- 1Institut de recherche sur les forêts, Université du Québec en Abitibi-Témiscamingue, Rouyn-Noranda, QC, Canada
- 2Département de biologie, chimie et géographie, Université du Québec à Rimouski, Rimouski, QC, Canada
Edge habitats resulting from the construction and maintenance of forest roads favour pioneer, shade-intolerant and disturbance-adapted plant species. The effect of roads on the spread of non-native species has been frequently studied, but few studies have focused upon their effects on native tree species. We studied the effect of forest roads on the expansion dynamics of trembling aspen (Populus tremuloides Michx.) in a boreal forest landscape of eastern Canada. We determined whether roads act as a habitat and dispersal corridor for trembling aspen, and whether populations that established along roads act as a starting point for aspen expansion into adjacent stands. We evaluated the effect of forest roads on the distribution of trembling aspen by surveying the vegetation along 694 km of roads. In 19 stands, we compared the density and age of individuals in 100 m transects established parallel and perpendicular to roads, to determine the role of roads. Trembling aspen is abundant along the forest road network. Forest roads act sometimes as habitat corridors for trembling aspen, but their effects on its density extend only over a short distance (10 m) on each side of the roads. The forest roads did not act as a starting point for the expansion of trembling aspen into adjacent stands. Forest roads are particularly favourable habitats for trembling aspen. Although roads did not act as a starting point for aspen dispersal away from roads, these habitats would be vulnerable to invasion following a disturbance that would reduce the thickness of the organic layer.
1 Introduction
The global road network has expanded rapidly over the past century, to the point that roads are currently one of the most widespread forms of natural landscape modification (Forman et al., 2003). Roads fragment the landscape and create edge effects that affect the physico-chemical environment and ecological processes, together with the dynamics, structure and composition of plant communities (Andrews, 1990; Murcia, 1995; Forman and Alexander, 1998; Ries et al., 2004). In forest ecosystems, the effects of road construction and maintenance include vegetation removal, soil disturbance, and changes in wind patterns, nutrient availability, and light in response to canopy opening (Forman et al., 2003; Harper et al., 2005). Edge habitats resulting from road construction typically differ in their plant composition compared to adjacent habitats, including a high abundance of shade-intolerant pioneer, nutrient-demanding and disturbance-adapted species (Trombulak and Frissell, 2000; Seiler, 2001). Roads can thus act as habitat corridors for these species by providing them with environments favourable to their establishment and growth, within a less suitable habitat matrix (Christen and Matlack, 2006; Huijser and Clevenger, 2006). Subsequently, these populations established along roads can act as a source of propagules and facilitate the expansion of some species into adjacent habitats away from roads, particularly following a disturbance (Tyser and Worley, 1992; Parendes and Jones, 2000). Road edges can also act as dispersal corridors for certain plant species, by facilitating their dispersal along the roads (e.g., Mortensen et al., 2009; Geng et al., 2017). For example, seeds can be transported preferentially along roads by anthropogenic (e.g., vehicles, clothing) (Clifford, 1959; Ross, 1986; Pickering et al., 2011; Taylor et al., 2012; Auffret and Cousins, 2013; von der Lippe et al., 2013) or by wildlife vectors (Suárez-Esteban et al., 2013a; Suárez-Esteban et al., 2013b), or through modifications of wind patterns within the road corridor (Damschen et al., 2014).
The effect of roads on the composition of plant communities has been widely studied in the context of non-native species propagation (e.g., Flory and Clay, 2006; Lu and Ma, 2006; Kalwij et al., 2008; Barnaud et al., 2013; Padmanaba and Sheil, 2014; Manee et al., 2015), and in the context of native species conservation (e.g., Tikka et al., 2001; Spooner et al., 2004; Deckers et al., 2005; Fekete et al., 2017). Comparatively few studies have focused upon the effects of roads on the expansion of native species (but see Buckley et al., 2003; Najafi et al., 2012; Avon et al., 2013; Gill et al., 2014; Picchio et al., 2018), especially trees. Nevertheless, populations of trembling aspen (Populus tremuloides Michx.) (Ackerman and Breen, 2016) and white spruce (Picea glauca [Moench] Voss) (Elsner and Jorgenson, 2009) have recently been observed along Arctic roads beyond the current northern limit of these species, thereby highlighting the role that roads can play in expanding native species distributions. In the boreal forest east of James Bay (Quebec, Canada), the distribution of trembling aspen (hereafter, referred to as aspen) appears to be concentrated near anthropogenic infrastructures, including roads (Whitbeck et al., 2016). Given that aspen is a shade-intolerant, fast-growing, disturbance-adapted species, which reproduces both asexually and sexually (Perala, 1990), it may be particularly effective in using road-edge habitats (Andrews, 1990). More specifically, forest roads can: 1) act as habitat for aspen; 2) act as a source of propagules to allow its expansion into adjacent habitats away from roads, if habitat conditions are favourable; 3) act as a dispersal corridor to facilitate its expansion along roads (Figure 1).
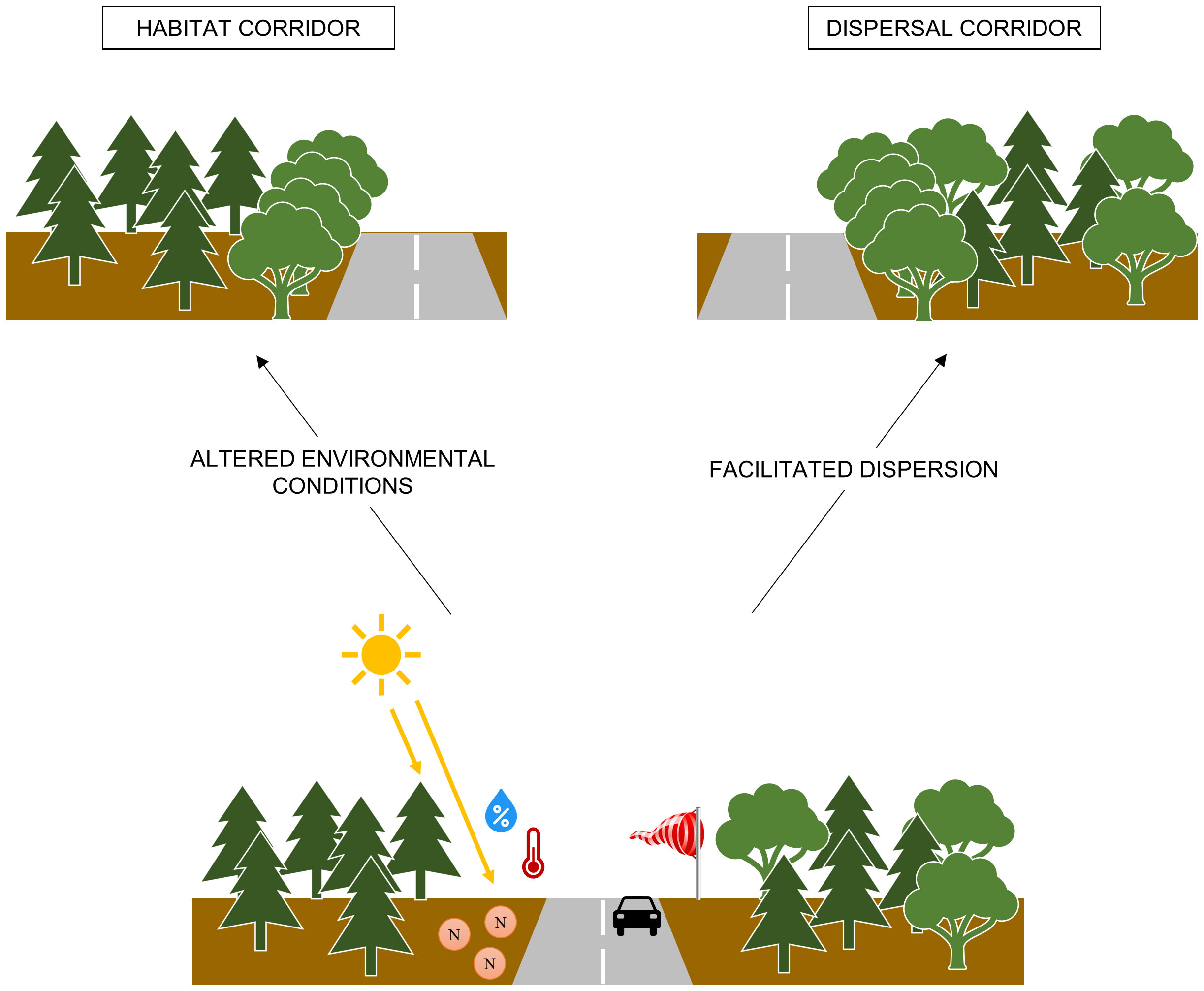
Figure 1 Habitat and dispersal corridor functions of roads. The roads can act as habitat corridors, providing a species with favourable edge habitat within an unfavourable habitat matrix. The roads can act as dispersal corridors, favouring dispersal in the road direction relative to other directions.
