- 1Department of Archaeology, University of Aberdeen, Aberdeen, Scotland, United Kingdom
- 2Institute of Marine Sciences, UC Santa Cruz, Santa Cruz, CA, United States
- 3National Marine Fisheries Service, Southwest Fisheries Science Center, Santa Cruz, CA, United States
- 4LDPL – Laboratoire Départemental de Préhistoire du Lazaret, Nice, France
- 5CEPAM UMR 7264 CNRS, Université Côte d’Azur, Nice, France
- 6Archéozoologie, Archéobotanique: Sociétés, Pratiques et Environnements, AASPE UMR 7209, CNRS/Muséum national d’Histoire naturelle, Paris, France
Zooarchaeological analysis is a useful means of exploring faunal palaeoecology, paleoclimate and past human behaviours. The Middle Pleistocene archaeological site Lazaret Cave, located in modern-day Nice, France, features a vast assemblage of faunal remains pertinent to the understanding of early Neanderthal subsistence behaviours as well as red deer (Cervus elaphus) ecology during MIS 6. This pilot study examines materials from archaeological layer UA25, a short-term occupation layer at Lazaret dating to ~150,000 years ka, which has revealed 28 early Neanderthal remains as well as thousands of faunal bones, of which red deer and ibex (Capra ibex) are most abundant. Molars from three red deer mandibles and a single ibex were analysed for strontium (87Sr/86Sr) isotopic analysis using laser ablation mass spectrometry to determine animal movements during tooth formation, combined with intra-tooth oxygen (δ18O) isotope analysis to determine seasonality. The isotope data was modelled within a local 87Sr/86Sr isoscape and computational spatial assignment was undertaken to reconstruct potential summer and winter ranges of red deer. Results from this pilot study show seasonal mobility within 20 km of Lazaret, identifying two possible summer and winter ranges for the red deer excavated from UA25. Both possible summer ranges are located at higher elevations further from Lazaret while winter ranges have been assigned to lower elevations closer to the coastline and closer to Lazaret. The ibex shows no 87Sr/86Sr variation throughout the first, second and third molar and the spatial assignment indicates it lived proximal to the site during the period of tooth formation. In addition to providing the first evidence of red deer spatial ecology in southern France during MIS 6, we also infer from the faunal isotope data that hominins at Lazaret Cave were likely hunting red deer in autumn and winter when they were closer to the cave site, while hunting in summer would have required up to 20 km of travel.
1. Introduction
The study of faunal remains from archaeological sites (zooarchaeology) is a vital part of modern archaeological investigation, illuminating past human subsistence habits and other behaviours. These assemblages are also becoming increasingly important for the study of faunal palaeoecology and the reconstruction of palaeoclimatic conditions. In comparison to palaeontological assemblages, faunal remains at archaeological sites are often directly associated with past human activites, allowing insight into the natural world as experienced by past hunter-gatherer groups. Isotopic analyses have proven to be a particularly effective means of exploring past climates and ecological behaviours using zooarchaeological remains, and isotope zooarchaeology is an area of research with increasing interdispcilinary relevance, from modern conservation ecology to palaeotemperature reconstruction (Jones and Britton, 2019; Pederzani et al., 2021a). Isotope zooarchaeological studies have now been conducted on numerous Late Pleistocene assemblages from site-based studies (Bocherens et al., 2016; Pilaar Birch et al., 2016; Wooller et al., 2021) to large-scale explorations of faunal isotope palaeoecology (Bocherens, 2015; Price et al., 2017). Applications from the penultimate glacial period (Marine Isotope Stage 6) are rare but have the potential to illuminate the palaeoecology of extinct and extant species in the deeper past and in a period of profound climatic change.
The penultimate glacial period, Marine Isotope Stage 6 (MIS 6), spans the period between ca. 185 and 135 ka, and is referred to as the Last Saalian glaciation in Europe (Margari et al., 2010; Roucoux et al., 2011). While there have been numerous studies to understand climate fluctuations in MIS 6 (e.g., Ponel, 1995; Margari et al., 2010; Elderfield et al., 2012; Wainer et al., 2013) there are relatively few studies which have attempted to understand contemporary faunal palaeoecology and spatial behaviours at the time. Species such as red deer, Cervus elaphus, were abundant in Europe throughout the Middle and Late Pleistocene, and – along with other ungulate species – were an important subsistence species for hominin groups, particularly in more southernly regions such as northern Spain and the south of France, and particularly at Lazaret Cave, which is the subject of this study (e.g., Valensi, 2000; Valensi and Psathi, 2004; Valensi et al., 2013), although they were found as far north as Britain during warmer interstadial events (Lister, 1984). Red deer are typically considered a forest-woodland-ecotone species, adapted to warmer, more productive climates in comparison to open tundra-steppe landscapes which were more likely to be occupied by Wapiti (Cervus canadensis) in Late Pleistocene Europe (Croitor and Obada, 2018; Croitor, 2020). However, today, red deer can be ecologically plastic when under pressure and can thrive in open-highlands and mountainsides, for example in the highlands of Scotland, as long as they are protected from wind and deep snow, which prevents the species from occupying more northernly latitudes like reindeer, Rangifer tarandus (Clutton-Brock et al., 1982; Geist, 1998; Steele, 2002; Croitor, 2020). Despite their prevalence, Middle Pleistocene red deer ecology – particularly their spatial ecology – is poorly characterised, and it is unknown if they undertook seasonal ranging behaviours.
Today, European red deer populations are known for partial migration, meaning some populations or individuals will seasonally migrate while other herds and individuals will remain in one location all year, in response to local conditions, but do not undertake long-distance movements (Langvatn and Albon, 1986; Albon and Langvatn, 1992; Mysterud et al., 2011, 2016; Qviller et al., 2013; Pilaar Birch et al., 2016). Seasonal movement in red deer is predominantly influenced by access to food sources and avoiding locations with high snow fall resulting in seasonal altitudinal migration (moving to lower altitudes in winter and higher altitudes in summer) and migrations between coastal and inland regions (moving to coastal regions in the winter and inland in the summer; Langvatn and Albon, 1986; Albon and Langvatn, 1992; Mysterud et al., 2011; Qviller et al., 2013). While red deer currently do not seem to participate in group, long-distance seasonal migration, this could be due to anthropogenic pressures and human construction reducing the amount of landscape which this species can inhabit, and it cannot be known whether this modern form of seasonal migration is the same as it would have been in the Pleistocene. Some scholars have proposed that North American elk might be a better analogy/comparator species behaviourally to Late Pleistocene red deer (Pilaar Birch et al., 2016; Steele, 2002), although as wapiti may too have been present in Late Pleistocene Europe this comparison to modern wapiti behaviour may also be problematic (Croitor and Obada, 2018; Croitor, 2020). The issues with the use of modern species inferring the behaviour of Late Pleistocene species highlights the need for direct methods. The Middle Pleistocene site, Lazaret Cave, located in southern France on the Mediterranean coast in the city of Nice (Figure 1) dating to MIS 6, offers a unique opportunity to better understand red deer ecology during the penultimate glacial period and possible relationships with hominin subsistence behaviours within a localised area.
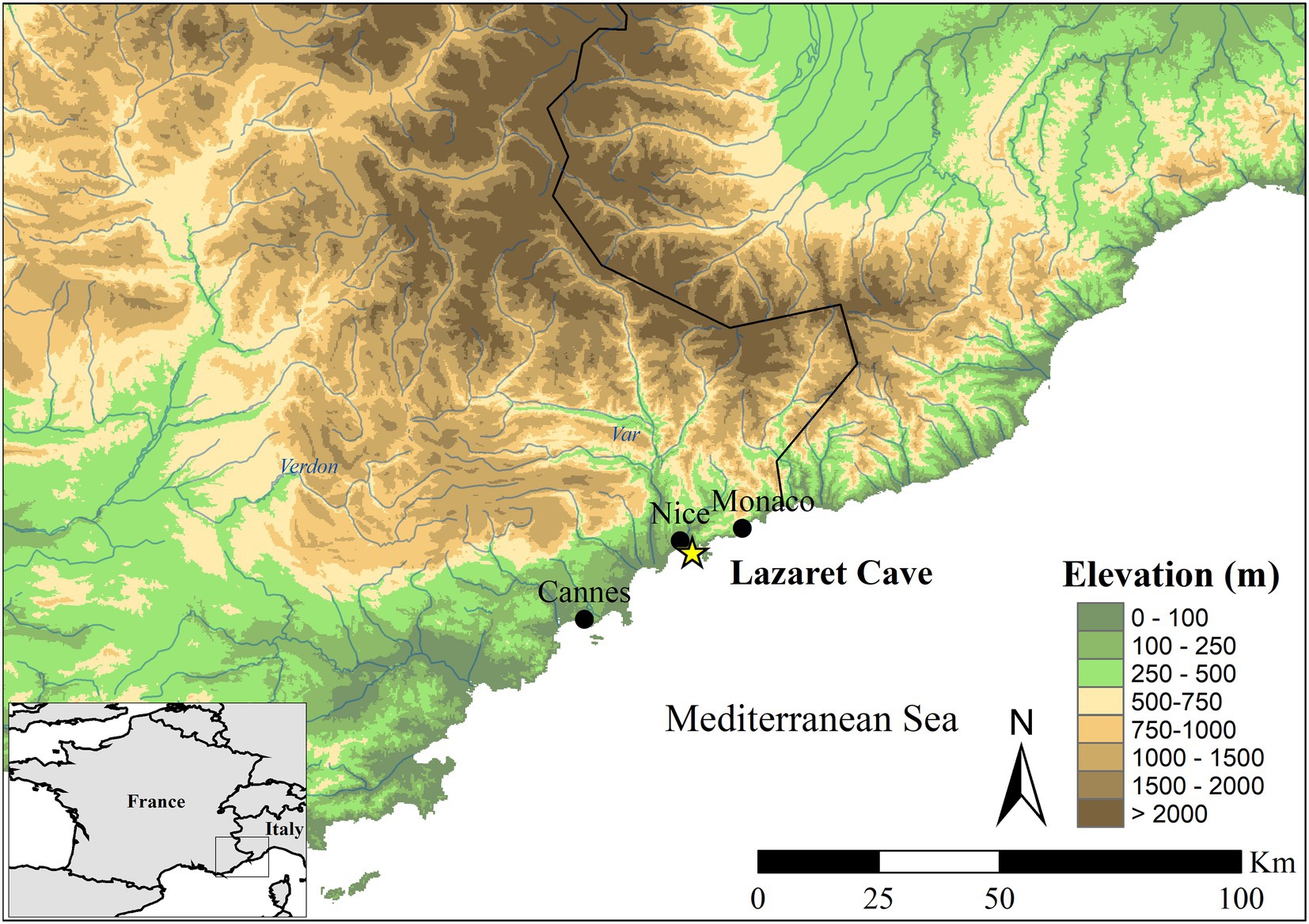
Figure 1. Elevation map of selected research area covered by the 87Sr/86Sr isoscape around Lazaret Cave (43°41′25″N, 7°17′42″E). Lazaret is indicated by a yellow star while modern cities are denoted by black circles (Elevation data source: Esri, 2015).
Over the past 20 years, many studies have been devoted to understanding the taphonomy, paleoenvironments and paleoecology of Lazaret Cave (e.g., de Lumley et al., 2004; Valensi et al., 2007; Desclaux et al., 2008; Hanquet et al., 2010; Hanquet, 2011; Desclaux, 2013; De Lumley, 2018; López-García et al., 2021). Deposits at Lazaret Cave consist of five Complexes (Figure 2), of which Complex C holds archaeological layers dating between 230 and 130 ka (Michel et al., 1996, 2009). Excavations in Complex C have revealed a rich assemblage including 28 hominin remains assigned to archaic Neanderthals (Homo neanderthalensis), thousands of faunal (vertebra and invertebrate) remains, and an abundant collection of lithic bifacial tools from a succession of different occupations attributed to the transition between the Acheulean and Mousterian periods (de Lumley, 1969; Michel et al., 1995, 2009; Valensi, 2000, 2009; Valensi and Psathi, 2004; Cauche, 2012; Valensi et al., 2013; Roussel et al., 2019).
