- 1U.S. Geological Survey, Western Ecological Research Center, Yosemite Field Station, Wawona, CA, United States
- 2Global Change Ecology Lab, Department of Biology, New Mexico State University, Las Cruces, NM, United States
- 3Department of Ecosystem and Conservation Science, University of Montana, Missoula, MT, United States
Soil seed banks help maintain species diversity through temporal storage effects and function as germination pools that can optimize fitness across varying environmental conditions. These characteristics promote the persistence of native plant communities, yet disturbances such as fire and associated invasions by non-native species can disrupt these reserves, fundamentally altering successional trajectories. This may be particularly true in deserts, where native plant communities are less adapted to fire. While studies of fire effects on desert plant communities are not uncommon, information regarding the short- and long-term effects of fire on seed banks is less available. To better understand the influence of fire and invasive species on desert seed banks, we investigated soil seed bank biodiversity from 30 wildfires that burned between 1972 and 2010 across the Mojave Desert ecoregion of North America. We assessed how characteristics of fire regimes (frequency, time since fire, and burn severity) interacted with climate and invasive plants on measures of α-, β-, and γ-diversities. Because β-diversity is a direct measure of community variability and reveals important information about biodiversity loss, we further examined the nestedness and turnover components of β-diversity. Mean α- and γ-diversities were generally higher for burned locations than in unburned reference sites, however individual fire variables had little influence on patterns of seed bank diversity. Burned area seed banks tended to be dominated by non-native invasive species, primarily two grasses, (Bromus rubens, Bromus tectorum), as well as an invasive forb (Erodium cicutarium). The most striking pattern we observed was a collective sharp decline in α-, β-, and γ-diversities with increased invasive species dominance, indicating the homogenization of seed bank communities with the colonization of invasive species after fire. Evidence of homogenization was further supported by reduced turnover and increased nestedness in burn areas compared to reference areas indicating potential biodiversity loss. Our findings highlight how biological processes such as plant invasions can combine with disturbance from fire to alter patterns of seed bank composition and diversity in desert ecosystems.
1 Introduction
Disturbance plays a pivotal role in influencing biodiversity across ecosystems (Huston, 1979; Sousa, 1984). Characterized by heterogeneity in time and space, disturbances create a patchwork of varying resources and habitats that directly contribute to the maintenance of diversity (Petraitis et al., 1989). Ultimately, disturbances moderate the balance among deterministic (environmental and biotic filtering) and stochastic (random dispersal and recruitment) assembly processes that shape local ecological community composition and structure, and determine levels of similarity and turnover in species pools across communities (Chesson, 2000; Kraft et al., 2008; Ferrenberg et al., 2013). In light of global change pressures, including novel species introductions, and climate and land-use change that can rapidly alter disturbance regimes, understanding the effects of disturbance on species diversity remains crucial (Pulsford et al., 2016).
In western North America, global change pressures are reshaping historical fire regimes, increasing the frequency, severity, and extent of fires (Abatzoglou and Williams, 2016). A large proportion of this region is composed of arid desert ecosystems (Barbour and Billings, 2000). Lacking frequent exposure to fire over evolutionary time, native desert vegetation is thought to be less adapted and more vulnerable to fire compared to plants of more mesic fire-prone environments (Chambers and Wisdom, 2009; Brooks et al., 2018). As such, changes to the historical fire regimes in deserts can result in a long-term transformation of native vegetation and a shift in the trajectory of recovering post-fire plant communities. This has been well documented in the Mojave and Great Basin deserts, where disturbance to native woody plant cover and surface soils from fire has led to post-fire communities dominated by herbaceous plants, often non-native annual grasses of genus Bromus. The spread of Bromus has combined with climate and land-use change to alter the fire regime and promote positive feedback resulting in a “grass/fire cycle” that lead can to further invasions (D’Antonio and Vitousek, 1992; Brooks et al., 2004).
Introductions of non-native species like Bromus complicate the relationship between disturbance and plant diversity. Disturbance often reduces the abundance of competitively dominant species and increases the availability of limiting resources (Petraitis et al., 1989), which can promote colonization by less competitive species and lead to higher levels of diversity (Chesson and Huntly, 1997; Cadotte, 2007). However, the rapid spread of invasive annual grasses after fire can suppress the recruitment of native species and reduce overall diversity (Brooks, 2000; DeFalco et al., 2007). While extensive research has explored the link between disturbance, invasive plants, and their combined effects on native plant communities (Hobbs and Huenneke, 1992; McIntyre and Lavorel, 1994; Keeley et al., 2005), most studies focus on aboveground plant assemblages, often overlooking the crucial role of soil seed banks (Faist, 2013; Vandvik et al., 2016; Hosna et al., 2023). Seed banks, through dormancy strategies, enable plants to disperse across space and time, contributing to the maintenance of diversity via temporal “storage effects” (Chesson, 2000; Anderson et al., 2012). This is an important strategy in arid ecosystems where climatic conditions that promote successful recruitment may be sporadic across growing seasons and years (Gremer and Venable, 2014). The impact of altered disturbance regimes, coupled with non-native species introductions, on seed banks remains unclear for most ecosystems including deserts.
In this study, we assess the interactive roles of fire frequency, time since fire, burn severity, climate, topography, and the abundance of invasives on plant diversity in seed banks in the Mojave Desert, USA (Figure 1). Vegetation cover across the Mojave Desert varies as a function of local climate regimes (Aronson and Shmida, 1992; Rodríguez-Moreno and Bullock, 2014), with high aridity producing sparse, heterogeneous vegetation cover which translates to high spatial variability in both plant community types, and the risk and severity of fire (Abella, 2009). Patterns of diversity in aboveground vegetation and belowground seed banks are likely to reflect such variation (Engel and Abella, 2011). However, given temporal storage effects, the rate at which seed banks come to reflect aboveground vegetation patterns remains unclear. Following fires, plant diversity in the Mojave Desert is expected to vary as a function of the initial community, the dispersal potential of species in local and regional pools, local environmental characteristics, and the severity and time since fire as successional processes take place (Safford and Harrison, 2004; Keeley et al., 2005). Collectively, the combination of these factors determines diversity across different spatial scales while simultaneously shaping variability among vegetation communities (β-diversity). Consequently, observed aboveground plant diversity patterns in both burned and unburned landscapes can vary greatly based on annual and seasonal rainfall (Winkler and Brooks, 2020). Therefore, evaluations of seed banks provide temporally integrated insights into local communities, offering a more representative measure of potential plant diversity than short-term aboveground observations. To better understand how seed bank diversity varies with disturbance we specifically ask:
1. What relationship does seed bank diversity have with climate and topography?
2. How is seed bank diversity affected by the frequency, severity, and time since fire?
3. How is seed bank diversity influenced by invasive species with and without fire disturbance?
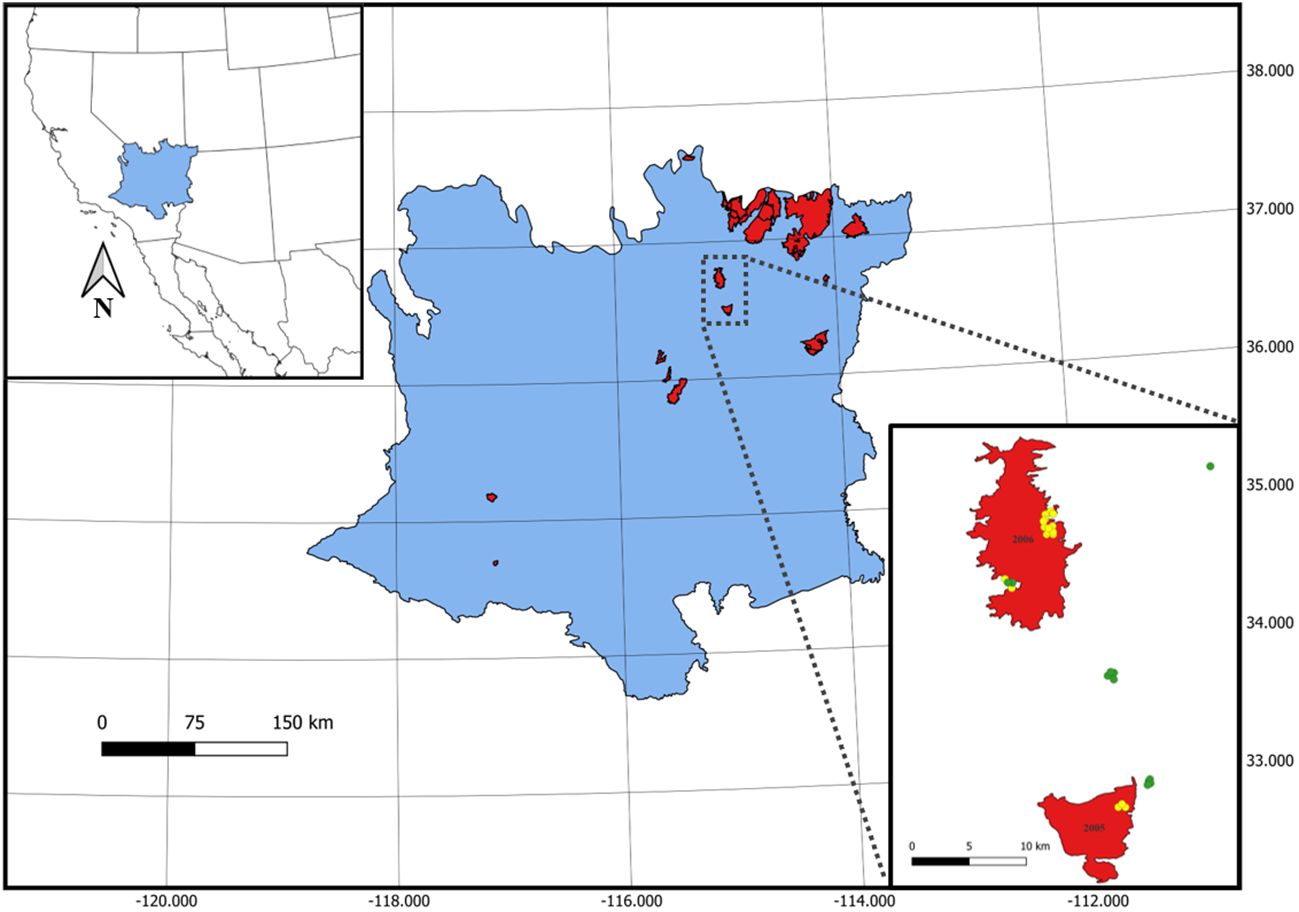
Figure 1 Burn perimeters (red) from 30 unique wildfires representing a chronosequence of fires that burned between 1972 and 2010 in the Mojave Desert of North America. Lower right inset panel shows an example of point locations for burned (yellow; total n = 432) and unburned (green; total n = 111) 0.1 ha plots where seed bank samples were collected. Mojave Desert Ecoregion and burn perimeter data provided by Klinger et al. (2022).
