- 1Department of Anatomy, New York Institute of Technology College of Osteopathic Medicine, Old Westbury, NY, United States
- 2Division of Paleontology, American Museum of Natural History, New York, NY, United States
- 3Quaternary Palaeontology Program, Royal Alberta Museum, Edmonton, AB, Canada
- 4Division of Dermatology, Department of Medicine, University of Calgary, Calgary, AB, Canada
- 5Department of Biological Sciences, University of Calgary, Calgary, AB, Canada
Mesowear is a dietary proxy that relates attritive wear and abrasive wear to the shape of worn tooth cusps of ungulates. Traditional mesowear methods categorize cusps according to relief and sharpness. A geometric morphometric approach has the potential to measure shape with higher precision and to discover unrecognized aspects of cusp shape, possibly improving the efficacy of mesowear. We quantified mesowear in extant Ruminantia, using a 2-D semilandmark outline technique on upper second molar metacones generated from photographs. Among the 91 species sampled, 65 were preassigned to dietary categories, browser, grazer, mixed feeder, and frugivore based on substantiated documentation of diet in the wildlife literature. Metacone cusp shape and metacone mesowear score were found to be independent of size. Principal component and discriminant function analyses of Procrustes transformed semilandmark coordinates revealed two diet-related components of cusp shape. The primary component is related to the traditional mesowear variables of cusp height and side steepness. The secondary shape component reveals variation in the mesiodistal symmetry of the metacone and may relate to a proal vector during the power stroke phase or the relative orientation of the cusps with respect to the chewing stroke vector. Discriminant function analysis of semilandmark data accurately classified the diets of species more frequently (67.2%) than the traditional mesowear method (56.1%). The semilandmark data successfully recognized the diets of grazing and browsing species with correct classification rates ranging from 69% to 95%. The diets of frugivorous and mixed feeding species were less frequently correctly recognized (33%–53%). Mixed feeding diets may be more difficult to recognize due to more heterogeneous diets when compared to browsers and grazers. Frugivores are more difficult to recognize because their rounded cusp apices resemble those of mixed feeders and grazers. We conclude that quantitative shape analysis improves the potential of mesowear. When used as a dietary proxy, we anticipate that mesowear analysis will correctly categorize the diets of most species. When misclassifications are made, they may most often be misclassifications of generalist mixed feeders and frugivores as either browsers or grazers.
Introduction
Mesowear analysis is a widely employed technique for testing hypotheses about the diets, feeding ecologies, and paleoenvironments of ungulates (Croft and Weinstein, 2008; Mihlbachler et al., 2011, 2017; Danowitz et al., 2016; Green and Croft, 2018; Jiménez-Hidalgo et al., 2019; Ackermans, 2020). Mesowear refers to the macroscopic morphologies of worn buccal cusp apices of the molar teeth of ungulates, which vary in ways that are correlated to the observed diets of extant species (e.g., Fortelius and Solounias, 2000; Franz-Odendaal and Kaiser, 2003; Fraser and Theodor, 2011; Louys et al., 2011, 2012; Fraser et al., 2014; Ackermans et al., 2020). Browsing species presumably have minimally abrasive diets and therefore experience predominantly attrition-dominated wear and maintain sharpened molar cusp apices with high amounts of occlusal relief as a consequence of their ability to maintain precise dental occlusion. Grazers, on the other hand, consume a greater abundance of highly-abrasive particles including opaline silicates, which are more abundant in grasses, and also higher amounts of geological particles (dust, silt, sand) as a consequence of feeding closer to the soil substrate (Sanson, 2006; Janis, 2008; Semprebon et al., 2019). The tendency for grazers to develop and maintain blunter and lower relief molar cusp apices has been explained as a result of more abrasion-dominated wear. Recent work has abundantly demonstrated tooth wear and occlusion to be a complex phenomenon whose causal factors are not easily unraveled (Sanson et al., 2018; Ackermans et al., 2020; Schulz-Kornas et al., 2020; Martin et al., 2021). While the general explanation of mesowear as a reflection of an attrition-abrasion gradient (Fortelius and Solounias, 2000) is, no doubt, an oversimplification (e.g., Erickson et al., 2016), there is, nonetheless, an abundantly demonstrated correlation between diet and apical cusp morphology among extant ungulates.
Fortelius and Solounias (2000) first evaluated molar cusp apical morphologies among ungulates and their relationship to diet using extant species. They found a strong association between diet (browsing, grazing, and mixed feeding) and the apical cusp morphology of worn buccal cusps of upper molars. They examined the buccal cusps of the second upper molars and categorized them based on relief and sharpness (Figure 1). Many mesowear studies have employed the original scoring technique or they have devised related scoring techniques that categorize cusps by some combination of relief and sharpness (Blondel et al., 2010; Semprebon and Rivals, 2010; Taylor et al., 2013; Ulbricht et al., 2015; Mihlbachler et al., 2017; Cohen et al., 2021). In its simplest representation (e.g., Mihlbachler et al., 2011), cusps are scored according to arbitrary stages along a shape continuum ranging from tall and sharp with steeply sloped sides, to low and dull with shallow sloping sides. Browsers and grazers occupy the extreme ends of this continuum and mixed feeders occupy the intermediate range. This conveniently tidy mesowear continuum is disrupted by frugivores which develop rounded cusp apices, presumably due to tip-crushing wear associated with mastication of hard objects (Janis, 1990; Fortelius and Solounias, 2000). The phenomenon of frugivore tooth wear has not been thoroughly studied in ruminants, nonetheless, small frugivorous ruminants maintain rounded cusp morphologies that resemble mixed feeders and grazers, thus rendering it difficult to differentiate the mesowear signatures of frugivorous diets from other diets in the fossil record (Mihlbachler and Solounias, 2006; Mihlbachler et al., 2011).
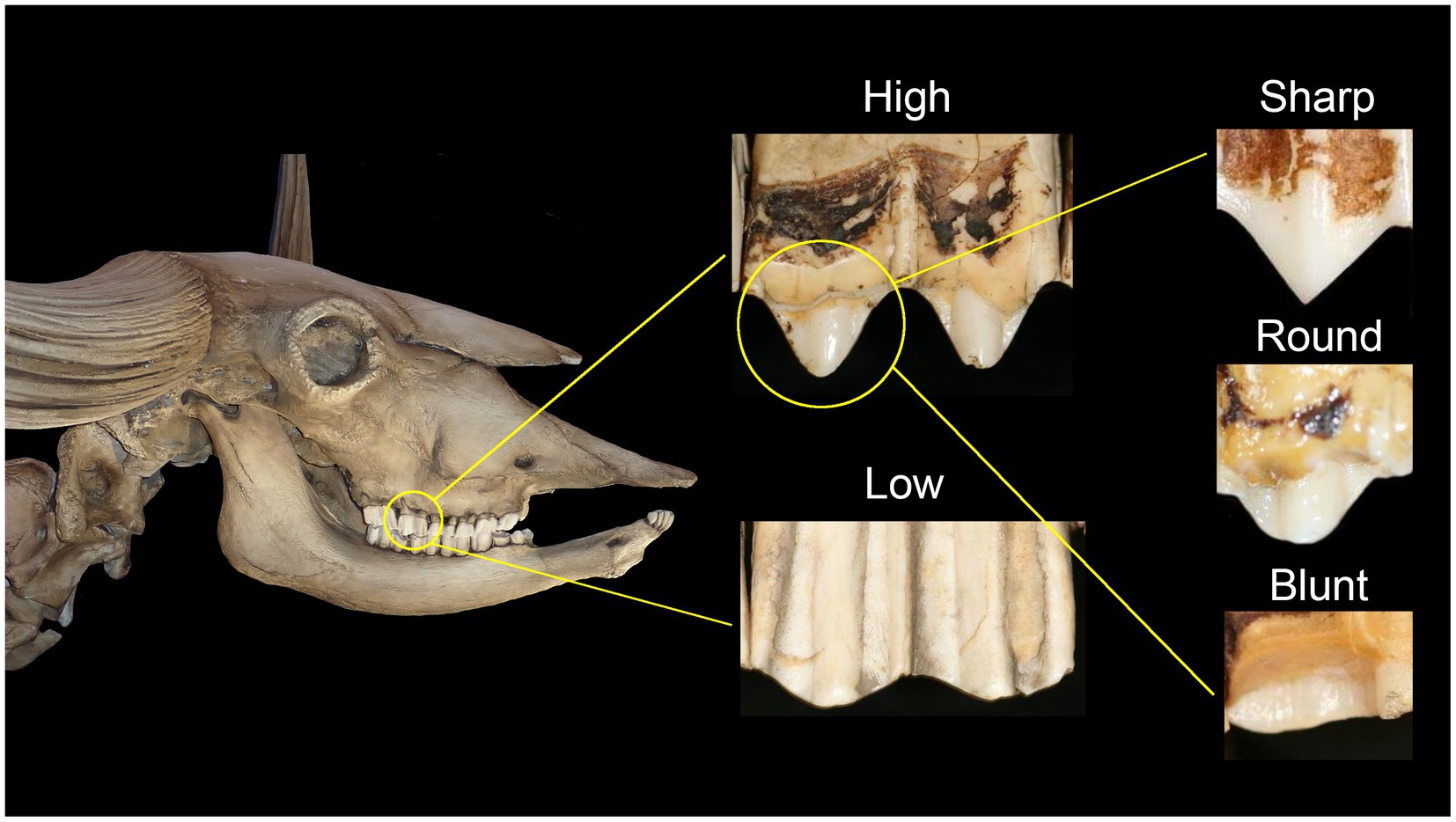
Figure 1. Traditional mesowear analysis uses a categorical approach to scoring cusp relief (high or low) and apical shape (sharp, round, or blunt). Browsing ungulates tend to have high and sharp cusp morphologies, whereas grazing ungulates tend to show low and blunt or rounded cusps, with mixed feeders showing intermediate morphologies.
Prior attempts to develop quantitative measures of mesowear involve measures of cusp heights and angles (Widga, 2006; Valli and Palombo, 2008; Fraser and Theodor, 2010; Loffredo and DeSantis, 2014; Saarinen et al., 2014; Jiménez-Manchón et al., 2021). However, as we reveal below, cusp shape varies in other ways. Geometric morphometric methods have the potential to shed light on more nuanced aspects of tooth mesowear and its relation to diet. To our knowledge, geometric morphometric methods have not been widely applied to mesowear (see Rødven et al., 2006 for an example). One concern is expediency. Unlike more laborious and costly methods, such as stable isotope analysis and dental microwear texture analysis, mesowear is a simple technique that facilitates the expedient compilation of large datasets (Mihlbachler et al., 2011; Semprebon et al., 2019). More laborious approaches of mesowear quantification, such as 3-D quantification (Hernesniemi et al., 2011) may offer finer-grained insights into the shapes of the occlusal facets of teeth, but such approaches also limit the potential for building large data sets thus removing one of the primary advantages of the technique.
In this paper, we describe an expedient 2-D geometric morphometric mesowear analysis using photographs in lateral view of upper molars and apply it to 91 species of extant ruminants. We hypothesize that quantitative measurement of cusp shape will provide additional insights into how cusp shape varies with diet. Secondly, we hypothesize that a more nuanced view of mesowear provided by morphometrics will sharpen the mesowear tool for predicting the diets of extinct species and other ancient populations.
