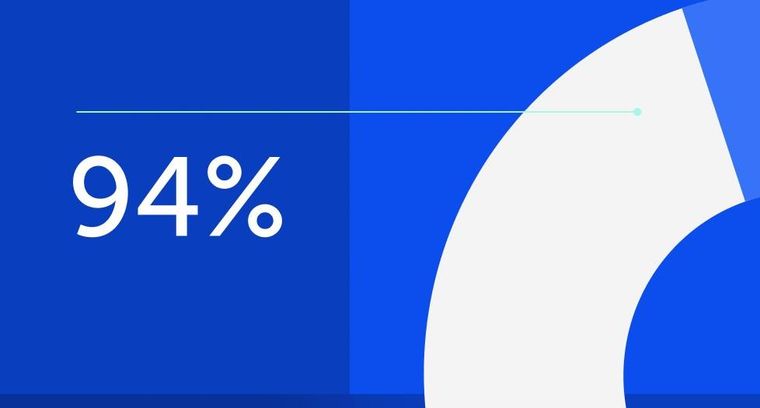
94% of researchers rate our articles as excellent or good
Learn more about the work of our research integrity team to safeguard the quality of each article we publish.
Find out more
ORIGINAL RESEARCH article
Front. Ecol. Evol., 06 November 2023
Sec. Conservation and Restoration Ecology
Volume 11 - 2023 | https://doi.org/10.3389/fevo.2023.1280303
Soil stoichiometric characteristics and aggregate stability are affected by vegetation restoration in degraded land. Yet, it is not known that how the aggregate stability is related to soil stoichiometric characteristics under different vegetation restorations. A 5-year in situ experiment was conducted to investigate the effects of vegetation restoration on the composition, stability and stoichiometric properties of soil aggregates. In the northwest part of Beijing, Bromus inermis Leyss. and Medicago sativa L. were planted in a typical area of desertification, and natural restoration was used as control. Boosted regression trees (BRTs) were applied to partition the factors that control aggregate stability. The results showed that the mean weight diameter (MWD) of soil water-stable aggregates under natural restoration (CK) and Medicago sativa L. sowing treatments (AF) was significantly higher than that under the restoration of the Bromus inermis Leyss. sowing treatments (SB). Compared with CK, AF significantly increased the geometric mean diameter (GMD) water stable aggregates, while SB showed the opposite result. AF significantly increased the proportion of soil aggregates >2 mm compared with CK. AF could improve the stability of soil aggregates by increasing the proportion of large aggregates. For the stoichiometric characteristics of the aggregates, AF increased significantly the value of C/P in 0.053−0.125 mm particle size aggregates in all soil layers. The MBC/MBN ratio aggregates at depths of 0–10 cm and 10–20 cm was also significantly increased in the treatment of AF. The BRTs indicated that stoichiometric ratio is the main factor driving the stability of soil aggregates rather than microbial community characteristics and soil nutrients. The C/P is the main driving factor affecting the MWD, in which the overall stoichiometric influence accounts for 46%, followed by the microbial influence of 36%. For the GMD, MBC/MBN is the main driving factor, and the stoichiometric influence accounts for 94%. Our findings indicate that AF is beneficial to the stability of deep soil aggregates, and their stoichiometric characteristics of soil are the key factors affecting the stability of soil aggregates.
A growing number of ecosystems are experiencing large-scale degradation due to the increasing impact of climate change and unsustainable land use (Mao et al., 2018; Xie et al., 2020; Bardgett et al., 2021). Land degradation poses a serious threat to biodiversity, land desertification, human living environment and sustainable development of social and economic systems (Winslow et al., 2011; Solomun et al., 2018; Liu et al., 2022a). Vegetation restoration can speed up the restoration process and is one of the common and effective ways to restore degraded land and ecosystem functions (Chen et al., 2005; Klopf et al., 2017; Miao et al., 2018; Hu et al., 2022).The purpose of vegetation restoration is not only to improve biodiversity, but also to improve soil health, including soil structure, soil nutrient and soil quality (Vaverkova et al., 2018; Zhao et al., 2020; Xu et al., 2021; Liu et al., 2022b).
Soil aggregates play an important role in soil structure and fertility (Pérès et al., 2013; Yao et al., 2019; Liu et al., 2023). Soil aggregate stability is one of the relevant indexes of broader soil stability. This refers to the ability of soil aggregates to resist damage when resulting from destructive force (water, wind), and is a good indicator of soil sensitivity to erosion (Barthes and Roose, 2002; Oztas and Fayetorbay, 2003). Soil mean weight diameter (MWD) and geometric mean diameter (GMD) are important indices of aggregate stability, and the larger the value of MWD and GMD, the stronger the stability of aggregates (Schaller and Stockinger, 1953; Padbhushan et al., 2016). Soil aggregation is a complex process, which is regulated by vegetation type (Duchicela et al., 2012; Dou et al., 2020), fertilization (Eviner and Chapin, 2002) and management measures (Du et al., 2022; Ren et al., 2022), etc. For example, the distances between alfalfa and jujube at 0.5 m can significantly increase the amounts of macro-aggregates, and improved soil mechanical properties and aggregate stability among the other treatments in herbage-fruit tree intercropping systems (Chen et al., 2023). Long-term fertilization for 26 years significantly increased MWD and GMD, which meant that the stability of soil aggregates was improved (Mustafa et al., 2020). Previous studies have shown microbial changes driven by the change of soil nutrient might be the major factor affecting soil surface aggregate stability in the process of degradation succession in typical grassland (Ren et al., 2022). Microorganisms can interact with soil particles through several ways such as mycelium entanglement, microbial secretion of metabolites, and accelerated decomposition of organic matter, thus contributing to the formation and stability of soil aggregates (Chenu et al., 2000; Chenu and Stotzky, 2001). In addition, in the process of vegetation restoration, the selection of different plants has different effects on soil aggregates. For example, studies have shown that the effects of vegetation restoration measures on the stability of soil aggregates were different and the MWD and GMD were both highest in two soil layers (0–20 cm and 20–40 cm) only under the natural shrub restoration on the Loess Plateau, China (Dou et al., 2020). Conversely, a previous study showed that natural pasture restoration improved soil physical properties and water stable aggregate stability better than planting species (Wang et al., 2012; Wu et al., 2017). In northern China, Medicago sativa L. (alfalfa) and Bromus inermis Leyss. (smooth brome) are often used a community building species for degraded land restoration (Huang et al., 2018). Unlike the fibrous roots of smooth brome, alfalfa is a premium perennial leguminous plant that may be an improvement in texture and nutrients in deeper soils (Hafner and Kuzyakov, 2016). Our previous studies indicated that short-term alfalfa planting could enhance surface soil fertility (Xu et al., 2022a). Previous studies have shown that the increase of organic matter and total nitrogen content in aged workers is conducive to the formation and stability of aggregates (Zhang et al., 2016; Ma et al., 2020; Lu et al., 2021). However, in the process of vegetation restoration, there are few reports on the influence of different herbaceous restoration methods on the soil aggregate stability and its driving factors (microbial community characteristics, stoichiometric characteristics and soil nutrients) in degraded land.