The main objective of this study was to assess the effect of forest roads on the distribution and abundance of aspen in a boreal landscape of eastern Canada (Quebec). The length of the permanent forest road network had tripled in the Province of Quebec between 1975 and 1999 (Coulombe et al., 2004). Between 2018 and 2019, nearly 6 000 new kilometres of forest roads were constructed (MFFP, 2020), compared to 4 000 to 5 000 km that were built annually in the early 2000s (Coulombe et al., 2004). Despite the strong development of the forest road network since the 1970s, few studies have focussed on their ecological impacts (Bourgeois et al., 2005). In the study landscape, clay deposits to the south are particularly favourable for aspen, while the great abundance of thick organic deposits to the north limits its presence (Blouin and Berger, 2005; Gewehr et al., 2014; Lafleur et al., 2015). However, industrial logging that has been carried out since the 1970s was accompanied by the construction of an extensive network of forest roads, which may have allowed expansion of aspen to the north (see Grondin and Cimon, 2003). If aspen is able to form well-established populations along roads, these may have acted as a starting point for its dispersal along roads as well as into adjacent stands away from roads, especially following clear-cutting (see Parendes and Jones, 2000). Aspen has indeed experienced a strong expansion in the study landscape since the 1970s (Marchais et al., 2022). While clear-cuts have favoured this expansion (Brumelis and Carleton, 1988; Carleton and MacLellan, 1994; Laquerre et al., 2009), the particular role played by forest roads remains unknown. The specific objectives of the study were thus to assess whether forest roads: 1) are favourable aspen habitats within a less suitable landscape matrix; 2) are dispersal corridors for aspen; and 3) acted as a starting point for the expansion of aspen into adjacent stands away from roads. We hypothesise that forest roads provide favourable habitat for aspen, and act as habitat corridor notably on organic deposits. We also hypothesise that aspen populations established along roads acted as a starting point for its dispersal into adjacent stands.
2 Materials and methods
2.1 Study landscape
The study landscape covers an area of 10930 km² (49°-49° 50’N; 78°-79° 30’W) in the Clay Belt of Quebec and Ontario (Figure 2), which was created during the drainage of proglacial Lake Ojibway, about 8000 years ago (Veillette, 1994). The main types of surface deposits are organic material (45%), clays (35%), and till (12%). The topography is flat and the climate is subpolar, subhumid continental (Blouin and Berger, 2005). Average annual precipitation is 909 mm, of which 29% falls as snow. Mean annual temperature is 0° C (± 2.9° C) (data from the Joutel weather station for the period 1981-2010 (EC, 2023)). The study landscape is located in the bioclimatic domain of the black spruce-feather moss forest of western Quebec (MFFP, 2021). The vegetation is largely dominated by black spruce (Picea mariana [Mill.] BSP.). Jack pine (Pinus banksiana Lamb.) and aspen are also abundant, forming pure stands or mixed stands with black spruce.
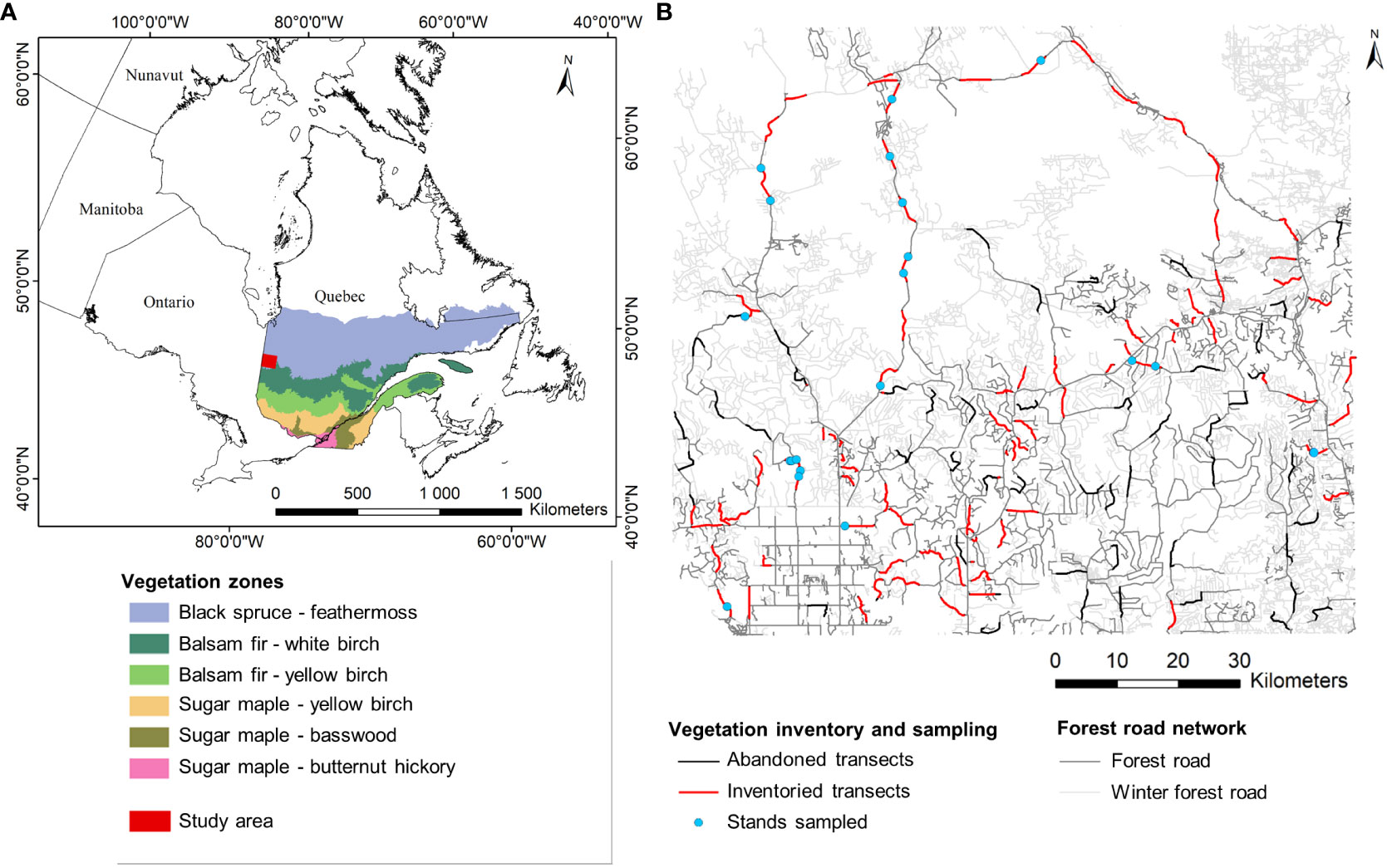
Figure 2 Location of the study landscape (i.e., study area) in the bioclimatic domain of the black spruce-feathermoss forest (A). Study area and location of the vegetation inventory carried out in 2018 along forest roads and trembling aspen stands sampled in 2019 (B).
The forest road network is highly developed, with 10493 km of roads, including 6971 km that are passable only in winter (i.e., winter forest roads) and 3522 km passable all-year-round (i.e., forest roads; Figure 2). Considering a right-of-way (i.e., width deforested during construction of the road) of 20 m for winter forest roads and 30 m for other forest roads (MFFP, 2021), the right-of-way of the forest road network extends over a total 245.1 km² and over 105.7 km² off-road in winter. Most of the regional road network, excluding winter forest roads, is constituted of class 3 forest roads (2174 km). The foundations of these class 3 forest roads consist of exogenous mineral soil with road surfaces of natural gravel. The planned period of use for class 3 forest roads is 10 to 15 years. The foundations of winter forest roads consist of materials in place (e.g., mineral soil, organic soil, woody debris) and the road surfaces are of compacted snow. The planned period of use for the winter forest roads is three months (MFFP, 2021).
2.2 Inventory of vegetation along forest roads
A vegetation inventory along forest roads was carried out in the summer of 2018, to quantify the distribution of aspen. Winter forest roads were not included in the inventory, given that they are not passable in summer with heavy vehicles. Portions of 5 km of roads (i.e., transects) were inventoried every 10 km in the northern part of the study landscape. In the southern part, given the more complex structure of the road network, points that were spaced at least 6 km apart were randomly placed to indicate the starting points of the transects. Given that we had no information on the accessibility of the road network, we initially selected a large number of transects to ensure that we had enough observations to have a representative image of the vegetation along the road edges. During the fieldwork, many roads became inaccessible (i.e., partially closed) or impassable (e.g., collapsed, flooded). Some transects were therefore partially or totally moved during the fieldwork, while others were inventoried over less than 5 km or were completely abandoned. Of the 130 transects of 5 km that had been planned at the start, 54 transects were completely inventoried, 36 were partially inventoried (length ranging from 100 m to 4600 m) and 40 had to be abandoned (Figure 2). In total, 347.2 km of transects were inventoried. Vegetation inventories were conducted by a single observer, from a vehicle travelling at reduced speed (≈ 10 km/h). Only tree species were inventoried. The composition was noted for the road-edge and for the adjacent stand, on both sides of the roads (i.e., 694.4 km of vegetation observations in total).
2.3 Selection and sampling of aspen stands
As aspen is naturally present in the study landscape, its presence in stands adjacent to roads may predate and be independent of their construction. However, the date of road construction is not available in our study landscape. As industrial logging began in the 1970s, most of the forest roads were built since this decade. Consequently, in order to limit the risk of sampling aspen stems established prior to road construction, buffer zones of 1 km were placed around each observation of vegetation carried out during the summer of 2018, and all observations containing aspen in these buffer zones on the forest map that had been produced by the Quebec government in the 1970s were excluded. Of the remaining observations from the 2018 summer, 19 aspen stands were randomly selected for sampling during the summer of 2019 (Figure 2). In each of these stands, a 100 m x 10 m transect was placed parallel to the forest road, along with a second that ran perpendicular to the first. The starting point of the two transects corresponds to the centre of the stand (i.e., L-shaped transects). Each transect was subdivided into 10 plots of 10 x 10 m in which the stems of each tree species were counted as saplings (DBH ≤ 8 cm) or trees (DBH > 8cm). In addition, the aspen with the largest DBH in each plot was selected for dendrochronological analysis, assuming that this tree was the oldest individual in the plot. Two core samples taken perpendicularly to one another, were collected at 30 cm from the ground surface on each selected individual. The samples were prepared and dated using standard dendrochronology methods (Payette and Filion, 2010).
