- Department of Biological Sciences, Idaho State University, Pocatello, ID, United States
Emergence of adult aquatic insects from rivers is strongly influenced by water temperature, and emergence timing helps to determine the availability of this ephemeral food resource for birds and other terrestrial insectivores. It is poorly understood how spatial heterogeneity in riverine habitat mediates the timing of emergence. Such spatiotemporal variation may have consequences for terrestrial insectivores that rely on aquatic-derived prey resources. We investigated emergence phenology of the giant salmonfly, Pteronarcys californica, at three spatial scales in two Idaho river networks. We examined the influence of tributary confluences on salmonfly emergence timing and associated insectivorous bird responses. Salmonfly emergence timing was highly variable at the basin-scale during the period we sampled (May–June). Within sub-drainage pathways not punctuated by major tributaries, emergence followed a downstream-to-upstream pattern. At the scale of reaches, abrupt changes in thermal regimes created by 10 major tributary confluences created asynchrony in emergence of 1–6 days among the 20 reaches bracketing the confluences. We observed 10 bird species capturing emerged salmonflies, including 5 species typically associated with upland habitats (e.g., American robin, red-tailed hawk, American kestrel) but that likely aggregated along rivers to take advantage of emerging salmonflies. Some birds (e.g., Lewis’s woodpecker, western tanager, American dipper) captured large numbers of salmonflies, and some of these fed salmonflies to nestlings. Emergence asynchrony created by tributaries was associated with shifts in bird abundance and richness which both nearly doubled, on average, during salmonfly emergence. Thermal heterogeneity in river networks created asynchrony in aquatic insect phenology which prolonged the availability of this pulsed prey resource for insectivorous birds during key breeding times. Such interactions between spatial and temporal heterogeneity and organism phenology may be critical to understanding the consequences of fluxes of resources that link water and land. Shifts in phenology or curtailment of life history diversity in organisms like salmonflies may have implications for these organisms, but could also contribute to mismatches or constrain availability of pulsed resources to dependent consumers. These could be unforeseen consequences, for both aquatic and terrestrial organisms, of human-driven alteration and homogenization of riverscapes.
Introduction
The ecology of aquatic and terrestrial habitats is intertwined (Hynes, 1975; Wallace et al., 1997; Baruch et al., 2021) by strong reciprocal fluxes of materials and organisms, referred to as “resource subsidies” (Polis et al., 1997, 2004), that link population, community and ecosystem processes across land-water boundaries (Baxter et al., 2005; Richardson et al., 2010; Marcarelli et al., 2011; Schindler and Smits, 2017). An important dimension of water-land connectivity is the emergence of adult aquatic insects, and numerous studies have shown the influence of this flux on terrestrial insectivores and food webs (Nakano and Murakami, 2001; Iwata et al., 2003; Power et al., 2004; Paetzold et al., 2005). These insects are important prey for a suite of terrestrial predators including spiders (Marczak and Richardson, 2008), lizards (Sabo and Power, 2002), bats (Fukui et al., 2006) and birds (Nakano and Murakami, 2001), and can contribute 25–100% of the energy used by populations of such animals (Baxter et al., 2005). For instance, in northern Japan, emergence supported more than 25% of the total annual energy budget of an entire forest bird assemblage, and more than 90% for some resident birds, and its timing created key feeding opportunities when terrestrial arthropod prey were less available (Nakano and Murakami, 2001). Thus, these insects may have profound effects on recipient consumers like birds, and their emergence timing may be crucial. Nevertheless, the effects of emergence timing have received less research attention.
Seasonality of emergence of aquatic insects may constrain use by terrestrial insectivores, but environmental heterogeneity has the potential to desynchronize this flux and extend its use. On the one hand, aquatic insect emergence events may represent resource pulses, resulting in times of scarcity interspersed with superabundance (Yang et al., 2008) such that predators may become satiated (Ims, 1990) or miss opportunities altogether depending on timing of their own life histories (Visser et al., 1998; Winder and Schindler, 2004). On the other hand, spatial heterogeneity in phenology of prey organisms can cause asynchronous resource pulses that may prolong foraging opportunities for consumers (Werner and Gilliam, 1984; Wilmshurst et al., 1999; Yang et al., 2008; Armstrong et al., 2016). Because aquatic insect life cycles are strongly influenced by water temperature (e.g., Sweeney, 1984), which can be heterogeneous over a variety of spatial scales (e.g., Fullerton et al., 2015), insect emergence may exhibit asynchronous peaks in quantity, quality and accessibility. However, even resource pulses that might be described as spatially and temporally turbulent could be exploited by terrestrial insectivores if these insectivores were highly mobile, behaviorally plastic, and capable of recognizing ephemeral pulses. Birds, as a group, fit this description of terrestrial insectivore, but few studies have examined the spatiotemporal complexity of aquatic insect emergence and simultaneously assessed the exploitation of these resource pulses by birds.
Asynchrony in space of pulsed resources may protract foraging opportunities for mobile consumers, such that a series of pulses, a phenomenon Armstrong et al. (2016) have referred to as a “resource wave,” may be tracked across a landscape. This may be important when a prey species is available to a given consumer only during a specific developmental stage (e.g., Deacy et al., 2016) such as an emergent adult insect. Several studies have illustrated how spatial variation in resource phenology can enhance the seasonal resource consumption for a variety of consumers that track and exploit asynchronous resource pulses across a landscape. For example, ungulates track spring vegetation to higher elevations (Bischof et al., 2012). Glaucous-winged gulls (Larus glaucescens) and coastal brown bears (Urus arctos) track migrations of spawning anadromous fishes (Schindler et al., 2013); surf scoters (Melanitta perspicillata) move northbound parallel to spawning migrations of herring (family Clupeidae) and consume energy-rich herring eggs (Lok et al., 2012; Armstrong et al., 2016). Emergence of aquatic insects has not been studied in this context, though it may occur as asynchronous pulses across the landscape or in river networks, allowing consumers such as birds extended foraging opportunities.
Habitat variation in rivers, including variation created by tributary confluences, can influence aquatic insect community composition and timing of life histories, with potential consequences for emergence timing that could create heterogeneity in resources for terrestrial insectivores. Characters of rivers such as discharge, channel form and temperature have long been studied in relation to aquatic insects. For example, the “River Continuum Concept” (Vannote et al., 1980) led to predictions that continuous longitudinal gradients of physical habitat should result in similar patterns in riverine biota, including insects. However, empirical investigations have revealed that physical and ecological shifts in river networks often are discontinuous (Montgomery, 1999; Poole, 2002), and that tributary confluences create such heterogeneity (Benda et al., 2004; Rice et al., 2008). Confluences can alter discharge, temperature, and other environmental conditions of the mainstem (Rice et al., 2008) that influence aquatic insects (Bruns et al., 1984; Rice et al., 2001). Confluences are important to the heterogeneity and dynamics of the network ecosystem (Benda et al., 2004), and their effects may include creating asynchrony in organism phenology and increasing life-history diversity of riverine organisms (Moore et al., 2015). Such spatial discontinuities can affect the flow of ecologically important materials with implications for consumers (Kiffney et al., 2006; Harris et al., 2015, 2018). Habitat heterogeneity at tributary confluences may result in phenological variation in the life histories and emergence timing of aquatic insects which may induce insectivore responses. Investigation of these kinds of relationships primarily has been conducted at the scales of a reach or smaller. For example, by increasing stream length along valleys, meandering channels were associated with increased the flux of emerging insects to forests and attendant increases in insectivorous bird abundance (Iwata et al., 2003). Spatial heterogeneity in stream water temperature within a river reach desynchronized the emergence timing of a large-bodied mayfly, leading to their increased availability to and influence on riparian spiders (Uno, 2016). Response of aquatic insect emergence to such habitat heterogeneity at larger scales in river networks has received little quantitative investigation. Nor has there been study of the potential consequence of this variation for vagile predators. In particular, tributary confluences have not been studied as mediators of aquatic insect emergence timing, and any subsequent effect of prolonging access to insect prey for birds.
Giant salmonflies, Pteronarcys californica (Order Plecoptera) (Figure 1), are large-bodied aquatic insects whose emergence is an iconic character of wild rivers of the western U.S.A., and that may serve as important prey for insectivores including birds. Salmonfly nymphs can reach 35 to 50 mm in length prior to emerging
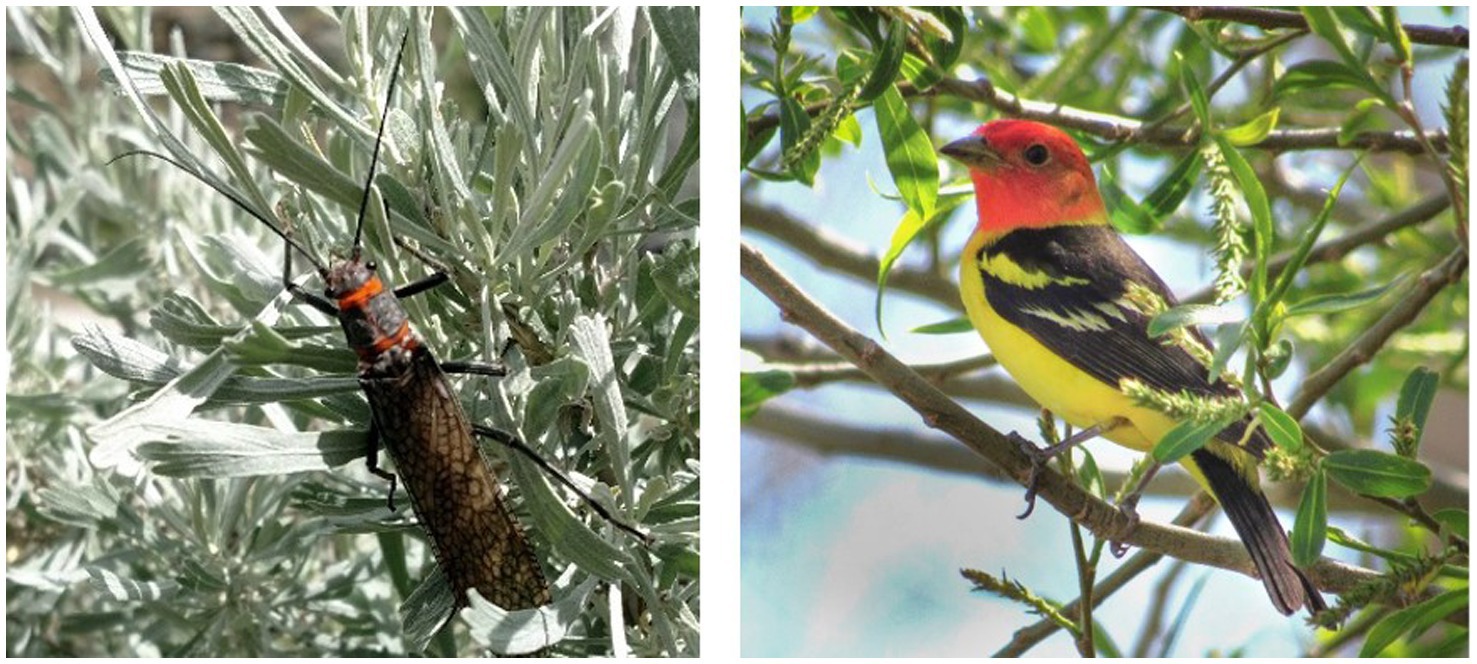
Figure 1. (Left) An adult giant salmonfly Pteronarcys californica recently emerged from the mainstem of the Salmon River, Idaho (photo by Colden Baxter). (Right) A male western tanager, Piranga ludoviciana, one of the species of birds frequently observed feeding on adult salmonflies in this study (photo by Austin Young).
Kauwe et al., 2004). Adults are distinctive, with a bright orange band behind their head and on the underside of their abdomen, as well as broad, flat wings that are darkly veined. The nymphal stage is 2–4 years (Stewart and Stark, 1993), with their rate of development influenced by water temperature (Baumann et al., 1977; Poole, 1981). To attain maturity, a cumulative regimen of development is required, customarily measured in degree-days, as is common for aquatic insects (Sweeney, 1984). Thus, salmonfly emergence appears to be water temperature dependent such that under warmer thermal regimes, emergence typically occurs earlier (Gregory et al., 2000; Anderson et al., 2019). Additionally, their emergence flux can be very large, contributing up to 250% of the predicted combined annual carbon flux of the rest of the insect assemblage in some rivers (Walters et al., 2018). Annually, salmonfly emergence at a given location is a highly synchronized event, often occurring over a matter of a few weeks or less in early summer, such that at different spatial and temporal scales, it may be a pulsed event (Walters et al., 2018). Because the timing of emergence is associated with water temperature, salmonflies are an interesting case for investigating the effect of abrupt changes in thermal regime created by tributary confluences, which may be expressed as asynchrony in their emergence timing. Timing of their emergence may have consequences for insectivores such as birds whose typical breeding seasons often span the weeks of salmonfly emergence. Although there have been anecdotal reports of birds feeding on salmonflies (e.g., Sheldon, 1999; Rockwell and Newell, 2009), salmonfly consumption has not been the focus of investigations. Due to the potential importance of aquatic insects in bird diets, particularly for breeding success (e.g., Twining et al., 2018), these highly mobile avian consumers may take advantage of asynchronous pulses of salmonfly emergence created by thermal discontinuities, by tracking extended availability of salmonflies resulting from emergence heterogeneity across spatial scales within a network.