Soil carbon (C), nitrogen (N) and phosphorus (P) stoichiometry has been extensively studied around the world in recent decades (Cleveland and Liptzin, 2007; Bai et al., 2012; Sun et al., 2022; Wang et al., 2022). Soil aggregate stability and its stoichiometric characteristics are the effective ways to evaluate the effect of soil restoration and various sized aggregates play different roles in the supply and transport of soil C, N, and P (Chen et al., 2021; Tang and Wang, 2022). Therefore, in order to better understand the effects of different vegetation restoration on soil structure and function, it is necessary to study the stoichiometric characteristics of soil C, N and P at the aggregate scale (Zhao et al., 2015; Smith et al., 2019; Xu et al., 2022c). In recent years, some studies have investigated the distribution of soil stoichiometric characteristics in aggregates. For example, the increases in soil aggregates C:N, C:P, and N:P ratios were accompanied by the decreasing aggregate size in Chinese fir plantations (Tang and Wang, 2022). The vegetation restoration only significantly reduced the value of N:P and C:P in different particle size aggregates and had no significant effect on the C:N in all particle size aggregates (Xu et al., 2022c). However, it is not known how the stability of soil aggregates is related to soil stoichiometric characteristics under different vegetation restorations. Therefore, we hypothesized that different vegetation restoration may change the soil stoichiometric characteristics of carbon and nitrogen, thus affecting the stability of aggregates.
The effects of planting alfalfa and brome after five years on soil aggregate composition, stoichiometry and stability in degraded wastelands in North China Plain were studied. The objectives of this study were to (1) analyze the influence of different vegetation restorations on the soil aggregate stability, (2) evaluate the changes of soil aggregate composition and structure after five years of vegetation restoration, and (3) explore the key driving factors (microbial community characteristics, stoichiometric characteristics and soil nutrients) affecting the soil aggregate stability.
The experiment was conducted at the Long-term Ecological Research Station of Degraded Land in Yanqing District, Beijing (40°27′53″ N, 115°50′23″ E, 492 m a.s.l.) (Figure S1). The area receives an average annual precipitation of approximately 600 mm, rainfall mainly occurs between June and September. The average annual temperature from 2015 to 2020 was 8.4 °C. The soil at the site is classified as intermediate between sandy soil and loam (Xu et al., 2022a). The soil is currently a cinnamon soil. The climate type of the region is warm temperate semi-humid and semi-arid monsoon climate. The relative biomass of dominant plant species observed at the experiment were Pennisetum centrasiaticum Tzvel. (55%), Artemisia capillaris Thunb (18%), and Melica scabrosa Trin. (15%).
In 2015, we conducted a randomized field experiment utilizing 12 plots in total. Each plot had an area of 100 m2 (10 m × 10 m), and we planted three different species: Medicago sativa (AF) in four plots, Bromus inermis Leyss. (SB) in four plots, and natural restoration (CK) in the remaining four plots (3 treatment and 4 plots). The seeds were selected based on the adaptation of these plants to the local environment. The seeds were sown without plowing in May 2015 at a density of 200 seeds per m2 for each species. Once the vegetation had become established, the area was fenced off. Medicago sativa (AF) and Bromus inermis Leyss. (SB) allow it to grow naturally without any interference.
In 2020, three quadrats of 0.5 m × 0.5 m were randomly placed in each plot. In each plot, aboveground biomass and litter weight were investigated in three randomly selected 0.5 m × 0.5 m quadrats. Three 0–10 cm, 10–20 cm, and 20–30 cm soil cores (in 5 cm diameter) were collected from each quadrat and then pooled to form one sample. The samples were passed through a 5 mm sieve to remove plant roots and rocks, and then air-dried. 100 g of the air-dried soil samples were utilized for wet sieving. The distribution of different particle size aggregates was determined using a nested sieve consisting of three sieve sizes with apertures of 2.00 mm, 0.25 mm, and 0.053 mm (Pohl et al., 2012; Cates et al., 2016). By utilizing wet sieving (Tang and Wang, 2022), four different size classes of soil aggregates were separated: i) >2000 μm (macro-aggregates), ii) 250–2000 μm (macro-aggregates), iii) 53–250 μm (micro-aggregates), and iv) < 53 μm (silt + clay particles). After wet sieving, all of the separated aggregates were dried at 65 °C and weighed. The particle size distribution of soil aggregates is determined by calculating the weight percentage of each aggregate size class relative to the total weight of all aggregates.
The organic carbon (TOC) content of aggregates with different particle sizes was determined by combustion method (Wallace and Purcell, 2003), which involves pre-treating the sample with dilute acid to remove carbonate carbon and then analyzing for total carbon using a TOC analyzer (Rapid CS Cube, Elementar, Langenselbold, Germany). The total nitrogen (TN) was measured with a C/N analyzer (Rapid CS Cube, Elementar, Langenselbold, Germany). Soil pH was detected through the glass electrode. Soil pH was measured by a potentiometer after shaking a soil water suspension (1:2.5 water/soil) for 30 min (Miao et al., 2018). Soil P content was measured by the approach of molybdenum blue colorimetric (Xu et al., 2022c). Microbial biomass carbon (MBC) and microbial biomass nitrogen (MBN) were quantified by comparing sets of chloroform-fumigated and non-fumigated soil samples (Xu et al., 2022b). The coefficients giving the extractable part of MBC and MBN were set at 0.45 and 0.54, respectively (Jaeger III et al., 1999). The microbial community’s genomic DNA was extracted from 0.25g of moist soil using the PowerSoil DNA Isolation Kit (MO BIO laboratories, Carlsbad, CA, USA) following a standardized protocol. High throughput sequencing was used to calculate the diversity of soil microbial, bacterial and fungal communities (Xu et al., 2022b). Information on primers for bacteria and fungi can be found in this study (Xu et al., 2022b).