2.4 Data analyses
To assess the effect of forest roads on aspen abundance at the landscape scale, we compared stem density (number of stems/ha) at different distances from roads using Friedman tests. Analyzes were performed separately for trees (DBH > 8cm) and saplings (DBH ≤ 8 cm) using the rstatix library (version 0.7.2) of R software (version 4.3.1, R Foundation for Statistical Computing, Vienna, AT). For both analyses, aspen density data were grouped into a single distance class for transects parallel to roads (0 m), while data from perpendicular transects were divided into four distance classes (10 m, 20 m, 30 m and more than 30 m from roads). These classes were considered because the right-of-way (i.e., area deforested during road construction) in the study landscape is generally 30 m, with cleared strips along the roadbed which are generally 11 m wide, but can vary between 0 and 27 m wide if the roadbed is off-centre due to operational or safety constraints (see MFFP, 2021). The use of a single distance class of 0-10 m corresponding to the common width of the cleared strips (i.e., 11 m), could therefore have prevented us from detecting situations where the cleared strips are wider (e.g., 20 m). Conversely, the use of a distance class of 0-30 m could have masked the effect of the right-of-way on aspen density, by including plots not located in the right-of-way if the latter is 11m wide.
Post hoc analyses were then carried out to identify between which pairs of distance classes aspen density differed significantly. Given that cleared strips along the roadbed never extend beyond 27 m, the >30 m distance class was used as “control” in post hoc tests, to increase tests power by minimising the number of pairwise comparisons. Aspen densities in all other distance classes were compared to aspen density in the >30 m distance class using Desmar’s post hoc tests in the R PMCMRplus library (version 1.9.7). Desmar’s tests were preferred to exact tests (Eisinga et al., 2017) and Nemenyi’s tests, as they allow both one-tailed testing and the use of the Benjamini-Hochberg correction method for multiple comparisons, thus increasing the power of the tests. One-tailed tests were preferred to two-tailed tests because we suppose that aspen density is higher near roads than far from them.
We used the method developed by Christen and Matlack (2006) to determine if forest roads are habitat corridor and if they are dispersal corridor for aspen in the study landscape. The relationship between the number of aspen stems and the distance to the centre of the stand was plotted for each sampled transect. If the number of aspen stems decreases at a lower rate (i.e., lower slope) along the transect parallel to the forest road compared to the perpendicular transect, this indicates facilitated dispersal along the forest road (i.e., dispersal corridor). If the Y-intercept is larger for the transect parallel compared to the transect perpendicular to the road, this indicates better habitat quality along the road (i.e., habitat corridor). For the analyses, the number of aspen stems was transformed using a logarithmic function and plotted in the R Stats library (version 4.0.0).
Finally, in order to determine if the forest roads acted as a starting point for the dispersal of aspen into adjacent stands, we used a linear mixed model followed by ANOVA to compare the maximum age of aspen stems at different distances from the roads. If aspen stems established along the forest roads before dispersing into adjacent stands, then individuals should be older near roads than far from them. For this analysis, the perpendicular transects were divided into four distance classes (10 m, 20 m, 30 m and more than 30 m from the forest road) to distinguish the effect of the right-of-way, while parallel transects form a single distance class (0 m). Homogeneity of the variance was checked using Levene’s test, while normality of the residuals was tested using a Shapiro-Wilk test. A Tukey’s post hoc test was used to identify pairs of distance classes in which the maximum age of aspen differed significantly. Only stands that had been severely disturbed after road construction, allowing the potential dispersal of aspen into adjacent stands, were included in this analysis (13 stands). The analyses were carried out in the R lme4, car and emmeans libraries (respectively, version 1.1-34, 3.1-2 and 1.8.8).
3 Results
3.1 Distribution of aspen along forest roads
Forest roads seem to influence the distribution and abundance of aspen in the study landscape, acting as dispersal and habitat corridors. Of the 694.4 km of vegetation observations made in 2018, aspen was present on 525.7 km (75.7%). Aspen was present only on the road-edges (≈ 5 m) along 312.1 km (45.0%), present on the road-edges plus the adjacent stand on 75.8 km (10.9%), and present only in the adjacent stands on 137.8 km (19.8%).
3.2 Role of forest roads
The 2019 survey confirms that distribution and abundance of aspen trees and saplings are influenced by forest roads. The density of trees (DBH > 8 cm) and saplings (DBH ≤ 8 cm) differ significantly between distance classes (Friedman tests; X² = 18.8, df = 4, p = 8.66 x 10-4 vs. X² = 27.7, df = 4, p = 1.43 x 10-5, respectively). The density of trees is significantly higher at 0 and 10 m from roads than at more than 30 m (Figure 3A). The median density of trees at 0 and 10 m is 400 stems/ha, compared to 0, 0 and 57 stems/ha at 20, 30 and more than 30 m from roads, respectively. The density of saplings is higher at 0 m than more than 30 m from roads (Figure 3B). However, saplings density is not higher at 10 m, indicating that the distribution of aspen saplings is more restricted in the immediate vicinity of forest roads than that of trees. The median density of saplings at 0 m is 100 stems/ha, compared to 0 stems/ha for all other distance classes.
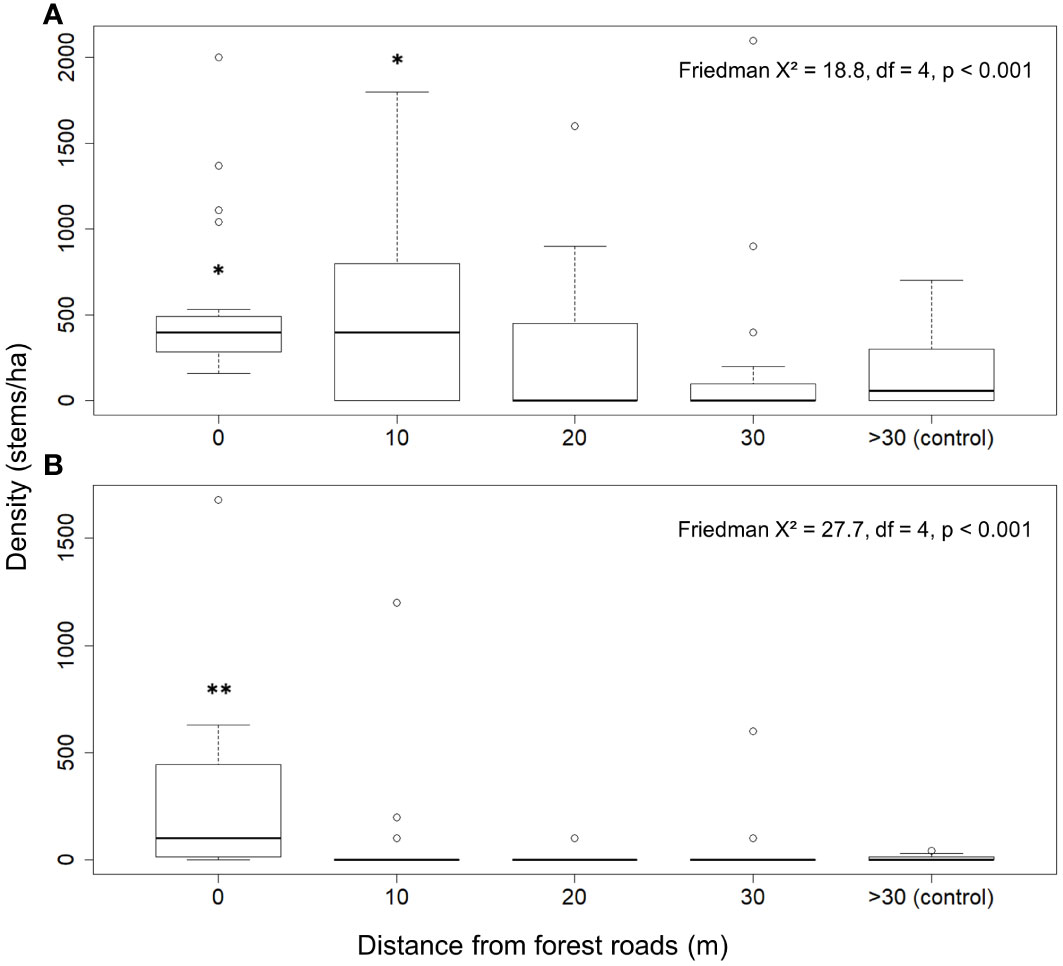
Figure 3 Trembling aspen density according to distance from forest roads for trees (DBH > 8 cm) (A), and saplings (DBH ≤ 8 cm) (B). The x-axis scale varies between graphs. Friedman test results are shown for trees and saplings. The transects parallel to forest roads form a single distance class (0 m). The transects perpendicular to the forest roads are divided into four classes of distance: 10 m, 20 m, 30 m and more than 30 m from the roads (>30). The symbol * indicates a statistically significant difference between the distance class and the control (> 30 m) at a threshold of α = 0.05, according to the results of Desmar’s post-hoc test with Benjamini-Hochberg correction. The symbol ** indicates a statistically significant difference at a threshold of α = 0.01.
The number of aspen stems according to the distance to the stand centres indicate that forest roads sometimes act as habitat corridors for aspen. Indeed, part of the study landscape is characterised by the presence of habitats adjacent to forest roads that are unsuitable for aspen, as evidenced by its absence on the perpendicular transects (Figure 4A; Supplementary Figure 1). Aspen distribution is channeled along forest roads, given that they provide favourable habitat for its establishment and growth, within a less favourable habitat matrix. This situation was observed in 8 of the 19 sampled stands (Supplementary Figure 1).
