- 1Department of Soil Science, “Luiz de Queiroz” College of Agriculture/University of São Paulo (ESALQ/USP), Piracicaba, Brazil
- 2Paul O’Neill School of Public and Environmental Affairs, Indiana University – Bloomington, Bloomington, IN, United States
- 3Department of Soil Science, Federal University of Ceará, Fortaleza, Ceará, Brazil
Land use change (LUC), specifically the cultivation of monoculture sugarcane, can negatively impact soil biodiversity, leading to a decline in soil health and ecosystem functioning. However, while studies focusing on macrofauna and microorganisms are more frequent in the literature, the impacts of LUC on mesofauna are still little known. Therefore, this study aimed to investigate the impacts of the predominant LUC for sugarcane production in Brazil on the diversity of edaphic mesofauna in soils with contrasting textures. In addition, we assessed correlations between biodiversity and soil properties chemical, biological, and physical attributes. We took samples from two sites (clayey and sandy soils) in southeastern Brazil. The sequence of LUC included i) native vegetation (NV), ii) pasture (PA), iii) sugarcane (SC), and iv) sugarcane ratoon (SCr). In the rainy season, monoliths (25 x 25 x 10 cm), soil samples were collected at 0-10, 10-20, and 20-30 cm to assess soil mesofauna, soil chemical (pH, soil organic matter, phosphorus, sulfur, potassium, calcium, magnesium, potential acidity, cation exchange capacity), physical (soil porosity) and biological (microbial biomass carbon and nitrogen) properties. The mesofauna taxonomic groups were quantified after the classification. Briefly, a total of 22 taxonomic groups were classified. The most predominant groups were non-oribatid mites, oribatid mites, and Collembola. Richness and abundance were lower in the three land uses studied (PA, SCr, SC) compared to the intercept (NV). In clayey soil, diversity decreased from NV to PA (-0.68 ± 0.27) and SC (-0.55 ± 0.27) but not to SCr. In sandy soil, land use significantly impacted the mesofauna diversity and evenness index, significantly reducing these indexes in SCr in relation to NV. Although land use change towards more intensified systems resulted in a loss of richness and abundance of soil mesofauna, sugarcane cultivation over the years can recover the diversity of mesofauna in clay-textured soils. These results provide a scientific background to better understand the LUC effects on sugarcane cultivation and support the establishment of sustainable practices that enhance soil health and biodiversity in different soil textures. This study highlights the need for tailored land management considering soil texture and biodiversity for improved ecosystem services.
1 Introduction
Soils host more than half of the Earth’s biodiversity (Anthony et al., 2023). Biodiversity comprises the three domains of life, Bacteria, Archaea, and Eukarya, which are indispensable drivers of the composition and ecosystem functions. They govern global biogeochemical cycles and directly influence climate change and human health (Crowther et al., 2019; Banerjee and van der Heijden, 2023).
Soil organisms can be classified according to size, divided into macrofauna, mesofauna, microfauna, and microorganisms (Lavelle et al., 2006). The mesofauna includes invertebrates, such as Oligochaeta (Enchytraeidae family), Symphyla (symphylans), Pauropoda (pauropods), Protura (proturans), Diplura (diplurans), Collembola (springtails), Arachnida and Insecta with a size between 0.2 and 2 mm (de Morais et al., 2010). These organisms have an essential role in the soil food web and consequently in the soil functioning, acting as predators of microorganisms, plants, and other invertebrates and performing crucial functions as decomposers such as the fragmentation of organic material, nutrient cycling, and soil formation (Pulleman et al., 2012). Furthermore, they are highly sensitive to land use practices, which makes them valuable indicators of terrestrial ecosystem health (Baretta et al., 2011; Duran-Bautista et al., 2020).
The diversity of mesofauna is intrinsically linked to the physical and chemical properties of the soils, making them valuable drivers of pore formation and nutrient cycling (Porre et al., 2016) but also as indicators of changes in soil properties (Pereira R de et al., 2012; Rieff et al., 2016). Their rapid response to environmental changes and sensitivity to pesticides and soil amendments make them suitable soil health indicators (Joimel et al., 2022). The Soil Biological Quality Index (BSQ) considers the presence/absence of soil mesofauna as a soil quality indicator (Parisi et al., 2005). Other studies have computed soil quality indices based on the combination of mesofauna abundance and specific characteristics (Yan et al., 2012). Abundances of Acari and Collembola have proven instrumental in elucidating biotic responses to land use intensity and impacts in ecosystems (Black et al., 2003; Rutgers et al., 2009; George et al., 2017). Despite ongoing tradeoff regarding the taxonomic resolution and specific characteristics of mesofauna, total mesofauna abundance is already acknowledged as a valuable soil quality indicator (Arboláez et al., 2023). In addition, reducing soil biodiversity directly impacts soil functions, nutrient cycling, and organic matter decomposition (Handa et al., 2014; De Graaff et al., 2015). Agricultural management can negatively impact the mesofauna community, whether through the use of mineral fertilizers (Cao et al., 2011; Su et al., 2015), pesticides (Roy et al., 2009), and tillage activities (Heisler and Kaiser, 1995). However, those effects are little known in tropical soils under LUC for large-scale agricultural expansion, such as those occurring for sugarcane in Brazil.
Previous studies conducted in Brazil have revealed the impacts of LUC and sugarcane cultivation on soil macrofauna (Franco et al., 2016; Menandro et al., 2019; Cherubin et al., 2021b; Vanolli et al., 2021; Vanolli et al., 2023) and microrganisms (Pimentel et al., 2019; Morais et al., 2020). However, the effects of management practices on soil mesofauna in Brazilian sugarcane fields remain unexplored. The impact of practices such as inadequate sugarcane straw removal and intensive soil management on soil health depends on variables such as climate and soil composition (Cherubin et al., 2021a). Researchers observed that sandy soils are more susceptible to degradation than clayey soils, indicating that straw removal should be avoided in sandy soils. Additionally, physical degradation has been identified as the primary factor contributing to declining soil health in clayey soils.
This study aims to link land management in different soil texture and biodiversity for improved ecosystem services. Therefore, our study aimed to analyze the impacts on the abundance and community of mesofauna in areas where native vegetation has been converted into extensive pasture and then into sugarcane plantations. In addition, two different soil classes were considered, clayey and sandy, in the southeastern region of Brazil. The underlying hypothesis is that sugarcane cultivation may represent an alternative for recovering degraded pasture areas based on the mesofauna’s dynamics and resilience after the land use change.
2 Materials and methods
2.1 Description of study sites
The study was carried out in the southeastern Brazil, the main sugarcane-producing region of the world, in Manduri (Latitude 23°0′10″ S, and Longitude 49°19’19” W) and Brotas (Latitude 22°17’4’’ S, Longitude: 48°7’39’’ W) municipalities, São Paulo state, Brazil (Figure 1). Manduri has a Cfa climate (Köppen and Geiger classification), with an average annual rainfall of 1249 mm and an average temperature of 19.8°C, while Brotas has a Cwa climate (Köppen and Geiger classification), with an annual rainfall of 1,337 mm, concentrated from October to March and an average temperature of 20.0°C (Alvares et al., 2013). The rainy season starts in the spring-summer transition and typically lasts between October and March, and the dry season in the fall and winter, from April to September. Manduri has clayey soil (average 576 g kg-1 for the 0-30 cm layer), while Brotas has sandy soil (average 87 g kg-1 for the 0-30 cm layer). In the taxonomic context, sandy soils are classified as Arenossolos (WRB, 2015) or Quartzipsamments (USDA-Natural Resources Conservation Service, 2014), while clayey soils fall into Ferralsols (WRB, 2015) or Oxisols (USDA-Natural Resources Conservation Service, 2014). A detailed chemical analysis of the soil was carried out in a previous study by (Vanolli et al., 2023).
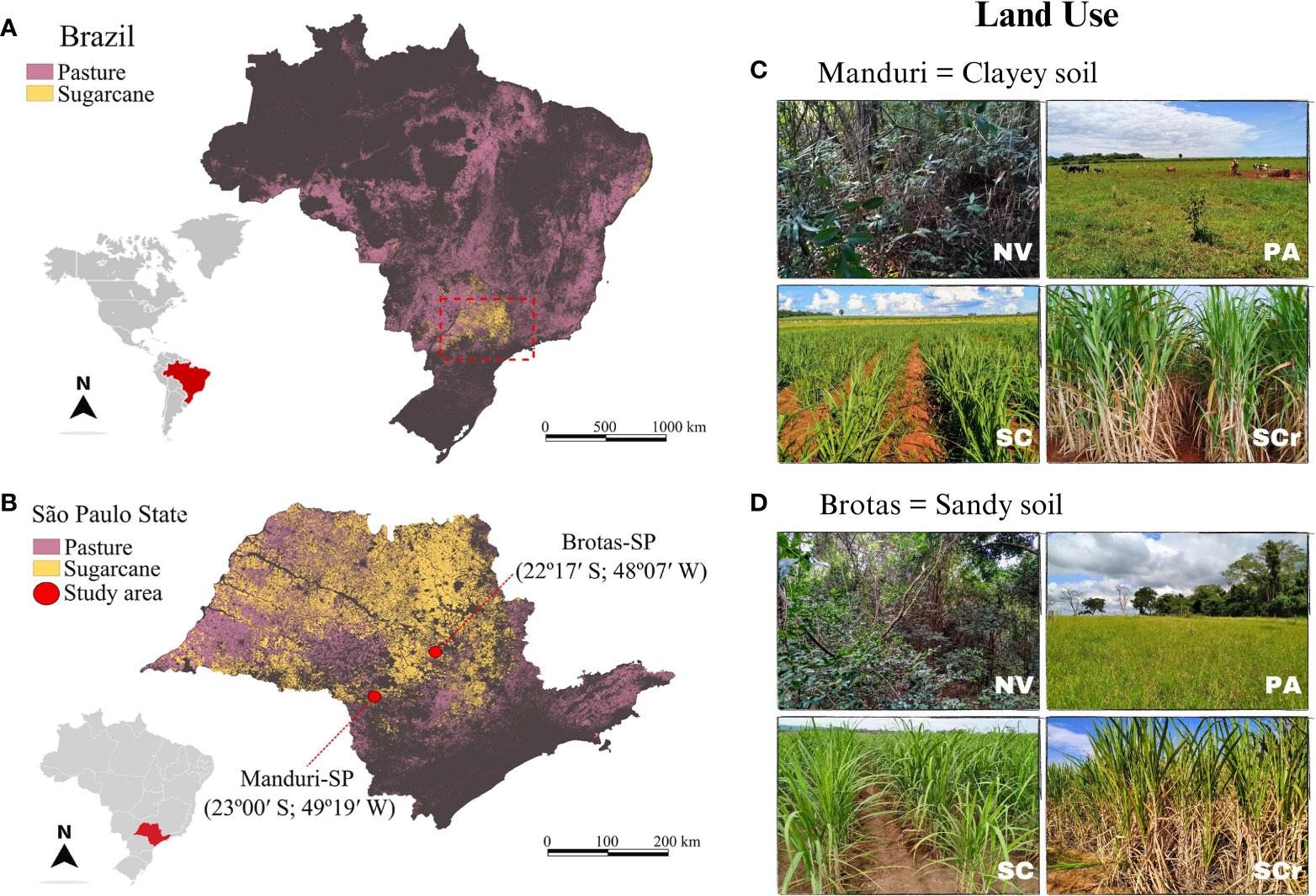
Figure 1 Geographical locations depicted for the study sites in Brazil (A) and the state of São Paulo (B), focusing on the cities of Manduri/SP (C) with clayey soil and Brotas/SP (D) with sandy soil. The figure highlights diverse experimental areas with distinct land uses: Native Vegetation Area (NV), Extensive Pasture (PA), Newly Planted Sugarcane (SC), and Sugarcane Ratoon (SCr).