Soil mean weight diameter (MWD, mm) value and geometric mean diameter (GMD, mm) were obtained by this formula:
In the formula, i (mm) indicates the average diameter associated with the ith size aggregates, and Wi (% in weight) represents the proportion associated with the ith size aggregates.
The normality and homogeneity of the data were checked using Shapiro–Wilk and Levene tests before any statistical analysis (Figure S2). One-way analysis of variance (ANOVA) was conducted for detecting how different restoration methods affected soil aggregate composition, stoichiometry and stability in R 3.5.2 (R Development Core Team, 2015). The Bray–Curtis method was used to further verify the effects of different vegetation restoration on bacterial and fungal community structure by non-metric multidimensional scaling (NMDS). The Duncan new repolarization difference method was used for significant differences at a significance level of P < 0.05. We used boosted regression trees (BRTs) to partition independent influences of microbial characteristic (α diversity and β diversity of bacteria and fungi, MBC and MBN), stoichiometric characteristics of aggregates (C/N, N/P, C/P, MBC/MBN) and soil conditions (TOC, TP, TN, pH) on MWD and GMD. The parameter combination returning the highest cross-validated model fit was selected, with a tree complexity of one, a learning rate of 0.001 and a bag fraction of 0.8. BRTs were fitted in R, using the dismo package (Elith et al., 2008; Guo et al., 2021).
The MWD of soil water-stable aggregates under natural restoration (CK) and the reseeding of AF was significantly higher than reseeding of SB (Figure 1A; P < 0.001). The GWD of soil water-stable aggregates was significantly increased by reseeding of AF compared to the CK, but the effect was opposite to the reseeding of SB (Figure 1B; P < 0.001). Through the analysis of the MWD of water-stable aggregates in different soil layers, AF significantly increased the MWD of water-stable aggregates in 20–30 cm soil layers (Figure 1C; P < 0.05). Through the analysis of the GWD of soil water-stable aggregates in different soil layers, it was found that the GWD of soil water-stable aggregates in 10–20 cm and 20–30 cm was significantly reduced by reseeding of SB (Figure 1D; P < 0.01). AF significantly increased the GWD of soil water-stable aggregates at 20–30 cm (Figure 1D; P < 0.01). By analyzing the composition of soil aggregates in the 0–10 cm, 10–20 cm and 20–30 cm soil layer, it was found that AF significantly increased the soil aggregate mass fraction of grain size >2.00 mm (Figure 2).
Figure 1 Effects of different restoration measures on soil mean weight diameter (A) and geometric mean diameter (B). soil mean weight diameter (C) and geometric mean diameter (D) response at different soil depth. CK, natural recovery; AF, Medicago sativa L sowing; and SB, Bromus inermis Leyss. sowing.
Figure 2 Effects of different restoration methods on soil aggregate composition. CK, natural recovery; AF, Medicago sativa L sowing; and SB, Bromus inermis Leyss. sowing.
In the 0–10 cm soil layer, different restoration methods had different effects on TN in soil aggregates under different particle sizes (Figure 3; P < 0.05). SB significantly increased TN content of soil with diameter of >2.00 mm. SB and AF significantly increased soil TN content of soil with diameter of 0.250–1 mm (Figure 3; P < 0.05). SB and AF significantly increased soil TOC content in aggregates of all particle sizes. Compared with soil total phosphorus, vegetation restoration significantly increased. SB and AF significantly increased soil TP content of soil with diameter of >2 mm and 0.053–0.250 mm. Both restoration methods were significantly improved the MBC content of >2 mm, 1~2 mm, 0.053~1 mm diameter. Only at 0.053–0.250 mm particle size, SB significantly improved the MBN content (Figure 3; P < 0.05).
Figure 3 Soil carbon, nitrogen and phosphorus content characteristics under different soil depth and aggregate particle size. CK, natural recovery; AF, Medicago sativa L sowing; and SB, Bromus inermis Leyss. sowing.
In the 10–20 cm soil layer, SB significantly increased TN content of >2.00 mm grain size (Figure 3; P < 0.05). SB and AF significantly increased TOC content in aggregates of all particle sizes. Vegetation restoration had no significant difference in soil TP content under all particle sizes. Both restoration methods improved significantly MBC content of >2.00 mm, 1~2 mm, 0.250–1 mm diameter. The MBN of SB was significantly higher than that of other treatments only at the size of 1–2 mm (Figure 3; P < 0.05).
In the 20–30 cm soil layer, there was no significant difference in TN among different particle sizes (Figure 3; P < 0.05). SB and AF significantly increased soil TOC content. For soil TP, AF significantly increased the soil TP content at 1~2 mm and 0.250–1 mm grain size (Figure 3; P < 0.05). Both restoration methods were significantly improved MBC content of >2 mm, 0.250–1 mm, 0.053–0.250 mm particle size. The content of MBN of AF was significantly higher than that of other treatments only at 0.250–1 mm diameter (Figure 3; P < 0.05).
In the 0–10 cm soil layer, AF and SB had significantly increased the C/N ratio of 0.053–0.1235mm particle size (Figure 4; P < 0.05). Vegetation restoration had no significant effect on N/P in any soil layer and particle size. At 0–10 cm, the C/P ratio of 0.125–1 mm and 0.053–0.125 mm was significantly increased by AF. The C/P ratio of 0.053–0.0125 mm in soil layers of 10–20 cm and 20–30 cm was also significantly increased by AF and SB (Figure 4; P < 0.05). AF significantly increased the MBC/MBN of all particle sizes 0–10 cm and 10–20 cm (Figure 5; P < 0.05). AF significantly increased the stoichiometric ratio of MBC/MBN of > 2 mm particle size, while SB significantly increased the stoichiometric ratio of MBC/MBN of 0.125–1 mm (Figure 5; P < 0.05).
Figure 4 Soil C/N, N/P and C/P under different soil depth and aggregate particle size. CK, natural recovery; AF, Medicago sativa L sowing; and SB, Bromus inermis Leyss. sowing. C/P: the ratio of carbon to phosphorus; C/N: the ratio of carbon to nitrogen; N/P: the ratio of nitrogen to phosphorus.
Figure 5 The effects of different vegetation restoration on microbial biomass carbon (MBC), microbial biomass nitrogen (MBN) and microbial biomass carbon nitrogen ratio (MBC/MBN) under different soil depth and different aggregate particle size. CK, natural recovery; AF, Medicago sativa L sowing; and SB, Bromus inermis Leyss. sowing.
