- 1Graduate School of Advanced Integrated Studies in Human Survivability, Kyoto University, Kyoto, Japan
- 2School of Geographical Sciences, East China Normal University, Shanghai, China
- 3Key Laboratory of Geographic Information Science, Ministry of Education, East China Normal University, Shanghai, China
- 4Lake Biwa Environmental Research Institute, Otsu, Japan
Lake Biwa, Japan represents a crucial example of the complex climatic and anthropogenic drivers influencing lake ecological transformations, vital to informing Sustainable Development Goals globally. This study utilizes 2002–2022 Landsat, MODIS and in situ Lake Biwa monitoring data to analyze surface layer spatiotemporal dynamics across interrelated vegetation, water quality and meteorological indicators—encompassing Normalized Difference Vegetation Index (NDVI), nitrogen (N), phosphorus (P), chlorophyll-a (Chl) and water temperature (W-TEM). Upward NDVI raster trends were found over 20 years alongside prevalent N, P and Chl declines—although some increases did occur spatially in P and Chl—while W-TEM mostly rose lakewide. Southwest–northeast gradients typified distributions. Further attribution analyses revealed W-TEM as the primary N, P and Chl driver, while agricultural expansion and urbanization mediated crucial N and P changes. Moreover, wind speed (WS), Crop, W-TEM, minimum temperature (TMMN), Chl and N constituted top NDVI raster influence factors respectively. These novel integrated models quantifying Lake Biwa ecological responses to multifaceted environmental change provide new perspectives to inform sustainable management of Lake Biwa itself and critical freshwater resources worldwide.
1 Introduction
Global climate change alongside rising human population growth and associated agricultural expansion, industrialization and urbanization have profoundly impacted lake and river ecosystems by altering hydrological balances and threatening critical water resources and ecological health (Ishtiaque et al., 2022; Jeppesen et al., 2014; Salk et al., 2022). As integrally interconnected carbon sinks and sources, shifts in lake ecology additionally drive wider carbon cycling changes (Meyers, 1997). Japan’s Lake Biwa represents an archetypal example of a lake ecosystem undergoing rapid transformations due to multifaceted human and climatic pressures (Xue et al., 2021). As an invaluable regional water resource and ecosystem underpinning key economic, ecological and societal services, Lake Biwa possesses immense significance. However, while its ecological disturbances mirror wider global lake trends, Lake Biwa further exhibits unique geographical and ecological characteristics molding localized responses (Kumagai, 2008; Le Moal et al., 2019). Therefore, this study seeks to deeply analyze Lake Biwa’s complex ecological transitions under interacting human and climatic influences through an interconnected surface layer indicator framework, in order to inform sustainable regional resource management pathways supporting global climate change mitigation efforts and Sustainable Development Goals.
Lake Biwa’s shifting ecology depends upon climatic, hydrological and environmental change drivers. Recent climate shifts combined with agricultural and urban encroachment have altered nutrient balances and water quality regimes (Reichwaldt and Ghadouani, 2012; Zhu et al., 2015; Zhou et al., 2022). Anthropogenic disruptions of nitrogen, phosphorus and water quality relationships cascade to impact vegetation dynamics and ecosystem functioning (Zhu et al., 2015; Xu et al., 2020; Lemenkova, 2022). Global population growth and intensifying urbanization since 1900 has amplified nutrient loads across watersheds worldwide (Bennett et al., 2001; Le Moal et al., 2019), evidenced in heavily populated regions including China’s eutrophic Lake Taihu (Zhang et al., 2016). Satellite sensors now provide unparalleled spatiotemporal visibility into global lake processes including water quality, algal blooms and carbon cycling to inform conservation priorities (Jarvie et al., 2018; Huo et al., 2021; Saravanan et al., 2021; Wu et al., 2023a). While Lake Biwa historically experienced 1990s–2000s eutrophication pressures, subsequent water quality and ecological improvements have occurred regionally (Nakamura, 2002; Nishino, 2012). Further research should now disentangle complex anthropogenic impact mechanisms affecting contemporary Lake Biwa amid continued environmental change.
Hydrological monitoring lends critical ecological insights through water levels, aquatic vegetation mapped via Normalized Difference Vegetation Indices (NDVIs), and phytoplankton abundances—key ecological health indicators (Kiage and Walker, 2009; Qing et al., 2020). Regional nitrogen, phosphorus, chlorophyll-a and water temperature profiles similarly inform surface layer health assessments (Dodds et al., 1998; Takamura et al., 2003; Filstrup and Downing, 2017; Liang et al., 2020). Prior Lake Biwa research has quantified hydrological cycle interdependencies with regional seismicity and climatic factors using integrated in situ gauge and satellite data (Xue et al., 2021). Relative nitrogen versus phosphorus controls over eutrophication also relate directly to depth and water quality regimes (Qin et al., 2020). Climate change driven regional warming trends further impact ecological functioning through lake temperature influences (Yamashiki et al., 2010; Nazari-Sharabian et al., 2018). Cascading temperature effects on stratification, nutrient cycling and phytoplankton productivity provide important mechanistic links to eutrophication (Elliott and Defew, 2012; Bhagowati and Ahamad, 2019; Salk et al., 2022). Thus, coordinated hydrological monitoring offers comprehensive eutrophication insights.
Meteorological shifts driven by climate change have also been observed across actual evapotranspiration, radiation, vapor pressure, air temperatures and wind patterns over Lake Biwa with ecological implications (Shao et al., 2015; Du et al., 2018; Zhou et al., 2022). Process-based models successfully reproduce Lake Biwa ecosystem responses to observed climate factor changes from 1955 to 2005 (Lemenkova, 2022; Zhou et al., 2022). These analyses clearly demonstrate meteorology linkages to ecological transformations, informing sustainable management needs under non-stationary climate change.
While previous research has generated critical insights about drivers of lake environmental change, recent studies underscore key knowledge gaps regarding multifaceted anthropogenic impacts on Lake Biwa across space and time in the context of climate change. This research encompasses an expansive set of interconnected parameters—spanning NVDI, water quality indicators (nitrogen, phosphorus, chlorophyll-a, temperature), meteorological variables (evapotranspiration, radiation, vapor pressure deficit, air temperature)—to unveil novel perspectives into relationships between regional meteorology and ecological transformations. This integrated assessment approach enables an improved theoretical framework for formulating adaptive management strategies responsive to intensifying environmental change pressures. Study outcomes are further intended to provide valuable references for promoting ecological sustainability and resilience of Lake Biwa and other comparable freshwater ecosystems moving forward.
This comprehensive 2002–2022 Lake Biwa assessment incorporating Landsat, MODIS and hydrological data offers unprecedented visibility into multidimensional ecological changes. The integrated framework assessing connections across NDVI, water quality indicators, meteorology and human pressures provides new perspectives for elucidating climate change and anthropogenic impacts on Lake Biwa. Study findings further offer scientifically grounded guidance to promote sustainable regional environmental management aligned with global priorities.