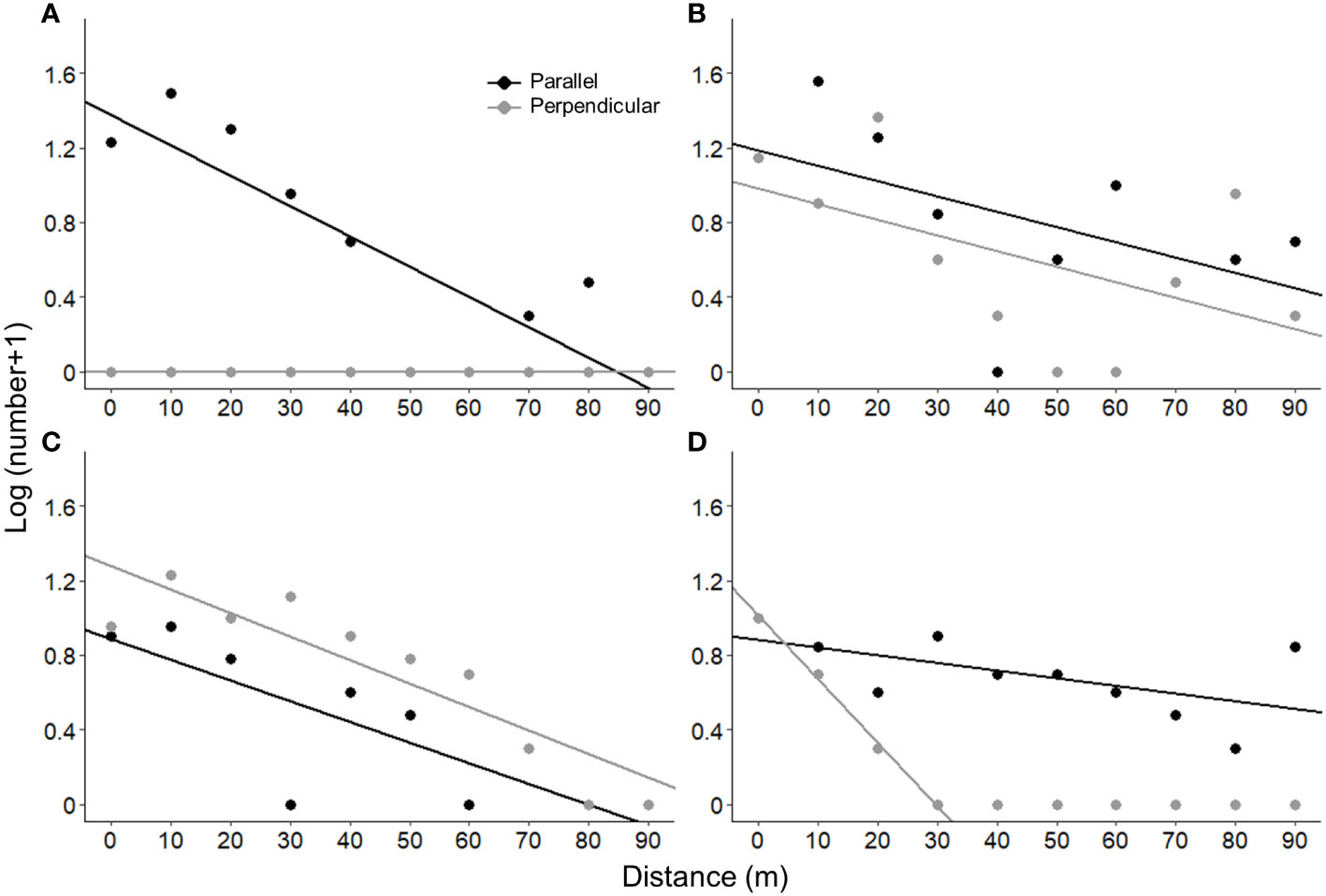
Figure 4 Relationships between the number of trembling aspen stems and the distance to the centre of the stands for transects parallel (in black) and perpendicular (in grey) to the forest roads. Only four “typical” stands are represented (for all the stands, see Supplementary Figure 1). The number of trembling aspen stems was transformed using a logarithmic function. Panels (A, B) show two examples of stands where forest roads act as a habitat corridor for trembling aspen. Panel (C) shows an example of a stand where forest roads play no particular role in the distribution of trembling aspen. Panel (D) shows a stand where the forest road appears to act as a dispersal corridor for trembling aspen.
The role of forest roads is more difficult to establish for stands with aspen in perpendicular transect. The distribution of aspen in five of these stands suggests that forest roads act as a habitat corridor (Supplementary Figure 1), because aspen established at greater densities close to the road than farther away (Figure 4B). Yet, in five other stands, the forest roads did not play a particular role in the distribution of the aspen and for three of them, the habitat seems to be of better quality away from the roads in the perpendicular transect than parallel to the roads (Figure 4C and Supplementary Figure 1). In the last stand, the road seems to act as a dispersal corridor (Figure 4D), but this result could reflect the presence of unsuitable habitat beyond the first 30 m of the perpendicular transect.
Contrary to our hypothesis, forest roads do not appear to have acted as a starting point for the dispersal of aspen into adjacent stands away from roads. Indeed, although the ANOVA indicates significant differences between the average maximum age of individuals according to distance classes (X² = 25.1, df = 4, p = 4.82 x 10-5), Tukey’s post hoc test does not indicate difference between distance classes 0 m, 30 m and >30 m from roads (Figure 5). However, aspen stems established at 10 and 20 m from roads are significantly younger than aspen stems established at 0 and more than 30 m from roads. Furthermore, although the age of aspen stems does not differ significantly between the 20, 30 and >30 m distance classes, individuals located at 30 m tend to be slightly younger than individuals located beyond 30 m, and slightly older than individuals located at 20 m. Thus, the colonisation of road-edges seems to have occurred gradually from aspen populations established both in the immediate vicinity of roads (0 m) and far from roads (>30 m).
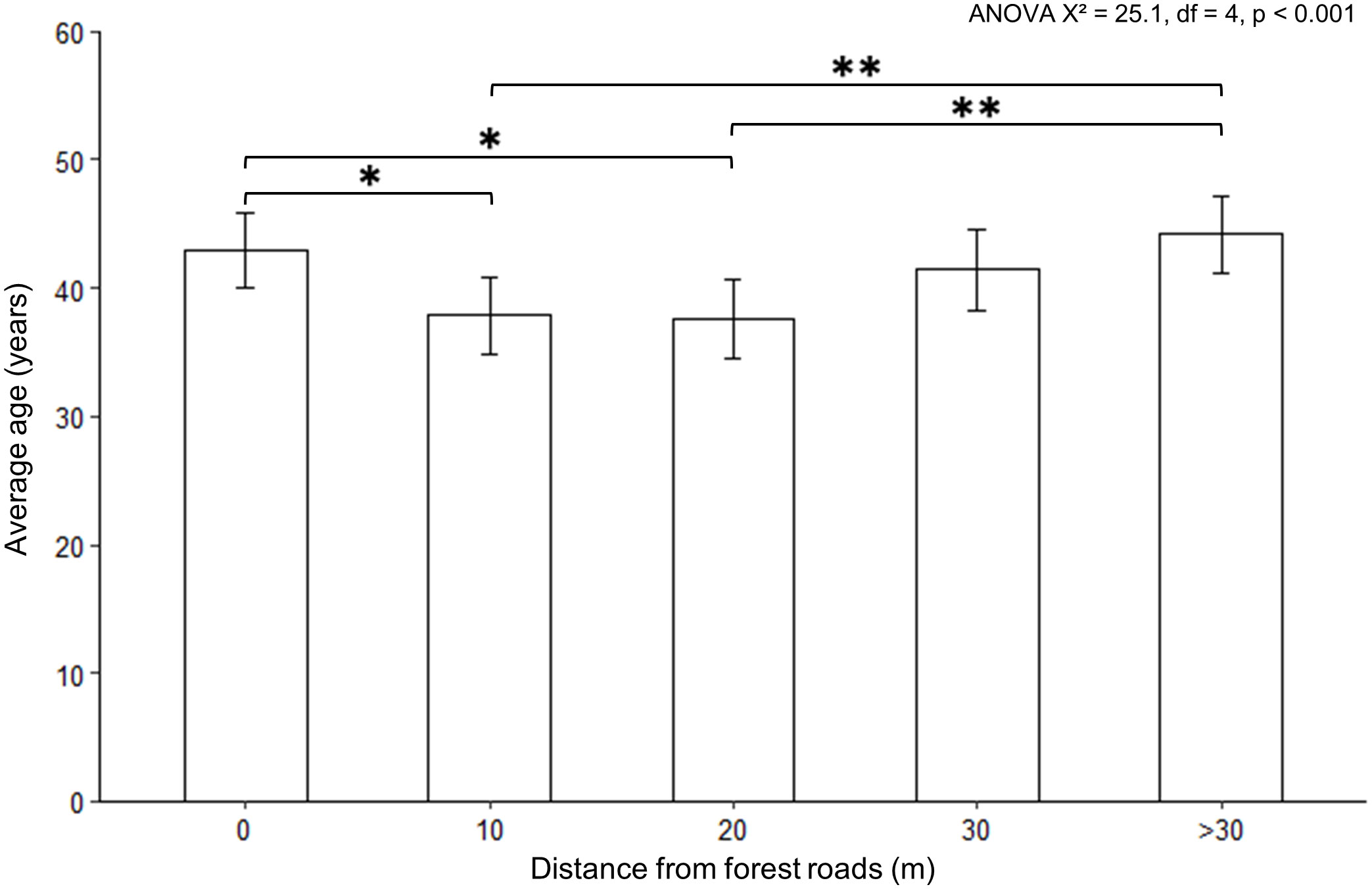
Figure 5 Average maximum age of trembling aspen estimated by the linear mixed model, according to distance from forest roads. Error bars are standard errors. The results of the ANOVA performed on the values estimated by the linear mixed model are shown. The transects parallel to forest roads form a single distance class (0 m). The transects perpendicular to the forest roads are divided into four classes of distance: 10 m, 20 m, 30 m and more than 30 m from the roads (>30). The symbol * indicates a statistically significant difference between the average age at a threshold of α = 0.05, according to the results of Tukey’s post-hoc test. The symbol ** indicates a statistically significant difference at a threshold of α = 0.01.
