, this study selectively uses the data of spring and summer, which are characterized by less cloud cover and better vegetation growth.
Using ENVI5.3 and ArcGIS10.7 software, the remote sensing images were preprocessed with radiometric calibration, atmospheric correction, etc. Support vector machine supervised classification was performed by considering the actual situation of the study area and the research needs, referring to the Google Earth images in the same period, and by using China’s 2017 land use classification standard (GB/T 21010-2017) as the classification standard, the established image decoding flag, and ENVI5.3 software (Yan et al., 2011). The landscape type of Shilin World Geopark was divided into six categories: cultivated land, forest land, grassland, water area, rocky desertification land, and building land, as shown in Figure 2 and Table 1. The supervised classification results of remote sensing images were validated by evaluating the overall accuracy and Kappa coefficient accuracy; the results are presented in Table 2.
3.2 Research method
Six types of landscape pattern indices, such as landscape fragmentation in Shilin World Geopark, were calculated using Fragstats4.2 software. By dividing the landscape ecological risk community of Shilin World Geopark and using the grid analysis method and kriging interpolation method, the landscape risk index of each risk community of the park across different time periods between 2000 and 2020 was calculated. In addition, the landscape ecological risk distribution map of Shilin World Geopark was obtained to analyze the dynamic changes in the landscape ecological risk distribution characteristics of the park.
3.2.1 Division of landscape ecological risk communities
In landscape ecology related studies, the area of the landscape sample is set to be 2–5 times the average area of the patch to better reflect the information of the landscape pattern around the sample area (Su and He, 2010). The average size of landscape patches in the study area is 0.388 km2, considering the total area of Shilin World Geopark of nearly 369.4 km2 and the research scale of this study, and referring to related studies (Zhao, 2013), the study area was divided into 1200 m ×1200 m grid cells, resulting in a total of 314 risk communities.
3.2.2 Construction of the landscape ecological risk index
Based on the structure of a landscape, landscape pattern indicators, which are related to disturbance and can describe the concept and processes of ecosystem, such as landscape dominance and landscape fragmentation, are selected to construct an ecological risk index. Landscape ecological risk index can be used to quantitatively analyze the changes and results of ecosystems in different landscape types under the influence of other factors. Accordingly, landscape pattern indices such as landscape fragmentation were selected in this study, and the damage degree reflecting the natural attributes of ecosystems in various landscape types under the influence of natural and human factors was obtained through superposition calculation of different indices (Jiao et al., 2006). In other words, the landscape loss index was obtained by synthesizing the landscape disturbance index and the landscape vulnerability index, and the landscape structure was linked with the regional ecological risk to describe the comprehensive ecological damage degree of each risk community. Six landscape pattern indices were selected to build a landscape ecological risk index (ERI) model, and their calculation method is shown in Table 3.
Using the landscape ERI model, the ERI value in each landscape ecological risk community was used as the ecological risk index of the central point of the corresponding risk community. Kriging interpolation was performed in ArcGIS software, and the landscape ecological risk distribution map of Shilin World Geopark was obtained.
3.2.3 Spatial analysis method
The landscape ecological risk value calculated through Kriging interpolation is a spatial variable, and this study uses spatial autocorrelation to explore and analyze the spatial differences of landscape ecological risk in the Shilin World Geopark (Xie, 2008). Details of the expression of the global spatial autocorrelation index Moran’s I and the local spatial autocorrelation index LISA used in this paper can be obtained from a previous study (Anselin, 1983).