2.2 Soil sampling
A synchronous approach was adopted, based on space-for-time substitution, using a chronosequence to evaluate different types of land use, namely: i) Native Vegetation (NV) - representing a fragment of Atlantic Forest as a natural ecosystem reference; ii) Extensive Pasture (PA) - subjected to continuous grazing management without the application of fertilizers or liming, using brachiaria as a forage plant; iii) Newly planted sugarcane (SC) - area converted to sugarcane cultivation in October 2018, from an area of extensive pasture, using conventional tillage techniques; and iv) Sugarcane ratoon (SCr) - areas cultivated with sugarcane in second ratoon (on sandy soil) and third ratoon (on clay soil), being subjected to conventional cultivation practices with mechanized harvesting and without straw removal. The four types of land use were established in contiguous areas to mitigate possible effects resulting from climatic, topographical, and soil variations. Detailed information on the land use history and concise descriptions of the management operations on the study sites can be found in (Vanolli et al., 2023).
The soil mesofauna was collected at the end of the rainy season between March and April 2019. In each land use area, with an approximate extension of 2.25 hectares, four sampling points were designated, 50 meters apart in each study site. Thus, the total number of sampling points was 16 per area (i.e., four types of land use multiplied by four sampling points). Samples of edaphic mesofauna were collected at three different depths: 0-10 cm, 10-20 cm, and 20-30 cm. Using the “Tropical Soil Biology and Fertility” (TSBF) methodology, monoliths 25 cm long, 25 cm wide, and 10 cm deep (Anderson and Ingram, 1989).
2.3 Extraction and counting of mesofauna
The mesofauna samples were extracted using Berlese-Tüllgren funnels, a method widely used due to its practicality and low cost. It involves transferring the soil into stainless steel funnels under 25W incandescent lamps over ten days (Freire et al., 2015). At the top of the funnels is a heat source, while at the bottom is a container with a 70% alcohol solution. As the soil absorbs heat, the arthropods migrate downwards and fall into the sampling container and are then selected through a 2 mm sieve (maximum body diameter for mesofauna organisms) located at the base of the funnel. The biological components of the mesofauna were quantified according to their size classification (organisms larger than 0.02 mm and smaller than 2 mm) and taxonomically identified down to phylum (Nematoda), class (Diplopoda, Chilopoda, Symphyla and Pauropoda), subclass (Oligochaeta), order (Acariformes non-oribatid, Collembola, Protura, Diplura, Araneae, Blattodea, Diptera, Coleoptera, Pseudoscorpionida, Lepidoptera, Thysanoptera and Psocodea), suborder (Isoptera, Heteroptera and Oribatid), and family (Formicidae) using optical stereoscopic microscopes with 40 x magnification. Therefore, microscopic organisms, such as nematodes, were only considered when larger than 0.02 mm. Deformed samples for chemical characterization, microbial biomass carbon and nitrogen, and undeformed samples for soil physical analysis were collected at the same points and times as the mesofauna data, and these data were used to verify correlations between soil chemical attributes and soil mesofauna.
2.4 Chemical, physical, and biological parameters of the soil
We quantified the microbial biomass carbon (MBC) and nitrogen (MBN) using the chloroform extraction method according to (Sparling and West, 1988) and (Vance et al., 1987). In the case of MBC, the values were calculated by subtracting the organic carbon from fumigated and non-fumigated soil samples, using a correction factor (kEC) of 0.33 (Sparling and West, 1988).
The concentrations of calcium (Ca2+), magnesium (Mg2+), potassium (K+), phosphorus (P), and sulfur (S-sulphate) were determined using analytical methods described by (Van Raij et al., 2001). Soil pH (pH CaCl2 0.01 mol L-1) and potential acidity (H+Al) were assessed using a SMP solution. In addition, base saturation (BS%) and potential cation exchange capacity (CECpH7) were calculated, as well as organic matter (OM), which was quantified using a colorimetric method with sulfuric acid (Van Raij et al., 2001).
Undeformed soil samples were collected at different depths to assess the physical properties of the soil using volumetric rings with a volume of approximately 100 cm³. Gravimetric moisture and soil bulk density (BD) were measured. The dry mass of the soil was divided by the volume of the ring to calculate BD. The volumetric water content (θ) was calculated based on the relationship between BD and gravimetric moisture. In addition, macro (MaP), meso (MeP), and microporosity (MiP) were calculated based on pore distribution, classifying macropores as those with a diameter >50 μm, mesopores with a diameter between 50 and 15 μm, and micropores with a diameter<15 μm and total porosity were also determined (Equation 1) (Cavalcanti et al., 2020).
Where TP represents the total porosity, PD is the particle density, θ30 h Pa and θ100 hPa are the volumetric water contents under tensions of 30 and 10 hPa, respectively.
2.5 Data analysis
We sought to evaluate if land use affects the biodiversity of soil mesofauna, combining various measurements, including the total density of organisms per square meter, taxonomic richness (number of mesofauna groups), and the Shannon diversity (H′) and Pielou evenness (E) indexes. The Shannon and Pielou indexes help assess the biodiversity of mesofauna communities’ biodiversity by considering the number of different species and the relative density of each species in the sample.
The Shannon index will be calculated using Equation 2.
Where: H’ = Shannon index. Σ = Total number of taxa found at the site. pi = Relative abundance (proportion) of species “i” in the sample.
The Pielou evenness index measures the evenness of the community and ranges from 0 to 1; it indicates how equally the proportions of species are distributed in the community. The Pielou index will be calculated using Equation 3.
Where E= Pielou index; H’= Shannon index; S = the number of species or groups; ln = logarithm in the natural base.
Overall, the Shannon and Pielou indexes are versatile tools that help us to understand the biodiversity of mesofauna to analyze the relationship between soil mesofauna variables (abundance, group richness, Shannon and Pielou indexes) and land use; we used linear mixed-effects models. The fixed effects are related to the response variable we are studying and the independent variables (land use and depth). The random effects capture the variation not explained by the fixed effects, and in this study, we consider the random variability between different sites as a random effect.
We calculated both the marginal R (proportion of variance explained by the independent variables) and the conditional R (also considering the random effect) for each model. To assess the significance of the effects, we compared full models with effects versus models without these effects, using p-values. These models allowed for a more direct approach to understanding the responses of mesofauna to these variables, providing specific interpretability and detailing individual effects. All analyses were carried out using R software, version 4.3.1, and specific packages, including nlme, vegan, and ggplot2.
The methodology used to evaluate non-oribatid mites, collembolans and oribatid mites in relation to land use change in the 0-30 cm soil layer involved all the individuals found in 1m2 for all soil layers summed together, submitting the evaluated data to the analysis of variance (ANOVA). The normality of the data was confirmed through the Shapiro-Wilk test, when the F value was significant (F<0.05), the data was compared using the Tukey mean comparison test with a probability of 5% (p<0,05). The effect of land use on the mesofauna community for each site was tested by perMANOVA test using the adonis function of R package vegan (Oksanen and Simpson, 2009). The principal component analysis (PCA) was performed by the fviz_pca_biplot function from the factoextra package to visualize these differences (Kassambara and Mundt, 2017). The ellipses represent the Euclidean distance from the centroid of each land use with a 95% confidence level. The PCAs were performed for each site aiming to visualize the differences in mesofauna community alone and the mesofauna community associated with the soil properties (pH CaCl2; soil organic matter content: OM; P: phosphor extracted with resin; S: sulfur; K: potassium; Ca: calcium; Mg: Magnesium; H+Al: potential acidity; BS: sum of bases; CEC: cation exchange capacity; BS: bases saturation; MBC: microbial biomass carbon; MBN: microbial biomass nitrogen; BD: bulk density; Porosity: total porosity; Macro, meso and micro-porosity) in the different sites and land uses.
3 Results
3.1 Description of organisms distribution across soil texture and land use
A total of 11950 individuals m-2 were collected, 5168 in clay soil and 6782 in sandy soil (Table 1). These individuals were grouped into 22 distinct taxonomic categories, including Oligochaeta, Acariformes (Non-Oribatid), Oribatid, Collembola, Protura, Diplura, Formicidae, Diplopoda, Chilopoda, Heteroptera, Blattodea, Diptera, Isoptera, Coleoptera, Nematoda, Symphyla, Pauropoda, Pseudoscorpionida, Lepidoptera, Thysanoptera and Psocodea. Sixteen of these taxonomic groups were found in both soil textures (Figure 2). However, five groups, Symphyla, Pauropoda, Pseudoscorpionida, Lepidoptera, and Thysanoptera, were found exclusively in clay soil, indicating a possible preference for this soil texture. On the other hand, the Psocodea group was only found in sandy soil (Figure 2).
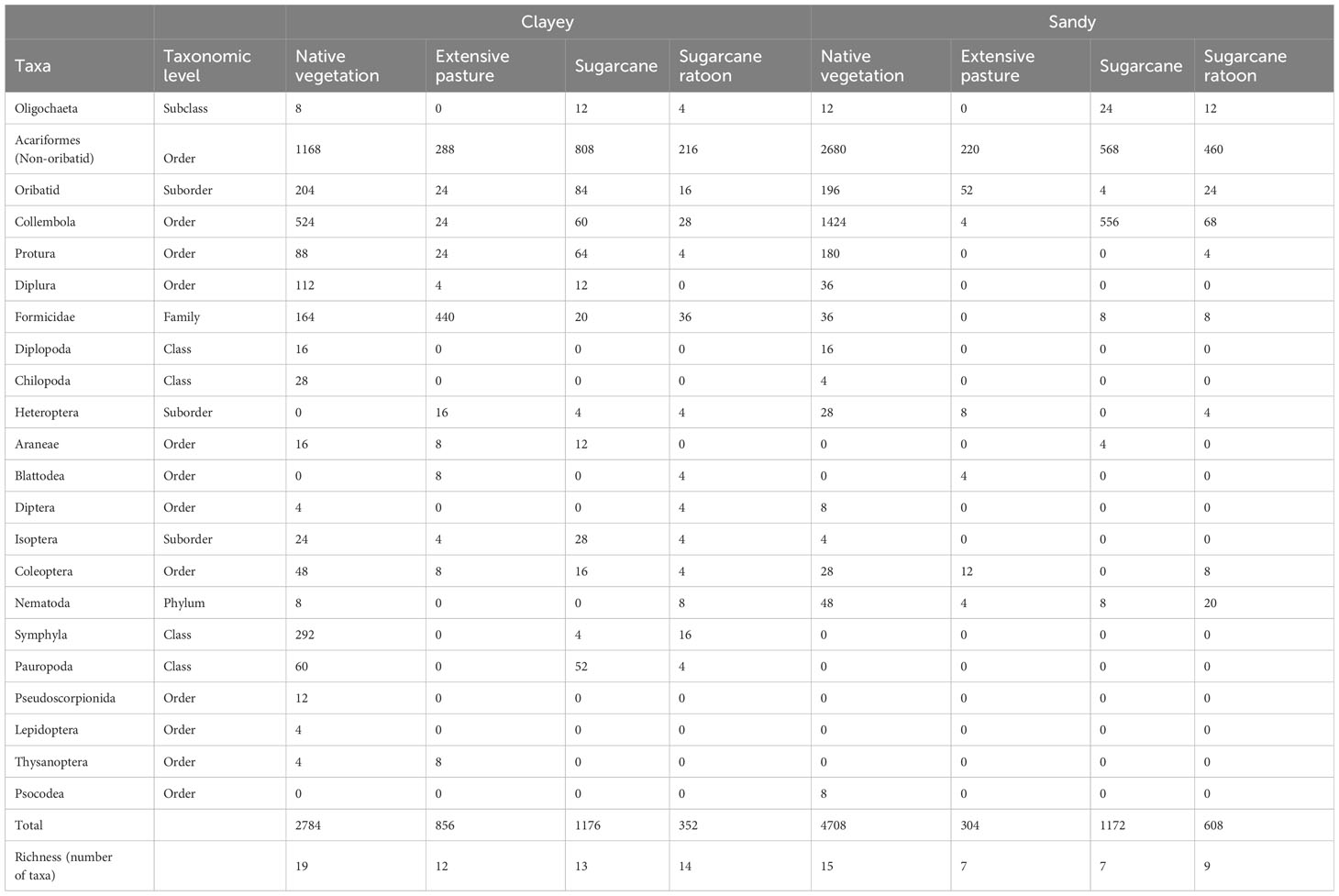
Table 1 Abundance (individuals m−2) and standard deviation of edaphic mesofauna groups in the 0–30 cm layer in different land uses, N = 4.
