- 1Northwest Fisheries Science Center, Fish Ecology Watershed Program, National Oceanic and Atmospheric Administration, Seattle, WA, United States
- 2Natural Resources Department, Lower Elwha Klallam Tribe, Port Angeles, WA, United States
- 3K. Denton and Associates LLC, Sequim, WA, United States
- 4Washington Department of Fish and Wildlife, Olympia, WA, United States
- 5Western Washington Fish and Wildlife Conservation Office, U.S. Fish and Wildlife Service, Lacey, WA, United States
- 6The Conservation Angler, Port Angeles, WA, United States
- 7National Park Service – Olympic National Park, Port Angeles, WA, United States
- 8U.S. Geological Survey, Western Fisheries Research Center, Seattle, WA, United States
Large dam removal is being used to restore river systems, but questions remain regarding their outcomes. We examine how the removal of two large dams in the Elwha River, coupled with hatchery production and fishing closures, affected population attributes of Chinook salmon (Oncorhynchus tshawytscha) and steelhead (O. mykiss). Initial responses by returning adult Chinook salmon and steelhead was an increase in the number and spatial extent of natural and hatchery origin fish. Although few naturally produced juvenile Chinook salmon and steelhead outmigrants were observed prior to and during dam removal, abundances increased three years after adult fish passage was restored, suggesting that impacts due to downstream sedimentation after dam removal were reduced. The Chinook salmon population demographics remain dominated by hatchery production, while increases in winter steelhead abundance included both hatchery and natural-origin spawners. The spatial expansion of winter steelhead upstream of former dam sites was predominantly by natural-origin spawners. We also observed a natural “reawakening” of summer steelhead that were in part derived from an up-river resident population that returned to the Upper Elwha. Our results showed that a combination of habitat, hatchery, and harvest actions can result in positive responses for salmonid populations.
1 Introduction
Dams are a major threat to the connectivity of river ecosystems across the world and have contributed to extinctions and imperiled status of migratory fishes (Pringle et al., 2000). However, many dams have now outlived their intended purposes and life spans, and consequently, over 1200 dams have been decommissioned and removed in the United States over the last two decades (O’Connor et al., 2015; Bellmore et al., 2016). Dam removal can lead to rapid ecosystem responses, such as downstream changes in spawning and rearing habitats, the re-emergence of river channels in former reservoirs, and restored fish passage (O’Connor et al., 2015; Tullos et al., 2016; Bellmore et al., 2019). Most removed dams to date have been small structures (< 8 m in height) (Bellmore et al., 2016), but removal of large dams (> 15 m) has gained momentum, particularly in the western United States (O’Connor et al., 2015).
Removing large dams that block the migration of anadromous salmon, trout, and char can reopen habitat and provide imperiled stocks an opportunity to increase their abundance and productivity as they expand their distribution and diversity across a reconnected watershed (Bellmore et al., 2019). This is a primary reason why dam removal is increasingly being considered and implemented to assist the recovery of depleted populations of Pacific salmonids (Oncorhynchus spp.) (Hare et al., 2019; Waldman and Quinn, 2022). Returning adult salmon can reoccupy either historically available or new habitats and increase their population size, even when initial abundance from donor populations is small (i.e., less than 100) or large (i.e., ~ 1 million) (Milner et al., 2007; Kiffney et al., 2009; Pess et al., 2012; Anderson et al., 2015). Salmon have generally responded favorably after removal of smaller dams (Hogg et al., 2015). However, large dams carry greater ecological tradeoffs. Large dam removals may provide access to a larger amount of habitat, but they also can store and potentially release more sediment that can exert a strong short-term impact on the productivity of affected downstream habitats and food webs (Morley et al., 2020). These sediment impacts can potentially impede the rate of movement into newly opened habitats upstream of former barriers and lead to short-term degraded conditions for fish occupying downstream habitats (Bellmore et al., 2019). While the long-term benefits to salmonids are expected to outweigh the short-term ecological costs, large dam removal is rare and consequently knowledge and peer-reviewed studies on the short- and long-term responses by Pacific salmon are scarce (e.g., Liermann et al., 2017).
In 1992, the Elwha River Ecosystem and Fisheries Restoration Act called for the “full restoration of the Elwha River ecosystem and native anadromous fisheries”, setting the stage for one of the largest planned dam removals in history (Winter and Crain, 2008). The Act authorized the Department of the Interior to acquire and remove the Elwha and Glines Canyon dams on the Elwha River, Washington State (Figure 1). Both dams blocked access to most of the potential anadromous fish habitat for all five species of Pacific salmon, steelhead (Oncorhynchus mykiss), coastal cutthroat trout (Oncorhynchus clarkii), and bull trout (Salvelinus confluentus) (Ward et al., 2008). The concurrent removal of both dams started in September 2011 and was completed in October 2014. Approximately 30 million metric tonnes (Mt) of impounded sediment were ultimately exposed to fluvial erosion, and over 65% of the stored sediment has eroded since dam removal (as of 2016), of which only ~10% was deposited in the fluvial system (Ritchie et al., 2018). The remaining ~90% of the released sediment was transported to the coast, expanding the delta by ~60 ha (Ritchie et al., 2018), significantly diversifying and improving the estuarine and nearshore environment (Foley et al., 2017; Shaffer et al., 2017; Perry et al., 2023; Rubin et al., 2023).
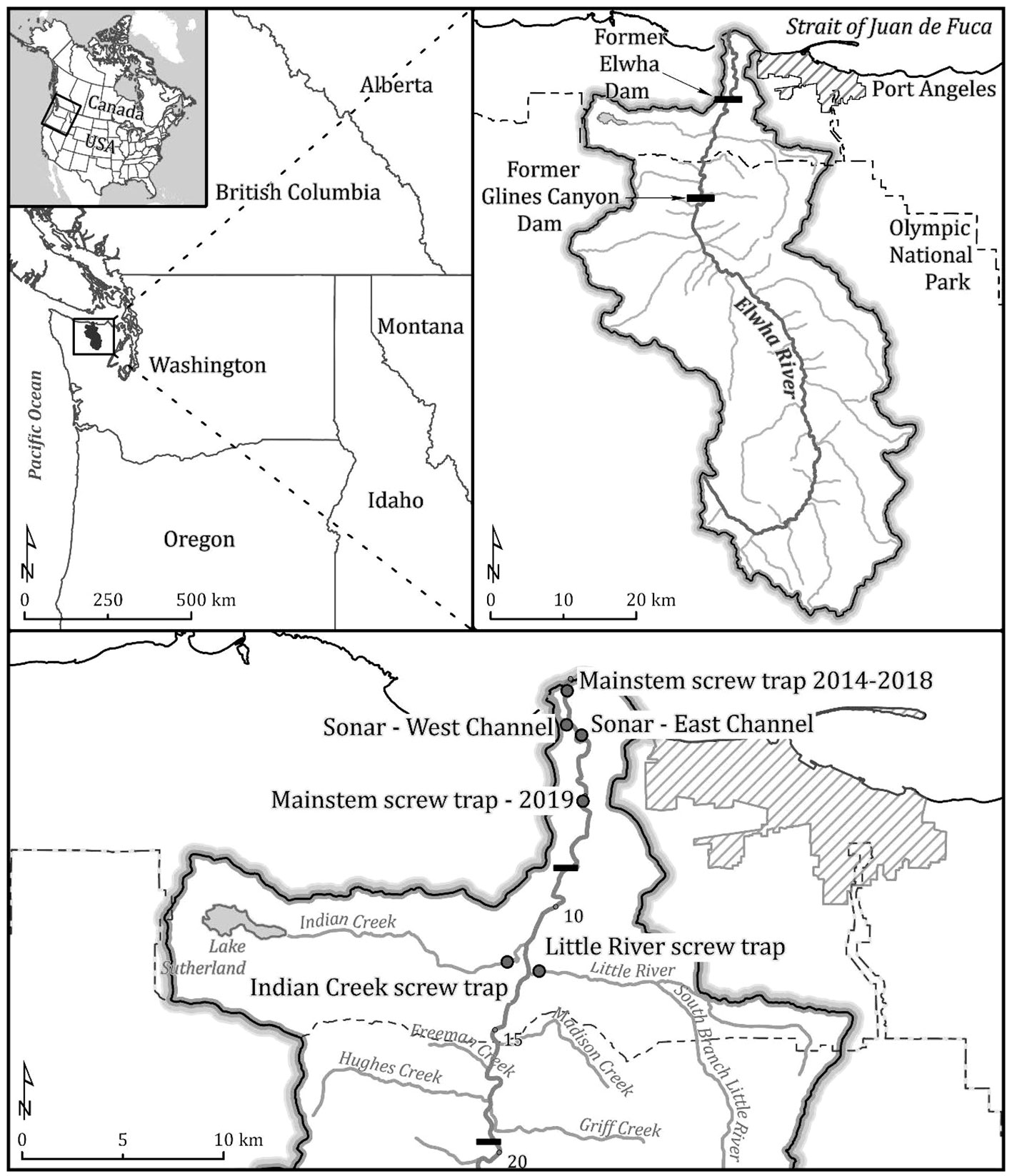
Figure 1 The Elwha River basin. Upper left is regional map of Elwha River, upper right is the entire Elwha River watershed. Lower map includes location of SONAR units and smolt screw traps. Map layer sources: National Park Service, Statistics Canada Census Program, United States Census Bureau’s TIGER/Line, United States Geological Survey, Washington State Department of Ecology. Map projections: Lambert Conformal Conic, WGS 1984 Web Mercator.
The Elwha River provides a unique opportunity to understand the geomorphic evolution of a river system and the short-term responses of anadromous salmonids during and after removal of two large dams (East et al., 2015). In addition to intense sediment impacts, the initial movement of fish into riverine habitats between and upstream of the former dams could depend on several factors, such as the size and origin of the source populations, the potential for strays from other adjacent populations, the types and characteristics of the newly accessible habitat, and life history diversity (Pess et al., 2014). Further, the homing phenomenon displayed by salmon could be important because straying and movement can contribute to initial occurrence, but homing in future generations will be necessary to maintain the population gains and contribute to further growth and expansion. Lastly, dam removal can potentially improve resilience by increasing diversity (e.g., Schindler et al., 2010) if sufficiently unique habitats exist above the dams (e.g., Beechie et al., 2006; Waples et al., 2008) and the adaptive genetic diversity to express those life histories is retained by the fish (Thompson et al., 2019). Short-term, post dam removal response and diversification of life histories in coho salmon (Oncorhynchus kisutch) (Liermann et al., 2017), bull trout (Quinn et al., 2017; Brenkman et al., 2019), and Pacific lamprey (Entosphenus tridentatus) (Hess et al., 2021) has already been documented in the Elwha River since dam removal. Additionally, Duda et al. (2021) reported increases in spatial extent of Pacific salmon and bull trout upstream of the former dam sites. We present the first evaluation of interannual trends in juvenile and adult Chinook salmon (Oncorhynchus tshawytscha) and steelhead abundances during and after dam removal, highlighting the relative contributions of hatchery and natural-origin individuals in the Elwha River.
Herein we focus on the short-term (i.e., less than 10 years) responses of hatchery and natural-origin Chinook salmon and steelhead populations during and after reconnection of the Elwha River. Leading up to the full removal of both dams, we expected an intense, short-term disturbance due to the large-scale increase and subsequent reduction in sediment supply, and eventual access to a large expanse of previously inaccessible pristine habitats. This combined with the current status (e.g., abundance), origin (i.e., hatchery or natural-origin), and diversity (i.e., resident and anadromous forms) of the populations prior to dam removal would exert a strong influence on Elwha River Chinook salmon and steelhead (Brenkman et al., 2008; Pess et al., 2008). A wide variety of monitoring approaches, ranging from SONAR to snorkel surveys, were implemented to evaluate these general hypotheses. Specific questions asked include:
1) What was the annual abundance and origin of returning adult Chinook salmon and winter steelhead during and after dam removal?
2) How many Chinook salmon migrants and steelhead smolts were produced and were annual estimates of juvenile abundance affected by potential streamflow and sediment impacts?
3) How far have Chinook salmon and steelhead expanded their spawning distribution?
4) Has dam removal given rise to the re-emergence of different life histories that were not present, or very rare, when migration into the Elwha River headwaters was blocked?
We used the data to compare and contrast the extent of reintroduction between the two species and in relation to prior research, review potential reasons underlying the responses, and discuss implications of our findings for other large dam removal projects.
2 Study area and salmonid populations of interest
The Elwha River is located on Washington State’s Olympic Peninsula, originating in Olympic National Park (Figure 1). The Elwha drains 833 km2 and flows 72 km from an elevation of 1,372 m at the headwaters to its mouth on the Strait of Juan de Fuca in the Pacific Ocean. The physical geography of the Elwha River system includes a series of alternating canyons and floodplains, which occur throughout the watershed (Pess et al., 2008). Two hydroelectric dams were built without fish passage facilities and eliminated upstream access to 95% of the Elwha River watershed (Brenkman et al., 2019), including Elwha Dam, which was constructed at river kilometer (rkm) 7.9 in 1912 and created Lake Aldwell reservoir, and Glines Canyon Dam, which was constructed at rkm 21.4 in 1927 and created Lake Mills reservoir (Figure 1). The 32‐m‐tall Elwha Dam was removed over an 8‐month period from September 2011 to April 2012, while the Glines Canyon Dam (64 m in height) was removed over a 3‐year period from 2011 to 2014 (Brenkman et al., 2019). In October 2014, shortly after the Glines Canyon Dam removal was complete, a large rockfall occurred in the canyon immediately downstream of the dam site near rkm 20.0 (Figure 1). The rockfall created a new barrier to upstream passage of adult salmonids, and consequently, removal of the boulders was initiated in October 2015, and completed in September 2016 (Brenkman et al., 2019; Ertle et al., 2019). Similar to previous Elwha publications, we refer to three main sections of the Elwha watershed (Figure 1). The Lower Elwha is downstream of the former Elwha Dam site to the river mouth (rkm 0.0–7.9). The Middle Elwha is between the two former dam sites, including the former Lake Aldwell Reservoir (rkm 7.9–21.7). Lastly, the Upper Elwha is upstream of the former Glines Canyon Dam, which included the former Mills Reservoir (rkm 21.7–61.6).
The Elwha River currently supports wild, natural‐origin, hatchery, and nonnative fishes (Brenkman et al., 2019), including Chinook salmon, coho salmon, chum salmon (O. keta), pink salmon, sockeye salmon (O. nerka), rainbow trout, summer and winter steelhead (anadromous form of rainbow trout), coastal cutthroat trout, bull trout, and nonnative brook trout (S. fontinalis). Although two distinct runs of Chinook salmon were historically present in the Elwha River, a spring run and a summer/fall run, only the latter was thought to persist prior to dam removal (Brannon and Hershberger, 1984). Two juvenile life history strategies of Chinook salmon – stream type (1+ year old outmigrants) and ocean type (0+ year olds outmigrants) – also both currently exist in the Elwha River, with a large majority being ocean type. A dedicated Chinook salmon hatchery program was initiated in 1930 from Elwha River origin stock (Brannon and Hershberger, 1984), and in recent years, Chinook salmon releases have been large (annual average number released 1985 to 2014 = 2.5 million). The current Chinook salmon hatchery program was deemed necessary because the population has been dependent upon hatchery production for multiple decades and dam removal was predicted to reduce survival and production of Chinook salmon in the main stem Elwha River below the dams (NMFS, 2012). The Chinook salmon hatchery program played an important role in maintaining the persistence of the unique genetic lineage of Elwha Chinook salmon during the century that the dams were in place (NMFS, 2012). Releases of hatchery winter run steelhead have occurred since 1965, and out-of-basin summer steelhead were released from 1968 to 2008 (Duda et al., 2018). Native Elwha winter steelhead persisted in low abundance downstream of the dams prior to dam removal, but as with Chinook salmon, dam removal presented a potential threat to their short-term viability due to the expected elevated suspended sediment concentrations from stored sediment being released during and after dam removal. Managers developed a winter steelhead hatchery program using native broodstock that started releasing smolts in 2011 and stopped prior releases of hatchery steelhead derived from an out-of-basin stock (NMFS, 2012). The initial release goal for the winter steelhead program was 175,000 age-2 smolts (LEKT 2012), but that was adjusted to 30,000 in 2023 based on entry timing, increased abundance, adult-to-adult productivity, and distribution (Peters et al., 2024). Summer run steelhead existed only at very low numbers and may have been extirpated prior to dam removal, but they were expected to rebuild and reoccupy their former historical habitats upstream of both dams (Ward et al., 2008). A moratorium on commercial and recreational fishing for all species within the Elwha River watershed and terminal nearshore area was implemented in 2012 and continued through summer of 2023. A limited ceremonial and subsistence-fishing season for coho salmon occurred in the fall of 2023. The only other exception to the moratorium has been an ongoing recreational fishery targeting kokanee (non-anadromous form of the sockeye salmon) in Lake Sutherland, the headwaters of Indian Creek.
