- 1Department of Biotechnology, Delhi Technological University, Delhi, India
- 2Biology Department, Silliman University, Dumaguete, Philippines
Being chemically inert and morphologically similar (<5 mm in size) to the natural prey of copepods, microplastics (MPs) affect them through entanglement, ingestion, and interference with their natural prey selection. The effects of external factors, such as MP, can be observed in the form of alterations in the functional and numerical responses of copepods. Functional response is explained as the relationship between the intake rate of a consumer and the amount of food available in a particular ecotope. Considered three types of functional response: (i) with increasing food density, when intake rate increases linearly until asymptote, called Type-I functional response; (ii) when the proportion of prey ingested by the consumer decreases monotonically with prey density, considered as a Type-II functional response; and (iii) when ingestion rate and food density show a sigmoid relationship, is considered as Type-III functional response. In the present study, we attempted to elucidate the effects of MP on the functional responses of brackish water, demersal, calanoid copepod, Pseudodiaptomus annandalei, feeding on the rotifer Brachionus rotundiformis, and the ciliate Frontonia microstoma. The ability of P. annandalei to survive environmental fluctuations and its nutritional value make it an ecologically important organism in estuarine ecosystems. The experiment for estimation of functional response included four setups with adult and copepodite stages of P. annandalei, each with six replicates at six different prey densities, in the presence and absence of MP particles. Without MPs, P. annandalei showed a typical Type-II functional response, where the proportion of prey consumed significantly declined with increasing prey density. In the MP-applied medium, the proportion of prey consumed did not exhibit a significant relationship with the initial prey density. The number of ciliates and rotifers ingested by copepodites and adults was significantly lower in MP-contaminated medium than in prey either monospecific or in combination with microalgae. Because of the comparable size of MP to the prey species and unspecialized feeding of P. annandalei in natural waters, we hypothesized reduced predation rates as a result of pseudo-satiation resulting from MP ingestion leading to malnutrition and MP storage in copepod biomass.
Highlights
● Microplastic (MP) has negative effects on prey consumption rates of Pseudodiaptomus annandalei
● P. annandalei exhibited type-II functional response to monospecific prey and in the presence of microalgae
● MPs per se caused deviation from Type-II functional response
● MP contamination caused a ~20%–95% reduction in prey consumption by P. annandalei copepodites and adults.
● MP imposed alterations in the functional response type and prey ingestion can be used as sensitive endpoints to establish the impact of MP pollution.
Introduction
Microplastic (MP) particles have been reported from surface waters to the deepest trenches in every ocean (Auta et al., 2017; Richon et al., 2022). The rise in oceanic contamination by these small (<5 mm) and sometimes down to the micro- to nanometer scale (Barnes et al., 2009; Ter Halle et al., 2017) anthropogenic debris may lead to widespread contamination of oceanic food webs. MP particles or fibers have become emergent pllutants in estuarine and marine ecosystems. Food-web contamination by MP has been observed in fish and other higher trophic levels (Neves et al., 2015; Ugwu et al., 2021; Nair and Perumal, 2022; Avignon et al., 2023).
Recent studies have found MPs in wild-caught and commercial fish stomachs and guts (Jabeen et al., 2017; Baalkhuyur et al., 2018; Halstead et al., 2018; Kumari et al., 2023b), indicating large-scale MP contamination of the oceanic food web caused by direct MP ingestion (Roch et al., 2020), indirect MP ingestion, such as consumption of contaminated prey, or MP entanglements (Setälä et al., 2014; Nelms et al., 2018; Zhang et al., 2019). The ubiquitous nature of MP pollution in air, water, and soil has become a major concern among environmental activists, scientists, and citizens alike (Cunningham et al., 2020). In estuarine and brackish water ecosystems, copepods and rotifers play an important role in linking nano-and microplankton to higher trophic levels (Beaugrand et al., 2003; Tseng et al., 2011). Food-web contamination may begin with MP ingestion by zooplankton, which is the primary consumer and constitutes the lowest heterotrophic trophic level of aquatic ecosystems (Kumar, 2005; Amélineau et al., 2016; Richon et al., 2022). Zooplankton contamination with MPs may also have several biological implications, such as decreased growth, reproduction, and lower survival rates (Cole et al., 2019; Isinibilir et al., 2023). Their position in the food web is at the interface between the primary producers and higher trophic levels (Kumar, 2005; Kerfoot and DeMott, 2019). Thus, MP ingestion by zooplankton may act as an entry point for MPs into the marine food web (Gunaalan et al., 2023).
The ingestion of inert particles such as plastic beads by calanoid copepods has been frequently documented (Bundy and Vanderploeg, 2002; Ferrao Filho et al., 2005). With the help of mechanoreception ability, calanoid copepods can detect boundary layer disturbances and ingest non-motile inert particles in proximity to the first antennae of copepods (Bundy et al., 1998; Ayukai, 2020). These copepods redirect and ingest large inert particles entrained in their feeding currents (Vanderploeg et al., 1990). MPs are chemically inert and morphologically similar to the natural food of copepods, and may affect them through entanglement, ingestion, and interference with other natural food organisms (Bhuyan, 2022). The smaller size and higher availability of MPs increases the probability of ingestion by marine organisms (Woods et al., 2020). The patchy heterogeneous distribution of MPs in estuaries (Akdogan et al., 2023; Rajan et al., 2023) and oceans (Pan et al., 2021) may also lead to zooplankton in some parts of the ocean being much more vulnerable to MPs than the rest. The extent of spread is also on the rise at an alarming rate, with more recent studies such as Kanhai et al. (2020) showing the presence of MPs even in the sea ice cores of the Arctic central basin. A range of zooplankton taxa have been shown to be able to ingest MPs of similar size to algal prey (7 μm–30 μm; 650 MP mL−1–3,000 MP mL−1), while smaller microplastics (3.8 μm; 40 MP mL−1 × 103 MP mL−1) can externally adhere to a copepod’s functional appendages (Cole et al., 2015). Ingestion of plastic or latex beads, used as mimics or tracers of prey, by copepods has been reported by Frost (1977); Paffenhöfer and Van Sant (1985), and Cole et al. (2015). The feeding capacity and metabolic rates of calanoids, Centropages typicus and Calanus helgolandicus were significantly reduced in the presence of 6 μm–12 μm polystyrene beads (>4,000 MPs mL−1) (Cole et al., 2013; Isinibilir et al., 2020). However, another recent study by Svetlichny et al. (2021) showed an increase in the consumption rate of pure microplastics in a mixture of microplastic beads (6μm diameter) and algae Rhodomonas salina (5 μm–10 μm size range) with equal concentrations of approximately 5,000 cells/beads mL−1. The presence of micro- and nano-sized polystyrene in the environment has been observed to alter the feeding behavior of diving beetle (Cybister japonicas) and crucian carp (Carassius carassius) (Kim et al., 2018). The negative effects of MPs on physiology, organ damage, and even mortality have been shown in the laboratory (Franzellitti et al., 2019; Isinibilir et al., 2023), but only limited information is available on the behavioral impacts, feeding response, and functional and numerical responses of brackish water copepods commonly occurring in regions with higher MP pollution (Franzellitti et al., 2019; Cunningham et al., 2021; Rajan et al., 2023).
The ingestion of MP by zooplankton not only affects the aquatic food web, but also the entire ecosystem (Alava et al., 2023). Isinibilir et al. (2020) highlighted the negative effects of microplastic intake on the energy metabolism and swimming frequency of the calanoid copepod C. helgolandicus. As feeding is fundamental to the energetic requirements of an organism, MPs are likely to negatively affect zooplankton health by inducing a false sense of satiety, bioaccumulation in organisms, and blocking gut passage. Since copepods are an important component of the fish diet (Kerfoot and DeMott, 2019), they can also lead to trophic transfer of MPs to higher trophic levels, thereby posing a threat to the ecological functioning of the entire aquatic ecosystem.
Most feeding studies related to food selection have shown that copepods can distinguish between algal-flavored and unflavored artificial particles (DeMott, 1986), algae and polystyrene microspheres of similar size (DeMott, 1988), live and dead prey of the same species (Paffenhöfer and Van Sant, 1985; DeMott, 1988), toxic and non-toxic algae (Fulton and Paerl, 1987; DeMott and Moxter, 1991; Kumar, 2003), nitrogen-sufficient and nitrogen-limited algae of the same species (Cowles et al., 1998), algae and ciliates (Hartmann et al., 1993), ciliates and rotifers (Rao and Kumar, 2002), and larger and smaller rotifers (Dhanker et al., 2012). In contrast, some studies have reported that calanoid copepods are unable to exercise selection in regions with higher levels of suspended solids (Arendt et al., 2011) and tidally influenced estuaries with high sediment concentrations.
Previous studies have shown the effect of algae on animal prey consumption rate and alterations in the functional response of copepods (Kumar and Rao, 1999; Enríquez-García et al., 2013). Being numerically dominant and a preferred live food for fish larvae, Psudodiaptomus annadalei exerts a significant impact on the estuarine food web in the Indo-Pacific. Recent studies have demonstrated the predation efficiency of P. annandalei in natural setups, and ciliates and rotifers are important food items in their diet (Dhanker et al., 2012). P. annandalei is a generalist species with a large dietary niche breadth ranging from algal size, i.e., 7 μm–20 μm (Lee et al., 2010; Beyrend-Dur et al., 2011; Dhanker et al., 2015), to the size (150 μm–220 μm) of a rotifer (Dhanker et al., 2012). P. annandalei is able to select similarly sized rotifer over ciliate diet and show prey switching behavior on the basis of relative abundance of prey species. Dhanker et al. (2012) determined that the predatory success of P. annandalei on rotifers and ciliates followed an inverse size function, as observed for other invertebrate predators (Rao and Kumar, 2002; Hwang et al., 2009). However, there is limited knowledge regarding its ecology and functional feeding response. These organisms are currently facing potential threats from rapidly increasing MP concentrations in their immediate surroundings. MPs have been recorded in various domains of marine and estuarine environments (Li et al., 2019), which are supposed to be the habitat of the calanoid copepod P. annandalei, in the tropical and subtropical Indo-Pacific region. Another calanoid copepod, C. helgolandicus, showed a gradual decrease in food consumption rate during the 6-day experiment, feeding on a mixture of the microalgae R. salina and MP (Isinibilir et al., 2020). In a short-term experiment to evaluate the effect of initial MP concentration on consumption rate, Isinibilir et al. (2020) recorded a linear increase in MP consumption rates in C. helgolandicus with increasing concentration of MP in the medium, showing a Type-I functional response. Recent studies have shown the ability of copepods to distinguish between the chemical properties of MPs with the help of their mouthparts (Xu et al., 2022); however, despite the study showing the preference of zooplankton for its natural prey when both MP and natural prey are present, they do not shed light on the effect of MPs on the overall functional response of zooplankton. However, it is worth noting that the functional response can be best assessed in the presence of alternative prey (Iyer and Rao, 1996; Chan et al., 2017) as the functional response elucidates predator feeding rates with varying food densities (Solomon, 1949; Smith and Smith, 2020), thus, providing an estimation of energy transfer across trophic levels, understanding the stability and mechanism of coexistence of prey and predators (Oaten and Murdoch., 1975; Kumar and Rao, 2001). Xu et al. (2022) compares the ingestion of natural prey to MPs, it does not attempt to address and compare the number of natural prey consumed in presence and absence of MP.