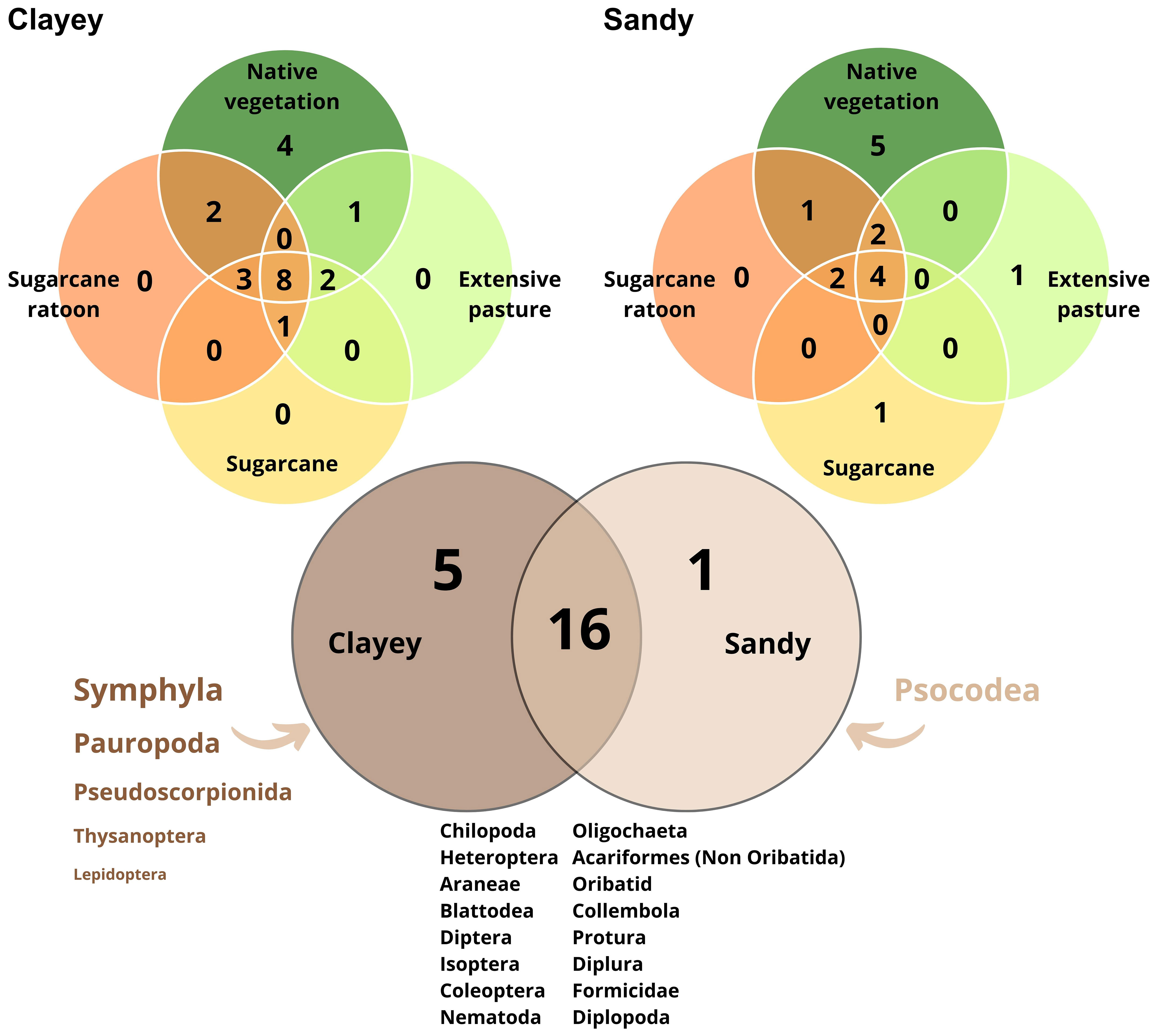
Figure 2 Venn diagram of the number of shared and unique soil mesofaunal taxa. The shared and unique numbers within the circles indicate the number of either shared taxa or unique taxa in the overlapping regions.
In both sites, there was a greater abundance of mesofauna in the areas of native vegetation, 2,784 individuals m-2 in the clay soil and 4,708 individuals m-2 in the sandy soil, as well as in the areas of sugarcane cultivation, with observations of 1176 individuals m-2 in the clay soil and 1172 individuals m-2 in the sandy soil (Table 1). When assessing the relative abundance of organisms, the Acariformes (Non-Oribatid) were the group with the highest relative abundance in all the areas studied, in clay soil NV 42.0%; PA 33.6%; SCp 68.7%; SCr 61.4%, in sandy soil NV 56.9%; PA 72.4%; SCp 48.5%; SCr 75.7% (Figure 3). The results showed that Collembola were the second most abundant group in the studied areas, except the PA. In clay soils, Collembola represented 19% of the mesofauna community in NV, 3% in PA, 5% in SC, and 8% in SCr, while in sandy soils, they were 30% in NV, 1% in PA, 47% in SC, and 11% in SCr (Figure 3). In the PA, the Formicidae group was the second most abundant in clay soils, with 51%, while in sandy soils, it was the Coleoptera (4%). The third most abundant group studied were oribatid mites, with higher abundance (17%) in the pasture area on sandy soil (Supplementary Table S1).
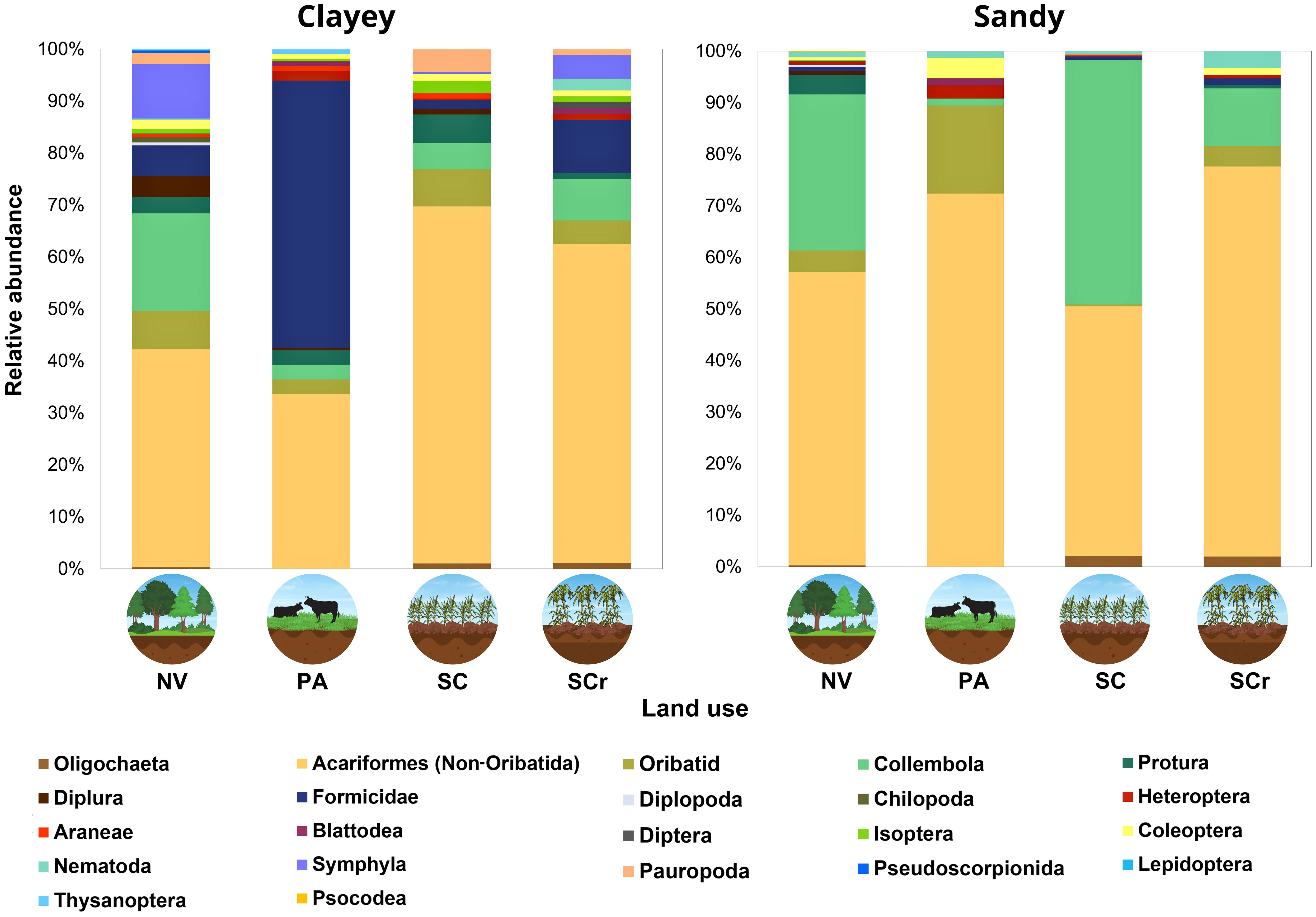
Figure 3 Taxonomic composition of soil mesofauna between land use: Native vegetation area (NV); Extensive pasture (PA); Newly planted sugarcane (SC) and sugarcane ratoon (SCr).
When analyzed separately, the effect of land use on the three main groups of mesofauna, Acari non-oribatid, Acari oribatid, and Collembola, higher abundance of non-oribatid mites was observed in NV, followed by a higher abundance of this group in SC in the clayey soil (Figure 4). For PA and SCr, no significant differences were found between them. Abundance was lower than in the NV and SC areas (Figure 4). Concerning oribatid mites, NV and SC had a higher abundance of this group, while PA and SCr had a lower abundance. For the sandy soil, there was a greater abundance of non-oribatid mites in NV, while in the less diversified systems, such as PA, SC, and SCr, there was a reduction of this group (Figure 4). The same occurred with oribatid mites, with a predominance in NV and a reduction in PA, SC, and SCr, with no significant difference between them. The Collembola were more abundant in NV and SC than in PA and SCr in both soil textures (Figure 4).