Materials and methods
This study consists of 834 skulls representing 91 extant species of Ruminantia, following the taxonomy of Groves and Grubb (2011), housed at the mammalogy collections of the American Museum of Natural History (New York, USA), the Royal Alberta Museum (Edmonton, Canada), and the National Museum of Natural History, Smithsonian Institution (Washington D.C., USA). We prioritized maximization of taxonomic and ecological diversity; therefore, we sampled specimens without regard for locality, as long as the specimen was collected from a non-feral wild population. Additional insights may be achieved from more focused population-level studies, but this approach also has limitations due to the finite coverage of natural history collections. The species are divided into two tables (Tables 1, 2) for reasons explained below. We attempted to sample a minimum number of 10 specimens when possible but the number of available specimens for many species was fewer than 10. Our sample size for each species ultimately ranged from 1 to 20 (Tables 1, 2).
This study focuses on the metacone of the upper second molar (M2). The cusps of the different tooth positions frequently have non-identical apical morphologies and there is most often a wear gradient among the molars. The M1 tends to be the most worn, while the M3 tends to be the least worn. M2 most often tends to be in an intermediate state of wear. This wear gradient is related to the different eruption times (Ungar, 2010) and possibly also to the variable spatial relationships that teeth have with the temporomandibular joint and muscles of mastication which may differentially influence occlusal dynamics at different positions along the tooth row. While it is likely that the general conclusions of this study about the relationship of tooth wear and diet can be generalized to other cusps along the molar row, we caution that any subsequent direct comparisons to our data would be most meaningful if based on the same M2 metacone.
The amount of tooth wear that an animal has accumulated during its lifetime is a consideration when selecting specimens for mesowear analysis. Experimental evidence suggests that mesowear is more of a general signal than a seasonal one (Ackermans et al., 2020). All but the most brachydont ruminants tend to maintain their cusp morphologies in a state of relative stasis as the teeth wear, beginning with the initial development of wear facets and ending with the mechanical senescence of the tooth (Rivals et al., 2007). Bearing these basic findings in mind, we followed typical inclusion criteria for mesowear studies where young and old individuals were excluded. Inclusion criteria included a fully erupted adult dentition with visible wear on the third molar. Dentally senescent individuals, defined as having nearly all of the first molar crown worn away, were excluded.
Each species was assigned to one of four dietary categories based on the percentage of dicotyledons, monocotyledons, and fruits in their diet as follows: browsers (>70% leafy, woody components of dicotyledons), frugivores (>70% fruits), grazers (>70% monocotyledons), and mixed feeders (species external to the parameters of other categories). Evidence for diet in the literature for 65 out of the 91 species (Table 1), was considered by us to be sufficient and these were used extensively in the analyses described below. The remaining 26 species (Table 2) were used more sparingly as we were less confident about their dietary assignments. In these instances, the literature-based evidence for the diet was scant or of low quality, due to incomplete information or discrepancies in the percentages of food types reported between different studies. Other species relegated to Table 2, (e.g., Rangifer tarandus) have abundantly documented wild diets, but consume foods, such as moss and lichens, that fall outside of the scope of the four dietary categories. The species of Table 2 were used to gain a heuristic understanding of how insufficient evidence of diet and peculiar diets not fitting typical criteria may complicate the use of mesowear as a dietary proxy.
Image acquisition
We photographed the upper left cheek tooth row with focus on the second molar (M2) using a Canon EOS5D or Nikon D200 digital camera. In instances when the left M2 was damaged or pathological, we photographed the right tooth row and horizontally inverted the image. The specimens were oriented so that the buccal wall of the M2 was perpendicular to the camera. If lingual parts of the molars were visible through the camera (Figure 2A), the specimen was rotated slightly so that these parts of the tooth were obscured (Figure 2B). The specimens were handheld by the photographer and aligned this way by eye. With this approach, we attempted to sufficiently standardize the orientation of the specimen without greatly compromising expediency. While more precise orientation of specimens would have been possible with anchoring devices, this would have further limited the potential to sample large numbers of specimens and would have constrained the sizes and shapes of skulls that could be photographed.
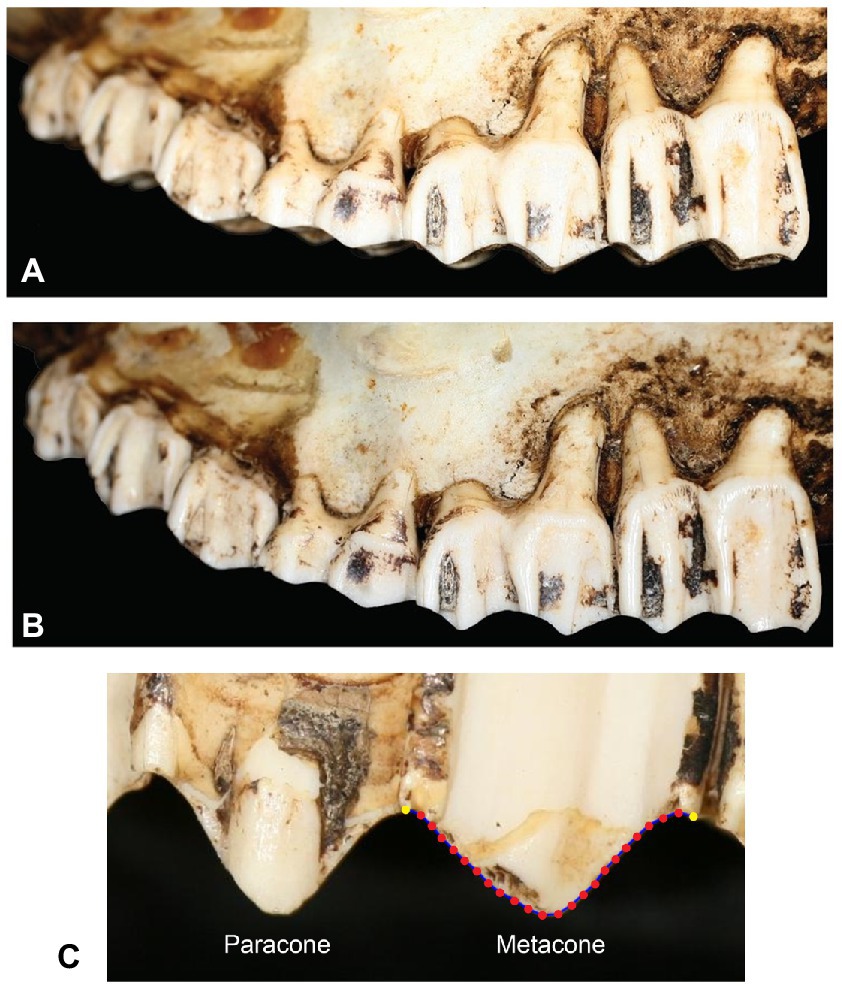
Figure 2. Left upper cheek tooth row of Cervus canadensis showing photographic angle. Specimens were oriented so the buccal wall of M2 was perpendicular to the camera lens. (A) When lingual sides of the cheek teeth were visible, (B) the specimen was rotated slightly until the lingual sides were obscured from view, and the photograph was taken. (C) Buccal view of the second upper molar (M2) of a specimen of Syncerus caffer (AMNH 53582) showing the landmarks (yellow) and semilandmarks (red) that were digitized on the occlusal outline of the metacone cusp. Digitization of the occlusal outline starts at the posterior end of the metacone (right side of the image) and ends at the metacone-paracone valley.
Landmark digitization and superimposition
Every image was renamed with a four-digit identifier generated at random, with the objective of mixing the sample of images and removing the identity of each image to minimize systematic biases during landmark digitization. We used tpsDig 2.32 (Rohlf, 2015) to digitize the outline of the occlusal edge of the metacone cusp, starting on the distal end of the metacone and finishing at the high point of the arch between the paracone and metacone. The resulting cusp outline was then resampled to obtain 24 evenly spaced points that were judged to adequately capture the metacone outline (Supplementary Table S1). Points 1 and 24 are regarded as landmarks and points 2–23 represent semilandmarks (Figure 2C). All of the specimens were digitized by the same researcher (CIB-O). Levels of photographing and digitization error were low (2.1%), as evidenced by Procrustes ANOVA performed on a subset of an earlier version of our dataset in which specimens were photographed and digitized twice (Barrón-Ortiz et al., 2013).
We superimposed the 834 landmark configurations in tpsRelw 1.75 (Rohlf, 2015) using a generalized least squares (GLS) Procrustes superimposition method in which semilandmarks were allowed to slide to minimize thin-plate spline bending energy. To accomplish this, we created a “sliders file” in tpsUtil 1.82 (Rohlf, 2015) to indicate that semilandmarks 2–23 were allowed to slide during GLS Procrustes superimposition. In standard GLS Procrustes superimposition, the configurations of landmarks are translated to the origin, scaled to unit centroid size (centroid size is the square root of the sum of squared distances of all the landmarks from their centroid), and rotated to minimize the summed square distances between homologous landmarks (Zelditch et al., 2004). A consensus (average) configuration is obtained and the deviation of each configuration of landmarks from the consensus yields the Procrustes residuals (Procrustes transformed coordinates). The standard GLS Procrustes superimposition is extended in the sliding semilandmarks method. In addition to translating, scaling, and rotating the configurations of landmarks, the sliding semilandmarks method allows semilandmarks to slide along lines tangent to the outline curve to optimize their position with respect to the average shape of the entire sample (Zelditch et al., 2004; Perez et al., 2006; Gunz and Mitteroecker, 2013). Semilandmarks are allowed to slide to either minimize thin-plate spline bending energy or to minimize Procrustes distance (Perez et al., 2006; Gunz and Mitteroecker, 2013). The sliding semilandmarks method attempts to reduce the amount of shape variation that results solely from the arbitrary spacing of semilandmarks; thus, improving the geometric or biological correspondence of the semilandmarks across specimens (Zelditch et al., 2004; Perez et al., 2006; Gunz and Mitteroecker, 2013).
Scoring of traditional mesowear variables
In order to compare traditional mesowear analysis and 2-D geometric morphometric mesowear analysis, we also scored the metacones from the photographs for every specimen in our dataset (Supplementary Table S1) using the categorical methodology introduced by Fortelius and Solounias (2000). This methodology uses two categories: (1) cusp height (high or low) and (2) cusp shape (sharp, rounded, or blunt) (Fortelius and Solounias, 2000). Cusps judged to be at the border between high and low were measured using ImageJ 1.53 s (Rasband et al., 2022) to assign them to one of these two categories. Categorization of these borderline specimens was accomplished by measuring the maximum depth of the valley formed between the paracone and metacone (by measuring the vertical distance from a line connecting the paracone and metacone cusps) and dividing this value by the length of the molar, as indicated by Fortelius and Solounias (2000). Ratios ≥0.15 were assigned as high, conversely ratios <0.15 were assigned to the low category. The same observer (CIB-O) evaluated all specimens in our dataset for traditional mesowear variables.