We investigated the emergence of the giant salmonfly in two Idaho river networks. We wanted to know how temperature differences at tributary-mainstem river confluences affected emergence timing and associated bird responses. We evaluated emergence patterns across time and space at three different scales within the thermally heterogeneous Salmon River Basin, Idaho, United States. We also investigated temporal patterns in salmonfly emergence associated with tributary confluences, and the feeding behavior and occurrence of birds at sites above and below confluences at the scale of river reaches, in the Salmon River Basin, as well as in another river network, the Henry’s Fork of the Snake River. With this combined approach, we addressed the following questions: (1) How does emergence of giant salmonflies vary across space and time at different scales within a river network? (2) Is asynchrony in salmonfly emergence timing associated with thermal discontinuities created by tributary confluences? (3) What bird species feed on adult salmonflies, and to what extent? and (4) Do birds exhibit responses in distribution (i.e., in local abundance and species richness) associated with asynchrony in salmonfly emergence at tributary confluences?
Methods
Study areas
We chose to conduct this study in the Salmon River Basin in central Idaho and the Henry’s Fork of the Snake River in southeastern Idaho, for several reasons, including the presence of robust populations of salmonflies. The Salmon River Basin has an overall network topology formed by a series of mid-sized rivers joining each other at major confluences. It is one of the largest (36,000 km2) free-flowing rivers in the conterminous United States. It drains a mixture of elevations, which combined with topographic complexity, contributes to significant thermal heterogeneity (Tang et al., 2012), potentially influencing the timing of salmonfly emergence. Because we wanted to expand the range of conditions over which we made observations above and below confluences, we also conducted investigations at a pair of confluences in the Henry’s Fork of the Snake River watershed, which allowed us address questions 2–4 in this context as well. The Henry’s Fork has abrupt changes in thermal regime associated with major spring water influences (Gregory et al., 2000), which could contribute to differences in emergence timing. Additionally, salmonfly emergence generally occurs weeks earlier in the Henry’s Fork than in the Salmon River Basin, which from a logistical standpoint allowed us to conduct measurements in both settings. Bird communities differed somewhat between the two study areas. For example, Lewis’s woodpeckers (Melanerpes lewis) were abundant and, as we report below, foraged on salmonflies in the Salmon River Drainage, whereas American robins (Turdus migratorius) were abundant and foraged on salmonflies in the Henry’s Fork watershed. Thus, salmonfly phenology and bird assemblages differed slightly between these river networks. However, because we did not detect any systematic differences between sites in the two basins with respect to results regarding questions 2–4 (see below), we judged that we could the include the observations from both these settings in these analyses.
Study design
To evaluate salmonfly emergence timing across spatial scales (question 1), we measured emergence at three scales in the Salmon River network: the basin, the sub-drainage, and the reach. We examined reaches immediately upstream and downstream of confluences, an experimental design that also allowed us to address thermal effects and avian responses (questions 2–4). We conducted our field measurements across several weeks from May through June, 2018. Accomplishing these measurements in this large basin was challenging, as sites were spread hundreds of kilometers and access to many involved traversing unimproved roads and/or hiking. Consequently, there were some tradeoffs between accomplishing measurements at this large suite of locations and the frequency or intensity of measurements that could be achieved at each.
We used a nested, hierarchical study design. First, we stratified tributary confluences by presence of salmonflies, selecting locations where salmonflies were known to occur up and downstream of a confluence. Second, we stratified the confluences within the Salmon River Basin by major sub-drainages, or forks of the river (e.g., mainstem, South Fork and Middle Fork). Third, in both the Salmon River and Henry’s Fork networks, we further stratified confluences by tributary discharge, identifying discharges large enough potentially to create ecologically meaningful (Benda et al., 2004) shifts in thermal regime. We then selected confluences across an elevation gradient, from the headwaters to the mainstem, encompassing hundreds of kilometers of river. All of the chosen confluences included a tributary within two orders of their mainstem, ranging from first to eighth-order, but were generally larger streams and mid-sized rivers (5th to 8th order), with the exception of those in the Henry’s Fork of the Snake River network. For the Henry’s Fork, the Warm River is a first-order stream, but is a large, spring-fed tributary that contributes 15.6% of the average May discharge of the mainstem Henry’s Fork. Similarly, Robinson Creek is a second-order stream, but contributes 18.6% of the average May discharge to the mainstem Henry’s Fork. We chose confluences that were not in urban areas or in areas of high land-use to avoid human impacts on salmonflies (Elder and Gaufin, 1973) or birds in riparian areas. Finally, sites were located within 1 day’s travel for logistical reasons. Overall, we identified 10 confluences (Figures 2A,B) that encompassed a range of stream size, gradient, aspect, and thermal heterogeneity, with study reaches bracketing each confluence upstream and downstream.
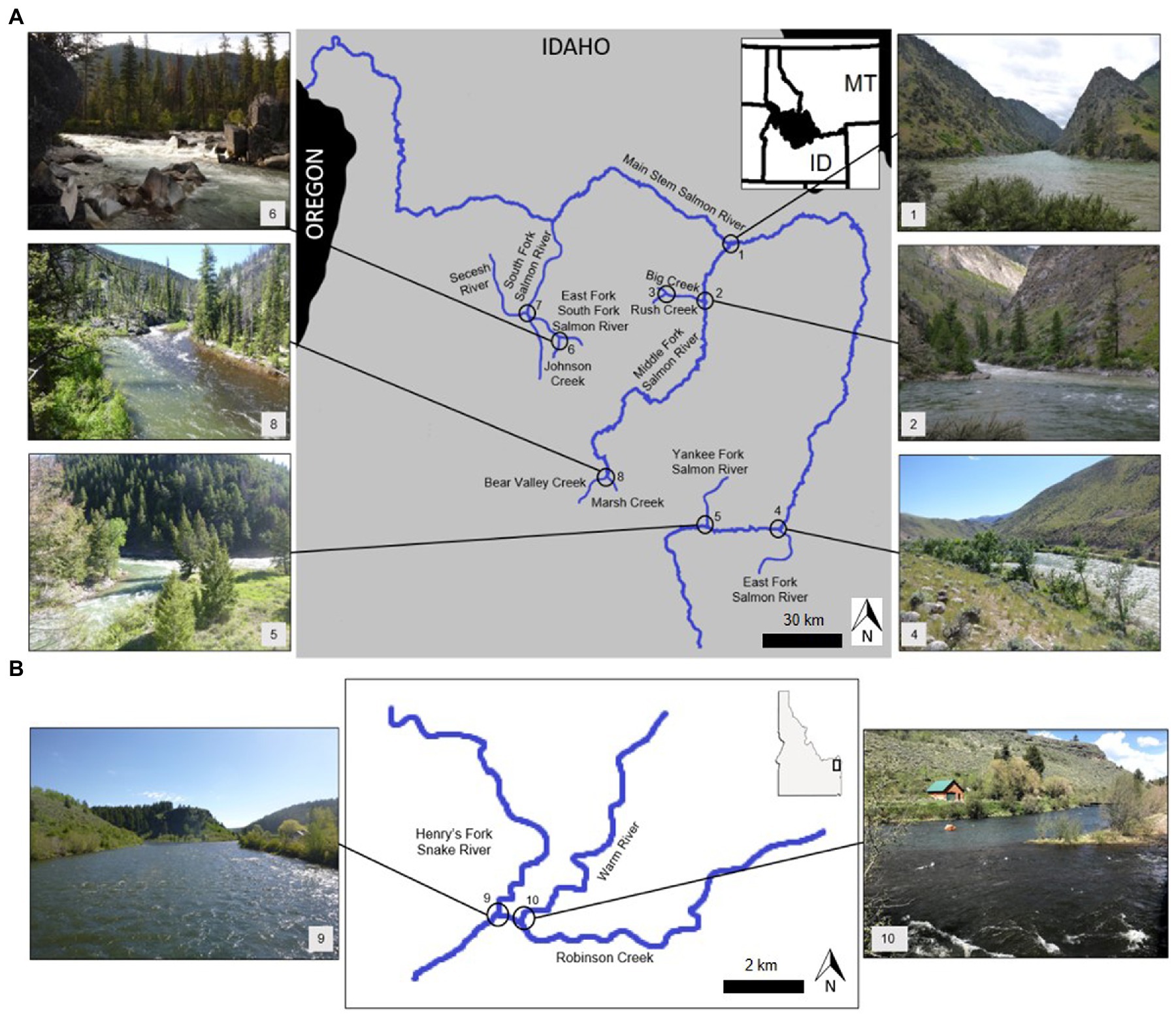
Figure 2. (A) Map of the study confluences, indicated by numbers 1–8, in the Salmon River Basin, Idaho, United States, which were surveyed in summer 2018. (B) Map of the study confluences, indicated by numbers 9–10, in the Henry’s Fork of the Snake River, Idaho, United States which were surveyed in summer 2018.
Timing of salmonfly emergence
To assess the timing of emergence of salmonflies, we conducted repeated counts of the shed exoskeletons of emergent nymphs (exuviae) along 20-m sections of the riverbank at a series of sites distributed throughout the Salmon River basin. At the basin scale, we conducted repeated counts of exuviae at 22 sites distributed throughout the Salmon River network (Figure 3B and Table 1), including sites bracketing confluences (see below) as well as supplementary locations located to encompass the longitudinal profile of major sub-basins. We used up to three crews of observers when emergences were concurrent among sampling locations. At this scale, we used publicly available, predicted values generated by the NorWeST Stream Temperature model (Isaak et al., 2016a,b). That model (Isaak et al., 2017) draws on an integrated collection of past summertime temperature logger data from sites in the Salmon River network to generate spatially continuous thermal maps for the basin, assigning values in 2°C increments to river segments (Figure 3A). As a relative index of temperature, we assumed the summertime thermal patterns described in the NorWeST map would be indicative of relative differences in river temperature at the basin scale. We used simple linear regression to evaluate if the general sequence of emergence was associated with the overall pattern of modeled stream temperature (coded as 4, 2-degree temperature levels, from 12 to 20°C).
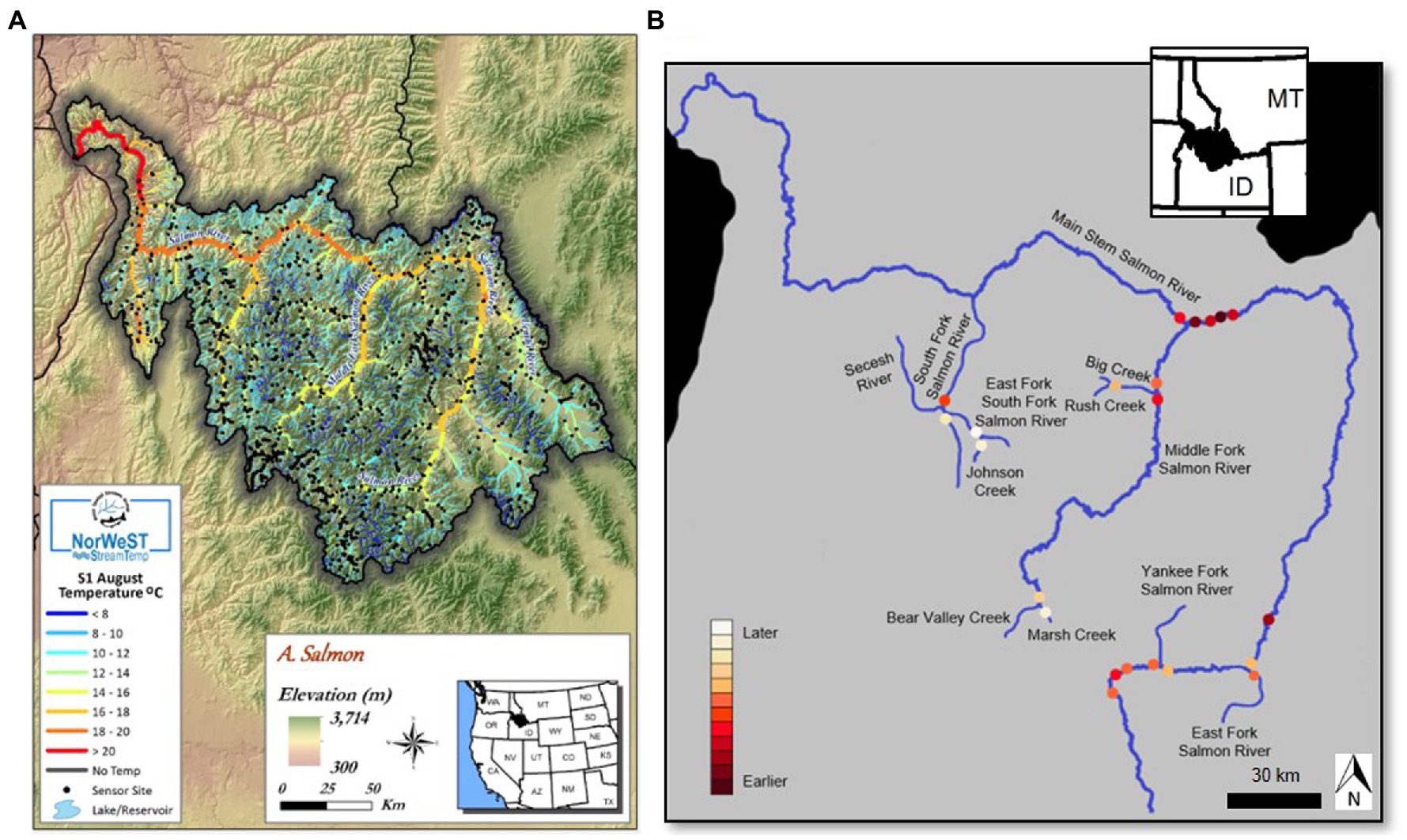
Figure 3. (A) Map of thermal heterogeneity in the Salmon River Basin, Idaho, United States, based on mean August temperatures from 1993 to 2011 (NorWeST temperature model; Isaak et al., 2017). (B) Salmonfly emergence across space and time in the Salmon River Drainage, Idaho, United States in June 2018. The color scale indicates the occurrence of emergence in 2018 from earliest (June 1st) to latest (June 14th).