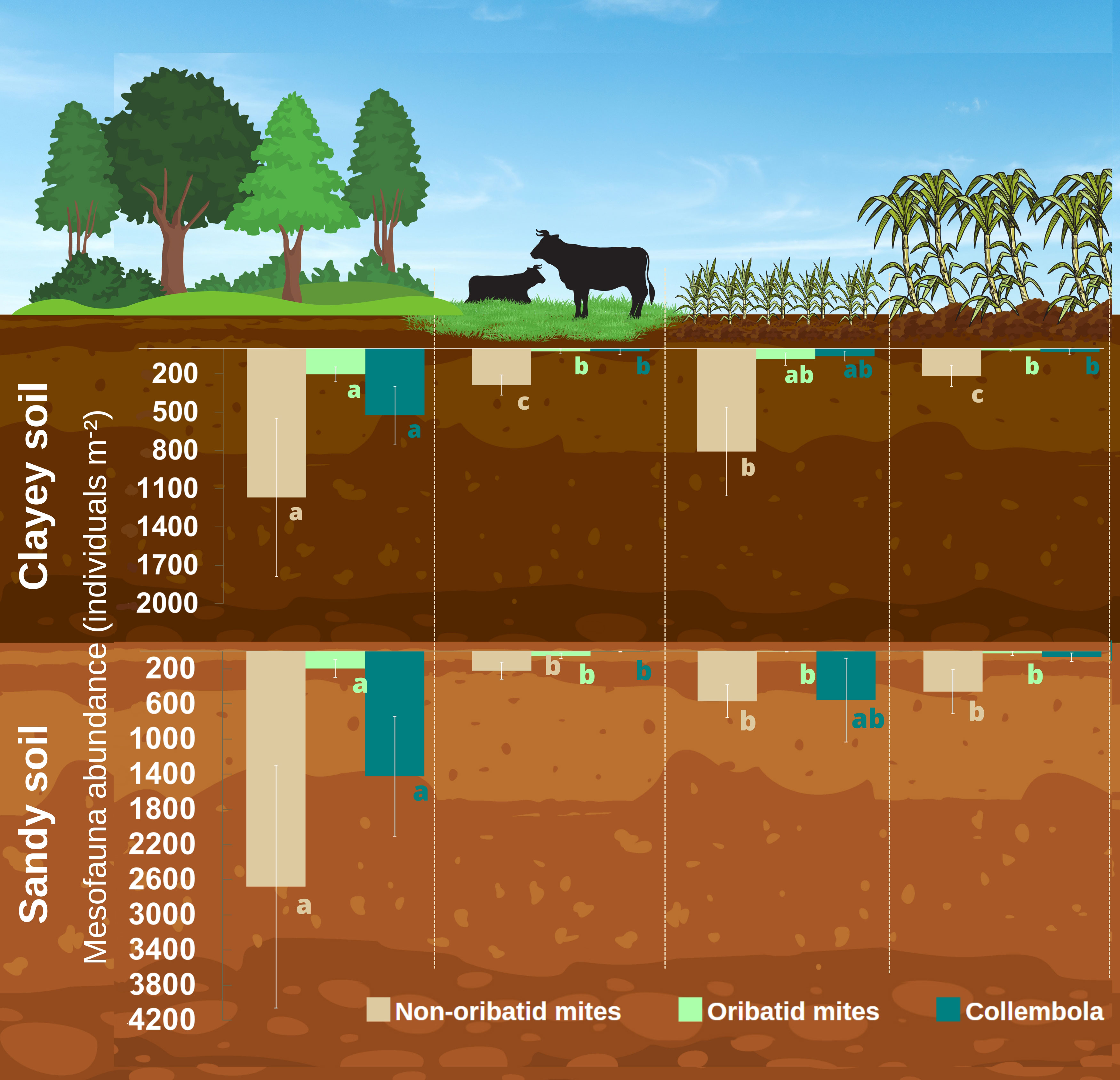
Figure 4 Arithmetic means and standard errors of Non-Oribatid mites, Oribatid mites and Collembola group in Different Land Uses (Native vegetation area; Extensive pasture; Newly planted sugarcane and sugarcane ratoon) in Clayey and Sandy Soil (n = 4). Means followed by the same letter do not differ statistically from each other in relation to land uses using the Tukey test at 5% probability.
3.2 Abundance, richness, diversity, and evenness of edaphic mesofauna
Land use change significantly affected the abundance, richness, diversity, and evenness of edaphic mesofauna in clay soil (Figure 5). The richness and abundance were lower for the three land uses (i.e., PA, SCr, SC) compared to the intercept (NV) (p<0.05). PA experienced a reduction of -4.25 taxonomic groups ±1.50 (standard error) in richness, SC reduced by -4.75 taxonomic groups ±1.50, and SCr reduced by -4.00 taxonomic groups ±1.50 (Figure 5). The abundance was also reduced due to land use change, with PA being unaffected, while SC showed a reduction of -820 individuals m-2 ± 354.3 and SCr showed a reduction of -812 individuals m-2 ± 354.3 (Figure 5). The Shannon index (H′) revealed lower indexes in PA (-0.68 ± 0.27) and a reduction in SC (-0.55 ± 0.27) (Figure 5). However, there were no significant changes in diversity for SCr compared to NV (p>0.05), indicating no major impact on the diversity of soil mesofauna in the SCr. The Pielou index (E), with PA showing a reduction of -0.22 ± 0.09, SC showing a reduction of -0.18 ± 0.09, and SCr not affecting the equality of the mesofauna population (p>0.05) (Figure 5). There was no interaction between land use and depth and no significant differences in the indexes across soil depths.
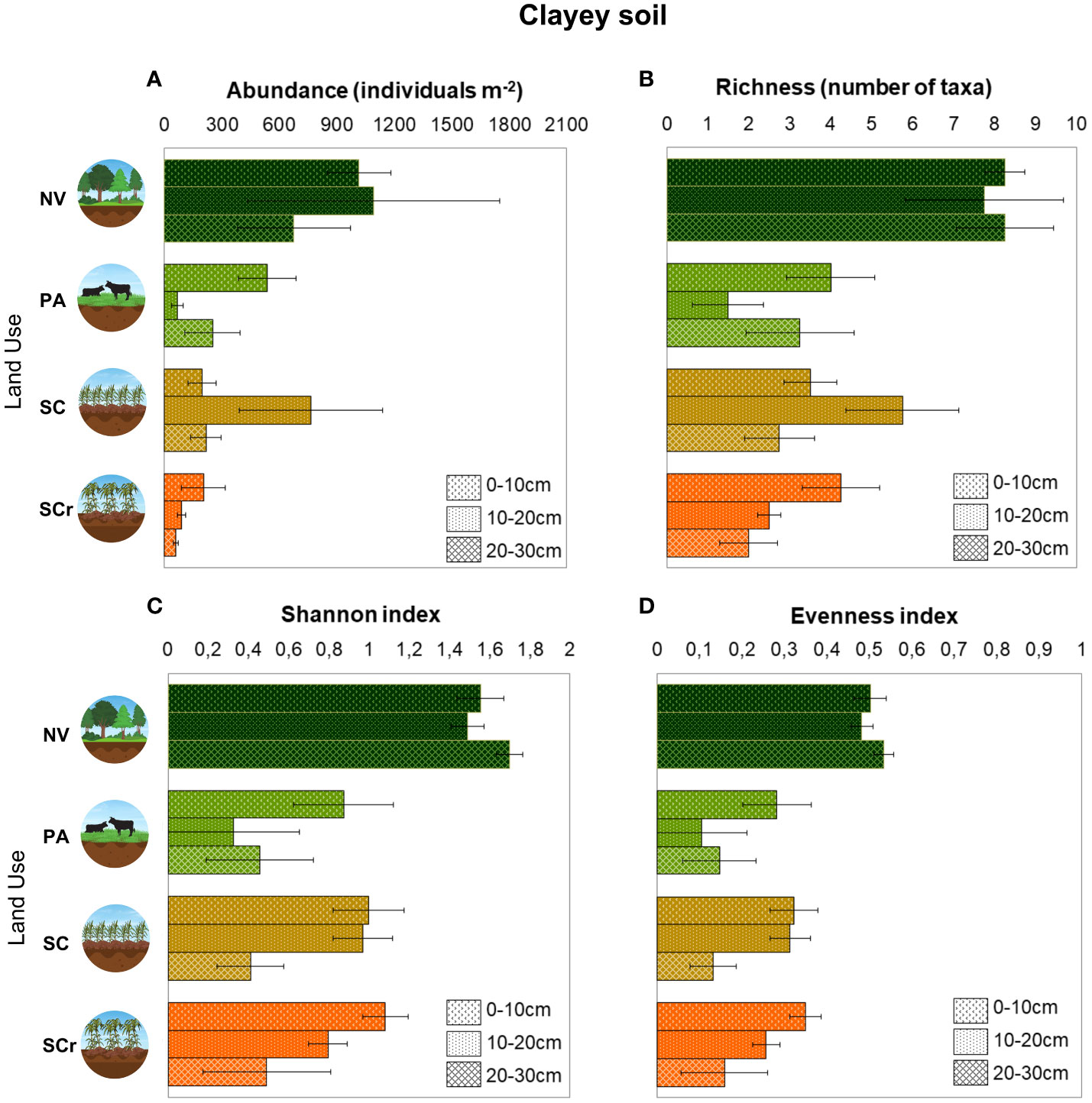
Figure 5 Arithmetic means and standard errors of total abundance (A) and taxa richness (B) Shannon diversity (C) and Pielou equability index (D) of edaphic mesofauna as a function of land use in Clayey soil (n = 4).
For the sandy soil, abundance (abundance r2marginal = 0.329, r2conditional = 0.416) and richness (r2marginal = 0.456, r2conditional = 0.526) were reduced with soil depth (p<0.05) (Figure 6). The abundance of soil mesofauna was low in the 10-20 cm (-1820 individuals m-2 ± 774.8) and 20-30 cm (-2256 individuals m-2 ± 774.8) layers compared to the 0-10 cm layer. However, there were no significant interactions between land use and soil depth. For taxa richness, in the 10-20 cm layer richness was not significantly affected (p>0.05), while in the 20-30 cm layer, there was a reduction of -2.50 taxonomic groups ± 1.26 compared to the surface layer (p<0.05) (Figure 6). Both the abundance and richness of mesofauna were reduced in PA (-2636 individuals m-2 ± 774.8; -3.25 ± 1.26), SC (-1844 individuals m-2 ± 774.8; -3.25 taxonomic groups ± 1.26), and SCr (-2392 individuals m-2 ± 774.8; -3.50 ± 1.26), with the SCr area showing the lowest diversity (Figure 6). The study found that land use had a significant impact on the diversity index (H′) and mesofauna equability (E) (p<0.05; r2marginal = 0.302, r2conditional = 0.392), with a significant reduction in these indexes in SCr, -0.626 ± 0.313 (p<0.05), there were no significant changes in PA and SC compared to NV (p>0.05) (Figure 6). Soil depth did not affect these indexes (p>0.05), and there was no interaction between land use and soil depth regarding their effects on the diversity index and mesofauna equability (p>0.05).