4 Discussion
Forest roads play an important role in the distribution of aspen in our study landscape, as evidenced by its presence on road-edges along 55.9% of the 694 km of forest roads that we inventoried in 2018. Road construction and maintenance creates edge habitats in which environmental conditions differ from those found in interior forests, which in turn influences plant composition (Andrews, 1990; Forman and Alexander, 1998; Trombulak and Frissell, 2000). Direct effects of forest road construction include vegetation removal and soil disturbance (Harper et al., 2005), together with altered nutrient availability (Santelmann and Gorham, 1988; Devlaeminck et al., 2005; Schmidt et al., 2017), wind and incident light patterns (Young and Mitchell, 1994; Damschen et al., 2014; Stern et al., 2018), and temperature and humidity of the air and soil (Chen et al., 1993; Chen et al., 1995; Pohlman et al., 2007; Pohlman et al., 2009). Consequently, edge habitats are generally favourable for pioneer, shade-intolerant and disturbance-adapted species (e.g., Honnay et al., 2002; Godefroid and Koedam, 2004; McDonald and Urban, 2006; Najafi et al., 2012; Picchio et al., 2018), such as aspen (Perala, 1990). For example, Harper et al. (2015) and Whitbeck et al. (2016) showed that in the boreal forest, edge habitats of natural (i.e., fire, lakes, wetlands) and anthropogenic origin (i.e., cutting, roads) favoured poplars (Populus spp.). Since winter forest roads were not considered in this study, their effects on the distribution of aspen remain unknown. Yet, winter forest roads are probably less favourable habitats for aspen, because the use of endogenous materials for road construction (e.g., organic soil) tends to limit the modification of edaphic conditions (Godefroid and Koedam, 2004; Bergès et al., 2013).
At the scale of our study landscape, the effect of road-edges on aspen regeneration and tree densities extends over a relatively short distance (i.e., respectively 0 and 10 m; Figure 3). These results are consistent with literature reports. Indeed, the effect of roads on the composition of vegetation has been reported to be limited to about 10 m in several forest ecosystems (Watkins et al., 2003; Hansen and Clevenger, 2005; Arévalo et al., 2008; Deljouei et al., 2017). The restriction of aspen regeneration to the immediate vicinity of roads (i.e., transect parallel to the road) could be due to insufficient solar irradiance beyond a few metres from the roadway (Buckley et al., 2003; Delgado et al., 2007; Avon et al., 2010; Deljouei et al., 2018), to allow the regeneration of this very shade-intolerant species. Our results are also consistent with the width of rights-of-way (i.e., the area deforested during road construction) in our study landscape (i.e., generally 30 m). These rights-of-way include an 8 m-wide roadbed, which is normally located at the centre of the right-of-way, plus an 11 m-wide cleared strip on either side of the roadway (i.e., road-edge habitats available for aspen) (MFFP, 2021). However, at the stand scale, the distance of the road-edge effect on aspen density is variable (Figure 3). The distance of the road-edge effect depends upon many factors such as the width of the road and the intensity of traffic (Angold, 1997; Forman et al., 2003; Zhou et al., 2020), the position of the road in relation to the slope and wind (Forman and Alexander, 1998), or the orientation of the edges, their age and the characteristics of the adjacent vegetation (Matlack, 1993; Matlack, 1994; Camargo and Kapos, 1995; Didham and Lawton, 1999; Harper and Macdonald, 2002; Dignan and Bren, 2003). In our study, the orientation of the edges and the characteristics of the adjacent stands (e.g., composition, age, height, surface deposit, drainage) differ among sites, which probably explains the observed variability in the response of aspen at the stand scale (see Murcia, 1995; Ries et al., 2004).
Forest roads do not appear to have acted as dispersal corridors for aspen in our study landscape (Figure 4). Nevertheless, the presence of aspen stands along the Dalton Highway in Alaska, beyond the northern range of the specie and the topographic barrier of the Brooks Range, highlights the role that vehicles can play as a dispersal vector for aspen seeds (Ackerman and Breen, 2016). According to von der Lippe and Kowarik (2012), road-edge species abundance and seed mass are the main factors affecting the magnitude and probability of dispersal by vehicles, with dispersal events of greater magnitude and frequency for abundant species with light seeds (≤ 0.1 mg). The high abundance of aspen on road edges (Figure 3), and the low weight of these seeds (≈ 0.1 mg) (Greene et al., 1999; Wolken et al., 2010), could therefore favour its dispersal by vehicles in our study area.
Forest roads sometimes act as habitat corridors for aspen in our study landscape (Figure 4A and Supplementary Figure 1), because its expansion appears to be channeled along the roads due to unsuitable or less favourable conditions in adjacent habitats (Christen and Matlack, 2006; Huijser and Clevenger, 2006). In our study landscape, edaphic conditions, especially the thickness of organic layers, appear to be the main factor limiting the presence of aspen away from roads. A thick organic layer (> 30 cm) is known to limit establishment of aspen through sexual reproduction and vegetative propagation (Gewehr et al., 2014; Lafleur et al., 2015), which can be attributed to low soil temperatures and low nutrient availability induced by organic matter accumulation (van Cleve et al., 1983; Simard et al., 2007), and to low carbohydrate reserves contained in small-sized aspen seeds (Greene et al., 1999; Greene et al., 2007). In our study, 7 out of the 8 stands where aspen is absent more than 10 m away from forest roads exhibit an organic layer thicker than 30 cm. In these stands, road construction has created favourable edge habitats for aspen, within a less favourable matrix. The use of gravel for road construction may be responsible for creating favourable conditions for aspen establishment in edge habitats, as observed in Alaska (Ackerman and Breen, 2016). Road dust transport due to wind, road traffic and runoff may have increased soil pH and nutrient availability along the roads, and limited moss cover, particularly Sphagnum spp. (Walker and Everett, 1987; Auerbach et al., 1997; Myers-Smith et al., 2006; Müellerová et al., 2011; Ackerman and Finlay, 2019). In addition, dust deposits may have created islands of mineral soil that favour aspen germination. Once established, aspen is able to exert a positive feedback on its environment by limiting sphagnum cover and improving nutrient cycling (Prescott et al., 2000; Fenton et al., 2005; Légaré et al., 2005; Laganière et al., 2009; Laganière et al., 2010), thereby facilitating its subsequent establishment along the roads.
In addition to suitable edaphic conditions, the establishment of aspen away from roads seems to require the occurrence of severe disturbance. Indeed, all stands where aspen is present perpendicular to the roads have been clear-cut (9 stands) or burned (2 stands) over the last 40 to 50 years. The vigorous regeneration of aspen after severe disturbance is a widely documented phenomenon (Bartos and Mueggler, 1982; Bella, 1986; Brown and Debyle, 1987; Bartos et al., 1991; Ilisson and Chen, 2009), and clear-cuts have played a major role in aspen expansion in the boreal forest (Brumelis and Carleton, 1988; Carleton and MacLellan, 1994; Friedman and Reich, 2005). As the practice of clear-cutting will continue in the future, some stands may be vulnerable to aspen expansion from populations that have established on the road-edges (see Parendes and Jones, 2000). According to our 2018 vegetation inventory, aspen is present only on the road-edges in 45% of our observations. Yet, severe disturbance does not appear to be systematic trigger of aspen establishment away from roads, as three out of the 8 stands where aspen is absent perpendicular to the roads have been clearcut. Given the high coverage of thick organic deposits in our study landscape (45% of the soils surface), the potential for aspen expansion from the roads could be lower than what our vegetation inventory suggests, if disturbances do not reduce sufficiently the thickness of organic layer to allow establishment of aspen. Conversely, if disturbances, such as clearcutting or fire, sufficiently reduce the thickness of the organic layer, these habitats could be particularly vulnerable to invasion by aspen.
Contrary to our hypothesis, aspen stands along forest roads do not appear to have acted as a starting point for dispersal into adjacent habitats. Indeed, the establishment of the aspen took place relatively synchronously near to and far from the roads (i.e., 0 m and more than 30 m, Figure 5). This result could be due to the synchronicity between road construction and clear-cutting. Given that forest roads have a planned use of 10 to 15 years (MFFP, 2021), logging must occur shortly after road construction. This hypothesis is supported by the fact that roads and cuts appear together on the governmental decadal forest map.
Construction of forest roads create edge habitats in which environmental conditions differ from those found in adjacent sites, which in turn influences vegetation composition. These edge habitats are particularly favourable to aspen in our study landscape, a frequent species along the road network. Soil disturbance, opening of the canopy, and the use of gravel for the construction of forest roads are likely important factors in explaining the high density of aspen along the roads. The influence of roads on aspen density extends over a short distance (10 m on each side of the roads) and roads act as a habitat corridor for aspen, due to the presence of unsuitable adjacent habitats. Although roads do not appear to have acted as a starting point for aspen dispersal away from roads, these habitats would be particularly vulnerable to invasion by aspen following a disturbance that would reduce the thickness of the organic layer.