Following Fortelius and Solounias (2000), we calculated the percentage of individuals with high, low, sharp, rounded, and blunt cusps for every species. We confined analysis of traditional mesowear data to species with sample sizes of 5 or more individuals (Supplementary Table S2). We also calculated mesowear scores for individual specimens (Supplementary Table S1) by assigning a score of 0 to specimens with high and sharp cusps, a score of 1 to specimens with high and rounded cusps, a score of 2 to specimens with low and rounded cusps, and a score of 3 to specimens with low and blunt cusps (Rivals and Semprebon, 2006; Rivals et al., 2007). Out of 834 specimens, 19 could not be scored because the paracone cusp was damaged preventing us from determining cusp height, and 26 specimens had peculiar metacone shapes that were difficult to score and were ignored; thus, we only report and analyze the mesowear score for the remaining 789 specimens (Supplementary Table S1).
Covariation between size and cusp shape/mesowear score
To test for allometry (covariation between size and shape), we performed multivariate regressions in MorphoJ 1.05f (Klingenberg, 2011), where size is the independent variable and the Procrustes transformed coordinates (Supplementary Table S1) are the dependent variables. Size was measured as either M2 ectoloph length (measured with a digital caliper accurate to 0.01), metacone cusp length (obtained by calculating the Euclidean distance between the extreme points of the cusp), or metacone ln centroid size (Supplementary Table S1). We also evaluated covariation between size and metacone mesowear score by performing linear regressions in PAST 4.12b (Hammer et al., 2001), where size (as quantified above) is the independent variable and mesowear score (Supplementary Table S1) is the dependent variable. For each analysis, we report the amount of cusp shape variation predicted by size and p-values of permutation tests (10,000 replicates) used to evaluate the null hypothesis of independence.
In order to evaluate covariation between metacone mesowear score and metacone cusp shape, we performed a multivariate regression in MorphoJ 1.05f (Klingenberg, 2011). In this analysis, mesowear score (Supplementary Table S1) is treated as the independent variable and Procrustes transformed coordinates (Supplementary Table S1) are the dependent variables.
Principal component analysis
To identify directions of maximal shape variation in our dataset, we performed a principal component analysis (PCA) on the variance–covariance matrix of the Procrustes transformed coordinates (Supplementary Table S1) using species in Tables 1, 2. PCA was performed in MorphoJ 1.05f (Klingenberg, 2011).
Discriminant function analysis
To test the efficacy of 2D cusp shape as a means of accurately classifying diets, stepwise discriminant function analyses (DFA) were run using SPSS v. 27 on the Procrustes transformed coordinates (Supplementary Table S1) using diet (browser, grazer, mixed feeder, frugivore) as the group variable (Table 1). In stepwise DFA, single variables are added to the discriminant model to optimize discrimination among groups according to predetermined inclusion criteria. We followed Meloro (2011) in using p ≤ 0.05 and p ≥ 0.1 for the probability of F for the entry and removal of variables, respectively (Supplementary Table S5). Attempts were made to match the dietary classifications of species made from the literature to dietary classifications generated from the DFAs. We refer to dietary classifications based on the literature as a priori and dietary classifications resulting from DFA of mesowear data as a posteriori.
DFAs were run including the individual specimens as observations. The analyses were done assuming equal prior classification probabilities for all dietary groups and using a within-groups covariance matrix. The modal (most common) a posteriori individual classification within each species was used to assign that species to an a posteriori dietary category. Modal classifications were not generated for species represented by fewer than three specimens.
The common method of DFA classification is resubstitution, where the DFA classifies the same specimens used to generate the discriminant model. A degree of circularity is inherent in this method because the resulting classifications are not independent of the observations (Lance et al., 2000) and the resulting apparent error rate of classification tends to be underestimated. To avoid this problem, we employed a leave-one-out jackknifing technique that maintains independence between the observations used to generate the discriminant function and the classifications (Lance et al., 2000; Louys et al., 2011). The leave-one-out method works by removing one observation from the data and then it classifies that observation based on the DFA of the remaining data. That observation is subsequently returned to the data set and the procedure is repeated for each observation. Because each classification is based on a model that excluded the observation, the resulting actual error rate is expected to be less biased than the apparent error rate generated from the resubstitution method. Species in Table 1 were used to generate the discriminant models. Species in Table 2 were entered into the analysis without a priori classifications and jackknifing was therefore not used for a posteriori classifications of these species’ diets.
To visualize cusp shape features that covary with discriminant functions that maximize the separation between dietary groups, we performed multivariate regressions in MorphoJ 1.05f (Klingenberg, 2011). In these analyses, discriminant function scores were the independent variables and the Procrustes transformed coordinates (Supplementary Table S1) of species in Table 1 were the dependent variables.
Two additional series of DFAs were run using only the species in Table 1 to account for potential complications of our dataset:
(1) Frugivores were excluded to examine the possibility that they interfere with discrimination of browsers, grazers, and mixed feeders, due to their potentially overlapping low-relief and rounded cusp morphologies.
(2) To compare the performance of 2D semilandmarks and the original categorical techniques, we ran standard DFAs (i.e., not stepwise DFAs) on the arcsine transformed traditional mesowear data we calculated (% high, % sharp, % round, and % blunt) (Supplementary Table S2).
Results
Covariation between size and cusp shape/mesowear score
Table 3 summarizes the results of the regression analyses. The amount of cusp shape variation predicted by size is minimal (values ranging from 0.13% to 0.34%) and none of the analyses produced a statistically significant result. Cusp shape does not appear to covary with either tooth size or cusp size. Similarly, the amount of mesowear score variation predicted by size is minimal (values ranging from 0.01% to 0.24%) and none of the analyses produced a statistically significant result.
Cusp shape significantly covaries with mesowear score. The amount of cusp shape variation predicted by mesowear score is 24.4%. Features of cusp shape that covary with mesowear score are primarily related to the traditional mesowear variables of cusp height and side steepness. Low mesowear scores are associated with high cusps and steep sides, whereas high mesowear scores are associated with low cusps and shallow sides (Figure 3).

Figure 3. Metacone cusp shape deformations obtained from multivariate regression analysis of metacone mesowear score and Procrustes transformed coordinates (Supplementary Table S1; n = 789). Cusp shape deformations at a mesowear score (MS) of 0, 1, 2, and 3.
Principal component analysis
The first two principal components (PCs) account for over 90% of the variation (Supplementary Table S3). The resulting PC1 is related to variation in the height of the cusp and steepness of the sides, with positive scores corresponding to low relief and shallower sides, and negative scores corresponding to high relief and steeper sides (Figure 4). Dietary groups are distributed on PC1 as we might predict, with browsers tending to be positioned at one end of the axis (tall and steep) and grazers and frugivores tending to be positioned at the other (low and shallow), with mixed feeders tending to occupy the intermediate range. PC2 reveals a component of variation in the degree of mesiodistal cusp asymmetry (Figure 4). Browsers are more frequently distributed at one end of the component where the cusp apex is more mesially positioned with a longer and shallower distal slope. At the other extreme, grazers and frugivores tend to have a more distally positioned cusp apex with a longer and shallower mesial slope. There is considerable overlap between the dietary groups in the PCA results, however, the group centroids of browsers, mixed feeders and grazers form a trend that is negatively associated with both PC1 and PC2. The frugivore group centroid departs slightly from this overall trend, as they tend to possess lower cusp relief than expected with respect to cusp symmetry.
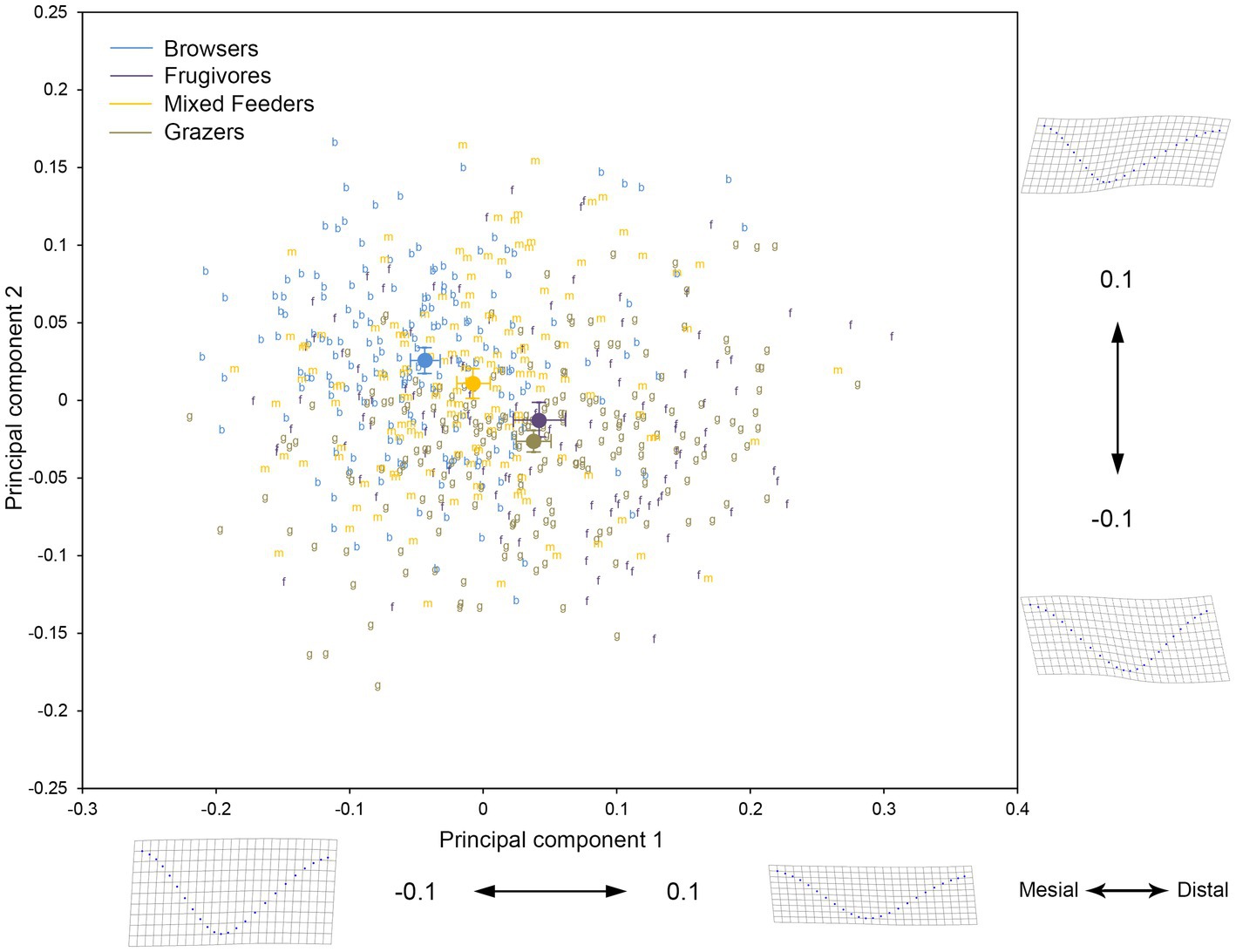
Figure 4. Scatter plot of the first two principal component scores derived from PCA of Procrustes transformed coordinates shown in Supplementary Table S1. Indicated in the plot are the centroids for each dietary group with corresponding 95% confidence intervals. The shape models located along the margins of the plot demonstrate the change in cusp shape along each axis. Abbreviations are as follows: b = browser, f = frugivore, g = grazer, and m = mixed feeder. Procrustes transformed coordinates for all 834 specimens were used in this analysis, but only specimens of species with high-quality dietary information (Table 1) are shown in the plot.