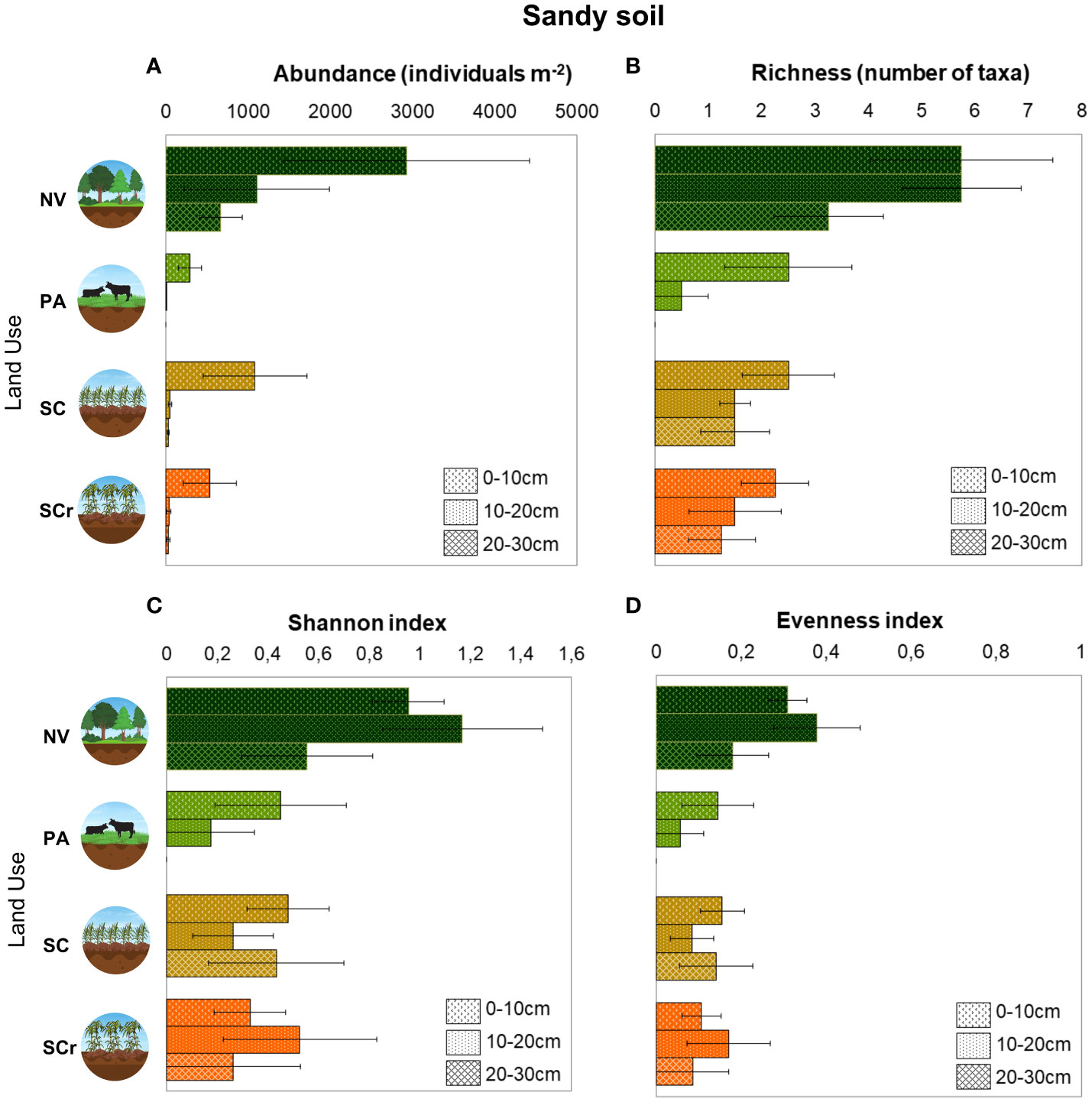
Figure 6 Arithmetic means and standard errors of total abundance (A) and taxa richness (B) Shannon diversity (C) and Pielou equability index (D) of edaphic mesofauna as a function of land use in Sandy soil (n = 4).
3.3 Correlation between mesofauna groups and soil chemical, physical, and biological attributes across land use
The mesofauna community was only affected by the land use in the clayey soil, where the NV was different from the others (Supplementary Table S2, Figure 8). When the principal component analysis was plotted with the soil properties, the PA differentiated from the sugarcane soil in the clayey soil. However, SC and SCr clustered together (Figure 7). SC with SCr was largely due to the soil’s chemical attributes, like those of corrected agricultural soil. In addition, some physical attributes, such as soil porosity, and biological attributes, such as microbial biomass carbon, were clustered with mesofauna groups, including Diplopoda, Oligochaeta, Chilopoda, and Protura. Soil organic matter clustered with Acari oribatid, Collembola, Symphyla, and Diplura. The pasture areas were grouped close to the Formicidae and Heteroptera groups (Figure 7).
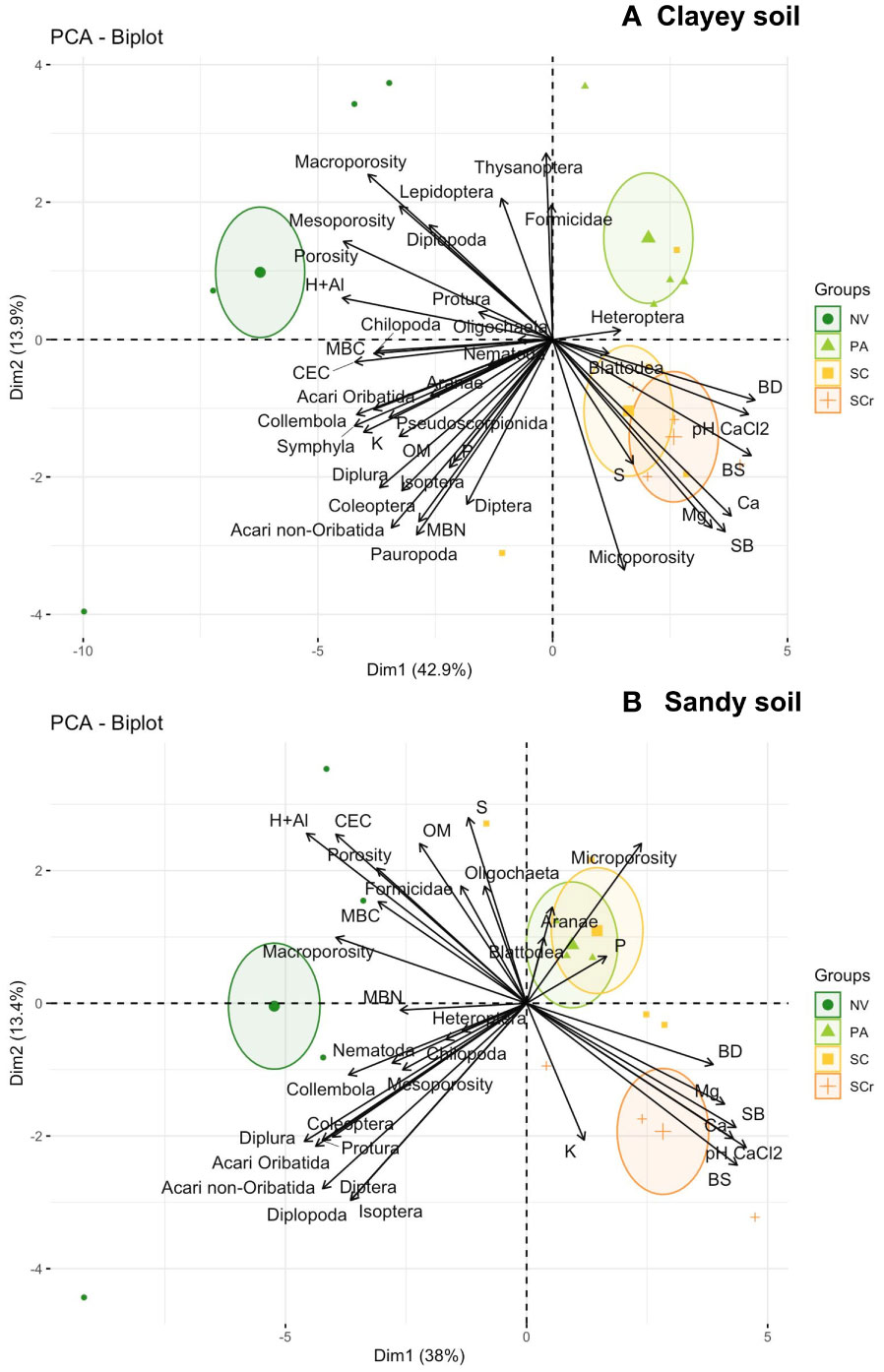
Figure 7 Principal component analysis of mesofauna community and soil properties from the soil (0-30 cm) in Clayey soil (A) and Sandy soil (B) under different land uses: Native vegetation area (NV); Extensive pasture (PA); Newly planted sugarcane (SC) and sugarcane ratoon (SCr).
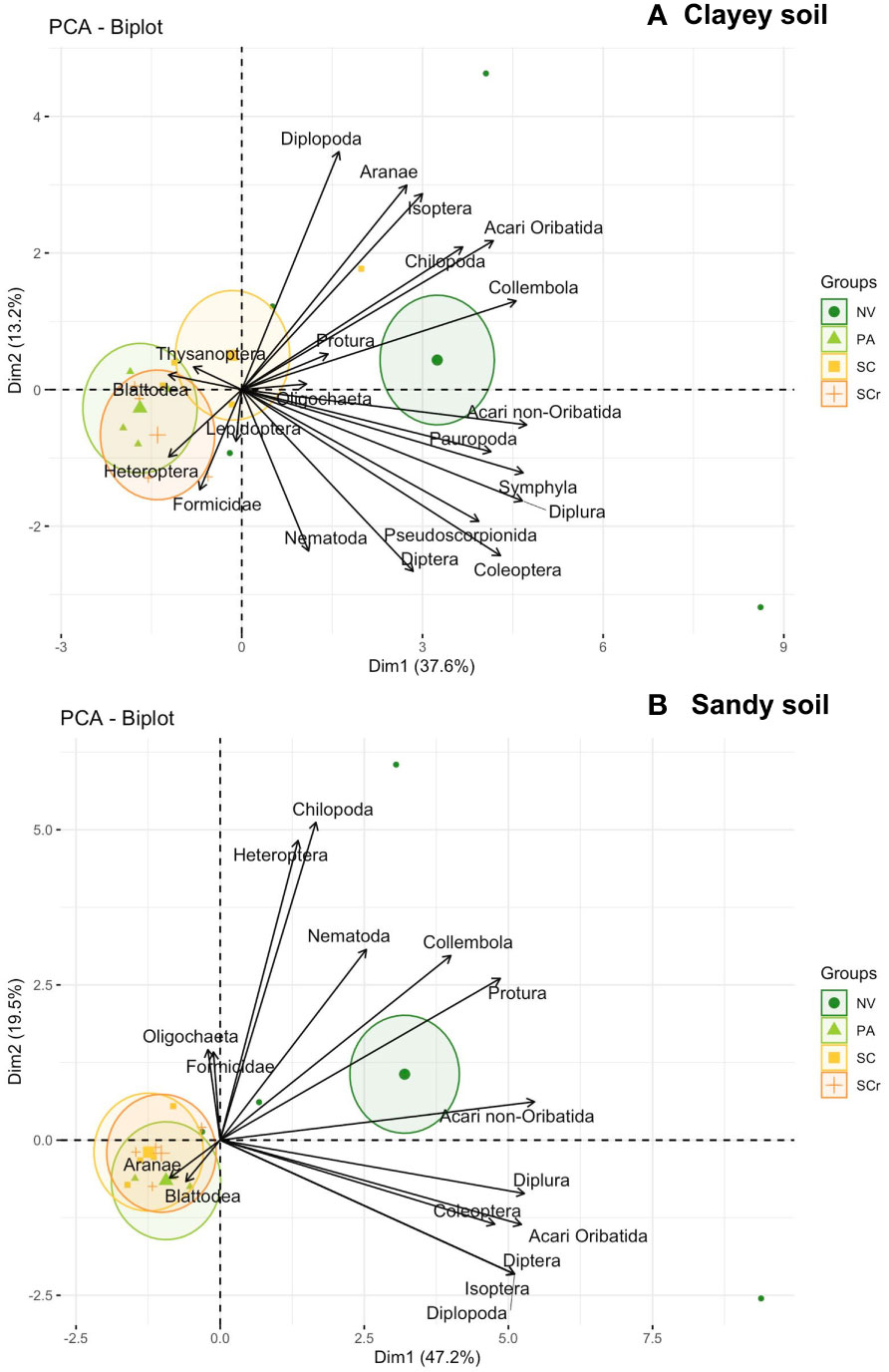
Figure 8 Principal component analysis of mesofauna community from the soil (0-30 cm) in Clayey soil (A) and Sandy soil (B) under different land uses: Native vegetation area (NV); Extensive pasture (PA); Newly planted sugarcane (SC) and sugarcane ratoon (SCr).
