- 1Ecological Restoration and Conservation on Forest and Wetland Key Laboratory of Sichuan Province, Sichuan Academy of Forestry Sciences, Chengdu, China
- 2Ecological Security and Protection Key Laboratory of Sichuan Province, Mianyang Normal University, Mianyang, China
Desertification has a significant impact on the Zoige meadow. Research efforts are directed towards the rehabilitation of the Zoige desertified alpine meadow, specifically in understanding the soil’s physical and chemical characteristics and the functional groupings of plants. However, the relationships between plant functional groups and soil properties remains uncertain. The primary objective of this study is to investigate the correlation between plant functional groups and soil nutrients across various stages of restoration. This study examined important value of plant functional groups, as well as variations in several soil characteristics, such as soil total nitrogen, total carbon, total phosphorus, available nitrogen, available potassium, available phosphorus, and soil pH during the stages of recovery. The results showed that the relative significance of importance values differed across distinct functional groups during different stages of recovery. The important value of legumes was found to have a strong negative correlation with the levels of soil available nitrogen and potassium. In contrast, there was a substantial positive correlation between the important value of grass and both soil total carbon and available potassium. Conversely, there was a strong negative relationship between the importance value of sedges and both total soil carbon and phosphorus. These findings indicate that the linkages between soil properties and plant functional groups varied across different recovery periods. This study would shed new light on the restoration in the Zoige desertified alpine meadows.
1 Introduction
The grassland ecosystems represent the most expansive terrestrial ecosystems globally, encompassing approximately 40% of the Earth’s land area. These ecosystems play a crucial and indispensable role in maintaining the stability of global ecosystem functions (Reeder and Schuman, 2002). The Tibet Plateau holds a pivotal position in atmospheric circulation and the regional monsoon climate, exerting notable influences on both local and global climatic conditions. Numerous studies have demonstrated the heightened vulnerability of plateau ecosystems to the impacts of global climate change, thereby positioning them as potential precursors for broader global environmental shifts (Zhang and Welker, 1996; Li and Zhou, 1998; Zheng et al., 2002). The Zoige alpine meadow is located in the eastern Tibet Plateau and has been experiencing significant desertification. This phenomenon has been particularly pronounced in recent years due to the combined effects of global climate change, excessive grazing practices, and the excavation of ditches (Hill, 2011; Jia et al., 2013). This has had a concerning impact on the proper functioning of ecosystem services (Liu et al., 2021). Plant communities, biodiversity, and soil properties in the Zoige meadow area exhibited a notable decline as desertification progressed (Lv and Lu, 2006). The desertification of certain regions within the Zoige alpine meadow has occurred as a result of climate change and anthropogenic disturbances (Li, 2008). After a treatment period exceeding a decade, there has been a reduction in the severity of desertification and a partial restoration of the impaired ecosystem functions (Jiang et al., 2011). Currently, the prevailing research has primarily focused on examining the nutrients of desertified soil and the integrated management framework for desertified grassland (Hu et al., 2012; Xiao et al., 2013).
During the recovery process, higher nutrient uptake generally occurs in the early stages of vegetation recovery, while higher nutrient output generally occurs in the later stages of vegetation recovery (Deng et al., 2019). The diversity and abundance of species may also be lower during the early stages of restoration due to disturbances caused by restoration, but as the restoration period lengthens, the plant community resumes succession, and both diversity and abundance will rise (Zhang et al., 2022). Significant increases in vegetation density, coverage, height, and biomass occurred together with a mutual promotion of soil and vegetation variables (Qian et al., 2014; Li et al., 2016). Restoration time affects vegetation restoration, and vegetation restoration has positive and negative effects on various physical and chemical properties of soil, and soil properties change differently at different restoration times (Sun et al., 2008).
Functional groups are assemblages of organisms that exhibit similar functions in ecosystem processes or display similar responses to environmental conditions. Functional groups can serve as a valuable and responsive approach for examining and forecasting alterations in the recovery of desertification, acting as a connection between ecosystems and physiology (Craine et al., 2001; Kleyer, 2002). Currently, a key area of investigation in the field of grassland ecology pertains to the correlation between grassland degradation and the diversity of grassland species. Additionally, researchers are examining the fluctuating patterns of species composition and functional groups within grassland communities. The intricate interplay between the material composition within a community and soil factors within the environment has long been a central focus in ecological research (Legendre and Fortin, 1989). Plant functional groups exhibit a high degree of adaptability in response to variations in soil factors, which are crucial determinants of plant growth and development (Reynolds et al., 2003; Begon and Townsend, 2006). However, there is a limited understanding of the patterns of plant functional groups and soil nutrition in response to restoration periods in Zoige desertification land.
This study focused on the Zoige desertification land as the subject of investigation. The research methodology employed involves field investigations, with the objective of using recovery time as a means to gain clarity on the subject matter. This study examined 1) the alterations in plant functional groups within Zoige desertification land across recovery time; 2) the alterations in soil physical and chemical properties across recovery time; and 3) the correlation between functional groups and soil physical and chemical factors. The objective is to analyze the dynamic changes in these factors and offer insights for vegetation restoration in Zoige desertification land and the recovery and sustainable utilization of desertified alpine meadow.
2 Materials and methods
2.1 Study site
Zoige alpine meadow is located on the eastern Tibetan Plateau, specifically on the Zoige Plateau, which situated in the northwestern region of Sichuan Province. The geographical coordinates of the area span from a longitude of 101°36’–103°55’E to a latitude of 32°20’–34°05’N. The average elevation ranges from 3400 m to 3900 m. This climate is characterized by prolonged winters and brief summers. The mean annual temperature is recorded at 1.73°C, while the annual precipitation measures 756 mm. Additionally, the average relative humidity throughout the year is approximately 69%, and there are no absolute frost-free periods. The prevailing soil types in the area consist of subalpine meadow soil and mountain meadow soil (Wu et al., 2021; Wang et al., 2023).
The local shepherds planted Salix takasagoalpina Koid on the desertified soil in an attempt to restore the desertified alpine meadow. The plant had a height of 2–3 m and a crown diameter of 1–1.5 m. The plant density was 1 per square meter. The restoration sites were surrounded by the wire fence that prohibited both grazing and human disturbance. No herbal seeds were artificially introduced throughout the restoration process.
2.2 Field survey
In the middle of August 2022, a total of eight sites were established to represent varying recovery time periods, including those less than ten years (3 sites), more than ten years but less than twenty years (3 sites), and more than twenty years (2 sites). (Table 1). Nature carried out the entire restoration process, aside from the initial act of planting the willow. All the study sites commenced under identical conditions. The investigation employed a random selection process to choose sample plots that exhibited flat slopes and maintained consistent habitat conditions within each plot (Figure 1).
In each site, we randomly selected six 5 m × 5 m plots to measure the composition of the shrubs. Additionally, within each 5 m × 5 m plot, one 1 m × 1 m plot is established specifically for herbaceous plants.
The investigation encompassed a period spanning from July 30 to August 15, 2022, during which an examination was undertaken to assess the attributes of vegetation and community dynamics. Throughout the investigation, data pertaining to the various types of species, their respective densities, and the extent of their coverage were meticulously documented. To identify unfamiliar plant species, it is recommended to collect specimens and subsequently transport them to a laboratory for identification purposes. The determination of density and coverage measurements in the sample plots was conducted using the visual estimate (VE) method (Bråkenhielm and Qinghong, 1995). Plant height was assessed by employing a ruler (Heady, 1957). The aerial portion of the herbaceous was severed using scissors, carefully enclosed within envelope bags, and subsequently transported to the laboratory. The aboveground biomass of a 1 m × 1 m herb sample was dried to constant a weight in an oven at 65°C. Biomass dry weight was obtained by weighing with a precision electronic balance of 0.001 g.
Simultaneously, soil samples were obtained within the depth range of 0 to 20 cm using a soil auger with a diameter of 10 cm. A single soil sample was obtained from each standard plot using the following prescribed procedure: a 5 m × 5 m quadrat was employed, within which a five-point sampling technique was utilized. This involved collecting five soil samples from the topsoil layer (0–20 cm) at specific locations, namely the four corners and the center of the quadrat, by means of a soil auger (Wang et al., 2021). The soil samples, which were of approximately equal mass, were carefully positioned in a plastic bag. Any extraneous materials, such as stones and plant roots, were eliminated from the samples. Approximately 500 g of soil samples were retained for packaging purposes. Simultaneously, undisturbed soil samples were obtained from the 0–20 cm layer using a ring knife and subsequently transported to the laboratory for the purpose of measuring soil porosity. Following the process of observation and sampling, the soil was subsequently reinstated in accordance with its initial stratification to mitigate any potential disruptions caused by human activities. A comprehensive set of 48 soil samples was gathered from the alpine meadows.
2.3 Determination of soil properties
The soil physical and chemical properties assessed in this study encompassed soil pH, total nitrogen (TN), total carbon (TC), total potassium (TK), available potassium (AK), available nitrogen (AN), available phosphorus (AP), carbon-to-nitrogen ratio (C/N), and nitrogen-to-phosphorus ratio (N/P). The soil pH was assessed using a Soil All-in-One Soil Meter, while the determination of TN and TC was conducted through the utilization of an elemental analyzer. The measurement of TP was accomplished using the sulfuric acid-perchloric acid digestion method. The determination of soil AN was carried out using the alkali hydrolysis diffusion method (Mulvaney and Khan, 2001). The AP content was measured employing the sodium bicarbonate extraction–molybdenum antimony colorimetric method (Holliday and Gartner, 2007). Lastly, the available potassium was determined through NH4OAC extraction and flame photometry.