2.1 Chinook salmon and steelhead adult relocation
To help jumpstart salmonid reintroduction, adult Chinook salmon and winter run steelhead were relocated to habitats above the dam sites during and immediately after dam removal (Tables 1, 2). Relocated adults were obtained via natural recruitment into hatchery facilities (Chinook salmon and steelhead), and netting and trapping in the river (Chinook salmon only). In five of ten years during and after dam removal, adult Chinook salmon were relocated into five locations of the Middle Elwha upstream of the Elwha Dam site, with the greatest number of fish being relocated in 2018 and 2019 (Table 1). However, because only surplus hatchery fish were used for Chinook salmon relocation, males were numerically dominant in all years except 2019. From 2012 to 2014 and again in 2016, adult winter steelhead were captured at the Lower Elwha Klallam Tribe’s (LEKT) hatchery trap and relocated into two Middle Elwha tributaries – Indian Creek and Little River (Figure 1; Table 2).
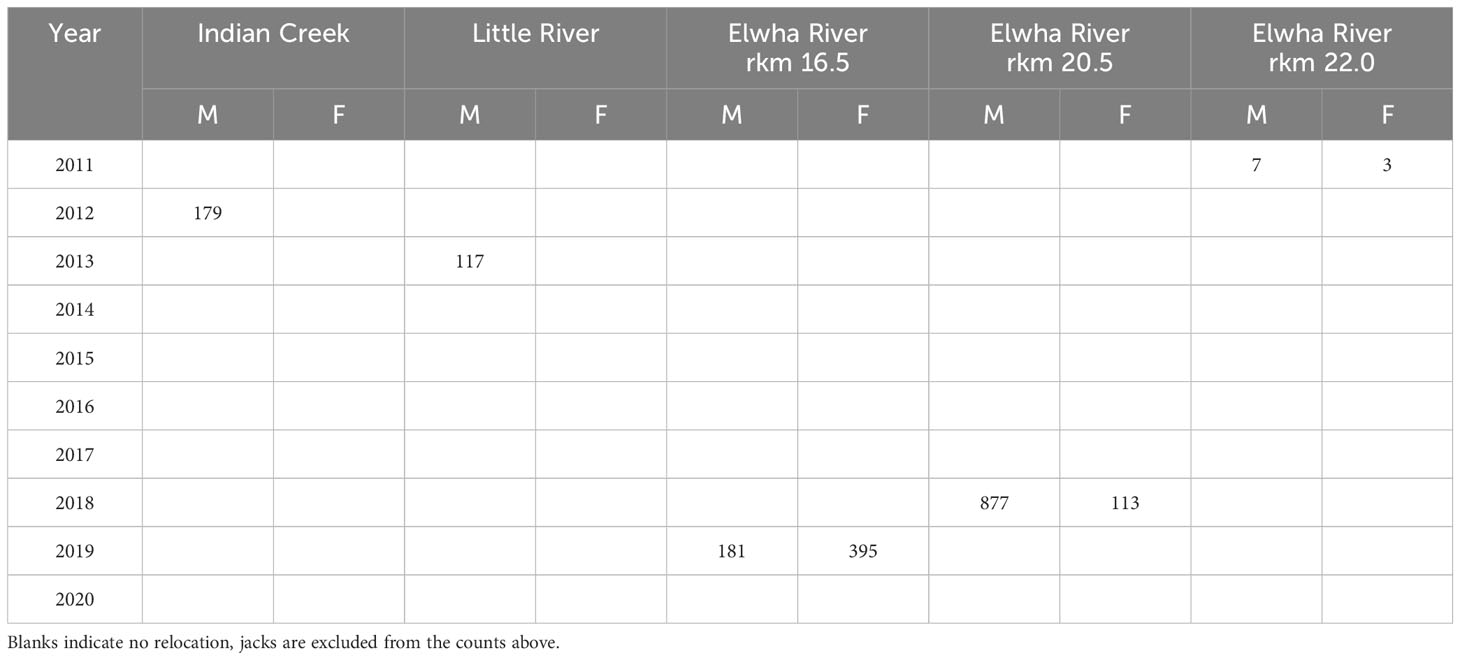
Table 1 Chinook salmon relocation by sex from the hatchery facilities in the Lower Elwha River to areas upstream of the former Elwha Dam site from 2011 to 2020.
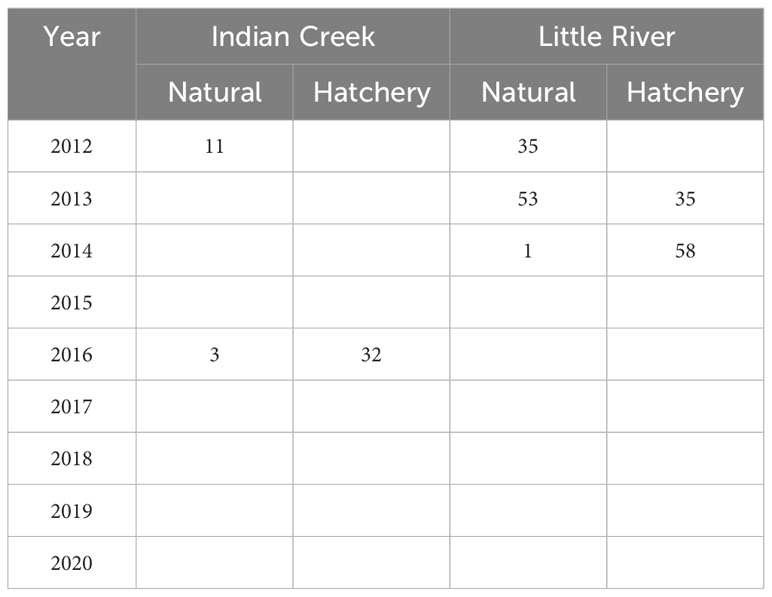
Table 2 Steelhead relocation from the Lower Elwha River to Indian Creek and Little River upstream of the former Lower Elwha Dam site from 2012 to 2020.
3 Methods
3.1 How do the questions link to the methods and results?
Multiple methods answered each of the four questions. Determining annual abundance of adult Chinook salmon and winter steelhead employed the use of SONAR units in the Lower Elwha. To determine the origin of returning adult Chinook salmon we evaluated carcasses of post-spawned fish for hatchery marks to estimate the proportion of hatchery-origin spawning Chinook salmon. For winter steelhead origin we utilized an adipose clip or coded-wire tag (CWT) (sometimes both could be present) to identify hatchery-origin adults. Individuals of both species were also captured during SONAR tangle netting in the Lower Elwha.
We utilized rotary screw traps to estimate the abundance of juvenile Chinook salmon and steelhead outmigrants. To determine if river conditions prior to, during, and following dam removal impacted production of juvenile Chinook salmon, we modeled the relationship between river discharge, sediment transport, and productivity of sub-yearling Chinook salmon (age-0 migrants/spawner), which includes data from both the SONAR and the smolt traps.
To determine the pattern of spawning distribution and expansion we conducted foot surveys to count spawning nests or “redds” to determine the distribution of spawning Chinook salmon and steelhead. We also conducted opportunistic snorkel surveys for adult summer steelhead because of our limited ability to enumerate them with other methods due to their apparent preference to immediately migrate upstream when returning to the Elwha between June and October. These surveys also gave us a relative indication of a unique life-history strategy that was not typically observed in the Lower Elwha prior to dam removal.
3.2 What is the annual abundance of returning adult Chinook salmon and winter steelhead during and after dam removal?
We used two methods to estimate the abundance of adult Chinook salmon and steelhead before and after dam removal (Figure 1). Prior to 2012 (before dam removal), adult abundance was estimated for both species by visually enumerating redds throughout the entire Lower Elwha and then multiplying the total count by an expansion factor (Chinook salmon 2.5 fish per redd, steelhead 1.8 fish per redd). For Chinook salmon redd-based estimates, the total number of visible redds was counted throughout the accessible river in weekly surveys during the spawning period as conditions permitted. The redd counts were then plotted against date and the area under the resulting curve was calculated. This area was divided by estimated redd life (21 days) and then multiplied by 2.5 to account for the sex ratio (English et al., 1992; Smith and Castle, 1994). For the redd-based steelhead estimates a similar sampling approach was used, but instead of using area under the curve, redds were individually flagged to avoid double counting, and the total number of unique redds was tallied at the end of the season and multiplied by 1.8 to account for the sex ratio.
From 2012 through 2020, during and after dam removal, adult Chinook salmon (starting in 2012) and steelhead (starting in 2014) were enumerated using two different multi-beam SONAR units, a DIDSON-LR (0.7/1.1 MHz) and an ARIS 1800 (1.1/1.8 MHz) (http://www.soundmetrics.com/Products, Sound Metrics Corp., Bellevue, WA). Multi-beam imaging SONARs acoustically ensonify the entire width and depth of a cross section of the river, producing movie-like imagery of fish swimming through the cross section. Two SONAR units were needed because the channel split near the mouth of the Elwha River (Figure 1). The SONAR units operated from late January or early February through September. The primary enumeration site was in the East Channel (EC) while a secondary site was in the West Channel (WC), with both sites located at rkm 0.8. SONAR site selection was based on four criteria: 1) almost all fish would pass the site; 2) the location was downstream of most spawning habitat; 3) the river channel was sufficiently narrow to accommodate the effective range of the SONAR; and 4) fish movement was primarily directed upstream with little milling (i.e., back and forth swimming) in the location of the SONAR. Depending on river discharge, the WC site was between 12 and 25 m wide and 1.3 m deep in the thalweg, while the EC site was 15 to 30 m wide and 2 m deep in the thalweg. We estimated that during Chinook salmon migration approximately 80% of the flow was in the EC, while the remaining 20% was in the WC. During the winter steelhead migration, the estimated proportion of flow was 60% EC and 40% WC. We did not account for or estimate fish spawning below the SONAR site.
3.2.1 Data analysis
We sub-sampled SONAR files to count returning adults and then modeled those data to estimate total annual abundance. For Chinook salmon, 20 minutes of each hour-long file was reviewed for fish passage at each SONAR site, which is on the upper end of the range of recommended subsampling regimes (Lilja et al., 2008). Due to relatively low spawner abundance during the steelhead season, the full hour was reviewed. Several variables were noted for each fish passage event, including the date, time, direction (upstream or downstream), distance from SONAR head, and body length (mm).
The net upstream fish passage count was tabulated by subtracting downstream passage events from upstream passage events (Xie et al., 2005). The method of estimation was slightly different for winter steelhead because we had to account for downstream migrating individuals that had migrated upstream and already spawned (kelts). Hence, we did not subtract downstream moving targets for any 24-hour period that had a net total downstream passage. This adjustment strikes a balance between accounting for kelts leaving the system that were not subtracted from the total escapement estimate versus subtracting downstream passage events due to milling or spawning behavior near the SONAR site. This adjustment increased the final escapement an average of 13% in any year. We were able to calculate this percentage because the Elwha River currently has a unimodal winter steelhead run timing with spawning concentrated in late-April through May.
To sum upstream and downstream passage events in each file, we also had to establish a minimum threshold length to distinguish adult Chinook salmon and winter steelhead from other species and life stages. We used field-measured lengths of fish captured during weekly or bi-weekly in-river tangle net sampling conducted at nine different sites within 1 km of the SONAR sites over the entire course of the SONAR season. The netting also allowed us to estimate the onset and completion of the Chinook salmon and steelhead run timing, and the proportion of each species present during the period when they overlapped. The size thresholds for adult Chinook salmon and winter steelhead were 550 mm and 500 mm, respectively. The 550 mm threshold effectively excluded Chinook salmon jacks (males that return after one year in the ocean and at smaller sizes than normal adults), smaller bodied bull trout, and pink salmon. For winter steelhead, we used 500 mm as the minimum size threshold, which excluded most bull trout. We then applied those length criteria to all SONAR measured targets so only adult steelhead and Chinook salmon were included in the simulation model. The 550 mm length cutoff for Chinook salmon included ~98% of all those field-measured each year. The 500 mm cutoff included ~95% of all field-measured steelhead.
3.2.2 Chinook salmon and winter steelhead
To estimate annual abundance, we used four-step (Chinook salmon) or three-step (winter steelhead) simulation models to adjust the total counts of the raw SONAR targets. In the first step, for Chinook salmon only, we expanded the 20-minute sub-sample counts to a full-hour (Lilja et al., 2008). Second, the raw targets exceeding the species-specific size thresholds were adjusted to species using a random draw from a binomial distribution populated by the number of target species in the tangle net sampling for that week and the total number of fish sampled that week. Third, we corrected the species-specific counts to account for observer error by using a linear regression analysis between the counting technician and a more experienced counter. Lastly, we filled in data gaps resulting from periods when the SONAR was not operating in order to expand the data to account for the entire run. The simulation also provided season- and year-specific coefficients of variation. Full methods utilized in this study including SONAR installation and simulation modelling are described in Supplementary Material Appendix A.
3.3 What is the proportion of hatchery-origin adult Chinook salmon and steelhead during and after dam removal?
We evaluated carcasses of post-spawned fish for hatchery marks to estimate the proportion of hatchery-origin spawning Chinook salmon. Chinook salmon carcasses were collected via stream surveys, a channel-spanning weir deployed from 2010–2013, and from the hatchery following spawning. We examined each carcass for four different hatchery marks, including a thermal otolith mark, fin clip, CWT, and scale analysis. The primary marking strategy employed in the Elwha with a marking-rate goal of 100% was a thermal otolith mark. A subset of hatchery Chinook salmon received adipose fin clips and CWT, which allowed us to detect Elwha-origin fish in (rare) cases where thermal otolith marks were not successfully applied, or to identify hatchery-origin fish from other watersheds. Finally, we classified a small number of fish as hatchery-origin based on scale analysis that indicated they had growth patterns indicative of hatchery rearing, despite not having other marks. We compared percent hatchery-origin Chinook salmon before and after dam removal using a binomial generalized linear model.
For winter steelhead, we used an adipose clip or CWT (sometimes both could be present) to identify hatchery-origin adults. Individuals were captured during SONAR tangle netting in the Lower Elwha River supplemented by limited sampling upstream of the former dam sites during 2014–2020. However, most winter steelhead were collected within 1 km of the LEKT hatchery (~rkm 2.4). Consequently, our samples were likely biased and therefore we only used those data to illustrate spatial differences in hatchery- and natural-origin proportions from 2014–2020. In 2019, a more intensive and spatially representative effort was undertaken to produce an unbiased estimate of basin-wide and reach-specific (Lower Elwha, Middle Elwha, and Upper Elwha) hatchery and natural proportions which considered spatial and temporal differences as well as differences in catch per unit effort (CPUEs) between sites (Peters et al., 2020).
3.4 How many Chinook salmon migrants and steelhead smolts were produced during and after dam removal?
We used rotary screw traps to estimate the abundance of juvenile Chinook salmon and steelhead outmigrants. Presumably, this effort could have also included some steelhead offspring destined to become summer run steelhead. Traps were in three locations – the Lower Elwha (rkm 0.3 and 3.3 in 2014–2018 and 4.0 in 2019–2020) and one each near the mouth of Little River (rkm 0.2) and Indian Creek (rkm 0.7). We report on two trap locations – the Lower Elwha and Indian Creek (Figure 1). The main stem trap was typically installed and operable by February 15th and removed by July 26th, although annual start and end dates varied due to river conditions and safety concerns. Indian Creek was installed and operable by January 27th and was completed by September 5th.
The trap in the main stem Lower Elwha was, on average, operational 73% (~118 days) of all potential days, compared to 95% (~211 days) of all potential days in Indian Creek. During the period of active dam removal and associated sediment transport (2012–2014), large amounts of sediment and organic debris (e.g., coarse wood) transported from the former reservoirs hindered the main stem trap operations. In 2013, no results were reported due to the trap being pulled or failing due to the amount and duration of debris effects. We report hatchery release numbers as a point of comparison for the abundance of natural-origin juvenile migrants, and for estimating smolt-to-adult return rate (SAR) of hatchery Chinook salmon.