2 Materials and methods
2.1 Study sites
This study occurred in the Mojave Desert ecoregion of North America (Mojave hereafter). The Mojave is the smallest North American desert (≈152,000 km2) but encompasses large variations in landforms and climate. Elevation ranges from −85 m to 3635 m with most of the ecoregion being between 600 m and 1600 m. A majority of precipitation, ~70%, occurs during the cool season between October and March with annual totals ranging from 50 to 130 mm in lower elevations to 250–750 mm at higher elevations. Temperatures range from minimums of −20°C (high elevation) to −5°C (low elevation) during the winter and highs of 30°C (high elevation) to 50° C (low elevation) in the summer (Hereford et al., 2006; Tagestad et al., 2016). This large variation in climate and landform results in distinct vegetation communities across climatic and soil gradients: lower elevations (< 1200 m) feature low-stature shrubs with sparse herbaceous cover, mid-elevations (1200 to 1800 m) exhibit denser shrubs and increased herbaceous cover, and high elevations (> 1800 m) host a mix of large shrubs and small trees, with limited herbaceous vegetation and bare interspaces (Barbour and Billings, 2000).
2.2 Experimental design and soil sampling
Our seed bank study was conducted concurrently with assessments of above-ground vegetation response to fire and detailed descriptions of methods for site selection are given in Klinger and Brooks (2017) and Klinger et al. (2021). In brief, we acquired fire perimeters and burn severity (differenced normalized burn ratio; dNBR) from the Monitoring and Trends in Burn Severity program (MTBS; https://www.mtbs.gov) for fires ≥ 405 ha (the MTBS minimum fire size) that occurred throughout the Mojave from 1972–2010 (Eidenshink et al., 2007). We then used a Geographic Information System (GIS; ESRI http://www.esri.com/) to map fire frequency (number of times a site burned since 1972) by overlaying the perimeters and calculating their overlap through time. We then used GIS to randomly select sites within (burned) or adjacent (unburned) to the perimeters of 53 fires across the Mojave Desert. While fires in the Mojave spanned a large range of elevations, most occurred in middle to high-elevation zones (Brooks et al., 2018). We accounted for differences that may occur due to topography, soils, and land use history by randomly selecting 1 km2 site polygons entirely within or outside the fire perimeters, then randomly selecting 3 to 7 plots within each site polygon. Each plot was 0.1 ha (32 m × 32 m) containing three randomly positioned, parallel 30 m transects. Five subplots of 1 m2 were spaced at 5 m intervals along the three transects for a total of 15 subplots per plot (Figure 2). Visual assessments were conducted in the field to ensure that no unburned plots had evidence of burning to avoid inaccurate burn perimeters or smaller fires not reported in the MTBS database.
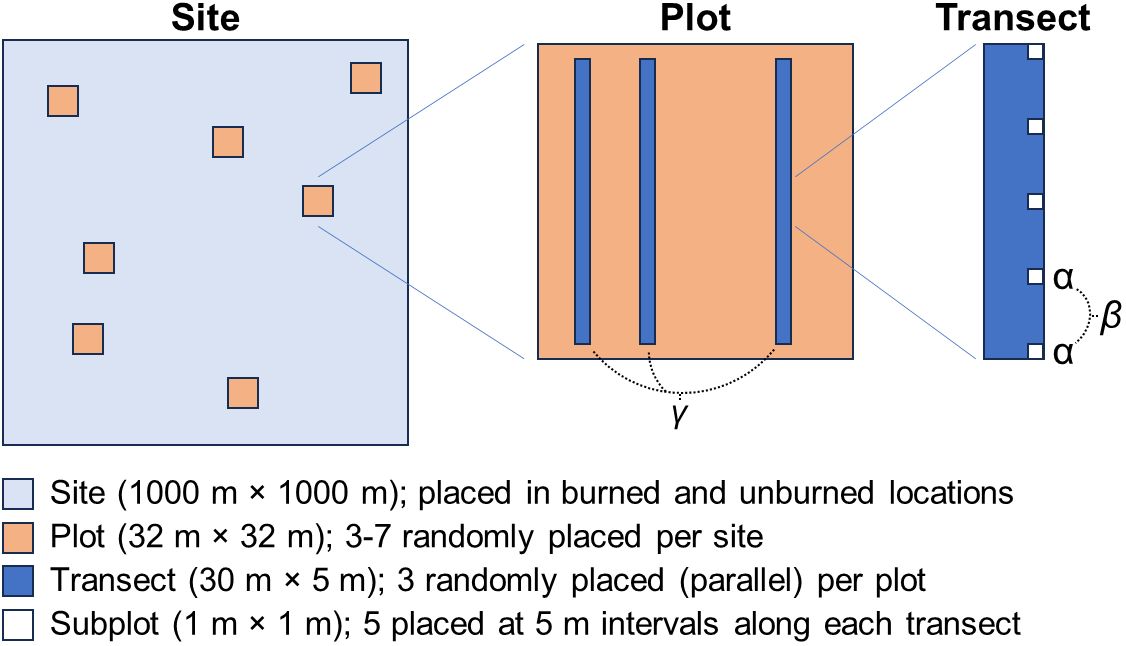
Figure 2 Conceptual diagram illustrating the scale and relationships between sampling sites, plots, transects, and subplots. Greek letters indicate the level at which each diversity metric was calculated, with alpha measuring species diversity of each subplot, beta diversity being calculated as the pairwise dissimilarities among the subplots, and gamma encompassing species diversity for plots.
Soil samples for seed bank assays were collected once from 432 burned plots across 30 unique fires and 111 unburned plots (N=543; Figure 1); this was a subset of the plots sampled in Klinger and Brooks (2017). Within each plot, we collected four samples from each subplot—one at each corner—that were pooled together. Each soil sample was collected using a cylindrical tin measuring 6.0 cm diameter × 4.4 cm deep (volume = 124.4 cm3). This approach focused on the top 4 cm of soil where the bulk of viable seeds are located in desert soils (Young and Evans, 1975; Kemp, 1989; Ferrandis et al., 2001). Soil samples were collected in the fall (October-November) of 2009, 2011, and 2012, when annual plants had senesced, and the majority of seed bank inputs for the year had occurred. Seed bank samples were then stored in a cool (temperatures ranging from −10 to 20 degrees C), dry, dark location for two to three months, to maintain viability and aid germination (Baskin and Baskin, 1998).
We used an emergence method, where germinated seedlings are used as a surrogate for counting seeds, to determine seed bank composition and abundance. Individual soil samples were homogenized by crushing soil aggregates, removing rocks and litter >1 cm diameter, and thoroughly mixing. Soil on rocks and litter was brushed back into the samples to capture any seeds before discarding them. Litter with crevices or potentially seed-bearing surfaces was placed back into the soil sample. One-half cup of soil (~118 cm3) from each sample was thoroughly mixed with ½ cup vermiculite to help with moisture regulation of the soils. Soil-vermiculite samples were then placed in plastic bulb pots (15.72 cm outside diameter × 9.21 cm height) and spread to approximately 2 cm deep on top of weed block fabric. Pots were arranged randomly on greenhouse benches and watered overhead using a gentle rain nozzle. Greenhouse temperature was maintained between 5 and 30°C.
Two consecutive germination trials, each lasting ~50 days, were completed with pots monitored every 1 to 3 days for seedlings and soil moisture. Pots were watered as needed (every 1 to 5 days) to maintain adequate moisture for seeds and seedling growth. Each trial was stopped once two weeks had passed with no new germination. Soils were then allowed to dry for three weeks between the first and second trials—an approach that simulates natural wetting from rainfall and is needed to break dormancy of some seeds (see Faist and Collinge, 2015; Haight et al., 2019). Seedlings were identified to species, counted, and plucked to quantify species abundance by subplot without allowing crowding or competition to reduce germination rates. Species not readily identifiable after germination were allowed to grow until features supported their identification. In cases where features remained non-descript, individuals were identified to Genus or higher taxonomic groups (this was the case for a very small number of seedlings). Because cheatgrass (Bromus tectorum) and red brome (Bromus rubens) are difficult to differentiate at the seedling stage, they were collectively identified as Bromus spp. Nomenclature for emerging species followed the U.S. Department of Agriculture, Natural Resource Conservation Service (plants.usda.gov; 2013).