2 Materials and methods
2.1 Study area
Japan’s largest freshwater ecosystem, the over 4-million-year-old Lake Biwa spans 670 square kilometers in Shiga Prefecture. Containing 27.5 billion cubic meters of water, Lake Biwa has sufficient capacity to meet the entire Yodo River basin population’s 11-year water demands. As a vital supply for 14.5 million downstream residents in Kyoto, Osaka and Hyogo via the Seta and Yodo tributaries, the lake underpins regional water security, economies, and sustainable development (Kumagai et al., 2003; Kawanabe et al., 2020). A biodiversity refuge with over 60 endemic species, ongoing environmental change threatens Lake Biwa’s ecological character and services.
Spatiotemporal 2002–2022 hydrological data from 17 Lake Biwa water monitoring stations (Figure 1) enables quantitative analysis of ecological transitions across this critical supply amidst intensifying climatic and human pressures on the lake and dependent communities. High-frequency water quality indicators were obtained from long-term Shiga Prefecture Lake Biwa Environmental Research Institute programs for 11 northern sites, 5 southern sites and 1 Seta River site, enabling pollution and eutrophication trend assessments via nitrogen, phosphorus, chlorophyll-a, and water temperature.
2.2 Data
2.2.1 Measured data
Lake Biwa Environmental Research Institute long-term monitoring (https://www.lberi.jp/investigate/long_term) provided 2002–2022 water quality and ecosystem health indicator data for 11 northern sites, 5 southern sites and 1 Seta River site at 0.5 m water depth (Figure 1). Incorporating nitrogen, phosphorus, chlorophyll-a and water temperature measurements, this extensive spatiotemporal sampling enables quantification of pollution, eutrophication and ecological variation trends across Lake Biwa.
2.2.2 Vegetation data
Landsat 5, 8 and 9 satellite 30 m resolution top-of-atmosphere reflectance data was extracted via Google Earth Engine (developers.google.com/earth-engine) to derive annual maximum value composites and per-pixel monthly NDVI time series from 2002–2022 across the study area (Table 1). This enables vegetation change detection across Lake Biwa through the decades-long Landsat record.
2.2.3 Climate data
Gridded monthly TerraClimate climate data (Abatzoglou et al., 2018) was utilized in this study for the Lake Biwa region from 2002 to 2022. TerraClimate integrates high-resolution climatological normals from WorldClim with time-varying data from CRU Ts4.0 and Japanese 55-year Reanalysis (JRA55) (Table 1). This enabled correlation analysis between regional meteorology and Lake Biwa ecology.
2.2.4 Supporting data
The 30-meter resolution digital elevation information from the NASA Shuttle Radar Topography Mission (Farr et al., 2007) provided terrain perspectives (Table 1) enabling analysis of topographic influences on spatial ecological gradients. Crop and City is the land area selected from the study area overview map (Figure 1), utilizing the area of Cropland and Cities from the IGBP classification of MODIS MCD12Q1 for 2002–2022 (Justice et al., 2002).
2.3 Methods
2.3.1 Calculation of normalized difference vegetation index
NDVI can be extracted by subtracting the ratio of a red band and near-infrared band plus a red band from the near-infrared band of the image.
Where: NIR represents the reflectance in the near-infrared band. R represents the reflectance in the visible red band. The NDVI values range from −1 to 1. Negative values typically correspond to water, values close to zero may indicate bare soil or very low vegetation cover, and positive values indicate vegetation, with higher values signifying denser vegetation.
2.3.2 Partial least squares regression
Partial least squares regression (PLSR) enables analysis of multivariate relationships in contexts with more predictor variables than observations and potential inter-variable multicollinearities (Geladi and Kowalski, 1986). This study leverages PLSR to construct empirical models quantifying connections between climatic factors and Lake Biwa vegetation area changes, selecting from nine candidates (evapotranspiration, radiation, vapor pressure deficit, wind speed, maximum temperature, minimum temperature, water temperature, crop area, city area). Careful tuning of the PLSR principal component parameter is critical to balance model accuracy with overfitting avoidance for valid analytics. By iteratively evaluating out-of-sample PLSR cross-validation root mean square errors across different principal component choices for each vegetation–climate pair, optimal selections with minimized validation errors can be identified, enhancing model reliability (Ma et al., 2023). The systematic procedure provides robust vegetation–climate models from the multivariate, collinear Lake Biwa dataset.
3 Results
3.1 Vegetation changes of Lake Biwa
Analysis of annual 2002–2022 NDVI pixels across Lake Biwa (excluding 2012) indicates uptrending vegetation over the past two decades. Categorizing pixels by NDVI thresholds (0.2–0.3, 0.3–0.4, 0.4–1) revealed higher 0.2–0.3 fractions, indirectly reflecting heightened satellite-detected greenness and eutrophication levels. Years 2008, 2013–2015 and 2021–2022 marked peak periods, while 2003 and 2018–2019 represented lower NDVI years (Figure 2).
3.2 Spatiotemporal variation of nitrogen, phosphorus, chlorophyll-a, and water temperature
Distributions and ranges were also quantified across depth-profiled 2002–2022 nitrogen (0.229–0.445 mg/L), phosphorus (0.007–0.030 mg/L), chlorophyll-a (2.268–8.745 μg/L) and water temperatures (16.73–17.56°C) averaged across 17 Lake Biwa stations. Kriging interpolation (Figure 3) depicted southwest-northeast declines in all parameters, highlighting significant regional water temperature influence.
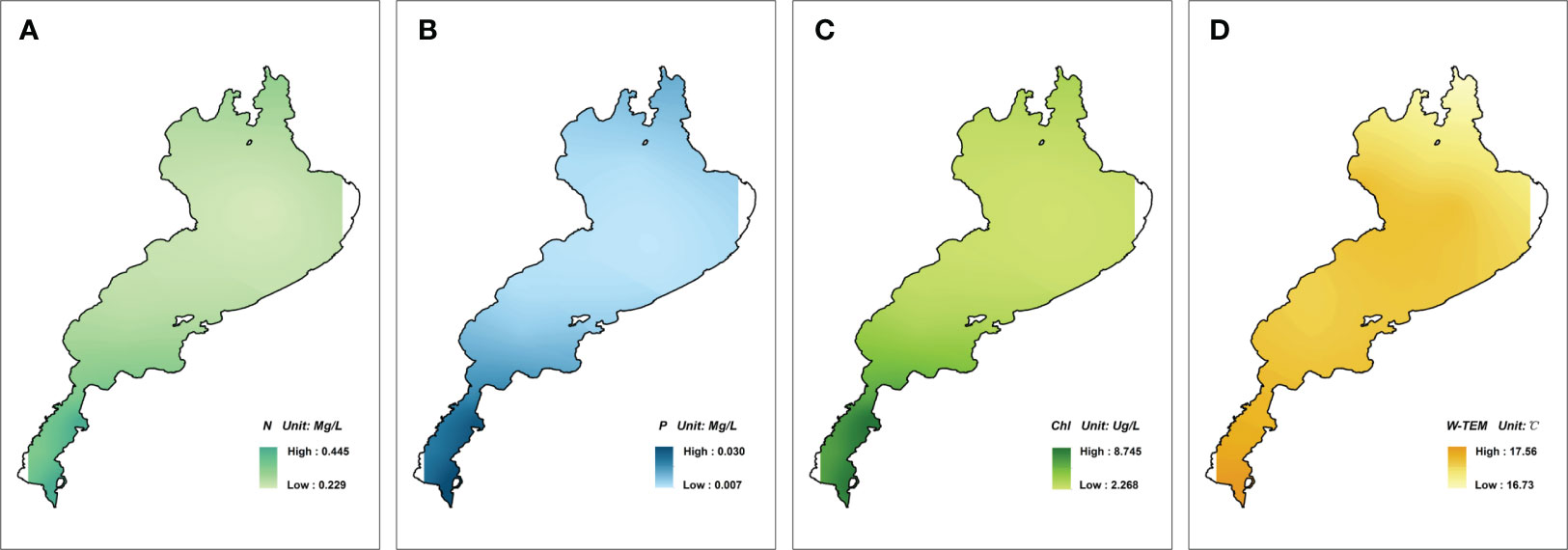
Figure 3 Spatial distribution of yearly mean (calculated by kriging difference from 17 sample points) indicators of (A) nitrogen, (B) phosphorus, (C) chlorophyll-a, and (D) water temperature from 2002 to 2022 in Lake Biwa.