4 Results and analysis
4.1 Dynamic changes of landscape pattern
The landscape pattern index (Table 4) of six types of landscape during five periods from 2000 to 2020 in the study area was calculated using Fragstats4.2 software. Natural and human factors have led to changes in the different types of landscape areas (Figure 3), which has changed the risk indices of corresponding landscape types (Gao et al., 2010). The main landscape types of Shilin World Geopark are cultivated land, forest land, and rocky desertification land. Cultivated land landscape area has decreased over the years, with the decrease in the cultivated land area being the most significant. Landscape separation and fragmentation indices have increased, resulting in increased landscape ERIs. During the early stages of research, the cultivated land landscape had a concentrated spatial distribution, which gradually transformed into a scattered small random distribution. During the study period, the area of forest land landscape first increased and then gradually decreased, with the overall change being slight. The landscape damage and separation indices also showed a fluctuating trend, and the landscape ERI increased. Grassland landscape area was the smallest, and its area fluctuated and changed considerably during the study period. The area of water area landscape and its variation range were both small, and the landscape fragmentation and separation indices were high and exhibited fluctuations. The grassland and water area landscape had a scattered and random spatial distribution. Rocky desertification landscape was the most dominant landscape in the park. Because of the increasing karst rocky desertification in the park, its area is increasing every year. The rocky desertification landscape had the highest fragility among the six landscape types. Landscape damage and separation showed a decreasing trend, and the spatial distribution changed from being small random scattered to concentrated contiguous distribution. With the economic and tourism development in the park, the landscape area of the building land has increased, and landscape fragmentation and separation indices have decreased, showing a more concentrated spatial distribution. Many factors, such as landscape type, fragility, and disturbance, affect the landscape ERI (Liu et al., 2018), which can be expressed in terms of landscape loss. In terms of landscape types, grassland and water area had the largest landscape loss index; however, owing to their small areas, their impact on the overall landscape ecological risk of the park was weak. The landscape loss index of the building land and forest land was the smallest. The loss index of the same landscapes also varied across different periods. Except for the loss index of building land landscape, which has gradually decreased over the past 20 years, the loss index of the other five types of landscapes fluctuated.
4.2 Landscape ecological risk analysis
Considering the landscape ecological risk values of 314 ecological risk communities in the study area as the value of the unit center, spatial interpolation of the evaluation unit was performed using kriging interpolation in the geostatistical analysis module of ArcGIS software, and the spatial distribution of the landscape ecological risk of Shilin World Geopark was obtained. According to the distribution characteristics of landscape ERI values of ecological risk assessment units in five periods, and referring to a previous study (Wang and Xu, 2017; Liu et al., 2018), different areas of Shilin World Geopark were divided based on the ecological risks into high-risk area (ERI≥0.38), higher-risk area (0.32≤ERI<0.38), medium-risk area (0.26≤ERI<0.32), lower-risk area (0.20≤ERI<0.26) and low-risk area (ERI<0.20) by using the equal-interval classification method.
4.2.1 Spatial distribution of landscape ecological risk
As shown in Figures 4, 5, from 2000 to 2020, the five types of landscape of Shilin World Geopark exhibited mainly medium and higher ecological risks, and the area of medium and higher-risk grades accounted for 19% and 41% of the total park area. In these 20 years, the overall landscape ecological risk in the study area has continued to increase, and the high-risk and higher-risk areas have also increased. By 2020, the high-risk areas reached 22% of the park area. Specifically, in 2000, the low-risk and lower-risk areas in the park accounted for 6% and 18% of the total area of the park, respectively. Among these, the low-risk areas were mainly distributed in the southwest and southeast. The area of forest land in the southwest of the park was large, and the landscape stability of forest land was higher than that of other landscape types. The degree of rocky desertification in this area was low because of less human activities. The lower-risk areas were concentrated in the southwest-southeast and middle of the park, and forest land landscape was the main landscape type in these areas. The areas with medium-risk and higher-risk levels accounted for 38% and 25% of the park area and were concentrated in the north-central and south, respectively. Rocky desertification land, cultivated land, forest land, and building land were the main landscape types in the north-central and southern parts of the park. The landscape itself had low stability, and human activities and rocky desertification contributed to the increased vulnerability of the landscape. The high-risk area accounted for 13% of the total area and was mainly distributed in the northwest and northeast of the park. The main landscape types were building land, cultivated land, and rocky desertification land, all of which were obviously affected by rocky desertification and human activities.