2.4 Data analysis
Based on how alpine meadow plants were used in the study area, four different functional groups were found. These groups were called grass, sedges, legumes, and forbs. The calculation of the importance value of herbaceous plants is determined by the following formula: The formula for calculating the importance value (IV) is derived from three variables: the relative coverage (RC), the relative height (RH), and the relative abundance (RA). The RC is determined by dividing the coverage rate of a specific species by the coverage rate of all species and then multiplying the result by 100%. Similarly, the RH is obtained by dividing the height of a particular species by the height of all species and multiplying the outcome by 100%. Lastly, the RA is computed by dividing the number of individuals in a given species by the sum of the number of individuals in all species, and then multiplying the quotient by 100%. After that, the IV is determined by adding the RC, RH, and RA, then dividing the total by 3 (Zuo et al., 2010).
The statistical analyses were conducted using the R software (R Core Team, 2022). The R software can be accessed at http://www.R-project.org/.
Before data analysis, the normality and homogeneity of the variance of the data were checked. For data that conformed to the normal distribution, the data was analyzed using generalized linear mixed models (GLMMs). The fixed effects in the study encompassed different recovery times, while the plot was considered a random effect. The soil physical and chemical properties, soil carbon to nitrogen ratio, and soil nitrogen to phosphorus ratio were modeled using a Gaussian model with an identity-link function. Next, we used a Gamma model (with a Logit-link) and the Laplace approximation to estimate parameters for a number of factors, such as the soil’s TN, TC, TP, AN, AK, AP, carbon-to-nitrogen ratio, nitrogen-to-phosphorus ratio, bulk density, and porosity. The parameters were estimated using the Laplace approximation method, specifically utilizing the glm.nb function in the ‘lme4’ package. Following the identification of a notable impact on the different recovery times, subsequent post-hoc LSD tests were employed to conduct pairwise comparisons between the different recovery times. The lares package in R was used to analyze soil environmental indicators, community characteristics, community stability, and functional group diversity, respectively (Bates, 2007).
3 Results
3.1 Effects of recovery time on the importance value of herbaceous functional groups
The Shannon-Wiener index, Simpson index, Pielou evenness index, and Margalef richness index did not show any statistically significant differences when comparing functional groupings of herbaceous plants (Table 1S).
The relative significance of importance values differed across distinct functional groups during different stages of recovery (Figure 2). The important value of the grass exhibited a higher level of significance compared to forbs, legumes, and sedges during time intervals of less than a decade. Over time periods of more than 20 years and within time periods ranging from 10 to 20 years, the values of forbs outweighed those of grass, legumes, and sedges. Overall, the essential value of herbs increased by 19.7% over a period ranging from less than ten years to more than twenty years. Conversely, the important value of grass declined by 17.45%, the important value of legumes decreased by 25.8%, and the important value of sedges decreased by 22.5%.
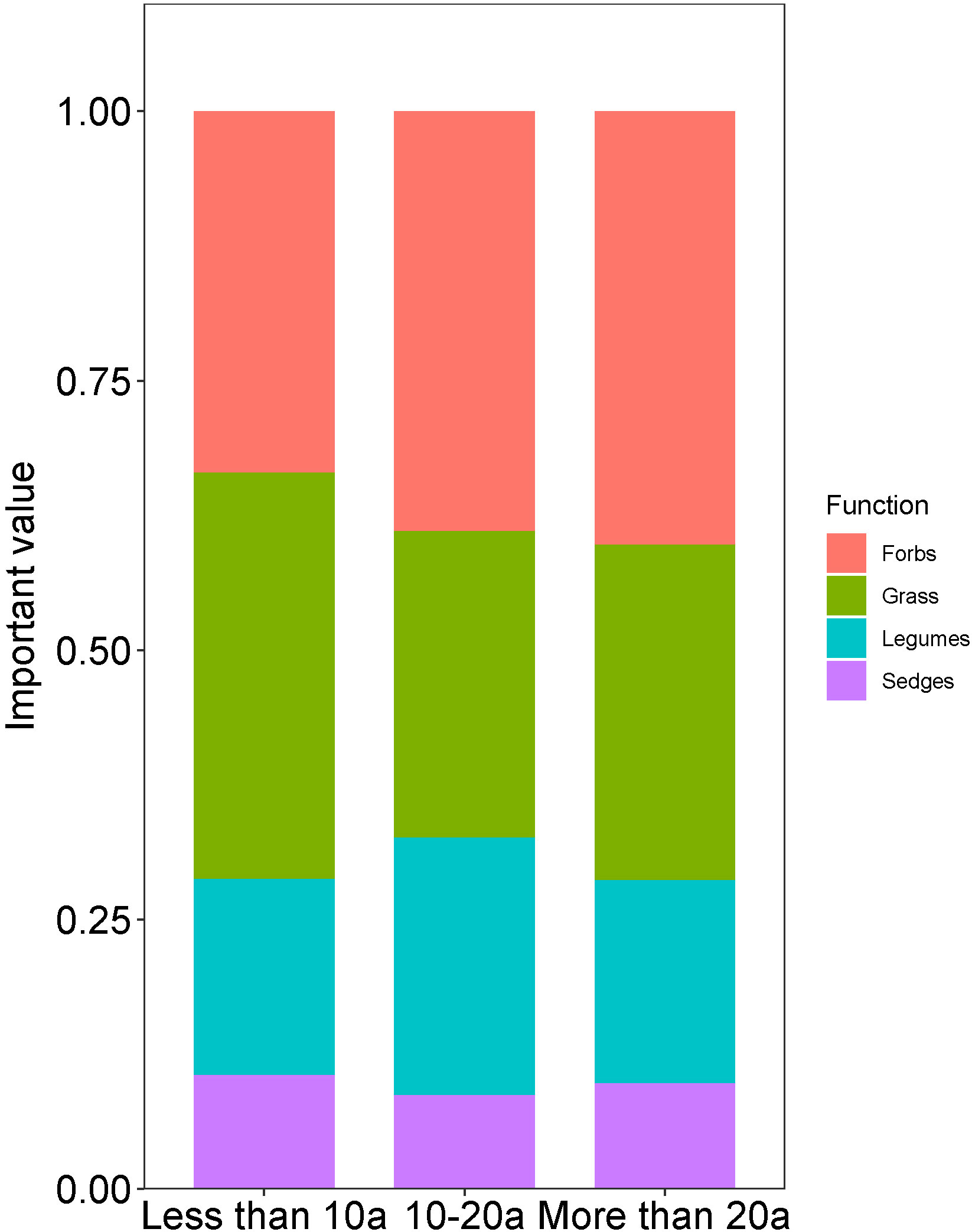
Figure 2 Comparison of the important values of plant functional groups with different recovery times. The abscissa represents important values, and the ordinate represents the recovery years.
3.2 Effects of recovery time on soil physicochemical properties
The duration of recovery had a significant impact on various soil parameters, including TN, TC, TP, AN, AP, and AK (Table S2). The levels of soil TN, AN, and AK exhibited a significant increase as the recovery time progressed (Figure 3). Specifically, the recovery time exceeding 20 years demonstrated the highest increase of TN, AN, and AK, followed by the recovery time ranging from 10 to 20 years, and finally, the recovery time less than 10 years exhibited the lowest increase of TN, AN, and AK. There were significant variations in the TN levels observed across different recovery periods. The soil TC displayed a pattern that included initial high levels during the recovery phase, a decline in the middle stage, and a notable increase in the later stage. TC in the plots with a recovery period exceeding 20 years exhibited a statistically significant increase compared to the plots with a recovery period ranging from 10 to 20 years. TP was found to be significantly higher in areas with a recovery time from 10 to 20 years compared to areas with a recovery time of less than 10 years. Conversely, AP in soil with a recovery time of less than 10 years and more than 20 years was significantly lower than that in soil with a recovery time ranging from 10 to 20 years.