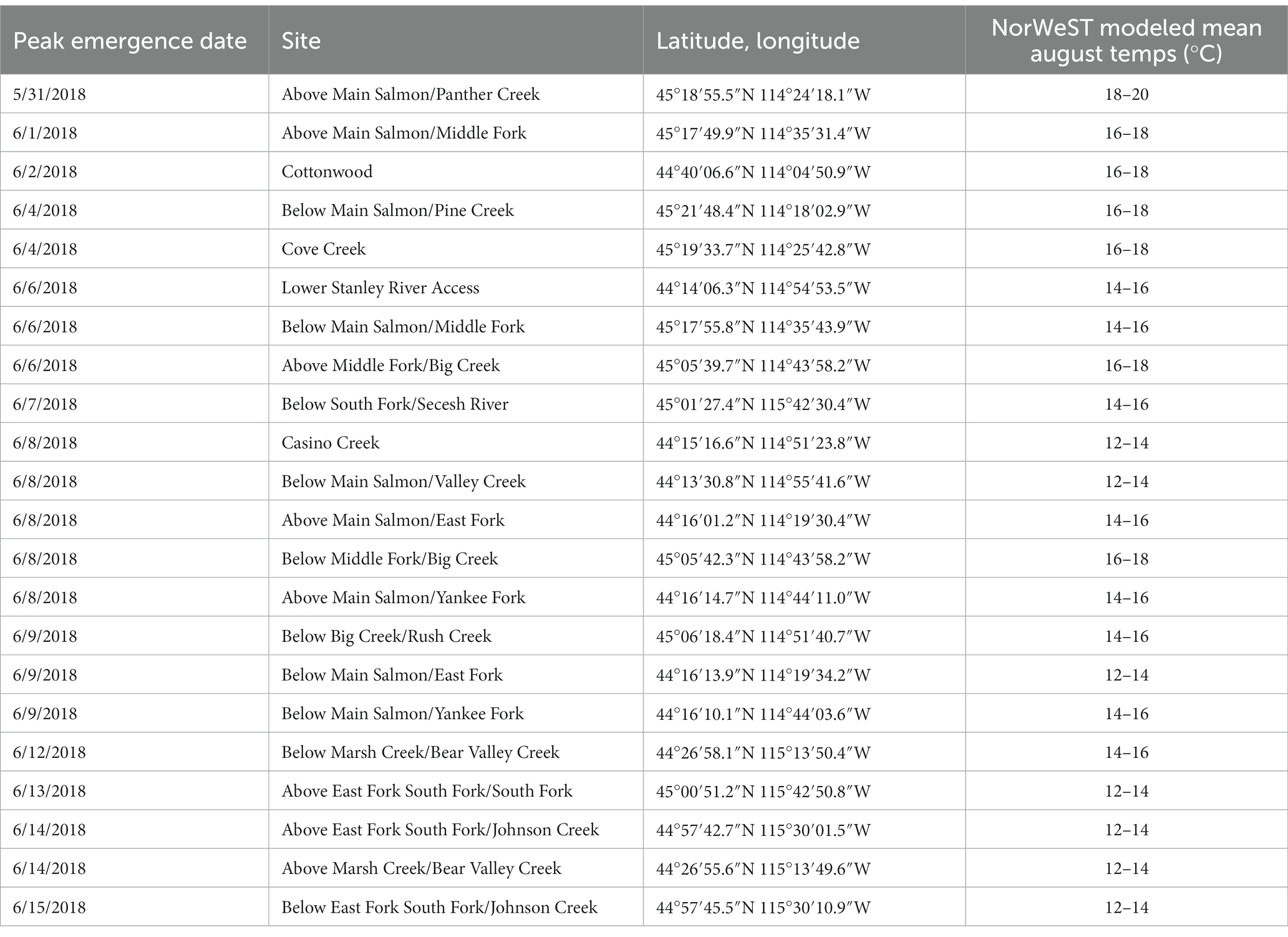
Table 1. Modeled NorWeST August temperatures and the date of peak emergence of salmonflies for 22 sites surveyed for timing of salmonfly emergence throughout the Salmon River Drainage, Idaho, United States.
At the reach scale, we used two complementary methods to survey salmonflies in 1 km reaches above and below each confluence (n = 20 reaches). First, within each reach, we conducted randomized, 20-m counts of exuviae within every 200 m sub-section, generating 5 measures per reach upon each visit. These were performed at locations within sections adjacent to riffles or runs (randomly assigned upon each repeated visit), as these represent the principal habitat for salmonflies and the expected areas where they would emerge (Elder and Gaufin, 1973; Bryce et al., 2010; Relyea et al., 2012). These counts were repeated until no further increases in exuviae (summed for the reach as a whole) were observed. These observations, along with counts of live salmonfly adults (see below), were used to identify a date on which emergence reached its highest observed level, which we refer to here as “date of peak emergence,” though in some cases this may have corresponded more closely to the end of peak of emergence. In any case, we judged this combined approach was sufficiently precise to assess broad patterns across a large number of locations and time periods. For the date on which the maximum number of adult, live salmonflies were observed in a reach, the exuviae counts for all 5 20-m transects were summed and used to estimate the mass of emergence as in terms of carbon flux (g C * [m bank]−1, adapted from Walters et al., 2018), which we also used to assess the relationship between bird response and C flux on that peak date and in the days leading up to it (below). Second, along each 1-km transect on the same days bird counts were performed (see below), we also conducted continuous, bank-side walking surveys of all live adult salmonflies, recording numbers every 200 m. This was done to gather a “snapshot” of adult insects that might be indicative of what was available to birds, to improve our assessment of the timing of peak emergence levels, and to do so at a larger, more reach-integrative scale than that at which the exuviae counts were performed. It was also done to help evaluate whether adult salmonflies might be emerging in one reach but disperse into the nearby reach where emergence was not occurring. We observed only a few cases of individual adult salmonflies that appeared to have made such extended movements, such that we judged we could make comparisons between paired reaches in our analyses without accounting for exchange of organisms between the reaches.
To investigate short-term patterns that might occur between temperature and emergence at the reach scale, we deployed HOBO Stowaway temperature loggers. The purpose of collecting these data was to measure relative differences in temperatures above versus below each confluence during the period encompassing emergence, not to estimate the seasonal or annual thermal conditions of each habitat. We measured water temperature above and below each confluence during the emergence process using temperature loggers positioned in riffles and far enough downstream from confluences that tributary waters had mixed. We evaluated these salmonfly and temperature data for any threshold associated with salmonfly emergence timing. In addition, we conducted a simple binomial test using program R (Aho, 2014; R Core Team, 2018, version 3.5.1) to evaluate the likelihood that the thermal difference of a tributary relative to its mainstem was associated with the directionality of emergence timing. The unit of replication for this analysis was the pair of reaches bracketing a confluence. Due to logistical challenges, we were unable to collect temperature and emergence data at all locations, creating some gaps in analyses among sites as reported (below). The purpose of our study was not to precisely or mechanistically assess the relationship between temperature and salmonfly emergence phenology, which would have required more temperature data as well as multi-factor analyses to which our limited dataset was not suited.
Focal bird feeding observations
To evaluate the extent to which birds were capturing adult salmonflies (question 3), we conducted avian foraging observations coincident with bird surveys that occurred when salmonflies were actively emerging. When this was the case, we conducted several focal bird surveys (n = 105 total, across reaches) at opportunistic locations throughout the study reach. The duration of the focal surveys ranged from 30 s to 20 min, and each focused on an individual bird. Time started once the focal bird was identified, and was stopped after the bird could no longer be seen, or after 2 min of inactivity. We recorded the species of bird, the total number of salmonflies captured and the total elapsed time. All salmonfly-consuming species observed were assigned habitat associations based on Sullivan and Vierling (2009). Foraging instances were only recorded when the observer was confident that it was a salmonfly that had been captured (194 instances). Distinguishing capture events of salmonflies was not difficult owing to salmonflies’ large size, unique coloration and flight behavior. Most of our observations of birds feeding on salmonflies were instances in which salmonflies were captured in flight, though we also recorded birds picking salmonflies from river banks or gleaning them from riparian vegetation. We judged that we likely underestimated the frequency of the latter, because bird foraging in vegetation was more difficult to detect and follow.
Bird count surveys
At the reach scale, we assessed whether changes in bird distribution were associated with the presence of adult salmonflies (question 4). These comparisons were limited to a total of 14 reaches (Supplementary Appendix 1), as sampling logistics prevented our completing bird surveys pre-salmonfly emergence at a few sites that were included in other study components. At these 14 sites, we conducted repeated point counts every 200 m along each 1 km transect, above and below each confluence when salmonflies were present and when salmonflies were absent, but within a week of peak emergence. We positioned points at 200 m intervals to ensure that the distance between each point was enough such that it minimized the double counting of birds (Reynolds et al., 1980). During each point count, the number of individuals of all bird species detected was recorded within a 50 m radius surrounding the observer. We recorded bird presence starting immediately upon arrival at each point for a duration of 10 min (Hutto et al., 1986).
Point counts of birds were supplemented by repeated, transect surveys which were conducted during the same period and along the same 1 km segments above and below confluences, while walking each 200-m section between the point counts (These were also the same 200 m transects along which live adult salmonflies were counted.). As with the point counts, these additional bird surveys were done when salmonflies were absent, and when salmonflies were actively emerging. Observers walked steadily along each transect at a pace of approximately 10 m/min (Ralph et al., 1993), consistently spending at least 10 min along all five survey transects. The principal aim of these surveys when adult salmonflies were present was to identify opportunities to make focal bird foraging activity observations. Secondarily, we used these walking observations to qualitatively assess whether bird species or large groups of birds were not detected via the point counts. In fact, we found point counts to be the more reliable technique for detecting species richness and abundance, so the point count data were used in our analyses of bird richness and abundance reported below. Birds were not surveyed on days with rain or strong winds. A single observer conducted the point counts, as well as the line transects, and all birds that were seen within the established area were recorded (Iwata et al., 2003).
We were unable to determine if the birds at each reach within a confluence pair of reaches were the same birds, and in this sense, bird counts from these reaches were not spatially independent of one another. The confluence pairs themselves, however, were more likely to be spatially independent of other confluence pairs due to the distance between them which ranged from 1 km to 100 s of kms. Regardless, with respect to any potential distributional response, we were interested in the overall change in abundance and species richness of birds associated with the presence of adult salmonflies. We conducted binomial tests to evaluate whether the presence of adult salmonflies was associated with an increase in the numbers of individual birds or avian species richness more frequently than would be expected by chance.
Because p-values describe a continuous measure of evidence and are influenced by small sample size (Gelman, 2013), we used a graded, weight of evidence approach to describe our certainty that results differed from what would be expected by chance alone (Wasserstein et al., 2019). Based upon this rationale, p-values <0.05 were considered significant, while those between 0.05 and 0.1 were considered marginally significant, but of potential ecological importance considering our study’s small sample sizes.