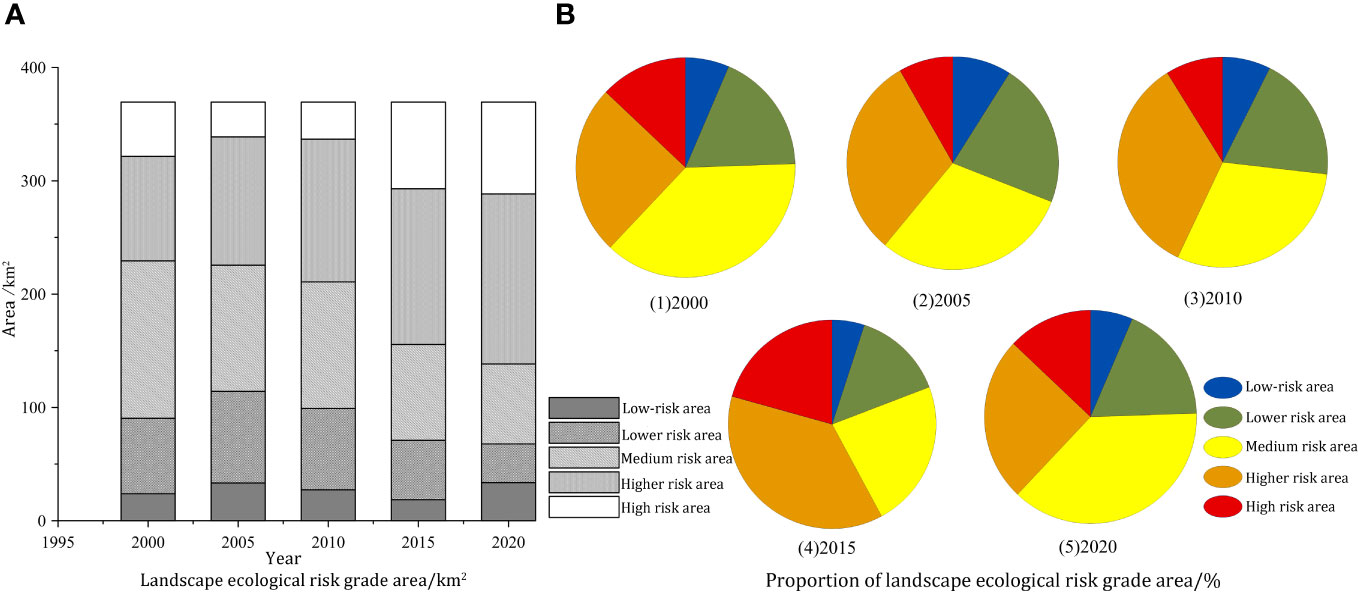
Figure 5 Landscape ecological risk grade area (A) and Proportion of landscape ecological risk grade area (B) of Shilin World Geopark.
In 2005, the areas with low and lower-risk levels accounted for 9% and 22%, respectively, and these were concentrated in the southwest-southeast and south parts of the park. The medium-risk grade area accounted for 30%, and it was mainly distributed in the central and southern parts of the park. The high-risk and higher-risk areas were mainly distributed in the central and northern parts of the park, accounting for 8% and 31% and, respectively.
In 2010, areas with low and lower-risk levels accounted for 7% and 19% of the total park area, respectively. Compared with 2005, low-risk areas in the southeast and south of the park disappeared, while low-risk areas in the middle increased. Medium-risk areas were concentrated in the central and northern parts of the park, accounting for 30% of the total park area. High and higher-risk grade areas gradually spread to the south of the park and were concentrated in the central, northern, and southern parts of the park, accounting for 21% and 37% of the total area, respectively.
In 2015, a small number of areas with low and lower-risk levels were concentrated in the central part of the park, mainly in the southwest-southeast part of the park, accounting for 5% and 14% of the total park area, respectively. During this period, the middle risk grade areas were distributed in the central and southern parts of the park. However, the areas with high and higher-risk levels gradually expanded to the central and southern parts of the park. Except for the contiguous areas from southwest to southeast, all areas had high and higher-risk levels, and they were mainly distributed in the central and northern parts of the park.
In 2020, in addition to the contiguous areas in the southwest and southeast of the park, the areas with low and lower-risk levels in the northwest and middle of the park increased and were more concentrated, whereas the building landscape was distributed in the northwest and middle. In addition, the areas of high and higher-risk levels increased significantly, reaching 22% and 41%, respectively. These areas were distributed in the whole park from north to south, especially in the central high-risk area, and the main landscape type was rocky desertification.
4.2.2 Spatial pattern change of the landscape ecological risk
The analysis of the landscape ecological risk levels of Shilin World Geopark in different periods showed that the area of different landscape ecological risk levels in the park has changed from 2000 to 2020. Low and lower-risk levels areas accounted for a small proportion and fluctuated during the study period. The spatial distribution of these two types of landscape ecological risk areas changed slightly, and they were mainly distributed in the southwest-southeast contiguous area of the park and a small part of the central area. In addition, lower-risk areas in the central area of the study area have increased. With the development of tourism and other industries in the park, the building land area has increased, and the landscape fragility and loss have decreased, resulting in lower landscape ecological risks in this area. During the study period, medium-risk areas changed most obviously, from a concentrated distribution in the central and southern parts of the park at the beginning of the study to a scattered distribution, with their spatial distribution concentrated around low-risk and lower-risk areas in 2020. High and higher-risk levels areas increased obviously during the study period. Furthermore, the spatial distribution gradually spread from the central and northern parts of the study area to the central and southern parts, especially in the central area, which increased rocky desertification in the park, thereby increasing the landscape ecological risk in this area.