2.3 Data analysis
2.3.1 Diversity indices
Using count data of species emergence from our subplot samples, we partitioned diversity into plot-scale α, β, and γ components and constructed diversity profiles for each based-on Hill numbers (Hill, 1973) of diversity using the ‘entropart’ package (Marcon and Hérault, 2015) for R. Hill numbers are a parametric family of diversity indices that incorporate species richness and relative abundance information to quantify diversity (Jost, 2007). These are broken into three primary orders (q): q0, species richness; q1, the exponential of Shannon entropy; and q2, the inverse of Gini-Simpson index. An advantage of assessing diversity via Hill numbers is that each diversity metric is expressed in units of effective numbers of species (i.e., the number of equally abundant species that would be needed to give an equivalent measure of diversity; Chao et al., 2014). We focused our assessment of diversity across the Mojave landscape at the order of q1, which is considered “true diversity” or the effective number of species in a community (Jost, 2013). We did this because these metrics are generalizable across samples and have intuitive interpretations of our dataset: α-diversity for q1 is the mean effective number of species across 1-m2 subplots within a plot; γ-diversity is the effective number of species for the entire 0.1 ha plot, and β-diversity represents the effective number of distinct localized communities within a plot, a direct measure of community variability (Jost, 2007; Silva Pedro et al., 2016).
Beta diversity was calculated following Whittaker (1972) multiplicative law (α × β = γ), which, when combined with Hill numbers, produces a β independent of the plot α values (Jost, 2007). Additionally, utilizing the subplots as individual samples, we decomposed β-diversity via Sørensen dissimilarity into plot-level nestedness (i.e., the degree to which species losses are a function of being a subset of a larger community) and turnover (i.e., species replacement across local assemblages) components using the ‘nestedbetasor’ function in the ‘vegan’ package (Oksanen et al., 2020). Separating β-diversity into various components may help determine whether local community variability is the result of environmental and dispersal processes thought to reflect species turnover versus patterns of local colonization and extinction that can influence levels of species nestedness (Soininen et al., 2018). We derived β at the 0.1 ha (plot) scale to characterize variability within the seed bank at a spatial scale relevant to potential biological processes and interactions (e.g., species competition, nurse plant effect). Furthermore, by having a measure of variability at the plot scale, we could then model β as a response to environmental, fire, and biotic factors.
2.3.2 Explanatory variables
We identified 10 explanatory variables across four categories influencing diversity in the Mojave landscape: climate, topography, biotic, and fire (see Table 1). Climate variables included 30-year normals (1984–2014) for mean annual precipitation (MAP) and maximum July temperature (tmax7) using PRISM data. (PRISM Climate Group, Oregon State University, http://prism.oregonstate.edu, accessed 19 August 2019). Topographic variables included elevation, hillshade (an index of solar radiation that utilizes aspect and slope) and a terrain ruggedness index (TRI; Wilson et al., 2007). Due to the strong correlation between elevation and temperature (r > 0.8; Appendix 1), we withheld temperature in favor of elevation in our analyses for its significant role in determining both climate and landscape position. Interactions among plant species can strongly influence pattern diversity in vegetation communities (McIntire and Fajardo, 2014; Lucero et al., 2020). To address this, we incorporated the relative cover of above-ground woody vegetation (shrub and tree cover) and the relative abundance of non-native plants from soil seed bank greenhouse trials as biotic factors. Woody cover plays a crucial role in shaping plant composition patterns in desert ecosystems (Schade et al., 2003; Haas-Desmarais and Lortie, 2023), and non-native species can impact biodiversity (Underwood et al., 2019). We classified each species as either native or non-native (alien), and calculated the relative abundance of non-native plants for each plot. Fire variables included burn severity (dNBR), years’ post-fire (YPF), and fire frequency (0,1 vs 2–3 times since 1972). YPF was transformed as 1 divided by the time since the last burn, establishing a gradient supporting theoretical successional states along a directional time axis. Unburned plots were assigned a YPF value of 0, facilitating the inclusion of burned and unburned plots in the same model along a time since disturbance gradient. Data on burn severity and fire history can be found in Klinger et al. (2022).
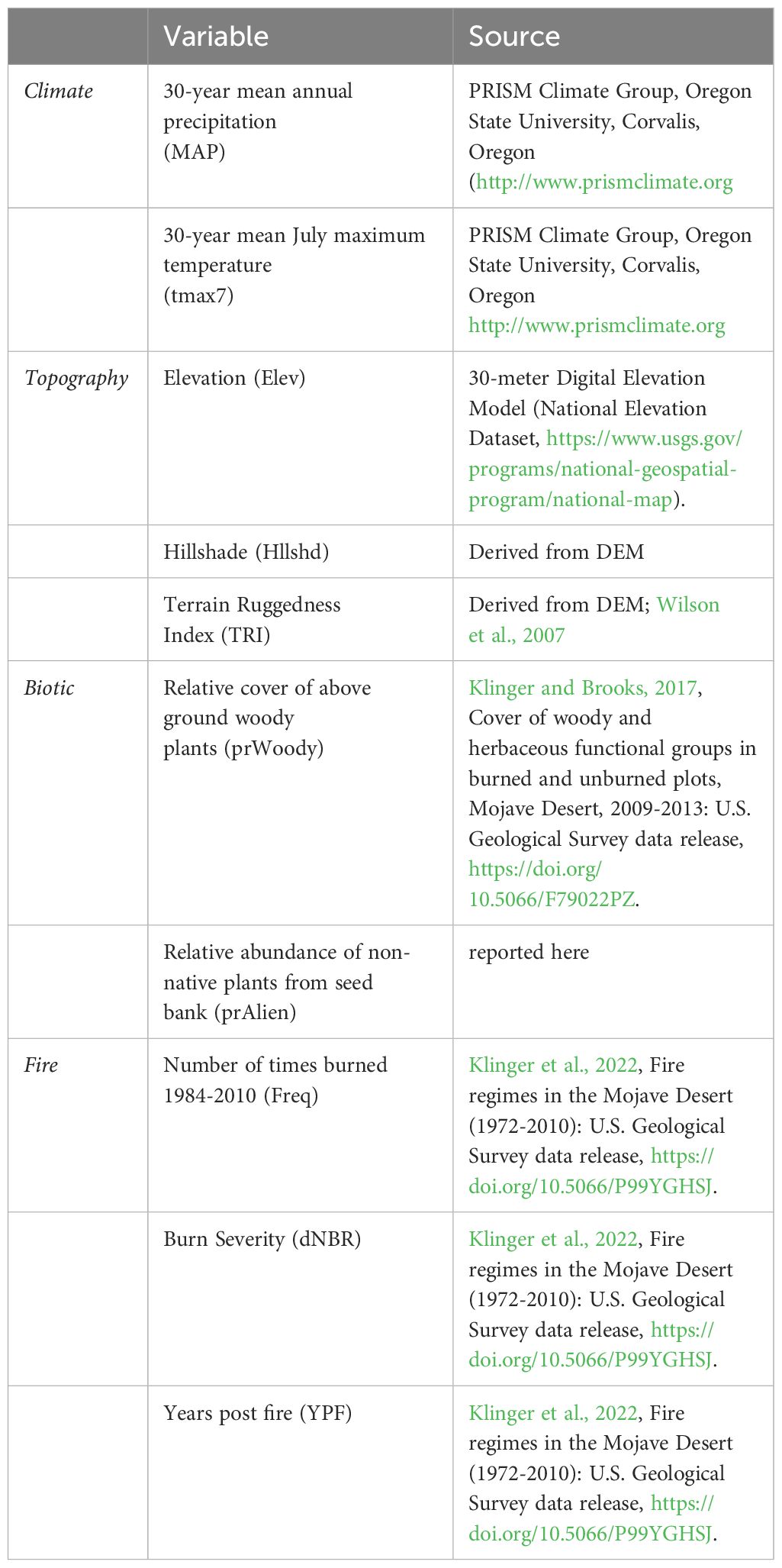
Table 1 Variables used to assess patterns of soil seed bank diversity across the Mojave Desert ecoregion, USA.
2.3.3 Diversity modelling
We used generalized linear mixed models (GLMMs) to examine the relationship between plot-level diversities and various climate, topography, biotic, and fire-related variables (Table 1). We constructed individual GLMMs incorporating a gamma distribution and log link function for the q1 order α-, β-, and γ-diversities, designating “site” as a random effect. Additionally, we developed individual GLMMs for the decomposed nestedness and turnover components of Sørensen dissimilarity, using a Gaussian distribution and identity link function, with site specified as a random effect. For each model, we employed model averaging using the MuMin package (Bartoń, 2020) to estimate 95% confidence intervals of standardized parameter estimates (β coefficients) for each explanatory variable. We considered variables ecologically significant if their 95% CIs did not overlap zero. The use of multi-model inference allowed us to assess the relative importance of each variable. To address non-linear relationships identified in preliminary analyses between diversity responses and the relative abundance of non-native species, as well as mean annual precipitation (MAP), we incorporated quadratic terms for these variables into each model. We used the lme4 package (Bates et al., 2015) to construct all GLMMs and model fits were checked (Appendices 2–4) using the DHARMa (Hartig, 2022) and performance packages (Lüdecke et al., 2020). We assessed possible collinearity issues between fixed effects by estimating the variance inflation factors (VIF) for each model (Appendix 5). VIF values were <5, indicating little collinearity among predictors (Akinwande et al., 2015). All analyses were run with R 4.3.1 (R Core Team, 2023).
3 Results
We recorded the emergence of 173 unique plant species from the soil seed bank, the majority of which were native annual plants. We documented 14 non-native species, the most abundant being the invasive annual grasses B. tectrorum and B. rubens and the annual forb Erodium cicutarium (Appendix Table 1). In general, plot level species richness (γ at q0) was higher in burned areas, 9.61 (95% CI = 8.50–10.57), than in unburned areas, 6.75 (95% CI = 6.00–7.37; Figure 3). In terms of true diversities (q1), a subset of explanatory variables tended to have significant influences on seed bank diversity at subplot (α) and plot (γ) scales, as well as plot-scale community variability (β), nestedness, and turnover. Additionally, all measures of diversity had a non-linear relationship with the relative abundance of non-native plants found within the seed bank, particularly in burned sites, where measures of diversity initially increased with non-native abundance before peaking and rapidly declining as non-native plants became dominant (Figure 4).