Results
Timing and magnitude of salmonfly emergence
The pattern of salmonfly emergence varied strongly across space and time at different scales within the Salmon River Basin, as did the magnitude of emergence. Counts of exuviae along study reaches (based on 5, 20-m transects along each) ranged from dozens to hundreds, corresponding to estimates of 0.1 to nearly 3 g of C-flux per meter of riverbank, and during emergence counts of live adult salmonflies along entire 1-km reaches ranged from hundreds to >1,000 (i.e., > 1 salmonfly per meter at this scale; Supplementary Appendix 1). Salmonfly emergence timing was highly variable across this large basin between May and June. At the largest scale, emergence occurred in discrete segments at varied locations throughout the basin (Figure 3 and Table 2). For example, emergence occurred at roughly the same time on the mainstem Salmon River below the Middle Fork confluence, on the Middle Fork above the Big Creek confluence approximately 50 river km away, and at the Lower Stanley river access site near Stanley, ID approximately 300 river km away. In 17 of the 22 observed sites, emergence happened earlier at warmer sites (Table 1). At the scale of a sub-drainage and following pathways beginning above confluences, emergence generally proceeded from downstream to upstream (Figure 4). For example, along the Middle Fork sub-drainage, emergence occurred over a period of approximately 8 days, starting at the confluence with the mainstem Salmon River and continuing upstream to the headwater confluence of Bear Valley and Marsh Creek, a total distance of approximately 108 river km. However, along the Middle Fork/Big Creek sub-drainage pathway, which is only ~50 river km in length, emergence occurred over a period of approximately 3 days. At these basin and sub-drainage scales, regression analysis revealed an association between the relative thermal patterns derived from the NorWeST temperature model map and the general timing of peak emergence of salmonflies (R2 = 0.67; Figure 5A). In addition, at the reach scale, the timing of the actual emergence event was associated with local temperature. Although we did not observe straightforward relationships with daily means or temperature fluctuations, we observed a minimum threshold of 8.4°C for emergence across all sites (Figure 5B), below which we never observed peak salmonfly emergence.
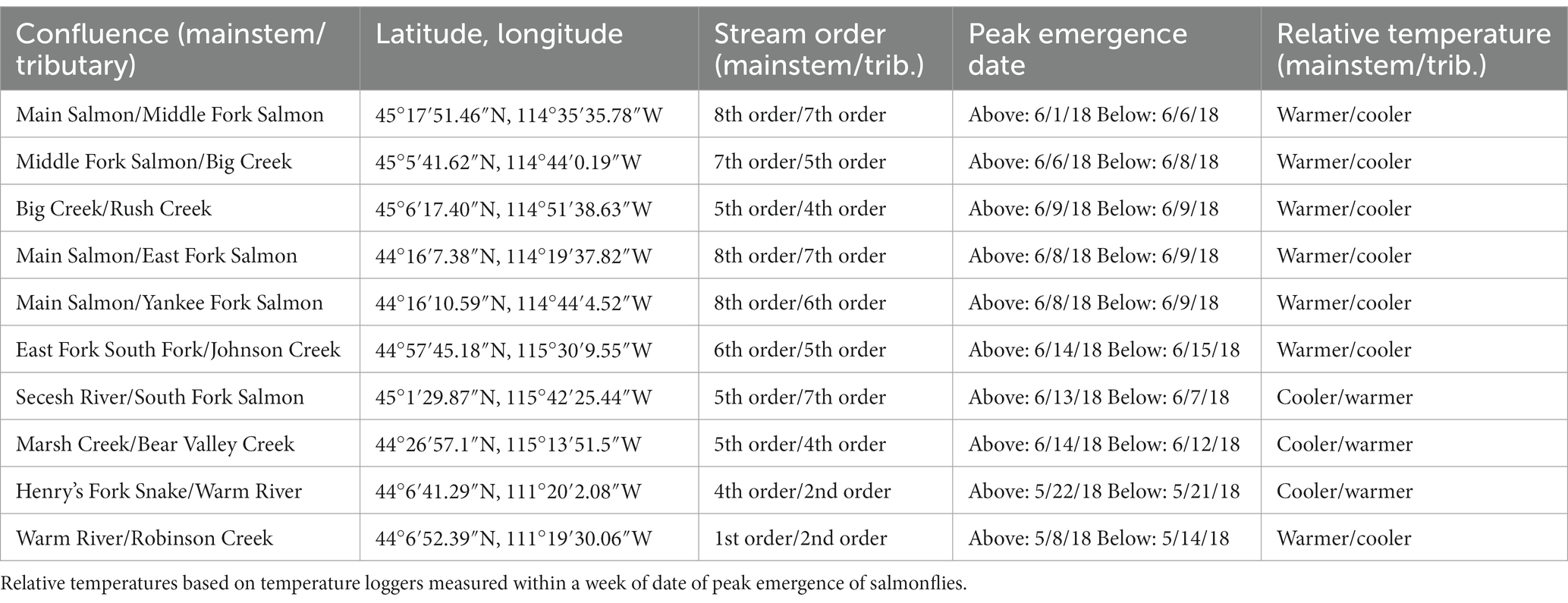
Table 2. Physical descriptions and locations for study sites in the Salmon River Basin and Henry’s Fork of the Snake River, Idaho, United States.
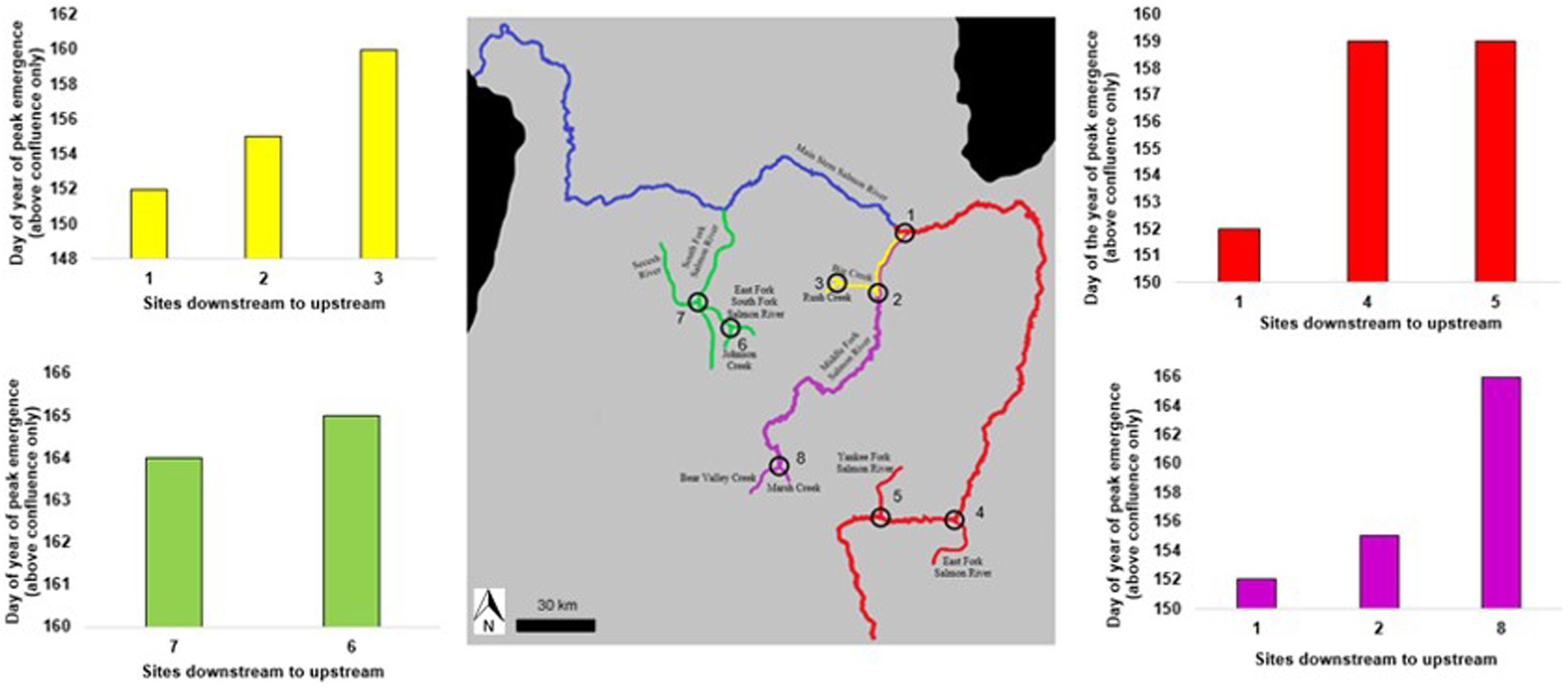
Figure 4. Timing of salmonfly emergence in the Salmon River sub-drainages, Idaho, United States in June 2018, following longitudinal pathways. At this sub-drainage scale, we started the downstream end of each pathway above respective confluences, which reveals how the pattern of emergence consistently appears to proceed from down to upstream when such confluence effects are excluded (as compared to what occurs at the scale of such confluences, see Figure 6). Numbers indicate confluences as labeled in Figure 2. Different colors indicate river sub-drainages.
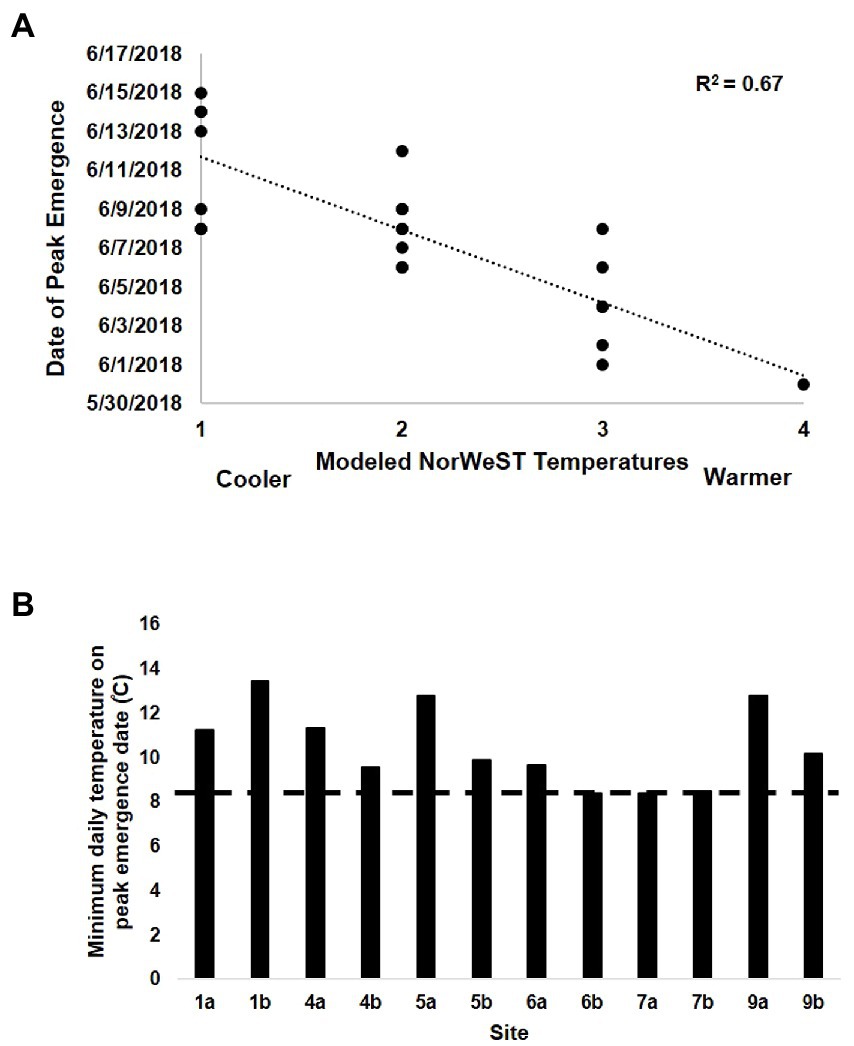
Figure 5. (A) Date of peak emergence across 22 sites in the Salmon River Basin as a function of modeled NorWeST temperatures. Temperatures ̊C are categorized based on color categorizations from the modeled map: 1 = 12–14; 2 = 14–16; 3 = 16–18; 4 = 18–20, and range from cooler to warmer. Note that 22 points are not visible because several points overlapped. (B) Minimum temperature threshold associated with timing of salmonfly emergence at each reach-scale site in the Salmon River Drainage and Henry’s Fork of the Snake River Drainage, Idaho, United States in May–June 2018. Across these sites, peak emergence never occurred if water temperatures were below 8.4 ̊C. Note that for this analysis, confluences 2, 3, 8, and 10 were excluded due to lack of temperature data on peak emergence date.
At the scale of reaches bracketing confluences, we analyzed data gathered in the Salmon River Basin, as well as in the Henry’s Fork of the Snake River. At this scale, thermal differences created by tributary confluences were associated with temporal asynchrony in emergence timing across all confluence reach pairs (n = 10 confluences; p = 0.002), with the offset between paired reaches ranging from 1 to 6 days. At the four confluences at which tributaries were colder relative to their mainstem, emergence occurred first above the confluence (Figure 6A). At confluences with the opposite thermal pattern, emergence occurred first at the downstream, warmer reaches (Figure 6B).