3.4.1 Field methods
Smolt traps were inspected and cleaned daily or every other day. All captured fish were removed from the trap box using dip nets and transferred to plastic buckets so that each fish could be individually examined and identified. A weekly subsample of all species caught was measured and weighed throughout the outmigration period. Most hatchery Chinook salmon (0+) were not externally marked (only otolith marked) and thus difficult to distinguish from natural-origin fish without sacrificing them. However, most natural-origin Chinook salmon (0+) tended to migrate past the trap prior to the hatchery releases in June. Trap operations during hatchery releases typically ceased for several days because it was too difficult to sample such large numbers of fish. Starting in 2019, the trap was moved upstream of the Chinook salmon hatchery and all fish were assumed to be of natural origin. While errors in the hatchery vs. natural origin designation could have added variability to the Chinook salmon (0+) estimates, any error would be small relative to the observed increase in abundance in the most recent years. Winter steelhead produced in hatcheries were all adipose fin clipped post 2013 and therefore distinguished from naturally produced fish at the trap. See Supplementary Material Appendix B for details on determining the origin of smolts.
We estimated trap catch efficiency (i.e., proportion of total outmigrants captured) using multiple mark recapture tests across the trapping season at all three trap sites. In the smaller tributaries, weekly samples of 50 to 100 fish, representative of the species migrating at any given time (i.e., Chinook salmon subyearlings or smolts, coho salmon parr or smolts), were given a distinctive mark (Bismarck Brown) and released approximately 100 m upstream of the trap site. For the main stem trap, we used small-bodied (0+) Chinook salmon or chum salmon obtained from the LEKT and Washington Department of Fish and Wildlife (WDFW) fish hatcheries; these were also given a distinctive mark (Bismarck Brown) and then released approximately 1000 m upstream to test efficiency. For the small-bodied fish, we typically attempted multiple trials between late March and late May (~6 trials/year, Standard Deviation (S.D.) ± 3 trials/year) and averaged 16 days (S.D. ± 8 days) between trials. We estimated efficiency for 1+ fish (steelhead smolts) using 0+ trials in all years, because there were only three years with efficiency trials using 1+ fish at the main stem trap. This allowed for consistency across years. For the three years with trials based on 1+ fish, we generated a second estimate to allow for comparison. The 1+ efficiencies were generated using 1+ coho salmon that were captured and marked at the Indian Creek trap and recaptured in the main stem trap (2016, 2017 and 2019) and 1+ hatchery coho salmon that were marked and released from the LEKT hatchery and recaptured at the main stem trap (2017).
3.4.2 Data analysis
We combined daily catch data with efficiency trials to estimate total annual production. To incorporate uncertainty due to periods of missing data and expansion based on trap efficiency, we applied a flexible Bayesian model. Daily passage was assumed to follow a negative binomial distribution with a mean constrained to change smoothly with time – a random walk. Catch was modeled as a binomial distribution where the probability of capture was estimated from efficiency trials. Period-specific efficiencies were assumed to be independent due to observed temporal trends in efficiency for some traps. The estimates only incorporated passage during the trap operation. Therefore, if the trap was not in place during fish passage, these fish were not included in the estimate. We summarize the results with the median and 95% credible interval for total passage. We also include the coefficient of variation (CV) and the geometric CV, which is more appropriate for skewed distributions. See Supplementary Material Appendix B (section 3) for details of the smolt data analysis.
3.5 How productive were Chinook salmon during and after dam removal?
We used a combination of in-river abundance from SONAR estimates, hatchery mark rate, and age structure to estimate productivity as the total number of adult recruits produced by each cohort of Chinook salmon that spawned naturally in the Elwha River from 2004–2015. To estimate adult recruits, we first estimated the number of naturally produced Chinook salmon by multiplying the abundance of adults returning to the river by the proportion of natural-origin adults (1 – hatchery mark rate). We calculated separate estimates of proportion hatchery-origin in the hatchery broodstock and Chinook salmon spawning naturally in the river beginning in 2013, when we began consistently obtaining a representative sample of the fish spawning in the river. Next, natural-origin adult returns were then allocated to spawning cohorts using scale-derived age data for individuals collected from 2007 to 2020 (median = 572 individuals per year, range = 216–1,104). Because we sampled so few unmarked, natural-origin Chinook salmon (≤ 50 each year), we assumed no difference in the age structure between hatchery-origin and natural-origin fish. This allowed us to increase our age structure sample size, and implicitly prioritized capturing age variation among years rather than age variation between hatchery-origin and natural-origin salmon.
We report productivity as the ratio of adult salmon returning to the river relative to the number of spawners that produced them, with a value of 1.0 indicating replacement. We provide separate productivity estimates for fish spawned at the hatchery and those that spawn naturally in the river in order to compare them. Our approach did not distinguish between natural mortality and harvest mortality. To compare survival in the marine environment between natural-origin and hatchery-origin fish, we also estimated SAR. Here, we divided the number of natural-origin salmon returning from each cohort by the corresponding juvenile abundance estimate from the smolt trap, and the number of hatchery-origin salmon returning from each cohort by the number released from the hatchery. We note that these productivity and SAR estimates encompassed the period before and a small portion during dam removal.
3.6 How were annual estimates of Chinook salmon abundance affected by streamflow and sediment impacts?
To determine if river conditions prior to, during, and following dam removal impacted production of Chinook salmon, we modeled the relationship between river discharge, sediment transport, and productivity of sub-yearling Chinook salmon (age-0 migrants/spawner) from 2011–2018. We used daily discharge data (2011–2018) from the U.S. Geological Survey (USGS) (12045500 Elwha River at McDonald bridge near Port Angeles, WA) and estimates of suspended and bedload sediment discharge (tonnes per day) (Ritchie et al., 2018) as explanatory variables. As a response variable, we divided the total number of naturally spawning Chinook salmon (total escapement estimate minus hatchery take) by the number of Chinook salmon outmigrating subyearlings to calculate yearly estimates of Chinook salmon subyearlings per spawner.
To evaluate streamflow events, we developed a flow index for stream discharge (annual flow index) that includes the number of days above 56.6 m3s−1, which is the estimated bankfull discharge where bedload is mobilized (Ritchie et al., 2018), between October 1st and December 31st, which is the primary incubation and emergence period for Chinook salmon (Greene et al., 2005). We then summed the number of days above 56.6 m3s−1 and multiplied that by the average discharge greater than 56.6 m3s−1. This allowed us to identify the overall duration and magnitude of events that could have affected egg-to-fry survival for the period of incubation.
Equation 1 assumes that the number of days and the amount of flow over the course of the entire incubation period would have the largest impact on egg-to-fry survival, a factor that can limit overall Chinook salmon productivity (Greene et al., 2005).
We developed a sediment transport index by summing the average total amount of sediment transport (TST) (tonnes) during the egg incubation and emergence period (Ritchie et al., 2018). Processed data were not available after September 30, 2016, so we estimated sediment transport from October 1, 2016, to December 31, 2016, using bedload data from bedload impact sensor plates located near rkm 4.9 available from the Bureau of Reclamation (Hilldale et al., 2015; Ritchie et al., 2018.). Based on prior years, the bedload sediment sensors quantified approximately 44% of the total estimated bedload transport. In addition, the daily bedload sediment (Sdailybed) is roughly 25% of the total sediment load (Stotal) mobilized. We used those data to estimate the overall total sediment discharge for the October 1, 2016, to December 31, 2016, period with Equation 2:
Our comparison of prior year estimates to measured sediment discharge produced an r2 of 0.89.
We calculated the flow-sediment index (Equation 3) for the egg incubation period (October 1st to December 31st) as the product of 1) the sum of annual flow index and 2) the total sediment transport during the same period:
After calculating the annual index values, we then fit a linear model to the relationship between the log of the flow sediment index (FlowSedIndex) and the log of Chinook subyearlings per spawner for each year from 2011 to 2018 (Equation 4). This is equivalent to the power law model on the un-logged scale,
where b is the slope of the log-log relationship. We used visual inspection of the relation on the log-log scale to confirm that the assumption of linearity was appropriate and that the variance was stable across the range of values.
3.7 How far have Chinook salmon and steelhead expanded their spawning distribution?
We conducted foot surveys to count redds to determine the distribution of spawning Chinook salmon and steelhead. We use the term steelhead here because we could not determine which portion, if any, of the redds were from summer run steelhead. To estimate each species’ spatial expansion, we delineated the Elwha River into three sections based on the presence of the former dams. Each individual redd was geolocated (latitude and longitude) with GPS (Garmin model GPSmap 60CSx). It is important to note that Elwha River Chinook salmon redd surveys are more readily apparent and feasibly surveyed than steelhead redd surveys in the roadless area above former Mills reservoir due to their seasonal low flow timing (i.e., late summer/early fall) compared to steelhead which spawn during higher winter and spring flows.
3.7.1 Chinook salmon redd counts
Each year in mid-September from 2012–2018, we conducted one- to five-day long peak redd counts in the main stem Elwha River, its larger floodplain channels, and several major tributaries. Survey timing was based on the estimated historical date of peak spawning activity for Elwha River Chinook salmon, approximately September 15th–September 25th. The Lower Elwha and Middle Elwha were surveyed in all years, while the Upper Elwha was surveyed in 2016–2018. Limited supplemental surveys were conducted in the Upper Elwha beginning in 2014 in the former Mills Reservoir area from the former Glines Canyon Dam (rkm 22) upstream to the entrance of Rica Canyon (rkm 25.7). Surveys did not cover major canyon areas of the Elwha River during peak surveys except for Rica Canyon in 2014 and 2015 (Duda et al., 2008; Brenkman et al., 2012). Additionally, no comprehensive surveys have occurred in larger tributaries to the Upper Elwha except Long Creek in 2018. Finally, river discharge and turbidity levels were greatest in 2012, and as a result, surveys were limited to above the Elwha Dam site where turbidity levels were much lower. In 2013, water clarity of the river improved enough to allow surveys below the former Elwha Dam, and 2014 conditions allowed for a full survey from the mouth to just above the former Glines Canyon Dam. Since 2015, turbidity has not been a factor during surveys in any reach, and during this period, we consider redd data reflective of the spatial distribution of spawning.
3.7.2 Steelhead redd counts
Between 2012 and 2018, we conducted weekly to bi-weekly redd counts from February through June or early July to determine the location and timing of adult steelhead spawning (Gallagher et al., 2007). Resident rainbow trout were the only other spring spawning salmonid and their redds were distinguished from steelhead redds based on size and substrate (McMillan et al., 2015). Most redd counts occurred in tributaries where water clarity was unaffected by dam removal and their small size allowed for surveys to safely occur. Surveys were completed in four Upper Elwha tributaries with the following percentages of potential steelhead spawning habitat surveyed: Cat Creek (100%), Long Creek (90%), Hurricane Creek (100%), and Boulder Creek (100%) and six Middle Elwha tributaries: Little River (50%), Indian Creek (25%), Griff (100%), Madison (100%), Campground (100%), and Hughes Creeks (100%). Surveys of the main stem were conducted as conditions allowed, but due to the timing of their spawning visual counts were severely limited by reduced water clarity that often made it impossible to identify and count redds. Steelhead redd data were used to document spawning in previously unavailable locations but may not accurately reflect the spatial distribution of redds due to difficulties surveying the main stem. Except for surveys in 2010 and 2013, when main stem water clarity was good (see section 3.2), we do not use steelhead redd survey data to estimate total spawners.
3.8 Has dam removal given rise to the re-emergence of different life histories that were not present, or very rare, when migration into the Elwha River headwaters was blocked?
3.8.1 Snorkel surveys
We conducted opportunistic snorkel surveys for adult Chinook salmon and adult summer steelhead in 2013 and 2016 in shorter sections of the Middle and Upper Elwha, followed by annual snorkel surveys from 2017–2020 in the Middle and Upper Elwha. The annual surveys covered most of the Middle and Upper Elwha except for canyons (see Brenkman et al., 2012). Snorkel counts were conducted in early- to mid-September to ensure the majority of adult summer steelhead had entered freshwater. Once in the water, divers moved downstream and would enumerate fish in each habitat unit and then relay those numbers to a bank recorder. Generally, the process consisted of two divers swimming downstream side-by-side, with some up river reaches covered with a single diver. Summer steelhead were distinguished from resident rainbow trout by their larger size, silvery coloration, presence of a strong sea line, and few spots below the lateral line. Divers also classified each adult steelhead as hatchery, wild, or unknown, depending on the presence of an adipose fin. For more details on the snorkel survey methods please refer to Brenkman et al. (2012) and Duda et al. (2021). We only surveyed a portion of the watershed therefore our resulting counts represent a lower bound on total abundance.
4 Results
4.1 What is the annual abundance of returning adult Chinook salmon and winter steelhead during and after dam removal?
Prior to dam removal (1986–2010), expanded redd count data showed an average annual return ± 1 SD of 2,827 (± 1,778) adult Chinook salmon in the Elwha River (Figure 2A). During (2011–2014) and following (2015–2020) dam removal, SONAR data showed average annual returns of 3,444 (± 1,125) and 4,734 (± 2,409) Chinook salmon respectively. We estimated the average number of naturally spawning Chinook salmon before, during, and after dam removal as 1,393 (± 1,218), 1,930 (± 747), and 3,523 fish (± 1,949), respectively (Figure 2A). The proportion of total returning adult Chinook salmon taken for hatchery breeding purposes before dam removal was 53% (± 15%), compared to 45% (± 6%) during dam removal and 31% (± 8%) following dam removal. Based on SONAR, the estimated number of returning adult winter steelhead to the Elwha River from 2014 to 2020 ranged between 890 and 1,985 fish (average 1,400 ± 350) (Figure 2B). Starting in 2016, the population has been increasing at approximately 10% annually except for a 10% decrease in 2019 (Figure 2B).
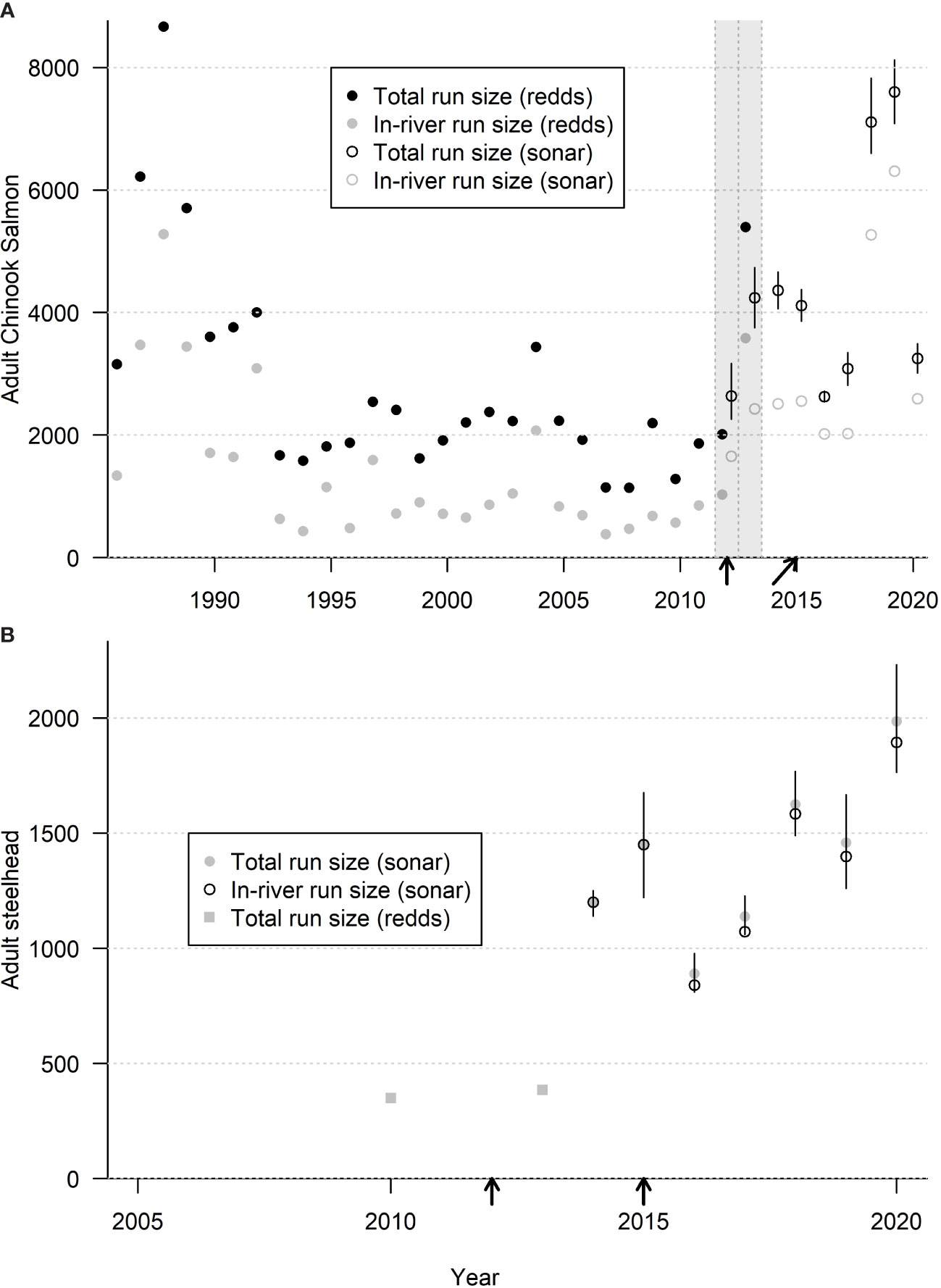
Figure 2 Interannual trends in abundance of (A) adult Chinook salmon and (B) adult steelhead in the Elwha River before, during, and after dam removal based on redd surveys and SONAR. Shaded areas denote estimates of Chinook salmon during years of simultaneous redd surveys and SONAR. Dark solid lines denote 95% confidence intervals. Arrows (straight and angled) denote the removal of the Elwha and Glines Canyon dams and associated rockfall blockages in Glines Canyon. Removals for hatchery broodstock account for the difference between total run size and in-river run size.
