- 1Biology Department, University of Massachusetts Boston, Boston, MA, United States
- 2Biology Department, Syracuse University, Syracuse, NY, United States
A long-standing problem in the study of mutualism is to understand the effects of non-mutualistic community members that exploit the benefits of mutualism without offering commodities in exchange (i.e., ‘exploiters’). Mutualisms are continually challenged by exploiters and their persistence may depend on the costliness of exploitation or on adaptations that allow mutualists to avoid the negative effects of exploiters. Coevolution could lead to changes in mutualists and exploiters that allow mutualisms to persist. Although coevolution is considered essential for mutualism persistence and resistance to disturbance, we have yet to obtain direct experimental evidence of the role of coevolution in resistance to exploitation. Additionally, resistance to exploitation via coevolutionary processes might vary with the degree of dependency between mutualistic partners, as facultative mutualisms are thought to be under weaker coevolutionary selection than obligate mutualisms. Here, we conducted an experimental evolution study using a synthetic yeast mutualism to test how coevolution in facultative and obligate mutualisms affects their resistance to exploitation. We found that naïve facultative mutualisms were more likely to breakdown under exploitation than naïve obligate mutualisms. After 15 weeks of coevolution, both facultative and obligate evolved mutualists were more likely to survive exploitation than naïve mutualists when we reassembled mutualist communities. Additionally, coevolved exploiters were more likely to survive with mutualists, whereas naïve exploiters frequently went extinct. These results suggest that coevolution between mutualists and exploiters can lead to mutualism persistence, potentially explaining why exploitation is ubiquitous but rarely associated with mutualism breakdown.
1 Introduction
Mutualistic interactions are ubiquitous and essential for many ecosystem processes. For instance, mutualisms are necessary for primary productivity of terrestrial ecosystems, as many plants depend on pollinators and seed dispersers for their reproduction and form mycorrhizae or rhizobia with belowground microorganisms for nutrient exchange. As mutualisms are prevalent, so is the exploitation of these interactions by organisms that do not provide benefits in return. Species that exploit mutualistic interactions, often termed exploiters or cheaters, are extremely common and have been identified in virtually every type of mutualism (Bronstein, 2001). Exploiters can evolve from previously mutualistic species, be mutualists that exploit their partners under certain conditions, or be members of the community that opportunistically use the resources offered by mutualists. Despite the presence of exploiters, mutualisms can persist and be stable over time (Bronstein, 2001; Frederickson, 2013; Jones et al., 2015). Thus, the persistence of mutualisms with exploitation poses a puzzling evolutionary question: how can the exchange of costly commodities be maintained if organisms that take advantage of this exchange might make the interaction disadvantageous to the mutualists?
Research aimed at understanding this long-standing question has suggested that the potential negative effects of exploitation can be circumvented by ‘controlling’ mechanisms (Bull and Rice, 1991; Herre et al., 1999). For example, plants can plastically adjust the amount of carbon directed to different root locations depending on the rate of receipt of benefits from their mycorrhizae, directing more carbon into the roots that receive the most reward (Kiers et al., 2011). Similarly, yucca plants abscise fruits that are heavily exploited by their pollinating moths (Pellmyr and Huth, 1994). However, controlling mechanisms that rely on partner choice or retaliation have been suggested to be rare (Weyl et al., 2010). Instead, mutualisms might have more general, intrinsic characteristics that facilitate persistence with exploiters.
Part of the challenge of understanding how mutualisms persist with exploitation stems from our perceptions of the costliness of exploitation. Initial work assumed that the negative effects of exploitation could drive mutualism breakdown (e.g., Bull and Rice, 1991; Ferriere et al., 2002). More recently, however, it has been argued that exploitation is perhaps common because it does not pose such a high cost to mutualists (Frederickson, 2013; Jones et al., 2015). For instance, nectar-robbers that take nectar from flowers without pollinating would have negligible costs because nectar is often a cheap commodity to make. Even so, the cost of exploitation will depend not only on the costliness of exploited commodities, but also on how much of the commodity is depleted by the exploiter, how it affects subsequent mutualist interactions, and if exploiters have additional direct negative effects on the mutualists. For example, nectar robbers can have negative effects on exploited plants if they deplete the nectar and subsequently reduce visitation by pollinators (Irwin, 2003). Considering direct negative effects, exploiters that lead to the death of mutualistic partners are arguably the ones associated with greater costs to the mutualism (e.g., Letourneau, 1990; Vidal et al., 2016), but these cases are rare. Given that death of mutualists due to exploitation is uncommon, and that mutualisms persist despite the potential impacts of exploiters on the exchange of commodities, the question remains why exploiters don’t have a more negative impact on mutualisms.
One important, but yet relatively understudied aspect of mutualism is how the coevolutionary process impacts interactions between mutualists and exploiters. Coevolutionary selection of mutualists and exploiters could lead to optimization of the exchange of commodities that would minimize the costs of exploitation. Although this idea remains experimentally untested, theoretical work examining coevolution of unexploited mutualisms suggests that mutualist partners should coevolve to fine-tune the mutualistic investment, reaching an optimal benefit to cost ratio (O’Brien et al., 2021). In exploited mutualisms, there may be limits to increasing investment, as too high of an investment can be detrimental to persistence with exploiters (Ferrière et al., 2007). If exploiters coevolve to exert reduced impacts on the mutualists or if the mutualists themselves reduce investment in the mutualism, the interaction between mutualists and exploiters could potentially be more stable through time (e.g., Ferrière et al., 2007). In situations where exploiters invade a mutualism before increased investment evolves, the coevolutionary trajectory of mutualists and exploiters might change such that mutualism investment remains low and mutualists and exploiters can coexist (‘evolutionary immunization’ hypothesis, Ferrière et al., 2007). Thus, we would predict that mutualists that coevolve with exploiters would be more resistant to exploitation than coevolved mutualists that evolved without exploiters.
To understand the role of coevolution in the persistence of mutualism with exploitation, we also need to consider an important factor that might influence coevolutionary strength: the dependency of the interaction. Mutualisms vary in dependency along a spectrum (Chomicki et al., 2020), where on one end are strictly obligate mutualisms formed by partners that require each other for survival and reproduction. On the other end of the spectrum are facultative mutualists that don’t need each other to achieve fitness but still benefit from the interaction. Because facultative mutualists can exist independently of their partners, it is predicted that there is weaker coevolutionary selection in these mutualisms (Chomicki et al., 2020). Weaker coevolutionary selection could make facultative mutualisms more vulnerable to exploitation and potentially reduce reciprocal evolutionary changes in mutualistic traits as compared to obligate mutualisms. If that is the case, we would expect coevolved facultative mutualists to be less resistant to exploitation than obligate mutualists. An experimental approach testing the likelihood of persistence of obligate and facultative mutualists with exploiters could clarify potential differences in resistance to exploitation for these types of mutualisms.
Here, we use a synthetic yeast mutualism to experimentally investigate how coevolution impacts the persistence of mutualisms with exploiters (Figure 1). In this system, there is an adenine mutualist (Ade mutualist) that produces excess adenine but cannot produce lysine and a lysine mutualist (Lys mutualist) that produces excess lysine but cannot make adenine. The overproduced products are released into the environment, making them available for uptake by other cells. Thus, these mutualists indirectly exchange adenine and lysine in order to survive and reproduce. We used a lysine exploiter (Lys exploiter) that needs to uptake lysine but does not contribute any additional resources. This exploiter competes with the Ade mutualist for lysine and is competitively superior to the Ade mutualist (Vidal et al., 2020). Because this exploiter was derived from the Ade mutualist, with only overproduction and a few genetic markers being different, it mimics exploiters that have evolved from a mutualist lineage. By artificially altering the amount of adenine and lysine that we add to the growth medium, we created comparable mutualisms that vary in dependency and in the presence or absence of exploiters. Using this system, we addressed the following questions: 1) Are coevolved mutualists more resistant to exploitation? 2) Are mutualists that coevolved with exploiters more resistant to exploitation than mutualists that evolved without exploiters? 3) Do exploiters evolve to become less harmful? 4) How does dependency influence the outcome of coevolution and resistance to exploitation?