All indexes were divided into microorganisms (α diversity and β diversity of bacteria and fungi, microbial biomass carbon and nitrogen), stoichiometry (C/N, N/P, C/P, MBC/MBN) and soil physicochemical properties (TOC, TP, TN, pH). Through enhanced regression tree analysis, the results show that C/P is the main driving factor affecting the MWD, in which the overall stoichiometric influence accounts for 46%, followed by the microbial influence of 36%. For the GMD, MBC/MBN is the main driving factor, and the stoichiometric influence accounts for 94%.
A previous study showed that the stability of aggregates at 0.15m of soil surface varies with different plant species, and that the stability of aggregates at 0.15–0.45 m is not affected by plant species (Eviner and Chapin, 2002). In this study, different plant types have different effects on the stability of deep soil aggregates. The MWD and GMD of water-stable aggregate were significantly reduced after 5 years of reseeding SB, but AF significantly increased GMD by 15.1% compared with CK treatment (Figures 1A, B). This was mainly because AF significantly increased the MWD and GMD in 20–30 cm (Figure 1D). On the other hand, AF can improve the stability of soil aggregates by increasing the content of large aggregates (>2 mm) in all soil layers (Figure 2). The higher the content of soil water-stable macroaggregates, the more stable soil aggregates, and the better the structural stability of soil (Celik, 2005; Xu et al., 2022c). This indicates that AF planting in degraded land can improve soil aggregate structure and enhance aggregate stability within 0–30 cm. However, SB had no significant effect on large aggregates, but significantly increased the proportion aggregates of 0.053–0.125 mm size at 0–10 cm (Figure 2). A previous study showed that the stability of soil aggregates in the surface layer was higher than that in the subsoil layer on the Loess Plateau (An et al., 2008), which could indirectly support our results. This may be because, unlike the deep roots of AF (Clement et al., 2022; Song et al., 2022; Wang et al., 2023), the shallow rhizomes of SB affect aggregate structure only in surface soil. This could also explain why the GMD of 10–20 cm and 20–30 cm in SB were decreased. The results of this study also showed that the proportion of >2 mm size of soil aggregates was a good index to evaluate the effect of vegetation restoration on soil aggregates (Huang et al., 2010). Therefore, AF has important potential as a recovery plant for restoring degraded land from the perspective of soil aggregate stability and soil quality improvement.
The C and N are structural elements of organic matter, so the distribution of C and N contents in aggregates will affect the change of soil organic matter content (Cooper et al., 2020). Vegetation restoration can promote the accumulation of organic carbon through biomass input, thus promoting the stability of soil aggregates (Tang et al., 2010). In addition, vegetation restoration reduced nutrient loss in soil aggregates and leads to a greater ability to collect organic matter (Zhang and Marschner, 2018). It has been found that the content of C and N varies with different aggregates sizes, and <0.25 mm particle size aggregates had higher C and N content (Egan et al., 2018; Tang and Wang, 2022; Wang et al., 2019), but C and N content were evenly distributed in various sized aggregates in our study. In addition, in our present research, both vegetation restoration measures significantly increased the TOC content of aggregates in all soil layers (Figure 3). Different from the increasing trend of carbon, only the TN content of large aggregates in 0–10 cm and 10–20 cm soil layers was increased under the treatment of AF seeding (Figure 3). This indicates that different restoration methods have different degrees of influence on TOC and TN content in different aggregates sizes and soil layers, which will significantly affect the C/N of different aggregates. Previous research had shown that the C/N ratio of soil aggregates was relatively stable in both Chinese fir forest restoration (Tang and Wang, 2022) and herbaceous plant restoration (Xu et al., 2022c). Unlike our results, only in the size of 0.053–0.125 mm, vegetation restoration significantly increased soil TOC content, but there was no significant difference in TN content among different treatments, which resulted in a significant increase in C/N by vegetation restoration in 20–30 cm soil layer (Figure 4). This indicated that the two vegetation restoration methods had insufficient ability to improve the nitrogen content of deep soil microaggregates (0.053–0.125 mm). N/P ratio is an effective indicator of nutrient restriction, because soil N and P are the most common limiting factors in plant growth (Khan et al., 2016). In our study, there was no significant difference in N/P ratio between different aggregates and soil depth (Figure 4). However, vegetation restoration significantly reduced the value of N:P in different particle size aggregates in a typical acid rain area in south China (Xu et al., 2022c). This is because the average P content in this area is about 0.19 g/kg (the P content in our study site was about 0.6 g/kg), and the soil aggregates were obviously limited by the P element. This indicated that the effect of vegetation restoration on soil N/P ratio was influenced by the P content in the background soil before restoration. Different from the study results that vegetation restoration significantly reduced C/P ratio under different aggregate sizes (Xu et al., 2022c), our study showed that AF significantly increased the C/P of small aggregates. The main reason is that different vegetation restoration has no significant effect on the P content in small aggregates, while the C content increased significantly (Figure 3). This differential response leads to a significant increase in C/P ratio. The MBC/MBN in soil strongly determines the adjustment of terrestrial ecosystem C and N cycling, which is very important for the stoichiometric balance of resources (Mooshammer et al., 2014). In general, the distribution of microbial biomass in soil aggregates is closely related to TOC content, which is similar to the present study (Six et al., 2004). The TOC and MBC content of aggregate particle size and soil depth were significantly increased by both the two restoration methods (Figures 3, 5). This may be because alfalfa has higher aboveground biomass (Figure S3A), which leads to more litter input (Figure S3B), and the increase of exogenous organic matter increases microbial biomass carbon.