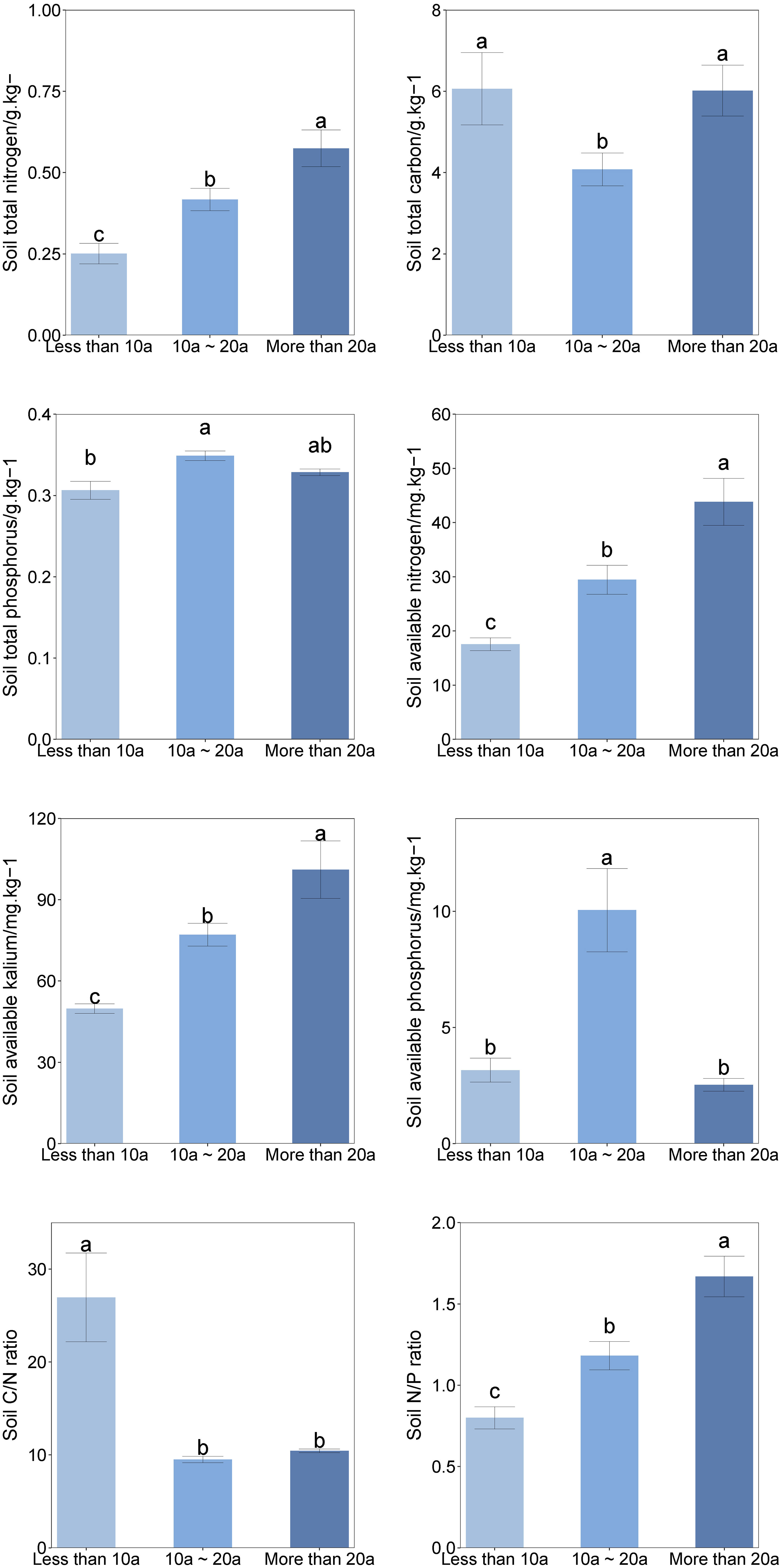
Figure 3 Comparison of soil physical and chemical properties at different recovery times. Ends of the boxes represent the 25th and 75th percentiles of the data, while ends of whiskers represent the minimum and maximum of the data. Line within the box represents the median of the responses and green circle within the box represent the mean of the responses. Different letters above the boxes denote significant differences among treatments (P < 0.05).
The observed correlation between an extended duration of recovery and a decline in the C/N ratio in soil was noted (Figure 4). There was a positive correlation observed between the N/P ratio in soil and recovery times, with statistically significant variations observed among different recovery times.
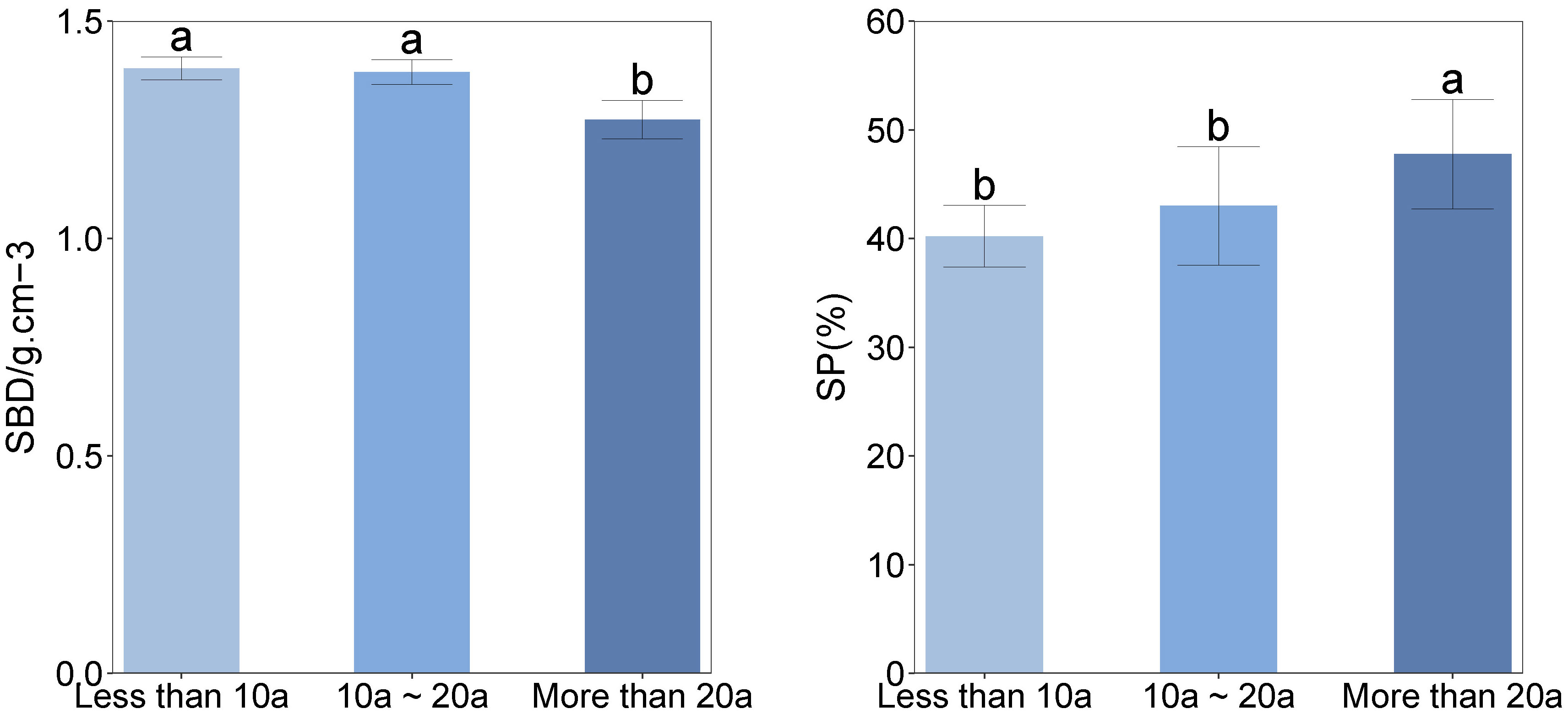
Figure 4 Soil carbon–nitrogen ratio and nitrogen–phosphorus ratio vary with different recovery times. SBD, Soil bulk density; SP, Soil porosity. Different lower-case letters above the boxes denote significance among treatments (P<0.05).
3.3 Correlation analysis between plant functional groups and soil factors at different recovery times
There was a significant negative correlation between herbaceous biomass and soil AN as well as TN when the recovery time was less than 10 years. Additionally, the important value of legumes was positively associated with soil AN (Figure 5). The important value of grass exhibited a negative correlation with soil AK when the duration of recovery ranged from 10 years to 20 years (Figure 6). There was a strong link between the important value of sedges and the levels of soil TC, soil TP, and soil AK when the recovery period was longer than 20 years (Figure 7). Conversely, the important value of forbs exhibited a negative correlation with soil AK and soil TC. Additionally, a significant positive correlation was found between the important value of legumes and the AK of soil (Figure 7).