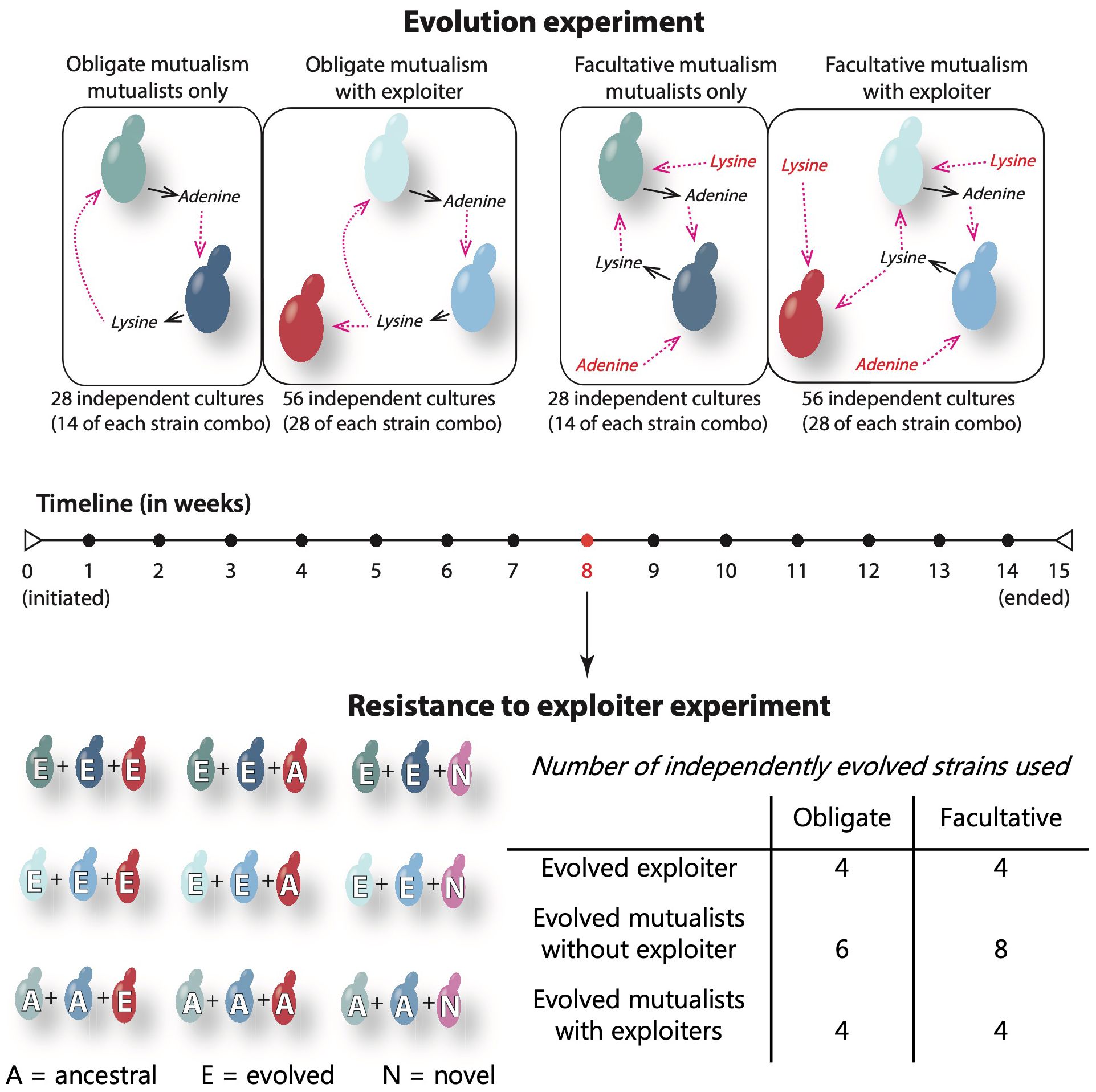
Figure 1 Representation of the yeast study system and experimental design. In this nutritional mutualism, the Ade mutualist (Ade) overproduces adenine but cannot make lysine, while the Lys mutualist (Lys) overproduces lysine but cannot produce adenine. Thus, when together, the yeast mutualists indirectly feed on each other’s resources. The lysine exploiter that evolved with the mutualists does not provide any resource to the community but uptake the lysine available, thus competing with the Ade mutualist. The obligate and facultative mutualisms are directly comparable, as they are similar in the strains involved, differing only in the artificial availability of adenine and lysine (represented in red in the facultative mutualism). Solid black arrows represent the release of the mutualistic resources (adenine and lysine) to the liquid medium and the dashed pink arrows represent the uptake of these resources by the mutualistic partner. The evolution experiment was run for 15 weeks, and the strains used to test the resistance of mutualists to exploiter have been coevolving for 8 weeks. We challenged mutualists that had evolved without exploiters (top row), mutualists that had evolved with exploiters (mid row), and ancestral mutualists (bottom row) with three types of exploiters: evolved, ancestral, and novel (uptake adenine instead of lysine).
2 Methods
2.1 Yeast system and experimental evolution
We engineered yeast strains as described in Vidal et al. (2020) that were originally adapted from the system described by Shou et al. (2007). We used two combinations of strains to create pairwise mutualisms. In one combination, we grew the Ade mutualist strain RY1069 with the Lys mutualist RY1051 with or without the Lys exploiter RY1060. In the other combination, we mixed the Ade mutualist RY1063 with the Lys mutualist RY1039 and grew them with or without the Lys exploiter RY1072 (more information about genotypes in Supplementary Materials). Because the interacting strains are genetically different and reproductively isolated, they function ecologically as species. Strains have highly similar niches; thus, exploiters should have strong negative effects on mutualists. Additionally, similar niches among strains should also drive stronger coevolutionary selection, particularly in obligate mutualisms.
The Lys and Ade mutualists do not produce adenine and lysine, respectively; thus, the amount of adenine and lysine that is artificially added to the medium determines the dependency between mutualists. We used the same approach as described in Vidal and Segraves (2021) for obligate mutualism, where we grew obligate mutualists in synthetic dextrose (SD) medium without lysine or adenine [0.15% (w/v) Difco yeast nitrogen base without amino acids or ammonium sulfate, 0.5% ammonium sulfate, 2% (w/v) dextrose, with supplemental amino acids]. The facultative medium contained a small amount of adenine and lysine (14.8μM adenine and 30.78μM lysine). This amount of adenine and lysine allowed mutualists to double their carrying capacity (yield) when in monoculture as compared to growth in medium lacking these nutrients. This level of growth in the facultative medium was substantially lower than in complete medium, thus it represents a facultative mutualism where mutualists are still fairly dependent on each other. To set up the cultures, we grew overnight cultures of each individual strain in yeast extract peptone dextrose (YPD) [1% (w/v) yeast extract, 2% (w/v) peptone, 2% (w/v) dextrose] and then washed them in sterile water. We started co-cultures at an optical density of 0.1 OD600 where for the mutualists-only communities, we added 0.05 OD600 of each mutualist type, whereas in the cultures with exploiters we added 0.04 OD600 of each mutualist type and 0.02 OD600 of the exploiter strain in a 2ml culture in SD medium in a 48-well deep-well plate. This ratio of mutualists to exploiters follows that observed in natural populations of the mutualism between yuccas and yucca moths where cheaters occur at lower densities than mutualists (K.A.S, unpublished data). We set up 14 independent cultures of mutualists-only communities and 28 independent cultures with all three strains (mutualists and exploiter) per strain combination per mutualist type (obligate or facultative) (Figure 1). These cultures were maintained for 15 weeks (~150-200 generations) on a rotating wheel at 30°C by transferring yeast to fresh medium every 48 hours, returning the cultures to a density of about 0.1 OD600. This density roughly corresponds to 106 cells/mL, a population size sufficiently large to reduce the effects of genetic drift. Each week we plated the cultures to assess persistence and froze stocks in 25% glycerol. We used time as a proxy for generations because we were unable to accurately estimate the generation time of each strain included in the communities, as they were mixtures of 2-3 strains. We acknowledge that the total number of generations for obligate mutualisms is likely lower than those for facultative mutualisms; however, the obligate mutualists are also likely under stronger coevolutionary selection, thus they may have greater potential for fixation of advantageous mutations than facultative mutualists.