Discriminant function analysis
The DFAs produced significant results (p < 0.001 in all cases). The first two discriminant functions (DFs) account for 95% of the variation among groups (Supplementary Table S4). DF1 tends to separate browsers from grazers, with mixed feeders and frugivores occupying intermediate positions (Figure 5). DF1 is related to cusp height, side steepness, and mesiodistal asymmetry. The metacones of browsers tend to be taller, steeper, and with an apex that is more mesially positioned with a longer and shallower distal slope. The cusps of grazers are lower, shallower, and with a more distally positioned apex with a longer and shallower mesial slope. Along DF2, frugivores separate most strongly from the other dietary groups (Figure 5). The shape transformation in DF2 is strongly related to cusp height with aforementioned cusp asymmetry only transforming very subtly.
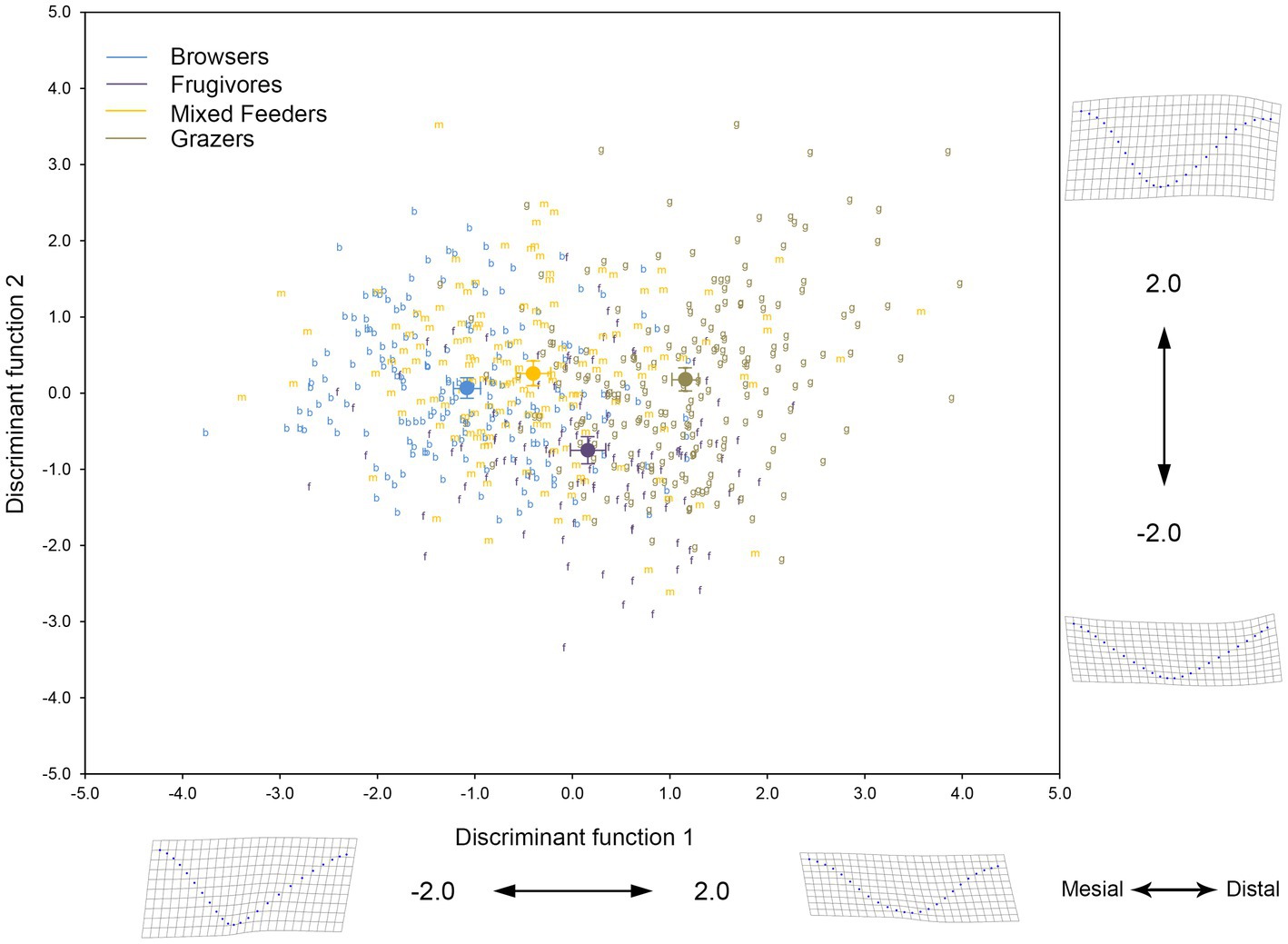
Figure 5. Scatter plot of the first two discriminant function scores derived from DFA of Procrustes transformed coordinates shown in Supplementary Table S1. Indicated in the plot are the centroids for each dietary group with corresponding 95% confidence intervals. The shape models located along the margins of the plot demonstrate the change in cusp shape along each axis. Abbreviations are as follows: b = browser, f = frugivore, g = grazer, and m = mixed feeder. Only Procrustes transformed coordinates for specimens of species with high-quality dietary information (Table 1) were used in this analysis (n = 649).
Supplementary Table S6 compares the a priori (literature) and a posteriori (DFA) dietary classifications of the species of Table 1, resulting in a match rate of 53% for individual cases (Table 4; Figure 6). The a posteriori classification of species correctly matched the mode based a priori classifications 67.2% of the time, and this rate is an improvement of 11 percentage points over match rates of species diets using traditional mesowear data (56.1%). Grazing diets were most frequently matched (73.7%) using the mode-based classifications, followed by browsers (68.8%), frugivores (50%) and mixed feeders (43.8%). Although the traditional data yielded lower frequency of correct match rates, the successful match rate for grazers was identical to that of the morphometric data (73.7%), but the traditional data yielded lower match rates for other diets in the same diminishing order: browsers (56.3%), frugivores (50%) and mixed feeders (33.3%). The order of the diminishing trend, common to both methods, seems to highlight a general problem with mesowear, and not a weakness of any particular method.
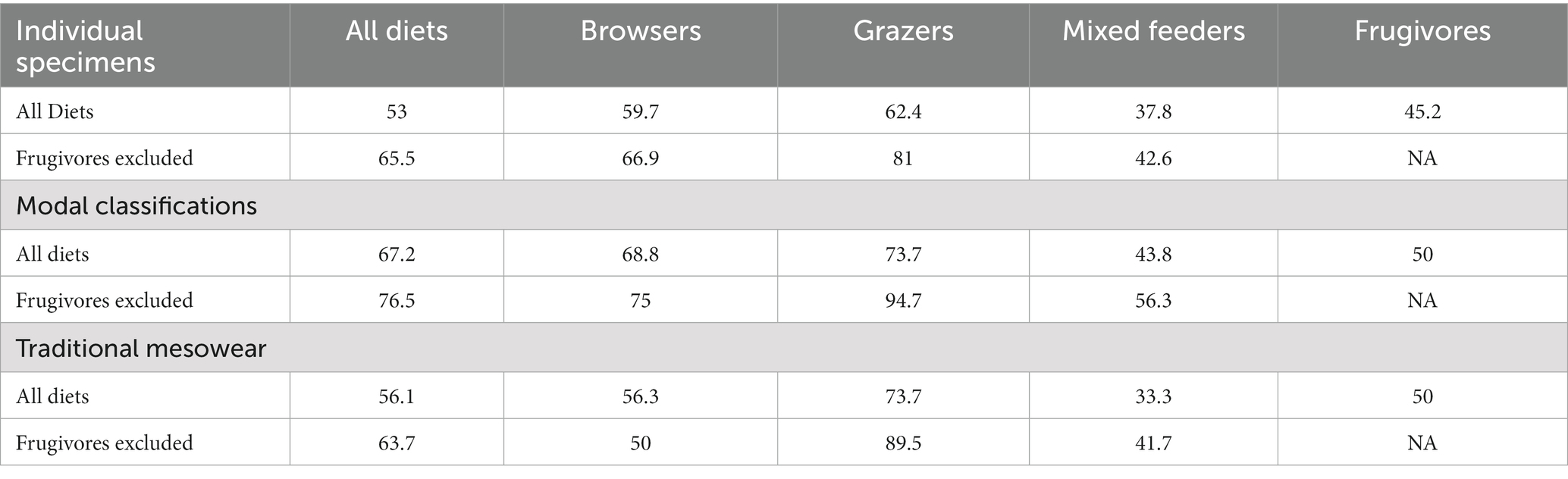
Table 4. Jackknifed percentages of correct a priori and a posteriori matches based on DFAs with high-quality data.
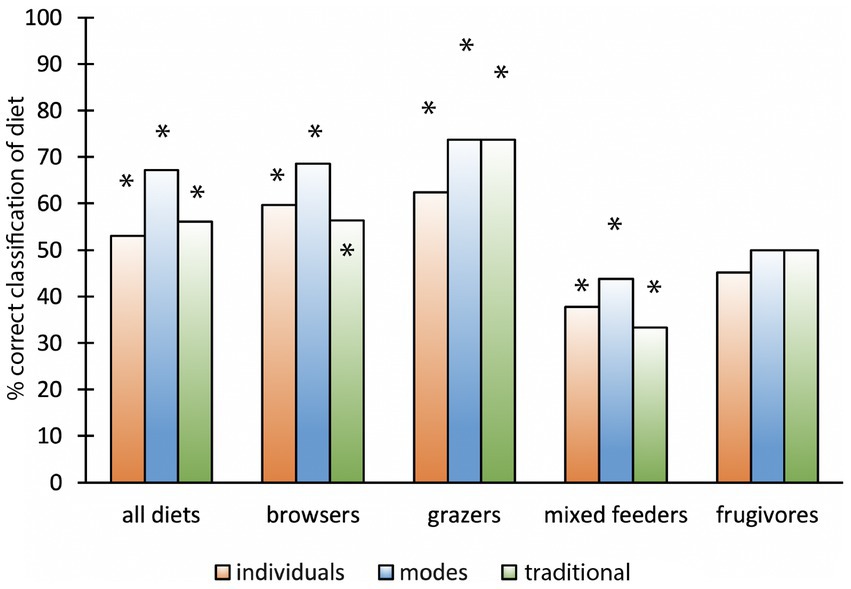
Figure 6. Percent of species whose a priori and a posteriori dietary assignments were correctly matched using the jackknifed DFA results. Asterisks (*) are the percent of correct matches in the analyses excluding frugivores.
The exclusion of frugivores from the analysis had the tendency of improving correct match rates of individual specimens (65.5%) and species (based on modes: 76.5%) for the remaining dietary groups (Table 4; Figure 6). The strongest improvement was among grazers (94.7%), with a diminishing magnitude of improvement in match rate among browsers (75%) and mixed feeders (56.3%). Exclusion of frugivores in the traditional mesowear data gave more mixed results with match rate improvements for grazers (89.5%) and mixed feeders (41.7%), but diminished match rates for browsers (50%). Mesowear most successfully identifies grazers and, to a lesser extent, browsers, but mesowear has more difficulty identifying mixed feeders and frugivores.