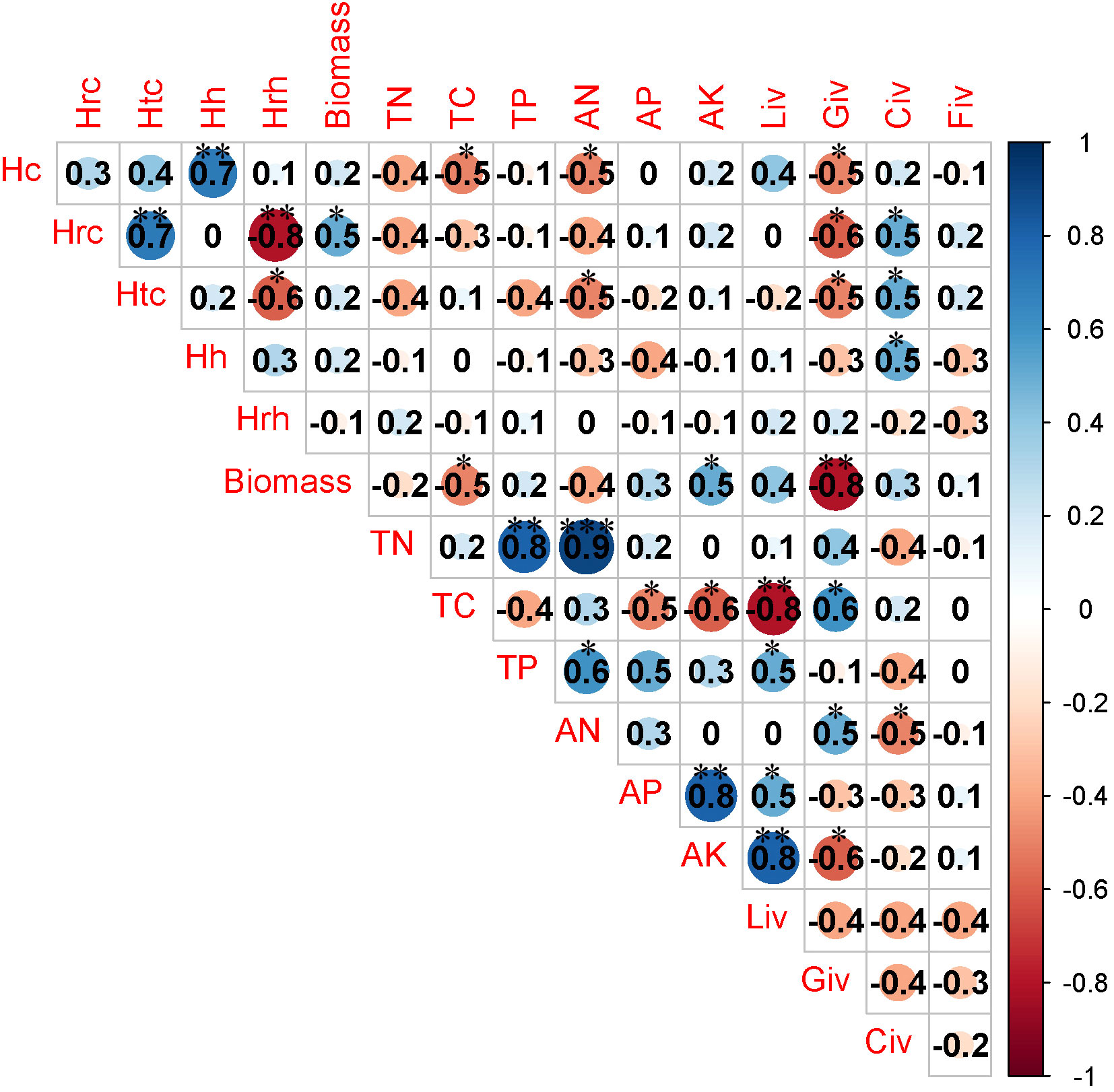
Figure 5 Correlation analysis between community traits and soil properties when recovery time is less than 10 years. TN, soil total nitrogen; TC, soil total carbon; TP, soil total phosphorus; AN, soil available nitrogen; AK, soil available potassium; AP, soil available phosphorus; Liv, important value of legumes; biomass, above ground biomass; Civ, important value of sedges; Fiv, important value of forbs; Giv, important value of grass; hrh, bush height; hh, herb height; hrc, Shrub cover; htc, total community cover; hhc, herb coverage. * P<0.05; ** P<0.01; *** P<0.001.
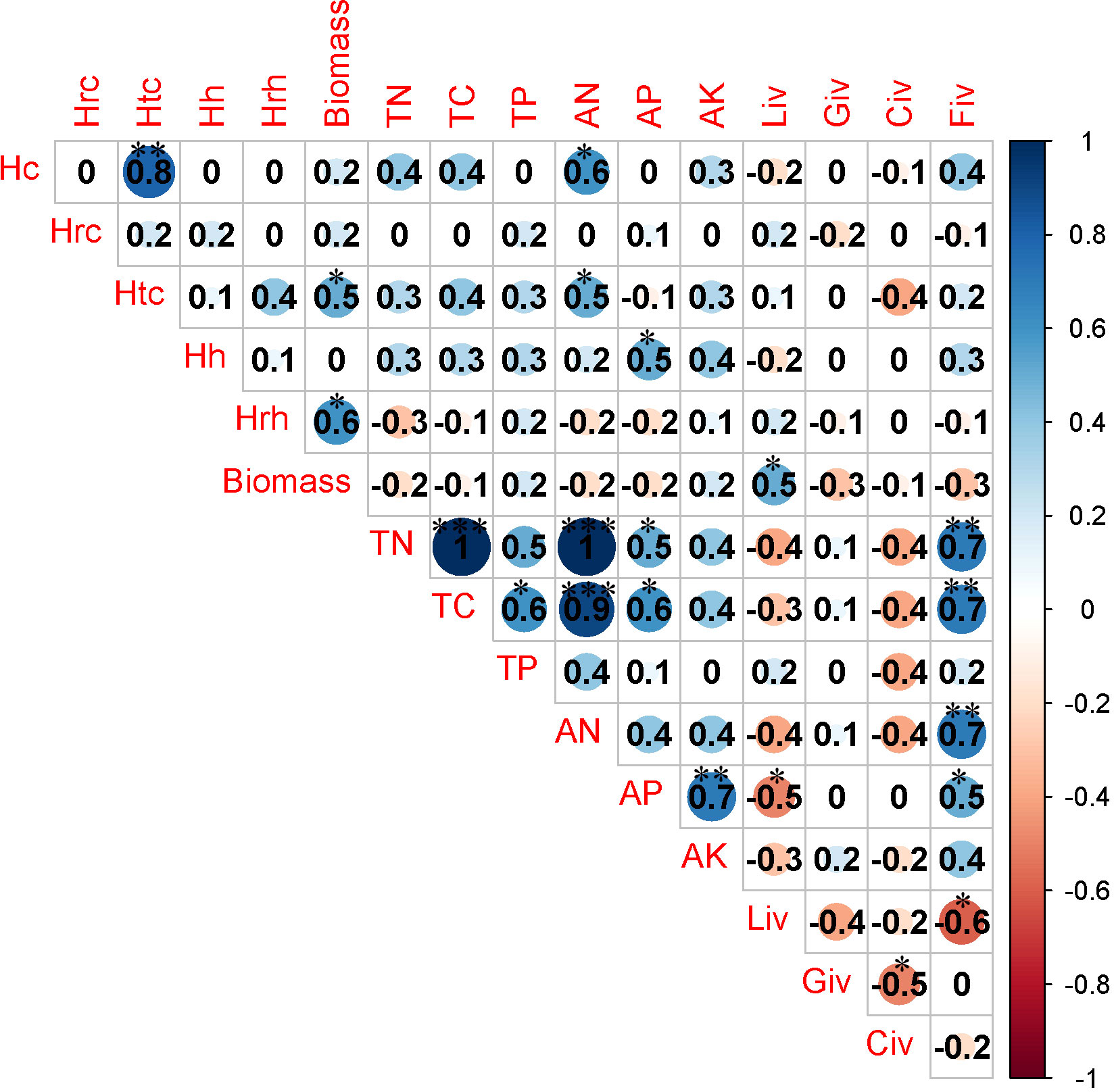
Figure 6 Correlation analysis between community traits and soil properties during recovery time between 10 and 20 years. * P<0.05; ** P<0.01; *** P<0.001.
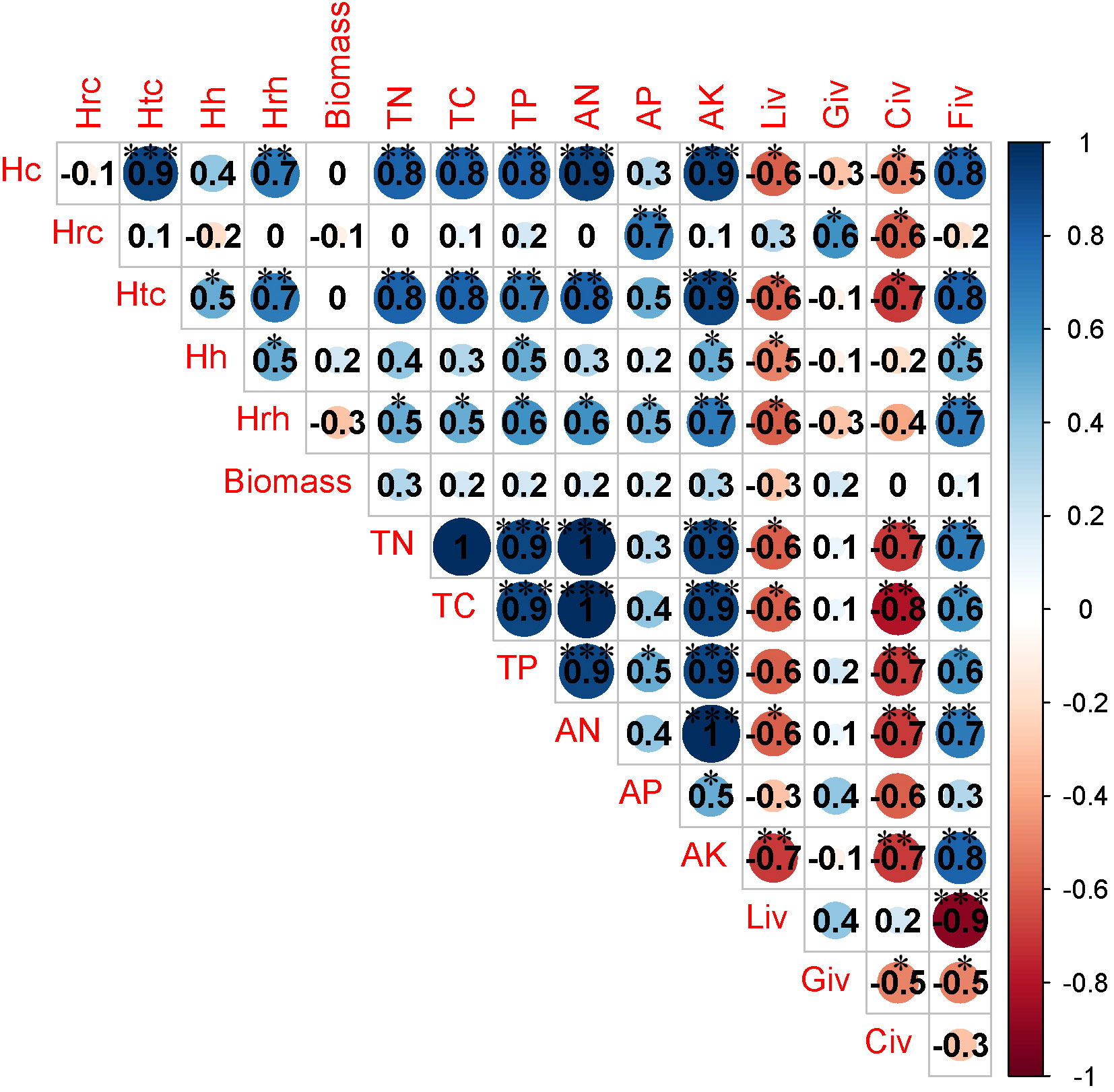
Figure 7 Correlation analysis between community traits and soil properties when recovery time is greater than 20 years. * P<0.05; ** P<0.01; *** P<0.001.