Data availability statement
The raw data supporting the conclusions of this article will be made available by the authors, without undue reservation.
Author contributions
MM: Conceptualization, Data curation, Formal analysis, Investigation, Methodology, Visualization, Writing – original draft. DA: Conceptualization, Project administration, Supervision, Validation, Writing – review & editing. YB: Conceptualization, Funding acquisition, Project administration, Supervision, Validation, Writing – review & editing.
Funding
The author(s) declare financial support was received for the research, authorship, and/or publication of this article. This project was financially supported by the Natural Sciences and Engineering Research Council of Canada (NSERC, Grant Number: CRSNG_SPS 380893-09) and by a practical field grant (Mitacs Accelerate, Grant Number: IT08209) in collaboration with the company Rayonier Advanced Materials.
Acknowledgments
We thank Todor Minchev and Joëlle Boutin for their help during data sampling. W.F.J. Parsons corrected the English.
Conflict of interest
The authors declare that the research was conducted in the absence of any commercial or financial relationships that could be construed as a potential conflict of interest.
The authors declare that this study received funding from the company Rayonier Advanced Materials Grant Number: IT08209. The funder was not involved in the study design, data collection, analysis and interpretation, the writing of this article or the decision to submit it for publication.
Publisher’s note
All claims expressed in this article are solely those of the authors and do not necessarily represent those of their affiliated organizations, or those of the publisher, the editors and the reviewers. Any product that may be evaluated in this article, or claim that may be made by its manufacturer, is not guaranteed or endorsed by the publisher.
Supplementary material
The Supplementary Material for this article can be found online at: https://www.frontiersin.org/articles/10.3389/fevo.2023.1336409/full#supplementary-material
References
Ackerman D., Breen A. (2016). Infrastructure development accelerates range expansion of trembling aspen (Populus tremuloides, Salicaceae) into the arctic. Arctic 69 (2), 130–136. doi: 10.14430/arctic4560
Ackerman D., Finlay J. (2019). Road dust biases NDVI and alters edaphic properties in Alaskan arctic tundra. Sci. Rep. 9 (1), 1–8. doi: 10.1038/s41598-018-36804-3
Andrews A. (1990). Fragmentation of habitat by roads and utility corridors: a review. Aust. Zoologist 26 (3-4), 130–141. doi: 10.7882/AZ.1990.005
Angold P. (1997). The impact of a road upon adjacent heathland vegetation: effects on plant species composition. J. Appl. Ecol. 34 (2), 409–417. doi: 10.2307/2404886
Arévalo J., Delgado J., Fernández-Palacios J. (2008). Changes in plant species composition and litter production in response to roads and trails in the laurel forest of Tenerife (Canary Islands). Plant Biosyst. 142 (3), 614–622. doi: 10.1080/11263500802410991
Auerbach N., Walker M., Walker D. (1997). Effects of roadside disturbance on substrate and vegetation properties in arctic tundra. Ecol. Appl. 7 (1), 218–235. doi: 10.1890/1051-0761(1997)007[0218:EORDOS]2.0.CO;2
Auffret A., Cousins S. (2013). Humans as long-distance dispersers of rural plant communities. PloS One 8 (5), 1–7. doi: 10.1371/journal.pone.0062763
Avon C., Bergès L., Dumas Y., Dupouey J.-L. (2010). Does the effect of forest roads extend a few meters or more into the adjacent forest? A study on understory plant diversity in managed oak stands. For. Ecol. Manage. 259 (8), 1546–1555. doi: 10.1016/j.foreco.2010.01.031
Avon C., Dumas Y., Bergès L. (2013). Management practices increase the impact of roads on plant communities in forests. Biol. Conserv. 159, 24–31. doi: 10.1016/j.biocon.2012.10.008
Barnaud A., Kalwij J., Berthouly-Salazar C., McGeoch M., Jansen van Vuuren B. (2013). Are road verges corridors for weed invasion? Insights from the fine-scale spatial genetic structure of Raphanus raphanistrum. Weed Res. 53 (5), 362–369. doi: 10.1111/wre.12033
Bartos D., Mueggler W. (1982). Early succession following clearcutting of aspen communities in Northern Utah. J. Range Manage. 35 (6), 764–768. doi: 10.2307/3898260
Bartos D., Mueggler W., Campbell R. (1991). Regeneration of aspen by suckering on burned sites in western Wyoming (Ogden, UT, USA: US Department of Agriculture, Forest Service, Intermountain Research Station).
Bella I. (1986). Logging practices and subsequent development of aspen stands in east-central Saskatchewan. Forestry Chronicle 62 (2), 81–83. doi: 10.5558/tfc62081-2
Bergès L., Chevalier R., Avon C. (2013). Influence of forest road, road-surfacing material and stand age on floristic diversity and composition in a nutrient-poor environment. Appl. Vegetation Sci. 16 (3), 470–479. doi: 10.1111/avsc.12019
Blouin J., Berger J.-P. (2005). Guide de reconnaissance des types écologiques de la région écologique 6a-Plaine du lac Matagami et 6b-Plaine de la baie de Rupert (Québec, QC, CA: Gouvernement du Québec, Ministère des Ressources naturelles et de la Faune, Direction des inventaires forestiers, Division de la classification écologique et productivité des stations).
Bourgeois L., Kneeshaw D., Boisseau G. (2005). Les routes forestières au Québec: Les impacts environnementaux, sociaux et économiques. VertigO 6 (2), 1–9. doi: 10.4000/vertigo.4352
Brown J., Debyle N. (1987). Fire damage, mortality, and suckering in aspen. Can. J. For. Res. 17 (9), 1100–1109. doi: 10.1139/x87-168
Brumelis G., Carleton T. (1988). The vegetation of postlogged black spruce lowlands in central Canada. I. Trees and tall shrubs. Can. J. For. Res. 18 (11), 1470–1478. doi: 10.1139/x88-226
Buckley D., Crow T., Nauertz E., Schulz K. (2003). Influence of skid trails and haul roads on understory plant richness and composition in managed forest landscapes in Upper Michigan, USA. For. Ecol. Manage. 175 (1-3), 509–520. doi: 10.1016/s0378-1127(02)00185-8
Camargo J., Kapos V. (1995). Complex edge effects on soil moisture and microclimate in central Amazonian forest. J. Trop. Ecol. 11 (2), 205–221. doi: 10.1017/S026646740000866X
Carleton T., MacLellan P. (1994). Woody vegetation responses to fire versus clear-cutting logging: a comparative survey in the central Canadian boreal forest. Ecoscience 1 (2), 141–152. doi: 10.1080/11956860.1994.11682238
Chen J., Franklin J., Spies T. (1993). Contrasting microclimates among clearcut, edge, and interior of old-growth Douglas-fir forest. Agric. For. Meteorology 63 (3-4), 219–237. doi: 10.1016/0168-1923(93)90061-L
Chen J., Franklin J., Spies T. (1995). Growing-season microclimatic gradients from clearcut edges into old-growth Douglas-fir forests. Ecol. Appl. 5 (1), 74–86. doi: 10.2307/1942053
Christen D., Matlack G. (2006). The role of roadsides in plant invasions: a demographic approach. Conserv. Biol. 20 (2), 385–391. doi: 10.1111/j.1523-1739.2006.00315.x
Clifford H. (1959). Seed dispersal by motor vehicles. J. Ecol. 47 (2), 311–315. doi: 10.2307/2257368
Coulombe G., Huot J., Arsenault J., Bauce É., Bernard J.-T., Bouchard A., et al. (2004). Commission d’étude sur la gestion de la forêt publique québécoise (Rapport Coulombe) (Québec, QC, CA: Bibliothèque nationale du Québec).
Damschen E., Baker D., Bohrer G., Nathan R., Orrock J., Turner J., et al. (2014). How fragmentation and corridors affect wind dynamics and seed dispersal in open habitats. Proc. Natl. Acad. Sci. United States America 111 (9), 3484–3489. doi: 10.1073/pnas.1308968111
Deckers B., De Becker P., Honnay O., Hermy M., Muys B. (2005). Sunken roads as habitats for forest plant species in a dynamic agricultural landscape: effects of age and isolation. J. Biogeography 32 (1), 99–109. doi: 10.1111/j.1365-2699.2004.01101.x
Delgado J., Arroyo N., Arévalo J., Fernández-Palacios J. (2007). Edge effects of roads on temperature, light, canopy cover, and canopy height in laurel and pine forests (Tenerife, Canary Islands). Landscape Urban Plann. 81 (4), 328–340. doi: 10.1016/j.landurbplan.2007.01.005
Deljouei A., Abdi E., Marcantonio M., Majnounian B., Amici V., Sohrabi H. (2017). The impact of forest roads on understory plant diversity in temperate hornbeam-beech forests of Northern Iran. Environ. Monit. Assess. 189 (8), 392–407. doi: 10.1007/s10661-017-6105-1
Deljouei A., Sadeghi S., Abdi E., Bernhardt-Römermann M., Pascoe E., Marcantonio M. (2018). The impact of road disturbance on vegetation and soil properties in a beech stand, Hyrcanian forest. Eur. J. For. Res. 137 (6), 759–770. doi: 10.1007/s10342-018-1138-8
Devlaeminck R., De Schrijver A., Hermy M. (2005). Variation in throughfall deposition across a deciduous beech (Fagus sylvatica L.) forest edge in Flanders. Sci. Total Environ. 337 (1-3), 241–252. doi: 10.1016/j.scitotenv.2004.07.005
Didham R., Lawton J. (1999). Edge structure determines the magnitude of changes in microclimate and vegetation structure in tropical forest fragments. Biotropica 31 (1), 17–30. doi: 10.1111/j.1744-7429.1999.tb00113.x
Dignan P., Bren L. (2003). Modelling light penetration edge effects for stream buffer design in mountain ash forest in southeastern Australia. For. Ecol. Manage. 179 (1-3), 95–106. doi: 10.1016/S0378-1127(02)00491-7
EC (2023) Normales climatiques canadiennes: Normales et moyennes climatiques de 1981-2010 (Government of Canada, Environnement Canada). Available at: https://climat.meteo.gc.ca/climate_normals/ (Accessed October 2023).