Current knowledge of MP-imposed impacts on zooplankton largely focuses on the potential of zooplankton to engulf plastics. Most studies have used ingestion rates of MP as a parameter to describe the effects of MP on copepods (Jeong et al., 2017; Li et al., 2019).
Scientific knowledge of the functional response to a gradient of food density in MP-contaminated environment is essential to elucidate the adaptability of P. annandalei in an MP-contaminated environment and its response to the differential density of natural food. To date, no information is available on the estuarine calanoid, P. annandalei, whose natural habitats are contaminated by MP. A thorough understanding of the effects of MPs on the feeding ecology and foraging strategy of micro-meso-sized organisms is relevant to understand the effects of MPs on ecosystem functioning. To elucidate the impact of MPs on ecosystem functioning and feeding ecology, it is important to address the effects on the functional and numerical responses of an organism at the base of the food chain. Herein, we demonstrated the effects of MPs falling within the dietary niche size of the copepod P. annandalei. We also compared the effects of microalgae as an alternative food type in the medium to determine whether MP-imposed effects are similar to, or different from, alternative natural diets.
Thus, in the present study, we investigated the effects of MPs on the functional responses of P. annandalei (adult and copepodid stages, C2–C3) feeding on the rotifer B. rotundiformis and ciliate F. microstoma. We hypothesized that the presence of MPs along with the rotifer or ciliate would negatively influence prey ingestion rates in the copepod, P. annandalei, by inducing a false sense of satiety upon uptake of MPs, which would be reflected as an alteration in its functional response.
Materials and methods
Culture of experimental organisms
All experimental organisms were cultured in the laboratory three months prior to the start of the experiment. The starter cultures of the copepod P. annandalei (Sewell, 1919) and the rotifer B. rotundiformis (Tshugunoff 1921) were originally obtained from a coastal brackish water pond (salinity ~18 psu–20 psu) by filtering water through a 70 µm mesh plankton net. Field-collected animals were acclimatized to laboratory culture conditions. Pure cultures of the rotifer B. rotundiformis were performed in a 2-L beaker at 18 psu-20 psu salinity and 28°C ± 1.5°C using food from the microalgae Isochrysis galbana Parke (length: 5 μm–8 μm; width: 2.5 μm–4 μm). The copepod cultures were expanded to 20 L round cylindrical plastic tanks. A mixture of I. galbana (6–10 × 104 cells mL−1) and rotifer B. rotundiformis was used as food for the copepods. The copepod culture was mildly aerated continuously to mix and maintain the food uniformly distributed in the culture tank. The culture medium was renewed with a mixture of filtered seawater (0.45 μm Millipore filters) and autoclaved freshwater twice a week. All cultures were maintained at ambient temperature (annual range: 20°C–30°C) under a 12L:12D. Mass cultures of the algae, I. galbana, were established in the laboratory. The algal culture was maintained in a 2 L borosilicate glass flask in Walne medium (Walne, 1970). Cultures of algae were maintained at 20–22 salinity at 25°C –32°C at 1,000 lx–20,000 lx under 12 h light:12 h dark photoperiodic conditions. The algae were harvested in the log phase of growth, sedimented by centrifugation, and resuspended in experimental medium. The ciliated protist Frontonia microstoma was continuously cultured at 20–22 salinity and 25°C using the algae I. galbana and adding Hay infusions (10%) to the culture medium. Table 1 lists the attributes of the grazer, prey type, and prey density used in the experiment.
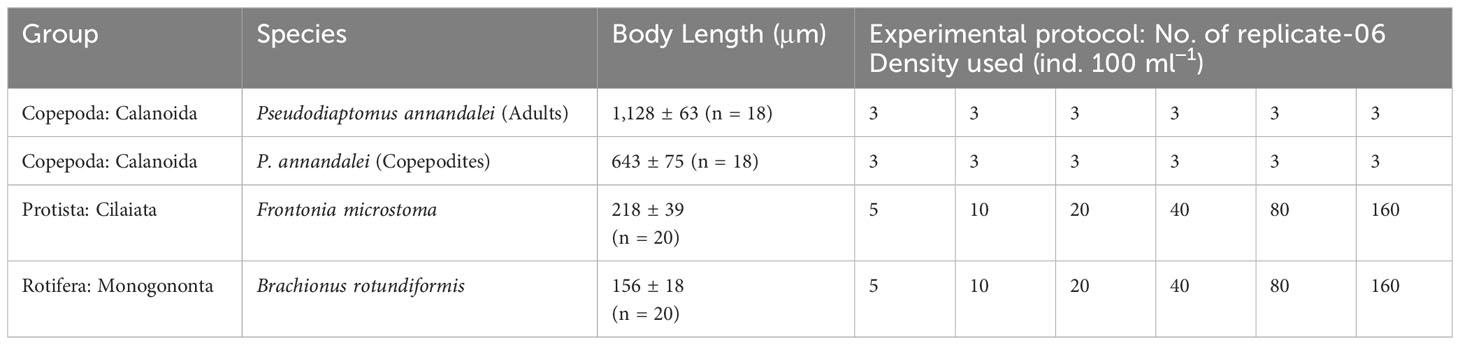
Table 1 Taxonomic group, body length (Mean ± SE) and density of experimental organisms, and experimental protocol followed of the functional response experiment.
MP preparation
Commercial polystyrene and polyethylene spheres were used in the experiment. The bead sizes (15 μm–30 μm) used in each experiment were selected to be comparable to the algal-food size range of P. annandalei. The encounter rate depends on the ratio of MP to zooplankton; therefore, our experimental protocol represented different ratios of ciliate and rotifer diets. In one set of treatments (i.e., prey ingestion in the presence of MP) with six replicates, MP particles were carefully counted and added to each treatment medium at a concentration of 100 particles mL−1 in each experimental vessel.
Experimental design
To understand the effect of MP on functional response of P. annandalei adult (females) and copepodites, following experiments were conducted (i) Feeding rates of copepodites (C3–C4 stage) on ciliate F. microstoma (ii) feeding rates of copepodites (C3–C4 stage) on rotifer B. rotundiformis, (iii) feeding rates of adult P. annandalei on ciliate F. microstoma, and (iv) feeding rates of adult P. annandalei on rotifer B. rotundiformis.
A batch of copepods (n = 180) of the same age group and previously exposed to the prey species to be used in the experiments were removed and kept in filtered water for 3 h for starvation and acclimation to the experimental volume to ensure uniform acclimation and hunger levels. Prior to the functional response experiment, the optimal feeding duration was standardized using a satiation time experiment. Based on the results obtained from the preliminary satiation time experiment, a 120-minute duration of feeding was followed for all the feeding trial experiments.
Functional response estimation
For both ciliate and rotifer prey types, six selected prey densities (Table 1) were offered to 3 h prestarved P. annandalei to elucidate the effects of MP on the functional response (response to changing prey density in the environment) of P. annandalei. Two sets of experiments were conducted in phases, consisting of (i) adult and (ii) copepodite stages of P. annandalei, each with three diet combinations (monospecific prey, presence of algae, and presence of MP), making a total of 108 (three prey combinations × six prey concentrations × six replicates) test jars of 150 ml capacity, containing 100 ml medium. For functional response studies involving copepodites, the experimental design was similar and copepodites at stages C3–C4 were used for the experiment. Prey concentrations set up in the geometric series (Table 1) were chosen based on preliminary experiments to cover the steeply rising and satiated portions of the functional response. All prey species were individually counted and introduced into the test jars at the desired density using a stereomicroscope. The experimental vessels were placed on black paper to provide contrast and minimize the prey stratification caused by uneven illumination. The copepods (three individuals per vessel) were then gently introduced into the experimental vessels and allowed to feed for 120 min. All the experimental vessels were covered with Perspex sheets, and light was provided by overhead fluorescent illumination. The number of prey eaten was estimated as the difference between the initial and final prey numbers after allowing copepods to feed for 120 min in the test jars. At the end of the experiment, copepods were anesthetized with 2-phenoxy ethanol and then preserved in buffered formalin for later gut content analysis and validation of the estimated number of prey eaten during the experimental period. The ciliate F. microstoma was kept in separate experimental vessels at the same density as that used for the experiment in quadruplicate to monitor any multiplication during the experimental period. Prey consumption by the predator was expressed as the number of prey items (rotifers and ciliates) consumed per 120 min.
Statistical analyses
The data on functional response were analyzed in two steps. First, to discriminate the type of functional response (Holling, 1959), the positive or negative sign of the linear coefficient was determined by logistic regression of the proportion of prey consumed (Na/Nt) as a function of prey density (Nt):
where Nt is the initial prey density, Na is the number of preys consumed, and Na/Nt is the probability of being eaten. P0, P1, P2, and P3 are the intercept, linear, quadratic, and cubic coefficients, respectively, estimated using the maximum likelihood method. Type-II functional response is described by P1 <0, as the proportion of prey consumed, declines gradually with increasing the initial prey density offered. If P1 >0 and P2 <0, the proportion of prey consumed is positively density-dependent, i.e., prey consumption increases with an increase in prey density, which indicates a Type-III functional response (Juliano, 2001).
The data were then transformed using the Michaelis–Menten equation as shown below (Lampert and Sommer, 1997; Sarma and Nandini, 2002)
Where, V = prey consumption rate, Vmax = saturation value of consumption rate, S = density of prey offered kt = the half saturation constant, the prey concentration at which is reached. Functional response curves were plotted using Excel. A Shapiro–Wilk test was used to assess the normal distribution.
Results
The presence of MPs significantly affected the functional response curves of adult P. annandalei feeding on either exclusive ciliate or exclusive rotifer diets. In general, P. annandalei adults exhibited a classical Type-II functional response to ciliates or rotifers when offered as monospecific or in combination with algae (Figure 1). Where no clear asymptote was observed, the proportion of prey consumed in relation to initial prey density declined significantly in the MP-contaminated medium (Figure 2). The prey ingestion rate initially increased with increasing prey density but reached a plateau, after which the prey ingestion rates slightly declined (Figures 1A, B). The maximum number of rotifers consumed at the highest prey density (160 ind. 100 mL−¹) in medium without MP was ~89.2 ± 5.51, while the maximum number of rotifers consumed by three adults P. annandalei at the highest prey density was (~40.4 ± 4.26) in the MP contaminated medium. The same prey species and density when offered in combination with MP showed a deviation from the Type-II functional response for copepodites (Figure 2), as the gradual decrease in the proportion of prey consumed was not significant (Pearson’s correlation; R2 = 0.018; P = 0.086) with increasing prey concentration (Figure 3) for copepodites (C3–C4).