Long-term assessments further demonstrated declines at most sampling points for nitrogen and phosphorus over 20 years. However localized phosphorus and chlorophyll-a increases emerged, often concurrently with predominant water temperature rises. Chlorophyll-a exhibited substantial interannual variability without directional trends (Figure 4), yet some synchronization was visible with 2003–2006 and 2015–2017 co-elevated, versus 2006–2007 and 2013–2016 co-suppressed phases. Intra-annual analysis revealed winter/spring nitrogen and chlorophyll peaks, contrasting with heights in summer water temperatures (Figure 5). These intricate spatiotemporal patterns underscore complex interdependent processes regulating Lake Biwa water quality.
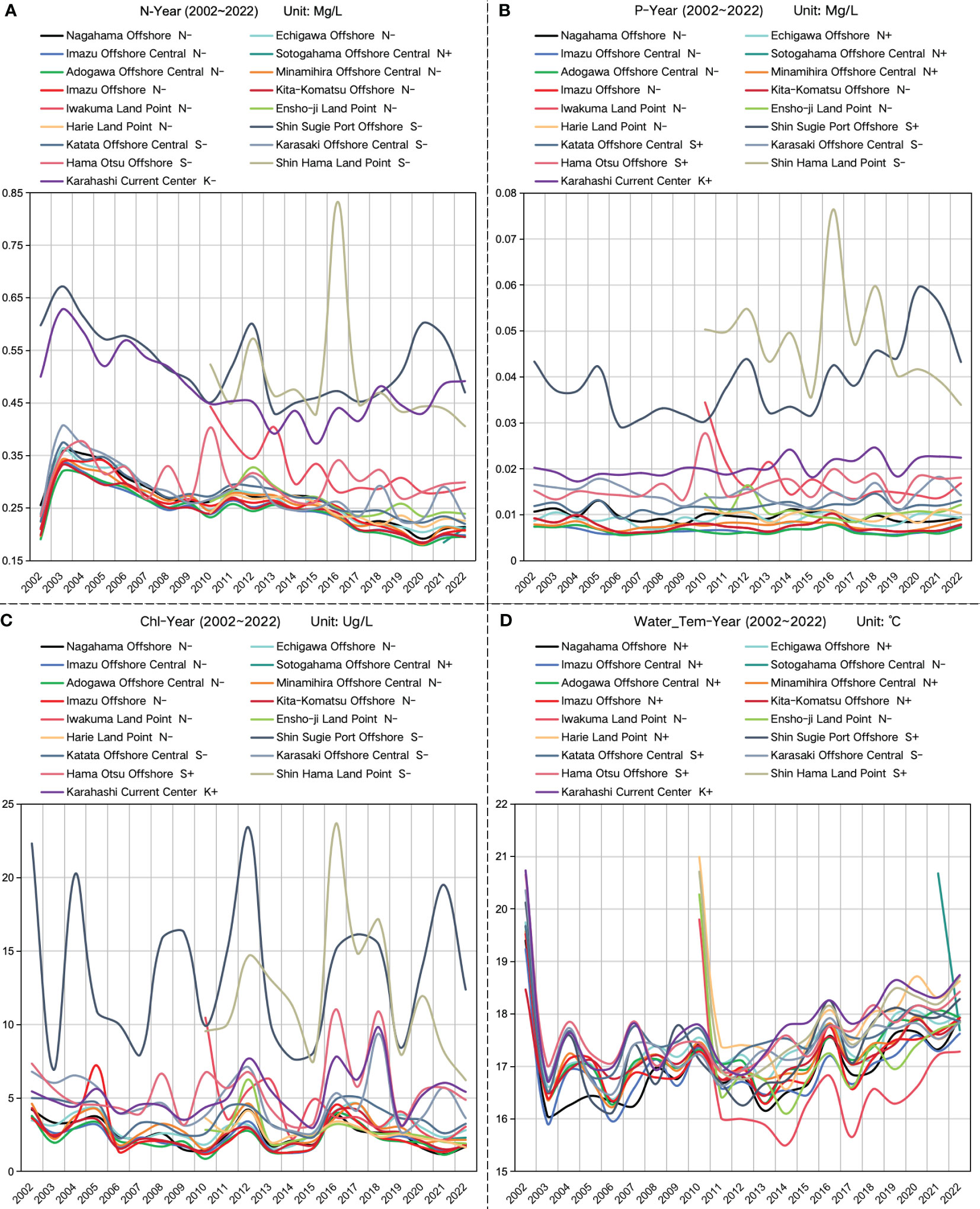
Figure 4 Time series of yearly mean indicators of (A) nitrogen, (B) phosphorus, (C) chlorophyll-a, and (D) water temperature from 2002 to 2022 in Lake Biwa. (N: Northern Lake, S: Southern Lake, K: Karahashi Current Center, +: Positive trend, −: Negative trend).