At the end of each week of growth, we plated the communities on selective agar media to assess the presence or absence of strains in each community. Each strain had a unique combination of genetic markers that allowed for positive selection such that each community could be plated onto a set of selective plates that would independently isolate all strains present in that community. For obligate mutualisms, communities experiencing community collapse lost the Ade mutualist due to competitive exclusion by the exploiter, and this eventually caused extinction of the community because the strains cannot survive without adenine provided by the Ade mutualist. In comparison, mutualism breakdown in facultative mutualisms was characterized by the competitive exclusion of the Ade mutualist followed by persistence of the Lys mutualist and Lys exploiter at similar population densities as observed when growing single strains in SD medium with minimal adenine and lysine.
2.2 Test of coevolution
To test for coevolution between the mutualistic partners that evolved without exploiters, we set up time-shift assays using different combinations of evolved and ancestral mutualist partners. The evolved mutualists were revived from frozen stocks saved during the evolution experiment explained above. These strains had grown together for 8 weeks in either an obligate or facultative mutualism. We conducted the time-shift assays on revived strains from week 8 of the experiment in order to make comparisons with mutualists that had evolved with exploitation possible (see below). We did this because extinction eliminated either all strains or the exploiters by the end of the experiment in the obligate mutualisms with exploitation.
The time-shift data for obligate mutualisms were published previously (Vidal and Segraves, 2021), and so we used the results from week eight of evolution. From this publication, the time-shift data represent three independent cultures from each obligate mutualism strain combination (Figure 1). To set up the time-shift assays for facultative mutualisms, we followed the same procedure as in Vidal and Segraves (2021), using the mutualist strains that had evolved without exploiters. Briefly, we tested for coevolution by comparing yield in time-shift assays that paired evolved strains with ancestral strains to assess whether each mutualist type evolved (Lys evolved with Ade ancestral: Lysevo + Adeanc, Lys ancestral with Ade evolved: Lysanc + Adeevo). We also compared the yield of cultures containing both ancestral partners (Lysanc + Adeanc) and cultures containing both evolved partners for each time-point tested (Lysevo + Adeevo). We used four independently evolved strain pairs from facultative mutualisms of each strain combination, totaling eight evolutionarily independent mutualisms, that represent a subset of the 28 cultures from the evolution experiment. We used a higher replication for facultative mutualisms because they were more variable in community density than obligate mutualisms. For this time-shift assay, we only used mutualists that had evolved without exploiters because comparing the growth of mutualists that had evolved with exploiters without having the exploiter present would not be representative of the condition they experienced during evolution. Evidence of coevolution is shown if the evolved strains (Lysevo + Adeevo) differ in yield as compared to the ancestral strains, and differences in the time-shift assays (evolved + ancestral pairings) show that the differences observed in the evolved pair is a result of both partners evolving. For instance, if the yield of both evolved strains is different from ancestral yield, and the time shift assays show Lysevo + Adeanc but not Lysanc + Adeevo being different from ancestral partners, then we can assume the difference in yield in the Lysevo + Adeevo pair is mainly due to evolution of Lys, but not Ade. By using a time-shift with the ancestral mutualist as the partner, we can show evidence of change in each evolved mutualist (Gaba and Ebert, 2009). This test of coevolution does not test for genetic correlations but, instead, provides evidence for the evolution of each partner in response to the environment, which is directly linked to the mutualist traits that alter nutrient availability.
We used a second set of time-shift assays to examine potential coevolution among the mutualists and exploiters. Because it was unfeasible to include all possible combinations of evolved and ancestral strains, we only time-shifted the mutualist pairs and exploiters. We compared the yield of cultures containing all the evolved strains together (Lysevo + Adeevo + Exploiterevo) with cultures with all ancestral strains together (Lysanc + Adeanc + Exploiteranc). Additionally, we compared yield of evolved mutualists with the ancestral exploiter (Lysevo + Adeevo + Exploiteranc), and cultures where the exploiter was evolved but was grown with ancestral mutualists (Lysanc + Adeanc + Exploiterevo). Because we rarely observed coexistence of mutualists with exploiters in the evolution experiment, we were able to use only four independent cultures for each dependency treatment. We predicted that, if mutualists and exploiter have coevolved, cultures with all evolved interacting species would have greater yield than any other combination. Additionally, if the mutualists coevolving with the exploiters have evolved to better resist exploitation regardless of shared evolutionary history, then the Lysevo + Adeevo + Exploiteranc cultures would also have higher yield than cultures with ancestral mutualists (Lysanc + Adeanc + Exploiterevo and Lysanc + Adeanc + Exploiteranc).
To statistically analyze the time-shift assay with mutualists that evolved without exploiters, we used a linear model where the response variable was the natural logarithm of the yield of pairs with evolved strains divided by the ancestral pair, and the fixed effect was the pair combinations, Lysevo + Adeanc, Lysanc + Adeevo, or Lysevo + Adeevo. Similarly, to test for differences in the yield of the cultures with mutualists and exploiters, we used linear mixed models with yield as the response variable and strain combination as the random effect, but the fixed effect was the interaction between exploiter type (evolved or ancestral) and mutualist type (evolved with exploiter or ancestral). We analyzed the data separately for facultative and obligate mutualisms. We used the function lmer from package lme4 (Bates et al., 2015) to run the models. We performed post-hoc pairwise comparisons using the function lsmeans from the package emmeans (Lenth, 2022). All statistical tests were performed in R (R Development Core Team, 2021).
2.3 Assays of coevolved mutualists and exploiters
To test the influence of coevolution on exploitation resistance, we set up an assay comparing persistence of coevolved and ancestral mutualists with three types of exploiters (Figure 1). We compared mutualists that had coevolved without exploitation to mutualists that had coevolved with exploiters, and we also compared evolved mutualists to ancestral mutualists. We tested the same cultures as used for the time-shift assays explained above (Figure 1). Each culture was replicated at least twice.
To test whether coevolution of the exploiter with the mutualists influenced the outcome of mutualists’ resistance to exploitation, we started mutualism cultures that contained one of three types of exploiters: 1) evolved exploiter, 2) ancestral exploiter, and 3) novel exploiter. The evolved exploiters were derived from the cultures that contained both mutualists and the exploiter. The ancestral exploiters were the original strains (RY1060 and RY1072) used to set up the original evolution experiment. These ancestral strains had not experienced any prior coevolutionary selection. The novel exploiters were a new genotype that had a different ecological role: they exploited adenine instead of lysine and thus did not compete directly with the Ade mutualists for lysine (strains RY1042 and RY1048, Supplementary Materials).
We set up the experiment by growing each strain individually overnight in YPD medium, washing them with sterile water and setting up the experiment with a 2:2:1 ratio of Ade mutualist: Lys mutualist: exploiter. Obligate mutualisms grew in SD medium without adenine and lysine, whereas facultative mutualisms grew in facultative SD medium with minimal adenine and lysine added (see above). We transferred the cultures to fresh medium every other day and placed them on selective agar plates to assess the presence/absence of each strain. When the Ade mutualist showed no growth on selective plates and the exploiters were still present, we scored it as mutualism breakdown. If the exploiters showed no growth on the agar plates and both mutualists were still present, we scored it as exploiter exclusion. Lastly, if all three strains remained in the cultures throughout the experiment, we scored it as coexistence. We ran the experiment for three weeks for obligate mutualism and four weeks for facultative mutualism, as the timing of exclusion events differed between the two dependency treatments.