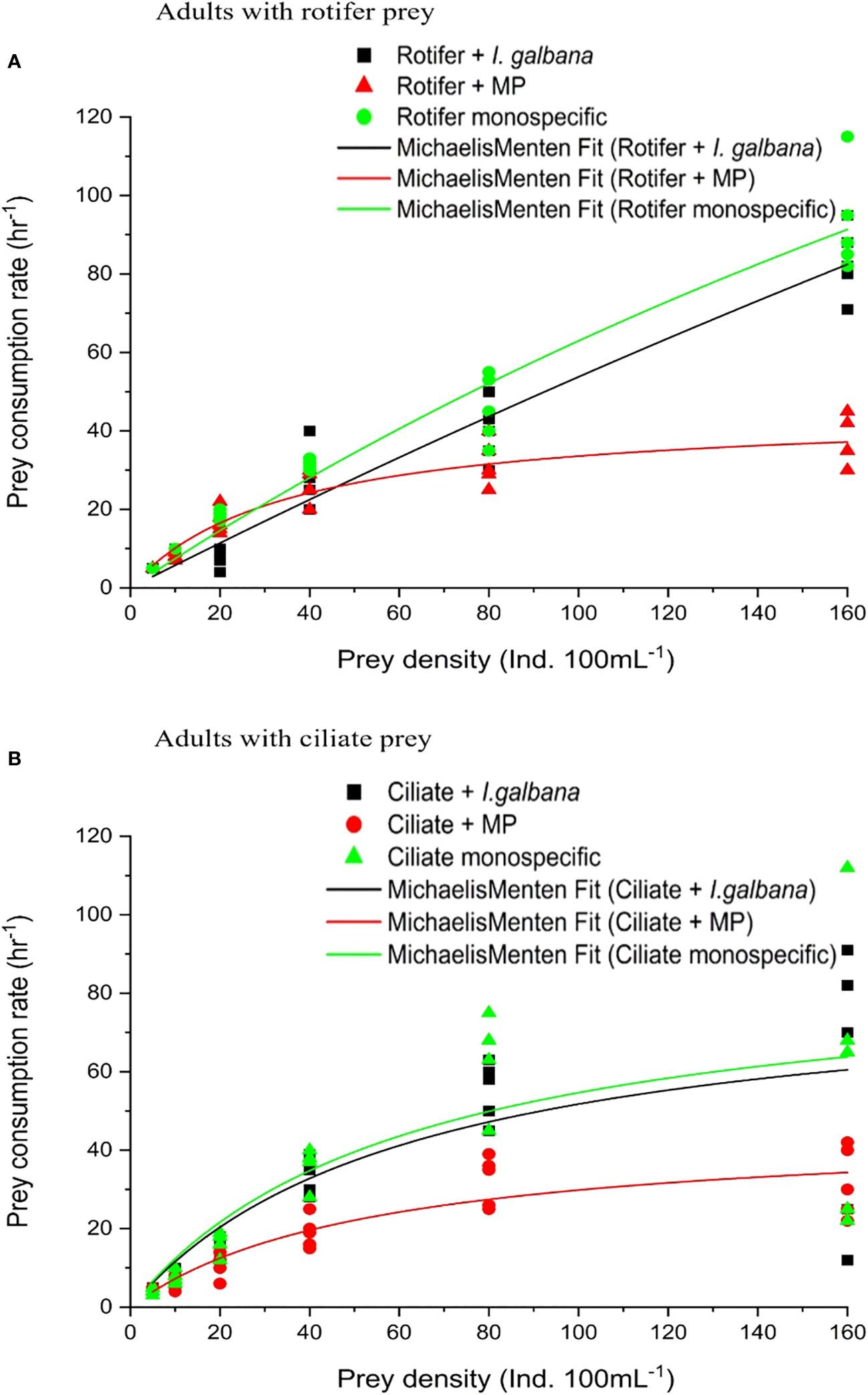
Figure 1 Functional response of P. annandalei adults offered monospecific prey, in the presence of microalgae I. galbana, and in the presence of Microplastic particles; (A) Rotifer prey B. rotundiformis, (B) Ciliate prey Frontonia microstoma. Shown are the actual prey consumption and the transformation of the curve using the Michaelis–Menten equation.
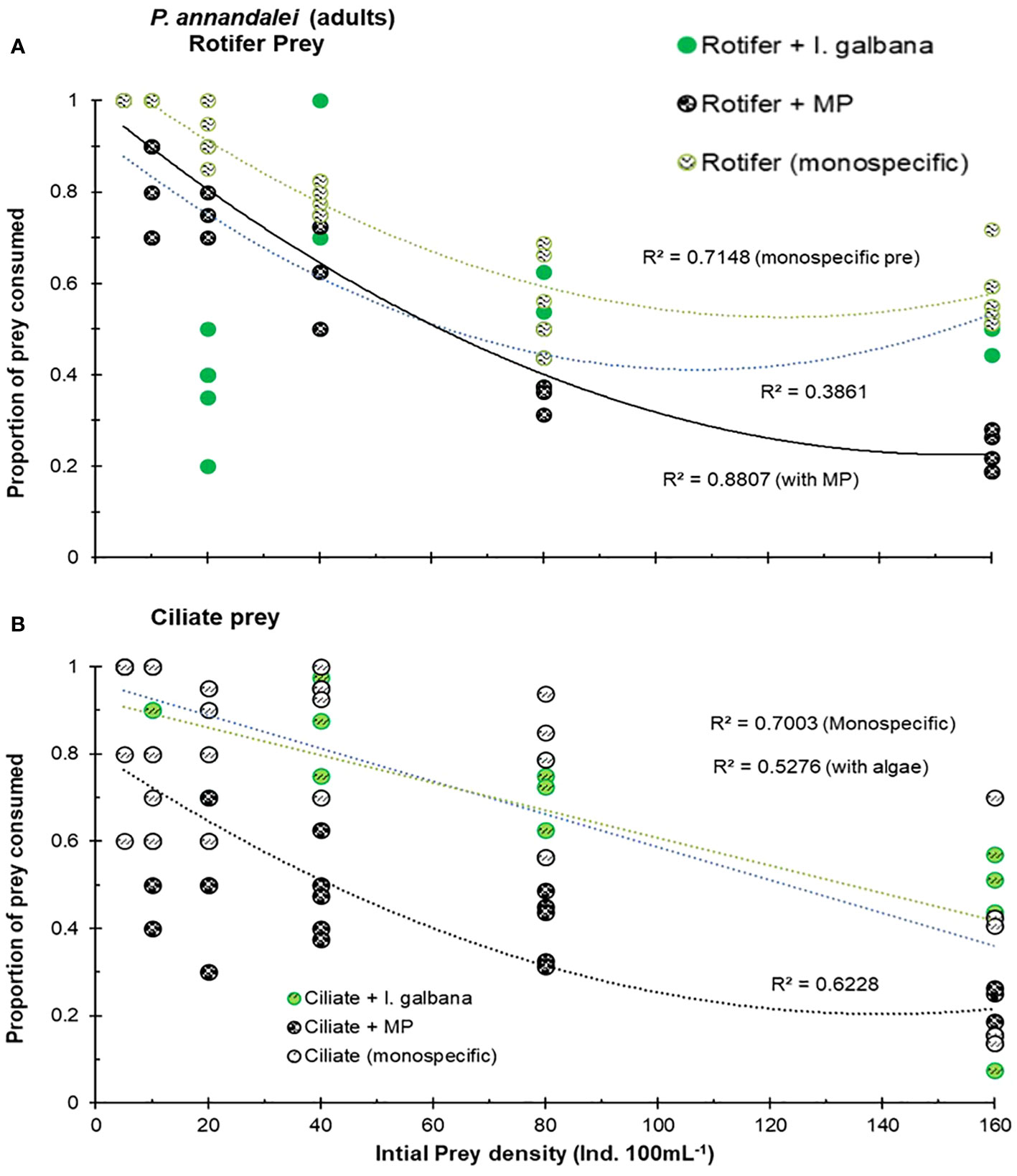
Figure 2 Proportion of initial prey consumed by the copepod P. annandalei adults, in the medium with monospecific prey, in the presence of microalgae I. galbana, and in the presence of MP particles (A) Rotifer prey B. rotundiformis, (B) Ciliate prey Frontonia microstoma.
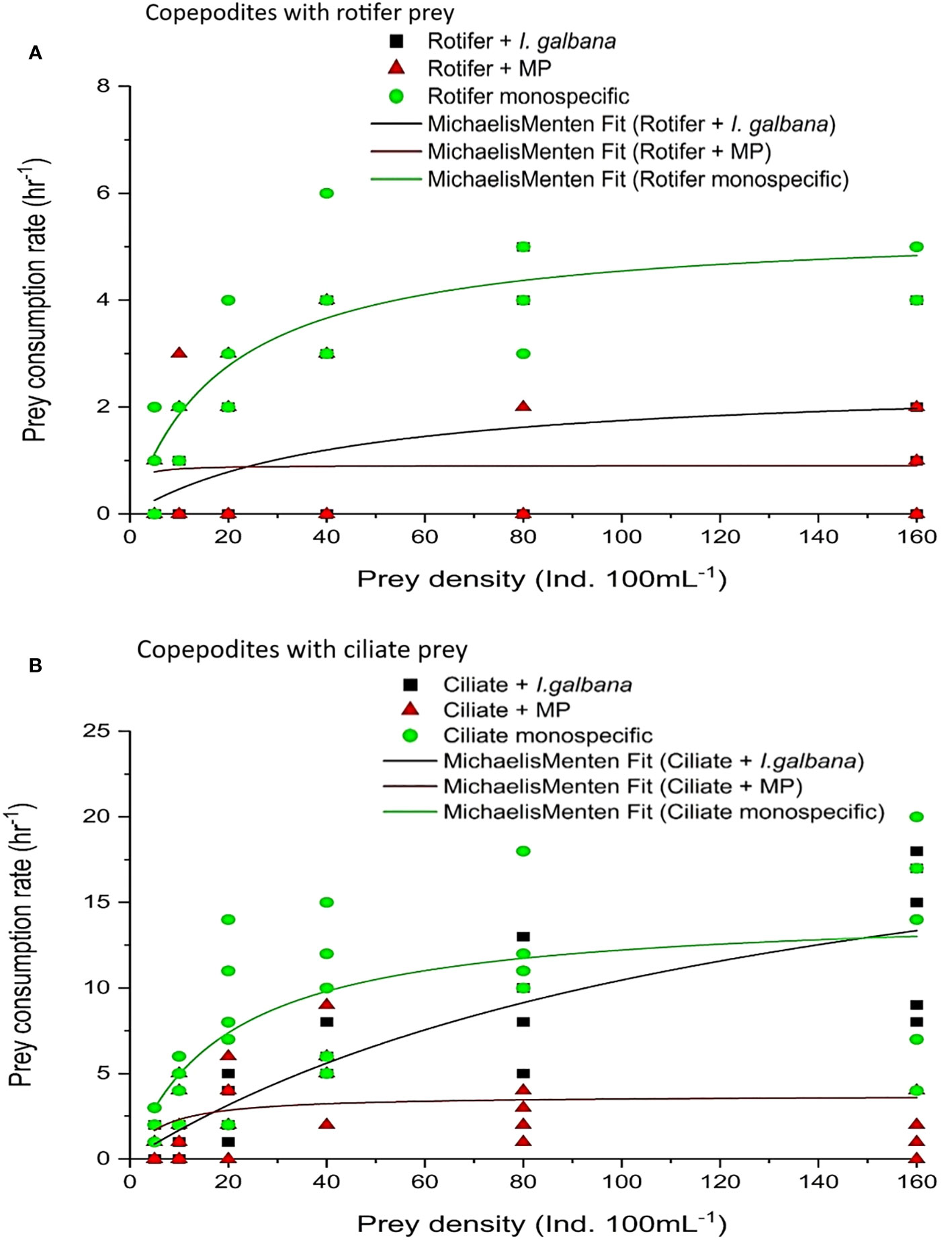
Figure 3 Functional response of P. annandalei copepodites (C3–C4) offered monospecific prey, in the presence of microalgae I. galbana, and in the presence of Microplastic particles; (A) Rotifer prey B. rotundiformis, (B) Ciliate prey Frontonia microstoma. Shown are the actual prey consumption and the transformation of the curve using the Michaelis–Menten equation.