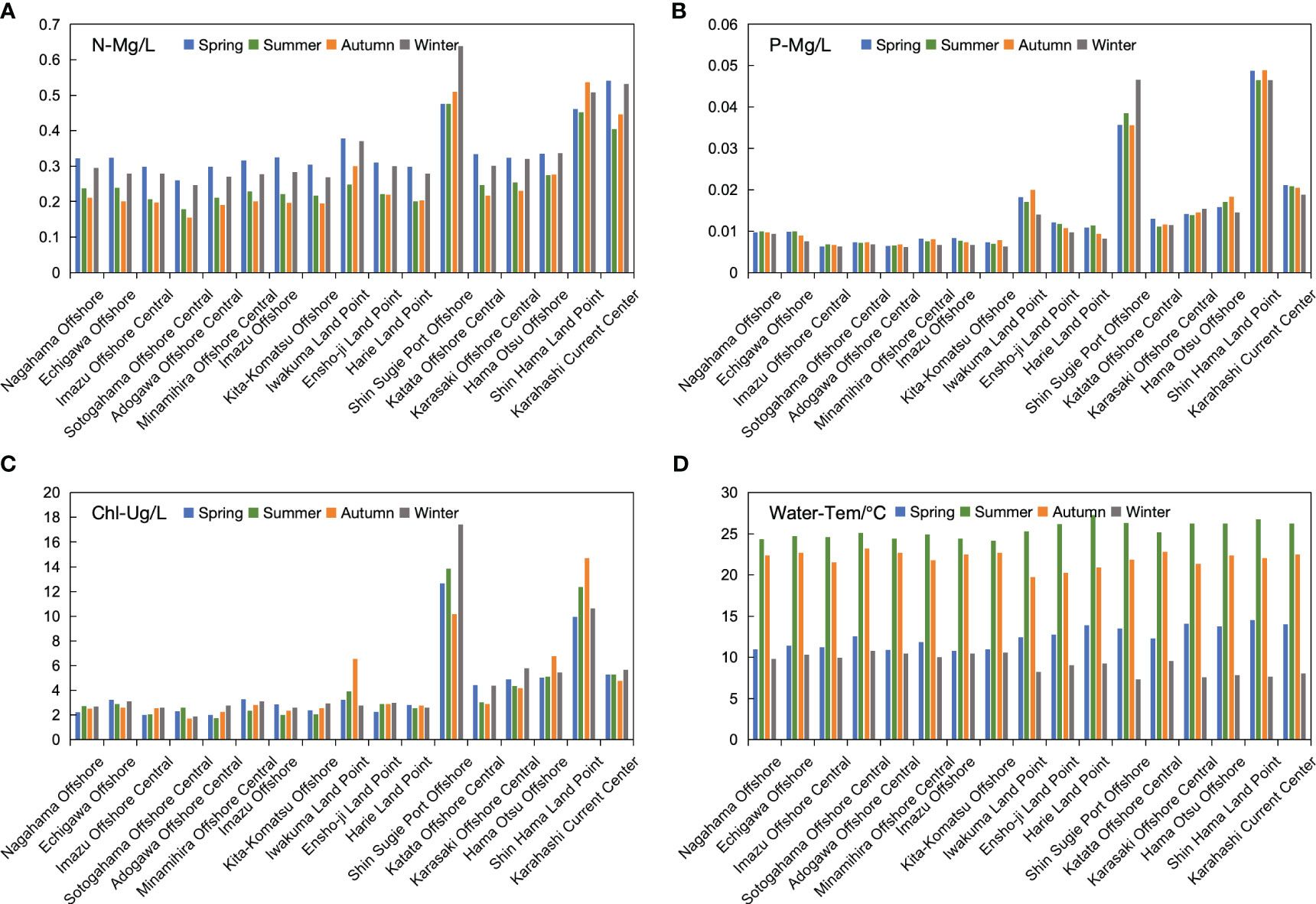
Figure 5 Seasonal changes of (A) nitrogen, (B) phosphorus, (C) chlorophyll-a, and (D) water temperature in Lake Biwa during 2002–2022.
3.3 Attribution of nitrogen, phosphorus, chlorophyll-a, and NDVI raster changes
Attribution assessments quantified relative contributions of regional meteorology, temperature, land use changes and human activities toward 2002–2022 fluctuations in Lake Biwa water quality and vegetation indices. Considering nitrogen dynamics, dominant factors included water temperature (43.3%), urban expansion (36.5%), vapor pressure deficit (10.6%), wind speed (7.0%) and maximum air temperatures (4.7%). Phosphorus variations were largely attributable to water temperature (40.1%), urban (37.6%) and agricultural land use changes (24.9%), alongside evapotranspiration (26.7%), radiation (19.1%) and maximum temperatures (21.2%). Water temperature (79.3%) also overwhelmingly explained chlorophyll-a shifts, followed by evapotranspiration (67.3%), radiation (44.4%) and vapor pressure deficit (42.4%). Finally, wind speed (55.5%), crops (34.3%), water temperature (33.3%), minimum air temperature (67.3%), chlorophyll-a (28.6%) and nitrogen (23.5%) constituted primary drivers of detected uptrends in Lake Biwa vegetative index raster counts (Figure 6).
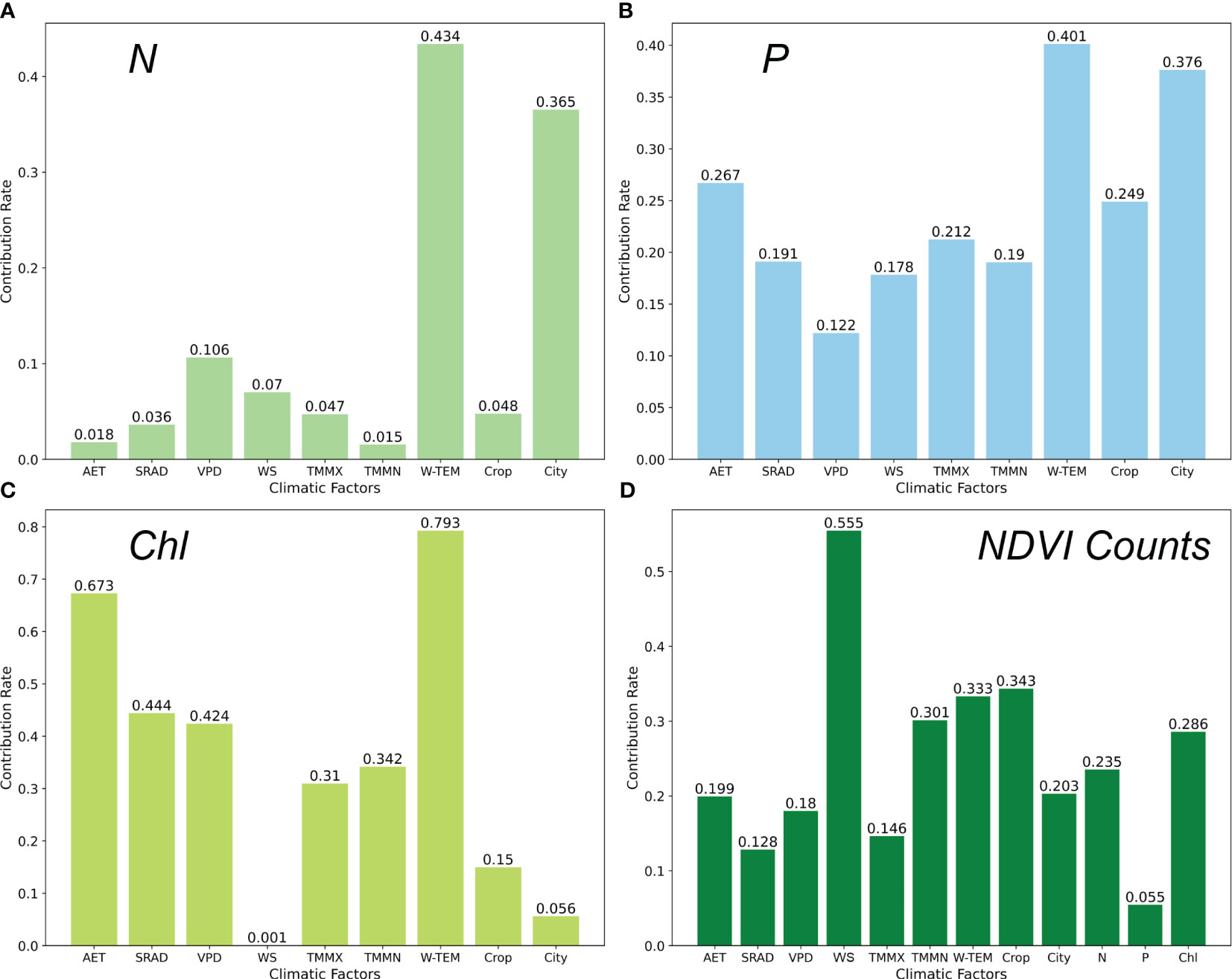
Figure 6 Contribution of climatic and anthropogenic factors to (A) nitrogen, (B) phosphorus, (C) chlorophyll-a, and (D) NDVI counts change in Lake Biwa.