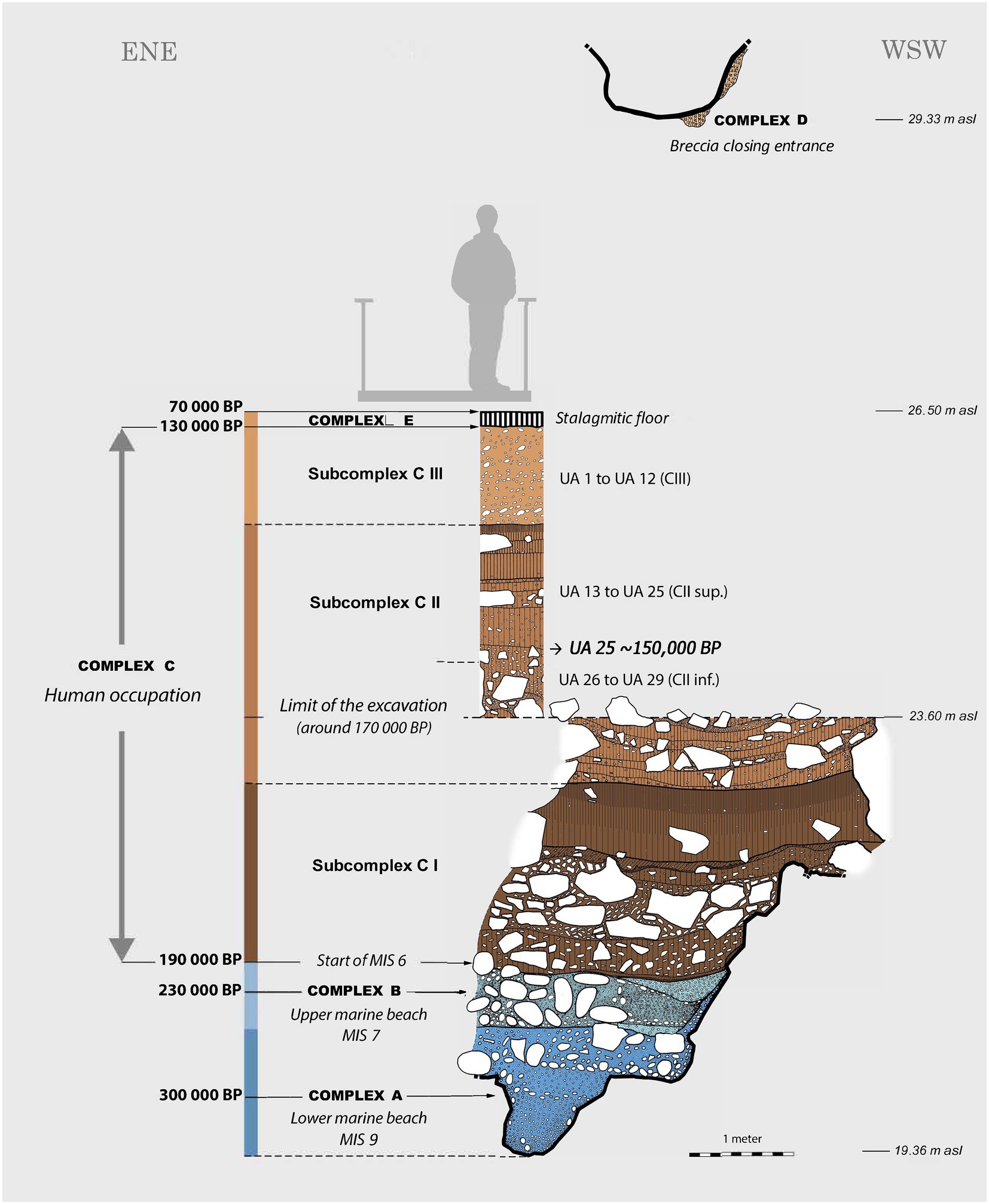
Figure 2. Profile of Lazaret Cave from excavations prior to 2015. Image adapted from Michel et al. (2009).
Dating to ~150 ka, archaeological layer UA25 contains evidence for human activity during the sites use as a short-term occupation camp (Valensi et al., 2013). Excavations from UA25 revealed over 3,000 large mammal remains, of which ~1,200 were identifiable to species. The faunal assemblage is dominated by herbivores including Cervus elaphus (red deer), Capra ibex (ibex), and Bos primigenius (aurochs; Valensi, 2000; Valensi et al., 2013; Hassani et al., 2017). The high relative abundance of ibex in UA25 suggests the climate was persistently colder and more arid than nowadays (Fiore et al., 2004; Valensi et al., 2007, 2013). All skeletal elements of red deer and ibex have been found within the cave site, while only elements with high meat and marrow indices and heads are found from larger herbivores, such as aurochs. Red deer is the most abundant species in all archaeological layers at Lazaret, including UA25, indicating red deer were a reliable source of meat throughout hominin occupation at Lazaret (Valensi, 2000; Steele, 2002). Based on zooarchaeological analysis of faunal remains from Lazaret UA25, it has been proposed that early hominins were selectively hunting red deer, alongside ibex, who were near the camp site in the autumn period and potentially using meat curing activities to preserve meat through the winter (Valensi, 2000; de Lumley et al., 2004; Valensi and Psathi, 2004; Valensi et al., 2013).
Focusing on remains from layer UA25 at Lazaret Cave, this study employs laser ablation strontium isotope (87Sr/86Sr) analysis, coupled with isoscape modelling and spatial assignment tools, to reconstruct red deer spatial palaeoecology in southern France during MIS 6. Oxygen isotope (δ18O) analysis was also used to provide a seasonal framework for data interpretation, allowing the inference of winter and summer ranges. Hominin hunting behaviours and landscape use around the site will then be re-interpreted in light of new evidence for the seasonal spatial ecology of this key subsistence species. Thus, the aims of this study are to:
1. Use 87Sr/86Sr and δ18O analysis combined with spatial assignment, to determine minimum, seasonal mobility ranges of red deer and ibex hunted at Lazaret Cave UA25, providing the first evidence of red deer spatial ecology during MIS 6.
2. Compare the new evidence for red deer seasonal spatial ecology to hominin hunting activities at the site, in order to better understand early Neanderthal subsistence behaviours.
2. Reconstructing faunal spatial palaeoecology using strontium and oxygen isotopes
Strontium (87Sr/86Sr) isotope analysis allows the investigation of past human and faunal movement through the comparison of 87Sr/86Sr ratios in archaeological teeth and bones and 87Sr/86Sr ratio in the environment. Within lithological units there are four naturally occurring strontium isotopes with varying abundances: 84Sr, 86Sr, 87Sr, and 88Sr. 87Sr is the only radiogenic isotope and is the daughter product of actively decaying rubidium isotope, 87Rb, which has a half-life of ~4.88 × 1010 years (Capo et al., 1998; Nielsen, 2004; Bentley, 2006). Therefore, in a closed system, 87Rb should decrease, 87Sr should increase, and all other strontium isotopes should remain the same, leading to an increase of the 87Sr/86Sr ratio. Bioavailable 87Sr/86Sr values in soils and plants, which absorb strontium from their surrounding environment, depend highly on the underlying bedrock, including the original amount of 87Rb and age of bedrock, with older lithologies and those which initially had higher levels of 87Rb having higher 87Sr/86Sr ratios (Capo et al., 1998). In herbivores, 87Sr/86Sr ratios of skeletal tissues directly relate to the 87Sr/86Sr ratio of plants ingested and thus to underlying soils. As strontium isotopes fractionation during diet uptake is small compared to the environmental variability, it can be used for geographic assignments. The sampling of incrementally formed tissues, such as tooth enamel, generates time-series 87Sr/86Sr, reflecting movements during the period of tissue formation, as demonstrated in modern migratory caribou (Britton et al., 2009). Since strontium isotope ratios may overlap amongst different geographic areas with similar geologies, they can be combined with additional isotope systems to improve the reconstruction of movements (Balasse, 2002; Evans et al., 2012).
The oxygen isotope value (δ18O) of tooth enamel is directly related to water consumed during enamel formation (Longinelli, 1984; Pederzani and Britton, 2019). In mid-northern latitudes δ18O is highly dependent on the rain out effect and local air temperature, with high δ18O values in warmer seasons and low δ18O values in cold periods (Pederzani and Britton, 2019). Based on the changes of δ18O values in relation to temperature, seasonal changes can be identified by sequentially sampling enamel for intratooth δ18O measurements. Depending on how long the enamel took to develop, multiple seasons and years can be detected using δ18O analysis within individual teeth and a timescale can be created by analysing and correlating δ18O values from multiple teeth in the same mandible (Dansgaard, 1964; Pederzani and Britton, 2019). Using these techniques, past human and faunal movements can be identified through the relating of variations in tooth enamel 87Sr/86Sr to strontium bioavailability maps (isoscapes; Capo et al., 1998; Bentley, 2006), and placed within a seasonal framework provided by co-measured δ18O data (e.g., Gigleux et al., 2019). Characterisation of movements can also employ spatial assignment tools to empirically identify probable winter and summer ranges (e.g., Wooller et al., 2021; Britton et al., 2023). By understanding movements of past fauna found at archaeological sites, archaeologists can recreate living landscapes encountered by early hominins, enhancing our understanding of past hunting strategies, landscape use, and other aspects of human palaeoecology.
3. Materials and methods
3.1. Tooth samples
First, second, and third molars from three red deer were selected for this pilot study, along with those from a single ibex (Figure 3). Samples were selected based on availability, and the presence of multiple teeth from single jaws to maximise time series geolocational information. Export of samples for analysis was accompanied by the official validation of the French Ministry of Culture (DRAC PACA, which also largely financed this analysis). The owner of the archaeological collections (Département des Alpes-Maritimes) gave directed permissions for all analyses to one of our authors, ED, the scientist responsible for the study of the paleontological collections from Lazaret Cave.
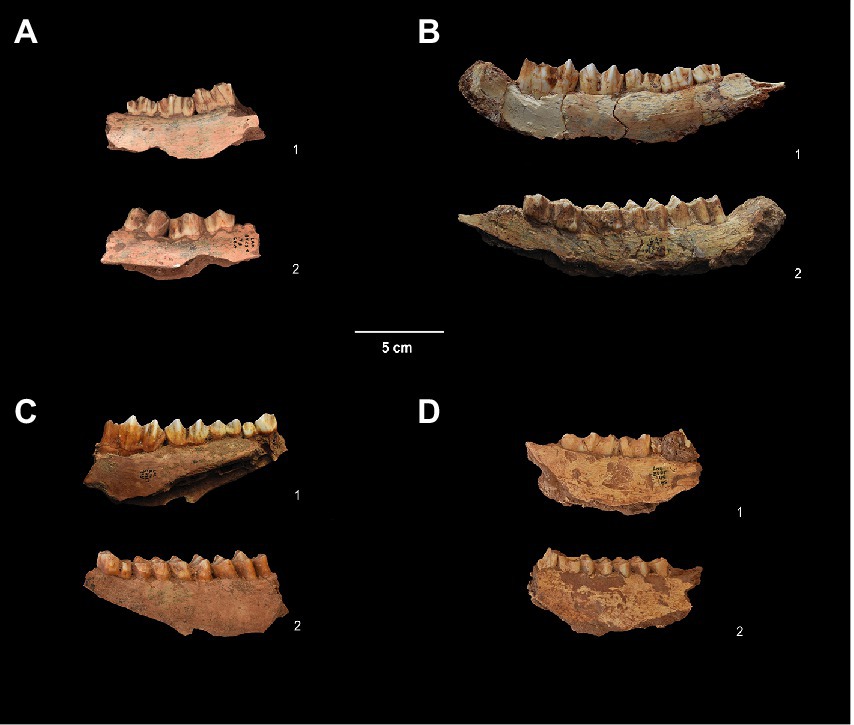
Figure 3. Analysed samples from UA 25 of Lazaret Cave. With: (A) Right mandible of Cervus elaphus with p/3-m/1 (T9 BT29T n°60, UA25), 1 = lingual view, 2 = buccal view; (B) Left mandible of Cervus elaphus with p/3-m/3 (S17 JE71T n°1873, UA25), 1 = lingual view, 2 = buccal view; (C) Left mandible of Cervus elaphus with p/4-m/3 (Q14 GA88T n°3,731, UA25), 1 = lingual view, 2 = buccal view; (D) Left mandible of Capra ibex with p/3-m/3 (U9 BU9T n°85, UA25), 1 = lingual view, 2 = buccal view.
Red deer permanent molariform teeth begin forming and mineralising in utero (M1), with crowns completed around the age of 4 months (Table 1; Brown and Chapman, 1991). This mineralisation period suggests that isotope analysis on M1 enamel should provide isotopic information on the calf and mother during late spring and the calf’s first summer of life following birth in May/June. The M2 mineralises between ~4 months and 9 months which includes the calf’s first autumn and winter towards the beginning of spring. Finally, the M3 shows consistent mineralisation between 13 and 26 months suggesting a full M3 without any wear should record isotopes between the late spring of the calf’s first year of life through to the third summer (Brown and Chapman, 1991). Depending on the age of the animal/degree of tooth wear, adjacent molars may have slight overlapping periods of growth; this should be detectable by oxygen and strontium isotope analysis.