The copepodites (C3–C4) of P. annandalei exhibited different responses to the prey type and density. The functional response curve for copepodites with ciliate diet showed a Type-II curve, where the rate of prey consumption increased with an increase in prey density but eventually levelled off and declined thereafter. Type-II functional responses of copepodites were recorded with monospecific rotifer and ciliate prey types (Figure 3). In the presence of microalgae, copepodites showed a stronger response to ciliate density (R2 = 0.77; P <0.001), exhibiting a Type-II functional response (Figure 3). The presence of MPs along with natural prey affected prey consumption in both adults and copepodites. The functional response curve of copepodites showed a Type-II functional response with rotifers both with a monospecific diet and with algae (Figure 2A), but the functional response curve in the presence of MPs showed linear regression with a negative slope (Figure 3A). The gradual decline in the proportion of prey consumed showed a highly significant correlation with the increasing initial prey density (Figure 4). Copepodites did not exhibit any response with rotifers or ciliates to the changed initial prey availability in the medium treated with MP (Figure 3). In the MP medium, the proportion of initial prey consumed by the copepodites did not correlate significantly (R2 = 0.02, MP-rotifer; R2 = 0.2, MP-ciliate) with increasing initial prey density (Figure 4).
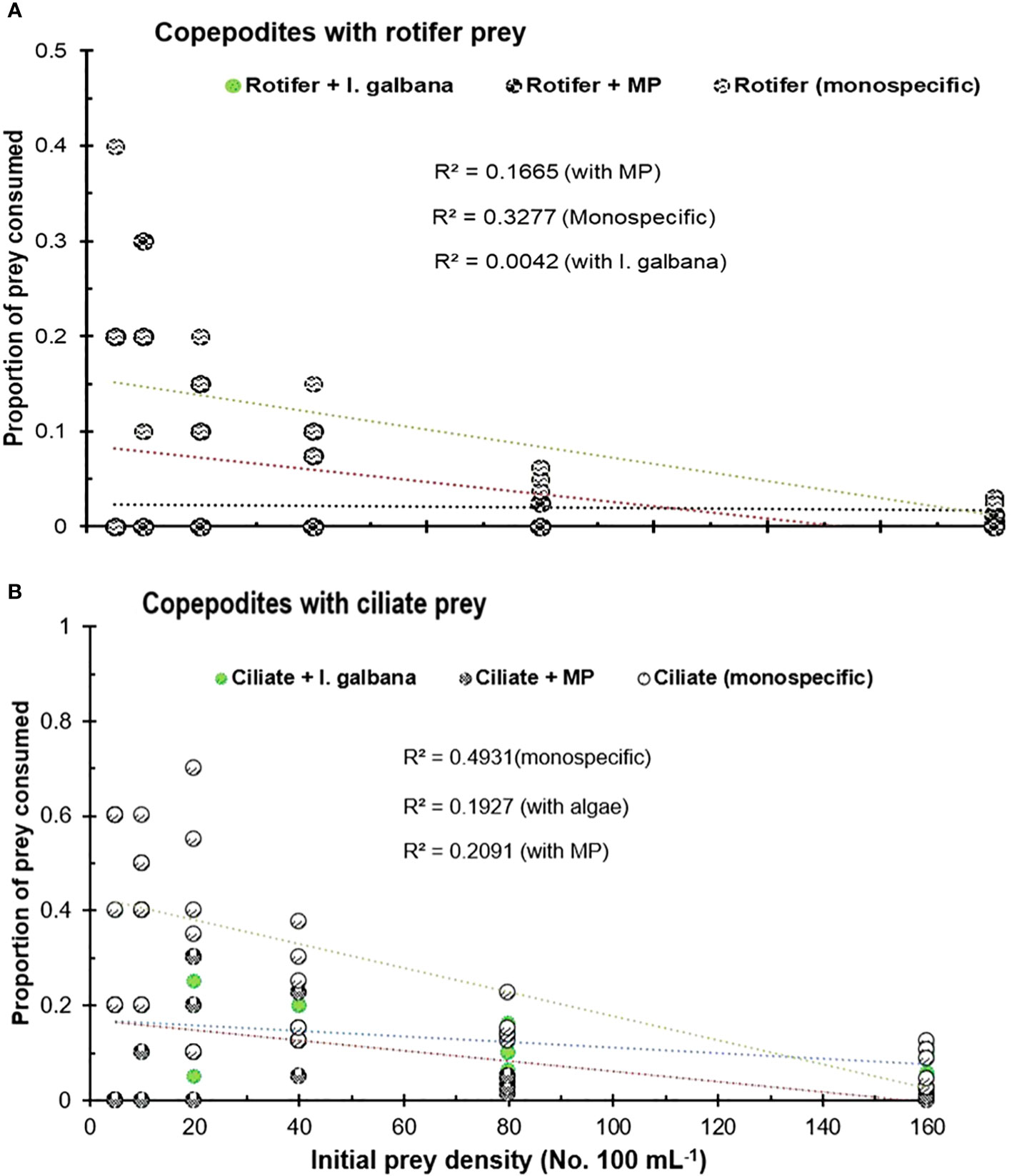
Figure 4 Proportion of initial prey consumed by the copepodid (C3–C4) stage of P. annandalei in the medium with monospecific prey, in the presence of microalgae I. galbana, and in the presence of MP particles. (A) Rotifer prey B. rotundiformis. (B) Ciliate prey Frontonia microstoma.
In the present study, adults in all cases ingested natural rotifer and ciliate prey, but the presence of MPs differentially affected ingestion depending on food type. Regardless of prey density, the prey ingestion rates were significantly lower (Figure 5; p <0.01; one-way ANOVA) for rotifer prey than for ciliate prey. The ingestion of natural prey by P. annandalei adults and copepodites varied significantly with prey density, MPs concentration, and their interaction (Figure 5; p <0.001; two-factor ANOVA). MP contamination did not affect prey ingestion in adults or copepodites at lower prey densities. The number of preys consumed in case of rotifer diet was maximum at the maximum prey density (160 ind. 100 mL−1) ~80.4 ± 2.7 which reduced by ~60% in presence of MPs. A similar decrease in prey consumption at maximum density was observed in the case of ciliate F. microstoma (maximum prey consumed with only ciliate prey was ~117 ± 4.55, while in the presence of MPs, ciliate consumption was reduced to ~39.8 ± 2.305 (Figure 5). At medium and high prey densities, we recorded a 40%–66% reduction in ciliate ingestion and 20%–60% reduction in rotifer ingestion by P. annandalei adults (Figure 5; Table 2). In the case of copepodites, we recorded a 40% to 98% reduction in ciliate consumption and a 50% to 91% reduction in rotifer consumption due to the presence of MP in the medium (Figure 5; Table 2).
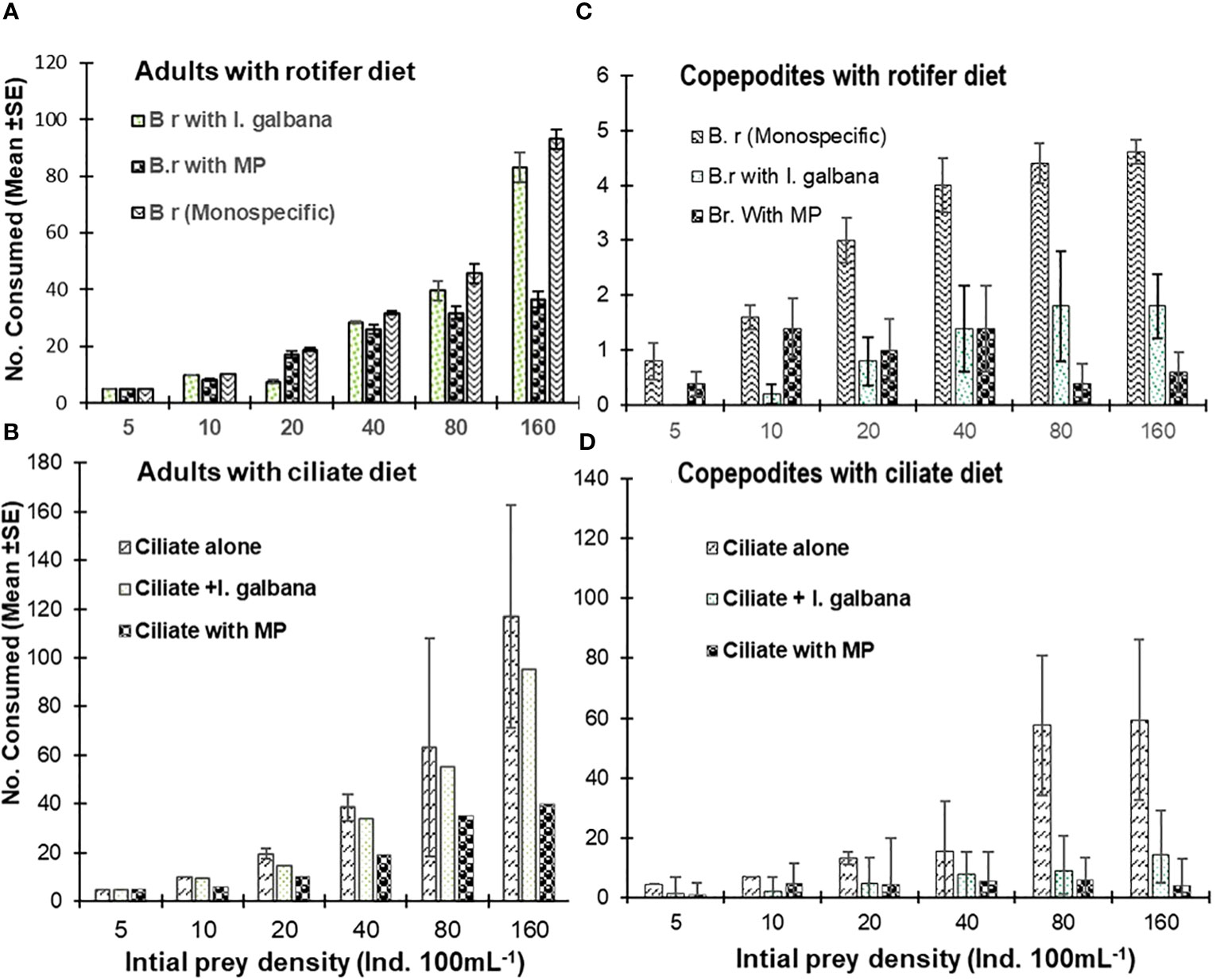
Figure 5 No. of prey consumed (mean ± SD) by the adults (A, B), and copepodites (C, D) of Pseudodiaptomus annandalei when offered as prey the rotifer Brachionus rotundiformis (A, C) and the ciliate Frontonia microstoma (B, D) as monospecific prey, in the medium containing either microalgae I. galbana or MP particle.