4 Discussion
4.1 Changes in the vegetation index of Lake Biwa
Uptrending 2002–2022 Lake Biwa NDVI rasters suggest intensification of regional human activities and global climate change vegetation impacts. Hsieh et al. (2010) found 1960s–1990s oligotrophic conditions from management intervention shifted post 1990s warming enabling vegetation expansion, though overall ecological health persists (Flaim et al., 2016).
Global analyses reveal combined climatic and anthropogenic NDVI drivers, including intensified agriculture expanding Iran’s Lake Urmia vegetation (Tootoonchi et al., 2020) while Yangtze River basin shifts were 79.29% attributable to human activities versus 20.71% climate change (Yi et al., 2022). Analogously, Lake Biwa has undergone complex eutrophication–oligotrophication transitions from intersecting nutrient, climatic and management feedbacks. Despite ubiquitous climate NDVI linkages, local human pressures remain equally influential.
4.2 Changes in nitrogen and phosphorus indicators in Lake Biwa
As nitrogen–phosphorus ratios strongly dictate algal development and lake ecosystem responses to nutrient perturbation, observed southwest–northeast gradients in Lake Biwa nitrogen, phosphorus, chlorophyll-a and temperature highlight southern zones facing enhanced eutrophication threats due to positional nutrient loading disparities.
Long-term nitrogen and phosphorus declines at most sampling sites imply agricultural and sewage treatment improvements (Nakanishi et al., 2022), however continued uncontrolled phosphorus inputs may sustain eutrophication risks (Nakakuni et al., 2022). Concurrent warming from global climate change appears to drive thermal structure shifts enabling summer algal proliferation (Yan et al., 2022). Dynamic chlorophyll-a lacking trends indicates seasonal and inlet–outlet biomass regulation (Liu et al., 2023; Wu et al., 2023b). Interactive shifts in nutrient availability and temperature differentially benefit specific phytoplankton groups (Shatwell and Köhler, 2019; Wurtsbaugh et al., 2019; Qin et al., 2020), highlighting nitrogen-phosphorus ratios as key indicators of lake transformations.
4.3 Attribution of ecological changes in Lake Biwa
This study revealed wind speed, agricultural extent, lake water temperature, air minimum temperature, chlorophyll-a and nitrogen as dominant factors driving detected uptrends in Lake Biwa NDVI raster area from 2002–2022. Furthermore, rising water temperatures most profoundly impacted observed nitrogen, phosphorus and chlorophyll-a shifts, while urban expansion was found to strongly influence detected nitrogen and phosphorus dynamics.
A variety of factors, including WS, Crop, W-TEM, TMMN, Chl and nitrogen levels, collectively influence the changes in NDVI raster area. Increased wind speed, especially during precipitation events, can cause changes in Chl concentration in coastal areas, thereby affecting NDVI values (Valentin et al., 2021). Climate change also impacts vegetation NDVI by altering air and water temperatures, with vegetation greenness variability primarily driven by climate, and vegetation of different land use types being sensitive to climate change (Revadekar et al., 2012; Bao et al., 2021). NDVI also responds differently to seasonal changes in temperature and precipitation patterns (Yan et al., 2022). Furthermore, studies indicate that nitrogen levels in lakes and oceans affect NDVI by influencing the growth of plants and algae, with the impact dependent on the supply, form, ratio, and environmental conditions of nitrogen (Nizzoli et al., 2018; Palacin-Lizarbe et al., 2020). The balance of nitrogen and phosphorus is crucial in managing eutrophication in aquatic ecosystems and maintaining clear water bodies. Therefore, changes in lake NDVI are the result of the interaction between climate and human activities.
W-TEM affects the levels of nitrogen, phosphorus, and Chl in aquatic ecosystems through various mechanisms. Research indicates that water temperature not only directly influences the growth rates of algae and aquatic plants but also indirectly alters nutrient cycling and ecosystem dynamics (Beisner et al., 2003; Zhang et al., 2020). Liang et al. suggest that under the backdrop of climate warming, elevated water temperatures can increase the prevalence of certain algae, especially when ample phosphorus nutrients are available, and may also change the patterns of nitrogen cycling in aquatic ecosystems (Liang et al., 2020). The impact of temperature on these ecosystem elements is complex and is influenced by a combination of ecosystem type, other environmental stresses, and local environmental characteristics (Zhang et al., 2020). Therefore, research assessing the impact of changes in W-TEM on lake nitrogen, phosphorus, and Chl is crucial to understand and predict ecosystem responses to climate change.
Changes in city area play a key role in the changes in nitrogen and phosphorus. Studies have shown that agricultural and impervious city lands produce higher levels of nitrogen and phosphorus than other land surfaces, severely affecting water quality in water bodies (Tong and Chen, 2002). Research has also found that climate and land use changes have a significant inhibitory effect on water retention, nitrogen emissions, and phosphorus emissions (Bai et al., 2019). These factors act together on lake ecosystems and may have profound effects on biological networks, chemical cycles, and their overall health. Therefore, protecting water quality requires integrated management strategies that consider climate and land use changes.
4.4 Uncertainty analysis and prospects
This paper investigates the ecological changes of the surface layer of Lake Biwa and its driving factors through Landsat 5/8/9 and MODIS remote sensing data, measured data, and reanalyzed data. There are inconsistencies in the spatiotemporal accuracy of remote sensing data and its products, as well as inaccuracies in the representation of ground objects. For example, the saturation defect of NDVI may lead to a loss of resolution in areas with high vegetation coverage, while errors in measured data, such as improper sensor calibration, inaccurate atmospheric correction, or inappropriate selection of ground sampling points, can affect the accuracy of the data. Although the reanalyzed data has a long time series, it has the defect of low spatial accuracy, which may affect the accuracy of data in medium and small-scale regions. At the same time, errors in measured data are also a factor affecting the accuracy of the research, including but not limited to issues such as sensor calibration, inaccurate atmospheric correction, representativeness of ground sampling points, and irregularities in human operation. However, these shortcomings do not affect the scientific nature and accuracy of the article. The study compares remote sensing data with empirical data and conducts detailed assessments to ensure the scientific nature and reliability of the results.
To overcome these limitations and improve the accuracy of future research, future studies need to improve data calibration accuracy, increase measured samples, use advanced algorithms to reduce analysis uncertainty, and monitor ecological changes over a longer time span to establish a comprehensive ecological model to enhance the understanding and protection of the Lake Biwa ecosystem. These measures will help to improve the overall quality of the research and ensure the scientific nature and accuracy of the results, even in the face of uncertainties and challenges.