We analyzed the outcome of the experiment separately for obligate and facultative mutualisms, but all models were similar for these treatments. We used a Bayesian generalized linear model with persistence (1 = persistence, 0 = breakdown) as the response variable, and the interaction between mutualism history of evolution (with or without exploiter) and exploiter type (evolved, ancestral, and or novel) as the fixed effect. To test if exploiter exclusion varied with the history of mutualist evolution and exploiter type, we used a similar Bayesian generalized linear model but with exploiter survival (1 = survived, 0 = excluded by mutualists) as the response variable. We excluded mutualisms that broke down, as we were interested in examining the frequency of exploiter survival with mutualists. Lastly, we used a linear model to test the time required for mutualists to remove exploiters. We used the number of days to exploiter exclusion as the response variable, and the interaction between exploiter type and mutualist history of evolution as the fixed effects. We included the strain combination as a covariate in all models. We excluded the interaction between fixed effects when it was not significant. For the persistence and exploiter exclusion models, we used a binomial distribution, and the tests were performed using function bayesglm of the package arm (Gelman and Su, 2022). We opted to use Bayesian glms for our binary data because most of the persistence data had treatments where all data were 0 or 1; thus, we had to account for quasi-complete separation (i.e., Hauck-Donner effect) where one group within the model could be completely explained by the predictor variable. We performed post-hoc pairwise comparisons using the function lsmeans from package emmeans (Lenth, 2022).
3 Results
3.1 Outcomes of experimental evolution
After 15 weeks of evolution, we found that all mutualisms without exploitation persisted, whereas mutualisms that experienced exploitation suffered 45-74% breakdown (Figure 2A). Facultative mutualisms with exploiters suffered more breakdown than obligate mutualisms with exploiters. Of the exploited mutualisms that persisted, facultative mutualisms showed similar rates of persistence of mutualists with exploiters and persistence via exclusion of exploiters. In comparison, obligate mutualisms persisted only in mutualisms where the exploiter was excluded. The timing of outcomes in exploited mutualisms also varied with mutualism dependency (Figures 2B, C). For instance, most cases of exploiter exclusion occurred between 6 and 10 weeks of evolution in obligate mutualisms, but generally occurred after 11 weeks of evolution in facultative mutualisms (Figure 2C).
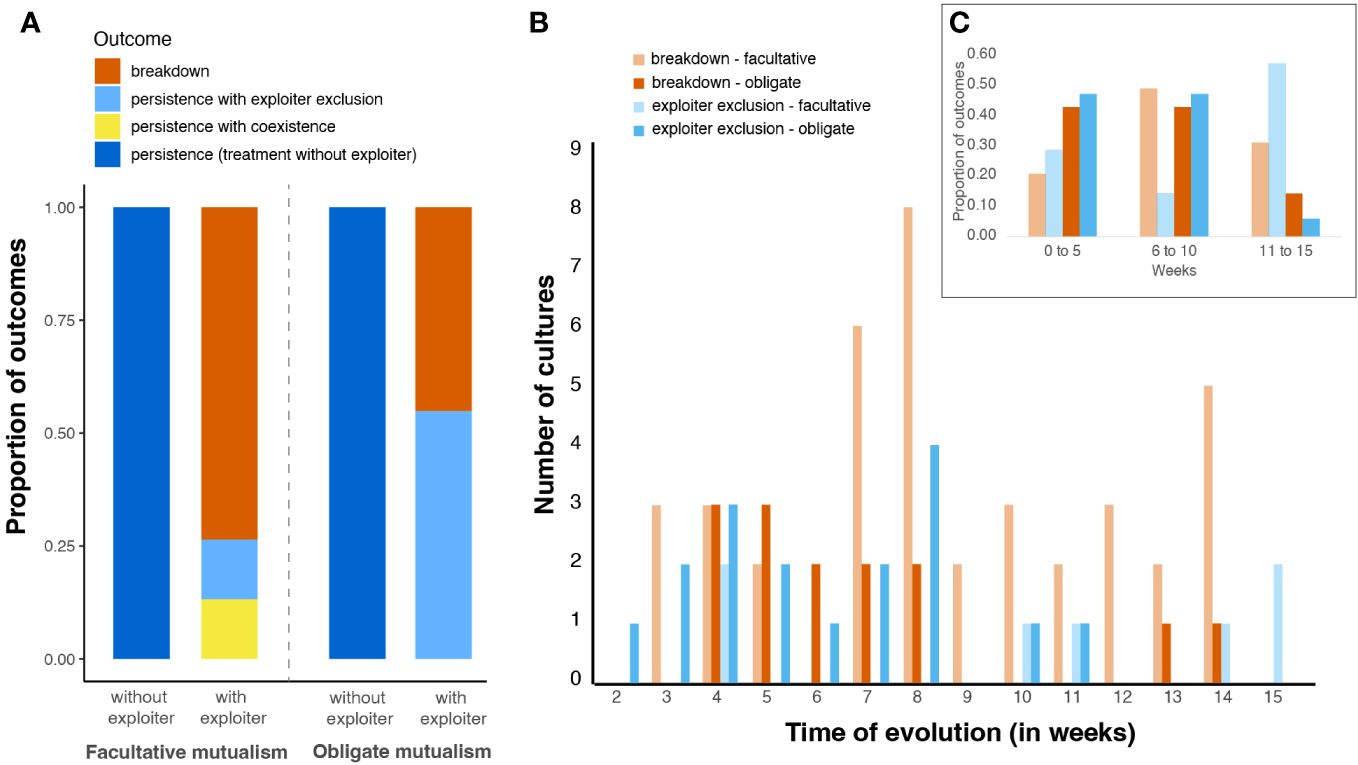
Figure 2 (A) Outcome of evolution experiment with and without exploiters for obligate and facultative mutualisms. (B) Number of independent cultures that experienced breakdown or exploiter exclusion per week of evolution experiment. (C) Proportion of outcome of independent cultures that experienced breakdown or exploiter exclusion per week of evolution experiment, color legend is the same as in (B).
3.2 Tests for coevolution
We used time-shift assays to test if the mutualists growing without exploiters had coevolved. We found that the time-shifted cultures containing obligate mutualists where the ancestral Ade mutualists were paired with evolved Lys mutualists (Lysevo + Adeanc) had lower yield than the ancestral mutualist pairs (Figure 3A, t=-4.16, P=0.0008). Additionally, evolved Ade with ancestral Lys (Lysanc + Adeevo) and both evolved mutualists (Lysevo + Adeevo) had higher yield than ancestral pairs (t=6.22, P<0.0001 and t=3.56, P=0.003, respectively). In contrast, none of the time-shifted and evolved pairs differed from the ancestral pair in facultative mutualisms (F3,25=0.01, P=0.99).