4.3 Spatial autocorrelation analysis of ecological risk in Shilin World Geopark
4.3.1 Global correlation analysis of ecological risk
Using spatial autocorrelation to analyze the distribution characteristics of a group of spatial variables is a suitable method for determining the correlation degree of spatial objects in a study area (Anselin, 1983). In this study, the landscape ecological risk value of Shilin World Geopark was determined using Moran’s I index. Spatial autocorrelation analysis was divided into global and local autocorrelation analyses. According to the spatial distribution data characteristics of landscape ecological risk in the park, the global autocorrelation analysis was performed using GeoDa software, and Moran’s I scatter plot was obtained (Figure 6). From 2000 to 2020, global Moran’s I values of the park landscape ecological risk values in the five periods were 0.520, 0.565, 0.486, 0.485, and 0.524, respectively, all of which are >0. This result shows that the landscape ecological risk values of Shilin World Geopark exhibited a positive spatial correlation and an agglomeration effect.
4.3.2 Local correlation analysis of ecological risk
Using the local autocorrelation method, the landscape ecological risk value of Shilin World Geopark was determined, and the local autocorrelation LISA aggregation map of the park was obtained (Figure 7). In the past 20 years, the ‘high–high’ risk areas of the park landscape ecological risk have been concentrated mainly in the central and northern parts of the park, with only a few being in the south. The degree of landscape ecological risk around high-risk areas has been high. The ‘low–low’ risk areas have been concentrated in the central part and the southwest-southeast area of the park. The degree of landscape ecological risk in the area around the low-risk area has been relatively low, with ‘low–high’ and ‘high–low’ risk areas being less distributed in the park.
Rocky desertification and cultivated land were the main landscape types in the concentrated ‘high–high’ risk areas, consistent with the main landscape types in areas with high landscape ecology and higher-risk levels. In the ‘high–high’ risk area, the building land, grassland, and forest land had scattered distribution, high landscape fragility and loss, and poor internal stability of the landscape. Forest land was the main landscape type in the ‘low–low’ risk area of the park, accounting for the low landscape fragility and landscape loss in this area as well as a low landscape ERI.
5 Conclusion
Landscape ecological risk analysis integrates many factors, rather than being a single-factor process. Based on the landscape pattern index, this study constructed a landscape ERI model to quantitatively analyze the landscape ecological risk of Shilin World Geopark. The study findings provide valuable insights into the ecological environment problems of Shilin World Geopark and guidance for the sustainable development and scientific management of the park. Five remote sensing images during the period 2000–2020 were used to examine the landscape pattern changes and spatial–temporal characteristics of landscape ecological risks in Shilin World Geopark. The results can be summarized as follows: (1) Cultivated land, forest land, and rocky desertification land are the main landscape types of Shilin World Geopark. Among these, the areas of cultivated land and forest land have decreased, whereas those of water area, rocky desertification land, and building land have increased, and the grassland landscape area exhibits marked fluctuations. (2) Spatial differences of the landscape ecological risks in Shilin World Geopark have been obvious. High and higher-risk grade areas were concentrated mainly in the north-central part of the park, with some distributed in the south, whereas low and lower-risk grade areas were mainly distributed in the southwest-southeast area of the park. (3) From 2000 to 2020, the landscape ecological risk of Shilin World Geopark showed an overall increasing trend, and its landscape ecological risk degree showed a positive spatial correlation.