5 Conclusion
This study, based on the infrared and near-infrared bands of Landsat and MODIS land use remote sensing data products, as well as reanalyzed and measured data, analyzes the ecological changes of the surface layer of Lake Biwa in Japan. It calculates and analyzes the high-precision NDVI vegetation index and explores the changes in the number of rasters and the spatiotemporal variations of surface nitrogen, phosphorus, chlorophyll-a, and water temperature in the lake area. The study attributes the changes in nitrogen, phosphorus, and chlorophyll-a in the lake area to climatic factors such as AET, SRAD, VPD, WS, TMMX, TMMN, W-TEM, and human activities such as changes in crop and city area, and ultimately integrates these factors to attribute the changes in NDVI comprehensively. We found that:
1. From 2002 to 2022, the number of NDVI rasters in Lake Biwa showed an upward trend, with the trend weakening as the NDVI value range increased.
2. The spatial distribution pattern of nitrogen, phosphorus, chlorophyll-a, and water temperature decreased from the southwest to the northeast of Lake Biwa at 17 sample points from 2002 to 2022, with nitrogen, phosphorus and chlorophyll-a showing a downward trend at most sample points over the past 20 years, but increasing trends in phosphorus and chlorophyll at some sites and water temperature at most sites.
3. In terms of factor contribution rates, WS (55.5%), Crop (34.3%), W-TEM (33.3%), TMMN (67.3%), Chl (28.6%), and N (23.5%) had the greatest impact on the change in the number of NDVI rasters. Among them, water temperature (N: 43.4%, P: 40.1%, Chl: 79.3%) had the greatest impact on the changes in nitrogen, phosphorus, and chlorophyll-a, followed by changes in city area (N: 36.5%, P: 37.6%) which played a key role in the changes in nitrogen and phosphorus.
This study provides new insights for the monitoring and application of ecological indicators in lakes or oceans, offers a scientific theoretical basis for the ecological protection management and decision-making of lakes or oceans, and contributes to the ecological and environmental protection and development of similar water bodies.
Data availability statement
The original contributions presented in the study are included in the article/supplementary material. Further inquiries can be directed to the corresponding author.
Author contributions
BG: Conceptualization, Data curation, Formal Analysis, Software, Visualization, Writing – original draft. MC: Investigation, Methodology, Writing – review & editing. HH: Conceptualization, Data curation, Formal Analysis, Methodology, Software, Visualization, Writing – original draft. YY: Funding acquisition, Supervision, Writing – review & editing. KI: Funding acquisition, Supervision, Writing – review & editing. CJ: Resources, Writing – review & editing. JC: Funding acquisition, Supervision, Writing – review & editing. SI: Validation, Writing – review & editing.
Funding
The author(s) declare financial support was received for the research, authorship, and/or publication of this article. This research was supported by (Research and Development Project on the Development of Earth Observation System Using Space Technology) of Mori Memorial Foundation, Restoring and evaluating the ecological condition of the shallow areas in Lake Biwa (LBERI-7-IA1), Science and Technology Research Partnership for Sustainable Development (SATREPS; grant number JPMJSA2005) and China Scholarship Council (CSC).
Acknowledgments
The authors acknowledge gratitude to all the staff who participated in this study.
Conflict of interest
The authors declare that the research was conducted in the absence of any commercial or financial relationships that could be construed as a potential conflict of interest.
Publisher’s note
All claims expressed in this article are solely those of the authors and do not necessarily represent those of their affiliated organizations, or those of the publisher, the editors and the reviewers. Any product that may be evaluated in this article, or claim that may be made by its manufacturer, is not guaranteed or endorsed by the publisher.
Abbreviations
AET, actual evapotranspiration; Chl, chlorophyll-a; City, area of cities; Crop, area of cropland; MODIS, Moderate-resolution Imaging Spectroradiometer; N, nitrogen; NDVI, Normalized Difference Vegetation Index; P, phosphorus; PLSR, partial least squares regression; RS, remote sensing; SDGs, Global Sustainable Development Goals; SRAD, surface shortwave radiation; TMMN, minimum temperature; TMMX, maximum temperature; VPD, vapor pressure difference; WS, wind speed at 10 meters; W-TEM, water temperature.
References
Abatzoglou J. T., Dobrowski S. Z., Parks S. A., Hegewisch K. C. (2018). TerraClimate, a high-resolution global dataset of monthly climate and climatic water balance from 1958–2015. Sci. Data 5, 1–12. doi: 10.1038/sdata.2017.191
Bai Y., Ochuodho T. O., Yang J. (2019). Impact of land use and climate change on water-related ecosystem services in Kentucky, USA. Ecol. Indic. 102, 51–64. doi: 10.1016/j.ecolind.2019.01.079
Bao Z., Zhang J., Wang G., Guan T., Jin J., Liu Y., et al. (2021). The sensitivity of vegetation cover to climate change in multiple climatic zones using machine learning algorithms. Ecol. Indic. 124, 107443. doi: 10.1016/j.ecolind.2021.107443
Beisner B. E., Dent C. L., Carpenter S. R. (2003). Variability of lakes on the landscape: roles of phosphorus, food webs, and dissolved organic carbon. Ecology 84, 1563–1575. doi: 10.1890/0012-9658(2003)084[1563:VOLOTL]2.0.CO;2
Bennett E. M., Carpenter S. R., Caraco N. F. (2001). Human impact on erodable phosphorus and eutrophication: a global perspective: increasing accumulation of phosphorus in soil threatens rivers, lakes, and coastal oceans with eutrophication. Bioscience 51, 227–234. doi: 10.1641/0006-3568(2001)051[0227:HIOEPA]2.0.CO;2
Bhagowati B., Ahamad K. U. (2019). A review on lake eutrophication dynamics and recent developments in lake modeling. Ecohydrol Hydrobiol 19, 155–166. doi: 10.1016/j.ecohyd.2018.03.002