Small sample sizes were a concern for some species, however, the exclusion of the 17 species with fewer than 8 observations in Table 1 suggests that improved sampling may not substantially improve the apparent efficacy of mesowear analysis. Modal match rates of species from Table 1 with greater than 8 observations were 69.6%, only a 2.4 percentage-point increase over the modal match rate found in the entire sample in Table 1. Within the set of results from the traditional mesowear data, discarding species with fewer than 8 observations actually diminished the rate of correct classification by about 2 percentage points. Elimination of the most poorly sampled species did not have notable effects on the match rate, suggesting that improved sampling may not greatly improve the results.
Species in Table 2 are those species whose a priori dietary classifications were more uncertain or problematic. The individual classifications correctly matched 33.5% of the time and modal classifications correctly matched 37.5% of the time (Supplementary Table S7). Traditional mesowear data correctly matched only 14.3% (Supplementary Table S7). These match rates are considerably lower than species in Table 1 that were deemed to have more substantiated literature-based diets. These lower match rates are most easily explained by the more questionable a priori categorizations of the diets of these species.
Discussion
Cusp shape variation and distribution of dietary groups in morphospace
Neither tooth size nor cusp size was found to significantly influence metacone cusp shape or metacone mesowear score (Table 3). Previously, body mass and mesowear score were not found to be correlated in extant ungulates (Kaiser et al., 2013). The semilandmark data was found to significantly covary with mesowear score. However, the amount of cusp shape variation predicted by mesowear score was less than 25% (Table 3), suggesting that there are aspects of cusp shape variation, discussed below that are not reflected by mesowear score.
The main shape transformations captured by the semilandmark data are related to the height of the cusp and steepness of the sides and the traditional mesowear variables cusp height and sharpness. These shape variables have been abundantly associated with relative amounts of abrasion and attrition and, thus far, this relationship has continued to serve as the theoretical basis for mesowear (Ackermans, 2020). A secondary shape component that has not yet been accounted for in mesowear analysis involves the relative position of the cusp apex along a mesiodistal axis and the differential slopes, lengths, and shapes of the mesial and distal sides of the cusp.
On average, the cusps of browsing species are taller, with steeper sides, and with cusp apices that are positioned more mesially. The distal slope of the cusp is longer than the mesial slope, has a shallower angle, and is more concave. Among grazers, the cusps are shorter, with shallower sides, with apices that are positioned slightly distally with the mesial slope of the cusp having a greater length, shallower slope, and more concave surface than the distal slope. Mixed feeders occupy an intermediate position and overlap all other dietary categories. Frugivores tend to resemble mixed feeders and grazers more frequently than browsers, but frugivores may have some subtle shape distinctions as suggested by DF2 (Figure 5).
In ungulates, the height of cusps and the complexity of the occlusal relief of the antagonistic dentition play an important role in guiding the movement of the jaw during food comminution (Fortelius, 1985; von Koenigswald et al., 2013). Attritional wear promotes precise occlusion and maintains the guiding effect of the cheek teeth during comminution thereby generating strongly defined attritional facets with high cusp relief and sharpened tips. Abrasion causes decreased tooth relief, thereby diminishing the guiding effect of the teeth, resulting in less precise chewing movements and less precise occlusion with rounded cusp apices. In browsers, attritional wear is the predominant mode of wear, while grazers experience high rates of abrasive wear.
Principal components are, by their very nature, uncorrelated. Because cusp relief is strongly reflected in PC1 (Figure 4) and has previously been associated with relative amounts of attrition and abrasion, it is difficult to explain the uncorrelated shape factor revealed by PC2, relating to cusp asymmetry, as also being a consequence of attrition and abrasion.
Cusp asymmetry may relate to differences in how ruminants chew or how their cusps are oriented with respect to the chewing direction that may have some correlation with diet. The power stroke of the ruminant chewing cycle occurs primarily in a transverse direction with a minor proal (from posterior to anterior) contribution (von Koenigswald et al., 2013). A proal vector during the power stroke may exert greater compressive loads and, consequently, more wear on the distal slopes of the upper cusps by the occluding lower teeth. This proal vector may play a role in creating asymmetrical cusp wear and could explain the condition observed more frequently among browsers (top of the PC2 axis in Figure 4; left of the DF1 axis in Figure 5) where the posterior slope of the metacone is more worn resulting in a mesial-ward shift in the position of the cusp apex.
Grazers tend to have more distally shifted cusp apices (bottom of the PC2 axis in Figure 4; right of the DF1 axis in Figure 5), suggesting the mesial and distal slopes of the cusp are subjected to slightly more wear on the anterior cusp slope causing the cusp apex to shift slightly distally. For frugivores, a greater vertical inclination during phase I of the power stroke and a “tip crushing” type of wear associated with mastication of hard objects may explain the shape of frugivore cusps, but in this case, the similarity of frugivore cusp morphology with those of mixed feeders and grazers would be due to a different type of wear process compared to other dietary categories where the power stroke is mostly transverse and optimized for shearing.
On the efficacy of mesowear as a dietary proxy
Studies that attempt to match mesowear patterns with literature-based dietary classifications of extant species agree that while mesowear is strongly related to diet, it also yields some frequency of erroneous dietary classifications (Fortelius and Solounias, 2000; Fraser and Theodor, 2011; Louys et al., 2011; this study). Nonetheless, despite its shortcomings, mesowear seems to generate higher rates of correct diet classification than dental microwear and craniodental measurements (Fraser and Theodor, 2011), therefore it remains a promising means for predicting diet.
Fortelius and Solounias (2000) and Louys et al. (2011) reported accurate diet classification rates ranging from 49% to 58% using similar statistical methods (DFA jackknifing) to analyze traditional categorical mesowear data. Geometric morphometric data presented here produced higher correct classification rates ranging from 67.2–76.5%, depending on whether or not frugivores were considered. The semilandmark data improved the correct classification rates of species’ diets by 12–13 percentage points in comparison to our results from traditional mesowear data. Geometric morphometric techniques seem capable of outperforming categorical techniques, but realistically, it seems that mesowear will inevitably lead to some proportion of erroneous dietary classifications.
The most widely recognized confounding variable when relating mesowear to diet is the relative impact of extrinsic geological material (e.g., dust, silt, sand) that is ingested along with food (Schulz et al., 2013; Wronski and Schulz-Kornas, 2015) and this additional source of abrasion may be responsible for some degree of dietary misclassification.
Intrinsic differences in the properties of the animals themselves may also obfuscate a simple relationship between mesowear and diet. Heritable (phylogenetic) differences in the dynamic aspects of mastication (bite force, occlusal vectors), functional morphology of the masticatory apparatus, and dental material properties cause teeth to wear differently regardless of diet. Phylogenetic methods are occasionally applied to mesowear analyses (Mihlbachler and Solounias, 2006; Mihlbachler et al., 2011; Kaiser et al., 2013; Fraser et al., 2014), although mesowear is most often assumed to be a taxon-free method (Fraser and Theodor, 2011; Ackermans, 2020). Nonetheless, phylogenetic influences on dental wear are demonstrable (Fraser et al., 2018). For example, dental microwear of ruminant artiodactyls differs from those of perissodactyls (Mihlbachler et al., 2016). Ruminants have highly specialized digestive adaptations allowing them to ingest, wash, and regurgitate their food before orally processing (ruminating) their food (Hatt et al., 2019, 2020, 2021). Ruminants adopt different chewing strategies than perissodactyls (Zhou et al., 2019) who must process their food fully during initial ingestion, and this may contribute to differential tooth wear.
Despite the great diversity of Ruminantia, phylogenetic influence may not strongly obfuscate dietary classifications generated from mesowear within this group. Captive ruminants consistently show different mesowear patterns from their wild conspecifics due to artificial zoo diets (Clauss et al., 2007; Kaiser et al., 2009), and experimental approaches to mesowear in goats show that mesowear responds to feeding treatments with different abrasive properties (Ackermans et al., 2018). Such studies demonstrate that among ruminants mesowear is highly sensitive to proximate ecological variables and these, rather than phylogenetic influence, may be the primary drivers of the morphology of worn cusps. Although there are presently no clear signs that phylogeny complicates use of mesowear data within Ruminantia, phylogenetic methods are needed to ascertain the contribution of phylogenetic influence to mesowear (Barr and Scott, 2014). Ruminants are the dominant clade of herbivorous ungulates today, and all datasets of extant ungulate mesowear are overwhelmingly comprised of ruminants. Phylogeny may exert a stronger influence on more disparate assemblages of species that may include non-ruminant artiodactyls, perissodactyls, marsupials, and other extinct clades, such as South American notoungulates (Croft and Weinstein, 2008).
The heterogeneous quantity and quality of evidence for extant species’ diets in the literature (Gagnon and Chew, 2000) is a third complication that may undermine the apparent efficacy of mesowear analysis. Incomplete, erroneous, or biased observations in the wildlife literature concerning species diets almost certainly explain, to some degree, the mismatches found in our DFAs and other similar analyses (e.g., Fortelius and Solounias, 2000; Fraser and Theodor, 2011; Louys et al., 2011). Likewise, highly specialized diets that are not easily pigeonholed into basic dietary categories may have similar effects. Consequently, the match rates for species with uncertain dietary classifications (in Table 2) was far lower than species with more certain a priori dietary classifications (in Table 1).
Opportunities to examine independent evidence of diet (such as stable isotopes) and mesowear, where both proxies are measured in the same specimens, are rare (Louys et al., 2012). Within a species, diet may vary between populations and between individuals, and possibly within individuals’ lifetimes. The literature and mesowear data may yield unmatched dietary classifications due to such ecological diversity. Mixed feeders are expected to have lower match rates than browsers and grazers as their diets are expected to be more heterogeneous in nature, possibly shifting seasonally or between populations in comparison to more specialized browsers and grazers. The mesowear data seem to reflect this expectation, as mixed feeders have lower match rates than browser and grazers.
We were able to correctly match literature-based and mesowear-based dietary classifications for species 67.2%–76.5% of the time, depending upon whether frugivores were excluded. However, these results underestimate, to some degree, the magnitude of error in species-level mesowear-based dietary classifications. Browsing, mixed feeding, and grazing are segments with arbitrary boundaries along a continuum of diets relating to the proportions of monocots and dicots consumed. It would be less erroneous to misclassify a browser as a mixed feeder than to misclassify a browser as a grazer. The former error still correctly acknowledges that the animal participates, to some degree, in one aspect of its diet. Many of the mismatches in our results are of the lesser kind. Eleven of twenty mismatches of species in Table 1 are assignments to an adjacent category in the continuum. Four other misclassifications are more egregious errors involving failure to differentiate grazers and mixed feeders from frugivores and vice versa.
Removing frugivorous species from consideration is a reasonable strategy for improving dietary classification in some instances. Frugivorous diets only occur among small-bodied ruminants (<30 kg) from tropical and subtropical rainforests (Pineda-Munoz et al., 2016). Medium and large species and species from other environments that do not facilitate frugivory are unlikely to be specialized frugivores and, in these instances, frugivores can be removed from consideration. When frugivores are excluded, all 12 misclassifications in the species of Table 1 are classifications to the adjacent category in the browser-grazer continuum. In no instances were species at the opposite ends of the dietary continuum (browsers and grazers) confused with one another.