6 Discussion
As a typical area in the highland mountain karst region, Shilin World Geopark has a highly fragile ecosystem, which, together with the interference of human activities, contributes to an increase in its landscape ecological risk. Due to the aggravation of problems such as rocky desertification, with the increase in the level of tourism development in the park as well as the influence of anthropogenic factors on the regional landscape, the frequency and intensity of the transformation of landscape types of Shilin World Geopark have increased. These problems have also led to an increase in the spatial-temporal heterogeneity of its landscape pattern and intensified the ecological risk of the landscape. The southwest–southeast zone of the park, where the forest land landscapes exhibit a concentrated distribution, has a small degree of landscape separation, fragility, and loss, which contributes to a reduction in the degree of landscape ecological risk of Shilin World Geopark. During the study period, the local management department implemented various rocky desertification control projects including “artificial afforestation of barren mountains,” “artificial afforestation of sloping cultivated land,” “forestation,” and “grass-fed animal husbandry project,” which led to a reduction in the cultivated land area in the park, except for the permanent basic cultivated land area. In addition, the forest land area underwent constant changes under the double influence of human interference and rocky desertification land, all of which played a pivotal role in changing the ecological risk of the park’s landscape. Some studies have shown that although the landscape of construction land has a low landscape ecological risk, the increase in the area of construction land landscape increases the landscape ecological risk of the surrounding area due to a high level of human activities around the construction land (Fang et al., 2014). The data also showed that rocky desertification land and grasslands exhibited high vulnerability and loss. Additionally, rocky desertification landscape has been the dominant landscape type in the park, contributing to the ecological risk of park landscape and resulting in the increased landscape ecological risk in Shilin World Geopark.
Relevant departments should scientifically plan the development and utilization of land resources in the Shilin World Geopark, and in the future, priority should be given to the protection of its ecological environment as far as possible by minimizing the damage caused by human activities. Moreover, the development and construction of the park should be based on the ecosystem carrying capacity of the park itself. It will require the planners and builders of the park to fully understand and recognize the ecological potential of the park and then implement the ecological development law to ensure that the Geopark’s land resources can be scientifically and reasonably planned, developed, and utilized, and the development of the tourism construction of the Shilin World Geopark can be coordinated with the ecological protection of the park.
To reduce the ecological risk of the park with future development and construction, based on the results of the landscape ecological risk analysis of the study area, this study proposes the following countermeasures for the maintenance and operation management of the Shilin World Geopark:
(1) In the arable land management planning, relevant departments should focus on protecting arable land and the permanent basic cultivated land; for example, if there is a need to withdraw from the area of permanent basic cultivated land within the Shilin World Geopark, the replenishment of Shilin County in the county administrative area should be optimized, and if it is not possible to replenish the county administrative area, the municipal administrative area in Kunming should be replenished; use of the barren or unused areas of cultivated land for construction purpose, as well as the change of the use of arable land for the construction of villages and townships should be prohibited; and other specific measures should be implemented for the protection of arable land.
(2) Within the scope of Shilin World Geopark, forest land is mainly used to provide forestry production, ecological environmental protection, and related services. Therefore, in the park’s forest land management planning, it is necessary to implement suitable measures such as prohibiting unauthorized transformation of forestry land, obtaining approval of the relevant management departments and management methods prior to using the land, prohibiting other land use from arbitrarily occupying forest land as well as indiscriminate logging and destruction of forests, and prohibiting all kinds of construction to take up soil and water conservation forests and water conservation forests as well as other protective forests. To reduce the vulnerability of the ecosystem of the park, appropriate measures should be taken to continuously improve the quality of forest land in the park, increase the proportion of forest land area in the total area of the park, and increase the percentage of forest cover in the park.
(3) The management of building land in the park should be formulated in strict accordance with the relevant national regulations, and the relevant departments should strictly control the scale of each building land, fully utilize the existing building land and vacant land, and revitalize the stock of land. Any unit or individual occupying land, constructing houses, or other works in the park should be examined, and approval of the management organization of Shilin World Geopark should be obtained according to the relevant regulations.
(4) The utilization of water should also be strictly controlled within the scope of Shilin World Geopark, and the Water Law, the Water Pollution Prevention and Control Law, and other regulations should be implemented to strengthen the management and protection of rivers and lakes such as Yunhu Lake, Heilongtan, Bajiang River, and Qingshui River. The enclosure of lakes to create land and the illegal occupation of waters should be prohibited. Measures should be taken to protect the water surface and prevent water pollution. Publicity efforts should be increased to make the residents aware of the importance of protecting, developing, and rationally utilizing aquatic life, while extinction fishing should be prohibited. Reclaiming lakes (reservoirs) and rivers without authorization by any individual or unit should be prohibited.
(5) Comprehensive management of rocky desertification should be intensified and promoted in strict accordance with the management methods such as “Project Management Measures for Rocky Desertification Comprehensive Management Project in Shilin Yi Autonomous County” issued by the Office of the People’s Government of Shilin County. This can further enhance the effectiveness of the comprehensive management of rocky desertification in the park and continuously improve the ecological environment of the Shilin World Geopark.