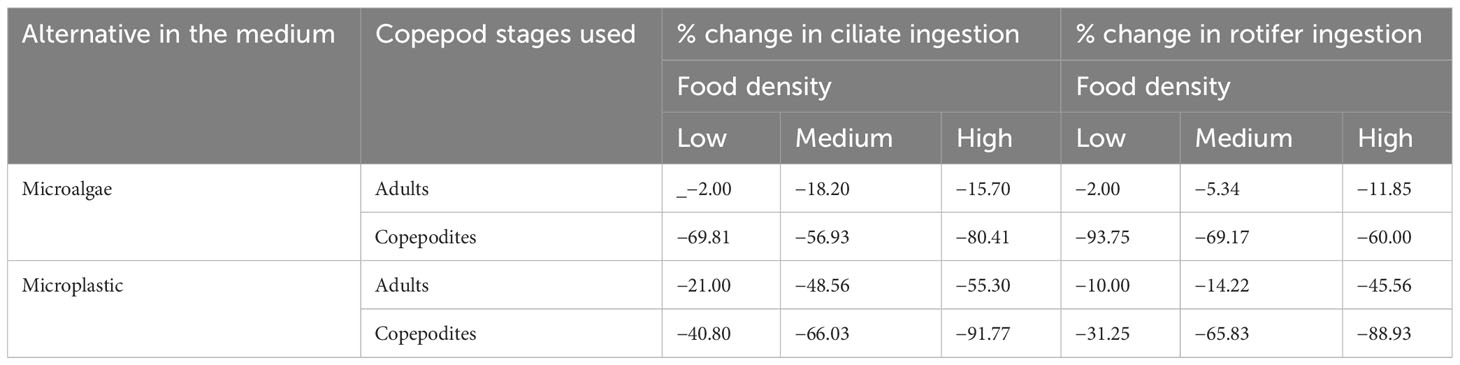
Table 2 MP induced changes in prey ingestion rates on natural prey (F. microstoma and B. rotundiformis) by adult and copepodid stages of P. annandalei at different food density levels.
Discussion
The main aim of this study was to obtain definitive information on the impact of MP contamination on feeding rates in P. annandalei, a common brackish water copepod species, with natural prey. In the natural setup, the distribution of prey species and MP (Rajan et al., 2023) are patchy, where copepods encounter an array of prey concentrations (Hwang et al., 2010). Various types of MP, similar to the size range of microzooplankton, are abundant in coastal and estuarine ecosystems (Cole et al., 2019; Rajan et al., 2023).
Evaluation of MP imposed alterations in consumer behavior is complex because of (i) patchy distribution and natural concentrations of MP, (ii) limited information on MP concentration-specific ingestion of natural diet, and (iii) lack of quantitative data on behavioral responses of zooplankton at varying MP concentrations. Unless colonized by biota or affected by external pollutants, MP are inert particles, and the main mode of effects on consumers is food dilution and behavioral responses, such as functional and numerical responses of consumers to MP, as the polymer structure and chemical properties of particles are less important (Mehinto et al., 2022). While food dilution outweighs other effects, the major response of organisms with a dietary niche size overlapping with MP would be the adjustment of feeding rates, i.e., functional response.
Our results on prey ingestion by P. annandalei adults and copepodites were within the ranges reported for related taxa (Hwang et al., 2009; Dhanker et al., 2012; He et al., 2020). Recent studies have also confirmed ingestion of MP by copepods (Desforges et al., 2015; Cheng et al., 2020; Bai et al., 2021); however, this is the first study to understand the effect of MP on natural prey ingestion in P. annandalei. Contributing to the size range, sorption potential, hydrophobicity, wide occurrence in the global ocean (Foekema et al., 2013; Mathalon and Hill, 2014; Ugwu et al., 2021), and bioavailability to zooplankton (Cole et al., 2013; Setälä et al., 2014), ichthyoplankton (Rochman et al., 2013; Batel et al., 2016), and other meso-macro-sized invertebrates (Browne et al., 2008; Graham and Thompson, 2009; Gray and Weinstein, 2017; Kumari et al., 2023a), MPs are likely to have large-scale effects on marine ecosystem functioning. Various experimental results have revealed lethal and sublethal effects on different organisms (von Moos et al., 2012; Rochman et al., 2013; Au et al., 2015; Gray and Weinstein, 2017).
P. annandalei showed a direct functional response to varying concentration of prey in the medium. Thus, the presence of MPs (size range 15 μm–30 μm diameter) in the environment of P. annandalei directly affects the functional response and feeding behavior of both adults and the developmental stages of copepods. The prey ingestion rate showed a decline in the presence of MPs in all cases, suggesting interference of MPs in the feeding behavior and feeding efficiency of P. annandalei. Copepods can ingest plastics by either selective grazing or by indiscriminately ingesting particles with natural prey (Traboni et al., 2023). Copepods can also actively refuse MPs or passively reduce MP intake by physical impacts/interference or chemical toxicity associated with plastics (leachates or sorbed pollutants) (Cássio et al., 2022). Similarly, the presence of plastics in the environment, along with natural prey, may obstruct predator–prey interactions, leading to a reduced prey ingestion rate (Turner and Tester, 1989). The linear increase in prey ingestion by copepods with increasing prey density suggests a Type-I functional response, which assumes a linear increase in food ingestion rate with food concentration, either for all initial food concentrations or only for the particular maximum concentration, beyond which the feeding rate is constant. This could be attributed to the adaptive feeding response of P. annandalei, as has also been reported for other copepods (Abrams, 1990; Kumar and Rao, 1999; Kerfoot and DeMott, 2019). The MP incurred reduction in ciliate and rotifer prey ingestion, recorded in this study, indicating the adaptive feeding strategy, as adults of P. annandalei did not exhibit any discernible reduction in prey consumption at lower concentrations; however, at higher concentrations, it showed a 20% to 60% reduction in rotifer ingestion and a 40% to 66% reduction in ciliate ingestion. Thus, the presence of a more satiating prey has the potential to reduce MP uptake by copepods. P. annandalie is mainly a suspension feeder copepod, though occasionally capture a food item through a surprise jerky movement of appendages. A previous study recorded different feeding modes for P. annandalei when dealing with different prey sizes and mobilities (Dhanker et al., 2013). MP-driven alterations in the behavioral and physiological responses of the calanoid copepods Acartia clausi and C. typicus have been attributed to differences in the feeding strategies of the two species (Svetlichny et al., 2021). Further comparative studies are required to understand the role of different feeding strategies in the uptake of MP by P. annandalei.
In the present study, copepods showed neither linear nor curvilinear relationships with the initial prey concentrations in the medium with MP. Conclusively P. annandalei was much less efficient in feeding B. rotundiformis or F. microstoma when MP was present, indicating a negative impact of MPs contamination. The ability of copepods, such as Temora longicornis, to selectively refuse MPs and their preference for natural prey has been previously demonstrated (Xu et al., 2022). Overall, copepods have been shown to be equipped with diverse sensors on their antennae, feeding appendages or body surfaces for detecting either hydromechanical or chemical signals emitted by their prey (Turner and Tester, 1989; Vanderploeg et al., 1990; DeMott, 1995). The reduced frequency of prey predator encounters in the presence of MPs may be a factor in changes in the functional response. In addition, accidental or voluntary intake of MPs by P. annandalei could induce false feelings of satiety that may reduce the hunger level of copepods (Gommer et al., 2018; Botterell et al., 2019). MPs acceptance and rejection by copepods create a challenging environment for copepods to extract natural food particles from the environment because of the morphological similarity of MPs with the natural diet (Nielsen et al., 2020) and microbial coating on MP surfaces as biofilms (Zimmermann et al., 2019). The purpose of this study was to evaluate the effect of MP on the functional response (prey consumption rate) of P. annandalei. The complete life cycle of this species has been studied on an exclusive algal diet (Beyrend-Dur et al., 2011; Nielsen et al., 2020), and lower availability of microalgae showed an increase in MP ingestion and retention in P. annandalei (Cheng et al., 2020); therefore, it is also relevant to study the effect of MP on the clearance rate.
Earlier reports suggest ingestion of inorganic particles of low nutritional value (Arendt et al., 2011) resulting in pseudo-satiation (Turner and Tester, 1989). However, a lack of nutrients adversely affects an organism’s reproductive potential and inclusive fitness (White and Dagg, 1989; Kang, 2012).