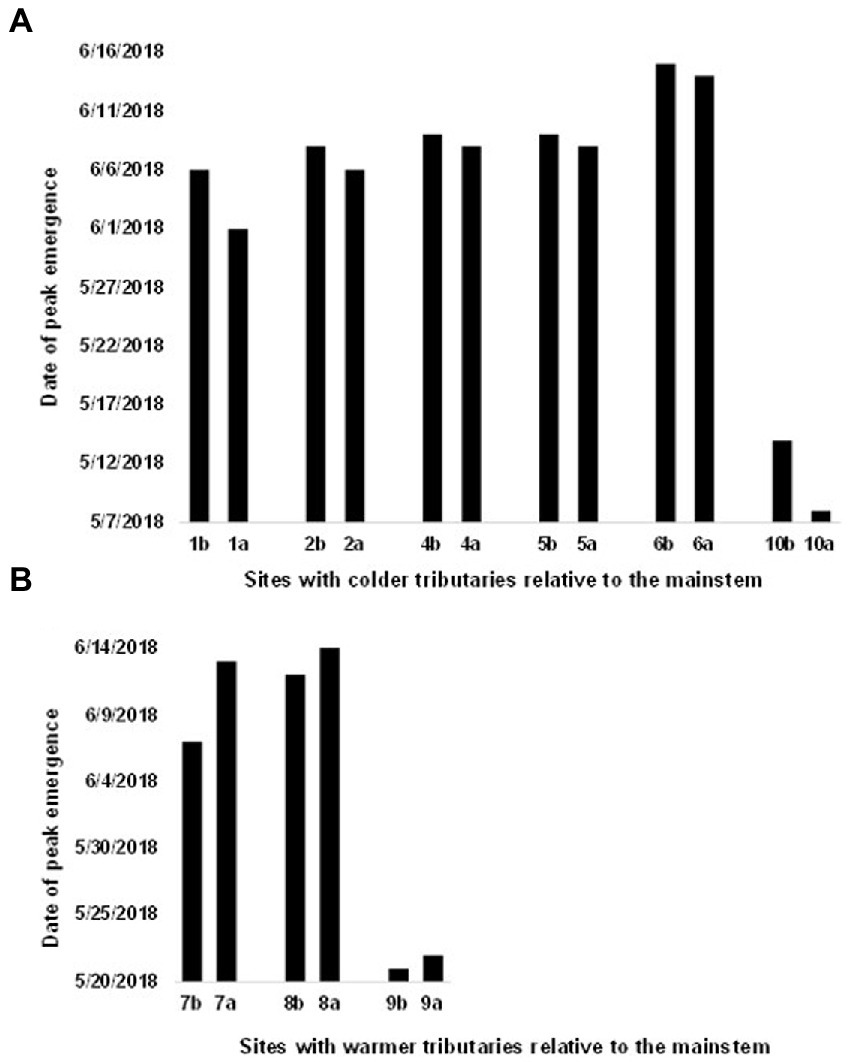
Figure 6. Comparison of timing of salmonfly emergence at paired reaches in the Salmon River basin [sites (1–8) and Henry’s Fork of the Snake River (sites 9 and 10)] located above and below confluences (a and b, respectively), Idaho, United States in June 2018. Thermal discontinuities created by tributary confluences were associated with temporal asynchrony in emergence timing (binomial test p = 0.002; a = above, b = below confluence). (A) Sites with colder tributaries relative to the mainstem. Emergence occurred above the confluence first then below. (B) Sites with warmer tributaries relative to the mainstem. Emergence occurred below the confluence first, then above. Temperature is based on measurements taken within a few days of peak emergence. Site 3 was excluded for this comparison due to lack of sufficient temperature data required to assess whether the waters above or below the confluence were warmer on the date of peak emergence.
Bird feeding behavior
We observed 10 bird species feeding on salmonflies across the 10 confluence pairs (Table 3 and Supplementary Appendix 1), which we henceforth refer to as “salmonfly-consuming birds.” Five (50%) of the bird species feeding on salmonflies were classified in the literature (Sullivan and Vierling, 2009) as associated with upland habitats (e.g., American kestrel Falco sparverius, Western tanager, American robin), four (40%) were associated with riparian habitats (e.g., Bullock’s oriole Icterus bullockii, Lewis’s woodpecker), and one (10%) was classified as a waterbird (i.e., the American dipper Cinclus mexicanus). Some of these species fed heavily on salmonflies. The capture rates of salmonflies we observed varied among the 10 species, with the most observations (n = 44) of Lewis’s woodpeckers and the fewest observations (n = 3) of Black-billed magpies (Pica hudsonia). During this period, some species were observed nesting in the riparian area, principally Lewis’s woodpeckers and American dippers. Accordingly, we observed these species, and especially Lewis’s woodpeckers, capturing salmonflies which were fed to their nestlings, based on direct observation or repeated transport of captured salmonflies into typical nesting cavities. On the Warm River above the confluence with Robinson Creek, we observed a pair of American dippers capturing salmonflies, killing them with repeated blows on a rock and feeding them to three nestlings. Between the two adult dippers, they captured four salmonflies in 10 min. We also consistently observed Western tanagers (Figure 1), which had recently arrived from their neotropical migration. Frequently, tanagers were in groups of up to 20 individuals in riparian zones and we recorded them capturing salmonflies by both hawking and gleaning. Though we had repeated foraging rate observations for the days leading up to and including peak emergence for only 7 reaches, in 6 of these 7 cases peak capture rates by all birds preceded peak emergence (average of 2 days earlier). For example, below the confluence of the Middle Fork and mainstem Salmon River, we observed an average rate of salmonfly capture by Lewis’s woodpeckers of 3.9 salmonflies/10 min on the day before peak emergence, and 3.0 salmonflies/10 min on the day of peak emergence.
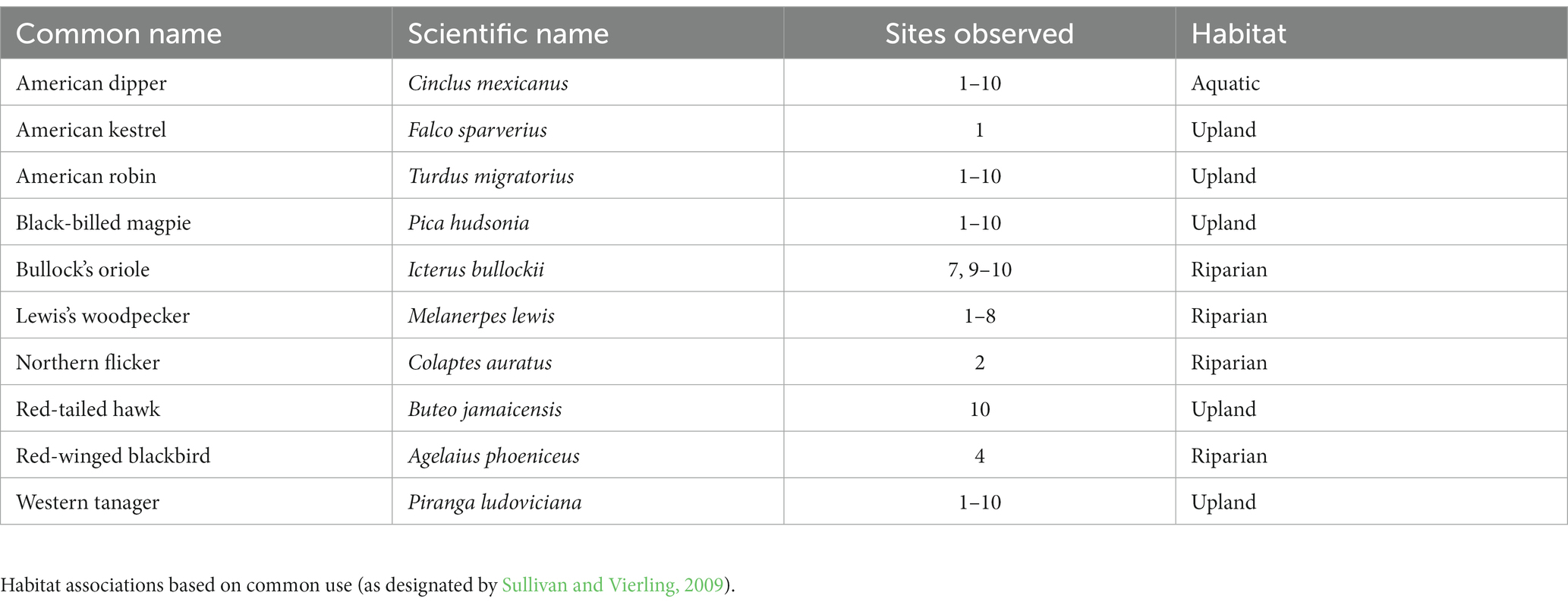
Table 3. Species observed capturing and feeding on adult giant salmonflies in the Salmon River Basin and Henry’s Fork of the Snake River, Idaho, United States in May–June 2018.
Bird distribution and abundance
Salmonfly emergence appeared to attract birds. Bird species richness and abundance nearly doubled, on average, during salmonfly emergence. The presence of emerging adult salmonflies was associated with an increase in the local species richness of salmonfly-consuming birds. The number of salmonfly-consuming bird species observed was greater when salmonflies were present, and this richness increased with salmonfly presence at 10 of the 14 reaches (p = 0.090; Figure 7A). Though these differences were only marginally significant, we note that in the remaining 4 reaches, species richness remained constant; it never decreased. The average percent change in bird species richness was 114%, ranging from 0 to 400%. The increase in the average number of individuals and species was comprised of different species at different sites (Supplementary Appendix 1). For example, Lewis’s woodpeckers were exploiting salmonflies primarily at the sites in the lower Salmon River drainage, whereas American robins were exploiting salmonflies primarily in the Henry’s Fork drainage.
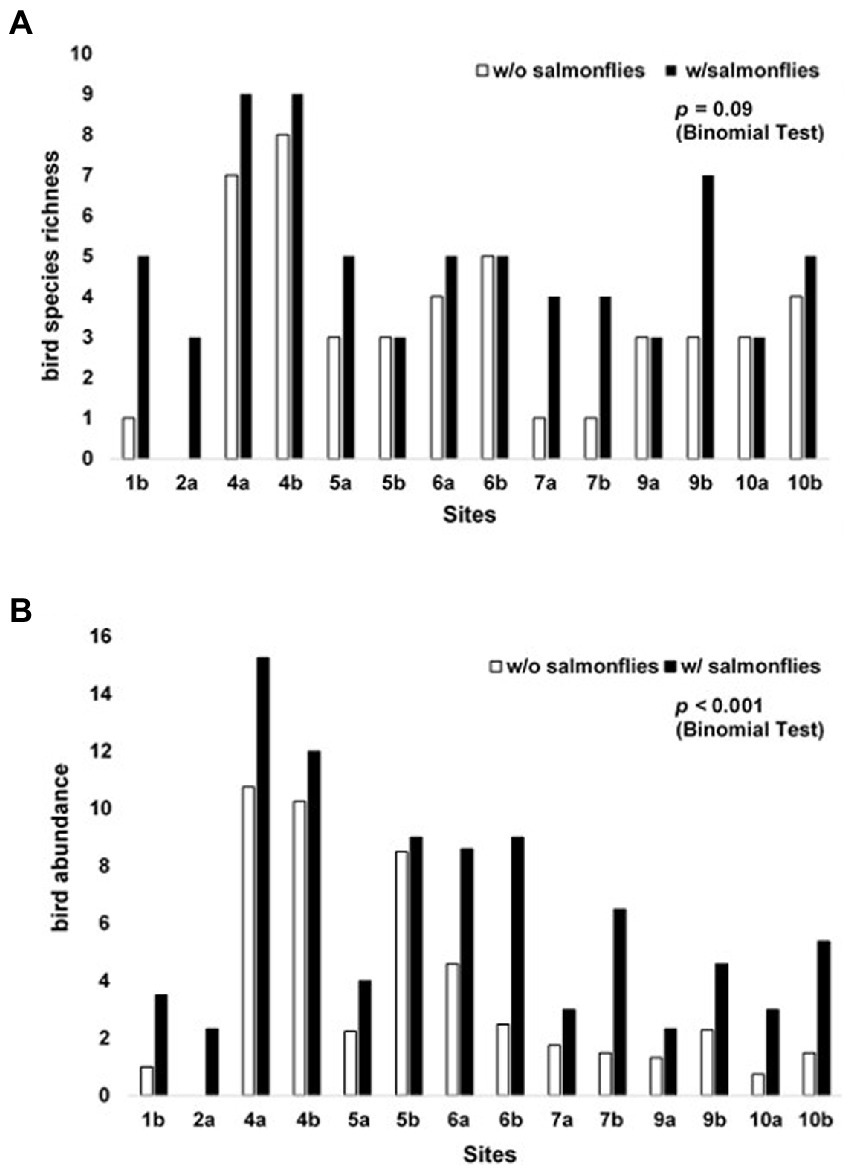
Figure 7. (A) Shifts in the average number of bird species (species richness), for the 10 species of birds observed feeding on salmonflies observed at each reach above and below the confluence when adult salmonflies were present vs. absent (binomial test p = 0.090) in the Salmon River Drainage and Henry’s Fork of the Snake River Drainage, Idaho, United States during May–June 2018. (B) Changes in average abundance of individual salmonfly-consuming birds among the 10 species of birds observed feeding on salmonflies at each reach above and below the confluence (denoted by a vs. b) when adult salmonflies were present vs. absent (binomial test p < 0.001) in the Salmon River Drainage (sites 1–8) and Henry’s Fork of the Snake River Drainage (sites 9 and 10), Idaho, United States during May–June 2018.