Dodds W. K., Jones J. R., Welch E. B. (1998). Suggested classification of stream trophic state: distributions of temperate stream types by chlorophyll, total nitrogen, and phosphorus. Water Res. 32, 1455–1462. doi: 10.1016/S0043-1354(97)00370-9
Du Q., Liu H. Z., Liu Y., Wang L., Xu L. J., Sun J. H., et al. (2018). Factors controlling evaporation and the CO2 flux over an open water lake in southwest of China on multiple temporal scales. Int. J. Climatol 38, 4723–4739. doi: 10.1002/joc.5692
Elliott J., Defew L. (2012). “Modelling the response of phytoplankton in a shallow lake (Loch Leven, UK) to changes in lake retention time and water temperature,” in Developments in Hydrobiology, vol 218. Eds. May L., Spears B. M. (Dordrecht: Springer), 105–116. doi: 10.1007/978-94-007-4333-5_10
Farr T. G., Rosen P. A., Caro E., Crippen R., Duren R., Hensley S., et al. (2007). The shuttle radar topography mission. Rev. Geophys. 45, 3915-3931. doi: 10.1029/2005RG000183
Filstrup C. T., Downing J. A. (2017). Relationship of chlorophyll to phosphorus and nitrogen in nutrient-rich lakes. Inland Waters 7, 385–400. doi: 10.1080/20442041.2017.1375176
Flaim G., Eccel E., Zeileis A., Toller G., Cerasino L., Obertegger U. (2016). Effects of re-oligotrophication and climate change on lake thermal structure. Freshw. Biol. 61, 1802–1814. doi: 10.1111/fwb.12819
Geladi P., Kowalski B. R. (1986). Partial least-squares regression: a tutorial. Anal. Chim. Acta 185, 1–17. doi: 10.1016/0003-2670(86)80028-9
Hsieh C. H., Ishikawa K., Sakai Y., Ishikawa T., Ichise S., Yamamoto Y., et al. (2010). Phytoplankton community reorganization driven by eutrophication and warming in Lake Biwa. Aquat Sci. 72, 467–483. doi: 10.1007/s00027-010-0149-4
Huo D., Gan N., Geng R., Cao Q., Song L., Yu G., et al. (2021). Cyanobacterial blooms in China: Diversity, distribution, and cyanotoxins. Harmful Algae 109, 102106. doi: 10.1016/j.hal.2021.102106
Ishtiaque A., Estoque R. C., Eakin H., Parajuli J., Rabby Y. W. (2022). IPCC’s current conceptualization of “vulnerability” needs more clarification for climate change vulnerability assessments. J. Environ. Manage 303, 114246. doi: 10.1016/j.jenvman.2021.114246
Jarvie H. P., Smith D. R., Norton L. R., Edwards F. K., Bowes M. J., King S. M., et al. (2018). Phosphorus and nitrogen limitation and impairment of headwater streams relative to rivers in Great Britain: A national perspective on eutrophication. Sci. Total Environ. 621, 849–862. doi: 10.2134/jeq2016.07.0248
Jeppesen E., Meerhoff M., Davidson T., Trolle D., Sondergaard M., Lauridsen T., et al. (2014). Climate change impacts on lakes: an integrated ecological perspective based on a multi-faceted approach, with special focus on shallow lakes. J. Limnol. 73. doi: 10.4081/jlimnol.2014.844
Justice C. O., Townshend J., Vermote E. F., Masuoka E., Wolfe R. E., Saleous N., et al. (2002). An overview of MODIS Land data processing and product status. Remote Sens Environ. 83, 3–15. doi: 10.1016/S0034-4257(02)00084-6
Kawanabe H., Nishino M., Maehata M. (2020). Lake Biwa: interactions between nature and people. Nature 1–29. doi: 10.1007/978-3-030-16969-5
Kiage L. M., Walker N. D. (2009). Using NDVI from MODIS to monitor duckweed bloom in Lake Maracaibo, Venezuela. Water Resour. Manage 23, 1125–1135. doi: 10.1007/s11269-008-9318-9
Kumagai M. (2008). Lake Biwa in the context of world lake problems. Internationale Vereinigung Für Theoretische Und Angewandte Limnol: Verhandlungen 30, 1–15. doi: 10.1080/03680770.2008.11902076
Kumagai M., Vincent W. F., Ishikawa K., Aota Y. (2003). “Lessons from Lake Biwa and other Asian lakes: Global and local perspectives,” in Freshwater Management. Eds. Kumagai M., Vincent W. F. (Tokyo: Springer). doi: 10.1007/978-4-431-68436-7_1
Le Moal M., Gascuel-Odoux C., Ménesguen A., Souchon Y., Étrillard C., Levain A., et al. (2019). Eutrophication: a new wine in an old bottle? Sci. Total Environ. 651, 1–11. doi: 10.1016/j.scitotenv.2018.09.139
Lemenkova P. (2022). Mapping climate parameters over the Territory of Botswana using GMT and gridded surface data from TerraClimate. ISPRS Int. J. Geo-Information 11, 473. doi: 10.3390/ijgi11090473
Liang Z., Soranno P. A., Wagner T. (2020). The role of phosphorus and nitrogen on chlorophyll a: Evidence from hundreds of lakes. Water Res. 185, 116236. doi: 10.1016/j.watres.2020.116236
Liu X., Liu H., Chen H., Liu Y., Xu Z., Cao X., et al. (2023). Spatiotemporal distribution and prediction of chlorophyll-a in Ulansuhai lake from an arid area of China. Front. Environ. Sci. 11. doi: 10.3389/fenvs.2023.1045464
Ma M., Wang Q., Liu R., Zhao Y., Zhang D. (2023). Effects of climate change and human activities on vegetation coverage change in northern China considering extreme climate and time-lag and-accumulation effects. Sci. Total Environ. 860, 160527. doi: 10.1016/j.scitotenv.2022.160527
Meyers P. A. (1997). Organic geochemical proxies of paleoceanographic, paleolimnologic, and paleoclimatic processes. Org. Geochem 27, 213–250. doi: 10.1016/S0146-6380(97)00049-1
Nakakuni M., Loassachan N., Ichimi K., Nagao S., Tada K. (2022). Biophilic elements in core sediments as records of coastal eutrophication in the Seto Inland Sea, Japan. Regional Stud. Mar. Sci. 50, 102093. doi: 10.1016/j.rsma.2021.102093
Nakamura M. (2002). Lake Biwa watershed transformation and the changed water environments. Internationale Vereinigung Für Theoretische Und Angewandte Limnol: Verhandlungen 28, 69–83. doi: 10.1080/03680770.2001.11902549
Nakanishi K., Yokomizo H., Fukaya K., Kadoya T., Shin-Ichiro S. M., Nishihiro J., et al. (2022). Inferring causal impacts of extreme water-level drawdowns on lake water clarity using long-term monitoring data. Sci. Total Environ. 838, 156088. doi: 10.1016/j.scitotenv.2022.156088
Nazari-Sharabian M., Ahmad S., Karakouzian M. (2018). Climate change and eutrophication: a short review. Engineering Technol. Appl. Sci. Res. 8, 3668. doi: 10.5281/zenodo.2532694
Nishino M. (2012). Lake Biwa: Interactions between Nature and People. Eds. Kawanabe H., Nishino M., Maehata M. (Dordrecht: Springer Netherlands), 155–238.