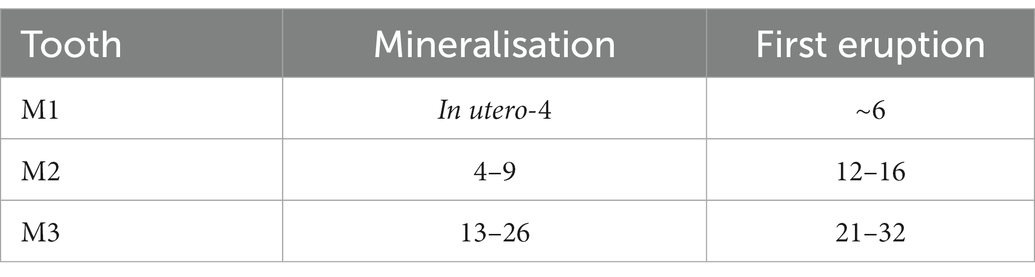
Table 1. Red deer crown estimated mineralisation and eruption periods in months (Brown and Chapman, 1991).
3.2. Laser ablation strontium isotope analysis
To analyse 87Sr/86Sr isotope ratios in red deer and ibex molars we used a dental drill with an ultra-fine diamond disc to cut narrow enamel slices from selected teeth (Table 2) and mounted them flat on petrographic glass slides, with 3 individual cross-sections per slide. This method exposes the dentine-enamel junction and offers an ideal trajectory to perform laser-ablation mass spectrometry to reconstruct the full time series of variation in strontium throughout the entire length of the enamel formation (Copeland et al., 2008, 2011; Willmes et al., 2016; Wooller et al., 2021).
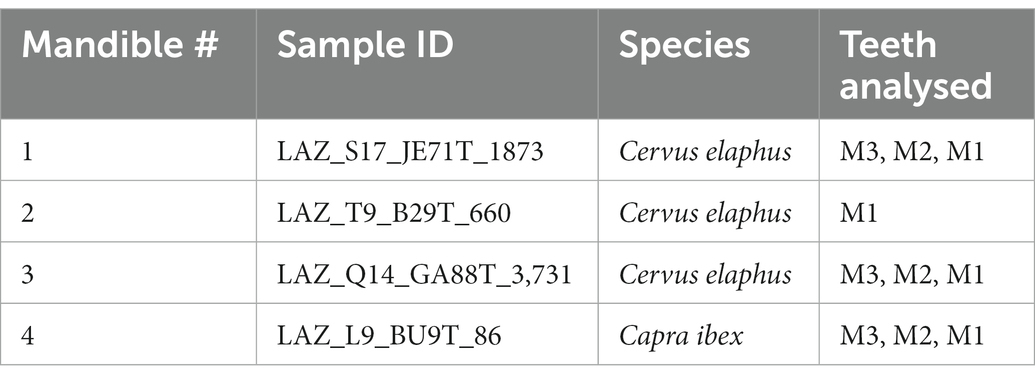
Table 2. Lazaret Cave red deer and ibex sample information including preserved teeth and teeth analysed for 87Sr/86Sr and δ18O.
87Sr/86Sr isotope ratios were measured by Laser Ablation Multi-Collector Inductively Coupled Plasma Mass Spectrometry (LA-MC-ICP-MS) at the University of California Davis, Interdisciplinary Centre for Plasma Mass Spectrometry. For the Sr. isotope analyses a Nd:YAG 213 nm laser (New Wave Research UP213) is coupled to a Nu Plasma HR MC-ICP-MS (Nu032). A line of laser spots with a 100 μm diameter and a spacing of 150 μm is used to sample across the entire tooth cross-section. The laser is operated using a 20-Hz frequency and 5–15 J/cm2 photon output.
Different methods have been used to account and correct for the different interferences and mass bias present when analysing strontium isotopes using LA-MC-ICP-MS (Vroon et al., 2008; Lewis et al., 2014; Lugli et al., 2020). The 87Sr/86Sr isotope ratio is normalized for instrumental mass discrimination by monitoring the 86Sr/88Sr isotope ratio (86Sr/88Sr = 0.1194) and 87Rb is corrected by monitoring the 85Rb signal and normalized by the same mass bias coefficient as Sr. The Rb correction is considered robust for samples with low 85Rb:88Sr ratios (<0.002), which included all samples in this study. Krypton interference (86Kr) originating from the argon supply is corrected using on-peak subtraction before each analysis. Potential analytical interferences caused by polyatomic molecules were monitored by measuring strontium oxide on mass 104 (88Sr + 16O) and 87Sr/86Sr isotope ratios of known marine reference materials. Processing of the geochemical data is performed using the IsoFishR application (Willmes et al., 2018a,b). In short, a 5-point average is applied to the raw data collected by the mass spectrometer with an integration time of 0.2 s resulting in 1 datapoint per second, and outliers were removed based on 2SD outlier criterion using a 10-point moving average window. Precision and reproducibility of the LA-MC-ICP-MS is evaluated using two in-house reference materials consisting of a modern marine aragonitic otolith from a White Seabass (Atractoscion nobilis) and a bioapatite Green Sturgeon (Acipenser medirostris) fin ray (Table 3). Repeated measures of these two in-house reference materials showed values of 0.70914 ± 13 (n = 54, ±2SD) for the White Seabass and 0.70916 ± 14 (n = 63, ±2SD) for the Green Sturgeon which are in good agreement with the average 87Sr/86Sr isotope ratio of the modern ocean 0.70918 within uncertainty.
Furthermore, seven rodent and two bird specimens from within Lazaret Cave were analysed for 87Sr/86Sr using solution-based strontium methods to find the local 87Sr/86Sr signature of the cave site itself. Rodents were chosen as they typically live in one location throughout life and do not have a large ranging territory meaning their 87Sr/86Sr value should accurately reflect the local 87Sr/86Sr signature. The bird specimens are rock-dwelling species which likely nested in the cave or nearby and taphonomic studies on these specimens show they fell prey to raptors, carnivores, or humans before being discarded inside Lazaret Cave. Of the nine specimens, six rodents and both birds came from archaeological layers while one rodent was a surficial find. This modern specimen was used to identify whether 87Sr/86Sr values within the cave has changed over time (Supplementary Table 1).
3.3. Oxygen isotopic analysis
Following strontium isotope analysis, second and third molars from red deer were chosen for carbonate analysis alongside 87Sr/86Sr analysis, except for red deer LAZ-T9 in which case the M1 was the only molar which was available for sampling. While oxygen isotope analysis of the phosphate component is often considered more desirable in Late Pleistocene studies (e.g., Britton et al., 2019, 2023; Pederzani et al., 2021a,b), due to the increased resistance to diagenesis, carbonate analysis is not uncommon (Sponheimer and Lee-Thorp, 2001; Rivals et al., 2015; Pilaar Birch et al., 2016; Price et al., 2017) and studies have demonstrated enamel (as opposed to bone) preserves in vivo signals in even very ancient samples (e.g., Iacumin et al., 1996). Here, we made the decision to undertake carbonate oxygen isotope analysis because, in addition to being more time- and cost-effective to undertake, it is sufficient to produce the intra-tooth profiles required to ‘anchor’ intra-tooth isotope data. However, due to the potential of diagenetic alteration (or even the slight shifting of isotopic ratios due to acetic acid pretreatment), we focus here only on intra-tooth trends and not on the use of specific values (e.g., for palaeotemperature reconstruction).
Prior to carbonate sampling, dirt and cementum were removed from the outside enamel layer using a tungsten carbide burr. A strip of enamel adjacent to the strontium sample, so as to collect carbonate data which directly relates to the same cusp and time of tooth development as the strontium data, was then removed using a circular diamond coated drill bit. Adhering dentine was then removed from the enamel sample strip using a tungsten carbide burr following methods outlined in Britton et al. (2009) to ensure only enamel would be analysed. Next, the strip of enamel was sectioned into smaller samples by approximately every 1.7 mm from the top of the crown to the apex of the tooth. Each section was cleaned in a MilliQ water super sonicated bath for 3 min before being ground into a powder using a mortar and pestle.
There is currently no consensus for which pretreatment method to use ahead of carbonate isotope analysis of whole tooth enamel from archaeological samples (Koch et al., 1997; Balasse, 2002; Crowley and Wheatley, 2014; Snoeck and Pellegrini, 2015; Pellegrini and Snoeck, 2016; Demény et al., 2019; Skippington et al., 2019; Wood et al., 2021). However, in older bone and tooth samples, particularly those originating from sedimentary deposits, secondary carbonates can form. Pretreatment techniques, such as using acetic acid, are often used in an attempt to remove secondary carbonates. However, such pretreatments also have the potential to influence structural carbonates, or cause recrystallisation, potentially altering isotopic signatures. Here, we selected a short 0.1 M acetic acid pretreatment protocol (10 min, after Pellegrini and Snoeck, 2016 and Skippington et al., 2019), followed by three rinses with MilliQ water with the goal of targeting secondary carbonates and minimising the influence on structural carbonates. Pre-treated samples were then freeze-dried, and subs-samples were analysed for their oxygen isotope composition at Iso-Analytical Ltd. (Cheshire, United Kingdom). Aliquots were weighed into clean Exetainer™ tubes and flushed with 99.995% helium. After flushing, phosphoric acid was added to the samples and left overnight to allow complete conversion of carbonate to CO2. Carbon dioxide gas was then analysed using Continuous Flow-Isotope Ratio Mass Spectrometry (CF-IRMS).
3.4. Isoscape modelling and spatial assignment
Plant and soil sample data were taken from published 87Sr/86Sr databases covering France (Willmes et al., 2014, 2018a) and northern Italy (Bataille et al., 2018; Willmes et al., 2018a; Lugli et al., 2022). We used kriging with external drift to model the bioavailble 87Sr/86Sr isoscape near Lazaret Cave, following the methods outlined in Willmes et al. (2018a). Kriging is a geostatistical approach to interpolate continuous distributions of 87Sr/86Sr values across the landscape between known data points. Kriging with external drift improves the accuracy of the isoscape by accounting for additional spatial trends defined by an auxiliary variable (Britton et al., 2020; Holt et al., 2021; Lazzerini et al., 2021), here, the underlying lithology. In order to define the auxiliary variable, we used the GLiM map (Global Lithological Map, Supplementary Figure 1; Hartmann and Moosdorf, 2012) which regroups geological formations into broader geological units (metamorphic rocks, volcanic/plutonic felsic/intermediate/mafic rocks, carbonate/siliclastic sedimentary rocks). We used 954 sampling location to build the 87Sr/86Sr isoscape (France: n = 516, northern Italy: n = 438). When several plant and soil samples were available at a given sampling location, we used the average 87Sr/86Sr value. The GLiM geological unit of each sampling location was retrieved using ArcGIS 10.5. Kriging was carried out with the gstat package in R (krige function, Gräler et al., 2016) using an exponential variogram model and a search neighbourhood set to min. = 5 and max. = 50 (Willmes et al., 2018a).
Spatial assignment was performed using the assignR package in R (Ma et al., 2020). In order to assess the most probable locality of summer and winter ranges, maximum and minimum 87Sr/86Sr values were determined for each red deer molars and we used the δ18O results to identify the season associated with these values (Supplementary Table 3). These time-correlated values were then assigned to the 87Sr/86Sr bioavailable isoscape within 100 km of Lazaret. We produced a posterior probabilities of origin raster for each sample. We then extracted the area corresponding to 90% of the probability density to identify possible locations of red deer in summer and winter periods identified in each tooth. Given the lack of the variation within the ibex M1-M3 samples, we used the mean 87Sr/86Sr value of the teeth to establish the year-round range of the individual.
4. Results
4.1. Bioavailable 87Sr/86Sr isoscape
The 87Sr/86Sr isoscape of Lazaret Cave shows the environmental bioavailability of 87Sr/86Sr across the landscape near the archaeological site (Figure 4). Lazaret is near the boundary of two 87Sr/86Sr ratios, one made of carbonate sediments which have a low 87Sr/86Sr value ≤0.710 and the other which is made of siliciclastic sediments and have a 87Sr/86Sr value ≥0.719. Majority of the surrounding area around Lazaret is made of the same carbonate sediments which Lazaret is on and similarly have 87Sr/86Sr values <0.712. Starting at 25 km and ending ~125 km southeast of Lazaret there is a large portion of coastline with values ranging from 0.716–0.722. Approximately 40 km to the northeast of Lazaret there is a second area with high 87Sr/86Sr values ≥0.720. 87Sr/86Sr values ≥0.750 are located ~75 km northeast in the Italian Alps. This isoscape was further verified by the 87Sr/86Sr analysis of seven rodents and two bird bones found within Lazaret Cave which showed the cave itself has an average 87Sr/86Sr value of 0.70847 ± 0.000228.