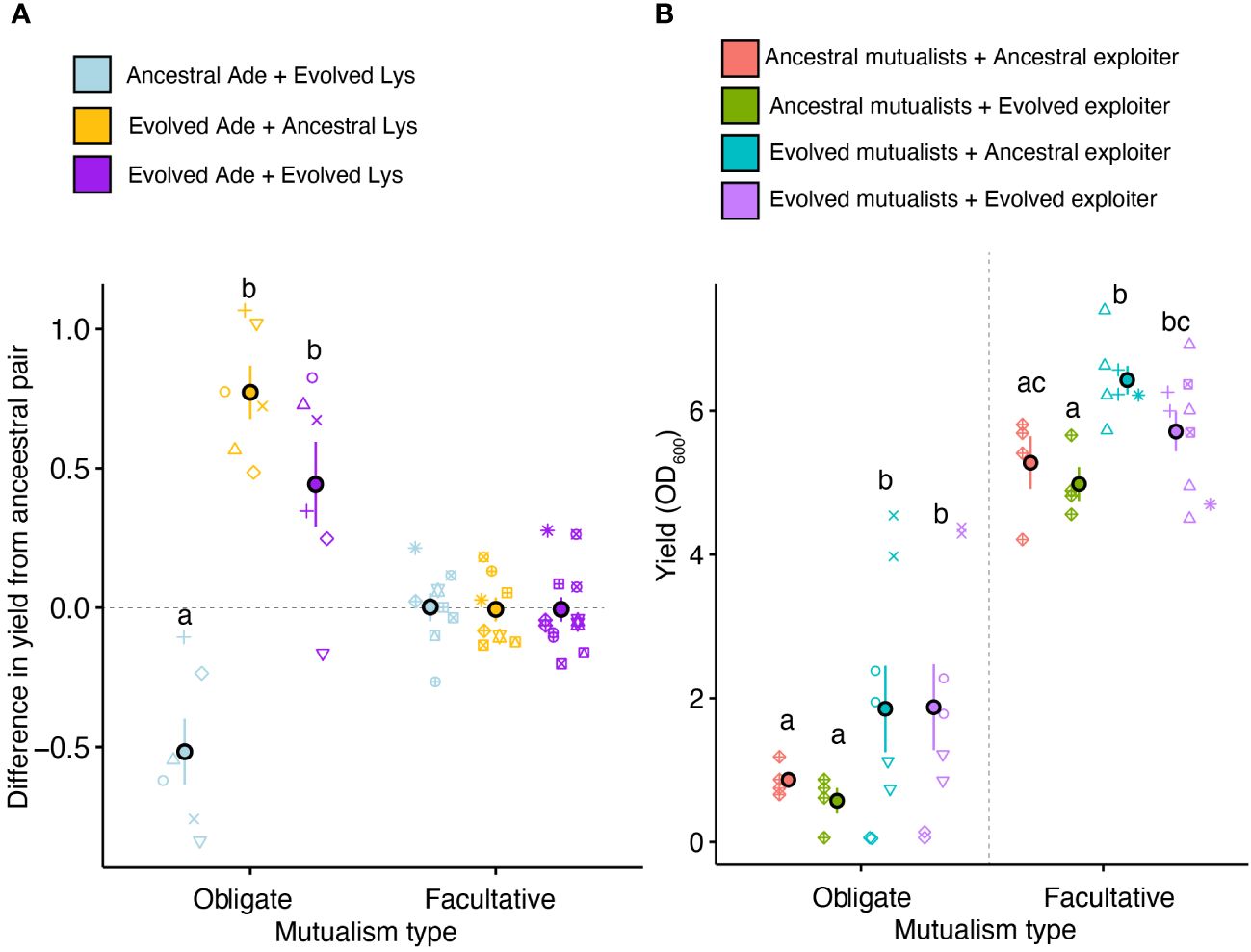
Figure 3 (A) Outcome of time-shift assays with mutualists that evolved without exploiters. Horizontal dashed grey line is crossing zero and represents differences from ancestral pairs. (B) Yield of cultures with mutualists and exploiters. Letters represent pairwise comparisons from Tukey’s HSD posthoc comparisons within each mutualism type. Error bars represent standard error. Icons represent independently evolved communities from which the evolved strains were sampled.
We also assessed if the cultures containing exploiters had coevolved by comparing the yield of the cultures with evolved mutualists and either evolved or ancestral exploiter to cultures where the ancestral mutualists were grown with ancestral exploiters or evolved exploiters. We found that cultures with ancestral mutualists had lower yield than cultures with evolved mutualists, for both obligate (F1,19=6.42, P=0.020) and facultative (F1,21=10.09, P=0.004) mutualisms (Figure 3B). There was no interaction between the evolutionary history of mutualists (evolved or ancestral) and the type of exploiter (evolved or ancestral) (obligate: F1,19=0.12, P=0.731; facultative: F1,20=0.5, P=0.488). For obligate mutualisms, evolved mutualists had greater yield with both ancestral and evolved exploiters as compared to ancestral mutualists (Tukey’s pairwise comparisons <0.01). In contrast, facultative mutualisms varied with exploiter evolutionary history (F1,21=4.31, P=0.05). The combination with Lysevo + Adeevo + Exploiteranc had greater yield than Lysanc + Adeanc + Exploiteranc, and Lysevo + Adeevo + Exploiterevo had greater yield than Lysanc + Adeanc + Exploiterevo.
3.3 Resistance to exploitation by coevolved mutualists
After the mutualists had coevolved, we challenged them with different exploiters and evaluated the persistence of mutualism. For these assays, we used three types of exploiters: exploiters that had coevolved with mutualists, ancestral exploiters, and novel exploiters. For both obligate and facultative mutualisms, we tested mutualists that evolved with exploiters and mutualists that evolved without exploiters, as well as naïve mutualists (i.e., ancestral strains).
We found no significant interaction between mutualist evolutionary history and exploiter type on explaining mutualism persistence (obligate: X2=4.76, df=4, P=0.313, facultative: X2=1.58, df=4, P=0.81); however, the type of exploiter influenced persistence in both mutualism types (obligate: X2=14.91, df=2, P=0.0006; facultative: X2=15.32, df=2, P=0.0004). Obligate mutualisms experienced more breakdown when mutualists were paired with evolved exploiters as compared to ancestral (z=2.84, P=0.013) or novel (z=-2.64, P=0.02) exploiters. Similarly, facultative mutualisms showed marginal differences in breakdown between evolved and ancestral (z=2.21, P=0.069) or novel (z=-2.20, P=0.057) exploiters. Additionally, the mutualists’ history of evolution influenced persistence of obligate mutualisms (X2=7.21, df=2, P=0.03), but not facultative mutualisms (X2=3.21, df=2, P=0.191). These changes in persistence in obligate mutualisms were likely due to the high breakdown that occurred when ancestral mutualists were paired with evolved exploiters (100% breakdown) (Figures 4A, D); however, pairwise comparisons yielded no significant differences.