Eisinga R., Heskes T., Pelzer B., Te Grotenhuis M. (2017). Exact p-values for pairwise comparison of Friedman rank sums, with application to comparing classifiers. BMC Bioinf. 18 (1), 1–18. doi: 10.1186/s12859-017-1486-2
Elsner W., Jorgenson J. (2009). White spruce seedling (Picea glauca) discovered north of the Brooks Range along Alaska’s Dalton Highway. Arctic 62 (3), 342–344.
Fekete R., Nagy T., Bodis J., Biro E., Loki V., Suveges K., et al. (2017). Roadside verges as habitats for endangered lizard-orchids (Himantoglossum spp.): Ecological traps or refuges? Sci. Total Environ., 607–608. doi: 10.1016/j.scitotenv.2017.07.037
Fenton N., Lecomte N., Légaré S., Bergeron Y. (2005). Paludification in black spruce (Picea mariana) forests of eastern Canada: Potential factors and management implications. For. Ecol. Manage. 213 (1), 151–159. doi: 10.1016/j.foreco.2005.03.017
Flory S., Clay K. (2006). Invasive shrub distribution varies with distance to roads and stand age in eastern deciduous forests in Indiana, USA. Plant Ecol. 184 (1), 131–141. doi: 10.1007/s11258-005-9057-4
Forman R., Alexander L. (1998). Roads and their major ecological effects. Annu. Rev. Ecol. Systematics 29 (1), 207–231. doi: 10.1146/annurev.ecolsys.29.1.207
Forman R., Sperling D., Bissonette J., Clevenger A., Cutshall C., Dale V., et al. (2003). Road ecology: science and solutions (Washington DC, USA: Island press).
Friedman S., Reich P. (2005). Regional legacies of logging: departure from presettlement forest conditions in northern Minnesota. Ecol. Appl. 15 (2), 726–744. doi: 10.1890/04-0748
Geng S.-L., Chen Q., Cai W.-L., Cao A.-C., Ou-Yang C.-B. (2017). Genetic variation in the invasive weed Mikania micrantha (Asteraceae) suggests highways as corridors for its dispersal in southern China. Ann. Bot. 119 (3), 457–464. doi: 10.1093/aob/mcw218
Gewehr S., Drobyshev I., Berninger F., Bergeron Y. (2014). Soil characteristics mediate the distribution and response of boreal trees to climatic variability. Can. J. For. Res. 44 (5), 487–498. doi: 10.1139/cjfr-2013-0481
Gill H., Lantz T., O’Neill B., Kokelj S. (2014). Cumulative impacts and feedbacks of a gravel road on shrub tundra ecosystems in the Peel Plateau, Northwest Territories, Canada. Arctic Antarctic Alpine Res. 46 (4), 947–961. doi: 10.1657/1938-4246-46.4.947
Godefroid S., Koedam N. (2004). The impact of forest paths upon adjacent vegetation: effects of the path surfacing material on the species composition and soil compaction. Biol. Conserv. 119 (3), 405–419. doi: 10.1016/j.biocon.2004.01.003
Greene D. F., Macdonald S. E., Haeussler S., Domenicano S., Noël J., Jayen K., et al. (2007). The reduction of organic-layer depth by wildfire in the North American boreal forest and its effect on tree recruitment by seed. Can. J. For. Res. 37 (6), 1012–1023. doi: 10.1139/X06-245
Greene D., Zasada J., Sirois L., Kneeshaw D., Morin H., Charron I., et al. (1999). A review of the regeneration dynamics of North American boreal forest tree species. Can. J. For. Res. 29 (6), 824–839. doi: 10.1139/x98-112
Grondin P., Cimon A. (2003). Les enjeux de biodiversité relatifs à la composition forestière (Québec, QC, CA: Gouvernement du Québec, Ministère des Ressources Naturelles, de la Faune et des Parcs, Direction de l’environnement forestier).
Hansen M., Clevenger A. (2005). The influence of disturbance and habitat on the presence of non-native plant species along transport corridors. Biol. Conserv. 125 (2), 249–259. doi: 10.1016/j.biocon.2005.03.024
Harper K., Macdonald S. (2002). Structure and composition of edges next to regenerating clear-cuts in mixed-wood boreal forest. J. Vegetation Sci. 13 (4), 535–546. doi: 10.1111/j.1654-1103.2002.tb02080.x
Harper K., Macdonald S., Burton P., Chen J., Brosofske K., Saunders S., et al. (2005). Edge influence on forest structure and composition in fragmented landscapes. Conserv. Biol. 19 (3), 768–782. doi: 10.1111/j.1523-1739.2005.00045.x
Harper K. A., Macdonald S. E., Mayerhofer M. S., Biswas S. R., Esseen P. A., Hylander K., et al. (2015). Edge influence on vegetation at natural and anthropogenic edges of boreal forests in Canada and Fennoscandia. J. Ecol. 103 (3), 550–562. doi: 10.1111/1365-2745.12398
Honnay O., Verheyen K., Hermy M. (2002). Permeability of ancient forest edges for weedy plant species invasion. For. Ecol. Manage. 161 (1-3), 109–122. doi: 10.1016/S0378-1127(01)00490-X
Huijser M., Clevenger A. (2006). “Habitat and corridor function of rights-of-way,” in The ecology of transportation: managing mobility for the environment. Ed. Davenport J.D. &J. L. (Dordrecht, NL: Springer), 233–254.
Ilisson T., Chen H. (2009). Response of six boreal tree species to stand replacing fire and clearcutting. Ecosystems 12 (5), 820–829. doi: 10.1007/s10021-009-9259-z
Kalwij J., Milton S., McGeoch M. (2008). Road verges as invasion corridors? A spatial hierarchical test in an arid ecosystem. Landscape Ecol. 23 (4), 439–451. doi: 10.1007/s10980-008-9201-3
Lafleur B., Cazal A., Leduc A., Bergeron Y. (2015). Soil organic layer thickness influences the establishment and growth of trembling aspen (Populus tremuloides) in boreal forests. For. Ecol. Manage. 347, 209–216. doi: 10.1016/j.foreco.2015.03.031
Laganière J., Paré D., Bradley R. (2009). Linking the abundance of aspen with soil faunal communities and rates of belowground processes within single stands of mixed aspen–black spruce. Appl. Soil Ecol. 41 (1), 19–28. doi: 10.1016/j.apsoil.2008.08.005
Laganière J., Paré D., Bradley R. (2010). How does a tree species influence litter decomposition? Separating the relative contribution of litter quality, litter mixing, and forest floor conditions. Can. J. For. Res. 40 (3), 465–475. doi: 10.1139/X09-208
Laquerre S., Leduc A., Harvey B. (2009). Augmentation du couvert en peuplier faux-tremble dans les pessières noires du nord-ouest du Québec après coupe totale. Ecoscience 16 (4), 483–491. doi: 10.2980/16-4-3252
Légaré S., Paré D., Bergeron Y. (2005). Influence of aspen on forest floor properties in black spruce-dominated stands. Plant Soil 275 (1-2), 207–220. doi: 10.1007/s11104-005-1482-6
Lu Z., Ma K. (2006). Spread of the exotic croftonweed (Eupatorium adenophorum) across southwest China along roads and streams. Weed Sci. 54 (6), 1068–1072. doi: 10.1614/ws-06-040r1.1
Manee C., Rankin W., Kauffman G., Adkison G. (2015). Association between roads and the distribution of Microstegium vimineum in Appalachian Forests of North Carolina. Southeastern Nat. 14 (4), 602–611. doi: 10.1656/058.014.0402
Marchais M., Arseneault D., Bergeron Y. (2022). The rapid expansion of Populus tremuloides due to anthropogenic disturbances in eastern Canada. Can. J. For. Res. 52 (7), 991–1001. doi: 10.1139/cjfr-2022-0082
Matlack G. (1993). Microenvironment variation within and among forest edge sites in the eastern United States. Biol. Conserv. 66 (3), 185–194. doi: 10.1016/0006-3207(93)90004-K
Matlack G. (1994). Vegetation dynamics of the forest edge - Trends in space and successional time. J. Ecol. 82 (1), 113–123. doi: 10.2307/2261391
McDonald R., Urban D. (2006). Edge effects on species composition and exotic species abundance in the North Carolina Piedmont. Biol. Invasions 8 (5), 1049–1060. doi: 10.1007/s10530-005-5227-5
MFFP (2020). Ressources et industries forestières du Québec - Portrait statistique 2019 (Québec, QC, CA: Gouvernement du Québec, Ministère des Forêts, de la Faune et des Parcs, Direction de la modernisation de l’industrie des produits forestiers).
MFFP (2021). Guide d’application du règlement sur l’aménagement durable des forêts du domaine de l’état (Québec, QC, CA: Gouvernement du Québec, Ministère des Forêts, de la Faune et des Parcs, Direction de la protection des forêts).