Previous studies have shown that soil aggregate stability is positively correlated with the content of MBC, MBN, OC and TN in forest ecosystems (Zhong et al., 2017; Xiao et al., 2019; Mao et al., 2021). This may be because organic matter plays a critical role in the cementing agents of soil aggregation, and it has an impact on soil aggregation stabilization (Tisdall and Oades, 1982). In addition, soil OC and TN contents have a significant positive correlation with soil aggregate stability (Zhong et al., 2017; Mao et al., 2021). TN had a positive effect on the stability of soil aggregates by affecting plant yield and root growth (Liu et al., 2019). Thus, the soil C/N ratio is considered the best predictor of soil aggregate stability, and the impacts of the soil C/N ratio on soil aggregate stability have been reported (He et al., 2023). For example, the C/N ratio of large-size macroaggregates had an important influence on the MWD of aggregates in a woodland system (Xu et al., 2020) and in subtropical China (He et al., 2023). Soil stoichiometry was a balanced coupling mechanism between soil organic carbon, nitrogen and phosphorus. The changes in the MBC:MBN strongly determined the adjustment of microbial N use efficiency (NUE) and C use efficiency (CUE), which are important for carbon (C) and nitrogen (N) cycling (Mooshammer et al., 2014). Previous research has also found that soil nutrient-driven microbial changes might be the dominant factor that caused the changes in the stability of the soil surface soil aggregate in typical grasslands (Ren et al., 2022). We found that vegetation restoration significantly changed the bacterial and fungal community structure, and SB significantly reduced the bacterial and fungal community diversity in soil surface (Figure S4).
However, through enhanced regression tree analysis, the results show that C/P is the main driving factor affecting the MWD, in which the overall stoichiometric influence accounts for 46%, followed by the microbial influence of 36%. For the GMD, MBC/MBN ratio is the main driving factor, and the stoichiometric influence accounts for 94% (Figure 6). The indicated that stoichiometric ratio is the main factor driving the stability of soil aggregates rather than microbial community characteristics and soil nutrients in the restoration of vegetation after five years.
Figure 6 Relative influence of different factors on decomposition of (A) soil mean weight diameter (MWD) and (B) geometric mean diameter (GMD). Barplots and donut charts indicate the relative influence of microbe (α diversity and β diversity of bacteria and fungi, MBC and MBN), stoichiometry (C/N, N/P, C/P, MBC/MBN) and soil physicochemical properties (TOC, TP, TN, pH) on MWD and GMD. FNMDS1: NMDS1 of fungi; BNMDS1: NMDS1 of bacteria; Fshannon: The Shannon index of fungi; Bshannon: The Shannon index of bacteria. TOC: organic carbon; TP: soil total phosphorus; TN: soil total nitrogen; C/P: the ratio of carbon to phosphorus; C/N: the ratio of carbon to nitrogen; N/P: the ratio of nitrogen to phosphorus; MBC: microbial biomass carbon; MBN: microbial biomass nitrogen; MBC/MBN: the ratio of MBC to MBN; pH: pH value.
After five years of vegetation restoration, alfalfa planting significantly improved the structure of large aggregates and thus improved the stability of soil aggregates. Our finding indicates that alfalfa is beneficial to the stability of deep soil aggregates, and the stoichiometric characteristics of soil are the key factors affecting the stability of soil aggregates. Therefore, soil stoichiometry can be regulated and soil aggregates can be improved by planting legumes, which has potential application value to rehabilitate degraded land. Also, it provided additional information on the relationship between global soil ecological stoichiometry and soil aggregates in terrestrial ecosystems.
The original contributions presented in the study are included in the article/Supplementary Material. Further inquiries can be directed to the corresponding author.
HK: Funding acquisition. HX: Writing – original draft. GZ: Data curation, Investigation, Writing – review & editing. CC: Data curation, Writing – review & editing. ZP: Data curation, Investigation, Writing – review & editing. XF: Funding acquisition, Validation, Writing – review & editing. JW: Funding acquisition, Investigation, Writing – original draft.
The author(s) declare financial support was received for the research, authorship, and/or publication of this article. This study was supported by the Special Project on Hi-Tech Innovation Capacity of Beijing Academy of Agriculture and Forestry Sciences (KJCX20230305; KJCX20200301); Youth Research Foundation of Beijing Academy of Agriculture and Forestry Sciences (QNJJ202333); Beijing Natural Science Foundation (5204031).
The authors declare that the research was conducted in the absence of any commercial or financial relationships that could be construed as a potential conflict of interest.
All claims expressed in this article are solely those of the authors and do not necessarily represent those of their affiliated organizations, or those of the publisher, the editors and the reviewers. Any product that may be evaluated in this article, or claim that may be made by its manufacturer, is not guaranteed or endorsed by the publisher.
The Supplementary Material for this article can be found online at: https://www.frontiersin.org/articles/10.3389/fevo.2023.1280303/full#supplementary-material
An S. S., Huang Y. M., Zheng F. L., Yang J.G. (2008). Aggregate characteristics during natural revegetation on the Loess plateau. Pedosphere 18, 809–816. doi: 10.1016/s1002-0160(08)60077-6
Bai Y. F., Wu J. G., Clark C. M., Pan Q.M., Zhang L.X., Chen S.P., et al. (2012). Grazing alters ecosystem functioning and c:N:P stoichiometry of grasslands along a regional precipitation gradient. J. Appl. Ecol. 49, 1204–1215. doi: 10.1111/j.1365-2664.2012.02205.x
Bardgett R. D., Bullock J. M., Lavorel S., Manning P., Schaffner U., Ostle N., et al. (2021). Combatting global grassland degradation. Nat. Rev. Earth Environ. 2, 720–735. doi: 10.1038/s43017-021-00207-2
Barthes B., Roose E. (2002). Aggregate stability as an indicator of soil susceptibility to runoff and erosion; validation at several levels. Catena 47, 133–149. doi: 10.1016/s0341-8162(01)00180-1
Cates A. M., Ruark M. D., Hedtcke J. L., Posner J.L. (2016). Long-term tillage, rotation and perennialization effects on particulate and aggregate soil organic matter. Soil Tillage Res. 155, 371–380. doi: 10.1016/j.still.2015.09.008
Celik I. (2005). Land-use effects on organic matter and physical properties of soil in a southern mediterranean highland of Turkey. Soil Tillage Res. 83, 270–277. doi: 10.1016/j.still.2004.08.001
Chen G. D., Fan W. X., Yin W., Fan Z.L., Wan S.M., Zhai Y.L., et al. (2023). Soil aggregates are governed by spacing configurations in alfalfa-jujube tree intercropping systems. Agronomy-Basel 13, 264. doi: 10.3390/agronomy13010264
Chen H., Zhou J. X., Cai Q. G., Yue Z.Q., Lu Z.C., Liang G.L., et al. (2005). The impact of vegetation restoration on erosion-induced sediment yield in the middle yellow river and management prospect. Sci. China Ser. D-Earth Sci. 48, 724–741. doi: 10.1360/04yd0048
Chen J., Zhu D., Chen H., Chen H. (2021). Soil aggregate stability and its stoichiometric characteristics in process of rocky desertification in plateau mountains. Guangxi Zhiwu/Guihaia 41, 715–725. doi: 10.11931/guihaia.gxzw2021m7052
Chenu C., Le Bissonnais Y., Arrouays D. (2000). Organic matter influence on clay wettability and soil aggregate stability. Soil Sci. Soc. America J. 64, 1479–1486. doi: 10.2136/sssaj2000.6441479x
Chenu C., Stotzky G. (2001). Interactions between microorganisms and soil particles: An overview (Chichester: John Wiley & Sons Ltd).