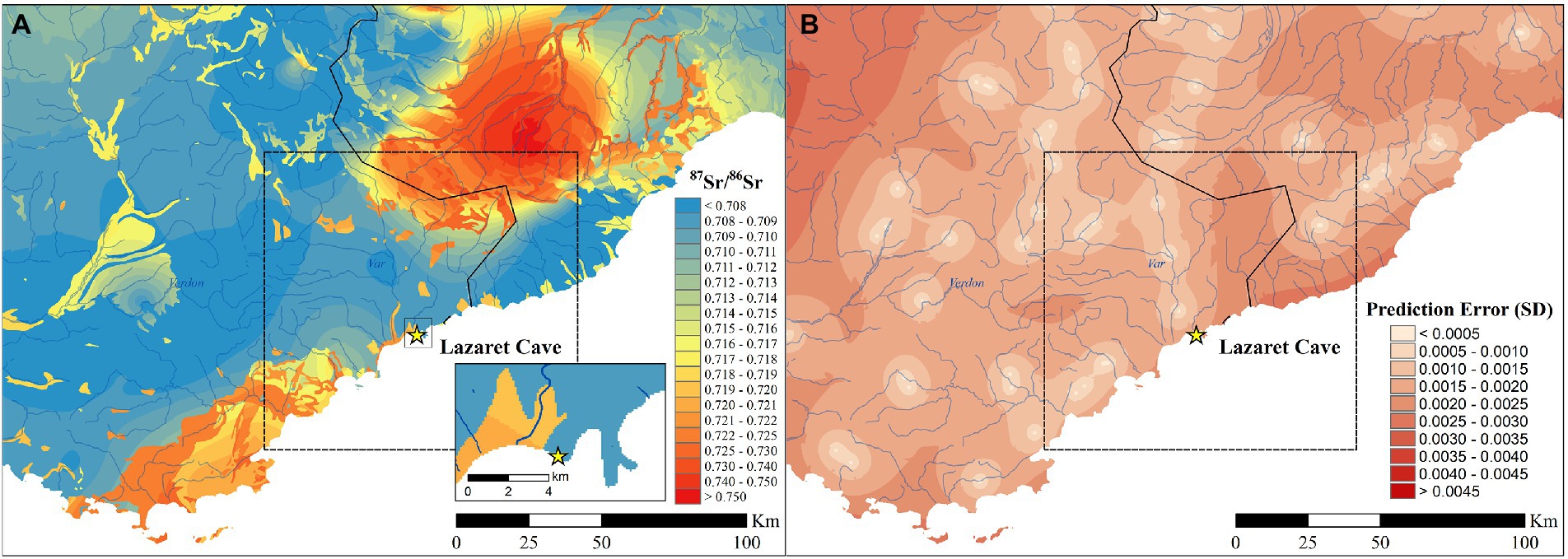
Figure 4. (A) bioavailable 87Sr/86Sr isoscape of the area around Lazaret Cave including an inset map focusing on the area directly surrounding Lazaret Cave, (B) associated spatial error map. The black dashed box in both images is the spatial assignment limit shown in Figures 7, 8.
4.2. Oxygen isotope evidence for seasonality of tooth mineralization
Carbonate results help distinguish time of tooth growth and therefore period of mobility (Supplementary Table 4). Red deer results show a cosinusoidal pattern where peaks are equivalent to summers and troughs represent winters between first, second, and third molars from three different red deer (Figure 5). Sample T9 is the only sample to represent the M1 and shows that this tooth begins mineralisation during summer and ends in autumn. Samples S17 and Q14 represent M2 and M3 growth and show both M2s beginning to form during the first winter of life and ending towards the second summer while M3s begin formation during the second summer of life and complete mineralisation during the second winter. Both S17 and Q14 have similar patterns and δ18O values suggesting red deer in this study are tracking δ18O changes better than we would expect to see in nonobligate drinkers.
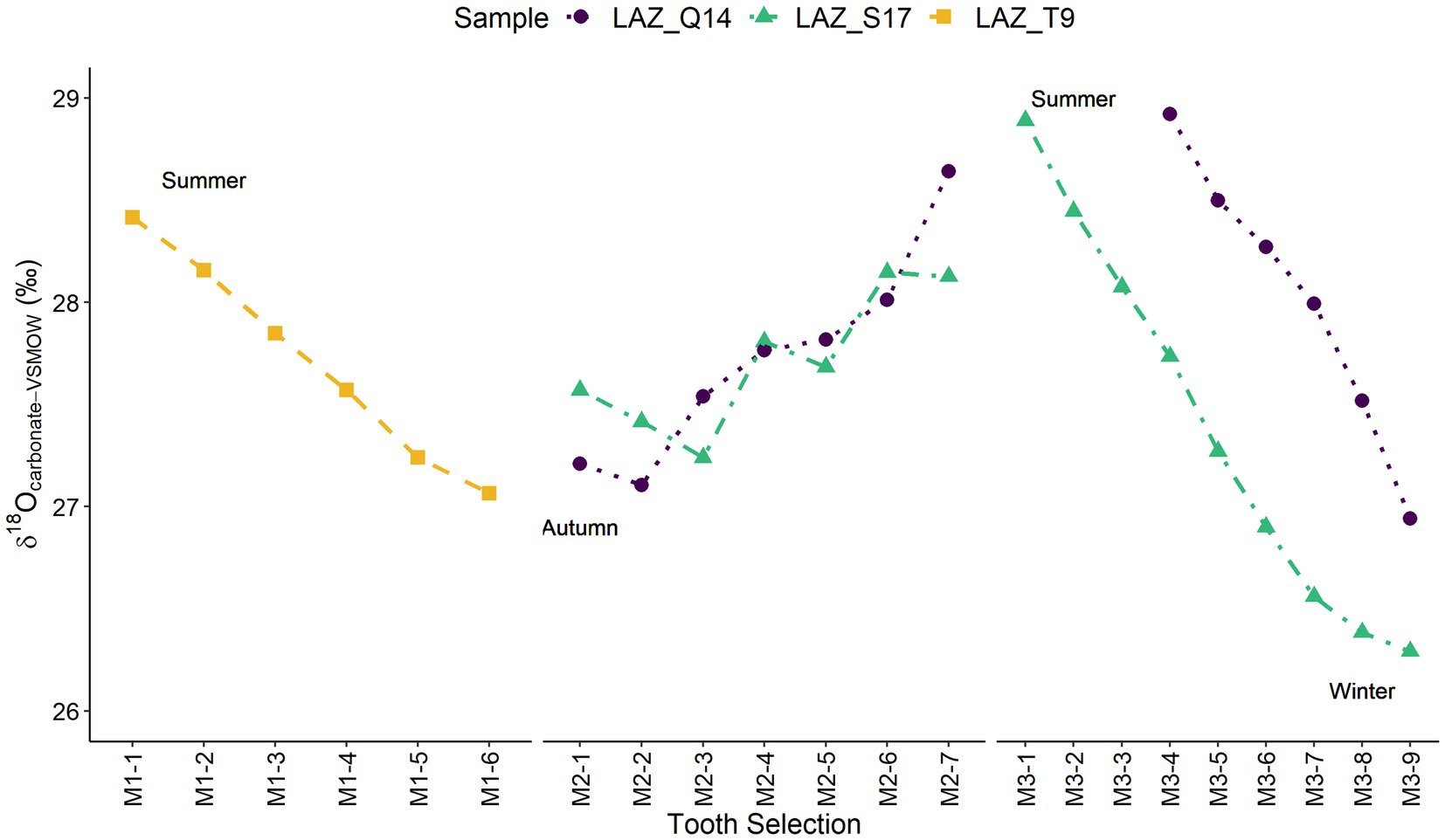
Figure 5. Plot of all δ18O data from enamel intra-tooth sections from first, second and third molars of three red deer from Lazaret.
4.3. Measured 87Sr/86Sr laser ablation and spatial assignment of lazaret fauna
Figure 6 shows 87Sr/86Sr results for three red deer and a single ibex. 87Sr/86Sr data from three red deer shows a similar start of M1 with a range between 0.712 and 0.714, possibly suggesting a similar calving location. All three individuals first molars’ show a peak in 87Sr/86Sr values between 0.715 and 0.716, before the end of the first molar mineralisation when the red deer is approaching their first autumn/early winter. The second molar is only shown by individuals S17 and Q14. Individual Q14 shows the largest range in 87Sr/86Sr values and reaches a peak close to 0.716 near the enamel-root-junction (ERJ); in comparison, S17 shows the most 87Sr/86Sr variation at the start of the tooth mineralisation period when the red deer is in the first winter. Finally, both individuals show clear 87Sr/86Sr variation in the beginning of the M3 during the end of the second summer which then homogenises around 0.713 towards the start of the second winter. In this phase of tooth mineralisation, two individual peaks are discernible in Q14 which would have not likely been discernible using traditional intra-tooth sampling and solution preparation methods. The ibex has a relatively stable 87Sr/86Sr value between 0.711 and 0.712 throughout the growth of the first, second and third molars.
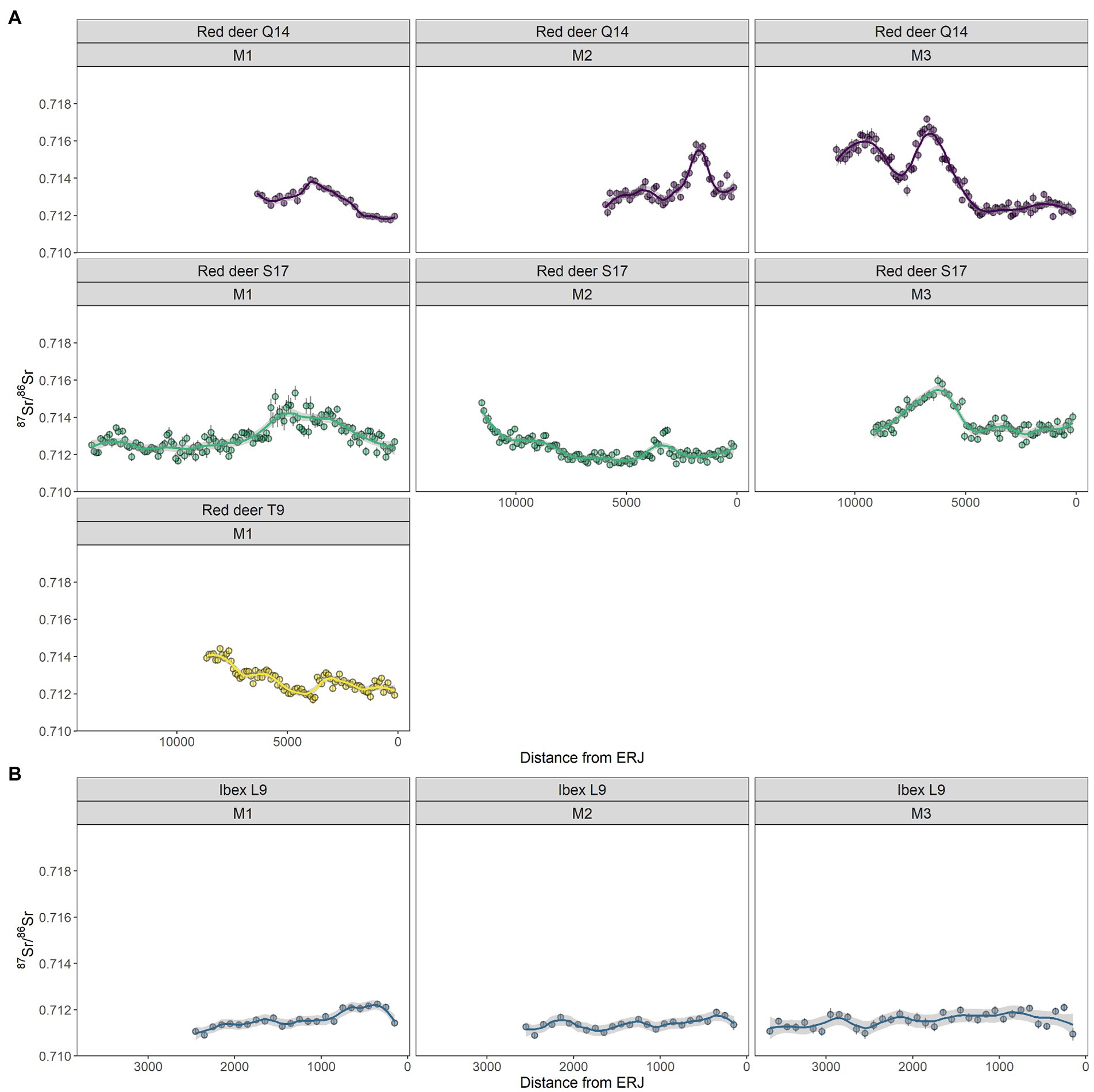
Figure 6. 87Sr/86Sr LA-MC-ICP-MS results for three red deer (shown in A) and an ibex (shown in B). Each fauna is represented by a different colour and displayed in rows. Columns represent different teeth (from left to right first, second and third molar). The x-axis represents the laser ablation distance from the ERJ. Graphs are read from left to right with the oldest section of tooth (crown) on the far left and the latest-forming portion of the tooth (enamel-root junction) located on the right-hand side of the graph. Individual dots represent a single 100 μm spot while the line follows the trend of the laser ablation data points.