4 Discussion
4.1 Implications of land use change on the abundance, richness, diversity, and evenness of edaphic mesofauna in contrasting soil textures
The study tackled the substantial impact of land use change and management practices on soil biological attributes. Our research systematically investigated alterations in the abundance, richness, and diversity of soil mesofauna resulting from the conversion of natural vegetation into pasture and sugarcane cultivation, specifically examining these dynamics independently of soil textures (i.e., clayey or sandy soils). This scientific inquiry yields interesting insights into the relationships between land use change and the abundance and diversity of edaphic mesofauna while concurrently revealing the interesting role played by soil texture in shaping these ecological dynamics.
Soil disturbance, such as the cultivation of sugarcane or extensive grazing, has the potential to induce significant environmental changes. These alterations impact not only the structural characteristics of the habitat but also the availability of essential resources, thereby leading to consequential shifts in the community of soil mesofauna (van Capelle et al., 2012; Howe and Smith, 2021). These disturbances may homogenize ecosystems compared to native vegetation, where niche diversification is more pronounced. The shift towards intensified sugarcane cultivation and extensive grazing may negatively impact the diversity and uniformity of soil invertebrate communities, potentially compromising essential ecosystem services such as nitrogen cycling (Gergócs et al., 2022), decomposition, nutrient cycling, and soil formation, thereby facilitating water supply and regulating local erosion and climate (Lavelle et al., 2006; Barrios, 2007; George et al., 2017).
In our study, we observed differences in the community of mesofauna community resulting from alterations in both land use and soil texture. Our results indicated that the impact of sugarcane cultivation on the structure of mesofauna community was comparatively lower in response to changes in land use within clayey soil, providing support for our hypothesis specific to this soil texture. In the clayey soil, SCr had no negative impact on the diversity and evenness of the mesofauna compared to NV, as observed for the Shannon and Pielou indexes, respectively. These results suggest that mechanized sugarcane harvesting, without burning and straw removal, can create a suitable environment for the survival and establishment of mesofauna, offering adequate habitat and resources. In addition, clayey soils can recover more quickly from disturbances caused by the land use change, providing an environment that is more stable for mesofauna. This contrasts with sandy soils, which typically possess limited aggregation and lower water and nutrient retention capabilities than clayey soils (Reichert et al., 2009; Reichert et al., 2016). Therefore, these results highlight the need to consider not only the type of disturbance but also the characteristics of the soil when assessing impacts on edaphic mesofauna.
When investigating the impact of the conversion of native vegetation into pasturelands, PA had a lower diversity of mesofauna compared to NV. Diversity loss can be attributed to several factors, among which animal trampling during extensive pasture management stands out, as it leads to soil compaction (Mayel et al., 2021). Tsiafouli et al. (2015), observed that agricultural intensification through extensive and intensive pasture rotations tends to consistently simplify the diversity of the soil food web, potentially impacting its overall functioning. As a result, pasture areas can induce alterations in the community of soil fauna, favoring more adapted groups to this habitat conditions, such as ants (Esquivel et al., 2019; Vanolli et al., 2023). The prevalence of the Formicidae family in pasture areas and under clayey soil accounts for the lower diversity and evenness observed in the mesofauna community.
In sandy soil, SC had a relatively milder impact on the reduction of total mesofauna abundance compared to PA and SCr, albeit with a prevailing dominance of Collembola and non-oribatid mites. Similarly, SCr had the lowest richness, with approximately 75% of the total abundance belonging to the same group (non-oribatid mites). The soil biota positively relates with pore spaces and nutrient cycling (Porre et al., 2016) affected by tillage intensity. However (Cherubin et al., 2021a), demonstrated that the soil health of Brazilian sugarcane fields is closely linked to variables such as soil texture (van Capelle et al., 2012). found that tillage intensity significantly impacts collembolans, with the effects depending on the soil texture. Collembola species that rely on a connected network of soil pores and have limited burrowing activity were found to be negatively affected by plough less tillage in clayey soils. Our results align with these findings, as we found that the recent planting of sugarcane did not alter the diversity of mesofauna in sandy soils.
Therefore, our study indicates that the impact of land use on the biodiversity of edaphic mesofauna depends on the specific functional traits of organisms and their preferences for habitat needs and food sources. Therefore, to make sugarcane cultivation favorable for maintaining mesofauna biodiversity, local conditions such as soil texture should be considered, where clayey soil could provide better conditions for mesofauna in long-term sugarcane cultivation.
4.2 Implications of land use change on the community of edaphic mesofauna
Mesofauna community was only affected by the land use in the clayey soil, where the NV was different from the others (Supplementary Table S2, Figure 8). Twenty-two taxonomic categories were identified, with over 70% found in both soil classes, indicating a wide distribution of several organisms. However, five groups were exclusively found in clayey soil, while one group was only found in sandy soil, suggesting a possible preference for specific soil textures. The total abundance of organisms was higher in sandy soil, with mites and Collembola being the most prominent groups. These findings highlight the ecological importance, adaptability, colonization capacity, and efficient reproduction of these organisms (Sanjuán et al., 2022). A plausible hypothesis to be considered is that the method of extracting mesofauna using the Berlese funnel may be less effective in clay soils, favoring the extraction of mesofauna in coarser-textured soils.
Symphyla and Pauropoda predominantly inhabit undisturbed soils characterized by high organic matter content and porosity (Bachelier, 1963; Domínguez Rodríguez, 1992). These organisms exhibit superior adaptation to humid environments, often being found in leaf litter and beneath tree bark. Consequently, the heightened prevalence of Pauropoda in native forests suggests that these ecosystems experience less soil compaction and degradation compared to managed or disturbed areas (Blasi et al., 2013). The predominance of the Symphyla group in a native forest area with clayey soil can be attributed to their preference for such environments, as clayey soils retain greater moisture and organic matter compared to sandy soils (Amooh and Bonsu, 2015) Although the nematodes are collected by our sampling method, it does not give the best representativeness of this specific group, so other methods such as 18S sequencing should be used to investigate in depth this group.
The Psocodea group of mesofauna was found exclusively in NV and only in sandy soil. These insects are specialized in parasitizing birds and are found mainly in the feathers and plumage of these animals (Brewer and Sweet, 2023). NV offers a more favorable environment for the presence of birds, with a greater diversity of species and availability of food resources and shelter (Maitima et al., 2009). However, these are only assumptions based on observations and studies of the ecology of these insects. To better understand why the Psocodea group is only found in native forest areas, more specific research is needed to investigate in detail the relationship between these insects, their host birds, and their environment.
The increased relative abundance led to a higher proportion of mites (non-oribatid) compared to other taxonomic groups, irrespective of land use. The prevalence of mites across various land uses (Menezes-Oliveira et al., 2021) and soil management practices (Menta et al., 2020), suggests their strong adaptability to diverse environmental conditions, underscoring their significant role in regulating soil biological processes. However, higher taxonomic resolution is essential to investigate the feeding habits and specific functions of these soil mites. A decrease in the abundance of mites (non-oribatid) was observed in NV compared to other land uses. The lack of soil disturbance in NV might have contributed to the higher abundance of mites, mainly due to the preserved physical structure and protection of these microarthropods (George et al., 2017), regardless of the soil texture. Oribatids exhibited a lower abundance compared to non-oribatids, aligning with recent research that demonstrated a reduction of oribatid mites to intensified land use (Wehner et al., 2021). This reduced abundance implies the responsiveness of oribatids to changes in land management, underscoring their sensitivity to environmental alterations associated with intensified land use. However, due to their slow development and low metabolic rates, they do not decrease in abundance immediately after disturbance (Behan-Pelletier, 1999), suggesting that the sampling time since the land use conversion in this study was sufficient to observe changes correlated with oribatid abundance.
Similarly, Collembola was more abundant in NV without differing from SC in the two soil textures but with lower relative abundance in the clayey texture. The lower density of Collembola in SCr and PA may decrease the ecosystem services provided by this key functional group (Yin et al., 2020). observed that the functional diversity of Collembola is highly vulnerable to land use management. The authors found that favoring the predominance of species with parthenogenetic reproduction and slow dispersal may justify the results found in intensive land uses (Yin et al., 2020). In addition, the lower abundance of Collembola in clayey soil can be explained by the difficulty these organisms have in digging up the soil due to their small size and the absence of specialized appendages for this function (Kanal, 2004). However, these associations can vary depending on other factors, such as the availability of food resources and the presence of predators.
Thus, conservation practices and proper management are essential to mitigate the negative effects of land use change on mesofauna and, by extension, the ecosystem functioning. Understanding the biodiversity of mesofauna is crucial to understanding and conserving terrestrial ecosystems, and further studies are needed to explore the complex ecological interactions between the different mesofauna groups.
4.3 Relationship between mesofauna groups and soil chemical, physical, and biological attributes across land use
Soil structure and fertility, as well as organic matter, were essential factors in determining the community and distribution of mesofauna (Chamorro-Martínez et al., 2022). The decomposition of litter and the stabilization of organic matter can be influenced by the soil organisms responsible for decomposition (Anderson and Ingram, 1989; Lavelle, 1997). Although soil microorganisms are responsible for most of the decomposition of organic matter, several studies have highlighted the influence of soil mesofauna on decomposition rates, mainly by affecting microbial activity (Cortet et al., 2003; Dervash et al., 2018; Frouz, 2018).
The Diplopoda (millipedes) and Oligochaeta (earthworms) had a direct relationship with soil porosity. Many studies have reported the generally favorable relationship of earthworms on soil porosity (Hallaire et al., 2000). These organisms actively construct tunnels and galleries within the soil, a process that significantly enhances its porosity. As a result, these channels facilitate the ingress of both air and water into the soil, contributing to improved soil aeration and moisture infiltration. On the other hand, Acari, Collembola, Symphyla, and Diplura are associated with potassium and organic matter in the soil. These organisms play an important role in decomposing organic matter and nutrient cycling. They consume decomposing organic matter and transform it into readily available plant nutrients, including potassium (Gunn and Cherrett, 1993; Fujii et al., 2014; Potapov et al., 2017). Kouakou et al. (2022), demonstrated that the total abundance of collembola is intrinsically linked to a substantial increase in organic matter in the soil. A large amount of organic matter creates a favorable microclimate for developing these small arthropods (Chauvat et al., 2007). Symphyla and Diplura remain relatively mysterious in the scientific literature on soil ecology, with little information available (Suárez et al., 2018). studied different land uses in the Colombian Amazon, identifying that the Diplura group shows a strong affinity with native forest areas, underlining their dependence on environments with reduced levels of disturbance. Furthermore, the ideal conditions for these organisms include deeper soil layers, characterized by stable water availability and temperature.
The grouping of SC with SCr and the distancing of PA in the analysis indicates that the pasture has distinct characteristics due to the management practices adopted in this area. At the same time, the sugarcane soils are more similar to each other, especially regarding fertilization and liming. The liming of the sugarcane soil has significantly impacted the mesofauna’s community in these land uses due to its impact on soil pH and nutrient availability (Nisa et al., 2021). Pasture areas have limited food resources and shelter, which favors specific mesofauna groups such as Formicidae and Heteropteran (Vanolli et al., 2021; Vanolli et al., 2023). In addition, some physical attributes, such as soil porosity and biological attributes, such as MBC, were correlated with specific mesofauna groups, including Diplopoda, Oligochaeta, Chilopoda, and Protura. This shows that these groups of organisms are influenced by the structure and fertility of the soil, as well as the presence of organic matter.