The average number of individual salmonfly-consuming birds observed was greater at all of the 14 reaches analyzed when salmonflies were present vs. when they were not (p < 0.001; Figure 7B). Across all reaches, we observed the average number of salmonfly-consuming birds increased when salmonflies were present, exhibiting a range of increase from 6 to 333% (Mainstem/Yankee Fork below and South Fork/Secesh below, respectively) and an average increase in bird abundance with salmonfly emergence of 148%.
Although we consistently observed increases in bird abundance when adult salmonflies were present, the degree of increase was not associated with our estimates of the magnitude of salmonfly emergence; there was no relationship with salmonfly C-flux, nor with the total number of live adult salmonflies observed at the time of a bird count (Supplementary Figure S1). Indeed, we observed the beginning of the emergence on the Henry’s Fork where we counted only six salmonflies, all of which were eaten within 10 min by four American robins. On the other hand, at the same site during the peak of the emergence, with hundreds of salmonflies present, we observed relatively little feeding activity by birds. Such a pattern was most evident at sites in the Henry’s Fork watershed where the magnitude of emergence was larger (average of 552 adult salmonflies on days of peak emergence) than at sites in the Salmon River Basin (average of 110 adult salmonflies on days of peak emergence).
Discussion
We found that variation in timing of the emergence of giant salmonflies was associated with spatial variation in river temperature, and the pattern of salmonfly emergence varied at different spatial scales. At the scale of the Salmon River basin, salmonfly emergence timing was highly variable across the network, from May–June. Thermal heterogeneity within the network helped to explain this variability, with emergence in warmer waters preceding emergence in cooler waters, as has been previously reported (e.g., Gregory et al., 2000; Anderson et al., 2019). The thermal relationship we assessed at the basin scale was based on the NorWeST Stream Temperature model (Isaak et al., 2017) which employed mean August temperatures. While August temperatures were a reasonable proxy for thermal heterogeneity of springtime water temperatures, a richer understanding of basin scale emergence may be possible if data were available for June temperatures when emergence actually occurs or for accumulated degree days prior to emergence. We did not conduct a mechanistic evaluation of drivers of salmonfly development, and it is likely that factors such as accumulated degree-days, food resources available for salmonflies, or other variables influence emergence timing at the basin scale. In fact, across such distances and elevation differences, within-basin differences in life cycle duration can occur (e.g., 3 vs. 4-year larval stage; Schultheis et al., 2008) that might also influence emergence timing. Nevertheless, the relative thermal patterns from August values indicated a clear and biologically reasonable association of temperature and emergence timing at the basin scale. The overall emergence pattern occurred sequentially from downstream to upstream, as might be expected in response to gradually cooler thermal regimes upstream, and as has been observed elsewhere (e.g., Anderson et al., 2019). Interestingly, this general pattern was most evident along extended segments not punctuated by major tributary confluences, as shown in the consistent down-to-upstream pattern we observed along sub-basin pathways when that path was begun upstream of a confluence. Hence, even at the basin scale we found a basis for recognizing confluences as biologically important features at smaller scales.
At the scale of reaches within river segments, our study revealed that the abrupt changes in thermal regimes created by tributary confluences resulted in temporal asynchrony of 1–6 days in emergence timing above and below confluences. Our observations at this scale are consistent conceptually with the view that rivers are punctuated by ecologically important discontinuities (Montgomery, 1999; Poole, 2002) and that confluences are an important form of discontinuity that introduce dynamics such as geomorphic and thermal heterogeneity, into networks (Benda et al., 2004; Rice et al., 2008; Fullerton et al., 2015). Because temperature drives aquatic insect development (Sweeney, 1984), and can affect the synchrony of aquatic insect life history events (Śniegula et al., 2016), it is reasonable that thermal discontinuities created by confluences are associated with nuanced differences in insect emergence timing. Importantly, the details of ecologically significant events like the emergence of a prey species transporting nutrients and energy to terrestrial systems was revealed through finer scale analysis, in this case at the sub-basin scale. Increasingly, the benefits of such multi-scale analyses are being recognized (Fausch et al., 2002; Torgersen et al., 2022). By moving to smaller scales, we observed ephemeral, disjunct pulses of a potentially valuable trophic resource throughout a river network. The pattern we observed is more nuanced than the concept of a “resource wave” (sensu Armstrong et al., 2016) moving progressively and systematically through the river system each year. One consequence of ephemeral, disjunct pulses being the pattern of emergence rather than a smoothly progressing wave is that to exploit salmonfly emergence as a bonanza, predators of salmonflies may need the capacity to employ mobility and behavioral flexibility. In our study, birds appeared to be able to recognize and exploit emerging salmonflies despite emergence heterogeneity.
Our observations of emergence within reaches, and of individual salmonfly behavior, indicate that there may be a minimum water temperature threshold that is associated with the timing of peaks in salmonfly emergence. We did not observe a relationship with the mean temperature leading up to emergence, as has been found in other studies of salmonflies (Gregory et al., 2000; Anderson et al., 2019), nor the daily thermal range, which has been associated with emergence timing of other aquatic insects (e.g., Uno, 2016). Rather, the minimum water temperature we recorded on the date of peak emergence at any site was 8.4°C. Such a minimum threshold may be an important cue to salmonflies that reduces mortality upon emergence that might result from low air temperatures (Rockwell and Newell, 2009). However, males typically emerge up to 3 days before females begin to emerge (Sheldon, 1999), which may increase their chances of mortality due to cold temperatures and predation. Our qualitative observations suggest that salmonflies that emerged earlier were predominantly male, as has been described by others (Sheldon, 1999). Emergence of females, and hence the subsequent peak of the overall emergence, may have lagged and required warmer conditions more conducive to mating and ovipositing, behaviors that we typically observed with greater frequency on warmer days. Future investigations are needed to determine if female salmonflies are more sensitive to colder temperatures for successful reproduction, or to evaluate other processes that might be responsible for the patterns we observed. Such studies may help address the importance of emergence timing and behavior to the maintenance of salmonfly populations which are increasingly imperiled (below), as well as reveal processes that mediate salmonfly interactions with predators like birds.
In the thermally heterogeneous river networks we studied, salmonfly emergence did not occur as a single synchronized pulse, but instead as an asynchronous set of pulses. If salmonfly emergence were synchronous across a basin or over long river segments, birds would have only a few days to locate and exploit the salmonflies. However, spatial variation in the phenology of emergence like we observed bracketing major tributary confluences, or that can occur at smaller scales within reaches (e.g., Gregory et al., 2000; Uno, 2016; Uno and Pneh, 2020), may allow mobile consumers to extend their foraging opportunities by foraging sequentially among localized pulses. In fact, our observations likely provide a conservative account of the potential for this phenomenon. For example, we focused on mainstem reaches bracketing tributary confluences, but did not include the tributaries themselves as a part of our study. This was due, in part, to logistical constraints, but also because many of the tributaries lacked salmonflies or possessed them in low abundance. Nevertheless, for contexts where the tributaries themselves also had substantial salmonfly populations, ours likely represent conservative estimates of the protracted nature of emergence and the prolonged availability of adult salmonflies to birds.
A diverse array of bird species foraged on salmonflies. Some may be drawn to riparian zones to take advantage of this opportunity, and certainly we observed individual birds intensively foraging on salmonflies and feeding salmonflies to nestlings. During emergence, we observed 10 species of birds capturing adult salmonflies, with individuals of some (e.g., Lewis’s woodpeckers) capturing at average rates of 1 salmonfly every 2 min. Salmonfly-consuming birds included several species typically associated with upland habitats (e.g., American kestrels, Western tanagers, American robins). Such behavioral aggregation by upland birds in riparian zones during periods of aquatic insect emergence has been reported in other settings (e.g., Gray, 1993; Uesugi and Murakami, 2007). The few published reports of birds feeding on salmonflies have been anecdotal, but are consistent with our observations [e.g., Western tanagers (Sheldon, 1999)] and include several others that we did not observe but that do occur in our study region [e.g., Stellar’s jay Cyanocitta stelleri (DeWalt and Stewart, 1995), Peregrine falcon Falco peregrinus and Common raven Corvus corax (Rockwell and Newell, 2009)]. We suspect that our observations resulted in a conservative estimate of the diversity of birds that feed on salmonflies in the study region, as a thorough assessment of this was not the focus of our study. We also frequently observed species that were nesting during this period in riparian zones, especially Lewis’s woodpeckers and American dippers, capturing and feeding salmonflies to their young. Not only are salmonflies large, energy and nutrient-rich prey (Walters et al., 2018; Wesner et al., 2019), but a recent analysis (Albertson et al., 2022) revealed that they are concentrated in highly unsaturated omega-3 fatty acids (HUFAs), for which aquatic insects in general may be a key source for birds that positively influence avian reproduction (Twining et al., 2018). Moreover, a pilot analysis we conducted of individual salmonflies collected from the Salmon River basin (Adams et al., unpublished data), indicated that adult salmonflies may also possess relatively high levels of several carotenoid compounds, the same compounds that female birds sequester into egg yolks to support embryonic development and which also have been linked to immune function and plumage features (McGraw et al., 2006; Eeva et al., 2010). We conjecture that salmonflies could be especially valuable nutritionally to birds and future investigations to directly evaluate this hypothesis seem warranted.
Our findings suggest birds may shift their spatial distributions to exploit salmonflies, including to take advantage of the asynchrony in salmonfly emergence created by thermal heterogeneity above and below confluences in river networks. Across all sites, both bird species richness and abundance nearly doubled, on average, during salmonfly emergence. We infer that birds were responding to the presence of salmonflies above and below a given confluence, as indicated by the 148% average increase in bird abundance in relation to emergence. Of course, many factors influence the distribution, abundance, and behavior of the birds we observed, and do so across scales. Even for those species that are riparian (e.g., Lewis’s woodpeckers) or aquatic oriented (e.g., American dippers) and are well known to feed on riverine insects (e.g., Abele et al., 2004; Sullivan and Vierling, 2012), other relationships of importance to these birds seem likely to mediate the overall role that may be played by a pulse of resources from salmonflies, and there is a need to evaluate whether the patterns we observed translate into fitness. Admittedly we did not track individual birds, and our results may in part be a function of changes in bird detectability, which might have increased when salmonflies were present if birds were more likely to be observed when they increased their foraging activities or focused their foraging effort on salmonflies. Regardless, because salmonflies are an ephemeral resource at any single location, individual birds that can shift their distributions among habitats to track this phenological variation may extend their access to this resource. In fact, this may extend to consumers other than birds, as animals such as snakes, frogs, spiders, and ground squirrels have also been documented to consume adult salmonflies (Muttkowski, 1925), as have indigenous peoples (Sutton, 1985). Overall, the patterns we observed in salmonfly emergence may prolong the seasonal opportunities for any animals that track and exploit these insects, as has been described for other resources and their consumers (Bischof et al., 2012; Lok et al., 2012; Schindler et al., 2013), and our findings are consistent with the idea that interactions between spatial and temporal heterogeneity and organism phenology may be critical to understanding the consequences of fluxes of resources that link water and land.
Our results contribute to growing evidence that phenological diversity, not simply abundance, is an important component of energy flow within upper trophic levels of food webs in seasonally dynamic ecosystems (Armstrong et al., 2016; Takimoto and Sato, 2020). For instance, we observed that the magnitude of the bird response was not associated with total salmonfly C flux, nor with the total number of adult salmonflies present in the reach, but was instead apparently more closely linked to timing of prey occurrence. This observation was somewhat surprising because we had expected that a large flux of salmonflies would elicit a stronger avian response. It is possible that the precision of our estimates regarding timing of emergence may not have been adequate to unequivocally assess these relationships. Moreover, the lack of evidence for a straightforward numerical response in this case does not necessarily mean that a functional response did not occur. However, observations made in the field suggested that the ratio of salmonflies to birds plays an important role in the feeding and capturing rate exhibited by birds. This may be a result of the evolutionary strategy of predator swamping, which is the hypothesis that individuals of prey species can improve their fitness by synchronizing various aspects of their life history, such as emergence (Tucker et al., 2007). Indeed, as described by Sweeney and Vannote (1982), this phenomenon may occur when the quantity of emerging aquatic insects outnumbers the potential number that can be captured by predators like birds, allowing remaining insects to survive and reproduce. Thus, as Uno (2016) showed for a large-bodied mayfly whose spatial variation in emergence had consequences for riparian spiders, phenological diversity in timing of emergence may be more important to the foraging efforts made by birds than overall abundance of emerging insects like salmonflies.