4.2 How many Chinook salmon migrants and steelhead smolts were produced during and after dam removal?
The number of subyearling and yearling Chinook salmon released from the hatchery prior to dam removal averaged 2,596,545 (± 801,861), which was higher than the 1,953,609 (± 808,897) released during and after dam removal. The number of natural-origin subyearling Chinook salmon from the Elwha River averaged 43,828 (± 47,932), 46,973 (± 39,798), and 323,764 (± 407,976), before, during, and after dam removal, respectively (Figure 3A). A dramatic increase in the estimated number of natural-origin subyearling Chinook salmon occurred when over 500,000 and almost 1 million subyearlings were produced in 2019 and 2020 (Figure 3A), respectively. The estimates for 2016, 2017, and 2020 yearlings were one to two orders of magnitude less than the subyearlings, the only years when trap efficiency was sufficient to allow estimates of yearling outmigrants (Table 3).
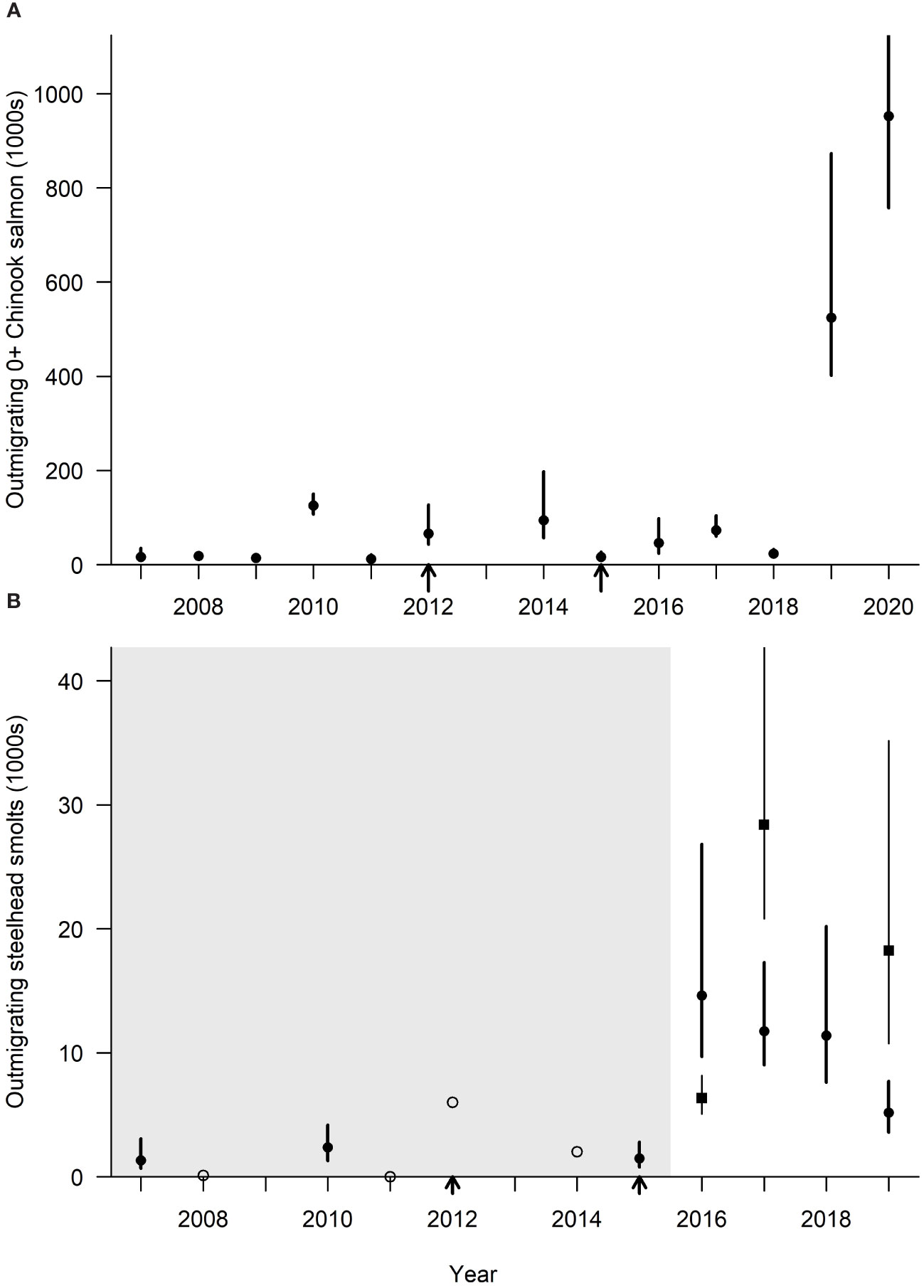
Figure 3 Interannual trends in abundance of outmigrating natural-origin subyearling juvenile Chinook salmon and natural-origin steelhead smolts from 2008 to 2020 in the Elwha River. (A) Outmigrating subyearling juvenile Chinook salmon estimated at the screw trap in the main stem Elwha River (rkm 0.3 and 3.3 in 2014–2018 and 4.0 in 2019–2020). The filled circles and vertical bars represent the median estimate and 95% credible interval. Arrows denote the removal of the Elwha and Glines Canyon dams and associated rockfall blockages in Glines Canyon. (B) Steelhead smolts estimated at the main stem screw trap (rkm 0.3 and 3.3 in 2014–2018 and 4.0 in 2019–2020). The open circles without credible intervals represent years in which the catch was less than 10. The black filled rectangles represent the separate estimates based on the independent large-bodied fish efficiency estimates. The gray region represents years in which the outmigrant estimates are believed to be under-estimated due to expansions based on 0+ salmon for this period.
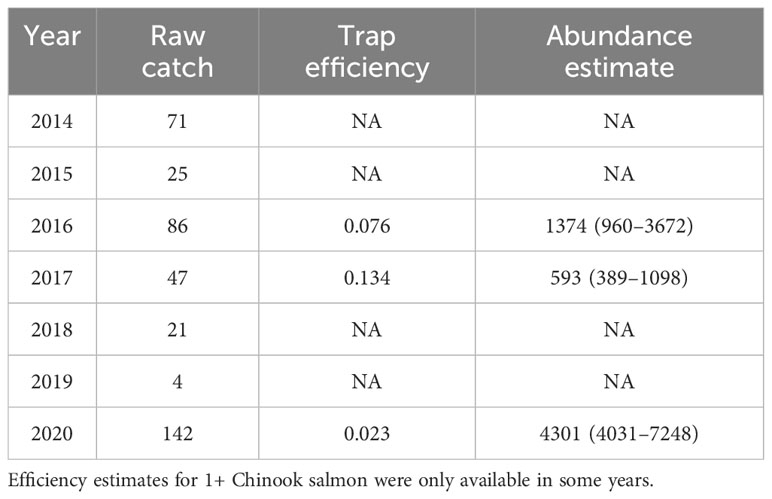
Table 3 Elwha River smolt trap catch data and abundance estimates for yearling Chinook salmon from 2014 to 2020.
The average hatchery releases of steelhead smolts during and after dam removal was approximately 122,596 (± 53,514) fish. The average annual estimate of natural-origin steelhead smolts during and after dam removal was 8,884 (± 5,380), but unlike Chinook salmon they did not display the same large increase in natural production in 2019 and 2020 (Figure 3B). Between 2013 and 2020, outmigrating subyearling Chinook salmon from Indian Creek, a tributary located at rkm 12.1 not impacted by the sediment supply changes from the dam removal, ranged between 1,188 and 129,759 and averaged 53,396. Between 2013 and 2020, the annual average number of steelhead smolts from Indian Creek was 1,523 fish with a low of 146 in 2014 and a high of 2,550 in 2019.
4.3 What is the proportion of hatchery-origin adult Chinook salmon and steelhead during and after dam removal?
Across return years 2009–2020, the median proportion of hatchery-origin returning adult Chinook salmon from all collection sources was 95.9% (range = 92.0–98.0%, Figure 4A). In 2016–2020, when some returning salmon might have been produced from parents that spawned upstream of the Elwha Dam site, the proportion of hatchery-origin Chinook salmon was not significantly different from 2009–2015, based on a binomial general linear model (p > 0.10, Figure 4A). However, restricting the analysis to only Chinook salmon spawning naturally in the Elwha River yielded a slightly lower proportion hatchery-origin in 2016–2020 (median = 93.8%) compared to 2009–2015 (median = 95.5%, glm p< 0.05). The proportion of hatchery winter steelhead caught during sampling from 2014–2018 was 0.85, but 0.54 in 2019 (Table 4). However, the proportion of hatchery winter steelhead caught upstream of former Elwha Dam was lower than that downstream of the dam during both periods (Table 4).
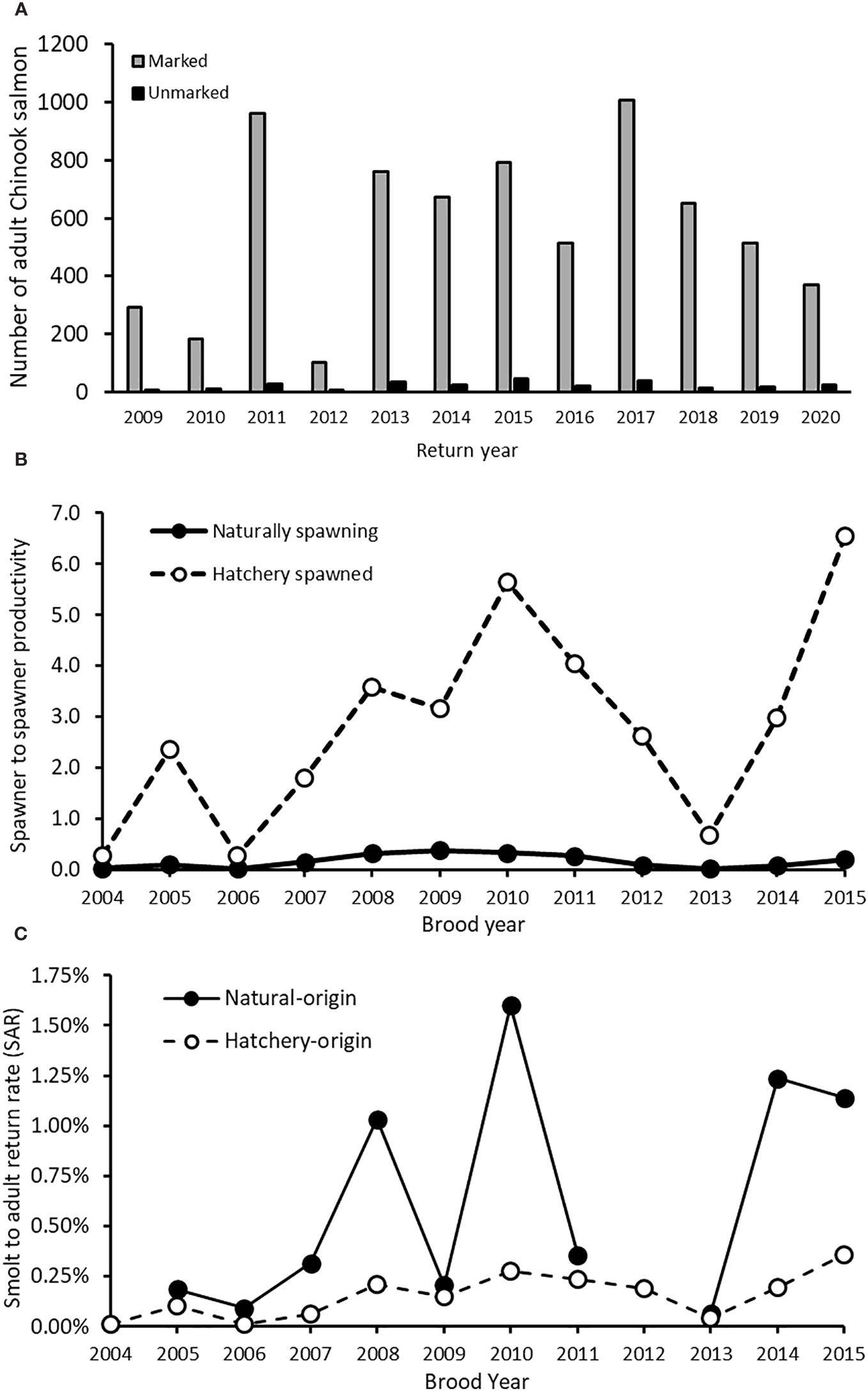
Figure 4 Elwha River Chinook salmon natural- and hatchery-origin metrics. (A) Number of hatchery marked and unmarked adults, (B) spawner-to-spawner productivity, and (C) Smolt-to-adult return rate (SAR) estimates for Elwha River Chinook salmon. In (C), for each brood year (BY), hatchery-origin juveniles include subyearling (BY + 1) and yearling (BY + 2) releases.

Table 4 Numbers and proportion of natural and hatchery origin adult winter steelhead observed during sampling in the Elwha River with 95% credible intervals from 2014 to 2019.
4.4 How productive were Chinook salmon during and after dam removal?
Estimates of naturally spawning Chinook salmon productivity (adult to adult) from 2004–2015 were ≤ 0.40 in all years and ≤ 0.20 in eight of 12 years, which is below the 1.0 replacement value (Figure 4B). Adult-to-adult productivity of Chinook salmon spawned in the hatchery was greater than that of naturally spawning salmon in all years, exceeding replacement in nine of 12 years (Figure 4B). However, SAR of natural-origin Chinook salmon was consistently greater than hatchery-origin Chinook salmon in the Elwha River (Figure 4C). Median SAR increased from the period prior to dam removal (brood years 2005–2010) to the period during dam removal (brood years 2011–2015), for both hatchery-origin (before = 0.13%; during = 0.19%) and natural-origin (before = 0.26%; during = 0.75%) Chinook salmon. However, the SAR were highly variable and one of the lowest values for both hatchery- and natural-origin Chinook salmon was observed during dam removal (Figure 4C).
4.5 How were annual estimates of Chinook salmon abundance affected by streamflow and sediment impacts?
Plotting values of subyearling Chinook salmon migrants per spawner against the flow-sediment index between 2011 and 2018 suggests an inverse relationship (Figure 5). This was supported by an estimated negative slope for the log-log linear fit (b = −0.44, 95%CI [−0.67,−0.20], R2 = 0.78). During and after dam removal, the years 2014, 2015, and 2017 had the highest flow-sediment index, and the lowest estimated Chinook salmon freshwater productivity (Figure 5).