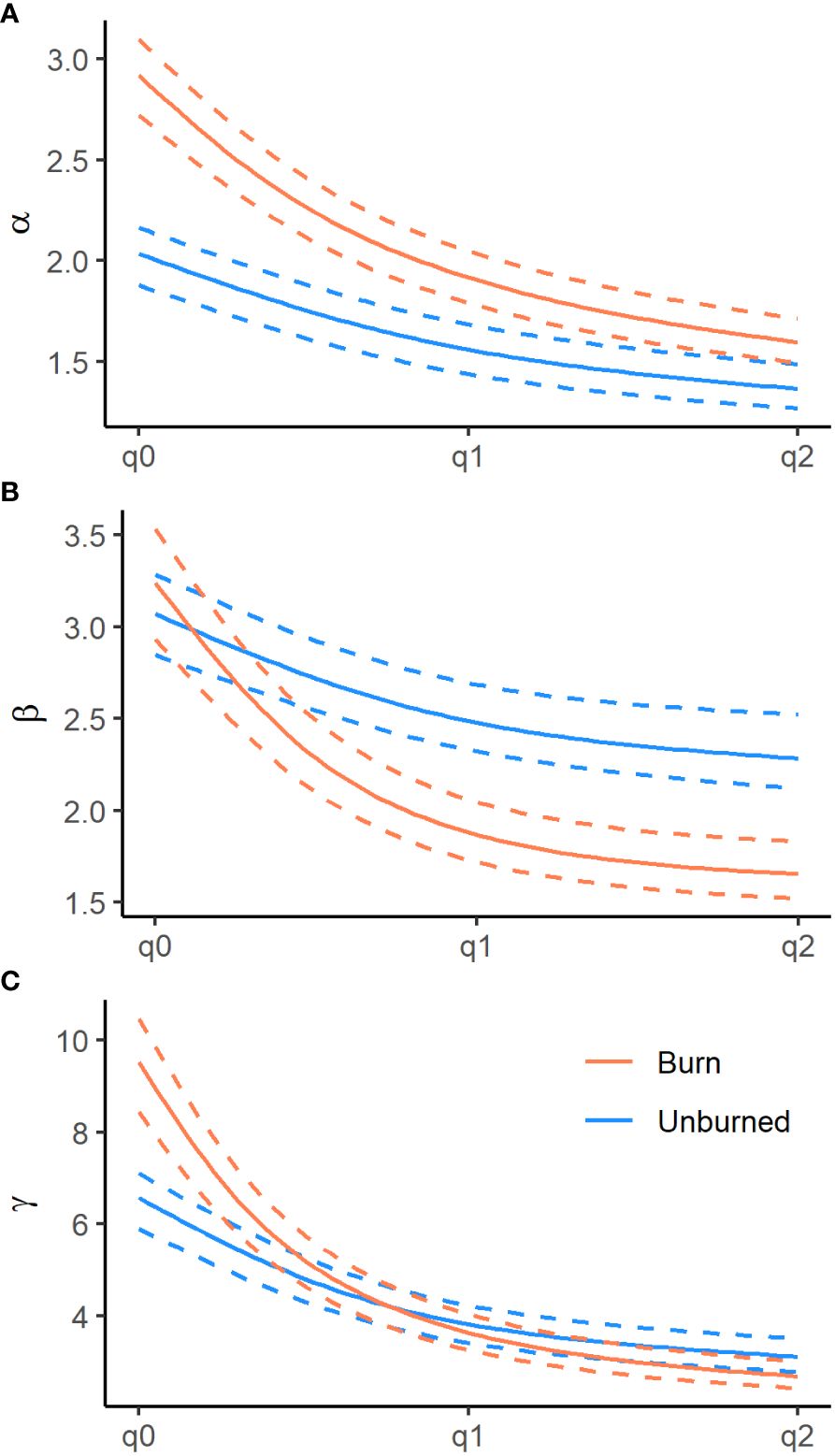
Figure 3 Diversity profiles for alpha (α, panel A), beta (β, panel B), and gamma (γ, panel C) diversities of burned (red ) and unburned (blue) plots from the Mojave Ecoregion. Diversity orders range across species richness (q0), exponent of Shannon’s entropy (q1) and the Gini-Simpson index (q2). Dashed lines indicate 95% confidence intervals.
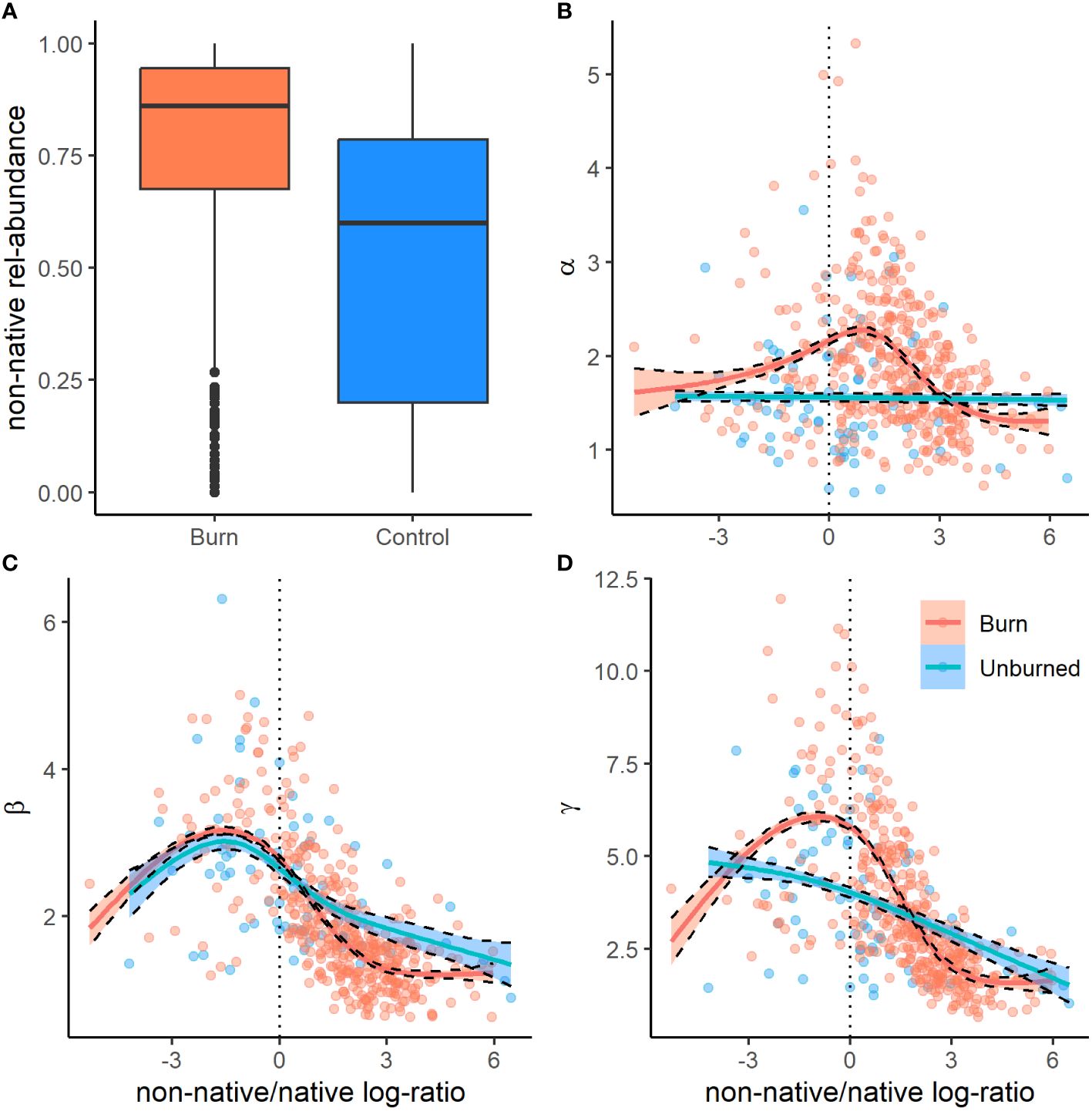
Figure 4 Box and whisker plots (A) of the relative abundance of non-native germinates from seed bank samples from burned (red) and unburned (blue) sites from across the Mojave Desert. Bold lines represent the median, boxes the upper and lower quartiles (interquartile range), whiskers 1.5× the interquartile range, and points outliers. Panels (B–D) show the relationship of α (B), β (C), and γ (D) diversity with the ratio (log) of non-native to native seed bank abundance.
3.1 Alpha diversity
At the local 1 m2 subplot scale, the effective number of species (α-diversity at q1) tended to be greater in areas that burned, 1.92 (95% CI = 1.79–2.05), than in unburned areas, 1.56 (95% CI = 1.45–1.68). Our GLMM was able to explain roughly half of the variation in α-diversity (R2 = 0.51). Alpha diversity had a significant positive relationship with topographic complexity (TRI) and burn frequency, and a negative relationship with relative cover of woody plants and elevation (Figure 3). However, the largest influence on local α-diversity was the relative abundance of non-native plants within the seed bank, which had a strong non-linear relationship with α-diversity across both burned and unburned locations (Figures 4, 5).