Clement C., Sleiderink J., Svane S. F., Smith A.G., Diamantopoulos E., Desbroll D.B. (2022). Comparing the deep root growth and water uptake of intermediate wheatgrass (kernza (r)) to alfalfa. Plant Soil 472, 369–390. doi: 10.1007/s11104-021-05248-6
Cleveland C. C., Liptzin D. (2007). C: N: P stoichiometry in soil: Is there a “redfield ratio” for the microbial biomass? Biogeochemistry 85, 235–252. doi: 10.1007/s10533-007-9132-0
Cooper J., Greenberg I., Ludwig B., Hippich L., Fischer D., Glaser B., et al. (2020). Effect of biochar and compost on soil properties and organic matter in aggregate size fractions under field conditions. Agric. Ecosyst. Environ. 295, 106882. doi: 10.1016/j.agee.2020.106882
Dou Y. X., Yang Y., An S. S., Zhu Z.L. (2020). Effects of different vegetation restoration measures on soil aggregate stability and erodibility on the Loess plateau, China. Catena 185, 104294. doi: 10.1016/j.catena.2019.104294
Du J. X., Liu K. L., Huang J., Han T.F., Zhang L., Anthonio C.K., et al. (2022). Organic carbon distribution and soil aggregate stability in response to long-term phosphorus addition in different land-use types. Soil Tillage Res. 215, 105195. doi: 10.1016/j.still.2021.105195
Duchicela J., Vogelsang K. M., Schultz P. A., Kaonongbua W., Middleton E.L., Bever J.D. (2012). Non-native plants and soil microbes: Potential contributors to the consistent reduction in soil aggregate stability caused by the disturbance of North American grasslands. New Phytol. 196, 212–222. doi: 10.1111/j.1469-8137.2012.04233.x
Egan G., Crawley M. J., Fornara D. A. (2018). Effects of long-term grassland management on the carbon and nitrogen pools of different soil aggregate fractions. Science of the Total Environment 613, 810–819. doi: 10.1016/j.scitotenv.2017.09.165
Elith J., Leathwick J. R., Hastie T. (2008). A working guide to boosted regression trees. J. Anim. Ecol. 77, 802–813. doi: 10.1111/j.1365-2656.2008.01390.x
Eviner V. T., Chapin F. S. (2002). The influence of plant species, fertilization and elevated co2 on soil aggregate stability. Plant Soil 246, 211–219. doi: 10.1023/A:1020657107687
Guo L., Deng M., Yang S., Liu W., Wang X., Wang J., et al. (2021). The coordination between leaf and fine root litter decomposition and the difference in their controlling factors. Global Ecol. Biogeogr. 30, 2286–2296. doi: 10.1111/geb.13384
Hafner S., Kuzyakov Y. (2016). Carbon input and partitioning in subsoil by chicory and alfalfa. Plant Soil 406, 29–42. doi: 10.1007/s11104-016-2855-8
He Y., Zhang Q., Jiang C., Lan Y., Zhang H., Ye S. (2023). Mixed planting improves soil aggregate stability and aggregate-associated c-n-p accumulation in subtropical China. Front. Forests Global Change 6. doi: 10.3389/ffgc.2023.1141953
Hu L., Li Q., Yan J., Liu C., Zhong J. (2022). Vegetation restoration facilitates belowground microbial network complexity and recalcitrant soil organic carbon storage in southwest China karst region. Sci. Total Environ. 820, 153137. doi: 10.1016/j.scitotenv.2022.153137
Huang L., Wang C. Y., Tan W. F., Hu H.Q., Cai C.F., Wang M.K. (2010). Distribution of organic matter in aggregates of eroded ultisols, central China. Soil Tillage Res. 108, 59–67. doi: 10.1016/j.still.2010.03.003
Huang Z., Liu Y., Cui Z., Fang Y., He H., Liu B.-R., et al. (2018). Soil water storage deficit of alfalfa (medicago sativa) grasslands along ages in arid area (China). Field Crops Res. 221, 1–6. doi: 10.1016/j.fcr.2018.02.013
Jaeger C. H. III, Monson R. K., Fisk M. C., et al. (1999). Seasonal partitioning of nitrogen by plants and soil microorganisms in an alpine ecosystem. Ecology 80, 1883–1891. doi: 10.1890/0012-9658(1999)080[1883:SPONBP]2.0.CO;2
Khan K. S., Mack R., Castillo X., Kaiser M., Joergensen R.G. (2016). Microbial biomass, fungal and bacterial residues, and their relationships to the soil organic matter c/n/p/s ratios. Geoderma 271, 115–123. doi: 10.1016/j.geoderma.2016.02.019
Klopf R. P., Baer S. G., Bach E. M., Six J. (2017). Restoration and management for plant diversity enhances the rate of belowground ecosystem recovery. Ecol. Appl. 27, 355–362. doi: 10.1002/eap.1503
Liu Y., Li S. Y., Shi J. J., Niu Y.L., Cui Z., Zhang Z.C., et al. (2022a). Effectiveness of mixed cultivated grasslands to reduce sediment concentration in runoff on hillslopes in the Qinghai-Tibetan plateau. Geoderma 422, 115933. doi: 10.1016/j.geoderma.2022.115933
Liu Z., Wang M., Zhou J., Chen Z., Xu X., Zhu Y., et al. (2023). Soil aggregation is more important than mulching and nitrogen application in regulating soil organic carbon and total nitrogen in a semiarid calcareous soil. Sci. Total Environ. 854, 158790. doi: 10.1016/j.scitotenv.2022.158790
Liu Y., Zhao L. R., Liu Y. F., Huang Z., Shi J.J., Wang Y.L., et al. (2022b). Restoration of a hillslope grassland with an ecological grass species (elymus tangutorum) favors rainfall interception and water infiltration and reduces soil loss on the Qinghai-Tibetan plateau. Catena 219, 106632. doi: 10.1016/j.catena.2022.106632
Liu R., Zhou X., Wang J., Shao J., Fu Y., Liang C., et al. (2019). Differential magnitude of rhizosphere effects on soil aggregation at three stages of subtropical secondary forest successions. Plant Soil 436, 365–380. doi: 10.1007/s11104-019-03935-z
Lu X., Hou E., Guo J., Gilliam F.S., Li J., Tang S., et al. (2021). Nitrogen addition stimulates soil aggregation and enhances carbon storage in terrestrial ecosystems of China: A meta-analysis. Global Change Biol. 27, 2780–2792. doi: 10.1111/gcb.15604