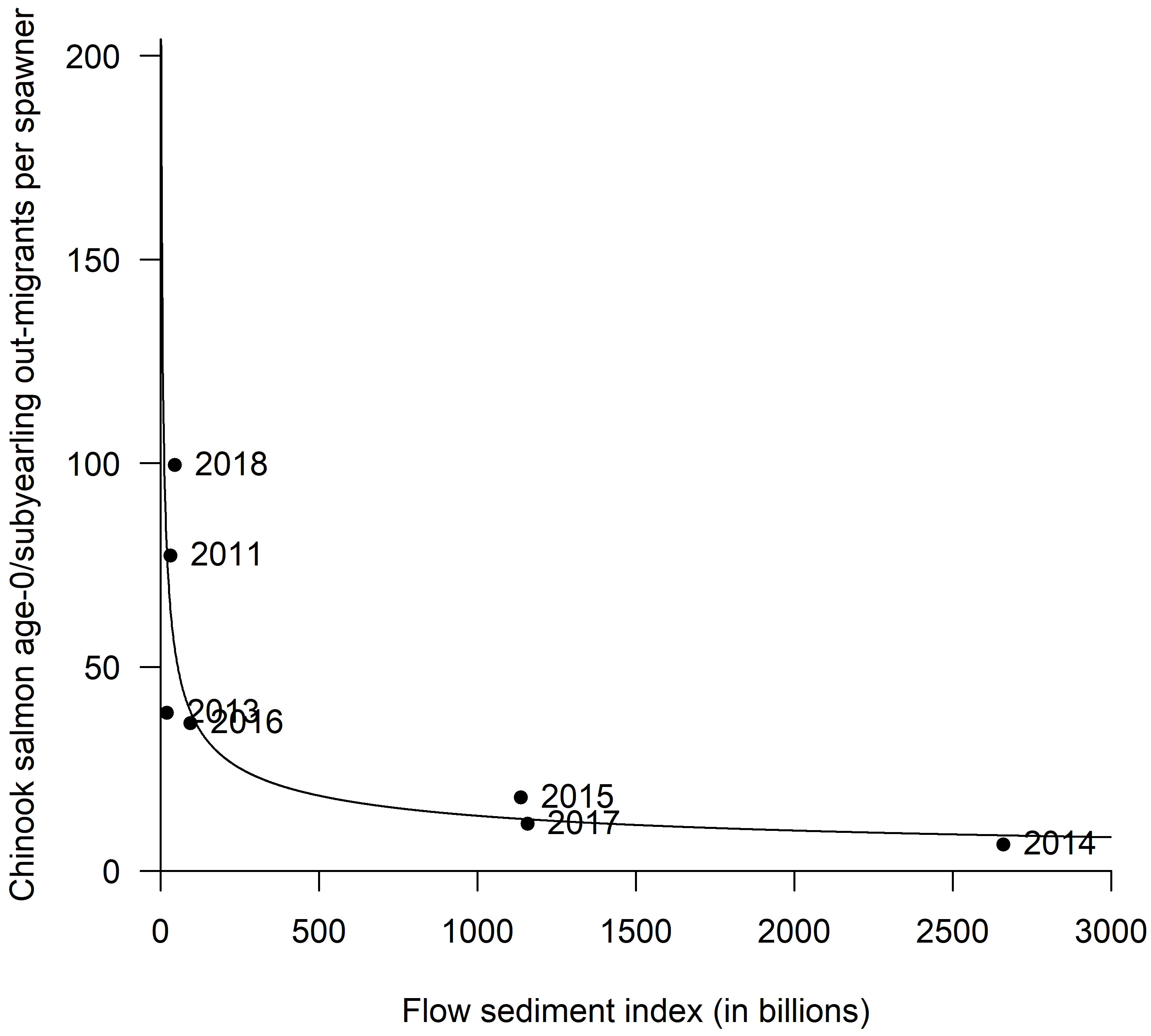
Figure 5 The relationship between the sum of the discharge of the number of days where flow is above bankfull discharge (56.6 cms) multiplied by the total sediment volume (tonnes) during incubation (September to December) vs. the number of subyearling juvenile Chinook salmon per spawner – 2011 to 2018.
4.6 How far have Chinook salmon and steelhead expanded their spawning distribution?
Although the spatial extent of our Chinook salmon redd counts varied and was limited in the main stem Elwha due to poor visibility, our surveys were able to document several patterns. First, we observed adult Chinook salmon in the Middle Elwha immediately after removal of the Elwha Dam (rkm 7.9) in April of 2012. Second, following that initial observation, from 2012–2018 the density of Chinook salmon redds in the Middle Elwha was similar or greater than the densities in the Lower Elwha (Figure 6). Third, following removal of the rockfall blockage in 2015 and 2016, Chinook salmon redds have been consistently observed above the former Glines Canyon Dam (rkm 22), with the former Lake Mills Reservoir (rkm 22–25) being the most intensively used spawning area in the Upper Elwha. Fourth, as a result of the expanding spatial distribution, the overall extent of redds (difference between furthest upstream and downstream) has ranged between 45 and 55 km upstream.
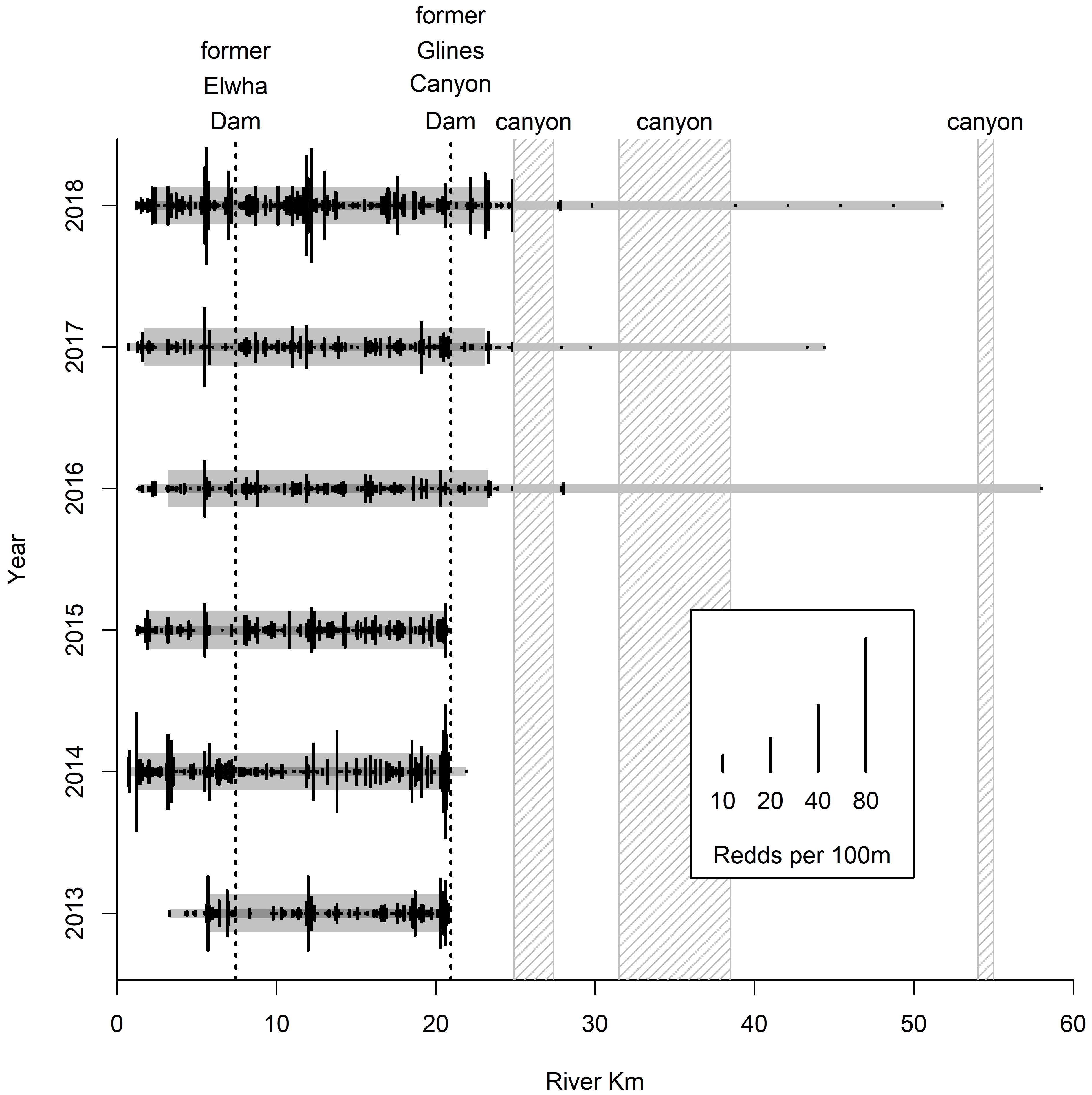
Figure 6 Number of Chinook salmon redds observed downstream, in-between, and upstream of former dam sites in the Elwha River from 2013 to 2018. Solid black lines denote Chinook salmon redd densities/100 m. Narrow grey lines denote the total extent of Chinook salmon redd distribution. Thicker grey line denotes the central 90% of Chinook salmon redd distribution.
Although based on surveys in tributaries rather than the main stem (due to poor visibility), we observed a similar pattern for steelhead redds. For example, following removal of the former Elwha Dam in 2011, spawning winter steelhead were immediately observed in two tributaries – Little River and Indian Creek – to the Middle Elwha that were unaffected by sediment from dam removal. Since that time, numerous steelhead have moved into and spawned in Little River, which contained the greatest cumulative number of redds among all tributaries for all years from 2012–2018 (Figure 7). Indian Creek, located immediately west of Little River, has also consistently supported spawning winter steelhead. Since 2014/2015, Hughes Creek, another tributary to the Middle Elwha, has been used by spawning steelhead and has supported the highest annual number of redds among tributaries in some of those years (Figure 7). Like Chinook salmon, once the rockfall blockage in Glines Canyon was removed, steelhead were immediately observed spawning in tributaries draining areas upstream of the former Glines Canyon Dam, with Boulder Creek generally containing the greatest number of redds (Figure 7). Overall, for Chinook salmon and steelhead, the spatial distribution of redds shifted from the Lower Elwha prior to dam removal to the Middle Elwha during and after dam removal.
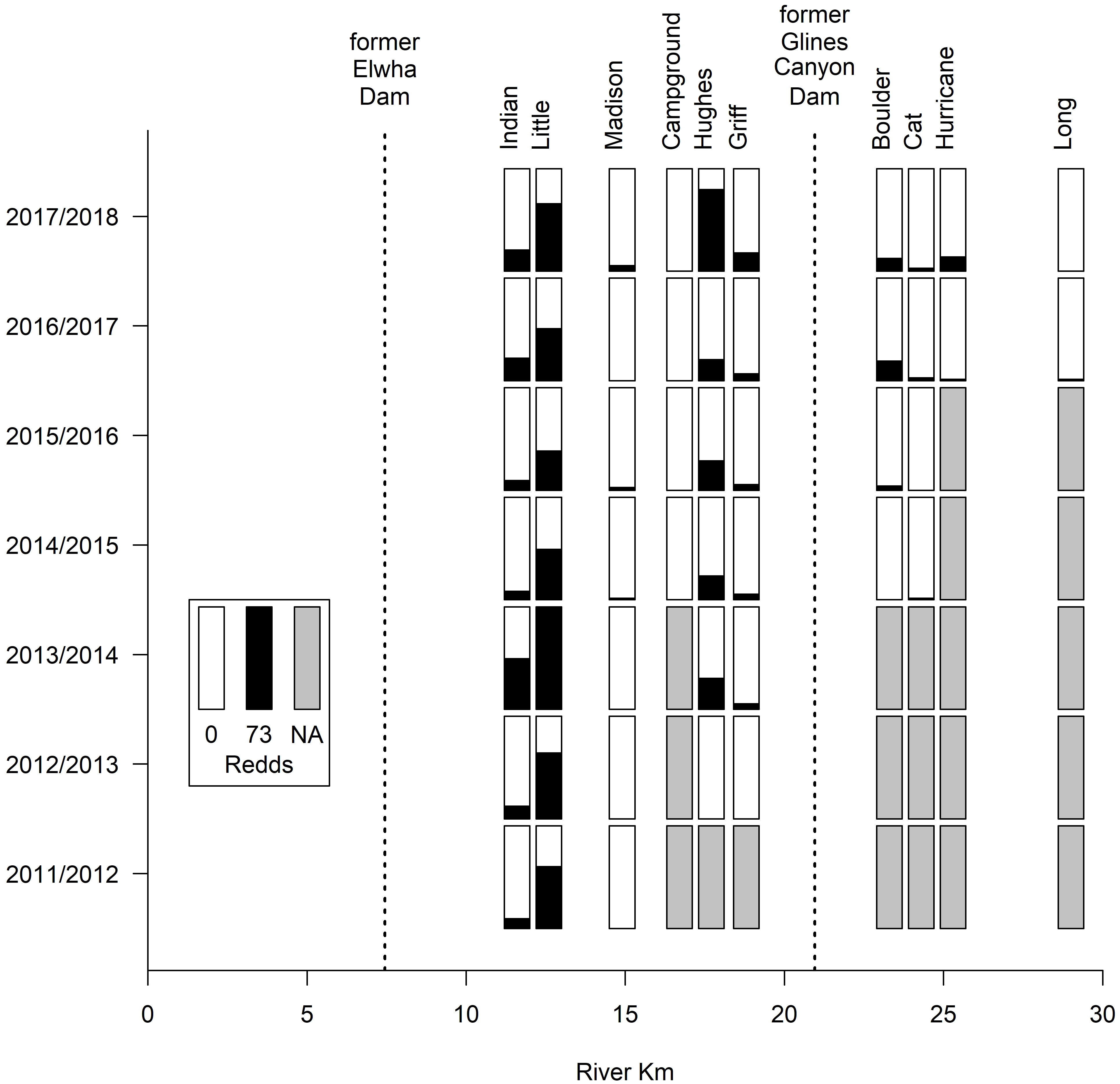
Figure 7 The number of steelhead redds in surveyed tributaries in the Middle and Upper Elwha River from 2011 to 2018. “NA” indicates no survey conducted. The black filled bars indicate the relative number of steelhead redds to the total maximum steelhead redds counted during this time period (73 in Little River in 2013/2014).
4.7 Has dam removal given rise to the re-emergence of different life histories that were not present, or very rare, when migration into the Elwha River headwaters was blocked?
Adult summer steelhead were first observed by snorkelers in 2013 (one adult) and 2016 (six adults) during two relatively short surveys of the Lower and Middle Elwha (rkm 5–18). Once surveys were expanded to systematically cover the same length of habitat each year, the number of adult summer steelhead observed during snorkel surveys in the Middle and Upper Elwha ranged between 74 and 318 between 2017 and 2020 (Table 5). The number of steelhead increased from 2017–2019, and then declined in 2020. Less than 1% of the summer steelhead counted via snorkel survey in the Middle and Upper Elwha were identified as hatchery steelhead via a clipped adipose fin.
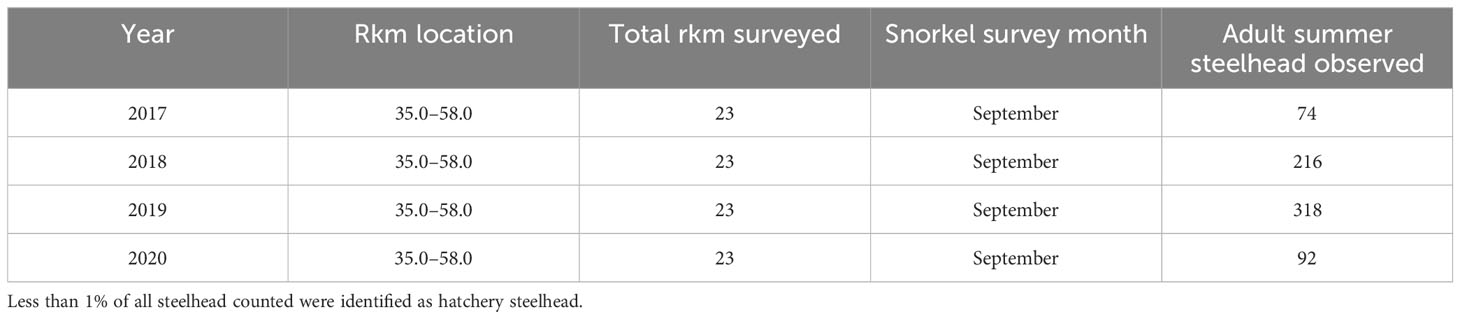
Table 5 Number of adult summer steelhead observed upstream of former dam sites in the Elwha River from 2017 to 2020 based on snorkel surveys.
5 Discussion
Dam removal can help recover and increase the abundance, productivity, spatial distribution, and diversity of imperiled populations of anadromous salmonids by restoring access to formerly productive habitats. However, removal of large dams is a relatively new conservation action and consequently, data on the potential benefits are scarce. We used multiple lines of evidence over an approximately 10-year period (2011–2020) to evaluate the short-term responses of Chinook salmon and steelhead to the removal of two large dams in the Elwha River. We found several results that are important to fish populations on the Elwha, as well as to future dam removal actions scheduled in other watersheds. First, estimates from spawning surveys or redd counts prior to dam removal and SONAR during and after dam removal indicate the number of returning Chinook salmon and winter steelhead has improved since the dams were removed. This was driven by increased SAR of hatchery Chinook salmon and increased abundance of hatchery- and natural-origin winter steelhead. Second, smolt trap data showed that the number of subyearling Chinook salmon migrants and steelhead smolts has also increased since dam removal. The increased production of natural-origin subyearling migrant Chinook salmon appears related to improved river conditions in recent years, based on a strong negative relationship between dam-removal induced sediment impacts (i.e., our flow-sediment index) and the abundance of natural-origin subyearling migrants during and immediately following dam removal when conditions in the main stem Elwha River were unfavorable for survival. Third, redd counts indicate the spatial distribution of spawning Chinook salmon and steelhead has expanded – including above both former dam sites – after dam removal. Last, snorkel surveys revealed the initial re-establishment of summer run steelhead, a life history that was only rarely observed in the years leading up to dam deconstruction (Duda et al., 2021). The combined results suggest dam removal and the associated management actions has already improved viable salmon population parameters for two ESA-listed species in the short term, and recent increases in productivity associated with stabilization of the main stem Elwha River below the dams offer hope for continued rebuilding of natural-origin populations over the long term.