Conclusion
Laterally oriented photographs of upper molar cusps capture aspects of 2D cusp shape variation that, in ruminants, are correlated with diet and not with size. Geometric morphometric analysis of cusp shape can be used, with some expected degree of error, to classify species according to their type of diet (browser, grazer, mixed feeder, frugivore) that may improve upon traditional categorical approaches to mesowear analysis. Aspects of shape variation related to cusp relief and sharpness, the fundamental traditional mesowear variables, were found to be the primary elements of shape variation in the semilandmark data. The semilandmark data revealed a secondary shape aspect related to mesiodistal cusp asymmetry. Browsers more frequently have mesially positioned cusp apices with longer, shallower distal cusp slopes. Grazers more frequently have cusp apices that are slightly shifted distally. Mixed feeders and frugivores tend to have intermediate cusp morphologies. Mesiodistal cusp asymmetry in all but frugivores may be both influenced by the attritive and abrasive wear process and intrinsic differences in the relative strength of vertical and proal vectors in the power stroke phase of the chewing cycle, or the orientation of cusps with respect to that cycle. The cusp morphology of frugivores may relate to a greater vertical inclination during phase I of the power stroke and a “tip crushing” type of wear.
While geometric morphometric techniques show potential for providing a higher rate of correct dietary classification compared to categorical methods, researchers can expect a certain misclassification rate with either approach. The semilandmark data correctly classified diet ~67% of the time but when small frugivorous species are not considered mesowear was able to correctly classify browsers, grazers, and mixed feeders more than 75% of the time. With frugivores eliminated, mesowear correctly identifies browsing and grazing diets more frequently than it correctly identifies mixed feeding diets. Therefore, while mesowear remains a promising dietary proxy, we caution that its primary weakness may be its tendency to underestimate the proportion of generalized mixed feeders.
Data availability statement
The original contributions presented in the study are included in the article/Supplementary material, further inquiries can be directed to the corresponding author.
Ethics statement
Ethical review and approval was not required for the animal study because we used museum specimens. No live animals were used in this study.
Author contributions
MM and CB-O conceived the study. MM, CB-O, and BR collected the data. MM and CB-O analyzed the data. JT provided supervision, materials and equipment. MM and CB-O prepared an earlier version of the manuscript. All authors contributed to the article and approved the submitted version.
Acknowledgments
We thank Eileen Westwig, Neil Duncan, Eleanor Hoeger (AMNH Mammalogy Dept.), John Ososky, Darrin Lunde (Smithsonian Institution), Mark A. Edwards, and Bill Weimann (Royal Alberta Museum) for providing access to specimens. Additional thanks go to Raúl Barrón-Corvera (Universidad Autónoma de Zacatecas) for assistance in data visualization and discussions on statistical analyses. We greatly appreciate the thorough and insightful comments we received from the Editor (Carlo Meloro) and the Reviewers (Marcus Clauss, Michael Berthaume, and Eline van Asperen); their comments significantly improved earlier versions of this manuscript.
Conflict of interest
The authors declare that the research was conducted in the absence of any commercial or financial relationships that could be construed as a potential conflict of interest.
Publisher’s note
All claims expressed in this article are solely those of the authors and do not necessarily represent those of their affiliated organizations, or those of the publisher, the editors and the reviewers. Any product that may be evaluated in this article, or claim that may be made by its manufacturer, is not guaranteed or endorsed by the publisher.
Supplementary material
The Supplementary material for this article can be found online at: https://www.frontiersin.org/articles/10.3389/fevo.2023.950463/full#supplementary-material
References
Ackermans, N. L. (2020). The history of mesowear: a review. PeerJ 8:e8519. doi: 10.1016/j.palaeo.2021.110360
Ackermans, N. L., Winkler, D. E., Martin, L. F., Kaiser, T. M., Clauss, M., and Hatt, J.-M. (2020). Dust and grit matter: abrasives of different size lead to opposing dental microwear textures in experimentally fed sheep (Ovis aries). J. Exp. Biol. 223:jeb220442. doi: 10.1242/jeb.220442
Ackermans, N. L., Winkler, D. E., Schulz-Kornas, E., Kaiser, T. M., Müller, D. W., Kircher, P. R., et al. (2018). Controlled feeding experiments with diets of different abrasiveness reveal slow development of mesowear signal in goats (Capra aegagrus hircus). J. Exp. Biol. 221:jeb186411. doi: 10.1242/jeb.186411
Ali, N. A. N. G., Abdullah, M. L., Nor, S. A. M., Pau, T. M., Kulaimi, N. A. M., and Naim, D. M. (2021). A review of the genus Rusa in the indo-Malayan archipelago and conservation efforts. Saudi J. Biol. Sci. 28, 10–26. doi: 10.1016/j.sjbs.2020.08.024
Baamrane, M. A. A., Shehzad, W., Ouhammou, A., Abbad, A., Naimi, M., Coissac, E., et al. (2012). Assessment of the food habits of the Moroccan dorcas gazelle in M’Sabih Talaa, west Central Morocco, using the trnL approach. PLoS One 7:e35643. doi: 10.1371/journal.pone.0035643
Barr, W. A., and Scott, R. S. (2014). Phylogenetic comparative methods compliment discriminant function analysis in ecomorphology. Am. J. Phys. Anthropol. 153, 663–674. doi: 10.1002/ajpa.22462
Barrio, J. (2013). Hippocamelus antisensis (Artiodactyla: Cervidae). Mamm. Species 901, 49–59. doi: 10.1644/901.1
Barrón-Ortiz, C., Mihlbachler, M., Rankin, B., and Theodor, J. (2013). Investigating the applicability of outline-based geometric morphometric techniques to the study of ungulate mesowear. J. Vertebrate Paleontol. 2013, 82A–83A.
Baskin, L., and Danell, K. (2003). Ecology of ungulates: a handbook of species in Eastern Europe and northern and Central Asia. New York: Springer Science & Business Media.
Blondel, C., Merceron, G., Androssa, L., Taisso, M. H., Vignaud, P., and Brunet, M. (2010). Dental mesowear analysis of late Miocene Bovidae from Toros-Menalla (Chad) and early hominid habitats in Central Africa. Palaeogeogr. Palaeoclimatol. Palaeoecol. 292, 184–191. doi: 10.1016/j.palaeo.2010.03.042
Campos-Arceiz, A., Takatsuki, S., and Lhagvasuren, B. (2004). Food overlap between Mongolian gazelles and livestock in Omnogobi, southern Mongolia. Ecol. Res. 19, 455–460. doi: 10.1111/j.1440-1703.2004.00658.x
Clauss, M., Franz-Odendaal, T. A., Brasch, J., Castell, J. C., and Kaiser, T. (2007). Tooth wear in captive giraffes (Giraffa camelopardalis): Mesowear analysis classifies free-ranging specimens as browsers but captive ones as grazers. J. Zoo Wildl. Med. 38, 433–445. doi: 10.1638/06-032.1
Clemente, F., Holechek, J. L., Valdez, R., and Mendoza, G. D. (2009). Influence of range condition on pronghorn (Antilocapra americana) and cattle diets in southern New Mexico. J. Appl. Anim. Res. 36, 13–16. doi: 10.1080/09712119.2009.9707021
Codron, D., Codron, J., Lee-Thorp, J. A., Sponheimer, M., de Ruiter, D., Sealy, J., et al. (2007). Diets of savanna ungulates from stable carbon isotopes composition of faeces. J. Zool. 273, 21–29. doi: 10.1111/j.1469-7998.2007.00292.x
Cohen, J. E., DeSantis, L. R., Lindsey, E. L., Meachen, J. A., O’Keefe, F. R., Southon, J. R., et al. (2021). Dietary stability inferred from dental mesowear analysis in large ungulates from rancho La Brea and opportunistic feeding during the late Pleistocene. Palaeogeogr. Palaeoclimatol. Palaeoecol. 570:110360. doi: 10.1016/j.palaeo.2021.110360
Cook, J. G. (2002). Nutrition and food. North American elk: ecology and management. Smithsonian Institution Press, Washington, DC, 259–349.
Croft, D. A., and Weinstein, D. (2008). The first application of the mesowear method to endemic south American ungulates (Notoungulata). Palaeogeogr. Palaeoclimatol. Palaeoecol. 269, 103–114. doi: 10.1016/j.palaeo.2008.08.007
Custodio, C. C., Lepiten, M. V., and Heaney, L. R. (1996). Bubalus mindorensis. Mamm. Species 43, 1–5. doi: 10.2307/3504276
Dagg, A. I. (2014). Giraffe: biology, behaviour and conservation. Cambridge: Cambridge University Press.
Danowitz, M., Hou, S., Mihlbachler, M. C., Hastings, V., and Solounias, N. (2016). A combined-mesowear analysis of late Miocene giraffids from north Chinese and Greek localities of the Pikermian biome. Palaeogeogr. Palaeoclimatol. Palaeoecol. 449, 194–204. doi: 10.1016/j.paleo.2016.02.026
Davis, N. E., Coulson, G., and Forsyth, D. M. (2008). Diets of native and introduced mammalian herbivores in shrub-encroached grassy woodland, South-Eastern Australia. Wildl. Res. 35, 684–694. doi: 10.1071/WR08042
Djagoun, C. A., Kassa, B., Mensah, G. A., and Sinsin, B. A. (2013). Seasonal habitat and diet partitioning between two sympatric bovid species in Pendjari biosphere reserve (northern Benin): waterbuck and western kob. Afr. Zool. 48, 279–289. doi: 10.1080/15627020.2013.11407594
Erickson, G. M., Sidebottom, M. A., Curry, J. F., Kay, D. I., Kuhn-Hendricks, S., Norell, M. A., et al. (2016). Paleo-tribology: development of wear measurement techniques and a three-dimensional model revealing how grinding dentitions self-wear to enable functionality. Surf. Topogr.Metrol. Properties 4:024001. doi: 10.1088/2051-672X/4/2/024001
Flores-Miyamoto, K., Clauss, M., Ortmann, S., and Sainsbury, A. W. (2005). Nutrition of captive lowland anoa (Bubalus depressicornis): a study on ingesta passage, intake, digestibility, and a diet survey. Zoo Biol. 24, 125–134. doi: 10.1002/zoo.20036
Fortelius, M. (1985). Ungulate cheek teeth: developmental, functional, and evolutionary interrelations. Acta Zool. Fenn. 180, 1–76.
Fortelius, M., and Solounias, N. (2000). Functional characterization of ungulate molars using the abrasion-attrition wear gradient: a new method for reconstructing paleodiets. Am. Mus. Novit. 3301, 1–36. doi: 10.1206/0003-0082(2000)301<0001:FCOUMU>2.0.CO;2
Franz-Odendaal, T. A., and Kaiser, T. M. (2003). Differential mesowear in the maxillary and mandibular cheek dentition of some ruminants (Artiodactyla). Ann. Zool. Fenn. 30, 395–410.