When the duration of recovery was less than 10 years, a notable and statistically significant positive relationship was observed between the availability of nitrogen in the soil and the coverage of herbaceous vegetation (Figure 5). A significant negative correlation was observed between herb height and the levels of AP and AK in the soil when the recovery time ranged from 10 years to 20 years (Figure 6). There was a significant negative correlation observed between the levels of AN and TC in the soil, as well as the coverage of herbaceous plants and community coverage (Figure 6). When the duration of recovery exceeded 20 years, there was a significant negative correlation between soil AK potassium and the coverage of herbaceous plants, as well as the overall coverage of the entire plant community and the height of shrubs (Figure 7). Additionally, there was a significant negative correlation between the availability of phosphorus in the soil and the coverage of shrubs (Figure 7). Furthermore, the TC content in the soil exhibited a significant negative correlation with the coverage of herbaceous plants and the overall coverage of the entire plant community (Figure 7). The data analysis revealed a substantial inverse relationship in coverage (Figure 7).
4 Discussion
Our findings revealed that during the process of rehabilitating desertified alpine meadows, there was a significant augmentation in soil TN, soil AN, soil AK, soil N/P ratio, and forbs. In contrast, a reduction was observed in the soil C/N ratio, soil bulk density, and pH value. The physical and chemical properties of the soil were found to have a strong positive relationship with the length of time it took to restore the area. This was especially true for herbaceous coverage, total community coverage, herbaceous biomass, and shrub coverage. Furthermore, there was a notable inverse relationship between the important value of legumes and the concentrations of AN and AK within the soil. In contrast, there was a noteworthy positive association between the important value of forbs and both TC and AK, highlighting its significance. Conversely, there was a notable inverse relationship observed between the important value of the sedges and the levels of TC and TP. Our findings would shed new light on the restoration in the Zoige desertified alpine meadows.
4.1 Influence of restoration time on plant functional groups
The restoration of degraded ecosystems is frequently evaluated based on the recovery of plant functional groups, which is widely recognized as a significant indicator (Winikoff et al., 2020). The analysis of functional groups encompasses not only the delineation of species classification and the challenges associated with species identification but also takes into account variations in species traits (Mermillod-Blondin et al., 2002). Furthermore, this analysis can be extended to encompass a more elevated level of organizational hierarchy, enabling the comparison of plant functions across a diverse array of plant taxa (Vamosi et al., 2009). As a result, it possesses an enhanced comprehension and prognostic framework pertaining to the performance of plants across varying durations of recuperation (Zhao et al., 2016). The present study employed a selection process to identify desertified alpine meadow areas in the Tibet meadows that had undergone varying durations of recovery. The primary objective was to investigate the temporal fluctuations in plant functional groups within these selected sites. The varying recovery times of functional groups had an impact on the community structure (Mu et al., 2020). So, learning about how rules about different types of plant functional groups during different recovery periods gives us a scientific reason for restoring sandy landscapes.
As the years of restoration increased, the dominant plant species within the community changed significantly (Figure 2). Overall, the importance of forbs increased, while that of grasses decreased. In addition, the importance value of legumes first increases and then decreases, and the importance value of sedges first decreases and then increases. Different functional group species exhibit notable variations in environmental adaptability, which can be the cause of the observed phenomenon (Loreau et al., 2001). Consequently, grasslands with varying recovery periods experience alterations in the abundance of functional group species as they strive to acclimate to specific survival conditions (Chapin et al., 1997; Mori et al., 2013). During the initial restoration phase of desertified alpine meadows, the dominant species are grass plants, while legumes facilitate the growth of sedges through the process of biological nitrogen fixation (Holzmann and Haselwandter, 1988). This, in turn, intensifies the competition for resources among grass plants. Nevertheless, as the duration of recovery time lengthens, the three entities will engage in a competitive struggle for essential nutrients derived from the soil, consequently impeding their individual growth (Qi et al., 2011). Throughout the process of desertified alpine meadows restoration, the significant value of forbs exhibited a continuous increase. The reason for the prevalence of forbs in desertified alpine meadows can be attributed to their adaptation to the arid conditions of the study area (Qi et al., 2011). The restricted availability of soil nutrients further exacerbates these conditions, which restrict plant growth. Forbs has exhibited robust stress resilience and adeptness in resource allocation (Craine et al., 2001). Consequently, forbs gradually expand their ecological niche during community succession, surpassing other functional groups in terms of competitiveness (Qi et al., 2011). The plasticity alterations observed in plant functional groups provide an objective measure of plants’ ability to adapt to varying recovery durations (Meng et al., 2007). Different ecological phenomena are linked to different combinations of functional groups, which has led to the development of different ecological strategies during the time progression of desertified meadow restoration. We found a notable pattern in the evenness metric, which initially increases and subsequently decreases as recovery time progresses (Table 1S). This suggests that as the number of recovery years increases, the distribution of functional groups becomes more evenly distributed, resulting in a reduction in landscape heterogeneity (Hu et al., 2019). With the exception of the Pielou evenness index, all other diversity indexes pertaining to plant functional groups exhibited a general upward trend as restoration time progressed (Table 1S). This phenomenon may be a sign that herbaceous communities are moving gradually towards a relatively stable stage with comparable levels of species richness and complexity. Consequently, the desertified alpine meadows ecosystem has progressively attained a more stable ecological impact (Hao et al., 2023).
4.2 Influence of recovery time on soil properties
The principal indicators of soil nutrients encompass nitrogen, phosphorus, potassium, and carbon (She et al., 2021). These nutrients are essential for supplying the requisite elements for the growth and development of vegetation (Ulrich, 1952). As a result, they exert a substantial influence on the composition and dynamics of plant communities (Huang et al., 2021). Prior studies have established that soil is a relatively stable component in alpine meadow ecosystems. Changes in soil composition often begin with alterations in its physical structure, which subsequently have a substantial impact on its chemical properties (Van der Putten et al., 2013; Li et al., 2020). Variations in soil properties resulting from different recovery durations exert an influence on the community structure (Auslander et al., 2003; Gong et al., 2008). The assessment of soil quality in grassland ecosystems is heavily influenced by soil factors (Nortcliff, 2002), which serve as fundamental determinants of sustainability. Therefore, gaining an understanding of the changes in plant functional groups during different stages of recovery provides a scientific basis for the strategic management and utilization of grasslands.
A positive correlation between recovery time and the levels of soil TN, AN, and AK (Figure 3). This relationship can be attributed to the restoration efforts in sandy areas of alpine meadows, which have led to an increase in vegetation cover and a decrease in bare areas. Consequently, the reduced exposure of soil surfaces to weathering and rain erosion has resulted in the retention of nutrients within the soil (Wei and Li, 2012; Yang et al., 2020). In contrast, the restoration of desertified alpine meadow resulted in an increase in the quantity of subterranean plant roots. Additionally, there was a gradual increase in the presence of litter and humus in the soil, as well as an increase in the number of microorganisms and soil enzyme activity. These factors collectively contributed to the enhancement of soil nutrition supply capacity and an overall improvement in soil quality (Li et al., 2018). The levels of TP and AP in the soil showed an initial increase and a subsequent decrease (Figure 3). The observed increase prior to the restoration procedure can be attributable to the interplay between soil TN and AN, as well as soil TP and AP. The prolonged duration of recovery negatively impacts the ability of soil TN and AN to facilitate the uptake of soil TP and AP (Vitousek et al., 2010). The soil’s TC content within 10 to 20 year recovery period was found to be lower compared to that during the recovery period of less than 10 years. This observation can potentially be attributed to the favorable conditions provided by moderately alkaline soil for the nitrification process carried out by nitrifying bacteria (Li et al., 2010; Yao et al., 2011). Consequently, this promotes the utilization of nitrogen by plants in the soil. Furthermore, as the recovery time continues to increase, there is an observed increase in soil clay content and a decrease in soil roughness. The soil TC exhibited a positive correlation with the increase in clay content. This relationship can be attributed to the enhanced carbon sequestration capacity of soils with finer textures, such as clay (Ingram and Fernandes, 2001; Wiesmeier et al., 2014). The results indicate a declining trend in the soil carbon-to-nitrogen ratio, while conversely, an increasing trend was observed in the nitrogen-to-phosphorus ratio. The decline in the ratio of carbon to nitrogen in soil commenced subsequent to a recovery period exceeding ten years. This phenomenon can be attributed to the more rapid reduction in soil carbon compared to nitrogen, suggesting an acceleration in mineralization and nitrogen release (Shen et al., 2012). This acceleration is advantageous for facilitating nutrient absorption by plants.