Salmonfly populations are declining or have been lost from many rivers of the western U.S. for reasons not well understood (Nehring et al., 2011; Birrell et al., 2019; Kowalski and Richer, 2020). This conservation concern provides added context for our findings for ecological, socio-cultural, and economic reasons. The salmonfly emergence is among the most iconic and anticipated in the country for trout anglers, and is therefore important for many local economies (Nehring et al., 2011). Moreover, as global temperatures increase, salmonfly emergence is occurring earlier in the year in locations like the Henry’s Fork of the Snake River (Gregory et al., 2000; R. Van Kirk, Henry’s Fork Foundation, personal communication). This may affect the overlap in timing between emergence and the timing of bird life histories, including the arrival in this region of migratory neotropical birds. If salmonflies represent an important resource to migratory birds breeding in western montane ecosystems, progressively earlier emergence may shift emergence to periods outside its utility to migratory birds, with unknown impact. For instance, the Western tanager is a neotropical migrant that arrives in Idaho during the timeframe of salmonfly emergence, and we repeatedly observed what appeared to be newly arriving tanagers feeding on salmonfly adults. Even shifts at the scale of days in salmonfly emergence timing could mean that this prey resource would be unavailable to these birds upon their arrival in the region, especially if salmonfly emergence timing also became more synchronized in response to habitat homogenization. Climate change and river habitat homogenization, driven by processes like flow regulation, channelization, or changes in riparian vegetation, are changing river thermalscapes, and this could threaten salmonfly persistence (Nehring et al., 2011; Birrell et al., 2019; Kowalski and Richer, 2020), change synchrony important to population processes and predator satiation, or alter the mechanisms underlying spatial variation in their phenology. For example, a more homogenous thermal regime might amplify the synchrony of salmonfly emergence, which could favor salmonflies if it increased satiation of predators. On the other hand, thermal heterogeneity might allow salmonflies to spread the risk in terms of emergence timing, not to mention prolong their access as prey to terrestrial insectivores. Future investigations are needed to understand how phenological diversity like that we observed for salmonfly emergence may contribute to the maintenance of biodiversity in food webs that link land and water, and to assess the consequences of climate change and river management policies not only for individual species, but for webs of interacting organisms associated with rivers and their surrounding landscapes.
Data availability statement
The datasets presented in this study can be found in online repositories. The names of the repository/repositories and accession number(s) can be found in the article/Supplementary material.
Ethics statement
Ethical review and approval was not required for the animal study because no handling of birds was required, only passive observation.
Author contributions
MA and CB conceived, designed, and led data collection for the study and writing of manuscript. MA analyzed the data. DD contributed input to study design and methods, advice on analysis and interpretation, and assisted in writing. All authors contributed to the article and approved the submitted version.
Funding
This work was supported through funding from the DeVlieg Foundation, the James Morris Stream Ecology Endowment, Idaho State University’s College of Science and Engineering and Department of Biological Sciences, and instrumentation of Idaho State University’s Center for Ecological Research and Education.
Acknowledgments
We thank the following individuals for field and lab assistance: Joel Adams, Kate Behn, Rachel Brinkley, Jeremy Brooks, Jennifer Hardy, Sophie Hill, Jade Ortiz, James Paris, and Justin and Laurie Seamons. We thank Sarah Godsey and the late G. Wayne Minshall for study input and manuscript review. We also thank Taylor Wilderness Research Station, the Idaho Outfitter Guiding Association, the US Forest Service and the Henry’s Fork Foundation for assistance. Finally, thanks to the “salmonfly working group,” including David Walters, Jeff Wesner, Lindsey Albertson, Wyatt Cross, and Cornelia Twining, for sharing their enthusiasm and ideas.
Conflict of interest
The authors declare that the research was conducted in the absence of any commercial or financial relationships that could be construed as a potential conflict of interest.
Publisher’s note
All claims expressed in this article are solely those of the authors and do not necessarily represent those of their affiliated organizations, or those of the publisher, the editors and the reviewers. Any product that may be evaluated in this article, or claim that may be made by its manufacturer, is not guaranteed or endorsed by the publisher.
Supplementary material
The Supplementary material for this article can be found online at: https://www.frontiersin.org/articles/10.3389/fevo.2023.804143/full#supplementary-material
References
Abele, S. C., Saab, V. A., and Garton, E. O. (2004). Lewis's woodpecker (Melanerpes lewis): A technical conservation assessment. Golden, CO: USDA Forest Service, Rocky Mountain Region. 50
Albertson, L. K., Briggs, M. A., Maguire, Z., Swart, S., Cross, W. F., Twining, C. W., et al. (2022). Dietary composition and fatty acid content of giant salmonflies (Pteronarcys californica) in two Rocky Mountain rivers. Ecosphere 13:e 3904. doi: 10.1002/ecs2.3904
Anderson, H. E., Albertson, L. K., and Walters, D. M. (2019). Water temperature drives variability in salmonfly abundance, emergence timing, and body size. River Res. Appl. 35, 1013–1022. doi: 10.1002/rra.3464
Armstrong, J. B., Takimoto, G., Schindler, D. E., Hayes, M. M., and Kauffman, M. J. (2016). Resource waves: phenological diversity enhances foraging opportunities for mobile consumers. Ecology 97, 1099–1112. doi: 10.1890/15-0554.1
Baruch, E. M., Bateman, H. L., Lytle, D. A., Merritt, D. M., and Sabo, J. L. (2021). Integrated ecosystems: linking food webs through reciprocal resource reliance. Ecology 102:e03450. doi: 10.1002/ecy.3450
Baumann, R. W., Gaufin, A. R., and Surdick, R. F. (1977). The stoneflies (Plecoptera) of the Rocky Mountains [USA]. Memoirs of the American Entomological Society (USA). no. 31. American Entomological Society, Philadelphia.
Baxter, C. V., Fausch, K. D., and Carl Saunders, W. (2005). Tangled webs: reciprocal flows of invertebrate prey link streams and riparian zones. Freshw. Biol. 50, 201–220. doi: 10.1111/j.1365-2427.2004.01328.x
Benda, L. E. E., Poff, N. L., Miller, D., Dunne, T., Reeves, G., Pess, G., et al. (2004). The network dynamics hypothesis: how channel networks structure riverine habitats. AIBS Bull. 54, 413–427. doi: 10.1641/0006-3568(2004)054[0413:TNDHHC]2.0.CO;2
Birrell, J. H., Meek, J. B., and Nelson, C. R. (2019). Decline of the giant salmonfly Pteronarcys californica Newport, 1848 (Plecoptera: Pteronarcyidae) in the Provo River, Utah, USA. Illiesia 15, 53–97. doi: 10.25031/2019/15.05
Bischof, R., Loe, L. E., Meisingset, E. L., Zimmermann, B., Van Moorter, B., and Mysterud, A. (2012). A migratory northern ungulate in the pursuit of spring: jumping or surfing the green wave? Am. Nat. 180, 407–424. doi: 10.1086/667590
Bruns, D. A., Minshall, G. W., Cushing, C. E., Cummins, K. W., and Brock, J. T. (1984). Tributaries as modifiers of the river continuum concept: analysis by poplar ordination and regression models. Archiv fur Hydrobiol, 99, 208–220.
Bryce, S. A., Lomnicky, G. A., and Kaufmann, P. R. (2010). Protecting sediment-sensitive aquatic species in mountain streams through the application of biologically based streambed sediment criteria. J. N. Am. Benthol. Soc. 29, 657–672. doi: 10.1899/09-061.1
Deacy, W., Leacock, W., Armstrong, J. B., and Stanford, J. A. (2016). Kodiak brown bears surf the salmon red wave: direct evidence from GPS collared individuals. Ecology 97, 1091–1098. doi: 10.1890/15-1060.1
DeWalt, R. E., and Stewart, K. W. (1995). Life histories of stoneflies (Plecoptera) in the Rio Conejos of southern Colorado. The Great Basin Naturalist, 1–18.
Eeva, T., Helle, S., Salminen, J. P., and Hakkarainen, H. (2010). Carotenoid composition of invertebrates consumed by two insectivorous bird species. J. Chem. Ecol. 36, 608–613. doi: 10.1007/s10886-010-9796-0
Elder, J. A., and Gaufin, A. R. (1973). Notes on the occurrence and distribution of Pteronarcys californica Newport (Plecoptera) within streams. Great Basin Naturalist 33, 218–220.
Fausch, K. D., Torgersen, C. E., Baxter, C. V., and Li, H. W. (2002). Landscapes to riverscapes: bridging the gap between research and conservation of stream fishes: a continuous view of the river is needed to understand how processes interacting among scales set the context for stream fishes and their habitat. BioScience 52, 483–498.
Fukui, D. A. I., Murakami, M., Nakano, S., and Aoi, T. (2006). Effect of emergent aquatic insects on bat foraging in a riparian forest. J. Anim. Ecol. 75, 1252–1258. doi: 10.1111/j.1365-2656.2006.01146.x
Fullerton, A. H., Torgersen, C. E., Lawler, J. J., Faux, R. N., Steel, E. A., Beechie, T. J., et al. (2015). Rethinking the longitudinal stream temperature paradigm: region-wide comparison of thermal infrared imagery reveals unexpected complexity of river temperatures. Hydrol. Process. 29, 4719–4737. doi: 10.1002/hyp.10506
Gelman, A. (2013). Commentary: P values and statistical practice. Epidemiology 24, 69–72. doi: 10.1097/EDE.0b013e31827886f7
Gray, L. J. (1993). Response of insectivorous birds to emerging aquatic insects in riparian habitats of a tallgrass prairie stream. Am. Midl. Nat. 129, 288–300. doi: 10.2307/2426510
Gregory, J. S., Beesley, S. S., and Van Kirk, R. W. (2000). Effect of springtime water temperature on the time of emergence and size of Pteronarcys californica in the Henry's fork catchment, Idaho, USA. Freshw. Biol. 45, 75–83. doi: 10.1046/j.1365-2427.2000.00619.x
Harris, H. E., Baxter, C. V., and Davis, J. M. (2015). Debris flows amplify effects of wildfire on magnitude and composition of tributary subsidies to mainstem habitats. Freshw. Sci. 34, 1457–1467. doi: 10.1086/684015
Harris, H. E., Baxter, C. V., and Davis, J. M. (2018). Wildfire and debris flows affect prey subsidies with implications for riparian and riverine predators. Aquat. Sci. 80, 1–14. doi: 10.1007/s00027-018-0589-9
Hutto, R. L., Pletschet, S. M., and Hendricks, P. (1986). A fixed-radius point count method for nonbreeding and breeding season use. Auk 103, 593–602. doi: 10.1093/auk/103.3.593
Hynes, H. B. N. (1975). The stream and its valley. Internationale Vereinigung für Theoretische und Angewandte Limnologie: Verhandlungen 19, 1–15
Ims, R. A. (1990). On the adaptive value of reproductive synchrony as a predator-swamping strategy. Am. Nat. 136, 485–498. doi: 10.1086/285109
Isaak, D.J., Wenger, S.J., Peterson, E.E., Ver Hoef, J.M., Hostetler, S.W., Luce, C.H., et al. (2016a). NorWeST modeled summer stream temperature scenarios for the western U.S. Fort Collins, CO: Forest Service Research Data Archive.
Isaak, D., Wenger, S., Peterson, E., Ver Hoef, J., Nagel, D., Luce, C., et al. (2017). The NorWeST summer stream temperature model and scenarios for the western U.S.: a crowd-sourced database and new geospatial tools foster a user community and predict broad climate warming of rivers and streams. Water Resour. Res. 53, 9181–9205. doi: 10.1002/2017WR020969
Isaak, D. J., Young, M. K., Luce, C. H., Hostetler, S. W., Wenger, S. J., Peterson, E. E., et al. (2016b). Slow climate velocities of mountain streams portend their role as refugia for cold-water biodiversity. Proc. Natl. Acad. Sci. 113, 4374–4379. doi: 10.1073/pnas.1522429113
Iwata, T., Nakano, S., and Murakami, M. (2003). Stream meanders increase insectivorous bird abundance in riparian deciduous forests. Ecography 26, 325–337. doi: 10.1034/j.1600-0587.2003.03355.x
Kauwe, J. S., Shiozawa, D. K., and Evans, R. P. (2004). Phylogeographic and nested clade analysis of the stonefly Pteronarcys californica (Plecoptera:Pteronarcyidae) in the western USA. J. N. Am. Benthol. Soc. 23, 824–838. doi: 10.1899/0887-3593(2004)023<0824:PANCAO>2.0.CO;2
Kiffney, P. M., Greene, C. M., Hall, J. E., and Davies, J. R. (2006). Tributary streams create spatial discontinuities in habitat, biological productivity, and diversity in mainstem rivers. Can. J. Fish. Aquat. Sci. 63, 2518–2530. doi: 10.1139/f06-138
Kowalski, D. A., and Richer, E. E. (2020). Quantifying the habitat preferences of the stonefly Pteronarcys californica in Colorado. River Res. Appl. 36, 2043–2050. doi: 10.1002/rra.3733
Lok, E. K., Esler, D., Takekawa, J. Y., Susan, W., Boyd, W. S., Nysewander, D. R., et al. (2012). Spatiotemporal associations between Pacific herring spawn and surf scoter spring migration: evaluating a ‘silver wave’ hypothesis. Mar. Ecol. Prog. Ser. 457, 139–150. doi: 10.3354/meps09692
Marcarelli, A. M., Baxter, C. V., Mineau, M. M., and Hall Jr, R. O. (2011). Quantity and quality: unifying food web and ecosystem perspectives on the role of resource subsidies in freshwaters. Ecology 92, 1215–1225. doi: 10.1890/10-2240.1
Marczak, L. B., and Richardson, J. S. (2008). Growth and development rates in a riparian spider are altered by asynchrony between the timing and amount of a resource subsidy. Oecologia 156, 249–258. doi: 10.1007/s00442-008-0989-y
McGraw, K. J., Crino, O. L., Medina-Jerez, W., and Nolan, P. M. (2006). Effect of dietary carotenoid supplementation on food intake and immune function in a songbird with no carotenoid coloration. Ethology 112, 1209–1216. doi: 10.1111/j.1439-0310.2006.01280.x
Montgomery, D. R. (1999). Process domains and the river continuum. J. Am. Water Resour. Assoc. 35, 397–410. doi: 10.1111/j.1752-1688.1999.tb03598.x
McGraw, J. W., Beakes, M. P., Nesbitt, H. K., Yeakel, J. D., Patterson, D. A., Thompson, L. A., et al. (2015). Emergent stability in a large, free‐flowing watershed. Ecology, 96, 340–347.