Mortensen D., Rauschert E., Nord A., Jones B. (2009). Forest roads facilitate the spread of invasive plants. Invasive Plant Sci. Manage. 2 (3), 191–199. doi: 10.1614/IPSM-08-125.1
Müellerová J., Vítková M., Vítek O. (2011). The impacts of road and walking trails upon adjacent vegetation: effects of road building materials on species composition in a nutrient poor environment. Sci. Total Environ. 409 (19), 3839–3849. doi: 10.1016/j.scitotenv.2011.06.056
Murcia C. (1995). Edge effects in fragmented forests: implications for conservation. Trends Ecol. Evol. 10 (2), 58–62. doi: 10.1016/S0169-5347(00)88977-6
Myers-Smith I., Arnesen B., Thompson R., Chapin F. (2006). Cumulative impacts on Alaskan arctic tundra of a quarter century of road dust. Ecoscience 13 (4), 503–510. doi: 10.2980/1195-6860(2006)13[503:CIOAAT]2.0.CO;2
Najafi A., Torabi M., Nowbakht A.-A., Moafi M., Eslami A., Sotoudeh Foumani B. (2012). Effect of forest roads on adjacent tree regeneration in a mountainous forest. Ann. Biol. Res. 3 (4), 1700–1703.
Padmanaba M., Sheil D. (2014). Spread of the invasive alien species Piper aduncum via logging roads in Borneo. Trop. Conserv. Sci. 7 (1), 35–44. doi: 10.1177/194008291400700108
Parendes L., Jones J. (2000). Role of light availability and dispersal in exotic plant invasion along roads and streams in the H. J. Andrews Experimental Forest, Oregon. Conserv. Biol. 14 (1), 64–75. doi: 10.1046/j.1523-1739.2000.99089.x
Payette S., Filion L. (2010). La dendroécologie: principes, méthodes et applications (Québec, QC, CA: Les Presses de l’Université Laval).
Perala D. (1990). “Populus tremuloides Michx. quaking aspen,” in Silvics of North America: Hardwoods. Eds. Burns R., Honkala B. (Washington DC, USA: US Department of Agriculture, Forest Service), 555–569.
Picchio R., Tavankar F., Venanzi R., Lo Monaco A., Nikooy M. (2018). Study of forest road effect on tree community and stand structure in three italian and Iranian temperate forests. Croatian J. For. Eng. 39 (1), 57–70.
Pickering C., Mount A., Wichmann M., Bullock J. (2011). Estimating human-mediated dispersal of seeds within an Australian protected area. Biol. Invasions 13 (8), 1869–1880. doi: 10.1007/s10530-011-0006-y
Pohlman C., Turton S., Goosem M. (2007). Edge effects of linear canopy openings on tropical rain forest understory microclimate. Biotropica 39 (1), 62–71. doi: 10.1111/j.1744-7429.2006.00238.x
Pohlman C., Turton S., Goosem M. (2009). Temporal variation in microclimatic edge effects near powerlines, highways and streams in Australian tropical rainforest. Agric. For. Meteorology 149 (1), 84–95. doi: 10.1016/j.agrformet.2008.07.003
Prescott C., Maynard D., Laiho R. (2000). Humus in northern forests: friend or foe? For. Ecol. Manage. 133 (1-2), 23–36. doi: 10.1016/S0378-1127(99)00295-9
Ries L., Fletcher R., Battin J., Sisk T. (2004). Ecological responses to habitat edges: mechanisms, models, and variability explained. Annu. Rev. Ecology Evolution Systematics 35, 491–522. doi: 10.1146/annurev.ecolsys.35.112202.130148
Ross S. (1986). Vegetation change on highway verges in south-east Scotland. J. Biogeography 13 (2), 109–117. doi: 10.2307/2844986
Santelmann M. V., Gorham E. (1988). The influence of airborne road dust on the chemistry of Sphagnum mosses. J. Ecol. 76 (4), 1219–1231. doi: 10.2307/2260644
Schmidt M., Jochheim H., Kersebaum K.-C., Lischeid G., Nendel C. (2017). Gradients of microclimate, carbon and nitrogen in transition zones of fragmented landscapes – a review. Agric. For. Meteorology 232, 659–671. doi: 10.1016/j.agrformet.2016.10.022
Seiler A. (2001). Ecological effects of roads: a review (Uppsala, SE: Swedish University of Agricultural Sciences).
Simard M., Lecomte N., Bergeron Y., Bernier P., Paré D. (2007). Forest productivity decline caused by successional paludification of boreal soils. Ecol. Appl. 17 (6), 1619–1637. doi: 10.1890/06-1795.1
Spooner P., Lunt I., Briggs S. (2004). Spatial analysis of anthropogenic disturbance regimes and roadside shrubs in a fragmented agricultural landscape. Appl. Vegetation Sci. 7 (1), 61–70. doi: 10.1111/j.1654-109X.2004.tb00596.x
Stern E., Riva F., Nielsen S. (2018). Effects of narrow linear disturbances on light and wind patterns in fragmented boreal forests in northeastern Alberta. Forests 9 (8), 486–498. doi: 10.3390/f9080486
Suárez-Esteban A., Delibes M., Fedriani J. (2013a). Barriers or corridors? The overlooked role of unpaved roads in endozoochorous seed dispersal. J. Appl. Ecol. 50 (3), 767–774. doi: 10.1111/1365-2664.12080
Suárez-Esteban A., Delibes M., Fedriani J. (2013b). Unpaved road verges as hotspots of fleshy-fruited shrub recruitment and establishment. Biol. Conserv. 167, 50–56. doi: 10.1016/j.biocon.2013.07.022
Taylor K., Brummer T., Taper M., Wing A., Rew L. (2012). Human-mediated long-distance dispersal: an empirical evaluation of seed dispersal by vehicles. Diversity Distributions 18 (9), 942–951. doi: 10.1111/j.1472-4642.2012.00926.x
Tikka P., Hogmander H., Koski P. (2001). Road and railway verges serve as dispersal corridors for grassland plants. Landscape Ecol. 16 (7), 659–666. doi: 10.1023/a:1013120529382
Trombulak S., Frissell C. (2000). Review of ecological effects of roads on terrestrial and aquatic communities. Conserv. Biol. 14 (1), 18–30. doi: 10.1046/j.1523-1739.2000.99084.x
Tyser R., Worley C. (1992). Alien flora in grasslands adjacent to road and trail corridors in Glacier National Park, Montana (USA). Conserv. Biol. 6 (2), 253–262. doi: 10.1046/j.1523-1739.1992.620253.x
van Cleve K., Dyrness C., Viereck L., Fox J., Chapin F., Oechel W. (1983). Taiga ecosystems in interior Alaska. Bioscience 33 (1), 39–44. doi: 10.2307/1309243
Veillette J. (1994). Evolution and paleohydrology of glacial lakes Barlow and Ojibway. Quaternary Sci. Rev. 13 (9), 945–971. doi: 10.1016/0277-3791(94)90010-8
von der Lippe M., Bullock J., Kowarik I., Knopp T., Wichmann M. (2013). Human-mediated dispersal of seeds by the airflow of vehicles. PloS One 8 (1), 1–10. doi: 10.1371/journal.pone.0052733
von der Lippe M., Kowarik I. (2012). Interactions between propagule pressure and seed traits shape human-mediated seed dispersal along roads. Perspect. Plant Ecol. Evol. Systematics 14 (2), 123–130. doi: 10.1016/j.ppees.2011.09.006
Walker D. A., Everett K. R. (1987). Road dust and its environmental impact on Alaskan taiga and tundra. Arctic Alpine Res. 19 (4), 479–489. doi: 10.1080/00040851.1987.12002630
Watkins R., Chen J., Pickens J., Brosofske K. (2003). Effects of forest roads on understory plants in a managed hardwood landscape. Conserv. Biol. 17 (2), 411–419. doi: 10.1046/j.1523-1739.2003.01285.x
Whitbeck K., Oetter D., Perry D., Fyles J. (2016). Interactions between macroclimate, microclimate, and anthropogenic disturbance affect the distribution of aspen near its northern edge in Quebec: Implications for climate change related range expansions. For. Ecol. Manage. 368, 194–206. doi: 10.1016/j.foreco.2016.03.013
Wolken J., Landhäusser S., Lieffers V., Dyck M. (2010). Differences in initial root development and soil conditions affect establishment of trembling aspen and balsam poplar seedlings. Botany 88 (3), 275–285. doi: 10.1139/B10-016
Young A., Mitchell N. (1994). Microclimate and vegetation edge effects in a fragmented podocarp-broadleaf forest in New Zealand. Biol. Conserv. 67 (1), 63–72. doi: 10.1016/0006-3207(94)90010-8
Keywords: habitat corridors, dispersal corridors, edge habitats, forest roads, road edge effects, Populus tremuloides, boreal forest
Citation: Marchais M, Arseneault D and Bergeron Y (2024) Forest roads act as habitat corridors for Populus tremuloides in the boreal forest of eastern Canada. Front. Ecol. Evol. 11:1336409. doi: 10.3389/fevo.2023.1336409
Received: 10 November 2023; Accepted: 26 December 2023;
Published: 12 January 2024.
Edited by:
Yixin Zhang, Soochow University, ChinaReviewed by:
Zdenka Krenova, Czech Academy of Sciences, CzechiaFrancis Isselin, UMR7324 Cités, Territoires, Environnement et Sociétés (CITERES), France
Copyright © 2024 Marchais, Arseneault and Bergeron. This is an open-access article distributed under the terms of the Creative Commons Attribution License (CC BY). The use, distribution or reproduction in other forums is permitted, provided the original author(s) and the copyright owner(s) are credited and that the original publication in this journal is cited, in accordance with accepted academic practice. No use, distribution or reproduction is permitted which does not comply with these terms.
*Correspondence: Mathilde Marchais, Mathilde.Marchais@uqat.ca