Fraser, D., Haupt, R. J., and Barr, W. A. (2018). Phylogenetic signal in tooth wear dietary niche proxies. Ecol. Evol. 8, 5355–5368. doi: 10.1002/ece3.4052
Fraser, D., and Theodor, J. M. (2010). The use of gross dental wear in dietary studies of extinct lagomorphs. J. Paleontol. 84, 720–729. doi: 10.1666/09-066.1
Fraser, D., and Theodor, J. M. (2011). Comparing ungulate dietary proxies using discriminant function analysis. J. Morphol. 272, 1513–1526. doi: 10.1002/jmor.11001
Fraser, D., Zybutz, T., Lightner, E., and Theodor, J. M. (2014). Ruminant mandibular tooth mesowear: a new scheme for increasing paleoecological sample sizes. J. Zool. 294, 41–48. doi: 10.1111/jzo.12149
Gagnon, M., and Chew, A. E. (2000). Dietary preferences in extant African Bovidae. J. Mammal. 81, 490–511. doi: 10.1644/1545-1542(2000)081<0490:DPIEAB>2.0.CO;2
Gayot, M., Henry, O., Dubost, G., and Sabatier, D. (2004). Comparative diet of the two forest cervids of the genus Mazama in French Guiana. J. Trop. Ecol. 20, 31–43. doi: 10.1017/S0266467404006157
Green, M. J. (1987). Diet composition and quality in Himalayan musk deer based on fecal analysis. J. Wildl. Manag. 51, 880–892. doi: 10.2307/3801755
Green, J. L., and Croft, D. A. (2018). “Using dental mesowear and microwear for dietary inference: a review of current techniques and applications” in Methods in paleoecology: reconstructing Cenozoic terrestrial environments and ecological communities. eds. D. A. Croft, D. F. Su, and S. W. Simpson (Switzerland: Springer Nature), 53–73.
Gunz, P., and Mitteroecker, P. (2013). Semilandmarks: a method for quantifying curves and surfaces. Hystrix 24, 103–109. doi: 10.4404/hystrix-24.1-6292
Hammer, Ø., Harper, D. A. T., and Ryan, P. D. (2001). PAST: paleontological statistics software package for education and data analysis. Palaeontol. Electron. 4:4A.
Hart, J. A., and Hart, T. B. (1989). Ranging and feeding behaviour of okapi (Okapia johnstoni) in the Ituri Forest of Zaire: food limitation in a rain-forest herbivore. in Symposium of the Zoological Society of London, 31–50.
Hatt, J.-M., Codron, D., Ackermans, N. L., Martin, L. F., Richter, H., Kircher, P. R., et al. (2020). The effect of the rumen washing mechanism in sheep differs with concentration and size of abrasive particles. Palaeogeogr. Palaeoclimatol. Palaeoecol. 550:109728. doi: 10.1016/j.palaeo.2020.109728
Hatt, J.-M., Codron, D., Müller, D. W., Ackermans, N. L., Martin, L. F., Kircher, P. R., et al. (2019). The rumen washes off abrasives before heavy-duty chewing in ruminants. Mamm. Biol. 97, 104–111. doi: 10.1016/j.palaeo.2020.109728
Hatt, J.-M., Codron, D., Richter, H., Kircher, P. R., Hummel, J., and Clauss, M. (2021). Preliminary evidence for a forestomach washing mechanism in llamas (Lama glama). Mamm. Biol. 101, 941–948. doi: 10.1007/s42991-021-00142-1
Heffelfinger, J. (2018). Deer of the southwest: a complete guide to the natural history, biology, and management of southwestern mule deer and white. College Station, TX: Texas A&M University Press.
Hejcmanová, P., Homolka, M., Antonínová, M., Hejcman, M., and Podhájecká, V. (2010). Diet composition of western Derby eland (Taurotragus derbianus derbianus) in the dry season in a natural and a managed habitat in Senegal using faecal analyses. Afr. J. Wildl. Res. 40, 27–34. doi: 10.3957/056.040.0105
Hejcmanová, P., Ortmann, S., Stoklasová, L., and Clauss, M. (2020). Digesta passage in common eland (Taurotragus oryx) on a monocot or a dicot diet. Comp. Biochem. Physiol. A Mol. Integr. Physiol. 246:110720. doi: 10.1016/j.cbpa.2020.110720
Hernesniemi, E., Blomstedt, K., and Fortelius, M. (2011). Multi-view stereo three-dimensional reconstruction of lower molars of recent and Pleistocene rhinoceroses for mesowear analysis. Palaeontol. Electron. 14, 1–15.
Hofmann, T., and Roth, H. (2003). Feeding preferences of duiker (Cephalophus maxwelli, C. rufilatus, and C. niger) in Ivory Coast and Ghana. Mamm. Biol. 68, 65–77. doi: 10.1078/1616-5047-00065
Ilyas, O., and Khan, J. A. (2003). Food habits of barking deer (Muntiacus muntjak) and goral (Naemorhedus goral) in Binsar wildlife sanctuary, India. Mammalia 67, 521–532. doi: 10.1515/mamm-2003-0406
Janis, C. M. (1990). “The correlation between diet and dental wear in herbivorous mammals, and its dental relationship to the determination of diets in extinct species” in Evolutionary Paleobiology of behavior and coevolution. ed. J. Boucot (Amsterdam: Elsevier), 241–260.
Janis, C. M. (2008). “An evolutionary history of browsing and grazing ungulates,” in The ecology of browsing and grazing, eds. Gordon, I. J., and Prins, H. H. T. (Berlin: Springer), 21–45.
Jiang, Z., Takatsuki, S., Li, J., Wang, W., Gao, Z., and Ma, J. (2002). Seasonal variations in foods and digestion of Mongolian gazelles in China. J. Wildl. Manag. 66:40. doi: 10.2307/3802869
Jiménez-Hidalgo, E., Carbot-Chanona, G., Guerrero-Arenas, R., Bravo-Cuevas, V. M., Holdridge, G. S., and Israde-Alcántara, I. (2019). Species diversity and paleoecology of late Pleistocene horses from southern México. Front. Ecol. Evol. 7:394. doi: 10.3389/fevo.2019.00394
Jiménez-Manchón, S., Blaise, É., Albesso, M., Gardeisen, A., and Rivals, F. (2021). Quantitative dental mesowear analysis in domestic caprids: a new method to reconstruct management strategies. J. Archaeol. Method Theory 29, 540–560. doi: 10.1007/s10816-021-09530-w
Johnsingh, A. J. T., and Sankar, K. (1991). Food plants of chital, sambar and cattle on Mundanthurai plateau, Tamil Nadu, South India. Mammalia 55, 57–66. doi: 10.1515/mamm.1991.55.1.57
Kaiser, T., Brasch, J., Castell, J. C., Schulz, E., and Clauss, M. (2009). Tooth wear in captive ruminant species differs from that of free-rangin conspecifics. Mamm. Biol. 74, 425–437. doi: 10.1016/j.mambio.2008.09.003
Kaiser, T., Müller, D. W. H., Fortelius, M., Schulz, E., Codron, D., and Clauss, M. (2013). Hypsodonty and tooth facet development in relation to diet and habitat in herbivorous ungulates: implications for understanding tooth wear. Mammal Rev. 43, 34–46. doi: 10.1111/j.1365-2907.2011.00203.x
Kingswood, S. C., and Blank, D. A. (1996). Gazella subgutturosa. Mammalian species 518, 1–10. doi: 10.2307/3504241
Klingenberg, C. P. (2011). MorphoJ: an integrated software package for geometric morphometrics. Mol. Ecol. Resour. 11, 353–357. doi: 10.1111/j.1755-0998.2010.02924.x
Lance, R. F., Kennedy, M. L., and Leberg, P. L. (2000). Classification bias in discriminant function analyses used to evaluate putatively different taxa. J. Mammal. 81, 245–249. doi: 10.1644/1545-1542(2000)081<0245:CBIDFA>2.0.CO;2
Leslie, D. M. (2008). Boselaphus tragocamelus (Artiodactyla: Bovidae). Mamm. Species 813, 1–16. doi: 10.1644/813.1
Leslie, D. M. Jr. (2011). Rusa unicolor (Artiodactyla: Cervidae). Mamm. Species 43, 1–30. doi: 10.1644/871.1
Leslie, D. M. Jr., Lee, D. N., and Dolman, R. W. (2013). Elaphodus cephalophus (Artiodactyla: Cervidae). Mamm. Species 904, 80–91. doi: 10.1644/904.1
Leslie, D. M., and Sharma, K. (2009). Tetracerus quadricornis (Artiodactyla: Bovidae). Mamm. Species 843, 1–11. doi: 10.1644/843.1
Loffredo, L. F., and DeSantis, L. R. G. (2014). Cautionary lessons from assessing dental mesowear observer variability and integrating paleoecological proxies of an extreme generalis Cormohipparion emsliei. Palaeogeogr. Palaeoclimatol. Palaeoecol. 395, 42–52. doi: 10.1016/j.palaeo.2013.12.020
Louys, J., Ditchfield, P., Meloro, C., Elton, S., and Bishop, L. (2012). Stable isotopes provide independent support for the use of mesowear variables for inferring diets in African antelopes. Proc. R. Soc. B 279, 4441–4446. doi: 10.1098/rspb.2012.1473
Louys, J., Meloro, C., Elton, S., Ditchfield, P., and Bishop, L. (2011). Mesowear as a means of determining diets in African antelopes. J. Archaeol. Sci. 38, 1485–1495. doi: 10.1016/j.jas.2011.02.011
Marin, V. C., Fernández, V. A., Dacar, M. A., Gutiérrez, D. G., Fergnani, D., and Pereira, J. A. (2020). Diet of the marsh deer in the Paraná River Delta, Argentina—a vulnerable species in an intensive forestry landscape. Eur. J. Wildl. Res. 66, 1–9. doi: 10.1007/s10344-019-1358-3
Martin, L. F., Ackermans, N. L., Richter, H., Kircher, P., Hummel, J., Codron, D., et al. (2021). Macrowear effects of external quartz abrasives of different size and concentration in rabbits (Oryctolagus cuniculus). J. Exp. Zool. B Mol. Dev. Evol. 338, 586–597. doi: 10.1002/jez.b.23104
Meloro, C. (2011). Feeding habits of Plio-Pleistocene large carnivores as revealed by the mandibular geometry. J. Vertebr. Paleontol. 31, 428–446. doi: 10.1080/02724634.2011.550357
Mihlbachler, M. C., Campbell, D., Ayoub, M., Chen, C., and Ghani, I. (2016). Comparative dental microwear of ruminant and perissodactyl molars: implications for paleodietary analysis of rare and extinct ungulate clades. Paleobiology 42, 98–116. doi: 10.1017/pab.2015.33
Mihlbachler, M. C., Campbell, D., Chen, C., Ayoub, M., and Kaur, P. (2017). Microwear-mesowear congruence and mortality bias in rhinoceros mass-death assemblages. Paleobiology 44, 131–154. doi: 10.1017/pab.2017.13
Mihlbachler, M. C., Rivals, F., Solounias, N., and Semprebon, G. M. (2011). Dietary change and evolution of horses in North America. Science 331, 1178–1181. doi: 10.1126/science.1196166
Mihlbachler, M. C., and Solounias, N. (2006). Coevolution of tooth crown height and diet in oreodonts (Merycoidodontidae, Artiodactyla) examined with phylogenetically independent contrasts. J. Mamm. Evol. 13, 11–36. doi: 10.1007/s10914-005-9001-3
Müller, D. W., Lackey, L. B., Streich, W. J., Fickel, J., Hatt, J.-M., and Clauss, M. (2011). Mating system, feeding type and ex situ conservation effort determine life expectancy in captive ruminants. Proc. R. Soc. B Biol. Sci. 278, 2076–2080. doi: 10.1098/rspb.2010.2275
O’shaughnessy, R., Cain, J. W. III, and Owen-Smith, N. (2014). Comparative diet and habitat selection of puku and lech we in northern Botswana. J. Mammal. 95, 933–942. doi: 10.1644/13-MAMM-A-301
Owen-Smith, N. (1997). Distinctive features of the nutritional ecology of browsing versus grazing ruminants. Zeitschrift fuer Saeugetierkunde (Germany). 62, 176–191.