Because of the long-time span and the difficulty of data collection, this study neither quantitatively analyzed the influence of population and economic factors on landscape ecological risk nor considered the interaction among factors. In the future, we aim to conduct in-depth quantitative research on the influencing factors to understand the interaction among different factors and the impact on the regional landscape ecological risk. In addition, considering the lack of accuracy of remote sensing data and possible errors in the calculation method, we aim to conduct subsequent research by employing suitable methods to improve the accuracy of the research results.
Data availability statement
The original contributions presented in the study are included in the article/supplementary material. Further inquiries can be directed to the corresponding author.
Author contributions
YS: Writing – original draft, Writing – review & editing. HG: Writing – review & editing. ST: Writing – review & editing. HQ: Writing – review & editing. ZT: Writing – review & editing. JM: Writing – review & editing. XZ: Writing – review & editing.
Funding
The author(s) declare financial support was received for the research, authorship, and/or publication of this article. This work was supported by Geological evolution of Yunnan Shilin and its aesthetic and scientific value exploration of Yunnan University (No. H2016073).
Conflict of interest
The authors declare that the research was conducted in the absence of any commercial or financial relationships that could be construed as a potential conflict of interest.
Publisher’s note
All claims expressed in this article are solely those of the authors and do not necessarily represent those of their affiliated organizations, or those of the publisher, the editors and the reviewers. Any product that may be evaluated in this article, or claim that may be made by its manufacturer, is not guaranteed or endorsed by the publisher.
References
Ai J., Yu K., Zeng Z., Yang L., Liu Y., Liu J. (2022). Assessing the dynamic landscape ecological risk and its driving forces in an island city based on optimal spatial scales: Haitan Island, China. Ecol. Indicators. 137, 108771. doi: 10.1016/j.ecolind.2022.108771
Anselin L. (1983). Spatial processes, models and applications by A. D. Cliff, J. K. Ord. Econ. Geogr. 59 (3), 322–325. doi: 10.2307/143420
Ayre K., Landis W. (2012). A bayesian approach to landscape ecological risk assessment applied to the upper grande ronde water areashed, oregon. Hum. AND Ecol. Risk ASSESSMENT. 18), 964–970. doi: 10.1080/10807039.2012.707925
Cai Y. (1996). Preliminary research on ecological reconstruction in karst mountain poverty areas of southwest China. Adv. Earth Sci. 11 (6), 602–606. doi: 10.11867/j.issn.1001-8166.1996.06.0602
Cao H., Shen Y. (1991). Brief rebiew: Ecological risk assessment research. Environ. Chem. 10 (3), 26–30.
Cao Q., Zhang X., Ma H., Wu J. (2018). Review of landscape ecological risk and an assessment framework based on ecological services: ESRISK. Acta GEOGRAPHICA SINICA. 73 (5), 843–855. doi: 10.11821/dlxb201805005
Chen J. (2018). Study on Evaluation of Tourism Ecological Security in Shilin County Based on Landscape Pattern (Kunming: Yunnan University). Master's thesis.
Chen J., Li T. (2017). Landscape ecological risk analysis for Jingzhou City based on PSR model and projection pursuit method. J. Peking Univ. 53 (4), 731–740. doi: 10.13209/j.0479-8023.2017.065
Chen J., Zhao L., Zhu S., Zhao J. (2018). Zoning and management orientation for the comprehensive management of rocky desertification in Shilin County of Yunnan Province. Subtropical Soil Water area Conser. 30 (1), 20–22. doi: 10.3969/j.issn.1002-2651.2018.01.006
Chen X., Xie G., Zhang J. (2021). Landscape ecological risk assessment of land use changes in the coastal area of Haikou City in the past 30 years. Acta ECOLOGICA SINICA. 41 (3), 975–986. doi: 10.5846/stxb201909211974
Chen Y. (2017). Dynamic Evaluation and Prediction of Landscape Ecological Security in Rocky Desertification Area. (Guiyang: Guizhou Normal University). Master's thesis.