5 Conclusion
Different groups of organisms prefer different habitats of soil type, showing how soil influences community. The most abundant groups studied were non-oribatid Acari, oribatid Acari, and Collembola. Land use change causes a loss of richness and abundance of soil mesofauna. However, soil texture is a crucial factor in the restructuring of this soil community since, in clayey soils, the cultivation of sugarcane over the years can recover the diversity of mesofauna in the soil. Managing agroecosystems for enhanced soil biodiversity, such as not burning (green harvesting systems), not removing post-harvest straw, and diversifying crops in the reform, are necessary if sugarcane cultivation is to be beneficial to the soil ecosystem. These results provided a strong understanding of the LUC effects on soil health, supporting the decisions for management practices that promote soil health, biodiversity, and related ecosystem services in different soil textures. We highlight the need for tailored land management considering soil texture and biodiversity for improved ecosystem services.
Data availability statement
The raw data supporting the conclusions of this article will be made available by the authors, without undue reservation.
Ethics statement
The manuscript presents research on animals that do not require ethical approval for their study.
Author contributions
BV: Writing – original draft, Writing – review & editing. NdA: Writing – original draft. LPC: Writing – original draft. AF: Writing – review & editing. AP: Writing – original draft. MC: Writing – original draft.
Funding
The author(s) declare financial support was received for the research, authorship, and/or publication of this article. This research was financed in part by the Coordination for the Improvement of Higher Education Personnel – Brazil (CAPES) – Finance Code 001 and by São Paulo Research Foundation (Process #2018/09845-7, #2019/16764-6, # 2022/14212-9 and 2023/08814-9). MC thanks the National Council for Scientific and Technological Development- CNPq for his Research Productivity Fellowship (311787/2021-5).
Acknowledgments
Sincere thanks are extended to Victor Ferreira Maciel for collaboration in the process of identifying soil mesofauna groups.
Conflict of interest
The authors declare that the research was conducted in the absence of any commercial or financial relationships that could be construed as a potential conflict of interest.
Publisher’s note
All claims expressed in this article are solely those of the authors and do not necessarily represent those of their affiliated organizations, or those of the publisher, the editors and the reviewers. Any product that may be evaluated in this article, or claim that may be made by its manufacturer, is not guaranteed or endorsed by the publisher.
Supplementary material
The Supplementary Material for this article can be found online at: https://www.frontiersin.org/articles/10.3389/fevo.2023.1305115/full#supplementary-material
References
Alvares C. A., Stape J. L., Sentelhas P. C., De Moraes Gonçalves J. L., Sparovek G. (2013). Köppen’s climate classification map for Brazil. Meteorol Zeitschrift. 22 (6), 711–728. doi: 10.1127/0941-2948/2013/0507
Amooh M. K., Bonsu M. (2015). Effects of Soil Texture and Organic Matter on Evaporative Loss of Soil Moisture. Shade Management Options in Cocoa Agroforestry in two ecological zones in Ghana. J. Glob Agric. Ecol. 3, 152–161.
Anderson J. M., Ingram J. S. I. (1989). Tropical soil biology and fertility: A handbook of methods. J. Ecol. 78 (2), 547. doi: 10.2307/2261129
Anthony M. A., Bender S. F., van der Heijden M. G. A. (2023). Enumerating soil biodiversity. Proc. Natl. Acad. Sci. 120 (33). doi: 10.1073/pnas.2304663120
Arboláez H. P. H., Hu J., Orozco Y. N., Gebremikael M. T., Alcantara E. A., Sleutel S., et al. (2023). Mesofauna as effective indicators of soil quality differences in the agricultural systems of central Cuba. Appl. Soil Ecol. 182, 104688. doi: 10.1016/j.apsoil.2022.104688
Banerjee S., van der Heijden M. G. A. (2023). Soil microbiomes and one health. Nat. Rev. Microbiol. 21 (1), 6–20. doi: 10.1038/s41579-022-00779-w
Baretta D., Santos J., Segat J. C., Geremia E. V. (2011). Fauna edáfica e qualidade do solo. Tópicos Em Ciec. Do Solo 7, 119–170.
Barrios E. (2007). Soil biota, ecosystem services and land productivity. Ecol. Econ. 64 (2), 269–285. doi: 10.1016/j.ecolecon.2007.03.004
Behan-Pelletier V. M. (1999). Oribatid mite biodiversity in agroecosystems: role for bioindication. Agric. Ecosyst. Environ. 74 (1–3), 411–423. doi: 10.1016/S0167-8809(99)00046-8
Black H. I. J., Parekh N. R., Chaplow J. S., Monson F., Watkins J., Creamer R., et al. (2003). Assessing soil biodiversity across Great Britain: national trends in the occurrence of heterotrophic bacteria and invertebrates in soil. J. Environ. Manage. 67 (3), 255–266. doi: 10.1016/S0301-4797(02)00178-0
Blasi S., Menta C., Balducci L., Conti F. D., Petrini E., Piovesan G. (2013). Soil microarthropod communities from Mediterranean forest ecosystems in Central Italy under different disturbances. Environ. Monit Assess. 185 (2), 1637–1655. doi: 10.1007/s10661-012-2657-2
Brewer P. J., Sweet A. D. (2023). Prevalence and diversity of parasitic bird lice (Insecta: Psocodea) in northeast Arkansas. Int. J. Parasitol. Parasites Wildl 22, 205–2015. doi: 10.1016/j.ijppaw.2023.06.007
Cao Z., Han X., Hu C., Chen J., Zhang D., Steinberger Y. (2011). Changes in the abundance and structure of a soil mite (Acari) community under long-term organic and chemical fertilizer treatments. Appl. Soil Ecol. 49 (1), 131–138. doi: 10.1016/j.apsoil.2011.06.003
Cavalcanti R. Q., Rolim M. M., de Lima R. P., Tavares U. E., Pedrosa E. M. R., Cherubin M. R. (2020). Soil physical changes induced by sugarcane cultivation in the Atlantic Forest biome, northeastern Brazil. Geoderma 370, 114353. doi: 10.1016/j.geoderma.2020.114353
Chamorro-Martínez Y., Torregroza-Espinosa A., Morenopallares M., Osorio D., Paternina A., Echeverría-González A. (2022). Soil macrofauna, mesofauna and microfauna and their relationship with soil quality in agricultural areas in northern Colombia: ecological implications. Rev. Bras. Ciec. do Solo 46, e0210132. doi: 10.36783/18069657rbcs20210132
Chauvat M., Wolters V., Dauber J., Chauvat M., Wolters V., Dauber J. (2007). Response of collembolan communities to land-use change and grassland succession. Ecography (Cop) 30 (2), 183–192. doi: 10.1111/j.0906-7590.2007.04888.x
Cherubin M. R., Bordonal R. O., Castioni G. A., Guimarães E. M., Lisboa I. P., Moraes L. A. A., et al. (2021a). Soil health response to sugarcane straw removal in Brazil. Ind. Crops Prod. 163, 113315. doi: 10.1016/j.indcrop.2021.113315
Cherubin M. R., Carvalho J. L. N., Cerri C. E. P., Nogueira L. A. H., Souza G. M., Cantarella H. (2021b). Land use and management effects on sustainable sugarcane-derived bioenergy. Land 10 (1), 72. doi: 10.3390/land10010072
Cortet J., Joffre R., Elmholt S., Krogh P. H. (2003). Increasing species and trophic diversity of mesofauna affects fungal biomass, mesofauna community structure and organic matter decomposition processes. Biol. Fertil Soils 37 (5), 302–312. doi: 10.1007/s00374-003-0597-2
Crowther T. W., van den Hoogen J., Wan J., Mayes M. A., Keiser A. D., Mo L., et al. (2019). The global soil community and its influence on biogeochemistry. Science (80-) 365 (6455). doi: 10.1126/science.aav0550
De Graaff M. A., Adkins J., Kardol P., Throop H. L. (2015). A meta-analysis of soil biodiversity impacts on the carbon cycle. SOIL 1 (1), 257–271. doi: 10.5194/soil-1-257-2015
de Morais J. W., Oliveira V dos S., Dambros C de S., Tapia-Coral S. C., Acioli A. N. S. (2010). Soil mesofauna in differents systems of land use soil in upper River Solimões, AM, Brazil. Neotrop Entomol 39 (2), 145–152. doi: 10.1590/S1519-566X2010000200001
Dervash M. A., Bhat R. A., Mushtaq N. (2018). Dynamics and importance of soil mesofauna. 7 (4), 2019–2019
Domínguez Rodríguez M. T. (1992) Shymphyla y Pauropoda (Myriapoda) de suelos de España. Available at: https://dialnet.unirioja.es/servlet/tesis?codigo=15544&info=resumen&idioma=SPA.
Duran-Bautista E. H., Armbrecht I., Serrão Acioli A. N., Suárez J. C., Romero M., Quintero M., et al. (2020). Termites as indicators of soil ecosystem services in transformed amazon landscapes. Ecol. Indic. 117, 106550. doi: 10.1016/j.ecolind.2020.106550
Esquivel D. M. S., Wajnberg E., de Menezes e Souza L. C., Acosta-Avalos D., Pinho M. B., Harada A. Y. (2019). Magnetic material diversity in Brazilian ants: displacement behaviour and environmental adaptability. Eur. Biophys. J. 48 (2), 161–171. doi: 10.1007/s00249-018-1343-x
Franco A. L. C., Bartz M. L. C., Cherubin M. R., Baretta D., Cerri C. E. P., Feigl B. J., et al. (2016). Loss of soil (macro)fauna due to the expansion of Brazilian sugarcane acreage. Sci. Total Environ. , 563–564:160–8. doi: 10.1016/j.scitotenv.2016.04.116
Freire L. R., Araújo E da S., Berbara R. L. L. (2015). Tempo de Captura de Organismos da Mesofauna do Solo e seus Reflexos na Interpretação de Índices da Comunidade Edáfica. Rev. Bras. Ciec. do Solo 39 (5), 1282–1291. doi: 10.1590/01000683rbcs20150117
Frouz J. (2018). Effects of soil macro- and mesofauna on litter decomposition and soil organic matter stabilization. Geoderma 332, 161–172. doi: 10.1016/j.geoderma.2017.08.039
Fujii S., Saitoh S., Takeda H. (2014). Effects of rhizospheres on the community composition of Collembola in a temperate forest. Appl. Soil Ecol. 83, 109–115. doi: 10.1016/j.apsoil.2014.03.018
George P. B. L., Keith A. M., Creer S., Barrett G. L., Lebron I., Emmett B. A., et al. (2017). Evaluation of mesofauna communities as soil quality indicators in a national-level monitoring programme. Soil Biol. Biochem. 115, 537–546. doi: 10.1016/j.soilbio.2017.09.022
Gergócs V., Flórián N., Tóth Z., Sipőcz L., Dombos M. (2022). Detangling ecosystem services: Open-field manipulation of soil-dwelling microarthropods provides new opportunities to investigate their effects on nitrogen cycling. Ecol. Evol. 12 (7), e9134. doi: 10.1002/ece3.9134
Gunn A., Cherrett J. M. (1993). The exploitation of food resources by soil meso- and macro invertebrates. Pedobiologia (Jena). 37 (5), 303–320.
Hallaire V., Curmi P., Duboisset A., Lavelle P., Pashanasi B. (2000). Soil structure changes induced by the tropical earthworm Pontoscolex corethrurus and organic inputs in a Peruvian ultisol. Eur. J. Soil Biol. 36 (1), 35–44. doi: 10.1016/S1164-5563(00)01048-7
Handa I. T., Aerts R., Berendse F., Berg M. P., Bruder A., Butenschoen O., et al. (2014). Consequences of biodiversity loss for litter decomposition across biomes. Nat 509 (7499), 218–221. doi: 10.1038/nature13247
Heisler C., Kaiser E. A. (1995). Influence of agricultural traffic and crop management on collembola and microbial biomass in arable soil. Biol. Fertil Soils 19 (2–3), 159–165. doi: 10.1007/BF00336153
Howe J. A., Smith A. P. (2021). “The soil habitat,” in Principles and applications of soil microbiology, eds. T. J. Gentry, J. J. Fuhrmann and D. A. Zuberer (Amsterdan: Elsevier), 23–55.