Using spatial assignment of minimum and maximum 87Sr/86Sr values combined with δ18O of each red deer molar, we identified distinct likely areas of origins for summer and winter periods (Figure 7). Summer ranges (shown in warm colours) for all red deer map in similar locations with two possible ranging areas within 20–30 km from Lazaret, one to the southwest and the other to the northeast. Red deer Q14 has summer value ranges closest to Lazaret Cave, specifically shown by the M3, but otherwise majority of summer ranges are located in areas of higher elevations. Winter ranges (shown in different shades of blue) are all much larger than summer rangers and map on lowlands within 5 km of Lazaret Cave. The first and second molar autumn to winter ranges all have a very similar pattern, only in red deer S17 is the M3 winter range slightly smaller and further away from Lazaret Cave. Across all teeth there is little to no overlap between summer and winter ranges. In addition to seasonal range locations, the M1 summer ranges can also designate possible calving locations as red deer are born in late spring/early summer.
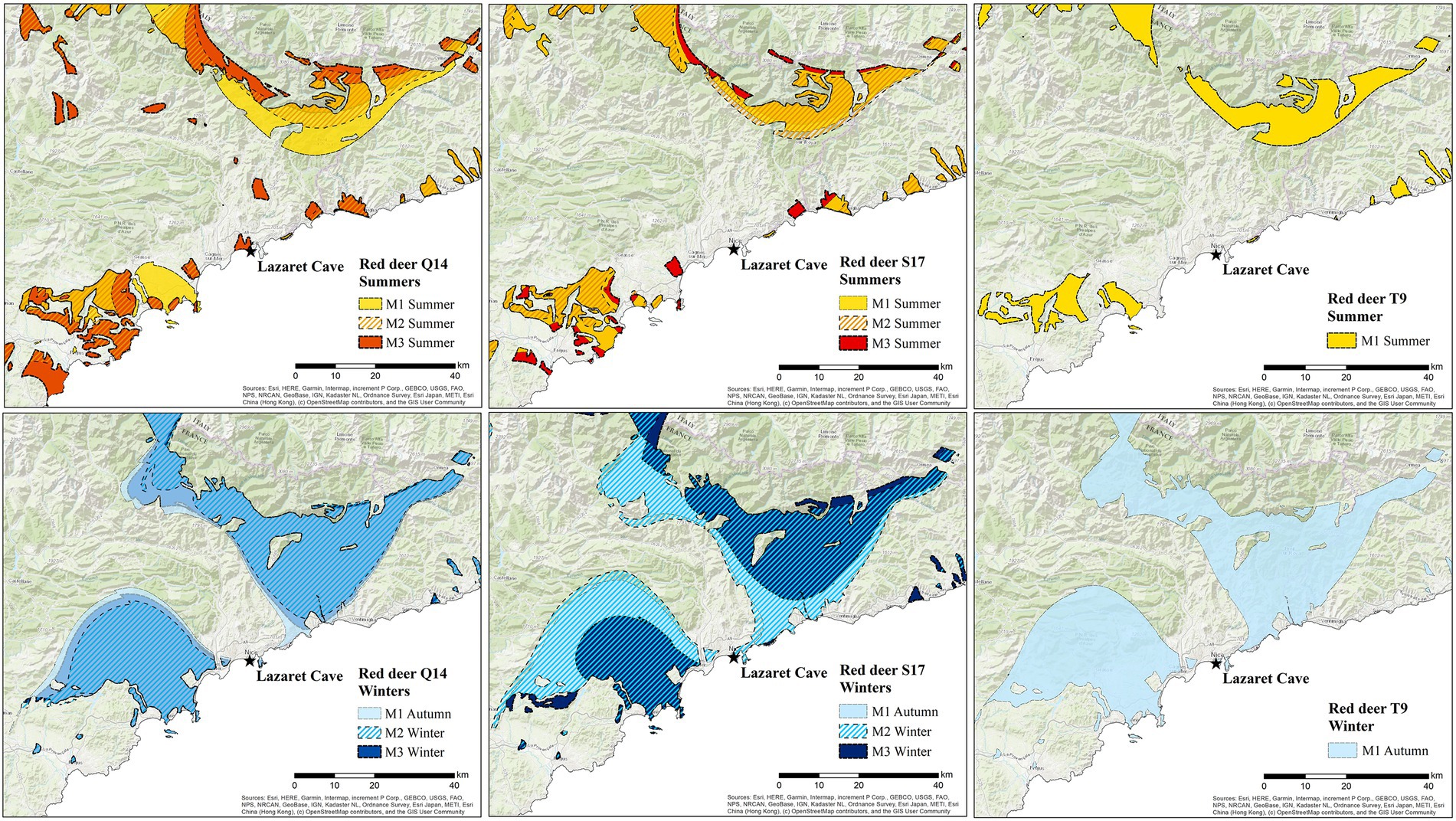
Figure 7. Spatial assignment of red deer remains analysed from Lazaret Cave showing likely summer and winter ranges of all red deer with a 90% probability of origin, meaning there is a 90% chance that the red deer were within these locations during the period of enamel formation/mineralisation. Individual teeth are shown in columns, while summer and winter ranges are shown in rows. Each tooth is assigned an individual colour, while M2s are shown with diagonal lines. The landscape shown in this image is within the dashed rectangle in Figure 4; Esri (2022).
A single ibex was analysed as a comparison to the red deer from Lazaret Cave as it is the second highest fauna found alongside hominin remains. Unlike the red deer which show 87Sr/86Sr variation in all molars, the ibex (L9-BU9T-86) has almost no 87Sr/86Sr variation across the first, second and third molars (Figure 5). The ibex 87Sr/86Sr values remain between 0.711–0.712 throughout its early life, suggesting this individual was either nonmigratory or moved only within locations with homogenous 87Sr/86Sr values. Spatial analysis of the mean 87Sr/86Sr values in each ibex molar shows a 90% probability of origin, this area does include the region close to Lazaret but also encompasses wider mountainous regions to the east, and flat lands and hills to the west near modern-day Cannes (Figure 8). As the 87Sr/86Sr values remain relatively constant throughout tooth formation, it is likely that the ibex was located within one region and did not cross between these eastern and western regions.
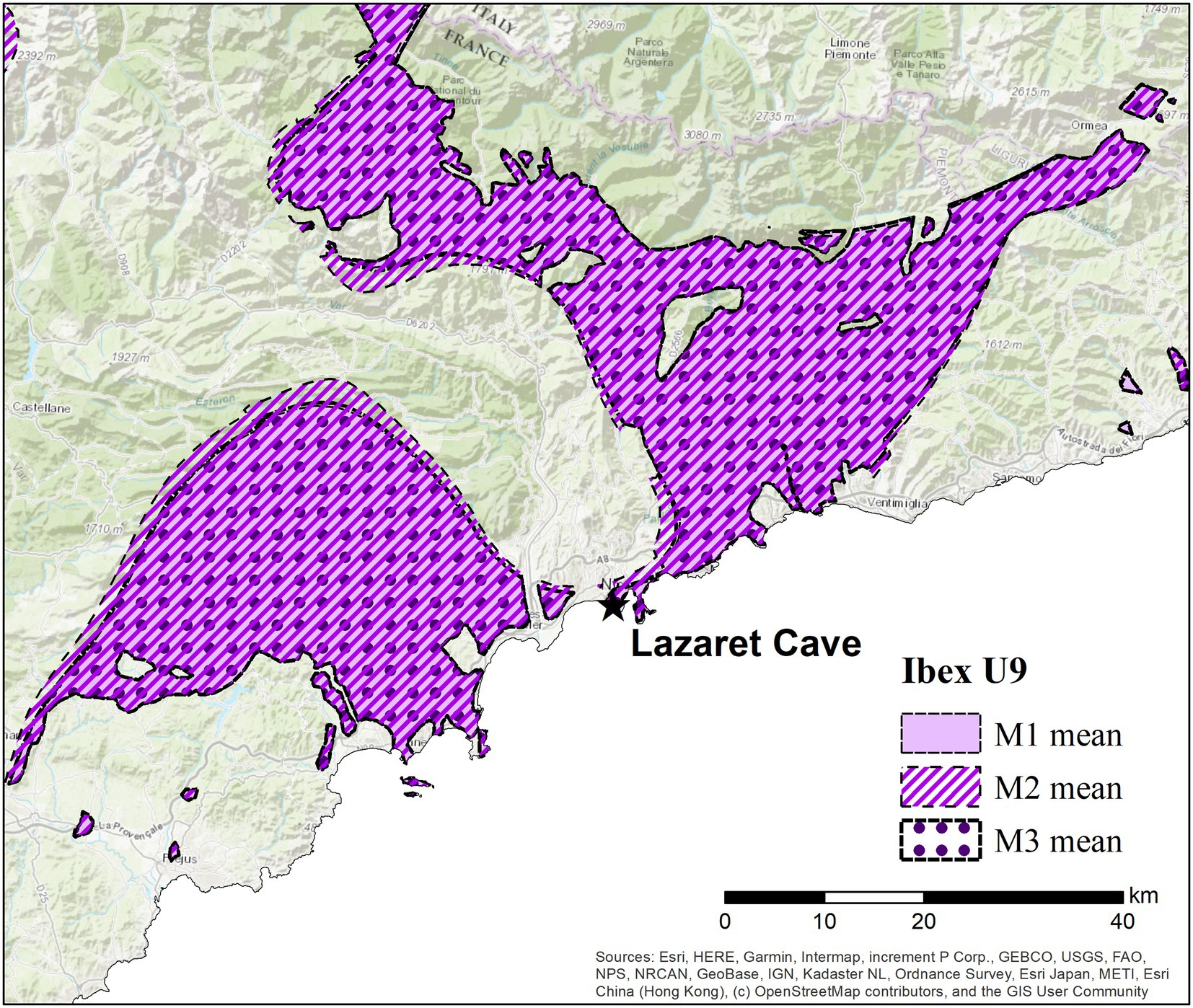
Figure 8. Spatial assignment of strontium isotope data from ibex first, second and third molars from Lazaret Cave. Star indicates location of Lazaret Cave. As there was no variation in 87Sr/86Sr values across the molars, the mean of each tooth was assigned. The M1 is show as a solid colour, while M2 is shown by diagonal lines and the M3 is represented by circles so that all overlapping rangers can be identified. The landscape shown in this image is within the dashed rectangle in Figure 4; Esri (2022).
5. Discussion
5.1. Red deer and ibex mobility
Through the combination of isoscape modelling, multi-isotopic analysis, and spatial assignment we empirically identify likely summer and winter ranges for red deer in relation to Lazaret, allowing us to better understand the spatial palaeoecology of red deer during MIS 6 and possible Neanderthal hunting ranges and/or behaviours. Based on spatial analysis we can conclude red deer could likely be found close to Lazaret (i.e., within ~20 km) for much of the year, well within known red deer migration limits (Mysterud et al., 2011; Kropil et al., 2015). The potential summer and winter ranges identified through the analysis of multiple molars analysed from the three red deer are similar, indicating either a herd structure (perhaps reflecting the contemporaniety of the individuals analysed and/or rapid accumulation of the material at the site) or conservation of movement behaviours throughout the period in which this phase of site formed. Whether considering the easterly or westerly ranges identified, seasonal differences are largely altitudinal, with winter ranges closer to the coastline on lowlands, and summer ranges located adjacent to winter ranges in higher elevations. This pattern of elevational seasonal migration is well known in modern red deer (Langvatn and Albon, 1986; Albon and Langvatn, 1992; Mysterud et al., 2011; Qviller et al., 2013). Additionally, spatial analysis shows two possible ranges which red deer could have occupied during summer and winter periods, one to the east towards Italy and the other area to the west near now Cannes. Possible localities shown by these results match with other Middle Palaeolithic sites located at the French-Italian border and in the western part of Liguria which also have high abundances of red deer, such as Grotte du Prince (MIS 5 to MIS 3), Grotte du Cavillon (MIS 4 to MIS 3), and Madonna dell’Arma (MIS 5) (Valensi and Psathi, 2004; Valensi, 2009; Moussous et al., 2014; de Lumley, 2016; Carrión and Walker, 2019). More work is needed to identify which range red deer found at Lazaret Cave were more likely to occupy during MIS 6.