Perez, S. I., Bernal, V., and Gonzalez, P. (2006). Differences between sliding semi-landmark methods in geometric morphometrics, with an application to human craniofacial and dental variation. J. Anat. 208, 769–784. doi: 10.1111/j.1469-7580.2006.00576.x
Pinder, L., and Grosse, A. P. (1991). Blastocerus dichotomus. Mamm. Species 1–4:1. doi: 10.2307/3504311
Pineda-Munoz, S., Evans, A. R., and Alroy, J. (2016). The relationship between diet and body mass in terrestrial mammals. Paleobiology 42, 659–669. doi: 10.1017/pab.2016.6
Prado, H. M. (2013). Feeding ecology of five Neotropical ungulates: a critical review. Oecologia Australis 17, 459–473. doi: 10.4257/oeco.2013.1704.02
Rasband, W., et al. (2022). ImageJ, image processing and analysis in Java, version 1.53s. Bethesda, MD: National Institutes of Health.
Renecker, L. A., and Schwartz, C. C. (1997). “Food habits and feeding behavior” in Ecology and Management of the North American Moose. eds. F. Aw and C. C. Schwartz (Boulder, CO: University of Colorado Press), 403–440.
Rivals, F., and Semprebon, G. M. (2006). A comparison of the dietary habits of a large sample of the Pleistocene pronghorn Stockoceros onusrosagris from the Papago Springs cave in Arizona to the modern Antilocapra americana. J. Vertebr. Paleontol. 26, 495–500. doi: 10.1671/0272-4634(2006)26[495:ACOTDH]2.0.CO;2
Rivals, F., Solounias, N., and Mihlbachler, M. C. (2007). Evidence for geographic variation in the diets of late Pleistocene and early Holocene bison in North America, and differences from the diets of recent bison. Quat. Res. 68, 338–346. doi: 10.1016/j.yqres.2007.07.012
Rødven, R., Yoccoz, N. G., Ims, R. A., and Wiig, Ø. (2006). Assessing dental wear in reindeer using geometric morphometrical methods. Rangifer 26, 29–34. doi: 10.7557/2.26.1.186
Rohlf, F. J. (2015). The tps series of software. Hystrix, the Italian Journal of Mammalogy 26, 9–12. doi: 10.4404/hystrix-26.1-11264
Saarinen, J., Karme, A., Cerling, T. E., Uno, K., Säilä, L., Kasiki, S., et al. (2014). A new tooth wear-based dietary analysis method for Proboscidea (Mammalia). J. Vertebr. Paleontol. 35:e918546. doi: 10.1080/02724634.2014.918546
Sanson, G. D. (2006). The biomechanics of browsing and grazing. Am. J. Bot. 93, 1531–1545. doi: 10.3732/ajb.93.10.1531
Sanson, G. D., Kerr, S., and Read, J. (2018). Dietary exogenous and endogenous abrasives and tooth wear in African buffalo. Biosurf. Biotribol. 3, 211–223. doi: 10.1016/j.bsbt.2017.12.006
Schaller, G. B. (1967). The deer and the Tiger: Study of wildlife in India. Chicago IL, University of Chicago Press.
Schulz, E., Fraas, S., Kaiser, T. M., Cunningham, P. L., Ismail, K., and Wronski, T. (2013). Food preferences and tooth wear in the sand gazelle (Gazella marica). Mamm. Biol. 78, 55–62. doi: 10.1016/j.mambio.2012.04.006
Schulz-Kornas, E., Winkler, D. E., Clauss, M., Carlsson, J., Ackermans, N. L., Martin, L. F., et al. (2020). Everything matters: molar microwear texture in goats (Capra aegagrus hircus) fed diets of different abrasiveness. Palaeogeogr. Palaeoclimatol. Palaeoecol. 552:109783. doi: 10.1016/j.palaeo.2020.109783
Sempéré, A. J., Sokolov, V. E., and Danilkin, A. A. (1996). Capreolus capreolus. Mamm. Species 1–9:1. doi: 10.2307/3504309
Semprebon, G. M., and Rivals, F. (2010). Trends in the paleodietary habits of fossil camels from the tertiary and quaternary of North America. Palaeogeogr. Palaeoclimatol. Palaeoecol. 295, 131–145. doi: 10.1016/j.palaeo.2010.05.033
Semprebon, G. M., Rivals, F., and Janis, C. M. (2019). The role of grass vs. exogenous abrasives in the paleodietary patterns of north American ungulates. Front. Ecol. Evol. 7:65. doi: 10.3389/fevo.2019.00065
Seri, H., Chammem, M., Silva, S., Rodrigues, M., Khorchani, T., and Ferreira, L. (2018). Assessment of diet composition of free-ranging addax antelopes (Addax nasomaculatus) by the combination of microhistological procedures and n-alkanes and long-chain alcohols as fecal markers. Can. J. Zool. 96, 1284–1289. doi: 10.1139/cjz-2017-0320
Taylor, L. A., Kaiser, T. M., Schwitzer, C., Müller, D. W. H., Codron, D., Clauss, M., et al. (2013). Detecting inter-cusp and inter-tooth wear patterns in rhinocerotids. PLoS One 8, 1–12. doi: 10.1371/journal.pone.0080921
Tomas, W. M., and Salis, S. M. (2000). Diet of the marsh deer (Blastocerus dichotomus) in the Pantanal wetland, Brazil. Stud. Neotropical Fauna Environ. 35, 165–172. doi: 10.1076/snfe.35.3.165.8861
Tripathi, S., Basumatary, S. K., Singh, Y. R., McDonald, H. G., Tripathi, D., and Singh, L. J. (2019). Multiproxy studies on dung of endangered Sangai (Rucervus eldii eldii) and hog deer (Axis porcinus) from Manipur, India: implications for paleoherbivory and paleoecology. Rev. Palaeobot. Palynol. 263, 85–103. doi: 10.1016/j.revpalbo.2019.01.008
Tuboi, C., and Hussain, S. A. (2016). Factors affecting forage selection by the endangered Eld’s deer and hog deer in the floating meadows of Barak-Chindwin basin of north-East India. Mamm. Biol. 81, 53–60. doi: 10.1016/j.mambio.2014.10.006
Ulbricht, A., Maul, L. C., and Schulz, E. (2015). Can mesowear analysis be applied to small mammals? A pilot-study on leporines and murines. Mamm. Biol. 80, 14–20. doi: 10.1016/j.mambio.2014.06.014
Ungar, P. (2010). Mammal teeth: origin, evolution, and diversity. Johns Hopkins University Press, Baltimore, MD. 320.
Valli, A. M., and Palombo, M. R. (2008). Feeding behaviour of middle-size deer from the upper Pliocene site of saint-Vallier (France) inferred by morphological and micro/mesowear analysis. Palaeogeogr. Palaeoclimatol. Palaeoecol. 257, 106–122. doi: 10.1016/j.palaeo.2007.09.006
von Koenigswald, W., Anders, U., Engels, S., Schulz, J. A., and Kullmer, O. (2013). Jaw movement in fossil mammals: analysis, description and visualization. Paläontol. Z. 87, 141–159. doi: 10.1007/s12542-012-0142-4
Watter, K., Baxter, G., Brennan, M., Pople, T., and Murray, P. (2020). Seasonal diet preferences of chital deer in the northern Queensland dry tropics, Australia. Rangeland J. 42, 211–220. doi: 10.1016/j.palaeo.2007.09.006
Wegge, P., Shrestha, A. K., and Moe, S. R. (2006). Dry season diets of sympatric ungulates in lowland Nepal: competition and facilitation in alluvial tall grasslands. Ecol. Res. 21, 698–706. doi: 10.1007/s11284-006-0177-7
Widga, C. (2006). Niche variability in late Holocene bison: a perspective from big bone lick, KY. J. Archaeol. Sci. 33, 1237–1255. doi: 10.1016/j.jas.2005.12.011
Wiles, G. J., Buden, D. W., and Worthington, D. J. (1999). History of introduction, population status, and management of Philippine deer (Cervus mariannus) on Micronesian Islands. Mammalia 63, 193–216. doi: 10.1515/mamm.1999.63.2.193
Wilson, D. E., and Mittermeier, R. A. (2011). Handbook of the mammals of the world: Hoofed mammals 2. Lynx Edicions, Barcelona.
Wronski, T., and Schulz-Kornas, E. (2015). The Farasan gazelle–a frugivorous browser in an arid environment? Mamm. Biol. 80, 87–95. doi: 10.1016/j.mambio.2014.12.002
Xu, F., Ma, M., Wu, Y., and Yang, W. (2012). Winter daytime activity budgets of Asiatic ibex Capra sibirica in Tomur National Nature Reserve of Xinjiang, China. Pak. J. Zool. 44, 389–392.
Zelditch, M. L., Swiderski, D. L., Sheets, D., and Fink, W. L. (2004). Geometric morphometrics for biologists: a primer. San Diego, CA: Elsevier Academic Press.
Zhang, Q., Yang, B., Fu, Q., Wang, L., Gong, X., and Zhang, Y. (2020). The winter diet of sambar (Rusa unicolor) in the Qionglai Mountains. Biodivers. Sci. 28, 1192–1201. doi: 10.17520/biods.2020063
Keywords: mesowear, tooth wear, paleodiet, Ruminantia, geometric morphometrics
Citation: Mihlbachler MC, Barrón-Ortiz CI, Rankin BD and Theodor JM (2023) Sharpening the mesowear tool: geometric morphometric analysis of cusp shape and diet in ruminants. Front. Ecol. Evol. 11:950463. doi: 10.3389/fevo.2023.950463
Edited by:
Carlo Meloro, Liverpool John Moores University, United KingdomReviewed by:
Marcus Clauss, University of Zurich, SwitzerlandEline Naomi Van Asperen, Durham University, United Kingdom
Copyright © 2023 Mihlbachler, Barrón-Ortiz, Rankin and Theodor. This is an open-access article distributed under the terms of the Creative Commons Attribution License (CC BY). The use, distribution or reproduction in other forums is permitted, provided the original author(s) and the copyright owner(s) are credited and that the original publication in this journal is cited, in accordance with accepted academic practice. No use, distribution or reproduction is permitted which does not comply with these terms.
*Correspondence: Christina I. Barrón-Ortiz, Y2hyaXN0aW5hLmJhcnJvbi1vcnRpekBnb3YuYWIuY2E=
†These authors have contributed equally to this work and share first authorship