Muttkowski, R. A. (1925). The food of trout in Yellowstone National Park. Roosevelt Wildlife Bullet. 2, 470–497.
Nakano, S., and Murakami, M. (2001). Reciprocal subsidies: dynamic interdependence between terrestrial and aquatic food webs. Proc. Natl. Acad. Sci. 98, 166–170. doi: 10.1073/pnas.98.1.166
Nehring, R. B., Heinhold, G., and Pomeranz, J. (2011). Colorado River aquatic resources investigations. Colorado Division of Wildlife Progress Report, Federal Aid Project F-237R-18, Fort Collins, CO.
Paetzold, A., Schubert, C. J., and Tockner, K. (2005). Aquatic-terrestrial linkages along a braided river: riparian arthropods feeding on aquatic insects. Ecosystems 8, 748–759. doi: 10.1007/s10021-005-0004-y
Polis, G. A., Anderson, W. B., and Holt, R. D. (1997). Toward an integration of landscape and food web ecology: the dynamics of spatially subsidized food webs. Annu. Rev. Ecol. Syst. 28, 289–316. doi: 10.1146/annurev.ecolsys.28.1.289
Polis, G. A., Power, M. E., and Huxel, G. R. (2004). Food webs at the landscape level. University of Chicago Press, Chicago.
Poole, W. C. (1981). The ecological bioenergetics of Pteronarcys californica (Newport). Ph.D. Dissertation, Idaho State University, Pocatello, IDaho, USA.
Poole, G. C. (2002). Fluvial landscape ecology: addressing uniqueness within the river discontinuum. Freshw. Biol. 47, 641–660. doi: 10.1046/j.1365-2427.2002.00922.x
Power, M. E., Rainey, W. E., Parker, M. S., Sabo, J. L., Smyth, A., Khandwala, S., et al. (2004). “River to watershed subsidies in an old-growth conifer forest,” in Food Webs at the Landscape Level, eds. G.A. Polis, M.E. Power and G.R. Huxel (Chicago, IL, U.S.A: The University of Chicago Press), 217–240.
R Core Team. (2018). R: A language and environment for statistical computing Vienna: R Foundation for Statistical Computing.
Ralph, C. J., Geupel, G. R., Pyle, P., Martin, T. E., and DeSante, D. F. (1993). Handbook of field methods for monitoring Landbirds. Albany, CA: USDA Forest Service/UNL Faculty Publications, 105.
Relyea, C. D., Minshall, G. W., and Danehy, R. J. (2012). Development and validation of an aquatic fine sediment biotic index. Environ. Manag. 49, 242–252. doi: 10.1007/s00267-011-9784-3
Reynolds, R. T., Scott, J. M., and Nussbaum, R. A. (1980). A variable circular-plot method for estimating bird numbers. The Condor 82, 309–313.
Rice, S. P., Greenwood, M. T., and Joyce, C. B. (2001). Tributaries, sediment sources, and the longitudinal organisation of macroinvertebrate fauna along river systems. Can. J. Fish. Aquat. Sci. 58, 824–840. doi: 10.1139/f01-022
Rice, S. P., Kiffney, P., Greene, C., and Pess, G. R. (2008). “The ecological importance of tributaries and confluences” in River confluences, tributaries and the fluvial network (West Sussex: John Wiley & Sons, Ltd), 209–242.
Richardson, J. S., Zhang, Y., and Marczak, L. B. (2010). Resource subsidies across the land–freshwater interface and responses in recipient communities. River Res. Appl. 26, 55–66. doi: 10.1002/rra.1283
Rockwell, I. P., and Newell, R. L. (2009). Note on mortality of the emerging stonefly Pteronarcys californica on the Jocko River, Montana, USA. Western N. Am. Nat. 69, 264–266. doi: 10.3398/064.069.0218
Sabo, J. L., and Power, M. E. (2002). Numerical response of lizards to aquatic insects and short-term consequences for terrestrial prey. Ecology 83, 3023–3036. doi: 10.1890/0012-9658(2002)083[3023:NROLTA]2.0.CO;2
Schindler, D. E., Armstrong, J. B., Bentley, K. T., Jankowski, K., Lisi, P. J., and Payne, L. X. (2013). Riding the crimson tide: mobile terrestrial consumers track phenological variation in spawning of an anadromous fish. Biol. Lett. 9:20130048. doi: 10.1098/rsbl.2013.0048
Schindler, D. E., and Smits, A. P. (2017). Subsidies of aquatic resources in terrestrial ecosystems. Ecosystems 20, 78–93. doi: 10.1007/s10021-016-0050-7
Schultheis, A. S., Booth, J. Y., Vinson, M. R., and Miller, M. P. (2008). Genetic evidence for cohort splitting in the merovoltine stonefly Pteronarcys californica (Newport) in blacksmith fork, Utah. Aquat. Insects 30, 187–195. doi: 10.1080/01650420801971350
Sheldon, A. L. (1999). Emergence patterns of large stoneflies (Plecoptera: Pteronarcys, Calineuria, Hesperoperla) in a Montana river. Great Basin Naturalist 59, 169–174.
Śniegula, S., Gołąb, M. J., and Johansson, F. (2016). Time constraint effects on phenology and life history synchrony in a damselfly along a latitudinal gradient. Oikos 125, 414–423. doi: 10.1111/oik.02265
Stewart, K.W., and Stark, B.P.S. (1993). Nymphs of north American stonefly genera (Plecoptera). University of North Texas Press, Denton, TX.
Sullivan, S. M. P., and Vierling, K. T. (2009). Experimental and ecological implications of evening bird surveys in stream-riparian ecosystems. Environ. Manage. 44, 789–799. doi: 10.1007/s00267-009-9318-4
Sullivan, S. M. P., and Vierling, K. T. (2012). Exploring the influences of multiscale environmental factors on the American dipper Cinclus mexicanus. Ecography 35, 624–636. doi: 10.1111/j.1600-0587.2011.07071.x
Sutton, M. Q. (1985). The California salmon fly as a food source in northeastern California. J. Calif. Gt. Basin Anthropol. 7, 176–182.
Sweeney, B. W. (1984). “Factors influencing life-history patterns of aquatic insects” in The ecology of aquatic insects (Praeger Scientific: New York, NY), 56–100.
Sweeney, B. W., and Vannote, R. L. (1982). Population synchrony in mayflies: a predator satiation hypothesis. Evolution 36, 810–821. doi: 10.2307/2407894
Takimoto, G., and Sato, T. (2020). Phenology in a community context: toward a better understanding of the causes and consequences of phenology in seasonal environments. Ecol. Res. 35, 442–444. doi: 10.1111/1440-1703.12124
Tang, C., Crosby, B. T., Wheaton, J. M., and Piechota, T. C. (2012). Assessing streamflow sensitivity to temperature increases in the Salmon River basin, Idaho. Glob. Planet. Chang. 88-89, 32–44. doi: 10.1016/j.gloplacha.2012.03.002
Torgersen, C. E., Le Pichon, C., Fullerton, A. H., Dugdale, S. J., Duda, J. J., Giovannini, F., et al. (2022). Riverscape approaches in practice: perspectives and applications. Biol. Rev. 97, 481–504. doi: 10.1111/brv.12810
Tucker, J. K., Paukstis, G. L., and Janzen, F. J. (2007). Does predator swamping promote synchronous emergence of turtle hatchlings among nests? Behav. Ecol. 19, 35–40. doi: 10.1093/beheco/arm097
Twining, C. W., Shipley, J. R., and Winkler, D. W. (2018). Aquatic insects rich in omega-3 fatty acids drive breeding success in a widespread bird. Ecol. Lett. 21, 1812–1820. doi: 10.1111/ele.13156
Uesugi, A., and Murakami, M. (2007). Do seasonally fluctuating aquatic subsidies influence the distribution pattern of birds between riparian and upland forests? Ecol. Res. 22, 274–281. doi: 10.1007/s11284-006-0028-6
Uno, H. (2016). Stream thermal heterogeneity prolongs aquatic-terrestrial subsidy and enhances riparian spider growth. Ecology 97, 2547–2553. doi: 10.1002/ecy.1552
Uno, H., and Pneh, S. (2020). Effect of source habitat spatial heterogeneity and species diversity on the temporal stability of aquatic-to-terrestrial subsidy by emerging aquatic insects. Ecol. Res. 35, 474–481. doi: 10.1111/1440-1703.12125
Vannote, R. L., Minshall, G. W., Cummins, K. W., Sedell, J. R., and Cushing, C. E. (1980). The river continuum concept. Can. J. Fish. Aquat. Sci. 37, 130–137. doi: 10.1139/f80-017
Visser, M. E., Van Noordwijk, A. J., Tinbergen, J. M., and Lessells, C. M. (1998). Warmer springs lead to mistimed reproduction in great tits (Parus major). Proc. R. Soc. Lond. B Biol. Sci. 265, 1867–1870. doi: 10.1098/rspb.1998.0514
Wallace, J. B., Eggert, S. L., Meyer, J. L., and Webster, J. R. (1997). Multiple trophic levels of a forest stream linked to terrestrial litter inputs. Science 277, 102–104. doi: 10.1126/science.277.5322.102
Walters, D. M., Wesner, J. S., Zuellig, R. E., Kowalski, D. A., and Kondratieff, M. C. (2018). Holy flux: spatial and temporal variation in massive pulses of emerging insect biomass from western US rivers. Ecology 99, 238–240. doi: 10.1002/ecy.2023
Wasserstein, R. L., Schirm, A. L., and Lazar, N. A. (2019). Moving to a world beyond “p < 0.05”. Am. Stat. 73, 1–19. doi: 10.1080/00031305.2019.1583913
Werner, E. E., and Gilliam, J. F. (1984). The ontogenetic niche and species interactions in size-structured populations. Annu. Rev. Ecol. Syst. 15, 393–425. doi: 10.1146/annurev.es.15.110184.002141
Wesner, J. S., Walters, D. M., and Zuellig, R. E. (2019). Pulsed salmonfly emergence and its potential contribution to terrestrial detrital pools. Food Webs 18:e00105. doi: 10.1016/j.fooweb.2018.e00105
Wilmshurst, J. F., Fryxell, J. M., and Colucci, P. E. (1999). What constrains daily intake in Thomson’s gazelles? Ecology 80, 2338–2347. doi: 10.1890/0012-9658(1999)080[2338:WCDIIT]2.0.CO;2
Winder, M., and Schindler, D. E. (2004). Climate change uncouples trophic interactions in an aquatic ecosystem. Ecology 85, 2100–2106. doi: 10.1890/04-0151
Keywords: giant salmonfly, Pteronarcys californica, aquatic insect emergence, riparian birds, avian insectivory, river-riparian linkages, resource subsidy, resource pulse
Citation: Adams MM, Baxter CV and Delehanty DJ (2023) Emergence phenology of the giant salmonfly and responses by birds in Idaho river networks. Front. Ecol. Evol. 11:804143. doi: 10.3389/fevo.2023.804143
Edited by:
Pablo Urrutia Cordero, Lund University, SwedenReviewed by:
Francis J. Burdon, University of Waikato, New ZealandFernando Chaguaceda, Swedish University of Agricultural Sciences, Sweden
Copyright © 2023 Adams, Baxter and Delehanty. This is an open-access article distributed under the terms of the Creative Commons Attribution License (CC BY). The use, distribution or reproduction in other forums is permitted, provided the original author(s) and the copyright owner(s) are credited and that the original publication in this journal is cited, in accordance with accepted academic practice. No use, distribution or reproduction is permitted which does not comply with these terms.
*Correspondence: Colden V. Baxter, coldenbaxter@isu.edu