Ma L., Wang Q., Shen S. (2020). Response of soil aggregate stability and distribution of organic carbon to alpine grassland degradation in Northwest Sichuan. Geoderma Regional 22, e00309. doi: 10.1016/j.geodrs.2020.e00309
Mao L., Tang L., Ye S., Wang S. (2021). Soil organic c and total n as well as microbial biomass c and n affect aggregate stability in a chronosequence of Chinese fir plantations. Eur. J. Soil Biol. 106, 103347. doi: 10.1016/j.ejsobi.2021.103347
Mao D., Wang Z., Wu B., Zeng Y., Luo L., Zhang B. (2018). Land degradation and restoration in the arid and semiarid zones of China: Quantified evidence and implications from satellites. Land Degrad. Dev. 29, 3841–3851. doi: 10.1002/ldr.3135
Miao R., Qiu X., Guo M., Musa A., Jiang D. (2018). Accuracy of space-for-time substitution for vegetation state prediction following shrub restoration. J. Plant Ecol. 11, 208–217. doi: 10.1093/jpe/rtw133
Mooshammer M., Wanek W., Hammerle I., Fuchslueger L., Hofhansl F., Knoltsch A., et al. (2014). Adjustment of microbial nitrogen use efficiency to carbon: Nitrogen imbalances regulates soil nitrogen cycling. Nat. Commun. 5, 3694. doi: 10.1038/ncomms4694
Mustafa A., Xu M., Shah S. A. A., Sun N., Wang B., Cai Z., et al. (2020). Soil aggregation and soil aggregate stability regulate organic carbon and nitrogen storage in a red soil of Southern China. J. Environ. Manage. 270, 110894. doi: 10.1016/j.jenvman.2020.110894
Oztas T., Fayetorbay F. (2003). Effect of freezing and thawing processes on soil aggregate stability. Catena 52, 1–8. doi: 10.1016/S0341-8162(02)00177-7
Padbhushan R., Rakshit R., Das A., Sharma R.P. (2016). Effects of various organic amendments on organic carbon pools and water stable aggregates under a scented rice-potato-onion cropping system. Paddy Water Environ. 14, 481–489. doi: 10.1007/s10333-015-0517-8
Pérès G., Cluzeau D., Menasseri S., Soussana J.F., Bessler H., Engels C., et al. (2013). Mechanisms linking plant community properties to soil aggregate stability in an experimental grassland plant diversity gradient. Plant Soil 373, 285–299. doi: 10.1007/s11104-013-1791-0
Pohl M., Graf F., Buttler A., Rixen C. (2012). The relationship between plant species richness and soil aggregate stability can depend on disturbance. Plant Soil 355, 87–102. doi: 10.1007/s11104-011-1083-5
R Development Core Team. (2015). R: a language and environment for statistical computing. Vienna: R Foundation for Statistical Computing.
Ren C., Liu K. S., Dou P. P., Shao X.Q., Zhang D.Y., Wang K.L., et al. (2022). Soil nutrients drive microbial changes to alter surface soil aggregate stability in typical grasslands. J. Soil Sci. Plant Nutr. 22, 4943–4959. doi: 10.1007/s42729-022-00972-z
Schaller F. W., Stockinger K. R. (1953). A comparison of five methods for expressing aggregation data. Soil Sci. Soc. Am. J. 17. doi: 10.2136/sssaj1953.03615995001700040002x
Six J., Bossuyt H., Degryze S., Denef K. (2004). A history of research on the link between (micro)aggregates, soil biota, and soil organic matter dynamics. Soil Tillage Res. 79, 7–31. doi: 10.1016/j.still.2004.03.008
Smith D. R., Jarvie H. P., Harmel R. D., Haney R.L. (2019). The role of field-scale management on soil and surface runoff c/n/p stoichiometry. J. Environ. Qual. 48, 1543–1548. doi: 10.2134/jeq2018.09.0338
Solomun M. K., Barger N., Cerda A., Keesstra S., Markovic M. (2018). Assessing land condition as a first step to achieving land degradation neutrality: A case study of the republic of srpska. Environ. Sci. Policy 90, 19–27. doi: 10.1016/j.envsci.2018.09.014
Song X., Fang C., Yuan Z.-Q., Li F.-M., Sardans J., Penuelas J. (2022). Long-term alfalfa (medicago sativa l.) establishment could alleviate phosphorus limitation induced by nitrogen deposition in the carbonate soil. J. Environ. Manage. 324, 116346. doi: 10.1016/j.jenvman.2022.116346
Sun Y., Wang X., Ma C., Zhang Q. (2022). Characteristics of carbon, nitrogen and phosphorus stoichiometry and nutrient reabsorption in alfalfa leaves with different fall-dormancy levels in northern Xinjiang, China. Agriculture-Basel 12, 2154. doi: 10.3390/agriculture12122154
Tang X., Liu S., Liu J., Zhou G. (2010). Effects of vegetation restoration and slope positions on soil aggregation and soil carbon accumulation on heavily eroded tropical land of southern China. J. Soils Sediments 10, 505–513. doi: 10.1007/s11368-009-0122-9
Tang L., Wang S. (2022). Dynamics of soil aggregate-related c-n-p stoichiometric characteristics with stand age and soil depth in Chinese fir plantations. Land Degrad. Dev. 33, 1290–1306. doi: 10.1002/ldr.4217
Tisdall J. M., Oades J. M. (1982). Organic matter and water-stable aggregates in soils. Eur. J. Soil. Sci. 33, 141–163. doi: 10.1111/j.1365-2389.1982.tb01755.x
Vaverkova M. D., Radziemska M., Barton S., Cerda A., Koda E. (2018). The use of vegetation as a natural strategy for landfill restoration. Land Degrad. Dev. 29, 3674–3680. doi: 10.1002/ldr.3119
Wallace B., Purcell M. (2003). The benefits of nitrogen and total organic carbon (toc) determination by high temperature combustion. Am. Lab. 35, 58–5+. doi: 10.1016/j.trac.2004.09.003
Wang L., Ali G., Wang Z. (2023). Deep soil water depletion and soil organic carbon and total nitrogen accumulation in a long-term alfalfa pasture. Land Degrad. Dev 29, 3674–3680. doi: 10.1002/ldr.4597