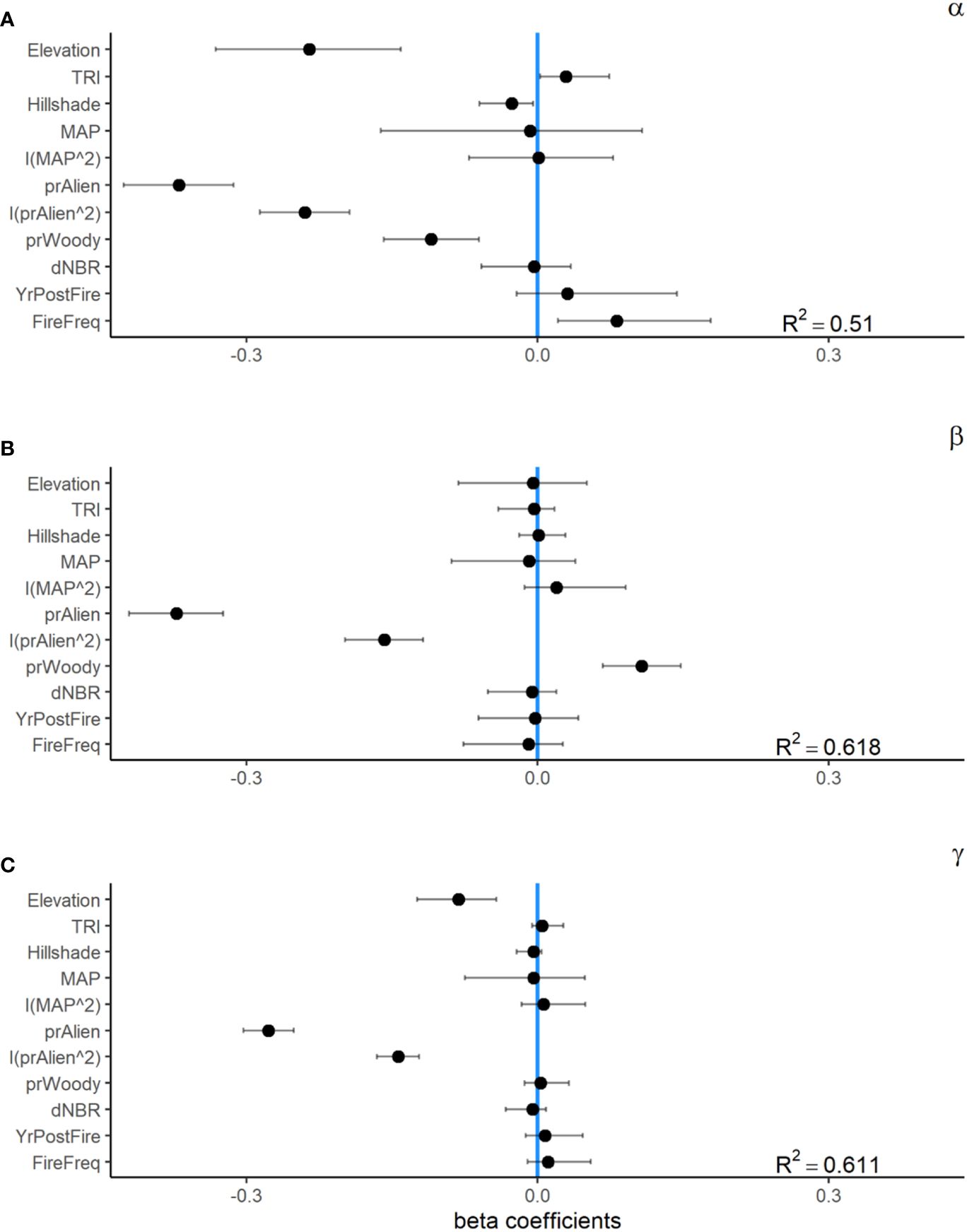
Figure 5 Standardized beta coefficients for fixed effects from generalized linear mixed effect models (GLMMs) for seed bank alpha (A), beta (B), and gamma (C) diversity from a 35-year chronosequence of burned locations from across the Mojave Desert. Error bars indicate 95% confidence intervals.
3.2 Beta diversity
Plot-scale variability in community composition among (β-diversity at q1) was generally lower in burned sites, 1.87 (95% CI = 1.72–2.05), than in unburned sites, 2.47(95% CI = 2.31–2.65; Figure 3). Our GLMM explained a relatively high amount of variation in β-diversity (R2 = 0.62). Beta diversity had a significant positive relationship with relative cover of woody vegetation and a negative relationship with burn frequency. Similar to α-diversity, β-diversity had a non-linear relationship with the relative abundance of non-native plants within the seed bank (Figure 4).
Plot-scale Sørensen dissimilarity was similar across burned (µ = 0.74, 95% CI = 0.65–0.83) and unburned (µ = 0.78, 95% CI = 0.65–0.90) sites, with the majority of dissimilarity contributed to by species turnover (losses and gains that appear as “replacements” in species composition) as opposed to nestedness (changes in abundance where local communities are subsets of the larger, regional or site-level pool). Species turnover was higher across unburned (87% of dissimilarity) than burned locations (72% of dissimilarity). Accordingly, species nestedness was twice as high in burned (28%) compared to unburned plots (13%), indicating that a greater proportion of observed differences in composition within burn plots was composed by variable subsets of local species pools with a shrinking role of replacement via turnover (Figure 6). The GLMMs explained slightly less of the total variation in plot-scale dissimilarity for both nestedness (R2 = 0.42) and turnover (R2 = 0.44) than standard measures of diversity. However, both turnover and nestedness had similar, non-linear relationships with the relative abundance of non-native plants (Figure 6). Turnover exhibited a negative relationship with burn frequency and a positive relationship with the relative cover of woody vegetation. Nestedness had a significant positive relationship with the relative cover of woody vegetation, and a negative relationship with burn frequency.
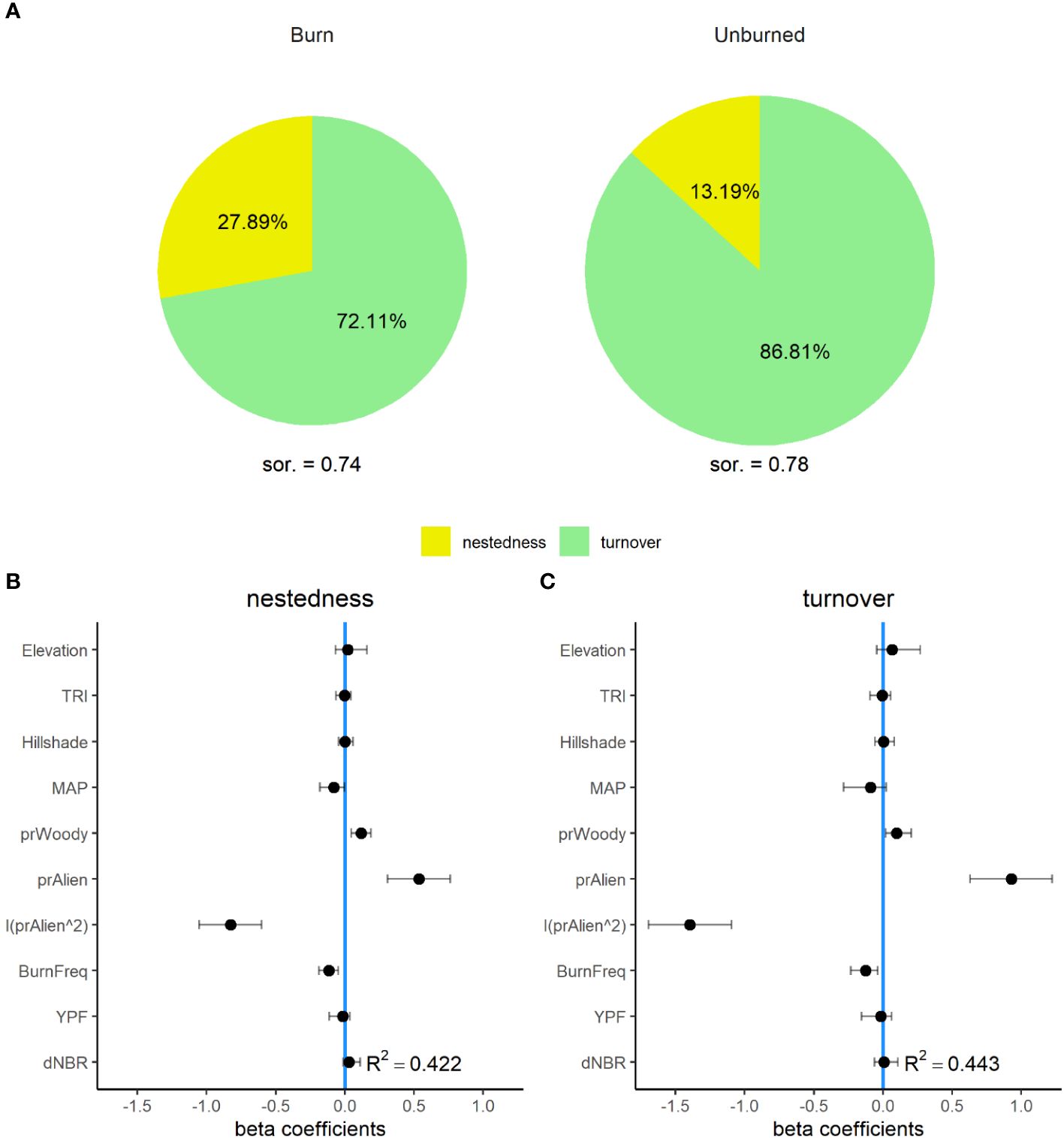
Figure 6 Nestedness and turnover components of beta diversity for burned and unburned sites across the Mojave Desert (A). Percent values within each pie chart represent the proportion of mean plot-level Sørensen dissimilarity (sor.) portioned into either nestedness (yellow) or turnover (green). Panels (B, C) are the standardized beta coefficients for fixed effects (see Table 1) from generalized linear mixed effect models (GLMMs) for seed bank nestedness (B), and turnover (C) from a 35-year chronosequence of burned locations from across the Mojave Desert. Error bars indicate 95% confidence intervals.
3.2 Gamma diversity
The effective number of species at the plot scale (γ at q1) was similar for burned and unburned sites. Mean γ-diversity was 3.63 (95% CI = 3.26–4.04) for burned and 3.82 (95% CI = 3.41–4.24) for unburned plots. Similar to α- and β-diversity models, the model explained a little over half of the variation in γ-diversity (R2 = 0.55; Figure 5). Gamma diversity was significantly and positively related with tmax7 and negatively related with Hillshade. Also similar to the other diversity measures, γ-diversity had a strong non-linear relationship with the relative abundance of non-native species.