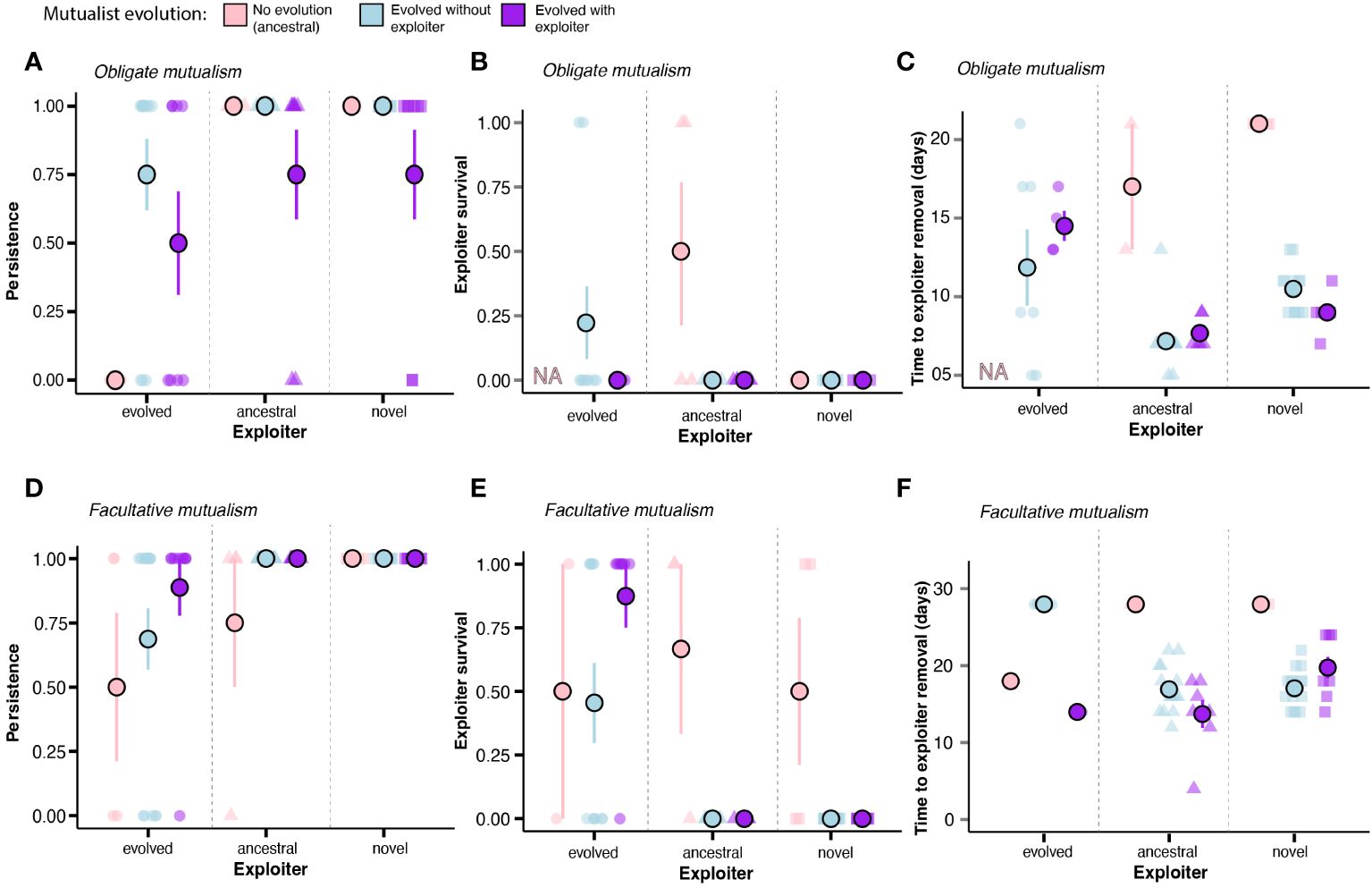
Figure 4 Resistance to exploitation by coevolved mutualists. (A) Persistence of obligate mutualisms challenged with each exploiter type. (B) Exploiter survival in obligate mutualism (evolved exploiter with ancestral mutualists not included because none of these mutualisms persisted). (C) Time took for mutualists to exclude exploiters in obligate mutualisms (evolved exploiter with ancestral mutualists not included because none of these mutualisms persisted). (D) Persistence of facultative mutualisms challenged with each exploiter type. (E) Exploiter survival in facultative mutualisms. (F) Time took for mutualists to exclude exploiters in facultative mutualisms. Error bars represent standard error.
In the mutualisms that persisted, we found that the type of exploiter influenced the likelihood of exploiter survival in both obligate and facultative mutualisms (obligate: X2 = 6.95, df=2, P=0.031; facultative: X2 = 41.85, df=2, P<0.0001; Figures 4B, E). In facultative mutualisms, evolved exploiters were more likely to survive than either ancestral (z=-3.27, P=0.003) or novel (z=3.2, P=0.004) exploiters. However, when we compared among the different exploiters in obligate mutualisms, there were no significant pairwise differences in survival of the exploiter in these mutualisms. Additionally, the history of mutualist evolution influenced the likelihood of exploiter survival in facultative (facultative: X2=8.20, df=2, P=0.017) but not obligate mutualism (X2=3.81, df=2, P=0.149; but note that all obligate mutualisms with ancestral mutualists paired with evolved exploiters brokedown and thus were not included in the analysis). In facultative mutualisms, exploiters were more likely to survive with ancestral mutualists than with mutualists that evolved without exploiters (z=2.66, P=0.021), whereas there was no difference between ancestral mutualists and mutualists that evolved with exploiters (z=1.55, P=0.266) or between mutualists that evolved with exploiters and mutualists that evolved without exploiters (z=-0.98, P=0.590). We again found no significant interaction between exploiter type and mutualist history of evolution (obligate: X2=0.664, df=4, P=0.956, facultative: X23.78, df=4, P=0.437).
Overall, the patterns of breakdown (X2=2.85, df=1, P=0.091) and exploiter survival (X2=0.55, df=1, P=0.457) were similar when comparing obligate and facultative mutualisms, even though mutualism persistence was marginally lower in obligate mutualisms (z=-1.66, P=0.097). There was, however, a difference in the time required for facultative and obligate mutualists to remove exploiters (F1,34.43=38.26, P<0.0001). Facultative mutualists took between 14 and 28 days to remove exploiters, regardless of exploiter type, whereas obligate evolved mutualists removed ancestral and novel exploiters in less than 10 days (Figures 4C, F). Additionally, there was a significant interaction between exploiter type and history of mutualist evolution for facultative mutualisms (F2,50.116=9.97, P=0.0002), but not obligate mutualisms (F3,46.34=1.18, P=0.328). For obligate mutualisms, the type of exploiter (F2,46.15=15.26, P<0.0001) and the mutualists’ evolutionary history (F2,46.032=32.69, P<0.0001) influenced the time to exclusion of exploiters. Ancestral mutualists took longer to exclude ancestral and novel exploiters than evolved mutualists, and evolved mutualists (evolving with or without exploiters) were the fastest to remove ancestral and novel exploiters. For facultative mutualisms, mutualists that evolved without exploiters took longer to exclude evolved exploiters, and ancestral mutualists also took longer to remove ancestral and novel exploiters. There was only one culture of ancestral facultative mutualists that survived exploitation by the evolved exploiter and none for obligate mutualisms, and so these were not included in the analysis.
4 Discussion
Exploitation of mutualisms is ubiquitous. As a result, understanding how mutualisms persist with exploiters has been a major question in the study of species interactions. Theoretical and empirical studies have shown that sanctions and other regulatory mechanisms can help to keep exploiters in check (e.g., Pellmyr and Huth, 1994; Kiers et al., 2011), and others have suggested that exploitation may not be as costly as initially expected (Frederickson, 2013; Jones et al., 2015). Yet, the question remains if there is a universal explanation that would apply to most, if not all mutualisms. Here we propose that coevolutionary selection enhances mutualist persistence with exploitation, which would operate in most mutualisms. We provide experimental evidence for the role of coevolution in promoting resistance to exploitation and suggest that mutualists and exploiters can coevolve in ways that facilitate mutualism persistence.
We first asked if coevolution changed the mutualists in a way that made them more resistant to exploitation. The results showed that coevolved mutualists, regardless of whether they evolved with exploiters or not, were indeed more resistant to exploitation than ancestral mutualists. Coevolved mutualists were generally better able to persist when challenged with exploiters (Figures 4A, D) and were more likely to outcompete exploiters (Figures 4B, E). When coevolved mutualists were challenged with naïve ancestral and novel exploiters, they were able to drive these exploiters to extinction every time. These results suggest that coevolution buffers mutualisms from breakdown caused by exploitation. This is also consistent with the evolutionary immunization hypothesis set forth by Ferrière and collaborators (Ferrière et al., 2007) where coevolution between mutualists could lead to resistance of coevolved mutualists to future invasion by new exploiters. This idea is supported by our results as both obligate and facultative coevolved mutualists were more resistant to exploiters that did not share an evolutionary history with them. Exclusion of ancestral and novel exploiters occurred in 100% of the mutualisms in the exploiter resistance experiment, compared to 12.5% in the facultative mutualism and 55% in the obligate mutualism during the 15-week experiment.