Joimel S., Chassain J., Artru M., Faburé J. (2022). Collembola are among the most pesticide-sensitive soil fauna groups: A meta-analysis. Environ. Toxicol. Chem. 41 (10), 2333–2341. doi: 10.1002/etc.5428
Kanal A. (2004). Effects of fertilisation and edaphic properties on soil-associated Collembola in crop rotation. Agron. Res. 2 (2), 153–168.
Kassambara A., Mundt F. (2017). Package ‘factoextra’. Extr Vis. results Multivar Data Anal. 76, 50–54.
Kouakou A. K., Cortet J., Kolo Y., Brauman A. (2022). Using trait-based approaches to assess the response of epedaphic collembola to organic matter management practices: A case study in a rubber plantation in South-Eastern Côte d’Ivoire. Insects 13 (10), 892. doi: 10.3390/insects13100892
Lavelle P. (1997). Faunal activities and soil processes: adaptive strategies that determine ecosystem function. Adv. Ecol. Res. 27 (C), 93–132. doi: 10.1016/S0065-2504(08)60007-0
Lavelle P., Decaëns T., Aubert M., Barot S., Blouin M., Bureau F., et al. (2006). Soil invertebrates and ecosystem services. Eur. J. Soil Biol. 42 (SUPPL. 1), S3–15. doi: 10.1016/j.ejsobi.2006.10.002
Maitima J. M., Mugatha S. M., Reid R. S., Gachimbi L. N., Majule A., Lyaruu H., et al. (2009). The linkages between land use change, land degradation and biodiversity across East Africa. Afr. J. Environ. Sci. Technol. 3 (10), 310–325.
Mayel S., Jarrah M., Kuka K. (2021). How does grassland management affect physical and biochemical properties of temperate grassland soils? A review study. Grass Forage Sci. 76 (2), 215–244. doi: 10.1111/gfs.12512
Menandro L. M. S., de Moraes L. O., Borges C. D., Cherubin M. R., Castioni G. A., Carvalho J. L. N. (2019). Soil macrofauna responses to sugarcane straw removal for bioenergy production. Bioenergy Res. 12 (4), 944–957. doi: 10.1007/s12155-019-10053-2
Menezes-Oliveira V. B., Bianchi M. O., Espíndola E. L. G. (2021). Changes in soil mesofauna structure due to different land use systems in south Minas Gerais, Brazil. Environ. Monit Assess. 193 (7), 1–9. doi: 10.1007/s10661-021-09214-8
Menta C., Conti F. D., Fondón C. L., Staffilani F., Remelli S. (2020). Soil arthropod responses in agroecosystem: implications of different management and cropping systems. Agron 10 (7), 982. doi: 10.3390/agronomy10070982
Morais M. C., Siqueira-Neto M., Guerra H. P., Satiro L. S., Soltangheisi A., Cerri C. E. P., et al. (2020). Trade-offs between sugarcane straw removal and soil organic matter in Brazil. Sustain 12 (22), 9363. doi: 10.3390/su12229363
Nisa R. U., Tantray A. Y., Kouser N., Allie K. A., Wani S. M., Alamri S. A., et al. (2021). Influence of ecological and edaphic factors on biodiversity of soil nematodes. Saudi J. Biol. Sci. 28 (5), 3049–3059. doi: 10.1016/j.sjbs.2021.02.046
Oksanen J., Simpson G. L. (2009) The vegan Package Ecological legacies in the Mesoamerican forest View project Tools for carbon neutral farming: the role of dugouts (agricultural reservoirs) in greenhouse gas capture View project. Available at: http://cran.r-project.org/.
Parisi V., Menta C., Gardi C., Jacomini C., Mozzanica E. (2005). Microarthropod communities as a tool to assess soil quality and biodiversity: a new approach in Italy. Agric. Ecosyst. Environ. 105 (1–2), 323–333. doi: 10.1016/j.agee.2004.02.002
Pereira R de C., Albanez J. M., Mamédio I. M. P. (2012). Diversity of meso and macrofauna in different management systems of land use in Cruz das Almas-Bahia. Magistra 24 (SINSECTA), 63–76.
Pimentel L. G., Cherubin M. R., Oliveira D. M. S., Cerri C. E. P., Cerri C. C. (2019). Decomposition of sugarcane straw: Basis for management decisions for bioenergy production. Biomass Bioenergy. 122, 133–144. doi: 10.1016/j.biombioe.2019.01.027
Porre R. J., van Groenigen J. W., De Deyn G. B., de Goede R. G. M., Lubbers I. M. (2016). Exploring the relationship between soil mesofauna, soil structure and N2O emissions. Soil Biol. Biochem. 96, 55–64. doi: 10.1016/j.soilbio.2016.01.018
Potapov A. M., Goncharov A. A., Semenina E. E., Korotkevich A. Y., Tsurikov S. M., Rozanova O. L., et al. (2017). Arthropods in the subsoil: Abundance and vertical distribution as related to soil organic matter, microbial biomass and plant roots. Eur. J. Soil Biol. , 82:88–82:97. doi: 10.1016/j.ejsobi.2017.09.001
Pulleman M., Creamer R., Hamer U., Helder J., Pelosi C., Pérès G., et al. (2012). Soil biodiversity, biological indicators and soil ecosystem services—an overview of European approaches. Curr. Opin. Environ. Sustain. 4 (5), 529–538. doi: 10.1016/j.cosust.2012.10.009
Reichert J. M., Amado T. J. C., Reinert D. J., Rodrigues M. F., Suzuki L. E. A. S. (2016). Land use effects on subtropical, sandy soil under sandyzation/desertification processes. Agric. Ecosyst. Environ. 233, 370–380. doi: 10.1016/j.agee.2016.09.039
Reichert J. M., Norton L. D., Favaretto N., Huang C., Blume E. (2009). Settling velocity, aggregate stability, and interrill erodibility of soils varying in clay mineralogy. Soil Sci. Soc. Am. J. 73 (4), 1369–1377. doi: 10.2136/sssaj2007.0067
Rieff G., Natal-da-Luz T., Sousa J., Science M. W.-C. (2016). Collembolans and Mites communities as a tool for assessing soil quality: effect of eucalyptus plantations on soil mesofauna biodiversity. Curr. Sci. 110 (4), 713–719. doi: 10.18520/cs/v110/i4/713-719
Roy M. L., Dey B., Srivastava R. C. (2009). Influence of soil application of growth-promoting diazotrophs and chemical fertilizers on biochemical parameters and biomass of a rice cultivar of Tripura. Proc. Natl. Acad. Sci. India Sect B Biol. Sci. 79 (3), 283–288.
Rutgers M., Schouten A. J., Bloem J., Van Eekeren N., De Goede R. G. M., Jagers Op Akkerhuis G. A. J. M., et al. (2009). Biological measurements in a nationwide soil monitoring network. Eur. J. Soil Sci. 60 (5), 820–832. doi: 10.1111/j.1365-2389.2009.01163.x
Sanjuán F. A. B., González L. C., Prado R de M. (2022). Edaphic mesofauna, some studies done: a review. INGE CUC 18 (2), 197–208. doi: 10.17981/ingecuc.18.2.2022.16
Sparling G. P., West A. W. (1988). A direct extraction method to estimate soil microbial C: calibration in situ using microbial respiration and 14C labelled cells. Soil Biol. Biochem. 20 (3), 337–343. doi: 10.1016/0038-0717(88)90014-4
Su J. Q., Ding L. J., Xue K., Yao H. Y., Quensen J., Bai S. J., et al. (2015). Long-term balanced fertilization increases the soil microbial functional diversity in a phosphorus-limited paddy soil. Mol. Ecol. 24 (1), 136–150. doi: 10.1111/mec.13010
Suárez L. R., Josa Y. T. P., Samboni E. J. A., Cifuentes K. D. L., Bautista E. H. D., Salazar J. C. S. (2018). Soil macrofauna under different land uses in the Colombian Amazon. Pesqui Agropecuária Bras. 53 (12), 1383–1391. doi: 10.1590/s0100-204x2018001200011
Tsiafouli M. A., Thébault E., Sgardelis S. P., de Ruiter P. C., van der Putten W. H., Birkhofer K., et al. (2015). Intensive agriculture reduces soil biodiversity across Europe. Glob Chang Biol. 21 (2), 973–985. doi: 10.1111/gcb.12752
USDA-Natural Resources Conservation Service (2014). “Keys to soil taxonomy,” in Soil Taxonomy, 12th ed Agriculture Department (Washington, DC: Agriculture Department).
van Capelle C., Schrader S., Brunotte J. (2012). Tillage-induced changes in the functional diversity of soil biota – A review with a focus on German data. Eur. J. Soil Biol. 50, 165–181. doi: 10.1016/j.ejsobi.2012.02.005
Vance E. D., Brookes P. C., Jenkinson D. S. (1987). An extraction method for measuring soil microbial biomass C. Soil Biol. Biochem. 19 (6), 703–707. doi: 10.1016/0038-0717(87)90052-6
Vanolli B. S., Canisares L. P., Franco A. L. C., Delabie J. H. C., Cerri C. E. P., Cherubin M. R. (2021). Epigeic fauna (with emphasis on ant community) response to land-use change for sugarcane expansion in Brazil. Acta Oecologica. 110, 103702. doi: 10.1016/j.actao.2021.103702
Vanolli B. S., Pereira A. P. A., Franco A. L. C., Cherubin M. R. (2023). Edaphic and epigeic macrofauna responses to land use change in Brazil. Eur. J. Soil Biol. 117, 103514. doi: 10.1016/j.ejsobi.2023.103514
Van Raij B., Andrade J. C., Cantarella H., Quaggio J. (2001). Análise química para avaliação da fertilidade de solos tropicais (Campinas: Instituto Agronômico), 285. Available at: www.iac.br.
Wehner K., Simons N. K., Blüthgen N., Heethoff M. (2021). Drought, windthrow and forest operations strongly affect oribatid mite communities in different microhabitats. Glob Ecol. Conserv. 30, e01757. doi: 10.1016/j.gecco.2021.e01757
Yan S., Singh A. N., Fu S., Liao C., Wang S., Li Y., et al. (2012). A soil fauna index for assessing soil quality. Soil Biol. Biochem. 47, 158–165. doi: 10.1016/j.soilbio.2011.11.014
Keywords: bioenergy, soil biodiversity, soil fauna, soil health, TSBF
Citation: Vanolli BS, de Andrade N, Canisares LP, Franco ALC, Pereira APA and Cherubin MR (2024) Edaphic mesofauna responses to land use change for sugarcane cultivation: insights from contrasting soil textures. Front. Ecol. Evol. 11:1305115. doi: 10.3389/fevo.2023.1305115
Received: 30 September 2023; Accepted: 18 December 2023;
Published: 08 January 2024.
Edited by:
Luís Carlos Iunes Oliveira Filho, Universidade do Estado de Santa Catarina, BrazilCopyright © 2024 Vanolli, de Andrade, Canisares, Franco, Pereira and Cherubin. This is an open-access article distributed under the terms of the Creative Commons Attribution License (CC BY). The use, distribution or reproduction in other forums is permitted, provided the original author(s) and the copyright owner(s) are credited and that the original publication in this journal is cited, in accordance with accepted academic practice. No use, distribution or reproduction is permitted which does not comply with these terms.
*Correspondence: Beatriz S. Vanolli, YmVhdHJpenZhbm9sbGlAdXNwLmJy