4 Discussion
Fire, while historically rare and infrequent across North American deserts (Wright and Bailey, 1982), has become an increasingly significant disturbance on the landscape. An examination of Mojave fires over the last four decades indicates a highly heterogeneous regime with varying frequency, severity, and size (Klinger et al., 2021). Shifts in fire frequency and severity can promote transitions toward communities dominated by herbaceous and non-native plants (Brooks et al., 2004; Klinger and Brooks, 2017). Notably, certain Bromus grass species, once introduced, exhibit explosive invasiveness in post-fire areas in the Mojave and other regions (Reid et al., 2006). The rapid alteration of local plant community dynamics by invasives raises concerns about the emergence of persistent alternative ecological states. Our findings in the Mojave indicate that fire can increase seed bank species richness (γ at q0) and the effective number of species (α & γ at q1) at relatively small subplot (1 m) and plot (0.1 ha) spatial scales. This likely underscores the crucial role of disturbance in promoting variability in resources that sustain plant diversity within this ecoregion. However, seed bank diversity was minimally influenced by measures of fire severity, frequency, and YPF. Instead, diversity was strongly shaped by the invasion severity of non-native plants and the cover of woody perennial plants—factors reflecting the long-term effects of climate and disturbance history on plant communities. Effectively, invasion of the Mojave ecoregion by non-native annual plants homogenizes the soil seed bank by rapidly driving a decline in native plant diversity beyond a critical threshold in non-native plant relative abundance, and a concomitant decrease in the compositional variability of the seed bank across the landscape (i.e., a decrease in β-diversity). While unburned locations also host non-native plants at lower abundance than burned, fire disturbances allow for a rapid increase in the relative abundance of non-native plants, driving more rapid losses of native diversity and resulting in greater homogenization across space. Further efforts to quantify this threshold will be pivotal for developing management strategies and designating urgent restoration zones to support native plant biodiversity in the Mojave system.
4.1 Disturbance and changes in native plant species diversity
Measures of q1 diversity (i.e., effective number of species) at the subplot (α; 1m2) and plot (γ; 0.1 ha) scale were higher in burned than unburned areas. The effective number of species in burned areas, however, was not significantly influenced by the severity, frequency, or years since fire such that areas that burned once differed little from sites that burned multiple times over the data timeline.
The seemingly weak influence of fire variables on the effective number of species at local subplot and plot scales may also be because the strongest influence we observed in determining diversity at both scales was the abundance of non-native alien species within the seed bank. This relationship was variable across unburned and burned sites but was non-linear and often parabolic. The relationship was consistent in that the effective diversity initially increased as the relative abundance of non-native species in the seed bank increased before reaching a threshold peak that was followed by a rapid loss of diversity at higher non-native seed abundance. This relationship was strongest in burned sites—an outcome that was expected given the positive influence of disturbance on the abundance of non-native plants in the Mojave and other ecoregions. While the common non-native plants of the Mojave benefit from disturbances, these species can still invade unburned sites where they persist at lower abundances than in more heavily disturbed locations. For example, the earlier emergence of B. tectoroum from the seed bank relative to native plants allows it to capture greater amounts of soil nitrogen, increasing its abundance while elevating resource competition that causes a decline in native plant diversity (Prevéy and Seastedt, 2014). Similar plant community responses and outcomes of soil nutrient pulses have been documented in other prolific plant invaders (Besaw et al., 2011). Bromus tectorum, and some other non-native annual plants, have also been shown to capitalize on resources provided by soil-surface biocrust communities (Ferrenberg et al., 2018; Havrilla et al., 2019) which are more common and widespread in less frequently disturbed locations of drylands (Weber et al. 2022). Fire effects on vegetation and soils typically cause short-duration pulses in soil inorganic nitrogen, which have been shown to amplify non-native plant invasion in the Mojave Desert (Esque et al., 2010).
We also found a negative relationship between the effective number of species and woody plant cover at the local, subplot scale. This result seems contrary to expectations of shrubs and trees acting as “nurse plants” that promote species diversity in arid ecosystems (Madrigal-González et al., 2020). However, an overwhelming majority of nurse plant studies report patterns derived from aboveground plant assemblages and are not measures based on the larger plant community found in soil seed banks which can harbor additional species diversity (Hosna et al., 2023). Woody plant cover is also correlated to local climate and disturbance histories; areas with more woody plants are likely to have experienced fewer or less severe disturbances over time (Sühs et al., 2020). In the context of our study, the presence of woody vegetation in burned areas likely reflects the natural heterogeneity in fire effects across study sites and transects, impacting local vegetation community structure and the average age/size of shrubs. This aligns with findings in the Chihuahuan Desert, where nurse plant effects depend on shrub size and species, emphasizing the role of both floristic composition and disturbance regimes in shaping the plant diversity of arid systems (Ferrenberg et al., 2023).
4.2 Fire as a homogenizing influence on soil seed banks
Disturbance is expected to alter β-diversity in several ways. In the short term, fire may increase β-diversity by altering environmental heterogeneity, reducing local α-diversity, and pushing fire intolerant species to be more clumped or in “patches” (Myers et al., 2015). Under classical post-disturbance recovery models, β-diversity would have a trajectory towards pre-disturbance levels as time passed. However, invasion can disrupt this process (e.g., Kuebbing et al., 2014). We observed that the abundance of native species represented in the seed bank declined linearly with non-native relative abundance at burned sites, but not at unburned sites. β-diversity similarly declined, although non-linearly, with the increase in relative abundance of invasives. This nonlinear relationship might reflect changes in biotic interactions (e.g., competitive or facilitative effects), environmental filtering, or responses by plants to localized resource pools. This relationship might also reflect stochastic influences on seed bank community assembly, particularly dispersal processes and the effects of fire disturbance on the probability of seed movements among heterogenous resource and vegetation patches (Applestein et al., 2022).
The decline in both α- and β-diversity indicates that the seed bank communities of this system are being homogenized by non-native plant invasion. Fire disturbance plays an important role in the progress of seed bank homogenization: we observed a relative reduction in species turnover and a greater than 200% increase in species nestedness in burned compared to unburned sites. Thus, across burned sites, seed banks experience less species turnover (replacements) while dwindling toward a subset of plants found in the more diverse γ-level communities. This increased similarity in community composition is produced by the loss of species from a community and the non-random replacement of those species by more widespread occurring species (Clavel et al., 2011). This phenomenon has been documented widely across biota and ecosystems in response to the proliferation of invasive non-native species (Olden and Poff, 2003). This occurs in the Mojave, where the suppression of recruitment of native species by invasive annual grass and forb species has been observed (Brooks, 2000; DeFalco et al., 2007). Meanwhile, the greatest difference in turnover was driven by fire frequency with two or more fires increasing turnover suggesting that ongoing increases in fire frequency are likely to produce alternate community states within soil seed banks.
Beta diversity is a crucial concept in ecology, representing variation in species composition between different ecological communities. Beta diversity is important as it provides insights into the mechanisms of community assembly and biodiversity patterns across different habitats or environmental gradients. For, instance, studies have shown that beta diversity can vary with elevation (Kraft et al., 2011) and shift in response to fire disturbance and time since fire (Ferrenberg et al., 2013). This variation in beta diversity across elevations or disturbed habitats can indicate the influence of various ecological processes, such as environmental filtering, species interactions, or spatial constraints on community composition. Understanding these patterns is vital, as it helps in identifying drivers of biodiversity and understanding the impact of environmental changes on plant communities.
5 Conclusions
Variability and maintenance of native species in local plant assemblages has important implications for the conservation and ecological integrity of Mojave Desert ecosystems. We measured the effects of fire severity, frequency, and history on seed bank species diversity. We found that invasive plants are driving a homogenization process of desert plant communities, which is amplified by the positive effects of fire disturbance on the abundance of key invaders, particularly annual Bromus grasses. Such homogenization could have profound impacts on the maintenance of regional biodiversity pools, including across trophic levels where numerous species, such as the threatened desert tortoise (Gopherus agassizii), are already taxed by habitat alteration. Understanding the mechanistic pathways through which plant invasion and disturbance combine to alter seed bank composition and abundance patterns, and what role direct and indirect effects of disturbance on soil systems play in seed bank homogenization, is crucial for identifying targets for restoration as well as conservation goals.
Data availability statement
The raw data supporting the conclusions of this article will be made available by the authors, without undue reservation.
Author contributions
SL: Data curation, Formal analysis, Investigation, Methodology, Supervision, Visualization, Writing – original draft, Writing – review & editing. RK: Conceptualization, Data curation, Funding acquisition, Methodology, Project administration, Resources, Supervision, Validation, Writing – review & editing. MB: Conceptualization, Funding acquisition, Investigation, Project administration, Resources, Supervision, Validation, Writing – review & editing. SF: Conceptualization, Investigation, Resources, Supervision, Writing – original draft, Writing – review & editing.
Funding
The author(s) declare financial support was received for the research, authorship, and/or publication of this article. Research was funded by the United States Strategic Environmental Research & Development Program (SERDP grant 10 SI01-016), U.S. Joint Fire Science Program (JFSP project number 10-1-04-21), and US Geological Survey Invasive Species Program.
Acknowledgments
We thank the Strategic Environmental Research & Development Program (SERDP, Department of Defense), Joint Fire Science Program (JFSP, Department of Interior), and U.S. Geological Survey Invasive Species Program for funding. We thank the USGS staff in Bishop, California for collecting soil seed bank samples, especially, Michael Cleaver, Sunny Sawyer, Robyn Lee, Laurel Triatik, Elizabeth Moore, Lindsay Swinger, and Stacey Huskins. Laurel Triatik helped immensely with the greenhouse work. Any use of trade, firm, or product names is for descriptive purposes only and does not imply endorsement by the U.S. Government.