To further explore the evolutionary immunization hypothesis, we asked if mutualists that evolved with exploiters were more resistant to exploitation than mutualists that evolved without exploitation. Even though we found resistance to invasion by ancestral and novel exploiters, partially supporting the evolutionary immunization hypothesis, our results suggest that coevolution of mutualists without exploiters was sufficient to ameliorate the negative effects of exploitation. Thus, coevolution resulted in increased resistance to exploitation whether or not an exploiter was present during the mutualists’ evolution. These changes in resistance to exploiters could be facilitated if the changes in mutualistic traits result in a decrease in the costs or an increase in the benefits derived from the mutualism, both of which are traits that could evolve in response to the mutualism with or without an exploiter. Indeed, our previous experiments in this yeast system have shown that obligate Ade mutualists can reduce production of adenine, which represents one of the costs associated with this mutualism (Vidal and Segraves, 2021). A reduction in investment in the interaction might reduce the asymmetry of competition between exploiters and mutualists (e.g., Ferrière et al., 2007) or may even give the Ade mutualist an advantage, as evolved mutualists frequently excluded ancestral and novel exploiters.
We were also curious if the exploiters were coevolving alongside the mutualist partners and if so, whether they evolved to be less harmful, thus explaining their increased persistence in mutualisms. When we compared the yield of cultures of mutualists with exploiters, we found consistent evidence that evolved mutualists grew more with exploiters than ancestral mutualists, providing evidence that the three interacting species were coevolving together. The coevolutionary change among all three species that led to increased community productivity could have come in the form of changes in many traits including growth rate, resource use efficiency, or reductions in costs, and these should be explored in the future. Changes in the traits related to resource dynamics could also be caused by culturing conditions, and may not be related to the mutualism; for example, the yeast strains could have evolved to grow better in an environment with fluctuating nutrients caused by nutrient consumption and scheduled transfers to new media. However, we have previously shown that obligate mutualists evolved changes in mutualism-related traits not associated with the media conditions (e.g., overproduction, Vidal and Segraves, 2021), indicating that these yeast mutualists can coevolve. Although the exploiters were evolving alongside the mutualists, contrary to our expectations, they actually became more harmful. Evolved exploiters became even stronger competitors as indicated by the quick extinction of ancestral mutualists via competitive exclusion by the evolved exploiters, and overall greater breakdown of mutualisms caused by evolved exploiters. Even though the exploiter evolved to be more harmful to ancestral mutualists, the evolved exploiters had a lower impact on the evolved mutualists. This outcome is most likely caused by evolutionary change in the mutualists that leveled the competitive landscape of mutualists and exploiters.
Lastly, we asked if the strength of the dependency between mutualist partners influenced the outcome of coevolution and resistance to exploitation. There was stronger evidence of coevolution in obligate than in facultative mutualisms, but all evolved mutualists showed similar resistance to exploitation. In our time-shift assays with only obligate mutualists, we found that evolved Lys mutualists paired with ancestral Ade (Lysevo + Adeanc) had lower yield than ancestral mutualists (Lysanc + Adeanc), whereas evolved Ade paired with ancestral Lys (Lysanc + Adeevo) had higher yield. In obligate mutualisms we observed higher yield when the two evolved mutualists were together (Lysevo + Adeevo) as compared to ancestral pairs, which, together with the time-shift results, suggests that both mutualists were changing to have complementary traits, such that they grew better together than they did with other partners. This pattern was not apparent in the facultative mutualists evolving without exploiters, as the yield of evolved pairs and time-shifted pairs were not different from ancestral pairs. Facultative mutualisms are likely under weaker coevolutionary selection than obligate mutualisms, which could result in slower and more variable evolutionary changes. Thus, our results do not necessarily indicate an absence of coevolution in facultative mutualisms, but they highlight that the evolutionary timing and variation and trait evolution of mutualists should be investigated to directly test coevolution in facultative mutualisms.
Although we predicted that coevolution might lead to changes in persistence with exploiters in mutualisms that differ in dependency, this prediction was not upheld. When we compared resistance of coevolved mutualists to novel exploiters, both facultative and obligate mutualists were similarly resistant. In contrast, facultative mutualisms had clear evidence of increased survival of evolved exploiters as compared to the ancestral and novel exploiters, whereas obligate, coevolved mutualists excluded exploiters in almost all the trials (Figures 4B, E). This result, together with the lower yield of evolved facultative mutualists with evolved exploiters (Figure 3B), suggests one of two possibilities. The first possibility is that the evolved exploiter from facultative mutualisms may have become a stronger competitor, thus increasing the chances that exploiters would persist in facultative mutualisms. Alternatively, the evolved facultative mutualists may not have evolved to be as resistant to exploitation as the obligate mutualists. In either case, it would seem that facultative mutualisms offered more opportunities for coexistence of mutualists and exploiters.
The greatest distinction between facultative and obligate mutualisms was in the time to remove exploiters. These differences could potentially be explained by obligate mutualists being under stronger coevolutionary selection than facultative mutualists, as the former completely depend on their partner for fitness (Raimundo et al., 2014; Chomicki et al., 2020). With stronger coevolutionary selection, we would expect to see greater changes in mutualistic traits in obligate mutualisms, and as a result of these changes, coevolved obligate mutualists would outcompete the exploiters faster and more frequently than the facultative mutualists. Indeed, the results showed that obligate coevolved mutualists were up to three times faster in their ability to remove exploiters than facultative mutualists. Even so, these results showed only modest differences between dependency treatments. One potential explanation of this outcome is that our facultative mutualisms were still relatively dependent on their partners for fitness gains, so they too may have experienced fairly strong selection. Facultative mutualisms with lower dependency than that tested here might show weaker coevolution and low resistance to exploiters. However, weaker dependency can also result in weaker competition for the mutualistic resource, potentially facilitating coexistence between exploiters and mutualists, even without coevolution.
The results also indicated that there are inherent differences in breakdown and exploiter exclusion between facultative and obligate mutualisms. We found that as mutualisms evolved during the 15-week experiment, facultative mutualisms were more prone to breakdown by the exploiter competitively excluding the Ade mutualist. Interestingly, in most of these cases, breakdown in the facultative mutualisms did not lead to co-extinction of both mutualists, a stark contrast to breakdown in obligate mutualisms where all members of the community went extinct. While facultative mutualisms were more likely to breakdown, they were also more likely to coexist with the exploiter than obligate mutualists. Johnson and Bronstein (2019) suggest that coexistence is not possible when the exploiter and mutualist compete solely for the shared mutualistic resource unless the competitors are also competing for another external resource. In our system, the Lys exploiter and Ade mutualist are strongly limited by lysine in obligate mutualisms. In contrast, the small amount of artificially available mutualistic resources in facultative mutualisms supports higher populations of mutualists and exploiters than in obligate mutualisms, suggesting that competition for other resources (e.g., dextrose) might come into play. If the conditions present in facultative mutualisms leads to stronger competition due to greater population density, this suggests a possible avenue for coexistence. In instances in which mutualists didn’t evolve to be better competitors, the result was competitive exclusion of the mutualist. Whereas in other cases, the mutualists may have evolved quickly enough to persist with the exploiter. These results indicate that mutualism dependency may create a complex landscape of competition that could have critical impacts on the persistence of mutualism under exploitation.
In conclusion, our study shows that coevolution leads to greater resistance to exploitation, and that mutualists and exploiters are potentially coevolving. These coevolutionary outcomes demonstrate an avenue for persistence of mutualists with exploiters that is likely mediated by the strength of competition and the resources for which mutualists and exploiters are competing. Furthermore, the consistent patterns observed between facultative and obligate mutualisms suggest that coevolution is important in mutualisms that vary in dependency even though the coevolutionary process itself likely differs between obligate and facultative mutualisms (Raimundo et al., 2014; Chomicki et al., 2020). Given that exploiters are present in virtually every mutualism and mutualisms persist over millions of years despite exploitation, coevolution among these interacting species could lead to persistence of mutualists with exploiters.