5.1 Responses of Chinook salmon and winter steelhead to dam removal
One of the primary goals of dam removal was to increase the long-term abundance and resilience of natural-origin Chinook salmon and winter steelhead in the Elwha River, both of which were highly depleted and protected under the ESA (Brenkman et al., 2008; Pess et al., 2008; Ward et al., 2008). The rate of dam removal was designed to be fast enough to affect only up to four brood years of salmonids, but slow enough that erosion and redistribution of the sediment stored in the former reservoirs would keep pace with dam removal and maintain conditions suitable to meet municipal water needs (Randle et al., 2015). Nonetheless, there was a great deal of uncertainty about how salmonids would respond because of the magnitude and rate of reservoir sediment erosion associated with dam removal.
We found the abundance of returning adult Chinook salmon generally increased, rather than declined, since the dams were removed, including some of the largest returns in the past several decades. However, the Chinook salmon population is demographically dominated by hatchery-origin fish (≥ 92% in all years, Figure 4A), and natural reproduction is well below replacement (Figure 4B). Nonetheless, the distribution of spawning adults has expanded into newly opened habitats, which is a common result of barrier removals (Kiffney et al., 2009; Pess et al., 2014; Anderson et al., 2015). Chinook salmon population stray rates range from less than 5% to up to 34%, averaging ~15% (Westley et al., 2013; Keefer and Caudill, 2014; Pearsons and O’Connor, 2020), so some of the initial occupiers may have originated from other river systems. Based on CWT information from the Elwha approximately 8.5% (23 out of 275) of the fish collected in 2017 were from nearby streams (Weinheimer et al., 2018). Regardless, our results indicate most Chinook salmon are hatchery-origin and that adult abundance fluctuations during and after dam removal are, in large part, due to hatchery production and survival of hatchery-reared juveniles.
Returns of winter steelhead have also increased since dam removal, from hundreds of fish in 2013 to almost 2,000 adults in 2020. Hatchery-origin steelhead derived from native Elwha River broodstock have significantly contributed to the overall increased abundance of winter steelhead, and they are particularly common in the Lower Elwha. Our observations of adults in the Middle and Upper Elwha suggest that expansion of the spatial distribution of winter steelhead is largely being driven by natural-origin fish. Although we did not genetically determine the origin of returning adults in this study, stray rates for steelhead range from less than 5% to 14% (Keefer and Caudill, 2014; Pess et al., 2014; Pearsons and O’Connor, 2020), though winter steelhead recipient stray rates are typically greater than donor stray rates (~29%) (Pearsons and O’Connor, 2020). However, a large contribution from strays seems unlikely, considering the immediately adjacent watersheds contain only small populations of steelhead and other, larger populations in Puget Sound overall are greatly depleted, with most having fewer returning adults than we documented in the Elwha River (Cram et al., 2018).
The limited spatial, numerical and life-history expansion of Chinook salmon compared to steelhead could be partly related to sediment impacts associated with differences in the temporal and spatial distribution of spawning adults and their associated redds. Although Chinook salmon and steelhead have moved upstream of both dams, most Chinook salmon spawn in the fall in the main stem Elwha River between or below the former dam sites. Sediment concentrations in those sections was consistently high during the Chinook salmon egg-to-fry incubation period from October through December of 2012–2015, due to the considerable increase in sediment supply mobilized via dam removal (Ritchie et al., 2018). Sediment transported from the former Mills Reservoir aggraded the streambed in the Middle Elwha by over 1.0 m and the Lower Elwha by 0.5 m after October of 2012 (Ritchie et al., 2018). High stream flow events in 2014 and 2015 created further aggradation and degradation (+/− 0.3 m), and as a result, over 3 Mt and 1.5 Mt of sediment was mobilized in 2014 and 2015, respectively (Ritchie et al., 2018).
Our model using annual flow-sediment index suggests the natural production of subyearling Chinook salmon migrants was strongly negatively impacted in years with high streamflow and sediment loads. Salmon survival during egg incubation and through emergence partly depends on egg burial depths exceeding the depth of streambed scour during the incubation period (Montgomery et al., 1996; DeVries, 1997). Salmonid egg burial depths can range from 0.03 m to 0.5 m depending upon the species, size of the female, substrate size, and other factors (DeVries, 1997). During and immediately following the dam removal years, aggradation and degradation in the main stem Middle and Lower Elwha River approached or exceeded these egg burial depths. We hypothesize streambed scour and fill associated with changes in sediment initially impacted egg-to-fry survival of naturally spawning Chinook salmon.
We did not see a similar pattern for natural steelhead smolt production. Although winter steelhead also spawned in the main stem, they did so in spring. The later spawn timing means their eggs and emergent juveniles, unlike Chinook salmon, were not exposed to peak flow events that occurred in fall and winter. Additionally, steelhead have also more frequently spawned in habitats that were not directly impacted by increased sediment, such as tributaries like Little River and Hughes Creek and the main stem Middle and Upper Elwha. Therefore, the timing and location of steelhead spawning and emergence likely reduced their vulnerability to potential sediment impacts from dam removal. Accordingly, as annual sediment loads stabilize to background levels (Ritchie et al., 2018), and conditions in the main stem become more favorable for spawning and egg incubation, we predict that the productivity of naturally spawning Chinook salmon will increase and become more similar to what we observed in 2018 and 2019.
Other factors may also have shaped the early response of natural-origin Chinook salmon and steelhead. Differences between the hatcheries, the status of each population prior to dam removal, and the presence of resident O. mykiss life histories between and above the dams may have contributed to the observed patterns. Major dam removal projects present tradeoffs, such as whether to use hatcheries or rely on natural-origin fish for reintroduction (Anderson et al., 2014). Hatcheries on the Elwha River were used to reduce the risk of population extinction during a period of heightened environmental impacts (largely from sediment) immediately following dam removal and to increase the abundance of Chinook salmon and steelhead populations (Ward et al., 2008; Peters et al., 2014). The original start time of Elwha hatchery operations varies with each species. The Elwha River Chinook salmon hatchery program was initiated in 1930 (Brannon and Hershberger, 1984) and almost all Chinook salmon production before dam removal was due to hatchery production. Thus, considering the long duration of Chinook salmon hatchery production, the associated potential risk of domestication selection for traits advantageous to the hatchery environment, and loss of fitness in the wild (Pess et al., 2014; Araki et al., 2008), are greater for Chinook salmon than steelhead in the Elwha. Some level of re-adaptation to the natural environment may be necessary for Chinook salmon to achieve sustained natural population growth and meet demographic thresholds linked to recovery (NMFS, 2012; Peters et al., 2014). Under this hypothesis, the naturally spawning population must have a level of reproductive isolation from the hatchery to observe any such readaptation, and reduced hatchery production could help achieve this goal. Whether the population retains suitable genetic material for re-wilding and the degree of reproductive isolation needed to achieve it are open questions.
Elwha steelhead had a much different hatchery history than Chinook salmon. Steelhead hatchery stocks released into the Elwha prior to 2011 were intended to be segregated from, rather than integrated with, the wild steelhead population in the Elwha River (sensu Mobrand et al., 2005). When these releases were terminated, a small but resilient population of wild steelhead remained more than 100 years after Elwha Dam was constructed. Beginning in 2011, releases from a new hatchery program derived from native Elwha steelhead was designed to increase abundance of fish harboring native genetic diversity using captive brood techniques (NMFS, 2012). Thus, the current steelhead program has a much shorter history than the Chinook salmon hatchery program.
The origin of the Chinook salmon and steelhead may also have affected the spatial distribution of spawning adults, which has implications for reintroduction success because it can influence productivity, habitat use and overall viability (Pess et al., 2012). For instance, hatchery Chinook salmon (Hoffnagle et al., 2008) and steelhead (Hayes et al., 2004; Feeken et al., 2019) may disproportionally return to areas near their release sites, resulting in a more downstream distribution compared to natural-origin fish (Hughes and Murdoch, 2017). These hatchery-influenced patterns are presumably related to the strong homing ability displayed by salmonids (Quinn, 1993). Thus, homing may help explain, in part, why the distribution of Chinook salmon and their redds was skewed towards the Lower and Middle Elwha, and why hatchery-origin winter steelhead were more common in the Lower Elwha, while natural-origin steelhead seem to be driving spatial expansion into the Middle and Upper Elwha. This behavioral tendency could limit access to lesser used, but higher quality habitats further upstream that were not impacted by dam removal. For example, the estimated numbers of recruits per spawner were two times greater for spawning pink salmon in the Fraser River above the former Hell’s Gate rockfall than below it during the peak time of reintroduction (Pess et al., 2014). Therefore, population productivity and growth rates of natural-origin Chinook salmon may also partly depend on their ability to expand their spatial distribution into habitats where they would have less competition and better conditions for spawning and egg incubation.
5.2 Re-emergence of summer run steelhead into headwaters of Elwha River
Finally, the response of steelhead, particularly the re-emergence of a summer run, has likely benefited from the abundant population of native resident rainbow trout present upstream of the former dams. Resident rainbow trout can produce anadromous offspring (Kendall et al., 2014) and represent a source of anadromous individuals, particularly when anadromous adult abundances are low (Losee et al., 2020). Populations isolated above barriers often retain both the genetic (Clemento et al., 2008) and physiological (Holecek et al., 2012) traits of anadromy. Residents can also mate with (McMillan et al., 2007) and contribute genes to their anadromous counterparts (Christie et al., 2011). Resident rainbow trout upstream of Glines Canyon Dam were producing migrants that were seawater tolerant and apparently capable of an anadromous life history as late as the early 1990’s (Hiss and Wunderlich, 1994). It thus appears dam removal not only opened up additional freshwater habitat for anadromous steelhead, but also provided access to the ocean and potential interbreeding between steelhead and resident trout, both of which can increase the number of breeders and genetic variation within a reconnected population (Weigel, 2013).
Increased life history diversity was a predicted response to the removal of the Elwha River dams (Brenkman et al., 2008; Pess et al., 2008). Adaptive management guidelines explicitly recognized the importance of life history diversification to the recovery of Chinook salmon and steelhead in the basin (Peters et al., 2014). Although spring Chinook salmon and summer steelhead life history types historically occurred in the Elwha River before the dams were constructed, thus far only summer steelhead have re-emerged after dam removal. Summer steelhead were rarely seen prior to dam removal (Duda et al., 2021), but rapidly increased their observed numbers from 2017 to 2020 (Table 5). This is likely due to reconnection with the favorable cold-water temperature regime and alternating canyon and floodplain geomorphology of the Elwha River basin upstream of the dams (Beechie et al., 2006; Brenkman et al., 2008; Pess et al., 2008). Additionally, there have not been any releases of hatchery summer steelhead during or after dam removal, with preliminary genetic analyses indicating that the summer run fish harbor alleles for early run timing and a large portion of the resident rainbow trout above the former dams harbor those alleles as well (Fraik et al., 2021). A similar response of increased anadromy, in addition to larger body size, was documented in Elwha River bull trout, which were common between and above the dams prior to removal (Brenkman et al., 2019; Duda et al., 2021). The “reawakening” of the summer steelhead life history strategy and increased abundance and condition of bull trout in the Elwha River further suggests salmonids can retain the ability to express anadromy after decades of isolation from the sea and highlights how species with sources of resident fish can respond to re-connectivity of a watershed (Thrower et al., 2004).
5.3 Implications for other dam removal projects
The management and monitoring of how salmonids respond to dam removal in the Elwha River has several implications for future dam removal projects. First, sediment impacts and large-scale changes to the river can occur from dam removal during and immediately following deconstruction (Ritchie et al., 2018). Given substantial sediment impacts from dam removal, reduced invertebrate prey availability for salmon can force them to temporarily adjust their foraging and diets (Morley et al., 2020). Although impacted sections of river can become productive for salmon relatively quickly, depending on discharge and sediment transport, species that most heavily rely on habitats impacted by dam removal, such as Chinook salmon in the main stem Elwha River, will likely struggle to maintain natural production until sediment levels return to a more natural level and regime.
Second, hatchery production was important for Chinook salmon and steelhead to persist through the most disruptive phase of dam removal. However, reintroduction into upstream areas has included hatchery, transplantation, and natural approaches. Chinook salmon, for example, have been almost entirely reliant on releases of large numbers of hatchery smolts. Reintroduction for other species, such as coho salmon, was jumpstarted by temporary relocation of hatchery adults to two tributaries to the Middle Elwha during the highest sediment impact periods before natural spawning started to occur, as well as consistent coho salmon hatchery production post dam removal (Liermann et al., 2017; Denton et al., 2022). Hatchery production was not used for bull trout (Quinn et al., 2017; Brenkman et al., 2019), Pacific lamprey (Hess et al., 2021), or summer steelhead (Fraik et al., 2021). These studies of other species, in combination with our results, suggest hatchery production may be particularly beneficial for species with an extensive history of hatchery operation that also are expected to rely heavily on the most impacted habitats. However, hatchery production may not be as needed for species with a long history of natural production, resident life histories, and the ability to access and spawn in unimpacted habitats.
Third, attempts to re-establish self-sustaining populations through barrier removals may be assisted by reducing or eliminating harvest. A fishing moratorium was enacted in the Elwha River watershed to protect fish and reduce the risk of extinction during dam removal and help to increase immediate abundance (Pess et al., 2012; Anderson et al., 2014; Bellmore et al., 2019). There was no commercial or recreational in-river Elwha River Chinook salmon fishery for decades prior to dam removal. There was a steelhead recreational and commercial fishery that varied in season length over the years up until 2012. Terminal harvest of Chinook salmon varies substantially across Puget Sound as a whole, but some populations experienced rates up to approximately 30% (PSIT and WDFW, 2022); avoiding this level of terminal harvest mortality has resulted in thousands of additional Chinook salmon spawners, equivalent to approximately an additional year of adult Chinook salmon returns following dam removal. Similarly, using the average steelhead harvest rate in Puget Sound for the same period of 7% (S.D. 6%) (Cram et al., 2018), would result in an additional 493 (+/−35) additional spawners in the Elwha since 2011. A similar approach of fishing closures may benefit susceptible species and stocks in watersheds where survival maybe dramatically impacted by dam removal.
Last, large dam removal can be disruptive, rendering many traditional monitoring approaches unviable, particularly in larger watersheds with multiple species of interest. High turbidity levels for extended periods of time forced a shift from redd counts to SONAR, which allowed annual estimates of Chinook salmon and steelhead abundance and associated run timing. Foot and boat surveys during peak spawning allowed us to continue tracking the spatial distribution of spawning adults in areas and years where river conditions allowed. Smolt trapping was also impacted by changes to the river throughout the study period (but in particular during and after dam removal) requiring adjustments to the mainstem trap location and operations. Together these adaptations to fish sampling approaches allowed us to capture important aspects of reintroduction that otherwise would not have been possible.
5.4 Conclusions
Fish reintroduction in larger watersheds can take up to 20 years or more (DOI, 1996; Milner et al., 2008; Pess et al., 2012), while smaller watersheds can establish self-sustaining salmon populations in five years or less (Bryant et al., 1999; Glen, 2002). It is too early to conclude that recovery of Chinook salmon and steelhead populations due to dam removal in the Elwha River has been successful. Nonetheless, in the short period since dam removal we observed several promising results, ranging from increased abundance and spatial distribution to the re-emergence of a unique life history, and were able to evaluate the effects of streamflow and sedimentation on the production of natural-origin juvenile Chinook salmon.