The ibex, with almost no 87Sr/86Sr variation, also shows a similar distribution to the east and west of Lazaret Cave, however it could not have occupied both spaces as there is no corridor which connects the two locations isotopically and no evidence of movement through areas of more diverse 87Sr/86Sr. Spatial assignment of ibex molars overlap with Lazaret Cave, however as the ibex 87Sr/86Sr values never reach 0.708 it is unlikely the ibex was living within the cave site during its early life. We therefore hypothesise that the single non-migratory ibex could have lived nearby Lazaret Cave and brought back to the cave after death.
Due to topographic differences, we suggest red deer and ibex may have been living and ranging in separate locations. The Meridional Alps to the northeast have highly fragmented topography with steep alpine massifs which form an ecological niche favourable to fauna such as ibex who are commonly found in cliff environments. In comparison, to the southwest and areas immediately surrounding Lazaret, the topography and climate is more coastal due to the Mediterranean Sea which forms a better environment for deer evolution. The corridor between the costal and alpine environment in this region is very narrow, between 30 and 50 km, which is well within the ibex’s easterly range seen in Figure 8. Additionally, preliminary results from dental micro- and meso-wear studies in red deer and ibex from Lazaret Cave suggest both species were mixed feeders occupying different ecological niches (Rivals, in Desclaux et al., 2021; Rivals, in progress).
Spatial assignment of 87Sr/86Sr data from the crowns of early forming teeth has also allowed us to identify possible locations of red deer birthing season. Modern red deer rutting season is in the autumn followed by the birthing season in late May to early June (Albon et al., 1987; Stevens et al., 2011). While red deer are not known to have designated birthing grounds, it can be helpful to know more about the individuals we are studying to recreate lifetime movement. First molar mineralisation begins in utero and finishes around 4 months, meaning early tooth formation (near the M1 crown) should help us identify possible birthing grounds. All first molars have similar 87Sr/86Sr values which align with the summer areas identified in Figure 7, this suggests red deer were born further away from Lazaret. This is supported by zooarchaeological results which have suggested an autumn/ winter occupation through identification of pregnant hinds/infant remains within Lazaret Cave (Valensi et al., 2013).
Palaeoclimatic studies have suggested that conditions in this part of southern France and northern Italy during MIS 6 were colder than today but also that the region may have been a glacial refugia with a relatively steady climate which did not rapidly fluctuate due to the surrounding environment being protected by mountains in the north and west and the Mediterranean Sea to the south (Valensi and Psathi, 2004; Desclaux, 2013; Valensi et al., 2013; López-García et al., 2021). With a relatively stable climate and no other outside pressures, we can postulate that red deer ranging behaviours may also have stayed relatively constant. Analysis of other remains in other phases of the site would enable the testing of this hypothesis and to determine any relationship between seasonality of site use and the movements of red deer.
Furthermore, through oxygen isotopic analysis of a single red deer M1 and two red deer M2 and M3s we observed slight differences with the tooth formation periods (identified by Brown and Chapman, 1991), specifically in the M3. Each molar analysed shows a clear pattern of seasonality; the M1 begins in summer and ends mineralisation prior to winter (4 months = September/October), the M2 begins mineralising in a colder period but ends mineralisation in a warm period, and the M3 shows a steep decline from a warm period and ends in a cold period. Based on Brown and Chapman’s (1991) assessment of modern red deer the M3 begins mineralisation around 13 months and the crown is completed by 26 months, however, from oxygen analysis in this study we suggest that the M3 in these archaeological red deer mineralises much quicker than 13 months and possibly in as short of time as 6–8 months. However, given the likely loss of at least some crown height due to wear in all individuals this period may be estimated to be slightly longer.
To further pinpoint whether red deer or ibex were primarily occupying the eastern or western range, further work is required – for example, using sulphur isotope analysis (collagen preservation permitting). Further zooarchaeological research in the wider region could also help to cross correlate species identification and abundance with spatial distribution data (as inferred by isotopes). Finally, complementary techniques such as aDNA analysis, geometric morphometrics, and dental microwear would also allow a better understanding of the spatial palaeoecology, niche feeding behaviours, and community structure of ungulates in this glacial refugia zone in MIS 6.
5.2. Neanderthal hunting
Based on the isotope data, and spatial assignment, it is possible that Neanderthals could have hunted ibex year-round and red deer in the winter when their location could have been closer to the cave site. This hypothesis is in agreement with Valensi et al. (2013, p. 128–131) who postulated that the hominins responsible for the deposits in layer UA25 utilised the site in late autumn/early winter. While these results fit for previous hypotheses in UA25, it is interesting to note that every layer of occupation holds more red deer remains than any other fauna, whether the layer was a summer or winter occupation (Valensi, 2000; Steele, 2002; Valensi and Psathi, 2004). This again supports the notion that, while there may have been marked summer and winter ranges, total red deer annual range may have been relatively small (i.e., they could be found within 20 km of the site all year round) and also relatively conservative throughout the period of site use.
While ibex territory may have been closer to Lazaret Cave throughout the year, the high abundance of red deer remains throughout all archaeological layers shows Neanderthal hunting preference. One possible reason for preferential red deer hunting versus ibex is the calorific intake versus energy spent hunting. A full red deer has a calorific value of 163,680 calories (muscles), while ibex have a much lower muscle calorific value of only 45,780 calories (Cole, 2017). Furthermore, the energy expenditure to hunt ibex in steep, cliff environments is potentially greater than hunting deer in flatter environments, especially when considering ibex anti-predator behaviours to escape to inaccessible terrain (Grignolio et al., 2007, 2019; Sarmento and Berger, 2020). This level of focus on hunting strategies suggests a high level of cognition in early Neanderthals living at Lazaret Cave.
6. Conclusion
This pilot study has explored mobility in three red deer and a single ibex from Lazaret Cave (layer UA25) using multi-isotopic analysis, isoscape modelling, and spatial assignment. LA-MC-ICP-MS 87Sr/86Sr data provides a high-resolution account of movements made during the first years of life for these different individuals and spatial assignment of seasonal peaks and troughs in the intra-tooth data identified two distinct regions which red deer and ibex could have occupied. Unlike ibex, red deer likely undertook seasonal movements albeit over a fairly restrictive total range and could have been within 20 km of Lazaret throughout the year. Mapping the seasonal range locations on an elevation map supports a distinct seasonal altitudinal migration in red deer, with red deer more likely to occupy higher elevations further from Lazaret in the summer and lowland areas closer to the cave site in winter. These findings agree with past zooarchaeological studies of Lazaret Cave which hypothesise a winter occupation in UA25 and hunting deer in late autumn to early winter. Furthermore, our preliminary results allow us to suggest that the Neanderthals at Lazaret Cave had seasonal and sustainable management practices, in terms of hunting activities, in the landscape surrounding Lazaret Cave. Further analyses will allow us to improve this hypothesis. Finally, results from this pilot study highlight the importance of isotope zooarchaeology not only in better understanding the archaeological record at Pleistocene sites, but also the value of utilising zooarchaeological remains to better understand the paleoecology of extinct and ancestral species.
Data availability statement
The original contributions presented in the study are included in the article/Supplementary material, further inquiries can be directed to the corresponding author.
Ethics statement
Ethical review and approval was not required for the animal study because this study involved palaeontological faunal remains.
Author contributions
SB undertook data analysis, oxygen sampling, spatial analysis modelling, and wrote the initial draft of manuscript. MC undertook data analyses including statistics, isoscape modelling, and spatial assignment. MW undertook strontium sampling and laser ablation data analysis. ED and MW designed study, with KB. ED, JC, and MV provided specialist archaeological/site-based knowledge. KB oversaw isotopic analysis and data interpretation, and edited the initial draft of the manuscript. All authors contributed to the article and approved the submitted version.
Funding
This research is part of the collective research project named “Paleoecology of the Lazaret Cave: human-environment interactions on the coast of the meridional Alps during the late Middle Pleistocene (MIS6),” granted by the DRAC PACA (French Ministry of Culture). SB thanks QUADRAT DTP NERC (NE/S007377/1) studentship for stipend support. KB and MC thank the Leverhulme Trust (RPG-2017-410 and PLP-2019-284) for support during the production of this paper.
Conflict of interest
The authors declare that the research was conducted in the absence of any commercial or financial relationships that could be construed as a potential conflict of interest.
Publisher’s note
All claims expressed in this article are solely those of the authors and do not necessarily represent those of their affiliated organizations, or those of the publisher, the editors and the reviewers. Any product that may be evaluated in this article, or claim that may be made by its manufacturer, is not guaranteed or endorsed by the publisher.
Supplementary material
The Supplementary material for this article can be found online at: https://www.frontiersin.org/articles/10.3389/fevo.2023.988837/full#supplementary-material
References
Albon, S. D., Clutton-Brock, T. H., and Guinness, F. E. (1987). Early development and population dynamics in Red Deer. II. density-independent effects and cohort variation. J. Anim. Ecol. 56, 69–81. doi: 10.2307/4800
Albon, S. D., and Langvatn, R. (1992). Plant phenology and the benefits of migration in a temperate ungulate. Oikos 65, 502–513. doi: 10.2307/3545568
Balasse, M. (2002). Reconstructing dietary and environmental history from enamel isotopic analysis: time resolution of intra-tooth sequential sampling. Int. J. Osteoarchaeol. 12, 155–165. doi: 10.1002/oa.601
Bataille, C. P., von Holstein, I. C. C., Laffoon, J. E., Willmes, M., Liu, X.-M., and Davies, G. R. (2018). A bioavailable strontium isoscape for Western Europe: a machine learning approach. PLoS One 13:e0197386. doi: 10.1371/journal.pone.0197386
Bentley, R. A. (2006). Strontium isotopes from the earth to the archaeological skeleton: a review. J. Archaeol. Method Theory 13, 135–187. doi: 10.1007/s10816-006-9009-x
Bocherens, H. (2015). Isotopic tracking of large carnivore palaeoecology in the mammoth steppe. Quat. Sci. Rev. 117, 42–71. doi: 10.1016/j.quascirev.2015.03.018
Bocherens, H., Díaz-Zorita Bonilla, M., Daujeard, C., Fernandes, P., Raynal, J. P., and Moncel, M. H. (2016). Direct isotopic evidence for subsistence variability in middle Pleistocene Neanderthals (payre, southeastern France). Quat. Sci. Rev. 154, 226–236. doi: 10.1016/j.quascirev.2016.11.004
Britton, K., Grimes, V., Dau, J., and Richards, M. P. (2009). Reconstructing faunal migrations using intra-tooth sampling and strontium and oxygen isotope analyses: a case study of modern caribou (Rangifer tarandus granti). J. Archaeol. Sci. 36, 1163–1172. doi: 10.1016/j.jas.2009.01.003
Britton, J., Jimenez, E.-L., le Corre, M., Pederzani, S., Daujeard, C., Jaouen, K., et al. (2023). Multi-isotope zooarchaeological investigations at Abri du Maras: the paleoecological and paleoenvironmental context of Neanderthal subsistence strategies in the Rhône Valley during MIS 3. J. Hum. Evol. 174:103292. doi: 10.1016/j.jhevol.2022.103292
Britton, K., le Corre, M., Willmes, M., Moffat, I., Grün, R., Mannino, M. A., et al. (2020). Sampling plants and malacofauna in 87Sr/86Sr bioavailability studies: implications for isoscape mapping and reconstructing of past mobility patterns. Front. Ecol. Evol. 8:579473. doi: 10.3389/fevo.2020.579473
Britton, K., Pederzani, S., Kindler, L., Roebroeks, W., Gaudzinski-Windheuser, S., Richards, M. P., et al. (2019). Oxygen isotope analysis of Equus teeth evidences early Eemian and early Weichselian palaeotemperatures at the middle Palaeolithic site of Neumark-Nord 2, Saxony-Anhalt, Germany. Quat. Sci. Rev. 226:106029. doi: 10.1016/j.quascirev.2019.106029
Brown, W. A. B., and Chapman, N. G. (1991). Age assessment of red deer (Cervus elaphus): from a scoring scheme based on radiographs of developing permanent molariform teeth. J. Zool. 225, 85–97. doi: 10.1111/j.1469-7998.1991.tb03803.x
Capo, R. C., Stewart, B. W., and Chadwick, O. A. (1998). Strontium isotopes as tracers of ecosystem processes: theory and methods. Geoderma 82, 197–225. doi: 10.1016/S0016-7061(97)00102-X
Carrión, J. S., and Walker, M. J. (2019). Background to Neanderthal presence in Western Mediterranean Europe. Quat. Sci. Rev. 217, 7–44. doi: 10.1016/J.QUASCIREV.2018.10.011
Cauche, D. (2012). Productions lithiques et comportements techno-économiques de groupes humains acheuléens et moustériens en région liguro-provençale. Comptes Rendus Palevol 11, 519–527. doi: 10.1016/J.CRPV.2011.12.008
Clutton-Brock, T. H., Guinness, F. E., and Albon, S. D. (1982). Red Deer: behavior and ecology of two sexes. Edinburgh: University of Chicago Press.