Nizzoli D., Bartoli M., Azzoni R., Longhi D., Castaldelli G., Viaroli P. (2018). Denitrification in a meromictic lake and its relevance to nitrogen flows within a moderately impacted forested catchment. Biogeochemistry 137, 143–161. doi: 10.1007/s10533-017-0407-9
Palacin-Lizarbe C., Camarero L., Hallin S., Jones C. M., Catalan J. (2020). Denitrification rates in lake sediments of mountains affected by high atmospheric nitrogen deposition. Sci. Rep. 10, 3003. doi: 10.1038/s41598-020-59759-w
Qin B., Zhou J., Elser J. J., Gardner W. S., Deng J., Brookes J. D. (2020). Water depth underpins the relative roles and fates of nitrogen and phosphorus in lakes. Environ. Sci. Technol. 54, 3191–3198. doi: 10.1021/acs.est.9b05858
Qing S., Runa A., Shun B., Zhao W., Bao Y., Hao Y. (2020). Distinguishing and mapping of aquatic vegetations and yellow algae bloom with Landsat satellite data in a complex shallow Lake, China during 1986–2018. Ecol. Indic. 112, 106073. doi: 10.1016/j.ecolind.2020.106073
Reichwaldt E. S., Ghadouani A. (2012). Effects of rainfall patterns on toxic cyanobacterial blooms in a changing climate: between simplistic scenarios and complex dynamics. Water Res. 46, 1372–1393. doi: 10.1016/j.watres.2011.11.052
Revadekar J., Tiwari Y. K., Kumar K. R. (2012). Impact of climate variability on NDVI over the Indian region during 1981–2010. Int. J. Remote Sens 33, 7132–7150. doi: 10.1080/01431161.2012.697642
Salk K. R., Venkiteswaran J. J., Couture R. M., Higgins S. N., Paterson M. J., Schiff S. L. (2022). Warming combined with experimental eutrophication intensifies lake phytoplankton blooms. Limnol. Oceanogr. 67, 147–158. doi: 10.1002/Ino.11982
Saravanan A., Kumar P. S., Jeevanantham S., Karishma S., Tajsabreen B., Yaashikaa P., et al. (2021). Effective water/wastewater treatment methodologies for toxic pollutants removal: Processes and applications towards sustainable development. Chemosphere 280, 130595. doi: 10.1016/j.chemosphere.2021.130595
Shao C., Chen J., Stepien C. A., Chu H., Ouyang Z., Bridgeman T. B., et al. (2015). Diurnal to annual changes in latent, sensible heat, and CO2 fluxes over a Laurentian Great Lake: A case study in Western Lake Erie. J. Geophysical Res: Biogeosci 120, 1587–1604. doi: 10.1029/2020EA001091
Shatwell T., Köhler J. (2019). Decreased nitrogen loading controls summer cyanobacterial blooms without promoting nitrogen-fixing taxa: Long-term response of a shallow lake. Limnol Oceanogr 64, S166–S178. doi: 10.1002/lno.11002
Takamura N., Kadono Y., Fukushima M., Nakagawa M., Kim B.-H. O. (2003). Effects of aquatic macrophytes on water quality and phytoplankton communities in shallow lakes. Ecol. Res. 18, 381–395. doi: 10.1046/j.1440-1703.2003.00563.x
Tong S. T., Chen W. (2002). Modeling the relationship between land use and surface water quality. J. Environ. Manage. 66, 377–393. doi: 10.1006/em.2002.0593
Tootoonchi R., Nourani V., Andaryani S., Tootoonchi F. (2020). Application of Mann-Kendall trend test and Normalized Difference Vegetation Index (NDVI) in hydroclimatological change detection – A Case Study of Urmia Lake watershed, Iran, EGU General Assembly 2020, Online, 4–8 May 2020, EGU2020-6904. doi: 10.5194/egusphere-egu2020-6904
Valentin J. L., Leles S. G., Tenenbaum D. R., Figueiredo G. M. (2021). Frequent upwelling intrusions and rainfall events drive shifts in plankton community in a highly eutrophic estuary. Estuar. Coast. Shelf Sci. 257, 107387. doi: 10.1016/j.ecss.2021.107387
Wu H., Nian T., Shan Z. (2023a). Investigation of landslide dam life span using prediction models based on multiple machine learning algorithms. Geomatics Natural Hazards Risk 14, 2273213. doi: 10.1080/19475705.2023.2273213
Wu H., Nian T.-K., Shan Z.-G., Li D.-Y., Guo X.-S., Jiang X.-G. (2023b). Rapid prediction models for 3D geometry of landslide dam considering the damming process. J. Mountain Sci. 20, 928–942. doi: 10.1007/s11629-022-7906-z
Wurtsbaugh W. A., Paerl H. W., Dodds W. K. (2019). Nutrients, eutrophication and harmful algal blooms along the freshwater to marine continuum. Wiley Interdiscip. Reviews: Water 6, e1373. doi: 10.1002/wat2.1373
Xu X., Zhang Y., Chen Q., Li N., Shi K., Zhang Y. (2020). Regime shifts in shallow lakes observed by remote sensing and the implications for management. Ecol. Indic. 113, 106285. doi: 10.1016/j.ecolind.2020.106285
Xue L., Fu Y., Johnson C. W., Otero Torres J. J., Shum C., Bürgmann R. (2021). Seasonal seismicity in the Lake Biwa Region of Central Japan moderately modulated by Lake Water storage changes. J. Geophysical Res: Solid Earth 126, e2021JB023301. doi: 10.1029/2021JB023301
Yamashiki Y., Kato M., Takara K., Nakakita E., Kumagai M., Jiao C. (2010). Sensitivity Analysis on Lake Biwa under the A1B SRES climate change scenario using Biwa-3D Integrated Assessment Model: part I–projection of lake temperature. Hydrol Res. Lett. 4, 45–49. doi: 10.3178/hrl.4.45
Yan X., Liu J., Rühland K. M., Smol J. P., Chen F. (2022). Climate change as the dominant driver of recent ecological changes in a semi-arid alpine lake from the Chinese Loess Plateau. J. Paleolimnol 68, 39–57. doi: 10.1007/s10933-020-00167-5
Yi L., Sun Y., Ouyang X., Yin S. (2022). Identifying the impacts of climate change and human activities on vegetation cover changes: A case study of the yangtze river basin, China. Int. J. Env Res. Public Health 19, 6239. doi: 10.3390/ijerph19106239
Zhang P., Kuramae A., Van Leeuwen C. H., Velthuis M., Van Donk E., Xu J., et al. (2020). Interactive effects of rising temperature and nutrient enrichment on aquatic plant growth, stoichiometry, and palatability. Front. Plant Sci. 11. doi: 10.3389/fpls.2020.00058
Zhang Y., Yao X., Qin B. (2016). A critical review of the development, current hotspots, and future directions of Lake Taihu research from the bibliometrics perspective. Environ. Sci. pollut. Res. 23, 12811–12821. doi: 10.1007/s11356-016-6856-1
Zhou J., Yoshida T., Kitazawa D. (2022). Numerical analysis of the relationship between mixing regime, nutrient status, and climatic variables in Lake Biwa. Sci. Rep. 12, 19691. doi: 10.1038/s41598-022-22124-0
Keywords: climate change, chlorophyll, human activities, Lake Biwa, NDVI, nitrogen, phosphorus
Citation: Gao B, Chen M, Hao H, Yamashiki YA, Ishikawa K, Jiao C, Cai J and Ismail S (2023) Recent mechanisms of surface ecological changes driven by climate change and human activities in Lake Biwa, Japan. Front. Ecol. Evol. 11:1335990. doi: 10.3389/fevo.2023.1335990
Received: 09 November 2023; Accepted: 27 November 2023;
Published: 11 December 2023.
Edited by:
Hao Wu, Nanjing Hydraulic Research Institute, ChinaCopyright © 2023 Gao, Chen, Hao, Yamashiki, Ishikawa, Jiao, Cai and Ismail. This is an open-access article distributed under the terms of the Creative Commons Attribution License (CC BY). The use, distribution or reproduction in other forums is permitted, provided the original author(s) and the copyright owner(s) are credited and that the original publication in this journal is cited, in accordance with accepted academic practice. No use, distribution or reproduction is permitted which does not comply with these terms.
*Correspondence: Haichao Hao, aGFvaGNAc3R1LmVjbnUuZWR1LmNu; Yosuke Alexandre Yamashiki, eWFtYXNoaWtpLnlvc3VrZS4zdUBreW90by11LmFjLmpw