Conflict of interest
The authors declare that the research was conducted in the absence of any commercial or financial relationships that could be construed as a potential conflict of interest.
Publisher’s note
All claims expressed in this article are solely those of the authors and do not necessarily represent those of their affiliated organizations, or those of the publisher, the editors and the reviewers. Any product that may be evaluated in this article, or claim that may be made by its manufacturer, is not guaranteed or endorsed by the publisher.
Supplementary material
The Supplementary Material for this article can be found online at: https://www.frontiersin.org/articles/10.3389/fevo.2024.1271824/full#supplementary-material
References
Abatzoglou J. T., Williams A. P. (2016). Impact of anthropogenic climate change on wildfire across Western US forests. Proc. Natl. Acad. Sci. U.S.A. 113, 11770–11775. doi: 10.1073/pnas.1607171113
Abella S. R. (2009). Post-fire plant recovery in the Mojave and Sonoran deserts of western North America. J. Arid Environ. 73, 699–707. doi: 10.1016/j.jaridenv.2009.03.003
Akinwande M.O., Dikko H.G., Samson A. (2015). Variance Inflation Factor: As a Condition for the Inclusion of Suppressor Variable(s) in Regression Analysis. Open J Stat. 5, 754.
Anderson T. M., Schütz M., Risch A.C. (2012). Seed germination cues and the importance of the soil seed bank across an environmental gradient in the Serengeti. Oikos 121, 306–312. doi: 10.1111/j.1600-0706.2011.19803.x
Applestein C., Caughlin T. T., Germino M. J. (2022). Post-fire seed dispersal of a wind-dispersed shrub declined with distance to seed source, yet had high levels of unexplained variation. AoB Plants 14, plac045. doi: 10.1093/aobpla/plac045
Aronson J., Shmida A. (1992). Plant species diversity along a Mediterranean-desert gradient and its correlaiton with interannual rainfall fluctuations. J. Arid Environ. 23, 235–247. doi: 10.1016/S0140-1963(18)30513-5
Barbour M. G., Billings W. D. (Eds.) (2000). North American terrestrial vegetation. 2nd ed. (Cambridge and New York: Cambridge University Press).
Bartoń K. (2020) MuMIn: multi-model inference. Available at: https://CRAN.R-project.org/package=MuMIn.
Baskin C. C., Baskin J. M. (1998). Seeds: ecology, biogeography, and evolution of dormancy and germination (San Diego, California, USA: Academic Press).
Bates D., Maechler M., Bolker B., Walker S. C. (2015). Fitting linear mixed-effects models using lme4. J. Stat. Software 67, 1–48. doi: 10.18637/jss.v067.i01
Besaw L. M., Thelen G. C., Sutherland S., Metlen K., Callaway R. M. (2011). Disturbance, resource pulses, and invasion: short-term shifts in competitive effects, not growth responses, favour exotic annuals. J. Appl. Ecol. 48, 998–1006. doi: 10.1111/jpe.2011.48.issue-4
Brooks M. L. (2000). Competition between alien annual grasses and native annual plants in the Mojave Desert. Am. Midland Nat. 144, pp.92–pp108. doi: 10.1674/0003-0031(2000)144[0092:CBAAGA]2.0.CO;2
Brooks M. L., D’Antonio C.M., Richardson D.M., Grace J.B., Keeley J.E., DiTomaso J.M., et al. (2004). Effects of invasive alien plants on fire regimes. BioSience 54, 677–688. doi: 10.1641/0006-3568(2004)054[0677:EOIAPO]2.0.CO;2
Brooks M. L., Minnich R., Matchett J. R. (2018). Southeastern deserts bioregion. Fire in California’s Ecosystems. 2nd. Ed. Eds. Wagtendonk J. W. V., Sugihara N. G., Stephens S. L., Thode A. E., Shaffer K. E., Fites-Kaufman (Berkeley, CA, USA: University of California Press).
Cadotte (2007). Concurrent niche and neutral processes in the competition-colonization model of species coexistence. Proc. R. Soc B. 274, 2739–2744. doi: 10.1098/rspb.2007.0925
Chambers J. C., Wisdom M. J. (2009). Priority research and management issues for the imperiled great basin of the western United States. Restor. Ecol. 17, 707–714. doi: 10.1111/j.1526-100X.2009.00588.x
Chao A., Gotelli N. J., Hsieh T. C., Sander E. L., Ma K. H., Colwell R. K., et al. (2014). Rarefaction and extrapolation with Hill numbers: a framework for sampling and estimation in species diversity studies. Ecol. Monogr. 84, 45–67. doi: 10.1890/13-0133.1
Chesson P. (2000). Mechanisms of maintenance of species diversity. Annu. Rev. Ecol. Syst. 31, 343–366. doi: 10.1146/annurev.ecolsys.31.1.343
Chesson P. L., Huntly N. (1997). The roles of harsh and fluctuating conditions in the dynamics of ecological communities. Am. Nat. 150, 519–553. doi: 10.1086/286080
Clavel J., Julliard R., Devictor V. (2011). Worldwide decline of specialist species: toward a global functional homogenization? Front. Ecol. Environ. 9, 222–228. doi: 10.1890/080216
D’Antonio C. M., Vitousek P. M. (1992). Biological invasions by exotic grasses, the grass/fire cycle and global change. Annu. Rev. Ecol. Syst. 23, 63–87. doi: 10.1146/annurev.es.23.110192.000431
DeFalco L. A., Fernandez G. C., Nowak R. S. (2007). Variation in the establishment of a non-native annual grass influences competitive interactions with Mojave Desert perennials. Biol. Invasions 9, 293–307. doi: 10.1007/s10530-006-9033-5
Eidenshink J., Schwind B., Brewer K., Zhu Z.-L., Quayle B., Howard S. (2007). A project for monitoring trends in burn severity. Fire Ecol. 3, 3–21. doi: 10.4996/fireecology.0301003
Engel E. C., Abella S. R. (2011). Vegetation recovery in a desert landscape after wildfires: influences of community type, time since fire and contingency effects. J. Appl. Ecol. 48, 1401–1410. doi: 10.1111/j.1365-2664.2011.02057.x
Esque T. C., Kaye J. P., Eckert S. E., DeFalco L. A., Tracy C. R. (2010). Short-term soil inorganic N pulse after experimental fire alters invasive and native annual plant production in a Mojave Desert shrubland. Oecologia 164, 253–263. doi: 10.1007/s00442-010-1617-1
Faist A. M. (2013). Banking on the past: seed banks as a reservoir for rare and native species in restored vernal pools. Aob Plants 5, plt043. doi: 10.1093/aobpla/plt043
Faist A. M., Collinge S. K. (2015). Seed bank composition varies along invasion and inundation gradients in vernal pool wetlands. Plant Ecol. 216, 553–564. doi: 10.1007/s11258-015-0458-8
Ferrandis P., Herranz J. M., Martinez-Sanches J. J. (2001). Response to fie of a predominantly transient seed bank in a Mediterranean weedy pasture (eastern-central Spain). Ecoscience 8, 211–219. doi: 10.1080/11956860.2001.11682647
Ferrenberg S., Faist A. M., Howell A., Reed S. C. (2018). Biocrusts enhance soil fertility and Bromus tectorum growth, and interact with warming to influence germination. Plant Soil 429, 77–90. doi: 10.1007/s11104-017-3525-1
Ferrenberg S., Faist A., Osborne B., Lee S. (2023). Quantifying potential abiotic drivers of the nurse-plant effect in two dominant shrub species of the northern Chihuahuan Desert. bioRxiv, 2023–2011. doi: 10.1101/2023.11.16.567462
Ferrenberg S., O’Neill S. P., Knelman J. E., Todd B., Duggan S., Bradley D., et al. (2013). Changes in assembly processes in soil bacterial communities following a wildfire disturbance. ISME J. 7, 1102–1111. doi: 10.1038/ismej.2013.11
Gremer J. R., Venable D. L. (2014). Bet hedging in desert winter annual plants: optimal germination strategies in a variable environment. Ecol. Lett. 17, 380–387. doi: 10.1111/ele.12241
Haas-Desmarais S., Lortie C. J. (2023). Direct and indirect interactions between shrubs and the flowering annual community in an arid ecosystem. Flora 298, 152202. doi: 10.1016/j.flora.2022.152202
Haight J. D., Reed S. C., Faist A. M. (2019). Seed bank community and soil texture relationships in a cold desert. J. Arid Environ. 164, 46–52. doi: 10.1016/j.jaridenv.2019.01.008
Hartig F. (2022). DHARMa: Residual diagnostics for hierarchical (multi-level /mixed) regression models. R package version 0.4.5.
Havrilla C. A., Chaudhary V. B., Ferrenberg S., Antoninka A. J., Belnap J., Bowker M. A., et al. (2019). Towards a predictive framework for biocrust mediation of plant performance: A meta-analysis. J. Ecol. 107, 2789–2807. doi: 10.1111/1365-2745.13269
Hereford R., Webb R. H., Longpre C. I. (2006). Precipitation history and ecosystem response to multidecadal precipitation variability in the Mojave Desert region 1893–2001. J. Arid Environ. 67, 13–34. doi: 10.1016/j.jaridenv.2006.09.019
Hill M. O. (1973). Diversity and evenness: A unifying notation and its consequences. Ecology 54, 427–432. doi: 10.2307/1934352
Hobbs R. J., Huenneke L. F. (1992). Disturbance, diversity, and invasion: implications for conservation. Conserv. Biol. 6, 324–337. doi: 10.1046/j.1523-1739.1992.06030324.x
Hosna R. K., Reed S. C., Faist A. M. (2023). Long-term relationships between seed bank communities and wildfire across four North American desert sites. Ecosphere 14, e4398. doi: 10.1002/ecs2.4398
Huston M. (1979). A general hypothesis of species diversity. Am. Nat. 113, 81–101. doi: 10.1086/283366
Jost L. (2007). Partitioning diversity into independent alpha and beta components. Ecology 88, 2427–2439. doi: 10.1890/06-1736.1
Jost L. (2013). “Effective number of species,” in The new synthesis of diversity indices and similarity measures. Available at: http://www.loujost.com/Statistics%20and%20Physics/Diversity%20and%20Similarity/EffectiveNumberOfSpecies.htm.