The brackish water, egg-carrying euryhaline copepod, P. annandalei, is distributed widely in tropical and subtropical Indo-Pacific with an ability to survive in altered marine and brackish waters (Beyrend-Dur et al., 2011; Beyrend-Dur et al., 2013; Blanda et al., 2015). This species is widely used as a live feed in the aquaculture industry; however, the harvest of P. annandalei in aquaculture ponds has declined in recent years (Blanda et al., 2015). The present results emphasize the need to assess MP contamination in aquaculture ponds and further large-scale studies of the numerical response of P. annandalei in the presence and absence of MP. The impact of MPs on the functional responses of P. annandalei is of particular concern because they have the potential for trophic transfer to fish and humans (Lee et al., 2010; Nelms et al., 2018; Ugwu et al., 2021). Furthermore, P. annandalei which is a filter-feeder, is more vulnerable to MPs ingestion. It is important to consider that a cocktail of various additives and chemical contaminants (Rochman et al., 2019; Zimmermann et al., 2019) are adsorbed by plastic surfaces and ingested along with microplastics, which further adds to toxicity and oxidative stress (Jeong et al., 2017; Qiao et al., 2019). Although the present study does not suggest any toxic effects of MPs, the effects on functional responses are suggestive of chronic effects on ecosystem functioning of planktonic organisms, particularly at the base of the pelagic food webs, hints at the knock-on alterations in ecosystem functioning, resulting in trophic cascades (Galloway et al., 2017; Botterell et al., 2019; Nair and Perumal, 2022). MPs are expected to become more prevalent in aquatic environments because of their extensive use and dumping in aquatic ecosystems (Zeynep et al., 2023). Their potential to transport and adsorb toxic materials, as well as their absorbance by living cells, tissues, and organs of aquatic organisms, and interfere with physiological functions, all cause significant health risks. Could the observed MP imposed alterations in functional responses have implications for the numerical responses of copepods? The predator’s functional response is hypothesized to be an important determinant of the population structure and numerical responses (Dhanker et al., 2012; Dhanker et al., 2015).
To support the generalizability of the present results, we recommend studying a range of brackish water zooplankton species used as live feed in the aquaculture industry, by applying different types of MP particles.
Conclusions
This paper reports significant changes in the functional response curve of the adult and copepodid stages of P. annandalei when MPs are present along with natural prey. These results clearly suggest that MP contamination adversely affects the feeding rates of P. annandalei adults and juveniles fed on their natural diets. The impact of MPs on the functional responses of P. annandalei and prey ingestion rates is of particular concern because they have the potential for trophic transfer to fish and humans. Ingestion of inert particles, such as MP, gives a sense of false satiety, which in turn results in an altered prey selection pattern (Gommer et al., 2018) and reduced saturation level prey intake. Further research is needed to fully understand the feeding modes in zooplankton species, prey behavior, prey selection patterns, and trophic transfer to fish in MP-contaminated environments.
Data availability statement
The original contributions presented in the study are included in the article/Supplementary Material. Further inquiries can be directed to the corresponding author.
Ethics statement
The manuscript presents research on animals that do not require ethical approval for their study.
Author contributions
JS: Conceptualization, Writing - review & editing. MR: Writing – original draft, Writing – review & editing. RG-o: Writing – original draft, Writing – review & editing.
Funding
The author(s) declare that no financial support was received for the research, authorship, and/or publication of this article.
Acknowledgments
The authors thank the guest editor for inviting us to submit this special issue. We thank Silliman University for providing the facility for conducting this research. MR thanks Delhi Technological University for the internship.
Conflict of interest
The authors declare that the research was conducted in the absence of any commercial or financial relationships that could be construed as a potential conflict of interest.
Publisher’s note
All claims expressed in this article are solely those of the authors and do not necessarily represent those of their affiliated organizations, or those of the publisher, the editors and the reviewers. Any product that may be evaluated in this article, or claim that may be made by its manufacturer, is not guaranteed or endorsed by the publisher.
Supplementary material
The Supplementary Material for this article can be found online at: https://www.frontiersin.org/articles/10.3389/fevo.2024.1277332/full#supplementary-material
References
Abrams P. A. (1990). The effects of adaptive behavior on the type-2 functional response. Ecology 71, 877–8 85. doi: 10.2307/1937359
Akdogan Z., Guven B., Kideys A. E. (2023). Microplastic distribution in the surface water and sediment of the Ergene River. Environ. Res. 234, 116500. doi: 10.1016/j.envres.2023.116500. ISSN 0013-9351.
Alava J. J., Báez M. M., McMullen K., Tekman M. B., Barrows A. P. W., Bergmann M., et al. (2023). Ecological Impacts of Marine Plastic Pollution, Microplastics’ Food web Bioaccumulation Modelling and Global Ocean Footprint: Insights into the Problems, the Management Implications and Coastal Communities Inequities. The Nippon Foundation-Ocean Litter Project, (2019-2023) (Main Mall, Vancouver, BC, V6T 1Z4, Canada: Institute for the Oceans and Fisheries, University of British Columbia). Working Paper 2023 – 01.
Amélineau F., Bonnet D., Heitz O., Mortreux V., Harding A. M. A., Karnovsky. N., et al. (2016). MP pollution in the Greenland Sea: Background levels and selective contamination of planktivorous diving seabirds. Environ. pollut. 219, 1131–1139. doi: 10.1016/j.envpol.2016.09.017
Arendt K. E., Dutz J., Jónasdóttir S. H., Jung-Madsen S., Mortensen J., Møller. E. F., et al. (2011). Effects of suspended sediments on copepods feeding in a glacial influenced sub-Arctic fjord. J. Plankton Res. 33, 10, 1526–1537. doi: 10.1093/plankt/fbr054
Au S. Y., Bruce T. F., Bridges W. C., Klaine S. J. (2015). Responses of Hyalella azteca to acute and chronic microplastic exposures. Environ. Toxicol. Chem. 34, 2564–2572. doi: 10.1002/etc.3093
Auta H. S., Emenike C. U., Fauziah S. H. (2017). Distribution and importance of MPs in the marine environment: A Review of the Sources, Fate, Effects, and Potential Solutions. Environ. Int. 2017, 165–176. doi: 10.1016/j.envint.2017.02.013
Avignon G. D., Hsu S. S. H., Gregory-Eaves I., Ricciardi A. (2023). Feeding behavior and species interactions increase the bioavailability of microplastics to benthic food webs. Sci. Total Environ. 896, 165261. doi: 10.1016/j.scitotenv.2023.165261
Ayukai T. (1987). Discriminate feeding of the calanoid copepod Acartia clausi in mixtures of phytoplankton and inert particles. Mar. Biol. 94, 579–587. doi: 10.1007/BF00431404
Baalkhuyur F. M., Dohaish E.-J. A., Elhalwagy M. E. A., Alikunhi N. M., Suwailem A. M., Røstad A., et al. (2018). MP in the gastrointestinal tract of fishes along the Saudi Arabian Red Sea coast. Mar. pollut. Bull. 131, 407–415. doi: 10.1016/j.marpolbul.2018.04.040
Bai Z., Wang N., Wang M. (2021). Effects of microplastics on marine copepods. Ecotoxicol Environ. Saf. 217, 112243. doi: 10.1016/j.ecoenv.2021.112243
Barnes D. K. A., Galgani F., Thompson R. C., Barlaz M. (2009). Accumulation and fragmentation of plastic debris in global environments. Phil. Trans. R. Soc B 364, 1526. doi: 10.1098/rstb.2008.0205
Batel A., Linti F., Scherer M., Erdinger L., Braunbeck T. (2016). Transfer of benzo[a]pyrene from microplastics to Artemia nauplii and further to zebrafish via a trophic food web experiment: CYP1A induction and visual tracking of persistent organic pollutants. Environ. Toxicol. Chem. 35 (7), 1656–1666. doi: 10.1002/etc.3361
Beaugrand G., Brander K. M., Alistair J. A., Souissi S., Reid P. C. (2003). Plankton effect on cod recruitment in the North Sea. Nature 426, 661–664. doi: 10.1038/nature02164
Beyrend-Dur D., Kumar R., Rao T. R., Souissi S., Cheng S. H., Hwang J. S. (2011). Demographic parameters of adults of Pseudodiaptomus annandalei (Copepoda: Calanoida): Temperature–salinity and generation effects. J. Exp. Mar. Biol. Ecol. 404 (1–2), 1–14. doi: 10.1016/j.jembe.2011.04.012
Beyrend-Dur D., Souissi S., Hwang J. S. (2013). Population dynamics of calanoid copepods in the subtropical mesohaline Danshuei Estuary (Taiwan) and typhoon effects. Ecol. Res. 28, 771–780. doi: 10.1007/s11284-013-1052-y
Bhuyan M. (2022). Effects of MPs on fish and in human health. Front. Environ. Sci. 10, 827289. doi: 10.3389/fenvs.2022.827289
Blanda E., Drillet G., Huang C.-C., Hwang J.-S., Jakobsen H. H., Rayner T. A., et al. (2015). Trophic interactions and productivity of copepods as live feed from tropical Taiwanese outdoor aquaculture ponds. Aquaculture 445, 11–21. doi: 10.1016/j.aquaculture.2015.04.003
Botterell Z. L. R., Beaumont N., Dorrington T., Steinke M., Thompson R. C., Lindeque P. K. (2019). Bioavailability and effects of microplastics on marine zooplankton: A review. Environ. pollut. 245, 98–110. doi: 10.1016/j.envpol.2018.10.065
Browne M. A., Dissanayake A., Galloway T. S., Lowe D. M., Thompson R. C. (2008). Ingested microscopic plastic translocates to the circulatory system of the mussel, Mytilus edulis (L). Environ. Sci. Technol. 42 (13), 5026–5031. doi: 10.1021/es800249a
Bundy M. H., Gross T. F., Vanderploeg H. A., Strickler J. R. (1998). Perception of inert particles by calanoid copepods: Behavioral observations and a numerical model. J. Plankton Res. 20, 2129–2152. doi: 10.1093/plankt/20.11.2129