4.3 Relationship between soil factors and plant functional groups at different recovery ages
Natural elements like vegetation, soil composition, precipitation, and temperature primarily affect the alteration of desertified alpine meadows. Additionally, climate and soil nutrient levels are closely associated with plant diversity and the distribution of plant functional groups, thereby exerting a direct influence on patterns of biodiversity (Bremer et al., 2007). The enhancement of the sandy soil environment has a direct impact on the process of vegetation succession. The accumulation of nutrients plays a crucial role in influencing vegetation coverage, plant functional group composition, and plant diversity (Zhang et al., 2022). The relationship between plant diversity and soil characteristics, such as physical and chemical properties, is significant. Additionally, the nutrient composition of soil plays a direct role in determining plant productivity (Wu et al., 2013). The assessment of soil fertility often relies on the examination of soil nutrient content, which serves as a key indicator (Hu et al., 2020). The fluctuations in soil nutrient content exhibit a strong correlation with vegetation growth.
When the recovery time was less than 10 year, the correlation analysis showed that there was a positive relationship between soil AN and herbaceous biomass but a negative relationship between soil AN and the importance value of legumes (Figure 5). Additionally, a significant positive correlation was found between herbaceous coverage and soil AN (Figure 5). This phenomenon can be attributed to the rise in soil nitrogen availability, which effectively mitigates the nitrogen constraint on vegetation, stimulates plant growth, and enhances plant biomass (Luo et al., 2004; Chen et al., 2022). In conditions where there was a significant amount of nitrogen available in the soil, legumes had a tendency to enhance the absorption of nitrogen by their roots. However, this increased nitrogen uptake comes at the expense of suppressing the development of root nodules and the process of biological nitrogen fixation, which requires a substantial amount of energy (Nacry et al., 2013; Schwember et al., 2019). This suggests that the rise in soil nitrogen availability is associated with a widespread occurrence of reduced importance value in legumes.
In the period of 10 to 20 years of recovery time, there was a notable positive correlation between the overall coverage of the community and the levels of AN and TN (Figure 6). The fact that plants produce more nitrogen-containing substances as they recover can help to explain this connection. This leads to more microbial and enzyme activity in both plants and soil (Zhu et al., 2021). Consequently, this promotes the cycling of soil nutrients, resulting in an increased production of nitrogen (An et al., 2021). There was a notable positive correlation between the height of shrubs and the presence of AN and phosphorus (Figure 6). This can be explained by the fact that shrubs grow slowly, which allows their root systems to grow and improves their ability to absorb nutrients in the rhizosphere (Raynaud et al., 2008; Hao et al., 2023).
There was a clear and statistically significant positive relationship between the amount of herbaceous vegetation, the amount of the ecological community as a whole, and the TC content when recovery took more than 20 years (Figure 7). This finding suggests that as the duration of recovery increases, there is a gradual improvement in the progression of vegetation community succession in a positive direction (Zhu et al., 2010). Additionally, the physical and chemical properties of the soil gradually improve, which promotes the process of carbon sequestration in degraded grassland soil (Zhu et al., 2010; Xu et al., 2017). There is a significant positive correlation between herbaceous coverage, total community coverage, and shrub height with AK and phosphorus (Figure 7). This association may be attributed to the overall augmentation of plant coverage in desertified alpine meadow, resulting in reduced susceptibility to wind erosion and enhanced preservation of soil nutrients (Li et al., 2009). The AK exhibited a significant negative correlation with the importance value of sedges and legumes, while it showed a significant positive correlation with the importance value of forbs (Figure 7). Additionally, the TC demonstrated a significant positive correlation with the importance value of forbs. On the other hand, both TC and TP displayed a significant negative correlation with the importance value of sedges (Figure 7). This means that raising the TC and AK levels in the soil can make forb species more important in the community of desertified alpine meadows. However, it is observed that TC, AK, and TP do not promote an increase in the importance value of sedges. To figure out the underlying mechanism, more research needs to be done, but it is thought that the regulation of soil nutrition status has an effect on how different species in a functional group compete with each other.
5 Conclusion
Overall, the length of the recovery time had a big effect on both the characteristics of the plant community and the soil properties in Zoige desertification land. During different stages of healing, the soil’s properties changed in noticeable ways. The correlation study showed that there were strong connections between some soil factors and plant functional groups. Our study gives a scientific explanation for the problem in Zoige desertification land and a basis for how to solve it. This study exclusively examines the impact of varying healing durations on plant community characteristics and soil properties, while also investigating the interrelationships between soil properties and functional groups. Our study demonstrate that desertified alpine meadows are achieved through the implementation of additional vegetation and modifications to soil dynamics. The phenomenon being referred to is commonly known as a reciprocal feedback process, which results in the amplification of certain effects. Further investigation is required to ascertain the impact of alterations in soil properties on the composition of functional groups within plant communities. Our findings would shed new light on the restoration in the Zoige desertified alpine meadows.
Data availability statement
The original contributions presented in the study are included in the article/Supplementary Materials. Further inquiries can be directed to the corresponding author.
Author contributions
RW: Investigation, Writing – original draft, Writing – review & editing. YL: Investigation, Writing – review & editing. LH: Investigation, Writing – review & editing. JC: Investigation, Writing – review & editing. YC: Investigation, Writing – review & editing. WY: Investigation, Writing – review & editing. DD: Funding acquisition, Writing – review & editing. JM: Investigation, Writing – review & editing.
Funding
The author(s) declare financial support was received for the research, authorship, and/or publication of this article. This study was funded by the National Natural Science Foundation of China (32371604), by the science and technology innovation project of the Sichuan Forestry and Grassland Bureau (510201202109731), and by the project of Zoige National Park (HX2022097).
Acknowledgments
We thank Rui Gao, Mengying He, Rui Zhao, Jiacheng Yu, Zhu Xin for the field and lab assistance.
Conflict of interest
The authors declare that the research was conducted in the absence of any commercial or financial relationships that could be construed as a potential conflict of interest.
Publisher’s note
All claims expressed in this article are solely those of the authors and do not necessarily represent those of their affiliated organizations, or those of the publisher, the editors and the reviewers. Any product that may be evaluated in this article, or claim that may be made by its manufacturer, is not guaranteed or endorsed by the publisher.
Supplementary material
The Supplementary Material for this article can be found online at: https://www.frontiersin.org/articles/10.3389/fevo.2023.1331618/full#supplementary-material
References
An Y., Gao Y., Liu X., Tong S., Liu B., Song T., et al. (2021). Soil organic carbon and nitrogen variations with vegetation succession in passively restored freshwater wetlands. Wetlands 41, 1–10. doi: 10.1007/s13157-021-01413-w
Auslander M., Nevo E., Inbar M. (2003). The effects of slope orientation on plant growth, developmental instability and susceptibility to herbivores. J. Arid. Environ. 55 (3), 405–416. doi: 10.1016/S0140-1963(02)00281-1
Bates D. (2007) Linear mixed-effects models using S4 classes. Available at: http://cran.r-project.org/src/contrib/Descriptions/lme4.html.
Begon M., Townsend C. R. (2006). Ecology: from individuals to ecosystems. John Wiley Sons., 661–663. doi: 10.1111/j.1365-2427.2006.01592.x
Bråkenhielm S., Qinghong L. (1995). Comparison of field methods in vegetation monitoring. Water Air Soil Pollut. 79, 75–87. doi: 10.1007/BF01100431
Bremer C., Braker G., Matthies D., Reuter A., Engels C., Conrad R. (2007). Impact of plant functional group, plant species, and sampling time on the composition of nirK-type denitrifier communities in soil. Appl. Environ. Microbiol. 73 (21), 6876–6884. doi: 10.1128/AEM.01536-07
Chapin III, F. S., Walker B. H., Hobbs R. J., Hooper D. U., Lawton J. H., Sala O. E., et al. (1997). Biotic control over the functioning of ecosystems. Science 277 (5325), 500–504. doi: 10.1126/science.277.5325.500
Chen H., Ju P., Zhu Q., Xu X., Wu N., Gao Y., et al. (2022). Carbon and nitrogen cycling on the Qinghai–Tibetan Plateau. Nat. Rev. Earth. Environ. 3 (10), 701–716. doi: 10.1038/s43017-022-00344-2
Craine J. M., Froehle J., Tilman D. G., Wedin D. A., Chapin III, F. S. (2001). The relationships among root and leaf traits of 76 grassland species and relative abundance along fertility and disturbance gradients. Oikos 93 (2), 274–285. doi: 10.1034/j.1600-0706.2001.930210.x
Deng L., Peng C., Huang C., Wang K., Liu Q., Liu Y., et al. (2019). Drivers of soil microbial metabolic limitation changes along a vegetation restoration gradient on the Loess Plateau, China. Geoderma 353, 188–200. doi: 10.1016/j.geoderma.2019.06.037
Gong X., Brueck H., Giese K. M., Zhang L., Sattelmacher B., Lin S. (2008). Slope aspect has effects on productivity and species composition of hilly grassland in the Xilin River Basin, Inner Mongolia, China. J. Arid. Environ. 72 (4), 483–493. doi: 10.1016/j.jaridenv.2007.07.001
Hao J. H., Xiao G., Ming C., Jun S. L. (20232023). Study on the characteristics of plant communities in different ecological restoration stages of Hulun Buir sandy grassland (in Chines). J. Environ. Eng. Techn. 13, 1–13. doi: 10.12153/j.issn.1674-991X.20220877
Heady H. F. (1957). The measurement and value of plant height in the study of herbaceous vegetation. Ecology 38 (2), 313–320. doi: 10.2307/1931691
Hill K. J. (2011). Research on preventing and remediating the dust storms of China: A case study investigating the development of salt water agriculture. OSU, 4–6.