While these results are encouraging, it is also important to remember that many challenges remain, particularly for Chinook salmon that are mostly hatchery-origin and have not distributed themselves as broadly, to this point, as expected. Whether dam removal in the Elwha River results in salmon, trout, and char populations that are more abundant, diverse, and resilient than prior to dam removal will be determined over the course of the next several decades. Although we cannot answer that question yet, the project has taught us many lessons about monitoring and managing salmonids and their habitat during and after dam removal (see also Peters et al., 2024). The response of Chinook salmon and steelhead to the removal of the Elwha River dams is not just about dam removal; rather, it is about a suite of cumulative management actions including use of hatchery production, and an in-river fishing and terminal nearshore moratorium. Succinctly characterizing this complexity during a period of extreme changes was challenging, which is why we relied on several different methods, such as SONAR to count fish and estimate run timing in river conditions that did not allow for visual surveys, and smolt traps to enumerate outmigrating juveniles. We complimented those stationary-point sampling methods with a spatial component that included systematic and opportunistic on-the-ground foot and snorkel surveys when and where visibility allowed. By being adaptive with our monitoring, we were thus able to use multiple methods and lines of evidence to track the short-term response of Chinook salmon and steelhead. Our findings improve existing knowledge about potential short-term salmonid responses to dam removal and offers insight into the complexity for those tasked with trying to quantify reintroduction of multiple species across remote watersheds with varying degrees of hatchery- and natural-origin salmon and steelhead populations.
Data availability statement
The original contributions presented in the study are included in the article/Supplementary Material, further inquiries can be directed to the corresponding author/s.
Ethics statement
Ethical approval was not required for the study involving animals in accordance with the local legislation and institutional requirements because we followed authorization of Federal and State sanctioned permits.
Author contributions
GP: conceptualization, methodology, formal analysis, investigation, resources, data curation, original draft, review and editing, visualization, supervision, project administration, funding acquisition. MM: conceptualization, methodology, investigation, resources, data curation, original draft, review and editing, supervision, project administration, funding acquisition. KD: methodology, formal analysis, investigation, resources, data curation, original draft, review and editing, visualization, supervision, project administration. JA: conceptualization, methodology, formal analysis, investigation, resources, data curation, original draft, review and editing, visualization, supervision, project administration, funding acquisition. ML: conceptualization, methodology, formal analysis, resources, data curation, original draft, review and editing, visualization. RP: conceptualization, methodology, investigation, resources, data curation, review and editing, supervision, project administration, funding acquisition. JM: conceptualization, methodology, formal analysis, investigation, resources, data curation, original draft, review & editing, visualization, supervision, project administration, funding acquisition. SB: conceptualization, methodology, investigation, resources, data curation, review and editing, supervision, project administration, funding acquisition. TB: conceptualization, methodology, formal analysis, investigation, resources, data curation, original draft, review and editing, visualization, supervision, project administration, funding acquisition. JD: conceptualization, investigation, resources, data curation, review and editing. KH: review and editing. All authors contributed to the article and approved the submitted version.
Funding
The author(s) declare financial support was received for the research, authorship, and/or publication of this article. Funding for these efforts, and loan of one SONAR unit to the Lower Elwha Klallam Tribe, was provided by the United States National Park Service – Olympic National Park to the Lower Elwha Klallam Tribe (contract nos. 1443PC00296, P14PC00364, and P16PC00005). The National Park Foundation also provided funds to the National Park Service and the Lower Elwha Klallam Tribe (contract no.140P2018R042). KD was funded by the Lower Elwha Klallam Tribe for all aspects of the SONAR and species composition work (contract nos. 1443PC00296, P14PC00364, and P16PC00005). Funding for general Chinook salmon and steelhead monitoring was also provided to the Lower Elwha Klallam Tribe by the Environmental Protection Agency/Puget Sound Partnership through the Northwest Indian Fisheries Commission (contract no. PA-01J64601–1). Funding for the Elwha River native steelhead captive broodstock program was provided by the Pacific Coast Salmon Restoration Fund/Hatchery reform funds (contract nos. 09–1749, 11–1653, 12–1950, 13–1551, and 14–2247). The National Park Service also funded the Bureau of Reclamation between 2012 and 2017 in support of the sediment management monitoring plan via interagency agreements. This funding was used for installing and operating reservoir stage gages, river stage gages, real-time monitoring and numerical modeling of reservoir sediment erosion and transport, and providing technical support for construction implementation of the dam removals and associated mitigation discussions. The Bureau of Reclamation, in support of the sediment management program, contracted to U.S. Geological Survey for collection of sediment turbidity, discharge, and river stage gage data at three locations, and contracted to Erdman Video Systems, Inc. for six time-lapse web camera installations, operation and maintenance. Additionally, the Bureau of Reclamation funded the U.S. Geological Survey to acquire aerial photographs over time (PlaneCam), the purchase of general monitoring equipment, and funding for one full-time on-site project geomorphologist. The National Park Service – Olympic National Park provided funding to the Washington Department of Fish and Wildlife in support of the collection of biological data during carcass surveys from adult Chinook salmon returns (contract nos. P13PC00291, P14PX01377, P15PX02717, P16PX02828, and others). The National Parks Foundation provided additional funding to the Washington Department of Fish and Wildlife in support of the tagging of juvenile Chinook salmon with coded wire tags (CWTs), the collection of the tags during spawning operations and spawning ground surveys for carcasses, and the subsequent reading and interpretation of the CWTs and otoliths. The United States Fish and Wildlife Service provided funding for a portion of the labor associated with adult and juvenile enumeration and the proportion of hatchery-origin steelhead (PHOS), which received additional funding from the Lower Elwha Klallam Tribe and the Bureau of Indian Affairs. Funding, in part, was provided by the Lower Elwha Klallam Tribe to the National Oceanic and Atmospheric Administration – Northwest Fisheries Science Center for the contributions of GP, TB, and ML (contract no. PA-01J64601–1). Oleksandr Stefankiv of AIS, Inc. (contractor to the Northwest Fisheries Science Center) provided assistance with SONAR enumeration and created Figure 1.
Acknowledgments
We thank Vanessa Castle, Mel Elofson, John Mahan, Allyce Miller, Raymond Moses, Rebecca Paradis, Ernest Sampson, McKenzi Taylor, Justin Stapleton, Wilson Wells, and Kim Williams of the Lower Elwha Klallam Tribe for leading efforts on the screw traps and spawner surveys, as well as assisting with the SONAR and species composition work. We also thank Joshua Weinheimer, Randy Cooper, Andrew Simmons, Chris O’Connell, Scott Williams, Troy Tisdale, Jeff Gufler, Kent Mayer, Michael Ackley, and Mike Mizell of the Washington Department of Fish and Wildlife for leading or helping with Chinook salmon carcass sampling collection and the fish weir, which was used for a brief period of time. National Park Service (NPS), United States Geologic Survey (USGS), and Bureau of Recreation (BOR) employees Pat Crain, Heidi Connor, Anna Geffre, Josh Geffre, Philip Kennedy, Alex Pavlinovic, James Starr, Kathryn Sutton (now with Washington Department of Fish and Wildlife), Andy Ritchie, Tim Randle, and Jennifer Bountry all provided assistance on various data collection tasks throughout the years. Numerous National Park Service volunteers also provided assistance, as did Dave Shreffler of Shreffler Environmental. We thank numerous United States Fish and Wildlife Service temporary and permanent employees who have assisted with multiple aspects of the adult and juvenile enumeration. Steve Corbett of the National Oceanic and Atmospheric Administration – Northwest Fisheries Science Center provided field assistance on all aspects of Chinook salmon and steelhead monitoring. The National Oceanic and Atmospheric Administration – Northwest Fisheries Science Center employees Tim Beechie, Morgan Bond, and Carl Ostberg of USGS reviewed early versions of this manuscript. We thank Craig Busack of NOAA Northwest Regional Office and John Mahan of the Tulalip Tribes who reviewed the most recent version of the manuscript.
Conflict of interest
Author KD was employed by the company K. Denton and Associates LLC.
The remaining authors declare that the research was conducted in the absence of any commercial or financial relationships that could be construed as a potential conflict of interest.
Publisher’s note
All claims expressed in this article are solely those of the authors and do not necessarily represent those of their affiliated organizations, or those of the publisher, the editors and the reviewers. Any product that may be evaluated in this article, or claim that may be made by its manufacturer, is not guaranteed or endorsed by the publisher.
Author disclaimer
Any use of trade, firm, or product names is for descriptive purposes only and does not imply endorsement by the U.S. Government.
Supplementary material
The Supplementary Material for this article can be found online at: https://www.frontiersin.org/articles/10.3389/fevo.2024.1241028/full#supplementary-material
References
Anderson J. H., Faulds P. L., Denton K. D., Koehler M. E., Atlas W. I., Quinn T. P. (2015). Dispersal and newly accessible habitat. Can. J. Fish. Aquat. Sci. 72, 454–465. doi: 10.1139/cjfas-2014-0180
Anderson J. H., Pess G. R., Carmichael R. W., Ford M. J., Cooney T. D., Baldwin C. M., et al. (2014). Planning Pacific salmon and steelhead reintroductions aimed at long-term viability and recovery. N. Am. J. Fish. Manage. 34, 72–93. doi: 10.1080/02755947.2013.847875
Araki H., Berejikian B. A., Ford M. J., Blouin M. S. (2008). Fitness of hatchery-reared salmonids in the wild. Evol. App. 1 (2), 342–355. doi: 10.1111/j.1752-4571.2008.00026.x
Beechie T., Buhle E., Ruckelshaus M., Fullerton A., Holsinger L. (2006). Hydrologic regime and the conservation of salmon life history diversity. Biol. Conserv. 130, 560–572. doi: 10.1016/j.biocon.2006.01.019
Bellmore J. R., Duda J. J., Craig L. S., Greene S. L., Torgersen C. E., Collins M. J., et al. (2016). Status and trends of dam removal research in the United States. WIREs Water 4(2):e1164. doi: 10.1002/wat2.1164
Bellmore J. R., Pess G. R., Duda J. J., O’Connor J. E., East A. E., Foley M. M., et al. (2019). Conceptualizing ecological responses to dam removal: If you remove it, what’s to come? BioScience 69, 26–39. doi: 10.1093/biosci/biy152
Brannon E. L., Hershberger W. K. (1984). “Elwha River fall Chinook salmon,” in Proceedings of the Olympic wild fish conference, 169–172 (Port Angeles, WA: Peninsula College).
Brenkman S. J., Duda J. J., Torgersen C. E., Welty E., Pess G. R., Peters R., et al. (2012). A riverscape perspective of Pacific salmonids and aquatic habitats prior to large-scale dam removal in the Elwha River, Washington, USA. Fish. Manage. Ecol. 19, 36–53. doi: 10.1111/j.1365-2400.2011.00815.x
Brenkman S. J., Pess G. R., Torgersen C. E., Kloehn K. K., Duda J. J., Corbett S. C. (2008). Predicting recolonization patterns and interactions between potamodromous and anadromous salmonids in response to dam removal in the Elwha River, Washington State, USA. Northwest Sci. 82 (sp1), 91–106. doi: 10.3955/0029-344X-82.S.I.91
Brenkman S. J., Peters R. J., Tabor R. A., Geffre J. J., Sutton K. T. (2019). Rapid recolonization and life history responses of bull trout following dam removal in Washington’s Elwha River. N. Am. J. Fish. Manage. 39, 560–573. doi: 10.1002/nafm.10291
Bryant M. D., Frenette B. J., McCurdy S. J. (1999). Colonization of a watershed by anadromous salmonids following the installation of a fish ladder in Margaret Creek, Southeast Alaska. N. Am. J. Fish. Manage. 19, 1129–1136. doi: 10.1577/1548-8675(1999)019<1129:COAWBA>2.0.CO;2
Christie M. R., Marine M. L., Blouin M. S. (2011). Who are the missing parents? Grandparentage analysis identifies multiple sources of gene flow into a wild population. Mol. Ecol. 20, 1263–1276. doi: 10.1111/j.1365-294X.2010.04994.x
Clemento A. J., Anderson E. C., Boughton D., Girman D., Garza J. C. (2008). Population genetic structure and ancestry of Oncorhynchus mykiss populations above and below dams in south-central California. Conserv. Genet. 10, 1321–1336. doi: 10.1007/s10592-008-9712-0
Cram J., Kendall N., Marshall A., Buehrens T., Seamons T., Leland B., et al. (2018). Steelhead at risk report: assessment of Washington’s steelhead populations. WDFW FPT 19-03, (Olympia, WA: Washington Department of Fish and Wildlife). Available at: https://wdfw.wa.gov/publications/02070.
Denton K., Stefankiv O., Urnes C. J. (2022). Estimating coho salmon escapement and mark rates in the Elwha River in 2021 using multi-beam SONAR technology. Report to the Lower Elwha Tribe (Port Angeles, WA: Lower Elwha Klallam Tribe), 33.
DeVries P. (1997). Riverine salmonid egg burial depths: review of published data and implications for scour studies. Can. J. Fish. Aquat. Sci. 54, 1685–1698. doi: 10.1139/f97-090
DOI (1996). Elwha River ecosystem restoration implementation, final environmental impact statement. NPS D-271A (Port Angeles, WA: Department of the Interior, National Park Service, Olympic National Park).
Duda J. J., Brenkman S. J., Crain P. (2018). “Ch. 4: Pacific salmonids,” in Natural resource condition assessment: Olympic National Park,” in Natural resource report NPS/OLYM/NRR—2018/1826. Eds. McCaffery R., Jenkins K. (National Park Service, Fort Collins), 123–167. Available at: https://www.researchgate.net/publication/358131197_Chapter_4_Pacific_Salmon-Natural_Resource_Condition_Assessment_Olympic_National_Park.
Duda J. J., Freilich J. E., Schreiner E. G. (2008). Baseline studies in the Elwha River ecosystem prior to dam removal: introduction to the special issue. Northwest Sci. 82(sp1), 1–12. doi: 10.3955/0029-344X-82.S.I.1
Duda J. J., Torgersen C. E., Brenkman S. J., Peters R. J., Sutton K. T., Connor H. A., et al. (2021). Reconnecting the Elwha River: spatial patterns of fish response to dam removal. Front. Ecol. Evol. 9. doi: 10.3389/fevo.2021.765488
East A. E., Pess G. R., Bountry J. A., Magirl C. S., Ritchie A. C., Logan J. B., et al. (2015). Large-scale dam removal on the Elwha River, Washington, USA: River channel and floodplain geomorphic change. Geomorphology 228, 765–786. doi: 10.1016/j.geomorph.2014.08.028
English K. K., Bocking R. C., Irvine J. R. (1992). A robust procedure for estimating salmon escapement based on the area-under-the-curve method. Can. J. Fisheries Aquat. Sci. 49, 1982–1989. doi: 10.1139/f92-220
Ertle C. W., Roth J. M., Judson J. S., Vankirk G. H. (2019). Rock demolition and hazardous debris removal for ecosystem restoration on the Elwha River ERCD/GSL TR19-21. (Vicksburg, MS: U.S. Army Engineer Research and Development Center). Available at: https://apps.dtic.mil/sti/pdfs/AD1081525.pdf.
Feeken S. F., Bowersox B. J., Dobos M. E., Corsi M. P., Quist M. C., Copeland T. (2019). Distribution and movement of steelhead and anglers in the Clearwater River, Idaho. N. Am. J. Fish. Manage. 39, 1056–1072. doi: 10.1002/nafm.10345
Foley M. M., Warrick J. A., Ritchie A., Stevens A. W., Shafroth P. B., Duda J. J., et al. (2017). Coastal habitat and biological community response to dam removal on the Elwha River. Ecol. Monogr. 87, 552–577. doi: 10.1002/ecm.1268
Fraik A. K., McMillan J. R., Liermann M., Bennett T., McHenry M. L., McKinney G. J., et al. (2021). The impacts of dam construction and removal on the genetics of recovering steelhead (Oncorhynchus mykiss) populations across the Elwha River watershed. Genes 12, 89. doi: 10.3390/genes12010089
Gallagher S. P., Hahn P. K., Johnson D. H. (2007). “Redd counts,” in Salmonid field protocols handbook: techniques for assessing status and trends in salmon and trout populations. Eds. Johnson D. H., Shrier B. M., O’Neal J. S., Knutzen J. A., Augerot X., O’Neil T. A., Pearsons T. N. (American Fisheries Society, Bethesda, MD, U.S.A), 197–234. doi: 10.47886/9781888569926.ch12
Glen D. (2002). “Recovery of salmon and trout following habitat enhancement works: review of case studies 1995–2002,” in Proceedings of the 13th International Salmonid Habitat Enhancement workshop, 93–112 (Dublin, Ireland: Central Fisheries Board).