Wang L., Mu Y., Zhang Q. F., Jia Z.K. (2012). Effects of vegetation restoration on soil physical properties in the wind-water erosion region of the northern loess plateau of China. Clean Soil Air Water 40, 7–15. doi: 10.1002/clen.201100367
Wang L., Xu H., Zhang H., Zhang Y. (2022). Grazing and mowing affect the carbon-to-nitrogen ratio of plants by changing the soil available nitrogen content and soil moisture on the meadow steppe, China. Plants 11, 286. doi: 10.3390/plants11030286
Wang S. Q., Li T. X., Zheng Z. C., Chen H. Y. H. (2019). Soil aggregate-associated bacterial metabolic activity and community structure in different aged tea plantations. Science of the Total Environment 654, 1023–1032. doi: 10.1016/j.scitotenv.2018.11.032
Winslow M. D., Vogt J. V., Thomas R. J., Sommer S., Martius C., Akhtar-Schuster M. (2011). Science for improving the monitoring and assessment of dryland degradation. Land Degrad. Dev. 22, 145–149. doi: 10.1002/ldr.1044
Wu G.-L., Liu Y., Yang Z., Cui Z., Deng L., Chang X.-F., et al. (2017). Root channels to indicate the increase in soil matrix water infiltration capacity of arid reclaimed mine soils. J. Hydrol. 546, 133–139. doi: 10.1016/j.jhydrol.2016.12.047
Xiao L., Zhang Y., Li P., Xu G., Shi P., Zhang Y. (2019). Effects of freeze-thaw cycles on aggregate-associated organic carbon and glomalin-related soil protein in natural-succession grassland and Chinese pine forest on the loess plateau. Geoderma 334, 1–8. doi: 10.1016/j.geoderma.2018.07.043
Xie H., Zhang Y., Wu Z., Lv T. (2020). A bibliometric analysis on land degradation: Current status, development, and future directions. Land 9, 28. doi: 10.3390/land9010028
Xu H., Chen C., Pang Z., Zhang G., Wu J., Kan H. (2022a). Short-term vegetation restoration enhances the complexity of soil fungal network and decreased the complexity of bacterial network. J. Fungi 8, 1122. doi: 10.3390/jof8111122
Xu H., Liu N., Zhang Y. (2022b). Short-term snow removal alters fungal but not bacterial beta diversity and structure during the spring snowmelt period in a meadow steppe of China. J. Fungi 8, 234. doi: 10.3390/jof8030234
Xu L., Xing X., Bai J., Li D. (2022c). Soil aggregate structure, stability, and stoichiometric characteristics in a smelter-impacted soil under phytoremediation. Front. Environ. Sci. 10, 900147. doi: 10.3389/fenvs.2022.900147
Xu H., Yuan H., Yu M., Cheng X. (2020). Large macroaggregate properties are sensitive to the conversion of pure plantation to uneven-aged mixed plantations. Catena 194. doi: 10.1016/j.catena.2020.104724
Xu S., Zhao Q., Qin C., Qin M., Lee J., Li C., et al. (2021). Effects of vegetation restoration on accumulation and translocation of heavy metals in post-mining areas. Land Degrad. Dev. 32, 2000–2012. doi: 10.1002/ldr.3861
Yao Y., Ge N., Yu S., Wei X., Wang X., Jin J., et al. (2019). Response of aggregate associated organic carbon, nitrogen and phosphorous to re-vegetation in agro-pastoral ecotone of northern China. Geoderma 341, 172–180. doi: 10.1016/j.geoderma.2019.01.036
Zhang Y., Marschner P. (2018). Respiration, microbial biomass and nutrient availability are influenced by previous and current soil water content in plant residue amended soil. J. Soil Sci. Plant Nutr. 18, 173–187. doi: 10.4067/S0718-95162018005000703
Zhang S., Wang R., Yang X., Sun B., Li Q. (2016). Soil aggregation and aggregating agents as affected by long term contrasting management of an anthrosol. Sci. Rep. 6. 39107. doi: 10.1038/srep39107
Zhao J., Feng X., Deng L., Yang Y., Zhao Z., Zhao P., et al. (2020). Quantifying the effects of vegetation restorations on the soil erosion export and nutrient loss on the loess plateau. Front. Plant Sci. 11. doi: 10.3389/fpls.2020.573126
Zhao F., Sun J., Ren C., Kang D., Deng J., Han X., et al. (2015). Land use change influences soil c, n, and p stoichiometry under “grain-to-green program” in China. Sci. Rep. 5, 10195. doi: 10.1038/srep10195
Keywords: soil aggregate stability, soil erodibility, vegetation restoration measures, stoichiometric characteristics, boosted regression trees (BRT)
Citation: Kan H, Xu H, Zhang G, Chen C, Pang Z, Fan X and Wu J (2023) Stoichiometric characteristics drive the soil aggregate stability after 5 years of vegetation restoration in China. Front. Ecol. Evol. 11:1280303. doi: 10.3389/fevo.2023.1280303
Received: 20 August 2023; Accepted: 23 October 2023;
Published: 06 November 2023.
Edited by:
Yu Liu, Northwestern Polytechnical University, ChinaReviewed by:
Xinqing Shao, China Agricultural University, ChinaCopyright © 2023 Kan, Xu, Zhang, Chen, Pang, Fan and Wu. This is an open-access article distributed under the terms of the Creative Commons Attribution License (CC BY). The use, distribution or reproduction in other forums is permitted, provided the original author(s) and the copyright owner(s) are credited and that the original publication in this journal is cited, in accordance with accepted academic practice. No use, distribution or reproduction is permitted which does not comply with these terms.
*Correspondence: Juying Wu, d3VqdXlpbmdAZ3Jhc3MtZW52LmNvbQ==
Disclaimer: All claims expressed in this article are solely those of the authors and do not necessarily represent those of their affiliated organizations, or those of the publisher, the editors and the reviewers. Any product that may be evaluated in this article or claim that may be made by its manufacturer is not guaranteed or endorsed by the publisher.
Research integrity at Frontiers
Learn more about the work of our research integrity team to safeguard the quality of each article we publish.