Holliday V. T., Gartner W. G. (2007). Methods of soil P analysis in archaeology. J. Archaeol. Sci. 34 (2), 301–333. doi: 10.1016/j.jas.2006.05.004
Holzmann H. P., Haselwandter K. (1988). Contribution of nitrogen fixation to nitrogen nutrition in an alpine sedge community (Caricetum curvulae). Oecologia 76, 298–302. doi: 10.1007/BF00379967
Hu L. T., Mao Y., Hu J. Y., Da W. L. M. (2019). Characteristics of plant community changes during the restoration of alpine desertified grassland in northwest Sichuan (in Chinese). J.Sichuan. For. Scuence. Techn. 40 (50), 11–16. doi: 10.16779/j.cnki.1003-5508.2019.05.003
Hu Q. W., Yao B., Zhen L., Li X. F., Ding M. J., Cao J. (2012). Characteristic of soil nutrients and direction of vegetation restoration on sandy hills area of Poyang Lake (in Chinese). Chin. J. Soil. Sci. 43 (3), 651–655. doi: 10.19336/j.cnki.trtb.2012.03.024
Hu J. J., Zhou Q. P., Lu Y. H., Lü Y. H., Hu J., Chen Y. J., et al. (2020). Comparison study to the effectiveness of typical ecological restoration measures in semi-humid sandy land in eastern Qinghai-Tibetan Plateau, China. Sheng Tai Xue Bao. 40 (20), 7410–7418. doi: 10.5846/stxb201910102111
Huang K., Kardol P., Yan X., Luo X., Guo H. (2021). Plant–soil biota interactions explain shifts in plant community composition under global change. Funct. Ecol. 35 (12), 2778–2788. doi: 10.1111/1365-2435.13940
Ingram J. S. I., Fernandes E. C. M. (2001). Managing carbon sequestration in soils: concepts and terminology. Agric. Ecosyst. Environ. 87 (1), 111–117. doi: 10.1016/S0167-8809(01)00145-1
Jia W., Zuo L., Cai Y., Deng D. Z., Yan W. X. (2013). An analysis of desertification status and its preventive strategies in Ruoergai County. J. Sichuan For. Sci.Techn. 34 (4), 42–46.
Jiang G., Liu M., Niu S., Li Y., Peng Y., Li G., et al. (2011). Ten-year-period demonstration project in Hunshandake Sandland and prospect for the future development of eco-stock farming industry. Sci. Technol. Rev. 29 (25), 19–25. doi: 10.3981/j.issn.1000-7857.2011.25.002
Kleyer M. (2002). Validation of plant functional types across two contrasting landscapes. J.Veg. Sci. 13 (2), 167–178. doi: 10.1111/j.1654-1103.2002.tb02036.x
Legendre P., Fortin M. J. (19891989). Spatial pattern and ecological analysis. Vegetatio 80, 107–138. doi: 10.1007/BF00048036
Li B. (2008). Driving factors of Zoige wetland desertification and countermeasures (in Chinese). Chin. Popul. Res. Environ. 18, 145–149. doi: 10.3969/j.issn.1002-2104.2008.02.028
Li Y., Cui J., Zhang T., Okuro T., Drake S. (2009). Effectiveness of sand-fixing measures on desert land restoration in Kerqin Sandy Land, northern China. Ecol. Eng. 35 (1), 118–127. doi: 10.1016/j.ecoleng.2008.09.013
Li Z. F., Gao J. X., Wang Y. P. (2016). Changes of soil physical and chemical properties with the development of artificial planted and natural invaded vegetation in southern hulunbeir sandy land. J. Nat. Res. 31 (10), 1739–1751. doi: 10.11849/zrzyxb.20151207
Li G., Li X., Chen W., Li J., Zhu H., Hu X., et al. (2020). Effects of degradation severity on the physical, chemical and mechanical properties of topsoil in alpine meadow on the Qinghai-Tibet Plateau, west China. Catena 187, 104370. doi: 10.1016/j.catena.2019.104370
Li C. J., Ma J., Li Y., Fan L. L. (2010). Effects of pH value on the morphological features and activity of roots of three life-form plant species. Arid Zone Res. 27 (06), 915–920. doi: 10.3724/SP.J.1077.2010.01263
Li J., Tong X., Awasthi M. K., Wu F., Ha S., Ma J., et al. (2018). Dynamics of soil microbial biomass and enzyme activities along a chronosequence of desertified land revegetation. Ecol. Eng. 111, 22–30. doi: 10.1016/j.ecoleng.2017.11.006
Li W., Zhou X. (1998). Ecosystem and optimal utilized pattern of Qinghai-Tibet Plateau (In Chinese). Guangdong Sci. Technol. Press Guangzhou China, 11–15. doi: 10.3724/SP.J.1077.2010.01263
Liu F., Liu F. G., Zhou Q., Chen Q., Wang S. Z., Guo R., et al. (2021). Ecological risk and regional differentiation in the Qinghai-Tibet Plateau. J. Nat. Resour. 36, 3232–3246. doi: 10.31497/zrzyxb.20211216
Loreau M., Naeem S., Inchausti P., Bengtsson J., Grime J. P., Hector A., et al. (2001). Biodiversity and ecosystem functioning: current knowledge and future challenges. Science 294 (5543), 804–808. doi: 10.1126/science.1064088
Luo Y., Su B. O., Currie W. S., Dukes J. S., Finzi A., Hartwig U., et al. (2004). Progressive nitrogen limitation of ecosystem responses to rising atmospheric carbon dioxide. Bioscience 54 (8), 731–739. doi: 10.1641/0006-3568(2004)054[0731:PNLOER]2.0.CO;2
Lv S., Lu X. (2006). Studies on the bio-diversity in the wind eroded and desertified vegetation of Hulunbier stepped (in Chinese). Chin. J. Grassl. 04), 6–10+23. doi: 10.3321/j.issn:1673-5021.2006.04.002
Meng T. T., NI J., Wang G. H. (2007). Plant functional traits, environments and ecosystem functioning. Chin. J. Plant Ecol. 31 (1), 150. doi: 10.17521/cjpe.2007.0019
Mermillod-Blondin F., Roy J., Spehn E., van Peer L. (2002). Species diversity, functional diversity, and ecosystem functioning. Bio. Ecosyst. Funct. Synth. Perspect. 195, 195.
Mori A. S., Furukawa T., Sasaki T. (2013). Response diversity determines the resilience of ecosystems to environmental change. Bio. Rev. 88 (2), 349–364. doi: 10.1111/brv.12004
Mu Z. J., Liu H. G., Gui R., Su H. L., Li Y. H., Liu L. H., et al. (2020). Dynamic changes of plant community structure at different recovery stages of aerial-seeding region in Otindag Sandy Land (in Chinese). J.Huazhong. Agric. Univ. 39 (4), 46–56. doi: 10.13300/j.cnki.hnlkxb.2020.04.007
Mulvaney R. L., Khan S. A. (2001). Diffusion methods to determine different forms of nitrogen in soil hydrolysates. Soil Sci. Soc. Am. J. 65 (4), 1284–1292. doi: 10.2136/sssaj2001.6541284x
Nacry P., Bouguyon E., Gojon A. (2013). Nitrogen acquisition by roots: physiological and developmental mechanisms ensuring plant adaptation to a fluctuating resource. Plant Soil. 370, 1–29. doi: 10.1007/s11104-013-1645-9
Nortcliff S. (2002). Standardisation of soil quality attributes. Agric. Ecosyst. Environ. 88 (2), 161–168. doi: 10.1016/S0167-8809(01)00253-5
Qi D., Chen W., Liu Z., Gong X., Jin B., Min X., et al. (2011). Population structure and niche dynamics characteristics of plants communities in the sandy succession of Maqu alpine meadow in first meander of Yellow River. Acta Botanica Boreali-Occidentalia Sin. 31 (12), 2522–2531. doi: 10.1093/mp/ssq070
Qian Z., Yun Y. C., Yu X. P., Gao H. D., Lv R., Zhang W. Y. (2014). Changes of vegetation characteristics and soil properties in Mu Us Sandy Land by aerial seeding afforestation. J. Cent. South Univ. For. Techn 34, 102–107. doi: 10.14067/j.cnki.1673-923x.2014.04.021
R Core Team (2022). R: A language and environment for statistical computing. R Foundation for Statistical Computing, Vienna, Austria.