Bundy M. H., Vanderploeg H. A. (2002). Detection and capture of inert particles by calanoid copepods: the role of the feeding current. J. Plankton Res. 24, 3, 215–223. doi: 10.1093/plankt/24.3.215
Cássio F., Batista D., Pradhan A. (2022). Plastic interactions with pollutants and consequences to aquatic ecosystems: what we know and what we do not know. Biomolecules 12 (6), 798. doi: 10.3390/biom1206079898
Chan K., Boutin S., Hossie T. J., Krebs C. J., O’Donoghue M., Murray D. L. (2017). Improving the assessment of predator functional responses by considering alternate prey and predator interactions. Ecology 98, 1787–17 96. doi: 10.1002/ecy.1828
Cheng Y., Wang J., Yi X., Li L., Liu X., Ru S. (2020). Low microalgae availability increases the ingestion rates and potential effects of microplastics on marine copepod Pseudodiaptomus annandalei. Mar. Poll. Bul. 152, 110919. doi: 10.1016/j.marpolbul.2020.110919
Cole M., Coppock R., Lindeque P. K., Altin D., Reed S., Pond D. W., et al. (2019). Effects of nylon MP on feeding, lipid accumulation, and moulting in a coldwater copepod. Environ. Sci. Technol. 53, 7075–7082. doi: 10.1021/acs.est.9b01853
Cole M., Lindeque P., Fileman E., Halsband C., Galloway T. S. (2015). The impact of polystyrene MPs on feeding, function and fecundity in the marine copepod Calanus helgolandicus. Environ. Sci. Technol. 49 (2), 1130–1137. doi: 10.1021/es504525u
Cole M., Lindeque P., Fileman E., Halsband C., Goodhead R., Moger J., et al. (2013). Microplastic ingestion by zooplankton. Environ. Sci. Technol. 47 (12), 6646–6655. doi: 10.1021/es400663f
Cowles T. J., Desiderio R. A., Carr M.-E. (1998). Small-scale planktonic structure: persistence and trophic consequences. Oceanography 11 (1), 4–9. doi: 10.5670/oceanog.1998.08
Cunningham E. M., Cuthbert R. N., Coughlan N. E., Kregting L., Cairnduff V., Dick J. T. A. (2021). Microplastics do not affect the feeding rates of a marine predator'. Sci. Total Environ. 779, 146487. doi: 10.1016/j.scitotenv.2021.146487
Cunningham E. M., Kiriakoulakis K., Dick J. T. A., Kregting L., Schuchert P., Sigwart J. D. (2020). Driven by speculation, not by impact - the effects of plastic on fish species. J. Fish Biol. 96 (6), 1294–1297. doi: 10.1111/jfb.14303
DeMott W. R. (1986). The role of taste in food selection by freshwater zooplankton. Oecologia 69, 334–340. doi: 10.1007/BF00377053
DeMott W. R. (1988). Discrimination between algae and artificial particles by freshwater and marine copepods. Limnol. Oceanogr. 33, 397–408. doi: 10.4319/lo.1988.33.3.0397
DeMott W. R. (1995). Optimal foraging by a suspension-feeding copepod: responses to short-term and seasonal variation in food resources. Oecologia 103 (2), 230–240. doi: 10.1007/BF00329085
DeMott W. R., Moxter F. (1991). Foraging cyanobacteria by copepods: responses to chemical defense and resource abundance. Ecology 72 (5), 1820–1834. doi: 10.2307/1940981
Desforges J. P., Galbraith M., Ross P. S. (2015). Ingestion of microplastics by zooplankton in the Northeast Pacific Ocean. Arch. Environ. Contam Toxicol. 69 (3), 320–330. doi: 10.1007/s00244-015-0172-5
Dhanker R., Kumar R., Hwang J. S. (2012). Predation by Pseudodiaptomus annandalei (Copepoda: Calanoida) on rotifer prey: size selection, egg predation and effect of algal diet. J. Exp. Mar. Biol. Ecol. 414–415, 44–53. doi: 10.1016/j.jembe.2012.01.011
Dhanker R., Kumar R., Tseng L. C., Hwang J. S. (2013). Ciliate (Euplotes sp.) predation by Pseudodiaptomus annandalei (Copepoda: Calanoida) and the effects of mono-algal and pluri-algal diets. Zool. Stud. 52, 34. doi: 10.1186/1810-522X-52-34
Dhanker R., Molinero J. C., Kumar R., Tseng L. C., Ianora A., Hwang J. S. (2015). Responses of the estuarine copepod Pseudodiaptomus annandalei to diatom polyunsaturated aldehydes: Reproduction, survival and postembryonic development. Harmful Algae 43, 74–81. doi: 10.1016/j.hal.2015.02.002
Enríquez-García C., Nandini S., Sarma S. S. S. (2013). Feeding behaviour of Acanthocyclops americanus (Marsh)(Copepoda: Cyclopoida). J. Nat. Hist. 47 (5-12), 853–862. doi: 10.1080/00222933.2012.747637
Ferrao Filho A. D. S., Demott W. R., Tessier A. J. (2005). Responses of tropical cladocerans to a gradient of resource quality. Freshwater Biol. 50 (6), 954–964.
Foekema E. M., De Gruijter C., Mergia M. T., van Franeker J. A., Murk A. J., Koelmans A. A. (2013). Plastic in North Sea fish. Environ. Sci. Technol. 47 (15), 8818–8824. doi: 10.1021/es400931b
Franzellitti S., Canesi L., Auguste M., Wathsala R. H., Fabbri E. (2019). Microplastic exposure and effects in aquatic organisms: A physiological perspective. Environ. Toxicol. Pharmacol. 68, 37–51. doi: 10.1016/j.etap.2019.03.009
Frost W. E. (1977). The food of Charr Salvelinus willughbii (Gunther) in Windermere. J. Fish Biol. 11, 531–547. doi: 10.1111/j.1095-8649.1977.tb05710.x
Fulton R. S., Paerl H. W. (1987). Toxic and inhibitory effects of the blue-green alga Microcystis aeruginosa in herbivorous zooplankton. J. Plankton Res. 9, 837–855. doi: 10.1093/plankt/9.5.837
Galloway T., Cole M., Lewis C. (2017). Interactions of microplastic debris throughout the marine ecosystem. Nat. Ecol. Evol. 1, 116. doi: 10.1038/s41559-017-0116
Gommer R., Bom R. A., Fijen T. P. M., van Gils J. A. (2018). Stomach fullness shapes prey choice decisions in crab plovers (Dromas ardeola). PloS One 13 (4), e0194824. doi: 10.1371/journal.pone.0194824
Graham E. R., Thompson J. T. (2009). Deposit and suspension- feeding sea cucumbers (Echinodermata) ingest plastic fragments. J. Exp. Mar. Biol. Ecol. 368, 22–29. doi: 10.1016/j.jembe.2008.09.007
Gray A. D., Weinstein J. E. (2017). Size- and shape-dependent effects of microplastic particles on adult daggerblade grass shrimp (Palaemonetes pugio). Environ. Toxicol. Chem. 36 (11), 3074–3080. doi: 10.1002/etc.3881
Gunaalan K., Almeda R., Lorenz C., Vianello A., Iordachescu L., Papacharalampos K., et al. (2023). Abundance and distribution of microplastics in surface waters of the Kattegat/ Skagerrak (Denmark). Environ. pollut. 318, 120853. doi: 10.1016/j.envpol.2022.120853
Halstead J. E., Smith J. A., Carter E. A., Lay P. A., Johnston E. L. (2018). Assessment tools for MPs and natural fibres ingested by fish in an urbanised estuary. Environ. pollut. 234, 552–561. doi: 10.1016/j.envpol.2017.11.085
Hartmann H. J., Taleb H., Aleya L., Lair N. (1993). Predation on ciliates by the suspension-feeding calanoid copepod Acanthodiaptomus denticornis. Can. J. Fish. Aquat. Sci. 50, 1382–1393. doi: 10.1139/f93-158
He X., Cheng F., Chen W. (2020). Effects of maternal phosphorus deficiency on reproductive allocation of the copepod Pseudodiaptomus annandalei Sewel. Hydrobiologia 847, 2035–2048. doi: 10.1007/s10750-020-04229-7
Holling C. S. (1959). The components of predation as revealed by a study of small-mammal predation of the European pine sawfly. Can. Entomologist. 91 (5), 293–320. doi: 10.4039/Ent91293-5
Hwang J. S., Kumar R., Hsieh C. W., Kuo A. Y., Souissi S., Hsu M. H., et al. (2010). Patterns of zooplankton distribution along the marine, estuarine and riverine portions of the Danshuei ecosystem in northern Taiwan. Zoological Studies 49 (3), 335–352.
Hwang J. S., Kumar R., Kuo C.-S. (2009). Impacts of predation by the copepod, mesocyclops pehpeiensis, on life table demographics and population dynamics of four cladoceran species: A comparative laboratory study. Zoological Stud. 48 (6), 738–752.
Isinibilir M., Eryalçın K. M., Kideys A. E. (2023). Effect of Polystyrene Microplastics in Different Diet Combinations on Survival, Growth and Reproduction Rates of theWater Flea (Daphnia magna). Microplastics 2, 27–38. doi: 10.3390/microplastics2010002
Isinibilir M., Svetlichny L., Mykitchak T., Türkeri E. E., Eryalçın K. M., Doğan O., et al. (2020). Microplastic consumption and its effect on respiration rate and motility of calanus helgolandicus from the Marmara Sea. Front. Mar. Science. 7. doi: 10.3389/fmars.2020.603321
Iyer N., Rao T. R. (1996). Responses of the predatory rotifer Asplanchna intermedia to prey species differing in vulnerability: laboratory and field studies. Freshw. Biol. 36 (3), 521–533. doi: 10.1046/j.1365-2427.1996.d01-510.x
Jabeen K., Su L., Li J., Yang D., Tong C., Mu J. (2017). MPs and mesoplastics in fish from coastal and fresh waters of China. Environ. pollut. 221, 141–149. doi: 10.1016/j.envpol.2016.11.055
Jeong C. B., Kang H. M., Lee M. C., Kim D. G., Han J., Hwang D. S., et al. (2017). Adverse effects of microplastics and oxidative stress-induced MAPK/Nrf2 pathway-mediated defense mechanisms in the marine copepod. Paracyclopina nana. Sci. Rep. 2017, 7. doi: 10.1038/srep41323
Juliano S. A. (2001). Nonlinear curve fitting: predation and functional response curves. Design Anal. Ecol. Experiments 2, 178–96. doi: 10.1093/oso/9780195131871.003.0010
Kang H. K. (2012). Effects of suspended sediments on reproductive responses of Paracalanus sp. (Copepoda: Calanoida) in the laboratory. J. Plankton Res. 34, 7, 626–635. doi: 10.1093/plankt/fbs033
Kanhai L. D. K., Gardfeldt K., Krumpen T., Thompson R. C., O’Connor I. (2020). MPs in sea ice and seawater beneath ice floes from the Arctic Ocean. Sci. Rep. 10 (1), 5004. doi: 10.1038/s41598-020-61948-6
Kerfoot C. W., DeMott W. R. (2019). Food web dynamics: dependent chains and vaulting. In Trophic interactions within aquatic ecosystems (pp. 347–382). Routledge.
Kim S. W., Kim D., Chae Y., An Y.–J. (2018). Dietary uptake, biodistribution, and depuration of microplastics in the freshwater diving beetle Cybister japonicus: Effects on predacious behavior. Environ. Poll 242, 839e844. doi: 10.1016/j.envpol.2018.07.071
Kumar R. (2003). Effects of mesocyclops thermocyclopoides (Copepoda: cyclopoida) predation on the population growth patterns of different prey species. J. Freshwat. Ecol. 18 (3), 383–393. doi: 10.1080/02705060.2003.9663974
Kumar R. (2005). Feeding modes and associated mechanisms in zooplankton, (In ecology of plankton ed: kumar, A). Daya Publ., 241–265.