Cole, J. (2017). Assessing the calorific significance of episodes of human cannibalism in the Palaeolithic. Sci. Rep. 7:44707. doi: 10.1038/srep44707
Copeland, S. R., Sponheimer, M., de Ruiter, D. J., Lee-Thorp, J. A., Codron, D., le Roux, P. J., et al. (2011). Strontium isotope evidence for landscape use by early hominins. Nature 474, 76–78. doi: 10.1038/nature10149
Copeland, S. R., Sponheimer, M., le Roux, P. J., Grimes, V., Lee-Thorp, J. A., de Ruiter, D. J., et al. (2008). Strontium isotope ratios (87Sr/86Sr) of tooth enamel: a comparison of solution and laser ablation multicollector inductively coupled plasma mass spectrometry methods. Rapid Commun. Mass Spectrom. 22, 3187–3194. doi: 10.1002/rcm.3717
Croitor, R. (2020). A new form of wapiti Cervus canadensis Erxleben, 1777 (Cervidae, Mammalia) from the late Pleistocene of France. Palaeoworld 29, 789–806. doi: 10.1016/j.palwor.2019.12.001
Croitor, R., and Obada, T. (2018). On the presence of late Pleistocene wapiti, Cervus canadensis Erxleben, 1777 (Cervidae, Mammalia) in the Palaeolithic site Climăuți II (Moldova). Contrib. Zool. 87, 1–10. doi: 10.1163/18759866-08701001
Crowley, B. E., and Wheatley, P. V. (2014). To bleach or not to bleach? Comparing treatment methods for isolating biogenic carbonate. Chem. Geol. 381, 234–242. doi: 10.1016/j.chemgeo.2014.05.006
Dansgaard, W. (1964). Stable isotopes in precipitation. Tellus 16, 436–468. doi: 10.3402/tellusa.v16i4.8993
de Lumley, H. (1969). A Palaeolithic camp at Nice. Sci. Am. 220, 42–50. doi: 10.1038/scientificamerican0569-42
de Lumley, H. (2016). “La Grotte du Cavillon sous la falaise des Baousse Rousse. Grimaldi, Vintimille, Italie,” in Éditions du Centre National de la Recherche Scientifique (Paris: CNRS éditions).
De Lumley, M. (2018). Les restes humains fossiles de la grotte du Lazaret. Nice, Alpes-Maritimes, France. Des Homo erectus européens évolués en voie de néandertalisation. (Paris: CNRS Publication).
de Lumley, H., Echassoux, A., Bailon, S., Cauche, D., de Marchi, M.-P., Desclaux, E., et al. (2004). Le sol d’occupation acheuléen de l’unité archéostratigraphique UA 25 de La Grotte du Lazaret. Edisud, Aix-en-Provence.
Demény, A., Gugora, A. D., Kesjár, D., Lécuyer, C., and Fourel, F. (2019). Stable isotope analyses of the carbonate component of bones and teeth: the need for method standardization. J. Archaeol. Sci. 109:104979. doi: 10.1016/j.jas.2019.104979
Desclaux, E. (2013). Evolution des communautés de mammifères dans les Alpes-Maritimes au cours du Pléistocène. Bulletin du Mus’ee d’Anthropologie pr’ehistorique de Monaco, suppl. n°4, 9–19.
Desclaux, E., Auguste, P., Blain, H.-A., Blasco, R., Bray, F., Cohen, J., et al. (2021). Projet Collectif de Recherche, Paléoécologie de la grotte du Lazaret, Interactions « Hommes-Milieu(x) » sur le littoral maralpin au cours du Pléistocène moyen final (MIS 6). Rapport d’étude, DRAC PACA.
Desclaux, E., Hazzazi, N. E. L., Vilette, P., and Dubar, M. (2008). Le contexte paléoenvironmental des occupations humaines. L’apport de la microfaune, des restes aviaries et de la malacofaune. Le site de payre-occupations humaines dand la vallée du Rhône à ña fin du Pléistocène moyen et au début du Pléistocène supérieur. Mémoire de la S.P.F, XLVI. Mem. French Prehist. Soc. 46, 91–106.
Elderfield, H., Ferretti, P., Greaves, M., Crowhurst, S., McCave, I. N., Hodell, D., et al. (2012). Evolution of ocean temperature and ice volume through the mid-Pleistocene climate transition. Science 337, 704–709. doi: 10.1126/SCIENCE.1221294
Esri (2022). “Topographic” [basemap]. Scale not given. “world topographic map”. Available at: https://www.arcgis.com/home/item.html?id=7dc6cea0b1764a1f9af2e679f642f0f5 (Accessed May 1, 2022).
Esri (2015). DeLorme, HERE, TomTom, Intermap, increment P Corp., GEBCO, USGS, FAO, NPS, NRCAN, GeoBase, IGN, Kadaster NL, ordnance survey, Esri Japan, METI, Esri China (Hong Kong), swisstopo, MapmyIndia, and the GIS user community.
Evans, J. A., Chenery, C. A., and Montgomery, J. (2012). A summary of strontium and oxygen isotope variation in archaeological human tooth enamel excavated from Britain. J. Anal. At. Spectrom. 27, 754–764. doi: 10.1039/c2ja10362a
Fiore, I., Gala, M., and Tagliacozzo, A. (2004). Ecology and subsistence strategies in the eastern Italian Alps during the middle Palaeolithic. Int. J. Osteoarchaeol. 14, 273–286. doi: 10.1002/oa.761
Geist, V. (1998). Deer of the world: their evolution, behaviour, and ecology. Mechanicsburg, PA: Stackpole Books.
Gigleux, C., Grimes, V., Tütken, T., Knecht, R., and Britton, K. (2019). Reconstructing caribou seasonal biogeography in little ice age (late Holocene) Western Alaska using intra-tooth strontium and oxygen isotope analysis. J. Archaeol. Sci. Rep. 23, 1043–1054. doi: 10.1016/j.jasrep.2017.10.043
Gräler, B., Pebesma, E., and Heuvelink, G. (2016). Spatio-temporal interpolation using gstat. Available at: https://cran.r-project.org/web/views/SpatioTemporal.html
Grignolio, S., Brivio, F., Sica, N., and Apollonio, M. (2019). Sexual differences in the behavioural response to a variation in predation risk. Ethology 125, 603–612. doi: 10.1111/eth.12887
Grignolio, S., Rossi, I., Bassano, B., and Apollonio, M. (2007). Predation risk as a factor affecting sexual segregation in alpine ibex. J. Mammal. 88, 1488–1497. doi: 10.1644/06-MAMM-A-351R.1
Hanquet, C. (2011). Évolution des paléoenvironnements et des paléoclimats au Pléistocène moyen, en Europe méridionale, d’après l’étude des faunes de micromammifères – TEL – Thèses en ligne, Archéologie et Préhistoire. Ph.D. Thesis, Université Paul Valéry- Montpellier III, HAL Id.
Hanquet, C., Valensi, P., Bailon, S., Desclaux, E., el Guennouni, K., Roger, T., et al. (2010). Caractérisation du climat et de la biodiversité au Pléistocène moyen final, d’après les faunes de vertébrés de la grotte du Lazaret (Nice, France). Quaternaire 21, 203–214. doi: 10.4000/QUATERNAIRE.5579
Hartmann, J., and Moosdorf, N. (2012). The new global lithological map database GLiM: A representation of rock properties at the earth surface. Geochem. Geophys. Geosyst 13, 1–37. doi: 10.1029/2012GC004370
Hassani, M., Valensi, P., Visweswara, S. C., Michel, V., Guennouni, K. E. L., and de Lumley, H. (2017). Les petits ongulés de la grotte acheuléano-moustérienne du Lazaret (Nice, France) – Origine anthropique ou carnivore? Anthropologie (France) 121, 367–393. doi: 10.1016/j.anthro.2017.10.007
Holt, E., Evans, J. A., and Madgwick, R. (2021). Strontium (87Sr/86Sr) mapping: a critical review of methods and approaches. Earth Sci. Rev. 216:103593. doi: 10.1016/j.earscirev.2021.103593
Iacumin, P., Bocherens, H., Mariotti, A., and Longinelli, A. (1996). Oxygen isotope analyses of co-existing carbonate and phosphate in biogenic apatite: a way to monitor diagenetic alteration of bone phosphate? Earth Planet. Sci. Lett. 142, 1–6. doi: 10.1016/0012-821X(96)00093-3
Jones, J., and Britton, K. (2019). Multi-scale, integrated approaches to understanding the nature and impact of past environmental and climatic change in the archaeological record, and the role of isotope zooarchaeology. J. Archaeol. Sci. Rep. 23, 968–972. doi: 10.1016/j.jasrep.2019.02.001
Koch, P. L., Tuross, N., and Fogel, M. L. (1997). The effects of sample treatment and diagenesis on the isotopic integrity of carbonate in biogenic Hydroxylapatite. J. Archaeol. Sci. 24, 417–429. doi: 10.1006/jasc.1996.0126
Kropil, R., Smolko, P., and Garaj, P. (2015). Home range and migration patterns of male red deer Cervus elaphus in Western Carpathians. Eur. J. Wildl. Res. 61, 63–72. doi: 10.1007/s10344-014-0874-4
Langvatn, R., and Albon, S. D. (1986). Geographic clines in body weight of Norwegian red deer: a novel explanation of Bergmann’s rule? Ecography 9, 285–293. doi: 10.1111/j.1600-0587.1986.tb01221.x
Lazzerini, N., Balter, V., Coulon, A., Tacail, T., Marchina, C., Lemoine, M., et al. (2021). Monthly mobility inferred from isoscapes and laser ablation strontium isotope ratios in caprine tooth enamel. Sci. Rep. 11:2277. doi: 10.1038/s41598-021-81923-z
Lewis, J., Coath, C. D., and Pike, A. W. G. (2014). An improved protocol for 87Sr/86Sr by laser ablation multi-collector inductively coupled plasma mass spectrometry using oxide reduction and a customised plasma interface. Chem. Geol. 390, 173–181. doi: 10.1016/j.chemgeo.2014.10.021
Lister, A. M. (1984). Evolutionary and ecological origins of British deer. Proc. R. Soc. Edinburgh Sect. B: Biol. Sci. 82, 205–229. doi: 10.1017/S0269727000003754
Longinelli, A. (1984). Oxygen isotopes in mammal bone phosphate: a new tool for paleohydrological and paleoclimatological research? Geochim. Cosmochim. Acta 48, 385–390. doi: 10.1016/0016-7037(84)90259-X
López-García, J. M., Blain, H. A., Sánchez-Bandera, C., Cohen, J., Lebreton, L., Montuire, S., et al. (2021). Multi-method approach using small vertebrate assemblages to reconstruct the marine isotope stage 6 climate and environment of the lazaret cave sequence (maritime Alps, Nice, France). Palaeogeogr. Palaeoclimatol. Palaeoecol. 577:110529. doi: 10.1016/j.palaeo.2021.110529
Lugli, F., Cipriani, A., Bruno, L., Ronchetti, F., Cavazzuti, C., and Benazzi, S. (2022). A strontium isoscape of Italy for provenance studies. Chem. Geol. 587:120624. doi: 10.1016/j.chemgeo.2021.120624
Lugli, F., Weber, M., Giovanardi, T., Arrighi, S., Bortolini, E., Figus, C., et al. (2020). Fast offline data reduction of laser ablation MC-ICP-MS Sr isotope measurements via the interactive excel-based spreadsheet ‘SrDR.’. J. Anal. At. Spectrom. 35, 852–862. doi: 10.1039/c9ja00424f
Ma, C., Vander Zanden, H. B., Wunder, M. B., and Bowen, G. J. (2020). assignR: an r package for isotope-based geographic assignment. Methods Ecol. Evol. 11, 996–1001. doi: 10.1111/2041-210X.13426
Margari, V., Skinner, L. C., Tzedakis, P. C., Ganopolski, A., Vautravers, M., and Shackleton, N. J. (2010). The nature of millennial-scale climate variability during the past two glacial periods. Nat. Geosci. 3, 127–131. doi: 10.1038/ngeo740
Michel, V., Ildefonse, P., and Morin, G. (1995). Chemical and structural changes in Cervus elaphus tooth enamels during fossilization (lazaret cave): a combined IR and XRD Rietveld analysis. Appl. Geochem. 10, 145–159. doi: 10.1016/0883-2927(95)00001-Z
Michel, V., Ildefonse, P., and Morin, G. (1996). Assessment of archaeological bone and dentine preservation from lazaret cave (middle Pleistocene) in France. Palaeogeogr. Palaeoclimatol. Palaeoecol. 126, 109–119. doi: 10.1016/S0031-0182(96)00074-0
Michel, V., Shen, G., Valensi, P., and de Lumley, H. (2009). ESR dating of dental enamel from middle Palaeolithic levels at lazaret cave, France. Quat. Geochronol. 4, 233–240. doi: 10.1016/j.quageo.2008.07.003
Moussous, A., Valensi, P., and Simon, P. (2014). Identification de l’ivoire de Proboscidiens des grottes de Balzi Rossi (Ligurie, Italie) à partir de la méthode des lignes de Schreger. Bull. Mus. Anthropo. Prehist. Monaco 54, 83–90.