Keeley J. E., Baer-Kelley M., Fotheringham C. J. (2005). Alien plant dynamics following fire in Mediterranean-climate California shrublands. Ecol. Appl. 15, 2109–2125. doi: 10.1890/04-1222
Kemp P. R. (1989). “Seed banks and vegetation processes in deserts,” in Ecology of soil seed banks. Eds. Leck M. A., Parker V. T., Hanneke (Academic, New York), 257–282.
Klinger R., Brooks M. (2017). Alternative pathways to landscape transformation: invasive grasses, burn severity and fire frequency in arid ecosystems. J. Ecol. 105, 1521–1533. doi: 10.1111/1365-2745.12863
Klinger R., Underwood E. C., McKinley R., Brooks M. L. (2021). Contrasting geographic patterns of ignition probability and burn severity in the Mojave Desert. Front. Ecol. Evol. 9, 79. doi: 10.3389/fevo.2021.593167
Klinger R. C., Underwood E. C., McKinley R., Brooks M. L. (2022). Fire regimes in the Mojave Desert (1972-2010): U.S. Geological Survey data release. doi: 10.5066/P99YGHSJ
Kraft N. J. B., Comita L. S., Chase J. M., Sanders N. J., Swenson N. G., Crist T. O., et al. (2011). Disentangling the drivers of beta diversity along latitudinal and elevational gradients. Science 333, 1755–1758. doi: 10.1126/science.1208584
Kraft N. J., Valencia R., Ackerly D. D. (2008). Functional traits and niche-based tree community assembly in an Amazonian Forest. Science 322, 580–582. doi: 10.1126/science.1160662
Kuebbing S. E., Souza L., Sanders N. J. (2014). Effects of co-occurring non-native invasive plant species on old-field succession. For. Ecol. Manage. 324, 196–204. doi: 10.1016/j.foreco.2013.10.031
Lucero J. E., Seifan M., Callaway R. M., Lortie C. J. (2020). Positive associations with native shrubs are intense and important for an exotic invader but not the native annual community across an aridity gradient. Divers. Distrib. 26, 1177–1197. doi: 10.1111/ddi.13111
Lüdecke D., Makowski D., Waggoner P., Patil I. (2020). performance: assessment of regression models performance. R Package Version 0.4. 7. Appl. Stat. Using R 440, 5.
Madrigal-González J., Cano-Barbacil C., Kigel J., Ferrandis P., Luzuriaga A. L. (2020). Nurse plants promote taxonomic and functional diversity in an arid Mediterranean annual plant community. J. Vegetation Sci. 31, pp.658–pp.666. doi: 10.1111/jvs.12876
Marcon E., Hérault B. (2015). entropart: an R package to measure and partition diversity. J. Stat. Software 67, 1–26. doi: 10.18637/jss.v067.i08
McIntire E. J. B., Fajardo A. (2014). Facilitation as a ubiquitous driver of biodiversity. New Phytol. 201, 403–416.-416. doi: 10.1111/nph.12478
McIntyre S., Lavorel S. (1994). Predicting richness of native, rare, and exotic plants in response to habitat and disturbance variables across a variegated landscape. Conserv. Biol. 8, 521–531. doi: 10.1046/j.1523-1739.1994.08020521.x
Myers J. A., Chase J. M., Crandall R. M., Jimenez I. (2015). Disturbance alters beta-diversity but not the relative importance of community assembly mechanisms. J. Ecol. 103, 1291–1299. doi: 10.1111/1365-2745.12436
Oksanen J., Blanchet F. G., Friendly M., Kindt R., Legendre P., McGlinn D., et al. (2020). Vegan: community ecology package version 2.5-7. R Project for Statistical Computing. Vienna, Austria.
Olden J. D., Poff L. (2003). Toward a mechanistic understanding and prediction of biotic homogenization. Am. Nat. 162, 442–460. doi: 10.1086/378212
Petraitis P. S., Latham R. E., Niesenbaum R. A. (1989). The maintenance of species-diversity by disturbance. Q. Rev. Biol. 64, 393–418. doi: 10.1086/416457
Prevéy J. S., Seastedt T. R. (2014). Seasonality of precipitation interacts with exotic species to alter composition and phenology of a semi-arid grassland. J. Ecol. 102, 1549–1561. doi: 10.1111/1365-2745.12320
Pulsford S. A., Lindenmayer D. B., Driscoll D. A. (2016). A succession of theories: purging redundancy from disturbance theory. Biol. Rev. 91, 148–167. doi: 10.1111/brv.12163
R Core Team (2023). R: A language and environment for statistical computing (Vienna, Austria: R Foundation for Statistical Computing). Available at: https://www.R-project.org.
Reid C. R., Goodrich S., Bowns J. E. (2006). “Cheatgrass and red brome; History and biology of two invaders,” in Proceedings–shrublands under fire: disturbance and recovery in a changing world (Fort Collins, Colorado: US Department of Agriculture, Forest Service, Rocky Mountain Research Station), 27–32.
Rodríguez-Moreno V. M., Bullock S. H. (2014). Vegetation response to hydrologic and geomorphic factors in an arid region of the Baja California Peninsula. Environ. Monit. Assess. 186, 1009–1021. doi: 10.1007/s10661-013-3435-5
Safford H., Harrison S. (2004). Fire effects on plant diversity in serpentine and sandstone chaparral. Ecology 85, 539–548. doi: 10.1890/03-0039
Schade J. D., Sponseller R., Collins S. L., Stiles A. (2003). The influence of Prosopis canopies on understorey vegetation: Effects of landscape position. J. Vegetation Sci. 14, 743–750. doi: 10.1111/j.1654-1103.2003.tb02206.x
Silva Pedro M., Rammer W., Seidl R. (2016). A disturbance-induced increase in tree species diversity facilitates forest productivity. Landscape Ecol. 31, 989–1004. doi: 10.1007/s10980-015-0317-y
Soininen J., Heino J., Wang J. (2018). A meta-analysis of nestedness and turnover components of beta diversity across organisms and ecosystems. Global Ecol. Biogeography. 27, 96–109. doi: 10.1111/geb.12660
Sousa W. P. (1984). The role of disturbance in natural communities. Annu. Rev. Ecol. Syst. 15, 353–391. doi: 10.1146/annurev.es.15.110184.002033
Sühs R. B., Giehl E. L. H., Peroni N. (2020). Preventing traditional management can cause 1468 grassland loss within 30 years in southern Brazil. Sci. Rep. 10, 783. doi: 10.1038/s41598-020-57564-z
Tagestad J., Brooks M., Cullinan V., Downs J., McKinley R. (2016). Precipitation regime classification for the Mojave Desert: implications for fire occurrence. J. Arid Environ. 124, 388–397. doi: 10.1016/j.jaridenv.2015.09.002
Underwood E. C., Klinger R. C., Brooks M. L. (2019). Effects of invasive plants on fire regimes and postfire vegetation diversity in an arid ecosystem. Ecol. Evol. 9, 12421–12435. doi: 10.1002/ece3.5650
Vandvik V., Klanderud K., Meineri E., Måren I. E., Töpper J. (2016). Seed banks are biodiversity reservoirs: species–area relationships above versus below ground. Oikos 125, 218–228. doi: 10.1111/oik.02022
Weber B., Belnap J., Büdel B., Antoninka A. J., Barger N. N., Chaudhary V. B., et al. (2022). What is a biocrust? A refined, contemporary definition for a broadening research community. Biol. Rev. 97, 1768–1785. doi: 10.1111/brv.12862
Whittaker R. H. (1972). Evolution and measurement of species diversity. Taxon 21, 213–251. doi: 10.2307/1218190
Wilson M. F. J., O’Connell B., Brown C., Guinan J. C., Grehan A. J. (2007). Multiscale terrain analysis of multibeam bathymetry data for habitat mapping on the continental slope. Mar. Geodesy 30, 3–35. doi: 10.1080/01490410701295962
Winkler D. E., Brooks E. (2020). Tracing extremes across iconic desert landscapes: socio-ecological and cultural responses to climate change, water scarcity, and wildflower superblooms. Hum. Ecol. 48, 211–233. doi: 10.1007/s10745-020-00145-5
Wright H. E., Bailey A. W. (1982). Fire ecology, United States and Canada (New York, USA: Wiley, New York).
Keywords: fire, invasives, seed banks, species diversity, desert, drylands, variability, beta diversity
Citation: Lee S, Klinger R, Brooks ML and Ferrenberg S (2024) Homogenization of soil seed bank communities by fire and invasive species in the Mojave Desert. Front. Ecol. Evol. 12:1271824. doi: 10.3389/fevo.2024.1271824
Received: 02 August 2023; Accepted: 02 April 2024;
Published: 09 May 2024.
Edited by:
Daniel de Paiva Silva, Goiano Federal Institute (IFGOIANO), BrazilReviewed by:
Luciana Ghermandi, National Scientific and Technical Research Council (CONICET), ArgentinaRafael Barbizan Sühs, Federal University of Santa Catarina, Brazil
Copyright © 2024 Lee, Klinger, Brooks and Ferrenberg. This is an open-access article distributed under the terms of the Creative Commons Attribution License (CC BY). The use, distribution or reproduction in other forums is permitted, provided the original author(s) and the copyright owner(s) are credited and that the original publication in this journal is cited, in accordance with accepted academic practice. No use, distribution or reproduction is permitted which does not comply with these terms.
*Correspondence: Steven Lee, c3JsZWVAdXNncy5nb3Y=