Data availability statement
The original contributions presented in the study are included in Supplementary Table 2. Further inquiries can be directed to the corresponding author/s.
Author contributions
KAS modified the yeast strains. MCV, RA, and KAS designed the experiments and synthesized reagents. MCV, RA, and KAS conducted the experiments and collected data. MCV analyzed the data and wrote the first draft. MCV, RA, and KAS edited the manuscript. All authors contributed to the article and approved the submitted version.
Funding
The author(s) declare financial support was received for the research, authorship, and/or publication of this article. NSF-DEB 1655544, 2137555, and 2137554.
Acknowledgments
We thank M. Comerford for help with the experiment, and M. Comerford and D. Althoff for insightful discussions. Funding was provided by NSF-DEB 1655544, 2137555, and 2137554. This publication is based on work conducted while KAS served at the National Science Foundation.
Conflict of interest
The authors declare that the research was conducted in the absence of any commercial or financial relationships that could be construed as a potential conflict of interest.
Publisher’s note
All claims expressed in this article are solely those of the authors and do not necessarily represent those of their affiliated organizations, or those of the publisher, the editors and the reviewers. Any product that may be evaluated in this article, or claim that may be made by its manufacturer, is not guaranteed or endorsed by the publisher.
Supplementary material
The Supplementary Material for this article can be found online at: https://www.frontiersin.org/articles/10.3389/fevo.2024.1113963/full#supplementary-material
References
Bates D., Maechler M., Bolker B., Walker S. (2015). Fitting Linear Mixed-Effects Models Using lme4. J. Stat. Software. 67 (1), 1–48. doi: 10.18637/jss.v067.i01
Bronstein J. L. (2001). The exploitation of mutualisms. Ecol. Lett. 4, 277–287. doi: 10.1046/j.1461-0248.2001.00218.x
Bull J. J., Rice W. R. (1991). Distinguishing mechanisms for the evolution of cooperation. J. Theoret. Biol. 149, 63–74. doi: 10.1016/S0022-5193(05)80072-4
Chomicki G., Kiers E. T., Renner S. S. (2020). The evolution of mutualistic dependence. Ann. Rev. Ecol., Evol., and Syst. 51, 409–432. doi: 10.1146/annurev-ecolsys-110218024629
Ferriere R., Bronstein J. L., Rinaldi S., Law R., Gauduchon M. (2002). Cheating and the evolutionary stability of mutualisms. Proc. R. Soc. London. Ser. B: Biol. Sci. 269, 773–780. doi: 10.1098/rspb.2001.1900
Ferrière R., Gauduchon M., Bronstein J. L. (2007). Evolution and persistence of obligate mutualists and exploiters: competition for partners and evolutionary immunization. Ecol. Lett. 10, 115–126. doi: 10.1111/j.1461-0248.2006.01008.x
Frederickson M. E. (2013). Rethinking mutualism stability: cheaters and the evolution of sanctions. Q. Rev. Biol. 88, 269–295. doi: 10.1086/673757
Gaba S., Ebert D. (2009). Time-shift experiments as a tool to study antagonistic coevolution. Trends Ecol. Evol. 24, 226–232. doi: 10.1016/j.tree.2008.11.005
Gelman A., Su Y. S. (2022). arm: Data Analysis Using Regression and Multilevel/Hierarchical Models. R package version 1.13-1. Available at: https://CRAN.R-project.org/package=arm.
Herre E. A., Knowlton N., Mueller U. G., Rehner S. A. (1999). The evolution of mutualisms: exploring the paths be-tween conflict and cooperation. Trends Ecol. Evol. 14, 49–53. doi: 10.1016/S0169-5347(98)01529-8
Irwin R. E. (2003). Impact of nectar robbing on estimates of pollen flow: conceptual predictions and empirical outcomes. Ecology 84, 485–495. doi: 10.1890/0012-9658(2003)084[0485:IONROE]2.0.CO;2
Johnson C. A., Bronstein J. L. (2019). Coexistence and competitive exclusion in mutualism. Ecology 100, e02708. doi: 10.1002/ecy.2708
Jones E. I., Afkhami M. E., Akçay E., Bronstein J. L., Bshary R., Frederickson M. E., et al. (2015). Cheaters must prosper: reconciling theoretical and empirical perspectives on cheating in mutualism. Ecol. Lett. 18, 1270–1284. doi: 10.1111/ele.12507
Kiers E. T., Duhamel M., Beesetty Y., Mensah J. A., Franken O., Verbruggen E., et al. (2011). Reciprocal rewards stabilize cooperation in the mycorrhizal symbiosis. science 333, 880–882. doi: 10.1126/science.1208473
1Lenth R. V. (2022). emmeans: Estimated Marginal Means, aka Least-Squares Means. R package version 1.7.5. Available at: https://CRAN.R-project.org/package=emmeans.
Letourneau D. K. (1990). Code of ant-plant mutualism broken by parasite. Science 248, 215–217. doi: 10.1126/science.248.4952.215
O’Brien A. M., Jack C. N., Friesen M. L., Frederickson M. E. (2021). Whose trait is it anyways? Coevolution of joint phenotypes and genetic architecture in mutualisms. Proc. R. Soc. B 288, 20202483. doi: 10.1098/rspb.2020.2483
Pellmyr O., Huth C. J. (1994). Evolutionary stability of mutualism between yuccas and yucca moths. Nature 372, 257–260. doi: 10.1038/372257a0
Raimundo R. L., Gibert J. P., Hembry D. H., Guimaraes P. R. Jr. (2014). Conflicting selection in the course of adaptive diversification: the interplay between mutualism and intraspecific competition. Am. Nat. 183, 363–375. doi: 10.1086/674965
R Development Core Team (2021). R: A Language and Environment for Statistical Computing (Vienna, Austria: R Foundation for Statistical Computing).
Shou W., Ram S., Vilar J. M. (2007). Synthetic cooperation in engineered yeast populations. Proc. Natl. Acad. Sci. 104, 1877–1882. doi: 10.1073/pnas.0610575104
Vidal M. C., Segraves K. A. (2021). Coevolved mutualists experience fluctuating costs and benefits over time. Evolution 75, 219–230. doi: 10.1111/evo.14155
Vidal M. C., Sendoya S. F., Oliveira P. S. (2016). Mutualism exploitation: predatory drosophilid larvae sugar-trap ants and jeopardize facultative ant-plant mutualism. Ecology 97, 1650–1657. doi: 10.1002/ecy.1441
Vidal M. C., Wang S. P., Rivers D. M., Althoff D. M., Segraves K. A. (2020). Species richness and redundancy promote persistence of exploited mutualisms in yeast. Science 370, 346–350. doi: 10.1126/science.abb6703
Keywords: facultative mutualism, obligate mutualism, yeast, experimental evolution, mutualism evolution, cheater
Citation: Vidal MC, Agarwal R and Segraves KA (2024) Coevolution and dependency influence resistance of mutualists to exploitation. Front. Ecol. Evol. 12:1113963. doi: 10.3389/fevo.2024.1113963
Received: 01 December 2022; Accepted: 28 February 2024;
Published: 14 March 2024.
Edited by:
Michael Charleston, University of Tasmania, AustraliaReviewed by:
Kristina Linnea Hillesland, University of Washington Bothell, United StatesKevin R. Theis, Wayne State University, United States
Copyright © 2024 Vidal, Agarwal and Segraves. This is an open-access article distributed under the terms of the Creative Commons Attribution License (CC BY). The use, distribution or reproduction in other forums is permitted, provided the original author(s) and the copyright owner(s) are credited and that the original publication in this journal is cited, in accordance with accepted academic practice. No use, distribution or reproduction is permitted which does not comply with these terms.
*Correspondence: Mayra C. Vidal, bWF5cmEuY2Fkb3JpbnZpZGFsQHVtYi5lZHU=