Kumar R., Rao T. R. (1999). Effect of algal food on animal prey consumption rates in the omnivorous copepod, mesocyclops thermocyclopoides. Internat. Rev. der gesamten Hydrobiologie und Hydrographie 84 (5), 419–426. doi: 10.1002/iroh.199900035
Kumar R., Rao T. R. (2001). Effect of the cyclopoid copepod Mesocyclops thermocyclopoides on the interactions between the predatory rotifer Asplanchna intermedia and its prey Brachionus calyciflorus and B. angularis. Hydrobiologia 453, 261–268. doi: 10.1023/A:1013105125857
Kumari N., Samantaray B. P., Patel A., Kumar R. (2023a). Microplastics affect rates of locomotion and reproduction via dietary uptake in globally invasive snail. Physa acuta. Water 15 (5), 928. doi: 10.3390/w15050928
Kumari N., Yadav D. K., Khan P. K., Kumar R. (2023b). Occurrence of plastics and their characterization in wild-caught fish species (Labeo rohita, Wallago attu, and Mystus tengara) of River Ganga (India) compared to a commercially cultured species (L. rohita). Environ. pollut. 334, 122141. doi: 10.1016/j.envpol.2023.122141
Lampert W., Sommer U. (1997). Limnoloecology: the ecology of lakes and streams (Oxford: Oxford University Press).
Lee C.-H., Dahms H.-U., Cheng S.-H., Souissi S., Schmitt F. G., Kumar R., et al. (2010). Predation of Pseudodiaptomus annandalei (Copepoda: Calanoida) by the grouper fish fry Epinephelus coioides under different hydrodynamic conditions. J. Exp. Mar. Bio. Ecol. 393 (1-2), 17–22. doi: 10.1016/j
Li D., Peng G., Lixin Z. (2019). Progress and prospects of marine microplastic research in China. Anthropocene Coasts. 2 (1), 330–339. doi: 10.1139/anc-2018-0014
Mathalon A., Hill P. (2014). Microplastic fibers in the intertidal ecosystem surrounding Halifax Harbor, Nova Scotia. Mar. pollut. Bull. 81 (1), 69–79. doi: 10.1016/j.marpolbul.2014.02.018
Mehinto A. C., Coffin S., Koelmans A. A., Brander S. M., Wagner M., Thornton Hampton L. M., et al. (2022). Risk-based management framework for microplastics in aquatic ecosystems. Micro Nanoplastics 2, 17. doi: 10.1186/s43591-022-00033-3
Nair H. T., Perumal S. (2022). Trophic transfer and accumulation of microplastics in freshwater ecosystem: risk to food security and human health. Internat. J. Ecol. 2022, 1234078. doi: 10.1155/2022/1234078
Nelms S. E., Galloway T. S., Godley B. J., Jarvis D. S., Lindeque P. K. (2018). Investigating MP trophic transfer in marine top predators. Environ. pollut. 238, 999–1007. doi: 10.1016/j.envpol.2018.02.016
Neves D., Sobral P., Ferreira J. L., Pereira T. (2015). Ingestion of MPs by commercial fish off the Portuguese coast. Mar. pollut. Bull. 101 (1), 119–126. doi: 10.1016/j.marpolbul.2015.11.008
Nielsen B. L. H., Gréve H. V. S., Hansen B. W. (2020). Cultivation success and fatty acid composition of the tropical copepods Apocyclops royi and Pseudodiaptomus annandalei fed on monospecific diets with varying PUFA profiles. Aquaculture Res. 52 (3), 1127–1138. doi: 10.1111/are.14970
Oaten A., Murdoch. W. W. (1975). Functional response and stability in predator-prey systems. Am. Nat. 109, 289–298. Available at: http://www.jstor.org/stable/2459696.
Paffenhöfer G.-A., Van Sant K. B. (1985). The feeding response of a marine planktonic copepod to quantity and quality of particles. Mar. Ecol. Prog. Ser. 27, 55–65. doi: 10.3354/meps027055
Pan D., Su F., Liu H., Liu C., Umar A., Castañeda L., et al. (2021). Research progress on catalytic pyrolysis and reuse of waste plastics and petroleum sludge. ES Mat Manuf 11, 3–15. doi: 10.30919/esmm5f415
Qiao R., Lu K., Deng. Y., Ren H., Zhang Y. (2019). Combined effects of polystyrene microplastics and natural organic matter on the accumulation and toxicity of copper in zebrafish. Sci. Total Environ. .682, 128–137. doi: 10.1016/j.scitotenv.2019.05.163
Rajan K., Khudsar F. A., Kumar R. (2023). Spatio-temporal patterns of microplastic contamination in surface waters of Hooghly River Estuary: Causes and consequences. Regional Stud. Mar. Sci. 65, 103111. doi: 10.1016/j.rsma.2023.103111
Rao T. R., Kumar R. (2002). Patterns of prey selectivity in the cyclopoid copepod Mesocyclops thermocyclopoides. Aquat. Ecol. 36, 411–424. doi: 10.1023/A:1016509016852
Richon C., Gorgues T., Paul-Pont I., Maes C. (2022). Zooplankton exposure to MPs at global scale: Influence of vertical distribution and seasonality. Front. Mar. Sci. 9. doi: 10.3389/fmars.2022.947309
Roch S., Friedrich C., Brinker A. (2020). Uptake routes of MPs in fishes: Practical and theoretical approaches to test existing theories. Sci. Rep. 10, 3896. doi: 10.1038/s41598-020-60630-1
Rochman C. M., Brookson C., Bikker J., Djuric N., Earn A., Bucci K., et al. (2019). Rethinking microplastics as a diverse contaminant suite. Environ. Toxicol. Chem. 38, 703–711. doi: 10.1002/etc.4371
Rochman C., Hoh E., Kurobe T., Teh S. J. (2013). Ingested plastic transfers hazardous chemicals to fish and induces hepatic stress. Sci. Rep. 3, 3263. doi: 10.1038/srep03263
Sarma S. S. S., Nandini S. (2002). Studies on functional response and prey selection using zooplankton in the anostracan Chirocephalus diaphanus Prevos. Hydrobiologia 486, 169–174. doi: 10.1023/A:1021398718074
Setälä O., Fleming-Lehtinen V., Lehtiniemi M. (2014). Ingestion and transfer of MPs in the planktonic food web. Environ. pollut. 185, 77–83. doi: 10.1016/j.envpol.2013.10.013
Smith B. E., Smith L. A. (2020). Multispecies functional responses reveal reduced predation at high prey densities and varied responses among and within trophic groups. Fish Fisheries 21, 891–905. doi: 10.1111/faf.12468
Solomon M. E. (1949). The natural control of animal populations. J. Anim. Ecol. 18, 1–35. doi: 10.2307/1578
Svetlichny L., İşinibilir M., Mykitchak T., Eryalçın K. M., Türkeri E. E., Yüksel E. (2021). Microplastic consumption and physiological response in Acartia clausi and Centropages typicus: possible roles of feeding mechanisms. Regional Stud. Mar. Sci. 43, 101650. doi: 10.1016/j.rsma.2021.101650
Ter Halle A., Ladirat L., Martignac. M., Mingotaud A. F., Boyron O., Perez E. (2017). To what extent are MPs from the open ocean weathered? Environ. pollut. 227, 167–174. doi: 10.1016/j.envpol.2017.04.051
Traboni C., Sarno D., Ribera d’Alcalà M., Mazzocchi M. G. (2023). Microplastics in the menu of Mediterranean zooplankton: Insights from the feeding response of the calanoid copepod Centropages typicus. Mar. Ecol. 00, e12760. doi: 10.1111/maec.12760
Tseng L. C., Kumar R., Chen Q. C., Hwang J. S. (2011). Summer distribution of noctiluca scintillans and mesozooplankton in the Western and Southern East China Sea prior to the three gorges dam operation. Hydrobiologia 666 (1), 239–256. doi: 10.1007/s10750-010-0587-y
Turner J., Tester P. (1989). Zooplankton feeding ecology: copepod grazing during an expatriate red tide. 359–374. doi: 10.1007/978-3-642-75280-3_21
Ugwu K., Herrera A., Gomez M. (2021). Microplastics in marine biota: A review. Mari Pol. Bul. 169, 112540. doi: 10.1016/j.marpolbul.2021.112540
Vanderploeg H. A., Paffenhöfer G. A., Liebig J. R. (1990). Concentration-variable interactions between calanoid copepods and particles of different food quality: observations and hypotheses. Behav. Mech. Food Selection 20, 595–613. ISBN: 978-3-642-75120-2.
von Moos N., Burkhardt-Holm P., Köhler A. (2012). Uptake and effects of microplastics on cells and tissue of the blue mussel Mytilus edulis L. after an experimental exposure. Environ. Sci. Technol. 46 (20), 11327–11335. doi: 10.1021/es302332w
Walne P. R. (1970). Studies on the food value of nineteen genera ofalgae to juvenile bivalves of the genera Ostrea, Crassostrea, Mercenaria,and Mytilis. Fishery Investigations 26, 1–62.
White J. R., Dagg M. J. (1989). Effects of suspended sediments on egg production of the calanoid copepod Acartia tonsa. Mar. Biol. 102, 315–319. doi: 10.1007/BF00428483
Woods M. N., Hong T. J., Baughman D., Andrews G., Fields D. M., Matrai P. A. (2020). Accumulation and effects of microplastic fibers in American lobster larvae (Homarus americanus). Marine Pollu. Bulletin 157, 111280.
Xu J., Rodríguez-Torres R., Rist S., Nielsen T. G., Hartmann N. B., Brun P., et al. (2022). Unpalatable plastic: efficient taste discrimination of MPs in planktonic copepods. Environ. Sci. Technol. 56 (10), 6455–6465. doi: 10.1021/acs.est.2c00322
Zhang F., Wang X., Xu J., Zhu L., Peng G., Xu P., et al. (2019). Food-web transfer of MPs between wild caught fish and crustaceans in East China Sea. Mar. pollut. Bull. 146, 173–182. doi: 10.1016/j.marpolbul.2019.05.061
Keywords: copepods, microplastics, functional response, foraging, ecosystem
Citation: Sharma J, Rai M and Guino-o RS II (2024) Microplastics influence the functional responses of a tropical estuarine calanoid Pseudodiaptomus annandalei. Front. Ecol. Evol. 12:1277332. doi: 10.3389/fevo.2024.1277332
Received: 14 August 2023; Accepted: 08 January 2024;
Published: 05 February 2024.
Edited by:
Jiang-Shiou Hwang, National Taiwan Ocean University, TaiwanReviewed by:
Devesh Kumar Yadav, Central University of South Bihar, IndiaAhmet Erkan Kideys, Middle East Technical University, Türkiye
Copyright © 2024 Sharma, Rai and Guino-o. This is an open-access article distributed under the terms of the Creative Commons Attribution License (CC BY). The use, distribution or reproduction in other forums is permitted, provided the original author(s) and the copyright owner(s) are credited and that the original publication in this journal is cited, in accordance with accepted academic practice. No use, distribution or reproduction is permitted which does not comply with these terms.
*Correspondence: Jaigopal Sharma, sharmajaigopal@dce.ac.in
†Present address: Malayaj Rai, Department of Microbiology, University of Hawai’i at Mānoa, Honolulu, HI, United States
‡These authors have contributed equally to this work