Fang G., Xiang B., Wang B., Jin X., Hu Y., Zhang L. (2014). Ecological risk assessment of human activity of rapid economic development regions in southern Jiangsu, China: A case study of Dantu District of Zhenjiang City. Chin. J. Appl. Ecol. 25 (4), 1076–1084. doi: 10.13287/j.1001-9332.2014.0084
Gao Y., Gao J., Xu Y. (2010). Response of landscape ecological risk to land use change in level I aquatic eco-functional regions in Taihu Lake water areashed. J. Nat. Resour. 25 (7), 1088–1096. doi: 10.11849/zrzyxb.2010.07.004
Gong J., Cao E., Xie Y., Xu C., Li H., Yan L. (2020). Integrating ecosystem services and landscape ecological risk into adaptive management: Insights from a western mountain—basin area, China. J. Environ. management. 281(555):111817. doi: 10.1016/j.jenvman.2020.111817
Huang M., Xiao Y., Xu J., Liu J., Wang Y., Gan S., et al. (2022). A Review on the supply-demand relationship and spatial flows of ecosystem services. J. Resour. Ecology. 13 (5), 925–935. doi: 10.5814/j.issn.1674-764x.2022.05.016
Jiao Y., Zhang P., Hu W. (2006). Progress of the human impacts on mountainious landscape. YUNNAN GEOGRAPHIC ENCIRONMENT Res. 05), 38–42+57. doi: 10.3969/j.issn.1001-7852.2006.05.008
Li Q., Zhang Z., Wan L., Yang C., Zhang J., Ye C., et al. (2019). Landscape pattern optimization in Ningjiang River Basin based on landscape ecological risk assessment. Acta GEOGRAPHICA SINICA. 74 (7), 1420–1437. doi: 10.11821/dlxb201907011
Liu X. (2020). Study on the Formation and Evolution Characteristics of Stone Forest in Shilin World Geo-park, Yunnan (Kunming: Yunnan University). Master's thesis.
Liu Y., Li J., Shi X., Yuan Q., Pu R., Yang L. (2016). Landscape ecological risk assessment in tampa bay water areashed of America during 1985-2015. Bull. Soil Water area Conserv. 36 (3), 125–130+135+369. doi: 10.13961/j.cnki.stbctb.2016.03.023
Liu C., Zhang J., Liu J. (2018). A long-term site study for the ecological risk migration of landscapes and its driving forces in the Sanjiang Plain from 1976 to 2013. Acta Ecol. Sin. 38 (11), 3729–3740. doi: 10.5846/stxb201707041208
Lou N., Wang Z., He S. (2020). Assessment on ecological risk of aha lake national wetland park based on landscape pattern. Res. Soil Water area Conser. 27 (1), 233–239. doi: 10.13869/j.cnki.rswc.2020.01.033
Ma K., Kong H., Guan W., Fu B. (2001). Ecosystem health assessment: methods and directions. Acta Ecol. Sin. 21 (12), 2106–2116. doi: 10.3321/j.issn:1000-0933.2001.12.020
Peng J., Dang W., Liu Y., Zong M., Hu X. (2015). Review on landscape ecological risk assessment. Acta Geogr. Sin. 70 (4), 664–677. doi: 10.11821/dlxb201504013
Peng J., Wang Y., Wu J., Zhang Y. (2007). Evaluation for regional ecosystem health: methodology and research progress. Acta Ecol. Sin. 27 (11), 4877–4885. doi: 10.1016/S1872-2032(08)60009-8
Qiao F., Bai Y., Xie L., Yang X., Sun S. (2021). Spatio—Temporal characteristics of landscape ecological risks in the ecological functional zone of the upper yellow river, China. Int. J. Environ. Res. Public Health 18 (24), 12943–12943. doi: 10.3390/ijerph182412943
Qiu P., Xu S., Xie G., Tang B., Bi H., Yu L. (2007). Analysis on the ecological vulnerability of the western Hainan Island based on its landscape pattern ecosystem sensitivity. Acta ECLOLGICA SINICA. 27 (04), 1257–1264. doi: 10.3321/j.issn:1000-0933.2007.04.001
Rosero C., Otero X., Bravo C., Frey C. (2023). Multitemporal incidence of landscape fragmentation in a protected area of central andean Ecuador. land. 12 (2), 500. doi: 10.3390/land12020500
Sahraoui Y., Leski C., Benot M., Revers F., Salles D., Van Halder D., et al. (2021). Integrating ecological networks modelling in a participatory approach for assessing impacts of planning scenarios on landscape connectivity. Landscape Urban Planning. 209, 104039. doi: 10.1016/j.landurbplan.2021.104039