Mysterud, A., Loe, L. E., Zimmermann, B., Bischof, R., Meisingset, E., Mysterud, A., et al. (2011). Partial migration in expanding red deer populations at northern latitudes – a role for density dependence? Oikos 120, 1817–1825. doi: 10.1111/j.1600-0706.2010.19439.x
Mysterud, A., Qviller, L., Meisingset, E. L., and Viljugrein, H. (2016). Parasite load and seasonal migration in red deer. Oecologia 180, 401–407. doi: 10.1007/s00442-015-3465-5
Nielsen, S. (2004). The biological role of strontium. Bone 35, 583–588. doi: 10.1016/j.bone.2004.04.026
Pederzani, S., Aldeias, V., Dibble, H. L., Goldberg, P., Hublin, J. J., Madelaine, S., et al. (2021a). Reconstructing late Pleistocene paleoclimate at the scale of human behavior: an example from the Neandertal occupation of La Ferrassie (France). Sci. Rep. 11:1419. doi: 10.1038/s41598-020-80777-1
Pederzani, S., and Britton, K. (2019). Oxygen isotopes in bioarchaeology: principles and applications, challenges and opportunities. Earth-Sci. Rev. 188, 77–107. doi: 10.1016/j.earscirev.2018.11.005
Pederzani, S., Britton, K., Aldeias, V., Bourgon, N., Fewlass, H., Lauer, T., et al. (2021b). Subarctic climate for the earliest Homo sapiens in Europe. Sci. Adv. 7, 1–10. doi: 10.1126/sciadv.abi4642
Pellegrini, M., and Snoeck, C. (2016). Comparing bioapatite carbonate pre-treatments for isotopic measurements: part 2 – impact on carbon and oxygen isotope compositions. Chem. Geol. 420, 88–96. doi: 10.1016/j.chemgeo.2015.10.038
Pilaar Birch, S. E., Miracle, P. T., Stevens, R. E., and O’Connell, T. C. (2016). Late Pleistocene/early Holocene migratory behavior of ungulates using isotopic analysis of tooth enamel and its effects on forager mobility. PLoS One 11:e0155714. doi: 10.1371/journal.pone.0155714
Ponel, P. (1995). Rissian, Eemian and Würmian Coleoptera assemblages from La Grande Pile (Vosges, France). Palaeogeography, Palaeoclimatology, Palaeoecology 114, 1–41. doi: 10.1016/0031-0182(95)00083-X
Price, T. D., Meiggs, D., Weber, M. J., and Pike-Tay, A. (2017). The migration of late Pleistocene reindeer: isotopic evidence from northern Europe. Archaeol. Anthropol. Sci. 9, 371–394. doi: 10.1007/s12520-015-0290-z
Qviller, L., Risnes-Olsen, N., Bærum, K. M., Meisingset, E. L., Loe, L. E., Ytrehus, B., et al. (2013). Landscape level variation in tick abundance relative to seasonal migration in red deer. PLoS One 8:e71299. doi: 10.1371/journal.pone.0071299
Rivals, F., Julien, M.-A., Kuitems, M., Van Kolfschoten, T., Serangeli, J., Drucker, D. G., et al. (2015). Investigation of equid paleodiet from Schöningen 13 II-4 through dental wear and isotopic analyses: archaeological implications. J. Hum. Evol. 89, 129–137. doi: 10.1016/j.jhevol.2014.04.002
Roucoux, K. H., Tzedakis, P. C., Lawson, I. T., and Margari, V. (2011). Vegetation history of the penultimate glacial period (marine isotope stage 6) at Ioannina, north-West Greece. J. Quat. Sci. 26, 616–626. doi: 10.1002/JQS.1483
Roussel, A., Lionel, G., Valensi, P., and Brugal, J.-P. (2019). Large game hunting strategies in middle Palaeolithic of southern France: contribution of dental cementum analysis through the study of the Canalettes rockshelter and lazaret cave sites. Bern, Switzerland: European Association of Archaeologists.
Sarmento, W., and Berger, J. (2020). Conservation implications of using an imitation carnivore to assess rarely used refuges as critical habitat features in an alpine ungulate. PeerJ 8:e9296. doi: 10.7717/peerj.9296
Skippington, J., Veth, P., Manne, T., and Slack, M. (2019). Preanalytical processing of archaeological mammal enamel apatite carbonates for stable isotope investigations: a comparative analysis of the effect of acid treatment on samples from Northwest Australia. Int. J. Osteoarchaeol. 29, 760–771. doi: 10.1002/oa.2787
Snoeck, C., and Pellegrini, M. (2015). Comparing bioapatite carbonate pre-treatments for isotopic measurements: part 1–impact on structure and chemical composition. Chem. Geol. 417, 394–403. doi: 10.1016/j.chemgeo.2015.10.004
Sponheimer, M., and Lee-Thorp, J. A. (2001). The oxygen isotope composition of mammalian enamel carbonate from Morea estate, South Africa. Oecologia 126, 153–157. doi: 10.1007/s004420000498
Steele, T. E. (2002). Red Deer: Their Ecolgy and how they were hunted by late Pleistocene hominids in Western Europe. PhD Thesis, Department of Anthropological Sciences Stanford University.
Stevens, R. E., Balasse, M., and O’Connell, T. C. (2011). Intra-tooth oxygen isotope variation in a known population of red deer: implications for past climate and seasonality reconstructions. Palaeogeogr. Palaeoclimatol. Palaeoecol. 301, 64–74. doi: 10.1016/j.palaeo.2010.12.021
Valensi, P. (2000). The archaeozoology of lazaret cave (Nice, France). Int. J. Osteoarchaeol. 10, 357–367. doi: 10.1002/1099-1212(200009/10)10:5<357::AID-OA561>3.0.CO;2-W
Valensi, P. (2009). Evolution des peuplements mammaliens en Europe méditerranéenne occidentale durant le Pléistocène moyen et supérieur. Un exemple régional: les Alpes du Sud françaises et italiennes. Quaternaire 20, 551–567. doi: 10.4000/QUATERNAIRE.5368
Valensi, P., Bailon, S., Michel, V., Desclaux, E., Rousseau, L., Onoratini, G., et al. (2007). invertebrate fauna Cadre climatique et environnemental des acheuléens de la grotte du Lazaret, à Nice. Données paléontologiques, biogéochimiques et radiométriques établies sur les faunes de vertébrés et d’invertébrés Climatic and environmental condit. ArcheoSciences Revue d’archéométrie 31, 137–150. doi: 10.4000/archeosciences.844
Valensi, P., Michel, V., el Guennouni, K., and Liouville, M. (2013). New data on human behavior from a 160,000 year old Acheulean occupation level at lazaret cave, south-East France: an archaeozoological approach. Quat. Int. 316, 123–139. doi: 10.1016/j.quaint.2013.10.034
Valensi, P., and Psathi, E. (2004). Faunal exploitation during the middle Palaeolithic in South-Eastern France and North-Western Italy. Int. J. Osteoarchaeol. 14, 256–272. doi: 10.1002/oa.760
Vroon, P. Z., van der Wagt, B., Koornneef, J. M., and Davies, G. R. (2008). Problems in obtaining precise and accurate Sr isotope analysis from geological materials using laser ablation MC-ICPMS. Anal. Bioanal. Chem. 390, 465–476. doi: 10.1007/s00216-007-1742-9
Wainer, K., Genty, D., Blamart, D., Bar-Matthews, M., Quinif, Y., and Plagnes, V. (2013). Millennial climatic instability during penultimate glacial period recorded in a south-western France speleothem. Palaeogeography, Palaeoclimatology, Palaeoecology 376, 122–131. doi: 10.1016/J.PALAEO.2013.02.026
Willmes, M., Bataille, C. P., James, H. F., Moffat, I., McMorrow, L., Kinsley, L., et al. (2018a). Mapping of bioavailable strontium isotope ratios in France for archaeological provenance studies. Appl. Geochem. 90, 75–86. doi: 10.1016/j.apgeochem.2017.12.025
Willmes, M., Kinsley, L., Moncel, M.-H. H., Armstrong, R. A., Aubert, M., Eggins, S., et al. (2016). Improvement of laser ablation in situ micro-analysis to identify diagenetic alteration and measure strontium isotope ratios in fossil human teeth. J. Archaeol. Sci. 70, 102–116. doi: 10.1016/j.jas.2016.04.017
Willmes, M., McMorrow, L., Kinsley, L., Armstrong, R., Aubert, M., Eggins, S., et al. (2014). The IRHUM (isotopic reconstruction of human migration) database – bioavailable strontium isotope ratios for geochemical fingerprinting in France. Earth Syst. Sci. Data 6, 117–122. doi: 10.5194/essd-6-117-2014
Willmes, M., Ransom, K. M., Lewis, L. S., Denney, C. T., Glessner, J. J. G., and Hobbs, J. A. (2018b). IsoFishR: an application for reproducible data reduction and analysis of strontium isotope ratios (87 Sr/86 Sr) obtained via laser-ablation MC-ICP-MS. PLoS One 13:e0204519. doi: 10.1371/journal.pone.0204519
Wood, R., Fleury, A. B. C., Fallon, S., Nguyen, T. M. H., and Nguyen, A. T. (2021). Do weak or strong acids remove carbonate contamination from ancient tooth enamel more effectively? The effect of acid pretreatment on radiocarbon and δ13C analyses. Radiocarbon 63, 935–952. doi: 10.1017/RDC.2021.32
Keywords: spatial palaeoecology, Middle Palaeolithic, Neanderthals, Saalian glaciation, isoscape, multi-isotope analysis, southern France
Citation: Barakat S, Le Corre M, Willmes M, Cohen J, Vuillien M, Desclaux E and Britton K (2023) Laser ablation strontium isotopes and spatial assignment show seasonal mobility in red deer (Cervus elaphus) at Lazaret Cave, France (MIS 6). Front. Ecol. Evol. 11:988837. doi: 10.3389/fevo.2023.988837
Edited by:
Peter David Roopnarine, California Academy of Sciences, United StatesReviewed by:
Roman Croitor, Academy of Sciences of Moldova (ASM), MoldovaMaciej Tomasz Krajcarz, Institute of Geological Sciences, Polish Academy of Sciences, Poland
Copyright © 2023 Barakat, Le Corre, Willmes, Cohen, Vuillien, Desclaux and Britton. This is an open-access article distributed under the terms of the Creative Commons Attribution License (CC BY). The use, distribution or reproduction in other forums is permitted, provided the original author(s) and the copyright owner(s) are credited and that the original publication in this journal is cited, in accordance with accepted academic practice. No use, distribution or reproduction is permitted which does not comply with these terms.
*Correspondence: Sarah Barakat, cy5iYXJha2F0LjIwQGFiZG4uYWMudWs=