Raynaud X., Jaillard B., Leadley P. W. (2008). Plants may alter competition by modifying nutrient bioavailability in rhizosphere: a modeling approach. Am. Nat. 171 (1), 44–58. doi: 10.1086/523951
Reeder J. D., Schuman G. E. (2002). Influence of livestock grazing on C sequestration in semi-arid mixed-grass and short-grass rangelands. Environ. pollut. 116 (3), 457–463. doi: 10.1016/S0269-7491(01)00223-8
Reynolds H. L., Packer A., Bever J. D., Clay K. (2003). Grassroots ecology: plant–microbe–soil interactions as drivers of plant community structure and dynamics. Ecology 84 (9), 2281–2291. doi: 10.1890/02-0298
Schwember A. R., Schulze J., Del Pozo A., Cabeza R. A. (2019). Regulation of symbiotic nitrogen fixation in legume root nodules. Plants 8 (9), 333. doi: 10.3390/plants8090333
She Y. D., Yang X. Y., Ma L., Zhang Z. H., Wang D. J., Huang X. T., et al. (2021). Study on the characteristics and interrelationship of plant community and soil in degraded alpine meadow. Acta Agrestia Sinica. 29 (S1), 62. doi: 10.11733/j.issn.1007-0435.2021.Z1.008
Shen F., Yuan Y., Fan H., Liu W., Liu Y. (2012). Effects of elevated nitrogen deposition on soil organic carbon mineralization and soil enzyme activities in a Chinese fir plantation. Sheng Tai Xue Bao. 32 (2), 517–527. doi: 10.5846/stxb201012011710
Sun C., Wang W., Dong L., Yin Z. (2008). Review on impact of vegetation restoration on soil properties (in Chinese). J.Yangtze River. Sci. Res. Inst. 25 (3), 6. doi: 10.3724/SP.J.1005.2008.01083
Ulrich A. (1952). Physiological bases for assessing the nutritional requirements of plants. Annu. Rev. Physlol. 3 (1), 207–228. doi: 10.1146/annurev.pp.03.060152.001231
Vamosi S. M., Heard S. B., Vamosi J. C., Webb C. O. (2009). Emerging patterns in the comparative analysis of phylogenetic community structure. Mol. Ecol. 18 (4), 572–592. doi: 10.1111/j.1365-294X.2008.04001.x
Van der Putten W. H., Bardgett R. D., Bever J. D., Bezemer T. M., Casper B. B., Fukami T., et al. (2013). Plant–soil feedbacks: the past, the present and future challenges. J. Ecol. 101 (2), 265–276. doi: 10.1111/1365-2745.12054
Vitousek P. M., Porder S., Houlton B. Z., Chadwick O. A. (2010). Terrestrial phosphorus limitation: mechanisms, implications, and nitrogen–phosphorus interactions. Ecol. Appl. 20 (1), 5–15. doi: 10.1890/08-0127.1
Wang R., Lou Y., Deng D., Che P., Zhao C., Yang Y., et al. (2023). Summer grazing change fitness in a Tibetan lotus. Global Ecol. Conserv. 44, e02505. doi: 10.1016/j.gecco.2023.e02505
Wang L., Wang X., Wang D., Qi B., Zheng S., Liu H., et al. (2021). Spatiotemporal changes and driving factors of cultivated soil organic carbon in northern China’s typical agro-pastoral ecotone in the last 30 years. Remote Sens. 13 (18), 3607. doi: 10.3390/rs13183607
Wei W. D., Li X. L. (2012). Characteristics of soil on different degraded grasslands on alpine meadow in source area of Lancang, Yellow and Yangtze River (in Chinese). Hubei. Agric. Sci. 51 (06), 1102–1106. doi: 10.14088/j.cnki.issn0439-8114.2012.06.056
Wiesmeier M., Hübner R., Spörlein P., Geuß U., Hangen E., Reischl A., et al. (2014). Carbon sequestration potential of soils in southeast Germany derived from stable soil organic carbon saturation. Global. Change. Biol. 20 (2), 653–665. doi: 10.1111/gcb.12384
Winikoff S. G., Larkin D. J., Meier S. L., Finlay J. C. (2020). Vegetation trajectories of restored agricultural wetlands following sediment removal. Restor. Ecol. 28 (3), 612–622. doi: 10.1111/rec.13128
Wu G. L., Ren G. H., Wang D., Shi Z. H., Warrington D. (2013). Above-and below-ground response to soil water change in an alpine wetland ecosystem on the Qinghai-Tibetan Plateau, China. J. Hydrol. 476, 120–127. doi: 10.1016/j.jhydrol.2012.10.031
Wu X., Wang Y., Sun S. (2021). Long-term fencing decreases plant diversity and soil organic carbon concentration of the Zoige alpine meadows on the eastern Tibetan plateau. Plant Soil. 458, 191–200. doi: 10.1007/s11104-019-04373-7
Xiao Q., Xin W., Hua Y., Yu G., Lin Z., Jun F. (2013). Discussion on comprehensive management modes of the grassland desertification in Ruoergai county in northwest Sichuan. J. Sichuan. For. Sci. Techn. 34 (5), 47–50.
Xu D., Xu X., Wang G., Tao L. (2017). Variations in soil organic carbon content and distribution during natural restoration succession on the desert steppe in Ningxia. Sheng Tai Xue Bao. 26 (8), 35–42. doi: 10.11686/cyxb2016440
Yang J., Liu Q. R., Wang X. T. (2020). Plant community and soil nutrient of alpine meadow in different degradation stages on the Tibetan Plateau, China (in Chinese). Chin.J.Appl. Ecol. 31 (12), 4067–4072. doi: 10.13287/j.1001-9332.202012.008
Yao H., Campbell C. D., Qiao X. (2011). Soil pH controls nitrification and carbon substrate utilization more than urea or charcoal in some highly acidic soils. Biol. Fert. Soils. 47, 515–522. doi: 10.1007/s00374-011-0554-4
Zhang Q. Q., Han G. X., Lu F., Zhou Y. F., Wang X. J., Li P. G., et al. (2022). Effects of different restoration ages on plant diversity and community stability of wet lands in the Yellow River Delta (in Chinese). Chi. J. Ecol. 41 (7), 1249–1257. doi: 10.1007/s11104-021-05287-z
Zhang L., Wang X., Wang J., Liao L., Lei S., Liu G., et al. (2022). Alpine meadow degradation depresses soil nitrogen fixation by regulating plant functional groups and diazotrophic community composition. Plant Soil. 473, 319–335. doi: 10.1007/s11104-021-05287-z
Zhang Y., Welker J. M. (1996). Tibetan alpine tundra responses to simulated changes in climate: aboveground biomass and community responses. Arct. Alp. Res. 28 (2), 203–209. doi: 10.2307/1551761
Zhao N., Zhao X. Q., Zhao L., Xu S. X., Zou X. Y. (2016). Progress in researches of the response of plant functional traits to grazing disturbance. Chin. J. Ecol. 35 (7), 1916. doi: 10.13292/j.1000-4890.201607.027
Zheng D., Lin Z., Zhang X. (2002). Progress in studies of Tibetan Plateau and global environmental change. Earth Sci. Front. 9 (1), 95–102. doi: 10.3321/j.issn:1005-2321.2002.01.012
Zhu B., Li Z., Li P., Liu G., Xue S. (2010). Soil erodibility, microbial biomass, and physical–chemical property changes during long-term natural vegetation restoration: a case study in the Loess Plateau, China. Ecol. Res. 25, 531–541. doi: 10.1007/s11284-009-0683-5
Zhu W. W., Wang P., Xu Y. X., Li C. H., Yu H. L., Huang J. Y. (2021). Soil enzyme activities and their influencing factors in a desert steppe of northwestern China under changing precipitation regimes and nitrogen addition. Chin. J. Plant Ecol. 45 (3), 309. doi: 10.17521/cjpe.2020.0264
Zuo X. A., Zhao X. Y., Zhao H. L., Guo Y. R., Zhang T. H., Cui J. Y. (2010). Spatial pattern and heterogeneity of soil organic carbon and nitrogen in sand dunes related to vegetation change and geomorphic position in Horqin Sandy Land, Northern China. Environ. Monit. Assess. 164, 29–42. doi: 10.1007/s10661-009-0872-2
Keywords: alpine meadows, restoration years, community stability, soil factors, functional groups
Citation: Wang R, Lou Y, He L, Chen J, Chen Y, Yan W, Deng D and Mu J (2023) Plant functional groups and soil properties vary with the restoration periods in Zoige desertification land. Front. Ecol. Evol. 11:1331618. doi: 10.3389/fevo.2023.1331618
Received: 10 November 2023; Accepted: 04 December 2023;
Published: 15 December 2023.
Edited by:
Dong Wang, Henan University, ChinaReviewed by:
Shuang Xiang, Chinese Academy of Sciences (CAS), ChinaBo Tan, Sichuan Agricultural University, China
Yinzhan Liu, Henan University, China
Copyright © 2023 Wang, Lou, He, Chen, Chen, Yan, Deng and Mu. This is an open-access article distributed under the terms of the Creative Commons Attribution License (CC BY). The use, distribution or reproduction in other forums is permitted, provided the original author(s) and the copyright owner(s) are credited and that the original publication in this journal is cited, in accordance with accepted academic practice. No use, distribution or reproduction is permitted which does not comply with these terms.
*Correspondence: Dongzhou Deng, dongzhoud@163.com