Greene C. M., Jensen D. W., Pess G. R., Steel E. A., Beamer E. (2005). Effects of environmental conditions during stream, estuary, and ocean residency on Chinook salmon return rates in the Skagit River, Washington. Trans. Am. Fish. Soc 134, 1562–1581. doi: 10.1577/T05-037.1
Hare J. A., Kocik J. F., Link J. S. (2019). Atlantic salmon recovery informing and informed by ecosystem-based fisheries management. Fisheries 44, 403–411. doi: 10.1002/fsh.10262
Hayes S. A., Bond M. H., Hanson C. V., MacFarlane R. B. (2004). Interactions between endangered wild and hatchery salmonids: can the pitfalls of artificial propagation be avoided in small coastal streams? J. Fish Biol. 65, 101–121. doi: 10.1111/j.0022-1112.2004.00547.x
Hess J. E., Paradis R. L., Moser M. L., Weitkamp L. A., Delomas T. A., Narum S. R. (2021). Robust recolonization of Pacific lamprey following dam removals. Trans. Am. Fish. Soc 150, 56–74. doi: 10.1002/tafs.10273
Hilldale R. C., Carpenter W. O., Goodwiller B., Chambers J. P., Randle T. J. (2015). Installation of impact plates to continuously measure bed load: Elwha River, Washington, USA. J. Hydraul. Eng. 141, 06014023. doi: 10.1061/(ASCE)HY.1943-7900.0000975
Hiss J. M., Wunderlich R. C. (1994). Salmonid availability and migration in the middle Elwha River system. Prepared for the National Park Service, Olympic National Park, Port Angeles, WA (Olympia, WA: US Fish and Wildlife Service, Western Washington Fishery Resource Office).
Hoffnagle T. L., Carmichael R. W., Frenyea K. A., Keniry P. J. (2008). Run timing, spawn timing, and spawning distribution of hatchery- and natural-origin spring Chinook salmon in the Imnaha River, Oregon. N. Am. J. Fish. Manage. 28, 148–164. doi: 10.1577/M05-145.1
Hogg R. S., Coghlan S. M. Jr., Zydlewski J., Gardner C. (2015). Fish community response to a small-stream dam removal in a Maine coastal river tributary. Trans. Am. Fish. Soc 144, 467–479. doi: 10.1080/00028487.2015.1007164
Holecek D. E., Scarnecchia D. L., Miller S. E. (2012). Smoltification in an impounded, adfluvial redband trout population upstream from an impassable dam: does it persist? Trans. Am. Fish. Soc 141, 68–75. doi: 10.1080/00028487.2011.651550
Hughes M. S., Murdoch A. R. (2017). Spawning habitat of hatchery spring Chinook Salmon and possible mechanisms contributing to lower reproductive success. Trans. Am. Fish. Soc 146, 1016–1027. doi: 10.1080/00028487.2017.1336114
Keefer M. L., Caudill C. C. (2014). Homing and straying by anadromous salmonids: a review of mechanisms and rates. Rev. Fish Biol. Fish. 24, 333–368. doi: 10.1007/s11160-013-9334-6
Kendall N. W., McMillan J. R., Sloat M. R., Buehrens T. W., Quinn T. P., Pess G. R., et al. (2014). Anadromy and residency in steelhead and rainbow trout (Oncorhynchus mykiss): a review of the processes and patterns. Can. J. Fish. Aquat. Sci. 72, 319–342. doi: 10.1139/cjfas-2014-0192
Kiffney P. M., Pess G. R., Anderson J. H., Faulds P., Burton K., Riley S. C. (2009). Changes in fish communities following recolonization of the Cedar River, WA, USA by Pacific salmon after 103 years of local extirpation. River Res. Appl. 25, 438–452. doi: 10.1002/rra.1174
Liermann M., Pess G., McHenry M., McMillan J., Elofson M., Bennett T., et al. (2017). Relocation and recolonization of coho salmon in two tributaries to the Elwha River: implications for management and monitoring. Trans. Am. Fish. Soc 146, 955–966. doi: 10.1080/00028487.2017.1317664
Lilja J., Ridley T., Cronkite G. M., Enzenhofer H. J., Holmes J. A. (2008). Optimizing sampling effort within a systematic design for estimating abundant escapement of sockeye salmon (Oncorhynchus nerka) in their natal river. Fish. Res. 90, 118–127. doi: 10.1016/j.fishres.2007.10.002
Losee J. P., Claiborne A. M., Madel G. M., Kungle M., Campbell L. (2020). Is marine survival for Puget Sound’s wild steelhead really that bad? A Nisqually River case study evaluating the estimates of productivity and survival of Oncorhynchus mykiss. Trans. Am. Fish. Soc 150, 160–174. doi: 10.1002/tafs.10275
McMillan J. R., Katz S. L., Pess G. R. (2007). Observational evidence of spatial and temporal structure in a sympatric anadromous (winter steelhead) and resident rainbow trout mating system on the Olympic Peninsula, Washington. Trans. Am. Fish. Soc 136, 736–748. doi: 10.1577/T06-016.1
McMillan J. R., Pess G. R., Liermann M., Morley S. A., McHenry M. L., Campbell L. A., et al. (2015). Using redd attributes, fry density, and otolith microchemistry to distinguish the presence of steelhead and rainbow trout in the Elwha River dam removal project. N. Am. J. Fish. Manage. 35, 1019–1033. doi: 10.1080/02755947.2015.1074965
Milner A. M., Fastie C. L., Chapin F. S., Engstrom D. R., Sharman L. C. (2007). Interactions and linkages among ecosystems during landscape evolution. BioScience 57, 237–247. doi: 10.1641/B570307
Milner A. M., Robertson A. L., Monaghan K. A., Veal A. J., Flory E. A. (2008). Colonization and development of an Alaskan stream community over 28 years. Front. Ecol. Environ. 6, 413–419. doi: 10.1890/060149
Mobrand L. E., Barr J., Blankenship L., Campton D. E., Evelyn T. T. P., Flagg T. A., et al. (2005). Hatchery reform in Washington State. Fisheries 30, 11–23. doi: 10.1577/1548-8446(2005)30[11:HRIWS]2.0.CO;2
Montgomery D. R., Buffington J. M., Peterson N. P., Schuett-Hames D., Quinn T. P. (1996). Stream-bed scour, egg burial depths, and the influence of salmonid spawning on bed surface mobility and embryo survival. Can. J. Fish. Aquat. Sci. 53, 1061–1070. doi: 10.1139/f96-028
Morley S. A., Foley M. M., Duda J. J., Beirne M. M., Paradis R. L., Johnson R. C., et al. (2020). Shifting food web structure during dam removal—Disturbance and recovery during a major restoration action. PloS One 16, e0246517. doi: 10.1371/journal.pone.0246517
NMFS (2012). Elwha channel hatchery summer/fall Chinook salmon fingerling and yearling, Lower Elwha fish hatchery steelhead, Lower Elwha fish hatchery coho salmon, Lower Elwha fish hatchery fall chum salmon, and Elwha River odd and even year pink salmon programs. Endangered Species Act (ESA) section 7 biological opinion and Magnuson-Stevens Fishery conservation and Management Act essential fish habitat (EFH) consultation. NMFS consultation NWR-2012-9426, National Marine Fisheries Service, Seattle, Washington.
O’Connor J. E., Duda J. J., Grant G. E. (2015). 1000 dams down and counting. Science 348, 496–497. doi: 10.1126/science.aaa9204
Pearsons T. N., O’Connor R. R. (2020). Stray rates of natural-origin Chinook salmon and steelhead in the upper Columbia River watershed. Trans. Am. Fish. Soc 149, 147–158. doi: 10.1002/tafs.10220
Perry L. G., Shafroth P. B., Alfieri S. J., Miller I. M. (2023). Coastal vegetation responses to large dam removal on the Elwha River. Front. Ecol. Evol. 11. doi: 10.3389/fevo.2023.1233903
Pess G. R., Hilborn R., Kloehn K., Quinn T. P. (2012). The influence of population dynamics and environmental conditions on pink salmon (Oncorhynchus gorbuscha) recolonization after barrier removal in the Fraser River, British Columbia, Canada. Can. J. Fish. Aquat. Sci. 69, 970–982. doi: 10.1139/f2012-030
Pess G. R., McHenry M. L., Beechie T. J., Davies J. (2008). Biological impacts of the Elwha River dams and potential salmonid responses to dam removal. Northwest Sci. 82 (sp1), 72–90. doi: 10.3955/0029-344X-82.S.I.72
Pess G. R., Quinn T. P., Gephard S. R., Saunders R. (2014). Re-colonization of Atlantic and Pacific rivers by anadromous fishes: linkages between life history and the benefits of barrier removal. Rev. Fish Biol. Fish. 24, 881–900. doi: 10.1007/s11160-013-9339-1
Peters R., Anderson J. H., Duda J. J., Johnson J. R., McHenry M., Pess G., et al. (2024). Challenges of implementing a multi-agency monitoring and adaptive management strategy for federally threatened Chinook salmon and steelhead trout during and after dam removal in the Elwha River. Front. Environ. Sci. 12. doi: 10.3389/fenvs.2024.1291265
Peters R. J., Denton K., Liermann M. (2020). Proportion of hatchery origin winter steelhead and broodstock collection in the Elwha River: 2019. U.S. Fish and Wildlife Service Report # 4837-1336 to the Lower Elwha Klallam Tribe. Available online at: https://purl.fdlp.gov/GPO/gpo172370.
Peters R. J., Duda J. J., Pess G. R., Zimmerman M., Crain P., Hughes Z., et al. (2014). Guidelines for monitoring and adaptively managing restoration of Chinook salmon (Oncorhynchus tshawytscha) and steelhead (O. mykiss) on the Elwha River (Lacey, WA: U.S. Fish and Wildlife Service), 111. Available at: https://www.researchgate.net/publication/342165354_Guidelines_for_Monitoring_and_Adaptively_Managing_Restoration_of_Chinook_Salmon_Oncorhynchus_tshawytscha_and_Steelhead_O_mykiss_on_the_Elwha_River_US_Fish_Wildlife_Service.
Pringle C. M., Freeman M. C., Freeman B. J. (2000). Regional effects of hydrologic alterations on riverine macrobiota in the new world: Tropical-temperate comparisons. BioScience 50, 807–823. doi: 10.1641/0006-3568(2000)050[0807:reohao]2.0.co;2
PSIT, WDFW (2022). Comprehensive management plan for Puget Sound Chinook: harvest management component. Available online at: https://wdfw.wa.gov/publications/02309.
Quinn T. P. (1993). A review of homing and straying of wild and hatchery-produced salmon. Fish. Res. 18, 29–44. doi: 10.1016/0165-7836(93)90038-9
Quinn T. P., Bond M. H., Brenkman S. J., Paradis R., Peters R. J. (2017). Re-awakening dormant life history variation: stable isotopes indicate anadromy in bull trout following dam removal on the Elwha River, Washington. Environ. Biol. Fishes. 100, 1659–1671. doi: 10.1007/s10641-017-0676-0
Randle T. J., Bountry J. A., Ritchie A., Wille K. (2015). Large-scale dam removal on the Elwha River, Washington, USA: Erosion of reservoir sediment. Geomorphology 246, 709–728. doi: 10.1016/j.geomorph.2014.12.045
Ritchie A. C., Warrick J. A., East A. E., Magirl C. S., Stevens A. W., Bountry J. A., et al. (2018). Morphodynamic evolution following sediment release from the world’s largest dam removal. Sci. Rep. 8, 13279. doi: 10.1038/s41598-018-30817-8
Rubin S. P., Foley M. M., Miller I. M., Stevens A. W., Warrick J. A., Berry H. D., et al. (2023). Nearshore subtidal community response during and after sediment disturbance associated with dam removal. Front. Ecol. Evol. 11. doi: 10.3389/fevo.2023.1233895
Schindler D. E., Hilborn R., Chasco B., Boatright C. P., Quinn T. P., Rogers L. A., et al. (2010). Population diversity and the portfolio effect in an exploited species. Nature 465, 609–612. doi: 10.1038/nature09060
Shaffer J. A., Juanes F., Quinn T. P., Parks D., McBride T., Michel J., et al. (2017). Nearshore fish community responses to large scale dam removal: implications for watershed restoration and fish management. Aquat. Sci. 79, 643–660. doi: 10.1007/s00027-017-0526-3
Smith C., Castle P. (1994). Puget Sound Chinook Salmon (Oncorhynchus tshawytscha) Escapement Estimates and Methods - 1991. Northwest Fishery Resource Bulletin Project Report Series No. 1 (Olympia WA: Washington Department of Fish and Wildlife), 50.
Thompson T. Q., Bellinger M. R., O’Rourke S. M., Prince D. J., Stevenson A. E., Rodrigues A. T., et al. (2019). Anthropogenic habitat alteration leads to rapid loss of adaptive variation and restoration potential in wild salmon populations. Proc. Natl. Acad. Sci. 116, 177–186. doi: 10.1101/310714
Thrower F., Guthrie Iii C., Nielsen J., Joyce J. (2004). A comparison of genetic variation between an anadromous steelhead, Oncorhynchus mykiss, population and seven derived populations sequestered in freshwater for 70 years. Environ. Biol. Fishes 69, 111–125. doi: 10.1023/B:EBFI.0000022880.52256.92
Tullos D. D., Collins M. J., Bellmore J. R., Bountry J. A., Connolly P. J., Shafroth P. B., et al. (2016). Synthesis of common management concerns associated with dam removal. J. Am. Water Resour. Assoc. 52, 1179–1206. doi: 10.1111/1752-1688.12450
Waldman J. R., Quinn T. P. (2022). North American diadromous fishes: Drivers of decline and potential for recovery in the Anthropocene. Sci. Adv. 8, eabl5486. doi: 10.1126/sciadv.abl5486
Waples R. S., Pess G. R., Beechie T. (2008). Evolutionary history of Pacific salmon in dynamic environments. Evol. Appl. 1, 189–206. doi: 10.1111/j.1752-4571.2008.00023.x
Ward L., Crain P., Freymond B., McHenry M., Morrill D., Pess G., et al. (2008). Elwha River Fish Restoration Plan–Developed pursuant to the Elwha River Ecosystem and Fisheries Restoration Act, Public Law 102-495 (U.S. Dept. Commer., NOAA Tech. Memo), 191. Available at: https://repository.library.noaa.gov/view/noaa/3569.
Weigel D. E. (2013). Colonization of steelhead (Oncorhynchus mykiss) after barrier removal in a tributary to the Methow River, Washington. Ph.D. Thesis. University of Idaho, Moscow. Available at: https://www.salmonrecovery.gov/Files/APR/Section%202%20Literature%20Cited/Weigel%202013_Dissertation.pdf.
Weinheimer J., Anderson J., Cooper R., Williams S., McHenry M., Crain P., et al. (2018). Age structure and hatchery fraction of Elwha River Chinook Salmon: 2017 carcass survey report (Olympia, WA: Washington Department of Fish and Wildlife, Fish Science Division).
Westley P. A., Quinn T. P., Dittman A. H. (2013). Rates of straying by hatchery-produced Pacific salmon (Oncorhynchus spp.) and steelhead (Oncorhynchus mykiss) differ among species, life history types, and populations. Can. J. Fish. Aquat. Sci. 70, 735–746. doi: 10.1139/cjfas-2012-0536
Winter B. D., Crain P. (2008). Making the case for ecosystem restoration by dam removal in the Elwha River, Washington. Northwest Sci. 82 (sp1), 13–28. doi: 10.3955/0029-344X-82.S.I.13
Keywords: dam removal, restoration, salmon, monitoring, salmon life-history diversity, hatcheries
Citation: Pess GR, McHenry ML, Denton K, Anderson JH, Liermann MC, Peters RJ, McMillan JR, Brenkman SJ, Bennett TR, Duda JJ and Hanson KM (2024) Initial responses of Chinook salmon (Oncorhynchus tshawytscha) and steelhead (Oncorhynchus mykiss) to removal of two dams on the Elwha River, Washington State, U.S.A.. Front. Ecol. Evol. 12:1241028. doi: 10.3389/fevo.2024.1241028
Received: 15 June 2023; Accepted: 24 June 2024;
Published: 17 July 2024.
Edited by:
Jaime A. Collazo, North Carolina State University, United StatesReviewed by:
Joseph Zydlewski, US Geological Survey, United StatesJames Peterson, United States Department of the Interior, United States
Copyright © 2024 Pess, McHenry, Denton, Anderson, Liermann, Peters, McMillan, Brenkman, Bennett, Duda and Hanson. This is an open-access article distributed under the terms of the Creative Commons Attribution License (CC BY). The use, distribution or reproduction in other forums is permitted, provided the original author(s) and the copyright owner(s) are credited and that the original publication in this journal is cited, in accordance with accepted academic practice. No use, distribution or reproduction is permitted which does not comply with these terms.
*Correspondence: George R. Pess, George.Pess@noaa.gov