Su H., He A. (2010). Analysis of land use based on RS and geostatistics in Fuzhou City. J. Nat. Resour. 25 (1), 91–99. doi: 10.11849/zrzyxb.2010.01.010
Wang J., Xu C. (2017). Geodetector: principle and prospective. Acta Geographica Sinica. 72 (01), 116–134. doi: 10.11821/dlxb201701010
Xiao L., Tian G. (2014). Eco-risk assessment of land use in Tianjin City. Chin. J. Ecol. 33 (2), 469–476. doi: 10.13292/j.1000-4890.2014.0031
Xie H. (2008). Regional eco-risk analysis ofbased on landscape structure and spatial statistics. Acta Ecol. Sin. 28 (10), 5020–5026. doi: 10.3321/j.issn:1000-0933.2008.10.048
Yan Y., Dong X., Li Y. (2011). The comparative study of remote sensing image supervised classification methods based on ENVI. Beijing Surv. Mapp. 3, 14–16. doi: 10.19580/j.cnki.1007-3000.2011.03.005
Yang L., Li Y., Jia L., Ji Y., Hu G. (2023). Ecological risk assessment and ecological security pattern optimization in the middle reaches of the Yellow River based on ERI+MCR model. J. Geographical Sci. 33), 1442–1460. doi: 10.1007/s11442-023-2108-8
Yang W., Wang R., Huang J., Li F., Chen Z. (2007). Ecological risk assessment and its research progress. Chin. J. Appl. Ecol. 19 (8), 1869–1876. doi: CNKI:SUN:YYSB.0.2007-08-033
Yu J., Tang B., Chen Y., Zhang L., Nie Y., Deng W. (2022). Landscape ecological risk assessment and ecological security pattern construction in landscape resource-based city: A case study of Zhangjiajie City. Acta Ecologica Sinica. 42 (4), 1290–1299. doi: 10.5846/stxb202012313341
Yuan D. (1997). On the environmental and geologic problems of karst and rocks in the south-west China. World Sci-Tech R&D. 5), 41–43. doi: 10.16507/j.issn.1006-6055.1997.05.010
Yuan D. (2001). World correlation of karst ecosystem: objectives and implementation plan. Adv. Earth Sci. 4), 461–466. doi: 10.11867/j.issn.1001-8166.2001.04.0461
Zeng D., Jiang F., Fan Z., Du X. (1999). Ecosystem health and sustainable development for human. Chin. J. Appl. Ecol. 10 (6), 751–756. doi: CNKI:SUN:YYSB.0.1999-06-027
Zhang Y., Cai X., Yang C., Song X., Li R., Li E. (2019). Driving force analysis of landscape pattern changes in Honghu Wetland Nature Reserve in recent 40 years. J. Lake Sci. 31 (1), 171–182. doi: 10.18307/2019.0116
Zhang Y., Fang Y., He F., Shao Q. (2016). Ecological risk dynamic assessment based on simulation of land use change. Geomatics Spatial Inf. Technol. 39 (1), 5–8,12. doi: 10.3969/j.issn.1672-5867.2016.01.002
Zhang Y., Lei G., Lin J., Zhang H. (2012). Spatiotemporal change and its ecological risk of landscape pattern in different spatial scales in Zhalong Nature Reserve. Chin. J. Ecology. 31 (05), 1250–1256. doi: 10.13292/j.1000-4890.2012.0193
Zhao C. (2013). Ecological Risk Assessment in the Bailongjiang Water areashed in Southern Gansu (Lanzhou: Lanzhou University). Master's thesis.
Keywords: landscape pattern, landscape ecological risk, landscape index, Shilin World Geopark, spatial autocorrelation
Citation: Shi Y, Gao H, Tan S, Qin H, Tian Z, Meng J and Zheng X (2024) Pattern change and ecological risk analysis of Shilin World Geopark landscape. Front. Ecol. Evol. 12:1341969. doi: 10.3389/fevo.2024.1341969
Received: 21 November 2023; Accepted: 19 January 2024;
Published: 05 February 2024.
Edited by:
Changchun Huang, Nanjing Normal University, ChinaReviewed by:
Liu Chunxue, Yunnan University of Finance And Economics, ChinaYuanmei Jiao, Yunnan Normal University, China
Copyright © 2024 Shi, Gao, Tan, Qin, Tian, Meng and Zheng. This is an open-access article distributed under the terms of the Creative Commons Attribution License (CC BY). The use, distribution or reproduction in other forums is permitted, provided the original author(s) and the copyright owner(s) are credited and that the original publication in this journal is cited, in accordance with accepted academic practice. No use, distribution or reproduction is permitted which does not comply with these terms.
*Correspondence: Shucheng Tan, shchtan@ynu.edu.cn